- 1Department of Pharmacology, School of Basic Medical Science, Xi’an Jiaotong University Health Science Center, Xi’an, Shaanxi, China
- 2Precision Medical Institute, The Second Affiliated Hospital of Xi’an Jiaotong University, Xi’an, Shaanxi, China
Helicobacter pylori (H. pylori) is a strict microaerophilic bacterial species that exists in the stomach, and H. pylori infection is one of the most common chronic bacterial infections affecting humans. Eradicating H. pylori is the preferred method for the long-term prevention of complications such as chronic gastritis, peptic ulcers, gastric mucosa-associated lymphoid tissue lymphoma, and gastric cancer. However, first-line treatment with triple therapy and quadruple therapy has been unable to cope with increasing antibacterial resistance. To provide an updated review of H. pylori infections and antibacterial resistance, as well as related treatment options, we searched PubMed for articles published until March 2024. The key search terms were “H. pylori”, “H. pylori infection”, “H. pylori diseases”, “H. pylori eradication”, and “H. pylori antibacterial resistance.” Despite the use of antimicrobial agents, the annual decline in the eradication rate of H. pylori continues. Emerging eradication therapies, such as the development of the new strong acid blocker vonoprazan, probiotic adjuvant therapy, and H. pylori vaccine therapy, are exciting. However, the effectiveness of these treatments needs to be further evaluated. It is worth mentioning that the idea of altering the oxygen environment in gastric juice for H. pylori to not be able to survive is a hot topic that should be considered in new eradication plans. Various strategies for eradicating H. pylori, including antibacterials, vaccines, probiotics, and biomaterials, are continuously evolving. A novel approach involving the alteration of the oxygen concentration within the growth environment of H. pylori has emerged as a promising eradication strategy.
1 Introduction
Helicobacter pylori is a spiral-shaped gram-negative bacterium. Its persistent infection is associated with severe gastric complications (Malfertheiner et al., 2023) and plays a significant role in the development of conditions such as gastric cancer, duodenal ulcers, and gastric ulcers, particularly contributing to the onset of gastric adenocarcinoma (Sung et al., 2021). Accurate diagnosis of H. pylori infection is essential for effective treatment. diagnostic methods include non-invasive and invasive approaches (Chen Y. C. et al., 2024). Non-invasive tests comprise the 13C-urea breath test, fecal antigen detection, serological tests, and molecular biology tests (e.g., PCR). Invasive tests involve endoscopy with tissue biopsy, the rapid urease test, and histopathology. Patients diagnosed with H. pylori infection typically develop chronic gastritis, making H. pylori gastritis an infectious disease that warrants eradication therapy (Ranjbar et al., 2017).
Since the discovery of H. pylori in 1983, its eradication treatments have evolved from single antibiotics (e.g., metronidazole or amoxicillin) to combinations of multiple antibiotics. Standard triple therapies, which include a proton pump inhibitor such as omeprazole along with two antibiotics like clarithromycin and amoxicillin or metronidazole, have been superseded by quadruple therapies. These quadruple therapies involve a proton pump inhibitor, bismuth agents such as bismuth potassium citrate, and two antibiotics (Malfertheiner et al., 2024). Addressing H. pylori infection requires appropriate antibacterial treatment. While the incidence of H. pylori infection has declined in developed countries (Megraud et al., 2021), it remains widespread in developing nations, with increasing drug resistance. In most cases, the infection is acquired during childhood (Malaty et al., 2002; Park et al., 2021; Yuan et al., 2022). The prevalence rate may also be influenced by local economic conditions and health care infrastructure.
Eradicating H. pylori requires a suitable treatment regimen. However, treating H. pylori infection presents challenges. Standard triple therapy (STT) comprises a proton pump inhibitor and two antibiotics. Owing to increasing antimicrobial resistance, STT is no longer effective for treating H. pylori infection (Ho et al., 2022; Boyanova et al., 2023). Consequently, the search for new H. pylori treatments and strategies to reduce antimicrobial resistance has become a primary focus.
This study comprehensively reviews H. pylori infection, antimicrobial treatment approaches, drug resistance, and the development of new anti-H. pylori drugs or novel ideas to eradicate H. pylori, which introduces novel perspectives that can optimize future treatment strategies.
2 The prevalence of Helicobacter pylori infection
Helicobacter pylori gastritis (Sugano et al., 2015) is an infectious disease that has been included in the 11th revision of the International Classification of Diseases (Malfertheiner et al., 2022). H. pylori infection has been widespread worldwide (Figure 1; Table 1), with an overall infection rate as high as 43.2% (95% CI: 40.3–45.9%) in 2011–2022 (Li et al., 2023). H. pylori infection is highly prevalent in East Asia. A systematic review and meta-analysis covering 1,377,349 individuals from 412 eligible studies indicated that the H. pylori infection rate in mainland China was 40% in 2015–2019 (Ren et al., 2022). The epidemiological landscape of H. pylori in Japan is evolving, with an infection rate of 70% in less developed areas and 40% in more developed regions (Inoue, 2017). In a Korean population of 23,770 people, the detection of anti-H. pylori IgG antibodies, indicating the presence of H. pylori infection, revealed a seroprevalence rate of 41.5% (Lim et al., 2018). A recent cross-sectional study in India, using 2,998 biopsy samples, indicated an overall prevalence of H. pylori infection of approximately 64% (Venkatesan et al., 2024).
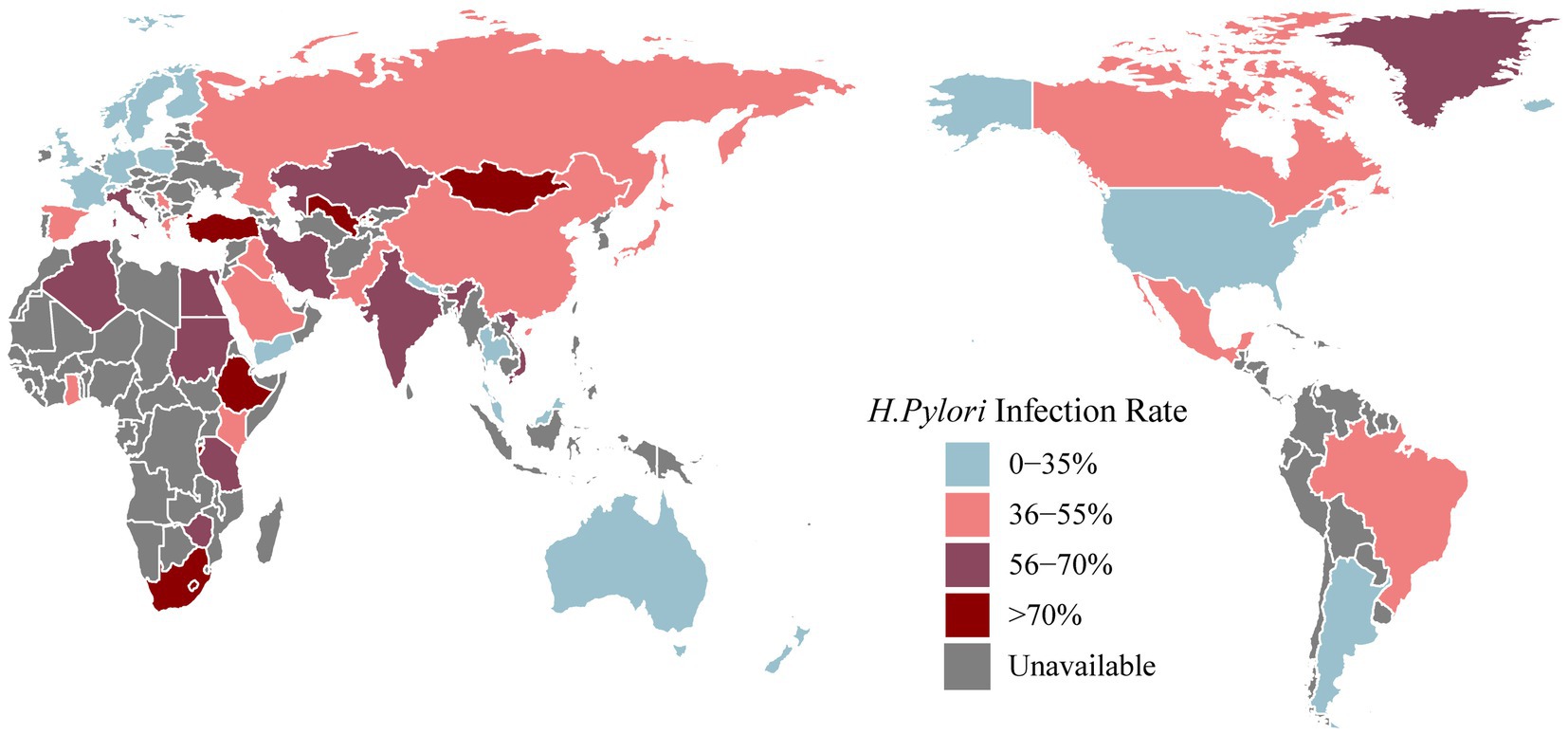
Figure 1. The latest prevalence of Helicobacter pylori infection [Antarctica removed (no data collected)].
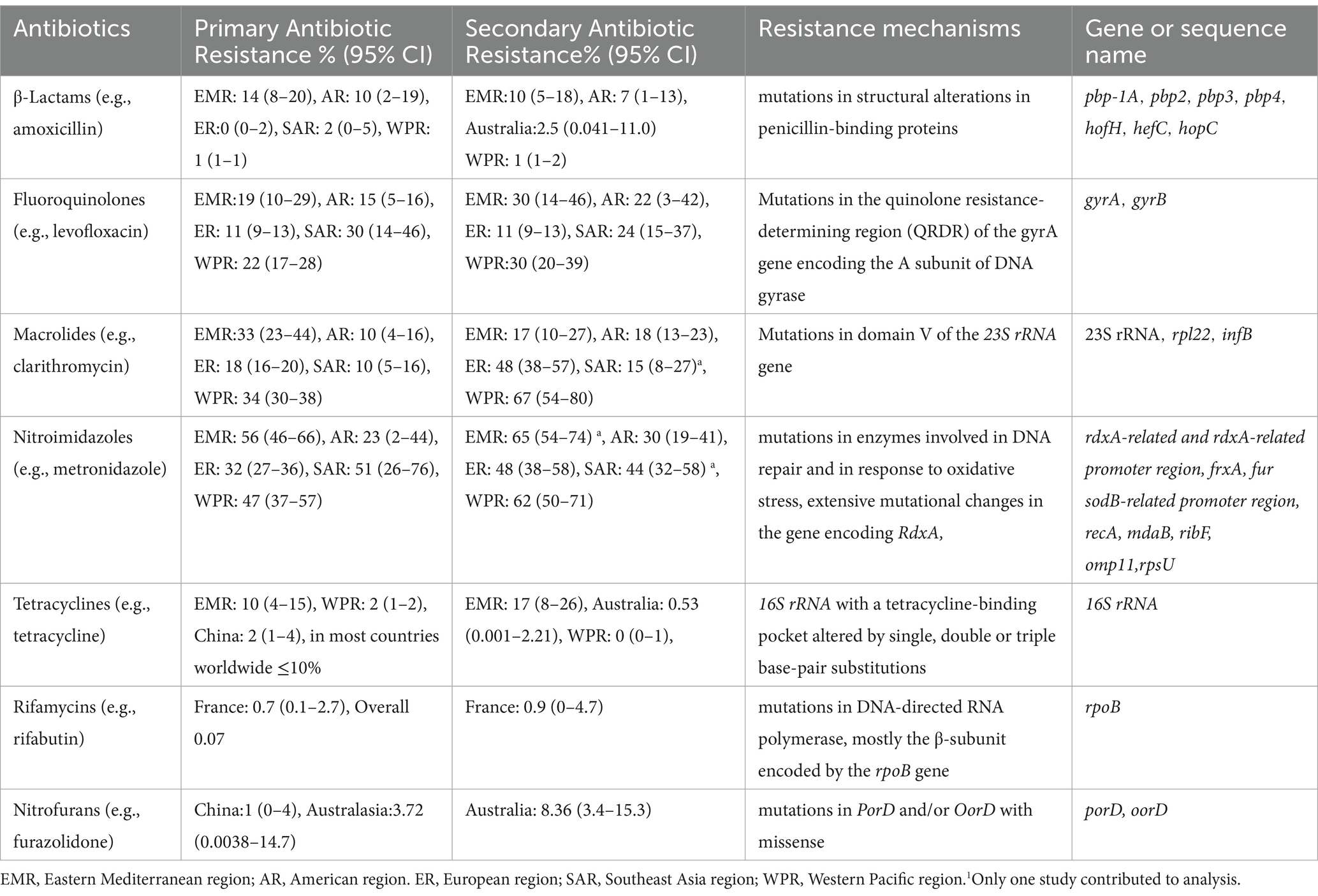
Table 1. The primary and secondary resistance rate and mechanism of antibiotics (Savoldi et al., 2018; Boyanova et al., 2020; Gisbert, 2020; Schubert et al., 2021; Chen et al., 2022; Borka Balas et al., 2023).
In North America, the prevalence of H. pylori infection from 1980 to 2022 was 36.2% (95% CI: 22.6–49.9%) (Li et al., 2023). A meta-analysis covering the years 1999–2018 reported a diagnosis rate of H. pylori infection of 25.8% in the USA (Shah et al., 2024). Willems et al. reported that the H. pylori infection rate in Canada was approximately 20–30% (Willems et al., 2020). Victor et al. collected dental plaque samples from 36 families in a southwestern Mexican community and reported an H. pylori infection rate of 41.7% (Urrutia-Baca et al., 2023). In a urease test report from Brazil, involving 852 individuals, the overall H. pylori infection rate was 35.4%, with a specific infection rate of 24.7% among children and adolescents (Toscano et al., 2018). The H. pylori infection rate in Argentina falls between the high incidence observed in African countries and the lower incidence observed in other countries in the Americas (Olmos et al., 2000).
The overall prevalence of H. pylori infection in Europe was reported to be 47.5% (95% CI: 43.0–52.1%) (Li et al., 2023). Bordin compared H. pylori infection rates across all Russian Federation communities in 2017 and 2019. Among untreated persons, the overall H. pylori infection rates were 41.8% in 2017 and 36.4% in 2019 (Bordin et al., 2022). In a seven-year prospective cross-sectional study examining the presence of H. pylori infection among 3,241 students in Gruzice, Poland, the positivity rate was 23.6% (Szaflarska-Poplawska and Soroczynska-Wrzyszcz, 2019). The reported prevalence of H. pylori infection in Germany in 2018 ranged from 20 to 40% (Fischbach and Malfertheiner, 2018). A recent meta-analysis reported infection rates of 28.6% in France, 18.8% in Denmark and 26.3% in the United Kingdom (Chen Y. C. et al., 2024).
In the representative countries of Oceania, namely, Australia and New Zealand, H. pylori infection rates vary. As reported in a global meta-analysis from 2010 to 2022, Australia recorded a rate of 24.8%, while New Zealand had a rate of 9.2% (Chen Y. C. et al., 2024). Biopsy samples collected from 12,482 individuals in South Australia from 1998 to 2017 revealed a positive H. pylori infection rate of 11.5% (Schubert et al., 2022).
The H. pylori infection rate in Africa was reported to be 52.6% (Chen Y. C. et al., 2024). Notably, the prevalence in Africa surpasses that in other continents and exhibits significant variability, depending on the geographical location. In a comprehensive review of H. pylori infection in the Middle East and North Africa, infection rates displayed wide-ranging disparities within regions, ranging from 7 to 50% in young children and from 36.8 to 94% in adults (Alsulaimany et al., 2020). A review by Smith revealed elevated infection rates, reaching 87.8% in northern Nigeria and 70.8% in Burundi and exceeding 80% in Ethiopia (Smith et al., 2022). A 2019 report from Egypt revealed an overall H. pylori infection rate of 66.1% (Galal et al., 2019).
3 Helicobacter pylori infectious diseases and its morphological form
After H. pylori infection, various gastrointestinal and systemic diseases ensue in the human body (Figure 2). H. pylori infection always causes chronic gastritis, and approximately 10–15% of infected individuals may develop peptic ulcers. Peptic ulcer disease has a lifetime risk of approximately 17% and may lead to serious complications, such as bleeding, perforation, and gastric cancer. Chronic atrophic gastritis is currently considered to be a precancerous lesion.
An increasing focus has been directed toward clinical extragastric manifestations following H. pylori infection. Research exploring the relationship between H. pylori infection and cardiovascular-related diseases has gained substantial attention. The presence of H. pylori infection in patients increases susceptibility to coronary atherosclerotic disease (Chen et al., 2013; Wang et al., 2021), stroke (Sun et al., 2023), and myocardial infarction (Wang et al., 2023). Moreover, H. pylori infection has been linked to neurological disorders, including Alzheimer’s disease (Kandpal et al., 2024) and multiple sclerosis (Arjmandi et al., 2022). Eye-related ailments such as open-angle glaucoma (Doulberis et al., 2020) and blepharitis (Sacca et al., 2006) may also result from infection. In children and young individuals, H. pylori infection has been associated with the onset of allergic systemic conditions like Asthma (Durazzo et al., 2021). H. pylori infection can induce alterations in the metabolic system, contributing to blood sugar disorders in individuals with diabetes (Chang et al., 2023). Additionally, stomach colonization by H. pylori has been implicated in accelerating liver fibrosis (Okushin et al., 2018) and mediating tumorigenesis. Encouragingly, the eradication of H. pylori has led to improvements in hematologic disorders, including iron deficiency anemia (Elbehiry et al., 2023) and primary immune thrombocytopenia (Wang et al., 2022).
The morphological form of H. pylori plays a pivotal role in disease development among H. pylori-infected patients. In human gastric biopsy specimens, H. pylori predominantly exists in a spiral form (Gladyshev et al., 2020). Under adverse conditions, such as high temperature, pH fluctuations or the use of antibacterial drugs, H. pylori can transform into a latent spherical form. The intermediate stages between the spiral and spherical forms are referred to as the C-shaped and/or U-shaped forms of H. pylori, also known as the dormant state. Dormancy is regarded as a reversible state in which bacterial cells exhibit reduced metabolic activity. When favorable culture conditions are restored, the cells regain metabolic activity and can be cultured (Ierardi et al., 2020). Various antibiotics (amoxicillin, clarithromycin, metronidazole, and tetracycline) at the minimum inhibitory concentrations (MICs) induce a spherical form. Furthermore, after exposing H. pylori to omeprazole (a proton pump inhibitor) at 0.5 MIC for 3, 6, and 9 days, the bacterium could revert to the spiral form after omeprazole removal from the culture medium (Krzyzek and Grande, 2020). Patients with H. pylori infection is susceptible to reinfection if the bacterium is not completely eradicated. Vasiliy et al. noted that after one year of anti-H. pylori treatment in patients with duodenal peptic ulcers, the identification of H. pylori suggested that the bacterium may have transitioned from the dormant state to an active state (Reshetnyak and Reshetnyak, 2017). Nikita et al. reported that the virulence of the spherical form was comparable to that of the spiral form and that the spherical form increased the risk of developing gastric cancer in patients (Gladyshev et al., 2020). In summary, when patients with H. pylori infection exhibit significant symptoms, such as peptic ulcers, eradication therapy should be initiated. However, incomplete eradication with antibiotics and proton pump inhibitors may lead to the conversion of the spherical form back to the active spiral form.
4 Helicobacter pylori colonization and its virulence mechanisms
Helicobacter pylori colonizes the gastric mucosa and is highly adapted to the acidic environment through complex molecular processes. Adhesion mechanisms are crucial for its sustained colonization. Two key outer membrane proteins, blood group antigen-binding adhesion (BabA) and salivary acid-binding adhesion (SabA), play significant roles (Malfertheiner et al., 2023). BabA, one of the earliest discovered H. pylori adhesins, recognizes and binds to various antigens, particularly the ABO group, facilitating survival and colonization in the stomach (Chang et al., 2023). SabA, also known as HopP, binds to sialylated Lewis x (sLex) antigens on gastric epithelial cells, promoting adhesion and survival despite the host’s immune response (Pang et al., 2014; Doohan et al., 2021). BabA initiates adhesion by binding to antigens, while SabA maintains persistent infection in an inflammatory environment. Their synergistic action and dynamic regulation enable H. pylori to efficiently colonize the host and cause disease.
Inflammasomes play a crucial role in the development of gastric inflammation caused by H. pylori (Kandpal et al., 2024). These important cytoplasmic multiprotein complexes, when activated, promote the release of pro-inflammatory cytokines such as IL-1β and IL-18 (Zheng et al., 2024). Among the various inflammasomes, the NLRP3 inflammasome is the most commonly studied in H. pylori infection. When exposed to intracellular stimuli, such as bacterial toxins and oxidative stress, or danger signaling molecules, including the loss of intracellular potassium ions and pH alterations (Mugengana et al., 2021), NLRP3 proteins are activated. This activation accelerates the development of peptic ulcers associated with H. pylori infection.
The primary virulence factors of H. pylori include Cytotoxin-associated gene A (CagA), Vacuolating cytotoxin A (VacA), Duodenal ulcer-promoting gene A (DupA), and Urease (Malfertheiner et al., 2023). CagA is a pathogenic protein delivered into host cells via the Type IV secretion system (T4SS) (Bhattacharjee et al., 2024). It activates multiple signaling pathways, such as NF-κB, MAPK/PI3K, and Wnt/β-catenin, affecting cell proliferation, motility, polarity, apoptosis, and inflammation (Ayana et al., 2014; Hayashi et al., 2017). VacA forms an anion-selective membrane channel upon contact with host cells, causing the formation of large intracellular vacuoles (Yahiro et al., 2015; Nejati et al., 2018), disrupting mitochondrial function, inducing apoptosis and autophagy, and inhibiting T and B cell proliferation (Cover and Blanke, 2005). DupA, associated with T4SS-related proteins, is implicated in urease regulation (Asimellis et al., 2005), ATPase-associated efflux pumps, and apoptotic pathways, potentially enhancing H. pylori virulence (Chen et al., 2018). Urease, composed mainly of the ureA and ureB genes and cofactors, including nickel, and regulated by the gastric microenvironment’s pH, hydrolyzes urea into ammonia and carbon dioxide. This reaction neutralizes gastric acid, creating a protective microenvironment that promotes colonization and evades the host immune system (Ha et al., 2001; Allen et al., 2023; Malfertheiner et al., 2023).
5 Antibacterial treatment of Helicobacter pylori infection
Advancements in the understanding of H. pylori infection have transformed peptic ulcer treatment from anti-acid therapies to antibacterial-based approaches (Figure 3). The eradication of H. pylori by antibacterial drugs promotes ulcer healing and reduces recurrence rates. In the late 20th century, antibacterial drugs were less effective in the acidic environment of the stomach. Therefore, treatments targeting both bacteria and stomach acid were needed. Omeprazole and amoxicillin were used to be a classical dual therapy, which was highly effective and well tolerated owing to its bactericidal and antacid effects (Labenz et al., 1994). Nevertheless, plans to eradicate H. pylori have shifted to triple therapy, mainly including bismuth +2 antibacterial drugs or proton pump inhibitors (PPIs) + 2 antibacterial drugs (consisting of amoxicillin and clarithromycin, metronidazole or levofloxacin).
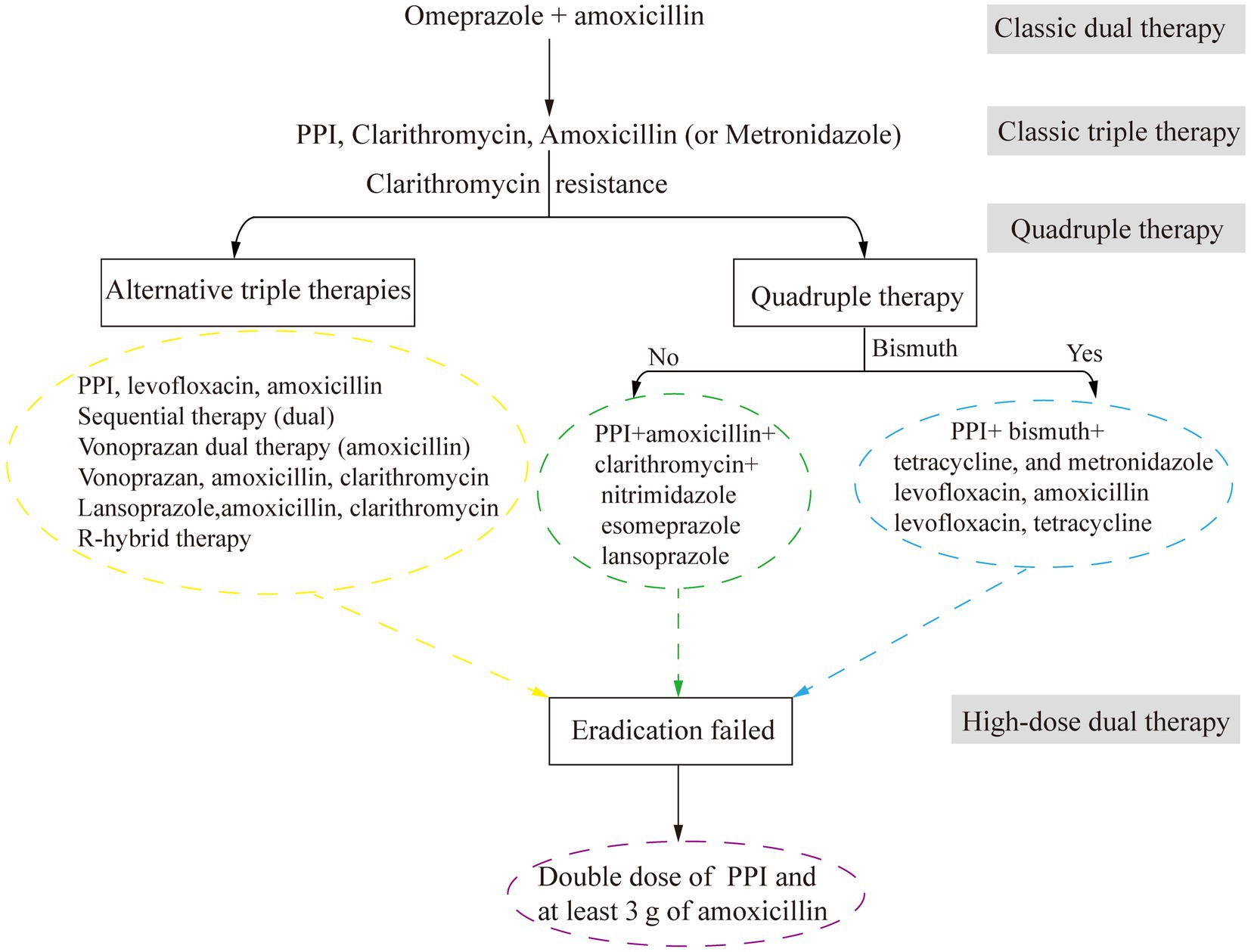
Figure 3. The development of antimicrobial treatment for H. pylori infection. PPI, proton pump inhibitors.
Increasing antibacterial resistance leads to a further reduction in treatment efficacy. For example, a successful 14-day concomitant therapy containing 1 g of metronidazole and clarithromycin produced 14,000 kg of unnecessary antibacterial agents per 1 million successful treatments (Graham, 2020). To address this issue, alternative therapies, such as Vono-triple therapy (a vonoprazan-containing therapy) and R-hybrid therapy (a reverse hybrid therapy), have been developed and have achieved eradication rates greater than 90% those of STT. However, while R-hybrid therapy has a high cure rate, it has failed to achieve significant comparative efficacy. One promising drug is vonoprazan, a potassium-competitive acid blocker (Rokkas et al., 2021).
In areas with high clarithromycin resistance, STT has been replaced with bismuth-containing quadruple therapy (BQT). The improved bismuth-containing quadruple therapy (mBCQT) consists of a PPI, bismuth, metronidazole, and tetracycline. The results showed that mBCQT achieved an H. pylori eradication rate of 88.2% after continuous treatment of patients for two weeks (Kim et al., 2019). In Greece, where clarithromycin resistance is greater than 20% and bismuth is not available, a 10-day nonbismuth-based quadruple concomitant therapy (esomeprazole, clarithromycin, amoxicillin, and metronidazole) is currently the first-line treatment for H. pylori eradication (Apostolopoulos et al., 2020). Hybrid therapy, which combines sequential therapy and concomitant therapy, is a novel two-step therapy (Chey et al., 2018). A 14-day hybrid therapy regimen (pantoprazole plus amoxicillin for 7 days, followed by pantoprazole plus amoxicillin, clarithromycin, and metronidazole for another 7 days) had an H. pylori eradication rate of 93.9% (154/164; 95% confidence interval [CI]: 90.3 to 97.5%). The H. pylori eradication rate of bismuth quadruple therapy (pantoprazole, bismuth subcitrate, tetracycline, and metronidazole for 14 days) was 92.8% (154/166; 95% CI: 88.9 to 96.7%). The H. pylori eradication rates of the two therapies are similar, but hybrid therapy has fewer adverse effects than bismuth quadruple therapy (Tsay et al., 2017). For patients allergic to penicillin who are infected with H. pylori for the first time, the triple therapy of bismuth (using bismuth potassium citrate and esomeprazole) with minocycline, cefuroxime, and full-dose metronidazole (pairwise) has the same satisfactory efficacy in H. pylori eradication, with a good safety and compliance (Zhang Y. X. et al., 2023). Nonbismuth or bismuth quadruple therapy is characterized by poor compliance and a high cost, similar to many other drugs.
High-dose dual therapy (HDDT) consisting of a PPI and amoxicillin has been proven to have a better H. pylori eradication rate and fewer adverse reactions than traditional triple or quadruple therapy (Yang et al., 2019; Zhu et al., 2020). The HDDT group (40 mg of esomeprazole and 100 mg of amoxicillin three times daily) and the bismuth-containing quadruple therapy group, which was called the TFEB group (40 mg of esomeprazole, 220 mg of bismuth potassium citrate, and 100 mg of furazolidone twice daily, combined with 500 mg of tetracycline three times daily), were treated continuously for 14 days, and the eradication rates of H. pylori reinfection in patients who failed eradication were similar in both groups. However, the incidence of adverse events in the HDDT group was significantly lower than that in the TFEB group (11.1% vs. 26.8%, p < 0.001), and the treatment compliance was good (Bi et al., 2022). The replacement of PPIs with vonoprazan was also proven to be effective. After 10 days of continuous administration, the eradication rates were 93.4% for VHA-dual therapy (20 mg of vonoprazan twice daily +750 mg of amoxicillin four times daily) and 90.9% for B-quadruple therapy (20 mg of esomeprazole +200 mg of bismuth +1,000 mg of amoxicillin +500 mg of clarithromycin twice daily). Although compliance was similar between the two therapies, the former had a lower rate of adverse events (Qian et al., 2023). VHA-dual therapy is expected to become the first-line therapy for H. pylori eradication.
In addition to the use of modifying drugs to improve eradication rates, patient compliance is also a factor that directly affects treatment success. Patients received telephone follow-ups on the 3rd, 14th, and 30th days of treatment, and the results showed that semiautomatic intensive follow-ups improved the H. pylori eradication rate and patient compliance (Chen et al., 2021). The use of technology-enhanced communication strategies, such as telephone re-education, text messaging, social media re-education, and structured patient counseling programs, enables patients to comprehensively understand the treatment process, leading to improved eradication rates and compliance (Zhao et al., 2020; Chua et al., 2022).
6 The resistance of Helicobacter pylori to antibacterial drugs
Helicobacter pylori colonizes the surface of the gastric mucosa and is protected by the gastric mucus layer, making it difficult to remove H. pylori from the stomach. Resistance of H. pylori gradually develops over the course of treatment owing to limited selection of antibacterial agents and the limited frequency of treatment. Furthermore, when patients who have previously used antibacterial drugs are treated, the resistance rate increases, as does the risk of treatment failure (Guo C. G. et al., 2022). Clarithromycin is one of the most important drugs for the treatment of H. pylori worldwide, but its primary resistance rate has significantly increased, from 17.2 to 19.7% or even 27.2% (Kasahun et al., 2020). Metronidazole shows a universal drug resistance pattern worldwide, with the resistance rate > 15% on all continents (Table 1; Savoldi et al., 2018; Boyanova et al., 2020; Gisbert, 2020; Schubert et al., 2021; Chen et al., 2022; Borka Balas et al., 2023). The primary resistance rates are expected to continue increasing in the future (Savoldi et al., 2018). Resistance rates are on the rise, affecting not only clarithromycin and metronidazole but also drugs such as levofloxacin, which are often deemed alternative therapies. Currently, the rates of H. pylori resistance to clarithromycin, metronidazole, and levofloxacin are 27.2, 39.7, and 22.5%, respectively, worldwide (Kasahun et al., 2020). In the United States, the rates of resistance to clarithromycin, metronidazole, and levofloxacin are 17.6, 43.6, and 57.8%, respectively (Hulten et al., 2021). Over a 10-year period, the resistance rate to clarithromycin increased from 17.5 to 21.4% in European countries. The H. pylori resistance was assessed, and 201 strains showed double resistance, most commonly to clarithromycin and metronidazole (Megraud et al., 2021). In the Asia Pacific region, overall resistance to clarithromycin increased from 7 to 21% over the same period (Jiang et al., 2022). In a study of 125 Vietnamese patients with peptic gastric ulcers, the percentages of patients who were resistant to amoxicillin, clarithromycin, metronidazole, levofloxacin, and tetracycline were 27.5, 50.0, 67.5, 35.0, and 5.0%, respectively. Additionally, 7.5% of the patients were sensitive to all five antibacterial drugs, 27.5% were single-drug resistant, 42.5% were double-drug resistant, 17.5% were triple-drug resistant, and 5% were quadruple-drug resistant (Vu et al., 2022).
Primary resistance rates for clarithromycin, metronidazole, levofloxacin, and amoxicillin vary across different regions (Table 2). Metronidazole generally showed higher resistance rates than the other three antibiotics, with rates reaching up to 91% in Africa and the lowest at approximately 25% in Europe. Clarithromycin also exhibited substantial resistance, peaking at around 37% in Asia. Resistance rates for levofloxacin were highest in Asia at about 25%, with other regions not exceeding 20%. Amoxicillin consistently had low resistance rates, maintaining around 1% in Europe.
Analysis of data from China revealed that the average resistance rate to clarithromycin was 24.0%, with single-, double-, triple-, and quadruple-drug resistance rates of 54.6, 29.0, 11.7, and 0.1%, respectively (Zhang et al., 2021). In the last 20 years, primary resistance to major antibacterial drugs (clarithromycin, metronidazole, levofloxacin, and amoxicillin) has been analyzed in China (Figure 4; Tables 1–3). Clarithromycin and metronidazole, which are commonly used as first-line treatments, have shown increased resistance, with the median rates having increased from 18.1 to 40.0% and from 43.1 to 79.9%, respectively. Since 2010, levofloxacin has been increasingly used as an alternative antibiotic. A trend toward resistance has emerged, with the primary resistance at 34.8% from 2018 to 2024. Recently, high-dose amoxicillin therapy has gained popularity as a novel treatment approach. While the median resistance rate to amoxicillin remains low at 5%, the variability in the data indicates that certain regions already exhibit relatively high resistance levels. The increasing trend of amoxicillin resistance appears to be unstoppable.
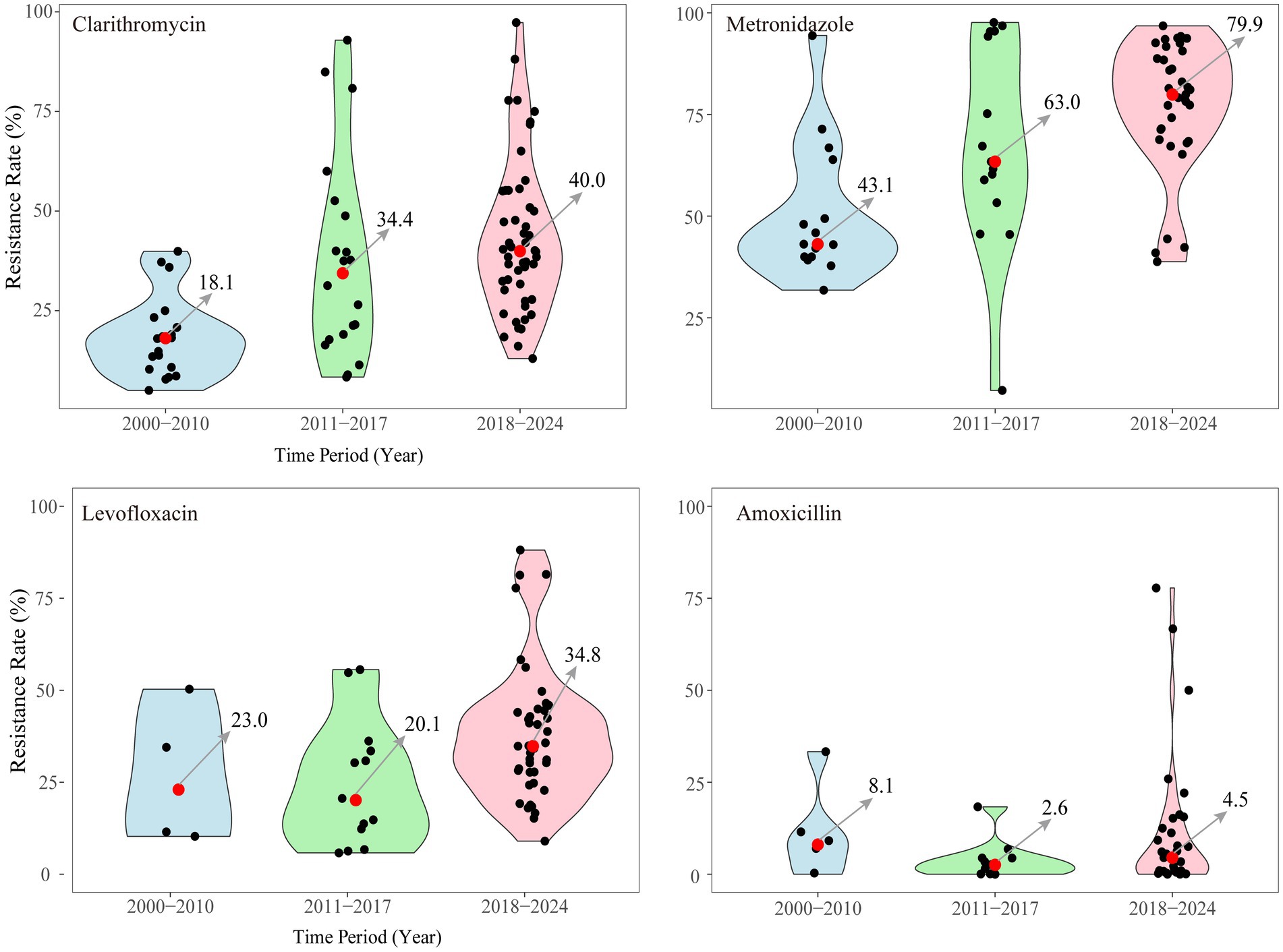
Figure 4. Primary resistance to 4 major antibacterial used of H. pylori infection in China from 2000 to 2024.
In recent years, antibacterial resistance in H. pylori-infected children has become a growing concern. Although the triple combination of PPI-CA (proton pump inhibitor, clarithromycin, and amoxicillin) is the preferred regimen, the eradication rates in children are still lower than those in adults owing to differences in susceptibility to and compliance with antimicrobial agents (Lai and Lai, 2022). Among Asian children, the primary resistance rate to clarithromycin in Southeast Asia is 10%, which is the same as that in the Americas but lower than that in Europe and the Eastern Mediterranean, where the resistance rate exceeds 15%. The primary resistance rates to metronidazole are 51% in Asia, 23% in the Americas, 91% in Africa, and 32% in Europe. As an alternative salvage therapy, levofloxacin has a primary resistance rate as high as 30% in Asia (Borka Balas et al., 2023). In Spain, 67.5% of 80 pediatric patients showed resistance to more than two antibacterial drugs, and 6.3% had double-drug resistance, with resistance to clarithromycin, metronidazole, levofloxacin, and amoxicillin being the main manifestations (Botija et al., 2021). In Germany, multidrug resistance in pediatric patients reaches 16% (Helmbold et al., 2022), and the rate of amoxicillin resistance in patients is as high as 20%, which is of great concern. In general, the drug resistance of H. pylori in children cannot be ignored. Owing to the low compliance of children, the eradication rate is greatly affected, and the possibility of developing multidrug resistance is greatly increased.
The effects of H. pylori eradication in older patients should also be considered. In a study of 264 patients diagnosed with H. pylori infection, the eradication rate in patients aged 40 years and older was greater than that in patients under 40 years of age (85.7% versus 54.7%, p = 0.002), and the eradication rate in patients older than 60 years was 100% (Tang et al., 2020). The eradication rate of the PPI-clarithromycin-amoxicillin regimen in the young and middle-aged group (≤50 years) was significantly lower than that in the aged group (>50 years) (Nishizawa et al., 2017). One possible reason for this is that younger patients have earlier exposure to clarithromycin and greater resistance, which may reduce the eradication rate. The success rates of esomeprazole treatment were significantly greater in patients over 65 years of age than in those under 65 years of age. Nonetheless, there was no significant difference in the clarithromycin resistance rates between the two groups receiving the new first-line triple therapy with vonoprazan (Saito et al., 2019), possibly due to the potent gastric acid inhibition by vonoprazan. However, another study from Japan showed that the eradication effectiveness of first-line triple therapy with amoxicillin, clarithromycin, and vonoprazan decreased with age, possibly due to reduced gastric acid secretion in older patients, reducing the need for maintenance therapy with vonoprazan (Kusunoki et al., 2019). In conclusion, the impact of age on H. pylori eradication is manifested through two primary mechanisms, namely, gastric acid secretion and premature exposure to antibiotic therapy. With an increasing age, there is a reduction in gastric acid secretion (Haruma et al., 2000). However, it is noteworthy that the predominant agents employed in the current first-line H. pylori treatment regimens often include PPIs. These agents exert a bactericidal effect by elevating the pH of gastric juice and synergizing with antibiotics (Scott et al., 1998).
In the older population, a frequent occurrence is an elevated incidence of gastric mucosal atrophy, which is coupled with diminished gastric acid secretion. Consequently, potent gastric acid inhibitors aimed at suppressing acid production are urgently needed. This context potentially elucidates the lack of discernible distinction between the efficacy of the novel gastric acid blocker vonoprazan and that of established agents, such as clarithromycin, metronidazole, or esomeprazole. Furthermore, it is important to highlight that insufficient eradication outcomes in young patients extend beyond merely premature antibiotic exposure. These suboptimal results are also intertwined with their personal habits and medication adherence (Shakya Shrestha et al., 2016).
7 Helicobacter pylori biofilm and efflux pump promoted resistance
There is a widespread consensus that the development of antibacterial resistance in H. pylori is linked to the complex physiological process of biofilm formation (Tshibangu-Kabamba and Yamaoka, 2021). H. pylori strategically forms biofilms as a defense mechanism against external factors such as pH and oxidative stress. These biofilms predominantly consist of static cell clusters enveloped by an extracellular matrix composed of proteins and extracellular DNA (Guzman-Soto et al., 2021). Stark provided the first evidence of the ability of H. pylori to create biofilms under specific conditions, particularly in supplemented 10% Brucella broth (Stark et al., 1999). The process of H. pylori biofilm formation encompasses stages such as bacterial adhesion, biofilm assembly, maturation, and dispersion. Within mature biofilms, the majority of bacterial cells assume a spherical shape, considered the dormant state of H. pylori, contributing to its resistance and disease induction potential (Elshenawi et al., 2023). H. pylori biofilms exhibit significantly greater antibacterial tolerance than their planktonic counterparts. Notably, the amoxicillin tolerance level was shown to be up to 1,000 times greater, while that to clarithromycin, levofloxacin, and metronidazole was 31, 16, and 8 times greater, respectively, in biofilms (Fauzia et al., 2020). However, the mechanism by which biofilms enhance H. pylori tolerance remains a subject of ongoing research. This phenomenon may be associated with the upregulation of genes involved in modifying the cell wall of H. pylori within the biofilm, such as UppS (Hathroubi et al., 2020; Rahman et al., 2020) and PdgA (Zhao et al., 2023).
Efflux pumps are a type of multidrug transporters found in the bacterial cell membrane (Hou et al., 2022). These pumps actively transport antibacterial drugs out of bacterial cells, thereby reducing the drug concentration within the cells and promoting drug resistance. In the case of H. pylori, efflux pumps play a central role in the development of multidrug resistance and mediate resistance to antibacterial agents such as amoxicillin, metronidazole, clarithromycin, and tetracycline (Cai et al., 2020). In clinical multidrug-resistant H. pylori strains, the expression levels of efflux pump genes are significantly increased. These efflux pump-encoding genes are notably upregulated within biofilms, further enhancing the tolerance of H. pylori to antibiotics (Ge et al., 2018). Yonezawa et al. demonstrated that the expression of outer membrane proteins from family 5 efflux pumps, including hefa (HP0605), hefd (HP0971), and hefG (HP1327), was significantly greater in biofilm-forming cells than in planktonic cells (Yonezawa et al., 2019). These findings confirm that efflux pumps can collaborate with biofilms to amplify drug resistance and play a pivotal role in enabling H. pylori to thrive within biofilms, which are unaffected by antibiotics.
While H. pylori cells residing within biofilms may shield themselves from antibacterial drugs and the immune system, the precise impacts of H. pylori biofilms on antibiotic resistance and immune system clearance in vivo remain to be fully elucidated. Existing evidence indicates that H. pylori can indeed form biofilms, and it has been observed that genes related to efflux pumps are upregulated within biofilms. However, the mechanisms through which biofilms influence efflux pumps remain unclear. Future research aimed at enhancing the efficacy of eradication therapy should prioritize exploring the yet uncharted role of biofilms in H. pylori infections.
8 New approaches
Drug resistance of H. pylori has a significant impact on therapeutic efficacy. Although continuous improvements of antibacterial drug combinations can make them effective in the short term, the efficacy tends to decline over time. This fact emphasizes that solving the problems of drug resistance and H. pylori eradication may be difficult if antimicrobial drugs continue to be the focus. It may be necessary to change the strategy and find a different approach to address this issue (Figure 5).
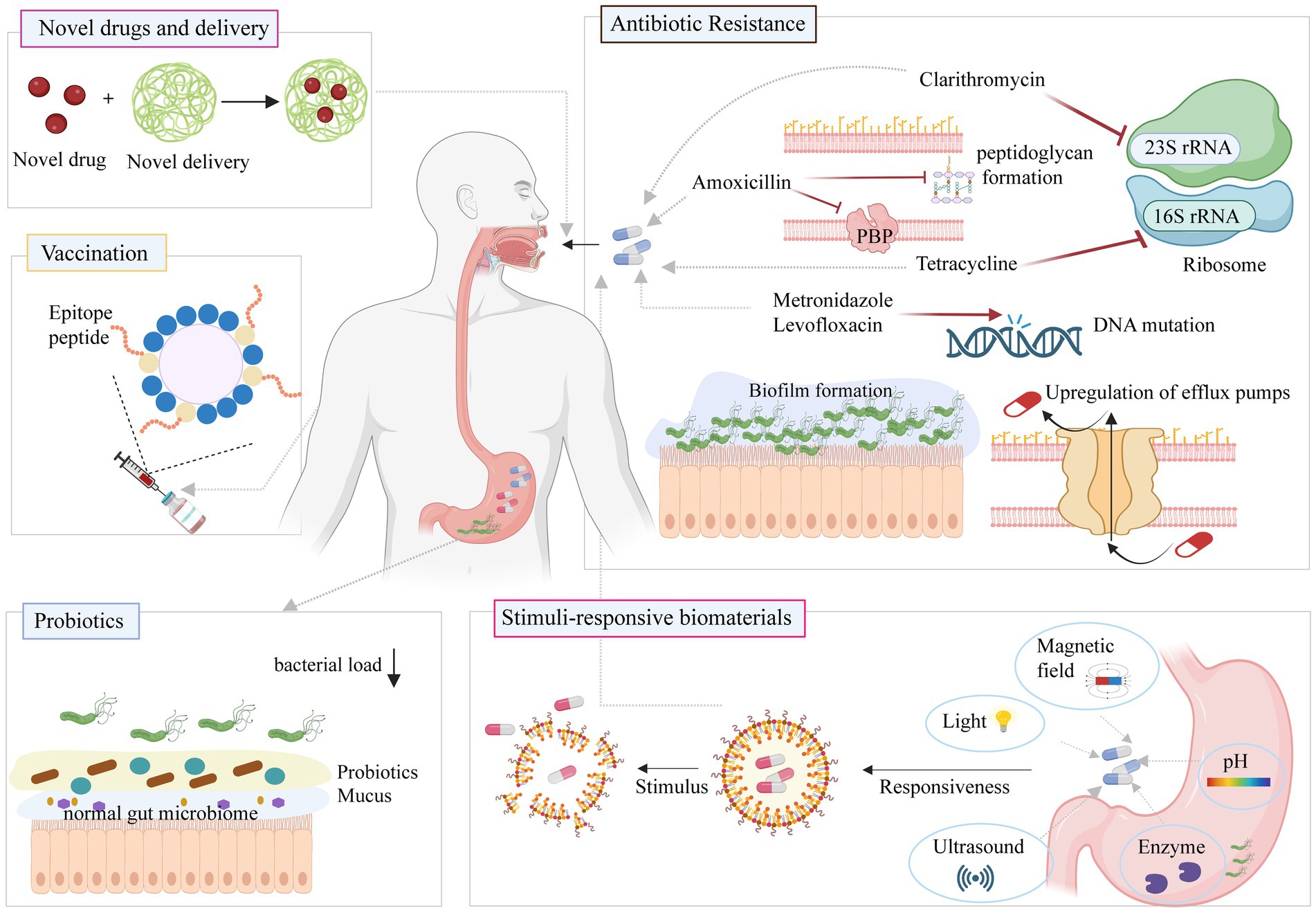
Figure 5. Classic and new approaches against H. pylori infection. Figure is created based on BioRender (BioRender.com).
8.1 Natural products
Eradication therapy for H. pylori remains a challenge. The development of new natural drugs continues to reduce side effects, improve patient compliance, and thus increase eradication rates (Hafeez et al., 2021). Natural products involving terpenoids, polyphenols, and alkaloids (Chen C. L. et al., 2023; Deng et al., 2024). Terpenoids like carvacrol disrupt bacterial membranes and reduce ATP synthesis, leading to bacterial death (Campestre et al., 2021). Polyphenols such as kaempferol inhibit reproductive enzymes, destabilize the cytoplasmic membrane, and regulate MAPK and NF-κB pathways to mitigate inflammatory damage (Bi et al., 2022). Alkaloids, such as coptisine, inhibit urease activity and disrupt cell membranes (Habimana et al., 2023). Combining plant foods with antibiotics enhances H. pylori eradication and offers potential for preventing associated gastric disorders (Liu et al., 2023).
Aminoglycosides such as gentamicin and netilmicin are currently considered new H. pylori eradication regimens. A gentamicin-intercalated montmorillonite hybrid remains in the gastric mucosal layer for up to 1 h, producing a local therapeutic effect against H. pylori, without side effects (Jeong et al., 2018). Animal experiments showed that topical aminoglycoside therapy with a montmorillonite delivery system was effective against H. pylori (Lee et al., 2019; Jeong et al., 2020). Tomokazu investigated the efficacy of intervenolin, a novel quinolone derivative, as a potential monotherapy for H. pylori infection. This study demonstrated that AS-1934 exhibited potent activity against various strains of H. pylori in vitro. In mice infected with H. pylori SS1, treatment with AS-1934 as a monotherapy resulted in a better eradication rate than treatment with triple therapy. Importantly, AS-1934 is stable at pH 2, but its activity could be blocked by a PPI (Ohishi et al., 2018).
8.2 Probiotic intervention
Compared with those in H. pylori-negative individuals, the main genera of gastric bacteria that were found to be abundant in H. pylori-positive individuals were Streptococcus, Neisseria, Prevosia, Roche, Clostridium, Vermicelli, and Haemophilus (Monstein et al., 2000; Bik et al., 2006). In addition to causing gastroduodenal disease, H. pylori infection may be involved in metabolic syndrome (Pellicano et al., 2020). Infection with H. pylori causes metabolic imbalances and disrupts host-gut microbiota interactions, leading to ecological dysregulation and triggering an inflammatory response (Behzadi et al., 2024). The metabolism of the intestinal microbiota is disrupted after H. pylori infection. The combination of Lactobacillus rhamnosus LGG-18 and Lactobacillus salivarius Chen-08 probiotics could reduce the severity of gastritis and inhibit precancerous lesions caused by H. pylori infection. However, it is clear that the supplementation with probiotics does not reduce the number of H. pylori cells (He et al., 2022a). After 14 days on bismuth-containing quadruple therapy combined with probiotics, 276 first-time H. pylori-positive patients had a lower incidence of gastrointestinal adverse events than did the placebo group (23.6% vs. 37.7%), but there was no significant difference in eradication rates (He et al., 2022b). The probiotic Miya-BM® was also used to assist in H. pylori eradication, and 468 H. pylori-positive patients were randomized to receive a PPI and amoxicillin or clarithromycin (PPI group); vonoprazan and amoxicillin or clarithromycin (vonoprazan group); or a PPI, amoxicillin or clarithromycin and the probiotic (probiotic group). The results showed no significant differences in adverse reactions among the three groups (Mukai et al., 2020). Fifty-six patients were randomly divided into quadruple therapy, probiotic supplement quadruple therapy, and probiotic monotherapy groups. The adjuvant probiotic therapy could partially help in the recovery of the gastric microbiota after H. pylori eradication; however, the results showed that the use of probiotics as a single eradication therapy not only had no benefit in patients infected with H. pylori but increased the possibility of potential infection with pathogenic bacteria such as Clostridium (Yuan et al., 2021). Several scholars have suggested combining inactive probiotics with triple therapy to eliminate H. pylori. To investigate the effectiveness of this approach, 200 untreated H. pylori-positive adult patients were randomly assigned to receive either inactive L. reuteri DSM 17648 (LR group) or a placebo for two weeks. After the initial two weeks, both groups underwent two weeks of triple therapy (esomeprazole, amoxicillin, and clarithromycin). The results showed that adding inactive L. reuteri DSM 17648 to triple therapy did not increase the eradication rate of H. pylori. Nevertheless, it helped establish a beneficial microbial spectrum and reduce side effects such as abdominal distension and diarrhea (Yang et al., 2021). Biofermin-R is a preparation of the multidrug-resistant lactic acid bacterium Enterococcus faecium 129 BIO 3B-R. When used in combination with vonoprazan, Biofermin-R can increase the eradication rate and help maintain species diversity in the intestinal microbiota (Kakiuchi et al., 2020). More research is needed to verify the effectiveness of this approach. Overall, the effectiveness of probiotic strains may depend not on their ability to colonize the gastrointestinal tract but rather on their ability to affect gene expression and metabolite levels and directly influence the intestinal barrier and immune cells. Although probiotics may play a role in eradicating H. pylori, further research is needed to confirm their effects.
8.3 Vaccination
Vaccination is a new strategy to address the rising problem of bacterial antibiotic resistance (Czinn and Blanchard, 2011; Dos Santos Viana et al., 2021). Vaccination is considered the best way to boost the production of secretory IgA (SIgA) in the stomach lining, which prevents bacterial colonization. H. pylori relies on virulence factors such as urease and heat shock protein A (HspA) to survive and colonize the stomach. Urease contains the UreA and UreB subunits, with UreB being a well-known antigen associated with H. pylori. Both subunits have been extensively studied for vaccines against H. pylori infection. Many promising H. pylori vaccine candidate antigens have been used, including UreB, VacA, CagA, HspA, neutrophil-activating protein (NAP), and rsLPS (Sutton and Boag, 2019; Yunle et al., 2024). Most of the vaccines are in the preclinical stage (Table 2). Paidamoyo modified Bacillus subtilis spores to present the protective antigens UreA and UreB from H. pylori on their surfaces. When these spore vaccines were orally administered to mice following H. pylori exposure, the H. pylori colony counts in the stomach decreased by approximately one log (Katsande et al., 2023). The immunogenicity and immunoprotection of the Shigella vector vaccine SH02 expressing an H. pylori UreB-HspA fusion protein were evaluated in a mouse model. The basic immunization consisted of either three oral administrations or two oral immunizations, followed by a third immunization involving a subcutaneous injection of rUreB-HspA for immune enhancement. Both approaches significantly reduced H. pylori colonization and gastric inflammation in mice (Zhang et al., 2022).
The EpiVax H. pylori vaccine, which is based on a multiepitope approach, is currently in the preclinical phase. This vaccine employs multiepitope DNA priming, followed by peptide boosting to enhance immunity. Notably, compared with that in mice that received either an empty plasmid or a complete H. pylori lysate intranasally as immunogens, H. pylori colonization was significantly reduced in mice that received this vaccine through intramuscular injection (Moss et al., 2011). Multivalent subunit vaccines that contain CagA, VacA, and NAP have proven effective in reducing H. pylori infections. Guo et al. actively contributed to the development of an H. pylori vaccine. They designed an immune adjuvant called NAP, along with three specific functional fragments (CagA 302–437, VacA 146, and VacA 332–494) derived from CagA and VacA. These components were incorporated into a multivalent epitope vaccine known as FVpE, which was derived from the urease multiepitope peptide fused with CTB. Oral therapeutic immunization with this vaccine reduced the numbers of H. pylori colonies in the stomachs of Mongolian gerbils (Gisbert and McNicholl, 2017; Guo et al., 2019).
Vaccines stimulating mucosal immune responses raise concerns when weakened viruses or pathogens are used because of the risk of virulence reversion. CD4+ T cells serve as crucial effector cells in the adaptive immune response against H. pylori. While this response is traditionally considered to be Th1-mediated, recent studies have introduced Th17 cell subsets into the context of H. pylori infections. Activation of both Th1 and Th17 cells leads to the production of cytokines such as IFN-γ, IL-17, and TNF-α, which collectively contribute to the defense against inflammatory H. pylori infections (Karkhah et al., 2019). Hence, their group enhanced the oral vaccine by creating a recombinant Lactococcus lactis vaccine, LL-plSAM-FVpE, which targets microfold cells more efficiently. The protective immunity provided by LL-plSAM-FVpE seems to be associated with specific sIgA and IgG antibodies, as well as with CD4+ T-cell responses against several important H. pylori virulence factors (such as urease, CagA, VacA, and NAP) (Guo L. et al., 2022). Further evaluation of this vaccine in animal models and clinical trials will be a research focus. Some scholars have suggested various methods, including targeting γ-glutamyl transpeptidase (Rossi et al., 2012), outer inflammatory protein A (Soudi et al., 2021), and H. pylori neutrophil-activating protein (Peng et al., 2018; Liu et al., 2020), for vaccine design. These studies need further experimental verification for the direct evaluation of H. pylori colonization and inflammation after treatment.
8.4 Stimulus-responsive biomaterials
Two main types of stimulus-responsive biological materials include those responsive to endogenous stimuli, such as pH and enzymes, and those responsive to exogenous stimuli, including magnetic fields, photodynamic forces, and ultrasound. An acidic gastric environment significantly influences the residence time and drug behavior of antibacterial agents. Jing et al. assessed pH-sensitive urea-conjugated chitosan/sodium tripolyphosphate nanoparticles specifically designed to combat H. pylori infections. The nanoparticles utilize urea as a targeted head group to enhance their affinity for H. pylori. Upon encapsulating amoxicillin at a concentration of 0.5 μg/mL within the nanoparticles and incubating them for 24 h, the inhibition rate against H. pylori exceeded 80%. However, further comprehensive in vivo experiments are necessary to validate the efficacy of these nanoparticles in eradicating H. pylori (Jing et al., 2016). Yang et al. employed magnetic field stimulation to prolong the gastric residence time of novel nanocarriers loaded with amoxicillin. These nanocarriers, composed of chitosan and polyacrylic acid, were specifically designed to deliver superparamagnetic iron oxide in response to an externally applied magnetic field. The rate of H. pylori eradication following oral administration of amoxicillin (33 mg/kg)-loaded nanoparticles, combined with 30 min of daily magnetic field treatment over a 7-day period reached 60%(Yang et al., 2020).
A cascade of potent oxidative molecules, including superoxide anions (O2−) and hydroxyl radicals (•OH), generated by exogenous reactive oxygen species (ROS), has the capacity to inflict damage upon bacterial lipids, proteins, and DNA, ultimately resulting in bacterial death (Hong et al., 2019). Yu et al. created a novel pH-responsive ROS nanogenerator, termed Fe-HMME@DHA@MPN. It comprises a metal–organic nanostructure (Fe-HMME) formed by coordinating the sonosensitizer hematoporphyrin monomethyl ether (HMME) with ferric iron. When loaded with dihydroartemisinin (DHA) as a hydroperoxidant, the nanogenerator has an acidic pH-sensitive metal shell. Following oral administration, it rapidly dissociates in the stomach and is activated by ultrasound. Then, DHA, which serves as a ROS source, is released through the Fenton reaction, generating •OH to kill H. pylori (Yu et al., 2023).
Zhou et al. developed a novel nanoparticle system capable of ultrasonically driven motion and ultrasonically excited afterglow emission (Zhou et al., 2022). Specifically, an enzyme-responsive polydopamine layer was applied to encapsulate the clarithromycin and luminescent particle complex, followed by fluorescence quenching. Upon oral administration, ultrasound activation of the nanoparticles facilitates their movement through mucus to the site of H. pylori infection. Bacterial secretion of phospholipase triggers the dissociation of responsive polydopamine layer, thereby releasing the encapsulated clarithromycin to eradicate the infection. In vivo experiments demonstrated that nanoparticle-mediated delivery of clarithromycin significantly reduced the bacterial burden at lower antibiotic doses, without causing notable surface degradation or erosion in the gastrointestinal tract.
8.5 Phage therapy
Phage therapy is a method of treating bacterial infections by employing phages, which are viruses that infect and reproduce within bacteria (Kushwaha et al., 2024). Once a bacterium is infected, the phage utilizes the bacterium’s metabolic machinery to replicate, ultimately leading to the bacterium’s lysis and the release of more phages, thereby exerting an anti-infective effect. There are two primary types of phages: virulent phages and temperate phages (Hatfull et al., 2022). Virulent phages infect the host bacteria rapidly, leading to bacterial lysis and death, making them suitable for rapidly clearing bacterial infections (Jonczyk-Matysiak et al., 2019). In contrast, temperate phages replicate more slowly and cause gentler bacterial killing, making them preferable for chronic bacterial infections with less inflammatory reaction and tissue damage (Hatfull et al., 2022).
In approximately 20% of H. pylori isolates, prophages like PhiHp33 from strain B45 are present (Munoz et al., 2020). However, the precise function of prophages remains unclear, and their link to disease has been scarcely explored. In 2008, a 100 nm-sized phage was isolated from human feces but wasn’t further investigated (Munoz et al., 2020). In 2013, two lysogenic phages (ΦHPE1 and ΦHPE2) were identified in wastewater, belonging to the Podoviridae and Siphoviridae families, yet their antimicrobial potential was not evaluated (Abdel-Haliem and Askora, 2013). Recently, H. pylori (Hp φ) was isolated from gastric biopsies-bound lactoferrin and adsorbed on hydroxyapatite nanoparticles, which effectively reduced bacterial colonization and inflammatory damage (Cuomo et al., 2020).
Phages show promise as a therapeutic avenue for H. pylori, yet the field remains in its early stages, with the phage genome sequence yet to be determined. Moving forward, efforts should be directed toward identifying and designing phages specifically targeting H. pylori.
8.6 Antimicrobial peptides
Due to the escalating issue of antibiotic resistance, antimicrobial peptides are emerging as an alternative therapy to antibiotics for combating bacterial infections. These peptides, known as natural antimicrobial peptides (AMPs), are innate immune molecules produced in nearly all organisms (Kumar et al., 2018). Predominantly of eukaryotic origin, AMPs exhibit direct bactericidal activity. Typically composed of 10–100 amino acids, AMPs are characterized as cationic or amphoteric (Mohaideen et al., 2023). They act by interacting with the negatively charged head groups of phospholipids on the cell membranes of both Gram-positive and Gram-negative microorganisms through electrostatic forces. This interaction results in the formation of pores or channels, disrupting the cell membrane and ultimately leading to cell lysis. Additionally, the phospholipids of Gram-positive microorganisms and the lipopolysaccharides of Gram-negative bacteria continuously provide negative charges to the bacterial surface, thereby further enhancing the interaction with AMPs (Mahlapuu et al., 2020).
Natural antimicrobial peptides exhibit instability in the gastrointestinal tract, poor absorption, distribution, and rapid excretion, leading to low bioavailability (Kumar et al., 2018). Xiong et al. synthesized pH-sensitive antimicrobial peptides with a transformable helix–helix conformation, demonstrating low toxicity at pH 6–8 and potent antimicrobial activity against H. pylori SS1 and drug-resistant strains at pH 2. Orally administered to C57BL/6 J mice, these peptides achieved a comparable eradication rate of H. pylori SS1 to the positive control triple drugs, with reduced side effects on intestinal bacteria.(Xiong et al., 2017). Fonseca et al. developed a novel microfluidic system for AMP-nanoparticles that is stable and exhibits rapid bactericidal activity in acidic environments. This microfluidic device is simple, quick, minimally toxic to cells, and shows great potential for in vivo experiments to validate its effectiveness in eradicating H. pylori intragastric colonization (Fonseca et al., 2024). Zhang et al. designed genetically encoded H. pylori-responsive bactericidal protein crystals to improve the bioavailability of antimicrobial peptide (LL-37) in the stomach. Oral administration of the LL-37 significantly decreased gastric colonization of H. pylori in mice, mitigated inflammatory injury, and had no impact on the intestinal microbiota (Zhang W. et al., 2023).
Antimicrobial peptides hold promise for treating infections, but face challenges in clinical application. The stomach’s acidity and GI tract enzymes degrade orally administered peptides. Further research is needed to understand their mechanisms, improve chemical properties, enhance stability, and reduce manufacturing costs. Increased investment in AMP-based drug development is necessary to advance medical practice.
8.7 Alteration of microaerobic environment
H. pylori has strict oxygen requirements. The optimal oxygen concentration range in air is 5 to 8% (Dai et al., 2022), which is equal to 1.7 to 2.0 mg/L in aqueous solutions at 37°C. When the oxygen concentration in the environment is outside this range, H. pylori cannot survive. We carried out a series of experiments that showed a good eradication effect of altering the oxygen concentration on H. pylori (Di et al., 2020). The addition of 0.02 to 2.0 mg/mL Hydrogen peroxide increased the oxygen content in an aqueous solution (or rabbit gastric fluid) 1.2 to 3.9 (4.2 to 5.2) times, from 6.7 (3.6) μg/mL to between 7.8 and 26.0 (15.2 to 18.7) g/mL, respectively. In Columbia blood agar with H. pylori special peptone, hydrogen peroxide significantly inhibited the growth of H. pylori. The inhibition potency of hydrogen peroxide was greater than that of colloidal bismuth subcitrate. Furthermore, similar to other antibacterial drugs, colloidal bismuth subcitrate easily promoted the development of resistant strains of H. pylori, which sharply increased the MIC and minimum bactericidal concentration of the antibacterial drug. Hydrogen peroxide did not easily promote resistance of H. pylori. In vivo experimental results showed no H. pylori colonization in the stomachs of Mongolian gerbils in the hydrogen peroxide group, suggesting that the killing effect of hydrogen peroxide on H. pylori was greater than that of triple drugs (Di et al., 2020). A low concentration of hydrogen peroxide does not cause gastric injury or inflammatory reactions and has no significant toxicity.
Donelli et al. (1998) confirmed that when the oxygen concentration was reduced to 4%, cultured H. pylori could still reproduce, and the number of bacterial cells increased with time. However, when the oxygen concentration was reduced to 2–3%, H. pylori did not reproduce, and the number of bacterial cells decreased with time. Moreover, when the oxygen concentration was reduced to 0–1%, the number of bacterial cells decreased by 90% after 24 h of culture, and all cells died within 7 days. Using this strategy, we recently reduced the oxygen level in water by adding vitamin C (a reducing agent) to reduce the oxygen concentration in gastric juice and showed that this resulted in an anti-H. pylori effect. It was reported that vitamin C could increase the clearance rate of H. pylori after antibacterial treatment (Hussain et al., 2018; Cai et al., 2021), which supports our results, but the effect was still unsatisfactory. If strongly reducing drugs are used to consume oxygen in gastric juice so that the oxygen concentration is greatly reduced or oxygen is eliminated, H. pylori will inevitably fail to survive, and its eradication will be achieved. Sodium sulfite is a potent reducing agent that reacts with dissolved oxygen in aqueous solutions, effectively depleting oxygen. It is a food additive that restricts bacterial contamination and can be safely consumed at concentrations up to 5000 ppm (Irwin et al., 2017). Our latest results showed that 1, 3, and 10 mg/mL sodium sulfite lowered the concentration of oxygen in rabbit gastric juice from 2 to 0.2 mg/L. Sodium sulfite significantly inhibited the growth of H. pylori (ATCC 43504 and SS1 strains) in both solid and liquid cultures in vitro. Subsequently, we used the Mongolian gerbil infection model and confirmed the superior efficacy of sodium sulfite in eradicating H. pylori. Additionally, in mice, we showed a lower recurrence rate with sodium sulfite than with triple control drugs following the eradication of H. pylori. We also conducted acute and long-term toxicity experiments, which revealed that the LD50 of sodium sulfite in Kunming mice was 6.8 g/kg. After 6 weeks of continuous administration to rats, no significant differences in blood biochemical indicators, the body weight, or food intake were detected between the 0.3 g/kg sodium sulfite group and the control group (Huang et al., 2023).
The Hcp protein is a cysteine-rich Helicobacter protein. HcpE contains 19 cysteines and is the most prominent member of the Hcp family (Dumrese et al., 2009). Mittl et al. verified an HcpE IgG antibody in patients with H. pylori infection and confirmed that the Hcp protein was specific to H. pylori, which makes it a potential therapeutic target (Mittl et al., 2003). Lester et al. demonstrated that HcpE acted as a substrate for a dimeric oxidoreductase (HP0231), which transfers electrons to the respiratory chain for reoxidation in the H. pylori oxidation pathway (Grzeszczuk et al., 2018). Knocking out HP0231 resulted in a decreased ability of H. pylori to maintain redox homeostasis (Lester et al., 2015). H. pylori adjusts its metabolism in response to hypoxic conditions. Under normal oxygen levels, it relies on microaerobic respiration for energy production (Kelly et al., 2001). In a hypoxic state, microaerophilic respiration is blocked in H. pylori, resulting in a sharp decrease in the ATP content and significant downregulation of the HcpE protein and antioxidant/reducing steady-state enzymes (catalase and superoxide dismutase). This leads to an increase in the ROS content, damaging the bacterial structure and eventually causing bacterial death due to energy exhaustion and structural destruction. Thus, regulating the oxygen environment for H. pylori growth may have antibacterial effects, with HcpE identified as a potential target (Huang et al., 2024).
Overall, altering the oxygen concentration in the gastric solution is an innovative and intriguing approach for eradicating H. pylori. This has shifted our focus away from antimicrobials and combinations of drugs. This concept may serve as a valuable source of inspiration for future H. pylori treatments.
8.8 Agonists and antagonists in anti-H. pylori infection
In addition to devising novel strategies for directly eradicating H. pylori, researches have also been toward counteracting the pathogenesis of H. pylori infection. When persistently infected with H. pylori, gastric mucosal epithelial cells (GES-1) exhibit reduced sensitivity of Toll-like receptor (TLR) 6 to H. pylori components, resulting in decreased expression of IL-1β and IL-8. Treatment with Pam2CSK4, a TLR6 agonist, restores TLR6 expression and reduces H. pylori colonization in gerbil gastric mucosa (Zhang et al., 2024). SHP1, the only homolog of the tyrosine phosphatase SHP2 expressed in gastric epithelial cells, negatively regulates the H. pylori virulence factor CagA (Saju et al., 2016). It inhibits H. pylori-induced migration and invasion of GES-1 and gastric cancer cells. Sorafenib, acting as an SHP1 agonist, inhibits H. pylori-induced proliferation and metastasis (Chen S. et al., 2024). A2BR, a functional protein in gastric mucosal wall cells, activates the p38MAPK pathway and exacerbates oxidative stress. Treatment with BAY60-6583 (an A2BR agonist) exacerbates gastric ulceration in rats, while PSB1115 (an A2BR antagonist) has the opposite effect. Incubation of GES-1 cells with agonists increased apoptosis, migration, and oxidative stress, effects that are reversed by inhibitors of the p38MAPK pathway (Tang et al., 2023). In summary, combating H. pylori infection can be approached from various angles, and agents such as A2BR antagonists, TLR6 agonists, and SHP1 agonists hold promise as novel therapeutic strategies against H. pylori infection in the future.
9 Conclusions and future perspectives
Antibacterial drugs show great therapeutic effects against H. pylori infection, but sometimes, they are not sufficient to eradicate H. pylori. In the past decade, approximately 10–30% of all first-and second-line eradication therapies recommended by global treatment guidelines have failed, which led to the development of triple therapy. Quadruple therapy, which involves adding another antimicrobial or replacing it with an acid inhibitor, was developed to overcome this problem. The wide range of antimicrobial agents extensively used for the eradication of H. pylori has led to an increase in drug resistance over time (Tshibangu-Kabamba and Yamaoka, 2021). As a result, retreatment and subsequent cure have become more difficult (Thung et al., 2016; Malfertheiner et al., 2017). To address this issue, new drugs and treatment strategies are necessary.
Notwithstanding, further study of the mechanisms of drug resistance in H. pylori is essential. Whole-genome sequencing predicted resistance in H. pylori and showed that antimicrobial resistance is primarily due to point mutations in the genome. For example, clarithromycin resistance in H. pylori is strongly associated with the presence of the A2146C, A2146G, or A2147G mutations in exon V of the 23S rRNA gene (Lauener et al., 2019). Mutations G94E in the pbp1A gene, C2173T and G2212A in the 23S rRNA gene, T239M in the gyrA gene, G122R in the rdxA gene, and A70T and A138V in the frxA gene have been found only in drug-resistant strains (Domanovich-Asor et al., 2020). The pbp1A gene mutation is a major factor causing amoxicillin resistance, while levofloxacin resistance may be mainly attributed to mutations in single or dual genes encoding DNA gyrase subunits A and B (gyrA and gyrB) in the quinolone resistance-determining region (QRDR). Mutations at codons 87 (N to K, I, or T) and 91 (D to Y, G, or H) in the QRDR of gyrA were found in 91% of resistant strains (Tshibangu-Kabamba et al., 2020). Although these studies have some value, it should be noted that gene mutations in H. pylori are not fixed in patients from different regions and some patients may have dual or multiple resistance. Therefore, the whole-genome sequencing results may not provide a representative conclusion.
Furthermore, new therapeutic strategies can be developed. In addition to traditional combination therapies, such as concomitant and sequential therapies, probiotics and vaccines have currently become popular new therapies. Probiotics can inhibit H. pylori colonization and produce antibacterial substances (Song et al., 2018). The eradication rate of H. pylori by using probiotics combined with the triple regimen was lower than that of the bismuth quadruple regimen, indicating that bismuth is irreplaceable and can inhibit the growth of probiotics. The acidic environment of the stomach may also affect the effect of probiotics. The eradication effects of different probiotics vary with treatment duration. Therefore, more detailed studies are needed on the use of probiotics as adjuvant therapy. An H. pylori vaccine was shown to be effective at preventing H. pylori infection (Zeng et al., 2015). However, one of the concerns regarding the treatment of H. pylori infection is whether the antibodies produced in the body can travel smoothly into the gut and not be destroyed by gastric acid or pepsin. Further evaluation is needed to confirm the long-term effectiveness of vaccines. Research on stimuli-responsive materials has shown promising potential against H. pylori; yet this field remains in its nascent stage. Responsive biomaterials pose challenges, including their complex compositions, intricate manufacturing processes, elevated costs, and complex treatment procedures. Future investigations should prioritize comprehensive in vivo verification of H. pylori clearance and further enhancement of biomaterial properties. Phage and antimicrobial peptide therapies are emerging for H. pylori eradication but are still in the early stages of development. Their antimicrobial mechanisms and effectiveness compared to first-line drugs require further investigation through in vivo experiments and preclinical validation.
A different path may lead to better results. Because a strictly microaerophilic environment is suitable for survival of H. pylori, a completely novel way to change the oxygen concentration that is required for the growth of H. pylori to a level at which H. pylori cannot survive (Di et al., 2020). has been proposed. Under normal microaerobic conditions in the stomach, H. pylori infection prompts the bacterium to employ various survival mechanisms. Upon reaching the mucus layer, urease aids in resisting the acidic gastric environment, while flagella facilitate movement toward mucosal epithelial cells for colonization and the release of pathogenic factors (such as NapA, CagA, and VacA) (Figure 6). However, in the hypoxic stomach environment, bacterial cells outside the mucus layer struggle to respire and metabolize because of limited oxygen availability. This leads to a sharp reduction in energy levels, causing a transition from the active rod-shaped forms to globular shapes, which is indicative of the dormancy status or eventual death. With diminished flagella and urease activities, bacterial cells are unable to penetrate the mucus layer and to colonize epithelial cells (Figure 6). The proposed method can efficiently eradicate H. pylori without generating drug resistance. This kind of clearance does not require drugs to enter cells and is easy to achieve. This strategy may be a good therapeutic option for eradicating H. pylori in the future.
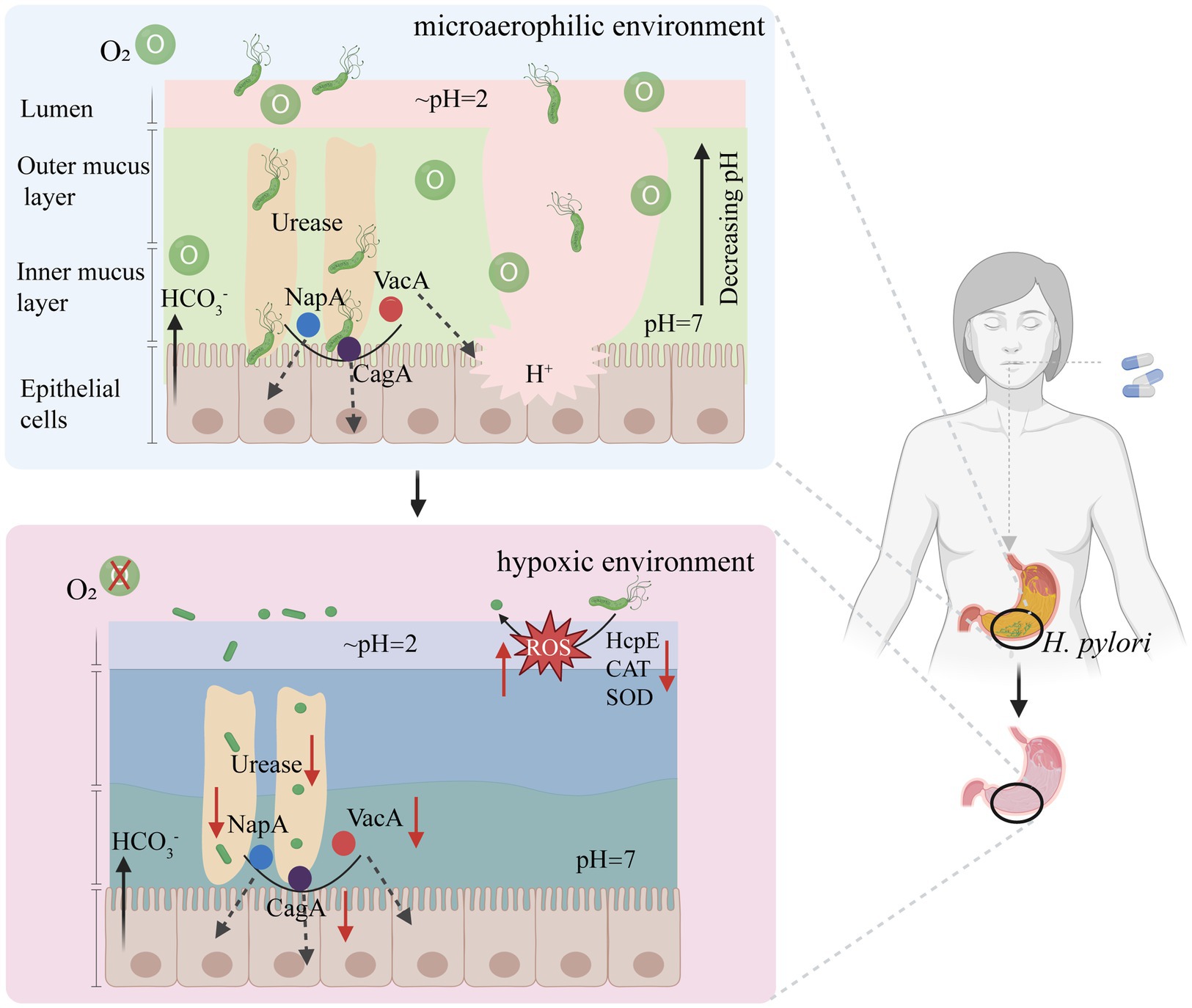
Figure 6. A new approach to eradicate H. pylori based on reducing intragastric oxygen concentration. HcpE, Helicobacter cysteine-rich protein; VacA, vacuolating toxin A; CagA, Cytotoxin-associated-gene A; NapA, neutrophil-activating protein A subunit; CAT, Catalase; SOD, Superoxide dismutase. Figure is created based on BioRender (BioRender.com).
Author contributions
T-TH: Data curation, Formal analysis, Investigation, Methodology, Software, Visualization, Writing – original draft, Writing – review & editing. Y-XC: Conceptualization, Project administration, Supervision, Validation, Writing – original draft, Writing – review & editing. LC: Conceptualization, Data curation, Formal analysis, Investigation, Project administration, Resources, Software, Supervision, Writing – original draft, Writing – review & editing.
Funding
The author(s) declare that no financial support was received for the research, authorship, and/or publication of this article.
Conflict of interest
The authors declare that the research was conducted in the absence of any commercial or financial relationships that could be construed as a potential conflict of interest.
Publisher’s note
All claims expressed in this article are solely those of the authors and do not necessarily represent those of their affiliated organizations, or those of the publisher, the editors and the reviewers. Any product that may be evaluated in this article, or claim that may be made by its manufacturer, is not guaranteed or endorsed by the publisher.
References
Abdel-Haliem, M. E. F., and Askora, A. (2013). Isolation and characterization of bacteriophages of Helicobacter pylori isolated from Egypt. Future Virol. 8, 821–826. doi: 10.2217/fvl.13.58
Allen, M. G., Bate, M. Y., Tramonte, L. M., Avalos, E. Y., Loh, J., Cover, T. L., et al. (2023). Regulation of Helicobacter pylori urease and acetone carboxylase genes by nitric oxide and the CrdRS two-component system. Microbiol. Spectr. 11:e0463322. doi: 10.1128/spectrum.04633-22
Alsulaimany, F. A. S., Awan, Z. A., Almohamady, A. M., Koumu, M. I., Yaghmoor, B. E., Elhady, S. S., et al. (2020). Prevalence of Helicobacter pylori infection and diagnostic methods in the Middle East and North Africa region. Medicina (Kaunas) 56:169. doi: 10.3390/medicina56040169
Apostolopoulos, P., Ekmektzoglou, K., Georgopoulos, S., Chounta, E., Theofanopoulou, A., Kalantzis, C., et al. (2020). 10-Day versus 14-day quadruple concomitant nonbismuth therapy for the treatment of Helicobacter pylori infection: results from a randomized prospective study in a high clarithromycin resistance country. J. Clin. Gastroenterol. 54, 522–527. doi: 10.1097/MCG.0000000000001328
Arjmandi, D., Abdollahi, A., Ardekani, A., Razavian, I., Razavian, E., Sartip, B., et al. (2022). Helicobacter pylori infection and risk of multiple sclerosis: an updated meta-analysis. Helicobacter 27:e12927. doi: 10.1111/hel.12927
Asimellis, G., Hamilton, S., Giannoudakos, A., and Kompitsas, M. (2005). Controlled inert gas environment for enhanced chlorine and fluorine detection in the visible and near-infrared by laser-induced breakdown spectroscopy. Spectrochimica Acta Part B-Atomic Spectroscopy 60, 1132–1139. doi: 10.1016/j.sab.2005.05.035
Ayana, S. M., Swai, B., Maro, V. P., and Kibiki, G. S. (2014). Upper gastrointestinal endoscopic findings and prevalence of Helicobacter pylori infection among adult patients with dyspepsia in northern Tanzania. Tanzan. J. Health Res. 16, 16–22. doi: 10.4314/thrb.v16i1.3
Behzadi, P., Dodero, V. I., and Golubnitschaja, O. (2024). “Systemic inflammation as the health-related communication tool between the human host and gut microbiota in the framework of predictive, preventive, and personalized medicine” in All around suboptimal health: Advanced approaches by predictive, preventive and personalised medicine for healthy populations. ed. W. Wang (Cham: Springer Nature Switzerland), 203–241.
Bhattacharjee, A., Sahoo, O. S., Sarkar, A., Bhattacharya, S., Chowdhury, R., Kar, S., et al. (2024). Infiltration to infection: key virulence players of Helicobacter pylori pathogenicity. Infection 52, 345–384. doi: 10.1007/s15010-023-02159-9
Bi, H., Chen, X., Chen, Y., Zhao, X., Wang, S., Wang, J., et al. (2022). Efficacy and safety of high-dose esomeprazole-amoxicillin dual therapy for Helicobacter pylori rescue treatment: a multicenter, prospective, randomized, controlled trial. Chin. Med. J. 135, 1707–1715. doi: 10.1097/CM9.0000000000002289
Bik, E. M., Eckburg, P. B., Gill, S. R., Nelson, K. E., Purdom, E. A., Francois, F., et al. (2006). Molecular analysis of the bacterial microbiota in the human stomach. Proc. Natl. Acad. Sci. USA 103, 732–737. doi: 10.1073/pnas.0506655103
Bordin, D., Morozov, S., Plavnik, R., Bakulina, N., Voynovan, I., Skibo, I., et al. (2022). Helicobacter pylori infection prevalence in ambulatory settings in 2017-2019 in RUSSIA: the data of real-world national multicenter trial. Helicobacter 27:e12924. doi: 10.1111/hel.12924
Borka Balas, R., Melit, L. E., and Marginean, C. O. (2023). Current worldwide trends in pediatric Helicobacter pylori antimicrobial resistance. Children (Basel) 10:403. doi: 10.3390/children10020403
Botija, G., Garcia Rodriguez, C., Recio Linares, A., Campelo Gutierrez, C., Perez-Fernandez, E., and Barrio Merino, A. (2021). Antibiotic resistances and eradication rates in Helicobacter pylori infection. An Pediatr. (Engl Ed) 95, 431–437. doi: 10.1016/j.anpede.2020.10.010
Boyanova, L., Hadzhiyski, P., Gergova, R., and Markovska, R. (2023). Evolution of Helicobacter pylori resistance to antibiotics: a topic of increasing concern. Antibiotics (Basel) 12:332. doi: 10.3390/antibiotics12020332
Boyanova, L., Markovska, R., Hadzhiyski, P., Kandilarov, N., and Mitov, I. (2020). Rifamycin use for treatment of Helicobacter pylori infection: a review of recent data. Future Microbiol. 15, 1185–1196. doi: 10.2217/fmb-2020-0084
Bujanda, L., Nyssen, O. P., Ramos, J., Bordin, D. S., Tepes, B., Perez-Aisa, A., et al. (2024). Effectiveness of Helicobacter pylori treatments according to antibiotic resistance. Am. J. Gastroenterol. 119, 646–654. doi: 10.14309/ajg.0000000000002600
Cai, X., Li, X., Jin, Y., Zhang, M., Xu, Y., Liang, C., et al. (2021). Vitamins and Helicobacter pylori: an updated comprehensive Meta-analysis and systematic review. Front. Nutr. 8:781333. doi: 10.3389/fnut.2021.781333
Cai, Y., Wang, C., Chen, Z., Xu, Z., Li, H., Li, W., et al. (2020). Transporters HP0939, HP0497, and HP0471 participate in intrinsic multidrug resistance and biofilm formation in Helicobacter pylori by enhancing drug efflux. Helicobacter 25:e12715. doi: 10.1111/hel.12715
Campestre, C., De Luca, V., Carradori, S., Grande, R., Carginale, V., Scaloni, A., et al. (2021). Carbonic anhydrases: new perspectives on protein functional role and inhibition in Helicobacter pylori. Front. Microbiol. 12:629163. doi: 10.3389/fmicb.2021.629163
Chaleshtori, Z. A., Rastegari, A. A., Nayeri, H., and Doosti, A. (2024). Chitosan-LeoA-DNA nanoparticles promoted the efficacy of novel LeoA-DNA vaccination on mice against Helicobacter pylori. Curr. Microbiol. 81:125. doi: 10.1007/s00284-024-03642-8
Chang, A., Chang, A., Chen, W. T., Chan, L., Hong, C. T., and Chien, L. N. (2023). Triple therapy for Helicobacter pylori eradication and the risk of hypoglycemia in patients with diabetes: a population-based cohort study. BMC Public Health 23:1772. doi: 10.1186/s12889-023-16689-2
Chen, P., Chen, M., Peng, C., Yan, J., Shen, X., Zhang, W., et al. (2024). In vitro anti-bactrical activity and its preliminary mechanism of action of the non-medicinal parts of Sanguisorba officinalis L. against Helicobacter pylori infection. J. Ethnopharmacol. 318:116981. doi: 10.1016/j.jep.2023.116981
Chen, J., Li, P., Huang, Y., Guo, Y., Ding, Z., and Lu, H. (2022). Primary antibiotic resistance of Helicobacter pylori in different regions of China: a systematic review and meta-analysis. Pathogens 11:786. doi: 10.3390/pathogens11070786
Chen, Y. C., Malfertheiner, P., Yu, H. T., Kuo, C. L., Chang, Y. Y., Meng, F. T., et al. (2024). Global prevalence of Helicobacter pylori infection and incidence of gastric Cancer between 1980 and 2022. Gastroenterology 166, 605–619. doi: 10.1053/j.gastro.2023.12.022
Chen, X., Shan, T., Ren, B., Zhang, L., Xu, H. H. K., Wang, N., et al. (2023). Dimethylaminododecyl methacrylate-incorporated dental materials could be the first line of defense against Helicobacter pylori. Int. J. Mol. Sci. 24:644. doi: 10.3390/ijms241713644
Chen, C. L., Wu, I. T., Wu, D. C., Lei, W. Y., Tsay, F. W., Chuah, S. K., et al. (2023). Independent risk factors predicting eradication failure of hybrid therapy for the first-line treatment of Helicobacter pylori infection. Microorganisms 12:10006. doi: 10.3390/microorganisms12010006
Chen, B. F., Xu, X., Deng, Y., Ma, S. C., Tang, L. Q., Zhang, S. B., et al. (2013). Relationship between Helicobacter pylori infection and serum interleukin-18 in patients with carotid atherosclerosis. Helicobacter 18, 124–128. doi: 10.1111/hel.12014
Chen, J., Ye, L., Jin, L., Xu, X., Xu, P., Wang, X., et al. (2018). Application of next-generation sequencing to characterize novel mutations in clarithromycin-susceptible Helicobacter pylori strains with A2143G of 23S rRNA gene. Ann. Clin. Microbiol. Antimicrob. 17:10. doi: 10.1186/s12941-018-0259-8
Chen, Y., Yuan, H., Ye, H., Shi, Z., Deng, X., Zhang, X., et al. (2021). Application of a semi-automatic, intensive follow-up for improving efficacy and adherence of Helicobacter pylori eradication therapy: a randomized controlled trial. Microbiology 10:e1172. doi: 10.1002/mbo3.1172
Chen, S., Zhao, H., Tian, Y., Wu, Q., Zhang, J., Liu, S., et al. (2024). Antagonizing roles of SHP1 in the pathogenesis of Helicobacter pylori infection. Helicobacter 29:e13066. doi: 10.1111/hel.13066
Chey, W. D., Leontiadis, G. I., Howden, C. W., and Moss, S. F. (2018). Correction: ACG clinical guideline: treatment of Helicobacter pylori infection. Am. J. Gastroenterol. 113:1102. doi: 10.1038/s41395-018-0132-6
Chua, B. Q. Y., Chong, V. W. S., Teng, T. Z. J., Chia, C. T. W., Aung, M. O., and Shelat, V. G. (2022). Does technology-enhanced communication improve Helicobacter pylori eradication outcomes?-a meta-analysis. Helicobacter 27:e12890. doi: 10.1111/hel.12890
Cover, T. L., and Blanke, S. R. (2005). Helicobacter pylori VacA, a paradigm for toxin multifunctionality. Nat. Rev. Microbiol. 3, 320–332. doi: 10.1038/nrmicro1095
Cuomo, P., Papaianni, M., Fulgione, A., Guerra, F., Capparelli, R., and Medaglia, C. (2020). An innovative approach to control H. pylori-induced persistent inflammation and colonization. Microorganisms 8:1214. doi: 10.3390/microorganisms8081214
Czinn, S. J., and Blanchard, T. (2011). Vaccinating against Helicobacter pylori infection. Nat. Rev. Gastroenterol. Hepatol. 8, 133–140. doi: 10.1038/nrgastro.2011.1
Dai, J., Zhao, J., Mao, L., Hu, Y., and Lv, B. (2022). Study on the value of antibiotic-resistant gene detection in Helicobacter pylori in China. Exp. Ther. Med. 23:228. doi: 10.3892/etm.2022.11153
Deng, R., Chen, X., Zhao, S., Zhang, Q., and Shi, Y. (2024). The effects and mechanisms of natural products on Helicobacter pylori eradication. Front. Cell. Infect. Microbiol. 14:1360852. doi: 10.3389/fcimb.2024.1360852
Di, J., Zhang, J., Cao, L., Huang, T. T., Zhang, J. X., Mi, Y. N., et al. (2020). Hydrogen peroxide-mediated oxygen enrichment eradicates Helicobacter pylori in vitro and in vivo. Antimicrob. Agents Chemother. 64:2192. doi: 10.1128/AAC.02192-19
Domanovich-Asor, T., Motro, Y., Khalfin, B., Craddock, H. A., Peretz, A., and Moran-Gilad, J. (2020). Genomic analysis of antimicrobial resistance genotype-to-phenotype agreement in Helicobacter pylori. Microorganisms 9:2. doi: 10.3390/microorganisms9010002
Donelli, G., Matarrese, P., Fiorentini, C., Dainelli, B., Taraborelli, T., Di Campli, E., et al. (1998). The effect of oxygen on the growth and cell morphology of Helicobacter pylori. FEMS Microbiol. Lett. 168, 9–15. doi: 10.1111/j.1574-6968.1998.tb13248.x
Doohan, D., Rezkitha, Y. A. A., Waskito, L. A., Yamaoka, Y., and Miftahussurur, M. (2021). Helicobacter pylori BabA-SabA key roles in the adherence phase: the synergic mechanism for successful colonization and disease development. Toxins (Basel) 13:485. doi: 10.3390/toxins13070485
Dos Santos Viana, I., Cordeiro Santos, M. L., Santos Marques, H., de Souza, L., Goncalves, V., Bittencourt de Brito, B., et al. (2021). Vaccine development against Helicobacter pylori: from ideal antigens to the current landscape. Expert Rev. Vaccines 20, 989–999. doi: 10.1080/14760584.2021.1945450
Doulberis, M., Papaefthymiou, A., Polyzos, S. A., Bargiotas, P., Liatsos, C., Srivastava, D. S., et al. (2020). Association between active Helicobacter pylori infection and Glaucoma: a systematic review and Meta-analysis. Microorganisms 8:894. doi: 10.3390/microorganisms8060894
Dumrese, C., Slomianka, L., Ziegler, U., Choi, S. S., Kalia, A., Fulurija, A., et al. (2009). The secreted Helicobacter cysteine-rich protein a causes adherence of human monocytes and differentiation into a macrophage-like phenotype. FEBS Lett. 583, 1637–1643. doi: 10.1016/j.febslet.2009.04.027
Durazzo, M., Adriani, A., Fagoonee, S., Saracco, G. M., and Pellicano, R. (2021). Helicobacter pylori and respiratory diseases: 2021 update. Microorganisms 9:2033. doi: 10.3390/microorganisms9102033
Elbehiry, A., Marzouk, E., Aldubaib, M., Abalkhail, A., Anagreyyah, S., Anajirih, N., et al. (2023). Helicobacter pylori infection: current status and future prospects on diagnostic, therapeutic and control challenges. Antibiotics (Basel) 12:191. doi: 10.3390/antibiotics12020191
Elshenawi, Y., Hu, S., and Hathroubi, S. (2023). Biofilm of Helicobacter pylori: life cycle, features, and treatment options. Antibiotics (Basel) 12:1260. doi: 10.3390/antibiotics12081260
Espinosa-Ramos, D., Caballero-Hernandez, D., Gomez-Flores, R., Trejo-Chavez, A., Perez-Limon, L. J., de la Garza-Ramos, M. A., et al. (2019). Immunization with a synthetic Helicobacter pylori peptide induces secretory IgA antibodies and protects mice against infection. Can. J. Infect. Dis. Med. Microbiol. 2019:8595487. doi: 10.1155/2019/8595487
Fauzia, K. A., Miftahussurur, M., Syam, A. F., Waskito, L. A., Doohan, D., Rezkitha, Y. A. A., et al. (2020). Biofilm formation and antibiotic resistance phenotype of Helicobacter pylori clinical isolates. Toxins (Basel) 12:473. doi: 10.3390/toxins12080473
Fischbach, W., and Malfertheiner, P. (2018). Helicobacter pylori infection. Dtsch. Arztebl. Int. 115, 429–436. doi: 10.3238/arztebl.2018.0429
Fonseca, D. R., Alves, P. M., Neto, E., Custodio, B., Guimaraes, S., Moura, D., et al. (2024). One-pot microfluidics to engineer chitosan nanoparticles conjugated with antimicrobial peptides using "Photoclick" chemistry: validation using the gastric bacterium Helicobacter pylori. ACS Appl. Mater. Interfaces 16, 14533–14547. doi: 10.1021/acsami.3c18772
Galal, Y. S., Ghobrial, C. M., Labib, J. R., and Abou-Zekri, M. E. (2019). Helicobacter pylori among symptomatic Egyptian children: prevalence, risk factors, and effect on growth. J. Egypt. Public Health Assoc. 94:17. doi: 10.1186/s42506-019-0017-6
Ge, X., Cai, Y., Chen, Z., Gao, S., Geng, X., Li, Y., et al. (2018). Bifunctional enzyme SpoT is involved in biofilm formation of Helicobacter pylori with multidrug resistance by upregulating efflux pump Hp1174 (gluP). Antimicrob. Agents Chemother. 62:957. doi: 10.1128/AAC.00957-18
Ghasemifar, S., Chabak, O., Piri-Gharaghie, T., and Doosti, A. (2024). PIRES2-EGFP/CTB-UreI vaccination activated a mixed Th1/Th2/Th17 immune system defense towards Helicobacter pylori infection in the BALB/c mice model. Vaccine. 1–11. doi: 10.1016/j.vaccine.2024.02.050
Ghotaslou, R., Leylabadlo, H. E., and Asl, Y. M. (2015). Prevalence of antibiotic resistance in Helicobacter pylori: a recent literature review. World J Methodol 5, 164–174. doi: 10.5662/wjm.v5.i3.164
Gisbert, J. P. (2020). Rifabutin for the treatment of Helicobacter pylori infection: a review. Pathogens 10:15. doi: 10.3390/pathogens10010015
Gisbert, J. P., and McNicholl, A. G. (2017). Optimization strategies aimed to increase the efficacy of H. pylori eradication therapies. Helicobacter 22:12392. doi: 10.1111/hel.12392
Gladyshev, N., Taame, M., and Kravtsov, V. (2020). Clinical and laboratory importance of detecting Helicobacter pylori coccoid forms for the selection of treatment. Prz Gastroenterol 15, 294–300. doi: 10.5114/pg.2020.101557
Graham, D. Y. (2020). Transitioning of Helicobacter pylori therapy from trial and error to antimicrobial stewardship. Antibiotics (Basel) 9:671. doi: 10.3390/antibiotics9100671
Grzeszczuk, M. J., Bocian-Ostrzycka, K. M., Banas, A. M., Roszczenko-Jasinska, P., Malinowska, A., Stralova, H., et al. (2018). Thioloxidoreductase HP0231 of Helicobacter pylori impacts HopQ-dependent CagA translocation. Int. J. Med. Microbiol. 308, 977–985. doi: 10.1016/j.ijmm.2018.08.002
Guo, L., Hong, D., Wang, S., Zhang, F., Tang, F., Wu, T., et al. (2019). Therapeutic protection against H. pylori infection in Mongolian gerbils by Oral immunization with a tetravalent epitope-based vaccine with polysaccharide adjuvant. Front. Immunol. 10:1185. doi: 10.3389/fimmu.2019.01185
Guo, C. G., Jiang, F., Cheung, K. S., Li, B., Ooi, P. H., and Leung, W. K. (2022). Timing of prior exposure to antibiotics and failure of Helicobacter pylori eradication: a population-based study. J. Antimicrob. Chemother. 77, 517–523. doi: 10.1093/jac/dkab415
Guo, L., Zhang, F., Wang, S., Li, R., Zhang, L., Zhang, Z., et al. (2022). Oral immunization with a M cell-targeting recombinant L. lactis vaccine LL-plSAM-FVpE stimulate protective immunity against H. pylori in mice. Front. Immunol. 13:918160. doi: 10.3389/fimmu.2022.918160
Guzman-Soto, I., McTiernan, C., Gonzalez-Gomez, M., Ross, A., Gupta, K., Suuronen, E. J., et al. (2021). Mimicking biofilm formation and development: recent progress in in vitro and in vivo biofilm models. iScience 24:102443. doi: 10.1016/j.isci.2021.102443
Ha, N. C., Oh, S. T., Sung, J. Y., Cha, K. A., Lee, M. H., and Oh, B. H. (2001). Supramolecular assembly and acid resistance of Helicobacter pylori urease. Nat. Struct. Biol. 8, 505–509. doi: 10.1038/88563
Habimana, J. D., Mukama, O., Chen, G., Chen, M., Amissah, O. B., Wang, L., et al. (2023). Harnessing enhanced CRISPR/Cas12a trans-cleavage activity with extended reporters and reductants for early diagnosis of Helicobacter pylori, the causative agent of peptic ulcers and stomach cancer. Biosens. Bioelectron. 222:114939. doi: 10.1016/j.bios.2022.114939
Hafeez, M., Qureshi, Z. A., Khattak, A. L., Saeed, F., Asghar, A., Azam, K., et al. (2021). Helicobacter pylori eradication therapy: still a challenge. Cureus 13:e14872. doi: 10.7759/cureus.14872
Haruma, K., Kamada, T., Kawaguchi, H., Okamoto, S., Yoshihara, M., Sumii, K., et al. (2000). Effect of age and Helicobacter pylori infection on gastric acid secretion. J. Gastroenterol. Hepatol. 15, 277–283. doi: 10.1046/j.1440-1746.2000.02131.x
Hatfull, G. F., Dedrick, R. M., and Schooley, R. T. (2022). Phage therapy for antibiotic-resistant bacterial infections. Annu. Rev. Med. 73, 197–211. doi: 10.1146/annurev-med-080219-122208
Hathroubi, S., Hu, S., and Ottemann, K. M. (2020). Genetic requirements and transcriptomics of Helicobacter pylori biofilm formation on abiotic and biotic surfaces. NPJ Biofilms Microbiomes 6:56. doi: 10.1038/s41522-020-00167-3
Hayashi, T., Senda, M., Suzuki, N., Nishikawa, H., Ben, C., Tang, C., et al. (2017). Differential mechanisms for SHP2 binding and activation are exploited by geographically distinct Helicobacter pylori CagA Oncoproteins. Cell Rep. 20, 2876–2890. doi: 10.1016/j.celrep.2017.08.080
He, C., Peng, C., Xu, X., Li, N., Ouyang, Y., Zhu, Y., et al. (2022a). Probiotics mitigate Helicobacter pylori-induced gastric inflammation and premalignant lesions in INS-GAS mice with the modulation of gastrointestinal microbiota. Helicobacter 27:e12898. doi: 10.1111/hel.12898
He, C., Xie, Y., Zhu, Y., Zhuang, K., Huo, L., Yu, Y., et al. (2022b). Probiotics modulate gastrointestinal microbiota after Helicobacter pylori eradication: a multicenter randomized double-blind placebo-controlled trial. Front. Immunol. 13:1033063. doi: 10.3389/fimmu.2022.1033063
Helmbold, L., Ghebremedhin, B., Bellm, A., Hopkins, M. A., Wirth, S., and Aydin, M. (2022). Increased antibiotic resistance in children with Helicobacter pylori infection: a retrospective study. Pathogens 11:178. doi: 10.3390/pathogens11020178
Ho, J. J. C., Navarro, M., Sawyer, K., Elfanagely, Y., and Moss, S. F. (2022). Helicobacter pylori antibiotic resistance in the United States between 2011 and 2021: a systematic review and Meta-analysis. Am. J. Gastroenterol. 117, 1221–1230. doi: 10.14309/ajg.0000000000001828
Hong, T. C., El-Omar, E. M., Kuo, Y. T., Wu, J. Y., Chen, M. J., Chen, C. C., et al. (2024). Primary antibiotic resistance of Helicobacter pylori in the Asia-Pacific region between 1990 and 2022: an updated systematic review and meta-analysis. Lancet Gastroenterol. Hepatol. 9, 56–67. doi: 10.1016/S2468-1253(23)00281-9
Hong, Y., Zeng, J., Wang, X., Drlica, K., and Zhao, X. (2019). Post-stress bacterial cell death mediated by reactive oxygen species. Proc. Natl. Acad. Sci. USA 116, 10064–10071. doi: 10.1073/pnas.1901730116
Hou, C., Yin, F., Wang, S., Zhao, A., Li, Y., and Liu, Y. (2022). Helicobacter pylori biofilm-related drug resistance and new developments in its anti-biofilm agents. Infect. Drug Resist. 15, 1561–1571. doi: 10.2147/IDR.S357473
Huang, T. T., Liu, Y. N., Huang, J. X., Yan, P. P., Wang, J. J., Cao, Y. X., et al. (2024). Sodium sulfite-driven Helicobacter pylori eradication: unraveling oxygen dynamics through multi-omics investigation. Biochem. Pharmacol. 222:116055. doi: 10.1016/j.bcp.2024.116055
Huang, T. T., Yan, P. P., Liu, Y. N., Di, J., Shi, Q. J., Cao, Y. X., et al. (2023). The effects of sodium sulfite on Helicobacter pylori by establishing a hypoxic environment. Toxicol. Appl. Pharmacol. 470:116549. doi: 10.1016/j.taap.2023.116549
Hulten, K. G., Lamberth, L. B., Kalfus, I. N., and Graham, D. Y. (2021). National and regional US antibiotic resistance to Helicobacter pylori: lessons from a clinical trial. Gastroenterology 161, 342–344.e1. doi: 10.1053/j.gastro.2021.03.045
Hussain, A., Tabrez, E., Peela, J., Honnavar, P. D., and Tabrez, S. S. M. (2018). Vitamin C: a preventative, Therapeutic Agent Against Helicobacter pylori. Cureus 10:e3062. doi: 10.7759/cureus.3062
Ierardi, E., Losurdo, G., Mileti, A., Paolillo, R., Giorgio, F., Principi, M., et al. (2020). The puzzle of coccoid forms of Helicobacter pylori: beyond basic science. Antibiotics (Basel) 9:293. doi: 10.3390/antibiotics9060293
Inoue, M. (2017). Changing epidemiology of Helicobacter pylori in Japan. Gastric Cancer 20, 3–7. doi: 10.1007/s10120-016-0658-5
Irwin, S. V., Fisher, P., Graham, E., Malek, A., and Robidoux, A. (2017). Sulfites inhibit the growth of four species of beneficial gut bacteria at concentrations regarded as safe for food. PLoS One 12:e0186629. doi: 10.1371/journal.pone.0186629
Jeong, S. J., Kim, J. H., Jung, D. H., Lee, K. H., Park, S. Y., Song, Y., et al. (2018). Gentamicin-intercalated smectite as a new therapeutic option for Helicobacter pylori eradication. J. Antimicrob. Chemother. 73, 1324–1329. doi: 10.1093/jac/dky011
Jeong, S. J., Lee, K. H., Kim, J. H., Park, S. Y., and Song, Y. G. (2020). Efficacy and gut Dysbiosis of gentamicin-intercalated Smectite as a new therapeutic agent against Helicobacter pylori in a mouse model. Antibiotics (Basel) 9:502. doi: 10.3390/antibiotics9080502
Jiang, F., Guo, C. G., Cheung, K. S., Li, B., Law, S. Y. K., and Leung, W. K. (2022). Age of eradication and failure rates of clarithromycin-containing triple therapy for Helicobacter pylori: a 15-year population-based study. Helicobacter 27:e12893. doi: 10.1111/hel.12893
Jing, Z. W., Jia, Y. Y., Wan, N., Luo, M., Huan, M. L., Kang, T. B., et al. (2016). Design and evaluation of novel pH-sensitive ureido-conjugated chitosan/TPP nanoparticles targeted to Helicobacter pylori. Biomaterials 84, 276–285. doi: 10.1016/j.biomaterials.2016.01.045
Jonczyk-Matysiak, E., Lodej, N., Kula, D., Owczarek, B., Orwat, F., Miedzybrodzki, R., et al. (2019). Factors determining phage stability/activity: challenges in practical phage application. Expert Rev. Anti-Infect. Ther. 17, 583–606. doi: 10.1080/14787210.2019.1646126
Kakiuchi, T., Mizoe, A., Yamamoto, K., Imamura, I., Hashiguchi, K., Kawakubo, H., et al. (2020). Effect of probiotics during vonoprazan-containing triple therapy on gut microbiota in Helicobacter pylori infection: a randomized controlled trial. Helicobacter 25:e12690. doi: 10.1111/hel.12690
Kandpal, M., Baral, B., Varshney, N., Jain, A. K., Chatterji, D., Meena, A. K., et al. (2024). Gut-brain axis interplay via STAT3 pathway: implications of Helicobacter pylori derived secretome on inflammation and Alzheimer's disease. Virulence 15:2303853. doi: 10.1080/21505594.2024.2303853
Karkhah, A., Ebrahimpour, S., Rostamtabar, M., Koppolu, V., Darvish, S., Vasigala, V. K. R., et al. (2019). Helicobacter pylori evasion strategies of the host innate and adaptive immune responses to survive and develop gastrointestinal diseases. Microbiol. Res. 218, 49–57. doi: 10.1016/j.micres.2018.09.011
Kasahun, G. G., Demoz, G. T., and Desta, D. M. (2020). Primary resistance pattern of Helicobacter pylori to antibiotics in adult population: a systematic review. Infect. Drug Resist. 13, 1567–1573. doi: 10.2147/IDR.S250200
Katsande, P. M., Nguyen, V. D., Nguyen, T. L. P., Nguyen, T. K. C., Mills, G., Bailey, D. M. D., et al. (2023). Prophylactic immunization to Helicobacter pylori infection using spore vectored vaccines. Helicobacter 28:e12997. doi: 10.1111/hel.12997
Kaveh-Samani, A., Dalali, S., Kaviani, F., Piri-Gharaghie, T., and Doosti, A. (2024). Oral administration of DNA alginate nanovaccine induced immune-protection against Helicobacter pylori in Balb/C mice. BMC Immunol. 25:11. doi: 10.1186/s12865-024-00602-6
Keikha, M., Eslami, M., Yousefi, B., Ghasemian, A., and Karbalaei, M. (2019). Potential antigen candidates for subunit vaccine development against Helicobacter pylori infection. J. Cell. Physiol. 234, 21460–21470. doi: 10.1002/jcp.28870
Kelly, D. J., Hughes, N. J., and Poole, R. K. (2001). “Microaerobic physiology: aerobic respiration, anaerobic respiration, and carbon dioxide metabolism” in Helicobacter pylori: Physiology and genetics. eds. H. L. T. Mobley, G. L. Mendz, and S. L. Hazell (Washington, DC: ASM Press).
Kim, S. J., Chung, J. W., Woo, H. S., Kim, S. Y., Kim, J. H., Kim, Y. J., et al. (2019). Two-week bismuth-containing quadruple therapy and concomitant therapy are effective first-line treatments for Helicobacter pylori eradication: a prospective open-label randomized trial. World J. Gastroenterol. 25, 6790–6798. doi: 10.3748/wjg.v25.i46.6790
Krzyzek, P., and Grande, R. (2020). Transformation of Helicobacter pylori into coccoid forms as a challenge for research determining activity of antimicrobial substances. Pathogens 9:184. doi: 10.3390/pathogens9030184
Kumar, P., Kizhakkedathu, J. N., and Straus, S. K. (2018). Antimicrobial peptides: diversity, mechanism of action and strategies to improve the activity and biocompatibility in vivo. Biomol. Ther. 8:4. doi: 10.3390/biom8010004
Kushwaha, S. O., Sahu, S. K., Yadav, V. K., Rathod, M. C., Patel, D., Sahoo, D. K., et al. (2024). Bacteriophages as a potential substitute for antibiotics: a comprehensive review. Cell Biochem. Funct. 42:e4022. doi: 10.1002/cbf.4022
Kusunoki, M., Yuki, M., Ishitobi, H., Kobayashi, Y., Nagaoka, M., Takahashi, Y., et al. (2019). Effect of age on effectiveness of vonoprazan in triple therapy for Helicobacter pylori eradication. Intern. Med. 58, 1549–1555. doi: 10.2169/internalmedicine.2233-18
Labenz, J., Ruhl, G. H., Bertrams, J., and Borsch, G. (1994). Medium-or high-dose omeprazole plus amoxicillin eradicates Helicobacter pylori in gastric ulcer disease. Am. J. Gastroenterol. 89, 726–730
Lai, H. H., and Lai, M. W. (2022). Treatment of pediatric Helicobacter pylori infection. Antibiotics (Basel) 11:757. doi: 10.3390/antibiotics11060757
Lauener, F. N., Imkamp, F., Lehours, P., Buissonniere, A., Benejat, L., Zbinden, R., et al. (2019). Genetic determinants and prediction of antibiotic resistance phenotypes in Helicobacter pylori. J. Clin. Med. 8:53. doi: 10.3390/jcm8010053
Le Thi, T. G., Werkstetter, K., Kotilea, K., Bontems, P., Cabral, J., Cilleruelo Pascual, M. L., et al. (2023). Management of Helicobacter pylori infection in paediatric patients in Europe: results from the EuroPedHp registry. Infection 51, 921–934. doi: 10.1007/s15010-022-01948-y
Lee, K. H., Park, S. Y., Jeong, S. J., Jung, D. H., Kim, J. H., Jeong, S. H., et al. (2019). Can aminoglycosides be used as a new treatment for Helicobacter pylori? In vitro activity of recently isolated Helicobacter pylori. Infect. Chemother. 51, 10–20. doi: 10.3947/ic.2019.51.1.10
Lester, J., Kichler, S., Oickle, B., Fairweather, S., Oberc, A., Chahal, J., et al. (2015). Characterization of Helicobacter pylori HP0231 (DsbK): role in disulfide bond formation, redox homeostasis and production of Helicobacter cystein-rich protein HcpE. Mol. Microbiol. 96, 110–133. doi: 10.1111/mmi.12923
Li, Y., Chen, Z., Ye, J., Ning, L., Luo, J., Zhang, L., et al. (2016). Antibody production and Th1-biased response induced by an epitope vaccine composed of cholera toxin B unit and Helicobacter pylori Lpp20 epitopes. Helicobacter 21, 234–248. doi: 10.1111/hel.12268
Li, Y., Choi, H., Leung, K., Jiang, F., Graham, D. Y., and Leung, W. K. (2023). Global prevalence of Helicobacter pylori infection between 1980 and 2022: a systematic review and meta-analysis. Lancet Gastroenterol. Hepatol. 8, 553–564. doi: 10.1016/S2468-1253(23)00070-5
Li, X., Xing, Y., Guo, L., Lv, X., Song, H., and Xi, T. (2014). Oral immunization with recombinant Lactococcus lactis delivering a multi-epitope antigen CTB-UE attenuates Helicobacter pylori infection in mice. Pathog. Dis. 72, 78–86. doi: 10.1111/2049-632X.12173
Lim, S. H., Kim, N., Kwon, J. W., Kim, S. E., Baik, G. H., Lee, J. Y., et al. (2018). Trends in the seroprevalence of Helicobacter pylori infection and its putative eradication rate over 18 years in Korea: a cross-sectional nationwide multicenter study. PLoS One 13:e0204762. doi: 10.1371/journal.pone.0204762
Liu, M., Gao, H., Miao, J., Zhang, Z., Zheng, L., Li, F., et al. (2023). Helicobacter pylori infection in humans and phytotherapy, probiotics, and emerging therapeutic interventions: a review. Front. Microbiol. 14:1330029. doi: 10.3389/fmicb.2023.1330029
Liu, M., Zhong, Y., Chen, J., Liu, Y., Tang, C., Wang, X., et al. (2020). Oral immunization of mice with a multivalent therapeutic subunit vaccine protects against Helicobacter pylori infection. Vaccine 38, 3031–3041. doi: 10.1016/j.vaccine.2020.02.036
Mahlapuu, M., Bjorn, C., and Ekblom, J. (2020). Antimicrobial peptides as therapeutic agents: opportunities and challenges. Crit. Rev. Biotechnol. 40, 978–992. doi: 10.1080/07388551.2020.1796576
Malaty, H. M., El-Kasabany, A., Graham, D. Y., Miller, C. C., Reddy, S. G., Srinivasan, S. R., et al. (2002). Age at acquisition of Helicobacter pylori infection: a follow-up study from infancy to adulthood. Lancet 359, 931–935. doi: 10.1016/S0140-6736(02)08025-X
Malfertheiner, P., Camargo, M. C., El-Omar, E., Liou, J. M., Peek, R., Schulz, C., et al. (2023). Helicobacter pylori infection. Nat. Rev. Dis. Primers 9:19. doi: 10.1038/s41572-023-00431-8
Malfertheiner, P., Megraud, F., O'Morain, C. A., Gisbert, J. P., Kuipers, E. J., Axon, A. T., et al. (2017). Management of Helicobacter pylori infection-the Maastricht V/Florence consensus report. Gut 66, 6–30. doi: 10.1136/gutjnl-2016-312288
Malfertheiner, P., Megraud, F., Rokkas, T., Gisbert, J. P., Liou, J. M., Schulz, C., et al. (2022). Management of Helicobacter pylori infection: the Maastricht VI/Florence consensus report. Gut 71, 1724–1762. doi: 10.1136/gutjnl-2022-327745
Malfertheiner, P., Schulz, C., and Hunt, R. H. (2024). H. pylori infection: a 40-year journey through shifting the paradigm to transforming the management. Dig. Dis., 1–10. doi: 10.1159/000538079
Megraud, F., Bruyndonckx, R., Coenen, S., Wittkop, L., Huang, T. D., Hoebeke, M., et al. (2021). Helicobacter pylori resistance to antibiotics in Europe in 2018 and its relationship to antibiotic consumption in the community. Gut 70, 1815–1822. doi: 10.1136/gutjnl-2021-324032
Mittl, P. R., Luthy, L., Reinhardt, C., and Joller, H. (2003). Detection of high titers of antibody against Helicobacter cysteine-rich proteins a, B, C, and E in Helicobacter pylori-infected individuals. Clin. Diagn. Lab. Immunol. 10, 542–545. doi: 10.1128/cdli.10.4.542-545.2003
Mohaideen, N. S. M. H., Vaani, S., and Hemalatha, S. (2023). Antimicrobial Peptides. Curr. Pharmacol. Rep. 9, 433–454. doi: 10.1007/s40495-023-00342-y
Monstein, H. J., Tiveljung, A., Kraft, C. H., Borch, K., and Jonasson, J. (2000). Profiling of bacterial flora in gastric biopsies from patients with Helicobacter pylori-associated gastritis and histologically normal control individuals by temperature gradient gel electrophoresis and 16S rDNA sequence analysis. J. Med. Microbiol. 49, 817–822. doi: 10.1099/0022-1317-49-9-817
Moss, S. F., Moise, L., Lee, D. S., Kim, W., Zhang, S., Lee, J., et al. (2011). HelicoVax: epitope-based therapeutic Helicobacter pylori vaccination in a mouse model. Vaccine 29, 2085–2091. doi: 10.1016/j.vaccine.2010.12.130
Mugengana, A. K., Vita, N. A., Brown Gandt, A., Moran, K., Agyapong, G., Sharma, L. K., et al. (2021). The discovery and development of Thienopyrimidines as inhibitors of Helicobacter pylori that act through inhibition of the respiratory complex I. ACS Infect. Dis. 7, 1044–1058. doi: 10.1021/acsinfecdis.0c00300
Mukai, R., Handa, O., Suyama, Y., Majima, A., and Naito, Y. (2020). Effectiveness of including probiotics to Helicobacter pylori eradication therapies. J. Clin. Biochem. Nutr. 67, 102–104. doi: 10.3164/jcbn.20-37
Munoz, A. B., Stepanian, J., Trespalacios, A. A., and Vale, F. F. (2020). Bacteriophages of Helicobacter pylori. Front. Microbiol. 11:549084. doi: 10.3389/fmicb.2020.549084
Nejati, S., Karkhah, A., Darvish, H., Validi, M., Ebrahimpour, S., and Nouri, H. R. (2018). Influence of Helicobacter pylori virulence factors CagA and VacA on pathogenesis of gastrointestinal disorders. Microb. Pathog. 117, 43–48. doi: 10.1016/j.micpath.2018.02.016
Nishizawa, T., Suzuki, H., Fujimoto, A., Kinoshita, H., Yoshida, S., Isomura, Y., et al. (2017). Effects of patient age and choice of antisecretory agent on success of eradication therapy for Helicobacter pylori infection. J. Clin. Biochem. Nutr. 60, 208–210. doi: 10.3164/jcbn.16-86
Oertli, M., Noben, M., Engler, D. B., Semper, R. P., Reuter, S., Maxeiner, J., et al. (2013). Helicobacter pylori gamma-glutamyl transpeptidase and vacuolating cytotoxin promote gastric persistence and immune tolerance. Proc. Natl. Acad. Sci. USA 110, 3047–3052. doi: 10.1073/pnas.1211248110
Ohishi, T., Masuda, T., Abe, H., Hayashi, C., Adachi, H., Ohba, S. I., et al. (2018). Monotherapy with a novel intervenolin derivative, AS-1934, is an effective treatment for Helicobacter pylori infection. Helicobacter 23:e12470. doi: 10.1111/hel.12470
Okushin, K., Tsutsumi, T., Ikeuchi, K., Kado, A., Enooku, K., Fujinaga, H., et al. (2018). Helicobacter pylori infection and liver diseases: epidemiology and insights into pathogenesis. World J. Gastroenterol. 24, 3617–3625. doi: 10.3748/wjg.v24.i32.3617
Olmos, J. A., Rios, H., and Higa, R. (2000). Prevalence of Helicobacter pylori infection in Argentina: results of a nationwide epidemiologic study. Argentinean Hp epidemiologic study group. J. Clin. Gastroenterol. 31, 33–37. doi: 10.1097/00004836-200007000-00008
Pang, S. S., Nguyen, S. T. S., Perry, A. J., Day, C. J., Panjikar, S., Tiralongo, J., et al. (2014). The three-dimensional structure of the extracellular adhesion domain of the sialic acid-binding adhesin SabA from Helicobacter pylori. J. Biol. Chem. 289, 6332–6340. doi: 10.1074/jbc.M113.513135
Park, J. S., Jun, J. S., Seo, J. H., Youn, H. S., and Rhee, K. H. (2021). Changing prevalence of Helicobacter pylori infection in children and adolescents. Clin Exp Pediatr 64, 21–25. doi: 10.3345/cep.2019.01543
Park, S. A., Lee, H. W., Hong, M. H., Choi, Y. W., Choe, Y. H., Ahn, B. Y., et al. (2006). Comparative proteomic analysis of Helicobacter pylori strains associated with iron deficiency anemia. Proteomics 6, 1319–1328. doi: 10.1002/pmic.200500293
Pellicano, R., Ianiro, G., Fagoonee, S., Settanni, C. R., and Gasbarrini, A. (2020). Review: Extragastric diseases and Helicobacter pylori. Helicobacter 25:e12741. doi: 10.1111/hel.12741
Peng, X., Zhang, R., Duan, G., Wang, C., Sun, N., Zhang, L., et al. (2018). Production and delivery of Helicobacter pylori NapA in Lactococcus lactis and its protective efficacy and immune modulatory activity. Sci. Rep. 8:6435. doi: 10.1038/s41598-018-24879-x
Qian, H. S., Li, W. J., Dang, Y. N., Li, L. R., Xu, X. B., Yuan, L., et al. (2023). Ten-Day vonoprazan-amoxicillin dual therapy as a first-line treatment of Helicobacter pylori infection compared with bismuth-containing quadruple therapy. Am. J. Gastroenterol. 118, 627–634. doi: 10.14309/ajg.0000000000002086
Rahman, M. M., Tikhomirova, A., Modak, J. K., Hutton, M. L., Supuran, C. T., and Roujeinikova, A. (2020). Antibacterial activity of ethoxzolamide against Helicobacter pylori strains SS1 and 26695. Gut Pathog. 12:20. doi: 10.1186/s13099-020-00358-5
Ranjbar, R., Behzadi, P., and Farshad, S. (2017). Advances in diagnosis and treatment of Helicobacter pylori infection. Acta Microbiol. Immunol. Hung. 64, 273–292. doi: 10.1556/030.64.2017.008
Ren, S., Cai, P., Liu, Y., Wang, T., Zhang, Y., Li, Q., et al. (2022). Prevalence of Helicobacter pylori infection in China: a systematic review and meta-analysis. J. Gastroenterol. Hepatol. 37, 464–470. doi: 10.1111/jgh.15751
Reshetnyak, V. I., and Reshetnyak, T. M. (2017). Significance of dormant forms of Helicobacter pylori in ulcerogenesis. World J. Gastroenterol. 23, 4867–4878. doi: 10.3748/wjg.v23.i27.4867
Rokkas, T., Gisbert, J. P., Malfertheiner, P., Niv, Y., Gasbarrini, A., Leja, M., et al. (2021). Comparative effectiveness of multiple different first-line treatment regimens for Helicobacter pylori infection: a network meta-analysis. Gastroenterology 161, 495–507.e4. doi: 10.1053/j.gastro.2021.04.012
Rossi, M., Bolz, C., Revez, J., Javed, S., El-Najjar, N., Anderl, F., et al. (2012). Evidence for conserved function of gamma-glutamyltranspeptidase in Helicobacter genus. PLoS One 7:e30543. doi: 10.1371/journal.pone.0030543
Sacca, S. C., Pascotto, A., Venturino, G. M., Prigione, G., Mastromarino, A., Baldi, F., et al. (2006). Prevalence and treatment of Helicobacter pylori in patients with blepharitis. Invest. Ophthalmol. Vis. Sci. 47, 501–508. doi: 10.1167/iovs.05-0323
Saito, Y., Konno, K., Sato, M., Nakano, M., Kato, Y., Saito, H., et al. (2019). Vonoprazan-based third-line therapy has a higher eradication rate against Sitafloxacin-resistant Helicobacter pylori. Cancers (Basel) 11:116. doi: 10.3390/cancers11010116
Saju, P., Murata-Kamiya, N., Hayashi, T., Senda, Y., Nagase, L., Noda, S., et al. (2016). Host SHP1 phosphatase antagonizes Helicobacter pylori CagA and can be downregulated by Epstein-Barr virus. Nat. Microbiol. 1:16026. doi: 10.1038/nmicrobiol.2016.26
Savoldi, A., Carrara, E., Graham, D. Y., Conti, M., and Tacconelli, E. (2018). Prevalence of antibiotic resistance in Helicobacter pylori: a systematic review and Meta-analysis in World Health Organization regions. Gastroenterology 155, 1372–1382.e17. doi: 10.1053/j.gastro.2018.07.007
Schubert, J. P., Gehlert, J., Rayner, C. K., Roberts-Thomson, I. C., Costello, S., Mangoni, A. A., et al. (2021). Antibiotic resistance of Helicobacter pylori in Australia and New Zealand: a systematic review and meta-analysis. J. Gastroenterol. Hepatol. 36, 1450–1456. doi: 10.1111/jgh.15352
Schubert, J. P., Warner, M. S., Rayner, C. K., Roberts-Thomson, I. C., Mangoni, A. A., Costello, S., et al. (2022). Increasing Helicobacter pylori clarithromycin resistance in Australia over 20 years. Intern. Med. J. 52, 1554–1560. doi: 10.1111/imj.15640
Scott, D., Weeks, D., Melchers, K., and Sachs, G. (1998). The life and death of Helicobacter pylori. Gut 43, S56–S60. doi: 10.1136/gut.43.2008.s56
Shah, S. C., Halvorson, A. E., Lee, D., Bustamante, R., McBay, B., Gupta, R., et al. (2024). Helicobacter pylori burden in the United States according to individual demographics and geography: a Nationwide analysis of the veterans healthcare system. Clin. Gastroenterol. Hepatol. 22, 42–50.e26. doi: 10.1016/j.cgh.2023.05.016
Shakya Shrestha, S., Bhandari, M., Thapa, S. R., Shrestha, R., Poudyal, R., Purbey, B., et al. (2016). Medication adherence pattern and factors affecting adherence in Helicobacter pylori eradication therapy. Kathmandu Univ. Med. J. (KUMJ) 14, 58–64
Shrestha, A. B., Pokharel, P., Sapkota, U. H., Shrestha, S., Mohamed, S. A., Khanal, S., et al. (2023). Drug resistance patterns of commonly used antibiotics for the treatment of Helicobacter pylori infection among south Asian countries: a systematic review and Meta-analysis. Trop med. Infect. Dis. 8:172. doi: 10.3390/tropicalmed8030172
Smith, S. I., Ajayi, A., Jolaiya, T., Onyekwere, C., Setshedi, M., Schulz, C., et al. (2022). Helicobacter pylori infection in Africa: update of the current situation and challenges. Dig. Dis. 40, 535–544. doi: 10.1159/000518959
Song, Z., Li, B., Zhang, Y., Li, R., Ruan, H., Wu, J., et al. (2020). Outer membrane vesicles of Helicobacter pylori 7.13 as adjuvants promote protective efficacy against Helicobacter pylori infection. Front. Microbiol. 11:1340. doi: 10.3389/fmicb.2020.01340
Song, H. Y., Zhou, L., Liu, D. Y., Yao, X. J., and Li, Y. (2018). What roles do probiotics play in the eradication of Helicobacter pylori? Current knowledge and ongoing research. Gastroenterol. Res. Pract. 2018:9379480. doi: 10.1155/2018/9379480
Soudi, H., Falsafi, T., Mahboubi, M., and Gharavi, S. (2021). Evaluation of Helicobacter pylori OipA protein as a vaccine candidate and propolis as an adjuvant in C57BL/6 mice. Iran. J. Basic Med. Sci. 24, 1220–1230. doi: 10.22038/ijbms.2021.56232.12579
Stark, R. M., Gerwig, G. J., Pitman, R. S., Potts, L. F., Williams, N. A., Greenman, J., et al. (1999). Biofilm formation by Helicobacter pylori. Lett. Appl. Microbiol. 28, 121–126. doi: 10.1046/j.1365-2672.1999.00481.x
Sugano, K., Tack, J., Kuipers, E. J., Graham, D. Y., El-Omar, E. M., Miura, S., et al. (2015). Kyoto global consensus report on Helicobacter pylori gastritis. Gut 64, 1353–1367. doi: 10.1136/gutjnl-2015-309252
Sun, L., Zheng, H., Qiu, M., Hao, S., Liu, X., Zhu, X., et al. (2023). Helicobacter pylori infection and risk of cardiovascular disease. Helicobacter 28:e12967. doi: 10.1111/hel.12967
Sung, H., Ferlay, J., Siegel, R. L., Laversanne, M., Soerjomataram, I., Jemal, A., et al. (2021). Global cancer statistics 2020: GLOBOCAN estimates of incidence and mortality worldwide for 36 cancers in 185 countries. CA Cancer J. Clin. 71, 209–249. doi: 10.3322/caac.21660
Sutton, P., and Boag, J. M. (2019). Status of vaccine research and development for Helicobacter pylori. Vaccine 37, 7295–7299. doi: 10.1016/j.vaccine.2018.01.001
Szaflarska-Poplawska, A., and Soroczynska-Wrzyszcz, A. (2019). Prevalence of Helicobacter pylori infection among junior high school students in Grudziadz, Poland. Helicobacter 24:e12552. doi: 10.1111/hel.12552
Tang, W., Guan, M., Li, Z., Pan, W., and Wang, Z. (2023). A2BR facilitates the pathogenesis of H. pylori-associated GU by inducing oxidative stress through p38 MAPK phosphorylation. Heliyon 9:e21004. doi: 10.1016/j.heliyon.2023.e21004
Tang, Y., Tang, G., Pan, L., Zhu, H., Zhou, S., and Wei, Z. (2020). Clinical factors associated with initial Helicobacter pylori eradication therapy: a retrospective study in China. Sci. Rep. 10:15403. doi: 10.1038/s41598-020-72400-0
Thung, I., Aramin, H., Vavinskaya, V., Gupta, S., Park, J. Y., Crowe, S. E., et al. (2016). Review article: the global emergence of Helicobacter pylori antibiotic resistance. Aliment. Pharmacol. Ther. 43, 514–533. doi: 10.1111/apt.13497
Tobias, J., Lebens, M., Wai, S. N., Holmgren, J., and Svennerholm, A. M. (2017). Surface expression of Helicobacter pylori HpaA adhesion antigen on Vibrio cholerae, enhanced by co-expressed enterotoxigenic Escherichia coli fimbrial antigens. Microb. Pathog. 105, 177–184. doi: 10.1016/j.micpath.2017.02.021
Toscano, E. P., Madeira, F. F., Dutra-Rulli, M. P., Goncalves, L. O. M., Proenca, M. A., Borghi, V. S., et al. (2018). Epidemiological and clinical-pathological aspects of Helicobacter pylori infection in Brazilian children and adults. Gastroenterol. Res. Pract. 2018:8454125. doi: 10.1155/2018/8454125
Tsay, F. W., Wu, D. C., Yu, H. C., Kao, S. S., Lin, K. H., Cheng, J. S., et al. (2017). A randomized controlled trial shows that both 14-Day hybrid and bismuth quadruple therapies cure most patients with Helicobacter pylori infection in populations with moderate antibiotic resistance. Antimicrob. Agents Chemother. 61:140. doi: 10.1128/AAC.00140-17
Tshibangu-Kabamba, E., Ngoma-Kisoko, P. J., Tuan, V. P., Matsumoto, T., Akada, J., Kido, Y., et al. (2020). Next-generation sequencing of the whole bacterial genome for tracking molecular insight into the broad-Spectrum antimicrobial resistance of Helicobacter pylori clinical isolates from the Democratic Republic of Congo. Microorganisms 8:887. doi: 10.3390/microorganisms8060887
Tshibangu-Kabamba, E., and Yamaoka, Y. (2021). Helicobacter pylori infection and antibiotic resistance – from biology to clinical implications. Nat. Rev. Gastroenterol. Hepatol. 18, 613–629. doi: 10.1038/s41575-021-00449-x
Urrutia-Baca, V. H., Gonzalez Brosig, K. I., Salazar-Garza, A. A., Gomez-Flores, R., Tamez-Guerra, P., and De La Garza-Ramos, M. A. (2023). Prevalence of Oral Helicobacter pylori infection in an indigenous Community in Southwest Mexico. Clin. Exp. Gastroenterol. 16, 173–180. doi: 10.2147/CEG.S424559
Venkatesan, A., Gonuguntla, A., Abraham, A. P., Janumpalli, K. K. R., and Lakshminarayana, B. (2024). Leveraging the multidimensional poverty index to estimate Helicobacter pylori prevalence in districts in Karnataka. India. Trop. Doct. 54, 16–22. doi: 10.1177/00494755231206850
Vu, T. B., Tran, T. N. Q., Tran, T. Q. A., Vu, D. L., and Hoang, V. T. (2022). Antibiotic resistance of Helicobacter pylori in patients with peptic ulcer. Medicina (Kaunas) 59:6. doi: 10.3390/medicina59010006
Wang, L., Cao, Z. M., Zhang, L. L., Dai, X. C., Liu, Z. J., Zeng, Y. X., et al. (2022). Helicobacter pylori and autoimmune diseases: involving multiple systems. Front. Immunol. 13:833424. doi: 10.3389/fimmu.2022.833424
Wang, Q., Liu, Y., Xu, Z., Wang, Z., Xue, M., Li, X., et al. (2023). Causality of anti-Helicobacter pylori IgG levels on myocardial infarction and potential pathogenesis: a Mendelian randomization study. Front. Microbiol. 14:1259579. doi: 10.3389/fmicb.2023.1259579
Wang, N., Zhou, F., Chen, C., Luo, H., Guo, J., Wang, W., et al. (2021). Role of outer membrane vesicles from Helicobacter pylori in atherosclerosis. Front. Cell Dev. Biol. 9:673993. doi: 10.3389/fcell.2021.673993
Willems, P., de Repentigny, J., Hassan, G. M., Sidani, S., Soucy, G., and Bouin, M. (2020). The prevalence of Helicobacter pylori infection in a quaternary Hospital in Canada. J. Clin. Med. Res. 12, 687–692. doi: 10.14740/jocmr4348
Xiong, M., Bao, Y., Xu, X., Wang, H., Han, Z., Wang, Z., et al. (2017). Selective killing of Helicobacter pylori with pH-responsive helix-coil conformation transitionable antimicrobial polypeptides. Proc. Natl. Acad. Sci. USA 114, 12675–12680. doi: 10.1073/pnas.1710408114
Yahiro, K., Hirayama, T., Moss, J., and Noda, M. (2015). Helicobacter pylori VacA toxin causes cell death by inducing accumulation of cytoplasmic connexin 43. Cell Death Dis. 6:e1971. doi: 10.1038/cddis.2015.329
Yang, J., Dai, L. X., Pan, X., Wang, H., Li, B., Zhu, J., et al. (2015). Protection against Helicobacter pylori infection in BALB/c mice by oral administration of multi-epitope vaccine of CTB-UreI-UreB. Pathog Dis 73:26. doi: 10.1093/femspd/ftv026
Yang, S.-J., Huang, C.-H., Yang, J.-C., Wang, C.-H., and Shieh, M.-J. (2020). Residence time-extended nanoparticles by magnetic field improve the eradication efficiency of Helicobacter pylori. ACS Appl. Mater. Interfaces 12, 54316–54327. doi: 10.1021/acsami.0c13101
Yang, C., Liang, L., Lv, P., Liu, L., Wang, S., Wang, Z., et al. (2021). Effects of non-viable Lactobacillus reuteri combining with 14-day standard triple therapy on Helicobacter pylori eradication: a randomized double-blind placebo-controlled trial. Helicobacter 26:e12856. doi: 10.1111/hel.12856
Yang, J., Zhang, Y., Fan, L., Zhu, Y. J., Wang, T. Y., Wang, X. W., et al. (2019). Eradication efficacy of modified dual therapy compared with bismuth-containing quadruple therapy as a first-line treatment of Helicobacter pylori. Am. J. Gastroenterol. 114, 437–445. doi: 10.14309/ajg.0000000000000132
Yonezawa, H., Osaki, T., Hojo, F., and Kamiya, S. (2019). Effect of Helicobacter pylori biofilm formation on susceptibility to amoxicillin, metronidazole and clarithromycin. Microb. Pathog. 132, 100–108. doi: 10.1016/j.micpath.2019.04.030
Yu, J., Guo, Z., Yan, J., Bu, C., Peng, C., Li, C., et al. (2023). Gastric acid-responsive ROS nanogenerators for effective treatment of Helicobacter pylori infection without disrupting homeostasis of intestinal flora. Adv. Sci. (Weinh) 10:e2206957. doi: 10.1002/advs.202206957
Yuan, C., Adeloye, D., Luk, T. T., Huang, L., He, Y., Xu, Y., et al. (2022). The global prevalence of and factors associated with Helicobacter pylori infection in children: a systematic review and meta-analysis. Lancet Child Adolesc Health 6, 185–194. doi: 10.1016/S2352-4642(21)00400-4
Yuan, Z., Xiao, S., Li, S., Suo, B., Wang, Y., Meng, L., et al. (2021). The impact of Helicobacter pylori infection, eradication therapy, and probiotics intervention on gastric microbiota in young adults. Helicobacter 26:e12848. doi: 10.1111/hel.12848
Yunle, K., Tong, W., Jiyang, L., and Guojun, W. (2024). Advances in Helicobacter pylori vaccine research: from candidate antigens to adjuvants-a review. Helicobacter 29:e13034. doi: 10.1111/hel.13034
Zeng, M., Mao, X. H., Li, J. X., Tong, W. D., Wang, B., Zhang, Y. J., et al. (2015). Efficacy, safety, and immunogenicity of an oral recombinant Helicobacter pylori vaccine in children in China: a randomised, double-blind, placebo-controlled, phase 3 trial. Lancet 386, 1457–1464. doi: 10.1016/S0140-6736(15)60310-5
Zhang, X., He, Y., Zhang, X., Fu, B., Song, Z., Wang, L., et al. (2024). Sustained exposure to Helicobacter pylori induces immune tolerance by desensitizing TLR6. Gastric Cancer 27, 324–342. doi: 10.1007/s10120-023-01461-7
Zhang, Y., Meng, F., Jin, J., Wang, J., Gu, B. B., Peng, J. B., et al. (2021). Ninety-four thousand-case retrospective study on antibacterial drug resistance of Helicobacter pylori. World J. Clin. Cases 9, 10838–10849. doi: 10.12998/wjcc.v9.i35.10838
Zhang, X., Sang, S., Guan, Q., Tao, H., Wang, Y., and Liu, C. (2022). Oral administration of a shigella 2aT32-based vaccine expressing UreB-HspA fusion antigen with and without parenteral rUreB-HspA boost confers protection against Helicobacter pylori in mice model. Front. Immunol. 13:894206. doi: 10.3389/fimmu.2022.894206
Zhang, Y. X., Suo, B. J., Tian, X. L., Zhang, H., Lu, H. P., Yao, X. Y., et al. (2023). New regimens as first-line eradication therapy for Helicobacter pylori infection in patients allergic to penicillin: a randomized controlled trial. Helicobacter 28:e12956. doi: 10.1111/hel.12956
Zhang, W., Yang, Z., Zheng, J., Fu, K., Wong, J. H., Ni, Y., et al. (2023). A bioresponsive genetically encoded antimicrobial crystal for the Oral treatment of Helicobacter pylori infection. Adv. Sci. (Weinh) 10:e2301724. doi: 10.1002/advs.202301724
Zhao, Y., Cai, Y., Chen, Z., Li, H., Xu, Z., Li, W., et al. (2023). SpoT-mediated NapA upregulation promotes oxidative stress-induced Helicobacter pylori biofilm formation and confers multidrug resistance. Antimicrob. Agents Chemother. 65:152. doi: 10.1128/AAC.00152-21
Zhao, Y., Ren, M., Wang, X., Lu, G., Lu, X., Zhang, D., et al. (2020). Telephone-based reeducation of drug administration for Helicobacter pylori eradication: a multicenter randomized controlled study. Gastroenterol. Res. Pract. 2020:8972473. doi: 10.1155/2020/8972473
Zheng, H., Xia, P., Fu, W., and Ding, S. (2024). Helicobacter pylori infection and inflammasomes. Helicobacter 29:e13043. doi: 10.1111/hel.13043
Zhou, Y., Zhang, Y., and Du, S. (2024). Antibiotic resistance in Helicobacter pylori among children and adolescents in East Asia: a systematic review and meta-analysis. Chin. Med. J. 1–14. doi: 10.1097/CM9.0000000000002884
Zhou, D., Zhang, Z., Qiu, B., Zhang, D., Xie, S., Huang, K., et al. (2022). Ultrasound-activated persistent luminescence imaging and Bacteria-triggered drug release for Helicobacter pylori infection Theranostics. ACS Appl. Mater. Interfaces 14, 26418–26430. doi: 10.1021/acsami.2c04683
Zhu, Y. J., Zhang, Y., Wang, T. Y., Zhao, J. T., Zhao, Z., Zhu, J. R., et al. (2020). High dose PPI-amoxicillin dual therapy for the treatment of Helicobacter pylori infection: a systematic review with meta-analysis. Ther. Adv. Gastroenterol. 13:1756284820937115. doi: 10.1177/1756284820937115
Glossary
Keywords: Helicobacter pylori , antibacterial resistance, probiotic, natural products, vaccine, oxygen, agonist, antagonist
Citation: Huang T-T, Cao Y-X and Cao L (2024) Novel therapeutic regimens against Helicobacter pylori: an updated systematic review. Front. Microbiol. 15:1418129. doi: 10.3389/fmicb.2024.1418129
Edited by:
Asad U. Khan, Aligarh Muslim University, IndiaReviewed by:
Payam Behzadi, Islamic Azad University, ShahreQods, IranMajid Validi, Shahrekord University of Medical Sciences, Iran
Emeka Nweze, University of Nigeria, Nsukka, Nigeria
Ifeanyi Mba, University of Nigeria, Nsukka, Nigeria, in collaboration with reviewer EN
Copyright © 2024 Huang, Cao and Cao. This is an open-access article distributed under the terms of the Creative Commons Attribution License (CC BY). The use, distribution or reproduction in other forums is permitted, provided the original author(s) and the copyright owner(s) are credited and that the original publication in this journal is cited, in accordance with accepted academic practice. No use, distribution or reproduction is permitted which does not comply with these terms.
*Correspondence: Lei Cao, bGVpY2FvQG1haWwueGp0dS5lZHUuY24=