- 1Innovative Anatomy Program, Faculty of Medicine, Srinakharinwirot University, Bangkok, Thailand
- 2Division of Cardiology, Department of Medicine, Chulabhorn Hospital, Chulabhorn Royal Academy, Bangkok, Thailand
- 3Princess Srisavangavadhana College of Medicine, Chulabhorn Royal Academy, Bangkok, Thailand
- 4Omics Sciences and Bioinformatics Center, Faculty of Science, Chulalongkorn University, Bangkok, Thailand
- 5Mod Gut Co., Ltd., Bangkok, Thailand
- 6Service of Cardiology, Lausanne University Hospital and University of Lausanne, Lausanne, Switzerland
- 7Center of Excellence in Probiotics, Srinakharinwirot University, Bangkok, Thailand
- 8Department of Microbiology, Faculty of Medicine, Srinakharinwirot University, Bangkok, Thailand
- 9Clinical Research Center, Faculty of Medicine, Srinakharinwirot University, Ongkharak, Thailand
Chronic coronary syndrome (CCS) has a high mortality rate, and dyslipidemia is a major risk factor. Atherosclerosis, a cause of CCS, is influenced by gut microbiota dysbiosis and its metabolites. The objective of this study was to study the diversity and composition of gut microbiota and related clinical parameters among CCS patients undergoing coronary angiography and dyslipidemia patients in comparison to healthy volunteers in Thailand. CCS patients had more risk factors and higher inflammatory markers, high-sensitivity C-reactive protein (hs-CRP) than others. The alpha diversity was lower in dyslipidemia and CCS patients than in the healthy group. A significant difference in the composition of gut microbiota was observed among the three groups. The relative abundance of Proteobacteria, Fusobacteria, Enterobacteriaceae, Prevotella, and Streptococcus was significantly increased while Roseburia, Ruminococcus, and Faecalibacterium were lower in CCS patients. In CCS patients, Lachnospiraceae, Peptostreptococcaceae, and Pediococcus were positively correlated with hs-CRP. In dyslipidemia patients, Megasphaera was strongly positively correlated with triglyceride (TG) level and negatively correlated with high-density lipoprotein cholesterol (HDL-C). The modification of gut microbiota was associated with changes in clinical parameters involved in the development of coronary artery disease (CAD) in CCS patients.
Introduction
Cardiovascular disease (CVD) is the world’s leading cause of mortality and one of Thailand’s significant health issues. This disease has several risk factors, including dyslipidemia (Hedayatnia et al., 2020), diabetes mellitus (Abdul-Ghani et al., 2017), hypertension (Dustan, 2000), obesity, insulin resistance, and metabolic syndrome (Tune et al., 2017). Currently, lifestyle changes like exercising, eating healthily, and consuming less salt to lower blood pressure can help to reduce the risk factors of CVD. These changes can be combined with blood-sugar-lowering drugs, statins, and antihypertensive drugs. However, morbidity and mortality rates, which are still very high all over the world, including Thailand, are impacted by CVD.
Even though there are other coronary artery disease (CAD) risk factors, dyslipidemia is a major one (Yusuf et al., 2004). Acute and chronic coronary syndromes are two subtypes of CAD that nare distinguished by the timing of the onset of the signs and symptoms. A phrase used to describe CAD as a chronic, progressive condition that can be stabilized is chronic coronary syndrome (CCS) (Knuuti et al., 2020).
More than 1,100 different bacterial species have been linked to numerous symptoms and diseases, including cancer, diabetes mellitus, obesity, and CVD (Afzaal et al., 2022), according to earlier research. Since metabolic syndrome is a cardiovascular condition, eating the proper amount of healthful food can both prevent and treat it. The majority of gastrointestinal tract microorganisms cannot be cultured. The genes of the microorganism, also known as the microbiome, must therefore be examined to determine the type and quantity of these microorganisms.
Gut microbiota is a microorganism that lives in the human digestive tract. The quantity and type of microorganisms in the gastrointestinal tract are determined and influenced by several external factors such as geographic origin, age, genetics, diet, and use of prebiotics and antibiotics (Thursby and Juge, 2017; Fan and Pedersen, 2021). The gastrointestinal tract contains a variety of microorganisms such as Firmicutes, Bacteroidetes, Actinobacteria, Proteobacteria, and Fusobacteria, in which their mechanisms of action and habitats are different. Crucial gastrointestinal bacteria that have been presented since birth include Lactobacillus and Bifidobacterium (Yang et al., 2019). Nowadays people pay attention to the diversity of gut microbiota, which affects the immune system, nutrient metabolism, and the stabilization of the lining of the gastrointestinal tract (gut epithelium). The gut microbiota is also responsible for digesting certain nutrients that cannot be digested in the stomach and also helps to synthesize vitamins and hormones in the body. The body and the gut microbiome have a complicated interaction through metabolites, including short-chain fatty acids (SCFAs), bile acid (BA), and trimethylamine-N-oxide (TMAO). Several organs may be affected by these metabolites’ effects. Atherosclerosis, a cause of CVD, is influenced by gut microbiota and metabolites (Pieczynska et al., 2020).
Recent studies have highlighted the role of gut dysbiosis in several diseases, including atherosclerosis and many CVDs. These microorganisms are inherited from the mother at birth, as well as from the environment, food, and medicine (Santacroce et al., 2021). The changing intake of macronutrients leads to metabolic syndrome, if there is an imbalance of microorganisms (dysbiosis) in the gastrointestinal tract. Alterations in gut microbiota will lead to an increase in various diseases and consuming a low-fiber, high-fat, and high-sugar diet affects the digestive system including the gut ecosystem. The increase of the abundance of lipopolysaccharides (LPS)-producing gram-negative bacteria, such as Escherichia, Shigella, Veillonella, Haemophilus, and Klebsiella, increased with the severity of CAD in contrast to the bacteria that produce butyric acids, such as Ruminococcaceae and Lachnospiraceae, decreased the onset of CAD. Moreover, several types of gut microbiota, including those from Bacteroidetes phylum, Roseburia intestinalis, Faecalibacterium prausnitzii, and Eubacterium rectale, are also protective factors against CAD (Jonsson and Bäckhed, 2017; Liu H. et al., 2019; Liu et al., 2020).
Sequencing of the 16S rRNA gene is a clustering-independent widely used technique in microbiome studies to analyze the composition and diversity of bacterial communities. This technique provides insights into the diversity, distribution, and relative abundance of bacteria, which are essential for understanding the role of microbiota in various environments, including the human body, soil, water, and other ecological systems (Lane et al., 1985; Wensel et al., 2022).
To the best of our knowledge, no study has been conducted in Thailand to determine if the gut microbiome is associated with the parameters of dyslipidemia and CCS patients undergoing coronary angiography. The purpose of this exploratory study is to identify potential relationships among relative gut microbiota composition and related parameters in patients with dyslipidemia and CCS patients undergoing coronary angiography in Thailand.
Materials and methods
Subject enrollment
Ninety-one patients between the ages of 35 and 70 who were admitted to Chulabhorn Hospital’s cardiovascular center between February and June of 2023 were enlisted. Using statistical matching techniques with age and sex, participants were separated into three groups: CCS patients undergoing coronary angiography, patients with cardiovascular risk factors, dyslipidemia, and healthy volunteers. This study protocol was approved by the ethics committee of Chulabhorn Hospital. All individuals provided written informed consent before participating in the study. The Schematic diagram showing the research workflow is in Figure 1.
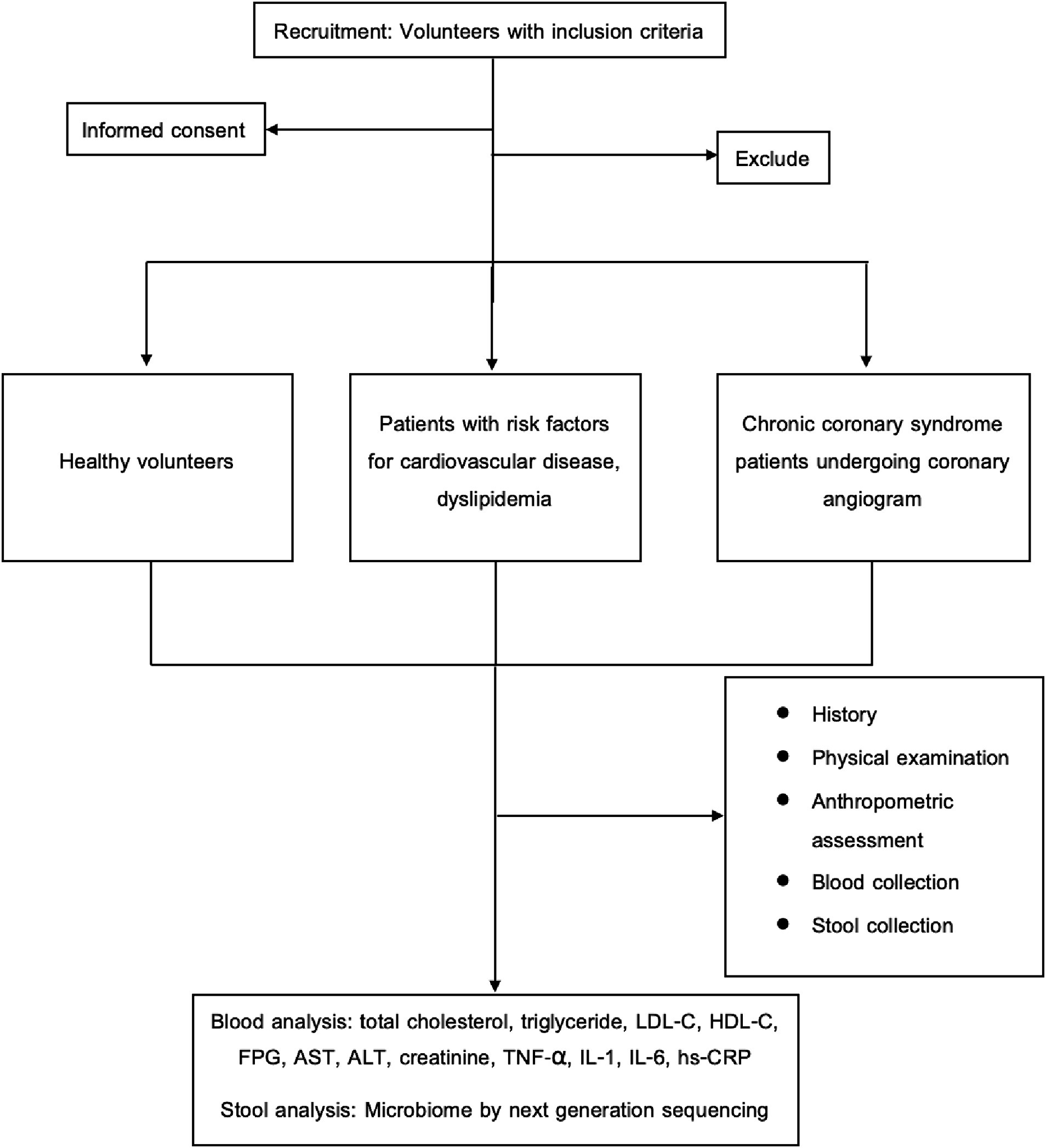
Figure 1. Schematic diagram showing the research workflow. ALT, alanine transaminase; AST, aspartate transaminase; FPG, fasting plasma glucose; HDL-C, high-density lipoprotein cholesterol; hs-CRP, high-sensitivity C-reactive protein; IL-1, interleukin-1; IL-6, interleukin-6; LDL-C, low-density lipoprotein cholesterol; TNF-α, tumor necrosis factor-alpha.
Inclusion criteria
In patients with CCS undergoing coronary angiography group, they must meet the following criteria: (1) patients with stable anginal symptoms (2) patients <1 year after an acute coronary syndrome (ACS) with stabilized symptoms after revascularization (3) CCS patients >1 year after initial diagnosis or revascularization (4) asymptomatic patients in whom CAD is found after screening (Knuuti et al., 2020). Coronary angiography was used to confirm the diagnosis of CCS in those with ≥70% stenosis in coronary arteries larger than 2.5 mm in one view; ≥ 50% stenosis in coronary arteries in two views; and ≥ 50% stenosis in the left main coronary artery (Jiangping et al., 2013); and they must assent by signing the consent forms.
In the group of dyslipidemia patients, they must only have one risk factor for CVD, dyslipidemia (Total cholesterol (TC) ≥ 200 mg/dL or triglyceride (TG) ≥ 150 mg/dL or low-density lipoprotein cholesterol (LDL-C) ≥ 160 mg/dL or patients on treatment) (Lin et al., 2018), without type 2 diabetes mellitus (T2DM) (fasting blood glucose (FBS) ≥ 126 mg/dL for 2 times separately or patients on treatment) (American Diabetes Association, 2014), hypertension (systolic blood pressure ≥ 140 mmHg and/or diastolic blood pressure ≥ 90 mmHg at least 2 different days or patients on treatment) (Williams et al., 2019), obesity (body mass index ≥30 kg/m2) (Samadoulougou et al., 2020), and metabolic syndrome [at least 3 of the following risk factors: waist circumference ≥ 90 cm for men and ≥ 80 cm for women, blood pressure ≥ 130/85 mmHg or on antihypertensive drugs, TG ≥ 150 mg/dL or on medication, high-density lipoprotein cholesterol (HDL-C) ≤ 40 mg/dL for men and ≤ 50 mg/dL for women and FBS ≥100 mg/dL or on medication] (Huang, 2009). Additionally, none of the aforementioned diseases must exist among the group of healthy volunteers.
Exclusion criteria
Patients who met the following criteria were excluded: (1) kidney disease (estimated glomerular filtration rate (eGFR) < 60 mL/min/1.73 m2 for more than 3 months), liver disease (Aspartate aminotransferase (AST), Alanine aminotransferase (ALT) > 5 times normal or jaundice), cancer, intestinal diseases such as inflammatory bowel disease, thyroid dysfunction, immunodeficiency such as human immunodeficiency virus (HIV); (2) use antibiotics, immunosuppressants, probiotics supplement, synbiotics, herbal supplements, antacids or laxatives within 4 weeks before participating in the study; (3) history of gastrointestinal disease and other infections within 4 weeks; (4) smoking; (5) alcoholism; (6) pregnant or lactating; (7) Covid-19 infection within 4 weeks.
Sample size calculation
The sample size calculation is based on “Hypothesis testing and power calculations for taxonomic-based human microbiome data,” in this research, the value of Alpha (α) = 5%, power = 80%, and the function of the number of sequence reads is equal to 50,000 with three groups. A total of 25 volunteers were obtained and it was determined that the dropout rate of 20%, resulting in a total of 30 volunteers per group, or 90 volunteers in total (La Rosa et al., 2012).
Sample collection and high-throughput sequencing
Each patient’s blood was collected to evaluate FBS, hemoglobin A1C (HbA1C), TC, TG, HDL-C, LDL-C, AST, ALT, creatinine, and high-sensitivity C-reactive protein (hs-CRP). The fresh stool was collected in DNA/RNA shield fecal collection tubes (Zymo Research, CA, United States) 1 day before an appointment and immediately frozen at-20°C for 48 h before further analysis. Using the QIAamp Stool Mini kit (Qiagen, United States), DNA was extracted from stool samples. Nanodrop and electrophoresis were used to evaluate the quantity and quality of DNA. Using 515\u00B0F and 806R primers and 2X KAPA hot-start ready mix, the V4 hypervariable region of the 16S rRNA gene was amplified by PCR. The PCR conditions included an initial denaturation at 94°C for 3 min, followed by 25 cycles of 98°C for 20 s., 55°C for 30 s., 72°C for 30 s., and a final extension step at 72°C for 5 min. The 16S amplicons were purified using AMPure XP beads and indexed using Nextera XT index kit, followed by 8 cycles of the aforementioned PCR condition. Finally, the PCR products were cleaned and pooled for cluster generation and 250-bp paired-end read sequencing on the Illumina® MiSeq™.
Sequencing data analysis
QIIME 22019.10 was used for microbiome bioinformatics (Bolyen et al., 2019). The raw sequence data was demultiplexed using the q2-demux plugin, and reads with expected errors (maxEE) higher than 3.0 were discarded by denoising software, DADA2 (via q2-dada2). A phylogeny was constructed using the SEPP q2-plugin, placing short sequences into sepp-refs-gg-13-8.qza reference phylogenetic tree. Alpha-diversity metric, beta-diversity metric, and Principle Coordinate Analysis (PCoA) were estimated using q2-diversity after samples were rarefied (subsampled without replacement) to a minimum read. Taxonomy was assigned to ASVs using the classify-sklearn naïve Bayes taxonomy classifier against the Greengenes 13_8 99% operational taxonomic units (OTUs) reference sequences. Statistical tests of alpha and beta diversity were performed using Kruskal-Wallis and permutational multivariate analysis of variance (PERMANOVA) (number of permutations = 999), respectively. Moreover, the significantly differential abundance analysis of microbiota was conducted using LEfSe (Segata et al., 2011) via the algorithm module on the Galaxy platform at http://huttenhower.sph.harvard.edu/galaxy. First, nonparametric factorial Kruskal-Wallissum-rank tests were applied to choose features differentially distributed among classes (p < 0.05). The linear discriminant analysis (LDA) model was used to estimate their effect sizes and supported by 30-fold bootstrapping (cutoff = logarithmic LDA score of ≥2.0). In addition, significant p-values associated with the microbial significantly differential features by LEfSe were corrected for multiple hypothesis testing using the Benjamini and Hochberg false discovery rate correction.
Statistical analysis and visualization
Stata/SE 16.1 software (StataCorp LP, College Station, TX, United States) was used to analyze the statistical data. Statistics were considered significant when p-value <0.05. All study variables were subjected to descriptive statistics analysis, which was provided as frequency (%) for categorical data and mean ± standard deviation (SD) or median for nonnormal quantitative data. One-way analysis of variance (ANOVA) statistic and post hoc analysis using the Scheffe test with p-value <0.05 were utilized if the distribution of the quantitative data, such as age and laboratory results, was normal. The Kruskal-Wallis test and post hoc Mann–Whitney U test with p-value <0.017 were used if the data distribution was not normal.
The following Spearman’s correlation coefficient was analyzed; (1) anthropometric measurements, including BMI and waist circumference (2) physical examination; blood pressure and (3) blood tests; FBS, HbA1C, TC, TG, LDL-C, HDL-C, AST, ALT, and hs-CRP. Correlation heat map visualization was performed using the ggplot2 R package. A p-value <0.05 was considered statistically significant and was labeled in the figure. In addition, the phylogenetic heat tree was visualized using the metacoder R package.
Results
Clinical characteristics
Ninety-one patients were included and divided into three groups: CCS patients undergoing coronary angiography, dyslipidemia patients, and healthy volunteers, with 30, 32, and 29 patients in each group, respectively. To be mentioned, 96 patients were recruited but the microbiota data of one dyslipidemia patient and four CCS patients were missed because stool samples could not be collected. So, we examined 91 samples of the gut microbiota. The patients were 43.96% female, with a mean age of 57.57 ± 8.35 years, and 26.37% had hypertension. There was no statistically significant difference in age or gender among the groups. Baseline characteristics are shown in Table 1.
The average age of the CCS patients was 59.60 ± 9.04 years and 56.67% of them had a history of CAD. In comparison to other groups, more patients had obesity, low HDL-C level, metabolic syndrome, hypertension, and diabetes mellitus at 43.33, 43.33, 33.33, 80, and 30% with statistically significant, respectively. Additionally, the levels of FBS, HbA1C, and hs-CRP were higher in this group than in others with statistical significance.
In dyslipidemia patients, the mean age was 57.44 ± 8.88 years. Diabetes mellitus and hypertension patients were omitted. There were 9.38% of the patients with impaired fasting glucose in this group however the average of FBS and HbA1C was in the normal range. TC and LDL-C were higher than others with statistical significance.
Unexpectedly, TC and LDL-C were found to be lowest in CCS patients compared to patients with dyslipidemia and healthy volunteers at 144.33 ± 41.24 and 77.11 ± 34.44 vs. 202.93 ± 43.67 and 129.96 ± 40.78 vs. 178.28 ± 22.28 and 111.70 ± 21.97, respectively. According to this study, 96.67% of CCS patients utilized statins, with a high-intensity statin rate of 93.10%. All patients took statins for a minimum of three months. Patients with dyslipidemia used statins at a rate of 90.63% with only high-intensity statins at 37.93%.
Diversity of the gut microbiota
A bacterial diversity analysis was performed to characterize the microbial community. Chao1 index was estimated to reflect the number of OTUs in a sample, the values of which are positively correlated with the species richness of the sample. Moreover, Shannon indicates it reflects the averaging or uniformity of the abundance of different species in a sample, which is positively correlated with the species diversity. Alpha diversity analyses, including the values of Chao1 and Shannon indexes, are comprehensive indicators of species richness and uniformity in community ecology (Figures 2A,B). From both Chao1 and Shannon indexes, there was a trend that showed lower diversity in dyslipidemia patients and CCS patients than in healthy volunteers.
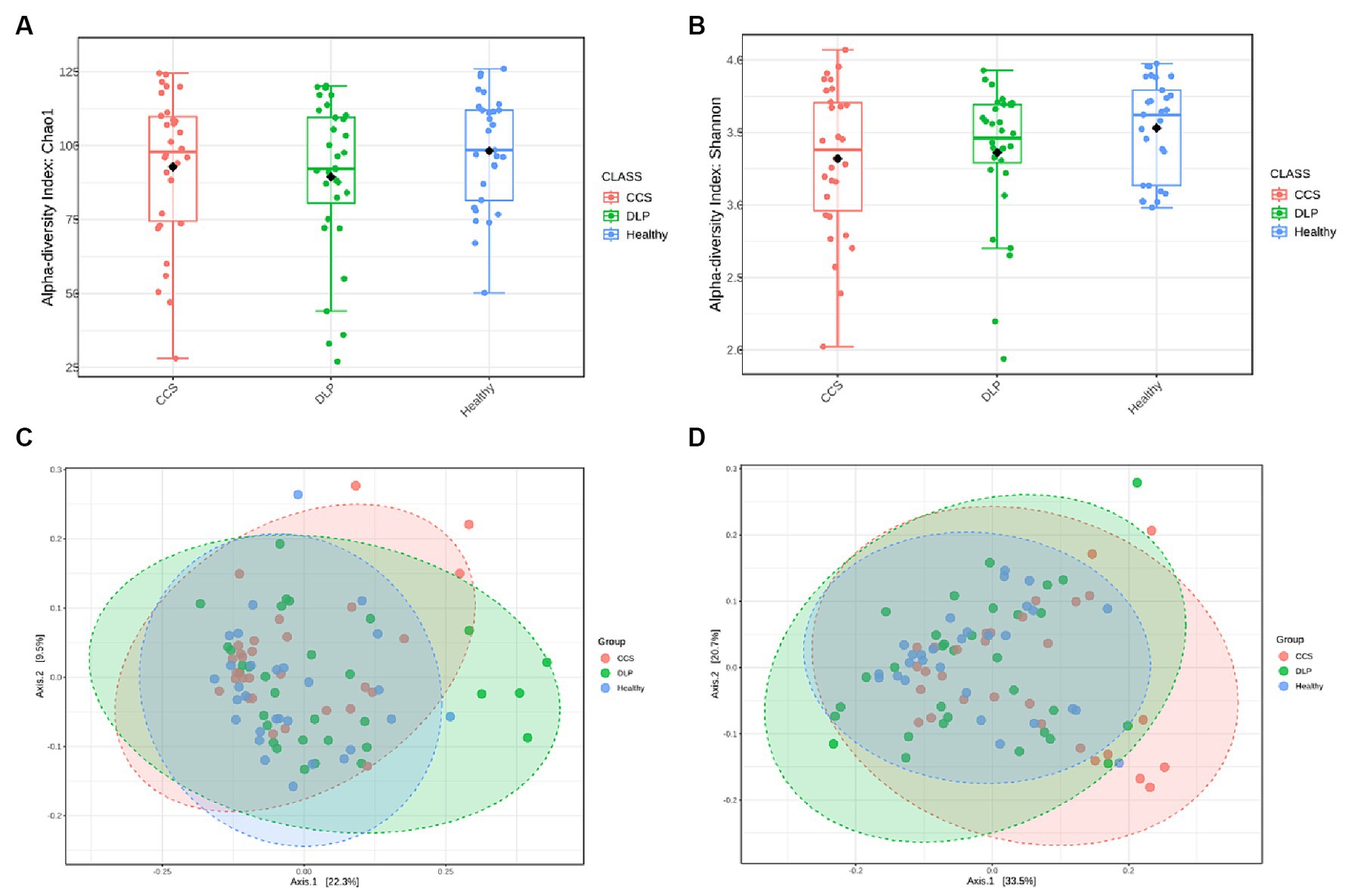
Figure 2. Analysis of alpha- and beta-diversity of microbial composition in the three patient groups. Diversity within bacterial communities was measured by the Chao1 index (A), the Shannon diversity index (B) the principal coordinate analysis (PCoA) of beta diversity based on the unweighted UniFrac distance (C) and the weighted UniFrac distance (D). Kruskal-Wallis H test was used in the statistical test of the alpha diversity (no statistically significant difference found). Permanova (Permutational multivariate analysis of variance) test was used in the statistical test of the beta diversity. Statistically significant differences found at p < 0.001. CCS, chronic coronary syndrome patients; DLP, dyslipidemia patients; Healthy, healthy volunteers.
Beta diversity refers to species differences between different environmental communities, and it also can be used to evaluate the overall heterogeneity of the species or the environmental community. Weighted and Unweighted UniFac distances were used in this study to determine the similarities and differences in the composition structure between the microbial communities. Principal coordinates analysis illustrated a statistically significant difference (PERMANOVA, p < 0.01) in the clustering of fecal samples between the gut microbiome of the dyslipidemia patients, CCS patients, and healthy volunteers (Figures 2C,D). The different colors in the PCoA analysis represent different samples, and the different shapes represent different groups. The closer the sample distance is, the more similar the microbial composition between the samples is, and the smaller the difference is.
Assessment of bacterial taxonomic composition of CCS and dyslipidemia patients and healthy volunteers
At the phylum level, among all three groups, the most common bacterial phyla were Firmicutes, Bacteroidetes, Proteobacteria, and Actinobacteria. The relative abundance of Proteobacteria was increased in CCS patients (the percentage of Proteobacteria in CCS patients, dyslipidemia patients, and healthy volunteers: 8.22, 4.79, and 4.16, respectively). Moreover, the relative abundance of Fusobacteria was also increased in CCS patients (the percentage of Fusobacteria in CCS patients, dyslipidemia patients, and healthy volunteers: 1.48, 1.07, and 0.89, respectively). On the other hand, in CCS patients, Actinobacteria and Verrucomicrobia had the lowest relative abundance proportion among the three groups. In terms of other phyla, there was no difference. The proportion of Firmicutes and Bacteroidetes was higher in the group of dyslipidemia patients than in the other groups (Figure 3A).
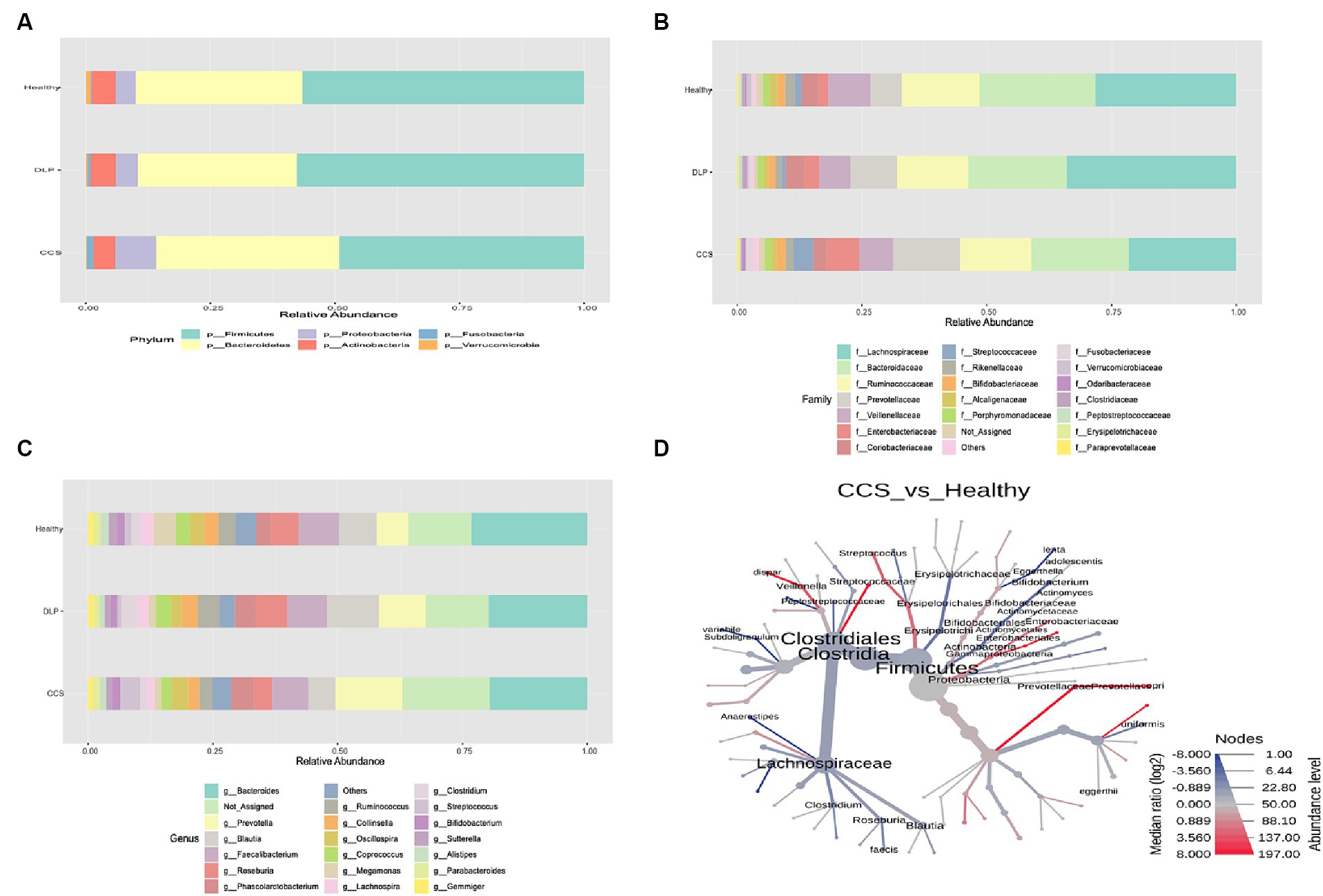
Figure 3. The relative abundance of bacterial taxonomic profile in the feces at the phylum (A) family (B) and genus (C) level. The phylogenetic heat tree (D) in comparison of bacterial microbiota between CCS and healthy groups shows the bacteria composition at the species level. The color of nodes and edges represents the mean change in operational taxonomic units (OTUs) richness at each taxonomic group, with red indicating greater richness in CCS and blue in healthy, while node size represents OTUs richness of each taxonomic group across the dataset. CCS, chronic coronary syndrome patients; DLP, dyslipidemia patients; Healthy, healthy volunteers.
At the family level, when the gut microbiota of the three groups was analyzed at this level, Lachnospiraceae and the Bacteroidaceae families were shown to be the two most common families. Compared to other groups, Prevotellaceae, Enterobacteriaceae and Streptococcaceae families were more prevalent proportionately in CCS patients (Figure 3B).
At the genus level, the highest abundance in each group belonged to the genus Bacteroides (the percentage of Bacteroides in CCS patients, dyslipidemia patients, and healthy volunteers: 19.02, 19.15, and 21.50, respectively). In the group of CCS patients, Prevotella and Streptococcus genera were more prevalent in abundance than other groups, with statistical significance following from Bacteroides genus (Figure 3C). Moreover, the relative abundance of Roseburia (the percentage of Roseburia in CCS patients, dyslipidemia patients and healthy volunteers: 3.17, 5.67, and 4.71, respectively), Ruminococcus (the percentage of Ruminococcus in CCS patients, dyslipidemia patients and healthy volunteers: 2.40, 4.54, and 3.79, respectively) and Faecalibacterium genera (the percentage of Faecalibacterium in CCS patients, dyslipidemia patients and healthy volunteers: 6.20, 6.98 and 7.08, respectively) were the lowest in CSS patients’ group than in the others.
At the species level, from the pairwise phylogenetic heat tree, the abundance of Streptococcus, Veillonella dispar, and Prevotella copri was higher in CCS patients than in healthy volunteers with statistical significance. On the other side, fewer Roseburia faecis, Subdoligranulum variabile, Bifidobacterium, and Anaerostipes were detected in this group (Figure 3D).
The characteristic of bacterial microbiota of CCS patients undergoing coronary angiography
In CCS patients, the relative abundance of Prevotellaceae, Enterobacteriaceae, Streptococcaceae, Clostridiaceae, and Paraprevotellaceae families had significantly risen at the family level compared with healthy volunteers, according to the linear discriminant analysis effect size (LEfSe). At the genus level, the LEfSe revealed that Prevotella, Streptococcus, Phascolarctobacterium, Dorea, Paraprevotella, and Veillonella genera had significantly higher relative abundances in CCS patients compared to healthy volunteers (Figure 4A).
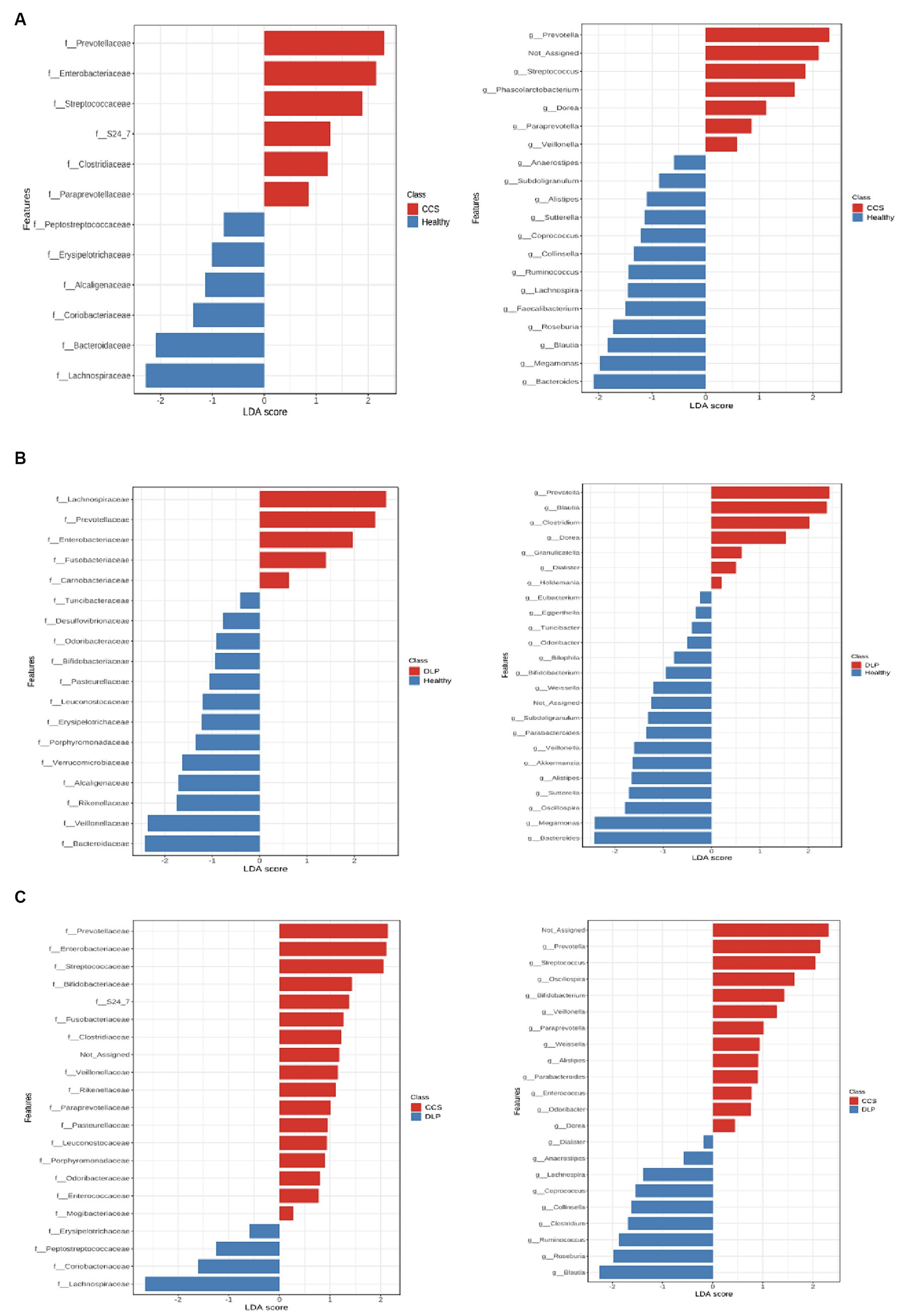
Figure 4. The linear discriminant analysis effect size (LEfSe) in comparison of bacterial microbiota between CCS patients and healthy volunteers (A), dyslipidemia patients and healthy volunteers (B), and CCS patients and dyslipidemia patients (C). CCS, chronic coronary syndrome patients; DLP, dyslipidemia patients; Healthy, healthy volunteers.
When comparing CCS patients with dyslipidemia patients, The result showed that the relative abundance of Prevotellaceae, Enterobacteriaceae, Streptococcaceae, Fusobacteriaceae, Clostridiaceae, Veillonellaceae, and Porphyromonadaceae families were higher in CCS patients (Figure 4C).
The characteristic of bacterial microbiota of dyslipidemia patients
In dyslipidemia patients, the relative abundance of Prevotellaceae, Enterobacteriaceae, and Fusobacteriaceae families had significantly risen at the family level compared with healthy volunteers, according to the LEfSe. At the genus level, Prevotella, Clostridium, and Dorea genera had significantly higher relative abundances in dyslipidemia patients compared to healthy volunteers (Figure 4B).
When we compared dyslipidemia patients with CCS patients, we found that the relative abundance of the Lachnospiraceae family was higher in dyslipidemia patients (Figure 4C).
Associations of risk factors with gut microbiome composition in CCS patients undergoing coronary angiography
We calculated the Spearman correlation coefficient between a range of clinical indicators that may be associated with the risk factors of CCS patients (Table 1) and gut microbiota at the genus level (Figure 5A). We found that Lachnospiraceae, Peptostreptococcaceae families, and Pediococcus genus were positively correlated with hs-CRP. Weissella genus was positively correlated with LDL-C while the Sutterella and Roseburia genera were negatively correlated with LDL-C.
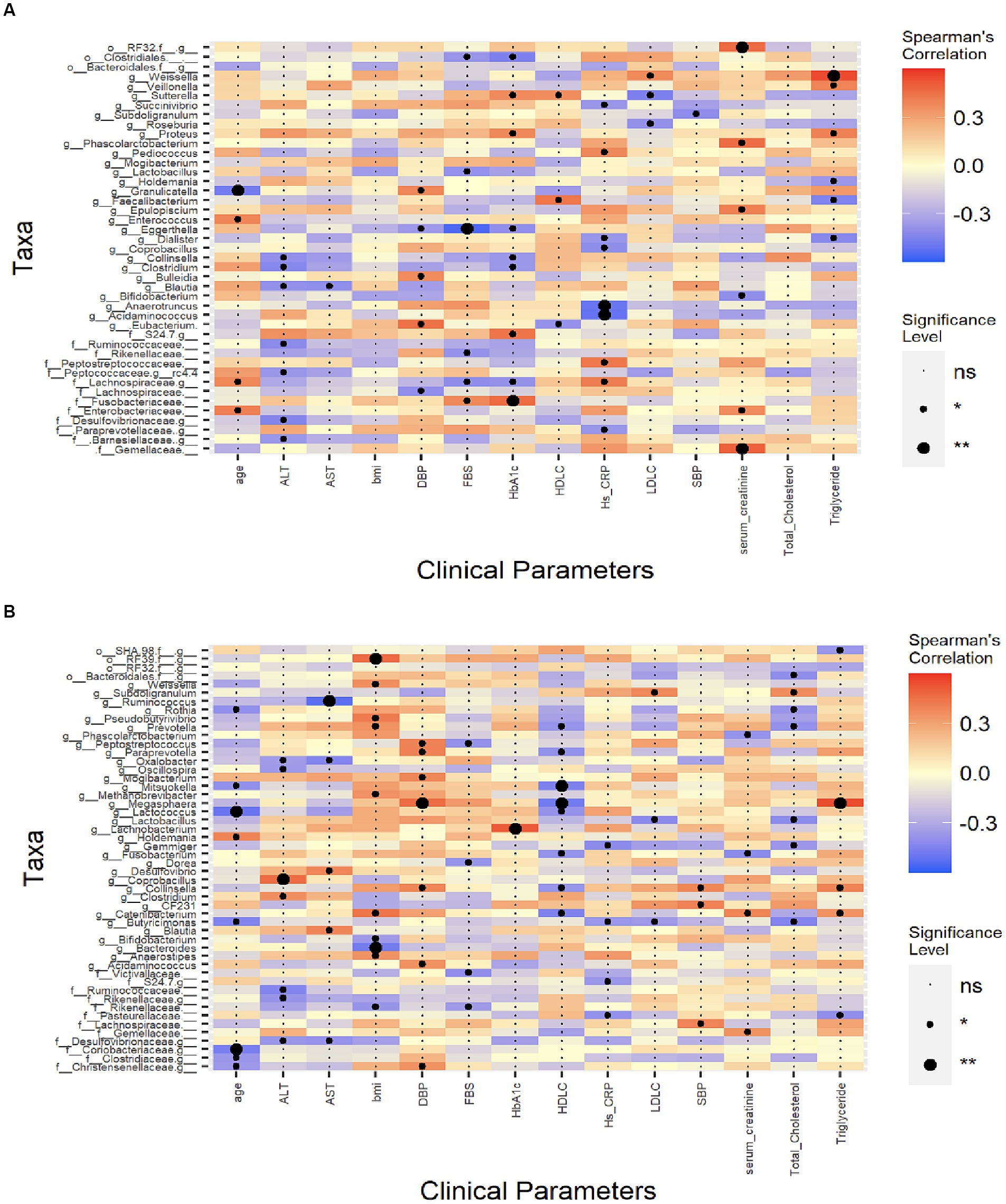
Figure 5. Spearman’s correlation analysis between the clinical indexes and the microbiota. CCS patients undergoing coronary angiography group (A), dyslipidemia patients’ group (B). The colour represents positive (red) or negative (blue) correlations, and *p < 0.05, **p < 0.01. ALT, alanine aminotransferase; AST, aspartate aminotransferase; BMI, body mass index; DBP, diastolic blood pressure; FBS, fasting blood sugar; HbA1C, hemoglobin A1C; hs-CRP, high-sensitivity C-reactive protein; HDL-C, high-density lipoprotein cholesterol; LDL-C, low-density lipoprotein cholesterol; SBP, systolic blood pressure.
Associations of risk factors with gut microbiome composition in dyslipidemia patients
In dyslipidemia patients, Pseudobutyrivibrio, Catenibacterium, Weissella, Prevotella, and Anaerostipes genera were positively correlated with body mass index (BMI) while Bacteroides and Bifidobacterium genera were negatively correlated with BMI. For this group’s lipid profile, genus Subdoligranulum was positively correlated with TC level; genus Megasphaera was strongly positively correlated and genera Collinsella and Catenibacterium were also positively correlated with TG level; genus Megasphaera was negatively correlated with HDL-C; genus Subdoligranulum was positively correlated with LDL-C level and genera Lactobacillus and Butyricimonas were negatively correlated with LDL-C level (Figure 5B).
Discussion
This is the first investigation on gut microbiota and associated factors in CCS patients undergoing coronary angiography in Thailand. In this study, the gut microbiome differed among the three groups. There was a reduction in diversity in dyslipidemia patients and CCS patients’ group, which correlated with other studies (Huttenhower et al., 2012; Fu et al., 2015; Sawicka-Smiarowska et al., 2021). This finding is consistent with other studies that patients suffering from various diseases have also reduced bacterial diversity, for instance, hypertension (Yang et al., 2015), Crohn’s disease (Hansen et al., 2012), psoriatic arthritis (Scher et al., 2015), metabolic syndrome (Arora and Bäckhed, 2016), diabetes mellitus, and obesity (Pascale et al., 2019).
In this study, the composition pattern of the bacterial microbiota differed significantly between the group of CCS patients and healthy volunteers. The most prevalent bacterial phyla were Firmicutes, Bacteroidetes, Proteobacteria, and Actinobacteria, which correlated with other studies (Zheng et al., 2020). Alterations in the ratio of the major phyla Firmicutes to Bacteroidetes have been proposed as a potential CAD risk factor, suggesting that changes in the microbiome’s composition may also contribute to the onset and progression of atherosclerosis and CAD (Emoto et al., 2016, 2017; Cui et al., 2017). Furthermore, it was established that a greater Firmicutes/Bacteroidetes ratio (F/B ratio) plays a significant impact on CAD patients (Cui et al., 2017). The F/B ratio is used to diagnose gut dysbiosis and the relationship between this ratio and numerous well-known cardiovascular risk factors, including age, sex, food, and BMI, has been demonstrated (Mariat et al., 2009; Holscher et al., 2015; Emoto et al., 2016; Canfora et al., 2017). However, in this study, dyslipidemia patients had the greatest F/B ratio whereas the CCS patients’ group did not.
Numerous pathogenic genera, including Escherichia, Salmonella, Vibrio, Yersinia, and Legionella, are members of the phylum Proteobacteria. In our study, the relative abundance proportion of Proteobacteria was the highest in CCS patients. According to Shin et al. study, a higher relative abundance of Proteobacteria is associated with gut dysbiosis and many diseases (obesity, T2DM, and cancers) in human (Shin et al., 2015). Mainly Proteobacteria, particularly Enterobacteriaceae and some Firmicutes is the abundance of bacteria producing TMAO precursor (Via et al., 2019).
Fusobacteria proportion was increased in the group of CCS patients, however, Actinobacteria and Verrucomicrobia exhibited the lowest relative abundance proportion in CCS patients in comparison with others. This finding is consistent with the study by Zhang et al. (2019) and Cui et al. (2017). Fusobacterium nucleatum may initially cause periodontal disease, which then causes CAD due to atherosclerosis via inflammation and lipid metabolism (Zhou et al., 2022). However, no research on the association between Fusobacterium and CAD has been published.
In addition, Streptococcus, Veillonella, and Prevotella genera were more common in abundance than other groups in the group of CCS patients, with statistical significance following from the Bacteroides genus. Previous studies have reported that Prevotella and Streptococcus genera had a close relationship with metabolic syndrome, atherosclerosis, and CAD (Abranches et al., 2009; Yakob et al., 2011; Madhogaria et al., 2022; Bazaz et al., 2023). It suggested that the changes in the abundance of Streptococcus, Veillonella, Prevotella were the characteristics of the bacterial microbiota of the CCS patients. The greatest associations were found for Streptococcus anginosus and Streptococcus oralis, according to Sayols-Baixeras et al.’s study of the correlation between Streptococcus spp. and subclinical coronary atherosclerosis (Sayols-Baixeras et al., 2023). The prevalence of various LPS-producing gram-negative bacteria, including Escherichia, Shigella, Veillonella, Klebsiella, and Haemophilus increased with the severity of the CAD, according to a study by Liu H. et al. (2019). High blood LPS levels were associated with a threefold increased risk of incident atherosclerosis (Mayr et al., 2006). Veillonella can be found in atherosclerosis plaque and is associated with cholesterol levels (Koren et al., 2011). In addition, Veillonella has a high relative abundance in CAD patients (Zhang et al., 2019; Bouzid et al., 2022). These findings suggested that the differences in bacterial abundance between the three groups may be linked to the progression of CAD and lipid metabolism in dyslipidemia patients.
The role of Prevotella in human health is controversial. Prevotella is a beneficial microbe, however, it is associated with chronic inflammation. Prevotella is linked to high complex carbohydrate diets from plants, fruits, and vegetables, whereas Bacteroides is connected to fat and protein diets (Wu et al., 2011). Prevotella can be found in healthy humans and is considered a commensal bacteria. According to De Filippis et al.’s research, vegetarians had the Mediterranean diet at a high level, which was connected with this bacteria strains and higher levels of SCFAs (De Filippis et al., 2016). According to Emoto et, Lactobacillus, Streptococcus, and Enterococcus increased in the gut microbiota of CAD patients whereas Bacteroides and Prevotella decreased (Emoto et al., 2016).
Prevotella strains’ genetic diversity may help to explain the variations in how they react to dietary and health conditions in different patients (Ley, 2016). For example, the study of Italian people’s gut microbiome showed that varying dietary choices could be responsible for P. copri strains with different functions, which resulted in different ways for human health (Filippis et al., 2019). P. copri increases the prevalence in non-Westernized people. They typically consume diets rich in fresh vegetables and fruits (Tett et al., 2019).
However, recent studies have connected increasing Prevotella abundance and certain strains to metabolic syndrome, obesity, hypertension, insulin resistance, non-alcoholic fatty liver disease (NAFLD), and low-grade systemic inflammation (Zhu et al., 2013; Pedersen et al., 2016; Madhogaria et al., 2022) due to augmentation mucosal helper T-cell (Th17) immune responses and stimulation epithelial cells to produce interleukin-1 (IL-1), IL-8, IL-6 and IL-23 (Larsen, 2017).
Prevotella copri was higher in CCS patients than in healthy volunteers in our study, which is correlated with many studies. Numerous diseases are linked with this microorganism to chronic inflammatory processes; for example, rheumatoid arthritis, periodontitis, HIV infection, metabolic syndrome, inflammatory bowel disease, CAD, and cardiac valve calcification. This bacterium has a role in the development of rheumatoid arthritis (RA) and is an immune-relevant (Pianta et al., 2017). In RA patients, gut dysbiosis may have a role in the early stage of RA as shown by the enrichment of P. copri (Alpizar-Rodriguez et al., 2019). Moreover, P. copri showed a high degree of genetic and functional diversity depending on the lifestyle of the patients and was associated with worse arthritis in these patients (Nii et al., 2023). In CVD patients, P. copri may be a major risk factor, particularly in cardiac valve calcification patients. This microorganism and LDL-C have a positive correlation, which likely supports its pro-inflammatory effects. It is a potential key pathogen implicated in CVDs because of its roles in immunity and inflammation (Liu Z. et al., 2019). Moreover, P. intermedia, P. nigrescens were periodontopathic bacteria in atherosclerotic plaques (Gaetti-Jardim et al., 2009).
In this investigation, Faecalibacterium genera were lower in the CSS patients’ group than in the others. This finding is correlated with Zhu et al. (2016), Faecalibacterium, Subdoligranulum, Roseburia, and Eubacterium rectale were decreased. There is a significant anti-inflammatory property of Faecalibacterium (Machiels et al., 2014).
The Bacteroides genus had the highest relative abundance when we compared the healthy volunteers’ group to the other groups, and the relative abundance of the Roseburia genus was higher in the healthy volunteers’ group than in the other groups in our study. Due to their ability to produce SCFAs, prior research indicated that Bacteroides and Bifidobacterium have a certain protective effect on metabolism and are primarily protective bacteria for CCS (Hoving et al., 2018; Kazemian et al., 2020; Abdi et al., 2022). In addition, Roseburia has been linked to improved glucose intolerance and weight loss in mice and in patients with atherosclerosis with comparatively high amounts of Collinsella, whereas the normal control group has a substantially larger abundance of Roseburia and Eubacterium (Zhu et al., 2018; Liu H. et al., 2019). A study by Liu H. et al. (2019) revealed that the prevalence of bacteria that produced butyric acid, such as Lachnospiraceae and Ruminococcaceae, decreased with the progression of CAD, this findings are correlated with our study that Lachnospiraceae and Ruminococcacea families were less proportion in CCS patients when compared to others.
SCFAs are metabolites of the fermentation of complex carbohydrates. Members of phylum Bacteroidetes can produce butyrate and acetate, whereas phylum Firmicutes can produce butyrate. SCFAs have a favorable correlation with Roseburia, Bacteroides spp., and Eubacterium rectale. They have been proposed as a protective effect on CCS patients and SCFA producers have decreased in some CCS patients. Additionally, they improve health by boosting the immunological response of the host, preserving the integrity of the intestinal barrier by controlling the expression of tight junction proteins, and reducing blood lipid levels by preventing the production of cholesterol (David et al., 2014; Krishnan et al., 2015; Chambers et al., 2018). SCFAs play a protective role in atherosclerosis, while LPS stimulates body inflammation and accelerates the formation of atherosclerosis (Vinolo et al., 2011).
When butyrate-producing bacteria disappear, the gut barrier may become damaged, making it easier for microbial toxins like LPS to leak and cause inflammation by binding to Toll-like receptors. Patients with CAD have been found to have a higher LPS biosynthesis in the microbiome, which is connected to insulin resistance and abdominal obesity (Trøseid et al., 2013; Brandsma et al., 2019).
The synthesis of TMAO, a strong risk factor for the development of CAD, involves dietary choline, betaine, phosphatidylcholine, L-carnitine, and lecithin which are obtained from a variety of sources, including egg, fish, red meat, soybean, peanuts (Tang et al., 2013; Zhu et al., 2016). By producing choline and the intermediary molecule trimethylamine (TMA), the gut bacteria also contribute to the synthesis of TMAO. The capacity of the gut microbiota to synthesize choline via the phospholipase D (PLD) enzyme has just recently been discovered. The flavin-containing monooxygenase (FMO) enzyme metabolizes the microbiome-derived TMA molecule into TMAO in hepatocytes (Phillips et al., 1995). Firmicutes, Proteobacteria, Actinobacteria, and Bacteroidetes are the TMA producers. The production of foam cells is stimulated by the impairment of cholesterol metabolism in macrophages caused by TMAO-dependent activation of macrophage scavenger receptors and CD36 expression. The higher the TMAO production, the higher the CAD risk (Wang et al., 2011). In the context of increased intestinal permeability, TMAO is also linked to C-reactive protein (CRP), endothelial dysfunction, and elevated blood levels of the LPS endotoxin. It can also cause platelet hyperreactivity, which has an impact on the CAD progression (Al-Obaide et al., 2017).
The LDL-C level was lowest in CCS patients. The reason for the differences in LDL-C levels was that the majority of patients (96.67%) were treated with statins. The treatment with statins is associated with a lower prevalence of gut microbiota dysbiosis (Vieira-Silva et al., 2020). In mice study, statins could moderate gut microbiota by increasing the abundance of Bacteroides, Butyricimonas, and Mucispirillum (Kim et al., 2019). Moreover, the clinical response to statin therapy in individuals with CAD is associated with the gut microbiota. For instance, poor statin response is associated with a significant reduction in the number of beneficial bacteria (Akkermansia muciniphila and Lactobacillus) and an increase in the number of bacteria (Holdemanella and Facecallibacterium) (Wang et al., 2021). Statins have also been linked to anti-inflammatory and immunomodulatory properties (Lee et al., 2016).
The genus Megasphaera was strongly positively correlated with TG level and negatively correlated with HDL-C. López-Montoya et al. (2022) investigation confirmed our results, showing Megasphaera and Escherichia-Shigella were highly associated with atherogenic dyslipidemia patients, defined as both hypertriglyceridemia and low HDL-C. Moreover, one study has shown that patients with symptomatic stroke had an altered gut microbiota and defined Megasphaera as opportunistic pathogens (Zeng et al., 2019). In obesity and overweight patients, Megasphaera significantly increased relative abundance and correlated with low physical activity (Palmas et al., 2021). Megasphaera is involved in a mechanism that generates ammonia, which has negative consequences (Anand et al., 2016).
Hs-CRP can help in identifying chronic inflammation. Periodontal disease was linked to CAD and low-grade inflammation, increased CRP, and fibrinogen, according to De Oliveira et al.’s research (De Oliveira et al., 2010). There is a correlation between hs-CRP and CAD severity in CCS patients (Habib and Masri, 2013). Patients with myocardial infarction with elevated hs-CRP level (≥ 2 mg/L) were at higher risk of major adverse cardiovascular events and death (Carrero et al., 2019). In CCS patients of our study, the Lachnospiraceae, Peptostreptococcaceae families, and Pediococcus genus were positively correlated with hs-CRP, a finding that has not been reported before.
This study had some limitations, one of which was that the functional roles of the gut microbiota were not examined using a shotgun sequencing approach yielded more information due to its broader gene list and capacity to profile metabolic pathways. Moreover, the cross-sectional nature of this study makes it difficult to determine a causal relationship between changes in the gut microbiome and the disease. Other possible confounding variables that might have influenced the study’s findings were obesity, impaired fasting glucose, and polypharmacy.
Conclusion
In conclusion, this study demonstrated a significant difference in the composition of gut microbiota between CCS patients, dyslipidemia patients, and healthy volunteers. The alpha diversity was lower in CCS and dyslipidemia patients than in healthy volunteers. The relative abundance of Proteobacteria, Fusobacteria, Prevotella, and Streptococcus was significantly increased while Roseburia, Ruminococcus, and Faecalibacterium were lower in CCS patients. In dyslipidemia patients, Megasphaera was strongly positively correlated with TG level and negatively correlated with HDL-C. Modification of gut microbiota is associated with changes in clinical parameters involved in the development of CAD in CCS patients.
Data availability statement
The original contributions presented in the study are publicly available. This data can be found at: https://www.ncbi.nlm.nih.gov/bioproject/; PRJNA1000984.
Ethics statement
The studies involving humans were approved by Ethics Committee of Chulabhorn Hospital and Srinakharinwirot University research ethics committees (IEC No: 174/2564, issued 22 July 2022 and SWUEC/E/M-100/2565E, issued 22 February 2023, respectively). The studies were conducted in accordance with the local legislation and institutional requirements. The participants provided their written informed consent to participate in this study.
Author contributions
WL: Conceptualization, Data curation, Formal analysis, Investigation, Methodology, Project administration, Resources, Software, Validation, Visualization, Writing – original draft, Writing – review & editing. PP: Conceptualization, Data curation, Formal analysis, Methodology, Project administration, Software, Supervision, Validation, Visualization, Writing – review & editing. VM: Conceptualization, Validation, Visualization, Writing – review & editing. DS: Conceptualization, Data curation, Methodology, Project administration, Visualization, Writing – review & editing. EE: Conceptualization, Data curation, Supervision, Validation, Visualization, Writing – review & editing. MT: Conceptualization, Data curation, Funding acquisition, Investigation, Methodology, Project administration, Resources, Supervision, Validation, Visualization, Writing – review & editing.
Funding
The author(s) declare financial support was received for the research, authorship, and/or publication of this article. This study was supported by the Faculty of Medicine, Srinakharinwirot University (grant number 505/2565) and the Center of Excellent in Probiotics Srinakharinwirot University (grant number 324/2565).
Conflict of interest
PP is employed by Mod Gut Co., Ltd.
The remaining authors declare that the research was conducted in the absence of any commercial or financial relationships that could be construed as a potential conflict of interest.
Publisher’s note
All claims expressed in this article are solely those of the authors and do not necessarily represent those of their affiliated organizations, or those of the publisher, the editors and the reviewers. Any product that may be evaluated in this article, or claim that may be made by its manufacturer, is not guaranteed or endorsed by the publisher.
References
Abdi, M., Hadi Esmaeili, G. G., and Ranjbar, R. (2022). Lactobacilli and Bifidobacterium as anti-atherosclerotic agents. Iran. J. Basic Med. Sci. 25, 934–946. doi: 10.22038/IJBMS.2022.63860.14073
Abdul-Ghani, M., DeFronzo, R. A., Del Prato, S., Chilton, R., Singh, R., and Ryder, R. E. J. (2017). Cardiovascular disease and type 2 diabetes: has the Dawn of a new era arrived? Diabetes Care 40, 813–820. doi: 10.2337/dc16-2736
Abranches, J., Zeng, L., Bélanger, M., Rodrigues, P. H., Simpson-Haidaris, P. J., Akin, D., et al. (2009). Invasion of human coronary artery endothelial cells by Streptococcus Mutans OMZ175: S. mutans endothelial cell invasion. Oral Microbiol. Immunol. 24, 141–145. doi: 10.1111/j.1399-302X.2008.00487.x
Afzaal, M., Saeed, F., Shah, Y. A., Hussain, M., Rabail, R., Socol, C. T., et al. (2022). Human gut microbiota in health and disease: unveiling the relationship. Front. Microbiol. 13, 1–14. doi: 10.3389/fmicb.2022.999001
Al-Obaide, M., Singh, R., Datta, P., Rewers-Felkins, K., Salguero, M., Al-Obaidi, I., et al. (2017). Gut microbiota-dependent trimethylamine-N-oxide and serum biomarkers in patients with T2DM and advanced CKD. J. Clin. Med. 6:86. doi: 10.3390/jcm6090086
Alpizar-Rodriguez, D., Lesker, T. R., Gronow, A., Gilbert, B., Raemy, E., Lamacchia, C., et al. (2019). Prevotella Copri in individuals at risk for rheumatoid arthritis. Ann. Rheum. Dis. 78, 590–593. doi: 10.1136/annrheumdis-2018-214514
American Diabetes Association (2014). Diagnosis and classification of diabetes mellitus. Diabetes Care 37, S81–S90. doi: 10.2337/dc14-S081
Anand, S., Kaur, H., and Mande, S. S. (2016). Comparative in silico analysis of butyrate production pathways in gut commensals and pathogens. Front. Microbiol. 7:1945. doi: 10.3389/fmicb.2016.01945
Arora, T., and Bäckhed, F. (2016). The gut microbiota and metabolic disease: current understanding and future perspectives. J. Intern. Med. 280, 339–349. doi: 10.1111/joim.12508
Bazaz, R., Marriott, H. M., Wright, C., Chamberlain, J., West, L. E., Gelsthorpe, C., et al. (2023). Transient increase in atherosclerotic plaque macrophage content following Streptococcus Pneumoniae pneumonia in ApoE-deficient mice. Front. Cell. Infect. Microbiol. 13:1090550. doi: 10.3389/fcimb.2023.1090550
Bolyen, E., Rideout, J. R., Dillon, M. R., Bokulich, N. A., Abnet, C. C., Al-Ghalith, G. A., et al. (2019). Reproducible, interactive, scalable and extensible microbiome data science using QIIME 2. Nat. Biotechnol. 37, 852–857. doi: 10.1038/s41587-019-0209-9
Bouzid, F., Gtif, I., Alfadhli, S., Charfeddine, S., Ghorbel, W., Abdelhédi, R., et al. (2022). A potential Oral microbiome signature associated with coronary artery disease in Tunisia. Biosci. Rep. 42:BSR20220583. doi: 10.1042/BSR20220583
Brandsma, E., Kloosterhuis, N. J., Koster, M., Dekker, D. C., Gijbels, M. J. J., Van Der Velden, S., et al. (2019). A proinflammatory gut microbiota increases systemic inflammation and accelerates atherosclerosis. Circ. Res. 124, 94–100. doi: 10.1161/CIRCRESAHA.118.313234
Canfora, E. E., Van Der Beek, C. M., Hermes, G. D. A., Goossens, G. H., Jocken, J. W. E., Holst, J. J., et al. (2017). Supplementation of diet with Galacto-oligosaccharides increases Bifidobacteria, but not insulin sensitivity, in obese prediabetic individuals. Gastroenterology 153, 87–97.e3. doi: 10.1053/j.gastro.2017.03.051
Carrero, J. J., Franko, M. A., Obergfell, A., Gabrielsen, A., and Jernberg, T. (2019). Hs-CRP level and the risk of death or recurrent cardiovascular events in patients with myocardial infarction: a healthcare-based study. J. Am. Heart Assoc. 8:e012638. doi: 10.1161/JAHA.119.012638
Chambers, E. S., Preston, T., Frost, G., and Morrison, D. J. (2018). Role of gut microbiota-generated short-chain fatty acids in metabolic and cardiovascular health. Curr. Nutr. Reports 7, 198–206. doi: 10.1007/s13668-018-0248-8
Cui, L., Zhao, T., Haibing, H., Zhang, W., and Hua, X. (2017). Association study of gut Flora in coronary heart disease through high-throughput sequencing. Biomed. Res. Int. 2017, 1–10. doi: 10.1155/2017/3796359
David, L. A., Maurice, C. F., Carmody, R. N., Gootenberg, D. B., Button, J. E., Wolfe, B. E., et al. (2014). Diet rapidly and reproducibly alters the human gut microbiome. Nature 505, 559–563. doi: 10.1038/nature12820
De Filippis, F., Pellegrini, N., Vannini, L., Jeffery, I. B., La Storia, A., Laghi, L., et al. (2016). High-level adherence to a Mediterranean diet beneficially impacts the gut microbiota and associated metabolome. Gut 65, 1812–1821. doi: 10.1136/gutjnl-2015-309957
De Oliveira, C., Watt, R., and Hamer, M. (2010). Toothbrushing, inflammation, and risk of cardiovascular disease: results from Scottish health survey. BMJ 340:c2451. doi: 10.1136/bmj.c2451
Dustan, H. P. (2000). Hypertension. J. Am. Coll. Cardiol. 35, 10B–12B. doi: 10.1016/S0735-1097(00)80042-X
Emoto, T., Yamashita, T., Kobayashi, T., Sasaki, N., Hirota, Y., Hayashi, T., et al. (2017). Characterization of gut microbiota profiles in coronary artery disease patients using data mining analysis of terminal restriction fragment length polymorphism: gut microbiota could be a diagnostic marker of coronary artery disease. Heart Vessel. 32, 39–46. doi: 10.1007/s00380-016-0841-y
Emoto, T., Yamashita, T., Sasaki, N., Hirota, Y., Hayashi, T., So, A., et al. (2016). Analysis of gut microbiota in coronary artery disease patients: a possible link between gut microbiota and coronary artery disease. J. Atheroscler. Thromb. 23, 908–921. doi: 10.5551/jat.32672
Fan, Y., and Pedersen, O. (2021). Gut microbiota in human metabolic health and disease. Nat. Rev. Microbiol. 19, 55–71. doi: 10.1038/s41579-020-0433-9
Filippis, D., Francesca, E. P., Tett, A., Tarallo, S., Naccarati, A., De Angelis, M., et al. (2019). Distinct genetic and functional traits of human intestinal Prevotella Copri strains are associated with different habitual diets. Cell Host Microbe 25, 444–453.e3. doi: 10.1016/j.chom.2019.01.004
Fu, J., Bonder, M. J., Cenit, M. C., Tigchelaar, E. F., Maatman, A., Dekens, J. A. M., et al. (2015). The gut microbiome contributes to a substantial proportion of the variation in blood lipids. Circ. Res. 117, 817–824. doi: 10.1161/CIRCRESAHA.115.306807
Gaetti-Jardim, E., Marcelino, S. L., Feitosa, A. C. R., Romito, G. A., and Avila-Campos, M. J. (2009). Quantitative detection of periodontopathic Bacteria in atherosclerotic plaques from coronary arteries. J. Med. Microbiol. 58, 1568–1575. doi: 10.1099/jmm.0.013383-0
Habib, S. S., and Al Masri, A. (2013). Relationship of high sensitivity C-reactive protein with presence and severity of coronary artery disease. Pak. J. Med. Sci. 29, 1425–1429. doi: 10.12669/pjms.296.3302
Hansen, R., Russell, R. K., Reiff, C., Louis, P., McIntosh, F., Berry, S. H., et al. (2012). Microbiota of De-novo Pediatric IBD: increased Faecalibacterium Prausnitzii and reduced bacterial diversity in Crohn’s but not in ulcerative colitis. Am. J. Gastroenterol. 107, 1913–1922. doi: 10.1038/ajg.2012.335
Hedayatnia, M., Asadi, Z., Zare-Feyzabadi, R., Yaghooti-Khorasani, M., Ghazizadeh, H., Ghaffarian-Zirak, R., et al. (2020). Dyslipidemia and cardiovascular disease risk among the MASHAD study population. Lipids Health Dis. 19:42. doi: 10.1186/s12944-020-01204-y
Holscher, H. D., Gregory Caporaso, J., Hooda, S., Brulc, J. M., Fahey, G. C., and Swanson, K. S. (2015). Fiber supplementation influences phylogenetic structure and functional capacity of the human intestinal microbiome: follow-up of a randomized controlled trial. Am. J. Clin. Nutr. 101, 55–64. doi: 10.3945/ajcn.114.092064
Hoving, L. R., Katiraei, S., Heijink, M., Pronk, A., Van Der Wee-Pals, L., Streefland, T., et al. (2018). Dietary mannan oligosaccharides modulate gut microbiota, increase Fecal bile acid excretion, and decrease plasma cholesterol and atherosclerosis development. Mol. Nutr. Food Res. 62:1700942. doi: 10.1002/mnfr.201700942
Huang, P. L. (2009). A comprehensive definition for metabolic syndrome. Dis. Model. Mech. 2, 231–237. doi: 10.1242/dmm.001180
Huttenhower, C., Gevers, D., Knight, R., Sahar Abubucker, H., Jonathan, B., Chinwalla, A. T., et al. (2012). Structure, function and diversity of the healthy human microbiome. Nature 486, 207–214. doi: 10.1038/nature11234
Jiangping, S., Zhe, Z., Wei, W., Yunhu, S., Jie, H., Hongyue, W., et al. (2013). Assessment of coronary artery stenosis by coronary angiography: a head-to-head comparison with pathological coronary artery anatomy. Circ. Cardiovasc. Interv. 6, 262–268. doi: 10.1161/CIRCINTERVENTIONS.112.000205
Jonsson, A. L., and Bäckhed, F. (2017). Role of gut microbiota in atherosclerosis. Nat. Rev. Cardiol. 14, 79–87. doi: 10.1038/nrcardio.2016.183
Kazemian, N., Mahmoudi, M., Halperin, F., Wu, J. C., and Pakpour, S. (2020). Gut microbiota and cardiovascular disease: opportunities and challenges. Microbiome 8:36. doi: 10.1186/s40168-020-00821-0
Kim, J., Lee, H., An, J., Song, Y., Lee, C.-K., Kim, K., et al. (2019). Alterations in gut microbiota by statin therapy and possible intermediate effects on Hyperglycemia and Hyperlipidemia. Front. Microbiol. 10:1947. doi: 10.3389/fmicb.2019.01947
Knuuti, J., Wijns, W., Saraste, A., Capodanno, D., Barbato, E., Funck-Brentano, C., et al. (2020). 2019 ESC guidelines for the diagnosis and Management of Chronic Coronary Syndromes. Eur. Heart J. 41, 407–477. doi: 10.1093/eurheartj/ehz425
Koren, O., Spor, A., Felin, J., Fåk, F., Stombaugh, J., Tremaroli, V., et al. (2011). Human Oral, gut, and plaque microbiota in patients with atherosclerosis. Proc. Natl. Acad. Sci. 108, 4592–4598. doi: 10.1073/pnas.1011383107
Krishnan, S., Alden, N., and Lee, K. (2015). Pathways and functions of gut microbiota metabolism impacting host physiology. Curr. Opin. Biotechnol. 36, 137–145. doi: 10.1016/j.copbio.2015.08.015
Lane, D. J., Pace, B., Olsen, G. J., Stahl, D. A., Sogin, M. L., and Pace, N. R. (1985). Rapid determination of 16S ribosomal RNA sequences for phylogenetic analyses. Proc. Natl. Acad. Sci. USA 82, 6955–6959. doi: 10.1073/pnas.82.20.6955
Larsen, J. M. (2017). The immune response to Prevotella Bacteria in chronic inflammatory disease. Immunology 151, 363–374. doi: 10.1111/imm.12760
La Rosa, P. S., Brooks, J. P., Deych, E., Boone, E. L., Edwards, D. J., Wang, Q., et al. (2012). Hypothesis testing and power calculations for taxonomic-based human microbiome data. PLoS One 7:e52078. doi: 10.1371/journal.pone.0052078
Lee, S., Lee, Y., Kim, J., An, J., Kim, K., Lee, H., et al. (2016). Atorvastatin and rosuvastatin improve physiological parameters and alleviate immune dysfunction in metabolic disorders. Biochem. Biophys. Res. Commun. 478, 1242–1247. doi: 10.1016/j.bbrc.2016.08.101
Ley, R. E. (2016). Prevotella in the gut: choose carefully. Nat. Rev. Gastroenterol. Hepatol. 13, 69–70. doi: 10.1038/nrgastro.2016.4
Lin, C.-F., Chang, Y.-H., Chien, S.-C., Lin, Y.-H., and Yeh, H.-Y. (2018). Epidemiology of Dyslipidemia in the Asia Pacific region. Int. J. Gerontol. 12, 2–6. doi: 10.1016/j.ijge.2018.02.010
Liu, H., Chen, X., Xiaomin, H., Niu, H., Tian, R., Wang, H., et al. (2019). Alterations in the gut microbiome and metabolism with coronary artery disease severity. Microbiome 7:68. doi: 10.1186/s40168-019-0683-9
Liu, Z., Li, J., Liu, H., Tang, Y., Zhan, Q., Lai, W., et al. (2019). The intestinal microbiota associated with cardiac valve calcification differs from that of coronary artery disease. Atherosclerosis 284, 121–128. doi: 10.1016/j.atherosclerosis.2018.11.038
Liu, H., Zhuang, J., Tang, P., Li, J., Xiong, X., and Deng, H. (2020). The role of the gut microbiota in coronary heart disease. Curr. Atheroscler. Rep. 22:77. doi: 10.1007/s11883-020-00892-2
López-Montoya, P., Cerqueda-García, D., Rodríguez-Flores, M., López-Contreras, B., Villamil-Ramírez, H., Morán-Ramos, S., et al. (2022). Association of gut Microbiota with atherogenic Dyslipidemia, and its impact on serum lipid levels after bariatric surgery. Nutrients 14:3545. doi: 10.3390/nu14173545
Machiels, K., Joossens, M., Sabino, J., De Preter, V., Arijs, I., and Eeckhaut, V. (2014). A decrease of the butyrate-producing species Roseburia hominis and Faecalibacterium Prausnitzii defines dysbiosis in patients with ulcerative colitis. Gut 63, 1275–1283. doi: 10.1136/gutjnl-2013-304833
Madhogaria, B., Bhowmik, P., and Kundu, A. (2022). Correlation between human gut microbiome and diseases. Inf. Med. 1, 180–191. doi: 10.1016/j.imj.2022.08.004
Mariat, D., Firmesse, O., Levenez, F., Vd Guimarăes, H., Sokol, J. D., Corthier, G., et al. (2009). The Firmicutes/Bacteroidetes ratio of the human microbiota changes with age. BMC Microbiol. 9:123. doi: 10.1186/1471-2180-9-123
Mayr, M., Kiechl, S., Tsimikas, S., Miller, E., Sheldon, J., Willeit, J., et al. (2006). Oxidized low-density lipoprotein autoantibodies, chronic infections, and carotid atherosclerosis in a population-based study. J. Am. Coll. Cardiol. 47, 2436–2443. doi: 10.1016/j.jacc.2006.03.024
Nii, T., Maeda, Y., Motooka, D., Naito, M., Matsumoto, Y., Ogawa, T., et al. (2023). Genomic repertoires linked with pathogenic potency of arthritogenic Prevotella Copri isolated from the gut of patients with rheumatoid arthritis. Ann. Rheum. Dis. 82, 621–629. doi: 10.1136/ard-2022-222881
Palmas, V., Pisanu, S., Madau, V., Casula, E., Deledda, A., Cusano, R., et al. (2021). Gut microbiota markers associated with obesity and overweight in Italian adults. Sci. Rep. 11:5532. doi: 10.1038/s41598-021-84928-w
Pascale, A., Marchesi, N., Govoni, S., Coppola, A., and Gazzaruso, C. (2019). The role of gut microbiota in obesity, diabetes mellitus, and effect of metformin: new insights into old diseases. Curr. Opin. Pharmacol. 49, 1–5. doi: 10.1016/j.coph.2019.03.011
Pedersen, H. K., Gudmundsdottir, V., Nielsen, H. B., Hyotylainen, T., Nielsen, T., Jensen, B. A. H., et al. (2016). Human gut microbes impact host serum metabolome and insulin sensitivity. Nature 535, 376–381. doi: 10.1038/nature18646
Phillips, I. R., Dolphin, C. T., Clair, P., Hadley, M. R., Hutt, A. J., McCombie, R. R., et al. (1995). The molecular biology of the flavin-containing monooxygenases of Man. Chem. Biol. Interact. 96, 17–32. doi: 10.1016/0009-2797(94)03580-2
Pianta, A., Arvikar, S., Strle, K., Drouin, E. E., Wang, Q., Costello, C. E., et al. (2017). Evidence of the immune relevance of Prevotella Copri, a gut microbe, in patients with rheumatoid arthritis. Arthritis Rheumatol. 69, 964–975. doi: 10.1002/art.40003
Pieczynska, M. D., Yang, Y., Petrykowski, S., Horbanczuk, O. K., Atanasov, A. G., and Horbanczuk, J. O. (2020). Gut microbiota and its metabolites in atherosclerosis development. Molecules 25:594. doi: 10.3390/molecules25030594
Samadoulougou, S., Idzerda, L., Dault, R., Lebel, A., Cloutier, A.-M., and Vanasse, A. (2020). Validated methods for identifying individuals with obesity in health care administrative databases: a systematic review. Obes. Sci. Pract. 6, 677–693. doi: 10.1002/osp4.450
Santacroce, L., Man, A., Charitos, I. A., Haxhirexha, K., and Topi, S. (2021). Current knowledge about the connection between health status and gut microbiota from birth to elderly. Front. Biosci. Landmark 26:135. doi: 10.52586/4930
Sawicka-Smiarowska, E., Bondarczuk, K., Bauer, W., Niemira, M., Szalkowska, A., Raczkowska, J., et al. (2021). Gut microbiome in chronic coronary syndrome patients. J. Clin. Med. 10:5074. doi: 10.3390/jcm10215074
Sayols-Baixeras, S., Dekkers, K. F., Baldanzi, G., Jönsson, D., Hammar, U., Lin, Y. T., et al. (2023). Streptococcus species abundance in the gut is linked to subclinical coronary atherosclerosis in 8973 participants from the SCAPIS cohort. Circulation 148, 1–14. doi: 10.1161/CIRCULATIONAHA.123.063914
Scher, J. U., Ubeda, C., Artacho, A., Attur, M., Isaac, S., Reddy, S. M., et al. (2015). Decreased bacterial diversity characterizes the altered gut microbiota in patients with psoriatic arthritis, resembling dysbiosis in inflammatory bowel disease: gut microbiota in PsA. Arthritis Rheumatol. 67, 128–139. doi: 10.1002/art.38892
Segata, N., Izard, J., Waldron, L., Gevers, D., Miropolsky, L., Garrett, W. S., et al. (2011). Metagenomic biomarker discovery and explanation. Genome Biol. 12:R60. doi: 10.1186/gb-2011-12-6-r60
Shin, N.-R., Whon, T. W., and Bae, J.-W. (2015). Proteobacteria: microbial signature of dysbiosis in gut microbiota. Trends Biotechnol. 33, 496–503. doi: 10.1016/j.tibtech.2015.06.011
Tang, W. H. W., Wang, Z., Levison, B. S., Koeth, R. A., Britt, E. B., Xiaoming, F., et al. (2013). Intestinal microbial metabolism of phosphatidylcholine and cardiovascular risk. N. Engl. J. Med. 368, 1575–1584. doi: 10.1056/NEJMoa1109400
Tett, A., Huang, K. D., Asnicar, F., Fehlner-Peach, H., Pasolli, E., Karcher, N., et al. (2019). The Prevotella Copri complex comprises four distinct clades underrepresented in westernized populations. Cell Host Microbe 26, 666–679.e7. doi: 10.1016/j.chom.2019.08.018
Thursby, E., and Juge, N. (2017). Introduction to the human gut microbiota. Biochem. J. 474, 1823–1836. doi: 10.1042/BCJ20160510
Trøseid, M., Nestvold, T. K., Rudi, K., Thoresen, H., Nielsen, E. W., and Lappegård, K. T. (2013). Plasma lipopolysaccharide is closely associated with Glycemic control and abdominal obesity. Diabetes Care 36, 3627–3632. doi: 10.2337/dc13-0451
Tune, J. D., Goodwill, A. G., Sassoon, D. J., and Mather, K. J. (2017). Cardiovascular consequences of metabolic syndrome. Transl. Res. 183, 57–70. doi: 10.1016/j.trsl.2017.01.001
Via, D., Alessandro, G. G., Taverniti, V., Rondini, G., Velardi, I., Gambaro, V., et al. (2019). Urinary TMAO levels are associated with the taxonomic composition of the gut microbiota and with the choline Tma-lyase gene (cutC) Harbored by Enterobacteriaceae. Nutrients 12:62. doi: 10.3390/nu12010062
Vieira-Silva, S., Falony, G., Belda, E., Nielsen, T., Aron-Wisnewsky, J., Chakaroun, R., et al. (2020). Statin therapy is associated with lower prevalence of gut microbiota dysbiosis. Nature 581, 310–315. doi: 10.1038/s41586-020-2269-x
Vinolo, M. A. R., Rodrigues, H. G., Hatanaka, E., Sato, F. T., Sampaio, S. C., and Curi, R. (2011). Suppressive effect of short-chain fatty acids on production of proinflammatory mediators by neutrophils. J. Nutr. Biochem. 22, 849–855. doi: 10.1016/j.jnutbio.2010.07.009
Wang, Z., Klipfell, E., Bennett, B. J., Koeth, R., Levison, B. S., DuGar, B., et al. (2011). Gut Flora metabolism of phosphatidylcholine promotes cardiovascular disease. Nature 472, 57–63. doi: 10.1038/nature09922
Wang, L., Zhou, W., Guo, M., Hua, Y., Zhou, B., Li, X., et al. (2021). The gut microbiota is associated with clinical response to statin treatment in patients with coronary artery disease. Atherosclerosis 325, 16–23. doi: 10.1016/j.atherosclerosis.2021.03.007
Wensel, C. R., Pluznick, J. L., Salzberg, S. L., and Sears, C. L. (2022). Next-generation sequencing: insights to advance clinical investigations of the microbiome. J. Clin. Invest. 132:e154944. doi: 10.1172/JCI154944
Williams, B., Mancia, G., Spiering, W., Rosei, E. A., Azizi, M., Burnier, M., et al. (2019). 2018 ESC/ESH guidelines for the management of arterial hypertension. Eur. Heart J. 77, 71–159. doi: 10.5603/KP.2019.0018
Wu, G. D., Chen, J., Hoffmann, C., Bittinger, K., Chen, Y.-Y., Keilbaugh, S. A., et al. (2011). Linking long-term dietary patterns with gut microbial enterotypes. Science 334, 105–108. doi: 10.1126/science.1208344
Yakob, M., Söder, B., Meurman, J. H., Jogestrand, T., Nowak, J., and Söder, P.-Ö. (2011). Prevotella Nigrescens and Porphyromonas Gingivalis are associated with signs of carotid atherosclerosis in subjects with and without periodontitis: periodontal microorganisms and atherosclerosis. J. Periodontal Res. 46, 749–755. doi: 10.1111/j.1600-0765.2011.01398.x
Yang, B., Chen, Y., Catherine Stanton, R., Ross, P., Lee, Y.-K., Zhao, J., et al. (2019). Bifidobacterium and Lactobacillus composition at species level and gut microbiota diversity in infants before 6 weeks. Int. J. Mol. Sci. 20:3306. doi: 10.3390/ijms20133306
Yang, T., Santisteban, M. M., Rodriguez, V., Li, E., Ahmari, N., Carvajal, J. M., et al. (2015). Gut dysbiosis is linked to hypertension. Hypertension 65, 1331–1340. doi: 10.1161/HYPERTENSIONAHA.115.05315
Yusuf, S., Hawken, S., Ôunpuu, S., Dans, T., Avezum, A., Lanas, F., et al. (2004). Effect of potentially modifiable risk factors associated with myocardial infarction in 52 countries (the INTERHEART study): case-control study. Lancet 364, 937–952. doi: 10.1016/S0140-6736(04)17018-9
Zeng, X., Xuxuan Gao, Y., Peng, Q. W., Zhu, J., Tan, C., Xia, G., et al. (2019). Higher risk of stroke is correlated with increased opportunistic pathogen load and reduced levels of butyrate-producing Bacteria in the gut. Front. Cell. Infect. Microbiol. 9:4. doi: 10.3389/fcimb.2019.00004
Zhang, Y., Jun, X., Wang, X., Ren, X., and Liu, Y. (2019). Changes of intestinal bacterial microbiota in coronary heart disease complicated with nonalcoholic fatty liver disease. BMC Genomics 20:862. doi: 10.1186/s12864-019-6251-7
Zheng, Y.-Y., Ting-Ting, W., Liu, Z.-Q., Li, A., Guo, Q.-Q., Ma, Y.-Y., et al. (2020). Gut microbiome-based diagnostic model to predict coronary artery disease. J. Agric. Food Chem. 68, 3548–3557. doi: 10.1021/acs.jafc.0c00225
Zhou, J., Liu, L., Peiyao, W., Zhao, L., and Yafei, W. (2022). Fusobacterium Nucleatum accelerates atherosclerosis via macrophage-driven aberrant proinflammatory response and lipid metabolism. Front. Microbiol. 13:798685. doi: 10.3389/fmicb.2022.798685
Zhu, L., Baker, S. S., Gill, C., Liu, W., Alkhouri, R., Baker, R. D., et al. (2013). Characterization of gut microbiomes in nonalcoholic steatohepatitis (NASH) patients: a connection between endogenous alcohol and NASH. Hepatology 57, 601–609. doi: 10.1002/hep.26093
Zhu, Q., Gao, R., Zhang, Y., Pan, D., Zhu, Y., Zhang, X., et al. (2018). Dysbiosis signatures of gut microbiota in coronary artery disease. Physiol. Genomics 50, 893–903. doi: 10.1152/physiolgenomics.00070.2018
Keywords: coronary artery disease, dyslipidemia, gut microbiome, gut microbiota, chronic coronary syndrome, cardiovascular disease
Citation: Luangphiphat W, Prombutara P, Muangsillapasart V, Sukitpunyaroj D, Eeckhout E and Taweechotipatr M (2024) Exploring of gut microbiota features in dyslipidemia and chronic coronary syndrome patients undergoing coronary angiography. Front. Microbiol. 15:1384146. doi: 10.3389/fmicb.2024.1384146
Edited by:
George Grant, Independent Researcher, Aberdeen, United KingdomReviewed by:
Abdullah Al Mamun, Lishui People’s Hospital, ChinaNikolaos Pagonas, University Hospital Ruppin-Brandenburg, Germany
Copyright © 2024 Luangphiphat, Prombutara, Muangsillapasart, Sukitpunyaroj, Eeckhout and Taweechotipatr. This is an open-access article distributed under the terms of the Creative Commons Attribution License (CC BY). The use, distribution or reproduction in other forums is permitted, provided the original author(s) and the copyright owner(s) are credited and that the original publication in this journal is cited, in accordance with accepted academic practice. No use, distribution or reproduction is permitted which does not comply with these terms.
*Correspondence:Malai Taweechotipatr, bWFsYWlAZy5zd3UuYWMudGg=