- 1State Key Laboratory of Biobased Material and Green Papermaking (LBMP), Qilu University of Technology (Shandong Academy of Sciences), Jinan, China
- 2Institute of Poultry Science, Shandong Academy of Agricultural Sciences, Jinan, China
Introduction: This study aimed to investigated the effects of 10-hydroxy-2-decenoic acid (10-HDA) on the growth performance, intestinal barrier, inflammatory response, oxidative stress, and gut microbiota of chickens challenged with lipopolysaccharide (LPS).
Methods: A total of 240 one-day-old chickens were randomly assigned to five treatment groups: (1) control group (basal diet + saline); (2) LPS group (basal diet + LPS); (3) Chlortetracycline (CTC) group (basal diet containing 75 mg/kg CTC + LPS); (4) 0.1% 10-HDA group (basal diet containing 1 g/kg 10-HDA + LPS); and (5) 0.5% 10-HDA group (basal diet containing 5 g/kg 10-HDA + LPS). All chickens were injected intraperitoneally with 0.5 mg/kg body weight of either LPS or saline at 17, 19, and 21 days of age.
Results: The results showed that dietary 10-HDA supplementation attenuated the loss in growth performance caused by the LPS challenge (p < 0.05). 10-HDA effectively alleviated LPS-induced intestinal mucosal injury, as evidenced by reduced bleeding, decreased serum diamine oxidase levels (p < 0.05), and increased villus/crypt ratios of the jejunum and ileum (p < 0.05). Dietary treatment with 0.1% 10-HDA reduced the concentrations of inflammatory cytokines (TNF-α, IL-1β, IL-6; p < 0.05), and increased immunoglobulin (IgA, IgG) and antioxidant enzyme levels (CAT, GSH-px, T-SOD) in the serum of LPS-challenged chickens (p < 0.05). These effects were similar to those observed in the CTC group. Moreover, 0.1% 10-HDA treatment reversed the LPS-induced variations in the mRNA expression of genes related to inflammation, antioxidant capacity, and intestinal tight junctions (p < 0.05). 16S rRNA analysis revealed that 10-HDA supplementation increased the relative abundance of Faecalibacterium and Clostridia_UCG-014 (p < 0.05). Additionally, it decreased the abundance of Clostridia_vadinBB60_group, Eubacterium_nodatum_group, and UC5-1-2E3 (p < 0.05). These changes were correlated with reduced inflammation and improved antioxidant capacity in the LPS-challenged chickens.
Conclusion: Collectively, dietary 10-HDA supplementation alleviated LPS-induced intestinal mucosal injury and the loss of growth performance through anti-inflammatory, antioxidant, and gut microbiota modulation activities in chickens. Moreover, 0.1% 10-HDA supplementation had comparable or even better protection for LPS-challenged chickens than supplementation with antibiotics or 0.5% 10-HDA. 10-HDA has the potential to be used as an alternative to antibiotics in protecting the intestinal health and improving the performance of poultry.
1. Introduction
The intestinal tract is not only the main organ for the digestion and absorption of nutrients in animals, but also acts as an innate barrier to maintain body homeostasis (Vancamelbeke and Vermeire, 2017). Modern intensive production makes poultry more susceptible to various stress factors, such as environmental, nutritional, bacterial, and toxin infections, which may destroy the intestinal barrier function and cause intestinal and systemic diseases in poultry (Yang et al., 2011; Adedokun and Olojede, 2019). Lipopolysaccharide (LPS) is an outer membrane component of Gram-negative bacteria. LPS-mediated intestinal barrier dysfunction increases intestinal permeability, causing systemic inflammatory responses and imbalances in the oxidative and antioxidant defense systems (Chen Y. P. et al., 2018; Zhang et al., 2020; Tao et al., 2022). Therefore, intraperitoneal injection of LPS in animals serves as an effective model to study intestinal mucosal damage and inflammation (Leshchinsky and Klasing, 2001). Antibiotics have been widely used in animal production as feed additives to reduce stress and promote growth (Mehdi et al., 2018). Nevertheless, prolonged antibiotic usage leads to the presence of antibiotic residues, drug resistance, and environmental pollution (Bacanli and Basaran, 2019; Robles-Jimenez et al., 2022). Hence, it is urgent to explore new feed additives that can replace antibiotics in the poultry industry.
10-Hydroxy-2-decenoic acid (10-HDA), an unsaturated medium-chain fatty acid with terminal hydroxylation, is the main fatty acid component in royal jelly. It has various physiological activities, such as antibacterial (Melliou and Chinou, 2005; Gao et al., 2022), anti-inflammatory (Chen Y. F. et al., 2018; You et al., 2020), antioxidant (Gui et al., 2018), and immunomodulation activities (Fan et al., 2020). In-vitro studies conducted on murine macrophages and human colon cancer cells have demonstrated that 10-HDA exerts anti-inflammatory activities through inhibition of the NF-κB pathway and reduction of proinflammatory cytokine secretion (Sugiyama et al., 2012; Yang et al., 2018). Furthermore, 10-HDA mitigates DSS-induced colitis in mice through regulation of the NLRP3 inflammasome-mediated pyroptotic pathway and enhancement of the colonic barrier function (Huang et al., 2022). 10-HDA can also resist oxidative stress by increasing antioxidant oxidase activity and reducing ROS production (Hu X. et al., 2022). Previously, the low yield and high cost of the physical extraction and chemical synthesis of 10-HDA have limited its application. Recently, Li et al. reported the production of 10-HDA by microbial whole-cell catalysis utilizing synthetic biology strategies (Li et al., 2022), which has laid the foundation for the application of 10-HDA in the poultry industry.
A previous study showed that 10-HDA improves the growth performance, immune function, and antioxidant capacity of broilers under non-stress conditions (Zhang et al., 2022). However, the effects of 10-HDA on the intestinal health and the gut microbiota composition in chickens under stressful conditions remain unclear. Therefore, this study aimed to determine the effects of dietary 10-HDA supplementation on the intestinal barrier, inflammatory responses, and oxidative stress, as well as to investigate the potential correlations with alterations in the gut microbiota in chickens subjected to LPS challenge. This study provides valuable references for the application of 10-HDA in protecting intestinal health and improving the performance of poultry.
2. Materials and methods
2.1. Experimental design
A total of 240 one-day-old male chickens (Hy-Line Brown) with similar body weights were randomly assigned to five groups, with six replicates per group and eight chickens per replicate. The five groups were as follows: (1) CON group, basal diet + saline challenge; (2) LPS group, basal diet + LPS challenge; (3) CTC group, basal diet containing 75 mg/kg chlortetracycline (CTC) + LPS challenge; (4) 0.1% 10-HDA group, basal diet containing 1 g/kg 10-HDA + LPS challenge; and (5) 0.5% 10-HDA group, basal diet containing 5 g/kg 10-HDA + LPS challenge. 10-HDA (purity ≥98%) was purchased from Shanghai Deepak Biotechnology Co., Ltd. (Shanghai, China). The Escherichia coli LPS was dissolved in 0.86% sterile saline. On day 17, 19, and 21, the chickens were intraperitoneally injected with LPS (0.5 mg/kg body weight) or an equivalent amount of saline. All chickens were housed in an environmentally controlled room. The temperature was maintained at 33 to 35°C in the first week, and then gradually decreased to 26°C at 21 days of age. Continuous light was provided throughout the experimental period. All chickens had ad-libitum access to feed and fresh water. The composition and nutrient levels of the basal diet are shown in Supplementary Table S1. The basal diet fulfilled the nutritional requirements of chickens (NY/T33-2004, China).
2.2. Growth performance measurement
The body weight and feed intake of all chickens were recorded on day 1 and 21 to determine the average daily gain (ADG) and the average daily feed intake (ADFI). The feed-to-gain ratio (F/G) was defined as ADFI:ADG.
2.3. Sample collection
Three hours after the LPS injection on day 21, one chicken whose body weight was close to the average body weight for each replicate (six chickens per treatment group) was selected for sampling. Blood was collected from the wing vein, and the serum was obtained via centrifugation at 3,000 × g for 15 min at 4°C. The chickens were euthanized via artificial cervical dislocation. Duodenum, jejunum, and ileum specimens were taken from the mesenteric tissue and photographed. An intestinal segment with a size of 2 cm was dissected from both the jejunum and ileum and fixed in 4% paraformaldehyde for histological analysis. Jejunal mucosa was scraped and stored at −80°C for gene expression analysis. Cecal contents were collected for subsequent 16S rRNA sequencing.
2.4. Intestinal morphometry
After fixation, the jejunal and ileal segments were dehydrated using an ethanol gradient, cleared using dimethylbenzene, and subsequently embedded in paraffin. The tissues were sliced and subjected to hematoxylin and eosin (HE) staining. Images were acquired using an Eclipse 80i microscope (Nikon Inc., Tokyo, Japan). The villus height (VH) and crypt depth (CD) were determined using the Image-Pro Plus 6.0 software, and the VH/CD ratios (VCR) were calculated.
2.5. Analysis of the serum biochemical status
The serum levels of diamine oxidase (DAO), tumor necrosis factor-α (TNF-α), interleukin (IL)-1β, IL-6, immunoglobulin (Ig) A, IgG, catalase (CAT), total superoxide dismutase (T-SOD), and glutathione peroxidase (GSH-px) were determined by chicken ELISA kits (Hengyuan Biotech. Co., Shanghai, China) according to the manufacturer’s protocols.
2.6. Real-time quantitative PCR (RT-qPCR)
Total RNA was extracted from the jejunal mucosa using TRI pure reagent (Aidlab Biotech. Co., Beijing, China). For each sample, cDNA was synthesized with 1 μg of total RNA using a SPARKscript II RT Plus Kit (SparkJade Biotech. Co., Jinan, China) following the manufacturer’s protocol. RT-qPCR was conducted using a real-time PCR system and SYBR Green qPCR Mix (Vazyme, Nanjing, China). The relative mRNA expression levels were analyzed using the 2-ΔΔCT method. The gene-specific primers are shown in Supplementary Table S2, and β-actin was used as the internal standard.
2.7. Analysis of the cecal microbiota composition
The microbial DNA of the cecal contents was extracted using the EZNA® soil DNA Kit (Omega Bio-tek, Norcross, GA, United States). The V3–V4 variable region of the bacterial 16S rRNA gene was amplified using primers 338F (5′-ACTCCTACGGGAGGCAGCAG-3′) and 806R (5′-GGACTACHVGGGTWTCTAAT-3′) (Liu et al., 2016). After purification, amplicons were collected and subjected to paired-end sequencing using an Illumina MiSeq PE300 platform (Illumina, CA, United States). The raw FASTQ files underwent quality filtering and merging using FLASH 1.2.11. Subsequently, operational taxonomic units (OTUs) were derived by clustering the optimized sequences at a 97% identity threshold. The taxonomy of the OTU sequences was determined using the RDP Classifier (version 2.13) and the 16S rRNA gene database. Bioinformatic analysis of the cecal microbiota was performed on the Majorbio Cloud platform.1
2.8. Statistical analysis
Data were analyzed using SPSS 26.0 (SPSS Inc., Armonk, NY, USA). Variations among groups were compared using one-way ANOVA, followed by Duncan’s multiple range test. Data are presented as the mean ± standard error of the mean (SEM). Differences were considered significant at p < 0.05.
3. Results
3.1. Growth performance
The effects of 10-HDA supplementation on the growth performance of LPS-challenged chickens are shown in Table 1. The LPS challenge resulted in a significant decrease in ADFI (p < 0.05) and ADG (p < 0.05), as well as in an increase in the feed to gain ratio of chickens from 1 to 21 days (p < 0.05) when compared to the control group. Dietary addition of 0.1 and 0.5% 10-HDA significantly reduced the feed-to-gain ratio of the LPS-challenged chickens (p < 0.05), but had no significant effects on ADFI or ADG during the experimental period (p > 0.05). The CTC supplementation did not show any significant effect on the LPS-induced decline in the growth performance of chickens (p > 0.05).
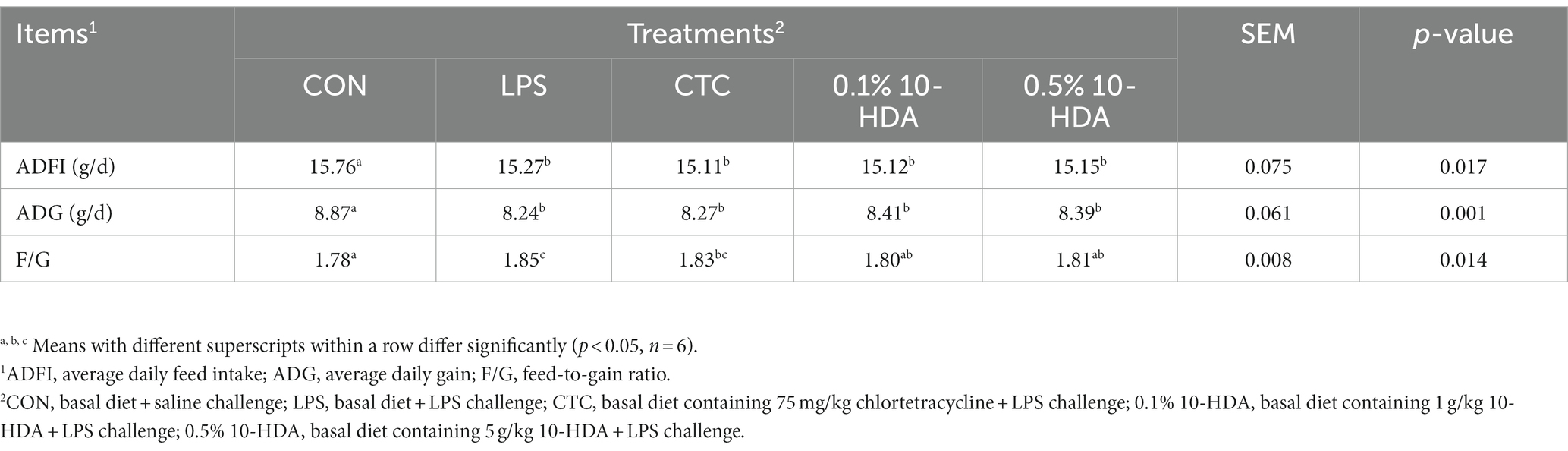
Table 1. Effects of dietary 10-HDA supplementation on the growth performance of LPS-challenged chickens.
3.2. Intestinal morphology and permeability
The intestinal morphology of chickens is shown in Figure 1A. After the LPS challenge, the duodenum, jejunum, and ileum showed obvious bleeding spots. Dietary addition of CTC or 10-HDA effectively alleviated the bleeding, and the 0.1% 10-HDA treatment appeared to be more effective than the CTC and 0.5% 10-HDA treatments. The HE staining results revealed that the epithelial villi of the jejunum and ileum were severely damaged, and a large area of the intestinal villi epithelium was shed in response to the LPS challenge, whereas the CTC or 10-HDA treatments alleviated this damage (Figure 1B). Moreover, the integrity of the intestinal barrier was determined by measuring the activity of DAO in the serum. The LPS challenge resulted in a notable increase in the serum DAO levels compared to those of the control (p < 0.05). In contrast, the addition of CTC or 10-HDA markedly reduced the LPS-induced elevation in the serum DAO levels (p < 0.05). Moreover, no significant differences were observed between the 10-HDA and CTC groups (p > 0.05; Table 2).
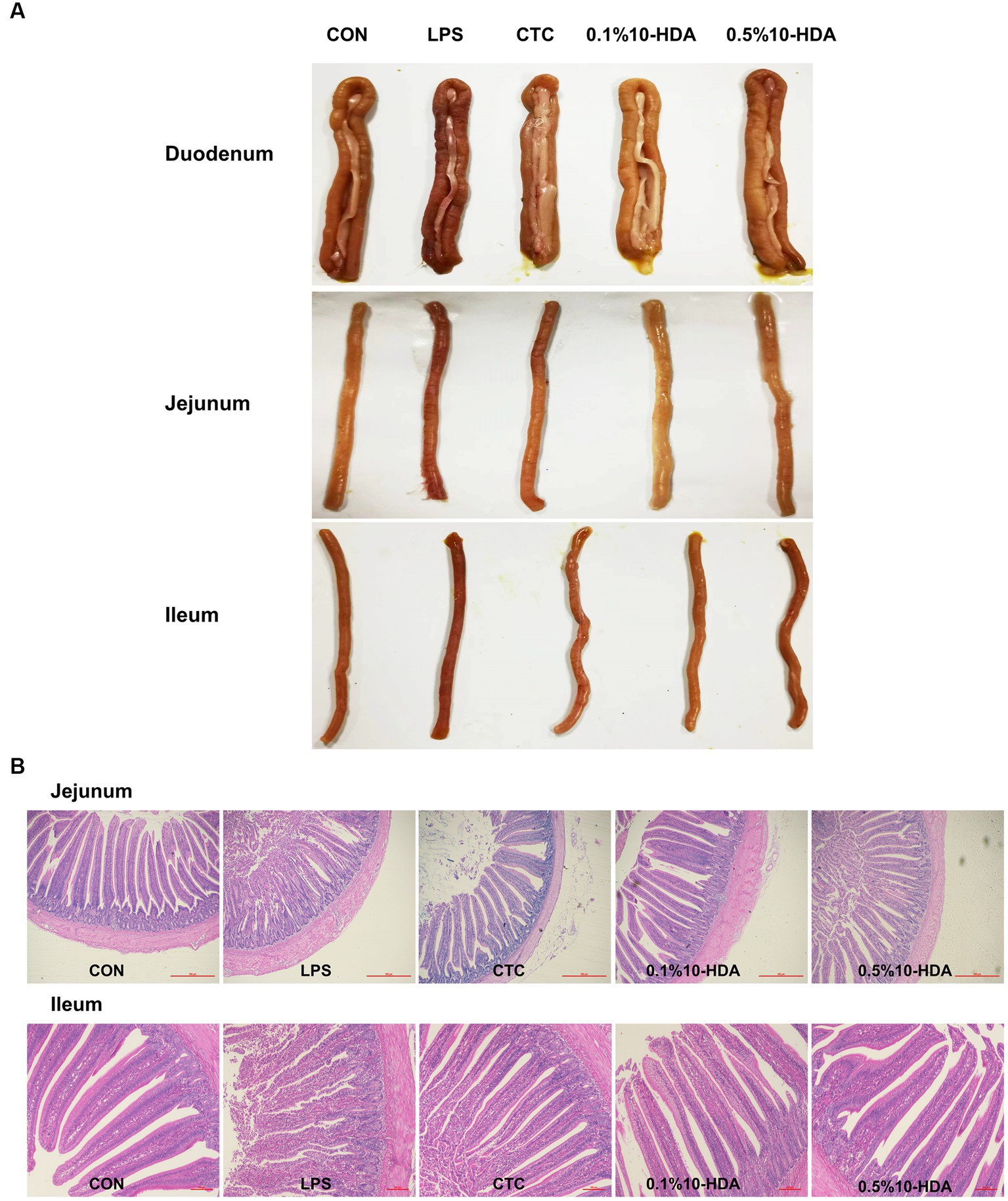
Figure 1. Effect of 10-HDA supplementation on the intestinal morphology of LPS-challenged chickens. (A) Photographs of the duodenum, jejunum, and ileum. (B) Morphological structure of the jejunum and ileum by HE staining. Scale bar 500 μm, 40×. Scale bar 100 μm, 100 ×. CON, basal diet + saline challenge; LPS, basal diet + LPS challenge; CTC, basal diet containing 75 mg/kg chlortetracycline + LPS challenge; 0.1% 10-HDA, basal diet containing 1 g/kg 10-HDA + LPS challenge; 0.5% 10-HDA, basal diet containing 5 g/kg 10-HDA + LPS challenge.
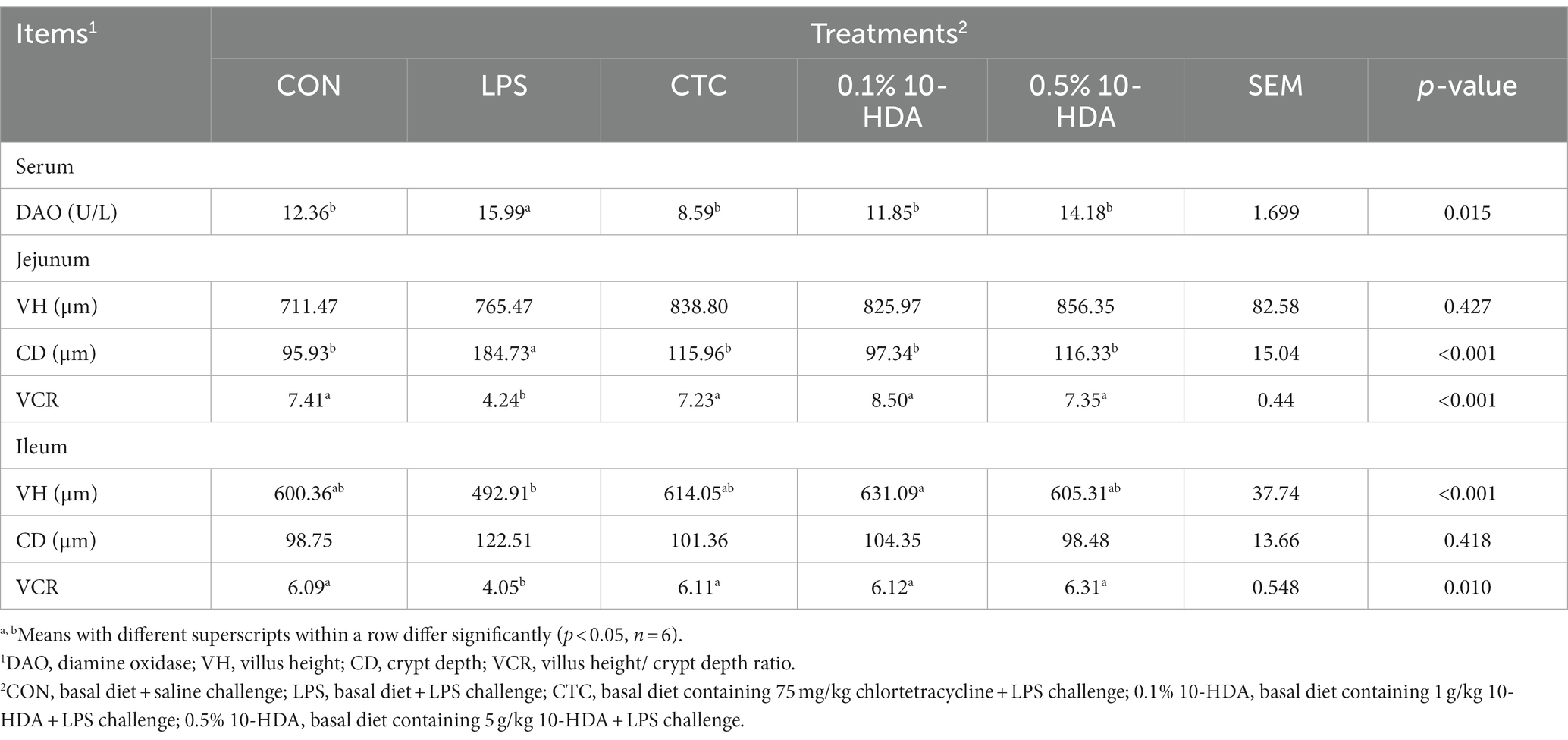
Table 2. Effects of dietary 10-HDA supplementation on serum DAO levels and the intestinal histomorphology of LPS-challenged chickens.
As shown in Table 2, LPS challenge significantly increased the CD in the jejunum (p < 0.05) and decreased the VCR of the jejunum and ileum (p < 0.05). In contrast, dietary 10-HDA supplementation significantly decreased the CD in the jejunum (p < 0.05) and increased the VCR of the jejunum and ileum compared to the LPS group (p < 0.05). These effects were similar to those observed in the CTC group (p > 0.05). Additionally, 0.1% 10-HDA supplementation significantly increased the ileal VH (p < 0.05). The results indicate that 10-HDA could replace antibiotics to alleviate LPS-induced injury to the intestinal mucosal structure.
3.3. Serum cytokines, immunoglobulin levels, and oxidation status
The effects of 10-HDA supplementation on the inflammatory cytokine and immunoglobulin levels and antioxidant enzyme activities in the serum of LPS-challenged chickens are shown in Figure 2. LPS challenge significantly increased the serum levels of cytokines TNF-α, IL-1β, and IL-6 (p < 0.05), whereas CTC or 0.1% 10-HDA supplementation significantly dampened the increase in the levels of these cytokines (p < 0.05). Moreover, no significant differences were observed between the 0.1% 10-HDA group and the CTC group (p > 0.05). Compared to the LPS group, the 0.5% 10-HDA treatment reduced IL-6 levels (p < 0.05), but had no significant effect on TNF-α and IL-1β levels (p > 0.05) (Figure 2A). Figure 2B shows that LPS challenge decreased the activities of CAT, GSH-px, and T-SOD in the serum (p < 0.05), whereas the CTC or 0.1% 10-HDA pretreatment significantly increased their activities (p < 0.05). Furthermore, treatment with 0.5% 10-HDA increased CAT and GSH-px activities (p < 0.05), but had no significant effect on T-SOD activity (p > 0.05). As shown in Figure 2C, LPS challenge reduced serum IgA and IgG concentrations (p < 0.05), whereas the CTC or 10-HDA treatments significantly reversed this decrease (p < 0.05). These results indicate that dietary supplementation with 10-HDA, particularly at a concentration of 0.1%, could effectively alleviate LPS-induced inflammation and oxidative stress in chickens.
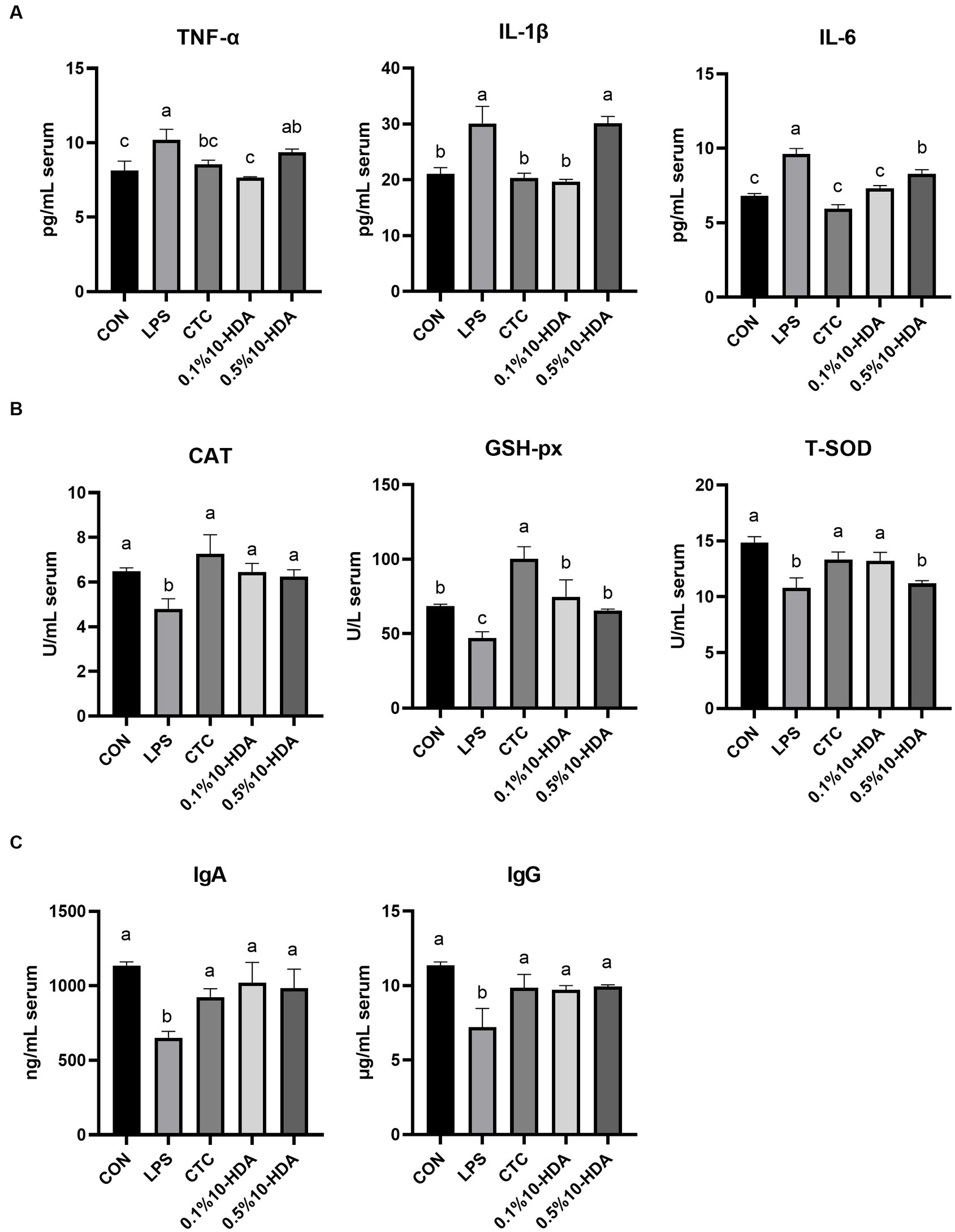
Figure 2. Effect of 10-HDA on the serum biochemical indices of LPS-challenged chickens. (A) Serum levels of inflammatory cytokines. (B) Activity of antioxidant enzymes. (C) Serum concentrations of immunoglobulins. Grouping information is the same as that in Figure 1. a, b, c Means with different superscripts are significantly different (p < 0.05).
3.4. Inflammation-related gene expression
Quantitative PCR was used to investigate the mRNA expression of inflammatory cytokines and apoptotic regulators in the chicken jejunum (Figure 3). The results revealed that LPS challenge dramatically enhanced the expression levels of TLR4, NF-κB, IL-1β, IL-6, TNF-α, and Caspase-3 (p < 0.05), whereas 0.1% 10-HDA supplementation mitigated the up-regulation of these genes (p < 0.05). CTC supplementation decreased the expression of TLR4, IL-6, and TNF-α compared to the LPS group (p < 0.05), but had no considerable effect on NF-κB and IL-1β expression (p > 0.05). Treatment with 0.5% 10-HDA decreased the expression of TNF-α (p < 0.05), increased the expression of NF-κB (p < 0.05), and had no significant effect on TLR4, IL-1β, IL-6, Bax, and Caspase-3 levels (p > 0.05). LPS challenge decreased the expression of the antiapoptotic gene Bcl2 (p < 0.05), whereas treatment with CTC or 10-HDA significantly increased Bcl2 expression (p < 0.05). These results further demonstrate the superiority of 0.1% 10-HDA supplementation in alleviating LPS-induced intestinal inflammation in chickens.
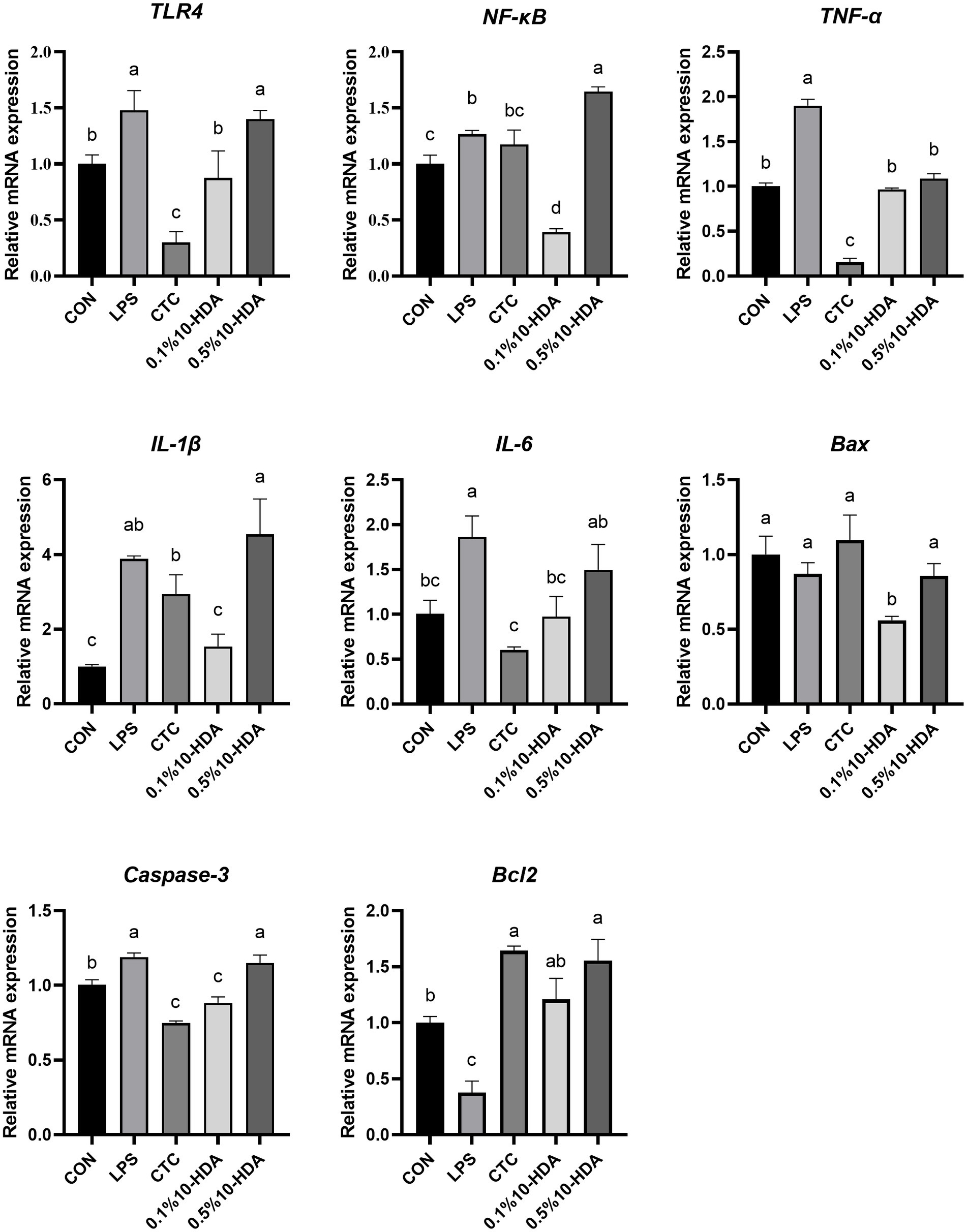
Figure 3. Effect of 10-HDA on the mRNA expression of inflammatory cytokines and apoptosis genes in the jejunum of chickens challenged with LPS. Grouping information is the same as that in Figure 1. a, b, c, d Means with different superscripts are significantly different (p < 0.05).
3.5. Expression of antioxidant and tight junction genes
The mRNA expression of antioxidant and tight junction genes in the jejunum of chickens is presented in Figure 4. LPS stimulation reduced the expression of CAT, SOD2, and OCLN (p < 0.05). Compared with those of the LPS group, the expression levels of CAT, SOD2, ZO-1, and OCLN were significantly increased by the supplementation with 0.1 and 0.5% 10-HDA (p < 0.05). The CTC treatment enhanced the expression levels of GSH-px, SOD2, ZO-1, and OCLN (p < 0.05).
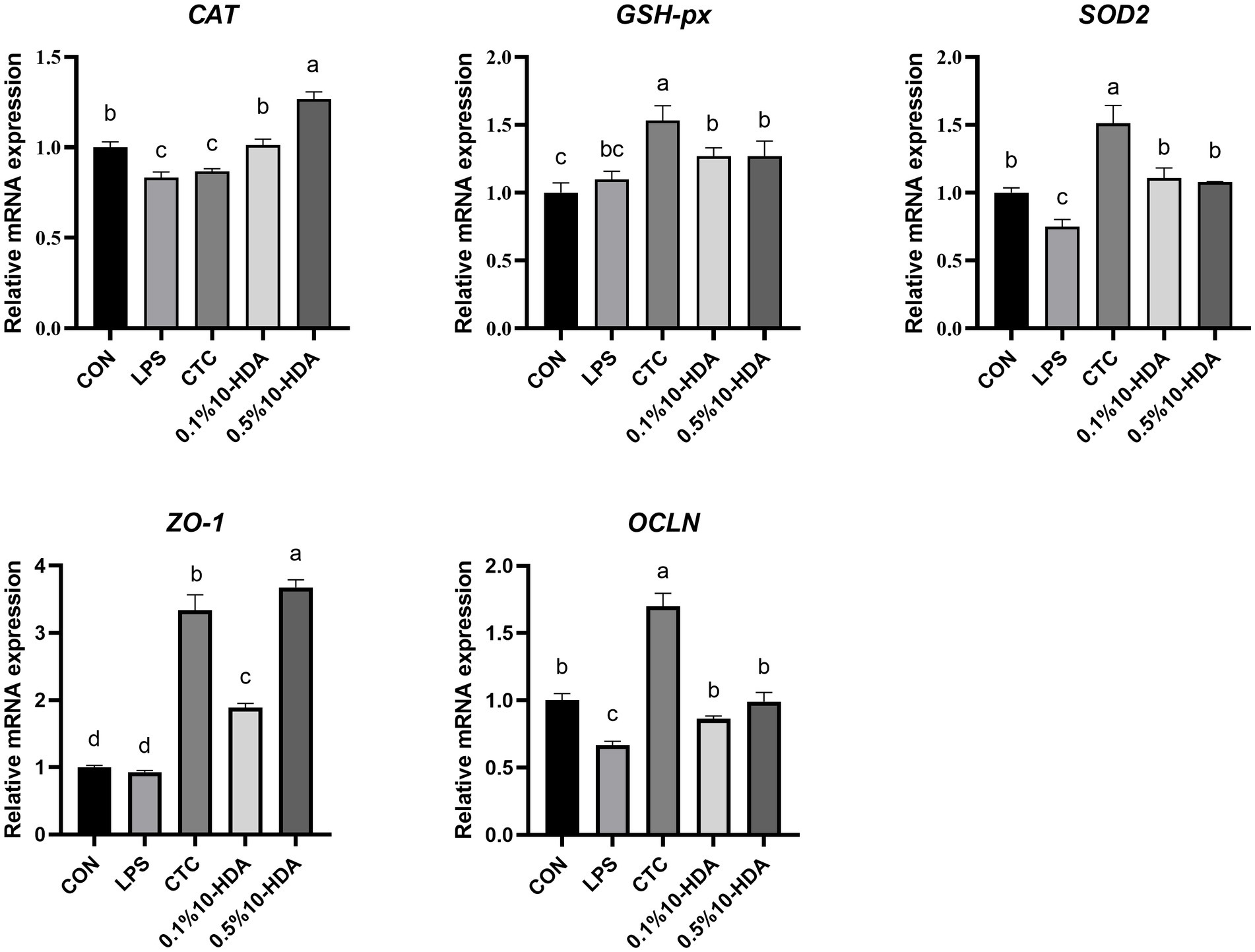
Figure 4. Effect of 10-HDA on the mRNA expression of antioxidant and tight junction genes in the jejunum of LPS-challenged chickens. Grouping information is the same as that in Figure 1. a, b, c, d Means with different superscripts are significantly different (p < 0.05).
3.6. Cecal microbial composition
We investigated the effects of 10-HDA on the cecal microbiota of chickens treated with LPS using 16S rRNA high-throughput sequencing. The Venn diagram in Figure 5A shows that the total OTUs in the CON, LPS, CTC, 0.1% 10-HDA, and 0.5% 10-HDA groups were 774, 844, 774, 798, and 758, respectively, and 460 mutual OTUs were detected among the five groups. The Chao1, Faith PD, Shannon, and Simpson indices were calculated to assess the alpha diversity of the samples. However, no significant variations were detected among the groups (Figure 5B). PCoA and PLS-DA analysis showed that the samples of the LPS group were clearly separated from those of the CON group, suggesting that the cecal microbial community of the LPS group was distinct from that of the CON group (Figures 5C,D). The distribution of samples in the 0.1% 10-HDA group was similar to that of the CON group.
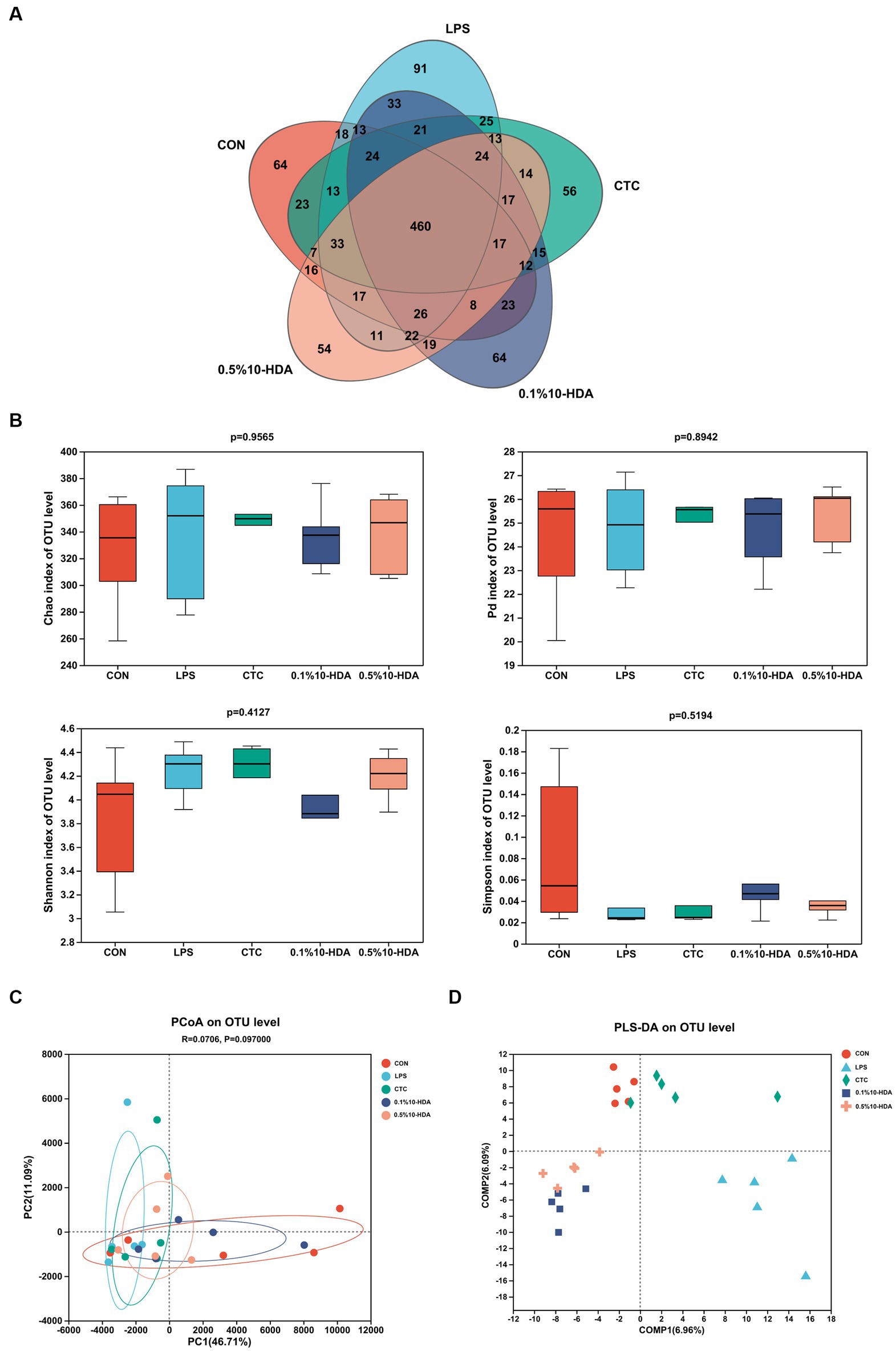
Figure 5. Effects of dietary 10-HDA supplementation on the cecal microbiota diversity of LPS-challenged chickens. (A) Venn diagram. (B) Alpha diversity index analysis. (C) PCoA based on the Euclidean distance. (D) Partial least squares discriminant analysis (PLS-DA). Grouping information is the same as that in Figure 1.
Figure 6 shows the differences in microbial composition at the phylum level. The results indicate that Firmicutes and Bacteroidetes were the predominant intestinal phyla in all groups. Dietary 0.1% 10-HDA supplementation decreased the proportion of Actinobacteria and Desulfobacterota in the LPS-challenged chickens (p < 0.05). At the genus level, we analyzed the abundance of the community of the 20 dominant genera within each group (Figure 7A). Compared with the LPS group, the 0.1% 10-HDA treatment significantly increased the abundance of Faecalibacterium (p < 0.05) and reduced the abundance of Clostridia_vadinBB60_group (p < 0.05) and Eubacterium_nodatum (p < 0.01). Dietary 0.5% 10-HDA increased the abundance of Faecalibacterium (p < 0.05) and Clostridia_UCG-014 (p < 0.05), and reduced the abundance of Eubacterium_nodatum (p < 0.05) and UC5-1-2E3 (p < 0.05) (Figure 7B). Furthermore, the Circos diagram illustrates that the proportion of Lactobacillus and Butyricicoccus in the 0.1 and 0.5% 10-HDA groups was higher than that in the LPS group. Alistipes was the dominant genus in the LPS group (Figure 7C).
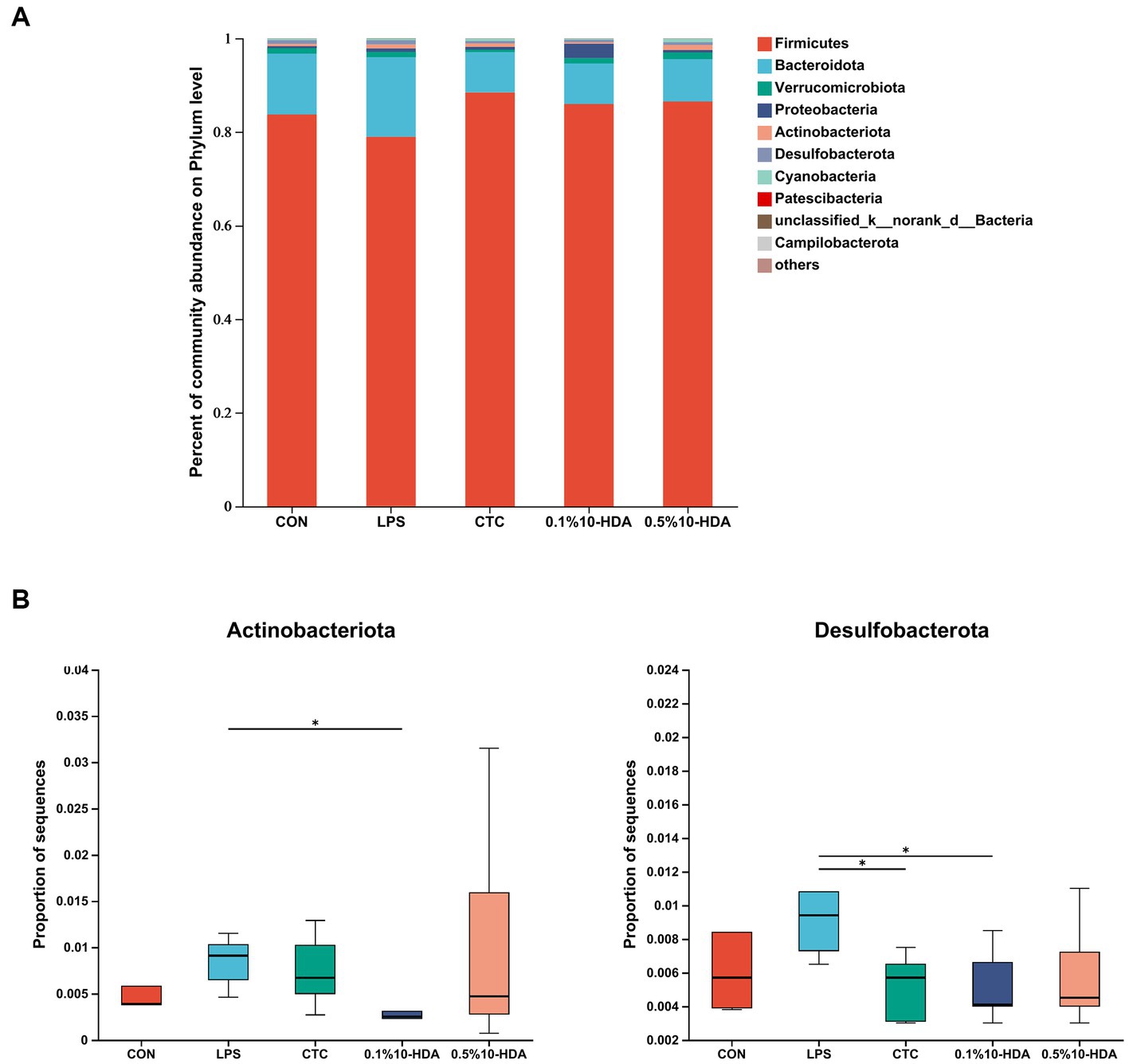
Figure 6. Effects of 10-HDA on the cecal microbiota composition in LPS-challenged chickens at the phylum level. (A) Gut microbiota composition. (B) Analysis of differences in abundance among groups. Grouping information is the same as that in Figure 1; *p < 0.05, **p < 0.01.
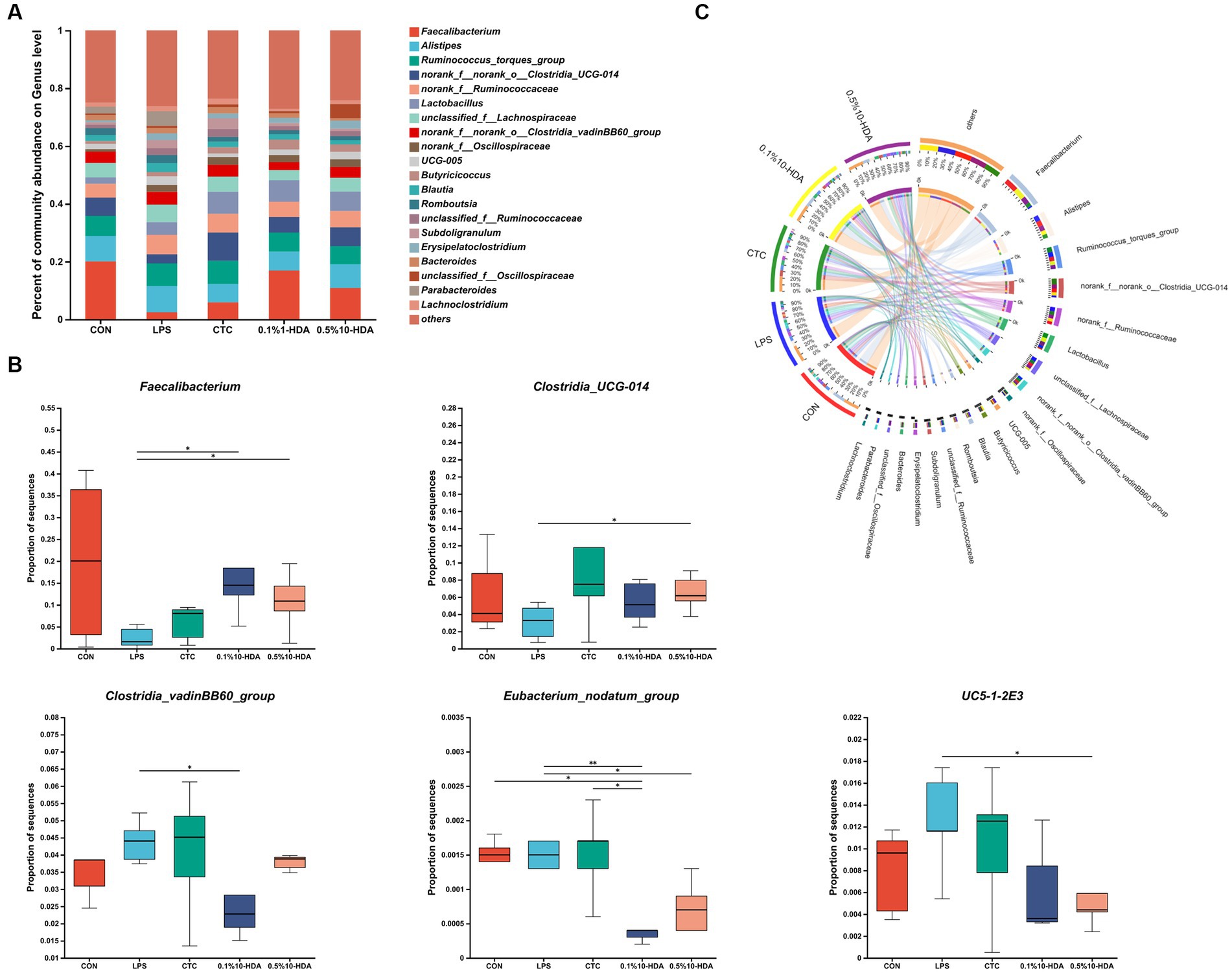
Figure 7. Effects of 10-HDA on the cecal microbiota composition of LPS-challenged chickens at the genus level. (A) Gut microbiota composition. (B) Analysis of differences in abundance among groups. (C) Circos diagram showing the dominant genera in each group. Grouping information is the same as that in Figure 1; *p < 0.05, **p < 0.01.
Spearman correlation analysis was performed to investigate the correlation between the gut microbiota and the expression of genes related to gut inflammation, oxidative stress, and intestinal barrier. As shown in Figure 8, the abundance of Faecalibacterium, Clostridia_UCG-014, Anaerofilum, Butyricicoccus, and Lactobacillus was positively correlated with the expression of antioxidant genes (CAT, GSH-px, SOD2), intestinal tight junction genes (ZO-1, OCLN), and anti-apoptotic genes (Bcl2), while exhibiting a negative correlation with the expression of inflammatory genes (IL-1β, TNF-α). The abundance of UCG-005, Bilophila, Eisenbergiella, Alistipes, Clostridia_vadinBB60_group, unclassified Ruminococcaceae, and DTU089 was positively correlated with the expression of inflammatory cytokines (TLR4, IL-6, TNF-α, NF-κB) and apoptotic genes (Caspase-3), and negatively associated with the expression of OCLN and SOD2.
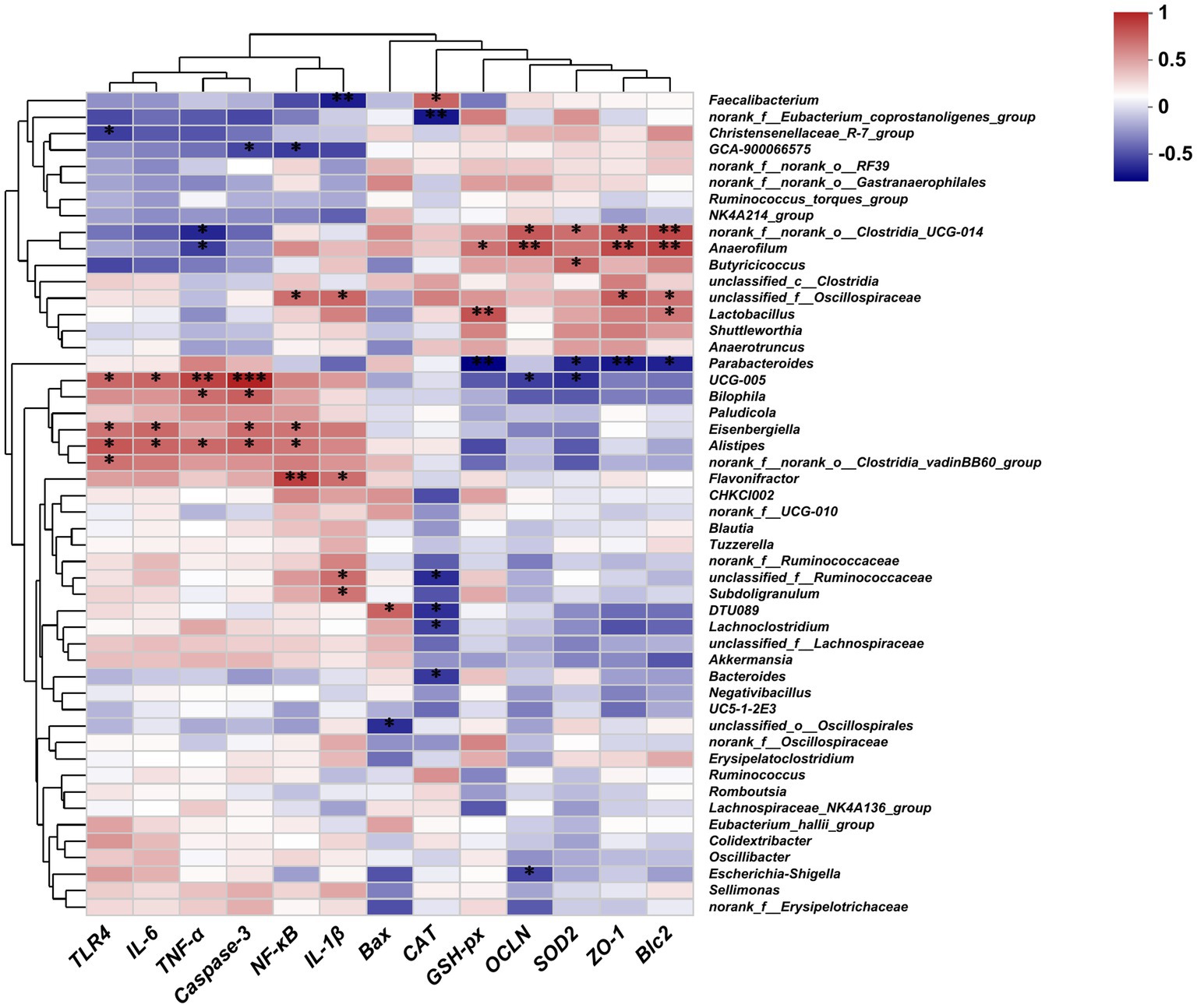
Figure 8. Heatmaps of the Spearman correlation between the gut microbiota and the expression of genes related to gut inflammation, oxidative stress, and the intestinal barrier; *p < 0.05, **p < 0.01, ***p < 0.001.
4. Discussion
Bacterial LPS challenge induces immune and oxidative stress in animals, which subsequently affects energy utilization and decreases animal growth performance (Liu et al., 2015; Shi et al., 2022). Similarly, we found that LPS challenge reduced the growth performance of chickens, as evidenced by the decreased ADFI and ADG, and the increased feed-to-gain ratio. Dietary 10-HDA supplementation reduced the feed-to-gain ratio and attenuated the growth performance loss induced by LPS in chickens; however, high supplementation doses of 10-HDA were not superior to low supplementation doses. A previous study demonstrated that 10-HDA supplementation improved the growth performance of broiler chickens by increasing the ADG on days 22–42 and 0–42 (Zhang et al., 2022). Here, the addition of 10-HDA did not have a significant effect on the ADFI and ADG in chickens challenged with LPS, which may be attributed to various factors such as the breed or age of the chickens, as well as the ingredients of their diet.
The intestinal mucosal barrier is essential for maintaining homeostasis and preventing intestinal inflammation (Okumura and Takeda, 2018). LPS can induce intestinal inflammation, disrupt the structural integrity of the intestinal wall, and impair intestinal barrier function (Wu et al., 2021). Damage of the intestinal mucosal barrier increases serum DAO activity, which is considered an intestinal permeability marker (Jiang et al., 2019). Here, dietary supplementation with CTC or 10-HDA effectively reduced the LPS-induced elevation of serum DAO levels. Moreover, intestinal tight junction proteins play key roles in the formation of the intestinal selective permeability barrier, contributing to maintaining the integrity of the intestinal structure (Ren et al., 2022). We found that supplementation with CTC or 10-HDA could ameliorate the downregulation of ZO-1 and OCLN expression in the chickens’ jejunum induced by LPS. The unique architecture of the villus crypt of the intestinal tract plays a crucial role in intestinal health (Tong et al., 2023). A higher VCR is associated with stronger digestive and absorptive capacities in the intestines (Saadatmand et al., 2019). Our results showed that the jejunal and ileal VCR decreased after LPS challenge, whereas supplementation with CTC or 10-HDA reversed this decline.
Cytokines are involved in the pathogenesis of inflammatory intestinal diseases (Neurath, 2014). LPS induces intestinal inflammatory responses in chickens by increasing the levels of proinflammatory cytokines (Wang et al., 2014; Shang et al., 2015; Zhang et al., 2021). TLR4 functions as a receptor for LPS, binding to LPS and initiating the TLR4/NF-κB signaling pathway, thereby facilitating the secretion of proinflammatory cytokines (Park and Lee, 2013). NF-κB is a crucial regulatory factor in inflammatory responses and essential in maintaining immune system homeostasis (Christian et al., 2016). Previous studies on murine macrophage cell lines indicated that 10-HDA inhibits the LPS-induced NF-κB pathway and reduces the release of downstream IL-6 (Sugiyama et al., 2012). Chen Y. F. et al. (2018) demonstrated that oral administration of 10-HDA lowered the serum levels of TNF-α, IL-6, and IL-10 in mice. In the current study, LPS stimulation up-regulated the mRNA expression of TLR4, NF-κB, TNF-α, IL-1β, and IL-6 in chicken jejunal mucosa, and elevated the serum concentration of TNF-α, IL-1β, and IL-6, whereas 0.1% 10-HDA supplementation effectively decreased the expression and secretion of these inflammatory cytokines. However, the mRNA expression of TLR4, NF-κB, IL-1β, IL-6, and the serum levels of TNF-α and IL-1β induced by LPS were not reversed by 0.5% 10-HDA supplementation. These results suggest that 0.1% 10-HDA supplementation can effectively inhibit intestinal inflammation to attenuate LPS-induced intestinal damage in chickens.
In addition, LPS challenge induces oxidative stress, leading to intestinal injury in chickens (Wu et al., 2013). Antioxidant enzymes could effectively inhibit oxidative damage and reflect the oxidative stress state of animals (Zheng Y. et al., 2020). This study revealed that LPS challenge reduced the activity of T-SOD, CAT, and GSH-px in the serum, and downregulated the expression of the genes encoding these antioxidant enzymes in the jejunal mucosa. Previous studies have shown that 10-HDA increases antioxidant enzyme activity in the liver of diabetic mice and reduces the production of free radicals and lipid peroxidation (Hu X. et al., 2022). Similarly, our results revealed that dietary 0.1% 10-HDA supplementation improved the activities of T-SOD, CAT, and GSH-px in LPS-challenged chickens, indicating that 10-HDA exerts an antioxidant function to maintain the intestinal health of chickens. No significant differences were observed in the activities of CAT and T-SOD between the 0.1% 10-HDA and CTC groups. Moreover, intestinal inflammation and oxidative stress can trigger excessive apoptosis in intestinal mucosal cells and inhibit cell proliferation, thereby destroying intestinal barrier function (Jiang et al., 2019; Chang et al., 2020). We found that the addition of 0.1% 10-HDA to the diet inhibited LPS-induced apoptosis by downregulating the mRNA expression of Caspase-3 and Bax and increasing Bcl2 expression.
The intestinal microbiota plays key roles in preventing pathogen invasion and regulating intestinal homeostasis, which is essential for maintaining the intestinal health of chickens (Carrasco et al., 2019). LPS stimulation disrupts the ecological balance of the gut microbiota (Lucke et al., 2018; He et al., 2022; Ye et al., 2023). Our PCoA and PLS-DA results demonstrated a significant separation of the gut microbiota between the LPS and control groups. However, the addition of 10-HDA attenuated these differences and improved the compositional structure of the cecal microbiota. 10-HDA treatment increased the abundance of Faecalibacterium and Clostridia_UCG-014, while reducing the abundance of Clostridia_vadinBB60_group, Eubacterium_nodatum, and UC5-1-2E3. Faecalibacterium is closely related to butyrate production in the chicken intestinal tract and plays a crucial role in anti-inflammation and recovery from intestinal injury (Singh et al., 2014; Breyner et al., 2017; Sun Y. et al., 2022). Our study revealed that the abundance of Faecalibacterium was negatively correlated with the expression of IL-1β and positively correlated with CAT levels. The probiotic genus Clostridia_UCG-014 is associated with tryptophan metabolism and inflammation. It has been shown to be beneficial in treating disorders of glucose and lipid metabolism (Hu Q. et al., 2022; Niu et al., 2022). Clostridia_vadinBB60_group is positively correlated with inflammatory markers and negatively correlated with anti-inflammatory markers (Sun J. et al., 2022). Eubacterium_nodatum is an opportunistic pathogen associated with chronic inflammation (Moen et al., 2006). It has been reported that a pro-inflammatory diet is linked to a higher abundance of Eubacterium_nodatum (Zheng J. et al., 2020). Furthermore, Eubacterium_nodatum is positively correlated with intestinal disorder indices in DSS-induced colitis mice (Ma et al., 2022). Circos analysis revealed that Alistipes was the dominant genus in the LPS group, and the addition of 10-HDA reduced its abundance. Alistipes is an opportunistic pathogen associated with inflammation and dysbiosis (Kong et al., 2019; Parker et al., 2020). Our study demonstrated a significant positive correlation between the abundance of Alistipes and the expression of TLR4, NF-κB, TNF-α, IL-6, and Caspase-3. Overall, the results indicate that 10-HDA supplementation can alter the cecal microbial composition in LPS-challenged chickens. This alteration may contribute to an enhancement in antioxidant capacity and a reduction in LPS-induced intestinal inflammation and injury.
5. Conclusion
In conclusion, 10-HDA alleviated the LPS-induced intestinal damage and growth performance loss in chickens through anti-inflammatory, antioxidant, and gut microbiota-modulating activities. The alterations in the cecal microbiota caused by 10-HDA supplementation might be related to the recovery of intestinal health in chickens subjected to LPS challenge. Moreover, dietary supplementation with 0.1% 10-HDA had comparable or even better protection for LPS-challenged chickens than supplementation with antibiotics or 0.5% 10-HDA. This study provides valuable references for the application of 10-HDA as an alternative to antibiotics in protecting the intestinal health and improving the performance of poultry.
Data availability statement
The datasets presented in this study can be found in online repositories. The names of the repository/repositories and accession number(s) can be found at: https://www.ncbi.nlm.nih.gov/, PRJNA1000644.
Ethics statement
The animal study was approved by Animal Ethics committee of Shandong Academy of Agricultural Sciences. The study was conducted in accordance with the local legislation and institutional requirements.
Author contributions
LH: Conceptualization, Methodology, Data curation, Investigation, Writing – original draft. MZ: Writing – original draft, Investigation, Validation, Writing – original draft. FL: Investigation, Conceptualization, Resources, Writing – review & editing. JS: Investigation, Validation, Writing – original draft. RW: Conceptualization, Resources, Supervision, Writing – review & editing. GL: Conceptualization, Data curation, Investigation, Writing – original draft. XY: Funding acquisition, Writing – review & editing, Conceptualization, Methodology, Project administration.
Funding
The author(s) declare financial support was received for the research, authorship, and/or publication of this article. This work was supported by the Shandong Provincial Natural Science Foundation (ZR2020MC049), the Shandong Province Science Small and Medium-Sized Sci-Tech Enterprises Innovation Capacity Promotion Project (2023TSGC0265), the Key Innovation Project of Qilu University of Technology (Shandong Academy of Sciences) (2022JBZ01-06), the Taishan Industrial Experts Program (TSCX202306046), and the Agricultural Breed Project of Shandong Province (2022LZGC013).
Conflict of interest
The authors declare that the research was conducted in the absence of any commercial or financial relationships that could be construed as a potential conflict of interest.
Publisher’s note
All claims expressed in this article are solely those of the authors and do not necessarily represent those of their affiliated organizations, or those of the publisher, the editors and the reviewers. Any product that may be evaluated in this article, or claim that may be made by its manufacturer, is not guaranteed or endorsed by the publisher.
Supplementary material
The Supplementary material for this article can be found online at: https://www.frontiersin.org/articles/10.3389/fmicb.2023.1285299/full#supplementary-material
Footnotes
References
Adedokun, S. A., and Olojede, O. C. (2019). Optimizing gastrointestinal integrity in poultry: the role of nutrients and feed additives. Front. Vet. Sci. 5:348. doi: 10.3389/fvets.2018.00348
Bacanli, M., and Basaran, N. (2019). Importance of antibiotic residues in animal food. Food Chem. Toxicol. 125, 462–466. doi: 10.1016/j.fct.2019.01.033
Breyner, N. M., Michon, C., de Sousa, C. S., Boas, P. B. V., Chain, F., Azevedo, V. A., et al. (2017). Microbial anti-inflammatory molecule (mam) from Faecalibacterium prausnitzii shows a protective effect on dnbs and DSS-induced colitis model in mice through inhibition of NF-kappa B pathway. Front. Microbiol. 8:114. doi: 10.3389/fmicb.2017.00114
Carrasco, J. M. D., Casanova, N. A., and Miyakawa, M. E. F. (2019). Microbiota, gut health and chicken productivity: what is the connection? Microorganisms 7:374. doi: 10.3390/microorganisms7100374
Chang, Y. C., Yuan, L., Liu, J. R., Ishfaq, M., Cao, C. B., Shi, C. X., et al. (2020). Dihydromyricetin attenuates Escherichia coli lipopolysaccharide-induced ileum injury in chickens by inhibiting NLRP3 inflammasome and TLR4/NF-kappa B signalling pathway. Vet. Res. 51:72. doi: 10.1186/s13567-020-00796-8
Chen, Y. F., You, M. M., Liu, Y. C., Shi, Y. Z., Wang, K., Lu, Y. Y., et al. (2018). Potential protective effect of trans-10-hydroxy-2-decenoic acid on the inflammation induced by lipoteichoic acid. J. Funct. Foods 45, 491–498. doi: 10.1016/j.jff.2018.03.029
Chen, Y. P., Zhang, H., Cheng, Y. F., Li, Y., Wen, C., and Zhou, Y. M. (2018). Dietary L-threonine supplementation attenuates lipopolysaccharide-induced inflammatory responses and intestinal barrier damage of broiler chickens at an early age. Br. J. Nutr. 119, 1254–1262. doi: 10.1017/s0007114518000740
Christian, F., Smith, E. L., and Carmody, R. J. (2016). The regulation of NF-kappa B subunits by phosphorylation. Cells 5:12. doi: 10.3390/cells5010012
Fan, P., Han, B., Hu, H., Wei, Q. H., Zhang, X. F., Meng, L. F., et al. (2020). Proteome of thymus and spleen reveals that 10-hydroxydec-2-enoic acid could enhance immunity in mice. Expert Opin. Ther. Targets 24, 267–279. doi: 10.1080/14728222.2020.1733529
Gao, K. K., Su, B., Dai, J., Li, P. W., Wang, R. M., and Yang, X. H. (2022). Anti-biofilm and anti-hemolysis activities of 10-hydroxy-2-decenoic acid against staphylococcus aureus. Molecules 27:1485. doi: 10.3390/molecules27051485
Gui, H., Song, I. B., Han, H. J., Lee, N. Y., Cha, J. Y., Son, Y. K., et al. (2018). Antioxidant activity of royal jelly hydrolysates obtained by enzymatic treatment. Korean J. Food Sci. Anim. Resour. 38, 135–142. doi: 10.5851/kosfa.2018.38.1.135
He, Z. T., Li, Y. J., Xiong, T. D., Nie, X. Y., Zhang, H. H., and Zhu, C. (2022). Effect of dietary resveratrol supplementation on growth performance, antioxidant capacity, intestinal immunity and gut microbiota in yellow-feathered broilers challenged with lipopolysaccharide. Front. Microbiol. 13:977087. doi: 10.3389/fmicb.2022.977087
Hu, X. Y., Liu, Z. G., Lu, Y. T., Chi, X. P., Han, K., Wang, H. F., et al. (2022). Glucose metabolism enhancement by 10-hydroxy-2-decenoic acid via the PI3K/AKT signaling pathway in high-fat-diet/streptozotocin induced type 2 diabetic mice. Food Funct. 13, 9931–9946. doi: 10.1039/d1fo03818d
Hu, Q., Wu, C. Y., Yu, J. T., Luo, J. M., and Peng, X. C. (2022). Angelica sinensis polysaccharide improves rheumatoid arthritis by modifying the expression of intestinal Cldn5, Slit3 and Rgs18 through gut microbiota. Int. J. Biol. Macromol. 209, 153–161. doi: 10.1016/j.ijbiomac.2022.03.090
Huang, S. S., Tao, R. R., Zhou, J. F., Qian, L. X., and Wu, J. (2022). Trans-10-hydroxy-2-decenoic acid alleviates dextran sulfate sodium-induced colitis in mice via regulating the inflammasome-mediated pyroptotic pathway and enhancing colonic barrier function. Mol. Nutr. Food Res. 66:e2100821. doi: 10.1002/mnfr.202100821
Jiang, J. L., Qi, L. N., Lv, Z. P., Jin, S., Wei, X. H., and Shi, F. X. (2019). Dietary stevioside supplementation alleviates lipopolysaccharide-induced intestinal mucosal damage through anti-inflammatory and antioxidant effects in broiler chickens. Antioxidants 8:575. doi: 10.3390/antiox8120575
Kong, C., Gao, R. Y., Yan, X. B., Huang, L. S., and Qin, H. L. (2019). Probiotics improve gut microbiota dysbiosis in obese mice fed a high fat or high-sucrose diet. Nutrition 60, 175–184. doi: 10.1016/j.nut.2018.10.002
Leshchinsky, T. V., and Klasing, K. C. (2001). Divergence of the inflammatory response in two types of chickens. Dev. Comp. Immunol. 25, 629–638. doi: 10.1016/S0145-305X(01)00023-4
Li, Y., Wang, J. Q., Wang, F., Wang, L., Wang, L. L., Xu, Z. Q., et al. (2022). Production of 10-hydroxy-2-decenoic acid from decanoic acid via whole-cell catalysis in engineered Escherichia coli. ChemSusChem 15:e202102152. doi: 10.1002/cssc.202102152
Liu, L., Qin, D. K., Wang, X. F., Feng, Y., Yang, X. J., and Yao, J. H. (2015). Effect of immune stress on growth performance and energy metabolism in broiler chickens. Food Agric. Immunol. 26, 194–203. doi: 10.1080/09540105.2014.882884
Liu, C. S., Zhao, D. F., Ma, W. J., Guo, Y. D., Wang, A. J., Wang, Q. L., et al. (2016). Denitrifying sulfide removal process on high-salinity wastewaters in the presence of Halomonas sp. Appl. Microbiol. Biotechnol. 100, 1421–1426. doi: 10.1007/s00253-015-7039-6
Lucke, A., Bohm, J., Zebeli, Q., and Metzler-Zebeli, B. U. (2018). Dietary deoxynivalenol contamination and oral lipopolysaccharide challenge alters the cecal microbiota of broiler chickens. Front. Microbiol. 9:804. doi: 10.3389/fmicb.2018.00804
Ma, L., Zhao, X., Liu, T., Wang, Y., Wang, J., Kong, L., et al. (2022). Xuanfei baidu decoction attenuates intestinal disorders by modulating NF-κB pathway, regulating T cell immunity and improving intestinal flora. Phytomedicine 101:154100. doi: 10.1016/j.phymed.2022.154100
Mehdi, Y., Letourneau-Montminy, M. P., Gaucher, M. L., Chorfi, Y., Suresh, G., Rouissi, T., et al. (2018). Use of antibiotics in broiler production: global impacts and alternatives. Anim. Nutr. 4, 170–178. doi: 10.1016/j.aninu.2018.03.002
Melliou, E., and Chinou, I. (2005). Chemistry and bioactivity of royal jelly from Greece. J. Agric. Food Chem. 53, 8987–8992. doi: 10.1021/jf051550p
Moen, K., Brun, J. G., Valen, M., Skartveit, L., Eribe, E. K. R., Olsen, I., et al. (2006). Synovial inflammation in active rheumatoid arthritis and psoriatic arthritis facilitates trapping of a variety of oral bacterial DNAs. Clin. Exp. Rheumatol. 24, 656–663.
Neurath, M. F. (2014). Cytokines in inflammatory bowel disease. Nat. Rev. Immunol. 14, 329–342. doi: 10.1038/nri3661
Niu, K. X., Bai, P. P., Yang, B. B., Feng, X. C., and Qiu, F. (2022). Asiatic acid alleviates metabolism disorders in Ob/Ob mice: mechanistic insights. Food Funct. 13, 6934–6946. doi: 10.1039/d2fo01069k
Okumura, R., and Takeda, K. (2018). Maintenance of intestinal homeostasis by mucosal barriers. Inflamm. Regen. 38:5. doi: 10.1186/s41232-018-0063-z
Park, B. S., and Lee, J. O. (2013). Recognition of lipopolysaccharide pattern by TLR4 complexes. Exp. Mol. Med. 45:e166:e66. doi: 10.1038/emm.2013.97
Parker, B. J., Wearsch, P. A., Veloo, A. C. M., and Rodriguez-Palacios, A. (2020). The genus Alistipes: gut bacteria with emerging implications to inflammation, cancer, and mental health. Front. Immunol. 11:906. doi: 10.3389/fimmu.2020.00906
Ren, Z. S., Fang, H. T., Zhang, J., Wang, R., Xiao, W. Y., Zheng, K. X., et al. (2022). Dietary aronia melanocarpa pomace supplementation enhances the expression of ZO-1 and occludin and promotes intestinal development in pigs. Front. Vet. Sci. 9:904667. doi: 10.3389/fvets.2022.904667
Robles-Jimenez, L. E., Aranda-Aguirre, E., Castelan-Ortega, O. A., Shettino-Bermudez, B. S., Ortiz-Salinas, R., Miranda, M., et al. (2022). Worldwide traceability of antibiotic residues from livestock in wastewater and soil: a systematic review. Animals 12:60. doi: 10.3390/ani12010060
Saadatmand, N., Toghyani, M., and Gheisari, A. (2019). Effects of dietary fiber and threonine on performance, intestinal morphology and immune responses in broiler chickens. Anim. Nutr. 5, 248–255. doi: 10.1016/j.aninu.2019.06.001
Shang, Y., Regassa, A., Kim, J. H., and Kim, W. K. (2015). The effect of dietary fructooligosaccharide supplementation on growth performance, intestinal morphology, and immune responses in broiler chickens challenged with salmonella enteritidis lipopolysaccharides. Poult. Sci. 94, 2887–2897. doi: 10.3382/ps/pev275
Shi, L. L., Jin, X., Xu, Y. Q., Xing, Y. Y., Yan, S. M., Guo, Y. F., et al. (2022). Effects of total flavonoids of artemisia ordosica on growth performance, oxidative stress, and antioxidant status of lipopolysaccharide-challenged broilers. Antioxidants 11:1985. doi: 10.3390/antiox11101985
Singh, N., Gurav, A., Sivaprakasam, S., Brady, E., Padia, R., Shi, H. D., et al. (2014). Activation of gpr109a, receptor for niacin and the commensal metabolite butyrate, suppresses colonic inflammation and carcinogenesis. Immunity 40, 128–139. doi: 10.1016/j.immuni.2013.12.007
Sugiyama, T., Takahashi, K., Tokoro, S., Gotou, T., Neri, P., and Mori, H. (2012). Inhibitory effect of 10-hydroxy-trans-2-decenoic acid on LPS-induced IL-6 production via reducing I kappa B-zeta expression. Innate Immun. 18, 429–437. doi: 10.1177/1753425911416022
Sun, J., Liu, J., Ren, G., Chen, X. T., Cai, H. H., Hong, J. H., et al. (2022). Impact of purple sweet potato (Ipomoea batatas l.) polysaccharides on the fecal metabolome in a murine colitis model. RSC Adv. 12, 11376–11390. doi: 10.1039/d2ra00310d
Sun, Y. G., Zhang, S. S., Nie, Q. X., He, H. J., Tan, H. Z., Geng, F., et al. (2022). Gut firmicutes: relationship with dietary fiber and role in host homeostasis. Crit. Rev. Food Sci. Nutr. 12, 1–16. doi: 10.1080/10408398.2022.2098249
Tao, W. J., Wang, G., Pei, X., Sun, W. J., and Wang, M. Q. (2022). Chitosan oligosaccharide attenuates lipopolysaccharide-induced intestinal barrier dysfunction through suppressing the inflammatory response and oxidative stress in mice. Antioxidants 11:1384. doi: 10.3390/antiox11071384
Tong, Y. C., Yu, C. Y., Chen, S., Zhang, X. L., Yang, Z. B., and Wang, T. (2023). Trans-anethole exerts protective effects on lipopolysaccharide-induced acute jejunal inflammation of broilers via repressing NF-κB signaling pathway. Poult. Sci. 102:102397. doi: 10.1016/j.psj.2022.102397
Vancamelbeke, M., and Vermeire, S. (2017). The intestinal barrier: a fundamental role in health and disease. Expert Rev.Gastroenterol.Hepatol. 11, 821–834. doi: 10.1080/17474124.2017.1343143
Wang, X. F., Shen, J., Li, S. Z., Zhi, L. H., Yang, X. J., and Yao, J. H. (2014). Sulfated astragalus polysaccharide regulates the inflammatory reaction in LPS-infected broiler chicks. Int. J. Biol. Macromol. 69, 146–150. doi: 10.1016/j.ijbiomac.2014.05.004
Wu, Y. P., Li, Q., Liu, J. S., Liu, Y. L., Xu, Y. L., Zhang, R. Q., et al. (2021). Integrating serum metabolome and gut microbiome to evaluate the benefits of lauric acid on lipopolysaccharide- challenged broilers. Front. Immunol. 12:759323. doi: 10.3389/fimmu.2021.759323
Wu, Q. J., Zhou, Y. M., Wu, Y. N., Zhang, L. L., and Wang, T. (2013). The effects of natural and modified clinoptilolite on intestinal barrier function and immune response to LPS in broiler chickens. Vet. Immunol. Immunopathol. 153, 70–76. doi: 10.1016/j.vetimm.2013.02.006
Yang, Y. C., Chou, W. M., Widowati, D. A., Lin, I. P., and Peng, C. C. (2018). 10-Hydroxy-2-decenoic acid of royal jelly exhibits bactericide and anti-inflammatory activity in human colon cancer cells. BMC Complement. Altern. Med. 18:202. doi: 10.1186/s12906-018-2267-9
Yang, X. J., Li, W. L., Feng, Y., and Yao, J. H. (2011). Effects of immune stress on growth performance, immunity, and cecal microflora in chickens. Poult. Sci. 90, 2740–2746. doi: 10.3382/ps.2011-01591
Ye, J. X., Yang, H. A., Hu, W. D., Tang, K. Y., Liu, A. F., and Bi, S. C. (2023). Changed cecal microbiota involved in growth depression of broiler chickens induced by immune stress. Poult. Sci. 102:102598. doi: 10.1016/j.psj.2023.102598
You, M. M., Miao, Z. N., Tian, J., and Hu, F. L. (2020). Trans-10-hydroxy-2-decenoic acid protects against LPS-induced neuroinflammation through FOXO1-mediated activation of autophagy. Eur. J. Nutr. 59, 2875–2892. doi: 10.1007/s00394-019-02128-9
Zhang, Y. X., Geng, S. X., Di, Y. T., Sun, Y. B., Liu, Y., Li, J. T., et al. (2022). 10-Hydroxy-trans-2-decenoic acid, a new potential feed additive for broiler chickens to improve growth performance. Animals 12:1846. doi: 10.3390/ani12141846
Zhang, J. F., Yang, Y. X., Han, H. L., Zhang, L. L., and Wang, T. (2021). Bisdemethoxycurcumin attenuates lipopolysaccharide-induced intestinal damage through improving barrier integrity, suppressing inflammation, and modulating gut microbiota in broilers. J. Anim. Sci. 99:skab296. doi: 10.1093/jas/skab296
Zhang, S. F., Zhou, Q. N., Li, Y. C., Zhang, Y. L., and Wu, Y. M. (2020). Mitoq modulates lipopolysaccharide-induced intestinal barrier dysfunction via regulating Nrf2 signaling. Mediat. Inflamm. 2020:3276148. doi: 10.1155/2020/3276148
Zheng, J. L., Hoffman, K. L., Chen, J. S., Shivappa, N., Sood, A., Browman, G. J., et al. (2020). Dietary inflammatory potential in relation to the gut microbiome: results from a cross-sectional study. Br. J. Nutr. 124, 931–942. doi: 10.1017/s0007114520001853
Zheng, Y. W., Zhang, J. Y., Zhou, H. B., Guo, Y. P., Ma, Q. G., Ji, C., et al. (2020). Effects of dietary pyrroloquinoline quinone disodium supplementation on inflammatory responses, oxidative stress, and intestinal morphology in broiler chickens challenged with lipopolysaccharide. Poult. Sci. 99, 5389–5398. doi: 10.1016/j.psj.2020.08.007
Keywords: 10-hydroxy-2-decenoic acid, lipopolysaccharide, intestinal barrier, inflammation, antioxidant, gut microbiota
Citation: Han L, Zhang M, Li F, Su J, Wang R, Li G and Yang X (2023) 10-hydroxy-2-decenoic acid alleviates lipopolysaccharide-induced intestinal mucosal injury through anti-inflammatory, antioxidant, and gut microbiota modulation activities in chickens. Front. Microbiol. 14:1285299. doi: 10.3389/fmicb.2023.1285299
Edited by:
Li Wang, Guangdong Academy of Agricultural Sciences (GDAAS), ChinaReviewed by:
Shuting Cao, Guangdong Academy of Agricultural Sciences, ChinaKan Xiao, Wuhan Polytechnic University, China
Copyright © 2023 Han, Zhang, Li, Su, Wang, Li and Yang. This is an open-access article distributed under the terms of the Creative Commons Attribution License (CC BY). The use, distribution or reproduction in other forums is permitted, provided the original author(s) and the copyright owner(s) are credited and that the original publication in this journal is cited, in accordance with accepted academic practice. No use, distribution or reproduction is permitted which does not comply with these terms.
*Correspondence: Xiaohui Yang, eWFuZ3hoOTE5QDEyNi5jb20=