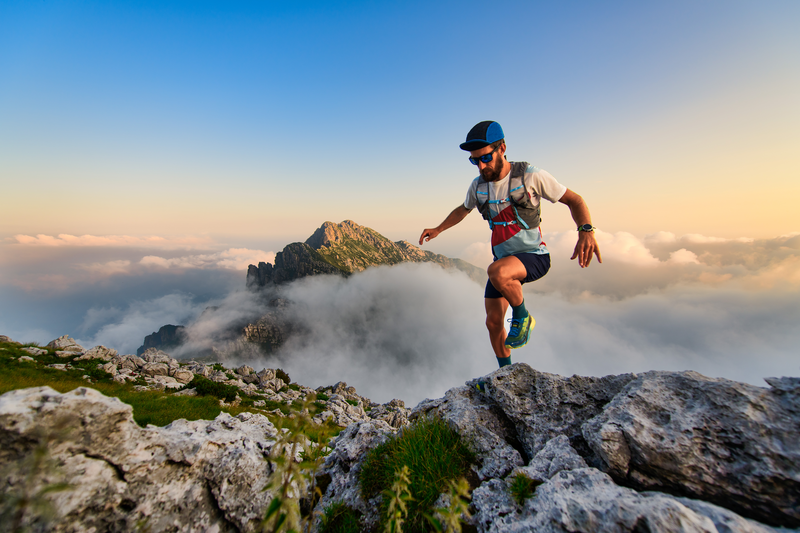
94% of researchers rate our articles as excellent or good
Learn more about the work of our research integrity team to safeguard the quality of each article we publish.
Find out more
REVIEW article
Front. Med. , 06 January 2025
Sec. Infectious Diseases: Pathogenesis and Therapy
Volume 11 - 2024 | https://doi.org/10.3389/fmed.2024.1523991
This article is part of the Research Topic Infection-Mediated Inflammation that Promotes Cancer Initiation and/or Progression View all 6 articles
One of the most prevalent human infections is Helicobacter pylori (H. pylori), which affects more than half of the global population. Although H. pylori infections are widespread, only a minority of individuals develop severe gastroduodenal disorders. The global resistance of H. pylori to antibiotics has reached concerning levels, significantly impacting the effectiveness of treatment. Consequently, the development of vaccines targeting virulence factors may present a viable alternative for the treatment and prevention of H. pylori infections. This review aims to provide a comprehensive overview of the current understanding of H. pylori infection, with a particular focus on its virulence factors, pathophysiology, and vaccination strategies. This review discusses various virulence factors associated with H. pylori, such as cytotoxin-associated gene A (cagA), vacuolating cytotoxin gene (vacA), outer membrane proteins (OMPs), neutrophil-activated protein (NAP), urease (ure), and catalase. The development of vaccines based on these virulence characteristics is essential for controlling infection and ensuring long-lasting protection. Various vaccination strategies and formulations have been tested in animal models; however, their effectiveness and reproducibility in humans remain uncertain. Different types of vaccines, including vector-based vaccines, inactivated whole cells, genetically modified protein-based subunits, and multiepitope nucleic acid (DNA) vaccines, have been explored. While some vaccines have demonstrated promising results in murine models, only a limited number have been successfully tested in humans. This article provides a thorough evaluation of recent research on H. pylori virulence genes and vaccination methods, offering valuable insights for future strategies to address this global health challenge.
Helicobacter pylori (H. pylori) is an ancient microbe that predates Columbus’s expeditions (1). It is a gram-negative, microaerophilic spiral bacterium first identified in the early 1980s by Australian physicians Barry Marshall and Robin Warren. In recognition of their discovery of H. pylori and its link to gastrointestinal disorders, including gastritis and peptic ulcers, they received the Nobel Prize in Physiology or Medicine from the Nobel Assembly at the Karolinska Institute in 2005 (2, 3). Research on H. pylori has advanced significantly, as scientists have strived to clarify the complexities of this infection. More than half of the global population is estimated to be chronically infected with H. pylori, a major public health concern because of its potential to contribute to severe health issues (4–7). The prevalence is 20 to 40% in high-income countries and 70 to 90% in low-income countries (6, 8, 9). H. pylori is a formidable pathogen known for causing chronic stomach infections that can last a lifetime (10, 11). Its remarkable adaptability to the acidic environment of the stomach has resulted in various host responses and pathogenic outcomes (12, 13). Initially, linked to peptic ulcers, H. pylori is now associated with gastritis, duodenal ulcers, stomach cancer, and multiple extragastric conditions, including neurological, ophthalmic, hematological, cardiovascular, and dermatological disorders (14–17). Millions of people worldwide suffer from these conditions, leading to substantial financial and medical burdens (18). The World Health Organization classifies H. pylori as a class I carcinogen, the primary cause of stomach cancer deaths globally (5, 19–21).
Virulence genes from related families, including flagella, ureases, membrane glycoproteins, and outer membrane proteins (OMPs), play a significant role in H. pylori pathogenicity (9, 22, 23). Four to six flagella per cell enhance mobility and gastric epithelium penetration (24). Urease secretion lowers the gastric pH and releases ammonia, creating a conducive environment for microbial colonization and potential ulceration (25). Lipopolysaccharide (LPS) improves the adherence of pathogens to the gastrointestinal mucosa, promoting infection (13, 26). OMPs are crucial for adhesion and pathogenicity, leading to inflammation (27). Sixty-four OMP gene family members, including iron-regulated OMPs and principal OMPs (Figure 1), such as Hop, Hor, Hof, and Hom, have been identified (28). Other OMPs, such as oipA, sabA, and babA, enhance gastric mucosa colonization (22, 29). Seo (30) reported that vaccines containing vacA, cagA, and NAP effectively prevented experimental infections in animal models.
Figure 1. Overview of H. pylori OMPs. The Hop family includes 22 genes (hopA–hopQ, hopU, hopZ, babA, babB), the Hor family has 12 genes (horA–horL), the Hof family consists of 8 genes (hofA–hofH), and the Hom family contains 4 genes (homA–homD). The FecA-like and FrpB-like families each have 3 genes (fecA-1, fecA-2, fecA-3, and frpB-1, frpB-2, frpB-3, respectively). The efflux pump family comprises 6 genes: hefA, hefD, hefG, flgH, palA, and lpp20.
Amoxicillin, clarithromycin, and metronidazole are commonly used to treat H. pylori-related gastric infections, often with proton pump inhibitors (29). Studies have shown that these antibiotics achieve an average eradication rate of approximately 80% (31); however, overuse may contribute to antimicrobial resistance. The resistance rates of H. pylori to various antibiotics were reported as follows: in the United States from 2011–2021, the rates were 42.1% for metronidazole, 31.5% for clarithromycin, 37.6% for levofloxacin, and 2.6% for amoxicillin (32). In Europe, during the period from 2008–2017, the resistance rates were 38.9% for metronidazole, 21.4% for clarithromycin, 15.8% for levofloxacin, and 0.2% for amoxicillin (33). In Africa, from 1986–2017, the resistance rates were 75.8% for metronidazole, 29.2% for clarithromycin, 17.4% for levofloxacin, and 72.6% for amoxicillin (34). Researchers are investigating innovative strategies for treating and preventing H. pylori infections, particularly through vaccine development. Recent studies have identified virulence determinants that may protect against infection and help eradicate bacteria in murine models (35). The evidence supports the potential use of these factors in the development of an effective human vaccine (9, 36–38). A vaccination program targeting these virulence factors could effectively manage or eliminate pathogenic strains (39, 40). Various vaccination regimens tested in animal models have shown positive results. This review explores the pathogenesis and virulence factors of H. pylori infection, along with the current research status and limitations of H. pylori vaccines.
A comprehensive literature search was conducted to review the virulence factors, pathogenesis, and vaccines associated with H. pylori. The inclusion criteria included original research articles, review articles, and clinical trials focused on pathogenicity, virulence factors, and vaccine development. The key topics addressed were mechanisms of pathogenesis; immune responses; and specific virulence factors, such as OMPs, cagA, vacA, NAP, ure, and catalase. Only English-language publications from 1989–2024 were included, whereas nonresearched materials, non-English publications, and duplicate studies were excluded. Searches were performed in databases such as PubMed, Web of Science, Scopus, and Google Scholar, using terms such as “H. pylori,” “pathophysiology,” “antimicrobial resistance,” “virulence factors,” “vector vaccine,” “subunit vaccine,” and “DNA vaccine.”
The interaction of the host immune system with bacterial components leads to an immunological response to H. pylori infection (41, 42). This triggers a complex local inflammatory response in the stomach, which is typical of H. pylori infections (7, 43, 44). During the innate immune response, H. pylori causes a persistent inflammatory reaction in the gastric mucosa (Figure 2). The relationship between LPS and peptidoglycan in the H. pylori cell wall is essential for this response, which is marked by the infiltration of immune cells such as neutrophils, macrophages, and lymphocytes and the release of proinflammatory cytokines such as IL-1β, IL-6, IL-8, and TNF-α (45, 46). Moreover, IL-17 plays a crucial role in the immune response to Helicobacter infections in both humans and mice (47, 48). In humans, IL-17 induces the secretion of IL-8 by activating the ERK 1/2 MAP kinase pathway, and the released IL-8 attracts neutrophils, promoting inflammation (49). IL-17 has two main roles: T regulatory cells modulate inflammation to support bacterial survival, and vaccination generates Helicobacter-specific memory T helper cells, increasing IL-17-mediated inflammation and assisting in bacterial clearance (49). The enhanced proinflammatory effects of IL-17 by CD4+ cells can significantly help eradicate bacteria in murine models (50, 51). Compared with unvaccinated mice, vaccinated mice exhibit higher IL-17 mRNA levels in their stomachs (52). Innate immune responses are activated by pattern recognition receptors, such as Toll-like receptors, which detect bacterial components such as LPS and peptidoglycan, triggering inflammatory reactions (53). The adaptive immune response follows, with CD4+ T helper (Th) cells stimulating a Th1 response that secretes interferon-gamma (IFN-γ). B lymphocytes produce antibodies against H. pylori, and regulatory T cells help modulate infection (54–56).
Figure 2. The pathogenesis of H. pylori is illustrated in the accompanying figure, which has been carefully edited and designed utilizing dynamic elements from BioRender. Created with BioRender.com
Bacteria use various immune evasion strategies, including urease synthesis and molecular mimicry, to avoid detection by T and B cells (57, 58). Prolonged H. pylori infection causes chronic inflammation and damage to the gastric mucosa. Increased turnover of gastric epithelial cells contributes to ulceration and mucosal injury (59). This compromises mucosal barrier integrity due to tight junction disruption. H. pylori penetrates the mucosal layers, facilitating rapid spread. The continuous release of inflammatory mediators and genomic instability foster an environment conducive to cancer development (60). H. pylori disrupts local immune responses, which may lead to malignancies in the gastric epithelium (61).
Certain virulence antigens are associated with the severity of symptoms and clinical outcomes in H. pylori infections. Antigens of H. pylori, such as cagA, vacA, NAP, OMPs (e.g., babA, sapA, oipA), urease, catalase, and Hsp60, are also considered potential candidates for vaccines. These antigens trigger both humoral and cellular immune responses during infection. This section provides an overview of their roles in invasion, survival, colonization, and inflammation in the gastric mucosa as well as their role in improving vaccine development.
The virulence factors cagA and vacA of H. pylori are crucial for cytotoxin production (62). These genes are part of the type IV secretion system (T4SS), which is vital for bacterial pathogenicity (63). T4SSs are complex structures that penetrate bacterial cell walls, aiding survival and protein or DNA translocation (64). Approximately 60–70% of H. pylori strains express the cagA protein, which produces a specific cytotoxin (65). Phosphorylation of tyrosine motifs in cagA allows its translocation into gastric epithelial cells via T4SSs. Research by Yamaoka et al. (61), Selbach et al. (66), and Stein et al. (67) indicates that variations in these motifs are linked to gastric degeneration and increased gastric cancer risk. Phosphorylated cagA triggers pathological responses in host cells, enhancing motility, actin polymerization, and cell stretching and disrupting physiological signals (68). cagA influences Th17 cell differentiation by interacting with the STAT3 protein, which is crucial for T and B lymphocyte development. It also interacts with NF-κB, a key regulator of innate immune responses (69). cagA activates Th1 and Th17 cells to eliminate H. pylori and promotes proinflammatory cytokine expression in gastric epithelial cells (70). H. pylori strains with cagA enhance IL-8 secretion (71). The high immunogenicity of cagA is linked to increased gastric inflammation (72), which negatively affects H. pylori survival (73). Furthermore, cagA promotes a Th1-polarized immune response that aids infection clearance (74). This dual role indicates that H. pylori must regulate cagA expression during gastric colonization. The cagA gene is vital for vaccine development beyond its pathogenic properties. Paydarnia et al. (75) studied the effects of mixed immunization with H. pylori LPS and recombinant cagA on immune responses in a murine model. The recombinant cagA protein, given with a cytosine phosphoguanine adjuvant, maintained its antigenic properties and triggered strong Th1-biased immune responses throughout the experiment. These findings suggest that cagA may be key to an effective vaccine for H. pylori infection.
Most H. pylori isolates contain the vacA gene, which targets epithelial and immune cells in the digestive tract (76, 77). Like the cagA gene, vacA is unique to type I H. pylori. The vacA protein not only facilitates intracellular vacuole formation but also has toxic effects on various cells (78, 79) and survives the acidity of the stomach via multiple exit routes (79). Although the exact mechanisms by which vacA induces autophagy are not fully understood, it has been shown that the autophagy triggered by vacA is dependent on its interaction with low-density lipoprotein receptor-related protein 1 (80). The vacA protein affects apoptotic signaling in host cells, limiting apoptosis. Its influence can be proapoptotic or antiapoptotic, depending on the cell type and environment. The vacA toxin also alters host cell morphology and function by inducing vacuole formation (78). Additionally, toxins hinder T cells and other immune cells, impacting the overall immune response (81, 82). In most H. pylori-infected patients, anti-vacA antibodies are found in their blood and gastric juice (83). The growth of CD4+ lymphocytes from the gastric epithelium is antigen dependent when vacA is present (84, 85). While vacA-induced T and B-cell responses are detectable, they do not eliminate H. pylori infection. However, these immune responses indicate that vacA is immunogenic in humans and may be a candidate for vaccines. Therefore, the vacA gene also plays a role as a protective factor against H. pylori infection (86). Moyat and Velin (85) reported that a vacA-based vaccine showed significant protective effects on infected mice that received therapeutic intragastric immunization with a nontoxic recombinant version of vacA and the LT mutant LTK63. For the majority of vaccinated individuals, it effectively eliminates H. pylori infection and reduces the risk of reinfection (87). Preventive vaccination in animal models has also shown promise, with recombinant vacA and mucosal adjuvants providing protection (88).
The interaction between the cagA and vacA proteins significantly contributes to H. pylori-associated gastric cancer. Abdullah et al. (89) reported that the absence of vacA allows the host immune system to degrade cagA, preventing its accumulation in gastric epithelial cells. H. pylori infection increases the risk of gastric cancer, posing a major public health challenge. There is a strong link between cancer progression and the growth of other gastric malignancies, driven by inflammation, genotoxic factors, and genomic instability (90). This relationship is influenced by host genetics, environmental conditions, and H. pylori virulence genes such as oipA, vacA, and cagA (62). Understanding these pathways is crucial for developing effective treatments and preventing future infections. Recent advancements are improving our knowledge of H. pylori-related diseases and aiding the development of innovative therapies, including potential vaccines.
In all strains of H. pylori, a 150 kDa multimeric protein, referred to as neutrophil-activating protein (HP-NAP), has been identified (5, 91, 92). Research indicates that HP-NAP enhances the penetration and generation of oxygen radicals and the adhesion of neutrophils and monocytes to gastric endothelial cells while also increasing their motility (93). This activity contributes to long-term inflammatory conditions in the gastrointestinal epithelium. HP-NAP activation leads to increased interleukin-12 (IL-12) production, triggering a T helper 1 (Th1) immune response (94). Immunodominant antigens associated with the H. pylori G27 strain were identified through two-dimensional gel electrophoresis in a patient suffering from various gastric diseases, with this protein being significantly recognized in the serum of infected individuals (95). Furthermore, animals immunized with HP-NAP have demonstrated immunity against subsequent infections, indicating that this virulent gene may serve as a promising candidate for vaccine development. Owing to its high antigenicity, HP-NAP is frequently incorporated into vaccines aimed at preventing H. pylori infection (94). In addition to its application in vaccines, HP-NAP may also hold potential as an immunotherapeutic agent in cancer treatment, as its immunomodulatory properties enable dendritic cells to promote Th1 responses and enhance the immune responses of recipients (94).
OMPs of H. pylori are essential for physiological processes, assisting in material transport and host interactions (96, 97). They are promising targets for vaccines and medications (9, 98, 99). H. pylori has diverse OMPs, such as lipoproteins, porins, and adhesins, which are vital for survival and pathogenicity (27). OMP expression varies among strains, contributing to pathogenicity through adherence, invasion, and immune evasion (26, 96). Genome sequencing revealed that approximately 4% of H. pylori genetic material encodes OMPs, which are categorized into five gene families: Hop, Hor, Hom, Hof, and iron-regulated proteins (27, 100, 101). This section summarizes recent advancements in understanding well-characterized OMPs.
Blood group antigen-binding adhesin (babA) is part of the Helicobacter Hop family and plays a crucial role in H. pylori adhesion (102, 103). Currently, three genetic variants of the bab gene have been identified: babA1, babA2, and babB (104). Three genetic variants of the bab gene exist: babA1, babA2, and babB (27, 62, 102). The babA2 gene encodes a significant adhesin that binds to Lewis b (Le-b) blood group antigens, aiding colonization and bacterial density. Strains with babA1 do not express babA, whereas those with babA2 can be poor or significant producers of babA, affecting adhesion to Le-b antigens. Genomic analysis revealed that babA and babB are unrelated, with their expression levels varying geographically (62). The prevalence of the babA2 gene ranges from 44.0% in Portugal to 79.7% in Iran, with only 9.8% of Western strains lacking babA (105). Studies have linked H. pylori to diseases such as gastric cancer, resulting in increased babA expression in affected patients, suggesting its role in disease severity (27, 106, 107). These observations suggest that babA may play a role in the severity of disease outcomes associated with H. pylori infection (108). Additionally, the T4SS may facilitate cagA penetration through the gastrointestinal epithelium via the interaction between babA and Le-b (109). babA is a crucial factor in H. pylori infections and could be further investigated as a potential preventative treatment and vaccine candidate (102, 110, 111). Bai et al. (112) successfully isolated recombinant babA2 from the serum of patients infected with babA2-positive H. pylori, as well as from BALB/c mice infected with recombinant babA. This discovery suggests that babA2 could be a promising vaccine antigen because of its immunogenic properties.
The sialic acid-binding adhesin (sabA) gene in H. pylori is the second most prevalent OMP (27) and has two alleles: sabA (HopP or OMP17) and sabB (HopO or OMP16), both of which are part of the Hop protein family (113). sabA, which is smaller than babA at approximately 70 kDa (102), detects and binds to sialylated glycans, particularly sialyl Le-x antigens (114). As a sialic acid-binding adhesin, sabA interacts with host cell receptors. H. pylori strains often carry both sabA and sabB, indicating preferential expression of sabA during colonization (115, 116). sabA is increasingly recognized as crucial in gastrointestinal disease pathogenesis (117). H. pylori infection likely begins with babA binding to fucosylated antigens related to the ABO blood group and the Le-b antigen (102). Furthermore, sialyl-Le-X expression increases during the host inflammatory response, enhancing H. pylori adhesion to the gastric mucosa alongside sabA activity (102, 118, 119). Research in developed and developing nations has linked sabA generation to severe gastrointestinal diseases, gastric atrophy, and gastric cancer (120). Further investigations into sabA are urgently needed, particularly in developing countries. sabA also mimics selectin, activating neutrophils and producing reactive oxygen species (ROS), which prolong inflammation (121).
H. pylori colonizes the gastric mucosa more readily in the presence of gamma-glutamyl transpeptidase (GGT), which induces programmed cell death in gastric epithelial cells (5, 122, 123). GGT also impairs dendritic cell development and T-cell-mediated immunity, enhancing resistance to infection. Additionally, H. pylori transports GGT in outer membrane vesicles, increasing hydrogen peroxide and interleukin-8 (IL-8) production in gastric epithelial cells (26, 93, 124). Multiepitope vaccines contain antigenic epitopes from the virulence factors of H. pylori, such as the sabA and babA genes (102). Urrutia-Baca et al. (111) developed an oral vaccine with 11 epitopes linked to pathogenicity and colonization, including babA and sabA. Modeling studies suggest that this vaccine candidate will show antigenicity, nonallergenicity, and solubility, with an appropriate molecular weight. A study by AlEraky et al. (125) identified antigenic peptides from H. pylori for vaccine development via an in silico proteomic method. Four peptides—cagA1, cagA2, vacA, and sabA—were further investigated through reverse vaccinology. After immunization with these peptides and Freund’s adjuvant, BALB/C mice were orally challenged with H. pylori. sabA-vaccinated mice presented significantly higher IgG and IL-4 levels than did the adjuvant-only group. Histopathological evaluations revealed a protective immune response in the vaccinated groups, particularly with the sabA antigen. However, further in vitro and in vivo studies are needed to assess its efficacy before its use in humans (111).
Gastric cancer development is linked to the outer membrane proteins of H. pylori, particularly outer inflammatory protein A (oipA), which includes HopB, HopQ, and HopH (27, 126, 127). oipA, encoded by the HopH gene, is associated with gastric mucosa inflammation. Compared with oipA-negative strains, H. pylori strains that are oipA positive provoke a stronger inflammatory response (124), which is correlated with a greater risk of gastric ulcers and cancer (128). oipA is more prevalent in gastric biopsies from cancer patients than in those from uncomplicated gastroenteritis patients (124). It also induces B-cell lymphoma-2 (Bcl-2) family proteins, contributing to apoptosis (93, 129), and upregulates inflammatory cytokines such as IL-6, IL-8, and IL-1. Additionally, Sukri et al. (130) reported that gastric cancer influences T cell, B cell, and dendritic cell development and IL-10 release, increasing cancer risk. A meta-analysis by Liu et al. (131) revealed a strong link between the presence of oipA and the risk of peptic ulcer disease, especially in Western countries. Several studies have evaluated oipA as a potential vaccine against H. pylori infection (3, 132). Soudi et al. (133) tested recombinant oipA with propolis as an adjuvant in a mouse model at doses of 10 μg/mL and 40 μg/mL. They reported that oipA effectively induces IFN-γ production and enhances the cellular immune response, with propolis acting as a beneficial adjuvant. Another study reported the production of anti-oipA IgA antibodies in C57BL/6 mice (134).
H. pylori urease (ure), comprising 10 to 15% of a bacterium’s total protein, consists of 12 heterodimers formed by the ureA and ureB enzymes (135). This catalytic enzyme hydrolyzes urea into carbon dioxide and ammonia, which neutralizes excess stomach acid, inhibits neutrophil activity (136–138), promotes the production of toxic ammonia-derived compounds (139), and disrupts stomach epithelial cell interactions (140), fostering bacterial colonization. The peroxynitrite anion can harm bacteria, but carbon dioxide mitigates this effect, aiding colonization (93, 141). The unique surface structure of the urease complex allows H. pylori to interact with host immune elements, ensuring its indefinite colonization. Inhibiting urease function prevents H. pylori from thriving, providing therapeutic and preventive strategies against infection (135). In numerous studies, urease has been identified as a potential antigen candidate for vaccine production. In a laboratory model of H. pylori infection, Nasr-Esfahani et al. (142) demonstrated that the recombinant plasmid pcDNA3.1 (+)-ureA could induce an immune response in murine models. Furthermore, the vaccination of mice with a recombinant ureB vaccine, which incorporates plant polysaccharides as adjuvants, was found to confer immunity against H. pylori infection. This protective effect may be attributed to the enhancement of Th1/Th17 CD4+ T-cell activation and the promotion of gastrointestinal-specific secretory immunoglobulin A (143). Most vaccines that have advanced to the clinical trial phase include the urease antigen (37, 135, 144–146).
Catalase is a key enzyme that protects bacteria from hydrogen peroxide. In H. pylori, this tetrameric protein makes up approximately 1% of the total protein and has an isoelectric point of 9.0–9.3 (135, 147). It shields H. pylori from host-produced reactive oxygen species and helps bacteria evade macrophages (148, 149). Its role in various pathological processes contributes to inflammation, apoptosis, and tumor formation, with mutagenesis occurring in the cytoplasm, periplasm, and occasionally on the surface (150). H. pylori catalase is one of its most highly expressed proteins and shows greater resistance to cyanide and amino triazole suppression than do catalases from other bacteria (124). Recent studies have provided detailed characterizations of immunodominant Th1 epitopes associated with catalase (151). Through the production of IFN-γ, seven novel catalase epitopes have been identified as potent inducers of a robust Th1 immune response (152). The LHUC vaccine is a multivalent epitope vaccine that incorporates the adjuvant heat-labile enterotoxin B subunit, along with five B-cell epitopes and three Th-cell epitopes (HpaA, ureB, and catalase), designed to create an effective multivalent epitope vaccine against H. pylori (135). Following the administration of the LHUC vaccine to mice, serum analysis revealed the presence of antibodies specific to the antigen, accompanied by a significant increase in the production of IFN-γ, IL-4, and IL-17 by lymphocytes. Studies have demonstrated that LHUC is highly effective in preventing H. pylori infections in murine models (153).
The increase in antibiotic resistance underscores the need to explore virulence factors as alternative vaccine targets for H. pylori infections. Understanding these factors is crucial for advancing vaccine development and effective therapies. While various vaccine types, such as vector-based, whole-cell, and subunit vaccines, have shown efficacy in animal models, few have reached human clinical trials. This section reviews recent H. pylori vaccine developments and the role of virulence genes in potential vaccine formulations.
Inactivated whole-cell vaccines for H. pylori are created by disrupting the bacteria with ultrasonic waves and inactivating them with formalin (29). These vaccines reduce H. pylori proliferation and elicit strong immune responses in the gastric mucosa. Kotloff et al. (154) suggested that the administration of an oral H. pylori whole-cell vaccine can effectively stimulate both mucosal and systemic immune responses in humans. Murine experiments performed with whole-cell vaccines demonstrated that these vaccines can elicit a dose-dependent response, including the production of cross-reactive IgG, against H. pylori. The high-dose Hp 26695 whole-cell vaccine group presented reduced bacterial colonization in challenge experiments with SS1 (155). Oral vaccination is preferred for its ease of use and high adherence rates (156–159). However, it requires higher antigen dosages than intramuscular injections do, which may cause immunological tolerance. Researchers often use lower antigen doses with mucosal adjuvants to improve efficacy (160, 161), especially against H. pylori (29).
Aluminum adjuvants are essential in vaccine formulations, enhancing systemic immunity and promoting a Th2-type response (162, 163). Cholera toxin is a potent mucosal adjuvant (164) that activates a Th2 response (165) but poses toxicity risks (35). Cholera toxin B is a safer alternative (157, 166). Oral immunization with cholera toxin and bacterial antigens increased antibody levels in a germ-free mouse model of H. felis infection (167), and Lee et al. (168) reported that this combination was more effective than the H. felis antigen or adjuvant alone. Holmgren’s et al. (164) developed a novel adjuvant with mutant cholera toxins for H. pylori infections. A formalin-inactivated whole-cell Helicobacter vaccine increased serum IgG, mucosal IgA, IFN-γ, and IL-17 levels while reducing H. pylori colonization (169, 170). Improving vaccine delivery methods is vital for effective mucosal vaccination (171). Techniques such as liposomes, viral vectors, and attenuated bacterial vectors offer unique benefits. However, no commercially available vaccine exists for inactivated whole-cell H. pylori, and enhancing the immune response while ensuring safe delivery is challenging.
Antigenic subunit vaccines use purified pathogen components to trigger a strong immune response (172). They contain only antigenic elements, enhancing safety by removing live components (173). These vaccines are suitable for individuals with compromised immune systems (174) and have a complex manufacturing process that often requires booster doses, adjuvants, and significant time to determine optimal antigen combinations (175). The development of a subunit vaccine for H. pylori is particularly challenging and costly (29). Genetic engineering improves the purification and large-scale production of specific antigens, enhancing vaccine efficacy (176, 177). Key antigens for an H. pylori vaccine include urease, catalase, cagA, babA, vacA, and fliD (110, 178, 179). Urease was one of the first antigens recognized as beneficial for vaccine development (145, 180). Urease subunits A and B (ureA and ureB) are vital for colonization and are therapeutic targets (110, 181). While ureB is well studied as a vaccine candidate (145, 182, 183), research on ureA is limited. ureA activates urease via interactions with HSP60, which is crucial for protein balance (184). Murine studies suggest that ureA-specific CD4+ T cells provide protective immunity (185), and oral immunization with Bacillus subtilis spores expressing ureA has shown protective effects in trials (186).
Michetti et al. (180) demonstrated that an oral H. pylori urease vaccine, along with Escherichia coli heat-labile enterotoxin as an adjuvant, elevated anti-urease serum immunoglobulin A titers in twenty-six H. pylori-infected participants. Zhong et al. (187) developed a recombinant fusion protein, ureA-ureB-NAP, as a preventive vaccine, showing improved protection in mice compared with a bacterial lysate vaccine. Skakic et al. (179) examined protein nanocapsules with the A subunit of H. pylori-ureA and reported that TiterMax with SC/MS nanocapsules significantly reduced gastric H. pylori infections in murine models, indicating effective immune response stimulation. The role of H. pylori oipA in promoting the proinflammatory cytokine IL-8 and its effect on inflammation has been studied (188). While oipA is vital for host protection against H. pylori (134), selecting an effective adjuvant is crucial for a strong immune response. Many vaccines still use oil emulsions or aluminum salts, but research into alternatives continues due to the adverse effects of oil-based adjuvants (189). Natural adjuvants such as propolis have shown promise in animal models (190) and may enhance vaccination strategies. A 2021 study revealed that propolis combined with recombinant oipA increased IFN-γ production and strengthened the immune response (133).
The H. pylori-NAP gene is a key virulence factor and a potential target for gastrointestinal disorder treatments (5, 191). Its immunological properties also suggest potential for cancer treatment and H. pylori infections (94). Guo et al. (192) studied multivalent epitope-based vaccines in Mongolian gerbils in 2017 and 2019 (193). The first vaccine combines H. pylori-NAP with various antigens, while the second includes epitopes from cagA, vacA, and urease, both of which effectively reduce bacterial colonization and gastritis. Liu et al. (194) developed a multivalent vaccine with H. pylori-NAP, a mucosal adjuvant, and ureA and ureB, which stimulate mucosal IgA and specific humoral immune responses. Chen et al. (195) reported that cyclic guanosine monophosphate-adenosine monophosphate provided protection against H. pylori at lower dosages via intranasal immunization. The immunogenic properties of H. pylori-NAP are promising for vaccine development (94), but mixed results indicate that more research is needed to understand H. pylori evasion of host antibody responses.
cagA is a potential antigen that triggers immune responses in clinical trials (196, 197). Like other H. pylori proteins, such as ureA, babA, sabA, and oipA, cagA is an effective vaccine antigen that inhibits H. pylori proliferation when combined with suitable adjuvants (198, 199). In animal models, vaccination with recombinant antigens such as cagA, vacA, and NAP has shown protective effects, enhancing T-cell memory and cell-mediated immune responses. A study of healthy volunteers vaccinated with a cagA-positive strain revealed limited protection after exposure (197). Paydarnia et al. (75) revealed that recombinant cagA and H. pylori LPS stimulate host immunity in murine models. The recombinant cagA protein with the CpG adjuvant maintained its antigenicity and induced strong Th1-biased immune responses. Other antigens, such as HpaA, FlaA, SOD, and Hsp, may also enhance the immune response to H. pylori infection.
Researchers are exploring ways to increase DNA vaccine efficacy through adjuvants, cytokines, chemokines, CpG incorporation, and electroporation (200). Nonmethylated CpG motifs in plasmid backbones increase vaccination success by stimulating immune responses in B cells and natural killer cells (201). The protective antigen of H. pylori is encoded by cDNA in an expression vector that is absorbed by host cells, activating the immune response (29). Kumari et al. (202) stated that the absence of cagW disrupts pilus formation, preventing cagA from entering the bacterial membrane. babA is crucial for H. pylori adherence to gastric epithelial cells and may worsen gastritis by promoting cagA translation (107), making it a promising vaccination target (29, 203, 204). Xue et al. (205) developed plasmid vaccines targeting cagA, vacA, and babA in albino mice, which showed potential anticancer properties for gastric cancer immunotherapy. Figure 3 presents recent advancements in H. pylori DNA vaccines, including cagW, cagA-vacA-babA (205), and flaA (206). However, challenges such as degradation by deoxyribonucleases, delivery issues, and limited immune responses in some primates hinder DNA vaccine efficacy. Future advancements are expected to improve clinical trial prospects for these vaccines.
Figure 3. H. pylori DNA vaccines, including cagW, cagA-vacA-babA, and flaA, have been developed to enhance immunological responses.
Both viruses and bacteria can harm human health. Modifying virulence-associated genes while preserving infectious properties at the mucosal barrier is one strategy to reduce pathogen effects. H. pylori-derived immunogens can stimulate an immune response when delivered to antigen-presenting cells. Vector-based vaccines can mimic natural infections and sustain immune activation, making live vectors a promising alternative to mucosal adjuvants in recombinant subunit vaccines (169). This section reviews vector vaccines for preventing H. pylori infection (see Figure 4).
Figure 4. Some vector (carrier) vaccines used in murine models in trials to protect against H. pylori.
Intramuscular administration of a replication-defective adenovirus vector can reduce H. felis infection spread (207); however, uncertainties remain about the immune response from poliovirus replicons with the ureB component (208). Research shows that intranasal or oral Salmonella enterica serovar Typhimurium-vectored vaccines effectively prevent H. pylori colonization (209–211). This method allows for needle-free vaccination and promotes the use of recombinant antigens and DNA vaccine vectors (212, 213). While effective in animal models (214, 215), these vaccines have shown limited efficacy in humans. There is a need for Salmonella strains that produce protective antigens for comprehensive host immunization. Ghasemi et al. (216) reported that a vaccine with hpaA, H. pylori-NAP, ureA, and ureB conferred protection against H. pylori SS1 in 70% of tested mice.
Nie et al. (217) developed the intranasal influenza A virus (IAV) vector vaccine IAV-NapA, which uses two live attenuated influenza viruses to express the H. pylori NapA A subunit. The strains WSN-NapA and PR8-NapA exhibited significant attenuation and strong immunogenicity in mice, inducing robust Th1 and Th17 immune responses, as well as antigen-specific humoral and mucosal responses. The vaccine effectively reduced H. pylori colonization and inflammation, suggesting its potential as a dual-purpose vaccine for influenza and H. pylori. Lactic acid bacteria serve as effective carriers for oral vaccines (218, 219), enhancing immunization due to their durability and resistance to gastric acid (220). Furthermore, the recombinant measles virus (MV) vaccine expressing the H. pylori HspA antigen has demonstrated significant cancer efficacy and strong immunogenicity (221), making MV a promising platform for vaccine development.
Vaccines using Lactococcus lactis (L. lactis) increase mucosal immunogenicity (222), and modified strains can trigger immune responses at both the mucosal and systemic levels (223). Zhang et al. (224) studied a recombinant L. lactis LL-plSAM-WAE vaccine in BALB/c mice, which expressed the SAM-WAE antigen. This vaccine induced antibodies against H. pylori virulence factors and activated T cells, indicating strong potential for H. pylori vaccine development in clinical trials. Bacillus subtilis (B. subtilis) spores are effective vectors for mucosal vaccination (186, 225, 226). Oral or nasal administration enhances mucosal immunity, particularly Th1 responses, and increases secretory immunoglobulin A (sIgA) production (227). These spores can germinate under suitable conditions and endure extreme environments, including gastric secretions (228). Thus, animals and humans are likely exposed to low levels of Bacillus (229). In a study by Katsande et al. (186), mice were given genetically modified B. subtilis spores expressing the H. pylori antigens ureA and ureB, leading to specific mucosal responses, increased fecal sIgA levels, and increased antibody production.
Saccharomyces cerevisiae (S. cerevisiae) is a promising candidate for immune studies against various pathogens (230). Cen et al. (231) used S. cerevisiae to express recombinant ureB and vacA, creating an oral vaccine that significantly reduced H. pylori infection in mice. Attenuated Listeria monocytogenes (L. monocytogenes) is an effective vector for enhancing antibody production against H. pylori (232). It stimulates immune responses, especially from CD4+ and CD8+ T lymphocytes (233), and is widely used as a vaccine carrier in immunotherapy for tumors and infectious diseases (234). The hly gene promoter (PhIy), encoding listeriolysin O, is often used for developing vaccine strains for foreign antigen production (235), but Ding et al. (236) reported that it is inadequate for optimal antigen expression and strong immune responses. Live attenuated bacteria must survive acidic environments such as macrophage phagosomes and the gastric cancer microenvironment (237). A key limitation is the lack of a well-characterized promoter array for regulating foreign antigen transcription in L. monocytogenes. Ma et al. (238) identified 21 potential promoters from L. monocytogenes cultured at pH 7.4 and 5.5, with seven intrinsic promoters outperforming Phelp and five constitutive promoters showing high activity in the production of ureB, an antigen against H. pylori.
The effectiveness and immunogenicity of human vaccines differ from those of animal vaccines, creating translational challenges. No H. pylori vaccine candidates have advanced to human clinical trials, with many discontinued (135). Factors affecting H. pylori include immune evasion, genetic variations, its intracellular presence, and limited funding. T-regulatory cells are essential for a nonharmful relationship with H. pylori, but their responses may inhibit Th1 and Th17 functions (239), potentially promoting H. pylori proliferation. Genetic variations account for approximately 30% of the heterogeneity among H. pylori isolates (240), leading to significant genotype variability among individuals and within the same patient. The intracellular presence of H. pylori is also a critical factor to consider (241). H. pylori is commonly found in the stomach layers, epithelium, and immune cells of patients with gastric disorders (242) and often outnumbers and prolongs the lifespan of immune cells (243). Mouse models are inadequate for studying H. pylori (244), and Amalia et al. (245) highlighted the need for better models, as murine immune responses do not reflect human responses (240). Domestic monkeys share similarities with humans regarding H. pylori, suggesting that larger mammals may be more suitable for vaccine studies (246). Despite the prevalence of H. pylori, major biopharmaceutical companies are reluctant to develop vaccines because of challenges in assessing immune protection, genetic diversity, and host responses, complicating manufacturing.
Research involving vulnerable populations requires a careful approach to prevent exploitation, especially in vaccine trials. This is critical in developing countries, where socioeconomic disadvantages can lead to coercion. Past ethical violations emphasize the need for sound design and ethical guidance. Inclusive design and ethical considerations are vital for advancing scientific knowledge while preserving human dignity. Informed consent is essential for ethical research and human rights, particularly for vulnerable populations, but it poses challenges in vaccine trials. These principles must be adapted to local conditions and cultural sensitivities.
H. pylori vaccines are vital for therapy and prevention, enhancing mucosal immunity and mixed immune responses. The main types are toxin-based and OMP vaccines, with OMP vaccines being safer and more effective because of their lower antigen and adjuvant requirements, potentially reducing antibiotic resistance in aging populations in developing countries. However, viable OMP candidates are limited. Prophylactic vaccination can eradicate bacteria, but challenges exist in identifying suitable antigens and developing effective delivery systems. Toxin vaccines also improve safety but face selection challenges. Innovations such as virus-like particle and DNA vaccines offer new research avenues, although significant hurdles remain before their clinical use. Vaccine development is crucial for preventing H. pylori infections and establishing herd immunity. Various vaccine types, including whole-cell, subunit, and epitope vaccines, have been developed, but their efficacy is uncertain. Researchers are also exploring DNA and live vector vaccines, which may activate antibodies or T cells for protective immunity, although the mechanisms are not well understood. Current vaccines do not meet ideal criteria, such as inducing systemic Th1-biased immune responses and stimulating mucosal immunity in gastric tissue. Future efforts should prioritize optimal protection with minimal side effects.
The development of H. pylori vaccination strategies requires further preclinical testing in suitable animal models before human trials. A reliable method for assessing vaccine efficacy would aid progress. Research has shown that mice immunized with combinations of five adjuvant H. pylori proteins achieve better bacterial clearance. Prime-boost vaccination strategies involving various H. pylori antigens have shown significant protective effects in murine models. However, an effective H. pylori vaccine has not yet been developed because of limited knowledge of antigens and immune responses. It remains uncertain whether vaccines alone can eradicate H. pylori or if antibiotics are needed. The link between H. pylori infection and upper gastrointestinal disorders has renewed interest in vaccine development, which could help prevent chronic gastritis, peptic ulcers, gastric cancer, and MALT lymphoma.
H. pylori is implicated in a range of gastric disorders, including peptic ulcers, stomach cancer, lymphoma, and gastritis. The pathogenicity of this bacterium is modulated by several virulence factors, including cagA, vacA, ure, HP-NAP, catalase, and OMPs. A comprehensive understanding of these factors is essential for the effective treatment and management of associated conditions. The prevalence of OMPs varies geographically, and all H. pylori strains possess these proteins, rendering them potential targets for vaccine development. Current clinical trials are exploring recombinant vaccines that incorporate various antigens, including the cagA, vacA, ure, babA, sabA, oipA, and porin proteins. Recent advancements in antigen detection have opened new avenues for vaccine development, emphasizing innovative delivery methods and adjuvants. Nevertheless, the majority of research remains in preliminary stages. Continued efforts are critical to the development of a safe and effective vaccine against H. pylori, which necessitates the identification of immune-suppressing mechanisms, the selection of effective antigens and adjuvants, and the enhancement of public awareness, particularly in developing countries.
AE: Data curation, Formal analysis, Methodology, Supervision, Validation, Visualization, Writing – original draft, Writing – review & editing. EM: Conceptualization, Data curation, Formal analysis, Methodology, Validation, Visualization, Writing – original draft, Writing – review & editing. AlA: Conceptualization, Data curation, Writing – original draft, Writing – review & editing. WS: Writing – original draft, Writing – review & editing. YA: Writing – original draft, Writing – review & editing. SA: Writing – original draft, Writing – review & editing. TAl: Writing – original draft, Writing – review & editing. NA: Writing – original draft, Writing – review & editing. TAL: Writing – original draft, Writing – review & editing. AdA: Writing – original draft, Writing – review & editing. FA: Writing – original draft, Writing – review & editing. AhA: Writing – original draft, Writing – review & editing. AD: Formal analysis, Supervision, Visualization, Writing – original draft, Writing – review & editing. AMA: Writing – original draft, Writing – review & editing. SNA: Writing – original draft, Writing – review & editing. AbA: Validation, Formal analysis, Writing – review & editing. AA-O: Writing – original draft, Writing – review & editing.
The author(s) declare that financial support was received for the research, authorship, and/or publication of this article. The researchers would like to thank the Deanship of Graduate Studies and Scientific Research at Qassim University for financial support (QU-APC-2024).
The authors declare that the research was conducted in the absence of any commercial or financial relationships that could be construed as a potential conflict of interest.
The authors declare that no Gen AI was used in the creation of this manuscript.
All claims expressed in this article are solely those of the authors and do not necessarily represent those of their affiliated organizations, or those of the publisher, the editors and the reviewers. Any product that may be evaluated in this article, or claim that may be made by its manufacturer, is not guaranteed or endorsed by the publisher.
1. Warren, JR, and Marshall, B. Unidentified curved bacilli on gastric epithelium in active chronic gastritis. Lancet. (1983) 321:1273–5. doi: 10.1016/S0140-6736(83)92719-8
2. Bordin, D, and Livzan, M. History of chronic gastritis: how our perceptions have changed. World J Gastroenterol. (2024) 30:1851–8. doi: 10.3748/wjg.v30.i13.1851
3. Wroblewski, LE, Peek, RM Jr, and Wilson, KT. Helicobacter pylori and gastric cancer: factors that modulate disease risk. Clin Microbiol Rev. (2010) 23:713–39. doi: 10.1128/CMR.00011-10
4. O’Connor, A, O’Morain, CA, and Ford, AC. Population screening and treatment of Helicobacter pylori infection. Nat Rev Gastroenterol Hepatol. (2017) 14:230–40. doi: 10.1038/nrgastro.2016.195
5. Reyes, VE. Helicobacter pylori and its role in gastric cancer. Microorganisms. (2023) 11:1312. doi: 10.3390/microorganisms11051312
6. Hooi, JK, Lai, WY, Ng, WK, Suen, MM, Underwood, FE, Tanyingoh, D, et al. Global prevalence of Helicobacter pylori infection: systematic review and meta-analysis. Gastroenterology. (2017) 153:420–9. doi: 10.1053/j.gastro.2017.04.022
7. Malfertheiner, P, Camargo, MC, El-Omar, E, Liou, J-M, Peek, R, Schulz, C, et al. Helicobacter pylori infection. Nat Rev Dis Primers. (2023) 9:19. doi: 10.1038/s41572-023-00431-8
8. Kotilea, K, Bontems, P, and Touati, E. Epidemiology, diagnosis and risk factors for Helicobacter pylori infection In: Helicobacter pylori in human diseases. Advances in experimental medicine and biology. Cham: Springer (2019). 17–33.
9. Sedarat, Z, and Taylor-Robinson, AW. Helicobacter pylori outer membrane proteins and virulence factors: potential targets for novel therapies and vaccines. Pathogens. (2024) 13:392. doi: 10.3390/pathogens13050392
10. Kusters, JG, van Vliet, AH, and Kuipers, EJ. Pathogenesis of Helicobacter pylori infection. Clin Microbiol Rev. (2006) 19:449–90. doi: 10.1128/CMR.00054-05
11. Reshetnyak, VI, Burmistrov, AI, and Maev, IV. Helicobacter pylori: commensal, symbiont or pathogen? World J Gastroenterol. (2021) 27:545–60. doi: 10.3748/wjg.v27.i7.545
12. Ansari, S, and Yamaoka, Y. Survival of Helicobacter pylori in gastric acidic territory. Helicobacter. (2017) 22:e12386. doi: 10.1111/hel.12386
13. Cheok, YY, Lee, CYQ, Cheong, HC, Vadivelu, J, Looi, CY, Abdullah, S, et al. An overview of Helicobacter pylori survival tactics in the hostile human stomach environment. Microorganisms. (2021) 9:2502. doi: 10.3390/microorganisms9122502
14. Engelsberger, V, Gerhard, M, and Mejías-Luque, R. Effects of Helicobacter pylori infection on intestinal microbiota, immunity and colorectal cancer risk. Front Cell Infect Microbiol. (2024) 14:1339750. doi: 10.3389/fcimb.2024.1339750
15. Campuzano-Maya, G. Cure of alopecia areata after eradication of Helicobacter pylori: a new association? World J Gastroenterol. (2011) 17:3165–70. doi: 10.3748/wjg.v17.i26.3165
16. Gravina, AG, Zagari, RM, De Musis, C, Romano, L, Loguercio, C, and Romano, M. Helicobacter pylori and extragastric diseases: a review. World J Gastroenterol. (2018) 24:3204–21. doi: 10.3748/wjg.v24.i29.3204
17. Elbehiry, A, Marzouk, E, Aldubaib, M, Abalkhail, A, Anagrayyah, S, Anajirih, N, et al. Helicobacter pylori infection: current status and future prospects on diagnostic, therapeutic and control challenges. Antibiotics. (2023) 12:191. doi: 10.3390/antibiotics12020191
18. Ghazanfar, H, Javed, N, Reina, R, Thartori, O, Ghazanfar, A, and Patel, H. Advances in diagnostic modalities for Helicobacter pylori infection. Life. (2024) 14:1170. doi: 10.3390/life14091170
19. Chmiela, M, Karwowska, Z, Gonciarz, W, Allushi, B, and Stączek, P. Host pathogen interactions in Helicobacter pylori related gastric cancer. World J Gastroenterol. (2017) 23:1521–40. doi: 10.3748/wjg.v23.i9.1521
20. Muzaheed,. Helicobacter pylori oncogenicity: mechanism, prevention, and risk factors. Sci World J. (2020) 2020:1–10. doi: 10.1155/2020/3018326
21. Piscione, M, Mazzone, M, Di Marcantonio, MC, Muraro, R, and Mincione, G. Eradication of Helicobacter pylori and gastric cancer: a controversial relationship. Front Microbiol. (2021) 12:630852. doi: 10.3389/fmicb.2021.630852
22. Ansari, S, and Yamaoka, Y. Helicobacter pylori virulence factors exploiting gastric colonization and its pathogenicity. Toxins. (2019) 11:677. doi: 10.3390/toxins11110677
23. Jarzab, M, Posselt, G, Meisner-Kober, N, and Wessler, S. Helicobacter pylori-derived outer membrane vesicles (OMVs): role in bacterial pathogenesis? Microorganisms. (2020) 8:1328. doi: 10.3390/microorganisms8091328
24. Lazar, V, Oprea, E, and Ditu, L. Resistance, tolerance, virulence and bacterial pathogen fitness—current state and envisioned solutions for the near future. Pathogens. (2023) 12:746. doi: 10.3390/pathogens12050746
25. Marcus, EA, and Scott, DR. Gastric colonization by H. pylori In: Helicobacter pylori. Singapore: Springer (2024). 25–37.
26. Chmiela, M, Walczak, N, and Rudnicka, K. Helicobacter pylori outer membrane vesicles involvement in the infection development and Helicobacter pylori-related diseases. J Biomed Sci. (2018) 25:1–11. doi: 10.1186/s12929-018-0480-y
27. Xu, C, Soyfoo, DM, Wu, Y, and Xu, S. Virulence of Helicobacter pylori outer membrane proteins: an updated review. Eur J Clin Microbiol Infect Dis. (2020) 39:1821–30. doi: 10.1007/s10096-020-03948-y
28. Alm, RA, Bina, J, Andrews, BM, Doig, P, Hancock, RE, and Trust, TJ. Comparative genomics of Helicobacter pylori: analysis of the outer membrane protein families. Infect Immun. (2000) 68:4155–68. doi: 10.1128/IAI.68.7.4155-4168.2000
29. Zhang, Y, Li, X, Shan, B, Zhang, H, and Zhao, L. Perspectives from recent advances of Helicobacter pylori vaccines research. Helicobacter. (2022) 27:e12926. doi: 10.1111/hel.12926
30. Seo, GS. Safety and immunogenicity of an intramuscular Helicobacter pylori vaccine in noninfected volunteers: a phase I study. Korean J Gastroenterol. (2009) 53:62–3.
31. Tshibangu-Kabamba, E, and Yamaoka, Y. Helicobacter pylori infection and antibiotic resistance—from biology to clinical implications. Nat Rev Gastroenterol Hepatol. (2021) 18:613–29. doi: 10.1038/s41575-021-00449-x
32. Ho, JJ, Navarro, M, Sawyer, K, Elfanagely, Y, and Moss, SF. Helicobacter pylori antibiotic resistance in the United States between 2011 and 2021: a systematic review and meta-analysis. Am J Gastroenterol. (2022) 117:1221–30. doi: 10.14309/ajg.0000000000001828
33. Megraud, F, Bruyndonckx, R, Coenen, S, Wittkop, L, Huang, T-D, Hoebeke, M, et al. Helicobacter pylori resistance to antibiotics in Europe in 2018 and its relationship to antibiotic consumption in the community. Gut. (2021) 70:1815–22. doi: 10.1136/gutjnl-2021-324032
34. Jaka, H, Rhee, JA, Östlundh, L, Smart, L, Peck, R, Mueller, A, et al. The magnitude of antibiotic resistance to Helicobacter pylori in Africa and identified mutations which confer resistance to antibiotics: systematic review and meta-analysis. BMC Infect Dis. (2018) 18:1–10. doi: 10.1186/s12879-018-3099-4
35. Anderl, F, and Gerhard, M. Helicobacter pylori vaccination: is there a path to protection? World J Gastroenterol. (2014) 20:11939–49. doi: 10.3748/wjg.v20.i34.11939
36. Das, S, Banerjee, S, Halder, P, Dutta, S, Mukhopadhyay, A, and Koley, H. A review for the prevention and management of Helicobacter pylori induced gastritis through development of novel vaccine candidates. Microbe. (2024) 4:100114. doi: 10.1016/j.microb.2024.100114
37. Ikuse, T, Blanchard, TG, and Czinn, SJ. Inflammation, immunity, and vaccine development for the gastric pathogen Helicobacter pylori In: Molecular mechanisms of inflammation: induction, resolution and escape by Helicobacter pylori. Cham: Springer (2019). 1–19.
38. Zhang, Z, Cui, M, Ji, X, Su, G, Zhang, YX, and Du, L. Candidate antigens and the development of Helicobacter pylori vaccines. Helicobacter. (2024) 29:e13128. doi: 10.1111/hel.13128
39. Hernández-Hernández, LC, Lazcano-Ponce, EC, López-Vidal, Y, and Aguilar-Gutiérrez, GR. Relevance of Helicobacter pylori virulence factors for vaccine development. Salud Publica Mex. (2009) 51:S447–54. doi: 10.1590/S0036-36342009000900010
40. Sousa, C, Ferreira, R, Azevedo, NF, Oleastro, M, Azeredo, J, Figueiredo, C, et al. Helicobacter pylori infection: from standard to alternative treatment strategies. Crit Rev Microbiol. (2022) 48:376–96. doi: 10.1080/1040841X.2021.1975643
41. Kronsteiner, B, Bassaganya-Riera, J, Philipson, C, Viladomiu, M, Carbo, A, Abedi, V, et al. Systems-wide analyses of mucosal immune responses to Helicobacter pylori at the interface between pathogenicity and symbiosis. Gut Microbes. (2016) 7:3–21. doi: 10.1080/19490976.2015.1116673
42. Abadi, ATB. Strategies used by Helicobacter pylori to establish persistent infection. World J Gastroenterol. (2017) 23:2870–82. doi: 10.3748/wjg.v23.i16.2870
43. White, JR, Winter, JA, and Robinson, K. Differential inflammatory response to Helicobacter pylori infection: etiology and clinical outcomes. J Inflamm Res. (2015) 8:137–47. doi: 10.2147/JIR.S64888
44. Bravo, D, Hoare, A, Soto, C, Valenzuela, MA, and Quest, AF. Helicobacter pylori in human health and disease: mechanisms for local gastric and systemic effects. World J Gastroenterol. (2018) 24:3071–89. doi: 10.3748/wjg.v24.i28.3071
45. Pachathundikandi, SK, Blaser, N, and Backert, S. Mechanisms of inflammasome signaling, microRNA induction and resolution of inflammation by Helicobacter pylori In: Molecular mechanisms of inflammation: induction, resolution and escape by Helicobacter pylori. Cham: Springer (2019). 267–302.
46. Charitos, IA, D’Agostino, D, Topi, S, and Bottalico, L. 40 years of Helicobacter pylori: a revolution in biomedical thought. Gastroenterol Insights. (2021) 12:111–35. doi: 10.3390/gastroent12020011
47. Scott Algood, HM, Gallo-Romero, J, Wilson, KT, Peek, RM Jr, and Cover, TL. Host response to Helicobacter pylori infection before initiation of the adaptive immune response. FEMS Immunol Med Microbiol. (2007) 51:577–86. doi: 10.1111/j.1574-695X.2007.00338.x
48. Khamri, W, Walker, MM, Clark, P, Atherton, JC, Thursz, MR, Bamford, KB, et al. Helicobacter pylori stimulates dendritic cells to induce interleukin-17 expression from CD4+ T lymphocytes. Infect Immun. (2010) 78:845–53. doi: 10.1128/IAI.00524-09
49. Kabir, S. The role of interleukin-17 in the Helicobacter pylori induced infection and immunity. Helicobacter. (2011) 16:1–8. doi: 10.1111/j.1523-5378.2010.00812.x
50. Blanchard, TG, Yu, F, Hsieh, C-L, and Redline, RW. Severe inflammation and reduced bacteria load in murine helicobacter infection caused by lack of phagocyte oxidase activity. J Infect Dis. (2003) 187:1609–15. doi: 10.1086/374780
51. Ismail, HF, Fick, P, Zhang, J, Lynch, RG, and Berg, DJ. Depletion of neutrophils in IL-10−/− mice delays clearance of gastric Helicobacter infection and decreases the Th1 immune response to Helicobacter. J Immunol. (2003) 170:3782–9. doi: 10.4049/jimmunol.170.7.3782
52. DeLyria, ES, Redline, RW, and Blanchard, TG. Vaccination of mice against H. pylori induces a strong Th-17 response and immunity that is neutrophil dependent. Gastroenterology. (2009) 136:247–56. doi: 10.1053/j.gastro.2008.09.017
53. Su, B, Ceponis, PJ, Lebel, S, Huynh, H, and Sherman, PM. Helicobacter pylori activates Toll-like receptor 4 expression in gastrointestinal epithelial cells. Infect Immun. (2003) 71:3496–502. doi: 10.1128/IAI.71.6.3496-3502.2003
54. O’Keeffe, J, and Moran, AP. Conventional, regulatory, and unconventional T cells in the immunologic response to Helicobacter pylori. Helicobacter. (2008) 13:1–19. doi: 10.1111/j.1523-5378.2008.00559.x
55. Walduck, AK, and Raghavan, S. Immunity and vaccine development against Helicobacter pylori In: Helicobacter pylori in human diseases: advances in microbiology, infectious diseases and public health. Cham: Springer (2019). 257–75.
56. Bergman, MP, and D’Elios, MM. Cytotoxic T cells in H. pylori-related gastric autoimmunity and gastric lymphoma. Biomed Res Int. (2010) 2010:104918. doi: 10.1155/2010/104918
57. Croitoru, G-A, Niculescu, A-G, Epistatu, D, Mihaiescu, DE, Antohi, AM, Grumezescu, AM, et al. Nanostructured drug delivery systems in immunotherapy: an updated overview of nanotechnology-based therapeutic innovations. Appl Sci. (2024) 14:8948. doi: 10.3390/app14198948
58. Reyes, VE, and Peniche, AG. Helicobacter pylori deregulates T and B-cell signaling to trigger immune evasion In: Molecular mechanisms of inflammation: induction, resolution and escape by Helicobacter pylori. Cham: Springer (2019). 229–65.
59. Kumar, S, Patel, GK, and Ghoshal, UC. Helicobacter pylori-induced inflammation: possible factors modulating the risk of gastric cancer. Pathogens. (2021) 10:1099. doi: 10.3390/pathogens10091099
60. Miftahussurur, M, Yamaoka, Y, and Graham, DY. Helicobacter pylori as an oncogenic pathogen, revisited. Expert Rev Mol Med. (2017) 19:e4. doi: 10.1017/erm.2017.4
61. Yamaoka, Y, Kodama, T, Kashima, K, Graham, DY, and Sepulveda, AR. Variants of the 3′ region of the cagA gene in Helicobacter pylori isolates from patients with different H. pylori-associated diseases. J Clin Microbiol. (1998) 36:2258–63. doi: 10.1128/JCM.36.8.2258-2263.1998
62. Šterbenc, A, Jarc, E, Poljak, M, and Homan, M. Helicobacter pylori virulence genes. World J Gastroenterol. (2019) 25:4870–84. doi: 10.3748/wjg.v25.i33.4870
63. Cover, TL, Lacy, DB, and Ohi, MD. The Helicobacter pylori cag type IV secretion system. Trends Microbiol. (2020) 28:682–95. doi: 10.1016/j.tim.2020.02.004
64. Bergé, C, Waksman, G, and Terradot, L. Structural and molecular biology of type IV secretion systems In: Type IV secretion in gram-negative and gram-positive bacteria. Cham: Springer (2017). 31–60.
65. Kim, JM. H. pylori virulence factors: toxins (CagA, VacA, DupA, OipA, IceA) In: Helicobacter pylori. Singapore: Springer (2024). 89–102.
66. Selbach, M, Moese, S, Hauck, CR, Meyer, TF, and Backert, S. Src is the kinase of the Helicobacter pylori CagA protein in vitro and in vivo. J Biol Chem. (2002) 277:6775–8. doi: 10.1074/jbc.C100754200
67. Stein, M, Bagnoli, F, Halenbeck, R, Rappuoli, R, Fantl, WJ, and Covacci, A. c-Src/Lyn kinases activate Helicobacter pylori CagA through tyrosine phosphorylation of the EPIYA motifs. Mol Microbiol. (2002) 43:971–80. doi: 10.1046/j.1365-2958.2002.02781.x
68. Begum, N, Hassan, SMA, Padmanabhan, P, Ahmed, SI, Bardia, A, and Khan, AA. Prevalence and comprehensive insights into molecular mechanisms in Helicobacter pylori pathogenesis. J Med Allied Sci. (2021) 11:105. doi: 10.5455/jmas.49688
69. Jafarzadeh, A, Larussa, T, Nemati, M, and Jalapour, S. T-cell subsets play an important role in the determination of the clinical outcome of Helicobacter pylori infection. Microb Pathog. (2018) 116:227–36. doi: 10.1016/j.micpath.2018.01.040
70. Li, N, Tang, B, Jia, Y-p, Zhu, P, Zhuang, Y, Fang, Y, et al. Helicobacter pylori CagA protein negatively regulates autophagy and promotes inflammatory response via c-Met-PI3K/Akt-mTOR signaling pathway. Front Cell Infect Microbiol. (2017) 7:417. doi: 10.3389/fcimb.2017.00417
71. Jang, S, Hansen, LM, Su, H, Solnick, JV, and Cha, J-H. Host immune response mediates changes in cagA copy number and virulence potential of Helicobacter pylori. Gut Microbes. (2022) 14:2044721. doi: 10.1080/19490976.2022.2044721
72. Bhat, N, Gaensbauer, J, Peek, RM, Bloch, K, Tham, K-T, Blaser, MJ, et al. Local and systemic immune and inflammatory responses to Helicobacter pylori strains. Clin Vaccine Immunol. (2005) 12:1393–400. doi: 10.1128/CDLI.12.12.1393-1400.2005
73. Ismail, HF, Zhang, J, Lynch, RG, Wang, Y, and Berg, DJ. Role for complement in development of Helicobacter-induced gastritis in interleukin-10-deficient mice. Infect Immun. (2003) 71:7140–8. doi: 10.1128/IAI.71.12.7140-7148.2003
74. Larussa, T, Leone, I, Suraci, E, Imeneo, M, and Luzza, F. Helicobacter pylori and T helper cells: mechanisms of immune escape and tolerance. J Immunol Res. (2015) 2015:981328. doi: 10.1155/2015/981328
75. Paydarnia, N, Mansoori, B, Esmaeili, D, Kazemi, T, Aghapour, M, Hajiasgharzadeh, K, et al. Helicobacter pylori recombinant CagA regulates Th1/Th2 balance in a BALB/c murine model. Adv Pharm Bull. (2020) 10:264–70. doi: 10.34172/apb.2020.031
76. Cover, TL, and Blanke, SR. Helicobacter pylori VacA, a paradigm for toxin multifunctionality. Nat Rev Microbiol. (2005) 3:320–32. doi: 10.1038/nrmicro1095
77. Rieder, G, Fischer, W, and Haas, R. Interaction of Helicobacter pylori with host cells: function of secreted and translocated molecules. Curr Opin Microbiol. (2005) 8:67–73. doi: 10.1016/j.mib.2004.12.004
78. Foegeding, NJ, Caston, RR, McClain, MS, Ohi, MD, and Cover, TL. An overview of Helicobacter pylori VacA toxin biology. Toxins. (2016) 8:173. doi: 10.3390/toxins8060173
79. Jarzab, M, and Skorko-Glonek, J. There are no insurmountable barriers: passage of the Helicobacter pylori VacA toxin from bacterial cytoplasm to eukaryotic cell organelle. Membranes. (2023) 14:11. doi: 10.3390/membranes14010011
80. Yahiro, K, Satoh, M, Nakano, M, Hisatsune, J, Isomoto, H, Sap, J, et al. Low-density lipoprotein receptor-related protein-1 (LRP1) mediates autophagy and apoptosis caused by Helicobacter pylori VacA. J Biol Chem. (2012) 287:31104–15. doi: 10.1074/jbc.M112.387498
81. Chauhan, N, Tay, ACY, Marshall, BJ, and Jain, U. Helicobacter pylori VacA, a distinct toxin exerts diverse functionalities in numerous cells: an overview. Helicobacter. (2019) 24:e12544. doi: 10.1111/hel.12544
82. Ahmed, S, and Belayneh, YM. Helicobacter pylori and duodenal ulcer: systematic review of controversies in causation. Clin Exp Gastroenterol. (2019) 12:441–7. doi: 10.2147/CEG.S228203
83. Perez-Perez, GI, Peek, RM Jr, Atherton, JC, Blaser, MJ, and Cover, TL. Detection of anti-VacA antibody responses in serum and gastric juice samples using type s1/m1 and s2/m2 Helicobacter pylori VacA antigens. Clin Diagn Lab Immunol. (1999) 6:489–93. doi: 10.1128/CDLI.6.4.489-493.1999
84. D’Elios, MM, Manghetti, M, De Carli, M, Costa, F, Baldari, CT, Burroni, D, et al. T helper 1 effector cells specific for Helicobacter pylori in the gastric antrum of patients with peptic ulcer disease. J Immunol. (1997) 158:962–7.
85. Moyat, M, and Velin, D. Use of VacA as a vaccine antigen. Toxins. (2016) 8:181. doi: 10.3390/toxins8060181
86. Manetti, R, Massari, P, Burroni, D, de Bernard, M, Marchini, A, Olivieri, R, et al. Helicobacter pylori cytotoxin: importance of native conformation for induction of neutralizing antibodies. Infect Immun. (1995) 63:4476–80. doi: 10.1128/iai.63.11.4476-4480.1995
87. Ghiara, P, Rossi, M, Marchetti, M, Di Tommaso, A, Vindigni, C, Ciampolini, F, et al. Therapeutic intragastric vaccination against Helicobacter pylori in mice eradicates an otherwise chronic infection and confers protection against reinfection. Infect Immun. (1997) 65:4996–5002. doi: 10.1128/iai.65.12.4996-5002.1997
88. Marchetti, M, Rossi, M, Giannelli, V, Giuliani, MM, Pizza, M, Censini, S, et al. Protection against Helicobacter pylori infection in mice by intragastric vaccination with H. pylori antigens is achieved using a nontoxic mutant of E. coli heat-labile enterotoxin (LT) as adjuvant. Vaccine. (1998) 16:33–7. doi: 10.1016/S0264-410X(97)00153-9
89. Abdullah, M, Greenfield, LK, Bronte-Tinkew, D, Capurro, MI, Rizzuti, D, and Jones, NL. VacA promotes CagA accumulation in gastric epithelial cells during Helicobacter pylori infection. Sci Rep. (2019) 9:38. doi: 10.1038/s41598-018-37095-4
90. Ali, A, and AlHussaini, KI. Helicobacter pylori: a contemporary perspective on pathogenesis, diagnosis and treatment strategies. Microorganisms. (2024) 12:222. doi: 10.3390/microorganisms12010222
91. Zanotti, G, and Cendron, L. Structural and functional aspects of the Helicobacter pylori secretome. World J Gastroenterol. (2014) 20:1402–23. doi: 10.3748/wjg.v20.i6.1402
92. Pero, R, Coretti, L, Nigro, E, Lembo, F, Laneri, S, Lombardo, B, et al. β-defensins in the fight against Helicobacter pylori. Molecules. (2017) 22:424. doi: 10.3390/molecules22030424
93. Toh, JW, and Wilson, RB. Pathways of gastric carcinogenesis, Helicobacter pylori virulence and interactions with antioxidant systems, vitamin C and phytochemicals. Int J Mol Sci. (2020) 21:6451. doi: 10.3390/ijms21176451
94. Fu, H-W, and Lai, Y-C. The role of Helicobacter pylori neutrophil-activating protein in the pathogenesis of H. pylori and beyond: from a virulence factor to therapeutic targets and therapeutic agents. Int J Mol Sci. (2022) 24:91. doi: 10.3390/ijms24010091
95. Kimmel, B, Bosserhoff, A, Frank, R, Gross, R, Goebel, W, and Beier, D. Identification of immunodominant antigens from Helicobacter pylori and evaluation of their reactivities with sera from patients with different gastroduodenal pathologies. Infect Immun. (2000) 68:915–20. doi: 10.1128/IAI.68.2.915-920.2000
96. Sharndama, HC, and Mba, IE. Helicobacter pylori: an up-to-date overview on the virulence and pathogenesis mechanisms. Braz J Microbiol. (2022) 53:33–50. doi: 10.1007/s42770-021-00675-0
97. Jan, AT. Outer membrane vesicles (OMVs) of gram-negative bacteria: a perspective update. Front Microbiol. (2017) 8:1053. doi: 10.3389/fmicb.2017.01053
98. Waskito, LA, Salama, NR, and Yamaoka, Y. Pathogenesis of Helicobacter pylori infection. Helicobacter. (2018) 23:e12516. doi: 10.1111/hel.12516
99. Pasala, C, Chilamakuri, CSR, Katari, SK, Nalamolu, RM, Bitla, AR, and Umamaheswari, A. An in silico study: novel targets for potential drug and vaccine design against drug resistant H. pylori. Microb Pathog. (2018) 122:156–61. doi: 10.1016/j.micpath.2018.05.037
100. Bauwens, E, Joosten, M, Taganna, J, Rossi, M, Debraekeleer, A, Tay, A, et al. In silico proteomic and phylogenetic analysis of the outer membrane protein repertoire of gastric Helicobacter species. Sci Rep. (2018) 8:15453. doi: 10.1038/s41598-018-32476-1
101. Joosten, M. Proteomic and phylogenetic analysis of the outer membrane protein repertoire of gastric Helicobacter species In: The zoonotic pathogen Helicobacter heilmannii from feline origin: aspects of virulence and gastric colonization. Merelbeke: Ghent University (2017). 149.
102. Doohan, D, Rezkitha, YAA, Waskito, LA, Yamaoka, Y, and Miftahussurur, M. Helicobacter pylori BabA–SabA key roles in the adherence phase: the synergic mechanism for successful colonization and disease development. Toxins. (2021) 13:485. doi: 10.3390/toxins13070485
103. Ong, L-L, and Lin, C-H. Adhesion, infection, and therapeutic treatment of Helicobacter pylori: a review on current aspects and future promise. Discov Appl Sci. (2024) 6:323. doi: 10.1007/s42452-024-05923-0
104. Moonens, K, Gideonsson, P, Subedi, S, Bugaytsova, J, Romaõ, E, Mendez, M, et al. Structural insights into polymorphic ABO glycan binding by Helicobacter pylori. Cell Host Microbe. (2016) 19:55–66. doi: 10.1016/j.chom.2015.12.004
105. Fujimoto, S, Ojo, OO, Arnqvist, A, Wu, JY, Odenbreit, S, Haas, R, et al. Helicobacter pylori BabA expression, gastric mucosal injury, and clinical outcome. Clin Gastroenterol Hepatol. (2007) 5:49–58. doi: 10.1016/j.cgh.2006.09.015
106. Shirvani, JS, Salehi, M, Majd, AR, Sadeghi, F, Ferdosi-Shahandashti, E, Khafri, S, et al. Expression assessment of the Helicobacter pylori babA and sabA genes in patients with peptic ulcer, duodenal ulcer and gastric cancer. Int J Mol Cell Med. (2023) 12:211. doi: 10.22088/IJMCM.BUMS.12.2.211
107. Bugaytsova, JA, Björnham, O, Chernov, YA, Gideonsson, P, Henriksson, S, Mendez, M, et al. Helicobacter pylori adapts to chronic infection and gastric disease via pH-responsive BabA-mediated adherence. Cell Host Microbe. (2017) 21:376–89. doi: 10.1016/j.chom.2017.02.013
108. Chang, W-L, Yeh, Y-C, and Sheu, B-S. The impacts of H. pylori virulence factors on the development of gastroduodenal diseases. J Biomed Sci. (2018) 25:1–9. doi: 10.1186/s12929-018-0466-9
109. Ishijima, N, Suzuki, M, Ashida, H, Ichikawa, Y, Kanegae, Y, Saito, I, et al. BabA-mediated adherence is a potentiator of the Helicobacter pylori type IV secretion system activity. J Biol Chem. (2011) 286:25256–64. doi: 10.1074/jbc.M111.233601
110. Keikha, M, Eslami, M, Yousefi, B, Ghasemian, A, and Karbalaei, M. Potential antigen candidates for subunit vaccine development against Helicobacter pylori infection. J Cell Physiol. (2019) 234:21460–70. doi: 10.1002/jcp.28870
111. Urrutia-Baca, VH, Gomez-Flores, R, De La Garza-Ramos, MA, Tamez-Guerra, P, Lucio-Sauceda, DG, and Rodríguez-Padilla, MC. Immunoinformatics approach to design a novel epitope-based oral vaccine against Helicobacter pylori. J Comput Biol. (2019) 26:1177–90. doi: 10.1089/cmb.2019.0062
112. Bai, Y, Zhang, Y-L, Chen, Y, Jin, J-F, Zhang, Z-S, and Zhou, D-Y. Cloning and expression and immunogenicity of Helicobacter pylori BabA2 gene. World J Gastroenterol. (2004) 10:2560–2. doi: 10.3748/wjg.v10.i17.2560
113. Bonsor, DA, and Sundberg, EJ. Roles of adhesion to epithelial cells in gastric colonization by Helicobacter pylori In: Helicobacter pylori in human diseases: advances in microbiology, infectious diseases and public health. Cham: Springer (2019). 57–75.
114. Kim, A, Servetas, SL, Kang, J, Kim, J, Jang, S, Cha, HJ, et al. Helicobacter pylori bab paralog distribution and association with cagA, vacA, and homA/B genotypes in American and South Korean clinical isolates. PLoS One. (2015) 10:e0137078. doi: 10.1371/journal.pone.0137078
115. O’Brien, V, Jackson, L, Frick, J, Rodriguez Martinez, A, Jones, D, Johnston, C, et al. Helicobacter pylori chronic infection selects for effective colonizers of metaplastic glands. mBio. (2023) 14:e03116–22. doi: 10.1128/mbio.03116-22
116. Åberg, A, Gideonsson, P, Bhat, A, Ghosh, P, and Arnqvist, A. Molecular insights into the fine-tuning of pH-dependent ArsR-mediated regulation of the SabA adhesin in Helicobacter pylori. Nucleic Acids Res. (2024) 52:5572–95. doi: 10.1093/nar/gkae188
117. Arnqvist, A. Roles of the BabA and the SabA Adhesins in gastroduodenal diseases In: Helicobacter pylori research. Tokyo: Springer (2016). 143–63.
118. Yamaoka, Y. Increasing evidence of the role of Helicobacter pylori SabA in the pathogenesis of gastroduodenal disease. J Infect Dev Ctries. (2008) 2:174–81. doi: 10.3855/jidc.259
119. Lindén, SK, Wickström, C, Lindell, G, Gilshenan, K, and Carlstedt, I. Four modes of adhesion are used during Helicobacter pylori binding to human mucins in the oral and gastric niches. Helicobacter. (2008) 13:81–93. doi: 10.1111/j.1523-5378.2008.00587.x
120. Jaroenlapnopparat, A, Bhatia, K, and Coban, S. Inflammation and gastric cancer. Diseases. (2022) 10:35. doi: 10.3390/diseases10030035
121. Unemo, M, Aspholm-Hurtig, M, Ilver, D, Jr, B, Borén, T, Danielsson, D, et al. The sialic acid binding SabA adhesin of Helicobacter pylori is essential for nonopsonic activation of human neutrophils. J Biol Chem. (2005) 280:15390–7. doi: 10.1074/jbc.M412725200
122. Ricci, V, Giannouli, M, Romano, M, and Zarrilli, R. Helicobacter pylori gamma-glutamyl transpeptidase and its pathogenic role. World J Gastroenterol. (2014) 20:630–8. doi: 10.3748/wjg.v20.i3.630
123. de Brito, BB, da Silva, FAF, Soares, AS, Pereira, VA, Santos, MLC, Sampaio, MM, et al. Pathogenesis and clinical management of Helicobacter pylori gastric infection. World J Gastroenterol. (2019) 25:5578–89. doi: 10.3748/wjg.v25.i37.5578
124. Baj, J, Forma, A, Sitarz, M, Portincasa, P, Garruti, G, Krasowska, D, et al. Helicobacter pylori virulence factors—mechanisms of bacterial pathogenicity in the gastric microenvironment. Cells. (2020) 10:27. doi: 10.3390/cells10010027
125. AlEraky, DM, Abuohashish, HM, Bugshan, AS, Abdelsalam, MM, AlHawaj, HA, AlKhamis, TT, et al. Potential antigenic candidates for the development of peptide-based vaccines to induce immunization against Helicobacter pylori infection in BALB/c mice. Int J Mol Sci. (2022) 23:12824. doi: 10.3390/ijms232112824
126. Cover, TL. Helicobacter pylori diversity and gastric cancer risk. mBio. (2016) 7:e01869. doi: 10.1128/mBio.01869-15
127. Braga, LLBC, Batista, MHR, de Azevedo, OGR, da Silva Costa, KC, Gomes, AD, Rocha, GA, et al. oipA “on” status of Helicobacter pylori is associated with gastric cancer in north-eastern Brazil. BMC Cancer. (2019) 19:1–7. doi: 10.1186/s12885-018-5249-x
128. Zhang, Z, Liu, J, Wang, Y, Zhang, L, Zhou, T, Huang, Y, et al. Toll-like receptor 4 signaling mediates gastritis and gastric cancer. Curr Mol Med. [ahead of print] (2024). doi: 10.2174/0115665240276139231206071742
129. He, Y, Wang, C, Zhang, X, Lu, X, Xing, J, Lv, J, et al. Sustained exposure to Helicobacter pylori lysate inhibits apoptosis and autophagy of gastric epithelial cells. Front Oncol. (2020) 10:581364. doi: 10.3389/fonc.2020.581364
130. Sukri, A, Hanafiah, A, and Kosai, NR. The roles of immune cells in gastric cancer: anticancer or pro-cancer? Cancers. (2022) 14:3922. doi: 10.3390/cancers14163922
131. Liu, J, He, C, Chen, M, Wang, Z, Xing, C, and Yuan, Y. Association of presence/absence and on/off patterns of Helicobacter pylori oipA gene with peptic ulcer disease and gastric cancer risks: a meta-analysis. BMC Infect Dis. (2013) 13:555. doi: 10.1186/1471-2334-13-555
132. Dossumbekova, A, Prinz, C, Gerhard, M, Brenner, L, Backert, S, Kusters, J, et al. Helicobacter pylori outer membrane proteins and gastric inflammation. Gut. (2006) 55:1360–1. doi: 10.1136/gut.2005.083014
133. Soudi, H, Falsafi, T, Mahboubi, M, and Gharavi, S. Evaluation of Helicobacter pylori OipA protein as a vaccine candidate and propolis as an adjuvant in C57BL/6 mice. Iran J Basic Med Sci. (2021) 24:1220–30. doi: 10.22038/ijbms.2021.56232.12579
134. Mahboubi, M, Falsafi, T, Sadeghizadeh, M, and Mahjoub, F. The role of outer inflammatory protein a (OipA) in vaccination of theC57BL/6 mouse model infected by Helicobacter pylori. Turk J Med Sci. (2017) 47:326–33. doi: 10.3906/sag-1505-108
135. Li, S, Zhao, W, Xia, L, Kong, L, and Yang, L. How long will it take to launch an effective Helicobacter pylori vaccine for humans? Infect Drug Resist. (2023) 16:3787–805. doi: 10.2147/IDR.S412361
136. Kappaun, K, Piovesan, AR, Carlini, CR, and Ligabue-Braun, R. Ureases: historical aspects, catalytic, and noncatalytic properties–a review. J Adv Res. (2018) 13:3–17. doi: 10.1016/j.jare.2018.05.010
137. Loharch, S, and Berlicki, Ł. Rational development of bacterial ureases inhibitors. Chem Rec. (2022) 22:e202200026. doi: 10.1002/tcr.202200026
138. da Silva, RMA. Evaluation of the antimicrobial potential of natural extracts on Helicobacter Pylori. Portugal: Universidade de Coimbra (2020).
139. Suzuki, M, Miura, S, Suematsu, M, Fukumura, D, Kurose, I, Suzuki, H, et al. Helicobacter pylori-associated ammonia production enhances neutrophil-dependent gastric mucosal cell injury. Am J Physiol. (1992) 263:G719–25. doi: 10.1152/ajpgi.1992.263.5.G719
140. Wroblewski, LE, Shen, L, Ogden, S, Romero-Gallo, J, Lapierre, LA, Israel, DA, et al. Helicobacter pylori dysregulation of gastric epithelial tight junctions by urease-mediated myosin II activation. Gastroenterology. (2009) 136:236–46. doi: 10.1053/j.gastro.2008.10.011
141. Handa, O, Naito, Y, and Yoshikawa, T. Redox biology and gastric carcinogenesis: the role of Helicobacter pylori. Redox Rep. (2011) 16:1–7. doi: 10.1179/174329211X12968219310756
142. Nasr-Esfahani, M, Doosti, A, and Sazegar, H. Evaluation of the immune response against Helicobacter pylori in infused BALB/c mice by pcDNA3.1(+)- ureA. Folia Med. (2020) 62:37–45. doi: 10.3897/folmed.62.e47932
143. Liu, P-F, Wang, Y, Ulrich, RG, Simmons, CW, VanderGheynst, JS, Gallo, RL, et al. Leaf-encapsulated vaccines: agroinfiltration and transient expression of the antigen staphylococcal endotoxin B in radish leaves. J Immunol Res. (2018) 2018:1–9. doi: 10.1155/2018/3710961
144. Sutton, P, and Boag, JM. Status of vaccine research and development for Helicobacter pylori. Vaccine. (2019) 37:7295–9. doi: 10.1016/j.vaccine.2018.01.001
145. Dos Santos, VI, Cordeiro Santos, ML, Santos Marques, H, de Souza, L, Goncalves, V, Bittencourt de Brito, B, et al. Vaccine development against Helicobacter pylori: from ideal antigens to the current landscape. Expert Rev Vaccines. (2021) 20:989–99. doi: 10.1080/14760584.2021.1945450
146. Blanchard, TG, and Czinn, SJ. Identification of Helicobacter pylori and the evolution of an efficacious childhood vaccine to protect against gastritis and peptic ulcer disease. Pediatr Res. (2017) 81:170–6. doi: 10.1038/pr.2016.199
147. Basu, M, Czinn, SJ, and Blanchard, TG. Absence of catalase reduces long-term survival of Helicobacter pylori in macrophage phagosomes. Helicobacter. (2004) 9:211–6. doi: 10.1111/j.1083-4389.2004.00226.x
148. Wu, S, Chen, Y, Chen, Z, Wei, F, Zhou, Q, Li, P, et al. Reactive oxygen species and gastric carcinogenesis: the complex interaction between Helicobacter pylori and host. Helicobacter. (2023) 28:e13024. doi: 10.1111/hel.13024
149. Gupta, N, Maurya, S, Verma, H, and Verma, VK. Unraveling the factors and mechanism involved in persistence: host-pathogen interactions in Helicobacter pylori. J Cell Biochem. (2019) 120:18572–87. doi: 10.1002/jcb.29201
150. Bauer, G, Bereswill, S, Aichele, P, and Glocker, E. Helicobacter pylori protects oncogenically transformed cells from reactive oxygen species-mediated intercellular induction of apoptosis. Carcinogenesis. (2014) 35:1582–91. doi: 10.1093/carcin/bgu074
151. Makvandi, M, Neissi, N, Tarighi, P, Makvandi, K, and Rashidi, N. Evaluation of the genes expression related to the immune system in response to Helicobacter pylori catalase epitopes. Mol Gen Microbiol Virol. (2020) 35:47–51. doi: 10.3103/S089141682001005X
152. Mohammadzadeh, R, Soleimanpour, S, Pishdadian, A, and Farsiani, H. Designing and development of epitope-based vaccines against Helicobacter pylori. Crit Rev Microbiol. (2022) 48:489–512. doi: 10.1080/1040841X.2021.1979934
153. Xie, W, Zhao, W, Zou, Z, Kong, L, and Yang, L. Oral multivalent epitope vaccine, based on UreB, HpaA, CAT, and LTB, for prevention and treatment of Helicobacter pylori infection in C57BL/6 mice. Helicobacter. (2021) 26:e12807. doi: 10.1111/hel.12807
154. Kotloff, KL, Sztein, MB, Wasserman, SS, Losonsky, GA, DiLorenzo, SC, and Walker, RI. Safety and immunogenicity of oral inactivated whole-cell Helicobacter pylori vaccine with adjuvant among volunteers with or without subclinical infection. Infect Immun. (2001) 69:3581–90. doi: 10.1128/IAI.69.6.3581-3590.2001
155. Suganya, K, Kumar, AP, Sekar, B, and Sundaran, B. Protection of mice against gastric colonization of Helicobacter pylori by therapeutic immunization with systemic whole cell inactivated vaccines. Biologicals. (2017) 45:39–46. doi: 10.1016/j.biologicals.2016.10.002
156. Ramirez, JEV, Sharpe, LA, and Peppas, NA. Current state and challenges in developing oral vaccines. Adv Drug Deliv Rev. (2017) 114:116–31. doi: 10.1016/j.addr.2017.04.008
157. Zhang, Y, Li, M, Du, G, Chen, X, and Sun, X. Advanced oral vaccine delivery strategies for improving the immunity. Adv Drug Deliv Rev. (2021) 177:113928. doi: 10.1016/j.addr.2021.113928
158. Kwong, KW-Y, Xin, Y, Lai, NC-Y, Sung, JC-C, Wu, K-C, Hamied, YK, et al. Oral vaccines: a better future of immunization. Vaccine. (2023) 11:1232. doi: 10.3390/vaccines11071232
159. Trincado, V, Gala, RP, and Morales, JO. Buccal and sublingual vaccines: a review on oral mucosal immunization and delivery systems. Vaccine. (2021) 9:1177. doi: 10.3390/vaccines9101177
160. Anggraeni, R, Ana, ID, and Wihadmadyatami, H. Development of mucosal vaccine delivery: an overview on the mucosal vaccines and their adjuvants. Clin Exp Vaccine Res. (2022) 11:235–48. doi: 10.7774/cevr.2022.11.3.235
161. Correa, VA, Portilho, AI, and De Gaspari, E. Vaccines, adjuvants and key factors for mucosal immune response. Immunology. (2022) 167:124–38. doi: 10.1111/imm.13526
162. Facciolà, A, Visalli, G, Laganà, A, and Di Pietro, A. An overview of vaccine adjuvants: current evidence and future perspectives. Vaccine. (2022) 10:819. doi: 10.3390/vaccines10050819
163. Friedrich, V, and Gerhard, M. Vaccination against Helicobacter pylori—an approach for cancer prevention? Mol Asp Med. (2023) 92:101183. doi: 10.1016/j.mam.2023.101183
164. Holmgren, J, Nordqvist, S, Blomquist, M, Jeverstam, F, Lebens, M, and Raghavan, S. Preclinical immunogenicity and protective efficacy of an oral Helicobacter pylori inactivated whole cell vaccine and multiple mutant cholera toxin: a novel and nontoxic mucosal adjuvant. Vaccine. (2018) 36:6223–30. doi: 10.1016/j.vaccine.2018.07.073
165. Sanchez, J, and Holmgren, J. Cholera toxin structure, gene regulation and pathophysiological and immunological aspects. Cell Mol Life Sci. (2008) 65:1347–60. doi: 10.1007/s00018-008-7496-5
166. Orosco, FL, and Espiritu, LM. Navigating the landscape of adjuvants for subunit vaccines: recent advances and future perspectives. Int J App Pharm. (2024) 10:18–32. doi: 10.22159/ijap.2024v16i1.49563
167. Czinn, SJ, Cai, A, and Nedrud, JG. Protection of germ-free mice from infection by Helicobacter felis after active oral or passive IgA immunization. Vaccine. (1993) 11:637–42. doi: 10.1016/0264-410X(93)90309-L
168. Lee, A, and Chen, M. Successful immunization against gastric infection with Helicobacter species: use of a cholera toxin B-subunit-whole-cell vaccine. Infect Immun. (1994) 62:3594–7. doi: 10.1128/iai.62.8.3594-3597.1994
169. Yunle, K, Tong, W, Jiyang, L, and Guojun, W. Advances in Helicobacter pylori vaccine research: from candidate antigens to adjuvants—a review. Helicobacter. (2024) 29:e13034. doi: 10.1111/hel.13034
170. Akhtar, M, Nizam, NN, Basher, SR, Hossain, L, Akter, S, Bhuiyan, TR, et al. dmLT adjuvant enhances cytokine responses to T-cell stimuli, whole cell vaccine antigens and lipopolysaccharide in both adults and infants. Front Immunol. (2021) 12:654872. doi: 10.3389/fimmu.2021.654872
171. Firdaus, FZ, Skwarczynski, M, and Toth, I. Developments in vaccine adjuvants In: Vaccine design. Methods in molecular biology. New York, NY: Humana (2022). 145–78.
172. Zuber, PL, Gruber, M, Kaslow, DC, Chen, RT, Giersing, BK, and Friede, MH. Evolving pharmacovigilance requirements with novel vaccines and vaccine components. BMJ Glob Health. (2021) 6:e003403. doi: 10.1136/bmjgh-2020-003403
173. Van der Weken, H, Cox, E, and Devriendt, B. Advances in oral subunit vaccine design. Vaccine. (2020) 9:1. doi: 10.3390/vaccines9010001
174. Foged, C. Subunit vaccines of the future: the need for safe, customized and optimized particulate delivery systems. Ther Deliv. (2011) 2:1057–77. doi: 10.4155/tde.11.68
175. Bobbala, S, and Hook, S. Is there an optimal formulation and delivery strategy for subunit vaccines? Pharm Res. (2016) 33:2078–97. doi: 10.1007/s11095-016-1979-0
176. Cid, R, and Bolívar, J. Platforms for production of protein-based vaccines: from classical to next-generation strategies. Biomol Ther. (2021) 11:1072. doi: 10.3390/biom11081072
177. Thakur, R, and Shankar, J. Strategies for gene expression in prokaryotic and eukaryotic system In: Metabolic engineering for bioactive compounds. Singapore: Springer (2017). 223–47.
178. Apolonio, JS, da Silva Júnior, RT, Cuzzuol, BR, Araújo, GRL, Marques, HS, de Souza, BI, et al. Syndemic aspects between COVID-19 pandemic and social inequalities. World J Methodol. (2022) 12:350–64. doi: 10.5662/wjm.v12.i5.350
179. Skakic, I, Francis, JE, Dekiwadia, C, Aibinu, I, Huq, M, Taki, AC, et al. An evaluation of urease a subunit nanocapsules as a vaccine in a mouse model of Helicobacter pylori infection. Vaccine. (2023) 11:1652. doi: 10.3390/vaccines11111652
180. Michetti, P, Kreiss, C, Kotloff, KL, Porta, N, Blanco, JL, Bachmann, D, et al. Oral immunization with urease and Escherichia coli heat-labile enterotoxin is safe and immunogenic in Helicobacter pylori—infected adults. Gastroenterology. (1999) 116:804–12. doi: 10.1016/S0016-5085(99)70063-6
181. Xiao, S, Shang, K, Li, W, and Wang, X. An efficient biosensor based on the synergistic catalysis of Helicobacter pylori urease b subunit and nanoplatinum for urease inhibitors screening and antagonistic mechanism analyzing. Sensors Actuators B Chem. (2022) 355:131284. doi: 10.1016/j.snb.2021.131284
182. Banga Ndzouboukou, JL, Lei, Q, Ullah, N, Zhang, Y, Hao, L, and Fan, X. Helicobacter pylori adhesins: HpaA a potential antigen in experimental vaccines for H. pylori. Helicobacter. (2021) 26:e12758. doi: 10.1111/hel.12758
183. Yang, J, Dai, L-x, Pan, X, Wang, H, Li, B, Zhu, J, et al. Protection against Helicobacter pylori infection in BALB/c mice by oral administration of multiepitope vaccine of CTB-UreI-UreB. Pathog Dis. (2015) 73:ftv026. doi: 10.1093/femspd/ftv026
184. Zhao, H, Wu, Y, Xu, Z, Ma, R, Ding, Y, Bai, X, et al. Mechanistic insight into the interaction between Helicobacter pylori urease subunit α and its molecular chaperone Hsp60. Front Microbiol. (2019) 10:153. doi: 10.3389/fmicb.2019.00153
185. Lucas, B, Bumann, D, Walduck, A, Koesling, J, Develioglu, L, Meyer, TF, et al. Adoptive transfer of CD4+ T cells specific for subunit A of Helicobacter pylori urease reduces H. pylori stomach colonization in mice in the absence of interleukin-4 (IL-4)/IL-13 receptor signaling. Infect Immun. (2001) 69:1714–21. doi: 10.1128/IAI.69.3.1714-1721.2001
186. Katsande, PM, Nguyen, VD, Nguyen, TLP, Nguyen, TKC, Mills, G, Bailey, DM, et al. Prophylactic immunization to Helicobacter pylori infection using spore vectored vaccines. Helicobacter. (2023) 28:e12997. doi: 10.1111/hel.12997
187. Zhong, Y, Chen, J, Liu, Y, Zhang, Y, Tang, C, Wang, X, et al. Oral immunization of BALB/c mice with recombinant Helicobacter pylori antigens and double mutant heat-labile toxin (dmLT) induces prophylactic protective immunity against H. pylori infection. Microb Pathog. (2020) 145:104229. doi: 10.1016/j.micpath.2020.104229
188. Tabassam, FH, Graham, DY, and Yamaoka, Y. OipA plays a role in Helicobacter pylori-induced focal adhesion kinase activation and cytoskeletal re-organization. Cell Microbiol. (2008) 10:1008–20. doi: 10.1111/j.1462-5822.2007.01104.x
189. Shi, S, Zhu, H, Xia, X, Liang, Z, Ma, X, and Sun, B. Vaccine adjuvants: understanding the structure and mechanism of adjuvanticity. Vaccine. (2019) 37:3167–78. doi: 10.1016/j.vaccine.2019.04.055
190. Fischer, G, Paulino, N, Marcucci, MC, Siedler, BS, Munhoz, LS, Finger, PF, et al. Green propolis phenolic compounds act as vaccine adjuvants, improving humoral and cellular responses in mice inoculated with inactivated vaccines. Mem Inst Oswaldo Cruz. (2010) 105:908–13. doi: 10.1590/S0074-02762010000700012
191. Codolo, G, Coletta, S, D’Elios, MM, and de Bernard, M. HP-NAP of Helicobacter pylori: the power of the immunomodulation. Front Immunol. (2022) 13:944139. doi: 10.3389/fimmu.2022.944139
192. Guo, L, Yang, H, Tang, F, Yin, R, Liu, H, Gong, X, et al. Oral immunization with a multivalent epitope-based vaccine, based on NAP, urease, HSP60, and HpaA, provides therapeutic effect on H. pylori infection in Mongolian gerbils. Front Cell Infect Microbiol. (2017) 7:349. doi: 10.3389/fcimb.2017.00349
193. Guo, L, Hong, D, Wang, S, Zhang, F, Tang, F, Wu, T, et al. Therapeutic protection against H. pylori infection in Mongolian gerbils by oral immunization with a tetravalent epitope-based vaccine with polysaccharide adjuvant. Front Immunol. (2019) 10:1185. doi: 10.3389/fimmu.2019.01185
194. Liu, M, Zhong, Y, Chen, J, Liu, Y, Tang, C, Wang, X, et al. Oral immunization of mice with a multivalent therapeutic subunit vaccine protects against Helicobacter pylori infection. Vaccine. (2020) 38:3031–41. doi: 10.1016/j.vaccine.2020.02.036
195. Chen, J, Zhong, Y, Liu, Y, Tang, C, Zhang, Y, Wei, B, et al. Parenteral immunization with a cyclic guanosine monophosphate-adenosine monophosphate (cGAMP) adjuvanted Helicobacter pylori vaccine induces protective immunity against H. pylori infection in mice. Hum Vaccin Immunother. (2020) 16:2849–54. doi: 10.1080/21645515.2020.1744364
196. Malfertheiner, P, Schultze, V, Rosenkranz, B, Kaufmann, SH, Ulrichs, T, Novicki, D, et al. Safety and immunogenicity of an intramuscular Helicobacter pylori vaccine in noninfected volunteers: a phase I study. Gastroenterology. (2008) 135:787–95. doi: 10.1053/j.gastro.2008.05.054
197. Malfertheiner, P, Selgrad, M, Wex, T, Romi, B, Borgogni, E, Spensieri, F, et al. Efficacy, immunogenicity, and safety of a parenteral vaccine against Helicobacter pylori in healthy volunteers challenged with a cag-positive strain: a randomized, placebo-controlled phase 1/2 study. Lancet Gastroenterol Hepatol. (2018) 3:698–707. doi: 10.1016/S2468-1253(18)30125-0
198. Chen, J, Lin, L, Li, N, and She, F. Enhancement of Helicobacter pylori outer inflammatory protein DNA vaccine efficacy by co-delivery of interleukin-2 and B subunit heat-labile toxin gene encoded plasmids. Microbiol Immunol. (2012) 56:85–92. doi: 10.1111/j.1348-0421.2011.00409.x
199. Prinz, C, Hafsi, N, and Voland, P. Helicobacter pylori virulence factors and the host immune response: implications for therapeutic vaccination. Trends Microbiol. (2003) 11:134–8. doi: 10.1016/S0966-842X(03)00024-6
200. Yu, YZ, Li, N, Ma, Y, Wang, S, Yu, WY, and Sun, ZW. Three types of human C p G motifs differentially modulate and augment immunogenicity of nonviral and viral replicon DNA vaccines as built-in adjuvants. Eur J Immunol. (2013) 43:228–39. doi: 10.1002/eji.201242690
201. Liu, Y, Luo, X, Yang, C, Yu, S, and Xu, H. Three CpG oligodeoxynucleotide classes differentially enhance antigen-specific humoral and cellular immune responses in mice. Vaccine. (2011) 29:5778–84. doi: 10.1016/j.vaccine.2011.05.087
202. Kumari, R, Shariq, M, Sharma, S, Kumar, A, and Mukhopadhyay, G. CagW, a VirB6 homolog interacts with Cag-type IV secretion system substrate CagA in Helicobacter pylori. Biochem Biophys Res Commun. (2019) 515:712–8. doi: 10.1016/j.bbrc.2019.06.013
203. Dayhimi, V, Ziadlou, F, Nafian, S, and Nafian, F. An immunoinformatic approach to designing a multiepitope vaccine against Helicobacter pylori with the VacA toxin and BabA adhesion. Curr Proteomics. (2024) 21:97–112. doi: 10.2174/0115701646302487240524103934
204. Bugaytsova, JA, Piddubnyi, A, Tkachenko, I, Rakhimova, L, Edlund, JO, Thorell, K, et al. (2023) Vaccination with Helicobacter pylori attachment proteins protects against gastric cancer. bioRxiv. Available at: https://doi.org/10.1101/2023.05.25.542131. [Epub ahead of preprint]
205. Xue, L-J, Mao, X-B, Liu, X-B, Gao, H, Chen, Y-N, Dai, T-T, et al. Activation of CD3+ T cells by Helicobacter pylori DNA vaccines in potential immunotherapy of gastric carcinoma. Cancer Biol Ther. (2019) 20:866–76. doi: 10.1080/15384047.2019.1579957
206. Ansari, H, Tahmasebi-Birgani, M, and Bijanzadeh, M. DNA vaccine containing Flagellin A gene induces significant immune responses against Helicobacter pylori infection: an in vivo study. Iran J Basic Med Sci. (2021) 24:796–804. doi: 10.22038/ijbms.2021.54415.12227
207. Jiang, B, Jordana, M, Xing, Z, Smaill, F, Snider, DP, Borojevic, R, et al. Replication-defective adenovirus infection reduces Helicobacter felis colonization in the mouse in a gamma interferon-and interleukin-12-dependent manner. Infect Immun. (1999) 67:4539–44. doi: 10.1128/IAI.67.9.4539-4544.1999
208. Novak, MJ, Smythies, LE, McPherson, SA, Smith, PD, and Morrow, CD. Poliovirus replicons encoding the B subunit of Helicobacter pylori urease elicit a Th1 associated immune response. Vaccine. (1999) 17:2384–91. doi: 10.1016/S0264-410X(99)00035-3
209. InE, C-T, Hopkins, S, Bachmann, D, Saldinger, PF, Porta, N, Haas, R, et al. Mice are protected from Helicobacter pylori infection by nasal immunization with attenuated Salmonella typhimurium phoPc expressing urease A and B subunits. Infect Immun. (1998) 66:581–6. doi: 10.1128/IAI.66.2.581-586.1998
210. Gomez-Duarte, OG, Lucas, B, Yan, Z-X, Panthel, K, Haas, R, and Meyer, TF. Protection of mice against gastric colonization by Helicobacter pylori by single oral dose immunization with attenuated Salmonella typhimurium producing urease subunits A and B. Vaccine. (1998) 16:460–71. doi: 10.1016/S0264-410X(97)00247-8
211. Zhang, S, Walters, N, Cao, L, Robison, A, and Yang, X. Recombinant Salmonella vaccination technology and its application to human bacterial pathogens. Curr Pharm Biotechnol. (2013) 14:209–19. doi: 10.2174/1389201011314020011
212. Clark-Curtiss, JE, and Curtiss, R. Salmonella vaccines: conduits for protective antigens. J Immunol. (2018) 200:39–48. doi: 10.4049/jimmunol.1600608
213. Liu, K-Y, Shi, Y, Luo, P, Yu, S, Chen, L, Zhao, Z, et al. Therapeutic efficacy of oral immunization with attenuated Salmonella typhimurium expressing Helicobacter pylori CagA, VacA and UreB fusion proteins in mice model. Vaccine. (2011) 29:6679–85. doi: 10.1016/j.vaccine.2011.06.099
214. Bumann, D, Metzger, WG, Mansouri, E, Palme, O, Wendland, M, Hurwitz, R, et al. Safety and immunogenicity of live recombinant Salmonella enterica serovar Typhi Ty21a expressing urease A and B from Helicobacter pylori in human volunteers. Vaccine. (2001) 20:845–52. doi: 10.1016/S0264-410X(01)00391-7
215. Amanna, IJ, and Slifka, MK. Contributions of humoral and cellular immunity to vaccine-induced protection in humans. Virology. (2011) 411:206–15. doi: 10.1016/j.virol.2010.12.016
216. Ghasemi, A, Wang, S, Sahay, B, Abbott, JR, and Curtiss, R III. Protective immunity enhanced Salmonella vaccine vectors delivering Helicobacter pylori antigens reduce H. pylori stomach colonization in mice. Front Immunol. (2022) 13:1034683. doi: 10.3389/fimmu.2022.1034683
217. Nie, L, Huang, Y, Cheng, Z, Luo, H, Zhan, Y, Dou, K, et al. An intranasal influenza virus vector vaccine protects against Helicobacter pylori in mice. J Virol. (2024) 98:e01923–3. doi: 10.1128/jvi.01923-23
218. Wu, J, Xin, Y, Kong, J, and Guo, T. Genetic tools for the development of recombinant lactic acid bacteria. Microb Cell Fact. (2021) 20:118. doi: 10.1186/s12934-021-01607-1
219. Guan, H, Ke, W, Yan, Y, Shuai, Y, Li, X, Ran, Q, et al. Screening of natural lactic acid bacteria with potential effect on silage fermentation, aerobic stability and aflatoxin B1 in hot and humid area. J Appl Microbiol. (2020) 128:1301–11. doi: 10.1111/jam.14570
220. Tarahomjoo, S. Development of vaccine delivery vehicles based on lactic acid bacteria. Mol Biotechnol. (2012) 51:183–99. doi: 10.1007/s12033-011-9450-2
221. Iankov, ID, Kurokawa, C, Viker, K, Robinson, SI, Ammayappan, A, Panagioti, E, et al. Live attenuated measles virus vaccine expressing Helicobacter pylori heat shock protein a. Mol Ther Oncolytics. (2020) 19:136–48. doi: 10.1016/j.omto.2020.09.006
222. Ren, C, Zhang, Q, Wang, G, Ai, C, Hu, M, Liu, X, et al. Modulation of peanut-induced allergic immune responses by oral lactic acid bacteria-based vaccines in mice. Appl Microbiol Biotechnol. (2014) 98:6353–64. doi: 10.1007/s00253-014-5678-7
223. Guo, S, Yan, W, McDonough, SP, Lin, N, Wu, KJ, He, H, et al. The recombinant Lactococcus lactis oral vaccine induces protection against C. difficile spore challenge in a mouse model. Vaccine. (2015) 33:1586–95. doi: 10.1016/j.vaccine.2015.02.006
224. Zhang, F, Ni, L, Zhang, Z, Luo, X, Wang, X, Zhou, W, et al. Recombinant L. lactis vaccine LL-plSAM-WAE targeting four virulence factors provides mucosal immunity against H. pylori infection. Microb Cell Fact. (2024) 23:61. doi: 10.1186/s12934-024-02321-4
225. Lv, P, Song, Y, Liu, C, Yu, L, Shang, Y, Tang, H, et al. Application of Bacillus subtilis as a live vaccine vector: a review. J Vet Med Sci. (2020) 82:1693–9. doi: 10.1292/jvms.20-0363
226. Zhou, Z, Gong, S, Li, X-M, Yang, Y, Guan, R, Zhou, S, et al. Expression of Helicobacter pylori urease B on the surface of Bacillus subtilis spores. J Med Microbiol. (2015) 64:104–10. doi: 10.1099/jmm.0.076430-0
227. Permpoonpattana, P, Hong, HA, Phetcharaburanin, J, Huang, J-M, Cook, J, Fairweather, NF, et al. Immunization with Bacillus spores expressing toxin A peptide repeats protects against infection with Clostridium difficile strains producing toxins A and B. Infect Immun. (2011) 79:2295–302. doi: 10.1128/IAI.00130-11
228. Nicholson, WL, Munakata, N, Horneck, G, Melosh, HJ, and Setlow, P. Resistance of Bacillus endospores to extreme terrestrial and extraterrestrial environments. Microbiol Mol Biol Rev. (2000) 64:548–72. doi: 10.1128/MMBR.64.3.548-572.2000
229. Ferreira, WT, Hong, HA, Adams, JR, Hess, M, Kotowicz, NK, Tan, S, et al. Environmentally acquired Bacillus and their role in C. difficile colonization resistance. Biomedicines. (2022) 10:930. doi: 10.3390/biomedicines10050930
230. Piewngam, P, De Mets, F, and Otto, M. Intestinal microbiota: the hidden gems in the gut? Asian Pac J Allergy Immunol. (2020) 38:215. doi: 10.12932/AP-020720-0897
231. Cen, Q, Gao, T, Ren, Y, Lu, X, and Lei, H. Immune evaluation of a Saccharomyces cerevisiae-based oral vaccine against Helicobacter pylori in mice. Helicobacter. (2021) 26:e12772. doi: 10.1111/hel.12772
232. Wang, S, Ma, J, Ji, Q, and Liu, Q. Evaluation of an attenuated Listeria monocytogenes as a vaccine vector to control Helicobacter pylori infection. Immunol Lett. (2021) 238:68–74. doi: 10.1016/j.imlet.2021.07.010
233. Guleria, I, and Pollard, JW. Aberrant macrophage and neutrophil population dynamics and impaired Th1 response to Listeria monocytogenes in colony-stimulating factor 1-deficient mice. Infect Immun. (2001) 69:1795–807. doi: 10.1128/IAI.69.3.1795-1807.2001
234. Stark, FC, Sad, S, and Krishnan, L. Intracellular bacterial vectors that induce CD8+ T cells with similar cytolytic abilities but disparate memory phenotypes provide contrasting tumor protection. Cancer Res. (2009) 69:4327–34. doi: 10.1158/0008-5472.CAN-08-3160
235. Yang, Y, Hou, J, Lin, Z, Zhuo, H, Chen, D, Zhang, X, et al. Attenuated Listeria monocytogenes as a cancer vaccine vector for the delivery of CD24, a biomarker for hepatic cancer stem cells. Cell Mol Immunol. (2014) 11:184–96. doi: 10.1038/cmi.2013.64
236. Ding, C, Liu, Q, Li, J, Ma, J, Wang, S, Dong, Q, et al. Attenuated Listeria monocytogenes protecting zebrafish (Danio rerio) against Vibrio species challenge. Microb Pathog. (2019) 132:38–44. doi: 10.1016/j.micpath.2019.03.040
237. Gallagher, FA, Kettunen, MI, Day, SE, Hu, DE, Ardenkjaer-Larsen, JH, Ri, Z, et al. Magnetic resonance imaging of pH in vivo using hyperpolarized 13C-labeled bicarbonate. Nature. (2008) 453:940–3. doi: 10.1038/nature07017
238. Ma, J, Ji, Q, Wang, S, Qiu, J, and Liu, Q. Identification and evaluation of a panel of strong constitutive promoters in Listeria monocytogenes for improving the expression of foreign antigens. Appl Microbiol Biotechnol. (2021) 105:5135–45. doi: 10.1007/s00253-021-11374-z
239. Oertli, M, Noben, M, Engler, DB, Semper, RP, Reuter, S, Maxeiner, J, et al. Helicobacter pylori γ-glutamyl transpeptidase and vacuolating cytotoxin promote gastric persistence and immune tolerance. Proc Natl Acad Sci USA. (2013) 110:3047–52. doi: 10.1073/pnas.1211248110
240. Maleki Kakelar, H, Barzegari, A, Dehghani, J, Hanifian, S, Saeedi, N, Barar, J, et al. Pathogenicity of Helicobacter pylori in cancer development and impacts of vaccination. Gastric Cancer. (2019) 22:23–36. doi: 10.1007/s10120-018-0867-1
241. Amieva, MR, Salama, NR, Tompkins, LS, and Falkow, S. Helicobacter pylori enter and survive within multivesicular vacuoles of epithelial cells. Cell Microbiol. (2002) 4:677–90. doi: 10.1046/j.1462-5822.2002.00222.x
242. Baj, J, Forma, A, Flieger, W, Morawska, I, Michalski, A, Buszewicz, G, et al. Helicobacter pylori infection and extragastric diseases—a focus on the central nervous system. Cells. (2021) 10:2191. doi: 10.3390/cells10092191
243. Mu, Q, Kirby, J, Reilly, CM, and Luo, XM. Leaky gut as a danger signal for autoimmune diseases. Front Immunol. (2017) 8:598. doi: 10.3389/fimmu.2017.00598
244. Solnick, JV, Canfield, DR, Hansen, LM, and Torabian, SZ. Immunization with recombinant Helicobacter pylori urease in specific-pathogen-free rhesus monkeys (Macaca mulatta). Infect Immun. (2000) 68:2560–5. doi: 10.1128/IAI.68.5.2560-2565.2000
245. Amalia, R, Panenggak, NSR, Doohan, D, Rezkitha, YAA, Waskito, LA, Syam, AF, et al. A comprehensive evaluation of an animal model for Helicobacter pylori-associated stomach cancer: fact and controversy. Helicobacter. (2023) 28:e12943. doi: 10.1111/hel.12943
Keywords: Helicobacter pylori, pathogenesis, virulence factors, vaccine immunogenicity, public health
Citation: Elbehiry A, Marzouk E, Abalkhail A, Sindi W, Alzahrani Y, Alhifani S, Alshehri T, Anajirih NA, ALMutairi T, Alsaedi A, Alzaben F, Alqrni A, Draz A, Almuzaini AM, Aljarallah SN, Almujaidel A and Abu-Okail A (2025) Pivotal role of Helicobacter pylori virulence genes in pathogenicity and vaccine development. Front. Med. 11:1523991. doi: 10.3389/fmed.2024.1523991
Received: 06 November 2024; Accepted: 13 December 2024;
Published: 06 January 2025.
Edited by:
Vivek P. Chavda, L. M. College of Pharmacy, IndiaReviewed by:
Holly Algood, Vanderbilt University, United StatesCopyright © 2025 Elbehiry, Marzouk, Abalkhail, Sindi, Alzahrani, Alhifani, Alshehri, Anajirih, ALMutairi, Alsaedi, Alzaben, Alqrni, Draz, Almuzaini, Aljarallah, Almujaidel and Abu-Okail. This is an open-access article distributed under the terms of the Creative Commons Attribution License (CC BY). The use, distribution or reproduction in other forums is permitted, provided the original author(s) and the copyright owner(s) are credited and that the original publication in this journal is cited, in accordance with accepted academic practice. No use, distribution or reproduction is permitted which does not comply with these terms.
*Correspondence: Ayman Elbehiry, YXIuZWxiZWhpcnlAcXUuZWR1LnNh
Disclaimer: All claims expressed in this article are solely those of the authors and do not necessarily represent those of their affiliated organizations, or those of the publisher, the editors and the reviewers. Any product that may be evaluated in this article or claim that may be made by its manufacturer is not guaranteed or endorsed by the publisher.
Research integrity at Frontiers
Learn more about the work of our research integrity team to safeguard the quality of each article we publish.