- 1Department of Pharmacy, Faculty of Medical Sciences, University of Kragujevac, Kragujevac, Serbia
- 2Department of Pharmacology and Toxicology, Faculty of Medical Sciences, University of Kragujevac, Kragujevac, Serbia
- 3Department of Clinical Pharmacology, University Clinical Centre Kragujevac, Kragujevac, Serbia
- 4Department of Infectious Diseases, Faculty of Medical Sciences, University of Kragujevac, Kragujevac, Serbia
- 5Infectious Diseases Clinic, University Clinical Centre Kragujevac, Kragujevac, Serbia
- 6Department of Internal Medicine, Faculty of Medical Sciences, University of Kragujevac, Kragujevac, Serbia
- 7Clinic for Haematology, University Clinical Centre Kragujevac, Kragujevac, Serbia
- 8Department of Genetics, Faculty of Medical Sciences, University of Kragujevac, Kragujevac, Serbia
- 9Department of Forensic Medicine, Faculty of Medical Sciences, University of Kragujevac, Kragujevac, Serbia
- 10Department of Forensic Medicine and Toxicology, University Clinical Center Kragujevac, Kragujevac, Serbia
- 11Centre for Molecular Medicine and Stem Cell Research, Faculty of Medical Sciences, University of Kragujevac, Kragujevac, Serbia
- 12Bioengineering Research and Development Center (BioIRC), Kragujevac, Serbia
- 13Department of Sciences Institute for Information Technologies Kragujevac, University of Kragujevac, Kragujevac, Serbia
- 14Department of Chemistry, Faculty of Sciences, University of Kragujevac, Kragujevac, Serbia
- 15Faculty of Engineering, University of Kragujevac, Kragujevac, Serbia
- 16Institute of Public Health Kragujevac, Kragujevac, Serbia
Introduction: ACE2 and TMPRSS2 represent the major gateways for SARS-CoV-2 cell entry. The presence of functional ACE2 and TMPRSS2 genetic polymorphisms that affect gene expression may affect the risk of severe form of COVID-19 and its fatal outcome.
Material and patients: This observational study enrolled 178 hospitalized patients diagnosed with SARS-CoV-2 infection at the University Clinical Centre of Kragujevac, Serbia. Demographic, clinical, and laboratory data were gathered at admission. Genotyping for single nucleotide polymorphisms of ACE2 (rs2106809 and rs2074192) and TMPRSS2 (rs2070788 and rs4818239) was performed using the Real-Time PCR method with TaqMan assays.
Results: Controlling for other factors of influence, such as CCI, N/L ratio, LDH level, and pO2, we showed that females with TMPRSS2 rs2070788 A/A genotype were less likely to develop severe COVID-19 (odds ratio [OR] [95% confidence interval (95% CI)]: 0.030 [0.001; 0.862]). Additionally, the likelihood of dying of SARS-CoV-2 infection was lower in female carriers of at least one ACE2 rs2106809 C allele (OR [95% CI]: 0.004 [0.000; 0.981]).
Conclusion: Our findings indicate TMPRSS2 rs2070788 and ACE2 rs2106809 polymorphisms as independent predictors of severity and outcome of COVID-19 in females.
1 Introduction
Since the beginning of the COVID-19 pandemic, angiotensin-converting enzyme 2 (ACE2) has emerged as one of the key proteins in the pathogenesis of this infectious disease. This transmembrane protein, predominantly expressed on alveolar lung cells (1), assumes the role of a receptor for the SARS-CoV-2 virus and enables its entry into human cells (2). Variations in ACE2 gene are known to affect the ability of ACE2 to bind to coronaviruses (3), and vast inter-ethnic differences in minor allele frequencies of its polymorphisms suggest their impact on the differences in development, course, and outcome of SARS-CoV-2 infection among populations. The possible significance of ACE2 genotype is further supported by recent preliminary reports of an association between severe COVID-19 (4). On the other hand, the clinical effects of some of the frequent and functional ACE2 SNPs, such as rs2106809 and rs2074192, have been associated with circulating ACE2 levels only in females (5). The observed difference in ACE2 expression in males as compared to females, which could be explained by on the X ACE2 gene location on the X chromosome (6), is expected to contribute to sex-related disparities in COVID-19 outcomes.
The fusion of SARS-CoV-2 with the host cell and the subsequent onset of infection is facilitated by several types of proteases, the most important being transmembrane serine protease (TMPRSS2) (7). Like ACE2, TMPRSS2 is mainly expressed on the alveolar lung cells surface (8), but also in many other tissues and organs that could become the loci of complications in severe forms of COVID-19 (9). The role of TMPRSS2 in COVID-19 is further confirmed by the fact that TMPRSS2 inhibitors approved for clinical use (such as camostat mesylate—a drug used in the treatment of chronic pancreatitis) can block SARS-CoV-2 virus infection (7). The TMPRSS2 gene is polymorphic, and some of its SNPs, such as rs2070788, have already been confirmed as a significant risk factor for influenza (10). Similarly, recent in silico analyses have introduced the possibility that many other TMPRSS2 polymorphisms, including rs4818239, may affect COVID-19 infection (11). Having in mind the difference between sexes in terms of COVID-19 susceptibility and outcome (12, 13), the possible role of TMPRSS2 genotype in this disease is further supported by its androgen-dependent regulation (14).
Given that ACE2 and TMPRSS2 represent the major gateways for SARS-CoV-2 cell entry, their sex-dependent expression strongly advocates for COVID-19 studies which would take sex into account when investigating the role of ACE2 and TMPRSS2 polymorphism. To meet these expectations, we evaluated the association of polymorphisms of ACE2 and TMPRSS2 genes with the likelihood of severe COVID-19 and fatal outcome, separately in females and males.
2 Material and patients
2.1 Study population
This prospective observational study included 178 COVID-19 patients, hospitalized at the University Clinical Center Kragujevac (UKCKG), Serbia. The sample size calculations were based on the study by Abdelsattar et al. (15), where TMPRSS2 gene polymorphism has been assessed as a potential determinate on COVID-19 infection severity. In this study, genotyping of COVID-19 patients for TMPRSS2 rs12329760 revealed significantly higher frequency of homozygous carriers of variant allele among severe as compared to mild cases (16.4 and 2.6%, respectively). Assuming type I error rate of 0.05 and 90% power level, minimum sample size for our study was thus estimated to 89 subjects per group, i.e., to 178 in total.
In this study, patients were diagnosed with COVID-19 by analysis of pharyngeal or nasopharyngeal swabs, using either reverse-transcriptase polymerase chain reaction (RT-PCR) as a gold-standard method (16), or rapid antigen test (RAT) as an alternative. Although sensitivity of 69.86% generally precludes the routine use of RAT for diagnosis and surveillance of COVID-19 (17), due to high specificity (99.61%) i.e., low rate of false positive results (18), it was considered valid and reliable to confirm SARS-CoV-2 infection in our study participants, including those who were asymptomatic. Patients included in the study were Caucasians of Serbian nationality, 18 years of age or older; pregnancy and breastfeeding served as exclusion criteria. A signed written informed consent was obtained from all patients or their legal representatives, and all necessary demographic and clinical data were taken from the electronic medical records. The study was conducted under the ethical standards outlined in the Declaration of Helsinki and Good Clinical Practice. The local Ethics Committee of UKCKG, Serbia, approved the study protocol by decision no. 01/20–405.
2.2 Data collection
The data for each individual were collected from hospital medical records, and included age, sex, symptoms and signs of COVID-19, radiological imaging results, and laboratory parameters. Pre-existing medical conditions (subsequently used to calculate the Charlson Comorbidity Index, CCI), as well as prior therapy, were assessed at admission.
Study patients were classified according to the World Health Organization (WHO) guidelines into either mild/moderate, or severe/critically severe COVID-19 cases, with the latter distinguished from the former by compromised respiratory function. All participants were followed up until hospital discharge or in-hospital death, with severity classification performed based on the worst clinical condition observed during hospitalization. Blood samples used for DNA extraction and genotyping was obtained during routine laboratory analyses.
2.3 DNA extraction and SNPs genotyping
Genomic DNA was extracted from 200 μL of whole EDTA blood using a commercial PureLink Genomic DNA Mini Kit (Invitrogen), according to the manufacturer’s recommendations. The quantity and quality of DNA samples were determined by spectrophotometry, using standard absorbance measurements at A260nm and A280nm on a Cary 300 UV–Vis spectrophotometer (Agilent Technologies). Samples having A260/A280 ratios ranging from 1.7 to 1.9 were suitable for genotyping (19).
Genotyping for ACE2 and TMPRSS2 gene polymorphisms was performed on Mic qPCR 48-well thermal cycler (BioMolecular Systems) using predesigned, commercially available TaqMan genotyping assay mix (20X; C__16098179_20 for rs2106809; C__16163821_10 for rs2074192; C___2592038_1_ for rs2070788 and C___3080270_20 for rs4818239; Applied Biosystems, Foster City, United States). TaqMan Genotyping Master Mix (2X; Applied Biosystems, Foster City, United States) was used to amplify DNA segments of interest. The final volume of the reaction mixture of 20 μL contained 10 μL TaqMan Genotyping Master Mix (2X), 0.5 μL TaqMan genotyping assay mix (20X), 0.5 μL deionized water, and 9 μL genomic DNA (or deionized water as non-template control). Two researchers independently determined the genotype calls, and repeated samples revealed no inconsistencies.
2.4 Statistical analyzes
Genotyping results were presented as absolute and relative frequencies of alleles and genotypes, using additive, dominant, and recessive genetic models to capture different inheritance pattern. To assess the consistency of TMPRSS2 genotype frequencies with Mendelian inheritance, testing for the deviations from Hardy–Weinberg equilibrium (HWE) was performed by the chi-square (χ2) test with one degree of freedom (df = 1). Due to its location on the X chromosome, ACE2 was tested for HWE using χ2—maximum-likelihood test (χ2-ML) with df = 1, which compares the observed with the expected genotype frequencies, where latter is calculated based on both male and female data (20). Testing for linkage disequilibrium (LD) between polymorphisms of the same gene was conducted using Haploview software, version 4.2 (Broad Institute, Cambridge, MA, United States), providing insight into non-random associations between loci.
Data were analyzed using the statistical program SPSS version 26 (IBM, Armonk, NY, United States). The association between independent variables and the risk of severe disease development or in-hospital death was first tested by univariable logistic regression; this analysis was used to evaluate the individual effect of each of the independent variables, and to select those deemed relevant to undergo subsequent analyses. To further assess the simultaneous effect of multiple factors on the outcomes of interest, the stepwise backward multivariable logistic regression was performed, with an aim to indentify predictors to be included in the statistical model that will provide best prediction of a probability of COVID-19 progression or death. To determine the strength of the observed association, odds ratios (OR) with corresponding 95% confidence intervals (CI) were calculated for each independent variable. Hosmer-Lemeshow goodness-of-fit test was used to assess the prediction model’s quality. The significance level (p) for all tests was set at less than 0.05.
3 Results
Genotyping of study participants for TMPRSS2 and ACE2 polymorphisms resulted in minor allele frequencies of 45.8, 46.9, 17.6, and 42.7% for rs2070788, rs4818239, rs2106809, and rs207419, respectively. Corresponding frequency distributions of alleles and genotype groups were separated according to sex, and presented in Supplementary Tables 1, 2. TMPRSS2 genotype frequencies were consistent with HWE for both rs2070788 (χ2(1) = 0.043, p = 0.836) and rs4818239 (χ2(1) = 0.303, p = 0.582). Similar was observed for ACE2 rs2074192 (χ2(1) = 2.705, p = 0.05), but not for rs2106809, which showed statistically significant difference between expected and observed genotype frequencies (χ2(1) = 9.255, p = 0.01). Testing for LD between polymorphisms at ACE2 and TMPRSS2 loci (Supplementary Figure 1) revealed very low and moderate linkage disequilibrium between rs2106809 and rs2074192, and between rs2070788 and rs4818239, respectively.
Tables 1–4 present the frequency distribution of allele and genotype groups of ACE2 and TMPRSS2 polymorphisms, stratified by sex, according to the severity of SARS-CoV-2 infection and its outcome. Univariate logistic regression did not detect any significant association of ACE2 alleles and genotype groups with either severity (Table 1) or outcome (Table 3) of COVID-19. Contrarily, TMPRSS2 rs2070788 A and rs4818239 C alleles were significantly associated with a two- to three-fold decrease in the likelihood of developing severe COVID-19 in both females and males (Table 2), while the odds for in-hospital death decreased in the presence of rs2070788 A and rs4818239 C in females and males, respectively (Table 4).
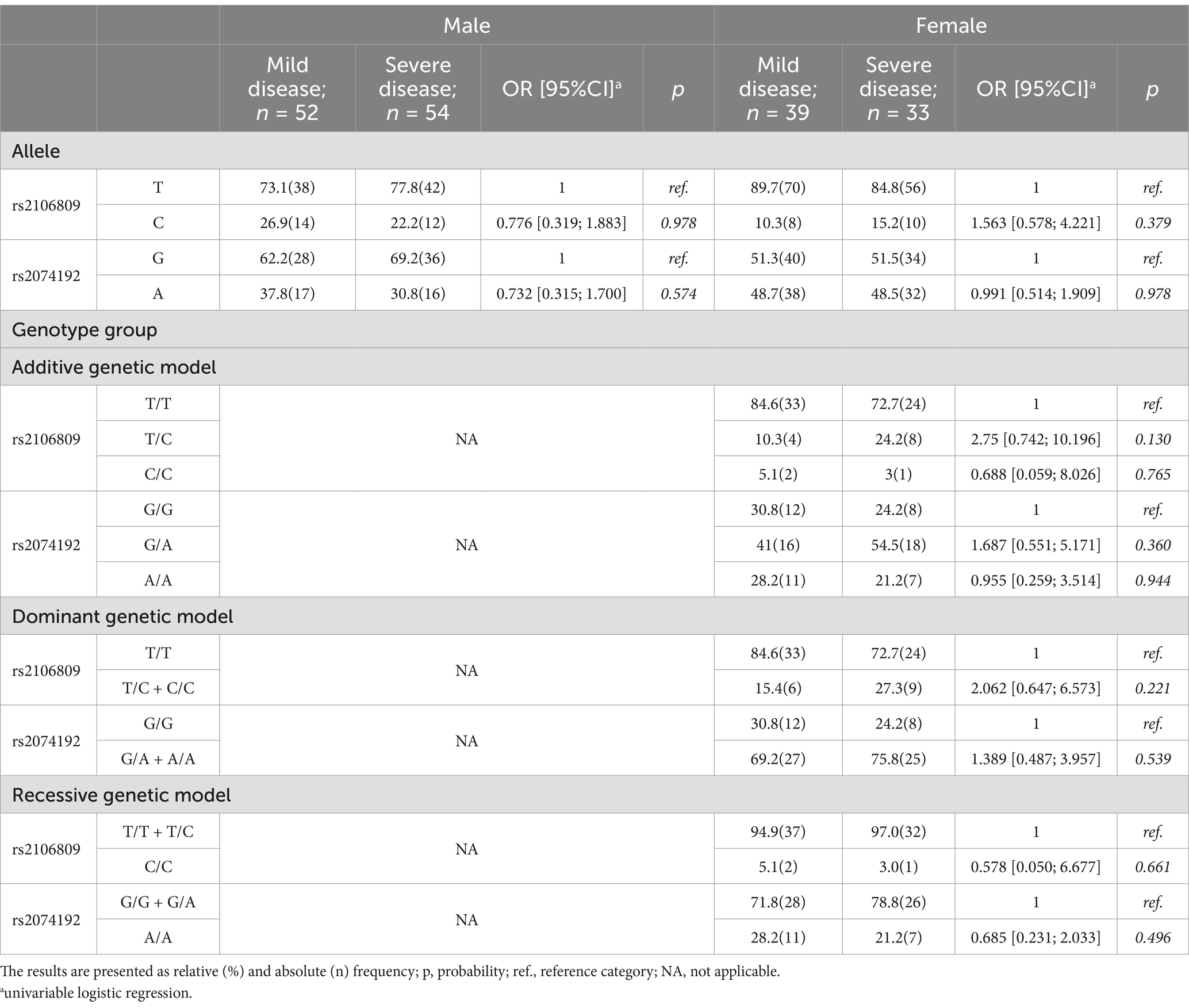
Table 1. ACE2 frequency of allele and genotype group in male and female according severity of SARS-CoV-2 infection.
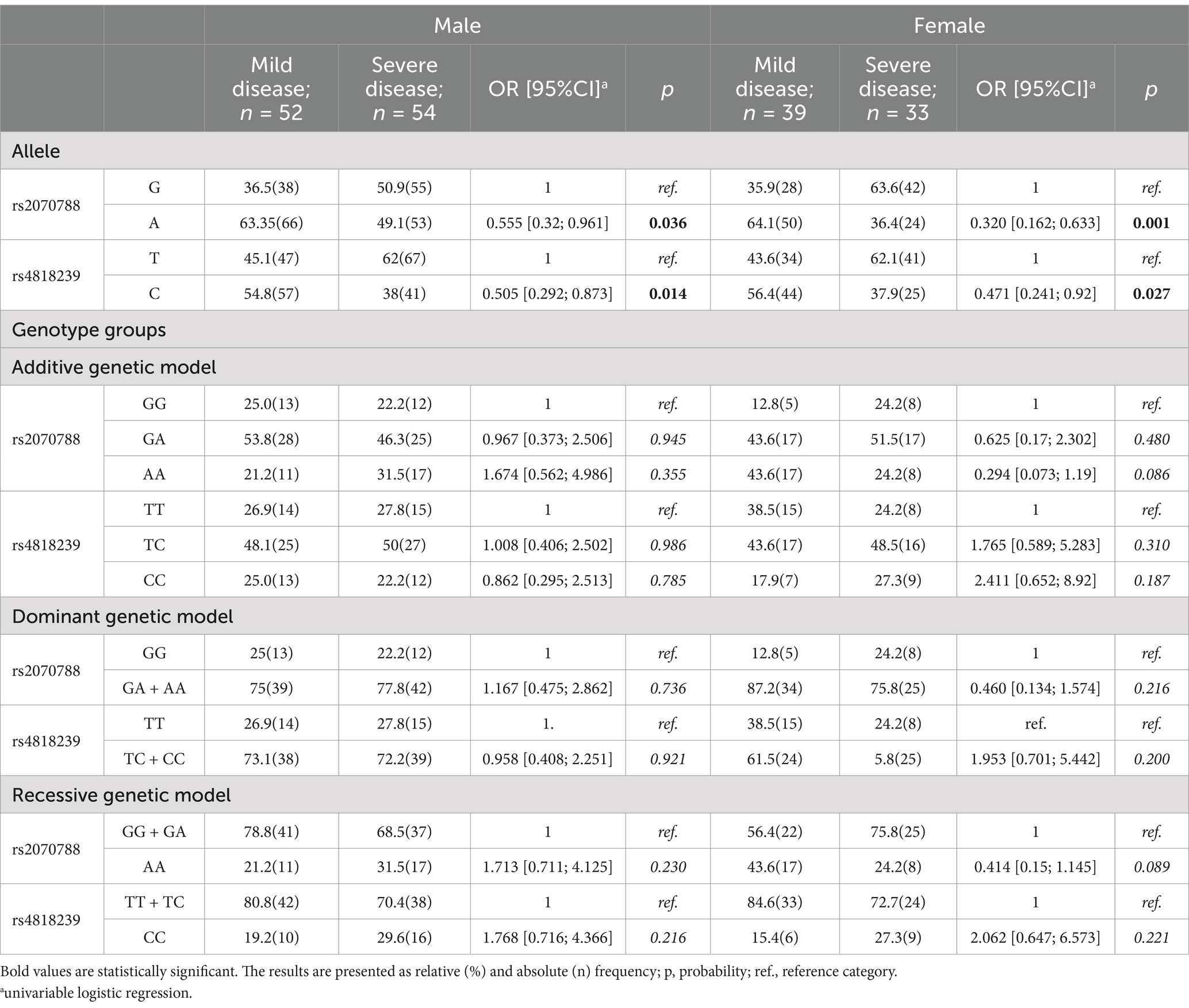
Table 2. TMPRSS2 frequency of allele and genotype groups in male and female according severity of SARS-CoV-2 infection.
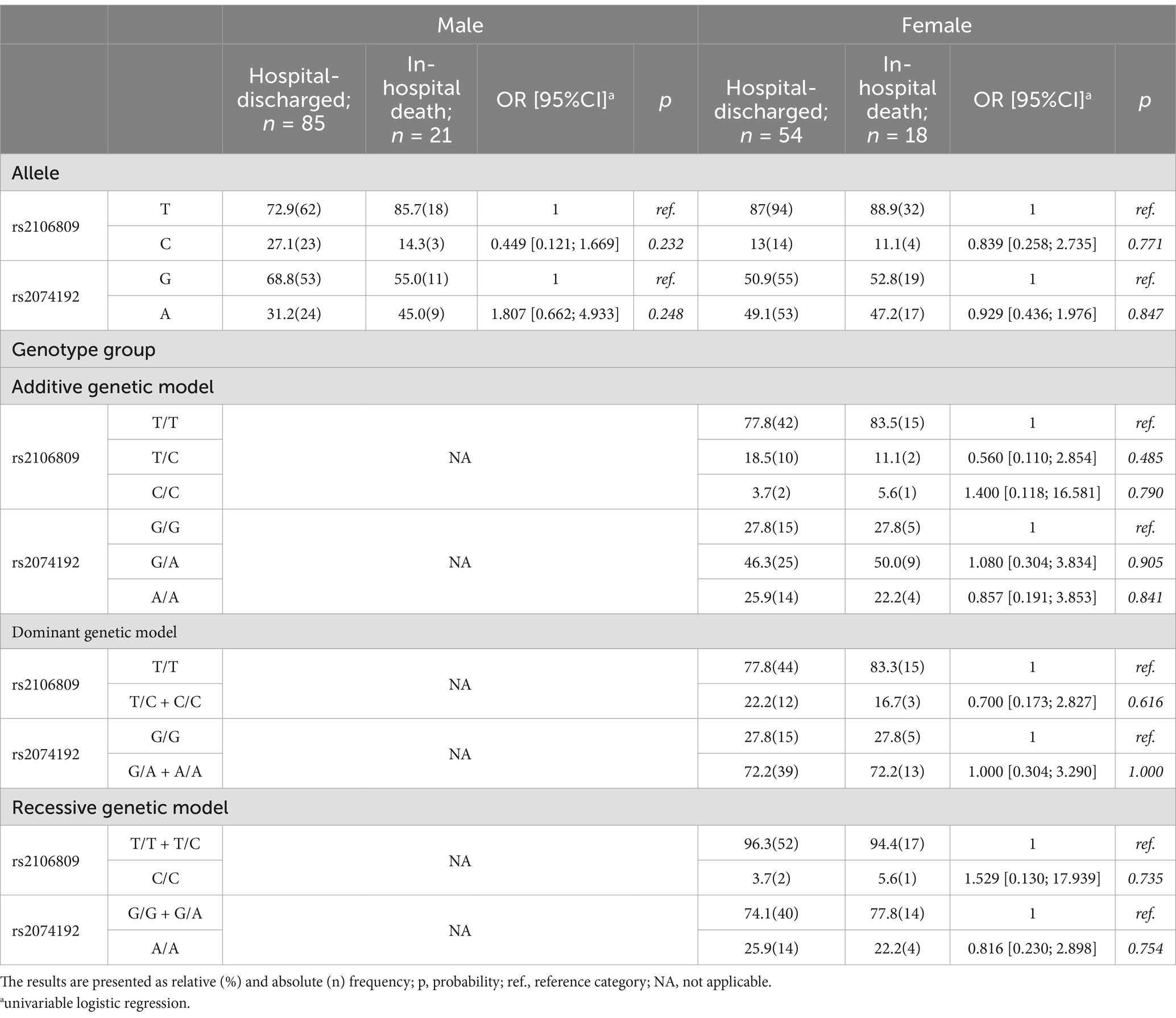
Table 3. ACE2 frequency of allele and genotype group in male and female according outcome of SARS-CoV-2 infection.
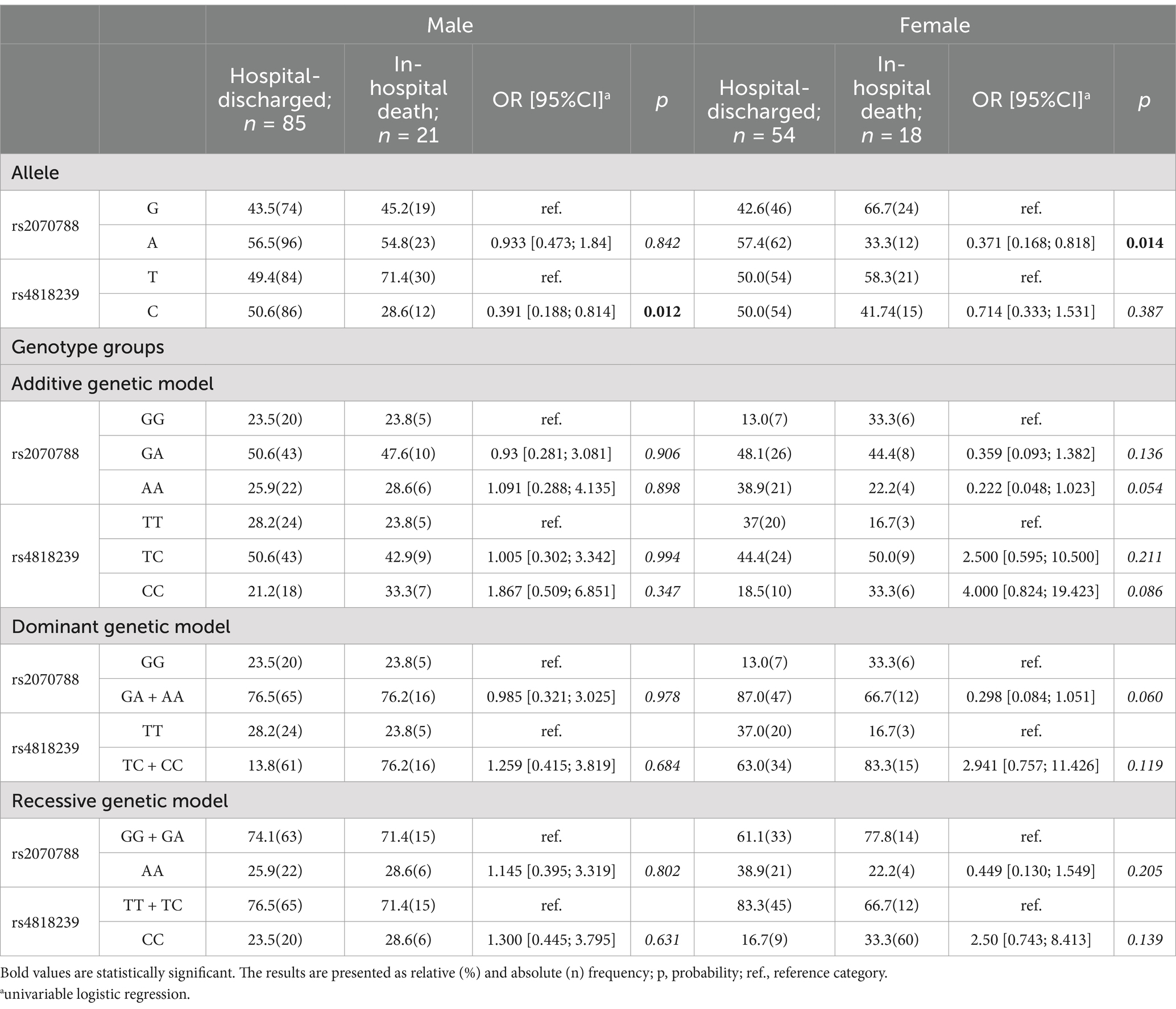
Table 4. TMPRSS2 frequency of allele and genotype groups in male and female according to outcome of SARS-CoV-2 infection.
Multivariable logistic models, developed to evaluate the association of genetic polymorphisms in ACE2 and TMPRSS2 genes, laboratory and clinical parameters at admission, and the likelihood of developing severe COVID-19 and lethal outcome in female and male patients separately (Table 5; Supplementary Table 3), revealed significance of tested polymorphisms only in female population. The final model of highest predictive quality (Hosmer-Lemeshow test: χ2(7) = 4.016, p = 0.778; Nagelkerke R2 = 75.2%, correctly classified 83.3% of cases) showed that females carrying both major alleles of TMPRSS2 rs2070788 were less likely to develop severe COVID-19. On the other hand, the best fitting model for outcome prediction (Hosmer-Lemeshow test: χ2(7) = 0.341, p = 1.000; Nagelkerke R2 = 77.2%; adequately classified 92.4% of cases) revealed that the likelihood of surviving SARS-CoV-2 infection was 95% higher in females carrying at least one minor allele of ACE2 rs2106809 compared to those carrying two major alleles.
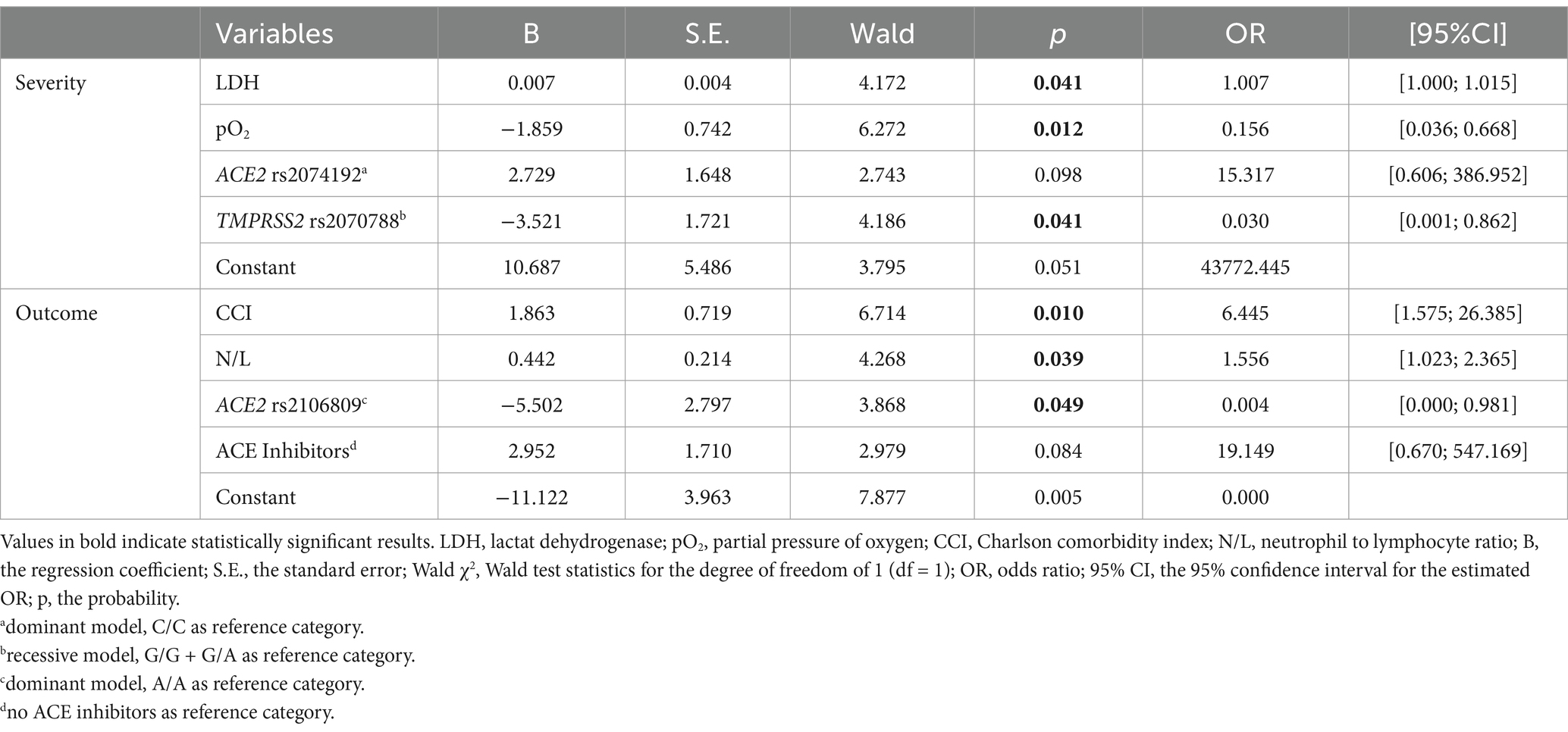
Table 5. Summary of variable estimates from the best fitting models of multiple logistic regression analysis regarding severity and outcome of SARS-CoV-2 infection in females.
4 Discussion
Although the WHO announced in May 2023 that COVID-19 no longer represents a public health emergency of international concern, the increase in the frequency of new subvariants of Omicron SARS-CoV-2 continue to attract public attention (21). These new strains, especially BA.2.86 (Pirola) and JN.1, are characterized by more than 30 mutations on the spike (S) protein (22), which heighten their ability to evade vaccine-induced immunity and increase the prevalence of COVID-19 worldwide (23). Therefore, even 4 years after the initial outbreak, the clinical presentation of SARS-CoV-2 infection is still variable, ranging from completely asymptomatic, to critically severe forms that are potentially life-threatening (24).
Despite well-established risk factors like age, sex, and chronic diseases, early identification of individuals at risk for severe viral infections remains important clinical challenge. Genetic variations, particularly of genes coding for receptors and enzymes responsible for viral entrance into the human organism, can significantly influence host response to SARS-CoV-2 infection (25). Our research highlights the potential of genetic markers associated with ACE2 and TMPRSS2 in predicting the severity and outcome of COVID-19, especially in females. Specifically, our results indicate that female carriers of both major A alleles of TMPRSS2 rs2070788 and at least one minor G allele of ACE2 rs2106809 have lower odds of severe COVID-19 and fatal outcome, respectively. To our knowledge, our study is the first to report the association of ACE2 rs2106809 with SARS-CoV-2 infection outcome, and one of the very few to describe the protective role of the TMPRSS2 rs2070788 A/A genotype on COVID-19 severity, both in females. Additionally, our study reaffirms the significance of certain clinical factors, such as CCI, N/L ratio (26), LDH level (27), and pO2 at admission, in predicting COVID-19 severity and mortality.
ACE2 gene maps to X chromosome and codes for transmembrane protein ACE2 (28). There are two known forms of human ACE2: membrane-bound (mACE2) and soluble (sACE2), latter arising from the former by ADAM17-mediated cleavage and subsequent shedding of its extracellular catalytic domain from the membrane (28, 29). In the context of SARS-CoV-2 infection, mACE2 is identified as a receptor (7) that triggers conformational change of the virion (30), fostering its TMPRSS2-mediated proteolytic cleavage and consequent entry into the target cell (31). At the same time, sACE2 competes with mACE2 for binding to SARS-CoV-2, but without prompting entrance to the cell; this reduces the number of viral particles that will attach to the cell surface, providing protective effect against SARS-CoV-2 infection (8, 31). In the context of COVID-19 severity and mortality, both mACE2 and sACE2 counteract the effects of its homolog angiotensin-converting enzyme (ACE) within renin-angiotensin-aldosterone system (RAAS) axis, decreasing the level of angiotensin (Ang) II in favor of Ang (1–7). This leads to an increased activation of MAS instead of AT1R receptor, creating vasodilatatory and anti-inflammatory effects that decrease the risk of severe form of the disease and its fatal outcome (32). Both of these cascades of events correspond well to the suggested considering COVID-19 as a dual phase phenomenon: in the first phase of infection, ACE2 promotes viral entry, but in the later phase, it acts protectively against respiratory failure and COVID-19-related death (33). However, upon infection, virion-bound mACE2 gets internalized along with the viral particle, decreasing the number of available ACE2 molecules on the cell membrane (34). Moreover, during the course of the disease, SARS coronaviruses, including SARS-CoV-2, downregulate ACE2 cell surface expression (35, 36). Within RAAS, reduction of mACE2 gives advantage to ACE, which shifts abovementioned Ang II/Ang (1–7) proportion toward the former, prompting its binding to ATR1. This further downregulates mACE2 (37), but also initiates a crucial mechanism of ADAM17 activation (38), which in turn triggers an increase in sACE2 level at the expense of mACE2 (28, 29). In line with these observations, previous studies reported elevated levels of sACE2 in COVID-19 patients that did not survive the infection, suggesting its potential role as an independent biomarker of COVID-19 mortality (39). The persistency of high sACE2 throughout hospitalization in SARS-CoV-2 non-survivors, as well as the clear disparity in its level observed between males and females, point toward the role of both ACE2 genetic polymorphism and sex in determining the proposed prognostic value of sACE2 in COVID-19.
ACE2 rs2106809 is an intronic variant that alters gene splicing efficiency (5, 40), with minor C allele being associated with increased ACE2 expression in multiple tissues (41). In female carriers of the same allele, reduced levels of sACE2, but higher levels of Ang (1–7) were previously detected (5), indicating an increase in mACE2/sACE2 ratio that is probably due to impediment in either ADAM17 docking on mACE2 cleavage site, or its efficacy in ectodomain shedding (29). In contrast to several earlier reports of rs2106809 C/C genotype connection with higher risk of hospitalization and ICU admission (42, 43), we failed to observe any significant association between this polymorphism and a severe form of COVID-19. However, our results suggest the presence of variant rs2106809 C allele as a prominent protective factor for in-hospital death due to COVID-19, but only among females. While this finding corresponds well to the expected genotype-related change in mACE2 and sACE2 levels, as well as to its inverse association with COVID-19 mortality, question arises why it applies to female population only. One of the plausible explanations we propose considers the location of ACE2 gene on the X chromosome (28) within a region of incomplete X-chromosome inactivation (XCI) (44). Namely, for most of the X-linked genes, one allele is normally inactivated to balance its expression between females and males (45). However, some of them, including ACE2 (44), escape XCI, resulting in female-biased ACE2 gene expression (46), and thus a more pronounced effect of any functional ACE2 genetic polymorphism, including rs2106809, in females. Finally, it should be noted that in our study ACE2 rs2106809 deviates from HWE. Although HW disequilibrium in genetic studies could indicate issues like selection bias, population stratification, or genotyping errors (47), we assume the departure we observed is due to location of ACE2 on the X chromosome, as it causes violation of one of the basic assumptions that HW principle relies on—that the locus should be autosomal (48).
TMPRSS2 gene is located on chromosome 21 and encodes transmembrane protease TMPRSS2 (7). This enzyme represents one of the key factors for penetration of various viruses, including SARS-CoV-2, into the cells: upon binding to the ACE2 receptor, TMPRSS2 initiates fusion machinery between the viral particle and cell phospholipid membranes, and delivers the virion into the cytoplasm (7). TMPRSS2 is expressed in many human organs and tissues (49–51), and in most it is colocalized with ACE2, making them more susceptible targets for SARS-CoV-2 (52). Previous observations of more severe forms of COVID-19 in men as compared to women (53), as well as of decreased risk of severe respiratory illness in SARS-CoV-2-infected infants and children (54), led to speculations about the critical influence of well-known androgen-dependent upregulation of TMPRSS2 expression (55) on the clinical course of this disease. However, TMPRSS2 expression is regulated in organ- and tissue-specific manner (56), with inducing effect of male hormones being mainly responsible for prostate localization (14). In lungs, on the other hand, TMPRSS2 displays less prominent androgen responsiveness (56), and gene expression to a significant extent depends on the presence of genetic polymorphisms (10, 57).
TMPRSS2 rs2070788 represents a regulatory intron variant, whose ancestral G allele leads to higher gene expression in lungs (57, 58). In line with the role of TMPRSS2 in SARS-CoV-2 infection, rs2070788 G allele has been repeatedly (10, 59, 60), although not consistently (61), associated with severe clinical presentation and increased mortality of several respiratory infectious diseases, including COVID-19. In our study, homozygous carriers of variant A allele were less likely to develop severe clinical presentation after SARS-CoV-2 infection. This finding corresponds well to the expected genotype effect, as well as to most of the previous studies investigating the risk of severe COVID-19 in the presence of variant rs2070788 allele. However, after controlling for other factors of influence, the genotype–phenotype association we observed remained significant only among females. Similar reports in the literature are not many, but the protective role of rs2070788 A/A genotype in female COVID-19 patients has been described previously (62). We believe the observed sex-related difference has a hormonal background: genetically determined decrease in TMPRSS2 levels appears more evident in females as compared to males, because females lack androgen-dependent upregulation of TMPRSS2 gene expression.
There are several limitations in our study. Firstly, it was designed as a single centre study, which included only one population of respondents. Furthermore, our relatively small sample size could have reduced statistical power, while other potentially functional ACE2 and TMPRSS2 SNPs, which we did not genotype for, could have affected our results. Most importantly, we could not collect data on confounding variables, such as level of viral exposure, viral load, patient health habits, environmental influences, and the use of other drugs, which could have influenced the outcome.
5 Conclusion
In conclusion, our study highlights association between the presence of TMPRSS2 rs2070788 and ACE2 rs2106809 polymorphisms, and the severity and outcome of COVID-19, respectively, among female patients. These genetic variations may serve as important markers for identifying individuals at higher risk for severe disease and unfavorable outcomes, potentially guiding personalized treatment approaches in the future.
Data availability statement
The raw data supporting the conclusions of this article will be made available by the authors, without undue reservation.
Ethics statement
The studies involving humans were approved by Ethics Committee of the University Clinical Center Kragujevac, Serbia. The studies were conducted in accordance with the local legislation and institutional requirements. The participants provided their written informed consent to participate in this study.
Author contributions
SM: Formal analysis, Investigation, Methodology, Writing – original draft. DM: Data curation, Validation, Writing – review & editing. ZM: Resources, Writing – review & editing. PD: Resources, Writing – review & editing. SS: Data curation, Formal analysis, Methodology, Validation, Writing – review & editing. DT: Formal analysis, Methodology, Writing – review & editing. KV: Investigation, Methodology, Writing – review & editing. VC: Investigation, Writing – original draft. SP: Formal analysis, Methodology, Writing – review & editing. NMD: Investigation, Writing – review & editing. MZ: Formal analysis, Methodology, Writing – review & editing. DS: Investigation, Writing – review & editing. SA: Resources, Writing – review & editing. NeDj: Resources, Writing – original draft. MV: Data curation, Validation, Writing – review & editing. NV: Data curation, Validation, Writing – review & editing. NF: Resources, Writing – review & editing. DB: Conceptualization, Formal analysis, Methodology, Project administration, Writing – original draft. NaDj: Conceptualization, Formal analysis, Methodology, Writing – original draft.
Funding
The author(s) declare that financial support was received for the research, authorship, and/or publication of this article. This research was funded by The Science Fund of the Republic of Serbia (grant no. 7749739, SVETOVID-19 project).
Conflict of interest
The authors declare that the research was conducted in the absence of any commercial or financial relationships that could be construed as a potential conflict of interest.
Publisher’s note
All claims expressed in this article are solely those of the authors and do not necessarily represent those of their affiliated organizations, or those of the publisher, the editors and the reviewers. Any product that may be evaluated in this article, or claim that may be made by its manufacturer, is not guaranteed or endorsed by the publisher.
Supplementary material
The Supplementary material for this article can be found online at: https://www.frontiersin.org/articles/10.3389/fmed.2024.1493815/full#supplementary-material
References
1. Zhang, H, Penninger, JM, Li, Y, Zhong, N, and Slutsky, AS. Angiotensin-converting enzyme 2 (ACE2) as a SARS-CoV-2 receptor: molecular mechanisms and potential therapeutic target. Intensive Care Med. (2020) 46:586–90. doi: 10.1007/S00134-020-05985-9
2. Li, W, Moore, MJ, Vasilieva, N, Sui, J, Wong, SK, Berne, MA, et al. Angiotensin-converting enzyme 2 is a functional receptor for the SARS coronavirus. Nature. (2003) 426:450–4. doi: 10.1038/NATURE02145
3. Li, W, Zhang, C, Sui, J, Kuhn, JH, Moore, MJ, Luo, S, et al. Receptor and viral determinants of SARS-coronavirus adaptation to human ACE2. EMBO J. (2005) 24:1634–43. doi: 10.1038/SJ.EMBOJ.7600640
4. Cheng, H, Wang, Y, and Wang, GQ. Organ-protective effect of angiotensin-converting enzyme 2 and its effect on the prognosis of COVID-19. J Med Virol. (2020) 92:726–30. doi: 10.1002/JMV.25785
5. Chen, YY, Zhang, P, Zhou, XM, Liu, D, Zhong, JC, Zhang, CJ, et al. Relationship between genetic variants of ACE2 gene and circulating levels of ACE2 and its metabolites. J Clin Pharm Ther. (2018) 43:189–95. doi: 10.1111/JCPT.12625
6. Patel, SK, Velkoska, E, Freeman, M, Wai, B, Lancefield, TF, and Burrell, LM. From gene to protein—experimental and clinical studies of ACE2 in blood pressure control and arterial hypertension. Front Physiol. (2014) 5:227–39. doi: 10.3389/FPHYS.2014.00227
7. Hoffmann, M, Kleine-Weber, H, Schroeder, S, Krüger, N, Herrler, T, Erichsen, S, et al. SARS-CoV-2 cell entry depends on ACE2 and TMPRSS2 and is blocked by a clinically proven protease inhibitor. Cell. (2020) 181:271–280.e8. doi: 10.1016/J.CELL.2020.02.052
8. Heurich, A, Hofmann-Winkler, H, Gierer, S, Liepold, T, Jahn, O, and Pöhlmann, S. TMPRSS2 and ADAM17 cleave ACE2 differentially and only proteolysis by TMPRSS2 augments entry driven by the severe acute respiratory syndrome coronavirus spike protein. J Virol. (2014) 88:1293–307. doi: 10.1128/JVI.02202-13
9. Strope, JD, PharmD, CHC, and Figg, WD. TMPRSS2: potential biomarker for COVID-19 outcomes. J Clin Pharmacol. (2020) 60:801–7. doi: 10.1002/JCPH.1641
10. Cheng, Z, Zhou, J, To KKWChu, H, Li, C, Wang, D, et al. Identification of TMPRSS2 as a susceptibility gene for severe 2009 pandemic a(H1N1) influenza and a(H7N9) influenza. J Infect Dis. (2015) 212:1214–21. doi: 10.1093/INFDIS/JIV246
11. Sharma, S, Singh, I, Haider, S, MDZ, M, Ponnusamy, K, and Rai, E. ACE2 Homo-dimerization, human genomic variants and interaction of host proteins explain high population specific differences in outcomes of COVID19. bioRxiv. (2020) 24:2020–04. doi: 10.1101/2020.04.24.050534
12. Klein, SL, Dhakal, S, Ursin, RL, Deshpande, S, Sandberg, K, and Mauvais-Jarvis, F. Biological sex impacts COVID-19 outcomes. PLoS Pathog. (2020) 16:e1008570. doi: 10.1371/JOURNAL.PPAT.1008570
13. Elgendy, IY, and Pepine, CJ. Why are women better protected from COVID-19: clues for men? Sex and COVID-19. Int J Cardiol. (2020) 315:105–6. doi: 10.1016/J.IJCARD.2020.05.026
14. Clinckemalie, L, Spans, L, Dubois, V, Laurent, M, Helsen, C, Joniau, S, et al. Androgen regulation of the TMPRSS2 gene and the effect of a SNP in an androgen response element. Mol Endocrinol. (2013) 27:2028–40. doi: 10.1210/ME.2013-1098
15. Abdelsattar, S, Kasemy, ZA, Ewida, SF, Abo-Elsoud, RAA, Zytoon, AA, Abdelaal, GA, et al. ACE2 and TMPRSS2 SNPs as determinants of susceptibility to, and severity of, a COVID-19 infection. Br J Biomed Sci. (2022) 79:10238. doi: 10.3389/BJBS.2021.10238
16. Oliveira, MC, Scharan, KO, Thomés, BI, Bernardelli, RS, Reese, FB, Kozesinski-Nakatani, AC, et al. Diagnostic accuracy of a set of clinical and radiological criteria for screening of COVID-19 using RT-PCR as the reference standard. BMC Pulm Med. (2023) 23:81. doi: 10.1186/S12890-023-02369-9
17. CDC. Coronavirus disease 2019 (COVID-19): interim guidance for antigen testing for SARS-CoV-2. Atlanta, GA: US Department of Health and Human Services, CDC (2021).
18. Peña, M, Ampuero, M, Garcés, C, Gaggero, A, García, P, Velasquez, MS, et al. Performance of SARS-CoV-2 rapid antigen test compared with real-time RT-PCR in asymptomatic individuals. Int J Infect Dis. (2021) 107:201–4. doi: 10.1016/J.IJID.2021.04.087
19. Vitošević, K, Todorović, M, Varljen, T, Slović, Ž, Matić, S, and Todorović, D. Effect of formalin fixation on pcr amplification of DNA isolated from healthy autopsy tissues. Acta Histochem. (2018) 120:780–8. doi: 10.1016/J.ACTHIS.2018.09.005
20. Graffelman, J, and Weir, BS. Testing for hardy-Weinberg equilibrium at biallelic genetic markers on the X chromosome. Heredity (Edinb). (2016) 116:558–68. doi: 10.1038/HDY.2016.20
21. Kupferschmidt, K, and Wadman, M. End of COVID-19 emergencies sparks debate. Science. (2023) 380:566–7. doi: 10.1126/SCIENCE.ADI6511
22. Rahimi, F, Darvishi, M, and Bezmin Abadi, AT. “The end” - or is it? Emergence of SARS-CoV-2 EG.5 and BA.2.86 subvariants. Future Virol. (2023) 18:823–5. doi: 10.2217/FVL-2023-0150
23. Yang, S, Yu, Y, Xu, Y, Jian, F, Song, W, Yisimayi, A, et al. Fast evolution of SARS-CoV-2 BA.2.86 to JN.1 under heavy immune pressure. Lancet Infect Dis. (2024) 24:e70–2. doi: 10.1016/S1473-3099(23)00744-2
24. Pung, R, Kong, XP, Cui, L, Chae, SR, Chen, MIC, Lee, VJ, et al. Severity of SARS-CoV-2 omicron XBB subvariants in Singapore. Lancet Reg Health West Pac. (2023) 37:100849. doi: 10.1016/J.LANWPC.2023.100849
25. Choudhary, S, Sreenivasulu, K, Mitra, P, Misra, S, and Sharma, P. Role of genetic variants and gene expression in the susceptibility and severity of COVID-19. Ann Lab Med. (2021) 41:129–38. doi: 10.3343/ALM.2021.41.2.129
26. Simadibrata, DM, Calvin, J, Wijaya, AD, and Ibrahim, NAA. Neutrophil-to-lymphocyte ratio on admission to predict the severity and mortality of COVID-19 patients: a meta-analysis. Am J Emerg Med. (2021) 42:60–9. doi: 10.1016/J.AJEM.2021.01.006
27. Martha, JW, Wibowo, A, and Pranata, R. Prognostic value of elevated lactate dehydrogenase in patients with COVID-19: a systematic review and meta-analysis. Postgrad Med J. (2022) 98:422–7. doi: 10.1136/POSTGRADMEDJ-2020-139542
28. Tipnis, SR, Hooper, NM, Hyde, R, Karran, E, Christie, G, and Turner, AJ. A human homolog of angiotensin-converting enzyme. Cloning and functional expression as a captopril-insensitive carboxypeptidase. J Biol Chem. (2000) 275:33238–43. doi: 10.1074/JBC.M002615200
29. Hong, PJ, Look, DC, Tan, P, Shi, L, Hickey, M, Gakhar, L, et al. Ectodomain shedding of angiotensin converting enzyme 2 in human airway epithelia. Am J Phys Lung Cell Mol Phys. (2009) 297:L84–96. doi: 10.1152/AJPLUNG.00071.2009
30. Li, F, Berardi, M, Li, W, Farzan, M, Dormitzer, PR, and Harrison, SC. Conformational states of the severe acute respiratory syndrome coronavirus spike protein ectodomain. J Virol. (2006) 80:6794–800. doi: 10.1128/JVI.02744-05
31. Chaudhary, M. COVID-19 susceptibility: potential of ACE2 polymorphisms. Egyptian J Med Human Genetics. (2020) 21:1–8. doi: 10.1186/S43042-020-00099-9
32. Beyerstedt, S, Casaro, EB, and Rangel, ÉB. COVID-19: angiotensin-converting enzyme 2 (ACE2) expression and tissue susceptibility to SARS-CoV-2 infection. Eur J Clin Microbiol Infect Dis. (2021) 40:905–19. doi: 10.1007/S10096-020-04138-6
33. González-Rayas, JM, Rayas-Gómez, AL, García-González, JJ, González-Yáñez, JM, Hernández-Hernández, JA, López-Sánchez, R, et al. COVID-19 and ACE -inhibitors and angiotensin receptor blockers-: the need to differentiate between early infection and acute lung injury. Revista Colombiana de Cardiología. (2020) 27:129–31. doi: 10.1016/J.RCCAR.2020.04.005
34. Sriram, K, and Insel, PA. A hypothesis for pathobiology and treatment of COVID-19: the centrality of ACE1/ACE2 imbalance. Br J Pharmacol. (2020) 177:4825–44. doi: 10.1111/BPH.15082
35. Lu, Y, Zhu, Q, Fox, DM, Gao, C, Stanley, SA, and Luo, K. SARS-CoV-2 down-regulates ACE2 through lysosomal degradation. Mol Biol Cell. (2022) 33:ar147. doi: 10.1091/mbc.E22-02-0045
36. Kuba, K, Imai, Y, Rao, S, Gao, H, Guo, F, Guan, B, et al. A crucial role of angiotensin converting enzyme 2 (ACE2) in SARS coronavirus–induced lung injury. Nat Med. (2005) 11:875–9. doi: 10.1038/NM1267
37. Koka, V, Xiao, RH, Chung, ACK, Wang, W, Truong, LD, and Lan, HY. Angiotensin II up-regulates angiotensin I-converting enzyme (ACE), but down-regulates ACE2 via the AT1-ERK/p38 MAP kinase pathway. Am J Pathol. (2008) 172:1174–83. doi: 10.2353/AJPATH.2008.070762
38. de Queiroz, TM, Lakkappa, N, and Lazartigues, E. ADAM17-mediated shedding of inflammatory cytokines in hypertension. Front Pharmacol. (2020) 11:535849. doi: 10.3389/FPHAR.2020.01154/BIBTEX
39. Fagyas, M, Fejes, Z, Sütő, R, Nagy, Z, Székely, B, Pócsi, M, et al. Circulating ACE2 activity predicts mortality and disease severity in hospitalized COVID-19 patients. Int J Infect Dis. (2022) 115:8–16. doi: 10.1016/J.IJID.2021.11.028
40. Huang, G, Wang, Y, Qin, L, Huang, B, and Yu, X. Association and functional analysis of angiotensin-converting enzyme 2 genetic variants with the pathogenesis of pre-eclampsia. Front Endocrinol (Lausanne). (2022) 13:926512. doi: 10.3389/FENDO.2022.926512
41. Luo, YS, Luo, L, Li, W, Chen, Y, Wu, GF, Chen, F, et al. Evaluation of a functional single nucleotide polymorphism of the SARS-CoV-2 receptor ACE2 that is potentially involved in long COVID. Front Genet. (2022) 13:931562. doi: 10.3389/fgene.2022.931562
42. Sabater Molina, M, Nicolás Rocamora, E, Bendicho, AI, Vázquez, EG, Zorio, E, Rodriguez, FD, et al. Polymorphisms in ACE, ACE2, AGTR1 genes and severity of COVID-19 disease. PLoS One. (2022) 17:e0263140. doi: 10.1371/JOURNAL.PONE.0263140
43. Mohammadi-Berenjestanaki, H, Mohammadali, E, Khasayesi, M, Rafiei, A, Kashi, Z, Mirzaei-Ilali, N, et al. Association between angiotensin-converting enzyme-2 gene polymorphism (rs2106809) with severity and outcome of COVID-19 infection. Mol Biol Rep. (2023) 50:6669–79. doi: 10.1007/S11033-023-08493-3
44. Tukiainen, T, Villani, AC, Yen, A, Rivas, MA, Marshall, JL, Satija, R, et al. Landscape of X chromosome inactivation across human tissues. Nature. (2017) 550:244–8. doi: 10.1038/nature24265
45. Heard, E, Chaumeil, J, Masui, O, and Okamoto, I. Mammalian X-chromosome inactivation: an epigenetics paradigm. Cold Spring Harb Symp Quant Biol. (2004) 69:89–102. doi: 10.1101/SQB.2004.69.89
46. Viveiros, A, Rasmuson, J, Vu, J, Mulvagh, SL, Yip, CYY, Norris, CM, et al. Sex differences in COVID-19: candidate pathways, genetics of ACE2, and sex hormones. Am J Physiol Heart Circ Physiol. (2021) 320:H296–304. doi: 10.1152/ajpheart.00755.2020
47. Namipashaki, A, Razaghi-Moghadam, Z, and Ansari-Pour, N. The essentiality of reporting hardy-Weinberg equilibrium calculations in population-based genetic association studies. Cell J. (2015) 17:187–92. doi: 10.22074/CELLJ.2016.3711
48. Lachance, J. Hardy–Weinberg equilibrium and random mating. Encyclopedia of Evolutionary Biol. (2016) 2:208–11. doi: 10.1016/B978-0-12-800049-6.00022-6
49. Chen, YW, Lee, MS, Lucht, A, Chou, FP, Huang, W, Havighurst, TC, et al. TMPRSS2, a serine protease expressed in the prostate on the apical surface of luminal epithelial cells and released into semen in prostasomes, is misregulated in prostate cancer cells. Am J Pathol. (2010) 176:2986–96. doi: 10.2353/AJPATH.2010.090665
50. Bertram, S, Heurich, A, Lavender, H, Gierer, S, Danisch, S, Perin, P, et al. Influenza and SARS-coronavirus activating proteases TMPRSS2 and HAT are expressed at multiple sites in human respiratory and gastrointestinal tracts. PLoS One. (2012) 7:e35876. doi: 10.1371/JOURNAL.PONE.0035876
51. Donaldson, SH, Hirsh, A, Li, DC, Holloway, G, Chao, J, Boucher, RC, et al. Regulation of the epithelial sodium channel by serine proteases in human airways. J Biol Chem. (2002) 277:8338–45. doi: 10.1074/JBC.M105044200
52. Matsuyama, S, Nao, N, Shirato, K, Kawase, M, Saito, S, Takayama, I, et al. Enhanced isolation of SARS-CoV-2 by TMPRSS2-expressing cells. Proc Natl Acad Sci USA. (2020) 117:7001–3. doi: 10.1073/PNAS.2002589117
53. Matic, S, Milovanovic, D, Mijailovic, Z, Djurdjevic, P, Sazdanovic, P, Stefanovic, S, et al. Its all about IFN-λ4: protective role of IFNL4 polymorphism against COVID-19-related pneumonia in females. J Med Virol. (2023) 95:e29152. doi: 10.1002/JMV.29152
54. Schuler, BA, Habermann, AC, Plosa, EJ, Taylor, CJ, Jetter, C, Negretti, NM, et al. Age-determined expression of priming protease TMPRSS2 and localization of SARS-CoV-2 in lung epithelium. J Clin Invest. (2021) 131:e140766. doi: 10.1172/JCI140766
55. Chen, Z, Song, X, Li, Q, Xie, L, Guo, T, Su, T, et al. Androgen receptor-activated enhancers simultaneously regulate oncogene TMPRSS2 and lncRNA PRCAT38 in prostate Cancer. Cells. (2019) 8:864–82. doi: 10.3390/CELLS8080864
56. Li, F, Han, M, Dai, P, Xu, W, He, J, Tao, X, et al. Distinct mechanisms for TMPRSS2 expression explain organ-specific inhibition of SARS-CoV-2 infection by enzalutamide. Nat Commun. (2021) 12:866. doi: 10.1038/S41467-021-21171-X
57. Irham, LM, Chou, WH, Calkins, MJ, Adikusuma, W, Hsieh, SL, and Chang, WC. Genetic variants that influence SARS-CoV-2 receptor TMPRSS2 expression among population cohorts from multiple continents. Biochem Biophys Res Commun. (2020) 529:263–9. doi: 10.1016/J.BBRC.2020.05.179
58. Pandey, RK, Srivastava, A, Singh, PP, and Chaubey, G. Genetic association of TMPRSS2 rs2070788 polymorphism with COVID-19 case fatality rate among Indian populations. Infect Genet Evol. (2022) 98:105206. doi: 10.1016/J.MEEGID.2022.105206
59. de Andrade, CC, Silva, ATP, Vasconcelos, LRS, Oliveira, PRS, de Souza, CDF, da Costa, AA, et al. A polymorphism in the TMPRSS2 gene increases the risk of death in older patients hospitalized with COVID-19. Viruses. (2022) 14:2557–65. doi: 10.3390/V14112557
60. Smail, SW, Babaei, E, and Amin, K. Demographic, clinical and genetic factors associated with COVID-19 disease susceptibility and mortality in a Kurdish population. Ann Saudi Med. (2023) 43:125–42. doi: 10.5144/0256-4947.2023.125
61. Schönfelder, K, Breuckmann, K, Elsner, C, Dittmer, U, Fistera, D, Herbstreit, F, et al. Transmembrane serine protease 2 polymorphisms and susceptibility to severe acute respiratory syndrome coronavirus type 2 infection: a German case-control study. Front Genet. (2021) 12:667231. doi: 10.3389/FGENE.2021.667231
Keywords: ACE2, TMPRSS2, COVID-19, severity, outcome
Citation: Matic S, Milovanovic D, Mijailovic Z, Djurdjevic P, Stefanovic S, Todorovic D, Vitosevic K, Canovic V, Popovic S, Dimitrijevic NM, Zivanovic M, Seklic D, Aleksic S, Djordjevic N, Vukic M, Vukovic N, Filipovic N, Baskic D and Djordjevic N (2024) ACE2 and TMPRSS2 genetic polymorphisms as potential predictors of COVID−19 severity and outcome in females. Front. Med. 11:1493815. doi: 10.3389/fmed.2024.1493815
Edited by:
Vivek P. Chavda, L M College of Pharmacy, IndiaReviewed by:
Crystell Guadalupe Guzmán Priego, Universidad Juárez Autónoma de Tabasco, MexicoRutvi Vaidya, Uka Tarsadia University, India
Copyright © 2024 Matic, Milovanovic, Mijailovic, Djurdjevic, Stefanovic, Todorovic, Vitosevic, Canovic, Popovic, Dimitrijevic, Zivanovic, Seklic, Aleksic, Djordjevic, Vukic, Vukovic, Filipovic, Baskic and Djordjevic. This is an open-access article distributed under the terms of the Creative Commons Attribution License (CC BY). The use, distribution or reproduction in other forums is permitted, provided the original author(s) and the copyright owner(s) are credited and that the original publication in this journal is cited, in accordance with accepted academic practice. No use, distribution or reproduction is permitted which does not comply with these terms.
*Correspondence: Dejan Baskic, ZGVqYW4uYmFza2ljQGdtYWlsLmNvbQ==