- Rheumatology Unit, University of Verona, Verona, Italy
Osteoporosis is a skeletal disorder characterized by impaired bone strength and increased risk of fragility fracture and is among the most relevant comorbidities of rheumatic diseases. The purpose of the present review is to discuss the pathogenesis of local and systemic bone involvement in inflammatory arthritides, especially Rheumatoid Arthritis, Psoriatic Arthritis, and Spondyloarthritides, as well as the effect of anti-rheumatic treatments and anti-osteoporotic medication on bone health and fracture incidence, including recent data on novel therapeutic perspective.
Introduction
Inflammatory arthritides are frequently associated to systemic skeletal complications, such as osteoporosis and fragility fractures. Herein, we presented a review of the available literature on osteoporosis in inflammatory arthritides, with a special focus on Rheumatoid Arthritis (RA), Psoriatic Arthritis (PsA), and Spondyloarthritides (SpA). We also concentrated on the effects of anti-osteoporotic medications on the skeletal involvement in such diseases and on the effects of anti-rheumatic treatments on bone heath and fracture incidence.
Rheumatoid Arthritis
Pathogenesis of Osteoporosis in Rheumatoid Arthritis
RA is a chronic systemic disease characterized by pain and joint inflammation with a prominent involvement of the small joints of the hands and feet, leading to joint deformity and functional loss (1).
Due to the presence of a variable grade of systemic inflammation, many comorbidities can accompany the joint involvement, such as extra-articular manifestations and osteoporosis (2–4).
Osteoporosis (OP) is a relevant comorbidity of RA and is caused by the concurrence of several factors such as systemic inflammation, cytokine secretion and circulating autoantibodies. Moreover, the frequent and chronic use of glucocorticoids in RA can also exacerbate the development of OP. Despite adequate strategies in OP primary prevention, such as vitamin D and calcium repletion, most patients will develop OP during their disease history (5).
There are several mechanisms involved in the pathogenesis of OP in RA patients, which is characterized by two main features: local and systemic bone loss (5).
Local bone loss, also called periarticular osteopenia, is characterized by both an impairment of trabecular and cortical bone. Indeed, RA patients have an increased cortical porosity with lower volumetric bone mineral density (vBMD) (6, 7). The cortical thinning has been demonstrated to affect the entire surface of the bone but it is particularly pronounced at the insertion of the synovia, a susceptibility that drives the genesis of bone erosions in RA (5, 8).
Periarticular bone loss, generally preempted by the presence of bone marrow edema (5), appears during an early stage of the disease and is independently associated with the development of bone erosions (9).
The pathogenesis of local bone loss is multifactorial, and many mechanisms contribute to it, such as proinflammatory cytokine secretion (es. IL-6 and TNF) (6, 7), T-cell derived receptor activator of nuclear factor kappa B ligand (RANKL) hyperexpression (10, 11) and a hypothesized direct effect of autoantibodies against citrullinated proteins (ACPA) (7, 12, 13). It was demonstrated that RA patients develop ACPA years before the clinical onset of the arthritis and frequently local bone loss is already present during the preclinical phase of the disease (14, 15). Moreover, the presence of local bone loss at the metacarpal site, detected with High Resolution peripheral Quantitative Computed Tomography (HRpQCT), was observed in RA patients with ACPA positivity, but not in ACPA-negative subjects or in other seronegative inflammatory arthritides such as psoriatic arthritis (PsA) (16). Indeed, ACPA positivity in PsA patients was associated with an erosive form of the disease, strengthening the theory of a direct role played by these antibodies in causing periarticular bone loss (17). This effect might be potentially mediated by a direct stimulation of autoantibodies and osteoclasts FcγR, enhancing osteoclasts maturation (18). In addition macrophages, stimulated by autoantibodies, release inflammatory cytokines which might be able to enhance osteoclast differentiation and function (18).
Osteopenia affects both trabecular and cortical bone, but cortical sites, such as femoral neck and distal radius, seem to be maximally involved (19). Several mechanisms contribute to the detrimental effect on bone homeostasis, overlapping their pathogenetic role on local bone loss.
Inflammatory cytokines such as TNF, IL-6, IL-1, and immune cell-derived RANKL stimulate osteoclastogenesis, while decreasing osteoblastogenesis (20). Moreover, higher levels of circulating senescent CD4+ CD28- T cells were found in RA patients with lower BMD. This subpopulation is known to present a major expression of RANKL compared to CD28+ T cells and to induce osteoclastogenesis in a more efficient way (11).
Furthermore, a subset of patients with RA develops functional antibodies to osteoprotegerin (OPG) and the presence of these antibodies has been associated to higher disease activity and increased bone resorption (21).
Metabolic factors have a central role as well. Dickkopf-related protein 1 (Dkk-1), a Wnt signaling inhibitor, is involved in bone remodeling and OP development (22) and greater serum levels of this inhibitor of bone formation were detected in RA patients compared to healthy controls (23, 24).
As previously discussed, ACPA positivity is an independent risk factor for local bone loss in RA patients, but their action seems to be detrimental also for systemic bone loss. Indeed, a correlation between the low BMD and ACPA serum titer has been demonstrated recently (25).
Systemic and local bone loss are strictly linked and the presence of systemic bone loss, found in almost 60% of early RA patients, is a strong predictor of radiographic joint damage (26). Moreover, a possible association between low systemic BMD and atlantoaxial subluxation occurrence was suggested in subjects affected by RA (27). This evidence suggests that OP could increase susceptibility to bone erosions in RA patients (28, 29).
Chronic treatment with glucocorticoids represents an independent risk factor for the premature onset of OP in RA patients, even if there is still some controversy regarding the safe dosage for bone health. Indeed low-dose and short-term treatment with glucocorticoids in patients with active RA may have a favorable effect by reducing bone resorption driven by systemic inflammation, as seen in many studies and meta-analyses that showed non-significative BMD changes in low-dose glucocorticoid users affected by active RA (30–33). Nevertheless, glucocorticoids have a well-documented detrimental effect on bone homeostasis (34–36) and other studies showed that even low-doses or intra-articular glucocorticoids may have a relevant role in increasing the risk of fracture in RA patients (37, 38).
Not surprisingly, post-menopause is a supplemental risk factor for OP in RA women (39).
Epidemiology of OP in RA
As previously discussed, RA patients have lower BMD levels at lumbar spine and femoral sites than healthy subjects and this difference can be detected also in an early phase of the disease.
The prevalence of osteoporosis and osteopenia is estimated to be doubled in RA patients as compared to healthy controls (40, 41) with a prevalence ranging from 30 to 50% (40, 42, 43). The risk of developing OP in RA is correlated with the duration and the severity of the disease (19, 44) and even RA pre-menopausal women or men are exposed to a greater risk of osteoporosis in comparison with age- and sex-matched healthy controls (45, 46). A prospective longitudinal study on 379 patients identified some biomarkers predictive of BMD change in patients with RA (47). It was found that the annual BMD change at the lumbar spine had a significant association with glucocorticoids use, bisphosphonate or vitamin D use, and homocysteine. On the other hand, BMD changes at the femur were associated with DAS28, C-reactive protein (CRP), and ACPA titer (47). These results further highlight that there is a strict association between cortical bone health (femoral site) and disease activity while trabecular bone is more affected by classical risk factors (i.e., vitamin D assumption, glucocorticoid use).
Albeit OP is a major problem in the management of RA patients, the percentage of patients receiving calcium and vitamin D supplementation is about 45% and only 5.4% are treated with bisphosphonates (48).
RA Therapy and OP
Several studies investigated the effect of conventional disease modifying anti-rheumatic drugs (cDMARDs) on bone turnover markers in RA patients (49). Overall, the body of evidence orients toward a decrease in osteoclastic activity induced by cDMARDs, leading to a positive effect on bone mineral density. However, it is not clear if such positive effect corresponds to a fracture risk reduction (50).
Again, controversial results have been found regarding the effect of biological DMARDs (bDMARDs) on BMD and bone markers changes, possibly in relation to the presence of confounding factors such as vitamin D deficiency or corticosteroid therapy (51–56). However, treatment with biologics seemed to be associated with a decrease in bone loss (52).
The first molecules to be studied among bDMARDs were TNF inhibitors, which were associated with a significant, although small, reduction of the vertebral fracture risk in RA patients (57).
A recently published study investigated the effect of TNF inhibitors on BMD and bone biomarkers in patients with RA and ankylosing spondylitis (AS) (58). It was demonstrated that 12 months treatment with anti-TNF drugs prevented further generalized bone loss at both lumbar spine and femoral neck. Moreover, it was found an inverse correlation of baseline C reactive protein (CRP) levels with BMD values, both at baseline measurement and after 12 months treatment, suggesting that baseline high-grade inflammation was associated with lower BMD (58).
IL-6 blocking agents showed an efficacy in reducing systemic bone resorption. As highlighted in a substudy of the OPTION trial, a decrease in bone degradation markers was found in patients treated with tocilizumab, an anti-IL6 agent, plus methotrexate (MTX) as compared to MTX plus placebo (59). In addition, the RADIATE study confirmed such finding in anti-TNF refractory patients (60). Moreover, IL-6 blocking agents were successful in reducing RA localized bone loss, as discussed in a study performed by Axmann et al. (61). Indeed, they found that IL-6 blockade reduced bone erosion in TNF-α transgenic murine models through a direct effect on osteoclast activity which was independent from the anti-inflammatory action (61). Other studies on human subjects further confirmed the positive effect of tocilizumab on bone erosions (62) and systemic bone loss, especially in ACPA positive patients (52, 63).
In 2012 the first Janus Kinases (JAK) Inhibitor was approved for the treatment of RA. The data on the efficacy of these small molecules in reducing systemic bone loss are scarce. A small pilot study showed that tofacitinib, possibly by halting inflammation, can regulate the secretion of serum RANKL and OPG, with a favorable effect on the RANKL/OPG ratio. The interesting speculation of the authors was that tofacitinib could regulate synovial hyperexpression of RANKL via the inhibition of the secretion of IL-17 and IL-6. These effects on RANKL promoted an increase in the osteocalcin levels from 6.9 ± 4.3 ng/mL at the baseline to 8.8 ± 6.1 after 12 weeks of treatment with a concomitant reduction in NTx serum levels (64).
OP Treatment in RA
Bisphosphonates (BPs) are widely used in post-menopausal OP treatment, because of their attested efficacy in fracture prevention with an acceptable safety profile (65, 66). The majority of data on fracture risk reduction in RA patients of these medications are available only from indirect evidence coming from glucocorticoid induced osteoporosis (GIOP) clinical trials (67). In a 2006 placebo-controlled RCT RA patients on glucocorticoids were randomized to receive placebo or daily doses of alendronate (68). A significant difference was found between the two groups in BMD increase at lumbar spine and femoral site (major in the alendronate group), although without any relevant difference in fracture reduction (68). However, the RCT was not powered to detect any fracture difference.
A recent study explored the effect of zoledronic acid on secondary osteoporosis in 66 RA patients (69). Patients were randomized into three groups: combination-therapy (zoledronic acid and methotrexate), zoledronic acid monotherapy and methotrexate monotherapy and followed over a 12 months period. A significant improvement in lumbar spine and hip BMD within the combination-therapy group was demonstrated.
Another recent study (RISOTTO study) investigated efficacy of sodium Risedronate for glucocorticoid-induced osteoporosis in patients with RA finding a significant increase in lumbar BMD in the Risedronate group compared to the placebo one after 6-month treatment, with no significant difference in femoral neck and total hip BMD (70).
Denosumab is a human monoclonal antibody against RANKL (51, 71).
A recent study showed that denosumab was superior in improving spine and hip BMD to risedronate in GIOP patients, 40% of them affected by RA (72). The 24 months extension of this study, further confirmed the superiority of denosumab over risedronate, albeit no significant differences were found in fracture incidence (73). However, as opposite to the FREEDOM study, which was performed on >7,000 patients followed for 3 years, this GIOP study didn't have an adequate statistical power to detect fracture differences between denosumab and risedronate (an active comparator) (71).
A clinical observational study, recently published, showed the results of denosumab therapy over a 3-years period within two groups of female patients, the former with RA, the latter with primary OP (74). The ΔBMD for the total hip, femoral neck and lumbar spine as well as ΔP1NP did not differ significantly between the two groups at any time points (1, 2, and 3 years assessment), hence, denosumab treatment for osteoporosis had a similar efficacy over 3 years among women with RA and OP.
Another randomized, placebo-controlled study investigated the effects of Denosumab combined with Methotrexate on bone erosion progression in Japanese patients with RA (75, 76). Denosumab significantly inhibited radiographic progression of the disease.
In a recently published study these data have been confirmed (77). This study, named DESIRABLE study, proved the efficacy of denosumab in reducing erosions when added to csDMARD therapy in RA patients. Since there was no improvement in disease activity level, it is reasonable presuming that denosumab action is driven only by a direct effect on bone metabolism rather than an additional indirect way on immune system cells (2). Moreover, denosumab prevented bone erosion but it did not prevent joint space narrowing (77–79), showing its effect in impairing bone destruction but not cartilage destruction. In addition, no differences in infection rate were found between the two groups (77).
Also combined with biological DMARDs (bDMARDs) denosumab was attested to be more efficient in reducing radiographic progression rather than bDMARDs alone (80, 81).
Moreover, switching from bisphosphonates to denosumab significantly reduced radiographic joint destruction compared to continuing bisphosphonates (82). In the same cohort, switching from bisphosphonates to teriparatide was associated with a worse outcome on bone erosions. This result was somehow expected given the detrimental effect of teriparatide on cortical bone health (83).
Romosozumab is a humanized monoclonal antibody that binding to sclerostin neutralizes its blocking effect on Wnt signaling bone formation pathway (84). So romosozumab increases bone formation and, differently from classical osteoanabolic agents, suppresses bone resorption. The role of romosozumab in RA patients or GIOP patients is still unclear. In particular, caution should be taken regarding sclerostin inhibition in RA patients. Indeed, sclerostin inhibition was shown to induce an exaggerated inflammatory response in an arthritis mouse model by promoting TNF secretion (85).
New suggestions for future treatment perspectives have been debated in a very recent review by Gambari et al. which explored the mechanisms regulating monocytes/macrophages fusion and multinucleation (M-FM), a key process to generate mature multinucleated cells such as giant cells (GCs) and osteoclasts (OCs), depending on environment features (86). As discussed in the article, targeting M-FM process in OP therapy might be an interesting alternative to decrease OCs function while preserving osteoblasts (OBs)-OCs communication. In this field, different molecules have been found, like miRNA, that can specifically inhibit M-FM and could be a challenging area to be explored. But targeting the process of monocyte-macrophage differentiation could have interesting implications also for RA therapy strategies. In fact, activated macrophages has an established role in feeding the inflammatory milieu at the synovium site in RA, leading to bone remodeling and erosions; indeed drugs targeting inflammatory cytokines such as TNF, IL-1, and IL-6 have a significative impact on osteoclastogenesis and prevent generalized and local bone loss. A new intriguing opportunity might be to modulate the activation status of macrophages by targeting macrophage fusion and multinucleation, instead of inflammatory cytokines, as a way for preventing an excessive OCs and GCs formation and inflammatory activation (86). In this perspective F-MF inhibition, a mechanism already listed for OP, might be helpful also for preventing bone loss in RA. However, albeit good promises as therapeutic strategy for OP and RA bone loss, clinical trials are mandatory to validate the efficacy, safety and potential superiority of multinucleation inhibitors to current drugs.
Psoriatic Arthritis and Spondyloarthritis
Psoriatic arthritis (PsA) is an inflammatory musculoskeletal disease commonly associated with psoriasis (PsO), that can be characterized by heterogeneous manifestations, including peripheral arthritis, axial involvement, enthesitis, and dactylitis (87). PsA has an estimated prevalence in the general population of 0.05–0.25%, with little discrepancy depending on the country examined (87, 88). Many comorbidities are associated with PsO/PsA such as increased cardiovascular risk, diabetes, osteoporosis, metabolic syndrome, ophthalmic disease, depression, and anxiety (89).
Epidemiology of OP in PsA and the Other Spondyloarthritides
Several observational studies have investigated the association between psoriasis or psoriatic arthritis and low BMD values or osteoporosis, with conflicting results.
A cross-sectional study performed on a large population sample with PsO or PsA found that these conditions were significantly associated with osteopenia, osteoporosis, and fragility fractures (90). Moreover, a 2013 population-based analysis showed a significant association between osteoporosis and previous diagnosis of psoriasis in both sexes (91).
On the contrary, a Norwegian study based on hospital-derived fractures data found no association between psoriasis and forearm/hip fracture risk or between psoriasis and osteoporosis (92). Moreover, no differences were found in lumbar spine BMD between Spanish patients with or without PsA (93) and similar findings were underlined during another study conducted in Norway (94).
In 2020, Xia et al. explored the correlation between psoriasis, psoriatic arthritis and osteoporosis considering potential confounding factors that could justify the aforementioned controversial results (95). Conditional regression analyses were performed, and three models were examined: model 0, which included age, height, weight, smoking, and drinking status, model 1, which included all the factors comprised in model 0 plus regular physical activity and model 2, which included all the factors of model 1 plus concomitant medications. In their analyses no estimated bone mineral density (eBMD) difference was highlighted between patients with psoriasis (without arthritis) and healthy controls, therefore psoriasis alone was not correlated to osteopenia and osteoporosis.
More interestingly, it was found that PsA was significantly associated with low eBMD levels even after adjustment for confounding factors in model 0 (age, sex, height, weight, smoking, and drinking status), but this significance became less strong when physical activity was included. Moreover, the association between PsA and low eBMD levels disappeared when conditioning on treatment with methotrexate or ciclosporin (model 2) (95). Therefore, PsA was associated with low eBMD and higher risk of osteopenia, but it seems that this association was somehow mediated by the medical treatment (such as methotrexate or ciclosporin). Furthermore, using a systematic Mendelian Randomization (MR) in order to explore the relationship between PsO/PsA and osteoporosis no causal effects for these factors on BMD score and viceversa were found, reinforcing the idea that the increased risk might be mediated by the treatment and not by the disease itself (95).
However, it's to be noted that all these data refer to quantitative ultrasound (QUS) estimated BMD at heel site, which has high specificity but whose sensitivity to predict BMD as defined by dual-energy x-ray absorptiometry (DEXA) can be significantly variable depending on QUS parameters.
A recently published study compared the incidence of fragility fractures in patients with PsA matched with controls by age and sex (96) and no differences were found in the overall fracture incidence rate. This result was true even for vertebral fractures, that were apparently more frequent in PsA patients compared to controls, but this difference did not reach the statistical significance (96).
Beyond PsA, data on the other Spondyloarthritides (SpA) with axial involvement (axSpA), such as Ankylosing Spondylitis (AS), are heterogeneous (51). Moreover, in patients with axSpA the proper evaluation of vertebral deformities and BMD levels is frequently altered by the presence of syndesmophytes and periosteal bone proliferation (97).
Nevertheless, several data confirm that axSpA patients have lower BMD levels as compared to healthy controls even in the early stage of the disease, independently from spine mobility and exercise (98, 99). The mechanism involved in this process is, at least in part, related to the prevalent inflammation, which can contribute to local bone loss especially in those sites interested by Bone Marrow Edema (BME)/osteitis (100, 101). In fact, inflammation leads to trabecular bone loss, which is associated with the presence of lesions defined as BME/osteitis on MRI that quintuplicate the risk of finding low spine BMD. Hence, the presence of inflammation signs on MRI represents the main risk factor associated with low BMD in axSpA.
Interestingly, the lumbar spine seemed to be more susceptible to bone loss in patient with non-radiographic axSpA (nr-axSpA) as compared to the femur (102).
In addition, the estimated prevalence of osteopenia and osteoporosis within 10 years from the onset of AS is about 51–54% and 13–16%, respectively (99).
Results from a primary care-based nested case-control study showed that AS patients had a higher risk of clinical vertebral fractures without an increased risk of non-vertebral fractures, further highlighting that AS is a risk factor for fractures, but limited to the axial skeleton (103).
In order to find more effective methods to predict the risk of fragility fractures in AS patients, a recent study investigated the usefulness of the trabecular bone score (TBS) in assessing bone strength in patients with AS compared with DEXA (104). TBS showed promising results in improving the ability to detect patients at high risk of fractures, especially in patients with normal or osteopenic BMD levels at standard DEXA (104).
Another recent study investigated the effect of vertebral ankylosis on scanographic bone attenuation coefficient (SBAC), measured from L1 to L5, in AS patients (105). It was found that patients with at least one bony bridge had lower SBAC values, while there was a correlation between the presence of full ankylosis and the probability of presenting SBAC ≤145 HU (fracture threshold), suggesting an impact of ankylosed vertebrae on trabecular bone deterioration.
Bone Remodeling in PsA and the Other Spondyloarthritides
In contrast to RA, PsA, and the other SpA, such as AS, are characterized by the simultaneous occurrence of both bone resorption and bone formation signs, the latter of which has not been observed in patients with RA (106).
While most of the mechanisms leading to bone erosions are well-understood and overlap those in RA (107), part of the mechanisms of bone formation in SpA remain unknown. Pro-inflammatory cytokines (such as TNF-α and IL-17) and signaling pathway (including Wnt pathway) have been shown to be involved (108). IL-17, the prevailing inflammatory cytokine in many patients with SpA (109), has been shown to promote both OC bone resorption and OB bone formation (109), while TNFα has a greater effect on promoting bone erosion and suppressing OBs function. Entheses, are poorly populated by OCs and when reached by the IL-17 inflammatory stimulus, bone formation may be induced in a way that can overcome bone resorption (109).
Nevertheless, despite several data, many features of this process remain still incompletely clarified.
IL-23 is another distinctive cytokine that is abundantly present in the affected tissues of PsA patients. IL-23 is generally overexpressed in PsA, leading to an upregulation of IL-22 and consequently to osteoblast-related genes induction, which eventually results in osteoblast expansion and enthesophytes formation (110). Moreover, a recent study exploring Wnt pathway regulators found significantly lower levels of Dkk-1 in patients with PsA rather than RA or healthy controls, suggesting another possible explanation for the different bone phenotype between RA (erosive) and PsA (erosive-proliferative) (111). Furthermore, possibly in relation to a negative feedback response, a rapid increase in Dkk-1 after 6 months of secukinumab, an anti-IL17 antibody, was recently observed (112). This finding might well-explain the promising results that IL-17 blockade had achieved in halting the syndesmophytes progression in AS. Indeed, in this setting, the raise of Dkk-1 might be even beneficial by stopping the bone proliferation induced by the Wnt pathway.
Moreover, a dysregulation in RANK-RANKL pathway had been reported in patients with AS. Increased expression of intracellular RANKL levels in CD4+ and CD8+ T cells and a significantly lower expression of membrane-bound RANKL were found in AS patients (113).
A 2016 study explored the association of Dkk-1 levels with low BMD and vertebral fracture prevalence among patients with AS (114). AS patients had lower levels of Dkk-1 compared to healthy controls; however Dkk-1 serum levels inversely correlated with lumbar spine Z-score BMD and higher serum levels of Dkk-1 were associated with a higher prevalence of 1 or more vertebral fractures. So serum Dkk-1 titer, even though lower in AS patients, than in healthy controls, appears to be associated with an increased risk of severe osteoporosis (114).
Furthermore, a correlation between Dkk-1 and PTH was observed, with higher levels of PTH and lower levels of Dkk-1 measured in AS patients rather than healthy controls (115). Then dividing the patients in two equal groups according to disease duration, the association between PTH and Dkk-1 remained only in the group with longer disease duration, where Dkk-1 levels were also correlated with higher CTX and lower BMD (Z-score ≤ – 1) (115).
In addition anti-OPG antibodies has been isolated in a cohort of SpA patients and a correlation with low BMD and fractures was found (116).
In summary, multiple players are involved in bone remodeling of PSA/SpA, with a combination of systemic effects mainly driven by inflammatory cytokines and metabolic factors.
SpA Therapy and OP
Non-steroidal anti-inflammatory drugs (NSAIDs) play a pivotal role in the treatment of SpA, especially in those with an axial involvement (axSpA), in whom NSAIDs represent the first line treatment (117). However, despite their wide use, few data have been collected about their possible effects on bone (51). A case-control study found a decreased risk of any clinical fracture in patients with AS treated with NSAIDs (103). Moreover, data from a large population-based public health database supported the protective role of NSAIDs on clinical fracture risk in SpA patients, highlighting a higher fracture risk in those not assuming chronic NSAIDs (118).
TNF inhibitors use was associated with an increase in lumbar spine and total hip BMD and maintenance of femoral neck BMD for up to 2 years in patients with AS (119). Another study investigated the effects of 4-years course with anti-TNFs in patients with long-term AS and albeit no differences were found in the incidence of fragility fractures the authors found an improvement in BMD levels during 4 years period (120).
Moreover, a single-center study explored the effect of the TNF-blocker infliximab vs. i.v. neridronate (a potent amino-bisphosphonate) on bone turnover and disease activity after 3–6 months treatment in AS patients (121). As regards bone metabolism, no significant BMD variations were observed at 6 months in the infliximab group, while a significant BMD increase at LS site, was found in the bisphosphonate arm (p<0.05 vs baseline and vs infliximab) (121). In addition i.v. neridronate was as effective as infliximab in controlling the leading symptoms (as assessed by BASDAI or BASFI) of AS even without, as expected, any changes in systemic inflammation parameter (CRP and ESR), suggesting that, at least in part, the symptoms of AS are related to bone metabolic factors.
In fact, as known (122), bisphosphonates reduce bone resorption, which is elevated mainly in the early stages of AS. Furthermore, through the coupling of osteoclast and osteoblast activity, this mechanisms leads to later inhibition also of bone formation and causes an increase in serum sclerostin, which is low in AS and negatively correlated to the development of syndesmophytes (123, 124).
As concerning PsA, a recent study compared the effects of methotrexate (MTX) with bDMARDs on bone structure and biomechanical properties (125). It was found that bDMARD-treated patients had higher bone mass and better bone strength than patients receiving MTX or no DMARDs (despite longer disease duration in bDMARDs-treated group) (125).
JAK-inhibitors had been recently approved for the treatment of SpA. While a reduction in articular bone erosion in RA and PsA patients has been shown during treatment with tofacitinib, still no data on BMD or osteoporosis has been published (110).
Conclusions
OP is a hallmark of inflammatory arthritides. Its pathogenesis is manly driven by the predominant inflammation, notwithstanding that, other metabolic factors are increasingly believed to play a crucial role in the development of OP and fragility fractures in such diseases. Novel therapeutic agents have been approved for the treatment of inflammatory arthritides and their role in preventing or even treating osteoporosis is becoming clearer.
In the figure above (Figure 1) the principal targets of bDMARDs and anti-osteoporotic drugs previously discussed are summarized.
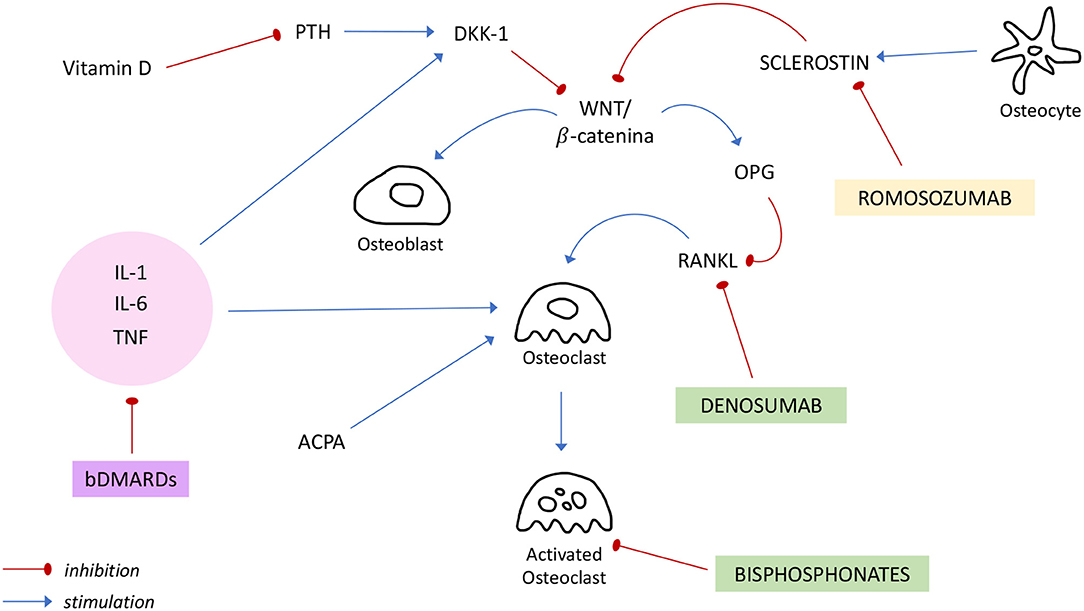
Figure 1. Regulation of bone metabolism and mechanisms of action of bDMARDs and anti-osteoporotic drugs. ACPA, anti-citrullinated protein antibody; bDMARDs, biologic Disease Modifying Anti Rheumatic Drugs; DKK-1, Dickkopf-1; IL, interleukin; OPG, osteoprotegerin; PTH, Parathormon; RANKL, receptor activator of NF-κB ligand; TNF, tumor necrosis factor.
Nevertheless, albeit clinicians can benefit from new treatment discovery, further research is needed to expand our knowledge about the interaction between bone metabolism and inflammation, in order to find shared mechanisms to target and expand the therapeutic armamentarium available in the clinical practice either to prevent or to treat the bone loss in arthritides.
Author Contributions
All authors listed have made a substantial, direct and intellectual contribution to the work, and approved it for publication.
Conflict of Interest
The authors declare that the research was conducted in the absence of any commercial or financial relationships that could be construed as a potential conflict of interest.
References
1. Smolen JS, Aletaha D, McInnes IB. Rheumatoid arthritis. Lancet. (2016) 388:2023–38. doi: 10.1016/S0140-6736(16)30173-8
2. Raterman HG, Lems WF. Pharmacological management of osteoporosis in rheumatoid arthritis patients: a review of the literature and practical guide. Drugs Aging. (2019) 36:1061–72. doi: 10.1007/s40266-019-00714-4
3. Symmons DPM. Epidemiology of rheumatoid arthritis: determinants of onset, persistence and outcome. Best Pract Res Clin Rheumatol. (2002) 16:707–22. doi: 10.1053/berh.2002.0257
4. Alamanos Y, Drosos AA. Epidemiology of adult rheumatoid arthritis. Autoimmun Rev. (2005) 4:130–6. doi: 10.1016/j.autrev.2004.09.002
5. Adami G, Saag KG. Osteoporosis pathophysiology, epidemiology, and screening in rheumatoid arthritis. Curr Rheumatol Rep. (2019) 21:34. doi: 10.1007/s11926-019-0836-7
6. Zhu TY, Griffith JF, Qin L, Hung VW, Fong TN, Au SK, et al. Alterations of bone density, microstructure, and strength of the distal radius in male patients with rheumatoid arthritis: a case-control study with HR-pQCT. J Bone Mineral Res. (2014) 29:2118–29. doi: 10.1002/jbmr.2221
7. Stach CM, Bäuerle M, Englbrecht M, Kronke G, Engelke K, Manger B, et al. Periarticular bone structure in rheumatoid arthritis patients and healthy individuals assessed by high-resolution computed tomography. Arthritis Rheum. (2010) 62:330–9. doi: 10.1002/art.27252
8. McInnes IB, Schett G. The pathogenesis of rheumatoid arthritis. N Engl J Med. (2011) 365:2205–19. doi: 10.1056/NEJMra1004965
9. Black RJ, Spargo L, Schultz C, Chatterton B, Cleland L, Lester S, et al. Decline in hand bone mineral density indicates increased risk of erosive change in early rheumatoid arthritis. Arthritis Care Res. (2014) 66:515–22. doi: 10.1002/acr.22199
10. Kato G, Shimizu Y, Arai Y, Suzuki N, Sugamori Y, Maeda M, et al. The inhibitory effects of a RANKL-binding peptide on articular and periarticular bone loss in a murine model of collagen-induced arthritis: a bone histomorphometric study. Arthritis Res Ther. (2015) 17:251. doi: 10.1186/s13075-015-0753-8
11. Fessler J, Husic R, Schwetz V, Lerchbaum E, Aberer F, Fasching P, et al. Senescent T-cells promote bone loss in rheumatoid arthritis. Front Immunol. (2018) 9:95. doi: 10.3389/fimmu.2018.00095
12. Hecht C, Englbrecht M, Rech J, Schmidt S, Araujo E, Engelke K, et al. Additive effect of anti-citrullinated protein antibodies and rheumatoid factor on bone erosions in patients with RA. Ann Rheum Dis. (2015) 74:2151–6. doi: 10.1136/annrheumdis-2014-205428
13. Kocijan R, Harre U, Schett G. ACPA and bone loss in rheumatoid arthritis. Curr Rheumatol Rep. (2013) 15:366. doi: 10.1007/s11926-013-0366-7
14. Nielen MMJ, van Schaardenburg D, Reesink HW, van de Stadt RJ, van de Horst-Bruinsma IE, de Koning MHMT, et al. Specific autoantibodies precede the symptoms of rheumatoid arthritis: a study of serial measurements in blood donors. Arthritis Rheum. (2004) 50:380–6. doi: 10.1002/art.20018
15. Kleyer A, Finzel S, Rech J, Manger B, Krieter M, Faustini F, et al. Bone loss before the clinical onset of rheumatoid arthritis in subjects with anticitrullinated protein antibodies. Ann Rheum Dis. (2014) 73:854–60. doi: 10.1136/annrheumdis-2012-202958
16. Simon D, Kleyer A, Englbrecht M, Stemmler F, Simon C, Berlin A, et al. A comparative analysis of articular bone in large cohort of patients with chronic inflammatory diseases of the joints, the gut and the skin. Bone. (2018) 116:87–93. doi: 10.1016/j.bone.2018.07.017
17. Behrens F, Koehm M, Thaçi D, Gnann H, Greger G, Maria Wittig B, et al. Anti-citrullinated protein antibodies are linked to erosive disease in an observational study of patients with psoriatic arthritis. Rheumatology. (2016) 55:1791–5. doi: 10.1093/rheumatology/kew229
18. Steffen U, Schett G, Bozec A. How autoantibodies regulate osteoclast induced bone loss in rheumatoid arthritis. Front Immunol. (2019) 10:1483. doi: 10.3389/fimmu.2019.01483
19. Haugeberg G, Helgetveit KB, Førre Ø, Garen T, Sommerseth H, Prøven A. Generalized bone loss in early rheumatoid arthritis patients followed for ten years in the biologic treatment era. BMC Musculoskelet Disord. (2014) 15:289. doi: 10.1186/1471-2474-15-289
20. Takayanagi H. New developments in osteoimmunology. Nat Rev Rheumatol. (2012) 8:684–9. doi: 10.1038/nrrheum.2012.167
21. Hauser B, Riches PL, Gilchrist T, Visconti MR, Wilson JF, Ralston SH. Autoantibodies to osteoprotegerin are associated with increased bone resorption in rheumatoid arthritis. Ann Rheum Dis. (2015) 74:1631–2. doi: 10.1136/annrheumdis-2014-207219
22. Diarra D, Stolina M, Polzer K, Zwerina J, Ominsky MS, Dwyer D, et al. Dickkopf-1 is a master regulator of joint remodeling. Nat Med. (2007) 13:156–63. doi: 10.1038/nm1538
23. Rossini M, Viapiana O, Adami S, Fracassi E, Idolazzi L, Dartizio C, et al. In patients with rheumatoid arthritis, dickkopf-1 serum levels are correlated with parathyroid hormone, bone erosions and bone mineral density. Clin Exp Rheumatol. (2015) 33:77–83.
24. Ma Y, Zhang X, Wang M, Xia Q, Yang J, Wu M, et al. The serum level of dickkopf-1 in patients with rheumatoid arthritis: a systematic review and meta-analysis. Int Immunopharmacol. (2018) 59:227–32. doi: 10.1016/j.intimp.2018.04.019
25. Orsolini G, Caimmi C, Viapiana O, Idolazzi L, Fracassi E, Gatti D, et al. Titer-dependent effect of anti-citrullinated protein antibodies on systemic bone mass in rheumatoid arthritis patients. Calcif Tissue Int. (2017) 101:17–23. doi: 10.1007/s00223-017-0253-8
26. Ziegelasch M, Forslind K, Skogh T, Riklund K, Kastbom A, Berglin E. Decrease in bone mineral density during three months after diagnosis of early rheumatoid arthritis measured by digital X-ray radiogrammetry predicts radiographic joint damage after one year. Arthritis Res Ther. (2017) 19:195. doi: 10.1186/s13075-017-1403-0
27. Han MH, Ryu JI, Kim CH, Kim JM, Cheong JH, Bak KH, et al. Influence of systemic bone mineral density on atlantoaxial subluxation in patients with rheumatoid arthritis. Osteoporos Int. (2017) 28:1931–8. doi: 10.1007/s00198-017-3972-9
28. Rossini M, Adami G, Viapiana O, Idolazzi L, Orsolini G, Fassio A, et al. Osteoporosis: an independent determinant of bone erosions in rheumatoid arthritis? J Bone Miner Res. (2017) 32:2142–3. doi: 10.1002/jbmr.3209
29. Rossini M, Adami G, Viapiana O, Idolazzi L, Gatti D. Denosumab, cortical bone and bone erosions in rheumatoid arthritis. Ann Rheum Dis. (2016) 75:e70. doi: 10.1136/annrheumdis-2016-210022
30. Sambrook PN, Eisman JA, Yeates MG, Pocock NA, Eberl S, Champion GD. Osteoporosis in rheumatoid arthritis: safety of low dose corticosteroids. Ann Rheum Dis. (1986) 45:950–3. doi: 10.1136/ard.45.11.950
31. Blavnsfeldt ABG, de Thurah A, Thomsen MD, Tarp S, Langdahl B, Hauge EM. The effect of glucocorticoids on bone mineral density in patients with rheumatoid arthritis: a systematic review and meta-analysis of randomized, controlled trials. Bone. (2018) 114:172–80. doi: 10.1016/j.bone.2018.06.008
32. Siu S, Haraoui B, Bissonnette R, Bessette L, Roubille C, Richer V, et al. Meta-analysis of tumor necrosis factor inhibitors and glucocorticoids on bone density in rheumatoid arthritis and ankylosing spondylitis trials. Arthritis Care Res. (2015) 67:754–64. doi: 10.1002/acr.22519
33. Santiago T, da Silva JAP. Safety of low- to medium-dose glucocorticoid treatment in rheumatoid arthritis: myths and reality over the years. Ann N Y Acad Sci. (2014) 1318:41–9. doi: 10.1111/nyas.12428
34. Amiche MA, Albaum JM, Tadrous M, Pechlivanoglou P, Lévesque LE, Adachi JD, et al. Fracture risk in oral glucocorticoid users: a Bayesian meta-regression leveraging control arms of osteoporosis clinical trials. Osteoporos Int. (2016) 27:1709–18. doi: 10.1007/s00198-015-3455-9
35. Balasubramanian A, Wade SW, Adler RA, Saag K, Pannacciulli N, Curtis JR. Glucocorticoid exposure and fracture risk in a Cohort of US patients with selected conditions. J Bone Miner Res. (2018) 33:1881–8. doi: 10.1002/jbmr.3523
36. Balasubramanian A, Wade SW, Adler RA, Lin CJF, Maricic M, O'Malley CD, et al. Glucocorticoid exposure and fracture risk in patients with new-onset rheumatoid arthritis. Osteoporos Int. (2016) 27:3239–49. doi: 10.1007/s00198-016-3646-z
37. Emkey RD, Lindsay R, Lyssy J, Weisberg JS, Dempster DW, Shen V. The systemic effect of intraarticular administration of corticosteroid on markers of bone formation and bone resorption in patients with rheumatoid arthritis. Arthritis Rheum. (1996) 39:277–82. doi: 10.1002/art.1780390215
38. van Staa TP, Geusens P, Bijlsma JWJ, Leufkens HGM, Cooper C. Clinical assessment of the long-term risk of fracture in patients with rheumatoid arthritis. Arthritis Rheum. (2006) 54:3104–12. doi: 10.1002/art.22117
39. Simon D, Kleyer A, Stemmler F, Simon C, Berlin A, Hueber AJ, et al. Age- and sex-dependent changes of intra-articular cortical and trabecular bone structure and the effects of rheumatoid arthritis. J Bone Miner Res. (2017) 32:722–30. doi: 10.1002/jbmr.3025
40. Haugeberg G, Uhlig T, Falch JA, Halse JI, Kvien TK. Bone mineral density and frequency of osteoporosis in female patients with rheumatoid arthritis: results from 394 patients in the Oslo County rheumatoid arthritis register. Arthritis Rheum. (2000) 43:522–30. doi: 10.1002/1529-0131(200003)43:3<522::AID-ANR7>3.0.CO;2-Y
41. Haugeberg G, Uhlig T, Falch JA, Halse JI, Kvien TK. Reduced bone mineral density in male rheumatoid arthritis patients: frequencies and associations with demographic and disease variables in ninety-four patients in the Oslo County rheumatoid arthritis register. Arthritis Rheum. (2000) 43:2776–84. doi: 10.1002/1529-0131(200012)43:12<2776::AID-ANR18>3.0.CO;2-N
42. Sinigaglia L, Nervetti A, Mela Q, Bianchi G, Del Puente A, Di Munno O, et al. A multicenter cross sectional study on bone mineral density in rheumatoid arthritis. Italian study group on bone mass in rheumatoid arthritis. J Rheumatol. (2000) 27:2582–9.
43. Hauser B, Riches PL, Wilson JF, Horne AE, Ralston SH. Prevalence and clinical prediction of osteoporosis in a contemporary cohort of patients with rheumatoid arthritis. Rheumatology. (2014) 53:1759–66. doi: 10.1093/rheumatology/keu162
44. Mori Y, Kuwahara Y, Chiba S, Kogre A, Baba K, Kamimura M, et al. Bone mineral density of postmenopausal women with rheumatoid arthritis depends on disease duration regardless of treatment. J Bone Miner Metab. (2017) 35:52–7. doi: 10.1007/s00774-015-0716-9
45. Fassio A, Idolazzi L, Jaber MA, Dartizio C, Viapiana O, Rossini M, et al. The negative bone effects of the disease and of chronic corticosteroid treatment in premenopausal women affected by rheumatoid arthritis. Reumatismo. (2016) 68:65–71. doi: 10.4081/reumatismo.2016.878
46. Kweon SM, Sohn DH, Park JH, Koh JH, Park EK, Lee HN, et al. Male patients with rheumatoid arthritis have an increased risk of osteoporosis: frequency and risk factors. Medicine. (2018) 97:e11122. doi: 10.1097/MD.0000000000011122
47. Tomizawa T, Ito H, Murata K, Hashimoto M, Tanaka M, Murakami K, et al. Distinct biomarkers for different bones in osteoporosis with rheumatoid arthritis. Arthritis Res Ther. (2019) 21:174. doi: 10.1186/s13075-019-1956-1
48. Richards JS, Cannon GW, Hayden CL, Amdur RL, Lazaro D, Mikuls TR, et al. Adherence with bisphosphonate therapy in US veterans with rheumatoid arthritis. Arthritis Care Res. (2012) 64:1864–70. doi: 10.1002/acr.21777
49. Fassio A, Rossini M, Viapiana O, Idolazzi L, Vantaggiato E, Benini C, et al. New strategies for the prevention and treatment of systemic and local bone loss; from pathophysiology to clinical application. Curr Pharm Des. (2017) 23:6241–50. doi: 10.2174/1381612823666170713104431
50. Barreira SC, Fonseca JE. The impact of conventional and biological disease modifying antirheumatic drugs on bone biology. Rheumatoid arthritis as a case study. Clin Rev Allergy Immunol. (2016) 51:100–9. doi: 10.1007/s12016-016-8547-6
51. Adami G, Fassio A, Rossini M, Caimmi C, Giollo A, Orsolini G, et al. Osteoporosis in rheumatic diseases. Int J Mol Sci. (2019) 20:5867. doi: 10.3390/ijms20235867
52. Zerbini CAF, Clark P, Mendez-Sanchez L, Pereira RMR, Messina OD, Uña CR, et al. Biologic therapies and bone loss in rheumatoid arthritis. Osteoporos Int. (2017) 28:429–46. doi: 10.1007/s00198-016-3769-2
53. Manara M, Sinigaglia L. Bone and TNF in rheumatoid arthritis: clinical implications. RMD Open. (2015) 1 (Suppl. 1):e000065. doi: 10.1136/rmdopen-2015-000065
54. Orsolini G, Fassio A, Rossini M, Adami G, Giollo A, Caimmi C, et al. Effects of biological and targeted synthetic DMARDs on bone loss in rheumatoid arthritis. Pharmacol Res. (2019) 147:104354. doi: 10.1016/j.phrs.2019.104354
55. Adami G, Orsolini G, Adami S, Viapiana O, Idolazzi L, Gatti D, et al. Effects of TNF inhibitors on parathyroid hormone and Wnt signaling antagonists in rheumatoid arthritis. Calcif Tissue Int. (2016) 99:360–4. doi: 10.1007/s00223-016-0161-3
56. Vis M, Havaardsholm EA, Haugeberg G, Uhlig T, Voskuyl AE, van de Stadt RJ, et al. Evaluation of bone mineral density, bone metabolism, osteoprotegerin and receptor activator of the NFkappaB ligand serum levels during treatment with infliximab in patients with rheumatoid arthritis. Ann Rheum Dis. (2006) 65:1495–9. doi: 10.1136/ard.2005.044198
57. Ozen G, Pedro S, Wolfe F, Michaud K. Medications associated with fracture risk in patients with rheumatoid arthritis. Ann Rheum Dis. (2019) 78:1041–7. doi: 10.1136/annrheumdis-2019-215328
58. Gulyás K, Horváth Á, Végh E, Pusztai A, Szentpétery Á, Pethö Z, et al. Effects of 1-year anti-TNF-α therapies on bone mineral density and bone biomarkers in rheumatoid arthritis and ankylosing spondylitis. Clin Rheumatol. (2020) 39:167–75. doi: 10.1007/s10067-019-04771-3
59. Garnero P, Thompson E, Woodworth T, Smolen JS. Rapid and sustained improvement in bone and cartilage turnover markers with the anti-interleukin-6 receptor inhibitor tocilizumab plus methotrexate in rheumatoid arthritis patients with an inadequate response to methotrexate: results from a substudy of the multicenter double-blind, placebo-controlled trial of tocilizumab in inadequate responders to methotrexate alone. Arthritis Rheum. (2010) 62:33–43. doi: 10.1002/art.25053
60. Karsdal MA, Schett G, Emery P, Harari O, Byrjalsen I, Kenwright A, et al. IL-6 receptor inhibition positively modulates bone balance in rheumatoid arthritis patients with an inadequate response to anti-tumor necrosis factor therapy: biochemical marker analysis of bone metabolism in the tocilizumab RADIATE study (NCT00106522). Semin Arthritis Rheum. (2012) 42:131–9. doi: 10.1016/j.semarthrit.2012.01.004
61. Axmann R, Böhm C, Krönke G, Zwerina J, Smolen J, Schett G. Inhibition of interleukin-6 receptor directly blocks osteoclast formation in vitro and in vivo. Arthritis Rheum. (2009) 60:2747–56. doi: 10.1002/art.24781
62. Finzel S, Rech J, Schmidt S, Engelke K, Englbrecht M, Schett G. Interleukin-6 receptor blockade induces limited repair of bone erosions in rheumatoid arthritis: a micro CT study. Ann Rheum Dis. (2013) 72:396–400. doi: 10.1136/annrheumdis-2011-201075
63. Chen YM, Chen HH, Huang WN, Liao TL, Chen JP, Chao WC, et al. Tocilizumab potentially prevents bone loss in patients with anticitrullinated protein antibody-positive rheumatoid arthritis. PLoS ONE. (2017) 12:e0188454. doi: 10.1371/journal.pone.0188454
64. Kitano M, Furukawa T, Yokohama Y, Sekiguchi M, Azuma N, Matsui K, et al. Early Effects of Tofacitinib on Bone Homeostasis in Patients with Rheumatoid Arthritis. Available online at: https://acrabstracts.org/abstract/early-effects-of-tofacitinib-on-bone-homeostasis-in-patients-with-rheumatoid-arthritis/ (accessed September 4, 2020).
65. Sanderson J, Martyn-St James M, Stevens J, Goka E, Wong R, Campbell F, et al. Clinical effectiveness of bisphosphonates for the prevention of fragility fractures: a systematic review and network meta-analysis. Bone. (2016) 89:52–8. doi: 10.1016/j.bone.2016.05.013
66. Rossini M, Adami G, Adami S, Viapiana O, Gatti D. Safety issues and adverse reactions with osteoporosis management. Expert Opin Drug Saf. (2016) 15:321–32. doi: 10.1517/14740338.2016.1136287
67. Allen CS, Yeung JH, Vandermeer B, Homik J. Bisphosphonates for steroid-induced osteoporosis. Cochrane Database Syst Rev. (2016) 10:CD001347. doi: 10.1002/14651858.CD001347.pub2
68. Lems WF, Lodder MC, Lips P, Bijlsma JWJ, Geusens P, Schrameijer N, et al. Positive effect of alendronate on bone mineral density and markers of bone turnover in patients with rheumatoid arthritis on chronic treatment with low-dose prednisone: a randomized, double-blind, placebo-controlled trial. Osteoporos Int. (2006) 17:716–23. doi: 10.1007/s00198-005-0037-2
69. Xie J, Li S, Xiao L, Ouyang G, Zheng L, Gu Y, et al. Zoledronic acid ameliorates the effects of secondary osteoporosis in rheumatoid arthritis patients. J Orthop Surg Res. (2019) 14:421. doi: 10.1186/s13018-019-1492-3
70. Fujieda Y, Horita T, Nishimoto N, Tanimura K, Amasaki Y, Kasahara H, et al. Efficacy and safety of sodium RISedronate for glucocorticoid-induced OsTeoporosis with rheumaTOid arthritis (RISOTTO study): a multicentre, double-blind, randomized, placebo-controlled trial. Mod Rheumatol. (2020). doi: 10.1080/14397595.2020.1812835. [Epub ahead of print].
71. Cummings SR, San Martin J, McClung MR, Siris ES, Eastell R, Reid IR, et al. Denosumab for prevention of fractures in postmenopausal women with osteoporosis. N Engl J Med. (2009) 361:756–65. doi: 10.1056/NEJMoa0809493
72. Saag KG, Wagman RB, Geusens P, Adachi JD, Messina OD, Emkey R, et al. Denosumab versus risedronate in glucocorticoid-induced osteoporosis: a multicentre, randomised, double-blind, active-controlled, double-dummy, non-inferiority study. Lancet Diabetes Endocrinol. (2018) 6:445–54. doi: 10.1016/S2213-8587(18)30075-5
73. Saag KG, Pannacciulli N, Geusens P, Adachi JD, Messina OD, Morales-Torres J, et al. Denosumab versus risedronate in glucocorticoid-induced osteoporosis: final results of a twenty-four-month randomized, double-blind, double-dummy trial. Arthritis Rheumatol. (2019) 71:1174–84. doi: 10.1002/art.40874
74. Mochizuki T, Yano K, Ikari K, Hiroshima R, Nasu Y, Okazaki K. Three-year results of denosumab treatment for osteoporosis in women with rheumatoid arthritis and primary osteoporosis: a clinical observational study. Mod Rheumatol. (2020). doi: 10.1080/14397595.2020.1812793. [Epub ahead of print].
75. Takeuchi T, Tanaka Y, Ishiguro N, Yamanaka H, Yoneda T, Ohira T, et al. Effect of denosumab on Japanese patients with rheumatoid arthritis: a dose-response study of AMG 162 (Denosumab) in patients with rheumatoid arthritis on methotrexate to validate inhibitory effect on bone Erosion (DRIVE)-a 12-month, multicentre, randomised, double-blind, placebo-controlled, phase II clinical trial. Ann Rheum Dis. (2016) 75:983–90. doi: 10.1136/annrheumdis-2015-208052
76. Ishiguro N, Tanaka Y, Yamanaka H, Yoneda T, Ohira T, Okubo N, et al. Efficacy of denosumab with regard to bone destruction in prognostic subgroups of Japanese rheumatoid arthritis patients from the phase II DRIVE study. Rheumatology. (2019) 58:997–1005. doi: 10.1093/rheumatology/key416
77. Takeuchi T, Tanaka Y, Soen S, Yamanaka H, Yoneda T, Tanaka S, et al. Effects of the anti-RANKL antibody denosumab on joint structural damage in patients with rheumatoid arthritis treated with conventional synthetic disease-modifying antirheumatic drugs (DESIRABLE study): a randomised, double-blind, placebo-controlled phase 3 trial. Ann Rheum Dis. (2019) 78:899–907. doi: 10.1136/annrheumdis-2018-214827
78. Tanaka Y. Clinical immunity in bone and joints. J Bone Miner Metab. (2019) 37:2–8. doi: 10.1007/s00774-018-0965-5
79. Tanaka S. RANKL is a therapeutic target of bone destruction in rheumatoid arthritis. F1000Res. (2019) 8. doi: 10.12688/f1000research.17296.1
80. Hasegawa T, Kaneko Y, Izumi K, Takeuchi T. Efficacy of denosumab combined with bDMARDs on radiographic progression in rheumatoid arthritis. Joint Bone Spine. (2017) 84:379–80. doi: 10.1016/j.jbspin.2016.05.010
81. Suzuki T, Nakamura Y, Kato H. Effects of denosumab on bone metabolism and bone mineral density with anti-TNF inhibitors, tocilizumab, or abatacept in osteoporosis with rheumatoid arthritis. Ther Clin Risk Manag. (2018) 14:453–9. doi: 10.2147/TCRM.S156350
82. Ebina K, Hirao M, Hashimoto J, Matsuoka H, Iwahashi T, Chijimatsu R, et al. Impact of switching oral bisphosphonates to denosumab or daily teriparatide on the progression of radiographic joint destruction in patients with biologic-naïve rheumatoid arthritis. Osteoporos Int. (2018) 29:1627–36. doi: 10.1007/s00198-018-4492-y
83. Adami G, Rossini M, Viapiana O, Fassio A, Idolazzi L, Orsolini G, et al. Lack of effect of teriparatide on joint erosions in rheumatoid arthritis is an expected result: comment on the article by Solomon et al. Arthritis Rheumatol. (2018) 70:475–6. doi: 10.1002/art.40385
84. Bandeira L, Lewiecki EM, Bilezikian JP. Romosozumab for the treatment of osteoporosis. Expert Opin Biol Ther. (2017) 17:255–63. doi: 10.1080/14712598.2017.1280455
85. Wehmeyer C, Stratis A, Pap T, Dankbar B. The role of the WNT inhibitor sclerostin in rheumatoid arthritis. Ann Rheum Dis. (2010) 69 (Suppl. 2):A21. doi: 10.1136/ard.2010.129593n
86. Gambari L, Grassi F, Roseti L, Grigolo B, Desando G. Learning from monocyte-macrophage fusion and multinucleation: potential therapeutic targets for osteoporosis and rheumatoid arthritis. Int J Mol Sci. (2020) 21:6001. doi: 10.3390/ijms21176001
87. Tiwari V, Brent LH. Psoriatic arthritis. In: StatPearls [Internet]. Treasure Island, FL: StatPearls Publishing.
88. Ogdie A, Weiss P. The epidemiology psoriatic arthritis. Rheum Dis Clin North Am. (2015) 41:545–68. doi: 10.1016/j.rdc.2015.07.001
89. Ogdie A, Schwartzman S, Husni ME. Recognizing and managing comorbidities in psoriatic arthritis. Curr Opin Rheumatol. (2015) 27:118–26. doi: 10.1097/BOR.0000000000000152
90. Kathuria P, Gordon KB, Silverberg JI. Association of psoriasis and psoriatic arthritis with osteoporosis and pathological fractures. J Am Acad Dermatol. (2017) 76:1045–53.e3. doi: 10.1016/j.jaad.2016.11.046
91. Keller JJ, Kang JH, Lin HC. Association between osteoporosis and psoriasis: results from the longitudinal health insurance database in Taiwan. Osteoporos Int. (2013) 24:1835–41. doi: 10.1007/s00198-012-2185-5
92. Modalsli EH, Åsvold BO, Romundstad PR, Langhammer A, Hoff M, Forsmo S, et al. Psoriasis, fracture risk and bone mineral density: the HUNT Study, Norway. Br J Dermatol. (2017) 176:1162–9. doi: 10.1111/bjd.15123
93. Busquets N, Vaquero CG, Moreno JR, Vilaseca DR, Narváez J, Carmona L, et al. Bone mineral density status and frequency of osteoporosis and clinical fractures in 155 patients with psoriatic arthritis followed in a University hospital. Reumatol Clin. (2014) 10:89–93. doi: 10.1016/j.reumae.2013.07.003
94. Gulati AM, Michelsen B, Diamantopoulos A, Grandaunet B, Salvesen Ø, Kavanaugh A, et al. Osteoporosis in psoriatic arthritis: a cross-sectional study of an outpatient clinic population. RMD Open. (2018) 4:e000631. doi: 10.1136/rmdopen-2017-000631
95. Xia J, Xie SY, Liu KQ, Xu L, Zhao PP, Gai SR, et al. Systemic evaluation of the relationship between psoriasis, psoriatic arthritis and osteoporosis: observational and Mendelian randomisation study. Ann Rheum Dis. (2020) 79:1460–7. doi: 10.1136/annrheumdis-2020-217892
96. Lo Giudice LF, Scolnik M, Pierini FS, Marin Zucaro NM, Jaramillo Gallego JF, Soriano ER. Fragility fractures in psoriatic arthritis patients: a matched retrospective cohort study. Clin Rheumatol. (2020) 39:3685–91. doi: 10.1007/s10067-020-05074-8
97. Sambrook PN, Geusens P. The epidemiology of osteoporosis and fractures in ankylosing spondylitis. Ther Adv Musculoskelet Dis. (2012) 4:287–92. doi: 10.1177/1759720X12441276
98. Will R, Palmer R, Bhalla AK, Ring F, Calin A. Osteoporosis in early ankylosing spondylitis: a primary pathological event? Lancet. (1989) 2:1483–5. doi: 10.1016/S0140-6736(89)92932-2
99. van der Weijden MAC, Claushuis TAM, Nazari T, Lems WF, Dijkmans BAC, van der Horst-Bruinsma IE. High prevalence of low bone mineral density in patients within 10 years of onset of ankylosing spondylitis: a systematic review. Clin Rheumatol. (2012) 31:1529–35. doi: 10.1007/s10067-012-2018-0
100. Maas F, Spoorenberg A, van der Slik BPG, van der Veer E, Brouwer E, Bootsma H, et al. Clinical risk factors for the presence and development of vertebral fractures in patients with ankylosing spondylitis. Arthritis Care Res. (2017) 69:694–702. doi: 10.1002/acr.22980
101. Briot K, Durnez A, Paternotte S, Miceli-Richard C, Dougados M, Roux C. Bone oedema on MRI is highly associated with low bone mineral density in patients with early inflammatory back pain: results from the DESIR cohort. Ann Rheum Dis. (2013) 72:1914–9. doi: 10.1136/annrheumdis-2012-201845
102. Akgöl G, Kamanli A, Ozgocmen S. Evidence for inflammation-induced bone loss in non-radiographic axial spondyloarthritis. Rheumatology. (2014) 53:497–501. doi: 10.1093/rheumatology/ket385
103. Vosse D, Landewé R, van der Heijde D, van der Linden S, van Staa TP, Geusens P. Ankylosing spondylitis and the risk of fracture: results from a large primary care-based nested case-control study. Ann Rheum Dis. (2009) 68:1839–42. doi: 10.1136/ard.2008.100503
104. Nam SW, Sung YK, Kim D, Cho SK, Song Y, Choi YY, et al. The usefulness of trabecular bone score in patients with ankylosing spondylitis. Korean J Intern Med. (2020). doi: 10.3904/kjim.2020.065. [Epub ahead of print].
105. Fauny M, Morizot C, Allado E, Verhoeven F, Albuisson E, Semaan M, et al. Consequences of spinal ankylosis on bone trabecular fragility assessed on CT scans in patients with ankylosing spondylitis. A retrospective study. Joint Bone Spine. (2020). doi: 10.1016/j.jbspin.2020.05.009. [Epub ahead of print].
106. Kocijan R, Finzel S, Englbrecht M, Engelke K, Rech J, Schett G. Decreased quantity and quality of the periarticular and nonperiarticular bone in patients with rheumatoid arthritis: a cross-sectional HR-pQCT study. J Bone Mineral Res. (2014) 29:1005–14. doi: 10.1002/jbmr.2109
107. Shaw AT, Gravallese EM. Mediators of inflammation and bone remodeling in rheumatic disease. Semin Cell Dev Biol. (2016) 49:2–10. doi: 10.1016/j.semcdb.2015.10.013
108. Paine A, Ritchlin C. Altered bone remodeling in psoriatic disease: new insights and future directions. Calcif Tissue Int. (2018) 102:559–74. doi: 10.1007/s00223-017-0380-2
109. Rossini M, Viapiana O, Adami S, Idolazzi L, Fracassi E, Gatti D. Focal bone involvement in inflammatory arthritis: the role of IL17. Rheumatol Int. (2016) 36:469–82. doi: 10.1007/s00296-015-3387-x
110. Orsolini G, Bertoldi I, Rossini M. Osteoimmunology in rheumatoid and psoriatic arthritis: potential effects of tofacitinib on bone involvement. Clin Rheumatol. (2020) 39:727–36. doi: 10.1007/s10067-020-04930-x
111. Fassio A, Idolazzi L, Viapiana O, Benini C, Vantaggiato E, Bertoldo F, et al. In psoriatic arthritis Dkk-1 and PTH are lower than in rheumatoid arthritis and healthy controls. Clin Rheumatol. (2017) 36:2377–81. doi: 10.1007/s10067-017-3734-2
112. Fassio A, Gatti D, Rossini M, Idolazzi L, Giollo A, Adami G, et al. Secukinumab produces a quick increase in WNT signalling antagonists in patients with psoriatic arthritis. Clin Exp Rheumatol. (2019) 37:133–6.
113. Stupphann D, Rauner M, Krenbek D, Patsch J, Pirker T, Muschitz C, et al. Intracellular and surface RANKL are differentially regulated in patients with ankylosing spondylitis. Rheumatol Int. (2008) 28:987–93. doi: 10.1007/s00296-008-0567-y
114. Rossini M, Viapiana O, Idolazzi L, Ghellere F, Fracassi E, Troplini S, et al. Higher level of dickkopf-1 is associated with low bone mineral density and higher prevalence of vertebral fractures in patients with ankylosing spondylitis. Calcif Tissue Int. (2016) 98:438–45. doi: 10.1007/s00223-015-0093-3
115. Orsolini G, Adami G, Rossini M, Ghellere F, Caimmi C, Fassio A, et al. Parathyroid hormone is a determinant of serum Dickkopf-1 levels in ankylosing spondylitis. Clin Rheumatol. (2018) 37:3093–8. doi: 10.1007/s10067-018-4205-0
116. Hauser B, Zhao S, Visconti MR, Riches PL, Fraser WD, Piec I, et al. Autoantibodies to osteoprotegerin are associated with low hip bone mineral density and history of fractures in axial spondyloarthritis: a cross-sectional observational study. Calcif Tissue Int. (2017) 101:375–83. doi: 10.1007/s00223-017-0291-2
117. Marino F, D'Angelo S, Masala IF, Gerratana E, Nucera V, La Corte L, et al. Toxicological considerations in the treatment of axial spondylo-arthritis. Expert Opin Drug Metab Toxicol. (2020) 16:663–72. doi: 10.1080/17425255.2020.1783240
118. Muñoz-Ortego J, Vestergaard P, Rubio JB, Wordsworth P, Judge A, Javaid MK, et al. Ankylosing spondylitis is associated with an increased risk of vertebral and nonvertebral clinical fractures: a population-based cohort study. J Bone Miner Res. (2014) 29:1770–6. doi: 10.1002/jbmr.2217
119. Barnabe C, Hanley DA. Effect of tumor necrosis factor alpha inhibition on bone density and turnover markers in patients with rheumatoid arthritis and spondyloarthropathy. Semin Arthritis Rheum. (2009) 39:116–22. doi: 10.1016/j.semarthrit.2008.04.004
120. Beek KJ, Rusman T, van der Weijden MAC, Lems WF, van Denderen JC, Konsta M, et al. Long-term treatment with tnf-alpha inhibitors improves bone mineral density but not vertebral fracture progression in ankylosing spondylitis. J Bone Miner Res. (2019) 34:1041–8. doi: 10.1002/jbmr.3684
121. Viapiana O, Gatti D, Idolazzi L, Fracassi E, Adami S, Troplini S, et al. Bisphosphonates vs infliximab in ankylosing spondylitis treatment. Rheumatology. (2014) 53:90–4. doi: 10.1093/rheumatology/ket321
122. Orsolini G, Adami G, Rossini M, Fassio A, Giollo A, Caimmi C, et al. Is the exposure to bisphosphonates or osteoporosis the predictor of spinal radiographic progression in ankylosing spondylitis? Arthritis Res Ther. (2018) 20:233. doi: 10.1186/s13075-018-1730-9
123. Gatti D, Viapiana O, Adami S, Idolazzi L, Fracassi E, Rossini M. Bisphosphonate treatment of postmenopausal osteoporosis is associated with a dose dependent increase in serum sclerostin. Bone. (2012) 50:739–42. doi: 10.1016/j.bone.2011.11.028
124. Appel H, Ruiz-Heiland G, Listing J, Zwerina J, Herrmann M, Mueller R, et al. Altered skeletal expression of sclerostin and its link to radiographic progression in ankylosing spondylitis. Arthritis Rheum. (2009) 60:3257–62. doi: 10.1002/art.24888
Keywords: osteoporosis, rheumatoid arthritis, psoriatic arthritis, spondyloarthritis, fractures, bone mineral density
Citation: Rotta D, Fassio A, Rossini M, Giollo A, Viapiana O, Orsolini G, Bertoldo E, Gatti D and Adami G (2020) Osteoporosis in Inflammatory Arthritides: New Perspective on Pathogenesis and Treatment. Front. Med. 7:613720. doi: 10.3389/fmed.2020.613720
Received: 03 October 2020; Accepted: 06 November 2020;
Published: 01 December 2020.
Edited by:
Barbara Ruaro, University of Trieste, ItalyReviewed by:
Antonio Del Puente, University of Naples Federico II, ItalyJoão Eurico Fonseca, University of Lisbon, Portugal
Copyright © 2020 Rotta, Fassio, Rossini, Giollo, Viapiana, Orsolini, Bertoldo, Gatti and Adami. This is an open-access article distributed under the terms of the Creative Commons Attribution License (CC BY). The use, distribution or reproduction in other forums is permitted, provided the original author(s) and the copyright owner(s) are credited and that the original publication in this journal is cited, in accordance with accepted academic practice. No use, distribution or reproduction is permitted which does not comply with these terms.
*Correspondence: Denise Rotta, denise.rotta.dr@gmail.com