- 1Department of Orthopedics, Hefei BOE Hospital, Teaching Hospital of Shanghai University Medical College, Hefei, Anhui, China
- 2Huaibei Miner General Hospital, Spinal and Trauma Surgery, Huaibei, Anhui, China
- 3The Second People’s Hospital of Hefei, Hefei Hospital Affiliated to Anhui Medical University, Hefei, Anhui, China
- 4School of Food and Biological Engineering, Hefei University of Technology, Hefei, Anhui, China
A novel acrylic monomer containing a nitrofuran motif, referred to as long-chain nitrofuran methacrylate (LNFMA), is reported. In comparison to the previously reported nitrofuran methacrylate (NFMA), LNFMA has a longer side chain, and when incorporated into bone cement, the resulting LNFMA bone cement exhibits improved mechanical strength. At the same concentration, NFMA-5% cement has only 21.6 ± 1.3 MPa, while LNFMA-5% cement has a compressive strength of 42.64 ± 0.94 MPa. LNFMA bone cements exhibit antimicrobial activity against Staphylococcus aureus, with LNFMA-30% cement reaching 57.38% ± 5.53%. Moreover, LNFMA cement demonstrates excellent biocompatibility both in vitro and in vivo. The results showed that LNFMA monomer had optimized mechanical strength compared with previously reported NFMA monomers, and LNFMA bone cement had good antibacterial activity and biocompatibility.
1 Introduction
Antibiotic-loaded bone cement (ALBC) is widely used in surgical procedures, and polymethyl methacrylate (PMMA) is known for its excellent injectability and mechanical strength, making it the gold standard for ALBC (Galvez-Lopez et al., 2014; Ali et al., 2015; Xu et al., 2022). However, ALBC has the drawback of burst antibiotic release, resulting in a relatively short antimicrobial duration (Moojen et al., 2008). Additionally, the sustained low-level release of residual antibiotics can lead to bacterial resistance (Berberich and Sanz-Ruiz, 2019; Schwarz et al., 2021). Therefore, there is a need to develop novel antimicrobial bone cements. To optimize the release of antibiotics and other bioactive agents, cutting-edge research is exploring innovative ways to enhance bone cement properties. These include doping with degradable components and utilizing biocomposites made from PMMA blended with either ceramic or metal materials (El-Ghannam, A et al., 2010; Wu, K et al., 2016; Ploux, L et al., 2016; Eltohamy, M et al., 2018; Xu, D et al., 2021; Chen, L et al., 2021; Garcia-Garcia, J et al., 2022; Al, T. Y et al., 2022; Świeczko-Żurek, B et al., 2022; Robu, A et al., 2022; Mistry, S et al., 2022; Batista Campos, L et al., 2023; Yang, L et al., 2023; Seidenstuecker, M et al., 2024).
In recent years, research on non-leaching antimicrobial bone cement (NLBC) has become increasingly widespread (Shi et al., 2021; Chen et al., 2022a; Chen et al., 2022b). NLBC combines antimicrobial motifs with bone cement through covalent bonding or physical adsorption, where the antimicrobial components are not released in an burst manner and exhibit long-lasting antimicrobial activity on the surface of the bone cement (Wright et al., 2019; Gao et al., 2023a). There are primarily two categories of antimicrobial motifs in NLBC, quaternary ammonium salts and cyclic organic molecules (Deb et al., 2008; Mei et al., 2012; Beyth et al., 2014; Sun et al., 2016; Zhu et al., 2018b; Fu et al., 2022). While quaternary ammonium salt-based NLBC exhibit broad-spectrum antimicrobial activity, research has shown that they possess significant hemolytic properties, limiting further applications (Zhu et al., 2018b). Cyclic organic antimicrobial compounds represent a well-studied and promising new motif, such as thiazoles, furanones and benzothiazoles, all of which have been demonstrated to have antimicrobial activity in NLBC (Zhu et al., 2021; Chen et al., 2022a). In our previous studies, we developed a nitrofuran methacrylate monomer (NFMA) and prepared nitrofuran-containing NLBC through solid-phase modification, showing excellent antimicrobial activity (Chu et al., 2022; Gao et al., 2023b). It is first necessary to copolymerize NFMA with MMA to prepare p (MMA-co-NFMA) with different antibacterial NFMA contents. After complex purification and grinding, a certain proportion of radiopaque agent and initiator are added to prepare the solid phase of bone cement. These complicated operations have limited the ability of laboratories interested in antibacterial bone cement to research and apply this promising antibacterial material.
Liquid-phase modification is a convenient strategy for bone cement modification. It only requires adding antibacterial monomers into the liquid phase and stirring evenly, then NLBC containing novel antibacterial agents can be prepared according to the normal preparation steps. We note that most reported NLBCs are prepared through liquid-phase modification (Abid et al., 2022; Wang et al., 2021). We conducted a study to investigate the potential of NFMA in the liquid-phase modification of bone cement. However, we observed a significant reduction in the compressive strength of the bone cement. This may be attributed to the steric hindrance of the furan within the side chain of NFMA, which has an adverse impact on the polymerization and consequently led to a decrease in the mechanical properties of the polymer. Therefore, we suspect that the mechanical strength of nitrofuran-containing NLBC can be improved through the optimization of the side chain structure of the antibacterial monomer.
In this study, we designed and synthesized a new antibacterial long-chain nitrofuran methacrylate (LNFMA) and used it for liquid-phase modification to create antimicrobial NLBC containing nitrofuran (Figure 1). We assessed the mechanical strength, antimicrobial activity, in vitro cytotoxicity and in vivo biocompatibility of this new type of bone cement. The hypothesis was that the LNFMA could enhance the compressive strength of the bone cement compared to the previously reported NFMA monomer.
2 Materials and methods
2.1 Materials
Methyl methacrylate (MMA), benzoyl peroxide (BPO) and N, N-Dimethyl-p-toluidine (DMPT) were purchased from J&K Scientific Ltd., China. (5-Nitrofuran-2-yl) methanol was purchased from Bide Pharmatech Ltd., China. 2-Isocyanatoethyl methacrylate was purchased from Shanghai Acmec Biochemical Co., Ltd., China. Barium sulfate was purchased from Macklin Biochemical Technology Co., Ltd., China. Poly (methyl methacrylate) (Mw = 120,000 by GPC) was a product of Sigma-Aldrich Co., USA. NMR solvent dimethyl sulfoxide (DMSO-d6) was purchased from Cambridge Isotope Laboratories, Inc. Staphylococcus aureus (ACTT 25923) was obtained from the Laboratory Department of The Second People’s Hospital of Hefei. MC3T3-E1 cells were provided by Fenghbio Ltd., China. BPO was dried under vacuum at room temperature for 24 h before being used. Grind PMMA powder to a particle size that is fine enough to pass through an 80-mesh screen but coarse enough to be retained on a 100-mesh screen before use. The other chemicals were used without further purification.
2.2 Synthesis of LNFMA monomer
First, (5-nitrofuran-2-yl) methanol (30 mmol, 1.0 eq) and methyl prop-2-ynoate isocyanate (36 mmol, 1.2 eq) was added to 50 mL DCM, followed by the addition of two drops of lauric acid. Stir the reaction mixture at room temperature overnight. Dilute the reaction mixture with DCM, and extract the organic layer with distilled water. Subsequently, extract the aqueous phase with DCM for a reverse-phase extraction. Dry the organic layer over sodium sulfate and process it to obtain the crude product through rotary evaporation. Purify the crude product by silica gel column chromatography to obtain the purified LNFMA monomer compound.
2.3 Preparation of LNFMA bone cement
The formulas of bone cements for each experiment are shown in Table 1. The mixture of solid and liquid components of each cement was prepared separately to ensure homogeneity. The two bone cement components were manually mixed at 23°C ± 1°C, then filled into molds of different sizes during the dough stage, and demoulded after 1 h. Cylindrical cement samples with a diameter of 6 mm and a height of 12 mm were used to study mechanical properties, antibacterial properties, and to prepare leach solutions for biocompatibility studies.
Place the cylindrical cement sample in a container filled with cell culture medium or physiological saline. The ratio of cement sample to liquid is 0.2 g/mL. Incubate the material in a constant temperature incubator at 37°C and with 5% CO2, 24 h for the cell culture medium group and 48 h for the physiological saline group. After incubation, remove the sample, adjust the pH value of the extract to 7.4, and store it in a 4°C refrigerator for later use. The cell culture medium extract is intended to be used for examining cell toxicity.
2.4 Characterization of LNFMA bone cement
The FTIR spectra for the functional group analysis of the composites were recorded using a spectrometer (BRUKER VECTOR-22) over the frequency range of 4,000–400 cm−1. The samples were prepared in the form of pellets after mixing with potassium bromide. The morphologies of the synthetic bone cement materials were characterized by Scanning electron microscope (SEM) (JSM 6700, OLYMPUS, Tokyo, Japan) after coating with gold particles in a sputter coater. The mixed bone cement is filled into the polymerization temperature measuring mold, and the time-temperature change during the polymerization process is recorded by an infrared thermal imager. The temperature of the polymerization reaction is recorded every 30 s. Each group of samples is tested three times, and the average value of the maximum polymerization temperature is calculated. The setting time is calculated according to the formula Tset = (Tmax + Tabm)/2. Tmax is the maximum polymerization temperature, and Tamb is the ambient temperature.
2.5 Measurement of mechanical strength
The compressive strength and the elastic modulus of the bone cement were also determined by ISO 5833. The cylindrical samples were polished with a sandpaper of 1,000 mesh to guarantee that the two surfaces are completely parallel and incubated at 37°C in 100% humidity for 1 day. The test was conducted using the computer control material testing machine (BOSE company in the United States) at a loading rate of 20 mm min−1 until sample failure at 23°C. For each cement formulation, five samples were tested. The compressive strength was obtained from the recorded stress–strain curves and the elastic modulus could be calculated from the slope of the linear section of the stress–strain curve.
2.6 Evaluation of antibacterial activity
The cylindrical bone cement sample, with a diameter of 6 mm and a height of 12 mm, was soaked in distilled water at a ratio of 0.2 g/mL−1 at 37 °C for 24 h to eliminate residual unreacted monomers. Subsequently, surface disinfection was performed using ultraviolet light for 60 min. The individual samples were immersed in 1 mL of S. aureus bacterial suspension (0.5 MacFarland turbidity standard) and cultured at 37°C for 6 h. Afterward, the samples were washed to remove non-adherent bacteria, and then subjected to ultrasonic shaking in 5 mL of physiological saline (180 W, 40 kHz) for 3 min to separate the bacteria attached to the sample surface. Next, the above bacterial solution was diluted 100 times with physiological saline, and 40 μL of the diluted bacterial solution was inoculated on agar culture medium. After incubating at 37°C in a constant temperature incubator for 24 h, the bacterial count was determined. Each group was tested with three samples. The formula for calculating the contact inhibition rate is as follows:
in the formula, A represents the colony-forming units of the PMMA bone cement group, and B represents the colony-forming units of the various experimental groups.
2.7 Measurement of cell toxicity (CCK-8 assay using mouse embryo osteoblast precursor cells (MC3T3-E1))
Approximately 5 × 103 cells (MC3T3-E1) were seeded per well in a 96-well plate and cultured in MEM supplemented with 10% FBS as described above. The original culture medium was then removed and one hundred microliters of the different dilutions of the test material extract were co-cultured with MC3T3-E1, in three replicates. Cells cultured with 10% serum alone (i.e., cells unexposed to test material) were considered as the cell control. The morphological changes, OD values, and relative growth rate (RGR) of the osteoblasts were observed and recorded on days 1, 3, and 5 to evaluate the in vitro biocompatibility of the cement. The absorbance at 450 nm of every well was measured by a microplate reader. RGR was calculated according to equation:
in the formula, ODT is the absorbance of the experimental group, ODN is the absorbance of the negative control group, and ODR is the absorbance of the cell-free medium.
2.8 Histopathological examination
Fifteen healthy SPF C57 mice of about 20 g were divided into three groups. Normal saline extracts of the PMMA, and LNFMA-20% cements were injected into the abdominal cavity at a dosage of 50 mL/kg, respectively, and saline was used as a negative control group. General state (temperature, respiration, appetite, and exercise, etc.), mice weight changes and toxic manifestations (vomiting, diarrhea, convulsions, etc.) of the animals were observed before administration and on day 1, 2, and 3 after administration. On the third day (72 h later), the animals were sacrificed, and the liver and kidney were taken out for pathological observation. Experimental procedures were approved by the Biomedical Ethics Committee of Hefei University of Technology (approval no. HFUT20220522-001; approval date. “22 May 2022”).
2.9 Statistical analysis
All the results were expressed in mean ± standard deviation and were statistically analyzed using one-way ANOVA at the significance level of p < 0.05 by SPSS13.0 (SPSS Inc., Chicago, CA, United States, 2004). Subsequent multiple comparisons were conducted using Duncan’s post hoc analysis. The use of different lowercase letters on the bar graphs in Figures 5A, B, Figures 6B, C, Figure 2A and Figure 7B signifies statistically significant differences (p < 0.05) in the test values among the various cements being compared.
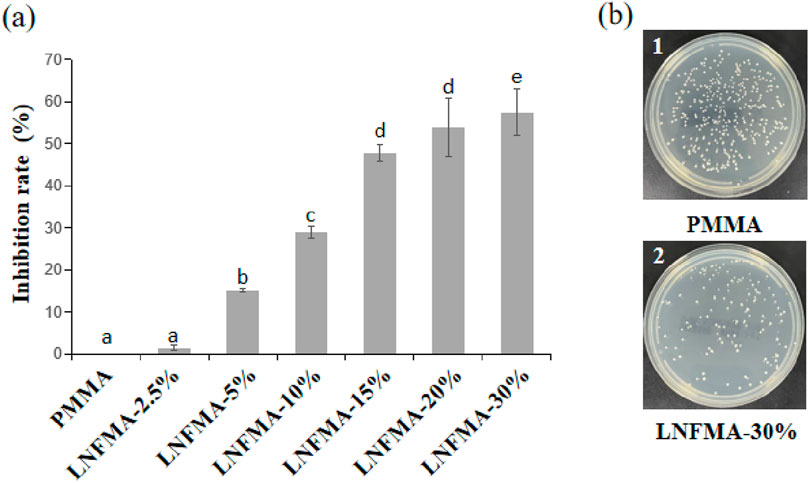
Figure 2. Antibacterial effect of PMMA and LNFMA cement on Staphylococcus aureus. (A) The inhibition rate of LNFMA cement at different concentrations; (B) Staphylococcus aureus colonies under the action of PMMA and LNFMA-30% cement, 1: PMMA cement group, 2: LNFMA-30% cement group. Different lowercase letters indicate significant differences in the inhibition rate of different cements (p < 0.05).
3 Results
3.1 Characterization of LNFMA bone cement
The FTIR spectra of PMMA cement and LNFMA cements containing different concentrations of LNFMA monomer are shown in Figure 3. The solid-phase of bone cement remains commercially available PMMA in solid phase modification. Therefore, we also observed characteristic peaks of acrylic bone cement in LNFMA bone cement. These samples had the same signal peaks at 2,950, 1730, and 1,244 cm−1 that represented the characteristic peaks of the acrylic bone cement. The peak around 811 cm−1 represented C–N stretching, and the peak at 1,353 and 1,507 cm−1 represented the O=N=O symmetric and asymmetric vibration modes, respectively. By comparing the FTIR spectra of the LNFMA cements, it can be observed that with the increase in the LNFMA monomer incorporation amount, the peak intensities of O=N=O and C–N in the furan ring were significantly enhanced (Figure 3). As depicted in Figure 4, the fracture surfaces produced by brittle fracture in liquid nitrogen were very smooth. As indicated by the arrows, all these types of bone cement contained uniformly shaped pores, which were air bubbles mixed in during the production of the cement. The scanning electron microscope (SEM) study of modified bone cement confirms that despite the increase in LNFMA monomer content, the microstructure remains largely unchanged.
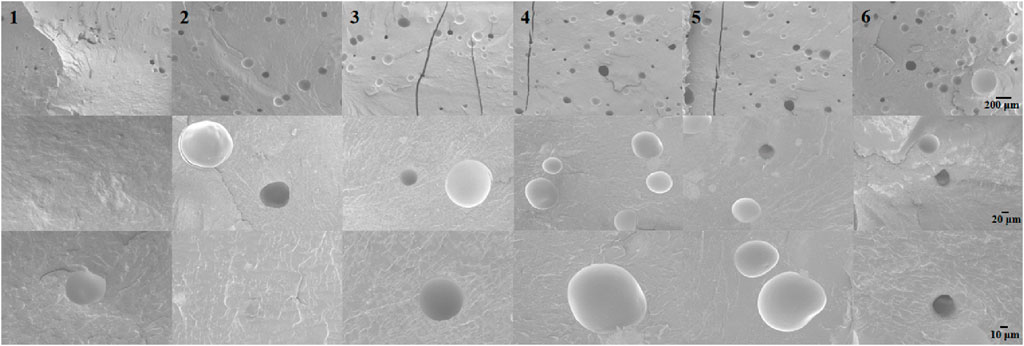
Figure 4. SEM images of the NFMA and LNFMA bone cements. 1: PMMA cement group, 2: LNFMA-2.5% cement group, 3: LNFMA-5% cement group, 4: NFMA-5% cement group, 5: LNFMA-15% cement group, 3: LNFMA-30% cement group.
As shown in Figure 5, both the polymerization temperature and the setting time decrease with the addition of LNFMA. We believe that this may be because the relative molecular mass of the LNFMA monomer is larger than that of MMA. Therefore, the molar amount of LNFMA is lower than that of MMA under the same mass, which makes the polymerization reaction less intense. At the same time, the steric hindrance of the side chain of LNFMA is relatively large, which further slows down the polymerization reaction rate.
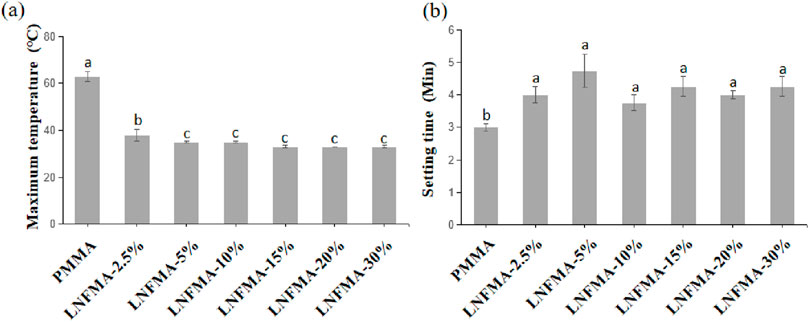
Figure 5. The polymerization properties of PMMA cement and LNFMA cement. (A) Maximum temperature; (B) Setting time. Different lowercase letters indicate significant differences in the maximum temperature or setting time among different cements (p < 0.05).
3.2 Mechanical strength
Based on our speculation, LNFMA with long side chains is less impactful on the compressive strength of bone cement during liquid-phase modification compared to NFMA with short side chains. As shown in Figure 6, we compared the compressive strengths of two types of bone cement, LNFMA-5% and NFMA-5%, and found that the compressive strength of LNFMA-5% cement is approximately 197% higher than that of NFMA-5% cement. Additionally, we tested bone cement with 2.5% and 10% LNFMA content, and discovered that the compressive strength and elastic modulus of bone cement decreases as the LNFMA content increases. The compressive strength of LNFMA-10% is only 19.8 ± 0.8 MPa. Since the compressive strength is already below the ISO 5833 standard, we did not proceed with further testing of the mechanical properties of bone cement with higher LNFMA content.
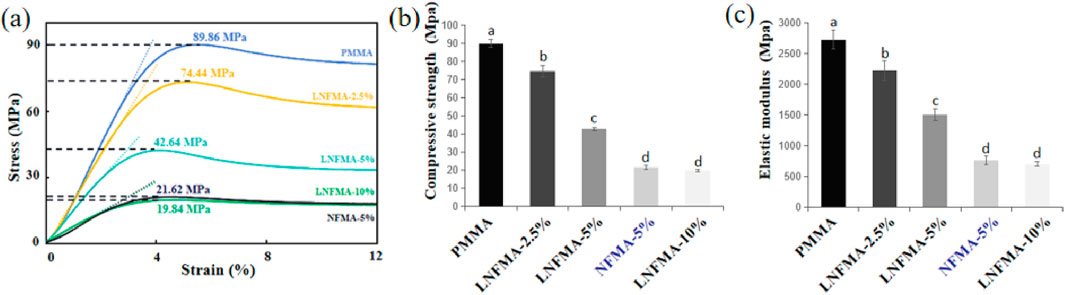
Figure 6. The mechanical properties of PMMA cement, LNFMA and NFMA cement. (A) Stress–strain curve; (B) Compressive strength; (C) Elastic modulus. Different lowercase letters indicate significant differences in the compressive strength or elastic modulus among different cements (p < 0.05).
3.3 Antibacterial activity
LNFMA-2.5% shows no significant inhibitory effect on S. aureus (p > 0.05). The inhibition rate of LNFMA-5% is 15.05% ± 0.5%, and the inhibition rate of LNFMA-10% is significantly higher than 5%, reaching 29%. When the addition amount of LNFMA is 20%, the inhibition rate is 53.9% ± 6.99%, and it increases to 57.38% ± 5.53% at 30% (Figure 2B). In summary, LNFMA bone cement exhibits good antimicrobial activity.
3.4 CCK-8 assay
The cell state of PMMA cement and bone cement with LNFMA content of 20% or less is normal (Figure 7A). However, under the microscope, most cells in the LNFMA-30% group are dead and in a suspended state. The CCK-8 test results (Figure 7) show that, except for the LNFMA-30% cement group, where the cell proliferation rate significantly decreases, exhibiting pronounced cytotoxicity (p < 0.05) with most cells dead and in a suspended state under the microscope; all other groups show no cytotoxicity, with good cell morphology.
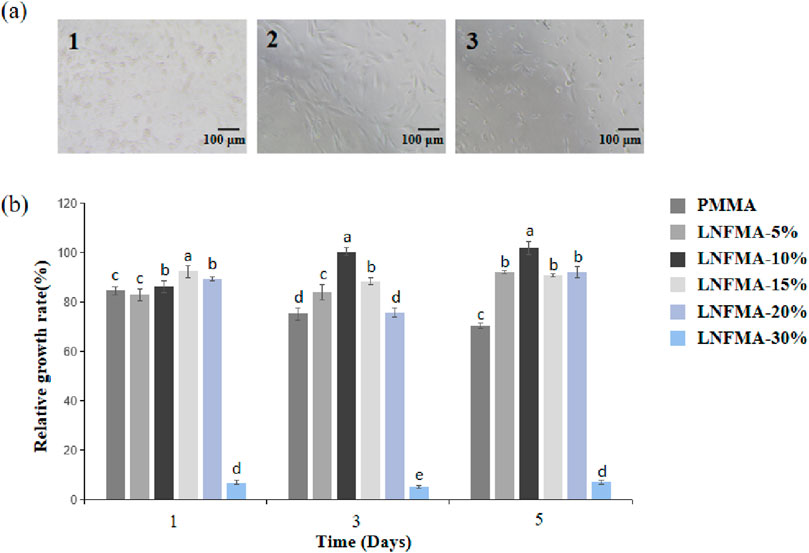
Figure 7. Cytotoxicity of the different fabricated cements. (A) Image of MC3T3-E1 cells incubated with different extracts (observed on the fifth day), 1: PMMA cement group, 2: LNFMA-20% cement group, 3: LNFMA-30% cement group; (B) Relative growth rate of cells. Different lowercase letters indicate significant differences in the relative growth rate of different cements (p < 0.05).
3.5 Histopathological examination
In the in vivo biocompatibility studies, we observed that mice in all groups were generally in good condition, showing no signs of toxicity or mortality. When sacrificing the mice, no abdominal adhesions were observed. Histological examination of the liver and kidneys of the mice revealed normal cellular and tissue morphology in all groups, with no apparent degeneration or necrosis (Figure 8).
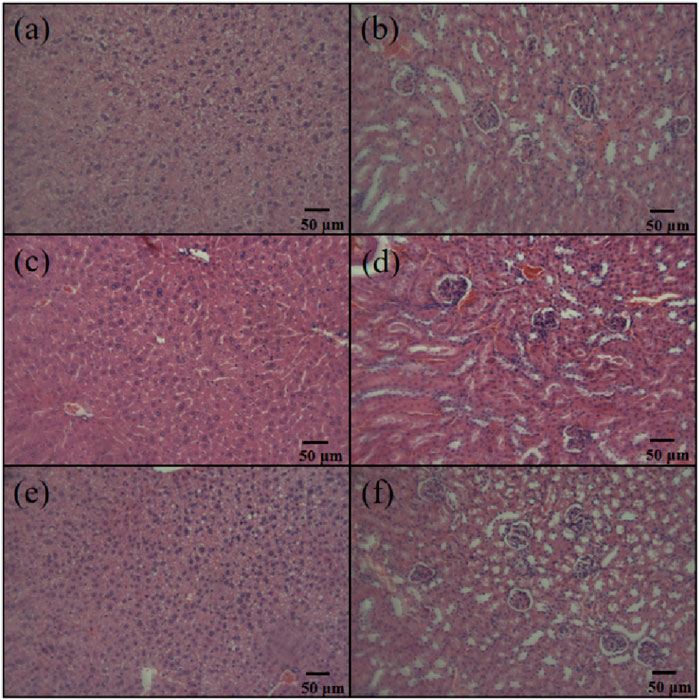
Figure 8. Liver and kidney sections of C57 mice injected with high-dose antibiotic-loaded NFBC extract. (A) Liver, PMMA cement group; (B) kidney, PMMA cement group; (C) liver, LNFMA-20% cement group; (D) kidney, LNFMA-20% cement group; (E) liver, negative control group; (F) kidney, negative control group.
4 Discussion
In order to overcome the burst release defects of traditional ALBC, researchers have optimized the release of antibiotics or other bioactive agents using technologies including nanotechnology, to create novel ALBC. As another complementary solution, NLBC has also been studied recently. The liquid-phase modification strategy provides a rapid and convenient way to obtain NLBC, among others, with enhanced biological activity (Deb et al., 2008; Mei et al., 2012; Beyth et al., 2014; Sun et al., 2016; Zhu et al., 2018a; Fu et al., 2022; Xu et al., 2024). We previously reported an antibacterial monomer NFMA, and we tried to use liquid-phase modification to produce antibacterial bone cement. However, we found that the mechanical strength of NFMA bone cement with liquid-phase modification is low. In this work, we designed a new antibacterial monomer LNFMA with a longer side chain structure. The longer side chain structure may help reduce the impact on mechanical strength.
Similar to the NFMA cement based on solid-phase modification reported previously, we observed some characteristic peaks of acrylic bone cement and nitrofuran in the FTIR spectra (Figure 3). Testing infrared requires grinding cement into powder, but cement containing LNFMA has low mechanical strength and is difficult to grind. Considering that the current experimental results can already explain that the cement contains nitrofuran groups, cement with LNFMA content exceeding 15% was not tested. Through SEM observation of the fracture surface of bone cement, we believe that the addition of LNFMA has little effect on the microstructure of bone cement. However, one observable change is the increase in the number of bubbles and, consequently, the enhanced porosity within the LNFMA cement. These oval inclusions are bubble cross sections of cement after liquid nitrogen brittle fracture treatment. In addition, we found that both the polymerization temperature and operating time decreased with the addition of LNFMA. We believe that this may be because the relative molecular mass of the LNFMA monomer is larger than that of MMA. Therefore, under the same mass, the molar amount of LNFMA is lower than that of MMA, which results in a less intense polymerization reaction. At the same time, the steric hindrance of the side chain of LNFMA is relatively large, which further slows down the polymerization reaction rate. Considering that the addition of LNFMA leads to a reduction in the polymerization rate, we believe that the increase in bubbles is also related to the incorporation of LNFMA. This could be attributed to the fact that with the addition of LNFMA, longer stirring times are required to facilitate setting, which results in the incorporation of more bubbles, thereby enhancing the porosity of the LNFMA bone cement.
According to ISO 5833, we tested the mechanical strength of LNFMA bone cement. We found that both LNFMA and NFMA monomers reduced the compressive strength and elastic modulus of acrylic cement (Figure 6). This is because they belong to the nitrofurantoin-based heterocyclic compounds, and the furan ring introduces certain steric hindrance during polymerization. The decrease in mechanical strength is proportional to the addition of antimicrobial monomers, possibly due to the alkyl chains in the monomers weakening intermolecular forces, promoting molecular mobility, and affecting cement polymerization, ultimately leading to the reduction in mechanical properties (Zhu et al., 2018a). In addition, we believe that there may be other factors influencing the mechanical strength with the increase of chain length. Zhu et al. believed that at the same mass, the number of monomer molecules in longer chains is smaller, which may reduce the adverse effects on the polymerization reaction, thereby improving the mechanical strength (Zhu et al., 2018b). Despite the reduction in mechanical strength caused by both LNFMA and NFMA, we observed that, at the same mass fraction (5%), the mechanical properties of LNFMA bone cement were significantly higher than NFMA bone cement. This improvement may be attributed to the smaller steric hindrance generated by LNFMA monomers with longer side chains during cement polymerization, reducing their impact on the polymerization reaction. Although LNFMA bone cement exhibits superior mechanical strength, the compressive strength and elastic modulus of bone cement decrease with the increase of LNFMA content (p < 0.05). When the LNFMA content is 2.5%, the compressive strength of the bone cement is 74.4 MPa. When the LNFMA content is 10%, the compressive strength is lower than the ISO 5833 standard, so we did not further test the mechanical strength of bone cement with higher LNFMA content. These results indicate that the LNFMA cement’s compressive strength generally struggles to reach 70 MPa, making it more suitable for clinical applications as a filler for bone defects or as a layer for implants rather than for use in weight-bearing bone (No et al., 2014).
Previously reported NFMA bone cement is a non-leaching antimicrobial bone cement, meaning that the antimicrobial motifs carried on the side chains do not leach out from the bone cement. The antimicrobial mechanism of NFMA bone cement may involve hydrophobic interactions between the nitrofuran structure and the bacterial membrane. It is also possible that the nitrofuran motif affects some enzymes on the surface of bacteria (Gao et al., 2023b; Chu et al., 2022; Alharthi et al., 2021).
In this study, we developed a long-chain LNFMA monomer with improved mechanical strength, and we need to further test the antibacterial activity of bone cement. Different detection methods have significant differences in presenting the antibacterial activity data. In our previous report, we used the direct contact test (DCT) as in literature X, to test the antibacterial activity. In this protocol, we dripped the liquid containing bacteria on the sample and incubated it for a period of time. Then we tested the number of bacteria in the droplet and compared it with the number of bacteria in the original bacterial liquid. However, when using this protocol to present the antibacterial activity data, PMMA bone cement also exhibits high antibacterial activity. Therefore, in subsequent studies, we proposed a new DCT protocol for testing the antibacterial activity (Gao et al., 2023a). In this protocol, PMMA is considered as a material with a antibacterial activity of 0, so this comparison makes the data more intuitive. To verify whether bone cement containing this new monomer possesses antimicrobial activity, we conducted a DCT protocol to observe the antibacterial activity of different LNFMA content bone cements against S. aureus which is a common clinical pathogenic bacterium. Among them, LNFMA-2.5% has almost no antibacterial activity, while LNFMA-30% has the strongest antibacterial activity. The antibacterial activity increases with the increase of LNFMA content. In summary, LNFMA bone cement exhibits good antimicrobial activity.
When an implant is applied clinically, the primary consideration is the biocompatibility of the material. It should meet requirements such as being non-toxic, free from carcinogenic or teratogenic risks, and not causing immune rejection (Helmus et al., 2008). The RGR of LNFMA-5%, 10%, 15%, and 20% are higher than that of PMMA bone cement, indicating no cytotoxicity. However, unexpectedly, the RGR of LNFMA-30% drop dramatically (Figure 7), exhibiting pronounced cytotoxicity (p < 0.05) with most cells dead and in a suspended state under the microscope. This may be because when the concentration of LNFMA is too high, the monomers are difficult to fully polymerize. Therefore, in the preparation of the bone cement leaching solution, residual monomers are released into the liquid, resulting in higher cell toxicity (Kostić et al., 2020). To validate this hypothesis, we conducted agar diffusion test on two types of LNFMA bone cement with 20% and 30% content, as shown in Supplementary Figure S2. Compared to LNFMA-20% cement, the LNFMA-30% cement samples exhibited a more pronounced partial inhibition zone against S. aureus, confirming the presence of unreacted monomers in samples with excessively high concentrations, which are released from the bone cement. Liquid-phase modification is easy to operate, but compared with solid-phase modification, it has to deal with the toxicity of unpolymerized monomers. Therefore, a careful assessment of the addition of novel monomers is necessary.
To further test the biocompatibility of the cement containing LNFMA monomer, we conducted histopathological examination. Since the unpolymerized monomer of LNFMA-30% caused higher cell toxicity, it was excluded from the in vivo study, and we conducted an in vivo experiment to compare the PMMA and LNFMA-20%. Liver and kidney cells and tissues in all groups displayed normal morphology, with no evident degeneration or necrosis, indicating the absence of acute toxicity in the experimental animals (Figure 8). At the same time, the body weight of mice in the LNFMA-20% group did not differ significantly from that of the PMMA group and the control group (Supplementary Table S1). These findings align with the results of the cytotoxicity study, confirming the good biocompatibility of LNFMA bone cement.
5 Conclusion
This study reported a long-chain nitrofuran methacrylate monomer and employed a liquid-phase modification strategy to modify acrylic bone cement, resulting in a new non-leaching antimicrobial LNFMA bone cement. In comparison to previously reported NFMA antimicrobial monomers, LNFMA exhibited optimized mechanical strength and demonstrated excellent antimicrobial activity. We found that the compressive strength of LNFMA-5% bone cement with long side chains is approximately 197% higher than that of previously reported NFMA-5% cement with short side chains. However, the compressive strength of LNFMA-5% cement and those with higher addition percentages falls below 70 MPa, indicating that it was suitable for non-bearing bones. We believe that the longer side chains of LNFMA may reduce the steric hindrance during the polymerization reaction, thereby promoting the elongation of the polymer. Additionally, when the same mass of new monomers is added, the molar number of long-chain monomers is smaller, making it easier for the monomers to diffuse and facilitate the occurrence of bone cement polymerization (Zhu et al., 2018a). These properties contribute to the enhanced compressive strength of LNFMA cement compared to NFMA cement. Biocompatibility studies in vitro revealed good biocompatibility when the LNFMA content did not exceed 20%.
To sum up, if there is a need for bone cement to balance both antibacterial activity and mechanical strength, we believe that LNFMA-5% is a suitable choice, with an antibacterial rate of 15.05% ± 0.5% and a compressive strength of 42.64 ± 0.94 MPa. If the primary consideration is solely the antibacterial property of the bone cement, we recommend using a higher LNFMA content, such as LNFMA-20%, which achieves an antibacterial rate of 53.9% ± 6.99% while also demonstrating good biocompatibility. This liquid-phase modification using the new LNFMA monomer represents a simple and efficient method for preparing antimicrobial bone cement, with potential clinical applications to prevent bacterial colonization on implant surfaces.
6 Limitation
In this study, we designed bone cement containing long-chain LNFMA monomer, which demonstrated improved mechanical strength compared to previously reported NFMA bone cement. However, at the concentration that exhibits good antibacterial activity, the compressive strength of LNFMA cement falls below the 70 MPa standard specified in the national standard (ISO 5833:2002). Future research will focus on enhancing the mechanical strength of this material. While the LNFMA cement in this study exhibited excellent antibacterial properties against S. aureus, which is the most common bacterial strain involved in orthopedic infections, other bacterial species such as Escherichia coli and Staphylococcus epidermidis are also prevalent in orthopedic infections. Unfortunately, the antibacterial activity of LNFMA cement against these bacteria was not tested in this study. Moreover, the investigation into the biocompatibility of this novel antibacterial bone cement remains preliminary. Further research is planned to explore its potential as an implant material in terms of its biocompatibility within animal models.
Data availability statement
The raw data supporting the conclusions of this article will be made available by the authors, without undue reservation.
Ethics statement
The animal study was approved by Biomedical Ethics Committee of Hefei University of Technology. The study was conducted in accordance with the local legislation and institutional requirements.
Author contributions
HL: Writing–original draft. ZG: Writing–original draft. L-YH: Writing–original draft. J-JC: Writing–review and editing, Writing–original draft. YX: Funding acquisition, Writing–review and editing, Writing–original draft. D-HS: Writing–review and editing, Writing–original draft, Funding acquisition.
Funding
The author(s) declare that financial support was received for the research, authorship, and/or publication of this article. The authors gratefully acknowledge the financial support from Anhui Provincial Natural Science Foundation (2308085QB47), the Health Research Project of Anhui Province (AHWJ2022b091), the Fundamental Research Funds for the Central Universities (JZ2023HGQA0114). Hefei Municipal Health Commission Applied Medical Project (Hwk2023zd011).
Acknowledgments
Thank the department of medical laboratory of Hefei Second People’s Hospital’s Xie Yang-Hu and the department of pathology’s Zhao Min for their guidance and assistance in the experiment.
Conflict of interest
The authors declare that the research was conducted in the absence of any commercial or financial relationships that could be construed as a potential conflict of interest.
Publisher’s note
All claims expressed in this article are solely those of the authors and do not necessarily represent those of their affiliated organizations, or those of the publisher, the editors and the reviewers. Any product that may be evaluated in this article, or claim that may be made by its manufacturer, is not guaranteed or endorsed by the publisher.
Supplementary material
The Supplementary Material for this article can be found online at: https://www.frontiersin.org/articles/10.3389/fmats.2024.1432482/full#supplementary-material
References
Abid, C. Z., Jain, S., Jackeray, R., Chattopadhyay, S., and Singh, H. (2017). Formulation and characterization of antimicrobial quaternary ammonium dendrimer in poly (methyl methcarylate) bone cement. J. Biomed. Mater. Res. Part B Appl. Biomaterials 105 (3), 521–530. doi:10.1002/jbm.b.33553
Al, T. Y., Khalil, R., Abdelghany, S., and Salem, M. S. (2022). Antimicrobial PMMA bone cement containing long releasing multi-walled carbon nanotubes. Nanomaterials 12 (8), 1381. doi:10.3390/nano12081381
Alharthi, S., Alavi, S. E., Moyle, P. M., and Ziora, Z. M. (2021). Sortase A (SrtA) inhibitors as an alternative treatment for superbug infections. Drug Discov. Today 26 (9), 2164–2172. doi:10.1016/j.drudis.2021.03.019
Ali, U., Karim, K. J. B. A., and Buang, N. A. (2015). A review of the properties and applications of poly (methyl methacrylate)(PMMA). Polym. Rev. 55 (4), 678–705. doi:10.1080/15583724.2015.1031377
Batista Campos, L., Kurihara, M. N. L., Santos, I. N. M., Dos Reis, F. B., and Salles, M. J. (2023). In vitro elution characteristics of antibiotic-loaded polymethylmethacrylate cement and a calcium sulfate bone substitute using staphylococci isolated from orthopedic implant-associated infections. J. Biomed. Mater. Res. Part B, Appl. biomaterials 111 (6), 1318–1327. doi:10.1002/jbm.b.35235
Berberich, C., and Sanz-Ruiz, P. (2019). Risk assessment of antibiotic resistance development by antibiotic-loaded bone cements: is it a clinical concern? EFORT Open Rev. 4 (10), 576–584. doi:10.1302/2058-5241.4.180104
Beyth, S., Polak, D., Milgrom, C., Weiss, E. I., Matanis, S., and Beyth, N. (2014). Antibacterial activity of bone cement containing quaternary ammonium polyethyleneimine nanoparticles. J. Antimicrob. Chemother. 69 (3), 854–855. doi:10.1093/jac/dkt441
Chen, L., Tang, Y., Zhao, K., Zha, X., Wei, M., Tan, Q., et al. (2021). Sequential release of double drug (graded distribution) loaded gelatin microspheres/PMMA bone cement. J. Mater. Chem. B 9 (2), 508–522. doi:10.1039/d0tb01452d
Chen, Y., Caneli, G., Almousa, R., and Xie, D. (2022b). A novel antibacterial zirconia-containing PMMA bone cement. J. Mech. Behav. Biomed. Mater. 129, 105135. doi:10.1016/j.jmbbm.2022.105135
Chen, Y., Caneli, G., and Xie, D. (2022a). A PMMA bone cement with improved antibacterial function and flexural strength. J. Biomaterials Sci. 33 (11), 1398–1414. doi:10.1080/09205063.2022.2056943
Chu, J., Li, C., Guo, J., Xu, Y., and Fu, Y. (2022). Preparation of new bio-based antibacterial acrylic bone cement via modification with a biofunctional monomer of nitrofurfuryl methacrylate. Polym. Chem. 13 (32), 4675–4683. doi:10.1039/d2py00235c
Deb, S., Doiron, R., DiSilvio, L., Punyani, S., and Singh, H. (2008). PMMA bone cement containing a quaternary amine comonomer with potential antibacterial properties. J. Biomed. Mater. Res. Part B Appl. Biomaterials An Official J. Soc. Biomaterials, Jpn. Soc. Biomaterials, Aust. Soc. Biomaterials Korean Soc. Biomaterials 85 (1), 130–139. doi:10.1002/jbm.b.30925
El-Ghannam, A., Jahed, K., and Govindaswami, M. (2010). Resorbable bioactive ceramic for treatment of bone infection. J. Biomed. Mater. Res. Part A 94 (1), 308–316. doi:10.1002/jbm.a.32705
Eltohamy, M., Kundu, B., Moon, J., Lee, H. Y., and Kim, H. W. (2018). Anti-bacterial zinc-doped calcium silicate cements: bone filler. Ceram. Int. 44 (11), 13031–13038. doi:10.1016/j.ceramint.2018.04.122
Fu, W., Liu, Q., Wang, L., Huang, X., Su, Z., Huang, Z., et al. (2022). Synthesis of polymerizable quaternary thiazole salts and their application as antibacterial agents for dental resin. J. Mech. Behav. Biomed. Mater. 130, 105183. doi:10.1016/j.jmbbm.2022.105183
Gálvez-López, R., Peña-Monje, A., Antelo-Lorenzo, R., Guardia-Olmedo, J., Moliz, J., Hernández-Quero, J., et al. (2014). Elution kinetics, antimicrobial activity, and mechanical properties of 11 different antibiotic loaded acrylic bone cement. Diagnostic Microbiol. Infect. Dis. 78 (1), 70–74. doi:10.1016/j.diagmicrobio.2013.09.014
Gao, Z., Kan, Y. C., Xie, Y. H., Guo, R., Li, C., Asilebieke, A., et al. (2023a). A review on non-leaching antibacterial bone cement for orthopedic surgery: from past to current insights. AIP Adv. 13 (10). doi:10.1063/5.0174845
Gao, Z., Xu, Y., Kan, Y., Li, H., Guo, R., Han, L., et al. (2023b). Comparison of antibacterial activity and biocompatibility of non-leaching nitrofuran bone cement loaded with vancomycin, gentamicin, and tigecycline. J. Orthop. Surg. Res. 18 (1), 569. doi:10.1186/s13018-023-04055-2
Garcia-Garcia, J., Azuara, G., Fraile-Martinez, O., Garcia-Montero, C., Alvarez-Mon, M. A., Ruiz-Diez, S., et al. (2022). Modification of the polymer of a bone cement with biodegradable microspheres of PLGA and loading with daptomycin and vancomycin improve the response to bone tissue infection. Polymers 14 (5), 888. doi:10.3390/polym14050888
Helmus, M. N., Gibbons, D. F., and Cebon, D. (2008). Biocompatibility: meeting a key functional requirement of next-generation medical devices. Toxicol. Pathol. 36 (1), 70–80. doi:10.1177/0192623307310949
Kostić, M., Stanojević, J., Tačić, A., Gligorijević, N., Nikolić, L., Nikolić, V., et al. (2020). Determination of residual monomer content in dental acrylic polymers and effect after tissues implantation. Biotechnol. and Biotechnol. Equip. 34 (1), 254–263. doi:10.1080/13102818.2020.1736952
Mei, L., Ren, Y., Loontjens, T. J., Van Der Mei, H. C., and Busscher, H. J. (2012). Contact-killing of adhering streptococci by a quaternary ammonium compound incorporated in an acrylic resin. Int. J. Artif. organs 35 (10), 854–863. doi:10.5301/ijao.5000149
Mistry, S., Roy, R., Jha, A. K., Pandit, N., Das, S., Burman, S., et al. (2022). Treatment of long bone infection by a biodegradable bone cement releasing antibiotics in human. J. Control. release official J. Control. Release Soc. 346, 180–192. doi:10.1016/j.jconrel.2022.04.018
Moojen, D. J. F., Hentenaar, B., Vogely, H. C., Verbout, A. J., Castelein, R. M., and Dhert, W. J. (2008). In vitro release of antibiotics from commercial PMMA beads and articulating hip spacers. J. arthroplasty 23 (8), 1152–1156. doi:10.1016/j.arth.2007.08.020
No, Y. J., Roohani-Esfahani, S. I., and Zreiqat, H. (2014). Nanomaterials: the next step in injectable bone cements. Nanomedicine 9 (11), 1745–1764. doi:10.2217/nnm.14.109
Ploux, L., Mateescu, M., Guichaoua, L., Valentin, J., Böhmler, J., Anselme, K., et al. (2016). New colloidal fabrication of bioceramics with controlled porosity for delivery of antibiotics. J. Mater. Sci. 51, 8861–8879. doi:10.1007/s10853-016-0133-z
Robu, A., Antoniac, A., Ciocoiu, R., Grosu, E., Rau, J. V., Fosca, M., et al. (2022). Effect of the antimicrobial agents peppermint essential oil and silver nanoparticles on bone cement properties. Biomimetics 7 (3), 137. doi:10.3390/biomimetics7030137
Schwarz, E. M., McLaren, A. C., Sculco, T. P., Brause, B., Bostrom, M., Kates, S. L., et al. (2021). Adjuvant antibiotic-loaded bone cement: concerns with current use and research to make it work. J. Orthop. Research® 39 (2), 227–239. doi:10.1002/jor.24616
Seidenstuecker, M., Hess, J., Baghnavi, A., Schmal, H., Voigt, D., and Mayr, H. O. (2024). Biodegradable composites with antibiotics and growth factors for dual release kinetics. J. Mater. Sci. Mater. Med. 35 (1), 40. doi:10.1007/s10856-024-06809-8
Shi, H., Zhuang, Q., Zheng, A., Zhan, P., Guan, Y., Wei, D., et al. (2021). Permanent antimicrobial polymethyl methacrylate prepared by chemical bonding with poly (hexamethylene guanidine hydrochloride). Polym. Adv. Technol. 32 (12), 4678–4689. doi:10.1002/pat.5459
Sun, X., Qian, Z., Luo, L., Yuan, Q., Guo, X., Tao, L., et al. (2016). Antibacterial adhesion of poly (methyl methacrylate) modified by borneol acrylate. ACS Appl. Mater. and Interfaces 8 (42), 28522–28528. doi:10.1021/acsami.6b10498
Świeczko-Żurek, B., Zieliński, A., Bociąga, D., Rosińska, K., and Gajowiec, G. (2022). Influence of different nanometals implemented in PMMA bone cement on biological and mechanical properties. Nanomater. 12 (5), 732. doi:10.3390/nano12050732
Wang, H., Maeda, T., and Miyazaki, T. (2021). Preparation of bioactive and antibacterial PMMA-based bone cement by modification with quaternary ammonium and alkoxysilane. J. Biomaterials Appl. 36 (2), 311–320. doi:10.1177/08853282211004413
Wright, Z. M., Pandit, A. M., Holt, B. D., and Sydlik, S. A. (2019). Therapeutic methacrylic comonomers for covalently controlled release from mechanically robust bone cement: kinetics and structure–function relationships. Macromolecules 52 (10), 3775–3786. doi:10.1021/acs.macromol.9b00368
Wu, K., Chen, Y. C., Hsu, Y. M., and Chang, C. H. (2016). Enhancing drug release from antibiotic-loaded bone cement using porogens. JAAOS-Journal Am. Acad. Orthop. Surg. 24 (3), 188–195. doi:10.5435/jaaos-d-15-00469
Xu, D., Song, W., Zhang, J., Liu, Y., Lu, Y., Zhang, X., et al. (2021). Osteogenic effect of polymethyl methacrylate bone cement with surface modification of lactoferrin. J. Biosci. Bioeng. 132 (2), 132–139. doi:10.1016/j.jbiosc.2021.04.006
Xu, T., Wu, K. L., and Jie, K. (2022). Comprehensive meta-analysis of antibiotic-impregnated bone cement versus plain bone cement in primary total knee arthroplasty for preventing periprosthetic joint infection. Chin. J. Traumatology 25 (06), 325–330. doi:10.1016/j.cjtee.2022.06.001
Xu, Y., Lin, H., Gao, Z., Guo, R., Kan, Y. C., Han, L. Y., et al. (2024). Injectable isoniazid-loaded bone cement based on hydrazone bonds achieving long-term release and decent mechanical properties. J. Mater. Chem. B 12, 4389–4397. doi:10.1039/d3tb02661b
Yang, L., Yergeshov, A. A., Al-Thaher, Y., Avdokushina, S., Statsenko, E., Abdullin, T. I., et al. (2023). Nanocomposite orthopaedic bone cement combining long-acting dual antimicrobial drugs. Biomater. Adv. 153, 213538. doi:10.1016/j.bioadv.2023.213538
Zhu, W., Lao, C., Luo, S., Liu, F., Huang, Q., He, J., et al. (2018a). Mechanical and antibacterial properties of benzothiazole-based dental resin materials. J. Biomaterials Sci. 29 (6), 635–645. doi:10.1080/09205063.2018.1429861
Zhu, W., Liu, F., and He, J. (2018b). Effect of polymerizable quaternary ammonium monomer MEIM-x's alkyl chain length and content on bone cement's antibacterial activity and physicochemical properties. J. Mech. Behav. Biomed. Mater. 87, 279–287. doi:10.1016/j.jmbbm.2018.08.004
Keywords: bone cement, antimicrobial monomers, liquid-phase modification, mechanical strength, bone implant
Citation: Lin H, Gao Z, Han L-Y, Chu J-J, Xu Y and Shen D-H (2024) Antibacterial bone cement modified by long-chain nitrofuran methacrylate using liquid-phase modification strategy. Front. Mater. 11:1432482. doi: 10.3389/fmats.2024.1432482
Received: 14 May 2024; Accepted: 17 September 2024;
Published: 03 October 2024.
Edited by:
Yan Huang, Brunel University London, United KingdomReviewed by:
Marcin Wekwejt, Gdansk University of Technology, PolandEyyup Murat Karakurt, Osmaniye Korkut Ata University, Türkiye
Copyright © 2024 Lin, Gao, Han, Chu, Xu and Shen. This is an open-access article distributed under the terms of the Creative Commons Attribution License (CC BY). The use, distribution or reproduction in other forums is permitted, provided the original author(s) and the copyright owner(s) are credited and that the original publication in this journal is cited, in accordance with accepted academic practice. No use, distribution or reproduction is permitted which does not comply with these terms.
*Correspondence: Dian-Hong Shen, 623222807@qq.com; Jian-Jun Chu, chujianj@mail.ustc.edu.cn; Yang Xu, xuyang@hfut.edu.cn