- 1School of Civil Engineering and Architecture, Guizhou Minzu University, Guiyang, China
- 2Shenzhen Huadi Geotechnical Engineering Co., Ltd., Shenzhen, China
During the preparation of asphalt mixtures, styrene-butadiene rubber (SBR) polymers are susceptible to thermal decomposition, which can significantly impair the binder’s low-temperature performance. This study explores the potential of combining warming agents with waste materials to enhance the low-temperature properties and aging resistance of the binder. Specifically, it examines the synergistic impact of Sasobit/recycled engine oil (Sasobit/REO) composites on the rheological and physical attributes of styrene-butadiene rubber asphalt binder (SBRAB). Utilizing fluorescence microscopy (FM), bending beam rheometer (BBR), and dynamic shear rheometer (DSR), the study assesses the aging resistance and modification mechanisms of Sasobit/REO on SBRAB. The findings indicate that the incorporation of Sasobit/REO composites more effectively reduces the mix preparation temperature than either component alone. The preparation of Sasobit/REO warm mix asphalt mixtures is feasible at temperatures 20°C lower than those required for traditional hot mixtures. These composites also enhance the performance of SBRAB at both high and low temperatures, counteracting the adverse effects associated with the individual use of Sasobit or REO. This reduced short-term aging temperature is beneficial in lessening the negative impact of high temperatures on SBRAB’s performance. Moreover, the addition of Sasobit/REO composites significantly improves the thermal cracking resistance of SBRAB mixtures. The study also demonstrates that Sasobit/REO enhances the short-term and long-term aging resistance of SBRAB, paving the way for the broader application of this novel warm mix additive in the asphalt industry.
1 Introduction
Asphalt binder (AB), renowned for its robust adhesive capabilities, is extensively employed as a bonding agent in road pavement construction. However, in colder climates, the AB’s propensity for increased brittleness and diminished flexibility can precipitate cracking within the asphalt matrix (Jain and Singh, 2021; Shanbara et al., 2021). The volatilization of lighter fractions and the concomitant formation of asphaltenes exacerbate the aging process, rendering cracking damage more pronounced (Canestrari and Ingrassia, 2020; Ziari et al., 2020). Therefore, the modification of AB to bolster its low-temperature performance is of paramount importance. Among various polymer modifiers, styrene-butadiene rubber (SBR) stands out for its efficacy in enhancing the low-temperature characteristics of AB (Hemmati et al., 2021; Wang et al., 2023). An optimal dosage of SBR facilitates the intertwining of polymer chains within the modified AB, forging a robust cross-linking network that significantly ameliorates the binder’s toughness and tensile capabilities in chilly conditions (Li et al., 2021a; Han et al., 2022).
Despite these advantages, the manufacturing of SBR-modified AB (SBRAB) in high-temperature settings inevitably induces intense oxidation and degradation reactions within the colloidal system. This, in turn, diminishes SBR’s capacity to combat thermally induced cracking in the modified AB (Liu C. et al., 2021; Liu F. et al., 2021). Studies have indicated that short-term aging can cause a more than 50% reduction in SBRAB’s low-temperature ductility compared to its pre-aged condition (Zhang and Hu, 2014). Furthermore, the limited thermal stability of SBRAB presents a significant challenge for its application in regions characterized by high and low temperatures (Vamegh et al., 2020). To surmount these obstacles, it is imperative to enhance SBRAB’s resistance to rutting and aging. This can be accomplished by incorporating additives that augment the physical or chemical properties of SBRAB, thereby elevating its performance and expanding its applicability in diverse climatic conditions.
Warm mix asphalt (WMA) technology enables the production of asphalt mixtures at temperatures 10°C–30°C lower than those traditionally employed for hot mix asphalt (HMA), thereby significantly reducing the extent of short-term aging experienced by the binder (Belc et al., 2021; Yousefi et al., 2021). To alleviate the thermal aging effects in SBR-modified asphalt, the modifier is frequently incorporated into the preparation of SBR-modified asphalt binder (SBRAB). Among the various WMA technologies, Sasobit stands out as a prevalent choice due to its ability to enhance the thermal elasticity of asphalt and mitigate the risks associated with thermal aging (Walther et al., 2019; Sobhi et al., 2020; Xu et al., 2020; Rezaeizadeh Herozi et al., 2022). For instance, the incorporation of 4% Sasobit has been shown to decrease the preparation temperature of SBRAB mixtures by 14°C and to elevate the softening point of SBRAB by 35% (Li et al., 2021b). However, this enhancement in thermal stability comes at the expense of a significant reduction in the low-temperature ductility of the asphalt, with a loss exceeding 80%. This reduction in low-temperature performance is attributed to the synthetic waxes present in Sasobit, which restrict the deformation capabilities of the asphalt at ambient and sub-ambient temperatures (Qin et al., 2014; Poulikakos et al., 2022; Liu et al., 2023). This insight underscores the need for a balanced approach in the design of WMA, ensuring that while thermal aging is minimized, the binder’s low-temperature performance is not adversely affected.
Research has demonstrated that recycled engine oil (REO), with its lubricating and elastic properties, can enhance the cracking resistance of asphalt, thereby mitigating the risk of cracking under fluctuating temperatures and traffic-induced loads (Luo et al., 2017; Fernandes et al., 2018; Yan et al., 2022). Moreover, investigations into the impact of REO on reclaimed asphalt have revealed that it promotes the homogeneous dispersion of asphaltene particles and the diffusion of reclaiming agents. The base oils present in REO improve the fluidity of asphalt, rendering it more manageable and facilitating paving operations. This enhancement in workability is particularly beneficial in maintaining asphalt fluidity at low temperatures, thereby boosting construction efficiency (Falchetto et al., 2018; Qurashi and Swamy, 2018; Eltwati et al., 2022). However, the presence of volatile components in REO can compromise its thermal stability, and its incorporation tends to diminish the high-temperature performance of modified asphalt (Wang W. et al., 2020; Liu et al., 2022a). As the addition of REO increases, so too do its adverse effects (Eleyedath et al., 2020; Liu et al., 2022b).
Prior studies have indicated that Sasobit, a conventional warm mix additive, can reduce the mixing temperature of asphalt mixtures and potentially enhance the aging resistance of the binder (Yan et al., 2022). However, this comes at the cost of a significant trade-off in low-temperature performance. To address these limitations, the addition of complementary modifiers is essential. While REO may be deleterious to the high-temperature properties of asphalt, it also exhibits a notable ability to improve asphalt ductility. This method suggests that composite modifiers, leveraging the synergistic effects of Sasobit and REO, can maintain low-temperature performance while enhancing the aging resistance of SBRAB. In this context, Sasobit is chosen as a representative warm mix component, with REO introduced to offset the negative impact on Sasobit’s low-temperature performance.
The present study aims to elucidate the mechanism and synergistic effects of Sasobit and REO on SBRAB. Physical and viscosity tests were conducted to ascertain the impact of various modifiers on the properties of SBRAB and the aging temperatures of different asphalt formulations. Subsequently, rheological tests were employed to investigate the aging characteristics of asphalts at diverse aging temperatures, and the short-term and long-term aging resistance was systematically evaluated. Ultimately, the synergistic mechanism was explored using fluorescence microscopy, providing valuable insights into the combined application of Sasobit and REO in the modification of SBRAB.
2 Testing materials and research methodology
2.1 Raw materials
2.1.1 Asphalt
In this study, the base asphalt of the 60/80 penetration grade, procured from China Jingbo Petrochemical Co., Ltd., was utilized as the fundamental material. The key characteristic parameters of this base asphalt are detailed in Table 1.
2.1.2 SBR
The anionic polymer modifier utilized in this investigation was liquid styrene-butadiene rubber (SBR) latex. The principal characteristics of the SBR are presented in Table 2.
2.1.3 Sasobit
In this research, Sasobit, a warm mix asphalt additive sourced from Henan Lupeng Transportation Technology Co., Ltd., was incorporated. The physical properties of Sasobit are detailed in Table 3.
2.1.4 REO
The REO employed in this study underwent a purification process. The critical technical specifications of the REO are delineated in Table 4.
2.2 Preparation processes of modified ABs
The preparation of the modified asphalt commenced with heating the base asphalt to a temperature of 140°C within an oven, followed by a shearing process for 20 min to ensure homogeneous distribution of the SBR, Sasobit, and REO within the base material. The proportions of SBR, Sasobit, and REO were determined to be 4 wt%, 4 wt%, and 6 wt%, respectively, drawing upon the modifier concentrations documented in existing literature about modified asphalt (Li et al., 2021a; Li et al., 2021b; Poulikakos et al., 2022). Post the incorporation of the modifiers, the mixture was subjected to shear mixing at 160°C for 40 min. Subsequently, the modified asphalt was allowed to develop at 120°C for 1 h. The resultant asphalt samples, categorized by their respective modifiers, are designated as SBRAB, SBRABS, SBRABR, and SBRABSR. A detailed illustration of the modified asphalt synthesis is provided in Figure 1.
2.3 Conventional tests
To evaluate the conventional properties of the Sasobit/REO modified asphalt binders, assessments of penetration, ductility, and softening point were executed. These tests are pivotal as they provide insights into the hardness, resistance to permanent deformation, and low-temperature flexibility of the binders. The rotational viscometer, a widely recognized instrument for generating viscosity-temperature profiles, was utilized in this study. This parameter is particularly significant as it delineates the workability of the asphalt binder under high-temperature mixing conditions. The viscosity measurements were conducted at discrete temperatures of 120°C, 135°C, 150°C, 165°C, and 180°C.
2.4 Aging tests
Considering the inherent variability in materials, the conventional short-term aging temperature fails to accurately replicate the aging dynamics of asphalt in practical applications (Wang F. et al., 2020; Cui et al., 2020). In light of the iso-viscosity principle, a reference temperature for short-term aging of asphalt is determined at the viscosity threshold of 0.17 Pas. Furthermore, to emulate the thermo-oxidative aging experienced by asphalt over extended service periods, the Pressure Aging Vessel (PAV) test is employed.
2.5 Bending beam rheometer (BBR) tests
Within the framework of the asphalt binder performance evaluation, the BBR test was deployed to quantify the binder’s stiffness at low temperatures. Before the BBR evaluation, all binders underwent aging using the PAV to simulate long-term thermal degradation. For each specimen, a triplicate of tests was conducted to ensure data reliability. The BBR test was conducted at −12°C and −18°C to evaluate the binder’s resistance to thermal cracking. For each sample, creep rate (m-values) and creep stiffness (S) were determined at these respective temperatures, providing a comprehensive assessment of the binder’s low-temperature performance characteristics.
2.6 Temperature sweep tests
The DSR was utilized to perform a temperature sweep test, aimed at characterizing the viscoelastic properties of the asphalt. A constant strain of 1% was applied, and the test was conducted over a temperature range of 40°C–85°C, with increments of 5°C to capture the temperature-dependent changes in the material’s response.
2.7 Fluorescence microscopy (FM) test
Upon absorption of light components within the asphalt matrix, the SBR domains can exhibit fluorescent emissions, which can be discerned through the SBR modifier (Che et al., 2022). Fluorescence microscopy (FM) offers a means to visualize the morphology of SBR within the asphalt matrix, both pre- and post-aging. For the analysis, a drop of molten asphalt was carefully placed onto a microscope slide and spread evenly under a coverslip to form a uniformly thin film. The experiments were conducted using a CAIKON-brand fluorescence microscope at a magnification of ×80, enabling a detailed examination of the SBR’s structural integrity and distribution within the matrix.
3 Results and discussion
3.1 Conventional test
As depicted in Figure 2, the conventional test outcomes for the modified asphalt specimens are presented. It is noted that the penetration and softening points of the asphalt binder and the SBRAB are strikingly similar. However, a marked enhancement in ductility of SBRAB is observed, with an increase from 6.1 cm to 58.3 cm, attributable to the formation of a cemented mesh structure by SBR, which significantly enhances the binder’s toughness and tensile characteristics at low temperatures. In contrast, the addition of Sasobit to SBRAB led to a substantial reduction in both penetration and ductility by 117.3 cm and 144.6 cm, respectively, and a 50% increase in the softening point compared to SBRAB. This suggests that the sole incorporation of Sasobit is detrimental due to its adverse impact on the low-temperature performance of the modified asphalt. The findings align with those of the prior study (Poulikakos et al., 2022). Conversely, the incorporation of REO resulted in a softening point decrease of 8°C for SBRAB, adversely affecting its high-temperature stability. Notably, the composite addition of Sasobit and REO led to a significant improvement in both softening point and penetration, ascribed to the synergistic interaction between Sasobit and REO. This enhancement in performance is evident across a broad temperature spectrum, encompassing both high- and low-temperature extremes. It is concluded that the Sasobit/REO composite modifier is distinctly advantageous over the individual modifiers, offering a superior balance of properties for SBRAB.
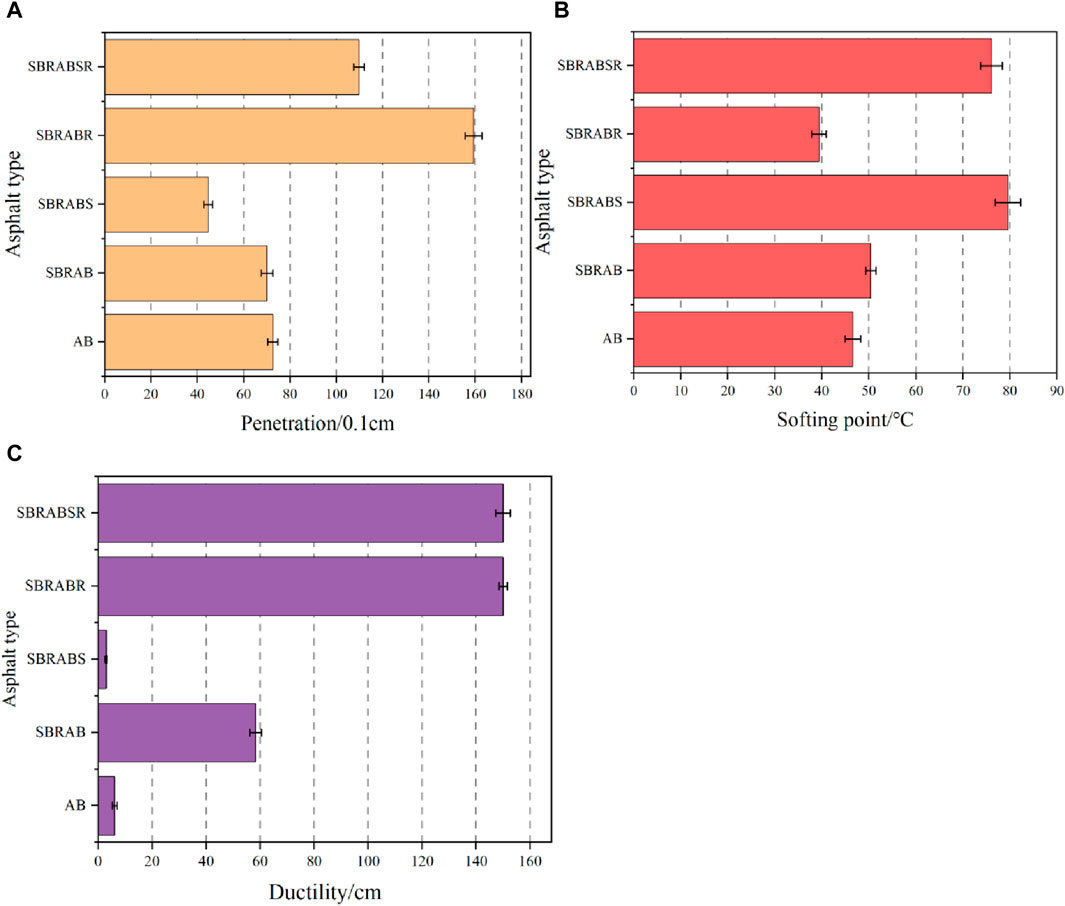
Figure 2. Conventional properties of different asphalts: (A) penetration (B) softening point (C) ductility.
3.2 Determination of aging temperature
Figure 3 presents the viscosity-temperature profiles for the various ABs, with the viscosity ranked in descending order as SBRAB > SBRABS > SBRABR > SBRABSR. The data demonstrate that, across the range of test temperatures, the viscosity of the modified asphalts—SBRABS, SBRABR, and SBRABSR—are consistently lower than those of SBRAB. This reduction in viscosity upon modification indicates the successful incorporation of additives designed to alleviate the viscosity of SBRAB. Two primary mechanisms are posited to explain this observation. Firstly, Sasobit, recognized as a conventional hot mix additive, effectively reduces binder viscosity, thereby facilitating the hot mixing process (Tong et al., 2022). Secondly, the principal constituents of REO are lightweight components that, when added in greater quantities, may amplify the softening effect on asphalt, thereby contributing to a reduction in viscosity (Wang W. et al., 2020).
The ideal mixing and compaction temperatures for asphalt are identified as corresponding to a viscosity of 0.17 Pas and 0.28 Pas, respectively. These specific viscosity provide a precise characterization of asphalt viscosity during production and construction phases (Luo et al., 2021). Table 5 delineates the mixing and compaction temperatures for the different modified asphalts, as derived from the viscosity-temperature curves. SBRAB exhibits the highest temperatures, while the incorporation of Sasobit and REO in SBRAB demonstrates a notable decrease in temperature, suggesting that these additives can significantly reduce the preparation temperature of SBRAB. Specifically, the composite modifier of Sasobit/REO reduced the mixing temperature of SBRAB by 20°C and by 14°C and 13°C for SBRABR and SBRABS, respectively. The mixing and compaction temperatures ascertained through viscosity-temperature testing offer a more accurate reflection of the asphalt’s viscosity traits. Consequently, in this study, the temperatures recommended in Table 5, in addition to the traditional aging temperatures, were employed as unconventional mixing and compaction temperatures to more precisely capture the viscosity characteristics of the asphalt.
3.3 Rheological performance
3.3.1 Temperature sweep results
Figure 4 illustrates the rutting factor (G*/sinδ) for the unaged asphalt specimens. It is observed that higher values of G*/sinδ at a given temperature correlate with enhanced rutting resistance. The rutting factors for the various asphalt types, in descending order, are SBRABS>SBRABSR>SBRAB>AB>SBRABR. The temperatures corresponding to 1.0 kPa and 2.2 kPa are commonly employed to distinguish the performance of unaged and aged asphalt (ASTM, 1999). Notably, the high-temperature critical temperatures for SBRABS, SBRABSR, SBRAB, AB, and SBRABR before aging are 85.1°C, 82.9°C, 75.9°C, 75.4°C, and 64.6°C, respectively. The combined use of Sasobit/REO is observed to significantly enhance the high-temperature rutting resistance of SBRAB. Furthermore, the difference in G*/sinδ between unaged AB and unaged SBRAB is minimal across all scanning temperatures, suggesting that SBR has a marginal impact on improving the rutting resistance of AB.
Figure 5 presents the G*/sinδ values for all asphalt types following RTFOT aging. The data indicates that the 158°C RSBRABS exhibits the highest G*/sinδ, implying superior resistance to high-temperature rutting. As the short-term aging temperature increases, the asphalt undergoes more advanced aging, resulting in reduced rutting resistance, with the G*/sinδ of 163°C RSBRABSR ranking just below that of 158°C RSBRABS and 152°C RSBRABSR. Additionally, the modified asphalt displays varying anti-aging properties after exposure to different RTFOT aging temperatures. Specifically, the critical temperatures for 152°C SBRABSR, 163°C SBRABSR, 172°C SBRAB, 163°C SBRAB, and 163°C AB are 82.2°C, 79.8°C, 71°C, 70.6°C, and 70.1°C, respectively. This suggests that both the incorporation of Sasobit/REO and the moderation of aging temperatures contribute positively to the rutting resistance of RTFOT-aged SBRAB. Moreover, the G*/sinδ differences between 163°C AB, 172°C SBRAB, and 163°C SBRAB are minimal, consistent with the findings for the unaged AB.
3.3.2 BBR test results
Figure 6 presents the BBR test outcomes for all PAV-aged modified asphalt binders at temperatures of −12°C and −18°C. The results indicate that the PAV-aged SBRABSR exhibits the lowest S-value across the board as shown in Figure 6A. This suggests that the incorporation of Sasobit/REO is effective in mitigating thermal stress within SBRAB, thereby reducing the susceptibility of SBRAB pavements to thermal cracking and minimizing the risk of pavement failure due to cracking. Furthermore, a decrease in the RTFOT aging temperature of SBRABSR results in a lower S-value for the asphalt post-long-term aging. For instance, a reduction in the aging temperature from 163°C to 152°C leads to a decrease in the S-value of PAV-aged SBRABSR at −18°C from 272 to 254. This observation implies that the synergistic interaction of Sasobit/REO, in conjunction with a reduction in short-term aging temperature, can influence the thermal stress experienced by SBRABSR to a notable extent.
In terms of m-value, the addition of Sasobit/REO successfully elevates the m-value of SBRAB, except for conditions at −18°C, indicating a positive impact on the stress relaxation characteristics of SBRAB. The S/m value ratio was utilized as a critical assessment criterion to more distinctly illustrate the influence of aging temperature on the low-temperature properties of different asphalt formulations. As depicted in Figure 6C, the S/m value of 152°C RP + SBRABSR is consistently lower than that of 172°C RP + SBRAB across all tested temperatures. This finding suggests that SBRABSR possesses superior resistance to low-temperature cracking compared to SBRAB.
3.4 Short- and long-term aging resistances
To delve into the aging resistance of composite-modified asphalt, this study conducted a comprehensive analysis of the alterations in the binder’s physical, rheological, and morphological attributes both before and after the aging process. Furthermore, the investigation extended to examining the impact of reduced aging temperatures on the performance characteristics of SBRAB.
3.4.1 Physical aging indices
The assessment of a binder’s aging resistance is typically facilitated by physical aging indices, including the aging residual ductility rate (ARDR), aging residual softening point (ARDP), and aging residual penetration (ARP) (Zhang et al., 2017). As illustrated in Figure 7, the ARDR for SBRAB and SBRABS at a temperature of 5°C is nil, indicative of their propensity for brittle fracture and consequent reduction in low-temperature ductility following RTFOT aging. In contrast, SBRABR and SBRABSR, subjected to RTFOT aging at 150°C, exhibited significantly higher ARDRs of 22% and 18% at 5°C, respectively. Furthermore, SBRABSR demonstrated a decrease in ductility, with an ARDR of 94% at 10°C after short-term aging at 163°C, compared to the ARDR of 100% post-RTFOT at 150°C. The ARDR outcomes suggest that employing a lower aging temperature can effectively mitigate the adverse effects of aging on the ductility of SBRAB.
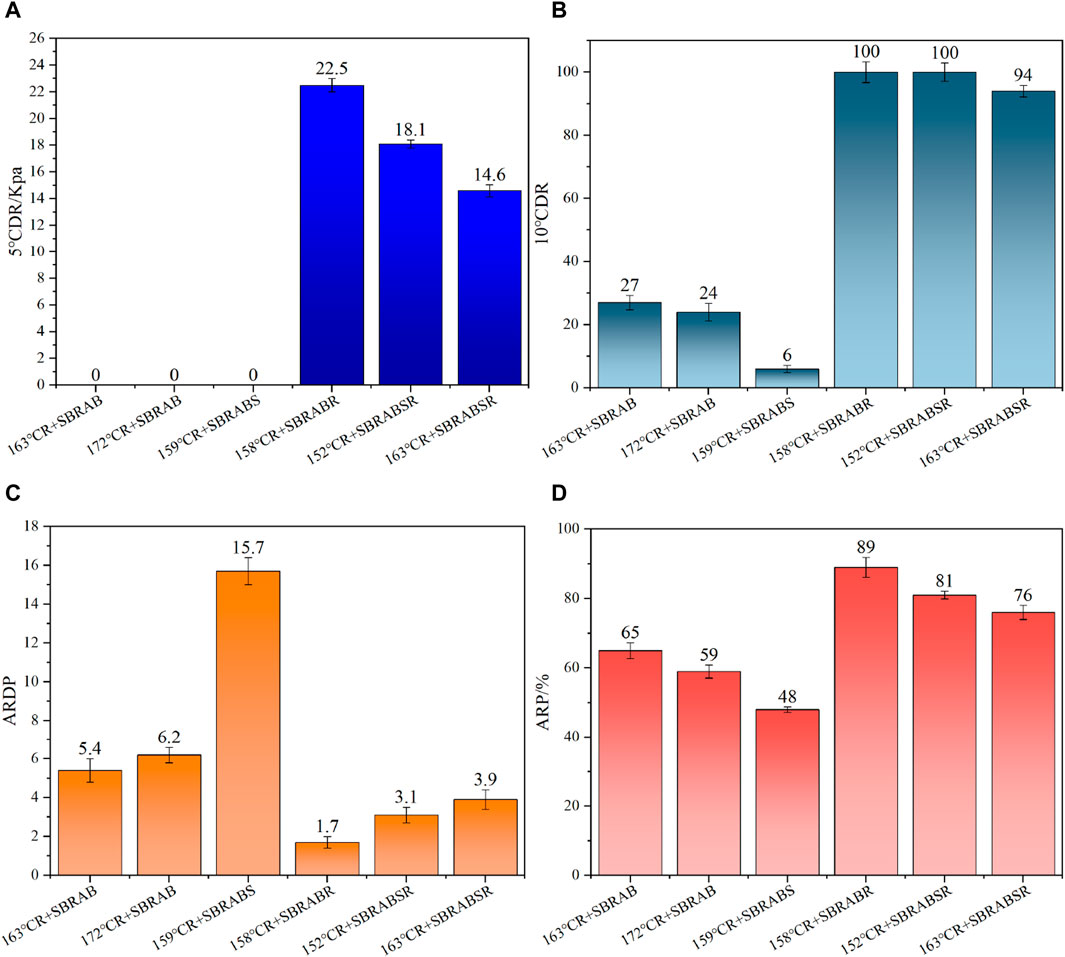
Figure 7. Physical aging indexes of modified ABs: (A) ARDR at 5°C; (B) ARDR at 10°C; (C) ARDP and (D) ARP.
It is also noteworthy that the ARDP for 169°C R + SBRAB, 156°C R + SBRABS, and 163°C R + SBRABSR are 6.2°C, 15.7°C, and 3.9°C, respectively, while the corresponding ARP values are 59%, 48%, and 76%. These results imply that the hardening impact of RTFOT on SBRAB is more pronounced than on SBRABSR. This indicates that the addition of Sasobit/REO can alleviate the hardening effects of RTFOT on SBRAB by reducing the aging temperature, thereby potentially enhancing its performance and longevity in practical applications.
3.4.2 Rheological aging indices
In this investigation, the influence of the Sasobit/REO additive on the short-term and long-term aging resistances of SBRAB is examined using established rheological aging indices (RAI), namely, complex modulus aging (CMA) and phase angle aging (PAA). The calculations are shown in Eqs 1 and 2, respectively. A lower CMA value is indicative of superior aging resistance in the asphalt binder, whereas a higher PAA value denotes greater anti-aging efficacy. The following descriptions are provided for these two rheological aging indices to clarify their significance in the assessment of binder performance:
Figures 8, 9 depict the RAI for the AB following RTFOT aging and PAV aging, respectively. As observed in Figure 8, the RTFOT-aged SBRABSR demonstrates a lower CMA curve and a higher PAA curve compared to the RTFOT-aged AB and RTFOT-aged SBRAB. This suggests that the composite modification with Sasobit and REO enhances the short-term aging resistance of SBRAB. Additionally, a comparison between the RTFOT-aged SBRABSR at 163°C and the variant aged at 150°C reveals a decrease in CMA and an increase in PAA for the latter, highlighting the positive impact of reduced aging temperatures on mitigating the short-term aging impacts on SBRAB.
An examination of Figure 9 reveals that both the 163°C RP + SBRABSR and 152°C RP + SBRABSR exhibit notably lower CMA values and significantly higher PAA values when compared to PAV-aged SBRAB. This trend indicates that SBRABSR, irrespective of the viscosity-lowering effect of the warm mix additive, possesses enhanced long-term aging resistance relative to SBRAB. Moreover, the distinctions in CMA and PAA between the 163°C RP + SBRABSR and 152°C RP + SBRABSR are relatively minimal, which may be attributed to the aging kinetics of the AB. As AB ages, the gradual depletion of light components and oxidizable substances, alongside an increase in stiffness, impedes the infiltration of oxygen atoms into AB, leading to a decelerating aging rate. Conversely, the 152°C RP + SBRABSR, due to the reduced short-term aging temperature, undergoes a less severe aging process. The advantages gained from lowering the RTFOT aging temperature become less distinct as the aging period extends, resulting in a convergence of aging performance over an extended aging duration.
The incorporation of Sasobit/REO may enhance the resistance of SBRAB to both short-term and long-term aging for several reasons. When Sasobit is combined with SBRAB, the wax crystals of Sasobit form a three-dimensional network that can encapsulate the light oil components of the AB. These light components become entrapped within the expanding crystal lattice, which inhibits their volatilization. Additionally, this interconnected and dense crystalline matrix reduces the interaction between oxygen atoms and the asphalt molecules, thereby mitigating the oxidation process.
3.4.3 Morphological analyses
The efficacy of SBRAB can be significantly compromised due to the degradation of SBR during the aging process. Therefore, a thorough examination of the morphological changes of SBR within the asphalt matrix, both before- and after-aging, is essential. Figure 10 presents fluorescence microscopy images of SBRAB and SBRABSR in their unaged state and after aging. The unaged SBRAB and SBRABSR both display a plethora of dense, porous, and swollen network structures, with the SBRABSR exhibiting a more compact mesh than the SBRAB. This observation suggests that the synergistic effect of Sasobit/REO bolsters the performance of SBRAB. However, upon RTFOT aging, the network structures in SBRAB dissipate, giving way to dispersed fragments, indicative of substantial SBR damage during aging. In contrast, the SBRABSR-modified asphalt, with its enhanced anti-aging capabilities, retains the integrity of the SBR-induced network structure. Furthermore, the 163°C short-term aged SBRABSR maintains the network structures essentially intact. A comparison with the aging images of SBRAB reveals that the reticulation in SBRAB becomes more delicate post-aging at 163°C, demonstrating that the incorporation of Sasobit and REO aids in diminishing the deleterious impacts of aging on SBR asphalt.
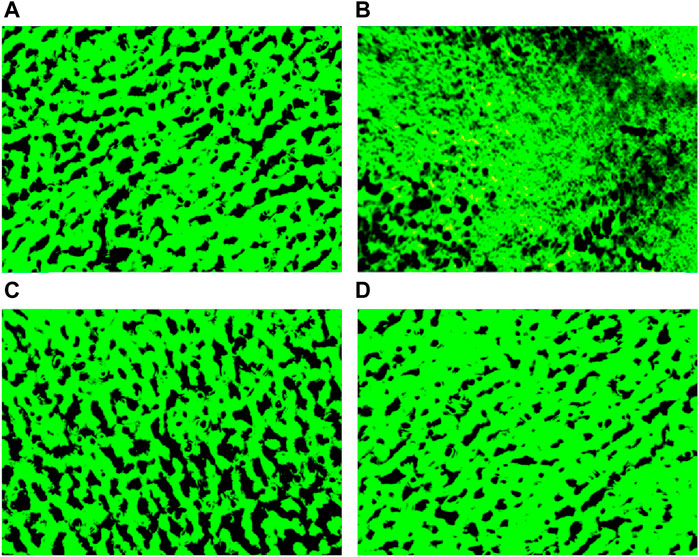
Figure 10. FM of SBRAB and SBRABSR before and after the aging. (A) Unaged SBRBA (B) RTFOT SBRBA (C) Unaged SBRBASR (D) RTFOT SBRBASR.
4 Conclusion
In this investigation, a comprehensive assessment of the effects of Sasobit/REO compounds on the physical, rheological, and aging characteristics of SBR-modified asphalt was conducted using a DSR, BBR, and conventional performance evaluations. The FM test was employed to elucidate the modification mechanisms. The key insights derived from the test results are summarized as follows:
(1) Across a wide temperature range, the synergistic combination of Sasobit/REO greatly improves the conventional features of SBRAB, such as needle penetration and softening points. When compared to using each modifier separately, Sasobit/REO combinations are noticeably more effective.
(2) The incorporation of the Sasobit/REO composite modifier resulted in a reduction of the SBRAB mixing temperature by 20°C, with SBRABR and SBRABS experiencing decreases of 14°C and 13°C, respectively.
(3) The addition of Sasobit/REO, in conjunction with the reduction of aging temperatures, collectively improves the rutting resistance of RTFOT-aged SBRAB.
(4) The combined effect of Sasobit/REO and the lowering of short-term aging temperatures can influence the thermal stress experienced by SBRABSR, with SBRABSR exhibiting enhanced resistance to low-temperature cracking.
(5) The inclusion of Sasobit/REO is likely to bolster the short-term and long-term aging resistance of SBRAB, offering potential benefits in terms of binder performance and longevity.
These findings underscore the potential of Sasobit/REO composites as effective modifiers for SBRAB, contributing to improved performance and resistance to both thermal and aging challenges.
5 Recommendations for future work
Systematic research on Sasobit/REO composite-modified asphalt can synthesize the advantages of various modifiers and improve the performance of asphalt. The following are suggestions for future research on Sasobit/REO composite-modified asphalt: 1) Study the composite effect of Sasobit and REO at different ratios to find the optimal ratio of components to achieve the best performance enhancement. 2) Conduct multi-scale performance evaluation, including macro performance, meso-performance, and micro performance, to comprehensively understand the performance of composite modified asphalt. 3) Optimize the production process to ensure that the preparation process of composite-modified asphalt is feasible and economically reasonable.
Data availability statement
The raw data supporting the conclusion of this article will be made available by the authors, without undue reservation.
Author contributions
ZLi: Conceptualization, Investigation, Writing–review and editing. ZLu: Formal Analysis, Methodology, Writing–original draft. XL: Software, Writing–original draft. JW: Resources, Validation, Writing–review and editing.
Funding
The author(s) declare that financial support was received for the research, authorship, and/or publication of this article. The study was supported by Foundation of Guizhou Provincial Education Department for Youth [Qianjiaoji, Grant No. (2022)154], Natural Science Foundation of Guizhou Minzu University [GZMUZK (2023) YB08].
Conflict of interest
Author ZL was employed by Shenzhen Huadi Geotechnical Engineering Co., Ltd.
The remaining authors declare that the research was conducted in the absence of any commercial or financial relationships that could be construed as a potential conflict of interest.
Publisher’s note
All claims expressed in this article are solely those of the authors and do not necessarily represent those of their affiliated organizations, or those of the publisher, the editors and the reviewers. Any product that may be evaluated in this article, or claim that may be made by its manufacturer, is not guaranteed or endorsed by the publisher.
References
Astm, D. (1999). Standard specification for performance graded asphalt binder. Annu. Book ASTM Stand. 4, 3–5. doi:10.1520/D6373-21A
Belc, A. L., Coleri, E., Belc, F., and Costescu, C. (2021). Influence of different warm mix additives on characteristics of warm mix asphalt. Materials 14 (13), 3534. doi:10.3390/ma14133534
Canestrari, F., and Ingrassia, L. P. (2020). A review of top-down cracking in asphalt pavements: causes, models, experimental tools and future challenges. J. Traffic Transp. Eng. Engl. Ed. 7 (5), 541–572. doi:10.1016/j.jtte.2020.08.002
Che, T., Pan, B., Li, Y., Ge, D., Jin, D., and You, Z. (2022). The effect of styrene-butadiene rubber modification on the properties of asphalt binders: aging and restoring. Constr. Build. Mater. 316, 126034. doi:10.1016/j.conbuildmat.2021.126034
Cui, B., Gu, X., Hu, D., and Dong, Q. (2020). A multiphysics evaluation of the rejuvenator effects on aged asphalt using molecular dynamics simulations. J. Clean. Prod. 259, 120629. doi:10.1016/j.jclepro.2020.120629
Eleyedath, A., and Swamy, A. K. (2020). “3 - use of waste engine oil in materials containing asphaltic components,” in Eco-efficient pavement construction materials. Editors F. Pacheco-Torgal, S. Amirkhanian, H. Wang, and E. Schlangen (Sawston, United Kingdom: Woodhead Publishing), 33–50.
Eltwati, A., Mohamed, A., Hainin, M. R., Jusli, E., and Enieb, M. (2022). Rejuvenation of aged asphalt binders by waste engine oil and SBS blend: physical, chemical, and rheological properties of binders and mechanical evaluations of mixtures. Constr. Build. Mater. 346, 128441. doi:10.1016/j.conbuildmat.2022.128441
Falchetto, A. C., Moon, K. H., Wang, D., and Riccardi, C. (2018). Investigation on the cooling medium effect in the characterization of asphalt binder with the bending beam rheometer (BBR). Can. J. Civ. Eng. 45 (7), 594–604. doi:10.1139/cjce-2017-0586
Fernandes, S. R. M., Silva, H. M. R. D., and Oliveira, J. R. M. (2018). Developing enhanced modified bitumens with waste engine oil products combined with polymers. Constr. Build. Mater. 160, 714–724. doi:10.1016/j.conbuildmat.2017.11.112
Han, Y., Tian, J., Ding, J., Shu, L., and Ni, F. (2022). Evaluating the storage stability of SBR-modified asphalt binder containing polyphosphoric acid (PPA). Case Stud. Constr. Mater. 17, e01214. doi:10.1016/j.cscm.2022.e01214
Hemmati, N., Yun, J., Mazumder, M., Lee, M.-S., and Lee, S.-J. (2021). Laboratory characterization of asphalt binders modified with styrene butadiene rubber (SBR). Materials 14 (24), 7666. doi:10.3390/ma14247666
Jain, S., and Singh, B. (2021). Cold mix asphalt: an overview. J. Clean. Prod. 280, 124378. doi:10.1016/j.jclepro.2020.124378
Li, Q., Zhang, H., and Chen, Z. (2021b). Improvement of short-term aging resistance of styrene-butadiene rubber modified asphalt by Sasobit and epoxidized soybean oil. Constr. Build. Mater. 271, 121870. doi:10.1016/j.conbuildmat.2020.121870
Li, Q., Zhang, H., Shi, C., and Chen, Z. (2021a). A novel warm-mix additive for SBR modified asphalt binder: effects of Sasobit/epoxidized soybean oil compound on binder rheological and long-term aging performance. J. Clean. Prod. 326, 129405. doi:10.1016/j.jclepro.2021.129405
Liu, C., Lv, S., Jin, D., and Qu, F. (2021a). Laboratory investigation for the road performance of asphalt mixtures modified by rock asphalt–styrene-butadiene rubber. J. Mater. Civ. Eng. 33 (3), 04020504. doi:10.1061/(asce)mt.1943-5533.0003611
Liu, F., Zheng, M., Fan, X., Li, H., Wang, F., and Lin, X. (2021b). Properties and mechanism of waterborne epoxy resin-SBR composite modified emulsified asphalt. Constr. Build. Mater. 274, 122059. doi:10.1016/j.conbuildmat.2020.122059
Liu, Z., Li, S., and Wang, Y. (2022a). Waste engine oil and polyphosphoric acid enhanced the sustainable self-healing of asphalt binder and its fatigue behavior. J. Clean. Prod. 339, 130767. doi:10.1016/j.jclepro.2022.130767
Liu, Z., Li, S., and Wang, Y. (2022b). Characteristics of asphalt modified by waste engine oil/polyphosphoric acid: conventional, high-temperature rheological, and mechanism properties. J. Clean. Prod. 330, 129844. doi:10.1016/j.jclepro.2021.129844
Liu, Z., Sun, L., Gu, X., Wang, X., Dong, Q., Zhou, Z., et al. (2023). Characteristics, mechanisms, and environmental LCA of WMA containing sasobit: an analysis perspective combing viscosity-temperature regression and interface bonding strength. J. Clean. Prod. 391, 136255. doi:10.1016/j.jclepro.2023.136255
Luo, H., Huang, X., Rongyan, T., Ding, H., Huang, J., Wang, D., et al. (2021). Advanced method for measuring asphalt viscosity: rotational plate viscosity method and its application to asphalt construction temperature prediction. Constr. Build. Mater. 301, 124129. doi:10.1016/j.conbuildmat.2021.124129
Luo, W., Zhang, Y., and Cong, P. (2017). Investigation on physical and high temperature rheology properties of asphalt binder adding waste oil and polymers. Constr. Build. Mater. 144, 13–24. doi:10.1016/j.conbuildmat.2017.03.105
Poulikakos, L. D., Pasquini, E., Tusar, M., Hernando, D., Wang, D., Mikhailenko, P., et al. (2022). RILEM interlaboratory study on the mechanical properties of asphalt mixtures modified with polyethylene waste. J. Clean. Prod. 375, 134124. doi:10.1016/j.jclepro.2022.134124
Qin, Q., Farrar, M. J., Pauli, A. T., and Adams, J. J. (2014). Morphology, thermal analysis and rheology of Sasobit modified warm mix asphalt binders. Fuel 115, 416–425. doi:10.1016/j.fuel.2013.07.033
Qurashi, I. A., and Swamy, A. K. (2018). Viscoelastic properties of recycled asphalt binder containing waste engine oil. J. Clean. Prod. 182, 992–1000. doi:10.1016/j.jclepro.2018.01.237
Rezaeizadeh Herozi, M., Valenzuela, W., Rezagholilou, A., Rigabadi, A., and Nikraz, H. (2022). New models for the properties of warm mix asphalt with sasobit. CivilEng 3 (2), 347–364. doi:10.3390/civileng3020021
Shanbara, H. K., Dulaimi, A., Al-Mansoori, T., Al-Busaltan, S., Herez, M., Sadique, M., et al. (2021). The future of eco-friendly cold mix asphalt. Renew. Sustain. Energy Rev. 149, 111318. doi:10.1016/j.rser.2021.111318
Sobhi, S., Yousefi, A., and Behnood, A. (2020). The effects of Gilsonite and Sasobit on the mechanical properties and durability of asphalt mixtures. Constr. Build. Mater. 238, 117676. doi:10.1016/j.conbuildmat.2019.117676
Tong, B., Song, X., Shen, J., Jiang, T., Chen, J., and Niu, J. (2022). Effect of Sasobit warm mix on micro properties of asphalt with different degrees of regeneration. Front. Mater. 9, 950550. doi:10.3389/fmats.2022.950550
Vamegh, M., Ameri, M., and Chavoshian Naeni, S. F. (2020). Experimental investigation of effect of PP/SBR polymer blends on the moisture resistance and rutting performance of asphalt mixtures. Constr. Build. Mater. 253, 119197. doi:10.1016/j.conbuildmat.2020.119197
Walther, A., Büchler, S., Cannone Falchetto, A., Wang, D., Riccardi, C., and Wistuba, M. P. (2019). Experimental investigation on asphalt mixtures prepared with reclaimed asphalt pavement and rejuvenators based on the BTSV method. Road Mater. Pavement Des. 20 (7), 1695–1708. doi:10.1080/14680629.2019.1594053
Wang, F., Xiao, Y., Cui, P., Lin, J., Li, M., and Chen, Z. (2020b). Correlation of asphalt performance indicators and aging Degrees: a review. Constr. Build. Mater. 250, 118824. doi:10.1016/j.conbuildmat.2020.118824
Wang, L., Liang, F., Li, Z., and Zhao, Q. (2023). Investigation on the rheological properties and microscopic characteristics of graphene and SBR composite modified asphalt. Coatings 13 (7), 1279. doi:10.3390/coatings13071279
Wang, W., Jia, M., Jiang, W., Lou, B., Jiao, W., Yuan, D., et al. (2020a). High temperature property and modification mechanism of asphalt containing waste engine oil bottom. Constr. Build. Mater. 261, 119977. doi:10.1016/j.conbuildmat.2020.119977
Xu, Z., Shi, J., Zhao, L., Yuan, Y., Akram, N., Shan, D., et al. (2020). A viscoelastic model and structural properties of Sasobit-modified asphalt. Petroleum Sci. Technol. 38 (5), 454–461. doi:10.1080/10916466.2020.1767646
Yan, S., Dong, Q., Chen, X., Zhou, C., Dong, S., and Gu, X. (2022). Application of waste oil in asphalt rejuvenation and modification: a comprehensive review. Constr. Build. Mater. 340, 127784. doi:10.1016/j.conbuildmat.2022.127784
Yousefi, A., Behnood, A., Nowruzi, A., and Haghshenas, H. (2021). Performance evaluation of asphalt mixtures containing warm mix asphalt (WMA) additives and reclaimed asphalt pavement (RAP). Constr. Build. Mater. 268, 121200. doi:10.1016/j.conbuildmat.2020.121200
Zhang, D., Zhang, H., and Shi, C. (2017). Investigation of aging performance of SBS modified asphalt with various aging methods. Constr. Build. Mater. 145, 445–451. doi:10.1016/j.conbuildmat.2017.04.055
Zhang, F., and Hu, C. (2014). Influence of aging on thermal behavior and characterization of SBR compound-modified asphalt. J. Therm. Analysis Calorim. 115 (2), 1211–1218. doi:10.1007/s10973-013-3338-2
Keywords: pavement, recycled engine oil, SBR-modified asphalt, Sasobit, aging property
Citation: Li Z, Lu Z, Liu X and Wang J (2024) Investigating the synergistic anti-aging effects of Sasobit and recycled engine oil in styrene-butadiene rubber modified asphalt. Front. Mater. 11:1412094. doi: 10.3389/fmats.2024.1412094
Received: 04 April 2024; Accepted: 08 May 2024;
Published: 04 June 2024.
Edited by:
Di Wang, University of Ottawa, CanadaReviewed by:
Yumei Liu, Shandong Jianzhu University, ChinaRuimeng Song, Nanyang Technological University, Singapore
Copyright © 2024 Li, Lu, Liu and Wang. This is an open-access article distributed under the terms of the Creative Commons Attribution License (CC BY). The use, distribution or reproduction in other forums is permitted, provided the original author(s) and the copyright owner(s) are credited and that the original publication in this journal is cited, in accordance with accepted academic practice. No use, distribution or reproduction is permitted which does not comply with these terms.
*Correspondence: Zhen Lu, a2VrZWxpbGxpQDE2My5jb20=