- 1School of Physical Science and Technology, Southwest University, Chongqing, China
- 2Analytical and Testing Center, Chongqing University, Chongqing, China
- 3Department of Physics, Pennsylvania State University, University Park, PA, United States
Sr4(Ru0.99Fe0.01)3O10 shows a ferromagnetic (FM) transition at TC ∼ 105 K with the magnetic easy axis in the ab plane, followed by a metamagnetic transition (MMT) at low temperatures when the magnetic field H is applied along the c axis, which is in sharp contrast to that of the pure Sr4Ru3O10, where the easy axis is along the c axis and the MMT is in the ab plane. Here, we studied the MMT in the Sr4(Ru0.99Fe0.01)3O10 nanosheet by the Hall effect. It was found that the ordinary Hall coefficient of Sr4(Ru0.99Fe0.01)3O10 is almost the same as that of the pure Sr4Ru3O10, while a sudden increase in the Hall resistance Rxy is observed below ∼50 K, above which the Rxy presents the conventional anomalous Hall effect up to TC. Analysis of the results indicates that the MMT has no direct correlation to the electronic structure but closely relates to the magnetic moment locking, where the magnetic-field-induced breakdown of the locked moments is responsible for the MMT.
Introduction
The 4d-electron-based perovskite ruthenium oxides Srn+1RunO3n+1 (n = 1, 2, ∞) with a strong inherent spin orbital coupling present rich and fascinating phenomena, such as unconventional superconductivity (Sr2RuO4, n = 1) (Mackenzie and Maeno, 2003), magnetic-field-tuned metamagnetic quantum criticality (Sr3Ru2O7, n = 2) (Grigera et al., 2001), and itinerant ferromagnetism (SrRuO3, n = ∞) (Koster et al., 2012). In contrast, the n = 3 member Sr4Ru3O10 is an exotic ferromagnetic (FM) metal (Crawford et al., 2002). It possesses an orthorhombic unit cell composed of triple layers of corner-shared RuO6 octahedra separated by double rock-salt Sr-O layers. The RuO6 octahedra in the outer two layers of each triple layer are rotated by an average of 5.25° around the c-axis, while the octahedra of the inner layers are rotated in the opposite sense by an average of 10.6° (Crawford et al., 2002). Sr4Ru3O10 undergoes an FM transition at a Curie temperature TC ∼ 105 K with the easy axis along the c axis, followed by a second transition at a critical temperature TM ∼ 50 K (Crawford et al., 2002; Cao et al., 2003). Below TM, a superlinear increase of magnetization, i.e., a metamagnetic transition (MMT), is observed when the magnetic field is applied in the ab plane (Cao et al., 2003).
The MMT in Sr4Ru3O10 has been widely investigated, but its nature remains elusive (Lin et al., 2004; Gupta et al., 2006; Mao et al., 2006; Jo et al., 2007; Schottenhamel et al., 2016). The magnetization and Raman spectrum studies suggest that the MMT is associated with a magnetic-field-induced breakdown of the antiferromagnetic (AFM) order in the ab plane below TM through a spin-flip process (Cao et al., 2003; Gupta et al., 2006). However, no AFM order in the ab plane is detected by neutron diffraction measurements (Granata et al., 2013; Zhu et al., 2018). On the other hand, systematical transport studies on the Sr4Ru3O10 nanosheets show that the MMT is likely an in-plane magnetic-field-induced spin-flop process from the c axis to the ab plane (Liu et al., 2018).
Interestingly, when the Ru is substituted by a small amount of Fe [Sr4(Ru0.99Fe0.01)3O10], the magnetic behavior is changed significantly (Liu et al., 2019). It is found that the magnetic easy direction is fully changed from the c axis to the ab plane below TC and that the second transition at TM, which usually occurs in pure Sr4Ru3O10 crystals, is not seen though the FM transition remains at 105 K. In addition, an MMT is observed when the magnetic field is applied along the c direction. The Fe doping opens a path to explore the nature of the MMT by using Sr4(Ru0.99Fe0.01)3O10 as a model system. Here, we perform a systematical Hall effect measurement of the MMT in the Sr4(Ru0.99Fe0.01)3O10 nanosheet, which contains both the ordinary Hall effect and the anomalous Hall effect. Results show that the MMT has less correlation to the electronic structure but depends on the magnetic moment locking.
Materials and Methods
The Sr4(Ru0.99Fe0.01)3O10 single crystals were grown by the floating zone method as described in Liu et al. (2019). The starting materials were SrCO3, Fe2O3, and RuO2. Twenty-five percent excess RuO2 was added to compensate the evaporation of RuO2 from the melting zone. The mixed powder was ground for ∼1 h, and then pressed into pellets, after which, the pellets were sintered at a temperature of about 900°C for 12 h. The sintered pellets were ground for ∼1 h again, and the ground powder was pressed to a rod and sintered at a temperature of 1,350°C for 4 h. The pressure in the quartz tube was ∼10 bar (10% O2 + 90% Ar), and the growth speed was about ∼15 mm/h.
The concentration of Fe was analyzed roughly by energy-dispersive spectrum (EDS, Oxford Instruments). Sr4(Ru0.99Fe0.01)3O10 nanosheets were obtained by the scotch tape-based micro-mechanical exfoliation method from the bulk single crystal and then transferred onto a SiO2 (300 nm)/Si substrate. Contacts were patterned using the electron-beam lithography technique followed by deposition of Ti/Au (5/100 nm). The scanning electron microscope (SEM) image of a device with patterned electrodes is shown in the top inset of Figure 1. The thickness of the nanosheet is about 45 nm, which is determined by the atomic force microscopy. The transport property of the nanosheet was measured by a physical property measurement system (PPMS, Quantum Design). The Hall resistance Rxy is determined from Rxy = [Rxy(H)−Rxy(−H)]/2 in order to subtract the component of the longitudinal resistance Rxx arising from the small misalignment of transverse contacts.
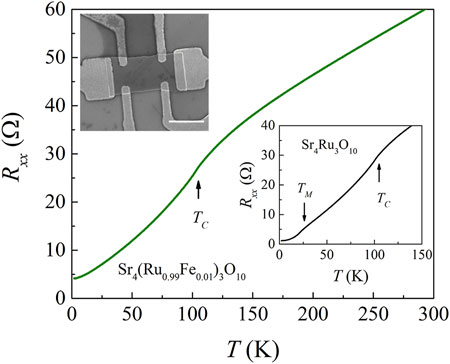
FIGURE 1. The temperature dependence of the zero-field resistance Rxx of a 45-nm-thick Sr4(Ru0.99Fe0.01)3O10 nanosheet. Insets (top) SEM image of the Sr4(Ru0.99Fe0.01)3O10 nanosheet with electrodes, scale bar: 3 μm. (Bottom) Rxx–T curve of a pure Sr4Ru3O10 nanosheet with the thickness of about 35 nm.
Results and Discussion
Figure 1 shows the temperature T-dependent longitudinal resistance Rxx of the Sr4(Ru0.99Fe0.01)3O10 nanosheet, which is measured at zero magnetic field. It is found that the Rxx decreases monotonously as the temperature decreases, indicating a metallic behavior of the nanosheet. An anomaly of the resistance near TC ∼ 105 K can be identified due to the FM transition, but no second anomaly at TM is seen, which is in sharp contrast to that observed in the pure Sr4Ru3O10 bulk or nanosheets, where two resistive anomalies at TC and TM can be found (Liu et al., 2018; Liu et al., 2017). For a comparison, the Rxx–T property of a pure Sr4Ru3O10 nanosheet with thickness of about 35 nm is presented in the bottom inset of Figure 1. Note that the TM of the pure nanosheet is about 25 K, which is smaller than that of the pure bulk due to the size effect (Liu et al., 2016).
Figure 2 shows the magnetic field H-dependent Hall resistance Rxy of the Sr4(Ru0.99Fe0.01)3O10 nanosheet measured at various temperatures, where H is applied perpendicular to the ab plane of the nanosheet. It is found that all the Rxy–H curves below TC ∼ 105 K can be well described by Nagaosa et al. (2010)
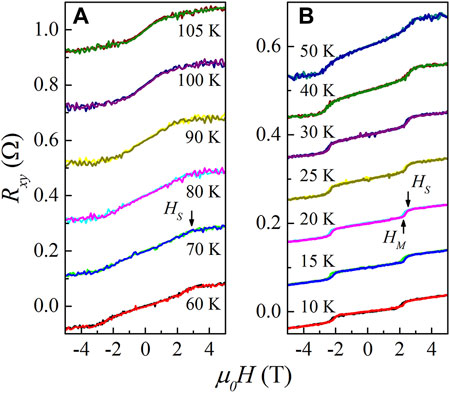
FIGURE 2. (A) and (B) Hall resistance Rxy of the 45-nm-thick Sr4(Ru0.99Fe0.01)3O10 nanosheet as a function of perpendicular magnetic field H at various temperatures. Data have been shifted vertically for clarity.
The Rxyo is the ordinary Hall resistance determined by the electronic structure and can be written as Rxyo = RHoH/t, with RHo and t being the ordinary Hall coefficient and the thickness of the sample, respectively; Rxya is the anomalous Hall resistance, which is proportional to the magnetization Mc along the c axis, i.e., Rxya = RHaMc/t, with RHa being the anomalous Hall coefficient. Therefore, the Hall effect measurement is a powerful tool to measure both the electronic structure and the magnetization Mc of the Sr4(Ru0.99Fe0.01)3O10 nanosheet. It is seen that
(1) The Rxy increases almost linearly as H increases in the temperature range of T* ∼ 50 K < T < TC (Figure 2A) and then turns to saturation at a magnetic field HS. This anomalous Hall effect is very similar to that observed in many other FM materials (Nagaosa et al., 2010). Since the magnetic easy axis of Sr4(Ru0.99Fe0.01)3O10 is in the ab plane, this behavior indicates that the magnetic moments at T* < T < TC are rotated collectively from the ab plane to the c axis by H.
(2) At T < T*, the Rxy first increases linearly as the magnetic field increases and then followed by a sharp increase at a magnetic field HM, which is slightly below the saturation field HS as indicated in Figure 2B. Above HS, the Rxy increases linearly. According to Eq. 1, this behavior demonstrates that there is a rapid increase of Mc at HM < H < HS, i.e., an MMT in the Sr4(Ru0.99Fe0.01)3O10 nanosheet as the sweeping of H along the c direction, which is in contrast to that of the pure Sr4Ru3O10, where the MMT is in the ab plane (Cao et al., 2003). The HM can thus be defined as the critical field of the MMT.
To better understand the MMT, we firstly focus on the ordinary Hall effect of the Sr4(Ru0.99Fe0.01)3O10 nanosheet. Figure 3A shows the ordinary Hall coefficient RHo as a function of temperature, which is extracted from the high field slope of the Hall isotherm at H = 5 T (>HS). The RHo is positive in the whole temperature range, indicating that the dominant carriers in the Sr4(Ru0.99Fe0.01)3O10 nanosheet are holes. Except for the RHo of the Sr4(Ru0.99Fe0.01)3O10 nanosheet at low temperatures being slightly smaller, the trace of the RHo–T curve here is very similar to that reported in the pure Sr4Ru3O10 nanosheet (Liu et al., 2016). Considering that the FM moment in Sr4(Ru0.99Fe0.01)3O10 is in the ab plane and the MMT is along the c direction, which is completely different to that in the pure Sr4Ru3O10, where the FM moment is along the c direction and the MMT is in the ab plane, the almost identical RHo indicates that the MMT has no direct correlation to the electronic structure, but only the spin-flop from the ab plane to the c direction.
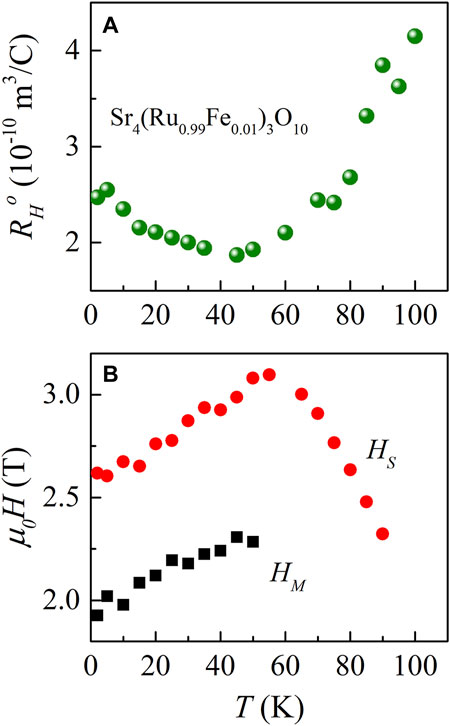
FIGURE 3. (A) The ordinary Hall coefficient RHo of the Sr4(Ru0.99Fe0.01)3O10 nanosheet as a function of temperature. (B) Temperature-dependent critical field HM of the MMT and the saturation field HS.
To give an insight of the MMT, we reinspect the Hall data shown in Figure 2. One can see that at 10 K, the slope of the Rxy–H curve below HM is almost the same as that at H > HS. The change of Rxy above HS is purely due to the orbital effect of H on carriers, i.e., the ordinary Hall resistance Rxyo, while that below HM is the sum of both the change of Rxyo and Rxya. The almost identical slope of the Rxy–H curve at H < HM and H > HS indicates that the Rxya is about zero (where Mc = 0) at H < HM. In other words, the magnetic moments are fully “locked” without any net moment along the c direction below HM. As the T increases, the slope of the Rxy–H curve at H < HM becomes larger than that at H > HS, indicating that some magnetic moments are unlocked due to the thermal activation. At T > T* ∼ 50 K, all the magnetic moments become a collective rotation under the magnetic field as mentioned above; hence, the MMT vanishes. This result indicates that the spin-flop is associated with a magnetic-field-induced breakdown of the locking moments, which is responsible for the MMT.
An unexpected feature seen in Figure 2 is that both HM and HS decrease with decreasing T below T*, which means intuitively that it is easier to align the magnetic moments along the c direction with decreasing temperature by the magnetic field. To see clearly, the temperature dependence of HM and HS is shown in Figure 3B. This behavior is in contrast to that of conventional magnetic materials, in which the magnetic moments are usually difficult to polarize to the magnetic hard axis at lower temperatures due to the reduction of thermal fluctuation.
We noticed that the T-dependent feature of the lattice parameter c of the pure Sr4Ru3O10 shows a minimum at T* (Granata et al., 2013; Schottenhamel et al., 2016), indicating a slightly negative expansion below T*. This negative expansion nicely echoes the anomaly in HM and RHo. As known previously (Liu et al., 2017; Liu et al., 2018), the shrinkage of the c axis will reduce the magnetocrystalline anisotropy energy and favors the magnetic moment off the c axis, such as the Fe-doping effect (Liu et al., 2019). The tiny expansion of the c axis below T* will favor the FM moment off the ab plane, resulting in the decrease of the pinning force for moment locking in the ab plane. The critical magnetic field HM is actually the competition between the moment locking force below T* and the c axis expansion-induced depinning effect. This is the reason that the HM decreases with decreasing T.
Finally, as a metamagnetic metal of Sr4(Ru0.99Fe0.01)3O10, we would like to present the scaling relation between the anomalous Hall conductivity σxy and the longitudinal conductivity σxx of σxy∝σxxφ, where φ is the scaling exponent, which is shown in Figure 4. The σxy is determined by σxy = ρxya/[(ρxx)2 + (ρxya)2] with ρxx being the longitudinal resistivity and ρxya the anomalous Hall resistivity at zero field, which is obtained by extrapolating the high-field linear term of the Hall data to the zero field shown in Figure 2. It is found that at T > 60 K, φ = 2.3, indicating that the side jump mechanism is dominant (Nagaosa et al., 2010). Below 40 K, φ = 0 with σxy = 130 S/cm, which can be attributed to the intrinsic dissipationless topological Berry-phase contribution (Nagaosa et al., 2010). The scaling relation is almost the same as many conventional FM materials (Nagaosa et al., 2010), indicating a regular FM nature of Sr4(Ru0.99Fe0.01)3O10 after being polarized.
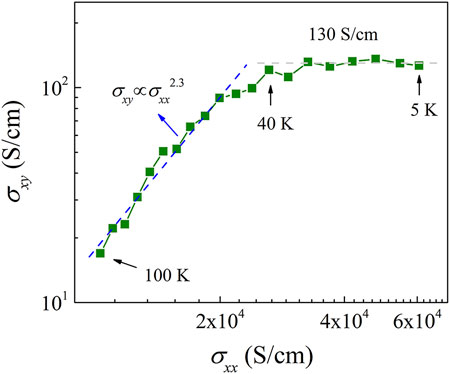
FIGURE 4. The scaling relation between the anomalous Hall conductivity σxy and the longitudinal conductivity σxx.
Conclusion
We have investigated the MMT of Sr4(Ru0.99Fe0.01)3O10 along the c axis by Hall effect. Results show that the ordinary Hall coefficient is almost the same as that of the pure Sr4Ru3O10. The magnetic moments are found to be fully locked without any net moments along the c direction at the ground state at 10 K and then gradually unlocked with increasing T. At about 50 K, the magnetic moments are fully depinned, and the MMT smears out. Our result indicates that the MMT has less correlation to the electronic structure but closely relates to the magnetic moment locking.
Data Availability Statement
The original contributions presented in the study are included in the article/Supplementary Material, further inquiries can be directed to the corresponding author.
Author Contributions
JW, YL, YW, and QW carried out the experiments of EBL and transport measurement. YW and ZM synthesized the sample. JW, JY, and JW wrote and reviewed the manuscript with the help of all authors.
Funding
This work was supported by the Fundamental Research Funds for the Central Universities (grant no. SWU019010), the Chongqing Municipal Program of Innovation and Entrepreneurship for Undergraduates (grant no. S202010635003), and the National Natural Science Foundation of China (grants nos. 11674323, 11804297, and U19A2093). The single-crystal growth effort was supported by the U.S. Department of Energy under EPSCoR Grant No. DE-SC0012432 with additional support from the Louisiana Board of Reagents.
Conflict of Interest
The authors declare that the research was conducted in the absence of any commercial or financial relationships that could be construed as a potential conflict of interest.
Publisher’s Note
All claims expressed in this article are solely those of the authors and do not necessarily represent those of their affiliated organizations, or those of the publisher, the editors and the reviewers. Any product that may be evaluated in this article, or claim that may be made by its manufacturer, is not guaranteed or endorsed by the publisher.
References
Cao, G., Balicas, L., Song, W. H., Sun, Y. P., Xin, Y., Bondarenko, V. A., et al. (2003). Competing Ground States in Triple-layeredSr4Ru3O10:Verging on Itinerant Ferromagnetism with Critical Fluctuations. Phys. Rev. B 68, 174409. doi:10.1103/physrevb.68.174409
Crawford, M. K., Harlow, R. L., Marshall, W., Li, Z., Cao, G., Lindstrom, R. L., et al. (2002). Structure and Magnetism of Single crystalSr4Ru3O10: A Ferromagnetic Triple-Layer Ruthenate. Phys. Rev. B 65, 214412. doi:10.1103/physrevb.65.214412
Granata, V., Capogna, L., Reehuis, M., Fittipaldi, R., Ouladdiaf, B., Pace, S., et al. (2013). Neutron Diffraction Study of Triple-Layered Sr4Ru3O10. J. Phys. Condens. Matter 25, 056004. doi:10.1088/0953-8984/25/5/056004
Grigera, S. A., Perry, R. S., Schofield, A. J., Chiao, M., Julian, S. R., Lonzarich, G. G., et al. (2001). Magnetic Field-Tuned Quantum Criticality in the Metallic Ruthenate Sr 3 Ru 2 O 7. Science 294, 329–332. doi:10.1126/science.1063539
Gupta, R., Kim, M., Barath, H., Cooper, S. L., and Cao, G. (2006). Field- and Pressure-Induced Phases in Sr4Ru3O10: a Spectroscopic Investigation. Phys. Rev. Lett. 96, 067004. doi:10.1103/physrevlett.96.067004
Jo, Y. J., Balicas, L., Kikugawa, N., Choi, E. S., Storr, K., Zhou, M., et al. (2007). Orbital-dependent Metamagnetic Response in Sr4Ru3O10. Phys. Rev. B 75, 094413. doi:10.1103/physrevb.75.094413
Koster, G., Klein, L., Siemons, W., Rijnders, G., Dodge, J. S., Eom, C.-B., et al. (2012). Structure, Physical Properties, and Applications ofSrRuO3thin Films. Rev. Mod. Phys. 84, 253–298. doi:10.1103/revmodphys.84.253
Lin, X. N., Bondarenko, V. A., Cao, G., and Brill, J. W. (2004). Specific Heat of Sr4Ru3O10. Solid State. Commun. 130, 151–154. doi:10.1016/j.ssc.2004.02.009
Liu, Y., Chu, W., Wang, Y., Yang, J., Du, H., Ning, W., et al. (2019). Fe-doping Induced Suppression of the Second Magnetic Transition in Sr4Ru3O10. Phys. Rev. B 99, 214418. doi:10.1103/physrevb.99.214418
Liu, Y., Chu, W. W., Yang, J. Y., Liu, G. Q., Du, H. F., Ning, W., et al. (2018). Magnetic Reversal in Sr4Ru3O10 Nanosheets Probed by Anisotropic Magnetoresistance. Phys. Rev. B 98, 024425. doi:10.1103/physrevb.98.024425
Liu, Y., Yang, J., Wang, W., Du, H., Ning, W., Ling, L., et al. (2017). Evidence of In-Plane Ferromagnetic Order Probed by Planar Hall Effect in the Geometry-Confined Ruthenate Sr4Ru3O10. Phys. Rev. B 95 (R), 161103. doi:10.1103/physrevb.95.161103
Liu, Y., Yang, J., Wang, W., Du, H., Ning, W., Ling, L., et al. (2016). Size Effect on the Magnetic Phase in Sr4Ru3O10. New J. Phys. 18, 053019. doi:10.1088/1367-2630/18/5/053019
Mackenzie, A. P., and Maeno, Y. (2003). The Superconductivity ofSr2RuO4and the Physics of Spin-Triplet Pairing. Rev. Mod. Phys. 75, 657–712. doi:10.1103/revmodphys.75.657
Mao, Z. Q., Zhou, M., Hooper, J., Golub, V., and O’Connor, C. J. (2006). Phase Separation in the Itinerant Metamagnetic Transition of Sr4Ru3O10. Phys. Rev. Lett. 96, 077205. doi:10.1103/physrevlett.96.077205
Nagaosa, N., Sinova, J., Onoda, S., MacDonald, A. H., and Ong, N. P. (2010). Anomalous Hall Effect. Rev. Mod. Phys. 82, 1539–1592. doi:10.1103/revmodphys.82.1539
Schottenhamel, W., Abdel-Hafiez, M., Fittipaldi, R., Granata, V., Vecchione, A., Hücker, M., et al. (2016). Dilatometric Study of the Metamagnetic and Ferromagnetic Phases in the Triple-Layered Sr4Ru3O10 System. Phys. Rev. B 94, 155154. doi:10.1103/physrevb.94.155154
Keywords: metamagnetic transition, Hall effect, Sr4(Ru0.99Fe0.01)3O10, magnetic moment locking, ferromagnetic transition
Citation: Wan J, Liu Y, Wan Y, Wu Q, Wang Y, Yang J, Mao Z and Wang J (2022) Hall Effect Study of the Metamagnetic Transition in the Sr4(Ru0.99Fe0.01)3O10 Nanosheet. Front. Mater. 9:856000. doi: 10.3389/fmats.2022.856000
Received: 16 January 2022; Accepted: 31 January 2022;
Published: 11 March 2022.
Edited by:
Xu Zuo, Nankai University, ChinaReviewed by:
Mingliang Tian, Hefei Institutes of Physical Science (CAS), ChinaLiang Li, Hefei Institutes of Physical Science (CAS), China
Copyright © 2022 Wan, Liu, Wan, Wu, Wang, Yang, Mao and Wang. This is an open-access article distributed under the terms of the Creative Commons Attribution License (CC BY). The use, distribution or reproduction in other forums is permitted, provided the original author(s) and the copyright owner(s) are credited and that the original publication in this journal is cited, in accordance with accepted academic practice. No use, distribution or reproduction is permitted which does not comply with these terms.
*Correspondence: Jiyong Yang, jyyang@swu.edu.cn