- State Key Laboratory of Organic-Inorganic Composites, Beijing University of Chemical Technology, Beijing, China
Covalent organic polymer (COPs) have emerged promising potentials for the fabrication of flexible supercapacitors. Numerous efforts are devoting to the promotion of processability of COPs and broaden their applications in practical devices. Herein we have developed a fully conjugated two-dimensional COP material (COPBTC-Mn) with nitrogen coordinated metal centers. The unique structures of COPBTC-Mn endow it with high solubility via an in-situ exfoliation pathway and the capability to adsorb onto carbon-based matrix by electrostatic force. COPBTC-Mn demonstrated superior processability, reliability, and repeatability for the fabrication of flexible electrodes of supercapacitors. Besides, superior to the electrodes based on traditional COPs, our as-prepared flexible electrodes exhibited a specific capacitance as high as 325 F g−1 with the current density of 0.2 A g−1 and excellent stability after charge/discharge cycles.
Introduction
Flexible supercapacitors are vital for the development of portable energy storage devices such as wearable electronics, bendable displays, and electronic papers (Lu et al., 2014; Chen et al., 2015). Recently, numerous efforts have been paid to explore the flexible supercapacitors with high power density, fast charge/discharge rate, and long lifetime (Peng et al., 2016; Wu et al., 2018). As the crucial part of flexible supercapacitors, the flexible electrodes determine the energy storage capability, working performance, and the fabrication of flexible devices (Chen and Dai, 2016). During the past research, various kinds of materials have been used to prepare the flexible electrode through methods such as in-situ growth method, tablet method, smear method etc. (Chen and Dai, 2014; Yu et al., 2014; Choi et al., 2016; Zhou et al., 2018). Despite the extensive efforts in the fabrication of electrodes, the rational design of supercapacitive electrodes material that meet large capacitance, high energy density, outstanding stability, and simple efficient processing method remains a substantial challenge (Kou et al., 2011; Chen et al., 2014, 2017; Li et al., 2017).
Covalent organic polymer (COPs) are a class of porous frameworks which have been identified as promising electrode materials for supercapacitors (DeBlase et al., 2015; Diercks and Yaghi, 2017; Ma et al., 2017; Wang et al., 2017). The long range ordered arrangement with high accessible surface area and tunable elemental compositions of COPs are beneficial for ion transport between the interface of electrode and electrolyte solution. Besides, the regular network structures of COPs lead to explicit incorporation of redox-active functionalities, holding great potentials for pseudocapacitive energy storage (Kandambeth et al., 2019). The common usage of COPs materials during the fabrication of flexible electrodes is daubing or in-situ growing active material on the support (Xu et al., 2015). During these years, COPs materials have enriched the relevant literature as promising supercapacitors, their superior processability, reliability, and repeatability for the fabrication of flexible electrodes are still limited (Halder et al., 2018). Therefore, a new kind COP show high performance electrode material with sizable electrolyte-accessible surface areas remains a huge challenge (Wang et al., 2019).
In our previous work, we have developed a fully conjugated two-dimensional COP material with rigid structure and uneven charged coordination single-atomic centers which demonstrated great solubility in many solutions through an in-situ charged exfoliation pathway (Peng et al., 2019a). Based on our previous outcomes, we synthesized through the assembly of benzene-1,2,4,5-tetracarbonitrile (BTC) with Mn riveted in the center and finally constructed into a fully closed conjugated planar COP system (termed as COPBTC-Mn). As expected, the COPBTC-Mn could be exfoliated into thin layers with positive charged metal centers. Driven by the intermolecular interactions, the COPBTC-Mn was able to be riveted on the carbon fiber through electrostatic adsorption with uniform distribution which could be directly used as flexible electrodes in supercapacitors (Peng et al., 2019b). Our approach to preparation flexible electrode much more convenient than the commonly used spin-casting method, in-situ synthesis and squash technique, holding promising potentials for industrial preparation (Guo et al., 2018). Moreover, the as-prepared flexible electrodes demonstrated outstanding capacitive performance (325 F g−1) and considerable galvanostatic charge/discharge (GCD) cyclic stability, exceeding 3,000 cycles without compromising the capacitance.
Experimental Section
Materials and Methods
All used chemicals in this work are analytical reagents without further treatment. Carbon fiber is supplied by Shanghai Hesen Company. Manganese(II) chloride (MnCl2) is bought from Saan chemical technology (Shanghai) Co., Ltd. Benzene-1,2,4,5- tetracarbonitrile (BTC) is got from Ark Pharm, Inc. N,N-Dimethylformamide (DMF), Ethylene glycol, and Sodium sulfate (Na2SO4) are both bought from Shanghai Macklin Biochemical Co., Ltd. Elemental analysis is performed on CARLO ERBA 1106. ICP-OES is performed on ICAP7400. X-ray photoelectron spectroscopy (XPS) analysis is performed on Thermo VG ESCALAB 250 equipped with an A1 Kα X-ray source. Scanning electron microscopic (SEM) images are obtained from a S4700 SEM instrument equipped with an energy dispersive spectrometer (EDS) analyzer. Fourier Transform Infrared Spectroscopy (FTIR) is tested by a Bruker AC-80 MHZ. Solid State Nuclear Magnetic Resonance (SSNMR) is measured on a Bruker AV300 spectrometer operating at 75.5 MHz for 13C.
Electrochemical Measurements
The electrochemical tests are performed in the standard three-electrode system using a CHI 660e electrochemistry workstation at room temperature. All potentials are calibrated. The obtained carbon felt and COPBTC-Mn/CF are directly used as working electrode. Platinum (Pt) loop is used as the counter electrode and silver chloride electrode is used as the reference electrode. The electrolyte is 1 M Na2SO4 (pH = 7). During the test, working electrode is performed by Cyclic Voltammetry (CV) with the potential set between −1 and 0 V at different sweep rate (10, 20, 50, 100, 200, and 400 mV/s, respectively). Galvanostatic charge/discharge (GCD) is used to evaluate the electrochemical performance of the developed flexible electrodes at different current density (0.2, 0.5, 1.0, 2.0, 5.0, and 10 A g−1, respectively).
Synthesis
Synthesis of COPBTC-Mn
Benzene-1,2,4,5-tetracarbonitrile is used as the monomers via a mild microwave process (150°C for 20 min) with 1,8-Diazabicyclo(5,4,0)undec-7-ene (DBU) as the catalysts. Typically, BTC, manganese (II) chloride, and DBU are dissolved in ethylene glycol and kept at 150°C for 20 min in a Discover SP-microwave synthesizer.
Synthesis of Flexible Electrode COPBTC-Mn/CF
Commercial carbon felt (CF) is trimmed into small pieces with the size of 1.5 × 1.0 cm. Then the CF pieces are immersed in ethyl alcohol under ultrasonication for three times. The cleaned CF pieces are dried at 75°C overnight. During the process of the electrodes, the CF was soaked into the DMF solution with COPBTC-Mn dissolved at different content. The flexible electrodes (termed as COPBTC-Mn/CF) were then obtained after evaporating the solvent (Figure 1A).
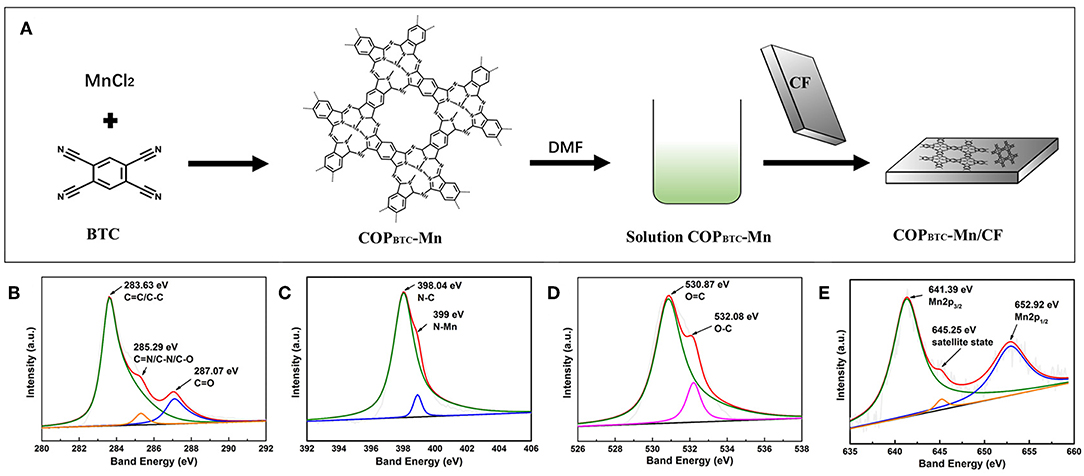
Figure 1. (A) Schematic illustration of the overall synthetic process of COPBTC-Mn and COPBTC-Mn/CF. The COPBTC-Mn XPS of (B) C, (C) N, (D) O, (E) Mn, respectively.
Results and Discussion
Material Characterization of the COPBTC-Mn and COPBTC-Mn/CF
COPBTC-Mn was synthesized according our previous work with nitrogen coordinated Mn in the center (Figure 1A). The structure of the COPBTC-Mn was confirmed by the 13C Solid-state NMR (Figure S1), which demonstrated four peaks at 44.43, 110.7, 137.52, and 168.11 ppm, attributing to the phthalocyanine macrocycles in the phthalocyanine-based conjugated polymer network. Fourier Transform Infrared Spectroscopy (FTIR) was also performed to further investigate the structure of the as-synthesized COPBTC-Mn (Figure S2), while the BTC monomer was used as the controlled sample. The peaks at 1,315, 1,616, and 1,760 cm−1 were the stretching vibration peaks of C=C and C=N on the framework of aromatic ring. Compared with the BTC monomer, new peaks at 742, 1,060, 1,125 cm−1 were found in the FTIR spectra of COPBTC-Mn, indicating the formation of phthalocyanine ring. Besides, cyano as the end group, the related characteristic peaks were found at 2,242 cm−1 in both BTC monomer and COPBTC-Mn, which further proved the synthetic route of COPBTC-Mn.
According to the previous reports, the abundant nitrogen coordinated Mn centers endowed the COPBTC-Mn with the capability to be dissolved and form intermolecular interactions with the carbon matrix. As analyzed by the X-ray photoelectron spectroscopy (XPS), the peaks for C, N, and Mn were generated during the synthesis (Figures 1B–E). The fitting curves in C 1 s spectrum were deconvoluted into three peaks corresponding to the typical “C-C/C=C” (283.63 eV), “C=N/C-N/C-O” (285.29 eV), and “C=O” (287.07.8 eV) bonds. The fitted peaks of N 1 s at 398.04 and 399 eV were attributable to “N-C” and “N-Mn,” respectively. The Mn 2p spectra demonstrated obvious peaks at 641.39, 645.25, and 652.92 eV, assigned to Mn 2p3/2, satellite state Mn, and Mn 2p1/2, which indicating the formation of nitrogen coordinated Mn as the centers. As controlled examinations, the chemical valence state of COPBTC-Mn/CF and CF were also performed (Figures S3, S4). The thermal stability of COPBTC-Mn was performed in Figure S5. The survey spectra revealed that CF only contains three elements of C, N, O while the C, N, O, Mn were found in COPBTC-Mn/CF, suggesting that the COPBTC-Mn was successfully introduce to the system. Elemental analysis (EA) and inductively coupled plasma (ICP) were performed to specifically explore the contents of CF and COPBTC-Mn/CF (Table S1). Consistently, the results proved that the COPBTC-Mn as the active sites were successfully riveted on the CF matrix and the COPBTC-Mn/CF electrodes were thus prepared.
The internal morphology and structure of the COPBTC-Mn/CF were revealed by SEM images. It was clear that the commercial carbon felt was composed of interconnected fibers, demonstrating a skeleton with smooth surface (Figure 2a). During the fabrication of electrodes, the COPBTC-Mn was adsorbed on CF through intermolecular interactions. Thus, the COPBTC-Mn/CF demonstrated a rough surface with uniformly distributed absorptions (Figure 2b). According to the energy-dispersive X-ray spectroscopy (EDS) tests, COPBTC-Mn/CF electrodes presented a homogeneous dispersion of N and Mn elements (Figure 2c), suggesting the efficiency of our developed method for the fabrication of flexible electrodes.
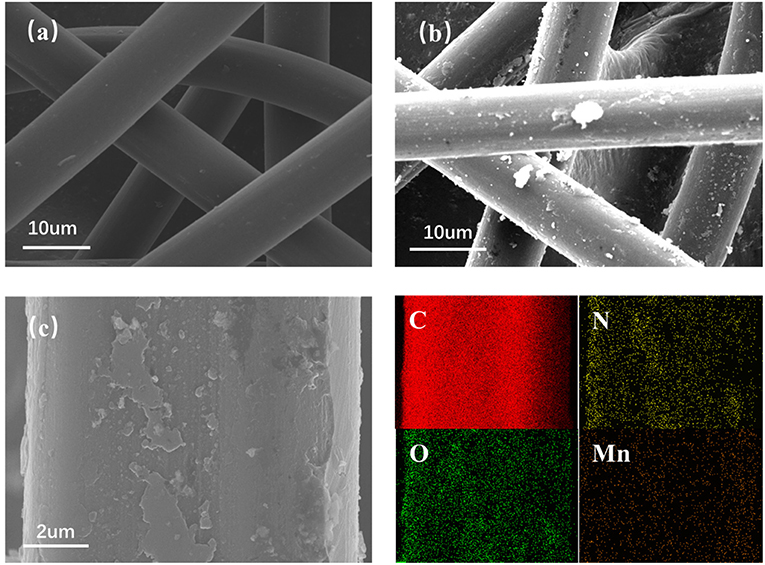
Figure 2. The scanning electron microscopy (SEM) images of (a) CF, (b) COPBTC-Mn/CF. (c) EDS spectra of C, N, O, and Mn elements in the COPBTC-Mn/CF. Corresponding elemental mapping images for (red) C, (yellow) N, (green) O, and (orange) Mn, respectively.
Electrochemical Performance of Flexible Electrode COPBTC-Mn/CF
To evaluate the electrochemical performance of the developed flexible electrodes, we performed the cyclic voltammetry (CV) and GCD. The as-prepared COPBTC-Mn/CF were directly used as the working flexible electrodes. Electrodes with different mass ratio of COPBTC-Mn were tested as comparison (marked as COPBTC-Mn/CF-α, where α was mass of COPBTC-Mn and equaled to 0, 1, 2, 3, and 4 mg, respectively). The CV curves of COPBTC-Mn/CF-α and CF in the voltage range of −1 to 0 V with different scanning rates were presented in Figure 3A and Figure S6. It could be found that the current of CF barely changed during the test, suggesting that CF was inactive for capacitive energy storage. Another feature was that none of obvious redox peaks were observed in the CV circles, indicating that the pseudocapacitance effect was small. The GCD test was performed at various current density from 0.2 to 10 A g−1 to further illuminate the electrochemical properties of the flexible COPBTC-Mn/CF-α electrodes. In Figure 3B and Figure S7, the as-prepared electrodes displayed typical charge-discharge curves. The voltage linearly increased with time in the charging process, while linearly decreased with time in the discharging process. Thus, the electrodes demonstrated symmetrical charge and discharge curves. Besides, with the increase of current density, the charge-discharge time shortened obviously. It should be noted that all of the COPBTC-Mn/CF-α electrodes was capable to be used at high current density of 10 A g−1. We further evaluated the specific capacitance from the discharge curve and found that the performance highly dependent on the mass of COPBTC-Mn, suggesting that COPBTC-Mn was the necessary active material (Figure 3C). Moreover, with COPBTC-Mn/CF-α as the electrodes, the capacitors demonstrated a superior specific capacitance to the state-of-art electrodes made up of COP and MOF (Xu et al., 2015; Khattak et al., 2016; Han et al., 2017; Sheberla et al., 2017; Sun et al., 2017; Halder et al., 2018; Liu et al., 2018; Table S2). The impedance plot of COPBTC-Mn/CF-1 was provided in Figure S8. The COPBTC-Mn/CF-α electrodes also demonstrated superiorly long stability. As shown in Figure 4A, during the cycling experiments, the COPBTC-Mn/CF-1 kept about 60% capacitance after 5,000 charge/discharge cycles with the current density as high as 10 A g−1. Meanwhile, the linearity and symmetry of the charge/discharge curves were well-retained (Figure 4B).
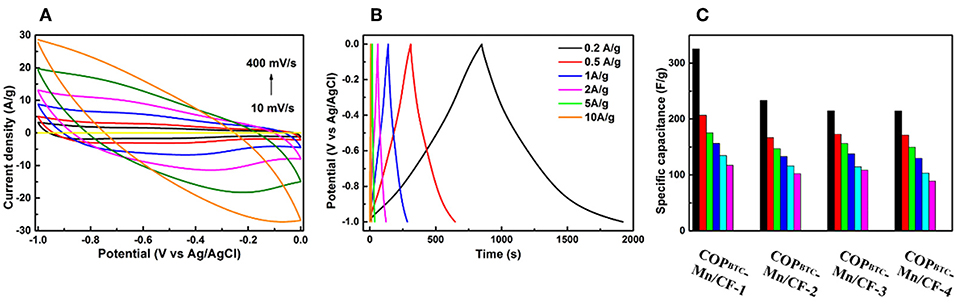
Figure 3. (A) The CV curves of COPBTC-Mn/CF-4 (α is quality of COPBTC-Mn, and α = 0, 1, 2, 3, and 4 mg, respectively.) in the voltage range of −1 to 0 V at scanning rate of 10 (black), 20 (red), 50 (blue), 100 (pink), 200 (green), and 400 mV s−1 (orange), and commercial carbon felt (CF) at a scan rate of 100 mV s−1 (yellow). (B) Charge–discharge profiles of COPBTC-Mn/CF-4 at current densities of 10 (orange), 5 (green), 2 (pink), 1 (blue), 0.5 (red), and 0.2 Ag−1 (black), respectively. (C) Capacitance of COPBTC-Mn/CF-α at different current densities.
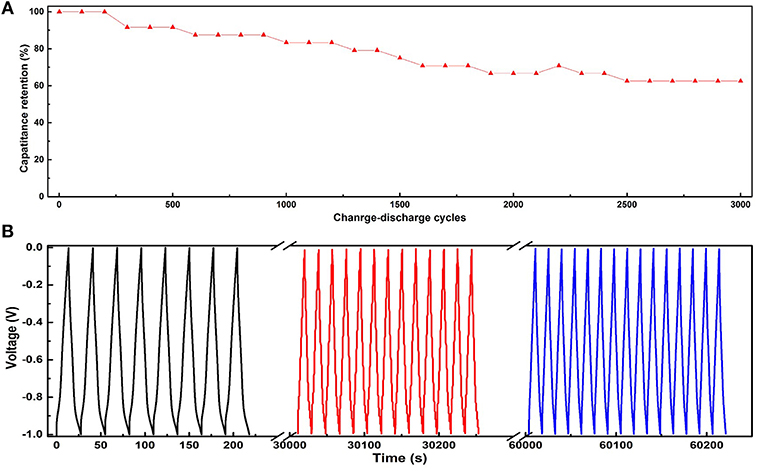
Figure 4. (A) COPBTC-Mn/CF-1 capacitance retention after 3,000 charge–discharge cycles at the high current density of 10 A/g. (B) The charge–discharge profiles of COPBTC-Mn/CF-1 during a test of 3,000 cycles.
Conclusion
In this work, we have developed a soluble COPBTC-Mn for supercapacitors. Benefited from the abundant nitrogen coordinated Mn centers, the COPBTC-Mn could be dissolved in DMF through an in-situ charge exfoliation pathway and form intermolecular interactions with the CF matrix. Combining the activity of COPBTC-Mn with the high surface area of carbon fiber skeleton, we developed a COPBTC-Mn/CF flexible electrode with considerable capacitance and superiorly long charge/discharge lifetime. Our findings paved a notable avenue to simply and repeatable prepare flexible electrodes for not only high-performance supercapacitors, but also many other flexible energy storage devices.
Data Availability Statement
The raw data supporting the conclusions of this manuscript will be made available by the authors, without undue reservation, to any qualified researcher.
Author Contributions
CM and PP performed synthesis, structural characterizations, electrochemical tests, and battery tests. CM wrote this paper. ZX supervised and led this project.
Funding
This work was supported by the National Key Research and Development Program of China (2017YFA0206500); the Natural Science Foundation of China (21676020); the Beijing Natural Science Foundation (17L20060); the Young Elite Scientists Sponsorship Program by CAST (2017QNRC001); the Talent cultivation and open project (OIC-201801007) of State Key Laboratory of Organic-Inorganic Composites; the Distinguished Scientist Program at BUCT (buctylkxj02); Double-First-Class construction projects (XK180301; XK1804-02) and the 111 Project of China (B14004).
Conflict of Interest
The authors declare that the research was conducted in the absence of any commercial or financial relationships that could be construed as a potential conflict of interest.
Supplementary Material
The Supplementary Material for this article can be found online at: https://www.frontiersin.org/articles/10.3389/fmats.2019.00242/full#supplementary-material
References
Chen, T., and Dai, L. (2016). Flexible and wearable wire-shaped microsupercapacitors based on highly aligned titania and carbon nanotubes. Energy Storage Mater. 2, 21–26. doi: 10.1016/j.ensm.2015.11.004
Chen, T., and Dai, L. M. (2014). Flexible supercapacitors based on carbon nanomaterials. J. Mater. Chem. A 2, 10756–10775. doi: 10.1039/c4ta00567h
Chen, T., Hao, R., Peng, H. S., and Dai, L. M. (2015). High-Performance, Stretchable, Wire-Shaped Supercapacitors. Angew. Chem. Int. Ed. 54, 618–622. doi: 10.1002/ange.201409385
Chen, T., Peng, H. S., Durstock, M., and Dai, L. M. (2014). High-performance transparent and stretchable all-solid supercapacitors based on highly aligned carbon nanotube sheets. Sci. Rep. 4:3612. doi: 10.1038/srep03612
Chen, X. L., Paul, R., and Dai, L. M. (2017). Carbon-based supercapacitors for efficient energy storage. Natl. Sci. Rev. 4, 453–489. doi: 10.1093/nsr/nwx009
Choi, C., Kim, K. M., Kim, K. J., Lepro, X., Spinks, G. M., Baughman, R. H., et al. (2016). Improvement of system capacitance via weavable superelastic biscrolled yarn supercapacitors. Nat. Commun. 7:13811. doi: 10.1038/ncomms13811
DeBlase, C. R., Hernandez-Burgos, K., Silberstein, K. E., Rodriguez-Calero, G. G., Bisbey, R. P., Abruna, H. D., et al. (2015). Rapid and efficient redox processes within 2D covalent organic framework thin films. ACS Nano 9, 3178–3183. doi: 10.1021/acsnano.5b00184
Diercks, C. S., and Yaghi, O. M. (2017). The atom, the molecule, and the covalent organic framework. Science 355:eaal1585. doi: 10.1126/science.aal1585
Guo, J. N., Lin, C. Y., Xia, Z. H., and Xiang, Z. H. (2018). Pyrolysis-free covalent organic polymer for oxygen reduction. Angew. Chem. Int. Ed. 57, 12567–12572. doi: 10.1002/anie.201808226
Halder, A., Ghosh, M., Khayum, M. A., Bera, S., Addicoat, M., Sasmal, H. S., et al. (2018). Interlayer hydrogen-bonded covalent organic frameworks as high-performance supercapacitors. J. Am. Chem. Soc. 140, 10941–10945. doi: 10.1021/jacs.8b06460
Han, Y., Zhang, Q., Hu, N. T., Zhang, X., Mai, Y. Y., Liu, J. Q., et al. (2017). Core-shell nanostructure of single-wall carbon nanotubes and covalent organic frameworks for supercapacitors. Chin. Chem. Lett. 28, 2269–2273. doi: 10.1016/j.cclet.2017.10.024
Kandambeth, S., Dey, K., and Banerjee, R. (2019). Covalent organic frameworks: chemistry beyond the structure. J. Am. Chem. Soc. 141, 1807–1822. doi: 10.1021/jacs.8b10334
Khattak, A. M., Ghazi, Z. A., Liang, B., Khan, N. A., Iqbal, A., Li, L. S., et al. (2016). A redox-active 2D covalent organic framework with pyridine moieties capable of faradaic energy storage. J. Mater. Chem. A 4, 16312–16317. doi: 10.1039/C6TA05784E
Kou, Y., Xu, Y. H., Guo, Z. Q., and Jiang, D. L. (2011). Supercapacitive energy storage and electric power supply using an Aza-fused pi-conjugated microporous framework. Angew. Chem. Int. Ed. 50, 8753–8757. doi: 10.1002/anie.201103493
Li, W. H., Ding, K., Tian, H. R., Yao, M. S., Nath, B., Deng, W. H., et al. (2017). Conductive metal-organic framework nanowire array electrodes for high-performance solid-state supercapacitors. Adv. Funct. Mater. 27:1702067. doi: 10.1002/adfm.201702067
Liu, W., Ulaganathan, M., Abdelwahab, I., Luo, X., Chen, Z. X., Tan, S. J. R., et al. (2018). Two-dimensional polymer synthesized via solid-state polymerization for high-performance supercapacitors. ACS Nano 12, 852–860. doi: 10.1021/acsnano.7b08354
Lu, X. H., Yu, M. H., Wang, G. M., Tong, Y. X., and Li, Y. (2014). Flexible solid-state supercapacitors: design, fabrication and applications. Energy Environ. Sci. 7, 2160–2181. doi: 10.1039/c4ee00960f
Ma, Y. X., Li, Z. J., Wei, L., Ding, S. Y., Zhang, Y. B., and Wang, W. (2017). A dynamic three-dimensional covalent organic framework. J. Am. Chem. Soc. 139, 4995–4998. doi: 10.1021/jacs.7b01097
Peng, P., Shi, L., Huo, F., Mi, C. X., Wu, X. H., Zhang, S. J., et al. (2019a). A pyrolysis-free path toward superiorly catalytic nitrogen-coordinated single atom. Sci. Adv. 5:eaaw2322. doi: 10.1126/sciadv.aaw2322
Peng, P., Shi, L., Huo, F., Zhang, S. J., Mi, C. X., Cheng, Y. H., et al. (2019b). In situ charge exfoliated soluble covalent organic framework directly used for Zn-air flow battery. ACS Nano 13, 878–884. doi: 10.1021/acsnano.8b08667
Peng, X., Liu, H. L., Yin, Q., Wu, J. C., Chen, P. Z., Zhang, G. Z., et al. (2016). A zwitterionic gel electrolyte for efficient solid-state supercapacitors. Nat. Commun.7:11782. doi: 10.1038/ncomms11782
Sheberla, D., Bachman, J. C., Elias, J. S., Sun, C. J., Shao-Horn, Y., and Dinca, M. (2017). Conductive MOF electrodes for stable supercapacitors with high areal capacitance. Nat. Mater. 16, 220–224. doi: 10.1038/nmat4766
Sun, B., Liu, J., Cao, A. M., Song, W. G., and Wang, D. (2017). Interfacial synthesis of ordered and stable covalent organic frameworks on amino-functionalized carbon nanotubes with enhanced electrochemical performance. Chem. Commun. 53, 6303–6306. doi: 10.1039/C7CC01902E
Wang, M. Y., Guo, H., Xue, R., Li, Q., Liu, H., Wu, N., et al. (2019). Covalent organic frameworks: a new class of porous organic frameworks for supercapacitor electrodes. ChemElectroChem 6, 2984–2997. doi: 10.1002/celc.201900298
Wang, S., Wang, Q. Y., Shao, P. P., Han, Y. Z., Gao, X., Ma, L., et al. (2017). Exfoliation of covalent organic frameworks into few-layer redox-active nanosheets as cathode materials for lithium-ion batteries. J. Am. Chem. Soc. 139, 4258–4261. doi: 10.1021/jacs.7b02648
Wu, X. J., Xu, Y. J., Hu, Y., Wu, G., Cheng, H. Y., Yu, Q., et al. (2018). Microfluidic-spinning construction of black-phosphorus-hybrid microfibres for non-woven fabrics toward a high energy density flexible supercapacitor. Nat. Commun. 9:4573. doi: 10.1038/s41467-018-06914-7
Xu, F., Xu, H., Chen, X., Wu, D. C., Wu, Y., Liu, H., et al. (2015). Radical covalent organic frameworks: a general strategy to immobilize open-accessible polyradicals for high-performance capacitive energy storage. Angew. Chem. Int. Ed. 54, 6814–6818. doi: 10.1002/anie.201501706
Yu, D. S., Goh, K., Wang, H., Wei, L., Jiang, W. C., Zhang, Q., et al. (2014). Scalable synthesis of hierarchically structured carbon nanotube-graphene fibres for capacitive energy storage. Nat. Nanotechnol. 9, 555–562. doi: 10.1038/nnano.2014.93
Keywords: soluble COPs, flexible electrode, supercapacitors, in-situ exfoliation, electrostatic force
Citation: Mi C, Peng P and Xiang Z (2019) Soluble Covalent Organic Polymer for the Flexible Electrode of Supercapacitors. Front. Mater. 6:242. doi: 10.3389/fmats.2019.00242
Received: 06 August 2019; Accepted: 18 September 2019;
Published: 02 October 2019.
Edited by:
Chunyi Zhi, City University of Hong Kong, Hong KongReviewed by:
Xiong Chen, Fuzhou University, ChinaMing Xu, Huazhong University of Science and Technology, China
Copyright © 2019 Mi, Peng and Xiang. This is an open-access article distributed under the terms of the Creative Commons Attribution License (CC BY). The use, distribution or reproduction in other forums is permitted, provided the original author(s) and the copyright owner(s) are credited and that the original publication in this journal is cited, in accordance with accepted academic practice. No use, distribution or reproduction is permitted which does not comply with these terms.
*Correspondence: Zhonghua Xiang, eGlhbmd6aCYjeDAwMDQwO21haWwuYnVjdC5lZHUuY24=