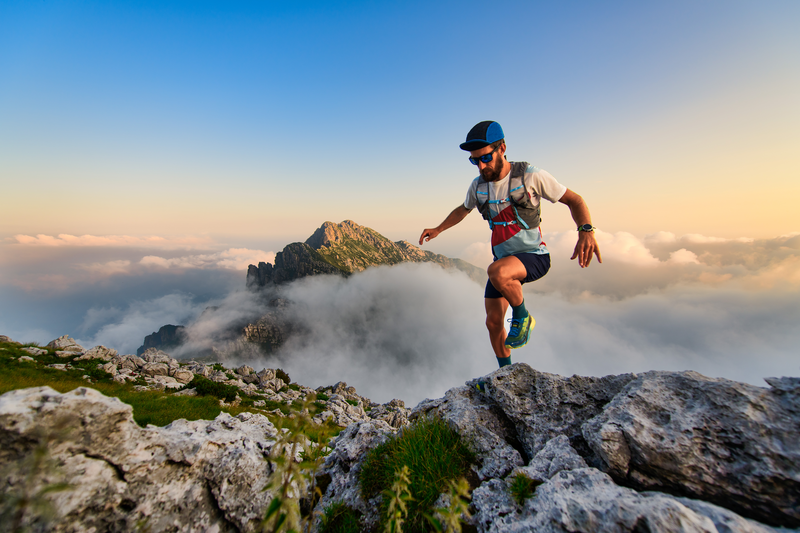
94% of researchers rate our articles as excellent or good
Learn more about the work of our research integrity team to safeguard the quality of each article we publish.
Find out more
ORIGINAL RESEARCH article
Front. Mar. Sci.
Sec. Marine Affairs and Policy
Volume 12 - 2025 | doi: 10.3389/fmars.2025.1442572
This article is part of the Research Topic Advances in Marine Environmental Protection: Challenges, Solutions and Perspectives View all 40 articles
The final, formatted version of the article will be published soon.
You have multiple emails registered with Frontiers:
Please enter your email address:
If you already have an account, please login
You don't have a Frontiers account ? You can register here
While previous research on environmental efficiency examines data from the annual reports of shipping companies, this study takes a novel approach. It applies data envelopment analysis (DEA) and backcasting theory to assess environmental efficiency and plan the transition to cleaner fuels among global container shipping companies. Companies are categorized as first movers, second movers, and last movers based on their environmental efficiency. This categorization provides a new perspective on strategic differentiation and improvement tactics for each group. It allows for the development of strategic frameworks tailored to the unique positions of different shipping companies, aiding them achieve the International Maritime Organization's net-zero target by 2050. The study's originality lies in its use of DEA to evaluate efficiency and backcasting for strategic planning. This combination provides policymakers and industry leaders with actionable insights and a robust methodological framework for promoting sustainable maritime management. The dual approach not only contributes to academic literature, but also aids in navigating the complexities of green transitions in the shipping industry.
Keywords: Environmental efficiency, strategic planning, data envelopment analysis, Backcasting theory, Net-zero emissions
Received: 02 Jun 2024; Accepted: 17 Feb 2025.
Copyright: © 2025 Sim, Lee, Kim, Jung and Chang Hee. This is an open-access article distributed under the terms of the Creative Commons Attribution License (CC BY). The use, distribution or reproduction in other forums is permitted, provided the original author(s) or licensor are credited and that the original publication in this journal is cited, in accordance with accepted academic practice. No use, distribution or reproduction is permitted which does not comply with these terms.
* Correspondence:
Min-seop Sim, Korea Maritime and Ocean University, Yeongdo District, Republic of Korea
Jeong-min Lee, Korea Maritime and Ocean University, Yeongdo District, Republic of Korea
Yul-seong Kim, Korea Maritime and Ocean University, Yeongdo District, Republic of Korea
Dong-ho Jung, Korea Research Institute of Ships & Ocean Engineering (KRISO), Daejeon, Republic of Korea
Lee Chang Hee, Korea Maritime and Ocean University, Yeongdo District, Republic of Korea
Disclaimer: All claims expressed in this article are solely those of the authors and do not necessarily represent those of their affiliated organizations, or those of the publisher, the editors and the reviewers. Any product that may be evaluated in this article or claim that may be made by its manufacturer is not guaranteed or endorsed by the publisher.
Research integrity at Frontiers
Learn more about the work of our research integrity team to safeguard the quality of each article we publish.