- 1University of Texas at Austin, Bureau of Economic Geology, Jackson School of Geosciences, Austin, TX, United States
- 2University of North Carolina at Chapel Hill, Department of Earth, Marine, and Environmental Sciences, Chapel Hill, NC, United States
- 3University of Texas at Austin, Department of Earth and Planetary Sciences, Jackson School of Geosciences, Austin, TX, United States
Coastal infrastructure projects, particularly the modification of coastal river channels, are becoming increasingly significant to economic activities worldwide as a response to climate-driven changes and urbanization. The benefits of channel modification projects can be realized quickly, but the altered movement of sediments in the river channel can lead to unintended geomorphic changes years or decades later. An example of this is the closure of the San Bernard River mouth, located on the central coast of Texas, which was clogged with sediments by the 1990s as a result of two major projects in the area: the diversion of the Brazos River channel (1929) and the construction of the Gulf Intracoastal Waterway (GIWW) (1940s). The objective of this study was to a) document the delayed geomorphic response to the projects using a GIS analysis of historical maps and aerial imagery, and b) provide a snapshot of altered flow pathways in the area using measurements collected in situ. Results showed that the GIWW was the main conduit for river flow as it bisects the San Bernard River 2 km inland of its river mouth, reducing discharge in the terminal limb of the river. Due to reduced flow, the river mouth became clogged with wave-transported sediment supplied by the still-adjusting Brazos River which had been diverted to within 6 km of the San Bernard River. With a limited connection to the sea, altered sediment and flow pathways have led to numerous hazards and costly corrective dredging projects surpassing $12 million to date. Optimizing the cost-effectiveness of channel modification projects requires considering their long-term impact as managers continue to adapt to ever-changing coastal zones.
1 Introduction
Fluvial-coastal transition zones are geomorphically dynamic areas that benefit both coastal economies and ecosystems (Reguero et al., 2014; Paola et al., 2011; Passalacqua and Moodie, 2022). Climate-driven stressors and urban development are expected to increase vulnerability along coastlines worldwide, making it increasingly important to address the interactions between natural and engineered processes (Davis et al., 2018; Marsooli et al., 2019). Modifications to coastal rivers, including bank stabilization, channel rerouting, and dredging, have been implemented to protect coastal areas from environmental hazards and enhance economic activity. These projects are often intended to make the coastal zone rigid and stable, which directly conflicts with a landscape defined by naturally occurring topographic and geomorphic changes. Furthermore, these engineering projects tend to focus on short-term solutions that provide immediate socioeconomic benefit (Cornwall, 2022; Coastal Protection and Restoration Authority of Louisiana, 2023), but can lead to unforeseen longer-term perturbations that prove costly and hazardous (Ndour et al., 2018; Barnard et al., 2009; Runyan and Griggs, 2005; Flatley et al., 2018; Boesch, 2020). In each one of these cases, the engineered structures were specifically designed to modify the flow of water with little-to-no consideration of potential impacts on the flow of sediment even though small changes to the sediment-transport field drive bed erosion and/or aggradation over time. Hydraulic adjustments to engineered changes are immediate while commensurate adjustments to the sediment-transport field drive geomorphic adjustment over considerably longer time spans (Roelvink, 2006).
Today, the mouth of the San Bernard River, located southwest of Freeport, Texas (Figure 1), is clogged with sediment as an unintended consequence of two channel modification projects implemented over the last century. These projects, decades after completion, led to a morphodynamic coupling of the San Bernard River (4,800 km2 drainage area, 5,840 m3/s annual discharge) with the adjacent Brazos River (118,000 km2 watershed, 79,570 m3/s annual discharge; Yao et al., 2022) which became the primary control on the dynamics of the San Bernard River mouth. First, in 1929 the US Army Corps of Engineers (USACE) diverted the lowermost 10 km of the Brazos River channel to a new location 10 km southwest of its natural mouth in order to construct the Port of Freeport, a major commercial and industrial hub (Fox, 1931; Fields et al., 1988; Carlin and Dellapenna, 2014). A new delta began to form at the outlet of the new Brazos River channel. The net transport of coastal and deltaic sediments by waves (longshore transport, or littoral drift; Bosboom and Stive, 2021) continued to be directed southwestward towards the mouth of the San Bernard River which now lay only ~6 km away from the new Brazos River delta. By ~1975, several decades later, the growing Brazos River delta began to encroach on the mouth of the San Bernard River, leading to its eventual closure in the late 1990s. The second project that contributed to the closure was the construction of the Gulf Intracoastal Waterway (GIWW). The GIWW was completed in 1943 as a nation-scale project to facilitate barge traffic, running parallel to the shoreline ~2 km inland of the coast and intersecting with the San Bernard River (USACE, 2022). Flow from the San Bernard River was disrupted at the intersection, as the GIWW essentially added two extra distributary channels that altered the hydrodynamics of the San Bernard River at its outlet into the Gulf of Mexico (Sanchez et al., 2001). Prior to 1929, mouths of the Brazos and San Bernard Rivers were separated by a sufficient distance that one did not affect the other. But in the present day, these channel modifications have coupled the hydrodynamic and sediment-transport regimes. Here, multiple sediment transport systems, including riverine, coastal, and engineered, have overlapped to create a unique example of the delayed geomorphic responses brought about by modifications to the coastal river system. Further background information on the geomorphic setting of the San Bernard and Brazos Rivers, the infrastructure projects, and the hazards associated with the clogged river mouth are provided in the supplemental text.
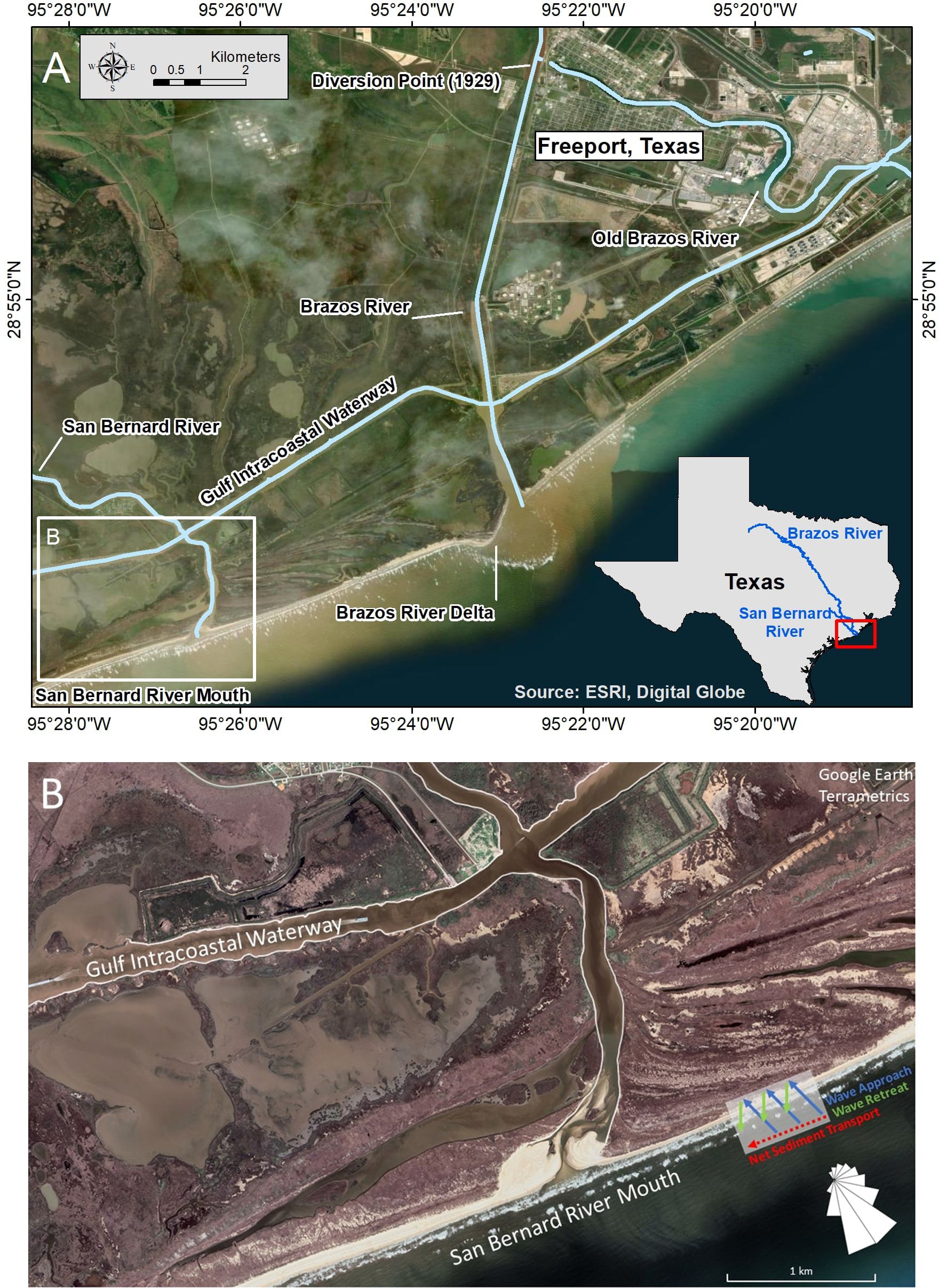
Figure 1. (A) Vicinity map of the study area showing the Brazos River delta system and the San Bernard River. (B) Aerial image of the closed mouth of the San Bernard River taken in 2024, including a wave-rose and a simplified cartoon depicting longshore transport (littoral drift) of coastal sediments by waves (bottom right).
Several negative impacts have arisen because of the clogging of the San Bernard River mouth. Enhanced backwater flooding during storm events (Sanchez et al., 2001), especially during Hurricane Harvey in 2017, severely damaged coastal communities and infrastructure nearby (Blake and Zelinsky, 2018). Currents in the GIWW frequently create hazards for barge traffic (Sanchez et al., 2001; Texas Department of Transportation (TxDOT), 2016), and deposition of fluvial sediments in the GIWW results in costly maintenance dredging (Hamilton et al., 2021). Nearby estuaries have also become fresher as a result of the lost connection to the sea (Kraus and Lin, 2002) which can negatively impact estuarine ecology (Palmer et al., 2011). Two dredging efforts were conducted to address this, in 2009 and again in 2021. Both of these efforts temporarily opened the mouth of the San Bernard River, but in both cases the mouth became once again clogged with coastal sediments within ~2 years. As a result, the continued closure of the San Bernard River has garnered significant attention from local residents, industry, and coastal engineers regarding possible long-term solutions (Kraus and Lin, 2002; Morris, 2016).
Coastal morphodynamics impact sizable portions of the global population and economies (Nicholls et al., 2007), and the need for sensible infrastructure is expected to increase as a result of climate-driven environmental changes to coastlines (Davis et al., 2018). As coastal modifications increase in importance, it is important to ensure that the projects are sustainable at timescales and spatial scales beyond the project-scope. The closure of the San Bernard River mouth highlights the need for regional, long-term planning of both hydrodynamic and sediment-transport fields. Although the dynamics of the San Bernard River system are well known by local residents and coastal engineers, the broader scientific community has paid little attention to these unintended negative consequences of coastal infrastructure. By documenting the delayed geomorphic responses to the infrastructure projects that impacted the San Bernard River, valuable context can be provided to coastal decision-makers to work toward more efficient solutions to emerging coastal problems.
The objective of this study was to present the case of the inter-linked San Bernard River, Brazos River, and engineered channels to highlight the sensitivity of sediment transport dynamics to modifications. Prior efforts focused on the evolution of the Brazos River channel and delta (Rodriguez et al., 2000; Carlin and Dellapenna, 2014) and occurred before recent dredging efforts and extreme storms have highlighted the complexity of the linked river systems. We expand on this work by evaluating a) the fluvial-coastal morphodynamic processes that led to the closure of the San Bernard River mouth, b) the sequence and magnitude of geomorphic responses, and c) the negative consequences that are presently driving costly corrective measures. To achieve this, historical aerial images were gathered from numerous sources and compiled into a timeline to highlight the morphodynamic evolution of the system. Using these images, a GIS analysis was conducted to investigate the changes to the geometry of the San Bernard River as coastal sediments began to fill the river mouth. Then, a field campaign was conducted to provide a snapshot of the flow regime where the San Bernard River intersects with the GIWW. This information provided a reconnaissance-level overview of the hydrodynamic and morphodynamic processes that led to the issues seen today, where negative consequences arose decades after the channels were modified.
2 Methods
First, a desktop analysis was paired with a snapshot of observational flow data to document the evolution of the San Bernard and Brazos rivers over time as a result of the channel modification projects, including their pre-modification conditions. This was done by a) compiling historical aerial/satellite imagery and nautical maps of the study area, b) digitizing the imagery to map changes to the position and geometry of the landforms over time, and c) comparing bathymetric surveys of the coastal “abandoned” limb of the San Bernard River. Second, in situ flow data were collected at the intersection of the San Bernard River and the GIWW (during low-flow conditions) to provide a snapshot of the flow field. The datasets and analytical techniques are described here.
The development of the Brazos delta and subsequent changes to the San Bernard River mouth were documented by compiling 52 historical aerial and satellite images, dating from 1938 to 2023, from publicly available sources such as the Texas Natural Resources Information System (TNRIS) repository, the Texas General Land Office, United States Geological Survey (USGS), Google Earth (derived from Quickbird, Digital Globe, and Terrametrics), and historical literature. Selected images were digitized and polygonized using ArcGIS. To evaluate the morphology of the original Brazos River Delta, historical nautical maps were sourced from the Historical Map and Chart Collection, managed by the National Oceanic and Atmospheric Administration’s Office of Coast Survey, which are publicly available online (https://historicalcharts.noaa.gov/).
A timeline of these images and maps shows the growth of the relocated Brazos delta, its encroachment on the San Bernard River, and the morphologic processes shaping this stretch of the coast. Using ESRI ArcGIS software, the position of the Brazos River delta alluvial ridges, San Bernard River mouth position, and San Bernard River pathways were manually estimated (by recording geographic coordinates) and mapped through time. The lengths of the San Bernard River channel (measured from the intersection with the GIWW to the mouth), river mouth positions, and channel mouth widths were then calculated in ArcGIS. The image files, shapefiles, and a Google Earth Engine timelapse video are publicly available and stored digitally in the Texas Data Repository (doi:10.18738/T8/INCGRW).
Furthermore, bathymetric surveys were conducted by the United States Army Corps of Engineers in 2014 and 2015 in the abandoned limb of the San Bernard River (between the intersection with the GIWW and the sea). These surveys are publicly available for download on the USACE GIWW Hydrographic Survey data portal, or available from the authors upon request. Difference mapping of the two bathymetric surveys provides insights into the sedimentation rates of the terminal stretch of the San Bernard River channel.
A secondary objective of this study was to provide a snapshot of calm-weather flow conditions at the intersection of the San Bernard River and the GIWW. Flow data (direction and magnitude of water flux) were collected using a surfboard-mounted Sontek Acoustic Doppler Current Profiler (ADCP) profiler. The survey was conducted during low discharge conditions in the summer (June 16, 2021). To minimize backwater effects from tidal flows, data were collected during an outgoing tide. Water flux was calculated by multiplying the depth-averaged flow velocity by the channel depth for each reading, resulting in units of m2/s. Water flux was calculated instead of discharge due to the a) inability of the survey-craft to span the full width of the channel given the shallow depth of the channel banks, and b) difficulty navigating due to wind and barge traffic affecting the craft. Thus, an estimate of the cross-sectional area could not be obtained. Measurements were taken in transects along and across the San Bernard River channel both upstream and downstream of its intersection with the GIWW, and along the GIWW east and west of the intersection. At United States Geological Survey station 08117705 in Sweeny, Texas, river discharge was less than 23 cubic meters per second, and the water level was controlled by the outgoing tide. A simple analysis of flow direction and magnitude is presented here to provide a preliminary understanding of the flow field at the intersection and within the relatively abandoned limb of the San Bernard River.
3 Results
3.1 Phased-evolution of the Brazos River Delta and interference with the San Bernard River mouth
To understand the evolution of the San Bernard River, the genesis and growth of the Brazos River delta must first be considered. The evolution of the Brazos River delta can be separated into three phases: 1) relocation and rapid growth, 2) shift to wave dominance, and 3) reworking into the San Bernard River mouth.
3.1.1 Relocation and rapid growth
Prior to the 1929 diversion of the Brazos River channel mouth, the Brazos delta lay 10 km to the northeast of its present position (Figure 2). Nautical maps dating back to the 19th century show that the morphology of the original Brazos River delta is similar to what is seen today. The original Brazos River delta had a cuspate shape and submerged channel bar on the western flank of the river mouth as a result of the net southwestward alongshore transport of sediments by waves.
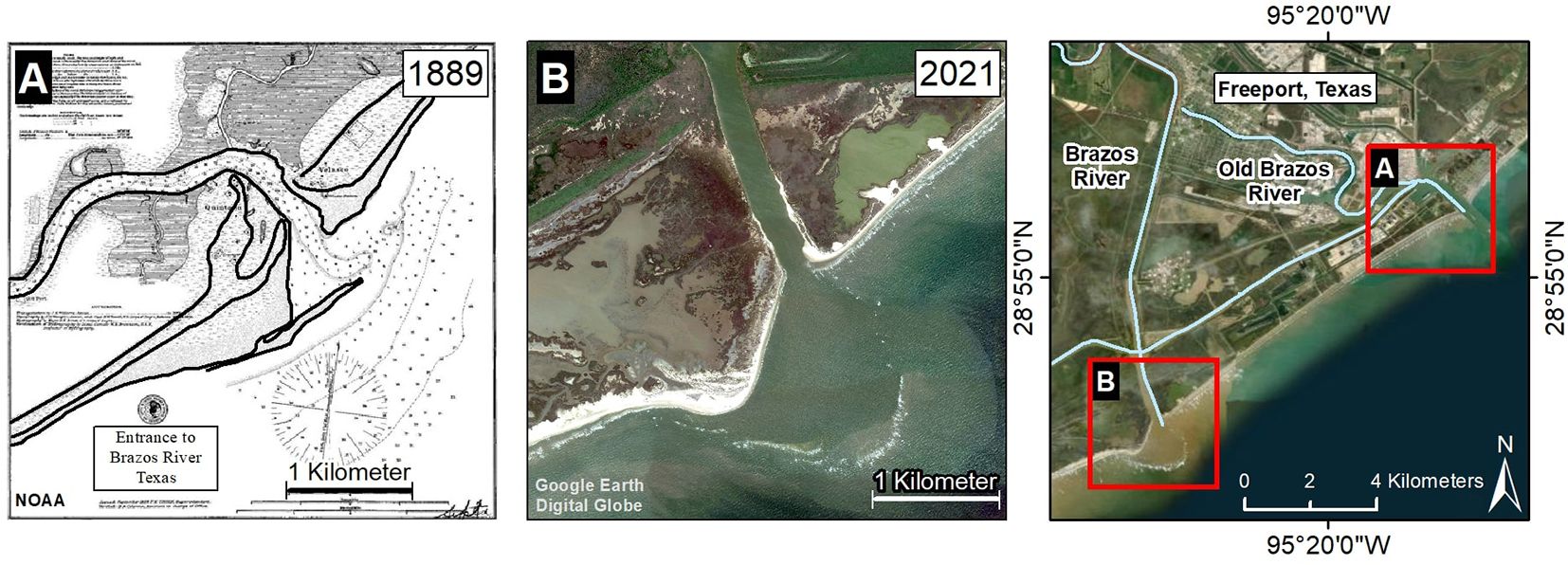
Figure 2. A nautical map from 1889 (A) shows the natural Brazos River before installation of jetties and diversion in 1929. An aerial image from 2021 (B) shows the new position of the Brazos delta 10 km southwest of the old delta. The morphology of both deltas is similar as a result of similar coastal sediment transport processes.
After the main channel was diverted in 1929, a new delta began to form, supplied by riverine sediments and wave-reworked sediments from the old delta top. While the original, abandoned delta began to erode under the influence of wave action, the new delta began to grow rapidly (Figure 3). Beginning in 1929, the delta took on a mostly symmetrical lobate shape that protruded outward into the Gulf of Mexico. The protruding flanks reached their peak size around 1948, extending 4 km seaward of the original 1929 shoreline.
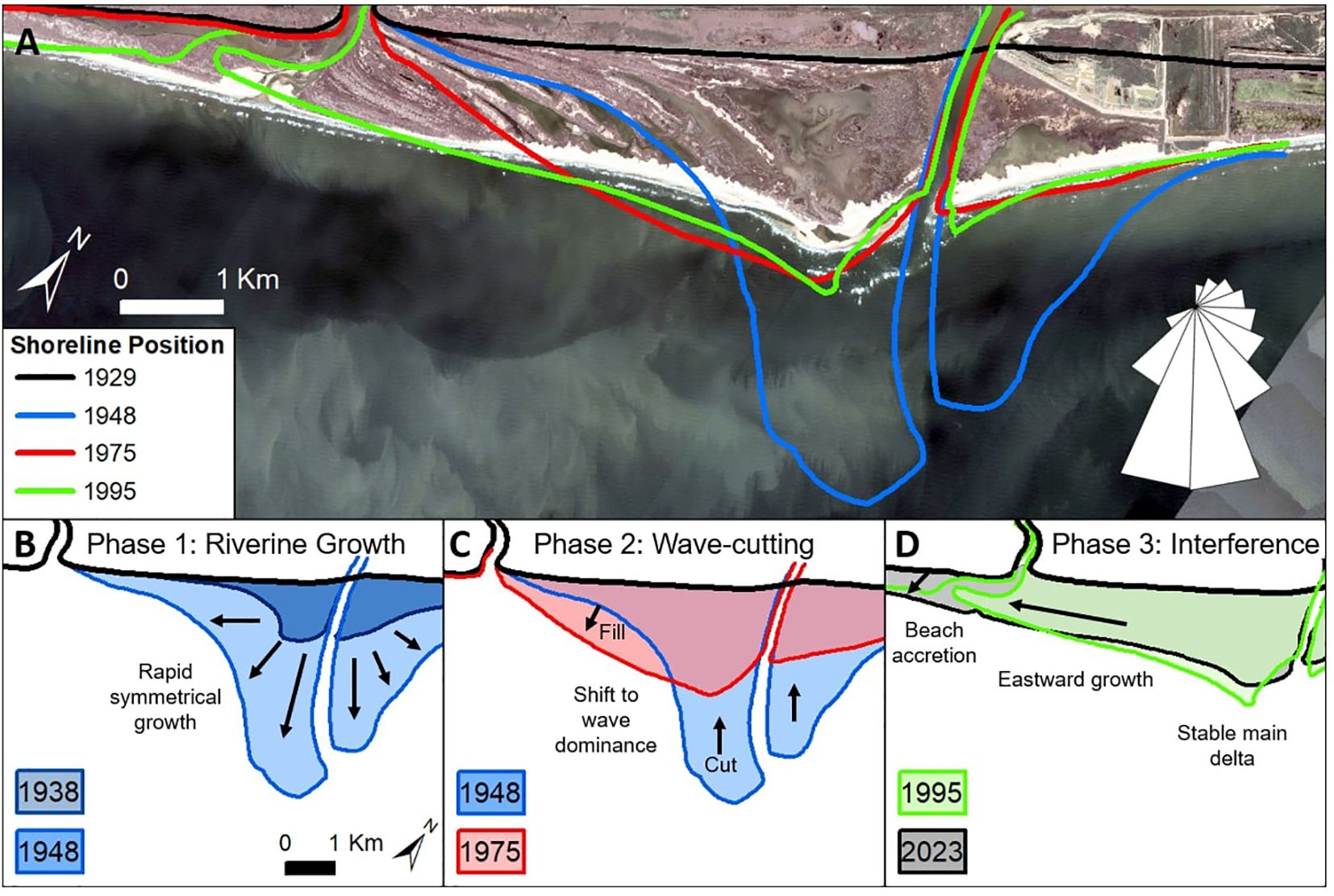
Figure 3. Shoreline position maps showing the position and extent of the Brazos River delta top as it evolved in multiple phases. (A) depicts shoreline positions at the major “checkpoints” in the phased-evolution of the delta, atop a 2024 aerial image. (B–D) provide details of the three phases of growth.
3.1.2 Shift to wave dominance
After 1948, the Brazos River delta top entered a phase of retrogradation and widening. Wave action drove sediment erosion from the protruding flanks and redistributed it to the western flank of the delta. This “cut and fill” regime resulted in rapid retreat of the delta flanks and subsequent westward growth, resulting in an overall flattening of the shoreline (Figure 3B). It was during this phase that the delta took on its asymmetrical shape.
Up until 1975, this redistribution occurred primarily between the main channel outlet and the San Bernard River mouth. The delta took on a form similar to its original one, having an asymmetrical shape favoring growth on the western flank. Growth of the delta became episodic during this time period, with long periods of relative stability sometimes interrupted by major flood events that produced beach ridges that are still visible in the surface architecture of the delta (Figures 3A, C).
3.1.3 Reworking into the San Bernard River mouth
Beginning in 1975 and continuing to the present-day, the Brazos River Delta has been characterized by westward extension that grew the Brazos River delta into the location of the San Bernard River mouth. In this time period the main protruding flanks have mostly remained the same size and shape. Growth sporadically occurred on the main flanks with the occasional emergence of a flood-associated channel mouth bar (Rodriguez et al., 2000) that was subsequently reworked by waves into a beach ridge over the following years (e.g., 1992 through 1995). In this phase the majority of the growth occurred in the form of spit accretion into the San Bernard River mouth (Figure 3D).
The first evidence of spit growth at the San Bernard River mouth occurred in 1975, expressed as a protrusion on the east side of the mouth. In its natural state (prior to the channel modification projects in 1929 and 1941) the San Bernard River flowed into the Gulf of Mexico with its channel oriented more or less perpendicular to the coast and a width of ~100 m (Figure 4). As early as 1975 the river mouth showed evidence of narrowing and steering by the growing Brazos delta. A spit began to form on the eastern flank of the San Bernard River mouth (the western flank of the Brazos River delta) and grew throughout the 1980s and 1990s. During this time, the San Bernard River channel was gradually steered parallel to the coast by the growing spit, which reached a length of ~2 km by 1995 and peaked at ~3.5 km in 2006 (Figure 4B).
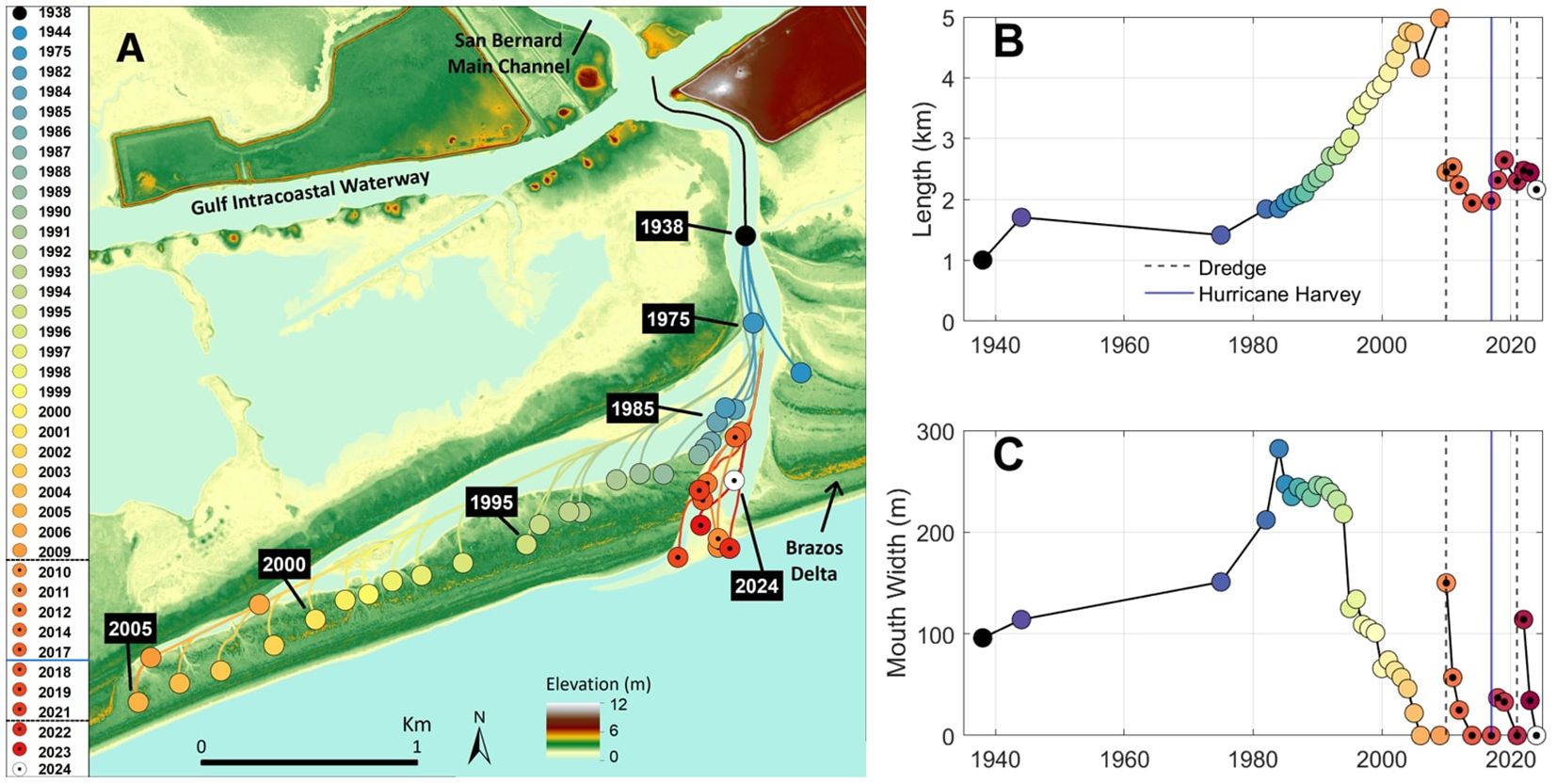
Figure 4. (A) The evolution of the San Bernard River mouth is shown atop a lidar-sourced digital elevation model. Colorful lines show the pathways of the terminal stretch of the San Bernard River channel through time, where the growing Brazos delta steered and closed the San Bernard River channel. (B) Time series of the length of terminal limb of the San Bernard channel from the natural, unaltered river mouth position (1938), to the present. (C) Time series of approximate channel widths at the mouth of the San Bernard River polar plot depicting the evolution of the length of the terminal limb of the San Bernard River. The dashed lines indicate dredging projects, and the blue line indicates Hurricane Harvey. Dots within the circles indicate that the position, mouth width, and length occurred after the 2009 dredge that restored the original location of the channel mouth.
The length of the San Bernard River channel, measured from the intersection with the GIWW, increased from approximately 1.4 km in 1975 to a maximum of 5.0 km in 2005, when the river mouth was completely disconnected from the Gulf of Mexico (Figure 4A, B). Further, the width of the channel mouth gradually increased to a peak of ~300 m in 1984 as erosion of the westward edge of the San Bernard River mouth occurred in tandem with spit growth on the eastern edge. However, after 1984 the spit growth on the eastern edge began to outpace the river mouth erosion on the westward end, and the width of the mouth gradually tapered until becoming fully closed in 2004 (Figure 4C).
3.2 Influence of the GIWW on flow in the San Bernard River
It has been well documented that the GIWW influences morphodynamic properties of features throughout the Gulf coast (Swarzenski and Perrien, 2015). The GIWW can carry sediment and interrupt the flow of the rivers it intersects, disrupting their typical conditions (Swarzenski, 2003). Combined with the dynamics of the Brazos River barge traffic, flows in the study area are observed to be complex in both fair-weather and high-discharge conditions (Sanchez et al., 2001; Mariotti and Boswell, 2023).
The GIWW interrupted the flow of the San Bernard River, providing two alternate pathways for river flow rather than only the original channel that emptied into the Gulf of Mexico. Here we provide a simple snapshot of the flow characteristics at the intersection of the San Bernard River and the GIWW from the summer of 2021. At the time of sampling, the channel mouth was open (~55 m). Results showed that the principal conduit for flow in the study area was the GIWW, with peak flow velocities greater than 35.0 cm/s, and flow was weakest on the abandoned limb of the San Bernard River channel (Figure 5). Flow down the GIWW was directed westward, away from the Brazos River. The west Brazos locks were open, potentially allowing the Brazos River to drive these flows. Fluxes increased downstream of the intersection with the San Bernard River, and a perturbation in the flow direction along the GIWW suggests that the San Bernard River interrupts and enhances its westward flow.
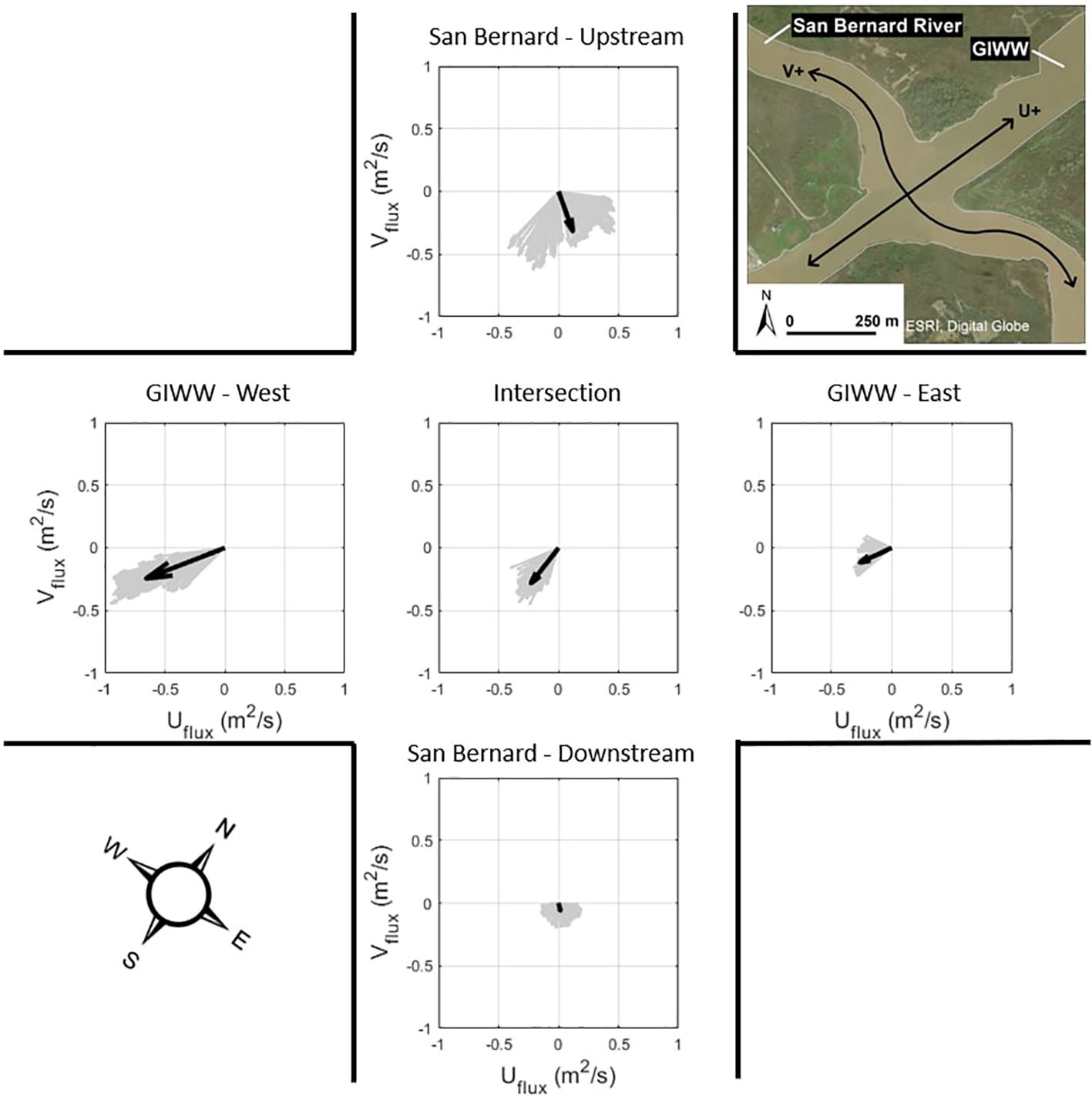
Figure 5. Observed directions and magnitudes of water flux at the intersection between the San Bernard River and GIWW during calm-weather conditions show that the GIWW is the main conduit for flow of the system. San Bernard River contributes discharge to GIWW flow, leading to reduced velocities in the terminal limb of the channel downstream of the intersection. Mean water flux vectors shown in black, individual vectors shown in gray.
In both the upstream and downstream portions of the San Bernard River, flows were directed seaward, with considerable directional spread due to the wind field at the time of sampling. Wind stress played a role in these data as our vessel was pushed around as the wind blew. Furthermore, small wind-waves were seen during gusts. Flow velocities in the San Bernard River were generally less than those of the GIWW and were more readily manipulated by the wind. Flow speeds in the upstream limb of the San Bernard River were generally between 10 and 20 cm/s. In the downstream limb of the San Bernard River, the flow was subdued relative to its upper limb, with speeds up to 12 cm/s (Figure 5).
Mean water fluxes, calculated by taking the average of measured flow velocities multiplied by channel depth, further highlight that the GIWW is the main conduit for flow in the system. The mean water flux for the GIWW was approximately 0.66 m2/s, while the upstream limb of the San Bernard River had a mean water flux of approximately 0.39 m2/s. In contrast, shallow depths (typically < 2 m) and relatively low flow velocities yielded a mean water flux of 0.15 m2/s in the downstream limb of the San Bernard River. Thus, San Bernard River flow appears to be captured more effectively by the GIWW rather than its own downstream limb.
The inactive limb of the San Bernard River channel (the “abandoned” channel, between the intersection with the GIWW and the Gulf of Mexico) has experienced a buildup of sediment behind the clogged river mouth. Using USACE bathymetric surveys taken in June 2014 and April 2015, 10 months’ worth of sedimentation is shown, typically between 20 and 50 cm with a maximum of 1 m (Figure 6). When compared to the approximate original depth of the San Bernard River channel, ~4.5 m, the total sedimentation ranged from 2.5 to 4.0 m since the mid-1940s when the GIWW canal was initially dug. Expressed as a simplified rate of change (between the period of 1945 to 2015), the deposition rate in this limb of the channel ranged from 3.6 cm/yr to 5.7 cm/yr. This rapid deposition of sediments coincided with the reduction of flow velocities in the abandoned channel while it steered and tapered down-drift of the Brazos River delta.
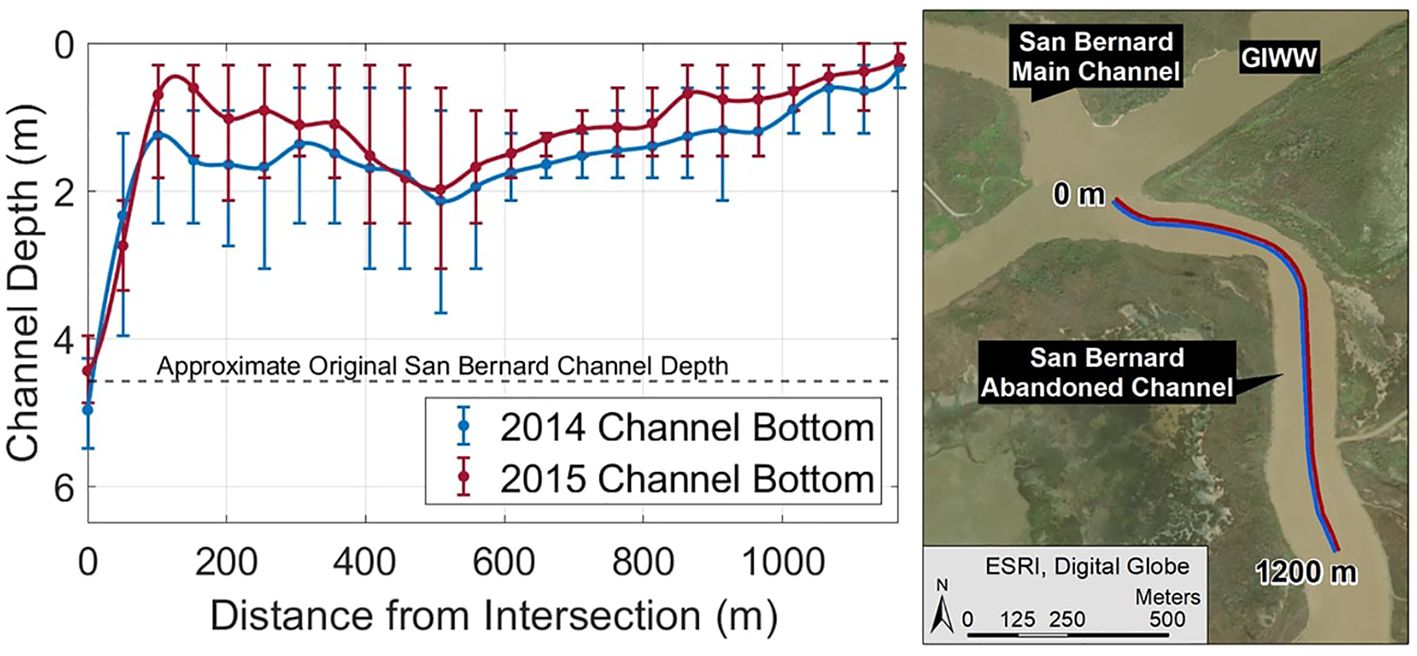
Figure 6. Bathymetric transects of the terminal limb of the San Bernard River in 2014 (blue) and 2015 (red) show rapid accumulation of sediments throughout, suggesting reduced riverine flow promoting sediment deposition. The solid line indicates the channel depth averaged across the cross-section of the channel and the bars indicate the minima and maxima of the cross-section.
3.3 Re-opening of the San Bernard River mouth
After this phase of steering and tapering, the San Bernard River mouth became completely disconnected from the Gulf of Mexico. In 2009, a dredging project briefly re-opened the mouth of the San Bernard River at its original position, to a width of 150 m (Figure 4). By 2012, however, a spit had re-grown on the eastern edge of the San Bernard River mouth and closed the channel once again by 2014, decreasing the width of the channel mouth at a rate of approximately 30 m per year (Figure 5). Flooding during Hurricane Harvey in August 2017 briefly re-opened the San Bernard River mouth to a width of ~37 m. This opening remained narrow, less than ~55 m, until 2021 when a new dredging project opened the mouth once again to ~100 m. By the summer of 2022, this new dredged channel mouth opening had been shut once again after the same spit accretion process occurred on the eastern edge of the mouth. Since 2010, the mouth of the San Bernard River has remained approximately in the same position, being periodically opened and closed by dredging projects and major storms. The geomorphic features of the channel mouth continue to be dynamic.
3.3.1 Dredging project in 2009
A $2.4 million dredging project in 2010 removed >250,000 m3 of material from the San Bernard River mouth (Edwards, 2013), but within 4 years the channel mouth was clogged once again. By 2011 beach sediments were reworked by wave action to form an elongated spit on the eastern flank of the new channel mouth. A spit formed and grew on the east side of the channel mouth, narrowing and steering the channel clockwise until it was once again closed (Figure 7). The dredged river mouth was closed by 2014 as a result of the same coastal processes that led to its initial closure in the late 1990s: a) accretion of a spit on the eastern flank by wave-driven transport of beach sediments, b) resultant steering of the San Bernard River channel down-drift of its dredged position, and c) tapering and closing of the river mouth.
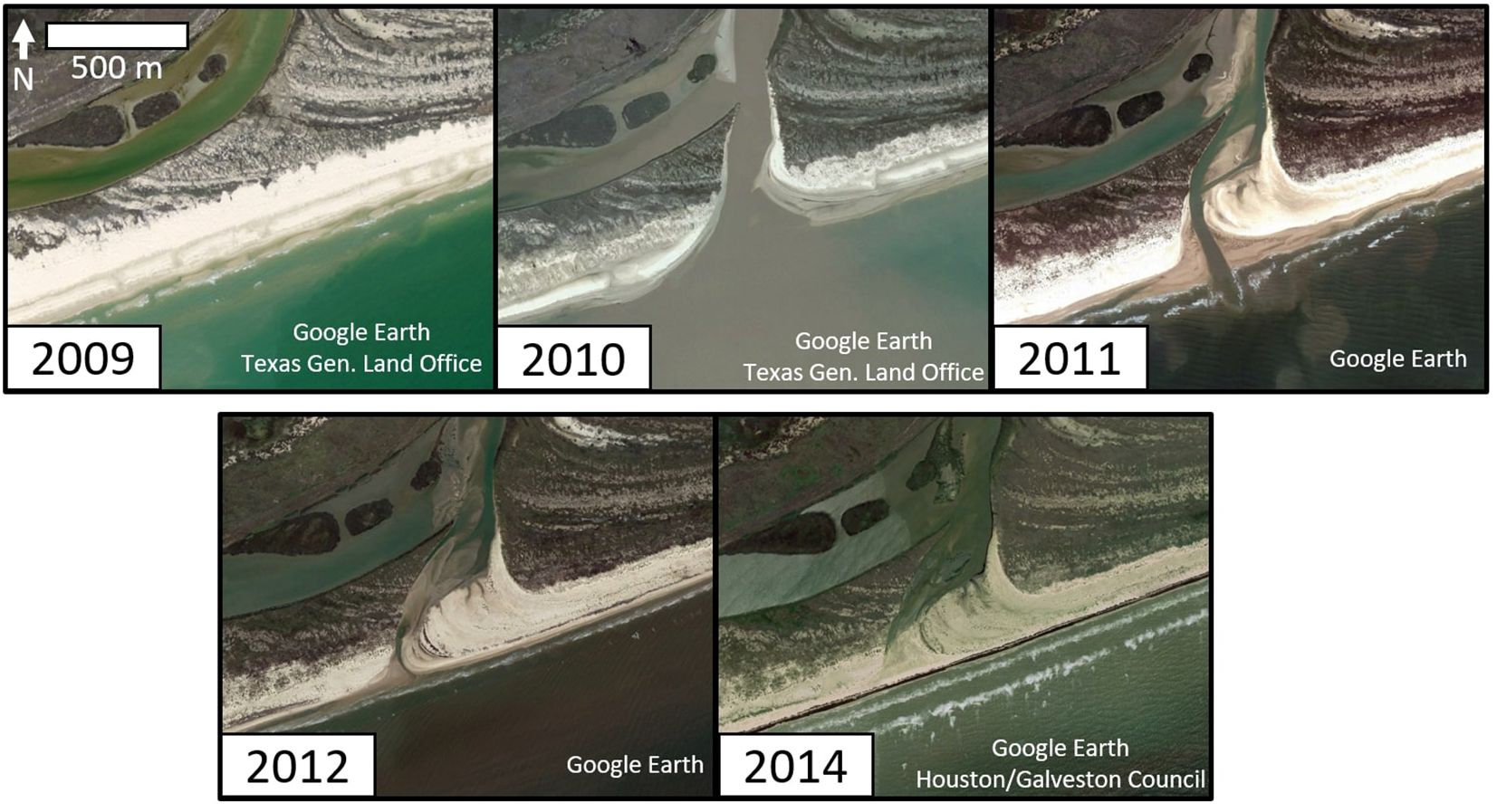
Figure 7. Series of aerial images that document the re-growth of the spit on the east flank of the San Bernard River mouth after being dredged open in 2009.
3.3.2 Hurricane Harvey impacts
The landfall of Hurricane Harvey in late August of 2017 was an extreme example of how the area responds to major flooding events. To better understand the dynamics of the area during these flooding episodes, aerial imagery taken shortly after Harvey helped reveal sediment and flow pathways around the San Bernard-Brazos River system under extreme conditions. The San Bernard River flooded to the highest stage ever recorded on the river, over 6 m higher than the next closest flooding event (Supplementary Figures S1, S2). If the San Bernard River were to ever gain enough erosive ability to cut through the sediment clogging its mouth, its strongest chance may have been during Hurricane Harvey.
Aerial images taken in the months after the hurricane reveal a brief breakthrough of the beach/dune complex at the San Bernard River mouth due to the erosive ability of the floodwaters (Figure 8). The flooding breached the ridges of the clogged river mouth at the location of the former natural and dredged channel mouths. The open channel has remained shallow and highly dynamic, with shoals and spits evolving on either side of the opening. A channel mouth bar on the eastern (up-drift) flank of the river mouth had formed by December, and by March a similar bar formed on the western side. Spits on either side of the river mouth were highly dynamic, with size and symmetry varying according to the most recent transport mechanisms (tidal flushing during fair weather, wave transport during energetic periods) (Figure 8). By the fall of 2019, an elongated spit on the eastern flank of the mouth had begun to steer the San Bernard River channel to the southwest, tapering and effectively closing the channel once again.
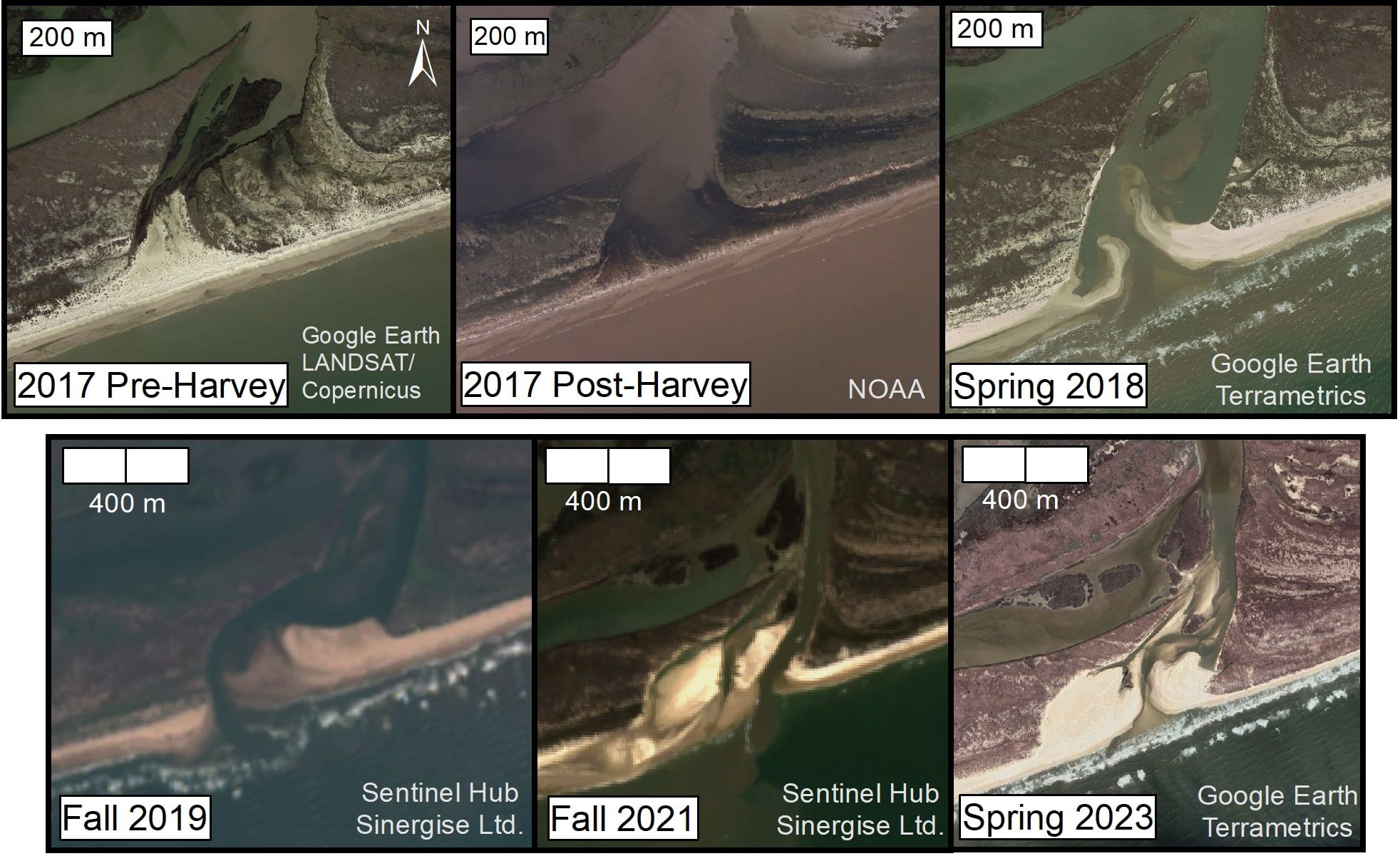
Figure 8. A series of aerial images show the brief breakthrough of the San Bernard River mouth after Hurricane Harvey flooding followed by formation of channel mouth bars and shallowing.
4 Discussion
4.1 Initial sediment response to the Brazos River Channel diversion
For the majority of the history of the Brazos River delta, the dominant mechanism of its evolution has been wave-driven transport of sediments. Only once in its history has the Brazos River delta (in both the old and present-day position) shown characteristics of river dominance. Between 1929 and 1948, the delta rapidly grew to a position approximately 4.31 km offshore of the original shoreline position (Figure 3), with symmetrical growth indicative of a coastal system dominated by riverine sediment delivery rather than erosive wave action (Nienhuis et al., 2015; Bosboom and Stive, 2021). Furthermore, this phase of growth did not show architectural signs of flood-associated pulses of sediment delivery, such as the rhythmic beach-lagoon complexes that developed during major floods in the 1990s (Rodriguez et al., 2000). Since 1948, the end of the riverine growth phase, the signs of flood-driven delta construction have included the development of a subaerial channel mouth bar that is reworked by wave action until welded to the shoreline, creating pairs of ridges and lagoons (Figure 3A).
If not supplied explicitly by flood events, where did this excess of riverine sediments come from? Documents from the USACE reveal that the 1929 Brazos diversion channel was intentionally dredged to smaller dimensions than that of the natural channel, allowing the Brazos river to scour its own river channel to equilibrium dimensions (Fox, 1931; USACE, 1929). The diversion channel was dredged to a depth of only ~3.81 m, with variable widths ranging from ~76 to ~121 m. Since then, the river channel has widened to approximately 180 m during normal flow conditions, with channel depths approaching and sometimes exceeding ~6 m (Fields et al., 1988; Morton and Pieper, 1975). Thus, the excess riverine sediments were sourced by the newly diverted river channel adjusting to its channel. Scouring of the bed and widening of the banks provided an ample, but finite, volume of sediments for delta construction. When the channel reached a state of equilibrium (~1948), the supply of riverine sediments waned, leading the delta to transition back to wave dominance (Mathewson and Minter, 1981). Designers of the project expected that the alterations to the river would change its hydrodynamics eventually, but were mostly concerned about the river re-establishing sinuosity and potential impacts on the upstream extent of tidal influence rather than sediment transport fields (Fox, 1931).
4.2 Morphological shifts of the Brazos River Delta contributed to the closure of the San Bernard River
The new Brazos River delta underwent several distinct phases of growth (Figure 4) dictated by ever-changing conditions of riverine sediment discharge and wave-driven transport of sediments (Seelig and Sorensen, 1973; Fields et al., 1988; Rodriguez et al., 2000; Carlin and Dellapenna, 2014). In the absence of major tidal forces, the plan-form shape of river deltas reveals a balance between sediment supply and the wave climate (Bosboom and Stive, 2021). This delta shape can be described by a fluvial dominance ratio, a relationship between the river sand flux (Qr) and the maximum wave-driven transport of sandy sediments at the river mouth (Qw; Nienhuis et al., 2015), with asymmetry driven by a shore-oblique wave climate (Li et al., 2015). When Qr exceeds Qw, a delta can be considered river-dominated, with its plan-view form characterized by large, rounded lobes protruding seaward often paired with a distributary channel network. If Qw exceeds Qr the delta can be considered wave-dominated, with large-scale arcuate (shaped like a bow) or cuspate (a pointed end where two curves meet) shapes, beach ridge complexes, and extensive barrier systems (Ashton and Giosan, 2011; Nienhuis et al., 2015).
Growth of the present-day Brazos River Delta began in 1929. The delta was river dominated, rapidly growing seaward with symmetrical lobes flanking the main river channel (Figure 4A). After achieving maximum growth in 1948, waves began to erode the protruding lobes of the delta, redistributing sediments primarily to the west flank while the delta flattened (Figure 4B). This shift to wave dominance was related to the decrease in riverine sediment supply, Qr, as the Brazos River channel had fully adjusted to its diversion (Figure 4B). By 1975, the main flanks of the delta were stable and have mostly maintained their size and shape to the present day (Figure 4D), implying equilibrium between Qr and Qw.
From 1975 onward, wave-driven transport of beach sediments has been the dominant mechanism of delta growth. Delta sands were transported directly into the mouth of the San Bernard River, manifesting as a spit that grew rapidly southwestward, contributing to the steering, tapering, and eventual closure of the river mouth (Figure 3A). River delta morphology is strongly linked with the predominant angle of wave approach, with the optimal angle for sediment transport occurring when the waves approach the shoreline at an angle of ~45° (Ashton and Giosan, 2011; Nienhuis et al., 2016). The self-optimization of wave-driven sediment transport may have occurred in 1975, accelerating the transport of sediments down-drift towards the still-open San Bernard river mouth. This rapid southwestward growth led to the steering and closure of the San Bernard River mouth, and influenced accretion/erosion dynamics downdrift (Paine et al., 2017).
4.3 Why did the San Bernard River mouth close completely?
It is not uncommon for coastal river discharge to “compete” with strong wave-driven transport of beach sediments at the river mouth. Nienhuis et al. (2016) suggest that channels discharging onto wave-dominated coasts migrate down-drift when there is a) significant littoral transport and b) bypassing of sediments across the river mouth is limited. Typically, rivers will steer alongshore until the river outlet has sufficient discharge to maintain a permanent river mouth (Nienhuis et al., 2015).
However, the San Bernard River lacks the discharge required to maintain its own river mouth given the excess supply of beach sediments from the Brazos river delta. At a gage station upstream of its intersection with the GIWW the average daily discharge of the San Bernard River is approximately 16 m3/s, ~13 times less than that of the Brazos River (218 m3/s; Yao et al., 2022). Furthermore, the average annual total suspended sediment load for the San Bernard River (0.04 million tons; MT) is approximately 150 times less than that of the Brazos River (6.3 MT; Yao et al., 2022). Sediment discharge from the San Bernard River is primarily driven by storm events and is insufficient to build permanent delta features at its mouth. The Brazos River, in contrast, discharges sediments year-round in addition to major storm events (Rodriguez et al., 2000; Yao et al., 2022), making it the primary supply of sediment controlling the morphology of the San Bernard River mouth. This contrast in discharge between the two rivers was exacerbated by the construction of the GIWW. At its intersection with the GIWW, flow from the San Bernard River can be routed through three distributary channels, the original river channel and/or in either direction along the GIWW (Figure 5). Thus, the construction of the GIWW artificially bifurcated the San Bernard River, routing flow through a distributary network rather than the single, natural channel. Rapid sedimentation in the inactive channel of the San Bernard River is likely related to this reduction in water flux downstream of the intersection with the GIWW (Figure 6). Shallowing of the San Bernard River channel downstream of the intersection may further divert river flow down the GIWW rather than towards the sea, promoting further deposition of sediments in the abandoned channel. The process of channel infilling coincided with changes to the plan-form geometry of the San Bernard River channel. The mouth of the San Bernard channel widened considerably, up to ~300 m in the late 1980s, before tapering shut over the next decade. Widening of river channels is commonly driven by more bed sediment entering a channel section than leaving it (Kim et al., 2006; Madej et al., 2009; Madej and Ozaki, 1996; Smith and Smith, 1984). This channel widening is associated with enhanced bed deposition and shallowing. In the case of the San Bernard River mouth, this sediment convergence was driven by a reduction in river discharge (Figure 5) and accretion of beach sediments on the seaward side of the river mouth, lowering the probability of the San Bernard River naturally reconnecting with the sea.
Similar morphodynamic processes have been observed in the absence of major engineering projects on the wave-dominated coast of North Canterbury, New Zealand. On the North Canterbury Bight, a coastline characterized by coarse sediments and a strong wave climate, river mouths are impounded by elongated spits controlled by alongshore drift processes, creating lagoon systems known as ‘hapua’ (Paterson et al., 2001; Measures et al., 2020). Typically, river mouth channels are steered parallel to the coastline in the direction of littoral drift (Paterson et al., 2001), leading to an offset between the main river channel and mouth (Hart, 2009). Akin to the San Bernard River mouth, the Waimakariri river mouth channel was silted shut and enhanced backwater flooding motivated a successful dredging effort in 1930 (Boyle, 2011). Major flood events have been observed to increase lagoon erosion and potentially breach the river mouth bar, providing the river with an outlet to the sea (Measures et al., 2020; Paterson et al., 2001).
However, the proximity of the San Bernard River to the Brazos River delta along with the bifurcation of its channel by the GIWW provide both an excess of littoral sediments to accrete at the river mouth and an artificial pathway for San Bernard River flow. These unique circumstances have led to the San Bernard River losing its connection with the sea entirely, contrary to the natural mechanisms by which a river mouth can “survive” in a wave dominated coast.
4.4 Fate of the San Bernard River
If the San Bernard River is ever to be restored to its natural state, ambitious and costly engineering projects are required. The two forces working against the San Bernard River - flow down the GIWW instead of the main channel and Brazos sediment shoaling at the river mouth- must be addressed. As shown by the quick failure of the 2009 San Bernard River dredging project (Figure 8), the longshore processes that transport Brazos River sediment towards the mouth must be blocked by engineered structures or frequent maintenance dredging must be done in order to keep the mouth open. However, the diversion of flow at the intersection with the GIWW will continue to reduce the flow volume and velocity down the terminal stretch of the San Bernard River, leading to continued sedimentation.
Since 2018, the governing institutions associated with the San Bernard River have been working toward achieving a long-term solution, garnering strong public support. Beginning in July 2021 and completed in the spring of 2022, the “Mouth of the San Bernard River Restoration Project” was intended to permanently widen and deepen the San Bernard River mouth channel, enhancing the rivers connection to the Gulf of Mexico. Material dredged in the abandoned channel was to be used to replenish marsh habitat in the San Bernard Wildlife Refuge nearby (NOAA, 2021). Immediate benefits were argued to include the reduction of flood hazard created by the backwater effect of the silted river mouth, calming of currents in the GIWW inhibiting barge traffic, and reduced sedimentation in the GIWW.
The proposed project was more substantial than the 2009 effort and suggested a dredge that created a channel of 100 m width at the channel surface and 3 m depth stretching > 5 km into the Gulf of Mexico (USACE, 2018; NOAA, 2021). In contrast to the dredging efforts of 2009, maintenance dredging will be performed every 3 – 7 years by the USACE to keep the river mouth free from excess sediment. Total cost estimates for the initial are around $10.7 million, with federal grant money being the source of funding. The Port of Freeport, and Brazoria County have agreed to split the cost of maintenance dredging, which is estimated to cost $2 million every 3-7 years (Callahan, 2016).
By October 2022 sedimentation had already made the outlet impassable to boat traffic as a result of low discharge and sedimentation over the previous summer (Holle, 2022). Sediment buildup at the river mouth can be expected to continue as the long and shallow channel continues to display the tendency to close (Kraus and Lin, 2002). This highlights the necessity of consistent maintenance dredging and shows that a “rigid coastline” approach is inherently at odds with the now-linked coastal system that is naturally mobile (Moodie and Nittrouer, 2021; Elsey-Quirk et al., 2019). Figure 9 depicts a timeline of the major events that led to the closure of the San Bernard River, highlighting the relatively slow but inevitable movement of sediments as the river systems became linked. As emphasized by Passalacqua et al. (2021), the sustainability of coastal systems hinges on the mobility of their channel networks and landforms, which creates challenges for coastal engineers tasked with managing these systems.
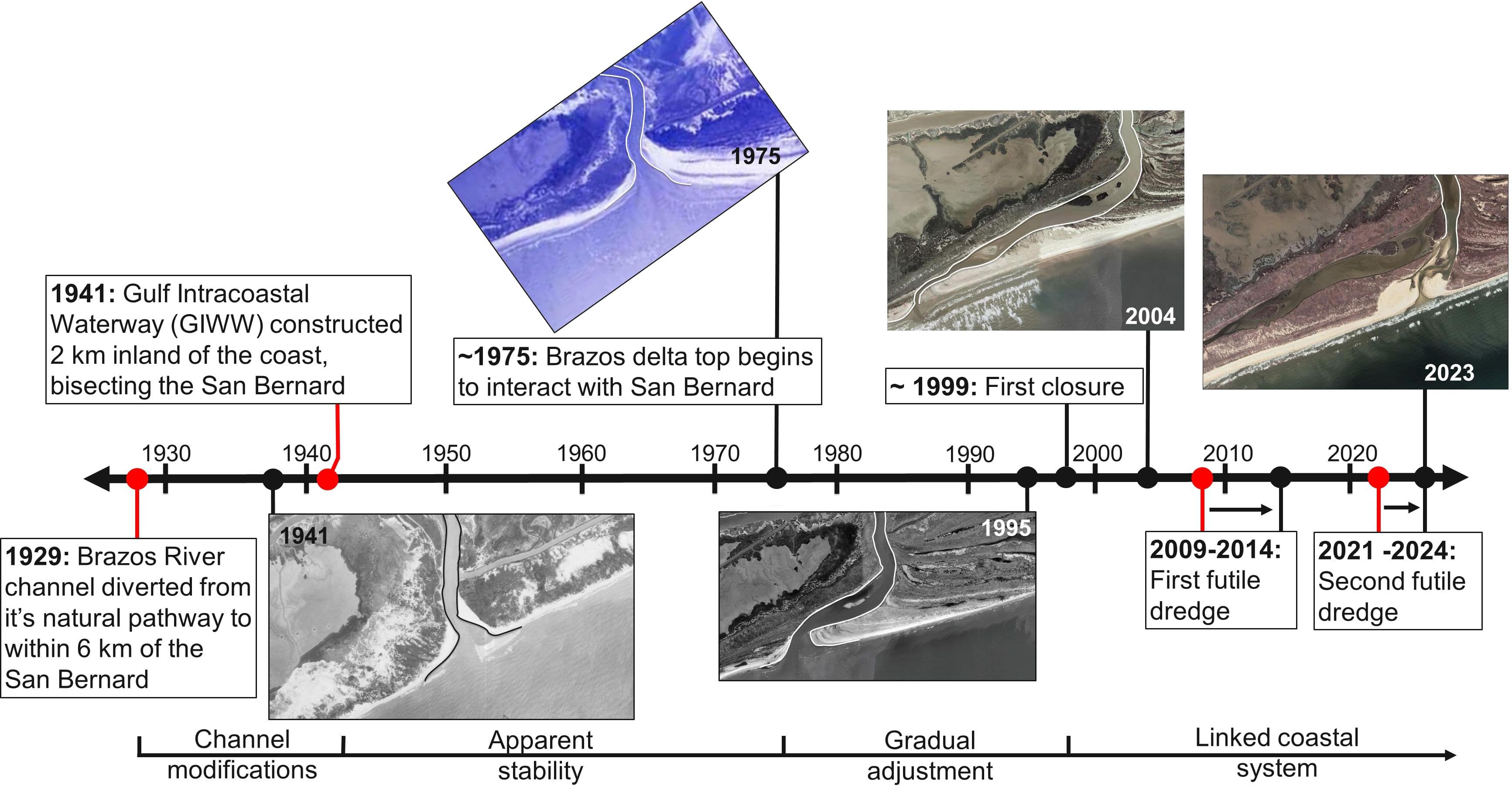
Figure 9. Annotated timeline that shows the key anthropogenic and geomorphic events that led to the coupling of the San Bernard and Brazos coastal river systems.
5 Conclusions
In this study, we focused on the unintended coupling of the San Bernard and Brazos coastal river systems in Texas, USA to provide a detailed example that engineering for rigidity and short-term benefits can lead to delayed geomorphic hazards. The closure of the natural pathway of the San Bernard River has had negative effects on barge traffic, estuarine ecology, and flooding hazards. A unique combination of coastal engineering projects - channel diversion and construction of a major barge canal - led to the San Bernard River mouth being clogged with sediments decades after the completion of the project.
Two formerly independent river systems became linked into one fluvial-coastal-engineered system after several decades of adjustment. A hydrodynamic equilibrium was achieved relatively quickly after the completion of the project, but the sediment transport field took decades to reach a new equilibrium. Simple estimates of the timeframes of sediment fluxes highlight this. The Brazos River channel was diverted by the USACE a distance of ~10 km in the course of 1 year. Then, the new delta top grew laterally 5 km over the next ~50 years (1929 to ~1975) until it began to interact with the mouth of the San Bernard River. When expressed as a linear rate of change, the channel modification (10 km/yr) was two full orders of magnitude greater than that of the resultant geomorphic adjustment (100 m/yr).
The difference in timescale between the hydrodynamic change and the resultant sediment transport response is considerable and can lead to unforeseen and problematic coastal change. One of the key challenges faced by coastal decision-makers is ensuring stability of built-structures in a coastal landscape that is geomorphically dynamic, but slow-moving. Understanding the interplay between coastal hydrodynamics, sediment transport, and human infrastructure can help guide future efforts to create sustainable coastal systems.
Data availability statement
The datasets presented in this study can be found in online repositories. The names of the repository/repositories and accession number(s) can be found below: Data files, aerial images, and shapefiles are publicly available and stored digitally at the Texas Data Repository (doi:10.18738/T8/INCGRW).
Author contributions
JM: Conceptualization, Data curation, Formal analysis, Investigation, Methodology, Visualization, Writing – original draft, Writing – review & editing. DM: Conceptualization, Funding acquisition, Investigation, Methodology, Project administration, Supervision, Writing – review & editing.
Funding
The author(s) declare that financial support was received for the research, authorship, and/or publication of this article. This research project was funded using Faculty Research Scientist Appointment funds (19-7958-2681) provided by the Jackson Endowment at the University of Texas at Austin.
Acknowledgments
We sincerely thank Jasmine Mason and Brandon Minton for their assistance with field data collection and logistics. We thank Jim Buttles for his help in cleaning the flow-field data. We also thank the Jackson School of Geosciences for use of their vehicles and lab space to conduct this research. Lastly, we thank Emily Eidam and the UNC coastal sediments lab group for their feedback on figures and presentations. I would also like to thank co-author DM for his continuous support and enthusiasm throughout the research process.
Conflict of interest
The authors declare that the research was conducted in the absence of any commercial or financial relationships that could be construed as a potential conflict of interest.
Publisher’s note
All claims expressed in this article are solely those of the authors and do not necessarily represent those of their affiliated organizations, or those of the publisher, the editors and the reviewers. Any product that may be evaluated in this article, or claim that may be made by its manufacturer, is not guaranteed or endorsed by the publisher.
Supplementary material
The Supplementary Material for this article can be found online at: https://www.frontiersin.org/articles/10.3389/fmars.2024.1492435/full#supplementary-material
References
Ashton A. D., Giosan L. (2011). Wave-angle control of delta evolution. Geophysical Res. Lett. 38 (13). doi: 10.1029/2011GL047630
Barnard P. L., Revell D. L., Hoover D., Warrick J., Brocatus J., Draut A. E., et al. (2009). Coastal processes study of Santa Barbara and Ventura counties, California. Geological Survey Open-File Report 2009-1029. Reston, VA: US Geological Survey, 904 p.
Blake E. S., Zelinsky D. A. (2018). National Hurricane center tropical cyclone report: Hurricane Harvey (Miami, FL: National Hurricane Center, National Oceanographic and Atmospheric Association).
Boesch D. F. (2020). “Managing risks in Louisiana’s rapidly changing coastal zone,” in Louisiana's response to extreme weather: a coastal state's adaptation challenges and successes (Cham, CH: Springer Nature), 35–62.
Bosboom J., Stive M. J. F. (2021). Coastal Dynamics (Delft, NL: TU Delft OPEN Publishing). doi: 10.5074/T.2021.001
Boyle T. (2011). An investigation into the southward migration of the Waimakariri River mouth (Christchurch, NZ: Environment Canterbury).
Callahan E. (2016). Support flows in for San Bernard (Clute, TX: The Facts). Available at: https://thefacts.com/news/article_5a6295a2-422d-510d-a3af-68bf3f1e95a4.html.
Carlin J. A., Dellapenna T. M. (2014). Event-driven deltaic sedimentation on a low-gradient, low-energy shelf: The Brazos River subaqueous delta, northwestern Gulf of Mexico. Mar. Geol. 353, 21–30. doi: 10.1016/j.margeo.2014.03.017
Coastal Protection and Restoration Authority of Louisiana (CPRAL) (2023). Louisiana comprehensive master plan for a sustainable coast (Baton Rouge, LA: Coastal Protection and Restoration Authority of Louisiana).
Cornwall W. (2022). Shelter from the storm. Sci. (New York NY) 378, 698–702. doi: 10.1126/science.adf8148
Davis R. A. Jr., Elko N., Wang P. (2018). Managing the Gulf Coast Using Geology and Engineering (Boulder, CO: Geological Society of America).
Edwards R. (2013). The San Bernard river mouth – one year after opening (Brazoria, TX: Friends of the San Bernard). Available at: https://www.sanbernardriver.com/info.php?pnum=55db9819cad7be.
Elsey-Quirk T., Graham S. A., Mendelssohn I. A., Snedden G., Day J. W., Twilley R. R., et al. (2019). Mississippi river sediment diversions and coastal wetland sustainability: Synthesis of responses to freshwater, sediment, and nutrient inputs. Estuarine Coast. Shelf Sci. 221, 170–183. doi: 10.1016/j.ecss.2019.03.002
Fields M. L., Weishar L. L., Clausner J. E. (1988). Analysis of sediment transport in the Brazos River diversion channel entrance region. Vicksburg, MS: Coastal Engineering and Research Center.
Flatley A., Rutherfurd I. D., Hardie R. (2018). River channel relocation: problems and prospects. Water 10, 1360. doi: 10.3390/w10101360
Fox M. (1931). Improving the Brazos river new outlet relieves freeport harbor of flood menace. Civil Eng. 1, 287–292.
Hamilton P. B., Lin L., Jones S. W. (2021). Investigation for Shoaling Reduction along the Gulf Intracoastal Waterway (GIWW) at Caney Creek (Texas: Sargent).
Hart D. E. (2009). Morphodynamics of non-estuarine rivermouth lagoons on high-energy coasts. J. Coast. Res. 56, 1355–1359.
Holle K. (2022). Silt happens: Drought already causing a return of San Bernard build-up (Clute, TX: The Facts). Available at: https://thefacts.com/news/silt-happens-drought-already-causing-a-return-of-san-bernard-build-up/article_5a6295a2-422d-510d-a3af-68bf3f1e95a4.html.
Kim W., Paola C., Swenson J. B., Voller V. R. (2006). Shoreline response to autogenic processes of sediment storage and release in the fluvial system. J. Geophys. Res. 111, F04013. doi: 10.1029/2006JF000470
Kraus N. C., Lin L. H. (2002). Coastal Processes Study of San Bernard River Mouth, Texas: Stability and Maintenance of Mouth (Vol. 2) (Vicksburg, MS: US Army Corps of Engineers, Engineer Research and Development Center, Coastal and Hydraulics Laboratory).
Li L., Walstra D. J. R., Storms J. E. (2015). The impact of wave-induced longshore transport on a delta–shoreface system. J. Sedimentary Res. 85, 6–20. doi: 10.2110/jsr.2014.95
Madej M. A., Ozaki V. (1996). Channel response to sediment wave propagation and movement, Redwood creek, California, USA. Earth Surface Processes Landforms 21, 911–927. doi: 10.1002/(SICI)1096-9837(199610)21:10<911::AID-ESP621>3.0.CO;2-1
Madej M. A., Sutherland D. G., Lisle T. E., Pryor B. (2009). Channel responses to varying sediment input: A flume experiment modeled after Redwood Creek, California. Geomorphology 103, 507–519. doi: 10.1016/j.geomorph.2008.07.017
Mariotti G., Boswell K. T. (2023). Barge-driven resuspension facilitates sediment bypass in the Gulf Intracoastal Waterway (Louisiana, USA). Coast. Eng. 183, 104326. doi: 10.1016/j.coastaleng.2023.104326
Marsooli R., Lin N., Emanuel K., Feng K. (2019). Climate change exacerbates hurricane flood hazards along US Atlantic and Gulf Coasts in spatially varying patterns. Nat. Commun. 10, 3785. doi: 10.1038/s41467-019-11755-z
Mathewson C. C., Minter L. L. (1981). Impact of water resource development on the hydrology and sedimentology of the Brazos River, Texas with implications on shoreline erosion. Bull. Assoc. Eng. Geologists 18, 39–53. doi: 10.2113/gseegeosci.xviii.1.39
Measures R. J., Hart D. E., Cochrane T. A., Hicks D. M. (2020). Processes controlling river-mouth lagoon dynamics on high-energy mixed sand and gravel coasts. Mar. Geol. 420, 106082. doi: 10.1016/j.margeo.2019.106082
Moodie A. J., Nittrouer J. A. (2021). Optimized river diversion scenarios promote sustainability of urbanized deltas. Proc. Natl. Acad. Sci. 118, e2101649118. doi: 10.1073/pnas.2101649118
Morris M. (2016). OUR VIEWPOINT: River dredging project worth it if it lasts (Clute, TX: The Facts). Available at: https://www.sanbernardriver.com/news_details.php?view=article&ref=archive&month=2&year=2016&id=775.
Morton R. A., Pieper M. J. (1975). Shoreline changes in the vicinity of the Brazos River Delta (San Luis Pass to Brown Cedar Cut): an analysis of historical changes of the Texas Gulf shoreline. The University of Texas at Austin, Bureau of Economic Geology, Geological Circular 75-4D. Austin, TX: Bureau of Economic Geology, 47 p. doi.org/10.23867/gc7504D
National Oceanic and Atmospheric Administration (NOAA) (2021). Mouth of the San Bernard river restoration project (Miami, FL: Gulf Spill Restoration Website). Available at: https://www.gulfspillrestoration.noaa.gov/restoration/give-us-your-ideas/view-submitted-projects.
Ndour A., Laïbi R. A., Sadio M., Degbe C. G., Diaw A. T., Oyédé L. M., et al. (2018). Management strategies for coastal erosion problems in West Africa: analysis, issues, and constraints drawn from the examples of Senegal and Benin. Ocean Coast. Manage. 156, 92–106. doi: 10.1016/j.ocecoaman.2017.09.001
Nicholls R. J., Wong P. P., Burkett V., Codignotto J., Hay J., McLean R., et al. (2007). Coastal systems and low-lying areas. Climate Change 2007: Impacts, Adaptation and Vulnerability. Contribution of Working Group II to the Fourth Assessment Report of the Intergovernmental Panel on Climate Change, Cambridge University Press, Cambridge, UK, 315–356. Available at: https://pubs.usgs.gov/publication/70204340
Nienhuis J. H., Ashton A. D., Giosan L. (2015). What makes a delta wave-dominated? Geology 43, 511–514. doi: 10.1130/G36518.1
Nienhuis J. H., Ashton A. D., Giosan L. (2016). Littoral steering of deltaic channels. Earth Planetary Sci. Lett. 453, 204–214. doi: 10.1016/j.epsl.2016.08.018
Paine J. G., Caudle T. L., Andrews J. R. (2017). Shoreline and sand storage dynamics from annual airborne LIDAR surveys, Texas Gulf Coast. J. Coast. Res. 33, 487–506. doi: 10.2112/JCOASTRES-D-15-00241.1
Palmer T. A., Montagna P. A., Pollack J. B., Kalke R. D., DeYoe H. R. (2011). The role of freshwater inflow in lagoons, rivers, and bays. Hydrobiologia 667, 49–67. doi: 10.1007/s10750-011-0637-0
Paola C., Twilley R. R., Edmonds D. A., Kim W., Mohrig D., Parker G., et al. (2011). Natural processes in delta restoration: Application to the Mississippi Delta. Annu. Rev. Mar. Sci. 3, 67–91. doi: 10.1146/annurev-marine-120709-142856
Passalacqua P., Giosan L., Goodbred S., Overeem I. (2021). Stable≠ sustainable: Delta dynamics versus the human need for stability. Earth's Future 9, e2021EF002121. doi: 10.1029/2021EF002121
Passalacqua P., Moodie A. J. (2022). Delta-scale solutions for human-scale needs. Science 376, 916–917. doi: 10.1126/science.abq1166
Paterson A., Hume T., Healy T. (2001). River mouth morphodynamics on a mixed sand-gravel coast. J. Coast. Res. (34), 288–294. doi: 10.1016/j.margeo.2013.08.001
Reguero B. G., Bresch D. N., Beck M., Calil J., Meliane I. (2014). Coastal risks, nature-based defenses and the economics of adaptation: An application in the Gulf of Mexico, USA. Coast. Eng. Proc. 1, 25. doi: 10.9753/icce.v34.management.25
Rodriguez A. B., Hamilton M. D., Anderson J. B. (2000). Facies and evolution of the modern Brazos Delta, Texas: wave versus flood influence. J. Sedimentary Res. 70, 283–295. doi: 10.1306/2DC40911-0E47-11D7-8643000102C1865D
Roelvink J. A. (2006). Coastal morphodynamic evolution techniques. Coast. Eng. 53, 277–287. doi: 10.1016/j.coastaleng.2005.10.015
Runyan K., Griggs G. (2005). “Implications of harbor dredging for the Santa Barbara Littoral cell,” in California and the World Ocean'02: Revisiting and Revising California's Ocean Agenda. Reston, VA: American Society of Civil Engineers, 121–135.
Sanchez J. A., Parchure T. M., Engineer research and development center vicksburg ms coastal and hydraulicslab (2001). Study of complex flows in the lower San Bernard River, Texas (Vicksburg, MS: ERDC/CHL TR-01-24, US Army Engineer Research and Development Center).
Seelig W. N., Sorensen R. M. (1973). Investigation of shoreline changes at Sargent Beach, Texas. Miami, FL: National Oceanic and Atmospheric Administration.
Smith N. D., Smith D. G. (1984). William River: An outstanding example of channel widening and braiding caused by bed-load addition. Geology 12, 78–82. doi: 10.1130/0091-7613(1984)12<78:WRAOEO>2.0.CO;2
Swarzenski C. M. (2003). Surface-water hydrology of the Gulf Intracoastal Waterway in south-central Louisiana 1996-99 (Reston, VA, USA: US Geological Survey), 51.
Swarzenski C. M., Perrien S. M. (2015). Discharge, suspended sediment, and salinity in the Gulf Intracoastal Waterway and adjacent surface waters in South-Central Louisiana 1997–2008 (No. 2015-5132) (Vicksburg, MS: US Geological Survey).
Texas Department of Transportation (TxDOT) (2016). GIWW Brazos River Floodgates and Colorado River Locks Feasibility Study. Texas Department of Transportation July 2016 Briefing, 1–4. Available online at: https://ftp.dot.state.tx.us/pub/txdot-info/mrt/brazos-colorado-feasibility.pdf.
United States Army Corps of Engineers (USACE) (1929). Report of the chief of engineers U. S. Army 1929 Part I. Annual Reports (War Department).
United States Army Corps of Engineers (USACE) (2018). Public notice permit application No. SWG-2015-00603 - Brazoria County - San Bernard river mouth - Brazoria county (Vicksburg, MS, Texas). Available at: https://www.swg.usace.army.mil/Media/Public-Notices/Article/3584757/swg-2015-00603-brazoria-county-san-bernard-river-mouth-brazoria-county-texas/.
United States Army Corps of Engineers (USACE) (2022). “Gulf Intracoastal Waterway Coastal Resilience Study,” in Texas Draft Integrated Feasibility Report and Environmental Assessment. Galveston, TX: U.S. Army Corps of Engineers, Galveston District, Southwestern Division.
Keywords: coastal infrastructure, coupled human-natural systems, coastal morphodynamics, hydrodynamics, river deltas, sediment transport
Citation: Malito J and Mohrig D (2024) Unintended consequences of modifying coastal river systems. Front. Mar. Sci. 11:1492435. doi: 10.3389/fmars.2024.1492435
Received: 06 September 2024; Accepted: 30 October 2024;
Published: 19 November 2024.
Edited by:
Haosheng Huang, Louisiana State University, United StatesCopyright © 2024 Malito and Mohrig. This is an open-access article distributed under the terms of the Creative Commons Attribution License (CC BY). The use, distribution or reproduction in other forums is permitted, provided the original author(s) and the copyright owner(s) are credited and that the original publication in this journal is cited, in accordance with accepted academic practice. No use, distribution or reproduction is permitted which does not comply with these terms.
*Correspondence: John Malito, am9obi5tYWxpdG9AYmVnLnV0ZXhhcy5lZHU=