- 1Key Laboratory of Microecological Resources and Utilization in Breeding Industry, Ministry of Agriculture and Rural Affairs, Guangdong Haid Group Co., Ltd., Guangzhou, Guangdong, China
- 2College of Fisheries and Life Science, Shanghai Ocean University, Shanghai, China
The mandarin fish (Siniperca chuatsi) is a typical carnivorous fish, which has been able to consume artificial diets after domestication in recent years. However, the potential health consequences of artificial diets in mandarin fish remain unclear. This study aimed to elucidate the molecular mechanisms underlying these concerns. Fish (initial weight: 25.1 ± 0.1 g) were fed with natural (CON group) or artificial diets (AF group) for 8 weeks. Each diet was randomly distributed to sextuplicate circular tanks (300 L) with 40 fish in each tank. The transcriptome analysis revealed significant changes in metabolism-related pathways, particularly those involved in lipid and carbohydrate metabolism. Further investigation confirmed that the artificial diets significantly increased hepatic triglyceride content and fatty acid synthase activity. The artificial diets also significantly increased hepatic glycogen and glucose-6-phosphatase activity. Furthermore, the artificial diets significantly increased hepatic malondialdehyde levels, indicating increased oxidative stress. Antioxidant defense enzyme activities and the expression of antioxidant stress-related genes were significantly decreased. Additionally, the artificial diets significantly increased the expression of proinflammatory genes, including interleukin 1 beta and interferon-gamma. These findings collectively demonstrated that the artificial diets disrupted hepatic lipid and glucose metabolism, leading to oxidative stress and inflammation, thus affecting the health status of mandarin fish.
Introduction
Mandarin fish (Siniperca chuatsi) is highly sought after for its delicious taste and rich nutritional value (Song et al., 2017; Li et al., 2019). With a thriving market and annual farming production exceeding 400,000 tons in China (2022), mandarin fish aquaculture significantly contributes to the country’s food security (Li et al., 2017). Mandarin fish have a very peculiar feeding habit, as they only accept live prey fish and refuse dead prey fish or artificial feed in the wild (Yi et al., 2013). Traditionally, mandarin fish have been fed with live prey fish. However, this method presents several challenges. The supply of live prey fish is unreliable, and the prey fish might be a source of pathogens or parasites (Tao et al., 2007), potentially jeopardizing the health of farmed fish. These limitations hinder the sustainable development of mandarin fish aquaculture. Exploring nutrient metabolism and related signaling pathways is essential in order to achieve precise nutrient regulation and meet the demand for fish health. Meanwhile, the level of domestication of mandarin fish in the juvenile stage has been greatly improved (Liang et al., 2001). Therefore, mandarin fish can be farmed with an artificial diet in recent years (Li et al., 2015). According to incomplete statistics, approximately 1/10 of mandarin fish in China have been fed with artificial diets. However, studies into the precise feed nutrition of mandarin fish have just started, and there are great differences in the nutritional composition of artificial diets from different companies. Moreover, concerns have emerged regarding its potential impact on fish health and potential farming failures during the feeding process.
The liver plays a central role in regulating lipid and glucose metabolism throughout the body (Watt et al., 2019). In mammals, metabolic imbalances leading to abnormal accumulation of lipids and glycogen in the liver can trigger the production of proinflammation cytokines, ultimately contributing to chronic liver inflammation (Badmus et al., 2022). Similar observations have been made in several fish species, such as large yellow croaker (Larimichthys crocea) (Fang et al., 2021), blunt snout bream (Megalobrama amblycephala) (Dai et al., 2019), spotted seabass (Lateolabrax maculatus) (Guo et al., 2023), and largemouth bass (Micropterus salmoides) (Zhao et al., 2024). In these cases, unsuitable artificial diets disrupt hepatic metabolism and induce oxidative stress and inflammation. However, the specific molecular mechanisms by which artificial diets affect mandarin fish remain unclear.
Therefore, this study aimed to investigate the effects and the underlying mechanisms of artificial and natural diets on the metabolism and immune response in the liver of mandarin fish. By designing an artificial diet formulated to completely replace live prey fish, we sought to establish a theoretical basis for optimizing mandarin fish feeds.
Materials and methods
Animals and diets
Four hundred eighty healthy and similar-sized mandarin fish juveniles (25.1 ± 0.1 g) were bought from Guangdong Bairong Aquatic Varieties Group Co., Ltd., and were divided into two groups. The control group (CON) was kept on live prey fish (Table 1), whereas the artificial diet group (AF) was kept on an artificial diet (Table 2), according to a previous study in our lab (Fang et al., 2024). White fish meal and Antarctic krill powder were the major protein sources. Fish oil was the major lipid source in the feed. All fish were fed twice daily for 8 weeks (at 6:00 a.m. and 6:00 p.m.). Each feeding session was limited to 30 min.
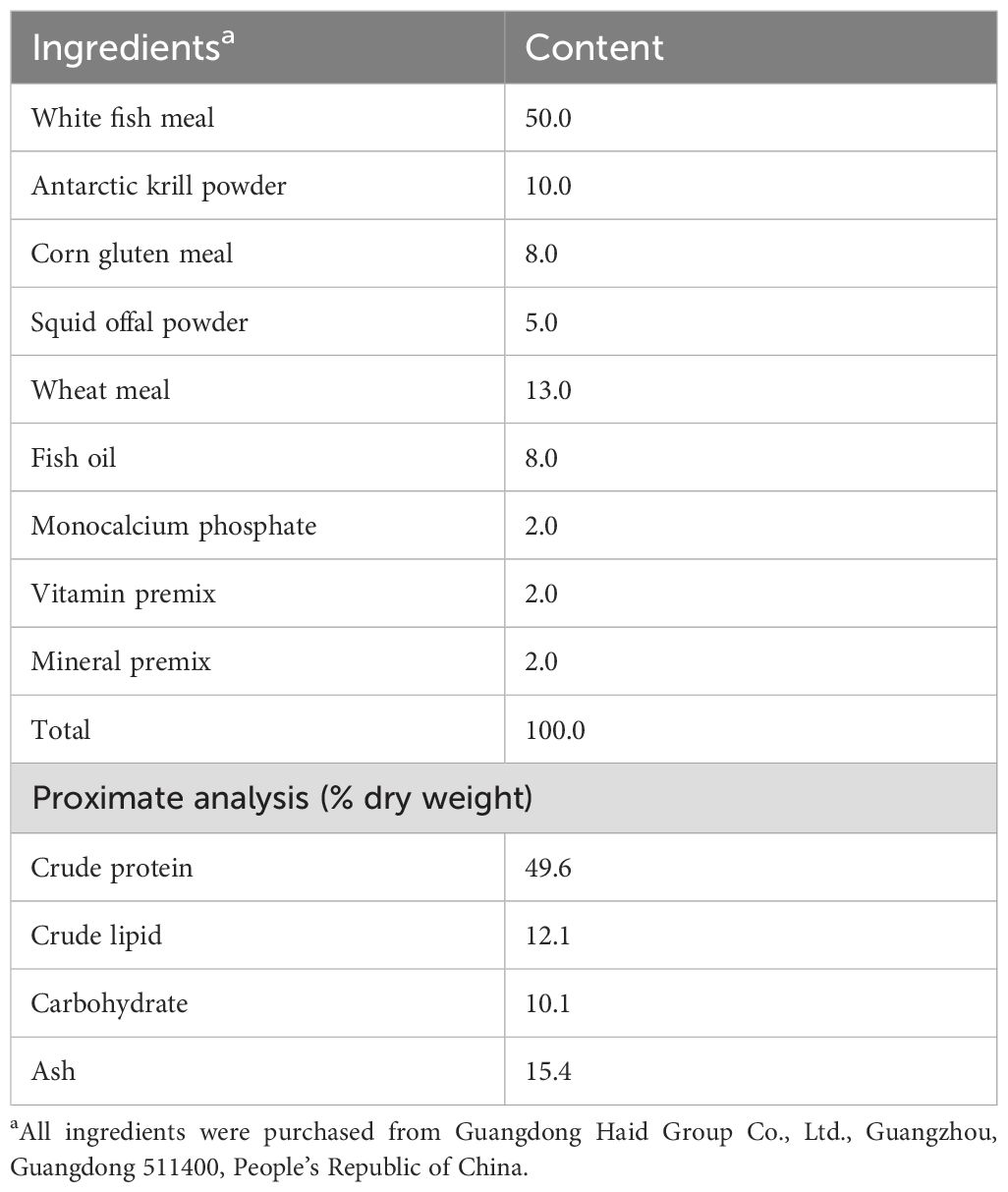
Table 2. Formulation and chemical proximate analysis of the experimental artificial feed (% dry weight).
Histological analysis
The fixed liver samples were soaked twice with distilled water. The samples were then dehydrated with xylene and 50%–100% ethanol and treated transparently with xylene. The transparent samples were embedded in molten paraffin and solidified into blocks. The paraffin was cut into 5 μm flakes using a slicer (Leica, Germany). Hematoxylin–eosin (H&E) staining was subsequently performed on the sections using an automatic staining instrument (Thermo Fisher Scientific, USA). Finally, the stained sections were examined under a light microscope (Nikon, Japan).
Biochemical analysis
The hepatic triglyceride (TG) and glycogen levels and antioxidant capacity were determined by commercially available kits, according to our previous study (Fang et al., 2024).
Gene expression
The total RNA of frozen liver issues was extracted with TRIzol reagent (TaKaRa, Japan). Then, cDNA was synthesized using RNA as a template using the PrimerScript™ RT reagent kit (TaKaRa, Japan). Primers for the target genes and the control gene beta-actin (β-actin) were designed with the NCBI Primer-BLAST, which are listed in Table 3. The gene levels were determined by RT-qPCR and calculated by the 2−△△CT method (Livak and Schmittgen, 2001).
Transcriptome sequencing and analysis
RNA-seq was performed by Majorbio Co., Ltd. (Shanghai, China). The differentially expressed genes (DEGs) were defined as P < 0.05. Furthermore, scatter plots, heatmap sequencing, etc. were processed and analyzed by an online software (www.majorbio.com).
Statistical methods
All experimental data were expressed as mean ± S.E.M. The significance of the difference between the two groups was analyzed using the independent t-test (P < 0.05) in SPSS 19.0 (IBM, USA).
Results
Effects of artificial diets on hepatic gene expression profiling
Transcriptome analysis was employed to identify DEGs. Compared with fish fed with the control diet, the livers of fish fed with an artificial diet exhibited 1,597 upregulated and 1,271 downregulated DEGs (Figure 1A). A heatmap was generated to visualize the expression patterns of these DEGs, with distinct subgroups identified based on expression levels (Figure 1B). GO enrichment indicated that most DEGs were associated with metabolic processes, including the cholesterol biosynthetic process and the lipid biosynthetic process (Figure 1C). Similarly, analysis of biological processes indicated significant enrichment for DEGs involved in cellular processes, biological regulation, and metabolism (Figure 1D). According to the KEGG enrichment analysis, a significant enrichment of DEGs within metabolism-related pathways was identified (Figure 1E). Notably, pathways associated with lipid and carbohydrate metabolism were significantly enriched (Figure 1F).
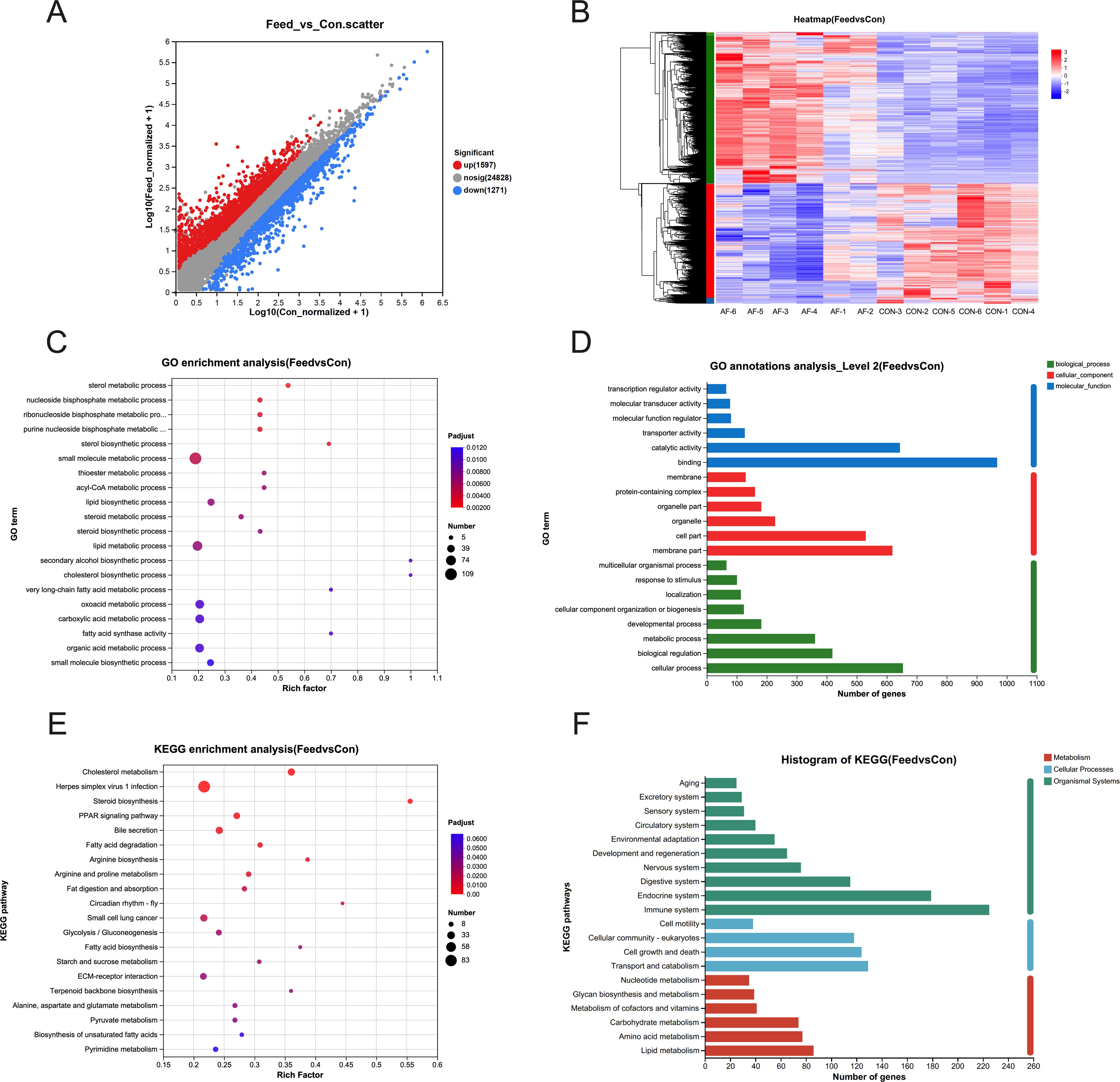
Figure 1. Effects of artificial diets on hepatic gene expression profiling. Scatter plot (A), heatmap sequencing (B), GO enrichment analysis (C), GO annotation analysis (D), KEGG enrichment analysis (E), and histogram of KEGG analysis (F) in the liver of mandarin fish after different treatments with different diets (n = 6).
Effects of artificial diets on hepatic lipid metabolism
H&E staining revealed that the vacuoles in the liver of mandarin fish fed with an artificial diet were larger and more numerous than those of the control group (Figure 2A). Meanwhile, the artificial diets significantly increased hepatic TG levels (P < 0.05) (Figure 2B). To confirm the effect, we examined related enzyme activity and gene expression of lipid metabolism. Artificial diets significantly increased the activity of fatty acid synthase (FAS) (P < 0.05) (Figure 2C). However, the mRNA levels of sterol regulatory element binding protein 1c (srebp1c), fas, diacylglycerol O-acyltransferase 2 (dgat2), and perilipin 2 (plin2) were significantly decreased in the fish fed with an artificial diet (P < 0.05) (Figure 2D). The mRNA levels of peroxisome proliferation-activated receptor alpha (pparα) and carnitine palmitoyltransferase 1 alpha (cpt1α) were not remarkably changed between the two groups (P > 0.05) (Figure 2E).
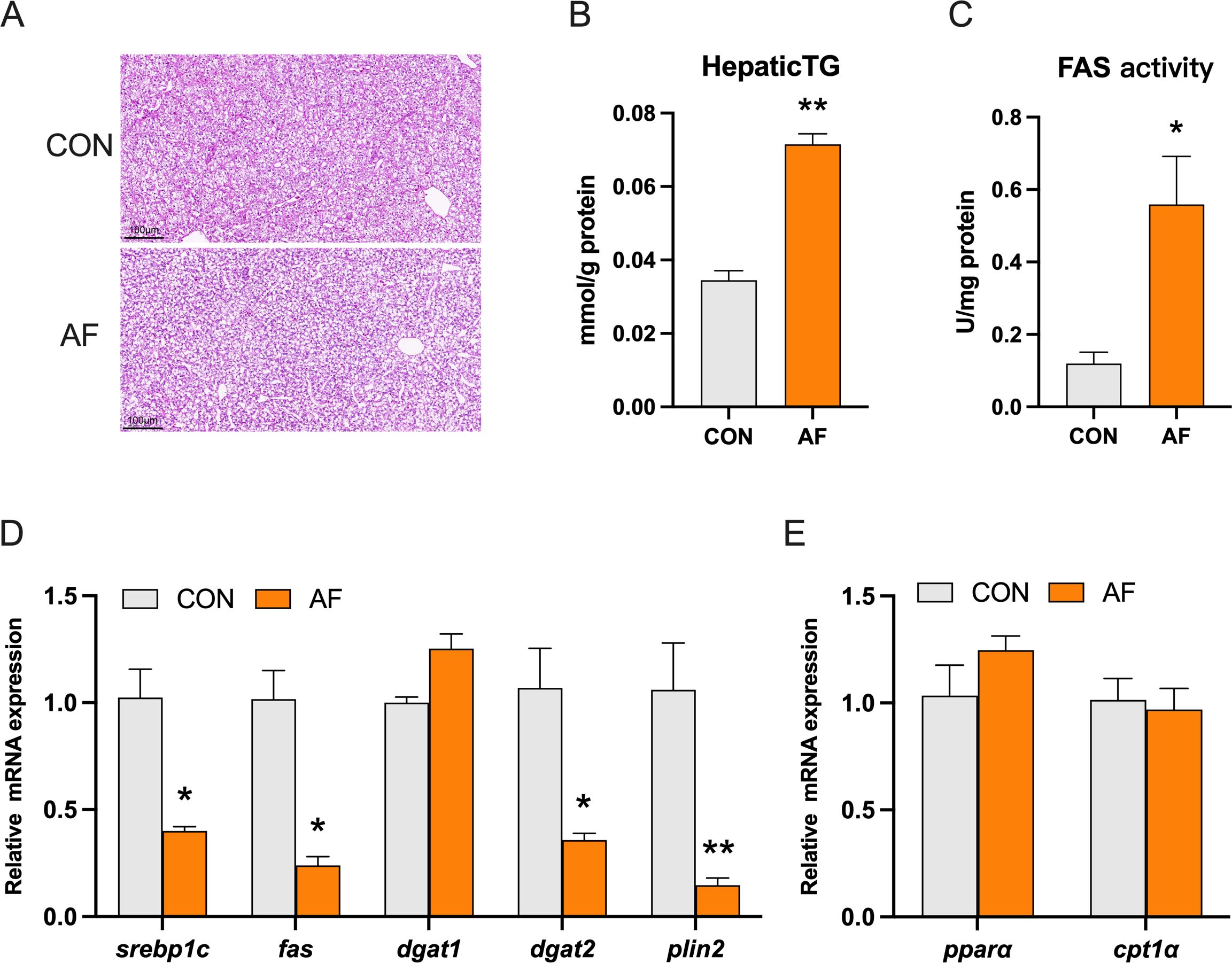
Figure 2. Effects of artificial diets on hepatic lipid metabolism. Hepatic H&E staining image (scale bar: 100 μm) (A), content of hepatic TG (B), and activity of FAS (C) of mandarin fish after different treatments with different diets. Relative mRNA expression of lipid synthesis (D) and fatty acid β-oxidation (E) in the liver of mandarin fish after different treatments with different diets. *P < 0.05, **P < 0.01 (n = 6).
Effects of artificial diets on hepatic glucose metabolism
The content of hepatic glycogen in the AF group was significantly upregulated (P < 0.05) (Figure 3A). The artificial diets significantly enhanced the activities of glucose-6-phosphatase (G6Pase) and pyruvate kinase (PK) (P < 0.05) (Figures 3B, C). Furthermore, the mRNA level of g6pase was significantly lower in the AF group (P < 0.05) (Figure 3D). The artificial diets upregulated the mRNA levels of genes related to glycolysis, including phosphofructokinase (pfk) and pk (P < 0.05) (Figure 3E).
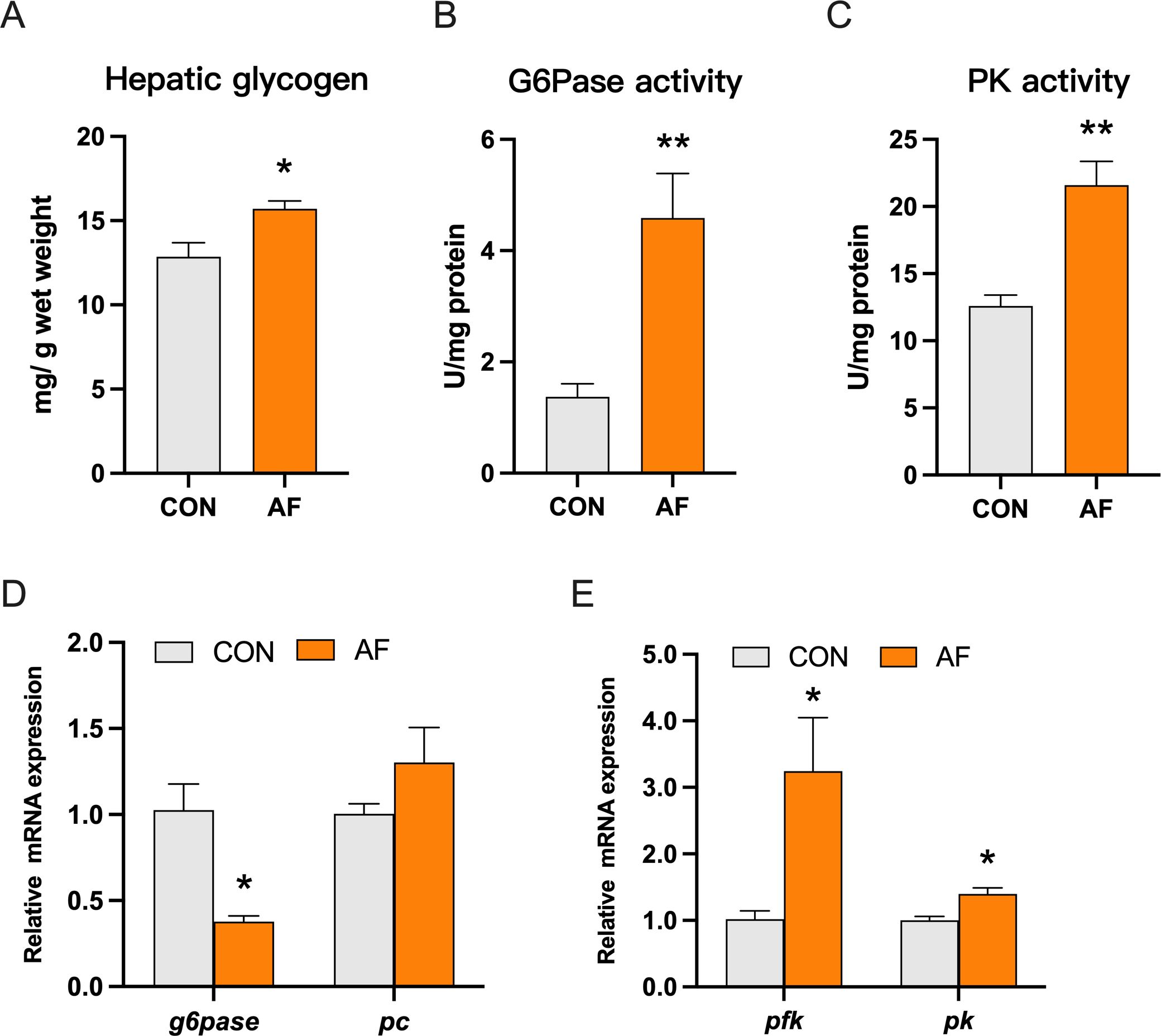
Figure 3. Effects of artificial diets on hepatic glucose metabolism. The content of hepatic glycogen (A) and activities of G6Pase (B) and PK (C) of mandarin fish after different treatments with different diets. Relative mRNA expression of gluconeogenesis (D) and glycolysis (E) in the liver of mandarin fish after different treatments with different diets. *P < 0.05, **P < 0.01 (n = 6).
Effects of artificial diets on hepatic antioxidative capacity and inflammation
Abnormal accumulation of hepatic lipids and glycogen has been previously linked to oxidative stress and inflammation. The levels of total antioxidant capacity (T-AOC) and reduced glutathione (GSH) were significantly decreased in the AF group (P < 0.05) (Figures 4A, B). The activities of superoxide dismutase (SOD) and catalase (CAT) were remarkably decreased in the AF group (P < 0.05) (Figures 4C, D). Moreover, the level of malondialdehyde (MDA) was remarkably increased in the AF group (P < 0.05) (Figure 4E). Furthermore, the expression of genes related to antioxidant stress, including Kelch-like ECH-associated protein 1 (keap1), cat, and glutathione peroxidase (gpx), was significantly decreased (P < 0.05) (Figure 4F). However, there was no significant change in the gene expression of nuclear factor erythroid2‐related factor 2 (nrf2) and sod (P > 0.05). In addition, we examined the mRNA expression of proinflammatory genes. Relative to the control group, the artificial diets significantly increased the gene expression of interleukin 1 beta (il-1β) and interferon-gamma (ifn-γ) (P < 0.05) (Figure 4G).
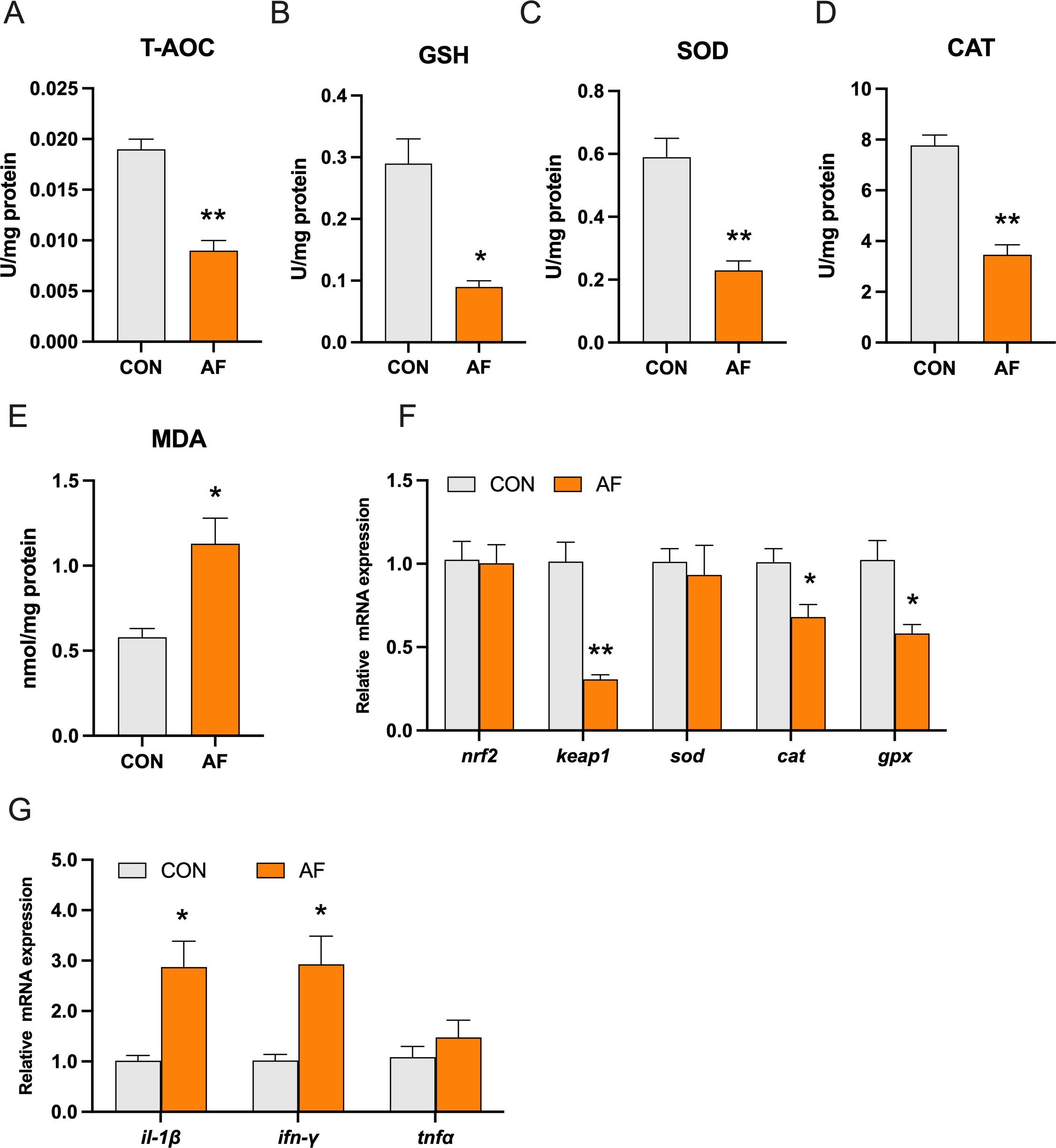
Figure 4. Effects of artificial diets on hepatic antioxidative capacity and inflammation. T-AOC (A), the content of reduced GSH (B), the activities of SOD (C) and CAT (D), the content of MDA (E), relative mRNA expression of antioxidative capacity (F), and inflammation (G) in the liver of mandarin fish after different treatments with different diets. *P < 0.05, **P < 0.01 (n = 6).
Discussion
In the past decade, advancements in mandarin fish feed nutrition research (Li et al., 2023; Xie et al., 2023) and improved domestication techniques for juveniles (Guan et al., 2020; Lu et al., 2023) have opened the door for using artificial diets in mandarin fish aquaculture. However, feeding artificial diets might affect the health status of mandarin fish and increase the probability of farming failure. The underlying mechanisms of these effects required further investigation.
This study therefore employed liver transcriptome analysis of mandarin fish fed with an artificial or natural diet to explore the dietary effects on metabolism and immunity. The transcriptome analysis revealed significant changes in the metabolism-related pathways after feeding different diets, particularly those involving lipid and carbohydrate metabolism. These findings were consistent with a previous study demonstrating altered transcript levels of several genes related to fatty acid biosynthesis pathway in hybrid mandarin fish fed with an artificial diet (Guan et al., 2020). The transcriptome analysis revealed that artificial diets affected the Toll-like receptor signaling pathway and NOD-like receptor signaling pathway. These receptors are responsible for recognizing and initiating the response of the immune system to defend against infectious pathogens (Dasari et al., 2008; Chu and Xu, 2020). Dysregulation of these receptors, along with their downstream signaling pathways, may lead to uncontrolled inflammation or pathogen transmission (Cook et al., 2004). According to the results of transcriptome analysis, in this study we systematically investigated the effects and mechanism of artificial diets on hepatic lipid and glucose metabolism and immunity response in mandarin fish.
In the present study, the artificial diets increased hepatic lipid accumulation by promoting FAS activity, which may be attributed to a potentially higher fat content in the artificial diets than in live prey fish. However, mRNA expression of lipid synthesis was inhibited in fish fed with an artificial diet, suggesting a potential feedback regulatory mechanism to protect against lipotoxicity. The results were similar to our previous study where artificial diets induced excessive TG accumulation in the muscle of mandarin fish (Fang et al., 2024). The regulation of lipid metabolism in an intertissue manner needs further study. In addition, previous studies have demonstrated that unsuitable artificial diets induced hepatic de-novo lipogenesis (Pang et al., 2021) and inhibited lipid secretion (Cao et al., 2019), leading to abnormal lipid accumulation. These results collectively indicated that artificial diets disrupt lipid metabolism in mandarin fish, leading to abnormal lipid accumulation in the liver.
Carbohydrates are an important non-protein energy source that are commonly incorporated into artificial diets (Hatlen et al., 2005). However, the ability of fish to utilize dietary carbohydrates varies, especially in carnivorous species, such as largemouth bass (Liu et al., 2017) and yellowtail kingfish (Seriola lalandi) (Booth et al., 2013). Carnivorous fish are characterized by continuous postprandial hyperglycemia after feeding with a high-carbohydrate diet (Stone, 2003). In the present study, the proportion of carbohydrates in artificial diets is higher than in live prey fish. The artificial diets induced excess glycogen accumulation in the liver by activating the gluconeogenesis pathway. However, the activity of PK and mRNA levels of genes was related to the glycolysis pathway, which might be a feedback regulation of the fish to decrease hepatic glycogen accumulation to some extent. These results indicated that the artificial diets disrupted glucose metabolism in the liver of mandarin fish.
Reactive oxygen species (ROS) are primarily generated in the mitochondria (Thannickal and Fanburg, 2000). While normal levels of ROS play a role in cellular functions (Lee et al., 2012), excessive production of ROS under stress conditions can induce oxidative stress, a highly toxic state for cells (Cichoż-Lach and Michalak, 2014). Previous studies have found that increased levels of hepatic lipid and glycogen will generate more substrates entering mitochondrial respiration, leading to increased production of ROS (Styskal et al., 2012; Sun et al., 2020). In the present study, long-term feeding of artificial diets significantly decreased the transcription level of antioxidative stress-related genes and the enzymatic activities of antioxidant defense systems and increased MDA content in the liver. MDA is an important biomarker of oxidative stress (Ding et al., 2015). Artificial diets significantly increased the hepatic MDA level in mandarin fish hybrid (Siniperca chuatsi ♀ × Siniperca scherzeri ♂) (Li et al., 2017). Thus, artificial diets destroyed the balance of oxidation and antioxidation, leading to oxidative stress in the liver. In addition, oxidative stress is largely thought to negatively affect lipid and glucose homeostasis, which might further aggravate metabolic homeostasis disorder (Sekiya et al., 2008; Zhang et al., 2024).
Numerous studies have found that oxidative stress induces inflammation by activating multiple pathways. The excessive ROS activate NF-κB, leading to TNF-α secretion (Anderson et al., 1994). ROS activate NOD-like receptor protein 3 (NLRP3) inflammasome, resulting in the release of more proinflammatory cytokines (Schroder and Tschopp, 2010; Zhou et al., 2011). In the present study, the artificial diets upregulated the il-1β and ifn-γ transcriptional levels. Previous studies have shown that the activities of alkaline phosphatase and lysozyme in the serum of mandarin fish hybrid fed with an artificial diet were lower than those in the serum of fish fed with a natural diet, which indicated that the artificial diets reduced the immunity of fish (Li et al., 2017). Collectively, the artificial diets induced inflammation in the liver of mandarin fish. Supplementation with suitable additives is an important way to improve the health of fish. For example, bile acid alleviated hepatic lipid accumulation and oxidative stress induced by high-fat diets (Ding et al., 2020). Supplementation with exogenous bile acids improved intestinal immune function in grass carp (Peng et al., 2019). Chinese herbal additives have been proven to have positive effects on improving fish health (Ji et al., 2021; Xia et al., 2022). Thus, in our future study, we will study several additives that can mitigate the issues caused by the replacement of live prey fish with artificial diets.
Conclusion
The artificial diets disrupted hepatic lipid and glucose metabolism, leading to oxidative stress and inflammation, thus affecting the health status of mandarin fish. Thus, the effects of artificial diets replacing live prey fish for the feeding of mandarin fish need further study, especially in metabolism, oxidative stress, inflammation, and additives.
Data availability statement
The original contributions presented in the study are included in the article/supplementary material. Further inquiries can be directed to the corresponding author.
Ethics statement
The animal study was approved by the experimental animal ethics committee of Shanghai Ocean University. The study was conducted in accordance with the local legislation and institutional requirements.
Author contributions
WF: Writing – review & editing, Writing – original draft. XL: Writing – review & editing. BY: Writing – review & editing. LW: Writing – review & editing. XQ: Writing – review & editing.
Funding
The author(s) declare financial support was received for the research, authorship, and/or publication of this article. This research was supported by the Panyu Innovation and Entrepreneurship Leading Team Project (2021-R01-4).
Conflict of interest
Authors WF, BY, LW, and XQ were employed by the company Guangdong Haid Group Co., Ltd.
The author declares that the research was conducted in the absence of any commercial or financial relationships that could be construed as a potential conflict of interest.
Publisher’s note
All claims expressed in this article are solely those of the authors and do not necessarily represent those of their affiliated organizations, or those of the publisher, the editors and the reviewers. Any product that may be evaluated in this article, or claim that may be made by its manufacturer, is not guaranteed or endorsed by the publisher.
References
Anderson M. T., Staal F. J., Gitler C., Herzenberg L. A., Herzenberg L. A. (1994). Separation of oxidant-initiated and redox-regulated steps in the NF-kappa B signal transduction pathway. Proc. Natl. Acad. Sci. U.S.A. 91, 11527–11531. doi: 10.1073/pnas.91.24.11527
Badmus O. O., Hillhouse S. A., Anderson C. D., Hinds T. D., Stec D. E. (2022). Molecular mechanisms of metabolic associated fatty liver disease (MAFLD): functional analysis of lipid metabolism pathways. Clin. Sci. (Lond) 136, 1347–1366. doi: 10.1042/CS20220572
Booth M. A., Moses M. D., Allan G. L. (2013). Utilisation of carbohydrate by yellowtail kingfish Seriola lalandi. Aquaculture 376, 151–161. doi: 10.1016/j.aquaculture.2012.11.024
Cao X. F., Dai Y. J., Liu M. Y., Yuan X. Y., Wang C. C., Huang Y. Y., et al. (2019). High-fat diet induces aberrant hepatic lipid secretion in blunt snout bream by activating endoplasmic reticulum stress-associated IRE1/XBP1 pathway. Biochim. Et Biophys. Acta-Molecular Cell Biol. Lipids 1864, 213–223. doi: 10.1016/j.bbalip.2018.12.005
Chu Q., Xu T. J. (2020). MicroRNA regulation of Toll-like receptor, RIG-I-like receptor and Nod-like receptor pathways in teleost fish. Rev. Aquaculture 12, 2177–2193. doi: 10.1111/raq.12428
Cichoż-Lach H., Michalak A. (2014). Oxidative stress as a crucial factor in liver diseases. World J. Gastroenterol. 20, 8082–8091. doi: 10.3748/wjg.v20.i25.8082
Cook D. N., Pisetsky D. S., Schwartz D. A. (2004). Toll-like receptors in the pathogenesis of human disease. Nat. Immunol. 5, 975–979. doi: 10.1038/ni1116
Dai Y. J., Cao X. F., Zhang D. D., Li X. F., Liu W. B., Jiang G. Z. (2019). Chronic inflammation is a key to inducing liver injury in blunt snout bream (Megalobrama amblycephala) fed with high-fat diet. Dev. Comp. Immunol. 97, 28–37. doi: 10.1016/j.dci.2019.03.009
Dasari P., Nicholson I. C., Zola H. (2008). Toll-like receptors. J. Biol. Regulators Homeostatic Agents 22, 17–26.
Ding T., Xu N., Liu Y. T., Du J. L., Xiang X. J., Xu D., et al. (2020). Effect of dietary bile acid (BA) on the growth performance, body composition, antioxidant responses and expression of lipid metabolism-related genes of juvenile large yellow croaker (Larimichthys crocea) fed high-lipid diets. Aquaculture 518, 734768. doi: 10.1016/j.aquaculture.2019.734768
Ding Z., Zhang Y., Ye J., Du Z., Kong Y. (2015). An evaluation of replacing fish meal with fermented soybean meal in the diet of Macrobrachium nipponense: Growth, nonspecific immunity, and resistance to Aeromonas hydrophila. Fish & Shellfish Immunol. 44, 295–301. doi: 10.1016/j.fsi.2015.02.024
Fang W., Chen Q., Cui K., Chen Q., Li X., Xu N., et al. (2021). Lipid overload impairs hepatic VLDL secretion via oxidative stress-mediated PKCδ-HNF4α-MTP pathway in large yellow croaker (Larimichthys crocea). Free Radical Biol. Med. 172, 213–225. doi: 10.1016/j.freeradbiomed.2021.06.001
Fang W., Leng X. J., Yun B., Wang L., Qian X. Q. (2024). Artificial diets affect glucose and lipid metabolism, antioxidant capacity, and inflammatory response in the muscle of mandarin fish (Siniperca chuatsi). Front. Mar. Sci. 11, 1445902. doi: 10.3389/fmars.2024.1445902
Guan W. Z., Qiu G. F., Feng L. (2020). Transcriptome analysis of the growth performance of hybrid mandarin fish after food conversion. PloS One 15, e0240308. doi: 10.1371/journal.pone.0240308
Guo J. R., Wang L., Song K., Lu K. L., Li X. S., Zhang C. X. (2023). Physiological response of spotted seabass (Lateolabrax maculatus) to different dietary available phosphorus levels and water temperature: changes in growth, lipid metabolism, antioxidant status and intestinal microbiota. Antioxidants 12, 2128. doi: 10.3390/antiox12122128
Hatlen B., Grisdale-Helland B., Helland S. J. (2005). Growth, feed utilization and body composition in two size groups of Atlantic halibut (Hippoglossus hippoglossus) fed diets differing in protein and carbohydrate content. Aquaculture 249, 401–408. doi: 10.1016/j.aquaculture.2005.03.040
Ji R. L., Xiang X. J., Li X. S., Mai K. S., Ai Q. H. (2021). Effects of dietary curcumin on growth, antioxidant capacity, fatty acid composition and expression of lipid metabolism-related genes of large yellow croaker fed a high-fat diet. Br. J. Nutr. 126, 345–354. doi: 10.1017/S0007114520004171
Lee J., Giordano S., Zhang J. (2012). Autophagy, mitochondria and oxidative stress: cross-talk and redox signalling. Biochem. J. 441, 523–540. doi: 10.1042/BJ20111451
Li H., Zeng Y., Zheng X., Wang G., Tian J., Gong W., et al. (2023). Dietary betaine attenuates high-carbohydrate-diet-induced oxidative stress, endoplasmic reticulum stress, and apoptosis in mandarin fish (Siniperca chuatsi). Antioxidants (Basel) 12, 1680. doi: 10.3390/antiox12101860
Li L., Fang J. G., Liang X. F., Alam M. S., Liu L. W., Yuan X. C. (2019). Effect of feeding stimulants on growth performance, feed intake and appetite regulation of mandarin fish, zSiniperca chuatsi. Aquaculture Res. 50, 3684–3691. doi: 10.1111/are.14327
Li Y., Li J. Z., Lu J. T., Li Z., Shi S. C., Liu Z. J. (2017). Effects of live and artificial feeds on the growth, digestion, immunity and intestinal microflora of mandarin fish hybrid (Siniperca chuatsi ♀ x Siniperca scherzeri ♂). Aquaculture Res. 48, 4479–4485. doi: 10.1111/are.2017.48.issue-8
Li Y., Shi J. H., Shi S. C., Liu Z. J., Li Z., Li J. Z. (2015). Effect of live, frozen and artificial feeds on digestive enzymes, aminotransferase, histology of liver and intestine in mandarin fish hybrid (Siniperca chuatsi ♀ x Siniperca scherzeri ♂). Israeli J. Aquaculture-Bamidgeh 67, 1185. doi: 10.46989/001c.20701
Liang X. F., Oku H., Ogata H. Y., Liu J., He X. (2001). Weaning Chinese perch Siniperca chuatsi (Basilewsky) onto artificial diets based upon its specific sensory modality in feeding. Aquaculture Res. 32, 76–82. doi: 10.1046/j.1355-557x.2001.00006.x
Liu Z., Chen N., Wang M., Lian X., Yan C., Yin J. (2017). Suitable dietary starch source and supplementation level for largemouth bass (Micropterus salmoides). J. Fishery Sci. China 24, 317–331. doi: 10.3724/SP.J.1118.2017.16111
Livak K. J., Schmittgen T. D. (2001). Analysis of relative gene expression data using real-time quantitative PCR and the 2-ΔΔCT method. Methods 25, 402–408. doi: 10.1006/meth.2001.1262
Lu H. L., Li L., Miao Y. L., Liang H., Zou J. M., You J. J., et al. (2023). Effects and regulatory pathway of proopinmelanocortin on feeding habit domestication in mandarin fish. Gene 878, 147581. doi: 10.1016/j.gene.2023.147581
Pang Y., Xu X., Xiang X., Li Y., Zhao Z., Li J., et al. (2021). High fat activates O-GlcNAcylation and affects AMPK/ACC pathway to regulate lipid metabolism. Nutrients 13, 1740. doi: 10.3390/nu13061740
Peng X. R., Feng L., Jiang W. D., Wu P., Liu Y., Jiang J., et al. (2019). Supplementation exogenous bile acid improved growth and intestinal immune function associated with NF-κB and TOR signalling pathways in on-growing grass carp (Ctenopharyngodon idella): Enhancement the effect of protein-sparing by dietary lipid. Fish Shellfish Immunol. 92, 552–569. doi: 10.1016/j.fsi.2019.06.047
Schroder K., Tschopp J. (2010). The inflammasomes. Cell 140, 821–832. doi: 10.1016/j.cell.2010.01.040
Sekiya M., Hiraishi A., Touyama M., Sakamoto K. (2008). Oxidative stress induced lipid accumulation via SREBP1c activation in HepG2 cells. Biochem. Biophys. Res. Commun. 375, 602–607. doi: 10.1016/j.bbrc.2008.08.068
Song S. L., Zhao J. L., Li C. H. (2017). Species delimitation and phylogenetic reconstruction of the sinipercids (Perciformes: Sinipercidae) based on target enrichment of thousands of nuclear coding sequences. Mol. Phylogenet. Evol. 111, 44–55. doi: 10.1016/j.ympev.2017.03.014
Stone D. A. J. (2003). Dietary carbohydrate utilization by fish. Rev. Fisheries Sci. 11, 337–369. doi: 10.1080/10641260390260884
Styskal J., Van Remmen H., Richardson A., Salmon A. B. (2012). Oxidative stress and diabetes: What can we learn about insulin resistance from antioxidant mutant mouse models. Free Radical Biol. Med. 52, 46–58. doi: 10.1016/j.freeradbiomed.2011.10.441
Sun X. D., Li X. B., Jia H. D., Wang H. Y., Shui G. H., Qin Y. L., et al. (2020). Nuclear factor E2-related factor 2 mediates oxidative stress-induced lipid accumulation in adipocytes by increasing adipogenesis and decreasing lipolysis. Antioxidants Redox Signaling 32, 173–192. doi: 10.1089/ars.2019.7769
Tao J. J., Gui J. F., zhang Q. Y. (2007). Isolation and characterization of a rhabdovirus from co-infection of two viruses in mandarin fish. Aquaculture 262, 1–9. doi: 10.1016/j.aquaculture.2006.09.030
Thannickal V. J., Fanburg B. L. (2000). Reactive oxygen species in cell signaling. Am. Jounal Physiology-Lung Cell. Mol. Physiol. 279, L1005–L1028. doi: 10.1152/ajplung.2000.279.6.L1005
Watt M. J., Miotto P. M., De Nardo W., Montgomery M. K. (2019). The liver as an endocrine organ-linking NAFLD and insulin resistance. Endocrine Rev. 40, 1367–1393. doi: 10.1210/er.2019-00034
Xia Y. T., Wu Q. Y., Hok-Chi Cheng E., Ting-Xia Dong T., Qin Q. W., Wang W. X., et al. (2022). The inclusion of extract from aerial part of Scutellaria baicalensis in feeding of pearl gentian grouper (Epinephelus fuscoguttatus♀ × Epinephelus lanceo-latus♂) promotes growth and immunity. Fish Shellfish Immunol. 127, 521–529. doi: 10.1016/j.fsi.2022.06.041
Xie R. P., Liang X. F., Peng D., Zhang Q. W., Wu D. L., Chen J. L., et al. (2023). Dietary supplementation of pyridoxine can enhance the growth performance and improve the protein, lipid utilization efficiency of mandarin fish (Siniperca chuatsi). Fish Physiol. Biochem. 49, 1063–1078. doi: 10.1007/s10695-023-01223-3
Yi T., Sun J., Liang X., He S., Li L., Wen Z., et al. (2013). Effects of polymorphisms in pepsinogen (PEP), amylase (AMY) and trypsin (TRY) genes on food habit domestication traits in mandarin fish. Int. J. Mol. Sci. 14, 21504–21512. doi: 10.3390/ijms141121504
Zhang Q. L., Shen X. T., Yuan X., Huang J., Zhu Y. L., Zhu T. T., et al. (2024). Lipopolysaccharide binding protein resists hepatic oxidative stress by regulating lipid droplet homeostasis. Nat. Commun. 15, 3213. doi: 10.1038/s41467-024-47553-5
Zhao X. Y., Chen W. J., Zhang Y. Y., Gao X. C., Huang Y., Ren H. T., et al. (2024). Dietary guar gum supplementation reduces the adverse effects of high-fat diets on the growth performance, antioxidant capacity, inflammation, and apoptosis of juvenile largemouth bass (Micropterus salmoides). Anim. Feed Sci. Technol. 308, 115881. doi: 10.1016/j.anifeedsci.2024.115881
Keywords: mandarin fish, lipid and glucose homeostasis, oxidative stress, inflammation, transcriptome
Citation: Fang W, Leng X, Yun B, Wang L and Qian X (2024) Effects of artificial diets on lipid and glucose metabolism, antioxidative capacity, and inflammation in the liver of mandarin fish (Siniperca chuatsi). Front. Mar. Sci. 11:1474836. doi: 10.3389/fmars.2024.1474836
Received: 02 August 2024; Accepted: 18 September 2024;
Published: 08 October 2024.
Edited by:
Jiamin Li, Jiangxi Agricultural University, ChinaReviewed by:
D. K. Meena, Central Inland Fisheries Research Institute (ICAR), IndiaGuanjie Yan, Nanyang Normal University, China
Lei Gan, Guizhou University, China
Jose Guadalupe Soñanez Organis, University of Sonora, Mexico
Min Jin, Ningbo University, China
Copyright © 2024 Fang, Leng, Yun, Wang and Qian. This is an open-access article distributed under the terms of the Creative Commons Attribution License (CC BY). The use, distribution or reproduction in other forums is permitted, provided the original author(s) and the copyright owner(s) are credited and that the original publication in this journal is cited, in accordance with accepted academic practice. No use, distribution or reproduction is permitted which does not comply with these terms.
*Correspondence: Xueqiao Qian, cXhxQGhhaWQuY29tLmNu