- 1Jiangsu Key Laboratory of Marine Bioresources and Environment, Jiangsu Ocean University, Lianyungang, China
- 2Co-Innovation Center of Jiangsu Marine Bio-industry Technology, Jiangsu Ocean University, Lianyungang, China
- 3College of Marine Ecology and Environment, Shanghai Ocean University, Shanghai, China
Recent years have seen the Ulva green tide and Sargassum golden tide become commonplace in the coastal waters of China. However, little is known on how the combination of ocean warming and eutrophication would affect the interaction of green and golden tides. In this study, we cultured the green tide alga Ulva prolifera and the golden tide alga Sargassum horneri under different temperatures (5, 10, 15, 20, 25, and 30°C) and two nutrient concentrations (Low nutrient, LN: 5 μM-nitrate and 0.5 μM-phosphate; High nutrient, HN: 500 μM-N and 50 μM-P) in both monoculture and coculture systems to investigate the physiological responses and their competitive relationships. In monocultures, the growth of U. prolifera and S. horneri, along with pigment concentrations and photosynthesis, increased with rising temperature, reaching a plateau at 15 - 25°C. However, when the temperature increased to 30°C, the growth of U. prolifera and S. horneri decreased abruptly, with S. horneri even suffering death. In coculture, the growth of both U. prolifera and S. horneri was inhibited compared to the monoculture, with the greatest decline observed in S. horneri at 25°C under two nutrient conditions. Our results show that U. prolifera would outcompete S. horneri under high temperature in coculture, suggesting that ocean warming would enhance the competitive advantage of green tide over golden tide under eutrophication in the future.
1 Introduction
Macroalgae, as important primary producers in the coastal zone, contribute about 1521 Tg C yr−1 of the global net primary production (Krause-Jensen and Duarte, 2016). Through carbon fixation, sequestration and habitat provision, they often play significantly structural roles in coastal ecosystems (Machado and Oliveira, 2024). Due to continued anthropogenic pressure on marine systems, many macroalgae species have been harmed by environmental changes and face an uncertain future, jeopardizing their important contributions to global productivity and ecosystem service (Hanley et al., 2024).
It is widely recognized that human-induced greenhouse gas emissions (CO2, methane, etc.) have been the primary driver of global warming since the industrial revolution (IPCC, 2019). Over 90% of the anthropogenic increase in heat is absorbed by the global ocean, leading to ocean warming (Durack et al., 2014). The average sea surface temperature has risen by 1.1°C up to now, and is predicted to further increase 1.9 – 5.8°C by the end of the century based on Representative Concentration Pathway (RCP) 8.5 (Gattuso et al., 2015; IPCC, 2019). In addition, macroalgae also experience diurnal and seasonal temperature variations in the nature (Martin and Gattuso, 2009). It is well established that temperature directly influences intracellular biochemical reactions and metabolic activities, thereby affecting their survival and growth (Zou and Gao, 2014b; Chen et al., 2018). In a suitable range, increased temperature is beneficial for the photosynthesis and growth of macroalgae (Fan et al., 2014; Zou and Gao, 2014a). However, temperatures below or above this range can slow down growth or even cause cellular damage and mortality. High temperatures could trigger the Ulva to generate more reactive oxygen species (ROS), resulting in oxidative damage to proteins, lipids, DNA within cells (Apel and Hirt, 2004). Macroalgae have also evolved ROS scavenging mechanisms to cope with the potential damage from ROS, such as superoxide dismutase (SOD), ascorbate peroxidase (APX), glutathione peroxidase (GPX) and catalase (CAT) (Apel and Hirt, 2004). A previous report found that temperatures exceed 35°C inhibit the photosynthetic performance of Ulva conglobate (Zou and Gao, 2014a). Moreover, tropical seaweeds such as Wurdemannia miniata and Valonia utricularis were found to be induced to death under extreme high temperatures (Pakker and Breeman, 1996). Moreover, ocean warming will expand the distribution of tropical and temperate species towards the poles (Díez et al., 2012), which was supported by a model prediction (Jueterbock et al., 2013).
Under the influence of prevalently industrial and agricultural activities, the anthropogenic input of nutrients (e. g. nitrogen and phosphorus) into coastal waters has continuously increased, leading to eutrophication, a trend that threatens the health of coastal ecosystems worldwide (Paerl et al., 2014; Malone and Newton, 2020). It has been shown that elevated nutrient concentrations reduce biodiversity, impact marine habitats, and alter ecosystem functions (Yang et al., 2005; Liu et al., 2009; Mineur et al., 2015). Nitrogen is a crucial component of many compounds, such as the photosynthetic enzyme, Rubisco (Dawes and Koch, 1990); and phosphorus is also an essential element in macroalgal cells for genetic replication, energy supply, and growth metabolism (Zer and Ohad, 2003). The increase in nitrogen and phosphorus concentrations could enhance the growth and biomass of macroalgae (Li et al., 2016). Moreover, temperature also influences algal growth rates by affecting nutrient uptake rates through the nitrate reductase activity (NRA) (Granbom et al., 2004). Feng et al. (2021) also reported that NRA of Ulva prolifera, associated with growth, decreased with the rising temperature while exceeded 15°C.
Macroalgae have competitive advantages due to its higher affinity with nutrients, leading to frequently outbreaks of macroalgae blooms (Luo et al., 2012). As an opportunistically growing macroalgal genera, Ulva is strongly adaptable to environment which has high tolerance for variable temperature, salinity, irradiance and nutrient concentrations (Taylor et al., 2001; Xiao et al., 2016). At optimal temperature conditions from April to June, detached green patches of Ulva species had grown rapidly and accumulated to form green tides, transporting northward into the Yellow Sea of China by monsoon winds and ocean currents (Sun et al., 2008; Liu et al., 2010, 2021b; Xia et al., 2024). Meanwhile, Large-scale drifting biomass of Sargassum horneri, known as golden tides, has been reported in the Yellow Sea since 2010 (Liu et al., 2018; Su et al., 2018; Wang et al., 2023). These drifting macroalgae originally grew on the rocky bottom. In spring, the increased buoyancy provided by their numerous sporophyte vesicles could keep the plants floating after detachment, forming the drifting biomass on the sea surface (Yoshida, 1963). Sargassum from the coastal region of Shandong Peninsula drifted southwards in winter months, while Sargassum along the coast of Zhejiang Province drifted northwards in summer, eventually reaching the largest Pyropia aquaculture area of China (Xing et al., 2017; Zhang et al., 2019; Liu et al., 2021a). In recent years, green and golden tides have frequently occurred simultaneously due to excessive nutrient inputs, resulting in a severely economic and ecological disaster in China’s coastal waters (Su et al., 2018; Xiao et al., 2020b).
Under the complex context of global climate changes coupled with regional eutrophication, harmful algal blooms are gradually increasing. In particular, the frequency of green and gold tides caused by Ulva and Sargassum has increased by years, replacing red tides as the main disasters in the coastal waters of China (Feng et al., 2024). However, few studies have been conducted to investigate the competition between Ulva and Sargassum under the combined effects of local stressor of eutrophication and global stressor of ocean warming. In this study, U. prolifera and S. horneri were selected and treated to explore the physiological responses and their competitive relationships of the typical harmful algae to high nutrients availability and temperature change scenarios in the Yellow Sea of China. Our results are expected to provide helpful insights into understanding the adaption mechanism and competitive relationships of two macroalgae species under ocean warming and eutrophication in the future.
2 Materials and methods
2.1 Sample collection and experiment design
Floating samples of U. prolifera and S. horneri were collected from Gaogong island, Lianyungang city, Jiangsu province (119.53°E; 34.91°N) and the nearshore sea of Dongtai, Yancheng city, Jiangsu province of China (121.33°E; 33.02°N) in early June of 2020, respectively. The in situ nutrient levels were 10.72 μmol L−1 nitrate and 0.42 μmol L−1 phosphate in coastal area of north Jiangsu in early summer (Wang et al., 2022). Considering that June is the end of the life cycle in S. horneri, the thalli should be a bit senescent. The thalli were transferred to the laboratory under low temperature conditions in a cool container within 2 hours. After removing the sediments and impurities using filtered and autoclaved seawater, healthy thalli were selected and pre-cultured in 1 L balloon flasks containing sterile seawater enriched with von Stosch’s enrichment (VSE) Medium (Ott, 1965), which was aerated continuously and changed every 2 days. The cultures were kept in an intelligent illumination incubator (Jiangnan GXZ-300C, Ningbo, China) at 20°C with a 12 h: 12 h (light/dark) photoperiod under 100 μmol photons m−2 s−1 light intensity.
After the pre-culture of one week, thalli samples with similar length and shape were chose and divided randomly to different treatments. Approximately 0.10 g (fresh weight, FW) thalli were cultured in 500 mL sterile seawater enriched with VSE medium. Six temperature treatments (5, 10, 15, 20, 25, and 30°C) were obtained using different incubators (same brand model to avoid the influence of light), while two levels of nutrient [Low nutrient, LN: 5 μmol L−1 N (nitrate) and 0.5 μmol L−1 P (phosphate); High nutrient, HN: 500 μmol L−1 N and 50 μmol L−1 P] were set based on VSE medium. The LN condition represented the low nutrient levels and HN condition was set as eutrophication (Wang et al., 2022). At the same time, we also selected three temperatures (15, 20, and 25°C) to study the competition between U. prolifera and S. horneri under eutrophication conditions. The initial biomass of U. prolifera and S. horneri were about 0.05 g (FW) in coculture, respectively. The medium was renewed every 3 d to maintain the abundance of nutrients. Triplicate cultures were conducted for two weeks and all the parameters were measured at the end of culture period.
2.2 Measurement of growth
The relative growth rates (RGR) of U. prolifera and S. horneri were estimated by changes in biomass (FW), which were performed according to the following formula:
where the W0 and Wt are the initial and final fresh weight of thalli after t days culture, respectively.
2.3 Measurement of photosynthetic pigments and soluble protein
To determine pigments content, about 0.02 g FW per sample were cut into pieces and extracted in absolute methanol and kept at 4°C for 24 h in darkness (Porra et al., 1989). The value of Chl a and Car might be low due to the incomplete extraction without grinding. After centrifugation (Centrifuge 5407, Eppondorf, Germany) at 5000×g for 10 min, the supernatant was scanned by a spectrophotometer (UV-1800, Shimadzu, Japan) at 470, 652, and 665 nm, respectively. The concentrations of Chlorophyll a (Chl a) and carotenoids (Car) were determined according to the methods of Wellburn (1994):
where the A470, A652, and A665 were the absorbance of samples at respective wavelength, V is the volume of methanol, and FW is the fresh weight of samples.
Soluble protein contents were measured according to the methods of Bradford (1976). Briefly, about 0.02 g FW thalli was homogenized in phosphate buffer (0.1 M, pH 6.8) and then centrifuged at 5000× g for 15 min at 4°C. The supernatant was mixed with Coomassie brilliant blue G-250 dye solution and scanned at 595 nm by spectrophotometer to calculate the soluble protein contents (SP, mg g FW−1) based on the standard curve of bovine serum albumin.
2.4 Measurement of photosynthesis and respiration
The photosynthetic oxygen evolution and respiration of these two species were measured with a Clark-type electrode (Oxygraph, Hansatech, UK) at the end of experiments. Samples of thalli were cut into 0.5 cm length segments and placed in culture conditions for about 2 h to alleviate cutting damage (Xu and Gao, 2012). During the middle light period (10:00−16:00), about 0.02 g FW thalli were transferred into the reaction chamber containing 5 ml fresh growth medium. The light (100 μmol photons m−2 s−1) and temperature condition were set at the same with every culture condition, and seawater in the chamber was stirred during the measurement to keep the oxygen signal steady. The decreased rate (in dark condition) and increased rate (in light condition) of oxygen concentrations were defined as net photosynthetic rate and dark respiration, respectively.
2.5 Assessment of superoxide dismutase activity
Superoxide dismutase (SOD) activity was were examined by using nitroblue tetrazolium (NBT) method (Merzbach and Obedeanu, 1975). Approximately 0.05 g of samples was homogenized in 4 mL phosphate buffer (0.05 M, pH 7.8) and then centrifuged at 5000× g for 10 min at 4°C. The supernatant of the crude extract of SOD was mixed with the NBT solution. After 20 min incubation under 80 µmol photons m−2 s−1 at 25°C, the absorbance at 560 nm was measured. The amount of SOD that reduces NBT by 50% is defined as the SOD activity.
where the Ac and As represent the absorbance of mixed NBT solution (V) with distilled water and sample enzyme, respectively. Vt is the crude extract of fresh weight (FW) thalli samples.
2.6 Statistical analysis
All data were expressed as mean of triplicate analysis ± standard deviations. Before performing parametric tests, data were tested for homogeneity of variance (Levene test, see Supplementary Table S1 in Supplementary Materials) and normality (Shapiro-Wilk test, Supplementary Table S2). Two-way ANOVA was performed to assess the interactive effects of temperature and nutrient levels. Three-way ANOVA employed to determine the effects of temperature, nutrient and coculture. One-way ANOVA was applied to analyze the statistical differences among different temperature treatments under LN and HN conditions. An independent-samples t-test were used to compare the differences between LN and HN within the same temperature treatment and differences between monoculture and coculture under the same condition. Considering that temperature treatments were achieved by different incubators (same brand model) and one incubator per temperature, it should be noted that all ANOVA analyses assessing temperature in this study assess the temperature plus incubator effects. Tukey’s honest significant difference (Tukey HSD) was used for ANOVA analysis and differences were termed significant when p < 0.05.
3 Results
3.1 Relative growth rate of U. prolifera and S. horneri
Two-way ANOVA analysis indicated that there were significant individual and interactive effects of temperature (i.e. the temperature and incubator effects) and nutrient on the relative growth rate (RGR) of U. prolifera (Table 1, p < 0.001, p < 0.001, p = 0.009),. The growth of thalli in both LN and HN conditions enhanced with the increased levels of temperature, peaking at 20°C (17.2 ± 1.0% for LN, 20.1 ± 3.0% for HN), and began decline at temperature above 25°C. RGR were significantly affected by HN at 5, 15, and 30°C (Figure 1A, p < 0.001, p = 0.009, p = 0.039). For S. horneri, temperature and the interaction with nutrient had significant effect on RGR of thalli (Table 1, p < 0.001, p < 0.001). In general, RGR of S. horneri showed an increased trend with temperatures (Figure 1B). Specially, RGR began to plunge at 30°C, and was even negative under HN condition.
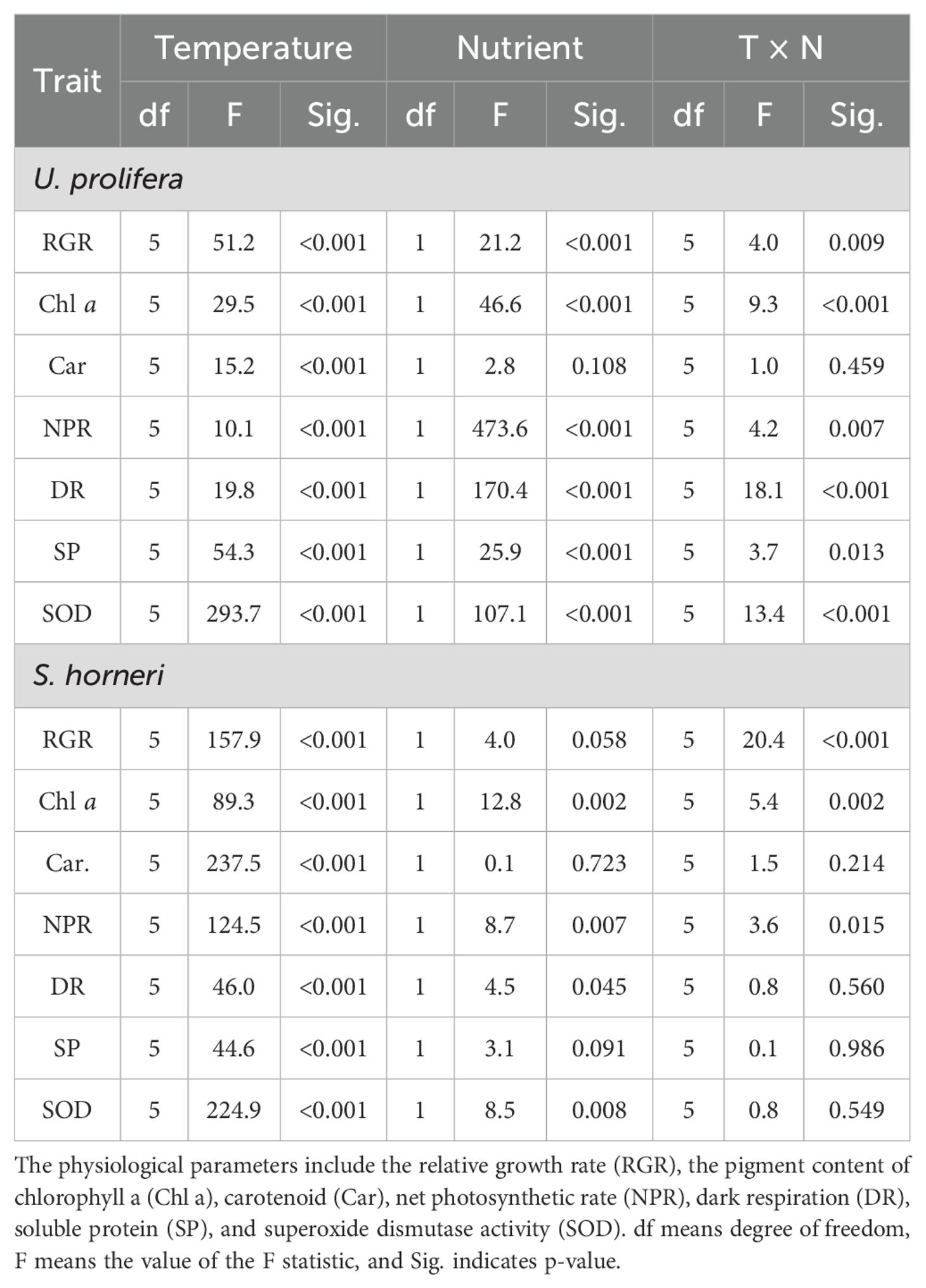
Table 1. Statistical analyses (two-way ANOVA) of physiological traits of Ulva prolifera and Sargassum horneri grown under various temperature and nutrient conditions in the monoculture.
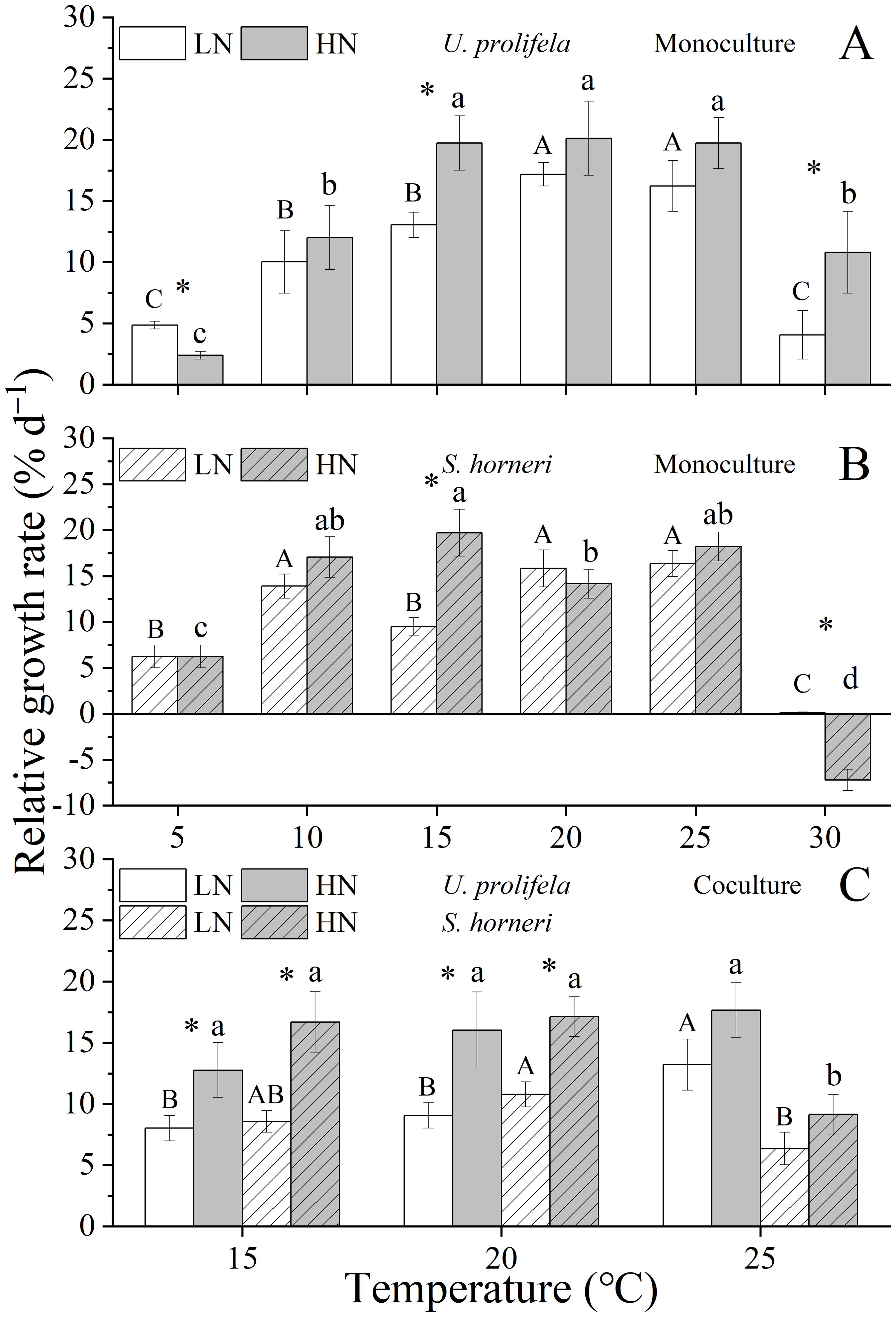
Figure 1. Relative growth rate (RGR) of Ulva prolifera and Sargassum horneri grown under various temperature and nutrient conditions in the monoculture (A, B) and coculture (C). Different uppercase letters represent significant differences among different temperature treatment under LN (p < 0.05), and different lowercase letters represent significant differences among different temperature treatment under HN (p < 0.05). Asterisk indicates whether there was a significant difference between LN and HN under the same temperature conditions (p < 0.05).
In coculture, RGR of both U. prolifera and S. horneri were declined compared to those in monoculture (Supplementary Table S3, Figure 1C, p = 0.002, p = 0.029). RGR of U. prolifera were significantly enhanced by HN compared to LN at 15 and 20°C (p = 0.029, p = 0.021), and S. horneri showed the same trend (p = 0.006, p = 0.005). Coculture with U. prolifera led to the obvious decline in RGR of S. horneri, which were decreased by 61.2% and 49.7% compared to monoculture at 25°C under LN and HN, respectively (p < 0.001, p = 0.002). Meanwhile, even though the RGR of U. prolifera were reduced by 18.6% and 10.4% compared to monoculture at 25°C under LN and HN, respectively, it was still highest in the coculture.
3.2 Pigment contents of U. prolifera and S. horneri
The Chlorophyll a (Chl a) content of U. prolifera was significantly influenced by temperature, nutrient, and the interaction between them (Table 1, p < 0.001, p < 0.001, p < 0.001). Meanwhile, the carotenoids (Car) content was only significantly influenced by temperature (p < 0.001). The Chl a of thalli was increased with rising temperature at the range of 5-25°C, especially under HN condition, and reached a maximum 309.4 ± 52.8 μg g−1 under LN at 30°C and 520.8 ± 46.8 μg g−1 under HN at 25°C, respectively (Figure 2A). HN significantly promoted the Chl a content of U. prolifera at 15, 20, and 25°C (p = 0.005, p = 0.018, p = 0.004). Similarly, the Car content was increased with the temperature up to 20°C, and declined at higher temperatures (25 and 30°C), which was in line with growth (Figure 3A).
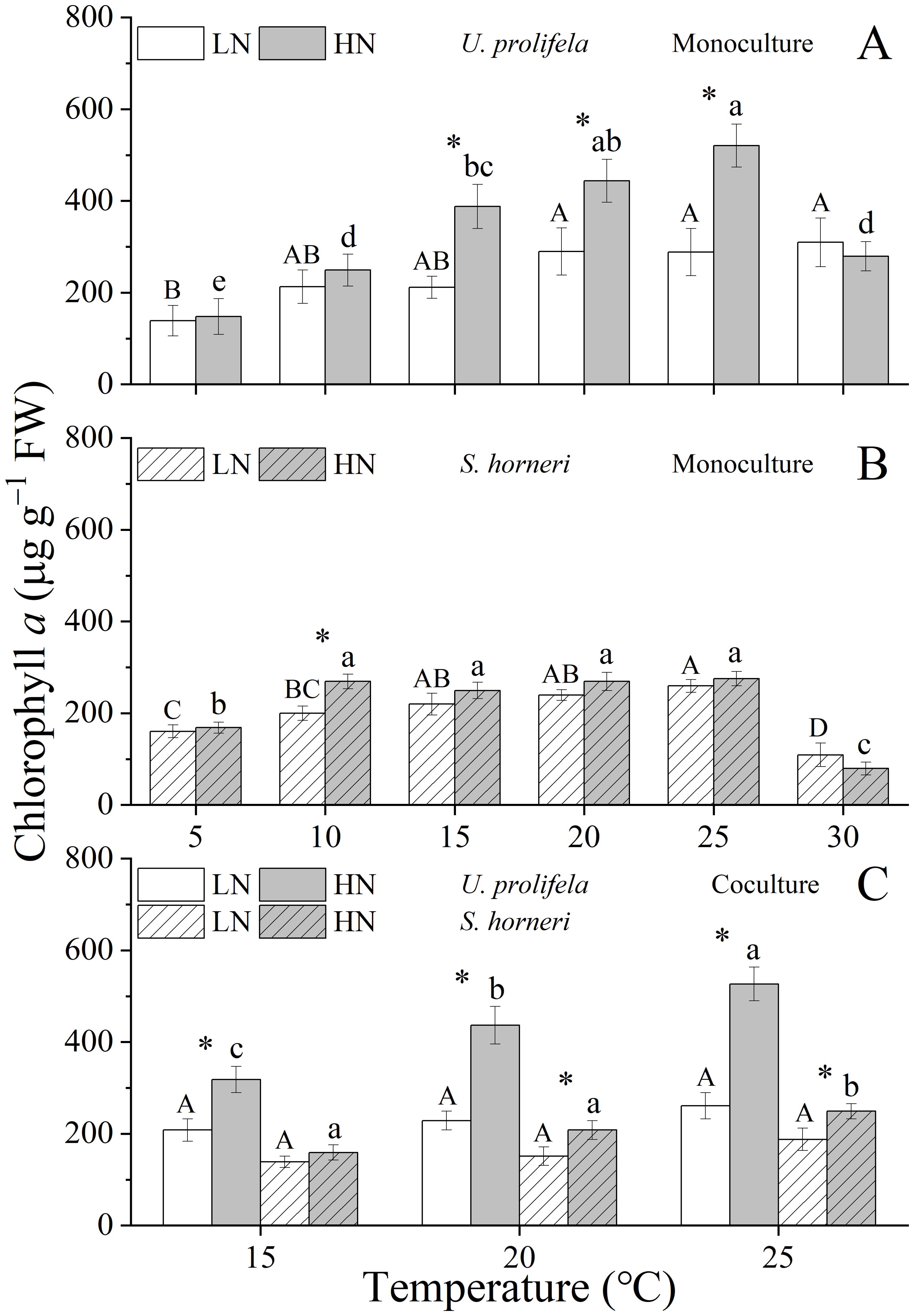
Figure 2. Content of Chlorophyll a in Ulva prolifera and Sargassum horneri grown under various temperature and nutrient conditions in the monoculture (A, B) and coculture (C). Different uppercase letters represent significant differences among different temperature treatment under LN (p < 0.05), and different lowercase letters represent significant differences among different temperature treatment under HN (p < 0.05). Asterisk indicates whether there was a significant difference between LN and HN under the same temperature conditions (p < 0.05).
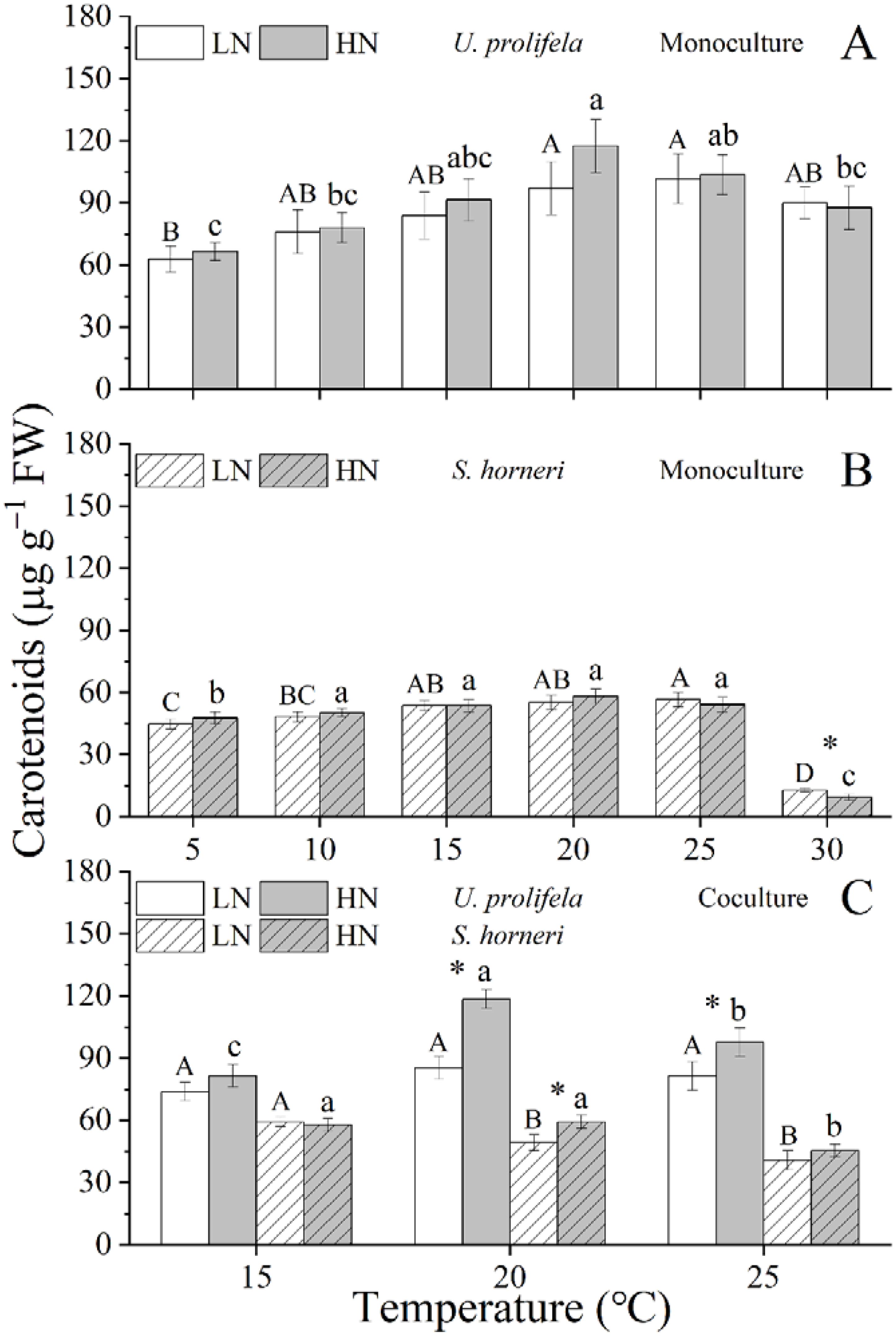
Figure 3. Content of Carotenoid in Ulva prolifera and Sargassum horneri grown under various temperature and nutrient conditions in the monoculture (A, B) and coculture (C). Different uppercase letters represent significant differences among different temperature treatment under LN (p < 0.05), and different lowercase letters represent significant differences among different temperature treatment under HN (p < 0.05). Asterisk indicates whether there was a significant difference between LN and HN under the same temperature conditions (p < 0.05).
Temperature and nutrient had individual and interactive effect on Chl a content of S. horneri (p < 0.001, p < 0.002, p = 0.002), and only temperature had an individual effect on Car content of thalli (Table 1, p < 0.001). The Chl a of S. horneri was enhanced with increased temperature but declined at 30°C under LN and HN conditions (Figure 2B). HN only increased significantly the Chl a at 10°C compared to LN (p = 0.006). Similarly, the Car of S. horneri was promoted slightly by temperature until up to 30°C, with a substantial decline (Figure 3B).
In coculture, both Chl a and Car contents of U. prolifera were decreased compared to those in monoculture (Supplementary Tables S4, S5, Figures 2C, 3C, p < 0.001, p < 0.001). Increased temperature only enhanced the Chl a of U. prolifera under HN condition. Meanwhile, HN promoted the Chl a of thalli at three temperatures compared to LN (p = 0.007, p = 0.001, p < 0.001). In addition, coculture with U. prolifera inhibited the Chl a of S. horneri at all treatments (p = 0.006, p = 0.003, p = 0.012 for 15, 20, 25 under LN and p = 0.003, p = 0.020, p = 0.116 for 15, 20, 25 under HN) but significantly enhanced the Car content of thalli by 10.3% at 15°C under LN (p = 0.047). HN only significantly enhanced the Chl a of S. horneri at 20 and 25°C (p = 0.027, p = 0.023).
3.3 Photosynthesis and respiration of U. prolifera and S. horneri
The net photosynthetic rate (NPR) and dark respiration rate (Rd) of U. prolifera were significantly influenced by temperature, nutrient and their interaction (Table 1, p < 0.001, p < 0.001, p = 0.007 for NPR, and p < 0.001, p < 0.001, p < 0.001 for Rd). NPR of U. prolifera thalli showed a relative stability regardless of temperature under LN and HN conditions (Figure 4A). However, the increased temperature enhanced the Rd of U. prolifera within a range of 5 - 25°C (Figure 5A). At the different temperatures, HN condition promoted both the NPR and Rd of thalli significantly except for the Rd under 5 and 10°C.
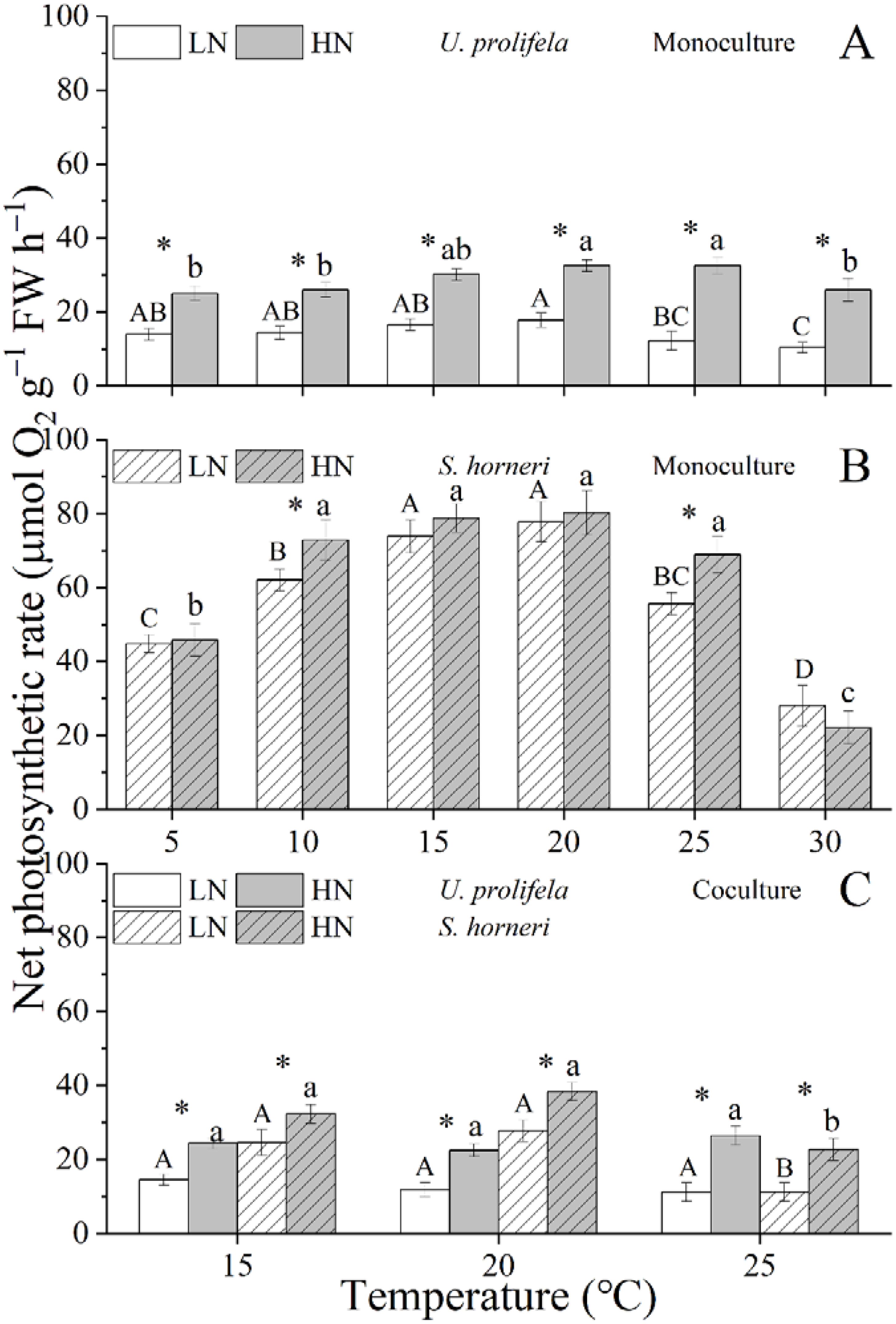
Figure 4. Net photosynthetic rate of Ulva prolifera and Sargassum horneri grown under various temperature and nutrient conditions in the monoculture (A, B) and coculture (C). Different uppercase letters represent significant differences among different temperature treatment under LN (p < 0.05), and different lowercase letters represent significant differences among different temperature treatment under HN (p < 0.05). Asterisk indicates whether there was a significant difference between LN and HN under the same temperature conditions (p < 0.05).
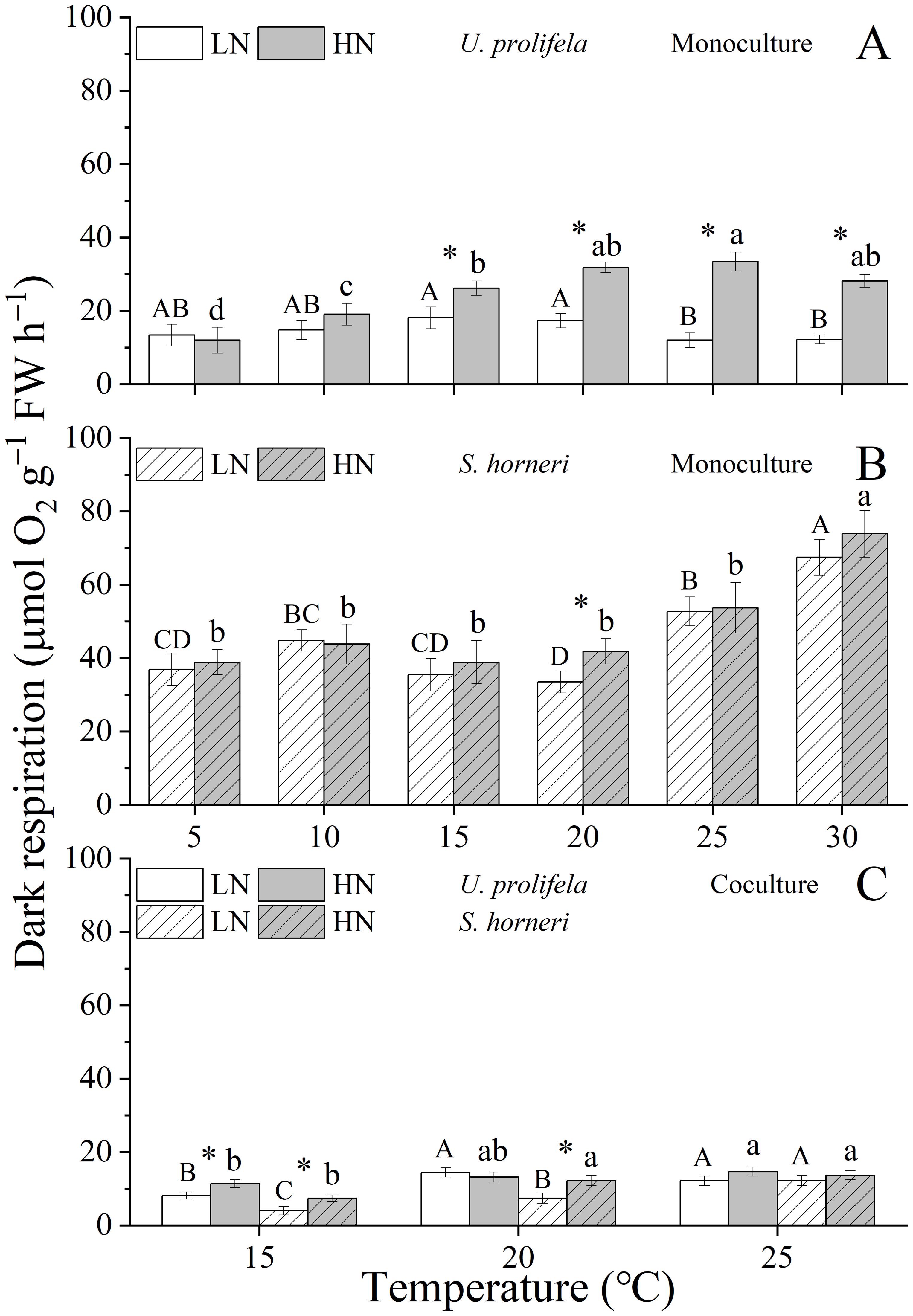
Figure 5. Dark respiration rate of Ulva prolifera and Sargassum horneri grown under various temperature and nutrient conditions in the monoculture (A, B) and coculture (C). Different uppercase letters represent significant differences among different temperature treatment under LN (p < 0.05), and different lowercase letters represent significant differences among different temperature treatment under HN (p < 0.05). Asterisk indicates whether there was a significant difference between LN and HN under the same temperature conditions (p < 0.05).
As for S. horneri, Temperature and nutrient had individual effects on NPR and Rd of thalli, and only had an interactive effect on NPR (Table 1, p < 0.001, p = 0.007, p = 0.015 for NPR, and p < 0.001, p = 0.045 for Rd). The NPR of S. horneri was enhanced with increased temperature within a range of 5-20°C, then decreased at temperature above 25 °C under LN and HN (Figure 4B). However, the Rd of S. horneri showed an increasing trend with the temperatures, and reached the highest value of 67.5 and 73.9 μmol O2 g−1 FW h−1 at 30°C under LN and HN condition, respectively (Figure 5B).
In coculture, NPR and Rd of both U. prolifera and S. horneri were decreased significantly compared to those in monoculture (Supplementary Tables S6, S7, Figure 4C and 5C, p < 0.001, p < 0.001 for NPR and Rd in U. prolifera, and p < 0.001, p < 0.001 in S. horneri). However, compared to monoculture, the highest drop of NPR in U. prolifera was about 33.0% and 30.8% under LN and HN at 20°C (p = 0.011, p = 0.002), while the highest drop in S. horneri was about 79.78% and 67.0% at 25 °C (p < 0.001, p < 0.001), respectively. Moreover, HN enhanced the NPR of thalli under all treatments. Similarly to the trend of Rd in U. prolifera and S. horneri under monoculture, temperature enhanced the Rd of U. prolifera and S. horneri in coculture.
3.4 Soluble protein content of U. prolifera and S. horneri
Significant individual and interactive effects of temperature and nutrient were observed on soluble protein content (SP) of U. prolifera (Table 1, p < 0.001, p < 0.001, p = 0.013). In general, the SP of U. prolifera showed a rising trend with the increased temperature except under HN at 30 °C. Compared to LN, HN promotes SP at all temperatures, with significant differences at 20 and 25°C and a maximum value of 2.7 ± 0.2 mg g−1 at 25°C (Figure 6A, p = 0.008, p = 0.010).
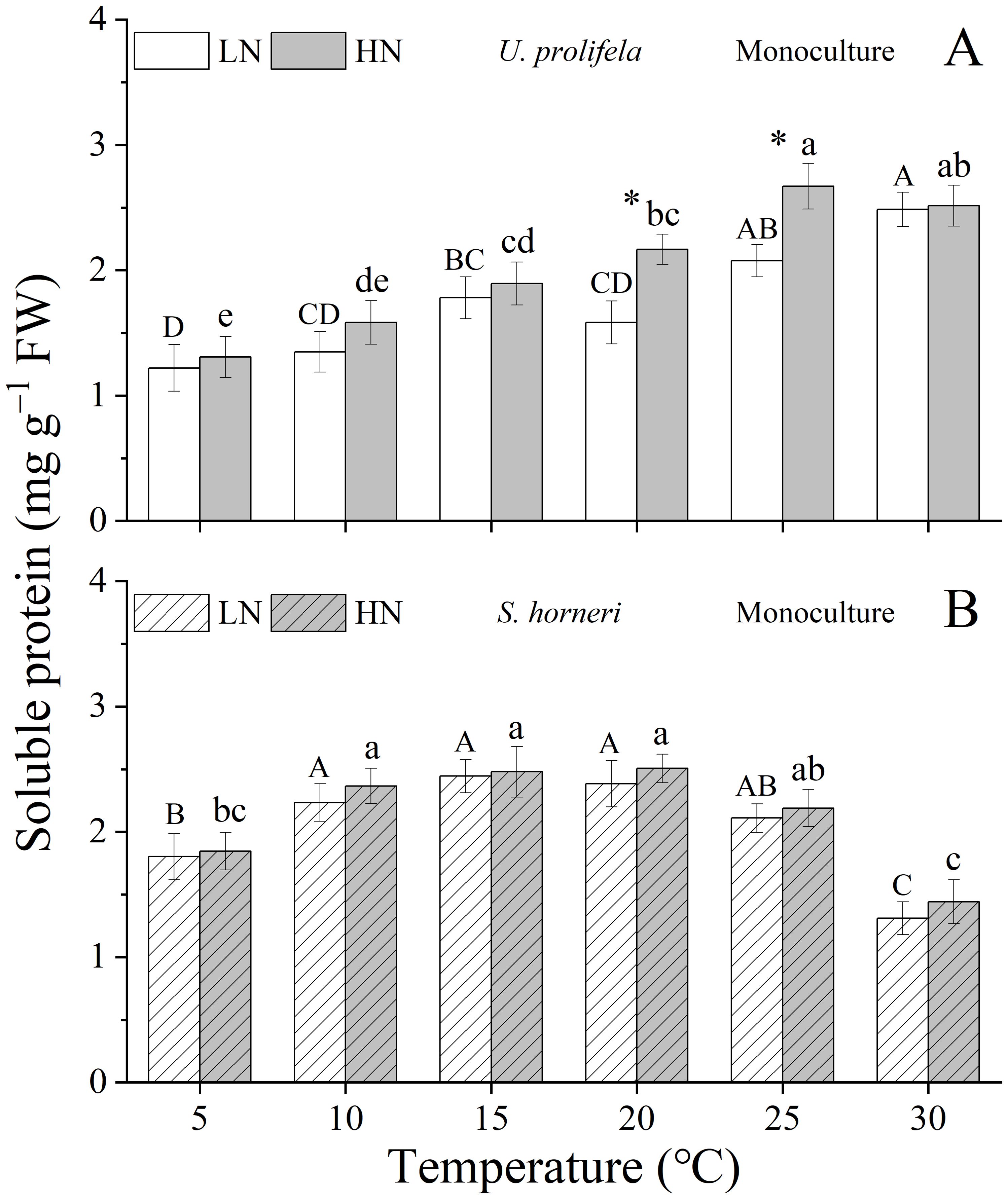
Figure 6. Content of soluble protein in Ulva prolifera (A) and Sargassum horneri (B) grown under various temperature and nutrient conditions in the monoculture. Different uppercase letters represent significant differences among different temperature treatment under LN (p < 0.05), and different lowercase letters represent significant differences among different temperature treatment under HN (p < 0.05). Asterisk indicates whether there was a significant difference between LN and HN under the same temperature conditions (p < 0.05).
As for S. horneri, only temperature had an individual effect on SP (Table 1, p < 0.001). The SP of thalli in both LN and HN treatments enhanced with the increased temperatures, peaking at 15 and 20°C, and thereafter declined at temperature above this optimal point. Compared to LN, HN enhanced SP, but there was no significant difference between them under all temperatures (Figure 6B).
3.5 Superoxide dismutase activity of U. prolifera and S. horneri
Temperature, nutrient, and the interaction between them had significant effect on SOD of U. prolifera (Table 1, p < 0.001, p < 0.001, p < 0.001). The SOD of thalli was enhanced with the temperature increased until 25°C, but decreased significantly at higher temperature (30°C) (Figure 7A). HN promotes SOD activity at all temperatures, but only was significant at 15, 20, and 25°C compared with LN condition (p = 0.032, p = 0.005, p < 0.001).
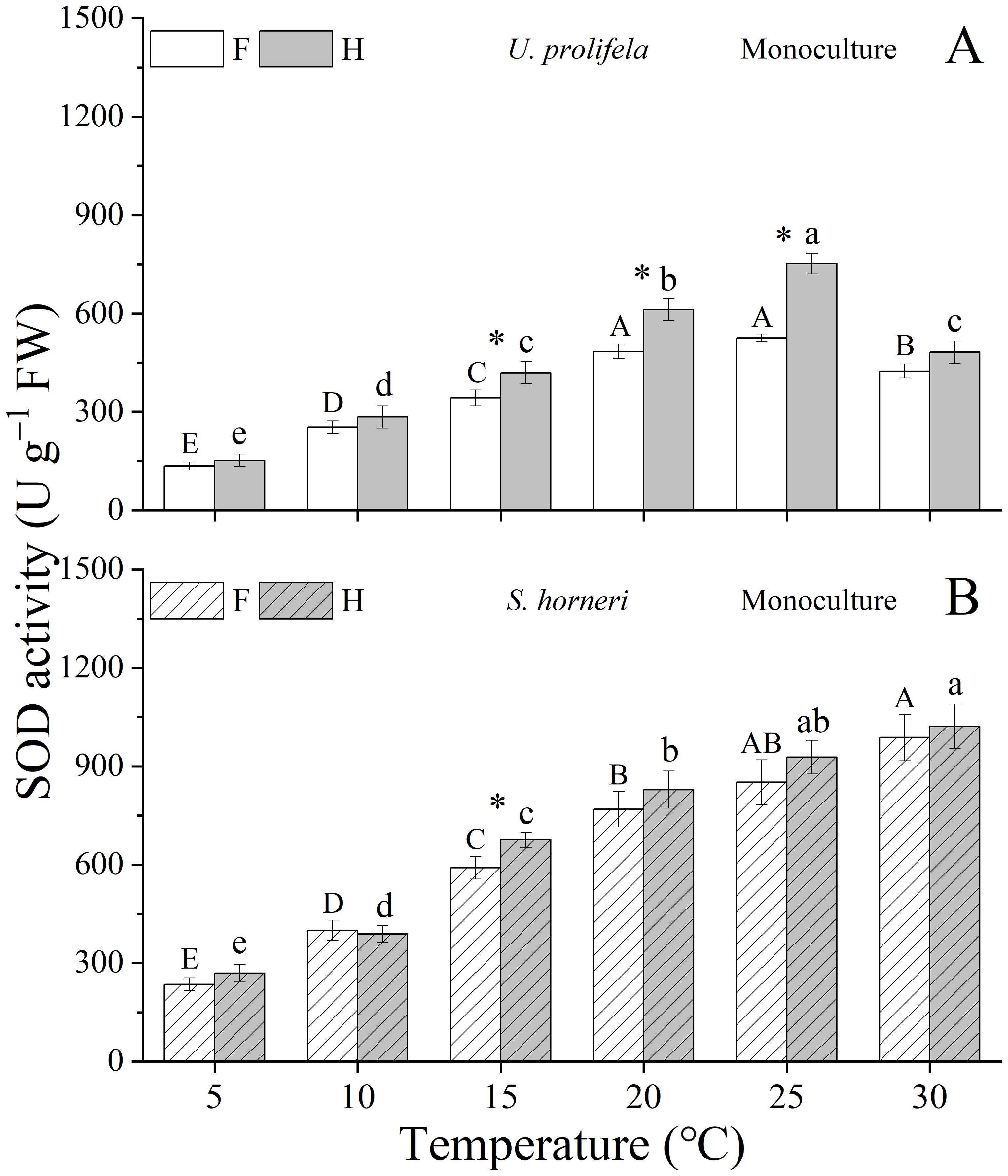
Figure 7. SOD activity of Ulva prolifera (A) and Sargassum horneri (B) grown under various temperature and nutrient conditions in the monoculture. Different uppercase letters represent significant differences among different temperature treatment under LN (p < 0.05), and different lowercase letters represent significant differences among different temperature treatment under HN (p < 0.05). Asterisk indicates whether there was a significant difference between LN and HN under the same temperature conditions (p < 0.05).
As for S. horneri, Temperature and nutrient had significant individual effect on SOD (Table 1, p < 0.001, p = 0.008). The SOD activity showed a significant increase by rising temperatures under both LN and HN conditions, with the maximum value of 987.8 ± 71.0 and 1021.8 ± 68.1 U g−1 FW at 30 °C, respectively (Figure 7B). HN enhanced the SOD significantly only at 15 °C compared with LN condition (p = 0.023).
4 Discussion
As main species of green and golden tides, U. prolifera and S. horneri both respond positively to temperature and eutrophication. In this study, the growth of U. prolifera and S. horneri, along with pigment concentrations and photosynthesis, increased with rising temperature, reaching a plateau at 15 − 25°C. However, when the temperature increased to 30°C, the growth of U. prolifera and S. horneri decreased abruptly, and the latter even suffered death. In cocultures, the growth of both U. prolifera and S. horneri was inhibited compared to the monocultures, with the greatest decrease in S. horneri at 25°C under two nutrient conditions.
4.1 Response of U. prolifera and S. horneri to temperature
Temperature is an important factor that limits the cellular enzymatic activities (Raven and Geider, 1988). In this study, proper warming promoted pigments synthesis in both U. prolifera and S. horneri in monoculture, which was reported in other macroalgae (Figures 2, 3) (Wu et al., 2022). The enhancement of Chl a and carotenoids in the two macroalgal genera allow the algae to absorb more light energy and maintain higher photosynthetic rates (Figure 4) (Jiang et al., 2016). In addition to its light-capture role, carotenoids can also act as auxiliary antioxidant that reduces damage caused by high temperature (Yoshiki et al., 2009). The high photosynthetic rate in PSII system, which provides more ATP and NADPH for subsequent physiological processes, improved the growth ultimately (Figure 1) (Jiang et al., 2016). Moreover, the rising temperature increased the mitochondrial respiration (Zou and Gao, 2014a). This similar phenomenon was observed for both algae at temperature from 5 to 25°C in this experiment (Figure 5). The increased consumption of carbon compound by respiration in nighttime could provide more ATP and carbon skeletons for the synthesis of pigment and soluble protein contents (Figures 2, 3, 6) (Zou and Gao, 2014a). Warming would cause algal cells to produce ROS, and algae can scavenge the increased reactive oxygen species (ROS) by activating antioxidant systems, one of which is superoxide dismutase (SOD), to prevent organelles from oxidative damage (Liu et al., 2017). The SOD activity of U. prolifera and S. horneri increased with rising temperature from 5 to 25°C (Figure 7). However, when temperature was up to 30°C, the scavenging efficiency of ROS by antioxidant in thalli was reduced, which led to the inhibition of all physiological parameters in both genera in this experiment. Although the SOD activity of U. prolifera was reduced at 30°C, it was still able to maintain low growth due to the highly environmental adaptability (Xiao et al., 2016). For S. horneri, the SOD activity was still at a high level at 30°C, but the thalli still suffered leaf shedding, which ultimately led to negative algal growth (Liu and Pang, 2010).
4.2 Effects of eutrophication on U. prolifera and S. horneri
In natural waters, Nitrogen and phosphorus are essential components for cellular metabolic synthesis and critical factors limiting algal primary productivity. Therefore, nutrient enrichment often enhances the physiological performance of Ulva spp (Kang et al., 2016; Li et al., 2016; Kang and Chung, 2017). In this study, pigment synthesis, soluble proteins were increased in both U. prolifera and S. horneri due to higher availability of nutrients, which ultimately improved their photosynthesis and growth (Figures 1-5). Furthermore, the morphology of Ulva spp. could enhance the nutrient uptake rates at elevated nutrient concentrations, affecting the metabolism of macroalgae, which could produce more Rubisco using nitrogen (Zer and Ohad, 2003). This is also verified by our results that nutrient enrichment promotes pigmentation and photosynthesis of U. prolifera more than that in S. horneri (Figures 2-4). Temperature plays a crucial role in the nutrient uptake, nitrate reductase activity of algae (Cade-Menun and Paytan, 2010; Gao et al., 2018). Our results also showed inconsistent enhancement effects of nutrient enrichment on the two macroalgal genera under various temperature conditions, indicating different nutrient requirements of macroalgae at different temperatures (Fan et al., 2014).
4.3 Competition between U. prolifera and S. horneri
In recent years, coexisting outbreaks of green and golden tides in coastal waters have occurred, yet have been little studied in laboratory (Xiao et al., 2020a; Zhao et al., 2021). In the present study, three temperature and two nutrient levels were selected to investigate the competition between U. prolifera and S. horneri. The results showed that the photosynthesis and growth of both U. prolifera and S. horneri in coculture were decreased compared to monoculture, suggesting ecological niche competition between the two genera (Figures 1, 4). Moreover, the pigment contents of U. prolifera did not change significantly in coculture compared to monoculture under both LN and HN conditions. However, an interesting finding is that the pigment contents of S. horneri were dramatically reduced, especially under nutrient-rich conditions (Figures 2, 3). Many factors could affect the coculture experiment, including shading, competition of nutrients, and allelopathy. The decline may be attributed to allelopathic effects from U. prolifera, as the abundant nutrients under HN condition are unlikely to be depleted given that the medium was renewed every 3 d to maintain nutrient levels. Additionally, the initial biomass of the thalli was consistent, with approximately 0.10 g FW for each species in monoculture and 0.05 + 0.05 g FW for both species in coculture. Furthermore, photosynthesis of both genera was declined compared to monoculture (Figure 4), suggesting that the allelopathic compounds may initially damage the photosynthetic apparatus, thereby inhibiting growth, as observed in other cocultures of macroalgae and microalgae (Ye and Zhang, 2013; Gao et al., 2019). Although the pigment contents of S. horneri was lower than those of U. prolifera, its photosynthesis was maintained at a higher level. Under these three temperature conditions, U. prolifera maintained a relatively stable photosynthetic rate, showing its stronger adaptability (Xiao et al., 2016). Meanwhile, at 15 and 20°C, S. horneri exhibited higher photosynthetic rates and lower respiration rates, resulted in more carbon accumulation for higher growth compared to U. prolifera under HN condition, suggesting that it was more competitive below 20°C (Figures 1, 4, 5). At 25°C, photosynthesis of S. horneri decreased and respiration increased dramatically, ultimately leading to a reduction in growth that was much lower than in monoculture (Figures 1, 3, 4). One reason for this phenomenon might be that temperature changes alter allelopathic efficiency and/or sensitivity (Semmouri et al., 2024). This suggests that coculture with U. prolifera weakened the resistance of S. horneri to high temperatures and exacerbated its apoptosis eventually. Further studies are required to confirm this conclusion, as allelopathic compounds were not directly measured in this study.
5 Conclusion
Our study investigated the combined impacts of ocean warming and eutrophication on the green tides and golden tides macroalgae and the interaction between them for the first time. As mentioned above, the temperatures in this study were achieved by different incubators, but one per temperature; therefore, the temperature effect is a combined temperature plus incubator effect. In conclusion, the findings demonstrate that the appropriate or seasonal temperature increases can promote the photosynthesis of U. prolifera and S. horneri. This effect is further exacerbated by eutrophication, which lead to the rapid blooms of Ulva and Sargassum and subsequently result in frequent outbreaks of green and golden tides. When green and gold tides occur simultaneously, the high environmental adaptivity of Ulva enables it to exacerbate the decline of Sargassum during periods of high temperatures. This suggests that green tides would outcompete golden tides in coastal waters under seasonal transition from spring to summer and even in future scenarios of ocean warming.
Data availability statement
The raw data supporting the conclusions of this article will be made available by the authors, without undue reservation.
Author contributions
HW: Conceptualization, Funding acquisition, Project administration, Supervision, Writing – original draft. JZ: Data curation, Formal analysis, Investigation, Methodology, Writing – original draft. HL: Writing – original draft, Writing – review & editing. SL: Formal analysis, Writing – original draft. CP: Data curation, Formal analysis, Investigation, Writing – original draft. LY: Formal analysis, Writing – review & editing. JX: Writing – review & editing. PH: Writing – review & editing.
Funding
The author(s) declare financial support was received for the research, authorship, and/or publication of this article. This study was supported by the Natural Science Foundation of Jiangsu Province (grant number BK20221398), National Natural Science Foundation of China (grant number 41706141), “333” project of Jiangsu Province, the Jiangsu Planned Projects for Postdoctoral Research Funds (grant number 2018K025A), “521 project” of Lianyungang city (grant number LYG06521202169), Open project of Jiangsu Institute of Marine Resources Development (grant number SH20231211), “Haiyan project” of Lianyungang city (grant number 2018-ZD-005).
Conflict of interest
The authors declare that the research was conducted in the absence of any commercial or financial relationships that could be construed as a potential conflict of interest.
Publisher’s note
All claims expressed in this article are solely those of the authors and do not necessarily represent those of their affiliated organizations, or those of the publisher, the editors and the reviewers. Any product that may be evaluated in this article, or claim that may be made by its manufacturer, is not guaranteed or endorsed by the publisher.
Supplementary material
The Supplementary Material for this article can be found online at: https://www.frontiersin.org/articles/10.3389/fmars.2024.1464511/full#supplementary-material
References
Apel K., Hirt H. (2004). Reactive oxygen species: metabolism, oxidative stress, and signal transduction. Annu. Rev. Plant Biol. 55, 373–399. doi: 10.1146/annurev.arplant.55.031903.141701
Bradford M. M. (1976). A rapid and sensitive method for the quantitation of microgram quantities of protein utilizing the principle of protein-dye binding. Anal. Biochem. 72, 248–254. doi: 10.1016/0003-2697(76)90527-3
Cade-Menun B. J., Paytan A. (2010). Nutrient temperature and light stress alter phosphorus and carbon forms in culture-grown algae. Mar. Chem. 121, 27–36. doi: 10.1016/j.marchem.2010.03.002
Chen B., Zou D., Du H., Ji Z. (2018). Carbon and nitrogen accumulation in the economic seaweed Gracilaria lemaneiformis affected by ocean acidification and increasing temperature. Aquaculture 482, 176–182. doi: 10.1016/j.aquaculture.2017.09.042
Dawes C. J., Koch E. W. (1990). Physiological responses of the red algae Gracllarla Verrucosa and G. Tikvahiae before and after nutrient enrichment. B. Mar. Sci. 46, 335–344.
Díez I., Muguerza N., Santolaria A., Ganzedo U., Gorostiaga J. (2012). Seaweed assemblage changes in the eastern Cantabrian Sea and their potential relationship to climate change. Estuar. Coast. Shelf S. 99, 108–120. doi: 10.1016/j.ecss.2011.12.027
Durack P. J., Gleckler P. J., Landerer F. W., Taylor K. E. (2014). Quantifying underestimates of long-term upper-ocean warming. Nat. Clim. Change 4, 999–1005. doi: 10.1038/nclimate2389
Fan X., Xu D., Wang Y., Zhang X., Cao S., Mou S., et al. (2014). The effect of nutrient concentrations, nutrient ratios and temperature on photosynthesis and nutrient uptake by Ulva prolifera: implications for the explosion in green tides. J. Appl. Phycol. 26, 537–544. doi: 10.1007/s10811-013-0054-z
Feng L., Shi X., Chen Y., Tang H., Wang L. (2021). Effects of temperature on the nitrate reductase activity and growth of Ulva prolifera. J. Phycol. 57, 955–966. doi: 10.1111/jpy.13141
Feng Y., Xiong Y., Hall-Spencer J. M., Liu K., Beardall J., Gao K., et al. (2024). Shift in algal blooms from micro-to macroalgae around China with increasing eutrophication and climate change. Global Change Biol. 30, e17018. doi: 10.1111/gcb.17018
Gao G., Clare A. S., Rose C., Caldwell G. S. (2018). Ulva rigida in the future ocean: potential for carbon capture, bioremediation and biomethane production. Gcb. Bioenergy 10, 39–51. doi: 10.1111/gcbb.12465
Gao G., Fu Q., Beardall J., Wu M., Xu J. (2019). Combination of ocean acidification and warming enhances the competitive advantage of Skeletonema costatum over a green tide alga, Ulva linza. Harmful Algae 85, 101698. doi: 10.1016/j.hal.2019.101698
Gattuso J.-P., Magnan A., Billé R., Cheung W. W., Howes E. L., Joos F., et al. (2015). Contrasting futures for ocean and society from different anthropogenic CO2 emissions scenarios. Science 349, aac4722. doi: 10.1126/science.aac4722
Granbom M., Chow F., De Oliveira M. C., Colepicolo P., De Paula E. J., et al. (2004). Characterisation of nitrate reductase in the marine macroalga Kappaphycus alvarezii (Rhodophyta). Aquat. Bot. 78, 295–305. doi: 10.1016/j.aquabot.2003.11.001
Hanley M. E., Firth L. B., Foggo A. (2024). Victim of changes? Marine macroalgae in a changing world. Ann. Bot-London 133, 1–16. doi: 10.1093/aob/mcad185
IPCC (2019). Special Report on the Ocean and Cryosphere in a Changing Climate. Eds. Pörtner H.-O., Roberts D. C., Masson-Delmotte V., Zhai P., Tignor M., Poloczanska E., Mintenbeck K., Alegría A., Nicolai M., Okem A., Petzold J., Rama B., Weyer N. M. (UK and New York, NY, USA: Cambridge).
Jiang H., Zou D., Li X. (2016). Growth, photosynthesis and nutrient uptake by Grateloupia livida (Halymeniales, Rhodophyta) in response to different carbon levels. Phycologia 55, 462–468. doi: 10.2216/16-11.1
Jueterbock A., Tyberghein L., Verbruggen H., Coyer J. A., Olsen J. L., Hoarau G. (2013). Climate change impact on seaweed meadow distribution in the North Atlantic rocky intertidal. Ecol. Evol. 3, 1356–1373. doi: 10.1002/ece3.541
Kang J. W., Chung I. K. (2017). The effects of eutrophication and acidification on the ecophysiology of Ulva pertusa Kjellman. J. Appl. Phycol. 29, 2675–2683. doi: 10.1007/s10811-017-1087-5
Kang E. J., Kim J.-H., Kim K., Kim K. Y. (2016). Adaptations of a green tide forming Ulva linza (Ulvophyceae, Chlorophyta) to selected salinity and nutrients conditions mimicking representative environments in the Yellow Sea. Phycologia 55, 210–218. doi: 10.2216/15-67.1
Krause-Jensen D., Duarte C. M. (2016). Substantial role of macroalgae in marine carbon sequestration. Nat. Geosci. 9, 737–742. doi: 10.1038/ngeo2790
Li S., Yu K., Huo Y., Zhang J., Wu H., Cai C.e., et al. (2016). Effects of nitrogen and phosphorus enrichment on growth and photosynthetic assimilation of carbon in a green tide-forming species (Ulva prolifera) in the Yellow Sea. Hydrobiologia 776, 161–171. doi: 10.1007/s10750-016-2749-z
Liu D., Keesing J. K., Xing Q., Shi P. (2009). World’s largest macroalgal bloom caused by expansion of seaweed aquaculture in China. Mar. pollut. Bull. 58, 888–895. doi: 10.1016/j.marpolbul.2009.01.013
Liu F., Liu X., Wang Y., Jin Z., Moejes F. W., Sun S. (2018). Insights on the Sargassum horneri golden tides in the Yellow Sea inferred from morphological and molecular data. Limnol. Oceanogr. 63, 1762–1773. doi: 10.1002/lno.10806
Liu F., Pang S. J. (2010). Stress tolerance and antioxidant enzymatic activities in the metabolisms of the reactive oxygen species in two intertidal red algae Grateloupia turuturu and Palmaria palmata. J. Exp. Mar. Biol. Ecol. 382, 82–87. doi: 10.1016/j.jembe.2009.11.005
Liu F., Pang S. J., Xu N., Shan T. F., Sun S., Hu X., et al. (2010). Ulva diversity in the Yellow Sea during the large-scale green algal blooms in 2008–2009. Phycol. Res. 58, 270–279. doi: 10.1111/j.1440-1835.2010.00586.x
Liu J., Xia J., Zhuang M., Zhang J., Sun Y., Tong Y., et al. (2021a). Golden seaweed tides accumulated in Pyropia aquaculture areas are becoming a normal phenomenon in the Yellow Sea of China. Sci. Total Environ. 774, 145726. doi: 10.1016/j.scitotenv.2021.145726
Liu J., Xia J., Zhuang M., Zhang J., Yu K., Zhao S., et al. (2021b). Controlling the source of green tides in the Yellow Sea: NaClO treatment of Ulva attached on Pyropia aquaculture rafts. Aquaculture 535, 736378. doi: 10.1016/j.aquaculture.2021.736378
Liu C., Zou D., Yang Y., Chen B., Jiang H. (2017). Temperature responses of pigment contents, chlorophyll fluorescence characteristics, and antioxidant defenses in Gracilariopsis lemaneiformis (Gracilariales, Rhodophyta) under different CO2 levels. J. Appl. Phycol. 29, 983–991. doi: 10.1007/s10811-016-0971-8
Luo M. B., Liu F., Xu Z. L. (2012). Growth and nutrient uptake capacity of two co-occurring species, Ulva prolifera and Ulva linza. Aquat. Bot. 100, 18–24. doi: 10.1016/j.aquabot.2012.03.006
Machado J. P., Oliveira V. P. (2024). Seaweed functional ecology models: a comprehensive review of theory and applications. J. Appl. Phycol., 1–16. doi: 10.1007/s10811-024-03293-z
Malone T. C., Newton A. (2020). The globalization of cultural eutrophication in the coastal ocean: causes and consequences. Front. Mar. Sci. 7. doi: 10.3389/fmars.2020.00670
Martin S., Gattuso J. P. (2009). Response of Mediterranean coralline algae to ocean acidification and elevated temperature. Global Change Biol. 15, 2089–2100. doi: 10.1111/j.1365-2486.2009.01874.x
Merzbach D., Obedeanu N. (1975). Standardisation of the nitroblue-tetrazolium test. J. Med. Microbiol. 8, 375–384. doi: 10.1099/00222615-8-2-375
Mineur F., Arenas F., Assis J., Davies A. J., Engelen A. H., Fernandes F., et al. (2015). European seaweeds under pressure: Consequences for communities and ecosystem functioning. J. Sea Res. 98, 91–108. doi: 10.1016/j.seares.2014.11.004
Ott F. D. (1965). Synthetic media and techniques for the xenic cultivation of marine algae and flagellate. Va. J. Sci. 16, 205–218.
Paerl H. W., Hall N. S., Peierls B. L., Rossignol K. L. (2014). Evolving paradigms and challenges in estuarine and coastal eutrophication dynamics in a culturally and climatically stressed world. Estuar. Coast. 37, 243–258. doi: 10.1007/s12237-014-9773-x
Pakker H., Breeman A. (1996). Temperature responses of tropical to warm-temperate Atlantic seaweeds. II. Evidence for ecotypic differentiation in amphi-Atlantic tropical-Mediterranean species. Eur. J. Phycol. 31, 133–141. doi: 10.1080/09670269600651301
Porra R. J., Thompson W. A., Kriedemann P. E. (1989). Determination of accurate extinction coefficients and simultaneous equations for assaying chlorophylls a and b extracted with four different solvents: verification of the concentration of chlorophyll standards by atomic absorption spectroscopy. BBA-Bioenergetics 975, 384–394. doi: 10.1016/S0005-2728(89)80347-0
Raven J. A., Geider R. J. (1988). Temperature and algal growth. New Phytol. 110, 441–461. doi: 10.1111/j.1469-8137.1988.tb00282.x
Semmouri I., Janssen C. R., Asselman J. (2024). Allelopathy in macroalgae: Ecological principles, research opportunities and pitfalls reviewed. J. Appl. Phycol. 36, 441–458. doi: 10.1007/s10811-023-03110-z
Su L., Shan T., Pang S., Li J. (2018). Analyses of the genetic structure of Sargassum horneri in the Yellow Sea: implications of the temporal and spatial relations among floating and benthic populations. J. Appl. Phycol. 30, 1417–1424. doi: 10.1007/s10811-017-1296-y
Sun S., Wang F., Li C., Qin S., Zhou M., Ding L., et al. (2008). Emerging challenges: Massive green algae blooms in the Yellow Sea. Nat. Precedings, 1–1. doi: 10.1038/npre.2008.2266.1
Taylor R., Fletcher R., Raven J. (2001). Preliminary studies on the growth of selected ‘green tide’algae in laboratory culture: effects of irradiance, temperature, salinity and nutrients on growth rate. Bot. Mar. 44, 327–336. doi: 10.1515/BOT.2001.042
Wang Z., Yuan C., Zhang X., Liu Y., Fu M., Xiao J. (2023). Interannual variations of Sargassum blooms in the Yellow Sea and East China Sea during 2017–2021. Harmful Algae 126, 102451. doi: 10.1016/j.hal.2023.102451
Wang Y., Zhang J., Wu W., Liu J., Ran X., Zhang A., et al. (2022). Variations in the marine seawater environment and the dominant factors in the Lianyungang coastal area. Reg. Stud. Mar. Sci. 52, 102276. doi: 10.1016/j.rsma.2022.102276
Wellburn A. R. (1994). The spectral determination of chlorophylls a and b, as well as total carotenoids, using various solvents with spectrophotometers of different resolution. J. Plant Physiol. 144, 307–313. doi: 10.1016/S0176-1617(11)81192-2
Wu H., Chen J., Feng J., Liu Y., Li X., Chen R., et al. (2022). Thermal fluctuations and nitrogen enrichment synergistically accelerate biomass yield of Pyropia haitanensis. Aquat. Bot. 179, 103501. doi: 10.1016/j.aquabot.2022.103501
Xia Z., Liu J., Zhao S., Sun Y., Cui Q., Wu L., et al. (2024). Review of the development of the green tide and the process of control in the southern Yellow Sea in 2022. Estuar. Coast. Shelf S. 30, 108772. doi: 10.1016/j.ecss.2024.108772
Xiao J., Fan S., Wang Z., Fu M., Song H., Wang X., et al. (2020a). Decadal characteristics of the floating Ulva and Sargassum in the Subei Shoal, Yellow Sea. Acta Oceanol. Sin. 39, 1–10. doi: 10.1007/s13131-020-1655-4
Xiao J., Wang Z., Song H., Fan S., Yuan C., Fu M., et al. (2020b). An anomalous bi-macroalgal bloom caused by Ulva and Sargassum seaweeds during spring to summer of 2017 in the western Yellow Sea, China. Harmful Algae 93, 101760. doi: 10.1016/j.hal.2020.101760
Xiao J., Zhang X., Gao C., Jiang M., Li R., Wang Z., et al. (2016). Effect of temperature, salinity and irradiance on growth and photosynthesis of Ulva prolifera. Acta Oceanol. Sin. 35, 114–121. doi: 10.1007/s13131-016-0891-0
Xing Q., Guo R., Wu L., An D., Cong M., Qin S., et al. (2017). High-resolution satellite observations of a new hazard of golden tides caused by floating Sargassum in winter in the Yellow Sea. IEEE Geosci. Remote S. 14, 1815–1819. doi: 10.1109/LGRS.2017.2737079
Xu J., Gao K. (2012). Future CO2-induced ocean acidification mediates the physiological performance of a green tide alga. Plant Physiol. 160, 1762–1769. doi: 10.1104/pp.112.206961
Yang H., Zhou Y., Mao Y., Li X., Liu Y., Zhang F. (2005). Growth characters and photosynthetic capacity of Gracilaria lemaneiformis as a biofilter in a shellfish farming area in Sanggou Bay, China. J. Appl.Phycol. 17, 199–206. doi: 10.1007/s10811-005-6419-1
Ye C. P., Zhang M. C. (2013). Allelopathic inhibitory effects of the dried macroalga Ulva pertusa on the photosynthetic activities of red tide-causing microalga Skeletonema costatum. Adv. Mater. Res. 726, 29–34. doi: 10.4028/www.scientific.net/AMR.726-731.29
Yoshida T. (1963). Studies on the distribution and drift of the floating seaweeds. Bull. Tohoku Reg. Fish. Res. Lab. 23, 141–186.
Yoshiki M., Tsuge K., Tsuruta Y., Yoshimura T., Koganemaru K., Sumi T., et al. (2009). Production of new antioxidant compound from mycosporine-like amino acid, porphyra-334 by heat treatment. Food Chem. 113, 1127–1132. doi: 10.1016/j.foodchem.2008.08.087
Zer H., Ohad I. (2003). Light, redox state, thylakoid-protein phosphorylation and signaling gene expression. Trends Biochem. Sci. 28, 467–470. doi: 10.1016/S0968-0004(03)00173-7
Zhang J., Ding X., Zhuang M., Wang S., Chen L., Shen H., et al. (2019). An increase in new Sargassum (Phaeophyceae) blooms along the coast of the East China Sea and Yellow Sea. Phycologia 58, 374–381. doi: 10.1080/00318884.2019.1585722
Zhao X., Zhong Y., Zhang H., Qu T., Hou C., Guan C., et al. (2021). Comparison of environmental responding strategies between Ulva prolifera and Sargassum horneri: an in-situ study during the co-occurrence of green tides and golden tides in the Yellow Sea, China in 2017. J. Oceanol. Limnol. 39, 2252–2266. doi: 10.1007/s00343-021-0397-2
Zou D., Gao K. (2014a). The photosynthetic and respiratory responses to temperature and nitrogen supply in the marine green macroalga Ulva conglobata (Chlorophyta). Phycologia 53, 86–94. doi: 10.2216/13-189.1
Keywords: competition, eutrophication, Sargassum horneri, temperature, Ulva prolifera
Citation: Wu H, Zhang J, Li H, Li S, Pan C, Yi L, Xu J and He P (2024) Ocean warming enhances the competitive advantage of Ulva prolifera over a golden tide alga, Sargassum horneri under eutrophication. Front. Mar. Sci. 11:1464511. doi: 10.3389/fmars.2024.1464511
Received: 14 July 2024; Accepted: 06 September 2024;
Published: 01 October 2024.
Edited by:
Christopher Edward Cornwall, Victoria University of Wellington, New ZealandCopyright © 2024 Wu, Zhang, Li, Li, Pan, Yi, Xu and He. This is an open-access article distributed under the terms of the Creative Commons Attribution License (CC BY). The use, distribution or reproduction in other forums is permitted, provided the original author(s) and the copyright owner(s) are credited and that the original publication in this journal is cited, in accordance with accepted academic practice. No use, distribution or reproduction is permitted which does not comply with these terms.
*Correspondence: Hailong Wu, hlwu@jou.edu.cn