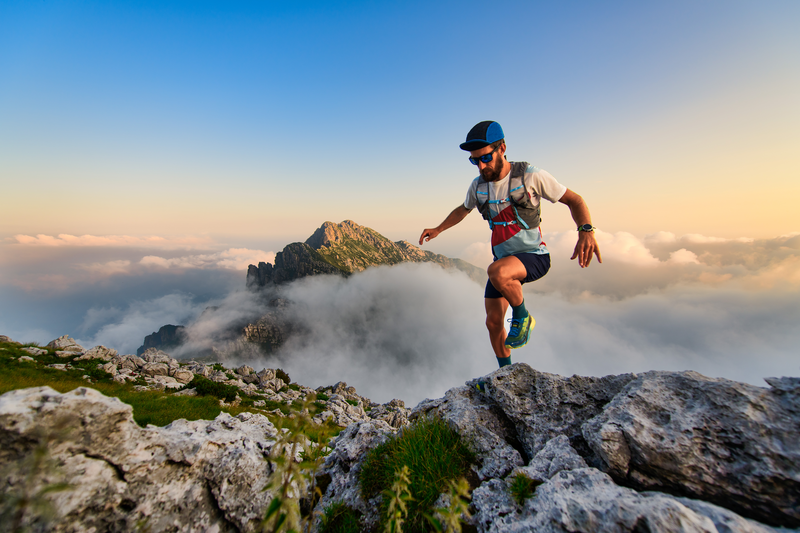
95% of researchers rate our articles as excellent or good
Learn more about the work of our research integrity team to safeguard the quality of each article we publish.
Find out more
ORIGINAL RESEARCH article
Front. Mar. Sci. , 17 January 2025
Sec. Coastal Ocean Processes
Volume 11 - 2024 | https://doi.org/10.3389/fmars.2024.1439266
This article is part of the Research Topic Carbon Cycle Vulnerability Across Coastal and Forested Wetlands in Response to Anthropogenic Perturbations View all 7 articles
Mangrove plantation is a fundamental approach for the sustainable management of tropical and subtropical coasts to capture and store atmospheric carbon. However, it is unknown whether the carbon accumulation potential of planted mangroves is as much as that of natural mangroves. Moreover, the effects of tree species, forest age, and hydrodynamic conditions on carbon storage are still unclear. This study investigated the carbon storage potential and influencing factors for planted mangroves in Kaozhouyang, Huidong County, Guangdong Province. The Vegetation carbon stock was calculated by community parameters collected from field investigation, and ecosystem carbon stock was calculated by the sum of vegetation and sediment. The results showed that mangrove plantation significantly increased the carbon stock of the vegetation and soil (vegetation carbon stock = 9.9645.06 t C/ha; soil carbon stock = 70.37-110.64 t C/ha) compared with the nonvegetation mudflat (63.73 t C/ha). However, the ecosystem carbon stock of the planting sites was still lower than that of natural Avicennia marina (282.86 t C/ha), with the significant difference mainly reflected on the soil carbon stock (p < 0.05). Further results revealed that carbon accumulation was affected by forest age, tree species, and tidal level. The vegetation biomass/carbon stock gradually increased with forest age (p < 0.05), but the difference was not significant for soil carbon stock, which indicated that the carbon accumulation was mainly concentrated on the vegetation at the early stage of mangrove restoration. In addition, suitable habitat conditions (landward) and fast-growing species (Sonneratia apetala) are more beneficial to carbon accumulation. Our results suggest that mangrove plantations can achieve carbon storage and sequestration in vegetation and soil for increasing carbon sinks with suitable species selection and management.
As a typical coastal blue carbon ecosystem, mangrove forests play an important role in the global carbon cycle (Atwood et al., 2017; Alongi et al., 2009; Alongi, 2012). Although mangrove forests occupy only 0.5% of the global coastal area, they account for 10%–15% of the coastal carbon storage (Duarte et al., 2005) and may be responsible for approximately 10% of the global terrestrial organic carbon (OC) exports to coastal oceans (Jennerjahn and Ittekkot, 2002; Perez et al., 2018; Peréz et al., 2022). Therefore, mangrove forests are among the most carbon-dense coastal vegetation ecosystems, with higher productivity and carbon capture efficiency in sediments than terrestrial ecosystems (Kauffman et al., 2016; Kauffman et al., 2020; Wang et al., 2019). However, approximately 35% of the world’s mangroves have been lost in the last century due to the harvesting of shrimp ponds, excessive timber harvesting, changing water flow patterns, environmental pollution, and increasing urbanization (Ren et al., 2010), with an estimated annual deforestation rate of 1%–3% (Alongi, 2012). Globally, tidal wetlands (e.g., tidal flats, tidal marshes, and mangroves) have lost nearly 4,000 km2 from 1999 to 2019 (Murray et al., 2022). The deforestation and the degradation of mangrove forests have accelerated the mineralization of OC in mangrove sediments, leading to massive greenhouse gas emissions into the atmosphere (Atwood et al., 2017).
Mangrove plantation is the most common approach to restoration and afforestation (Lee et al., 2019). By 2020, the total area of mangrove forests in China has been estimated to be 28,900 ha, with 33% being newly planted mangroves (Wang et al., 2021). Furthermore, the area will be increased by 18,800 ha through cultivation and restoration by 2025, according to “the Special Action Plan for Mangrove Protection and Restoration (2020–2025)” issued by the Chinese government. However, although planting mangroves to offset carbon emissions has been increasingly valued, there is limited understanding of whether this would contribute to blue carbon services similar to natural forests.
Mangrove plantation is crucial to mitigating global climate change (Ahmed et al., 2022). Many studies have indicated that planting mangroves can increase the vegetation and soil carbon storage (Ren et al., 2010; Osland et al., 2012; Lunstrum and Chen, 2014; Dung et al., 2016; Chen et al., 2018; He et al., 2018; Feng et al., 2019). The vegetation biomass could reach maturity after planting 20 years (20a), but the soil carbon storage needs to take 70a or more to reach maturity (Osland et al., 2012). Carbon storage is affected by many factors, including forest age, tree species/density, tidal level, growth mode, and other biological and abiotic factors (Aye et al., 2023). Tree species are considered as important factors affecting growth and biomass accumulation (Xiong et al., 2019), and growth rates from high to low are observed in fast-growing arbor, arbor, and shrubs (Hu et al., 2019a, 2019b). For example, the ecosystem carbon stock of Sonneratia apetala (262.03 t C/ha) was higher than that of native species such as Avicennia marina (212.88 t C/ha) in Yingluo Bay, Guangdong Province, South China (Wang et al., 2013). Another study also found that the biomass of Kandelia obovata in arbor form was higher than that of A. marina in shrub form of the same age in Luoyang River Estuary, South Fujian, China (Chen et al., 2021a). In addition, forest age also significantly affected the carbon sequestration, and the vegetation carbon accumulation rate (CAR) was higher than that of soil in the early stage of mangrove plantation (Osland et al., 2012). In addition, the carbon accumulation in mangrove ecosystems was also affected by tidal levels, with mangroves at high tide generally having higher carbon stocks (Wang et al., 2013). However, only a few studies have comprehensively considered the influencing factors of carbon accumulation in planted mangroves.
As one of the four 10,000-acre mangrove demonstration areas in Guangdong Province, Kaozhouyang has conducted mangrove restoration and plantation since 2011. Currently, more than 3,000 acre of mangroves have been planted in Kaozhouyang, and the tree species mainly included Rhizophora stylosa, K. obovata, A. marina, and S. apetala. In addition, natural A. marina are also present in Kaozhouyang, with a forest age of over 60 years. In this study, the carbon storage rates of planted and natural A. marina were systemically compared to assess the carbon storage potential of planted mangroves and to further explore the effects of tree species, forest age, and tidal level on carbon sequestration at the early stage of mangrove restoration.
The study area is located at the “10,000-acre mangrove Demonstration Zone of Kaozhouyang” in Huidong County, Huizhou City, Guangdong Province. It was historically rich in mangrove forests, most of which were destroyed before 2000 due to aquaculture, seawall construction, and water pollution. Fortunately, the Department of Natural Resources of Huizhou Bureau organized and managed the plantation of native species such as K. obovata, R. stylosa, and A. marina, as well as exotic species of S. apetala, since 2011. At present, the mangrove forests in Kaozhouyang are growing well, with the main tree species including R. stylosa, A. marina, Aegiceras corniculatum, Bruguiera gymnorrhiza, K. obovata, S. apetala, and other true and semi-mangrove plants.
The study area has a subtropical marine monsoon climate, with an average annual temperature of 23.1°;C, average rainfall of 1,266.5 mm, average wind speed of 2.7 m/s, and a total sunshine time of 2,335.3 h.
Field data [e.g., tree species, height, and diameter at breast height (DBH)] were collected from six survey sites to estimate the vegetation biomass and the carbon stock. The distribution of the survey sites is shown in Figure 1. A mudflat without any vegetation was used as a reference for pre-restoration. Three 10 m × 10 m quadrats were investigated at each survey site as repetitions. All trees were identified, and the number of trees of each species, the tree height, the DBH/basal diameter, and the survival status (healthy or dead) were recorded in each quadrat. No dead trees were found during this survey. Vertical differences were measured to record the microtopographic features using real-time kinematic (RTK), and the forest age was determined based on historical remote sensing images and the data provided by the local government. All field investigations were completed in August and September 2023. The elevation, location, and community composition of the survey sites are shown in Figure 2 and in Tables 1, 2.
Figure 1. Locations of the survey sites in this study. (A) Guangdong Province. (B) Huidong County. (C) Survey sites at Kaozhouyang.
Figure 2. Mangrove communities in the different survey sites. 3a/6a/11a represent the forest age of the mangrove communities. High and low tides are distinguished by the elevation at the sampling site.
The aboveground biomass (AGB) and the belowground biomass (BGB) were calculated using the allometric growth equations of specific tree species based on DBH/D0 and tree height (Table 3) (Ren et al., 2010; Wang et al., 2013). The total forest biomass (TFB) is equal to the sum of AGB and BGB. The carbon conversion coefficients of AGB and BGB were set at 0.45 and 0.39, respectively, to calculate the above- and belowground vegetation carbon stocks according to the coastal blue carbon accounting guidelines published by the International Union for Conservation of Nature (IUCN) (Kauffman et al., 2011; Peng et al., 2023).
CAR can be calculated according to the difference in the carbon stock before and after mangrove restoration. The calculation formula for vegetation is as follows:
where TCBt2 represents the current vegetation carbon stocks and TCBt1 represents the carbon stock before restoration, which is 0 t C/ha in this study. t2 and t1 represent the different monitoring times.
Three soil samples down to 50 cm were collected within each quarter using a PVC pipe of 7 cm diameter, as the carbon accumulation of newly planted mangroves is mainly concentrated on the soil surface. Moreover, another three soil samples were also collected from the mudflat far away from the mangroves. The soil samples were then divided into five layers of 0–10, 10–20, 20–30, 30–40, and 40–50 cm for bulk density and soil organic carbon (SOC) analyses. The soil samples were freeze-dried and sieved to 0.15 mm to remove plant residues and roots. Subsequently, the SOC was determined with the potassium dichromate (K2Cr2O7) oxidation–external heating method, while bulk density was determined by dividing the dry weight of the sample by the volume. All parameters were assessed in triple repeated analysis.
The average SOC stock of the surface 50 cm was calculated using the following formula (Yu et al., 2021; Zhu et al., 2012):
where SOCS is the soil stock of soil layer i, Di represents the bulk density of soil layer i, Mi is the SOC content of soil layer i, and Hi represents the depth interval of soil layer i.
Due to the negligible amount of litter in this investigation, the ecosystem carbon stock was equal to the sum of the vegetation (both aboveground and underground) and soil carbon stocks. Assessment of the carbon stocks of restored mangroves will contribute to marketable carbon stocks in China’s blue carbon transactions (Macreadie et al., 2022).
The vegetation biomass, SOC content, SOC stock, and ecosystem carbon stock were statistically analyzed using one-way analysis of variance (ANOVA) with SPSS 27.0 software. Duncan’s method was used to evaluate significant differences between the mean values of the different mangrove communities at the 95% confidence level.
In this study, the carbon stocks in the planting sites obviously increased compared with that in the mudflat (63.73 ± 9.27 t C/ha). Among them, the low-tide 6a-R. stylosa (6-year-old R. stylosa) and 11a-S. apetala had the largest carbon storage, with ecosystem carbon stocks of 133.60 ± 7.42 and 144.01 ± 27.87 t C/ha, respectively (Table 4, Figure 3). In addition, most of the carbon was concentrated in the soil layers, accounting for 66.27% and 76.83%, respectively. The carbon stocks of 3a-R. stylosa and 6a-R. stylosa at high tide and of 11a-K. obovata were 86.44 ± 4.27, 100.93 ± 13.16, and 94.96 ± 8.90 t C/ha, respectively, while the proportions of soil carbon stock were 85.72%, 69.72%, and 89.51%, respectively.
Table 4. Summary of the aboveground biomass/carbon stock (AGB/AGBC), belowground biomass/carbon stock (BGB/BGBC), total biomass (TBC), soil carbon stock (SOCD), and ecosystem carbon stock (TCS) at the different survey sites.
Figure 3. Ecosystem carbon stocks of the different mangrove communities. Capital letter represents survey sites.
However, the ecosystem carbon stocks of the five planting sites were obviously lower than that of natural A. marina (p < 0.05), and the differences were mainly reflected in the vegetation and soil layers. The vegetation, soil, and ecosystem carbon stocks of natural A. marina were 43.49 ± 2.89, 239.37 ± 35.25, and 284.93 ± 32.55 t C/ha, respectively, and nearly 85% of the carbon was stored in the soil. Furthermore, the soil carbon stocks in all planting sites only accounted for 37%–46% of natural A. marina, but the vegetation carbon stocks in 11a-S. apetala (33.37 ± 5.22 t C/ha) and the high-tide 6a-R. stylosa (45.06 ± 6.29 t C/ha) were relatively close to that of natural A. marina (43.49 ± 2.89 t C/ha) (Table 4, Figure 4).
Figure 4. Biomass and carbon stock of vegetation at the different investigation sites. Capital letter represents survey sites.
Variations of the ecosystem carbon stocks with forest age were determined by comparing 0a-mudflat, high-tide 3a-R. stylosa, and 6a-R. stylosa (Table 4, Figures 3, 4). The results showed that the ecosystem carbon stock of 0a-mudflat (63.73 ± 9.27 t C/ha) was much lower than that of 3a-R. stylosa (86.44 ± 4.27 t C/ha) and 6a-R. stylosa (133.60 ± 7.42 t C/ha; p < 005), and the proportion of soil carbon stock gradually decreased from 100% to 66.27% with increasing forest age. Furthermore, the vegetation carbon stock of 6a-R. stylosa (45.06 ± 6.29 t C/ha) was significantly higher than that of 3a-R. stylosa (12.34 ± 3.99 t C/ha; p < 0.05), and the vegetation carbon stock increased by nearly three times with forest age from 3a to 6a. However, the soil carbon stock only increased slightly from 3a to 6a (from 74.10 ± 2.69 to 88.54 ± 2.52 t C/ha; p > 0.05).
The results showed that the vegetation, soil, and ecosystem carbon stocks increased gradually with forest age (p < 0.05) at the early stage of mangrove restoration. Furthermore, the vegetation carbon stock increased by nearly three times with forest age from 3a to 6a, while the soil carbon storage only slightly increased.
The effect of tree species on carbon storage was determined by comparing 11a-K. obovata and 11a-S. apetala at similar tidal levels (Table 4, Figure 3). The vegetation, soil, and ecosystem carbon stocks of 11a-K. obovata (9.96 ± 2.54, 85.00 ± 6.73, and 94.96 ± 8.90 t C/ha, respectively) were significantly lower than those of 11a-S. apetala (33.37 ± 3.10, 110.64 ± 22.67, and 144.01 ± 27.87 t C/ha, respectively; p < 0.05). As a pioneer species, S. apetala has been introduced since 2011 due to its fast growth rate; therefore, the proportion of vegetation carbon stock of 11a-S. apetala was higher than that of 11a-K. obovata.
The effect of tidal level on carbon storage was determined by comparing 6a-R. stylosa at high tide and low tide (Table 4, Figure 3). The carbon stock of high-tide 6a-R. stylosa (133.60 ± 7.42 t C/ha) was much higher than that of low-tide 6a-R.stylosa (100.93 ± 13.16 t C/ha; p < 0.05). In addition, the vegetation and soil carbon stocks of low-tide 6a-R. stylosa were 30.56 ± 5.22 t C/ha (aboveground/belowground vegetation carbon stock: 20.34 ± 4.90/10.22 ± 0.38 t C/ha) and 71.84 ± 8.47 t C/ha, respectively, and the percentage of soil carbon stock was close to 70%. In high-tide 6a-R. stylosa, the carbon stocks of vegetation and soil were 45.06 ± 6.29 t C/ha (aboveground: 30.65 ± 4.74 and 14.41 ± 1.57 t C/ha) and 88.54 ± 2.52 t C/ha, respectively, and the proportion of soil carbon stock was 66%.
Mangrove plantations can significantly increase the mangrove area, vegetation biomass/stock, soil carbon stock, and soil accretion (Ren et al., 2010; Lunstrum and Chen, 2014; Dung et al., 2016; Pham et al., 2017; Chen et al., 2018; Wang et al., 2021). The carbon storage of five planting sites was higher than that of the mudflat, particularly that of 6a-R. stylosa and 11a-S. apetala, indicating that mangrove plantation is beneficial for carbon storage. Among the selected tree species, the carbon stock of 11a-S. apetala was higher than that of 11a-K. obovata and 6a-R. stylosa, indicating that the selected tree species affected the carbon storage (discussed in Section 4.3). In addition, the average biomass CAR (4.13 t C ha−1 a−1) was higher than that of the soil (2.98 t C ha−1 a−1) in the present study. Osland et al. (2012) found that the biomass CAR (global average = 4.56 t C ha−1 a−1) was higher than that of soil (global average = 2.64 t C ha−1 a−1) below 20a, which is consistent with the current study. Therefore, the CAR of vegetation is higher than that of soil at the early stage of mangrove plantation, and the selection of suitable habitat conditions, tree species, and planting measures at the initial stage of mangrove restoration can significantly enhance the carbon sequestration effect and mitigate global climate change (Chen et al., 2020).
However, the ecosystem carbon stocks of planted mangroves were much lower than that of natural A. marina, and the differences were mainly reflected in the soil layers. The soil carbon stock of mature mangrove forests is approximately 230 t C/ha (Ouyang and Lee, 2020), which is similar to that of the natural A. marina in this study. A previous study found that more than 70a would be required for soil layers for mangroves to reach maturity, with an average carbon sequestration rate of approximately 1.74 t C ha−1 a−1 (Alongi, 2014). In this study, the soil carbon storage in most planted sites was less than 100 t C/ha, indicating that the soil still has significant carbon sequestration potential.
Carbon storage is mainly reflected in the vegetation and sediment carbon pool of a mangrove ecosystem, while the carbon accumulation in litter and dead wood is relatively low (Ouyang and Lee, 2020; Chen et al., 2021a). The results showed that the aboveground, belowground, and vegetation carbon stocks of 6a-R. stylosa were higher than that of 3a-R. stylosa, and the vegetation biomass/stock was close to that of the natural A. marina forest, indicating that the vegetation biomass accumulated much faster before maturity.
Carbon sequestration in a mangrove ecosystem is affected by many factors (Duke, 2001; Pan et al., 2004; Damschroder et al., 2009), of which forest age can largely determine the accumulation of biomass and SOC (Daniel et al., 2009; Hu et al., 2022). Several studies have suggested that the increase of tree mass with forest age is accompanied by the accumulation of biomass (Song et al., 2024). Zakaria et al. (2023) demonstrated that the ecosystem carbon stock in different ages (i.e., 1a, 2a, 5a, 9a, 10a, and 21a) of planted mangroves increased with forest age, with the 10a mangrove having the highest ecosystem carbon stock (875.79 t C/ha). Yu et al. (2020) compared the differences in the vegetation biomass/carbon stocks of S. apetala of different forest ages (i.e., 1a, 4a, 9a, and 15a) and of native 40a-K. obovata and found a positive and linear relationship between the vegetation biomass/carbon stock and forest age of S. apetala, with 15a-S. apetala having a similar biomass/carbon stock to that of natural 40a-K. obovata. Song et al. (2024) demonstrated that the mangrove vegetation and root carbon stock in Qinzhou Bay gradually increased with forest age and reached maturity at 15a. The global average carbon stock of mature mangrove vegetation is approximately 200 t C/ha, which needs to take approximately 20a to reach maturity. Therefore, the mangrove ecosystem in Kaozhouyang still has a large carbon sequestration potential.
Although the SOC stocks for 3a-R. stylosa and 6a-R. stylosa were higher than that of the mudflat, there was no significant difference between the three sites, which may be due to a hysteresis effect of soil carbon accumulation and the relatively shorter monitoring time. The balance between carbon input and carbon consumption/decomposition generally determines the carbon sequestration and storage of mangrove ecosystems. In addition, the reason for the unchanged SOC stock during the study period could be that the SOC input was basically offset by carbon consumption/decomposition (Lu et al., 2014). A previous study showed that the soil develops more slowly than vegetation in the early stage of mangrove plantation and that the accumulation of SOC takes a longer time than vegetation (Osland et al., 2012). Chen et al. (2021b) found that the sequestered OC was considerably less in soil than in biomass before the vegetation has reached maturity between 12a-K. obovata and 12a-A. corniculatum, and the accumulation of OC in mangrove soil required 40a or longer to reach equilibrium (237.4 t C/ha) at a global scale (Ouyang and Lee, 2020). Therefore, this study indicates that the accumulation of OC is mainly concentrated in vegetation and that carbon accumulation in soil is slower before the mangroves reach maturity.
The vegetation and soil carbon stocks of 11a-S. apetala were much higher than those of the 11a-K. obovata in this study, indicating that the tree species significantly affected carbon storage. The growth rates of the different mangrove tree species varied, and arborous species or fast-growing mangroves showed higher growth rates than shrubs (Chen et al., 2021b). S. apetala has characteristics of high biomass, energy accumulation, and high return, and its productivity level is at a higher level in China’s mangrove communities. Therefore, non-native S. apetala plantations have been widely recommended for China’s mangrove restoration (Wang et al., 2021). Recent estimates have suggested that more than half of the total mangrove restoration area in China is S. apetala (Ren et al., 2010; He et al., 2018).
In this study, the total biomass and the biomass carbon stock of 11a-S. apetala were 76.15 t/ha and 43.49 t C/ha, respectively, which were higher than those of other planted native species such as K. obovata and R. stylosa (Table 3). Previous studies have shown that the total biomass of S. apetala in Yingluo Bay (294.32 t/ha) was much higher than that of local native species such as K. obovata, R. stylosa, A. marina, and B. gymnorrhiza (64.48–107.36 t C/ha) (Wang et al., 2019a, Wang et al., 2019b, Wang et al., 2021), but these studies did not mention whether the forest age of the different tree species differed. However, another study showed that the carbon stock of K. obovata (106.6 ± 1.4 t C/ha) was much larger than that of S. apetala monocultures, which is contrary to our results (He et al., 2020). Therefore, tree species should be selected according to the hydrological conditions of the restoration area.
However, despite the higher biomass and biomass carbon stock of 11a-S. apetala compared with 11a-K. obovata, there was no significant difference in the soil carbon stock between the two species, indicating that the soil carbon accumulation was relatively slow in the early stage of mangrove plantation (discussed in Section 4.1). In addition, the ecosystem carbon stock of 11a-S. apetala was still relatively lower than that in other study areas. For example, Wang et al. (2019a) demonstrated that the average ecosystem carbon stocks of S. apetala at Dongzhai Harbor, Yingluo Bay, and Dongjiang Estuary were 351.35, 306.93, and 162.04 t/ha, respectively, which were higher than the current results in this study. This may be due to the younger forest age of S. apetala and the SOC storage accounting for more than 85% of ecosystem carbon stock being only estimated on the top 50 cm sediment in this study. Therefore, the introduced S. apetala still has high carbon storage potential in this study.
In this study, the vegetation carbon stock of 6a-R. stylosa at high tide (45.06 ± 6.29 t C/ha) was significantly higher than that of 6a-R. stylosa at low tide (30.56 ± 5.55 t C/ha). Previous studies found that the ecosystem carbon stock was significantly influenced by the hydrogeomorphic settings associated with tidal hydrodynamics, and the higher biomass carbon storage in landward mangrove communities could be mainly attributed to the more stable geomorphic settings and nutrient-richer soils (Wang et al., 2019a; Hu et al., 2022). Similar results were also found in three estuarine mangrove forests (Dongzhai Harber, Yingluo Bay, and Zhangjiang Estuary) in China (Wang et al., 2019), Shenzhen in South China (Mao et al., 2012), in Micronesia (Kauffman et al., 2011), and in Vietnam (Tue et al., 2012, Tue et al., 2018). For example, Mao et al. (2012) showed that the mangrove carbon stocks in Shenzhen Futian Reserve decreased from 674.37 to 230.55 t C/ha along the landward-to-seaward gradient. Tue et al. (2012) suggested that the ABG and BGB carbon stocks ranged from 90.2 ± 15.8 to 115.2 ± 19.3 t C/ha and from 629.0 ± 32.5 to 687.0 ± 29.2 t C/ha, respectively, and slightly increased from the fringe toward the interior forest in mangroves. Therefore, landward optimum habitats are more conducive to mangrove growth.
Furthermore, although there was no significant difference in the soil carbon stocks between the high- and low-tide survey sites, 6a-R. stylosa at high tide had a higher SOC stock (88.54 ± 2.52) than 6a-R. stylosa at low tide (70.37 ± 8.21). Wang et al. (2013) suggested that the soil carbon stocks ranged from 188.07 to 279.04 t C/ha from low tide to high tide in Yingluo Bay, Guangdong Province, which is consistent with our results. The decomposition rate of SOC is accelerated under anaerobic and sub-oxidation conditions, which leads to a relatively lower soil carbon stock (Feng et al., 2019; Walcker et al., 2019). On the contrary, the tidal erosion in a mangrove ecosystem is less at the inner area than at the outer or middle area, which is more beneficial to the accumulation of vegetation biomass and SOC (Mao et al., 2012; Wang et al., 2019; Wang et al., 2021). There may be two possible reasons. One is that an increased productivity along tidal gradients leads to more litterfall, root exudates, and root necromass being imported into anaerobic soils (Mao et al., 2012; He et al., 2022). Secondly, the extensive root system in the middle/high tide zone and the isolation of the high tide zones from estuaries not only promote the retention of organic materials but also accelerate the retention of fine soil components with high OC concentrations (Sebastian and Chacko, 2006). Furthermore, increased plant litter and fine root biomass at mid- and high-tide levels further results in nutrient-rich sediments with high OC, which would be beneficial for mangrove growth.
The present study investigated the carbon storage potential and the influencing factors of young mangrove plantations in Kaozhouyang, Huidong County, Guangdong Province. The results showed that the mangrove plantation significantly increased the carbon sequestration/storage relative to the non-vegetation mudflat. The vegetation biomass/carbon stock increased rapidly with forest age (p < 0.05), but the soil carbon storage increased slowly from planting R. stylosa for 3a to 6a. The ecosystem carbon stock of 11a-S. apetala was higher than that of the native K. obovata (p < 0.05), which may be due to the high adaptability and rapid carbon accumulation of S. apetala. In addition, the vegetation biomass and the carbon stock of 6a-R. stylosa at high tide were obviously higher than those at low tide (p < 0.05), indicating that a stable hydrological environment is more conducive to carbon accumulation. The current results showed that the optimal afforestation plan is to cultivate S. apetala and R. stylosa at high tide, with carbon storage of 144.01 and 133.60 t C/ha, respectively. However, ecological risks could occur with planting S. apetala. Our results further revealed that mangrove plantation has a significant carbon sink effect and that the plantation should be at a high tidal level, particularly in aquaculture ponds where mangroves are converted.
The original contributions presented in the study are included in the article/supplementary material. Further inquiries can be directed to the corresponding author.
KL: Writing – review & editing, Writing – original draft, Visualization, Validation, Supervision, Software, Resources, Project administration, Methodology, Investigation, Funding acquisition, Formal analysis, Data curation, Conceptualization. HH: Writing – review & editing, Visualization, Validation, Supervision, Software, Resources, Project administration, Methodology, Investigation, Funding acquisition, Formal analysis, Data curation, Conceptualization. RY: Writing – review & editing, Data curation. SZ: Writing – review & editing, Data curation. DD: Writing – review & editing, Supervision. BP: Writing – review & editing, Data curation.
The author(s) declare financial support was received for the research, authorship, and/or publication of this article. This work was supported by the open fund of “Key Laboratory of Marine Environmental Survey Technology and Application, Ministry of Natural Resources” (MESTA-2023-B003). The Marine Economy Special Proiect of the Guangdong Province (GDNRC[2024]36); The open fund of “Key Laboratory of Marine Ecological Conservation and Restoration, Ministry of Natural Resources/Fujian Provincial Key Laboratory of Marine Ecological Conservation and Restoration (EPR2024002); the Science and Technology Project of Guangdong Forestry Administration (2024).
The authors declare that the research was conducted in the absence of any commercial or financial relationships that could be construed as a potential conflict of interest.
All claims expressed in this article are solely those of the authors and do not necessarily represent those of their affiliated organizations, or those of the publisher, the editors and the reviewers. Any product that may be evaluated in this article, or claim that may be made by its manufacturer, is not guaranteed or endorsed by the publisher.
Ahmed S., Kamruzzaman M., Rahman M. S., Sakib N., Azad M. S., Dey T. (2022). Stand structure and carbon storage of a young mangrove plantation forest in coastal area of Bangladesh: the promise of a natural solution. Nature-Based Solutions. 2, 100025. doi: 10.1016/j.nbsj.2022.100025
Alongi D. M. (2012). Carbon sequestration in mangrove forests. Carbon Manage. 3, 313–322. doi: 10.4155/cmt.12.20
Alongi D. M. (2014). Carbon cycling and storage in mangrove forests. Annu. Rev. Mar. Sci. 6, 195–219. doi: 10.1146/annurev-marine-010213-135020
Alongi D. M., McKinnon A. D., Brinkman R. (2009). The fate of organic matter derived from small-scale fish cage aquaculture in coastal waters of Sulawesi and Sumatra, Indonesia. Aquaculture. 295, 60–75. doi: 10.1016/j.aquaculture.2009.06.025
Atwood T. B., Connolly R. M., Almahasheer H. (2017). Global patterns in mangrove soil carbon stocks and losses. Nat. Clim. Change. 7, 523–528. doi: 10.1038/nclimate3326
Aye W. N., Tong X., Li J. (2023). Assessing the carbon storage potential of a young mangrove plantation in Myanmar. Forests. 14, 824. doi: 10.3390/f14040824
Chen G., Gao M., Pang B. (2018). Top-meter soil organic carbon stocks and sources in restored mangrove forests of different ages. For. Ecol. Manage. 422, 87–94. doi: 10.1016/j.foreco.2018.03.044
Chen J., Chen G., Gu Y., et al. (2020). Fate of leaf litter in restored Kandelia obovata (SL) mangrove forests with different ages in Jiulong River Estuary, China. Restoration Ecol. 28 (2), 369–377.
Chen S., An W. S., Chen B., Chen G. (2021a). Decisive factors impacting the carbon sequestration in mangrove ecological restoration. J. Appl. Oceanography. 40, 34–42.
Chen S., Chen B., Chen G. (2021b). Higher soil organic carbon sequestration potential at a rehabilitated mangrove comprised of Aegiceras corniculatum compared to Kandelia obovata. Sci. Total Environ. 752, 142279. doi: 10.1016/j.scitotenv.2020.142279
Comley B. W. T., McGuinness K. A. (2005). Above and below-ground biomass, and allometry of four common northern Australian mangroves. Aust. J. Bot. 53, 431–436. doi: 10.1071/BT04162
Damschroder L. J., Aron D. C., Keith R. E. (2009). Fostering implementation of health services research findings into practice: a consolidated framework for advancing implementation science. Implement Sci. 4, 1–15. doi: 10.1186/1748-5908-4-50
Daniel M., Donato D., Kauffman J. B., et al. (2009). Carbon storage in mangrove and peatland ecosystems: A preliminary account from plots in Indonesia.
Duarte C. M., Middelburg J. J., Caraco N. (2005). Major role of marine vegetation on the oceanic carbon cycle. Biogeosciences. 2, 1–8. doi: 10.5194/bg-2-1-2005
Duke N. C. (2001). Gap creation and regenerative processes driving diversity and structure of mangrove ecosystems. Wetl. Ecol. Manag. 9, 267–279. doi: 10.1023/A:1011121109886
Dung L. V., Tue N. T., Nhuan M. T., Omori K. (2016). Carbon storage in a restored mangrove forest in Can Gio Mangrove Forest Park, Mekong Delta, Vietnam. For. Ecol. Manag 380, 31–40. doi: 10.1016/j.foreco.2016.08.032
Feng J., Cui X., Zhou J. (2019). Effects of exotic and native mangrove forests plantation on soil organic carbon, nitrogen, and phosphorus contents and pools in Leizhou, China. Catena. 180, 1–7. doi: 10.1016/j.catena.2019.04.018
Fromard F., Puig H., Mougin E. (1998). Structure above-ground biomass and dynamics of mangrove ecosystems:new data from French Guiana. Oecologia 115, 39–53. doi: 10.1007/s004420050489
He Q. F., Zheng W., Huang X. R. (2017). Carbon storage and distribution characteristics of mangroves in Qinzhou Bay, Guangxi. J. Cent. South Univ. Forestry Technology. 37, 121–126.
He Z., Peng Y., Guan D., et al. (2018). Appearance can be deceptive: shrubby native mangrove species contributes more to soil carbon sequestration than fast-growing exotic species. Plant and Soil. 432, 425–436.
He Z., Sun H., Peng Y., Hu Z., Cao Y., Lee S. Y. (2020). Colonization by native species enhances the carbon storage capacity of exotic mangrove monocultures. Carbon Balance Management. 15, 1–11. doi: 10.1186/s13021-020-00165-0
He Z., Yen L., Huang H. (2022). Linkage between mangrove seedling colonization, sediment traits, and nitrogen input. Front. Mar. Science. 9, 793818. doi: 10.3389/fmars.2022.793818
Hu J., Loh P. S., Pradit S. (2022). Assessing the effect of age and geomorphic setting on organic carbon accumulation in high-latitude human-planted mangroves. Forests. 13, 105. doi: 10.3390/f13010105
Hu Y. K., Xu Y. W., Xue C. Q. (2019a). Studies on carbon storages of Sonneratia apetala forest vegetation and soil in Guangdong Province. J. South China Agric. University. 40, 95–103.
Hu Y., Zhu N., Liao B., You Y., Tang H. (2019b). Carbon density and carbon fixation rate of mangroves of different restoration types in Qi’ao island. (Chinese verison) J. Cent. South Univ. Forestry Technology. 12, 014.
Jennerjahn T. C., Ittekkot V. (2002). Relevance of mangroves for the production and deposition of organic matter along tropical continental margins. Naturwissenschaften. 89, 23–30. doi: 10.1007/s00114-001-0283-x
Kauffman J. B., Adame M. F., Arifanti V. B., SChile-Beers L. M., Bernardino A. F., Bhomia P. K., et al. (2020). Total ecosystem carbon stocks of mangroves across broad global environmental and physical gradients. Ecol. Monogr. 90, e01405. doi: 10.1002/ecm.v90.2
Kauffman J. B., Heider C., Cole T. G. (2011). Ecosystem carbon stocks of Micronesian mangrove forests. Wetlands. 31, 343–352. doi: 10.1007/s13157-011-0148-9
Kauffman J. B., Hernandez Trejo H., del Carmen Jesus Garcia M., et al. (2016). Carbon stocks of mangroves and losses arising from their conversion to cattle pastures in the Pantanos de Centla, Mexico. Wetlands Ecol. Management. 24 (2), 203–216.
Lee S. Y., Hamilton S., Barbier E. B., Primavera J., Lewis R. R. (2019). Better restoration policies are needed to conserve mangrove ecosystems. Nat. Ecol. Evol. 3, 870–872. doi: 10.1038/s41559-019-0861-y
Lu W., Yang S., Chen L. (2014). Changes in carbon pool and stand structure of a native subtropical mangrove forest after inter-planting with exotic species Sonneratia apetala. PLoS One 9, e91238. doi: 10.1371/journal.pone.0091238
Lunstrum A., Chen L. (2014). Soil carbon stocks and accumulation in young mangrove forests. Soil Biol. Biochem. 75, 223–232. doi: 10.1016/j.soilbio.2014.04.008
Macreadie P. I., Robertson A. I., Spinks B. (2022). Operationalizing marketable blue carbon. One Earth. 5, 485–492. doi: 10.1016/j.oneear.2022.04.005
Mao Z., Yang X., Zhao Z. (2012). Preliminary study on mangrove ecosystem carbon cycle of Kandelia candel in Futian nature reserve, Shenzhen, China. Ecol. Envir. Sci. 21, 1189–1199.
Murray N. J., Worthington T. A., Bunting P. (2022). High-resolution map of losses and gains of Earth’s tidal wetlands. Science. 376, 744–749. doi: 10.1126/science.abm9583
Osland M. J., Spivak A. C., Nestlerode J. A., Almario A. E., Heitmuller P. T., Russell M. J., et al. (2012). Ecosystem development after mangrove wetland creation: plant–soil change across a 20-year chronosequence. Ecosystems. 15, 848–866. doi: 10.1007/s10021-012-9551-1
Ouyang X., Lee S. Y. (2020). Improved estimates on global carbon stock and carbon pools in tidal wetlands. Nat. Commun. 11, 317. doi: 10.1038/s41467-019-14120-2
Pan Y., Luo T., Birdsey R. (2004). New estimates of carbon storage and sequestration in China’s forests: effects of age–class and method on inventory-based carbon estimation. Climatic Change. 67, 211–236. doi: 10.1007/s10584-004-2799-5
Peng Y. S., Zhuang X. Y., Zhao L. L., Wang Z. H., Gao J. C., Wang B. X., et al. (2023). Influence of species choice and tidal flat elevation on the carbon sequestration of early mangrove restoration. Acta Scientiarum Naturalium Universitatis Sunyatseni. 62, 37–46.
Pérez A., Escobedo R., Castro R., Jesus R., Cardich J., Romero P. E., et al. (2022). Carbon and nutrient burial within Peruvian coastal marsh driven by anthropogenic activities. Mar. Pollut. Bull. 181, 113948. doi: 10.1016/j.marpolbul.2022.113948
Perez A., Libardoni B. G., Sanders C. J. (2018). Factors influencing organic carbon accumulation in mangrove ecosystems. Biol. Letters. 14 (10), 20180237.
Pham V. H., Luu V. D., Nguyen T. T. (2017). Will restored mangrove forests enhance sediment organic carbon and ecosystem carbon storage? Reg. Stud. Mar. Sci. 14, 43–52. doi: 10.1016/j.rsma.2017.05.003
Ren H., Chen H., Li Z., Han W. (2010). Biomass accumulation and carbon storage of four different aged Sonneratia apetala plantations in Southern China. Plant Soil. 327, 279–291. doi: 10.1007/s11104-009-0053-7
Saenger P., Snedaker S. C. (1993). Pantropical trends in mangrove above-ground biomass and annual litterfall. Oecologia 96, 293–299. doi: 10.1007/BF00317496
Sebastian R., Chacko J. (2006). Distribution of organic carbon in tropical mangrove sediments (Cochin, India). Intern. J. Environment. Stud. 63 (3), 303–311.
Song W., Hou Y., Zhu W. (2024). Enhancement effects of mangrove restoration on blue carbon storage in Qinzhou Bay. Front. For Glob Change 7, 1328783. doi: 10.3389/ffgc.2024.1328783
Tamai S., Iampa P. (1988). Establishment and growth of mangrove seedling in mangrove forests of southern Thailand. Ecol. Res. 3, 227–238. doi: 10.1007/BF02348582
Tue N. T., Ngoc N. T., Quy T. D. (2012). A cross-system analysis of sedimentary organic carbon in the mangrove ecosystems of Xuan Thuy National Park, Vietnam. J. Sea Res. 67, 69–76. doi: 10.1016/j.seares.2011.10.006
Tue N. T., Nguyen P. T., Quan D. M. (2018). Sedimentary composition and organic carbon sources in mangrove forests along the coast of northeast Vietnam. Reg. Stud. Mar. Sci. 17, 87–94. doi: 10.1016/j.rsma.2017.12.001
Walcker R., Laplanche C., Herteman M. (2019). Damages caused by hurricane Irma in the human-degraded mangroves of Saint Martin (Caribbean). Sci. Rep-Uk. 9, 18971. doi: 10.1038/s41598-019-55393-3
Wang G., Guan D., Peart M. R. (2013). Ecosystem carbon stocks of mangrove forest in Yingluo Bay, Guangdong Province of South China. For. Ecol. Manage. 310, 539–546. doi: 10.1016/j.foreco.2013.08.045
Wang G., Guan D., Xiao L. (2019a). Ecosystem carbon storage affected by intertidal locations and climatic factors in three estuarine mangrove forests of South China. Regional Environ. Change 19, 1701–1712. doi: 10.1007/s10113-019-01515-6
Wang G., Guan D., Xiao L. (2019b). Changes in mangrove community structures affecting sediment carbon content in Yingluo Bay of South China. Mar. pollut. Bull. 149, 110581. doi: 10.1016/j.marpolbul.2019.110581
Wang F., Kroeger K. D., Gonneea M. E., Tang J. (2019). Water salinity and inundation control soil carbon decomposition during salt marsh restoration: An incubation experiment. Ecol. Evol. 9, 1911–1921. doi: 10.1002/ece3.2019.9.issue-4
Wang F., Tang J., Ye S., Liu J. H. (2021). Blue carbon sink function of Chinese coastal wetlands and carbon neutrality strategy. Bull. Chin. Acad. Sci. (Chinese Version). 36, 241–251.
Wang G., Zhang Y., Guan D. (2021). The potential of mature Sonneratia apetala plantations to enhance carbon stocks in the Zhanjiang Mangrove National Nature Reserve. Ecol. Indic. 133, 108415. doi: 10.1016/j.ecolind.2021.108415
Xiong Y. M., Cakir R., Phan S. M. (2019). Global patterns of tree stem growth and stand aboveground wood production in mangrove forests. For. Ecol. Manage. 444, 382–392. doi: 10.1016/j.foreco.2019.04.045
Yu C., Feng J., Liu K. (2020). Changes of ecosystem carbon stock following the plantation of exotic mangrove Sonneratia apetala in Qi'ao Island, China. Sci. Total Environ. 717, 137142. doi: 10.1016/j.scitotenv.2020.137142
Yu C., Guan D., Gang W., et al. (2021). Development of ecosystem carbon stock with the progression of a natural mangrove forest in Yingluo Bay, China. Plant Soil. 460, 391–401.
Zakaria R. M., Heng T. J., Halim A. (2023). Does age matter? Unravelling the effects of replanted mangrove forests on the total ecosystem carbon stocks. Reg. Stud. Mar. Sci. 66, 103155. doi: 10.1016/j.rsma.2023.103155
Keywords: mangrove plantation, ecosystem carbon stock, forest age, tree species, tidal gradient
Citation: Kang L, Huamei H, Ran Y, Shengpeng Z, Di D and Bo P (2025) Carbon storage potential and influencing factors of mangrove plantation in Kaozhouyang, Guangdong Province, South China. Front. Mar. Sci. 11:1439266. doi: 10.3389/fmars.2024.1439266
Received: 27 May 2024; Accepted: 15 November 2024;
Published: 17 January 2025.
Edited by:
Marta Marcos, University of the Balearic Islands, SpainReviewed by:
Yisheng Peng, Sun Yat-sen University, ChinaCopyright © 2025 Kang, Huamei, Ran, Shengpeng, Di and Bo. This is an open-access article distributed under the terms of the Creative Commons Attribution License (CC BY). The use, distribution or reproduction in other forums is permitted, provided the original author(s) and the copyright owner(s) are credited and that the original publication in this journal is cited, in accordance with accepted academic practice. No use, distribution or reproduction is permitted which does not comply with these terms.
*Correspondence: Huang Huamei, c2NzbWVlaUAxNjMuY29t
Disclaimer: All claims expressed in this article are solely those of the authors and do not necessarily represent those of their affiliated organizations, or those of the publisher, the editors and the reviewers. Any product that may be evaluated in this article or claim that may be made by its manufacturer is not guaranteed or endorsed by the publisher.
Research integrity at Frontiers
Learn more about the work of our research integrity team to safeguard the quality of each article we publish.