- 1School of Engineering, Huzhou University, Huzhou, China
- 2Institute of Agricultural Bio-Environmental Engineering, College of Biosystems Engineering and Food Science, Zhejiang University, Hangzhou, China
Large-mouth bass originally lived in rivers and lakes and now is often raised in recirculating aquaculture systems (RAS). However, the shortcoming of RAS is that there is very limited space for swimming fish in a high-density culture environment, thus leading to a lack of exercise. To investigate the effects of exercise training on growth performance, digestive enzymes, stress, and antioxidant capacity of large-mouth bass in a RAS, three training water flow velocities with three replicates were used in the present study: low water velocity: 0.5 body length per second (bl/s) (LV); medium water velocity: 1 bl/s (MV); and high water velocity: 1.5 bl/s (HV). 270 large-mouth bass (average initial body weight 47.51 ± 1.44 g, initial body length 12.71 ± 0.06 cm) were randomly divided into nine tanks (30 fish per tank). After 27 days experiment, the results showed that the growth performance of large-mouth bass, including feed intake, weight gain rate, and specific growth rate, in the HV group was significantly higher compared to the other groups. On the physiological level, the lipase and amylase activities were significantly increased in the HV group compared to the other groups. For the stress response parameters, the cortisol, glucose and lactate concentrations in the HV group were significantly increased, which may indicate higher stress in the HV group. Regarding the antioxidant enzyme activities, the activities of catalase (CAT) and glutathione peroxidase (GSH-PX) in the LV group were significantly higher than those in the HV group, which indicated that fish suffered higher oxidative stress in the LV group. Regarding the immune-related parameters, LZM and ACP activities had no significant differences among the groups, while AKP activities in the MV and HV groups were significantly higher than that in the LV group. Overall, the results demonstrated that exercise training had significant effects on the growth, digestion, stress, and immune response of large-mouth bass. It is suggested that exercise training with 1.5 bl/s can enhance fish growth, however, it also presents a potential risk of inducing stress in fish. Therefore, in rearing large-mouth bass in RAS, the setting of water flow velocity should not only focus on the growth of large-mouth bass but also consider its welfare.
1 Introduction
The large-mouth bass (Micropterus salmoides) has gained significant prominence in freshwater fish owing to its exceptional reproductive capabilities, rapid growth rate, and delectable flesh (Cai et al., 2011; Chen et al., 2021). This predatory species originated in North American lakes and rivers (Coyle et al., 2010), and it was initially introduced into the Chinese mainland in 1983 (Harimana et al., 2019). The cultivation of large-mouth bass in China has traditionally been conducted in ponds. Nonetheless, this approach is characterized by a significant reliance on manual labor and a lack of control over the breeding environment. Recently, the adoption of recirculating aquaculture systems (RAS) has witnessed substantial growth owing to its numerous benefits, including reduced water consumption, consistent water quality, and decreased pollution levels (Martins et al., 2010). Nevertheless, a drawback of RAS is the constrained area available for fish to swim in a densely populated culture setting, thus leading to lower exercise levels of captive fish compared to wild fish (Palstra and Planas, 2011). Furthermore, Palstra and Planas (2011) discovered that numerous fish species possess an inherent tendency to swim in opposition to the current, and the absence of flowing water can hinder the cultivation of this instinct, ultimately leading to diminished exercise-related benefits such as decreased physical capacity and muscle mass.
In response to the above-mentioned problems in a RAS, people generally adjust the water flow velocity in the tank. On the one hand, it helps clean the feces and residual bait in the aquaculture tank, and on the other hand, it can induce fish to exercise and maintain good growth and physiological status. Regarding exercise-induced growth, it is believed that when the velocity of water flow closely matches the swimming speed, the cost of transportation is minimized, and energy efficiency is maximized (Tucker, 1970; Palstra and Planas, 2011). This specific velocity represents the optimal swimming speed (Uopt). Therefore, at swimming velocities below Uopt, a significant amount of energy is expended as a result of increased spontaneous activity. Conversely, velocities exceeding Uopt render swimming unsustainable and induce stress, leading to anaerobic metabolism, elevated lactate levels, oxygen debt accumulation, and eventual fatigue (Davison, 1997; Palstra et al., 2010). Previous studies have demonstrated that exercise training serves as a growth-promoting factor in various fish species, including Nile tilapia (Oreochromis niloticus), gilthead seabream (Sparus aurata), Qingbo (Spinibarbus sinensis), as evidenced by investigations conducted by Moya et al. (2019); Obirikorang et al. (2019) and Li et al. (2016). In addition, researches have indicated that too high water flow velocity can result in increased stress levels in fish, ultimately compromising their welfare (Li et al., 2019; Timmerhaus et al., 2021).
According to Timmerhaus et al. (2021), training intensities for fish are often defined by the water velocity in relation to the fish’s body length and previous research has traditionally categorized medium velocities as around one body length per second of water velocity in the tank (BL/s). In addition, as fish grow, their body length changes, necessitating adjustments to water flow velocity to accommodate these changes. However, according to our knowledge, the impact of flow velocity on large-mouth bass predominantly centers on constant flow rates (Chen et al., 2021; Zhao et al., 2023), leaving the effects of water flow velocity based on body length variation on large-mouth bass unexplored. Furthermore, in a previous study conducted by our research team, three different flow velocities (4 cm/s, 0.90 bl/s; 11 cm/s, 2.45 bl/s; and 18 cm/s, 4 bl/s) were utilized for rearing juvenile fish with an initial body length of 4.5 cm and an average weight of 1.92 g in a RAS. This rearing period lasted for 66 days, during which the fish grew to a body length of 14.5 cm and a weight of 37.5 g. The results indicated that the high flow velocity of 18 cm/s, corresponding to 4 bl/s, resulted in the most optimal growth rate, reaching approximately 1.2 bl/s during the final phase of the experiment (Chen et al., 2021). On this basis, the impact of exercise training on the growth of larger fingerlings, with an average weight of 47.5 g and a body length of 12.71 cm, was investigated to offer new insights for implementing real RAS production.
Therefore, three training water flow velocities represented as low (LV), medium (MV) and high (HV) based on body length variation were adopted in the present study. The HV was set at 1.5 bl/s, approximately close to 18 cm/s, which was suggested by Chen et al. (2021). The MV was set at 1.0 bl/s, according to Timmerhaus et al. (2021). Timmerhaus et al. (2021) mentioned that previous research traditionally categorized medium velocities as around one body length per second of water velocity in the tank. In the LV group, the water velocity was set at 0.5 bl/s as a control, which was a minimum velocity to ensure self-cleaning of the tanks. Overall, this study aims to investigate whether exercise training at different water flow velocities has different effects on growth performance, feed intake, chronic stress, and antioxidant capacity of large-mouth bass reared in a RAS.
2 Materials and methods
2.1 Ethics statement
The Zhejiang University Animal Research and Ethics Committees gave their consent and approval of this study. No species that were listed as endangered or protected were included in the field research.
2.2 Experimental animals
Large-mouth bass juveniles were purchased from Hangzhou Weikang Agricultural Development Co., Ltd and were used in this experiment. During the experiment, 300 fish were randomly divided into two groups and subjected to a two-week acclimation period. During the acclimation period, the juvenile large-mouth basses were fed twice daily, at 9:00 and 17:00. The fish tank was kept at a temperature of 22 ± 1°C, with dissolved oxygen of around 6.5-8.5 mg/L, nitrogen concentration was maintained below 0.5 mg/L, and a photoperiod of 12 hours in light and 12 hours in darkness.
2.3 Experimental recirculating aquaculture systems
The current study utilized three experimental RAS, each consisting of three rearing tanks. Each tank had a capacity of 150L, with dimensions of 0.65 m in diameter, 0.45 m in height, and a water volume of 0.13 m3. Mechanical and biological filters were used, as well as a water pump, a thermostat, and other parts that are necessary for the function of a RAS. The biological filter had a working volume of 300 L and a suspended porous filter with a filling rate of 60%. The experiment was conducted at Yuya Technology Co., Ltd., Huzhou, China. According to Arbeláez-Rojas and Moraes, (2013), the tangential water velocity gradually decreases towards the center and Figure 1 shows the experimental schematic of the rearing tank. A column of plastic screen, measuring 1/3 of the total diameter of the tank, was employed to restrict fish access to the central water region in this study.
2.4 Experimental design and velocity adjustment
At the end of the acclimation phase, a cohort of 270 fish, all of similar dimensions, was randomly allocated into nine tanks. Subsequently, the water velocity in each tank was standardized to 0.5 body length per second (bl/s). In the initial week, the water velocity in each tank was incrementally modified to attain the targeted water velocity. The experimental procedure was conducted according to the method reported previously elsewhere (Timmerhaus et al., 2021). The desired water velocities in the three groups were: LV (low water velocity): 0.5 bl/s, MV (medium water velocity): 1 bl/s, HV (high water velocity): 1.5 bl/s. As the duration of the experiment progressed, the fish exhibited an increase in length, prompting a weekly adjustment of water velocity to align with the average body length in the respective tanks. The water velocity in each tank was quantified using a velocimeter (the LSH10-1M miniature Doppler flow meter) at distances of 15 cm and 20 cm from the center of the tank, and at depths of 15 cm and 30 cm. The average velocity across these depths was deemed representative of the overall water velocity in the tank.
During the experimental period, juvenile large-mouth bass were fed to satiety twice daily at 09:00 and 17:00 for 27 days. The dissolved oxygen levels in the fish tank were consistently maintained around 6.5-8.5 mg/L, while the water temperature was carefully regulated at 22 ± 1°C. Additionally, the nitrogen concentration in the tank did not exceed 0.5 mg/L, and a photoperiod of 12 hours in light and 12 hours in darkness was implemented.
2.5 Sampling and analysis protocol
Following the completion of the experiment, 24 hours of fasting was implemented for the fish. At the predetermined sampling interval, a random selection of fifteen fish from each tank underwent deep anesthesia using MS-222 at a 120 mg/L concentration. The fifteen fish from each tank were measured for body weight. Subsequently, blood samples were drained from the caudal vein of fifteen fish per tank. During this process, we randomly mixed the blood of five fish into one sample, and thus, n = 3 for each tank. The blood was placed in the refrigerator at 4°C overnight, centrifuged at a speed of 4000 rpm for 10 min, and the supernatant was collected and stored in the refrigerator at -80°C. After completing the blood sampling, the fish were quickly dissected under sterile conditions, and their intestinal and liver tissues were collected. During this process, we randomly divided 15 fish from the same tank into 3 groups, obtaining 3 groups of intestinal tissue samples and 3 groups of liver tissue samples, respectively. The collected intestinal and liver tissues were immediately placed in liquid nitrogen for later detection of intestinal digestive enzymes and detection of liver antioxidant enzymes and immune related indicators.
Digestive enzyme activities, including trypsin, lipase, and amylase activities, in the intestine were measured by assay kits (reference codes: A080-2-2, A054-1-1, C016-1-1) following the instruction provided by the manufacturer. The serum concentrations of cortisol, glucose, and lactate (reference codes H094, F006-1-1, A019-2-1) were determined using commercial kits according to the methods given by the manufacturer. Testing kits (reference codes A001-1-2, A005-1-2, and A007-1-1) were employed to determine the activities of superoxide dismutase (SOD), glutathione peroxidase (GSH-PX), catalase (CAT) in the liver, following the manufacturer’s protocols. The activities of lysozyme (LZM), alkaline phosphatase (AKP), and acid phosphatase (ACP) (reference codes A050-1-1, A060-1-1, and A059-1-1) in the liver were determined using commercial kits. All assay kits were procured from Nanjing Jiancheng Institute in Nanjing, China.
Additionally, the growth parameters of weight gain rate, specific growth rate and feed conversion were calculated by Equations 1, 2 and 3, respectively:
where W1 is initial body weight (g), W2 is final body weight (g), t is the rearing time (d).
2.6 Statistical analysis
Data related to growth and physiology of the fish are presented as a mean ± standard deviation (SD). An analysis of the data was carried out using the software SPSS 20.0. All data were reviewed for normality and homogeneity prior to analysis. The comparison of all indexes within each group was carried out using a one-way analysis of variance (ANOVA) and Turkey’s test. A significance level of 0.05 was established.
3 Results
3.1 Impact of exercise training on growth performance and feed intake
Table 1 presents the impact of exercise training on the growth performance of large-mouth bass. The results indicated that there were significant differences in growth performance parameters between the LV and HV groups. The final body weight, weight gain rate, and specific growth rate in the HV group were significantly higher than those in the LV and MV groups (P < 0.05). In terms of final weight, final body length, weight gain rate, and specific growth rate, there were no significant differences between the LV and MV groups (P > 0.05). Compared to the LV and MV groups, the HV group had a significantly lower feed conversion ratio (P < 0.05). No significant difference was found between the LV and MV groups in feed conversion ratio (P > 0.05).
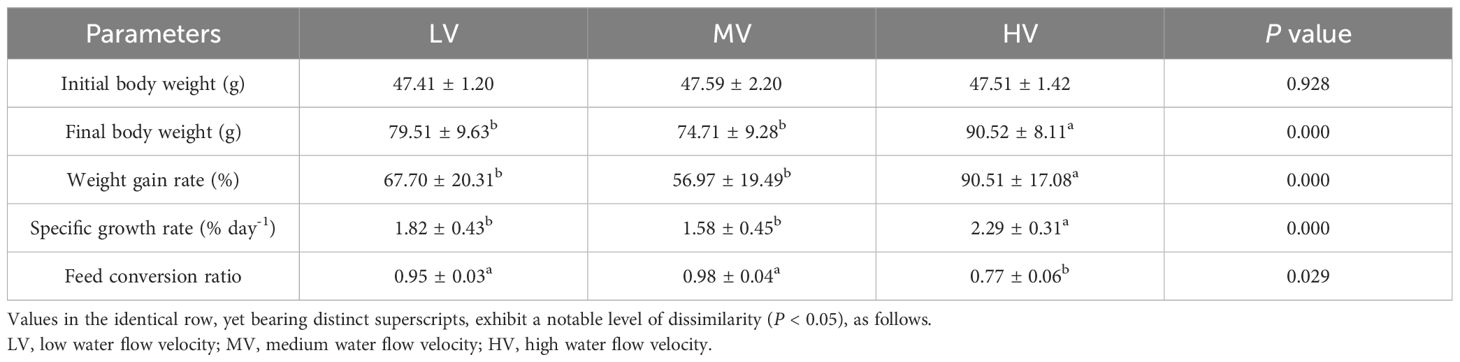
Table 1 Impact of exercise training on the development performance of large-mouth bass after 27 days.
Figure 2 displays the cumulative feed intake for each group. Notably, the HV group displayed the highest cumulative feed intake, compared to the LV and MV groups, at the end of the experiment. Commencing from day 22, the cumulative feed intake of the HV group surpassed that of the LV and MV groups.
3.2 Effect of exercise training on digestive enzyme activity
Figure 3 shows the effect of exercise training on digestive enzyme activity parameters of large-mouth bass under different water velocities. Compared to the MV group, lipase activity in the HV and LV groups was considerably higher (P < 0.05). No noticeable differences were found between the three groups regarding trypsin activity (P > 0.05). Nevertheless, as the water velocity increased, the trypsin activity also increased. As for amylase activity, the HV group showed significantly higher levels than the other groups.
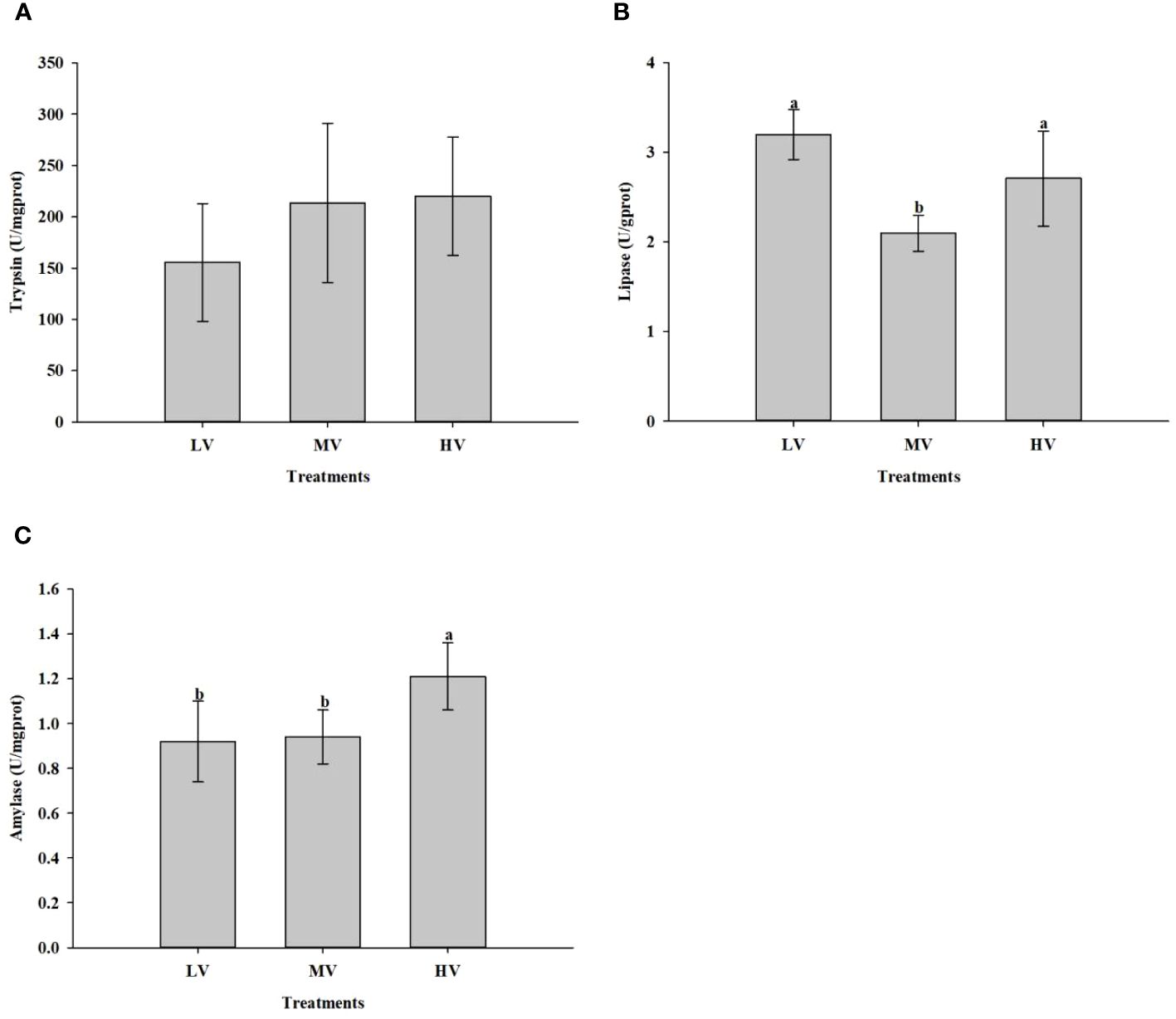
Figure 3 Effect of exercise training on digestive enzyme activity. (A) Trypsin, (B) Lipase, (C) Amylase. Values are presented as mean ± SD from three replicates. Different letters on the bar chart indicate significant differences (P < 0.05).
3.3 Effect of exercise training on stress response
The effect of exercise training on stress response is presented in Figure 4. The results showed that the exercise training exhibited an appreciable effect on cortisol, glucose, and lactate concentrations. The serum cortisol level in the HV group was significantly higher than those in the LV and MV groups (P < 0.05), but no significant difference was observed between the MV and LV group (P > 0.05). In the HV group, glucose concentration was higher compared to the LV group (P < 0.05), while no significant difference was found between the MV and LV group (P > 0.05). In the HV group, the lactate concentrations were significantly higher than those in the LV and MV groups (P < 0.05), while in the LV and MV groups, there was no significant difference (P > 0.05).
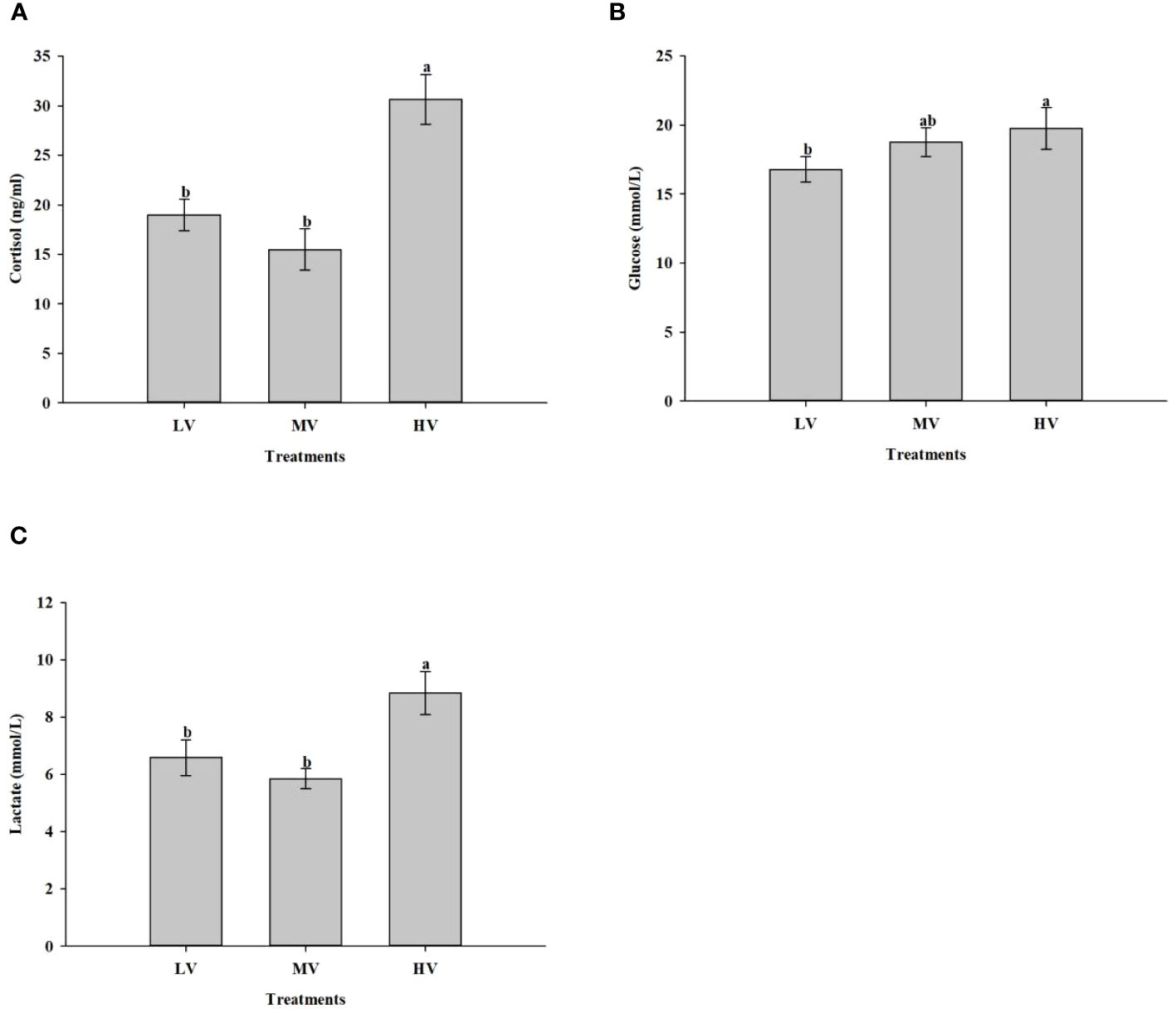
Figure 4 Exercise training effect on stress response. (A) Cortisol, (B) Glucose, (C) Lactate. Values are presented as mean ± SD from three replicates. Different letters on the bar chart indicate significant differences (P < 0.05).
3.4 Effect of exercise training on antioxidant enzyme activities and immune-related parameters
The antioxidant enzyme activity of large-mouth bass subjected to each group were listed in Figure 5. It was found that the exercise training had a crucial impact on SOD, CAT, and GSH-PX activities. There was a significant increase in SOD activity in the HV and MV groups compared to the LV group (P < 0.05), but no significant differences were observed among the MV and HV groups (P > 0.05). The HV and MV groups showed significantly lower CAT activity than the LV group (P < 0.05), but no significant difference was observed between the MV and HV groups (P > 0.05). There was a significant reduction in GSH-PX activity in the HV group compared to the LV group (P < 0.05), but no significant difference was found between the LV and MV groups (P > 0.05). The activity of AKP in the MV and HV groups were significantly higher than that in the LV group (P < 0.05). Regarding the LZM and ACP activities, no significant differences were observed among the groups (P > 0.05).
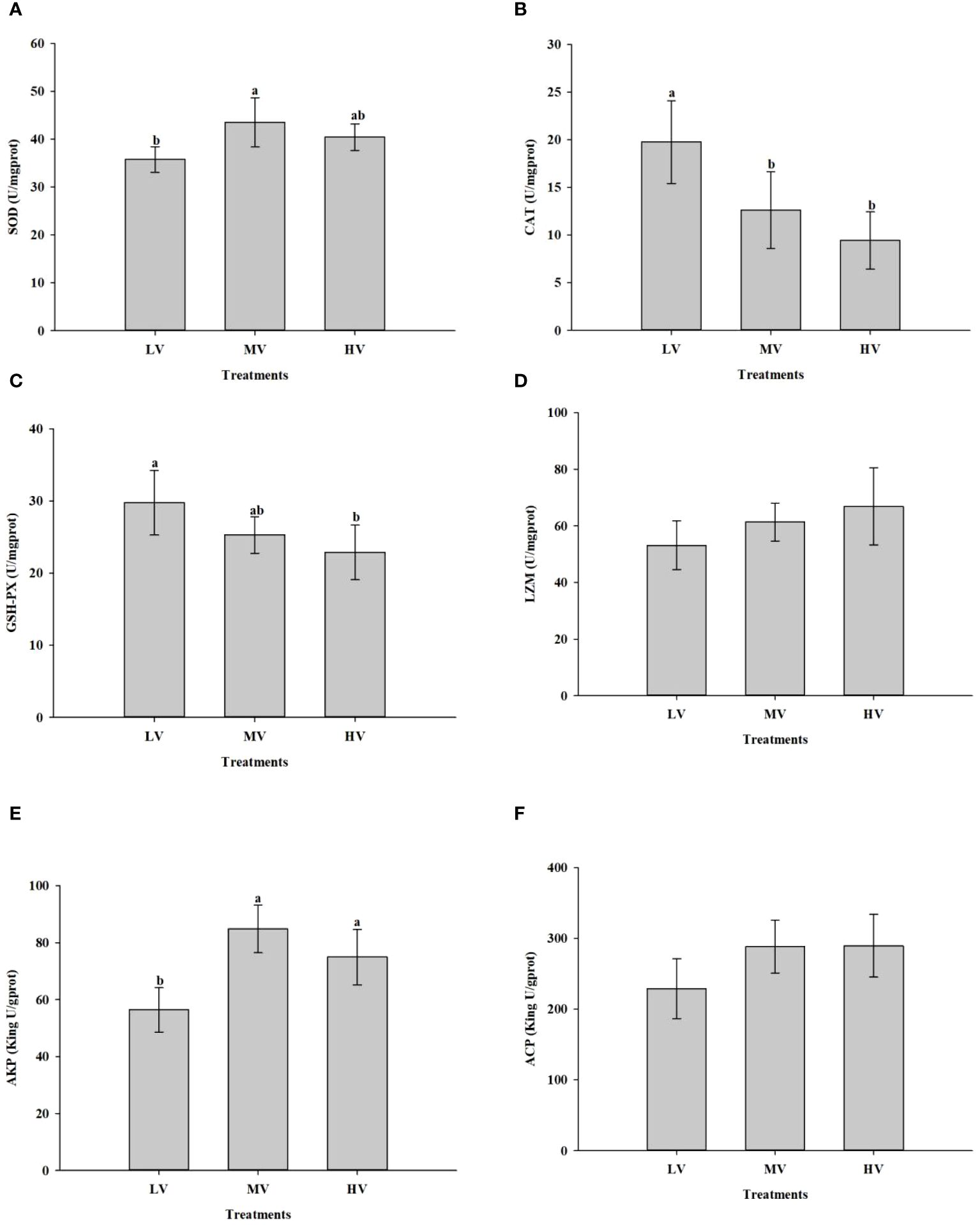
Figure 5 Effect of exercise training on antioxidant enzyme activities and immune-related parameters. (A) SOD superoxide dismutase, (B) CAT catalase, (C) GSH-PX glutathione peroxidase, (D) LZM lysozyme, (E) AKP alkaline phosphatase, (F) ACP acid phosphatase. Values are presented as mean ± SD from three replicates. Different letters on the bar chart indicate significant differences (P < 0.05).
4 Discussion
4.1 Growth performance
Exercise training can impact the growth performance of fish and may be influenced by various factors such as exercise intensity, employed methodology, and the lifestyle of the fish. The majority of scientific literature suggests that exercise training can have negative effects on certain inactive fish, such as the Japanese flounder (Paralichthys olivaceus) (Ogata and Oku, 2000) and rabbitfish (Siganus rivulatus) (Ghanawi et al., 2010). While in some publications, there was evidence that fish growth can be positively influenced by exercise training, such as Atlantic salmon (Salmo salar) (Castro et al., 2011), gilthead sea bream (Sparus aurata L.) (Blasco et al., 2015), yellowtail kingfish (Serbia lalandi) (Palstra et al., 2015), Schizothorax prenanti (Liu et al., 2018), and largemouth bass (Chen et al., 2021). In the present study, the HV group had significantly higher final body weights, weight gain rates, and specific growth rates compared to LV and MV groups. Chen et al. (2021) obtained similar results with juvenile large-mouth bass (1.9-37.5g), which agreed with the findings of this study. According to their study, the weight gain rate and specific growth rate of large-mouth bass were significantly higher at high flow (18cm/s) than at low (4cm/s) and middle flows (11cm/s). All the above results indicated that exercise training with high water flow velocity might directly promote the growth of large-mouth bass. According to Timmerhaus et al. (2021), fish in the high water flow velocity displayed a more static swimming position in relation to the tank, whereas fish in the low water flow velocity displayed a larger degree of locomotor activity, swimming more dynamically and freely. Based on the research of Waldrop et al. (2018), swimming exercise has been shown to potentially enhance growth performance in fish by facilitating ram ventilation in faster water flow. This process allows for increased water flow over the gills, reducing the need for active ventilation through the branchial pump and enabling the countercurrent oxygen exchange process to operate more efficiently with reduced energy expenditure. Therefore, exercise training with high velocity may result in lower energy consumption for ventilation.
Actually, several studies suggested that training fish improved their growth by increasing their feed intake and improving their feed conversion ratio (Li et al., 2013). Based on our research, we found that the outcome was consistent for the large-mouth bass. Compared to the other groups, the HV group had a significantly lower feed conversion ratio, which indicated that the fish exposed to the high water flow velocity performed better food utilization efficiency. Furthermore, during the period from the 22nd day until the end of the experiment, HV group had the highest cumulative feed intake out of all the groups. Based on the results mentioned above, it can be inferred that exercise training with high water velocity increases the feed intake of fish, resulting in better food utilization and, hence, a faster growth rate (Huang et al., 2007; Liu et al., 2018).
4.2 Digestive enzymes
Digestion enzymes have been commonly used to assess the nutritional status and growth performance of various fish species under diverse environmental conditions (Suzer et al., 2006; Zeng et al., 2012; Li et al., 2013, 2020). According to Ueberschär (1993), the activity of digestive enzymes can indicate fish’s digestive capacity and nutritional status. Furthermore, digestive enzyme activity is commonly measured as a method for assessing digestive capability, and it is a reliable physiological indicator (Shan et al., 2008). Consequently, the growth rate of fish is intricately linked to the activity of digestive enzymes, as supported by the studies conducted by Lemieux et al. (1999) and Hakim et al. (2006). Overall, increased digestive enzyme activity indicates improved digestion (Li et al., 2020). In this study, as compared to MV group, the activities of lipase and amylase were significantly higher in HV group. A significantly higher level of amylase activity was observed in HV group than in LV group. Based on these results, the observed elevation in enzyme activities could potentially facilitate digestion and increase feed absorption, thereby contributing to the promotion of large-mouth bass growth. Higher amylase and lipase activities further explained the reason why the large-mouth bass in the HV group had better growth performance.
4.3 Stress response
The hormone cortisol serves various functions in fish, including development, behavior and reproduction (Mommsen et al., 1999). According to Khansari et al. (1990), stress often affects blood cortisol levels. When cortisol levels are high, fish experience greater stress. Furthermore, fish experience an increase in their plasma glucose and lactate levels when they undergo stressful situations (Silbergeld, 1974; Strange and Schreck, 1978; Wei et al., 2019). The cortisol level in the HV group was significantly greater than in the LV and MV groups, as witnessed in the current investigation. However, there was no apparent difference in cortisol levels between the LV and MV groups. According to the aforementioned findings, it indicated that the fish in the HV group experienced more stress than those in the LV and MV groups. Additionally, the fish in the HV group had substantially greater amounts of lactate and glucose than the fish in the LV group. However, no significant changes were discovered between the LV and MV groups. It was further shown that fish in the HV group may be under higher stress.
4.4 Oxidative stress and immune response
Fish have a range of antioxidant mechanisms to defend themselves against reactive oxygen species (ROS) in reaction to abrupt changes in their environment (Pandey et al., 2003; Gao et al., 2016). In general, antioxidant enzymes that have been activated often eliminate ROS (Pandey et al., 2003). Antioxidant enzymes such as glutathione peroxidase (GSH-PX), catalase (CAT), and superoxide dismutase (SOD) are involved in endogenous antioxidant processes (Hansen et al., 2006). These antioxidant enzymes function via the subsequent pathways: SOD transforms O2- into H2O2, while GSH-PX and CAT then convert the hazardous H2O2 into water and molecular oxygen (O2), so removing any harmful effects (Bagnyukova et al., 2007). In the present study, a higher level of SOD activity was detected in the MV and HV groups compared with the LV group. It is possible that fish in the MV and HV groups may consume more oxygen due to higher water velocity, which could result in an increase in O2- production. SOD, an important enzyme, helps in converting O2- into H2O2, which is the primary and most crucial defense mechanism (Kohen and Nyska, 2002; Duan et al., 2015). Therefore, the SOD activity was elevated in the MV and HV groups. Furthermore, when compared to the fish in the other groups, the LV group’s fish had noticeably higher levels of CAT and GSH-PX activities. These high levels of antioxidant enzyme activities indicated that fish exposed to the low water flow velocity suffered more oxidative stress compared to other groups. This can be explained by the fact that antioxidant enzyme activities, such as those of CAT and GSH-PX, were boosted in fish under oxidative stress to get rid of harmful superoxide radicals and shield the cells from oxidative damage (Li et al., 2019).
Regarding immune-related metrics, it has been shown that AKP contributes significantly to disease defense and may enhance macrophage phagocytic activity (Li et al., 2019). A notable increase in serum AKP activity serves as an indicator of the stimulation of innate immunity (Yi et al., 2018). In the current study, the AKP activity in the MV and HV groups was significantly higher than in the LV group. This result revealed that a higher water velocity environment could improve the innate immune response of large-mouth bass.
5 Conclusions
In conclusion, the results demonstrated that exercise training had significant effects on the growth, digestion, stress and immune response of large-mouth bass. Exercise training with high water velocity remarkably promoted the growth performance and feed intake of large-mouth bass compared to the other groups. Large-mouth bass in the MV group had lower concentration of cortisol, lower enzyme activities of CAT and GSH-PX, and higher AKP activity than that in the LV group. However, exercise training with high water flow velocity may cause stress as the highest concentration of cortisol in large-mouth bass. Overall, exercise training with water velocity of 1.5 bl/s can promote the growth performance of the large-mouth bass, but it is necessary to take the fish welfare into account, particularly to prevent the stress response from high water flow velocity.
Data availability statement
The raw data supporting the conclusions of this article will be made available by the authors, without undue reservation.
Ethics statement
The animal study was approved by Zhejiang University Animal Research and Ethics Committees. The study was conducted in accordance with the local legislation and institutional requirements.
Author contributions
HL: Conceptualization, Data curation, Formal analysis, Investigation, Methodology, Software, Writing – original draft. MJ: Conceptualization, Data curation, Formal analysis, Funding acquisition, Methodology, Supervision, Writing – review & editing, Investigation, Software.
Funding
The author(s) declare financial support was received for the research, authorship, and/or publication of this article. This research was financially supported by the Key Program of Science and Technology of Zhejiang Province (Grant No. 2023C02050); the Rural Revitalization Project of Huzhou (No.2021ZD2039); and the Fundamental Research Funds for the Central Universities (Grant No. 2019QNA6005).
Conflict of interest
The authors declare that the research was conducted in the absence of any commercial or financial relationships that could be construed as a potential conflict of interest.
Publisher’s note
All claims expressed in this article are solely those of the authors and do not necessarily represent those of their affiliated organizations, or those of the publisher, the editors and the reviewers. Any product that may be evaluated in this article, or claim that may be made by its manufacturer, is not guaranteed or endorsed by the publisher.
References
Arbeláez-Rojas G. A., Moraes G. (2013). Effect of water velocity on intermediary metabolism of juvenile matrinxã fish (brycon amazonicus). Rev. Colomb. Cienc. Pec. 26, 288–299.
Bagnyukova T. V., Lushchak O. V., Storey K. B., Lushchak V. I. (2007). Oxidative stress and antioxidant defense responses by goldfish tissues to acute change of temperature from 3°C to 23°C. J. Therm. Biol. 32, 227–234. doi: 10.1016/j.jtherbio.2007.01.004
Blasco J., Moya A., Millán-Cubillo A., Vélez E. J., Capilla E., Pérez-Sánchez J., et al. (2015). Growth-promoting effects of sustained swimming in fingerlings of gilthead sea bream (sparus aurata L.). J. Comp. Physiol. B 185, 859–868. doi: 10.1007/s00360-015-0933-5
Cai L., Bai J., Quan Y., Li S., Chen K. (2011). Advances in genetics and breeding of largemouth bass. Guangdong Agric. Sci. 38, 96–99.
Castro V., Grisdale-Helland B., Helland S. J., Kristensen T., Jørgensen S. M., Helgerud J., et al. (2011). Aerobic training stimulates growth and promotes disease resistance in atlantic salmon (salmo salar). Comp. Biochem. Phys. A 160, 278–290. doi: 10.1016/j.cbpa.2011.06.013
Chen Z., Ye Z., Ji M., Zhou F., Ding X., Zhu S., et al. (2021). Effects of flow velocity on growth and physiology of juvenile largemouth bass (micropterus salmoides) in recirculating aquaculture systems. Aquac. Res. 52, 3093–3100. doi: 10.1111/are.15153
Coyle S. D., Tidwell J. H., Webster C. D. (2010). Response of largemouth bass micropterus salmoides to dietary supplementation of lysine, methionine, and highly unsaturated fatty acids. J. World. Aquacult. Soc 31, 89–95. doi: 10.1111/j.1749-7345.2000.tb00702.x
Davison W. (1997). The effects of exercise training on teleost fish, a review of recent literature. Comp. Biochem. Physiol. A 117, 67–75. doi: 10.1016/S0300-9629(96)00284-8
Duan Y., Zhang J., Dong H., Wang Y., Liu Q., Li H. (2015). Oxidative stress response of the black tiger shrimp penaeus monodon to vibrio parahaemolyticus challenge. Fish Shellfish Immun. 46, 354–365. doi: 10.1016/j.fsi.2015.06.032
Gao X., Li X., Li M., Song C., Liu Y. (2016). Effects of light intensity on metabolism and antioxidant defense in Haliotis discus hannai Ino. Aquaculture. 465, 78–87. doi: 10.1016/j.aquaculture.2016.08.010
Ghanawi J., Mohanna C., Saoud I. P. (2010). Effect of continuous water movement on growth and body composition of juvenile rabbitfish, siganus rivulatus. J. World Aquacult. Soc 41, 834–839. doi: 10.1111/j.1749-7345.2010.00427.x
Hakim Y., Uni Z., Hulata G., Harpaz S. (2006). Relationship between intestinal brush border enzymatic activity and growth rate in tilapias fed diets containing 30% or 48% protein. Aquaculture. 257, 420–428. doi: 10.1016/j.aquaculture.2006.02.034
Hansen B. H., Rømma S., Garmo Ø.A., Olsvik P. A., Andersen R. A. (2006). Antioxidative stress proteins and their gene expression in brown trout (salmo trutta) from three rivers with different heavy metal levels. Comp. Biochem. Phys. C 143, 263–274. doi: 10.1016/j.cbpc.2006.02.010
Harimana Y., Tang X., Xu P., Xu G., Karangwa E., Zhang K., et al. (2019). Effect of long-term moderate exercise on muscle cellularity and texture, antioxidant activities, tissue composition, freshness indicators and flavor characteristics in largemouth bass (micropterus salmoides). Aquaculture. 510, 100–108. doi: 10.1016/j.aquaculture.2019.05.051
Huang N. Y., Cheng Q. Q., Gao L. J., Yao Z. L., Xia L. J. (2007). Effect of water current and temperature on growth of juvenile acipenser baeri. Shuichan Xuebao. 31, 31–37.
Khansari D. N., Murgo A. J., Faith R. E. (1990). Effects of stress on the immune system. Immunol. Today. 11, 170–175. doi: 10.1016/0167-5699(90)90069-L
Kohen R., Nyska A. (2002). Oxidation of biological systems: Oxidative stress phenomena, antioxidants, redox reactions, and methods for their quantification. Toxicol. Pathol. 30, 620–650. doi: 10.1080/01926230290166724
Lemieux H., Blier P., Dutil J. (1999). Do digestive enzymes set a physiological limit on growth rate and food conversion efficiency in the atlantic cod (gadus morhua)? Fish Physiol. Biochem. 20, 293–303. doi: 10.1023/A:1007791019523
Li X., Ji L., Wu L., Gao X., Li X., Li J., et al. (2019). Effect of flow velocity on the growth, stress and immune responses of turbot (scophthalmus maximus) in recirculating aquaculture systems. Fish Shellfish Immun. 86, 1169–1176. doi: 10.1016/j.fsi.2018.12.066
Li X. M., Yuan J. M., Fu S. J., Zhang Y. G. (2016). The effect of sustained swimming exercise on the growth performance, muscle cellularity and flesh quality of juvenile qingbo (Spinibarbus sinensis). Aquaculture. 465, 287–295. doi: 10.1016/j.aquaculture.2016.09.021
Li X., Yu L., Wang C., Zeng L., Cao Z., Fu S., et al. (2013). The effect of aerobic exercise training on growth performance, digestive enzyme activities and postprandial metabolic response in juvenile qingbo (spinibarbus sinensis). Comp. Biochem. Phys. A 166, 8–16. doi: 10.1016/j.cbpa.2013.04.021
Li H., Zhao J., Ji B., Zhang Y., Ye Z., Zhu S. (2020). Effects of photoperiod on growth, digestive enzyme activity, stress, and oxidative status of juvenile Chinese soft-shelled turtles (pelodiscus sinensis) in a greenhouse. Trans. ASABE. 63, 1787–1793. doi: 10.13031/trans.13828
Liu G., Wu Y., Qin X., Shi X., Wang X. (2018). The effect of aerobic exercise training on growth performance, innate immune response and disease resistance in juvenile schizothorax prenanti. Aquaculture. 486, 18–25. doi: 10.1016/j.aquaculture.2017.12.006
Martins C. I. M., Eding E. H., Verdegem M. C. J., Heinsbroek L. T. N., Schneider O., Blancheton J. P., et al. (2010). New developments in recirculating aquaculture systems in europe: A perspective on environmental sustainability. Aquacult. Eng. 43, 83–93. doi: 10.1016/j.aquaeng.2010.09.002
Mommsen T. P., Vijayan M. M., Moon T. W. (1999). Cortisol in teleosts: Dynamics, mechanisms of action, and metabolic regulation. Rev. Fish Biol. Fisher. 9, 211–268. doi: 10.1023/A:1008924418720
Moya A., Torrella J. R., Fernandez-Borras J., Rizo-Roca D., Millan-Cubillo A., Velez E. J., et al. (2019). Sustained swimming enhances white muscle capillarisation and growth by hyperplasia in gilthead sea bream (Sparus aurata) fingerlings. Aquaculture. 501, 397–403. doi: 10.1016/j.aquaculture.2018.10.062
Obirikorang K. A., Agbo N. W., Obirikorang C., Adjei-Boateng D., Ahiave S. E., Skov P. V. (2019). Effects of water flow rates on growth and welfare of Nile tilapia (Oreochromis niloticus) reared in a recirculating aquaculture system. Aquacult. Int. 27, 449–462. doi: 10.1007/s10499-019-00342-0
Ogata H. Y., Oku H. (2000). Effects of water velocity on growth performance of juvenile Japanese flounder Paralichthys olivaceus. J. World Aquacult. Soc 31, 225–231. doi: 10.1111/j.1749-7345.2000.tb00357.x
Palstra A. P., Mes D., Kusters K., Roques J. A. C., Flik G., Kloet K., et al. (2015). Forced sustained swimming exercise at optimal speed enhances growth of juvenile yellowtail kingfish (Seriola lalandi). Front. Physiol. 5. doi: 10.3389/fphys.2014.00506
Palstra A. P., Planas J. V. (2011). Fish under exercise. Fish Physiol. Biochem. 37, 259–272. doi: 10.1007/s10695-011-9505-0
Palstra A. P., Tudorache C., Rovira M., Brittijn B., Burgerhout E., van den Thillart G. E. E. J. M., et al. (2010). Establishing zebrafish (Danio rerio) as a novel exercise model: swimming economy, swimming-enhanced growth and regulation of muscle growth marker gene expression. PloS One. 5, e14483. doi: 10.1371/journal.pone.0014483
Pandey S., Parvez S., Sayeed I., Haque R., Bin-Hafeez B., Raisuddin S. (2003). Biomarkers of oxidative stress: A comparative study of river yamuna fish wallago attu (bl. & schn.). Sci. Total Environ. 309, 105–115. doi: 10.1016/S0048-9697(03)00006-8
Shan X., Xiao Z., Huang W., Dou S. (2008). Effects of photoperiod on growth, mortality and digestive enzymes in miiuy croaker larvae and juveniles. Aquaculture. 281, 70–76. doi: 10.1016/j.aquaculture.2008.05.034
Silbergeld E. K. (1974). Blood glucose: A sensitive indicator of environmental stress in fish. B. Environ. Contam. Tox. 11, 20–25. doi: 10.1007/BF01685023
Strange R. J., Schreck C. B. (1978). Anesthetic and handling stress on survival and cortisol concentration in yearling chinook salmon (Oncorhynchus tshawytscha). Can. J. Fish Aquat. Sci. 35, 345–349. doi: 10.1139/f78-061
Suzer C., Saka Ş., Firat K. (2006). Effects of illumination on early life development and digestive enzyme activities in common pandora Pagellus erythrinus L. larvae. Aquaculture. 260, 86–93. doi: 10.1016/j.aquaculture.2006.06.025
Timmerhaus G., Lazado C. C., Cabillon N. A. R., Reiten B. K. M., Johansen L. (2021). The optimum velocity for atlantic salmon post-smolts in RAS is a compromise between muscle growth and fish welfare. Aquaculture. 532. doi: 10.1016/j.aquaculture.2020.736076
Tucker V. A. (1970). Energetic cost of locomotion in animals. Comp. Biochem. Physiol. 34, 841. doi: 10.1016/0010-406X(70)91006-6
Ueberschär B. (1993). “Measurement of proteolytic enzyme activity: significance and application in larval fish research,” in Physiological and Biochemical Aspects of Fish Development. Eds. Walter B. T., Fyhn H. J. (University of Bergen, Norway), 233–239.
Waldrop T., Summerfelt S., Mazik P., Good C. (2018). The effects of swimming exercise and dissolved oxygen on growth performance, fin condition and precocious maturation of early-rearing atlantic salmon salmo salar. Aquac. Res. 49, 801–808. doi: 10.1111/are.13511
Wei H., Li H., Xia Y., Liu H., Han D., Zhu X., et al. (2019). Effects of light intensity on phototaxis, growth, antioxidant and stress of juvenile gibel carp (Carassius auratus gibelio). Aquaculture. 501, 39–47. doi: 10.1016/j.aquaculture.2018.10.055
Yi Y., Zhang Z., Zhao F., Liu H., Yu L., Zha J., et al. (2018). Probiotic potential of Bacillus velezensis JW: antimicrobial activity against fish pathogenic bacteria and immune enhancement effects on Carassius auratus. Fish Shellfish Immunol. 78, 322–330. doi: 10.1016/j.fsi.2018.04.055
Zeng L., Li F., Li X., Cao Z., Fu S., Zhang Y. (2012). The effects of starvation on digestive tract function and structure in juvenile southern catfish (Silurus meridionalis Chen). Comp. Biochem. Phys. A 162, 200–211. doi: 10.1016/j.cbpa.2012.02.022
Keywords: water flow velocity, large-mouth bass, growth, physiology, recirculating aquaculture system
Citation: Ji M and Li H (2024) Effects of exercise training on growth and physiology of large-mouth bass (Micropterus salmoides) reared in a recirculating aquaculture system. Front. Mar. Sci. 11:1423146. doi: 10.3389/fmars.2024.1423146
Received: 25 April 2024; Accepted: 03 July 2024;
Published: 22 July 2024.
Edited by:
Yafei Duan, South China Sea Fisheries Research Institute, ChinaReviewed by:
Shijing Liu, Chinese Academy of Fishery Sciences (CAFS), ChinaChunsen Xu, Changjiang Water Resources Protection Institute, China
Copyright © 2024 Ji and Li. This is an open-access article distributed under the terms of the Creative Commons Attribution License (CC BY). The use, distribution or reproduction in other forums is permitted, provided the original author(s) and the copyright owner(s) are credited and that the original publication in this journal is cited, in accordance with accepted academic practice. No use, distribution or reproduction is permitted which does not comply with these terms.
*Correspondence: Haijun Li, lhjahau@zju.edu.cn