- 1Integrative Science Center of Germplasm Creation in Western China (CHONGQING) Science City, Key Laboratory of Freshwater Fish Reproduction and Development (Ministry of Education), Key Laboratory of Aquatic Science of Chongqing, College of Fisheries, Southwest University, Chongqing, China
- 2Technology Innovation Center of Ecological Fishery Industrialization, Chongqing University of Arts and Sciences, Chongqing, China
- 3The Agricultural Technology Service Center of Pengshui Miao and Tujia Autonomous County, Chongqing, China
An 8-week feeding trial was conducted to investigate the effects of dietary fucoidan levels (0 mg/kg, 500 mg/kg, 1,000 mg/kg, 1,500 mg/kg, and 2,000 mg/kg) on the hepatic ultrastructure and the transcriptomic landscape within the liver tissue of common carp. The results revealed that the addition of 1,000 mg/kg fucoidan to the diet significantly altered the ultrastructure of hepatocytes. Notably, the quantities of lipid droplets, autolysosomes, enlarged mitochondria, and endoplasmic reticulum were increased in the hepatocytes. The liver transcriptome analysis revealed that DEGs were notably abundant in the 1,000-mg/kg fucoidan group in glucolipid metabolism signaling pathways, including eukaryotes and nicotinate and nicotinamide metabolism, steroid biosynthesis, and PPAR signaling pathways. The quantitative real-time PCR (qPCR) results showed an excellent agreement on those of RNA-seq for both up- and down-regulated genes (a total of 13 genes selected for validation). Furthermore, fucoidan significantly influenced hepatic glucolipid metabolism of common carp at the mRNA level. Notably, the inclusion of lower concentrations of fucoidan in the diet, specifically at 500 mg/kg and 1,000 mg/kg, was found to significantly enhance the expression of mRNA for genes involved in glycolysis, including hk, gk, and pk, as well as genes linked to lipogenesis, such as srebf1, fasn, elovl5, acsl3a, scd, fads2, and cyp24a1. Conversely, higher concentrations of dietary fucoidan, at 1,500 mg/kg and 2,000 mg/kg, were observed to markedly upregulate the mRNA expression of genes associated with gluconeogenesis, including pck1, pck2, gs, gsk3a, gsk3b, and g6pca.1, along with genes related to lipolysis, such as hadhb, acadl, ecil, acacba, cpt1, fabp1, and fabp3. In summary, varying levels of fucoidan intake appear to exert differential influences on the hepatic glucolipid metabolism of common carp, suggesting a complex dose-dependent regulatory mechanism. For the first time, this research has established that fucoidan possesses a pronounced regulatory influence on the hepatic glucolipid metabolism in fish. This discovery not only underscores its potential as an immunostimulant but also opens new avenues for its application as a feed additive, with implications for modulating the nutritional metabolism of aquatic species.
Introduction
Recently, a certain amount of lipids or carbohydrates replacing protein showed the advantages of promoting fish growth and reducing nitrogen and phosphorus emissions in intensive aquaculture (Shi et al., 2022; Yan et al., 2024). So far, the replacement of feed protein with lipids or carbohydrates has been studied in a variety of fish species, such as Ctenopharyngodon idella (Wei et al., 2024), Acipenser baerii (Yu et al., 2024), Oreochromis niloticus (Jia et al., 2024), Cyprinus carpio (Mi et al., 2024), Sparus aurata (Pecino et al., 2024), Micropterus salmoides (Zhao et al., 2021; 2024), and Oncorhynchus mykiss (Liu et al., 2017). However, the appropriate amount of lipids and carbohydrates in different fish diet is not very clear, and the molecular mechanism of fish adaptation to high-lipid and high-carbohydrates diets is still unelucidated, so blindly replacing protein sources with lipids or carbohydrates may cause adverse effects on fish (Wang et al., 2022b; Abasubong et al., 2023). Therefore, it is important to explore green and safe feed additives with the function of regulating glucose and lipid metabolism for aquatic animals.
Fucoidan is a type of natural active polysaccharide mainly extracted from marine brown algae containing sufficient L-fucose and sulfate eater groups. Given the potential of fucoidan in various biological activity, such as immune regulation (Apostolova et al., 2020), anticancer (Wang et al., 2022a), radiation protection (Wu et al., 2020), antiviral (Fitton et al., 2020), antioxidant (Subramanian et al., 2019), and controlling chronic metabolic diseases (Shan et al., 2016; Lee et al., 2020), it has been well studied in mammals and even human beings. However, the research on the application of fucoidan on aquatic animal is very limited, which has mainly focused on restricted species, such as Penaeus monodon (Sivagnanavelmurugan et al., 2014), Marsupenaeus japonicus (Traifalgar et al., 2010), Litopenaeus vannamei (Setyawan et al., 2018), Pagrus major (Sony et al., 2019), Oreochromis niloticus (Mahgoub et al., 2020), Larimichthys crocea (Yin et al., 2022), and Astronotus ocellatus (Khanzadeh et al., 2024). However, these studies mainly focused on the growth promotion and immune enhancement of fucoidan, and whether it has other physiological effects on aquatic animal is unknown.
Cyprinus carpio, as a kind of freshwater economic fish, provides a high-quality protein source for people all over the world. In order to better cultivate and exploit this species, many studies have been carried out on carp, including growth (Khorshidi et al., 2022), disease resistance (Ahmadifar et al., 2022) and stress resistance (Xue et al., 2022). Previously, our research has found that fucoidan as a feed additive (500 mg/kg~1,500 mg/kg) for carp can significantly improve growth performance and ameliorate hepatic morphology, but the high dosage (2,000 mg/kg) probably caused damage to the liver (Li et al., 2023). As we all know, fish liver is an important organ that controls metabolism. Therefore, we speculated that fucoidan may regulate the glucolipid metabolism of fish primarily by acting on liver. Therefore, the present study aimed to investigate how fucoidan regulated the lipid and glucose metabolism in liver of carp. The results can provide a new view to reveal the mechanisms of fucoidan in regulating glucose and lipid metabolism and promote the further application of fucoidan to aquatic animals.
Materials and methods
Ethics statement
Animal experiments in this study were performed under the guidelines and approval of the Institutional Animal Care and Use Committee of Southwest University (No. IACUC-20220225-01).
Diet formulation and preparation
Fucoidan (purity ≥98%, Xi’an Risen Biotechnology Co., Ltd., China) was added to a basal diet at five levels (0 mg/kg, 500 mg/kg, 1,000 mg/kg, 1,500 mg/kg, and 2,000 mg/kg) (Li et al., 2023) as diet 1, diet 2, diet 3, diet 4, and diet 5. The dietary formulation and composition of five isonitrogenous (38%) and isolipidic (6.6%) experimental diets is listed in Supplementary Table S1. All feed ingredients were ground and passed through a 60-mesh sieve and then mixed by stepwise expansion method to make pellet feed with a diameter of 1 mm, which was dried naturally and stored at −20°C until feeding (Li et al., 2023).
Experimental fish and feeding management
Before the feeding trail, juvenile Cyprinus carpio were acclimated in a cement pond (6 m × 6 m × 0.8 m) with commercial feed. After 14-day acclimation, a total of 450 healthy fish with an average initial weight of (35.83 ± 0.24 g) were randomly assigned to 15 outdoors cement ponds (2.1 m × 1.2 m × 0.8 m) at the aquaculture base of Southwest university (Chongqing, China), each with 30 fish species. Fish in triplicate groups were fed with apparent satiations at 8:00 and 17:00 artificially. The culture conditions during feeding trial were set as follows: dissolved oxygen, <6.5 mg/L; water temperature, 25°C–28°C; total ammonia nitrogen and nitrites, <0.05 mg/L and pH, 7.4–8.2, which were tested by multiparameter water quality analyzer (HYDROLAB HL7). Meanwhile, the natural photoperiod was applied.
Sampling collection
After the feeding trial, carps were fasted for 24 h before sampling. There were 12 fish that were caught randomly from each group (30 fish per pond, 90 fish per group) and anesthetized by 100 mg/L tricaine methane sulfonate (MS-222, Sigma, USA). Among them, nine fish per group were selected to collect the liver for freezing in liquid nitrogenin and then stored in an ultra-low temperature refrigerator for analysis of transcriptome (diets 1 and 3) and gene expression (diets 1–5). Moreover, three fish per group from diets 1 and 3 were washed with precooled phosphate buffer and then fixed in 4% glutaraldehyde solution (4°C) for transmission electron microscope observation.
Hepatic transmission electron microscope analysis
Following a previous method (Li et al., 2021a), liver specimens were fixed in 1% osmic tetroxide for 2 h, dehydrated in alcohol and acetone, and then embedded in resin. The tissue was cut into 60-nm slices and stained with lead citrate and uranyl acetate. Finally, the stained sections were examined and photographed using transmission electron microscopy (TEM) (Hitachi 7500) and Gatan 780 CCD.
Total RNA extraction, cDNA library construction, and sequencing
Total RNA from common carp liver in diet 1 and diet 3 was isolated using TRIzol reagent (Invitrogen, Carlsbad, CA, USA) for RNA-seq analysis. The RNA integrity was visualized by agarose gel and then evaluated by 2100 Bioanalyzer (Agilent Technologies). An aliquot of 6 μg RNA was used for constructing cDNA library. Three separate biological replicates were performed in each dietary treatment. The cDNA libraries were then sequenced using an Illumina HiSeq platform by Novogene Bioinformatics Technology Co., Ltd.
Bioinformatic analysis of RNA-seq data
Firstly, the raw reads were filtered to remove the adaptor sequences. Low-quality reads (Q < 20) and >10% of N-containing reads were trimmed from both ends of the reads and filtered with Phred quality score (Q ≥ 20) and read length (≥25 bp). Next, these preprocessed reads were designed as clean reads which were uploaded to NCBI (https://www.ncbi.nlm.nih.gov/sra/PRJNA1139972) and aligned to common carp reference genome (https://ftp.ncbi.nlm.nih.gov/genomes/all/GCF/018/340/385/GCF_018340385.1_ASM1834038v1/) using HISAT2 software (Li et al., 2020). Transcriptome assembly was completed by Trinity (Grabherr et al., 2011) with the min_kmer_cov value set to 2 by default. Gene transcription levels were expressed as FPKM (Lu et al., 2019). Significantly DEGs relative to the control group were identified with P value <0.05 and |log2 (fold change)| > 1. Gene functions were annotated based on GO terminology (http://www.geneontology.org/). KEGG enrichment analysis was performed to identify associated pathways of the DEGs.
Quantitative real-time PCR
In order to validate the DEGs, 13 candidate DEGs were selected for qPCR analysis, including kcnk1a (potassium two pore domain channel subfamily k member 1), elfn1b (extracellular leucine-rich repeat and fibronectin type III domain containing 1), heatr1 (heat repeat containing 1), stox1 (storkhead box 1), wdr6 (WD repeat domain 6), lrch1 (leucine-rich repeats and calponin homology domain containing 1), klf15 (KLF transcription factor 15), fdps (farnesyl diphosphate synthase), fam20cb (FAM20C golgi associated secretory pathway kinase), DVL1 (disheveled segment polarity protein 1), greb1l (GREB1-like retinoic acid receptor coactivator), cyb5r2 (cytochrome b5 reductase 2), and cd9 (CD9 molecule). In addition, 24 genes involved in glucose and lipid metabolism, including glycolysis genes (hk (hexokinase), gk (glucokinase), and pk (pyruvate kinase)), gluconeogenesis genes (pck1 (phosphoenolpyruvate carboxykinase 1), pck2 (phosphoenolpyruvate carboxykinase 2), gs (glycogen synthase), gsk3a (glycogen synthase kinase 3 alpha), gsk3b (glycogen synthase kinase 3 beta), and g6pca.1 (glucose-6-phosphatase catalytic subunit 1)), lipolysis-related genes (hadhb (hydroxyacyl-coa dehydrogenase trifunctional multienzyme complex subunit beta), acadl (acyl-coa dehydrogenase), ecil (enoyl-coa delta isomerase 1), acacb (acetyl-coa aarboxylase beta), cpt1 (carnitine almitoyltransferase 1), fabp1 (fatty acid binding protein 1), fabp3 (fatty acid binding protein 3)), and lipogenesis-associated genes (srebf1 (sterol regulatory element binding transcription factor 1), fasn (fatty acid synthase), elovl5 (ELOVL fatty acid elongase 5), acsl3a (acyl-coa synthetase long chain family member 3), scd (acyl-coa desaturase), fads2 (fatty acid desaturase 2), and cyp24a1 (1,25-dihydroxyvitamin d (3) 24-hydroxylase)) were selected to detect the mRNA expression level.
The qPCR experiment was performed as described in the previous study (Li et al., 2021b). Total RNA was prepared from carps liver of diet 1 to diet 5. cDNA was synthesized utilizing the superscript III cDNA synthesis kit (Invitrogen) and then stored at −20°C until used. A 20-μL qPCR reaction containing 0.4 μL of PCR forward/reverse primers, 1 μL of cDNA template, and 10 μL of 2× SYBR® Green Premix Ex Taq™ II (Tli RNase H Plus) was prepared. The thermocycling conditions were as follows: 95°C for 5 min, followed by 40 cycles consisting of 95°C for 15 s and 60°C for 30 s. The expression level of target genes was normalized to housekeeping gene β-actin and calculated using the 2−ΔΔCt method (Li et al., 2021b). The primer sequences for these genes are listed in Supplementary Table S2.
Statistical analysis
All of the data were expressed as the mean ± SEM. The data were analyzed through one-way analysis of variance and Tukey’s multiple range test using SPSS 19.0 (SPSS Inc., Chicago, IL, USA). Differences were considered significant at P < 0.05. The histograms were drawn using Origin 8 (Electronic Arts Inc., California, USA).
Results
Ultrastructural observations
The ultrastructure of liver of common carp exhibited regular nucleus, normal bile canaliculus, mitochondria, moderate amounts of glycogen, degradative lipid droplets, rough endoplasmic reticulum, autophagic vacuole, and autolysosome (Figure 1A). Obviously, the amount of lipid droplets (Figure 1B), expansive and vacuolated mitochondria (Figure 1C), and rough endoplasmic reticulum and autolysosome (Figure 1D) was increased in the hepatocyte of common carp feeding with 1,000 mg/kg fucoidan in diet.
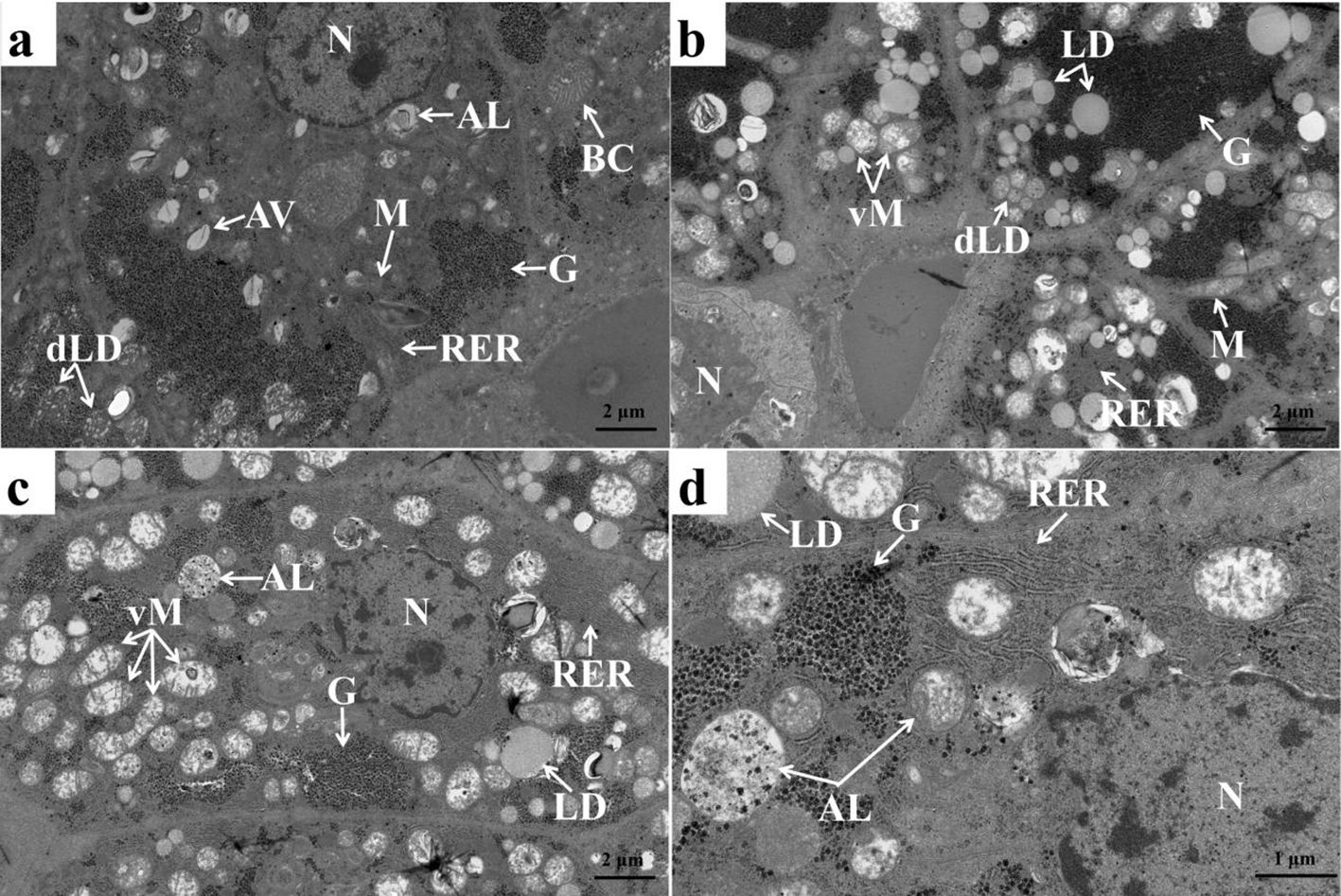
Figure 1. Ultrastructure of the liver of juvenile Cyprinus carpio in diet 1 and diet 3. (A) Liver of juvenile Cyprinus carpio in diet 1, showing bile canaliculus (BC), mitochondria (M), autophagic vacuole (AV), autolysosome (AL), glycogen (G), nucleus (N), degradative lipid droplet (dLD), and rough endoplasmic reticulum (RER) in hepatocyte. (B–D) Liver of Cyprinus carpio in diet 3, showing increased lipid droplet (LD), vacuolated mitochondria (vM), autolysosome (AL), and rough endoplasmic reticulum (RER).
RNA sequencing data
As shown in Supplementary Table S3, the total number of raw reads ranged from 43.19 to 46.44 million and obtained over 96.52% of clean reads. 73.51%–77.30% of clean reads were mapped to the common carp reference genome, and 60.60%–65.52% of the alignment was unique in the genome.
The differentially expressed genes (DEGs) between diet 1 and diet 3 were displayed in Figure 2. The number of up- and down-regulated genes were 307 and 154, respectively. Genes such as mgst2 (microsomal glutathione s-transferase 2), kcnk1a (potassium two pore domain channel subfamily k member 1), elfn1b (extracellular leucine rich repeat and fibronectin type III domain containing 1), zbtb16a (zinc finger and btb domain containing 16), and usp28 (ubiquitin-specific peptidase 28) were significantly highly up-regulated under dietary fucoidan treatment. While per1b (period circadian regulator 1), rock1 (rho associated coiled-coil containing protein kinase 1), esyt1a (extended synaptotagmin 1), dhcr24 (24-dehydrocholesterol reductase), hmgcs1 (3-hydroxy-3-methylglutaryl-coa synthase 1), and apc (apc regulator of the wnt signaling pathway) were representative genes inhibited by dietary fucoidan. Moreover, all of DEGs that up- and down-regulated are listed in Supplementary Table S4. Among the DEGs, 59 DEGs (38 up- and 21 down-regulated) were associated with tumor and disease; 25 DEGs (25 up- and 10 down-regulated) were related to metabolism; 11 DEGs (8 up- and 3 down-regulated) were relevant to immunity and stress; 10 DEGs (8 up- and 2 down-regulated) were assigned to growth and reproduction; 10 DEGs (9 up- and 1 down-regulated) were attributable to transcription, translation, and cell cycle; and 6 DEGs (five up- and one down-regulated) were belonged to homeostasis (Supplementary Table S5).
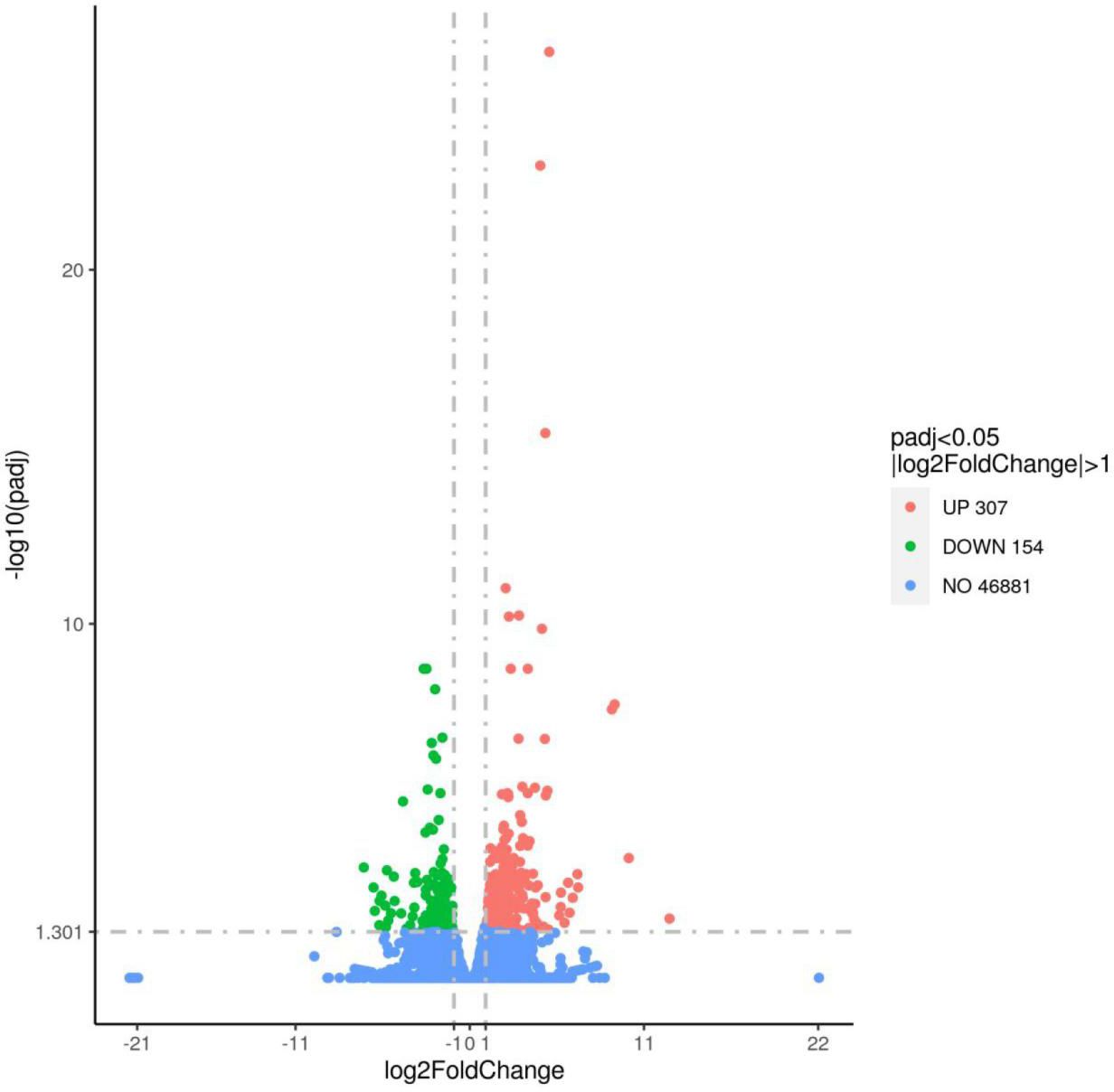
Figure 2. Volcano plot of differentially expressed genes between diet 1 and diet 3. Up- and down-regulated differentially expressed genes are in red and green, respectively. Genes not regulated by fucoidan treatment are in blue.
GO enrichment analysis was performed to reveal the DEGs in biological processes. As shown in Supplementary Figure S1 and Supplementary Table S6, nearly no functional categories were significantly enriched. Nevertheless, it can be seen that DEGs were mainly enriched in the following GO terms such as cellular biosynthetic process, cellular metabolic process, small-molecule metabolic process, organic substance biosynthetic process, cellular lipid metabolic process, and biosynthetic process.
All of the DEGs were mapped to the KEGG database (Supplementary Figure S2; Supplementary Table S7). Pathways including ribosome biogenesis in eukaryotes and nicotinate and nicotinamide metabolism were enriched among up-regulated genes. In contrast, pathways including steroid biosynthesis, terpenoid backbone biosynthesis, and PPAR signaling pathway were overrepresented among down-regulated genes. Notably, ribosome biogenesis in eukaryotes was the top significantly enriched pathway, in which 12.33% (18/146) of the associated genes were up-regulated. Steroid biosynthesis was the top significantly enriched pathway, in which 7.53% (11/146) of the associated genes were down-regulated.
Quantitative real-time PCR validation
To validate the RNA-seq data, 13 genes with different expression patterns were measured by qPCR. As shown in Supplementary Figure S3 and Supplementary Table S8, the data from qPCR and RNA-seq exhibited an excellent agreement on both up- and down-regulated genes. In addition, the high correlation between qPCR and RNA-seq was observed (R2 = 0.9154). These data indicate the reliability of RNA-seq data.
Expression patterns of lipid metabolism genes and glucose metabolism genes
As shown in Figure 3, the mRNA expression of lipolysis-related genes such as hadhb, acacb, cpt1, and fabp1 genes were significantly up-regulated in diet 4 and Diet 5 (P < 0.05). Similarly, eci1 and fabp3 genes were only significantly up-regulated in diet 5. In addition, the acadl gene was significantly up-regulated in diet 3 and diet 4. Meanwhile, the acacb gene was significantly down-regulated in diet 3 and diet 4. As shown in Figure 4, the mRNA expression of lipogenesis-associated genes, including srebf1, fasn, elovl5, acsl3a, scd, and cyp24a1 genes were significantly down-regulated in diet 4 and diet 5 (P < 0.05). Nevertheless, acsl3a and scd genes were significantly up-regulated in diet 2 and diet 3 (P < 0.05). In addition, fads2 was only significantly up-regulated in diet 2 and down-regulated in diet 5 (P < 0.05).
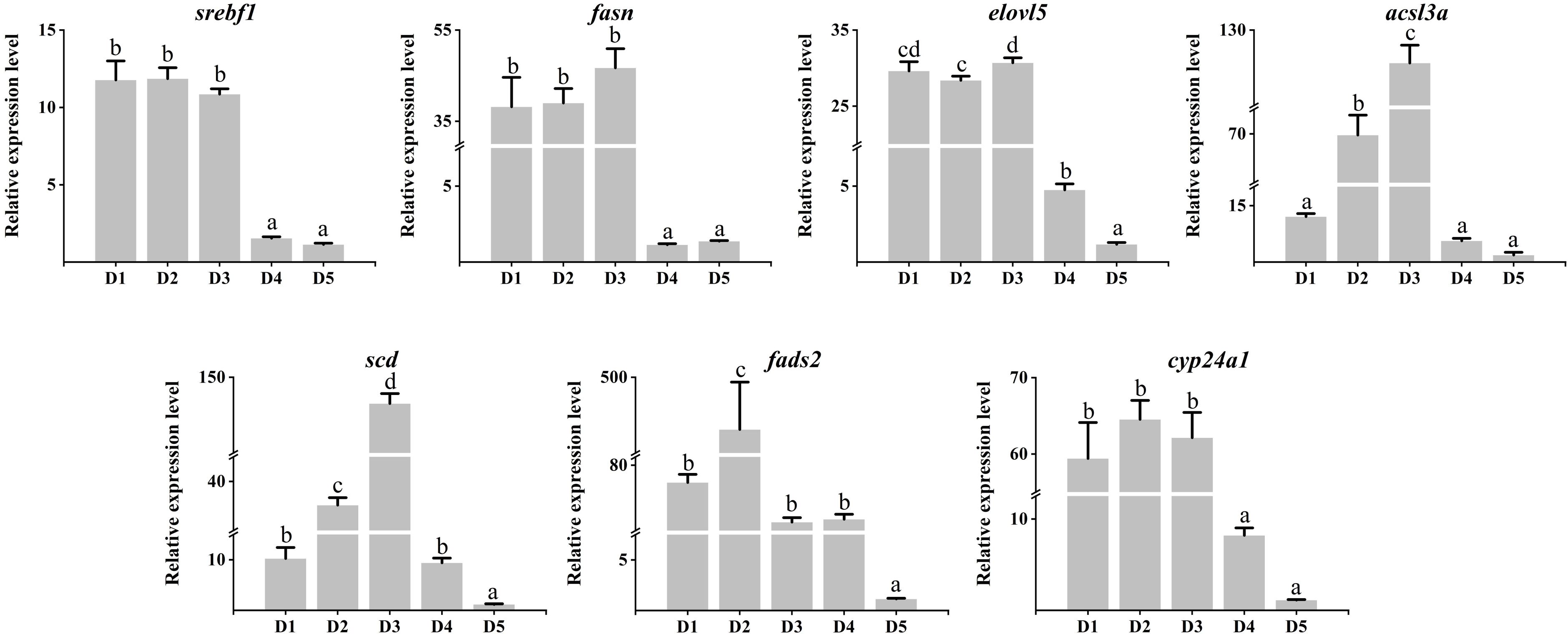
Figure 3. Relative expression levels of lipolysis genes in the liver of juvenile Cyprinus carpio fed experimental diets supplemented with different levels of fucoidan after an 8-week feeding trail. Different letters above the bars show significant difference among the treatments (P < 0.05).
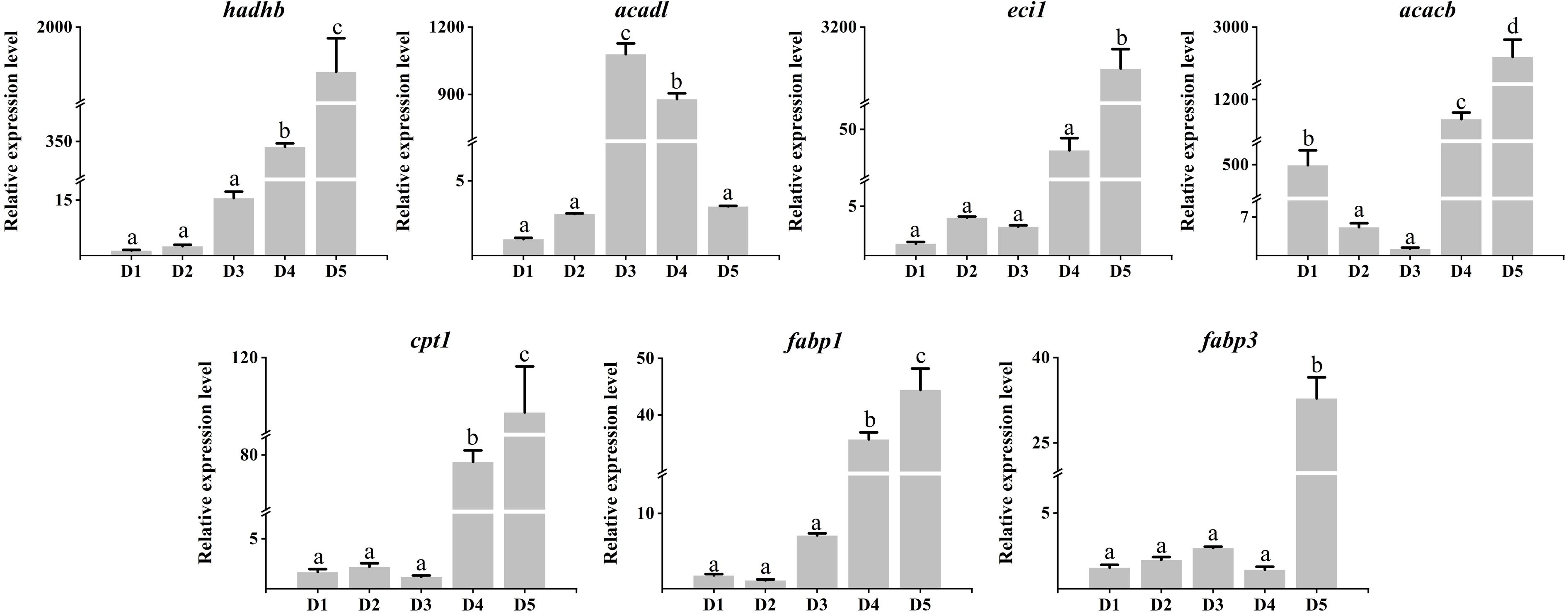
Figure 4. Relative expression levels of lipogenesis genes in the liver of juvenile Cyprinus carpio fed experimental diets supplemented with different levels of fucoidan after an 8-week feeding trail. Different letters above the bars show significant difference among the treatments (P < 0.05).
As shown in Figure 5, the mRNA expression of glycolysis genes such as hk, gk, and pk genes were significantly down-regulated in the liver of fish in diet 4 and diet 5 (P < 0.05). However, gk was significantly up-regulated in diet 3, both gk and pk were significantly up-regulated in diet 4 (P < 0.05). As shown in Figure 6, the relative expression levels of gluconeogenesis genes, including pck1, pck2, g6pca.1, gs, gsk3a, and gsk3b genes were significantly up-regulated in the liver of common carp in diet 4 and diet 5 (P < 0.05). Nevertheless, gs was significantly down-regulated in diet 2 and diet 3, and gsk3b was significantly down-regulated in diet 2 (P < 0.05).
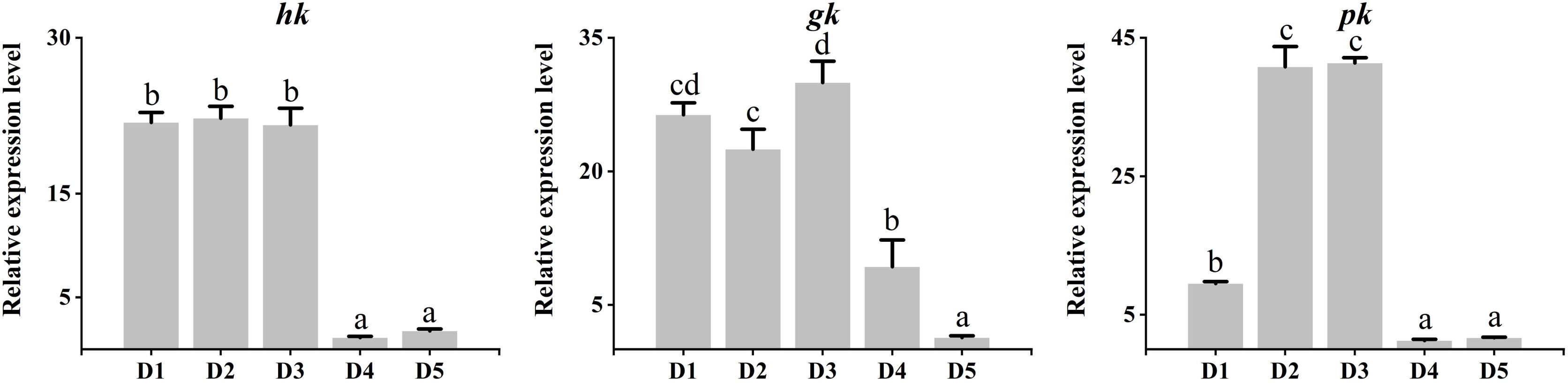
Figure 5. Relative expression levels of glycolysis genes in the liver of juvenile Cyprinus carpio fed experimental diets supplemented with different levels of fucoidan after an 8-week feeding trail. Different letters above the bars show significant difference among the treatments (P < 0.05).
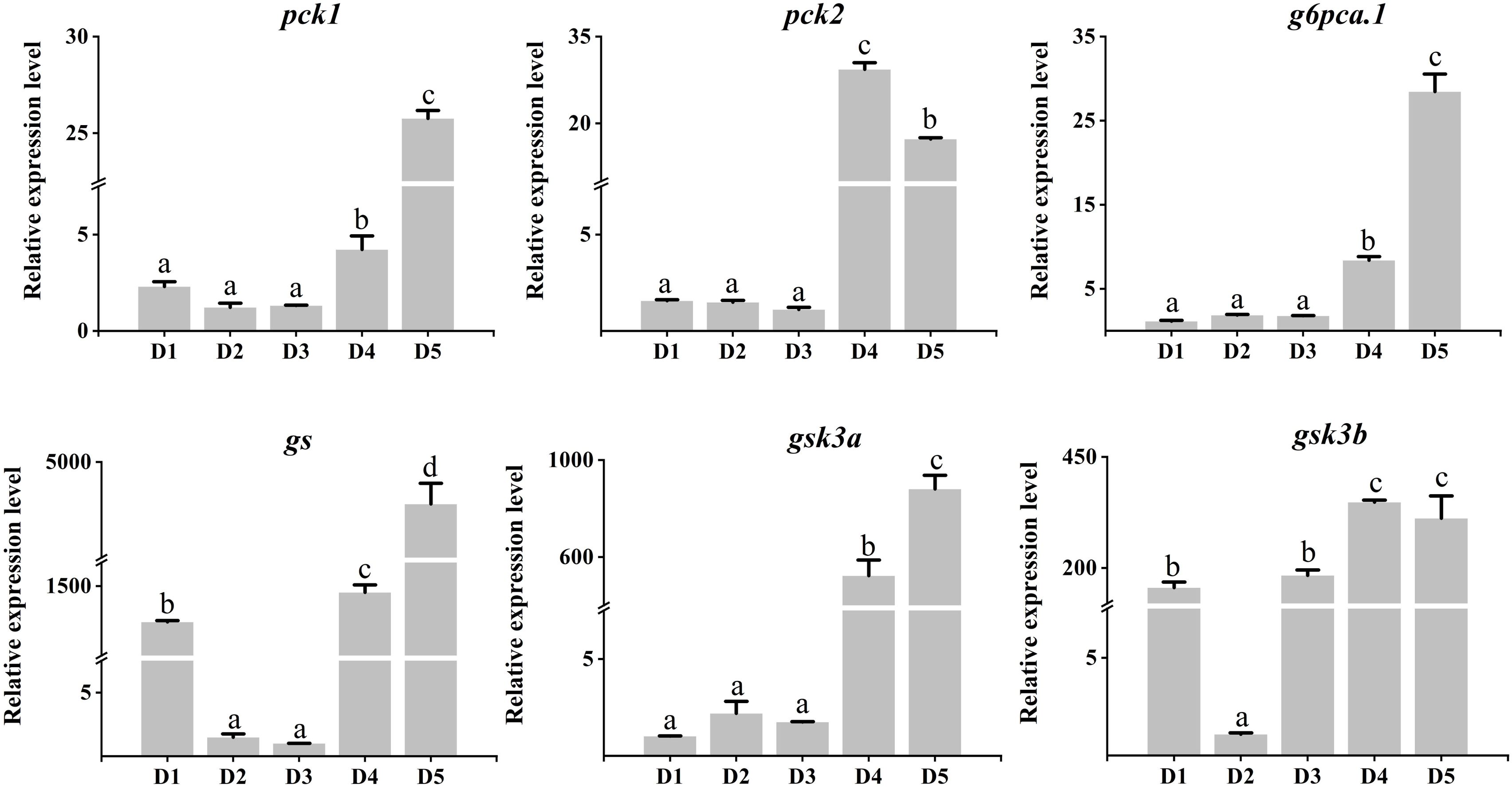
Figure 6. Relative expression levels of gluconeogenesis genes in the liver of juvenile Cyprinus carpio fed experimental diets supplemented with different levels of fucoidan after an 8-week feeding trail. Different letters above the bars show significant difference among the treatments (P < 0.05).
Discussion
The supplementation of fucoidan can effectively promote the growth, enhance antioxidant capacity, and improve immunity of aquatic animals (Subramanian et al., 2019; Apostolova et al., 2020; Li et al., 2023). In addition, the results showed that fucoidan can significantly change hepatocyte ultrastructural characteristics and cause the differential expression of many genes especially the glucose and lipid metabolism genes of liver in the present study.
Liver is an important organ for regulating energy metabolism. Glycogen and lipid droplets in liver can reflect the energy metabolism of the body to a certain extent (Sharma et al., 2022). In the present study, more lipid droplets were significantly stored in hepatocyte, indicating that lipid synthesis is vigorous in the 1,000-mg/kg fucoidan group. In addition, it was also found that mitochondria and RER were expand and their numbers increased, which indicated that hepatocytes were in an active metabolic state (Hu et al., 2019; Triolo et al., 2023). The orderly progression of active metabolic activity in the liver depends on hepatic homeostasis. Therefore, we can see that the number of autolysosome increased, which means that the liver may maintain cell homeostasis through autophagy (Kyung et al., 2023).
RNA-seq technology was used to clarify the fucoidan regulated gene expressions and molecular regulatory networks in common carp liver between diet 1 and diet 3. Differentially expressed profiles revealed that 461 DEGs were screened out (307 up-regulated and 154 down-regulated) based on |log2 (fold change)| > 1 and padj < 0.05. Further analysis indicated that GO terms were enriched in cellular biosynthetic process and cellular metabolic process, KEGG pathways were mainly enriched in eukaryotes and nicotinate and nicotinamide metabolism, steroid biosynthesis, and PPAR signaling pathway. Niacinamide and niacin have better physical stability and regulation effects on glucose and lipid metabolism than vitamins (Shi et al., 2020). PPARα can directly regulate the content of protein required for fatty acid uptake, and PPARγ is a key transcriptional regulator of adipogenesis (Yan et al., 2017; Wang et al., 2020). Previous studies have shown that the fucoidan can increase the protein expression of PPARα and PPARγ at the same time, and its combined effect on PPARs can help to reduce the content of blood lipid by regulating lipid metabolism of liver (Yin et al., 2019). Similarly, the expression of genes in eukaryotes and nicotinate and nicotinamide metabolism and PPAR signaling pathway were significantly affected by fucoidan in the present study. The results were similar to the study of acetylferulic paeonol ester (Yu et al., 2022), bile acids (Li et al., 2024), limonene, and thymol (Aanyu et al., 2020) as a feed additive applied to aquatic animals. In addition, the genes in the steroid synthesis signaling pathway were significantly down-regulated by fucoidan. As we all know, steroids including cholesterol, bile acids, and steroid hormones, are also closely related to metabolic regulation (Nishimura, 2020). Therefore, we speculated that fucoidan may also indirectly participate in regulation of lipid metabolism by influencing steroid synthesis, but this needs further study.
Liver plays essential roles in the synthesis and utilization of lipids in fish, and is a major site where lipolysis and lipogenesis occur. Lipid metabolism involved many crucial genes, including fatty acid oxidation-related genes (hadhb, acadl, ecil, and cpt1) (Sun et al., 2021), fatty acid uptake, transport and metabolism regulation genes (acacb, fabp1, and fabp3) (Zhang et al., 2023b), and fatty acid synthase genes (fasn, scd, fads2, and srebf1) (Shrivastav et al., 2022). In the present study, fucoidan can not only regulate hepatic lipid metabolism by activating eukaryotes and nicotinate and nicotinamide metabolism, steroid synthesis, and PPAR signaling pathways but also alter the expression of lipid metabolism-related genes, including lipolysis genes (hadhb, acadl, ecil, acacb, cpt1, fabp1, and fabp3) and lipogenesis genes (srebf1, fasn, elovl5, acsl3a, scd, fads2, and cyp24a1). Notably, most of the lipogenesis genes were significantly overexpressed in the 500-mg/kg and 1,000-mg/kg fucoidan groups, whereas most of the lipolysis genes were significantly up-regulated in the 1,500-mg/kg and 2,000-mg/kg fucoidan groups. Previous studies indicated that the fucoidan played a crucial part in lipid-lowering activity through reducing fat cell production or regulating cholesterol reverse transport and metabolism (Dong, 2021; Sharma and Baskaran, 2021; Chaves et al., 2022). However, this study found that fucoidan might promote fat synthesis at a low dose. It suggests that the precise dose of fucoidan applied to animals is worthy for further study.
The effect of fucoidan on glucose metabolism is also very significant. Studies have shown that 500 mg of fucoidan orally taken daily in obese patients can significantly increase insulin secretion and improve insulin resistance, thus maintaining blood glucose homeostasis (Vaughan et al., 2022). Gluconeogenesis and glycolysis are opposing metabolic pathways that regulate glucose metabolism, and many key genes involved in the two pathways, including hk, gk, pk, pck1, pck2, g6pca.1, gs, gsk3a, and gsk3b (Sagar et al., 2019). In the present study, low-dose (5,00 mg/kg–1,000 mg/kg) fucoidan in the diet promoted the expression of glycolysis gene, whereas high-dose (1,500 mg/kg–2,000 mg/kg) fucoidan promoted the expression of gluconeogenesis gene in the liver. Similarly, feed additives such as acetylferulic paeonal ester (Yu et al., 2022), bile acids (Wang et al., 2023), nanosized magnesium oxide (Zhang et al., 2023a), and prebiotics (Chen et al., 2023) can significantly regulate the expression of glucose metabolism genes of fish liver.
Conclusion
In this study, we discovered that fucoidan significantly modulates hepatic gene expression associated with glucose and lipid metabolism in common carp. At lower concentrations, fucoidan promotes glycolysis and lipogenesis, whereas at elevated concentrations, it induces gluconeogenesis and lipolysis. These insights are invaluable for the strategic use of fucoidan to ameliorate metabolic imbalances in aquatic species. However, a thorough understanding of the dose–response dynamics and the intricate molecular pathways through which fucoidan exerts its regulatory influence on glucose and lipid metabolism remains an area ripe for future research.
Data availability statement
The data presented in the study are deposited in the NCBI repository, accession numbers SAMN42622844-SAMN42622855 (BioProject number: PRJNA1139972).
Ethics statement
The animal studies were approved by the Committee of Laboratory Animal Experimentation at Southwest University. The studies were conducted in accordance with the local legislation and institutional requirements. Written informed consent was obtained from the owners for the participation of their animals in this study.
Author contributions
FL: Formal analysis, Investigation, Methodology, Supervision, Writing – original draft, Writing – review & editing. HS: Funding acquisition, Project administration, Resources, Supervision, Writing – review & editing, Writing – original draft. JM: Investigation, Resources, Validation, Writing – original draft. YD: Data curation, Investigation, Methodology, Writing – original draft. GH: Data curation, Investigation, Methodology, Writing – original draft. CZ: Conceptualization, Writing – review & editing. XX: Conceptualization, Writing – review & editing.
Funding
The author(s) declare financial support was received for the research, authorship, and/or publication of this article. This work was funded by Chongqing Aquatic Science and Technology Innovation Alliance Science and Technology Innovation Key Project (CQFTIU2024-10).
Acknowledgments
The authors thank to Professor Z.J. Wang’s lab for providing site support and personnel help for this experiment.
Conflict of interest
The authors declare that the research was conducted in the absence of any commercial or financial relationships that could be construed as a potential conflict of interest.
Publisher’s note
All claims expressed in this article are solely those of the authors and do not necessarily represent those of their affiliated organizations, or those of the publisher, the editors and the reviewers. Any product that may be evaluated in this article, or claim that may be made by its manufacturer, is not guaranteed or endorsed by the publisher.
Supplementary material
The Supplementary Material for this article can be found online at: https://www.frontiersin.org/articles/10.3389/fmars.2024.1415341/full#supplementary-material
References
Aanyu M., Betancor B. M., Monroig Ó. (2020). The effects of combined phytogenics on growth and nutritional physiology of Nile tilapia Oreochromis niloticus. Aquaculture 519, 734867. doi: 10.1016/j.aquaculture.2019.734867
Abasubong K. P., Jiang G. Z., Guo H. X., Wang X., Huang Y. Y., Li X. F., et al. (2023). Effects of a high-fat and high-carbohydrate diet on appetite regulation and central AMPK in the hypothalamus of blunt snout bream (Megalobrama amblycephala). J. Anim. Physiol. Anim. Nutr. 2023, 13908. doi: 10.1111/JPN.13908
Ahmadifar E., Mohammadzadeh S., Kalhor N., Yousefi M., Moghadam M. S., Naraballobh W., et al. (2022). Cornelian cherry (Cornus mas L.) fruit extract improves growth performance, disease resistance, and serum immune-and antioxidant-related gene expression of common carp (Cyprinus carpio). Aquaculture 558, 738372. doi: 10.1016/J.AQUACULTURE.2022.738372
Apostolova E., Lukova P., Baldzhieva A., Katsarov P., Nikolova M., Iliev I., et al. (2020). Immunomodulatory and anti-inflammatory effects of fucoidan: A review. Polymers (Basel) 12, 2338–2363. doi: 10.22038/IJBMS.2023.67466.14783
Chaves F. G. P., Batista L. A. N. C., de, Medeiros S. R. B., Rocha H. A. O., Moreira S. M. G. (2022). Sulfated glucan from the green seaweed Caulerpa sertularioides inhibits adipogenesis through suppression of adipogenic and lipogenic key factors. Mar. Drugs 20, 3319–3336. doi: 10.3390/MD20080470
Chen X. M., Zhang J. S., Li M. Y., Tian J. X., Niu X. T., Shan X. F., et al. (2023). Liver transcriptome analysis and identification of differentially expressed immune gene response to Aeromonas veronii infection in Channa argus. Aquacult. Int. 31, 1195–1211. doi: 10.1007/S10499-023-01079-7
Dong C. D. (2021). Isolation and purification of brown algae fucoidan from Sargassum siliquosum and the analysis of anti-lipogenesis activity. Biochem. Eng. J. 165, 107798–107806. doi: 10.1016/j.bej.2020.107798
Fitton J. H., Park A., Karpiniec S. S., Stringer D. N. (2020). Fucoidan and lung function: Value in viral infection. Mar. Drugs 19, 4–11. doi: 10.3390/MD19010004
Grabherr M. G., Haas B. J., Yassour M., Levin J. Z., Thompson D. A., Amit I., et al. (2011). Full-length transcriptome assembly from RNA-Seq data without a reference genome. Nat. Biotechnol. 29, 644–652. doi: 10.1038/nbt.1883
Hu L., Li H. Y., Chen W. Q., Lao S. X., Luo Q. (2019). Ultrastructure characteristics of different Chinese medicine syndromes of helicobacter pylori-correlated dastric diseases. Chin. J. Integr. Med. 25, 917–921. doi: 10.1007/s11655-019-3019-5
Jia R., Hou Y., Zhang L., Li B., Zhu J. (2024). Effects of berberine on lipid metabolism, antioxidant status, and immune response in liver of tilapia (Oreochromis niloticus) under a high-fat diet feeding. Antioxidants 13, 50548. doi: 10.3390/ANTIOX13050548
Khanzadeh M., Hoseinifar H. S., Zargari A., Tabibi H., Doan V., Rabetimarghezar N. (2024). Fucoidan derived from Sargassum ilicifolium affects growth and hemato-immunological parameters and antioxidant defense in Oscar (Astronotus ocellatus). Front. Mar. Sci. 11. doi: 10.3389/FMARS.2024.1370871
Khorshidi Z., Paknejad H., Sodagarm M. D., Hajimoradloo A., Shekarabi S. P. H. (2022). Effect of a commercial multi-effect additive (Biotronic® Top3) on growth performance, digestive enzymes, and intestinal barrier gene expression in common carp (Cyprinus carpio). Aquaculture 560, 738588. doi: 10.1016/J.AQUACULTURE.2022.738588
Kyung E. K., Hyun J. S., Yeajin J., Youngae J., Hyeong S. A., So J. L., et al. (2023). Intermittent fasting attenuates metabolic-dysfunction-associated steatohepatitis by enhancing the hepatic autophagy-lysosome pathway. Nutrients 15, 4574. doi: 10.3390/NU15214574
Lee S., Goodson M., Vang W., Kalanetra K., Barile D., Raybould H. (2020). 2’-fucosyllactose supplementation improves gut-brain signaling and diet-induced obese phenotype and changes the gut microbiota in high fat-fed mice. Nutrients 12, 1003–1022. doi: 10.3390/nu12041003
Li F., Liu X. H., Ge H. L., Huang J., Zhang Y. G., Wang Z. J. (2021b). Transcriptome profiling and differential expression analysis of the immune-related genes during the early phase of acute infection with Aeromonas hydrophila in the Chinese sucker (Myxocyprinus asiaticus). Aquaculture 545, 737258. doi: 10.1016/J.AQUACULTURE.2021.737258
Li F., Sun H. C., Li Y., He D., Ren C. Y., Zhu C. K., et al. (2023). Effects of fucoidan on growthperformance, immunity, antioxidant ability, digestive enzyme activity, and hepatic morphology in juvenile common carp (Cyprinus carpio). Front. Mar. Sci. 10. doi: 10.3389/fmars.2023.1167400
Li F., Zhao J. R., Zhao Y. Y., Liu X. H., Huang J., Zhang Y. G., et al. (2021a). Pathological findings of Chinese sucker (Myxocyprinus asiaticus) infected with virulent Aeromonas hydrophila. Aquac. Rep. 21, 100884. doi: 10.1016/J.AQREP.2021.100884
Li L., Liu T. Y., Li J. R., Yang Y. C., Liu H. Y., Zhang P. Y. (2024). Effectiveness of bile acids as a feed supplement to improve growth performance, feed utilization, lipid metabolism, digestive enzymes, and hepatic antioxidant status in aquaculture animals: A meta-analysis. Aquacult. Rep. 36, 102121. doi: 10.1016/J.AQREP.2024.102121
Li Y. C., Shen J. D., Lu S. F., Zhu L. L., Wang B. Y., Bai M., et al. (2020). Transcriptomic analysis reveals the mechanism of sulfasalazine-induced liver injury in mice. Toxicol. Lett. 321, 12–20. doi: 10.1016/j.toxlet.2019.12.011
Liu J., Karine D., Elisabeth P., Vincent V., Stephane P., Lucie M. (2017). Long-term programming effect of embryonic hypoxia exposure and high-carbohydrate diet at first feeding on glucose metabolism in juvenile rainbow trout. J. Exp. Biol. 220, 3686–3694. doi: 10.1242/jeb.161406
Lu X., Wen H., Li Q., Wang G., Li P., Chen J., et al. (2019). Comparative analysis of growth performance and liver transcriptome response of juvenile Ancherythroculter nigrocauda fed diets with different protein levels. Comp. Biochem. Phys. D 31, 100592. doi: 10.1016/j.cbd.2019.05.002
Mahgoub H. A., El-Adl M. A. M., Ghanem H. M., Martyniuk C. J. (2020). The effect of fucoidan or potassium permanganate on growth performance, intestinal pathology, and antioxidant status in Nile tilapia (Oreochromis niloticus). Fish Physiol. Biochem. 46, 2109–2131. doi: 10.1007/s10695-020-00858-w
Mi J., Liu D., Qin C., Yan X., Yang L., Xu X., et al. (2024). (–)-Epigallocatechin-3-O-gallate or (–)-epicatechin enhances lipid catabolism and antioxidant defense in common carp (Cyprinus carpio L.) fed a high-fat diet: mechanistic insights from the AMPK/Sirt1/PGC-1α signaling pathway. Aquaculture 587, 740876. doi: 10.1016/J.AQUACULTURE.2024.740876
Nishimura T. (2020). Feedforward regulation of glucose metabolism by steroid hormones drives a developmental transition in drosophila. Curr. Biol. 30, 3624–3632. doi: 10.1016/j.cub.2020.06.043
Pecino B. S., Montblanch M., Moll R. E., Fernandez V. G., Meilan I. G., Fontanillsa R., et al. (2024). Impact of hydroxytyrosol-Rich extract supplementation in a high-fat diet on gilthead sea bream (Sparus aurata) lipid metabolism. Antioxidants 13, 40403. doi: 10.3390/ANTIOX13040403
Sagar V., Sahu N. P., Pal A. K., Hassaan M., Jain K. K., Salim H. S., et al. (2019). Effect of different stock types and dietary protein levels on key enzyme activities of glycolysis-gluconeogenesis, the pentose phosphate pathway and amino acid metabolism in Macrobrachium rosenbergii. J. Appl. Ichthyol. 35, 1016–1024. doi: 10.1111/jai.13931
Setyawan A., Isnansetyo A., Murwantoko I. S., Handayani C. R. (2018). Comparative immune response of dietary fucoidan from three Indonesian brown algae in white shrimp Litopenaeus vannamei. AACL Bioflux 11, 1707–1723. Available at: www.bioflux.com.ro/aacl.
Shan X. D., Liu X., Hao J. J., Cai C., Dun Y. L., Zhao X. L., et al. (2016). In vitro and in vivo hypoglycemic effects of brown algal fucoidans. Int. J. Biol. Macromol. 82, 249–255. doi: 10.1016/j.ijbiomac.2015.11.036
Sharma K., Sharma P., Dhiman S. K., Chadha P., Saini H. S. (2022). Biochemical, genotoxic, histological and ultrastructural effects on liver and gills of fresh water fish Channa punctatus exposed to textile industry intermediate 2 ABS. Chemosphere 287, 132103. doi: 10.1016/J.CHEMOSPHERE.2021.132103
Sharma P. P., Baskaran V. (2021). Polysaccharide (laminaran and fucoidan), fucoxanthin and lipids as functional components from brown algae (Padina tetrastromatica) modulates adipogenesis and thermogenesis in diet-induced obesity in C57BL6 mice. Algal. Res. 54, 102187–102201. doi: 10.1016/J.ALGAL.2021.102187
Shi H. J., Li X. F., Xu C., Zhang D. D., Zhang L., Xia S. L., et al. (2020). Nicotinamide improves the growth performance, intermediary metabolism and glucose homeostasis of blunt snout bream Megalobrama amblycephala fed high-carbohydrate diets. Aquac. Nutr. 26, 1311–1328. doi: 10.1111/anu.13088
Shi Y., Zhong L., Zhong H., Zhang J., Che C., Fu G., et al. (2022). Taurine supplements in high-fat diets improve survival of juvenile Monopterus albus by reducing lipid deposition and intestinal damage. Aquaculture 547, 737431. doi: 10.1016/J.AQUACULTURE.2021.737431
Shrivastav A. K., Kumar G., Mittal P., Tocher D. R., Glencross B. D., Charkrabarti R., et al. (2022). Effect of greater duckweed Spirodela polyrhiza supplemented feed on growth performance, digestive enzymes, amino and fatty acid profiles, and expression of genes involved in fatty acid biosynthesis of juvenile common carp Cyprinus carpio. Front. Mar. Sci. 9. doi: 10.3389/FMARS.2022.788455
Sivagnanavelmurugan M., Thaddaeus B. J., Palavesam A., Immanuel G. (2014). Dietary effect of Sargassum wightii fucoidan to enhance growth, prophenoloxidase gene expression of Penaeus monodon and immune resistance to Vibrio parahaemolyticus. Fish Shellfish Immunol. 39, 439–449. doi: 10.1016/j.fsi.2014.05.037
Sony N. M., Ishikawa M., Hossain M. S., Koshio S., Yokoyama S. (2019). The effect of dietary fucoidan on growth, immune functions, blood characteristics and oxidative stress resistance of juvenile red sea bream, Pagrus major. Fish Physiol. Biochem. 45, 439–454. doi: 10.1007/s10695-018-0575-0
Subramanian P., Manoharan V., Periyannan R., Ravichandran A., Ganesan S., Thangapandi M., et al. (2019). Antibacterial efficacy of a fucoidan fraction (Fu-F2) extracted from Sargassum polycystum. Int. J. Biol. Macromol. 125, 485–495. doi: 10.1016/j.ijbiomac.2018.12.070
Sun J., Wu W. Y., Ji H. (2021). Effect of overwintering on body composition, antioxidant enzyme activities, fatty acid composition, glucose and lipid-metabolic related gene expression of grass carp (Ctenopharyngodon idellus). Aquaculture 545, 737125. doi: 10.1016/J.AQUACULTURE.2021.737125
Traifalgar R. F., Kira H., Tung H. T., Michael F. R., Yokoyama A. L. S., Ishikaw M. A., et al. (2010). Influence of dietary fucoidan supplementation on growth and immunological response of juvenile Marsupenaeus japonicus. J. World Aquac. Soc 41, 235–244. doi: 10.1111/j.1749-7345.2010.00363.x
Triolo M., Wade S., Baker N., Khacho M. (2023). Evaluating mitochondrial length, volume, and cristae ultrastructure in rare mouse adult stem cell populations. STAR Protoc. 4, 102107. doi: 10.1016/J.XPRO.2023.102107
Vaughan K., Ranawana V., Cooper D., Aceves M. M. (2022). Effect of brown seaweed on plasma glucose in healthy, at-risk, and type 2 diabetic individuals: systematic review and meta-analysis. Nutr. Rev. 80, 1194–1205. doi: 10.1093/NUTRIT/NUAB069
Wang L., Sagada G., Wang C. Y., Liu R. C., Li Q., Zhang C., et al. (2023). Exogenous bile acids regulate energy metabolism and improve the health condition of farmed fish. Aquaculture 562, 738852. doi: 10.1016/J.AQUACULTURE.2022.738852
Wang Q. Y., Lu C., Peng C. N., Zhong J. Y., Ling N. (2022a). New progress in research on antitumor activity of seaweed fucoidan. J. Food Saf. Qual. 13, 2043–2050. doi: 10.19812/j.cnki.jfsq11-5956/ts.2022.07.002
Wang T., Xu R., Qiao F., Du Z. Y., Zhang M. L. (2022b). Effects of mannan oligosaccharides (MOS) on glucose and lipid metabolism of largemouth bass (Micropterus salmoides) fed with high carbohydrate diet. Anim. Feed Sci. Tech. 292, 115449. doi: 10.1016/J.ANIFEEDSCI.2022.115449
Wang Y., Chen X., Huang Z., Chen D., Yu B., Yu J., et al. (2020). Dietary Ferulic acid supplementation improves antioxidant capacity and lipid metabolism in weaned piglets. Nutrients 12, 3811. doi: 10.3390/NU12123811
Wei M., Yuan X., Song L., Li H., Ji H., Sun J. (2024). Comparative proteomic analysis of pathological characterization of adipose tissue remodeling induced by high-fat diet and high-carbohydrate diet in grass carp (Ctenopharyngodon idellus). Aquaculture 590, 741079. doi: 10.1016/J.AQUACULTURE.2024.741079
Wu S. Y., Yang W. Y., Cheng C. C., Hsiao M. C., Tsai S. L., Lin H. K., et al. (2020). Low molecular weight fucoidan prevents radiation-induced fibrosis and secondary tumors in a zebrafish model. Cancers (Basel) 12, 1608–1629. doi: 10.3390/cancers12061608
Xue S., Xia B., Zou Y., Li L., Zhang B., Shen Z., et al. (2022). Dandelion extract on growth performance, immunity, stress and infection resistance in common carp. Aquac. Rep. 26, 101330. doi: 10.1016/J.AQREP.2022.101330
Yan J., Liao K., Mai K., Ai Q. (2017). Dietary lipid levels affect lipoprotein clearance, fatty acid transport, lipogenesis and lipolysis at the transcriptional level in muscle and adipose tissue of large yellow croaker (Larimichthys crocea). Aquac. Res. 48, 3925–3934. doi: 10.1111/are.13219
Yan X., Pang P., Qin C. B., Mi J. L., Yang L. P., Yang B. W., et al. (2024). Improvement of sea buckthorn (Hippophae rhamnoides L.) flavonoids on the antioxidant and immune performance of yellow river carp (Cyprinus carpio L.) fed high-carbohydrate diet. Fish Shellfish Immun. 144, 109289. doi: 10.1016/J.FSI.2023.109289
Yin J. Y., Wang J., Li F. H., Yang Z. X., Yang X. Q., Sun W. L., et al. (2019). The fucoidan from the brown seaweed Ascophyllum nodosum ameliorates atherosclerosis in apolipoprotein E-deficient mice. Food Funct. 10, 5124–5139. doi: 10.1039/c9fo00619b
Yin Z., Gong Y., Liu Y., He Y., Yao C., Huang W., et al. (2022). Fucoidan improves growth, digestive tract maturation, and gut microbiota in large yellow croaker (Larimichthys crocea) larvae. Nutrients 14, 4504. doi: 10.3390/NU14214504
Yu H., Li Y., Li T., Xu G., Xing W., Jiang N., et al. (2024). Spiral valve intestinal barrier functions of juvenile Siberian sturgeon (Acipenser baerii) were changed by low or high-lipid diet. Aquacult. Rep. 36, 102101. doi: 10.1016/J.AQREP.2024.102101
Yu L. J., Zhang C. X., Xue Z. Y., Lu X., Wen H., Jiang M. (2022). Acetylferulic paeonol ester: a new feed additive reduces lipid accumulation in the liver of Nile tilapia (Oreochromis niloticus) by modulating lipid and glucose metabolism. Aquaculture 2022, 561. doi: 10.1016/J.AQUACULTURE.2022.738671
Zhang L., Liu Z. S., Deng Y., He C. F., Liu W. B., Li X. F. (2023a). The benefits of nanosized magnesium oxide in fish Megalobrama amblycephala: evidence in growth performance, redox defense, glucose metabolism, and magnesium homeostasis. Antioxidants (Basel Switzerland) 12, 1350. doi: 10.3390/ANTIOX12071350
Zhang L., Tan P., Zhang P., Chen R. Y., Wang L. G., Xu D. D. (2023b). A comparative study of dietary lipid sources on growth performance, body composition, fillet quality, and nutrient retention in juvenile yellow drum (Nibea albiflora). Aquaculture 574, 739630. doi: 10.1016/J.AQUACULTURE.2023.739630
Zhao L., Liang J., Chen F., Tang X., Liao L., Liu Q., et al. (2021). High carbohydrate diet induced endoplasmic reticulum stress and oxidative stress, promoted inflammation and apoptosis, impaired intestinal barrier of juvenile largemouth bass (Micropterus salmoides). Fish Shellfish Immun. 119, 308–317. doi: 10.1016/J.FSI.2021.10.019
Zhao X., Chen W., Zhang Y., Gao X., Huang Y., Ren H., et al. (2024). Dietary guar gum supplementation reduces the adverse effects of high-fat diets on the growth performance, antioxidant capacity, inflammation, and apoptosis of juvenile largemouth bass (Micropterus salmoides). Anim. Feed Sci. Tech. 308, 115881. doi: 10.1016/J.ANIFEEDSCI.2024.115881
Keywords: fucoidan, common carp, liver, ultrastructure, glycolipid metabolism
Citation: Li F, Sun H, Mei J, Deng Y, Hu G, Zhu C and Xiang X (2024) Fucoidan changes lipid accumulation in the liver of common carp (Cyprinus carpio) by modulating lipid and glucose metabolism. Front. Mar. Sci. 11:1415341. doi: 10.3389/fmars.2024.1415341
Received: 15 April 2024; Accepted: 30 July 2024;
Published: 23 August 2024.
Edited by:
Rui Jia, Chinese Academy of Fishery Sciences (CAFS), ChinaReviewed by:
Kenneth Prudence Abasubong, University of South Bohemia, CzechiaBasanta Kumar Das, Central Inland Fisheries Research Institute (ICAR), India
Copyright © 2024 Li, Sun, Mei, Deng, Hu, Zhu and Xiang. This is an open-access article distributed under the terms of the Creative Commons Attribution License (CC BY). The use, distribution or reproduction in other forums is permitted, provided the original author(s) and the copyright owner(s) are credited and that the original publication in this journal is cited, in accordance with accepted academic practice. No use, distribution or reproduction is permitted which does not comply with these terms.
*Correspondence: Hanchang Sun, c3VuaGFuY2hhbmcxOTlAMTYzLmNvbQ==