- 1School of Environmental and Municipal Engineering, Qingdao University of Technology, Qingdao, China
- 2Department of Ocean Science, The Hong Kong University of Science and Technology, Hong Kong, Hong Kong SAR, China
Eroded soils sustain a substantial part of organic matter in tidal rivers adjacent to estuaries, and photochemical transformations of soils in tidal rivers would influence estuarine elemental cycles. However, complex aquatic environments and diverse soil sources complicate the enrichment of dissolved organic matter (DOM) photoreleased from soils. Here, we conducted a 7-day irradiation experiment for seven kinds of soils from the lower basin of Dagu River (DGR) in the laboratory to study the influence of salinity and soil properties on DOM chemistry by characterizing the content and optical properties of DOM. Results showed that light cultures had higher amount of DOM and humic-like components than dark cultures. Principal component analysis (PCA) and Mantel’s analysis found that salinity and soil properties significantly influence the production of photoreleased DOM, especially humic-like components. Salinity could inhibit the photodissolution of soils, and aged soils with low δ13CSOM released more DOM and humic-like components. Although the DGR is impacted by intruded seawater, high content of photoreleased DOM in seawater cultures still pointed out the important contribution of soil photodissolution to the DOM reservoir of tidal rivers. Considering high proportion of humic-like components in photoreleased DOM, photochemical transformations of soils in tidal rivers would promote the export flux of carbon from estuaries to open seas. This study emphasizes the importance of soil photodissolution of tidal rivers in the carbon transfer from lands to oceans.
1 Introduction
Natural organic matter (NOM), a ubiquitous matrix of organic materials and the largest reduced carbon reservoir, plays an important role in elemental cycles and ecosystem functions of aquatic environments (Massicotte et al., 2017). Tidal rivers are an emerging hot spot of estuarine elemental cycles and ecosystems, where organic matter (OM) composition is controlled by complex hydrological and biogeochemical processes (Bianchi and Allison, 2009; Hoitink and Jay, 2016). Eroded soils have contributed to the OM pool of tidal rivers due to land utilization and hydraulic erosion (Balthazar et al., 2013; Hurni et al., 2015). Part of soil organic matter (SOM) could be buried in estuarine environments through flocculation and sedimentation, forming a sink for geochemical elements. However, strong tidal oscillations drive the erosion and resuspension of sediments, causing the low burial rate of SOM in the sedimentary environment of tidal rivers (Sun et al., 2020), and a long hydraulic retention time supported subsequent biogeochemical transformations of SOM in the water column (Downing-Kunz and Schoellhamer, 2013; Xu et al., 2021), which influences the role of tidal rivers in estuarine elemental cycles and ecosystem functions.
Photochemistry is one important mechanism processing the OM of tidal rivers, which would induce the mineralization and dissolution of resuspended SOM in the water column (Mayer et al., 2006; Schiebel et al., 2015; He et al., 2016). Although generated inorganic carbon could escape from tidal rivers reducing estuarine carbon storage (Cory et al., 2007; Medeiros et al., 2015), some dissolved organic matter (DOM) could be released through the photochemical transformation of SOM, adding the complexity and uncertainty of estuarine elemental cycles (Estapa et al., 2012; Hu et al., 2020; Harfmann et al., 2021). Previous studies found that the characteristics and content of photoreleased DOM depend on particulate sources and size (Mayer et al., 2012; Helms et al., 2014). Traditionally, fine terrestrial particles released more humic-like DOM through photochemical reactions than plant residues (Lee et al., 2019, Lee et al., 2023). Driven by land utilization, diverse SOM would converge in tidal rivers and undergo the photochemical transformation to release DOM with different properties (Fellman et al., 2009; Ward et al., 2017). Moreover, aquatic ions also impact the photochemical transformation of OM by participating in photochemical reactions (Parker and Mitch, 2016; He et al., 2022). Recent studies revealed that halogen ions could react with intermediates to enhance the photolysis of DOM (Zhang et al., 2018). The effect of salinity on the decomposition and dispersion of SOM was also observed in tidal rivers (Wong et al., 2010; Qu et al., 2019; Zhang et al., 2023), and Marton et al. (2012) found that salinity can alter short-term carbon dynamics of tidal wetlands. Tidal rivers experienced dynamic variations of the ionic environment due to seawater intrusion (Hoitink and Jay, 2016), which could be another important factor modulating the photorelease of DOM from SOM. Moreover, intrinsic characteristics of photoreleased DOM would account for their persistence and biogeochemical cycles in tidal rivers (Bélanger et al., 2006; Canuel and Hardison, 2016; Cao et al., 2018). Compared with protein-like components, humic-like components as the photo-product could resist biodegradation to recycle throughout estuaries (Zhao et al., 2023). Thus, to get a comprehensive understanding about the role of tidal rivers in estuarine elemental cycles, it is necessary to explore the influence of soil sources and hydrological conditions on the photochemical transformation of SOM and the composition of photoreleased DOM.
Downstream Dagu River (DGR) adjacent to Jiaozhou Bay is a typical tidal river that processed and transported a large amount of OM, modulating estuarine carbon cycles (Yu et al., 2019). Previous studies showed that eroded soils have a major contribution to sediment OM of the DGR (Dong et al., 2020) and were repeatedly stirred and uplifted by strong tidal oscillations in the dry season (Chen et al., 2019). Furthermore, long water retention time prolonged the exposure of resuspended SOM to sunlight (Wang H. et al., 2014), stimulating their photochemical transformations in the water column and influencing the role of the DGR in elemental cycles. A significant correlation between aquatic DOM and land utilization was also observed in the DGR (Ding et al., 2022). However, anthropogenic activities shape the DGR basin with diverse land types including forest land, reeds, cropland, grassland, and aquaculture (Li et al., 2020; Gao et al., 2023). Moreover, tidal oscillations modulate the content of seawater intrusion in the DGR (Liu et al., 2019; Zhu et al., 2020), altering the hydrological condition. Dynamic hydrologic conditions and multiple sources of SOM add complexities and uncertainties to the photochemical transformation of SOM to DOM (He et al., 2016). In this study, seven types of soils along the tidal reach of the DGR were collected to employ light simulation experiments in different hydrological conditions (freshwater and artificial seawater). The variance in spectroscopic properties of DOM from SOM photolysis was also characterized by the absorption-fluorescence spectrum while featuring the geochemical properties of SOM. The objectives of this study were to (1) evaluate the qualitative and quantitative characteristics of photoreleased DOM from suspended soils in the DGR, (2) explore the influence of sources and environmental conditions (salinity) on the photochemical transformation of SOM, and (3) suggest the role of resuspended SOM photolysis in modulating estuarine elemental cycle.
2 Materials and methods
2.1 Soil collection and preparation
The DGR was located in the inner coastal area of Jiaozhou Bay, which covers an area of 6,131.3 km2 (Jiang et al., 2021). The downstream land of the DGR was cultivated for various purposes (Dong et al., 2020). Seven sampling sites were set along the downstream DGR to collect different types of SOM on 15 April 2022 (T1–T7, Figure 1). These representative sampling sites were located on both sides of the tidal section of the DGR, containing reeds (T1), cropland (T2), saline land (T3), aquaculture (T4), forest land (T5), grassland (T6), and unutilized land (T7) (Figure 1 and Table 1). We collected diverse soils with ca. 0- to 15-cm depths with different properties using a Luoyang shovel. All collected samples were transported within 24 h to the laboratory in an icebox and then freeze-dried. We ground and sieved freeze-dried soils (100 mesh) to prepare for photochemical simulation experiments under different hydrological conditions.
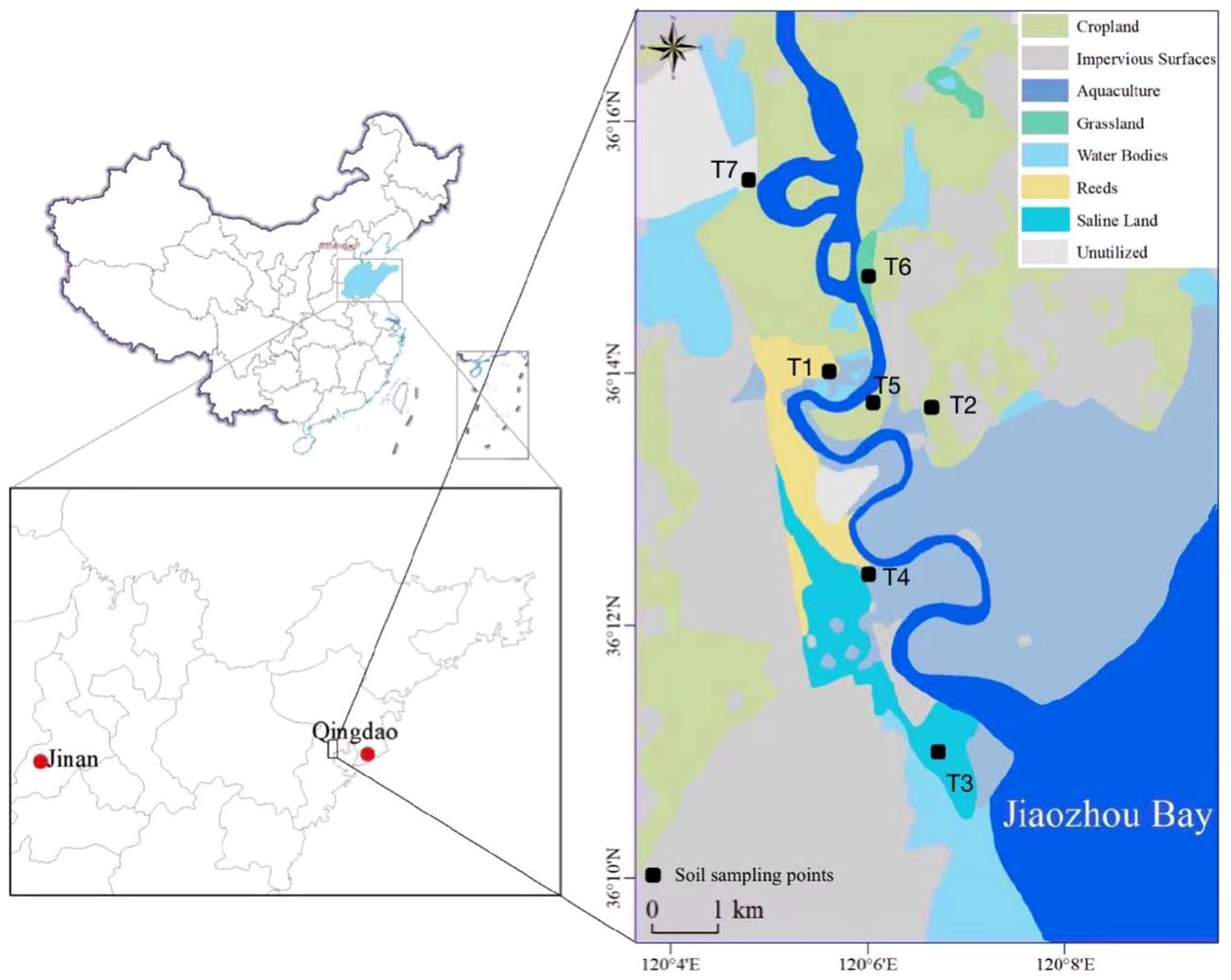
Figure 1 Study area and sampling sites in the Dagu River (DGR). Seven sites (T1-T7) were selected to collected representative soils (Reeds-T1, agricultural land-T2, saline land-T3, aquaculture-T4, forest land-T5, grassland-T6, unutilized land-T7).

Table 1 Land types, organic carbon content (TOCSOM%) and inorganic carbon content (TICsoil%), and organic carbon isotope composition (δ13CSOM‰) of seven soils in the tidal river basin.
2.2 Photochemical experiments of resuspended SOM
Photochemical experiments of resuspended SOM were conducted based on SOM sources and water salinity. SOM resuspension was prepared by immersing seven processed soils in acid-pretreated HDPE bottles with ultrapure water at solid concentrations of 5,000 mg/L, respectively. After stirring at 100 rpm for sample homogenization, the resuspension of seven soils was quartered, respectively; two sub-samples underwent photo-irradiation, while others were kept in the dark to serve as control cultures by covering them with aluminum foil. In order to intuitively reflect the influence of salinity in the photolysis of SOM through altering ionic strength and main ion concentration (Cl−), two sub-samples were artificially amended with solid sodium chloride (burned at 450°C for 4 h). The salinity of simulation experiments was set at 10 based on the salinity gradient of the DGR ranging from 0.6 to 27 (Supplementary Figure S1). For each soil sample, four experimental units were set up including freshwater with photo-irradiation (Light-F), artificial seawater with photo-irradiation (Light-S), freshwater without photo-irradiation (Dark-F), and artificial seawater without photo-irradiation (Dark-S). All sets of cylindrical acrylic reactors containing each resuspension solution were placed on the rooftop, where they could be exposed to direct sunlight. Maximum light intensity was approximately 120,000 lx, and maximum UV irradiation intensity was approximately 6,000 μW/cm2 as shown in Supplementary Table S1. In all cultures, resuspensions were continuously stirred at 100 rpm and incubated for 168 h with periodic ventilation.
2.3 Sample collection and basic chemical analysis
The carbon content of soils was characterized including total organic carbon content (TOCSOM) and total inorganic carbon content (TICsoil). Approximately 1 g of soil was acidified using 3 M HCl (30 mL) (60°C) to remove carbonate and then acid-treated soils were repeatedly rinsed with ultrapure water to pH = 7. The carbon content of acid-treated soils and original soils was featured by an elemental analyzer (Thermo Scientific FLASH2000 Series CNS, accuracy ±0.3%). Finally, an isotope ratio mass spectrometer (Thermal Scientific) with a precision and accuracy of ±0.5‰ was employed to determine the stable carbon isotopic composition (δ13CSOM) of acidified soils.
Resuspension samples at each experiment unit were collected at 6, 12, 24, 96, 120, and 168 h. All water samples were immediately centrifuged at 4,000 rpm for 30 min. The supernatant fluid was filtered through pre-combusted 0.7-μm membrane filters (450°C, 4 h) (47 mm, Whatman GF/F), then followed by filtration by pre-washed 0.2-μm membrane filters (47 mm, Isopore) for nutrient and DOM analysis. Nutrient concentration including phosphate (PO4-P), silicate (SiO3-Si), and dissolved inorganic nitrogen (DIN, NH4-N, NO3-N, and NO2-N) of water samples was measured according to Grasshoff et al. (2009) using a Bran and Luebbe 5-channel AAIII (Germany) segmented flow colorimetric auto-analyzer (NH4-N, NO3-N, NO2-N, PO4-P, and SiO3-Si at ±0.1 μM, ± 0.3 μM, ± 0.02 μM, ± 0.02 μM, and ±0.2 μM, respectively). Dissolved organic carbon (DOC) concentration of filtrates was determined by a TOC analyzer (Shimadzu TOC-L) (± 2%) to characterize DOM content.
2.4 Optical analysis
An Aqualog absorption-fluorescence spectrometer (Horiba) was used to measure UV-Vis and 3D-EEMs of filtrates according to Wang et al. (2021). Milli-Q water was used as blank, and the scan was conducted from 240 nm to 600 nm (increments, 3 nm; scan integration time, 1 s). Specific ultraviolet absorbance at 254 nm (SUVA254) was calculated to quantify the content of aromatic DOM (Weishaar et al., 2003), and the spectral slope ratio (SR; 275–295 nm slope: 350–400 nm slope), which is related to DOM molecular weight (MW) (Helms et al., 2008) and humification index (HIX) for the humification degree of DOM (Ohno, 2002), was also calculated. To perform a semi-quantitative analysis of DOM, the PARAFAC model (Stedmon and Bro, 2008) was used to identify three fluorescence components including component 1 (C1, Ex/Em, 240/430 nm), component 2 [C2, Ex/Em, (240, 276)/325 nm], and component 3 (C3, Ex/Em, 273/509 nm) (Supplementary Figure S2). C1 was similar to fluorescence peak A/C that was traditionally defined in terrestrial DOM (Supplementary Figure S2A; Burdige et al., 2004; Bernal et al., 2018) and C3 was similar to the terrestrial humic-like fluorescence peak A (Supplementary Figure S2C; Murphy et al., 2008). Component C2 was an autochthonous protein-like substance in tryptophan-like fluorescence peak T (Supplementary Figure S2B; Coble et al., 1998; Murphy et al., 2008).
2.5 Statistical analyses
The Student’s t-test was conducted using SPSS 16.0 to evaluate the significant differences between different cultures. A principal component analysis (PCA) of DOM and nutrient parameters in all samples was also introduced to analyze potential mechanisms modulating the photochemical transformation of SOM using Canoco 5.0. To study the correlation between the photochemical production of soils and different environmental factors, multivariate statistical analyses were performed in all samples. The Mantel’s analysis of all geochemical parameters and different environmental factors was conducted using the “LinkET” package in R, and Spearman’s correlation between different geochemical parameters was also conducted in R. Finally, structural equation modeling was used to detect the potential pathways in tidal rivers by which soil properties may cause total DOM content changes via dissolution and photorelease alterations based on the DOC concentration and humic-like component content of seawater cultures on 168 h. Moreover, dark cultures indicate the physical and microbial dissolution of soils, and the difference in DOM content between dark cultures and light cultures indicates the photorelease of soils.
3 Results
3.1 Geochemical properties of soils
Soils of the DGR show obvious regional differences in OM content (TOCSOM) and composition (δ13CSOM, Table 1) due to land utilization and vegetation destruction. All soils showed a higher content of organic carbon than inorganic carbon (TOCSOM 0.48 ± 0.22%; TICsoil 0.11 ± 0.07%). The organic carbon content of soils (TOCSOM) ranged from 0.18% to 0.91%. Station T2 covered by cropland had the highest organic content (0.91%), while T1 covered by reeds had the lowest organic content (0.18%). The δ13CSOM values related to SOM compositions varied from −24.6‰ to −20.5‰. The highest value of −20.5‰ was shown in T4 with aquaculture and the lowest value of −24.6‰ was shown in T7 with unutilized land.
3.2 Changes in nutrient concentration and DOM properties in photochemical experiments
Dynamic variations in DOM and nutrient concentration of water samples under different conditions were observed as shown in Figure 2. DOC concentration of all cultures showed an increased trend along with the culture time lasting and higher DOC concentration was observed in station T2 with cropland and station T7 with unutilized land (T2, 6.78 ± 0.67 mg/L; T7, 5.76 ± 0.68 mg/L; others, 3.82 ± 0.41 mg/L, Figure 2A). Light cultures showed a DOC concentration of 5.22 ± 1.84 mg/L, which was significantly higher than that of dark cultures (3.78 ± 1.17 mg/L; Figures 2A, 3A). There is also a significant difference between Light-F cultures and Light-S cultures (p < 0.01, Figure 3A), and Light-F cultures have a higher DOC concentration (Light-F, 5.52 ± 0.68 mg/L; Light-S, 4.92 ± 0.82 mg/L; Figures 2A, 3A). Silicate concentration (SiO3-Si) also showed an increased trend (Figure 2D), and cultures exposed to sunlight had a higher SiO3-Si concentration than dark cultures (light, 30.7 ± 13.0 μM; dark, 17.6 ± 8.6 μM; Figure 3D). However, DIN and PO4-P concentrations decreased during long-term culture of soils (Figures 2B, C). Cultures exposed to sunlight showed a significant decrease in DIN and PO4-P concentration, and their decreasing ratios (DIN, 95.2% ± 11%; PO4-P, 81.1 ± 13%) were much higher than those of dark cultures (DIN, 46.5% ± 23.1%; PO4-P, 58.5 ± 45.4%). Under light conditions, mean DIN (20.6 ± 4.7 μM) was lower than that of dark cultures (49.7 ± 5.1 μM; Figures 2B and 3B), while PO4-P concentration among cultures showed an opposite trend (light, 0.76 ± 0.49 μM; dark, 0.33 ± 0.39 μM; Figures 2C and 3C). Meanwhile, DIN and PO4-P of Light-F cultures showed values significantly lower than Light-S cultures (Light-F, DIN 18.9 ± 9.8 μM, PO4-P 0.68 ± 0.46 μM; Light-S, DIN 22.2 ± 10.9 μM, PO4-P 0.83 ± 0.57 μM; Figures 3B, C).
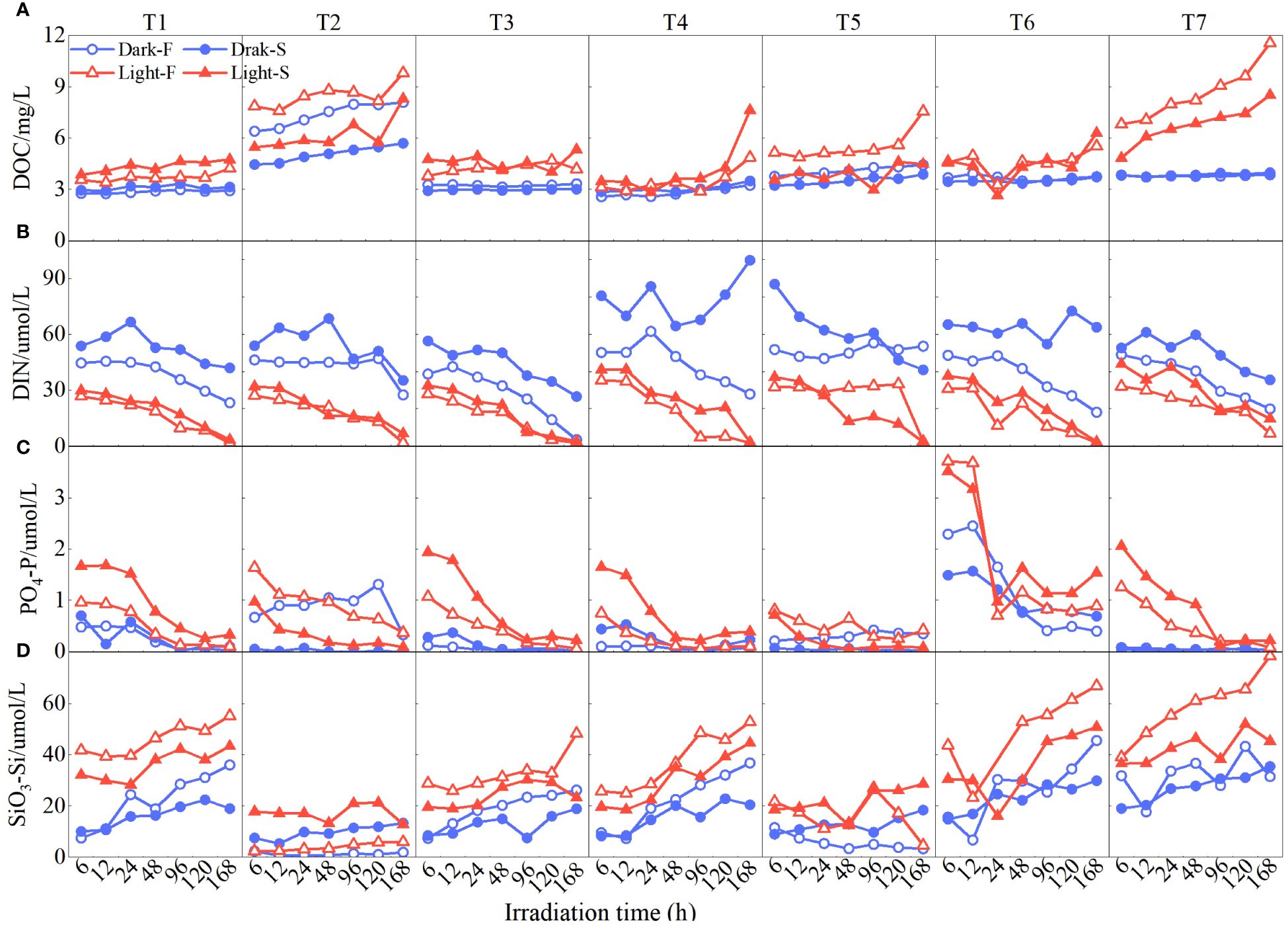
Figure 2 Temporal changes in DOC (A) and nutrient concentration (B, DIN; C, PO4-P; D, SiO3-Si) of water samples under different conditions (blue dots represent seawater dark conditions Dark-S, blue circles represent freshwater with dark conditions Dark-F, red solid triangles represent seawater with light conditions Light-S, and red triangles represent freshwater with light conditions Light-F).
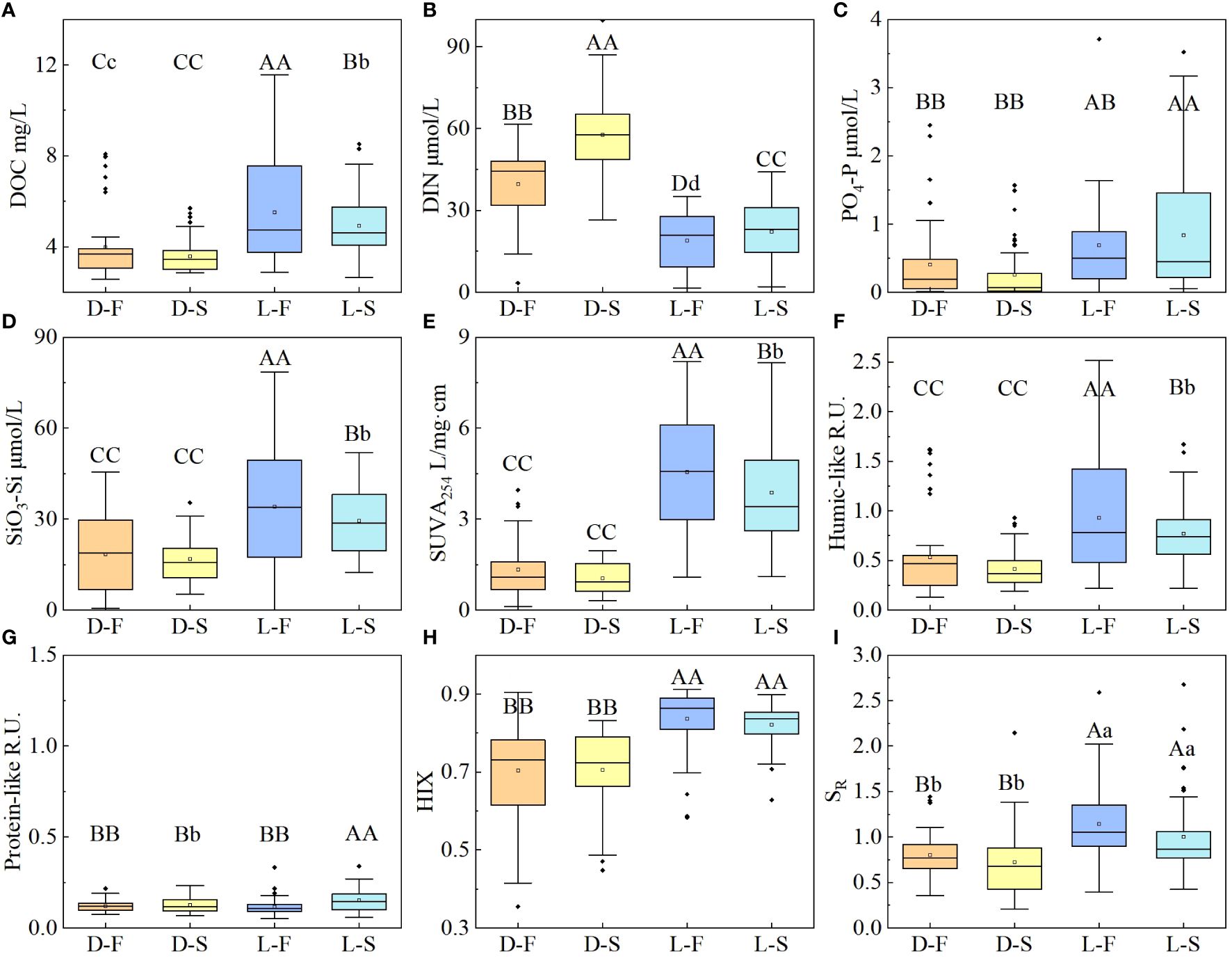
Figure 3 Boxplots of DOC (A), nutrient concentration (B DIN, C PO4-P, D SiO3-Si) and optical parameters (E SUVA254, F Humic-like components C1+C3, G Protein-like components C2, H HIX, I SR) under different conditions (yellow boxes represent seawater with dark conditions D-S, orange boxes represent freshwater with dark conditions D-F, blue boxes represent seawater with light conditions L-S, green boxes represent freshwater with light conditions L-F). Different letters indicate significant differences between different cultures (capital letters indicating p<0.01, and lower-case letter indicating p< 0.05).
The optical properties of DOM also displayed dynamic variations among cultures as shown in Figure 4. SUVA254, indicating the content of aromatic compounds (Figure 4A) and humic-like components (C1+C3, Figure 4B), showed an increased trend especially at stations T2 and T7 (T2, SUVA254, 38.07% ± 30%; humic-like, 51.03% ± 22%; T7, SUVA254, 20.23% ± 40%; humic-like, 45.52% ± 54%), while the protein-like component (C2) was relatively constant with low values (0.10 ± 0.04 R.U.; Figure 4C). Moreover, light cultures had a higher content of aromatic compounds (SUVA254) and humic-like components (SUVA254, light, 4.22 ± 1.54 mg/L·cm; dark, 1.19 ± 0.63 mg/L·cm; humic-like, light, 1.13 ± 0.21 R.U.; dark, 0.87 ± 0.13 R.U.), which were significantly different from those of dark cultures (p < 0.01; Figures 3E, F). Furthermore, freshwater cultures exposed to sunlight showed higher values in SUVA254 and humic-like components than seawater cultures exposed to sunlight (Light-F, SUVA254, 4.55 ± 0.92 mg/L·cm, humic-like, 0.93 ± 0.17 R.U.; Light-D, SUVA254, 3.88 ± 0.98 mg/L·cm, humic-like, 0.77 ± 0.17 R.U.). Correspondingly, HIX representing the humification degree of DOM also increased along with culture time lasting, and higher values were shown in Light-F cultures (Light-F, 0.86 ± 0.13; Light-S, 0.83 ± 0.1; Dark-F, 0.75 ± 0.03; Dark-S, 0.73 ± 0.04; Figures 4D, 3H). Moreover, SR being negatively correlated to the molecular weight of DOM randomly fluctuated over time, and higher values occurred in light cultures (light, 0.99 ± 0.34; dark, 0.86 ± 0.46; Figures 4E, 3I).
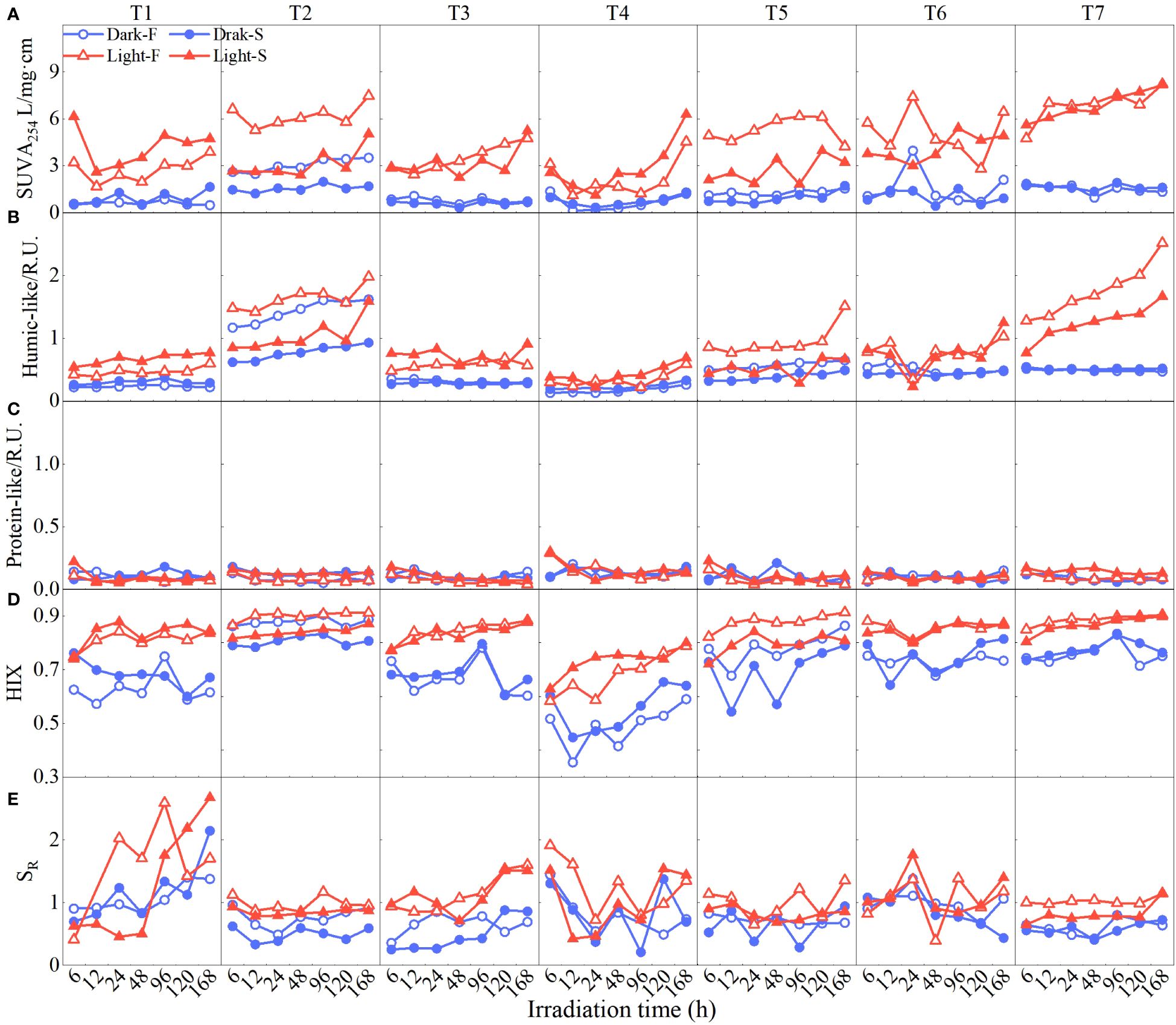
Figure 4 Temporal changes in optical parameters (A SUVA254, B Humic-like components C1+C3, C Protein-like components C2, D HIX, E SR) of water samples under different conditions (blue dots represent seawater with dark conditions Dark-S, blue circles represent freshwater with dark conditions, Dark-F, red solid triangles represent seawater with light conditions Light-S, and red triangles represent freshwater with light conditions Light-F).
3.3 Statistical analysis
To understand the influence of soil sources and aquatic environments in the photorelease of DOM from soils, PCA and Mantel’s analysis were performed based on geochemical parameters and environmental factors in all samples. The first and second principal components (PC1 and PC2) explain 52.8% and 14.5% of the variance in DOM composition, respectively (Figure 5A). Water samples in light cultures show DOM characteristics different from dark cultures, and light samples are located on the positive loading of PC1, which is positively correlated with all parameters except for DIN and protein-like components. However, diverse samples cultured under different conditions converge in the positive loading of PC2. For Mantel’s analysis, DOC showed significantly positive correlations with PO4-P, SUVA254, humic-like component, and HIX (r > 0), while it exhibited negative correlations with DIN, protein-like component, and SR (r < 0, Figure 5B). Environmental factors displayed different correlations with geochemical parameters. Light has significant correlations with all parameters except protein-like components (p < 0.05), and nutrient concentration was mainly correlated with light. DOC shows significant correlations with light, TOCSOM and δ13CSOM (p < 0.05), and humic-like components and HIX are significantly correlated with all environmental factors (p < 0.05).
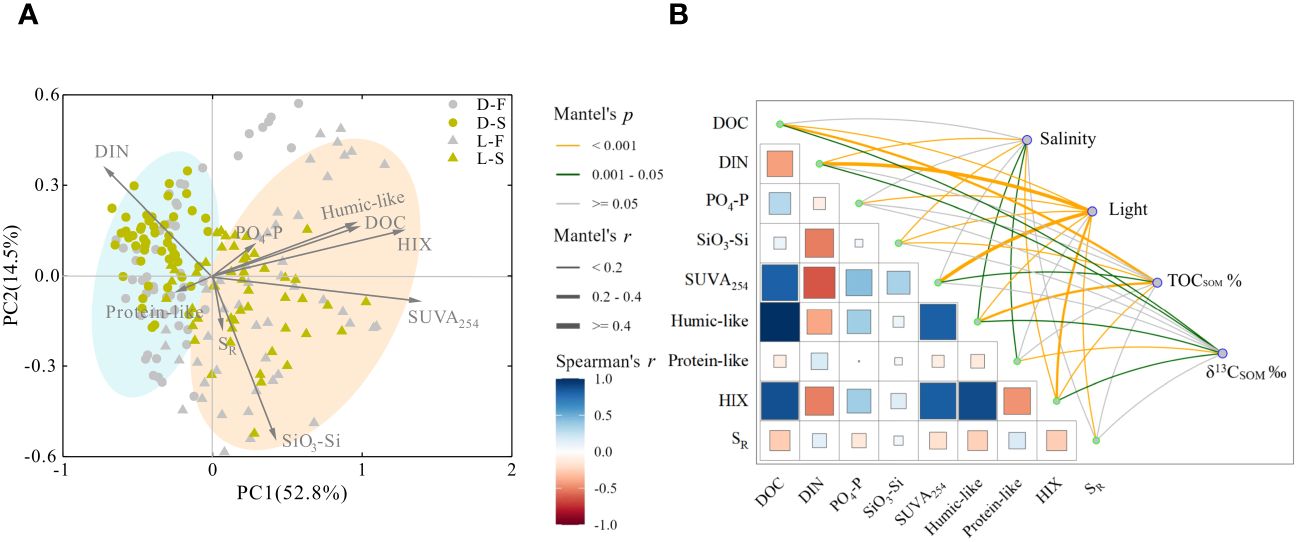
Figure 5 (A) Principal component analysis based on DOC, TOCSOM nutrients (DIN, PO4-P, SiO3-Si), and optical parameters (SUVA254, HIX, SR, humic-like components, and protein-like components) of all cultures. Yellow dots represent seawater cultures without light Dark-S, grey dots represent freshwater cultures without light Dark-F, yellow triangles represent seawater cultures with light Light-S, and grey triangles represent freshwater cultures with light Light-F). (B) Mantel analysis of geochemical parameters (DOC, DIN, PO4-P, SiO3-Si, SUVA254, HIX, SR, humic-like components, and protein-like components) and environmental factors (light, salinity, TOCSOM, δ13CSOM) in all samples. Among two parameters, the square represents correlation coefficient of geochemical parameters. Lines represent the correlation between environmental factors and different geochemical parameters (the color of lines represents p values, and the thickness of lines represents r values). p>0.05 means no significant difference; p<0.05 means significant difference.
4 Discussion
4.1 Photochemistry serves as an important factor driving DOM release from SOM in the aquatic environment
In the aquatic environment, suspended soils are processed by diverse biogeochemical processes, and numerous mechanisms including physical dissolution/desorption, biodegradation, and photodissolution account for DOM release from soils (He et al., 2016; Lee et al., 2023). Inorganic nutrient concentration and DOM content in the dark cultures present physical dissolution/desorption and microbial release of soils (Figures 2, 4). Furthermore, DOM enrichment during physical and microbial processes is modulated by the OM content of soils as indicated by the high DOC concentration in dark cultures of carbon-enriched soils at station T2 (Table 1; Figure 2A). Moreover, no significant differences between Dark-F and Dark-S cultures of all soils (Figure 3A) could suggest a few influences of water salinity on the physical/microbial release of DOM from soils. Previous studies also pointed out the resuspension-induced repartition of OM between solid and liquid phases in a tidal estuary with seawater intrusion (Komada and Reimers, 2001). Nonetheless, the higher content of DOM that appeared in light cultures (Figures 2, 4) could indicate the facilitation of photochemistry for DOM release from soils, which is also supported by significant differences between dark cultures and light cultures (Figures 3, 5A). Helms et al. (2014) and Schiebel et al. (2015) also found photochemistry accelerating the OM exchange between water columns and suspended sediments. The photorelease of DOM from particulate materials is thought to be driven by two mechanisms, namely, direct photo-assisted desorption and indirect photochemical reactions via radical oxygen species (Dong et al., 2021; Harfmann et al., 2021). Hu et al. (2020) found that indirect photochemical reactions play a dominant role in particulate matter photodissolution and NO3− could facilitate indirect process. Although microorganisms could consume nutrients inducing the decrease of DIN and PO4-P in all cultures (Figures 2B, C), the lower DIN concentration in light cultures could suggest the occurrence of DOM photorelease via indirect photochemical reactions of soils being involved in the participation of NO3− (Mostofa et al., 2012), which was also supported by the negative correlation between DIN and DOC (Figure 5B). Although some photoreleased DOM could be further remineralized into CO2 (Mopper et al., 2015), the increase in DOC concentration (Figure 2A) and the content of related compounds (Figures 4A, B) in light cultures display the continuous enrichment of DOM, underlining the important contribution of photochemical transformations of soils to the aquatic DOM pool.
4.2 Photochemical enrichment of DOM from SOM depending on soil sources and salinity
Given that the photodissolution of particulate materials in aquatic environments is one chemical reaction driven by irradiation, the photochemical enrichment of DOM from SOM would be affected by substrate properties and water environments (Helms et al., 2014; Appiani and McNeill, 2015; Hu et al., 2022). The SOM of tidal rivers has diverse sources, which combined with hydrological conditions to complicate DOM photorelease from suspended soils (Ward et al., 2017). PCA and Mantel’s analysis also showed that light exposure is only part of the reason for DOM variations among all experimental cultures, and soil properties and salinity also impact DOM content and composition (Figure 5). Because of land utilization and anthropogenic activities, soils of the DGR have high primary productivity as indicated by a δ13CSOM of −23.3‰ to −20.5‰ except for undeveloped T7 with aged soils (Table 1; Gao et al., 2023). Nonetheless, when suspended soils were exposed to sunlight, more aromatic (SUVA254) and humic-like compounds were released into the water column in all experimental cultures (Figures 4A, B, 3E, F), increasing the humification degree of DOM (Figures 4D, 3H). Lee et al. (2019) and Shank et al. (2011) also found that photoreleased DOM from suspended sediments and soils was dominated by humic-like components. As reported by previous studies, dissolved aromatic compounds are photochemically active to form reactive intermediates (Hu et al., 2022; Cai et al., 2023), and thus photochemistry could also preferentially process particulate aromatic OM releasing related intermediates. Although the SOM of stations T7 and T6 had a similar carbon content (Table 1), the higher content of aromatic compounds and humic-like components photoreleased from aged soils of station T7 could underline the propensity of photochemical transformations for humified SOM (Figures 4A, B), which is also supported by the significant correlation between δ13CSOM and specific components (SUVA254 and humic-like, Figure 5B). Helms et al. (2014) also found that the photodissolution of humified sediments released more DOM. Furthermore, the production of DOM photoreleased from soils also depends on the organic carbon content of soils as indicated by the higher DOM content in light cultures of station T2 (Figure 2) and significant correlations between TOCSOM and DOM parameters (DOC, SUVA254, humic-like, Figure 5B; Liu and Shank, 2015).
Water salinity is another factor modulating the photodissolution of suspended particulate matter (He et al., 2016). For all sunlight experiments, seawater cultures showed lower values in DOC concentration, SUVA254, and humic-like components than freshwater cultures (Figure 3). Previous studies found that salinity inhibited the photodegradation of DOM through the participation of halogen ions in reacting with photochemical intermediates (Minor et al., 2006; Zhang et al., 2020). Thus, chloride ions could also react with photochemical intermediates from soils to reduce the production of photoreleased DOM. Moreover, salinity improved the flocculation of suspended particulate matter from a fine to a coarse state (Mietta et al., 2009). Although all cultures of this study were stirred, salinity-induced flocculation could also occur, which is supported by the flocculation of particulate matter in tidal estuaries with strong hydrodynamic forcing (Zhang et al., 2021). Considering the specific surface area of different particulate matter, fine particulate matter may have a large specific surface area to experience irradiation (Atkinson et al., 2009; Jung et al., 2012). Although there is no significant difference in dissolved DOM composition between seawater cultures and freshwater cultures in the dark environment (Figure 3), the significant correlation between salinity and silicate concentration (Figure 5B) could suggest photochemical transformations amplifying the influence of particulate matter size on soil dissolution. The process would disrupt the photorelease of DOM from soils as indicated by the significant correlation between salinity and humic-like components (Figure 5B). Lee et al. (2023) also found that fine particulate OM releases more humic-like substances by photodissolution than coarse particulate matter.
4.3 Implications for estuarine elemental cycles and further considerations
Tidal rivers serve as a part of estuarine ecosystems with diverse OM sources and complex biogeochemical processes, modulating the role of estuaries in global elemental cycles (Bianchi and Allison, 2009; Hoitink and Jay, 2016). The photochemical transformation of soils is one key process concerning estuarine elemental cycles (Schiebel et al., 2015). Although results of culture experiments showed that intruded seawater inhibited the photochemical dissolution of SOM in the tidal river, photoreleased DOM in the seawater environment is still considerable (Figures 2–5). Based on the results of 168 h in seawater cultures, this study constructed a structural equation model (SEM) of soil properties causing total DOC concentration and humic-like component content changes via physical dissolution and photorelease alterations in seawater (Figure 6). The SEM shows that soil properties account for the production of humic-like components (p < 0.05, Figure 6B), and the photomineralization of humic-like components under irradiation could cause a low correlation coefficient between soil properties and photoreleased production of DOM and humic-like components (Figure 6; Lu et al., 2016). Nonetheless, the photolysis of soils is predominant in the DOM reservoir as indicated by high correlation coefficients between total DOM content and photoreleased production (Figure 6). When some inorganic nutrients physically released from soils were consumed during photochemical and microbial transformations of SOM (Figure 2), high DOM production from soil photodissolution would contribute a large number of organic nutrients fueling estuarine ecosystems (Southwell et al., 2011; Glibert et al., 2023). As the largest reduced carbon reservoir, dynamic variations of OM between different phases also have important implications for estuarine carbon cycle (Bianchi and Allison, 2009). Based on the DOC concentration of 168 h in seawater cultures of different soils (Figure 2), the average production of DOM via dissolution and photorelease of soils is calculated to be approximately 1.29 ± 0.31 mg per gram of soil (Figure 6A). The soil erosion modulus of the DGR basin is approximately 308 t/(km2·year), and the export flux is approximately 9.60 × 105 t/year (Zhang et al., 2013). Based on the drainage area of the DGR (4,631.3 km2) and the conversion rate of terrestrial sediments (85%) (Huang et al., 2024), the maximum production of DOM via dissolution and photorelease of soils is approximately 1.10 ×105 gC·km−2·year−1 (1.29 mg·g-1× (308 t·km−2·year−1×4,631.3 km2 − 9.60×105 t·year−1)/4,631.3 km2×0.85). Kong (2014) estimated a DOM export flux of 1.6 ×105 gC·km−2·year−1 all year round in the DGR. Although our estimation ignored the contribution proportion of different soils and the remineralization rate of soils, this result underlines the important contribution of soils through dissolution and photorelease to DOM reservoir of tidal rivers. Moreover, the promoted production of DOM from soil photolysis has also been observed in the aquatic environment (An et al., 2023). Furthermore, the content of humic-like components from dissolution and photorelease per gram of soils is approximately 0.22 ± 0.08 R.U. (Figure 6B), and the content of protein-like components is approximately 0.023 ± 0.004 R.U. Thus, some DOM with low biological activity from the photodissolution of soils would be transported into open seas participating in marine carbon cycles.
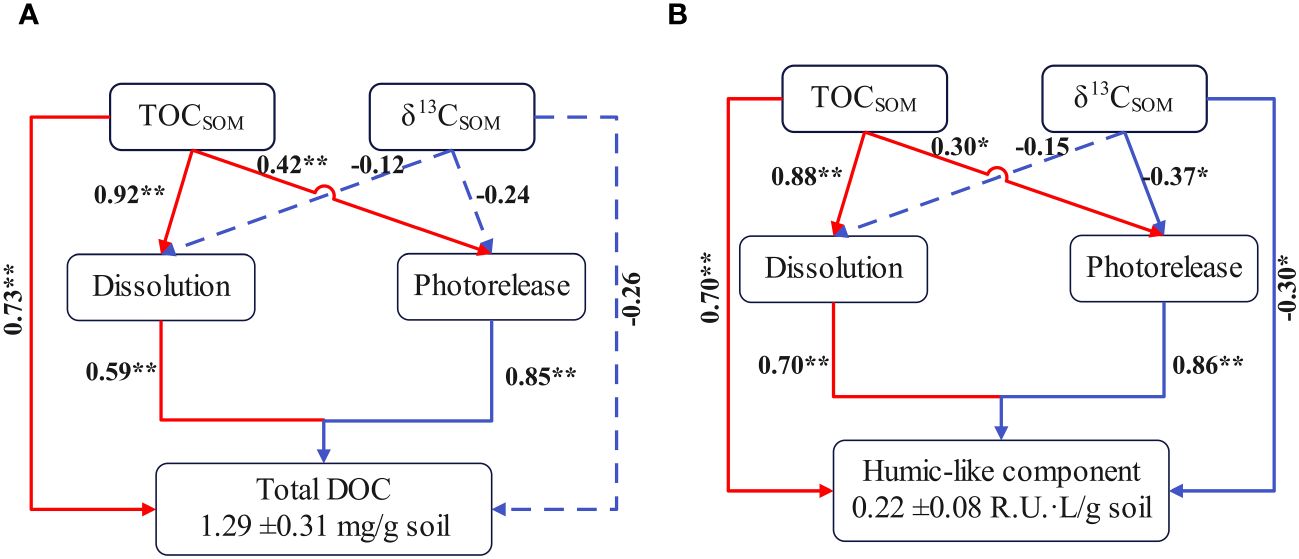
Figure 6 Structural equation model of soil properties may cause total DOC concentration (A) and humic-like component content (B) changes via physical dissolution and photorelease alterations in seawater cultures based on DOC concentration and humic-like component content of 168h. Single-headed arrows indicate the hypothesized direction of causation. Red arrows indicate the influence of organic matter content of soils (TOCSOM), and blue arrows indicate the influence of organic matter composition of soils (δ13CSOM). * and ** represent p < 0.05, p < 0.01 respectively. Numbers represent spearman’s r.
Although we carried out experimental cultures based on our purposes, limitations still exist. First, the influence of field seawater in the photochemical transformations of suspended particulate matters is complex. In this study, we recognized that artificial seawater is simple. The difference in results between freshwater and artificial seawater cultures could still clarify the influence of salinity on photochemical transformations of suspended particulate matter through altering ionic strength and the main ion concentration (Cl−). Second, DOM is a matrix of organic elements, and analytical techniques of this study are limited to clarifying complex biogeochemical transformations of different elements, and FT-ICR MS could provide an informational elemental composition of DOM (Zhou et al., 2022; Guo et al., 2023). Even though further efforts are needed to make up for the above limitations, this is the first study to introduce irradiation experiments of different soils from the tidal river basin to clarify the influence of salinity and soil properties on DOM enrichment. Although current analytical techniques are insufficient to address all issues related to estuarine elemental cycles, the important production of DOM from photochemical transformations of soils in tidal rivers is pointed out. Considering the intensification of soil erosion by anthropogenic activities and climate change and the critical role of soil transformation in estuarine elemental cycles (Wuepper et al., 2020), it is necessary to employ more analytical techniques, including FT-ICR MS, to comprehensively study different elemental cycles of tidal rivers under light irradiation.
5 Conclusions
This work carried out laboratory-controlled irradiation experiments to investigate the response of DOM content and composition to various soil photolysis under different aquatic environments. Resuspended soils were photodegraded in the water column and released some DOM, especially humic-like components. Diverse factors, including salinity and soil properties, modulate the production of DOM photoreleased from soils, and salinity could inhibit the photodissolution of soils. Furthermore, soils with a high organic carbon content and aged soils with low δ13CSOM released more DOM and humic-like components. Although the DGR is impacted by intruded seawater and receives diverse soils, photoreleased DOM from soils in tidal rivers is still considerable. These results underline the important contribution of photoreleased DOM from suspended soils to DOM reservoirs of tidal rivers, which promoted carbon export flux of estuaries to open seas.
Data availability statement
Data to support this study are available in the main text and are also available in Figshare: https://doi.org/10.6084/m9.figshare.26075602.
Author contributions
YPZ: Conceptualization, Data curation, Formal analysis, Funding acquisition, Investigation, Methodology, Project administration, Resources, Software, Supervision, Validation, Visualization, Writing – original draft, Writing – review & editing. YXZ: Conceptualization, Data curation, Formal analysis, Investigation, Methodology, Writing – original draft. SJ: Data curation, Investigation, Methodology, Writing – original draft. DM: Conceptualization, Data curation, Formal analysis, Investigation, Methodology, Writing – review & editing. YP: Conceptualization, Formal analysis, Methodology, Writing – review & editing. YX: Funding acquisition, Writing – review & editing.
Funding
The author(s) declare financial support was received for the research, authorship, and/or publication of this article. This research is supported by the Natural Science Foundation of Shandong Province (No. ZR2023MD094), the National Key Research and Development Program (No. 2021YFC3201004), the Taishan Scholar Foundation of Shandong Province (No. tsqn201909126), and the National Natural Science Foundation of China (No. 42103029).
Conflict of interest
The authors declare that the research was conducted in the absence of any commercial or financial relationships that could be construed as a potential conflict of interest.
The reviewer CH declared a past co-authorship with the author YZ to the handling editor.
Publisher’s note
All claims expressed in this article are solely those of the authors and do not necessarily represent those of their affiliated organizations, or those of the publisher, the editors and the reviewers. Any product that may be evaluated in this article, or claim that may be made by its manufacturer, is not guaranteed or endorsed by the publisher.
Supplementary material
The Supplementary Material for this article can be found online at: https://www.frontiersin.org/articles/10.3389/fmars.2024.1400196/full#supplementary-material
References
An S., Mao Z., Chen M., Huang X., Shi L., Xing P., et al. (2023). Sunlight irradiation promotes both the chemodiversity of terrestrial DOM and the biodiversity of bacterial community in a subalpine lake. Environ. Res. 227, 115823. doi: 10.1016/j.envres.2023.115823
Appiani E., McNeill K. (2015). Photochemical production of singlet oxygen from particulate organic matter. Environ. Sci. Technol. 49, 3514–3522. doi: 10.1021/es505712e
Atkinson C. L., Golladay S. W., Opsahl S. P., Covich A. P. (2009). Stream discharge and floodplain connections affect seston quality and stable isotopic signatures in a coastal plain stream. J. North Am. Benthological Soc. 28, 360–370. doi: 10.1899/08-102.1
Balthazar V., Vanacker V., Girma A., Poesen J., Golla S. (2013). Human impact on sediment fluxes within the Blue Nile and Atbara River basins. Geomorphology 180, 231–241. doi: 10.1016/j.geomorph.2012.10.013
Bélanger S., Xie H., Krotkov N., Larouche P., Vincent W. F., Babin M. (2006). Photomineralization of terrigenous dissolved organic matter in Arctic coastal waters from 1979 to 2003: Interannual variability and implications of climate change. Global Biogeochemical Cycles 20 (4), 1–13. doi: 10.1029/2006GB002708
Bernal S., Lupon A., Catalán N., Castelar S., Martí E. (2018). Decoupling of dissolved organic matter patterns between stream and riparian groundwater in a headwater forested catchment. Hydrology Earth System Sci. 22, 1897–1910. doi: 10.5194/hess-22-1897-2018
Bianchi T. S., Allison M. A. (2009). Large-river delta-front estuaries as natural “recorders” of global environmental change. Proc. Natl. Acad. Sci. 106, 8085–8092. doi: 10.1073/pnas.0812878106
Burdige D. J., Kline S. W., Chen W. (2004). Fluorescent dissolved organic matter in marine sediment pore waters. Mar. Chem. 89, 289–311. doi: 10.1016/j.marchem.2004.02.015
Cai T., Zhang X., Zhang S., Ming Y., Zhang Q. (2023). Photochemical behaviors of dissolved organic matter in aquatic environment: Generation, characterization, influencing factors and practical application. Environ. Res. 231 (2), 116174. doi: 10.1016/j.envres.2023.116174
Canuel E. A., Hardison A. K. (2016). Sources, ages, and alteration of organic matter in estuaries. Annu. Rev. Mar. Sci. 8, 409–434. doi: 10.1146/annurev-marine-122414-034058
Cao X., Aiken G. R., Butler K. D., Huntington T. G., Balch W. M., Mao J., et al. (2018). Evidence for major input of riverine organic matter into the ocean. Organic Geochemistry 116, 62–76. doi: 10.1016/j.orggeochem.2017.11.001
Chen X. Y., Liu D. H., Yin P., Liu J. Q., Cao K., Gao F. (2019). Temporal and spatial evolution of surface sediments characteristics in the Dagu River estuary and their dynamic response mechanism. China Geology 2, 325–332. doi: 10.31035/cg2018092
Coble P. G., Del Castillo C. E., Avril B. (1998). Distribution and optical properties of CDOM in the Arabian Sea during the 1995 Southwest Monsoon. Deep Sea Res. Part II: Topical Stud. Oceanography 45, 2195–2223. doi: 10.1016/S0967-0645(98)00068-X
Cory R. M., McKnight D. M., Chin Y. P., Miller P., Jaros C. L. (2007). Chemical characteristics of fulvic acids from Arctic surface waters: Microbial contributions and photochemical transformations. J. Geophysical Research: Biogeosciences 112. doi: 10.1029/2006JG000343
Ding B., Jiang D., Li X., Xia Y. (2022). Tempo-spatial distribution and its influencing factors of dissolved carbon in the Dagu River, Shandong Province. J. Agro-Environment Sci. 41, 670–680. doi: 10.11654/jaes.2021-0166
Dong Y., Li Y., Kong F., Zhang J. (2020). Source, structural characteristics and ecological indication of dissolved organic matter extracted from sediments in the primary tributaries of the Dagu River. Ecol. Indic. 109, 105776. doi: 10.1016/j.ecolind.2019.105776
Dong Y., Peng W., Liu Y., Wang Z. (2021). Photochemical origin of reactive radicals and halogenated organic substances in natural waters: A review. J. Hazardous Materials 401, 123884. doi: 10.1016/j.jhazmat.2020.123884
Downing-Kunz M. A., Schoellhamer D. H. (2013). Seasonal variations in suspended-sediment dynamics in the tidal reach of an estuarine tributary. Mar. Geology 345, 314–326. doi: 10.1016/j.margeo.2013.03.005
Estapa M. L., Mayer L. M., Boss E. (2012). Rate and apparent quantum yield of photodissolution of sedimentary organic matter. Limnology Oceanography 57, 1743–1756. doi: 10.4319/lo.2012.57.6.1743
Fellman J. B., Hood E., D’amore D. V., Edwards R. T., White D. (2009). Seasonal changes in the chemical quality and biodegradability of dissolved organic matter exported from soils to streams in coastal temperate rainforest watersheds. Biogeochemistry 95, 277–293. doi: 10.1007/s10533-009-9336-6
Gao W., Dai A., Wu J., Li Y., Hou J., Wang X., et al. (2023). Hydrological status of the Dagu River Basin and management suggestions based on soil and water assessment tool multi-station calibration. Appl. Water Sci. 13, 97. doi: 10.1007/s13201-023-01900-x
Glibert P. M., Heil C. A., Madden C. J., Kelly S. P. (2023). Dissolved organic nutrients at the interface of fresh and marine waters: flow regime changes, biogeochemical cascades and picocyanobacterial blooms—the example of Florida Bay, USA. Biogeochemistry 164, 229–255. doi: 10.1007/s10533-021-00760-4
Grasshoff K., Kremling K., Ehrhardt M. (Eds.) (2009). Methods of seawater analysis (Weimheim, Germany: John Wiley & Sons).
Guo M., Li X., Wang Y., Zhang Y., Fu Q., Huguet A., et al. (2023). New insights into the mechanism of phosphate release during particulate organic matter photodegradation based on optical and molecular signatures. Water Res. 236, 1199545. doi: 10.1016/j.watres.2023.119954
Harfmann J. L., Avery G. B. Jr., Rainey H. D., Mead R. N., Skrabal S. A., Kieber R. J., et al. (2021). Composition and lability of photochemically released dissolved organic matter from resuspended estuarine sediments. Organic Geochemistry 151, 104164. doi: 10.1016/j.orggeochem.2020.104164
He W., Chen M., Schlautman M. A., Hur J. (2016). Dynamic exchanges between DOM and POM pools in coastal and inland aquatic ecosystems: A review. Sci. Total Environ. 551, 415–428. doi: 10.1016/j.scitotenv.2016.02.031
He H., Lin Y., Yang X., Zhu X., Xie W., Lai C., et al. (2022). The photodegradation of 17 alpha-ethinylestradiol in water containing iron and dissolved organic matter. Sci. Total Environ. 814, 152516. doi: 10.1016/j.scitotenv.2021.152516
Helms J. R., Glinski D. A., Mead R. N., Southwell M. W., Avery G. B., Kieber R. J., et al. (2014). Photochemical dissolution of organic matter from resuspended sediments: Impact of source and diagenetic state on photorelease. Organic geochemistry 73, 83–89. doi: 10.1016/j.orggeochem.2014.05.011
Helms J. R., Stubbins A., Ritchie J. D., Minor E. C., Kieber D. J., Mopper K. (2008). Absorption spectral slopes and slope ratios as indicators of molecular weight, source, and photobleaching of chromophoric dissolved organic matter. Limnology oceanography 53, 955–969. doi: 10.4319/lo.2008.53.3.0955
Hoitink A. J. F., Jay D. A. (2016). Tidal river dynamics: Implications for deltas. Rev. Geophysics 54, 240–272. doi: 10.1002/2015RG000507
Hu B., Wang P., Bao T., Shi Y. (2020). The photochemical release of dissolved organic matter from resuspended sediments: Insights from fluorescence spectroscopy. Chemosphere 257, 127161. doi: 10.1016/j.chemosphere.2020.127161
Hu B., Wang P., Wang C., Bao T. (2022). Photogeochemistry of particulate organic matter in aquatic systems: A review. Sci. Total Environ. 806, 150467. doi: 10.1016/j.scitotenv.2021.150467
Huang T., Liu K., Li L., Xiao X. (2024). Sources, preservation, and influencing factors of organic carbon from the Dagu River in Shandong Peninsula. China Environ. Sci. 2, 1–16. (In chinese)
Hurni K., Zeleke G., Kassie M., Tegegne B., Kassawmar T., Teferi E., et al. (2015). Soil degradation and sustainable land management in the rainfed agricultural areas of Ethiopia: an assessment of the economic implications. Rep. economics land degradation initiative 62. doi: 10.13140/RG.2.1.1357.6807
Jiang D., Li Z., Luo Y., Xia Y. (2021). River damming and drought affect water cycle dynamics in an ephemeral river based on stable isotopes: The Dagu River of North China. Sci. Total Environ. 758, 143682. doi: 10.1016/j.scitotenv.2020.143682
Jung B. J., Lee H. J., Jeong J. J., Owen J., Kim B., Meusburger K., et al. (2012). Storm pulses and varying sources of hydrologic carbon export from a mountainous watershed. J. hydrology 440, 90–101. doi: 10.1016/j.jhydrol.2012.03.030
Komada T., Reimers C. E. (2001). Resuspension-induced partitioning of organic carbon between solid and solution phases from a river–ocean transition. Mar. Chem. 76, 155–174. doi: 10.1016/S0304-4203(01)00055-X
Kong F. (2014). Study on temporal-spatial distribution and the flux of dissolved organic carbon in Dagu River (Qingdao, China: Qingdao University).
Lee H. S., Hur J., Lee M. H., Brogi S. R., Kim T. W., Shin H. S. (2019). Photochemical release of dissolved organic matter from particulate organic matter: Spectroscopic characteristics and disinfection by-product formation potential. Chemosphere 235, 586–595. doi: 10.1016/j.chemosphere.2019.06.127
Lee H. S., Hur J., Shin H. S. (2023). Photochemical and microbial transformation of particulate organic matter depending on its source and size. Sci. Total Environ. 857, 159506. doi: 10.1016/j.scitotenv.2022.159506
Li M., Kong F., Li Y., Zhang J., Xi M. (2020). Ecological indication based on source, content, and structure characteristics of dissolved organic matter in surface sediment from Dagu River estuary, China. Environ. Sci. pollut. Res. 27, 45499–45512. doi: 10.1007/s11356-020-10456-1
Liu Q., Shank G. C. (2015). Solar radiation-enhanced dissolution (photodissolution) of particulate organic matter in Texas estuaries. Estuaries Coasts 38, 2172–2184. doi: 10.1007/s12237-014-9932-0
Liu J., Yin P., Chen X., Cao K. (2019). Distribution, enrichment and transport of trace metals in sediments from the Dagu River Estuary in the Jiaozhou Bay, Qingdao, China. Minerals 9, 545. doi: 10.3390/min9090545
Lu C. J., Benner R., Fichot C. G., Fukuda H., Yamashita Y., Ogawa H. (2016). Sources and transformations of dissolved lignin phenols and chromophoric dissolved organic matter in Otsuchi Bay, Japan. Front. Mar. Sci. 3, 85. doi: 10.3389/fmars.2016.00085
Marton J. M., Herbert E. R., Craft C. B. (2012). Effects of salinity on denitrification and greenhouse gas production from laboratory-incubated tidal forest soils. Wetlands 32, 347–357. doi: 10.1007/s13157-012-0270-3
Massicotte P., Asmala E., Stedmon C., Markager S. (2017). Global distribution of dissolved organic matter along the aquatic continuum: Across rivers, lakes and oceans. Sci. Total Environ. 609, 180–191. doi: 10.1016/j.scitotenv.2017.07.076
Mayer L. M., Schick L. L., Skorko K., Boss E. (2006). Photodissolution of particulate organic matter from sediments. Limnology Oceanography 51, 1064–1071. doi: 10.4319/lo.2006.51.2.1064
Mayer L. M., Thornton K. R., Schick L. L., Jastrow J. D., Harden J. W. (2012). Photodissolution of soil organic matter. Geoderma 170, 314–321. doi: 10.1016/j.geoderma.2011.11.030
Medeiros P. M., Seidel M., Powers L. C., Dittmar T., Hansell D. A., Miller W. L. (2015). Dissolved organic matter composition and photochemical transformations in the northern North Pacific Ocean. Geophysical Res. Lett. 42, 863–870. doi: 10.1002/2014GL062663
Mietta F., Chassagne C., Winterwerp J. C. (2009). Shear-induced flocculation of a suspension of kaolinite as function of pH and salt concentration. J Colloid Interface Sci. 336 (1), 134–141. doi: 10.1016/j.jcis.2009.03.044
Minor E. C., Pothen J., Dalzell B. J., Abdulla H., Mopper K. (2006). Effects of salinity changes on the photodegradation and ultraviolet-visible absorbance of terrestrial dissolved organic matter. Limnology Oceanography 51, 2181–2186. doi: 10.4319/lo.2006.51.5.2181
Mostofa K. M., Yoshioka T., Mottaleb A., Vione D. editors. (2012). Photobiogeochemistry of organic matter: principles and practices in water environments. (New York, USA: Springer Science & Business Media)
Mopper K., Kieber D. J., Stubbins A. (2015). Marine photochemistry of organic matter: processes and impacts. Biogeochemistry Mar. dissolved organic matter, 389–450. doi: 10.1016/B978-0-12-405940-5.00008-X
Mostofa K. M., Yoshioka T., Mottaleb A., Vione D. (Eds.) (2012). Photobiogeochemistry of organic matter: principles and practices in water environments (Springer Science & Business Media).
Murphy K. R., Stedmon C. A., Waite T. D., Ruiz G. M. (2008). Distinguishing between terrestrial and autochthonous organic matter sources in marine environments using fluorescence spectroscopy. Mar. Chem. 108, 40–58. doi: 10.1016/j.marchem.2007.10.003
Ohno T. (2002). Fluorescence inner-filtering correction for determining the humification index of dissolved organic matter. Environ. Sci. Technol. 36, 742–746. doi: 10.1021/es0155276
Parker K. M., Mitch W. A. (2016). Halogen radicals contribute to photooxidation in coastal and estuarine waters. Proc. Natl. Acad. Sci. 113, 5868–5873. doi: 10.1073/pnas.1602595113
Qu W., Li J., Han G., Wu H., Song W., Zhang X. (2019). Effect of salinity on the decomposition of soil organic carbon in a tidal wetland. J. Soils Sediments 19, 609–617. doi: 10.1007/s11368-018-2096-y
Shank G. C., Evans A., Yamashita Y., Jaffé R. (2011). Solar radiation–enhanced dissolution of particulate organic matter from coastal marine sediments. Limnol Oceanogr. 56 (2), 577–588. doi: 10.4319/lo.2011.56.2.0577
Schiebel H. N., Wang X., Chen R. F., Peri F. (2015). Photochemical release of dissolved organic matter from resuspended salt marsh sediments. Estuaries coasts 38, 1692–1705. doi: 10.1007/s12237-014-9893-3
Southwell M. W., Mead R. N., Luquire C. M., Barbera A., Avery G. B., Kieber R. J., et al. (2011). Influence of organic matter source and diagenetic state on photochemical release of dissolved organic matter and nutrients from resuspendable estuarine sediments. Mar. Chem. 126, 114–119. doi: 10.1016/j.marchem.2011.04.005
Stedmon C. A., Bro R. (2008). Characterizing dissolved organic matter fluorescence with parallel factor analysis: a tutorial. Limnology Oceanography: Methods 6, 572–579.
Sun X., Fan D., Liao H., Tian Y. (2020). Fate of organic carbon burial in modern sediment within Yangtze River Estuary. J. Geophysical Research: Biogeosciences 125. doi: 10.1029/2019JG005379
Wang H., Guan D., Zhang R., Chen Y., Hu Y., Xiao L. (2014). Soil aggregates and organic carbon affected by the land use change from rice paddy to vegetable field. Ecol. Eng. 70, 206–211. doi: 10.1016/j.ecoleng.2014.05.027
Wang K., Li P., He C., Shi Q., He D. (2021). Density currents affect the vertical evolution of dissolved organic matter chemistry in a large tributary of the Three Gorges Reservoir during the water-level rising period. Water Res. 204, 117609. doi: 10.1016/j.watres.2021.117609
Ward N. D., Bianchi T. S., Medeiros P. M., Seidel M., Richey J. E., Keil R. G., et al. (2017). Where carbon goes when water flows: carbon cycling across the aquatic continuum. Front. Mar. Sci. 4, 7. doi: 10.3389/fmars.2017.00007
Weishaar J. L., Aiken G. R., Bergamaschi B. A., Fram M. S., Fujii R., Mopper K. (2003). Evaluation of specific ultraviolet absorbance as an indicator of the chemical composition and reactivity of dissolved organic carbon. Environ. Sci. Technol. 37, 4702–4708. doi: 10.1021/es030360x
Wong V. N., Greene R. S. B., Dalal R. C., Murphy B. W. (2010). Soil carbon dynamics in saline and sodic soils: a review. Soil Use Manage. 26, 2–11. doi: 10.1111/j.1475-2743.2009.00251.x
Wuepper D., Borrelli P., Finger R. (2020). Countries and the global rate of soil erosion. Nat. sustainability 3, 51–55. doi: 10.1038/s41893-019-0438-4
Xu X., Wei H., Barker G., Holt K., Julian S., Light T., et al. (2021). Tidal freshwater zones as hotspots for biogeochemical cycling: sediment organic matter decomposition in the lower reaches of two South Texas rivers. Estuaries Coasts 44, 722–733. doi: 10.1007/s12237-020-00791-4
Yu X., Zhang J., Kong F., Li Y., Li M., Dong Y., et al. (2019). Identification of source apportionment and its spatial variability of dissolved organic matter in Dagu River-Jiaozhou Bay estuary based on the isotope and fluorescence spectroscopy analysis. Ecol. Indic. 102, 528–537. doi: 10.1016/j.ecolind.2019.03.004
Zhang Y., Gong L., Jin B., Dong Z. (2013). Preliminary estimate of soil erosion modulus in the daguhe river basin using (137) CS tracing method. Mar. Geology Quaternary Geology 33, 165. doi: 10.3724/SP.J.1140.2013.06165
Zhang Y., Ren J., Zhang W., Wu J. (2021). Importance of salinity-induced stratification on flocculation in tidal estuaries. J. Hydrology 596, 126063. doi: 10.1016/j.jhydrol.2021.126063
Zhang Y. N., Wang J., Chen J., Zhou C., Xie Q. (2018). Phototransformation of 2, 3-dibromopropyl-2, 4, 6-tribromophenyl ether (DPTE) in natural waters: important roles of dissolved organic matter and chloride ion. Environ. Sci. Technol. 52, 10490–10499. doi: 10.1021/acs.est.8b03258
Zhang P., Shao Y., Xu X., Huang P., Sun H. (2020). Phototransformation of biochar-derived dissolved organic matter and the effects on photodegradation of imidacloprid in aqueous solution under ultraviolet light. Sci Total Environ. 724, 137913. doi: 10.1016/j.scitotenv.2020.137913
Zhang J., Wang J. J., Xiao R., Deng H., DeLaune R. D. (2023). Effect of salinity on greenhouse gas production and emission in marsh soils during the decomposition of wetland plants. J. Soils Sediments 23, 131–144. doi: 10.1007/s11368-022-03334-5
Zhao C., Hou Y., Wang Y., Li P., He C., Shi Q., et al. (2023). Unraveling the photochemical reactivity of dissolved organic matter in the Yangtze river estuary: Integrating incubations with field observations. Water Res. 245, 120638. doi: 10.1016/j.watres.2023.120638
Zhou Y., Zhao C., He C., Li P., Wang Y., Pang Y., et al. (2022). Characterization of dissolved organic matter processing between surface sediment porewater and overlying bottom water in the Yangtze River Estuary. Water Res. 215, 118260. doi: 10.1016/j.watres.2022.118260
Keywords: photochemical transformation, soils, tidal rivers, carbon export, estuaries
Citation: Zhou Y, Zhu Y, Jiang S, Meng D, Pang Y and Xiao Y (2024) Photochemical enrichment of dissolved organic matter from different soils of a tidal river basin: significance to estuarine carbon cycle. Front. Mar. Sci. 11:1400196. doi: 10.3389/fmars.2024.1400196
Received: 13 March 2024; Accepted: 06 June 2024;
Published: 28 June 2024.
Edited by:
Bhaskar Mitra, The James Hutton Institute, United KingdomReviewed by:
Chen He, China University of Petroleum, Beijing, ChinaGuisheng Song, Tianjin University, China
Copyright © 2024 Zhou, Zhu, Jiang, Meng, Pang and Xiao. This is an open-access article distributed under the terms of the Creative Commons Attribution License (CC BY). The use, distribution or reproduction in other forums is permitted, provided the original author(s) and the copyright owner(s) are credited and that the original publication in this journal is cited, in accordance with accepted academic practice. No use, distribution or reproduction is permitted which does not comply with these terms.
*Correspondence: Yuping Zhou, zhouyupingtt@163.com