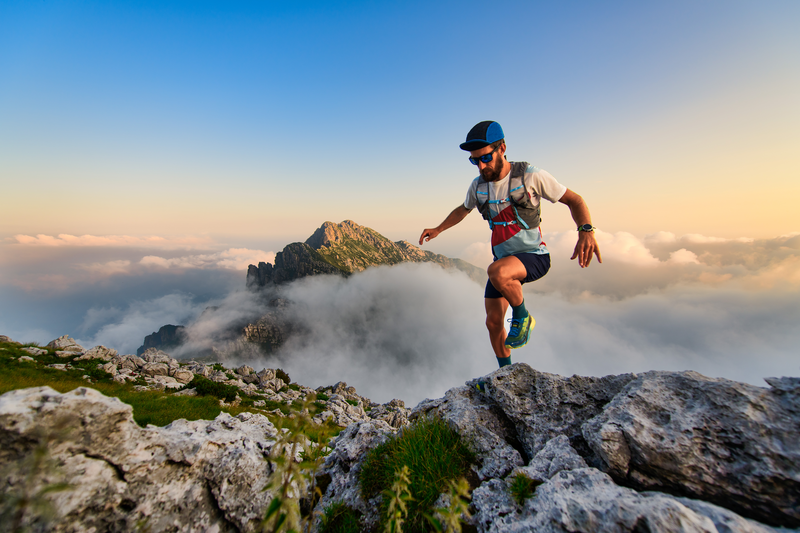
95% of researchers rate our articles as excellent or good
Learn more about the work of our research integrity team to safeguard the quality of each article we publish.
Find out more
ORIGINAL RESEARCH article
Front. Mar. Sci. , 27 November 2023
Sec. Marine Ecosystem Ecology
Volume 10 - 2023 | https://doi.org/10.3389/fmars.2023.1278810
This article is part of the Research Topic Turning with the Tide and Time in the Salish Sea: Change in Estuary and Nearshore Habitats and Species Dependent on Them View all 9 articles
Water temperature is a key dimension of estuaries that can influence important biological processes including organismal growth, survival, and habitat use. For example, juvenile Pacific salmon rely on temperature-mediated growth opportunities in estuaries during seaward migration in the spring and summer. However, oncoming climate change is warming estuary temperatures and transforming growth potential. Yet, it is likely that estuary water temperatures are complex and dynamic across space and time. Here we investigated spatial and temporal patterns of water temperature across two contrasting estuaries on Vancouver Island, BC, and used these data to simulate juvenile Chinook salmon growth potential under both present conditions and a simplified scenario of climate warming. Summer temperatures were warmer and more spatially homogeneous across the Englishman River estuary relative to the Salmon River estuary. Within each system, temperature was variable across habitats and sites, appearing to be driven by a combination of local climate, river and ocean temperatures, tidal fluctuations, and habitat features. This shifting mosaic of temperatures generated a complex portfolio of growth opportunities for juvenile Chinook salmon. There were broad patterns of increasing growth potential across both systems as temperatures warmed in early summer. However, excessively hot late summer temperatures drove steep declines in growth potential across the Englishman River estuary, while positive trends continued through August in the Salmon River estuary. A simple climate change scenario revealed that estuaries and habitats may have differing vulnerabilities to increasing temperatures—with climate warming, favorable growth opportunities were even more constricted to early summer in the Englishman River estuary, but expanded across most habitats and times in the Salmon River estuary given lower baseline temperatures and higher habitat complexity. Collectively, this work underscores the importance of habitat complexity and local climate for maintaining diverse growth opportunities across estuaries, which may confer resilience to the nursery function of estuaries as temperature mosaics warm.
Estuaries are mosaics of interconnected habitats at the interface between watersheds and oceans. Therefore, they are shaped by complex interactions between terrestrial, marine, and atmospheric processes (Talley et al., 2003; Ray, 2005; Sheaves, 2009). These interacting processes generate spatio-temporal variability in key habitat conditions (Beck et al., 2001; Sheaves et al., 2015). Numerous habitat conditions, such as salinity, water temperature, vegetation, and geomorphology, vary across multiple spatial scales (Peterson, 2003; Fulford et al., 2011). For example, salinity profiles are often characterized estuary-wide (i.e. salt-wedge or well-mixed) given dominant river-tide interactions, but may also show significant local variability (i.e. between channels) due to differences in stratification and mixing processes throughout the estuary (Giddings et al., 2011). Many of these spatial patterns also change over time, and shifts can range from daily fluctuations to interannual variation (Peterson et al., 2007). For example, daily tidal fluxes influence water quality patterns (i.e. temperature, salinity, and dissolved oxygen), while interannual cycles such as the El Niño Southern Oscillation can impact long-term estuarine mixing and temperature regimes (Wooldridge and Deyzel, 2012; Sutherland and O’Neill, 2016; Possamai et al., 2018). Together, these spatio-temporal processes shape estuarine habitats into highly complex and dynamic systems.
Water temperature is a key dimension of the estuarine habitat mosaic (Fulford et al., 2011). Many processes govern estuarine water temperature—including river flows and temperature, meteorologic conditions, and ocean temperature and tides—which together drive dynamic spatio-temporal patterns (Schumann, 2000; Brown et al., 2016; Marin Jarrin et al., 2022). Across aquatic systems, water temperature patterns can influence many biological processes including organismal physiology, species distributions, and community composition (Sanford, 1999; Perry et al., 2005; Eliason et al., 2011). For example, the growth potential and physiological performance of fishes is temperature dependent—growth increases as temperatures rise, but declines precipitously when temperatures exceed thermal optima (Beauchamp, 1989; Hanson et al., 1997). Within river networks, cold-water fishes have been shown to track optimal growth temperatures between seasons, exploiting different habitats over the year, and even complete daily migrations across temperature gradients (Armstrong et al., 2013; Armstrong et al., 2021, Hahlbeck et al., 2022). These and other studies have increasingly revealed how fish rely on both broad- and fine-scaled spatio-temporal variability in freshwater temperatures; however, few studies have examined these temperature dynamics in estuaries (Welsh et al., 2001; Kaylor et al., 2021). Investigating fine-scaled temperature variation across both space and time is therefore an important step towards understanding and predicting key biological processes in estuaries (Wagner et al., 2011; Marin Jarrin et al., 2022).
Estuarine systems can support many important species, including anadromous fishes like Pacific salmon (Oncorhynchus spp.). Juvenile salmon transit through estuaries during seaward migration in the spring, but may also rely on these systems as nursery grounds prior to ocean entry (Magnusson and Hilborn, 2003; Weitkamp et al., 2014; Moore et al., 2016; Sharpe et al., 2019; Woo et al., 2019). The duration of estuary residency varies considerably within and between species. For example, Chinook salmon (O. tshawytscha) are known to rear extensively in estuaries, while pink salmon (O. gorbuscha) typically pass through rapidly (Bottom et al., 2005; Moore et al., 2016; Quinn, 2018; Chalifour et al., 2021). During estuarine residency, spatio-temporal temperature dynamics mediate key physiological and ecological processes for juvenile salmon including growth, survival, movement, and prey availability (Cordell et al., 2011; David et al., 2014; Davis et al., 2019; Davis et al., 2022). Estuarine growth can be relatively rapid compared to upstream habitat, and this growth can have subsequent benefits for marine survival (Bond et al., 2008; Duffy and Beauchamp, 2011, Sawyer et al., in press). For example, Sawyer et al., (in press) estimated that estuarine growth improved marine survival by 31-47% for juvenile coho salmon (O. kisutch) on the Central Coast of British Columbia and that growth was substantially higher in a year with warmer temperatures. On the other hand, observations from an estuary lagoon in California suggest that excessively high estuary temperatures can limit rearing habitat and drive diel movements for juvenile steelhead and coho salmon (Osterback et al., 2018; Bond et al., 2022). Thus, navigating mosaics of temperature in estuaries and the associated growth opportunities can have important consequences for juvenile salmon during estuary rearing and seaward migration.
Understanding the spatial and temporal dynamics of temperature in estuaries is particularly important given oncoming climate change. Depending on the emissions scenario, sea-surface temperatures are expected to increase anywhere from 1.3-4.4°C in the northeast Pacific Ocean between 2010-2099 (Hoegh-Guldberg et al., 2014). In addition, global river temperatures are projected to increase by several degrees by 2100 relative to 1971-2000, also dependent on emissions, location, and river water source (van Vliet et al., 2013) (e.g., up to 6°C increase in annual maximum temperature by the 2080s relative to the 1980s in the Pacific Northwest, Mantua et al., 2010). These shifts, along with changes to other climatological processes like precipitation, will likely drive increases in estuarine water temperature (Wagner et al., 2011; Cho and Lee, 2012; Brown et al., 2016; Davis et al., 2022). Shifting temperature mosaics will transform growth opportunities for fishes across estuarine systems (Harley et al., 2006). For juvenile salmon, this could have cascading impacts including changes in marine survival and migration phenology (Pörtner and Peck, 2010; Gillanders et al., 2011; Wilson et al., 2021; Davis et al., 2022; Wilson et al., 2023). Therefore, predicting shifts in growth potential is a key step towards understanding Pacific salmon vulnerability to increased estuarine temperatures, which can in turn help inform conservation and management strategies (Griffiths and Schindler, 2012; Davis et al., 2022; Spanjer et al., 2022).
Here we aim to quantify estuary temperature mosaics and their implications for nursery function for salmon in this era of climate warming. Specifically, we (1) assess spatial and temporal patterns of summer temperatures across different habitats within two contrasting estuaries on Vancouver Island, BC, (2) use this data to explore juvenile Chinook salmon growth potential across each system using bioenergetics modeling, and (3) investigate changes in growth potential under a simple scenario of increased temperature. Collectively, we discovered high variation in water temperatures and associated growth opportunities across both space and time that suggests key linkages between estuarine habitat complexity and oncoming vulnerability to climate warming.
We performed this study in the estuaries of two salmon-bearing rivers, the Englishman and Salmon, on the eastern side of Vancouver Island, British Columbia, Canada. This work is a part of a large-scale collaborative initiative—Estuary Resilience project—led by The Nature Trust of British Columbia (NTBC), in collaboration with coastal First Nations and other organizations. Both the Englishman and Salmon Rivers and estuaries support all five species of Pacific salmon in addition to sea-run dolly varden (Salvelinus malma), cutthroat trout (Oncorhynchus clarkii), and anadromous steelhead trout (Oncorhynchus mykiss) (Silvestri, 2007; Lament et al., 2015). These two estuaries contrast in their size, local climate setting, and degree of anthropogenic alteration. The Salmon River estuary is larger and relatively intact with cooler and less variable climatic patterns. Contrastingly, the Englishman River estuary is smaller and highly altered by residential developments, flow modification, and land-use conversion, and experiences warmer and more seasonally variable climatic conditions.
The Salmon River estuary is located on the ancestral territory of the K’ómoks First Nation, who know this area as X’wésam. The estuary encompasses approximately 8.4 km2 of wetlands, bordering the small town of Sayward to the west, forested mountains to the east, and draining into Johnstone Straight (GPS: 50.39N, 125.95W) (Figure 1). Johnstone Strait is a deep (e.g. over 500 m in some sections) and narrow (e.g. 4.5-2.5 km wide) channel that experiences rapid tidal streams and high winds. Thus, water is vertically well-mixed and typically remains relatively cool year round (Thomson, 1981). The Salmon River watershed is 1210 km2, and the river is 208 km long (Atagi, 1994). This is a rainfall-dominated system, with the highest discharge in fall and winter (Environment and Climate Change Canada (ECCC), 2023a). The estuary sits within the Coastal Western Hemlock (CWH) biogeoclimatic zone, which is characterized by cool summers and mild winters (Pojar et al., 1991). The Salmon River watershed has a long history of logging, and a log-sort continues to operate in the northwest corner of the estuary, separated from the system by a narrow jetty (Baldwin and Bradfield, 2010).
Figure 1 (A) Map of the Salmon River estuary. Temperature logger locations are indicated by the shapes, with each shape corresponding to a habitat type as indicated by the key. Sites are numbered 1-3 within each habitat type. The white dot on the inset indicates the location of the Salmon River estuary on Vancouver Island. (B) Map of the Englishman River estuary. Temperature logger locations are indicated by the shapes (triangles are beach habitats, squares are marsh habitats, and circles are meadow habitats). Sites are numbered 1-3 within each habitat type. The white dot on the inset indicates the location of the Englishman River estuary on Vancouver Island.
The Englishman River estuary is in southeastern Vancouver Island on the ancestral territory of the Snaw’naw’as First Nation, who know this area as Kw’a’luxw. The system sits within the city of Parksville and includes about 3.7 km2 of wetlands that are almost entirely surrounded by residential developments (GPS: 49.33N, -124.29W) (Figure 1). Thus, the estuary is less than half the size of the Salmon River estuary. The Englishman watershed is 319 km2, and the river runs for 40 km before entering the estuary and Strait of Georgia (Bocking and Gaboury, 2001). The Strait of Georgia is shallower (e.g. average depth is 155 m) and wider (e.g. average width is 28 km) in comparison to Johnstone Strait, with strong year round vertical stratification. In the Strait of Georgia, water temperature in the upper layer displays strong seasonal variation, with summer temperatures exceeding 20°C in some places (Thomson, 1981). The Englishman River is rainfall-dominated and is generally characterized by lower discharges than the Salmon River (Environment and Climate Change Canada (ECCC), 2023b). A dam operates 34 km above the estuary in the headwaters. The estuary is also within the CWH biogeoclimatic zone (Pojar et al., 1991). However, the system typically experiences higher summer temperatures and less annual precipitation in comparison to the Salmon River estuary (Environment and Climate Change Canada-Meteorological Service of Canada (ECCC-MSC), 2023a; Environment and Climate Change Canada-Meteorological Service of Canada (ECCC-MSC), 2023b). Many land-use activities impact the Englishman River watershed, including logging, agriculture, and residential developments (Boom and Bryden, 1994). Similarly, the estuary has been altered substantially by development, although there have been recent restoration efforts.
To obtain temperature data across a range of estuarine habitat conditions, we deployed ten temperature loggers (HOBO TidbiT v2) in each estuary from May-August, 2022. Logger sites were chosen to cover three broad estuarine habitat types—beach, marsh, and meadow (Figure 1). These classifications span a range of vegetation, geomorphology, and tidal influence, thus representing a mosaic of available juvenile salmon estuarine habitat (Levings, 2016; Woo et al., 2019). More specifically, beach habitats are predominantly marine influenced with sandy and rocky shorelines and some marine macroalgae (e.g., Fucus spp.). Estuarine marshes are diurnally tidally influenced and dominated by salt tolerant plants such as Lyngbye’s sedge (Carex lyngbyei). Last, meadow habitats are generally highest and furthest upstream in the estuary, experiencing less frequent tidal inundation and featuring diverse plant communities (MacKenzie and Moran, 2004). We chose three sites within each habitat type based on accessibility and variability in characteristics such as water flow, depth, and vegetation. The tenth logger was placed upstream in the mainstem river channel above tidal influence to record freshwater inflow temperatures. Loggers were set to record at fifteen-minute intervals and deployed at every site between April 30-May 4, 2022. Rebar was hammered into the substrate, and loggers were connected using zip-ties to sit 15cm above the bottom. Although loggers were not deployed within a protective housing, research suggests that loggers at sites with continuous flow, turbid water, and/or shading (i.e. riparian or aquatic vegetation) experience much less heating from direct solar radiation. All of our deployment sites were characterized by at least one of these conditions, so we suggest that the lack of housings does not significantly impact our results (Johnson and Wilby, 2013; Caissie and El-Jabi, 2020). We downloaded and redeployed all loggers at the beginning of July with final retrieval on August 27-28, 2022. Throughout this field work, members of the K’ómoks and Snaw’naw’as First Nations joined us to share guidance on site selection and assist with field operations.
Temperature recordings taken during dewatered periods were removed from each dataset. This included readings taken during initial deployment, the mid-summer logger check, and final retrieval. In addition, two loggers in the Salmon River estuary (Marsh 2 and Beach 3) were found dewatered when retrieved in August due to lower water levels. We visually inspected these temperature time-series for increased daily variance, which indicates that the loggers were recording air temperature, and removed data accordingly. Data from all other loggers was also examined to check for dewatered periods, but none were identified. During the mid-summer check, one logger in the Salmon River estuary was missing (Meadow 2). A new logger was deployed at this time, so data for this site is limited to July-August.
We used generalized additive models (GAMs) to quantify variation in summer water temperatures between habitat types across each estuary. For each system, we modeled daily average water temperature against a factor-smooth interaction between day (an integer ranging from 1-119) and habitat type (beach, marsh, and meadow) using a Gaussian distribution. Factor-smooth interactions fit separate smooths for each level of a factor, which allows for post-hoc comparisons between these different levels (Pedersen et al., 2019). We fit the factor-smooth interaction between day and habitat type using thin-plate regression splines with forty knots. Habitat type was also added as a random effect to allow for varying intercepts. In addition, site was included as a random effect to account for variation between temperature loggers. Both models were fit with restricted maximum likelihood estimation (REML) using the R software package “mgcv” (R Core Team, 2022, R version 4.2.1 [2022-06-23], Wood, 2017). After models were fit, we used the “plot_differences” function from the R package “gratia” to examine pairwise differences between habitat-level smooths for each model (Simpson, 2023). This function calculates pairwise difference smooths with 95% credible intervals for every combination of factors in a factor-smooth interaction. We concluded that daily water temperature differed significantly between habitat types when the 95% credible interval for the difference smooth did not include zero.
For further data visualization and analysis, we used tide predictions from the nearest Fisheries and Oceans Canada stations, regional ocean temperatures from the nearest Fisheries and Oceans Canada lighthouse stations, and air temperature data from the nearest Meteorological Service of Canada weather stations (Department of Fisheries and Oceans Canada (DFO), 2023c—Tides, currents, and water levels for Kelsey Bay-08215 [data for the Salmon River estuary], Department of Fisheries and Oceans Canada (DFO), 2023d—Tides, currents, and water levels for Nanoose Bay-07930 [data for the Englishman River estuary], Department of Fisheries and Oceans Canada (DFO), 2023b—British Columbia Lightstation Sea-Surface Temperature and Salinity Data (Pacific), 1914-present for Pine Island [data for the Salmon River estuary], Department of Fisheries and Oceans Canada (DFO), 2023a—British Columbia Lightstation Sea-Surface Temperature and Salinity Data (Pacific), 1914-present for Departure Bay [data for the Englishman River estuary], Environment and Climate Change Canada-Meteorological Service of Canada (ECCC-MSC) (2023a) [data for the Salmon River estuary], Environment and Climate Change Canada-Meteorological Service of Canada (ECCC-MSC) (2023b) [data for the Englishman River estuary]).
We used bioenergetics models to explore the implications of temperature mosaics on potential growth of juvenile salmon given simple assumptions. Specifically, we used the Wisconsin Bioenergetics model to simulate growth of juvenile Chinook salmon at each temperature logger site from May-August (Hewett and Johnson, 1987; Hewett and Johnson, 1992; Hanson et al., 1997). We focused on Chinook salmon given their cultural importance, imperiled conservation status, estuary-dependent life-histories, and available bioenergetic parameters (Plumb and Moffitt, 2015; Price et al., 2017; Atlas et al., 2021; Chalifour et al., 2021). The Wisconsin model is an energy balance equation where energy consumed by a fish is balanced against metabolism, waste losses, and growth (Deslauriers et al., 2017). Model inputs of water temperature, prey energy density (EDprey), predator energy density (EDfish), starting weight, and the proportion of maximum consumption (Pcmax) can be used to estimate fish growth. We ran models on a daily time-step with inputs of daily-averaged water temperature and constant values of Pcmax, EDprey, EDfish, and starting weight with an output of weight per day. A separate model was run with each full temperature logger dataset to examine differences in juvenile Chinook salmon growth under different thermal regimes across the two estuaries. We did not run a model for the Salmon River estuary site with a July-August dataset (Meadow 2) to maintain consistency in start timing.
A starting weight of 5 grams was chosen to represent sub-yearling fish entering the estuary in early May (Davis et al., 2019). EDprey was set to 4000J based on recent values from bomb-calorimetry studies of juvenile Chinook salmon estuarine diets (David et al., 2014; Davis et al., 2019). EDfish was calculated as a function of body mass (Stewart et al., 1983). We set Pcmax to 0.6, which is an ecologically plausible consumption rate for juvenile Chinook salmon in estuaries (Davis et al., 2019, Kaylor et al., 2021). Physiological parameters for juvenile Chinook salmon were used in all simulations, which sets the thermal optimum for consumption at 20.93°C (Stewart and Ibarra, 1991; Plumb and Moffitt, 2015). All models were run with the R shinyApp program Fish Bioenergetics 4 (Deslauriers et al., 2017). The model outputs of daily weight were used to calculate daily growth rate potential (GRP) as follows, where t1 and t2 are on daily time increments:
We calculated average monthly GRP for each site from May-August to compare relative growth between sites over the summer.
An increase of 2°C was applied to all temperature datasets to simulate a simplistic end-of-century climate change scenario. We chose 2°C given end-of-century predictions for sea-surface (1.3-4.4°C from 2010-2099 in the northeast Pacific Ocean) and river (several degrees) temperature increases, although we recognize the limitations and assumptions of this method, as further detailed in the discussion (Mantua et al., 2010; van Vliet et al., 2013; Hoegh-Guldberg et al., 2014). Keeping all other parameters constant, we re-ran the bioenergetics models for each logger site under the increased temperature scenarios to investigate shifts in juvenile Chinook salmon GRP patterns.
Water temperatures generally increased from May-July across both estuaries, although the Englishman River estuary reached substantially warmer temperatures than the Salmon River estuary. At all Englishman River estuary sites, water temperature peaked in late July before decreasing slightly and roughly plateauing through August (Figure 2). For example, at a marsh site (Marsh 2), weekly mean temperature increased from 11.6°C in early May (May 5-11) to 22.9°C in late July (July 25-31). Mean weekly temperature at the end of August (Aug 20-26) was 21.7°C (Supplementary Figure 1). In comparison to the Englishman River estuary, Salmon River estuary temperatures followed a similar pattern, although May-July increases were generally smaller (Figure 2). For example, at a marsh site (Marsh 3), weekly mean temperature increased from 9.4°C (May 5-11) to 14.0°C (July 25-31). August temperatures in the Salmon River estuary displayed a steady trend with a mean of 13.5°C in late August (Aug 20-26) (Supplementary Figure 1). Thus, estuary temperatures generally increased during the spring to summer, but reached substantially warmer temperatures in the Englishman River estuary relative to the Salmon River estuary.
Figure 2 May-August water temperature for each temperature logger site in the Salmon and Englishman River estuaries. Each panel displays temperature from one temperature logger. Water temperature is color-coded by value, with the scale bar to the right of the panels. The horizontal gray line in each panel indicates the 20°C isoline to ease comparability between each plot.
Temperatures varied across different estuary habitats through time, although this thermal diversity was greater in the Salmon River estuary compared to the Englishman River estuary. The Salmon River estuary GAM and pairwise difference smooths indicated that all habitat-level smooths differed significantly from each other at more than one time throughout the summer (Figure 3). Broadly, meadow habitat had significantly lower early-summer temperatures and significantly higher late-summer temperatures compared to both beach and marsh habitats. Differences between marsh and beach habitat temperatures were smaller, but marsh temperatures were significantly lower in parts of May-June and significantly higher in parts of July. As an example, at the end of July (July 25-31), weekly mean temperatures were 11.8°C (beach), 13.4°C (marsh), and 17.9°C (meadow) (Supplementary Figure 1). In the Englishman River estuary, the GAM and pairwise difference smooths showed that only meadow habitat differed significantly from the beach and marsh habitats over the summer (Figure 3). Meadow habitat had significantly lower temperatures in parts of May-June, and significantly higher temperatures in parts of July-Aug compared to marsh and beach habitat, although differences were smaller than in the Salmon. For example, weekly mean temperatures at the end of July (July 25-31) were 22.9°C (beach), 24.0°C (marsh), and 23.6°C (meadow) (Supplementary Figure 1). Therefore, different habitats had diverse temperatures that were asynchronous through time, with the Englishman River estuary having a warmer and more homogenous temperature mosaic than the Salmon River estuary.
Figure 3 Difference smooths for each pairwise habitat type combination from the factor-smooth interactions of day and habitat type in the GAMs for the Salmon and Englishman River estuaries. Each panel displays the difference between the two habitat-level smooths indicated in the title from May-August, with the 95% credible interval included as a gray ribbon. Pairwise differences from the Salmon River estuary are displayed in the first row, with pairwise differences from Englishman River estuary below. Where the credible interval does not include zero, the pair of habitats have significantly different temperatures for that date.
Estuary temperature dynamics across time appeared to be driven in part by two key processes: first, there was a seasonal reversal in the temperature differences between ocean and freshwater inputs; second, tidal fluctuations controlled fine-scaled temperature patterns. Across both estuaries, the relationship between tide height and water temperature displayed a clear daily pattern that shifted seasonally at some sites. In early summer, high tide corresponded with warmer temperatures, but by mid-late summer, cooler temperatures were observed at high tides. This pattern suggests that sea-surface temperature (SST) is initially warmer than river temperature—thus, tidal influxes bring warmer water into the system—before reversing at some point over the summer. Indeed, this reversal is evident through a comparison of regional ocean and river temperature data for both systems, where river temperatures are initially cooler in early summer before exceeding more stable ocean temperatures (Supplementary Figure 3). Interestingly, the timing of this switch differed between estuaries. For example, a beach site in the Salmon River estuary (Beach 3) displayed the change about a month earlier than an Englishman River estuary beach site (Beach 1), reflecting differences in river and ocean temperature trends between the systems (Figure 4, Supplementary Figure 2, Supplementary Figure 3). On a larger spatial scale, between-habitat temperature differences in both estuaries also illustrate this shifting pattern between ocean and river temperatures—in early summer, river-dominated meadow habitat was generally cooler than beach and marsh habitats before switching in July (Figure 3). However, this seasonally shifting pattern was not evident across all sites. For some sites with limited freshwater influence (e.g. Sal-Beach 1 and Eng-Beach 3), high tide always corresponded with lower water temperatures. In addition, not all sites showed a clear link between water temperature and tide (e.g. Eng-Marsh 3). Instead, water temperature at these sites consistently peaked in the afternoon alongside air temperature, suggesting that these sites are likely more isolated from tidal flushing. Together, these complex temporal patterns of estuary temperatures suggest that rivers switch from being a source of cooler water to warmer water compared with the more stable ocean water temperatures over the summer, and that the magnitude and timing of tidal ebb and flow drives temperatures on a fine scale, all mediated by habitat complexity.
Figure 4 May-June water temperature (blue line) and tide height (black line) for site Beach 3 in the Salmon River estuary. Tide height data is from the nearest Fisheries and Oceans Canada station (Department of Fisheries and Oceans Canada (DFO), 2023c—Tides, currents, and water levels for Kelsey Bay-08215). (A) Temperature and tide height over the full May-June period. The grey bars indicate the time periods displayed in (B–D). (B) Temperature and tide height from May 1-3. Low tide corresponds with lower water temperatures. High and low tides are indicated by light and dark blue bars in (B–D). (C) Temperature and tide height from May 31-June 2. Water temperature shows little variation with tide height. (D) Temperature and tide height from June 28-30. Water temperature is higher at low tides.
The complex temperature mosaics within estuaries led to diverse growth opportunities for juvenile Chinook salmon across space and time, with temperatures ranging from colder-than-optimal, optimal, and excessively hot. Under present conditions, juvenile Chinook salmon GRP generally increased through early summer across both estuaries as temperatures warmed, but declined sharply in the Englishman River estuary over mid-late summer when temperatures got too hot (Figure 5). In the Englishman River estuary, average GRP was highest in June before dropping to negative values in late-July and early-August as summer temperatures peaked. This broad temporal trend was consistent estuary-wide, but spatio-temporal temperature patterns within the system generated variable growth opportunities between habitats and sites. For example, on a monthly timescale, average GRP was highest in marsh habitat in May, but highest in beach habitat from June-August. In contrast, growth opportunities in the Salmon River estuary continued to increase into July and remained stable through August as water temperatures never exceeded optimal growth conditions. GRP patterns between habitats were more consistent in the Salmon River estuary, with the highest average monthly GRP in meadow habitat across all months. Comparing growth potential between the two estuaries, GRP was generally higher across the Englishman River estuary from May-June. This trend switched from July-August, with higher GRP throughout the Salmon River estuary. Thus, spatio-temporal temperature patterns led to variable growth opportunities within and between estuaries, with warmer temperatures in the Englishman River estuary leading to higher early-summer GRP but substantial declines by late-summer, while lower temperatures in the Salmon River estuary created positive, stable GRP trends through the summer.
Figure 5 Juvenile Chinook salmon growth rate potential (GRP) for the Salmon and Englishman River estuaries under present and +2°C scenarios from May-August. Present GRP trends are shown in the top two panels for each estuary, while +2°C GRP trends are displayed below. The individual lines represent GRP at each temperature logger site, and these lines are color-coded by habitat type with the key to the right of the panels.
The simple climate change scenario had variable impacts between estuaries, with GRP increases across most habitats and times in the Salmon River estuary, but consistent GRP declines through the Englishman River estuary from June-August (Figure 5). Growth opportunities in the Salmon River estuary improved across all habitats from May-June, as an increase of 2°C pushed temperature mosaics closer to optimal conditions. Average GRP in beach and marsh habitats continued to increase in July-August, while meadow habitat GRP declined in late-summer as temperatures became excessively hot. These shifting patterns drove changes in relative GRP between habitats over space and time—average growth potential was highest in meadow habitat from May-June, but switched to marsh habitat from July-August. In the Englishman River estuary, warmer temperatures drove higher GRP across habitats in May, but temperatures began to exceed optimal growth conditions by June, causing GRP declines estuary-wide through mid-late summer. Relative GRP between habitats also changed in the Englishman River estuary as temperatures reached and exceeded optimal temperatures asynchronously. GRP was highest in beach and marsh habitats in May, meadow habitat in June, beach and meadow habitat in July, and beach habitat in August. By this final month, average GRP was negative across all habitats, effectively limiting the rearing capacity of the estuary to earlier in the summer. Between the two estuaries, growth potential was similar between the Englishman and Salmon River estuaries in May-June, but consistently higher in the Salmon River estuary from July-August. Therefore, increasing temperatures by 2°C largely expanded growth opportunities across space and time in the Salmon River estuary, but constricted growth opportunities to early-summer in the Englishman River estuary, illustrating how the nursery capacity of the Salmon River estuary may be more resilient to oncoming climate warming relative to the Englishman River estuary.
This study reveals how complex spatio-temporal summer water temperature patterns within and between two estuaries on Vancouver Island, BC provide rich mosaics of growth opportunities for juvenile Chinook salmon that may be challenged by climate warming. Different habitats had different water temperatures. Multiple processes likely drove these temperature patterns, including local climate setting, tidal influence, seasonally changing river and ocean temperatures, and habitat processes that together generated asynchronous temperature dynamics between habitats and sites over time. These dynamic temperature patterns drove shifting mosaics of growth opportunities. In the smaller, more degraded estuary with warmer and more variable climatic patterns (Englishman), temperatures reached excessively hot temperatures at some habitats and times, leading to substantial declines in growth opportunities over the summer. These declines were exacerbated in the simplified climate change scenario, further limiting favorable growth opportunities to early-summer. Water temperatures in the larger, more intact system with cooler and more stable climatic influences (Salmon) never exceeded optimal growth conditions, creating a mosaic of positive growth opportunities across the summer that was largely enhanced by increasing temperatures. Therefore, this study illustrates how modest levels of climate change may increasingly limit estuarine nursery capacity across some habitats and times—but expand it in others—dependent on the underlying spatio-temporally complex temperature patterns and associated portfolios of growth opportunities.
The dynamic temperature patterns across both systems implicate several key underlying processes. First, at the interface between rivers and oceans, estuarine temperatures are shaped by interactions between ocean and river temperatures (Thomson, 1981; Wooldridge and Deyzel, 2012; Khadami et al., 2022). In both the Salmon and Englishman River estuaries, this was evident when predominantly river-influenced meadow habitats were coolest in early summer but warmest in late summer, reflecting a pattern where river temperatures warm and eventually exceed more stable ocean temperatures through the summer (Hall et al., 2018). The timing of this pattern varied between systems, likely driven by differing river and regional ocean temperatures. For example, mean weekly stream temperature from May 5-11 was 6.6°C in the Salmon River vs. 7.2°C in the Englishman River, and regional ocean temperature for the same period was 9.65°C in Johnstone Strait and 10.17°C in the Strait of Georgia (Department of Fisheries and Oceans Canada (DFO), 2023b—British Columbia Lightstation Sea-Surface Temperature and Salinity Data (Pacific), 1914-present for Pine Island [data for the Salmon River estuary], Department of Fisheries and Oceans Canada (DFO), 2023a—British Columbia Lightstation Sea-Surface Temperature and Salinity Data (Pacific), 1914-present for Departure Bay [data for the Englishman River estuary]). Regardless, the seasonal reversal in temperature gradient across estuaries is an intriguing and likely widespread phenomenon at these latitudes. Second, tidal inundation interacted with these river and ocean temperature patterns to shape daily temperature fluctuations (Schumann, 2000; McKay and Iorio, 2008). At some sites, cyclic patterns reflected river and ocean temperature dynamics where lower temperatures occurred at high tide before reversing later in the summer, but this only occurred where there was substantial oceanic and freshwater mixing. In addition, temperature variability was likely also influenced by local habitat and site-specific features including flow patterns, aquatic and riparian vegetation, and channel connectivity (McKay and Iorio, 2008; Fogarty et al., 2018). For example, one of the Englishman River estuary meadow sites (Mead 3) is in a pool that disconnects from the channel at low tide—therefore resulting in stagnant flows—which may have contributed to higher temperatures relative to the other meadow sites that are both in higher flowing freshwater tributaries. Last, meteorological patterns obviously controlled water temperatures. For example, a heat dome occurred across Vancouver Island in late July, driving air temperatures in the Englishman River estuary up to 32°C and resulting in water temperatures in excess of 30°C at some estuary sites (Environment and Climate Change Canada-Meteorological Service of Canada (ECCC-MSC), 2023b). In comparison, air temperatures in the Salmon River estuary reached 22°C and water temperatures at some sites exceeded 20°C (ECCC-MSC 2023—Historical Climate Data for Fanny Island, British Columbia). Thus, these data reveal the underlying processes that generate spatio-temporal temperature dynamics within each system, working alongside key between-estuary differences in climate, oceanography, complexity, and degradation.
One of the striking patterns in this study was the degree to which one estuary (Englishman) had warmer and more homogenous water temperatures than the other (Salmon), which remained cool and more variable over space. We recognize that a comparison of only two estuaries offers limited inference, but suggest that these patterns bear careful consideration. First, the Englishman River estuary experiences warmer and more variable climatic patterns relative to the Salmon River estuary, which is likely driven in part by differing oceanographic patterns between the systems. Johnstone Strait (Salmon) has relatively cool and stable year-round temperatures with consistent vertical mixing, while the Strait of Georgia (Englishman) displays strong seasonal variability, year round vertical stratification, and warmer summer temperatures. These differences likely influence local climate patterns including air temperatures and fog frequency (Thomson, 1981, Supplementary Figure 3). Therefore, marine and climatic influences in each system vary considerably. In addition, the Englishman River has warmer water temperatures and lower discharge in the spring-summer relative to the Salmon River, which together brings warmer freshwater inputs into the Englishman River estuary [Supplementary Figure 3; Environment and Climate Change Canada (ECCC), 2023b); Environment and Climate Change Canada (ECCC) (2023a)]. Together, these differing atmospheric, terrestrial, and marine inputs may help explain the cooler summer water temperatures across the Salmon (Thomson, 1981; Vaz and Dias, 2008; Roegner et al., 2011; Cho and Lee, 2012). Additionally, the Salmon River estuary is a larger and more complex system, with over twice the area of the Englishman River estuary and more intact estuary habitats. Estuary size and morphology are known to impact mixing patterns and heat dispersion, where smaller systems are usually more well-mixed (McKay and Iorio, 2008, Webb, 2020). The Englishman River estuary is also more degraded, with a dammed and heavily urbanized upstream river that often runs at minimum baseflows throughout the summer that could more easily reach radiative equilibrium with the atmosphere, agriculture and logging across the watershed, and encroaching residential developments constraining the estuary (Boom and Bryden, 1994, Environment and Climate Change Canada (ECCC), 2023b); Sutherland, 2014). These characteristics have been linked to warmer and more homogenous temperature regimes in river systems (Olden and Naiman, 2010; Somers et al., 2013; Juracek et al., 2017; Cunningham et al., 2023). We suggest that understanding the impact of watershed and estuary degradation on estuary temperature mosaics is a key research priority for future work.
The complex summer temperature patterns across both estuaries generated shifting spatio-temporal mosaics of growth opportunities for juvenile Chinook salmon. Water temperatures fluctuated above and below the thermal optimum for consumption (20.9°C) at differing magnitudes and times between estuaries, habitats, and sites—thus, while growth opportunities shared similar seasonal trends across each system, they also varied at smaller habitat and site-level scales (Plumb and Moffitt, 2015). These multiple scales of variability generated portfolios of potential growth across the estuaries, which would be expected to drive several important responses in juvenile Chinook salmon (Greene et al., 2021; Davis et al., 2022). In both freshwater and estuarine systems, for example, water temperature has been shown to direct movement and habitat use patterns, where juvenile salmon will select for metabolically favorable conditions (Armstrong and Schindler, 2013; Matsubu et al., 2017; Morrice et al., 2020). In addition, estuarine water temperatures have been linked to the duration of estuary residency (Greene et al., 2021). For example, when temperatures exceeded tolerable rearing conditions, juvenile Chinook salmon have been found to spend less time in estuarine habitats before outmigrating and entered the ocean at smaller sizes (Munsch et al., 2019). These changes could have cascading impacts on subsequent marine survival (Sawyer et al. in press). Thus, our study serves as a first step towards understanding and predicting these key physiological and ecological responses to temperature mosaics, which may have important consequences for juvenile salmon during this life-history transition to sea.
The simplified warming scenario illustrated how forthcoming climate warming may have diverging impacts on estuarine growth opportunities for juvenile Pacific salmon, dependent on baseline temperatures and habitat complexity (Nielsen et al., 2013; Zhang et al., 2019). Our study was performed with a single year of temperature data and simple warming assumptions—climate change will undoubtedly manifest with more unpredictability across time given complex climatic processes and ecological patterns. That being said, our study did reveal several key findings that we anticipate will be robust to these study limitations. First, excessively warm temperatures have already made some estuarine habitats unsuitable at certain times, and climate warming will further constrict growth opportunities for cold water fishes such as salmon over space and time. Second, estuaries and habitats have differing vulnerabilities to these impacts—in some cases, rising temperatures may actually expand summer growth opportunities across cooler and more heterogenous systems like the Salmon River estuary over the next few decades, depending on climate change. However, these gains will have a finite temporal window—as temperatures continue to rise beyond a few degrees and exceed thermal tolerances, portfolios of growth opportunities will collapse. As temperature mosaics approach and exceed these tolerances, juvenile salmon will likely face several physiological and ecological consequences. For example, Marine and Cech (2004) found that juvenile Chinook salmon experimentally reared in 21-24°C experienced significantly decreased growth, impaired smoltification indices, and increased predation vulnerability in estuaries compared to fish reared at lower temperatures. In addition, the timing and duration of estuary migration could start to shift as temperatures rise, which may lead to phenological mismatches in marine prey availability (Visser and Both, 2005; Crozier et al., 2008; Wilson et al., 2021; Wilson et al., 2023). Our study suggests that juvenile salmon in warmer systems like the Englishman River estuary may face these consequences more acutely and rapidly, while growth opportunities may increase before losses occur in systems like the Salmon River estuary that are not as far along in this trajectory of warming.
Our bioenergetics modeling approach entailed assumptions and simplifications that are important to consider. Throughout all simulations, we held prey energy density and the proportion of maximum consumption at constant literature-obtained values given our lack of empirical data. However, numerous studies have shown that prey communities vary over space and time across estuaries (Arbeider et al., 2019; Davis et al., 2019; Woo et al., 2019; Weil et al., 2020). In addition, prey community dynamics are expected to shift under climate change, which we did not account for in our climate change scenario (Daufresne et al., 2004; Hallett et al., 2018; Woodland et al., 2021). Given this variability in prey resources, the proportion of maximum consumption for juvenile salmon could also vary spatio-temporally, and potentially shift with climate change (Beauchamp, 2009; Davis et al., 2019; Kaylor et al., 2021). Therefore, further work is needed to understand how prey mosaics shape present and future growth opportunities across these systems, but we suggest that the reasonable prey and consumption parameters we selected nonetheless generated useful estimates of growth potential. Our approach of applying a 2°C increase to temperature across all sites based on a single year of temperature data for the climate change scenario is also a major simplifying assumption. While this methodology allowed for a simplistic evaluation of potential impacts on juvenile Chinook salmon GRP, water temperature will not increase uniformly over space and time in estuaries. Process-based and statistical models of water temperature have the ability to account for some of this variability, and could therefore be used to improve estimates of future GRP (Wagner et al., 2011; Brown et al., 2016; Davis, 2019; Des et al., 2020).
In addition to water temperature, climate change will drive shifts in other key estuarine conditions that may impact the nursery quality of estuaries for juvenile salmon. Over the coming century, sea level rise (SLR) is projected to transform estuarine habitat mosaics, and systems constrained by coastal development may disappear entirely without the ability to migrate inland (Craft et al., 2009; SChile et al., 2014; Thorne et al., 2018). These changes are expected to impact habitat availability, connectivity, and growth opportunities for juvenile salmon (Fulford et al., 2014; Torio and Chmura, 2015; Flitcroft et al., 2018). For example, Davis et al. (2022) found that under end-of-century SLR and temperature scenarios, juvenile Chinook salmon are predicted to experience decreased summer growth in the Nisqually River estuary, WA, USA. In addition to rising sea levels, many other climate change-induced shifts including ocean acidification, extreme river flows, and more frequent and intense storm activity will potentially impact estuarine habitat structure and quality (Lee et al., 2016; Munsch et al., 2019; Scanes et al., 2020; Lonsdale et al., 2022). These climatic changes will occur alongside growing anthropogenic pressures such as pollution and habitat alteration, which together may have cumulative or interacting effects on estuarine nursery capacity (Lotze et al., 2006; Healey, 2011; Jorgensen et al., 2013; Toft et al., 2018; Hodgson et al., 2020; Tulloch et al., 2022). Thus, investigating changes in individual conditions such as water temperature is an important piece of the puzzle, but future work is needed to continue building an understanding of more comprehensive shifts in estuary quality and function for juvenile salmon with global change (Hodgson et al., 2020).
Our study demonstrates how variability in a key estuarine habitat condition, water temperature, shapes diverse growth opportunities for juvenile Chinook salmon in two contrasting estuaries. Across both systems, growth potential shifted across habitats and sites through the summer, suggesting that juvenile Chinook salmon could increase their growth by using different estuarine habitats over time (Greene et al., 2021). These portfolios of growth and habitat use may also confer resiliency to estuarine systems, as high GRP in some habitats can compensate for lower GRP across others (Harley et al., 2006; Peirson et al., 2015; Greene et al., 2021). However, we illustrate that as temperature increases across a smaller system with more homogenous spatial temperature patterns like the Englishman River estuary, growth opportunities faced consistent declines estuary-wide. In contrast, when temperatures were increased across a cooler and more complex system like the Salmon River estuary, only some sites faced decreased growth opportunities while others remained stable or increased given higher spatial heterogeneity within the estuary. Together, this underscores the importance of maintaining diverse temperature mosaics across estuaries, which will be especially important for creating and sustaining thermal refuges as temperatures continue to rise (Waples et al., 2009; Wade et al., 2013). Therefore, looking ahead, conservation and management initiatives that aim to maintain spatio-temporal habitat complexity and connectivity among these habitats can serve to support diverse growth opportunities and other key ecological functions for important species like Pacific salmon in this era of climate change (Beck et al., 2001; Schindler et al., 2010; Nagelkerken et al., 2015; Sheaves et al., 2015; Moore and Schindler, 2022).
The datasets presented in this study can be found in online repositories. The names of the repository/repositories and accession number(s) can be found below: https://doi.org/10.5281/zenodo.8033874.
PG: Conceptualization, Data curation, Formal Analysis, Investigation, Methodology, Project administration, Validation, Visualization, Writing – original draft, Writing – review & editing. JG: Investigation, Methodology, Writing – review & editing. DS: Investigation, Methodology, Writing – review & editing. JM: Conceptualization, Funding acquisition, Investigation, Methodology, Project administration, Resources, Supervision, Validation, Writing – original draft, Writing – review & editing. CoF: Investigation, Methodology, Writing – review & editing. CeF: Investigation, Methodology, Writing – review & editing. CM: Investigation, Methodology, Writing – review & editing. CB: Investigation, Methodology, Writing – review & editing.
The author(s) declare financial support was received for the research, authorship, and/or publication of this article. This project was supported by a BC Salmon Restoration and Innovation Fund grant to NTBC, with additional financial support from the Sitka Foundation to Simon Fraser University.
We would like to thank Sydney Berridge, Julie Charbonneau, April Chong, Jessie Everson, Marta Ulaski and Brian Ulaski for supporting field work. We appreciate additional feedback and guidance from Dr. Chelsea Little and Dr. Brett van Poorten.
The authors declare that the research was conducted in the absence of any commercial or financial relationships that could be construed as a potential conflict of interest.
All claims expressed in this article are solely those of the authors and do not necessarily represent those of their affiliated organizations, or those of the publisher, the editors and the reviewers. Any product that may be evaluated in this article, or claim that may be made by its manufacturer, is not guaranteed or endorsed by the publisher.
The Supplementary Material for this article can be found online at: https://www.frontiersin.org/articles/10.3389/fmars.2023.1278810/full#supplementary-material
Supplementary Figure 1 | Violin plots illustrating the distribution of water temperature data from every temperature logger site over three one-week periods between May-August. Abbreviations E and S indicate the Englishman and Salmon River estuaries, respectively, and abbreviations B, Ma, and Me indicate beach, marsh, and meadow habitat types, respectively. (A) Water temperature distributions from May 5-11 for each site. (B) Water temperature distributions from July 25-31 for each site. (C) Water temperature distributions from August 20-26 for each site. Across all panels, the black dot indicates the mean temperature for each site for the respective week.
Supplementary Figure 2 | May-August water temperature (blue line) and tide height (black line) for site Beach 1 in the Englishman River estuary. Tide height data is from the nearest Fisheries and Oceans Canada station (Department of Fisheries and Oceans Canada (DFO), 2023d—Tides, currents, and water levels for Nanoose Bay-07930). (A) Temperature and tide height over the full May-August period. The grey bars indicate the time periods displayed in (B–D). (B) Temperature and tide height from May 11-13. Low tide corresponds with lower water temperatures. High and low tides are indicated by light and dark blue bars in (B–D). (C) Temperature and tide height from July 13-15. Water temperature shows little variation with tide height. (D) Temperature and tide height from August 15-17. Water temperature is higher at low tides.
Supplementary Figure 3 | Air temperature (red lines), river temperature (light blue lines), regional ocean temperature (dark blue lines) and river discharge (green lines) for the Englishman (left) and Salmon (right) River estuaries. River and regional ocean temperature data is plotted together to illustrate the seasonal reversal in relative temperature. Air temperature data is from the nearest Meteorological Service of Canada weather stations (Environment and Climate Change Canada-Meteorological Service of Canada (ECCC-MSC), 2023a [data for the Salmon River estuary], Environment and Climate Change Canada-Meteorological Service of Canada (ECCC-MSC), 2023b [data for the Englishman River estuary]), regional ocean temperature data is from the nearest Department of Fisheries and Oceans lighthouse stations (Department of Fisheries and Oceans Canada (DFO), 2023b—British Columbia Lightstation Sea-Surface Temperature and Salinity Data (Pacific), 1914-present for Pine Island [data for the Salmon River estuary], Department of Fisheries and Oceans Canada (DFO), 2023a—British Columbia Lightstation Sea-Surface Temperature and Salinity Data (Pacific), 1914-present for Departure Bay [data for the Englishman River estuary]), and river discharge data is from Environment and Climate Change Canada maintained hydrometric stations (Environment and Climate Change Canada (ECCC), 2023b [data for the Englishman River estuary], Environment and Climate Change Canada-Meteorological Service of Canada (ECCC-MSC), 2023a [data for the Salmon River estuary).
Arbeider M., Sharpe C., Carr-Harris C., Moore J. (2019). Integrating prey dynamics, diet, and biophysical factors across an estuary seascape for four fish species. Mar. Ecol. Prog. Ser. 613, 151–169. doi: 10.3354/meps12896
Armstrong J. B., Fullerton A. H., Jordan C. E., Ebersole J. L., Bellmore J. R., Arismendi I., et al. (2021). The importance of warm habitat to the growth regime of cold-water fishes. Nat. Clim. Change 11, 354–361. doi: 10.1038/s41558-021-00994-y
Armstrong J. B., Schindler D. E. (2013). Going with the flow: spatial distributions of juvenile coho salmon track an annually shifting mosaic of water temperature. Ecosystems 16, 1429–1441. doi: 10.1007/s10021-013-9693-9
Armstrong J. B., Schindler D. E., Ruff C. P., Brooks G. T., Bentley K. E., Torgersen C. E. (2013). Diel horizontal migration in streams: Juvenile fish exploit spatial heterogeneity in thermal and trophic resources. Ecology 94, 2066–2075. doi: 10.1890/12-1200.1
Atagi D. Y. (1994). Estuarine use by juvenile coho salmon (Oncorhynchus kisutch): is it a viable life history strategy? (Vancouver (BC: The University of British Columbia).
Atlas W. I., Ban N. C., Moore J. W., Tuohy A. M., Greening S., Reid A. J., et al. (2021). Indigenous systems of management for culturally and ecologically resilient pacific salmon (Oncorhynchus spp.) fisheries. BioScience 71, 186–204. doi: 10.1093/biosci/biaa144
Baldwin L. K., Bradfield G. E. (2010). Resilience of bryophyte communities in regenerating matrix forests after logging in temperate rainforests of coastal British Columbia. Botany 88, 297–314. doi: 10.1139/B10-002
Beauchamp D. A. (1989). Corroboration of a bioenergetics model for sockeye salmon. Trans. Am. Fisheries Soc. 118, 597–607. doi: 10.1577/1548-8659(1989)1180597:COABMF2.3.CO;2
Beauchamp D. A. (2009). “Bioenergetic Ontogeny: Linking Climate and Mass- specific Feeding to Life-Cycle Growth and Survival of salmon,” in Pacific salmon: ecology and management of western alaska’s populations. Eds. Zimmerman C., Krueger C. C. (Bethesda, MD: American Fisheries Society Symposium), 53–72.
Beck M. W., Heck K. L., Able K. W., Childers D. L., Eggleston D. B., Gillanders B. M., et al. (2001). The identification, conservation, and management of estuarine and marine nurseries for fish and invertebrates. BioScience 51, 633. doi: 10.1641/0006-3568(2001)051[0633:TICAMO]2.0.CO;2
Bocking R., Gaboury M. (2001). Englishman river watershed recovery plan (Sidney, BC: LGL Limited environmental research associates).
Bond M. H., Hayes S. A., Hanson C. V., MacFarlane R. B. (2008). Marine survival of steelhead (Oncorhynchus mykiss) enhanced by a seasonally closed estuary. Can. J. Fish. Aquat. Sci. 65, 2242–2252. doi: 10.1139/F08-131
Bond R. M., Kiernan J. D., Osterback A.-M. K., Kern C. H., Hay A. E., Meko J. M., et al. (2022). Spatiotemporal variability in environmental conditions influences the performance and behavior of juvenile steelhead in a coastal california lagoon. Estuaries Coasts 45, 1749–1765. doi: 10.1007/s12237-021-01019-9
Boom A., Bryden G. (1994). Englishman river: water allocation plan. Ed. Nanaimo B. C. (Ministry of Environment, Lands and Parks, Vancouver Island Region: Province of British Columbia).
Bottom D. L., Jones K. K., Cornwell T. J., Gray A., Simenstad C. A. (2005). Patterns of Chinook salmon migration and residency in the Salmon River estuary (Oregon). Estuarine Coast. Shelf Sci. 64, 79–93. doi: 10.1016/j.ecss.2005.02.008
Brown C. A., Sharp D., Mochon Collura T. C. (2016). Effect of climate change on water temperature and attainment of water temperature criteria in the Yaquina Estuary, Oregon (USA). Estuarine Coast. Shelf Sci. 169, 136–146. doi: 10.1016/j.ecss.2015.11.006
Caissie D., El-Jabi N. (2020). The importance of cross-calibration and protecting water temperature sensors against direct solar radiation heating in hydrological studies. Hydrological Sci. J. 65, 102–111. doi: 10.1080/02626667.2019.1688818
Chalifour L., Scott D. C., MacDuffee M., Stark S., Dower J. F., Beacham T. D., et al. (2021). Chinook salmon exhibit long-term rearing and early marine growth in the Fraser River, British Columbia, a large urban estuary. Can. J. Fish. Aquat. Sci. 78, 539–550. doi: 10.1139/cjfas-2020-0247
Cho H.-Y., Lee K.-H. (2012). Development of an air–water temperature relationship model to predict climate-induced future water temperature in estuaries. J. Environ. Eng. 138, 570–577. doi: 10.1061/(ASCE)EE.1943-7870.0000499
Cordell J. R., Toft J. D., Gray A., Ruggerone G. T., Cooksey M. (2011). Functions of restored wetlands for juvenile salmon in an industrialized estuary. Ecol. Eng. 37, 343–353. doi: 10.1016/j.ecoleng.2010.11.028
Craft C., Clough J., Ehman J., Joye S., Park R., Pennings S., et al. (2009). Forecasting the effects of accelerated sea-level rise on tidal marsh ecosystem services. Front. Ecol. Environ. 7, 73–78. doi: 10.1890/070219
Crozier L. G., Hendry A. P., Lawson P. W., Quinn T. P., Mantua N. J., Battin J., et al. (2008). Potential responses to climate change in organisms with complex life histories: evolution and plasticity in Pacific salmon: Evolutionary responses to climate change in salmon. Evolutionary Appl. 1, 252–270. doi: 10.1111/j.1752-4571.2008.00033.x
Cunningham D. S., Braun D. C., Moore J. W., Martens A. M. (2023). Forestry influences on salmonid habitat in the North Thompson River watershed, British Columbia. Can. J. Fish. Aquat. Sci. 80, 1053–1070. doi: 10.1139/cjfas-2022-0255
Daufresne M., Roger M. C., Capra H., Lamouroux N. (2004). Long-term changes within the invertebrate and fish communities of the Upper Rhône River: effects of climatic factors: Climate change and freshwater communities. Global Change Biol. 10, 124–140. doi: 10.1046/j.1529-8817.2003.00720.x
David A. T., Ellings C. S., Woo I., Simenstad C. A., Takekawa J. Y., Turner K. L., et al. (2014). Foraging and growth potential of juvenile chinook salmon after tidal restoration of a large river delta. Trans. Am. Fisheries Soc. 143, 1515–1529. doi: 10.1080/00028487.2014.945663
Davis M. J. (2019). Dynamic habitat models for estuary-dependent Chinook salmon: informing management in the face of climate change impacts (Seattle, Washington: The University of Washington).
Davis M. J., Woo I., Ellings C. S., Hodgson S., Beauchamp D. A., Nakai G., et al. (2019). Freshwater tidal forests and estuarine wetlands may confer early life growth advantages for delta-reared chinook salmon. Trans. Am. Fish Soc. 148, 289–307. doi: 10.1002/tafs.10134
Davis M. J., Woo I., Ellings C. S., Hodgson S., Beauchamp D. A., Nakai G., et al. (2022). A climate-mediated shift in the estuarine habitat mosaic limits prey availability and reduces nursery quality for juvenile salmon. Estuaries Coasts 45, 1445–1464. doi: 10.1007/s12237-021-01003-3
Department of Fisheries and Oceans Canada (DFO) (2023a) British columbia lightstation sea-surface temperature and salinity data (Pacific), 1914-present for departure bay. Available at: https://open.Canada.ca/data/en/dataset/719955f2-bf8e-44f7-bc26-6bd623e82884 (Accessed October 14, 2023).
Department of Fisheries and Oceans Canada (DFO) (2023b) British columbia lightstation sea-surface temperature and salinity data (Pacific), 1914-present for pine island. Available at: https://open.Canada.ca/data/en/dataset/719955f2-bf8e-44f7-bc26-6bd623e82884 (Accessed October 14, 2023).
Department of Fisheries and Oceans Canada (DFO) (2023c) Tides, currents, and water levels for Kelsey Bay-08215. Available at: https://tides.gc.ca/en/stations/08215.
Department of Fisheries and Oceans Canada (DFO) (2023d) Tides, currents, and water levels for Nanoose Bay-07930. Available at: https://tides.gc.ca/en/stations/07930 (Accessed May 30, 2023).
Des M., Martínez B., deCastro M., Viejo R. M., Sousa M. C., Gómez-Gesteira M. (2020). The impact of climate change on the geographical distribution of habitat-forming macroalgae in the Rías Baixas. Mar. Environ. Res. 161, 105074. doi: 10.1016/j.marenvres.2020.105074
Deslauriers D., Chipps S. R., Breck J. E., Rice J. A., Madenjian C. P. (2017). Fish Bioenergetics 4.0: An Rbased modeling application. Fisheries 42, 586–596. doi: 10.1080/03632415.2017.1377558
Duffy E. J., Beauchamp D. A. (2011). Rapid growth in the early marine period improves the marine survival of Chinook salmon ( Oncorhynchus tshawytscha ) in Puget Sound, Washington. Can. J. Fish. Aquat. Sci. 68, 232–240. doi: 10.1139/F10-144
Eliason E. J., Clark T. D., Hague M. J., Hanson L. M., Gallagher Z. S., Jeffries K. M., et al. (2011). Differences in thermal tolerance among sockeye salmon populations. Science 332, 109–112. doi: 10.1126/science.1199158
Environment and Climate Change Canada (ECCC) (2023a) Real-time hydrometric data graph for salmon river near sayward (08HD0060) [BC]. Available at: https://wateroffice.ec.gc.ca/report/real_time_e.html?stn=08HD006 (Accessed May 30, 2023).
Environment and Climate Change Canada (ECCC) (2023b) Real-time hydrometric data graph for englishman river near parksville (08HB002) [BC]. Available at: https://wateroffice.ec.gc.ca/report/real_time_e.html?stn=08HB002 (Accessed May 30, 2023).
Environment and Climate Change Canada-Meteorological Service of Canada (ECCC-MSC) (2023a) Historical climate data for fanny island, british columbia. Available at: https://climate.weather.gc.ca/climate_data/hourly_data_e.html?hlyRange=2000-04-04%7C2023-05-29&dlyRange=2000-04-01%7C2023-05-28&mlyRange=2000-04-01%7C2007-02-01&StationID=29733&Prov=BC&urlExtension=_e.html&searchType=stnName&optLimit=yearRange&StartYear=1840&EndYear=2023&selRowPerPage=25&Line=0&searchMethod=contains&txtStationName=fanny+island&timeframe=1&time=LST&time=LST&Year=2022&Month=5&Day=1 (Accessed May 30, 2023).
Environment and Climate Change Canada-Meteorological Service of Canada (ECCC-MSC) (2023b) Historical climate data for qualicum beach airport, british columbia. Available at: https://climate.weather.gc.ca/climate_data/hourly_data_e.html?hlyRange=2006-12-11%7C2023-05-29&dlyRange=2006-11-01%7C2023-05-28&mlyRange=2006-11-01%7C2006-11-01&StationID=45627&Prov=BC&urlExtension=_e.html&searchType=stnName&optLimit=yearRange&StartYear=1840&EndYear=2023&selRowPerPage=25&Line=5&searchMethod=contains&txtStationName=qualicum&timeframe=1&time=LST&time=LST&Year=2023&Month=5&Day=1 (Accessed May 30, 2023).
Flitcroft R., Clinton P., Christiansen K. (2018). Adding to the toolbox for tidal-inundation mapping in estuarine areas. J. Coast. Conserv. 22, 745–753. doi: 10.1007/s11852-018-0605-1
Fogarty M. C., Fewings M. R., Paget A. C., Dierssen H. M. (2018). The influence of a sandy substrate, seagrass, or highly turbid water on albedo and surface heat flux. J. Geophys. Res. Oceans 123, 53–73. doi: 10.1002/2017JC013378
Fulford R. S., Peterson M. S., Grammer P. O. (2011). An ecological model of the habitat mosaic in estuarine nursery areas: Part I—Interaction of dispersal theory and habitat variability in describing juvenile fish distributions. Ecol. Model. 222, 3203–3215. doi: 10.1016/j.ecolmodel.2011.07.001
Fulford R. S., Peterson M. S., Wu W., Grammer P. O. (2014). An ecological model of the habitat mosaic in estuarine nursery areas: Part II—Projecting effects of sea level rise on fish production. Ecol. Model. 273, 96–108. doi: 10.1016/j.ecolmodel.2013.10.032
Giddings S. N., Fong D. A., Monismith S. G. (2011). Role of straining and advection in the intratidal evolution of stratification, vertical mixing, and longitudinal dispersion of a shallow, macrotidal, salt wedge estuary. J. Geophys. Res. 116, C03003. doi: 10.1029/2010JC006482
Gillanders B. M., Elsdon T. S., Halliday I. A., Jenkins G. P., Robins J. B., Valesini F. J. (2011). Potential effects of climate change on Australian estuaries and fish utilising estuaries: a review. Mar. Freshw. Res. 62, 1115–1131. doi: 10.1071/MF11047
Greene C. M., Beamer E., Chamberlin J., Hood G., Davis M., Larsen K., et al. (2021). Landscape, density-dependent, and bioenergetic influences upon Chinook salmon in tidal delta habitats: Comparison of four Puget Sound estuaries. ESRP Report 13-1508. (Seattle WA: NOAA Northwest Fisheries Science Center Watershed Program).
Griffiths J. R., Schindler D. E. (2012). Consequences of changing climate and geomorphology for bioenergetics of juvenile sockeye salmon in a shallow Alaskan lake: Changing climate and geomorphology affect juvenile sockeye salmon bioenergetics. Ecol. Freshw. Fish 21, 349–362. doi: 10.1111/j.1600-0633.2012.00555.x
Hahlbeck N., Tinniswood W. R., Sloat M. R., Ortega J. D., Wyatt M. A., Hereford M. E., et al. (2022). Contribution of warm habitat to cold-water fisheries. Conserv. Biol. 36. doi: 10.1111/cobi.13857
Hall J. E., Khangaonkar T. P., Rice C. A., Chamberlin J., Zackey T., Leonetti F., et al. (2018). Characterization of salinity and temperature patterns in a large river delta to support tidal wetland habitat restoration. Northwest Sci. 92, 36–52. doi: 10.3955/046.092.0105
Hallett C. S., Hobday A. J., Tweedley J. R., Thompson P. A., McMahon K., Valesini F. J. (2018). Observed and predicted impacts of climate change on the estuaries of south-western Australia, a Mediterranean climate region. Reg. Environ. Change 18, 1357–1373. doi: 10.1007/s10113-017-1264-8
Hanson P. C., Johnson T. B., Schindler D. E., Kitchell J. F. (1997). Fish bioenergetics 3.0 software for Windows. Madison, Wisconsin: University of Wisconsin Center for Limnology, Sea Grant Institute, Technical Report WISCU-T97-001. 116.
Harley C. D. G., Randall Hughes A., Hultgren K. M., Miner B. G., Sorte C. J. B., Thornber C. S., et al. (2006). The impacts of climate change in coastal marine systems: Climate change in coastal marine systems. Ecol. Lett. 9, 228–241. doi: 10.1111/j.1461-0248.2005.00871.x
Healey M. (2011). The cumulative impacts of climate change on Fraser River sockeye salmon ( Oncorhynchus nerka ) and implications for management. Can. J. Fish. Aquat. Sci. 68, 718–737. doi: 10.1139/f2011-010
Hewett S. W., Johnson B. L. (1987). A generalized bioenergetics model of fish growth for microcomputers (Madison, Wisconsin: University of Wisconsin Sea Grant Institute), 47.
Hewett S. W., Johnson B. L. (1992). A generalized bioenergetics model of fish growth for microcomputers (Madison, Wisconsin. UW Sea Grant Tech. Rep.WIS-SG-92-250: University of Wisconsin Sea Grant Institute), 79.
Hodgson E. E., Wilson S. M., Moore J. W. (2020). Changing estuaries and impacts on juvenile salmon: A systematic review. Glob. Change Biol. 26, 1986–2001. doi: 10.1111/gcb.14997
Hoegh-Guldberg O., Cai R., Poloczanska E. S., Brewer P. G., Sundby S., Hilmi K., et al. (2014). “The ocean,” in Climate change 2014: impacts, adaptation, and vulnerability. Part B: regional aspects. Contribution of working group II to the fifth assessment report of the intergovernmental panel on climate change. Eds. Barros V. R., Field C. B., Dokken D. J., Mastrandrea M. D., Mach K. J., Bilir T. E., Chatterjee M., Ebi K. L., Estrada Y. O., Genova R. C., Girma B., Kissel E. S., Levy A. N., MacCracken S., Mastrandrea P. R., White L. L. (Cambridge, UK and New York, USA: Cambridge University Press), 1655–1731.
Johnson M., Wilby R. (2013). Shield or not to shield: effects of solar radiation on water temperature sensor accuracy. Water 5, 1622–1637. doi: 10.3390/w5041622
Jorgensen J. C., Mcclure M. M., Sheer M. B., Munn N. L. (2013). Combined effects of climate change and bank stabilization on shallow water habitats of chinook salmon: climate change and riverbank stabilization. Conserv. Biol. 27, 1201–1211. doi: 10.1111/cobi.12168
Juracek K. E., Eng K., Carlisle D. M., Wolock D. M. (2017). Streamflow alteration and habitat ramifications for a threatened fish species in the Central United States. River Res. Appl. 33, 993–1003. doi: 10.1002/rra.3148
Kaylor M. J., Justice C., Armstrong J. B., Staton B. A., Burns L. A., Sedell E., et al. (2021). Temperature, emergence phenology and consumption drive seasonal shifts in fish growth and production across riverscapes. J. Anim. Ecol. 90, 1727–1741. doi: 10.1111/1365-2656.13491
Khadami F., Kawanisi K., Al Sawaf M. B., Gusti G. N. N., Xiao C. (2022). Spatiotemporal response of currents and mixing to the interaction of tides and river runoff in a mesotidal estuary. Ocean Sci. J. 57, 37–51. doi: 10.1007/s12601-022-00056-0
Lament J., Adair M., Desrosiers L., Morrison D. (2015). Salmon river estuary acquisition final report FWCP project no. 14.L.CBR.01 (Vancouver, British Columbia: The Nature Trust of British Columbia).
Lee S.-Y., Hamlet A. F., Grossman E. E. (2016). Impacts of climate change on regulated streamflow, hydrologic extremes, hydropower production, and sediment discharge in the skagit river basin. Northwest Sci. 90, 23–43. doi: 10.3955/046.090.0104
Levings C. D. (2016). Ecology of salmonids in estuaries around the world (Vancouver, British Columbia: UBC Press).
Lonsdale J.-A., Leach C., Parsons D., Barkwith A., Manson S., Elliott M. (2022). Managing estuaries under a changing climate: A case study of the Humber Estuary, UK. Environ. Sci. Policy 134, 75–84. doi: 10.1016/j.envsci.2022.04.001
Lotze H. K., Lenihan H. S., Bourque B. J., Bradbury R. H., Cooke R. G., Kay M. C., et al. (2006). Depletion, degradation, and recovery potential of estuaries and coastal seas. Science 312, 1806–1809. doi: 10.1126/science.1128035
MacKenzie W. H., Moran J. R. (2004). Wetlands of British Columbia: a guide to identification (Victoria, BC: British Columbia, Ministry of Forests, Forest Science Program).
Magnusson A., Hilborn R. (2003). Estuarine influence on survival rates of coho (Oncorhynchus kisutch) and chinook salmon (Oncorhynchus tshawytscha) released from hatcheries on the U.S. Pacific coast. Estuaries 26, 1094–1103. doi: 10.1007/BF02803366
Mantua N., Tohver I., Hamlet A. (2010). Climate change impacts on streamflow extremes and summertime stream temperature and their possible consequences for freshwater salmon habitat in Washington State. Climatic Change 102, 187–223. doi: 10.1007/s10584-010-9845-2
Marin Jarrin M. J., Sutherland D. A., Helms A. R. (2022). Water temperature variability in the Coos Estuary and its potential link to eelgrass loss. Front. Mar. Sci. 9. doi: 10.3389/fmars.2022.930440
Marine K. R., Cech J. J. (2004). Effects of high water temperature on growth, smoltification, and predator avoidance in juvenile sacramento riverChinook salmon. North Am. J. Fisheries Manage. 24, 198–210. doi: 10.1577/M02-142
Matsubu W., Simenstad C. A., Horton G. E. (2017). Juvenile steelhead locate coldwater refugia in an intermittently closed estuary. Trans. Am. Fisheries Soc. 146, 680–695. doi: 10.1080/00028487.2017.1301993
McKay P., Iorio D. D. (2008). Heat budget for a shallow, sinuous salt marsh estuary. Continental Shelf Res. 28, 1740–1753. doi: 10.1016/j.csr.2008.04.008
Moore J., Gordon J., Carr-Harris C., Gottesfeld A., Wilson S., Russell J. (2016). Assessing estuaries as stopover habitats for juvenile Pacific salmon. Mar. Ecol. Prog. Ser. 559, 201–215. doi: 10.3354/meps11933
Moore J. W., Schindler D. E. (2022). Getting ahead of climate change for ecological adaptation and resilience. Science 376, 1421–1426. doi: 10.1126/science.abo3608
Morrice K. J., Baptista A. M., Burke B. J. (2020). Environmental and behavioral controls on juvenile Chinook salmon migration pathways in the Columbia River estuary. Ecol. Model. 427, 109003. doi: 10.1016/j.ecolmodel.2020.109003
Munsch S. H., Greene C. M., Johnson R. C., Satterthwaite W. H., Imaki H., Brandes P. L. (2019). Warm, dry winters truncate timing and size distribution of seaward-migrating salmon across a large, regulated watershed. Ecol. Appl. 29, e01880. doi: 10.1002/eap.1880
Nagelkerken I., Sheaves M., Baker R., Connolly R. M. (2015). The seascape nursery: a novel spatial approach to identify and manage nurseries for coastal marine fauna. Fish Fish 16, 362–371. doi: 10.1111/faf.12057
Nielsen J. L., Ruggerone G. T., Zimmerman C. E. (2013). Adaptive strategies and life history characteristics in a warming climate: Salmon in the Arctic? Environ. Biol. Fish 96, 1187–1226. doi: 10.1007/s10641-012-0082-6
Olden J. D., Naiman R. J. (2010). Incorporating thermal regimes into environmental flows assessments: modifying dam operations to restore freshwater ecosystem integrity: Incorporating thermal regimes in environmental flows assessments. Freshw. Biol. 55, 86–107. doi: 10.1111/j.1365-2427.2009.02179.x
Osterback A.-M. K., Kern C. H., Kanawi E. A., Perez J. M., Kiernan J. D. (2018). The effects of early sandbar formation on the abundance and ecology of coho salmon ( Oncorhynchus kisutch ) and steelhead trout ( Oncorhynchus mykiss ) in a central California coastal lagoon. Can. J. Fish. Aquat. Sci. 75, 2184–2197. doi: 10.1139/cjfas-2017-0455
Pedersen E. J., Miller D. L., Simpson G. L., Ross N. (2019). Hierarchical generalized additive models in ecology: an introduction with mgcv. PeerJ 7, e6876. doi: 10.7717/peerj.6876
Peirson W., Davey E., Jones A., Hadwen W., Bishop K., Beger M., et al. (2015). Opportunistic management of estuaries under climate change: A new adaptive decision-making framework and its practical application. J. Environ. Manage. 163, 214–223. doi: 10.1016/j.jenvman.2015.08.021
Perry A. L., Low P. J., Ellis J. R., Reynolds J. D. (2005). Climate change and distribution shifts in marine fishes. Science 308, 1912–1915. doi: 10.1126/science.1111322
Peterson M. S. (2003). A conceptual view of environment-habitat-production linkages in tidal river estuaries. Rev. Fisheries Sci. 11, 291–313. doi: 10.1080/10641260390255844
Peterson M. S., Weber M. R., Partyka M. L., Ross S. T. (2007). Integratingin situ quantitative geographic information tools and size-specific, laboratory-based growth zones in a dynamic river-mouth estuary. Aquat. Conserv: Mar. Freshw. Ecosyst. 17, 602–618. doi: 10.1002/aqc.823
Plumb J. M., Moffitt C. M. (2015). Re-estimating temperature-dependent consumption parameters in bioenergetics models for juvenile chinook salmon. Trans. Am. Fisheries Soc. 144, 323–330. doi: 10.1080/00028487.2014.986336
Pojar J., Klinka K., Demarchi D. A. (1991). “Chapter 6: coastal western hemlock zone in ecosystems of british columbia,” in Ecosystems of British Columbia. Eds. Meidinger D. V., Pojar J. (BC: Ministry of Forests), 95–111.
Pörtner H. O., Peck M. A. (2010). Climate change effects on fishes and fisheries: towards a cause-and-effect understanding. J. Fish Biol. 77, 1745–1779. doi: 10.1111/j.1095-8649.2010.02783.x
Possamai B., Vieira J. P., Grimm A. M., Garcia A. M. (2018). Temporal variability, (1997-2015) of trophic fish guilds and its relationships with El Niño events in a subtropical estuary. Estuarine Coast. Shelf Sci. 202, 145–154. doi: 10.1016/j.ecss.2017.12.019
Price M. H. H., English K. K., Rosenberger A. G., MacDuffee M., Reynolds J. D. (2017). Canada’s Wild Salmon Policy: an assessment of conservation progress in British Columbia. Can. J. Fish. Aquat. Sci. 74, 1507–1518. doi: 10.1139/cjfas-2017-0127
Quinn T. P. (2018). The behavior and ecology of pacific salmon and trout (2nd ed.) (Seattle, USA: University of Washington Press).
Ray G. C. (2005). Connectivities of estuarine fishes to the coastal realm. Estuarine Coast. Shelf Sci. 64, 18–32. doi: 10.1016/j.ecss.2005.02.003
R Core Team (2022). R: A language and environment for statistical computing (Vienna, Austria: R Foundation for Statistical Computing). Available at: https://www.R-project.org/.
Roegner G. C., Seaton C., Baptista A. M. (2011). Climatic and tidal forcing of hydrography and chlorophyll concentrations in the columbia river estuary. Estuaries Coasts 34, 281–296. doi: 10.1007/s12237-010-9340-z
Sanford E. (1999). Regulation of keystone predation by small changes in ocean temperature. Science 283, 2095–2097. doi: 10.1126/science.283.5410.2095
Sawyer A. C., Atlas W. I., Seitz K. M., Wilson S. M., Moore J. W. in press . State-dependent estuary stopover boosts juvenile salmon growth: implications for marine survival. Ecosphere.
Scanes E., Scanes P. R., Ross P. M. (2020). Climate change rapidly warms and acidifies Australian estuaries. Nat. Commun. 11 1803. doi: 10.1038/s41467-020-15550-z
SChile L. M., Callaway J. C., Morris J. T., Stralberg D., Parker V. T., Kelly M. (2014). Modeling tidal marsh distribution with sea-level rise: evaluating the role of vegetation, sediment, and upland habitat in marsh resiliency. PloS One 9, e88760. doi: 10.1371/journal.pone.0088760
Schindler D. E., Hilborn R., Chasco B., Boatright C. P., Quinn T. P., Rogers L. A., et al. (2010). Population diversity and the portfolio effect in an exploited species. Nature 465, 609–612. doi: 10.1038/nature09060
Schumann E. H. (2000). Oceanic exchanges and temperature variability in the Knysna Estuary. Trans. R. Soc. South Afr. 55, 123–128. doi: 10.1080/00359190009520438
Sharpe C., Carr-Harris C., Arbeider M., Wilson S. M., Moore J. W. (2019). Estuary habitat associations for juvenile Pacific salmon and pelagic fish: Implications for coastal planning processes. Aquat. Conserv: Mar. Freshw. Ecosyst. 29, 1636–1656. doi: 10.1002/aqc.3142
Sheaves M. (2009). Consequences of ecological connectivity: the coastal ecosystem mosaic. Mar. Ecol. Prog. Ser. 391, 107–115. doi: 10.3354/meps08121
Sheaves M., Baker R., Nagelkerken I., Connolly R. M. (2015). True value of estuarine and coastal nurseries for fish: incorporating complexity and dynamics. Estuaries Coasts 38, 401–414. doi: 10.1007/s12237-014-9846-x
Silvestri S. M. (2007). Fish habitat rehabilitation in the englishman river 2006. Surrey, British Columbia: BC conservation foundation, greater Georgia basin steelhead recovery plan.
Simpson G. (2023) gratia: Graceful ggplot-Based Graphics and Other Functions for GAMs Fitted using mgcv. R package version 0.8.1. Available at: https://gavinsimpson.github.io/gratia/.
Somers K. A., Bernhardt E. S., Grace J. B., Hassett B. A., Sudduth E. B., Wang S., et al. (2013). Streams in the urban heat island: spatial and temporal variability in temperature. Freshw. Sci. 32, 309–326. doi: 10.1899/12-046.1
Spanjer A. R., Gendaszek A. S., Wulfkuhle E. J., Black R. W., Jaeger K. L. (2022). Assessing climate change impacts on Pacific salmon and trout using bioenergetics and spatiotemporal explicit river temperature predictions under varying riparian conditions. PloS One 17, e0266871. doi: 10.1371/journal.pone.0266871
Stewart D. J., Ibarra M. (1991). Predation and production by salmonine fishes in lake michigan 1978–88. Can. J. Fisheries Aquat. Sci. 48, 909–922. doi: 10.1139/f91-107
Stewart D. J., Weininger D., Rottiers D. V., Edsall T. A. (1983). An energetics model for lake trout, Salvelinus namaycush: Application to the Lake Michigan population. Can. J. Fisheries Aquat. Sci. 40, 681–698. doi: 10.1139/f83-091
Sutherland C. (2014). TM #2B – arrowsmith lake reservoir water supply (REVISED-FINAL). CH2MHILL. (Victoria, British Columbia: Kerr Wood Leidal Associates Ltd.)
Sutherland D. A., O’Neill M. A. (2016). Hydrographic and dissolved oxygen variability in a seasonal Pacific Northwest estuary. Estuarine Coast. Shelf Sci. 172, 47–59. doi: 10.1016/j.ecss.2016.01.042
Talley D. M., North E. W., Juhl A. R., Timothy D. A., Conde D., deBrouwer J. F. C., et al. (2003). Research challenges at the land–sea interface. Estuarine Coast. Shelf Sci. 58, 699–702. doi: 10.1016/j.ecss.2003.08.010
Thomson R. E. (1981). Oceanography of the british columbia coast (Ottawa: Department of Fisheries and Oceans).
Thorne K., MacDonald G., Guntenspergen G., Ambrose R., Buffington K., Dugger B., et al. (2018). U.S. Pacific coastal wetland resilience and vulnerability to sea-level rise. Sci. Adv. 4, eaao3270. doi: 10.1126/sciadv.aao3270
Toft J. D., Munsch S. H., Cordell J. R., Siitari K., Hare V. C., Holycross B. M., et al. (2018). Impact of multiple stressors on juvenile fish in estuaries of the northeast Pacific. Glob Change Biol. 24, 2008–2020. doi: 10.1111/gcb.14055
Torio D. D., Chmura G. L. (2015). Impacts of sea level rise on marsh as fish habitat. Estuaries Coasts 38, 1288–1303. doi: 10.1007/s12237-013-9740-y
Tulloch V. J. D., Adams M. S., Martin T. G., Tulloch A. I. T., Martone R., Avery-Gomm S., et al. (2022). Accounting for direct and indirect cumulative effects of anthropogenic pressures on salmon- and herring-linked land and ocean ecosystems. Phil. Trans. R. Soc B 377, 20210130. doi: 10.1098/rstb.2021.0130
van Vliet M. T. H., Franssen W. H. P., Yearsley J. R., Ludwig F., Haddeland I., Lettenmaier D. P., et al. (2013). Global river discharge and water temperature under climate change. Global Environ. Change 23, 450–464. doi: 10.1016/j.gloenvcha.2012.11.002
Vaz N., Dias J. M. (2008). Hydrographic characterization of an estuarine tidal channel. J. Mar. Syst. 70, 168–181. doi: 10.1016/j.jmarsys.2007.05.002
Visser M. E., Both C. (2005). Shifts in phenology due to global climate change: the need for a yardstick. Proc. R. Soc B. 272, 2561–2569. doi: 10.1098/rspb.2005.3356
Wade A. A., Beechie T. J., Fleishman E., Mantua N. J., Wu H., Kimball J. S., et al. (2013). Steelhead vulnerability to climate change in the P acific N orthwest. J. Appl. Ecol. 50, 1093–1104. doi: 10.1111/1365-2664.12137
Wagner R. W., Stacey M., Brown L. R., Dettinger M. (2011). Statistical models of temperature in the sacramento–san joaquin delta under climate-change scenarios and ecological implications. Estuaries Coasts 34, 544–556. doi: 10.1007/s12237-010-9369-z
Waples R. S., Beechie T., Pess G. R. (2009). Evolutionary history, habitat disturbance regimes, and anthropogenic changes: what do these mean for resilience of pacific salmon populations? E&S 14, art3. doi: 10.5751/ES-02626-140103
Webb P. (2020). ‘Estuaries’, in Introduction to oceanography (Rebus community) (Montreal, Quebec: Pressbooks), pp. 323–329.
Weil J., Duguid W., Juanes F. (2020). Fine-scale taxonomic and temporal variability in the energy density of invertebrate prey of juvenile Chinook salmon Oncorhynchus tshawytscha. Mar. Ecol. Prog. Ser. 655, 185–198. doi: 10.3354/meps13513
Weitkamp L. A., Goulette G., Hawkes J., O’Malley M., Lipsky C. (2014). Juvenile salmon in estuaries: comparisons between North American Atlantic and Pacific salmon populations. Rev. Fish Biol. Fisheries 24, 713–736. doi: 10.1007/s11160-014-9345-y
Welsh H. H., Hodgson G. R., Harvey B. C., Roche M. F. (2001). Distribution of juvenile coho salmon in relation to water temperatures in tributaries of the mattole river, california. North Am. J. Fisheries Manage. 21, 464–470. doi: 10.1577/1548-8675(2001)021<0464:DOJCSI>2.0.CO;2
Wilson S. M., Buehrens T. W., Fisher J. L., Wilson K. L., Moore J. W. (2021). Phenological mismatch, carryover effects, and marine survival in a wild steelhead trout Oncorhynchus mykiss population. Prog. Oceanography 193, 102533. doi: 10.1016/j.pocean.2021.102533
Wilson S. M., Moore J. W., Ward E. J., Kinsel C. W., Anderson J. H., Buehrens T. W., et al. (2023). Phenological shifts and mismatch with marine productivity vary among Pacific salmon species and populations. Nat. Ecol. Evol. 7, 852–861. doi: 10.1038/s41559-023-02057-1
Woo I., Davis M. J., Ellings C. S., Hodgson S., Takekawa J. Y., Nakai G., et al. (2019). A mosaic of estuarine habitat types with prey resources from multiple environmental strata supports a diversified foraging portfolio for juvenile chinook salmon. Estuaries Coasts 42, 1938–1954. doi: 10.1007/s12237-019-00613-2
Wood S. N. (2017). Generalized additive models: an introduction with R. 2nd (Boca Raton, Florida: Chapman and Hall/CRC). doi: 10.1201/9781315370279
Woodland R. J., Buchheister A., Latour R. J., Lozano C., Houde E., Sweetman C. J., et al. (2021). Environmental drivers of forage fishes and benthic invertebrates at multiple spatial scales in a large temperate estuary. Estuaries Coasts 44, 921–938. doi: 10.1007/s12237-020-00835-9
Wooldridge T., Deyzel S. (2012). Variability in estuarine water temperature gradients and influence on the distribution of zooplankton: a biogeographical perspective. Afr. J. Mar. Sci. 34, 465–477. doi: 10.2989/1814232X.2012.689622
Keywords: estuary, water temperature, nursery habitat, bioenergetics, oncorhynchus, climate change
Citation: Gross PL, Gan JCL, Scurfield DJ, Frank C, Frank C, McLean C, Bob C and Moore JW (2023) Complex temperature mosaics across space and time in estuaries: implications for current and future nursery function for Pacific salmon. Front. Mar. Sci. 10:1278810. doi: 10.3389/fmars.2023.1278810
Received: 16 August 2023; Accepted: 06 November 2023;
Published: 27 November 2023.
Edited by:
Alberto Basset, University of Salento, ItalyReviewed by:
Wei Cheng, University of Washington, United StatesCopyright © 2023 Gross, Gan, Scurfield, Frank, Frank, McLean, Bob and Moore. This is an open-access article distributed under the terms of the Creative Commons Attribution License (CC BY). The use, distribution or reproduction in other forums is permitted, provided the original author(s) and the copyright owner(s) are credited and that the original publication in this journal is cited, in accordance with accepted academic practice. No use, distribution or reproduction is permitted which does not comply with these terms.
*Correspondence: Phoebe L. Gross, cGhvZWJlX2dyb3NzQHNmdS5jYQ==
Disclaimer: All claims expressed in this article are solely those of the authors and do not necessarily represent those of their affiliated organizations, or those of the publisher, the editors and the reviewers. Any product that may be evaluated in this article or claim that may be made by its manufacturer is not guaranteed or endorsed by the publisher.
Research integrity at Frontiers
Learn more about the work of our research integrity team to safeguard the quality of each article we publish.