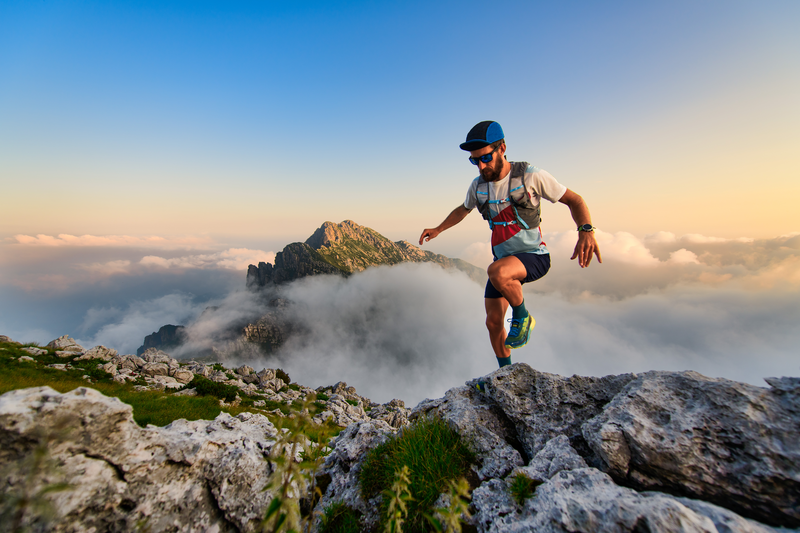
94% of researchers rate our articles as excellent or good
Learn more about the work of our research integrity team to safeguard the quality of each article we publish.
Find out more
BRIEF RESEARCH REPORT article
Front. Mar. Sci. , 13 November 2023
Sec. Marine Megafauna
Volume 10 - 2023 | https://doi.org/10.3389/fmars.2023.1223298
We report the first case series of osteofluorosis in free ranging marine mammals and documentation of baseline bone fluoride concentrations in this population; however, the exact source is not known. A stranded subadult male California sea lion, one subadult male carcass, and one partial carcass, presumably adult male, demonstrated osseous (bony) proliferations on various appendicular and axial bones. The most severe lesions were from the index case with symmetrical segmental circumferential periosteal new bone proliferation on the diaphysis of both femurs and humeri. This case, and the second case, also had significant segmental periosteal new bone proliferation on the mandibles as well as increased tooth wear with dull enamel. Total fluoride concentrations in various bones of the cases ranged from 3,700 to 9,700 ppm dw, with a mean of 5,431 ppm dw, approximately twice the concentration in adult cattle with osteofluorosis. Ribs from four control California sea lions of various ages and both sexes had an average total fluoride of 1,575 ppm dw, which is three times as high as the bone fluoride concentration of normal adult cattle, but four times lower than the mean rib fluoride concentrations in the cases. This is the first report of osteofluorosis in free ranging marine mammals and documentation of baseline bone fluoride concentrations in this population. The exact source of the excessive fluoride levels is not known as male California sea lions have a large range and varied diet.
California sea lions (Zalophus californianus, CSL) are endemic to the west coast of North America with five genetically distinct populations in California and Mexico. For the United States population (US or Pacific Temperate) breeding occurs mainly on the Channel Islands in the Southern California Bight (Laake et al., 2018). Reproductive females, from four years of age, remain close to the rookeries to give birth from June through July, and forage locally while nursing their pup, a process that can take almost a year. Breeding age males also spend the breeding season at the rookery sites but thereafter, in normal years, they disperse widely to forage off the coasts of northern California, Oregon, Washington and British Columbia and travel as far as southern Alaska (Laake et al., 2018). In warm water years (El Niño events), adult females may also disperse northwards in search of prey if not lactating (Hernández-Camacho et al., 2021). Sea lions are generalist foragers preying on a wide variety of pelagic fish such as sardines (Sardinops sagax), anchovies (Engraulis mordax), North pacific hake (Merluccinus productus), rockfish (Sebastes spp.), mackerel (Scomber japonicus), and to a lesser extent, invertebrates such as octopus and squid (Loligo opalescens) (Orr et al., 2011; Adame et al., 2020). They have also been described as opportunistic feeders or plastic specialists “that switch between a limited number of schooling … species” whose diet is influenced by life history and prey movement as well as climate changes (Weise and Harvey, 2008; Lowry et al., 2022). However, most CSL diet studies have been conducted on female fecal samples due to availability of sampling at rookeries.
As a keystone species and top predator in the marine environment, CSLs are regarded as sentinel species for ocean health. Their health has been greatly influenced through the bioaccumulation of toxins through the food chain such as biotoxins from harmful algal blooms like domoic acid (Bernstein et al., 2021; Elorriaga-Verplancken et al., 2022; McClain et al., 2023; Sandoval-Belmar et al., 2023). Furthermore, the sediments, and consequently the food chain in the waters around the Channel Islands, are heavily contaminated by persistent organochlorines such as dichlorodiphenyltrichloroethanes (DDTs) and polychlorinated biphenyls (PCBs). High levels of these lipid soluble pollutants are found in the blubber of sea lions and are associated with an extremely high prevalence of urogenital carcinoma (Randhawa et al., 2015; Lahvis, 2018; Gulland et al., 2020). While osteopathy has not been reported in organochlorine contaminated marine mammals from Californian waters, high levels of similar chemicals have been proposed as the cause of symmetric craniomandibular lesions in grey seals (Halichoerus grypus) from Sweden and the UK (Bergman et al., 1992). It is likely several other undocumented environmental toxins also greatly influence the health of CSLs, and one such toxin may be fluoride.
Fluoride toxicosis has been documented in terrestrial wildlife and domestic ungulates through ingestion of plants growing in soils or waters with naturally high concentrations of fluoride or contaminated by industry (Boulton et al., 1994; Death et al., 2015; Hufschmid et al., 2015; Maxie, 2016; Kelly et al., 2020; Ciosek et al., 2021). Fluoride accumulates in bones altering mineralization and mechanical properties of pre-existing bone causing disruption in bone formation and resorption (Maxie, 2016; Ciosek et al., 2021). Fluoride toxicosis has also been documented to impact joint cartilage leading to degenerative joint disease and in growing animals it impacts growth plate and tooth development leading to physeal and dental lesions (Boulton et al., 1994; Death et al., 2015; Hufschmid et al., 2015; Maxie, 2016; Zhang et al., 2016; Death et al., 2018).
Most studies on water fluoride concentrations and the effects on aquatic organisms have been conducted in freshwaters such as those in Yellowstone National Park or experimentally (Warrington, 1990; Camargo, 2003). However, there have been few studies indicating marine aquatic animals can be affected by excess fluoride and bioaccumulate in polluted waters (Pankhurst et al., 1980; Warrington, 1990). Fluoride toxicity has not been definitively demonstrated in marine mammals but has been hypothesized as the etiology of dental and bone lesions in a population of beluga whales (Delphinapterus leucas) in the St. Lawrence River, Canada (Mikaelian et al., 1999). Therefore, it is the aim of this case series to describe previously undocumented skeletal and dental lesions associated with high concentrations of fluoride from free ranging CSLs.
Case 1 and Controls 1-4 were obtained through The Marine Mammal Center’s marine mammal response and Cases 2 and 3 were carcasses examined as part of California Academy of Science’s dead marine mammal field response in collaboration with the Noyo Center. The estimated age class was assigned for all cases and control animals based on skull crest formation and standard length. When external genitalia and gonads were available for examination, appropriate sex was assigned. Sex and age class are summarized for the cases and controls in Table 1. Stranding or carcass locations are indicated on the map in Figure 1.
Table 1 Fluoride concentrations in parts per million dry weight within various bones and teeth from cases and controls.
Figure 1 Map of Central California with stranding locations indicated for Case 1 and Controls 1-4, and the carcass locations for Cases 2 and 3.
Case 1 was a subadult male that stranded at Noyo Beach, Mendocino County in Northern California (39.4251°N, 123.7994°W), in December 2020. Case 2 was a subadult male sea lion carcass with moderate decomposition found on a beach in February 2022, at Fort Bragg, Mendocino County (39.3900°N, 123.8183°W). Case 3 was a partial carcass/skeleton from a presumably adult male sea lion (based on size of bones, especially the scapula) with marked decomposition found in May 2022 at Bowling Ball Beach, Mendocino County (38.8694°N, 123.6564°W). Control cases, or unaffected animals lacking fluoride associated bone lesions, were selected based on time of stranding related to Case 1, sex and age class representation, as well as those with a complete necropsy and similar sampled tissues for total fluoride concentration measurements. Control 1 was a subadult male CSL, which stranded (36.5833°N, 121.9723°W) in February 2021, due to blunt force trauma. Control 2 was a yearling male CSL that stranded (36.9708°N, 121.9132°W) in May 2021, due to leptospirosis. Control 3 was a juvenile male CSL that stranded in March 2021 due to pleuropneumonia (36.6146°N, 121.9451°W) and Control 4 was a subadult female CSL that stranded in April 2021 for suspected domoic acid toxicosis. Anesthesia, when performed, included 0.015mg/kg Medetomidine IM (ZooPharm), 0.2mg/kg Butorphanol IM (ZooPharm), 0.2mg/kg Midazolam IM (ZooPharm), and 0.15mg/kg Atipamezole IM (ZooPharm), followed by maintenance of 1-3% isoflurane. A dosage of 0.12mg/kg Buprenorphine SQ (ZooPharm) was administered for analgesia. Case 1 died after administration of Telazol IM (Zoetis), while all controls were euthanized with 5mg/kg Telazol IM followed by 0.1mL/lb sodium pentobarbital IV (VetOne).
Case 1 and all control animals received a full physical examination including radiography (MinXray, HF100+), ultrasonography (Sonosite) and routine hematology and serum chemistry (SCIL Vet America) at The Marine Mammal Center (Sausalito CA). Serum from Case 1 was analyzed for selected vitamins, minerals, and hormones (Michigan State University Veterinary Diagnostic Laboratory) and serology was carried out for protozoa (Sarcocystis neurona and Toxoplasma gondii) and Leptospira interrogans serovar Pomona (University of California, Davis and California Animal Health and Food Safety Laboratory respectively).
Case 1 and the control animals had a complete necropsy performed by pathologists at the Center. As cases 2-3 were carcasses found in various states of decomposition, only the bones were examined. Select bones (skull, mandible, humerus, femur, tibia) from Control 1 and Case 1, and all bones collected from Cases 2 and 3 had the soft tissue macerated by warm water technique for additional examination by pathologists at the Center.
Based on the presence of lesions and little decomposition, only Case 1 had histopathology evaluated on the affected bones. A range of tissues were fixed with 10% neutral buffered formalin, trimmed, and routinely processed for paraffin embedding. Formalin fixed bones were rinsed with saline and decalcified using Decal stat (StatLab Medical Products) for ~48 hours. Histologic sections were prepared at 5 µm and stained with hematoxylin and eosin at the University of California, Davis, College of Veterinary Medicine. Immunohistochemistry for pan muscle actin (Cat #:M0635, Clone : HHF35, Dilution: 1:200, monoclonal mouse anti-human, Agilent Dako, Santa Clara, CA, USA) using a secondary mouse-on-canine HRP Polymer antibody was performed at University of California, Davis, College of Veterinary Medicine using negative, secondary only and isotype antibody controls with interpretation of canine positive control slides as well as internal controls on the submitted slide.
Various bones from cases and controls were measured for fluoride concentrations (Table 1). As reference values for fluoride in CSL bones are unknown, bones from stranded CSLs (controls) were grossly examined and processed for fluoride concentration measurement. At necropsy or after collection of bones from the field, 50g of various bones and teeth (premolars) were collected, stored at -80°C, and shipped to California Animal Health and Food Safety Laboratory (Davis, CA). The analysis of fluoride was performed by ion-selective electrode with internal standard operating protocols using bone or tooth that was cleaned, dried and ashed at 600°C for 12 hr (Fluoride Quantitation by Ion-Selective Electrode, ID 1952, CAHFS). Then 0.01g to 0.02g of ash was weighed and dissolved with 1ml 1M HCl. This solution was then neutralized with NaOH solution and brought to 5ml with 18-mega-ohm water and mixed with an equal volume of TISAB III (a buffer) and analyzed with an Orion combination fluoride electrode. Fluoride was quantitated by comparison with external calibration solutions. Quality control materials analyzed with the samples included a method blank, a laboratory control spike and if the sample amount permitted, a matrix over spike.
The index case (Case 1) was a subadult male sea lion, between four and seven years that stranded in Mendocino County in Northern California. He was observed in the region for over a month prior to rescue, including observations of him drinking water from drainage pipes. On admission to The Marine Mammal Center hospital, he was lethargic with a fractured mandibular canine tooth. Hematology revealed mild leukopenia, mild azotemia, mild hypernatremia, hyperphosphatemia and hypokalemia (Supplemental Table 1). Serum calcium and albumin were within reference range for the species (Gulland et al., 2018). Serum concentrations of several vitamins, minerals, and hormones were within normal limits or mildly to moderately different to the ranges for other adult mammalian species. The fractured canine tooth was extracted, and subsequent radiographs taken to monitor the surgery site revealed periosteal new bone proliferation on the mandible and both humeri in comparison to age-matched controls (Supplemental Figures 1A, B). Despite care, the animal became anorexic, lethargic and non-ambulatory on the forelimbs necessitating euthanasia.
At necropsy the femurs, humeri, mandibular rami, cranial sagittal crest, and tympanic bullae had a symmetric distribution of abundant white, hard, irregular to multinodular tissue on the surface of the cortical bone (periosteal new bone (PNB) proliferation). The proliferative PNB on the long bones was segmentally circumferential and focally extensive replacing most of the periosteal surface (Figure 2A; Supplemental Figure 1C). On cross-section at mid diaphysis of the long bones, the new bone was three to four times the depth of the original cortical bone and had a radiating pattern (Figure 2A inset). There was a large cavity at the location of the extracted right mandibular canine with PNB proliferation on the lateral alveolar surface, as well as at the base of the rami. All teeth were variably worn with significant adherent calculi. Most teeth had mildly dull enamel and were stained brown with variable wear and mild pitting of the enamel on the canines (Figure 2B). Total fluoride concentrations from multiple bones (tibia, fermur, humerus, rib, calvarium) were markedly elevated ranging from 4,000 to 9,700 ppm dw with a mean of 7,200 ppm dw (Table 1).
Figure 2 Select gross and histologic lesions from osteofluorosis cases. (A) Case 1 femur (cleaned) with segmental and circumferential hyperostosis. Inset- cross section of mid-diaphyseal femur with radiating trabeculae of periosteal new bone formation (bracket) that is 3-4x thicker than the normal cortical bone (asterisk). (B) Case 1 mandible (uncleaned) with dull brown enamel (arrow) and pitting on canine. Inset- Case 1 maxillary incisors and canines with variable and increased wear. (C) Case 2 ribs with diaphyseal hyperostosis. (D) Histology (H&E) of case 1 humerus with thick radiating and proliferating periosteal new bone formation (bracket) with thinner similar inner trabeculae with intertrabecular fibrous tissue. Inset- Higher magnification of bracket area comprised of osseous trabeculae formed by endochondral ossification comprised of chondrocytes and cartilaginous matrix transitioning into poorly mineralized osteoid matrix.
On histopathology, there were numerous anastomosing trabeculae of woven bone radiating perpendicular to the cortex (Figure 2D). Adjacent to the PNB were multiple atrophied and regenerating skeletal myofibers (polyphasic myopathy). Externally, there was a thick outer layer of periosteum (both cambium and fibrous layers) that transitioned into poorly mineralized osteoid of the thicker outer trabeculae (intramembranous ossification). The outer thicker trabeculae contained central chondrocytes and cartilage that transitioned to poorly mineralized osteoid and osteocytes (endochondral ossification, Figure 2D inset). Occasionally the outer trabeculae formed nodules that encircled or encompassed foci of collapsed connective tissue, vessels, and entrapped variably sized multinucleated myofibers (atrophied and regenerating myocytes) that were pan muscle actin positive. The inner two-thirds of woven bone trabeculae were separated by abundant spindle cells and large vessels. The trabeculae were characterized by haphazard poorly mineralized osteoid with numerous reversal lines and lined by plump osteoblasts with occasional osteoclasts within Howship’s lacunae (bone resorption, Supplemental Figure 2D). There were multiple infractions under various stages of repair. The cancellous bone within the bone marrow and the laminar bone of the cortex had regions of poorly mineralized osteoid. Histologically, the teeth had variable ameloblast clusters but unremarkable dentin, odontoblasts, and cementum. Therefore, bilateral hyperostosis of multiple bones was diagnosed.
In support of the initial clinical diagnosis, there was acute monophasic polyrhabdomyositis likely due to protozoal associated immune mediated rhadbomyositis. (Seguel et al., 2019) The Sarcocystis neurona antibody titer was high (1:20,480). In addition, there was lymphoplasmacytic tubulointerstitial nephritis consistent with a resolving Leptospira spp. infection supported by a Leptospira interrogans serovar Pomona antibody titer of 1:800. (Colagross-Schouten et al., 2002)
Case 2 was a subadult male sea lion, based on morphology as described, carcass with moderate decomposition found on a beach in Mendocino County. The skull, baculum, ribs and sacrum were collected and after soft tissue maceration were examined. Focally extensive nodules of PNB proliferation were markedly present on the medial, and lesser degree on the lateral, surface of the left rostral mandible (Supplemental Figure 2B), as well as mid-diaphysis of 8 ribs (Figure 2C). The tympanic bullae and proximal (moderate) and distal (mild) os penis (Supplemental Figure 2C) were less affected by coalescing dozens to hundreds of ~0.5cm in diameter nodules of proliferative PNB. The mandibular, and especially the maxillary, teeth were variably and markedly worn with dull enamel that were stained brown (Supplemental Figure 2B). Total fluoride concentrations from multiple bones were moderately elevated ranging from 3,700 to 5,000 ppm dw with a mean of 4,180 ppm dw (Table 1). The cause of death for Case 2 could not be determined due to the state of decomposition.
Case 3 was a partial carcass/skeleton from a presumably adult male sea lion from Mendocino County. The scapula, humerus, ribs, pelvis, and vertebrae were collected for examination. The scapula had the most PNB formation, though a mild lesion, with proliferative nodules on the dorsal border and the spinous process. There were dozens of <0.5cm in diameter periosteal osseous (bony) nodules on the deltoid tuberosity of the humerus and the pelvis. The vertebrae had multiple large regions of osteolysis. Total fluoride concentrations from multiple bones were moderately elevated ranging from 4,300 to 4,600 ppm dw with a mean of 4,475 ppm dw (Table 1). The cause of death for Case 3 could not be determined due to the state of decomposition.
As reference values for fluoride in CSL bones are unknown, bones from four stranded CSLs with unaffected bones were grossly examined and processed for fluoride concentration measurement. All four control cases were found stranded along the north-central California coast. Control 1 was a subadult male CSL, sex- and age-matched control for Cases 1 and 2. Bones and teeth from all control CSLs were unremarkable (Supplemental Figures 1D, 2A). The age- and sex-matched control (Control 1) bone total fluoride concentrations ranged from 1,500 to 3,800 ppm dw (mean 2,425 ppm dw, Table 1), and the mean fluoride concentration for all control ribs was 1,575 ppm dw (1,400 to 2,000 ppm dw).
Fluorosis has been documented most often in herbivores, specifically cattle, where chronic fluoride toxicosis may have a variable presentation including osteosclerosis, osteoporosis, hyperostosis, osteophytosis and/or osteomalacia (Maxie, 2016). There have been cases of fluorosis in wild species including eastern grey kangaroos (Macropus giganteus) and other marsupials in Australia foraging adjacent to an aluminum smelter (Death et al., 2015; Hufschmid et al., 2015). In kangaroos, fluoride toxicosis has been associated with cartilage ulceration and periarticular osteophytosis (Hufschmid et al., 2015), though no such changes were present in the current cases. Fluoride has also been documented to alter immune function (Singh et al., 2017; Wei et al., 2019), which may have been a factor in the clinical decline of Case 1 with concurrent polyphasic rhabdomyositis and leptospirosis. However, as fluoride is excreted in the urine, the renal disease due to leptospirosis may have contributed to the accumulation of fluoride within the musculoskeletal system, exacerbated fluorosis, and the associated lesions.
In growing animals, excess fluoride can prevent mineralization of physeal cartilage and damage ameloblasts and odontoblasts resulting in rickets-like chondrodystrophy and poorly mineralized and hypoplastic teeth (Boulton et al., 1994; Death et al., 2015; Maxie, 2016; Zhang et al., 2016). The current cases did not have physeal lesions and the two cases with teeth available for examination had mild (Case 1) to marked (Case 2) dental lesions with tooth fluoride concentrations approximately 1.5x higher than the age- and sex-matched control. Therefore, it appears likely that these CSLs ingested small amounts of fluoride compounds over a long period time mostly later in life, but also during growth. Although osteopathy was a striking lesion in Case 1, the animal ultimately declined due to nephropathy and myositis rather than osteofluorosis.
Total fluoride concentrations in various bones of the cases ranged from 3,700 to 9,700 ppm dw while the mean fluoride concentrations in the three cases of all bones (5,431 ppm dw) were markedly higher (2.5x) than for the control animals (2,060 ppm dw) or normal domestic cattle and even approximately twice the concentrations in cattle with clinical osteofluorosis (2,500 to 3,000 ppm) (Maxie, 2016). When comparing consistently sampled bones, such as ribs, fluorosis cases had four times higher mean fluoride concentrations (6,400 ppm dw, 4,500-9,700 ppm dw) compared to control CSLs (1,575 ppm dw, 1,400-2,000 ppm dw). However, more surprising was that the control CSL fluoride concentrations were nearly three times that reported from normal adult cattle (600 to 900 ppm) (Maxie, 2016). The higher baseline fluoride concentrations in the control CSLs of our study is similar to the findings for normal beluga whales (mean 10,365 ppm dw) harvested in Hudson’s Bay, Canada (Mikaelian et al., 1999), North Atlantic fin whales (mean 8,300 and 9,800 ppm) harvested in Iceland, and Antarctic seals (range 878-4442 ppm dw) (Adelung et al., 1985). Further studies are needed to fully characterize baseline fluoride levels in various marine mammals.
Marine animals appear to be more tolerant of higher fluoride concentrations, including possible prey items of sea lions, which could lead to further fluoride bioaccumulation in marine mammals. Studies on beluga whales and fin whales (Balaenoptera physalus), reported that fluoride concentrations are dependent on age, sex, location, and correlated fluoride concentrations in prey (e.g. krill for fin whales) (Landy et al., 1991; Mikaelian et al., 1999; Vighi et al., 2015). There was evidence of bioaccumulation of fluoride based on a positive correlation between bone fluoride concentrations and age as well as fluoride concentrations measured in the krill sampled from stomach contents in fin whales (Landy et al., 1991). Previous studies have also documented natural fluoride concentrations in seawater as 1.2-1.4 mg/L, and in marine fish like mullet (Mugil cephalus) at around 1.8 mg/kg (Warner, 1971; Rose and J. R, 1977; Warrington, 1990). However, in polluted waters from agriculture runoff (fertilizer, herbicides, pesticides) and emissions from smelters and fertilizer plants, fluoride concentrations have been reported to reach up to a mean of 25.4 mg/L, and mullet in polluted waters were reported to have four to five times greater fluoride concentrations than those in unpolluted water (Rose and J. R, 1977; Warrington, 1990). Biomagnification occurring up the food chain with fluoride, and that fish often bioaccumulate high concentrations of fluoride from the environment, are both reasons why government institutions recommend water fluoride concentrations do not exceed 1.5 mg/L (Stewart et al., 1974; Warrington, 1990). In general, marine fish appear to be more resilient to fluoride than freshwater fish, and it was estimated that a 100kg seal or sea lion likely ingests 2.5mg/kg fluoride through fish a day, whereas the recommended daily fluoride intake for an adult human is 3-4mg a day (Warrington, 1990; Camargo, 2003). If a man ingested that level of fluoride in the diet, it would cause acute toxicosis (Adelung et al., 1985; Warrington, 1990).
Therefore, it is exceptional that these three cases of CSLs developed fluorosis, though the source of the exposure is still unknown (Laake et al., 2018). Historically in domestic ungulates, fluorosis has been attributed to rock phosphates, contamination from mineral ore refineries, or less commonly volcanic ash (Maxie, 2016). In wildlife, studies of fluorosis in small rodents and marsupials have been attributed to smelters and chemical works (Boulton et al., 1994; Death et al., 2015; Hufschmid et al., 2015; Death et al., 2018), though there have also been studies in marine animals where industrial fluoride did not impact environmental or animal fluoride concentrations (Wright and Davison, 1974; Ares et al., 1983). Therefore, it is possible that the source of fluoride is industrial, though municipal sewage and agricultural runoff have also been found to contribute to higher fluoride water concentrations too (Warrington, 1990). However, California was also a site of historical mining, and a significant natural source of fluoride is the earth’s crust. Mining practices have also been suggested to contribute to heavy metal concentrations in various marine animals near Baja California Sur, Mexico (Frías-Espericueta et al., 2014; Jonathan et al., 2015; Martínez-Ayala et al., 2022). California also has volcanoes and thermal activity which also could contribute to adjacent elevated water fluoride levels. The fact that all three cases were found along the same stretch of Californian coast, Mendocino County, may indicate a source of fluoride in this region that warrants additional investigation. However, due to the large geographic range and generalist diet of this sex and age class (Weise and Harvey, 2008; Orr et al., 2011; Laake et al., 2018; Lowry et al., 2022), exposure could have occurred anywhere along the North American Pacific coast. The mechanisms and pathophysiology of fluoride exposure and accumulation in marine mammals is not well understood. Therefore, more directed studies are needed to understand baseline physiologic fluoride metabolism in marine mammals and determine pathways for exposure in the marine food chain.
These unusual and striking cases document hyperostosis induced by fluoride toxicosis in free ranging pinnipeds and provides preliminary evidence that baseline exposure to fluoride in free ranging marine mammals may be significantly greater than for terrestrial mammals.
The original contributions presented in the study are included in the article/Supplementary Material. Further inquiries can be directed to the corresponding author.
The animal study was approved by The Marine Mammal Center IACUC. The study was conducted in accordance with the local legislation and institutional requirements.
MM: study conception and design, data collection, analysis and interpretation of results, and manuscript preparation. MR: data collection, and manuscript preparation. CS: data collection, analysis and interpretation of results, and manuscript preparation. JI: data collection. SG: data collection. RP: study conception and design, analysis and interpretation of results, and manuscript preparation. PD: study conception and design, data collection, analysis and interpretation of results, and manuscript preparation. All authors contributed to the article and approved the submitted version.
The authors would like to thank the staff and volunteers of The Marine Mammal Center who contributed to the care and diagnostic work up of this case, in particular B. Halaska, C. Rios, L. Hortensius, and C. Field. We thank the donors that support the medical and pathology research at the Center. We would also like to thank the staff of California Academy of Sciences for their collaboration on the case series. NOAA permits # 24359 and 18786.
The authors declared no potential conflicts of interest with respect to the research, authorship, and/or publication of this article.
All claims expressed in this article are solely those of the authors and do not necessarily represent those of their affiliated organizations, or those of the publisher, the editors and the reviewers. Any product that may be evaluated in this article, or claim that may be made by its manufacturer, is not guaranteed or endorsed by the publisher.
The Supplementary Material for this article can be found online at: https://www.frontiersin.org/articles/10.3389/fmars.2023.1223298/full#supplementary-material
Adame K., Elorriaga-Verplancken F. R., Beier E., Acevedo-Whitehouse K., Pardo M. A. (2020). The demographic decline of a sea lion population followed multi-decadal sea surface warming. Sci. Rep. 10, 10499. doi: 10.1038/s41598-020-67534-0
Adelung D., Bößmann K., Rößler D. (1985). The distribution of fluoride in some antarctic seals. Polar Biol. 5, 31–34. doi: 10.1007/BF00446042
Ares J. O., Villa A., Gayosot A. M. (1983). Chemical and biological indicators of fluoride input in the marine environment near an industrial source (Argentina). Arch. Environ. Contam. Toxicol. 12, 589–602. doi: 10.1007/BF01056556
Bergman A., Olsson M., Reiland S. (1992). Skull-bone lesions in the Baltic grey seal (Halichoerus grypus). Ambio. 21, 517–519.
Bernstein S., Ruiz-Cooley R. I., Kudela R., Anderson C. R., Dunkin R., Field J. C. (2021). Stable isotope analysis reveals differences in domoic acid accumulation and feeding strategies of key vectors in a California hotspot for outbreaks. Harmful Algae 110, 102117. doi: 10.1016/j.hal.2021.102117
Boulton I. C., Cooke J. A., Johnson M. S. (1994). Fluoride accumulation and toxicity in wild small mammals. Environ. pollut. 85, 161–167. doi: 10.1016/0269-7491(94)90082-5
Camargo J. A. (2003). Fluoride toxicity to aquatic organisms: a review. Chemosphere 50, 251–264. doi: 10.1016/s0045-6535(02)00498-8
Ciosek Ż., Kot K., Kosik-Bogacka D., Łanocha-Arendarczyk N., Rotter I. (2021). The effects of calcium, magnesium, phosphorus, fluoride, and lead on bone tissue. Biomolecules 11, 506. doi: 10.3390/biom11040506
Colagross-Schouten A. M., Mazet J. A. K., Gulland F. M. D., Miller M. A., Hietala S. (2002). Diagnosis and seroprevalence of leptospirosis in California sea lions from coastal California. J. Wildl. Dis. 38, 7–17. doi: 10.7589/0090-3558-38.1.7
Death C., Coulson G., Kierdorf U., Kierdorf H., Morris W. K., Hufschmid J. (2015). Dental fluorosis and skeletal fluoride content as biomarkers of excess fluoride exposure in marsupials. Sci. Total Environ. 533, 528–541. doi: 10.1016/j.scitotenv.2015.06.054
Death C., Coulson G., Kierdorf U., Kierdorf H., Ploeg R., Firestone S., et al. (2018). Chronic excess fluoride uptake contributes to degenerative joint disease (DJD): Evidence from six marsupial species. Ecotoxicol. Environ. Saf. 162, 383–390. doi: 10.1016/j.ecoenv.2018.06.062
Elorriaga-Verplancken F. R., Hernandez-Camacho C. J., Alvarez-Santamaria L., Paniagua-Mendoza A., Robles-Hernandez R., Rebolledo-Villa F., et al. (2022). Largest mortality event to date of California Sea Lions in Mexico might be linked to a harmful algal bloom. Aquat. Mamm 48, 59–67. doi: 10.1578/AM.48.1.2022.59
Frías-Espericueta M. G., Cardenas-Nava N. G., Márquez-Farías J. F., Osuna-López J. I., Muy-Rangel M. D., Rubio-Carrasco W., et al. (2014). Cadmium, copper, lead and zinc concentrations in female and embryonic Pacific sharpnose shark (Rhizoprionodon longurio) tissues. Bull. Environ. Contam. Toxicol. 93, 532–535. doi: 10.1007/s00128-014-1360-0
Gulland F. M. D., Dierauf L. A., Whitman K. L. (2018). CRC Handbook of Marine Mammal Medicine. 3rd ed. (Boca Raton, FL: Taylor & Francis Group).
Gulland F. M. D., Hall AJ Y. G., Colegrove K. M., Norris T. ,. D. P., Halaska B., A. W. K., et al. (2020). Persistent contaminants and herpesvirus otHV1 are positively associated with cancer in wild California sea lions (Zalophus californianus). Front. Mar. Sci. 7. doi: 10.3389/fmars.2020.602565
Hernández-Camacho C. J., Pelayo-González L., Rosas-Hernández M. P. (2021). California sea lion (Zalophus californianus, Lesson 1828). Ecol. Conserv. pinnipeds Latin America, 119–143. Springer International Publishing. doi: 10.1007/978-3-030-63177-2_7
Hufschmid J., Beveridge I., Coulson G., Walker G., Shen P., Reynolds E., et al. (2015). Skeletal pathology of eastern grey kangaroos (Macropus giganteus) exposed to high environmental fluoride levels in South-Eastern Australia. J. Comp. Pathol. 153, 167–184. doi: 10.1016/j.jcpa.2015.06.002
Jonathan M. P., Aurioles-Gamboa D., Villegas L. E. C., Bohórquez-Herrera J., Hernández-Camacho C. J., Sujitha S. B. (2015). Metal concentrations in demersal fish species from Santa Maria Bay, Baja California Sur, Mexico (Pacific coast). Mar. pollut. Bull. 99, 356–361. doi: 10.1016/j.marpolbul.2015.07.032
Kelly L. H., Uzal F. A., Poppenga R. H., Kinde H., Hill A. E., Wilson W. D., et al. (2020). Equine dental and skeletal fluorosis induced by well water consumption. J. Vet. Diagn. Invest. 32, 942–947. doi: 10.1177/1040638720962746
Laake J. L., Lowry M. S., DeLong R. L., Melin S. R., Carretta J. V. (2018). Population growth and status of california sea lions. J. Wildl. Manage. 82, 583–595. doi: 10.1002/jwmg.21405
Lahvis M. A. (2018). Vertical screening distances for total petroleum hydrocarbon for vapour intrusion risk assessment at petroleum underground storage tank sites. Q. J. Eng. Geol. Hydrogeol. 51, 3–12. doi: 10.1144/qjegh2017-009
Landy R. B., Lambertsen R. H., Palsson P. A., Krook L., Nevius A., Eckerlin R. (1991). Fluoride in the bone and diet of fin whales, Balaenoptera physalus. Mar. Environ. Res. 31, 241–247. doi: 10.1016/0141-1136(91)90014-Y
Lowry M. S., Nehasil S. E., Moore J. E. (2022). Spatio-temporal diet variability of the California sea lion Zalophus californianus in the southern California current ecosystem. Mar. Ecol. Prog. Ser. 692, 1–21. doi: 10.3354/meps14096
Martínez-Ayala J. C., Galván-Magaña F., Tripp-Valdez A., Marmolejo-Rodríguez A. J., Piñón-Gimate A., Huerta-Diaz M. A., et al. (2022). Heavy metal concentrations in the Pacific sharpnose shark Rhizoprionodon longurio from the Santa Rosalia mining zone, Baja California Sur, Mexico. Mar. pollut. Bull. 182, 114018. doi: 10.1016/j.marpolbul.2022.114018
McClain A. M., Field C. L., Norris T. A., Borremans B., Duignan P. J., Johnson S. P., et al. (2023). The symptomatology and diagnosis of domoic acid toxicosis in stranded California sea lions (Zalophus californianus): A review and evaluation of 20 years of cases to guide prognosis. bioRxiv 10, 2007–2023. doi: 10.3389/fvets.2023.1245864
Mikaelian I., Qualls C. W. J., de Guise S., Whaley M. W., Martineau D. (1999). Bone fluoride concentrations in beluga whales from Canada. J. Wildl. Dis. 35, 356–360. doi: 10.7589/0090-3558-35.2.356
Orr A. J., VanBlaricom G. R., DeLong R. L., Cruz-Escalona V. H., Newsome S. D. (2011). Intraspecific comparison of diet of California sea lions (Zalophus californianus) assessed using fecal and stable isotope analyses. Can. J. Zool 89, 109–122. doi: 10.1139/Z10-101
Pankhurst N. W., Boyden C. R., Wilson J. B. (1980). The effect of a fluoride effluent on marine organisms. Environ. pollut. Ser. A Ecol. Biol. 23, 299–312. doi: 10.1016/0143-1471(80)90072-0
Randhawa N., Gulland F., Ylitalo G. M., DeLong R., Mazet J. A. K. (2015). Sentinel California sea lions provide insight into legacy organochlorine exposure trends and their association with cancer and infectious disease. One Health 1, 37–43. doi: 10.1016/j.onehlt.2015.08.003
Sandoval-Belmar M., Smith J., Moreno A. R., Anderson C., Kudela R. M., Sutula M., et al. (2023). A cross-regional examination of patterns and environmental drivers of Pseudo-nitzschia harmful algal blooms along the California coast. Harmful Algae 126, 102435. doi: 10.1016/j.hal.2023.102435
Seguel M., Colegrove K. M., Field C., Whoriskey S., Norris T., Duignan P. (2019). Polyphasic rhabdomyositis in California sea lions (Zalophus californianus): pathology and potential causes. Vet. Pathol. 56, 619–629. doi: 10.1177/0300985819829526
Singh R., Khatri P., Srivastava N., Jain S., Brahmachari V., Mukhopadhyay A., et al. (2017). Fluoride exposure abates pro-inflammatory response and induces in vivo apoptosis rendering zebrafish (Danio rerio) susceptible to bacterial infections. Fish Shellfish Immunol. 63, 314–321. doi: 10.1016/j.fsi.2017.02.022
Stewart D. J., Manley T. R., White D. A., Harrison D. L., Stringer E. A. (1974). Natural fluorine levels in the Bluff area, New Zealand. I. Concentrations in wildlife and domestic animals. NZJ Sci.;(New Zealand) 17, 105–113.
Vighi M., García-Nisa I., Borrell A., Aguilar A. (2015). The fin whale, a marine top consumer, exposes strengths and weaknesses of the use of fluoride as ecological tracer. Chemosphere 127, 229–237. doi: 10.1016/j.chemosphere.2015.02.023
Warner T. B. (1971). Normal fluoride content of seawater. Deep Sea Res. Oceanogr. Abst. 18, 1255–1263. doi: 10.1016/0011-7471(71)90030-1
Warrington P. (1990). Ambient Water Quality Criteria for Fluoride (Victoria, BC). Available at: https://www2.gov.bc.ca/assets/gov/environment/air-land-water/water/waterquality/water-quality-guidelines/approved-wqgs/fluoride-tech.pdf.
Wei W., Pang S., Fu X., Tan S., Wang Q., Wang S., et al. (2019). The role of PERK and IRE1 signaling pathways in excessive fluoride mediated impairment of lymphocytes in rats’ spleen in vivo and in vitro. Chemosphere 223, 1–11. doi: 10.1016/j.chemosphere.2019.02.031
Weise M. J., Harvey J. T. (2008). Temporal variability in ocean climate and California sea lion diet and biomass consumption: implications for fisheries management. Mar. Ecol. Prog. Ser. 373, 157–172. doi: 10.3354/meps07737
Wright D. A., Davison A. W. (1974). Fluoride in marine animals. Mar. pollut. Bull. 5, 119–121. doi: 10.1016/0025-326X(74)90144-1
Keywords: California sea lion, Zalophus californianus, fluoride toxicosis, hyperostosis, osteofluorosis, dental fluorosis
Citation: Martinez ME, Rivard M, Sykes C, Isbell J, Grimes SW, Poppenga R and Duignan PJ (2023) Osteofluorosis in free ranging California sea lions (Zalophus californianus). Front. Mar. Sci. 10:1223298. doi: 10.3389/fmars.2023.1223298
Received: 15 May 2023; Accepted: 07 September 2023;
Published: 13 November 2023.
Edited by:
Todd Atwood, U.S. Geological Survey, Alaska, United StatesReviewed by:
Salvatore Siciliano, Fundação Oswaldo Cruz (Fiocruz), BrazilCopyright © 2023 Martinez, Rivard, Sykes, Isbell, Grimes, Poppenga and Duignan. This is an open-access article distributed under the terms of the Creative Commons Attribution License (CC BY). The use, distribution or reproduction in other forums is permitted, provided the original author(s) and the copyright owner(s) are credited and that the original publication in this journal is cited, in accordance with accepted academic practice. No use, distribution or reproduction is permitted which does not comply with these terms.
*Correspondence: Margaret E. Martinez, bWFydGluZXptQHRtbWMub3Jn
Disclaimer: All claims expressed in this article are solely those of the authors and do not necessarily represent those of their affiliated organizations, or those of the publisher, the editors and the reviewers. Any product that may be evaluated in this article or claim that may be made by its manufacturer is not guaranteed or endorsed by the publisher.
Research integrity at Frontiers
Learn more about the work of our research integrity team to safeguard the quality of each article we publish.