- 1Alaska Department of Fish and Game, Division of Wildlife Conservation, Juneau, AK, United States
- 2Marine Mammal Laboratory, US National Oceanic and Atmospheric Administration (NOAA), National Marine Fisheries Service, Alaska Fisheries Science Center, Seattle, WA, United States
- 3Department of Science, Alaska SeaLife Center, Seward, AK, United States
The North Pacific marine heatwave of 2014–2016 (PMH), one of the most geographically-extensive and severe marine heatwaves on record, resulted in widespread and persistent perturbation of the Gulf of Alaska and California Current ecosystems. Negative effects of the PMH on marine mammals have been observed, but are not yet well understood. The endangered Steller sea lion Eumetopias jubatus is an important top predator in the Gulf of Alaska that is also particularly vulnerable to sudden or severe ecosystem shifts. We examined survival of 4,178 known-aged Steller sea lions marked from 2000 to 2016 from Kodiak Island through Southeast Alaska, using mark-recapture models and 12,811 resightings collected from 2001 to 2021. Survival of adult females aged 3–15 was reduced -0.05 to -0.23 during the PMH in the areas east, but not west, of Cook Inlet. Survival of Kodiak females was unaffected by the PMH, but survival of Sugarloaf females aged 5–8 was reduced -0.13 from summer 2015 to summer 2016. Lowest survival in Southeast Alaska occurred from summer 2016 to summer 2017, but was also reduced from summer 2014 to summer 2016. Reduced survival continued post-PMH in Kenai Peninsula/Prince William Sound, but not in Southeast Alaska. Survival of adult males was insensitive to the PMH, except in Southeast Alaska where male survival was reduced -0.25 from summer 2016 to summer 2017. Prolonged or intermittent high adult female mortality may reduce population growth and initiate regional declines. Survival response of Steller sea lions to the PMH varied regionally despite similar patterns of ocean warming throughout our study area, suggesting areas east versus west of Cook Inlet were affected differently by the PMH, perhaps due to habitat and oceanographic differences.
1 Introduction
Ocean warming has significantly impacted marine ecosystems and marine mammal populations worldwide, and marine mammal populations in the North Pacific Ocean are expected to be particularly vulnerable to future impacts due to particularly high predicted ocean temperature change in the North Pacific and increased vulnerabilities of many species due to habitat and foraging specializations (Albouy et al., 2020). Significant changes in arctic and subarctic environments include loss of sea ice habitat (Laidre et al., 2015), poleward shifts in distribution of species (van Weelden et al., 2021), and increased vessel traffic and risk of exposure to human-caused pollution including noise (Moore et al., 2012; Hauser et al., 2018), and food web shifts and reduced energy transfer efficiencies of food webs (Kortsch et al., 2015; du Pontavice et al., 2020). Additional effects include novel and increased exposure to pathogens (Van Wormer et al., 2019; Sanderson and Alexander, 2020) and biotoxins associated with harmful algal blooms (Lefebvre et al., 2016). Abrupt and extreme temperature events, such as marine heatwaves, are also expected to increase in frequency and magnitude in conjunction with long-term warming trends (Joh and Di Lorenzo, 2017; Oliver et al., 2018). The global annual average of marine heatwave days increased 54% between 1925 and 2016, with an accelerated rate of increase over the past several decades (Oliver et al., 2018). Significant biological effects of heatwaves include sudden and persistent regime shifts in community and ecosystem structure (Wernberg et al., 2016). Understanding the effects of marine heatwaves on marine ecosystems is therefore critical to effective management and conservation of marine species in the coming years.
When the North Pacific marine heatwave of 2014–2016 (PMH) occurred, it was the most anomalous heatwave on record in terms of geographic extent, magnitude and persistence. The PMH developed during the 2013–2014 winter in surface waters along the axis of the North Pacific Current in the Gulf of Alaska, with peak temperatures in 2015–2016. High temperatures persisted in all seasons in surface waters until summer/fall 2016 (Bond et al., 2015; Di Lorenzo and Mantua, 2016; Jacox et al., 2018; Walsh et al., 2018). Warm surface waters reached the coast of Alaska by May 2014 and spread south along the entire US West Coast by September 2014 (Bond et al., 2015; Gentemann et al., 2017). Surface temperatures cooled in 2017, but increased again to extreme heatwave state in spring 2019 through at least fall of 2021 in some areas (Thompson et al., 2019; Amaya et al., 2020; Chen et al., 2021; Barkhordarian et al., 2022). Although nearshore surface waters warmed in 2014, deeper waters remained cool until warming quickly in January 2015 in the northern Gulf of Alaska (Danielson et al., 2022). High temperatures occurred throughout the entire water column over the continental shelf in 2015–2016, and deep water temperatures remained above normal from 2017 to 2019 after surface waters had cooled (Jackson et al., 2018; Phillips et al., 2022; Suryan et al., 2021; Danielson et al., 2022).
The PMH of 2014–2016 resulted in widespread and persistent perturbation of the Gulf of Alaska and California Current ecosystems from phytoplankton to top predators and in important commercial fisheries (Cavole et al., 2016; Peterson et al., 2017; Barbeaux et al., 2020; Suryan et al., 2021). Although distinct shifts to new ecosystem states have not yet been observed in the Gulf of Alaska (Litzow et al., 2020), of particular concern were the many negative responses across multiple levels of the food web perhaps eroding ecological resiliency, coupled with the lagged response in returning to pre-heatwave conditions by many species (Suryan et al., 2021). With respect to upper trophic levels, increased metabolic demands due to warm temperatures coupled with reduced energy content and abundance of some forage fish (particularly Pacific herring Clupea pallasi, Pacific capelin Mallotus catervarius and Pacific sand lance Ammodytes personatus) and adult groundfish populations (Arimitsu et al., 2021; Barbeaux et al., 2020; Piatt et al., 2020) are likely contributing factors to the apparent negative effects of the PMH on some populations of top predators (pinnipeds, whales and piscivorous seabirds) in the Gulf of Alaska (Suryan et al., 2021).
Effects of the PMH on marine mammals in Alaska are not yet well understood, but negative effects on humpback whales Megaptera novaeangliae, killer whales Orcinus orca and Steller sea lions Eumetopias jubatus have been observed east of Cook Inlet and in Southeast Alaska (SEAK, Moran and Straley, 2021; Suryan et al., 2021; Gabriele et al., 2022). Steller sea lions are important top predators in the Gulf of Alaska that are also particularly vulnerable to sudden or severe shifts in the marine environment. The climate shift to a warm regime in 1976/1977 in the North Pacific (Hare and Mantua, 2000; Litzow and Mueter, 2014) resulted in a restructuring of marine biological communities (Anderson and Piatt, 1999) and was implicated in pinniped population declines (Pitcher, 1990; Anderson and Piatt, 1999). Most affected were Steller sea lions that suffered severe population declines (>70%) between the late 1970s to 2000–2003 resulting in listing of the species range-wide under the U.S. Endangered Species Act in 1990 (Trites and Larkin, 1996; Fritz et al., 2016). Definitive links between altered prey fields and sea lion population dynamics remain uncertain with available data, but the severity of the decline suggested the vulnerability of this species to rapid shifts in conditions over relatively short time intervals (at its worst, population declines averaged -16% per year over ~5 years; Goodman, 2008).
Evidence from count data suggested that abundance of Steller sea lion pups and nonpups may have been reduced by the PMH, as well as a possible failure to recover post-heatwave (Suryan et al., 2021; Sweeney et al., 2022). We relied on vital rates data based on longitudinal observations of uniquely marked individuals to address demographic processes underlying population trend and to account for resighting probabilities (which encompass haul-out probability and other factors) for important subgroups (e.g., breeding adult females or juveniles). This provided greater power to detect change in population status than using count data alone. Over 9,000 Steller sea lion pups have been individually marked and systematically resighted by various agencies over the past two decades providing a large and geographically-broad sample to assess changes in vital rates associated with the PMH (Hastings et al., 2011; Fritz et al., 2014; Maniscalco, 2014; Altukhov et al., 2015; Wright et al., 2017). Here we assessed whether survival was reduced during and following the PMH using long-term data on individually marked animals, and how survival effects varied among demographic groups and regions within the Gulf of Alaska.
2 Materials and methods
2.1 Data collection and dataset preparation
From 2000 to 2016, Steller sea lions were hot-branded at ~3 weeks of age in late June-early July with unique 2–5 digit alpha-numeric combinations at 8 rookeries in the Gulf of Alaska by the Alaska SeaLife Center (ASLC), Alaska Department of Fish and Game (ADFG), and the Marine Mammal Laboratory (MML)/National Marine Fisheries Service (NMFS, Figure 1, see Supplemental Table 1 for samples sizes marked by year and rookery). Branded sea lions were resighted and photographed annually during summer from 2001 to 2021 via field camps and standardized regional boat trips ranging from SEAK to Kodiak Island, Alaska (Figure 1; Hastings et al., 2011; Fritz et al., 2014; Maniscalco, 2014). Standardized and geographically-broad annual surveys provided extensive spatial and temporal coverage of our study area (all major haulouts and rookeries in SEAK and Prince William Sound; and all major haulouts around Chiswell, Sugarloaf and Marmot rookeries). In addition, an extensive multi-agency effort provided observations of branded Steller sea lions from California through the Gulf of Alaska, Russia and the Bering Sea, covering the range of the species such that survival probabilities were very unlikely to be biased by emigration (Pendleton et al., 2006; Hastings et al., 2011; Fritz et al., 2014; Wright et al., 2017). Methods for marking and resighting sea lions were approved by Institutional Animal Care and Use Committees and through permits issued by the NMFS.
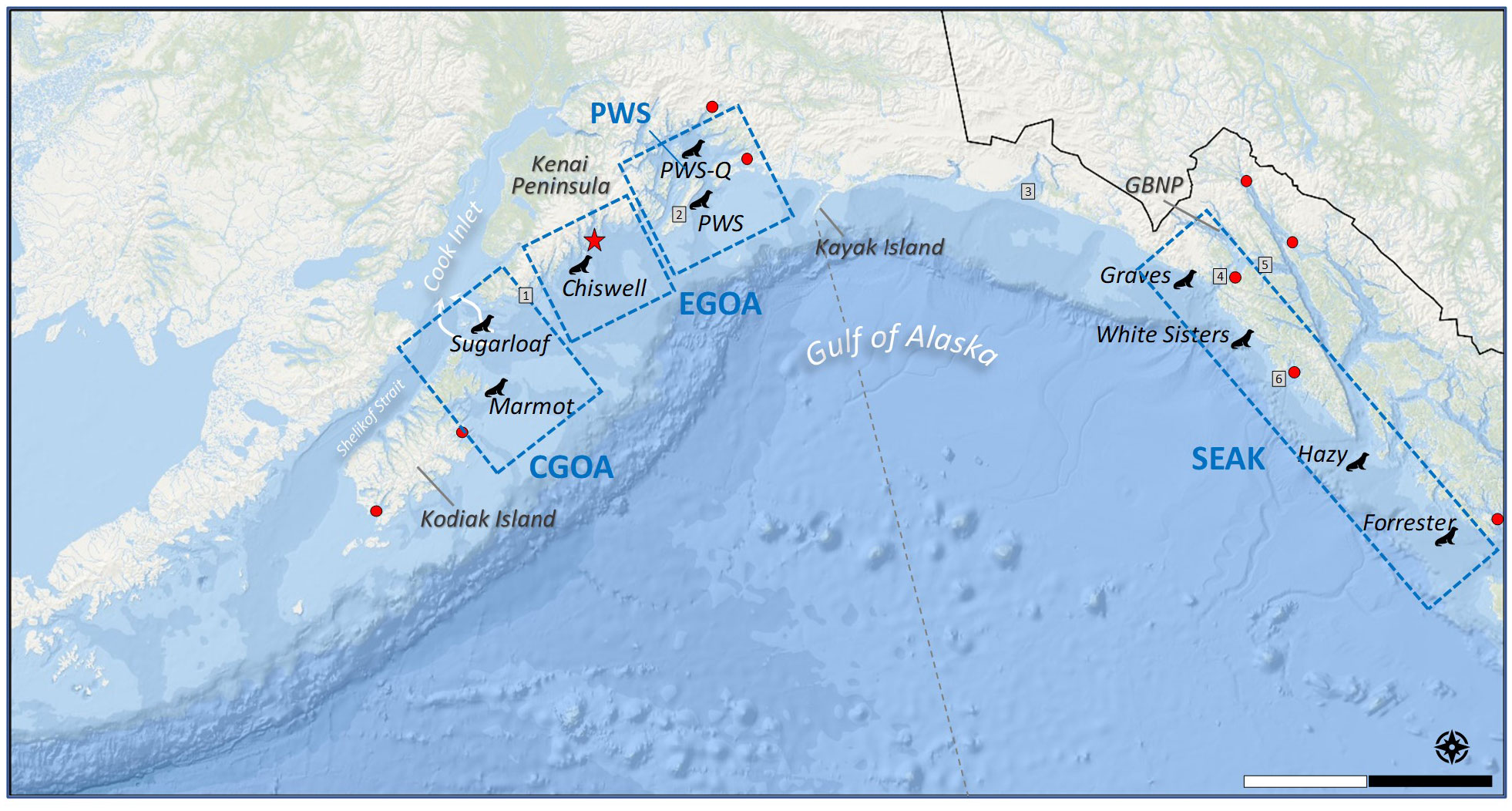
Figure 1 Map of Steller sea lion rookeries where pups were branded from 2000–2016. SEAK, Southeast Alaska; CGOA, Central Gulf of Alaska (Sugarloaf and Marmot); EGOA, Eastern Gulf of Alaska (Chiswell, Prince William Sound). PWS, Prince William Sound. PWS-Q: juveniles dive-captured in PWS (natal rookery unknown). Red dots are tide gauge stations where nearshore water temperatures were measured by the NOAA Center for Operational Oceanographic Products, https://tidesandcurrents.noaa.gov/. Stations from south to north in SEAK: Ketchikan, Sitka, Elfin Cove, Juneau, Skagway; and from east to west: Cordova, Valdez, Kodiak, Alitak. Red star is the nearshore permanent oceanographic mooring GAK1 operated by the University of Alaska Fairbanks and Gulf Watch Alaska (http://research.cfos.uaf.edu/gak1/). Boxed areas = regions used for satellite-based SST data. Scale = 150 km. GBNP, Glacier Bay National Park. Grey boxes = 1: Gore Point, 2: Montague Island, 3: Yakutat, 4: Cross Sound, 5: Icy Strait, 6: St. Lazaria Island. Grey dotted line is at 144°W, the division of eastern and western stocks of Steller sea lions.
We created annual capture histories for all marked individuals based on photograph-confirmed resightings collected during May–August (‘summer’) from 2000 to 2021. Two main datasets were created, which were analyzed separately but followed a similar protocol: one for sea lions marked at natal rookeries in SEAK (Forrester, Hazy, White Sisters, Graves) and one for those marked in the eastern and central Gulf of Alaska (E–CGOA: Prince William Sound [Seal and Fish], Sugarloaf, and Marmot Island; Figure 1). Since 2000, the numbers marked in SEAK and E–CGOA were 2,065 and 1,471, respectively (Supplemental Table 1). In the SEAK dataset, we also included data from 180 branded animals born at Forrester in 1994–1995, which entered the dataset the first time they were seen ≥2001 and we included their age at entry (all others entered at age 0). Maximum ages possible for the (1) old SEAK cohorts, (2) the new SEAK cohorts and Prince William Sound, and (3) Sugarloaf/Marmot were (1) 27, (2) 20, and (3) 21, respectively; and the oldest individuals observed were 25 (female) and 19 (male; Supplemental Table 1). Because no surveys were conducted in 2020, the last survival interval was a two-year interval (2019–2021) modeled as average annual survival over the two years.
Finally, we included separately two additional small datasets in the E–CGOA dataset. First, we added capture histories (annual resightings from May-August each year) of branded sea lions born at Chiswell off the Kenai Peninsula in the EGOA (Figure 1). From 2005 to 2016, 247 pups born at Chiswell were branded in 5 cohorts (2005 = 26, 2007 = 51, 2008 = 62, 2010 = 60, 2016 = 48; Maniscalco, 2014). During 2014-2016, ages of Chiswell animals ranged from 4 to 12 (Supplemental Table 1); resightings of Chiswell non-pups were >30 individuals/year starting in 2009 and sample sizes per age were small after age 10 and 8 for female and males, respectively (Supplemental Tables 1 and 2).
Second, we added capture histories of juveniles (0–2 yrs of age) that were captured in Kodiak (n = 13) and Prince William Sound (n = 202) using SCUBA and an underwater noosing technique to ensnare animals for marking and sampling (Raum-Suryan et al., 2004). These animals were captured outside the breeding season (fall–spring) from March 2001 to November 2007 (Supplemental Table 1), and their natal rookery was unknown (“Prince William Sound–Q” because these were given a brand beginning with “=“ + a unique number). Because releases occurred during non-summer months, the non-summer release occasions were added to the capture histories created for the E–CGOA analysis and resighting probability was fixed at 0 for these occasions (releases-only). Time intervals were adjusted to account for partial year intervals, and actual age was calculated based on age of first release (146, 56, 10, 2, and 1 were released at ages 0–4, respectively). Age of dive-captured juveniles was estimated based on coat type (pup vs nonpup), canine tooth length, tooth eruption patterns, and by stable isotope patterns in the whisker (King et al., 2007; Rea et al., 2015).
2.2 Mark-recapture modeling protocol
We used R package marked (v. 1.2.6) to fit and compare models and to estimate survival and resighting probabilities using the Cormack-Jolly-Seber model (Laake et al., 2013; R Core Team, 2022). We tailored our modeling protocol (detailed in Table 1) to best determine if survival of adult females, males and juveniles was reduced during and after the PMH, our primary research question. Previous analyses of these data determined demographic factors that shape survival and resighting probabilities particularly age, sex, and natal rookery that must be accounted for; also, year effects on survival had little support but resighting probabilities varied strongly with year in most datasets (Hastings et al., 2011; Fritz et al., 2014; Maniscalco, 2014; Hastings et al., 2018; Hastings et al., 2021). Our modeling protocol was (see Table 1): first, we determined preliminary base models of survival and resighting probability from previous analyses to reduce the number of models fit. Second, to form a final base survival model, we added a PMH effect on survival for prime-aged females (aged 3–15, Hastings et al., 2018), males and juveniles (3 additional dummy variables were added to the model) such that survival before and after summer 2014 would be estimated separately. For the final base survival and resighting models we also added any other potentially important interactions or variables that may have influenced demographic effects given the longer time series compared to previous analyses (detailed below for each dataset, Table 1).
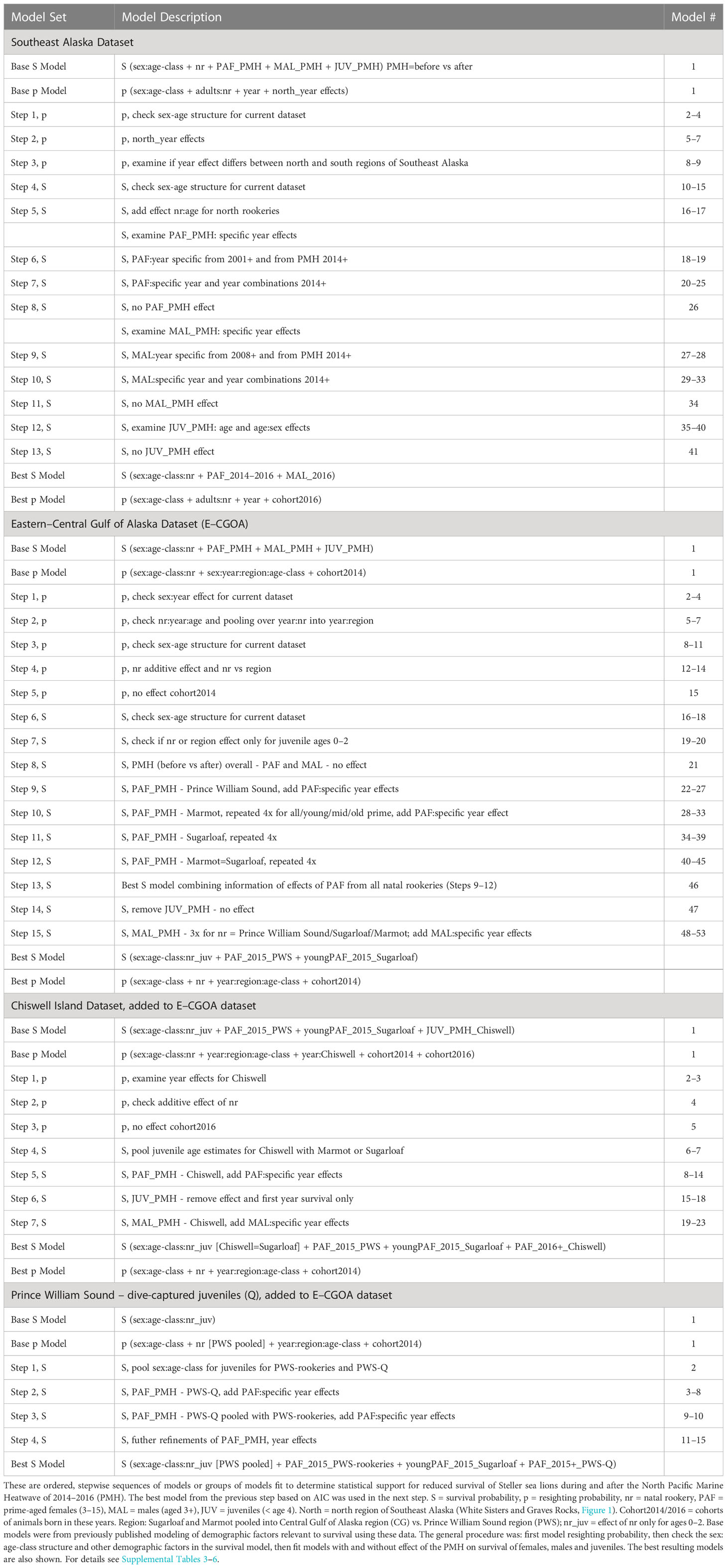
Table 1 Model selection procedure for four Steller sea lion mark-recapture datasets collected in the Gulf of Alaska.
Third, after establishing final base models, we used a stepwise procedure to simplify the base models (i.e., sequentially fit a series of a priori models with fewer parameters than the base model) to streamline the number of models fit. A more complex model (i.e., the model with greater number of parameters) was retained if the AIC of the model was > 2 AIC units lower than a simpler model, otherwise the simpler model was chosen (Akaike, 1973; Burnham and Anderson, 2002). AIC, rather than AICc or QAIC, was appropriate for our data because the ratio of observations N to parameters K in models was high for all datasets (N/K>>40, Burnham and Anderson, 2002) and our models were more complex than previously fit for these data where goodness-of-fit was judged adequate (Hastings et al., 2011; Fritz et al., 2014; Maniscalco, 2014).
Groups of simpler models were fit and the best models from the group were used in the next step of the procedure (Table 1). Stepwise fitting began by modeling resighting probability, first checking the sex-age-class structure determined in previous analyses was still appropriate (several alternative, plausible age-sex structures were fit in different models and the most parsimonious model was chosen, see Supplemental Table 3 for example). We then simplified newly-included demographic effects on resighting probability, and then used the resulting best resighting model, when simplifying the survival model. For survival, we first re-examined the age-sex-structure of the base model and then any other newly included demographic effects. Finally, our primary questions were addressed by fitting models with and without the effect of the PMH on survival for females, males and juveniles. At this stage, we fit models with survival different for single years or groups of years of interest, but we also considered the overall annual pattern for the full year series to determine if point estimates also appeared anomalous from the full year-specific model. We did this to guard against spurious results that could result from picking single years out of a series and comparing that value to the average of all other years.
Of particular concern was the ability to distinguish senescence from reduced survival during the PMH at the end of the time series. For animals marked in SEAK (new cohorts) and Prince William Sound, age and time were somewhat confounded because marking of most new cohorts was clumped in time (2001–2005 not including a small sample in 2016 in SEAK; animals were aged 9–18 ≥ 2014). In contrast, marking of other groups were intermittent over many years (e.g., Marmot/Sugarloaf: 2000–2014; Chiswell: 2005–2010, 2016). Past analyses determined senescence in females occurs at ~16–17 in Russia and SEAK (Altukhov et al., 2015; Hastings et al., 2018). To account for potential senescent effects, we fit full age-effects as in Hastings et al. (2018) including data of the 1994–1995 Forrester cohorts (who had reached old age before the PMH) and focused our models on effects on prime-aged females (3–15), after verifying survival was still constant for these ages for the newer cohorts (Supplemental Figure 1). This robust treatment was also assisted by mid- to young-aged females from the new cohorts that were alive during the PMH (females were 9–13 in 2014). All models included senescence in survival prior to comparing models with and without PMH effects.
We fit models in this manner separately to the four datasets mentioned previously: SEAK, E–CGOA, E–CGOA + Chiswell, and E–CGOA + Prince William Sound-Q. A few potentially unique demographic issues for the longer time series varied by dataset. For SEAK, we included resighting models with potential year:natal rookery interactions due to potentially greater effort in the north than south and especially reduced effort at Forrester/Hazy in latest years, and with an effect of the 2016 cohort at Graves Rocks because the use of time-lapse cameras in northern SEAK in later years may have increased resighting probabilities (Table 1; Supplemental Table 3). We reexamined natal rookery:age effects on survival for SEAK data because preliminary results suggested that a larger sample sizes for Graves (70 added in 2016 to the previous 93 individuals) may have improved power to detect this interaction compared to previous analyses (Hastings et al., 2011). Potential juveniles in SEAK during the PMH years were only from the 2016 cohort at Graves Rocks.
For the E–CGOA dataset, we included resighting models with an effect of the youngest cohort (2014) because we hypothesized reduced effort in the CGOA in later years may have lowered resighting probabilities particularly of juveniles (Table 1). We re-evaluated several demographic effects on resighting probability particularly interactions between sex, age, natal rookery and year (age = 2 age-classes, juvenile vs adult) for which best models varied in previous analyses (Fritz et al., 2014; Hastings et al., 2021). For this dataset, we also could examine PMH effect on survival by more specific age ranges within the prime-aged category for females (young-prime = 5–8, mid-prime = 8–12, old-prime = 11–15). We chose a start age of 5 for young-prime because few females produced pups < age 5 (Warlick et al., 2022) and therefore only potentially breeding females were included. For Marmot and Sugarloaf, only the 2014 cohort could have experienced the PMH as juveniles (the next youngest cohort was age 4 in 2014).
Additional dataset 1 (Chiswell): For these data, the initial model was the best model from the E–CGOA analysis and included effects of the 2016 cohort on resighting and survival (the next youngest cohort, 2010, was age 4 in 2014). For resighting probability, we included models with and without year effects and refit the sex-age class:natal rookery interaction (Table 1). For survival, we first determined if juvenile survival estimates for Chiswell could be pooled with those for Marmot/Sugarloaf; data of Chiswell and Prince William Sound animals were not pooled because high first-year survival of Chiswell animals compared to Prince William Sound animals is well documented (Fritz et al., 2014; Maniscalco, 2014). We then fit PMH effects in survival separately for Chiswell animals in the same manner as the E–CGOA analysis. Because the Chiswell dataset was small, the most complex year-specific model included years 2011+ to allow 3 cohorts to reach at least age 3 for all occasions.
Additional dataset 2 (Prince William Sound-Q): We fit a single resighting model (Table 1). Because animals from Prince William Sound (rookeries) and Prince William Sound-Q (dive-captured away from rookeries) likely frequented the same areas with similar survey effort, the year pattern in resighting probability was pooled for these groups. For survival, we first determined if age- and sex-specific survival varied between the two Prince William Sound groups, and then fit year specific patterns in survival as in the main E-CGOA analysis to Prince William Sound-Q separately and pooled with Prince William Sound (rookeries). We examined overall annual patterns in survival for years 2008+, after all cohorts released could contribute to the prime-aged female data. Very few Prince William Sound-Q animals were resighted after summer 2017 (Supplemental Table 2) and so year-specific estimates were not possible at the end of the time series. PMH effects were not fit for Prince William Sound-Q males due to very few observations after 2014 (n<13/year; Supplemental Table 2).
2.3 Annual water temperature anomalies in sea lion areas
In the Gulf of Alaska, Steller sea lions are much less commonly observed offshore of the continental shelf and often occur nearshore year-round (Merrick and Loughlin, 1997; Lander et al., 2020; Kapsar, 2022 but see Himes Boor and Small, 2012). To describe regional variation in water temperature anomalies at similar spatial and temporal scales to our sea lion data, we used R package heatwaveR (v. 0.4.6, Schlegel and Smit, 2018) to calculate the number of days in heatwave state per annual period (summer year i to summer year i + 1) for localized regions over the continental shelf around areas where sea lions were marked (boxed areas in Figure 1). For this, we used the U. S. National Oceanic and Atmospheric Administration’s (NOAA) daily Optimum Interpolation Sea Surface Temperature data (OISST, https://www.ncei.noaa.gov/products/optimum-interpolation-sst) for the time period 1 January 1982 to 31 December 2021. This is a global ¼° gridded interpolated dataset based on satellite-derived sea surface temperatures (Reynolds et al., 2007). For each boxed region: (1) daily temperatures were averaged across grids within the region, (2) daily climatologies were constructed using available data up to 30 years (i.e., for each day of the year, mean and 90th percentile threshold temperatures were calculated), and (3) heatwave events (≥ 5 days in length) were detected (Hobday et al., 2016). We then summed the number of days during heatwave events from 1 July to 30 June each annual period. The first of July was the midpoint of the period over which summer data were collected and was approximately the peak of when the majority of resightings were collected. We repeated this procedure for 2005–2019 temperature data from the deepest depth bin (~250 m) from the permanent oceanographic mooring GAK1 operated by the University of Alaska Fairbanks and Gulf Watch Alaska (http://research.cfos.uaf.edu/gak1/).
Synchrony in sea surface temperatures across distant regions based on OISST data during and following the 2014–2016 PMH was expected, and conditions in the nearshore frequented by sea lions may not adequately be represented by OISST data, particularly for inside waters (ocean waters separated from the open ocean by large islands) of SEAK and Prince William Sound not covered by that dataset (Danielson et al., 2022). Therefore, we also described regional nearshore water temperatures (~2–4 m depth below MLLW) using in situ measurements from coastal tide gauge stations operated by the NOAA Center for Operational Oceanographic Products and Services (https://tidesandcurrents.noaa.gov/). We calculated daily and then weekly average water temperatures from 13 May 1999 to 12 May 2022 separately for each of 9 stations (Figure 1). For each station, the annual average weekly anomaly was then calculated where years were 1 July to 30 June. Annual anomalies were averaged over some stations to cover SEAK South (stations Ketchikan and Sitka), SEAK North (Elfin Cove), SEAK Inside North (Juneau and Skagway), Prince William Sound (Cordova and Valdez), and Kodiak (Kodiak and Alitak, Figure 1). Data were averaged by weeks and across stations to impute missing data in these datasets. Determination of number of heatwave days per year with these data were not possible due to missing data in the time series (Schlegel and Smit, 2018).
3 Results
3.1 Southeast Alaska analysis
The best resighting model (Supplemental Table 3: model 6) was very similar to past analyses (age, sex and natal rookery variation in resighting probability is shown in Supplemental Figure 2A). Variation in resighting probability in later years north vs. south was not supported, but as expected, resighting probability was higher (+0.266) for the 2016 Graves cohort than for the 2002/2005 Graves cohorts (Supplemental Table 3: model 6 vs 7, ΔAIC ~ 20 [where ΔAIC = the difference in AIC of the simpler model compared to the more complex model; ΔAIC ≥ 2 indicated the factor unique to the complex model had statistical support and should not be removed from the model; the magnitude of ΔAIC above 2 indicated the strength of support). Resighting probabilities declined beginning in 2015 (e.g., for prime-aged females: from ~0.80 to ~0.67 in 2015–2017), which mirrored a decline in effort (Figure 2A, see figure caption for definition of effort). For survival, the best sex-age structure had six categories for females (0,1,2,3–15,16–17,18+) and males (0,1,2,3–8,9–10,11+) and this structure was preferred to the basis-spline (df=4) fit to Age for females and males separately (Supplemental Table 3, see comparison of estimates in Supplemental Figure 1). The age-specific pattern and age at senescence were very similar to past analyses, strongly supporting a drop in survival at age 16 (Supplemental Table 3, model 11 vs 12, ΔAIC ~ 33, Hastings et al., 2018; Supplemental Figure 1). The age pattern varied for Graves but not White Sisters, compared to Forrester/Hazy (Supplemental Table 3: models 16-17 vs 13) due to particularly high survival at ages 1 and 2 for Graves compared to other rookeries (Supplemental Figure 3).
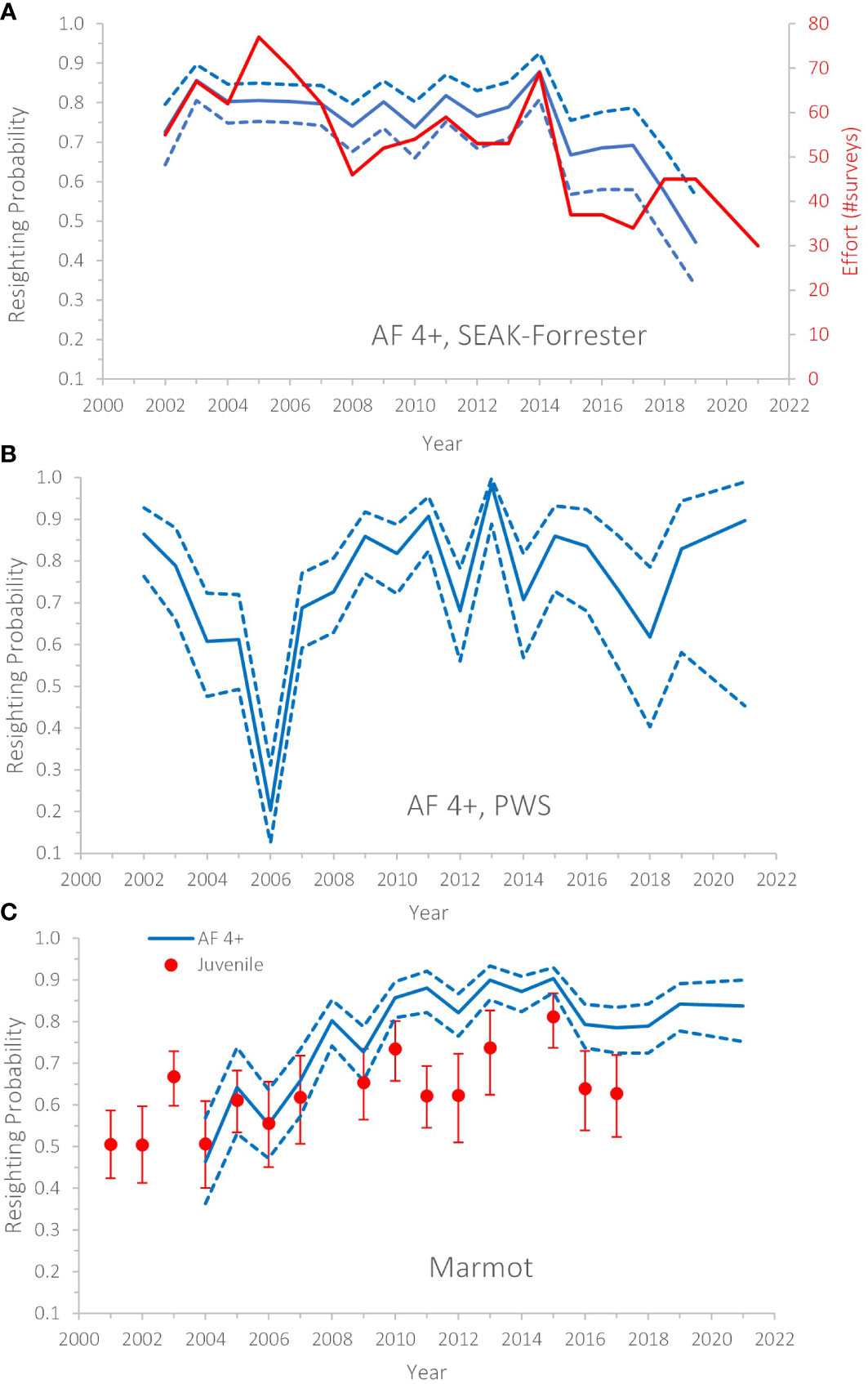
Figure 2 Annual resighting probabilities (May–August) of Steller sea lions in the Gulf of Alaska 2001–2021, by age-class and natal rookery. (A) Forrester Islands, Southeast Alaska; (B) Prince William Sound; (C) Marmot Island, see Figure 1). AF 4+, adult females 4+ years of age; SEAK, Southeast Alaska; PWS, Prince William Sound. Juvenile, < age 4. Error bars/dashed lines are 95% CI. Annual survey effort (red in a) = number of unique surveys (date*time*site) conducted per year during summer boat-based resighting trips in SEAK (excluding sites at Forrester Island Complex where a field camp was conducted).
Annual variation in survival for prime-aged females was not supported for all years (Supplemental Table 3) but a change in survival beginning in summer 2014 was strongly supported (Supplemental Table 3: models 16 or 19 vs 26, ΔAIC ~ 23), with anomalous estimates particularly apparent during the warming (Figure 3A). For prime-aged females, examination of specific years supported reduced survival for 2 years* 2014 and 2015 (years* refer to periods from summer to the next summer; e.g., 2014 = summer 2014 to summer 2015 and 2015 = summer 2015 to summer 2016), and especially in 2016 (Supplemental Table 3: model 22), but estimates at the end of the times series were very imprecise (Figure 3A) due to reduced probability of resighting coupled with dwindling sample sizes of this age class (see caption Figure 2A). Survival estimates were reduced by ~0.04 in 2014 and 2015 and by 0.16 in 2016 (Table 2). For males, reduced survival in 2016 was supported (Supplemental Table 3: models 31 vs 34, ΔAIC ~ 5). Male survival at age 11+ was reduced ~ 0.25 in this year (Table 2, overall annual pattern for males is shown in Figure 3B). Reduced juvenile survival for the 2016 cohort was not supported (Supplemental Table 3: models 41 vs 31, ΔAIC = 0.2), but sample size for this cohort was small (n = 70) and so we examined the point estimates from this model. Although not a preferred model, point estimates of survival of the 2016 cohort were -0.12 and ~-0.03 lower at ages 0 and at 1 and 2, respectively, when compared to the average from the 2002 and 2005 Graves Rocks cohorts (from Supplemental Table 3, model 36).
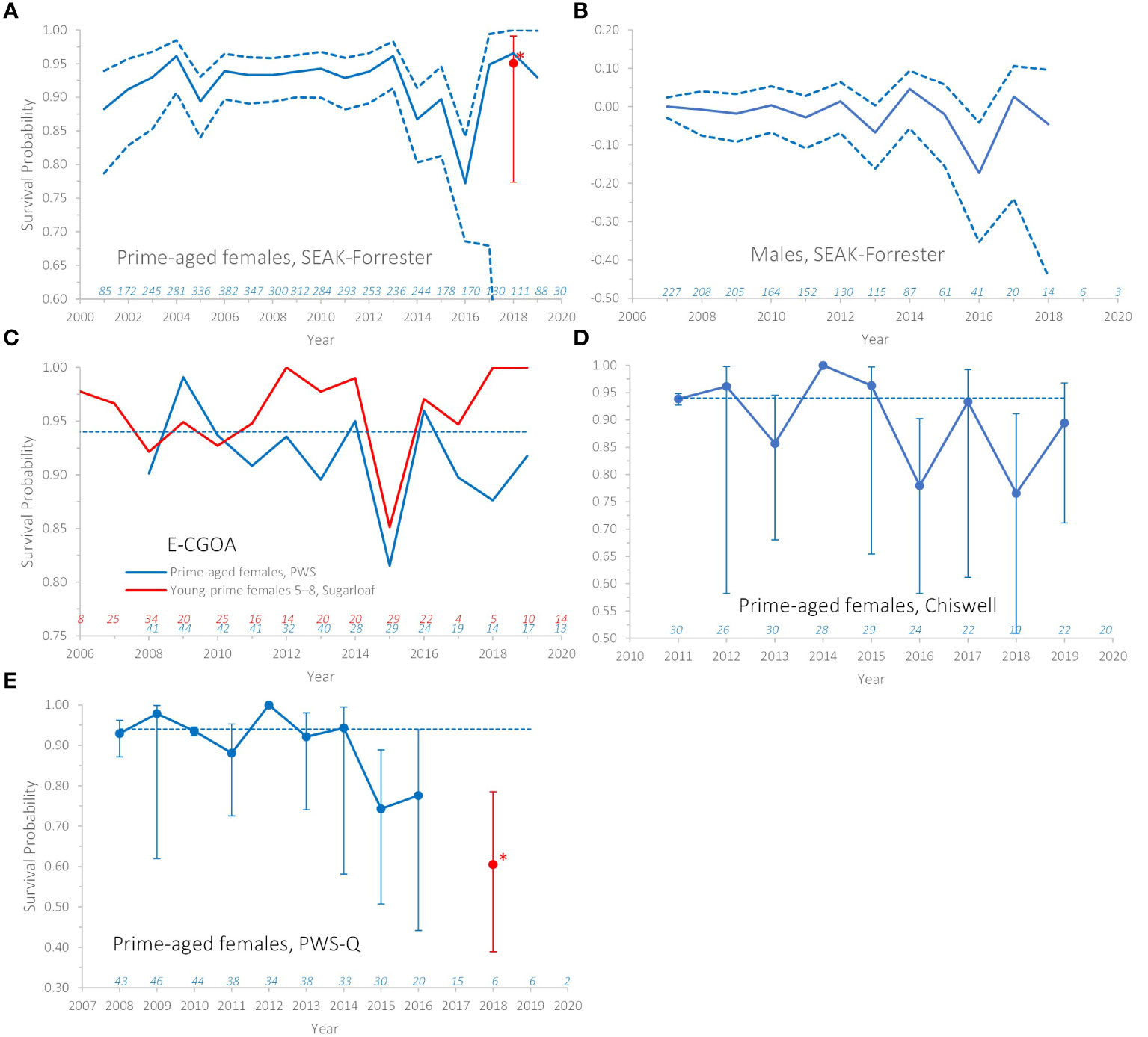
Figure 3 Annual survival probabilities of Steller sea lions by age-sex class and natal rookery. (A, B, Forrester Islands; C, Prince William Sound and Sugarloaf; D, Chiswell Island; E, dive-captured animals in Prince William Sound; see Figure 1). Annual intervals over which survival was estimated are indicated on the x-axis by the start of the interval, e.g., 2015= summer 2015 to summer 2016. Models with full year-specific estimates were not supported but were examined to verify that years of poor survival after 2013 also appeared anomalous within the series. Prime-aged females = age 3–15, for which survival was best modeled as constant with age (see Supplemental Figures 1, 3 for age-specific patterns). Young-prime females were age 5–8. SEAK, Southeast Alaska; E–CGOA, eastern–central Gulf of Alaska; PWS, Prince William Sound. Error bars/dashed lines are 95% CI. In (C–E), dotted lines = the average value across years (0.94). Only point estimates in (C) are shown because precision of annual estimates was poor. Numbers just above the x-axis are sample sizes of that group observed in that year. Average estimates from 2017–2019 in (A, E) are in red with *. Annual variation in (B) is plotted as the difference from the 2007 estimate for a single age-class.
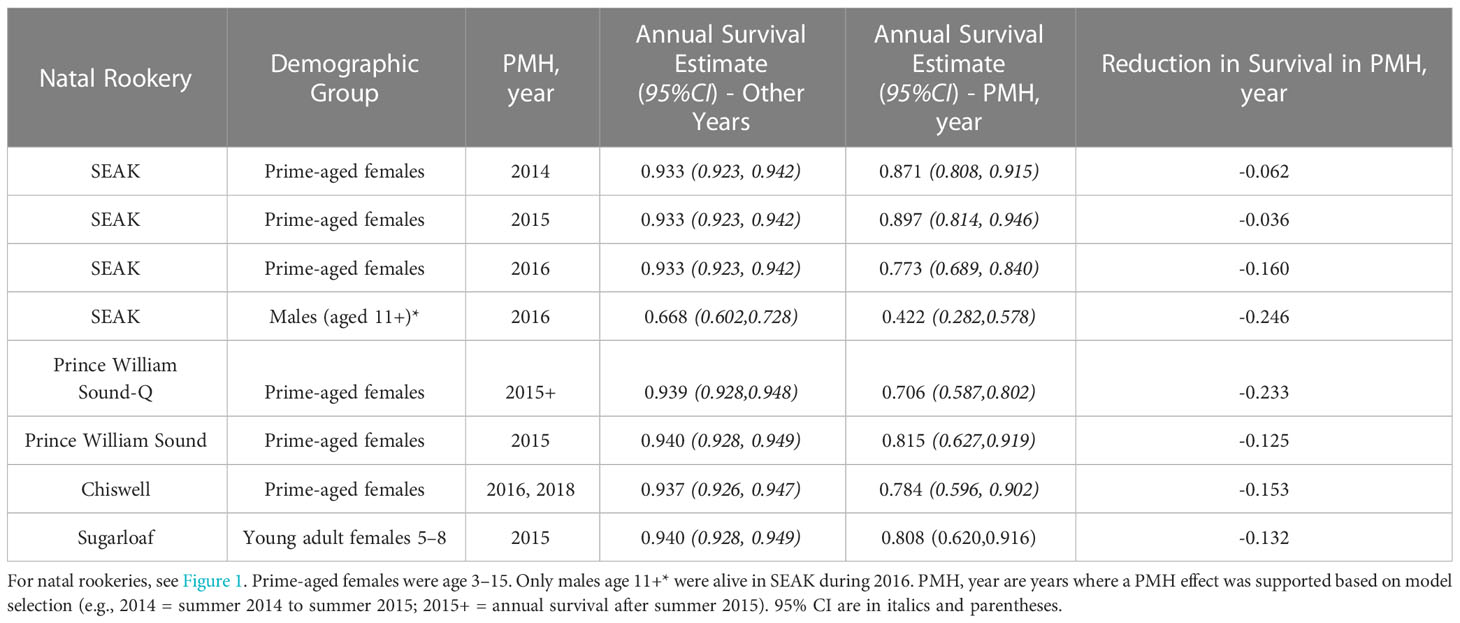
Table 2 Summary of potential effects on annual survival of Steller sea lions from Southeast Alaska (SEAK) and the Eastern–Central Gulf of Alaska, due to ocean warming during the Pacific Marine Heatwave of 2014–2016, and on-going.
3.2 Eastern-Central Gulf of Alaska analysis
The best resighting model (Supplemental Table 4, model 12) included sex and age with additive effect of natal rookery (estimates are shown in Supplemental Figure 2B). Differences were small among groups (ranged 0.680–0.833 for the oldest age-class) but were statistically supported; resighting probability was slightly higher for the mid-ages than for younger and older ages, and for females than males at some ages, and was highest for Chiswell- and Marmot-born animals (Supplemental Figure 2B). Resighting probability was particularly low in 2006 for Prince William Sound animals (Figure 2B). Annual variation differed among juveniles and adults in the CGOA (Figure 2C; Supplemental Table 4: model 4 vs 6, ΔAIC ~ 18). As expected, resighting probability was reduced by an average of -0.249 for the 2014 cohort compared to older CGOA cohorts (Supplemental Table 4, model 15 vs 12, ΔAIC ~ 37).
For survival, the oldest ages with sufficient sample size were 18 for females and 13 for males (Supplemental Table 1), and so sex-age class structures possible for survival did not include the category for females 20+ (Supplemental Table 4). It is well documented that the pattern in survival with age as juveniles differs between Prince William Sound and the CGOA (Fritz et al., 2014) and so the additive model (sex-age class + natal rookery) was not fit. The best age structure for survival was consistent with past work with regional variation in survival during juvenile years (0–2), but now with lower juvenile survival at Sugarloaf than Marmot also apparent (Supplemental Figure 3; Supplemental Table 4: model 20 vs 19, ΔAIC ~ 4). Senescence in male and female survival (at 16+ for females) was supported (Supplemental Figure 3; Supplemental Table 4: for females - model 17 vs 16, ΔAIC ~ 14).
Annual variation in prime-aged female survival (or for smaller age ranges within that category) were not supported for all years (Supplemental Table 4). For prime-aged females from Prince William Sound, reduced survival in 2015 was supported (Supplemental Table 4: model 25 vs 21, ΔAIC = 2), and this year appeared anomalous among annual estimates (Figure 3C). Anomalous years were not apparent among the annual estimates for Sugarloaf or Marmot females aged 3–15. For smaller age ranges within the prime age category, reduced survival for young-prime aged 5–8 in 2015 was supported for Sugarloaf (Supplemental Table 4: model 31 vs 21, ΔAIC = 2.4) and this year appeared anomalous within that series (Figure 3C). For these groups in 2015, survival of Sugarloaf and Prince William Sound females was reduced by ~0.13 (Table 2). Reduced survival for old-prime females from Marmot after summer 2014 also had support (Supplemental Table 4: model 39 vs 21, ΔAIC ~ 3), due to anomalously low survival in 2017, but this year was not within our primary years of interest (2014–2016). Reduced survival of the 2014 CGOA cohort was not supported (Supplemental Table 4: model 47 vs 46, AIC lower for the simpler model), even when the effect only in the first year or juvenile years was fit (not shown in Supplemental Table 4). A post-hoc model with all CGOA cohorts varying in first year survival was fit and was inferior to the no-effect model (ΔAIC < 2) and point estimates also did not indicate anomalous survival for the 2014 cohort compared to other cohorts. Reduced survival of males in years 2014–2016 from any rookery in the E–CGOA was not supported (Supplemental Table 4: models 48–53).
Additional dataset 1 (Chiswell): Annual variation in resighting probabilities for Chiswell animals was not supported nor was natal rookery*sex-age class (Supplemental Table 5: model 4). Estimates for Chiswell by sex-age class were nearly identical to those of Marmot (Supplemental Figure 2B). For females and males, respectively: resighting probabilities were 0.713 (0.661, 0.760; 95%CI) and 0.689 (0.635, 0.739) at age 1–3; and 0.868 (0.837, 0.894) and 0.830 (0.790, 0.863) at age 4+. Reduced resighting probability for the 2016 cohort was not supported (Supplemental Table 5: model 4 vs 5) but its retention produced a lower AIC and so it was conservatively retained for modeling survival (point estimates suggested a potential average reduction in resighting probability of -0.132 per age).
For juvenile survival, Chiswell was best pooled with Sugarloaf rather than Marmot (Supplemental Table 5: model 7), and this also was apparent from point estimates when Chiswell was estimated separately from other groups (Supplemental Figure 3). Annual variation in survival of prime-aged females was strongly supported due to reduced survival in 2016 and later (Figure 3D; Supplemental Table 5: models 12 and 14, ΔAIC > 4). Based on estimates from the model with year-specific estimates (Supplemental Table 5: model 9) and from post-hoc models (Supplemental Table 5: model 14), survival was also significantly lower in 2018 (Figure 3D). In these years, prime-aged female survival was reduced by ~ 0.15 (Table 2). Reduced juvenile survival of the 2016 cohort was not supported (Supplemental Table 5: models 15/16 vs 12/14), however, only 48 pups were released with brands from this cohort. Point estimates of first-year survival for this cohort were -0.116 lower than other cohorts (from Supplemental Table 5: model 17).
Additional dataset 2 (Prince William Sound-Q): The age-sex-specific pattern in survival was similar between the two Prince William Sound groups (Q and rookeries; Supplemental Table 6: model 1 vs 2). However, annual patterns in survival varied between these two groups (Supplemental Table 6: model 3/4 vs 9/10, ΔAIC ≥ 10). Annual variation in survival was strongly supported for Prince William Sound-Q (Supplemental Table 6: model 3/4 vs 2, ΔAIC ≥ 15) due to an abrupt drop in survival starting in summer 2015 which remained low through summer 2021 (Figure 3E; Supplemental Table 6: post-hoc model 15), although likely very few animals in the sample were alive after summer 2017. The best model pooled annual survival after summer 2015 (Supplemental Table 6: model 15) and produced an estimated reduction in annual female survival after summer 2015 of ~ 0.23 (Table 2).
3.3 Annual water temperature anomalies in sea lion areas
The pattern in the number of days satellite-derived sea surface temperatures at the outer coast and primarily over the continental shelf were in heatwave state were similar among sea lion areas and similar to large-scale patterns observed in the Gulf (Figure 4A; Danielson et al., 2022). Number of heatwave days was highest for the 3 years summer 2014 to summer 2017 and high again from summer 2018 to summer 2019 (or summer 2020 in some areas, Figure 4A). The number of heatwave days based on coastal sea surface temperatures may have been higher and occurred earlier in SEAK compared to other sea lion areas; number of heatwave days was highest from summer 2016 to summer 2017 at the ocean bottom at GAK1 (Figure 4A). Nearshore measurements, including inside waters of Prince William Sound and SEAK, demonstrated a similar temporal pattern (in terms of intensity rather than duration) with higher anomalies observed during summer 2014 to summer 2017, even for inside waters (Figure 4B, see also Vandersea et al., 2018; Danielson et al., 2022; Gabriele et al., 2022). Warm waters from summer 2018 to summer 2020 may not have penetrated all nearshore waters except on the outer coast of Kodiak Island, with temperatures also elevated somewhat in outer coast waters of southern SEAK and inside waters of Prince William Sound (Figure 4B). These general patterns suggest sea lions in all regions experienced anomalously warm conditions in the nearshore and continental shelf habitat they frequent, including the inside waters of SEAK and Prince William Sound.
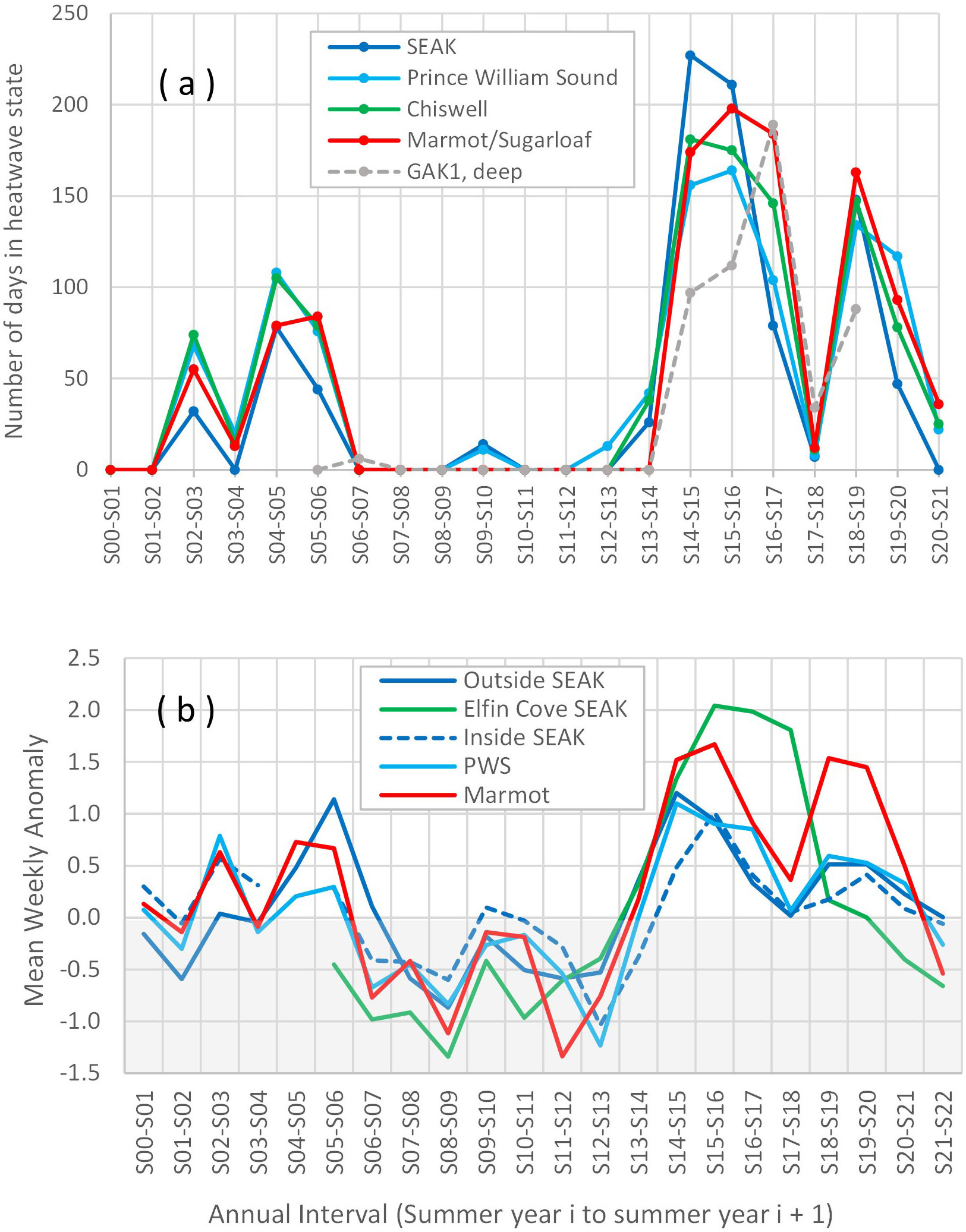
Figure 4 Regional annual (summer to summer) ocean temperature anomalies in areas where Steller sea lions were marked. (A) Number of days in heatwave state (≥ 90th percentile for heatwave events ≥ 5 days in duration) based on OISST satellite-derived sea surface temperature data for boxed regions in Figure 1, and the GAK1 oceanographic mooring (bottom temperature, see Figure 1). (B) Nearshore in situ data: Annual mean weekly near surface (~2-4 m below MLLW) water temperature anomalies (in °C) based on coastal tide gauge stations for Outside South Southeast Alaska (SEAK, stations Ketchikan and Sitka averaged), Elfin Cove (North SEAK), Inside North SEAK (Juneau and Skagway), Prince William Sound (PWS, Cordova and Valdez) and Kodiak (Kodiak and Alitak, Figure 1). Annual intervals were from 1 July – 30 June each year. Regions in (A) extend offshore and do not cover inside waters of Prince William Sound and Southeast Alaska; very nearshore waters at specific locations on the outer and inside coasts are represented in (B).
4 Discussion
Annual survival of prime-aged adult female Steller sea lions was reduced during the PMH from -0.05 to -0.23 below non-PMH average values (Table 2). This observation is particularly relevant to population status of Steller sea lions in the Gulf of Alaska because adult female survival strongly determines population trend in long-lived vertebrates (Eberhardt, 2002). Population trend may be resilient to short and intermittent periods (1–2 years) of poor survival of this important demographic group, but the more prolonged periods (5+ years) of high mortality observed in some areas of our study will likely reduce population growth rates and could lead to severe regional declines (Barbraud and Weimerskirch, 2001). We found no conclusive evidence for reduced survival of juveniles using our marked sample but point estimates of first-year survival from this small sample were ~ 0.12 lower during the PMH east of Cook Inlet, in the area where population effects were observed (Table 1; Suryan et al., 2021). More study is required to determine effects of the PMH on juvenile sea lions; currently no marked juveniles are available within our study area. Adult males were more resilient than females to the warm water event: we observed only males born in SEAK suffered particularly high mortality (-0.25), and only from summer 2016 to summer 2017, also the year of highest mortality for adult females in SEAK (Table 2). Males may have avoided or alleviated mortality risks by ranging widely and/or more offshore compared to females (Jemison et al., 2018).
As population stability depends most on adult female survival, this demographic group is expected to be less susceptible to detrimental changes in food supply than juveniles, given they are able to respond correctly and quickly to environmental cues that allow proper buffering of their own survival versus reproduction (Gaillard et al., 2000; Forcada et al., 2008). Juveniles are expected to suffer greater mortality during food shortages than adults, due to their smaller body size and reduced breath-hold capacity, higher mass-specific metabolic rates, and reduced foraging experience and efficiency compared to adults (Schreer and Kovacs, 1997; Winship et al., 2002; Pitcher et al., 2005). However, marked vulnerability of adults to abrupt or severe reductions in food supply due to ocean warming has been observed in some polar and subpolar birds and mammals due to combinations of: requirements for rich, predictable food resources near breeding areas or haulouts, high site fidelity, lack of behavioral plasticity, and particularly high metabolic demands (Barbraud and Weimerskirch, 2001; Forcada et al., 2008; Piatt et al., 2020). Mismatch between environmental conditions and life history buffers for adults may also occur when super-abundance of food resources occur seasonally, and mortality risk differs among seasons (Clutton-Brock et al., 1991).
The lack of statistically-supported evidence for lower survival of juveniles from the 2014 and 2016 cohorts was unexpected, even though sample sizes were small in 2016. It is unusual in large mammal species for adult females to be affected by poor food supply but not juveniles. Steller sea lion females have a particularly energetically-demanding life history owing to very high metabolic costs of lactation, and an extended offspring dependency period potentially lasting > 2 years where females may nutritionally support large juveniles (Pitcher and Calkins, 1981). Energy requirements may be 70% greater for lactating than for nonlactating females (Winship et al., 2002). Although proximate mechanisms for reduced female survival are currently unknown, detrimental changes in the prey field likely contributed to reduced adult female sea lion survival, given that the changes in the Gulf of Alaska food web were wide-spread and severe during the PMH (Suryan et al., 2021). If so, we expect juveniles were also likely impacted and/or that adult females with dependent young in some regions may have been particularly impacted by their high dietary requirements and restriction to the nearshore. Preliminary analyses suggest reproduction may also have been depressed during the PMH in SEAK and at Chiswell (ADFG and ASLC unpublished data). Juvenile Steller sea lion mortality was high during the years of severe population decline (York, 1994) but periods of high adult female mortality were also observed (Loughlin and Nelson, 1986; Pendleton et al., 2006) suggesting vulnerability of adult females to mortality risks. More study is required to determine effects of marine heatwave on juveniles versus adult females.
The observed regional variation in the sea lion population response to the PMH may reflect regional differences in oceanography, the response of the food web to warm conditions, and sea lion life history. Food web effects were particularly severe east of Cook Inlet to ~Kayak Island (Suryan et al., 2021) and Common Murre Uria aalge carcasses were particularly abundant in this area (Piatt et al., 2020). Also in this area, adult female sea lion survival was reduced, particularly off the Kenai Peninsula (Chiswell) and in Prince William Sound, where poor survival continued post-heatwave (Table 2). A long-term study of naturally-marked and branded females that use Chiswell also documented a severe and prolonged reduction in annual adult female survival after summer 2015, coupled with a potential modest drop in reproductive rate, with females possibly enduring a higher cost of reproduction and an extended period of offspring dependency during the PMH (ASLC unpublished data).
In our study, the sample with the greatest mortality following the PMH were sea lions captured as juveniles (most were pups and yearlings) in the nonbreeding season (fall–spring) in Prince William Sound (Prince William Sound-Q, Figure 3E). If seasonal movement patterns of individuals are consistent from year to year and/or learned as juveniles (ADFG unpublished data), females that used Prince William Sound and the Kenai coast to the west during the non-breeding season may have fared particularly poorly. Of Prince William Sound-Q females seen during adulthood, many used the area between Prince William Sound and Sugarloaf; 71% were seen only in Prince William Sound, 2% were seen in northern SEAK, and 27% were seen west of Prince William Sound along the eastern Kenai coast or at Sugarloaf (including 18% pupping at Sugarloaf, ADFG unpublished data). In contrast, females from Prince William Sound rookeries on the outer coast (Seal and Fish, Figure 1) fared better than those from Prince William Sound-Q and may have recovered somewhat post-heatwave (Figure 3C). Of Prince William Sound (rookeries) females, none were seen west of Prince William Sound and of those that reached adulthood, 27% were observed using the northern coast of SEAK, including 13% (n = 7) pupping there (ADFG unpublished data, Jemison et al., 2018).
Summer 2016 to summer 2017 was a particularly poor year for sea lions in SEAK, but adult female survival was also reduced to a lesser extent from summer 2014 to summer 2016, showing the earliest response to the PMH (Table 1). SEAK females may have recovered quickly from effects of the PMH (by summer 2017), based on the remaining small sample of prime-aged females (Figure 3A). A comprehensive summary of the impacts to the SEAK food web is not yet available, but biological effects may include reductions of zooplankton and preponderance of small warm-water-associated, lipid-poor copepods in Icy Strait, and poor Rhinoceros auklet Cerorhinca monocerata chick growth and/or production at St. Lazaria Island after 2014 (Fergusson et al., 2020; Ferriss and Zador, 2021). Humpback whales in Glacier Bay National Park (northern SEAK, Figure 1) were particularly affected by the PMH with reduced adult survival, calf survival, reproductive rate, and body condition from 2014 to 2018, with limited recovery in 2019 and 2020; in 2020, population abundance was ~50% of that in 2013 (Gabriele et al., 2022). Although an acute but significant increase in adult female Steller sea lion mortality occurred from summer 2014 to summer 2017, the current status of females in SEAK is uncertain because only a few marked females remain. Nonpup counts during the 2021 SEAK aerial survey were particularly low (Sweeney et al., 2022).
Similar to results suggesting greater resiliency of some species in areas west of Cook Inlet (Suryan et al., 2021), survival of the large sample of females marked at Marmot was not reduced during the PMH. A significant reduction (-0.131) in survival for young prime-aged females (ages 5–8) from Sugarloaf from summer 2015 to summer 2016 had marginal statistical support, but detrimental effects on juveniles from Sugarloaf were not obvious that year (when the 2014 cohort were yearlings). Sugarloaf Island is within an island group (the Barren Islands) located mid-way within the northeast entrance to Cook Inlet (Figure 1). Sea lions born at Sugarloaf use areas west and east of their natal site (Raum-Suryan et al., 2002; Jemison et al., 2018), but they have a greater tendency to use sites to the east (Kenai coast and Prince William Sound) rather than to the west (e.g., Kodiak, Figure 13, Jemison et al., 2018). The potential greater vulnerability of Sugarloaf than Marmot females to the warming may be due to different movement patterns and foraging areas, and/or poorer early-life conditions for Sugarloaf animals that had lower first-year survival (Supplemental Figure 3; Supplemental Table 4), lower weaning probabilities at ages 1–2, and a slightly higher survival cost of weaning at ages 1–2 than Marmot animals (Hastings et al., 2021).
We observed significant regional variation in sea lion survival and response to the PMH, yet the temporal pattern and intensity of warming was similar throughout our study area based on satellite and in situ measurements in the nearshore and inside waters (Figure 4; Danielson et al., 2022). Regional variation in biological response suggests the warming may have affected the food webs differently east versus west of Cook Inlet (Suryan et al., 2021), perhaps due to habitat, ecosystem and oceanographic differences (Dickson and Baker, 2016; Ormseth et al., 2019). Our study region is oceanographically diverse. Prince William Sound and the inside waters of SEAK contain complex coastlines includes narrow channels, inlets, glaciers and deep water fjords with seasonally high levels of freshwater discharge and limited points of exchange with outer coast waters (Weingartner et al., 2009, Campbell, 2018). The continental shelf in SEAK is particularly distinguished from the shelf in the northern Gulf by its narrow width, and by reduced mixing over the shelf caused by the reduction and interruption of the flow of the Alaska Coastal Current along the SEAK coast (Stabeno et al., 2016b). This important north-flowing current is interrupted by deep canyons in the SEAK shelf, many of which cut across the entire shelf such as at Cross Sound, and by reduced downwelling favorable winds between Cross Sound and Yakutat; the current reforms near Montague Island and builds to its strongest at Gore Point near the entrance to Cook Inlet (Figure 1; Stabeno et al., 2004; Stabeno et al., 2016a). Highest levels of vertical mixing and productivity, and freshest waters occur around Kodiak Island, whose productive shelf waters are assisted by high flow and enhanced vertical mixing over and at the edges of complex and extensive banks (Cheng et al., 2012; Stabeno et al., 2016a). In contrast, vertical mixing is weaker along the eastern Kenai Peninsula coast and productivity is lower (Stabeno et al., 2016a).
These habitat and oceanographic differences resulted in regional variation in fish abundance as measured by a multidecadal summer bottom trawl survey: fish abundance was higher and diversity lower around Kodiak compared to the shelf off Prince William Sound and SEAK, which were similar in fish composition (Mueter and Norcross, 2002). This shift towards lower fish abundance and higher fish diversity east of Kodiak may begin near the entrance to Cook Inlet along the Kenai coast, although whether this is truly a transition area was not formally addressed in past studies (Figure 5 in Mueter and Norcross, 2002). These patterns suggest that greater vulnerability of top predators to warming in the area east of Cook Inlet may be due to lower overall productivity, lower rates of vertical mixing, and/or other habitat and oceanographic differences in this region.
How prey abundance and composition influence Steller sea lion population resiliency has been a central research question since the severe population decline west of 144° W began over 40 years ago but it remains largely unanswered due to the complexity of this problem and to lack of comprehensive data specifically on sea lion prey. Coincident with declining population trends in the CGOA from 1975 to 1993 were reduced diet diversity (Merrick et al., 1997), reduced prevalence of energy-dense forage fish (i.e., capelin) and increased dominance of walleye pollock Gadus chalcogrammus and smaller sizes of pollock in the diet suggesting detrimental shifts in the fish community, which together with reduced body sizes of sea lions, suggested reduced carrying capacity (Calkins and Pitcher, 1982; Calkins and Goodwin, 1988; Merrick et al., 1997). From 1993 to 1997, diet diversity and prevalence of forage fish in the diet was also high in SEAK, where long-term population trend was positive (Trites et al., 2007; see also Scherer et al., 2015: dominance of forage fish in the diet was also observed here in gestating females from 1998 to 2011). Moderate population growth in Kodiak from 1999 to 2009 coincided with an increase in diet diversity, reduced reliance on pollock, and dominance of Pacific sand lance in sea lion diets (Sinclair and Zeppelin, 2002; McKenzie and Wynne, 2008; Sinclair et al., 2013).
Pollock is an important source of nutrition throughout the Gulf of Alaska including SEAK, historically and in recent years, but researchers hypothesized that resiliency may rely on diet diversity, and similarly habitat and oceanographic heterogeneity (Lander et al., 2009), and on some amount of energy-dense forage fish available to sea lions (Merrick et al., 1997; Trites et al., 2007). The fact that pollock increased in abundance and was the dominant groundfish in the Gulf at the time of the decline (the 1970s–1980s, Mueter and Norcross, 2002), also suggested diet composition may be more important than simply prey abundance. Diet diversity per se may not simply reflect the status of the prey field to sea lions as simple measures of diet diversity may be unrelated to population trends (Fritz et al., 2019) and switching from rich, preferred prey to unpreferred and more diverse prey during poor conditions may also occur (Lowry et al., 2022). Increased diet diversity also occurred after the PMH in the winter diet of Steller sea lions around Chiswell, due to a strong reduction in capelin coupled with an increased utilization of a variety of benthic and poorer-quality prey (Maniscalco, 2023).
Comparison of sea lion diet composition in our study region, between Kodiak, Kenai, and Prince William Sound, is limited, but the diet of gestating adult females, as determined by stable isotopes in their whiskers from 1998 to 2011, suggested a mixed diet for Kodiak females (forage fish, pollock, and salmon) and a potential greater reliance on arrowtooth flounder Atheresthes stomias and salmon for Prince William Sound females than for females in other areas (Scherer et al., 2015). Arrowtooth flounder female spawning biomass declined during the PMH, as did capelin, herring, pollock and the commercial harvest of Chinook and sockeye salmon in Prince Willian Sound or east of Cook Inlet (Suryan et al., 2021). In contrast, sand lance, an important diet component for Kodiak females (McKenzie and Wynne, 2008) appeared little affected or positively affected by the PMH (Suryan et al., 2021). An historical study (1975–1978, ~pre-decline, Trites and Larkin, 1996) based on stomach contents that assessed sea lion diet along the coast from Prince William Sound to Kodiak documented more squid and herring in Prince William Sound than other areas (prior to the herring population collapse in Prince William Sound after 1993), and greater reliance on salmon and capelin in Kodiak than other areas (Calkins and Pitcher, 1982). Comparison among studies is limited by sampling and methodological biases and inconsistencies, but together this information suggests sea lion diet composition likely varied among regions in our study and may shift through time due to interactions with regionally-varying habitat and oceanographic conditions, with consequences for sea lion population resiliency. Current diet composition information based on standard and robust methodology throughout our study region is needed to address this issue.
Steller sea lion life histories also differ east versus west of Cook Inlet. Sea lions born at Sugarloaf and west are larger than those born at the major rookeries to the east of Sugarloaf (Merrick et al., 1995; Brandon et al., 2005; Sweeney et al., 2015; Rea et al., 2016; Hastings et al., 2021). A similar spatial pattern was also noted in harbor seals Phoca vitulina, which were larger in the CGOA than in Prince William Sound and SEAK (Pitcher and Calkins, 1979). Regional differences in body size did not reflect current regional population trends, but instead large body size was correlated with a faster life history including faster body growth, a shorter time to weaning, and a potential trade-off of juvenile for adult survival (Hastings et al., 2021). The smaller body size, slower growth and extended weaning period for sea lions in Prince William Sound and SEAK may reflect long-term adaptation to a less productive environment requiring different foraging tactics to exploit less predictable and diverse prey, such that adult females here may operate at the edge of their biological capacity for supporting dependent offspring (Hastings et al., 2021). Sea lions west of Cook Inlet may benefit from a productive environment with abundant, predictable prey; large body size may allow them to employ greater capital in their life history strategy, such as for exploiting very seasonally abundant prey or enduring long fasts during winter (Hastings et al., 2021). A less productive environment and a stronger food web response east of Cook Inlet (Suryan et al., 2021), may have overwhelmed female sea lions in this area, especially given the energetically-demanding life history tactics for adult females with dependent young in this area. Data from 11 adult females satellite-tagged in the falls of 2018–2019 in Prince William Sound, Cook Inlet and Kodiak suggested females in Kodiak may extensively use the productive shelf south of Kodiak, and the success of this strategy may be assisted by large body size of females and offspring; in contrast, females at sites in Cook Inlet and Prince William Sound foraged in different and distinct areas east of Kodiak and many of them may have focused effort highly in the nearshore and near tagging sites (ADFG unpublished data, see Figure 5.2 Kapsar, 2022). Diet studies are currently being conducted to examine regional variation in sea lion diet composition post-PMH.
In addition to potential effects of prey and nutritional status of females, harmful algal blooms associated with warm water, more frequent interactions with fisheries, and disease may have contributed to reduced female survival, perhaps as cumulative effects of additional stressors (Orr et al., 2020). Levels of harmful algal biotoxins (HABs) produced by phytoplankton, which accumulate up the food chain and may cause neurological damage and death in marine species, increase with water temperature and are expected to increase in occurrence along the Alaskan coastline with ocean warming (Gobler et al., 2017). HABs were detected widely in Alaska marine mammals from 2004 to 2013, including Steller sea lions (Lefebvre et al., 2016). Biotoxins were prevalent year-round in Steller sea lion scats in Washington state USA from 2011 to 2013, where their presence was also observed with consumption of benthic fish, particularly walleye pollock (Akmajian et al., 2017). Increased levels of HABs, particularly in bays, occurred during the PMH in Cook Inlet (Vandersea et al., 2018) but were not obviously elevated during the PMH in the inside waters of northern SEAK (Tobin et al., 2019) or outside of bays in Cook Inlet (Vandersea et al., 2018). HABs were commonly detected in seabirds, forage fish and invertebrates in Prince William Sound and Cook Inlet during the PMH at low to moderate levels (Van Hemert et al., 2020).
Increased frequency of interaction with fisheries and coastal hazards may also have occurred during the PMH. A sharp increase in whale entanglements along the continental US west coast during the PMH (from 10/yr < 2014 to up to 50/yr during 2014–2016) resulted from increased numbers of whale-fisheries interactions due to the contraction of the upwelling zone to the nearshore, and reduced krill abundance offshore coupled with high abundance of northern anchovy Engraulis mordax nearshore (Santora et al., 2020). Adult females may interact with commercial fishing vessels to obtain prey (Loughlin and Nelson, 1986). Although entanglement rates in the population are currently low (Raum-Suryan et al., 2009), shifts in distribution and behavior under nutritional stress may increase the frequency of negative interactions. Finally, increased incidence and novel disease in marine mammals, such as Phocine distemper virus recently arriving from the Atlantic to sea lions in the Gulf of Alaska (Van Wormer et al., 2019), is expected with ocean warming (Burek et al., 2008; Sanderson and Alexander, 2020). The role of these additional factors, and whether adult females were particularly vulnerable to them, requires further study.
Our study indicates the need to incorporate temporal variation in adult female survival in Steller sea lion population models considering various plausible scenarios of marine heatwave occurrence in the north Pacific in future years. This vulnerability in such an important demographic group will likely reduce population viability in regions that are most affected. Reconsideration of recovery criteria may be required in light of this information, and also any other new information on population level effects not yet determined (e.g., reproduction), in a regional context. Given habitat and oceanographic characteristics and current prey community structures, populations east of Cook Inlet and in Southeast Alaska may show greatest vulnerability within the central and eastern Gulf of Alaska. Pinniped populations that live in the eastern equatorial Pacific and bordering temperate zones regularly experience every 3–7 years detrimental ecosystem changes that may last 9 months to 2 years due to ocean warming from the El Niño Southern Oscillation (Trillmich and Ono, 1991). These populations may be limited in size and also show large temporal variation in population age structure due to very high pup and juvenile mortality, reduced reproduction and elevated mortality of adults depending on the severity of the event (Trillmich and Limberger, 1985; Trillmich and Ono, 1991). Pinniped populations in the North Pacific are adapted to much less temporally variable conditions in a highly seasonal environment, and population response to abrupt and severe changes in local conditions as they increase in frequency is uncertain. Our study together with other marine mammal studies during the PMH (Suryan et al., 2021; Gabriele et al., 2022; Maniscalco, 2023) indicate the effect of marine heatwaves on marine mammals may be greater than previously observed during recent marine heatwave events worldwide (Smale et al., 2019).
Data availability statement
The raw data supporting the conclusions of this article will be made available by the authors, without undue reservation.
Ethics statement
The animal study was reviewed and approved by Institutional Animal Care and Use Committees and under permits issued by the NMFS to the ADFG, the ASLC and the MML.
Author contributions
KH is the lead author and analyzed the data. DJ and GP advised and reviewed statistical analysis. TG, LJ, RT, and JM oversaw long-term branding and resighting studies. RT, KH and JM created and maintained databases. All authors contributed to the article and approved the submitted version.
Funding
This research was funded by the NMFS, Alaska Region, through awards: NA17FX1079, NA04NMF4390170, NA08NMF4390544, NA11NMF4390200, NA15NMF4390170, NA16NMF4390029, NA19NMF4390084, and NA21NMF4720035 to the ADFG; and awards NA05NMF4391148, NA07NMF4390312, NA09NMF4390169, NA12NMF4390066, NA16NMF4390030 and NA19NMF4390082 to the ASLC. The NMFS also provided funding to the MML. Funding was also provided to the ADFG from the State of Alaska.
Acknowledgments
We thank the many individuals who marked and resighted Steller sea lions in Alaska over several decades. For many years leading these brand-resighting programs, we especially thank: L. Fritz, K. Chumbley, T. Loughlin, D. Calkins, P. Parker, K. Pitcher and K. Raum-Suryan. For significant contributions to field studies, we also especially thank: B. Fadely, T. Gage, P. Gearin, S. Goodglick, J. Jenniges, C. Kaplan, J. King, S. Lewis, D. McCallister, M. Rehberg, G. Snedgen, K. Sweeney, J. Thomason, A. Trites and B. Van Burgh. The Oregon Department of Fish and Wildlife, Glacier Bay National Park, and members of the public also provided valuable resighting data. This manuscript benefited greatly from discussions with R. Suryan and S. Danielson and journal reveiwers. This research was approved by the Institutional Animal Care and Use Committees and under permits issued by the NMFS to the ADFG, the ASLC and the MML. Work by the ADFG was permitted through US Marine Mammal Permits 358-1564, 358-1888, 14325, 18537, 22298, with additional permits granted by the U.S. National Park Service, the U. S. Fish and Wildlife Service–Alaska Maritime National Wildlife Refuge, and the Department of Fisheries and Oceans Canada. Research by the MML was conducted under permits 782-1532, 782-1768, 782-1889, and 14326. Work by the ASLC was conducted under permits 881-1890-02, 14324, 18438, and 22293.
Conflict of interest
The authors declare that the research was conducted in the absence of any commercial or financial relationships that could be construed as a potential conflict of interest.
Publisher’s note
All claims expressed in this article are solely those of the authors and do not necessarily represent those of their affiliated organizations, or those of the publisher, the editors and the reviewers. Any product that may be evaluated in this article, or claim that may be made by its manufacturer, is not guaranteed or endorsed by the publisher.
Author disclaimer
The findings and conclusions in this paper are those of the author(s) and do not necessarily represent the views of the NMFS.
Supplementary material
The Supplementary Material for this article can be found online at: https://www.frontiersin.org/articles/10.3389/fmars.2023.1127013/full#supplementary-material
References
Akaike H. (1973). Information theory as an extension of the maximum likelihood principle. 267–281 in Petrov B. N., Csaki F. (eds). Second International Symposium on Information Theory. Akademiai Kiado, Budapest. doi: 10.1007/978-1-4612-0919-5_38
Akmajian A. M., Scordino J. J., Acevedo-Gutiérrez A. (2017). Year-round algal toxin exposure in free-ranging sea lions. Mar. Ecol. Prog. Ser. 583, 243–258. doi: 10.3354/meps12345
Albouy C., Delattre V., Donati G., Frolicher T. L., Albouy-Boyer S., Rufino M., et al. (2020). Global vulnerability of marine mammals to global warming. Nat. Sci. Rep. 10, 548. doi: 10.1038/s41598-019-57280-3
Altukhov A. V., Andrews R. D., Calkins D. G., Gelatt T. S., Gurarie E. D., Loughlin T. R., et al. (2015). Age specific survival rates of Steller sea lions at rookeries with divergent population trends in the Russian far East. PLoS One 10 (5), e0127292. doi: 10.1371/journal.pone.0127292
Amaya D. J., Miller A. J., Xie S., Kosaka Y. (2020). Physical drivers of the summer 2019 North Pacific marine heatwave. Nat. Commun. 11, 1903. doi: 10.1038/s41467-020-15820-w
Anderson P. J., Piatt J. F. (1999). Community reorganization in the Gulf of Alaska following ocean climate regime shift. Mar. Ecol. Prog. Ser. 189, 117–123. doi: 10.3354/meps189117
Arimitsu M. L., Piatt J. F., Hatch S., Suryan R. M., Batten S., Bishop M. A., et al. (2021). Heatwave-induced synchrony within forage fish portfolio disrupts energy flow to top pelagic predators. Glob. Change Biol. 27, 1859–1878. doi: 10.1111/gcb.15556
Barbeaux S. J., Holsman K., Zador S. (2020). Marine heatwave stress test of ecosystem-based fisheries management in the Gulf of Alaska Pacific cod fishery. Front. Mar. Sci. 7, 703. doi: 10.3389/fmars.2020.00703
Barbraud C., Weimerskirch H. (2001). Emperor penguins and climate change. Nature 441, 183–186. doi: 10.1038/35075554
Barkhordarian A., Nielsen D. M., Johanna Baehr J. (2022). Recent marine heatwaves in the North Pacific warming pool can be attributed to rising atmospheric levels of greenhouse gases. Nat. Commun. Earth Environ. 3, 131. doi: 10.1038/s43247-022-00461-2
Bond N. A., Cronin M. F., Freeland H., Mantua N. (2015). Causes and impacts of the 2014 warm anomaly in the NE Pacific. Geophys. Res. Lett. 42, 3414–3420. doi: 10.1002/2015GL063306
Brandon E. A. A., Calkins D. G., Loughlin T. R., Davis R. W. (2005). Neonatal growth of Steller sea lion (Eumetopias jubatus) pups in Alaska. Fish. Bull. (Wash. D. C.) 103, 246–257.
Burek K. A., Gulland F. M., O’Hara T. M. (2008). Effects of climate change on arctic marine mammal health. Ecol. Appl. 18 (2), S126–S134. doi: 10.1890/06-0553.1
Burnham K. P., Anderson D. R. (2002). Model selection and inference: a practical information-theoretic approach, 2nd ed (New York, NY, USA: Springer).
Calkins D., Goodwin E. (1988). Investigating of the declining sea lion population in the Gulf of Alaska. (Alaska, 99518, USA: Report of the Alaska Department of Fish and Game).
Calkins D. G., Pitcher K. W. (1982). Population assessment, ecology, and trophic relationships of Steller sea lions in the Gulf of Alaska. (333 Raspberry Road, Anchorage, Alaska, USA: Report of Alaska Department of Fish and Game).
Campbell R. W. (2018). Hydrographic trends in Prince William Sound, Alaska 1960–2016. Deep Sea Res. Part II 147, 43–57. doi: 10.1016/j.dsr2.2017.08.014
Cavole L. M., Demko A. M., Diner R. E., Giddings A., Koester I., Pagniello C. M.L.S., et al. (2016). Biological impacts of the 2013–2015 warm-water anomaly in the Northeast Pacific: winners, losers, and the future. Oceanography 29, 273–285. doi: 10.5670/oceanog.2016.32
Chen Z., Shi J., Liu Q., Chen H., Li C. (2021). A persistent and intense marine heatwave in the Northeast Pacific during 2019–2020. Geophys. Res. Lett. 48, e2021GL093239. doi: 10.1029/2021GL093239
Cheng W., Hermann A. J., Coyle K. O., Dobbins E. L., Kachel N. B., Stabeno P. J. (2012). Macro- and micro-nutrient flux to a highly productive submarine bank in the Gulf of Alaska: a model-based analysis of daily and interannual variability. Prog. Oceanogr. 101, 63–77. doi: 10.1016/j.pocean.2012.01.001
Clutton-Brock T. H., Price O. F., Albon S. D., Jewell P. A. (1991). Persistent instability and population regulation in Soay sheep. J. Anim. Ecol. 60, 593–608. doi: 10.2307/5300
Danielson S., Hennon T. D., Monson D. H., Suryan R. M., Campbell R. W., Baird S. J., et al. (2022). Temperature variations in the northern Gulf of Alaska across synoptic to century-long time scales. Deep Sea Res. Part II 203, 105155. doi: 10.1016/j.dsr2.2022.105155
Dickson D. M., Baker M. R. (2016). Introduction to the North Pacific Research Board Gulf of Alaska Integrated Ecosystem Research Program (GOAIERP): volume I. Deep Sea Res. Part II 132, 1–5. doi: 10.1016/j.dsr2.2016.08.005
Di Lorenzo E., Mantua N. (2016). Multi-year persistence of the 2014/15 North Pacific heatwave. Nat. Climate Change 6, 1042–1047. doi: 10.1038/nclimate3082
du Pontavice H., Didier G., Reygondeau G., Maureaud A., Cheung W. W. L. (2020). Climate change undermines the global functioning of marine food webs. Glob. Change Biol. 26, 1306–1318. doi: 10.1111/gcb.14944
Eberhardt L. L. (2002). A paradigm for population analysis of long-lived vertebrates. Ecology 83, 2841–2854. doi: 10.1890/0012-9658(2002)083[2841:APFPAO]2.0.CO;2
Fergusson E., Miller T., McPhee M. V., Fugate C., Schultz H. (2020). Trophic responses of juvenile Pacific salmon to warm and cool periods within inside marine waters of Southeast Alaska. Prog. Oceanogr. 186, 102378. doi: 10.1016/j.pocean.2020.102378
Ferriss B. E., Zador S. (2021). Ecosystem status report 2021: Gulf of Alaska, stock assessment and fishery evaluation report (1007 West Third, Suite 400, Anchorage, Alaska 99501, USA: North Pacific Fishery Management Council).
Forcada J., Trathan P. N., Murphy E. J. (2008). Life history buffering in Antarctic mammals and birds against changing patterns of climate and environmental variation. Glob. Change Biol. 14, 2473–2488. doi: 10.1111/j.1365-2486.2008.01678.x
Fritz L., Brost B., Laman E., Luxa K., Sweeney K., Thomason J., et al. (2019). A re-examination of the relationship between Steller sea lion (Eumetopias jubatus) diet and population trend using data from the Aleutian islands. Can. J. Zool. 97, 1137–1155. doi: 10.1139/cjz-2018-0329
Fritz L., Sweeney K., Towell R., Gelatt T. (2016). Aerial and ship-based surveys of Steller sea lions (Eumetopias jubatus) conducted in Alaska in June-July 2013 through 2015, and an update on the status and trend of the western distinct population segment in Alaska (7600 Sand Point Way NE, Seattle, Washington, 99185, USA: NOAA Technical Memorandum NMFS-AFSC-321).
Fritz L. W., Towell R., Gelatt T. S., Johnson D. S., Loughlin T. R. (2014). Recent increases in survival of western Steller sea lions in Alaska and implications for recovery. Endanger. Species Res. 26, 13–24. doi: 10.3354/esr00634
Gabriele C. M., Amundson C. L., Neilson J. L., Straley J. M., Baker C. S., Danielson S. L. (2022). Sharp decline in humpback whale (Megaptera novaeangliae) survival and reproductive success in southeastern Alaska during and after the 2014–2016 Northeast Pacific marine heatwave. Mamm. Biol. 102, 1113–1131. doi: 10.1007/s42991-021-00187-2
Gaillard J.-M., Festa-Bianchet M., Yoccoz N. G., Loison A., Toigo C. (2000). Temporal variation in fitness components and population dynamics of large herbivores. Annu. Rev. Ecol. System. 31, 367–393. doi: 10.1146/annurev.ecolsys.31.1.367
Gentemann C. L., Fewings M. R., Garcia-Reyes M. (2017). Satellite sea surface temperatures along the West Coast of the United States during the 2014-2016 Northeast Pacific marine heat wave. Geophys. Res. Lett. 44, 312–319. doi: 10.1002/2016GL071039
Gobler C. J., Doherty O. M., Hattenrath-Lehmann T. K., Griffith A. W., Kang Y., Litaker R. W. (2017). Ocean warming since 1982 has expanded the niche of toxic algal blooms in the North Atlantic and North Pacific oceans. Proc. Natl. Acad. Sci. 114, 4975–4980. doi: 10.1073/pnas.1619575114
Goodman D. (2008). A PVA model for evaluating recovery criteria for the western Steller sea lion population. Report to the Steller Sea Lion Recovery Team, March 30, 2006. appendix in NMFS 2008: Recovery Plan of the Steller Sea Lion (Eumetopias jubatus). revision (Silver Spring, MD: US National Marine Fisheries Service).
Hare S. R., Mantua N. J. (2000). Empirical evidence for North Pacific regime shifts in 1977 and 1989. Prog. Oceanogr. 47, 103–145. doi: 10.1016/S0079-6611(00)00033-1
Hastings K. K., Jemison L. A., Gelatt T. S., Laake J. L., Pendleton G. W., King J. C., et al. (2011). Cohort effects and spatial variation in age-specific survival of Steller sea lions from southeastern Alaska. Ecosphere 2, 111. doi: 10.1890/ES11-00215.1
Hastings K. K., Jemison L. A., Pendleton G. W. (2018). Survival of adult Steller sea lions in Alaska: senescence, annual variation and covariation with male reproductive success. R. Soc. Open Sci. 5, 170665. doi: 10.1098/rsos.170665
Hastings K. K., Johnson D. S., Pendleton G. W., Fadely B. S., Gelatt T. S. (2021). Investigating life history traits of Steller sea lions with multistate hidden Markov mark-recapture models: age at weaning and body size effects. Ecol. Evol. 11, 714–734. doi: 10.1002/ece3.6878
Hauser D. D. W., Laidre K. L., Stern H. L. (2018). Vulnerability of Arctic marine mammals to vessel traffic in the increasingly ice-free Northwest Passage and Northern Sea Route. Proc. Natl. Acad. Sci. 115, 7617–7622. doi: 10.1073/pnas.1803543115
Himes Boor G. K., Small R. J. (2012). Steller sea lion spatial-use patterns derived from a Bayesian model of opportunistic observations. Mar. Mammal Sci. 28, E375–E403. doi: 10.1111/j.1748-7692.2011.00541.x
Hobday A. J., Alexander L. V., Perkins S. E., Smale D. A., Straub S. C., Oliver E. C.J., et al. (2016). A hierarchical approach to defining marine heatwaves. Prog. Oceanogr. 141, 227–238. doi: 10.1016/j.pocean.2015.12.014
Jackson J. M., Johnson G. C., Dosser H. V., Ross T. (2018). Warming from recent marine heatwave lingers in deep British Columbia fjord. Geophys. Res. Lett. 45, 9757–9764. doi: 10.1029/2018GL078971
Jacox M. G., Alexander M. A., Mantua N. J., Scott J. D., Hervieux G., Webb R. S., et al. (2018). Forcing of multiyear extreme ocean temperatures that impacted California Current living marine resources. In “Explaining extreme events of 2016 from a climate perspective”. Bull. Am. Meteorol. Soc. 99, S27–S33. doi: 10.1175/BAMS-D-17-0119.1
Jemison L. A., Pendleton G. W., Hastings K. K., Maniscalco J. M., Fritz L. W. (2018). Spatial distribution, movements, and geographic range of Steller sea lions (Eumetopias jubatus) in Alaska. PLoS One 13, e0208093. doi: 10.1371/journal.pone.0208093
Joh Y., Di Lorenzo E. (2017). Increasing coupling between NPGO and PDO leads to prolonged marine heatwaves in the Northeast Pacific. Geophys. Res. Lett. 44, 11,663–11,671. doi: 10.1002/2017GL075930
Kapsar K. (2022). The metacoupled Arctic and North Pacific: analyzing the spatiotemporal patterns and impacts of marine vessel traffic in coupled human and natural systems. Ph.D. dissertation (East Lansing, Michigan, USA: Michigan State University).
King J., Gelatt T. S., Pitcher K. W., Pendleton G. W. (2007). A field-based method for estimating age in free-ranging Steller sea lions (Eumetopias jubatus) less than twenty-four months of age. Mar. Mammal Sci. 23, 262–271. doi: 10.1111/j.1748-7692.2007.00108.x
Kortsch S., Primicerio R., Fossheim M., Dolgov A. V., Aschan M. (2015). Climate change alters the structure of arctic marine food webs due to poleward shifts of boreal generalists. Proc. R. Soc. B 282, 20151546. doi: 10.1098/rspb.2015.1546
Laake J. L., Johnson D. S., Conn P. B. (2013). marked: an R package for maximum likelihood and Markov Chain Monte Carlo analysis of capture-recapture data. Methods Ecol. Evol. 4, 885–890. doi: 10.1111/2041-210X.12065
Laidre K. L., Stern H., Kovacs K. M., Lowry L., Moore S. E., Regehr E. V., et al. (2015). Arctic marine mammal population status, sea ice habitat loss, and conservation recommendations for the 21st century. Conserv. Biol. 29, 724–737. doi: 10.1111/cobi.12474
Lander M. E., Loughlin T. R., Logsdon M. G., VanBlaricom G. R., Fadely B. S., Fritz L. W. (2009). Regional differences in the spatial and temporal heterogeneity of oceanographic habitat used by Steller sea lions. Ecol. Appl. 19, 1645–1659. doi: 10.1890/08-0159.1
Lander M. E., Fadely B. S., Gelatt T. S., Sterling J. T., Johnson D. S., Pelland N. A. (2020). Mixing it up in Alaska: habitat use of adult female Steller sea lions reveals a variety of foraging strategies. Ecosphere 11, e03021. doi: 10.1002/ecs2.3021
Lefebvre K. A., Quakenbush L., Frame E., Burek Huntington K., Sheffield G., Stimmelmayr R., et al. (2016). Prevalence of algal toxins in Alaskan marine mammals foraging in a changing arctic and subarctic environment. Harmful Algae 55, 13–24. doi: 10.1016/j.hal.2016.01.007
Litzow M. A., Mueter F. J. (2014). Assessing the ecological importance of climate regime shifts: an approach from the North Pacific Ocean. Prog. Oceanogr. 120, 110–119. doi: 10.1016/j.pocean.2013.08.003
Litzow M. A., Hunsicker M. E., Ward E. J., Anderson S. C., Gaoc J., Zadorf S. G., et al. (2020). Evaluating ecosystem change as Gulf of Alaska temperature exceeds the limits of preindustrial variability. Prog. Oceanogr. 186, 102393. doi: 10.1016/j.pocean.2020.102393
Loughlin T., Nelson R. (1986). Incidental mortality of northern sea lions in Shelikof Strait. Mar. Mammal Sci. 2, 14–33. doi: 10.1111/j.1748-7692.1986.tb00025.x
Lowry M. S., Nehasil S. E., Moore J. E. (2022). Spatio-temporal diet variability of the California sea lion Zalophus californianus in the southern California Current Ecosystem. Mar. Ecol. Prog. Ser. 692, 1–92. doi: 10.3354/meps14096
Maniscalco J. M. (2014). The effects of birth weight and maternal care on survival of juvenile Steller sea lions (Eumetopias jubatus). PLoS One 9, e96328. doi: 10.1371/journal.pone.0096328
Maniscalco J. M. (2023). Changes in the overwintering diet of Steller sea lions (Eumetopias jubatus) in relation to the 2014–2016 Northeast Pacific marine heatwave. Glob. Ecol. Conserv. 43, e02427. doi: 10.1016/j.gecco.2023.e02427
McKenzie J., Wynne K. M. (2008). Spatial and temporal variation in the diet of Steller sea lions in the Kodiak archipelago 1999 to 2005. Mar. Ecol. Prog. Ser. 360, 265–283. doi: 10.3354/meps07383
Merrick R. L., Brown R., Calkins D. G., Loughlin T. R. (1995). A comparison of Steller sea lion, Eumetopias jubatus, pup masses between rookeries with increasing and decreasing populations. Fish. Bull. (Wash. D. C.) 93, 753–758.
Merrick R. L., Chumbley M. K., Byrd G. V. (1997). Diet diversity of Steller sea lions (Eumetopias jubatus) and their population decline in Alaska: a potential relationship. Can. J. Fish. Aquat. Sci. 54, 1342–1348. doi: 10.1139/f97-037
Merrick R. L., Loughlin T. R. (1997). Foraging behavior of adult female and young-of-the-year Steller sea lions in Alaskan waters. Can. J. Zool. 75, 776–786. doi: 10.1139/z97-099
Moore S. E., Reeves R. R., Southall B. L., Ragen T. J., Suydam R. S., Clark C. W. (2012). A new framework for assessing the effects of anthropogenic sound on marine mammals in a rapidly changing Arctic. BioScience 62, 289–295. doi: 10.1525/bio.2012.62.3.10
Moran J., Straley J. (2021). “Fall surveys of humpback whales in Prince William Sound,” in Ecosystem Status Report 2021: Gulf of Alaska, stock assessment and fishery evaluation report. Eds. Ferriss B. E., Zador S. (1007 West Third, Suite 400, Anchorage, Alaska 99501, USA: North Pacific Fishery Management Council), Pp 206–Pp 207.
Mueter F. J., Norcross B. L. (2002). Spatial and temporal patterns in the demersal fish community on the shelf and upper slope regions of the Gulf of Alaska. Fish. Bull. (Wash. D. C.) 100, 559–581.
Oliver E. C. J., Donat M. G., Burrows M. T., Moore P. J., Smale D. A., Alexander L. V., et al. (2018). Longer and more frequent marine heatwaves over the past century. Nat. Commun. 9, 1324. doi: 10.1038/s41467-018-03732-9
Ormseth O., Baker M. M., Hopcroft R. R., Ladd C., Mordy C. W., Moss J. M., et al. (2019). Introduction to understanding ecosystem processes in the Gulf of Alaska, volume 2. Deep Sea Res. Part II 165, 1–6. doi: 10.1016/j.dsr2.2019.06.019
Orr J. A., Vinebrooke R. D., Jackson M. C., Kroeker K. J., Kordas R. L., Mantyka-Pringle C., et al. (2020). Towards a unified study of multiple stressors: divisions and common goals across research disciplines. Proc. R. Soc. B 287, 20200421. doi: 10.1098/rspb.2020.0421
Pendleton G. W., Pitcher K. W., Fritz L. W., York A. E., Raum-Suryan K. L., Loughlin T. R., et al. (2006). Survival of Steller sea lions in Alaska: a comparison of increasing and decreasing populations. Can. J. Zool. 84, 1163–1172. doi: 10.1139/z06-103
Peterson W. T., Fisher J. L., Strub P. T., Du X., Risien C., Peterson J., et al. (2017). The pelagic ecosystem in the northern California current off Oregon during the 2014–2016 warm anomalies within the context of the past 20 years. J. Geophys. Res. Oceans 122, 7267–7290. doi: 10.1002/2017JC012952
Phillips E. M., Chu D., Gauthier S., Parker-Stetter S. L., Shelton A. O., Thomas R. E. (2022). Spatiotemporal variability of euphausiids in the California Current Ecosystem: insights from a recently developed time series. ICES J. Mar. Sci. 79, 1312–1326. doi: 10.1093/icesjms/fsac055
Piatt J. F., Parrish J. K., Renner H. M., Schoen S. K., Jones T. T., Arimitsu M. L., et al. (2020). Extreme mortality and reproductive failure of common murres resulting from the Northeast Pacific marine heatwave of 2014–2016. PLoS One 15, e0226087. doi: 10.1371/journal.pone.0226087
Pitcher K. W. (1990). Major decline in number of harbor seals, Phoca vitulina richardsi, on Tugidak Island, Gulf of Alaska. Mar. Mammal Sci. 6, 121–134. doi: 10.1111/j.1748-7692.1990.tb00234.x
Pitcher K. W., Calkins D. G. (1979). Biology of the harbor seal, Phoca vitulina richardsi, in the Gulf of Alaska. Final report to the Outer Continental Shelf Environmental Assessment Program, 03-5-002-69 (333 Raspberry Rd., Anchorage, Alaska, 99518, USA: Report of the Alaska Department of Fish and Game).
Pitcher K. W., Calkins D. G. (1981). Reproductive biology of Steller sea lions in the Gulf of Alaska. J. Mammal. 62, 599–605. doi: 10.2307/1380406
Pitcher K. W., Rehberg M. J., Pendleton G. W., Raum-Suryan K. L., Gelatt T. S., Swain U. G., et al. (2005). Ontogeny of dive performance in pup and juvenile Steller sea lions in Alaska. Can. J. Zool. 83, 1214–1231. doi: 10.1139/z05-098
Raum-Suryan K., Pitcher K. W., Calkins D. G., Sease J. L., Loughlin T. R. (2002). Dispersal, rookery fidelity, and metapopulation structure of Steller sea lions (Eumetopias jubatus) in an increasing and decreasing population in Alaska. Mar. Mammal Sci. 18, 746–764. doi: 10.1111/j.1748-7692.2002.tb01071.x
Raum-Suryan K., Rehberg M. J., Pendleton G. W., Pitcher K. W., Gelatt T. S. (2004). Development of dispersal, movement patterns, and haul-out use by pup and juvenile Steller sea lions. Mar. Mammal Sci. 20, 823–850. doi: 10.1111/j.1748-7692.2004.tb01195.x
Raum-Suryan K. L., Jemison L. A., Pitcher K. W. (2009). Entanglement of Steller sea lions (Eumetopias jubatus) in marine debris: identifying causes and finding solutions. Mar. Pollut. Bull. 58, 1487–1497. doi: 10.1016/j.marpolbul.2009.06.004
R Core Team (2022). R: a language and environment for statistical computing (Vienna, Austria: R Foundation of Statistical Computing). Available at: https://www.R-project.org/.
Rea L. D., Christ A. M., Hayden A. B., Stegall V. K., Farley S. D., Stricker C. A., et al. (2015). Age-specific vibrissae growth rates: a tool for determining the timing of ecologically important events in Steller sea lions. Mar. Mammal Sci. 31, 1213–1233. doi: 10.1111/mms.12221
Rea L. D., Fadely B. S., Farley S. D., Avery J. P., Dunlap-Harding W. S., Stegall V. K., et al. (2016). Comparing total body lipid content of young-of-the-year Steller sea lions among regions of contrasting population trends. Mar. Mammal Sci. 32, 1200–1218. doi: 10.1111/mms.12327
Reynolds R. W., Smith T. M., Liu C., Chelton D. B., Casey K. S., Schlax M. G. (2007). Daily high resolution-blended analyses for sea surface temperature. J. Climate 20, 5473–5496. doi: 10.1175/2007JCLI1824.1
Sanderson C. E., Alexander K. A. (2020). Unchartered waters: climate change likely to intensify infectious disease outbreaks causing mass mortality events in marine mammals. Glob. Change Biol. 26, 4284–4301. doi: 10.1111/gcb.15163
Santora J. A., Mantua N. J., Schroeder I. D., Field J. C., Hazen E. L., Bograd S. J., et al. (2020). Habitat compression and ecosystem shifts as potential links between marine heatwave and record whale entanglements. Nat. Commun. 11, 536. doi: 10.1038/s41467-019-14215-w
Scherer R. D., Doll A. C., Rea L. D., Rea, Christ A. M., Stricker C. A., Witteveen B., et al. (2015). Stable isotope values in pup vibrissae reveal geographic variation in diets of gestating Steller sea lions Eumetopias jubatus. Mar. Ecol. Prog. Ser. 527, 261–274. doi: 10.3354/meps11255
Schlegel R. W., Smit A. J. (2018). heatwaveR: a central algorithm for the detection of heatwaves and cold-spells. J. Open Source Softw. 3, 821. doi: 10.21105/joss.00821
Schreer J. F., Kovacs K. M. (1997). Allometry of diving capacity in air-breathing vertebrates. Can. J. Zool. 75, 339–358. doi: 10.1139/z97-044
Sinclair E. H., Johnson D. S., Zeppelin T. K., Gelatt T. S. (2013). Decadal variation in the diet of Western stock Steller sea lions (Eumetopias jubatus) (7600 Sand Point Way NE, Seattle, Washington, 99185, USA: NOAA Technical Memorandum NMFSAFSC-248).
Sinclair E. H., Zeppelin T. K. (2002). Seasonal and spatial differences in diet in the western stock of Steller sea lions (Eumetopias jubatus). J. Mammal. 83, 973–990. doi: 10.1644/1545-1542(2002)083<0973:SASDID>2.0.CO;2
Smale D. A., Wernberg T., Oliver E. C. J., Thomsen M., Harvey B. P., Straub S. C., et al. (2019). Marine heatwaves threaten global biodiversity and the provision of ecosystem service. Nat. Climate Change 9, 306–312. doi: 10.1038/s41558-019-0412-1
Stabeno P. J., Bond N. A., Hermann A. J., Kachel N. B., Mordy C. W., Overland J. E. (2004). Meteorology and oceanography of the northern Gulf of Alaska. Cont. Shelf Res. 24, 859–897. doi: 10.1016/j.csr.2004.02.007
Stabeno P. J., Bond N. A., Kachel N. B., Ladd C., Mordy C. W., Strom S. L. (2016a). Southeast Alaskan shelf from southern tip of Baranof Island to Kayak Island: currents, mixing and chlorophyll-a. Deep Sea Res. Part II Topical Stud. Oceanogr. 132, 6–23. doi: 10.1016/j.dsr2.2015.06.018
Stabeno P. J., Bell S., Cheng W., Danielson S., Kachel N. B., Mordy C. W. (2016b). Long-term observations of Alaska Coastal Current in the northern Gulf of Alaska. Deep Sea Res. Part II 132, 24–40. doi: 10.1016/j.dsr2.2015.12.016
Suryan R. M., Arimitsu M. L., Coletti H. A., Hopcroft R. R., Lindeberg M. R., Barbeaux S. J., et al. (2021). Ecosystem response persists after a prolonged marine heatwave. Nat. Sci. Rep. 11, 6235. doi: 10.1038/s41598-021-83818-5
Sweeney K., Birkemeier B., K. Luxa K., Gelatt T. (2022). Results of Steller sea lion surveys in Alaska, June-July 2021. Memorandum to the record, February 7, 2022 (7600 Sand Point Way NE, Seattle, WA, 98115, USA: US National Marine Fisheries Service, Alaska Fisheries Science Center, Marine Mammal Laboratory).
Sweeney K. L., Shertzer K. W., Fritz L. W., Read A. J. (2015). A novel approach to compare pinniped populations across a broad geographic range. Can. J. Fish. Aquat. Sci. 72, 175–185. doi: 10.1139/cjfas-2014-0070
Thompson A. R., Schroeder I. D., Bograd S. J., Hazen E. L., Jacox M. G., Leising A., et al. (2019). State of the California current 2018-19: a novel anchovy regime and a new marine heat wave? Calif. Cooperative Oceanic Fish. Invest. Rep. 60, 1–65.
Tobin E. D., Wallace C. L., Crumpton C., Johnson G., Eckert G. L. (2019). Environmental drivers of paralytic shellfish toxin producing Alexandrium catenella blooms in a fjord system of northern Southeast Alaska. Harmful Algae 88, 101659. doi: 10.1016/j.hal.2019.101659
Trillmich F., Limberger D. (1985). Drastic effects of El Niño on Galapagos pinnipeds. Oecologia 67, 19–22. doi: 10.1007/BF00378445
Trillmich F., Ono K. (1991). Pinnipeds and El Niño: responses to environmental stress (New York: Springer-Verlag).
Trites A. W., Calkins D. G., Winship A. J. (2007). Diets of Steller sea lions (Eumetopias jubatus) in Southeast Alaska, 1993–1999. Fish. Bull. (Wash. D. C.) 105, 234–248.
Trites A. W., Larkin P. A. (1996). Changes in the abundance of Steller sea lions (Eumetopias jubatus) in Alaska from 1956 to 1992: how many were there? Aquat. Mamm. 22, 153–166.
Vandersea M. W., Kibler S. R., Tester P. A., Holderied K., Hondolero D. E., Powell K., et al. (2018). Environmental factors influencing the distribution and abundance of Alexandrium catenella in Kachemak Bay and lower Cook Inlet, Alaska. Harmful Algae 77, 81–92. doi: 10.1016/j.hal.2018.06.008
Van Hemert C., Schoen S. K., Litaker R. W., Smith M. M., Arimitsu M. L., Piatt J. F., et al. (2020). Algal toxins in Alaskan seabirds: evaluating the role of saxitoxin and domoic acid in a large-scale die-off of common murres. Harmful Algae 92, 101730. doi: 10.1016/j.hal.2019.101730
van Weelden C., Towers J. R., Bosker T. (2021). Impacts of climate change on cetacean distribution, habitat and migration. Climate Change Ecol. 1, 100009. doi: 10.1016/j.ecochg.2021.100009
Van Wormer E., Mazet J. A. K., Hall A., Gill V. A., Boveng P. L., London J. M., et al. (2019). Viral emergence in marine mammals in the North Pacific may be linked to Arctic sea ice reduction. Nat. Sci. Rep. 9, 15569. doi: 10.1038/s41598-019-51699-4
Walsh J. E., Thoman R. L., Bhatt U. S., Bieniek P. A., Brettschneider B., Brubaker M., et al. (2018). The high latitude marine heat wave of 2016 and its impacts on Alaska. In Explaining extreme events of 2016 from a climate perspective. Bull. Am. Meteorol. Soc. 99, S39–S43. doi: 10.1175/BAMS-D-17-0105.1
Warlick A. J., Johnson D. S., Gelatt T. S., Converse S. J. (2022). Environmental drivers of demography and potential factors limiting the recovery of an endangered marine mammal. Ecosphere 13, e4325. doi: 10.1002/ecs2.4325
Weingartner T., Eisner L., Eckert G. L., Danielson S. (2009). Southeast Alaska: oceanographic habitats and linkages. J. Biogeogr. 36, 387–400. doi: 10.1111/j.1365-2699.2008.01994.x
Wernberg T., Bennett S., Babcock R. C., de Bettignies T., Cure K., Depczynski M., et al. (2016). Climate-driven regime shift of a temperate marine ecosystem. Science 353, 6295. doi: 10.1126/science.aad8745
Winship A. J., Trites A. W., Rosen D. A. S. (2002). A bioenergetic model for estimating the food requirements of Steller sea lions Eumetopias jubatus in Alaska. Mar. Ecol. Prog. Ser. 229, 291–312. doi: 10.3354/meps229291
Wright B. E., Brown R. F., DeLong R. L., Gearin P. J., Riemer S. D., Laake J. L., et al. (2017). Survival rates of Steller sea lions from Oregon and California. J. Mammal. 98, 885–894. doi: 10.1093/jmammal/gyx033
Keywords: population dynamics, ocean warming, vital rates, pinniped, Eumetopias jubatus, marine mammals, climate change, survival
Citation: Hastings KK, Gelatt TS, Maniscalco JM, Jemison LA, Towell R, Pendleton GW and Johnson DS (2023) Reduced survival of Steller sea lions in the Gulf of Alaska following marine heatwave. Front. Mar. Sci. 10:1127013. doi: 10.3389/fmars.2023.1127013
Received: 19 December 2022; Accepted: 26 April 2023;
Published: 25 May 2023.
Edited by:
Jose Carlos Báez, Spanish Institute of Oceanography (IEO), SpainReviewed by:
Kristen B. Gorman, University of Alaska Fairbanks, United StatesBarbara Louise Chilvers, Massey University, New Zealand
Copyright © 2023 Hastings, Gelatt, Maniscalco, Jemison, Towell, Pendleton and Johnson. This is an open-access article distributed under the terms of the Creative Commons Attribution License (CC BY). The use, distribution or reproduction in other forums is permitted, provided the original author(s) and the copyright owner(s) are credited and that the original publication in this journal is cited, in accordance with accepted academic practice. No use, distribution or reproduction is permitted which does not comply with these terms.
*Correspondence: Kelly K. Hastings, a2VsbHkuaGFzdGluZ3NAYWxhc2thLmdvdg==