- Institute for Marine and Antarctic Studies, University of Tasmania, Hobart, TAS, Australia
Predator–prey interactions are key drivers in structuring communities, with the potential to substantially impact the whole ecosystem when important predators and prey are involved. Squid are voracious predators and also important prey for other top predators. To date, the available data suggests that under current and projected ocean warming, the behaviour of ectotherms could be modified (for example, through individual movement, predator avoidance and escape speed), yet little is known of the influence of temperature on the predatory behaviour of cephalopods. Here, the predatory behaviour of adult southern calamari (Sepioteuthis australis) under different thermal scenarios was examined demonstrating that squid exhibited different behaviour and performance capabilities across temperature treatments. Overall, attempts of squid to capture prey were faster and more persistent at higher temperature treatments (25°C), suggesting that individuals need to increase their food consumption rate, presumably associated with the higher energetic costs of living at elevated temperatures. However, we also observed a possible decrease in capture efficiency and increased prey handling time at higher temperatures suggesting that implications for energetic balance are not straightforward and that trade-offs need to be carefully explored. As cephalopods are ecologically important species acting as key links in food webs around the world, the results here could have important implications for the dynamics of many marine ecosystems in future.
1 Introduction
Marine ecosystems across the world are being affected by climate change, with impacts ranging from changes in species’ life histories (e.g. growth and development), distribution and interactions, through to shifts in ecosystem composition, stability and function (Hoegh-Guldberg and Bruno, 2010; Doney et al., 2012; Vergés et al., 2014; Payne et al., 2016; Pecl et al., 2017; Vergés et al., 2019). Ocean warming, induced by anthropogenic CO2 emissions, is expected to increase by between 2 and 4°C by the end of the century (IPCC, 2022), yet the velocity and magnitude of change differs regionally around the world.
In south-eastern Australia, ocean warming is occurring almost four times faster than the global average due to the intensification and poleward extension of the East Australian Current (Ridgway, 2007; Oliver et al., 2015), creating a regional ‘hotspot’. Such fast-warming regions can act as natural laboratories for climate change as climate-driven biological and ecological changes may be accelerated, occurring ahead of such changes in other regions (Hobday and Pecl, 2014). Across those waters, particularly Tasmania, extensive alteration of marine ecosystems has been linked with both heatwaves and trends of temperature increases, for example in the loss of habitat-forming species like kelp and the range extensions of many ‘new’ species (Johnson et al., 2011; Last et al., 2011; Pecl et al., 2019). However, most of the research effort has focused on understanding species current and projected distributional responses to warming, whereas very little work has been undertaken on how species might perform within their existing ranges and interact with existing or with novel species in response to warming waters. In this part of the world, squid have been identified as having a strong effect on ecosystems, acting as a link between food webs in different habitats (de la Chesnais et al., 2019). Consequently, squid performance under different thermal regimes could alter the stability and strength of the biotic interactions, leading into modification of whole marine community dynamics under warming conditions across waters.
Worldwide, cephalopods are considered marine keystone species, serving as important predators and prey (Villanueva et al., 2017; Chen et al., 2022). They are voracious carnivores and opportunistic animals, feeding on a wide variety of live prey including fish, cephalopods, gastropods, bivalves or crustaceans (Hanlon and Messenger, 2018). Furthermore, as fast-growing animals with the potential to respond quickly to environmental changes (Steer et al., 2003; Pecl et al., 2004), they are likely to play a significant role in the response of marine ecosystems to climate change (Pecl and Jackson, 2008; de la Chesnais et al., 2019). Therefore, the study of how temperature affects behavioural performance (e.g. predation and locomotion) (Gilman et al., 2010), in ecologically important species such as squid, could improve our capacity to more accurately forecast the dynamics of their associated ecosystems.
Ectotherms are particularly vulnerable to ocean warming, as temperature plays a vital role in physiological regulation and behavioural performance (Amarasekare, 2015) with the potential to impact ecological communities by altering the strength and stability of trophic interactions (Rall et al., 2010; Grigaltchik et al., 2012; Gilbert et al., 2014). Modification of these interactions could have significant consequences for whole marine communities when important predator or prey species are affected. As interactions of key species can determine the flux of nutrients among individuals through communities and ecosystems (Dell et al., 2014; Horwitz et al., 2020).
Predation is a key process in structuring communities with cascading effects across trophic levels (Steffan and Snyder, 2010; Warren et al., 2017). Furthermore, predation pressure can alter prey morphology, and regulate population size, as well as prey species composition by trophic cascades (Warren et al., 2017). Any encounter between predator and prey depends on a complex interaction of physiological and behavioral capacities such as locomotion, escape speed and foraging patterns (Lienart et al., 2014; Öhlund et al., 2015). Different factors may affect the motivation to make an attack, including predation risk, hunger or prey availability (Sentis et al., 2012). Yet, the behavioural functional response still mainly depends on two parameters – search rate and handling time (the time that a predator takes to kill and consume the prey), and both parameters are directly related to the water temperature (Sentis et al., 2012). Handling time is the time taken to capture and kill the prey, and the rate of gut clearance (Jeschke et al., 2002), whereas searching is an active predator’s behaviour, directly associated with locomotor performance. For example, in Stylocheilus striatus (sea hare), an increase in water temperature (from 28 to 31°C) leads to a reduction in locomotion speed (Horwitz et al., 2020), and in the predator Macquaria novemaculeata (Australian bass), the number of attacks increases under warm conditions (25°C) in comparison with cold acclimation (15°C) (Grigaltchik et al., 2012).
In previous studies of fish and marine invertebrates, predator behaviour has been shown to be affected by temperature (Kidawa et al., 2010; Grigaltchik et al., 2012; Sentis et al., 2012; Horwitz et al., 2020), and some species of squid have responded to other environmental stressors like acidification (Spady et al., 2018), yet the behavioural responses of cephalopods to warming waters have been under-represented in the literature (Higgins et al., 2012). To date, studies have examined how changes in temperature affect the anti-predatory behaviour or escape speeds of cephalopods (Neumeister et al., 2000). However, to the best of our knowledge, no studies have examined the effect of acclimation temperature on their predatory behaviour. This is an important gap in our understanding of how future warming conditions could affect ecologically important species, with potential flow-on effects for community stability.
The main objective of this study was to examine the effect of acclimation temperature on the predatory behaviour of southern calamari (Sepioteuthis australis). Specifically, the predatory interaction with a common prey, Australian salmon (Arripis trutta), under different thermal scenarios, simulating current (13°C, 16°C, 19°C) and possible future (22°C, and 25°C) environmental conditions was examined. Southern calamari are a large loliginid species, endemic to southern Australia and northern New Zealand. They are commonly found in shallow waters (< 20m deep) over seagrass meadows or sandy habitats with a lifespan of an approximately one year (Moltschaniwskyj and Steer, 2004; Pecl, 2004). Australian salmon can be found sharing a similar geographical distribution to southern calamari across south-eastern Australia, from western Victoria and Tasmania to north New South Wales, although Australian salmon distribution extends around southern Queensland (Paulin, 1993). Within their geographical Tasmania range, both species experiences average summer temperatures of 19°C with a maximum of 21°C, while in winter the average temperature is 12.5°C with a minimum of 10°C. Still, Australian salmon could encounter water temperatures as high as 24.6°C on their northern distribution. The study of how environmental temperature affects a species’ behavioural performance in key predator–prey interactions is crucial to potentially identify any climate-driven major changes in species interactions, which may in turn influence future population trends and ecosystem dynamics in response to climate change.
2 Materials and methods
2.1 Squid collection and holding conditions
A total of 100 adult squid were caught (mantle length 110–247 mm with a mean of 244 ± 91.67 g in weight) by hand-jigging from the south-east coast of Tasmania (43°00’27.0”S 147°19’32.5”E) from December 2018 to May 2019, with groups of 10 individuals captured within 24-hour periods. Collection depths varied from 5 to 15 m, as southern calamari are a largely inshore species. For acclimation to temperature and the experimental procedures, squid were transported to the IMAS Taroona research facilities at the University of Tasmania. During the animals’ transport, as well as their initial time in the holding tanks, water temperature was maintained at the same temperature as that of collection (± 0.5°C), which ranged between 14°C and 19°C over the capture months. In total, five temperature treatments were conducted (13°C, 16°C, 19°C, 22°C, and 25°C), representing current and possible future conditions, with 20 squid acclimated per treatment.
Once at the research facility, 10 individuals were placed in a holding tank (2.2 m diameter x 0.8 m high, two per temperature treatment) connected to a recirculating system supplied with ocean water subjected to multiple stages of filtration (drum filter, foam fractionator, biofilter, and UV treatment) and a heater/chiller unit (Aquahort heat pump LWH030SC). After the first 12 hours of the squid being in the holding tank, temperatures were increased/decreased progressively by 1°C every 12 hours until the treatment temperature was reached to avoid any possible thermal shock to the animals. Squid were acclimated to the treatment conditions for a full week prior to any procedures being undertaken. Water quality parameters in the holding tanks (checked 3 times per day) were kept constant and at a suitable level for the squid; salinity 34–36‰; NO3 <10 mg L-1; NO2 <0.1 mg L-1; NH4< 0.25mg L-1; and oxygen saturation < 110%, and the water delivery was maintained at a constant 30 ± 2 L/h. Additionally, a photoperiod cycle of 13 hr/11 hr light–dark was established, with 30 minutes each of programmed sunset and sunrise. Squid were fed daily (approximately 1:30 pm) with small live fish (body size >150 mm), including locally caught Australian salmon (Arripis trutta) or garfish (Hyporhamphus melanochir).
2.2 Experimental set-up and trial
The experimental behavioural arena (Figure 1) consisted of a circular tank (1.0 m diameter and 0.5m high) receiving water supply at ~ 20 l/h. Temperature in the arena was controlled by a heater/chiller unit (Aquahort heat pump VL130R), and an air stone kept the dissolved oxygen at around 100%. The arena was divided by a transparent acrylic window, with a GoPro HERO 4 camera mounted behind the window to record a lateral view of the squid during trials. A GoPro HERO 5 was mounted on top of the tank at a height of 0.5 m and two lamps (10 W RGB LED) were mounted parallel to but 5 cm higher than the GoPro HERO 5 to eliminate the shadow of the squid. The arena was completely covered with a black curtain, to isolate it from any external stimuli.
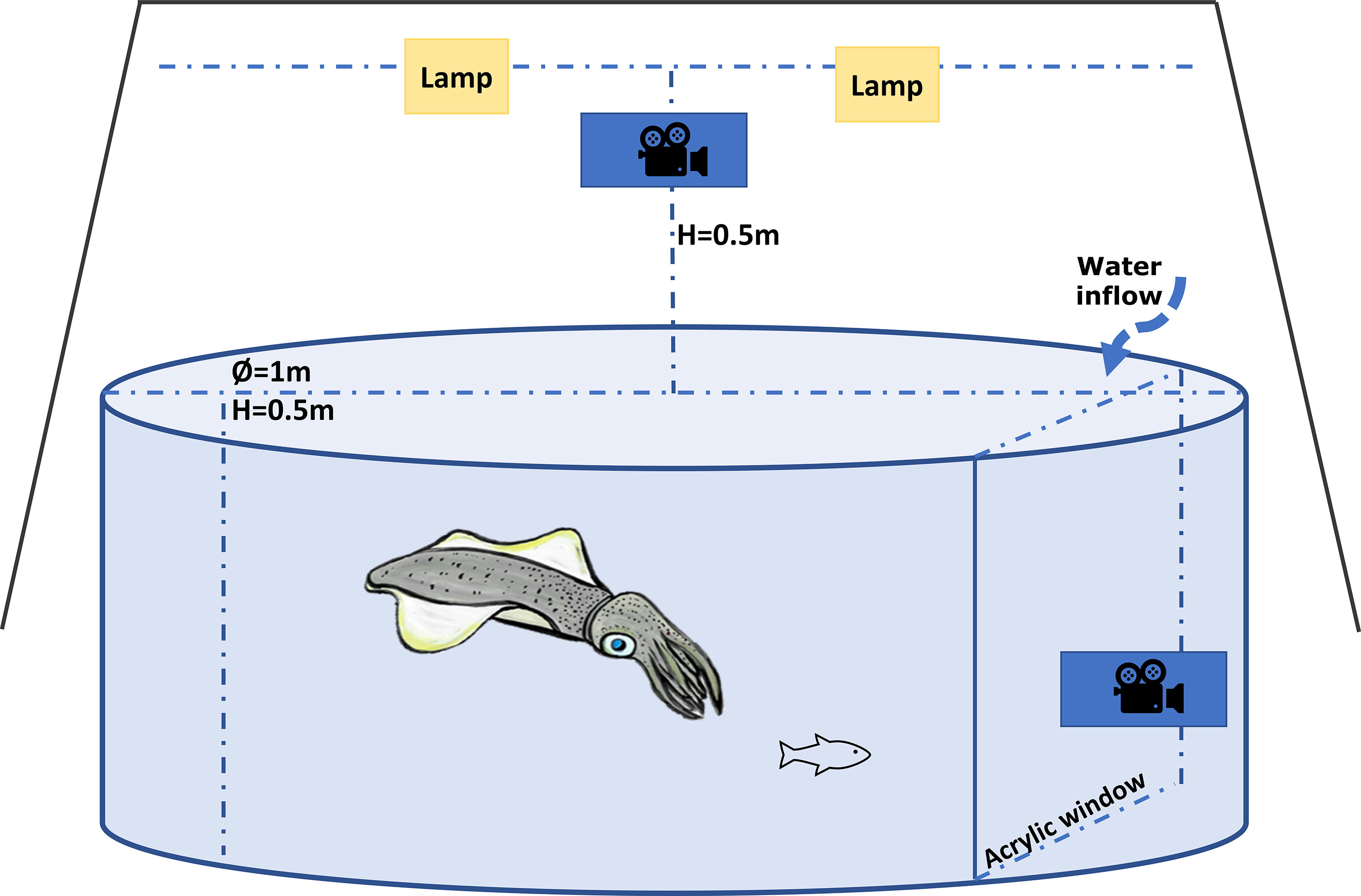
Figure 1 Diagram of the experimental arena used to measure predatory behaviour in southern calamari (examples of the video recording of interaction predatory-prey trial can be found in the Supplementary Materials).
Prior to behavioural trials, squid were exposed to metabolic activity trials for 22 hours refer to Peinado (2021) for more information on the methodology. During these trials, squid were exhausted in order to obtain the maximum metabolic rate (MMR), with squid swimming until they could no longer maintain equilibrium. As a result, mortality increased at higher temperature treatments, reducing the sample size to 38 squid across all the treatments, resulting in an unbalanced design (n = 6, 10, 10, 5 and 7 individuals at 13°C, 16°C 19°C, 22°C and 25°C, respectively). Additionally, individuals that showed unusual behaviour in the arena, such as curled arms or resting on the bottom for periods longer than two minutes were discarded from the trials.
To begin each trial, a single squid was moved from the respirometry chamber, placed in the behavioural arena and allowed to acclimate for 1 hour. Predatory trials started within 13-14 hours after exhaustive exercise was conducted. Furthermore, to increase hunger levels they were fasted for 47 hours (24 h starvation + 22 h of metabolic experiments + 1 h acclimation) prior the predator–prey trial commencing. Cameras began recording two minutes before the prey was introduced to record squid activity. A single live prey (Australian salmon) was then placed into the arena, and the resulting interaction was recorded for a further 15 minutes. Individual fish were always introduced into the tank in the same position with less than a second of air-exposure before the individual was placed in the behavioural arena. Once the fish was introduced into the tank, squid could immediately attack the prey. In the event that a squid did not eat the prey during this period, it was removed immediately and placed back into the holding tank. This period was chosen due to our previous observations as well as other studies (Sugimoto and Ikeda, 2013; Spady et al., 2018), where squid are generally able to capture their prey within 10 minutes. All the Australian salmon (103 ± 27.6 mm in length) used in the experiments were acclimated for one week to the same water temperature and conditions as the particular squid treatment in question.
2.3 Behavioural analysis
The behavioural parameters chosen for this study were established in previous studies as good proxies of squid predation behaviour (Jantzen and Havenhand, 2003; Sugimoto and Ikeda, 2013; Spady et al., 2018).
Individual behavioural parameters observed included:
● Number of attacks – number of attempts the squid made to capture the prey.
● Latency time to attack (min) – time between the introduction of the prey in the arena and the first attack.
● Attack distance (mm) – the distance between the end of the arms (immediately before the tentacle’s extension) and the prey (Figure 2).
● Tentacle elongation (mm) – the length of the tentacles when fully extended to capture the prey. To measure tentacle elongation and attack distances ImageJ software was use it. For that, images were extracted for the videos and three measures of the distance were taken. The data present here is the mean of the three replication measures.
● Attack direction/position – the orientation of the squid’s body in relation to the prey when the attack occurred. Attack position was classified as ‘horizontal arms’, ‘downward pointing’ and ‘upward pointing’. The ‘Horizontal arms’ position is when the arms and tentacles are held together in a horizontal body plan. ‘Downward pointing’ and ‘upward pointing’ postures are defined as the orientation when the whole body exceeded 45° from the horizontal plane in the corresponding direction (Jantzen and Havenhand, 2003) (Figure 2).
● Body pattern – display of body pigmentation once in the attack position, categorised as ‘clear (transparent)’, where chromatophores are reduced in size, and ‘dark’, where most of the chromatophores are expanded (Jantzen and Havenhand, 2003; York and Bartol (2016)).
● Handling time – the time that squid took to kill the prey.
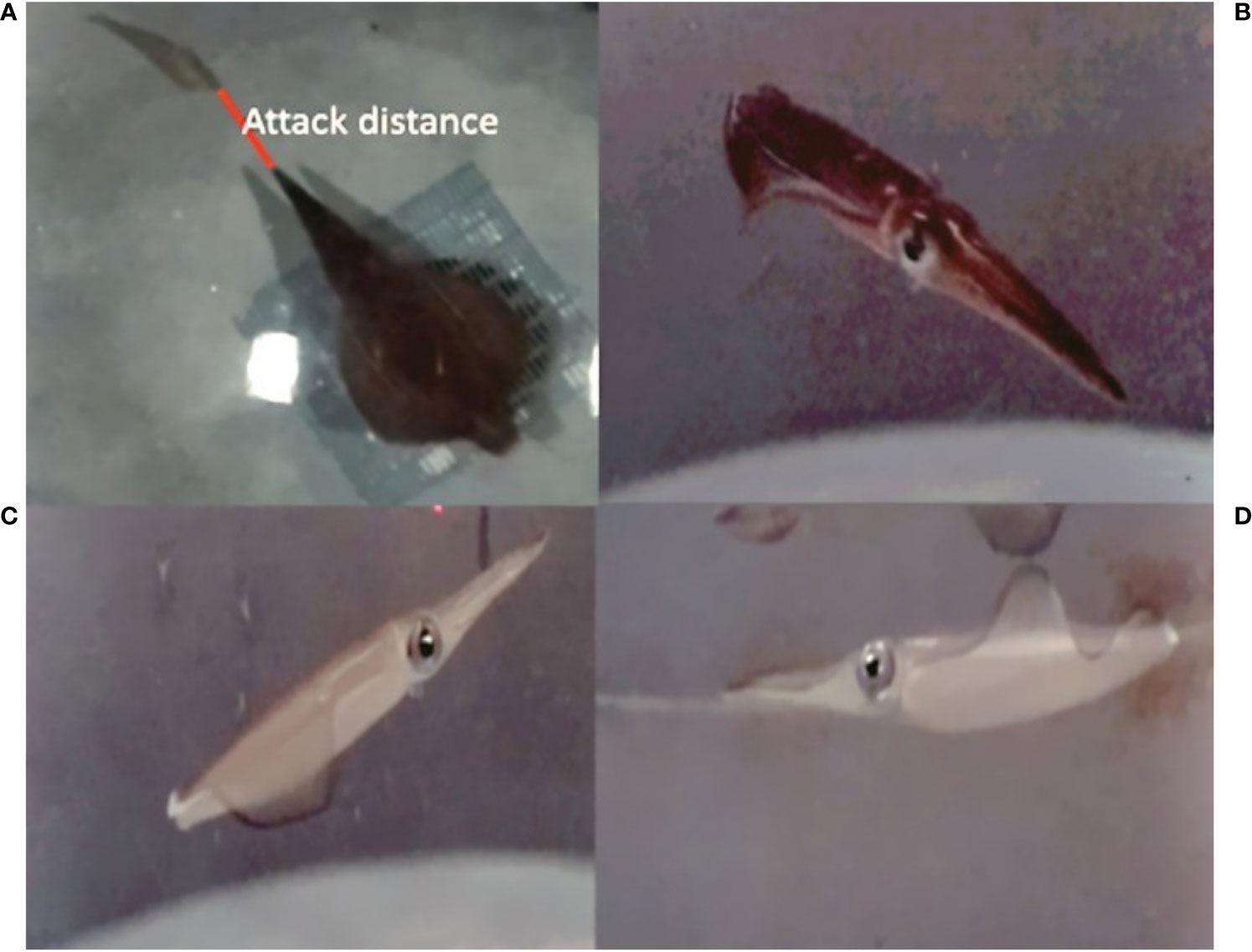
Figure 2 Southern calamari (Sepioteuthis australis) parameters observed in the experimental arena; (A) Attack distance and body pattern ‘dark’, (B) ‘downward pointing’ attack position, (C) ‘upward pointing’ attack position, (D) ‘horizontal arms’ attack position.
Additionally, the proportion of squid that attacked the prey, captured the prey, and captured the prey on the first attempt were recorded.
2.4 Data analysis
R software (www.r-project.org) was used to perform all the statistical analysis in this study. Generalized linear models (GLMs) with a binomial distribution and a logic link function were used to investigate the relationship between acclimation temperature (13°C, 16°C, 19°C, 22°C and 25°C) and the proportion of squid that attacked the prey and the capture success. GLMs with a negative binomial distribution were used to compare the effect of acclimation temperature on the number of strike attempts. Preliminary analyses were performed for those variables using generalized linear mixed models (GLMM), including capture group (date and location caught) and acclimation days as random effects. The variance and standard deviation were zero and consequently, they were dropped from the model, fitting simple GLM models.
For the remainder of the behavioural performance measures (attack position, distance, body pattern, latency and handling time) results were only examined for the individuals that successfully captured the prey. As a result of the small numbers of squid attacking the prey (n=1) at lower temperature treatments (13°C and 16°C), both these treatments were necessarily eliminated from further statistical analysis. GLMs with a binomial logistic regression were used to examine the relationship between acclimation temperature, the ability to capture the prey at the first strike, and body pattern, where the attack position was examined using a multinomial logistic regression. To investigate the relationship between attack distance, and tentacle elongation, GLMs with a gaussian distribution and a log link were used, and GLMs with a gamma distribution and a log link were used to model latency and handling time.
Additionally, squid mantle length and total weight were included together with the acclimation temperature as a factor as well as the relation between predator and prey size in all models. Those factors show no statistical differences across variables. Akaike information criterion (AIC) values were used to establish the best fit of the model for each measure of performance (data not shown), and model assumptions were verified by examining residuals compared to the fitted values by inspection of the residual-fit plots.
3 Results
Acclimation temperature had a significant effect on the probability of a squid making an attack (X2 = 12.4; df=1, P <0.0001), as well as the capture success (X2 = 7.1; df= 1; P <0.001). The proportion of squid that attacked the prey increased substantially between treatments. Around 30% of the squid acclimated to lower temperatures (13°C and 16°C) made an attack strike, increasing to 80% at 19°C and 22°C, and reaching 100% at 25°C (Figure 3). Similar percentages were observed in the proportion of squid that successfully captured the prey, with 16.6% and 10% of the squid capturing the prey at 13°C and 16°C respectively, compared to 50% at 19°C, 80% at 22°C and 100% at 25°C. The number of strikes also increased significantly with acclimation temperature (X2 = 4.8; df=1, P <0.05) (Figure 3), where 71.4% of the individuals at 25°C strike the prey more than twice in compared with 16% at 13°C, 20% at 16°C and 22°C and 40% at 19°C.
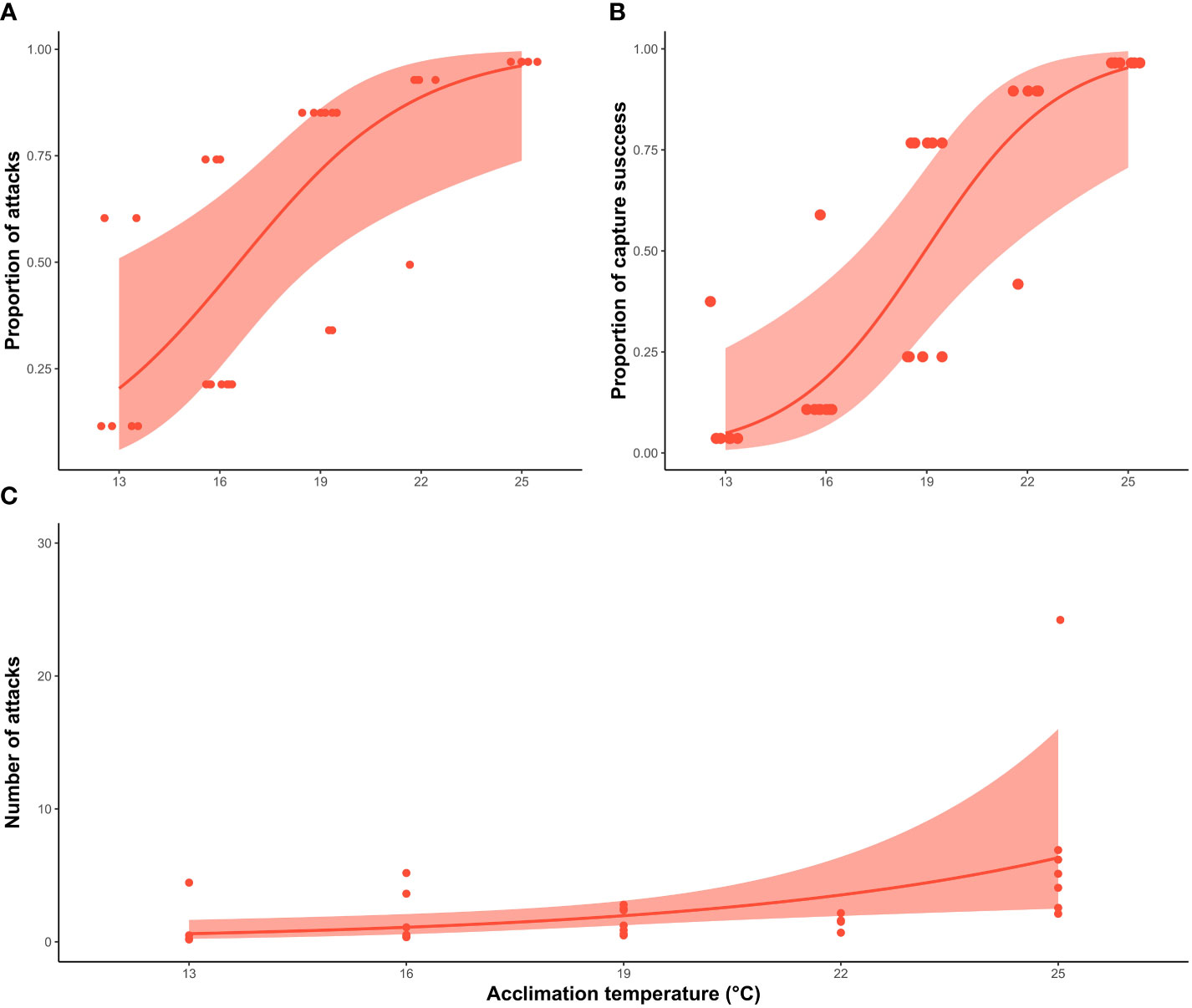
Figure 3 Relationship between behavioural responses of southern calamari (Sepioteuthis australis) and the five different acclimation temperatures (13, 16, 19, 22 and 25°C). (A) GLM with binomial distribution of the probability that individual squid will make an attack. (B) GLM with binomial distribution, of capture success of squid per treatment (C) GLM with a negative binomial distribution modelling the total number of strike attempts, including when squid were not successful. The thick line represents the expected value of the model for the variable measured. The shadow band corresponds to the 95% confidence interval, and the individual squid values are represented by the red points.
For individuals that caught the prey at 19°C, 22°C and 25°C, there were no statistical differences in the proportion of squid that successfully captured the fish at the first strike attempt (X2 = 1.4; df=1, P >0.05). Although an overall decrease occurred with temperature from 60% (3 out 5 individual) at 19°C, and 75% (3 out 4 individuals) at 22°C, to 28.5% (2 out 7 squid) at 25°C. The lack of statistical significance could be related to the low sample size. Latency and handling time were also different among treatments (X2 = 57.3; df=1, P <0.001; X2 = 24.3; df=1, P <0.001). At higher temperatures (22°C and 25°C), the time that squid took to initiate the attack was reduced compared to individuals acclimated at 19°C, in contrast to the increase in handling time at higher temperatures (Figure 4). At 19°C, squid took between 1 and 11 min to attack, whereas squid at 22°C and 25°C started the attack only seconds after the prey had been placed in the arena (0.15 min ± 0.08; 0.07 min ± 0.01, respectively). Furthermore, individuals at 19°C only handled the prey for a mean of 0.5 min (± 0.3 SD), rising to 1.5 min (± 0.6 SD) and 2.2 min (± 0.7 SD) in squid acclimated to 22°C and 25°C, respectively. The distance from which squid started the attack, and the elongation of tentacles, also significantly differed across temperature treatments (X2 = 10.3; df=1, P <0.01; X2 = 8.7; df=1, P <0.01), increasing with acclimation temperature (Figure 5). Individuals at 19°C chose to be closer to the prey when the attack occurred (66.1 ± 30 mm), compared with individuals at 22°C (96.5 ± 30.9 mm) and 25°C (106.1 ± 24.3 mm). Moreover, the elongation of the tentacles was also shorter at 19°C (78.9 ± 36.1 mm), while it was 102.1 mm (± 24.6) at 22°C and 108.5 mm (± 14.6) at 25°C.
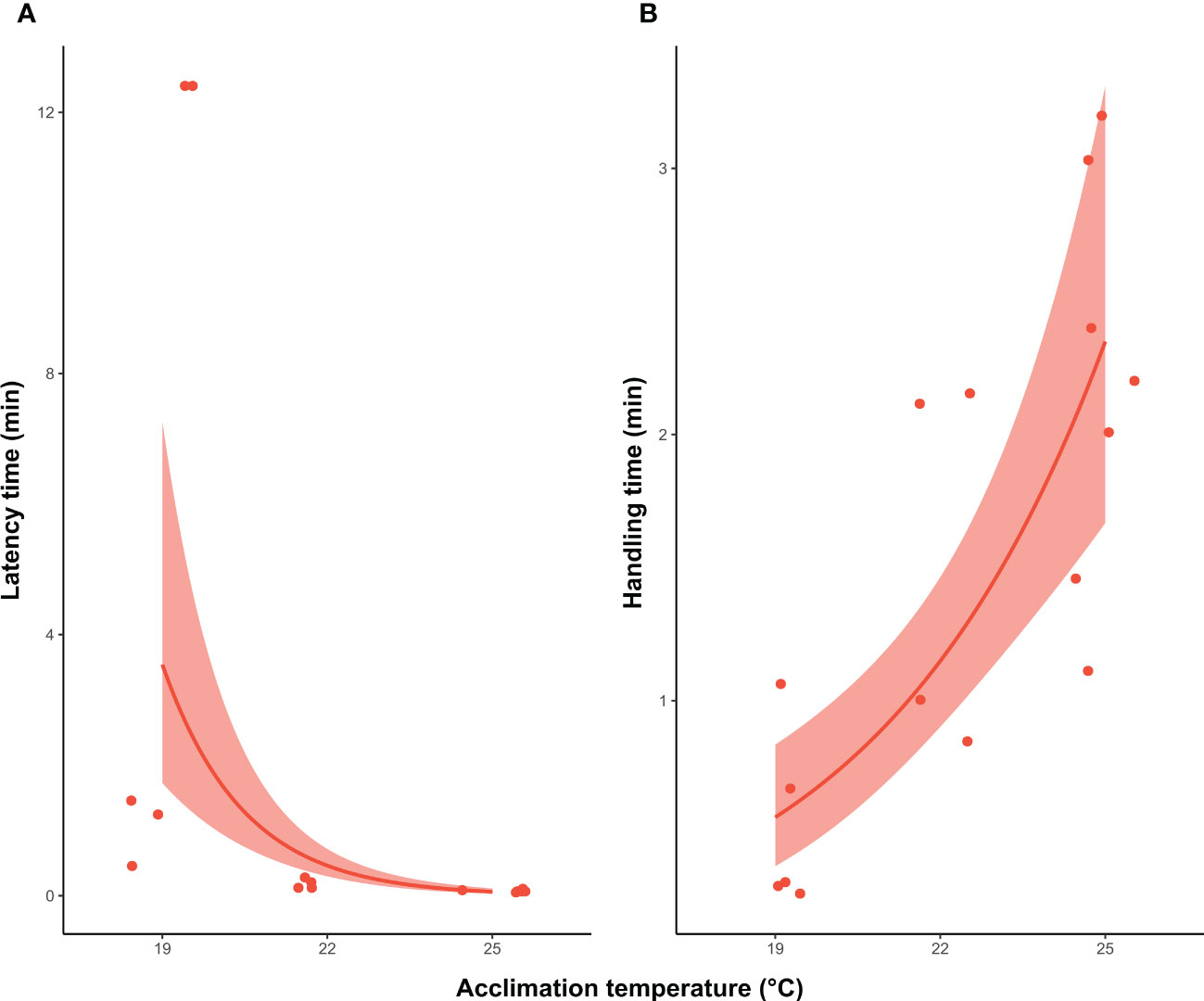
Figure 4 Southern calamari (Sepioteuthis australis) which successfully attack prey at different temperatures of acclimation (19, 22 and 25°C). (A) GLM model with a gamma distribution of latency time (min) that squid took to make the first attack (B) GLM with a gamma distribution of the handling time (min) to capture and kill the prey. Thick line represents the expected value from the model for the variable measure. The shadow band corresponds to the 95% confidence interval, and the individual values are represented by the red points.
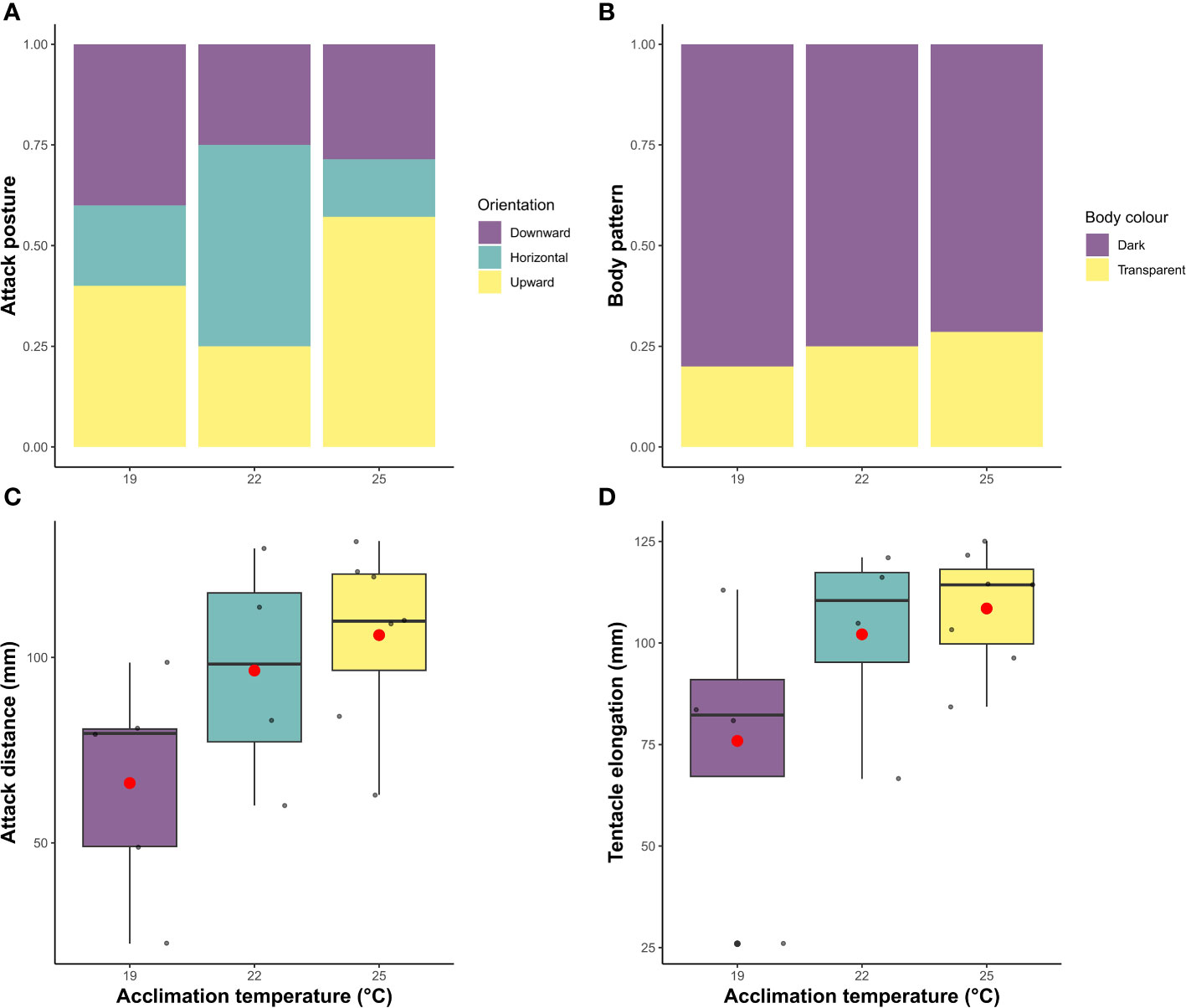
Figure 5 Behavioural measures of southern calamari (Sepioteuthis australis) that successfully attacked prey at different temperatures of acclimation (19, 22 and 25°C). (A) the proportion of squid exhibiting the different positions of attack (downward, horizontal arms, and upward). (B) the proportion of squid exhibiting different body display patterns (dark and transparent). (C) boxplots of the attack distance (mm) and (D) tentacle elongation (mm). In the boxplot, points indicate individual values and boxes represent the first and third quartiles. Within each box, the median is represented by the solid line and the mean by the red point.
Neither attack position nor body color choice differed between treatments (X2 = 2.0; df=2 P=0.7; X2 = 0.11; df=1 P=0.73). However, the preferred body pattern of southern calamari during the attack, across the three treatments, was the dark color display, with 80%, 75% and 71% of the squid choosing it at 19°C, 22°C, and 25°C respectively (Figure 5). Additionally, there was no effect of mantle length or squid body weight for any of the behavioural parameters (data not shown).
4 Discussion
Southern calamari predatory behaviour differed depending on acclimation temperature, suggesting a potentially major influence of temperature on predator-prey dynamics under current and future climate change. Two of the most notable differences between temperature treatments was the proportion of squid attacking the prey and the capture success, with both measures increasing with the temperature of acclimation. All individuals acclimated to 25°C successfully attacked and captured 100% of prey provided, whereas at low temperatures (13°C and 16°C) only 30% of squid attacked the prey and with less than 16% success. These results suggest that environmental temperature has a profound effect on the predatory behaviour of southern calamari, with greater consumption rate at higher acclimation temperatures. This could be a function of physiological changes that affect the squid’s ability to recognize and strike prey, however, it is likely linked to increased metabolic demand at higher temperatures. Cephalopods are well known for having a high metabolic rate (O’Dor and Webber, 1986; O’Dor et al., 2002), and in a previous study, metabolic activity (routine metabolic rates) of southern calamari increased with temperature by 30% at 25°C (471.1 ± 91.7 mg O2 h-1 kg-, mean ± SD) compared to 13 and 16°C (312.2 ± 112.8 and 324.3 ± 37.1 mg O2 h-1 kg-1, respectively) (Peinado, 2021). Squid will thus be expected to have a higher energetic requirement to support basal and maintenance processes under future warming conditions (Peinado, 2021) and the predatory behaviour results observed here in the current study suggest that squid will need to increase food consumption rates to compensate for the energetic costs associated with living at elevated temperatures. Similar results have been found in other species of marine fish (Bethea et al., 2007; Grigaltchik et al., 2012) and invertebrates (Morón Lugo et al., 2020), where individuals at elevated acclimation temperatures were more motivated to attack than those at lower temperatures.
The decrease in number of individuals that attack at lower temperature (13°C and 16°C) may also be a consequence of a reduction decreased (~ 30%) in southern calamari metabolism (Peinado, 2021), having reduced energy/feeding requirements and possibly saving energy by not trying to capture the prey. It has also been suggested that similar reductions in other species of squid could be linked to the depression of metabolic rates due to environmental factors (Spady et al., 2018). Additionally, even if southern calamari across Tasmania experience minimum temperatures of 10°C, 13°C could be at the lower end of their thermal window which might limit their fitness and other physiological performances (e.g., olfactory clues or vision), triggering the no or slow response of squid here. Yet, due to the limited understanding of the lower thermal limits and their associated performance in cephalopods, it is difficult establish the main cause of the attack decrease. Future research should consider the role of cold adaptation in squid to identify if cephalopods respond with the same magnitude to cold or warm acclimation temperatures.
Australian salmon were also acclimated to the same temperature as the squid, which might influence the prey performance during the experiments, as temperature could influence locomotion in ectotherms (Angilletta et al., 2002) as well as escape responsiveness (Preuss and Faber, 2003). Here, we attempted to measure prey response to the squid attack, however, in many of the cases, the squid strike was successful at the very first attempt eliminating the opportunity for the prey to react and consequently the potential to record meaningful prey response data.
Even with the limitations of our experiment, behavioural differences were found in the squid which attacked the prey at the different temperature treatments (19°C, 22°C and 25°C). An increase in acclimation temperature greatly reduced the latency time of squid to attack the prey. At 22°C and 25°C, individuals strike the prey in seconds, whereas at 19°C squid took between 1 and 11 min to attack. Time until first attack could be interpretated as measure of predation motivation (Grigaltchik et al., 2012), consequently results here suggest the motivation of squid to make an attack is directly influenced by temperature. In contrast, the handling time that squid needed to kill the prey increased with temperature, rising to over a min at 22°C and above 2 min at 25°C compared with a 0.5 min at 19°C. Furthermore, the capacity of squid to successfully strike the prey on the first attempt was also reduced at 25°C, with only 28% (2 out of 7) of squid capturing the prey in the first attempt compared with 60% and 75% at 19°C and 22°C, respectively. These results indicate that the physical capabilities of squid might be impacted at elevated temperatures, reducing their ability to handle (capture and kill) the prey effectively, although at this time, any potential prey co-response cannot be assessed. In other marine invertebrates, for example the Antarctic sea star (Odontasted validus), some physical abilities such as motor coordination or speed were also reduced at increased temperatures (Kidawa et al., 2010). Findings of a possible decrease in capture efficiency and increased prey handling time suggest that further research should consider the energetic balances and trade-offs involved in the need for increased predation among squid at elevated temperatures. Nevertheless, the effect of acclimation temperature on the prey could potentially be linked with the increase in handling time and the success rate of strikes on the first attempt, as higher temperatures may increase prey mobility. Future studies exploring these effects could examine how changes in water conditions affect the prey, to determine if those effects could be influencing squid ability to capture their prey.
Attack distance and tentacle elongation of squid increased at higher temperatures; this could also be related to the impatience of squid to capture the prey instead of a predatory strategy per se. Yet, greater attack distance could be beneficial for the squid if prey mobility is increased due to warmer waters by better disguising the incoming attack and reducing the chances of escape. In some cephalopod species, environmental stresses like acidification also lead to an increase in striking distances (Spady et al., 2018). Regarding their other predatory tactics, neither the attack direction nor body pattern showed differences between temperature treatments. However, across treatments 19°C, 22°C and 25°C, the most preferable body pattern was a dark color. This pattern (banded or totally dark) possibly acts as a disrupting coloration, distracting the attention from the extending tentacles (York and Bartol, 2016; Hanlon and Messenger, 2018) which might confuse the prey, and confer an advantage to the squid.
As the metabolic demands of squid are likely to increase due to ocean warming, in situ individuals might have different options for maintaining their energetic balance and overall fitness including, for example, consuming prey with higher energetic/nutritional content or increasing their feeding rates (Horwitz et al., 2020). If the individuals choose the latter option, squid may be more willing to actively search for prey, perhaps resulting in bolder behaviour and exposing them to a higher predation risk (Biro and Stamps, 2010; Killen et al., 2011; Careau and Garland, 2012; Cornwell et al., 2020). If individuals are more active, more energy would be allocated to locomotion, limiting energy available for other important traits and processes such as reproduction or growth. Furthermore, the metabolic thermal optimum (Topt) indicated by the aerobic scope for this species is between 19°C and 22°C (Peinado, 2021), beyond that point individual performance maybe be limited (Peck et al., 2009; Pörtner and Peck, 2010).
Modifications to predatory performance could alter the outcome of predator–prey interactions, potentially resulting in a cascade effect throughout food webs (Warren et al., 2017). Cephalopods are important and opportunistic predators, where changes in their feeding rates could have significant consequences for the structure of ecosystems (Spady et al., 2018; de la Chesnais et al., 2019). Southern calamari are likely to increase feeding intake rates as ocean warming continues in Tasmanian waters, putting greater pressure on their prey and potentially triggering a cascade effect on the food web, altering community and ecosystem stability.
To date, in an ocean warming context, information on feeding rates (especially in the wild), energy budgets and prey preferences in cephalopods is limited and future research could further examine these key concepts to better understand species performance under the various climate change scenarios. In the experiments reported here, trends across treatments were clear and consistent. However, further work could expand our results by exploring differences with sex, life stages and growth rate. This would be influential in more accurately predicting the responses of this critical animal group to future environmental challenges. Moreover, marine ecosystems are not only being altered by ocean warming, including changes in size structures (Audzijonyte et al., 2020), but also acidification, pollution, changes in productivity, and oxygen content, as well as overfishing (Hoegh-Guldberg and Bruno, 2010; Payne et al., 2016). The physical capacities of other species of squid to capture their prey were reportedly not impacted by environmental stressors such as acidification, although their decision-making processes and strategies have been shown to be affected (Spady et al., 2014; Spady et al., 2018) However, we have an extremely limited understanding of how multiple stressors will combine to impact the predatory behaviour of this group – an important gap in our knowledge of how future squid populations will respond to climate change over coming decades(Pecl and Jackson, 2008).
5 Conclusion
Ocean warming will modify ecosystems by affecting the interactions of existing predator–prey combinations, as well as creating novel ones as new species enter regions and existing species depart (Pecl et al., 2017; Bonebrake et al., 2018), In this context, it is important to examine how species predatory performance alters due to environmental changes (Twiname et al., 2020). Our study demonstrated that environmental temperature has a major impact on the behaviour of southern calamari. Overall, our findings indicate that elevated temperatures, in line with those predicted for the future, could alter the predatory behaviour of southern calamari by increasing consumption rates, as well as potentially increasing the number of attempts they need to capture their prey, and their prey handling times. Individuals would then need to be more active to capture prey to maintain energetic balance and as a result they will be more exposed to predation risk, as well as experiencing greater trade-offs.
Data availability statement
The original contributions presented in the study are includedin the article/Supplementary Material. Further inquiries can bedirected to the corresponding authors.
Ethics statement
The animal study was reviewed and approved by University of Tasmania Animal Ethics Committee (A0017463).
Author contributions
PP, QF, JS, ST, and GP designed the study. PP conducted the experiments, analyzed the data and drafted the first manuscript. QF, JS, ST, and GP reviewed and edited the manuscript. All authors contributed to the article and approved the submitted version.
Funding
This work was supported by the Australian Research Council (FT140100596 to GP).
Conflict of interest
The authors declare that the research was conducted in the absence of any commercial or financial relationships that could be construed as a potential conflict of interest.
Publisher’s note
All claims expressed in this article are solely those of the authors and do not necessarily represent those of their affiliated organizations, or those of the publisher, the editors and the reviewers. Any product that may be evaluated in this article, or claim that may be made by its manufacturer, is not guaranteed or endorsed by the publisher.
Supplementary material
The Supplementary Material for this article can be found online at: https://www.frontiersin.org/articles/10.3389/fmars.2023.1113984/full#supplementary-material
Table S.1 | Data form this study.
References
Amarasekare P. (2015). Effects of temperature on consumer-resource interactions. J. Anim. Ecol. 84 (3), 665–679. doi: 10.1111/1365-2656.12320
Angilletta M. J., Niewiarowski P. H., Navas C. A. The evolution of thermal physiology in ectotherms. J. Thermal Biol. (2002) 27 (4), 249–268. doi: 10.1016/S0306-4565(01)00094-8
Audzijonyte A., Richards S. A., Stuart-Smith R. D., Pecl G., Edgar G. J., Barrett N. S., et al. Fish body sizes change with temperature but not all species shrink with warming. Nat. Ecol. Evol. (2020) 4 (6), 809–814. doi: 10.1038/s41559-020-1171-0
Bethea D. M., Hale L., Carlson J. K., Cortés E., Manire C. A., Gelsleichter J. (2007). Geographic and ontogenetic variation in the diet and daily ration of the bonnethead shark, sphyrna tiburo, from the eastern gulf of Mexico. Mar. Biol. 152 (5), 1009–1020. doi: 10.1007/s00227-007-0728-7
Biro P. A., Stamps J. A. (2010). Do consistent individual differences in metabolic rate promote consistent individual differences in behavior? Trends Ecol. Evol. 25 (11), 653–659. doi: 10.1016/j.tree.2010.08.003
Bonebrake T. C., Brown C. J., Bell J. D., Blanchard J. L., Chauvenet A., Champion C., et al. (2018). Managing consequences of climate-driven species redistribution requires integration of ecology, conservation and social science. Biol. Rev. 93 (1), 284–305. doi: 10.1111/brv.12344
Careau V., Garland T. Jr (2012). Performance, personality, and energetics: Correlation, causation, and mechanism. Physiol. Biochem. Zoology 85 (6), 543–571. doi: 10.1086/666970
Chen R. S., Portner E. J., Choy C. A. (2022). Gelatinous cephalopods as important prey for a deep-sea fish predator. Mar. Biol. 169 (12), 155. doi: 10.1007/s00227-022-04116-w
Cornwell T. O., McCarthy I. D., Biro P. A. (2020). Integration of physiology, behaviour and life history traits: personality and pace of life in a marine gastropod. Anim. Behav. 163, 155–162. doi: 10.1016/j.anbehav.2020.03.009
de la Chesnais T., Fulton E. A., Tracey S. R., Pecl G. T. (2019). The ecological role of cephalopods and their representation in ecosystem models. Rev. Fish Biol. Fisheries 29 (2), 313–334. doi: 10.1007/s11160-019-09554-2
Dell A. I., Pawar S., Savage V. M. (2014). Temperature dependence of trophic interactions are driven by asymmetry of species responses and foraging strategy. J. Anim. Ecol. 83, 70–84. doi: 10.1111/1365-2656.12081
Doney S. C., Ruckelshaus M., Emmett Duffy J., Barry J. P., Chan F., English C. A., et al. (2012). Climate change impacts on marine ecosystems. Annu. Rev. Mar. Sci. 4, 11–37. doi: 10.1146/annurev-marine-041911-111611
Gilbert B., Tunney T. D., McCann K. S., Delong J. P., Vasseur D. A., Savage V., et al. (2014). A bioenergetic framework for the temperature dependence of trophic interactions. Ecol. Lett. 17 (8), 902–914. doi: 10.1111/ele.12307
Gilman S. E., Urban M. C., Tewksbury J., Gilchrist G. W., Holt R. D. (2010). A framework for community interactions under climate change. Trends Ecol. Evol. 25 (6), 325–331. doi: 10.1016/j.tree.2010.03.002
Grigaltchik V. S., Ward A. J. W., Seebacher F. (2012). Thermal acclimation of interactions: Differential responses to temperature change alter predator-prey relationship. Proc. R. Soc. B: Biol. Sci. 279 (1744), 4058–4064. doi: 10.1098/rspb.2012.1277
Hanlon R. T., Messenger J. B. (2018). “Feeding and foraging,” in Cephalopod behaviour, 2 ed. Eds. Messenger J. B., Hanlon R. T. (Cambridge: Cambridge University Press), 74–96.
Higgins F. A., Bates A. E., Lamare M. D. (2012). Heat tolerance, behavioural temperature selection and temperature-dependent respiration in larval octopus huttoni. J. Thermal Biol. 37 (1), 83–88. doi: 10.1016/j.jtherbio.2011.11.004
Hobday A. J., Pecl G. T. (2014). Identification of global marine hotspots: sentinels for change and vanguards for adaptation action. Rev. Fish Biol. Fisheries 24 (2), 415–425. doi: 10.1007/s11160-013-9326-6
Hoegh-Guldberg O., Bruno J. F. (2010). The impact of climate change on the world's marine ecosystems. Science 328 (5985), 1523–1528. doi: 10.1126/science.1189930
Horwitz R., Norin T., Watson S. A., Pistevos J. C. A., Beldade R., Hacquart S., et al. (2020). Near-future ocean warming and acidification alter foraging behaviour, locomotion, and metabolic rate in a keystone marine mollusc. Sci. Rep. 10 (1), 5461. doi: 10.1038/s41598-020-62304-4
IPCC (2022). Climate change 2022: Impacts, adaptation, and vulnerability. contribution of working group II to the sixth assessment report of the intergovernmental panel on climate change. Pörtner H.-O., Roberts D. C., Tignor M., Poloczanska E. S., Mintenbeck K., Alegría A. , editors. Cambridge, UK and New York, NY, USA: Cambridge University Press (2022). p. 3056. doi: 10.1017/9781009325844
Jantzen T. M., Havenhand J. N. (2003). Reproductive behavior in the squid sepioteuthis australis from south Australia: Ethogram of reproductive body patterns. Biol. Bull. 204 (3), 290–304. doi: 10.2307/1543600
Jeschke J. M., Kopp M., Tollrian R. (2002). Predator functional responses: Discriminating between handling and digesting prey. Ecol. Monogr. 72 (1), 95–112. doi: 10.1890/0012-9615(2002)072[0095:PFRDBH]2.0.CO;2
Johnson C. R., Banks S. C., Barrett N. S., Cazassus F., Dunstan P. K., Edgar G. J., et al. (2011). Climate change cascades: Shifts in oceanography, species' ranges and subtidal marine community dynamics in eastern Tasmania. J. Exp. Mar. Biol. Ecol. 400 (1-2), 17–32. doi: 10.1016/j.jembe.2011.02.032
Kidawa A., Potocka M., Janecki T. (2010). The effects of temperature on the behaviour of the Antarctic sea star odontaster validus. Polish Polar Res. 31 (3), 273–284. doi: 10.2478/v10183-010-0003-3
Killen S. S., Marras S., McKenzie D. J. (2011). Fuel, fasting, fear: routine metabolic rate and food deprivation exert synergistic effects on risk-taking in individual juvenile European sea bass. J. Anim. Ecol. 80 (5), 1024–1033. doi: 10.1111/j.1365-2656.2011.01844.x
Last P. R., White W. T., Gledhill D. C., Hobday A. J., Brown R., Edgar G. J., et al. (2011). Long-term shifts in abundance and distribution of a temperate fish fauna: a response to climate change and fishing practices. Global Ecol. Biogeography 20 (1), 58–72. doi: 10.1111/j.1466-8238.2010.00575.x
Lienart G. D. H., Mitchell M. D., Ferrari M. C. O., McCormick M. I. (2014). Temperature and food availability affect risk assessment in an ectotherm. Anim. Behav. 89, 199–204. doi: 10.1016/j.anbehav.2013.12.031
Moltschaniwskyj N. A., Steer M. A. (2004). Spatial and seasonal variation in reproductive characteristics and spawning of southern calamary (Sepioteuthis australis): Spreading the mortality risk. ICES J. Mar. Sci. 61 (6), 921–927. doi: 10.1016/j.icesjms.2004.06.007
Morón Lugo S. C., Baumeister M., Nour O. M., Wolf F., Stumpp M., Pansch C. (2020). Warming and temperature variability determine the performance of two invertebrate predators. Sci. Rep. 10 (1), 6780. doi: 10.1038/s41598-020-63679-0
Neumeister H., Ripley B., Preuss T., Gilly W. F. (2000). Effects of temperature on escape jetting in the squid loligo opalescens. J. Exp. Biol. 203 (3), 547–557. doi: 10.1242/jeb.203.3.547
O’Dor R. K., Webber D. M. The constraints on cephalopods: why squid aren't fish. Can. J. Zoology (1986) 64 (8), 1591–1605. doi: 10.1139/z86-241
O’Dor R. K., Adamo S., Aitken J. P., Andrade Y., Finn J., Hanlon R. T., et al. Currents as environmental constraints on the behavior, energetics and distribution of squid and cuttlefish. Bull. Mar. Sci. (2002) 71 (2), 601–617.
Öhlund G., Hedström P., Norman S., Hein C. L., Englund G. (2015). Temperature dependence of predation depends on the relative performance of predators and prey. Proc. R. Soc. B: Biol. Sci. 282 (1799), 20142254. doi: 10.1098/rspb.2014.2254
Oliver E. C. J., O'Kane T. J., Holbrook N. J. (2015). Projected changes to Tasman Sea eddies in a future climate. J. Geophysical Research: Oceans 120 (11), 7150–7165. doi: 10.1002/2015JC010993
Paulin C. (1993). Review of the Australasian fish family arripididae (Percomorpha), with the description of a new species. Mar. Freshw. Res. 44 (3), 459–471. doi: 10.1071/MF9930459
Payne J. L., Bush A. M., Heim N. A., Knope M. L., McCauley D. J. (2016). Ecological selectivity of the emerging mass extinction in the oceans. Science 353 (6305), 1284–1286. doi: 10.1126/science.aaf2416
Peck L. S., Clark M. S., Morley S. A., Massey A., Rossetti H. (2009). Animal temperature limits and ecological relevance: effects of size, activity and rates of change. Funct. Ecol. 23 (2), 248–256. doi: 10.1111/j.1365-2435.2008.01537.x
Pecl G. (2004). The in situ relationships between season of hatching, growth and condition in the southern calamary, sepioteuthis australis. Mar. Freshw. Res. 55 (4), 429–438. doi: 10.1071/MF03150
Pecl G. T., Araújo M. B., Bell J. D., Blanchard J., Bonebrake T. C., Chen I. C., et al. (2017). Biodiversity redistribution under climate change: Impacts on ecosystems and human well-being. Science 355 (6332). doi: 10.1126/science.aai9214
Pecl G. T., Jackson G. D. (2008). The potential impacts of climate change on inshore squid: biology, ecology and fisheries. Rev. Fish Biol. Fisheries 18, 373–385. doi: 10.1007/s11160-007-9077-3
Pecl G. T., Moltschaniwskyj N. A., Tracey S. R., Jordan A. R. (2004). Inter-annual plasticity of squid life history and population structure: Ecological and management implications. Oecologia 139 (4), 515–524. doi: 10.1007/s00442-004-1537-z
Pecl G. T., Ogier E., Jennings S., van Putten I., Crawford C., Fogarty H., et al. (2019). Autonomous adaptation to climate-driven change in marine biodiversity in a global marine hotspot. Ambio 48 (12), 1498–1515. doi: 10.1007/s13280-019-01186-x
Peinado P. (2021). Integrating physiology, behaviour and molecular mechanisms to understand impacts of ocean warming on southern calamari (Sepioteuthis australis) (University of Tasmania). Available at: https://eprints.utas.edu.au/45959/.
Pörtner H. O., Peck M. A. (2010). Climate change effects on fishes and fisheries: Towards a cause-and-effect understanding. J. Fish Biol. 77 (8), 1745–1779. doi: 10.1111/j.1095-8649.2010.02783.x
Preuss T., Faber D. S. (2003). Central cellular mechanisms underlying temperature-dependent changes in the goldfish startle-escape behavior. J. Neurosci. 23 (13), 5617–5626. doi: 10.1523/jneurosci.23-13-05617.2003
Rall B. C., Vucic-Pestic O., Ehnes R. B., EmmersoN M., Brose U. (2010). Temperature, predator-prey interaction strength and population stability. Global Change Biol. 16 (8), 2145–2157. doi: 10.1111/j.1365-2486.2009.02124.x
Ridgway K. R. (2007). Long-term trend and decadal variability of the southward penetration of the East Australian current. Geophysical Res. Lett. 34 (13). doi: 10.1029/2007GL030393
Sentis A., Hemptinne J. L., Brodeur J. (2012). Using functional response modeling to investigate the effect of temperature on predator feeding rate and energetic efficiency. Oecologia 169 (4), 1117–1125. doi: 10.1007/s00442-012-2255-6
Spady B. L., Munday P. L., Watson S.-A. (2018). Predatory strategies and behaviours in cephalopods are altered by elevated CO2. Global Change Biol. 24 (6), 2585–2596. doi: 10.1111/gcb.14098
Spady B. L., Watson S. A., Chase T. J., Munday P. L. (2014). Projected near-future CO2 levels increase activity and alter defensive behaviours in the tropical squid idiosepius pygmaeus. Biol. Open 3 (11), 1063–1070. doi: 10.1242/bio.20149894
Steer M. A., Pecl G. T., Moltschaniwskyj N. A. (2003). Are bigger calamary sepioteuthis australis hatchlings more likely to survive? a study based on statolith dimensions. Mar. Ecol. Prog. Ser. 261, 175–182. doi: 10.3354/meps261175
Steffan S. A., Snyder W. E. (2010). Cascading diversity effects transmitted exclusively by behavioral interactions. Ecology 91 (8), 2242–2252. doi: 10.1890/09-0787.1
Sugimoto C., Ikeda Y. (2013). Comparison of the ontogeny of hunting behavior in pharaoh cuttlefish (Sepia pharaonis) and oval squid (Sepioteuthis lessoniana). Mar. Biol. Lab. 225, 50–59. doi: 10.1086/BBLv225n1p50
Twiname S., Audzijonyte A., Blanchard J. L., Champion C., de la Chesnais T., Fitzgibbon Q. P., et al. (2020). A cross-scale framework to support a mechanistic understanding and modelling of marine climate-driven species redistribution, from individuals to communities. Ecography 43 (12), 1764–1778. doi: 10.1111/ecog.04996
Vergés A., McCosker E., Mayer-Pinto M., Coleman M. A., Wernberg T., Ainsworth T., et al. (2019). Tropicalisation of temperate reefs: Implications for ecosystem functions and management actions. Funct. Ecol. 33 (6), 1000–1013. doi: 10.1111/1365-2435.13310
Vergés A., Steinberg P. D., Hay M. E., Poore A. G. B., Campbell A. H., Ballesteros E., et al. (2014). The tropicalization of temperate marine ecosystems: Climate-mediated changes in herbivory and community phase shifts. Proc. R. Soc. B: Biol. Sci. 281 (1789), 20140846. doi: 10.1098/rspb.2014.0846
Villanueva R., Perricone V., Fiorito G. (2017). Cephalopods as predators: A short journey among behavioral flexibilities, adaptions, and feeding habits. Front. Physiol. 8 (AUG). doi: 10.3389/fphys.2017.00598
Warren D. T., Donelson J. M., McCormick M. I. (2017). Extended exposure to elevated temperature affects escape response behaviour in coral reef fishes. PeerJ 5 (8), e3652. doi: 10.7717/peerj.3652
Keywords: acclimation, cephalopods, ocean warming, predation, species interaction, squid
Citation: Peinado P, Fitzgibbon QP, Semmens JM, Tracey S and Pecl GT (2023) Understanding species responses in a changing world by examining the predatory behaviour of southern calamari to changes in temperature. Front. Mar. Sci. 10:1113984. doi: 10.3389/fmars.2023.1113984
Received: 02 December 2022; Accepted: 24 March 2023;
Published: 19 April 2023.
Edited by:
Rui Rosa, University of Lisbon, PortugalReviewed by:
Lloyd Trueblood, La Sierra University, United StatesManuel Nande, University of Porto, Portugal
Copyright © 2023 Peinado, Fitzgibbon, Semmens, Tracey and Pecl. This is an open-access article distributed under the terms of the Creative Commons Attribution License (CC BY). The use, distribution or reproduction in other forums is permitted, provided the original author(s) and the copyright owner(s) are credited and that the original publication in this journal is cited, in accordance with accepted academic practice. No use, distribution or reproduction is permitted which does not comply with these terms.
*Correspondence: Patricia Peinado, patricia.peinado@utas.edu.au