- 1College of Life and Environmental Sciences, University of Exeter Biosciences, Exeter, Devon, United Kingdom
- 2Operation Wallacea, Wallace House, Old Bolingbroke, Horncastle, Lincolnshire, United Kingdom
- 3School of Biological Sciences, University of Bristol, Bristol, United Kingdom
- 4Department of Applied Science, University of South Wales, Glyntaff Campus, Pontypridd, Rhondda Cynon Taff, United Kingdom
- 5Department of Biological Sciences, University of Alabama, Tuscaloosa, AL, United States
- 6Dauphin Island Sea Lab, Dauphin Island, AL, United States
Recreational SCUBA diving is widespread and increasing on coral reefs worldwide. Standard open-circuit SCUBA equipment is inherently noisy and, by seeking out areas of high biodiversity, divers inadvertently expose reef communities to an intrusive source of anthropogenic noise. Currently, little is known about SCUBA noise as an acoustic stressor, and there is a general lack of empirical evidence on community-level impacts of anthropogenic noise on coral reefs. Here, we conducted a playback experiment on Caribbean reefs to investigate impacts of SCUBA noise on fish communities and interspecific cooperation at ecologically important cleaning stations of the Pederson’s cleaner shrimp Ancylomenes pedersoni. When exposed to SCUBA-noise playback, the total occurrence of fishes at the cleaning stations decreased by 7%, and the community and cleaning clientele compositions were significantly altered, with 27% and 25% of monitored species being affected, respectively. Compared with ambient-sound playback, SCUBA-noise playback resulted in clients having to wait 29% longer for cleaning initiation and receiving 43% less cleaning; however, cheating, signalling, posing and time spent cleaning were not affected by SCUBA-noise playback. Our study is the first to demonstrate experimentally that SCUBA noise can have at least some negative impacts on reef organisms, confirming it as an ecologically relevant pollutant. Moreover, by establishing acoustic disturbance as a likely mechanism for known impacts of diver presence on reef animals, we also identify a potential avenue for mitigation in these valuable ecosystems.
1 Introduction
SCUBA diving is a multibillion-dollar industry and is one of the largest and fastest growing recreational sports globally, with over 28 million certified divers and one million new divers being certified annually (Lück, 2016; PADI, 2021). Because divers seek out areas of high biodiversity, and many reef organisms are small and visibility rarely exceeds 30 m, divers often move close to habitat and site-attached animals, meaning that this popular pastime can have negative impacts on coral reefs (Davenport and Davenport, 2006). SCUBA divers can cause physical damage to reef habitat (Hawkins and Roberts, 1993; Zakai and Chadwick-Furman, 2002; Giglio et al., 2020), but the mere presence of divers can also elicit stress and behavioural changes in marine mammals, fishes and invertebrates, thus affecting aquatic communities and disrupting ecosystem services (Curtin and Garrod, 2008; Lindfield et al., 2014; Titus et al., 2015a; Giglio et al., 2022). However, the mechanisms underpinning these detrimental diver-presence effects have not been established. Given that standard open-circuit SCUBA equipment is inherently noisy (Lobel, 2005; Radford et al., 2005), acoustic disturbance is a plausible but untested reason for organismal responses to diver presence.
Anthropogenic noise from a wide range of sources (e.g., pile-driving, sonar, shipping, motorboats) pervades almost all aquatic ecosystems (Duarte et al., 2021), with increasing evidence demonstrating a suite of negative impacts across many taxa (see reviews: Shannon et al., 2016; Cox et al., 2018; Kunc and Schmidt, 2019; Duarte et al., 2021). However, most of the research to date focuses on how underwater noise affects individual animals; there has been little investigation of how noise effects scale up to interspecific interactions and community compositions (Kunc and Schmidt, 2019). For example, only one aquatic study that we know of has considered community-level demographics (Nedelec et al., 2017), and only a small handful of studies have demonstrated that noise can alter interspecific relationships among fishes, such as predator–prey interactions (Simpson et al., 2016; Ferrari et al., 2018) and cooperative mutualisms (Nedelec et al., 2017).
While there is a paucity of investigations into community-level responses to noise in aquatic ecosystems, terrestrial anthropogenic noise (e.g., traffic noise near roads) has been shown to have a range of effects on avian communities, including to abundance, species richness and community structure (Francis et al., 2009; Slabbekoorn and Halfwerk, 2009; Herrera-Montes and Aide, 2011; Cooke et al., 2020). That body of work includes experimental application of traffic noise (a ‘phantom road’) to a roadless landscape, identifying noise as the principal mechanism for the negative impacts of roads on avian populations and communities (McClure et al., 2013, Ware et al., 2015; McClure et al., 2017). Whilst early studies suggested overall population reductions in response to road traffic (Reijnen and Foppen, 1994; Reijnen et al., 1995), more recent investigations show that community-level changes can be more complex, as species can respond differently to noise (Cooke et al., 2020; Senzaki et al., 2020). Applying this foundational knowledge (i.e., acoustic stressors driving community-level responses) to aquatic ecosystems, noise might underpin previously documented impacts of diver presence on coral reefs. For example, the presence of divers has been shown to affect coral reef fishes (Benevides et al., 2019; Branconi et al., 2019; Giglio et al., 2022) and fish communities, including species-specific changes to diversity and abundance (Lindfield et al., 2014; Andradi-Brown et al., 2017); in these studies, SCUBA noise was suggested as a potential contributing factor but was not evaluated experimentally in isolation.
To investigate impacts of SCUBA noise on coral reefs, we focused on ecologically important cleaning stations, considering potential changes to the local community composition and disruption to cooperative interactions between cleaners and clients. Mutualistic services play an integral part in the complex web of interactions that help maintain ecosystem health and function (Grutter et al., 2003; Clague et al., 2011; Waldie et al., 2011). On coral reefs, cleaning symbioses are iconic interspecific mutualisms between cleaners, such as gobies, wrasse and shrimp, and a diverse range of client fishes (Grutter, 1999; Becker and Grutter, 2004; Vaughan et al., 2017). These complex and highly developed associations positively impact the health of individual fishes and influence community-wide diversity (McCammon et al., 2010; Clague et al., 2011; Waldie et al., 2011). Furthermore, cleaner species are thought to modify movement patterns, habitat choice, activity and local abundance of reef fishes (Grutter et al., 2003), and may also play a role in determining the distribution of territorial fishes (Whiteman et al., 2002). Typically, a cleaner species will occupy discrete microhabitats that serve as cleaning stations and are visited by clients. During cleaning interactions, client fish will pose motionless, making them vulnerable to predation while cleaners inspect, remove and ingest ectoparasites and dead tissue. Conversely, cleaner species often service clients that would otherwise be natural predators. Because cleaning imposes costs and potential risk to participants (Cheney and Côté, 2001; Chapuis and Bshary, 2009), involves multiple species that are likely to differ in their sensitivity to stressors (Vaughan et al., 2017), and is important for ecosystem function (Losey, 1972), cleaning symbioses are ideal interactions for testing hypotheses about how anthropogenic stressors, such as noise, can have impacts beyond those on individual species.
Here, we experimentally assessed the effects of SCUBA noise on the local community structure and cooperative interactions at the cleaning stations of a well-studied cleaner shrimp species, the Pederson’s cleaner shrimp Ancylomenes pedersoni (Titus et al., 2015a, Titus et al., 2015b; Titus et al., 2019). Pederson’s cleaner shrimp are obligate cleaners (i.e., species that clean throughout juvenile and/or adult life), with their cleaning stations visited by over 20 reef fish families (Huebner and Chadwick, 2012a; Titus et al., 2015b; Gilpin and Chadwick, 2017; Huebner et al., 2019). These established locations facilitate observation of important interspecific mutualistic behaviours, allowing experimental exposure of cleaners and clients to different acoustic treatments to test for a mechanism underpinning previously documented impacts of diver presence on coral reef organisms. We conducted a playback experiment at A. pedersoni cleaning stations to evaluate the impacts of SCUBA noise on: 1) community structure near the stations, 2) clientele composition, and 3) interspecific behaviour during cleaning interactions.
2 Methods
2.1 Experimental overview
We conducted a playback experiment at 40 Ancylomenes pedersoni cleaning stations on Coral View Reef (N 16° 05’ 17.87” W 86° 54’ 38.56”) on the Bay Island of Utila, Honduras, which is located at the southern end of the Mesoamerican Barrier Reef. Coral View Reef is a fringing reef on the southern coast of the island that slopes from ca. 3 to 30 m depth and is a typical contemporary Caribbean reef in terms of oligotrophic nutrient conditions, coral cover, fish abundance and reef community structure (Titus et al., 2019). This site has been visited regularly by snorkelers and SCUBA divers for more than 20 years (Titus et al., 2015a). On Caribbean reefs, Pederson’s cleaner shrimps inhabit corkscrew sea anemones Bartholomea annulata to form mutualistic and ecologically important cleaning stations. Reef fish use sea anemones as visual cues to locate cleaning stations and engage in cleaning interactions with resident shrimp (Huebner and Chadwick, 2012b; Gilpin and Chadwick, 2017). Similarly, for research purposes, seeking B. annulata facilitates the finding and observing of cleaning stations. We located and monitored 40 B. annulata cleaning stations occupied by A. pedersoni at depths of 4–18 m across a continuous stretch of reef (ca. 600 m2 study area), and with a minimum distance of 5 m between experimental stations. We monitored cleaning activity and numbers of shrimp at all cleaning stations throughout the field season using a rotation of static cameras every 5–7 days.
At each cleaning station, we administered two acoustic treatments: playback of local reef soundscapes (ambient sound) and playback of local reef soundscapes with added noise from SCUBA (SCUBA noise), presented in a counterbalanced, repeated-measures design. By comparing responses to playback of local reef soundscapes with those to the soundscapes with SCUBA noise, we isolated SCUBA noise as the experimental stressor without any visual presence of divers, and also controlled for any influences of the acoustic playback itself and/or electromagnetic interference from the loudspeakers. To avoid disturbance by observers, and to allow analysis of replicate trials while blind to the treatment, we used video cameras to record the local fish community and cleaning activity during each deployment.
2.2 Playback tracks and sound analysis
We made field acoustic recordings using a digital recorder (H6-BLACK field recorder, sampling rate 48 kHz; Zoom Corporation, Tokyo, Japan). This was connected to an omnidirectional hydrophone (HTI-96-MIN with inbuilt preamplifier, High Tech Inc., Gulfport MS; manufacturer calibrated sensitivity -164.3 dB re 1 V μPa-1; frequency range 0.2–30 kHz) to measure sound pressure, and to a triaxial accelerometer (M20-040: sensitivity following a curve over the frequency range 0–3 kHz; calibrated by manufacturers; Geospectrum Technologies, Dartmouth, Canada) to measure particle acceleration. We took recordings in sea states between 0 and 2 on the Beaufort Scale in the absence of rain, with recording equipment suspended approximately 1 m above the seabed using a submerged stand.
We made three 5-min daytime recordings each of ambient coral reef soundscapes and of open-circuit SCUBA noise at coral reefs. In each SCUBA-noise recording, a pair of divers approached the recorder, remained stationary approximately 1 m from the recorder for 4 min, and then swam away from the recorder to simulate a recreational visit to inspect/observe/photograph a cleaning station. We used the original field recordings to create experimental playback tracks for each 45-min trial using Audacity 2.2.1 (http://audacity.sourceforge.net/). We constructed three replicate tracks per treatment and used those in rotation to avoid pseudoreplication on single exemplars for each treatment. Each replicate used a different recording of ambient sound or SCUBA noise and was played on a loop. For the SCUBA-noise treatment, this resulted in six SCUBA disturbances per trial, at randomised intervals of 4 ± 1 min (mean ± SD). We re-recorded, analysed and compared playback tracks to original recordings.
We analysed recordings using PAMGuide (sound pressure; Merchant et al., 2015) and paPAM (particle acceleration; Nedelec et al., 2016a) in MATLAB R2017b across a frequency range of 0–2 kHz, which covers the likely auditory range of coral reef fishes (Wright et al., 2011; Ladich and Fay, 2013) and decapods (Popper et al., 2001; Roberts and Elliot, 2017). We calculated spectrograms, power spectral densities (PSD), root-mean-square levels (SPLrms and SALrms) and cumulative sound exposure levels (SELcum and AELcum) in both the sound-pressure and particle-acceleration domains. Calculations were made over batch-processed 30-s subsamples of the recordings (n = 3 per recording type) for each of the four recording types (original ambient-sound recording, ambient-sound playback, original SCUBA-noise recording and SCUBA-noise playback; Figures 1, 2, and Table 1).
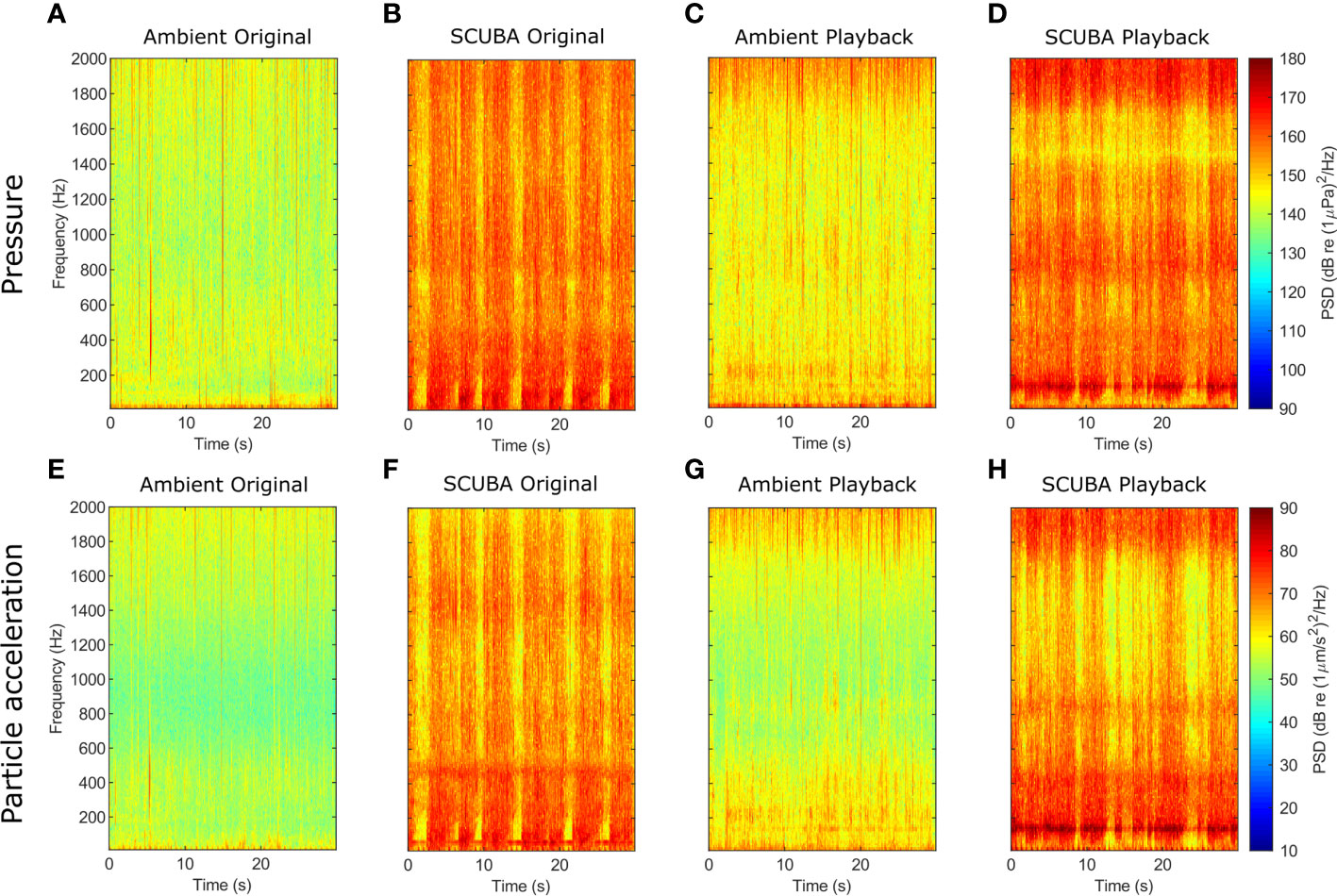
Figure 1 Spectrograms of sound-pressure (A–D) and triaxial particle-acceleration levels (E–H) for original ambient-sound (A, E) and SCUBA-noise (B, F) recordings, and playback tracks for ambient-sound (C, G) and SCUBA-noise (D, H) treatments. For these comparisons, 30-s subsamples of one SCUBA-diving pair and one ambient recording were analysed (fft window length = 4048, Hamming filter, 75% overlap, 0–2 kHz).
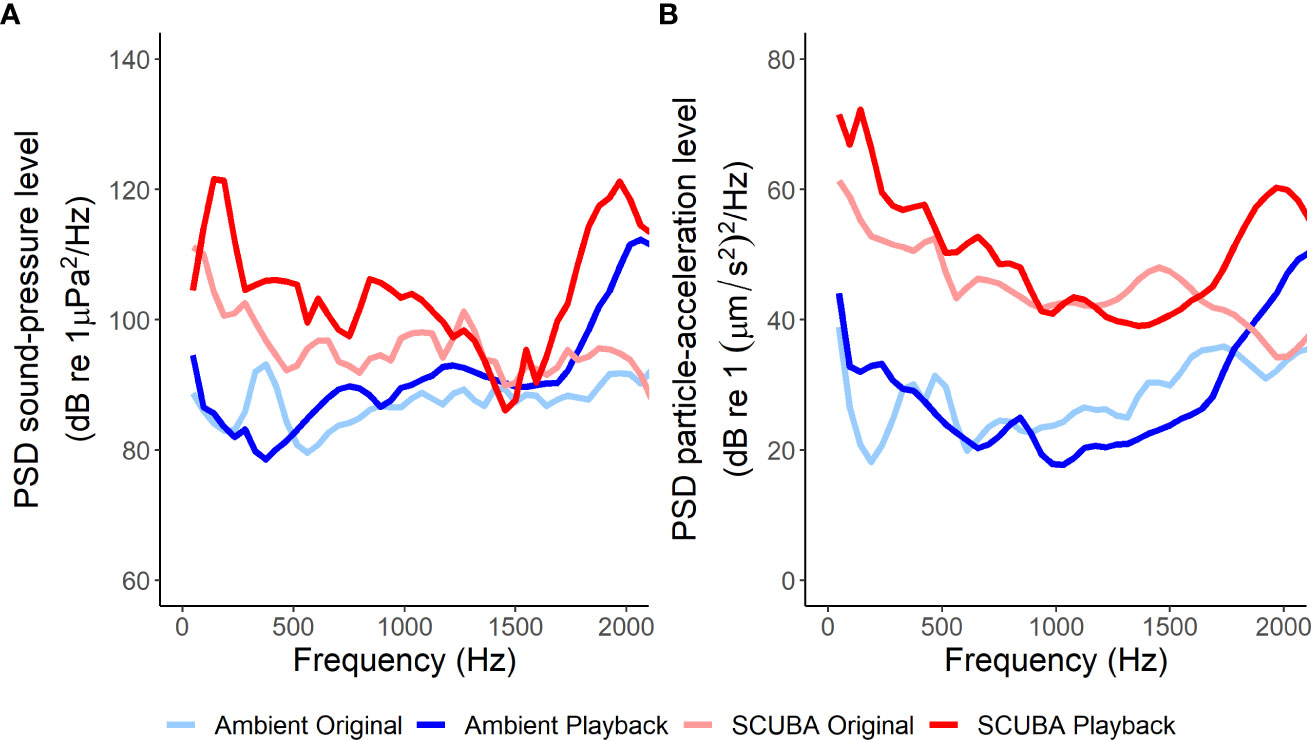
Figure 2 Mean power spectral densities (PSD) of the original ambient-sound recordings, ambient-sound playback tracks, original SCUBA-noise recordings and SCUBA-noise playback tracks in terms of sound pressure (A) and particle acceleration (B), giving an average sound profile for each treatment (fft length = 1024, Hamming evaluation window, 50% window overlap, 0–2 kHz).

Table 1 Root-mean-square and cumulative sound-exposure levels in both sound pressure (SPLrms and SELcum) and triaxial particle acceleration (SALrms and AELcum) for each recording type.
2.3 Experimental procedure
For experimental playbacks, we used recreational SCUBA to reach experimental stations and place equipment. Underwater loudspeakers (University Sound UW-30; max output level 156 dB re 1 μPa at 1 m, frequency response 0.1–10 kHz; Lubell Labs), kept in position using custom-made stands (PVC piping with loudspeaker attached using elastic bungee cord), were placed ca. 0.5 m away from and facing focal cleaning stations. Each loudspeaker was powered by an amplifier (M033N, 18 W, frequency response 0.40–20 kHz; Kemo Electronic GmbH), an MP3 player (SanDisk Clip Jam) and a battery (12V 12Ah sealed lead-acid) housed at the surface in a waterproof barrel. For each trial, we also placed a GoPro Hero 5 camera at 1 m from the focal cleaning station (Supplementary Figure S1). We administered both acoustic treatments (ambient-sound and SCUBA-noise playback) to a cleaning station on the same day, and two stations were treated simultaneously (with random allocation of one station to each treatment order). Trials were completed between 0800 and 1300 h, with previous research showing that cleaning interactions at A. pedersoni stations on this same study reef do not change predictably throughout the day (Titus et al., 2015b).
Our study was designed to evaluate noise as an underpinning mechanism behind previously identified impacts of SCUBA diver presence on interspecific interactions at A. pedersoni cleaning stations, using the same study system at the same location (Titus et al., 2015a). While we used SCUBA to access the cleaning stations, the first 10 min of a trial consisted of silent playback to allow the local fish and resident A. pedersoni to resume normal behaviour following disturbance from placing equipment (Titus et al., 2015a; Nedelec et al., 2016b; Nanninga et al., 2017); this was double the acclimation period from the previous work on the study system, to ensure a return to pre-disturbance behaviour (Titus et al., 2015a). There followed the administration of each treatment (SCUBA noise and ambient sound) over two 45-min segments separated by a 10-min gap of silent playback between the first and second treatment. We video-recorded both treatments at 40 cleaning stations over 20 non-consecutive days.
We cropped all videos collected in the field using ffmpeg 4.13 (ffmpeg.org). For each treatment, we cut 45-min segments and saved them with coded file names. Videos were watched with no sound so that the observer (K.P.M.) was blind to the acoustic condition. We scored community assessments and individual behaviours from the videos using the behavioural observation software BORIS 7.6.1 (Friard and Gamba, 2016).
2.4 Community-wide assessment and analysis
To test for impacts of SCUBA noise on the local community at A. pedersoni cleaning stations, we collected data on the frequency of fishes passing directly over the cleaning station and identified individual fish to species level during each trial. Analyses of the local fish communities were carried out for 39 of the possible 40 cleaning stations; one station was removed due to unintended interference by passing SCUBA divers. Similarly, we identified to species level all fish cleaned by A. pedersoni (hereafter clientele), and limited assessment of clientele composition to stations where at least one clean was observed (n = 22 stations). To analyse local community and clientele composition, we removed species with extremely low occurrences (< 1 % of total individuals; nine species from surrounding community: Acanthurus chirurgugs, Acanthurus coeruleus, Chaetodon capistratus, Chaetodon striatus, Emblemariopsis diaphana, Haemulon flavolineatum, Lutjanus jocu, Serranus tigrinus, and Stegastes viride; six species from clientele composition: E. diaphana, H. flavolineatum, Hypoplectrus unicolor, L. jocu, Scarus taeniopterus, and Stegastes leucostictus). We performed multivariate analyses in R v4.0.0 (R Core Team, 2020) using the Vegan 2.5-7 package (Oksanen et al., 2020), and conducted univariate analyses using generalised linear mixed models (GLMMs) fitted with AICc selection using the lme4 1.1-26 package (Bates et al., 2015). Levels of significance were determined for fixed effect terms via comparisons to null models without the term of interest (i.e., sound treatment). Test assumptions were checked by visualising and evaluating model residuals for normality, homogeneity of variance, collinearity and influence of outliers with Cook’s distance.
We measured total fish occurrence, recorded as the total number of fish for each species observed in the videos. This video-based method precludes a complete assessment of abundance, because it is possible that the same fish can re-enter the frame of view and any fish out of frame cannot be counted, but it avoids disturbance caused by observers in the water. We used species ID and measures of occurrence to calculate species composition for each station, and assessed these using GLMMs with a Poisson distribution; we included acoustic treatment and station as fixed and random factors, respectively. We compared species assemblages between ambient-sound and SCUBA-noise playback using unrestricted one-way nested PERMANOVA (maximum permutations = 9999), with acoustic treatment as a fixed factor and cleaning station as a random factor. Variation in fish species assemblages between ambient-sound and SCUBA-noise treatments was visualised using non-metric multidimensional scaling (nMDS) based on a Bray-Curtis similarity matrix. Lastly, we assessed species-level variation between the two treatments in separate GLMMs with either Poisson or negative binomial distributions (dependent on model fit). Our analyses were conducted across 15 species for local community analysis and eight species for clientele analysis, after the removal of those with < 1 % occurrence, using False Discovery Rate (FDR) to correct for multiple test comparisons.
2.5 Cleaning behaviour assessment and analysis
To investigate the impact of SCUBA noise on interspecific interactions at cleaning stations, we collected data on several cooperative behaviours of A. pedersoni and their clients: time that the shrimp was visible within the camera view (i.e., ‘in-frame’), ‘antenna whipping’ by the shrimp (hereafter signalling; Caves et al., 2018), fish ‘poses’ at the cleaning station (Titus et al., 2017; Caves et al., 2018), time to initiate a cleaning interaction (hereafter delay; Nedelec et al., 2017), cleaning rate and time, and cheating rates (Titus et al., 2019; Table 2). First, we determined whether acoustic treatment (SCUBA-noise or ambient-sound playback) affected the likelihood that each of signalling, posing and cleaning occurred, using separate McNemar’s tests for paired binomial data from all 39 stations. For sites where cameras recorded at least one cleaning interaction in either treatment (n = 22 stations; 113 cleaning interactions in total), we then determined whether acoustic treatment affected the rate (for counts) or activity-budget proportion (for durations) of each cleaning-related behaviour; paired t-tests or Wilcoxon signed-ranks tests were used, depending on whether the data met the assumptions for parametric testing. In some cases, behavioural measures are dependent on the occurrence of another behaviour and therefore only cleaning stations where the latter behaviour occurred were included in analyses. For example, cheating and cleaning delays are functions of cleaning interactions, and therefore analyses require that both treatments experienced at least one cleaning interaction (n = 8). These considerations were made to ensure statistical robustness, emphasise biological context and relevance, and maintain confidence and conservativeness in the resulting conclusions.
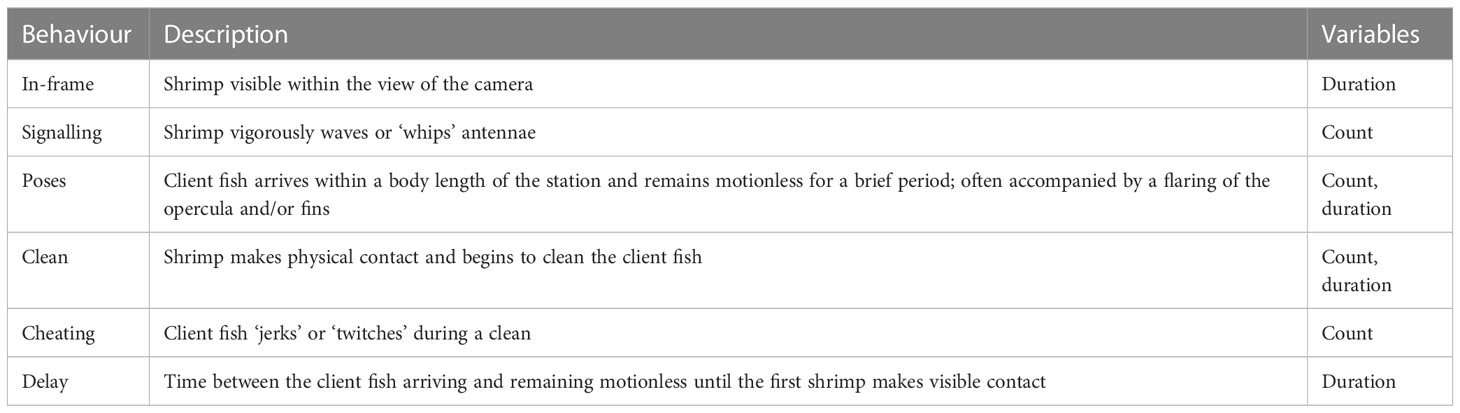
Table 2 Ethogram for the recorded interspecific behaviours by Ancylomenes pedersoni and client fishes.
We analysed all data using R v4.0.0 (R Core Team, 2020). Statistical significance was assumed where p < 0.05. We also derived effect sizes for significant results using the rstatix 0.6.0 package: Cohen’s d for t-tests and Wilcoxon’s effect size r for Wilcoxon tests (Kassambara, 2020).
3 Results
3.1 Local fish community
Fishes passed over the cleaning stations at a mean ± SE rate of 0.73 ± 0.09 events per min during the 45-min trials. For the local fish community, there was no significant difference in species richness between acoustic treatments (GLMM: X21 = 0.24, p = 0.62, treatment = −0.05 ± 0.11, intercept = 1.51 ± 0.08, station ID = 0 ± 0). However, there was a 7% lower total occurrence of fishes during SCUBA-noise playback compared to the ambient-sound control (X21 = 4.23, p = 0.04, treatment = 0.08 ± 0.04, intercept = 3.29 ± 0.12, station ID = 0.48 ± 0.70; Figure 3A), and the species composition of the local fish communities was significantly different between treatments (PERMANOVA: Pseudo-F = 0.77, df = 1, p = 0.03, 9999 permutations; Figure 3B). Three species were present significantly less during SCUBA-noise playback compared to ambient-sound playback (Figure 4): 22% fewer bicolour damselfish Stegastes partitus (GLMM: X21 = 7.34, FDR-adjusted p = 0.025), 61% fewer cocoa damselfish Stegastes varibilis (X21 = 19.01, FDR-adjusted p < 0.001) and 80% fewer bluehead wrasse Thalassoma bifasciatum (X21 = 30.14, FDR-adjusted p < 0.001). Conversely, one species was present significantly more during SCUBA-noise playback: 259% more beaugregory Stegastes leucostictus (X21 = 26.34, FDR-adjusted p < 0.001; Figure 4). None of the other species were found to differ significantly between the acoustic treatments (Supplementary Table S1).
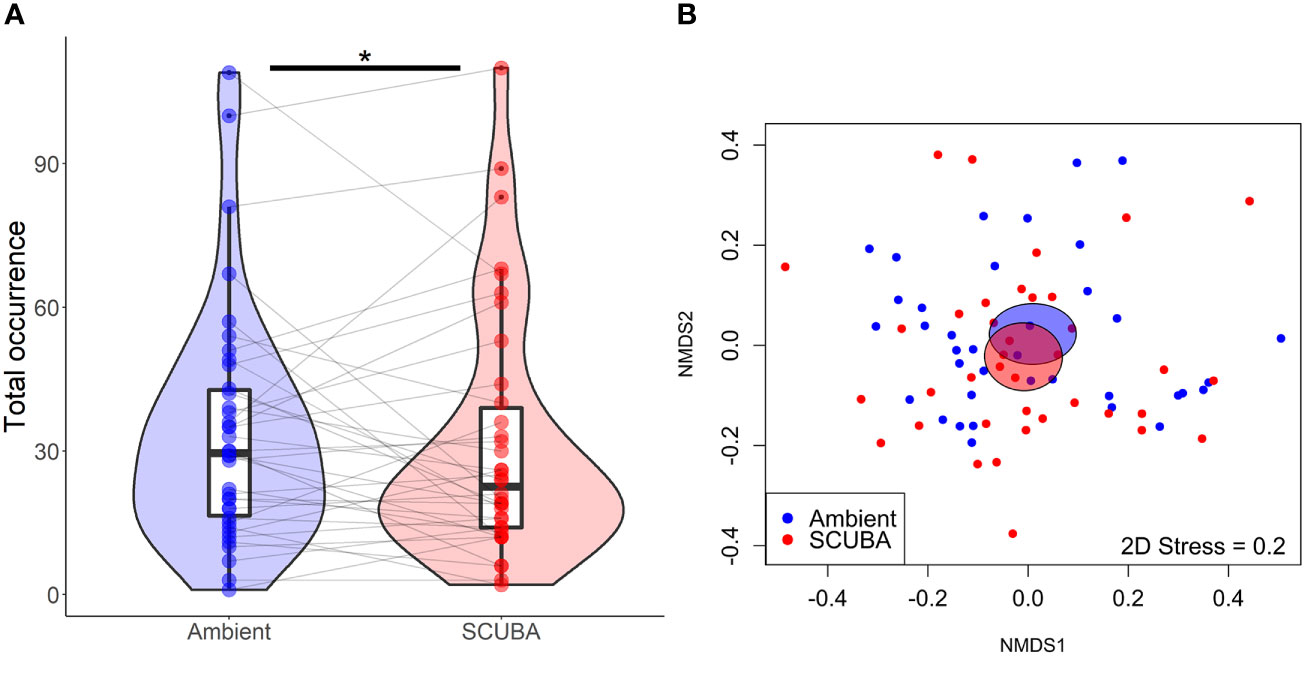
Figure 3 Community-level differences in total fish occurrence between the two acoustic treatments (playback of ambient sound or SCUBA noise). (A) Total fish occurrence. Boxes show median and interquartile range; violin plots show the kernel probability density of the data at different values; coloured points show treatment responses; grey lines join paired data from the same cleaning stations. *p < 0.05. N = 39 cleaning stations. (B) Nonmetric multidimensional scaling (nMDS) ordination showing variation in fish community. Individual dots show replicates at cleaning stations (n = 39); shaded ellipses represent the standard error of the weighted average for each treatment.
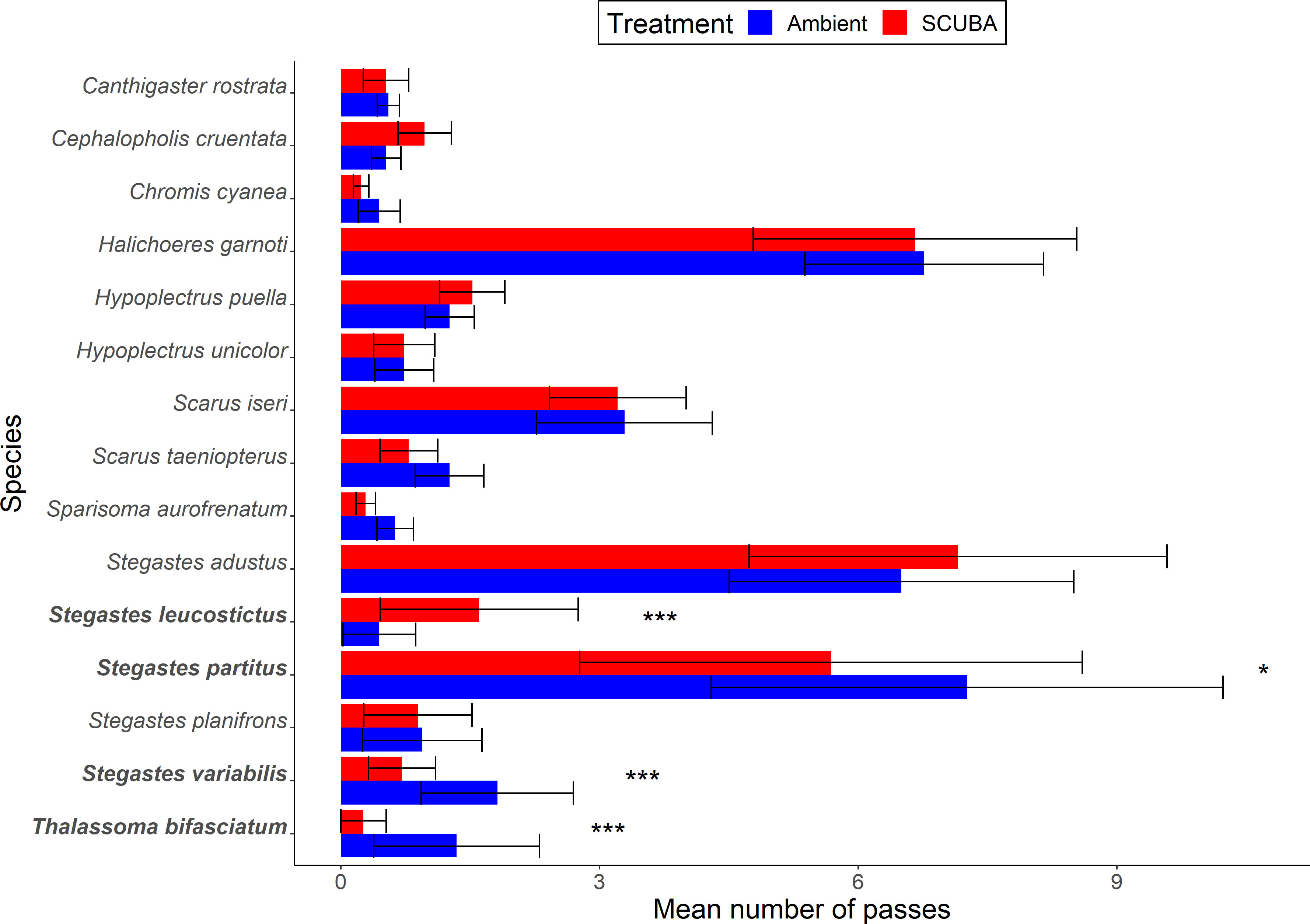
Figure 4 Occurrence of fish species in the surrounding community during the acoustic treatments (playback of ambient sound or SCUBA noise). Shown are mean ± SE number of passes by fishes for those species above the occurrence threshold (> 1%). *p < 0.05, ***p < 0001. N = 39 cleaning stations.
3.2 Clientele community
There was no significant difference between acoustic treatments in the overall composition of clientele across all eight species at cleaning stations (PERMANOVA: Pseudo-F = 0.16, df = 1, p = 0.90, 9999 permutations). However, when considering individual species, two were present significantly less during SCUBA-noise playback compared to the ambient-sound control (Figure 5): 89% fewer Caribbean sharp-nose puffer Canthigaster rostrata (GLMM: X21 = 7.36, FDR-adjusted p = 0.034) and 71% fewer dusky damselfish Stegastes adustus (X21 = 6.92, FDR-adjusted p = 0.034). None of the other species were found to differ significantly between the acoustic treatments (Supplementary Table S2).
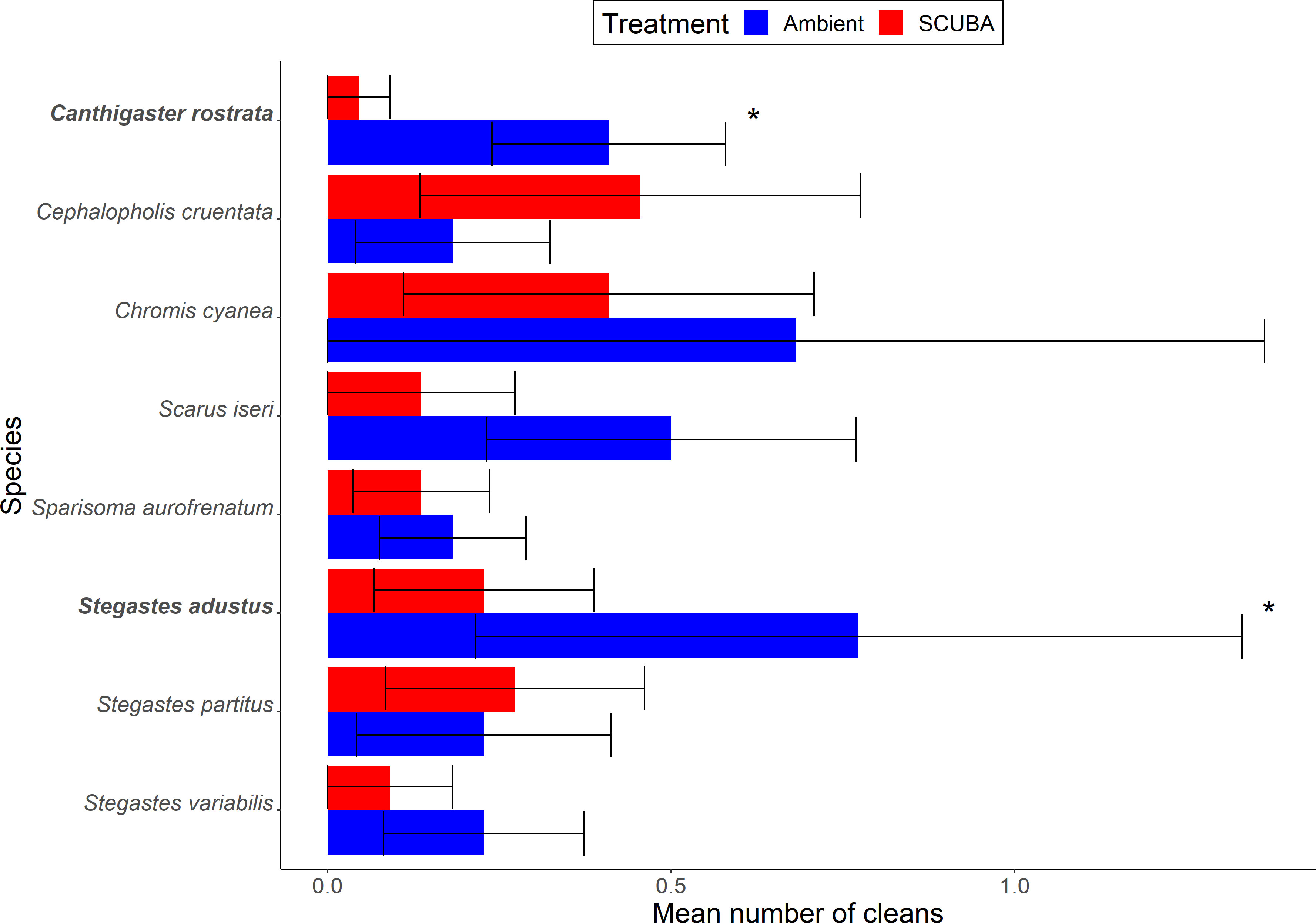
Figure 5 Client occurrences, identified to species level, during the two acoustic treatments (playback of ambient sound or SCUBA noise). Shown are mean ± SE number of cleans of fishes for species above the occurrence threshold (> 1%). *p < 0.05. N = 22 cleaning stations.
3.3 Cleaning behaviour
There were no significant differences between the two acoustic treatments (SCUBA-noise and ambient-sound playback) in the likelihood that any of the three cleaning-related behaviours occurred: signalling by Ancylomenes pedersoni (McNemar's test: X21 = 1.13, p = 0.29, n = 39 pairs), poses by client fishes (X21 = 0, p = 1, n = 39 pairs) and cleaning interactions between A. pedersoni and clients (X21 = 1.79, p = 0.18, n = 39 pairs) .
There was no significant difference between the two acoustic treatments in the total time that A. pedersoni spent in-frame at the focal cleaning stations (mean ± SE: 43 ± 1 min; Wilcoxon test: V22 = 128, p = 0.98). There was also no significant treatment difference in the signalling rate by A. pedersoni (23:9 ± 0:5 events per hour for time spent within view; V22 = 161; p = 0:28), nor any significant difference between the two treatments in posing behaviour by client fishes posing behaviour by client fishes (posing rate: 4.9 ± 0.1 events per hour, V22 = 110, p = 0.56; total posing time: 60 ± 3.8 s, V22 = 119; p = 0.82).
Acoustic treatment did significantly affect the delay to initiate cleaning when a client fish arrived at the station (mean ± SE: 1.9 ±0.5 s; Wilcoxon test:V8 = 0, p = 0.008, d = 0.89); delay times were 29% greater when there was SCUBA noise compared to ambient sound (Figure 6A). Acoustic treatment also significantly affected the cleaning rate of A. pedersoni (3.4 ± 0.1 events per hour; V22 = 151, p = 0.02, d = 0.52), with a 43% lower cleaning rate in the SCUBA-noise treatment compared to the ambient-sound control (Figure 6B). There was, however, no significant treatment difference in either the average clean time (7.4 ± 1.5 s; V22 = 127, p=0.70) or the rate of cheating by A. pedersoni (2.92 ± 1.06 events per min of cleaning; V8 = 3, p = 0.08).
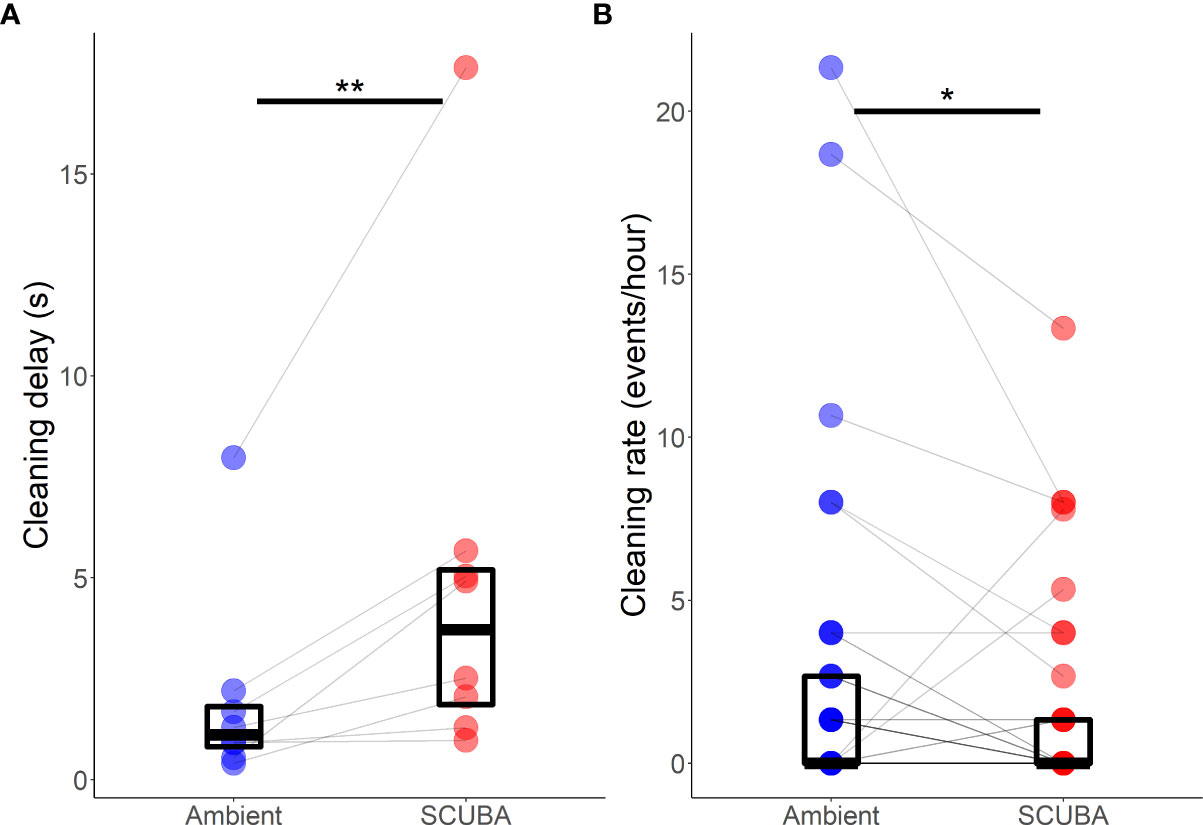
Figure 6 Difference in Ancylomenes pedersoni (A) delay to initiate cleaning and (B) cleaning rate between the two acoustic treatments (playback of ambient sound or SCUBA noise). Boxes show median and interquartile range; coloured points show data from individual cleaning stations; grey lines join paired data from the same cleaning stations. *p < 0.05, **p < 0.01. N = 8 cleaning stations for (A); and n = 22 cleaning stations for (B).
4 Discussion
While responses were varied, our experimental findings suggest that noise generated by open-circuit SCUBA diving can impact Caribbean coral reef communities and interspecific cooperation. Specifically, we found that SCUBA-noise playback altered community composition around cleaning stations, and affected cleaning interactions between the common Caribbean cleaner-shrimp species Ancylomenes pedersoni and client fishes. At the community level, the prevalence of four out of 15 common Caribbean reef fish species differed when exposed to SCUBA-noise playback compared to ambient-sound playback, with changes in the occurrence of these species driving differences in overall fish community composition between the two acoustic treatments. However, overall species richness was not affected by SCUBA noise. The significant effects on species prevalence at the community level were not uniform, with three species showing a reduction in occurrence during SCUBA noise, but one species showing an increase. Additionally, our results showed altered clientele composition of fishes cleaned by A. pedersoni, with two out of eight fish species being cleaned less during the SCUBA-noise treatment. However, these species-specific changes to clientele occurrence did not lead to a change in the overall clientele composition between the two acoustic treatments. Regarding individual cleaning behaviour, SCUBA-noise playback resulted in longer delays in cleaning initiation and fewer cleaning interactions between A. pedersoni and client fishes. SCUBA noise did not affect several other behaviours, such as signalling, posing, time spent cleaning and cheating. Overall, we believe that our study provides the first demonstration of the impacts of SCUBA noise on coral reef communities and interspecific interactions, highlighting SCUBA noise as a potentially harmful pollutant in coral reef ecosystems.
When exposed to SCUBA-noise playback, the occurrence of fishes near A. pedersoni cleaning stations was 7% lower and the overall community composition of fishes was significantly altered. These results mirror those from terrestrial studies where longer-term experimental playback of traffic noise along ‘phantom roads’ reduced overall bird abundance and altered community structures (McClure et al., 2013, McClure et al., 2017). The observed interspecific variation in noise effects (i.e., responses observed in some species but not others) is not surprising given that species differ in, for example, ecology (Kunc and Schmidt, 2019), life history (de Jong et al., 2020), prior exposure (Harding et al., 2018), hearing ability (Popper and Hawkins, 2019) and vocal behaviour (Radford et al., 2014), all of which may influence their responses to noise. For instance, noise can induce physiological stress (Wale et al., 2013; Celi et al., 2016; Mills et al., 2020), which may subsequently alter decision-making processes and behaviour during disturbance (Purser and Radford, 2011; Voellmy et al., 2014), but species differ considerably in their susceptibility to stress (Pottinger, 2010). Furthermore, inter- and intra-specific variation in tolerance, sensitisation/desensitisation and/or habituation to anthropogenic noise remain unclear (Harding et al., 2019; Stasso et al., 2023). In fact, previous research using the same study system and location observed a difference in the strength of responses between frequently dived and un-dived locations (Titus et al., 2015a)—a comparison that was logistically beyond the scope of our study. Consequently, local history of diving at this site may have already altered susceptibility to SCUBA noise impacts by some species and/or individuals but not others, potentially resulting in only 27% and 25% of monitored species being affected by noise exposure at the community and clientele levels, respectively. Lastly, because anthropogenic noise has the potential to mask acoustic cues and signals, soniferous species, such as damselfish, may be particularly vulnerable to noise disturbance (Radford et al., 2014; Weilgart, 2018). Interspecific variation in noise effects may also arise through knock-on consequences. We found that three of four species affected by SCUBA noise were members of the same damselfish genus, Stegastes: two species (S. partitus and S. variables) occurred less during SCUBA-noise playback, while a third species (S. leucostictus) occurred more. It is possible that S. partitus and S. variables moved away, sought refuge more or exhibited less territorial behaviour (Benevides et al., 2019) during SCUBA-noise playback, which, in turn, created an opportunity through competitor release for S. leucostictus to encroach on territories and resources (Robertson, 1996).
Our finding that SCUBA-noise playback altered cleaning interactions between the cleaner shrimp A. pedersoni and its clients, with a 29% longer delay to initiate cleaning and a 43% lower cleaning rate compared to the ambient-sound control, may be due to distraction (Chan et al., 2010) or stress (Pottinger, 2010; Wale et al., 2013; Mills et al., 2020) in cleaners and/or clients. Either way, the results establish acoustic disturbance as a potential mechanism for the previously documented impacts of diver presence on cleaning by A. pedersoni (Titus et al., 2015a), and are in line with work showing that motorboat-noise playback can disrupt mutualistic cleaning behaviour by the Indo-Pacific bluestreak cleaner wrasse Labroides dimidiatus (Nedelec et al., 2017). Similar to these previous studies, we also found that only some measured behaviours were impacted by exposure to anthropogenic noise; some individual behaviours and social interactions may be more susceptible to disruption than others. Regardless, a decrease in cleaning activity suggests a trade-off, with avoidance of the potential risk and/or cost associated with SCUBA noise occurring at the expense of parasite removal for client fishes and dietary intake for A. pedersoni (Cheney and Côté, 2001). While not assessed here, noise negatively affects physiology (Wale et al., 2013; Filiciotto et al., 2014), stress-related behaviour (Filiciotto et al., 2014, Filiciotto et al., 2016) and biochemical regulation (Celi et al., 2015; Filiciotto et al., 2016) in crustaceans, and therefore may be similarly affecting A. pedersoni. For clients, cleaning symbioses improve fitness (Grutter, 1999; Becker and Grutter, 2004); therefore, SCUBA noise could lead to a negative impact on the reproductive success and longevity of clients that lose out on cleaning opportunities. Where cleaning stations fail altogether, reef communities can be affected in the form of reduced abundance and species richness, lower growth rates and survivorship, and diminished larval recruitment (Waldie et al., 2011).
Care is needed when extrapolating results from short-term noise experiments to fitness consequences, given that there can be increased tolerance and/or habituation, and compensation during quieter periods (Nedelec et al., 2016b; Radford et al., 2016). However, popular dive sites can receive multiple visits per day, which may equally result in cumulative noise effects. Similar caution is advised about assuming lasting community-level impacts from short-term experiments, although longer-term terrestrial studies have revealed sustained changes in the composition and interactions of species in noisy areas (Francis et al., 2009; Slabbekoorn and Halfwerk, 2009; Barber et al., 2010). Ultimately, extended experimental tests are needed in aquatic ecosystems if we are to understand the full impact of noise pollution.
While our study identifies SCUBA noise as a stressor to coral reef inhabitants, it also suggests a potential avenue for mitigating the impact of SCUBA diving. Managing acoustic disturbance has the potential to reduce the broad-ranging effects of divers on coral reef ecosystems (Lindfield et al., 2014; Titus et al., 2015a; Andradi-Brown et al., 2017; Benevides et al., 2019), without requiring a reduction or cessation of diving activity or the widespread uptake of expensive closed-circuit rebreathers. Instead, divers and the dive industry can adopt simple alterations to dive protocols that reduce the amount of noise exposure to coral reefs, which is a mitigation strategy that has been shown to negate biological responses to other sources of noise (Jain-Schlaepfer et al., 2018; McCormick et al., 2018, McCormick et al., 2019; McCloskey et al., 2020; Nedelec et al., 2022). For example, increasing the distance between a source and the vulnerable site has been shown to be an effective means of reducing the amount of noise exposure to wildlife, alleviating noise-induced behavioural responses (MacLean et al., 2020; McCloskey et al., 2020; Nedelec et al., 2022). Furthermore, tourism and dive operators might consider rotating and/or including more dive sites to avoid concentrating noise exposure and disturbance to a few locations. This concept of managing noise exposure to protect wildlife has been successfully implemented and enforced to safeguard at-risk marine mammal populations, including the critically endangered southern resident killer whale Orcinus orca (Williams et al., 2019). While it would require further experimentation to test the benefits of specific temporal, spatial and behavioural management recommendations, we believe that simple guidelines could be developed that mitigate the negative impacts of anthropogenic noise on coral reef habitats, especially given that coral reefs are areas of high biodiversity (Roberts et al., 2002), provide nutrition and livelihoods for millions of people (Cinner, 2014), and have high socio-economic importance and value (de Groot et al., 2012).
Data availability statement
The datasets presented in this study can be found in online repositories. The names of the repository/repositories and accession number(s) can be found below: https://datadryad.org/stash/share/YPdRHTvM6DCGWVVVkMzARh5D61DEjuy4ljYyuc43kNo.
Ethics statement
Experimental protocols were approved by the University of Exeter Animal Ethics Committee (Application, eCLESBio000295). The research was conducted under a research permit (ICF-508-2019) issued to Operation Wallacea by the Honduran government.
Author contributions
KPM, ANR, BMT, DAE and SDS designed the research. KPM, AR, GC, and NL carried out the fieldwork and collected videos and acoustic recordings. KPM extracted data from videos. KPM analysed the data with advice from ANR, EW, BMT, DAE, and SDS. KPM analysed the acoustic data. KPM wrote the manuscript with revisions and advice from ANR, BMT, DAE, and SDS. All authors contributed to the article and approved the submitted version.
Funding
This work was supported by funding from Operation Wallacea, a University of Exeter Vice-Chancellor Scholarship for Postgraduate Research (to KPM), and a Natural Environment Research Council Research Grant (NE/P001572/1 to SDS and ANR).
Acknowledgments
We thank the Operation Wallacea staff and volunteers that enabled this project to be completed, with special thanks to Alec Atherton and Lydia Woods for their assistance in the field; the staff at Coral View Beach Resort & Dive Center (Tonia Woods, Calvin Woods Sr, Calvin Woods Jr, Taylor McField, Adelle Thompson, Rich Astley and Hans Grauer) for logistical support and accommodation during fieldwork; and Harry Harding and Lucille Chapuis for assistance with statistical and acoustic analyses.
Conflict of interest
The authors declare that they have no known competing financial interests or personal relationships that could have appeared to influence the work reported in this paper.
Publisher’s note
All claims expressed in this article are solely those of the authors and do not necessarily represent those of their affiliated organizations, or those of the publisher, the editors and the reviewers. Any product that may be evaluated in this article, or claim that may be made by its manufacturer, is not guaranteed or endorsed by the publisher.
Supplementary material
The Supplementary Material for this article can be found online at: https://www.frontiersin.org/articles/10.3389/fmars.2023.1058414/full#supplementary-material
References
Andradi-Brown D., Fund W. W., Gress E., Laverick J. H., Monfared M. (2017). ‘Wariness of reef fish to passive diver presence with varying dive gear type across a coral reef depth gradient’. J. Mar. Biol. Assoc. UK 98, 1733–1743. doi: 10.1017/S0025315417001278
Barber J. R., Crooks K. R., Fristrup K. M. (2010). ‘The costs of chronic noise exposure for terrestrial organisms’. Trends Ecol. Evol. 25, 180–189. doi: 10.1016/j.tree.2009.08.002
Bates D., Maechler M., Bolker B., Walker S. (2015). ‘Fitting linear mixed-effects models using lme4’. J. Stat. Soft. 67 (1), 1–48. doi: 10.18637/jss.v067.i01
Becker J. H., Grutter A. S. (2004). ‘Cleaner shrimp do clean’. Coral Reefs 23, 515–520. doi: 10.1007/s00338-004-0429-3
Benevides L. J., Cardozo-Ferreira G. C., Ferreira C. E. L., Pereira P. H. C., Pinto T. K., Sampaio C. L. S. (2019). ‘Fear-induced behavioural modifications in damselfishes can be diver-triggered’. J. Exp. Mar. Bio. Ecol. 514–515, 34–40. doi: 10.1016/j.jembe.2019.03.009
Branconi R., Wong M. Y. L., Buston P. M. (2019). ‘Comparison of efficiency of direct observations by scuba diver and indirect observations via video camera for measuring reef-fish behaviour’. J. Fish Biol. 94, 490–498. doi: 10.1111/jfb.13921
Caves E. M., Green P. A., Johnsen S. (2018). ‘Mutual visual signalling between the cleaner shrimp Ancylomenes pedersoni and its client fish’. Proc. R. Soc B Biol. Sci. 285, 20180800. doi: 10.1098/rspb.2018.0800
Celi M., Filiciotto F., Maricchiolo G., Genovese L., Quinci E. M., Maccarrone V., et al. (2016). ‘Vessel noise pollution as a human threat to fish: Assessment of the stress response in gilthead sea bream (Sparus aurata, Linnaeus 1758)’. Fish Physiol. Biochem. 42, 631–641. doi: 10.1007/s10695-015-0165-3
Celi M., Filiciotto F., Vazzana M., Arizza V., Maccarrone V., Ceraulo M., et al. (2015). ‘Shipping noise affecting immune responses of European spiny lobster (Palinurus elephas).’ Can. J. Zool. 93, 113–121. doi: 10.1139/cjz-2014-0219
Chan A. A. Y. H., Giraldo-Perez P., Smith S., Blumstein D. T. (2010). ‘Anthropogenic noise affects risk assessment and attention: The distracted prey hypothesis’. Biol. Lett. 6, 458–461. doi: 10.1098/rsbl.2009.1081
Chapuis L., Bshary R. (2009). ‘Strategic adjustment of service quality to client identity in the cleaner shrimp, Periclimenes longicarpus’. Anim. Behav. 78, 455–459. doi: 10.1016/j.anbehav.2009.06.001
Cheney K. L., Côté I. M. (2001). ‘Are Caribbean cleaning symbioses mutualistic? Costs and benefits of visiting cleaning stations to longfin damselfish’. Anim. Behav. 62, 927–933. doi: 10.1006/anbe.2001.1832
Cinner J. (2014). ‘Coral reef livelihoods’. Curr. Opin. Environ. Sustain. 7, 65–71. doi: 10.1016/j.cosust.2013.11.025
Clague G. E., Cheney K. L., Goldizen A. W., McCormick M. I., Waldie P. A., Grutter A. S. (2011). ‘Long-term cleaner fish presence affects growth of a coral reef fish.’ Biol. Lett. 7, 863–865. doi: 10.1098/rsbl.2011.0458
Cooke S. C., Balmford A., Johnston A., Newson S. E., Donald P. F. (2020). ‘Variation in abundances of common bird species associated with roads’. J. Appl. Ecol. 57, 1271–1282. doi: 10.1111/1365-2664.13614
Cox K., Brennan L. P., Gerwing T. G., Dudas S. E., Juanes F. (2018). ‘Sound the alarm: A meta-analysis on the effect of aquatic noise on fish behavior and physiology’. Glob. Change Biol. 24, 3105–3116. doi: 10.1111/gcb.14106
Curtin S., Garrod B. (2008). “Vulnerability of marine mammals to diving tourism activities,” in New frontiers in marine tourism, vol. p . Eds. Garrod B., Gössling S. (Amsterdam: the Netherlands: Elsevier), 93–113.
Davenport J., Davenport J. L. (2006). ‘The impact of tourism and personal leisure transport on coastal environments: A review’. Estuar. Coast. Shelf Sci. 67, 280–292. doi: 10.1016/j.ecss.2005.11.026
de Groot R., Brander L., van der Ploeg S., Costanza R., Bernard F., Braat L., et al. (2012). ‘Global estimates of the value of ecosystems and their services in monetary units’. Ecosyst. Serv. 1, 50–61. doi: 10.1016/j.ecoser.2012.07.005
de Jong K., Forland T. N., Amorim M. C. P., Rieucau G., Slabbekoorn H., Sivle L. D. (2020). ‘Predicting the effects of anthropogenic noise on fish reproduction’. Rev. Fish Biol. Fish. 30, 245–268. doi: 10.1007/s11160-020-09598-9
Duarte C. M., Chapuis L., Collin S. P., Costa D. P., Devassy R. P., Eguiluz V. M., et al. (2021). ‘The soundscape of the anthropocene ocean’. Science 371, eaba4658. doi: 10.1126/science.aba4658
Ferrari M. C. O., Mccormick M. I., Meekan M. G., Simpson S. D., Nedelec S. L., Chivers D. P. (2018). ‘School is out on noisy reefs: The effect of boat noise on predator learning and survival of juvenile coral reef fishes’. Proc. R. Soc B Biol. Sci. 285, 20180033. doi: 10.1098/rspb.2018.0033
Filiciotto F., Vazzana M., Celi M., Maccarrone V., Ceraulo M., Buffa G., et al. (2016). ‘Underwater noise from boats: Measurement of its influence on the behaviour and biochemistry of the common prawn (Palaemon serratus, pennant 1777).’ J. Exp. Mar. Biol. Ecol. 478, 24–33. doi: 10.1016/j.jembe.2016.01.014
Filiciotto F., Vazzana M., Celi M., Maccarrone V., Ceraulo M., Buffa G., et al. (2014). ‘Behavioural and biochemical stress responses of Palinurus elephas after exposure to boat noise pollution in tank.’ Mar. Pollut. Bull. 84, 104–114. doi: 10.1016/j.marpolbul.2014.05.029
Francis C. D., Ortega C. P., Cruz A. (2009). ‘Noise pollution changes avian communities and species interactions’. Curr. Biol. 19, 1415–1419. doi: 10.1016/j.cub.2009.06.052
Friard O., Gamba M. (2016). ‘BORIS: A free, versatile open-source event-logging software for video/audio coding and live observations’. Methods Ecol. Evol. 7, 1325–1330. doi: 10.1111/2041-210X.12584
Giglio V. J., Blumstein D. T., Motta F. S., Pereira-Filho G. H. (2022). ‘Diver presence increases egg predation on a nesting damselfish’. J. Exp. Mar. Bio. Ecol. 549, 151694. doi: 10.1016/j.jembe.2022.151694
Giglio V. J., Luis O. J., Ferreira C. E. L. (2020). ‘Ecological impacts and management strategies for recreational diving: A review’. J. Exp. Environ. Manage. 256, 109949. doi: 10.1016/j.jenvman.2019.109949
Gilpin J. A., Chadwick N. E. (2017). ‘Life-history traits and population structure of pederson cleaner shrimps ancylomenes pedersoni’. Biol. Bull. 233, 190–205. doi: 10.1086/695802
Grutter A. S., Murphy J. M., Choat J. H. (2003). ‘Cleaner fish drives local fish diversity on coral reefs’. Curr. Biol. 13, 64–67. doi: 10.1016/S0960-9822(02)01393-3
Harding H. R., Gordon T. A. C., Eastcott E., Simpson S. D., Radford A. N., Simmons L. (2019). ‘Causes and consequences of intraspecific variation in animal responses to anthropogenic noise.’ Behav. Ecol. 30, 1501–1511. doi: 10.1093/beheco/arz114
Harding H. R., Gordon T. A. C., Hsuan R. E., Mackaness A. C. E., Radford A. N., Simpson S. D. (2018). ‘Fish in habitats with higher motorboat disturbance show reduced sensitivity to motorboat noise’. Biol. Lett. 14, 20180441. doi: 10.1098/rsbl.2018.0441
Hawkins J. P., Roberts C. M. (1993). ‘Effects of recreational scuba diving on coral reefs: trampling on reef-flat communities’. J. Appl. Ecol. 30, 25–30. doi: 10.2307/2404267
Herrera-Montes M. I., Aide T. M. (2011). ‘Impacts of traffic noise on anuran and bird communities. Urban Ecosyst.’ 14, 415–427. doi: 10.1007/s11252-011-0158-7
Huebner L. K., Chadwick N. E. (2012a). ‘Patterns of cleaning behaviour on coral reef fish by the anemoneshrimp Ancylomenes pedersoni’. J. Mar. Biol. Assoc. 92, 1557–1562. doi: 10.1017/S0025315411001822
Huebner L. K., Chadwick N. E. (2012b). ‘Reef fishes use sea anemones as visual cues for cleaning interactions with shrimp’. J. Exp. Mar. Bio. Ecol. 416–417, 237–242. doi: 10.1016/j.jembe.2012.01.004
Huebner L. K., Shea C. P., Schueller P. M., Terrell A. D., Ratchford S. G., Chadwick N. E. (2019). ‘Crustacean symbiosis with Caribbean sea anemones Bartholomea annulata: Occupancy modeling, habitat partitioning, and persistence’. Mar. Ecol. Prog. Ser. 631, 99–116. doi: 10.3354/meps13160
Jain-Schlaepfer S., Fakan E., Rummer J. L., Simpson S. D., McCormick M. I. (2018). ‘Impact of motorboats on fish embryos depends on engine type’. Conserv. Physiol. 6, coy014. doi: 10.1093/conphys/coy014
Kassambara A. (2020)‘rstatix: Pipe-friendly framework for basic statistical tests’. In: Rstatix: R package version 0.6.0. Available at: https://CRAN.R-project.org/package=rstatix (Accessed 02/06/2020).
Kunc H. P., Schmidt R. (2019). ‘The effects of anthropogenic noise on animals: A meta-analysis’. Biol. Lett. 15, 20190649. doi: 10.1098/rsbl.2019.0649
Ladich F., Fay R. R. (2013). ‘Auditory evoked potential audiometry in fish.’ Rev. Fish Bio. Fish. 23, 317–364. doi: 10.1007/s11160-012-9297-z
Lindfield S. J., Harvey E. S., Mcilwain J. L., Halford A. R. (2014). ‘Silent fish surveys: Bubble-free diving highlights inaccuracies associated with SCUBA-based surveys in heavily fished areas’. Methods Ecol. Evol. 5, 1061–1069. doi: 10.1111/2041-210X.12262
Lobel P. S. (2005). “Scuba bubble noise and fish behavior: a rationale for silent diving technology,” in Diving for science: Proceedings of the American academy of underwater sciences (Groton, CT, USA: University of Connecticut at Avery Point), vol. 24, 49–59.
Losey G. S. (1972). ‘The ecological importance of cleaning symbiosis’, am. Soc Ichthyol. Herpetol. 1972, 820–833.
Lück M. (2016). ‘Scuba diving tourism’. Ann. Leis. Res. 19, 259–261. doi: 10.1080/11745398.2015.1070103
MacLean K., Prystay T. S., Lawrence M. J., Zolderdo A. J., Gutowsky L. F. G., Staaterman E., et al. (2020). ‘Going the distance: Influence of distance between boat noise and nest site on the behavior of paternal smallmouth bass’. Water. Air. Soil Pollut. 231, 151. doi: 10.1007/s11270-020-04470-9
McCammon A., Sikkel P. C., Nemeth D. (2010). ‘Effects of three Caribbean cleaner shrimps on ectoparasitic monogeneans in a semi-natural environment’. Coral Reefs 29, 419–426. doi: 10.1007/s00338-009-0583-8
McCloskey K. P., Chapman K. E., Chapuis L., McCormick M. I., Radford A. N., Simpson S. D. (2020). ‘Assessing and mitigating impacts of motorboat noise on nesting damsel fish’. Environ. Pollut. 266, 115376. doi: 10.1016/j.envpol.2020.115376
McClure C. J. W., Ware H. E., Carlisle J. D., Barber J. R. (2017). ‘Noise from a phantom road experiment alters the age structure of a community of migrating birds.’ Anim. Conserv. 20, 164–172. doi: 10.1111/acv.12302
McClure C. J. W., Ware H. E., Carlisle J., Kaltenecker G., Barber J. R. (2013). ‘An experimental investigation into the effects of traffic noise on distributions of birds: Avoiding the phantom road.’ Proc. R. Soc B Biol. Sci. 280, 20132290. doi: 10.1098/rspb.2013.2290
McCormick M. I., Allan B. J. M., Harding H., Simpson S. D. (2018). ‘Boat noise impacts risk assessment in a coral reef fish but effects depend on engine type’. Sci. Rep. 8, 3847.
McCormick M. I., Fakan E. P., Nedelec S. L., Allan B. J. M. (2019). ‘Effects of boat noise on fish fast-start escape response depend on engine type’. Sci. Rep. 9, 6554. doi: 10.1038/s41598-019-43099-5
Merchant N. D., Fristrup K. M., Johnson M. P., Tyack P. L., Witt M. J., Blondel P., et al. (2015). ‘Measuring acoustic habitats.’ Methods Ecol. Evol. 6, 257–265. doi: 10.1111/2041-210X.12330
Mills S. C., Beldade R., Henry L., Laverty D., Nedelec S. L., Simpson S. D., et al. (2020). ‘Hormonal and behavioural effects of motorboat noise on wild coral reef fish’. Environ. pollut. 262, 114250. doi: 10.1016/j.envpol.2020.114250
Nanninga G. B., Côté I. M., Beldade R., Mills S. C. (2017). ‘Behavioural acclimation to cameras and observers in coral reef fishes’. Ethology 123, 705–711. doi: 10.1111/eth.12642
Nedelec S. L., Campbell J., Radford A. N., Simpson S. D., Merchant N. D. (2016a). ‘Particle motion: The missing link in underwater acoustic ecology’. Methods Ecol. Evol. 7, 836–842. doi: 10.1111/2041-210X.12544
Nedelec S. L., Mills S. C., Lecchini D., Nedelec B., Simpson S. D., Radford A. N. (2016b). ‘Repeated exposure to noise increases tolerance in a coral reef fish’. Environ. pollut. 216, 428–436. doi: 10.1016/j.envpol.2016.05.058
Nedelec S. L., Mills S. C., Radford A. N., Beldade R., Simpson S. D., Nedelec B., et al. (2017). ‘Motorboat noise disrupts co-operative interspecific interactions’. Sci. Rep. 7. doi: 10.1038/s41598-017-06515-2
Nedelec S. L., Radford A. N., Gatenby P., Davidson I. K., Jimenez L. V., Travis M., et al. (2022). ‘Limiting motorboat noise on coral reefs boosts fish reproductive success’. Nat. Commun. 13, 2822. doi: 10.1038/s41467-022-30332-5
Oksanen J., Blanchet F. G., Friendly M., Kindt R., Legendre P., McGlinn D., et al. (2020)‘vegan: Community ecology package’. In: Vegan: R package version 2.5-7. Available at: https://CRAN.R-project.org/package=vegan (Accessed 02/06/2020).
PADI (2021). 2021 worldwide corporate statistics: Data for 2015-2020 (Rancho Santa Margarita, Califorinia, USA: PADI Headquarters).
Popper A. N., Hawkins A. D. (2019). ‘An overview of fish bioacoustics and the impacts of anthropogenic sounds on fishes’. J. Fish Biol. 94, 692–713. doi: 10.1111/jfb.13948
Popper A. N., Salmon M., Horch K. W. (2001). ‘Acoustic detection and communication by decapod crustaceans.’ J. Comp. Physiol. A 187, 83–89. doi: 10.1007/s003590100184
Pottinger T. G. (2010). ‘A multivariate comparison of the stress response in three salmonid and three cyprinid species: Evidence for inter-family differences.’ J. Fish Biol. 76, 601–621. doi: 10.1111/j.1095-8649.2009.02516.x
Purser J., Radford A. N. (2011). ‘Acoustic noise induces attention shifts and reduces foraging performance in three-spined sticklebacks (Gasterosteus aculeatus)’. PLoS One 6, e17478. doi: 10.1371/journal.pone.0017478
R Core Team. (2020). R: A language and environment for computing. Vienna, Austria: R Foundation for Statistical Computing. Retrieved from http://www.R-project.org.
Radford C. A., Jeffs A. G., Tindle C. T., Cole R. G., Montgomery J. C. (2005). ‘Bubbled waters: The noise generated by underwater breathing apparatus’. Mar. Freshw. Behav. Physiol. 38, 259–267. doi: 10.1080/10236240500333908
Radford A. N., Kerridge E., Simpson S. D. (2014). ‘Acoustic communication in a noisy world: can fish compete with anthropogenic noise?’ Behav. Ecol. 25, 1022–1030. doi: 10.1093/beheco/aru029
Radford A. N., Purser J., Bruintjes R., Voellmy I. K. (2016). “Beyond a simple effect: Variable and changing responses to anthropogenic noise,” in Effects of noise on aquatic life II. advances in experimental medicine and biology, vol. p . Eds. Popper A. N., Hawkins A. (New York: NY: Springer), 901–907.
Reijnen R., Foppen R. (1994). ‘The effects of car traffic on breeding bird populations in woodland. I. evidence of reduced habitat quality for willow warblers (Phylloscopus trochilus) breeding close to a highway’. J. Appl. Ecol. 31, 85–94. doi: 10.2307/2404601
Reijnen R., Foppen R., Ter Braak C., Thissen J. (1995). ‘The effects of car traffic on breeding bird populations in woodland. III. reduction of density in relation to the proximity of main roads’. J. Appl. Ecol. 32, 187–202. doi: 10.2307/2404428
Roberts L., Elliott M. (2017). ‘Good or bad vibrations? Impacts of anthropogenic vibration on the marine epibenthos’. Sci. Total Environ. 595, 255–268. doi: 10.1016/j.scitotenv.2017.03.117
Roberts C. M., McClean C. J., Veron J. E. N., Hawkins J. P., Allen G. R., McAllister D. E., et al. (2002). ‘Marine biodiversity hotspots and conservation priorities for tropical reefs’. Science 295, 1280–1284. doi: 10.1126/science.1067728
Robertson D. R. (1996). ‘Interspecific competition controls abundance and habitat use of territorial caribbean damselfishes’. Ecology 77, 885–899. doi: 10.2307/2265509
Senzaki M., Barber J. R., Phillips J. N., Carter N. H., Cooper C. B., Ditmer M. A., et al. (2020). ‘Sensory pollutants alter bird phenology and fitness across a continent’. Nature 587, 605–609. doi: 10.1038/s41586-020-2903-7
Shannon G., Mckenna M. F., Angeloni L. M., Crooks K. R., Fristrup K. M., Brown E., et al. (2016). ‘A synthesis of two decades of research documenting the effects of noise on wildlife’. Biol. Rev. 91, 982–1005. doi: 10.1111/brv.12207
Simpson S. D., Radford A. N., Nedelec S. L., Ferrari M. C. O., Chivers D. P., Mccormick M. I., et al. (2016). ‘Anthropogenic noise increases fish mortality by predation’. Nat. Commun. 7, 10544. doi: 10.1038/ncomms10544
Slabbekoorn H., Halfwerk W. (2009). ‘Behavioural ecology: Noise annoys at community level’. Curr. Biol. 19, R693–R695. doi: 10.1016/j.cub.2009.07.002
Stasso J. J., Pieniazek R. H., Higgs D. M. (2023). ‘Interspecific variation in the repsonse of fish to anthropogenic noise’. Freshw. Biol. 68, 25–32. doi: 10.1111/fwb.14006
Titus B. M., Daly M., Exton D. A. (2015a). ‘Do reef fish habituate to diver presence? evidence from two reef sites with contrasting historical levels of SCUBA intensity in the Bay islands, Honduras’. PLoS One 10, e0119645. doi: 10.1371/journal.pone.0119645
Titus B. M., Daly M., Exton D. A. (2015b). ‘Temporal patterns of pederson shrimp (Ancylomenes pedersoni chace 1958) cleaning interactions on Caribbean coral reefs’. Mar. Biol. 162, 1651–1664. doi: 10.1007/s00227-015-2703-z
Titus B. M., Daly M., Vondriska C., Hamilton I., Exton D. A. (2019). ‘Lack of strategic service provisioning by Pederson’s cleaner shrimp (Ancylomenes pedersoni) highlights independent evolution of cleaning behaviors between ocean basins’, Sci. Rep. 9, 629. doi: 10.1038/s41598-018-37418-5
Titus B. M., Vondriska C., Daly M. (2017). ‘Comparative behavioural observations demonstrate the ‘cleaner’ shrimp Periclimenes yucatanicus engages in true symbiotic cleaning interactions’. R. Soc Open Sci. 4, 170078. doi: 10.1098/rsos.170078
Vaughan D. B., Grutter A. S., Costello M. J., Hutson K. S. (2017). ‘Cleaner fishes and shrimp diversity and a re-evaluation of cleaning symbioses’. Fish Fish. 18, 698–716. doi: 10.1111/faf.12198
Voellmy I. K., Purser J., Flynn D., Kennedy P., Simpson S. D., Radford A. N. (2014). ‘Acoustic noise reduces foraging success in two sympatric fish species via different mechanisms’. Anim. Behav. 89, 191–198. doi: 10.1016/j.anbehav.2013.12.029
Waldie P. A., Blomberg S. P., Cheney K. L., Goldizen A. W., Grutter A. S. (2011). ‘Long-term effects of the cleaner fish Labroides dimidiatus on coral reef fish communities’. PLoS One 6, e21201. doi: 10.1371/journal.pone.0021201
Wale M. A., Radford A. N., Simpson S. D. (2013). ‘Size-dependent physiological responses of shore crabs to single and repeated playback of ship noise’. Biol. Lett. 9, 20121194. doi: 10.1098/rsbl.2012.1194
Ware H. E., McClure C. J. W., Carlisle J. D., Barber J. R., Daily G. C. (2015). ‘A phantom road experiment reveals traffic noise is an invisible source of habitat degradation.rsquo;. Proc. Natl. Acad. Sci. U. S. A. 112, 12105–12109. doi: 10.1073/pnas.1504710112
Weilgart L. S. (2018). “The impact of ocean noise pollution on fish and invertebrates,” in Report for OceanCare (OceanCare, Switzerland: Switzerland).
Whiteman E. A., Côté I. M., Reynolds J. D. (2002). ‘Do cleaning stations affect the distribution of territorial reef fishes?’ Coral Reefs 21, 245–251. doi: 10.1007/s00338-002-0241-x
Williams R., Veirs S., Veirs V., Ashe E., Mastick N. (2019). ‘Approaches to reduce noise from ships operating in important killer whale habitats’ . Mar. pollut. Bull. 139, 459–469. doi: 10.1016/j.marpolbul.2018.05.015
Wright K. J., Higgs D. M., Leis J. M. (2011). ‘Ontogenetic and interspecific variation in hearing ability in marine fish larvae’. Mar. Ecol. Prog. Ser. 424, 1–13. doi: 10.3354/meps09004
Keywords: anthropogenic noise, SCUBA, community-level impacts, cleaning mutualism, coral reefs, marine invertebrates, reef fishes, interspecific behaviour
Citation: McCloskey KP, Radford AN, Rose A, Casiraghi G, Lubbock N, Weschke E, Titus BM, Exton DA and Simpson SD (2023) SCUBA noise alters community structure and cooperation at Pederson’s cleaner shrimp cleaning stations. Front. Mar. Sci. 10:1058414. doi: 10.3389/fmars.2023.1058414
Received: 30 September 2022; Accepted: 10 March 2023;
Published: 25 April 2023.
Edited by:
Marta Solé, BarcelonaTech (UPC), SpainReviewed by:
Marta Picciulin, National Research Council (CNR), ItalyZan Hammerton, Southern Cross University, Australia
Christopher W. McKindsey, Fisheries and Oceans Canada (DFO), Canada
Copyright © 2023 McCloskey, Radford, Rose, Casiraghi, Lubbock, Weschke, Titus, Exton and Simpson. This is an open-access article distributed under the terms of the Creative Commons Attribution License (CC BY). The use, distribution or reproduction in other forums is permitted, provided the original author(s) and the copyright owner(s) are credited and that the original publication in this journal is cited, in accordance with accepted academic practice. No use, distribution or reproduction is permitted which does not comply with these terms.
*Correspondence: Stephen D. Simpson, cy5zaW1wc29uQGJyaXN0b2wuYWMudWs=
†Present address: Kieran P. McCloskey, Lincolnshire Wildlife Trust, Banovallum House, Spilsby, Lincolnshire, United Kingdom