- 1State Key Laboratory of Marine Resource Utilization in South China Sea, Hainan University, Haikou, China
- 2Ocean College, Hainan University, Haikou, China
Sea cucumber Stichopus monotuberculatus is one species of tropical sea cucumbers with high recognition and economic value. While advances have been made in the nursery rearing of the sea cucumber, influence of environmental factors on its locomotion behavior remains less understood, which restricts the establishment of mariculture technologies. In the present study, locomotion behavior of S. monotuberculatus under different temperatures and flow velocities were examined through controlled simulation experiments. Results showed that the creeping activities were obviously affected by temperature, and the most active movement and feeding behavior were recorded at relatively high temperatures. Diurnal variation of locomotion and feeding activities indicated that S. monotuberculatus displayed an evident nocturnal activity pattern, being the most active at night, exhibiting intermediate activity at dusk, and minimal activity during the daytime. The movement velocity decreased with the increasing flow speed and was only 1.65 ± 1.35cm·min-1 in the highest flow rate group (20.8 ± 3.4cm/s). Moreover, the sea cucumber S. monotuberculatus displayed positive rheotaxis behavior of moving downstream at all flow velocities. Overall, the sea cucumber S. monotuberculatus exhibited high locomotor and feeding activities at night of relatively high temperature, and its favoured flow regime was downstream and low water velocity area. These findings may assist the sea ranching and aquaculture development of the tropical commercial sea cucumber species.
1. Introduction
Sea cucumbers (Holothurians) are considered to be one of the most conspicuous marine invertebrates species belonging to class Holothuroidea of phylum Echinodermata. While resource managers struggle to address pandemic overfishing of tropical sea cucumber stocks, mariculture and sea ranching of some tropical species ascends as a viable prospect to subsidise waning captures (Bell et al., 2005; Yang et al., 2015). Tropical sea cucumber mariculture has potential to become a profitable industry and contribute towards natural population replenishment.
Movement of holothurians is mainly related to feeding, reproduction and searching for optimal environmental conditions (Uthicke, 1999; Navarro et al., 2013; Sun et al., 2018a; Hamel et al., 2019). Knowledge about the behavioral features of sea cucumbers under different environmental conditions is beneficial not just to understand the species’ behavior in wild but also to improve the rearing techniques for sea cucumbers. Sea cucumbers are mostly slow-moving or sedentary, and the feature makes their distribution and behavior susceptible to environmental drivers (Hair et al., 2020; Sun et al., 2020), such as substrate type (Woodby et al., 2000), salinity (Kashenko, 2002), light cycles (Dong et al., 2011) and so on. Temperature, as an essential environmental factor, can directly affect the locomotion (Sun et al., 2018b), circadian rhythm (Kato and Hirata, 1990), burying and feeding (Wolkenhauer, 2008; Purcell, 2010) behavior of sea cucumbers. Also, water flow is an important ecological factor for aquatic organisms, and researches on the effect of water flow on aquatic organisms mainly focuses on fishes (Pavlov et al., 2000; Liao and Cotel, 2013; Li et al., 2018). Water current can affect the movement, orientation, feeding, and growth of shellfish (Wildish and Kristmanson, 1988; Wildish and Saulnier, 1992; Pilditch, 1999; Sakurai and Seto, 2000). Movement pattern and feeding behavior in response to variable water currents have been observed in echinoderms, including sea urchin (Dumont et al., 2007), sea lily (Kitazawa and Oji, 2014), and numerous species of sea cucumbers. According to Lin (2014), A japonicus moved more distance at the slow flow (5 cm/s) than in the still water, but almost stationary in the riptide (30 cm/s); Qiu et al. (2014) considered that water current is a key factor that influences substrate selection by A. japonicus. In addition, water flow can elicit displacement and feeding behavior changes in suspension-feeding sea cucumber Cucumaria frondosa (Sun et al., 2018a).
Sea cucumber Stichopus monotuberculatus (previously named as Stichopus variegatus, Fan et al., 2012) is a tropical species that colonize benthic habitats in the Indian Ocean from the Red Sea and Madagascar to Easter Island, and in the Pacific Ocean from Japan to Australia (Massin, 1996; Purcell et al., 2012b). As a large edible sea cucumber with thick body wall and well mouthfeel, it is one of the species with high recognition and economic value among tropical economic sea cucumbers (Conand, 1993; Liao, 1997). Due to its nutritional and medicinal value, previous researches mainly focused on the nutritional components and biologically active components which have anticoagulant, lipid-lowering, anti-tumor, and other physiological pharmacological activities (Wang et al., 2010; Zhong et al., 2015; Huang et al., 2020). In recent years, the biological function of a series of immune related proteins such as ferritin, translationally controlled tumor protein, serine protease inhibitors, gamma-interferon-inducible lysosomal thiol reductase and poly-U-binding factor 60 kDa were characterized in sea cucumber S. monotuberculatus (Ren et al., 2014a; Ren et al., 2014b; Ren et al., 2015a; Ren et al., 2015b; Yan et al., 2016). Genetic structure of different populations of S. montuberculatus in the South China Sea were characterized (Yuan et al., 2013; Wen et al., 2018).As one widely accepted species in international bêche-de-mer markets, the artificial breeding of S. monotuberculatus has been successfully broken through in China (Hu et al., 2010; Wang et al., 2017; Cheng et al., 2021). Technology for culturing a few of the tropical species has advanced in recent years, but a number of diverse impediments currently undermine profitability and reliability of mariculture and present challenging research opportunities (Purcell et al., 2012a). However, the effect of important environmental factors, including water temperature and water flow, on the locomotor behaviors of S. monotuberculatus has received less attention.Water temperature and flows represent the critical aspects of the environment affecting sea cucumbers. They are inextricably linked to the distribution (Pan et al, 2015), locomotion behavior, ingestion rate, and diel rhythm of sea cucumber (Sun et al., 2018b), which were closely related to the area selection of sea ranching and the setting of suitable breeding conditions. For example, an inappropriately high flow rate would lead to feed waste, and sea cucumbers had to consume more energy to increase the adsorption force of the tube feet in order to maintain body stability. Well-designed experiments and analyses are needed to fill critical knowledge gaps if its mariculture is to expand in the tropics as the temperate sea cucumber A. japonicus has presented in Asia. The current study was carried out under controlled laboratory conditions to ensure that the effects of temperature or flow velocity were separate from other environmental factors. We used video technology and behavior analysis software to investigate the behavior of S. monotuberculatus under different water temperatures or flow velocity. Quantitative indices were applied to analyze the experimental data. We examined locomotion behavior, diel feeding activity of S. monotuberculatus under different temperatures, and behavior responses of S. monotuberculatus to flow velocity variation. Such research may be helpful for understanding the distribution as well as the feeding and locomotor behaviors of S. monotuberculatus in response to various stimuli. We hope our study can provide a reference for stock management in identifying the most suitable sea ranching areas and assist in the eventual development of aquaculture programs.
2. Materials and Methods
2.1 Specimen Collection and Maintenance
Sea cucumbers were collected from Dingda Breeding limited company, in Wenchang city of Hainan Province, China, and transported to the State Key Laboratory of Marine Resource Utilization in South China Sea. All individuals were acclimated in tanks (1000 L) at 26.0 ± 1.0°C, salinity 32-35, a Ph of 8.0-8.3. During the acclimation period, the light condition is natural without artificial light sources, and the sea cucumbers were fed using an artificial feed (sea mud: Sargasso algal powder =7:3, weight ratio) once at 09:00 h each day. Healthy and undamaged sea cucumbers (about 10g) were selected for the experiments.
2.2 Experimental Design
2.2.1 Effect of Temperature
Four temperatures (22, 25, 28, and 31°C) were selected, approximately representing the overall temperature range (20-33° C) found in the natural habitat of S. monotuberculatus, to study their effects on the sea cucumber’s behavior. After acclimation, a group of five sea cucumbers were introduced into each of the four opaque experimental tanks (100×100×150cm, height × width × depth). The water temperature was then adjusted to the desired temperature at a rate of 1°C day-1, and the sea cucumbers were maintained at the experimental temperatures for 7 days. The water depth was maintained at 30 cm during the experiment. Each trial was run for 5 days in the experimental condition. The animals were fed once per day, and half of the water was exchanged at 09: 00 h. Charge-coupled device cameras (Hikvision, DS-2CC11A2P-IR3, China) with the infrared system and a video recorder were used to record the behaviors of sea cucumbers. Video images were analyzed using Tracker Video Analysis and Modeling Tool software (Open Source Physics Project, https://physlets.org/tracker/, Vasquez et al., 2018; Vasquez et al., 2020). The experimental indexes include movement distance, cumulative motion time and movement velocity of the sea cucumber. Moving distance means the distance the sea cucumber traveled in the experimental area. Cumulative motion time refers to the total time of sea cucumber moved during the day or night. Movement velocity means the average velocity of the sea cucumber crept in each day.
Feeding behavior was determined by the sea cucumber’s position (if or not near the food), behavior (Sea cucumbers move their heads from side to side in order to scratch for food by their tentacles, rather than moving straight with their heads close to the ground) and the variation of feed on the aquarium bottom (Sun et al., 2018b). The proportion of feeding sea cucumbers were determined every 2 h based on the videos. The observation of locomotion is based on the analysis of the distance of sea cucumber every three minutes.In the present study, 06:00 h to 18:00 h was defined as ‘day’, and 18:00 h to 06:00 h was defined as ‘night’. The sea cucumbers were fed on the same diet as during the acclimation period for all studies.
2.2.2 Effect of Water Flow
The experiment was conducted in an annulus tank (1.2 m × 0.45 m) with a 0.2m wide region for water flow (Figure 1). A water pump was used to circulate water through the tank, and a frequency converter connected to the water pump was used to adjust water velocity. Water velocity was measured using a current meter (LS1206B, Xiangruide Inc., China).
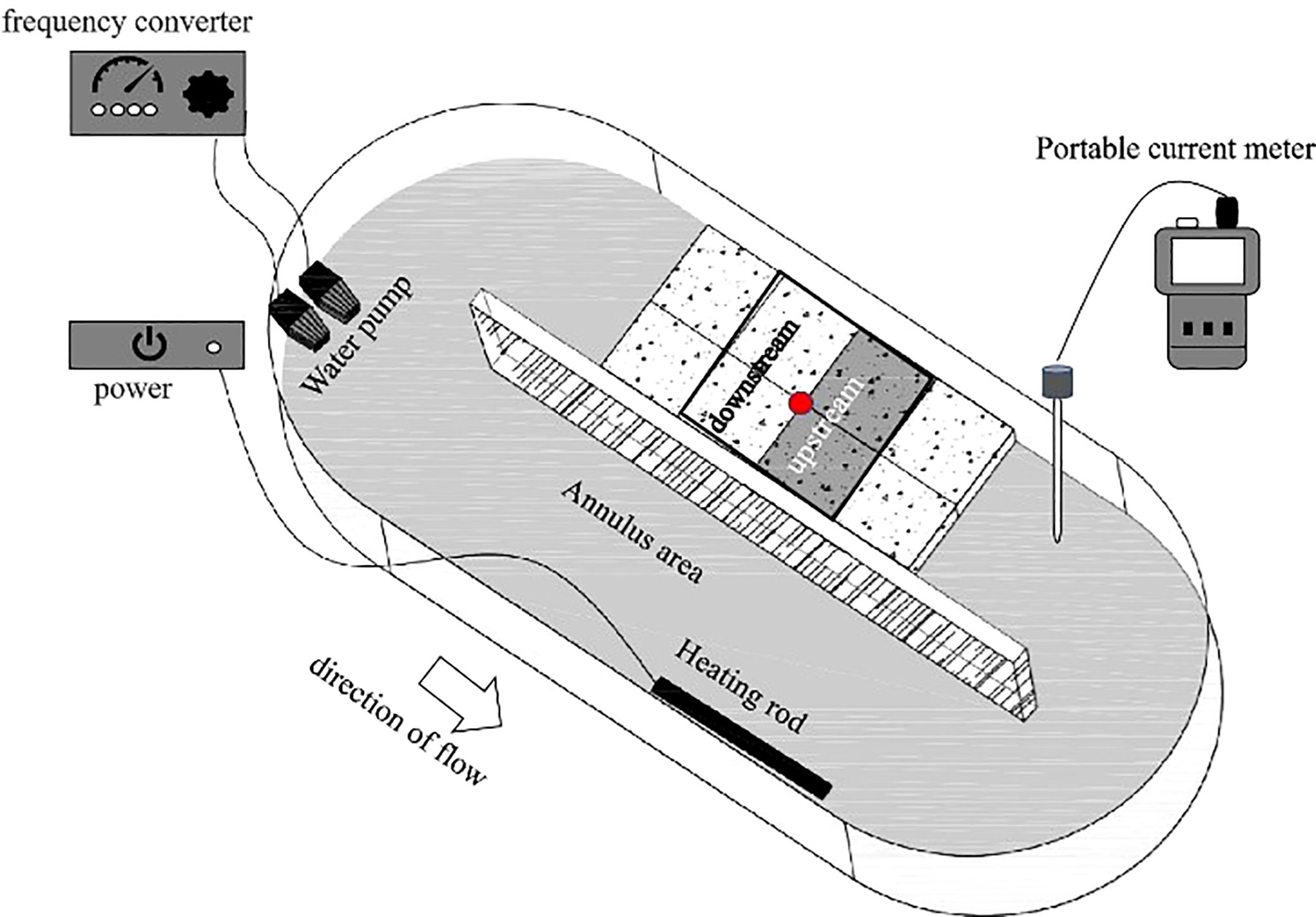
Figure 1 The tank used in the water flow experiment. The red dot is the starting point of the sea cucumbers.
The locomotion of S. monotuberculatus was investigated at four flow rates, 0 cm/s (S), 4.7 ± 1.2 cm/s (L), 10.5 ± 1.7 cm/s (M), and 20.8 ± 3.4 cm/s (H). Ten replicate trials were run for each of the four treatments, and each trial was conducted by different sea cucumber individuals. In each experiment, one sea cucumber was placed in the center of a rectangular arena (20 cm × 20 cm) in the straight section of the tank, so the arena was divided into two zones, the upstream area and the downstream area (Figure 1). Each trial was run until the experimented sea cucumber moved out of the arena or the time for the trial reached 0.5 h.
Charge-coupled device cameras (Hikvision, DS-2CC11A2P-IR3, China) were used to record the activities of S. monotuberculatus at 10s intervals. Video images were analyzed using Tracker software to record the tracks of S. monotuberculatus at four flow rates. The experimental indexes include movement distance, movement velocity, and cumulative residence time of the sea cucumber. The calculation of movement distance and movement velocity is the same with the index in part 2.2.1. Cumulative residence time means the time spent between the start of the experiment and the time the sea cucumber left the experimental area.
2.3 Statistical Analysis
All statistical analysis was performed with the SPSS 19.0 for Windows statistical package. One-way ANOVA followed by post hoc multiple comparisons with Tukey’s test was performed to test the distance, time, and speed over environmental factors. The differences between day and night of the distance, time, and speed of sea cucumbers were compared using an independent sample t-test. The probability level of 0.05 was used to reject of the null hypothesis, and all data were presented as means ± s. d.
3. Results
3.1 Effects of Temperature on Locomotion Behavior
3.1.1 Movement Distance
The movement distance of S. monotuberculatus was significantly affected by water temperature (ANOVA, F=15.39; P<0.001). At every temperature tested, the movement distance at night (82.58-321.12 cm) was significantly higher than that in the daytime (25.47-105.17 cm) (Figure 2, P<0.05). The movement distance during the day decreased as the temperature increased from 22°C to 25°C, then increased from 25°C and reached a peak at 31°C. At night, the movement distance decreased as the temperature increased from 22°C to 28°C, then increased above 28°C. No significant difference was observed in the movement distance at night at water temperatures of 22, 25, or 28°C. However, at 31°C, the movement distance at night was significantly higher than in other treatment groups (P<0.05).
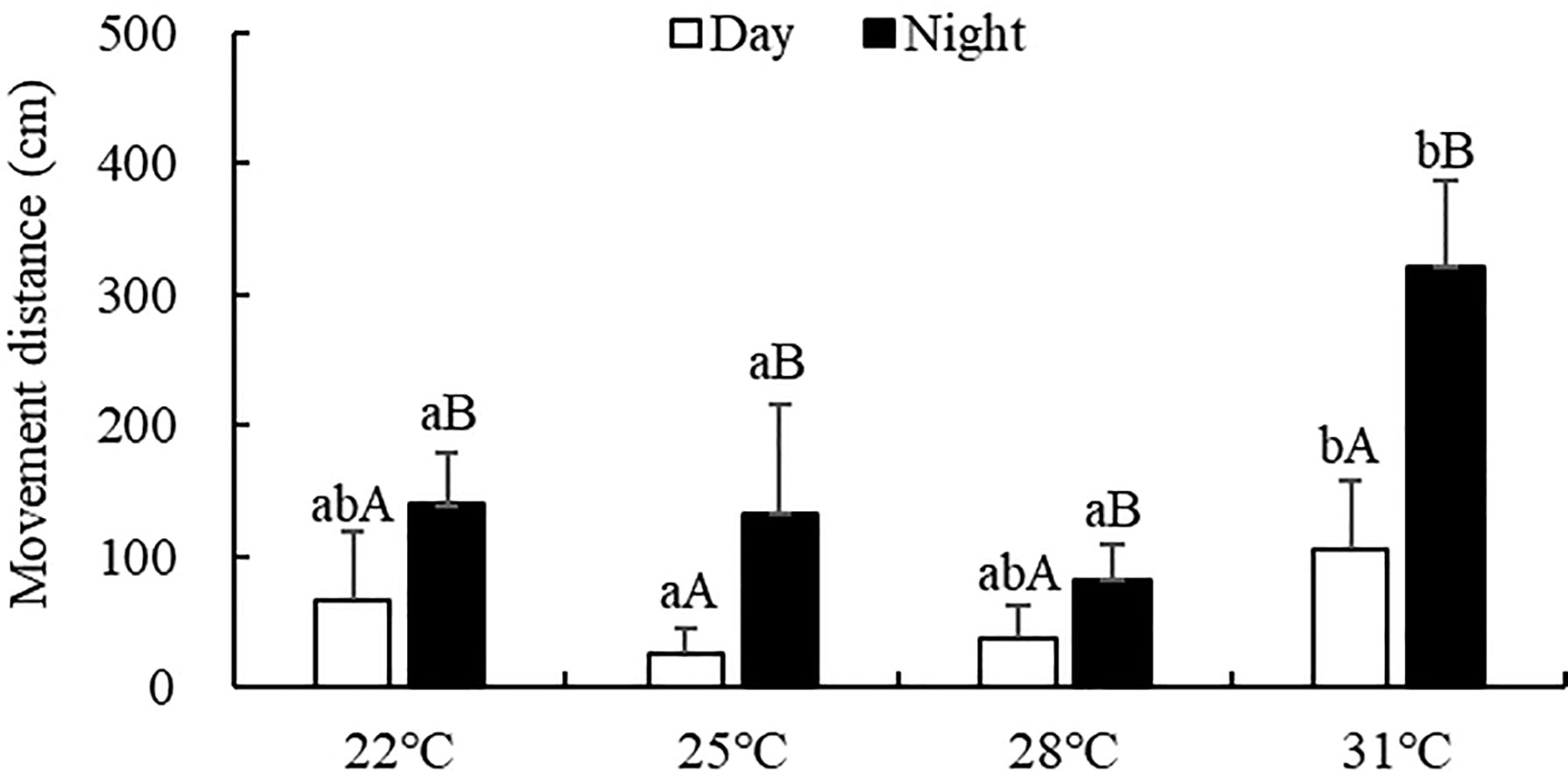
Figure 2 Movement distance of S. monotuberculatus at four temperature conditions. Marks of lowercase letters indicate that there is a significant difference at different temperatures (P < 0.05); marks of uppercase letters indicate that there is a significant difference between day and night (P < 0.05).
3.1.2 Cumulative Motion Time
The cumulative motion time of S. monotuberculatus was significantly affected by water temperature (ANOVA, F=12.87, P<0.001). At every temperature tested, the cumulative motion time was significantly higher at night (69.96-195.00 min) than that in the daytime (18.12-73.32 min) (P<0.05; Figure 3). During the day, S. monotuberculatus decreased its cumulative motion time as the temperature changed from 22 to 25°C, then increased above 25°C. The maximum movement distance in the day was observed at 31°C, which was significantly higher than the other groups (P<0.05). No significant differences in the cumulative motion time during the day were observed between 22, 25, and 28°C treatments (P>0.05). At night, the cumulative motion time decreased as the temperature increased from 22°C to 28°C, then increased above 28°C. At 31°C, the cumulative motion time at night was significantly higher with respect to other treatment groups (P<0.05 for all).
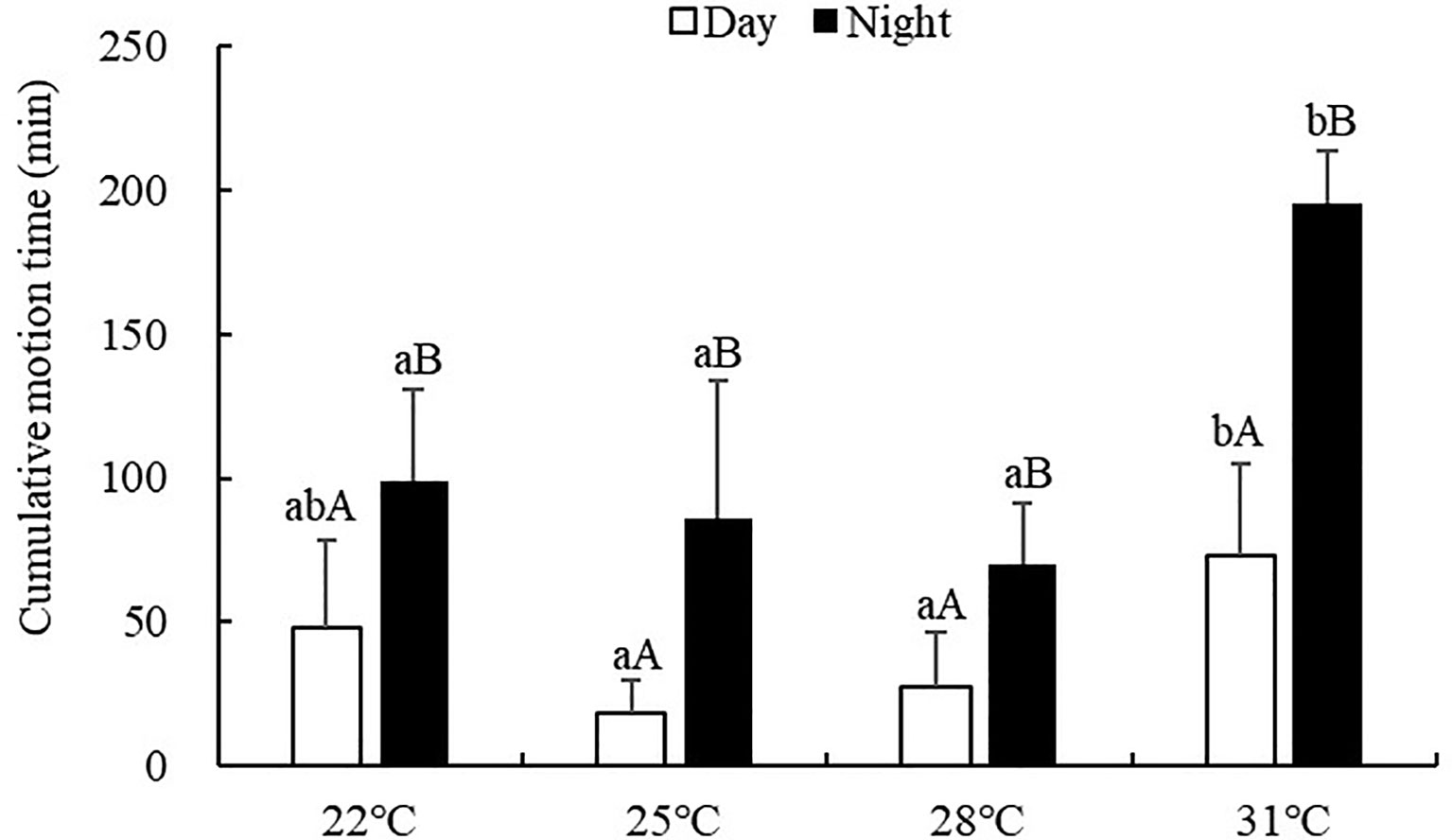
Figure 3 Cumulative motion time of S. monotuberculatus at four temperature conditions. Marks of lowercase letters indicate that there is a significant difference at different temperatures (P<0.05); marks of uppercase letters indicate that there is a significant difference between day and night (P<0.05).
3.1.3 Movement Velocity
Movement velocity of S. monotuberculatus was 0.85-1.91 cm/min during the experiment period. The movement velocity was also significantly affected by water temperature (ANOVA, F=4.89, P=0.01) (Figure 4). Significant differences were observed between day and night at 25 and 31°C temperature treatments (P<0.05). During the day, no significant difference was observed among all treatment groups (P>0.05). However, at night, the movement velocity at 31°C treatment was significantly higher than the other treatments (P<0.05).
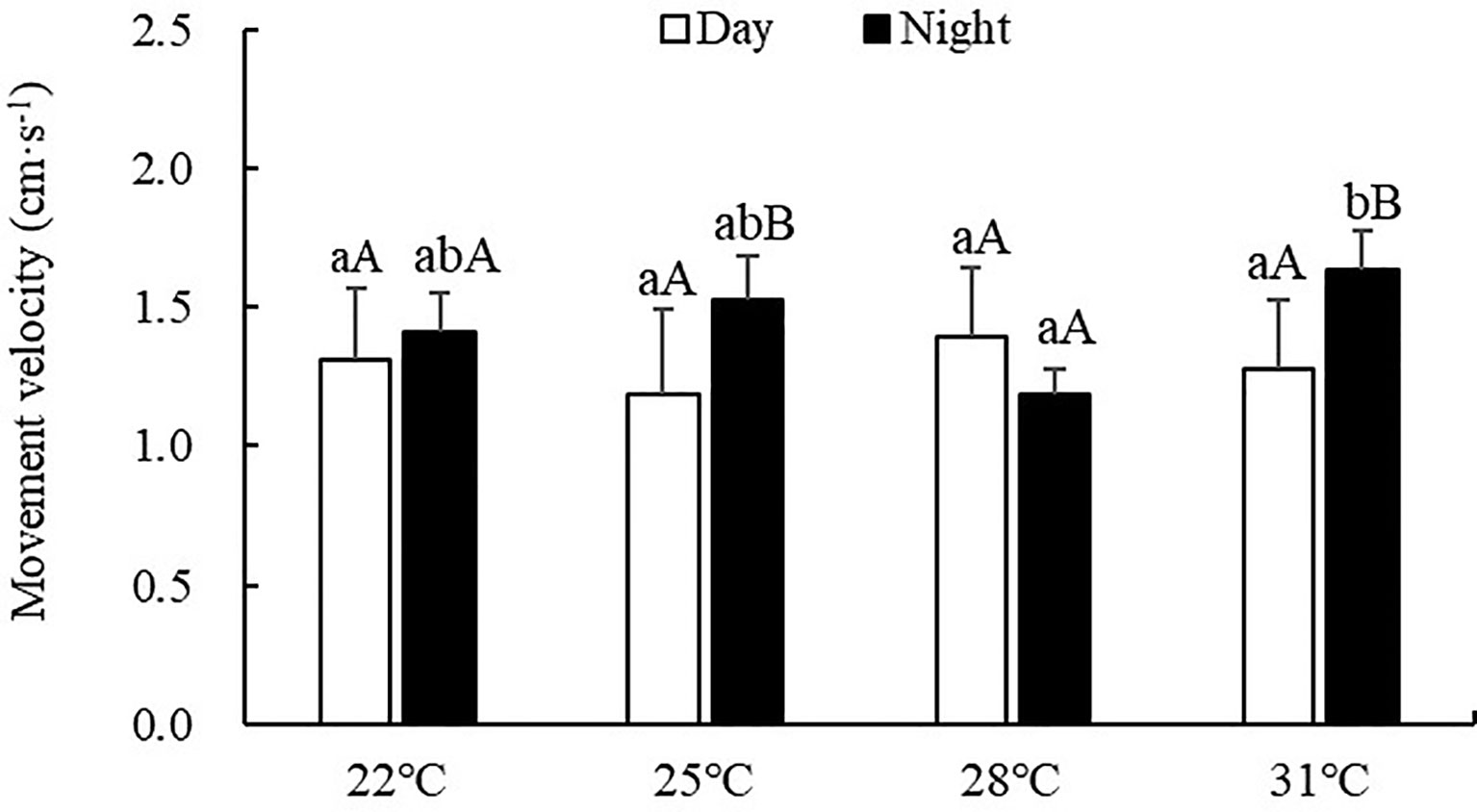
Figure 4 Movement velocity of S. monotuberculatus at four temperature conditions. Marks of lowercase letters indicate that there is a significant difference at different temperatures (P < 0.05); marks of uppercase letters indicate that there is a significant difference between day and night (P< 0.05).
3.1.4 Diel Motor Rhythm
All temperature treatments displayed a similar locomotion pattern, sea cucumbers traveled more at night than during the day (Figure 5). The motion peak appeared from 20:00 to 06:00 of the next day, while the through period was from 12:00 to 18:00. While comparing between different temperature treat groups, the sea cucumbers at 31°C showed higher locomotion activity, especially at night, with the movement distances at 31°C were significantly higher than all the other groups from 20:00 to 04:00 (P<0.05).
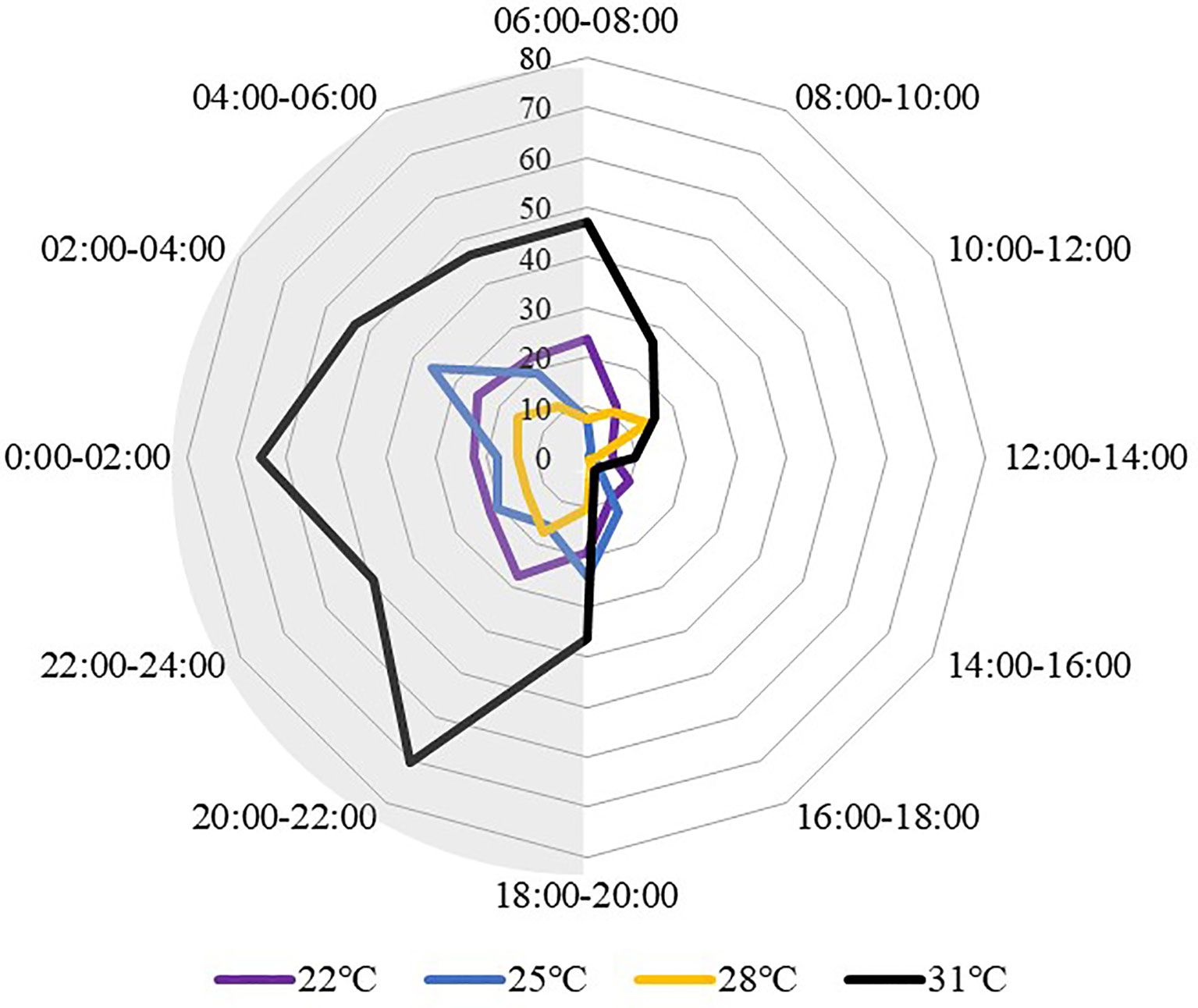
Figure 5 Movement distance of S. monotuberculatus changed with time at four temperature conditions. Grey areas correspond to periods of night.
3.2 Effects of Temperature on Diel Feeding Rhythm
Sea cucumbers showed a nocturnal feeding rhythm (Figure 6). The proportion of feeding sea cucumber did not change significantly within one day, but had a higher feeding proportion at night than during the day. The feeding proportion in the daytime was generally lower, then had an obvious rising trend after 18:00 under all temperature treatments, occurred peaks at 18:00 to 20:00 and 0:00 to 04:00. The feeding peak appeared at 00:00-02:00 at 31°C with an ingestion ratio of 60.00± 14.00%, and the lowest ingestion ratio appeared at 08:00-10:00 at 25°C (4.00 ± 2.20%). At 22°C and 28°C, sea cucumbers have a similar feeding rhythm. The 22°C and 25°C treatments showed a feeding peak at 18:00 to 20:00, whereas other treatments occurred during 0:00 to 02:00.
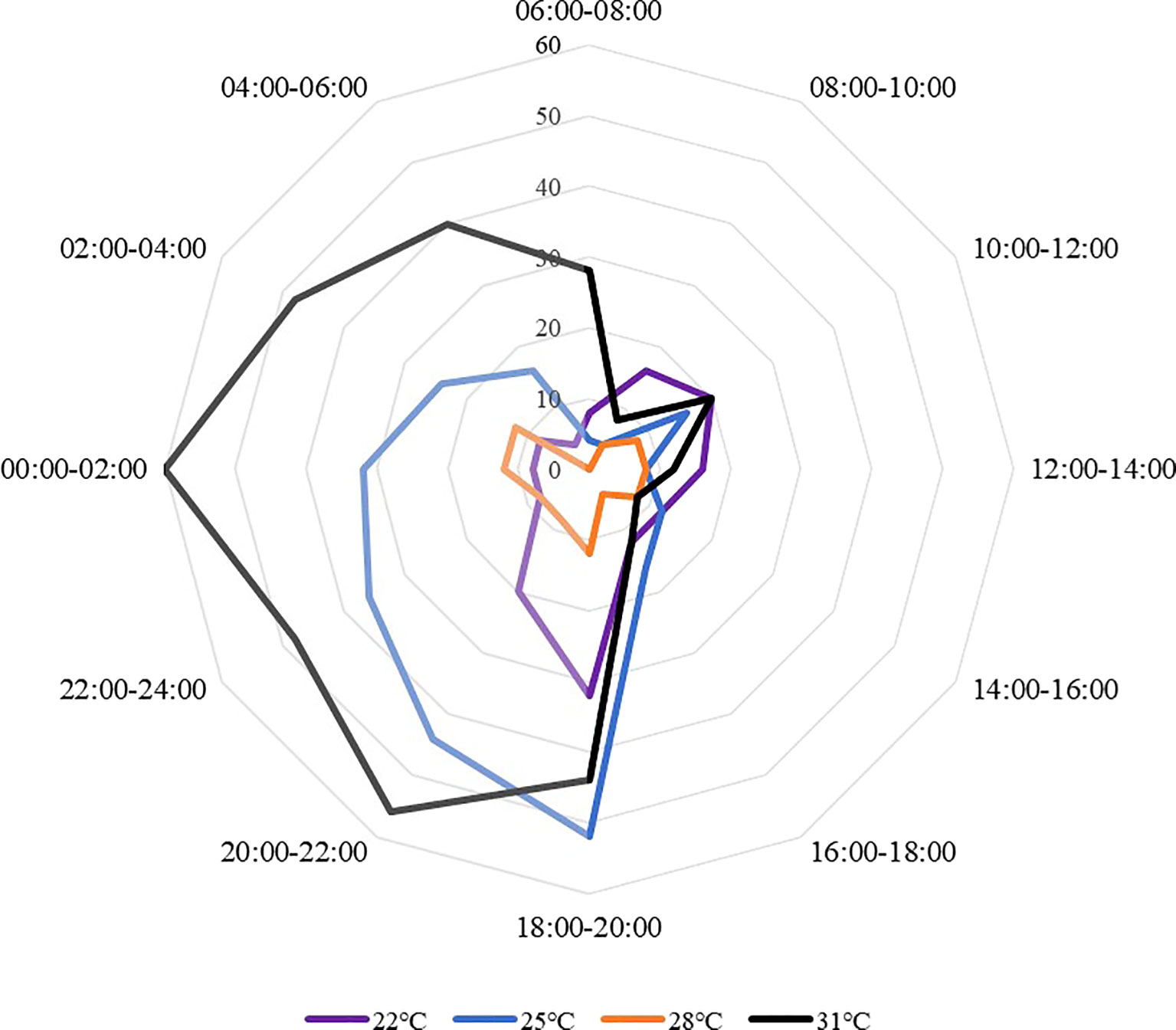
Figure 6 The feeding proportions of S. monotuberculatus changed with time at four temperature conditions.
3.3 Effects of Flow on Locomotion Behavior
3.3.1 Movement Velocity
The flow rate had a significant influence on the movement velocity of sea cucumbers (F=9.486; P<0.001). The movement velocity of S. monotuberculatus was 4.33 ± 2.29 cm/min, 5.46 ± 3.41 cm/min, 4.17 ± 1.87 cm/min, and 1.63 ± 1.35 cm/min for the still, low, medium, and high flow rate respectively, which showed a trend of increasing first and then decreasing with the further increasing flow velocity. The sea cucumber’s movement velocity was significantly lower at high flow rates (20.8 ± 3.4 cm/s) compared with that at the other three flow rates (P<0.05) (Figure 7).
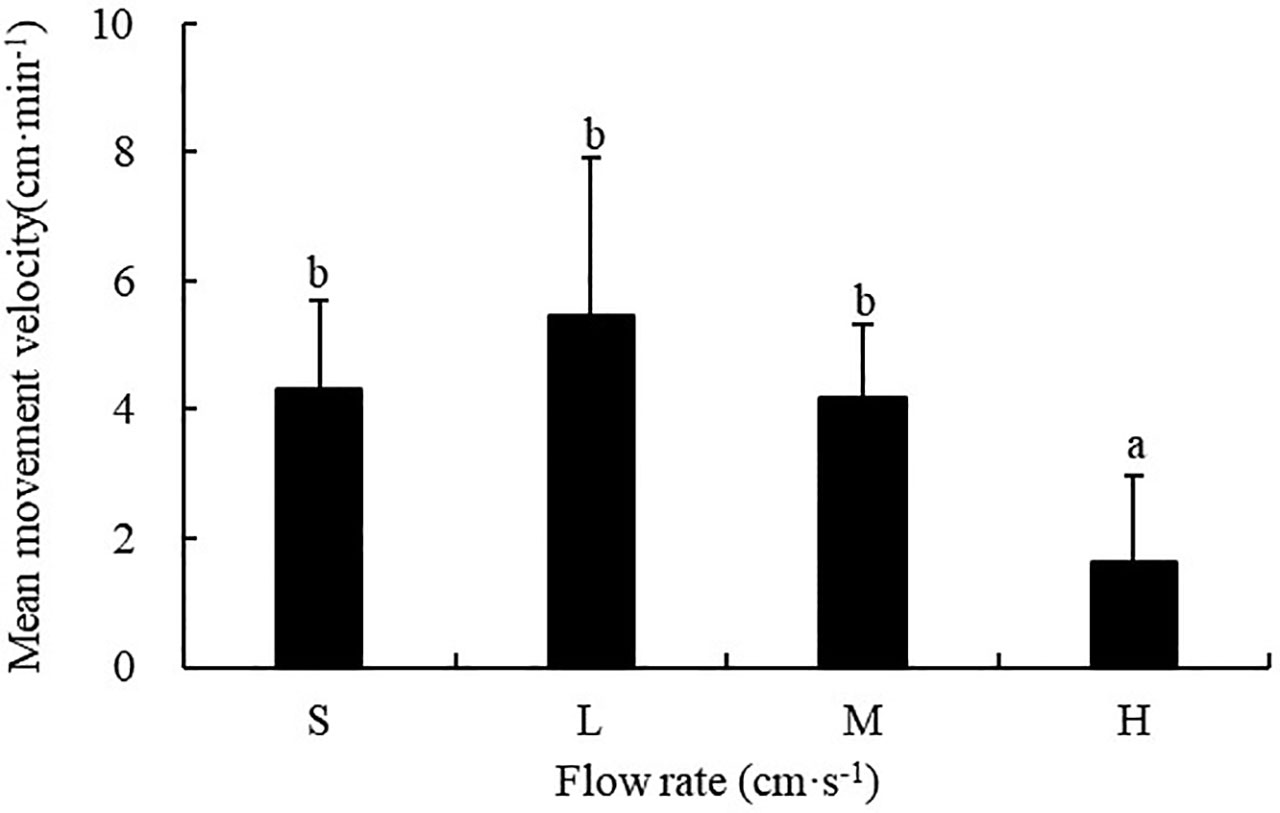
Figure 7 Movement velocity of S. monotuberculatus at four different flow rates. S, L, M, and H represent the four flow rates: 0 cm/s, 4.7 ± 1.2 cm/s, 10.5 ± 1.7 cm/s, and 20.8 ± 3.4 cm/s respectively.
3.3.2 Cumulative Residence Time
The flow rate had a significant influence on the cumulative residence time of sea cucumbers (F=45.186, P<0.001). The cumulative residence time of S. monotuberculatus was 4.88 ± 1.71 min, 7.58 ± 3.87 min, 10.28 ± 4.24 min and 26.6 ± 7.01 min for the still, low, medium, and high flow rate respectively, which showed a sustained rising tendency with the augment of flow rate. The cumulative residence time at high flow rates of 20.8 ± 3.4cm/s was significantly higher than those at the other three flow rates (P<0.05) (Figure 8).
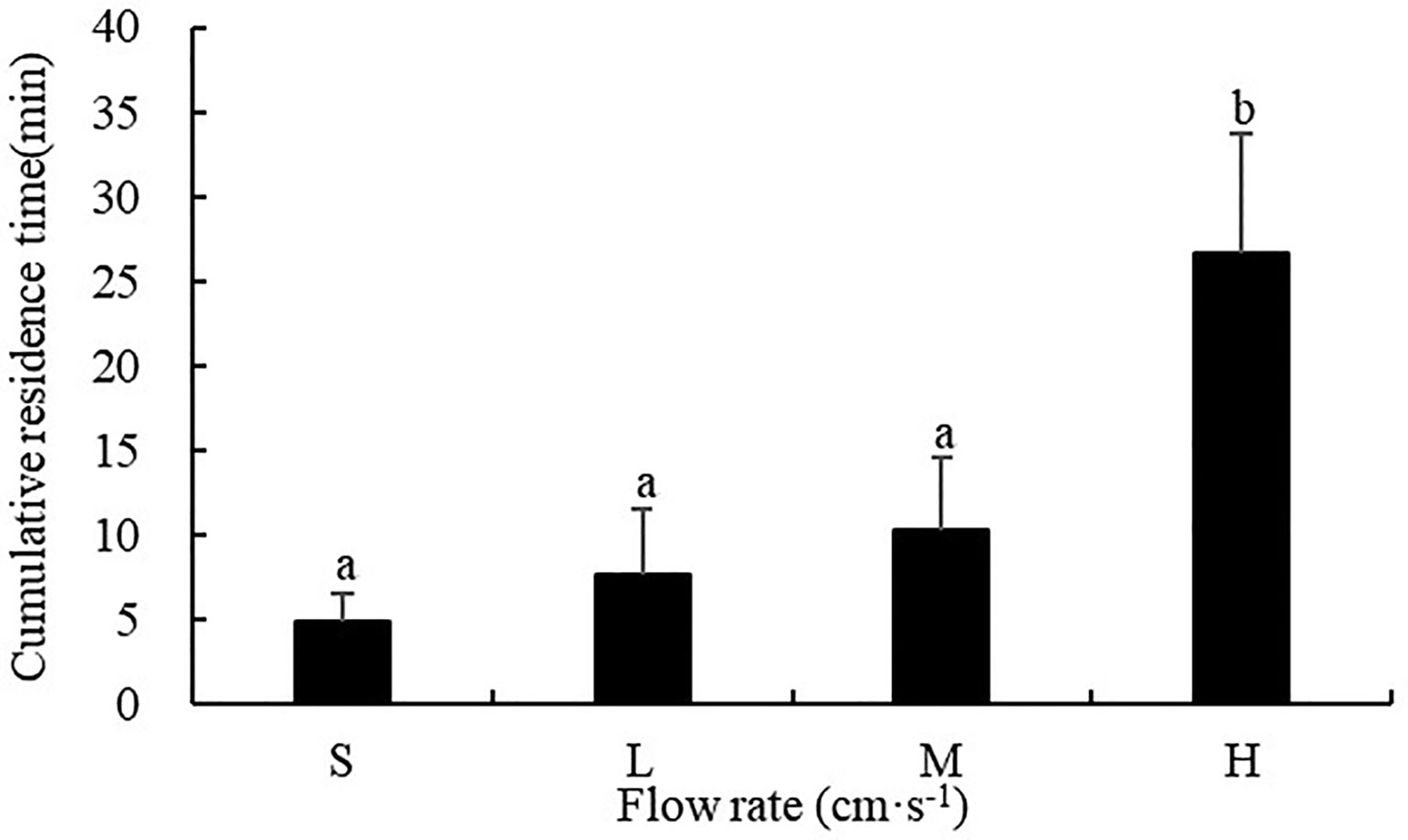
Figure 8 Cumulative residence time of S. monotuberculatus at four different flow rates. S, L, M, and H represent the four flow rates: 0 cm/s, 4.7 ± 1.2 cm/s, 10.5 ± 1.7 cm/s, and 20.8 ± 3.4 cm/s respectively.
3.3.3 Cumulative Residence Time in the Downstream and Upstream Area
At every flow rate tested, the cumulative residence time of S. monotuberculatus was longer in the downstream area compared with the upstream area (Figure 9). At the low and fastest flow rate, 4.7 ± 1.2 cm/s and 20.8 ± 3.4 cm/s, the cumulative residence time in the downstream area was significantly longer than in the downstream area (P<0.05) (Figure 3). At the other two flow rates, there were no significant differences in cumulative residence time between the two zones (P>0.05) (Figure 9).
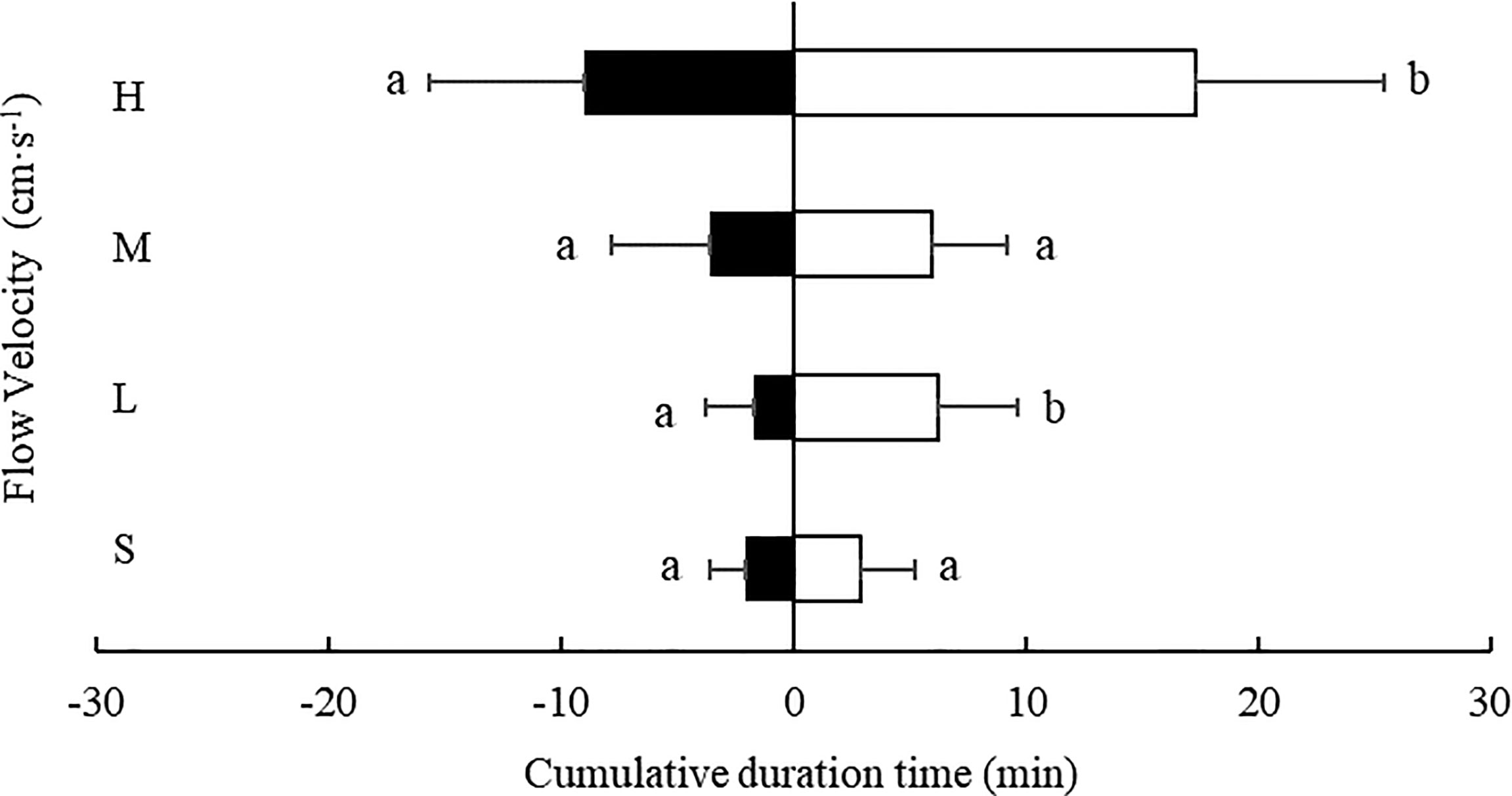
Figure 9 Cumulative residence time of S. monotuberculatus at four water velocities in the downstream (white) and upstream zones (black) at four different flow. S, L, M, and H represent the four flow rates: 0 cm/s, 4.7 ± 1.2 cm/s, 10.5 ± 1.7 cm/s, and 20.8 ± 3.4 cm/s respectively.
3.3.4 Movement Velocity in the Downstream and Upstream Area
Except for the low flow rate (4.7 ± 1.2 cm/s), at all other flow rates tested, the movement velocity of S. monotuberculatus was higher in the downstream area than in the upstream area (Figure 10). However, at every flow rate tested, there was no significant difference in the movement velocity of sea cucumber in the two zones (P>0.05).
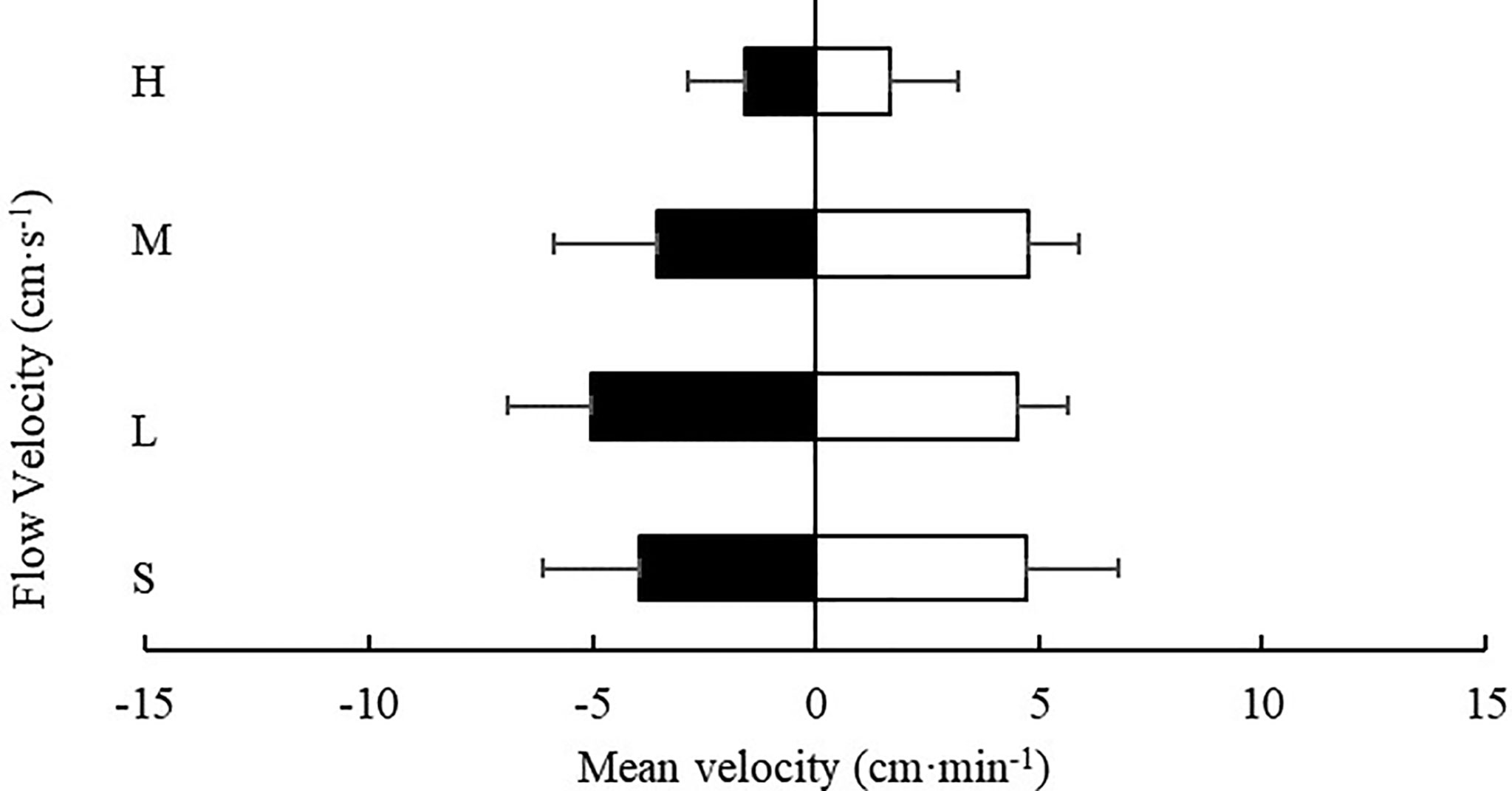
Figure 10 Movement velocity of S. monotuberculatus at four water velocities in the downstream (white) and upstream zones (black) at four different flows. S, L, M, and H represent the four flow rates: 0 cm/s, 4.7 ± 1.2 cm/s, 10.5 ± 1.7 cm/s, and 20.8 ± 3.4 cm/s respectively.
4. Discussion
The factors that influence aquatic animal distribution and behaviors have been studied in many respects to understand better habitat requirements, community dynamics, and species-specific aquaculture prerequisites (Lovatelli et al., 2004; Sun et al., 2018a). Researches on the locomotion behavior of sea cucumbers can be traced back to 1990, and an automatic apparatus was designed to quantitatively study the movement of sea cucumbers Stichopus japonicus (Kato and Hirata, 1990). In recent years, sea cucumber behavior studies mainly focus on the effects of substrate or artificial reefs on distribution (Zhang et al., 2014; Sun et al., 2020), the influence of light and phytoplankton on the behavior rhythm and feeding (Zhang et al., 2006; Dong et al., 2010; Dong et al., 2011; Sun et al., 2015; Sun et al., 2020), and the effects of water temperature and flow velocity on the feeding and locomotion behavior (Pan et al., 2015; Sun et al., 2018a; Sun et al., 2018b). The objects of previous researches mostly involved temperate deposit-feeding sea cucumber A. japonicus and suspension-feeding species C. frondosa. In the present study, the influences of environmental factors, including temperature and water flow, on the behavior of the tropical deposit-feeding sea cucumber S. monotuberculatus were studied under controlled laboratory conditions.
4.1 Effects of Temperature on Behavior
Water temperature is a crucial factor in the living condition of aquatic organisms (Buentello et al., 2000). It is closely linked to the growth (Dong and Dong, 2006; Dong et al., 2006; Ji et al., 2008), metabolism (Dong et al., 2006; Wang et al., 2008; Gao et al., 2009), and behavior of sea cucumbers (Wolkenhauer, 2008; Sun et al., 2018b). A. japonicus is one species of most studied temperate holothurian. Under low temperatures in winter or high temperatures in summer, A. japonicus reduces movement, metabolism, and feeding activities, entering hibernation when temperatures are lower than 3°C, commencing estivation at high temperature >20°C (Bao et al., 2010). The creeping activity of the sea cucumber A. japonicus decreased with increasing temperature from peak 52.3 m day-1 at 16°C to 21.6 m day-1 at 18°C (Kato and Hirata, 1990), and its highest feeding proportion was also at 16°C (Sun et al., 2018b). Studies of tropical sea cucumber species have also shown the effect of temperature on their behavior. The buried periods of sea cucumber H. scabra increased with decreasing temperature from 6.7 h per day at 24°C to 14.5 h per day at 17°C, and feeding activity decreased from 9.8 h a day at 24°C to 0.8 h per day at 17°C (Wolkenhauer, 2008). Mercier et al. (2000) found that most adult H. scabra did not follow their usual burying cycle when the water temperature increased to more than 30°C.
The present results showed that the creeping activities of S. monotuberculatus were also obviously affected by temperature. However, it was different from A. japonicus and H. scabra, and the highest movement distance and the cumulative motion time were recorded at the temperature of 31°C. The evolution of regional behavior may explain the differences in locomotion behavior among different species in response to temperature changes. Previous studies supported this point by Buccheri et al. (2019), which considered that different tropical sea cucumber species responded differently to temperature fluctuations, even though the testing sea cucumber species were collected from the same sea area. For instance, they found Holothuria atra had faster righting times at warmer temperatures (29°C and 33°C) than at 23°C, but Stichopus chloronotus had optimal righting times at 29°C and slower and more varied righting times at cooler and warmer temperatures (23°C and 33°C), while Holothuria edulis had righting times that were seemingly unaffected by the temperature changes. S. monotuberculatus is a tropical sea cucumber widely distributed from 1m to 30m depth in the coral reefs through the Indo-Pacific Ocean (Massin, 1996; Purcell et al., 2012b). In China, S. monotuberculatus has been recorded in the coastal shallow of the Xisha Islands, Hainan Island and the Leizhou Peninsula in the South China Sea (Liao, 1997). In Leizhou Peninsula sea area, S. monotuberculatus could survive in a temperature range of 14-32°C (Liang, 1987). Our results imply that this species of sea cucumber has the ability to live and feed at high water temperatures in the habitat. Additionally, one of the primary reasons for the movement of sea cucumbers is seeking for diets (Uthicke, 1999; Mercier et al., 1999), and high environmental temperatures resulted in an increased energetic cost and oxygen consumption (Dong and Dong, 2006; Dong et al., 2006). The sea cucumbers might augment movements to search for enough food meeting metabolic needs.Recent studies of other large tropical holothuroids, Stichopus herrmanni, B. argus and T. ananas, showed that they moved 9-15, 2-8 and 5-9 m d-1, respectively (Purcell et al., 2016; Wolfe and Byrne, 2017). These species exhibited site fidelity and long-term home ranging behaviour (Purcell et al., 2016; Wolfe and Byrne, 2017). The present results showed the movement distance of S. monotuberculatus was 1.19-4.26 m·d-1 in different temperature conditions. Thus, S. monotuberculatus may also display home range affinity. This behavior character highlights their vulnerability to overharvesting, with the potential risk of suffering significant declines, local extinctions and poor recovery (Uthicke et al., 2004). This finding has important implications for fisheries management regarding connectivity between recruitment and nearby adult habitats within the same area (Gillanders et al., 2003). In addition, artificial fishing reefs can be cast according to the movement distance of sea cucumbers or evoke reserve.
4.2 Diel Cycles
Many species of holothurians exhibited daily activity rhythms, with some ones having diurnal activity pattern (e.g., Pearsonothuria graeffei, Wheeling et al., 2007; Holothuria whitmaei, Shiell, 2006), some having nocturnal activity pattern (e.g., H. edulis, Wheeling et al., 2007; A. japonicus, Dong et al., 2011; H. scabra, Mercier et al., 1999; Mercier et al., 2000), or crepuscular activity pattern (e.g., Actinopyga mauritiana, Graham and Battaglene, 2004). In all the temperature treatments of the current study, the locomotion and feeding behavior of S. monotuberculatus displayed an evident diel cycle, being the most active at night, exhibiting intermediate activity at dusk, and minimal or no activity during the daytime, which showed a nocturnal activity pattern. Purcell et al. (2012b) also reported similar results that S. monotuberculatus hides during the day in crevices and under rubble, emerging at night on the reef flat, lagoons and reef slope. Nocturnal activity is thought to be a behavioral strategy that sea cucumbers evolved to increase their chances of survival in the nature, avoiding diurnal predation (Sun et al., 2018b). While water temperature significantly affected the movement activity of S. monotuberculatus, it did not alter diel locomotion rhythm. The observation of creeping activity at different temperatures showed that S. monotuberculatus exhibited a higher motor activity from 20:00 h to 04:00 h, and the minimum movement activity occurred at 12:00-18:00. Meanwhile, at all test temperatures, nocturnal feeding behavior of S. monotuberculatus was also evident. This suggests that the major factor influencing the diel locomotion rhythm of S. monotuberculatus, might be stress (Purcell et al., 2006), spring tides (Skewes et al., 2000), or predation (Dance et al., 2003), instead of temperature. Conversely, the high temperature (>24°C) may alter the diel locomotion rhythm of A. japonicus and became relatively inactive (Sun et al., 2018b); adult H. scabra did not follow their usual burying cycle when the water temperature was more than 30°C (Mercier et al., 2000). Future research would be required to aim specifically to exclude other variables to find a key factor be related to diel locomotion rhythm.
4.3 Effects of Flow on Behavior
As one of the most important environmental drivers, water flow can dramatically influence the locomotor behavior of marine organisms. Aquatic animals can adjust their activities with various strategies to adapt to the water flow. Like many other aquatic biotas, sea cucumbers also have distinct reactions to water flow. Qiu et al. (2014) found that water current is a key factor influencing sea cucumber distribution on the bare mud substrate, with 90% of juvenile sea cucumbers being unable to keep still in a current speed of 0.115 m·s-1 for 10 min. For suspension-feeding sea cucumber C. frondosa depending upon currents to bring food particles, the flow regime favoured by C. frondosa was proved to be between 21 and 40 cm s−1, under which a balance aquired between energy expenditure for attachment to the bottom and efficient food capture, but individuals passively rolled at flows >40 cm s−1 (Sun et al., 2018a). The present study demonstrated that S. monotuberculatus had the lowest velocity and the highest residence time at the highest flow rate (20.8 ± 3.4 cm/s). Sea cucumber A. japonicus also showed the lowest movement velocity while the experimental flow rate was the highest (29.3 ± 3.7 cm s−1, Pan et al., 2015). Pan et al. (2015) divided the holothurian’s movement into three steps, 1) grasping and adhesion to the substrate at the present position, 2) the tube feet are lifted from the substrate and migrate to a new position and 3) grasping and adhesion to the substrate at a new position. If the water flow rate was too high, more energy and time would be needed to grasp the substrate at the present position, which might result in especially less or slower movements.
Water motion could influence the distribution and locomotion of benthic echinoderms (Fankboner, 1978; McKenzie, 1991; Liao, 1997; Zhao, 1998). Sea urchin Echinometra oblonga dominates where water flow is vigorous and Echinometra mathaei dominates in calmer water (Russo, 1977). Dense individuals of the dendrochirotid Aslia lefevrei were commonly found in areas of moderately strong water movement (Costelloe and Keegan, 1984). Pan et al. (2015) observed that the sea cucumber A. japonicus did not show positive rheotaxis, but did move downstream at the fast current rates. In the present study, the sea cucumber Stichopus monotuberculatus displayed positive rheotaxis behavior of moving downstream at all flow velocities. The most obvious rheotaxis was observed at the highest water flow rates (20.8 ± 3.4cm·s-1). Rheotaxis is a directed behavioral response to flow direction and velocity involving locomotion or muscular turning of body parts. This behavior change is intended to ensure that the body remains relatively stable during the feeding process, allowing for efficient filter-feeding (Kitazawa and Oji, 2014), and can save energy used to move against the flow. Sea cucumbers respire mainly by taking up oxygen across the general body surface and drawing and expelling water through the cloaca, in and out of the respiratory tree (Woodby et al., 2000). Perhaps, the authors suggest, rheotaxis can reduce the pressure of water flow on respiratory tree during relaxation. For the sea star Asterias vulgaris, macroscale flow is an essential condition for locating distant prey, and they oriented themselves upstream and 70% succeeded in finding the prey in a flume existed current and prey odors (Morissette and Himmelman, 2000). The sea urchins Echinometra oblonga and E. mathaei generally moved along the water flow, rarely in a perpendicular direction, because the tube feet are too weak to stretch across the flow (Russo, 1977). The sea cucumber S. monotuberculatus moved both across the flow, like the sea stars, and along the flow axis, like the sea urchins. This finding is in line with the previous study, which showed that A. japonicus could display a search pattern in their motor behavior, moving diagonally across the direction of flow, and curve the body, turning in a new direction across flow (Pan et al., 2015).Overall, the sea cucumber S. monotuberculatus exhibited high locomotor and feeding activities at night of relatively high temperature, and its favored flow regime was downstream and low water velocity area. According to the present results, this species is suitable for being cultured in still or running water with a relatively low flow rate, and adequate feed should be supplied at night. The motion and feeding of S. monotuberculatus peaked at 31°C, yet the optimum growth temperature might not be determined as the lack of energy budget and growth rate data. While sea ranching, a reasonable releasing time and site is helpful to improve the survival rate, and juvenile S. monotuberculatus is recommended to be released in the high-temperature season and the slow flow region. Or, artificial reefs can be deployed to adjust water flow in sea areas with complex currents.
5. Conclusion
The behavioral reaction of tropical commercial sea cucumber S. monotuberculatus to different temperature and water flow were investigated using quantitative indices. S. monotuberculatus exhibited a comparatively higher locomotion and feeding activity at high test environmental temperature, and this is a converse behavioral feature with temperate sea cucumber, such as the most studied A. japonicus. Also, S. monotuberculatus showed a distinct nocturnal pattern at all temperature treatments, which may be a behavioral strategy, sea cucumbers have evolved in long history, in order to avoid diurnal predators. The movement velocity decreased with increasing flow current, which led to the increased staying time in the study area. Moreover, S. monotuberculatus displayed an “escape” behavior response to water flow, with higher levels of motor activity at all flow speeds tested than that in the experiment on the effect of temperature, while suspension-feeding sea cucumbers had their own favoured flow regime. Knowledge of the motor behavior habits of S. monotuberculatus at different water temperatures or flow rates would be conducive to optimize aquaculture environments and techniques of tropical commercial holothurian species.
Conflict of terest
The authors declare that the research was conducted in the absence of any commercial or financial relationships that could be construed as a potential conflict of interest.
Data Availability Statement
The original contributions presented in the study are included in the article/Supplementary Material. Further inquiries can be directed to the corresponding authors.
Author Contributions
FG, QX, and SS: conceptualization. FG, QX, and SS: methodology. FG, SS, and MC analyzed the data and wrote the original draft. All authors discussed the results and contributed to the writing the manuscript
Funding
This research was financially supported by the National Natural Science Foundation of China (Nos. 41766005, 42166005) and the National Key Research and Development Program of China (No. 2019YFD0901304).
Publisher’s Note
All claims expressed in this article are solely those of the authors and do not necessarily represent those of their affiliated organizations, or those of the publisher, the editors and the reviewers. Any product that may be evaluated in this article, or claim that may be made by its manufacturer, is not guaranteed or endorsed by the publisher.
Acknowledgments
We thank all researchers and funding agencies.
Supplementary Material
The Supplementary Material for this article can be found online at: https://www.frontiersin.org/articles/10.3389/fmars.2022.931430/full#supplementary-material
References
Bao J., Dong S. L., Tian X. L., Wang F., Gao Q. F., Dong Y. W. (2010). Metabolic Rates and Biochemical Compositions of Apostichopus japonicus (Selenka) Tissue During Periods of Inactivity. Chin. J. Oceanol. Limn. 28 (2), 218–223. doi: 10.1007/s00343-010-9016-3
Bell J. D., Rothlisberg P. C., Munro J. L., Loneragan N. R., Nash W. J., Ward R. D., et al. (2005). Restocking and Stock Enhancement of Marine Invertebrate Fisheries. Adv. Mar. Bio. 49, 1–374. doi: 10.1016/S0065-2881(05)49010-0
Buentello J. A., Gatlin D. M., Neill W. H. (2000). Effects of Water Temperature and Dissolved Oxygen on Daily Feed Consumption, Feed Utilization and Growth of Channel Catfish (Ictalurus punctatus). Aquaculture 182 (3-4), 339–352. doi: 10.1016/S0044-8486(99)00274-4
Buccheri E., Foellmer M. W., Christensen B. A., Langis P, Freeman A. S. (2019). Variation in righting times of Holothuria atra, Stichopus chloronotus and Holothuria edulis in response to increased seawater temperatures on Heron Reef in the Southern GBR. J. Mar. Biol. 2019, 1-6. doi: 10.1155/2019/6179705
Cheng C. H., Wu F. F., Ren C. H., Jiang X., Zhang X., Li X. M., et al. (2021). Aquaculture of the Tropical Sea Cucumber, Stichopus monotuberculatus: Induced Spawning, Detailed Records of Gonadal and Embryonic Development, and Improvements in Larval Breeding by Digestive Enzyme Supply in Diet. Aquaculture 540, 736690. doi: 10.1016/j.aquaculture.2021.736690
Conand C. (1993). Ecology and Reproductive Biology of Stichopus Variegatus an Indo-Pacific Coral Reef Sea Cucumber (Echinodermata: Holothuroidea). B. Mar. Sci. 52 (3), 970–981.
Costelloe J., Keegan B. F. (1984). Feeding and Related Morphological Structures in the Dendrochirote Aslia Lefevrei (Holothuroidea: Echinodermata). Mar. Biol. 84, 135–142. doi: 10.1007/BF00392998
Dance S. K., Lane I., Bell J. D. (2003). Variation in Short-Term Survival of Cultured Sandfish (Holothuria scabra) Released in Mangrove-Seagrass and Coral Reef Flat Habitats in Solomon Islands. Aquaculture 220 (1-4), 495–505. doi: 10.1016/S0044-8486(02)00623-3
Dong Y. W., Dong S. L. (2006). Growth and Oxygen Consumption of the Juvenile Sea Cucumber Apostichopus japonicus (Selenka) at Constant and Fluctuating Water Temperatures. Aquac. Res. 37 (13), 1327–1333. doi: 10.1111/j.1365-2109.2006.01570.x
Dong G. C., Dong S. L., Tian X. L., Wang F. (2011). Effects of Photoperiod on Daily Activity Rhythm of Juvenile Sea Cucumber, Apostichopus japonicus (Selenka). Chin. J. Oceanol. Limn. 29, 1015. doi: 10.1007/s00343-011-0204-6
Dong Y. W., Dong S. L., Tian X. L., Wang F., Zhang M. Z. (2006). Effects of Diel Temperature Fluctuations on Growth, Oxygen Consumption and Proximate Body Composition in the Sea Cucumber Apostichopus Japonicus (Selenka). Aquaculture 255 (1-4), 514–521. doi: 10.1016/j.aquaculture.2005.12.013
Dong G. C., Dong S. L., Wang F., Tian X. L. (2010). Effects of Light Intensity on Daily Activity Rhythm of Juvenile Sea Cucumber, Apostichopus Japonicus (Selenka). Aquac. Res. 41 (11), 1640–1647. doi: 10.1111/j.1365-2109.2010.02534.x
Dumont C. P., Himmelman J. H., Robinson S. M. C. (2007). Random Movement Pattern of the Sea Urchin Strongylocentrotus droebachiensis. J. Exp. Mar. Biol. Ecol. 340 (1), 80–89. doi: 10.1016/j.jembe.2006.08.013
Fan S. G., Hu C. Q., Zhang L. P., Sun H. Y., Wen J., Luo P. (2012). Complete Mitochondrial Genome of the Sea Cucumber Stichopus Sp. And its Application in the Identification of This Species. Aquac. Res. 43 (9), 1306–1316. doi: 10.1111/j.1365-2109.2011.02934.x
Fankboner P. V. (1978). Suspension-Feeding Mechanisms of the Armoured Sea Cucumber Psolus chitinoides Clark. J. Exp. Mar. Biol. Ecol. 31 (1), 11–25. doi: 10.1016/0022-0981(78)90133-8
Gao F., Yang H. S., Xu Q., Wang F. Y., Liu G. B. (2009). Effect of Water Temperature on Digestive Enzyme Activity and Gut Mass in Sea Cucumber Apostichopus japonicus (Selenka), With Special Reference to Aestivation. Chin. J. Oceanol. Lim. 27, 714–722. doi: 10.1007/s00343-009-9202-3
Gillanders B. M., Able K. W., Brown J. A., Eggleston D. B., Sheridan P. F. (2003). Evidence of Connectivity Between Juvenile and Adult Habitats for Mobile Marine Fauna: An Important Component of Nurseries. Mar. Ecol. Prog. Ser. 247, 281–295. doi: 10.3354/meps247281
Graham J. C. H., Battaglene S. C. (2004). Periodic Movement and Sheltering Behavior of Actinopyga mauritiana (Holothuroidea: Aspidochirotidae) in Solomon Islands. SPC Beche-de-mer Inf. Bull. 19, 23–30.
Hair C., Militz T., Daniels N., Southgate P. C. (2020). Comparison of Survival, Growth and Burying Behavior of Cultured and Wild Sandfish (Holothuria scabra) Juveniles: Implications for Ocean Mariculture. Aquaculture 526, 735355. doi: 10.1016/j.aquaculture.2020.735355
Hamel J. F., Sun J., Gianasi B. L., Montgomery E. M., Kenchington E. L., Burel B., et al. (2019). Active Buoyancy Adjustment Increases Dispersal Potential in Benthic Marine Animals. J. Anim. Ecol. 88 (6), 820–832. doi: 10.1111/1365-2656.12943
Huang L. H, Pang Q. J, Zhong S. P, Liu Y. H, Pan C. Y. (2020). Analysis on Morphological Character of Ossicles and Nutritive Content of Holothuria leucospilota and Stichopus variegatus in Beibu Gulf. Guangxi Sci. 27(5), 578–584. doi: 10.13656/j.cnki.gxkx.20201214.018
Hu C. Q., Xu Y. H., Wen J., Zhang L. P., Fan S. G., Su T. (2010). Larval Development and Juvenile Growth of the Sea Cucumber Stichopus Sp. (Curry Fish). Aquaculture 300, 73–79. doi: 10.1016/j.aquaculture.2009.09.033
Ji T. T., Dong Y. W., Dong S. L. (2008). Growth and Physiological Responses in the Sea Cucumber, Apostichopus japonicus Selenka: Aestivation and Temperature. Aquaculture 283 (1-4), 180–187. doi: 10.1016/j.aquaculture.2008.07.006
Kashenko S. D. (2002). Reactions of the Larvae of the Sea Cucumber Apostichopus japonicus to Sharp Desalination of Surface Water: A Laboratory Study. SPC Beche-de-Mer Inf. Bull. 16, 15–21.
Kato A., Hirata H. (1990). Effects of Water Temperature on the Circadian Rhythm of the Sea-Cucumber, Stichopus japonicus in Culture. Aquaculture Science. 38 (1), 75–80. doi: 10.11233/aquaculturesci1953.38.75
Kitazawa K., Oji T. (2014). Active Feeding Behavior of and Current Modification by the Sea Lily Metacrinus rotundus (Echinodermata: Crinoidea). J. Exp. Mar. Biol. Ecol. 453, 13–21. doi: 10.1016/j.jembe.2013.12.017
Liang G. Y. (1987). Preliminary Investigation of Three Sea Cucumber Resources in Guangxi Coastal Area. J. Guangxi Agric. Sci. 4, 54–56.
Liao Y. L. (1997). Fauna Sinica, Phylum Echinodermata, Class Holothuroidea (Beijing: Science Press).
Liao J. C., Cotel A. (2013). “Effects of Turbulence on Fish Swimming in Aquaculture,” in Swimming Physiology of Fish: Towards Using Exercise to Farm a Fit Fish in Sustainable Aquaculture. Eds. Palstra A. P., Planas J. V. (Berlin, Heidelberg: Springer Berlin Heidelberg), 109–127.
Lin C. G. (2014). Effects of Four Physical Environment Factors on the Movement and Feeding Behavior of Sea Cucumber Apostichopus japonicus (Selenka). doctor’s degree]. [Beijing]: University of Chinese Academy of Sciences. (in Chinese with English abstract)
Li M. N., Zhu H. F., Jin Z. J., Luo J., Xu J. W., Li, et al. (2018). The Effect of Accelerating Flow on Swimming Behavior of Juvenile Aristichthys nobilis and Hypophthalmichthys molitrix During Downstream Migration. Acta Hydrobiologica Sinica. 42 (3), 571–577. doi: 10.7541/2018.071
Lovatelli A., Conand C., Purcell S., Uthicke S., Hamel J. F., Mercier A. (2004). “Advances in Sea Cucumber Aquaculture and Management,” in Fisheries and Aquaculture Technical Paper No. 516, vol. 425. (Rome: FAO).
McKenzie J. D. (1991). The Taxonomy and Natural History of North European Dendrochirote Holothurians (Echinodermata). J. Nat. Hist. 25, 123–171. doi: 10.1080/00222939100770091
Mercier A., Battaglene S. C., Hamel J. F. (1999). Daily Burrowing Cycle and Feeding Activity of Juvenile Sea Cucumbers Holothuria scabra in Response to Environmental Factors. J. Exp. Mar. Biol. Ecol. 239, 125–156. doi: 10.1016/S0022-0981(99)00034-9
Mercier A., Battaglene S. C., Hamel J. F. (2000). Periodic Movement, Recruitment and Size-Related Distribution of the Sea Cucumber Holothuria scabra in Solomon Islands. Hydrobiologia 440, 81–100. doi: 10.1023/A:1004121818691
Morissette S., Himmelman J. H. (2000). Decision of the Asteroid Leptasterias polaris to Abandon its Prey When Confronted With its Predator, the Asteroid Asterias Vulgaris. J. Exp. Mar. Biol. Ecol. 252 (2), 151–157. doi: 10.1016/S0022-0981(00)00229-X
Navarro P. G., Garcia-Sanz S., Barrio J. M., Tuya F. (2013). Feeding and Movement Patterns of the Sea Cucumber Holothuria sanctori. Mar. Bio. 160, 2957–2966. doi: 10.1007/s00227-013-2286-5
Pan Y., Zhang L. B., Lin C. G., Sun J. M., Kan R. T., Yang H. S. (2015). Influence of Flow Velocity on Motor Behavior of Sea Cucumber Apostichopus japonicus. Physiol. Behav. 144, 52–59. doi: 10.1016/j.physbeh.2015.02.046
Pavlov D.S., Lupandin A. , Skorobogatov M. A. (2000). The Effects of Flow Turbulence on the Behavior and Distribution of Fish.J. Ichthyol. 20, 232–261.
Pilditch C. A. (1999). Effect of Variations in Flow Velocity and Phytoplankton Concentration on Sea Scallop (Placopecten magellanicus) Grazing Rates. J. Exp. Mar. Biol. Ecol. 240, 111–136. doi: 10.1016/S0022-0981(99)00052-0
Purcell S. W. (2010). Diel Burying by the Tropical Sea Cucumber Holothuria sabra: Effects of Environmental Stimuli, Handling and Ontogeny. Mar. Biol. 157, 663–671. doi: 10.1007/s00227-009-1351-6
Purcell S. W., Blockmans B. F., Agudo N. N. S. (2006). Transportation Methods for Restocking of Juvenile Sea Cucumber, Holothuria scabra. Aquaculture 251, 238–244. doi: 10.1016/j.aquaculture.2005.04.078
Purcell S. W., Conand C., Uthicke S., Byrne M. (2016). Ecological Roles of Exploited Sea Cucumbers. Oceanogr. Mar. Biol. 54, 367–386. doi: 10.1201/9781315368597-8
Purcell S. W., Hair C. A., Mills D. J. (2012a). Sea Cucumber Culture, Farming and Sea Ranching in the Tropics: Progress, Problems and Opportunities. Aquaculture 368-369, 68–81. doi: 10.1016/j.aquaculture.2012.08.053
Purcell S. W., Samyn Y., Conand C. (2012b). Commercially Important Sea Cucumbers of the World (Rome, Italy: FAO).
Qiu T. L., Zhang L. B., Zhang T., Yang H. S. (2014). Effects of Mud Substrate and Water Current on the Behavioral Characteristics and Growth of the Sea Cucumber Apostichopus japonicus in the Yuehu Lagoon of Northern China. Aquac. Int. 22 (2), 423–433. doi: 10.1007/s10499-013-9650-9
Ren C. H., Chen T., Jiang X., Luo X., Wang Y. H., Hu C. Q. (2015a). The First Echinoderm Gamma-Interferon-Inducible Lysosomal Thiol Reductase (GILT) Identified From Sea Cucumber (Stichopus monotuberculatus). Fish. Shellfish. Immunol. 42, 41–49. doi: 10.1016/j.fsi.2014.10.024
Ren C. H., Chen T., Jiang X., Wang Y. H., Hu C. Q. (2014a). Identification and Functional Characterization of a Novel Ferritin Subunit From the Tropical Sea Cucumber, Stichopus monotuberculatus. Fish. Shellfish. Immunol. 38, 265–274. doi: 10.1016/j.fsi.2014.03.022
Ren C. H., Chen T., Jiang X., Wang Y., Hu C. Q. (2014b). The First Characterization of Gene Structure and Biological Function for Echinoderm Translationally Controlled Tumor Protein (Tctp). Fish. Shellfish. Immunol. 41, 137–146. doi: 10.1016/j.fsi.2014.08.030
Ren C. H., Chen T., Sun H. Y., Jiang X., Hu C. Q., Qian J., et al. (2015b). The First Echinoderm Poly-U-Binding Factor 60 kDa (PUF60) From Sea Cucumber (Stichopus monotuberculatus): Molecular Characterization, Inducible Expression and Involvement of Apoptosis. Fish. Shellfish. Immunol. 47, 196–204. doi: 10.1016/j.fsi.2015.09.001
Russo A. R. (1977). Water Flow and the Distribution and Abundance of Echinoids (Genus echinometra) on an Hawaiian Reef. Mar. Freshw. Res. 28 (6), 693–702. doi: 10.1071/MF9770693
Sakurai I., Seto M. (2000). Movement and Orientation of the Japanese Scallop Patinopecten Yessoensis (Jay) in Response to Water Flow. Aquaculture 181, 269–279. doi: 10.1016/S0044-8486(99)00242-2
Shiell G. R. (2006). Effect of Invasive Tagging on the Activity of Holothuria Whitmaei [Echinodermata: Holothuroidea]: A Suitable Mark-Recapture Method for Short-Term Weld Studies of Holothurian Behavior. Mar. Fresh. Behav. Physiol. 39, 153–162. doi: 10.1080/10236240600688789
Skewes T, Dennis D., Burridge C. M. (2000). Survey of Holothuria scabra (Sandfish) on Warrior Reef, Torres Strait. CSIRO Marine Research Final Report(Brisbane)
Sun J., Hamel J. F., Mercier A. (2018a). Influence of Flow on Locomotion, Feeding Behavior and Spatial Distribution of a Suspension-Feeding Sea Cucumber. J. Exp. Biol. 221 (20), jeb189597. doi: 10.1242/jeb.189597
Sun J. M., Hamel J. F., Stuckless B., Small T. J., Mercier A. (2020). Effect of Light, Phytoplankton, Substrate Types and Colour on Locomotion, Feeding Behaviour and Microhabitat Selection in the Sea Cucumber Cucumaria frondosa. Aquaculture 526, 735369. doi: 10.1016/j.aquaculture.2020.735369
Sun J., Zhang L. B., Pan Y., Lin C. G., Wang F., Kan R. T., et al. (2015). Feeding Behavior and Digestive Physiology in Sea Cucumber Apostichopus japonicus. Physiol. Behav. 139, 336–343. doi: 10.1016/j.physbeh.2014.11.051
Sun J., Zhang L. B., Pan Y., Lin C. G., Wang F., Yang H. S. (2018b). Effect of Water Temperature on Diel Feeding, Locomotion Behaviour and Digestive Physiology in the Sea Cucumber Apostichopus japonicus. J. Exp. Biol. 221 (9), jeb177451. doi: 10.1242/jeb.177451
Uthicke S. (1999). Sediment Bioturbation and Impact of Feeding Activity of Holothuria (Halodeima) Atra and Stichopus Chloronotus, Two Sediment Feeding Holothurians, at Lizard Island, Great Barrier Reef. Bull. Mar Sci. 64, 129–141.
Uthicke S., Welch D., Benzie J. A. H. (2004). Slow Growth and Lack of Recovery in Overfished Holothurians on the Great Barrier Reef: Evidence From DNA Fingerprints and Repeated Large-Scale Surveys. Conserv. Biol. 18, 1395–1404. doi: 10.1111/j.1523-1739.2004.00309.x
Vasquez H. E., Zheng X., Zhan X., Gu Z. F., Wang A. M. (2018). The Effect of Light on the Locomotion and Byssal Reattachment of Winged Pearl Oyster Pteria Penguin (Rding 1798) Juveniles. J. Shellfish Res. 37 (5), 1061–1066. doi: 10.2983/035.037.0517
Vasquez H. E., Zheng X., Zhan X., Gu Z., Wang A. M. (2020). Byssal Re-Attachment Behavior in the Winged Pearl Oyster Apostichopus japonicus. in Response to Low Salinity Levels. J. World. Aquac. Soc 52, 457–465. doi: 10.1111/jwas.12744
Wang H. H., Yan J. X., Feng Y. Q., Fang Z., Wang S., Zhou Y., et al. (2017). Embryonic and Larval Development of Sea Cucumber Stichopus herrmanni. Fish. Sci. 36 (05), 606–611. doi: 10.16378/j.cnki.1003-1111.2017.05.011
Wang F. Y., Yang H. S., Gao F., Liu G. B. (2008). Effects of Acute Temperature or Salinity Stress on the Immune Response in Sea Cucumber, Apostichopus japonicus. Comp. Biochem. Physiol. Part A: Mol. Integr. Physiol. 151, 491–498. doi: 10.1016/j.cbpa.2008.06.024
Wang Y. H., Yu M. M., Wang D. Y., Lv Z. (2010). Analysis of Nutritional Components of Stichopus variegates, Thelenota ananas and Stichopus chloronotus Boandt. Acta Nutrimenta Sinica. 32 (04), 397–398. doi: 10.13325/j.cnki.acta.nutr.sin.2010.04.016
Wen J., Fan S. G., Li H. P., Hu C. Q. (2018). Genetic Diversity in Four Wild Populations of Sea Cucumber Stichopus monotuberculatus in South China Sea. Fish. Sci. 37 (3), 404–408. doi: 10.16378/j.cnki.1003-1111.2018.03.020
Wheeling R. J., Verde E. A., Nestler J. R. (2007). Diel Cycles of Activity, Metabolism, and Ammonium Concentration in Tropical Holothurians. Mar. Biol. 152, 297–305. doi: 10.1007/s00227-007-0683-3
Wildish D., Kristmanson D. D. (1988). Growth Response of Giant Scallops to Periodicity of Flow. Mar. Ecol. Prog. Ser. 42, 163–169. doi: 10.3354/meps042163
Wildish D. J., Saulnier A. M. (1992). The Effect of Velocity and Flow Direction on the Growth of Juvenile and Giant Scallops. Exp. Mar. Biol. Ecol. 133, 133–143. doi: 10.1016/0022-0981(92)90032-6
Wolfe K., Byrne M. (2017). Biology and Ecology of the Vulnerable Holothuroid, Stichopus herrmanni, on a High-Latitude Coral Reef on the Great Barrier Reef. Coral Reefs., 36: 1143- 1156. doi: 10.1007/s00338-017-1606-5
Wolkenhauer S. M. (2008). Burying and Feeding Activity of Adult Holothuria scabra (Echinodermata: Holothuroidea) in a Controlled Environment. SPC Beche Mer Inf. Bull. 27, 25–28.
Woodby D., Smiley S., Larson R. (2000). Depth and Habitat Distribution of Parastichopus californicus Near Sitka, Alaska. Alaska Fishery Res. Bull., 7 (1), 22–32. http://146.63.61.200/static/home/library/PDFs/afrb/woodbyv7.pdf
Yang H. S., Hamel J-F., Mercier A. (2015). The Sea Cucumber Apostichopus japonicus: History, Biology and Aquaculture (Landon: Academic Press).
Yan A. F., Ren C. H., Chen T., Jiang X., Sun H. Y. (2016). Identification and Functional Characterization of a Novel Antistasin/WAP-Like Serine Protease Inhibitor From the Tropical Sea Cucumber, Stichopus Monotuberculatus. Fish Shellfish Immunol. 59, 203–212. doi: 10.1016/j.fsi.2016.10.038
Yuan L. H., Hu C. Q., Zhang L. P., Xia J. J. (2013). Population Genetics of a Tropical Sea Cucumber Species (Stichopus monotuberculatus) in China. Conserv. Biol. 14, 1279–1284. doi: 10.1007/s10592-013-0506-7
Zhang S., Chen Y., Sun M. C. (2006). Effects of Light Intensity on the Behavior Characteristics of Sea Cucumbers and the Collection Effect of Artificial Reef Model. Fishery Sci. China. 1, 20–27.
Zhang L. B., Zhang T., Xu Q. Z., Qiu T. L., Yang H. S., Liu S. L. (2014). An Artificial Oyster-Shell Reef for the Culture and Stock Enhancement of Sea Cucumber, Apostichopus japonicus, in Shallow Seawater. Aquac. Res. 46 (9), 2260–2269. doi: 10.1111/are.12383
Keywords: Stichopus monotuberculatus, water temperature, flow velocity, locomotion, feeding
Citation: Chen M, Sun S, Xu Q, Gao F, Wang H and Wang A (2022) Influence of Water Temperature and Flow Velocity on Locomotion Behavior in Tropical Commercially Important Sea Cucumber Stichopus monotuberculatus. Front. Mar. Sci. 9:931430. doi: 10.3389/fmars.2022.931430
Received: 29 April 2022; Accepted: 09 June 2022;
Published: 11 July 2022.
Edited by:
Chenghua Li, Ningbo University, ChinaReviewed by:
Yingbin Wang, Zhejiang Ocean University, ChinaXiutang Yuan, Chinese Academy of Sciences (CAS), China
Copyright © 2022 Chen, Sun, Xu, Gao, Wang and Wang. This is an open-access article distributed under the terms of the Creative Commons Attribution License (CC BY). The use, distribution or reproduction in other forums is permitted, provided the original author(s) and the copyright owner(s) are credited and that the original publication in this journal is cited, in accordance with accepted academic practice. No use, distribution or reproduction is permitted which does not comply with these terms.
*Correspondence: Fei Gao, gaofeicas@126.com