- State Key Laboratory of Marine Resource Utilization in South China Sea, College of Marine Sciences, Hainan University, Haikou, China
Sea ranching of tropical edible sea cucumbers is an effective way to relieve the overfishing stress on their natural resources and protect the coral reef ecosystem, yet only a few species have been applied in the sea ranching practice based on hatchery-reared juveniles around the world. In this study, an 8-month (April to December) sea ranching study for hatchery-reared edible sea cucumber Stichopus monotuberculatus juveniles was carried out at a tropical coral reef island area in Sanya, China. Several growth performance indexes and basal nutritional components were monitored. Results revealed that the sea cucumbers had a growth rate of 0.35~0.78 mm day-1 during the experimental period, reaching 15.9 cm long before winter. The weight gain reached 491.13% at the end, and most sea cucumbers were able to grow to the commercial size (over 150 g WW) in the first year of sea ranching. The overall specific growth rate (SGR) and survival rates were 0.73 and 27.5%. Most of the death occurred in the first month after release (25.0%–37.5%), and this is probably due to inadaptation to the sudden change of the environment from the hatchery to the wild, which is proved by the remarkable decrease in nutritional indexes (amino acids, total lipid, and crude protein). Stable isotope and lipid biomarkers revealed that the food source mainly comes from water deposits (with microbes), Sargassum sanyaense seaweed debris, phytoplankton, and coral mucus-derived organics. The study proved the feasibility of the sea ranching of the hatchery-reared S. monotuberculatus juveniles in the tropical coral reef island area. Also, it is highly recommended that appropriate acclimation operation before release should be carried out to improve the survival rate of this species.
1 Introduction
In recent years, the market demand for sea cucumbers is increasing due to their high nutritional and medicinal value (Eriksson and Clarke, 2015). Meanwhile, wild sea cucumber resource has shown continuous depletion because of overfishing (Pakoa and Bertram, 2013). Therefore, the sea ranching of high-value sea cucumbers developed rapidly since the early 21st century, especially in China (Chen, 2004; Eriksson and Clarke, 2015). In addition to the temperate species of Stichopus japonicus, which is widely cultured in North China, most of the high-valued tropical sea cucumber species exploitation relies on wild catching around the world, such as Stichopus monotuberculatus, Stichopus horrens, Thelenota ananas, and Holothuria fuscogilva (Purcell et al., 2018). Only Holothuria scabra is widely cultured in the pond or the coastal area. The development of tropical sea cucumber sea ranching is mostly restricted by the lack of techniques (Purcell et al., 2012; Zamora et al., 2018). There is an urgent need to develop sea ranching techniques for new sea cucumber species to promote the production of artificial released tropical sea cucumber population and subsequently relieve the fishery stress on other depleted wild species.
S. monotuberculatus is a tropical detritus-feeding sea cucumber species with high economic value. It prefers sandy sediment, fragmented coral, coral rubble, and seagrass bed between the depth of 0 and 30 m (Conand, 1993; Desurmont, 2003). The hatchery technique has been developed for years (Hu et al., 2010). Pond culture mode used to be tested on a small scale, but the risk of mass mortality caused by extremely low water temperature (below 16°C) was encountered during occasional cold waves in winter. Sea ranching is the most feasible way to solve the problem, yet many theoretical and practical aspects are still not clear, such as appropriate density, juvenile size, and release procedure.
S. monotuberculatus is a native species in the coral reef area in the southern part of Hainan Island and the Sansha area, China (Hu et al., 2010). However, since 2000, the natural resource depleted remarkably due to overfishing. Aiming to recover the sea cucumber resource and develop sea ranching technique, we carried out an 8-month study at Wuzhizhou Island, an inshore coral reef island in the southern part of Hainan Island. More than 3,000 hatchery-reared S. monotuberculatus juveniles were released, and the growth index, survival rate (SR), and nutrition accumulation were evaluated. Seasonal diet composition was also quantified to understand the food availability in the habitat, using a lipid biomarker and stable isotope method. The sea ranching feasibility of the hatchery-reared juveniles in the tropical coral reef area was verified based on these results.
2 Materials and Methods
2.1 Study Area
Wuzhizhou Island is located in the southern part of Hainan Island (the South China Sea, 18°18′30″N–109°45′40″E) (Figure 1) with an area of 1.48 km2. It has a tropical monsoon climate, with the dry season from November to April and the rainy season from May to October (Huang et al., 2020). It is affected by seasonal wind and waves, especially the upwelling event on the eastern coast of Hainan Island during summer, which brings cool water to keep the corals away from a high-temperature threat (Huang et al., 2003; Li et al., 2015). The island is also a famous tourism site with site-seeing and scuba diving projects. The sea area is well protected by the tourism company, and the collection of all the marine creatures is banned except for scientific research purposes.
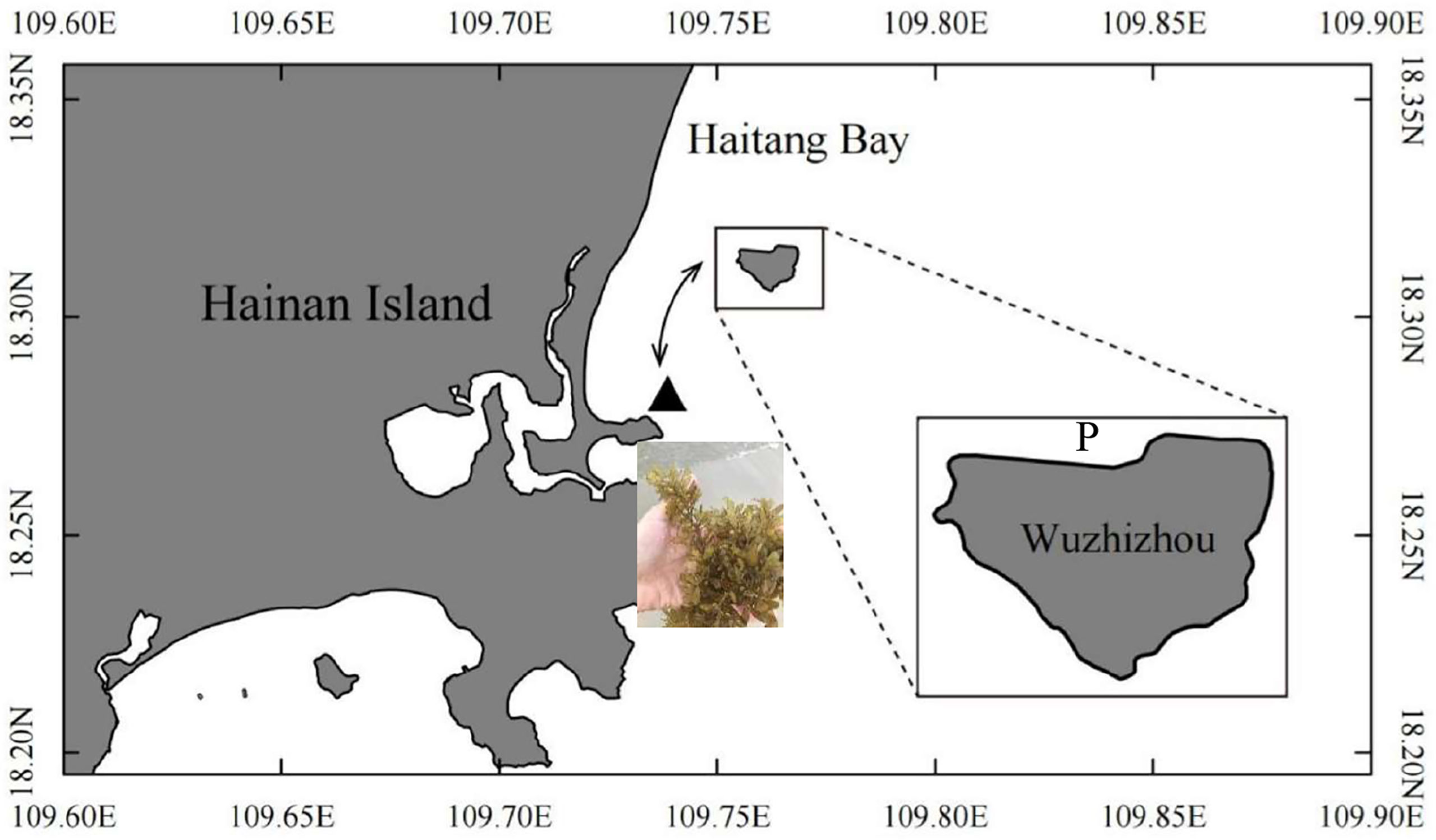
Figure 1 Sea cucumber Stichopus monotuberculatus sea ranching area at Wuzhizhou Island, Hainan Province, China. P, juvenile release site; ▲, the Sargassum sanyaense seaweed bed (photographed by Qiang Xu). The arrow shows the tidal current direction.
2.2 Juvenile Acquisition, Transportation, and Release
The experimental area was set in the northern part of Wuzhizhou Island, which is covered with fine sand, coral reef, and volcanic rocky artificial reefs (Figures 1, 2A). A total of over 3,000 sea cucumber juveniles (wet weight 17.36 ± 4.20 g, body length 5.6 ± 1.3 cm) were acquired from a hatchery in Wenchang (Hainan Province) in April 2019 (Figure 2B). The water temperature and salinity in the hatchery were 25.2°C and 32–33 ppt, respectively, and those in the sea ranching area were 26.7°C and 33 ppt, respectively. All the juveniles were carefully packed in plastic bags with water and oxygen, put in cool boxes with ice, and transported from the hatchery to the sea ranching area within 5 h. On the boat, the sea cucumbers were put into soft chinlon mesh bags (20 × 30 cm, 120 ind. each) and taken to the sea bottom by scuba divers. A stone was placed on each bag (mouth opened), and the juveniles crawled out by themselves in 2 days (Figure 2C).
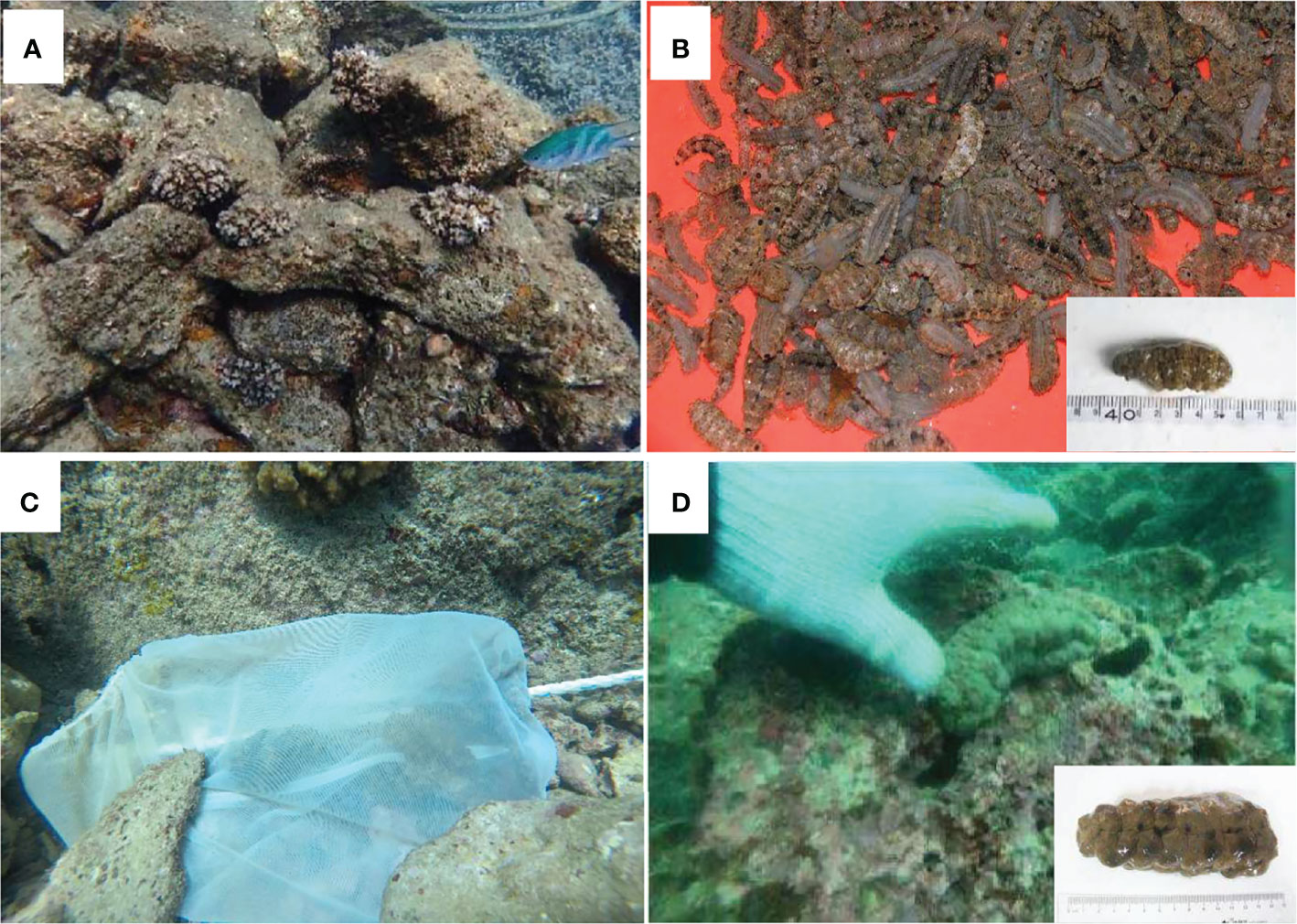
Figure 2 The deployed volcano rocky artificial reefs with settled corals (A). Sea cucumber Stichopus monotuberculatus juveniles in the hatchery (B). The self-release mesh bag with juveniles in it (C). A sea-ranched individual 8 months after release (D). (photographed by Qiang Xu).
2.3 Growth Performance and SurvivalRate Monitor
The growth index was monitored periodically (May, June, July, September, October, and December 2019). The water temperature and salinity at a depth of 1 m were also measured by a multi-parameter sonder (YSI—Model 6600 V2, Xylem, USA) from January to December 2019 (Figure 3). At each month, samples (n = 4–6) were collected from the released population by scuba divers and immediately taken in water to the laboratory on the island to measure the wet weight (including total and body wall) and the body length. The total length (TL) from mouth to anus was measured to the nearest 0.1 cm. Before being weighted, gentle pressure was applied to the sea cucumber body to enable the expulsion of the maximum amount of water from the respiratory tree. The body surface was blotted dry with a paper towel, and the total weight (TW) was recorded (Sánchez-Tapia et al., 2018). Then the sea cucumber was dissected, and the body wall was also weighted.
For the SR evaluation, eighty sea cucumber juveniles were selected from the total released population in April 2019 and placed into two steel-frame bottom culture cages set on the same sea area (2.0 × 2.0 × 0.6 m, area 4 m2; 40 ind·. each, density 10 ind· m−2). The cages are covered with a plastic net (mesh size 0.5 cm) except for the bottom so that sea cucumbers can live directly on the seafloor. To prevent escape, a circle of soft nylon net curtain (mesh size 0.5 cm, 20 cm high) was fixed on the lower part of the cage, folded inside, and then buried into the sediment. Several coral rocks were placed into the cage as the shelter. The cages were anchored on the seafloor with nylon ropes. In May, July, and October 2019, the SR was calculated by counting sea cucumbers observed in the cages. During the counting process, all the rocky substrates in the cages were not moved and checked so as to avoid the destruction of the habitat and possibly hurting the sea cucumbers. Unfortunately, one of the cages was destroyed in a storm in late October. At the end of the experiment in December, all the rocks in the remaining cages were moved out, the surviving ones were counted, and the cage was also carefully checked, so the data reflect the overall SR of the 8-month study.
2.4 Sample Collection for Nutrition and Food Source Analyses
The initial sea cucumber samples (n = 5) were collected from the hatchery prior to mass release in April 2019. During the field study, samples (n = 3–5) were collected by divers in May, July, October, and December. In the laboratory, sea cucumbers were dissected, and the body wall was washed with ultrapure water and then frozen and freeze-dried for 48 h. The samples were pulverized using a glass mortar and pestle and stored under the dry condition at −20°C for further analysis.
After referring to previous studies on other sea cucumber species (Apostichopus japonicus, Sun et al., 2013) and the character of the coral reef habitat (Coffroth, 1984; Wild et al., 2004; Wild et al., 2005), five potential food sources were selected from the pelagic and benthic habitats: phytoplankton, benthic microalgae, water deposit, Sargassum sanyaense, and coral mucus. Net-towed phytoplankton samples (mesh size 76 μm) were obtained by hauling them horizontally in the water. Benthic microalgae were collected using an in situ cultivation method: a polythene net was placed on a coral rock at a shallow area (3–5-m depth) with sufficient sunlight. After 5–10 days, the net was taken back and cleaned with filtered seawater. The cleaning water was filtered, and the microalgae sample was collected on Whatman GF/F glass-fiber filters (pre-combusted under 450°C for 4 h). Water deposit samples (n = 3) were acquired with three polythene cylinder trappers (Φ = 30 cm, 30-cm height), which were deployed for 5 days on the bottom. After the samples were taken back to the lab, the deposit was resuspended and filtered on pre-combusted Whatman GF/F glass-fiber filters for further analysis. Preliminary fatty acid biomarker results indicated brown algae organic source in the sea cucumber’s diet, but field investigation only found two small Phaeophyta species, Padina sp. and Turbinaria ornata, with tough blades and sporadic distribution. Considering their extremely low biomass and difficulty in detritus release, they are unlikely to be the diet source for the sea cucumbers. It is reported that there is a seasonal Sargassum sp. seaweed bed at the opposite coastal area 3~4 km from the island (Li et al., 2020), and a supplemental investigation on this area in April 2021 was performed, revealing a Sargassum seaweed bed of about 30 ha with S. sanyaense as the dominant species in high biomass (3.7 kg m−2, Figure 1, site ▲). The algae were sampled and added to the potential food sources. The specific isotope values of coral mucus come from the reference (Naumann et al., 2010).
2.5 Nutrition Composition Analysis
2.5.1 Crude Protein and Total Lipid
The content of crude protein was determined by the Kjeldahl method (AOAC, 1990). Lipids were extracted using a modified Folch extraction procedure (Folch et al., 1957; Parrish, 1999).
2.5.2 Amino Acid Analysis
Amino acid analysis was carried out based on literature methods (Block et al., 2016): the sample was hydrolyzed at 110°C for 22 h with 6 mol/L of HCl; following hydrolysis, 1 ml of hydrolyzate was withdrawn and evaporated to dryness under vacuum at 45°C to remove HCl. The hydrolyzate was dissolved in 5 ml of 0.02 mol/L of HCl, then centrifuged at 5,000 rpm, and filtered. Supernatant measuring 1 μl was used for amino acid analysis. Detection wavelengths were set at UV 570 and 440 nm. The amino acids’ identity and quantity were assessed by comparison with retention times and peak areas of standard amino acids. The amino acid score was calculated using the following formula: amino acid score = amount of amino acid per test protein (mg/g)/amount of amino acid per protein in reference pattern (mg/g) × 100.
2.5.3 Fatty Acid Composition Analysis
Extracted total lipid was evaporated to near dryness under a gentle nitrogen stream. Fatty acid methyl esters (FAMEs) were prepared by esterification using 2% sulfuric acid methanol as described by (Xu, 2007). Gas chromatography (GC) was performed with Agilent Technologies (Santa Clara, CA, USA) 6890N GC equipped with a DB-FFAP capillary column (30 m × 0.25 mm × 0.25 μm). Helium was the carrier gas (99.999%, purity). The heating program consisted of an initial temperature of 100°C, holding at 100°C for 5 min, a temperature increase to 240°C at a rate of 4°C/min, and then holding at 240°C for 15 min. The inlet temperature was 250°C, and the detector temperature was 250°C. Individual components were identified using mass spectral data and by comparing retention time data with those obtained for authentic and laboratory standards (Cod liver oil FAMEs (C2294-5G), Sigma-Aldrich, Germany). The peak area was quantified and expressed as a percentage of total fatty acids.
2.6 Stable C and N Isotope Analysis
The water deposit and benthic microalgae samples were acidified by exposing the filters to HCl vapor for 12 h to remove inorganic carbonates before analysis. δ15N and δ13C values of the sea cucumber and potential food sources were determined by a stable isotope ratio mass spectrometry (IRMS; Delta V Advantage, Thermo Fisher, Waltham, MA, USA) combined with an element analyzer (HT2000, Thermo, USA). About 5 mg of powdered samples was measured by the Millionth Scale (P6, Mettler, Schwerzenbach, Switzerland) and wrapped in a tin capsule. Then the capsule was closed with tweezers and placed into the automatic sampler of the elemental analyzer. The encapsulated samples were combusted and reduced at 1,020°C, and the flow rate of He (99.999%, purity) was 50 ml/min in a reactor and converted into CO2 and N2. Water was subsequently removed by anhydrous Mg(ClO4)2. The resulting gas was separated on a carbon sieve GC column (70°C) and then gradually entered into the ion source of the IRMS.
Results of the isotope ratios were expressed in standard δ-unit notation, as follows:
where X is 13C or 15N and R is 13C:12C ratio for C or 15N:14N ratio for N. The values were reported relative to the Vienna Pee Dee Belemnite (PDB) standard for C and air N2 for N.
2.7 Statistical Analysis
2.7.1 Growth Index Estimation
The calculation of size growth rate (GR; mm day−1), specific GR (SGR; % day−1), and weight gain (WG; %) are based on samples from the released population, whereas the SR (%) was quantified by counting the individuals in the cages. The growth index was calculated at four months (May, July, October, and December). Growth estimations are determined using the following expressions (Sánchez-Tapia et al., 2018):
where L1 and Li are the average body lengths at the beginning (April 2019) and the course of the evaluation, respectively; W1 and Wi are the TW of juveniles at the beginning and the course of the experiment, respectively; and Ti is the length of the experiment in days.
The SR was expressed as follows:
where Ni is the number of juveniles observed in the cages and N1 is the number of juveniles at the beginning of the experiment (N1 = 40).
The result was presented as mean values ± SD. The difference in the months of the content of δ13C, δ15N, amino acids, crude protein, total lipid, and relative percentages of the total pool of fatty acids was tested with one-way ANOVA. Significant differences (p< 0.05) were determined by using Tukey’s honestly significant difference (HSD) post-hoc test.
2.7.2 Food Composition Calculation
The Bayesian mixed-model SIMMR of R (version 4.1.2) was used for the evaluation of the relative contribution of each food source to the sea cucumber (Parnell et al., 2013). Compared with the software “Isosource,” SIMMR can incorporate supplementary features such as uncertainties, concentration dependence, and a greater quantity of sources (Lionel et al., 2018). For stable C and N isotopes, the average fractionation effects of 1‰ for 13C (McClelland and Valiela, 1998; McCutchan Jr. et al., 2003; Sun et al., 2013) and 3.4‰ for 15N (Sherwood & Rose, 2005; Carlier et al., 2007) were used to correct stable isotope shifts for each trophic level. By using the Markov chain Monte Carlo (MCMC) method, the model was run for 10,000 iterations with a burn rate of 1,000 to obtain the posterior distribution (Jiménez-Arias et al., 2020). SIMMR package checks whether the model works normally through the Gelman–Rubin diagnosis (Saccò et al., 2019). The results show that the model converges and runs normally. The average standard error of the mean for replicates was 0.11‰ for δ13C and 0.15‰ for δ15N (Sherwood & Rose, 2005).
3 Results
3.1 Growth and Survival Rate of Stichopus monotuberculatus
During the study period, the salinity and water temperature varied at 32.58°C–34.44°C and 23.76°C–29.31°C, respectively (Figure 3). A temperature drop of 3°C (28.31°C to 25.33°C) was observed from June to July due to the impact of the upwelling event on the east coast of Hainan Island.
During the routine investigation, the sea cucumbers’ activity showed a remarkable diurnal rhythm. Using an underwater time-lapse camera, we found that most of them hide in the reef during the daytime and come out to feed at night. The SR of juveniles in cage 1 over 8 months was 27.5% (Table 1). The SR of cage 2 in December was not available because it was destroyed by a typhoon storm in late October, so the SR in cage 2 over 6 months was 52.5%. The death rate of juveniles was 25.0%–37.5% in the first month after release (April to May), which is much higher than in other months.
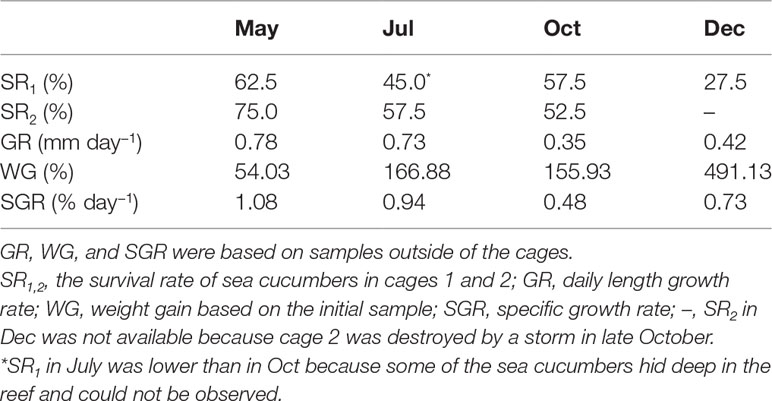
Table 1 Survival rate and growth performance of sea ranched Stichopus monotuberculatus in 2019 (n = 4–6 for GR, WG, and SGR).
The juvenile’s mean length increased from 5.6 ± 1.3 to 15.9 ± 2.2 cm at the end, and the total body weight was from 17.36 ± 4.20 to 102.62 ± 50.76 g (Figures 2D, 4). After 8 months’ growth, the maximum length and weight of adult individuals were 177.86 g and 19.0 cm, respectively. The final wet weight was about 4.9 times its initial value (WG 491.13%, Table 1). The highest WG occurred between October and December, but the GR showed an individual variation. The body wall wet weight grew from 12.94 ± 2.24 to 96.11 ± 20.91 g, 7.43 times the initial (Figures 4A, B). The juveniles grew faster from April to July (GR 0.78–0.73 mm day−1; SGR 1.08–0.94% day−1) compared to other months (GR 0.35–0.42 mm day−1; SGR 0.48–0.73% day−1), whereas a slight weight loss occurred from July to October (Table 1). SGR reached its lowest level in October (0.48% day−1) but recovered in December (0.73% day−1).
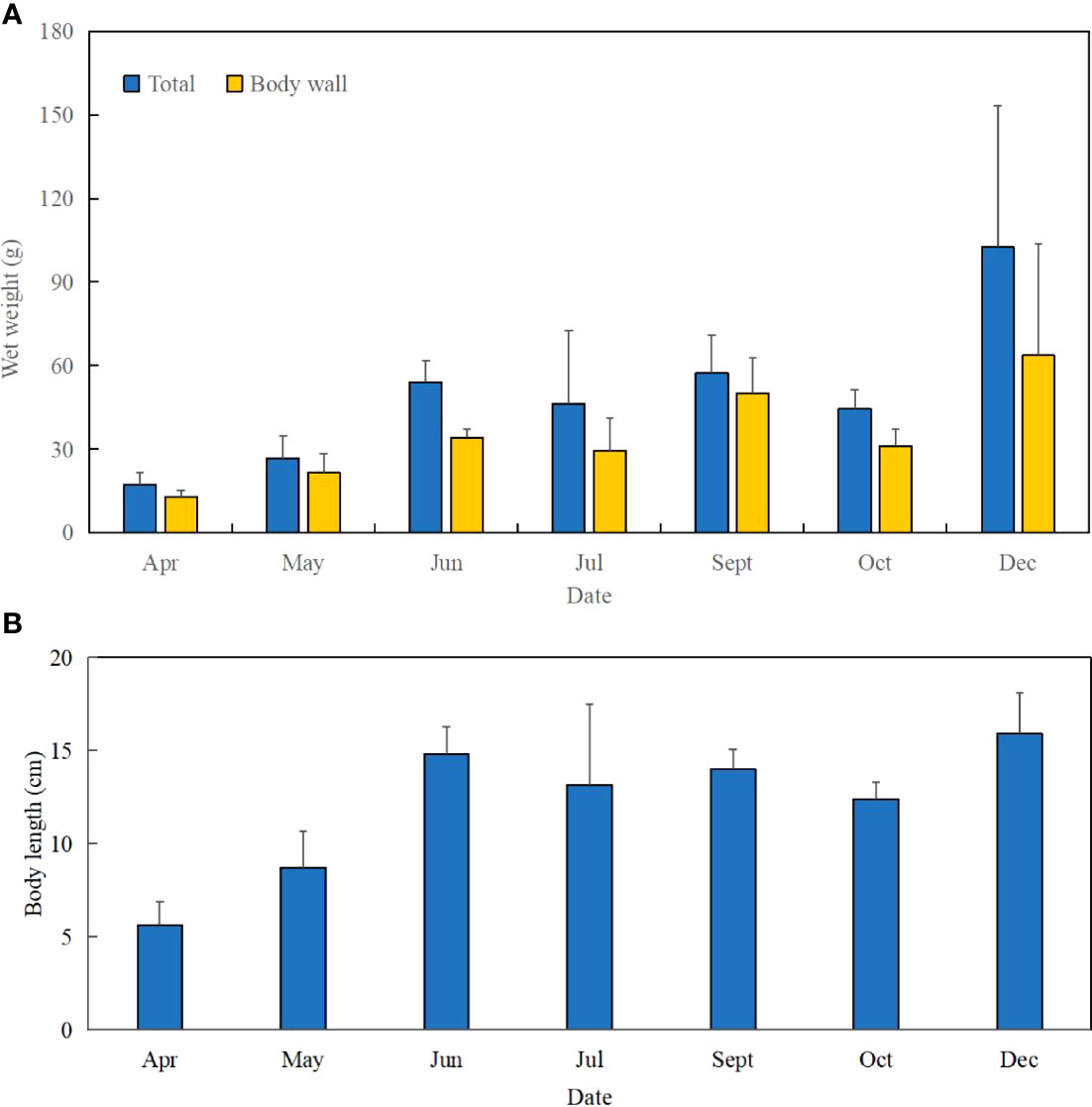
Figure 4 Increase of wet weight and body length of S. monotuberculatus from April to December 2019. (A) Change of wet weight of S. monotuberculatus from April to December 2019. (B) Change of body length of S. monotuberculatus from April to December 2019.
3.2 Nutritional Composition
3.2.1 Crude Protein and Total Lipid
The crude protein content in the body wall of S. monotuberculatus ranged from 18.13 ± 1.19 to 28.87 ± 1.82 g/100 g, and total lipid content was from 1.98 ± 0.04 to 2.95 ± 0.10 g/100 g (Table 2). During the first month after release, both the protein and lipid contents decreased significantly (p< 0.05). After that, the crude protein and lipid accumulated gradually, with the highest value reached in December and July, respectively.

Table 2 Crude protein and total lipid of Stichopus monotuberculatus body wall from April to December 2019.
3.2.2 Amino Acid Profiles
There were 16 amino acids detected in the body wall (Table 3). The total content of amino acids was 22.3 ± 0.97~29.19 ± 1.77 g/100 g throughout the experimental period, with the essential amino acid content varying from 4.67 ± 0.24 to 5.94 ± 0.36 g/100 g. Of all the amino acids, Gly and Glu are the most abundant, which accounted for 16.40%~18.10% and 16.30%~16.80% of the content, respectively. As for the eight essential amino acids for humans, seven of them were detected in the sea cucumber body, including Thr, Val, Ile, Leu, Phe, Lys, and Arg, whereas Trp was absent. Thr was the dominant essential amino acid, with the content ranging from 1.18 ± 0.09 to 1.53 ± 0.08 g/100 g. Surprisingly, all amino acid levels decreased during the first month after release, which is in line with the results of crude protein and total lipid content. From May to December, total and essential amino acid contents increased gradually, which is in accordance with the crude protein dynamic.
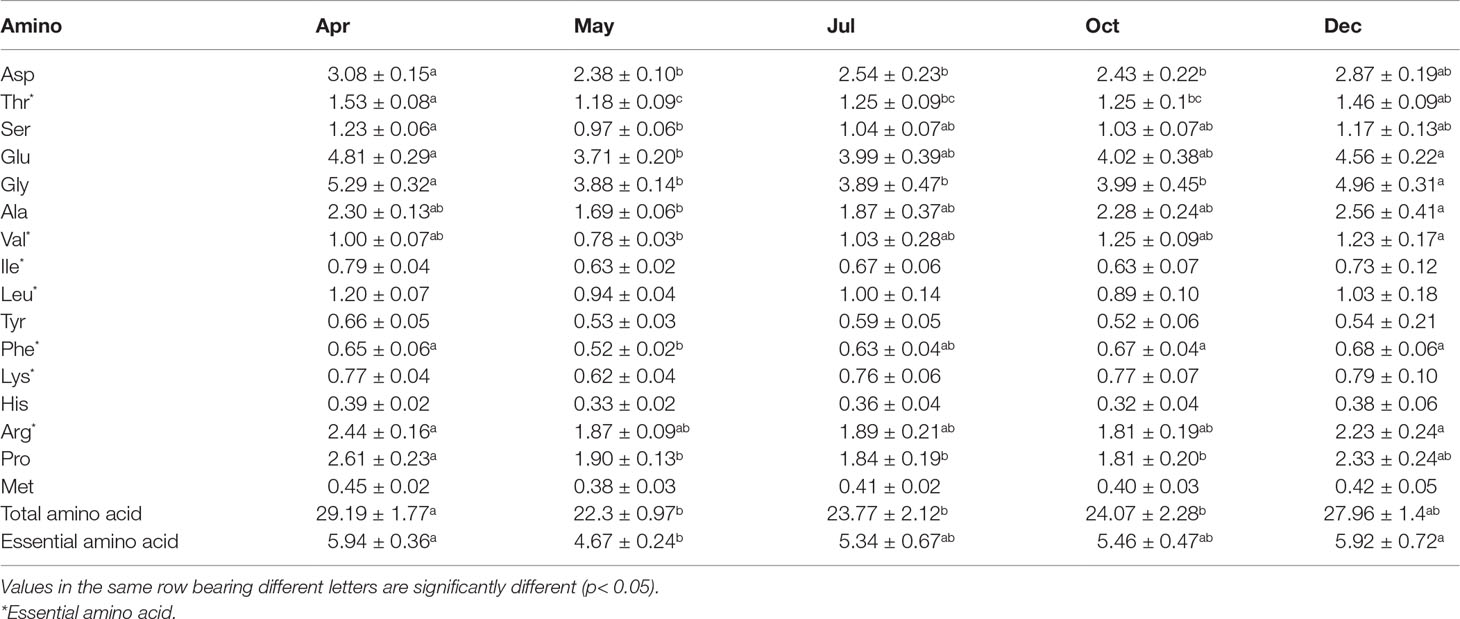
Table 3 Amino acid content in body wall of Stichopus monotuberculatus in different months (g/100 g).
3.2.3 Fatty Acid Composition
In this study, 33 fatty acids from 14 to 22 carbons were detected in the sea cucumber’s body wall (Table 4). Polyunsaturated fatty acids (PUFAs) were the dominant lipid class in all months, followed by monounsaturated fatty acids (MUFAs) and saturated fatty acids (SFAs). Branched-chain fatty acids (BFAs) were the lowest. SFAs were mainly composed of C16:0 and C18:0 and accounted for more than half of the total SFAs (Table 4). In December, the content of C16:0 was significantly lower than that in other months (p< 0.05), and the C18:0 percentage increased gradually after April (p< 0.05). The dominant MUFA was C20:1(n−9), which accounted for over 40% of the total MUFAs, and was followed by C22:1(n−9); both dropped to the lowest level in July (Table 4). The percent of C18:1(n−9) dropped remarkably after the release of the juveniles (from 4.87% to 0.66%). C20:4(n−6) was always the dominant PUFA throughout the experimental period, which accounted for over 40% of total PUFA. The percent of C20:4(n−6) reached the lowest in July and then increased gradually. EPA [C20:5(n−3)] content increased from 0.93% before release to 3.44% in the end, whereas DHA [C22:6(n−3)] content varied from 2.68% to 3.62% and peaked in May (Table 4).
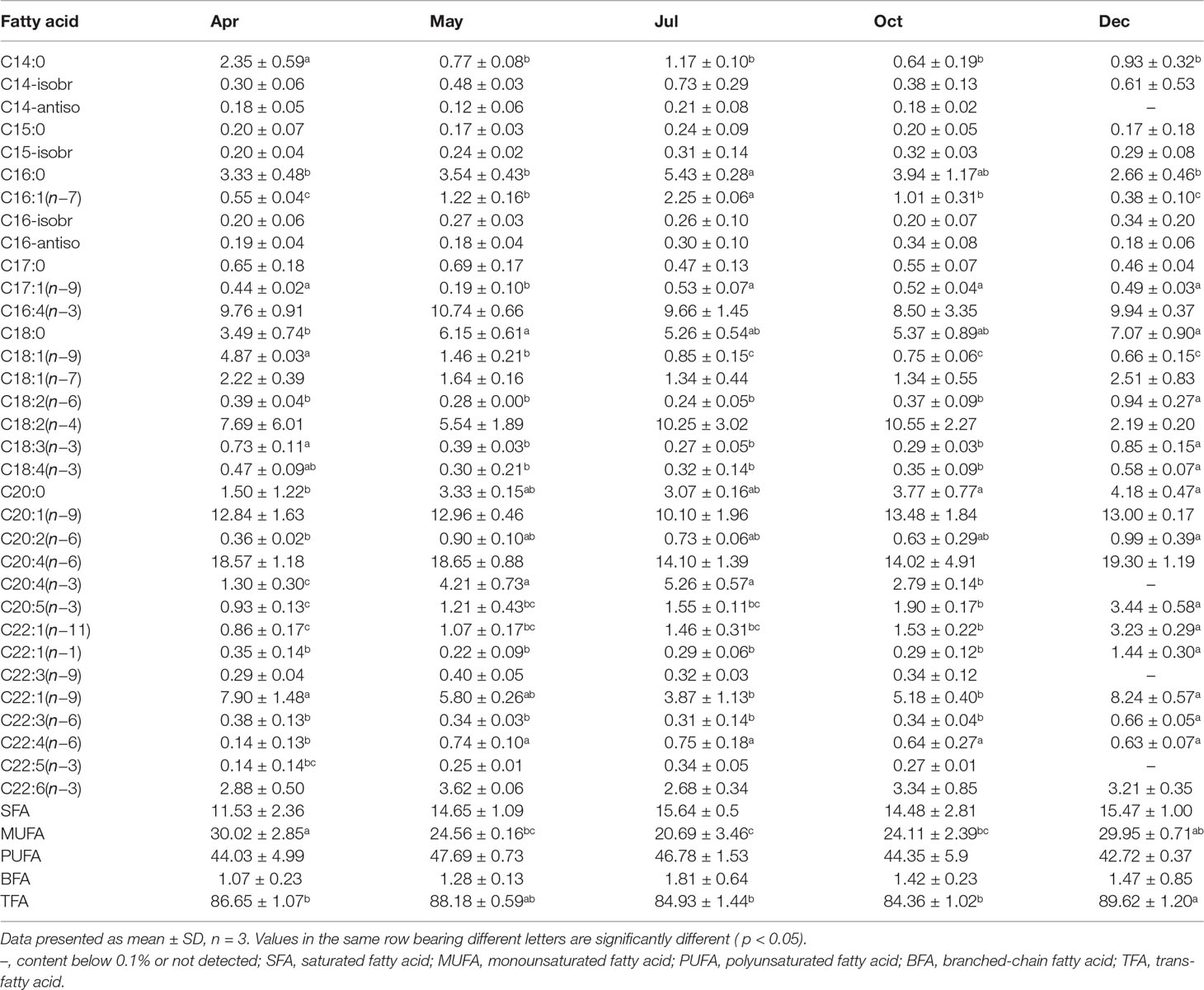
Table 4 Fatty acid composition of the body wall of Stichopus monotuberculatus in different months, 2019 (%).
3.3 Fatty Acid Biomarkers
In this study, seven fatty acid biomarkers were selected to indicate 6 potential food sources (Table 5; Figure 5). As for the two traditional diatom biomarkers C16:1(n−7)/C16:0 ratio and EPA content, the former was especially lower than 1.0 (0.15~0.42), whereas the latter was also very low from April to October (0.93%–1.90%), which only slightly raised to 3.44% in winter (Figures 5A, B). It indicates that diatoms may not be the prominent food source of the sea cucumber. The flagellate algae and protozoan’s biomarker DHA content fluctuated from 2.68% to 3.62% in different months, showing a varied contribution to the diet (Figure 5C). The high level of C20:4 (n−6) throughout the study (14.02% to 19.30%) indicates the important contribution of brown algae-derived food sources (Figure 5D).
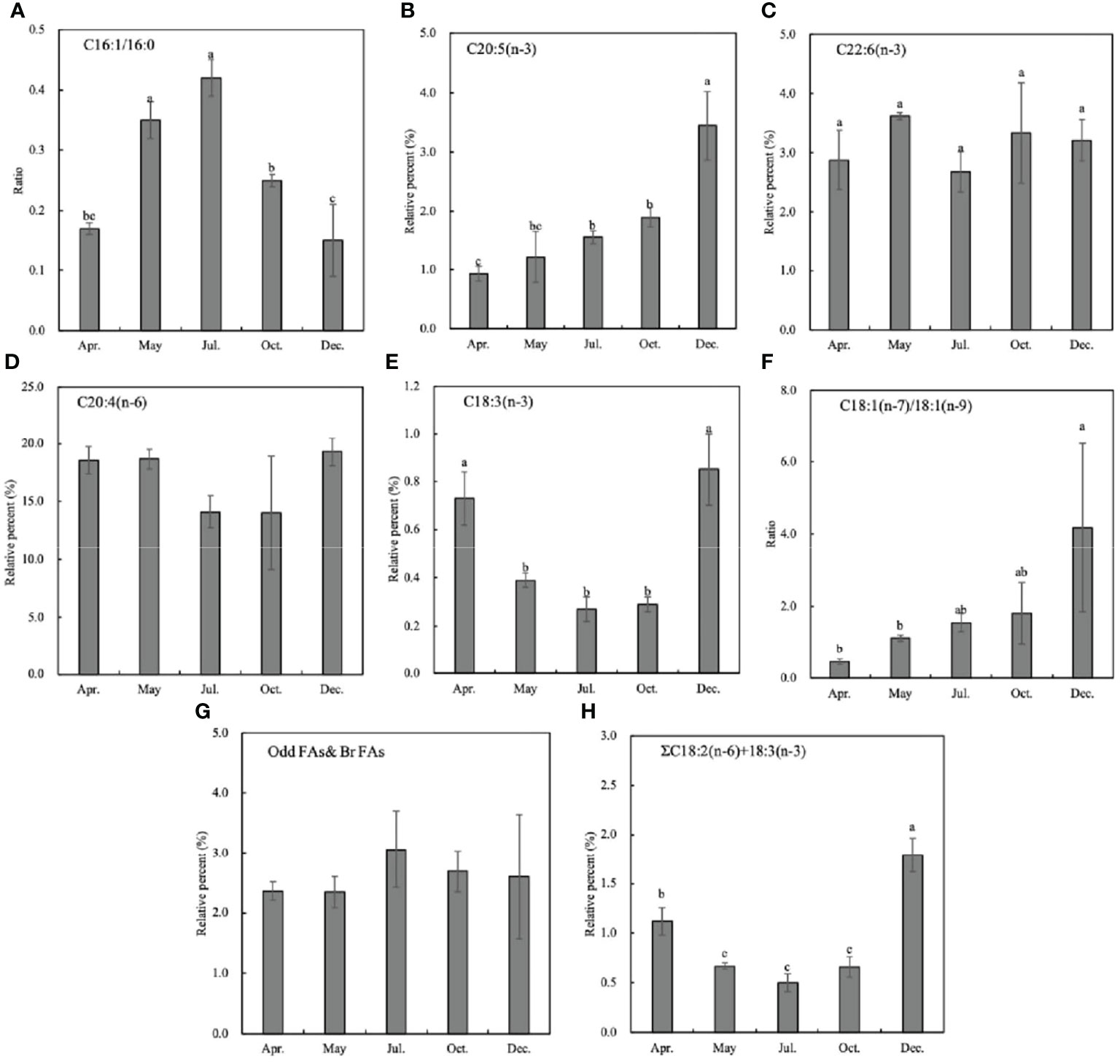
Figure 5 Fatty acid biomarkers of Stichopus monotuberculatus at different months. Food sources are indicated by different fatty acid markers: (A, B) diatoms; (C) flagellate algae or protozoan; (D) brown algae; (E, F) green algae; (G) heterotrophic bacteria; (H) terrestrial organic matter. No letters indicate that there is no significant difference between different months.
The biomarker C18:1(n−7)/C18:1(n−9) > 1 can indicate the green algae and bacterial organic matter (OM) together; hence, the data may give an ambiguous result. Considering the low level of another green alga biomarker 18:3(n−3) (below 1%, Figure 5E), it can be inferred that the increase of the C18:1(n−7)/C18:1(n−9) ratio indicates the bacterial food source to the sea cucumber (Figure 5F). After juveniles were released into the wild, the C18:1(n−7)/C18:1(n−9) ratio increased gradually, which indicates the elevated contribution of bacteria-derived OM (Figure 5F, p< 0.05). The bacterial odd and branched FA biomarker levels were abundant and stable from spring to winter, which coincides with the C18:1(n−7)/C18:1(n−9) biomarker (Figure 5G). ΣC18:2(n−6) + C18:3(n−3) > 2.5 indicates the terrestrial OM, and it stays at a low level (below 1%) except for a slightly high value of 1.79% in December (Figure 5H, p< 0.05), which indicates the less importance of the terrestrial organics to the sea cucumber.
3.4 Stable Isotope Values of Sea Cucumber and Potential Food Sources
The stable C and N isotopic values of the sea cucumber from May to December showed different variations (Table 6). The δ¹³C value had no remarkable seasonal change (−13.08‰ to −12.01‰, p > 0.05), whereas the δ¹⁵N varied from 8.04‰ to 10.98‰, with the lowest value appearing in December (p< 0.05). Both the carbon and nitrogen contents of the sea cucumber decreased remarkably in the first month after release, yet they were relatively stable during the field study (Table 6). The stable isotope values of the five potential food sources are shown in Table 7. The 13C and 15N isotopic dual plot clearly shows that the isotopic value of the sea cucumber gradually moved toward the center part of the closed region bounded by values of different potential food sources from May to December, which indicates that the diet shifted during the sea ranching period (Figure 6).
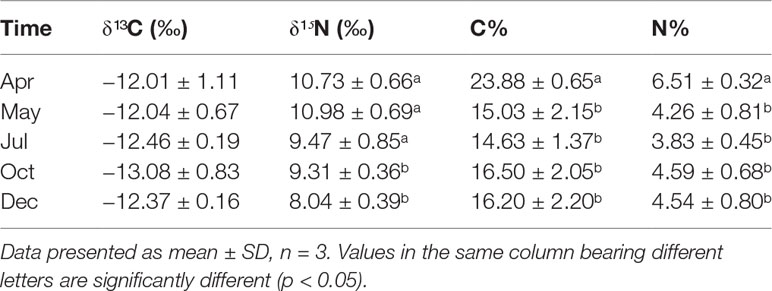
Table 6 The isotopic composition of Stichopus monotuberculatus body wall from April to December 2019.
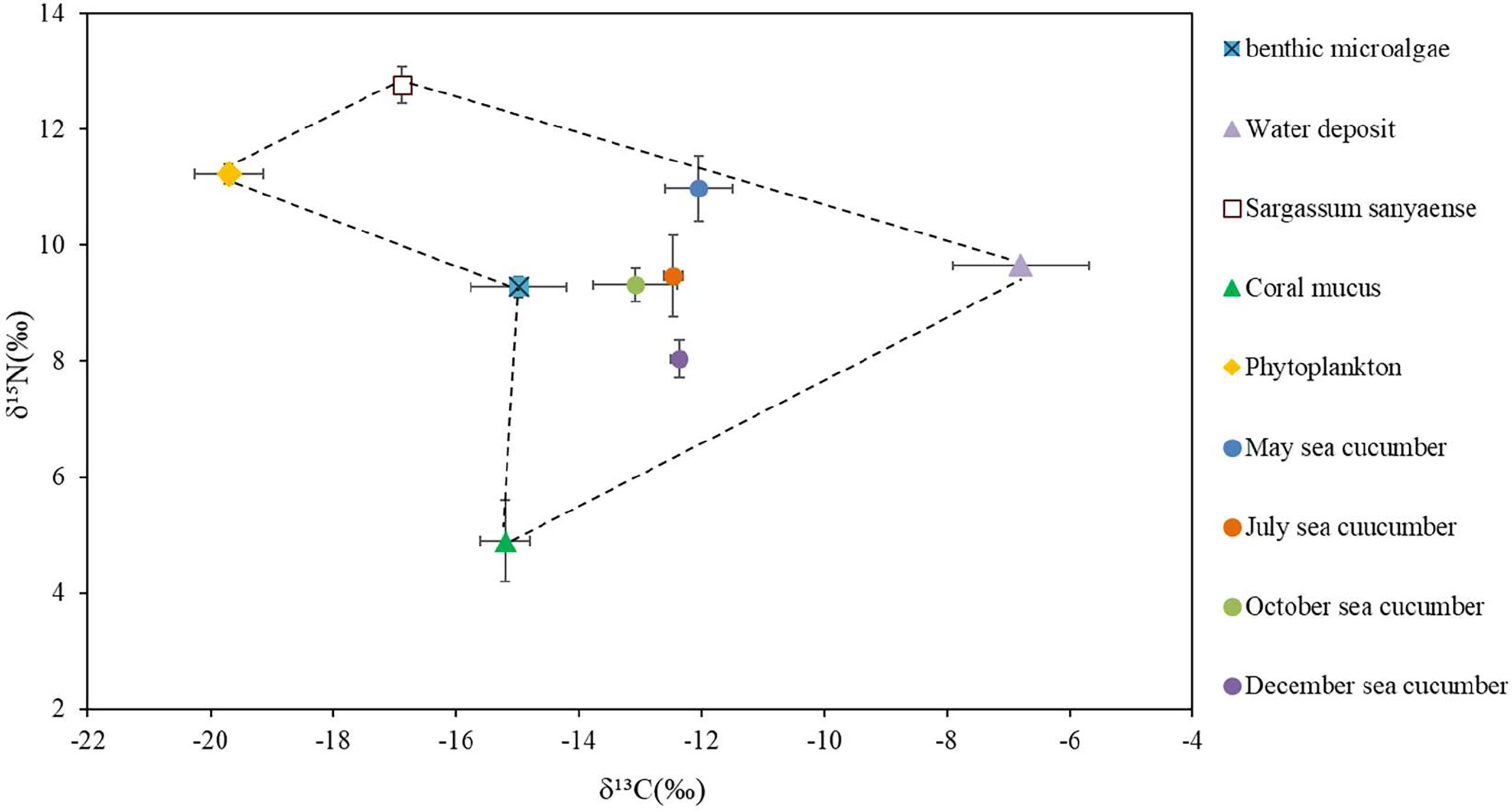
Figure 6 Dual stable isotope plots of δ13C and δ15N for the sea cucumber Stichopus monotuberculatus and its potential food sources in two sampling seasons, from May to December 2019. The stable isotope ratios of sea cucumber were corrected with the average fractionation effects of 1.0‰ and 3.4‰ for C and N, respectively. Note: the values of δ13C and δ15N for coral mucus are −16.2 ± 0.4 and 1.5 ± 0.7 (Naumann et al., 2010).
3.5 Food Composition Evaluation
The food source mixing model gave a result of the food composition of the sea cucumber in the four sampling months (Figure 7). Water deposit OM was the predominant diet part, which accounted for 33.4%–44.3% of the total. The organic proportion from Phaeophyta S. sanyaense peaked in May (41.4%) and then dropped gradually (11.3% to 6.0%) from July to December. The coral mucus-derived OM contribution was 13.1%–15.9% in July and October and peaked in December (37.2%), but in May, the contribution was only 2.7%. Benthic microalgae had a contribution in July and October (18.6%–23.2%) to the diet, but in May and December, the contribution was only 4.5% and 10.3%, respectively. Phytoplankton-derived organic sources had the same contribution of 11.2% to the diet in July and October, but in May and December, the contribution was only 5.1%–5.9%.
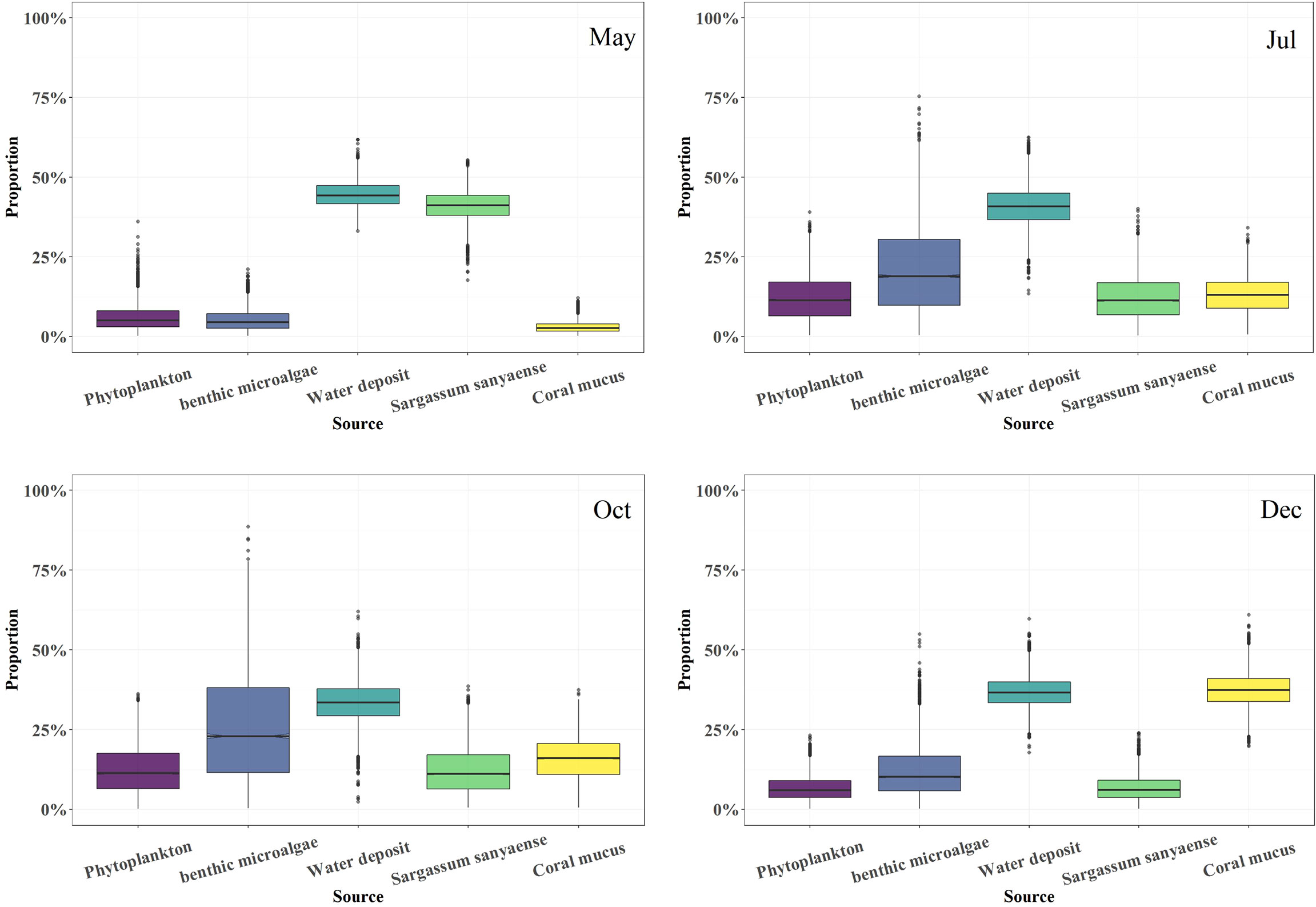
Figure 7 Food composition of the cultured sea cucumber Stichopus monotuberculatus from May to December 2019.
4 Discussion
4.1 The Growth and Survival of Stichopus monotuberculatus
This study provides the evaluation results on the growth performance of the sea-ranched S. monotuberculatus juveniles in the coral reef area. Our study found that the overall GR of the sea cucumber was 0.42 mm day−1 and SGR was 0.73% day−1 throughout the year. The sea cucumber grew fast in May–July and November–December, although there was a slowdown from August to October, which may be caused by the frequent influence of typhoons during this period (Table 1). It is reported that the widely cultured tropical species H. scabra showed an SR of 14% with an SGR of 0.66% day−1 during a 6-month experiment in the New Caledonia Népoui region (Purcell and Simutoga, 2008). The growth and survival of S. monotuberculatus, compared with H. scabra, are much better. Bottom cultured temperate A. japonicus juveniles (WW 40.35 g) achieved an average SGR of 0.15%~0.54% day−1 over a 5-month culture period under a fish farm in the northern part of the South China Sea (Yu et al., 2014), which is also lower than our result. Under pond culture conditions, sea cucumber juveniles tend to acquire a higher GR. Another tropical sea cucumber, Stichopus sp. (curry fish) juveniles (body length 4 cm), cultured in a pond showed a GR of 0.76 mm day−1 during 210 days, which is similar to our study (Hu et al., 2010). The individual growth variation (especially in December) of the S. monotuberculatus may result from the difference in the foraging efficiency and assimilation ability.
The final SR of S. monotuberculatus was 27.5%, which is lower than that of the pond cultured curry fish Stichopus sp. (30%~50%) (Hu et al., 2010) but higher than the bottom cultured H. scabra (less than 20%) (Purcell and Simutoga, 2008). There were 25%–37% of juveniles who died in the first month after being released, and this probably relates to the inability of the sea cucumber to adapt to the sudden change of living conditions from the indoor tank to the wild. Environmental factors such as water current and temperature variation together with natural food availability have been proved to affect the feeding behavior, metabolism, growth, and survival of sea cucumber juveniles (Zamora and Jeffs, 2012; Wang et al., 2015) (Zhou et al., 2013; Yu et al., 2016a; Montgomery et al., 2017; Montgomery et al., 2018; Sun et al., 2020). Considering the relatively low SR, necessary acclimation rearing should be carried out before samples are deployed into the wild. The sea ranching area should also be carefully selected for optimal physical and food conditions (such as mild wave and current, sufficient reefs for hiding, and abundant detritus food) to ensure better adaptation of the juveniles.
4.2 The Nutritional Composition
Protein and lipid nutrition is essential for the living of animals. Fatty acids and amino acids are crucial for the growth of sea cucumbers, as they are needed for energy conversion and body health (Wen et al., 2010; Omran, 2013; Rasyid, 2018). The dynamic change of the nutritional composition also gives insight into the growth performance. In the early stage of sea ranching, the contents of crude protein, total lipid, and total amino acids decreased significantly from April to May (Tables 2, 3). Total C and N contents also dropped remarkably in this period (Table 6). These indicated the large energy consumption of the sea cucumber and related closely with the acclimation process after release. Two months later, the nutrition required for growth began to accumulate, such as glutamic acid and essential amino acids. Meanwhile, the SR also remained stable, showing that the sea cucumber has adapted to the wild.
The amino acid composition of S. monotuberculatus, especially the predominant Gly and Glu (Table 3), is similar to that of other sea cucumber species such as Thelenota anax, H. fuscogilva, Holothuria fuscopunctata, Actinopyga mauritiana, Bohadschia argus, and Actinopyga caerulea (Wen et al., 2010). The fatty acid profile of the S. monotuberculatus mostly consists of PUFAs, and this is consistent with other sea cucumber species (Yu et al., 2015; Gianasi et al., 2017). The arachidonic acid [ARA, 20:4(n−6)], which is the predominant PUFA in S. monotuberculatus, plays a significant role in the growth and development of sea cucumbers (Yu et al., 2016b; King and Smith, 2018). Previous studies also proved that ARA accounts for a large proportion of unsaturated fatty acids in almost all tropical sea cucumbers (Wen et al., 2010; Rasyid, 2018).
4.3 The Potential Food Sources
The results of the lipid biomarker and stable isotope mixing model indicate that the diet of the sea cucumber changed gradually after release. Water deposits occupied the maximum part of the diet (33.4%–44.3%) throughout the year, proving its importance for the deposit feeder in the coral reef ecosystem. The organic composition of the water deposit may be complex, which includes debris and various kinds of microbes such as bacteria and protozoa (Lange et al., 2018). The considerable part of the microbial food source of the sea cucumber indicated by the flagellate/protozoa and bacterial fatty acid biomarkers may have a close relation to the organic debris from the water deposit.
Both the isotope model and lipid biomarker proved the importance of the adjacent S. sanyaense seaweed bed OM to the sea cucumber’s diet (41.4% at most). Although the seaweed bed is on the opposite side to the island, algal derived detritus may probably be transported to the sea ranching area by the strong tidal current in the bay (current direction marked in Figure 1), and this can be confirmed by the seaweed branches frequently found at the beach around the island in spring and early summer. It means that protecting the coastal seaweed bed can bring supplemental food sources to the sea ranching sea cucumber. Previous research proved that the temperate (A. japonicus) and tropical (S. horrens) sea cucumber species prefer Sargassum sp. as a habitat and food source (Wen et al., 2016; Palomar-Abesamis et al., 2017). Seaweed-derived food source for other sea cucumber species (A. japonicus) was also confirmed in the pond culture mode, which is as high as 54.4% in spring (Sun et al., 2013). Furthermore, the food contributions of benthic microalgae and phytoplankton to S. monotuberculatus are higher than the pond-cultured A. japonicus (2.8%–4.2% and 2.8%–6.5% for benthic microalgae and particulate OM (POM), respectively; Sun et al., 2013).
Coral mucus OM also has a varied contribution to the diet. Its contribution was high in December (37.2%), and it proved the importance of coral productivity to the sea cucumber in the coral reef ecosystem, especially in winter. Coral mucus can act as an important energy and nutrient carrier via particle trapping in benthic–pelagic coupling processes while serving as a food source for various reef-dwelling benthic organisms including deposit-feeding sea cucumbers (Coffroth, 1984; Wild et al., 2004; Wild et al., 2005).
5 Conclusion
This study proved the feasibility of sea ranching for the hatchery-reared S. monotuberculatus juveniles in the tropical coral reef area in China. Compared with other cultured tropical sea cucumber species, S. monotuberculatus had a higher growth performance (SGR 0.73) and SR (27.5%). The death rate of the juveniles was much higher in the first month after release, mostly because of the inadaptation to the environment transition from the indoor tank to the wild. The food source of the sea cucumber mostly comes from the OM of water deposits and brown algae debris combined with various microbes. Coral mucus-derived organics, benthic microalgae, and phytoplankton also showed their contribution in some seasons. Considering the high death rate in the first month after release, suitable acclimation operation such as pond domestication rearing is highly recommended before sea ranching. Meanwhile, more research is needed to optimize the release strategy so as to support a higher survival and GR of the species in tropical sea ranching areas.
Data Availability Statement
The original contributions presented in the study are included in the article/supplementary material. Further inquiries can be directed to the corresponding author.
Author Contributions
QX: Study design and paper writing; PW: Field experiment, sample analysis and paper writing; DH: Field experiment; YX: Food source quantification; XW: Field experiment; JX: Field experiment; WM: Field experiment; FG: Project raising, paper revise and sponsor; AW: Field study adviser. All authors contributed to the article and approved the submitted version.
Funding
The research was sponsored by the National Key R&D Project of China (No. 2019YFD0901304), National Natural Science Foundation of China (No. 42076097, 31760757), and National Natural Science Foundation of Hainan Province, China (No. 2019RC070).
Conflict of Interest
The authors declare that the research was conducted in the absence of any commercial or financial relationships that could be construed as a potential conflict of interest.
Publisher’s Note:
All claims expressed in this article are solely those of the authors and do not necessarily represent those of their affiliated organizations, or those of the publisher, the editors and the reviewers. Any product that may be evaluated in this article, or claim that may be made by its manufacturer, is not guaranteed or endorsed by the publisher.
Acknowledgments
The authors are grateful to Wang Fengguo and Zhou Zhigang for their help in the fieldwork.
References
Ackman R. G., Tocher C. S., McLachlan, (1968). Marine Phytoplankton Fatty Acids. J. Fisheries. Board. Canada. 25, 1603–1620. doi: 10.1139/f68-145
AOAC (1990). Official Methods of Analysis (Washington DC: Association of official analytical chemists).
Bachok Z., Mfilinge P. L., Tsuchiya M. (2003). The Diet of the Mud Clam Geloina Coaxans (Mollusca, Bivalvia) as Indicated by Fatty Acid Markers in a Subtropical Mangrove Forest of Okinawa, Japan. J. Exp. Mar. Biol. Ecology. 292, 187–197. doi: 10.1016/S0022-0981(03)00160-6
Block R. J., Durrum E. L., Zweig G. (2016). A Manual of Paper Chromatography and Paper Electrophoresis (Elsevier: New York).
Budge S. M., Parrish C. C., McKenzie C. H. (2001). Fatty Acid Composition of Phytoplankton, Settling Particulate Matter and Sediments at a Sheltered Bivalve Aquaculture Site. Mar. Chem. 76, 285–303. doi: 10.1016/S0304-4203(01)00068-8
Carlier A., Riera P., Amouroux J. M., Bodiou J. Y., Grémare A. (2007). Benthic Trophic Network in the Bay of Banyuls-Sur-Mer (Northwest Mediterranean, France): An Assessment Based on Stable Carbon and Nitrogen Isotopes Analysis. Estuarine. Coast. Shelf. Science. 72, 1–15. doi: 10.1016/j.ecss.2006.10.001
Chen J. X. (2004). ““Present Status and Prospects of Sea Cucumber Industry in China,”,” in Advances in Sea Cucumber Aquaculture and Management. Eds. Lovatelli A., Conand C., Purcell S., Uthicke S., Hamel J. F., Mercie A. (Rome: FAO), 25–38.
Coffroth M. A. (1984). Ingestion and Incorporation of Coral Mucus Aggregates by a Gorgonian Soft Coral. Mar. Ecol. Prog. series. 17, 193–199. doi: 10.3354/meps017193
Conand C. (1993). Ecology and Reproductive Biology of Stichopus Variegatus an Indo-Pacific Coral Reef Sea Cucumber (Echinodermata: Holothuroidea). Bull. Mar. Science. 52, 970–981.
Cook E. J., Bell M. V., Black K. D., Kelly M. S. (2000). Fatty Acid Compositions of Gonadal Material and Diets of the Sea Urchin, Psammechinus Miliaris: Trophic and Nutritional Implications. J. Exp. Mar. Biol. Ecology. 255, 261–274. doi: 10.1016/S0022-0981(00)00301-4
Desurmont A. (2003). Papua New Guinea Sea Cucumber and Beche-De-Mer Identification Cards. SPC. Beche-de-mer. Inf. Bulletin. 9, 12–15.
Eriksson H., Clarke S. (2015). Chinese Market Responses to Overexploitation of Sharks and Sea Cucumbers. Biol. Conserv. 184, 163–173. doi: 10.1016/j.biocon.2015.01.018
Findlay R. H., Trexler M. B., Guckert J. B., White D. C. (1990). Laboratory Study of Disturbance in Marine Sediments: Response of a Microbial Community. Mar. Ecol. Prog. series. 62, 121–133. doi: 10.3354/meps062121
Folch J., Less M., Stanley G. H. (1957). A Simple Method for the Isolation and Purification of Total Lipids From Animal Tissues. Biol. Chem. 226, 497–509. doi: 10.1016/s0021-9258(18)64849-5
Gianasi B. L., Parrish C. C., Hamel J. F., Mercier A. (2017). Influence of Diet on Growth, Reproduction and Lipid and Fatty Acid Composition in the Sea Cucumber Cucumaria Frondosa. Aquaculture. Res. 48, 3413–3432. doi: 10.1111/are.13168
Huang L. M., Tan Y. H., Song X. Y., Huang X. P., Wang H. K., Zhang S., et al. (2003). The Status of the Ecological Environment and a Proposed Protection Strategy in Sanya Bay, Hainan Island, China. Mar. pollut. Bull. 47, 180–186. doi: 10.1016/S0025-326X(03)00070-5
Huang J. Z., Wang F. X., Zhao H. W., Xu H. L., Li S., Xu Q., et al. (2020). Reef Benthic Composition and Coral Communities at the Wuzhizhou Island in the South China Sea: The Impacts of Anthropogenic Disturbance. Estuarine. Coast. Shelf. Sci. 243,106863. doi: 10.1016/j.ecss.2020.106863
Hu C. Q., Xu Y. H., Wen J., Zhang L. P., Fan S. G., Su T. (2010). Larval Development and Juvenile Growth of the Sea Cucumber Stichopus Sp. (Curry Fish). Aquaculture. 300, 73–79. doi: 10.1016/j.aquaculture.2009.09.033
Jiménez-Arias J. L., Morris E., Rubio-de-Inglés M. J., Peralta G., Robledo E. G., Corzo A., et al. (2020). Tidal Elevation is the Key Factor Modulating Burial Rates and Composition of Organic Matter in a Coastal Wetland With Multiple Habitats. Sci. Total. Environment. 724, 1–15. doi: 10.1016/j.scitotenv.2020.138205
Khotimchenko S. V., Vaskovsky V. E., Titlyanova T. V. (2002). Fatty Acids of Marine Algae From the Pacific Coast of North California. Botanica Marina. 45, 17–22. doi: 10.1515/BOT.2002.003
King J., Smith S. (2018). Arachidonic Acid Content in the Feed on the Growth Performance, Antioxidant Capacity and Fatty Acid Generation of Sea Cucumber. CCAMLR. Science. 25, 121–133.
Lange G., Haynert K., Dinter T., Scheu S., Kröncke I. (2018). Adaptation of Benthic Invertebrates to Food Sources Along Marine-Terrestrial Boundaries as Indicated by Carbon and Nitrogen Stable Isotopes. J. Sea. Res. 131, 12–21. doi: 10.1016/j.seares.2017.10.002
Li X. C., Fan X., Han L. J., Yan X. J., Lou Q. X. (2002). Fatty Acids of Common Marine Macrophytes From the Yellow and Bohai Seas. Oceanologia. Limnologia. Sinica. 33, 215–224. doi: 10.1016/S0031-9422(01)00437-X
Lionel M., Max G., Modou M., Katrin M., Arsenio T., Christian R., et al. (2018). Novel Application of Compound Specific Stable Isotope (CSSI) Techniques to Investigate on-Site Sediment Origins Across Arable Fields. Geoderma. 316, 19–26. doi: 10.1016/j.geoderma.2017.12.008
Li X. B., Titlyanov E. A., Titlyanova T. V., Belous O. S., Xia J. Q., Huang H. (2020). An Inventory and Seasonal Changes in the Recent Benthic Flora of Coral Reefs of Wuzhizhou Island, Haitang Bay, South China Sea (China). Russian J. Mar. Biol. 46, 485–492. doi: 10.1134/S1063074020310028
Li X. B., Wang D. R., Huang H., Zhang J., Lian J. S., Yuan X. C., et al. (2015). Linking Benthic Community Structure to Terrestrial Runoff and Upwelling in the Coral Reefs of Northeastern Hainan Island. Estuarine. Coast. Shelf. Science. 156, 92–102. doi: 10.1016/j.ecss.2014.09.021
McClelland J. W., Valiela I. (1998). Changes in Food Web Structure Under the Influence of Increased Anthropogenic Nitrogen Inputs to Estuaries. Mar. Ecol. Prog. Series. 168, 259–271. doi: 10.3354/meps168259
McCutchan J. H. Jr., Lewis W. M. Jr., Kendall C., McGrath C. C. (2003). Variation in Trophic Shift for Stable Isotope Ratios of Carbon, Nitrogen, and Sulfur. OIKOS. 102, 378–390. doi: 10.1034/j.1600-0706.2003.12098.x
Montgomery E. M., Hamel J. F., Mercier A. (2017). Ontogenetic Shifts in Swimming Capacity of Echinoderm Propagules: A Comparison of Species With Planktotrophic and Lecithotrophic Larvae. Mar. Biol. 164, 43. doi: 10.1007/s00227-017-3072-6
Montgomery E. M., Hamel J. F., Mercier A. (2018). Ontogenetic Variation in Photosensitivity of Developing Echinoderm Propagules. J. Exp. Mar. Biol. Ecology. 500, 63–72. doi: 10.1016/j.jembe.2017.12.003
Naumann M. S., Mayr C., Struck U., Wild C. (2010). Coral Mucus Stable Isotope Composition and Labeling: Experimental Evidence for Mucus Uptake by Epizoic Acoelomorph Worms. Mar. Biol. 157, 2521–2531. doi: 10.1007/s00227-010-1516-3
Omran N.E.S.E.S. (2013). Nutritional Value of Some Egyptian Sea Cucumbers. Afr. J. Biotechnol. 12, 5466–5472. doi: 10.5897/AJB2013.13020
Pakoa K, Bertram I (2013) BECHE-DE-MER Information Bulletin. Australia, France and New Zealand Secretariat of the Pacific Community 33, 49–52.
Palomar-Abesamis N., Abesamis R. A., Juinio-Meñez M. A. (2017). Distribution and Microhabitat Associations of the Juveniles of a High-Value Sea Cucumber, Stichopus Cf. Horrens, in Northern Philippines. Aquat. Ecol. 51 (1), 17–31. doi: 10.1007/s10452-016-9591-2
Parnell A. C., Phillips D. L., Bearhop S., Semmens B. X., Ward E. J., Moore J. W., et al. (2013). Bayesian Stable Isotope Mixing Models. Environmetrics 24, 387–399. doi: 10.1002/env.2221
Parrish C. C. (1999). ““Determination of Total Lipid, Lipid Classes, and Fatty Acids in Aquatic Samples,”,” in Lipids in Freshwater Ecosystems. Eds. Arts M., Wainman B. C. (New York: Springer), 4–20. doi: 10.1007/978-1-4612-0547-0_2
Purcell S. W., Hair C. A., Mills D. J. (2012). Sea Cucumber Culture, Farming and Sea Ranching in the Tropics: Progress, Problems and Opportunities. Aquaculture. 368, 68–81. doi: 10.1016/j.aquaculture.2012.08.053
Purcell S. W., Simutoga M. (2008). Spatio-Temporal and Size-Dependent Variation in the Success of Releasing Cultured Sea Cucumbers in the Wild. Rev. Fisheries. Sci. 16, 204–214. doi: 10.1080/10641260701686895
Purcell S. W., Williamson D. H., Ngaluafe P. (2018). Chinese Market Prices of Beche-De-Mer: Implications for Fisheries and Aquaculture. Mar. Policy. 91, 58–65. doi: 10.1016/j.marpol.2018.02.005
Rasyid A. (2018). Amino Acid and Fatty Acid Compositions of Sea Cucumber Stichopus Vastus From Salemo Island Waters, Indonesia. Squalen. Bull. Mar. Fisheries. Postharvest. Biotechnol. 13, 9–15. doi: 10.15578/squalen.v13i1.285
Saccò M., Blyth A. J., Humphreys W. F., Kuhl A., Mazumder D., Smith C., et al. (2019). Elucidating Stygofaunal Trophic Web Interactions via Isotopic Ecology. PloS One 14, e0223982. doi: 10.1371/journal.pone.0223982
Sánchez-Tapia I. A., Slater M., Miguel A. Olvera-Novoa M. A. (2018). Evaluation of the Growth and Survival Rate of the Caribbean Sea Cucumber, Isostichopus Badionotus (Selenk), Early Juveniles Produced in Captivity. J. World Aquaculture. Soc. 50, 763–773. doi: 10.1111/jwas.12568
Sargent J. R., Parkes R. J., Mueller-Harvey I., Henderson R. J., Parkes J. R. (1987). Lipid Biomarkers in Marine Ecology. (Ellis Horwood, Chichester, UK : Microbes in the Sea). 1987, 119–138.
Sherwood G. D., Rose G. A. (2005). Stable Isotope Analysis of Some Representative Fish and Invertebrates of the Newfoundland and Labrador Continental Shelf Food Web. Estuarine. Coast. Shelf. Science. 63, 537–549. doi: 10.1016/j.ecss.2004.12.010
Sun Z. L., Gao Q. F., Dong S. L., Shin P. K., Wang F. (2013). Seasonal Changes in Food Uptake by the Sea Cucumber Apostichopus Japonicus in a Farm Pond: Evidence From C and N Stable Isotopes. J. Ocean. Univ. China. 12, 160–168. doi: 10.1007/s11802-012-1952-z
Sun J. M., Hamel J. F., Stuckless B., Small T. J., Mercier A. (2020). Effect of Light, Phytoplankton, Substrate Types and Colour on Locomotion, Feeding Behaviour and Microhabitat Selection in the Sea Cucumber Cucumaria Frondosa. Aquaculture. 526, 735369. doi: 10.1016/j.aquaculture.2020.735369
Volkman J. K., Johns R. B., Gillan F. T., Perry G. J., Bavor H. J. (1980). Microbial Lipids of an Intertidal Sediment—I. Fatty Acids and Hydrocarbons. Geochimica. Cosmochimica. Acta 44, 1133–1143. doi: 10.1016/0016-7037(80)90067-8
Wang Q. L., Yu S. S., Dong Y. W. (2015). Parental Effect of Long Acclimatization on Thermal Tolerance of Juvenile Sea Cucumber Apostichopus Japonicus. PloS One 10, e0143372. doi: 10.1371/journal.pone.0143372
Wen B., Gao Q. F., Dong S. L., Hou Y. R., Yu H. B., Li W. D. (2016). Uptake of Benthic Matter by Sea Cucumber Apostichopus Japonicus (Selenka): Insights From Carbon Stable Isotopes and Fatty Acid Profiles. J. Exp. Mar. Biol. Ecol. 474, 46–53. doi: 10.1016/j.jembe.2015.10.003
Wen J., Hu C. Q., Fan S. (2010). Chemical Composition and Nutritional Quality of Sea Cucumbers. J. Sci. Food Agriculture. 90, 2469–2474. doi: 10.1002/jsfa.4108
Wild C., Rasheed M., Jantzen C., Cook P., Struck U., Huettel M., et al. (2005). Benthic Metabolism and Degradation of Natural Particulate Organic Matter in Carbonate and Silicate Reef Sands of the Northern Red Sea. Mar. Ecol. Prog. Series. 298, 69–78. 3354/meps298069. doi: 10.3354/meps298069
Wild C., Rasheed M., Werner U., Franke U., Johnstone R., Huettel M. (2004). Degradation and Mineralization of Coral Mucus in Reef Environments. Mar. Ecol. Prog. Series. 267, 159–171. doi: 10.3354/meps267159
Xu Q. (2007). Food sources composition of the bivalves in the seaweed-bivalve polyculture system. Ph D. thesis, Postgraduate school of Chinese Academy of Sciences (Institute of Oceanology), Qingdao, China. (in Chinese with English abstract).
Yu H. B., Gao Q. F., Dong S. L., Wen B. (2015). Changes in Fatty Acid Profiles of Sea Cucumber Apostichopus Japonicus (Selenka) Induced by Terrestrial Plants in Diets. Aquaculture. 442, 119–124. doi: 10.1016/j.aquaculture.2015.03.002
Yu H. B., Zhang C., Gao Q. F., Dong S. L., Ye Z., Tian X. L. (2016a). Impact of Water Temperature on the Growth and Fatty Acid Profiles of Juvenile Sea Cucumber Apostichopus Japonicus (Selenka). J. Thermal. Biol. 60, 155–161. doi: 10.1016/j.jtherbio.2016.07.011
Yu H. B., Gao Q. F., Dong S. L., Zhou J. S., Ye Z., Lan Y. (2016b). Effects of Dietary N-3 Highly Unsaturated Fatty Acids (HUFAs) on Growth, Fatty Acid Profiles, Antioxidant Capacity and Immunity of Sea Cucumber Apostichopus Japonicus (Selenka). Fish. Shellfish. Immunol. 54, 211–219. doi: 10.1016/j.fsi.2016.04.013
Yu Z. H., Zhou Y., Yang H. S., Hu C. Q. (2014). Survival, Growth, Food Availability and Assimilation Efficiency of the Sea Cucumber Apostichopus Japonicus Bottom-Cultured Under a Fish Farm in Southern China. Aquaculture. 426-427, 238–248. doi: 10.1016/j.aquaculture.2014.02.013
Zamora L. N., Jeffs A. G. (2012). Feeding, Metabolism and Growth in Response to Temperature in Juveniles of the Australasian Sea Cucumber, Australostichopus Mollis. Aquaculture. 358-359, 92–97. doi: 10.1016/j.aquaculture.2012.06.024
Zamora L. N., Yuan X. T., Carton A. G., Slater M. J. (2018). Role of Deposit-Feeding Sea Cucumbers in Integrated Multitrophic Aquaculture: Progress, Problems, Potential and Future Challenges. Rev. Aquaculture. 10, 57–74. doi: 10.1111/raq.12147
Keywords: Stichopus monotuberculatus, growth performance, nutritional quality, food source composition, sea ranching
Citation: Xu Q, Wu P, Huang D, Xiao Y, Wang X, Xia J, Ma W, Gao F and Wang A (2022) Sea Ranching Feasibility of the Hatchery-Reared Tropical Sea Cucumber Stichopus monotuberculatus in an Inshore Coral Reef Island Area in South China Sea (Sanya, China). Front. Mar. Sci. 9:918158. doi: 10.3389/fmars.2022.918158
Received: 12 April 2022; Accepted: 06 June 2022;
Published: 22 July 2022.
Edited by:
Marie Antonette Juinio-Meñez, University of the Philippines Diliman, PhilippinesReviewed by:
Anselmo Miranda-Baeza, State University of Sonora, MexicoLuis R. Martinez-Cordova, University of Sonora, Mexico
Nadia Palomar Abesamis, Silliman University, Philippines
Copyright © 2022 Xu, Wu, Huang, Xiao, Wang, Xia, Ma, Gao and Wang. This is an open-access article distributed under the terms of the Creative Commons Attribution License (CC BY). The use, distribution or reproduction in other forums is permitted, provided the original author(s) and the copyright owner(s) are credited and that the original publication in this journal is cited, in accordance with accepted academic practice. No use, distribution or reproduction is permitted which does not comply with these terms.
*Correspondence: Fei Gao, gaofeicas@126.com