- 1College of Marine Sciences, Beibu Gulf University, Qinzhou, China
- 2Guangxi Key Laboratory of Marine Disaster in the Beibu Gulf, Beibu Gulf University, Qinzhou, China
- 3College of Resources and Environment, Beibu Gulf University, Qinzhou, China
The invasion of Spartina alterniflora (S. alterniflora) has changed the carbon cycle process of local ecosystems. In order to clarify the effect of S. alterniflora invasion on coastal soil carbon pool in Northern Beibu Gulf, the distribution characteristics and influencing factors of soil organic carbon (SOC) and SOC storage (SOCS) at different intrusion stages were investigated and analyzed. The results showed that the SOC content in S. alterniflora wetlands (2.65–21.54 g/kg) was higher than that in mudflats (0.85–1.19 g/kg). SOC content in 0–20 cm depth was highest than that in 20–40 cm and 40–60 cm depth. The total SOCS increased by 72.11%, 78.45%, 77.56%, 80.42%, and 90.63% in 3a, 12a, 15a, 16–19a, and 26a compared with mudflats, respectively. S. alterniflora invasion increased SOC and SOCS both in surface soil and deep soil. SOCS increased rapidly during the initial stage of invasion, and remained in a relatively stable and continuous growth state after 12–15 years. The distributions and accumulation of SOC and SOCS were affected mainly by soil texture, soil bulk density, moisture content, total nitrogen and total phosphorus. The source of SOC from S. alterniflora was increasing with invasion ages and would be mainly input in 26a, while marine sources was mainly imported in other invasion ages. Our data indicated that S. alterniflora continuously enhances the SOC sequestration over the years in coastal wetland ecosystems.
Introduction
Coastal wetlands play an important role in the global cycle by acting as a valuable carbon sink due to their high sedimentation rates and burial of organic carbon (Drake et al., 2015), which store at least 44.6 Tg C yr−1 globally (Chmura et al., 2003). In coastal ecosystem, wetland soils were the important carbon pool as well as the environmental media of carbon accumulation from vegetation biomass and litter. The capacity of soil carbon sequestration in coastal wetlands is dynamically changing and often threatened by the impacts of non-native species invasion and climate change worldwide (Simas et al., 2001; Yang, 2019). Therefore, it is of great importance to understanding the response mechanisms of soil organic carbon (SOC) dynamics under invasive species.
Spartina alterniflora (S. alterniflora) is an aquatic plant that is native to the Atlantic coast of the United States and the Gulf of Mexico. In the late 1970s, S. alterniflora was introduced into China for coastal restoration and sediment stabilization (Lu and Zhang, 2013; Ge et al., 2015; Pan et al., 2015), then spread in coastal areas and replaced native plants rapidly due to its strong adaptability and competitiveness. Its distribution area has reached 546 km2 in 2015 (Mao et al., 2019), and its invasion scale in China is much larger than that in other area worldwide (An et al., 2007). After years of ecological succession, it has rapidly expanded in many introduced areas and become a dominant species that has an important impact on the structure and function of intertidal ecosystems (Adams et al., 2016).
There has been extensive research to determine the impact by S. alterniflora invasion on soil carbon sequestration in coastal wetlands (Yang et al., 2013; Kulawardhana et al., 2015; Li et al., 2016), indicating that S. alterniflora invasion would induce more SOC storage (SOCS) in coastal wetlands (Yang, 2019; Zhang et al., 2021a). This process is influenced by S. alterniflora biomass input in soils, the deposition rate of the tidal salt marsh, carbon turnover, and organic carbon stability in coastal wetlands, which are generally reported to be associated with the accumulation and decomposition of organic carbon (Negrin et al., 2011; Snedden et al., 2015; Gao et al., 2016; Unger et al., 2016; Yang et al., 2016). Previous researches indicate that soil physicochemical properties, such as bulk density (BD), pH, moisture content and salinity, as well as soil texture played crucial roles in changing surface SOC variation (Bai et al., 2016; Wang et al., 2016; Yang and Guo, 2018). However, the major control mechanism of SOC vertical distribution in response to S. alterniflora invasion may be different and complex in coastal wetlands (Yang, 2019). Therefore, it is necessary to address the driving mechanism of SOC both in surface and in depth soils in order to understand the multiple interactions of carbon dynamics between invasive species and soils.
Moreover, the research on the S. alterniflora SOC pool in the coastal wetlands of China mostly focuses on the areas with a concentrated distribution of S. alterniflora, mainly in Yangtze River estuary, and the coastal areas of Jiangsu, Zhejiang, and Fujian provinces, but few in Guangxi province. After introducing into Guangxi, the distribution area of S. alterniflora had spread from 0.94 hm2 to 686.48 hm2 with rapid invasion ratio (Pan et al., 2016), but the respond characteristics and mechanism of S. alterniflora invasion on SOC and SOCS has not been known well yet in this process. Thus, the aims of the present work are: (1) to determine the changing characteristics of SOC contents and SOCS both in surface and depth samples among different S. alterniflora invasion stages; (2) to explore the effects of multiple environmental factors on the distributions and accumulations of SOC and SOCS among different S. alterniflora invasion stages. We hope to provide a theoretical basis for an in-depth understanding of the ecological role of S. alterniflora in soil improvement and the carbon cycle in coastal wetlands.
Materials and Methods
Study Area
The Beihai coastal wetlands are located north of Beibu Gulf, China. Beibu Gulf is a closed bay with a total coastline of 1628 km that experiences a subtropical marine monsoon climate, with an annual average temperature of 22.0–23.4°C, annual sunshine of 1406–2050 h, and annual precipitation of 1250–2755 mm. A diurnal tide occurs with an average tidal range of 2–3 m. S. alterniflora was first introduced into the Dandou Bay wetlands in Beibu Gulf, Guangxi in 1979, and gradually spread along the adjacent coastline to cover an increasing area (Pan et al., 2016). S. alterniflora wetlands with different invasion years in Dandouhai and Yingpan Town in Beibu Gulf were selected in this study area, and their invasion age range was determined through Google Earth, Landsat5/6/7 image data, and GPS positioning. A basic overview of the sample plot obtained through fieldwork is shown in Table 1.
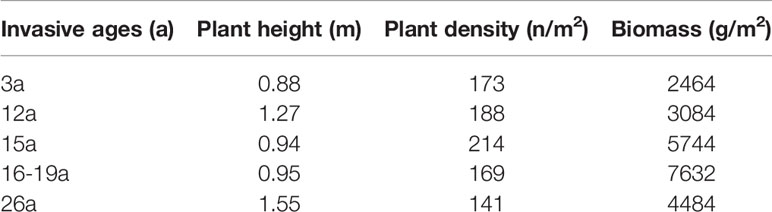
Table 1 The average plant height, density and biomass of Spartina alterniflora sample plots in different invasive ages.
Sediment Sampling and Sample Treatment
Sample plots of the S. alterniflora community comprising the Beibu Gulf coastal wetlands at different invasion stages were established in June 2020 (Figure 1). The plots of SA3, SA12, SA15, SA16-19, SA26, and MF represent the area of S. alterniflora and mudflats invading 3a, 12a, 15a, 16-19a, and 26a respectively. Six small sample plots of 0.25 m2 were established for each year. Then, the entire S. alterniflora plant was harvested in the small sample plots, the soil impurities were removed, and the samples were placed into polyethylene bags that were sealed and immediately transported to the laboratory to determine their fresh and dry weights. In each sample plot, 0–60 cm soil columns were collected with a soil sampler, consisting of 0–20 cm, 20–40 cm, and 40–60 cm samples that were sealed in polyethylene bags and immediately transported to the laboratory. Some samples were dried in a cool and ventilated place indoors, and then, indexes such as SOC, particle size, total nitrogen (TN), and total phosphorus (TP) were measured. A portion of the sample was preserved in the refrigerator for determining the microbial biomass carbon.
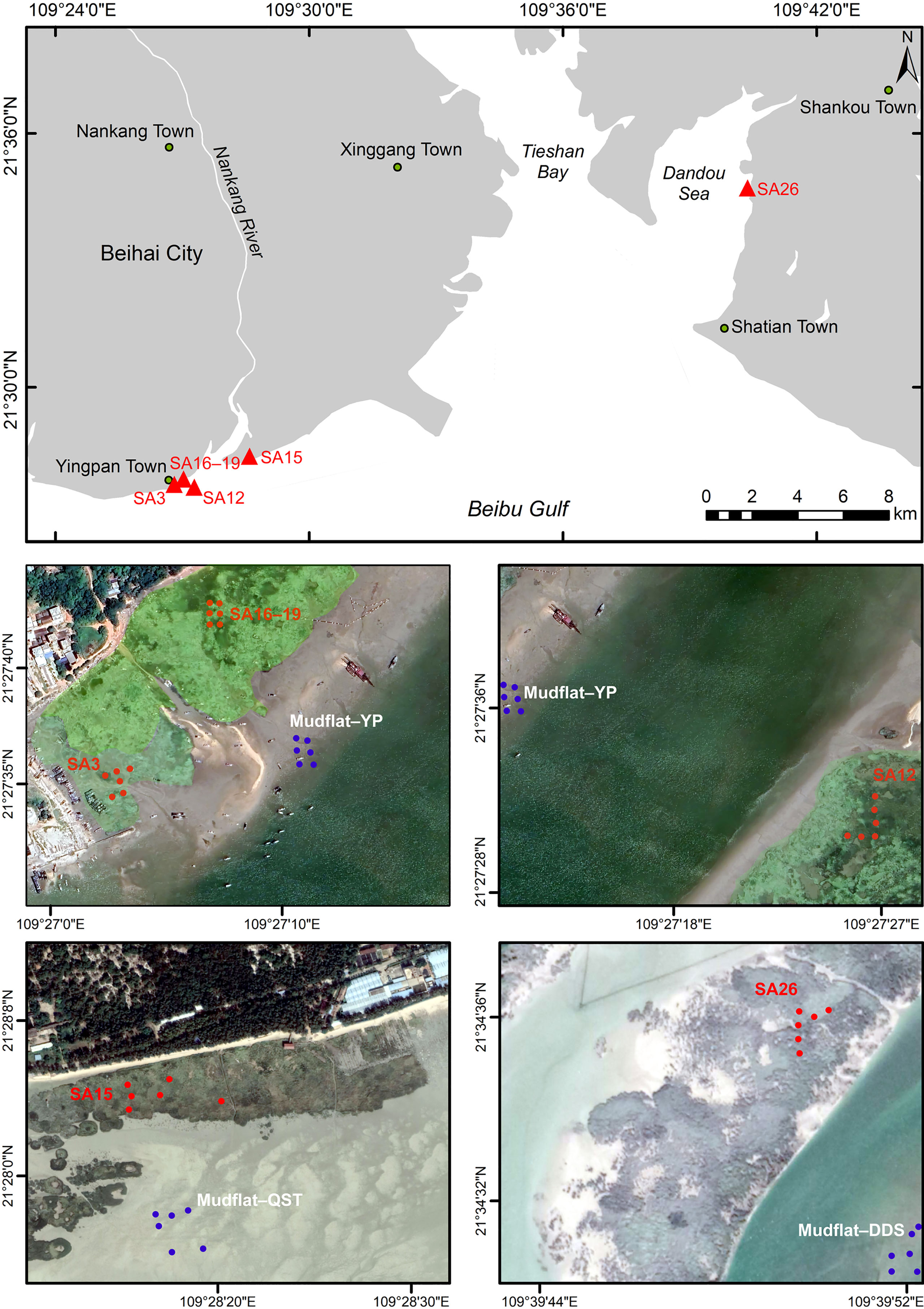
Figure 1 Locations of study sites and sampling areas in the Spartina alterniflora wetlands (number behind SA means the invasion ages; MF, mudflat).
The SOC content was determined by the potassium dichromate external heating oxidation method (NY/T 1121.6-2006, Ministry of Agriculture, PRC, 2006). The TN and TP were determined by the Kjeldahl method and molybdenum antimony resistance spectrophotometry (ly/T 1228-2015 and GB/T 9837-1988). Soil particle size was determined by an a-weight hydrometer (NY/T 1121.3-2006, Ministry of Agriculture, PRC, 2006). The classification of particle size is based on the international soil particle size classification standard, with 3–2 mm designated for gravel, 2–0.2 mm for coarse sand, 0.2–0.02 mm for fine sand, 0.020–0.002 mm for silt, and < 0.002 mm for clay (Tsukada et al., 2008). Microbial biomass carbon was determined by chloroform fumigation extraction (CFE).
Data Analysis
Soil bulk density (BD, g/cm3) is computed using the following equation:
Where g denotes the fresh weight of ring knife soil (g); v denotes the ring knife volume (100 cm³); MC denotes the moisture content of the sample (%), which is expressed as:
Where FW denotes the soil fresh weight; DW denotes the soil dry weight.
SOCS (t/hm2) is expressed as:
Where Ci denotes carbon mass fraction in i soil depth (g/kg); Di denotes the soil BD in i soil depth (g/cm3); Ei denotes thickness of soil in i soil depth (cm); n denotes the number of soil depth layers.
Microsoft Excel 2010 and SPSS v23.0 statistical software were utilized for the data analysis, ANOVA with Tukey HSD post hoc test was used to analyze the differences of SOC and SOCS among different soil depth and invasion ages (α = 0.05), and Pearson correlation analysis was used to determine the correlation among different indicators (α = 0.05).
Results
SOC Content in S. alterniflora Wetlands at Different Invasion Ages
SOC content in the S. alterniflora wetlands (2.65–21.54 g/kg) in all years was higher than that those in mudflats (0.85–1.19 g/kg). In 0-20 cm depth, SOC content in S. alterniflora wetlands in 3-26a was higher than that in mudflats with significantly difference, and the SOC content increased with the years except for 12a (Figure 2). SOC content in 20–40 cm depth increased with years in different stages except for 3a, and there was a significant difference between 26a and the mudflats, 3a, 12a, and 15a. There was an obvious accumulation of SOC content over time in soil in 40–60 cm depth, and there was a significant difference between 26a and the mudflats, 3a, 12a, and 15a.
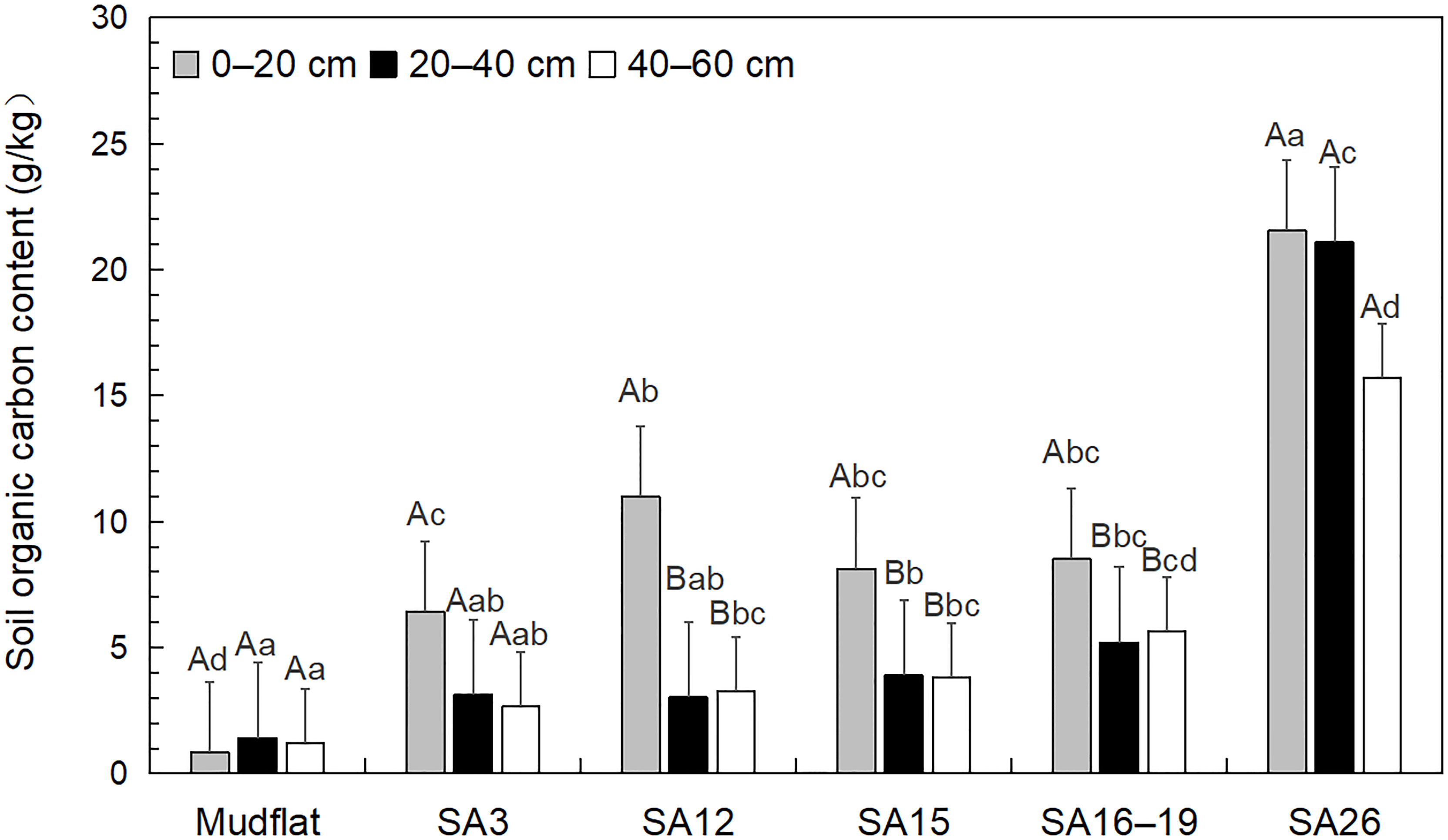
Figure 2 Distribution characteristics of organic carbon content in the soils from different Spartina alterniflora wetlands. Different lowercase letters indicate that there are significant differences at the same soil depth in different invasion ages. Different capital letters indicate that there are significant differences among different soil depths in the same invasion age (P < 0.05).
In each invasion age, SOC content in surface was the highest with significantly difference among 3a, 12a, 15a versus 16–19a, respectively (Figure 2). The content of SOC in 3a, 15a, and 26a decreased with increasing soil depth. There was a significant difference between SOC content in 0–20 cm and those in 20–40 cm, 40–60 cm depth at 12a, 15a, and 16–19a.
SOCS in S. alterniflora Wetlands at Different Invasion Ages
After 3, 12, 15, 16–19, and 26 years of invasion, the total SOCS increased by 72.11%, 78.45%, 77.56%, 80.42%, and 90.63% compared with those in mudflats, respectively, In 0-20 cm depth, SOCS at five different S. alterniflora invasion ages was higher than those in the mudflats with significantly difference (Figure 3). SOCS in 20–40 cm and 40–60 cm showed an increasing trend with invasion ages except for those in 20–40 cm of 12a. There were significant differences among SOCS in 20–40 cm and 40–60 cm depth of mudflats, 3a, and 12a versus those of 15a, 16–19a and 26a.
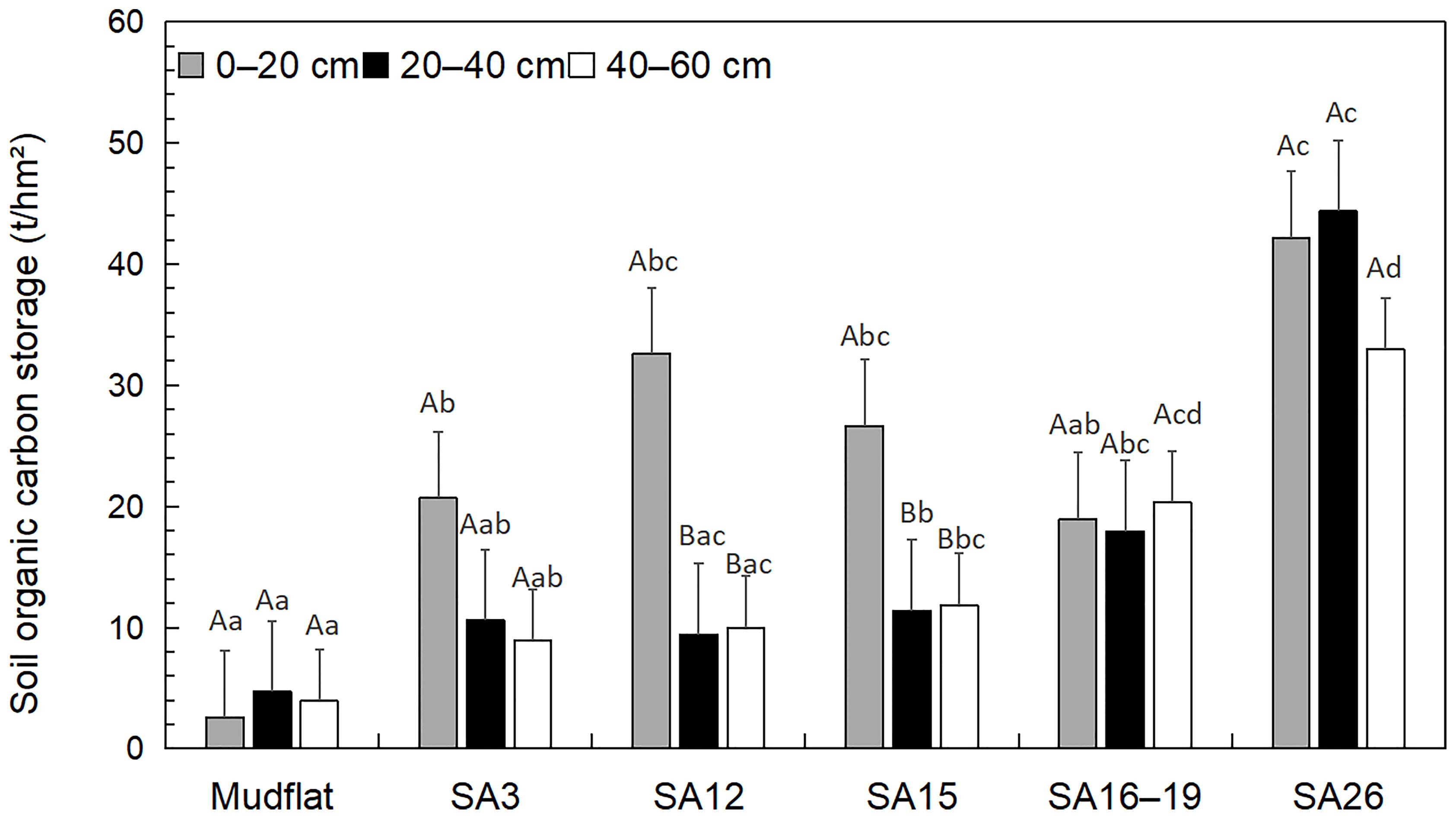
Figure 3 Distribution characteristics of organic carbon storage in the soils from different Spartina alterniflora wetlands. Different lowercase letters indicate that there are significant differences at the same soil depth in different invasion ages. Different capital letters indicate that there are significant differences among different soil depths in the same invasion age (P < 0.05).
Vertically, there was an apparent aggregation trend in the soil surface for the SOCS in each invasion age except for 26a (Figure 3). The lowest SOCS were found in 20–40 cm depth of 12a, 15a, and 16–19a but not in those of 0–20 cm or 40–60 cm. Moreover, the highest SOCS in 26a were in 20–40 cm, followed by those in 0–20 cm and 40–60 cm. There were significant differences among SOCS in 0–20 cm versus those in 20–40 cm and 40–60 cm of 12a and 15a.
Correlation Between SOCS and Physical And Chemical Factors in S. alterniflora Wetlands
The physical and chemical properties for the soils and their correlations with SOC, SPCS were displayed in Table 2 and Table 3 respectively. There was a significant positive correlation between the SOCS in S. alterniflora wetlands and the MC and SOC content, total nitrogen (TN), total phosphorus (TP), the molar ratio of soil organic carbon to total nitrogen (C/N), silt, and clay, and a significant negative correlation with BD and CS. The SOC content had significant positive correlation with the MC, TP, C/N, FS, silt, clay and SOCS, and significant negative correlation with BD and CS (Table 3).
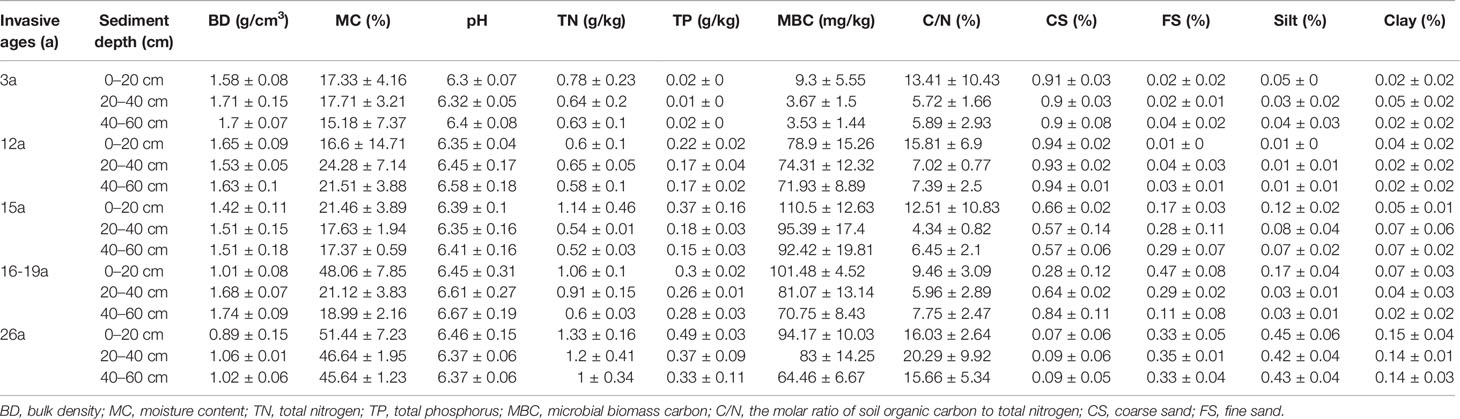
Table 2 Statistical results (Mean ± SD) of physical and chemical properties for the soils from Spartina alterniflora wetlands with different invasive ages and depth.
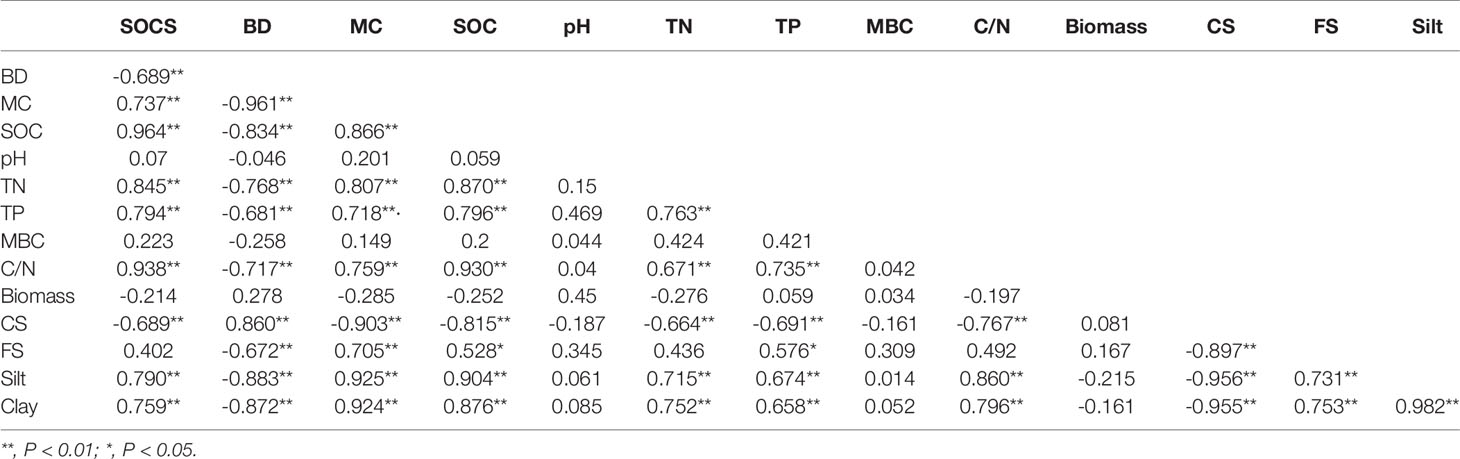
Table 3 Correlation matrix of soil organic carbon storage with other soil physical and chemical factors of Spartina alterniflora.
Further principal component analysis (PCA) showed that the first and second principal components contributed 60.9% and 12.8% of the cumulative variance, respectively (Figure 4). In the first principal component, the factor loads of SOC content, silt, clay and MC were higher. In the second principal component, the factor loads of pH, FS, and microbial biomass carbon (MBC) were higher. Figure 4 shows that physical and chemical factors such as silt, clay, MC, TN, TP, and C/N in the soil have an obvious driving effect on the distribution of SOCS in the area with the longest invasion age (26a). BD and CS play important roles in driving the change in SOCS in the 3-15a area with a short invasion age.
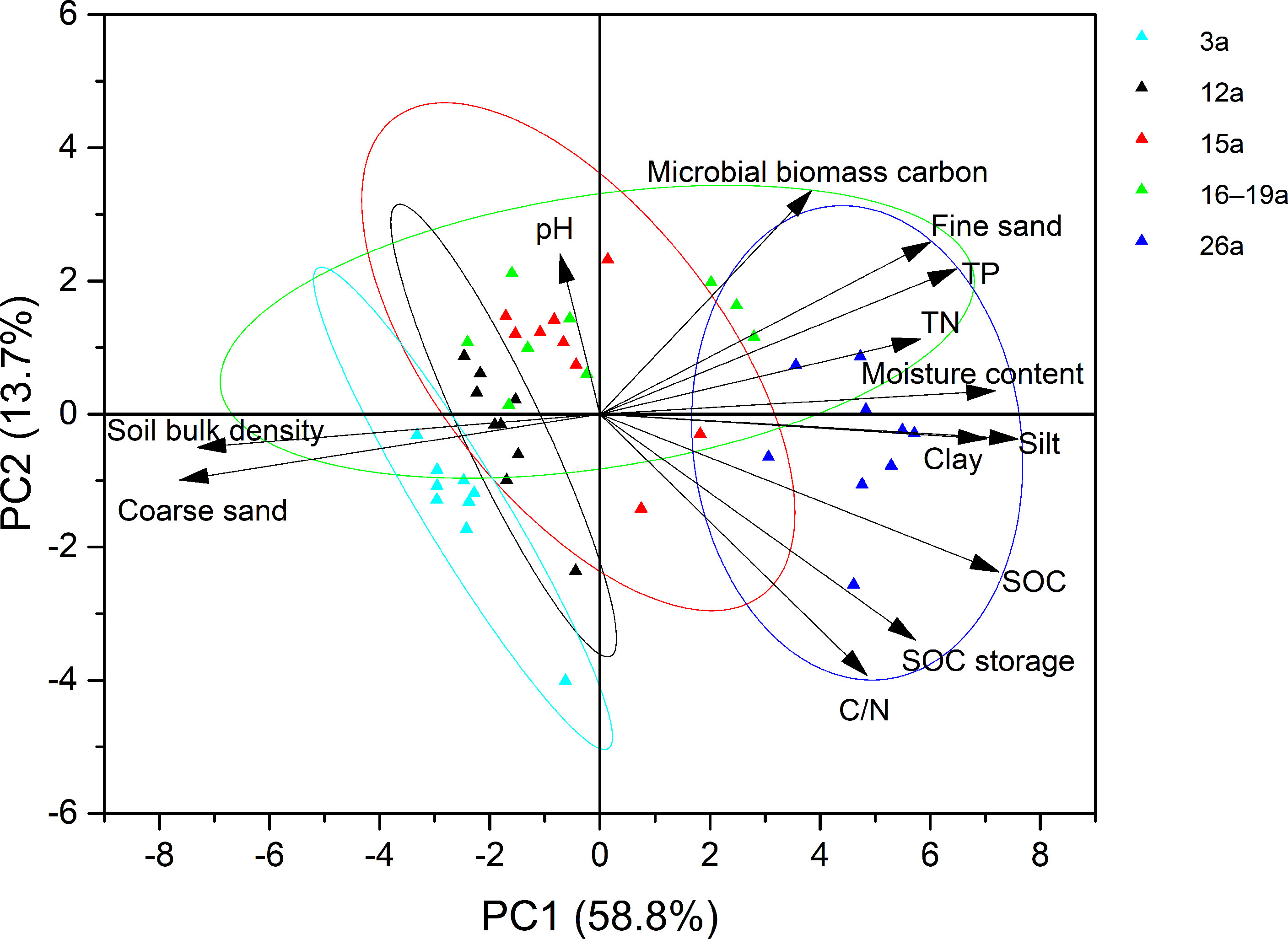
Figure 4 Results of PCA analysis of soil organic carbon and other physical and chemical factors among different soils from Spartina alterniflora wetlands with different invasion age.
Discussion
Effects of S. alterniflora Invasion on SOC and SOCS
Compared with previous researches, SOC content in S. alterniflora wetlands in this study was significantly higher than those in Yancheng, and the Yangtze River Estuary (Liu et al., 2007; Zhang et al., 2010) in similar invasion ages, and lower than that in Minjiang River estuary wetlands (Tong et al., 2011; Jin et al., 2016a). SOC content in surface soil of S. alterniflora in 3a (6.43 g/kg) was much larger than that in Xinyang port, Yancheng (1.55 g/kg) (Wang et al., 2013), but smaller than that in Minjiang estuary (16.9–14.3 g/kg) (Jin et al., 2016a).
The invasion of S. alterniflora changes the carbon cycle process of the ecosystem (He et al., 2016). In this study, SOCS in 12a was 4.63 times that in mudflats, and the mean and total reserves of SOCS in this study exhibited a cumulative effect with the extension of invasion age except for 12a, which is consistent with the research results of the SOCS in S. alterniflora in wetlands at Galveston Island, Texas (Kulawardhana et al., 2015), USA, and the Yellow River Estuary (Zhang et al., 2018). SOCS increased at the initial stage of S. alterniflora invasion and remained in a relatively stable and continuous growth state after 12–15a in this study, which is consistent with the results reported by Zhang et al. (2010) and Jin et al. (2016a). But the stable time of SOCS is different due to the regional differences of accumulation of SOC is different study areas. SOCS reached the highest value in 26a but did not attain the saturation state in this study. Craft et al. (1999) reported that saturation of SOCS had not been reached over 25 years with S. alterniflora invasion in North Carolina, USA. But in Yancheng, China, Wang et al. (2013) reported that SOCS tended to be saturated only for 5 years with S. alterniflora invasion.
Although the sedimentation rate of S. alterniflora wetlands in this study was not determined, but it could be inferred still that the depth of 0-20 cm soil were deposited in the study area over past 20-30 years (Xia et al., 2011; Gan et al., 2013; Xu et al., 2019). Therefore, surface accumulation history of SOC was consist with the invasion years of S. alterniflora basically. Generally, surface soil is greatly affected by the disturbance caused by human disturbance, while deep soilcan reflect the time accumulation characteristics of SOC reserves because of less disturbing of human beings (Feng et al., 2015). The results from most sample plots in this study showed that the SOCS first decreased and then increased with the soil depth. The SOCS in soil with a depth of 40–60 cm in 12a, 15a, and 16–19a was higher than that in 20–40 cm.
The SOC of S. alterniflora is derived from the periodic withered organs of plants and floating particles in the sea. The root system of S. alterniflora is deeply distributed and can even reach a depth of 100 cm, and the biomass of deep roots provides the source of SOC for deep soil (Liao et al., 2007). Additionally, the influence of tidal hydrodynamic forces and the anoxic state of the bottom environment are not conducive to the decomposition of SOC by aerobic microorganisms, and the deep soil is less disturbed by human beings, which promotes the more stable storage of bottom soil carbon (Feng et al., 2015). The SOCS in topsoil for each year was high (except for 26a), and the accumulation of SOC in the surface soil was the highest. This is related to the rich source of topsoil SOC, including the input of endogenous carbon from plants and exogenous carbon from seawater (Zhang et al., 2008; Feng et al., 2016).
The C/N of soil can be utilized to assess the source of organic carbon in wetland soil (Thornton and McManus, 1994). In this study, the C/N in 26a of this study (15.72–16.18) is greater than 15, which indicates that the SOC source in S. alterniflora wetlands is mainly from self-input after years of colonization. In contrast, the C/N in other invasion years was between 2.8–13, indicating marine sources, which is consistent with the results of source apportionment of SOC in S. alterniflora wetland by isotope method in Yangtze Estuary (Wang et al., 2015). The SOC mainly originated from marine sources at the initial stage of S. alterniflora settlement. With the extension of invasion age, the SOC accumulation from the plants themselves was gradually strengthened. Withering and decay from the roots, stems, and leaves of S. alterniflora become an important source of SOC, which also shows that the longer the plant settlement time, the more conducive the area is to the accumulation of soil nutrients.
Totally, S. alterniflora invasion increased SOCS both in surface soil and deep soil due to the fast reproduction speed, high biomass, high vegetation coverage and fast carbon sequestration effect (Hou et al., 2016; Liu et al., 2017; Bu et al., 2018). Many domestic scholars have reported that the SOCS in S. alterniflora wetland is higher than those in native salt marsh plants (Hang et al., 2014; Zhang et al., 2012; Zhang et al., 2014), such as Phragmites and Suaeda salsa in the Yellow River Delta (Du et al., 2022) and Liaohe River (Zhang et al., 2021c). But in our work, the average SOCS of S. alterniflora (63.75 t/hm2) and the maximum SOCS of 26a (119.48 t/hm2) are lower than those in mangrove in Qinglan port, Hainan (302.81 t/hm2) (Lin et al., 2015) and Qinzhou Bay, Guangxi (181.03 t/hm2) (Tao et al., 2020). Therefore, it is still necessary to control S. alterniflora invasion and strengthening native mangrove protection in northern Beibu Gulf, China.
Effects of Soil Physicochemical Factors on Soil Carbon Storage in the S. alterniflora Wetlands at Different Invasion Stages
The SOC in wetlands is derived from siltation, soil humus, plant litter, and microbial and root exudates, and is the result of the dynamic balance between input and output under the influence of various environmental factors (Jin et al., 2016a). In this study, BD and coarse sand play an important role in driving the change in SOCS in the early stage of S. alterniflora invasion while TN, TP, C/N, silt, clay, and water content have an obvious driving effect on the distribution of SOCS with the extension of settlement time.
BD is used to measure soil compactness and a reference for the calculation of SOCS. When there is a high amount of decomposed and semi-decomposed products from roots and other withered organs and the granules decompose and promote siltation, then BD is lower, indicating that the soil is loose, porous, and higher permeable (Jin et al., 2016a). Then, BD affects the decomposition of organic matter by affecting the permeability of the soil, thus further affecting the SOC content. In this study, especially with the development process of S. alterniflora plants and soil, the permeability of the surface soil was higher than that in the deep soil (Hou et al., 2016). Besides, BD is significantly correlation with soil texture in our study, indicating that the higher BD would be with fewer fine-grained soils. Some studies have shown that the SOCS of S. alterniflora is affected by the particle size effect, which increases with the increase in fine-grained sediments because of their large surface area (Gao et al., 2016). Usually, there is high adsorption in small particles, and it is easier for them to bind to root exudates and humus products, which will increase SOCS (Xia et al., 2021).
Wetland soil has a high water-holding capacity, which is 2–8 times that of general soil (Xu et al., 2015). In this study, the MC gradually increases with the years of S. alterniflorain invasion but decreased with the soil depth. The MC affected the mineralization and decomposition of organic carbon by affecting the permeability of the soil, which subsequently affected the SOC content (Zhao et al., 2018). With the increase of water in the soil, the activity of aerobic microorganisms was inhibited, and thus, the decomposition of SOC was inhibited which is conducive to the accumulation of SOC (Chen et al., 2018; Ren et al., 2022).
There is a significant positive correlation between SOC reserves and TN and TP as shown in Table 3, which indicated that there is a certain growth and decline relationship between SOC, nitrogen, and phosphorus. The increase in nitrogen and phosphorus can promote the primary productivity of plants, thus increasing the accumulation of SOC, and the decomposition process of SOC can promote the release of nitrogen and phosphorus in soil (Shi et al., 2019). The high C/N will reduce soil microbial activity, reduce the turnover rate of the activated carbon pool, reduce the oxidation and loss of SOC, and finally accelerate the accumulation of SOC and improve the quality of SOC (Wang et al., 2014).
Most previous studies indicated that SOC derived from plant biomass of S. alterniflora could increase SOC pool in coastal wetlands (Li et al., 2009; Jin et al., 2016b; Gao et al., 2016; Yang et al., 2017). But in this study, there was no significant correlation between S. alterniflora biomass and SOC (Table 3). Although the invasion of S. alternifolia would enhance soil dissolved organic matter (DOM) due to the greater amount of biomass (Zhang et al., 2019), the contribution of S. alterniflora biomass triggering SOC pool improvement is site-specific and depends on multiple environmental factors (Zhang et al., 2021a). Estuary and coast has the complex environment with periodic hydrological and hydrodynamic conditions, which would carry organic matter from both the terrestrial and marine sources (Dong et al., 2020). In northern Beibu Gulf, suspended and sedimentary organic matter (OM) mainly derive from freshwater and marine phytoplankton (Kaiser et al., 2014), while terrestrial matter, autochthonous inputs include roots and litters of S. alternifolia, and marine phytoplankton can be considered as the potential endmembers of the sedimentary OC sources (Zhang et al., 2021b). Therefore, it is necessary to clear the sources and their corresponding contribution in order to better understand the carbon sequestration capacity of S. alterniflora in coastal wetland ecosystem.
Conclusion
The changes of SOC and SOCS in S. alterniflora wetlands at different invasion stages in northern Beibu Gulf was studied by substituting space for time in this study. The total SOCS increased with the years, which showed that it rapidly increased in the early stage of invasion with a relatively stable and continuous growth state after 12–15 years of invasion. The SOC located below 20 cm increased with the years, which illustrated that the invasion of S. alterniflora not only increased the surface soil but also the SOCS of deep soil. Soil physical and chemical properties, including MC, TN, TP, C/N, silt, and clay, were the main driving factors for the occurrence of SOC and SOCS in different depth and invasion ages. The SOC source would be mainly input by plants themselves in 26a but was imported by marine sources in other invasion ages. Conclusions derived from this study are limited by the lack of sources of SOC, including DOM and POM, and accurate sedimentation rates. Therefore, it is necessary to establish long-term and comprehensive observation to track the succession and development process of S. alterniflora, and focus on the action mechanism of factors relevant to SOC.
Data Availability Statement
The original contributions presented in the study are included in the article/supplementary material. Further inquiries can be directed to the corresponding authors.
Author Contributions
YT and XW designed the study, coordinated the team. XH, YT and XW wrote the first draft of the manuscript. XW and YD performed the statistical analysis and the figures. HL, CL and YL contributed the experiments and helped with the writing. All authors contributed to manuscript revision, read, and approved the submitted version
Funding
This research was supported by the National Natural Science Foundation of China (31960251, 41901226, 1907320) and Science and technology base and talent special Foundation of Guangxi Region (2020AC19064).
Conflict of Interest
The authors declare that the research was conducted in the absence of any commercial or financial relationships that could be construed as a potential conflict of interest.
Publisher’s Note
All claims expressed in this article are solely those of the authors and do not necessarily represent those of their affiliated organizations, or those of the publisher, the editors and the reviewers. Any product that may be evaluated in this article, or claim that may be made by its manufacturer, is not guaranteed or endorsed by the publisher.
Acknowledgments
We are very grateful to the technicians of the Guangxi Mangrove Research Center for their technical assistance with the fieldwork.
References
Adams J., van Wyk E., Riddin T. (2016). First Record of Spartina alterniflora in Southern Africa Indicates Adaptive Potential of This Saline Grass. Biol. Inv. 18 (8), 2153–2158. doi: 10.1007/s10530-015-0957-5
An S. Q., Gu B. H., Zhou C. F., Wang Z. S., Deng Z. F., Zhi Y. B., et al. (2007). Spartina Invasion in China: Implications for Invasive Species Management and Future Research. Weed. Res. 47 (3), 183–191. doi: 10.1111/j.1365-3180.2007.00559.x
Bai J., Zhang G., Zhao Q., Lu Q., Jia J., Cui B., et al. (2016). Depth-Distribution Patterns and Control of Soil Organic Carbon in Coastal Salt Marshes With Different Plant Covers. Sci. Rep. 6 (1), 1–12. doi: 10.1038/srep34835
Bu N., Yang X., Li G., Ma X., Song Y., Ma F., et al. (2018). Effects of Spartina alterniflora Invasion on Soil Carbon Dynamics in Wetlands of the Yangtze River Estuary. China Environ. Sci. 38 (7), 2671–2679. doi: 10.19674/j.cnki.issn1000-6923.2018.0278
Chen X., Xu Y., Gao H. J., Mao J., Chu W., Thompson M. L. (2018). Biochemical Stabilization of Soil Organic Matter in Straw-Amended, Anaerobic and Aerobic Soils. Sci. Total. Environ. 625, 1065–1073. doi: 10.1016/j.scitotenv.2017.12.293
Chmura G. L., Anisfeld S. C., Cahoon D. R., Lynch J.C. (2003). Global Carbon Sequestration in Tidal, Saline Wetland Soils. Glob. Biogeochem. Cycle 17 (4), 1111. doi: 10.1029/2002GB001917
Craft C., Reader J., Sacco J. N., Broome S. W. (1999). Twenty-Five Years of Ecosystem Development of Constructed Spartina alterniflora (Loisel) Marshes. Ecol. Appl. 9 (4), 1405–1419. doi: 10.1890/1051-0761(1999)009[1405:TFYOED]2.0.CO;2
Dong Y., Li Y., Kong F., Zhang J., Xi M. (2020). Source, Structural Characteristics and Ecological Indication of Dissolved Organic Matter Extracted From Sediments in the Primary Tributaries of the Dagu River. Ecol. Indic. 109, 105776. doi: 10.1016/j.ecolind.2019.105776
Drake K., Halifax H., Adamowicz S. C., Craft C. (2015). Carbon Sequestration in Tidal Salt Marshes of the Northeast United States. Environ. Man. 56 (4), 998–1008. doi: 10.1007/s00267-015-0568-z
Du S., Bai J., Jia J., Guan Y, Zhang G., Chen G., Zhang S., Gai L. (2022). Changes of Soil Organic Carbon Storage in Phragmites Australis Wetlands Along a Salinity Gradient in the Yellow River Delta. Acta Sci. Circumstantiae 42 (1), 80–87. doi: 10.13671/j.hjkxxb.2021.0520
Feng Z., Gao J., Chen L., Wang Y., Gao J., Bai F. (2015). The Response of Organic Carbon Content to Biomass Dynamics in Spartina alterniflora Mash . Acta Ecol. Sin. 35 (7), 2038–2047. doi: 10.5846/stxb201404090685
Feng Z., Gao J., Chen L., Wang Y., Gao J., Bai F. (2016). Impact of Spartina alterniflora Biomass Variation on Content and Sources of Organic Carbon Fractions in Salt Marshes: A Case Study of Tidal Salt Marsh of Wanggang Estuary, Jiangsu Province. Geochimica 45 (1), 87–97. doi: 10.19700/j.0379-1726.2016.01.007
Gan H., Lin J., Liang K., Xia Z. (2013). Selected Trace Metals (As, Cd and Hg) Distribution and Contamination in the Coastal Wetland Sediment of the Northern Beibu Gulf, South China Sea. Mar. Pollut. Bull. 66 (1–2), 252–258. doi: 10.1016/j.marpolbul.2012.09.020
Gao J., Feng Z., Chen L., Wang Y., Bai F., Li J. (2016). The Effect of Biomass Variations of Spartina alterniflora on the Organic Carbon Content and Composition of a Salt Marsh in Northern Jiangsu Province, China. Ecol. Eng. 95, 160–170. doi: 10.1016/j.ecoleng.2016.06.088
Ge Z. M., Guo H. Q., Zhao B., Zhang L. Q. (2015). Plant Invasion Impacts on the Gross and Net Primary Production of the Salt Marsh on Eastern Coast of China: Insights From Leaf to Ecosystem. J. Geophys. Res.: Biogeosci. 120 (1), 169–186. doi: 10.1002/2014JG002736
Hang Z., Wang G., Liu J., Wang G., Wang H. (2014). Characterization of Soil Organic Carbon Fractions at Spartina alterniflora Salt Marsh in North Jiangsu. Acta Ecol. Sin. 34 (15), 4175–4182. doi: 10.5846/stxb201212141802
He Y., Widney S., Ruan M., Herbert E., Li X., Craft C. (2016). Accumulation of Soil Carbon Drives Denitrification Potential and Lab-Incubated Gas Production Along a Chronosequence of Salt Marsh Development. Estua. Coast. Shelf. Sci. 172, 72–80. doi: 10.1016/j.ecss.2016.02.002
Hou D., He D., Yan J., Wang R., Ji C., Wang P., et al. (2016). Effect of Invasive Spartina Alterniflora on Soil Organic Carbon in Eastern Fujian Coastal Wetlands. Trans. Oceanol. Limnol. 1, 68–76. doi: 10.13984/j.cnki.cn37-1141.2016.01.010
Jin B., Gao D., Yang P., Wang W., Zeng C. (2016a). Change of Soil Organic Carbon With Different Years of Spartina alterniflora Invasion in Wetlands of Minjiang River Estuary. J. Nat. Resour. 31 (4), 608–619. doi: 10.11849/zrzyxb.20150425
Jin B., Yan H., Zhang L., Zeng C. (2016b). Spatial-Temporal Variations and Theirs Influence Factors of Soil Organic Carbon Under the Spartina alterniflora Wetland in China. Ecol. Environ. Sci. 25 (12), 2021–2027. doi: 10.16258/j.cnki.1674-5906.2016.12.018
Kaiser D., Unger D., Qiu G. (2014). Particulate Organic Matter Dynamics in Coastal Systems of the Northern Beibu Gulf. Continent. Shelf. Res. 82, 99–118. doi: 10.1016/j.csr.2014.04.006
Kulawardhana R. W., Feagin R. A., Popescu S. C., Boutton T. W., Yeager K. M., Bianchi T. S. (2015). The Role of Elevation, Relative Sea-Level History and Vegetation Transition in Determining Carbon Distribution in Spartina alterniflora Dominated Salt Marshes. Estua. Coast. Shelf. Sci. 154, 48–57. doi: 10.1016/j.ecss.2014.12.032
Liao C., Luo Y., Jiang L., Zhou X., Wu X., Fang C., et al. (2007). Invasion of Spartina alterniflora Enhanced Ecosystem Carbon and Nitrogen Stocks in the Yangtze Estuary, China. Ecosystems 10 (8), 1351–1361. doi: 10.1007/s10021-007-9103-2
Li B. O., Liao C. H., Zhang X. D., Chen H. L., Wang Q., Chen Z. Y., et al. (2009). Spartina alterniflora Invasions in the Yangtze River Estuary, China: An Overview of Current Status and Ecosystem Effects. Ecol. Eng. 35 (4), 511–520. doi: 10.1016/j.ecoleng.2008.05.013
Lin H., Zeng S., Wang G., Liang D., Li M.. (2015). Carbon Density and Allocation of Bruguiera sexangula and Hibiscus tiliaceus Mangrove Ecosystem in Qinglan Harbor of Wenchang City, Hainan Province. J. Central South Univ. Forest. Tech. 35 (11), 99–103. doi: 10.14067/j.cnki.1673-923x.2015.11.018
Liu J. E., Han R. M., Su H. R., Wu Y. P., Zhang L. M., Richardson C. J., et al. (2017). Effects of Exotic Spartina alterniflora on Vertical Soil Organic Carbon Distribution and Storage Amount in Coastal Salt Marshes in Jiangsu, China. Ecol. Eng. 106, 132–139. doi: 10.1016/j.ecoleng.2017.05.041
Liu J., Zhou H., Qin P., Zhou J. (2007). Effects of Spartina alterniflora Salt Marshes on Organic Carbon Acquisition in Intertidal Zones of Jiangsu Province, China. Ecolo. Eng. 30 (3), 240–249. doi: 10.1016/j.ecoleng.2007.01.010
Li J., Zhang Q., Zhang L., Tong C. (2016). Effect of Spartina alterniflora Invasion Sequence on Soil Carbon and Nitrogen Distribution in a Cyperus Malaccensis Marsh of the Min River Estuary in Spring. Acta Ecol. Sin. 36 (12), 3628–3638. doi: 10.5846/stxb201510092040
Lu J., Zhang Y. (2013). Spatial Distribution of an Invasive Plant Spartina alterniflora and its Potential as Biofuels in China. Ecol. Eng. 52, 175–181. doi: 10.1016/j.ecoleng.2012.12.107
Mao D., Liu M., Wang Z., Li L., Man W., Jia M., et al. (2019). Rapid Invasion of Spartina alterniflora in the Coastal Zone of Mainland China: Spatiotemporal Patterns and Human Prevention. Sensors 19 (10), 2308. doi: 10.3390/s19102308
Negrin V. L., Spetter C. V., Asteasuain R. O., Perillo G. M., Marcovecchio J. E. (2011). Influence of Flooding and Vegetation on Carbon, Nitrogen, and Phosphorus Dynamics in the Pore Water of a Spartina alterniflora Salt Marsh. J. Environ. Sci. 23 (2), 212–221. doi: 10.1016/S1001-0742(10)60395-6
Pan L., Shi X., Tao Y., Fan H., Mo Z. (2016). Distribution and Expansion of Spartina alterniflora in Coastal Tidal Zone, Guangxi. Wetl. Sci. 14 (04), 464–470.
Pan T., Zeng L., Zeng C., Wang W. (2015). Effects of Spartina alterniflora Invasion on Soil Organic Carbon in the Bare Tidal Flat Wetland of Minjiang River Estuary. Sci. Soil Water Conserv. 13 (1), 84–90. doi: 10.16843/j.sswc.2015.01.013
Ren Q., Yuan J., Wang J., Liu X., Ma S., Zhou L., et al. (2022). Water Level has Higher Influence on Soil Organic Carbon and Microbial Community in Poyang Lake Wetland Than Vegetation Type. Microorganisms 10 (1), 131. doi: 10.3390/microorganisms10010131
Shi Y., Huang H., Ran S., Liu J., Su H., Yu P. (2019). Effects of Nitrogen and Phosphorus Addition on Photosynthesized Carbon Allocation in Spartina Alterniflora-Soil System. J. Ecol. Rural Environ. 35 (5), 627–633. doi: 10.19741/j.issn.1673-4831.2018.0605
Simas T., Nunes J. P., Ferreira J. G. (2001). Effects of Global Climate Change on Coastal Salt Marshes. Ecol. Modell. 139 (1), 1–15. doi: 10.1016/S0304-3800(01)00226-5
Snedden G. A., Cretini K., Patton B. (2015). Inundation and Salinity Impacts to Above-and Belowground Productivity in Spartina Patens and Spartina alterniflora in the Mississippi River Deltaic Plain: Implications for Using River Diversions as Restoration Tools. Ecol. Eng. 81, 133–139. doi: 10.1016/j.ecoleng.2015.04.035
Tao Y., Huang X., Wang X., Zhong Q., Kang Z. (2020). Spatial Distribution of Soil Carbon and Nitrogen Dtocks in Mangrove Wetland of Xiandao Park and Shajing in Guangxi. Prog. Fish. Sci. 41 (5), 38–45. doi: 10.19663/j.issn2095-9869.20190629001
Thornton S. F., McManus J. (1994). Application of Organic Carbon and Nitrogen Stable Isotope and C/N Ratios as Source Indicators of Organic Matter Provenance in Estuarine Systems: Evidence From the Tay Estuary, Scotland. Estua. Coast. Shelf. Sci. 38 (3), 219–233. doi: 10.1006/ecss.1994.1015
Tong C., Zhang L., Wang W., Gauci V., Marrs R., Liu B., et al. (2011). Contrasting Nutrient Stocks and Litter Decomposition in Stands of Native and Invasive Species in a Sub-Tropical Estuarine Marsh. Environ. Res. 111 (7), 909–916. doi: 10.1016/j.envres.2011.05.023
Tsukada H., Takeda A., Hisamatsu S. I., Inaba J. (2008). Concentration and Specific Activity of Fallout 137Cs in Extracted and Particle-Size Fractions of Cultivated Soils. J. Environ. Radioact. 99 (6), 875–881. doi: 10.1016/j.jenvrad.2007.11.014
Unger V., Elsey-Quirk T., Sommerfield C., Velinsky D. (2016). Stability of Organic Carbon Accumulating in Spartina alterniflora-Dominated Salt Marshes of the Mid-Atlantic Us. Estua. Coast. Shelf. Sci. 182, 179–189. doi: 10.1016/j.ecss.2016.10.001
Wang Q., Wang Y., Wang S., He T., Liu L. (2014). Fresh Carbon and Nitrogen Inputs Alter Organic Carbon Mineralization and Microbial Community in Forest Deep Soil Layers. Soil Biol. Biochem. 72, 145–151. doi: 10.1016/j.soilbio.2014.01.020
Wang G., Yang W., Wang G., Liu J., Hang Z. (2013). The Effects of Spartina alternifora Seaward Invasion on Soil Organic Carbon Fractions, Sources and Distribution. Acta Ecol. Sin. 33 (8), 2474–2483. doi: 10.5846/stxb201201100056
Wang D., Zhang R., Xiong J., Guo H., Zhan B. (2015). Contribution of Invasive Species Spartina alterniflora to Soil Organic Carbon Pool in Coastal Wetland: Stable Isotope Approach. Chin. J. Plant Ecol. 39 (10), 941–949.
Wang C., Pei X., Yue S., Wen Y. (2016). The Response of Spartina alterniflora Biomass to Soil Factors in Yancheng, Jiangsu Province, PR China. Wetlands 36, 229–235. doi: 10.1007/s13157-016-0732-0
Xia P., Meng X., Yin P., Cao Z., Wang X. (2011). Eighty-Year Sedimentary Record of Heavy Metal Inputs in the Intertidal Sediments From the Nanliu River Estuary, Beibu Gulf of South China Sea. Environ. Pollut. 159 (1), 92–99. doi: 10.1016/j.envpol.2010.09.014
Xia S., Wang W., Song Z., Kuzyakov Y., Guo L., Van Zwieten L., et al. (2021). Spartina alterniflora Invasion Controls Organic Carbon Stocks in Coastal Marsh and Mangrove Soils Across Tropics and Subtropics. Global Change Biol. 27 (8), 1627–1644. doi: 10.1111/gcb.15516
Xu X., Zhang Q., Tan Z., Li Y., Wang X. (2015). Effects of Water-Table Depth and Soil Moisture on Plant Biomass, Diversity, and Distribution at a Seasonally Flooded Wetland of Poyang Lake, China. Chin. Geogr. Sci. 25 (6), 739–756. doi: 10.1007/s11769-015-0774-x
Xu D., Wang R., Wang W., Ge Q., Zhang W., Chen L., Chu F. (2019). Tracing the Source of Pb Using Stable Pb Isotope Ratios in Sediments of Eastern Beibu Gulf, South China Sea. Mar. Pollut. Bull. 141, 127–136. doi: 10.1016/j.marpolbul.2019.02.028
Yang R. (2019). Mechanisms of Soil Organic Carbon Storage Response to Spartina alterniflora Invasion and Climate Change. Sci. Total. Environ. 690, 7–15. doi: 10.1016/j.scitotenv.2019.06.472
Yang W., An S., Zhao H., Xu L., Qiao Y., Cheng X. (2016). Impacts of Spartina alterniflora Invasion on Soil Organic Carbon and Nitrogen Pools Sizes, Stability, and Turnover in a Coastal Salt Marsh of Eastern China. Ecol. Eng. 86, 174–182. doi: 10.1016/j.ecoleng.2015.11.010
Yang R., Guo W. (2018). Exotic Spartina alterniflora Enhances the Soil Functions of a Coastal Ecosystem. Soil Sci. Soc. Am. J. 82 (4), 901–909. doi: 10.2136/sssaj2017.12.0411
Yang W., Zhao H., Chen X., Yin S., Cheng X., An S. (2013). Consequences of Short-Term C4 Plant Spartina alterniflora Invasions for Soil Organic Carbon Dynamics in a Coastal Wetland of Eastern China. Ecol. Eng. 61, 50–57. doi: 10.1016/j.ecoleng.2013.09.056
Yang W., Zhao H., Leng X., Cheng X., An S. (2017). Soil Organic Carbon and Nitrogen Dynamics Following Spartina alterniflora Invasion in a Coastal Wetland of Eastern China. Catena 156, 281–289. doi: 10.1016/j.catena.2017.03.021
Zhang Y., Wang Y., Li R., Zhu H., Zhang F., Huang B., Peng X. (2012). Effects of Spartina Alterniflora Invasion on Soil Active Organic Carbon Components in Tidal Flat Doil. Chinese J. of Soil Sci. 43 (1), 102–106. doi: 10.19336/j.cnki.trtb.2012.01.020
Zhang G., Bai J., Jia J., Zhuang T., Wang X., Wang W., et al. (2018). Impact of Spartina alterniflora Invasion on Spatial Distribution of Dissolved Organic Carbon in Salt Marsh Soils of the Yellow River Estuary, China. J. Beijing Norm. Univ. (Nat. Sci.) 54 (1), 90–97. doi: 10.16360/j.cnki.jbnuns.2018.01.012
Zhang G., Cai Y., Yan J., Ma Y., Gong W., Wang C. (2021c). Study on Carbon Sequestration Potential of Coastal Wetland Ecosystem and Suggestions for Carbon Neutrality: A Case Study of Salt Marsh in Liaohe Delta. Environ. Impact. Assess. 43 (5), 18–22. doi: 10.14068/j.ceia.2021.05.004
Zhang Y., Ding W., Luo J., Donnison A. (2010). Changes in Soil Organic Carbon Dynamics in an Eastern Chinese Coastal Wetland Following Invasion by a C4 Plant Spartina alterniflora. Soil Biol. Biochem. 42 (10), 1712–1720. doi: 10.1016/j.soilbio.2010.06.006
Zhang H., Li Y., Pang M., Xi M., Kong F. (2019). Responses of Contents and Structure of DOM to Spartina alterniflora Invasion in Yanghe Estuary Wetland of Jiaozhou Bay, China. Wetlands 39 (4), 729–741. doi: 10.1007/s13157-019-01124-3
Zhang Y., Meng X., Xia P., Zhang J., Liu D., Li Z., et al. (2021b). Spatiotemporal Variations in the Organic Carbon Accumulation Rate in Mangrove Sediments From the Yingluo Bay, China, Since 1900. Acta Oceanol. Sin. 40 (8), 65–77. doi: 10.1007/s13131-021-1864-5
Zhang X., Shi S., Pan G., Li L., Zhang X., Li Z. (2008). Changes in Eco-Chemical Properties of a Mangrove Wetland Under Spartina alterniflora Invasion From Zhangjiangkou, Fujian, China. Adv. Earth Sci. 23 (9), 974–981. doi: 1001-8166(2008)09-0974-07
Zhang L., Tong C., Marrs R., Wang T., Zhang W., Zeng C. (2014). Comparing Litter Dynamics of Phragmites Australis and Spartina alterniflora in a Sub-Tropical Chinese Estuary: Contrasts in Early and Late Decomposition. Aquat. Bot. 117, 1–11. doi: 10.1016/j.aquabot.2014.03.003
Zhang X., Zhang Z., Li Z., Li M., Wu H., Jiang M. (2021a). Impacts of Spartina Alterniflora Invasion on Soil Carbon Contents and Stability in the Yellow River Delta, China. Sci. Total. Environ. 775, 145188. doi: 10.1016/j.scitotenv.2021.145188
Keywords: Spartina alterniflora, invasion age, soil organic carbon (SOC), carbon sequestration, Northern Beibu Gulf
Citation: Huang X, Duan Y, Tao Y, Wang X, Long H, Luo C and Lai Y (2022) Effects of Spartina alterniflora Invasion on Soil Organic Carbon Storage in the Beihai Coastal Wetlands of China. Front. Mar. Sci. 9:890811. doi: 10.3389/fmars.2022.890811
Received: 06 March 2022; Accepted: 09 May 2022;
Published: 16 June 2022.
Edited by:
Shan Jiang, East China Normal University, ChinaReviewed by:
Xi Min, Qingdao University, ChinaLei Wang, Tongji University, China
Jianxiang Feng, Sun Yat-sen University, China
Copyright © 2022 Huang, Duan, Tao, Wang, Long, Luo and Lai. This is an open-access article distributed under the terms of the Creative Commons Attribution License (CC BY). The use, distribution or reproduction in other forums is permitted, provided the original author(s) and the copyright owner(s) are credited and that the original publication in this journal is cited, in accordance with accepted academic practice. No use, distribution or reproduction is permitted which does not comply with these terms.
*Correspondence: Yuhua Tao, MTUxNzgyNjUzQHFxLmNvbQ==; Xueping Wang, eHB3YW5nQGJiZ3UuZWR1LmNu