- 1Norwegian Polar Institute, Framcentre, Tromsø, Norway
- 2Department of Vertebrate Ecology and Zoology, Faculty of Biology, University of Gdańsk, Gdańsk, Poland
- 3Institute for Marine research, Framcentre, Tromsø, Norway
- 4Department of Biology, Pomona College, Claremont, CA, United States
- 5BioConsult SH, Husum, Germany
- 6Département de biologie, chimie et géographie & Centre d’études nordiques, Université du Québec à Rimouski, QC, Canada
- 7Institute of Oceanology, Polish Academy of Sciences, Sopot, Poland
- 8UNIS, The University Centre in Svalbard, Longyearbyen, Norway
Global warming, combined with an increasing influence of Atlantic Waters in the European Arctic, are causing a so-called Atlantification of the Arctic. This phenomenon is affecting the plankton biomass and communities with potential consequences for the upper trophic levels. Using long-term data (2005-2020) from a high Arctic zooplanktivorous seabird, the little auk (Alle alle), we tested the hypothesis that the Atlantification affects its diet, body condition and demography. We based our study on data collected in three fjords in West Spitsbergen, Svalbard, characterized by distinct oceanographic conditions. In all three fjords, we found a positive relationship between the inflow of Atlantic Waters and the proportion of Atlantic prey, notably of the copepod Calanus finmarchicus, in the little auk chick diet. A high proportion of Atlantic prey was negatively associated with adult body mass (though the effect size was small) and with chick survival (only in one fjord where chick survival until 21 days was available). We also found a negative and marginally significant effect of the average proportion of Atlantic prey in the chick diet on chick growth rate (data were available for one fjord only). Our results suggest that there are fitness costs for the little auk associated with the Atlantification of West Spitsbergen fjords. These costs seem especially pronounced during the late phase of the chick rearing period, when the energetic needs of the chicks are the highest. Consequently, even if little auks can partly adapt their foraging behaviour to changing environmental conditions, they are negatively affected by the ongoing changes in the Arctic marine ecosystems. These results stress the importance of long-term monitoring data in the Arctic to improve our understanding of the ongoing Atlantification and highlight the relevance of using seabirds as indicators of environmental change.
Introduction
The Arctic is warming faster than the rest of the globe (Cohen et al., 2020) and there is an increasing influence of warm and saline Atlantic waters entering the Arctic from the south (Pavlov et al., 2013; Skogseth et al., 2020). These changes strongly affect both the terrestrial and marine ecosystems (Parmesan, 2006; Poloczanska et al., 2013; Csapó et al., 2021) and are leading to shifts in biological communities with northern temperate species potentially replacing endemic Arctic species (Descamps and Strøm, 2021). This process is called “borealization”, or “Atlantification” in the marine Atlantic section of the Arctic (Ingvaldsen et al., 2021). Borealization has already been observed at different trophic levels, and in several regions of the Arctic, including the Barents Sea (see review in Ingvaldsen et al., 2021), Greenland (e.g., Møller and Nielsen, 2020), Canada (e.g., Ferguson et al., 2010; Tape et al., 2018) and the Chukchi and Beaufort Seas (e.g., Divoky et al., 2015; Gall et al., 2017).
In marine systems, the warming of the ocean is associated with changes in the distribution and/or abundance of key species. For example, the polar cod Boreogadus saida is a central species in Arctic marine food webs preyed upon by many different predators (Hop and Gjøsaeter, 2013). It has seen its distribution shrinking in the Barents Sea due to ocean warming and associated loss of habitat (Eriksen et al., 2015; Fossheim et al., 2015; Huserbråten et al., 2019), and is being gradually replaced by temperate species like the Atlantic cod Gadus morhua and capelin Mallotus villosus. Copepods from the Calanus genus are lipid-rich organisms (Hagen and Auel, 2001) that are key prey for fish, seabirds and mammals in the North Atlantic and Arctic ecosystems (Falk-Petersen et al., 2009). The proportion of the Arctic species, Calanus glacialis, has declined in the Barents Sea and West Greenland in the last decades while the proportion of the temperate species, C. finmarchicus, has increased (Aarflot et al., 2018; Møller and Nielsen, 2020; Tarling et al., 2022). Such a transition towards potentially smaller and less lipid-rich Atlantic copepods could affect the energy flow throughout the food web and have consequences on higher trophic levels (Falk-Petersen et al., 2009; Renaud et al., 2018). The ongoing borealization of Arctic food webs may also affect top- or meso- predators like seabirds (Descamps and Strøm, 2021; Stempniewicz et al., 2021b) through an effect on their foraging and thus reproductive performances (Ramírez et al., 2017) but very little is known about such consequences.
The little auk Alle alle is the most abundant seabird in the North Atlantic (Barrett et al., 2006) breeding almost exclusively at high latitudes (Descamps and Strøm, 2021; Wojczulanis-Jakubas et al., 2021). It has a strong influence on both marine and terrestrial ecosystem functioning and acts as an ecosystem engineer by consuming large amounts of zooplankton and by transporting vast amounts of nutrients from sea to land (Barrett et al., 2006; Zwolicki et al., 2016; González-Bergonzoni et al., 2017). In Svalbard during the breeding season, the little auk feeds primarily on the Arctic copepod, C. glacialis, but the Atlantic C. finmarchicus may also represent an important part of its diet (e.g., Karnovsky et al., 2003; Jakubas et al., 2011; Hovinen et al., 2014; Jakubas et al., 2016; Jakubas et al., 2020).
Along the west coast of Svalbard, the plankton communities are affected by the Atlantic water inflow (Kubiszyn et al., 2014; Gluchowska et al., 2016; Balazy et al., 2018; Hop et al., 2019). The influence of Atlantic water in this area has increased during the last decades (Muckenhuber et al., 2016; Nilsen et al., 2016; Tverberg et al., 2019; Skogseth et al., 2020; Bloshkina et al., 2021; Strzelewicz et al., 2022), which has contributed to an increase in the availability of North Atlantic prey such as C. finmarchicus (Aarflot et al., 2018; Hop et al., 2019). Little auks breeding in West Spitsbergen thus represent an ideal study system to assess the consequence of a shift from an Arctic to an Atlantic prey community on an endemic Arctic predator.
Several previous studies in western Svalbard fjords have suggested a link between the proportion of warm Atlantic Water (or more generally the sea temperature) and little auk foraging behaviour, chick diet and/or reproduction (Karnovsky et al., 2003; Jakubas et al., 2007; Kwasniewski et al., 2010; Jakubas et al., 2011; Kidawa et al., 2015). Results collectively indicate a negative effect of warm waters on little auk foraging (e.g. longer foraging trip duration, lower chick feeding frequency, lower energy value of the food delivered to the chick) and breeding performance (lower chick survival). However, all these studies were based on a limited time-scale (one to three years). Studies based on longer time-series are needed to unravel the potential consequences of the ongoing Atlantification. Such long-term data on little auk chick diet and life-history are now available from several western Svalbard fjords (Hornsund, Isfjorden and Kongsfjorden), characterized by distinct oceanographic conditions, with Kongsfjorden being most influenced by Atlantic Water, followed by Isfjorden and Hornsund (Gluchowska et al., 2016; Promińska et al., 2017).
Our study aimed primarily at testing for a relationship between the proportion of Atlantic Water in Svalbard fjords and the little auk chick diet composition and then to assess the consequences on their body condition and reproduction. More specifically, we hypothesized that an increase of the Atlantic Water inflow has detrimental effects on little auk body condition and reproduction. First, we predicted that the proportion of Atlantic prey in the little auk chick diet would be positively associated with the proportion of Atlantic Water in the fjord. Therefore the proportion would be highest on average in the fjords most influenced by the Atlantic Water (i.e. highest in Kongsfjorden, followed by Isfjorden and Hornsund) and higher in years of high Atlantic inflow. Second, we predicted that an increase in the proportion of Atlantic prey (in the chick diet) should be associated with a lower adult body condition as breeding birds may increase their foraging effort in such suboptimal foraging conditions (Kwasniewski et al., 2010; Karnovsky et al., 2011; Grémillet et al., 2012; Kidawa et al., 2015). This increased effort may lead to a sustained chick survival and/or growth rate (i.e. independent of the Atlantic water inflow), but a lower adult body condition (Harding et al., 2011). Alternatively, parents may not jeopardize their own condition by increasing their foraging effort to feed their chick when environmental conditions deteriorate and optimal food (i.e. Arctic prey) is less available (e.g., Welcker et al., 2009). In such a case, an increase in the contribution of Atlantic prey in the diet should be associated with lower chick survival and/or growth rate (Jakubas et al., 2007; Kwasniewski et al., 2010; Kidawa et al., 2015) but without a strong effect on adult body condition.
Material and Methods
Study Sites and Species
The little auk is a high Arctic colonial seabird, weighing between 130 and 190 grams and laying a single-egg clutch, generally in early June on Svalbard with hatching occurring in early/mid-July (Stempniewicz, 2001; Ramírez et al., 2017). Chicks usually fledge when they are between 3 and 4 weeks of age (Gaston and Jones, 1998). Their life history is characterized by a high adult survival (Descamps et al., 2021) and delayed age at first breeding (Del Hoyo et al., 1996; Stempniewicz, 2001). On Svalbard, the population size is uncertain but may be as high as a million breeding pairs (Anker-Nilssen et al., 2015; Keslinka et al., 2019), and may be declining in some colonies (Descamps and Strøm, 2021). The little auk is a zooplanktivorous species, primarily utilizing the Arctic copepods, with C. glacialis generally representing its main prey on Svalbard during the breeding period (Stempniewicz, 2001).
From 2005 to 2020, a sample of adult little auks have been captured annually (see details in Table 1) in three different colonies during the chick-rearing period (Ariekammen colony in Hornsund, Bjørndalen colony in Isfjorden and Feiringfjellet colony in Kongsfjorden; Figure 1). Captures started every year when the first sign of chick feeding (i.e. birds with a full gular pouch) have been observed. These birds were captured either by hand in the nest chamber (Hornsund only) and/or using passive traps (noose carpets) deployed on rocks in the colony (all three fjords) or mist-nets (Hornsund only). Such captures with noose carpets or mist-nets could not target breeding birds specifically and it is possible that a few non-breeders (or failed breeders) were also captured. All these birds were ringed, and most were measured (total head length, to the nearest 0.1 mm) and weighed (to the nearest 5 g). Adults transport the food for their chick in a sublingual gular pouch and this food can thus be easily sampled at capture for further prey identification (details below). The diet results presented in this study thus correspond to the chick diet (which is likely the same as adult diet, see Fort et al., 2010).
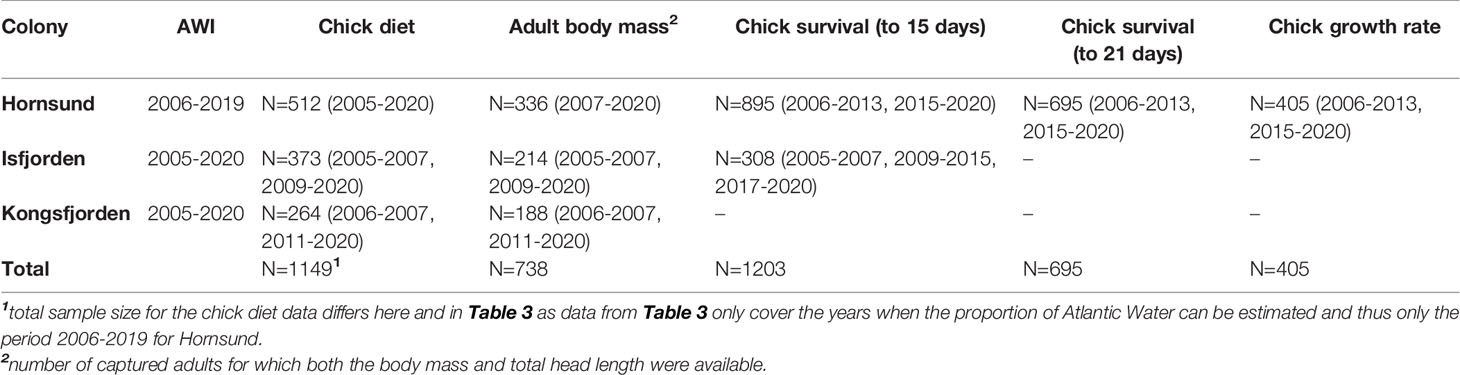
Table 1 Sample sizes of, and time periods covered by, the datasets on the Atlantic Water Index (AWI), proportion of Atlantic prey in little auk chick diet, adult body mass, chick survival and chick growth rate for three western Svalbard fjords (Hornsund, Isfjorden and Kongsfjorden).
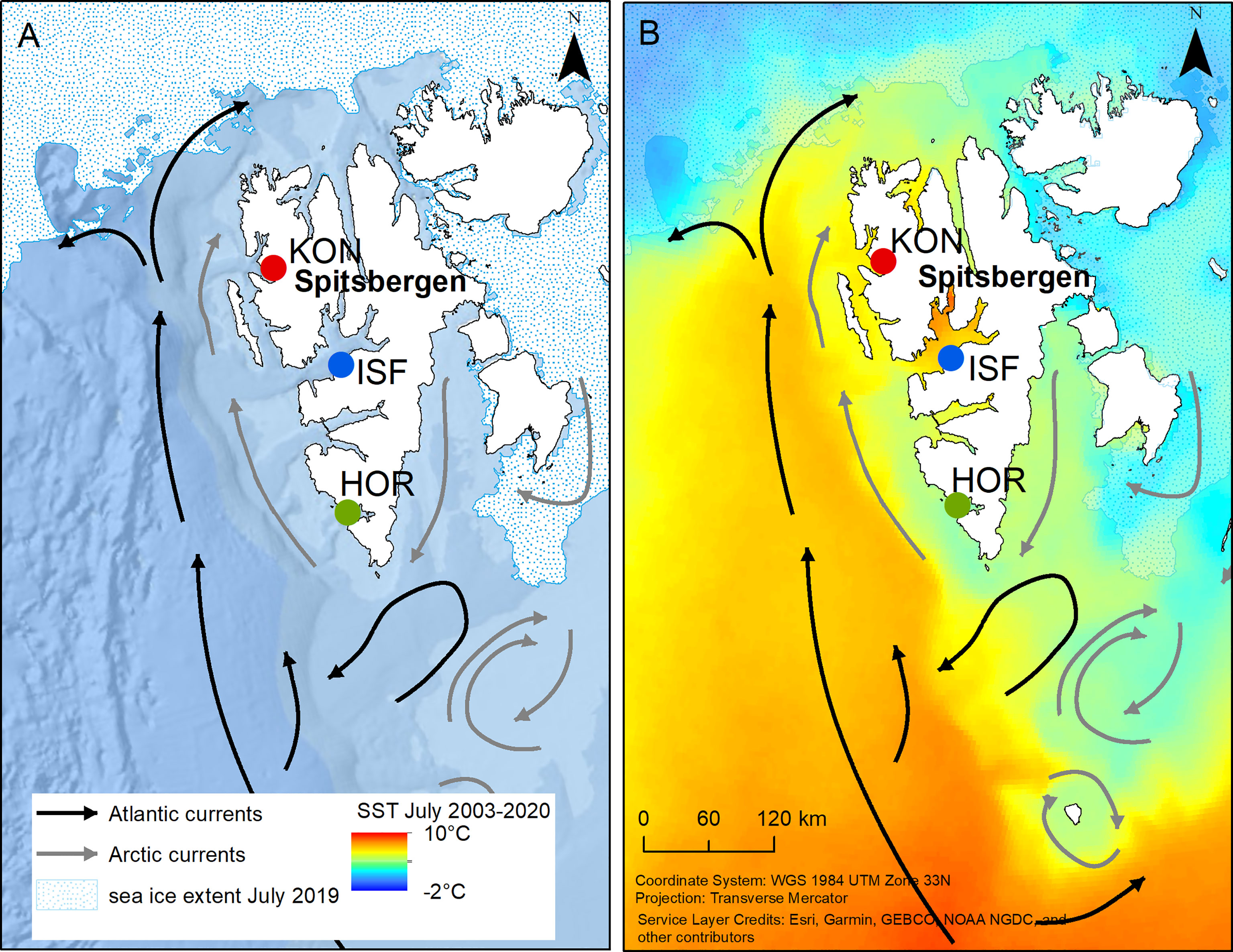
Figure 1 Study sites on Svalbard. A map showing studied little auk colonies (KON – Kongsfjorden, ISF – Isfjorden, HOR – Hornsund), bathymetry (source: Arctic Ocean Base, https://services.arcgisonline.com/arcgis/rest/services/Polar/Arctic_Ocean_Base/MapServer) and July sea ice extent [example for July 2019; source: Multisensor Analyzed Sea Ice Extent - Northern Hemisphere (MASIE-NH), Version 1 with 4 km grid cell size (National Ice Center (NIC) and NSIDC, 2010)] (A) and SST in July in 2003-2020 (source: Moderate-resolution Imaging Spectroradiometer (MODIS) Aqua satellite data from Ocean Color Data webpage, https://oceandata.sci.gsfc.nasa.gov/MODIS-Aqua) and the main Atlantic and Arctic currents in West Spitsbergen (after Loeng and Drinkwater, 2007) (B).
In Hornsund and Isfjorden, a group of nests (64 and 24 nests/year on average, with a range of [10, 155] and [9, 40], at Hornsund and Isfjorden respectively; Table 1) was monitored two to three times a week every season from approximately mid- (Isfjorden) or late- (Hornsund) incubation until the chicks were 15 (Isfjorden) or 21 (Hornsund) days old. At each visit, the status of the nest (egg, chick or empty) was recorded, and for a sample of nests at Hornsund, the chicks were weighed every 3 days.
Chick Diet Sample Analyses
Diet samples were analysed according to the procedures described in Harris et al. (2000). Organisms were enumerated and identified from subsamples until at least 300 individuals were counted. All Calanus specimens from copepodite stage (C1) to adult females (AF) were measured and identified on the basis of their prosome lengths, using size distribution tables that are established for Svalbard waters (Kwasniewski et al., 2003). Prey species were characterized as Arctic or Atlantic following the classification in Hop et al. (2019) and the total proportion of Atlantic and Arctic prey was calculated for each diet sample. A few species could not be clearly categorized as Arctic or Atlantic, but these species represented only approximately 5% of the little auk chick diet. Each diet sample was thus characterized by three proportions (i.e., % Arctic species, % Atlantic species and % species with undetermined distribution).
Atlantic Water Inflow
The Atlantic Water inflow was quantified as the percent contribution of Atlantic Water following the procedure introduced in Hop et al. (2019). Atlantic Water (AW) was defined as water with practical salinity at least 34.65 and potential temperature ≥ 3°C following the definition from Cottier et al. (2005). Temperature-salinity data collected using onboard CTDs (i.e. instruments measuring conductivity, temperature and depth) in July-September were extracted from multiple sources. The UNIS Hydrographic Database was used as the main source for data up to 2019 (described in Skogseth et al., 2020). This dataset was complemented with data from the Norwegian Polar Institute, Institute of Oceanology Polish Academy of Sciences, Joint Norwegian-Russian Ecosystem Survey for Barents Sea (Eriksen et al., 2018), the University Centre in Svalbard and UiT the Arctic University of Norway for 2019-2020. In total, these sources provided 945 (59.1 per year on average), 1156 (72.2), and 1085 (77.5) CTD casts to estimate an Atlantic Water Index (AWI) in the main basins of Kongsfjorden, Isfjorden and Hornsund respectively, without side fjords and ending at the fjord mouth. Only one CTD cast from the same position, measured using the available coordinates, per day was included in the AWI estimation. Even though little auks do not always forage inside the fjords during chick rearing, they are regularly observed there (at least in Hornsund, Stempniewicz et al., 2021a; Stempniewicz et al., 2021b) and generally do short trips to find food for their offspring and use areas located <50 km away from the colony (e.g., Welcker et al., 2009; Jakubas et al., 2020). We thus expect the within-fjord AWI to be representative of the level of “Atlantification” in the foraging areas used by breeding little auks for finding food for their chicks.
The Atlantic Water Index (AWI) was quantified as the average proportion of AW in CTD cast s between July and September as:
Annual AWI for a year y and region r was calculated as the average of all casts for the given year and fjord:
Where n is the number of stations per year (y) and fjord (r). Casts to shallower depths than 100 m were excluded to reduce the bias surface waters would cause to the AWI. Data between July and September were used because these months are oceanographically comparable in the studied fjords (Cottier et al., 2005; Skogseth et al., 2020).
Statistical Analyses
All data were analysed with R software version 4.1.1 (R Development Core Team, 2021). Except when specified otherwise, visual inspection of model residual distributions indicated no heteroscedasticity and no auto-correlation for all models considered.
For analysing the inter-fjord and inter-annual variations in the proportion of Atlantic prey in the little auk chick diet (n=1096 diet samples when considering only the years where the AWI could be estimated in all the three fjords), we used beta regression mixed models (with the glmmTMB function of package glmmTMB, Brooks et al. (2017)) with a beta distribution and a logit link function. Year was included as a random factor in all models. Since the models require the dependent variable to be in the open interval (0,1), data were transformed as where y is the dependent variable (proportion of Atlantic prey in each little auk diet sample) and n total number of diet samples available (Smithson and Verkuilen, 2006; Cribari-Neto and Zeileis, 2010). We adopted a model comparison approach based on the Akaike’s Information Criterion or AIC (Burnham and Anderson, 2002) to determine whether or not the % of Atlantic prey varied among fjords and/or varied (linearly) with the AWI. We also considered the Fjord × AWI interaction to test whether the relationships between % Atlantic prey and AWI varied among colonies. The most general model for this analysis was thus of the form: %Atlantic prey (in chick diet) ~ AWI + Fjord + AWI × Fjord +(1|Year).
Then, we tested for an effect of the proportion of Atlantic prey in the chick diet on adult body mass. We considered the mass of adults weighed between mid-July and mid-August, which corresponds to chick rearing period (n=738). We used a two-step approach: first, we considered the effect of the annual average proportions of Atlantic prey on individual body mass, and second, the effect of the proportion of Atlantic prey in individual diet samples on individual body mass. A significant effect of the proportion of Atlantic prey at both the inter-annual and inter-individual level would indicate that (i) seasons with high % Atlantic prey are associated with lower bird body mass on average, and that (ii) within a given year, individuals foraging more on Atlantic prey are characterized by a lower body mass. Such combined results would give support to a causal (i.e. mechanistic) relationship between the % Atlantic prey and bird body mass. To test these relationships, we used linear mixed models (lmer function of package lme4, Bates et al. (2015)) with a Gaussian error distribution and a random effect of the year. We also adopted a model comparison approach based on AIC (using maximum-likelihood estimation as all models had the same random structure) to assess if the bird body mass varied among fjords and/or varied (linearly) with the % Atlantic prey. We also considered the Fjord × % Atlantic prey interaction to test if the relationships between % Atlantic prey and body mass varied among colonies. The body size (i.e. total head length of little auks) was included in all models so that results could be interpreted in terms of body condition (i.e. body mass independent of body size) as these two traits are significantly correlated in little auks (Wojczulanis-Jakubas et al., 2014a; Wojczulanis-Jakubas et al., 2014b). Preliminary analyses indicated that the date of capture had no effect on little auk mass (between mid-July and mid-August) and was thus not included in our models. The most general model for this analysis was thus of the form: Adult body mass ~ Body size (total head length) + % Atlantic prey (annual mean or individual level data) + Fjord + %Atlantic prey × Fjord + (1|Year).
We then tested for an effect of the proportion of Atlantic prey on little auk chick survival (Hornsund and Isfjorden) and growth rate (Hornsund). Data on chick survival and growth rate could not be linked to the specific diet brought by their parents and we thus only tested for a relationship at the inter-annual level (i.e. tested for an effect of the annual average proportion of Atlantic prey on chick survival and growth rate). For analysing the chick survival, we considered mixed models with a binomial error distribution (the dependent variable following a Bernoulli distribution) and a logit link function using the glmer function of package lme4 (Bates et al., 2015). In Hornsund, 39% of the monitored nests and 24% in Isfjorden had chick survival data for multiple years. However, this dependency (i.e. repeated measures of chick survival for some nests) did not affect our results; results were identical whatever the method used (e.g. randomly sampling only one measure per nest or including the nest identity, nested within fjord, as a random effect). Therefore, we only present results from simple mixed models with year as a random factor). We first considered the chick survival until 15 days of age (both fjords; n=1203) and then until 21 days of age (Hornsund only, n=695). For 15 day-survival analyses, we used AIC to compare models and to assess if the chick survival was affected by the chick diet and if this relationship was the same in both fjords. The most general model for this analysis was thus of the form: Chick survival to 15 days ~ % Atlantic prey (annual mean) + Fjord + %Atlantic prey × Fjord + (1|Year). For the 21 day-survival analysis, data were only available for Hornsund and we thus considered a single model Chick survival ~ %Atlantic prey+(1|Year).
The chick growth rates were calculated as the average gain in body mass per day. We only considered the first 16 days after hatching when the growth was linear (see Figure SM-1) and only chicks weighed at least twice during this period were included (n=405). Chick growth rates were analysed with a linear mixed model and a Gaussian error distribution. We used the lmer function (from the lme4 package), and included the year as a random factor. Data were available for one fjord only and we considered a single model Chick growth rate ~ %Atlantic prey + (1|Year).
For each analysis, we assessed the proportion of variance in the data explained by the different models as the squared correlation between the observed and predicted values (Zheng and Agresti, 2000). To represent the uncertainty around the predicted relationships between the different variables considered, we calculated the 95% bootstrapped confidence intervals of the predicted values using the percentile method (i.e., using the 0.025 and 0.975 quantiles of 1000 replicates).
Results
Inter-Fjord and Inter-Annual Variations in Little Auk Chick Diet
The average proportion of Atlantic prey in the little auk chick diet was 0.17 (± 0.18 SD), 0.23 (± 0.19 SD) and 0.23 (± 0.21 SD) in Hornsund, Kongsfjorden and Isfjorden, respectively, when considering only the years where data were available for all three fjords (2006, 2007, 2011-2020). The Atlantic prey was mainly dominated by C. finmarchicus (stage CV; Table 2) while the Arctic prey was dominated by C. glacialis (stage CV; Table 2). The differences in Atlantic prey among fjords were significant (even though they explained only a small fraction of the variance in diet; see Table 3) and Atlantic prey species were less present in Hornsund little auk diets while the difference between Isfjorden and Kongsfjorden was not significant (Table 3; results from model %Atlantic prey ~ Fjord with Isfjorden as the reference level: estimate of the difference between Kongsfjorden and Isfjorden on a logit scale: 0.11 ± 0.08 SE, z=1.39, p=0.16, estimate of the difference between Hornsund and Isfjorden on a logit scale: -0.20 ± 0.07 SE, z=-2.91, p=0.004).
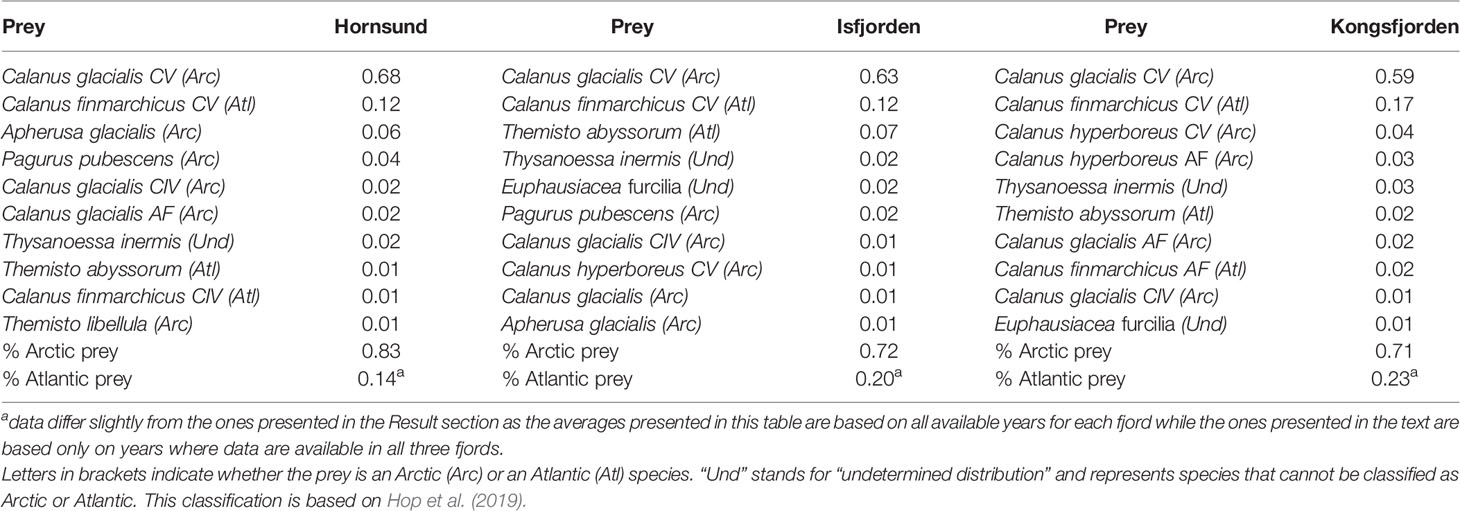
Table 2 Proportion of the ten main prey items in the little auk chick diet in three western Svalbard fjords (based on data from 2005-2020 for Hornsund and Isfjorden and from 2006-2020 for Kongsfjorden).
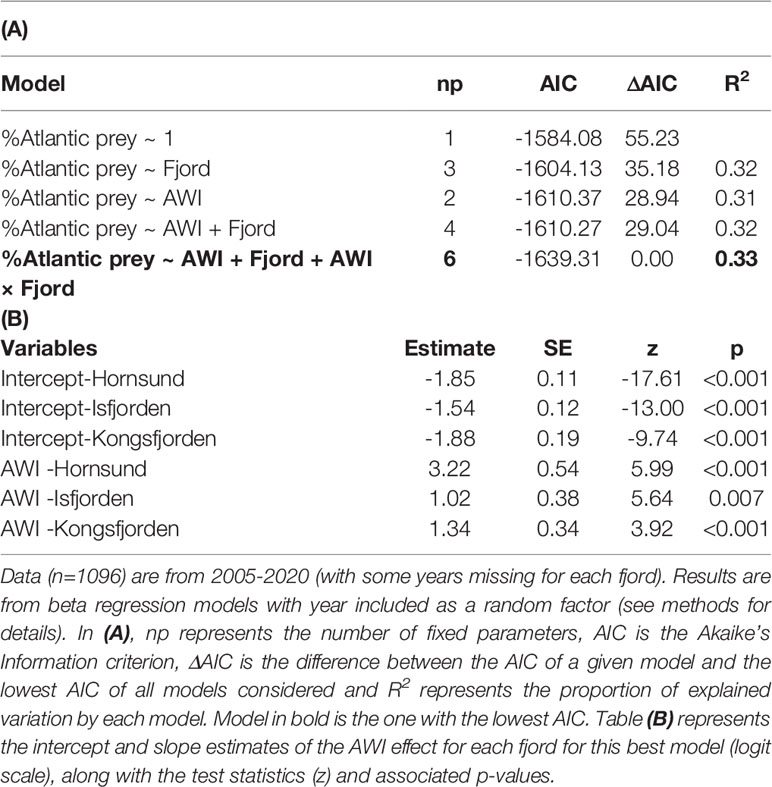
Table 3 Effect of the Atlantic Water Index (AWI) in three western Svalbard fjords (Hornsund, Isfjorden and Kongsfjorden) on the proportion of Atlantic prey in the little auk chick diet.
The proportion of Atlantic prey species showed large inter-annual fluctuations and these fluctuations were highly synchronous between Isfjorden and Kongsfjorden (Pearson’s r=0.93, p<0.001), but not between Hornsund and Isfjorden or between Hornsund and Kongsfjorden (Pearson’s r<0.4 and p>0.2 in both cases; Figure 2). Even though inter-annual changes in the proportion of Atlantic prey were not synchronized between Hornsund and the other fjords, some years were characterized by very high (e.g. 2014) or very low (e.g. 2018) proportions of Atlantic prey in all three fjords (Figure 2). The proportion of Atlantic species in the little auk chick diet has increased in all three fjords during the study period with no obvious difference in trend among fjords (Figure SM-2; Table SM-1).
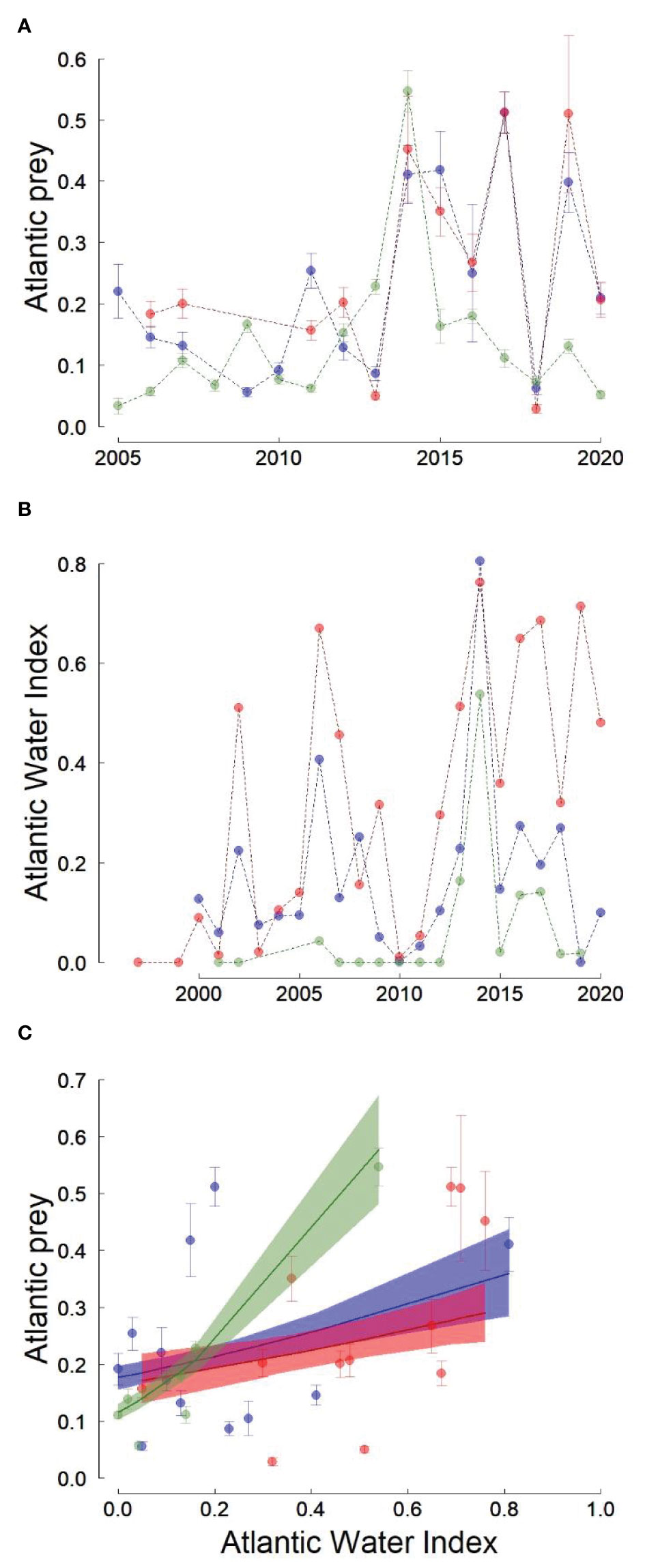
Figure 2 Proportion of Atlantic prey in little auk chick diet (A) and Atlantic water Index (B) in three western Svalbard fjords (green: Hornsund, blue: Isfjorden, red: Kongsfjorden). (C) represents the relationships between the % Atlantic prey and the AWI and the lines are the predicted relations from beta regressions. The shaded areas represent bootstrapped 95% confidence intervals of the predicted values. Error bars represent the standard errors of the average proportions of Atlantic prey.
The inter-annual changes in the proportion of Atlantic prey in the little auk chick diet were positively associated with the AWI (Table 3). This relationship varied among fjords and the slope of the AWI effect was steepest in Hornsund (Table 3; Figure 2). These relationships were independent of the long-term trends in AWI and in the % Atlantic prey (i.e. they remained the same, whether or not we kept a Trend × Fjord interaction in the models; Table SM-2; FigureSM-2).
Proportion of Atlantic Prey and Little Auk Body Mass
Body mass of adult little auks (controlled for body size) was similar among fjords but showed inter-annual variations that were negatively associated with the inter-annual fluctuations in the average proportion of Atlantic prey (slope of the effect: -6.29± 2.71 SE; Table 4; Figure 3A). This relationship was also significant when an individual body mass was linked to the proportion of Atlantic prey in its own chick diet (slope of the effect: -5.61 ± 1.85 SE; Table 5 and Figure 3B). However, even though the effect of the proportion of Atlantic prey was significant, it explained only a negligible proportion of the inter-annual variations in little auk adult body mass (Tables 4, 5) and had a small effect size (i.e., an increase of 50% in the proportion of Atlantic prey only led to a decline in body mass of approx. 3 grams meaning ~2% of little auk body mass; Figure 3). The effect of the proportion of Atlantic prey was similar in all three fjords (i.e., the best models did not include a %Atlantic prey × Fjord interaction; Tables 4, 5) but appeared weaker in Hornsund (Tables 4, 5; Figure 3). The relationship between the proportion of Atlantic prey and little auk body mass was not confounded by any potential linear trend common in both variables (i.e. the effect of the proportion of Atlantic prey remained significant even if we included a Trend × Fjord interaction in the model; see Table SM-3).
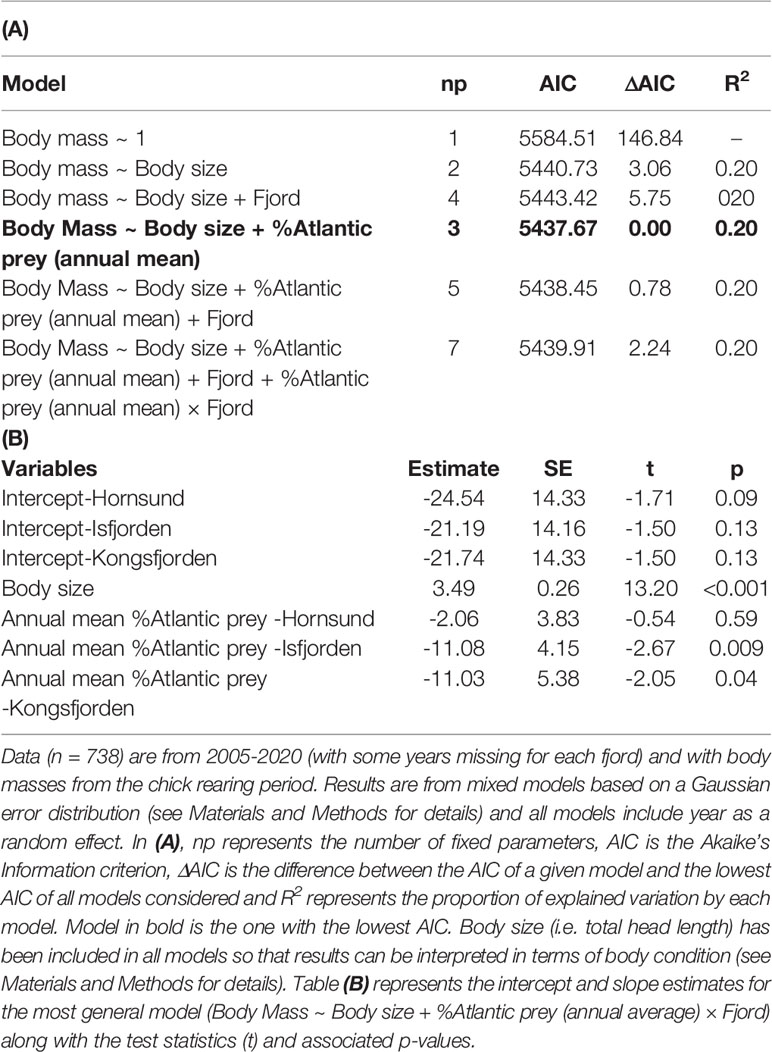
Table 4 Effect of the mean annual proportion of Atlantic prey on body mass of adult little auks breeding in three western Svalbard fjords (Hornsund, Isfjorden and Kongsfjorden).
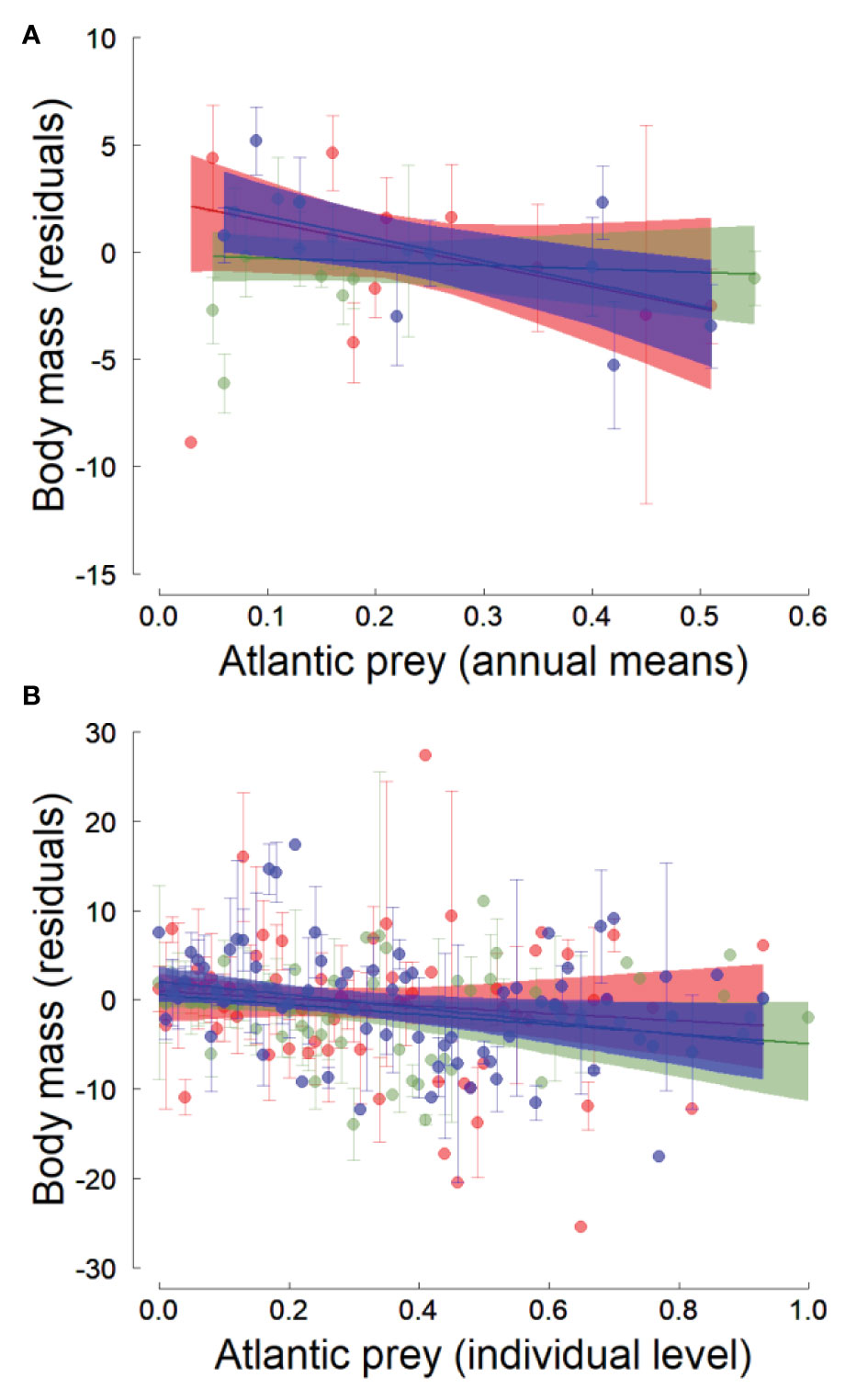
Figure 3 Effect of the proportion of Atlantic prey in little auk chick diet on adult residual body mass in three western Svalbard fjords (green: Hornsund, blue: Isfjorden, red: Kongsfjorden). The residual body mass is the body mass adjusted for body size (i.e. residuals from a linear regression), which can be interpreted in terms of body condition. In (A), the relation is between the average annual proportions of Atlantic prey and the little auk body mass, while in (B) the relation is at the individual level and represents the link between the individual proportion of Atlantic prey and the bird body mass. The lines are the regression lines from linear regressions and the shaded areas represent the bootstrapped 95% confidence intervals of the predicted values. Error bars represent the standard errors of the average body masses.
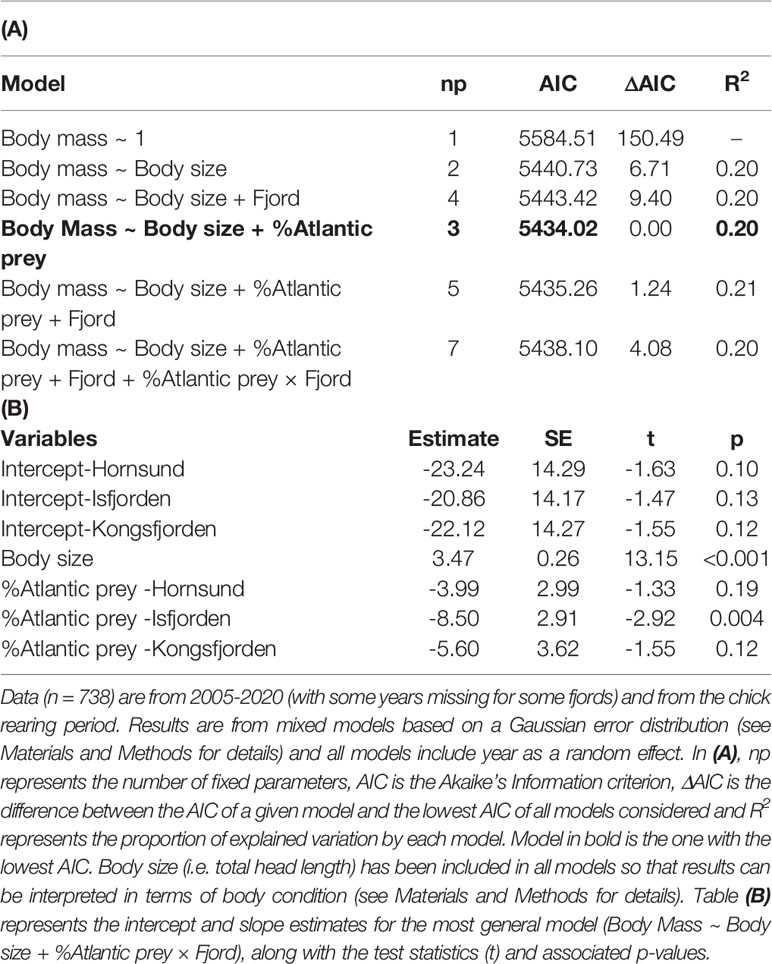
Table 5 Effect of the individual proportion of Atlantic prey in the little auk (chick) diet on body mass of adult little auk breeding in three western Svalbard fjords (Hornsund, Isfjorden and Kongsfjorden).
Proportion of Atlantic Prey, Little Auk Chick Survival and Chick Growth Rate
The chick survival rate (until 15 days of age) averaged 0.92 ± 0.07 SD in Hornsund (2006-2020) and 0.84 ± 0.10 SD in Isfjorden (2005-2020) and the difference was significant (Table 6). Overall, there was no clear effect of the proportion of Atlantic prey in the diet on chick survival in either of the fjords, but this effect was much stronger (and marginally significant) in Hornsund than in Isfjorden (Table 6; Figure 4A).
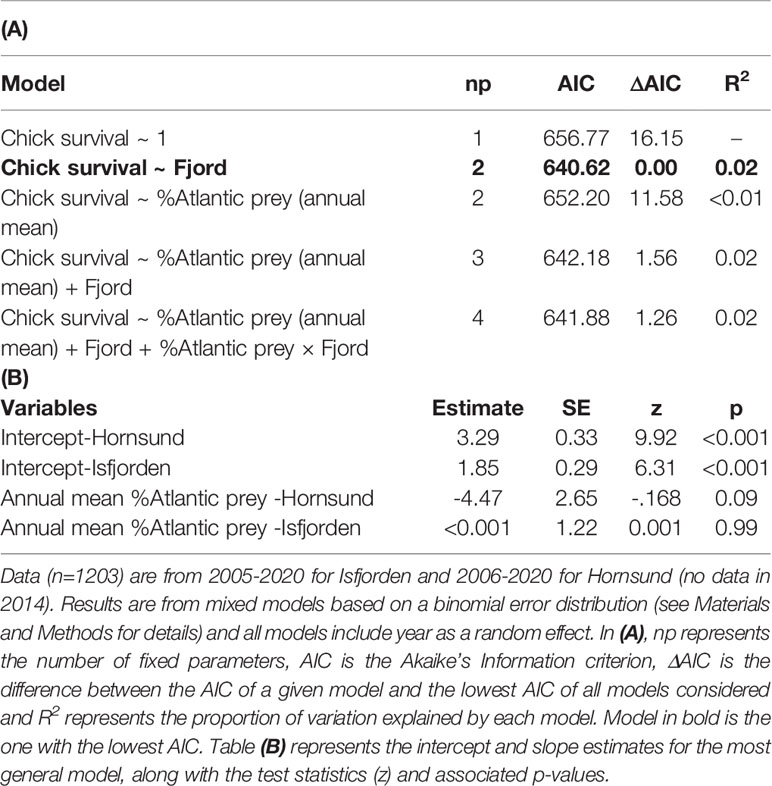
Table 6 Effect of the individual proportion of Atlantic prey in the little auk (chick) diet on chick survival (to 15 days) in two western Svalbard fjords (Hornsund and Isfjorden).
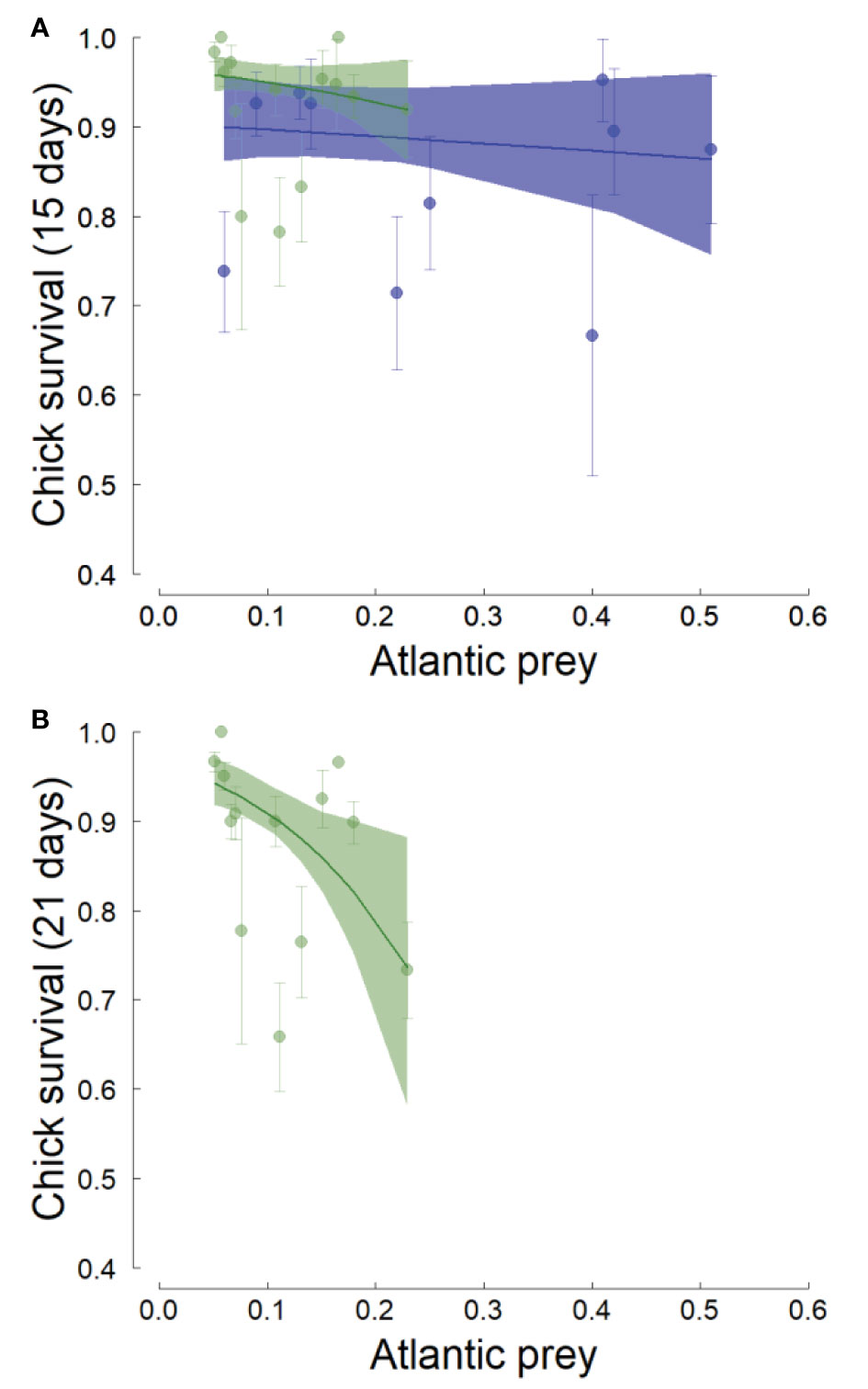
Figure 4 Effect of the average annual proportion of Atlantic prey in the little auk chick diet on chick survival in two western Svalbard fjords (green: Hornsund, blue: Isfjorden). The chick survival was defined as a binary variable describing whether or not the chick has survived until 15 (A) or 21 (B) days of age (data on chick survival until 21 days were not available in Isfjorden). The lines are the regression lines from linear models and the shaded areas represent the bootstrapped 95% confidence intervals of the predicted values. Error bars represent the standard errors of the average chick survivals.
When considering the chick survival until 21 days (Hornsund only), the average chick survival dropped to 0.81 ± 0.25 SD and the effect of the proportion of Atlantic prey became highly significant even though it explained a small amount of variation in chick survival (z=-3.51, p=0.04, R2 = 0.04, slope ± SE = -9.92 ± 4.95; Figure 4B).
During the first 16 days of life (linear phase of growth), chicks gained an average of 4.6 grams per day at Hornsund in 2006-2020. This growth rate was negatively affected by the average proportion of Atlantic prey in the chick diet; the effect was only marginally significant (n=405, t=-1.89, p=0.08, R2 = 0.25, slope ± SE = -11.53 ± 6.09; Figure 5).
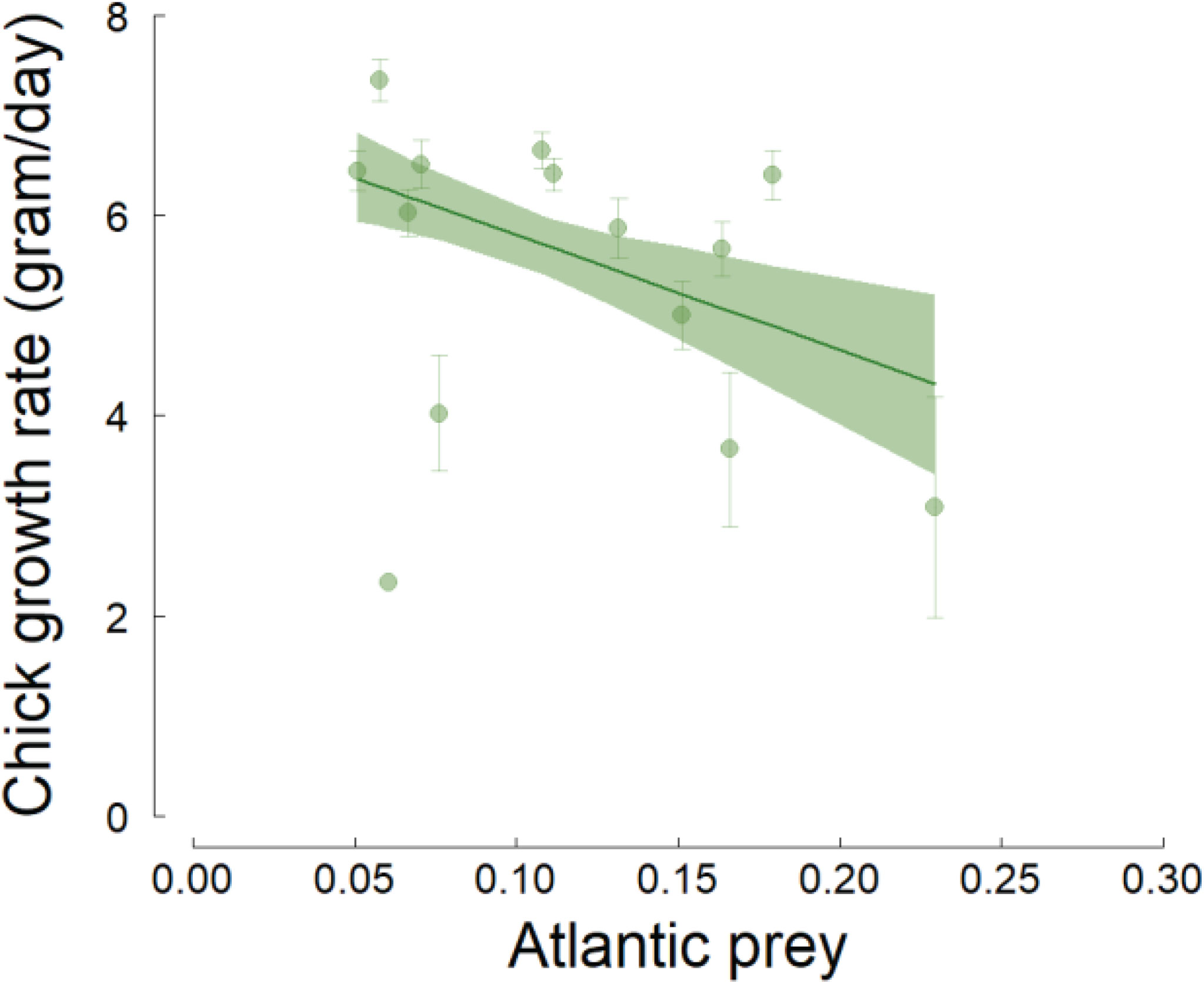
Figure 5 Effect of the average annual proportion of Atlantic prey in the little auk chick diet on chick growth rate in Hornsund. The chick growth rate represents the number of grams per day gained by the chicks during their first 16 days of life (i.e. when the growth is linear). The lines are the regression lines from linear models and the shaded areas represent the bootstrapped 95% confidence intervals of the predicted values. Error bars represent the standard errors of the average chick growth rates.
The effects of the proportion of Atlantic prey on chick survival or growth rate at Hornsund were not confounded by any potential linear trend common in both variables (i.e. the effect of the proportion of Atlantic prey remained the same even if we include a Trend covariate in the model; see Table SM-4).
Discussion
Our study adds to the growing body of evidence that the ongoing Atlantification impacts the Arctic environment and in particular the marine ecosystem in West Svalbard (e.g., Gluchowska et al., 2016; Vihtakari et al., 2018; Griffith et al., 2019; Hop et al., 2019). Using long-term data from three Svalbard fjords differing in environmental conditions, we show a positive relationship between the Atlantic Water Index, a proxy of Atlantic Water inflow, in all fjords and the amount of Atlantic prey in the little auk chick diet. These Atlantic prey items were generally more abundant in the more Atlantic Kongsfjorden and Isfjorden fjords as compared to the more Arctic Hornsund. While Arctic prey items were dominant in most years, little auk chick diet contained more Atlantic prey, and notably more C. finmarchicus, during years of high Atlantic Water inflow. The proportion of Atlantic prey had a significant negative impact on adult body mass, but the effect size was small resulting in only a few grams reduction in years with high AWI. Moreover, the proportion of Atlantic prey was negatively associated with the chick survival though only when considering the survival until 21 days of age (data available for Hornsund only). When considering the survival until 15 days of age (in Hornsund and Isfjorden), the effect was non-significant. Finally, the chick growth rate (data available for Hornsund only) was also negatively related to the proportion of Atlantic prey in the chick diet (though the effect was only marginally significant) with the growth rate decreasing from approximately 6 grams/day to less than 5 grams/day when the inflow increased from 5 to 25%. Our results thus indicate potential fitness costs associated with the Atlantification of the western Svalbard fjords. These costs seemed especially pronounced during the later part of the chick-rearing period (between 15 and 21 days of age) when the energetic needs of the chicks are at their highest (Konarzewski et al., 1993). It remains unknown, however, whether these costs were similar in all fjords. Even though our results indicate comparable relationship between the Atlantic Water inflow, the proportion of Atlantic prey in the chick diet and the decrease in adult body condition in all three fjords, this does not imply that similar effects on chick growth and survival, and thus similar fitness costs, should be expected. Additional data on chick growth and/or on chick survival in the later part of the chick rearing period would be needed in the other fjords to assess whether or not the Atlantification bears the same costs for all little auk colonies.
Our study identified negative relationships between the average proportion of Atlantic prey in chick diet in a given year and chick growth and survival. Testing for such relationships with diet data at the individual level (i.e. testing for a relationship between the diet of a given chick and its body condition or survival probability) could be potentially useful to confirm the potential mechanism linking the Atlantic Water inflow, the chick diet and their survival probability or growth rate. Such data would require sampling the food brought back to the chick by adults breeding in known nests and where chicks would be accessible.
Suboptimal Atlantic Prey
Our results confirm previous observations made in Svalbard fjords that were generally based on the comparison of two types of years (“warm” versus “cold”), or two types of fjords (one strongly influenced by the Atlantic Water inflow and one not). These earlier observations have shown that the proportion of C. glacialis in the chick diet is higher and the chick meals have higher energy content in Hornsund compared to Kongsfjorden (Karnovsky et al., 2011) and Magdalenefjorden, a fjord north of Kongsfjorden, largely influenced by Atlantic Water (Kwasniewski et al., 2010; Kidawa et al., 2015). Furthermore, the food loads delivered to the chicks are generally less energy-rich in Hornsund in warmer seasons (Jakubas et al., 2007; Kidawa et al., 2015; but see Wojczulanis-Jakubas et al., 2010) which in turn may be associated with a lower chick survival (Kidawa et al., 2015). All these results indicate that the little auk chick diet is more energy-rich when C. glacialis is more abundant (Kwasniewski et al., 2012). Chick diet composition of little auks from the main breeding colonies in Greenland and Svalbard shows that Atlantic prey, and more specifically C. finmarchicus, serve as a sub-optimal prey for little auks (Karnovsky et al., 2003; Frandsen et al., 2014) requiring a higher foraging effort from the parents (Karnovsky et al., 2010; Karnovsky et al., 2011). Our results support these findings and indicate that the adult little auks were in poorer condition when the Atlantic Water inflow was high, and that this may be a consequence of a higher foraging effort. Searching for the preferred prey, C. glacialis, among abundant but less favoured C. finmarchicus, may indeed require more time and energy (Jakubas et al., 2011; Karnovsky et al., 2011). Alternatively, the apparent negative effect of the Atlantic inflow on little auk body mass could be driven, at least partly, by changes in the proportion of non-breeders (including failed breeders) in our samples of captured birds. This would, however, assume that these birds have a lower body mass that breeders and that the proportion of non-breeders captured increased with a higher Atlantic inflow. We have no evidence for this and additional data on non-breeder body mass and on the probability to breed as a function of environmental conditions would be needed.
Adult Little Auks Prioritize Self-Maintenance
The potential increase in the little auk foraging effort when Atlantic waters, and thus Atlantic prey, are more prevalent is supported by the negative association between adult body mass and the proportion of Atlantic prey in the chick diet. However, even though this relationship was significant, the effect size was small and a large increase in the proportion of Atlantic prey in the chick diet only slightly decreased adult body mass. This indicates that, when foraging conditions deteriorate, even if there is a slight cost in terms of lower body condition, breeding little auks may prioritize their self-maintenance, and thus own condition, which could be at the cost of a lower chick growth and survival. This would represent the expected resource allocation strategy for such a long-lived species (Stearns, 1992) and fits with previous observations (Welcker et al., 2009; Kidawa et al., 2015). Therefore, little auks may increase their foraging effort to adjust to deteriorating environmental conditions (i.e. when the inflow of Atlantic Waters increases) but they would do it only until a certain extent that is unlikely to jeopardize their own survival. The fitness costs of the Atlantification of Svalbard may thus be mostly paid by the chicks. Our results from Hornsund fit such interpretation but additional data would be needed to confirm such potential costs, and to better understand the shapes of such relationships between Atlantic inflow, foraging behaviour and reproductive investment, that are likely complex.
Conclusions
The Atlantification is not only influencing Svalbard, but similar changes are occurring in the other parts of the Arctic (Ferguson et al., 2010; Møller and Nielsen, 2020; Polyakov et al., 2020; Ingvaldsen et al., 2021). Even though inter-annual variations have been very large, there has been a positive trend in the inflow of Atlantic Water into the Arctic in the last decades (Ingvaldsen et al., 2021). The little auk, an endemic Arctic species, is negatively affected by such Atlantification and even if they can be plastic and to some extent adapt their behaviour to new environmental conditions (e.g., Grémillet et al., 2012; Gremillet et al., 2015; Jakubas et al., 2017), they may still pay important fitness costs when the marine environment and associated prey become more “boreal” (i.e. north temperate). This may ultimately lead to population decline and local extinctions. The southernmost little auk population breeding in Iceland was extirpated at the end of the 20th century, possibly as a consequence of both ocean warming (that has affected the abundance and distribution of little auk prey) and human harvest (Jakubas et al., 2022). However, our work also showed that a large part of the inter-annual variations in little auk chick diet, body mass or chick growth/survival could not be accounted for by our Atlantic Water inflow index. Two explanations can be proposed to explain this low explanatory power.
First, this index was defined in our study as the proportion of Atlantic waters inside the fjords. However, little auk may also use areas outside the fjords to forage where oceanographic conditions and the influence of Atlantic water masses may be different. Creating an AWI index based on the actual foraging areas of the little auks for each specific colony would require identifying these foraging areas every year (through GPS-tracking for example). Although possible, this would be a very challenging study.
Second, the Atlantic Water inflow is only one among many factors that have the potential to affect little auk foraging and life-history. Other environmental parameters like the sea-ice (Amélineau et al., 2016; Ramírez et al., 2017; Amélineau et al., 2019; Jakubas et al., 2020), or the weather conditions (Konarzewski and Taylor, 1989) also affect their foraging behaviour or chick growth rate. All these parameters may interact with each other and also have non-linear and complex effects. Disentangling their respective role should be a priority in future research, and this can only be achieved through the use of long-term data. Such data are essential to improve our understanding of the ongoing Atlantification, or borealization, and its consequences on Arctic endemic species like the little auk.
Data Availability Statement
The raw data supporting the conclusions of this article will be made available by the authors, without undue reservation.
Ethics Statement
The animal study was reviewed and approved by Mattilsynet (Norwegian Food Safety Anthority, in charge of animal welfare).
Author Contributions
SD: conception, statistical analyses and draft the first version of the manuscript. SD, KW-J, DJ, HS, JW, KB-S, and PB: design of the study. MV: construction of the AWI index and contributed in data collection. KB-S and RB: diet data analyses. SD, KW-J, DJ, HS, JW, JH, AS, RS, NK, and DK: data collection. All authors revised the manuscript critically and had substantial impact on the final draft of the manuscript.
Funding
This study was conducted in the frame of the project FACE-IT (The Future of Arctic Coastal Ecosystems – Identifying Transitions in Fjord Systems and Adjacent Coastal Areas). FACE-IT has received funding from the European Union’s Horizon 2020 research and innovation programme under grant agreement No 869154. This research was also partly funded by the program SEAPOP (http://www.seapop.no/en/) through the Norwegian Research Council grant number 192141, the program MOSJ (http://www.mosj.no/en/), the Svalbard Environmental Fund (project 18/42) and a grant from Norway through the Norwegian Financial Mechanism (ALKEKONGE, PNRF-234-AI-1/07). This study was also supported in part by U.S. National Science Foundation Grants #0612504 and #0301469 to NK. CTD data from Hornsund were collected under a long - term monitoring program as a contribution to IO PAN statutory research areas (I.4). Additionally, this study received also support from the Polish Ministry of Science and Higher Education for co-funding project SEAPOP II (3605/SEAPOP/2016/2) for years 2016–2022.
Conflict of Interest
The reviewer MC declared a past co-authorship with one of the authors SD to the handling Editor. Author JW was employed by the company BioConsult SH.
The remaining authors declare that the research was conducted in the absence of any commercial or financial relationship that could be construed as a potential conflict of interest.
Publisher’s Note
All claims expressed in this article are solely those of the authors and do not necessarily represent those of their affiliated organizations, or those of the publisher, the editors and the reviewers. Any product that may be evaluated in this article, or claim that may be made by its manufacturer, is not guaranteed or endorsed by the publisher.
Acknowledgments
We thank all the field workers that helped in collecting the data and in particular Benjamin Merkel, Delphin Ruché, Saga Svavarsdóttir, Iñigo López Sarasa, Antonio Vilches, Charlotte Lassen, Eirik Grønningsæter, Lars Korslund, Anna Sandven and Ingrid Wathne for Kongsfjorden and Isfjorden fieldwork, and Mateusz Barcikowski, Liliana Shönberger (Keslinka), Lech Stempniewicz, Jan Samołyk, Madaleine Stigner, Antoine Grissot, Marion Devogel, Martyna Cendrowska, Adam Nawrot, Monika Kulpińska-Chamera, Izabela Kulaszewicz, Allison Bailey, Laurel McFadden, Nell Baldwin, Zachary Brown, Derek Buchner, Derek Young and Julia Gleichman for Hornsund fieldwork. We also thank the two referees for useful comments that helped improving our study.
Supplementary Material
The Supplementary Material for this article can be found online at: https://www.frontiersin.org/articles/10.3389/fmars.2022.878746/full#supplementary-material
References
Aarflot J. M., Skjoldal H. R., Dalpadado P., Skern-Mauritzen M. (2018). Contribution of Calanus Species to the Mesozooplankton Biomass in the Barents Sea. ICES J. Mar. Sci. 75, 2342–2354. doi: 10.1093/icesjms/fsx221
Amélineau F., Gremillet D., Bonnet D., Le Bot T., Fort J. (2016). Where to Forage in the Absence of Sea Ice? Bathymetry as a Key Factor for an Arctic Seabird. PloS One 11, e0157764. doi: 10.1371/journal.pone.0157764
Amélineau F., Grémillet D., Harding A. M., Walkusz W., Choquet R., Fort J. (2019). Arctic Climate Change and Pollution Impact Little Auk Foraging and Fitness Across a Decade. Sci. Rep. 9, 1014. doi: 10.1038/s41598-018-38042-z
Anker-Nilssen T., Barrett R., Lorentsen S., Strøm H., Bustnes J., Christensen-Dalsgaard S., et al. (2015). SEAPOP. De Ti Første Årene. Nøkkeldokument 2005-2014 (Trondheim, Tromsø: SEAPOP, Norsk Institutt for Naturforskning, Norsk Polarinstitutt & Tromsø Museum–Universitetsmuseet).
Balazy K., Trudnowska E., Wichorowski M., Błachowiak-Samołyk K. (2018). Large Versus Small Zooplankton in Relation to Temperature in the Arctic Shelf Region. Pol. Res. 37, 1427409. doi: 10.1080/17518369.2018.1427409
Barrett R. T., Chapdelaine G., Anker-Nilssen T., Mosbech A., Montevecchi W. A., Reid J. B., et al. (2006). Seabird Numbers and Prey Consumption in the North Atlantic. ICES J. Mar. Sci. 63, 1145–1158. doi: 10.1016/j.icesjms.2006.04.004
Bates D., Mächler M., Bolker B., Walker S. (2015). Fitting Linear Mixed-Effects Models Using Lme4. J. Stat. Softw. 67, 1–48. doi: 10.18637/jss.v067.i01
Bloshkina E. V., Pavlov A. K., Filchuk K. (2021). Warming of Atlantic Water in Three West Spitsbergen Fjords: Recent Patterns and Century-Long Trends. Pol. Res. 40, 5392. doi: 10.33265/polar.v40.5392
Brooks M. E., Kristensen K., Van Benthem K. J., Magnusson A., Berg C. W., Nielsen A., et al. (2017). glmmTMB Balances Speed and Flexibility Among Packages for Zero-Inflated Generalized Linear Mixed Modeling. R. J. 9, 378–400. doi: 10.32614/RJ-2017-066
Burnham K. P., Anderson D. R. (2002). Model Selection and Multimodel Inference: A Practical Information-Theoretic Approach (New York: Springer-Verlag).
Cohen J., Zhang X., Francis J., Jung T., Kwok R., Overland J., et al. (2020). Divergent Consensuses on Arctic Amplification Influence on Midlatitude Severe Winter Weather. Nat. Climate Change 10, 20–29. doi: 10.1038/s41558-019-0662-y
Cottier F., Tverberg V., Inall M., Svendsen H., Nilsen F., Griffiths C. (2005). Water Mass Modification in an Arctic Fjord Through Cross-Shelf Exchange: The Seasonal Hydrography of Kongsfjorden, Svalbard. J. Geophys. Res.: Ocean. 110, C12005. doi: 10.1029/2004JC002757
Cribari-Neto F., Zeileis A. (2010). Beta Regression in R. J. Stat. Softw. 34, 1–24. doi: 10.18637/jss.v034.i02
Csapó H. K., Grabowski M., Węsławski J. M. (2021). Coming Home-Boreal Ecosystem Claims Atlantic Sector of the Arctic. Sci. Total. Environ. 144817. doi: 10.1016/j.scitotenv.2020.144817
Del Hoyo J., Elliott A., Sargatal J. (1996). Handbook of the Birds of the World, Vol. 3: Hoatzin to Auks (Barcelona, Spain: Lynx Edicions).
Descamps S., Merkel B., Strøm H., Choquet R., Steen H., Fort J., et al. (2021). Sharing Wintering Grounds Does Not Synchronize Annual Survival in a High Arctic Seabird, the Little Auk. Mar. Ecol. Prog. Ser. 676, 233–242. doi: 10.3354/meps13400
Descamps S., Strøm H. (2021). As the Arctic Becomes Boreal: Ongoing Shifts in a High-Arctic Seabird Community. Ecology 102, e03485. doi: 10.1002/ecy.3485
Development Core Team R. (2021). "R: A Language and Environment for Statistical Computing" (Vienna, Austria: R Foundation for Statistical Computing). Available at: http://www.R-project.org/.
Divoky G. J., Lukacs P. M., Druckenmiller M. L. (2015). Effects of Recent Decreases in Arctic Sea Ice on an Ice-Associated Marine Bird. Prog. Oceanogr. 136, 151–161. doi: 10.1016/j.pocean.2015.05.010
Eriksen E., Gjøsæter H., Prozorkevich D., Shamray E., Dolgov A., Skern-Mauritzen M., et al. (2018). From Single Species Surveys Towards Monitoring of the Barents Sea Ecosystem. Prog. Oceanogr. 166, 4–14. doi: 10.1016/j.pocean.2017.09.007
Eriksen E., Ingvaldsen R., Nedreaas K., Prozorkevich D. (2015). The Effect of Recent Warming on Polar Cod and Beaked Redfish Juveniles in the Barents Sea. Region. Stud. Mar. Sci. 2, 105–112. doi: 10.1016/j.rsma.2015.09.001
Falk-Petersen S., Mayzaud P., Kattner G., Sargent J. R. (2009). Lipids and Life Strategy of Arctic Calanus. Mar. Biol. Res. 5, 18–39. doi: 10.1080/17451000802512267
Ferguson S. H., Loseto L. L., Mallory M. L. (2010). A Little Less Arctic: Top Predators in the World's Largest Northern Inland Sea, Hudson Bay (Dordrecht Heidelberg London New York: Springer).
Fort J., Cherel Y., Harding A. M., Welcker J., Jakubas D., Steen H., et al. (2010). Geographic and Seasonal Variability in the Isotopic Niche of Little Auks. Mar. Ecol. Prog. Ser. 414, 293–302. doi: 10.3354/meps08721
Fossheim M., Primicerio R., Johannesen E., Ingvaldsen R. B., Aschan M. M., Dolgov A. V. (2015). Recent Warming Leads to a Rapid Borealization of Fish Communities in the Arctic. Nat. Climate Change 5, 673–677. doi: 10.1038/nclimate2647
Frandsen M., Fort J., Rigét F., Galatius A., Mosbech A. (2014). Composition of Chick Meals From One of the Main Little Auk (Alle alle) Breeding Colonies in Northwest Greenland. Pol. Biol. 37, 1055–1060. doi: 10.1007/s00300-014-1491-0
Gall A. E., Morgan T. C., Day R. H., Kuletz K. J. (2017). Ecological Shift From Piscivorous to Planktivorous Seabirds in the Chukchi Sea 1975–2012. Pol. Biol. 40, 61–78. doi: 10.1007/s00300-016-1924-z
Głuchowska M., Kwasniewski S., Prominska A., Olszewska A., Goszczko I., Falk-Petersen S., et al. (2016). Zooplankton in Svalbard Fjords on the Atlantic–Arctic Boundary. Pol. Biol. 39, 1785–1802. doi: 10.1007/s00300-016-1991-1
González-Bergonzoni I., Johansen K. L., Mosbech A., Landkildehus F., Jeppesen E., Davidson T. A. (2017). Small Birds, Big Effects: The Little Auk (Alle Alle) Transforms High Arctic Ecosystems. Proc. R. Soc. B.: Biol. Sci. 284, 20162572. doi: 10.1098/rspb.2016.2572
Gremillet D., Fort J., Amelineau F., Zakharova E., Le Bot T., Sala E., et al. (2015). Arctic Warming: Nonlinear Impacts of Sea-Ice and Glacier Melt on Seabird Foraging. Global Change Biol. 21, 1116–1123. doi: 10.1111/gcb.12811
Grémillet D., Welcker J., Karnovsky N. J., Walkusz W., Hall M. E., Fort J., et al. (2012). Little Auks Buffer the Impact of Current Arctic Climate Change. Mar. Ecol. Prog. Ser. 454, 197–206. doi: 10.3354/meps09590
Griffith G. P., Hop H., Vihtakari M., Wold A., Kalhagen K., Gabrielsen G. W. (2019). Ecological Resilience of Arctic Marine Food Webs to Climate Change. Nat. Climate Change 9, 868–872. doi: 10.1038/s41558-019-0601-y
Hagen W., Auel H. (2001). Seasonal Adaptations and the Role of Lipids in Oceanic Zooplankton. Zoology 104, 313–326. doi: 10.1078/0944-2006-00037
Harding A. M., Welcker J., Steen H., Hamer K. C., Kitaysky A. S., Fort J., et al. (2011). Adverse Foraging Conditions may Impact Body Mass and Survival of a High Arctic Seabird. Oecologia 167, 49–59. doi: 10.1007/s00442-011-1971-7
Harris R., Wiebe P., Lenz J., Skjoldal H.-R., Huntley M. (2000). ICES Zooplankton Methodology Manual (Elsevier).
Hop H., Gjøsaeter H. (2013). Polar Cod (Boreogadus Saida) and Capelin (Mallotus Villosus) as Key Species in Marine Food Webs of the Arctic and the Barents Sea. Mar. Biol. Res. 9, 878–894. doi: 10.1080/17451000.2013.775458
Hop H., Wold A., Vihtakari M., Daase M., Kwasniewski S., Gluchowska M., et al. (2019). “Zooplankton in Kongsfjorden, (1996–2016) in Relation to Climate Change,” in The Ecosystem of Kongsfjorden, Svalbard (Cham: Springer), 229–300.
Hovinen J. E. H., Wojczulanis-Jakubas K., Jakubas D., Hop H., Berge J., Kidawa D., et al. (2014). Fledging Success of Little Auks in the High Arctic: Do Provisioning Rates and the Quality of Foraging Grounds Matter? Pol. Biol. 37, 665–674. doi: 10.1007/s00300-014-1466-1
Huserbråten M. B. O., Eriksen E., Gjøsæter H., Vikebø F. (2019). Polar Cod in Jeopardy Under the Retreating Arctic Sea Ice. Commun. Biol. 2, 1–8. doi: 10.1038/s42003-019-0649-2
Ingvaldsen R. B., Assmann K. M., Primicerio R., Fossheim M., Polyakov I. V., Dolgov A. V. (2021). Physical Manifestations and Ecological Implications of Arctic Atlantification. Nat. Rev. Earth Environ. 2, 874–889. doi: 10.1038/s43017-021-00228-x
Jakubas D., Gluchowska M., Wojczulanis-Jakubas K., Karnovsky N. J., Keslinka L., Kidawa D., et al. (2011). Foraging Effort Does Not Influence Body Condition and Stress Level in Little Auks. Mar. Ecol. Prog. Ser. 432, 277–290. doi: 10.3354/meps09082
Jakubas D., Iliszko L. M., Strøm H., Darecki M., Jerstad K., Stempniewicz L. (2016). Foraging Behavior of a High-Arctic Zooplanktivorous Alcid, the Little Auk, at the Southern Edge of Its Breeding Range. J. Exp. Mar. Biol. Ecol. 475, 89–99. doi: 10.1016/j.jembe.2015.11.010
Jakubas D., Wojczulanis-Jakubas K., Iliszko L. M., Kidawa D., Boehnke R., Błachowiak-Samołyk K., et al. (2020). Flexibility of Little Auks Foraging in Various Oceanographic Features in a Changing Arctic. Sci. Rep. 10, 1–18. doi: 10.1038/s41598-020-65210-x
Jakubas D., Wojczulanis-Jakubas K., Iliszko L. M., Strøm H., Stempniewicz L. (2017). Habitat Foraging Niche of a High Arctic Zooplanktivorous Seabird in a Changing Environment. Sci. Rep. 7, 16203. doi: 10.1038/s41598-017-16589-7
Jakubas D., Wojczulanis-Jakubas K., Petersen A. (2022). A Quiet Extirpation of the Breeding Little Auk Alle alle Population in Iceland in the Shadow of the Famous Cousin Extermination. Sci. Total. Environ. 808, 152167. doi: 10.1016/j.scitotenv.2021.152167
Jakubas D., Wojczulanis-Jakubas K., Walkusz W. (2007). Response of Dovekie to Changes in Food Availability. Waterbirds 30, 421–428. doi: 10.1675/1524-4695(2007)030[0421:RODTCI]2.0.CO;2
Karnovsky N. J., Brown Z. W., Welcker J., Harding A. M., Walkusz W., Cavalcanti A., et al. (2011). Inter-Colony Comparison of Diving Behavior of an Arctic Top Predator: Implications for Warming in the Greenland Sea. Mar. Ecol. Prog. Ser. 440, 229–240. doi: 10.3354/meps09351
Karnovsky N., Harding A., Walkusz W., Kwaśniewski S., Goszczko I., Wiktor J. Jr., et al. (2010). Foraging Distributions of Little Auks Alle alle Across the Greenland Sea: Implications of Present and Future Arctic Climate Change. Mar. Ecol. Prog. Ser. 415, 283–293. doi: 10.3354/meps08749
Karnovsky N. J., Kwasniewski S., Weslawski J. M., Walkusz W., Beszczynska-Moller A. (2003). Foraging Behavior of Little Auks in a Heterogeneous Environment. Mar. Ecol.-Progr. Ser. 253, 289–303. doi: 10.3354/meps253289
Keslinka L. K., Wojczulanis-Jakubas K., Jakubas D., Neubauer G. (2019). Determinants of the Little Auk (Alle alle) Breeding Colony Location and Size in W and NW Coast of Spitsbergen. PloS One 14, e0212668. doi: 10.1371/journal.pone.0212668
Kidawa D., Jakubas D., Wojczulanis-Jakubas K., Stempniewicz L., Trudnowska E., Boehnke R., et al. (2015). Parental Efforts of an Arctic Seabird, the Little Auk Alle alle, Under Variable Foraging Conditions. Mar. Biol. Res. 11, 349–360. doi: 10.1080/17451000.2014.940974
Konarzewski M., Taylor J. R. (1989). The Influence of Weather Conditions on Growth of Little Auk Alle alle Chicks. Ornis. Scandin. 20, 112–116. doi: 10.2307/3676877
Konarzewski M., Taylor J. R., Gabrielsen G. W. (1993). Chick Energy Requirements and Adult Energy Expenditures of Dovekies (Alle Alle). Auk. 110, 343–353. doi: 10.1093/auk/110.2.343
Kubiszyn A., Piwosz K., Wiktor J. Jr., Wiktor J.J.J.O.P.R. (2014). The Effect of Inter-Annual Atlantic Water Inflow Variability on the Planktonic Protist Community Structure in the West Spitsbergen Waters During the Summer. J. Plankt. Res. 36, 1190–1203. doi: 10.1093/plankt/fbu044
Kwasniewski S., Gluchowska M., Jakubas D., Wojczulanis-Jakubas K., Walkusz W., Karnovsky N., et al. (2010). The Impact of Different Hydrographic Conditions and Zooplankton Communities on Provisioning Little Auks Along the West Coast of Spitsbergen. Prog. Oceanogr. 87, 72–82. doi: 10.1016/j.pocean.2010.06.004
Kwasniewski S., Gluchowska M., Walkusz W., Karnovsky N. J., Jakubas D., Wojczulanis-Jakubas K., et al. (2012). Interannual Changes in Zooplankton on the West Spitsbergen Shelf in Relation to Hydrography and Their Consequences for the Diet of Planktivorous Seabirds. ICES J. Mar. Sci. 69, 890–901. doi: 10.1093/icesjms/fss076
Kwasniewski S., Hop H., Falk-Petersen S., Pedersen G. (2003). Distribution of Calanus Species in Kongsfjorden, a Glacial Fjord in Svalbard. J. Plankt. Res. 25, 1–20. doi: 10.1093/plankt/25.1.1
Loeng H., Drinkwater K. (2007). An Overview of the Ecosystems of the Barents and Norwegian Seas and Their Response to Climate Variability. Deep. Sea. Res. Part II.: Top. Stud. Oceanogr. 54, 2478–2500. doi: 10.1016/j.dsr2.2007.08.013
Møller E. F., Nielsen T. G. (2020). Borealization of Arctic Zooplankton—Smaller and Less Fat Zooplankton Species in Disko Bay, Western Greenland. Limnol. Oceanogr. 65, 1175–1188. doi: 10.1002/lno.11380
Muckenhuber S., Nilsen F., Korosov A., Sandven S. (2016). Sea Ice Cover in Isfjorden and Hornsund, Svalbard, (2000–2014) From Remote Sensing Data. Cryosph. 10, 149–158. doi: 10.5194/tc-10-149-2016
National Ice Center (NIC) and NSIDC (2010). Multisensor Analyzed Sea Ice Extent – Northern Hemisphere. IMS Daily Northern Hemisphere Snow and Ice Analysis at 4 km Resolution Data Set Developed by F. Fetterer, M. Savoie, S. Helfrich, and P. Clemente-Colón. Boulder, Colorado USA
Nilsen F., Skogseth R., Vaardal-Lunde J., Inall M. (2016). A Simple Shelf Circulation Model: Intrusion of Atlantic Water on the West Spitsbergen Shelf. J. Phys. Oceanogr. 46, 1209–1230. doi: 10.1175/JPO-D-15-0058.1
Parmesan C. (2006). Ecological and Evolutionary Responses to Recent Climate Change. Annu. Rev. Ecol. Evol. Syst. 37, 637–669. doi: 10.1146/annurev.ecolsys.37.091305.110100
Pavlov A. K., Tverberg V., Ivanov B. V., Nilsen F., Falk-Petersen S., Granskog M. A. (2013). Warming of Atlantic Water in Two West Spitsbergen Fjords Over the Last Century, (1912-2009). Pol. Res. 32, 11206. doi: 10.3402/polar.v32i0.11206
Poloczanska E. S., Brown C. J., Sydeman W. J., Kiessling W., Schoeman D. S., Moore P. J., et al. (2013). Global Imprint of Climate Change on Marine Life. Nat. Climate Change 3, 919. doi: 10.1038/nclimate1958
Polyakov I. V., Alkire M. B., Bluhm B. A., Brown K. A., Carmack E. C., Chierici M., et al (2020). Borealization of the Arctic Ocean in Response to Anomalous Advection From Sub-Arctic Seas. Front. Mar. Sci. 7. doi: 10.3389/fmars.2020.00491
Promińska A., Cisek M., Walczowski W. (2017). Kongsfjorden and Hornsund Hydrography–Comparative Study Based on a Multiyear Survey in Fjords of West Spitsbergen. Oceanologia 59, 397–412. doi: 10.1016/j.oceano.2017.07.003
Ramírez F., Tarroux A., Hovinen J., Navarro J., Afán I., Forero M. G., et al. (2017). Sea Ice Phenology and Primary Productivity Pulses Shape Breeding Success in Arctic Seabirds. Sci. Rep. 7, 4500. doi: 10.1038/s41598-017-04775-6
Renaud P. E., Daase M., Banas N. S., Gabrielsen T. M., Søreide J. E., Varpe Ø., et al. (2018). Pelagic Food-Webs in a Changing Arctic: A Trait-Based Perspective Suggests a Mode of Resilience. ICES J. Mar. Sci. 75, 1871–1881. doi: 10.1093/icesjms/fsy063
Skogseth R., Olivier L. L., Nilsen F., Falck E., Fraser N., Tverberg V., et al. (2020). Variability and Decadal Trends in the Isfjorden (Svalbard) Ocean Climate and Circulation–An Indicator for Climate Change in the European Arctic. Prog. Oceanogr. 187, 102394. doi: 10.1016/j.pocean.2020.102394
Smithson M., Verkuilen J. (2006). A Better Lemon Squeezer? Maximum-Likelihood Regression With Beta-Distributed Dependent Variables. psychol. Methods 11, 54. doi: 10.1037/1082-989X.11.1.54
Stempniewicz L. (2001). “Alle alle Little Auk in BWP Update,” in The Journal of the Birds of the Western Palearctic. Ed. Ogilvie M. (Oxford: Oxford University Press), 175–201.
Stempniewicz L., Goc M., Głuchowska M., Kidawa D., Węsławski J. M. (2021a). Abundance, Habitat Use and Food Consumption of Seabirds in the High-Arctic Fjord Ecosystem. Pol. Biol. 44, 739–750. doi: 10.1007/s00300-021-02833-4
Stempniewicz L., Weydmann-Zwolicka A., Strzelewicz A., Goc M., Głuchowska M., Kidawa D., et al. (2021b). Advection of Atlantic Water Masses Influences Seabird Community Foraging in a High-Arctic Fjord. Prog. Oceanogr. 193, 102549. doi: 10.1016/j.pocean.2021.102549
Strzelewicz A., Przyborska A., Walczowski W. (2022). Increased Presence of Atlantic Water on the Shelf South-West of Spitsbergen With Implications for the Arctic Fjord Hornsund. Prog. Oceanogr. 200, 102714. doi: 10.1016/j.pocean.2021.102714
Tape K. D., Jones B. M., Arp C. D., Nitze I., Grosse G. (2018). Tundra be Dammed: Beaver Colonization of the Arctic. Global Change Biol. 24, 4478–4488. doi: 10.1111/gcb.14332
Tarling G. A., Freer J. J., Banas N. S., Belcher A., Blackwell M., Castellani C., et al. (2022). Can a Key Boreal Calanus Copepod Species Now Complete Its Life-Cycle in the Arctic? Evidence and Implications for Arctic Food-Webs. Ambio 51, 333–344. doi: 10.1007/s13280-021-01667-y
Tverberg V., Skogseth R., Cottier F., Sundfjord A., Walczowski W., Inall M. E., et al. (2019). “The Kongsfjorden Transect: Seasonal and Inter-Annual Variability in Hydrography,” in The Ecosystem of Kongsfjorden, Svalbard. Eds. Hop H., Wiencke C. (Cham: Springer), 49–104.
Vihtakari M., Welcker J., Moe B., Chastel O., Tartu S., Hop H., et al. (2018). Black-Legged Kittiwakes as Messengers of Atlantification in the Arctic. Sci. Rep. 8, 1178. doi: 10.1038/s41598-017-19118-8
Welcker J., Harding A. M., Karnovsky N. J., Steen H., Strøm H., Gabrielsen G. W. (2009). Flexibility in the Bimodal Foraging Strategy of a High Arctic Alcid, the Little Auk Alle Alle. J. Avian Biol. 40, 388–399. doi: 10.1111/j.1600-048X.2008.04620.x
Wojczulanis-Jakubas K., Jakubas D., Karnovsky N. J., Walkusz W. (2010). Foraging Strategy of Little Auks Under Divergent Conditions on Feeding Grounds. Polar. Res. 29, 22–29. doi: 10.1111/j.1751-8369.2009.00145.x
Wojczulanis-Jakubas K., Jakubas D., Chastel O. (2014a). Different Tactics, One Goal: Initial Reproductive Investments of Males and Females in a Small Arctic Seabird. Behav. Ecol. Sociobiol. 68, 1521–1530. doi: 10.1007/s00265-014-1761-4
Wojczulanis-Jakubas K., Jakubas D., Kulaszewicz I., Kidawa D., Taylor J. R. (2014b). Influence of Primary Reproductive Investments on Blood Biochemistry, Leukocyte Profile, and Body Mass in a Small Arctic Seabird. Auk.: Ornithol. Adv. 131, 743–755. doi: 10.1642/AUK-14-62.1
Wojczulanis-Jakubas K., Jakubas D., Stempniewicz L. (2021). The Little Auk Alle alle: An Ecological Indicator of a Changing Arctic and a Model Organism. Pol. Biol. 25, 1–14. doi: 10.1007/s00300-021-02981-7
Zheng B. Y., Agresti A. (2000). Summarizing the Predictive Power of a Generalized Linear Model. Stat Med. 19, 1771–1781. doi: 10.1002/1097-0258(20000715)19:13<1771::AID-SIM485>3.0.CO;2-P
Keywords: Alle alle, borealization, copepod, Calanus, fitness costs, little auk, Spitsbergen
Citation: Descamps S, Wojczulanis-Jakubas K, Jakubas D, Vihtakari M, Steen H, Karnovsky NJ, Welcker J, Hovinen J, Bertrand P, Strzelewicz A, Skogseth R, Kidawa D, Boehnke R and Błachowiak-Samołyk K (2022) Consequences of Atlantification on a Zooplanktivorous Arctic Seabird. Front. Mar. Sci. 9:878746. doi: 10.3389/fmars.2022.878746
Received: 18 February 2022; Accepted: 13 May 2022;
Published: 20 June 2022.
Edited by:
Mark Jessopp, University College Cork, IrelandReviewed by:
Manon Clairbaux, University College Cork, IrelandAshley Bennison, British Antarctic Survey (BAS), United Kingdom
Copyright © 2022 Descamps, Wojczulanis-Jakubas, Jakubas, Vihtakari, Steen, Karnovsky, Welcker, Hovinen, Bertrand, Strzelewicz, Skogseth, Kidawa, Boehnke and Błachowiak-Samołyk. This is an open-access article distributed under the terms of the Creative Commons Attribution License (CC BY). The use, distribution or reproduction in other forums is permitted, provided the original author(s) and the copyright owner(s) are credited and that the original publication in this journal is cited, in accordance with accepted academic practice. No use, distribution or reproduction is permitted which does not comply with these terms.
*Correspondence: Sébastien Descamps, c2ViYXN0aWVuLmRlc2NhbXBzQG5wb2xhci5ubw==