- 1Yellow Sea Fisheries Research Institute, Chinese Academy of Fishery Sciences, Laboratory for Marine Fisheries Science and Food Production Processes, Pilot National Laboratory for Marine Science and Technology, Qingdao, China
- 2College of Marine Science and Fisheries, Jiangsu Ocean University, Lianyungang, China
- 3College of Fisheries and Life Science, Shanghai Ocean University, Shanghai, China
- 4Tianyuan Fisheries Co., Ltd, Yantai, China
Offshore cephalopods usually experience a short and extreme senescence process at the end of their lives. Unique behaviors such as brooding and fasting occur during this period. The cause of senescent cephalopod death is still unknown. Excessive energy consumption under prolonged starvation is one possible reason for death. A comparison analysis between normal starvation and senescence may provide valuable information to deepen our understanding of the senescence process of cephalopods. In this study, a comparison of behavior, histology and expression of a candidate senescence-specific gene (Imaginal morphogenesis protein-Late 2, ImpL2) of female Octopus sinensis under normal starvation and senescence was conducted. The females in the starvation group had similar behaviors, histology and ImpL2 expression level to the control group. The females in the senescence group exhibited typical behaviors and symptoms of senescent octopus, including fasting and unhealed lesions on the skin. Moreover, swimming and grasping ability was weakened in senescent individuals. The muscle tissue in the mantle and arm of senescent females showed a histolytic appearance. The secretory cells and their microvilli in the digestive gland gradually degraded and disappeared during senescence. The expression level of ImpL2 gene significantly changes in multiple organs of senescent females. The results showed that O. sinensis underwent different physiological and molecular processes during starvation and senescence. Starvation alone could not lead to senescent symptoms in the octopus. Tissue degeneration in the mantle, arm and digestive gland would increase the death risk of the octopus.
Introduction
The majority of shallow-water cephalopod species experience a short and extreme senescence process at the end of their lives. The senescence of female octopuses usually begins with spawning, and they tend to their clutches of eggs and blow water to clean and oxygenate the eggs (Gabe, 1975; Cosgrove, 1993). During this brooding period, females rarely leave their clutches and abstain from feeding (Mangold, 1987). Females usually die when their eggs hatch (Anderson et al., 2002). Symptoms such as retraction of skin around the eyes, undirected or uncoordinated activity and unhealed lesions on the skin also occur in senescent females (Anderson et al., 2002; Wang and Ragsdale, 2018; Roumbedakis and Guerra, 2019). The male octopuses do not go through the brooding process, but the aforementioned senescent conditions will gradually appear from sexual maturity, and they die approximately the same time as the females (Hanlon, 1983; Mangold, 1983; Van Heukelem, 1983).
The cause of senescent cephalopod death is still unclear. A significant loss of body weight often occurs in senescent females. In the wild, female giant Pacific octopus (Enteroctopus dofleini) lost 50% to 71% of their body weight during the brooding period (Cosgrove, 1993). In captivity, female common octopus (Octopus vulgaris) lost 50% of their body weight (O’Dor and Wells, 1987); big blue octopus (Octopus cyanea), 36% (Van Heukelem, 1976); and Octopus mimus, 25% (Cortez et al., 1995). Therefore, excessive energy consumption under prolonged starvation may lead to the death of cephalopods. However, female octopuses under normal starvation and senescence have different behaviors. Compare with the drastic behavioral changes found in senescent female octopuses, starving females remain alert and active even after 38 days of fasting with substantial body weight loss (Wells and Wells, 1975). This implies that the octopus may undergo completely different physiological processes during starvation and senescence. However, little is known about this physiological difference. Most studies have focused on either the starvation or senescence process (Castro et al., 1992; Jackson and Mladenov, 1994; Zamora and Olivares, 2004; Roumbedakis et al., 2018), but the comparison analysis of these two conditions is limited.
At present, the molecular mechanism of cephalopod senescence is largely unknown. The optic gland has been found to play an important role in prompting senescence (Wodinsky, 1977). The transcriptional level of the Imaginal morphogenesis protein-Late 2 gene (ImpL2) is significantly up-regulated in the optic gland of the California two-spot octopus (Octopus bimaculoides) during senescence, making it a candidate senescence-specific gene in cephalopods (Wang and Ragsdale, 2018). However, the role of ImpL2 in cephalopod senescence is still unclear.
To gain insight into the mechanisms underlying cephalopod senescence, a comparative analysis based on behavior, histology and gene expression (ImpL2) between starvation and senescence in the East Asian common octopus (Octopus sinensis) was conducted in this study.
Materials and Methods
Octopus Collection and Management
A total of 146 O. sinensis (70 females and 76 males, body weight 300 g~600 g) were caught from the coastal waters of Lianjiang (Fujian Province, China) in June 2020. The octopuses were used for larvae rearing purposes, and they provided a sampling pool. Only the individuals that met the experimental requirements were included in this study. They were separated into 3 tanks (6 m×6 m×1 m; dark yellow wall and white bottom). Each tank placed approximately 50 individuals with a 1:1 male/female sex ratio. Each tank placed 40 artificial dens at the bottom. The clams (Ruditapes philippinarum) and Zhikong scallops (Chlamys farreri) were provided twice a day, and the daily feed amount was approximately 15% of the octopus body weight. The same amount of live swimming crabs (Charybdis japonica) were offered every two days. The daily water exchange rates of the tanks were 200%. Water parameters were as follows: temperature, 19.5~23.1°C; salinity, 28~32 ppt; pH, 7.8~8.2; illumination intensity, 600~1000 Lux; and dissolved oxygen above 5 mg/L. A 14 h light:10 h dark photoperiod was maintained during the experiments. The octopuses were cultivated for two weeks, and mating frequently occurred during this period.
Experimental Design and Sample Collection
Females were chosen to conduct the tests in this study, because it was easier to identify their senescence stage. They were separated into fiberglass buckets (diameter-120 cm, height-70 cm), and each bucket contained one individual. The water parameters during the tests were as follows: temperature, 19.2~23.5°C; salinity, 28~32 ppt; pH, 7.8~8.2; and dissolved oxygen above 5 mg/L. The daily water exchange rate was 200%. The octopuses were divided into control, starvation and senescence groups. The control group included females that had not laid eggs with a feed supply. The starvation group included females that had not laid eggs without feed supply. The senescence group included females that had laid eggs. The octopuses in the control and starvation groups were chosen randomly from the tanks. They were kept undisturbed for one day to habituate to the bucket environment. Then the live clams (approximately 10% of the body weight) were provided for three days to test the condition of each octopus. The individuals that fed actively were kept to conduct subsequent experiments. The individuals in the control group were fed clams for another 3 days, and the samples were collected on the third day. No live prey items were provided in the starvation group, and the samples were collected on the 15th and 30th days after starvation. The octopuses in the senescence group were the females that just spawned in a day. The artificial dens were checked at 9 am every morning. Once the female was found to lay eggs, both the female and the artificial den were transported from the tank to the experimental bucket. The clams were offered every morning and cleaned on the next morning. The feeding amount of the senescent individuals was investigated by counting the empty shells of clams in the morning. The samples of the senescence group were collected on the 1st, 15th, 30th and 45th days after spawning. Three replicate samples were taken at each time point. A total of 21 females were used to conduct real-time quantitative PCR (qPCR) and histological analysis. The remaining individuals were used for larvae rearing. A diagram of the experimental flow can be found in Figure S1.
Octopuses were submerged in 20 g/L magnesium chloride seawater solution for at least 20 min to achieve deep anesthesia, which was certified by loss of reaction to all stimuli (Zhu et al., 2020). Once anesthetized, the individual was placed in a clean tray, and the body weight (BW) was recorded. The optic gland was first collected for qPCR test. Then the individual was immediately euthanized by destroying the brain to confirmation of death as method of human killing (Fiorito et al., 2015). The digestive gland weight (DGW) and ovary weight (OW) of each individual were recorded. The digestive gland index (DGI) was calculated using , and the calculation of gonadosomatic index (GSI) was carried out using (Castro et al., 1992; Sieiro et al., 2014). The mantle muscle, arm muscle, digestive gland, ovary, kidney, systemic heart and gill were collected for qPCR analysis and histological observation. The mantle samples were the muscle tissue near the funnel. The arm samples for qPCR were the muscle tissue corresponding to the 4th-6th row of suckers distal from the mouth on the second arm on the right side. The arm samples for histological analysis were the muscle tissue corresponding to the 7th-8th row of suckers on the same arm. The histological samples were fixed in Davidson fixative solution, and the qPCR samples were frozen in liquid nitrogen and stored at −80°C until RNA extraction. All of the animal experiments in this study were approved by the Animal Care and Use Committee of the Chinese Academy of Fishery Sciences (IACUC-2020-03).
Behavioral Observation During Starvation and Senescence
The females in the starvation and senescence treatments were also used for behavioral observation. They were observed three times daily (9 am-10 am, 2 pm-3 pm, 7 pm-8 pm). An underwater camera (Hero 5 Black, GoPro, USA) was used to record the behaviors. To record the predation behaviors of the females in the starvation group, they were fed empty shells of clams. The individuals in the senescence group rarely left their dens. To record their appearance and behaviors, several females were driven out of their home dens.
Histological Analysis During Starvation and Senescence
The samples were fixed in Davidson fixative solution for 48 hours. The fixed samples were dehydrated in a sequence of 75%, 85%, 95% and 100% ethanol solutions, and 95% and 100% ethanol dehydration was conducted twice. Each dehydration process lasted 20 min. The dehydrated samples were then cleared and impregnated with paraffin wax. The paraffin-embedded sections were cut into 6 μm sections using a microtome (RM2235, Leica, Germany), and sections were mounted onto glass slides. Then, the samples were dried at 45°C for 4 hours, followed by hematoxylin eosin staining. The stained samples were examined under a light microscope (MD4000 B, Leica, Germany).
RNA Extraction, Reverse Transcription, and Real-Time Quantitative PCR
Total RNA was extracted using an RNA extraction kit (DP419, TIANGEN, China). The quality and quantity of total RNA were detected by UV spectrophotometry (NanoDrop 2000, Thermo Scientific, USA) and 1.2% agarose gel electrophoresis. First strand cDNA was synthesized using the Evo M-MLV Mix Kit with gDNA Clean for qPCR (AG11728, Accurate Biology, China) according to the manufacturer’s instructions. The primers for ImpL2 were designed based on our genomic data of O. sinensis (Li et al., 2020), and the elongation factor 1-α (EF) gene was used as the internal reference (Sirakov et al., 2009; García-Fernández et al., 2016). The primer sequences (5’-3’) were as follows: ImpL2-F: TTCTAACGGAATGGGAGC, ImpL2-R:TCGGCAACTTAACTGGACAGC; EF-F:ACGAAGGCTGGGAAATTGA,EF-R: TGGTCTCTCCGTTGGTCTCT (Sirakov et al., 2009). The ImpL2 expression level of the optic gland in the control, starvation and senescence groups was detected using real-time quantitative PCR. Moreover, the ImpL2 expression levels of different tissues in the control and senescence groups (30 days after spawning) were also assessed. The qPCR was conducted using ChamQ SYBR Color qPCR Master Mix (Q411-02, Vazyme, China) and ABI StepOne Plus Sequence Detection System (Applied Biosystems, Foster City, CA, USA). A total of 20 μL of the PCR reaction volume contained 10 μL of 2×ChamQ SYBR Color qPCR Master Mix (Low ROX Premixed), 0.4 μL of 50×ROX Reference Dye I, 0.4 μL of forward and reverse primers (10 μM each), 1 μL of diluted cDNA templates, and 7.8 μL of sterile distilled water. The thermal cycling program was as follows: 95°C for 30 s, 40 cycles of 95°C for 10 s and 60°C for 30 s. A melting curve analysis was performed following the cycling stage to confirm the presence of a single PCR product. Each sample was detected in triplicate. The expression level of the EF gene remained stable across different samples. Relative gene expression levels were normalized to EF and quantified as the fold change relative to the expression levels in the control group according to the 2-ΔΔCT method (Livak and Schmittgen, 2001).
Statistical Analysis
Statistical analysis was carried out with SPSS Statistics 21 (IBM, USA). Data were first tested for normality (Shapiro-Wilk test) and variance homogeneity (Levene’s test). The ImpL2 expression level in the optic gland was analyzed with one-way analysis of variance (ANOVA). Multiple comparison was carried out using LSD test. The Kruskal-Wallis and Dunnett’s T3 tests were conducted for DGI, GSI and feeding amount data. The ImpL2 expression level in different tissues was analyzed using independent t test. All data are presented as the mean ± standard deviation (SD). Statistical significance was considered a p value of< 0.05.
Results
Behavioral Observation of O. sinensis in Different Groups
Control group: The females in the control group were active predators and spent time outside of their home dens (Figure 1A). They were able to change body color according to environmental variations. The females hunted clams through a swift pounce, and the prey were captured under the interbrachial web (Figure 1B) (Movie-Control group feeding). The clams were consumed fast due to the good appetite of the females.
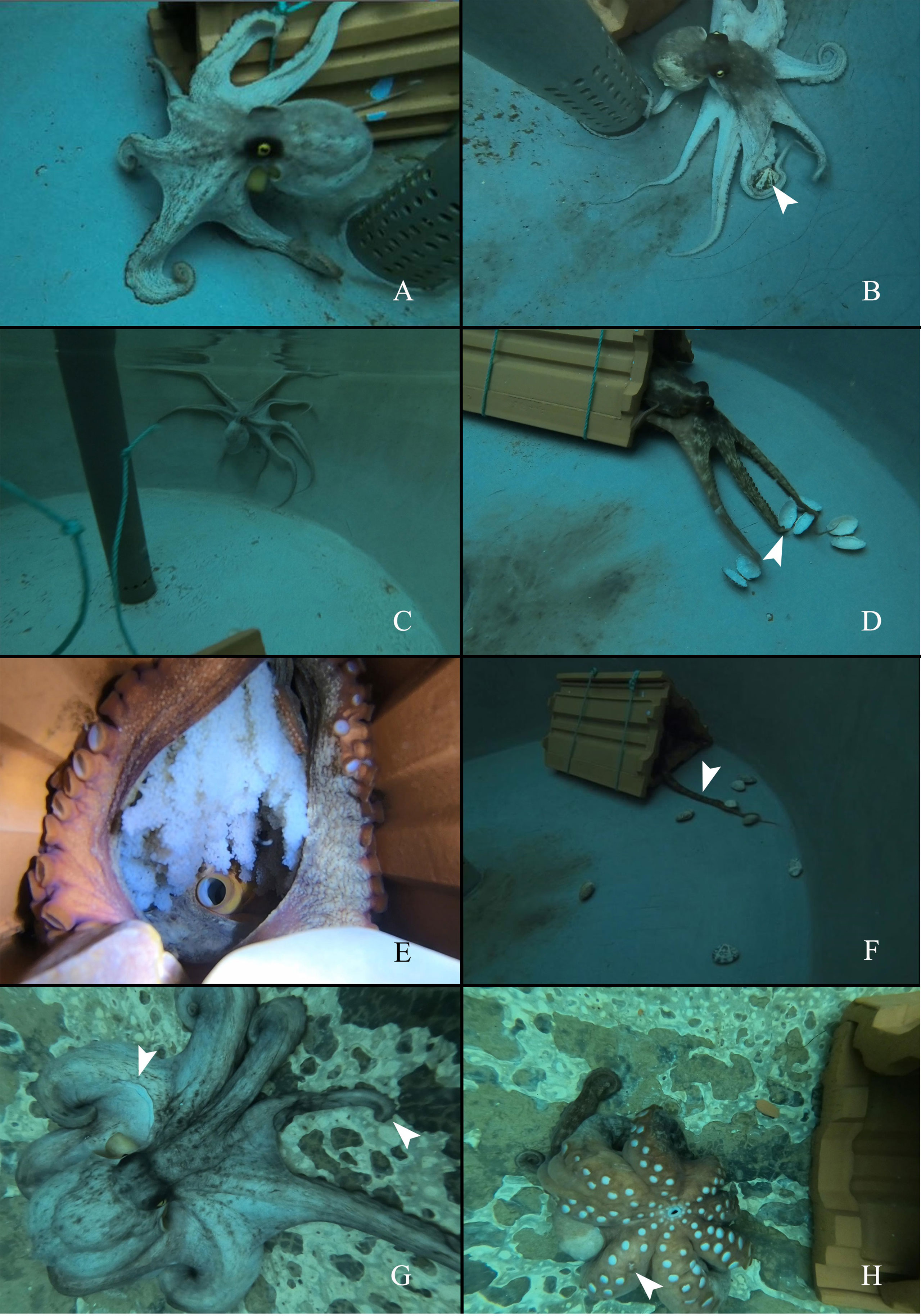
Figure 1 The appearance and behaviors of O.sinensis in control, starvation and senescence groups. (A) O.sinensis in control group spends time outside of its home den. (B) O.sinensis in control group attacks the clams (several clams are restrained under the interbranchial web). White arrow head indicates the clam. (C) O.sinensis in starvation group is active outside of its home den. (D) O.sinensis in starvation group attacks the empty shells of clams. White arrow head indicates the missing arm tip. (E) A brooding female. (F) O.sinensis in senescence group stays inside their home den, uses arm to grasp clams. White arrow head indicates the arm stretches out the den. (G) The appearance of a senescent female. White arrow heads indicate a missing patch of skin and a missing arm tip. (H) A senescent female cannot turn over (one day before death). White arrow head indicates a missing sucker.
Starvation group: The females in the starvation group began laying eggs on the 35th day, and the last spawning was found on the 39th day. Thus, behavioral observation was carried out for 39 days. They had similar behaviors to those in the control group and spent quite a long time outside of their dens (Figure 1C). The females kept attacking the empty shells during the 39-day starvation experiment (Figure 1D) (Movie-Starvation group feeding). Some individuals had missing arm tip (Figure 1D).Senescence group: In the senescence group, the food intake decreased gradually from 7-8 clams per day to 1-2 clams per day, and eventually the females stopped feeding (Supplementary Figure S2). There were feeding amount variations between different individuals (Supplementary Figure S3). The females had brooding activity, and they rarely left their home dens (Figure 1E). Instead of capturing prey by pouncing, the females used one arm to grab the clam back to the den (Figure 1F) (Movie-Senescence group feeding). One female was found to leave its clutch to obtain the prey (Movie-Senescent individual goes out). During this short period, she did not pounce on the clams; instead, she grabbed and carried the prey back to its den. Moreover, the grasping ability of the arms became weaker, and the clams could not be held by the arms very well. Unhealed wounds and missing arm tips were found in the senescent females (Figure 1G) (Movie-Appearance and behaviors before death). The females seemed to lose swimming ability before death, and they could only crawl on the bottom of the tank (Figure 1H) (Movie- Appearance and behaviors before death).
Histological Analysis of Different Tissues During Starvation and Senescence
In the present study, only mantle muscle, arm muscle, digestive gland and ovary showed histological variations during senescence. The kidney, systemic heart, and gill exhibited similar histological structures in the control, starvation and senescence groups. The mantle muscle of the females in the control and starvation groups had similar structures, and bundles of circular muscle fibers were separated by thin layers of radial muscle fibers. The muscle tissue on 15, 30 and 45 DAS (days after spawning) showed a histolytic appearance, the muscle fibers were broken and dissolved, the radial muscle fibers became incompact, and the gaps between the fibers were enlarged (Figures 2A–C; Supplementary Figure S4). A similar histological change pattern was observed in the arm muscle. Both the control and starvation groups possessed arms with a typical array of longitudinal muscle and trabeculae fibers. However, rather than since 15 DAS, the breakdown and disorganization of muscle fibers had occurred since 30 DAS. The histological structure on 15 DAS was the same as that of the control group (Figures 2D–F; Supplementary Figure S5). The digestive gland in the control and starvation groups exhibited a similar histological structure, with characteristic secretory cells and microvilli. The microvilli at the top of secretory cells began to disappear on 15 DAS. In the senescence group, the secretory cells were found to shrank and disappear on 30 DAS, while most of the secretory cells disappeared on 45 DAS (Figures 2G–I; Supplementary Figure S6). The digestive gland index of the starvation, 30 DAS and 45 DAS groups was significantly lower than that of the control group (Supplementary Figure S7). A large number of mature follicles were observed in the ovaries of the control and starvation groups. The epithelium of follicles infolded to form elongated crests. Most of the follicles degraded and disappeared on 15, 30 and 45 DAS (Figures 2J–L, Supplementary Figure S8). The gonadosomatic index of the 30 DAF (days after fasting) and 1 DAS groups was significantly higher than that of the control group, while the index of the 15, 30 and 45 DAS groups was significantly lower than that of the control group (Supplementary Figure S9).
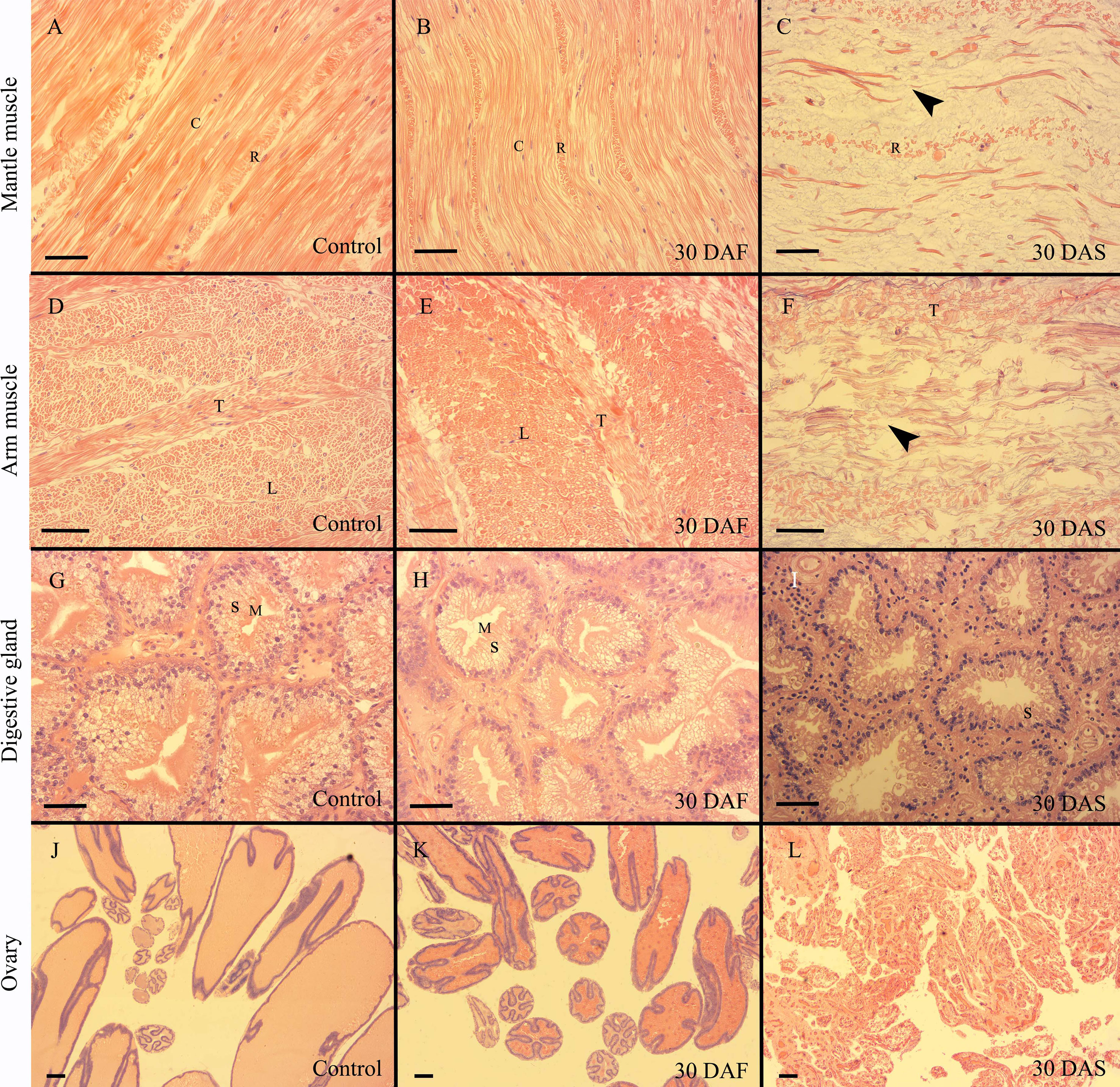
Figure 2 Histology of O.sinensis mantle muscle, arm muscle, digestive gland and ovary. (A–C) Longitudinal sections of mantle muscle. (D, E) Transverse sections of arm muscle. (F) Longitudinal section of arm muscle. (G–I) Digestive gland. (J–L) Ovary. Black arrow heads indicate the broken circular muscle fibers. C, circular muscle fiber; DAF, days after fasting; DAS, days after spawning; L, longitudinal muscle fiber; M, microvilli; R, radial muscle fiber; S, secretory cell; T, trabeculae; Scale bar (A–I):50 μm. Scale bar (J–L):100 μm.
Expression of ImpL2 During Starvation and Senescence
The ImpL2 expression level in the optic gland of starvation and control groups was significantly lower than that in the senescence group, and the peak expression level was found on 30 DAS (Figure 3). The ImpL2 expression level of optic gland, arm muscle, mantle muscle and ovary on 30 DAS was significantly higher than that of the control group (Figure 4). The largest difference was found in the optic gland, followed by the ovary and arm muscle (Figure 4). The down-regulation of ImpL2 expression was found in kidney and gill in the senescence group (Figure 4). While the ImpL2 expression level in digestive gland and systemic heart was similar between the control group and senescence group (Figure S4).
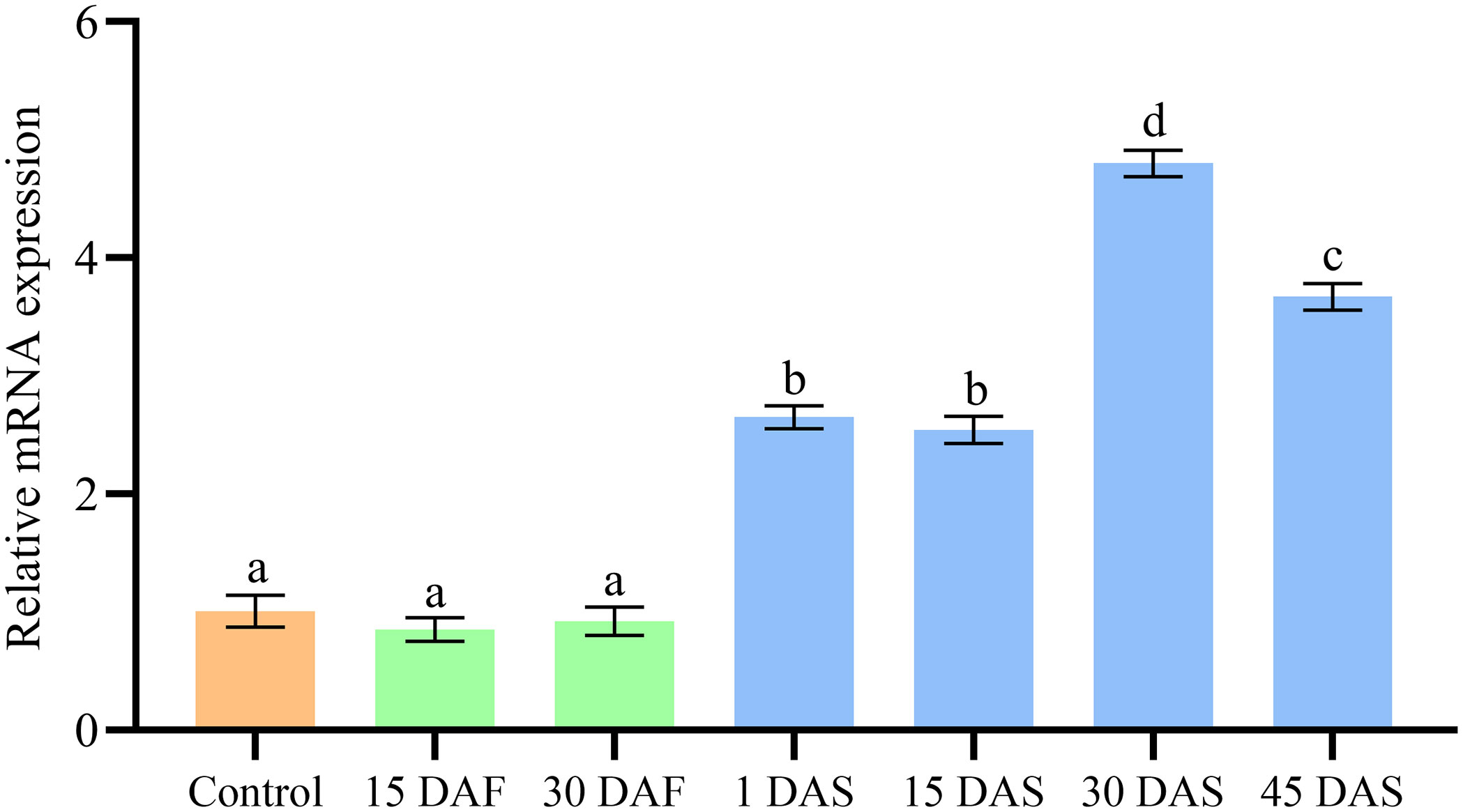
Figure 3 Expression profile ofImpL2 in optic gland in the control, starvation and senescence group. Data were normalized to the abundance of EF expressed in the same samples and are presented as the mean ± SD (n = 3). Groups with different letters are significantly different from each other (p<0.05, ANOVA followed by LSD test). DAF, days after fasting; DAS, days after spawning.
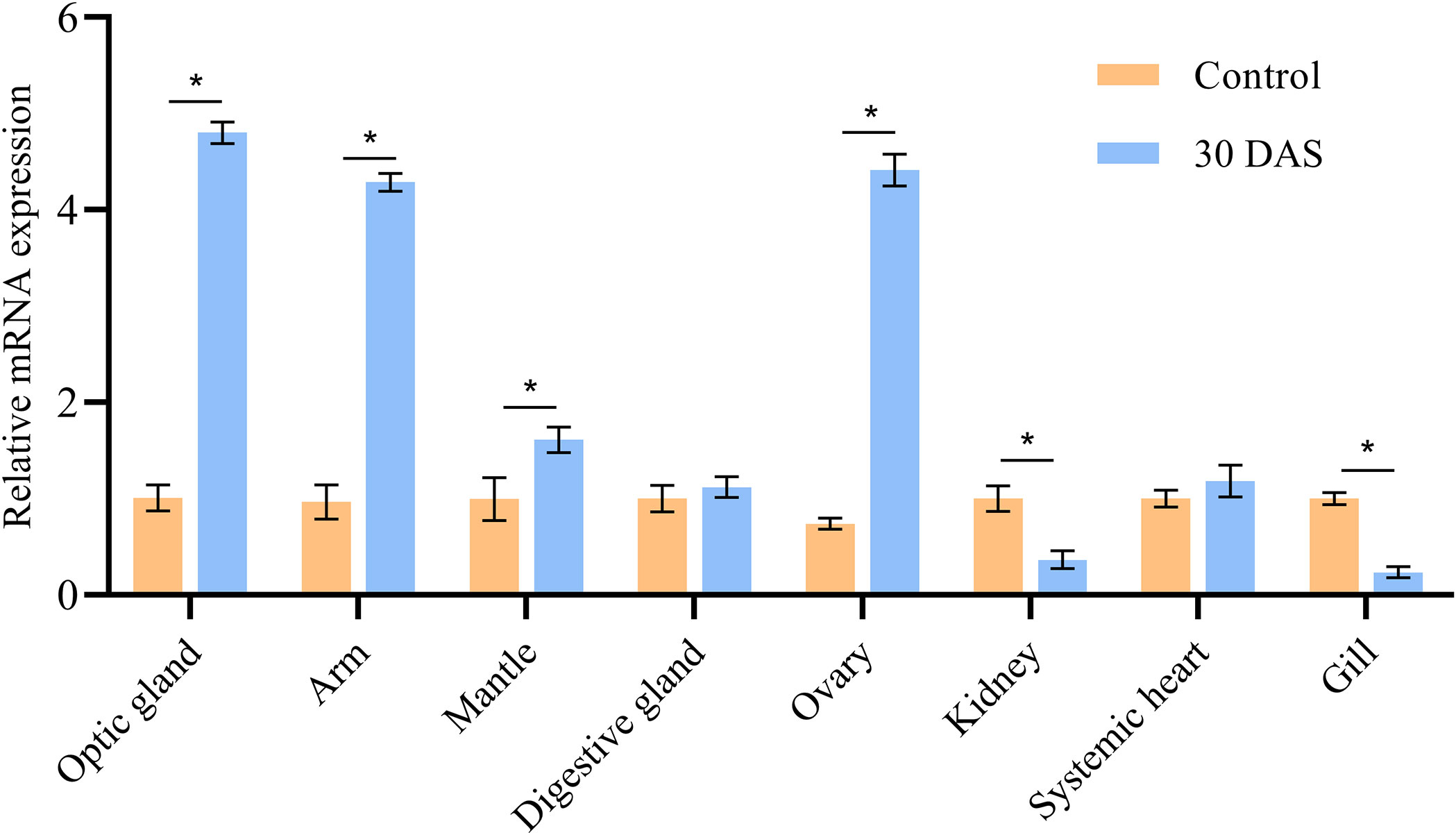
Figure 4 Expression profile of ImpL2 in different tissues in control group and senescence group (30 DAS). Data were normalized to the abundance of EF expressed in the same samples and are presented as the mean ± SD (n = 3). *p<0.05, independent t test. DAF, days after fasting; DAS, days after spawning.
Discussion
Behaviors of O. sinensisDuring Starvation and Senescence
The females in the control and starvation groups had similar behaviors. In general, octopuses can easily learn to recognize empty shells and stop capturing them. Interestingly, the individuals in the starvation group kept attacking the empty shells during the 39-day starvation experiment. Thus, it may be a better strategy for starving individuals to capture all potential prey rather than spending time identifying them. Overall, the octopus did not show obvious behavioral and appearance changes after nearly 40 days of starvation and remained alert and active, similar to the findings in O. vulgaris (Wells and Wells, 1975).
Compared to the control and starvation groups, the females in the senescence group exhibited completely different behaviors. First, the females rarely left their home dens after spawning. They had brooding activity before egg hatching, which is typical in octopus (Gabe, 1975; Cosgrove, 1993; Anderson et al., 2002; Hanlon and Messenger, 2018; Wang and Ragsdale, 2018). The individuals continued to stay in their home dens after eggs hatched; even if they were driven out, they would soon crawl back to their dens. However, O. bimaculoides has been shown to spend most of the time outside of the dens before death (Wang and Ragsdale, 2018). This indicated that senescent behaviors are variable in different species. Both O. sinensis and O. bimaculoides lost most of their motor ability after hatching. In the wild, slow-moving octopuses are easy targets for predators when they leave their dens. Thus, O. bimaculoides is likely to be captured by predators before the senescence process kills it.
Second, senescent females lost their appetite and abstained from feeding, which is also a typical symptom in octopus (Van Heukelem, 1977; Wodinsky, 1977; Cortez et al., 1995; Anderson et al., 2002). The females in the senescence group also exhibited different predatory behaviors; rather than pursuing prey, the individuals stayed in their home dens and used one arm to grab the clams. The predation of O. bimaculoides before spawning occurs through a visually directed jet-propelled “pounce”, while brooding females grab crabs as they move past the den (Wang and Ragsdale, 2018). The senescent octopus changes from an active predator to a lurker that can only wait for prey.
Third, the females in the senescence group showed weakened motor ability. Individuals rarely swam before death; they could only crawl on the bottom of the tank. The pupils of senescent O. bimaculoides have been found to gradually lose their horizontal orientation with respect to gravity, and the females spend time sitting on the bottom of the tank (Wang and Ragsdale, 2018). Moreover, the arms were less flexible in grabbing clams than the control group. This would further decrease their predatory ability to obtain prey.
Fourth, the females in the senescence group had unhealed wounds, missing arm tips and suckers. Similar symptoms have also been found in senescent O. bimaculoides (Wang and Ragsdale, 2018). In addition, arm regeneration may not occur in injured senescent European Cuttlefish (Sepia officinalis) (Féral, 1988). Resistance against parasite infection may be impaired in senescent O. vulgaris (Pascual et al., 2010). Thus, the senescence process of cephalopods is likely accompanied by a reduction in immunity.
Histological Variations During Senescence
The muscle tissue in the mantle and arm of O. sinensis had a high degree of muscle histolysis during senescence. In the post-spawning stage of deep-sea squid Moroteufhis ingens, the thick, elastic mantle becomes thin, inelastic and gelatinous, and the muscle tissue has a very disorganized structure and histolytic appearance, consisting predominantly of scattered collagen fibers with considerable gaps between (Jackson and Mladenov, 1994). In O. mimus, the arm muscle fibers of senescent individuals present atrophy and histolysis, with a great enlargement of the gaps between the fibers (Zamora and Olivares, 2004). The breakdown of muscle structure inevitably leads to a decrease in motor ability. The grabbing and swimming ability was reduced in O. sinensis, which would lower their chance to capture prey and escape from predators, eventually increasing the risk of death. There was no such muscle tissue wasting in the starvation group, indicating that starvation would not lead to muscle dissolution, and there must be other reasons for muscle histolysis in the senescent octopus.
The digestive gland index (wet weight) gradually decreased in the starvation and senescence groups, indicating that the digestive gland is one of the main energy reserve organs during these two processes. A similar phenomenon could be found in other cephalopods (Castro et al., 1992; Zamora and Olivares, 2004). The digestive gland structure of O. sinensis in the starvation group remained intact, while the secretory cells and microvilli in the senescence group continually degraded and disappeared. In the post-spawning stage, the secretory cells of the digestive gland in O. mimus lose their apical portion and are reduced to cubic cells (Zamora and Olivares, 2004). The degeneration of the digestive gland will destroy the digestion and absorption function, which would lead to death by starvation.
Expression Pattern of ImpL2 During Senescence
In the present study, most of the tissues showed ImpL2 expression variations during senescence, suggesting that ImpL2 plays important role in senescent octopus. The ImpL2 expression level of optic gland, arm muscle, mantle muscle and ovary in the senescence group was higher than that in the control group. IMPL2 is a homolog of insulin-like growth factor binding protein (IGFBP) that serves as a suppressor of the insulin/IGF signaling pathway in Drosophila (Honegger et al., 2008; Kwon et al., 2015; Roed et al., 2018). The up-regulated expression of ImpL2 in Drosophila has been shown to induce muscle tissue wasting (Figueroa-Clarevega and Bilder, 2015; Kwon et al., 2015), which is similar to the muscle histolysis found in senescent octopus. The function of ImpL2 in octopus is still unknown, but the similar histological changes imply a conserved function between octopus and Drosophila. As an important endocrine organ, the ImpL2 expression level in the optic gland increased significantly during senescence. This result is consistent with the findings in O. bimaculoides (Wang and Ragsdale, 2018). There was no ImpL2 expression difference between the starvation and control groups, indicating different insulin/IGF signaling regulation between normal and senescent octopus. Down-regulation of ImpL2 expression was found in kidney and gill during senescence, indicating different functions of ImpL2 played in these tissues. Only limited studies have been conducted on ImpL2, its function in different tissues during senescence needs further investigation in the future.
Conclusion
Based on behavioral, histological and gene expression analyses, it was found that O. sinensis underwent different physiological and molecular processes during starvation and senescence. Prolonged starvation alone could not lead to senescent symptoms in the octopus. Histological evidence corresponding to some senescent symptoms was found in the present study. The reduced motor ability of the senescent octopus was related to muscle tissue wasting in the mantle and arm, and the degeneration of secretory cells in the digestive gland weakened digestive function. The expression level of ImpL2 significantly changes in multiple tissues during senescence, implying its function in promoting senescence. We plan to focus on the muscle wasting of senescent octopus in the future. The regulation mechanism of ImpL2 on Insulin/IGF pathway and the function of ImpL2-Insulin/IGF signaling pathway on muscle wasting will be investigated through molecular biology techniques such as recombinant protein stimulation, RNA interference and pathway inhibitor/activator treatment.
Data Availability Statement
The original contributions presented in the study are included in the article/Supplementary Material. Further inquiries can be directed to the corresponding author.
Ethics Statement
This study was reviewed and approved by Animal Care and Use Committee of the Chinese Academy of Fishery Sciences.
Author Contributions
LB conducted the sample collection, data curation and draft writing. FL performed the histological analysis. QC conducted the writing-review and editing. CL and XL performed the molecular work. JT conducted the statistical analysis. SZ performed the data visualization. ML, YS and RX were involved in the sample collection. SC contributed to the concept and design of the study. All authors contributed to the article and approved the submitted version.
Funding
This work was supported by the China Agriculture Research System of MOF and MARA.
Conflict of Interest
Author RX is employed by Tianyuan Fisheries Co., Ltd.
The remaining authors declare that the research was conducted in the absence of any commercial or financial relationships that could be construed as a potential conflict of interest.
Publisher’s Note
All claims expressed in this article are solely those of the authors and do not necessarily represent those of their affiliated organizations, or those of the publisher, the editors and the reviewers. Any product that may be evaluated in this article, or claim that may be made by its manufacturer, is not guaranteed or endorsed by the publisher.
Acknowledgments
We would like to thank Xin Lusheng at Yellow Sea Fisheries Research Institute for providing advice on the data analysis.
Supplementary Material
The Supplementary Material for this article can be found online at: https://www.frontiersin.org/articles/10.3389/fmars.2022.874777/full#supplementary-material
References
Anderson R. C., Wood J. B., Byrne R. A. (2002). Octopus Senescence: The Beginning of the End. J. Appl. Anim. Welf. Sci. 5 (4), 275–283. doi: 10.1207/S15327604JAWS0504_02
Castro B. G., Garrido J. L., Sotelo C. G. (1992). Changes in Composition of Digestive Gland and Mantle Muscle of the Cuttlefish Sepia Officinalis During Starvation. Mar. Biol. 114 (1), 11–20. doi: 10.1007/BF00350851
Cortez T., Castro B. G., Guerra A. (1995). Reproduction and Condition of Female Octopus Mimus (Mollusca: Cephalopoda). Mar. Biol. 123 (3), 505–510. doi: 10.1007/BF00349229
Cosgrove J. (1993). In Situ Observations of Nesting Female Octopus Dofleini (Wülker 1910). J. Ceph. Biol. 2 (2), 33–45.
Féral J. P. (1988). Wound Healing After Arm Amputation in Sepia Officinalis (Cephalopoda: Sepioidea). J. Invertebr. Pathol. 52 (3), 380–388. doi: 10.1016/0022-2011(88)90049-3
Figueroa-Clarevega A., Bilder D. (2015). Malignant Drosophila Tumors Interrupt Insulin Signaling to Induce Cachexia-Like Wasting. Dev. Cell 33 (1), 47–55. doi: 10.1016/j.devcel.2015.03.001
Fiorito G., Affuso A., Basil J., Cole A., de Girolamo P., D’Angelo L., et al. (2015). Guidelines for the Care and Welfare of Cephalopods in Research-A Consensus Based on an Initiative by CephRes, FELASA and the Boyd Group. Lab. Anim. 49 (2 Suppl), 1–90. doi: 10.1177/0023677215580006
Gabe S. H. (1975). Reproduction in the Giant Octopus of the North Pacific, Octopus Dofleini Martini. Veliger 18 (2), 146–150.
García-Fernández P., Castellanos-Martínez S., Iglesias J., Otero J. J., Gestal C. (2016). Selection of Reliable Reference Genes for RT-qPCR Studies in Octopus Vulgaris Paralarvae During Development and Immune-Stimulation. J. Invertebr. Pathol. 138, 57–62. doi: 10.1016/j.jip.2016.06.003
Hanlon R. (1983). Octopus Briareus in Cephalopod Life Cycles:Species Accounts. Ed. B P. R. (London: London Academic Press), 251–256.
Hanlon R. T., Messenger J. B. (2018). Cephalopod Behaviour (Cambridge: Cambridge University Press). doi: 10.1017/9780511843600
Honegger B., Galic M., Köhler K., Wittwer F., Brogiolo W., Hafen E., et al. (2008). Imp-L2, a Putative Homolog of Vertebrate IGF-Binding Protein 7, Counteracts Insulin Signaling in Drosophila and is Essential for Starvation Resistance. J. Biol. 7 (3), 10. doi: 10.1186/jbiol72
Jackson G. D., Mladenov P. V. (1994). Terminal Spawning in the Deepwater Squid Moroteuthis Ingens (Cephalopoda: Onychoteuthidae). J. Zool. 234 (2), 189–201. doi: 10.1111/j.1469-7998.1994.tb06067.x
Kwon Y., Song W., Droujinine I. A., Hu Y., Asara J. M., Perrimon N. (2015). Systemic Organ Wasting Induced by Localized Expression of the Secreted Insulin/IGF Antagonist Impl2. Dev. Cell 33 (1), 36–46. doi: 10.1016/j.devcel.2015.02.012
Li F., Bian L., Ge J., Han F., Liu Z., Li X., et al. (2020). Chromosome-Level Genome Assembly of the East Asian Common Octopus (Octopus Sinensis) Using PacBio Sequencing and Hi-C Technology. Mol. Ecol. Resour. 20 (6), 1572–1582. doi: 10.1111/1755-0998.13216
Livak K. J., Schmittgen T. D. (2001). Analysis of Relative Gene Expression Data Using Real-Time Quantitative PCR and the 2–ΔΔct Method. Methods 25 (4), 402–408. doi: 10.1006/meth.2001.1262
Mangold K. (1983). Octopus Vulgaris in Cephalopod Life Cycles:Species Accounts. Ed. Boyle P. R. (London: London Academic Press), 335–364.
Mangold K. (1987). Reproduction in Cephalopod Life Cycles: Comparative Reviews. Ed. Boyle P. R. (London: London Academic Press), 157–200.
O’Dor R., Wells M. (1987). Energy and Nutrient Flow in Cephalopod Life Cycles. Ed. PR B. (London: London Academic Press), 109–133.
Pascual S., González A. F., Guerra A. (2010). Coccidiosis During Octopus Senescence: Preparing for Parasite Outbreak. Fish. Res. 106 (2), 160–162. doi: 10.1016/j.fishres.2010.05.013
Roed N. K., Viola C. M., Kristensen O., Schluckebier G., Norrman M., Sajid W., et al. (2018). Structures of Insect Imp-L2 Suggest an Alternative Strategy for Regulating the Bioavailability of Insulin-Like Hormones. Nat. Commun. 9 (1), 3860. doi: 10.1038/s41467-018-06192-3
Roumbedakis K., Guerra Á. (2019). Cephalopod Senescence and Parasitology in Handbook of Pathogens and Diseases in Cephalopods. Eds. Gestal C., Pascual S., Guerra Á., Fiorito G., Vieites J. M. (Cham: Springer International Publishing), 207–211. doi: 10.1007/978-3-030-11330-8_16
Roumbedakis K., Mascaró M., Martins M. L., Gallardo P., Rosas C., Pascual C. (2018). Health Status of Post-Spawning Octopus Maya (Cephalopoda: Octopodidae) Females From Yucatan Peninsula, Mexico. Hydrobiologia 808 (1), 23–34. doi: 10.1007/s10750-017-3340-y
Sieiro P., Otero J., Guerra Á. (2014). Contrasting Macroscopic Maturity Staging With Histological Characteristics of the Gonads in Female Octopus Vulgaris. Hydrobiologia 730 (1), 113–125. doi: 10.1007/s10750-014-1826-4
Sirakov M., Zarrella I., Borra M., Rizzo F., Biffali E., Arnone M. I., et al. (2009). Selection and Validation of a Set of Reliable Reference Genes for Quantitative RT-PCR Studies in the Brain of the Cephalopod Mollusc Octopus Vulgaris. BMC Mol. Biol. 10 (1), 70. doi: 10.1186/1471-2199-10-70
Van Heukelem W. F. (1976). Growth, Bioenergetics and Life-Span of Octopus Cyanea and Octopus Maya (Honolulu: University of Hawaii at Manoa).
Van Heukelem W. F. (1977). Laboratory Maintenance, Breeding, Rearing, and Biomedical Research Potential of the Yucatan Octopus (Octopus Maya). Lab. Anim. Sci. 27 (5 Pt 2), 852–859.
Van Heukelem W. F. (1983). Octopus Maya in Cephalopod Life Cycles: Species Accounts. Ed. B P. R. (London: London Academic Press), 311–323.
Wang Z. Y., Ragsdale C. W. (2018). Multiple Optic Gland Signaling Pathways Implicated in Octopus Maternal Behaviors and Death. J. Exp. Biol. 221 (19), jeb185751. doi: 10.1242/jeb.185751
Wells M. J., Wells J. (1975). Optic Gland Implants and Their Effects on the Gonads of Octopus. J. Exp. Biol. 62 (3), 579–588. doi: 10.1242/jeb.62.3.579
Wodinsky J. (1977). Hormonal Inhibition of Feeding and Death in Octopus: Control by Optic Gland Secretion. Science 198 (4320), 948–951. doi: 10.1126/science.198.4320.948
Zamora M., Olivares A. (2004). Variaciones Bioquímicas E Histológicas Asociadas Al Evento Reproductivo De La Hembra De Octopus Mimus (Mollusca: Cephalopoda). Int. J. Morphol 22 (3), 207–216. doi: 10.4067/S0717-95022004000300006
Keywords: octopus, senescence, starvation, behavior, histology, ImpL2
Citation: Bian L, Li F, Chang Q, Liu C, Tan J, Zhang S, Li X, Li M, Sun Y, Xu R and Chen S (2022) Comparison of Behavior, Histology and ImpL2 Gene Expression of Octopus sinensis Under Starvation and Senescence Conditions. Front. Mar. Sci. 9:874777. doi: 10.3389/fmars.2022.874777
Received: 13 February 2022; Accepted: 22 June 2022;
Published: 20 July 2022.
Edited by:
Enric Gisbert, Institute of Agrifood Research and Technology (IRTA), SpainReviewed by:
Eduardo Almansa, Spanish Institute of Oceanography (IEO), SpainKhor Waiho, University of Malaysia Terengganu, Malaysia
Camino Gestal, Spanish National Research Council (CSIC), Spain
Copyright © 2022 Bian, Li, Chang, Liu, Tan, Zhang, Li, Li, Sun, Xu and Chen. This is an open-access article distributed under the terms of the Creative Commons Attribution License (CC BY). The use, distribution or reproduction in other forums is permitted, provided the original author(s) and the copyright owner(s) are credited and that the original publication in this journal is cited, in accordance with accepted academic practice. No use, distribution or reproduction is permitted which does not comply with these terms.
*Correspondence: Siqing Chen, Y2hlbnNxQHlzZnJpLmFjLmNu