- 1Freshwater Fisheries Research Institute of Jiangsu Province, Nanjing, China
- 2Biology Program, School of Distance Education, Universiti Sains Malaysia, Penang, Malaysia
- 3Institute of Marine Biology, College of Oceanography, Hohai University, Nanjing, China
- 4Southern Marine Science and Engineering Guangdong Laboratory (Zhuhai), Zhuhai Key Laboratory of Marine Bioresources and Environment, Guangdong Provincial Key Laboratory of Marine Resources and Coastal Engineering, School of Marine Sciences, Sun Yat-Sen University, Guangzhou, China
- 5Nanjing University of Information Science and Technology, Nanjing, China
- 6Jiangsu Key Laboratory for Molecular and Medical Biotechnology, College of Life Sciences, Nanjing Normal University, Nanjing, China
Berberine hydrochloride is an isoquinoline alkaloid, which has antitumoral, antibacterial, and antiviral activities in vivo and in vitro. Charybdis japonica is one of the main economic species of crab in Southeast Asia. We studied the molecular mechanism of oxidative stress in berberine hydrochloride-treated C. japonica infected with Aeromonas hydrophila. C. japonica were infected with A. hydrophila after being submerged in different concentrations (0, 100, 200, and 300 mg/L) of berberine hydrochloride for 48 h. The full-length cDNA of Prx6 and the ORFs of Prx5 and PXL2A were cloned. Prx6 and PXL2A each have one conserved domain, Cys44, and Cys81. The Prx5 conserved domain contains three important Cys loci, Cys75, Cys100, and Cys76. Prx6 was different from Prx5 and PXL2A in the Peroxiredoxin family. The transcription levels of PXL2A infected with A. hydrophila were all higher than the control. The transcription levels of C. japonica were further increased by adding berberine hydrochloride and were increased the highest at a concentration of 300 mg/L. The activities of glutathione peroxidase, superoxide dismutase, and catalase in the hepatopancreas of berberine hydrochloride-treated C. japonica infected with A. hydrophila were significantly increased compared with those only infected with A. hydrophila and the control group. The glutathione transferase activity in the hepatopancreas was significantly increased in berberine hydrochloride-treated C. japonica. The results of this study provide a new understanding of the potential role of berberine hydrochloride on the oxidative stress mechanisms of C. japonica.
Introduction
Berberine hydrochloride is a natural isoquinoline alkaloid and the main active ingredient of several herbal medicines commonly used clinically, such as Coptis chinensis and Phellodendri (Habtemariam, 2016) berberine hydrochloride is the most important bioactive component in Phellodendron chinensis, accounting for about 0.6% of Phellodendron chinensis extract and 1.6% of berberine (Anna et al., 1995; Lu-Yang et al., 2014). It has been studied widely in biochemistry and pharmaceutical chemistry and found to have antitumoral, antibacterial, and antioxidant (Domadia et al., 2008) activities both in vivo and in vitro. Berberine hydrochloride has certain bacteriostatic and bactericidal effects on Candida albicans, Trichophyton mentagrophytes, and Staphylococcus aureus (Zhang et al., 2013; Zorić et al., 2017; Xiao et al., 2019). Berberine can inhibit the formation of extracellular amyloid peptides in bacteria, thus, interfering with the formation and stability of biofilms (Peng et al., 2015; Wang et al., 2018). In addition, berberine can also affect the integrity and permeability of bacterial cell membranes, and bind to some proteins on the cell membrane, thereby affecting the structure and function of proteins. It also affects the expression of bacterial DNA and binds to DNA (Kang et al., 2015; Du et al., 2020).
Importance
With the rapid development of the C. japonica farming industry, investors, in pursuit of economic benefits, have encountered problems such as the high density of crab farming, environmental degradation, and frequent outbreaks of various diseases, resulting in high mortality and huge economic losses (Jithendran et al., 2010). Bacterial infection of crabs in the process of culture is one of the main causes (Xia et al., 2010; Bonami and Zhang, 2011). The open water circulation system can give rise to several crab bacterial diseases, including black gill syndrome, shell disease, vibriosis, and edema disease (Wang, 2011). Among these, Aeromonas hydrophila of the Vibrionaceae is a pathogenic bacterium affecting fish and crustaceans, which leads to huge economic losses (Gonzalez-Escalona et al., 2006; Igbinosa et al., 2012).
Oxidative response in innate immunity is an important means to resist environmental stress and the invasion of pathogens (Liu et al., 2020). All organisms are equipped with a wide range of antioxidant proteins to maintain intracellular redox homeostasis, including superoxide dismutase (SOD), catalase (CAT), glutathione peroxidase (GPx), glutathione transferase (GST), oxidized glutathione (GSSG), glutathione (GSH), and peroxidase-reducing proteins (Fridovich, 1972).
Peroxiredoxins (Prxs) are a ubiquitous family of antioxidant enzymes that can prevent oxidative damage and regulate intracellular signal transduction (Jithendran et al., 2010). Prxs reduce peroxides by using a redox-active cysteine and are divided into three subgroups according to the number of cysteine residues involved in the catalysis (Wood et al., 2003). The Prx family has six subtypes, which can be divided into two subgroups, including five 2-Cys Prxs (from Prx1 to Prx5) and 1-Cys Prx (Prx6). In addition, depending on whether 70 conserved cysteine residues form either intermolecular or intramolecular disulfide bond bridges (Fridovich, 1972), 2-Cys Prxs are divided into typical and atypical categories. PRXL2A was identified during fetal liver development and was activated in monocytes stimulated by M-CSF (Yu et al., 2001). This protein protects cells from oxidative stress. For example, it appears to be one of the antioxidants involved in high-altitude adaptation in humans living in the Andes of South America (Guido et al., 2015). Prx5 was amplified in Macrobrachium rosenbergii (Arockiaraj et al., 2012), Exopalaemon carinicauda (Duan et al., 2013), and the crab Scylla paramamosain (Tu et al., 2017). Prx6 has been cloned in Procambarus clarkii (Wu et al., 2017).
In recent years, the treatment of bacterial diseases in aquatic animals has depended on the use of a large number of antibiotics. However, negative effects such as drug resistance, and cross infection of pathogenic bacteria have appeared, which endanger human health and pollute soil and water sources when released into the environment (Castanon, 2007; Dupont, 2007; Martínez, 2009; Seal et al., 2013). Secondary metabolites isolated from different plants can be beneficial to both humans and animals (Lazreg Aref et al., 2011). Therefore, berberine hydrochloride was considered as a bacterium drug to reduce side effects.
The molecular mechanism of oxidative stress in C. japonica infected with A. hydrophila and exposed to berberine hydrochloride was studied. The main objectives of this study are: (1) To study the mRNA expression of Prx5, Prx6, and PRXL2A genes in hepatopancreas, heart, intestine, muscle, and gills; (2) To characterize Prx5, Prx6, and PRXL2A, as well as oxidative active sites and functional properties; (3) To detect the oxidative stress response of Prx5, Prx6, and PRXL2A genes at the mRNA level in C. japonica immersed in berberine hydrochloride and infected with A. hydrophila; (4) To determine the oxidative stress response of C. japonica immersed in berberine hydrochloride and infected with A. hydrophila by the activities of antioxidant enzymes.
Materials and Methods
Experiment Design
The C. japonica used in this study weighed 82 ± 1.4 g, were 9.3 ± 0.9 cm long, and 6 ± 1.8 cm wide. C. japonica were obtained from the waters around Malaysia. There were seven treatment conditions and controls, each with three repeat tanks, each tank contained 12 C. japonica that were added with the same nets. PVC pipes were provided as shelter for the animals to prevent cannibalism. The experiment lasted for 2 weeks. During domestication, all the crabs were fed commercial feed (9812; Shanghai Harmony Feed Co., Ltd., Shanghai, China) at 7:00 and 20:00 daily. The daily feed was 5% of the crab’s body weight. The crabs were exposed to seawater using an artificial sea salt cycle (a salinity of 28 psu). Water temperature was controlled at 25 ± 1°C, pH 8.0 ± 0.2, dissolved oxygen concentration 5.0 mg L–1, and light/dark period was 12 h. There were 252 samples. The seven treatments were: C. japonica treated with 100, 200, and 300 mg/L of acid berberine and injected with 105 CFU/L of A. hydrophila; C. japonica injected with 105 CFU/L of A. hydrophila; C. japonica soaked in berberine hydrochloride 100, 200, and 300 mg/L. Two weeks after acclimation, water quality remained at the same level as during domestication. The C. japonica were not fed for 24 h before the experiment. According to the preliminary experiment, 300 mg/L is harmless to C. japonica. C. japonica were soaked in berberine hydrochloride in nine tanks containing either 100, 200, or 300 mg/L, and 48 h later, 105 CFU/L of A. hydrophila was injected into the fourth leg. According to the preliminary experiment, A. hydrophila 105 CFU/L could cause death in C. japonica after 24 h. Nine C. japonica not soaked in berberine hydrochloride were injected with 105 CFU/L A. hydrophila into the fourth leg as a control. After 72 h, 252 crabs were anesthetized on ice, and the tissues were collected.
RNA Extraction and Full-Length cDNA Cloning
Total RNA was extracted from the prepared samples using TRIzol Reagent (Aidlab Biotech Co., Beijing, China) according to the manufacturer’s instructions. Gene fragments of Prx5, Prx6, and PXL2A from C. japonica were obtained and verified in the transcriptome database of our laboratory (unpublished). BLAST comparison and open reading frame (ORF) analysis showed that Prx5 and PXL2A had complete ORF regions. After obtaining full-length genes, full-length primers were designed and validated, and full-length fluorescent quantitative primers PRX5f, PRX5R, PXL2A F, and PXL2A R were designed (Table 1). ST comparison and ORF analysis revealed partial cDNA regions of Prx6 gene fragments. RACE primers (3′ and 5′) were designed by Primer31. Full-length fluorescent quantitative primers Prx6 F1, Prx6 F2, Prx6 F3, Prx6 R1, and Prx6 F2 R2 were designed (Table 1).
RNA Extraction and Full-Length cDNA Cloning
The cDNA end amplification step, the full length of the cDNA sequence Prx6 expression products were negative according to SMARTer RACE 5′/3′ kit instructions. The PCR product of amplified Prx6 was detected by agarose gel electrophoresis to check the clarity of the band and the length of the fragment. The PCR products of full-length Prx5 and PXL2A were detected by agarose gel electrophoresis to check the clarity of the bands and the length of the fragments.
Full-length Prx6 sequences were obtained by splicing the terminal and intermediate sequences with DNAMan software. The primers were designed for PCR based on the spliced full-length fragments (Table 1) and sent to the Shanghai Baibaishun Biotechnology Co., Ltd. for sequencing. The results were compared through the NCBI database to verify the accuracy of the complementary DNA sequences.
Bioinformatics Analysis
This experiment adopted the ORF finder2 to predict ORF interval homologous proteins were analyzed using BLASTP, and protein hydrophobic water using ExPASy ProtScale3 analysis. ExPASy was used for ProtParam analysis of the amino acid composition, molecular weight, and isoelectric point. SignalP 5.1 Server4 was used to predict signal peptides. Protein domains were analyzed by SMART, and secondary and tertiary structures were analyzed by PSIRED Protein Structure Prediction Server and Swiss-model Server, respectively. DNAMan was used for multiple sequence alignment, and MEGA-X was used to construct a phylogenetic tree to compare gene sequence homology. In the process of phylogenetic tree construction, the evolutionary history was inferred by the neighbor-joining method, and the evolutionary distance was calculated by the Poisson correction method. Amino acid sequence alignment of species was performed using the NCBI database.
Quantitative Real-Time PCR Analysis
RNA was extracted from the hepatopancreas, gills, muscle, heart, and intestine for real-time fluorescence quantitative PCR analysis. Rt-qPCR was performed using CFX96 RT-PCR (BioRad, Hercules, CA, United States) and TransStart Top Green qPCR SuperMix (TransGen, Beijing, China). The primers qPCR-PRX6F, QPCR-PRx6r can be found in Table 1.
Enzyme Activities
Catalase, glutathione peroxidase, superoxide dismutase, glutathione transferase, oxidized glutathione, and glutathione were measured by kits provided by the Nanjing Jiangchen Bioengineering Research Institute. SOD activity was determined by the Peskin and Winterbourn methods. The ratio of autoxidation rate was measured at 450 nm for samples with or without serum. The activity was assessed in terms of U/mL. Determination of CAT activity was according to the method; H2O2 was determined by measuring residual at 405 nm. GSSG was detected at an absorbance of 405 nm, GSH was detected at 405 nm, and GPX was detected at 340 nm. An 0.01 increase in the absorbance of the reaction system resulting from 1 min reaction of GPX at 37°C per milliliter of serum was defined as one unit TAC. The amount of enzyme that reduced the GSH concentration to 1 mmol L–1 per minute in the reaction system was defined as one GPx unit, and the unit of glutathione disulfide reductase (GR) activity was defined as the amount of enzyme that consumed 1 mmol NADPH within 1 min, using 1-chloro-2 and 4-dinitrobenzene as substrates. GST was measured by absorbance at 340 nm.
Data Analysis
The experimental data were statistically analyzed by Microsoft Office Excel 2010 and SPSS 19.0, and the results were expressed as the mean ± standard deviation (SD). The relative mRNA levels of target genes were analyzed using 2–ΔΔCt. One-way analysis of variance (ANOVA) was used to test the significance of the difference (P < 0.05), and the Duncan test was used for multiple comparisons.
Results
Full-Length Sequence Analysis
The complete mRNA sequences for Prx5, Prx6, and PXL2A have been submitted to GenBank with accession numbers MZ611745, MZ611746, and MZ611747. The full-length mRNA of Prx5, Prx6, and PXL2A contained 498,660 and 651 bp ORFs, and the full-length cDNA of Prx6 was 1114 bp. The polypeptides of Prx5, Prx6, and PXL2A were 207, 219, and 216 amino acids long with predicted molecular weights of 18.2, 24.1, and 18.2 kDa and theoretical isoelectric points of 6.14, 5.36, and 6.13, respectively.
Multiple Sequence Alignment
Figures 1, 2 show the alignment of C. japonica PRDX5, PRDX6, and PXL2A with other homologous sequences. The PRDX5 subfamily protein contains a mitochondrial transmembrane transport signal peptide at the N-terminal, which is consistent with the prediction of a protein with subcellular localization. It also has a PRX5_like conserved domain, which contains three important Cys sites. There is a possible active site (red box), where Cys75 is associated with antioxidant activity and S-palmitoylation. If this site is modified by S-palmitoylation, it will lose antioxidant activity. Cys100 is not a key amino acid and has nothing to do with antioxidant activity and S-palmitoylation. Cys176 can form an internal disulfide bond with the Cys75 subunit, which is related to antioxidant activity and is independent of S-palmitoylation. PRDX6 subfamily proteins all have a PRX_1CYS domain, and the TxxCxxR (red box) is an important active site in the protein, which may have a cysteine sulfenic acid (-SOH) intermediate. Cys44 may form a disulfide for cysteine or small mercaptan molecules of other proteins. PXL2A subfamily proteins all have PRX_like2 domains, and only one Cys (Cys81 in the red box) is an important active site in the protein.
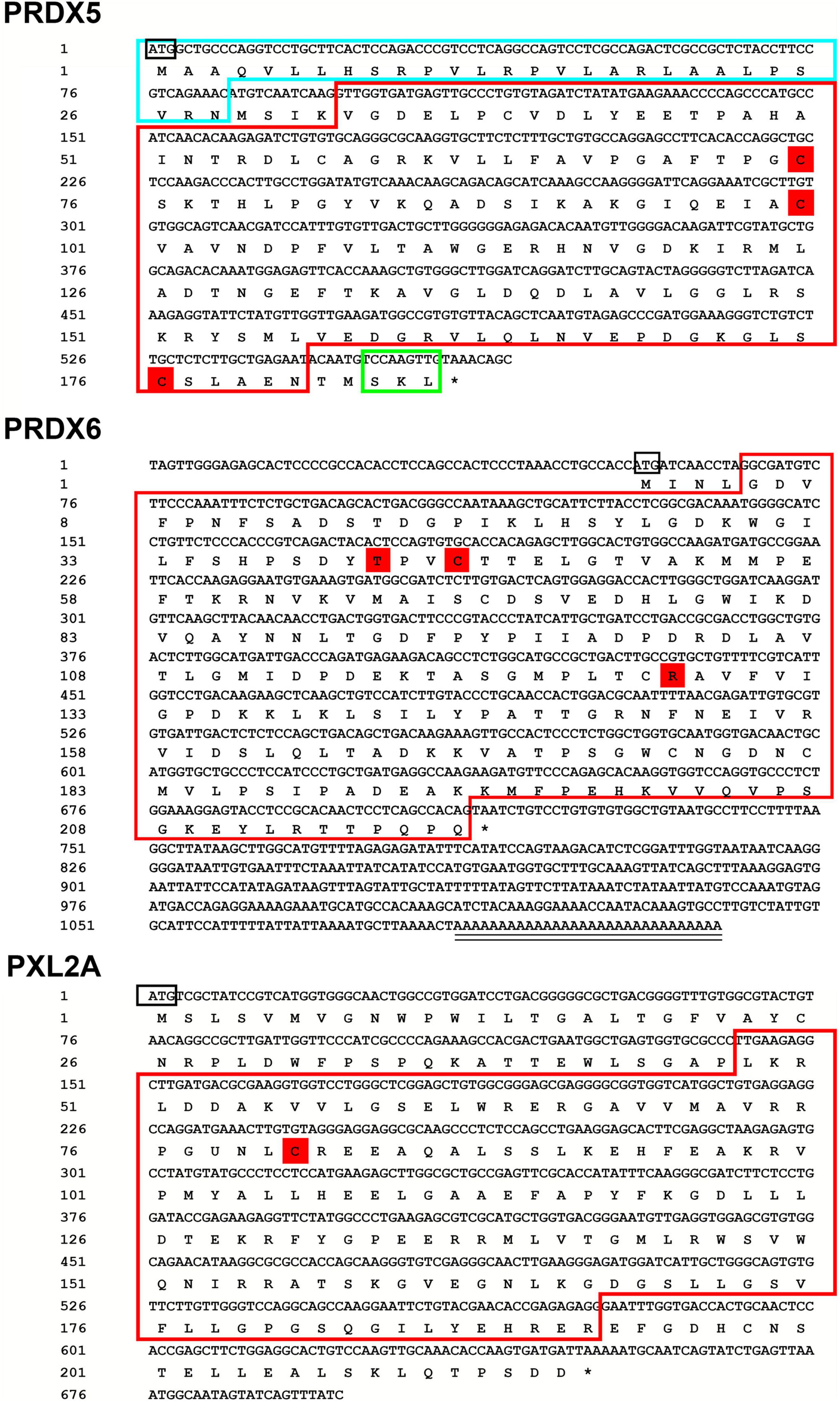
Figure 1. Sequencing results and amino acid sequence analysis of PRDX5, PRDX6, and PXL2A in C. japonica. The start (ATG) and end (TGA) codons are highlighted with a black box. *Denotes the termination codon. The blue boxes indicate possible mitochondrial signaling peptides. The red boxes represent the redoxin domain. The red shade indicates the likely active site, cysteine sulfenic acid (-SOH) intermediate, for peroxidase activity.
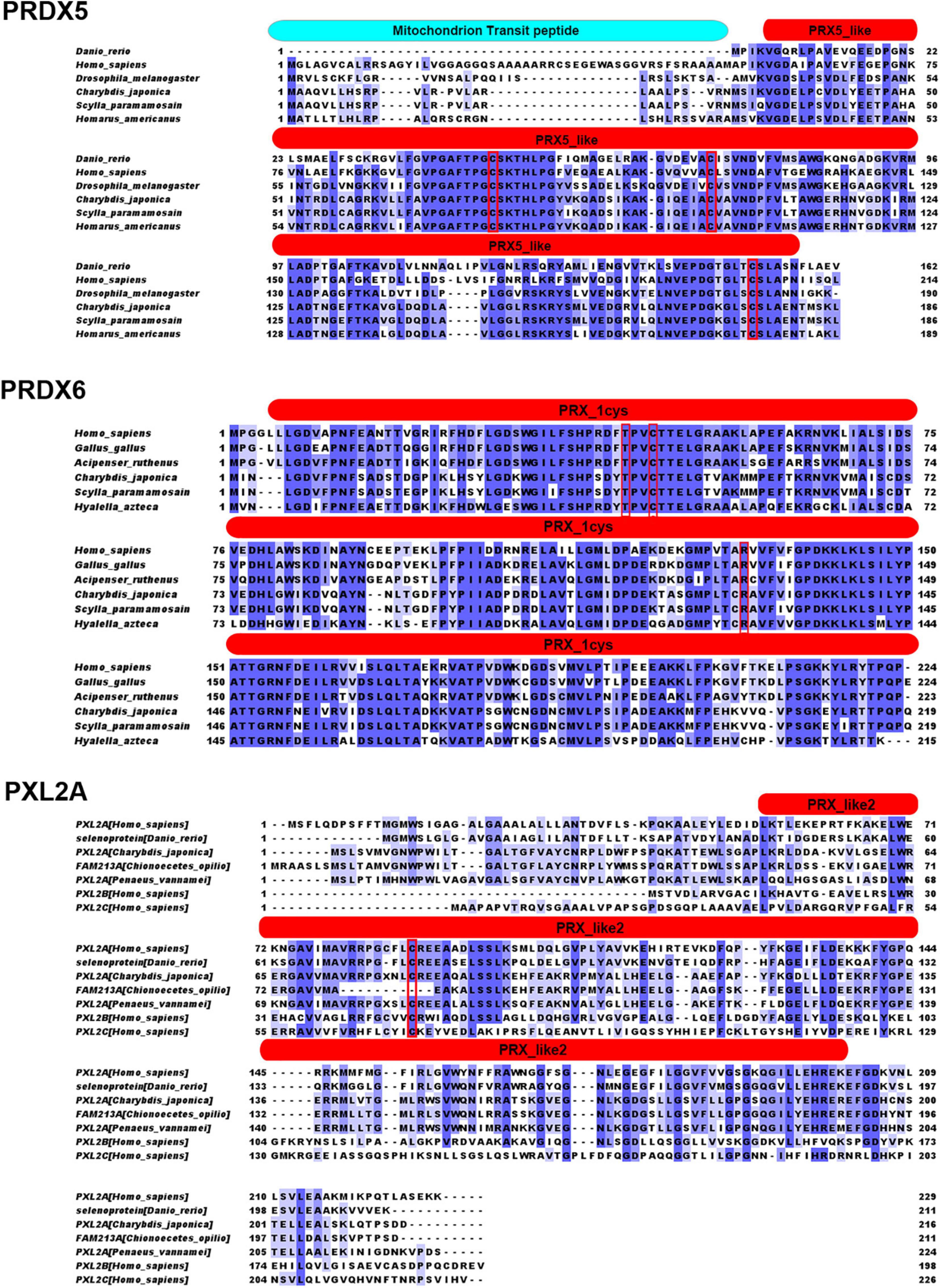
Figure 2. Results of the similarity analysis of PRDX5, PRDX6, and PXL2A. The red box is The important active site (R, T, and C), cysteine sulfenic acid (-SOH) intermediate, for peroxidase activity. The red entity box is located in the conservative domains of redoxin, PRX_1cys, and PRX_like2.
Phylogenetic Tree Construction
The amino acid sequences of PRDX5 and PRDX6 genes from C. japonica were compared with those from other representative organisms, and the amino acid sequences of PRDX5 and PRDX6 were constructed by the NJ method (Figure 3). PRDX5 from C. japonica was clustered with PRDX5 from Scylla paramamosain and with PRDX5 from other Malacostraca-like species in a branch, closest to insects, and classified into a large evolutionary division. PRDX5 of fishes and mammals was another evolutionary branch of clustering. It is most closely related to PRDX6 of other fungi. The PRDX6 from C. japonica is very close to PRDX5 in the evolutionary tree and has the highest similarity with the PRDX6 sequence of Scylla paramamosain and is grouped into a branch with PRDX6 of other Malacostraca species and is in an evolutionary relationship with insects and Demospongiae. It is classified with PRDX6 of fish, amphibians, birds, and mammals in another large branch of evolutionary clustering and is most closely related to the PRDX6 of other fungi.
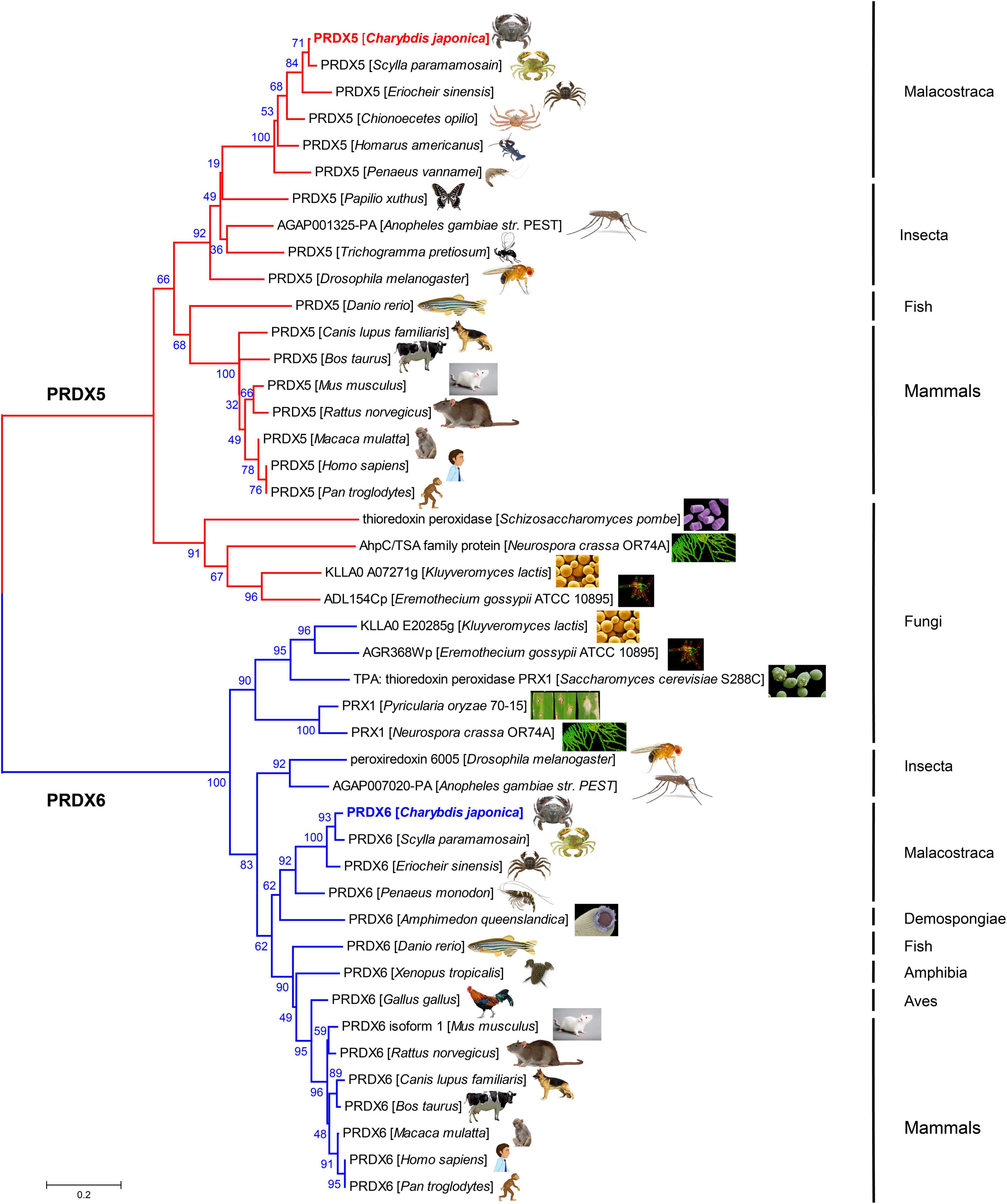
Figure 3. Evolutionary relationships of PRDX5 and PRDX6 amino acid sequence of C. japonica and other species.
The amino acid sequences of the PXL2A gene from C. japonica were compared with PXL2A amino acid sequences from other representative organisms, PXL2B and PXL2C, and the amino acid sequences of the PXL2A gene were constructed by the NJ method (Figure 4). As can be seen from the results of the evolutionary tree, PXL2A from C. japonica has the highest similarity with PXL2A from Chionoecetes opilio and is clustered into the same group with other Malacostraca species. The PXL2A of gastropods, bivalves, polyps, fish, amphibians, birds, and mammals are in another evolutionary branch of clustering. The evolutionary relationship with PXL2A of hexanauplia is the farthest. In contrast, PXL2B and PXL2C were clustered separately into two evolutionary branches, constituting the outgroups of PXL2A lineal homologs.
Tertiary Structure
The amino acid sequences of Prx5, Prx6, and PXL2A were deduced, as shown in Figure 5. Peroxiredoxins (Prxs) are an important class of peroxidases that are involved in antioxidant protection and redox signaling. The third-order structure of PRDX5 of C. japonica was constructed using the third-order structure detection results from PRDX5 of Alvinella pompejana (2xHF.1) Then, the results of tertiary structure human PRDX5 (2vL2.2) were used to predict the possible active site of PRDX5 in C. japonica. The results showed that the active site of C. japonica PRDX5 was a conserved redox-active cysteine residue, i.e., cysteine peroxide [C (P)], which subjects the peroxide substrate to nucleophilic activity. Hydrogen peroxide oxidizes C (P)-SH to cysteine sulfonic acid (C (P)-SOH), which then forms a disulfide bond with another cysteine residue (decomposing cysteine (C (R)). The disulfide is then reduced by the appropriate electron donor to complete the catalytic cycle. In this atypical 2-cys-PRx, C (R) is present in the same subunit to form an intramolecular disulfide. The disulfide is then reduced by thioredoxin.
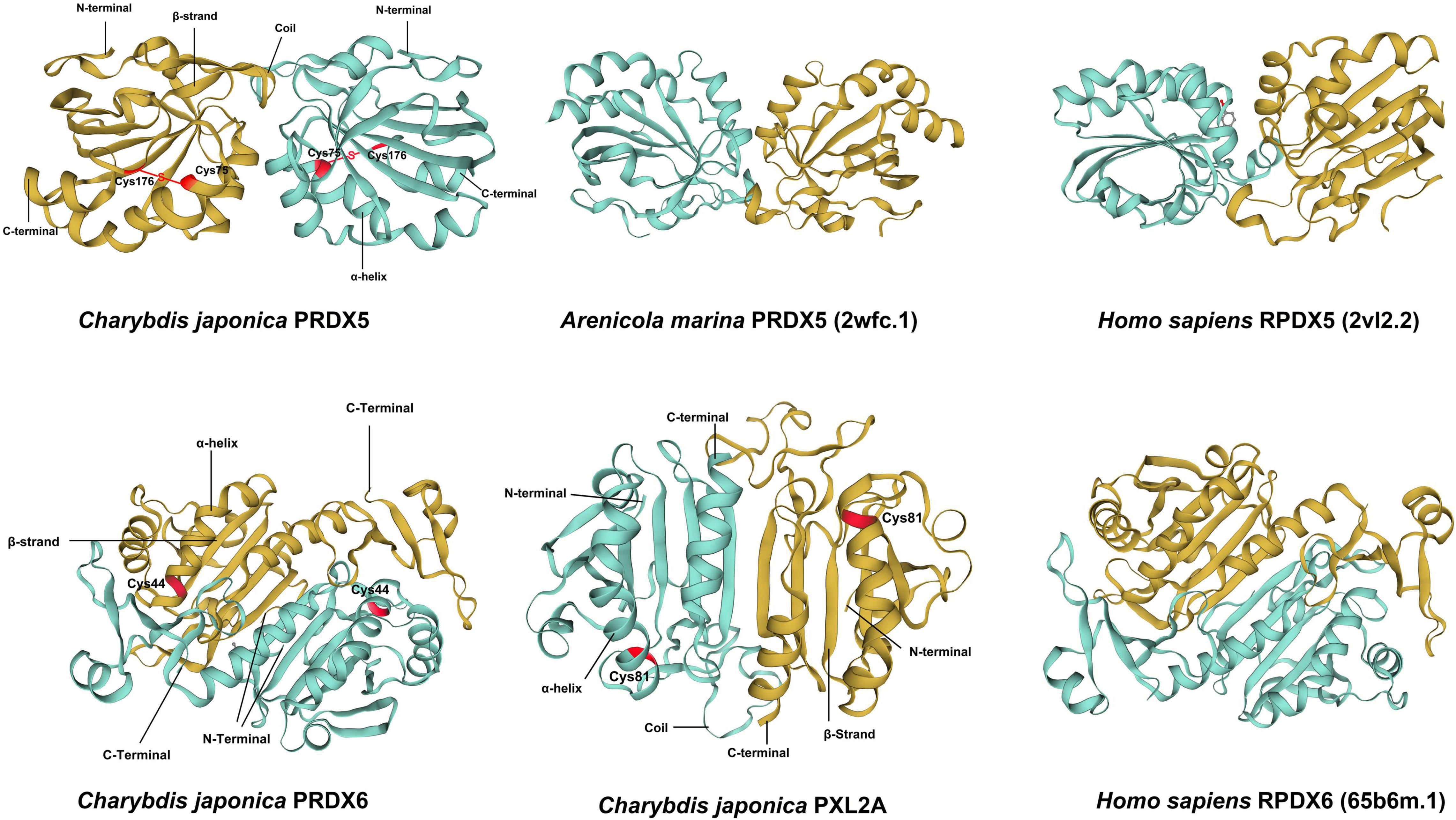
Figure 5. The predicted spatial structures of C. japonica PRDX5, C. japonica PRDX6, C. japonica PXL2A, Arenicola marina PRDX5, Homo sapiens PRDX6, and Homo sapiens PRDX5.
In the process of building the tertiary structure prediction models of C. japonica PRDX6 and PXL2A (Figure 5), we found that there were few relevant reference models. We finally selected the tertiary structure detection results of human PRDX6 (65b6m.1) to build the tertiary junction of C. japonica PRDX6 and PXL2A. The active sites were also predicted. The results showed that C. japonica PRDX6 and PXL2A were unique 1-cys-p. Unlike some 2-cys-PRx, it is found in all organs and all species from bacteria to humans.
Peroxiredoxin Family
The Peroxiredoxin family can be divided into seven subfamilies named PRDX1∼6 and peroxiredoxin-like 2 (Figure 6). The subfamilies of Peroxiredoxin-like 2 include PXL2A, PXL2B, and PXL2C. In C. japonica, we found PRDX1∼6 and PXL2A through omics data comparison. The expression levels of PRDX5, PRDX6, and PXL2A were relatively high, and the C. japonica infected with A. hydrophila soaked with berberine hydrochloride was significantly upregulated. The evolutionary tree of the cloned genes is shown in Figure 7.
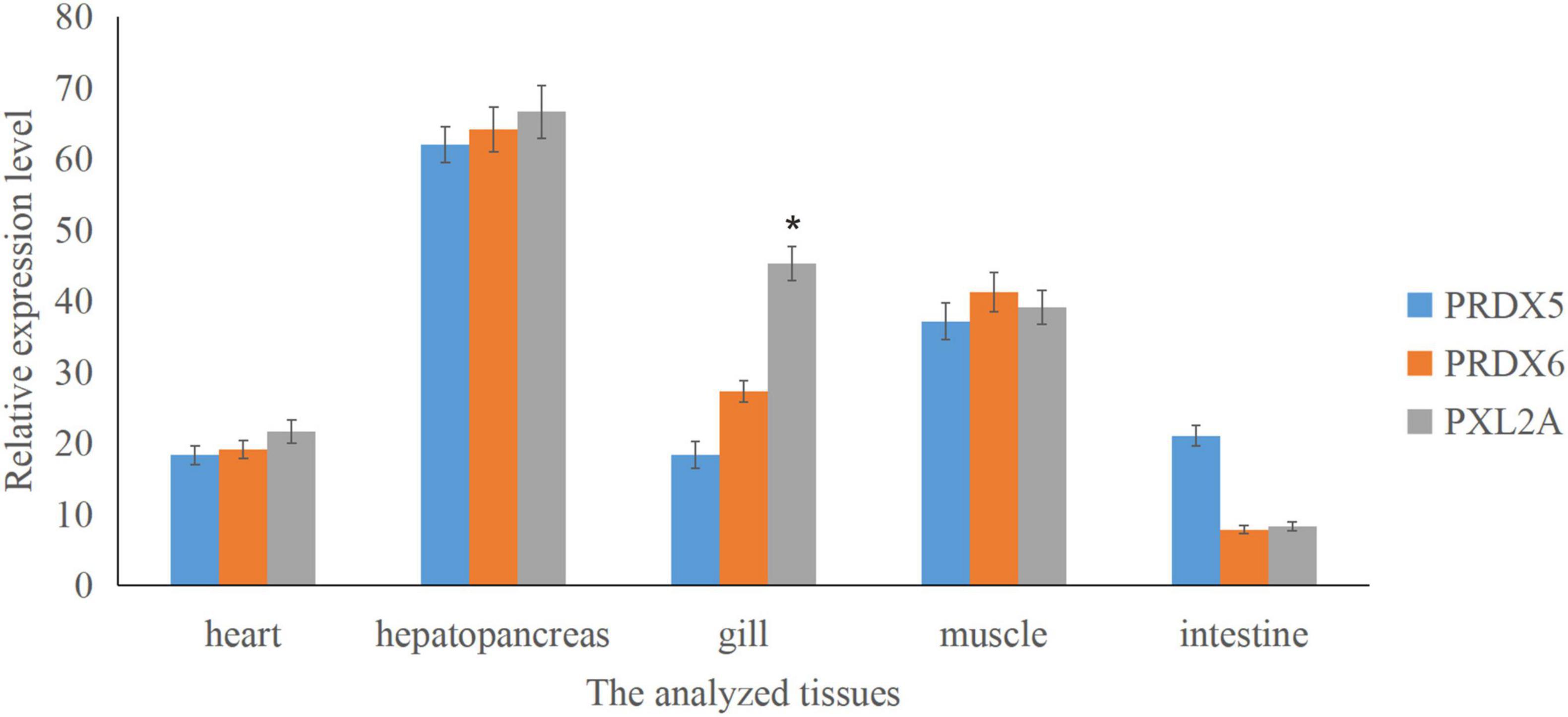
Figure 7. Relative expression of Prx5, Prx6, and PXL2A genes in different tissues of C. japonica is shown. The abscissa is the heart, hepatopancreas, gills, muscles, and intestines. The bar chart shows significant differences (*P < 0.05).
Tissue Expression Profile of Peroxiredoxins
Real-time quantitative PCR expression analysis showed that the transcription levels of Prx5, Prx6, and PXL2A were generally expressed in all the tested tissues, and the expression patterns were different in different tissues. As shown in Figure 7, transcription levels of Prx5, Prx6, and PXL2A are higher in the gills, hepatopancreas, and muscles, and lower in the intestine and heart. The hepatopancreas is the immune organ of C. japonica. In Figure 8, the transcription levels of Prx5, Prx6, and PXL2A increased stepwise in 100, 200, and 300 mg/L berberine hydrochloric acid-soaked hepatopancreas of C. japonica. In 300 mg/L berberine hydrochloric acid, the C. japonica infected with A. hydrophila had lower levels of Prx5 and Prx6 than the control group, while transcriptional level of PXL2A was just the opposite. The transcription levels of Prx5, Prx6, and PXL2A in the hepatopancreas of C. japonica soaked with 100, 200, and 300 mg/L berberine hydrochloride and injected with 105 CFU/L A. hydrophila, increased incrementally.
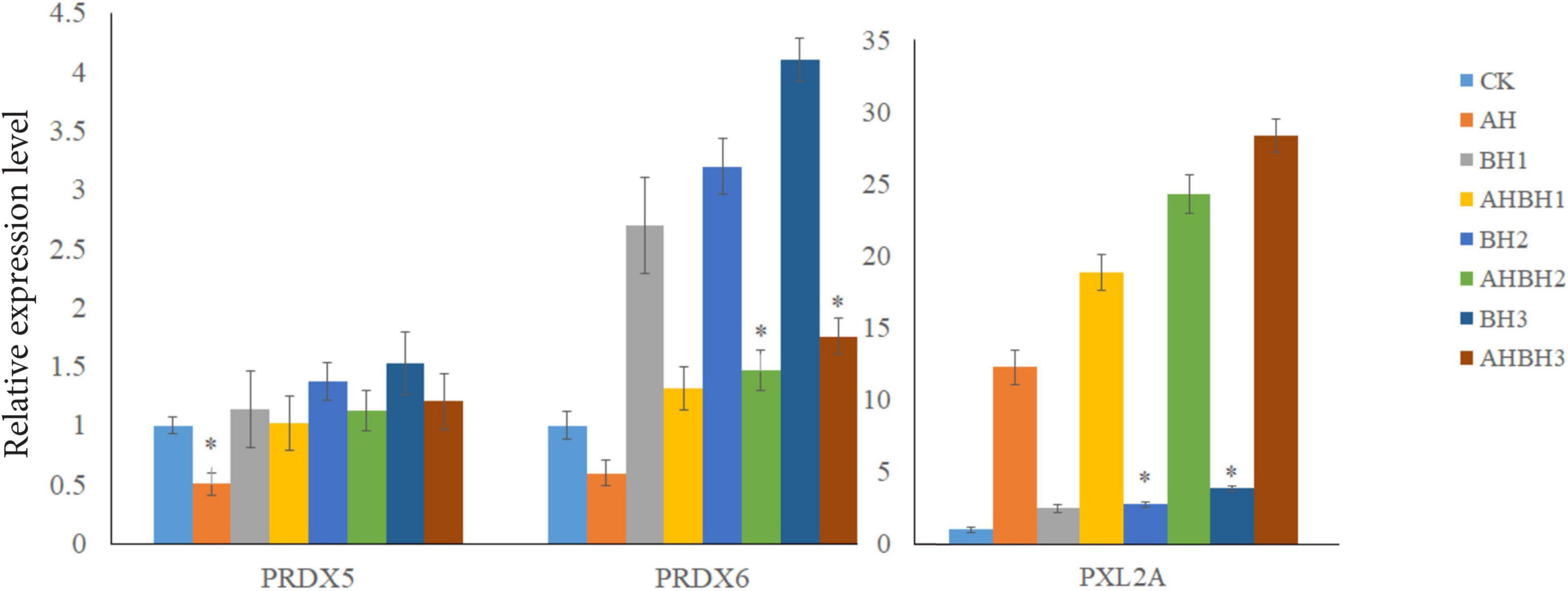
Figure 8. Relative expression of Prx5, Prx6, and PXL2A genes in the hepatopancreas of C. japonica. Infected with A. hydrophila (AH), C. japonica immersed in berberine hydrochloride at 100 mg/L (BH1) and 200 mg/L (BH2), C. japonica immersed in berberine hydrochloride at 300 mg/L (BH3), C. japonica immersed in berberine hydrochloride at 100 mg/L, and injected with A. hydrophila 1 (BHAH1), C. japonica immersed in berberine hydrochloride at 200 mg/L and injected with A. hydrophila of 105 CFU/L (BHAH2), C. japonica immersed in berberine hydrochloride at 300 mg/L and injected with A. hydrophila (BHAH3), and the control group (CK) at 105 CFU/L. The bar chart shows significant differences (*P < 0.05).
Results of Enzyme Activities of Glutathione Peroxidase, Catalase, Superoxide Dismutase, Glutathione Transferase, Oxidized Glutathione, and Glutathione
As shown in Figure 9, 100, 200, and 300 mg/L berberine hydrochloride soaked C. japonica infected with A. hydrophila significantly increased the activities of SOD, GPX, and CAT in hepatopancreas compared with C. japonica only infected with A. hydrophila and the control group. Supplementation of berberine hydrochloride at 100, 200, and 300 mg/L significantly increased the activity of GST in the hepatopancreas. The activity of GST and GSSG in hepatopancreas was maximized by soaking with 300 mg/L berberine hydrochloride. Supplementation of berberine hydrochloride at 100, 200, and 300 mg/L could affect the GSH content. A high GSH/GSSG ratio was maintained in the experimental group of 200 and 300 mg/L berberine hydrochloride and A. hydrophila at the same time.
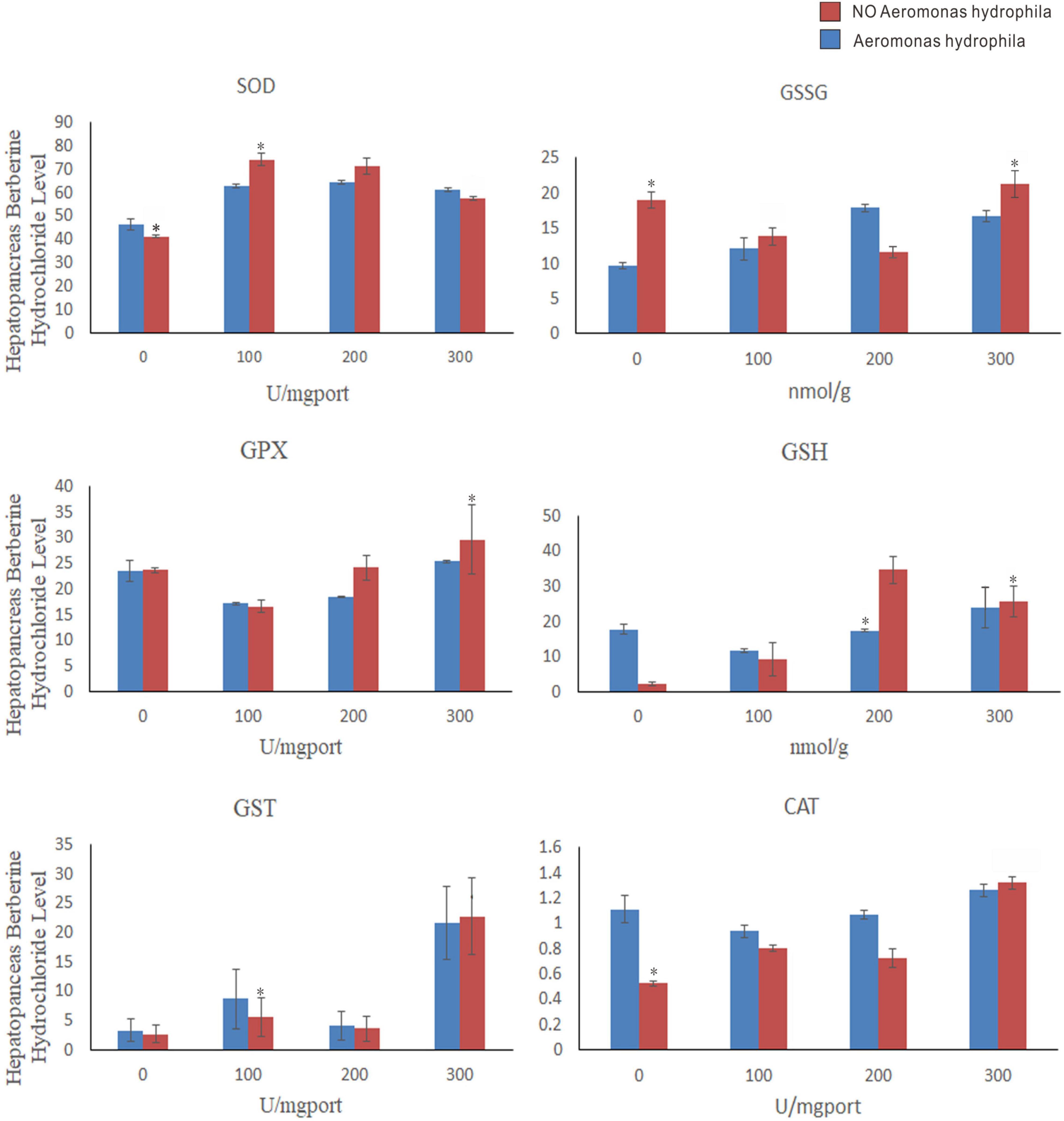
Figure 9. Charybdis japonica (AH) infected with A. hydrophila, crabs immersed in berberine hydrochloride 100 mg/L (BH1), 200 mg/L (BH2), and 300 mg/L berberine hydrochloride (BH3), 100 mg/L berberine hydrochloride and injected with 1 A. hydrophila (BHAH1), 200 mg/L berberine hydrochloride and injected with 105 CFU/L A. hydrophila (BHAH2). Crabs immersed in berberine hydrochloride 300 mg/L and injected with A. hydrophila (BHAH3) and control group CK at 105 CFU/L. Hepatopancreas were used to measure the enzymes and their contents. Each value represents the mean ± SEM (n = 4 for A. hydrophila injection group; N = 4 for the control). *Significantly different (P < 0.05) from control values.
Discussion
Peroxiredoxins (Prxs) are an important class of peroxidases that are involved in antioxidant protection and redox signaling (Ledgerwood et al., 2017). They use conserved Cys residues to reduce the peroxide substrate. Prxs have very high catalytic efficiency, making them major players in cell-wide hydrogen peroxide reduction. Prxs play an important role in a variety of diseases because reactive oxygen species are involved in many cellular metabolic and signaling processes. In this study, the Prx family members PRDX5, PRDX6, and PXL2A were studied. The likely active site in PRDX5 is indicated by a red circle in Figure 6. The results showed that the active site of C. japonica PRDX5 was a conserved redox-active cysteine residue Cys75, i.e., cysteine peroxide [C (P)], which subjected the peroxide substrate to nucleophilic activity. Hydrogen peroxide oxidizes C (P) -SH to cysteine sulfonic acid [C (P) -SOH], which then forms a disulfide bond with another cysteine residue Cys176 decomposing cysteine [C (R)]. The cys75-s-cys176 disulfide is then reduced by the appropriate electron donor to complete the catalytic cycle (Declercq et al., 2001). In this atypical 2-cys-PRx, C (R) is present in the same subunit to form an intramolecular disulfide. The disulfide is then reduced by thioredoxin (Hall et al., 2010). Both conserved Cys residues are required for their catalytic function, suggesting that Prx5 can be used as a reducing agent to reduce cell metabolism and oxidative stress (Wood et al., 2003). PRDX6 has a possible active site (red circle, Figure 6). Of the six Prxs, Prx6 has 1-cys-PRx, and unlike the 2-cys-PRx of PRDX5, it is present in all organs and all species from bacteria to humans (Poole and Nelson, 2016). In addition, Prx6 is unique in that it has Ca20 + -independent phospholipase A2 (PLA2) activity; Ser32, His26, and Asp140 of Prx6 are believed to be involved in PLA2 activity. Ser32 is involved in liposome binding and hydrolysis, while His26 is only involved in binding, and Asp140 is involved in catalysis (Manevich et al., 2007). Phosphorylation of Prx6 at Thr177 in the C-terminal domain may lead to necessary structural changes. When phospholipids bind to the monomer Prx6, the phospholipids points toward the PLA2 active site and the acyl chain points toward the peroxide active site (Fisher, 2010). PXL2A is an antioxidant molecule that protects cells from oxidative damage (Gong et al., 2013). PXL2A has a key region that activates thioredoxin, an antioxidant protein that protects cells from oxidative stress (Yu et al., 2001; Chen et al., 2016). In this experiment, Prx5 and Prx6 of C. japonica infected with A. hydrophila had lower transcriptional levels and the antioxidant capacity in hepatopancreas was also lower than the control group. The hepatopancreatic transcription level of C. japonica was significantly improved after the addition of 100, 200, and 300 mg/L berberine hydrochloride. The transcription levels of C. japonica PXL2A infected with A. hydrophila were all increased compared with the control group, while the hepatopancreatic transcription levels of C. japonica were further increased by adding 100, 200, and 300 mg/L berberine hydrochloride. From the above conclusions and considerations, berberine hydrochloride can improve the hepatopancreas expression of Prx5, Prx6, and PXL2A genes in C. japonica to enhance resistance to oxidative stress. Enhance oxidative stress by activating 1-Cys-Prx and 2-Cys-prx active sites. The strongest ability to oxidize stress was observed at 300 mg/L berberine hydrochloride.
Glutathionesylated Prx6 complexes are regenerated by glutathione into a new active form (Manevich et al., 2004; Ralat et al., 2006). A study that detected Prx6 and GST amino acid fragments by fluorescence titration found that Pro40-Cys47 and Leu148-PHE157 of Prx6 interact with GST (Kim et al., 2016). The Prx6 active site may be involved in the GST interaction, making it easier for GSH to enter the active site in the active state. The active site of PRDX6 was Cys44, and that of PXL2A was Cys81. C(P) is reactivated by glutathione s-transferase PI-mediated glutathione conversion, followed by spontaneous reduction of the enzyme by glutathione (Kim et al., 2016). Physiological activation of 1-Cysprx requires the reaction of its heterodimer with GST, then oxidized glutathione to cys-44, and then the heterodimer is separated to obtain glutamate mercaptosylated protein, which is catalyzed by spontaneous reduction of glutathione to complete 1-Cysprx activation (Manevich et al., 2004). Glutathione is used for detoxification by GST and glutathione peroxidase (GPx) is used for H2O2 degradation (Winston and Giulio, 1991). High levels of GSH are maintained through de novo synthesis and GR-dependent GSSG cycling back to GSH (Zhu et al., 2013). In this study, immersion of the crabs in berberine hydrochloride at 100, 200, and 300 mg/L could significantly increase the activity of GST in the hepatopancreas of C. japonica. It was found that 300 mg/L berberine hydrochloride in C. japonica infected with A. hydrophila maximized the activity of GST and GSSG in hepatopancreas. Different concentrations of berberine hydrochloride could affect the content of GSH. GSH/GSSG provides antioxidant protection in various disease models. A high GSH/GSSG ratio maintains normal cell function and prevents oxidative stress damage (Zhu et al., 2013). A higher GSH/GSSG ratio was maintained in the experimental group of C. japonica infected with A. hydrophila by immersion of berberine hydrochloride at 200 and 300 mg/L in this experiment. The activities of GST,GSSG and GSH in hepatopancreas of Acipenser japonicus soaked with berberine hydrochloride at 100 mg/L, 200 mg/L and 300 mg/L were significantly increased.
Superoxide dismutase and catalase are involved in a variety of physiological and metabolic reactions, especially scavenging free radicals and preventing biomolecular damage (Simon et al., 1974). GPX is an intracellular and cytoplasmic enzyme that degrades most hydrogen peroxide and, in the presence of reducing glutathione, converts it to oxidizing glutathione and water as well as catalase (CAT) (Leitão and Alcantara-Gomes, 1994). In general, CAT is an enzyme that converts high concentrations of H2O2 into water and oxygen. However, when H2O2 is present at low concentrations, GPX can control this transformation (Halliwell, 1992). GPX can eliminate H2O2 at low concentrations, while CAT can only eliminate H2O2 at high concentrations (Kang et al., 2005). Therefore, in our study, berberine hydrochloride immersion of C. japonica infected with A. hydrophila and the control group (CK), significantly increased the activities of SOD, CAT, and GPX in the hepatopancreas. It was found that 300 mg/L berberine hydrochloride can maximize the activation of GPX and CAT to help C. japonica fight against A. hydrophila infection and can degrade high and low concentrations of H2O2 in the crab. In this study, 100, 200, and 300 mg/L berberine hydrochloride significantly increased SOD activity in the hepatopancreas compared with the control group. Berberine hydrochloride can affect the activity of SOD, to improve its antioxidant capacity, and help C. japonica to challenge the A. hydrophila infection process.
Conclusion
In summary, the full-length cDNA sequence of Prx6 and the ORF sequence of Prx5 and PXL2A were obtained to analyze the evolutionary relationships of the Peroxiredoxin family. Berberine hydrochloride can enhance the expression of Prx5, Prx6, and PXL2A genes in the hepatopancreas of C. japonica to enhance the antioxidant ability. It was found that berberine hydrochloride could increase the activities of GPX, CAT, and GST in the hepatopancreas of C. japonica. The results of this study provide a new understanding of the role of berberine hydrochloride on the oxidative stress mechanism in crustaceans and a new scientific basis for the potential use of berberine hydrochloride in protecting crabs against bacterial infection and its application in aquaculture. In addition, secondary metabolites may provide an alternative to antibiotics in the treatment of aquatic animal bacterial diseases.
Data Availability Statement
The datasets presented in this study can be found in online repositories. The names of the repository/repositories and accession number(s) can be found below: https://www.ncbi.nlm.nih.gov/, MZ611745, MZ611746, and MZ611747.
Ethics Statement
The animal study was reviewed and approved by Charybdis japonica is a crustacean. Written informed consent was obtained from the owners for the participation of their animals in this study.
Author Contributions
FJ and YL designed the study and wrote the manuscript. CZ, TG, and ZZ conducted the feeding trial. XS and QJ did the qPCR studies. All authors read and approved the final version of the manuscript.
Funding
This work was supported by the National Key R&D Program of China (2020YFD0900305), Science Foundation of Jiangsu (No. BK20191488) in China, Major Project of Hydrobios Resources in Jiangsu Province (ZYHB16-3), and Agricultural Major New Variety Creation Project in Jiangsu Province (PZCZ201743).
Conflict of Interest
The authors declare that the research was conducted in the absence of any commercial or financial relationships that could be construed as a potential conflict of interest.
Publisher’s Note
All claims expressed in this article are solely those of the authors and do not necessarily represent those of their affiliated organizations, or those of the publisher, the editors and the reviewers. Any product that may be evaluated in this article, or claim that may be made by its manufacturer, is not guaranteed or endorsed by the publisher.
Acknowledgments
The authors would like to thank Mengling Sun for help with sampling.
Footnotes
- ^ http://bioinfo.ut.Ee/primer3-0.4.0
- ^ http://www.ncbi.nlm.nih.gov/gorf/orfig.cgi
- ^ https://web.expasy.org/protscale/
- ^ http://www.cbs.dtu.dk/services/SignalP/
References
Anna, V. D. K., Viktor, K., and Jaroslav, T. (1995). Superoxide radical and superoxide dismutases. Annu. Rev. Biochem. 64, 97–112. doi: 10.1146/annurev.bi.64.070195.000525
Arockiaraj, J., Easwvaran, S., Vanaraja, P., Singh, A., Othman, R. Y., and Bhassu, S. (2012). Immunological role of thiol-dependent peroxiredoxin gene in Macrobrachium rosenbergii. Fish Shellfish Immunol. 33, 121–129. doi: 10.1016/j.fsi.2012.04.010
Bonami, J. R., and Zhang, S. (2011). Viral diseases in commercially exploited crabs: a review. J. Invertebr. Pathol. 106, 6–17. doi: 10.1016/j.jip.2010.09.009
Castanon, J. (2007). History of the use of antibiotic as growth promoters in European poultry feeds. Poul. Sci. 86, 2466–2471. doi: 10.3382/ps.2007-00249
Chen, Y.-F., Yang, C.-C., Kao, S.-Y., Liu, C.-J., and Lin, S.-C. (2016). MicroRNA-211 enhances the oncogenicity of carcinogen-induced oral carcinoma by repressing TCF12 and increasing antioxidant activity. Can. Res. 76, 4872–4886. doi: 10.1158/0008-5472.CAN-15-1664
Declercq, J.-P., Evrard, C., Clippe, A., Vander Stricht, D., Bernard, A., and Knoops, B. (2001). Crystal structure of human peroxiredoxin 5, a novel type of mammalian peroxiredoxin at 1.5 Å resolution. J. Mol. Biol. 311, 751–759. doi: 10.1006/jmbi.2001.4853
Domadia, P. N., Bhunia, A., Sivaraman, J., Swarup, S., and Dasgupta, D. (2008). Berberine targets assembly of Escherichia coli cell division protein FtsZ. Biochemistry 47, 3225–3234. doi: 10.1021/bi7018546
Du, G.-F., Le, Y.-J., Sun, X., Yang, X.-Y., and He, Q.-Y. (2020). Proteomic investigation into the action mechanism of berberine against Streptococcus pyogenes. J. Proteom. 215:103666. doi: 10.1016/j.jprot.2020.103666
Duan, Y., Liu, P., Li, J., Li, J., Gao, B., and Chen, P. (2013). cDNA cloning, characterization and expression analysis of peroxiredoxin 5 gene in the ridgetail white prawn Exopalaemon carinicauda. Mol. Biol. Rep. 40, 6569–6577. doi: 10.1007/s11033-013-2702-4
Dupont, H. L. (2007). The growing threat of foodborne bacterial enteropathogens of animal origin. Clin. Infect. Dis. 45, 1353–1361. doi: 10.1086/522662
Fisher, A. B. (2010). Peroxiredoxin 6: a bifunctional enzyme with glutathione peroxidase and phospholipase A 2 Activities. Antioxid. Redox Signal. 15, 831–844. doi: 10.1089/ars.2010.3412
Gong, J., Zhang, J., Li, B., Zeng, C., You, K., Chen, M., et al. (2013). MicroRNA-125b promotes apoptosis by regulating the expression of Mcl-1, Bcl-w and IL-6R. Oncogene 32, 3071–3079. doi: 10.1038/onc.2012.318
Gonzalez-Escalona, N., Blackstone, G. M., and Depaola, A. (2006). Characterization of a Vibrio alginolyticus strain, isolated from alaskan oysters, carrying a hemolysin gene similar to the thermostable direct hemolysin-related hemolysin gene (trh) of Vibrio parahaemolyticus. Appl. Environ. Microbiol. 72, 7925–7929. doi: 10.1128/AEM.01548-06
Guido, V., Hang, Z., Sebastian, L., Cesare, D. F., Tang, K., David, L. H., et al. (2015). A novel candidate region for genetic adaptation to high altitude in andean populations. PLoS One 10:e0125444. doi: 10.1371/journal.pone.0125444
Habtemariam, S. (2016). Berberine and inflammatory bowel disease: a concise review. Pharmacol. Res. 113, 592–599. doi: 10.1016/j.phrs.2016.09.041
Hall, A., Parsonage, D., Poole, L. B., and Karplus, P. A. (2010). Structural evidence that peroxiredoxin catalytic power is based on transition-state stabilization. J. Mol. Biol. 402, 194–209. doi: 10.1016/j.jmb.2010.07.022
Halliwell, B. (1992). Reactive oxygen species and the central nervous system. J. Neurochem. 59, 1609–1623.
Igbinosa, I. H., Igumbor, E. U., Aghdasi, F., Tom, M., and Okoh, A. I. (2012). Emerging aeromonas species infections and their significance in public health. Sci. World J. 2012:625023. doi: 10.1100/2012/625023
Jithendran, K., Poornima, M., Balasubramanian, C., and Kulasekarapandian, S. (2010). Diseases of mud crabs (Scylla spp.): an overview. Indian J. Fish 57, 55–63.
Kang, K. W., Lee, S. J., and Kim, S. G. (2005). Molecular mechanism of nrf2 activation by oxidative stress. Antioxid. Redox Signal. 7, 1664–1673. doi: 10.1089/ars.2005.7.1664
Kang, S., Li, Z., Yin, Z., Jia, R., Song, X., Li, L., et al. (2015). The antibacterial mechanism of berberine against Actinobacillus pleuropneumoniae. Nat. Prod. Res. 29, 2203–2206. doi: 10.1080/14786419.2014.1001388
Kim, K. H., Lee, W., and Kim, E. K. (2016). Crystal structures of human peroxiredoxin 6 in different oxidation states. Biochem. Biophys. Res. Commun. 477, 717–722. doi: 10.1016/j.bbrc.2016.06.125
Lazreg Aref, H., Gaaliche, B., Fekih, A., Mars, M., Aouni, M., Pierre Chaumon, J., et al. (2011). In vitro cytotoxic and antiviral activities of Ficus carica latex extracts. Nat. Prod. Res. 25, 310–319. doi: 10.1080/14786419.2010.528758
Ledgerwood, E. C., Marshall, J., and Weijman, J. F. (2017). The role of peroxiredoxin 1 in redox sensing and transducing. Arch. Biochem. Biophys. 617, 60–67. doi: 10.1016/j.abb.2016.10.009
Leitão, A., and Alcantara-Gomes, R. (1994). Radiobiologia e Fotobiologia: Respostas Celulares às Lesões Induzidas por Agentes Físicos e Químicos. Rio de Janeiro: Universidade Federal do Rio de Janeiro.
Liu, J.-D., Liu, W.-B., Zhang, D.-D., Xu, C.-Y., Zhang, C.-Y., Zheng, X.-C., et al. (2020). Dietary reduced glutathione supplementation can improve growth, antioxidant capacity, and immunity on Chinese mitten crab, Eriocheir sinensis. Fish Shellfish Immunol. 100, 300–308. doi: 10.1016/j.fsi.2020.02.064
Lu-Yang, L. I., Long, W. F., Wan, D. R., Zhang, F., Ding, Q., and Mei, Z. N. (2014). Quantitative Determination of Total Alkaloids and Berberine in Five Medicinal Berberis Plants. Chinese Journal of Experimental Traditional Medical Formulae. Berlin: Springer.
Manevich, Y., Feinstein, S. I., and Fisher, A. B. (2004). Activation of the antioxidant enzyme 1-CYS peroxiredoxin requires glutathionylation mediated by heterodimerization with πGST. Proc. Natl. Acad. Sci. U.S.A. 101, 3780–3785. doi: 10.1073/pnas.0400181101
Manevich, Y., Reddy, K. S., Shuvaeva, T., Feinstein, S. I., and Fisher, A. B. (2007). Structure and phospholipase function of peroxiredoxin 6: identification of the catalytic triad and its role in phospholipid substrate binding. J. Lipid Res. 48, 2306–2318. doi: 10.1194/jlr.M700299-JLR200
Martínez, J. (2009). Antibiotics and antibiotic resistance genes in natural environments. Science 321, 365–367. doi: 10.1126/science.1159483
Peng, L., Kang, S., Yin, Z., Jia, R., Song, X., Li, L., et al. (2015). Antibacterial activity and mechanism of berberine against Streptococcus agalactiae. Int. J. Clin. Exp. Pathol. 8:5217.
Poole, L. B., and Nelson, K. J. (2016). Distribution and features of the six classes of peroxiredoxins. Mol. Cells 39, 53–59. doi: 10.14348/molcells.2016.2330
Ralat, L. A., Manevich, Y., Fisher, A. B., and Colman, R. F. (2006). Direct evidence for the formation of a complex between 1-cysteine peroxiredoxin and glutathione S-transferase pi with activity changes in both enzymes. Biochemistry 45, 360–372. doi: 10.1021/bi0520737
Seal, B. S., Lillehoj, H. S., Donovan, D. M., and Gay, C. G. (2013). Alternatives to antibiotics: a symposium on the challenges and solutions for animal production. Anim. Health Res. Rev. 14, 78–87. doi: 10.1017/s1466252313000030
Simon, L. M., Fatrai, Z., Jonas, D. E., and Matkovics, B. (1974). Study of peroxide metabolism enzymes during the development of phaseolus vulgaris1). Biochem. Physiol. Pflanzen 166, 387–392. doi: 10.1016/s0015-3796(17)30073-2
Tu, D. D., Jiang, M., Gu, W. B., Zhou, Y. L., Zhu, Q. H., Zhou, Z. K., et al. (2017). Identification and characterization of atypical 2-cysteine peroxiredoxins from mud crab Scylla paramamosain the first evidence of two peroxiredoxin 5 genes in non-primate species and their involvement in immune defense against pathogen infection. Fish Shellfish Immunol. 69, 119–127. doi: 10.1016/j.fsi.2017.07.040
Wang, W. (2011). Bacterial diseases of crabs: a review. J. Invertebr. Pathol. 106, 18–26. doi: 10.1016/j.jip.2010.09.018
Wang, X., Feng, S., Ding, N., He, Y., Li, C., Li, M., et al. (2018). Anti-inflammatory effects of berberine hydrochloride in an LPS-induced murine model of mastitis. Evid. Based Complement. Alternat. Med. 2018:5164314. doi: 10.1155/2018/5164314
Winston, G. W., and Giulio, R. (1991). Prooxidant and antioxidant mechanisms in aquatic organisms. Aquat. Toxicol. 19, 137–161. doi: 10.1016/0166-445x(91)90033-6
Wood, Z. A., Schröder, E., Harris, J. R., and Poole, L. B. (2003). Structure, mechanism and regulation of peroxiredoxins. Trends Biochem. Sci. 28, 32–40. doi: 10.1016/s0968-0004(02)00003-8
Wu, L., Zhou, Y., Abbas, M. N., Kausar, S., Chen, Q., Jiang, C. X., et al. (2017). Molecular structure and functional characterization of the peroxiredoxin 5 in Procambarus clarkii following LPS and Poly I:C challenge. Fish Shellfish Immunol. 71, 28–34. doi: 10.1016/j.fsi.2017.09.072
Xia, X. A., Wu, Q. Y., and Li, Y. Y. (2010). Isolation and identification of two bacterial pathogens from mixed infection mud crab Scylla serrata and drug therapy. J. Trop. Oceanogr. 29, 103–110.
Xiao, C. W., Liu, Y., Wei, Q., Ji, Q. A., Li, K., Pan, L. J., et al. (2019). Inhibitory Effects of berberine hydrochloride on Trichophyton mentagrophytes and the underlying mechanisms. Molecules 24:742. doi: 10.3390/molecules24040742
Yu, Y., Zhang, C., Zhou, G., Wu, S., Qu, X., Wei, H., et al. (2001). Gene expression profiling in human fetal liver and identification of tissue- and developmental-stage-specific genes through compiled expression profiles and efficient cloning of full-length cDNAs. Genome Res. 11, 1392–1403. doi: 10.1101/gr.175501
Zhang, S. L., Chang, J. J., Damu, G. L. V., Geng, R. X., and Zhou, C. H. (2013). Berberine azoles as antimicrobial agents: synthesis, biological evaluation and their interactions with human serum albumin. Med. Chem. Commun. 4, 839–846. doi: 10.1016/j.bmc.2013.05.007
Zhu, H., Wang, J., Santo, A., and Li, Y. (2013). “Downregulation of antioxidants and phase 2 proteins,” in Molecular Basis of Oxidative Stress: Chemistry, Mechanisms, and Disease Pathogenesis, ed. F. A. Villamena (Hoboken, NJ: John Wiley & Sons, Inc), 113–122. doi: 10.1002/9781118355886.ch5
Keywords: Prx6, C. japonica, peroxiredoxins, A. hydrophila, berberine hydrochloride
Citation: Han M, Gao T, Liu Y, Zuraini Z, Zhu C, Zhang T, Ji F, Jiang Q and Sun X (2021) Molecular Characterization of Prdx Family and Response of Antioxidant Enzymes in Berberine Hydrochloride-Treated Charybdis japonica Infected With Aeromonas hydrophila. Front. Mar. Sci. 8:784205. doi: 10.3389/fmars.2021.784205
Received: 27 September 2021; Accepted: 19 November 2021;
Published: 08 December 2021.
Edited by:
Li Zhang, South China Sea Institute of Oceanology, Chinese Academy of Sciences (CAS), ChinaReviewed by:
Huang Wei, Second Institute of Oceanography, Ministry of Natural Resources, ChinaXiaodan Wang, East China Normal University, China
Copyright © 2021 Han, Gao, Liu, Zuraini, Zhu, Zhang, Ji, Jiang and Sun. This is an open-access article distributed under the terms of the Creative Commons Attribution License (CC BY). The use, distribution or reproduction in other forums is permitted, provided the original author(s) and the copyright owner(s) are credited and that the original publication in this journal is cited, in accordance with accepted academic practice. No use, distribution or reproduction is permitted which does not comply with these terms.
*Correspondence: Qichen Jiang, qichenjiang@live.cn; Xian Sun, sunx27@mail.sysu.edu.cn