- 1University of South Bohemia in České Budějovice, Faculty of Fisheries and Protection of Waters, South Bohemian Research Center of Aquaculture and Biodiversity of Hydrocenoses, Institute of Aquaculture and Protection of Waters, České Budějovice, Czechia
- 2Department of Chemistry, Biology and Biotechnology, University of Perugia, Perugia, Italy
- 3Department of Veterinary Science, University of Torino, Turin, Italy
- 4Department of Agricultural, Forest and Food Sciences, University of Torino, Turin, Italy
- 5Institute of Sciences of Food Production, National Research Council, Turin, Italy
Effects of feeding dietary defatted black soldier fly (Hermetia illucens) larvae meal (HI) on intestine microbiota, and on histomorphology, oxidative enzyme activities in liver and intestine of pikeperch (Sander lucioperca) were investigated. Four isoproteic (45% crude protein) and isolipidic (18% ether extract) diets were formulated to include 0% (CO), 9% (HI9), 18% (HI18) and 36% (HI36) of HI as replacement for fishmeal at 0, 25, 50, and 100%, respectively, and were fed to triplicate groups of juvenile pikeperch (initial body weight, 68.7 ± 7.1 g) for 84 days. No adverse effects were detected on the intestine of pikeperch fed diet groups, in terms of histomorphology (P > 0.05), while fish fed free or low levels of HI (≤ 9% in diet) showed significant liver degeneration (P < 0.05). Dietary HI significantly affected the oxidative enzyme activities of catalase and glutathione peroxidase in the liver, and glutathione S-transferase in the intestine (P < 0.05), while activity of superoxide dismutase in both liver and intestine was HI-dose independent (P > 0.05). Feeding HI-containing diets positively modulated the richness and diversity of intestinal microbiota, especially for HI18 group (P < 0.05). Inclusion HI up to 18% (50% fishmeal replacement) in pikeperch diets increased abundance of Clostridium, Oceanobacillus, Bacteroides, and Faecalibacterium genera, whereas the predominant bacterium, Cetobacterium was found in control and HI36 groups. This study reveals the potential of HI as an immune and health booster for juvenile pikeperch.
Introduction
Aquaculture is the largest global consumer of fishmeal production, accounting for 68–73% (Shepherd and Jackson, 2013; Tacon and Metian, 2015). Fishmeal is mainly derived from marine capture fisheries (70% in 2018) (FAO, 2020a), which has reached a plateau since the 2000s (Shepherd and Jackson, 2013) and has been projected that the ecological limits of stock will be reached by 2037 (Froehlich et al., 2018a). Therefore, the current fastest growth of aquaculture in food-producing sectors (FAO, 2020a) and the continuous increasing trend, requires the development of novel aquafeed ingredients. Terrestrial crops have been used in aquafeeds more than other alternatives until recent (Tacon et al., 2011; Tacon and Metian, 2015) and, by 2050, the use of these feedstuffs in aquaculture will rise to twice the current level in a business-as-usual scenario, reaching 91 million tonnes (Froehlich et al., 2018b). However, crop-based feeds for aquatic animals introduce concerns regarding their nutritional properties and environmental consequences. An unbalanced essential amino acid profile, low palatability, and the presence of anti-nutritional substances could impair their inclusion in aquafeeds (Gatlin et al., 2007). Moreover, the expansion and intensification of the production of terrestrial crops will lead to tremendous environmental burdens pertaining to climate change, biodiversity loss, and increasing demand for arable land and water. Among such burdens, land use is considered the one that entails the greatest pressures on the planet (Foley et al., 2005, 2011; Boissy et al., 2011). Beyond terrestrial plant ingredients, fishery by-products and insect meals have shown the greatest potential to be protein-supplied to aquafeeds in the coming years (Hua et al., 2019; Gasco et al., 2020a). Although approximately 34% of the world’s fishmeal production will be derived from fish by-products by 2030 (FAO, 2020a), this potential protein source will still not be able to meet the projected aquafeed demand by 2050 (Froehlich et al., 2018a). The efficiency of insect meal as a future aquafeed ingredient has already been identified, especially concerning the feasibility of costs, scalability, and processing technology (Hua et al., 2019). Globally, insect production is on the rise, and will reach approximately 1.2 million tonnes by 2025 and become price-competitive with fishmeal by 2023 (Hua et al., 2019; Gasco et al., 2020a). In addition, the development of production facilities and processing techniques would help to improve the environmental performance of insect meal as a sustainable aquafeed ingredient (van Huis and Oonincx, 2017). The use of seven insect species (two flies, two mealworms, and three cricket species) in fish diets has been authorised by the European Commission (Regulation No. 2017/893). Among these species, black soldier fly (Hemertia illucens), which belongs to the Diptera order, has received the most research interest (Hua, 2021). Hemertia illucens larvae meal possesses important nutritional profiles, especially amino acid profile which is close to that of fishmeal (Nogales-Mérida et al., 2019). As far as environmental impact is concerned, H. illucens production, if obtained using non-valorised substrates, entails significantly less arable land and water use than soybean meal (Smetana et al., 2019; Gasco et al., 2020b). Moreover, H. illucens meal-containing diets have shown lower environmental impacts associated with abiotic depletion, acidification potential, eutrophication potential, climate change, human toxicity potential, and marine aquatic ecotoxicity potential for Arctic char (Salvelinus alpinus) (Smárason et al., 2017) and lower water use for European perch (Perca fluviatilis) (Stejskal et al., 2020) than insect-free diets.
The substitution of fishmeal with H. illucens meal in aquafeeds for the largest fishmeal consumers has already been investigated, and substitution levels have been achieved that do not delay growth production of the tested species, including, white leg shrimp (Litopenaeus vannamei) (60% plausible substitution) (Cummins et al., 2017), Atlantic salmon (Salmon salar) (85–100%) (Lock et al., 2016; Belghit et al., 2018, 2019), European seabass (Dicentrarchus labrax) (45%) (Magalhães et al., 2017), barramundi (Lates calcarifer) (50%) (Katya et al., 2017), and rainbow trout (Oncorhynchus mykiss) (45%) (Sealey et al., 2011; Renna et al., 2017; Dumas et al., 2018). In addition, dietary H. illucens meal has been proved to modulate bacterial diversity and richness, which play essential roles in nutrition, immunology, and health status of fish, such as rainbow trout (O. mykiss) (Bruni et al., 2018; Huyben et al., 2019; Rimoldi et al., 2019; Terova et al., 2019; Rimoldi et al., 2021), and zebrafish (Danio rerio) (Zarantoniello et al., 2020b). The gut health benefit of insect-fed fish has been confirmed to be suitable for species that naturally feed on insect (Antonopoulou et al., 2019; Gasco et al., 2020c).
Pikeperch (Sander lucioperca) is one of the main percid species that has drawn a great deal of attention in aquaculture (Schulz et al., 2006). Aquaculture production of pikeperch reached 1557 tonnes in 2018, which was doubled that of 2009 (750 tonnes) (FAO, 2020b), and has mainly been established in intensive recirculation systems (Dalsgaard et al., 2013). However, pikeperch and other percid fish have so far received very little attention from feed manufacturers (Bochert, 2020). Although some commercial aquafeeds for percids have become available, salmonids-targeted feeds are more widely used in practice (Stejskal et al., 2016). Since European pikeperch aquaculture is moving toward an established freshwater aquaculture sector (Policar et al., 2019), it will be necessary to develop suitable and sustainable feeds for aforementioned sector. Dietary protein requirements of at least 43% have been reported for appropriate growth performance and feed utilization of pikeperch fingerling (Nyina-Wamwiza et al., 2005). In the nature, aquatic insects, i.e., larvae of lake flies (Chironomidae) (Diptera order), play an important role as food sources for the early ontogenetic stages of pikeperch (Vinni et al., 2009; Ginter et al., 2011; Kashinskaya et al., 2018; Huuskonen et al., 2019). Therefore, the use of H. illuces larvae meal has been hypothesised to be suitable for pikeperch aquaculture. The aim of present study is to investigate the effects of dietary defatted black soldier fly (H. illucens) (HI) on the diets of juvenile pikeperch (S. lucioperca) on intestinal microbiota, histomorphology, and oxidative enzyme activities. The outputs could provide information in the choice of an alternative aquafeed ingredient for the emerging percid farming industry in Europe.
Materials and Methods
Ethics Statement
The experimental procedures were performed under European Communities Directive (No. 2010/63/EU) on the protection of animals used for scientific purposes and have been approved by the Czech Ministry of Health (MSMT-6744/2018-2).
Experimental Diets, Rearing Facilities, and Feeding Procedures
The feeding trial was conducted at the wet laboratory of the Faculty of Fisheries and Protection of Waters, University of South Bohemia in České Budějovice, Czech Republic. Defatted HI was obtained from a commercial source (Hermetia Geschäftsführungs GmbH, Baruth/Mark, Germany). Four isoproteic (approximately 45% crude protein) and isolipidic (approximately 18% ether extract) diets were formulated, comprising one fishmeal-based diet (CO) and three other diets, where HI was included at 9% (HI9), 18% (HI18), and 36% (HI36) to replace fishmeal at 25, 50, and 100%, respectively (Table 1). Experimental diets were prepared by a commercial feed producer (Exot Hobby s.r.o., Černá v Pošumaví, Czech Republic) using a dual-screw extruder (Saibainuo, China). Chemical composition of HI and experimental diets as well fatty acid (FA) composition of experimental diets are reported in Tables 1, 2, respectively.
The feeding experiment was conducted in a recirculation aquaculture system (total volume 11400 L), consisting of fifteen 250-L round conical plastic tanks (black walls, white bottom) connected to a mechanical drum filter (AEM 15, AEM-Products V.O.F., Lienden, Netherlands), sedimentation tanks (total volume 2600 l), a series of filtration sections (Bioakvacit PPI10), and a moving bed bio-filter (volume 4700 l, media BT10 Ratz Aqua & Polymer Technik, Remscheid, Germany), under controlled rearing conditions, with water temperature of 23.1 ± 1.0°C, photoperiod of 12h light – 12h dark, light intensity of 20–35 Lux, oxygen saturation of 98.4 ± 15.2%, and pH of 6.98 ± 0.28. Moreover, the concentration of nitrite-N, nitrate-N, and ammonia-N concentration were maintained at 0.42 ± 0.24, 48.8 ± 21.3, and 1.89 ± 0.58 mg/l, respectively.
The prepared diets were fed to triplicate groups of juvenile pikeperch (initial body weight 68.7 ± 7.1 g, with 50 individuals per tank) for 84 days. A combined feeding protocol of four meals per day, provided at 07.00, 09.00, 11.00, 13.00, by automatic feeders (EHEIM Twins, Deizisau, Germany), and one hand feeding, at 15.00 was adopted during the trial. Any unconsumed feeds were collected by siphoning and dried in an oven to calculate the exact feed intake.
Sampling Procedures
Fish Biometry
At the start and the end of the feeding trial, fish were individually weighed to calculate weight gain (WG) and feed conversion ratio (FCR):
WG (g) = final body weight-initial body weight
FCR = total feed supplied (g, Dry Matter)/WG
Antioxidative Enzyme and Histo-Morphological Analysis
After 84 days of the experiment, a total of 45 fish (3 individuals/tank) were randomly sampled, after 24 h of feed deprivation, and were euthanised by means of overdose anaesthesia (MS222, 125 mg/l).
Dissected livers and intestines from 15 fish/group were stored at −80°C for further antioxidative enzyme analysis. A similar number of samples, taken from another 15 fish/group, were fixed by immersion in a 10% buffered formalin solution for histo-morphological analysis.
Intestinal Microbiota
At the end of the experiment, three fish were randomly taken from each tank and euthanised by means of overdose anaesthesia (MS222, 125 mg/l). In order to ensure that all sampled fish had digesta throughout the intestinal tract, fish were deprived of feeds 12 h prior to sampling time. Fish exterior was wiped with 70% ethanol before abdomen was opened, whole intestine from each fish was removed from the abdominal cavity and digesta from proximal to distal intestine was squeezed gently into a 1.5 ml aseptic Eppendorf and immediately stored at −80°C for further analysis.
Analytical Methods
Diet Chemical Composition
Analysis of HI defatted meal and experimental diets for dry matter, crude protein, crude lipid, ash, and fatty acids (FAs) were performed as described elsewhere (Tran et al., 2021). Gross energy was determined by mean of a calorimetric bomb (IKA C7000, Stufen, Germany).
Oxidative Stress in Livers and Intestines
Oxidative stress biomarkers were evaluated in liver and intestine of each fish sample by means of spectrophotometer analysis (Varian Cary spectrophotometer, Santa Clara, CA, United States) as previously described by Elia et al. (2018). Briefly, superoxide dismutase (SOD) activity was measured in 50 mM Na2CO3, pH 10, 0.1 mM EDTA, 500 mM cytochrome C, and 1 mM hypoxanthine and xanthine oxidase. Reduction of cytochrome C by the xanthine/hypoxanthine system was measured versus a standard curve of SOD units at 550 nm. Catalase (CAT) activity was measured as the decrease in absorbance at 240 nm due to the consumption of H2O2. The assay was performed in an NaH2PO4 + Na2HPO4 buffer (100 mM, pH 7) and 12 mM H2O2. Glutathione peroxidase (SeGPx’s) activities were measured by following the oxidation of NADPH at 340 nm and using 0.6 mM H2O2 or 0.8 mM cumene hydroperoxides (tot GPx) as substrates. Glutathione S-transferase (GST) was measured at 340 nm using as a substrate 1-chloro-2,4-dinitrobenzene (CDNB).
Histo-Morphological Analysis of Intestine and Liver
Samples of the anterior intestine were excised and flushed with a 0.9% saline solution to remove all the content. The collected samples were fixed in a 10% buffered formalin solution, routinely embedded in paraffin wax blocks, sectioned at a 5 μm thickness, mounted onto glass slides and stained with Haematoxylin & Eosin (HE). One slide per intestinal segment was examined by means of light microscopy and captured with a Nikon DS-Fi1 digital camera, coupled to a Zeiss Axiophot microscope, using a 2.5× objective lens. NIS-Elements F software was used to capture images.
Morphometric analysis was performed using Image®-Pro Plus software on ten well-oriented and intact villi. The evaluated morphometric indices were villi height (from the villus tip to submucosa) and villi width (across the base of the villus, but not including the brush border).
The observed histopathological findings were evaluated in all the organs, using a semi-quantitative scoring system as follows: absent (score = 0), mild (score = 1), moderate (score = 2), and severe (score = 3). Histopathological findings in intestine were assessed separately for each segment for mucosa (inflammatory infiltrates) and submucosa [inflammatory infiltrates and Gut-Associated Lymphoid Tissue (GALT) activation]. The total score of each gut segment was obtained by adding to the mucosa and submucosa scores. All the slides were blind assessed by two independent observers, and any discordant cases were re-examined, using a multi-head microscope, until unanimous consensus was reached.
Microbiome Analysis
DNA Extraction and 16S rRNA Amplicon Target Sequencing
Nucleic acid was extracted from the intestine content (500 mg as starting materials). Total DNA from the samples was extracted using a RNeasy Power Microbiome KIT (Qiagen, Milan, Italy), according to the manufacturer’s instructions. One microlitre of RNase (Illumina Inc, San Diego, CA, United States) was added to digest the RNA in the DNA samples for an incubation period of 1 h at 37°C. DNA was quantified using Qubit ds and standardised at 5 ng/μl.
DNA extracted directly from digesta samples was used to assess the microbiota, through amplification of the V3–V4 region of the 16S rRNA gene (Klindworth et al., 2012). The PCR products were purified according to the Illumina metagenomic standard procedure (Illumina Inc, San Diego, CA, United States). Sequencing was performed with an MiSeq Illumina instrument, with V3 chemistry, and 250 bp paired-end reads were generated according to the manufacturer’s instructions.
Statistical Analysis
All data for antioxidative enzyme activities were tested for homogeneity of variance using Cochran, Hartley, Bartlett test. The effects of diet on oxidative stress in different organs were analysed separately, by means of one-way ANOVA, followed by Tukey test. Statistical analyses were performed using STATISTICA 12.0, with P-value < 0.05 as the significant difference.
Raw reads of microbiota were first joined, after sequencing, using FLASH software (Magoč and Salzberg, 2011), with default parameters, and were filtered, using QIIME 1.9.0 software and the pipeline as recently described (Biasato et al., 2018). Briefly, shorter reads (<300 bp) were discarded, using Prinseq. USEARCH software (version 8.1) was used for chimera filtering, and the Operational Taxonomic Units (OTUs) were picked, at a threshold of 97% similarity, using UCLUST algorithms. Taxonomy was assigned against 16S rRNA from Greengenes. The OTU table was rarefied at 10,144 sequences/sample. The OTU table displays the highest taxonomy resolution that was reached. When the taxonomy assignment was not able to reach the genus level, the family or phyla were displayed. R software was used to calculate the alpha diversity, while Weighted and Unweighted UniFrac distance matrix and OTUs table were used to find differences between samples, using permutational multivariate analysis of variance (Anosim) and analysis of similarity (Adonis) statistical test, considering the same function in R environment. Pairwise Wilcoxon test were used to determine any significant differences in alpha diversity or OTU abundance as a function of dietary insect meal. Principal component analysis (PCA) were plotted, using the dudi.pca function, through the made4 package of R environment. Non-normally distributed variables were presented as median values (interquartile range, IR), and box plots represented the interquartile range between the first and the third quartile, with the error bars showing the lowest and the highest value. Pairwise Kruskal-Wallis tests were used to find any significant differences in microbial taxa abundance according to the dietary treatment. P-values were adjusted for multiple testing, and a false discovery rate (FDR) < 0.05 was considered as significant. The data generated from sequencing were deposited in the NCBI Sequence Read Archive (SRA) and are available under the BioProject Accession Number PRJNA704237.
GraphPad Prism® software (version 8.0) was used to perform statistical analysis, for histo-morphometrical investigations. The Shapiro–Wilk test was used to test the normality of the data distribution before statistical analyses. Data were described by mean and standard deviation (SD), or median and IR depending on data distribution. Bivariate analysis was performed, by means of one way-ANOVA or Kruskall Wallis tests, to compare the intestine morphology and organs histopathology among different diet groups. P-values < 0.05 were considered statistically significant.
Results
Diet Composition and Growth Production of Pikeperch
Formulated diets had a similar proximate composition, except for chitin which increased with the increase of HI inclusion (Table 1). The inclusion of dietary HI significantly altered the FA profile of experimental diets. As regards saturated FAs (SFA), lauric (C12:0), myristic (C14:0), and palmitic acid (C16:0) significantly increased with the increase of HI inclusion (P < 0.05). Monounsaturated FAs (MUFA), dominated by palmitoleic acid (C16:1), C18:1n9 and C18:1n7, were found to be significantly higher in CO than H36 (P < 0.05), while MUFAs in H9 and H18 remained comparable (P > 0.05). Increasing inclusion level of HI significantly reduced polyunsaturated FAs (PUFA) (P < 0.05). A similar trend was observed for EPA, DHA, linoleic acid, alpha-linolenic acid (P < 0.05) (Table 2).
At the end of the feeding trial, WG in fish fed HI36 (62.8, mean value) was significantly lower than the control group (85.3 g) (P < 0.05), whereas pikeperch fed HI9 (84.8 g) and HI18 (83.2 g) did not show significant difference with CO (P > 0.05). FCR of the CO group (1.27) was comparable with that of HI9 (1.28) and HI18 (1.29) (P > 0.05), but significantly lower than HI36 (1.81) (P < 0.05) (Table 3).
Oxidative Stress in Liver and Intestine
The results of oxidative biomarkers, SOD, CAT, SeGPx, and GST, in liver and intestine of pikeperch fed experimental diets are depicted in Figure 1. Dietary HI did not alter the SOD activities in either liver or intestine, CAT activities in liver, SeGPx activities in intestine, or GST activities in liver of pikeperch (P > 0.05). No significant difference was observed across experimental groups (P > 0.05) for liver, as regards CAT activities, whereas this biomarker was significantly lower in HI18 and HI36 than in HI9 (P < 0.05), but remained similar to CO (P > 0.05) in intestine. Even if did not differ from the CO group, among fish fed HI-containing diets, HI9 produced highest SeGPx activity in liver (P < 0.05), while the lowest activity was found in HI36 group (P < 0.05). A significant increase in the GST concentration was observed in intestine of pikeperch fed HI-containing diets, compared to CO (P < 0.05). Of the different insect-fed groups, HI9 showed a higher GST than HI18 (P < 0.05), while HI36 was remained intermediate position.
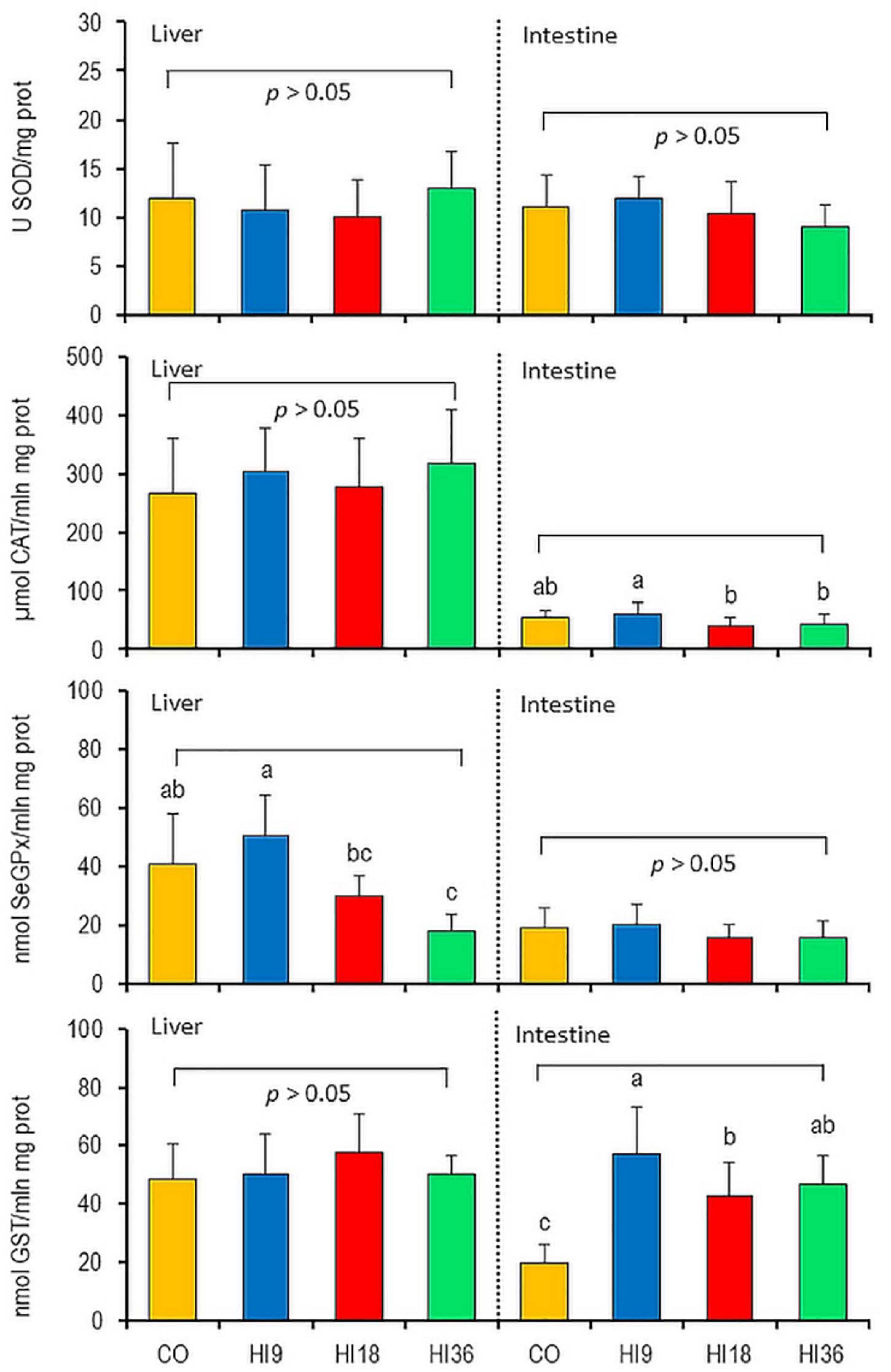
Figure 1. Oxidative biomarkers in liver and intestine of pikeperch (Sander lucioperca) fed experimental diets. Values are presented as means ± standard deviation. Means with different letters are significantly different (P < 0.05) from each other.
Histo-Morphology
Data regarding histopathological evaluation are reported in Table 3. Only few differences were observed for morphometry and histopathology of intestine among diet groups. Although there was no significant difference, a trend could be observed (P = 0.065) with HI36 group recording wider villi than the other groups. Thus, dietary HI inclusion did not induce any significant morphological changes in the pikeperch intestine, thereby suggesting no negative influence of such dietary HI on the physiological development of intestine.
Mild to severe multifocal to diffuse liver vacuolar degeneration was recorded in all treatments, and it was found to be greater in CO and HI9 group than in the HI18 and HI36 ones. Dietary HI did not show any evidence of inflammation of the liver of pikeperch (Table 3 and Figure 2).
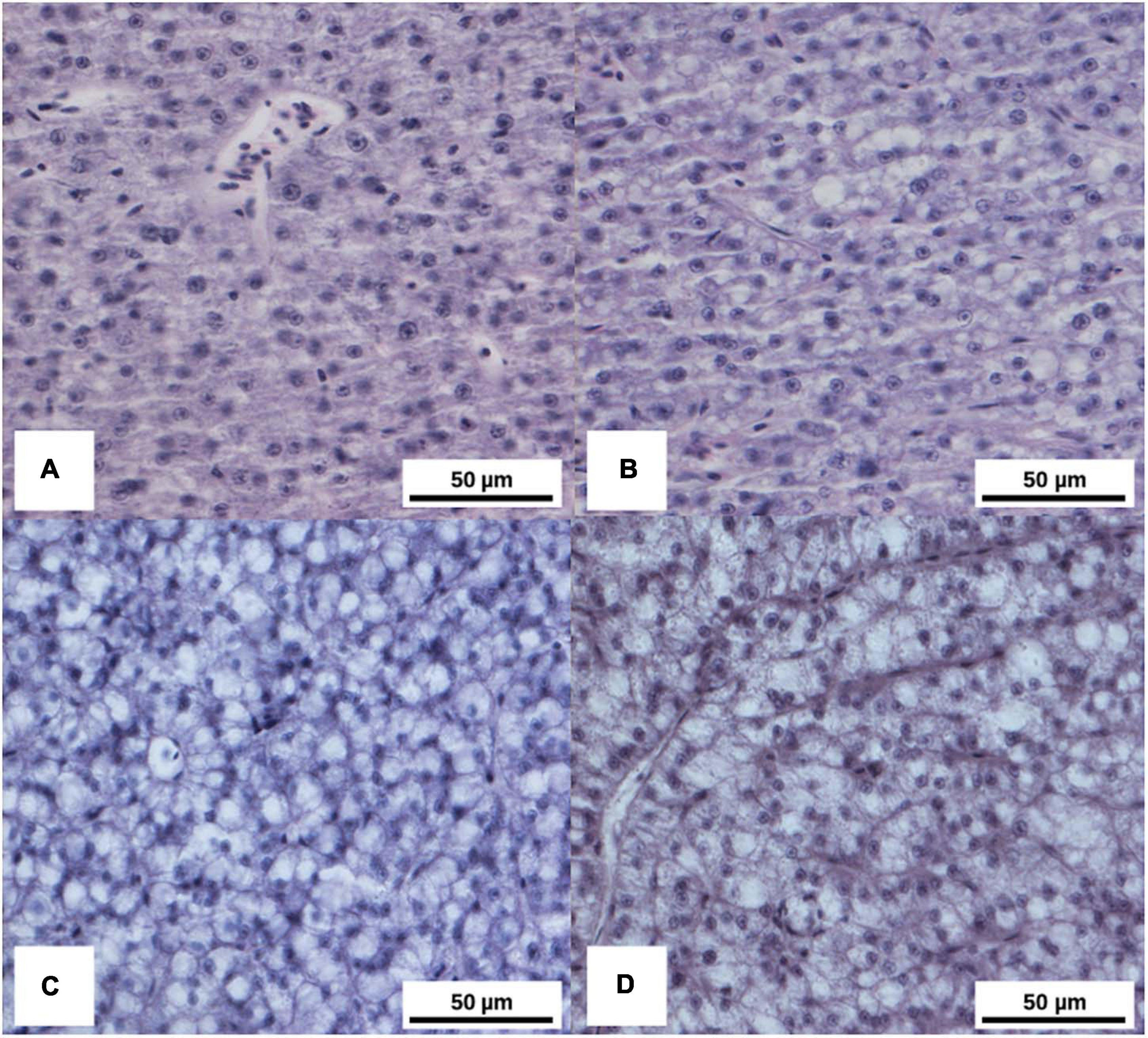
Figure 2. Histopathological alteration of liver of pikeperch considered in the present study. (A) Normal liver, Haematoxylin & Eosin (H-e) stain, 40× magnification, for HI36 diet. (B) Mild and multifocal vacuolar degeneration (grade 1), H-e, 40× magnification, for HI18 diet. (C) Moderate and multifocal vacuolar degeneration (grade 2), H-e, 40× magnification, for the HI9 diet. (D) Severe and diffuse vacuolar degeneration (grade 3), H-e, 40× magnification, for CO diet.
Microbiota
The total number of high-quality paired-end sequences obtained from 16S rRNA sequencing reached 1.916.822 raw reads. After the filtering, 1.295.693 reads passed the filters applied by QIIME, with a median value of 37.559 ± 15.565 reads/sample, and a mean sequence length of 443 bp. The rarefaction analysis and Good’s coverage, expressed as a median percentage (97%), also indicated satisfactory coverage of all samples.
The result of the OTUs analysis showed that there was no significant difference in Shannon index (P > 0.05) among diet groups, while alpha-diversity of intestinal bacteria, associated with Chao1 and observed OTUs, in fish fed HI18 significantly increased relative to CO diet (P < 0.05) (Figure 3).
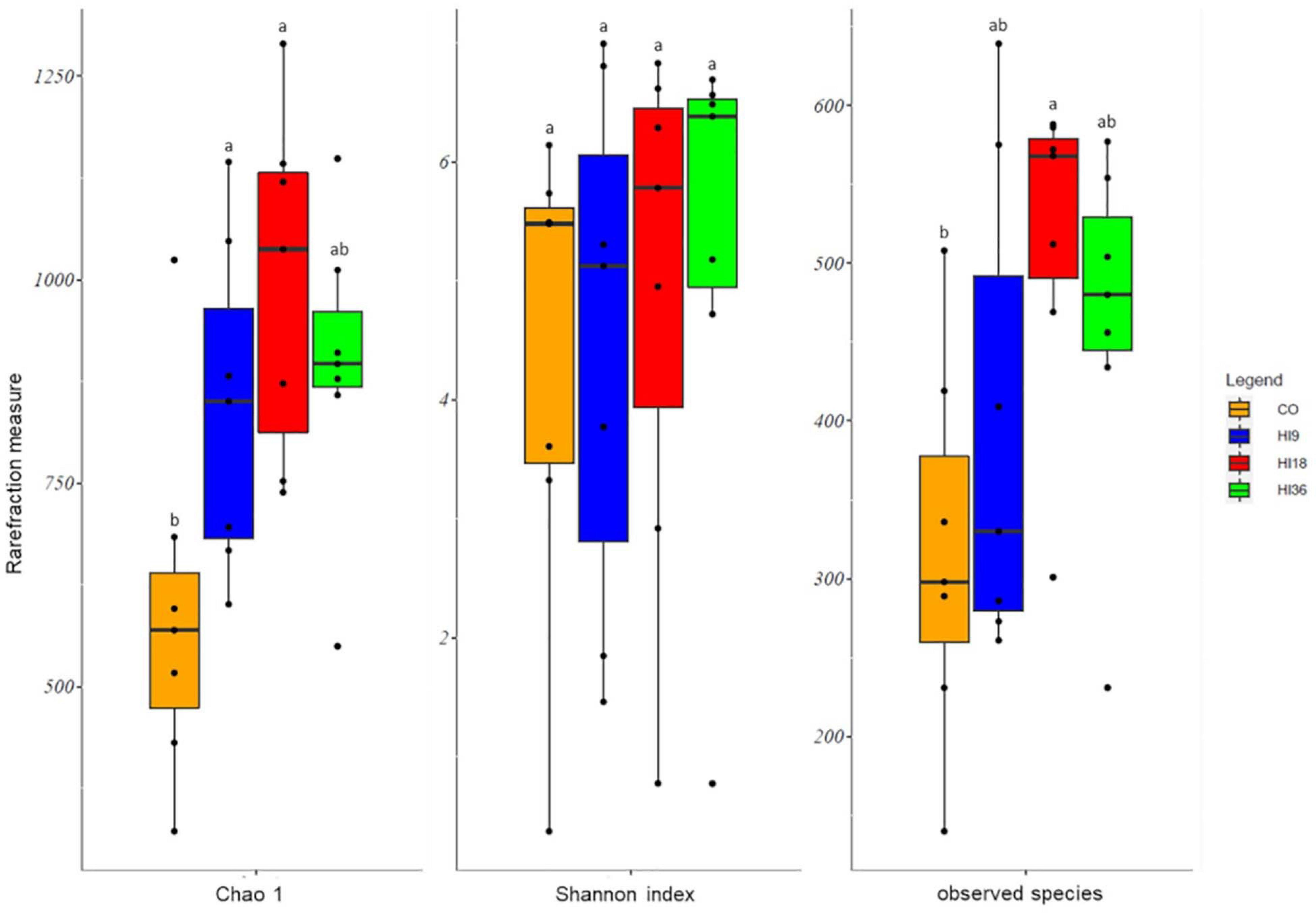
Figure 3. Boxplots showing the alpha diversity rarefaction index across experimental diets. The boxes represent the interquartile range (IR) between the first and third quartiles, and the line inside the boxes represents the median. Whiskers denote the lowest and the highest values within 1.56 IR from the first and third quartiles, respectively. Circles represent outliers beyond the whiskers. Different superscripts within boxplot indicate significant differences.
Adonis and Anosim statistical tests, based on weighted and on unweighted UniFrac distance matrix using the OTUs table, showed significant differences between diet groups as a administration of HI (P < 0.002). These differences were also observed when the PCA plot was produced at a genus level (Figure 4). It was also possible to observe a certain degree of separation, following diet groups. Microbiota of CO diet was near to the insect meal inclusion of 9%, while the microbiota of fish fed with 18 and 36% of HI was well separated (Figure 4).
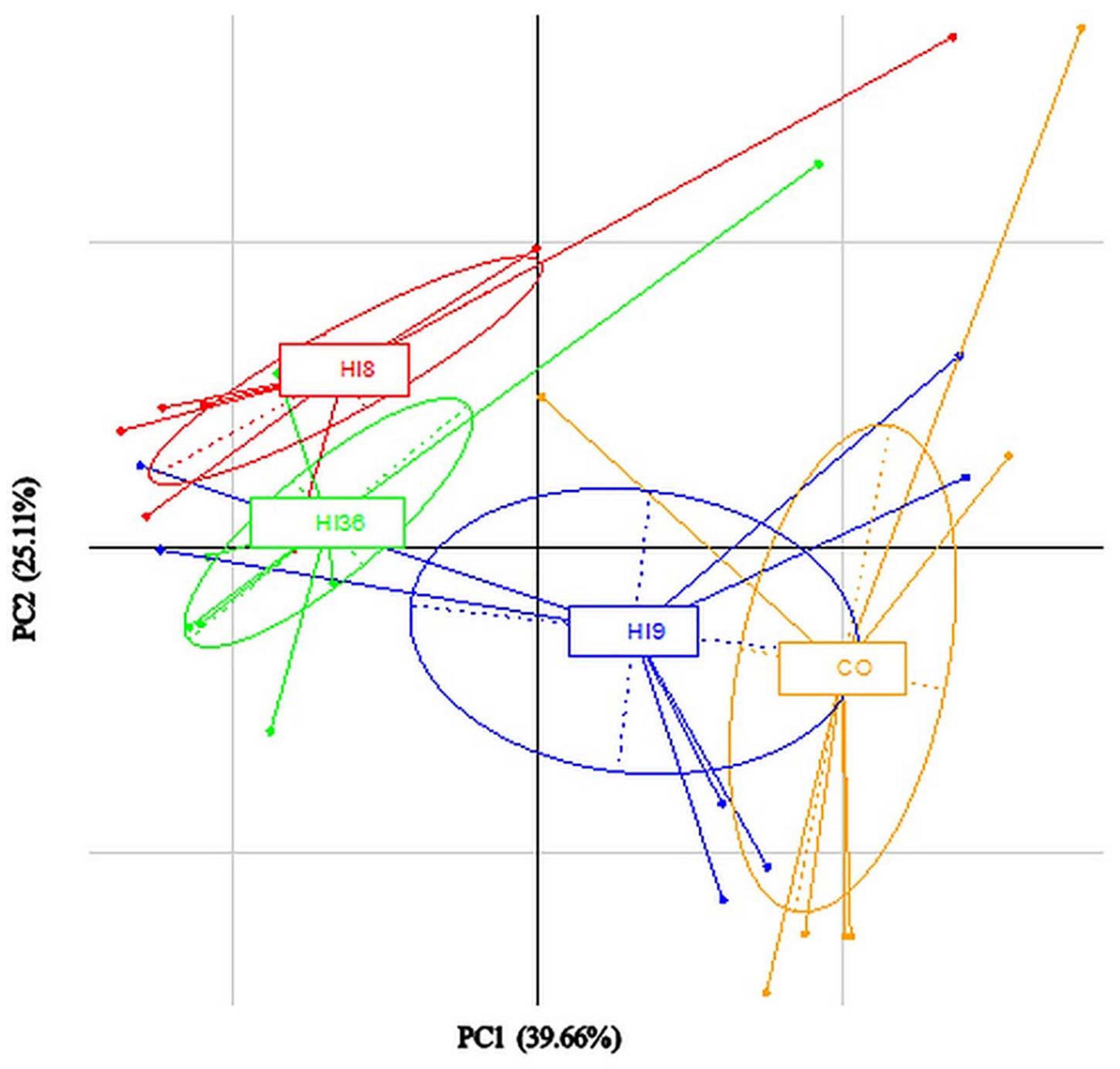
Figure 4. Microbiota composition (PCA plots) in intestine of pikeperch (Sander lucioperca) fed experimental diets.
The dominant OTUs, at the phyla level, were Firmicutes (mean values, 45–75%), regardless to dietary HI. Perch fed CO diet was enriched with Proteobacteria (26%), while Bacteroidetes (7–13%) was the prevalent phyla in fish fed HI-containing diets. As a result, Clostridiaceae, Enterococcaceae, and Bacillaceae were found to be the predominant families across fish fed diet groups. Clostridium, Acetobacter, Cetobacterium, Plesiomonas, Acetobacter, Peptostreptococcaceae, Bacteroides, and Oceanobacillus were, at the genus level, the most abundant genera found in intestine of perch considered in our study (Figure 5).
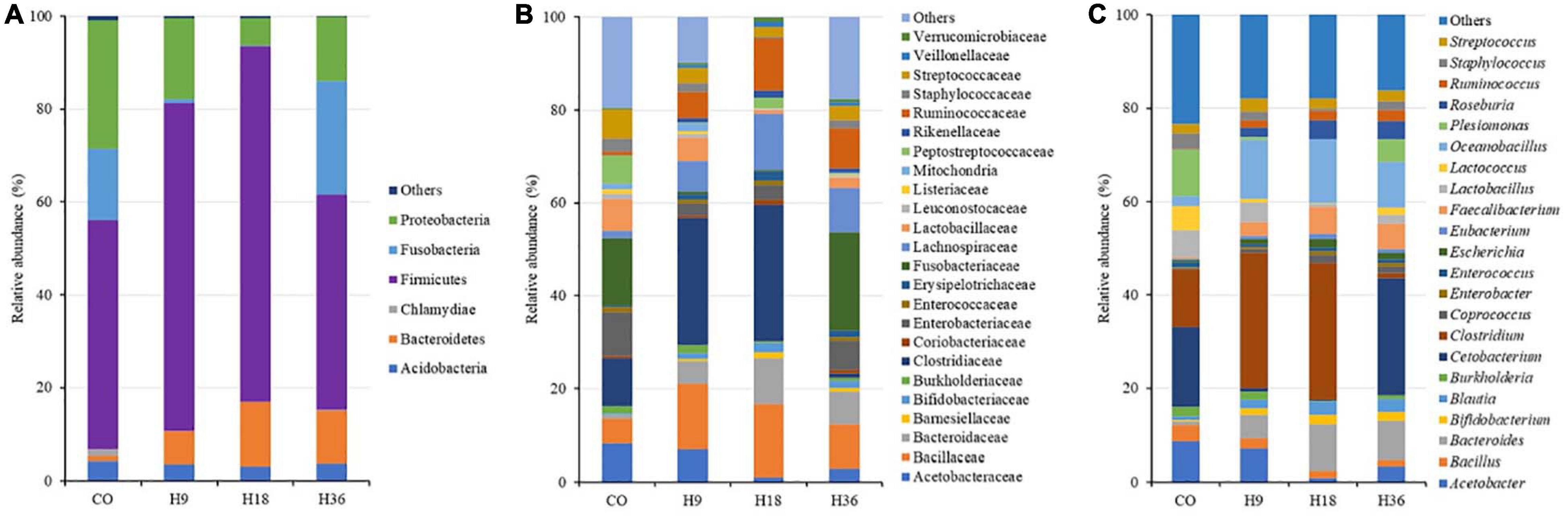
Figure 5. Relative abundance (%) of the OTUs in the intestine of pikeperch fed experimental diets at phyla (A), family (B), and genus (C) level. Only bacteria with an overall abundance of ≥ 1% and ≥ 0.5% at phylum and family/genus level, respectively, were presented. The bacteria were pool as “Others,” when lower than aforementioned abundance.
Dietary HI positively affected relative abundance of almost OTUs, compared with CO (FDR < 0.05), excepted for Bacillus, Burkholderia, and Sporosarcina, which were dominant in the CO group (Figure 6).
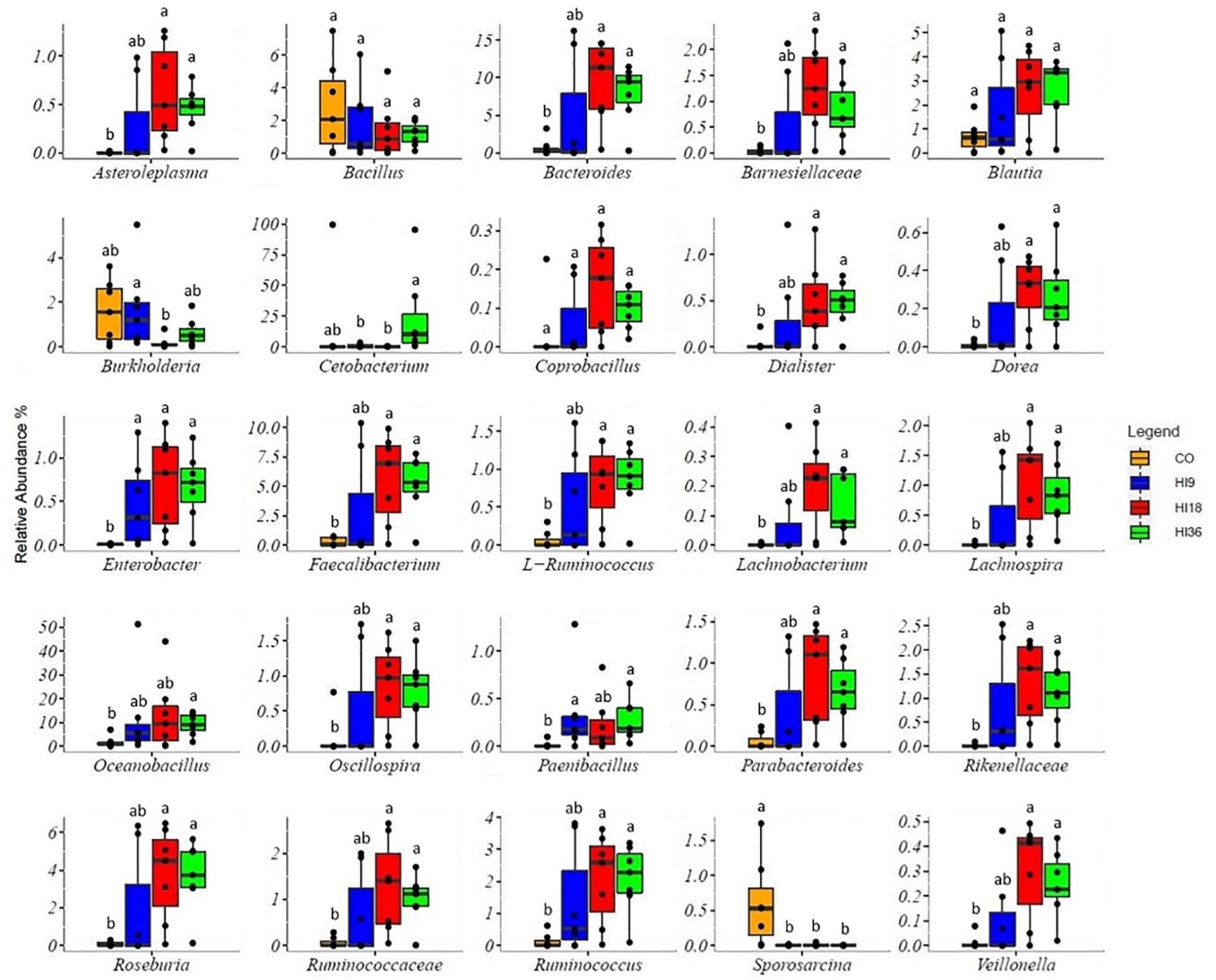
Figure 6. Boxplots showing the relative abundance at the genus level of the OTUs in the intestine of perch fed experimental diets. Means with different letters are significantly different (FDR < 0.05) from each other.
Discussion
Oxidative Enzymes
Reactive oxygen species (ROS) is the production of aerobic metabolism processes, including superoxide, hydrogen peroxide, and lipid peroxides (Buetler et al., 2004). Excessive ROS compounds cause cellular and tissue damages (Rosa et al., 2008). The balance of ROS production ensures the normal physical function of any organism and is regulated by antioxidant systems (Rosa et al., 2008) involving two mechanisms, (i) enzymes that remove ROS, including SOD, CAT, and SeGPx; and (ii) antioxidative compounds, i.e., ascorbate, glutathione, scavenge free radicals (Passi et al., 2002). Antioxidative enzyme activities were documented to be tissue-specific in pikeperch, and liver was the most sensitive organ to the diet manipulation under recirculating aquaculture system (Policar et al., 2016). In the case of detoxification in the intestine, however, certain enzymes such as SOD were known to play a vital role (Tang et al., 2013). This study indicates that in liver of pikeperch dietary HI did not alter the SOD, CAT, or GST oxidative enzymes, while significantly reduced SeGPx activity, a result that is in agreement with those of previous study (Elia et al., 2018), who performed a trial on rainbow trout fed dietary HI. The significant reduction in the catalytic SeGPx efficiency in liver of pikeperch fed dietary HI could be explained by the presence of chitin (Elia et al., 2018). Indeed, increasing inclusion levels of HI increased chitin levels in diets (Table 1). In addition, declining in SeGPx activities, as a result of increasing dietary HI, could be attributed to different dietary PUFA levels (Table 2), which are highly susceptible to oxidation. In fact, Tocher et al. (2002) reported that a high dietary PUFA content increased lipid peroxidation in fish tissues, and consequently the SeGPx enzyme activity involved in reducing peroxides, including FA hydroperoxides and hydrogen peroxide, will be also high (Passi et al., 2002).
The present study indicates that the CAT activity in intestine of pikeperch was significantly higher for HI9 than for HI18 and HI36 groups. A similar phenomenon was reported for CAT activity in the intestine of rainbow trout fed insect meal (T. molitor), where a substitution level of 25% fishmeal displayed higher activity than the 50% level (Henry et al., 2018a). The CAT and SeGPx activities in the present study were similar for CO and HI9, and lower than for HI18, HI36 groups. This result indicates that substantial substitution of fishmeal with HI reduced antioxidant enzyme activities in pikeperch. This is in line with a previous finding pertaining to rainbow trout (O. mykiss) (Elia et al., 2018). The decline of these biomarkers in HI18 and HI36 groups could be related to an imbalance between ROS production and antioxidant capacity. A suitable concentration of antioxidants, such as chitin and other bioactive compounds (Ngo and Kim, 2014), may support antioxidant enzyme activities in HI9 compared to the other HI-contained diets (Henry et al., 2018a).
Glutathione S-transferase plays an essential role in scavenging free radicals and xenobiotics detoxification (Aksnes and Njaa, 1981; Li et al., 2010). Increased glutathione S-transferase activity in intestine, but not liver, was observed across diet groups in the present study (Figure 1), thus implicating that some of the compounds in HI may have stimulated the biotransformation pathway in intestine of pikeperch, which was also found in liver of tilapia (Oreochromis niloticus) fed cricket-based feeds (Ogunji et al., 2007). In fact, insect meals may contain harmful substances, i.e., heavy metals and pesticides (van der Spiegel et al., 2013). The absence of an alteration of the hepatic GST activities after administration of HI could be the result of factors other than xenobiotics (Collier and Varanasi, 1991) or tissue-specific response (Martínez-Álvarez et al., 2005).
We also observed numerically higher oxidative biomarkers in liver of pikeperch than in intestine (Figure 1), which was in agreement with recent findings (Policar et al., 2016), reporting that liver was one of the most susceptible tissue in response to artificial nutrition and controlled conditions.
Histo-Morphology
Dietary HI in our study did not induce any morphological or inflammatory changes in the intestine of pikeperch, a result that is in agreement with previous studies conducted on different fish species fed dietary insect meals (Elia et al., 2018; Zarantoniello et al., 2019; Zarantoniello et al., 2020a). The absence of intestinal and hepatic inflammation could be linked to anti-inflammatory properties regulated by dietary saturated fatty acids content, especially lauric acid (C12:0) and chitin component (Henry et al., 2018b; Vargas-Abundez et al., 2019; Zarantoniello et al., 2019; Gasco et al., 2020b,c) which were found to be particularly high in HI and HI-containing diets in the present study. Although there were no significant differences (at P-value < 0.05), the villi were more expanded in the HI36 group than in the other groups (Table 3), and this was attributed to the presence of chitin. Chitin could stimulate the growth of villi thickness in tilapia (O. niloticus), probably due to its viscosity and water holding capacity (Kihara and Sakata, 1997). Chitin also induced the production of short-chain fatty acids, such as acetate, propionate and n-butyrate, and n-butyrate in particular was observed in intestine of tilapia (Kihara and Sakata, 1997), thereby increasing intestinal histo-morphology of fish, e.g., villi length and weight (Dawood, 2021). The large quantity of Paenibacillus genus in intestinal digesta of fish fed HI36 (Figure 6) could act as a probiotic for aquatic animal species (Midhun et al., 2017; Chen et al., 2019; Amoah et al., 2020), consequently enhancing intestinal health indices, including histomorphology (Dawood, 2021).
In contrast to recent findings, which reported that an increasing inclusion of insect meals induced a higher degree of hepatic vacuolization degeneration in fish (Li et al., 2017; Zarantoniello et al., 2019), the present study indicates that feeding pikeperch with ≤ 9% HI caused more severe hepatic degeneration than 18 or 36% did (Table 3), which could be related to a fatty liver status. Schulz et al. (2005) reported that a low level of palmitic acid (C16:0) yielded a higher hepatic lipid content. In the present study, the significantly lower palmitic acid in the control group than in the HI-containing groups could partly explain the hepatocellular vacuolization phenomenon. The mechanism to which palmitic acid affecting hepatic tissues remained to be elucidated. However, this FA promotes hepatocyte proliferation (Wang et al., 2011) and possess anti-inflammatory and antiviral effects (Librán-Pérez et al., 2019). On the other hand, the high content of dietary lauric acid (C12:0), high oxidation and low tissue deposition, was found to decrease liver lipid storage in freshwater Atlantic salmon (Belghit et al., 2019). This could explain the reduction in the adipose liver in pikeperch fed HI18 and HI36, compared to the control and HI9 diets (Table 3). Two FAs, linoleic and oleic acids, were confirmed to induce the occurrence of hepatic steatosis in sea bream (Sparus aurata) (Caballero et al., 2004). Moreover, owing to large molecular weight, oleic acid could produce a large lipid droplet while inrush hepatocyte (Bradbury, 2006). These FAs were found to be significantly higher in CO than in HI18, HI36 (Table 2), which could indicate severe steatosis in livers of the former group (Table 3). High intakes of eicosapentaenoic acid (EPA) and docosahexaenoic acid (DHA) are known to an inhibitor of lipid accumulation in livers of sea bream (S. aurata) (Caballero et al., 2004). Therefore, the change in the percentage of the different FAs in the experimental diets, due to the inclusion of HI, could further explain the severity of hepatic vacuolization degeneration observed in perch fed CO and H9 diets.
Microbiota
The present study reveals that dietary HI enhanced microbial biodiversity indices in intestine of pikeperch, compared with insect-free diet, a result that is in line with recent findings on rainbow trout (Bruni et al., 2018; Huyben et al., 2019; Rimoldi et al., 2019; Terova et al., 2019), thereby contributing to gut health and health status of the host.
In agreement with previous studies on intestinal microbiota of percid fish and freshwater species, the present study reveals that Firmicutes, Proteobacteria, Bacteroidetes were the most dominant phyla in the intestine of pikeperch, regardless of the HI inclusion level (Li et al., 2014; Kashinskaya et al., 2018; Terova et al., 2019).
Our results show an abundance of Clostridium genus in fish fed HI9 and HI18, which was even greater than those fed CO and HI36. Members of the Clostridium genus are common effective microorganism used as probiotics in aquaculture (Nayak, 2010a,b). Clostridium butyricum has been shown to possess a pathogenic inhibition capacity in farmed fishes (Pan et al., 2008a,b; Gao et al., 2013), improve feed efficiency in shrimp (Duan et al., 2017; Li et al., 2019), and to be suitable for use as probiotics in farmed fish (Hai, 2015; Zorriehzahra et al., 2016). The greater prevalence of Clostridium and other probiotic-used bacteria in HI9, such as Lactobaccillus and Bacillus genera, than in HI36, could explain the difference in feed conversion ratio between these diets in present study. The Bacteroides and Clostridium genera are known to be the main taxa involved in production of fatty acids and vitamins (Balcázar et al., 2006). The abundant presence of these taxa could partially compensate for nutritional insufficiencies in HI-containing diets, and consequently resulted in a comparable growth rate among control, HI9 and HI18 diets, yet the offset may be not efficient for HI36 group.
It is worth noting that Cetobacterium, the most predominant bacterium in intestine of natural pikeperch (Kashinskaya et al., 2018) and other freshwater fish (Larsen et al., 2014), was detected in our captive pikeperch fed dietary HI. Similar findings were also observed in rainbow trout (Etyemez and Balcázar, 2015), common carp (van Kessel et al., 2011), and giant arapaima (Ramírez et al., 2018) fed commercial aquafeeds. It seems relevant that Cetobacterium is among the core bacteria in pikeperch.
Insect meal, in general, is a chitin-rich ingredient. The degradation and digestion of this substance require binary enzymes, including chitinase and β−N−acetylglucosaminidase, and involve various microbacteria derived from digestive tract of fish with a chitinase-produced capacity (Ray et al., 2012; Ringø et al., 2012). Among these chitin-degraded bacteria, the Plesiomonas and Bacillus genus were detected across treatment groups at a particularly low abundance (Figure 4). This finding implicates that pikeperch may not be able to degrade chitin. A limited presence of chitinase-producing bacteria was also observed in rainbow trout (Bruni et al., 2018; Huyben et al., 2019; Rimoldi et al., 2019; Terova et al., 2019) and this may help to explain the low or absent chitin digestibility in this species (St-Hilaire et al., 2007; Henry et al., 2015; Renna et al., 2017; Caimi et al., 2020).
In conclusion, HI, fed as a partial or total replacement of fishmeal did not induce any inflammation of liver or intestine, or any intestine degeneration, but did show signs of severe hepatic steatosis of pikeperch fed CO and HI9 groups. Dietary HI promotes antioxidative enzyme activities of CAT, GPx and GST, but not of SOD, in liver and, to a lesser extent, in intestine of pikeperch. The inclusion of HI up to 18% or 50% fishmeal replacement in pikeperch diets increased abundance of Clostridium, Oceanobacillus, Bacteroides, and Faecalibacterium, whereas the predominant bacterium, Cetobacterium was found in the control and HI36 groups. Because of the absence of inflammation in tissues, the evolution of antioxidative enzyme, and modification of the favourable microbiota observed in the present study, it is possible to assume that defatted HI could have an immunological effect on juvenile pikeperch. Further study on immune response and disease resistance of pikeperch fed insect meal could help to explore this efficiency.
Data Availability Statement
The original contributions presented in the study are included in the article, further inquiries can be directed to the corresponding author.
Ethics Statement
The animal study was reviewed and approved by the Czech Ministry of Health (MSMT-6744/2018-2).
Author Contributions
LG and VS: planning the experiment and editing the manuscript. HT: data analysis, writing, and editing of the manuscript. MP, MZ, and TG: wrote the manuscript. AE, EC, IF, CC, and FG: analysis and the first draft. All authors contributed to the article and approved the submitted version.
Author Disclaimer
This output only reflects the authors’ views, and the European Union cannot be held responsible for any use that may be made of the information contained herein.
Conflict of Interest
The authors declare that the research was conducted in the absence of any commercial or financial relationships that could be construed as a potential conflict of interest.
Publisher’s Note
All claims expressed in this article are solely those of the authors and do not necessarily represent those of their affiliated organizations, or those of the publisher, the editors and the reviewers. Any product that may be evaluated in this article, or claim that may be made by its manufacturer, is not guaranteed or endorsed by the publisher.
Funding
The study was financially supported by the Ministry of Agriculture of the Czech Republic, project NAZV (QK1810296). This JU-IAPW research manuscript is part of a project that has received funding from the European Union’s Horizon 2020 Research and Innovation Programme under grant agreement No. 652831 (AQUAEXCEL2020), the TNA project ID number: AE070026.
References
Aksnes, A., and Njaa, L. R. (1981). Catalase, glutathione peroxidase and superoxide dismutase in different fish species. Comp. Biochem. Physiol. B Biochem. Mol. Biol. 69, 893–896. doi: 10.1016/0305-0491(81)90402-8
Amoah, K., Huang, Q.-C., Dong, X.-H., Tan, B.-P., Zhang, S., Chi, S.-Y., et al. (2020). Paenibacillus polymyxa improves the growth, immune and antioxidant activity, intestinal health, and disease resistance in Litopenaeus vannamei challenged with Vibrio parahaemolyticus. Aquaculture 518:734563. doi: 10.1016/j.aquaculture.2019.734563
Antonopoulou, E., Nikouli, E., Piccolo, G., Gasco, L., Gai, F., Chatzifotis, S., et al. (2019). Reshaping gut bacterial communities after dietary Tenebrio molitor larvae meal supplementation in three fish species. Aquaculture 503, 628–635. doi: 10.1016/j.aquaculture.2018.12.013
Balcázar, J. L., Blas, I. D., Ruiz-Zarzuela, I., Cunningham, D., Vendrell, D., and Múzquiz, J. L. (2006). The role of probiotics in aquaculture. Vet. Microbiol. 114, 173–186. doi: 10.1016/j.vetmic.2006.01.009
Belghit, I., Liland, N. S., Waagbø, R., Biancarosa, I., Pelusio, N., Li, Y., et al. (2018). Potential of insect-based diets for Atlantic salmon (Salmo salar). Aquaculture 491, 72–81. doi: 10.1016/j.aquaculture.2018.03.016
Belghit, I., Waagbo, R., Lock, E. J., and Liland, N. S. (2019). Insect-based diets high in lauric acid reduce liver lipids in freshwater Atlantic salmon. Aquacult. Nutr. 25, 343–357. doi: 10.1111/anu.12860
Biasato, I., Ferrocino, I., Biasibetti, E., Grego, E., Dabbou, S., Sereno, A., et al. (2018). Modulation of intestinal microbiota, morphology and mucin composition by dietary insect meal inclusion in free-range chickens. BMC Vet. Res. 14:383. doi: 10.1186/s12917-018-1690-y
Bochert, R. (2020). Comparative performance, biochemical composition, and fatty acid analysis of Eurasian perch (Perca fluviatilis) during grow-out in RAS fed different commercial diets. J. Appl. Aquacult. 2020, 1–15. doi: 10.1080/10454438.2020.1828217
Boissy, J., Aubin, J., Drissi, A., van der Werf, H. M. G., Bell, G. J., and Kaushik, S. J. (2011). Environmental impacts of plant-based salmonid diets at feed and farm scales. Aquaculture 321, 61–70. doi: 10.1016/j.aquaculture.2011.08.033
Bradbury, M. W. (2006). Lipid metabolism and liver inflammation. I. Hepatic fatty acid uptake: possible role in steatosis. Am. J. Physiol. Gastrointest. Liver Physiol. 290, 194–198. doi: 10.1152/ajpgi.00413.2005
Bruni, L., Pastorelli, R., Viti, C., Gasco, L., and Parisi, G. (2018). Characterisation of the intestinal microbial communities of rainbow trout (Oncorhynchus mykiss) fed with Hermetia illucens (black soldier fly) partially defatted larva meal as partial dietary protein source. Aquaculture 487, 56–63. doi: 10.1016/j.aquaculture.2018.01.006
Buetler, T. M., Krauskopf, A., and Ruegg, U. T. (2004). Role of superoxide as a signaling molecule. Physiology 19, 120–123. doi: 10.1152/nips.01514.2003
Caballero, M. J., Izquierdo, M. S., Kjørsvik, E., Fernández, A. J., and Rosenlund, G. (2004). Histological alterations in the liver of sea bream, Sparus aurata L., caused by short- or long-term feeding with vegeFig, oils. Recovery of normal morphology after feeding fish oil as the sole lipid source. J. Fish Dis. 27, 531–541. doi: 10.1111/j.1365-2761.2004.00572.x
Caimi, C., Renna, M., Lussiana, C., Bonaldo, A., Gariglio, M., Meneguz, M., et al. (2020). First insights on Black Soldier Fly (Hermetia illucens L.) larvae meal dietary administration in Siberian sturgeon (Acipenser baerii Brandt) juveniles. Aquaculture 515:734539. doi: 10.1016/j.aquaculture.2019.734539
Chen, S.-W., Liu, C.-H., and Hu, S.-Y. (2019). Dietary administration of probiotic Paenibacillus ehimensis NPUST1 with bacteriocin-like activity improves growth performance and immunity against Aeromonas hydrophila and Streptococcus iniae in Nile tilapia (Oreochromis niloticus). Fish Shellfish Immunol. 84, 695–703. doi: 10.1016/j.fsi.2018.10.059
Collier, T. K., and Varanasi, U. (1991). Hepatic activities of xenobiotic metabolizing enzymes and biliary levels of xenobiotics in English sole (Parophrys vetulus) exposed to environmental contaminants. Arch. Environ. Contam. Toxicol. 20, 462–473. doi: 10.1007/bf01065834
Cummins, V. C., Rawles, S. D., Thompson, K. R., Velasquez, A., Kobayashi, Y., Hager, J., et al. (2017). Evaluation of black soldier fly (Hermetia illucens) larvaemeal as partial or total replacement of marine fish meal in practical diets for Pacific white shrimp (Litopenaeus vannamei). Aquaculture 473, 337–344. doi: 10.1016/j.aquaculture.2017.02.022
Dalsgaard, J., Lund, I., Thorarinsdottir, R., Drengstig, A., Arvonen, K., and Pedersen, P. B. (2013). Farming different species in RAS in Nordic countries: Current status and future perspectives. Aquacult. Eng. 53, 2–13. doi: 10.1016/j.aquaeng.2012.11.008
Dawood, M. A. O. (2021). Nutritional immunity of fish intestines: important insights for sustainable aquaculture. Rev. Aquac. 13, 642–663. doi: 10.1111/raq.12492
Duan, Y., Zhang, Y., Dong, H., Wang, Y., Zheng, X., and Zhang, J. (2017). Effect of dietary Clostridium butyricum on growth, intestine health status and resistance to ammonia stress in Pacific white shrimp Litopenaeus vannamei. Fish Shellfish Immunol. 65, 25–33. doi: 10.1016/j.fsi.2017.03.048
Dumas, A., Raggi, T., Barkhouse, J., Lewis, E., and Weltzien, E. (2018). The oil fraction and partially defatted meal of black soldier fly larvae (Hermetia illucens) affect differently growth performance, feed efficiency, nutrient deposition, blood glucose and lipid digestibility of rainbow trout (Oncorhynchus mykiss). Aquaculture 492, 24–34. doi: 10.1016/j.aquaculture.2018.03.038
Elia, A. C., Capucchio, M. T., Caldaroni, B., Magara, G., Dörr, A. J. M., Biasato, I., et al. (2018). Influence of Hermetia illucens meal dietary inclusion on the histological traits, gut mucin composition and the oxidative stress biomarkers in rainbow trout (Oncorhynchus mykiss). Aquaculture 496, 50–57. doi: 10.1016/j.aquaculture.2018.07.009
Etyemez, M., and Balcázar, J. L. (2015). Bacterial community structure in the intestinal ecosystem of rainbow trout (Oncorhynchus mykiss) as revealed by pyrosequencing-based analysis of 16S rRNA genes. Res. Vet. Sci. 100, 8–11. doi: 10.1016/j.rvsc.2015.03.026
FAO (2020a). The State of World Fisheries and Aquaculture. Sustainability in action. Rome: FAO, doi: 10.4060/ca9229en
Finke, M. D. (2007). Estimate of chitin in raw whole insects. Zoo Biol. 26, 105–115. doi: 10.1002/zoo.20123
Foley, J. A., DeFries, R., Asner, G. P., Barford, C., Bonan, G., Carpenter, S. R., et al. (2005). Global Consequences of Land Use. Sci 309:570. doi: 10.1126/science.1111772
Foley, J. A., Ramankutty, N., Brauman, K. A., Cassidy, E. S., Gerber, J. S., Johnston, M., et al. (2011). Solutions for a cultivated planet. Nature 478, 337–342. doi: 10.1038/nature10452
Froehlich, H. E., Jacobsen, N. S., Essington, T. E., Clavelle, T., and Halpern, B. S. (2018a). Avoiding the ecological limits of forage fish for fed aquaculture. Nat. Sustain. 1, 298–303. doi: 10.1038/s41893-018-0077-1
Froehlich, H. E., Runge, C. A., Gentry, R. R., Gaines, S. D., and Halpern, B. S. (2018b). Comparative terrestrial feed and land use of an aquaculture-dominant world. Proce. Natl. Acad. Sci. 115, 5295–5300. doi: 10.1073/pnas.1801692115
Gao, Q., Xiao, Y., Sun, P., Peng, S., Yin, F., Ma, X., et al. (2013). In vitro protective efficacy of Clostridium butyricum against fish pathogen infections. Indian J. Microbiol. 53, 453–459. doi: 10.1007/s12088-013-0394-z
Gasco, L., Acuti, G., Bani, P., Dalle Zotte, A., Danieli, P. P., De Angelis, A., et al. (2020a). Insect and fish by-products as sustainable alternatives to conventional animal proteins in animal nutrition. Ital. J. Anim. Sci. 19, 360–372. doi: 10.1080/1828051X.2020.1743209
Gasco, L., Biancarosa, I., and Liland, N. S. (2020b). From waste to feed: A review of recent knowledge on insects as producers of protein and fat for animal feeds. Curr. Opin. Green Sustain. Chem. 23, 67–79. doi: 10.1016/j.cogsc.2020.03.003
Gasco, L., Józefiak, A., and Henry, M. (2020c). Beyond the protein concept: health aspects of using edible insects on animals. J. Insects Food Feed 0, 1–28. doi: 10.3920/jiff2020.0077
Gatlin, D. M., Barrows, F. T., Brown, P., Dabrowski, K., Gaylord, T. G., Hardy, R. W., et al. (2007). Expanding the utilization of sustainable plant products in aquafeeds: A review. Aquacult. Res. 38, 551–579. doi: 10.1111/j.1365-2109.2007.01704.x
Ginter, K., Kangur, K., Kangur, A., Kangur, P., and Haldna, M. (2011). Diet patterns and ontogenetic diet shift of pikeperch, Sander lucioperca (L.) fry in lakes Peipsi and Võrtsjärv (Estonia). Hydrobiologia 660, 79–91. doi: 10.1007/s10750-010-0393-6
Hai, N. V. (2015). The use of probiotics in aquaculture. J. Appl. Microbiol. 119, 917–935. doi: 10.1111/jam.12886
Henry, M. A., Gai, F., Enes, P., Peréz-Jiménez, A., and Gasco, L. (2018a). Effect of partial dietary replacement of fishmeal by yellow mealworm (Tenebrio molitor) larvae meal on the innate immune response and intestinal antioxidant enzymes of rainbow trout (Oncorhynchus mykiss). Fish Shellfish Immunol. 83, 308–313. doi: 10.1016/j.fsi.2018.09.040
Henry, M. A., Gasco, L., Chatzifotis, S., and Piccolo, G. (2018b). Does dietary insect meal affect the fish immune system? The case of mealworm, Tenebrio molitor on European sea bass, Dicentrarchus labrax. Dev. Comp. Immunol. 81, 204–209. doi: 10.1016/j.dci.2017.12.002
Henry, M., Gasco, L., Piccolo, G., and Fountoulaki, E. (2015). Review on the use of insects in the diet of farmed fish: Past and future. Anim. Feed Sci. Technol. 203, 1–22. doi: 10.1016/j.anifeedsci.2015.03.001
Hua, K. (2021). A meta-analysis of the effects of replacing fish meals with insect meals on growth performance of fish. Aquaculture 530:735732. doi: 10.1016/j.aquaculture.2020.735732
Hua, K., Cobcroft, J. M., Cole, A., Condon, K., Jerry, D. R., Mangott, A., et al. (2019). The future of aquatic protein: Implications for protein sources in aquaculture diets. One Earth 1, 316–329. doi: 10.1016/j.oneear.2019.10.018
Huuskonen, H., Piironen, J., Syväranta, J., Eronen, R., Biasi, C., Kiiskinen, P., et al. (2019). Diet and movements of pikeperch (Sander lucioperca) in a large oligotrophic lake with an exceptionally high pikeperch yield. Ecol. Freshwat. Fish 28, 533–543. doi: 10.1111/eff.12495
Huyben, D., Vidaković, A., Werner Hallgren, S., and Langeland, M. (2019). High-throughput sequencing of gut microbiota in rainbow trout (Oncorhynchus mykiss) fed larval and pre-pupae stages of black soldier fly (Hermetia illucens). Aquaculture 500, 485–491. doi: 10.1016/j.aquaculture.2018.10.034
Kashinskaya, E. N., Simonov, E. P., Kabilov, M. R., Izvekova, G. I., Andree, K. B., and Solovyev, M. M. (2018). Diet and other environmental factors shape the bacterial communities of fish gut in an eutrophic lake. J. Appl. Microbiol. 125, 1626–1641. doi: 10.1111/jam.14064
Katya, K., Borsra, M. Z. S., Ganesan, D., Kuppusamy, G., Herriman, M., Salter, A., et al. (2017). Efficacy of insect larval meal to replace fish meal in juvenile barramundi, Lates calcarifer reared in freshwater. Int. Aquat. Res. 9, 303–312. doi: 10.1007/s40071-017-0178-x
Kihara, M., and Sakata, T. (1997). Fermentation of dietary carbohydrates to short-chain fatty acids by gut microbes and its influence on intestinal morphology of a detritivorous teleost tilapia (Oreochromis niloticus). Comp. Biochem. Physiol. Part A Mol. Integr. Physiol. 118, 1201–1207. doi: 10.1016/S0300-9629(97)00052-2
Klindworth, A., Pruesse, E., Schweer, T., Peplies, J., Quast, C., Horn, M., et al. (2012). Evaluation of general 16S ribosomal RNA gene PCR primers for classical and next-generation sequencing-based diversity studies. Nucleic Acids Res. 41:gks808. doi: 10.1093/nar/gks808
Larsen, A. M., Mohammed, H. H., and Arias, C. R. (2014). Characterization of the gut microbiota of three commercially valuable warmwater fish species. J. Appl. Microbiol. 116, 1396–1404. doi: 10.1111/jam.12475
Li, G., Xie, P., Li, H., Chen, J., Hao, L., and Xiong, Q. (2010). Quantitative profiling of mRNA expression of glutathione S-transferase superfamily genes in various tissues of bighead carp (Aristichthys nobilis). J. Biochem. Mol. Toxicol. 24, 250–259. doi: 10.1002/jbt.20333
Li, H., Tian, X., Zhao, K., Jiang, W., and Dong, S. (2019). Effect of Clostridium butyricum in different forms on growth performance, disease resistance, expression of genes involved in immune responses and mTOR signaling pathway of Litopenaeus vannamai. Fish Shellfish Immunol. 87, 13–21. doi: 10.1016/j.fsi.2018.12.069
Li, J., Ni, J., Li, J., Wang, C., Li, X., Wu, S., et al. (2014). Comparative study on gastrointestinal microbiota of eight fish species with different feeding habits. J. Appl. Microbiol. 117, 1750–1760. doi: 10.1111/jam.12663
Li, S., Ji, H., Zhang, B., Zhou, J., and Yu, H. (2017). Defatted black soldier fly (Hermetia illucens) larvae meal in diets for juvenile Jian carp (Cyprinus carpio var. Jian): Growth performance, antioxidant enzyme activities, digestive enzyme activities, intestine and hepatopancreas histological structure. Aquaculture 477, 62–70. doi: 10.1016/j.aquaculture.2017.04.015
Librán-Pérez, M., Pereiro, P., Figueras, A., and Novoa, B. (2019). Antiviral activity of palmitic acid via autophagic flux inhibition in zebrafish (Danio rerio). Fish Shellf. Immunol. 95, 595–605. doi: 10.1016/j.fsi.2019.10.055
Lock, E. R., Arsiwalla, T., and Waagbø, R. (2016). Insect larvae meal as an alternative source of nutrients in the diet of Atlantic salmon (Salmo salar) postsmolt. Aquacult. Nutr. 22, 1202–1213. doi: 10.1111/anu.12343
Magalhães, R., Sánchez-López, A., Leal, R. S., Martínez-Llorens, S., Oliva-Teles, A., and Peres, H. (2017). Black soldier fly (Hermetia illucens) pre-pupae meal as a fish meal replacement in diets for European seabass (Dicentrarchus labrax). Aquaculture 476, 79–85. doi: 10.1016/j.aquaculture.2017.04.021
Magoč, T., and Salzberg, S. L. (2011). FLASH: fast length adjustment of short reads to improve genome assemblies. Bioinformatics 27, 2957–2963. doi: 10.1093/bioinformatics/btr507
Martínez-Álvarez, R. M., Morales, A. E., and Sanz, A. (2005). Antioxidant defenses in fish: Biotic and abiotic factors. Rev. Fish Biol. Fish. 15, 75–88. doi: 10.1007/s11160-005-7846-4
Midhun, S. J., Neethu, S., Vysakh, A., Arun, D., Radhakrishnan, E. K., and Jyothis, M. (2017). Antibacterial activity and probiotic characterization of autochthonous Paenibacillus polymyxa isolated from Anabas testudineus (Bloch, 1792). Microb. Pathog. 113, 403–411. doi: 10.1016/j.micpath.2017.11.019
Nayak, S. K. (2010a). Role of gastrointestinal microbiota in fish. Aquacult. Res. 41, 1553–1573. doi: 10.1111/j.1365-2109.2010.02546.x
Nayak, S. K. (2010b). Probiotics and immunity: A fish perspective. Fish Shellfish Immunol. 29, 2–14. doi: 10.1016/j.fsi.2010.02.017
Ngo, D.-H., and Kim, S.-K. (2014). “Chapter two - Antioxidant effects of chitin, chitosan, and their derivatives,” in Marine Carbohydrates: Fundamentals and Applications, Part B, ed. S.-K. Kim (Amsterdam: Elsevier Inc.), 15–31.
Nogales-Mérida, S., Gobbi, P., Józefiak, D., Mazurkiewicz, J., Dudek, K., Rawski, M., et al. (2019). Insect meals in fish nutrition. Rev. Aquac. 11, 1080–1103. doi: 10.1111/raq.12281
Nyina-Wamwiza, L., Xu, X. L., Blanchard, G., and Kestemont, P. (2005). Effect of dietary protein, lipid and carbohydrate ratio on growth, feed efficiency and body composition of pikeperch Sander lucioperca fingerlings. Aquacult. Res. 36, 486–492. doi: 10.1111/j.1365-2109.2005.01233.x
Ogunji, J. O., Nimptsch, J., Wiegand, C., and Schulz, C. (2007). Evaluation of the influence of housefly maggot meal (magmeal) diets on catalase, glutathione S-transferase and glycogen concentration in the liver of Oreochromis niloticus fingerling. Comp. Biochem. Physiol. Part A Mol. Integr. Physiol. 147, 942–947. doi: 10.1016/j.cbpa.2007.02.028
Pan, X., Wu, T., Song, Z., Tang, H., and Zhao, Z. (2008a). Immune responses and enhanced disease resistance in Chinese drum, Miichthys miiuy (Basilewsky), after oral administration of live or dead cells of Clostridium butyrium CB2. J. Fish Dis. 31, 679–686. doi: 10.1111/j.1365-2761.2008.00955.x
Pan, X., Wu, T., Zhang, L., Song, Z., Tang, H., and Zhao, Z. (2008b). In vitro evaluation on adherence and antimicrobial properties of a candidate probiotic Clostridium butyricum CB2 for farmed fish. J. Appl. Microbiol. 105, 1623–1629. doi: 10.1111/j.1365-2672.2008.03885.x
Passi, S., Cataudella, S., Di Marco, P., De Simone, F., and Rastrelli, L. (2002). Fatty acid composition and antioxidant levels in muscle tissue of different mediterranean marine species of fish and shellfish. J. Agric. Food Chem. 50, 7314–7322. doi: 10.1021/jf020451y
Policar, T., Blecha, M., Křišt’an, J., Mráz, J., Velíšek, J., Stará, A., et al. (2016). Comparison of production efficiency and quality of differently cultured pikeperch (Sander lucioperca L.) juveniles as a valuable product for ongrowing culture. Aquacult. Int. 24, 1607–1626. doi: 10.1007/s10499-016-0050-9
Policar, T., Schaefer, F. J., Panana, E., Meyer, S., Teerlinck, S., Toner, D., et al. (2019). Recent progress in European percid fish culture production technology–tackling bottlenecks. Aquacult. Int. 27, 1151–1174. doi: 10.1007/s10499-019-00433-y
Ramírez, C., Coronado, J., Silva, A., and Romero, J. (2018). Cetobacterium is a major component of the microbiome of giant amazonian fish (Arapaima gigas) in Ecuador. Animals 8:189. doi: 10.3390/ani8110189
Ray, A. K., Ghosh, K., and Ringø, E. (2012). Enzyme-producing bacteria isolated from fish gut: A review. Aquacult. Nutr. 18, 465–492. doi: 10.1111/j.1365-2095.2012.00943.x
Renna, M., Schiavone, A., Gai, F., Dabbou, S., Lussiana, C., Malfatto, V., et al. (2017). Evaluation of the suitability of a partially defatted black soldier fly (Hermetia illucens L.) larvae meal as ingredient for rainbow trout (Oncorhynchus mykiss Walbaum) diets. J. Anim. Sci. Biotechnol. 8:57. doi: 10.1186/s40104-017-0191-3
Rimoldi, S., Antonini, M., Gasco, L., Moroni, F., and Terova, G. (2021). Intestinal microbial communities of rainbow trout (Oncorhynchus mykiss) may be improved by feeding a Hermetia illucens meal/low-fishmeal diet. Fish Physiol. Biochem. 2021, 918–911. doi: 10.1007/s10695-020-00918-1
Rimoldi, S., Gini, E., Iannini, F., Gasco, L., and Terova, G. (2019). The effects of dietary insect meal from Hermetia illucens prepupae on autochthonous gut microbiota of rainbow trout (Oncorhynchus mykiss). Animals 9:143. doi: 10.3390/ani9040143
Ringø, E., Zhou, Z., Olsen, R. E., and Song, S. K. (2012). Use of chitin and krill in aquaculture – the effect on gut microbiota and the immune system: a review. Aquacult. Nutr. 18, 117–131. doi: 10.1111/j.1365-2095.2011.00919.x
Rosa, C. E., Figueiredo, M. A., Lanes, C. F. C., Almeida, D. V., Monserrat, J. M., and Marins, L. F. (2008). Metabolic rate and reactive oxygen species production in different genotypes of GH-transgenic zebrafish. Comp. Biochem. Physiol. B Biochem. Mol. Biol. 149, 209–214. doi: 10.1016/j.cbpb.2007.09.010
Schulz, C., Günther, S., Wirth, M., and Rennert, B. (2006). Growth performance and body composition of pike perch (Sander lucioperca) fed varying formulated and natural diets. Aquacult. Int. 14, 577–586. doi: 10.1007/s10499-006-9056-z
Schulz, C., Knaus, U., Wirth, M., and Rennert, B. (2005). Effects of varying dietary fatty acid profile on growth performance, fatty acid, body and tissue composition of juvenile pike perch (Sander lucioperca). Aquacult. Nutr. 11, 403–413. doi: 10.1111/j.1365-2095.2005.00369.x
Sealey, W. M., Gaylord, T. G., Barrows, F. T., Tomberlin, J. K., McGuire, M. A., Ross, C., et al. (2011). Sensory analysis of rainbow trout, Oncorhynchus mykiss, fed enriched black soldier fly prepupae, Hermetia illucens. J. World Aquacult. Soc. 42, 34–45. doi: 10.1111/j.1749-7345.2010.00441.x
Shepherd, C. J., and Jackson, A. J. (2013). Global fishmeal and fish-oil supply: Inputs, outputs and marketsa. J. Fish Biol. 83, 1046–1066. doi: 10.1111/jfb.12224
Smárason, B. O., Ogmundarson, O., Arnason, J., Bjornsdottir, R., and Daviosdottir, B. (2017). Life cycle assessment of Icelandic arctic char fed three different feed types. Turkish J. Fish. Aquat. Sci. 17, 79–90. doi: 10.4194/1303-2712-v17_1_10
Smetana, S., Schmitt, E., and Mathys, A. (2019). Sustainable use of Hermetia illucens insect biomass for feed and food: Attributional and consequential life cycle assessment. Resour. Conserv. Recycl. 144, 285–296. doi: 10.1016/j.resconrec.2019.01.042
Stejskal, V., Kouřil, J., Policar, T., and Svobodová, Z. (2016). Splenic lipidosis in intensively cultured perch, Perca fluviatilis L. J. Fish Dis. 39, 87–93. doi: 10.1111/jfd.12327
Stejskal, V., Tran, H. Q., Prokesova, M., Gebauer, T., Giang, P. T., Gai, F., et al. (2020). Partially defatted Hermetia illucens larva meal in diet of Eurasian perch (Perca fluviatilis) juveniles. Animals 10:1876. doi: 10.3390/ani10101876
St-Hilaire, S., Sheppard, C., Tomberlin, J. K., Irving, S., Newton, L., McGuire, M. A., et al. (2007). Fly prepupae as a feedstuff for rainbow trout, Oncorhynchus mykiss. J. World Aquacult. Soc. 38, 59–67. doi: 10.1111/j.1749-7345.2006.00073.x
Tacon, A. G. J., and Metian, M. (2015). Feed matters: Satisfying the feed demand of aquaculture. Rev. Fish. Sci. Aquac. 23, 1–10. doi: 10.1080/23308249.2014.987209
Tacon, A. G., Hasan, M. R., and Metian, M. (2011). Demand and supply of feed ingredients for farmed fish and crustaceans: Trends and prospects. FAO Fisheries and aquaculture technical paper, Vol. 564. Rome: FAO, doi: 10.4172/2155-9546.1000234
Tang, Q. Q., Feng, L., Jiang, W. D., Feng, W. D., Jiang, Y., Liu, J., et al. (2013). Effects of dietary copper on growth, digestive, and brush border enzyme activities and antioxidant defense of hepatopancreas and intestine for young grass carp (Ctenopharyngodon idella). Biol. Trace. Elem. Res. 155, 370–380. doi: 10.1007/s12011-013-9785-6
Terova, G., Rimoldi, S., Ascione, C., Gini, E., Ceccotti, C., and Gasco, L. (2019). Rainbow trout (Oncorhynchus mykiss) gut microbiota is modulated by insect meal from Hermetia illucens prepupae in the diet. Rev. Fish Biol. Fish. 29, 465–486. doi: 10.1007/s11160-019-09558-y
Tocher, D. R., Mourente, G., Van Der Eecken, A., Evjemo, J. O., Diaz, E., Bell, J. G., et al. (2002). Effects of dietary vitamin E on antioxidant defence mechanisms of juvenile turbot (Scophthalmus maximus L.), halibut (Hippoglossus hippoglossus L.) and sea bream (Sparus aurata L.). Aquacult. Nutr. 8, 195–207. doi: 10.1046/j.1365-2095.2002.00205.x
Tran, H. Q., Van Doan, H., and Stejskal, V. (2021). Does dietary Tenebrio molitor affect swimming capacity, energy use, and physiological responses of European perch Perca fluviatilis? Aquaculture 539:736610. doi: 10.1016/j.aquaculture.2021.736610
van der Spiegel, M., Noordam, M. Y., and van der Fels-Klerx, H. J. (2013). Safety of novel protein sources (insects, microalgae, seaweed, duckweed, and rapeseed) and legislative aspects for their application in food and feed production. Compr. Rev. Food Sci. Food Saf. 12, 662–678. doi: 10.1111/1541-4337.12032
van Huis, A., and Oonincx, D. G. A. B. (2017). The environmental sustainability of insects as food and feed. Rev. Agron. Sustainable Dev. 37:43. doi: 10.1007/s13593-017-0452-8
van Kessel, M. A. H. J., Dutilh, B. E., Neveling, K., Kwint, M. P., Veltman, J. A., Flik, G., et al. (2011). Pyrosequencing of 16S rRNA gene amplicons to study the microbiota in the gastrointestinal tract of carp (Cyprinus carpio L.). AMB Express 1:41. doi: 10.1186/2191-0855-1-41
Vargas-Abundez, A. J., Randazzo, B., Foddai, M., Sanchini, L., Truzzi, C., Giorgini, E., et al. (2019). Insect meal based diets for clownfish: Biometric, histological, spectroscopic, biochemical and molecular implications. Aquaculture 498, 1–11. doi: 10.1016/j.aquaculture.2018.08.018
Vinni, M., Lappalainen, J., Malinen, T., and Lehtonen, H. (2009). Stunted growth of pikeperch Sander lucioperca in lake Sahajọrvi, Finland. J. Fish Biol. 74, 967–972. doi: 10.1111/j.1095-8649.2009.02181.x
Wang, X., Liu, J. Z., Hu, J. X., Wu, H., Li, Y. L., Chen, H. L., et al. (2011). ROS-activated p38 MAPK/ERK-Akt cascade plays a central role in palmitic acid-stimulated hepatocyte proliferation. Free Radic. Biol. Med. 51, 539–551. doi: 10.1016/j.freeradbiomed.2011.04.019
Zarantoniello, M., Randazzo, B., Gioacchini, G., Truzzi, C., Giorgini, E., Riolo, P., et al. (2020a). Zebrafish (Danio rerio) physiological and behavioural responses to insect-based diets: A multidisciplinary approach. Sci. Rep. 10:10648. doi: 10.1038/s41598-020-67740-w
Zarantoniello, M., Randazzo, B., Truzzi, C., Giorgini, E., Marcellucci, C., Vargas-Abúndez, J. A., et al. (2019). A six-months study on Black Soldier Fly (Hermetia illucens) based diets in zebrafish. Sci. Rep. 9:8598. doi: 10.1038/s41598-019-45172-5
Zarantoniello, M., Zimbelli, A., Randazzo, B., Compagni, M. D., Truzzi, C., Antonucci, M., et al. (2020b). Black Soldier Fly (Hermetia illucens) reared on roasted coffee by-product and Schizochytrium sp. as a sustainable terrestrial ingredient for aquafeeds production. Aquaculture 518:734659. doi: 10.1016/j.aquaculture.2019.734659
Keywords: pikeperch, alternative ingredient, Hermetia illucens, microbiota, histomorphology, antioxidative
Citation: Tran HQ, Prokešová M, Zare M, Gebauer T, Elia AC, Colombino E, Ferrocino I, Caimi C, Gai F, Gasco L and Stejskal V (2021) How Does Pikeperch Sander lucioperca Respond to Dietary Insect Meal Hermetia illucens? Investigation on Gut Microbiota, Histomorphology, and Antioxidant Biomarkers. Front. Mar. Sci. 8:680942. doi: 10.3389/fmars.2021.680942
Received: 15 March 2021; Accepted: 07 July 2021;
Published: 27 July 2021.
Edited by:
Fotini Kokou, Wageningen University and Research, NetherlandsReviewed by:
Luisa M. P. Valente, University of Porto, PortugalZhenyan Cheng, Tianjin Agricultural University, China
Copyright © 2021 Tran, Prokešová, Zare, Gebauer, Elia, Colombino, Ferrocino, Caimi, Gai, Gasco and Stejskal. This is an open-access article distributed under the terms of the Creative Commons Attribution License (CC BY). The use, distribution or reproduction in other forums is permitted, provided the original author(s) and the copyright owner(s) are credited and that the original publication in this journal is cited, in accordance with accepted academic practice. No use, distribution or reproduction is permitted which does not comply with these terms.
*Correspondence: Laura Gasco, bGF1cmEuZ2FzY29AdW5pdG8uaXQ=