- 1LSAqua (Lambers-Seghers NV), Hooglede, Belgium
- 2Nutrigenomics and Fish Growth Endocrinology Group, Institute of Aquaculture Torre de la Sal (IATS-CSIC), Castellón, Spain
- 3Fish Pathology Group, Institute of Aquaculture Torre de la Sal (IATS-CSIC), Castellón, Spain
The present study aimed to unravel the effects of partial (50%; 50LSAqua) and total (100%; 100LSAqua) replacement of fish meal (FM) by a commercial protein source (LSAqua SusPro) made of bacterial and processed animal proteins (PAP) in farmed juveniles of gilthead sea bream (Sparus aurata). The trial lasted 8 weeks, and the feasibility of replacement was assessed in terms of growth performance, histopathological scoring and composition of mucosal adherent microbiota from anterior intestine (AI). Specific growth rates (SGR) of 50LSAqua fish were undistinguishable from the CTRL group, whereas a slight but significant growth impairment was found with the total replacement. Histological signs of inflammation across the intestine were more evident at the highest level of FM replacement, and the total concentration of short chain fatty acids (SCFA) in stripped feces decreased in a dose dependent manner. Illumina sequencing of gut mucosal microbiota yielded a mean of 130,439 reads per sample assigned to 1,567 OTUs at 97% identity threshold. The bacterial richness was similar in all groups, but a significantly higher Simpson diversity index was found in 100LSAqua fish. At the phylum level, Proteobacteria were the most abundant in all groups, whereas Firmicutes decreased and Actinobacteria increased with the FM replacement. At a closer look, pro-inflammatory Gammaproteobacteria of the genus Psychrobacter, and Acinetobacter decreased with FM replacement, whereas the anti-inflammatory Paracoccus, Arthrobacter, and Actinomycetales increased, with a remarkable presence of the Propioniciclava genus in LSAqua groups. The inferred metagenome analysis suggested that these discriminant bacteria could be implicated in a counter-regulatory anti-inflammatory response. It also pointed to an over-representation of mucosal microbes that can potentially be involved in the natural production of antimicrobials in fish fed the experimental diets.
Introduction
Over the last two decades, the aquaculture industry is growing faster (∼7% annual growth rate) than any animal protein sector, with feed conversion ratios (FCR, dry feed intake/weight gain) (1.1–1.6) lower than in poultry (1.4–1.8), pork (2.6–4.4), and cattle (3.5–9) production (Clark and Tilman, 2017; Ritchie and Roser, 2019). However, feed is a major cost in farmed fish production and the reliance of marine aquaculture on marine feed ingredients still remains high (Tacon and Metian, 2015; Ytrestøyl et al., 2015). Historically, fish meal (FM) has been used as the main protein source of most farmed fish feeds because of its high protein content and excellent amino acids balance, but it is also a good source of vitamins, essential fatty acids, calcium, phosphorous and other minerals, which makes high replacement levels by alternative raw materials difficult (Naylor et al., 2009; Olsen and Hasan, 2012; Oliva-Teles et al., 2015). Traditionally, plant-based diets have been considered the most suitable alternative, and high levels of combined FM and fish oil (FO) replacement can be achieved in Atlantic salmon (Salmo salar) and in typically marine fish, such as European sea bass (Dicentrarchus labrax) and gilthead sea bream (Sparus aurata) (Espe et al., 2006; Torrecillas et al., 2017; Simó-Mirabet et al., 2018; Aas et al., 2019; Perera et al., 2019). Nevertheless, these substitution diets need to be adequately supplemented with phosphorus, lysine, sulfur amino acids, protein hydrolysates, or nutraceuticals to meet the nutrient requirements for growth (Simó-Mirabet et al., 2018; Egerton et al., 2020), also preventing pro-inflammatory processes and impaired intestinal barrier permeability (Estensoro et al., 2016; Piazzon et al., 2017). However, even with diminished inclusion levels of FM, a shortage ranging from 0.4 to 1.32 million metric tons of FM could occur by 2050, significantly impairing the growth of the aquaculture industry (Jones et al., 2020). Thus, there is an urgent demand for suitable protein ingredients to maintain farmed fish performance, and stabilize the supply of feed ingredients during the expansion of aquaculture.
Among others, insect proteins, processed animal proteins (PAP) and single cell proteins (SCP) have the potential to play a major role in the development of future fish feeds (Nogales-Mérida et al., 2018; Révész and Biró, 2019; Turchini et al., 2019; Glencross et al., 2020). Certainly, in European sea bass, it is feasible to substitute up to 80% of FM meal by defatted Tenebrio molitor larvae meal without detrimental effects on nutrient digestibility, growth performance and markers of lipid/energy metabolism (Basto et al., 2021). PAPs from different animal by-products (feather meal, blood meal, meat, and bone meal from non-ruminants) also represent an excellent alternative to FM after the re-approval by the European Union of the use of PAPs in aquafeeds (EC Regulation No 56/2013). Indeed, its excellent palatability, competitive price, and high protein content with fewer carbohydrates than plant proteins make the locally produced agrifood by-products good candidates for more sustainable aquafeeds. However, a number of recent studies in gilthead sea bream highlighted that the maximum level of replacement is closely linked to diet composition and quality of processed PAPs (Moutinho et al., 2017; Davies et al., 2019; Karapanagiotidis et al., 2019; Psofakis et al., 2020; Fontinha et al., 2021). Likewise, SCP products prepared from different microbial sources, including microalgae, yeast and bacteria will contribute to the success of low FM diets in marine aquaculture (Jones et al., 2020). This was supported by studies conducted in Carangidae fish, Florida pompano (Trachinotus carolinus), where complete replacement of FM (12.82% of bacterial-SCP) was achieved with no signs of impaired growth performance (Rhodes et al., 2015). Recently, a high partial FM replacement with phototrophic bacteria was also proved with success in Asian sea bass (Lates calcarifer) (Delamare-Deboutteville et al., 2019). In gilthead sea bream, FM substitution with fungi or microalgae SCP has also been tested (Vizcaíno et al., 2014; Rimoldi et al., 2020; Zamani et al., 2020), but the use of bacterial SCP as a main dietary protein source in this species is less evaluated. In any case, the long-term suitability of high inclusion levels of alternative protein sources is often questioned in aquafeeds, because the potential use of new feed ingredients requires the use of conventional methodologies, but also cutting-edge tools for unraveling the interactions between diets, host metabolism and gut microbiota, which has co-evolved with the host to develop a mutualistic relationship (Zhang and Davies, 2016; Agus et al., 2018). Thus, in fish and gilthead sea bream in particular, this living ecosystem is affected not only by intrinsic factors such as sex, age, genetics, or developmental stage, but also by external agents like diet, trophic level, season or captive state (Estruch et al., 2015; Piazzon et al., 2017, 2019, 2020; Egerton et al., 2018; Rimoldi et al., 2020).
Taking in mind this complex trade-off, the aim of the present study was to evaluate, in a high valuable farmed fish such as gilthead sea bream, the effects of the partial and total replacement of FM by a commercial product (LSAqua SusPro; LSAqua, Lambers-Seghers, Dendermonde, Belgium) made by a combination of PAP and bacterial SCP. The suitability of this protein concentrate was assessed successfully in shrimp (Litopenaeus vannamei) (Van Nguyen et al., 2020), and herein we aimed to test an improved formulation to go further in fishless aquafeeds for marine farmed fish with a high economic value for the European aquaculture. In that respect, gilthead sea bream is the main cultured fish in the Mediterranean basin and the third most important produced species in Europe1. In the current study, attention was focused on the growth performance and indicators of gut health, including histopathological scoring of anterior and posterior intestine (PI) sections, measurements of concentration of lactic acid and short chain fatty acids (SCFA) in stripped feces, and in-depth analysis of autochthonous microbiota from the anterior intestine (AI).
Materials and Methods
Ethics Statement
Fish manipulation and tissue collection were carried out according to the Spanish (Royal Decree RD53/2013) and the current EU (2010/63/EU) legislations on the handling of experimental fish. All procedures were approved by the Ethics and Animal Welfare Committees of Institute of Aquaculture Torre de la Sal (IATS-CSIC, Castellón, Spain), CSIC (Permit number 112/2020) and “Generalitat Valenciana” (permit number 2020/VSC/PEA/0064).
Animals
Juveniles of gilthead sea bream (March 2020) were purchased from a Mediterranean hatchery (Piscimar, Burriana, Spain), and adapted for 2 months to the indoor experimental facilities of IATS-CSIC under natural photoperiod and temperature conditions (40°5′N; 0°10′E). Seawater was pumped ashore (open system), and water parameters were daily monitored. Oxygen content of water effluents was measured with an Oxyguard polarographic electrode (OxyGuard, Farum, Denmark) and it was always above 85% saturation. Unionized ammonia remained below 0.02 mg/L, as determined by means of a dissolved ammonia monitor Q46N (Analytical Technology, Inc., Delph, United Kingdom). During the acclimation and experimental period (May–July), water temperature increased from 18°C in May to 25°C in July.
Diets
Three extruded isoproteic and isolipidic diets were formulated by LSAqua, and produced by Research Diet Services BV (RDS, Wijk bij Duurstede, Netherlands) following current industry practices (Table 1 and Supplementary Table 1). The inclusion level of FM in the control (CTRL) diet was 15%. In the other two experimental diets, FM was partially (50%, 50LSAqua) or totally replaced (100%, 100LSAqua) by LS-Aqua SusPro, a mix of PAPs (66%) and bacterial SCP (33%). Both LS-Aqua diets were conveniently supplemented with L-lysine, DL-methionine and calcium bicarbonate phosphate in order to reach the same concentrations present in the control diet and meet the nutrient species requirements.
Feeding Trial
In May 2020, fish of 22–26 g were randomly distributed in nine 500 L tanks to establish triplicate groups of 40 fish each. All fish were tagged into the dorsal skeletal muscle with passive integrated transponders (PIT) (ID-100A 1.25 Nano Transponder, Trovan, Madrid, Spain) and were individually weighed and measured at initial, intermediate and final sampling points (every 4 weeks), using a FR-200 Fish Reader W (Trovan) for data capture and pre-processing. The trial lasted 8 weeks, and fish were fed by hand once daily (12:00 h), six days per week, near to visual satiety with CTRL or experimental diets for the entire duration of the trial. Feed intake was registered daily, and normal fish behavior was assessed routinely by camera monitoring. No mortalities were registered throughout all the experimental period.
Sample Collection
At the end of the feeding trial and following two fasting days, 9 fish per diet (3 fish/tank) were anaesthetized with 0.1 g/L of tricaine-methasulfonate (MS-222, Sigma-Aldrich) and sacrificed by cervical section. Liver, intestine (excluding the pyloric caeca) and mesenteric fat were weighed and length measured (intestine) to calculate the hepatosomatic index (HSI), mesenteric fat index (MSI), and intestine weight (IWI) and length (ILI) indices. Tissue portions (∼0.4 cm) of liver, AI (immediately after the pyloric caeca) and PI (immediately before the anal ampoule) were fixed in 10% neutral buffered formalin for subsequent histological analyses. The remaining AI was opened and gently washed with sterile Hank’s balanced salt solution to remove non-adherent bacteria. Intestinal mucus was scrapped off using the blunt edge of a sterile scalpel and collected into sterile 1.5 mL tubes. Mucus samples were kept on ice and DNA extraction was performed immediately after the sampling exactly as previously described (Piazzon et al., 2020). The anterior intestine portion was selected due to its importance in fish nutrient absorption and metabolism. This procedure targets the autochthonous bacteria, because these populations are capable of colonizing the mucosal surface, directly impacting the fish physiology. The allochthonous bacteria (not resident) cannot colonize these habitats under normal conditions and have a more transient impact on the host (Hao and Lee, 2004). Additional fish (10 fish per diet) were sampled 8 h after feeding for the analysis of intestinal lactic acid and SCFA. Briefly, fish were anesthetized and sacrificed by cervical section, intestine was cut out, and the intestinal content was collected by stripping. In the two sampling days corresponding to fasting and postprandial sample collection, all samples were obtained in a short-period lasting 2–3 h, alternating among replicates of each dietary group to avoid biases due to sampling time.
Histological Analysis
Formalin fixed pieces of liver, AI, and PI were embedded in paraffin, 4 μm-sectioned and stained with Giemsa and periodic acid-Schiff (PAS) following standard procedures. Tissue sections were analyzed under a Leitz Dialux 22 light microscope connected to an Olympus DP70 camera and representative microphotographs taken. The histological alterations observed were scored according to semiquantitative scales in order to evaluate the intensity of the inflammatory response, the intestinal epithelial lesions, and changes of the mucus secretion. Intestinal inflammatory markers included the degree of hyperplasia in the lamina propria-submucosa, scored from 0 (absence) to 3 (severe), as well as eosinophilic granular cells (EGCs) and intraepithelial lymphocytes (IELs) abundance, which were scored ranging from 0 (absence) to 3 (very abundant, meaning 25–30 cells/microscope field at 500× magnification). Intestinal epithelial lesions included the degree of epithelial necrosis and desquamation and the degree of lipid vacuolization in enterocytes, both scored from 0 (absence) to 3 (severe). Cell abundance of differentially stained goblet cells (light- or dark-stained with Giemsa) was used to assess dietary-induced changes of the mucus secretion, and scored as previously described for the other cell markers. In liver sections, the degree of lipid and glycogen storage in hepatocytes was scored from 0 (absence) to 3 (pervasive) by Giemsa or PAS staining, respectively. In addition, the abundance of melanomacrophage centers and of lymphocyte aggregates was quantified in the liver.
Lactic Acid and SCFA Determinations
Intestinal contents (200 mg) were homogenized with 0.1% peptone solution with 0.85% NaCl (500 μL) and centrifuged at 10,000 × g for 5 min at 4°C. The supernatant was filtered and 0.2 μL were injected on a HPLC system (Jasco, Tokyo, Japan) equipped with a UV-975 detector. SCFA were separated using a Rezex ROA Organic Acids column (Phenomenex, Macclesfield, United Kingdom) following the method described by Sanz et al. (2005). The mobile phase was a linear gradient of 0.005 M sulfuric acid in HPLC grade water, and flow rate was 0.6 mL/min. The elution profile was monitored at 210 nm and peak identification was carried out by comparing the retention times of target peaks with those of standards. Calibration curves of formic acid, acetic acid, propionic acid, butyric acid, and lactic acid were prepared in the concentration range of 1–100 mM.
Illumina MiSseq Sequencing and Bioinformatics Analysis
The V3–V4 region of the 16S rRNA gene (reference nucleotide interval 341–805 nt) was sequenced using the Illumina MiSeq system (2 × 300 paired-end run) at the Genomics Unit from the Madrid Science Park Foundation (FPCM). The details on the PCR and sequencing of amplicons were previously described (Piazzon et al., 2019). Raw sequence data from this experiment were uploaded to the Sequence Read Archive (SRA) under Bioproject accession number PRJNA713764 (BioSample accession numbers: SAMN18260336-62). Raw forward and reverse reads were quality filtered using FastQC2 and pre-processed using Prinseq (Schmieder and Edwards, 2011). Terminal N bases were trimmed in both ends and sequences with > 5% of total N bases were discarded. Reads that were < 150 bp long, with Phred quality score < 28 in both of the sequence ends and with a Phred average quality score < 26 were excluded. Then, forward and reverse reads were merged using fastq-join (Aronesty, 2013).
Bacteria taxonomy assignment was performed using the Ribosomal Database Project (RDP) release 11 as a reference database (Cole et al., 2014). Reads were aligned with a custom-made pipeline using VSEARCH and BLAST (Altschul et al., 1990; Rognes et al., 2016). Alignment was performed establishing high stringency filters (≥90% sequence identity, ≥90% query coverage). Taxonomic assignment results were filtered and data were summarized in an operational taxonomic units (OTUs) table. Sample depths were normalized by total sum scaling and then made proportional to the total sequencing depth, following the recommendations previously described (McKnight et al., 2019).
Inferred Metagenome and Pathway Analysis
Piphillin was used to normalize the amplicon data by 16S rRNA gene copy number and to infer metagenomic contents (Iwai et al., 2016). Piphillin predicts the gene inventory of the analyzed OTUs and estimates potential expression based on OTU count values, but does not estimate gene activity. In addition, some predictions are performed at the genus level, not considering the variability among species. Thus, this analysis contains a good degree of uncertainty, but it is helpful to evaluate the potential transcriptomic capacity of a subset of bacteria. The analysis was performed with the OTUs significantly driving the separation by diets in the PLS-DA analysis (described in the Statistics section). A sequence identity cut-off of 97% was implemented, and the inferred metagenomic functions were assigned using the Kyoto Encyclopaedia of Genes and Genomes database (KEGG, October 2018 Release). Raw KEGG pathway output from Piphillin was analyzed with the R Bioconductor package DESeq2 using default parameters, after flooring fractional counts to the nearest integer (Love et al., 2014; Bledsoe et al., 2016; Piazzon et al., 2020).
Statistics
Data of the growth were analyzed by one-way ANOVA using SigmaPlot v14 (Systat Software Inc., San Jose, CA, United States). Normality of the data was verified by Shapiro-Wilk test, and Dunn’s post-test was used for multiple comparisons among groups. Analysis of semiquantitative and quantitative histological data was carried out with the non-parametric Kruskal–Wallis test, followed by Dunn’s post-test for multiple comparisons. SCFA results were analyzed by one-way ANOVA followed by Holm-Sidak post-test. Rarefaction curves (plotting the number of observed taxonomic assignations against the number of sequences), species richness estimates and alpha diversity indices were obtained using the R package phyloseq (McMurdie and Holmes, 2013). To determine the coverage for the microbial communities, the ratio between observed and expected OTUs (determined by the Chao1 index) was calculated. Differences in species richness, diversity indices, and phylum abundance were determined by Kruskal–Wallis test using the Dunn’s post-test, with a significance threshold of P < 0.05. Beta diversity across groups was tested with permutational multivariate analysis of variance (PERMANOVA) using the non-parametric method adonis from the R package Vegan with 10,000 random permutations. To study the separation among groups, supervised partial least-squares discriminant analysis (PLS-DA) and hierarchical clustering of samples were sequentially applied using EZinfo v3.0 (Umetrics, Umeå, Sweden) and R package ggplot2, respectively. Values of normalized counts of OTUs present in 5 or more samples were included in the analyses. The contribution of the different genes to the group separation was determined by the minimum Variable Importance in the Projection (VIP) values achieving the complete clustering of the conditions with a VIP value of 1. Hotelling’s T2 statistic was calculated by the multivariate software package EZinfo v3.0 and points above 95% confidence limit for T2 were excluded. The quality of the PLS-DA model was evaluated by the parameters R2Y (cum) and Q2 (cum), which indicate the fit and prediction ability, respectively. To assess whether the supervised model was being over-fitted, a validation test consisting on 500 random permutations was performed using SIMCA-P+ (v11.0, Umetrics). The inferred metagenomics pathways were considered differentially represented using a FDR-corrected significance threshold of 0.05.
Results
Growth Performance
Data on the growth performance are shown in Table 2. All fish grew efficiently during the trial reaching an overall FCR of 1.09–1.19. However, daily specific growth rates (SGR) varied significantly from 2.31 in the CTRL fish to 2.19 in the 100LSAqua fish, with intermediate values (2.27) very close to CTRL values in fish fed the 50LSAqua diet. As a result of this, final body weight and condition factor were significantly lower (P < 0.001) in the 100LSAqua fish than in CTRL and 50LSAqua groups. Regarding organosomatic indices, no statistically significant differences were found in HSI, MSI and IWI, but overall the intestine of the LSAqua fish was shortened and the lowest ILI was observed in the 50LSAqua group.
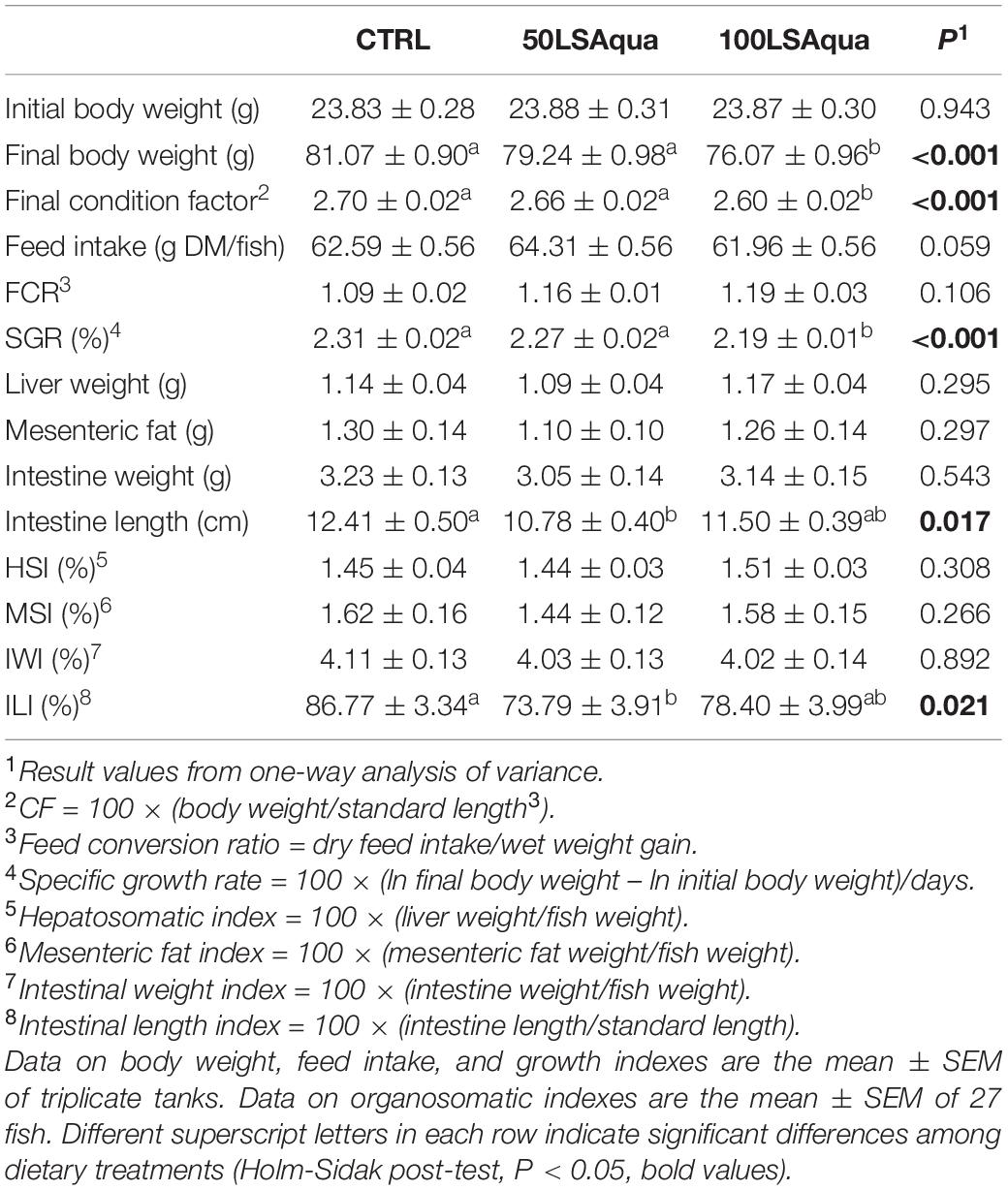
Table 2. Effects of dietary treatment on growth performance of gilthead sea bream juveniles fed to visual satiety from May to July (8 weeks) with CTRL, 50LSAqua and 100LSAqua diets.
Histological Scoring
Both replacement diets had prevailing pro-inflammatory effects at histological level in the two examined intestine portions (Figures 1, 2). In these fish, intestines presented inflammatory hyperplasia with intense EGC and lymphocyte recruitment at both AI and PI segments, and thus mean scoring for all inflammatory markers was lower in CTRL fish. At the AI, abundance of IELs was only significantly higher for the 100LSAqua diet, whereas submucosal hyperplasia was significantly severer for both 50LSAqua and 100LSAqua diets. No differences were found in the distribution pattern of goblet cells in the AI. In this intestinal segment, epithelial lesions were almost absent (score < 0.5), and no lipid vacuolization occurred (score 0). At the PI, the inflammatory reaction triggered by the dietary intervention was more acute. 50LSAqua and 100LSAqua groups showed a significant increase of EGC abundance as well as a significantly higher submucosal hyperplasia, compared to the CTRL fish. Furthermore, in fish fed the extreme substitution diet 100LSAqua, the scoring for submucosal hyperplasia was significantly severer than in the 50LSAqua fish. This intestinal segment presented extensive lipid vacuolization of enterocytes, which was not affected by the diet, and though not significant, epithelial lesions in the PI increased with the degree of protein replacement. Abundance of light-stained goblet cells (with Giemsa) in the PI significantly increased in the 100LSAqua fish, and this cell type presented a PAS + staining pattern indicative of neutral mucins. The inflammatory effect was also noted at hepatic level, where a significant increase of lymphocyte aggregates was observed for the 50LSAqua diet, which was concomitant with an increase in hepatic fat depots. No dietary-induced differences were found for hepatic glycogen storage, and the changes in melanomacrophage centers were not significant.
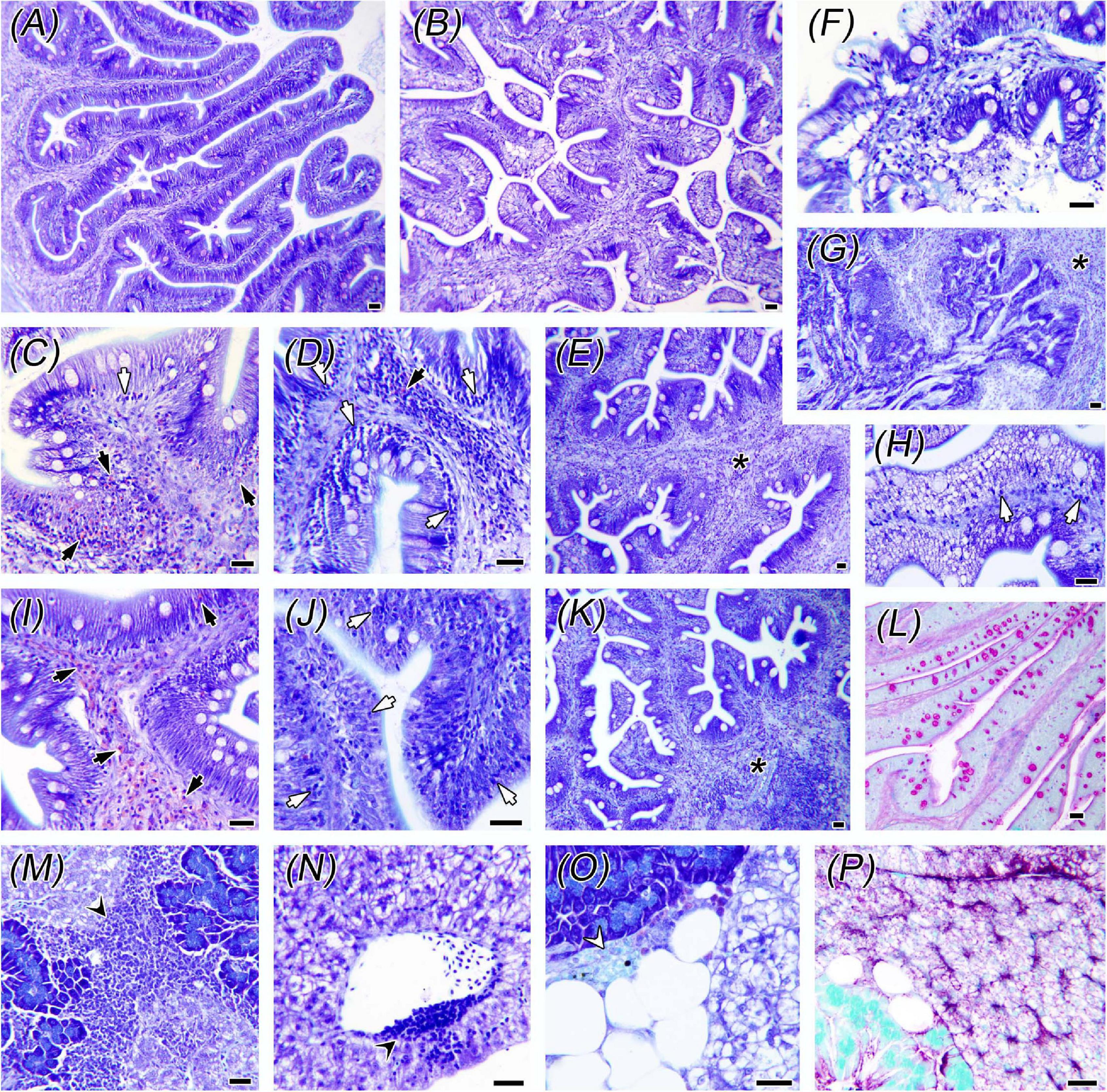
Figure 1. Histological alterations in intestine and liver. Control fish anterior (AI) and posterior (PI) intestines are shown in panels (A,B), respectively. Panels (C–H,L) correspond to PI of fish fed the 50LSAqua diet, (I-K) correspond to AI of the 100LSAqua diet, and (M–P) to livers of the 50LSAqua diet. Note the inflammatory reaction in both intestinal segments consisting of eosinophilic granular cells (EGCs) (black arrows), intraepithelial lymphocytes (IELs) (white arrows), and submucosal hyperplasia (asterisks). Epithelial necrosis and desquamation (F–G), together with lipid vacuolization in enterocytes (H) and higher abundance of PAS + Goblet cells (L) were found in 100LSAqua-PI. Livers presented lymphocyte aggregates (black arrowheads) close to pancreatic acini (M) and blood vessels (N), early melanomacrophage centers (white arrowhead) and large lipid depots (O), and moderate glycogen storage (P). All images are Giemsa-stained sections, except (L) and (P) PAS-stained. Scale bars = 20 μm.
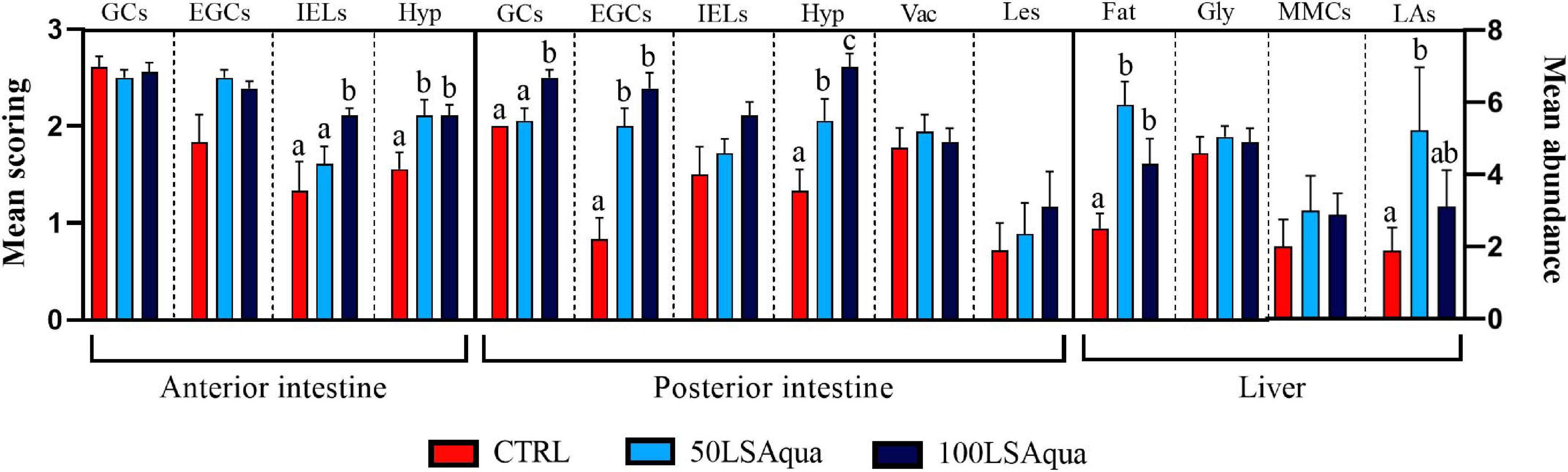
Figure 2. Scoring of histological alterations in fish fed with control (CTRL), 50LSAqua and 100LSAqua diets. Mean semiquantitative scoring (+SEM) from 0 (absence) to 3 (very abundant) is shown for abundance of PAS + Goblet cells (GCs), eosinophilic granular cells (EGCs), and intraepithelial lymphocytes (IELs). Mean semiquantitative scoring (+SEM) from 0 (absence) to 3 (severe) is shown for the degree of hyperplasia in the submucosa (Hyp), vacuolization of enterocytes (Vac), epithelial lesions (Les), hepatic fat storage (Fat) and hepatic glycogen storage (Gly). Total melanomacrophage centers (MMCs) and lymphocyte aggregates (LAs) were quantified in livers (mean abundance + SEM). Different letters within each alteration indicate statistically significant differences among diets (P < 0.05).
Intestinal Lactic Acid and SCFA
No significant differences among groups were found for the measured concentrations of lactic, formic, propionic, and acetic acids but they all showed a trend to be decreased with high FM replacement, except for propionic acid. Thus, the total concentration of SCFA decreased significantly with the FM replacement from 18.91 μmol/g in the CTRL fish to 14.05 μmol/g in the 50LSAqua fish, and 11.3 μmol/g in the 100LSAqua fish (Figure 3).
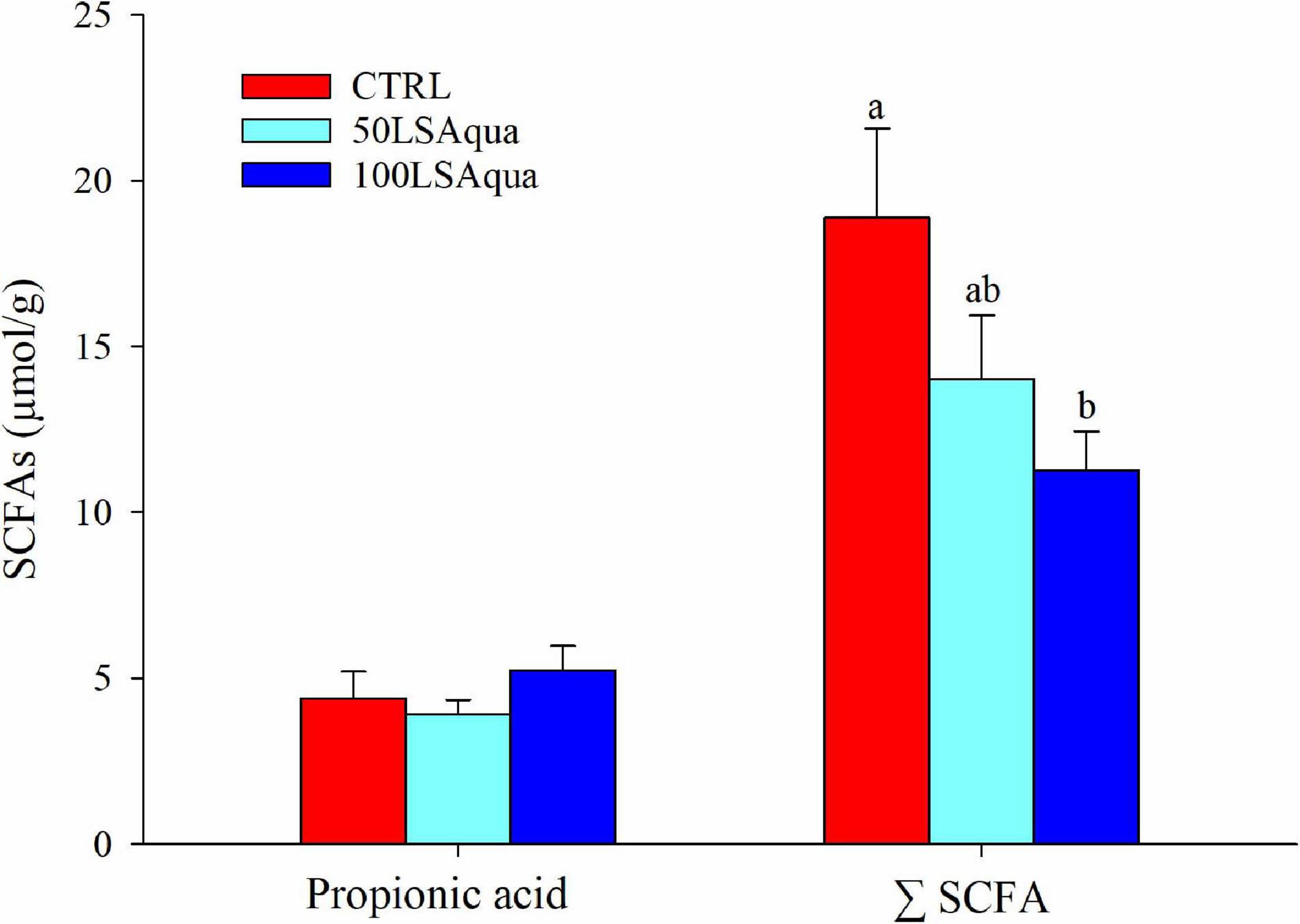
Figure 3. Concentration of intestinal propionic acid and total short chain fatty acids (ΣSCFA) in fish fed CTRL (red bars), 50LSAqua (light blue bars), and 100LSAqua (dark blue bars) diets. Means + SEM are represented (n = 10 fish per group). Significant differences (one-way ANOVA, Holm-Sidak post-test, P < 0.05) are indicated by different letters, which correspond to pairwise comparisons among dietary groups.
Alpha Diversity and Microbial Composition
Illumina sequencing of the 27 samples yielded 3,521,879 high quality reads, with a mean of 130,439 reads per sample, ranging from 81,080 to 198,602 (Supplementary Table 2). The reads were assigned to 1,567 OTUs at 97% identity threshold. Almost half of these OTUs (48.3%) were classified up to the level of species, 87.2% to the level of genus, and more than 95% to the level of family (95.1%), order (97.5%), class (98.8%), and phylum (99.9%). Rarefaction analysis showed most of the curves approximating saturation (horizontal asymptote) (Supplementary Figure 1) but to ensure the adequateness of the samples, the coverage in terms of richness achieved in our assay was calculated. This analysis helped us to unravel two samples with fewer predicted OTUs than expected, which can be considered as potential outliers. The rest of the samples showed a good coverage of the prokaryotic community, with an average value of 67.3% (Supplementary Table 2).
When comparing the bacterial diversity and composition of the CTRL and the LSAqua-based diets (Table 3), no significant differences were found in Chao1 and ACE richness indices, but a higher (P < 0.05) Simpson diversity index was found in the 100LSAqua group, with the 50LSAqua fish showing intermediate values. At the phylum level (Figure 4), Proteobacteria were the most abundant bacteria constituting more than 50% of the total microbiota in all groups, with no significant changes. In parallel, a significant increase in the phylum Actinobacteria was found in both 50LSAqua and 100LSAqua groups (22.1–24.1%) in comparison to the CTRL fish (12.1%). The phylum Firmicutes decreased significantly in 100LSAqua fish (8.6%) in comparison to the CTRL group (23.7%), with intermediate values for the 50LSAqua fish (11.9%). Finally, the less abundant Bacteroidetes phylum accounted for ∼3–6% in all groups.
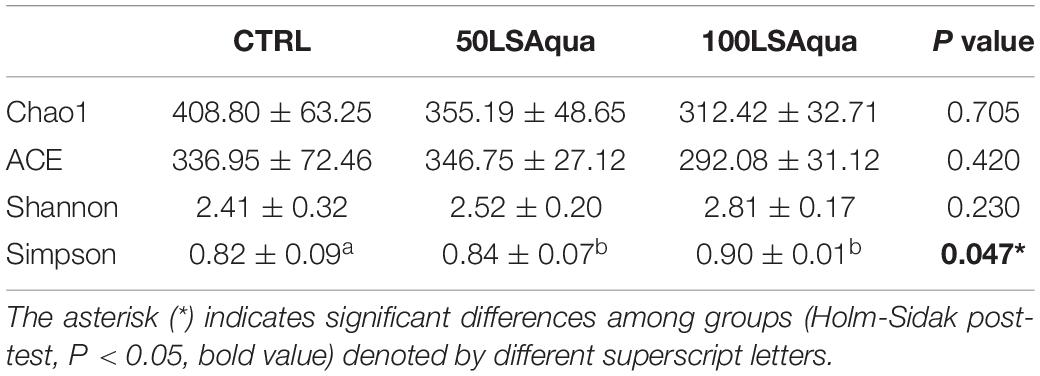
Table 3. Species richness estimators (Chao1 and ACE) and diversity indexes (Shannon and Simpson) of 9 fish of CTRL, 50LSAqua and 100LSAqua groups.
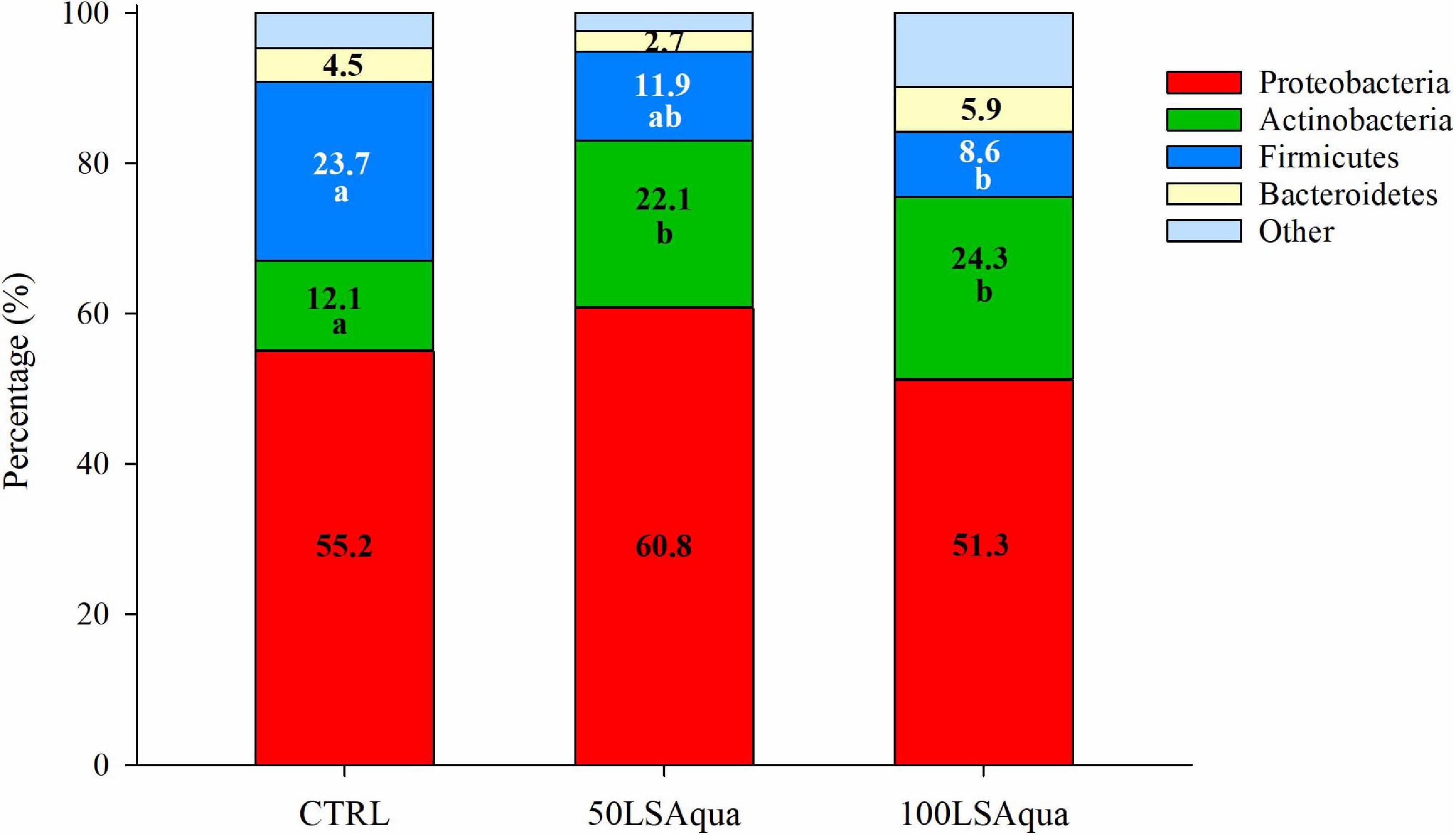
Figure 4. Relative abundance of bacterial phyla in the anterior intestine of fish fed CTRL, 50LSAqua, and 100LSAqua diets. Significant differences (Kruskal–Wallis test, Dunn’s post-test, P < 0.05) are indicated by different letters which correspond to pairwise comparisons within each phylum among dietary groups.
Microbiota Discriminant Analysis
Permutational multivariate analysis of variance test was used to evaluate differences in bacterial composition by dietary group but no statistical differences were detected when comparing animals fed the three different diets (P = 0.211, F = 1.1320, R2 = 0.0404). However, statistical differences were detected when comparing fish fed with CTRL and LSAqua-based diets (50/100LSAqua) (P = 0.032, F = 1.1820, R2 = 0.0531). To validate and study in more detail these differences, a PLS-DA model (R2Y = 98%, Q2 = 41%) was constructed and statistically validated. During the construction of the model, a fish from the 100LSAqua group and a fish from the CTRL group were identified as outliers and discarded from the model. These outliers coincided with the samples with low coverage ratios, formerly calculated in Supplementary Table 2. The remaining points were used to construct a PLS-DA model (Figure 5A) showing a clear separation of fish fed 50/100LSAqua diets along component 1 (89.02%) from fish fed CTRL diets. The PLS-DA model was successfully validated with a permutation test discarding the possibility of over-fitting of the supervised model (Supplementary Figure 2). These results highlight that the diet has a significant impact on the composition of the adherent bacterial communities of the anterior intestine. To determine which groups of bacteria were driving these separations with the diet changes, a more detailed analysis of the variable importance in projection (VIP) was performed in the all-group PLS-DA throughout a heatmap representation (Figure 5B). Hierarchical clustering of samples was applied and the minimum VIP values significantly driving the separation of the groups in the model were calculated. The OTUs within these values were selected for further analysis. Differences in dietary groups were mainly changing 112 OTUs (VIP ≥ 1), which can be accessed in Supplementary Table 3.
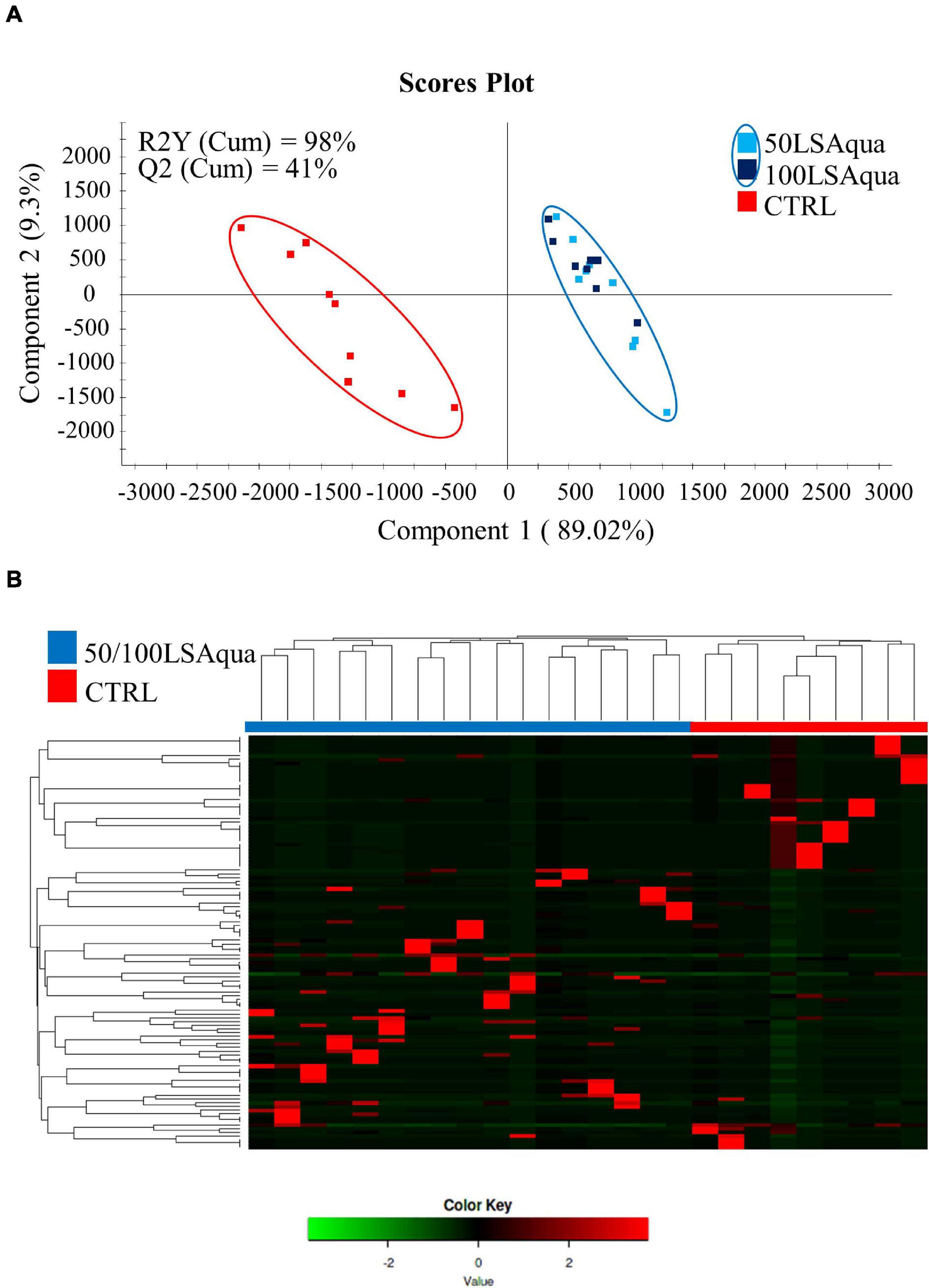
Figure 5. (A) Two-dimensional PLS-DA scores plot constructed using the variable diet with all groups, representing the distribution of the samples between the first two components in the model. The validation by the permutation test can be found in Supplementary Figure 2. (B) Heatmap representing the abundance distribution (Z-score) of the OTUs identified to be driving the separation by diet among all groups.
Figure 6 shows the most abundant bacteria (>1% in at least one group; 46 OTUs) of those that exclusively drove the separation by dietary group, ordered by the corresponding type of response retrieved by the clustering. A first type of response included 29 OTUs increasing with the introduction of LSAqua SusPro. Verrucomicrobia (Luteolibacter genus) and Chlamydiae (Parachlamydiaceae family) phyla were exclusively found in this first type of response, as well as several species of the Betaproteobacteria class. Some species of the Rhodospirillales, Rhizobiales, Salinisphaerales, Xanthomonadales, and Enterobacteriales families were increased in LSAqua-based diets. Lastly, at a genus level, the presence of Paracoccus, Omithinimicrobium, Tetrasphaera, Rubellimicrobium, Dietzia, Propioniclava, and Butyrivibrio was also characteristic of this response. The second type of response grouped 17 OTUs with a significantly higher proportion in the CTRL group. Bacteroidetes of the Flavobacteriaceae family appeared in this group, as well as certain OTUs from the Chromatiales, Bacillales, and Methylococcales orders. The genera exclusively changing in this type of response were Aggregatibacter, Clostridium sensu stricto, Acinetobacter, Rhodovulum, Novosphingobium, Albimonas, and Psychrobacter.
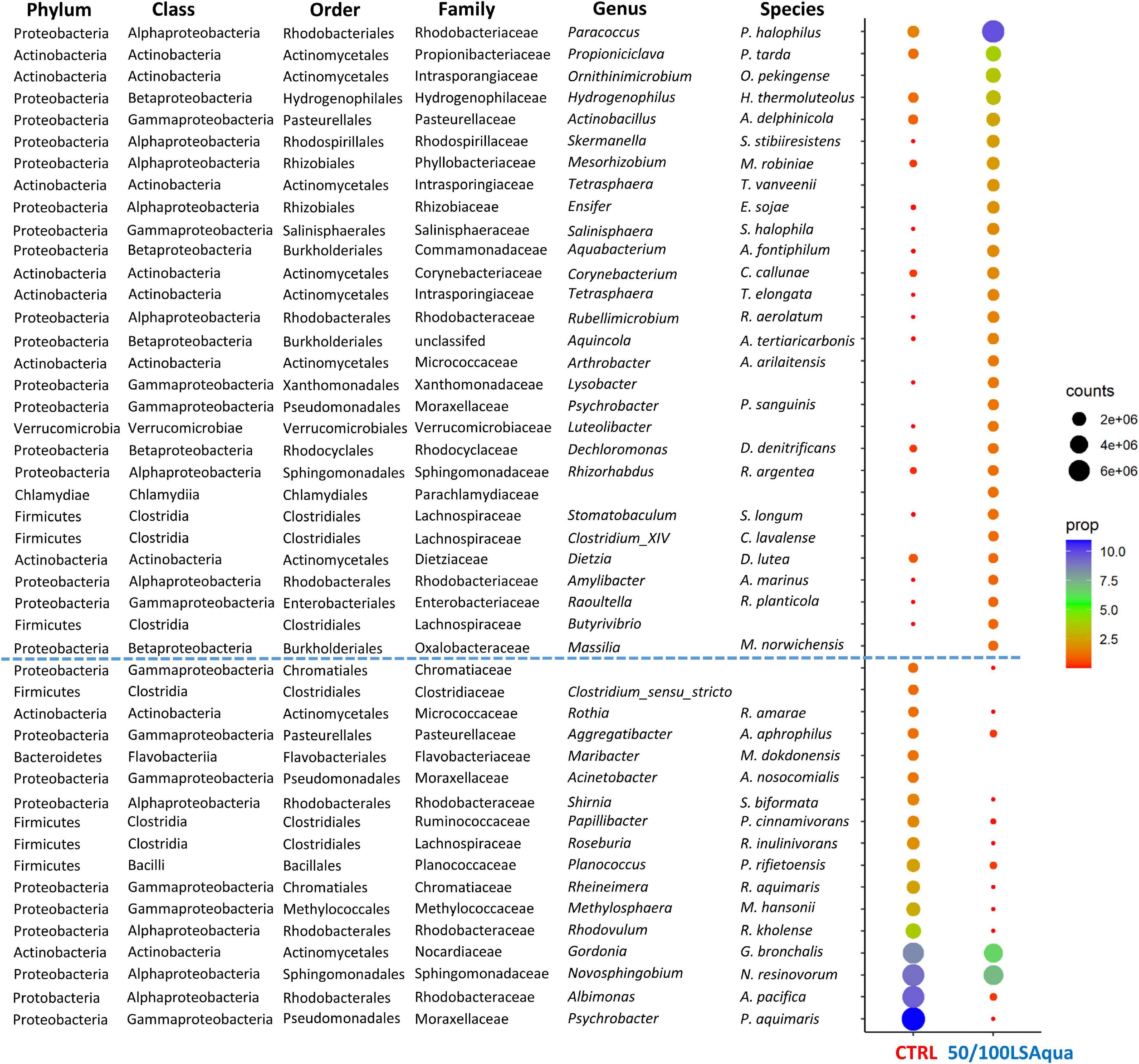
Figure 6. Dotplot map depicting the OTUs with more than 1% of proportion in at least one dietary group. The size of the dots represents the normalized counts in each group. The color scale represents the mean abundance, in percentage, of each OTU within each group. CTRL and 50/100LSAqua refers to control and combined LSAqua-based diets, respectively. OTUs above the dotted blue line showed an higher abundance in 50/100LSAqua group; OTUs below the blue dotted line showed an higher abundance in CTRL group.
Inferred Metagenome and Pathway Analysis
In an attempt to evaluate the biological significance of the differences induced by diets in the microbiota of the different groups of families, pathway analysis was performed using the inferred metagenomes of the 112 OTUs driving the separation by diet (Figure 7). The results showed that 27 pathways could be significantly changing, taking into account the gene inventory of the discriminant OTUs, in the comparison between fish fed 50/100LSAqua diets and fish fed CTRL diets.
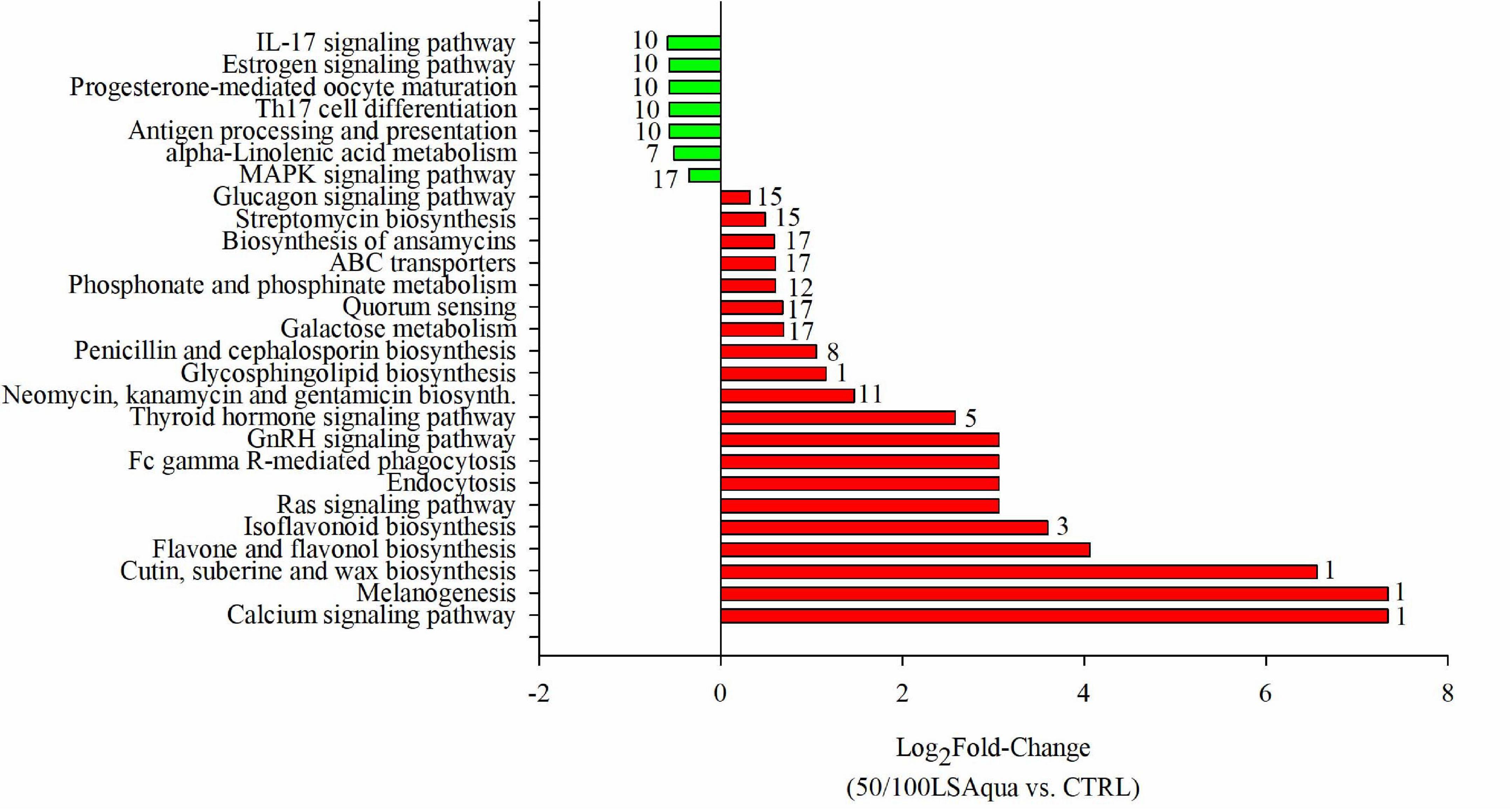
Figure 7. Pathway analysis from predicted metagenome. Values represent the Log2 fold change of the comparison between 50/100LSAqua vs. CTRL groups (FDR < 0.05). Numbers in bars indicate the number of abundant OTUs (VIP > 1; > 1%) related with the expression of genes involved in each pathway.
Pathways related to immune response (IL-17 signaling pathway, Th17 cell differentiation and antigen processing and presentation), hormonal processes (estrogen signaling pathway and progesterone-mediated oocyte maturation) and alpha-linoleic acid metabolism were under-represented in LSAqua-based diets. The over-represented pathways in LSAqua groups were those related with calcium signaling pathway, melanogenesis, cutin, suberin, and wax biosynthesis, flavonoid biosynthesis, quorum sensing and Ras signaling pathway. Hormonal regulation was also retrieved here, with the over-representation of thyroid hormone signaling and GnRH signaling pathways. A remarkable presence of up to four pathways related with antimicrobials production (streptomycin biosynthesis, biosynthesis of ansamycins, penicillin and cephalosporin biosynthesis and nemoycin, kanamycin and gentamicin biosynthesis) was predicted. Inferred metagenome analysis also displayed a total of 17 predominant (VIP ≥ 1; > 1% in abundance) OTUs whose genomes could be associated to the expression of genes involved in the differentially represented pathways (FDR < 0.05). The list of bacteria related to each pathway can be found in Supplementary Table 4. Of note, we have to consider that this information was obtained from in silico inference and only reflects what could be potentially occurring, but it is still of value to estimate the putative metabolic capability of the bacterial populations.
Discussion
Aquaculture intensification and sustainability led to new challenges since there is a need to adapt not only to the inclusion of new dietary ingredients, but also to cope with the challenges arising from intensive fish production. The use of wild fish as aquaculture feeds is becoming unsustainable and new substitution strategies with alternative and sustainable ingredients are being conducted for an efficient production of low FM or FM-free diets (Peixoto et al., 2019; Ramos-Pinto et al., 2019; Reis et al., 2021). Among these strategies, PAP and SCP are promising materials to develop future fish feeds. However, the effect of these new materials on the host physiology and its associated microbiota, and how these two systems interact, remain largely unknown. The present study suggested that the reshaping of gut microbiota is perhaps a permissive, but also necessary, process for the long-term preservation of growth performance and gut homeostasis in a model of a marine fish fed diets devoid of FM.
At a closer look, growth performance of fish fed with 50LSAqua was almost undistinguishable from the CTRL group. Yet, a slight but statistically significant decrease of SGR (5%) was found with the total replacement of FM by LSAqua SusPro. The achievement of statistically significant differences was favored by the low variability between replicate tanks through the feeding trial. Certainly, the growth performance in all dietary groups in the present study was in the upper range for the class of size and the rearing conditions given for gilthead sea bream (Simó-Mirabet et al., 2018; Martos-Sitcha et al., 2019). However, as reviewed by Glencross et al. (2020), the success of FM replacement by PAP and SCP products is highly variable in aquafeeds. Indeed, the partial and total replacement of FM by yeast (Zhao et al., 2017) or bacterial proteins (Hardy et al., 2018) is highly feasible in shrimp. Likewise, up to 40–50% can be replaced without growth impairment in tilapia (Oreochromis mossambicus) and European sea bass (Davies and Wareham, 1988; Oliva-Teles and Gonçalves, 2001). The replacement of FM by bacterial proteins seems to be more limited in Atlantic halibut (Hippoglossus hippoglossus) (Aas et al., 2007). Similarly, partial but no total replacement was feasible in Asian sea bass (Delamare-Deboutteville et al., 2019). Therefore, the optimal level of FM replacement needs to be assessed for each SCP, fish species, developmental stage and rearing condition. Apparently, the success of FM replacement by PAP is overall less restrictive. This is in fact extensive to gilthead sea bream, in which total or high levels of FM replacement (>75%) are feasible during short-term trials (Moutinho et al., 2017: Sabbagh et al., 2019; Fontinha et al., 2021). In agreement with this, only a slight impairment of growth performance was found herein with the use of a mix of poultry PAP and bacterial proteins. However, this will be limited by a pro-inflammatory condition that was evidenced in a dose-dependent manner by the histopathological scoring of AI and PI segments of fish fed LSAqua feeds. Indeed, inflammation is a common sign of nutritionally mediated metabolic derangements in gilthead sea bream juveniles fed practical diets with a high level of replacement of FM/FO by plant ingredients (Ballester-Lozano et al., 2015).
Short chain fatty acids are end products of protein and fiber microbial fermentation that serve as a primary energy source for enterocytes, but also to preserve intestinal homeostasis through a complex trade-off of anti-inflammatory activities (Donohoe et al., 2011; Corrêa-Oliveira et al., 2016; Morrison and Preston, 2016; Abdel-Latif et al., 2020; Abdel-Tawwab et al., 2021). Their beneficial effects have been demonstrated in gilthead sea bream, where dietary SCFA supplementation helped to reverse the pro-inflammatory condition of fish fed plant-based diets (Robles et al., 2013; Benedito-Palos et al., 2016; Piazzon et al., 2017). Otherwise, there is now evidence that dietary heptanoate, a medium chain fatty acid, contributed to improve the feed efficiency and swimming performance in gilthead sea bream (Martos-Sitcha et al., 2018). In the current study, the intestinal content of total SCFA decreased in a dose-dependent manner with FM replacement, being this feature associated with changes in the growth and pro-inflammatory status. In the same species, the dietary supplementation with protein extracts of Navicula microalgae triggered inflammatory markers (Reyes-Becerril et al., 2013). Likewise, the total substitution of FM with poultry by-product meal had a clear hepatic inflammatory action in tench (Tinca tinca) (Panicz et al., 2017), and increased lipid deposition rates with a negative impact on the growth and immune response of largemouth bass (Micropterus salmoides) (Subhadra et al., 2006). Taking all this in mind, it could be hypothesized that supplementation of 100LSAqua diets with SCFA, like butyrate or propionate, could counteract some of the observed drawback effects in these fish, though future studies are needed to answer this specific question as further discussed below.
Gut microbiota regulates feeding, digestive and metabolic processes, and is crucial for immune development and training (Feng et al., 2018; Butt and Volkoff, 2019), being dietary factors one of the most important drivers of intestinal microbial diversity (Moschen et al., 2012). In fact, the core intestinal microbiota is clearly different among carnivorous, omnivorous, and herbivorous fish species (Liu et al., 2016). In our study, an increase of the Simpson diversity index was disclosed in both 50LSAqua and 100LSAqua groups (Table 3), which suggests that the intestine of fish fed with FM-substitution diets contained a wider range of bacteria capable of enhancing a more diverse number of host functions, as previously reported in trout upon the use of algal SCP (Lyons et al., 2017). Despite this, the typical microbiota of marine fish with a high abundance (>90%) of Proteobacteria, Firmicutes, Actinobacteria, and Bacteroidetes phyla was found herein in all dietary groups in agreement with previous gilthead sea bream studies (Kormas et al., 2014; Estruch et al., 2015; Piazzon et al., 2019). However, LSAqua diets had an impact on microbiota beta-diversity, and changes in gut bacterial communities were already found at the phylum level (Figure 4), supporting the fact that intestinal microbiota is highly influenced by dietary nutrients. In this regard, discriminant analysis (Figure 5) disclosed the significant change of 46 dominant OTUs (VIP ≥ 1; > 1% in abundance) (Figure 6), which allowed discovering differences in the gut microbial communities at all taxonomic levels. These changes together with the predictions of the inferred metagenome (Figure 7) suggest that the microbiome structure is shifting to deal with the alternative fish feed formulations used in the present study. This assumption is further supported by a recent study in European sea bass using PAP and SCP as FM replacers (Pérez-Pascual et al., 2020). The exact nature of the main source of variation of bacterial OTUs cannot be deduced by comparing experiments performed in different species. However, it is likely that the protein source is an important factor modulating intestinal microbial communities.
Proteobacteria, as gram-negative facultative anaerobic organisms, commonly dominate aquatic niches due to their highly flexible metabolic properties (Ikeda-Ohtsubo et al., 2018), being one of the most abundant symbionts in marine fish (Tarnecki et al., 2017). In the present study, the most abundant OTU belonging to this phylum in LSAqua-fish was Paracoccus halophilus, present in < 3% in the CTRL group and raising up to ∼9.5% in fish fed the experimental diets. Supplementation with Paracoccus marcusii was recently described to decrease intestinal inflammation by suppressing TLR4 signaling, with a positive impact on the growth performance and intestinal function of juvenile grass carp (Ctenopharyngodon idella) (Xue et al., 2020). However, Proteobacteria, as gram negatives, are generally related to increased intestinal inflammation and its increase is very often related with an inflammatory condition in the human gut (Mukhopadhya et al., 2012; Satokari, 2015). One of the main drivers of the inflammatory action of gram negative bacteria is the lipopolysaccharide, an endotoxin molecule that induces the release of the pro-inflammatory chemokine IL-8 at the intestinal local level (Teghanemt et al., 2005; Hiippala et al., 2020). The lipopolysaccharides of different gram-negative bacteria differ structurally, and those from the Proteobacteria class Gammaproteobacteria present specific modifications that showed to increase the inflammatory effects, at least in mammals (Teghanemt et al., 2005). Interestingly, in our experimental model, the class Gammaproteobacteria was significantly reduced from a ∼21% in the CTRL fish to 8.5% in 50/100LSAqua fish. More precisely, the decrease of Gammaproteobacteria was mainly due, to a ∼10% decrease of the Psychrobacter genus. Some species of this genus have been described as activators of NF-kB transcription factor, capable of inducing the transcription of several pro-inflammatory cytokine genes (Chow et al., 1999; Korneev et al., 2014). Indeed, this genus has been recently described to be phylogenetically close to pathobionts (Welter et al., 2021). In addition, Acinetobacter, only present in the CTRL fish in our study, is also a known enhancer of the production of reactive oxygen species (ROS), inducing oxidative stress at the cellular level (Schieber and Chandel, 2014; Ajiboye et al., 2018). Thus, the detected changes in these OTUs could have a potential anti-inflammatory role that needs to be verified by host transcriptomic analyses.
The second and the third most abundant phyla in all groups were the gram-positive bacteria Firmicutes and Actinobacteria, which shared an altered equilibrium among groups. Actinobacteria significantly increased in the LSAqua-based diets in concomitancy with a decrease of Firmicutes. This imbalance does not seem to be senseless, as Actinobacteria, and specifically Actinomycetes, are considered a source of pharmaceutically important secondary metabolites (Dholakiya et al., 2017). Marine Actinomycetes have been found to possess in vitro anti-oxidant and anti-inflammatory potential (Lavanyalatha et al., 2014). All the predominant Actinobacteria in our experiment were assigned to the order Actinomycetales, which represented ∼10% in the CTRL group and ∼20% in LSAqua-based diets, again suggesting a potential shift to an anti-inflammatory gut microbiota which contradicts the histologically observed inflammatory profile. It can be hypothesized that the shift toward and anti-inflammatory microbial structure is a host-driven change of the bacterial populations in an attempt to balance the diet-induced inflammation, but more studies are required to unravel the real metabolic potential of these populations and the basis of this regulation. The most abundant genus within the Actinobacteria of LSAqua-based diets was Propioniciclava (∼6%), previously described as propionate-producing bacteria (Sugawara et al., 2011; Zhang et al., 2017). This feature might contribute to maintain the amount of propionic acid in stripped feces of LSAqua fish relatively constant despite the overall decreased concentration of total SCFA.
Another interesting result of the inferred metagenome was the over-representation of quorum sensing and several pathways related to the biosynthesis of antimicrobials. All the 17 abundant OTUs identified by Phiphillin were related to the quorum sensing pathway, key for effective signaling among bacteria (Jiménez and Sperandio, 2019). Among other processes, the synthesis of antimicrobial substances can be controlled by quorum sensing (Duerkop et al., 2009). It is clear that antibiotics affect bacterial community composition, but a high proportion of the fish intestinal microbiome seems to be able to persist despite antibiotic intake (Kokou et al., 2020). The production of antimicrobials in complex bacterial communities, such as the ones found in the gut, are key in competition or antagonism in bacteria with overlapping niches, but are also signaling facilitators between individual strains, helping to maintain the balance of these communities (García-Gutiérrez et al., 2019). Again, these results are based on predictions of potential functions within the observed bacterial populations, thus, metatranscriptomic or metabolomic approaches must be performed to identify actual functional metabolic differences between groups. In any case, the current results seem to suggest that the observed remodeling of microbiota in LSAqua-fed fish is, at least in part, directly driven by the gut bacteria through the production of antimicrobial substances.
In summary, in terms of the growth performance, the partial and total FM replacement by LSAqua SusPro is highly feasible in short term gilthead sea bream trials. Some detrimental effects involving changes in gut health markers are found, especially with the highest level of replacement resulting in a FM-free diet. This is a common constraining feature in fishless feeds and supplementation with pro- or prebiotics emerge as key strategies to induce an adaptive response of the gut microbiota to produce robust farmed fish with a high capacity to grow efficiently with low FM/FO diets. This opens new research opportunities for fish physiologists and breeders to promote a more ethical and sustainable farmed fish production, according to the criteria of circular economy.
Data Availability Statement
The datasets presented in this study can be found in online repositories. The names of the repositories and accession number(s) can be found below: https://www.ncbi.nlm.nih.gov/, PRJNA713764; https://www.ncbi.nlm.nih.gov/, SAMN18260336-62.
Ethics Statement
The animal study was reviewed and approved by the Ethics and Animal Welfare Committees of Institute of Aquaculture Torre de la Sal (IATS-CSIC, Castellón, Spain), CSIC (Permit number 112/2020), and “Generalitat Valenciana” (permit number 2020/VSC/PEA/0064).
Author Contributions
PS-J, FN-C, MCP, IE, AS-B, and JP-S: formal analysis. PS-J, FN-C, MCP, and JP-S: writing-original manuscript. PS-J, AS-B, DV, and JP-S: conceptualization. All authors involved in experimental investigation, writing-review and editing, and read and approved the final manuscript.
Conflict of Interest
PS-J and DV were employed by the company Lambers-Seghers NV (Hooglede, Belgium).
The remaining authors declare that the research was conducted in the absence of any commercial or financial relationships that could be construed as a potential conflict of interest.
Funding
This work was supported by the EU H2020 Research Innovation Program under the TNA Program (project AE170009) at IATS-CSIC Research Infrastructure within AQUAEXCEL2020 Project (652831). This output reflects only the author’s view and the European Union cannot be held responsible for any use that may be made of the information contained herein. Additional funding was obtained by a Spanish MICINN project (Bream-AquaINTECH, RTI2018–094128-B-I00). MCP was funded by a Ramón y Cajal Postdoctoral Research Fellowship [RYC2018-024049-I/AEI/10.13039/501100011033 co-funded by the European Social Fund (ESF) & ACOND/2020 Generalitat Valenciana].
Acknowledgments
We thank P. Simó-Mirabet and E. Rosell-Moll for their assistance in the fish handling and feeding during the trial, and J. Monfort and L. Rodríguez from the Histology Service of the IATS-CSIC for histological processing. The contribution of T. Requena and M. Miguel in SCFA analysis is also appreciated. We acknowledge support of the publication fee by the CSIC Open Access Publication Support Initiative through its Unit of Information Resources for Research (URICI).
Supplementary Material
The Supplementary Material for this article can be found online at: https://www.frontiersin.org/articles/10.3389/fmars.2021.705041/full#supplementary-material
Supplementary Figure 1 | Rarefaction curves obtained from the sequencing data of the 27 samples included in this study.
Supplementary Figure 2 | Validations (permutation tests) of the PLS-DA model shown in this study.
Supplementary Table 1 | Amino acid profile of experimental diets.
Supplementary Table 2 | Table showing the detailed sequencing data obtained in this study.
Supplementary Table 3 | List of OTUs with minimum VIP values (VIP ≥ 1) responsible for the separation of samples by diet in the different dietary groups.
Supplementary Table 4 | List of abundant OTUs (VIP ≥ 1; > 1%) with the genomic potential to express genes involved in differential (FDR < 0.05) pathways.
Footnotes
References
Aas, T. S., Hatlen, B., Grisdale-Helland, B., Terjesen, B. F., Penn, M., Bakke-McKellep, A. M., et al. (2007). Feed intake, growth and nutrient utilization in Atlantic halibut (Hippoglossus hippoglossus) fed diets containing a bacterial protein meal. Aquac. Res. 38, 351–360. doi: 10.1111/j.1365-2109.2007.01672.x
Aas, T. S., Ytrestøyl, T., and Åsgård, T. (2019). Utilization of feed resources in the production of Atlantic salmon (Salmo salar) in Norway: an update for 2016. Aquacult. Rep. 15:100216. doi: 10.1016/j.aqrep.2019.100216
Abdel-Latif, H. M. R., Abdel-Tawwab, M., Dawood, M. A. O., Menanteau-Ledouble, S., and El-Matbouli, M. (2020). Benefits of dietary butyric acid, sodium butyrate, and their protected forms in aquafeeds: a review. Rev. Fish. Sci. Aquac. 28, 421–448. doi: 10.1080/23308249.2020.1758899
Abdel-Tawwab, M., Shukry, M., Farrag, F. A., El-Shafai, N. M., Dawood, M. A. O., and Abdel-Latif, H. M. R. (2021). Dietary sodium butyrate nanoparticles enhanced growth, digestive enzyme activities, intestinal histomorphometry, and transcription of growth-related genes in Nile tilapia juveniles. Aquaculture 536:736467. doi: 10.1016/j.aquaculture.2021.736467
Agus, A., Planchais, J., and Sokol, H. (2018). Gut microbiota regulation of tryptophan metabolism in health and disease. Cell Host Microbe 23, 716–724. doi: 10.1016/j.chom.2018.05.003
Ajiboye, T. O., Skiebe, E., and Wilharm, G. (2018). Contributions of ferric uptake regulator fur to the sensitivity and oxidative response of Acinetobacter baumannii to antibiotics. Microb. Pathog. 119, 35–41. doi: 10.1016/j.micpath.2018.03.060
Altschul, S. F., Gish, W., Miller, W., Myers, E. W., and Lipman, D. J. (1990). Basic local alignment search tool. J. Mol. Biol. 215, 403–410.
Aronesty, E. (2013). Comparison of sequencing utility programs. Open Bioinforma. J. 7, 1–8. doi: 10.2174/1875036201307010001
Ballester-Lozano, G. F., Benedito-Palos, L., Estensoro, I., Sitjà-Bobadilla, A., Kaushik, S., and Pérez-Sánchez, J. (2015). Comprehensive biometric, biochemical and histopathological assessment of nutrient deficiencies in gilthead sea bream fed semi-purified diets. Br. J. Nutr. 114, 713–726. doi: 10.1017/s0007114515002354
Basto, A., Calduch-Giner, J., Oliveira, B., Petit, L., Sá, T., Maia, M. R., et al. (2021). The use of defatted Tenebrio molitor larvae meal as a main protein source is supported in European sea bass (Dicentrarchus labrax) by data on growth performance, lipid metabolism and flesh quality. Front. Physiol. 12:659567.
Benedito-Palos, L., Ballester-Lozano, G. F., Simó, P., Karalazos, V., Ortiz, Á, Calduch-Giner, J., et al. (2016). Lasting effects of butyrate and low FM/FO diets on growth performance, blood haematology/biochemistry and molecular growth-related markers in gilthead sea bream (Sparus aurata). Aquaculture 454, 8–18. doi: 10.1016/j.aquaculture.2015.12.008
Bledsoe, J. W., Peterson, B. C., Swanson, K. S., and Small, B. C. (2016). Ontogenetic characterization of the intestinal microbiota of channel catfish through 16S rRNA gene sequencing reveals insights on temporal shifts and the influence of environmental microbes. PLoS One 11:e0166379. doi: 10.1371/journal.pone.0166379
Butt, R. L., and Volkoff, H. (2019). Gut microbiota and energy homeostasis in fish. Front. Endocrinol. 10:9.
Chow, J. C., Young, D. W., Golenbock, D. T., Christ, W. J., and Gusovsky, F. (1999). Toll-like receptor-4 mediates lipopolysaccharide-induced signal transduction. J. Biol. Chem. 274, 10689–10692. doi: 10.1074/jbc.274.16.10689
Clark, M., and Tilman, D. (2017). Comparative analysis of environmental impacts of agricultural production systems, agricultural input efficiency, and food choice. Environ. Res. Lett. 12:064016. doi: 10.1088/1748-9326/aa6cd5
Cole, J. R., Wang, Q., Fish, J. A., Chai, B., McGarrell, D. M., Sun, Y., et al. (2014). Ribosomal database project: data and tools for high throughput rRNA analysis. Nucleic Acids Res. 42, D633–D642.
Corrêa-Oliveira, R., Fachi, J. L., Vieira, A., Sato, F. T., and Vinolo, M. A. R. (2016). Regulation of immune cell function by short-chain fatty acids. Clin. Transl. Immunol. 5:e73. doi: 10.1038/cti.2016.17
Davies, S. J., and Wareham, H. (1988). A preliminary evaluation of an industrial single cell protein in practical diets for tilapia (Oreochromis mossambicus). Aquaculture 73, 189–199. doi: 10.1016/0044-8486(88)90053-1
Davies, S. J., Laporte, J., Gouveia, A., Salim, H. S., Woodgate, S. M., Hassan, M. S., et al. (2019). Validation of processed animal proteins (mono-PAPS) in experimental diets for juvenile gilthead sea bream (Sparus aurata L.) as primary fish meal replacers within a European perspective. Aquac. Nutr. 25, 225–238. doi: 10.1111/anu.12846
Delamare-Deboutteville, J., Batstone, D. J., Kawasaki, M., Stegman, S., Salini, M., Tabrett, S., et al. (2019). Mixed culture purple phototrophic bacteria is an effective fishmeal replacement in aquaculture. Water Res. X 4:100031. doi: 10.1016/j.wroa.2019.100031
Dholakiya, R. N., Kumar, R., Mishra, A., Mody, K. H., and Jha, B. (2017). Antibacterial and antioxidant activities of novel Actinobacteria strain isolated from gulf of Khambhat, Gujarat. Front. Microbiol. 8:2420.
Donohoe, D. R., Garge, N., Zhang, X., Sun, W., O’Connell, T. M., Bunger, M. K., et al. (2011). The microbiome and butyrate regulate energy metabolism and autophagy in the mammalian colon. Cell Metab. 13, 517–526. doi: 10.1016/j.cmet.2011.02.018
Duerkop, B. A., Varga, J., Chandler, J. R., Peterson, S. B., Herman, J. P., Churchill, M. E. A., et al. (2009). Quorum-sensing control of antibiotic synthesis in Burkholderia thailandensis. J. Bacteriol. 191, 3909–3918. doi: 10.1128/jb.00200-09
Egerton, S., Culloty, S., Whooley, J., Stanton, C., and Ross, R. P. (2018). The gut microbiota of marine fish. Front. Microbiol. 9:873.
Egerton, S., Wan, A., Murphy, K., Collins, F., Ahern, G., Sugrue, I., et al. (2020). Replacing fishmeal with plant protein in Atlantic salmon (Salmo salar) diets by supplementation with fish protein hydrolysate. Sci. Rep. 10:4194.
Espe, M., Lemme, A., Petri, A., and El-Mowafi, A. (2006). Can Atlantic salmon grow on a diet devoid of fish meal? Aquaculture 255, 255–262. doi: 10.1016/j.aquaculture.2005.12.030
Estensoro, I., Ballester-Lozano, G., Benedito-Palos, L., Grammes, F., Martos-Sitcha, J. A., Mydland, L. T., et al. (2016). Dietary butyrate helps to restore the intestinal status of a marine teleost (Sparus aurata) fed extreme diets low in fish meal and fish oil. PLoS One 11:e0166564. doi: 10.1371/journal.pone.0166564
Estruch, G., Collado, M. C., Peñaranda, D. S., Tomás Vidal, A., Jover Cerdá, M., Pérez Martínez, G., et al. (2015). Impact of fishmeal replacement in diets for gilthead sea bream (Sparus aurata) on the gastrointestinal microbiota determined by pyrosequencing the 16S rRNA gene. PLoS One 10:e0136389. doi: 10.1371/journal.pone.0136389
Feng, Q., Chen, W. D., and Wang, Y. D. (2018). Gut microbiota: an integral moderator in health and disease. Front. Microbiol. 9:151.
Fontinha, F., Magalhães, R., Moutinho, S., Santos, R., Campos, P., Serra, C. R., et al. (2021). Effect of dietary poultry meal and oil on growth, digestive capacity, and gut microbiota of gilthead seabream (Sparus aurata) juveniles. Aquaculture 530:735879. doi: 10.1016/j.aquaculture.2020.735879
García-Gutiérrez, E., Mayer, M. J., Cotter, P. D., and Narbad, A. (2019). Gut microbiota as a source of novel antimicrobials. Gut Microbes 10, 1–21. doi: 10.1080/19490976.2018.1455790
Glencross, B. D., Huyben, D., and Schrama, J. W. (2020). The application of single-cell ingredients in aquaculture feeds-a review. Fishes 5, 1–39.
Hao, W. L., and Lee, Y. K. (2004). Microflora of the gastrointestinal tract: a review. Methods Mol. Biol. 268, 491–502. doi: 10.1385/1-59259-766-1:491
Hardy, R. W., Patro, B., Pujol-Baxley, C., Marx, C. J., and Feinberg, L. (2018). Partial replacement of soybean meal with Methylobacterium extorquens single-cell protein in feeds for rainbow trout (Oncorhynchus mykiss). Aquac. Res. 49, 2218–2224. doi: 10.1111/are.13678
Hiippala, K., Kainulainen, V., Suutarinen, M., Heini, T., Bowers, J. R., Jasso-Selles, D., et al. (2020). Isolation of anti-inflammatory and epithelium reinforcing Bacteroides and Parabacteroides spp. from a healthy fecal donor. Nutrients 12:935. doi: 10.3390/nu12040935
Ikeda-Ohtsubo, W., Brugman, S., Warden, C. H., Rebel, J. M. J., Folkerts, G., and Pieterse, C. M. J. (2018). How can we define “optimal microbiota?”: a comparative review of structure and functions of microbiota of animals, fish, and plants in agriculture. Front. Nutr. 5:90.
Iwai, S., Weinmaier, T., Schmidt, B. L., Albertson, D. G., Poloso, N. J., Dabbagh, K., et al. (2016). Piphillin: improved prediction of metagenomic content by direct inference from human microbiomes. PLoS One 11:e0166104. doi: 10.1371/journal.pone.0166104
Jiménez, A. G., and Sperandio, V. (2019). “Quorum sensing and the gut microbiome,” in Quorum Sensing: Molecular Mechanism and Biotechnological Application, ed. G. Tommonaro (London, EN: Elsevier), 151–169. doi: 10.1016/b978-0-12-814905-8.00006-x
Jones, S. W., Karpol, A., Friedman, S., Maru, B. T., and Tracy, B. P. (2020). Recent advances in single cell protein use as a feed ingredient in aquaculture. Curr. Opin. Biotech. 61, 189–197. doi: 10.1016/j.copbio.2019.12.026
Karapanagiotidis, I. T., Psofakis, P., Mente, E., Malandrakis, E., and Golomazou, E. (2019). Effect of fishmeal replacement by poultry by-product meal on growth performance, proximate composition, digestive enzyme activity, haematological parameters and gene expression of gilthead seabream (Sparus aurata). Aquac. Nutr. 25, 3–14. doi: 10.1111/anu.12824
Kokou, F., Sasson, G., Mizrahi, I., and Cnaani, A. (2020). Antibiotic effect and microbiome persistence vary along the European seabass gut. Sci. Rep. 10:10003.
Kormas, K. A., Meziti, A., Mente, E., and Frentzos, A. (2014). Dietary differences are reflected on the gut prokaryotic community structure of wild and commercially reared sea bream (Sparus aurata). Microbiologyopen 3, 718–728. doi: 10.1002/mbo3.202
Korneev, K. V., Kondakova, A. N., Arbatsky, N. P., Novototskaya-Vlasova, K. A., Rivkina, E. M., Anisimov, A. P., et al. (2014). Distinct biological activity of lipopolysaccharides with different lipid a acylation status from mutant strains of Yersinia pestis and some members of genus Psychrobacter. Biochemical 79, 1333–1338. doi: 10.1134/s0006297914120062
Lavanyalatha, Y., Rashmi, H. K., and Mahesawari Devi, P. (2014). In vitro anti-oxidant and anti-inflammatory potential of marine Actinomycetes. Int. J. Eng. Res. 5:12.
Liu, H., Guo, X., Gooneratne, R., Lai, R., Zeng, C., Zhan, F., et al. (2016). The gut microbiome and degradation enzyme activity of wild freshwater fishes influenced by their trophic levels. Sci. Rep. 6:24340.
Love, M. I., Huber, W., and Anders, S. (2014). Moderated estimation of fold change and dispersion for RNA-seq data with DESeq2. Genome Biol. 15:550.
Lyons, P. P., Turnbull, J. F., Dawson, K. A., and Crumlish, M. (2017). Effects of low-level dietary microalgae supplementation on the distal intestinal microbiome of farmed rainbow trout Oncorhynchus mykiss. Aquac. Res. 48, 2438–2452. doi: 10.1111/are.13080
Martos-Sitcha, J. A., Simó-Mirabet, P., de Las Heras, V., and Calduch-Giner, J. À, and Pérez-Sánchez, J. (2019). Tissue-specific orchestration of gilthead sea bream resilience to hypoxia and high stocking density. Front. Physiol. 10:840.
Martos-Sitcha, J. A., Simó-Mirabet, P., Piazzon, M. C., de las Heras, V., Calduch-Giner, J. A., Puyalto, M., et al. (2018). Dietary sodium heptanoate helps to improve feed efficiency, growth hormone status and swimming performane in gilthead sea bream (Sparus aurata). Aquac. Nutr. 24, 1638–1651. doi: 10.1111/anu.12799
McKnight, D. T., Huerlimann, R., Bower, D. S., Schwarzkopf, L., Alford, R. A., and Zenger, K. R. (2019). Methods for normalizing microbiome data: an ecological perspective. Methods Ecol. Evol. 10, 389–400. doi: 10.1111/2041-210x.13115
McMurdie, P. J., and Holmes, S. (2013). phyloseq: an R package for reproducible interactive analysis and graphics of microbiome census data. PLoS One 8:e61217. doi: 10.1371/journal.pone.0061217
Morrison, D. J., and Preston, T. (2016). Formation of short chain fatty acids by the gut microbiota and their impact on human metabolism. Gut Microbes 7, 189–200. doi: 10.1080/19490976.2015.1134082
Moschen, A. R., Wieser, V., and Tilg, H. (2012). Dietary factors: major regulators of the gut’s microbiota. Gut Liver 6, 411–416. doi: 10.5009/gnl.2012.6.4.411
Moutinho, S., Martínez-Llorens, S., Tomás-Vidal, A., Jover-Cerdá, M., Oliva-Teles, A., and Peres, H. (2017). Meat and bone meal as partial replacement for fish meal in diets for gilthead seabream (Sparus aurata) juveniles: growth, feed efficiency, amino acid utilization, and economic efficiency. Aquaculture 468, 271–277. doi: 10.1016/j.aquaculture.2016.10.024
Mukhopadhya, I., Hansen, R., El-Omar, E. M., and Hold, G. L. (2012). IBD-what role do Proteobacteria play? Nat. Rev. Gastroenterol. Hepatol. 9, 219–230. doi: 10.1038/nrgastro.2012.14
Naylor, R. L., Hardy, R. W., Bureau, D. P., Chiu, A., Elliott, M., Farrell, A. P., et al. (2009). Feeding aquaculture in an era of finite resources. Proc. Natl. Acad. Sci. U. S. A. 106:18040. doi: 10.1073/pnas.0910577106
Nogales-Mérida, D., Gobbi, P., Jozefiak, D., Mazurkiewicz, J., Dudek, K., Rawski, M., et al. (2018). Insect meal in fish nutrition. Rev. Aquac. 11, 080–1103.
Oliva-Teles, A., and Gonçalves, P. (2001). Partial replacement of fishmeal by brewers yeast (Saccaromyces cerevisae) in diets for sea bass (Dicentrarchus labrax) juveniles. Aquaculture 202, 269–278. doi: 10.1016/s0044-8486(01)00777-3
Oliva-Teles, A., Enes, P., and Peres, H. (2015). “Replacing fishmeal and fish oil in industrial aquafeeds for carnivorous fish,” in Feed and Feeding Practices in Aquaculture, ed. D. Allen Davis (Amsterdam: Elsevier), 203–233. doi: 10.1016/b978-0-08-100506-4.00008-8
Olsen, R. L., and Hasan, M. H. (2012). A limited supply of fishmeal: impact on future increases in global aquaculture production. Trends Food Sci. Technol. 27, 120–128. doi: 10.1016/j.tifs.2012.06.003
Panicz, R., Żochowska-Kujawska, J., Sadowski, J., and Sobczak, M. (2017). Effect of feeding various levels of poultry by-product meal on the blood parameters, filet composition and structure of female tenches (Tinca tinca). Aquac. Res. 48, 5373–5384. doi: 10.1111/are.13351
Peixoto, M. J., Ferraz, R., Magnoni, L. J., Pereira, R., Gonçalves, J. F., Calduch-Giner, J., et al. (2019). Protective effects of seaweed supplemented diet on antioxidant and immune responses in European seabass (Dicentrarchus labrax) subjected to bacterial infection. Sci. Rep. 9:16134.
Perera, E., Simó-Mirabet, P., SukShin, H., Rosell-Moll, E., Naya-Català, F., De las Heras, V., et al. (2019). Selection for growth is associated in gilthead sea bream (Sparus aurata) with diet flexibility, changes in growth patterns and higher intestine plasticity. Aquaculture 507, 349–360. doi: 10.1016/j.aquaculture.2019.04.052
Pérez-Pascual, D., Estellé, J., Dutto, G., Rodde, C., Bernardet, J.-F., Marchand, Y., et al. (2020). Growth performance and adaptability of European sea bass (Dicentrarchus labrax) gut microbiota to alternative diets free of fish products. Microorganisms 8:1346. doi: 10.3390/microorganisms8091346
Piazzon, M. C., Calduch-Giner, J. A., Fouz, B., Estensoro, I., Simó-Mirabet, P., Puyalto, M., et al. (2017). Under control: how a dietary additive can restore the gut microbiome and proteomic profile, and improve disease resilience in a marine teleostean fish fed vegetable diets. Microbiome 5:164.
Piazzon, M. C., Naya-Català, F., Perera, E., Palenzuela, O., Sitjà-Bobadilla, A., and Pérez-Sánchez, J. (2020). Genetic selection for growth drives differences in intestinal microbiota composition and parasite disease resistance in gilthead sea bream. Microbiome 8:168.
Piazzon, M. C., Naya-Català, F., Simó-Mirabet, P., Picard-Sánchez, A., Roig, F. J., Calduch-Giner, J. A., et al. (2019). Sex, age, and bacteria: How the intestinal microbiota is modulated in a protandrous hermaphrodite fish. Front. Microbiol. 10:2512.
Psofakis, P., Karapanagiotidis, I. T., Malandrakis, E. E., Golomazou, E., Exadactylos, A., and Mente, E. (2020). Effect of fishmeal replacement by hydrolyzed feather meal on growth performance, proximate composition, digestive enzyme activity, haematological parameters and growth-related gene expression of gilthead seabream (Sparus aurata). Aquaculture 521:735006. doi: 10.1016/j.aquaculture.2020.735006
Ramos-Pinto, L., Martos-Sitcha, J. A., Reis, B., Azeredo, R., Fernández-Boo, S., Pérez-Sánchez, J., et al. (2019). Dietary tryptophan supplementation induces a transient immune enhancement of gilthead seabream (Sparus aurata) juveniles fed fishmeal-free diets. Fish Shellfish Immunol. 93, 240–250. doi: 10.1016/j.fsi.2019.07.033
Reis, B., Ramos-Pinto, L., Martos-Sitcha, J. A., Machado, M., Azeredo, R., Fernández-Boo, S., et al. (2021). Health status in gilthead seabream (Sparus aurata) juveniles fed diets devoid of fishmeal and supplemented with Phaeodactylum tricornutum. J. Appl. Phycol. 33, 979–996. doi: 10.1007/s10811-021-02377-4
Révész, N., and Biró, J. (2019). Recent trends in fish feed ingredients – mini review. Acta Agrar. Kaposváriensis 23, 32–47.
Reyes-Becerril, M., Guardiola, F., Rojas, M., Ascencio-Valle, F., and Esteban, M. Á (2013). Dietary administration of microalgae Navicula sp. affects immune status and gene expression of gilthead seabream (Sparus aurata). Fish Shellfish Immunol. 35, 883–889. doi: 10.1016/j.fsi.2013.06.026
Rhodes, M. A., Zhou, Y., and Davis, D. A. (2015). Use of dried fermented biomass as a fish meal replacement in practical diets of Florida pompano. Trachinotus carolinus. J. Appl. Aquac. 27, 29–39. doi: 10.1080/10454438.2014.959834
Rimoldi, S., Gini, E., Koch, J. F. A., Iannini, F., Brambilla, F., and Terova, G. (2020). Effects of hydrolyzed fish protein and autolyzed yeast as substitutes of fishmeal in the gilthead sea bream (Sparus aurata) diet, on fish intestinal microbiome. BMC Vet. Res. 16:118.
Ritchie, H., and Roser, M. (2019). ∗Meat and Seafood Production and Consumption. Available Onlinew at: https://ourworldindata.org/meat-production.
Robles, R., Lozano, A. B., Sevilla, A., Márquez, L., Nuez-Ortín, W., and Moyano, F. J. (2013). Effect of partially protected butyrate used as feed additive on growth and intestinal metabolism in sea bream (Sparus aurata). Fish Physiol. Biochem. 39, 1567–1580. doi: 10.1007/s10695-013-9809-3
Rognes, T., Flouri, T., Nichols, B., Quince, C., and Mahé, F. (2016). VSEARCH: a versatile open source tool for metagenomics. PeerJ 4:e2584. doi: 10.7717/peerj.2584
Sabbagh, M., Schiavone, R., Brizzi, G., Sicuro, B., Zilli, L., and Vilella, S. (2019). Poultry by-product meal as an alternative to fish meal in the juvenile gilthead seabream (Sparus aurata) diet. Aquaculture 511:734220. doi: 10.1016/j.aquaculture.2019.734220
Sanz, M. L., Polemis, N., Morales, V., Corzo, N., Drakoularakou, A., Gibson, G. R., et al. (2005). In vitro investigation into the potential prebiotic activity of honey oligosaccharides. J. Agric. Food Chem. 53, 2914–2921. doi: 10.1021/jf0500684
Satokari, R. (2015). Contentious host-microbiota relationship in inflammatory bowel disease-can foes become friends again? Scand. J. Gastroenterol. 50, 34–42. doi: 10.3109/00365521.2014.966320
Schieber, M., and Chandel, N. S. (2014). ROS function in redox signaling and oxidative stress. Curr. Biol. 24, R453–R462.
Schmieder, R., and Edwards, R. (2011). Quality control and preprocessing of metagenomic datasets. Bioinformatics 27, 863–864. doi: 10.1093/bioinformatics/btr026
Simó-Mirabet, P., Felip, A., Estensoro, I., Martos-Sitcha, J. A., de las Heras, V., Calduch-Giner, J., et al. (2018). Impact of low fish meal and fish oil diets on the performance, sex steroid profile and male-female sex reversal of gilthead sea bream (Sparus aurata) over a three-year production cycle. Aquaculture 490, 64–74. doi: 10.1016/j.aquaculture.2018.02.025
Subhadra, B., Lochmann, R., Rawles, S., and Chen, R. (2006). Effect of fish-meal replacement with poultry by-product meal on the growth, tissue composition and hematological parameters of largemouth bass (Micropterus salmoides) fed diets containing different lipids. Aquaculture 260, 221–231. doi: 10.1016/j.aquaculture.2006.06.029
Sugawara, Y., Ueki, A., Abe, K., Kaku, N., Watanabe, K., and Ueki, K. (2011). Propioniciclava tarda gen. nov., sp. nov., isolated from a methanogenic reactor treating waste from cattle farms. Int. J. Syst. Evol. Microbiol. 61, 2298–2303. doi: 10.1099/ijs.0.027482-0
Tacon, A., and Metian, M. (2015). Feed matters: satisfying the feed demand of aquaculture. Trends Food Sci. Technol. 23, 1–10. doi: 10.1080/23308249.2014.987209
Tarnecki, A. M., Burgos, F. A., Ray, C. L., and Arias, C. R. (2017). Fish intestinal microbiome: diversity and symbiosis unravelled by metagenomics. J. Appl. Microbiol. 123, 2–17. doi: 10.1111/jam.13415
Teghanemt, A., Zhang, D., Levis, E. N., Weiss, J. P., and Gioannini, T. L. (2005). Molecular basis of reduced potency of underacylated endotoxins. J. Immunol. 175, 4669–4676. doi: 10.4049/jimmunol.175.7.4669
Torrecillas, S., Mompel, D., Caballero, M. J., Montero, D., Merrifield, D., Rodiles, A., et al. (2017). Effect of fishmeal and fish oil replacement by vegetable meals and oils on gut health of European sea bass (Dicentrarchus labrax). Aquaculture 468, 386–398. doi: 10.1016/j.aquaculture.2016.11.005
Turchini, G. M., Trushenski, J. T., and Glencross, B. D. (2019). Thoughts for the future of aquaculture nutrition: realigning perspectives to reflect contemporary issues related to judicious use of marine resources in aquafeeds. N. Am. J. Aquac. 81, 13–39. doi: 10.1002/naaq.10067
Van Nguyen, N., Van Mullem, D., Hoang, L., Van Khanh, T., Thanh, N., and Solé-Jiménez, P. (2020). Fishmeal substitution with a protein concentrate for the whiteleg shrimp Litopenaeus vannamei. AQUAculture Asia Pacific 16, 30–32.
Vizcaíno, A. J., López, G., Sáez, M. I., Jiménez, J. A., Barros, A., Hidalgo, L., et al. (2014). Effects of the microalga Scenedesmus almeriensis as fishmeal alternative in diets for gilthead sea bream, Sparus aurata, juveniles. Aquaculture 431, 34–43. doi: 10.1016/j.aquaculture.2014.05.010
Welter, D. K., Ruaud, A., Henseler, Z. M., De Jong, H. N., van Coeverden, de Groot, P., et al. (2021). Free-living, psychrotrophic bacteria of the genus Psychrobacter are descendants of pathobionts. mSystems 6:e00258-21.
Xue, J., Shen, K., Hu, Y., Hu, Y., Kumar, V., Yang, G., et al. (2020). Effects of dietary Bacillus cereus, B. subtilis, Paracoccus marcusii, and Lactobacillus plantarum supplementation on the growth, immune response, antioxidant capacity, and intestinal health of juvenile grass carp (Ctenopharyngodon idellus). Aquacult. Rep. 17:100387. doi: 10.1016/j.aqrep.2020.100387
Ytrestøyl, T., Aas, T. S., and Åsgård, T. (2015). Utilisation of feed resources in production of Atlantic salmon (Salmo salar) in Norway. Aquaculture 448, 365–374. doi: 10.1016/j.aquaculture.2015.06.023
Zamani, A., Khajavi, M., Nazarpak, M. H., and Gisbert, E. (2020). Evaluation of a bacterial single-cell protein in compound diets for rainbow trout (Oncorhynchus mykiss) fry as an alternative protein source. Animals 10:1676. doi: 10.3390/ani10091676
Zhang, L. S., and Davies, S. S. (2016). Microbial metabolism of dietary components to bioactive metabolites: opportunities for new therapeutic interventions. Genome Med. 8:46.
Zhang, L., Li, Q., Chen, C., Li, X., Li, M., Hu, J., et al. (2017). Propioniciclava sinopodophylli sp. Nov., isolated from leaves of Sinopodophyllum hexandrum (royle) ying. Int. J. Syst. Evol. Microbiol. 67, 4111–4115. doi: 10.1099/ijsem.0.002265
Keywords: fish meal, processed animal proteins, single cell proteins, gut microbiota, host defense, inflammation, Sparus aurata
Citation: Solé-Jiménez P, Naya-Català F, Piazzon MC, Estensoro I, Calduch-Giner JÀ, Sitjà-Bobadilla A, Van Mullem D and Pérez-Sánchez J (2021) Reshaping of Gut Microbiota in Gilthead Sea Bream Fed Microbial and Processed Animal Proteins as the Main Dietary Protein Source. Front. Mar. Sci. 8:705041. doi: 10.3389/fmars.2021.705041
Received: 04 May 2021; Accepted: 10 June 2021;
Published: 01 July 2021.
Edited by:
Fotini Kokou, Wageningen University and Research, NetherlandsReviewed by:
Konstantinos (Kostas) Ar. Kormas, University of Thessaly, GreeceMohsen Abdel-Tawwab, Agricultural Research Center, Egypt
Olav Vadstein, Norwegian University of Science and Technology, Norway
Copyright © 2021 Solé-Jiménez, Naya-Català, Piazzon, Estensoro, Calduch-Giner, Sitjà-Bobadilla, Van Mullem and Pérez-Sánchez. This is an open-access article distributed under the terms of the Creative Commons Attribution License (CC BY). The use, distribution or reproduction in other forums is permitted, provided the original author(s) and the copyright owner(s) are credited and that the original publication in this journal is cited, in accordance with accepted academic practice. No use, distribution or reproduction is permitted which does not comply with these terms.
*Correspondence: Jaume Pérez-Sánchez, jaime.perez.sanchez@csic.es
†These authors have contributed equally to this work