- 1Scottish Association for Marine Science, Scottish Marine Institute, Oban, United Kingdom
- 2Marine Scotland Science, Aberdeen, United Kingdom
An increasing number of pipelines and associated protective materials in the North Sea are reaching the end of their operational life and require decommissioning. Identifying the optimal decommissioning option from an environmental perspective requires an understanding of ecological interactions; currently there is little knowledge as to species associations with pipelines and associated protective materials. This study utilises industry ROV footage from the North Sea to quantify these interactions. A total of 58 taxa were identified, including 41 benthic taxa and 17 fish taxa. Taxa were grouped into seven groups for analysis including four groups for benthic epifauna: grazers, suspension/filter feeders, decapods, and colonial/encrusting taxa. Fish were organised into three groups: pollock, other fish, and other gadoids. Using zero-inflated generalised linear mixed models, we show that abundances of benthic epifauna and fish vary between types of protective structure (e.g., concrete mattresses, rock dump), depth, levels of fishing effort and proximity to oil and gas platforms. Six taxa groups exhibited higher abundances on concrete mattresses than bare pipelines with benthic epifaunal decapods showing the highest difference at 3.04 (1.83, 4.84, 95% CrI) times higher on mattresses compared to bare pipelines. Six groups were higher in abundance within the 500 m fisheries exclusion zone around platforms, compared to outside of the zone, with other gadoids showing the highest difference at 1.83 times (1.09, 2.89, 95% CrI) times higher inside zones. Five groups decreased in abundance with an increase in fishing effort, with the biggest effect observed on grazers which decreased in abundance by 28% (14 – 40, 95% CrI) per 50 h of fishing. We show that pipelines and protective materials are operating as artificial reefs, and our results suggest that removal of infrastructure could result in the loss of habitat and species.
Introduction
Offshore oil and gas production is declining with basins maturing and operations becoming less economically viable. As a consequence, many of the subsea structures supporting oil and gas production are reaching the end of their operational lifespan and require decommissioning. Subsea pipelines are an essential feature of offshore oil and gas operations and are present in all major hydrocarbon basins (Guo et al., 2005). Pipelines are either laid on the surface of the seafloor (surface laid) or partially/completely buried (BEIS, 2018). Protective materials are installed over pipelines to safeguard them from dropped objects, hydrodynamic erosion, and potential snagging by fishing gear. Pipeline protections can include quarried rock deposited on top of the pipeline (referred to as “rock dump”), or concrete blocks linked together with polypropylene rope laid on top of pipelines (referred to as “concrete mattresses”) (Oil and Gas UK, 2013). In the North Sea (NS), over 200 fields are forecast for decommissioning before 2028, including 6,234 km of subsea pipelines (Oil and Gas UK, 2019) and approximately 16,033 concrete mattresses, at a predicted cost of ∼€1.3 billion (Oil and Gas UK, 2019).
The NE Atlantic protocol from the Oslo and Paris Commission (OSPAR 98/3) requires installations to be removed as part of decommissioning where technically feasible. To date, the OSPAR commission has made no recommendations as to the decommissioning of pipelines or protections. Potential decommissioning scenarios include leave in situ, wherein pipelines are buried using sediment or rock dump, or complete or partial removal (BEIS, 2018). In the absence of specific guidance under OSPAR 98/3, the UK recommendations (Oil and Gas UK, 2013) are to remove surface laid, un-trenched, small pipelines (< 16″ diameter; BEIS, 2018) and, where safe, recover mattresses.
In the UK sector, proposed decommissioning of oil and gas infrastructure is assessed through a formalised “comparative assessment” (CA) (Ekins et al., 2006). The CA is a cost-benefit analysis and the Department of Energy and Climate Change (DECC) stipulates five main criteria to be considered during the CA process: economical, technological, safety, environmental, and societal (DECC, 2011). To consider these criteria, the operator relies on an evidence base, in the case of environmental criteria this evidence is supported by scientific studies.
Oil and gas operators are striving to operate within a circular economy, wherein a structure that is removed is repurposed or re-used in another capacity (Mearns and Hinze, 2015). The re-use options for protective structures associated with pipelines within the marine environment have not, to date, been fully explored. In the absence of enough evidence to support either re-cycling protective structures or leaving them in situ, most concrete mattresses from the North Sea are being recovered and disposed of on land (Cumming, 2015). There is increasing scrutiny as to the appropriateness of removing structures at the end of their lives, as under both economic and environmental interests, there are arguments for leaving certain structures in place in the NS (Aabel et al., 1997; Picken et al., 2000; Jensen, 2002; Sayer and Baine, 2002; Jørgensen, 2012). The potential benefits of re-using or retaining pipeline protections in situ have been shown by several successful rigs to reefs programmes where platforms are re-used to create artificial reefs to benefit benthic habitat conservation (Macreadie et al., 2011; Bergmark and Jorgensen, 2014), enhance fishery resources (Dauterive, 1999; Sayer and Baine, 2002), and reduce costs for the oil and gas industry (Kaiser and Pulsipher, 2005). Identifying and measuring the current benefits of in situ pipeline protections to benthic and fish species, as well as exploring the artificial reef creation potential of the structures, is essential in providing the evidence base to stakeholders during the decommissioning process.
Offshore platforms, renewable energy structures, shipwrecks, and other installations act as artificial reefs by providing hard substratum for the colonisation of marine organisms (see review; Svane and Petersen, 2001). Although not placed in the environment with the primary intention of creating artificial reefs, oil and gas installations act as artificial reefs and support marine flora and fauna (Techera and Chandler, 2015) to varying extents. In the NS, platforms act as artificial reefs (Forteath et al., 1982; Whomersley and Picken, 2003; Guerin et al., 2007), and can support species of conservation interest such as the cold-water coral species Lophelia pertusa (Gass and Roberts, 2006).
Subsea pipelines are beneficial to fish and shellfish populations (Love and York, 2005; McLean et al., 2017), including species of commercial value (Bond et al., 2018), whilst Glaholt (2008) showed that pipelines provided a suitable habitat for Dungeness Crab (Metacarcinus magister, Milne-Edwards, 1862). In the NS, fishing is an ecologically and economically important activity, with pelagic, demersal, and crustacean species being targeted by trawling, seine nets, static gear, dredging, and line fishing (Rogers and Stocks, 2001; Thorpe et al., 2016). Platforms in the NS are surrounded by a 500 m exclusion zone, within which fishing is prohibited, however, pipelines outside of 500 m zones are not protected by such zones. Rouse et al. (2018) estimated that 36.1% of fishing trips in the Scottish demersal fleet fished within 200 m of a pipeline within a 5-year period. implying modest aggregation of fishing around pipelines.
Only three studies have focused on NS pipelines from an ecological context with only two of these looking at benthic interactions with NS pipelines (Rouse et al., 2019; Lacey and Hayes, 2020). These studies suggest that northern North Sea pipelines can support species of conservation interest (Rouse et al., 2019) and that epifaunal communities in the central and NNS are driven by depth and latitude, with more complex pipeline structures hosting the highest number of taxa (Lacey and Hayes, 2020). However, no study has targeted fish species associated with pipelines and pipeline protections, nor looked at benthic or fish interactions within the entire NS region.
The previous lack of research regarding pipeline protections is partly due to the difficulty in obtaining data related to oil and gas structures. Recently, ecological studies on oil and gas infrastructure have become more achievable due to the increased use of Remotely Operated Vehicles (ROVs) offshore in the last 20 years (Shukla and Karki, 2016). ROVs carry out tasks including underwater survey, inspection, repair, welding, cutting, object recovery and sample collection (Whitcomb, 2000). Oil and gas operators hold an extensive library of ROV inspection footage collated across decades on a spatial scale ordinarily unobtainable for researchers (McLean et al., 2017; Thomson et al., 2018). This footage has been highlighted as invaluable for observing benthic and fish communities (Whomersley and Picken, 2003; McLean et al., 2020) and has the potential to provide insight into interactions between the marine environment and offshore pipelines (Macreadie et al., 2018; McLean et al., 2018).
Research into the environmental interactions of benthic and fish species with pipelines is limited; the studies in the North Sea (Russell et al., 2014; Rouse et al., 2019; Lacey and Hayes, 2020) have all been on a relatively limited scale, i.e., only covering the NNS. Rouse et al. (2019) highlighted the requirement for analysis to be conducted on the whole NS region, as the results presented from studies focusing on small scale areas will not represent the variations in epifaunal communities driven by regional differences in substratum type, fishing effort, current regime and stability of pipelines between the NNS and SNS. Our study provides the largest-scale ROV based study (globally) focusing on benthos and fish interactions with pipelines, and the first of its kind to place significant emphasis on pipeline protections. We used industry ROV pipeline inspection footage, from across the NS, to quantify the benthic and fish associations with pipelines and pipeline protections. These data contribute to the CA evidence base, assisting operators and regulators in decision making processes regarding the optimal decommissioning scenario of pipelines and pipeline protections.
Methods
Study Site Description
The North Sea (NS) is a shallow shelf sea, with an average depth of 90 m, reaching a maximum depth of over 700 m in the Norwegian Channel (Paramor et al., 2009). The NS can be split into two regions based on the physical environment, southern (SNS) and northern (NNS) (Otto et al., 1990). The SNS is much shallower than northern areas (Ducrotoy et al., 2000) with depths rarely exceeding 50 m (Paramor et al., 2009). Sediments in the NS are currently dominated by soft, mobile sediments, such as sand and mud, with areas of coarse sand, gravel and rock (Paramor et al., 2009).
ROV Footage
North Sea pipeline inspection footage from 55 pipelines, recorded in standard definition, during the day and night between 2013 and 2018, was obtained from operators in the NS. All pipelines were installed prior to 2007, however, data on the exact age of all pipelines and protective structures were unavailable. Chrysaor provided footage of pipelines in the SNS, while footage of pipelines in the NNS was provided by Repsol Sinopec and another anonymous operator (Figure 1).
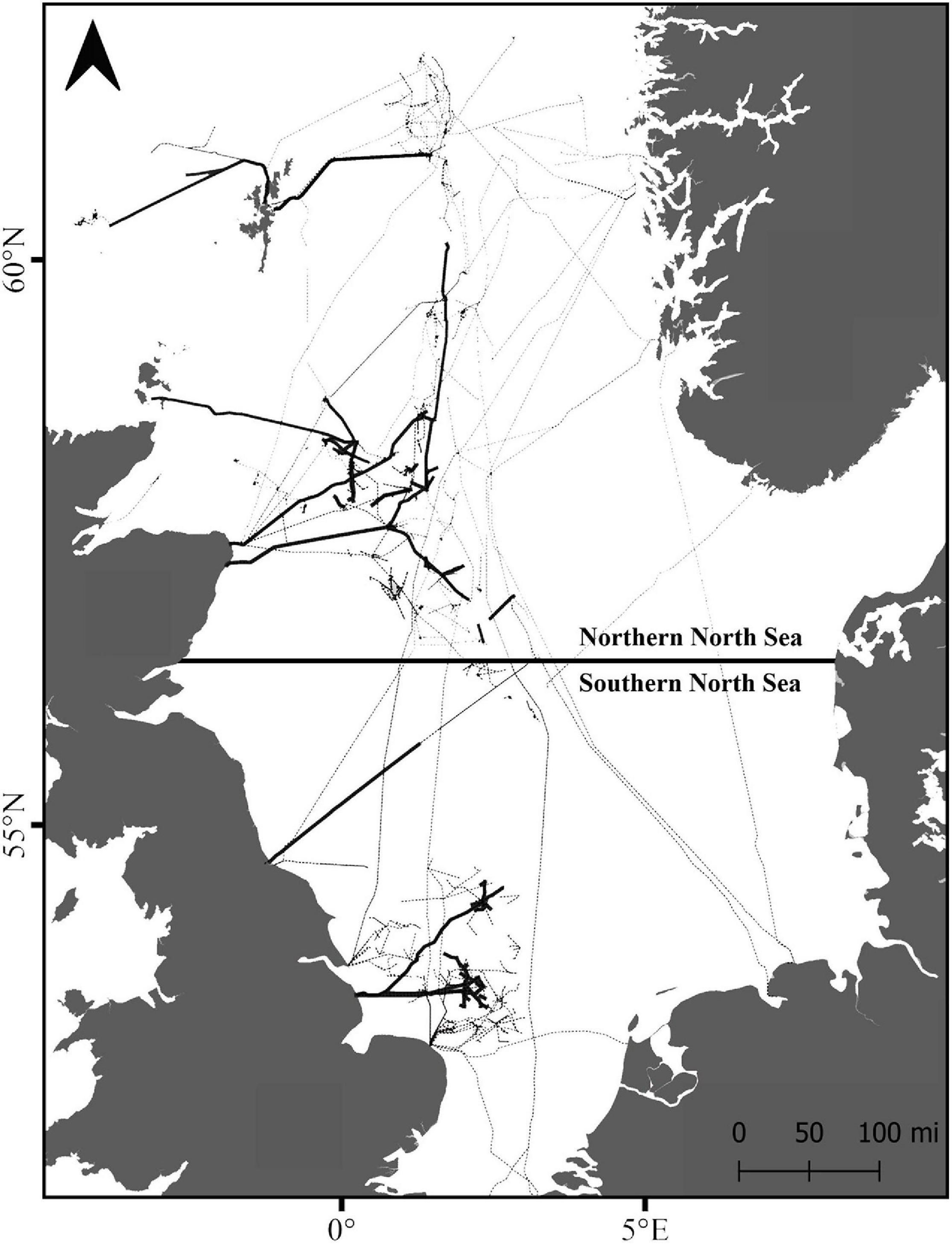
Figure 1. Map of North Sea study area with line showing divide between the northern North Sea and southern North Sea. Dotted lines represent pipelines, solid black lines indicate pipelines sampled in this study.
During a pipeline inspection a ROV travels directly above a pipeline with mounted cameras, providing top and side views of the pipelines (central, port, and starboard, see Figure 2), and records video footage. This footage was reviewed using specialised industry ROV viewing software, VisualReview (VisualSoft Suite, 2019), allowing all three cameras to be viewed simultaneously. The time, date, depth, altitude of ROV, and a kilometre point (KP) value, which provides a distance travelled along a pipeline from the nearest platform, were overlaid on the footage.
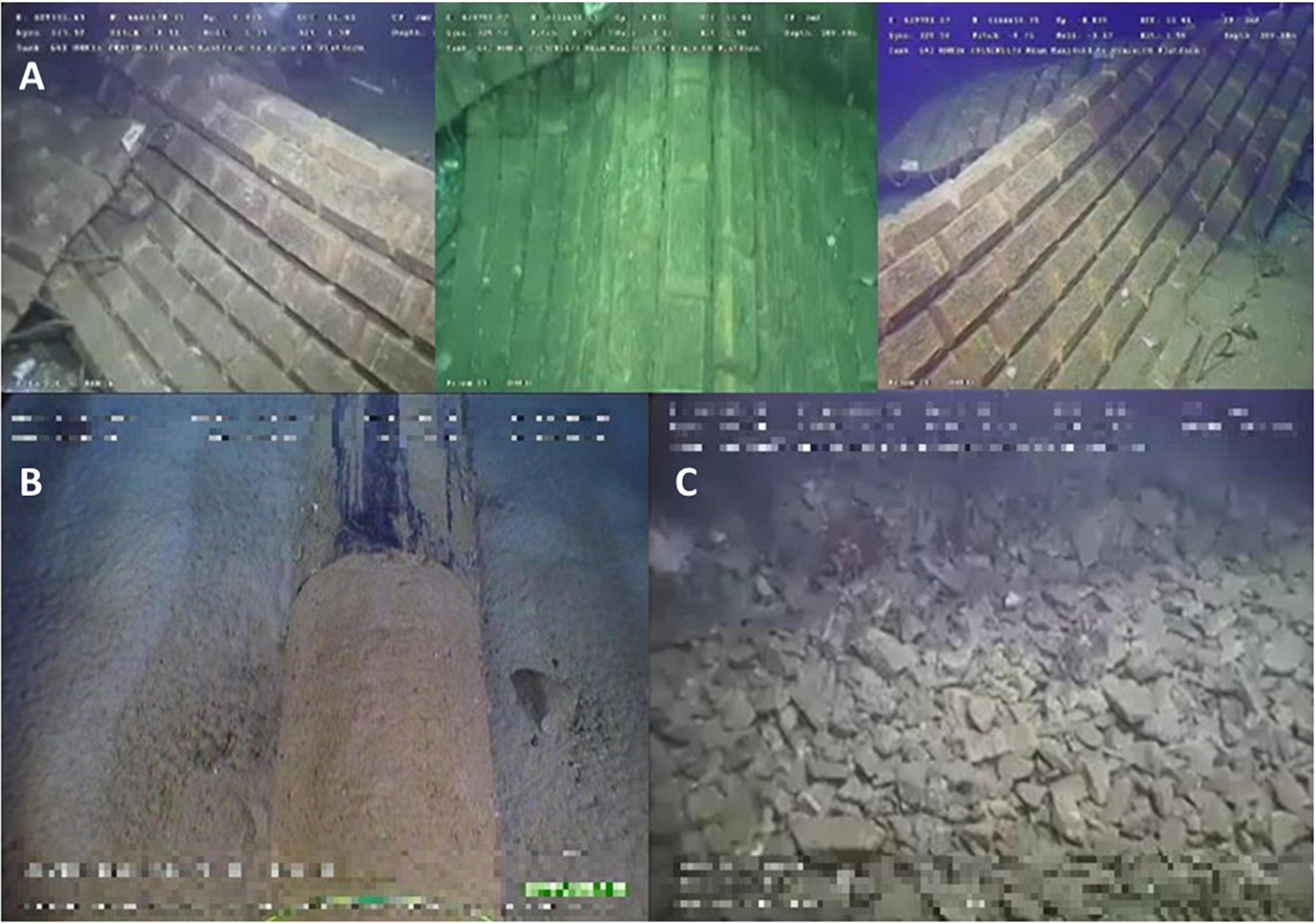
Figure 2. (A) Format of ROV footage playback with three camera views (top three images left to right; port, central, and starboard) observed in synchronisation. (B) Bare pipeline and (C) rock dump.
Sampling
Prior to analysis, all footage was reviewed to create an inventory of the locations of concrete mattresses, bare pipeline, and rock dump, and to familiarise the observer with footage characteristics. The footage from all three operators was collected for the same purpose (pipeline inspection), and as such the field of view provided by the video captured remained relatively standard. Any footage that exhibited low lighting or poor visibility was excluded along with pipelines or protections that were buried or partially buried (over 50%). Depths for each pipeline and protection in the inventory were obtained by overlaying the pipeline/protection locations with bathymetry layers in ArcGIS (Stevenson, 2012).
To analyse benthic epifauna taxa, 1,512 sample locations were randomly selected from this inventory with a minimum of 15 m between each. To analyse fish taxa 600 transect start locations were selected, with a minimum of distance of 15 m between each. Samples and transects were randomly selected independently of one another and according to the region of the NS (northern and southern), four depth bands (0–30, 30–60, 70–110, and 110–150 m), protection types (concrete mattress, rock dump, bare pipeline), and whether inside or outside of 500 m fisheries exclusion zones surrounding platforms. All samples and transects were equally distributed except for bare pipeline samples/transects inside and outside of zones in the SNS where pipelines are often trenched and buried.
At each of the 1,512 benthic epifauna sample locations a count of all species present within a 1 m2 area was conducted, colonial/encrusting taxa species were recorded as an estimate of percentage cover. At each of the 600 fish transect start locations, every fish within the field of view along a 5 m length was recorded. Benthic 1 m2 sample dimensions were approximated using known sizes of objects including concrete mattress dimensions, pipeline diameters and average rock dump sizes. 5 m transects for fish counts were approximated using the known sizes of objects and KP values. The analysis of each sample or transect included viewing the sample area in real-time several times, and then pausing the footage at small increments and examining the still images. Species were identified to the lowest possible taxonomic level, however, due to poor resolution of fine-scale taxonomic details some individuals had to be grouped. For example, where a fish could be identified as a gadoid, but could not be classified further due to factors such as the size or speed of the individual, they were classified as an “unidentified gadoid.” If footage of a sample or transect featured low lighting or poor visibility, it was discarded and another random sample or transect was selected.
To examine any possible impact of fishing, an estimate of fishing effort in the vicinity of the pipeline/pipeline protection was obtained by spatially overlaying the centre point of the sample section with standardised data layers of fishing effort in ArcGIS for vessels operating mobile demersal gear, for the 2 years preceding the footage recording date (ICES, 2017). A category of time of day for each sample/transect was assigned using historical sunrise and sunset data. The three categories were relatively equally distributed between day, night, and the crepuscular period (dawn and dusk).
Data Analysis
To allow count data to be modelled, taxa were organised in to seven groups to act as response variables in statistical analysis (Supplementary Material). The following groupings were selected to provide a biologically meaningful interpretation of the taxa present, however, they were not selected to represent known trophic classes/guilds. Benthic epifaunal species were grouped as grazers, decapods, suspension/filter feeders, and colonial/encrusting taxa species (Supplementary Material). Colonial/encrusting taxa were grouped together abundance of these taxa were recorded as percentage cover and as such, required analysis separate to groups featuring counts of taxa. Fish taxa were organised as pollock, other gadoids, and other fish (Supplementary Material). Pollock were assigned as a separate group as they were both the most abundant fish species and the most accurately identifiable from the footage, however, it should be noted that other gadoids could include pollock that were unidentifiable to a species level. These groups also allowed for data to be modelled by reducing inappropriate zero inflation while remaining logical and retaining less commonly observed species.
Generalised linear mixed models (GLMMs) were used to model variations in groups of species in relation to six exploratory variables; depth [continuous (m)], fishing effort [continuous (Total Hours)], protection type (3 levels), inside or outside 500 m fisheries exclusion zone (2 levels), time of day (3 levels), and region (2 levels). Three random effect variables were considered to account for: pipeline number (55 levels for benthic epifaunal models and 43 for fish models), month (6 levels), and year of footage (6 levels). Prior to analysis, data were checked for outliers, collinearity of variables, and trends in the response variable via exploratory, draftsman, and model diagnostic plots (Zuur et al., 2010). Seven GLMM’s were fitted to account for each of the seven response variables (taxa groups). The optimal model for each response variable was selected from a series of candidate models, including Poisson and negative binomial distributions, with and without zero-inflation components. The decision to include terms was based on assessing the model with and without variables using Widely Applicable Information Criterion [WAIC (Watanabe, 2010)] and Leave One Out Cross Validation (LOOCV, Korner-Nievergelt et al., 2015).
Selection of random effects was carried out first, conditional on including all fixed effects and biologically meaningful interactions. Where random effects did not improve model fit (lower WAIC values by more than 2), they were removed. Following the selection of random effects, biological meaningful interactions and fixed effects were tested in the same way, with variables and interactions being dropped if they did not improve model fit (reduce WAIC values by more then 2). If a variable was marginally close to be accepted in a model (reduced WAIC by less than 2 but more than 1) then posterior predictive checks (PP checks) were conducted for models with and without the variable in question. PP checks visually compare the raw data with 50 simulated datasets from the posterior distribution (as density plots or histograms) and were conducted using the “pp_check” function of the R package “rstanarm” (Goodrich et al., 2018). The model which best predicted the raw data was then selected.
Following model selection, the optimal models were created, using non-informative priors, in the Stan computational framework1 accessed with the brms package (Bürkner, 2017). To obtain the joint posterior distribution, samples were simulated using the Hamilton Monte Carlo method by running four independent chains for 4,000 iterations and discarding the first 2,000 iterations of each as warm-up. Model convergence was assessed via Markov Chain Monte Carlo (MCMC) trace plots, and R-hat values (potential scale reduction factor; Gelman et al., 2013). Model performance was assessed using posterior predictive checks to visually compare the raw data with 50 simulated datasets from the posterior distribution. Credible intervals and parameter estimates from the posterior distribution were accessed using the “posterior_samples” function of the R package “brms” (Bürkner, 2017). The 0.025 and 0.975 quantiles of the simulated parameter estimates defined the 95% credible intervals within which there is a 95% certainty that the true parameter lies.
The variable “region” was removed from model selection due to strong collinearity with “depth,” with samples and transects in the SNS not exceeding 60 m and not falling below 70 m in the NNS. All models, except the colonial/encrusting taxa group, included the fixed effects of depth, protection type, inside or outside 500 m exclusion zone, time of day and fishing effort. The colonial/encrusting taxa group included the same fixed effects except for time of day. All models included the random effect of pipeline, with month and year being excluded as they did not improve model fit. The colonial/encrusting taxa and suspension/filter feeder models included an interaction of zone and depth, all other models included the interaction of protection type and depth. The decapod group was modelled using a zero inflated Poisson, whereas all other groups were modelled using zero inflated negative binomials (Supplementary Material). All models converged with no warnings, with all r-hat (potential scale reduction factor) values for each model <1.1 (Gelman et al., 2013), indicating that it was safe to make inferences from the parameter estimates.
Results
A total of 41 benthic taxa and 17 fish taxa were observed (Supplementary Material). The most frequently observed benthic taxa were anemones, which included Actinauge richardi (Marion, 1882), Plumose anemones (Metridium sp., De Blaine, 1824), Dahlia anemones (Urticina sp., Ehrenber, 1834) and deeplet anemones (Bolocera tuediae, Johnston, 1832), as well as many individuals unidentifiable to a species level. Starfish species were also abundant and included the common starfish (Asterias rubens, Linnaeus, 1758), common brittle star (Ophiothrix fragilis, Müller and Troschel, 1840), and common sunstar (Crossaster papposus, Linnaeus, 1767), as well as many individuals unidentifiable to a species level. The most observed species in the SNS was the soft coral Alcyonium digitatum (Linnaeus, 1758). The soft coral was found in high abundances on concrete mattresses and rock dump, whilst also present on bare pipelines, albeit in lower abundances. During footage review prior to analysis a species of conservation interest, Lophelia pertusa, was observed in the NNS, although this species was not captured within any of the random sample locations. The most abundant fish species observed during footage analysis was pollock (Pollachius pollachius, Linnaeus, 1758). Individuals of other gadoid species were also observed including cod (Gadus morhua, Linnaeus, 1758), and ling (Molva molva, Linnaeus, 1758). Other species observed included the Atlantic wolffish (Anarhichas lupus, Linnaeus, 1758), and several species of flatfish including the common sole (Solea solea, Linnaeus, 1758). There were also many individuals unidentifiable to a genus or species level.
Overview
The highest differences in taxa group abundance were driven by protection type with six of the seven taxa groups higher in abundance on concrete mattresses compared to bare pipelines and rock dump. Five of the seven taxa groups exhibited different rates of change with depth depending on protection type with grazer abundance having the highest rate of increase with depth on concrete mattresses. An increase in total hours of fishing effort was shown to reduce the predicted abundance of five of the seven taxa groups. Grazers, suspension/filter feeders, decapods, colonial/encrusting taxa, and pollock all decreased in abundance with an increase in total fishing effort, however, the rate at which abundance decreased varied. The largest effect was observed on grazers and pollock. Grazers were also the only group to be higher inside 500 m fisheries exclusion zones than outside, although the difference between inside and outside these zones was relatively small for all taxa groups. There were also differences in abundances in relation to time of day, although these differences were not high. The highest differences were observed in pollock and benthic epifaunal decapods where abundances were higher during the night and crepuscular period than the day.
Protection Type
The abundance of grazers, suspension/filter feeders, decapods, colonial/encrusting taxa, pollock, and other fish were higher on mattresses than bare pipelines. The largest difference observed within the benthic epifaunal groups was decapods that were on average 3.04 times (1.83, 4.84, 95% CrI) higher on mattress than bare pipelines, and 1.74 times (1.06, 2.69, 95% CrI) higher on mattresses than rock dump. Suspension/filter feeders were on average 1.16 (0. 801, 1.67, 95% CrI) times higher on mattress than bare pipelines and 1.58 (1.06, 2.27, 95% CrI) times higher than rock dump. In contrast, they were found to be 1.38 times (0.902, 2.01, 95% CrI) higher on bare pipeline than rock dump. The highest difference in fish was observed in the other fish group with mattresses exhibiting on average 4.12 times (2.18, 7.22, 95% CrI) higher abundances than bare pipelines, and 1.61 times (0.904, 2.66, 95% CrI) higher than rock dump. In contrast, other gadoids were higher in abundance on bare pipelines at 1.48 times (0.775, 2.63, 95% CrI) higher than rock dump and 1.27 times (0.756, 1.99, 95% CrI) higher than concrete mattresses.
Depth
The differences in abundances between protection types varied according to the depth for grazers, suspension/filter feeders, decapods, pollock, other gadoids, and other fish. Predicted grazer abundance had the highest rate of change (Figure 3) with depth on concrete mattresses with abundance increasing by 18.42% (9.05, 28.79, 95% CrI) per 10 m increase in depth, grazers on rock dump increased at a slower rate of 6.10% (−2.98, 16.35, 95% CrI) per 10 m increase in depth, in contrast abundances on bare pipelines decreased with depth at −11.84% (−19.93, −3.58, 95% CrI) per 10 m increase in depth.
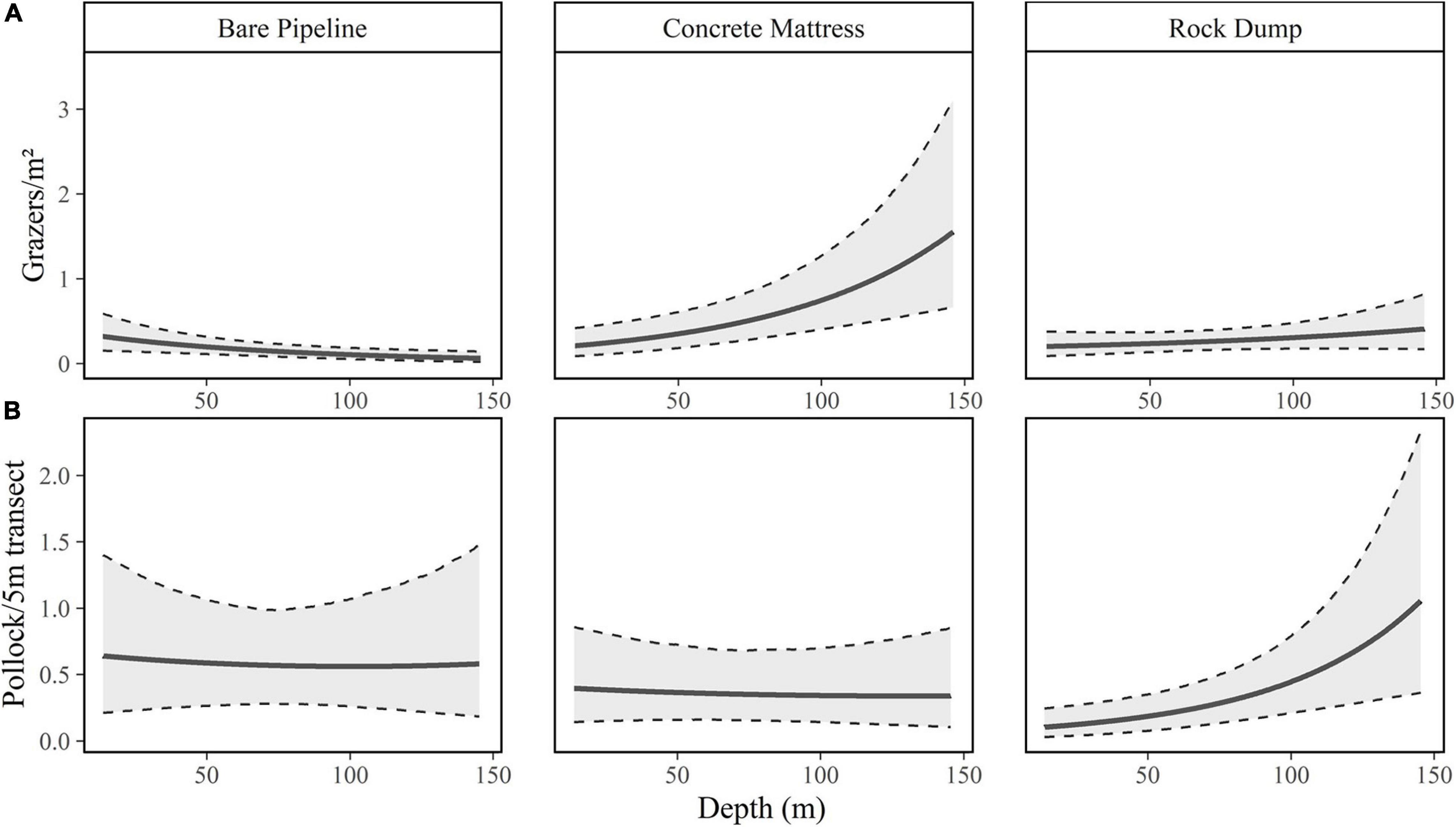
Figure 3. Modelled relationships and 95% CrI’s between (A) grazer abundance per 1 m2, (B) pollock abundance per 5 m transect with depth (m) according to protection type.
Predicted pollock abundances also exhibited varied rates of change between protection types with depth (Figure 3). Concrete mattresses and bare pipelines exhibited low rates of change at −1.06% (−10.02, 8.96, 95% CrI) and −1.09% (−11.43, 11.44, 95% CrI) per 10 m increase in depth, respectively. In contrast pollock abundances on rock dump exhibited a higher rate of change at 18.66% (7.10, 32.64, 95% CrI) per 10 m increase in depth. The rates of change did differ between protection types with depth for other taxa groups, however, the differences in rates were not as high (Table 1).

Table 1. Percent rate of change (with 95% CrI) in relation to a 10 m increase in depth, depending on protection type.
The difference in abundances inside and outside of 500 m exclusion zones varied depending on depth for suspension/filter feeders and colonial/encrusting taxa (Table 2). Colonial/encrusting taxa were found to decrease with depth inside 500 m zones at a rate of −6.15% (−16.53, 6.48, 95% CrI) per 10 m increase in depth, in contrast their abundance were found to increase outside of zones with depth at a rate of 0.37% (−11.12, 12.87, 95% CrI) per 10 m depth. Suspension/filter feeders were found to increase with depth both inside and outside of zones, however outside zones the rate of change was higher at 10.90% (3.66, 19.09, 95% CrI) per 10 m depth compared to 3.13% (−3.27, 10.19, 95% CrI) inside.
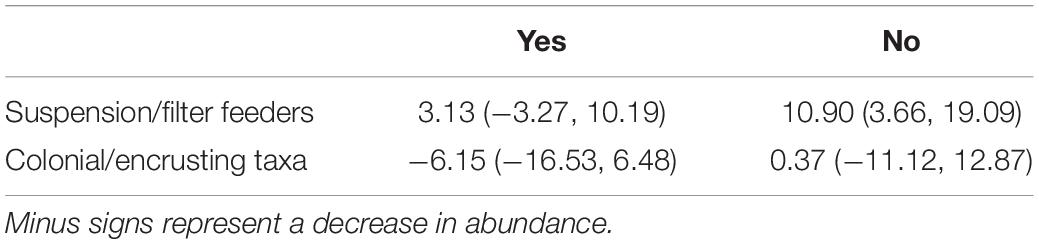
Table 2. Percent rate of change (with 95% CrI) in relation to a 10 m increase in depth, depending on whether a sample was inside or outside of a 500 m zone.
Fisheries Exclusion Zone
Grazers were the only taxa group observed to be higher outside 500 m zones compared to inside, although this difference was very small at an average of 1.01 times (0. 759, 1.34, 95% CrI) higher outside than inside. Decapods exhibited the highest difference of the benthic epifauna groups at an average of 1.31 (0.896, 1.88, 95% CrI) times higher inside zones compared to outside. In terms of fish groups, although all were higher inside zones, the biggest difference was observed in other gadoids at an average of 1.83 times (1.09, 2.89, 95% CrI) higher inside when compared to outside zones.
Fishing Effort
Grazers, suspension/filter feeders, colonial/encrusting taxa, decapods, and pollock all decreased in abundance with an increase in total fishing effort, however, the rate at which abundance decreased varied. Grazers decreased at the highest rate of −28.01% (−39.91, −13.88, 95% CrI) per 50 h increase of fishing effort. Decapods decreased at a rate of −16.89% (−32.48, 1.09, 95% CrI) per 50 h of fishing, and pollock decreased at a rate of −17.29% (−39.69, 16.09, 95% CrI). In contrast, other fish and other gadoids increased with an increase in fishing, other fish at a rate of 12.07% (−16.18, 48.68, 95% CrI), and other gadoids at a lower rate of 0.52% (−22.95, 25.47, 95% CrI) per 50 h of fishing (Table 3).
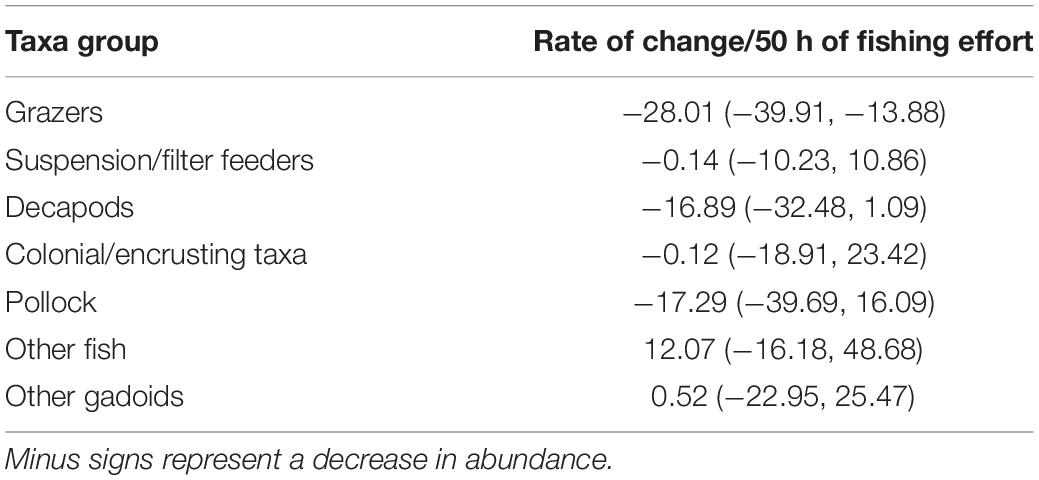
Table 3. Percent rate of change in relation to a 50 h increase in total fishing effort, with 95% CrI.
Time of Day
The time of day at which the ROV footage was recorded indicated that on average abundances were higher during the day for grazers at 1.22 (0.821, 1.74, 95% CrI) times higher during the day compared to at night, and 1.40 (0.791, 2.26, 95% CrI) times higher during the crepuscular period than at night. Suspension/filter feeders were 1.26 (0.749, 1.97, 95% CrI) times higher during the night compared to the crepuscular period, 1.24 (0.759, 1.91) higher during the day compared to the crepuscular period, and 1.02 (0.747, 1.37, 95% CrI) higher during the night compared to the day. Benthic epifaunal decapods were higher at night than during the day at 1.10 (0.677, 1.69, 95% CrI) times higher, however, they were higher during the crepuscular period than both day and night at 2.09 (1.20, 3.35, 95% CrI) and 1.96 (1.06, 3.31, 95% CrI) times higher, respectively.
Within the fish groups the highest differences were observed in the other fish group which were on average 1.74 (1.06, 2.74, 95% CrI) higher at night than during the day. Pollock were also higher at night at an average of 1.66 (0.982, 2.71, 95% CrI) times higher than during the day. In contrast, other gadoids were 1.12 (0.644, 2.04, 95% CrI) times higher during the day than the night, and 1.51 (0.536, 2.67, 95% CrI) times higher during the crepuscular period than at night.
Discussion
This study provides the first basin scale assessment of benthic and fish interactions with pipelines and pipeline protections and provides an evidence base which can be used to support decommissioning practices and policies. To make evidence-based decommissioning decisions, oil and gas operators and regulators require knowledge of the environmental impact of either removing or leaving infrastructure in place. The interactions of benthic epifauna and fish species with pipelines and associated protective structures in the NS, must be considered when selecting the optimal decommissioning approach. The taxa observed in this study were typical of NS hard substratum communities (Reiss et al., 2010), and similar species compositions have been observed on NS oil and gas platforms (De Mesel et al., 2015; Van Der Stap et al., 2016). We showed that pipelines and pipeline protections act as de facto artificial reefs, providing substrata for organisms that would otherwise be absent on the predominantly mobile sediment seafloor, and supporting fish species, some of which are commercially valuable (e.g., pollock).
We showed that concrete mattresses are associated with a higher abundance of grazers, decapods, suspension/filter feeders, pollock, and other fish in comparison to bare pipelines and rock dump. Concrete mattresses provided the most habitat variety and a larger surface area, with bare pipelines markedly homogenous in comparison. Rock dump will also provide a complex habitat, although, at a finer scale and lacking the larger sized void spaces observed on mattresses. The physical complexity of a structure, and increased environmental heterogeneity, have been shown to affect species abundance on other artificial structures through provision of a variety of niches and crevices for species to occupy (Hacker and Steneck, 1990; Frazer and Lindberg, 1994; Hunter and Sayer, 2009). The variety of spaces available on artificial reefs allow organisms to use the same structure at different stages of ontogeny (Taniguchi and Tokeshi, 2004), although, there is a species-specific response to increased complexity, and not all species will benefit (Langhamer and Wilhelmsson, 2009). Some species may benefit from more homogeneous structures, for example suspension/filter feeders were more abundant on bare pipelines than rock dump, although this could be due to the lower numbers of predators present on the less complex structures (i.e., bare pipelines).
Fish occur in consistently greater numbers around seabed features such as reefs compared with “featureless areas” (Bohnsack, 1989). In this study, pollock and other fish were found in higher abundances on mattresses than on both rock dump and bare pipelines, conversely other gadoids were found to be in higher abundance on bare pipelines. The complexity of the concrete mattresses (at more varied scales) is likely a driving factor in the higher abundances observed, by providing increased shelter provision, by means of a variety of space sizes, for both predators, and prey species (Hixon and Beets, 1989; Moreau et al., 2008). The higher complexity of concrete mattresses may be reducing competition by providing more refuges and a wider range of resources (Shulman, 1984), reducing predation risk (Ross et al., 2008), and allowing prey species populations to increase despite the presence of predators.
The effects of finer scale complexity may not be captured by this study due to the inaccuracy/difficulty in recording smaller species of <5 cm in size from ROV footage. For example, species that may be utilising fine scale complexity, such as small invertebrates, were not identifiable from the footage. It is also possible that taxa are more readily observable on bare pipelines in comparison to the more complex mattresses and rock dump where there is a higher probability that taxa are hidden from view of the ROV. The camouflage provided by the different structures also appeared to be resulting in species specific habitat choices. Common sole (Solea solea, Linnaeus, 1758) was observed on sediment adjacent to pipelines and protection structures, or in some cases, on mattresses where camouflage appeared to be playing an important role in the substrate choice. Monkfish species (Lophius sp. Linnaeus, 1758) were also observed in areas providing camouflage, predominantly on rock dump, although also rarely observed on concrete mattresses.
The location of a structure on the seabed determines the environmental conditions it experiences, the pool of potential colonising species, and can drive the structure of communities (Sammarco et al., 2004; Zintzen et al., 2008). Depth influences the pressure, temperature, nutrient availability and light present on a structure, and exerts a strong influence on the distribution of benthic fauna (Sammarco et al., 2004; Perkol-Finkel and Benayahu, 2005; Zintzen et al., 2008). Our study showed that abundances of taxa group varied with depth and that this relationship was different depending on either protection type or whether a sample was inside or outside of a 500 m exclusion zone. For example, we found that colonial/encrusting taxa inside 500 m exclusion zones decreased in abundance with an increase in depth, and this is likely due to the high abundance of Alcyonium digitatum in the southern North Sea. In contrast they increased with depth outside of 500 m zones, although this change was minimal.
The differences in abundances of taxa groups between protection types and at different depths may be due to the altered localised hydrodynamics caused by the structures themselves. Although hydrodynamics were not measured in this study, general observations indicated detritus building up on only one side of some pipelines, suggesting a reduction in local current speeds in the wake of the pipeline, which could have an impact on the benthic community (Glaholt, 2008) and fish assemblages. Other gadoids were found to be in slightly higher abundance on bare pipelines and within the footage, ling (Molva Molva), and cod (Gadus morhua, Linnaeus, 1758), were observed behind/under pipelines, potentially resting in the wake created by localised hydrodynamics behind pipelines. This suggests the abundance of other gadoids could be attributed to individuals deriving energetic savings by using current shadows or lee waves generated by the presence of a structure (Bohnsack, 1989).
Localised changes in the current regime around a structure such as an artificial reef could have implications for both the reef and the receiving environment (Wilding, 2006, 2014). Decreases in current speed allow the settlement of fine material including organic particles with a subsequent decrease in mean particle size and associated nutrient enrichment (Guiral et al., 1996). Artificial reefs with enhanced water flow are associated with higher growth of filter feeders and reduced mortality (Lenihan, 1999), and the same may apply to taxa within this study. The hydrodynamic flow regimes of different locations will affect the availability of potential colonists and the availability of food for suspension/filter feeding species, which will in turn reduce the amount of available food higher up the food chain (Baynes and Szmant, 1989; Guichard et al., 2001).
We found that grazers, decapods, suspension/filter feeders, colonial/encrusting species and pollock decreased in abundance with an increase in fishing intensity. Despite the structures posing threats to snagging fishing gear, it has been shown that vessels do target pipelines (Rouse et al., 2018), to potentially benefit from the artificial reef effects created. Studies have shown that larger and later maturing species are less able to withstand fishing mortality than their earlier maturing counterparts (Jennings et al., 1998). The results from this study do not indicate this effect, with pollock reaching maturity at 2–3 years of age (Cohen et al., 1990) and species within the other gadoid and other fish groups reaching maturity at similar ages, for example cod at 3 years, and flatfish at 2–4 years (Daan et al., 1990). The effect of bottom fisheries on demersal fish and benthic epifaunal species depends on the action of fishing gear in relation to the vertical distribution of the species, otter trawls catch mainly demersal fish and epifaunal species whereas beam trawls also catch infauna (Creutzberg et al., 1987). Investigations by means of experimental trawling showed that bottom fisheries increase the mortality not only of both target and by-catch species (Creutzberg et al., 1987; Kaiser and Spencer, 1996), but also of benthic epifaunal species that are not caught in the nets but damaged by the passing fishing gear (Bergman and Hup, 1992; Eleftheriou and Robertson, 1992; Currie and Parry, 1996; Bergman and Van Santbrink, 2000). These impacts could be responsible for changes to the benthic composition on the sediment surrounding pipelines and associated protective structures and subsequently the abundances of the taxa groups on the structures.
The resolution of the fishing intensity data used in this study, although the best available, did not capture the 500 m zones, and as such samples within zones were still attributed total fishing hour values. Platform 500 m exclusion zones are no fishing zones, it is possible that the lack of fishing activity in the areas is contributing to higher abundances of certain species. Decapods were 1.31 (0.896, 1.88, 95% CrI) times higher inside zones compared to outside zones, in contrast grazers were observed in higher abundances outside platform 500 m zones, although this difference was almost negligible at 1.01 times (0. 759, 1.34, 95% CrI) higher. All the fish groups were found to be higher in abundance inside platform 500 m exclusion zones. Although the lack of fishing within these 500 m zones is likely the driver for these observations, another possible mechanism which may be responsible for the attraction of fish will be the enhanced food availability (Page et al., 2007) on the platforms themselves. For example, pouting (Trisopterus luscus, Linnaeus, 1758) has been reported at elevated abundances around offshore wind turbines in the SNS and this was attributed to the enhanced provision of prey species that live on the structures (Reubens et al., 2013). Higher abundances of benthic species on concrete mattresses could be partly responsible for the increase in pollock and other fish on the structures. The proximity of a structure to other marine surfaces/sediment has also been shown to affect fish recruitment and colonisation rates by improving habitat connectivity (Fernández et al., 2008). The results from this study indicate that pipelines are contributing, at least to some extent, to the network of connecting hard substratum in the NS.
Platforms extend through the water column, from the sea floor beyond the surface, and experience a range of different environmental conditions and hence accommodate multiple assemblages (Van Der Stap et al., 2016), increasing overall diversity (Forteath et al., 1982; Perkol-Finkel and Benayahu, 2005). This increase in overall diversity has the potential to shape the benthic communities on the surrounding pipelines and protections. Oil and gas platforms act as “stepping-stones” facilitating the dispersion of larvae of coral (Sammarco et al., 2004) and facilitating range expansions of species such as Caprella linearis (Linnaeus, 1767; Coolen et al., 2015). In the NS, oil and gas platforms and wind turbines, have been described as a network of connected hard substrata (Hyder et al., 2017; Henry et al., 2018), however, to date research has excluded pipelines from connectivity models. It is plausible that pipelines are acting as habitat corridors between the “stepping-stone” platforms, with species in higher abundances nearer to the larger “stepping-stones” being dispersed outwards across pipelines. It is possible that with species in higher abundance on hard substrates such as pipelines and protections that a spillover effect may be observed in surrounding areas (Rabaoui et al., 2015), wherein the areas surrounding a pipeline experience increases in abundances. The concept of man-made structures as stepping-stones often raises questions as to the facilitation of movement for invasive species (Coolen et al., 2016). We did not observe any non-native species, however, most of the non-native species in the NS are small invertebrates (Eno et al., 1997; Coolen et al., 2016) and would not have been observable from ROV footage, if present on pipelines or protections.
While industry ROV footage provides data on an ordinarily unobtainable spatial and temporal scale (McLean et al., 2017), there are some limitations associated with the footage in comparison to scientific ROV footage. There are also challenges in obtaining the footage from operators, where time constraints to collate, catalogue, and provide the footage complicate access (Macreadie et al., 2018). The ability to accurately identify taxa to a species level is altered by several physical attributes of the footage itself and the limitations of reusing video footage, originally obtained for pipeline inspection, have previously been reported by Gormley et al. (2018); Macreadie et al. (2018), and Rouse et al. (2019). The use of large industry ROVs can also have an impact on the behaviour of fish making it difficult to obtain fine taxonomic resolution and record accurate counts, with much of this attraction or avoidance of fish occurring outside the field of view of cameras and/or observers (Stoner et al., 2008). Future work should focus on quantifying the effects of the physical qualities of ROV footage on an observer’s ability to accurately identifying species.
In the current study, the groupings used allowed for broader taxonomic classifications to be made, for example, where the species of an individual starfish was in doubt it could be included as an unidentified starfish. The inferences from this study can only be applied to the groups used, although other groupings could be achieved using the data provided in Supplementary Material. It is important to highlight that several methods have been used to analyse industry ROV footage for ecological purposes, and these differences should be considered when making comparisons in inference.
Simple adaptions to industry ROV surveys could provide benefits to ecological analysis, for example, the addition of scaling lasers or scaling bars to the ROVs would provide a useful tool to estimate taxa sizes and percentage cover calculations. The addition of such scaling would allow for accurate measurements of metrics such as biomass, which would unlock valuable information regarding the productivity of assemblages on pipelines and protections. The ideal solution to many of the limitations of industry collated footage would be to collect the data using a smaller scientific ROV with complete control over the footage characteristics, including lighting and video quality. By controlling the physical features of the footage, finer taxonomic detail could be extracted, allowing the effective use of metrics such as species richness. However, if scientific ROVs were used it would be extremely difficult to achieve the same spatial and temporal scales due to costs, time, and geographic restrictions.
What Does This Mean for Decommissioning?
This study improves our understanding of the ecological consequences of decommissioning decisions and can assist the CA process regarding pipeline and protection decommissioning. Operators and regulators must be sure to account for environmental impacts when determining best practices for decommissioning. Potential pipeline decommissioning scenarios include leave in situ options, such as burial via sediment or rock dump, or complete/partial removal. The current guidance in the UKCS is that that surface laid, un-trenched, small (< 16″) diameter pipelines are removed (BEIS, 2018).
This study has shown that pipelines and associated structures support benthic epifauna and fish, and complete removal of these structures will consequently remove available habitat as well as species that have already colonised. Although pipelines only represent a small amount (<0.01%) of the total area of the NS (Rouse et al., 2018), this study has shown that they may be facilitating the connectivity of hard substrates, and removal of the structures should be considered on this larger scale. If pipelines are decommissioned in situ, without intervention, then the level of disturbance to existing epifauna and fish would be minimal. If the decision is made to decommission in situ via means of burial via rock dump, the epifauna and fish currently interacting with structures would initially be displaced, although the rock dump would ultimately provide additional hard substrate which could become colonised with benthic epifauna. The burial of a pipeline with sediment could lead to the removal of all the species present on the structures, and although the hard substrate will be removed, it could be expected that soft-sediment communities would recover (Dernie et al., 2003).
If pipelines are decommissioned in situ, further research into comparisons between exposed and trenched/buried pipelines currently in situ would provide valuable insights into potential consequences. It is likely that pipeline/protection construction material, and burial status are likely to influence the community composition and abundance of epifauna and although the data obtained in this study were insufficient to explore this relationship, future work could focus on obtaining these details. If the decision is made to remove a pipeline and the associated protective structures this study highlights the potential re-use of the structures elsewhere in the marine environment to act as artificial reefs. Further evidence as to the suitability for the manipulation and re-use of the structures elsewhere would benefit the decommissioning evidence base further, for example the use of decommissioned mattresses as scour protections (and de facto reefs) or as aquaculture reefs targeting specific species such as Homarus gammarus (Linnaeus, 1758).
Although this study was conducted in the North Sea, many of the findings are globally relevant. In all major hydrocarbon basins, as operators continue to decommission infrastructure, this study supports the potential operation of pipelines and pipeline protections as artificial reefs and aims to prompt operators to consider these findings when making decisions into the decommissioning of such structures.
Data Availability Statement
The datasets presented in this study can be found in online repositories. The names of the repository/repositories and accession number(s) can be found in the article/Supplementary Material.
Author Contributions
MR, TW, SR, and PH conceived the presented study, developed the theory and methodology. MR conducted data collection and data analysis. TW, SR, and PH provided advice, assisted in the development of data analysis, and supervised the findings of this work. MR wrote the final manuscript, which was critically reviewed and edited by TW and SR. All authors contributed to the article and approved the submitted version.
Funding
This work was funded by the European Social Fund and Scottish Funding Council as part of Developing Scotland’s Workforce in the Scotland 2014–2020 European Structural and Investment Fund Programme.
Conflict of Interest
The authors declare that the research was conducted in the absence of any commercial or financial relationships that could be construed as a potential conflict of interest.
Acknowledgments
We gratefully acknowledge the in-kind provision of ROV footage by Chrysaor and Repsol Sinopec.
Supplementary Material
The Supplementary Material for this article can be found online at: https://www.frontiersin.org/articles/10.3389/fmars.2021.652630/full#supplementary-material
Footnotes
References
Aabel, J. P., Cripps, S. J., Jensen, A. C., and Picken, G. (1997). Creating Artificial Reefs from Decommissioned Platforms in the North Sea: Review of Knowledge and Proposed Programme of Research. Los Angeles, CA: Dames & Moore Group.
Baynes, T. W., and Szmant, A. M. (1989). Effect of current on the sessile benthic community structure of an artificial reef. Bull. Mar. Sci. 44, 545–566.
BEIS (2018). Guidance Notes Decommissioning of Offshore Oil and Gas Installations and Pipelines. Aberdeen: BEIS, 125.
Bergman, M. J. N., and Hup, M. (1992). Direct effects of beamtrawling on macrofauna in a sandy sediment in the southern North Sea. ICES J. Mar. Sci. 49, 5–11. doi: 10.1093/icesjms/49.1.5
Bergman, M. J. N., and Van Santbrink, J. W. (2000). Mortality in megafaunal benthic populations caused by trawl fisheries on the Dutch continental shelf in the North Sea in 1994. ICES J. Mar. Sci. 57, 1321–1331. doi: 10.1006/jmsc.2000.0917
Bergmark, P., and Jorgensen, D. (2014). Lophelia pertusa conservation in the North Sea using obsolete offshore structures as artificial reefs. Mar. Ecol. Prog. Ser. 516, 275–280. doi: 10.3354/meps10997
Bohnsack, J. A. (1989). Are high densities of fishes at artificial reefs the result of habitat limitation or behavioral preference? Bull. Mar. Sci. 44, 631–645.
Bond, T., Partridge, J. C., Taylor, M. D., Langlois, T. J., Malseed, B. E., Smith, L. D., et al. (2018). Fish associated with a subsea pipeline and adjacent seafloor of the North West Shelf of Western Australia. Mar. Environ. Res. 141, 53–65. doi: 10.1016/j.marenvres.2018.08.003
Bürkner, P.-C. (2017). brms: an R package for bayesian multilevel models using stan. J. Statist. Softw. 80, 1–28. doi: 10.18637/jss.v080.i01
Cohen, D. M., Inada, T., Iwamoto, T., and Scialabba, N. (1990). FAO Species Catalogue. v. 10: Gadiform Fishes of the World (Order Gadiformes). Rome: FAO Fisheries Synopsis (FAO).
Coolen, J. W., Lengkeek, W., Degraer, S., Kerckhof, F., Kirkwood, R. J., and Lindeboom, H. J. (2016). Distribution of the invasive Caprella mutica Schurin, 1935 and native Caprella linearis (Linnaeus, 1767) on artificial hard substrates in the North Sea: separation by habitat. Aquat. Invas. 11, 437–449. doi: 10.3391/ai.2016.11.4.08
Coolen, J. W., Lengkeek, W., Lewis, G., Bos, O. G., Van Walraven, L., and Van Dongen, U. (2015). First record of Caryophyllia smithii in the central southern North Sea: artificial reefs affect range extensions of sessile benthic species. Mar. Biodivers. Rec. 8:e140. doi: 10.1017/S1755267215001165
Creutzberg, F., Duineveld, G. C. A., and Van Noort, G. J. (1987). The effect of different numbers of tickler chains on beam-trawl catches. ICES J. Mar. Sci. 43, 159–168. doi: 10.1093/icesjms/43.2.159
Cumming, D. (2015). Mattress Solutions. Prepared by Jee Ltd. on Behalf of Zero Waste Scotland and Decom North Sea. Aberdeen: Decom North Sea, 94.
Currie, D. R., and Parry, G. D. (1996). Effects of scallop dredging on a soft sediment community: a large-scale experimental study. Mar. Ecol. Prog. Ser. 134, 131–150. doi: 10.3354/meps134131
Daan, N., Bromley, P. J., Hislop, J. R. G., and Nielsen, N. A. (1990). Ecology of North sea fish. Netherlands J. Sea Res. 26, 343–386. doi: 10.1016/0077-7579(90)90096-y
Dauterive, L. (1999). “Rigs-to reefs policy, progress, and perspective,” in Proceedings of the SPE/EPA Exploration & Production Environmental Conference, New York, NY. doi: 10.2118/52709-MS
De Mesel, I., Kerckhof, F., Norro, A., Rumes, B., and Degraer, S. (2015). Succession and seasonal dynamics of the epifauna community on offshore wind farm foundations and their role as stepping stones for non-indigenous species. Hydrobiologia 756, 37–50. doi: 10.1007/s10750-014-2157-1
DECC (2011). Decommissioning of Offshore Oil and Gas Installations and Pipelines Under the Petroleum Act 1998. Guidance Notes. Aberdeen: Department of Energy and Climate Change, doi: 10.1007/bf02298024
Dernie, K. M., Kaiser, M. J., and Warwick, R. M. (2003). Recovery rates of benthic communities following physical disturbance. J. Anim. Ecol. 72, 1043–1056.
Ducrotoy, J. P., Elliott, M., and de Jonge, V. N. (2000). The north sea. Mar. Pollut. Bull. 41, 5–23. doi: 10.1016/S0025-326X(00)00099-0
Ekins, P., Vanner, R., and Firebrace, J. (2006). Decommissioning of offshore oil and gas facilities: a comparative assessment of different scenarios. J. Environ. Manag. 79, 420–438. doi: 10.1016/j.jenvman.2005.08.023
Eleftheriou, A., and Robertson, M. R. (1992). The effects of experimental scallop dredging on the fauna and physical environment of a shallow sandy community. Netherlands J. Sea Res. 30, 289–299. doi: 10.1016/0077-7579(92)90067-o
Eno, N. C., Clark, R. A., and Sanderson, W. G. (eds) (1997). Non-Native Marine Species in British Waters: A Review And Directory. Peterborough: Joint Nature Conservation Committee.
Fernández, T. V., D’Anna, G., Badalamenti, F., and P rez-Ruzafa, A. (2008). Habitat connectivity as a factor affecting fish assemblages in temperate reefs. Aquat. Biol. 1, 239–248.
Forteath, G. N. R., Picken, B., Ralph, R., and Williams, J. (1982). Marine growth studies on the North sea oil platform montrose Alpha. Mar. Ecol. Prog. Ser. 8, 61–68. doi: 10.3354/meps008061
Frazer, T. K., and Lindberg, W. J. (1994). Refuge spacing similarly affects reef-associated species from three phyla. Bull. Mar. Sci. 55, 388–400.
Gass, S. E., and Roberts, J. M. (2006). The occurrence of the cold-water coral Lophelia pertusa (Scleractinia) on oil and gas platforms in the North Sea: colony growth, recruitment and environmental controls on distribution. Mar. Pollut. Bull. 52, 549–559. doi: 10.1016/j.marpolbul.2005.10.002
Gelman, A., Carlin, J. B., Stern, H. S., Dunson, D. B., Vehtari, A., and Rubin, D. B. (2013). Bayesian Data Analysis. Boca Raton, FL: CRC press.
Glaholt, R. D. (2008). “Investigation of the potential effects of marine pipelines on Dungeness crab movement and benthic ecology,” in Proceedings of the Environment Concerns in Rights-of-Way Management 8th International Symposium, Cambridge, MA, 679–692. doi: 10.1016/B978-044453223-7.50076-9
Goodrich, B., Gabry, J., Ali, I., and Brilleman, S. (2018). rstanarm: Bayesian Applied Regression Modeling via Stan. R Package Version 2.17.4.
Gormley, K., Mclellan, F., Mccabe, C., Hinton, C., Ferris, J., Kline, D., et al. (2018). Automated image analysis of offshore infrastructure marine biofouling. J. Mar. Sci. Eng. 6:2. doi: 10.3390/jmse6010002
Guerin, A. J., Jensen, A. C., and Jones, D. (2007). “Artificial reef properties of North Sea oil and gas production platforms,” in Proceedings of the OCEANS 2007 - Europe, Aberdeen. doi: 10.1109/OCEANSE.2007.4302338
Guichard, F., Bourget, E., and Robert, J. L. (2001). Scaling the influence of topographic heterogeneity on intertidal benthic communities: alternate trajectories mediated by hydrodynamics and shading. Mar. Ecol. Prog. Ser. 217, 27–41. doi: 10.3354/meps217027
Guiral, D., Gourbault, N., and Helleouet, M. N. (1996). Sediment nature and meiobenthos of an artificial reef (Acadja) used for extensive aquaculture. Oceanogr. Literat. Rev. 9:942.
Guo, B., Song, S., Ghalambor, A., and Chacko, J. (2005). Offshore Pipelines. Burlington, VT: Elsevier.
Hacker, S. D., and Steneck, R. S. (1990). Habitat architecture and the abundance and body-size-dependent habitat selection of a phytal amphipod. Ecology 71, 2269–2285. doi: 10.2307/1938638
Henry, L. A., Mayorga-Adame, C. G., Fox, A. D., Polton, J. A., Ferris, J. S., McLellan, F., et al. (2018). Ocean sprawl facilitates dispersal and connectivity of protected species. Sci. Rep. 8, 1–11. doi: 10.1038/s41598-018-29575-4
Hixon, M. A., and Beets, J. P. (1989). Shelter characteristics and Caribbean fish assemblages: experiments with artificial reefs. Bull. Mar. Sci. 44, 666–680.
Hunter, W. R., and Sayer, M. D. J. (2009). The comparative effects of habitat complexity on faunal assemblages of northern temperate artificial and natural reefs. ICES J. Mar. Sci. 66, 691–698. doi: 10.1093/icesjms/fsp058
Hyder, K., Van Der Molen, J., Garcia, L., Callaway, A., Posen, P., Wright, S., et al. (2017). Assessing the Ecological Connectivity Between Man-made Structures in the North Sea (EcoConnect). Norwich: CEFAS Laboratory.
ICES (2017). Spatial Data Layers of Fishing Intensity/Pressure per Gear Type for Surface and Subsurface Abrasion, for the Years 2009 to 2016 in the Ospar Area. Copenhagen: ICES.
Jennings, S., Reynolds, J. D., and Mills, S. C. (1998). Life history correlates of responses to fisheries exploitation. Proc. R. Soc. Lond. Ser. B Biol. Sci. 265, 333–339. doi: 10.1098/rspb.1998.0300
Jensen, A. (2002). Artificial reefs of Europe: perspective and future. ICES J. Mar. Sci. 59, 3–13. doi: 10.1006/jmsc.2002.1298
Jørgensen, D. (2012). OSPAR’s exclusion of rigs-to-reefs in the North. Ocean Coast. Manag. 58, 57–61. doi: 10.1016/j.ocecoaman.2011.12.012
Kaiser, M. J., and Pulsipher, A. G. (2005). Rigs-to-reef programs in the Gulf of Mexico. Ocean Dev. Intern. Law 36, 119–134. doi: 10.1080/00908320590943990
Kaiser, M. J., and Spencer, B. E. (1996). The effects of beam-trawl disturbance on infaunal communities in different habitats. J. Anim. Ecol. 65, 348–358. doi: 10.2307/5881
Korner-Nievergelt, F., Roth, T., Von Felten, S., Guélat, J., Almasi, B., and Korner-Nievergelt, P. (2015). Bayesian Data Analysis in Ecology Using Linear Models with R, BUGS, and Stan. Cambridge, MA: Academic Press. doi: 10.1016/B978-0-12-801370-0.00004-6
Lacey, N. C., and Hayes, P. (2020). Epifauna associated with subsea pipelines in the North Sea. ICES J. Mar. Sci. 77, 1137–1147. doi: 10.1093/icesjms/fsy196
Langhamer, O., and Wilhelmsson, D. (2009). Colonisation of fish and crabs of wave energy foundations and the effects of manufactured holes - A field experiment. Mar. Environ. Res. 68, 151–157. doi: 10.1016/j.marenvres.2009.06.003
Lenihan, H. S. (1999). Physical–biological coupling on oyster reefs: how habitat structure influences individual performance. Ecol. Monogr. 69, 251–275. doi: 10.2307/2657157
Love, M. S., and York, A. (2005). A comparison of the fish assemblages associated with an oil/gas pipeline and adjacent seafloor in the Santa Barbara Channel, Southern California Bight. Bull. Mar. Sci. 77, 101–117.
Macreadie, P. I., Fowler, A. M., and Booth, D. J. (2011). Rigs-to-reefs: will the deep sea benefit from artificial habitat? Front. Ecol. Environ. 9, 455–461. doi: 10.1890/100112
Macreadie, P. I., McLean, D. L., Thomson, P. G., Partridge, J. C., Jones, D. O., Gates, A. R., et al. (2018). Eyes in the sea: unlocking the mysteries of the ocean using industrial, remotely operated vehicles (ROVs). Sci. Total Environ. 634, 1077–1091. doi: 10.1016/j.scitotenv.2018.04.049
McLean, D. L., Macreadie, P., White, D. J., Thomson, P. G., Fowler, A., Gates, A. R., et al. (2018). “Understanding the global scientific value of industry ROV data, to quantify marine ecology and guide offshore decommissioning strategies,” in Proceedings of the Offshore Technology Conference Asia, Houston, TX. doi: 10.4043/28312-MS
McLean, D. L., Parsons, M. J., Gates, A. R., Benfield, M. C., Bond, T., Booth, D. J., et al. (2020). Enhancing the scientific value of industry remotely operated vehicles (ROVs) in our oceans. Front. Mar. Sci. 7:220. doi: 10.3389/fmars.2020.00220
McLean, D. L., Partridge, J. C., Bond, T., Birt, M. J., Bornt, K. R., Langlois, T. J., et al. (2017). Using industry ROV videos to assess fish associations with subsea pipelines. Continent. Shelf Res. 141, 76–97. doi: 10.1016/j.csr.2017.05.006
Mearns, E., and Hinze, D. (2015). Re-designing a more circular Scottish economy. Fraser Allander Instit. Econ. Comment. 39, 122–136.
Moreau, S., Peron, C., Pitt, K. A., Connolly, R. M., Lee, S. Y., and Méziane, T. (2008). Opportunistic predation by small fishes on epibiota of jetty pilings in urban waterways. J. Fish Biol. 72, 205–217. doi: 10.1111/j.1095-8649.2007.01705.x
Otto, L., Zimmerman, J. T. F., Furnes, G. K., Mork, M., Saetre, R., and Becker, G. (1990). Review of the physical oceanography of the North Sea. Netherlands J. Sea Res. 26, 161–238. doi: 10.1016/0077-7579(90)90091-T
Page, H. M., Dugan, J. E., Schroeder, D. M., Nishimoto, M. M., Love, M. S., and Hoesterey, J. C. (2007). Trophic links and condition of a temperate reef fish: comparisons among offshore oil platform and natural reef habitats. Mar. Ecol. Prog. Ser. 344, 245–256. doi: 10.3354/meps06929
Paramor, O. A. L. K. A., Allen, M., Aanesen, C., Armstrong, G. J., Piet, R., van Hal, L. J. W., et al. (2009). MEFEPO North Sea Atlas. Liverpool: University of Liverpool, 80.
Perkol-Finkel, S., and Benayahu, Y. (2005). Recruitment of benthic organisms onto a planned artificial reef: shifts in community structure one decade post-deployment. Mar. Environ. Res. 59, 79–99. doi: 10.1016/j.marenvres.2004.03.122
Picken, G., Baine, M., Heaps, L., and Side, J. (2000). “Rigs to reefs in the North Sea,” in Artificial Reefs in European Seas, eds A. C. Jensen, K. J. Collins, and A. P. M. Lockwood (Dordrecht: Springer), 331–342. doi: 10.1007/978-94-011-4215-1_20
Rabaoui, L., Lin, Y. J., Qurban, M. A., Maneja, R. H., Franco, J., Joydas, T. V., et al. (2015). Patchwork of oil and gas facilities in Saudi waters of the Arabian Gulf has the potential to enhance local fisheries production. ICES J. Mar. Sci. 72, 2398–2408. doi: 10.1093/icesjms/fsv072
Reiss, H., Degraer, S., Duineveld, G. C., Kröncke, I., Aldridge, J., Craeymeersch, J. A., et al. (2010). Spatial patterns of infauna, epifauna, and demersal fish communities in the North Sea. ICES J. Mar. Sci. 67, 278–293. doi: 10.1093/icesjms/fsp253
Reubens, J. T., Braeckman, U., Vanaverbeke, J., Van Colen, C., Degraer, S., and Vincx, M. (2013). Aggregation at windmill artificial reefs: CPUE of Atlantic cod (Gadus morhua) and pouting (Trisopterus luscus) at different habitats in the Belgian part of the North Sea. Fish. Res. 139, 28–34. doi: 10.1016/j.fishres.2012.10.011
Rogers, S., and Stocks, R. (2001). North Sea Fish and Fisheries. Technical Report Production for Strategic Environmental Assessment. Rome: Fisheries Research services.
Ross, P. M., Thrush, S. F., Montgomery, J. C., Walker, J. W., and Parsons, D. M. (2008). Habitat complexity and predation risk determine juvenile snapper (Pagrus auratus) and goatfish (Upeneichthys lineatus) behaviour and distribution. Mar. Freshw. Res. 58, 1144–1151. doi: 10.1071/mf07017
Rouse, S., Kafas, A., Catarino, R., and Hayes, P. (2018). Commercial fisheries interactions with oil and gas pipelines in the North Sea: considerations for decommissioning. ICES J. Mar. Sci. 75, 279–286. doi: 10.1093/icesjms/fsx121
Rouse, S., Lacey, N. C., Hayes, P., and Wilding, T. A. (2019). Benthic conservation features and species associated with subsea pipelines: considerations for decommissioning. Front. Mar. Sci. 6:200. doi: 10.3389/fmars.2019.00200
Russell, D. J., Brasseur, S. M., Thompson, D., Hastie, G. D., Janik, V. M., Aarts, G., et al. (2014). Marine mammals trace anthropogenic structures at sea. Curr. Biol. 24, R638–R639. doi: 10.1016/j.cub.2014.06.033
Sammarco, P. W., Atchison, A. D., and Boland, G. S. (2004). Expansion of coral communities within the Northern Gulf of Mexico via offshore oil and gas platforms. Mar. Ecol. Prog. Ser. 280, 129–143. doi: 10.3354/meps280129
Sayer, M. D. J., and Baine, M. S. P. (2002). Rigs to reefs: a critical evaluation of the potential for reef development using decommissioned rigs. Underw. Technol. 25, 93–97. doi: 10.3723/175605402783219181
Shukla, A., and Karki, H. (2016). Application of robotics in offshore oil and gas industry-A review Part II. Robotics and autonomous systems. Robot. Autonom. Syst. 75, 508–524. doi: 10.1016/j.robot.2015.09.013
Shulman, M. J. (1984). Resource limitation and recruitment patterns in a coral reef fish assemblage. J. Exper. Mar. Biol. Ecol. 74, 85–109. doi: 10.1016/0022-0981(84)90039-x
Stevenson, A. (2012). The European marine observation and data network: geological data. Baltica 25, 87–90. doi: 10.1111/geb.12729
Stoner, A. W., Ryer, C. H., Parker, S. J., Auster, P. J., and Wakefield, W. W. (2008). Evaluating the role of fish behavior in surveys conducted with underwater vehicles. Canad. J. Fisher. Aquat. Sci. 65, 1230–1243.
Svane, I., and Petersen, J. K. (2001). On the problems of epibioses, fouling and artificial reefs, a review. Mar. Ecol. 22, 169–188. doi: 10.1046/j.1439-0485.2001.01729.x
Taniguchi, H., and Tokeshi, M. (2004). Effects of habitat complexity on benthic assemblages in a variable environment. Freshw. Biol. 49, 1164–1178. doi: 10.1111/j.1365-2427.2004.01257.x
Techera, E. J., and Chandler, J. (2015). Offshore installations, decommissioning and artificial reefs: do current legal frameworks best serve the marine environment? Mar. Policy 59, 53–60. doi: 10.1016/j.marpol.2015.04.021
Thomson, P. G., Fowler, A. M., Davis, A. R., Pattiaratchi, C. B., and Booth, D. J. (2018). Some old movies become classics–A case study determining the scientific value of ROV inspection footage on a platform on Australia’s North West Shelf. Front. Mar. Sci. 5:471. doi: 10.3389/fmars.2018.00471
Thorpe, R. B., Dolder, P. J., Reeves, S., Robinson, P., and Jennings, S. (2016). Assessing fishery and ecological consequences of alternate management options for multispecies fisheries. ICES J. Mar. Sci. 73, 1503–1512. doi: 10.1093/icesjms/fsw028
Van Der Stap, T., Coolen, J. W. P., and Lindeboom, H. J. (2016). Marine fouling assemblages on offshore gas platforms in the southern North Sea: effects of depth and distance from shore on biodiversity. PLoS One 11:e0146324. doi: 10.1371/journal.pone.0146324
Watanabe, S. (2010). Asymptotic equivalence of Bayes cross validation and widely applicable information criterion in singular learning theory. J. Mach. Learn. Res. 11, 3571–3594.
Whitcomb, L. L. (2000). “Underwater robotics: out of the research laboratory and into the field,” in Proceedings of the IEEE International Conference on Robotics and Automation. Symposia Proceedings (Cat. No. 00CH37065), San Francisco, CA.
Whomersley, P., and Picken, G. B. (2003). Long-term dynamics of fouling communities found on offshore installations in the North Sea. J. Mar. Biol. Assoc. U.K. 83, 897–901. doi: 10.1017/s0025315403008014h
Wilding, T. A. (2006). The benthic impacts of the Loch Linnhe artificial reef. Hydrobiologia 555, 345–353. doi: 10.1007/s10750-005-1130-4
Wilding, T. A. (2014). Effects of man-made structures on sedimentary oxygenation: extent, seasonality and implications for offshore renewables. Mar. Environ. Res. 97, 39–47. doi: 10.1016/j.marenvres.2014.01.011
Zintzen, V., Norro, A., Massin, C., and Mallefet, J. (2008). Spatial variability of epifaunal communities from artificial habitat: Shipwrecks in the Southern Bight of the North Sea. Estuar. Coast. Shelf Sci. 76, 327–344. doi: 10.1016/j.ecss.2007.07.012
Keywords: North Sea, oil and gas, pipeline protections, ROV, artificial reef, fish, benthic epifauna, decommissioning
Citation: Redford M, Rouse S, Hayes P and Wilding TA (2021) Benthic and Fish Interactions With Pipeline Protective Structures in the North Sea. Front. Mar. Sci. 8:652630. doi: 10.3389/fmars.2021.652630
Received: 12 January 2021; Accepted: 29 March 2021;
Published: 20 April 2021.
Edited by:
Susana Carvalho, King Abdullah University of Science and Technology, Saudi ArabiaReviewed by:
Matt Streich, Texas A&M University Corpus Christi, United StatesLotfi Rabaoui, King Fahd University of Petroleum and Minerals, Saudi Arabia
Euan S. Harvey, Curtin University, Australia
Copyright © 2021 Redford, Rouse, Hayes and Wilding. This is an open-access article distributed under the terms of the Creative Commons Attribution License (CC BY). The use, distribution or reproduction in other forums is permitted, provided the original author(s) and the copyright owner(s) are credited and that the original publication in this journal is cited, in accordance with accepted academic practice. No use, distribution or reproduction is permitted which does not comply with these terms.
*Correspondence: Michael Redford, TWljaGFlbC5SZWRmb3JkQHNhbXMuYWMudWs=