- 1MARE - Marine and Environmental Sciences Centre, Agência Regional para o Desenvolvimento da Investigação Tecnologia e Inovação (ARDITI), Edifício Madeira Tecnopolo, Madeira, Portugal
- 2MARE - Marine and Environmental Sciences Centre, Faculdade de Ciências, Universidade de Lisboa, Lisbon, Portugal
- 3Departamento de Biologia Vegetal, Faculdade de Ciências, Universidade de Lisboa, Lisbon, Portugal
- 4Smithsonian Environmental Research Center, Edgewater, MD, United States
Marine forests ecosystems are typical of temperate rocky benthic areas. These systems are formed by canopy-forming macroalgae (Laminariales, Tilopteridales, and Fucales) of high ecological value that provide numerous ecosystem services. These key species are also indicators of good environmental status. In recent decades, marine forests have been threatened by different impacts of local and global origin, putting their stability and survival in question. On a global scale, in many temperate areas of the planet, marine forests have been replaced by “sea-urchins barrens.” We present a general overview of sea-urchins’ population status in the archipelagos of Azores, Webbnesia (Madeira, Selvagens, and Canary Islands) and Cabo Verde, focusing on their role in the maintenance of the so-called “alternate stable state.” After an in-depth evaluation of the different anthropogenic and environmental pressures, we conclude that sea-urchins population explosion has been facilitated in the benthic habitats of Madeira and Canary Islands, preventing the recovery of canopy-forming macroalgae assemblages and being one of the main drivers in maintaining a stable barren state. Diadema africanum is the main barrens-forming species in Webbnesia, where it reaches high densities and strongly impacts macroalgal assemblages. On the other hand, in the most pristine areas, such as the Selvagens Islands and other Marine Protected Areas from the Canary Islands, the density of D. africanum is up to 65% lower than in the nearby Madeira Island, and macroalgal communities are preserved in good status. This information is critical for marine environmental management, highlighting the urgent need for implementation of appropriate control mechanisms and restoration actions headed to the conservation of marine forests in Macaronesian archipelagos.
Introduction
Canopy-forming algae (Laminariales, Tilopteridales, and Fucales) have been widely recognized as the underwater forests of temperate rocky systems (Dayton, 1985). These key species are characteristic of mature high productive systems, since they are late-colonizers, with the ability to increase the structural complexity of ecosystems providing habitat and food for many associated species (Bray and Ebeling, 1975; Steneck et al., 2002; Filbee-Dexter and Scheibling, 2014). Furthermore, its important contribution to the benthic carbon cycle has been demonstrated (Duarte and Cebrián, 1996), highlighting the capacity of the macroalgal beds to act as atmospheric CO2 sinks (Watanabe et al., 2019). The loss or reduction of these underwater forests has multiple negative effects, with particularly strong effects on the composition, structure and biodiversity of the associated benthic assemblages (Steneck et al., 2002; Ling et al., 2015), producing undesirable consequences at the ecosystem level (Worm et al., 2006; Smale et al., 2013). By contrast, the presence of canopy-forming algae is considered a clear indicator of good state for rocky benthic communities (Hernández et al., 2008a). The loss and even disappearance of canopy-forming algae communities have been reported almost everywhere from various impacts, such as overgrazing (Tuya et al., 2004a; Hereu Fina, 2007; Gianni et al., 2017), sedimentation (Airoldi, 2003; Schiel and Gunn, 2019), pollution (Rodríguez Prieto and Polo Albertí, 1996; Soltan et al., 2001), coastal urbanization (Scherner et al., 2013), and climate change effects (Arafeh-Dalmau et al., 2020; Smale, 2020).
The role of sea-urchins as modifying agents for erect algae community structure is well known (Watanabe and Harrold, 1991; Filbee-Dexter and Scheibling, 2014; Miller et al., 2018). Although there are other herbivores recognized as relevant grazing agents, their functional activity is lower or insignificant compared to sea-urchins (Cordeiro et al., 2020). The intense browsing activity of these species can cause canopy-forming macroalgal communities to collapse, transforming large extensions of rocky bottoms in the named “sea-urchin barrens,” a status dominated by turf and crustose coralline algae (CCA) (Sala et al., 1998; Goreau et al., 2000; Guidetti et al., 2005). When benthic bottoms reach this alteration point, they are in an “alternate stable state,” presenting a high resistance to return to its starting point (Figure 1; Knowlton, 2004; Filbee-Dexter and Scheibling, 2014; Melis et al., 2019). This has been verified in different regions of the planet, where the extent of sea-urchin grazing exerts a major control on the structure and functioning of the coastal benthic ecosystems (Hill et al., 2003; Tuya et al., 2004a; Hereu Fina, 2007; Eklöf et al., 2008; Gianni et al., 2017; Yorke et al., 2019). For example, in the Mediterranean Sea, algal cover increased up to 40–50% in patches where the sea-urchin Arbacia lixula was removed, while in control patches their cover never exceeded 10% (Bulleri et al., 1999). Cover of macroalgae returned to original levels only 6 months after the removal of Paracentrotus lividus also in the Northwest Mediterranean (Bulleri et al., 1999). Sea-urchins significantly affect habitat structure, even in communities with low echinoid densities (Palacín et al., 1998). At a global level and from an ecological view, the leading causes that alter the balance between sea-urchin barrens and erect algae communities can be divided into: (1) top-down (loss of predation), (2) bottom-up (nutrient enrichment), and (3) “side-in” (temperature, etc.) mechanisms. Moreover, these drivers do not act in isolation, so the additive or synergistic effects of multiple co-occurring mechanisms may contribute to the maintenance of overgrazing over time (Eklöf et al., 2008).
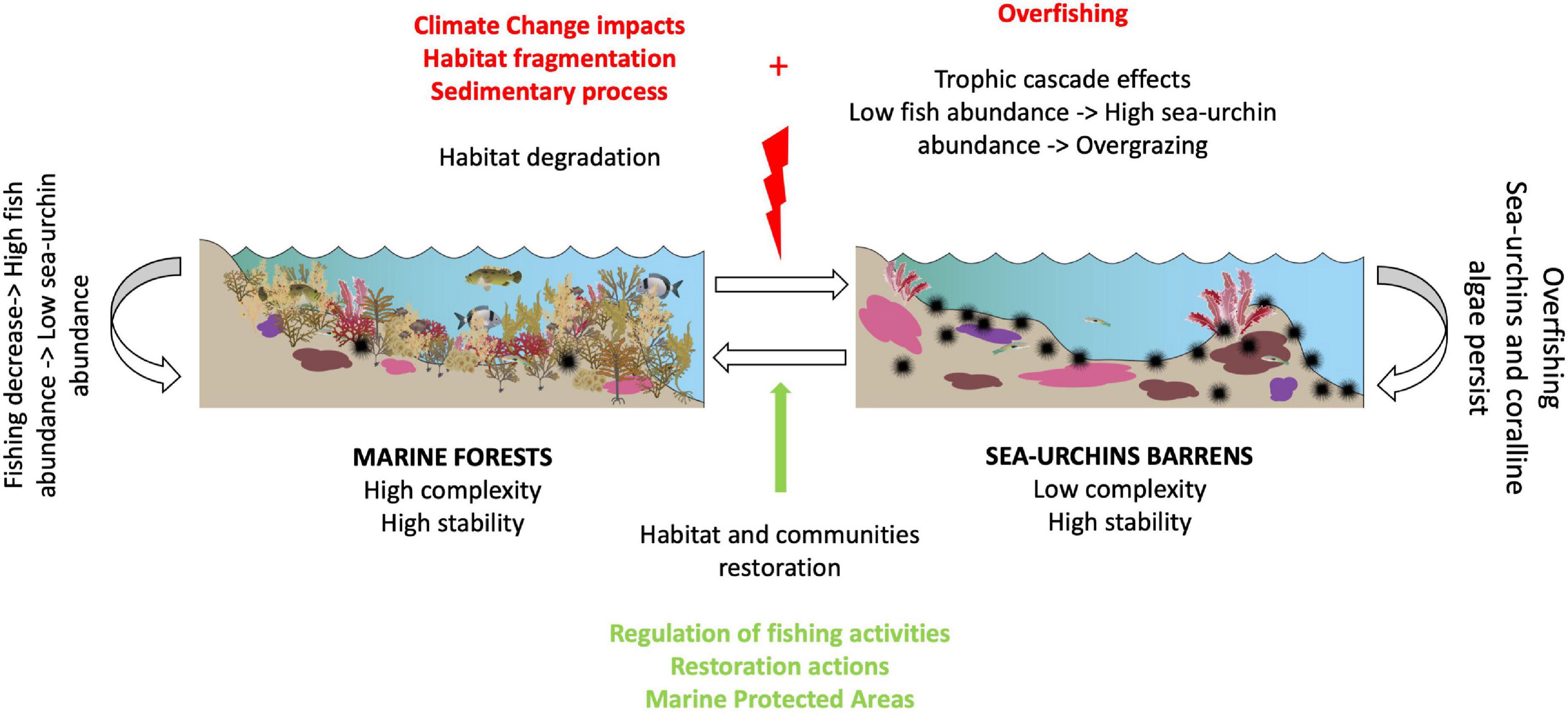
Figure 1. Diagram illustrating the two-system rocky benthic bottoms: marine forests and sea-urchins barrens. Transition processes between states depending on fishing activities and habitat degradations impacts (modified from Sala et al., 1998) (Symbols courtesy of the Integration and Application Network, University of Maryland Center for Environmental Science (ian.umces.edu/symbols/).
The Macaronesian islands, a group of archipelagos located in the Northeast (NE) Atlantic Ocean (Figure 2), have recently been redefined, based on extensive analysis encompassing numerous phyla (fish, echinoderms, macroalgae, among others) (Freitas et al., 2019). Now the marine biogeographic unit of Macaronesia, is consider as four distinct ecoregions: The South European Atlantic Shelf, the Saharan Upwelling, the Azores ecoregion and a new ecoregion herein named Webbnesia (formed by Madeira, Selvagens and Canary Islands). In addition, Cabo Verde has been proposed as an isolated ecoregion characterized as an important hotspot of tropical reef biodiversity (Roberts et al., 2002; Freitas et al., 2019). The altitudinal range of the various islands across these archipelagos, coupled with their isolation from the mainland result in climates that are atypical for their latitudinal locations (Martín et al., 2012). But, in general, their climate is framed by the semi-permanent presence of a high-pressure system from the Azores, prevailing from the northeast trade winds and the surrounding ocean, including the Azores and the Canary currents (Cropper, 2013).
The major guild of grazing sea-urchins inhabiting coastal rocky bottoms in the Azores, Webbnesia and Cabo Verde is well represented by Arbacia luxila (Linnaeus, 1758), Arbaciella elegans (Mortensen, 1910), Centrostephanus longispinus (Philippi, 1845), Eucidaris tribuloides (Lamarck, 1816), Heliophora orbicularis (Linnaeus, 1758), Paracentrotus lividus (Lamarck, 1816), Sphaerechinus granularis (Lamarck, 1816), and Diadema africanum (Rodríguez et al., 2013). Local primary production, recruitment, settlement of new individuals or topographic and substrate complexity are considered the main natural factors driving their abundance patterns (Clemente and Hernández, 2008). Moreover, ocean circulation, salinity, or sea surface temperature (Stobberup et al., 2004; Lázaro et al., 2005) are frequently recognized as additional key factors that specifically affect large-scale geographic echinoid assemblages. However, sea-urchin population explosions have been detected over the last decades, with the consequent loss of macroalgal covers from the Sargassaceae family as dominant habitat-forming species of the region (Tuya et al., 2004a, b). These losses are particularly evident when high-density populations of both A. lixula and D. africanum are reached due to their strong capacity of modifying the benthic communities (Alves et al., 2003). Although various authors have hypothesized that the overfishing of natural predators of sea-urchins is the main factor that favors their proliferation in the area (Tuya et al., 2004b), there has been no holistic approach conducted to set a basis for better understanding the different potential causes of sea-urchin proliferation (Sangil et al., 2018; Gizzi et al., 2020).
Thus, the present study aims to provide the first comprehensive compilation of the existing information on the potential threats affecting macroalgal forests of Azores, Webbnesia and Cabo Verde, focusing on the role of sea-urchins in the maintenance of the so-called “alternate stable state.” Evidence about the main anthropogenic pressures and other environmental factors involved in the sea-urchin population explosion historically detected are discussed and pondered across NE Atlantic archipelagos.
Sea-Urchin Barrens and Macroalgal Forests in NE Atlantic Archipelagos
Azores
The Azorean archipelago (38.5°N,–28°E) is a group of nine volcanic islands with coasts formed by cliffs and rocky shores interspersed with sandy beaches (Borges, 2004). Waters surrounding the Azores are mesotrophic with sea surface temperature (SST) usually range between 15.5 and 23.2°C (Amorim et al., 2017; Sangil et al., 2018).
The Azores’ rocky coasts are mainly characterized by patchy algae-based communities (Martins et al., 2005). The diversity of algal species is much lower than that of the remaining archipelagos, being Zonaria tournefortii and Dictyota dichotoma dominant species (Neto et al., 2014; Sangil et al., 2018). The diversity of herbivores is also low compared with the rest of the archipelagos (Sangil et al., 2018). The sea-urchins population is composed of A. lixula, P. lividus, and S. granularis (Hawkins et al., 2000), species with remarkably lower voracity than D. africanum (Bulleri et al., 1999; Alves et al., 2001). The species P. lividus and A. lixula do not appear to coexist in the archipelago (Hawkins et al., 2000). P. lividus was always found in shallow waters, while A. lixula was present from depths of 10–18 m, suggesting that this species could be responsible for the Azorean barrens. Those areas with low densities of A. lixula showed well-established algae communities. These covers were substantially reduced, or non-existent, when the echinoid density surpassed 15 individuals m–2 (Marques, 1984). Consequently, the area below the low tide mark has experienced zoning patterns from bottoms dominated by red algae in the upper layers, giving way to A. lixula, S. granularis and CCA in the low tidal zones (Hawkins et al., 2000). Although D. africanum has been sporadically found on the southern island of Santa Maria (Minderlein and Wirtz, 2014), it has not been detected in the rest of the Azorean archipelago.
Webbnesia: Madeira, Selvagens, and Canary Islands
Madeiran archipelago (32.4°N,–17°E) is formed by the islands of Madeira, Porto Santo, Desertas, and the distant southern Selvagens. The coastline in this archipelago is predominantly rocky, with a mix of platforms, boulders, and very few interspersed sandy-pebble beaches. The waters are oligotrophic, and SST typically ranges between 17.0 and 23.5°C (Schäfer et al., 2019).
Sea-urchin species known to occur in Madeira archipelago include P. lividus, A. lixula, S. granularis, D. africanum, with lower abundances of A. elegans and C. longispinus (Wirtz, 1995). Since the early 90’s the D. africanum populations in Madeira Island are in full expansion (Abreu et al., 1995). The high density of D. africanum appears to be reflected in the reduction of canopy-forming algae abundance, transforming macroalgal communities to mainly patchy cover of turf (Friedlander et al., 2017). The high-density population of D. africanum has been partly explained by the low control capacity of top-down processes associated with the absence or very low predator densities (Alves et al., 2001, 2003). Particularly, Alves et al. (2001) found high population densities of D. africanum (8–17 ind. m–2) reaching a maximum record of 68 ind. m–2 in the southern coast of Madeira, contrasting with low densities along the northwest coast. The north and west coast of Madeira is often exposed to rough waters, and thus lower recreational fishing pressure, which may be responsible for these observed differences in D. africanum density (Clemente et al., 2014; Friedlander et al., 2017). Similarly, densities reported on Porto Santo Island (33.06°N,–16.35°E) were also much lower, possibly due to its geomorphological features characterized by large sandy accumulations (Hernández et al., 2008b). Moreover, some sea-urchin specimens were found at lower depths than in Madeira Island (i.e., 1 m at Porto Santo pier), which is likely related to a lower wave exposure (Alves et al., 2001).
The Selvagens Islands (30.14°N,–15.86°E) form an isolated group of small islands and reefs located south of Madeira Island (290 km approx.) and north of the Canary Islands (170 km approx.). This sub-archipelago has been a Marine Protected Area (MPA) for over 40 years being one of the most pristine places in Webbnesia and one of the few intact marine ecosystems in the NE Atlantic Ocean. Selvagens benthic bottoms are dominated by turfs, erect algae and canopy-forming species (Friedlander et al., 2017). Although D. africanum is the most common mobile invertebrate in the Selvagens, its abundance is 65% lower than in Madeira (2.7 ind. m–2 vs. 4–7 ind. m–2) (Friedlander et al., 2017).
The Canary Islands (28.53°N,–15.74°E), located near the Northwest African Coast, are composed of seven islands that present high geological and topographic variability. The waters are oligotrophic, affected by a nutrient increase through the upwelling, and annual variation in SST ranging from 18.0 to 24.0°C (Valdazo et al., 2017; Sangil et al., 2018).
The most abundant sea-urchins’ species in coastal rocky habitats of the Canary Islands are: P. lividus, A. lixula, S. granularis and D. africanum (Hernández et al., 2013; Sangil et al., 2018). However, the barrens generated by D. africanum are more widely spread throughout the archipelago (Brito, 2004; Tuya et al., 2004b; Hernández et al., 2005, 2008a). D. africanum barrens extend up to 50 m in depth, and occupy approximately 70% of the rocky bottoms habitats (Barquín-Diez et al., 2005). The densities of D. africanum range between 6 and 12 ind. m–2 (Clemente et al., 2014) and, although it presents high spatial variability, is correlated with human activities (Hernández et al., 2008a; Clemente et al., 2011). The highest densities of D. africanum are found on Tenerife Island, which also supports the highest fishing pressure, population density and tourism development (Clemente et al., 2014). Densities of D. africanum higher than 2 ind. m–2 forms barrens through all the Canary Islands, reducing the cover of non-crustose algae below 50% (Hernández et al., 2008b; Sangil et al., 2011). More severe reductions (i.e., below 30% of algal cover) occurs when the density of sea-urchins increases to 4 ind. m–2, where the ecosystem shifts and becomes D. africanum dominated (Clemente et al., 2007).
In the Canary Islands, MPAs have proven to be a successful tool for the conservation of marine forests and the control of D. africanum populations. For example, the Marine Reserve of Mar de Las Calmas (El Hierro Island) and the MPA of the southern coast of La Palma Island. Both of the aforementioned areas are dominated by erect algal communities in the shallow infralittoral and circalittoral beds (Hernández et al., 2005, 2008a, Hernández, 2009; Sangil et al., 2012). Especially in the MPA of La Palma where the densities of D. africanum are lower than those found in the Selvagens, reaching maximum densities of 0.5 ind. M–2 at depths of 10–15 m (Sangil et al., 2012). Interestingly, individuals are smaller in these protected areas, related to lower availability of food resources (Clemente et al., 2007; Hernández et al., 2007) and higher abundances and biomass of predatory sea-urchin fishes, which exert direct pressure on the larger population sizes (Sangil et al., 2012).
Cabo Verde
Cabo Verde (14.91°N,–23.50°E) is an archipelago with an arid climate located in the mid-Atlantic Ocean (570 km off the west coast of Africa). SST usually ranges between 20 and 25°C with nutrient-rich waters supported by strong upwelling from the coast of Senegal and Mauritania (Freitas et al., 2019).
A total of 4 species of sea-urchins appear in the Cabo Verde’s coastal systems: A. elegans, D. africanum, E. tribuloides, and H. orbicularis. Benthic ecosystems in Cabo Verde, as a tropical region, have more complex trophic structures than that of the remaining archipelagos, in addition to a greater diversity of predators and herbivores. Shallow benthic systems of the archipelago are dominated by algae, anthozoan communities and D. africanum densities are low in the region [<1 ind. m–2 (Entrambasaguas et al., 2008)], with no reports of barrens originating from its grazing activities.
Coastal Features and Human Pressures
Wave Exposure, Depth and Bottom Complexity
Wave exposure and depth have been reported as important and interacting environmental factors in determining sea-urchins’ abundances (Hernández et al., 2020). In strictly sheltered areas, densities of D. africanum increased with water depth reaching a maximum density at 15–20 m (Sangil et al., 2011). Also, in Madeira Island, Friedlander et al. (2017) found a 35% increase in D. africanum density at 20 m compared to 10 m. Some sheltered coasts of the Canary Islands are still covered by erect algae in the shallow levels, while on exposed coasts, the higher densities of sea-urchins appear at greater depths, just below the shallow band of macroalgae (Hernández et al., 2008a).
Substrate complexity is an important driver for D. africanum, as this sea-urchin seemed to be associated with large and medium-sized rocky habitats, which may be related to particular behavior and feeding size (Entrambasaguas et al., 2008). The presence of boulders allows D. africanum to feed on epi- and endolytic algae, depleting erect algae and leading to barrens (Tuya et al., 2004b). Habitats with high topographic relief have denser populations of D. africanum than less suitable flat areas (Hernández et al., 2006a; Clemente et al., 2007), where low complexity is related with macroalgae dominance (Hernández et al., 2006a, 2008b; Clemente et al., 2007). Specifically, this species needs holes for refuge due to its very large spines (up to 500 mm long) and, therefore, it would be more exposed to predators in rocky platforms (Hendler et al., 1995).
Overfishing
Marine forests owe their existence to the balance reached by the different parts of the ecosystem, but the stressors derived from human activities can alter their ecological status. The disappearance of top predators due to overfishing has been linked to the shift from macroalgal forest to sea-urchin barrens (Tuya et al., 2004a, 2005; Clemente et al., 2007, 2010, 2011; Sangil et al., 2011). Fishing pressure is particularly high on coastal ecosystems of areas densely populated, which implies a lower abundance of D. africanum predators, and, through a cascade effect, a hyperabundance of sea-urchins, causing the disappearance of macroalgal forests (Hernández et al., 2008b). Recent studies also reported strong recreational fishing pressures, reaching annual catches of almost 12,000 t in the Canary Islands (Gordoa, 2019) and 1,500 t in Madeira (Martínez-Escauriaza et al., 2020a, b). In Madeira, many recreational fishermen have reported a decrease in catches, and attribute this to both (i) the generalized degradation of coastal systems, and (ii) the fishing of individuals below the minimum landing size (Hermida and Delgado, 2016; Martínez-Escauriaza et al., 2020a). Fish biomass is more than three times higher in Selvagens than Madeira (Friedlander et al., 2017). Species known to feed and control sea-urchins’ populations (e.g., Balistes capriscus, B. scrofa, Canthidermis sufflamen, and S. cretense) were abundant in the Selvagens compared to Madeira (Clemente et al., 2010; Friedlander et al., 2017). These patterns are very similar to those found in the Canary Islands, which show clear differences between MPAs and unprotected areas about abundance and biomass of these predatory species (Sangil et al., 2013).
Coastal Urbanization
Successful conservation and implementation of MPAs promote stability and positive growth of macroalgal communities. In contrast, poor macroalgal cover results from unprotected, and consequently more human-impacted areas (Sangil et al., 2012, 2018; Friedlander et al., 2017). In fact, the number of inhabitants has been positively correlated with the density of D. africanum, highlighting the strong effect of the anthropogenic pressure on the coastal bottoms of the region (Hernández et al., 2008b). Some densely populated areas are exposed to high levels of habitat loss and fragmentation, a threat that is considered to affect global coastal diversity (Airoldi and Beck, 2007). Modification of continuous natural rocky platforms can reduce the development of macroalgae and reduce the habitat available for canopies colonization (Cacabelos et al., 2016). Vertical substratum slope, pollution and urbanization are the main drivers involved in the distribution of Fucales (Fabbrizzi et al., 2020), although in the Azores, limited-dispersal capacity from adults has been also cited as a relevant factor limiting its ability to colonize artificial coastal defense structures (Cacabelos et al., 2016). In fact, in Madeira, extensive development and construction in marine and coastal systems (the so-called “ocean sprawl”) has destroyed some of the scarce natural rocky platforms in the island, diminishing the suitable habitat available for canopy-forming macroalgae communities. These human-related perturbations can lastly lead to local changes in the light and turbidity regimes due to modifications in coastal hydrodynamics (Tuya et al., 2002), producing adverse effects on benthic communities. Changes in land use, both inland and along the coasts of the islands can increase loads of sediments, pollutant and nutrients charges (Airoldi and Beck, 2007; Baioni et al., 2011; Smith, 2016). Specially, sedimentation can affect the survival and recruitment of erect algae and sea-urchins, and favoring the dominance of turf (Airoldi, 2003; Schiel and Gunn, 2019). Long-term data on water turbidity and sediment load is necessary to evaluate the effects of these stressors across our study region and the particular consequences for the conservation of their coastal benthic communities.
Climate Change-Related Effects
Ocean Warming
In the actual context of climate change, ocean warming implies a global impact to be taken into account to study coastal benthic communities (Steneck et al., 2002; Martín et al., 2012; Smale, 2020). The increase of NE Atlantic’s water temperatures in recent decades has been higher than expected, especially for the Azores, Webbnesia and Cabo Verde (Cropper and Hanna, 2014). This increase was higher than other areas with similar climatic and geographical characteristics (Cropper and Hanna, 2014). This is reflected in the increase of the SST since 1982, with an approximate increase of 0.3°C per decade, being highest in the Azores (Figure 3). Experimental studies have shown the positive relationship of increasing temperatures on recruitments, larval survival and development of D. africanum (Fuentes et al., 2013). Previous studies in the Canary Islands have shown the positive trend between the increase in temperatures and the settlement of D. africanum, becoming exponential above 23°C (Hernández et al., 2006a, b). Warming also allows tropical species to colonize new areas, the so-called tropicalization process (Brito, 2004; Bianchi, 2007; Afonso et al., 2013; Ribeiro et al., 2019; Schäfer et al., 2019). The detection of D. africanum in the island of Santa Maria (Minderlein and Wirtz, 2014), located in the south-eastern limit of the Azores, could be an example of this tropicalization. The possible expansion of tropical affinity species in the region seems to be favored by the recent increase in water temperatures (Afonso et al., 2013; Ribeiro et al., 2019). However, as only two individuals have been recorded in 2010, the establishment of a resident population of D. africanum in the Azorean archipelago seems unlikely at present. For macroalgae formations, the increase in temperatures has a negative impact (Wernberg et al., 2011). Various studies have shown that macroalgae, especially canopy-forming species, are threatened by rising temperatures (Smale, 2020). Furthermore, marine heatwaves have also shown significant impacts on coastal macroalgal formations (Frölicher and Laufkötter, 2018; Filbee-Dexter et al., 2020; Verdura et al., 2021). These extreme events are likely given the projected climate change scenarios (Filbee-Dexter et al., 2020). In the context of tropicalization, which the region currently finds itself (Freitas et al., 2019), the role of rising temperatures and marine heatwaves could be one of the main impacts for marine forests.
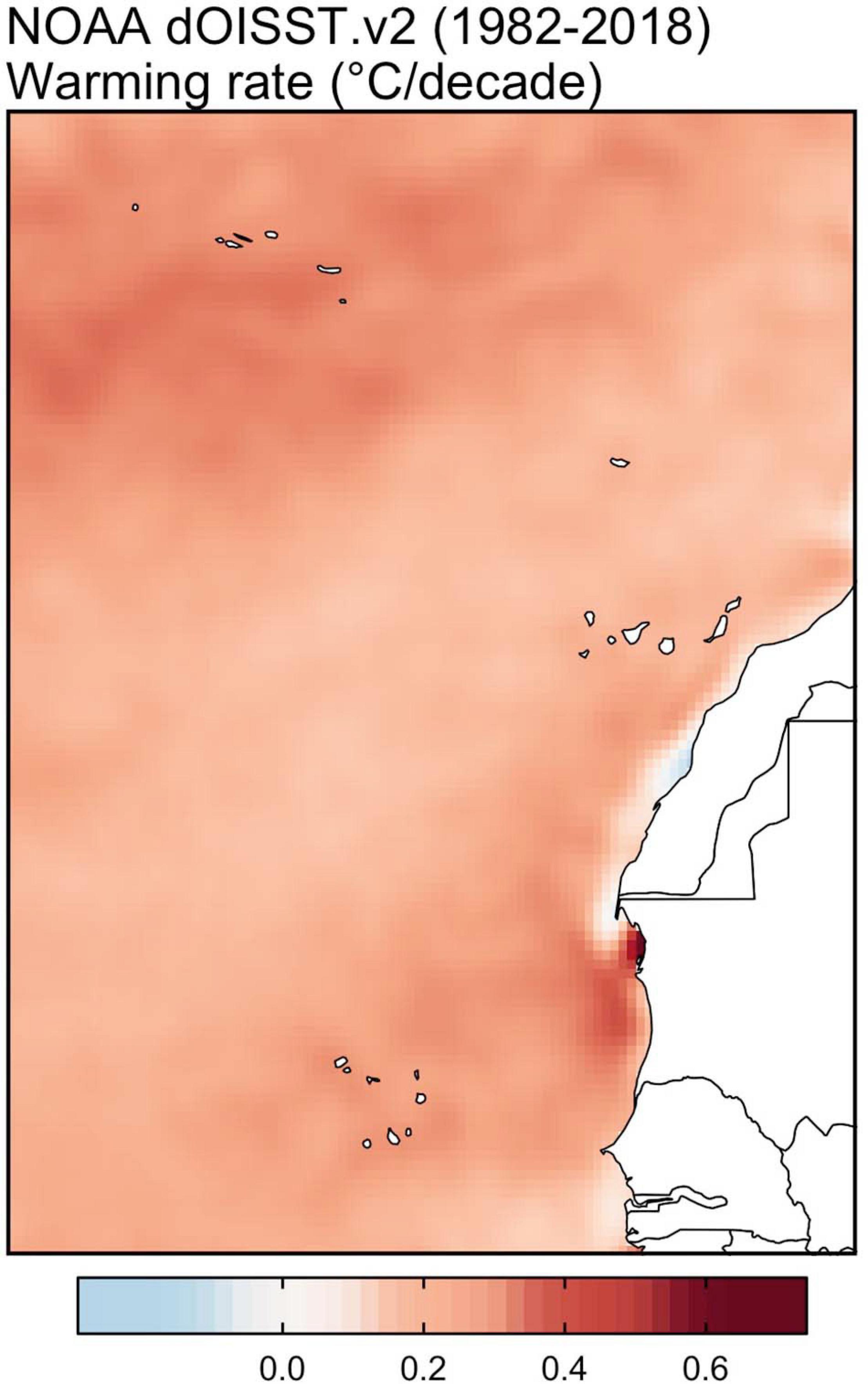
Figure 3. Rates of sea surface warming in NE Atlantic archipelagos, based on 37 year of daily sea surface temperatures (SSTs; 1982–2018) from National Oceanic and Atmospheric Administration (NOAA) 1/4 arc-degree Daily Optimum.
Ocean Acidification
Acidification of the oceans and coastal areas is another process related to climate change. In different experimental studies, sea-urchins are shown to be especially vulnerable to ocean acidification (OA) (Johnson et al., 2012; Byrne et al., 2013; Calosi et al., 2013; Hall-Spencer and Allen, 2015). OA seems to negatively affect their settlement and larval development (Pecorino et al., 2014). Recent studies with species from the region suggest that intertidal species (A. lixula and P. lividus) are less vulnerable than subtidal species (D. africanum and S. granularis) to future scenarios of acidification (García et al., 2018). Furthermore, OA increases the feeding rates of juveniles of P. lividus, yet differences in feeding rates of D. africanum on algae reared under low pH were not found (Rodríguez et al., 2018). Future scenarios of OA can have indirect effects over marine forests, increasing herbivorous pressure in and affecting the growth of macroalgae (Rodríguez et al., 2018). Many macroalgae species have been tolerant to long-term increases in CO2 levels, but habitats are significantly altered as pH decreases, being a long-term impact (Porzio et al., 2011). Long-term effects need to be explored in depth for NE Atlantic archipelagos, testing the combined effect of increased temperatures and acidification of coastal systems.
Stochastic Weather Events
Massive D. africanum mortality events have occurred in the last decade in the region, affecting particularly to Madeira and the Canary Islands (Clemente et al., 2014; Gizzi et al., 2020). The first mass mortality event was recorded between October 2009 and April 2010 (Clemente et al., 2014), and the second in February 2018 (Gizzi et al., 2020; Hernández et al., 2020). These massive mortality events have been attributed to different impacts or environmental conditions such as changes in temperature, salinity or pH that favor the proliferation of disease-causing pathogens (Scheibling and Stephenson, 1984; Girard et al., 2011; Gizzi et al., 2020). According to the “killer storm” hypothesis (Scheibling and Hennigar, 1997; Scheibling and Lauzon-Guay, 2010), strong storms would have generated a pronounced movement of underwater sediments and large-scale vertical mixing of particles. This mixing could promote the vertical movement of infectious agents, as the amoeba Paramoeba brachiphila, and its subsequent sedimentation in sea-urchins, causing infections and mortality (Hernández et al., 2020). The killer storms were also described in other latitudes typically involving tropical storms (Scheibling and Hennigar, 1997; Feehan et al., 2016). After the decrease in the abundance of sea-urchins at specific points, a slow colonization by fucoids has been observed, persisting for at least 2 years (Clemente et al., 2014), but further observations will be necessary to fully address the potential re-growth. The low frequency of these storms in the region can explain the persistence for decades of sea-urchin barrens in the Canary Islands and Madeira (Hernández, 2017). However, this scenario could change in the coming decades, as the magnitude and frequency of extreme events are expected to increase in the North Atlantic (Pardowitz, 2015). For this reason, attention should focus on possible new massive mortality events as a consequence of meteorological processes, as well as the consequences for the benthic macroalgal communities due to the sudden mortality of sea-urchins.
Conclusion and Considerations for Future Restoration Actions
In this study we have chosen a series of factors and impacts available in the literature (Table 1). We assume that there are other important drivers, not explored in the region yet, in the development and maintenance of sea-urchin’s barrens that should be studied in future works in the NE Atlantic archipelago (e.g., nutrient regimes, bio-invasions, light pollution). Most of the information identified as evidenced in this work comes from studies developed in the most studied areas of the region, the archipelago of the Canary Islands and the archipelago of Madeira. Even so, it is necessary to expand the study of these drivers in the region, in order to identify the main impacts and highlighting those that currently suppose correlative factors and hypotheses.
In general terms, the macroalgal communities of the NE Atlantic archipelagos have suffered a dramatic decline due to the interaction of different factors. An increase in grazer activity as a consequence of the explosion of sea-urchin populations due to the cascading effect of overfishing, seems to be a relevant factor in maintaining these habitats in the “barren” state (Figure 4). However, it is important to highlight that this has not been a common pattern throughout the coast of the different archipelagos, since there are still areas in the region where we can find well-developed macroalgal communities. Clearly, it is a consequence of the high variability on the conditions and the interplay of multiple environmental factors (e.g., level of protection, sedimentary processes, coastal urbanization, substrate complexity, climate change effects, extreme events) of the areas that modulate the sea-urchin population dynamics (Figure 4). In fact, the disappearance of canopy-forming species, especially in the Webbnesia, can have different reasons, with overfishing, habitat fragmentation, or processes related to climate change acting in different ways. Degradation of these ecosystems created a perfect scenario for sea-urchins proliferation, boosted by the elimination of their predators by overfishing and creating alternative stable barren systems. The recovery of key predatory fishes has been identified as the more efficient mechanism for the regulation of sea-urchin populations (Clemente et al., 2010). Regulation of fisheries can lead the recovery of macroalgal forests by cascade effects, promoting the conservation of predatory fishes to maximize predation across the size spectrum of D. africanum (Clemente et al., 2010). The MPA in La Palma Island is a clear successful example of how to recover an area dominated by urchin barrens and achieve well-developed macroalgae habitats. In addition, it shows how a well-managed protected area can reach these status changes in a short period of time 4 years (Sangil et al., 2012). Another example of success are the remote Selvagens Islands, currently representing one of the most pristine ecosystems of the NE Atlantic (Friedlander et al., 2017). However, other cases have shown how protection as an isolated passive action has not produced the desired consequences in the food chains in a long term, and even the biomass of sea-urchins increase directly (Malakhoff and Miller, 2021). The success of future actions for restoration of marine forests need to consider this reality and assume that passive restoration efforts are probably not enough (Figure 4). We suggest that to achieve the recovery of the marine forests in those degraded areas of the region, it is necessary to carry out a series of active actions, such as fishing regulation (Sangil et al., 2012), sea-urchin complete eradication and macroalgal revegetation (Medrano et al., 2020). In those more degraded areas, where the pressure of propagules of canopy-forming macroalgae is not enough, it will be necessary to carry out revegetation actions from source populations (Medrano et al., 2020). Furthermore, Cystoseira/Gongolaria/Ericaria are genus with low-dispersal capacity without planktonic stages (Susini et al., 2007). For this reason, the main restoration techniques focus on the translocation of fertile branches or individuals that can directly release the propagules where they are placed (Verdura et al., 2018; Medrano et al., 2020). Due to its dispersal strategy there may be limitations of connectivity, and as a consequence, smaller population gene pools and sizes render populations more vulnerable to threats (Buonomo et al., 2017). In future climate change scenarios, it may be necessary to use genetic techniques allowing the optimal selection of individuals or populations that are more resistant to the resulting impacts, thus increasing the resilience and adaptive capacity of the restored populations (Coleman et al., 2020). But these measures can be insufficient if they are not combined with the establishment of well-enforced No-Take areas (Medrano et al., 2020). The creation of marine reserves without active protection planning does not reach the minimum levels for the maintenance of the eco-services that these systems provide (Sangil et al., 2012; Figure 4). Recovery will definitely depend on first establishing important ecological protection rules, e.g., the successful MPAs in the region. However, the combination of multiple human pressures left the coastal system in a point of no return where the effectiveness of restoration actions will inevitably pass by the implementation of additional active management interventions, in order to help the system to overcome the tipping point and reach a long-term re-establishment of the macroalgal forests.
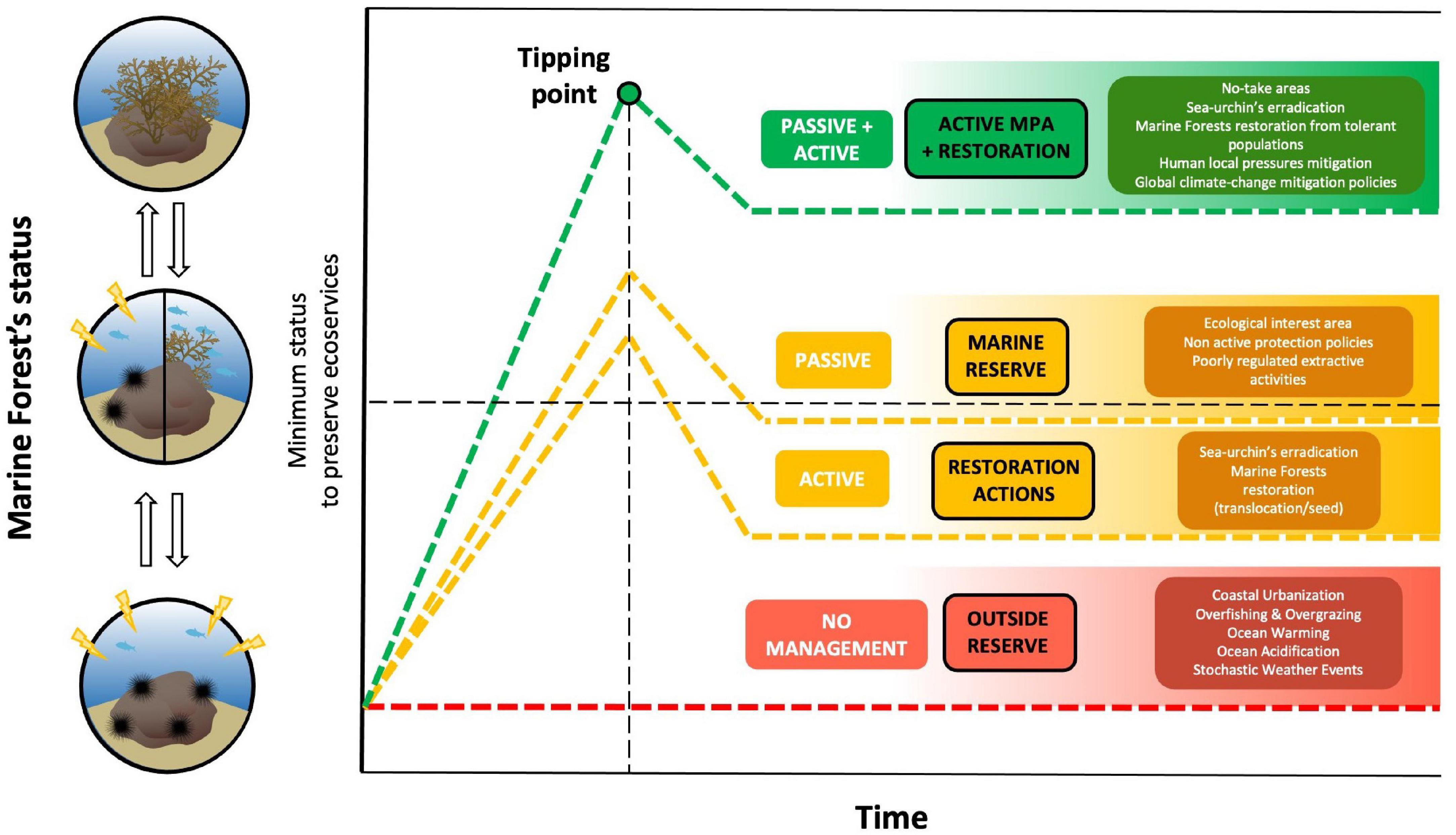
Figure 4. Different theoretical scenarios of restoration efforts: () MPA: no-take areas and restoration actions (passive + active, e.g., sea-urchin’s eradication, revegetation, human local pressures mitigation, and climate-change mitigation policies), (
) marine reserve (passive: non-active protection), (
) restoration actions (active), and (
) outside reserve (no management) with the hypothetical tipping point to overcome. For those highly degraded communities, only a combined management of active and passive activities is suggested as the option able to have a long-term recovery scenario. MPA, marine protected area. Symbols courtesy of the Integration and Application Network, University of Maryland Center for Environmental Science (ian.umces.edu/symbols/).
Author Contributions
AB-I, IG, and EC: conceptualization, methodology, and investigation. AB-I: formal analysis and writing—original draft preparation. AB-I, IG, EC, and RM: writing—review and editing. All authors have read and agreed to the published version of the manuscript.
Funding
AB-I, EC, and IG were financially supported by pre- and post-doctoral grants in the framework of the 2015 ARDITI Grant Program Madeira 14-20 (Project M1420-09-5369-FSE-000002). This study was supported by the Portuguese government grant Fundo Azul under the Seaforest Portugal Project (FA_06_2017_067). Finally, this study also had the support of Fundação para a Ciência e Tecnologia (FCT), Portugal, through the strategic project UID/MAR/04292/2020 granted to MARE UI&I.
Conflict of Interest
The authors declare that the research was conducted in the absence of any commercial or financial relationships that could be construed as a potential conflict of interest.
Acknowledgments
We thank to Ashlie J. McIvor for the English language editing and review. We thank to Dan Smale and Rui Seabra for their help to generate Figure 4. This is contribution 76 from the Smithsonian’s MarineGEO and Tennenbaum Marine Observatories Network.
References
Abreu, A. D., Araújo, R., Biscoito, M. J., Malaquias, M. A., and Gomes, P. N. (1995). Fauna Marinha Da Madeira. Funchal: Jornal da Madeira Magazine.
Afonso, P., Porteiro, F. M., Fontes, J., Tempera, F., Morato, T., Cardigos, F., et al. (2013). New and rare coastal fishes in the Azores islands: occasional events or tropicalization process? J. Fish Biol. 83, 272–294. doi: 10.1111/jfb.12162
Airoldi, L. (2003). The effects of sedimentation on rocky coast assemblages. Oceanogr. Mar. Biol. an Annu. Rev. 41, 161–236.
Airoldi, L., and Beck, M. (2007). Loss, status and trends for coastal marine habitats of Europe. Oceanogr. Mar. Biol. 7, 345–405. doi: 10.1201/9781420050943.ch7
Alves, F. M. A., Chícharo, L. M., Serrao, E., and Abreu, A. D. (2001). Algal cover and sea urchin spatial distribution at Madeira Island (NE Atlantic). Sci. Mar. 65, 383–392. doi: 10.3989/scimar.2001.65n4383
Alves, F. M. A., Chícharo, L. M., Serrao, E., and Abreu, A. D. (2003). Grazing by Diadema antillarum (Philippi) upon algal communities on rocky substrates. Sci. Mar. 67, 307–311. doi: 10.3989/scimar.2003.67n3307
Amorim, P., Perán, A. D., Pham, C. K., Juliano, M., Cardigos, F., Tempera, F., et al. (2017). Overview of the ocean climatology and its variability in the azores region of the north atlantic including environmental characteristics at the Seabed. Front. Mar. Sci. 4:56. doi: 10.3389/fmars.2017.00056
Arafeh-Dalmau, N., Schoeman, D. S., Montaño-Moctezuma, G., Micheli, F., Rogers-Bennett, L., Olguin-Jacobson, C., et al. (2020). Marine heat waves threaten kelp forests. Science 367:635.
Baioni, D., Castaldini, D., and Cencetti, C. (2011). Human activity and damaging landslides and floods on Madeira Island. Nat. Hazards Earth Syst. Sci. 11, 3035–3046. doi: 10.5194/nhess-11-3035-2011
Barquín-Diez, J., González-Lorenzo, G., Martín-García, L., Gil-Rodríguez, M. C., and Brito-Hernández, A. (2005). Distribución espacial de las comunidades bentónicas submareales de los fondos someros de Canarias I: las comunidades de sustrato blando de las costas de Tenerife. Vieraea 33, 435–438.
Bianchi, C. N. (2007). Biodiversity issues for the forthcoming tropical Mediterranean Sea. Hydrobiologia 580, 7–21. doi: 10.1007/978-1-4020-6156-1_1
Borges, P. (2004). Ambientes Litorais Nos Grupos Central e Oriental do Arquipélago dos Açores, Conteúdos e Dinâmica de Microescala. Ponta Delgada: Universidade dos Açores.
Bray, R. N., and Ebeling, A. W. (1975). Food, activity, and habitat of 3 picker-yype microcarnivorous fishes in kelp forests off Santa Barbara (California). Fish. Bull. 73:815.
Brito, A. (2004). Sobre la Tropicalicazión Reciente de la Ictiofauna Litoral de las Islas Canarias y su Relación con Cambios Ambientales y Actividades Antrópicas. Doctoral dissertation. Angra do Heroísmo: Universidade dos Açores.
Bulleri, F., Benedetti-Cecchi, L., and Cinelli, F. (1999). Grazing by the sea urchins Arbacia lixula L. and Paracentrotus lividus Lam. in the Northwest Mediterranean. J. Exp. Mar. Biol. Ecol. 241, 81–95. doi: 10.1016/S0022-0981(99)00073-78
Buonomo, R., Assis, J., and Fernandes, F. (2017). Habitat continuity and stepping-stone oceanographic distances explain population genetic connectivity of the brown alga Cystoseira amentacea. Mol. Ecol. 26, 766–780. doi: 10.1111/mec.13960
Byrne, M., Lamare, M., Winter, D., Dworjanyn, S. A., and Uthicke, S. (2013). The stunting effect of a high CO2 ocean on calcification and development in sea urchin larvae, a synthesis from the tropics to the poles. Philos. Trans. R. Soc. B Biol. Sci. 368:20120439. doi: 10.1098/rstb.2012.0439
Cacabelos, E., Martins, G. M., Thompson, R., Prestes, A. C., and Azevedo, S. (2016). Factors limiting the establishment of canopy-forming algae on artificial structures. Estua. Coast. Shelf Sci. 181, 277–283. doi: 10.1016/j.ecss.2016.08.036
Calosi, P., Rastrick, S. P. S., Graziano, M., Thomas, S. C., Baggini, C., Carter, H. A., et al. (2013). Distribution of sea urchins living near shallow water CO2 vents is dependent upon species acid-base and ion-regulatory abilities. Mar. Pollut. Bull. 73, 470–484. doi: 10.1016/j.marpolbul.2012.11.040
Clemente, S., and Hernández, J. C. (2008). Influence of wave exposure and habitat complexity in determining spatial variation of the sea urchin Diadema aff. antillarum (Echinoidea: Diadematidae) populations and macroalgal cover (Canary Islands - Eastern Atlantic Ocean). Rev. Biol. Trop. 56:26. doi: 10.1007/s10152-003-0164-0
Clemente, S., Hernández, J. C., and Brito, A. (2011). Context-dependent effects of marine protected areas on predatory interactions. Mar. Ecol. Prog. Ser. 437, 119–133. doi: 10.3354/meps09243
Clemente, S., Hernández, J. C., Rodríguez, A., and Brito, A. (2010). Identifying keystone predators and the importance of preserving functional diversity in sublittoral rocky-bottom areas. Mar. Ecol. Prog. Ser. 413, 55–67. doi: 10.3354/meps08700
Clemente, S., Hernández, J. C., Toledo, K., and Brito, A. (2007). Predation upon Diadema aff. antillarum in barren grounds in the Canary Islands. Sci. Mar. 71, 745–754. doi: 10.3989/scimar.2007.71n4745
Clemente, S., Lorenzo-Morales, J., Mendoza, J. C., López, C., Sangil, C., Alves, F., et al. (2014). Sea urchin Diadema africanum mass mortality in the subtropical eastern Atlantic: role of waterborne bacteria in a warming ocean. Mar. Ecol. Prog. Ser. 506, 1–14. doi: 10.3354/meps10829
Coleman, M. A., Wood, G., Filbee-Dexter, K., Minne, A. J., Goold, H. D., Vergés, A., et al. (2020). Restore or redefine: future trajectories for restoration. Front. Mar. Sci. 7:237. doi: 10.3389/fmars.2020.00237
Cordeiro, C. A. M. M., Harborne, A. R., and Ferreira, C. E. L. (2020). The biophysical controls of macroalgal growth on subtropical reefs. Front. Mar. Sci. 7:488. doi: 10.3389/fmars.2020.00488
Cropper, T. (2013). The weather and climate of Macaronesia: past, present and future. Weather 68, 300–307. doi: 10.1002/wea.2155
Cropper, T. E., and Hanna, E. (2014). An analysis of the climate of Macaronesia, 1865-2012. Int. J. Climatol. 34, 604–622. doi: 10.1002/joc.3710
Dayton, P. (1985). Ecology of kelp communities. Annu. Rev. Ecol. Syst. 16, 215–245. doi: 10.1146/annurev.es.16.110185.001243
Duarte, C. M., and Cebrián, J. (1996). The fate of marine autotrophic production. Limnol. Oceanogr. 41, 1758–1766. doi: 10.4319/lo.1996.41.8.1758
Eklöf, J. S., de la Torre-Castro, M., Gullström, M., Uku, J., Muthiga, N., Lyimo, T., et al. (2008). Sea urchin overgrazing of seagrasses: a review of current knowledge on causes, consequences, and management. Estuar. Coast. Shelf Sci. 79, 569–580. doi: 10.1016/j.ecss.2008.05.005
Entrambasaguas, L., Pérez-Ruzafa, Á, García-Charton, J. A., Stobart, B., and Bacallado, J. J. (2008). Abundance, spatial distribution and habitat relationships of echinoderms in the Cabo Verde Archipelago (eastern Atlantic). Mar. Freshw. Res. 59, 477–488. doi: 10.1071/MF07109
Fabbrizzi, E., Scardi, M., Ballesteros, E., Benedetti-Cecchi, L., Cebrian, E., Ceccherelli, G., et al. (2020). Modeling macroalgal forest distribution at Mediterranean Scale: present status, drivers of changes and insights for conservation and management. Front. Mar. Sci. 7:20. doi: 10.3389/fmars.2020.00020
Feehan, C. J., Scheibling, R. E., Brown, M. S., and Thompson, K. R. (2016). Marine epizootics linked to storms: mechanisms of pathogen introduction and persistence inferred from coupled physical and biological time-series. Limnol. Oceanogr. 61, 316–329. doi: 10.1002/lno.10217
Filbee-Dexter, K., and Scheibling, R. (2014). Sea urchin barrens as alternative stable states of collapsed kelp ecosystems. Mar. Ecol. Prog. Ser. 495, 1–25. doi: 10.3354/meps10573
Filbee-Dexter, K., Wernberg, T., Grace, S. P., Thormar, J., Fredriksen, S., Narvaez, C. N., et al. (2020). Marine heatwaves and the collapse of marginal North Atlantic kelp forests. Sci. Rep. 10, 1–11.
Freitas, R., Romeiras, M., Silva, L., Cordeiro, R., Madeira, P., González, J. A., et al. (2019). Restructuring of the ‘Macaronesia’ biogeographic unit: a marine multi-taxon biogeographical approach. Sci. Rep. 9:15792. doi: 10.1038/s41598-019-51786-51786
Friedlander, A. M., Ballesteros, E., Clemente, S., Gonçalves, E. J., Estep, A., Rose, P., et al. (2017). Contrasts in the marine ecosystem of two Macaronesian islands: a comparison between the remote Selvagens Reserve and Madeira Island. PLoS One 12:e0187935. doi: 10.1371/journal.pone.0187935
Frölicher, T. L., and Laufkötter, C. (2018). Emerging risks from marine heat waves. Nat. Commun. 9:650.
Fuentes, W., Dupont, S., Casties, I., Turon Barrera, X., and Palacín Cabañas, C. (2013). Some like it hot: temperature and pH modulate larval development and settlement of the sea urchin Arbacia lixula. J. Exp. Mar. Biol. Ecol. 449, 304–311. doi: 10.1016/j.jembe.2013.10.007
García, E., Hernández, J. C., and Clemente, S. (2018). Robustness of larval development of intertidal sea urchin species to simulated ocean warming and acidification. Mar. Environ. Res. 139, 35–45. doi: 10.1016/j.marenvres.2018.04.011
Gianni, F., Bartolini, F., Pey, A., Laurent, M., Martins, G. M., Airoldi, L., et al. (2017). Threats to large brown algal forests in temperate seas: the overlooked role of native herbivorous fish. Sci. Rep. 7:6012. doi: 10.1038/s41598-017-06394-6397
Girard, D., Clemente, S., Toledo-Guedes, K., Brito, A., and Hernandez, J. C. (2011). A mass mortality of subtropical intertidal populations of the sea urchin Paracentrotus lividus: analysis of potential links with environmental conditions. Mar. Ecol. 33, 377–385. doi: 10.1111/j.1439-0485.2011.00491.x
Gizzi, F., Jiménez, J., Schäfer, S., Castro, N., Costa, S., Lourenço, S., et al. (2020). Before and after a disease outbreak: tracking a keystone species recovery from a mass mortality event. Mar. Environ. Res. 156, 1–8. doi: 10.1016/j.marenvres.2020.104905
Gordoa, A. (2019). Recreational fishing in Spain: first national estimates of fisher population size, fishing activity and fisher social profile. Fish. Res. 211, 1–12. doi: 10.1016/j.fishres.2018.10.026
Goreau, T., McClanahan, T., Hayes, R., and Strong, A. L. (2000). Conservation of coral reefs after the 1998 global bleaching event. Conserv. Biol. 14, 5–15. doi: 10.1046/j.1523-1739.2000.00011.x
Guidetti, P., Bussotti, S., and Boero, F. (2005). Evaluating the effects of protection on fish predators and sea urchins in shallow artificial rocky habitats: a case study in the northern Adriatic Sea. Mar. Environ. Res. 59, 333–348. doi: 10.1016/j.marenvres.2004.05.008
Hall-Spencer, J., and Allen, R. (2015). The impact of CO2 emissions on ‘nuisance’ marine species. Res. Rep. Biodivers. Stud. 2015, 33–46. doi: 10.2147/RRBS.S70357
Hawkins, S. J., Corte-Real, H. B. S. M., Pannacciulli, F. G., Weber, L. C., and Bishop, J. D. D. (2000). Thoughts on the ecology and evolution of the intertidal biota of the Azores and other Atlantic islands. Hydrobiologia 440, 3–17. doi: 10.1023/A:1004118220083
Hendler, G., Miller, J. E., Pawson, D. L., and Kier, P. M. (1995). Echinoderms of Florida and the Caribbean: Sea Stars, Sea Urchins, and Allies. Washington, DC: Smithsonian Institution.
Hereu Fina, B. (2007). The Role of Trophic Interactions Between Fishes, Sea Urchins and Algae in the Northwestern Mediterranean Rocky Infralittoral. Doctoral dissertation. Catalanes: Universitat de Barcelona.
Hermida, M., and Delgado, J. (2016). High trophic level and low diversity: would Madeira benefit from fishing down? Mar. Policy 73, 130–137. doi: 10.1016/j.marpol.2016.07.013
Hernández, J. C. (2009). Estrategia Reproductiva de la Población Canaria de Erizo Diadema aff. Antillarum (Philippi), 1845: Maduración Gonadal, Asentamiento Larvario y Reclutamiento. Doctoral dissertation. Santa Cruz de Tenerife: Universidad de La Laguna, Servicio de Publicaciones.
Hernández, J. C. (2017). Influencia humana en las fluctuaciones poblacionales de erizos de mar. Rev. Biol. Trop. 65, (Suppl. 1), S23–S34.
Hernández, J. C., Brito, A., Cubero, E., García, N., Girard, D., González-Lorenzo, G., et al. (2006a). Temporal patterns of larval settlement of Diadema antillarum (echinodermata: echinoidea) in the Canary Islands using an experimental larval collector. Bull. Mar. Sci. 78:9.
Hernández, J. C., Brito, A., García, N., Gil-Rodríguez, M. C., Herrera, G., Cruz-Reyes, A., et al. (2006b). Spatial and seasonal variation of the gonad index of Diadema antillarum (Echinodermata: Echinoidea) in the Canary Islands. Sci. Mar. 70, 689–698. doi: 10.3989/scimar.2006.70n4689
Hernández, J. C., Clemente, S., Brito, A., Falcón, J. M., García, N., and Barquín, J. (2005). Estado de las poblaciones de Diadema antillarum (Echinoidea: Diadematidae) y del recubrimiento de macroalgas en las Reservas Marinas de Canarias: patrones de distribución espacial. Vieraea 33, 367–383.
Hernández, J. C., Clemente, S., Sangil, C., and Brito, A. (2008a). Actual status of the sea urchin Diadema aff. antillarum populations and macroalgal cover in marine protected areas compared to a highly fished area (Canary Islands - Eastern Atlantic Ocean). Aquat. Conserv. Mar. Fres. Ecol. 18, 1091–1108. doi: 10.1002/aqc.903
Hernández, J. C., Clemente, S., Sangil, C., and Brito, A. (2008b). The key role of the sea urchin Diadema aff. antillarum in controlling macroalgal assemblages throughout the Canary Islands (eastern subtropical Atlantic): a spatio-temporal approach. Mar. Environ. Res. 66, 259–270. doi: 10.1016/j.marenvres.2008.03.002
Hernández, J. C., Clemente, S., Tuya, F., Pérez-Ruzafa, A., and Sangil, C. (2013). Echinoderms of the Canary Islands, Spain. Echinoderm Research and Diversity in Latin America. Berlin: Springer, 471–510.
Hernández, J. C., Gil-Rodríguez, M. C., Herrera-López, G., and Brito, A. (2007). Diet of the “key herbivore” Diadema antillarum in two contrasting habitats in the Canary Islands (Eastern-Atlantic). Vieraea 35, 109–120.
Hernández, J. C., Sangil, C., and Lorenzo-Morales, J. (2020). Uncommon southwest swells trigger sea urchin disease outbreaks in Eastern Atlantic archipelagos. Ecol. Evol. 10, 7963–7970. doi: 10.1002/ece3.6260
Hill, N. A., Blount, C., Poore, A. G. B., Worthington, D., and Steinberg, P. D. (2003). Grazing effects of the sea urchin Centrostephanus rodgersii in two contrasting rocky reef habitats: effects of urchin density and its implications for the fishery. Mar. Freshw. Res. 54, 691–700. doi: 10.1071/MF03052
Johnson, V. R., Russell, B. D., Fabricius, K. E., Brownlee, C., and Hall-Spencer, J. M. (2012). Temperate and tropical brown macroalgae thrive, despite decalcification, along natural CO2 gradients. Glob. Chang. Biol. 18, 2792–2803. doi: 10.1111/j.1365-2486.2012.02716.x
Knowlton, N. (2004). Multiple ‘stable’ states and the conservation of marine ecosystems. Prog. Ocean. 60, 387–396. doi: 10.1016/j.pocean.2004.02.011
Lázaro, C., Fernandes, M. J., Santos, A. M. P., and Oliveira, P. (2005). Seasonal and interannual variability of surface circulation in the Cape Verde region from 8 years of merged T/P and ERS-2 altimeter data. Rem. Sen. Env. 98, 45–62. doi: 10.1016/j.rse.2005.06.005
Ling, S. D., Scheibling, R. E., Rassweiler, A., Johnson, C. R., Shears, N., Connell, S. D., et al. (2015). Global regime shift dynamics of catastrophic sea urchin overgrazing. Philos. Trans. R. Soc. B Biol. Sci. 370:20130269. doi: 10.1098/rstb.2013.0269
Malakhoff, K. D., and Miller, R. J. (2021). After 15 years, no evidence for trophic cascades in marine protected areas. Proc. R. Soc. B 288:20203061. doi: 10.1098/rspb.2020.3061
Marques, V. M. (1984). Effects of Arbacia lixula (L.)(Echinoidea) on the algal communities of Sao Miguel and Graciosa Islands (Azores Archipielago, Portugal). Arq. Museu Bocage Ser. B Notas Supl. 2, 103–108.
Martín, J. L., Bethencourt, J., and Cuevas-Agulló, E. (2012). Assessment of global warming on the island of Tenerife, Canary Islands (Spain). Trends in minimum, maximum and mean temperatures since 1944. Clim. Change 114, 343–355. doi: 10.1007/s10584-012-0407-7
Martínez-Escauriaza, R., Hermida, M., Villasante, S., Gouveia, L., Gouveia, N., and Pita, P. (2020a). Importance of recreational shore angling in the archipelago of Madeira, Portugal (northeast Atlantic). Sci. Mar. 84, 331–334. doi: 10.3989/scimar.05046.30A
Martínez-Escauriaza, R., Vieira, C., Gouveia, L., Gouveia, N., and Hermida, M. (2020b). Characterization and evolution of spearfishing in Madeira archipelago, Eastern Atlantic. Aquat. Living Resour. 33:15. doi: 10.1051/alr/2020015
Martins, G. M., Wallenstein, F. F. M., Álvaro, N. V., Neto, A. I., and Costa, A. C. (2005). Sampling strategies for biotope definition: minimal sampling area for selected groups of macroinvertebrates in the rocky subtidal of São Miguel. Azores. Helgol. Mar. Res. 59, 219–223. doi: 10.1007/s10152-005-0222-x
Medrano, A., Hereu, B., Cleminson, M., Pagès-Escolà, M., and Rovira, E. (2020). From marine deserts to algal beds: Treptacantha elegans revegetation to reverse stable degraded ecosystems inside and outside a No-Take marine reserve. Restoration Ecol. 28, 632–644. doi: 10.1111/rec.13123
Melis, R., Ceccherelli, G., Piazzi, L., and Rustici, M. (2019). Macroalgal forests and sea urchin barrens: structural complexity loss, fisheries exploitation and catastrophic regime shifts. Ecol. Complex 37, 32–37. doi: 10.1016/j.ecocom.2018.12.005
Miller, R. J., Lafferty, K. D., Lamy, T., Kui, L., Rassweiler, A., and Reed, D. C. (2018). Giant kelp, Macrocystis pyrifera, increases faunal diversity through physical engineering. Proc. R. Soc. B Biol. Sci. 285:20172571. doi: 10.1098/rspb.2017.2571
Minderlein, R., and Wirtz, P. (2014). A sea urchin (Diadema africanum Rodriguez et al., 2013) and a pipe fish (Syngnathus phlegon Risso, 1827): two new records for the Azores. Arquipélago. Life Mar. Sci. 31, 2–4.
Neto, A. I., Viera, M. A., and Haroun, R. (2014). A synthetic overview of marine phycological studies in the Macaronesian archipelagos. Silva Lusitana 22, 217–244.
Palacín, C., Giribet, G., Carner, S., Dantart, L., and Turon, X. (1998). Low densities of sea urchins influence the structure of algal assemblages in the western Mediterranean. J. Sea Res. 39, 281–290. doi: 10.1016/S1385-1101(97)00061-60
Pardowitz, T. (2015). Anthropogenic Changes in The Frequency and Severity of European Winter Storms: Mechanisms, Impacts and Their Uncertainties. Doctoral dissertation. Berlin: Free University.
Pecorino, D., Barker, M. F., Dworjanyn, S. A., Byrne, M., and Lamare, M. D. (2014). Impacts of near future sea surface pH and temperature conditions on fertilisation and embryonic development in Centrostephanus rodgersii from northern New Zealand and northern New South Wales, Australia. Mar. Biol. 161, 101–110. doi: 10.1007/s00227-013-2318-2311
Porzio, L., Buia, M. C., and Hall-Spencer, J. M. (2011). Effects of ocean acidification on macroalgal communities. J. Exp. Mar. Bio. Ecol. 400, 278–287. doi: 10.1016/j.jembe.2011.02.011
Ribeiro, C., Neto, A. I., Moreu, I., Haroun, R., and Neves, P. (2019). A new signal of marine tropicalization in the Macaronesia region: first record of the mesophotic macroalga Avrainvillea canariensis A. Gepp & ES Gepp in the Madeira archipelago. Aquat. Bot. 153, 40–43. doi: 10.1016/j.aquabot.2018.11.008
Roberts, C. M., McClean, C. J., Veron, J. E. N., Hawkins, J. P., Allen, G. R., McAllister, D. E., et al. (2002). Marine biodiversity hotspots and conservation priorities for tropical reefs. Science 295, 1280–1284. doi: 10.1126/science.1067728
Rodríguez, A., Clemente, S., Brito, A., and Hernández, J. C. (2018). Effects of ocean acidification on algae growth and feeding rates of juvenile sea urchins. Mar. Env. Res. 140, 382–389. doi: 10.1016/j.marenvres.2018.07.004
Rodríguez Prieto, C., and Polo Albertí, L. (1996). Effects of the sewage pollution in the structure and dynamics of the community of Cystoseira mediterranea (Fucales, Phaeophyceae). Sci. Mar. 60, 226–253.
Sala, E., Boudouresque, C. F., and Harmelin-Vivien, M. (1998). Fishing, trophic cascades, and the structure of algal assemblages: evaluation of an old but untested paradigm. Oikos 82, 425–439. doi: 10.2307/3546364
Sangil, C., Clemente, S., Martín-García, L., and Hernández, J. C. (2012). No-take areas as an effective tool to restore urchin barrens on subtropical rocky reefs. Estua. Coast. Shelf Sci. 112, 207–215. doi: 10.1016/j.ecss.2012.07.025
Sangil, C., Martín-García, L., Hernández, J. C., Concepción, L., Fernández, R., and Clemente, S. (2013). Impacts of fishing and environmental factors driving changes on littoral fish assemblages in a subtropical oceanic island. Estua. Coast. Shelf Sci. 128, 22–32. doi: 10.1016/j.ecss.2013.04.023
Sangil, C., Martins, G. M., Hernández, J. C., Alves, F., Neto, A. I., Ribeiro, C., et al. (2018). Shallow subtidal macroalgae in the North-eastern Atlantic archipelagos (Macaronesian region): a spatial approach to community structure. Eur. J. Phycol. 53, 83–98. doi: 10.1080/09670262.2017.1385098
Sangil, C., Sansón, M., and Afonso-Carrillo, J. (2011). Spatial variation patterns of subtidal seaweed assemblages along a subtropical oceanic archipelago: thermal gradient vs herbivore pressure. Estua. Coast. Shelf Sci. 94, 322–333. doi: 10.1016/j.ecss.2011.07.004
Sansón, M., Sangil, C., Orellana, S., and Afonso-Carrillo, J. (2013). “Do the size shifts of marine macroalgae match the warming trends in the Canary Islands?,” in XIX Simposio de Botanica Criptogamica, 24–28 June, Las Palmas de Gran Canaria.
Schäfer, S., Monteiro, J., Castro, N., Rilov, G., and Canning-Clode, J. (2019). Cronius ruber (Lamarck, 1818) arrives to Madeira Island: a new indication of the ongoing tropicalization of the northeastern Atlantic. Mar. Biodivers. 49, 2699–2707. doi: 10.1007/s12526-019-00999-z
Scheibling, R. E., and Hennigar, A. W. (1997). Recurrent outbreaks of disease in sea urchins Strongylocentrotus droebachiensis in Nova Scotia: evidence for a link with large-scale meteorologic and oceanographic events. Mar. Ecol. Prog. Ser. 152, 155–165. doi: 10.3354/meps152155
Scheibling, R. E., and Lauzon-Guay, J.-S. (2010). Killer storms: North Atlantic hurricanes and disease outbreaks in sea urchins. Limnol. Oceanogr. 55, 2331–2338. doi: 10.4319/lo.2010.55.6.2331
Scheibling, R. E., and Stephenson, R. L. (1984). Mass mortality of Strongylocentrotus droebachiensis (Echinodermata: Echinoidea) off Nova Scotia, Canada. Mar. Biol. 78, 153–164. doi: 10.1007/bf00394695
Scherner, F., Horta, P. A., de Oliveira, E. C., Simonassi, J. C., Hall-Spencer, J. M., Chow, F., et al. (2013). Coastal urbanization leads to remarkable seaweed species loss and community shifts along the SW Atlantic. Mar. Pollut. Bull. 76, 106–115. doi: 10.1016/j.marpolbul.2013.09.019
Schiel, D. R., and Gunn, T. D. (2019). Effects of sediment on early life history stages of habitat-dominating fucoid algae. J. Exp. Mar. Bio. Ecol. 516, 44–50. doi: 10.1016/j.jembe.2019.04.005
Smale, D. A. (2020). Impacts of ocean warming on kelp forest ecosystems. New Phytol. 225, 1447–1454. doi: 10.1111/nph.16107
Smale, D. A., Burrows, M. T., Moore, P., O’Connor, N., and Hawkins, S. J. (2013). Threats and knowledge gaps for ecosystem services provided by kelp forests: a northeast Atlantic perspective. Ecol. Evol. 3, 4016–4038. doi: 10.1002/ece3.774
Smith, M. E. (2016). Effects of proximity to stormwater on the sandy-beach macrofaunal assemblages of metropolitan Adelaide, South Australia. Mar. Environ. Res. 9, 76–84. doi: 10.1016/j.marenvres.2016.09.009
Soltan, D., Verlaque, M., Boudouresque, C. F., and Francour, P. (2001). Changes in macroalgal communities in the vicinity of a Mediterranean sewage outfall after the setting up of a treatment plant. Mar. Pollut. Bull. 42, 59–70. doi: 10.1016/s0025-326x(00)00116-8
Steneck, R. S., Graham, M. H., Bourque, B. J., Corbett, D., Erlandson, J. M., Estes, J. A., et al. (2002). Kelp forest ecosystems: biodiversity, stability, resilience and future. Environ. Conserv. 29, 436–459. doi: 10.1017/S0376892902000322
Stobberup, K. A., Ramos, V. D. M., and Coelho, M. L. (2004). “Ecopath model of the Cape Verde coastal ecosystem,” in West African Marine Ecosystems, eds M. L. D. Palomares and D. Pauly (Washington, DC: NOAA).
Susini, M. L., Thibaut, T., Meinesz, A., and Forcioli, D. (2007). A preliminary study of genetic diversity in Cystoseira amentacea (C. agardh) Bory var. stricta Montagne (Fucales, Phaeophyceae) using random amplified polymorphic DNA. Phycologia 46, 605–611. doi: 10.2216/06-100.1
Tuya, F., Boyra, A., Sanchez-Jerez, P., Barbera, C., and Haroun, R. (2004a). Can one species determine the structure of the benthic community on a temperate rocky reef? The case of the long-spined sea-urchin Diadema antillarum (Echinodermata: Echinoidea) in the eastern Atlantic. Hydrobiologia 519, 211–214. doi: 10.1023/b:hydr.0000026599.57603.bf
Tuya, F., Boyra, A., Sánchez-Jerez, P., Barbera, C., and Haroun, R. J. (2004b). Relationships between rocky-reef fish assemblages, the sea urchin Diadema antillarum and macroalgae throughout the Canarian Archipelago. Mar. Ecol. Prog. Ser. 278, 157–169. doi: 10.3354/meps278157
Tuya, F., Haroun, R. J., Boyra, A., and Sanchez-Jerez, P. (2005). Sea urchin Diadema antillarum: different functions in the structure and dynamics of reefs on both sides of the Atlantic. Mar. Ecol. Prog. Ser. 302, 307–310. doi: 10.3354/meps302307
Tuya, F., Martín, J. A., and Luque, A. (2002). Impact of a marina construction on a seagrass bed at Lanzarote (Canary Islands). J. Coast. Conserv. 8:157. doi: 10.1652/1400-0350(2002)008[0157:ioamco]2.0.co;2
Valdazo, J., Viera-Rodríguez, M. A., Espino, F., Haroun, R., and Tuya, F. (2017). Massive decline of Cystoseira abies-marina forests in Gran Canaria Island (Canary Islands, eastern Atlantic). Sci. Mar. 81, 499–507. doi: 10.3989/scimar.04655.23a
Verdura, J., Sales, M., Ballesteros, E., Cefalì, M. E., and Cebrian, E. (2018). Restoration of a canopy-forming alga based on recruitment enhancement: methods and long-term success assessment. Front. Plant Sci. 9:1832.
Verdura, J., Santamaría, J., Ballesteros, E., Smale, D. A., Cefalì, M. E., Golo, R., et al. (2021). Local-scale climatic refugia offer sanctuary for a habitat-forming species during a marine heatwave. J. Ecol. 109, 1758–1773. doi: 10.1111/1365-2745.13599
Watanabe, J. M., and Harrold, C. (1991). Destructive grazing by sea urchins Strongylocentrotus spp. in a central California kelp forest: potential roles of recruitment, depth, and predation. Mar. Ecol. Prog. Ser. Oldendorf. 71, 125–141. doi: 10.3354/meps071125
Watanabe, K., Yoshida, G., Hori, M., Umezawa, Y., Moki, H., and Kuwae, T. (2019). Macroalgal metabolism and lateral carbon flows can create significant carbon sinks. Biogeosciences 17, 2425–2440. doi: 10.5194/bg-17-2425-2020
Wernberg, T., Russell, B. D., Thomsen, M. S., Gurgel, C. F. D., Bradshaw, C. J. A., Poloczanska, E. S., et al. (2011). Seaweed communities in retreat from ocean warming. Curr. Biol. 21, 1828–1832. doi: 10.1016/j.cub.2011.09.028
Worm, B., Barbier, E. B., Beaumont, N., Duffy, J. E., Folke, C., Halpern, B. S., et al. (2006). Impacts of biodiversity loss on ocean ecosystem services. Science 314, 787–790.
Keywords: canopy-forming macroalgae, Diadema africanum, NE Atlantic archipelagos, coastal pressures, climate-change effects
Citation: Bernal-Ibáñez A, Cacabelos E, Melo R and Gestoso I (2021) The Role of Sea-Urchins in Marine Forests From Azores, Webbnesia, and Cabo Verde: Human Pressures, Climate-Change Effects and Restoration Opportunities. Front. Mar. Sci. 8:649873. doi: 10.3389/fmars.2021.649873
Received: 05 January 2021; Accepted: 17 May 2021;
Published: 15 June 2021.
Edited by:
Jesper H. Andersen, NIVA Denmark Water Research, DenmarkReviewed by:
Ricardo Haroun, Instituto Universitario de Acuicultura Sostenible y Ecosistemas Marinos (IU-ECOAQUA), SpainFabio Bulleri, University of Pisa, Italy
Hartvig Caspar Christie, Norwegian Institute for Water Research (NIVA), Norway
Copyright © 2021 Bernal-Ibáñez, Cacabelos, Melo and Gestoso. This is an open-access article distributed under the terms of the Creative Commons Attribution License (CC BY). The use, distribution or reproduction in other forums is permitted, provided the original author(s) and the copyright owner(s) are credited and that the original publication in this journal is cited, in accordance with accepted academic practice. No use, distribution or reproduction is permitted which does not comply with these terms.
*Correspondence: Alejandro Bernal-Ibáñez, YWxlamFuZHJvLmJlcm5hbEBtYXJlLWNlbnRyZS5wdA==