- 1Centro de Investigacións Científicas Avanzadas (CICA) e Departamento de Química, Facultade de Ciencias, AE CICA-INIBIC, Universidade da Coruña, A Coruña, Spain
- 2Unit of Infectious Diseases, Microbiology and Preventive Medicine, Virgen del Rocío University Hospital/Institute of Biomedicine of Seville (IBiS), Seville, Spain
- 3Departamento de Biología Marina, Campus de Ciencias Biológicas y Agropecuarias, Universidad Autónoma de Yucatán, Mérida, Mexico
- 4Fundación MEDINA, Centro de Excelencia en Investigación de Medicamentos Innovadores en Andalucía, Granada, Spain
- 5Unidad Académica de Ecología y Biodiversidad Acuática, Instituto de Ciencias del Mar y Limnología, Universidad Nacional Autónoma de México, Mexico City, Mexico
Viral infections are one of the main human health problems in recent decades and the cancer remains one of the most lethal diseases worldwide. The development of new antiviral drugs for the treatment of human adenovirus (HAdV) infections continues to be a challenging goal for medicinal chemistry. There is no specific antiviral drug approved to treat infections caused by HAdV so far and the off-label treatments currently available show great variability in their effectiveness. In relation to cancer, most of the available drugs are designed to act on specific targets by altering the activity of involved transporters and genes. Taking into account the high antiviral and antiproliferative activity against tumor cell lines displayed by some marine natural products reported in the literature, sixty five marine organisms were selected: 51 sponges (Porifera), 13 ascidians (Chordata), and 1 gorgonian (Cnidaria), collected from Yucatan Peninsula, Mexico, to evaluate their antiviral activity against human adenovirus type 5 (HAdV5) and their anticancer properties against five human tumor cell lines, namely human lung carcinoma (A549), human skin melanoma (A2058), hepatocyte carcinoma (HepG2), breast adenocarcinoma (MCF7), and pancreas carcinoma (MiaPaca-2). Eleven extracts displayed anti-HAdV activity being the organic extracts of Dysidea sp., Agelas citrina, Chondrilla sp., Spongia tubulifera, and Monanchora arbuscula the five most active ones. On the other hand, 24 extracts showed antiproliferative activity against at least one tumor cell line, being the extracts of the ascidian Eudistoma amanitum and the sponge Haliclona (Rhizoniera) curacaoensis the most active ones. This work constitutes the first wide antiviral and antiproliferative screening report of extracts from the marine sponges, ascidians, and a gorgonian collected from the Yucatan Peninsula, Mexico.
Introduction
Human adenoviruses (HAdV) are non-enveloped viruses with an icosahedral capsid containing a linear double-stranded DNA whose size ranges from 34 to 37 kb in size (Lion, 2014). Currently, more than 100 serotypes have been identified and grouped into 7 HAdV species (HAdV-A to -G) in Mastadenovirus genus (Qiu et al., 2018; HAdV Working Group, 2019). HAdV infections are common in the human population, as indicated by the high seroprevalence of anti-adenovirus antibodies (ranging from 80 to 90% in sub-Saharan Africa, and from 30 to 70% in Europe and North America), but in otherwise healthy adults, these infections are generally mild and self-limited (Grosso et al., 2017; Inturi et al., 2018). On the other hand, with the advances in molecular techniques of diagnosis, HAdV have been found to be increasingly involved in occasional cases and outbreaks of community-acquired pneumonia (CAP) in healthy population (Yu et al., 2015; Kajon and Ison, 2016; Tan et al., 2016; Jonnalagadda et al., 2017; Yoon et al., 2017). In immunocompromised patients, HAdV infections occur with a wide clinical symptomatology including pneumonia, colitis, hepatitis, hemorrhagic cystitis, tubule-interstitial nephritis or encephalitis, which could result in disseminated disease with high morbidity and mortality in this population especially in pediatric units (Lion, 2014; Sulejmani et al., 2018).
Despite HAdV significant clinical impact, there is currently not an approved drug to treat these infections and the off-label antiviral drugs currently available such as ribavirin, ganciclovir and cidofovir show high variability in their clinical efficacy and their use is also limited by their poor bioavailability and side effects (nephrotoxicity or bone marrow suppression). Brincidofovir (CMX001), a lipidic conjugate of cidofovir, that finished a phase III clinical trial in 2016 with no reported results so far (ClinicalTrials.gov Identifier: NCT02087306) and is now being evaluated for the treatment of serious HAdV infection or disease (ClinicalTrials.gov Identifier: NCT02596997), represents the only potential alternative to be used for the treatment of HAdV infections (Toth et al., 2008; Paolino et al., 2011). Based on this scenario, the research on additional drugs with increased anti-HAdV efficacy is thus necessary.
On the other hand, cancer remains one of the most life-threatening disease and an economic burden worldwide (Bray et al., 2018). Cancer is an abnormal growth of cells and tissues, mainly influenced by the environmental and genetic factors of each individual. More than 277 types of cancer have been identified and diagnosed among which prostate, breast, lung, colon, rectum, bronchus, and urinary bladder cancers are the predominant ones (Wogan et al., 2004; Kumar and Adki, 2018; Khalifa et al., 2019). In 2018, approximately 18 million new cases of cancer were reported globally, resulting in approximately 10 million deaths (Vogelstein and Kinzler, 2004; Bray et al., 2018). Currently four cancer treatments are available, which include: surgery, radiotherapy, chemotherapy, and immunotherapy (Topalian et al., 2012; Bray et al., 2018; June et al., 2018). Unlike surgery and radiotherapy, which are treatment methods mainly indicated for solid tumors, chemotherapy is a treatment that interferes with the process of growth and cell division in tumor cells (Ma and Wang, 2009). Although tumor recurrence and metastasis are usual in some cases, several drugs for cancer chemotherapy are currently in use with a considerably high therapeutic success (Kuczynski et al., 2013; Widmer et al., 2014).
In this regard, society has become more and more reliant upon the availability of safe and efficacious pharmaceutical products with fewer side effects. Considering that the marine world provides approximately half of the total biodiversity on earth (Aneiros and Garateix, 2004; Vo and Kim, 2010), and of course the vast expanse of the ocean, this underwater environment would represent an exceptional opportunity for the search of new chemical compounds (Bhadury et al., 2006) with biological activities for the development of new anticancer and antiviral therapies. Today, around 29,000 new compounds have been reported from marine species, such as sponges, ascidians, corals, and bacteria, and they represent a huge structural diversity of secondary metabolites with very promising candidates to be developed as new drugs (Blunt et al., 2017; Pye et al., 2017). Up to date, agencies such as United States Food and Drug Administration (FDA), European Medicines Agency (EMEA), Japanese Ministry of Health or Australia’s Therapeutic Goods Administration have approved only 8 compounds from marine origin as therapeutic drugs, and 22 drug candidates are in phases I, II, or III clinical trials (Pereira, 2019). Five out of the approved drugs are used in cancer therapies, namely Cytarabine (ara-C), Trabectedin, Eribulin mesylate, Brentuximab vedotin, and plitidepsin (dehydrodidemnin B), while just one is used to treat viral infections, which is the Vidarabine (ara-A) (Jiménez, 2018). In addition, due to the current SARS-CoV-2 pandemic situation, marine compounds have acquired special interest as a potential source of antiviral candidates (Gentile et al., 2020; Khan et al., 2020).
The coasts of Mexico extend along 11,122 km of maritime littorals from the Pacific Ocean to the Caribbean Sea and the Gulf of Mexico, where a rich marine flora and fauna can be found (Morales et al., 2006). Even so, the underwater Mexican ecosystems remains largely unexplored. Particularly, the Yucatan Peninsula (YP), with 1,500 km of coastline, which includes the Mexican States of Campeche, Yucatan and Quintana Roo (Herrera-Silveira et al., 2004), that extends along approximately 14% of total Mexican coast and it harbors a great biological diversity in the shore and the ocean (Bye et al., 1995). All along the western and northern coasts of the YP, extends a region known as the Campeche Bank (CB) with abundant coral reef ecosystems either well offshore (>100 km, such as Alacranes reef, Cayo Arenas, Cayo Arcas, among others) or closer to the shore of the Yucatan state (such as Sisal, Madagascar, and Serpiente); both have been recognized as important biodiversity hotspots (Jordán-Dahlgren, 2002; Tunnell et al., 2007; Ortiz-Lozano et al., 2013; Zarco-Perelló et al., 2013). Additionally, the eastern coast of YP is part of the Mesoamerican Reef, which contains the largest barrier reef in the Western Hemisphere, stretching nearly 700 miles from the northern tip of the YP down through the Honduran Bay Islands (Villela et al., 2003). The potential of Mexican marine resources along the coasts of the YP has not been intensively investigated. Most of the few reports are limited to the evaluation of the biological activity of their organic extracts and there are very few studies on the chemistry of the natural products (Pech-Puch et al., 2020).
As far as we know, the only study of the antiviral activity in extracts of marine organisms from the YP was the report about the high activity of the L-carrageenan polysaccharide obtained from the red algae Solieria filiformis (Peñuela et al., 2018). In relation to antiproliferative activity of the marine extracts of YP, there are only two reports corresponding to the evaluation of 30 extracts obtained exclusively from seaweeds (Moo-Puc et al., 2009; Caamal-Fuentes et al., 2014a) which yielded, so far, four compounds with antiproliferative activity: the diterpene dictyol B acetate, the steroid fucosterol (Caamal-Fuentes et al., 2014b) and the triterpenoid saponins stichloroside B2 and astichoposide C (Graniel-Sabido et al., 2016).
In our constant search for new biological compounds, the decision to explore the marine biodiversity of YP waters was made. In this work, we report the most comprehensive study undertaken to date on antiviral and antiproliferative screening of marine invertebrate species collected along the coasts of the YP, including a total of 65 organic extracts from sponges, ascidians and gorgonians.
Materials and Methods
Animal Collection and Identification
Sixty five marine organisms (51 sponges, 13 ascidians, and 1 gorgonian) were collected by snorkeling and scuba diving in two different ecosystems in the Yucatan Peninsula, coral reef and mangroves, during three different periods of time: September–December 2016, January–March 2017, and September 2018. The selected species were collected from two different regions of the Yucatan Peninsula: Mexican Caribbean (Cozumel Island, Rio Indio, Mahahual and Bermejo, Quintana Roo) and Campeche Bank (Alacranes Reef and Progreso, Yucatan) in areas that were chosen based on their rich biological diversity present in coral reefs, islands and mangroves (Figure 1).
The samples were labeled with a code according to the collection site, stored in plastic bags and chilled on ice during transport to the laboratory. Voucher specimens of sponges were deposited in the Phylum Porifera Gerardo Green National Collection of the Institute of Marine Sciences and Limnology (ICMyL) at the National Autonomous University of Mexico (UNAM), Mexico City, while voucher specimens of ascidians and gorgonian were deposited in the Marine Biology Collection at the Autonomous University of Yucatan (UADY) in Yucatan, Mexico.
The sponges were identified at the ICMyL-UNAM (Mexico) while the ascidians were identified at the University of Vigo (Spain), the Autonomous University of Yucatan (Mexico) and the University of A Coruña, Spain. Information about the taxonomic identification of all the selected marine organisms, code numbers, site of collection, weight of each organic extract along with the antiviral and antiproliferative activity previously reported for each studied species are shown in Table 1. Figures 2, 3 show the structures of compounds with antiviral and antiproliferative activities, respectively, previously reported from the marine organisms present in this study.
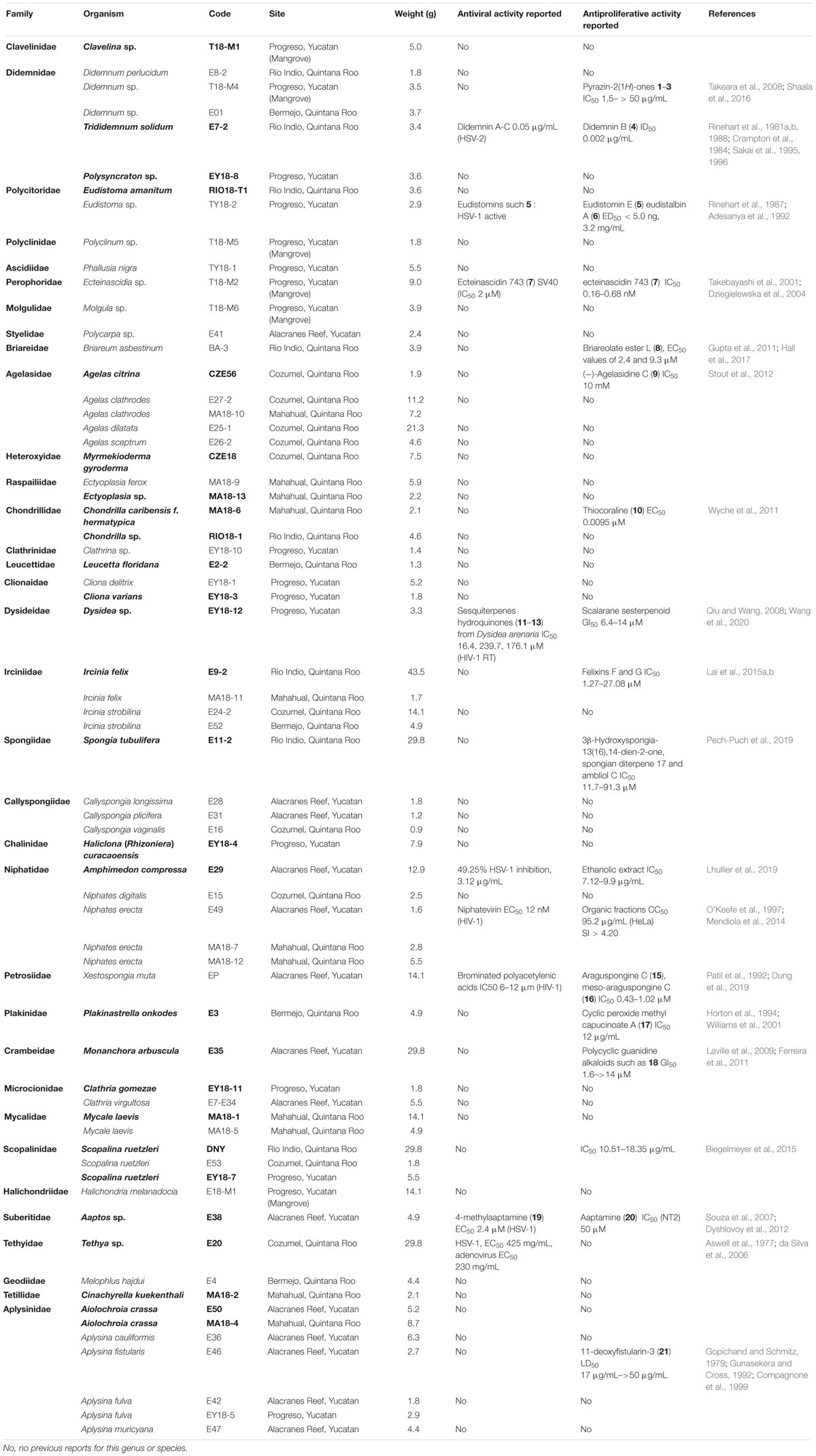
Table 1. Taxonomic information, voucher numbers, site of collection, weight of the organic extract and previously activity reported for the species studied.
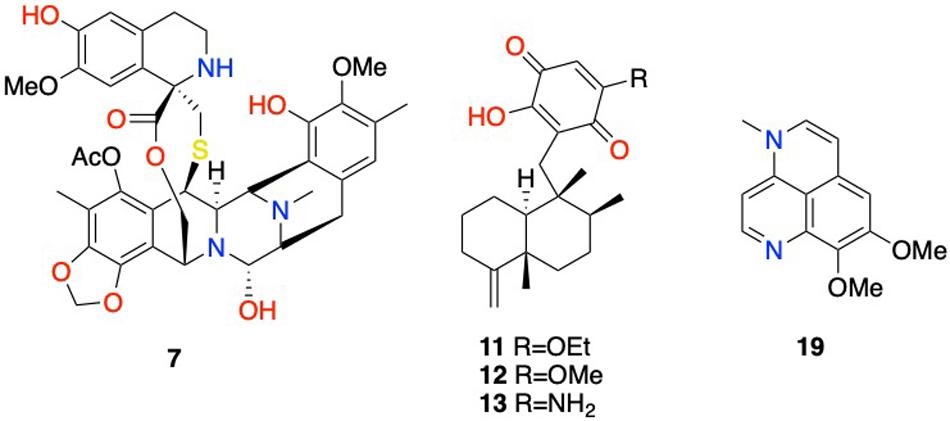
Figure 2. Selected structures of compounds with reported antiviral activities displayed in Table 1. 7: ecteinascidin 743; 11: isosemnonorthoquinone; 12: ilimaquinone; 13: smenospongine; 19: 4-methylaaptamine.
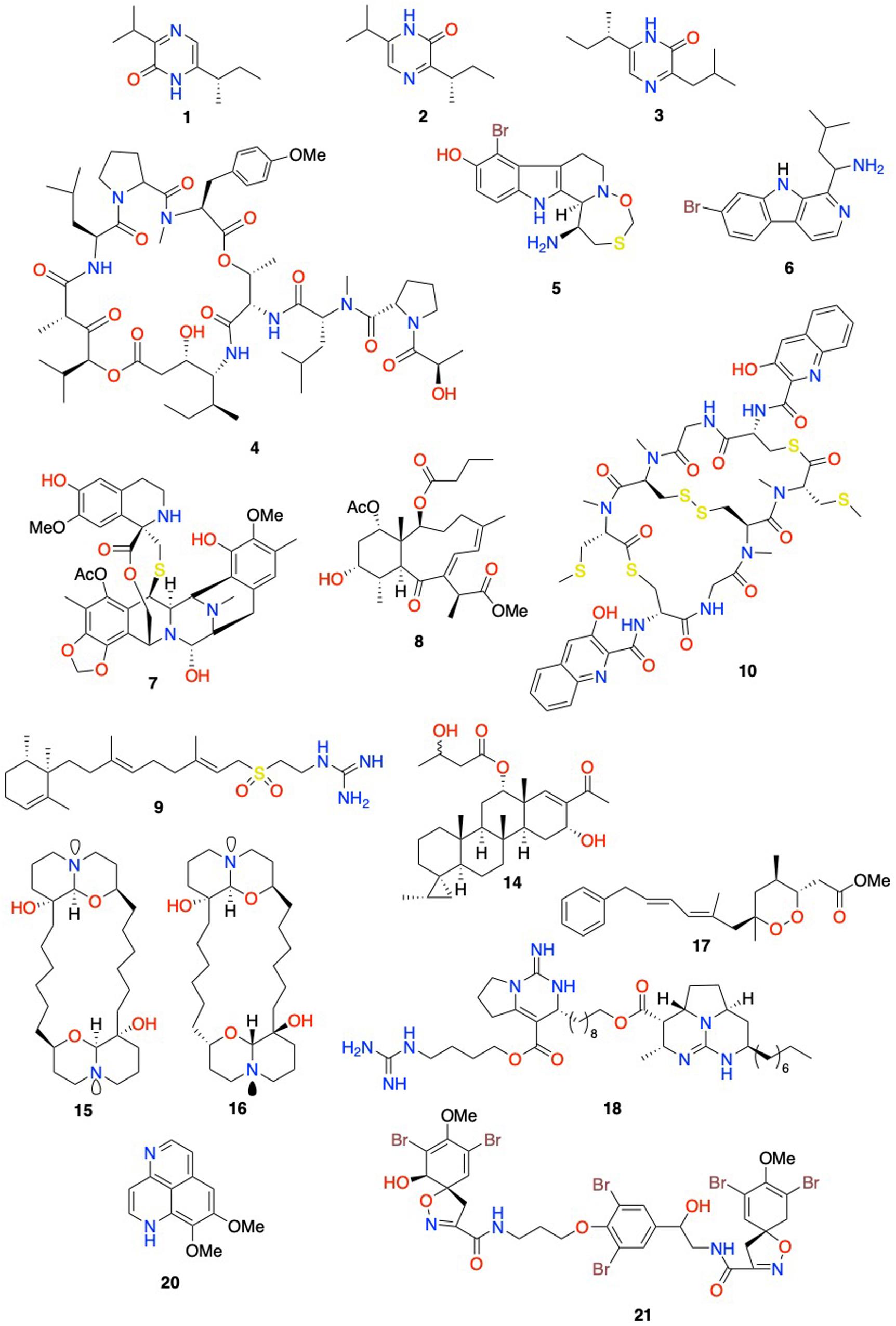
Figure 3. Selected structures of compounds with reported antiproliferative activities displayed in Table 1. 1: (S)-6-(sec-butyl)-3-isopropylpyrazin-2(1H)-one; 2: (S)-3-(sec-butyl)-6-isopropylpyrazin-2(1H)-one; 3: (S)-6-(sec-butyl)-3-isobutylpyrazin-2(1H)-one; 4: didemnin B; 5: eudistomin E; 6: eudistalbin A; 7: ecteinascidin 743; 8: briareolate ester L; 9: (−)-agelasidine C; 10: thiocoraline; 14: scalarane sesterpenoid; 15: araguspongine C; 16: meso-araguspongine C; 17: cyclic peroxide methyl capucinoate A; 18: norbatzelladine A; 20: aaptamine; 21: 11-deoxyfistularin-3.
Preparation of the Organic Extracts
Sliced bodies of each species were exhaustively extracted with the mixture of dichloromethane-methanol (1:1), three times with 500 mL (1.5 L total volume) at 25°C for 24 h for each extraction. After filtration, the solvent was then removed by rotatory evaporator at 40°C and the crude extract stored at −20°C in tightly sealed glass vials.
Antiviral Assays
Viruses and Cells
Human A549 (human lung carcinoma) and 293 (human embryonic kidney) cell lines were obtained from the American Type Culture Collection (ATCC, Manassas, VA, United States). The 293β5 stable cell line overexpressing the human β5 integrin subunit was kindly provided by Dr. Glen Nemerow (Nguyen et al., 2010). Both cell lines were propagated in Dulbecco’s modified Eagle medium (DMEM, Life Technologies/Thermo Fisher) supplemented with 10% fetal bovine serum (FBS) (Omega Scientific, Tarzana, CA, United States), 10 mM HEPES, 4 mM L-glutamine, 100 U/mL penicillin, 100 μg/mL streptomycin, and 0.1 mM non-essential amino acids (complete DMEM). Wild-type HAdV5 was obtained from ATCC. The HAdV5-GFP showed in this work is a replication-defective virus with a CMV promoter-driven enhanced green fluorescent protein (eGFP) reporter gene cassette in place of the E1/E3 region (Nepomuceno et al., 2007). HAdV were propagated in 293β5 cells and isolated from the cellular lysate by cesium chloride (CsCl) density gradient combined with ultracentrifugation. Virus concentration, in mg/mL, was calculated with the Bio-Rad protein assay (Bio-Rad Laboratories) and converted to virus particles/mL (vp/mL) using 4 × 1012 vp/mg.
Plaque Assay
Natural extracts were tested using low multiplicity of infection (MOI) (0.06 vp/cell) and at concentration of 10 μg/mL in a plaque assay. Organic extracts that showed HAdV inhibition greater than 70% were tested in a dose-response assay ranging from 10 to 0.625 μg/mL in plaque assay. Briefly, 293β5 cells were seeded in 6-well plates at a density of 4 × 105 cells per well in duplicate for each condition. When cells reached 80–90% confluency, they were infected with HAdV5-GFP (0.06 vp/cell) and rocked for 2 h at 37°C. After incubation, the inoculum was removed and the cells were washed once with phosphate buffered saline (PBS). The cells were then carefully overlaid with 4 mL/well of equal parts of 1.6% (water/vol) Difco Agar Noble (Becton, Dickinson and Co., Sparks, MD, United States) and 2× EMEM (Minimum Essential Medium Eagle, BioWhittaker) supplemented with 2× penicillin/streptomycin, 2× L-glutamine, and 10% fetal bovine serum (FBS). The mixture also contained the extracts in concentrations ranging from 10 to 0.625 μg/mL. Following incubation for 7 days at 37°C, plates were scanned with a Typhoon 9410 imager (GE Healthcare Life Sciences) and plaques were quantified with ImageJ (Schneider et al., 2012). This assay was performed in duplicate.
Cytotoxicity Assay
The cytotoxicity of the extracts was analyzed by the use of the commercial kit AlamarBlue® (Invitrogen, Ref. DAL1025). A549 cells at a density of 5 × 103 cells/well in 96-well plates were seeded. Decreasing concentrations of each extract (100, 80, 60, 40, 30, 20, 10, 5, 2.5, 1.25, and 0 μg/mL) were diluted in 100 μL of Dulbecco’s Modified Eagle Medium (DMEM). Cells were then incubated at 37°C for 48 h following the manufacture’s indications. The cytotoxic concentration 50 (CC50) value was calculated using the statistical package GraphPad Prism. This assay was performed in duplicate.
Entry Assay
The anti-HAdV activity was initially measured in an entry assay using human A549 epithelial cells (3 × 105 cells/well in corning black wall, clear bottom 96-well plates) infected with HAdV5-GFP (2,000 vp/cell) in the presence of 12.5 μg/mL of each extract and in a dose-response assay. A standard infection curve was generated in parallel by infecting cells in the absence of extracts using serial twofold dilutions of the virus. All reactions were done in triplicate. Cells, viruses, and extracts were incubated for 48 h at 37°C and 5% CO2. Infection, measured as HAdV5-mediated GFP expression, was analyzed using a Typhoon 9410 imager (GE Healthcare Life Sciences) and quantified with ImageQuantTL (GE Healthcare Life Sciences).
Statistical Analyses
Statistical analyses were performed with the GraphPad Prism 5 suite. Data are presented as the mean of duplicate/triplicate samples ± standard deviation (SD).
Antiproliferative Assays
Colorimetric MTT [3-(4,5-Dimethylthiazol-2-yl)-2,5-diphenyltetrazolium bromide] assays were carried out to assess the cell viability of the samples against a panel of five different tumor cell lines (i.e., human lung carcinoma A549 ATCC® CCL-185TM, human skin melanoma A2058 ATCC® CRL-11147TM, hepatocyte carcinoma HepG2 ATCC® HB-8065TM, breast adenocarcinoma MCF7 ATCC® HTB-22TM and pancreas carcinoma MiaPaca-2 ATCC® CRL-1420TM). All cells were obtained from the American Type Culture Collection (ATCC, Manassas, VA, United States). A549 cells were grown in Ham’s F12K medium with 2 mM Glutamine, 10% FBS, 100 U/mL penicillin and 100 μg/mL streptomycin. A2058 and HepG2 were grown in ATCC formulated Eagle’s M essential medium (MEM) with 10% FBS, 2 mM L-glutamine, 1 mM sodium pyruvate and 100 μM MEM non-essential amino acids. MCF-7 cells were grown in the previous medium supplemented with 0.01 mg/mL of bovine insulin. MiaPaca-2 cells were grown in DMEM with 10% FBS, 100 U/mL penicillin and 100 μg/mL streptomycin (Audoin et al., 2013). The antiproliferative activity was assessed after 48 h of treatment with extracts at concentrations of 30, 15, and 7.5 μg/mL.
Results and Discussion
The antiviral and antiproliferative activity of the organic extracts from 65 marine organisms, corresponding to 51 sponges, 13 ascidians, and 1 gorgonian, collected from two different ecosystems in the Yucatan Peninsula, coral reef and mangroves, were evaluated. Around 17% of the extracts showed antiviral activity against HAdV and 37% of them displayed antitumor activity against one or more tumor cell lines.
Antiviral Screening
Marine organisms are invaluable sources of bioactive natural products, some of them being highly significant hits for drug development against pathogenic bacteria, viruses, and fungi (Sagar et al., 2010). A current interest in developing antiviral drugs has been increased since viral diseases have become major human health problems (Sagar et al., 2010).
The results of the antiviral evaluation of marine organic extracts are shown in Table 2. Eleven extracts displayed significant in vitro antiviral activity against HAdV (Table 2), in particular extracts from the ascidian Clavelina sp. and 10 sponges which include: Agelas citrina, Myrmekioderma gyroderma, Ectyoplasia sp., Chondrilla sp., Dysidea sp., Ircinia felix (collected from Rio Indio, Quintana Roo), Spongia tubulifera, Monanchora arbuscula, Aaptos sp., and Cinachyrella kuekenthali.
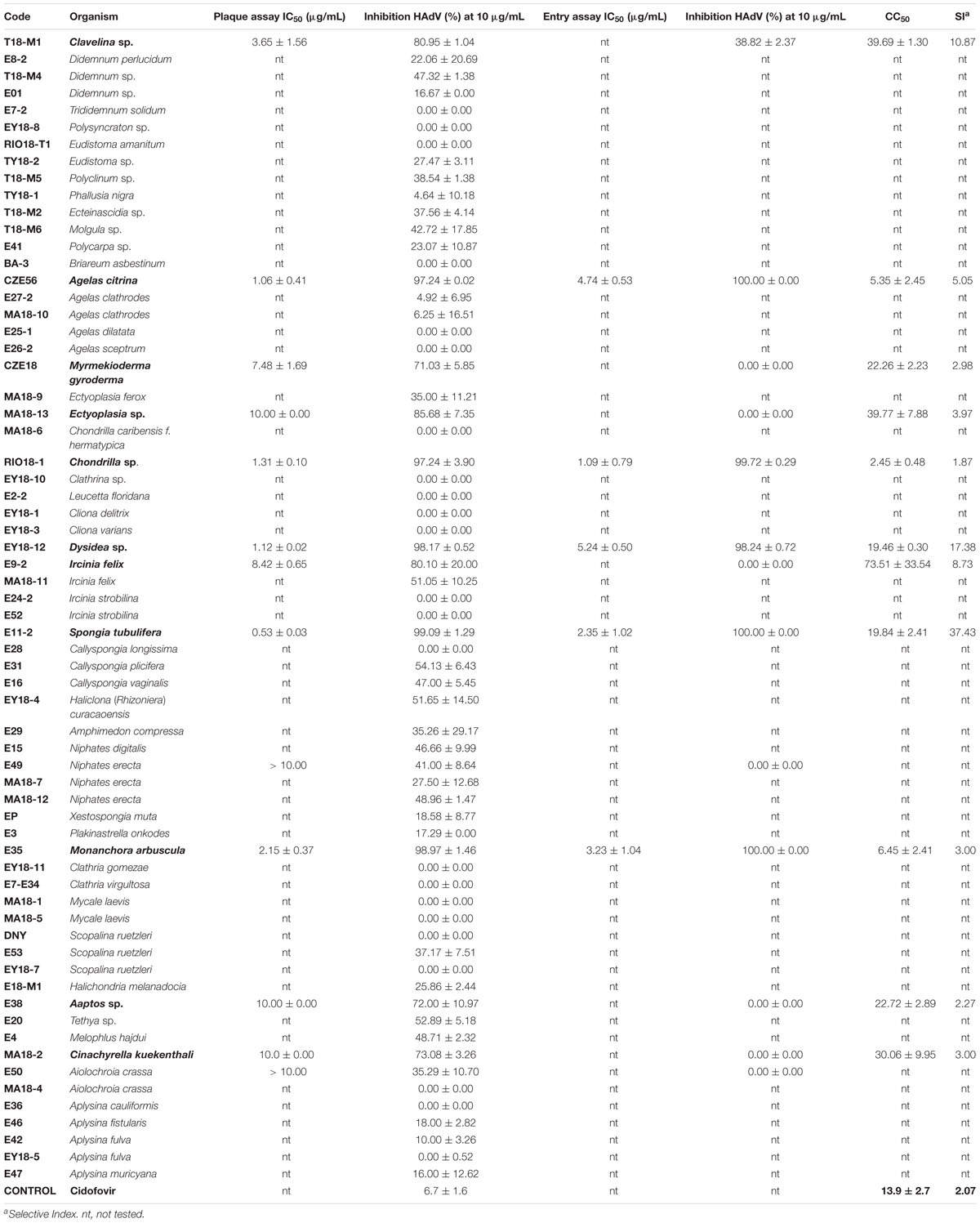
Table 2. IC50, CC50, SI, % inhibition of HAdV infection and % inhibition of HAdV entry of organic extracts of marine organisms from the Yucatan Peninsula.
All the extracts were first screened in plaque assay at the concentration of 10 μg/mL to quantify their ability to inhibit HAdV plaque formation. The active extracts screened out (inhibition >70%) were further evaluated to characterize their antiviral activity (IC50) in plaque assay and their cytotoxicity (CC50 values).
The extracts of the sponges Dysidea sp., A. citrina, Chondrilla sp., S. tubulifera, and M. arbuscula showed the higher activity with an inhibition > 97% at 10 μg/mL concentration and IC50 values between 0.53 and 2.15 μg/mL in the plaque assays (Table 2). The same sponges species showed >98% inhibition in the entry assay at 12.5 μg/mL and IC50 values of 5.24 μg/mL for Dysidea sp., 4.74 μg/mL for A. citrina, 3.23 μg/mL for M. arbuscula, 2.35 μg/mL for S. tubulifera, and 1.09 μg/mL for Chondrilla sp. The CC50 values were 19.84 μg/mL for S. tubulifera, 19.46 μg/mL for Dysidea sp., 6.45 μg/mL for M. arbuscula, 5.35 μg/mL for A. citrina and 2.45 μg/mL for Chondrilla sp. The sponge S. tubulifera displayed the best selectivity index (SI = 37.43), followed by Dysidea sp. (SI = 17.38), A. citrina (SI = 5.05), M. arbuscula (SI = 3.00), and finally Chondrilla sp. (SI = 1.87) (Table 2).
The ascidian Clavelina sp. displayed more than 80% inhibition of HAdV5 infection at 10 μg/mL and an IC50 of 3.65 μg/mL in the plaque assay. In the entry assay, it showed 39% of inhibition at 12.5 μg/mL and in addition, it showed a CC50 value of 39.69 μg/mL and the third best selectivity index (10.87).
The sponges M. gyroderma, Ectyoplasia sp., C. kuekenthali, Aaptos sp., and M. arbuscula showed an inhibition of HAdV5 infection ranging from 71 to 98.97% at 10 μg/mL, and IC50 values between 2.15 and 10 μg/mL. The CC50 values were 22.26 μg/mL for M. gyroderma, 39.77 μg/mL for Ectyoplasia sp., 30.06 μg/mL for C. kuekenthali, 22.72 μg/mL for Chondrilla sp. and 6.45 μg/mL for M. arbuscula. The selectivity index for the five sponges showed values between 2.27 and 3.97.
For some of the organisms included in this work, the antiviral activity of the extract had been previously described (Table 1) as well as the activity of some of their constituent compounds (Figure 2). That is the case of the species Trididemnum solidum, Eudistoma sp., Amphimedon compressa, or Aaptos sp., which extracts display significant anti-HSV or anti-SV40 activities with IC50 ranging between 0.05 and 3.12 μg/mL (Table 1). However, the antiviral activity showed for the former extracts did not always correlate with their corresponding anti-HAdV activity. Indeed, T. solidum, Eudistoma sp., and A. compressa extracts showed very low or any anti-HAdV activity (Table 2). Moreover, although the Thetya sp. extract displayed anti-HSV-1 activity, its antiviral activity against HAdV was only reached at high concentrations (Tables 1, 2).
On the contrary, the extract of the species Aaptos sp. showed both potent anti-HSV-1 (Table 1) and significant anti-HAdV activity (Table 2 and Figure 2). On the other hand, the anti-HAdV activity showed by the extract from Dysidea sp. was significantly higher than the anti-HIV activity, however, the Niphates erecta extract was significantly more active against HIV-1 than against HAdV (Tables 1, 2). The anti-HIV activity of both extracts from Dysidea sp. and N. erecta was previously reported.
These data are in line with previous studies from other groups which showed a wide variability in virus inhibition of extracts from marine sponges and cnidarian products (Cheung et al., 2014). Despite the fact that many authors published results of screening of marine organisms for antiviral activity (Donia and Hamann, 2003), there are no many screenings of marine extracts centered on detecting anti-HAdV activity, thus these results highlight the importance of studying further marine organisms extracts against HAdV as sources of new antiviral drugs.
Regarding the possible mechanism of action for the extracts from Dysidea sp. and S. tubulifera, depending on the HAdV entry inhibition assay and cytotoxic concentrations, it may be related to the first steps during HAdV entry into the cell host. On the other hand, the antiviral activity of the extracts from Clavelina sp., Aaptos sp. and Cinachyrella kuekenthali would be associated with later steps after the entry of HAdV genomes into the nucleus. The significant antiviral activity showed by Aaptos sp. against both HSV-1 and HAdV suggests a potential broad-spectrum mechanism of activity that will require further study. The very similar IC50 values of the entry assay with those of CC50 makes difficult to hypothesize the potential mechanism of action for A. citrina, Chondrilla sp., and M. arbuscula extracts.
Antiproliferative Screening
The results of the antiproliferative evaluation of marine organic extracts are shown in Table 3 as well as the extracts (Table 1) and compounds (Figure 3) previously described for their antiproliferative activity. Twenty-four extracts showed growth inhibition for one or more tumor cell lines, namely those obtained from 4 ascidians (Clavelina sp., T. solidum, Polysyncraton sp., and E. amanitum) and 20 sponges (A. citrina, M. gyroderma, Chondrilla caribensis f. hermatypica, Leucetta floridana, Cliona varians, Dysidea sp., S. tubulifera, Haliclona (Rhizoniera) curacaoensis, A. compressa, Plakinastrella onkodes, Monanchora arbuscula, Clathria gomezae, Mycale laevis (collected from Mahahual, Quintana Roo), and Scopalina ruetzleri (collected from Progreso, Yucatan and Rio Indio, Quintana Roo), Aaptos sp., Tethya sp., C. kuekenthali, and Aiolochroia crassa (collected from Alacranes Reef, Yucatan and Mahahual, Quintana Roo). Interestingly, the extracts of two organisms, the ascidian E. amanitum and the sponge H. (Rhizoniera) curacaoensis, displayed the most potent antiproliferative activities with a complete growth inhibition in all the cell lines at all concentrations tested (Table 3). No previous studies reporting the antiproliferative activity in extracts from these two species have been published, highlighting the value of these two organisms as a potential source of new antiproliferative compounds (Table 1).
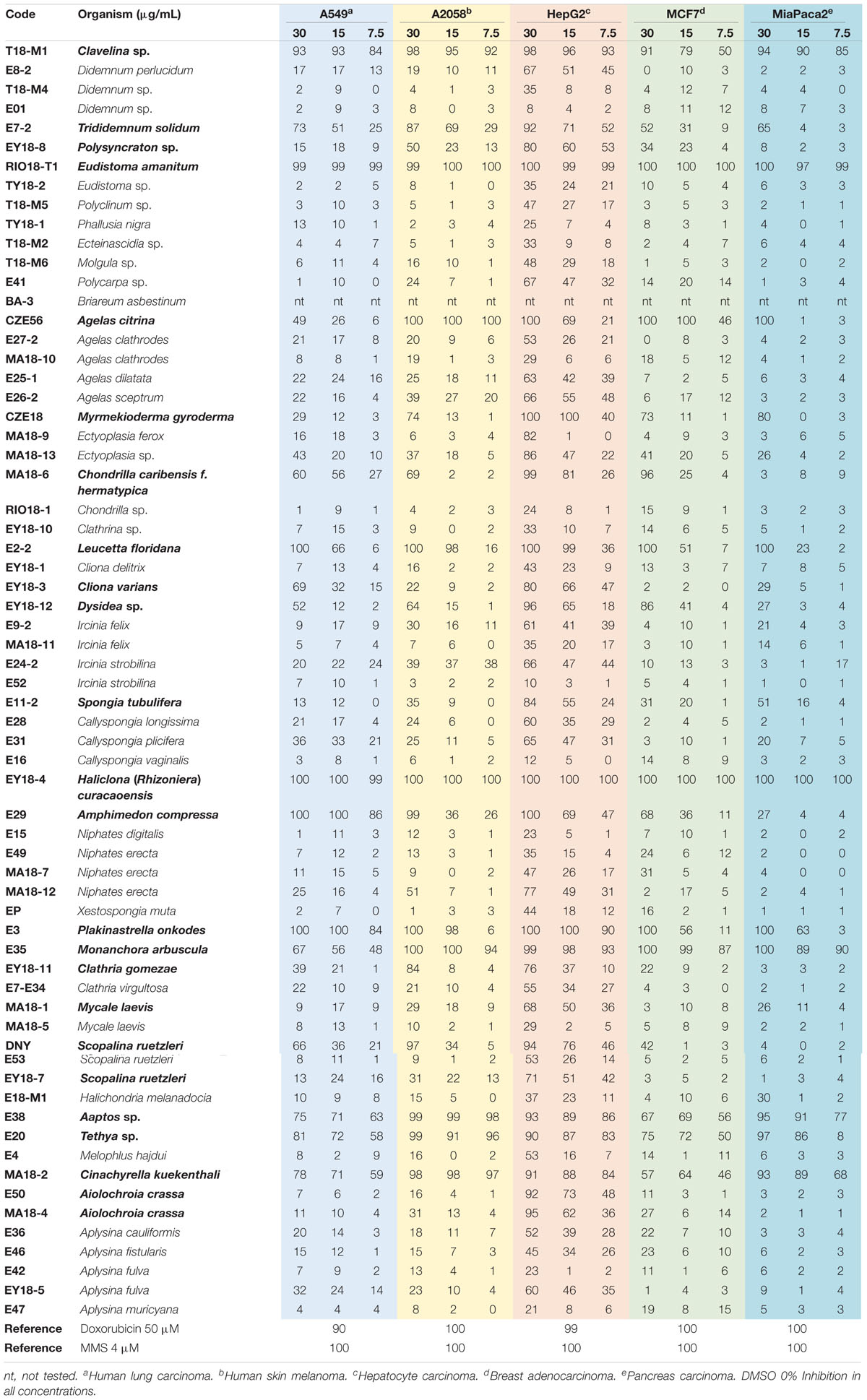
Table 3. Antiproliferative activity in % of inhibition of organic extracts at different concentrations (in μg/mL) of marine organisms from Yucatan Peninsula.
The extracts of seven additional organisms, the ascidian Clavelina sp. and the sponges P. onkodes, M. arbuscula, Aaptos sp., Tethya sp., L. floridana, and C. kuekenthali, also showed good activity against all the cell lines, but with variable potency according to the concentration tested (Table 3). Nonetheless, all of them except L. floridana, which only displays a 23% inhibition at 15 mg/mL against the MiaPaca-2 cell line, still meet the National Cancer Institute (NCI) guidelines to be considered as antiproliferative, i.e., inhibition higher than 50% at a concentration of 20 mg/mL (Hostettman, 1991; Boik, 2001). Two extracts of the organisms, Clavelina sp. and P. onkodes, showed more than 84% growth inhibition of the A549 cell line at all concentrations while the six extracts displayed an almost complete growth inhibition of the A2058 cell line at all concentrations, except the extract of the sponge P. onkodes that did not show any antiproliferative activity at the lowest concentration tested. In the particular case of the cell line HepG2, the six extracts showed more than 83% growth inhibition at all concentrations. Regarding the MCF7 cell line, only the sponge M. arbuscula displayed an almost complete growth inhibition at all concentrations tested. Finally, extracts of all the organisms except L. floridana showed antiproliferative activity according to the NCI guidelines against the cell line MiaPaca-2. Out of this group of seven organisms, the sponges C. kuekenthali and L. floridana stood out as the most interesting ones due to the lack of previous reports on the chemical composition and antiproliferative bioactivity of their extracts. On the contrary, previous reports on the cytotoxic properties of compounds isolated from extracts from P. onkodes, M. arbuscula, and Aaptos sp. make these samples less interesting for the identification of new cytotoxic molecules, although chemical analyses should be performed to discard the presence of other bioactive components not previously reported in extracts of these species.
The extracts of the sponges A. citrina, M. gyroderma, A. compressa, C. caribensis f. hermatypica, and Dysidea sp., were active against four cell lines, being selective against some types of cancer. The three most active extracts were those of the sponge A. citrina that showed 100% of growth inhibition of the A2058 cell line at all concentrations tested, the HepG2 at 30 μg/mL, the MCF7 at 30 and 15 μg/mL, and the MiaPaca-2 at 30 μg/mL, followed by the sponge M. gyroderma extract that showed 100% of growth inhibition of the HepG2 at 30 and 15 μg/mL, and more than 73% of growth inhibition against A2058, MCF7, and MiaPaca-2 cell lines at 30 μg/mL. Finally, the extract of the sponge A. compressa showed more than 86% of growth inhibition of the cell line A549 at all concentrations tested, it also showed more than 99% of growth inhibition of A2058 and HepG2 cell lines at 30 μg/mL and more than 68% growth inhibition of HepG2 and MCF7 cell lines at 15 μg/mL and at 30 μg/mL, respectively. The other two sponges, C. caribensis f. hermatypica showed more than 56% of growth inhibition of A549 (30 and 15 μg/mL), A2058 (30 μg/mL), HepG2 (30 and 15 μg/mL) and MiaPaca-2 (30 μg/mL) and the sponge Dysidea sp. showed more than 51% of growth inhibition against A549, A2058, HepG2, and MCF7 cell lines to 30 μg/mL. Despite the moderate activity found in most extracts, M. gyroderma is perhaps the most interesting sponge of this group due to the lack of reports on its antiproliferative activity. In the cases of A. citrina, A. compressa, C. caribensis f. hermatypica, and Dysidea sp., once again chemical analyses of the extracts will be necessary to asses the novelty of their components and their potential interest for further studies.
The extracts of the rest of the organisms displayed bioactivity to a lesser extension, hitting only a few cell lines of the panel tested. Thus, the sponge S. ruetzleri showed more than 66% growth inhibition of A549, A2058, and HepG2 cell lines at 30 μg/mL. On the other hand, the extract of sponge C. gomezae showed more than 76% growth inhibition of A2058 and HepG2 cell lines at 30 μg/mL. C. gomezae seems to be the most interesting of these two sponges due to the lack of previous reports on cytotoxic activity of its extracts, although a preliminar chemical investigation by LC/MS should also be performed on the extract of S. ruetzleri before discarding the sample for further studies. Even though Didemnum sp. had been previous shown antiproliferative activity (Table 1) in our experience it only showed very little activity at the highest concentration against the HepG2 cell line, perhaps indicating that the specimens collected by us do not contain didemnins or produce very low levels of these potent molecules. Finally, it is worth mentioning that more than 50% of the extracts tested showed antiproliferative activity against the cell line HepG2, 41 extracts exhibited at least more than 50% growth inhibition at 30 μg/mL concentration, and the organisms, Polysyncraton sp., C. varians, S. tubulifera, M. laevis (collected from Mahahual, Quintana Roo), S. ruetzleri (collected from Progreso, Yucatan) and A. crassa (collected from Alacranes Reef, Yucatan and Mahahual, Quintana Roo), only showed activity against the cell line HepG2.
Conclusion
Sixty-five marine organisms, corresponding to fifty-one sponges (Porifera), thirteen ascidians (Chordata) and one gorgonian (Cnidaria), were collected along the coast of Yucatan Peninsula in Mexico. They were selected on the basis of chemotaxonomical criteria. They were extracted with organic solvents and each extract was screened for its in vitro antiviral and antiproliferative activity against HAdV and five tumor cell lines, respectively. Evaluation through plaque assays showed a significant antiviral activity for 11 extracts corresponding to 10 sponges [A. citrina, M. gyroderma, Ectyoplasia sp., Chondrilla sp., Dysidea sp., M. arbuscula, Aaptos sp., C. kuekenthali, I. felix (collected from Rio Indio, Quintana Roo), and S. tubulifera] and one ascidian (Clavelina sp.). The extracts of the sponges Dysidea sp., A. citrina, Chondrilla sp., S. tubulifera, and M. arbuscula showed the best antiviral activity. The observed IC50 values of these extracts were lower than those shown by cidofovir (IC50 = 6.7 ± 1.6 μg/mL; CC50 = 13.9 ± 2.7), which is the drug of choice to treat HAdV infections. However, the high cytotoxicity displayed by A. citrina (5.35 ± 2.45 μg/mL. SI = 5.05) or Chondrilla sp. (2.45 ± 0.48 μg/mL, SI = 1.87) generated low SI values similar to those for cidofovir (SI = 2.07).
The high entry inhibition value registered for Dysidea sp. and S. tubulifera suggested that the antiviral action mechanism could be related with early steps in the HAdV replicative cycle involving the binding, internalization by clatrin-mediated endocytosis, endosomal escape and microtubular transport of the viral particles to the nuclear pores of the host cell. In contrast, the mechanism of action for the extracts from Clavelina sp., Aaptos sp., and C. kuekenthali would be associated with later steps after the entry of HAdV genomes into de nucleus which could be related with the transcription of the HAdV immediate early gene E1A or the HAdV DNA replication process, as in the case of cidofovir, a nucleoside analog that inhibit HAdV DNA polymerase. A. citrina, Chondrilla sp., and M. arbuscula did not show clear data to suggest a potential mechanism of action.
Twenty-four extracts showed antiproliferative activity that corresponded to twenty sponges [A. citrina, M. gyroderma, C. caribensis f. hermatypica, L. floridana, C. varians, Dysidea sp., S. tubulifera, H. (Rhizoniera) curacaoensis, A. compressa, P. onkodes, M. arbuscula, C. gomezae, M. laevis (collected from Mahahual, Quintana Roo), S. ruetzleri (collected from Progreso, Yucatan and Rio Indio, Quintana Roo), Aaptos sp., Tethya sp., C. kuekenthali and A. crassa (collected from Alacranes Reef, Yucatan and Mahahual, Quintana Roo) and four ascidians (Clavelina sp., T. solidum, Polysyncraton sp. and E. amanitum)]. Two organisms, the ascidian E. amanitum and the sponge H. (Rhizoniera) curacaoensis, showed the best antiproliferative activity. Additionally, more than 50% of the extracts showed antiproliferative activity against the hepatocyte carcinoma cell line (HepG2). According to the results reported in this study, extracts of the tunicate E. amanitum and those of the sponges H. (Rhizoniera) curacaoensis, C. kuekenthali, and L. floridana proved to be the most interesting for future studies due to their high potency against most of the cell lines tested and the lack of previous reports on their chemical composition.
Data Availability Statement
The datasets presented in this article are not readily available because the raw data supporting the conclusions of this article will be made available by the authors upon request. Requests to access the datasets should be directed to JS-C,anNhbmNoZXotaWJpc0B1cy5lcw==; CJ,Y2FybG9zLmppbWVuZXpAdWRjLmVz; JR,amFpbWUucm9kcmlndWV6QHVkYy5lcw==.
Author Contributions
DP-P and MP-P were responsible for the recollection of organisms and preparation of the marine extracts. JB-C and JS-C made the antiviral assays. FR and BC did the antiproliferative assays. PG, DP-P, HV-H, and SG-H performed taxonomic identification. DP-P, CJ, and JR wrote the original draft. DP-P, JR, CJ, FR, JP, and JS-C wrote, reviewed, and edited the manuscript. All authors contributed to the article and approved the submitted version.
Funding
This work was supported by Grants RTI2018-093634-B-C22 and RTC-2016-4611-1 (AEI/FEDER, EU) from the State Agency for Research (AEI) of Spain, both co-funded by the FEDER Programme from the European Union, BLUEBIOLAB (0474_BLUEBIOLAB_1_E), Programme INTERREG V A of Spain-Portugal (POCTEP). The study was also funded by projects GRC2018/039 and Agrupación Estratégica CICA-INIBIC ED431E 2018/03 (Consejería de Educación, Universidad y Formación Profesional de la Junta de Galicia) from the Xunta de Galicia (autonomous government of the region). DP-P received a fellowship from the program National Council of Science and Technology (CONACYT) of Mexico and the Secretariat of Research, Innovation and Higher Education (SIIES) of Yucatan (Mexico). Also supported by Instituto de Salud Carlos III, Ministerio de Economía, Industria y Competitividad, Spanish Network for Research in Infectious Diseases (REIPI RD16/0016/0009) – co-financed by “A way to achieve Europe” ERDF, the Instituto de Salud Carlos III, Proyectos de Desarrollo Tecnológico en Salud (DTS17/00130 and PI18/01191), and the Spanish Adenovirus Network (AdenoNet, BIO2015/68990-REDT). JS-C is a researcher belonging to the program “Nicolás Monardes” (C-0059-2018), Servicio Andaluz de Salud, Junta de Andalucía, Spain. The antiproliferative studies were financed with internal funds from Fundación MEDINA.
Conflict of Interest
The authors declare that the research was conducted in the absence of any commercial or financial relationships that could be construed as a potential conflict of interest.
References
Adesanya, S. A., Chbani, M., Pais, M., and Debitus, C. (1992). Brominated β-carbolines from the marine tunicate Eudistoma album. J. Nat. Prod. 55, 525–527. doi: 10.1021/np50082a025
Aneiros, A., and Garateix, A. (2004). Bioactive peptides from marine sources: pharmacological properties and isolation procedures. J. Chromatogr. B Anal. Technol. Biomed. Life Sci. 803, 41–53. doi: 10.1016/j.jchromb.2003.11.005
Aswell, J. F., Allen, G. P., Jamieson, A. T., Campbell, D. E., and Gentry, G. A. (1977). Antiviral activity of arabinosylthymine in herpesviral replication: mechanism of action in vivo and in vitro. Antimicrob. Agents Chemother. 12, 243–254. doi: 10.1128/AAC.12.2.243
Audoin, C., Bonhomme, D., Ivanisevic, J., Cruz, M., Cautain, B., Monteiro, M., et al. (2013). Balibalosides, an original family of glucosylated sesterterpenes produced by the mediterranean sponge Oscarella balibaloi. Mar. Drugs 11, 1477–1489. doi: 10.3390/md11051477
Bhadury, P., Mohammad, B. T., and Wright, P. C. (2006). The current status of natural products from marine fungi and their potential as anti-infective agents. J. Ind. Microbiol. Biotechnol. 33, 325–337. doi: 10.1007/s10295-005-0070-3
Biegelmeyer, R., Schröder, R., Rambo, D. F., Dresch, R. R., Stout, E. P., Carraro, J. L. F., et al. (2015). Cytotoxic effects on tumour cell lines of fatty acids from the marine sponge Scopalina ruetzleri. J. Pharm. Pharmacol. 67, 746–753. doi: 10.1111/jphp.12366
Blunt, J. W., Copp, B. R., Keyzers, R. A., Munro, M. H. G., and Prinsep, M. R. (2017). Marine natural products. Nat. Prod. Rep. 34, 235–294. doi: 10.1039/c6np00124f
Boik, J. (2001). Natural Compounds in Cancer Therapy, 1st Edn, ed. S. Farnel, (Princenton, MN: Oregon Medical Press).
Bray, F., Ferlay, J., Soerjomataram, I., Siegel, R. L., Torre, L. A., and Jemal, A. (2018). Global cancer statistics 2018: GLOBOCAN estimates of incidence and mortality worldwide for 36 cancers in 185 countries freddie. CA Cancer J. Clin. 68, 394–424. doi: 10.3322/caac.21492
Bye, R., Linares, E., and Estrada, E. (1995). “Biological diversity of medicinal plants in México,” in Phytochemistry of Medicinal Plants, eds J. T. Arnason, R. Mata, and J. T. Romeo, (Boston, MA: Springer), 65–82. doi: 10.1007/978-1-4899-1778-2_4
Caamal-Fuentes, E., Chale-Dzul, J., Moo-Puc, R., Freile-Pelegrin, Y., and Robledo, D. (2014a). Bioprospecting of brown seaweed (Ochrophyta) from the Yucatan Peninsula: cytotoxic, antiproliferative, and antiprotozoal activities. J. Appl. Phycol. 26, 1009–1017. doi: 10.1007/s10811-013-0129-x
Caamal-Fuentes, E., Moo-Puc, R., Freile-Pelegrín, Y., and Robledo, D. (2014b). Cytotoxic and antiproliferative constituents from Dictyota ciliolata, Padina sanctae-crucis and Turbinaria tricostata. Pharm. Biol. 52, 1244–1248. doi: 10.3109/13880209.2014.886273
Cheung, R. C. F., Wong, J. H., Pan, W. L., Chan, Y. S., Yin, C. M., Dan, X. L., et al. (2014). Antifungal and antiviral products of marine organisms. Appl. Microbiol. Biotechnol. 98, 3475–3494. doi: 10.1007/s00253-014-5575-0
Compagnone, R. S., Avila, R., Suárez, A. I., Abrams, O. V., Rangel, H. R., Arvelo, F., et al. (1999). 11-deoxyfistularin-3, a new cytotoxic metabolite from the caribbean sponge Aplysina fistularis insularis. J. Nat. Prod. 62, 1443–1444. doi: 10.1021/np9901938
Crampton, S. L., Adams, E. G., Kuentzel, S. L., Li, L. H., Badiner, G., and Bhuyan, B. K. (1984). Biochemical and cellular effects of Didemnins A and B. Cancer Res. 44, 1796–1801.
da Silva, A. C., Kratz, J. M., Farias, F. M., Henriques, A. T., dos Santos, J., Leonel, R. M., et al. (2006). In vitro antiviral activity of marine sponges collected off Brazilian Coast. Biol. Pharm. Bull. 29, 135–140. doi: 10.1248/bpb.29.135
Donia, M., and Hamann, M. T. (2003). Marine natural products and their potential applications as anti-infective agents. Lancet Infect. Dis. 3, 338–348. doi: 10.1016/S1473-3099(03)00655-8
Dung, D. T., Hang, D. T. T., Yen, P. H., Quang, T. H., Nhiem, N. X., Tai, B. H., et al. (2019). Macrocyclic bis-quinolizidine alkaloids from Xestospongia muta. Nat. Prod. Res. 33, 400–406. doi: 10.1080/14786419.2018.1455043
Dyshlovoy, S. A., Naeth, I., Venz, S., Preukschas, M., Sievert, H., Jacobsen, C., et al. (2012). Proteomic profiling of germ cell cancer cells treated with aaptamine, a marine alkaloid with antiproliferative activity. J. Proteome Res. 11, 2316–2330. doi: 10.1021/pr300170p
Dziegielewska, B., Kowalski, D., and Beerman, T. A. (2004). SV40 DNA replication inhibition by the monofunctional DNA alkylator Et743. Biochemistry 43, 14228–14237. doi: 10.1021/bi049407x
Ferreira, E. G., Wilke, D. V., Jimenez, P. C., de Oliveira, J. R., Pessoa, O. D. L., Silveira, E. R., et al. (2011). Guanidine alkaloids from Monanchora arbuscula: chemistry and antitumor potential. Chem. Biodivers. 8, 1433–1445. doi: 10.1002/cbdv.201000161
Gentile, D., Patamia, V., Scala, A., Sciortino, M. T., Piperno, A., and Rescifina, A. (2020). Putative inhibitors of SARS-COV-2 main protease from a library of marine natural products: a virtual screening and molecular modeling study. Mar. Drugs 18, 225. doi: 10.3390/md18040225
Gopichand, Y., and Schmitz, F. J. (1979). Marine natural products: fistularin-1, -2 and -3 from the sponge Aplysina fistularis forma fulva. Tetrahedron Lett. 20, 3921–3924. doi: 10.1016/S0040-4039(01)86465-0
Graniel-Sabido, M. J., Mirón-López, G., León-Deniz, L. V., Moo-Puc, R. E., Quintal-Novelo, C. J., Quijano, L., et al. (2016). Total NMR assignment of a new antiproliferative triterpene oligoglycoside from the sea cucumber Astichopus multifidus. Tetrahedron Lett. 57, 4375–4378. doi: 10.1016/j.tetlet.2016.08.051
Grosso, F., Stoilov, P., Lingwood, C., Brown, M., and Cochrane, A. (2017). Suppression of adenovirus replication by cardiotonic steroids. J. Virol. 91:e01623-16. doi: 10.1128/jvi.01623-16
Gunasekera, S. P., and Cross, S. S. (1992). Fistularin 3 and 11-ketofistularin 3. Feline leukemia virus active bromotyrosine metabolites from the marine sponge Aplysina archeri. J. Nat. Prod. 55, 509–512. doi: 10.1021/np50082a020
Gupta, P., Sharma, U., Schulz, T. C., Sherrer, E. S., McLean, A. B., Robins, A. J., et al. (2011). Bioactive diterpenoid containing a reversible “spring-loaded” (E,Z)-dieneone Michael acceptor. Org. Lett. 13, 3920–3923. doi: 10.1021/ol201443k
HAdV Working Group (2019). HAdV Working Group. Available online at: http://hadvwg.gmu.edu/ (accessed June 18, 2020).
Hall, A. J., Roche, S. P., and West, L. M. (2017). Synthesis of briarane diterpenoids: biomimetic transannular oxa-6π electrocyclization induced by a UVA/UVC photoswitch. Org. Lett. 19, 576–579. doi: 10.1021/acs.orglett.6b03689
Herrera-Silveira, J. A., Comin, F. A., Aranda-Cirerol, N., Troccoli, L., and Capurro, L. (2004). Coastal water quality assessment in the Yucatan Peninsula: management implications. Ocean Coast. Manag. 47, 625–639. doi: 10.1016/j.ocecoaman.2004.12.005
Horton, P. A., Longley, R. E., Kelly-Borges, M., McConnell, O. J., and Ballas, L. M. (1994). New cytotoxic peroxylactones from the marine sponge, Plakinastrella onkodes. J. Nat. Prod. 57, 1374–1381. doi: 10.1021/np50112a006
Hostettman K. (ed.) (1991). Methods in Plant Biochemistry: Assays for Bioactivity (Vol. 6 in Methods in Plant Biochemistry). San Diego, CA: Academic Press Ltd.
Inturi, R., Mun, K., Singethan, K., Schreiner, S., and Punga, T. (2018). Human adenovirus infection causes cellular E3 ubiquitin ligase MKRN1 degradation involving the viral core protein pVII. J. Virol. 92:e01154-17. doi: 10.1128/jvi.01154-17
Jiménez, C. (2018). Marine natural products in medicinal chemistry. ACS Med. Chem. Lett. 9, 959–961. doi: 10.1021/acsmedchemlett.8b00368
Jonnalagadda, S., Rodríguez, O., Estrella, B., Sabin, L. L., Sempértegui, F., and Hamer, D. H. (2017). Etiology of severe pneumonia in Ecuadorian children. PLoS One 12:e0171687. doi: 10.1371/journal.pone.0171687
Jordán-Dahlgren, E. (2002). Gorgonian distribution patterns in coral reef environments of the Gulf of Mexico: evidence of sporadic ecological connectivity? Coral Reefs 21, 205–215. doi: 10.1007/s00338-002-0226-9
June, C. H., O’Connor, R. S., Kawalekar, O. U., Ghassemi, S., and Milone, M. C. (2018). CAR T cell immunotherapy for human cancer. Science 359, 1361–1365. doi: 10.1126/science.aar6711
Kajon, A. E., and Ison, M. G. (2016). Severe infections with human adenovirus 7d in 2 adults in family, Illinois, USA, 2014. Emerg. Infect. Dis. 22, 730–733. doi: 10.3201/eid2204.151403
Khalifa, S. A. M., Elias, N., Farag, M. A., Chen, L., Saeed, A., Hegazy, M.-E. F., et al. (2019). Marine natural products: a source of novel anticancer drugs. Mar. Drugs 17:491. doi: 10.3390/md17090491
Khan, M. T., Ali, A., Wang, Q., Irfan, M., Khan, A., Zeb, M. T., et al. (2020). Marine natural compounds as potents inhibitors against the main protease of SARS-CoV-2—a molecular dynamic study. J. Biomol. Struct. Dyn. [Epub ahead of print] doi: 10.1080/07391102.2020.1769733
Kuczynski, E. A., Sargent, D. J., Grothey, A., and Kerbel, R. S. (2013). Drug rechallenge and treatment beyond progression-implications for drug resistance. Nat. Rev. Clin. Oncol. 10, 571–587. doi: 10.1038/nrclinonc.2013.158
Kumar, M. S., and Adki, K. M. (2018). Marine natural products for multi-targeted cancer treatment: a future insight. Biomed. Pharmacother. 105, 233–245. doi: 10.1016/j.biopha.2018.05.142
Lai, Y.-Y., Chen, L.-C., Wu, C.-F., Lu, M.-C., Wen, Z.-H., Wu, T.-Y., et al. (2015a). New cytotoxic 24-homoscalarane sesterterpenoids from the sponge Ircinia felix. Int. J. Mol. Sci. 16, 21950–21958. doi: 10.3390/ijms160921950
Lai, Y.-Y., Lu, M.-C., Wang, L.-H., Chen, J.-J., Fang, L.-S., Wu, Y.-C., et al. (2015b). New scalarane sesterterpenoids from the formosan sponge Ircinia felix. Mar. Drugs 13, 4296–4309. doi: 10.3390/md13074296
Laville, R., Thomas, O. P., Berrué, F., Marquez, D., Vacelet, J., and Amade, P. (2009). Bioactive guanidine alkaloids from two caribbean marine sponges. J. Nat. Prod. 72, 1589–1594. doi: 10.1021/np900244g
Lhullier, C., Moritz, M. I. G., Tabalipa, E. O., Sardá, F. N., Schneider, N. F. Z., Moraes, M. H., et al. (2019). Biological activities of marine invertebrates extracts from the northeast brazilian coast. Brazilian J. Biol. 80, 393–404. doi: 10.1590/1519-6984.213678
Lion, T. (2014). Adenovirus infections in immunocompetent and immunocompromised patients. Clin. Microbiol. Rev. 27, 441–462. doi: 10.1128/CMR.00116-13
Ma, X., and Wang, Z. (2009). Anticancer drug discovery in the future: an evolutionary perspective. Drug Discov. Today 14, 1136–1142. doi: 10.1016/j.drudis.2009.09.006
Mendiola, J., Regalado, E. L., Díaz-García, A., Thomas, O. P., Fernández-Calienes, A., Rodríguez, H., et al. (2014). In vitro antiplasmodial activity, cytotoxicity and chemical profiles of sponge species of Cuban coasts. Nat. Prod. Res. 28, 312–317. doi: 10.1080/14786419.2013.861835
Moo-Puc, R., Robledo, D., and Freile-Pelegrín, Y. (2009). Actividad citotóxica y antiproliferativa in vitro de macroalgas marinas de Yucatán, México. Ciencias Mar. 35, 345–358. doi: 10.7773/cm.v35i4.1617
Morales, J. L., Cantillo-Ciau, Z. O., Sánchez-Molina, I., and Mena-Rejón, G. J. (2006). Screening of antibacterial and antifungal activities of six marine macroalgae from coasts of Yucatán Peninsula. Pharm. Biol. 44, 632–635. doi: 10.1080/13880200600897569
Nepomuceno, R. R., Pache, L., and Nemerow, G. R. (2007). Enhancement of gene transfer to human myeloid cells by adenovirus-fiber complexes. Mol. Ther. 15, 571–578. doi: 10.1038/sj.mt.6300048
Nguyen, E. K., Nemerow, G. R., and Smith, J. G. (2010). Direct evidence from single-cell analysis that human-defensins block adenovirus uncoating to neutralize infection. J. Virol. 84, 4041–4049. doi: 10.1128/jvi.02471-09
O’Keefe, B. R., Beutler, J. A., Cardellina, J. H., Gulakowski, R. J., Krepps, B. L., Mcmahon, J. B., et al. (1997). Isolation and characterization of niphatevirin, a human-immunodeficiency-virus-inhibitory glycoprotein from the marine sponge Niphates Erecta. Eur. J. Biochem. 245, 47–53. doi: 10.1111/j.1432-1033.1997.t01-1-00047.x
Ortiz-Lozano, L., Pérez-España, H., Granados-Barba, A., González-Gándara, C., Gutiérrez-Velázquez, A., and Martos, J. (2013). The reef corridor of the Southwest Gulf of Mexico: challenges for its management and conservation. Ocean Coast. Manag. 86, 22–32. doi: 10.1016/j.ocecoaman.2013.10.006
Paolino, K., Sande, J., Perez, E., Loechelt, B., Jantausch, B., Painter, W., et al. (2011). Eradication of disseminated adenovirus infection in a pediatric hematopoietic stem cell transplantation recipient using the novel antiviral agent CMX001. J. Clin. Virol. 50, 167–170. doi: 10.1016/j.jcv.2010.10.016
Patil, A. D., Kokke, W. C., Cochran, S., Francis, T. A., Tomszek, T., and Westley, J. W. (1992). Brominated polyacetylenic acids from the marine sponge Xestospongia muta: inhibitors of HIV protease. J. Nat. Prod. 55, 1170–1177. doi: 10.1021/np50087a002
Pech-Puch, D., Pérez-Povedano, M., Lenis-Rojas, O. A., Rodríguez, J., and Jiménez, C. (2020). Marine natural products from the Yucatan peninsula. Mar. Drugs 18, 59. doi: 10.3390/md18010059
Pech-Puch, D., Rodríguez, J., Cautain, B., Sandoval-Castro, C. A., and Jiménez, C. (2019). Cytotoxic furanoditerpenes from the Sponge Spongia tubulifera collected in the Mexican Caribbean. Mar. Drugs 17, 416. doi: 10.3390/md17070416
Peñuela, A., Robledo, D., Bourgougnon, N., Bedoux, G., Hernández-Núñez, E., and Freile-Pelegrín, Y. (2018). Environmentally friendly valorization of Solieria filiformis (Gigartinales, Rhodophyta) from IMTA using a biorefinery concept. Mar. Drugs 16:487. doi: 10.3390/md16120487
Pereira, F. (2019). Have marine natural product drug discovery efforts been productive and how can we improve their efficiency? Expert Opin. Drug Discov. 14, 717–722. doi: 10.1080/17460441.2019.1604675
Pye, C. R., Bertin, M. J., Lokey, R. S., Gerwick, W. H., and Linington, R. G. (2017). Retrospective analysis of natural products provides insights for future discovery trends. Proc. Natl. Acad. Sci. U.S.A. 114, 5601–5606. doi: 10.1073/pnas.1614680114
Qiu, F. Z., Shen, X. X., Zhao, M. C., Zhao, L., Duan, S. X., Chen, C., et al. (2018). A triplex quantitative real-time PCR assay for differential detection of human adenovirus serotypes 2, 3 and 7. Virol. J. 15, 81. doi: 10.1186/s12985-018-0983-x
Qiu, Y., and Wang, X. (2008). A new sesquiterpenoid hydroquinone from the marine sponge Dysidea arenaria. Molecules 13, 1275–1281. doi: 10.3390/molecules13061275
Rinehart, K. L., Gloer, J. B., Cook, J. C., Mizsak, S. A., and Scahill, T. A. (1981a). Structures of the didemnins, antiviral and cytotoxic depsipeptides from a Caribbean Tunicate. J. Am. Chem. Soc. 103, 1857–1859. doi: 10.1021/ja00397a055
Rinehart, K. L., Gloer, J. B., Hughes, R. G., Renis, H. E., Patrick McGovren, J., Swynenberg, E. B., et al. (1981b). Didemnins: antiviral and antitumor depsipeptides from a Caribbean tunicate. Science 212, 933–935. doi: 10.1126/science.7233187
Rinehart, K. L., Kishore, V., Bible, K. C., Sakai, R., Sullins, D. W., and Li, K. I. M. (1988). Didemnins and tunichlorin: novel natural products from the marine tunicate Trididemnum solidum. J. Nat. Prod. 51, 1–21. doi: 10.1021/np50055a001
Rinehart, K. L., Kobayashi, J., Harbour, G. C., Gilmore, J., Mascal, M., Holt, T. G., et al. (1987). Eudistomins A-q, β-carbolines from the antiviral Caribbean Tunicate Eudistoma olivaceum. J. Am. Chem. Soc. 109, 3378–3387. doi: 10.1021/ja00245a031
Sagar, S., Kaur, M., and Minneman, K. P. (2010). Antiviral lead compounds from marine sponges. Mar. Drugs 8, 2619–2638. doi: 10.3390/md8102619
Sakai, R., Rinehart, K. L., Kishore, V., Kundu, B., Faircloth, G., Gloer, J. B., et al. (1996). Structure-activity relationships of the didemnins. J. Med. Chem. 39, 2819–2834. doi: 10.1021/jm960048g
Sakai, R., Stroh, J. G., Sullins, D. W., and Rinehart, K. L. (1995). Seven new didemnins from the marine Tunicate Trididemnum solidum. J. Am. Chem. Soc. 117, 3734–3748. doi: 10.1021/ja00118a010
Schneider, C. A., Rasband, W. S., and Eliceiri, K. W. (2012). NIH Image to ImageJ: 25 years of image analysis. Nat. Methods 9, 671–675. doi: 10.1038/nmeth.2089
Shaala, L., Youssef, D., Badr, J., and Harakeh, S. (2016). Bioactive 2(1H)-pyrazinones and diketopiperazine alkaloids from a tunicate-derived actinomycete Streptomyces sp. Molecules 21:1116. doi: 10.3390/molecules21091116
Souza, T. M. L., Abrantes, J. L., Epifanio, R. D. A., Fontes, C. F. L., and Frugulhetti, I. C. P. P. (2007). The alkaloid 4-methylaaptamine isolated from the sponge Aaptos aaptos impairs Herpes simplex virus type 1 penetration and immediate-early protein synthesis. Planta Med. 73, 200–205. doi: 10.1055/s-2007-967109
Stout, E. P., Yu, L. C., and Molinski, T. F. (2012). Antifungal diterpene alkaloids from the caribbean sponge Agelas citrina: unified configurational assignments of Agelasidines and Agelasines. Eur. J. Org. Chem. 2012, 5131–5135. doi: 10.1002/ejoc.201200572
Sulejmani, N., Nagai, S., Safwan, M., Rizzari, M. D., Raoufi, M., Abouljoud, M. S., et al. (2018). Brincidofovir as salvage therapy for adenovirus disease in intestinal transplant recipients. Pharmacother. J. Hum. Pharmacol. Drug Ther. 38, 470–475. doi: 10.1002/phar.2094
Takeara, R., Jimenez, P. C., Wilke, D. V., Odorico de Moraes, M., Pessoa, C., Peporine Lopes, N., et al. (2008). Antileukemic effects of Didemnum psammatodes (Tunicata: Ascidiacea) constituents. Comp. Biochem. Physiol. Part A Mol. Integr. Physiol. 151, 363–369. doi: 10.1016/J.CBPA.2007.02.011
Takebayashi, Y., Pourquier, P., Zimonjic, D. B., Nakayama, K., Emmert, S., Ueda, T., et al. (2001). Correction: antiproliferative activity of ecteinascidin 743 is dependent upon transcription-coupled nucleotide-excision repair. Nat. Med. 7:1255. doi: 10.1038/nm1101-1255c
Tan, D., Zhu, H., Fu, Y., Tong, F., Yao, D., Walline, J., et al. (2016). Severe community-acquired pneumonia caused by human adenovirus in immunocompetent adults: a multicenter case series. PLoS One 11:e0151199. doi: 10.1371/journal.pone.0151199
Topalian, S. L., Hodi, F. S., Brahmer, J. R., Gettinger, S. N., Smith, D. C., McDermott, D. F., et al. (2012). Safety, activity, and immune correlates of anti–PD-1 antibody in cancer. N. Engl. J. Med. 366, 2443–2454. doi: 10.1056/NEJMoa1200690
Toth, K., Spencer, J. F., Dhar, D., Sagartz, J. E., Buller, R. M. L., Painter, G. R., et al. (2008). Hexadecyloxypropyl-cidofovir, CMX001, prevents adenovirus-induced mortality in a permissive, immunosuppressed animal model. Proc. Natl. Acad. Sci. U.S.A. 105, 7293–7297. doi: 10.1073/pnas.0800200105
Tunnell, J. W., Chávez, E. A., Withers, K., and Earle, S. (eds) (2007). Coral Reefs of the Southern Gulf of Mexico. Texas, TX: Texas A&M University Press.
Villela, P. C., Sale, P., Gold-Bouchot, G., and Kjerfve, B. (2003). Mesoamerican Barrier Reef Systems Project: Manual of Method for the MBRS Synoptic Monitoring Program. Selected Methods for Monitoring Physical and Biological Parameters for Use in the Mesoamerican Region. Belize: Mesoamerican Barrier Reef Systems Project (MBRS).
Vo, T.-S., and Kim, S.-K. (2010). Potential Anti-HIV agents from marine resources: an overview. Mar. Drugs 8, 2871–2892. doi: 10.3390/md8122871
Vogelstein, B., and Kinzler, K. W. (2004). Cancer genes and the pathways they control. Nat. Med. 10, 789–799. doi: 10.1038/nm1087
Wang, Q., Sun, Y., Yang, L., Luo, X., de Voogd, N. J., Tang, X., et al. (2020). Bishomoscalarane sesterterpenoids from the sponge Dysidea granulosa collected in the South China Sea. J. Nat. Prod. 83, 516–523. doi: 10.1021/acs.jnatprod.9b01202
Widmer, N., Bardin, C., Chatelut, E., Paci, A., Beijnen, J., Levêque, D., et al. (2014). Review of therapeutic drug monitoring of anticancer drugs part two - targeted therapies. Eur. J. Cancer 50, 2020–2036. doi: 10.1016/j.ejca.2014.04.015
Williams, D. E., Allen, T. M., Van Soest, R., Behrisch, H. W., and Andersen, R. J. (2001). Glánvillic acids A and B and methyl capucinoate A, new metabolites isolated from the Caribbean sponges Plakortis halichondrioides and Plakinastrella onkodes. J. Nat. Prod. 64, 281–285. doi: 10.1021/np000417i
Wogan, G. N., Hecht, S. S., Felton, J. S., Conney, A. H., and Loeb, L. A. (2004). Environmental and chemical carcinogenesis. Semin. Cancer Biol. 14, 473–486. doi: 10.1016/j.semcancer.2004.06.010
Wyche, T. P., Hou, Y., Braun, D., Cohen, H. C., Xiong, M. P., and Bugni, T. S. (2011). First natural analogs of the cytotoxic thiodepsipeptide thiocoraline a from a marine Verrucosispora sp. J. Org. Chem. 76, 6542–6547. doi: 10.1021/jo200661n
Yoon, B. W., Song, Y. G., and Lee, S. H. (2017). Severe community-acquired adenovirus pneumonia treated with oral ribavirin: a case report. BMC Res. Notes 10:1–4. doi: 10.1186/s13104-016-2370-2
Yu, H. X., Zhao, M. M., Pu, Z. H., Wang, Y. Q., and Liu, Y. (2015). Clinical data analysis of 19 cases of community-acquired adenovirus pneumonia in immunocompetent adults. Int. J. Clin. Exp. Med. 8, 19051–19057.
Keywords: antiviral, antiproliferative, Yucatan Peninsula, marine organisms extracts, sponges, ascidians, gorgonian
Citation: Pech-Puch D, Berastegui-Cabrera J, Pérez-Povedano M, Villegas-Hernández H, Guillén-Hernández S, Cautain B, Reyes F, Pachón J, Gómez P, Rodríguez J, Jiménez C and Sánchez-Céspedes J (2020) Antiviral and Antiproliferative Potential of Marine Organisms From the Yucatan Peninsula, Mexico. Front. Mar. Sci. 7:607. doi: 10.3389/fmars.2020.00607
Received: 24 April 2020; Accepted: 01 July 2020;
Published: 20 August 2020.
Edited by:
Maria Angeles Esteban, University of Murcia, SpainReviewed by:
Daniel Robledo, Center for Research and Advanced Studies of the National Polytechnic Institute (CINVESTAV), MexicoPerumal Karthick, Sea6 Energy Pvt. Ltd., India
Copyright © 2020 Pech-Puch, Berastegui-Cabrera, Pérez-Povedano, Villegas-Hernández, Guillén-Hernández, Cautain, Reyes, Pachón, Gómez, Rodríguez, Jiménez and Sánchez-Céspedes. This is an open-access article distributed under the terms of the Creative Commons Attribution License (CC BY). The use, distribution or reproduction in other forums is permitted, provided the original author(s) and the copyright owner(s) are credited and that the original publication in this journal is cited, in accordance with accepted academic practice. No use, distribution or reproduction is permitted which does not comply with these terms.
*Correspondence: Jaime Rodríguez, amFpbWUucm9kcmlndWV6QHVkYy5lcw==; Carlos Jiménez, Y2FybG9zLmppbWVuZXpAdWRjLmVz; Javier Sánchez-Céspedes, anNhbmNoZXotaWJpc0B1cy5lcw==
†These authors have contributed equally to this work and share first authorship