- 1Centre for Ecology and Conservation, University of Exeter, Penryn, UK
- 2Department of Environment and Maritime Affairs, South Caicos, Turks and Caicos Islands
- 3Marine Conservation Society, Ross on Wye, UK
Disease in wildlife populations is often controlled through culling. But when healthy individuals are removed and diseased individuals are left in the population, it is anticipated that prevalence of disease increases. Although this scenario is presumably common in exploited populations where infected individuals are less marketable, it is not widely reported in the literature. We describe this scenario in a marine turtle fishery in the Turks and Caicos Islands (TCI), where green turtles are harvested for local consumption. During a 2-year period, we recorded the occurrence of fibropapillomatosis (FP) disease in green turtles (Chelonia mydas) captured during in-water surveys and compared it with that of turtles landed in the fishery. 13.4% (n = 32) of turtles captured during in-water surveys showed externally visible signs of FP. FP occurred at specific geographic locations where fishing also occurred. Despite the disease being prevalent in the size classes selected by fishers, FP was not present in any animals landed by the fishery (n = 162). The majority (61%) of fishers interviewed expressed that they had caught turtles with FP. Yet, 82% of those that had caught turtles with the disease chose to return their catch to the sea, thereby selectively harvesting healthy turtles and leaving those with the disease in the population. Our study illustrates that fisher choice may increase the prevalence of FP disease and highlights the importance of this widely neglected driver in the disease dynamics of exploited wildlife populations.
Introduction
It is thought that emerging infectious diseases of wildlife are increasing globally, with consequences to human, animal and ecosystem health (Cohen, 2000; Daszak et al., 2000; Ward and Lafferty, 2004; Jones et al., 2008). Causes of disease emergence are varied, complex and difficult to study but frequently implicate anthropogenic impacts (McCallum and Dobson, 1995; Daszak et al., 2001; Plowright et al., 2008). The consequences of wildlife exploitation on disease dynamics in host species, however, are widely neglected in resource management (Choisy and Rohani, 2006). What happens when non-diseased animals are exploited and diseased individuals are left in the population is a central question in this paper. Models have shown that predation (and likely harvesting) may increase disease prevalence when only immune or non-diseased individuals are selected (Choisy and Rohani, 2006; Holt and Roy, 2007). Such a scenario is presumably common, for example, in fisheries when disease renders fish less marketable (Dobson and May, 1987; Kuris and Lafferty, 1992; Wood et al., 2010). However, there are few empirical examples of this in marine ecosystems.
Harvesting may alter disease prevalence through preferential removal of non-infected animals (Choisy and Rohani, 2006). When this results in an increase in the disease, it may present a serious threat to wildlife conservation, particularly in the management of species of conservation concern. In several crustacean fisheries around the world, where diseased products are unpalatable or unmarketable and tend not to be landed in the fishery, the incidence of disease has been directly correlated with fishing effort (Kuris and Lafferty, 1992; Stentiford and Shields, 2005; Freeman and Macdiarmid, 2009; Bateman et al., 2011).
Fibropapillomatosis (FP) is a disease characterized by external and internal tumors (fibropapillomas) and has been found in most species of sea turtle, but primarily in green turtle (Chelonia mydas) (Herbst, 1994) (Figure 1). Since its discovery in the 1930s (Smith and Coates, 1938), FP has become a global pandemic (Williams et al., 1994) and has received much attention in the popular media and scientific literature. FP is considered one of the most significant neoplastic (the process of tumor formation) diseases in reptiles (Herbst, 1994). Although fibropapillomas appear to be benign, their location, size and frequency may be debilitating to the host by impeding vision, feeding, swimming, and internal organ function. Some studies, however, have documented the regression of infection, even in advanced cases (Chaloupka et al., 2009).
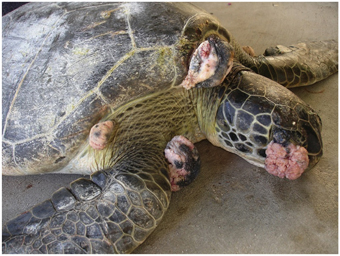
Figure 1. Green turtle showing externally visible signs of fibropapillomatosis (FP). This image was shown to fishers during interviews (Photo: A. Sanghera).
Many green turtle populations have been depleted by exploitation for food, leading to their globally endangered status (IUCN, 2010). It is believed that FP might impair recovery of such depleted populations (Herbst, 1994; Ene et al., 2005) because there is particularly high prevalence of the disease in immature animals, which is thought to impact the long-term survival of green turtle populations (Greenblatt et al., 2005). It is widely reported that turtle size is a risk factor for FP, such that larger juveniles are more prone to the disease than other size classes (Chaloupka and Balazs, 2005; Foley et al., 2005; Chaloupka et al., 2008; Van Houtan et al., 2010). Geographic location is also frequently implicated as a risk factor (Van Houtan et al., 2010). FP tends to be more prevalent in near-shore habitats such as lagoons and bays, especially those impacted by agricultural, industrial or urban developments, possibly due to poor water exchange (Herbst, 1994; Foley et al., 2005; dos Santos et al., 2010; Van Houtan et al., 2010). FP has consequently been associated with various environmental factors such as contaminants/pollutants, bio-toxins from algae and water temperature (Herbst and Klein, 1995; Arthur et al., 2008; Van Houtan et al., 2010, 2014). There is strong evidence that FP is caused by a herpes virus (Lackovich et al., 1999; Lu et al., 2000; Quackenbush et al., 2001; Herbst et al., 2004; Patricio et al., 2012; Alfaro-Núñez et al., 2014). However, its method of transmission is yet to be verified, although ectoparasite vectors have been implicated (Greenblatt et al., 2004).
In the historically and currently exploited Caribbean population of green turtles, where multiple nations take turtles for domestic consumption (Humber et al., 2014), the dynamics of FP prevalence are unknown. The Turks and Caicos Islands (TCI) has one of the largest legal marine turtle fisheries in the Caribbean region (Stringell et al., 2013). This setting provided an ideal opportunity to quantify and assess the impact of harvest on marine turtle populations. The presence of FP in the green turtle population of TCI, first documented in 2002 (Godley et al., 2004; Richardson et al., 2009), made this a suitable system in which to study the effect of selective harvest on FP prevalence in green turtles. In this study, we ask three broad questions: (1) do we observe different levels of FP prevalence in turtles landed by the fishery and those sampled during independent in-water surveys; (2) are turtle size and location risk factors for FP; and (3) are fishers selectively harvesting diseased animals?
Materials and Methods
This study was approved by the University of Exeter Ethical Committee and adhered to the Code of Human Research Ethics set out by the British Psychological Society. Informed consent was obtained from all subjects and identities remained anonymous. The ethics committee approved the in-water animal handling, sampling and tagging based on the Guidelines for the Treatment of Animals in Behavioral Research and Teaching from The Association of the Study of Animal Behavior. Research permission was granted by the Department of Environment and Maritime Affairs, TCI.
Study Site
This study took place over two years between November 2008 and December 2010 in the TCI, a UK Overseas Territory in the Caribbean (21°45N, 71°35W). Here, there are minor hawksbill turtle (Eretmochelys imbricata) and minor green turtle nesting populations (Stringell et al., 2015), yet regionally significant foraging aggregations of both species (Richardson et al., 2009). The islands have numerous creeks and shallow muddy-sand banks that offer extensive seagrass habitat ideal for foraging green turtles. The research team was stationed on South Caicos, the main fishing center of TCI, for the duration of this study. Regular visits were made to the islands of Grand Turk and Providenciales, the two main population centers, and several visits were made to North and Middle Caicos (Figure 2).
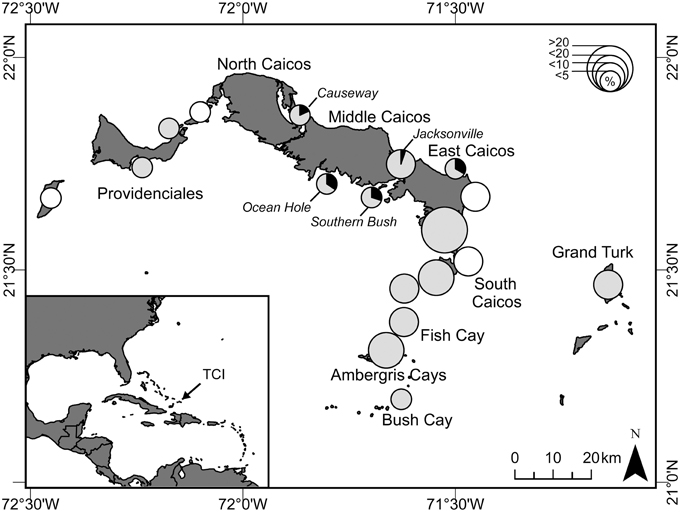
Figure 2. Map of Turks and Caicos Islands (TCI) showing locations (pies) where green turtles were harvested, and prevalence of fibropapillomatosis (FP). Size of pies indicates the relative percentage of the total harvest (< 5, < 10, < 20, and >20%; n = 233 turtles) during 25 months of survey (November 2008 to December 2010). Shaded pies indicate areas where we also conducted capture-mark-recapture (CMR) surveys, and the prevalence of fibropapillomatosis (black) in turtles caught in these surveys is shown. White circles indicate locations where turtles were harvested but no CMR surveys were conducted.
Monitoring Methods
In-water Surveys and Turtle Measurements
We used past information (Godley et al., 2004; Richardson et al., 2009) and local knowledge (Hall and Close, 2007) to select survey locations that reflected a range of turtle fishing intensities. We surveyed 14 locations in TCI in an extensive in-water capture-mark-recapture (CMR) program to sample foraging green turtles. We hand-captured 239 individual turtles via a combination of “free-diving” and “rodeo-style” (Ehrhart and Ogren, 1999), the commonest methods used by TCI fishers to catch turtles. We used turtle fishers and their boats for most surveys, with the fishers themselves making most of the captures. In general, each location was visited at least four times per year to provide a seasonal spread of effort, but logistics and weather occasionally constrained this.
Both CMR and harvested turtles were measured by curved carapace length (CCL cm: Bolten, 1999), weighed using Kern digital scales for turtles under 50 kg (±0.05 kg) or Salter analog scales for those weighing over 50 kg (±0.5 kg), and visually assessed for presence or absence of lesions typical of external FP tumors (Figure 1). With the exception of three individuals that developed FP between recaptures (where final capture measurements were used), we used data from the first capture of turtles in our analyses (CCL, weight, and location). Size at first capture provided the most data given that most turtles were captured only once (n = 355); there were 46 recaptures during our study (38 recaptured once, six recaptured twice and two turtles recaptured three times), and it was not always possible to measure recaptures landed by fishers. For in-water surveys, capture location was recorded by handheld GPS. The capture location of butchered turtles was approximated after discussion with fishers.
Turtle Fishery
We observed the turtle fishery at eight key fish-landing docks throughout the TCI (see Stringell et al., 2013 for details). All landings were recorded at South Caicos on 544 days of the 2-year study, 77 days at Grand Turk and 68 days at Providenciales. From these surveys, we recorded a total of 233 green turtles being butchered, with 162 visually assessed for the presence of FP. From discussion with fishers, we recorded capture location for 89% (n = 208) of all butchered turtles and for 92% (n = 149) of those assessed for FP. An index of turtle fishing intensity was created from the proportion of turtles harvested at each location during the survey period (Figure 2).
Fisher Interviews
The authors (AS, QP, TS) carried out interviews with 28 fishers at South Caicos (n = 13), Providenciales (n = 6), Grand Turk (n = 5), and North Caicos (n = 4). This represented approximately 10% of licensed fishers in TCI (287 licenses for fishing year 2009/10) and 18% of TCI's turtle fishers, and included full-time and part-time fishers and a variety of ages and ethnicities. The top 10 turtle fishers in the TCI (all of which were interviewed) contributed to the majority (63%) of the turtle catch. Subjects were selected purposively rather than randomly. That is, we intentionally sought people who we knew to be engaged in directed or incidental take of marine turtles. These people were selected through existing networks and “snowball” sampling where interviewees were asked to identify further people to approach. Having conducted an extended ethnographic study of the fishing community of South Caicos over 2 years, field staff had an excellent knowledge of fishing practices based on observation as well as an established rapport with fishers. This investment in relationship-building engendered confidence in the quality of information that was shared with researchers, which was supported by observations during accompanied fishing trips.
During the interview, a set of questions related to FP in turtles and harvesting practice (see Supplementary Table 1) was explored and an image of a green turtle with FP was shown (Figure 1). Interviews were conducted in English with all respondents individually in a semi-structured manner: discussion was not limited to the questions and respondents were encouraged to share as much information as they wanted to. Interviews generally lasted between 5 and 10 min but frequently ran over as discussions developed, and the subject was revisited over the sampling period to substantiate claims and verify answers. No fishers declined to interview.
Statistical Analyses
The actual number of turtles harvested with FP was compared to a null distribution of the number of turtles with FP expected in the fishery. The null model was created by: (1) restricting turtle size to that landed in the fishery (28.8–88.0 cm CCL; this removed 11 turtles from the 239 captured during CMR surveys); (2) using harvesting locations where FP was recorded in our CMR surveys (n = 5 sites, n = 140 turtles); and (3) taking the proportion of FP prevalence at these locations, as determined from the CMR data, multiplied by the number of turtles harvested from these locations. The data were sampled with replacement and a distribution of 10,000 means was generated, from which an expected 95% confidence interval was obtained. A p-value was calculated from the number of means that were equal to or worse than the observed number of turtles harvested with FP divided by the number of simulations. Two-sample t-tests were used to test differences in average CCL between groups (CMR vs. butchered turtles, with and without FP). These analyses were conducted in R v 2.13.0 (R Development Core Team, 2011). We then investigated the risk factors of location, size and their interaction on FP incidence using a two-way crossed mixed-effects permutational ANOVA using PERMANOVA+ (Anderson et al., 2008) and PRIMER v6 (Clarke and Gorley, 2006). CCL was the response variable, location a random factor with 13 levels (one location was excluded as an outlier) and FP a binary fixed factor. Where significant differences existed, we investigated pairwise comparisons of CCL of the FP factor at locations where FP occurred.
Results
Fishery vs. CMR
Of the 239 green turtles captured and released during our in-water CMR surveys, 13.4% (n = 32) showed externally visible signs of lesions consistent with FP. None of the turtles captured in the fishery that we assessed (n = 162) appeared to have the disease. The absence of FP in harvested turtles departed significantly (P < 0.001) from the expected number of FP-infected turtles captured in the fishery (null model). The null distribution had a mean of 0.98 (95% CI: 0.08–2.52).
FP Prevalence and Body Size
Turtles with FP caught in CMR surveys were significantly larger (mean CCL = 54.0 cm, SD = 10.2, n = 32) than those without FP (mean CCL = 42.7 cm, SD = 11.4, n = 207) [t(43.7) = 5.71, P < 0.001] (Figure 3), with FP most prevalent in turtles of 65–70 cm CCL (Figure 3A). A prevalence value of 50% at the 80–85 cm size range is probably an artifact of small sample size (n = 2). Turtles with FP did not differ in terms of body condition (weight vs. CCL) to those that were FP free (Supplementary Figure 1). Turtles captured by the fishery averaged 52.6 cm CCL (SD = 12.3, n = 136; Figure 3B), similar to turtles captured in CMR surveys with FP [t(54.16) = 0.6639, P = 0.510]. These results indicate that FP is present in the size classes of turtles selected by the fishers and that we would expect to find some harvested turtles to have FP.
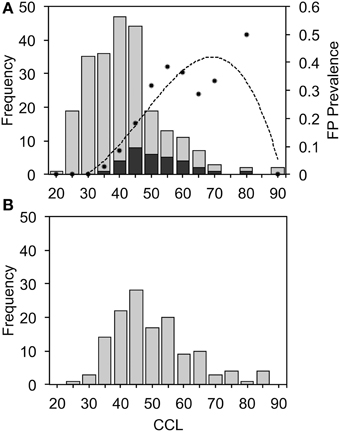
Figure 3. Curved carapace length (CCL, cm) of green turtles captured during capture-mark-recapture surveys (A) or in the fishery (B), showing external signs of fibropapillomatosis (FP) (stacked black bars) or no FP (gray bars). Dots in (A) indicate FP prevalence within each size-class and the dashed line indicates a 3-order polynomial fit (R2 = 0.84) of FP prevalence by size.
FP prevalence and Location
FP occurred only at central island locations, where prevalence varied from 5 to 34% (Figure 2). About 10% (n = 23 of 233) of turtles were harvested at these locations (Figure 2). Fishers therefore exploit turtles from areas where FP occurs and are likely to encounter them. There was a strong spatial effect to turtle size, with turtles generally being larger in central locations [Random factor: F(12) = 5.54, P = 0.001], and the incidence of FP tended to follow this pattern: four out of the five locations that had turtles with FP had the largest average turtle size (Supplementary Figure 2). Although overall, turtles with FP were larger than those without, when we included location in our model, this difference was only significant [Fixed factor: F(1) = 21.31, P = 0.002] at two locations [Causeway: t(1) = 2.3, P = 0.03, n = 22; Jacksonville: t(1) = 2.2, P = 0.024, n = 38], and not at those locations that had the highest prevalence of FP [Ocean Hole: t(1) = 1.53, P = 0.138, n = 32; Southern Bush: t(1) = 1.26, P = 0.216, n = 47]. These results imply that both size and location may be important risk factors for FP.
Fisher Selection and FP Prevalence
The absence of turtles with FP landed by the fishery is likely due to fisher choice. Of the 28 fishers interviewed, 21 (75%) were active turtle fishers at the time of the interviews; the remainder were once, or had worked closely with, turtle fishers (Table 1). Most fishers (61%) had seen or captured green turtles with FP in TCI and 82% of these fishers had returned the turtles to the sea because they did not want to eat diseased meat. Only three fishers reported having harvested turtles with FP and typically cut FP tumors off and sold the meat on to restaurants. The majority of fishers (90%) expressed that they would not harvest turtles with FP in the future. Just two of the 21 fishers stated that, in the future, they would harvest turtles with FP for food, one of which stated that he would eat meat from a turtle with FP, although he had not knowingly done so before.
Discussion
In our study, fishers encounter turtles with FP. However, from our dockside surveys and fisher questionnaires it is clear that fisher choice and harvest practice explains the absence of the disease in their landed catch. The fishery selectively harvests healthy turtles and leaves those with the disease in the population, thereby likely increasing the relative survivorship of turtles with FP through reduced fishing-mortality. This empirical example suggests that harvest and fisher choice may increase the proportion of the population exhibiting FP in green turtle foraging areas in TCI. These results highlight the potential importance of fisher choice as a widely neglected driver in the disease dynamics of exploited wildlife populations.
Although the majority of fishers in TCI would discard turtles that had external signs of FP, a few fishers indicated that they might remove small tumors before selling the turtle. This is presumably to make the turtle more marketable, although we did not observe such practice and would have likely seen such wounds on those whole turtles we inspected in the fishery. Although potential buyers are unlikely to know or detect that tumors have been removed from already-butchered and prepared meat prior to selling, it is unlikely that diseased turtle meat would be sold in TCI; the community is close-knitted and such practice would likely be considered unscrupulous. Indeed, most fishers interviewed stated that they would not want to eat turtle that had FP. Despite FP being pandemic (Williams et al., 1994) and green turtles being taken for food in artisanal fisheries throughout the world, both historically and at present (Humber et al., 2014), comprehensive studies on the human health impact of consuming FP-infected turtles are lacking. Although it is unlikely that there is a human health concern, the perceived risk of consuming diseased turtle meat may be an important reason why fishers choose not to land these turtles and would benefit from further study.
In our study, we estimated FP prevalence from fishery-independent in-water surveys. If observations of FP in harvested turtles had been used to estimate prevalence in the population, then we would have assumed that FP did not exist, when in fact the prevalence of FP in the population was around 13%. Adnyana et al. (1997) calculated the prevalence of FP in green turtles from slaughterhouses in Indonesia to be 21.5%, assuming this was representative of wild stocks in Indonesian seas. Clearly, if fisher selectivity also played a part in the slaughterhouses of Indonesia, then the wild prevalence of FP could have been much greater than that reported. It is, therefore, important to obtain reliable estimates of disease prevalence in wild stocks from fishery-independent surveys. Furthermore, differential catchability of infected animals may bias estimates of disease prevalence rates from harvested stocks (Conner et al., 2000). For example, FP may make turtles more susceptible to capture, because tumors can restrict mobility and impair vision (Herbst, 1994). In our study, it was clear that, despite the possibility that diseased turtles might have been easier to catch, fishers were evidently not choosing to retain them in their catch.
Our in-water survey results indicate that geographic location and turtle size are risk factors that interact; that is, there was a higher prevalence of FP in larger (65–75 cm) turtles and the proportion of these diseased animals was related to location, suggesting turtle size might be partitioned by location. This may be due to water depth at the sampled sites. Larger turtles can forage at greater depth and so at some deeper water sites only larger turtles were present (or caught); small animals tended to be present at shallower sites. The absence of FP in small size-classes (< 35 cm CCL) in the present study may indicate that FP is acquired after turtles recruit to coastal foraging pastures (e.g., Ene et al., 2005), and rarity in large size-classes (>80 cm CCL) may suggest either mortality or tumor regression (e.g., Chaloupka et al., 2009).
FP was only present at central island locations and green turtles at some of these sites had long residence times, as indicated from recapture histories (min. 1 year, n = 5) and satellite tagging data (min. 200 days, n = 7) (Authors' unpublished data). There were also short development times of tumors at these sites: three turtles recaptured during the study developed extensive FP in < 12 months. These two factors are likely to exacerbate the spread of the disease. However, the transmission mode of FP is currently unknown, although density dependence has been suggested (Herbst and Klein, 1995). In density-dependent transmission in parasite-host systems, removal of uninfected hosts may reduce host density, which can lead to a reduction in disease prevalence (Dobson and May, 1987; Wood et al., 2010). Conversely, Holt and Roy (2007) demonstrate a pronounced increase in disease prevalence with increasing take of immune hosts in modeled scenarios of non-regulatory pathogens with density-dependent transmission. In frequency-dependent transmission, removal of uninfected individuals by fishing may result in an increase in the relative proportion of infected hosts and an increase in the prevalence of disease (Wood et al., 2010). Transmission of FP is likely to be more complicated than simple contact transmission (Van Houtan et al., 2010) and further studies on its transmission mode are critically needed.
To better understand the disease and the impact of harvest on FP prevalence, we need to understand the mode of transmission, likely causes of the disease and potential anthropogenic influences. Causal agents of FP in turtles have not been unequivocally isolated (e.g., Work et al., 2014), likely because of the influence of multiple contributory factors. In many aquatic diseases, causal factors are difficult to isolate due to the complexity of interactions. However, anthropogenic factors such as pollution and movements of pathogens into new geographic locations as a result of human activity are commonly implicated (Daszak et al., 2001). Harvesting can have unintended consequences on disease prevalence. For example, overfishing of the food source of harp seals and subsequent seal movement to find alternative prey has indirectly been linked in the spread of phocine distemper virus from infected harp seals to European harbor seals, leading to a massively depleting epizootic in the species (Dietz et al., 1989; Heide-Jorgensen et al., 1992; Härkönen et al., 2006). An increase in FP disease prevalence might be an unintended consequence of preferentially taking non-diseased turtles in the TCI turtle fishery.
High wildlife disease incidence is often associated with environments heavily contaminated with anthropogenic chemicals (McAloose and Newton, 2009). For example, fish diseases have been linked to contaminant burdens and are used to assess health status under European directives (Stentiford et al., 2009, 2010; Lyons et al., 2010). Incidence of FP in green turtles may also prove to be a prime indicator of ecosystem health (Aguirre and Lutz, 2004) because, as juveniles, turtles remain in coastal feeding grounds where they may reflect their local environment and adopt keystone roles in tropical seagrass habitats (Bjorndal and Jackson, 2003). Therefore, surveillance of FP in turtles of TCI might be a useful monitoring tool in this regard. Further research on the influence of environmental conditions (water quality, contaminants etc.) on FP prevalence in TCI, and at other global locations where FP is present, is needed for furthering our knowledge of the multiple factors that contribute to this disease. This may provide insight in how best to reduce the disease burden in marine turtles.
With limited monitoring capacity in TCI, it remains to be seen whether the prevalence of FP in TCI will increase in the coming decade as a result of the turtle fishery. To further understand how harvesting might affect FP prevalence in marine turtles, monitoring some of the world's last remaining turtle fisheries (e.g., Humber et al., 2014) and gaining fishery independent assessments of FP prevalence at these locations is required. Rapid increases in FP have been reported in Hawaii (Chaloupka et al., 2009) and Florida (Foley et al., 2005) since the 1980s and 1930s, respectively. Both of these regions had substantial historical harvests and fisher choice may have played a part in the emergence of the disease in these locations. More widely, the effect of harvesting on disease emergence in fisheries and other exploitation scenarios needs more research. Knowledge of this neglected driver may prove invaluable in informing how exploitation might influence wildlife disease, especially in the control of epizootics of threatened species.
Author Contributions
AB, WC, BG, SR, and PR instigated the program of work and obtained funding. TS and AB conceived the study. QP, PR, AS, and TS conducted the fieldwork. TS led the analysis and writing with input from all authors.
Conflict of Interest Statement
The authors declare that the research was conducted in the absence of any commercial or financial relationships that could be construed as a potential conflict of interest.
Acknowledgments
The Turks and Caicos Islands Turtle Project is a collaborative project between the Department of Environment and Maritime Affairs, TCI (DEMA, formerly the Department of Environment and Coastal Resources, DECR); Marine Conservation Society (MCS), UK; University of Exeter, UK; Duke University, USA; and The School for Field Studies, Center for Marine Resource Studies, TCI (SFS). It was established in November 2008 to assess marine turtle populations and their use in TCI with a view to improving the management of the Islands' turtle fishery. This work was funded by: Natural Environment Research Council (NERC) CASE PhD studentship to TS, with the MCS as CASE partners (Ref: NE/F01385X/1). Simon and Anne Notley provided additional support and DEMA and SFS gave in-country support. We thank Marta Calosso, John Claydon, Flora Kent, SFS staff and students for fieldwork assistance, and Xavier Harrison for advice on randomization. Special thanks to the fishers of South Caicos, and interview participants throughout TCI who made this study possible. We thank the reviewers who helped improve this paper.
Supplementary Material
The Supplementary Material for this article can be found online at: http://journal.frontiersin.org/article/10.3389/fmars.2015.00057
References
Adnyana, W., Ladds, P. W., and Blair, D. (1997). Observations of fibropapillomatosis in green turtles (Chelonia mydas) in Indonesia. Aust. Vet. J. 75, 737–742. doi: 10.1111/j.1751-0813.1997.tb12258.x
Aguirre, A. A., and Lutz, P. L. (2004). Marine turtles as sentinels of ecosystem health: is fibropapillomatosis an indicator? EcoHealth 1, 275–283. doi: 10.1007/s10393-004-0097-3
Alfaro-Núñez, A., Bertelsen, M. F., Bojesen, A. M., Rasmussen, I., Zepeda-Mendoza, L., Olsen, M. T., et al. (2014). Global distribution of Chelonid fibropapilloma-associated herpesvirus among clinically healthy sea turtles. BMC Evol. Biol. 14:206. doi: 10.1186/s12862-014-0206-z
Anderson, M. J., Gorley, R. N., and Clarke, K. R. (2008). PERMANOVA+ for PRIMER: Guide to Software and Statistical Methods. Plymouth: PRIMER-E.
Arthur, K., Limpus, C., Balazs, G., Capper, A., Udy, J., Shaw, G., et al. (2008). The exposure of green turtles (Chelonia mydas) to tumour promoting compounds produced by the cyanobacterium Lyngbya majuscula and their potential role in the aetiology of fibropapillomatosis. Harmful Algae 7, 114–125. doi: 10.1016/j.hal.2007.06.001
Bateman, K. S., Hicks, R. J., and Stentiford, G. D. (2011). Disease profiles differ between non-fished and fished populations of edible crab (Cancer pagurus) from a major commercial fishery. ICES J. Mar. Sci. 68, 2044–2052. doi: 10.1093/icesjms/fsr148
Bjorndal, K. A., and Jackson, J. B. C. (2003). “Roles of sea turtles in marine ecosystems: reconstructing the past,” in The Biology of Sea Turtles, Vol. II, eds P. L. Lutz, J. A. Musick, and J. Wyneken (Florida: CRC Press), 259–273.
Bolten, A. B. (1999). “Techniques for measuring sea turtles,” in Research and Management Techniques for the Conservation of Sea Turtles, eds K. L. Eckert, K. A. Bjorndal, F. A. Abreu-Grobois, and M. Donnelly (Washington, DC: IUCN/SSC Marine Turtle Specialist Group), 110–114.
Chaloupka, M., and Balazs, G. (2005). Modelling the effect of fibropapilloma disease on the somatic growth dynamics of Hawaiian green sea turtles. Mar. Biol. 147, 1251–1260. doi: 10.1007/s00227-005-0026-1
Chaloupka, M., Balazs, G. H., and Work, T. M. (2009). Rise and fall over 26 years of a marine epizootic in hawaiian green sea turtles. J. Wildl. Dis. 45, 1138–1142. doi: 10.7589/0090-3558-45.4.1138
Chaloupka, M., Work, T., Balazs, G., Murakawa, S. K., and Morris, R. (2008). Cause-specific temporal and spatial trends in green sea turtle strandings in the Hawaiian Archipelago (1982–2003). Mar. Biol. 154, 887–898. doi: 10.1007/s00227-008-0981-4
Choisy, M., and Rohani, P. (2006). Harvesting can increase severity of wildlife disease epidemics. Proc. R. Soc. B Biol. Sci. 273, 2025–2034. doi: 10.1098/rspb.2006.3554
Cohen, M. L. (2000). Changing patterns of infectious disease. Nature 406, 762–767. doi: 10.1038/35021206
Conner, M., McCarty, C., and Miller, M. (2000). Detection of bias in harvest-based estimates of chronic wasting disease prevalence in mule deer. J. Wildl. Dis. 36, 691–699. doi: 10.7589/0090-3558-36.4.691
Daszak, P., Cunningham, A. A., and Hyatt, A. D. (2000). Wildlife ecology—emerging infectious diseases of wildlife—threats to biodiversity and human health. Science 287, 443–449. doi: 10.1126/science.287.5452.443
Daszak, P., Cunningham, A. A., and Hyatt, A. D. (2001). Anthropogenic environmental change and the emergence of infectious diseases in wildlife. Acta Trop. 78, 103–116. doi: 10.1016/S0001-706X(00)00179-0
Dietz, R., Ansen, C. T., Have, -P., and Heide-Jørgensen, M. P. (1989). Clue to seal epizootic? Nature 338, 627. doi: 10.1038/338627a0
Dobson, A. P., and May, R. M. (1987). The effects of parasites on fish populations - theoretical aspects. Int. J. Parasitol. 17, 363–370. doi: 10.1016/0020-7519(87)90111-1
dos Santos, R. G., Martins, A. S., Torezani, E., Baptistotte, C., da Nóbrega, F. J., Horta, P. A., et al. (2010). Relationship between fibropapillomatosis and environmental quality: a case study with Chelonia mydas off Brazil. Dis. Aquat. Org. 89, 87–95. doi: 10.3354/dao02178
Ehrhart, L. M., and Ogren, L. H. (1999). “Studies in foraging habitats: capturing and handling turtles,” in Research and Management Techniques for the Conservation of Sea Turtles, eds K. L. Eckert, K. A. Bjorndal, F. A. Abreu-Grobois, and M. Donnelly (Washington, DC: IUCN/SSC Marine Turtle Specialist Group Publication No. 4), 61–64.
Ene, A., Su, M., Lemaire, S., Rose, C., Schaff, S., Moretti, R., et al. (2005). Distribution of chelonid fibropapillomatosis-associated herpesvirus variants in florida: molecular genetic evidence for infection of turtles following recruitment to neritic developmental habitats. J. Wildl. Dis. 41, 489–497. doi: 10.7589/0090-3558-41.3.489
Foley, A. M., Schroeder, B. A., Redlow, A. E., Fick-Child, K. J., and Teas, W. G. (2005). Fibropapillomatosis in stranded green turtles (Chelonia mydas) from the eastern United States (1980–98): trends and associations with environmental factors. J. Wildl. Dis. 41, 29–41. doi: 10.7589/0090-3558-41.1.29
Freeman, D. J., and Macdiarmid, A. B. (2009). Healthier lobsters in a marine reserve: effects of fishing on disease incidence in the spiny lobster, Jasus edwardsii. Mar. Freshw. Res. 60, 140–145. doi: 10.1071/MF08091
Godley, B., Broderick, A. C., Campbell, L., Ranger, S., and Richardson, P. (2004). “Chapter 9. An assessment of the status and exploitation of marine turtles in the Turks and Caicos islands,” in An Assessment of the Status and Exploitation of Marine Turtles in the UK Overseas Territories in the Wider Caribbean (Final Project Report for the Department of Environment, Food and Rural Affairs and the Foreign and Commonwealth Office.), 180–222. Available online at: http://www.seaturtle.org/mtrg/projects/tcot/finalreport/
Greenblatt, R. J., Work, T. M., Balazs, G. H., Sutton, C. A., Casey, R. N., and Casey, J. W. (2004). The Ozobranchus leech is a candidate mechanical vector for the fibropapilloma-associated turtle herpesvirus found latently infecting skin tumors on Hawaiian green turtles (Chelonia mydas). Virology 321, 101–110. doi: 10.1016/j.virol.2003.12.026
Greenblatt, R. J., Work, T. M., Dutton, P., Sutton, C. A., Spraker, T. R., Casey, R. N., et al. (2005). Geographic variation in marine turtle fibropapillomatosis. J. Zoo Wildl. Med. 36, 527–530. doi: 10.1638/04-051.1
Hall, G. B., and Close, C. H. (2007). Local knowledge assessment for a small-scale fishery using geographic information systems. Fish. Res. 83, 11–22. doi: 10.1016/j.fishres.2006.08.015
Härkönen, T., Dietz, R., Reijnders, P., Teilmann, J., Harding, K., Hall, A., et al. (2006). The 1988 and 2002 phocine distemper virus epidemics in European harbour seals. Dis. Aquat. Org. 68, 115–130. doi: 10.3354/dao068115
Heide-Jorgensen, M. P., Harkonen, T., Dietz, R., and Thompson, P. M. (1992). Retrospective of the 1988 European seal epizootic. Dis. Aquat. Org. 13:37. doi: 10.3354/dao013037
Herbst, L., Ene, A., Su, M., Desalle, R., and Lenz, J. (2004). Tumor outbreaks in marine turtles are not due to recent herpesvirus mutations. Curr. Biol. 14, R697–R699. doi: 10.1016/j.cub.2004.08.040
Herbst, L. H. (1994). Fibropapillomatosis of marine turtles. Annu. Rev. Fish Dis. 4, 389. doi: 10.1016/0959-8030(94)90037-X
Herbst, L. H., and Klein, P. A. (1995). Green turtle fibropapillomatosis: challenges to assessing the role of environmental cofactors. Environ. Health Perspect. 103(Suppl. 4), 27–30. doi: 10.1289/ehp.95103s427
Holt, R. D., and Roy, M. (2007). Predation can increase the prevalence of infectious disease. Am. Nat. 169, 690–699. doi: 10.1086/513188
Humber, F., Godley, B. J., and Broderick, A. C. (2014). So excellent a fishe: a global overview of legal marine turtle fisheries. Divers. Distrib. 20, 579–590. doi: 10.1111/ddi.12183
IUCN. (2010). IUCN Red List of Threatened Species. Version 2010.1. Available online at: www.iucnredlist.org [Online]. [Accessed 14 March 2013].
Jones, K. E., Patel, N. G., Levy, M. A., Storeygard, A., Balk, D., Gittleman, J. L., et al. (2008). Global trends in emerging infectious diseases. Nature 451, 990–993. doi: 10.1038/nature06536
Kuris, A. M., and Lafferty, K. D. (1992). Modelling crustacean fisheries: effects of parasites on management strategies. Can. J. Fish. Aquat. Sci. 49, 327. doi: 10.1139/f92-037
Lackovich, J. K., Brown, D. R., Homer, B. L., Garber, R. L., Mader, D. R., Moretti, R. H., et al. (1999). Association of herpesvirus with fibropapillomatosis of the green turtle Chelonia mydas and the loggerhead turtle Caretta caretta in Florida. Dis. Aquat. Org. 37, 89–97. doi: 10.3354/dao037089
Lu, Y., Wang, Y., Yu, Q., Aguirre, A. A., Balazs, G. H., Nerurkar, V. R., et al. (2000). Detection of herpesviral sequences in tissues of green turtles with fibropapilloma by polymerase chain reaction. Arch. Virol. 145, 1885–1893. doi: 10.1007/s007050070063
Lyons, B. P., Thain, J. E., Stentiford, G. D., Hylland, K., Davies, I. M., and Vethaak, A. D. (2010). Using biological effects tools to define good environmental status under the European union marine strategy framework directive. Mar. Pollut. Bull. 60, 1647–1651. doi: 10.1016/j.marpolbul.2010.06.005
McAloose, D., and Newton, A. L. (2009). Wildlife cancer: a conservation perspective. Nat. Rev. Cancer 9, 517–526. doi: 10.1038/nrc2665
McCallum, H., and Dobson, A. P. (1995). Detecting disease and parasite threats to endangered species and ecosystems. Trends Ecol. Evol. 10, 190. doi: 10.1016/S0169-5347(00)89050-3
Patrício, A. R., Herbst, L. H., Duarte, A., Vélez-Zuazo, X., Santos Loureiro, N., Pereira, N., et al. (2012). Global phylogeography and evolution of the chelonid fibropapilloma-associated herpesvirus. J. Gen. Virol. 93, 1035–1045. doi: 10.1099/vir.0.038950-0
Plowright, R. K., Sokolow, S. H., Gorman, M. E., Daszak, P., and Foley, J. E. (2008). Causal inference in disease ecology: investigating ecological drivers of disease emergence. Front. Ecol. Environ. 6, 420–429. doi: 10.1890/070086
Quackenbush, S. L., Casey, R. N., Murcek, R. J., Paul, T. A., Work, T. M., Limpus, C. J., et al. (2001). Quantitative analysis of herpesvirus sequences from normal tissue and fibropapillomas of marine turtles with real-time PCR. Virology 287, 105–111. doi: 10.1006/viro.2001.1023
R Development Core Team. (2011). R: A Language and Environment for Statistical Computing. Vienna: R Foundation for Statistical Computing.
Richardson, P., Broderick, A., Bruford, M., Campbell, L., Clerveaux, W., Formia, A., et al. (2009). Marine turtles in the Turks and Caicos Islands: remnant rookeries, regionally significant foraging stocks and a major turtle fishery. Chelonian Conserv. Biol. 8, 192–207. doi: 10.2744/CCB-0871.1
Smith, G., and Coates, C. (1938). Fibro-epithelial growths of the skin in large marine turtles Chelonia mydas (Linnaeus). Zoologica 23, 93–98.
Stentiford, G. D., Bignell, J. P., Lyons, B. P., and Feist, S. W. (2009). Site-specific disease profiles in fish and their use in environmental monitoring. Mar. Ecol. Prog. Ser. 381, 1–15. doi: 10.3354/meps07947
Stentiford, G. D., Bignell, J. P., Lyons, B. P., Thain, J. E., and Feist, S. W. (2010). Effect of age on liver pathology and other diseases in flatfish: implications for assessment of marine ecological health status. Mar. Ecol. Prog. Ser. 411, 215–230. doi: 10.3354/meps08693
Stentiford, G. D., and Shields, J. D. (2005). A review of the parasitic dinoflagellates Hematodinium species and Hematodinium-like infections in marine crustaceans. Dis. Aquat. Org. 66:47. doi: 10.3354/dao066047
Stringell, T. B., Calosso, M. C., Claydon, J. A., Clerveaux, W., Godley, B. J., Lockhart, K. J., et al. (2013). Marine turtle harvest in a mixed small-scale fishery: evidence for revised management measures. Ocean Coast. Manag. 82, 34–42. doi: 10.1016/j.ocecoaman.2013.05.004
Stringell, T., Clerveaux, W., Godley, B., Phillips, Q., Ranger, S., Richardson, P., et al. (2015). Protecting the breeders: research informs legislative change in a marine turtle fishery. Biodivers. Conserv. 24, 1775–1796. doi: 10.1007/s10531-015-0900-1
Van Houtan, K. S., Hargrove, S. K., and Balazs, G. H. (2010). Land use, macroalgae, and a tumor-forming disease in marine turtles. PLoS ONE 5:e12900. doi: 10.1371/journal.pone.0012900
Van Houtan, K. S., Smith, C. M., Dailer, M. L., and Kawachi, M. (2014). Eutrophication and the dietary promotion of sea turtle tumors. PeerJ 2:e602. doi: 10.7717/peerj.602
Ward, J. R., and Lafferty, K. D. (2004). The elusive baseline of marine disease: are diseases in ocean ecosystems increasing? PLoS Biol. 2:E120. doi: 10.1371/journal.pbio.0020120
Williams, E. H. Jr., Bunkley-Williams, L., Peters, E. C., Pinto-Rodriguez, B., Matos-Morales, R., Mignucci-Giannoni, A. A., et al. (1994). An epizootic of cutaneous fibropapillomas in green turtles Chelonia mydas of the Caribbean: part of a panzootic? J. Aquat. Anim. Health 6, 70–78.
Wood, C. L., Lafferty, K. D., and Micheli, F. (2010). Fishing out marine parasites? Impacts of fishing on rates of parasitism in the ocean. Ecol. Lett. 13, 761–775. doi: 10.1111/j.1461-0248.2010.01467.x
Keywords: fisher choice, harvest, marine turtles, disease prevalence, fibropapillomatosis (FP)
Citation: Stringell TB, Clerveaux WV, Godley BJ, Phillips Q, Ranger S, Richardson PB, Sanghera A and Broderick AC (2015) Fisher choice may increase prevalence of green turtle fibropapillomatosis disease. Front. Mar. Sci. 2:57. doi: 10.3389/fmars.2015.00057
Received: 16 December 2014; Accepted: 30 July 2015;
Published: 27 August 2015.
Edited by:
Loren McClenachan, Colby College, USAReviewed by:
Sarah M. Buckley, University of Queensland, AustraliaTara Sayuri Whitty, Center for Marine Biodiversity and Conservation, USA
Copyright © 2015 Stringell, Clerveaux, Godley, Phillips, Ranger, Richardson, Sanghera and Broderick. This is an open-access article distributed under the terms of the Creative Commons Attribution License (CC BY). The use, distribution or reproduction in other forums is permitted, provided the original author(s) or licensor are credited and that the original publication in this journal is cited, in accordance with accepted academic practice. No use, distribution or reproduction is permitted which does not comply with these terms.
*Correspondence: Thomas B. Stringell and Annette C. Broderick, Centre for Ecology and Conservation, College of Life and Environmental Sciences, University of Exeter, Penryn TR10 9FE, UK,dG9tc3RyaW5nZWxsQGdtYWlsLmNvbQ==;YS5jLmJyb2Rlcmlja0BleGV0ZXIuYWMudWs=