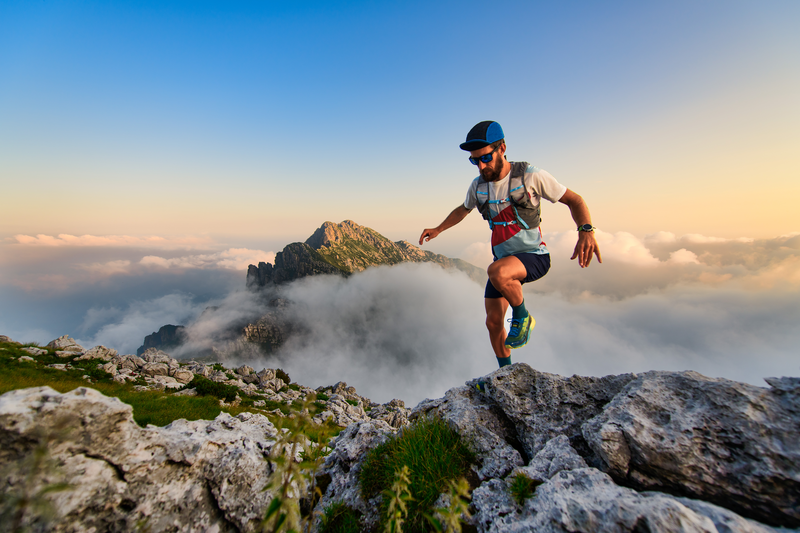
94% of researchers rate our articles as excellent or good
Learn more about the work of our research integrity team to safeguard the quality of each article we publish.
Find out more
MINI REVIEW article
Front. Immunol. , 22 January 2025
Sec. Cancer Immunity and Immunotherapy
Volume 16 - 2025 | https://doi.org/10.3389/fimmu.2025.1548527
This article is part of the Research Topic Harnessing Molecular Insights for Enhanced Drug Sensitivity and Immunotherapy in Cancer View all 22 articles
Pediatric osteosarcoma, the most prevalent primary malignant bone tumor in children, is marked by aggressive progression and a generally poor prognosis. Despite advances in treatment, including multi-agent chemotherapy, survival rates remain suboptimal, with metastasis, particularly to the lungs, contributing significantly to mortality. The tumor microenvironment plays a crucial role in osteosarcoma progression, with immune cells such as tumor-associated macrophages and T lymphocytes significantly influencing tumor behavior. The immunosuppressive environment, dominated by M2 macrophages, contributes to immune evasion and poor therapeutic outcomes, though recent findings suggest the potential for reprogramming these cells to enhance immune responses. This review provides a comprehensive overview of the immune landscape in pediatric osteosarcoma, with a focus on the role of immune cells and their interactions within the tumor microenvironment (TME). It examines the impact of immune checkpoints, genetic mutations, and inflammatory pathways on osteosarcoma progression, highlighting their contribution to tumor immune evasion and disease advancement. Additionally, emerging immunotherapeutic strategies, such as immune checkpoint inhibitors, macrophage reprogramming, and antibody-based therapies, are summarized in detail, showcasing their potential to improve therapeutic outcomes.
Pediatric osteosarcoma is the most common primary malignant bone tumor in children, representing a major challenge in pediatric oncology (1). It typically originates in the metaphyseal regions of long bones, notably the distal femur (43%), proximal tibia (23%), and humerus (10%) (2). The disease is highly aggressive, progressing rapidly with poor prognosis and high mortality rates despite treatment advancements. Pulmonary metastasis is the predominant site of distant spread, occurring in over 85% of metastatic cases, followed by bone metastases (3). Recurrence and metastasis are the main factors contributing to mortality and poor outcomes in pediatric osteosarcoma (3). Over the past four decades, multi-agent chemotherapy has yielded only modest survival improvements (4–7). Although risk factors for recurrence, such as tumor location and histological response to neoadjuvant chemotherapy, have been identified (2, 8, 9), risk-adapted therapies have largely failed to enhance outcomes (5, 10, 11). Consequently, there is an urgent need to identify new, modifiable prognostic factors to guide treatment strategies and develop novel therapies (12, 13).
Inflammation is a key feature in cancer biology, with the tumor microenvironment (TME) central to tumor progression and metastasis (14, 15). Immune cell interactions within the TME are pivotal, as evidenced in melanoma and breast cancer, where immune profiles correlate with patient outcomes and therapy responses (16, 17). The osteosarcoma TME comprises immune cells, osteoblasts, endothelial cells, stromal cells, extracellular matrix, and signaling molecules (14). Predominantly, tumor-associated macrophages (TAMs) with an M2 phenotype are present, alongside T lymphocytes, myeloid cells, and dendritic cells (18, 19). While M2 TAMs generally associate with poor 5-year event-free survival, some studies suggest they may reduce metastasis and improve survival in specific contexts (20). Thus, targeting immune components in the osteosarcoma TME emerges as a promising therapeutic strategy (21).
This review summarizes the current understanding of the immune microenvironment in pediatric osteosarcoma, exploring the potential immunotherapies and prognostic biomarkers that may guide future treatment approaches. By elucidating the role of immune cells, and their influence on disease progression, this review provides new insights into the pathogenesis of osteosarcoma and highlight opportunities for developing more effective and individualized therapies.
The immune system plays a crucial role throughout all stages of diseases (22, 23), with immune dysregulation significantly contributing to cancer initiation and progression (24). In OS, the immune system interacts with the TME in a complex manner. Tumor-infiltrating T cells, which are integral to anti-tumor immune responses, are activated within the TME (25, 26). However, these T cells often upregulate inhibitory receptors on their surface. When these receptors bind to corresponding ligands expressed by tumor cells, immune activity is suppressed. This immune suppression leads to a diminished anti-tumor immune response, allowing tumor cells to escape immune surveillance (27). Immune checkpoint blockade (ICB) therapy, which aims to disrupt these receptor-ligand interactions, has shown promise in enhancing T cell function and improving the immune system’s ability to target and eliminate cancer cells (28). Numerous clinical studies have demonstrated the efficacy of ICB in treating a variety of cancers (29–31), further stimulating research into the immune landscape of osteosarcoma. These findings underscore the importance of the TME in osteosarcoma, as it is closely associated with clinical outcomes, prognosis, and the response to immunotherapy (32, 33). Understanding the immune system’s role within the TME of osteosarcoma is critical for advancing therapeutic strategies aimed at improving outcomes for patients with this aggressive bone tumor (34).
The immune microenvironment in osteosarcoma is complex, characterized by diverse immune cell infiltration, though complete characterization remains elusive (35–37). Macrophages and T cells are the predominant immune cells within osteosarcoma (38–41). Elevated levels of infiltrating macrophages and CD8+ T cells correlate with reduced metastasis and improved survival in osteosarcoma patients (42–44). Conversely, increased infiltration of antigen-presenting cells, such as dendritic cells, correlates with poorer clinical outcomes (45). Natural killer (NK) cells play a critical role in innate immunity by directly killing tumor cells through stress-induced ligands and absence of MHC class I molecules on tumor surfaces (46). However, the osteosarcoma microenvironment often impairs NK cell function through various immunosuppressive mechanisms, including the secretion of inhibitory cytokines and the expression of immune checkpoint molecules (47). Enhancing NK cell activity through cytokine therapy or adoptive cell transfer is being explored as a potential therapeutic strategy in pediatric osteosarcoma (46).
Besides, osteosarcoma cells release PD-L1 to suppress immune responses (48) and indoleamine 2,3-dioxygenase (IDO) inhibiting neoantigen generation by dendritic cells (DCs), facilitating immune escape (49). DCs are essential for antigen presentation and T cell initiation but are compromised by the tumor-induced immunosuppressive environment, such as TGF-β and IL-10, which inhibit DC maturation and antigen-presenting capacity, reducing effective T cell activation (50). Strategies to restore DC function, including DC vaccines and agents blocking immunosuppressive signals, are under investigation to enhance anti-tumor immunity in osteosarcoma (50). Additionally, all-trans retinoic acid (ATRA) suppresses macrophage M2 polarization, inhibiting lung metastasis (51). Signaling pathways like VEGF, IL-10A, TGF-β, and STAT3 modulate the immunosuppressive microenvironment by affecting suppressive cells, macrophages, and stromal fibroblasts (52). These findings underscore the pivotal role of the immune microenvironment in osteosarcoma prognosis and therapeutic outcomes.
Further analysis highlights both homogeneity and heterogeneity within the osteosarcoma immune microenvironment (53). This may partly explain the limited efficacy of current immunotherapies in osteosarcoma, as the TME is dominated by immunosuppressive M2 or non-functional M0 macrophages, hindering effective immune responses. Patients with CD8+ T cell infiltration may derive greater survival benefits from immunotherapies. Thus, inducing the transition of M0 or M2 macrophages to the pro-inflammatory M1 phenotype represents a promising strategy to enhance anti-tumor immune responses in pediatric osteosarcoma (54). Understanding immune cell dynamics within the osteosarcoma TME offers new avenues for more effective immunotherapeutic approaches.
Understanding the immune-related mechanisms is crucial for developing targeted immunotherapies for pediatric osteosarcoma. Recent studies have identified differential expression of immune-related genes (IR-DEGs) within the TME, predominantly upregulated and enriched in various immune pathways (27). Macrophages play a pivotal role in the TME by interacting with other immune cells, promoting tumor development and progression while also contributing to tumor suppression through phagocytosis (55). Furthermore, T-cell cytotoxicity is vital for eliminating tumor cells, with tumor-infiltrating macrophages modulating T-cell activity, thereby impacting cancer prognosis and immunotherapy efficacy (56–58). Genetic mutations in key tumor suppressor genes, such as TP53, ATRX, and RB1 are extensively associated with osteosarcoma (59–61). Wu et al. found that mutations in these genes are more frequent in low-immunity groups, correlating with increased metastasis (27). Sexual dimorphism in immune responses has been observed, with significant differences in pathways related to macrophages, T-cells, B-cells, Th1, Th2, and the complement system (62, 63). Additionally, sex-specific differences are noted in the PD-1/PD-L1 immunotherapy pathway and signaling pathways such as calcium, p53, and cell cycle regulation, suggesting gender-tailored therapies may enhance outcomes (62).
Long non-coding RNAs (lncRNAs) are crucial regulators in osteosarcoma, affecting immune cell infiltration in the TME by modulating immune-related gene expression. A novel modeling algorithm (64) indicated that metastatic osteosarcoma patients have reduced activation of memory CD4+ T cells, monocytes, mast cells, and neutrophils compared to localized cases. irlncRNAs) may influence immune responses by regulating chemokine receptors like CXCR2 in neutrophils (65), and facilitating non-classical monocyte migration to the lungs, a primary metastasis site (66, 67). Hypoxia, common in malignant tumors, significantly impacts osteosarcoma progression by inducing immune cell death and impairing immune responses within the TME (68), including T-cell and NK cell activation, thereby fostering an immunosuppressive environment (69, 70). Moreover, hypoxia enhances VEGF expression, promoting tumor advancement (71). In conclusion, the immune landscape of pediatric osteosarcoma is complex, involving macrophages, T-cells, genetic mutations, gender differences, hypoxia, and lncRNAs, collectively contributing to tumor progression and immune evasion, thus offering novel strategies for improving osteosarcoma prognosis (Figure 1).
Osteosarcoma treatment traditionally encompasses chemotherapy, surgery, and radiation, with methotrexate-based chemotherapy forming the cornerstone of systemic therapy, supplemented by agents such as doxorubicin, and cisplatin. Immunotherapy, an innovative approach in cancer treatment, aims to bolster the immune system’s capability to combat cancer progression and establishment (72).
The most compelling evidence for immune modulation in osteosarcoma comes from the use of mifamurtide [liposomal muramyl tripeptide phosphatidylethanolamine (L-MTP-PE)] (72). Approved for use with standard treatment regimens in non-metastatic osteosarcoma, it raised the 6-year survival rate from 70% with chemotherapy alone to 78% (73–75). L-MTP-PE activates macrophages and monocytes, leveraging the immune system’s cancer-fighting capabilities (74). Punzo et al. observed a slowdown in OS progression through the activation of macrophages by L-MTP-PE and notably, its direct action on MG63 cells. They demonstrated not only the anti-tumor activity of L-MTP-PE in OS but also induced a shift in macrophage phenotype from M1/M2, promoting a balance between pro-inflammatory and immunoregulatory functions (76). L-MTP-PE also influences bone metabolism, inducing anti-osteoporotic effects in children undergoing chemotherapy for osteosarcoma (77).
Antibody therapies like olaratumab, in combination with doxorubicin, has been approved as first-line for soft tissue sarcomas (78), and carotuximab with pazopanib in Phase III trials for angiosarcomas (79), are gaining ground in osteosarcoma treatment. Glembatumumab-vedotin targets osteoactive substances overexpressed on osteosarcoma cells, showing cytotoxic effects in both osteosarcoma and breast cancer (80–83). Trastuzumab, initially for HER2+ breast cancer, targets HER2 tyrosine kinase activity critical for cell proliferation, which is present also in osteosarcoma cells, indicating its potential for osteosarcoma therapy (84, 85). Research with nivolumab in humanized mouse models has revealed that while the primary tumor volume and growth rate of osteosarcoma matched the control group, the rate of lung metastases was significantly reduced, underscoring the potential of targeting the tumor microenvironment with immunotherapy (54).
Recent advances in immunotherapy have shown promise in the treatment of pediatric osteosarcoma, leveraging both innovative antibody technologies and the enhancement of immune system responses. A novel approach involves the use of bispecific antibodies, specifically anti-CD3 x anti-GD2, which recruit T cells to significantly enhance the tumor-killing effects of anti-GD2 antibodies (86). Additionally, the combination of chemotherapy and immunotherapy, such as irinotecan and temozolomide with denosumab, has demonstrated significant activity in neuroblastoma, suggesting its potential applicability in osteosarcoma (87, 88).
CAR-T therapy represents a cutting-edge immunotherapeutic approach that has revolutionized the treatment of certain hematological malignancies and is now being explored in solid tumors, including pediatric osteosarcoma. CAR-T therapy involves the genetic modification of a patient’s T cells to express receptors that specifically target tumor-associated antigens (89). Potential targets for CAR-T therapy include HER2, GD2, and B7-H3, which are overexpressed on osteosarcoma cells (90). Preclinical studies have demonstrated the efficacy of CAR-T cells in recognizing and eliminating osteosarcoma cells in vitro and in vivo (91). Clinical trials are currently underway to evaluate the safety and efficacy of CAR-T cell therapy in pediatric osteosarcoma patients (92). Ongoing research is focused on enhancing the persistence and infiltration of CAR-T cells within the TME in osteosarcoma, as well as overcoming the immunosuppressive barriers that limit their efficacy (92).
Recent strategies include liposomal and aerosolized drug formulations such as sustained-release lipid inhalation targeting cisplatin and aerosolized granulocyte–monocyte colony-stimulating factor (GM-CSF) alongside NK cell infusions and aerosol IL-2, enhancing local chemotherapy efficacy for lung metastases in metastatic osteosarcoma (93). IL-2 activates lymphocytes into lymphokine-activated killer (LAK) cells, effective against multidrug-resistant cells and targeting lung metastasis sites (94, 95), underlining the potential of IL-2 and LAK/NK cell-based therapies in managing pediatric osteosarcoma lung metastases. In addition, denosumab, targeting the receptor activation of nuclear factor kappa-β ligand (RANKL), reduces fracture risks in tumor metastases and showed a 99% inhibition in giant bone cell tumor progression in Phase II studies (96, 97). The RANK-L/RANK/osteoprotegerin (OPG) pathway plays a critical role in osteosarcoma, with studies indicating its involvement in tumor progression and potential as a therapeutic target (98–101). Despite these advances, challenges remain, particularly in managing the drug’s impact on standard chemotherapy effectiveness and deciphering the mechanisms behind resistance to immunotherapy (102–104). Thus, there is a pressing need to identify effective combinations of immunotherapy and conventional treatments to overcome resistance pathways and enhance therapeutic outcomes in pediatric osteosarcoma.
Accurate prediction of prognosis is related to determining the best treatment plan for an individual (105, 106). Therefore, accurate prognostic indicators are crucial for optimal treatment strategies in pediatric osteosarcoma. Traditional markers like Enneking surgical standards and alkaline phosphatase often show variability across the same tumor stages, however lacking precision (107–110). Histological subtype and tumor stage are considered inaccurate and inadequate prognostic parameters (3). Critical adverse prognostic factors include age, gender, tumor size, metastases at diagnosis, and poor chemotherapy response, highlighting the insufficiency of traditional prognostic methods (111–115). Notably, metastasis at diagnosis is a major independent prognostic factor (116, 117). Other reported adverse factors include high serum alkaline phosphatase levels, involvement of the visceral pleura. Additionally, the histological response to neoadjuvant chemotherapy is recognized as a prognostic factor for both metastatic and non-metastatic patients (93). Delay in time to completion of chemotherapy is independently associated with poor prognosis in children with osteosarcoma (118).
The immunological characteristics of the osteosarcoma TME are valuable for prognosis. TAMs and T cells play a key role in determining cancer prognosis and the efficacy of immunotherapies (27). Inflammation markers such as the Systemic Immune-Inflammation Index (SII) are potent predictors of tumor prognosis, offering new directions for predicting survival at different time points and improving long-term survival rates (3). A higher Immune-Score correlates with better survival due to greater immune infiltration, whereas higher Tumor-Purity correlates with lower survival rates (27). Metabolic factors also significantly impact prognosis. Ma et al. have shown that preoperative platelet-to-albumin ratio (PAR) and apolipoprotein B-to-apolipoprotein ratio (ApoB/ApoA1) are independent prognostic factors for 5-year overall survival (105). On the genetic front, Yang et al. identified 69 DEGs related to metastasis and immune infiltration, with GATA3, LPAR5, EVI2B, RIAM and CFH demonstrating prognostic potential (119). Mutations in TP53 and high levels of WNT6, resulting from low DNA methylation, are associated with poor pediatric osteosarcoma prognosis (120, 121). Additionally, circulating tumor DNA (ctDNA) detected by next-generation sequencing (NGS) offers novel prognostic insights for localized bone tumors (122). The multivariate Cox model identified miR-29b and miR-422 as independent prognostic markers, where their downregulation predicts poor outcomes, underscoring their value in pediatric osteosarcoma prognosis (123).
In addition, the presence of a four-gene signature associated with hypoxia (EFNA1, P4HA1, STC2, and MAFF) correlates with clinical and molecular features and is an important prognostic predictor in pediatric osteosarcoma patients (71). Human osteosarcoma cells have exosomes that express specific metalloproteinases (MMP-1 and -13) that are involved in cell recruitment and cancer cell colonization and are therefore good therapeutic targets or good biomarkers for prognosis (72). There is a strong correlation between circulating angiogenic factors and poor prognosis (124). In a Cox proportional hazards model, VEGF-A expression in biopsy samples was confirmed to be an independent prognostic factor for poor survival in osteosarcoma (125) (Table 1). Integrating clinical, immune, inflammatory, metabolic, and genetic prognostic factors enables personalized treatments and better risk stratification for pediatric osteosarcoma. Challenges include standardizing biomarker assessments, advanced bioinformatics, interdisciplinary collaboration, large-scale validation, ethical issues, and cost-effectiveness. Overcoming these barriers is essential for enhancing patient outcomes through targeted therapies and immunotherapies.
In conclusion, pediatric osteosarcoma remains a challenging malignancy with poor prognosis despite advances in treatment. The TME, particularly the immune landscape, plays a crucial role in disease progression and metastasis. TAMs, T lymphocytes, and other immune cells interact within the TME, influencing both immune evasion and therapeutic response. Emerging immunotherapies, including immune checkpoint inhibitors and novel antibody treatments, show promise in enhancing anti-tumor immunity. However, overcoming immunosuppressive factors, such as M2 macrophage polarization and immune checkpoint upregulation, is essential for improving outcomes. Identifying prognostic biomarkers within the TME could guide individualized treatment strategies and ultimately improve survival rates in pediatric osteosarcoma patients. Furthermore, future research should prioritize optimizing specific immunotherapeutic strategies, such as enhancing the efficacy of immune checkpoint inhibitors and macrophage-targeted therapies; developing personalized treatment plans tailored to individual patient’s immune profiles and genetic backgrounds; and designing robust clinical trials to evaluate the safety and effectiveness of these novel approaches.
LZ: Writing – original draft. HJ: Writing – original draft. HM: Writing – original draft, Writing – review & editing.
The author(s) declare that no financial support was received for the research, authorship, and/or publication of this article.
The authors declare that the research was conducted in the absence of any commercial or financial relationships that could be construed as a potential conflict of interest.
The author(s) declare that no Generative AI was used in the creation of this manuscript.
All claims expressed in this article are solely those of the authors and do not necessarily represent those of their affiliated organizations, or those of the publisher, the editors and the reviewers. Any product that may be evaluated in this article, or claim that may be made by its manufacturer, is not guaranteed or endorsed by the publisher.
1. Ritter J, Bielack SS. Osteosarcoma. Ann Oncol. (2010) 21 Suppl 7:vii320–325. doi: 10.1093/annonc/mdq276
2. Bielack SS, Kempf-Bielack B, Delling G, Exner GU, Helmke K, Kotz R, et al. Prognostic factors in high-grade osteosarcoma of the extremities or trunk: an analysis of 1,702 patients treated on neoadjuvant cooperative osteosarcoma study group protocols. J Clin Oncol. (2002) 20:776–90. doi: 10.1200/JCO.2002.20.3.776
3. Ouyang H, Wang Z. Predictive value of the systemic immune-inflammation index for cancer-specific survival of osteosarcoma in children. Front Public Health. (2022) 10:879523. doi: 10.3389/fpubh.2022.879523
4. Bielack SS, Smeland S, Whelan JS, Marina N, Jovic G, Hook JM, et al. Methotrexate, doxorubicin, and cisplatin (MAP) plus maintenance pegylated interferon alfa-2b versus MAP alone in patients with resectable high-Grade osteosarcoma and good histologic response to preoperative MAP: first results of the EURAMOS-1 good response randomized controlled trial. J Clin Oncol. (2015) 33:2279–87. doi: 10.1200/JCO.2014.60.0734
5. Marina NM, Smeland S, Bielack SS, Bernstein M, Jovic G, Krailo MD, et al. Comparison of MAPIE versus MAP in patients with a poor response to preoperative chemotherapy for newly diagnosed high-grade osteosarcoma (EURAMOS-1): an open-label, international, randomized controlled trial. Lancet Oncol. (2016) 17:1396–408. doi: 10.1016/S1470-2045(16)30214-5
6. Luetke A, Meyers PA, Lewis I, Juergens H. Osteosarcoma treatment - where do we stand? A state of the art review. Cancer Treat Rev. (2014) 40:523–32. doi: 10.1016/j.ctrv.2013.11.006
7. Bielack SS, Hecker-Nolting S, Blattmann C, Kager L. Advances in the management of osteosarcoma. F1000Res. (2016) 5:2767. doi: 10.12688/f1000research
8. Smeland S, Bielack SS, Whelan J, Bernstein M, Hogendoorn P, Krailo MD, et al. Survival and prognosis with osteosarcoma: outcomes in more than 2000 patients in the EURAMOS-1 (European and American Osteosarcoma Study) cohort. Eur J Cancer. (2019) 109:36–50. doi: 10.1016/j.ejca.2018.11.027
9. Bacci G, Longhi A, Versari M, Mercuri M, Briccoli A, Picci P. Prognostic factors for osteosarcoma of the extremity treated with neoadjuvant chemotherapy: 15-year experience in 789 patients treated at a single institution. Cancer. (2006) 106:1154–61. doi: 10.1002/cncr.v106:5
10. Boye K, Del Prever AB, Eriksson M, Saeter G, Tienghi A, Lindholm P, et al. High-dose chemotherapy with stem cell rescue in the primary treatment of metastatic and pelvic osteosarcoma: final results of the ISG/SSG II study. Pediatr Blood Cancer. (2014) 61:840–5. doi: 10.1002/pbc.24868
11. Bacci G, Forni C, Ferrari S, Longhi A, Bertoni F, Mercuri M, et al. Neoadjuvant chemotherapy for osteosarcoma of the extremity: intensification of preoperative treatment does not increase the rate of good histologic response to the primary tumor or improve the final outcome. J Pediatr Hematol Oncol. (2003) 25:845–53. doi: 10.1097/00043426-200311000-00006
12. Shao W, Lin Z, Xiahou Z, Zhao F, Xu J, Liu X, et al. Single-cell RNA sequencing reveals that MYBL2 in Malignant epithelial cells is involved in the development and progression of ovarian cancer. Front Immunol. (2024) 15:1438198. doi: 10.3389/fimmu.2024.1438198
13. Zhao Z, Luo Q, Liu Y, Jiang K, Zhou L, Dai R, et al. Multi-level integrative analysis of the roles of lncRNAs and differential mRNAs in the progression of chronic pancreatitis to pancreatic ductal adenocarcinoma. BMC Genomics. (2023) 24:101. doi: 10.1186/s12864-023-09209-4
14. Hanahan D, Weinberg RA. Hallmarks of cancer: the next generation. Cell. (2011) 144:646–74. doi: 10.1016/j.cell.2011.02.013
15. Diakos CI, Charles KA, McMillan DC, Clarke SJ. Cancer-related inflammation and treatment effectiveness. Lancet Oncol. (2014) 15:e493–503. doi: 10.1016/S1470-2045(14)70263-3
16. Lee N, Zakka LR, Mihm MC Jr., Schatton T. Tumor-infiltrating lymphocytes in melanoma prognosis and cancer immunotherapy. Pathology. (2016) 48:177–87. doi: 10.1016/j.pathol.2015.12.006
17. Wu Y, Yi M, Niu M, Mei Q, Wu K. Myeloid-derived suppressor cells: an emerging target for anticancer immunotherapy. Mol Cancer. (2022) 21:184. doi: 10.1186/s12943-022-01657-y
18. Gay LJ, Felding-Habermann B. Contribution of platelets to tumor metastasis. Nat Rev Cancer. (2011) 11:123–34. doi: 10.1038/nrc3004
19. Mantovani A, Cassatella MA, Costantini C, Jaillon S. Neutrophils in the activation and regulation of innate and adaptive immunity. Nat Rev Immunol. (2011) 11:519–31. doi: 10.1038/nri3024
20. Stegner D, Dutting S, Nieswandt B. Mechanistic explanation for platelet contribution to cancer metastasis. Thromb Res. (2014) 133 Suppl 2:S149–157. doi: 10.1016/S0049-3848(14)50025-4
21. Bambace NM, Holmes CE. The platelet contribution to cancer progression. J Thromb Haemost. (2011) 9:237–49. doi: 10.1111/j.1538-7836.2010.04131.x
22. Zhang H, Xia T, Xia Z, Zhou H, Li Z, Wang W, et al. KIF18A inactivates hepatic stellate cells and alleviates liver fibrosis through the TTC3/Akt/mTOR pathway. Cell Mol Life Sci. (2024) 81:96. doi: 10.1007/s00018-024-05114-5
23. Zhang J, Peng G, Chi H, Yang J, Xie X, Song G, et al. CD8 + T-cell marker genes reveal different immune subtypes of oral lichen planus by integrating single-cell RNA-seq and bulk RNA-sequencing. BMC Oral Health. (2023) 23:464. doi: 10.1186/s12903-023-03138-0
24. Zhang X, Zhang P, Cong A, Feng Y, Chi H, Xia Z, et al. Unraveling molecular networks in thymic epithelial tumors: deciphering the unique signatures. Front Immunol. (2023) 14:1264325. doi: 10.3389/fimmu.2023.1264325
25. Sun L, Shao W, Lin Z, Lin J, Zhao F, Yu J. Single-cell RNA sequencing explored potential therapeutic targets by revealing the tumor microenvironment of neuroblastoma and its expression in cell death. Discovery Oncol. (2024) 15:409. doi: 10.1007/s12672-024-01286-5
26. Deng Y, Shi M, Yi L, Naveed Khan M, Xia Z, Li X. Eliminating a barrier: Aiming at VISTA, reversing MDSC-mediated T cell suppression in the tumor microenvironment. Heliyon. (2024) 10:e37060. doi: 10.1016/j.heliyon.2024.e37060
27. Wu A, Yang ZK, Kong P, Yu P, Li YT, Xu JL, et al. Exploring osteosarcoma based on the tumor microenvironment. Front Immunol. (2024) 15:1423194. doi: 10.3389/fimmu.2024.1423194
28. Xia Z, Chen S, He M, Li B, Deng Y, Yi L, et al. Editorial: Targeting metabolism to activate T cells and enhance the efficacy of checkpoint blockade immunotherapy in solid tumors. Front Immunol. (2023) 14:1247178. doi: 10.3389/fimmu.2023.1247178
29. Lipson EJ, Drake CG. Ipilimumab: an anti-CTLA-4 antibody for metastatic melanoma. Clin Cancer Res. (2011) 17:6958–62. doi: 10.1158/1078-0432.CCR-11-1595
30. Paddock LE, Lu SE, Bandera EV, Rhoads GG, Fine J, Paine S, et al. Skin self-examination and long-term melanoma survival. Melanoma Res. (2016) 26:401–8. doi: 10.1097/CMR.0000000000000255
31. Sharon E, Streicher H, Goncalves P, Chen HX. Immune checkpoint inhibitors in clinical trials. Chin J Cancer. (2014) 33:434–44. doi: 10.5732/cjc.014.10122
32. Petitprez F, de Reynies A, Keung EZ, Chen TW, Sun CM, Calderaro J, et al. B cells are associated with survival and immunotherapy response in sarcoma. Nature. (2020) 577:556–60. doi: 10.1038/s41586-019-1906-8
33. Oike N, Kawashima H, Ogose A, Hotta T, Hatano H, Ariizumi T, et al. Prognostic impact of the tumor immune microenvironment in synovial sarcoma. Cancer Sci. (2018) 109:3043–54. doi: 10.1111/cas.2018.109.issue-10
34. Xiong K, Fang Y, Qiu B, Chen C, Huang N, Liang F, et al. Investigation of cellular communication and signaling pathways in tumor microenvironment for high TP53-expressing osteosarcoma cells through single-cell RNA sequencing. Med Oncol. (2024) 41:93. doi: 10.1007/s12032-024-02318-4
35. Liu T, Li C, Zhang J, Hu H, Li C. Unveiling efferocytosis-related signatures through the integration of single-cell analysis and machine learning: a predictive framework for prognosis and immunotherapy response in hepatocellular carcinoma. Front Immunol. (2023) 14:1237350. doi: 10.3389/fimmu.2023.1237350
36. Wang Y, Ma L, He J, Gu H, Zhu H. Identification of cancer stem cell-related genes through single cells and machine learning for predicting prostate cancer prognosis and immunotherapy. Front Immunol. (2024) 15:1464698. doi: 10.3389/fimmu.2024.1464698
37. Li C, Liu T, Liu Y, Zhang J, Zuo D. Prognostic value of tumor microenvironment-related genes by TCGA database in rectal cancer. J Cell Mol Med. (2021) 25:5811–22. doi: 10.1111/jcmm.v25.12
38. Wolf-Dennen K, Gordon N, Kleinerman ES. Exosomal communication by metastatic osteosarcoma cells modulates alveolar macrophages to an M2 tumor-promoting phenotype and inhibits tumoricidal functions. Oncoimmunology. (2020) 9:1747677. doi: 10.1080/2162402X.2020.1747677
39. Koirala P, Roth ME, Gill J, Chinai JM, Ewart MR, Piperdi S, et al. HHLA2, a member of the B7 family, is expressed in human osteosarcoma and is associated with metastases and worse survival. Sci Rep. (2016) 6:31154. doi: 10.1038/srep31154
40. Koirala P, Roth ME, Gill J, Piperdi S, Chinai JM, Geller DS, et al. Immune infiltration and PD-L1 expression in the tumor microenvironment are prognostic in osteosarcoma. Sci Rep. (2016) 6:30093. doi: 10.1038/srep30093
41. Zhai X, Zhang H, Xia Z, Liu M, Du G, Jiang Z, et al. Oxytocin alleviates liver fibrosis via hepatic macrophages. JHEP Rep. (2024) 6:101032. doi: 10.1016/j.jhepr.2024.101032
42. Gomez-Brouchet A, Illac C, Gilhodes J, Bouvier C, Aubert S, Guinebretiere JM, et al. CD163-positive tumor-associated macrophages and CD8-positive cytotoxic lymphocytes are powerful diagnostic markers for the therapeutic stratification of osteosarcoma patients: An immunohistochemical analysis of the biopsies from the French OS2006 phase 3 trial. Oncoimmunology. (2017) 6:e1331193. doi: 10.1080/2162402X.2017.1331193
43. Dumars C, Ngyuen JM, Gaultier A, Lanel R, Corradini N, Gouin F, et al. Dysregulation of macrophage polarization is associated with the metastatic process in osteosarcoma. Oncotarget. (2016) 7:78343–54. doi: 10.18632/oncotarget.13055
44. Buddingh EP, Kuijjer ML, Duim RA, Burger H, Agelopoulos K, Myklebost O, et al. Tumor-infiltrating macrophages are associated with metastasis suppression in high-grade osteosarcoma: a rationale for treatment with macrophage activating agents. Clin Cancer Res. (2011) 17:2110–9. doi: 10.1158/1078-0432.CCR-10-2047
45. Corre I, Verrecchia F, Crenn V, Redini F, Trichet V. The osteosarcoma microenvironment: A complex but targetable ecosystem. Cells. (2020) 9:976. doi: 10.3390/cells9040976
46. Omer N, Nicholls W, Ruegg B, Souza-Fonseca-Guimaraes F, Rossi GR. Enhancing natural killer cell targeting of pediatric sarcoma. Front Immunol. (2021) 12:791206. doi: 10.3389/fimmu.2021.791206
47. Liang H, Cui M, Tu J, Chen X. Advancements in osteosarcoma management: integrating immune microenvironment insights with immunotherapeutic strategies. Front Cell Dev Biol. (2024) 12:1394339. doi: 10.3389/fcell.2024.1394339
48. Wang J, Zhang H, Sun X, Wang X, Ren T, Huang Y, et al. Exosomal PD-L1 and N-cadherin predict pulmonary metastasis progression for osteosarcoma patients. J Nanobiotechnology. (2020) 18:151. doi: 10.1186/s12951-020-00710-6
49. Troyer RM, Ruby CE, Goodall CP, Yang L, Maier CS, Albarqi HA, et al. Exosomes from Osteosarcoma and normal osteoblast differ in proteomic cargo and immunomodulatory effects on T cells. Exp Cell Res. (2017) 358:369–76. doi: 10.1016/j.yexcr.2017.07.011
50. Tian H, Cao J, Li B, Nice EC, Mao H, Zhang Y, et al. Managing the immune microenvironment of osteosarcoma: the outlook for osteosarcoma treatment. Bone Res. (2023) 11:11. doi: 10.1038/s41413-023-00246-z
51. Zhou Q, Xian M, Xiang S, Xiang D, Shao X, Wang J, et al. All-trans retinoic acid prevents osteosarcoma metastasis by inhibiting M2 polarization of tumor-associated macrophages. Cancer Immunol Res. (2017) 5:547–59. doi: 10.1158/2326-6066.CIR-16-0259
52. Chen C, Xie L, Ren T, Huang Y, Xu J, Guo W. Immunotherapy for osteosarcoma: Fundamental mechanism, rationale, and recent breakthroughs. Cancer Lett. (2021) 500:1–10. doi: 10.1016/j.canlet.2020.12.024
53. Xia Y, Rao L, Yao H, Wang Z, Ning P, Chen X. Engineering macrophages for cancer immunotherapy and drug delivery. Adv Mater. (2020) 32:e2002054. doi: 10.1002/adma.202002054
54. Gong L, Sun X, Jia M. New gene signature from the dominant infiltration immune cell type in osteosarcoma predicts overall survival. Sci Rep. (2023) 13:18271. doi: 10.1038/s41598-023-45566-6
55. Zhou L, Zhao T, Zhang R, Chen C, Li J. New insights into the role of macrophages in cancer immunotherapy. Front Immunol. (2024) 15:1381225. doi: 10.3389/fimmu.2024.1381225
56. Yuan Y, Li J, Chen J, Han L, Wang L, Yue Y, et al. Characterization of a novel T cell-engaging bispecific antibody for elimination of L1CAM-positive tumors. BioMed Pharmacother. (2024) 174:116565. doi: 10.1016/j.biopha.2024.116565
57. Zheng C, Wang J, Zhou Y, Duan Y, Zheng R, Xie Y, et al. IFNalpha-induced BST2(+) tumor-associated macrophages facilitate immunosuppression and tumor growth in pancreatic cancer by ERK-CXCL7 signaling. Cell Rep. (2024) 43:114088. doi: 10.1016/j.celrep.2024.114088
58. Li Y, Wang Z, Lu F, Miao Y, Feng Q, Zhu W, et al. Novel T cell exhaustion gene signature to predict prognosis and immunotherapy response in thyroid carcinoma from integrated RNA-sequencing analysis. Sci Rep. (2024) 14:8375. doi: 10.1038/s41598-024-58419-7
59. Lang GA, Iwakuma T, Suh YA, Liu G, Rao VA, Parant JM, et al. Gain of function of a p53 hot spot mutation in a mouse model of Li-Fraumeni syndrome. Cell. (2004) 119:861–72. doi: 10.1016/j.cell.2004.11.006
60. Zoumpoulidou G, Alvarez-Mendoza C, Mancusi C, Ahmed RM, Denman M, Steele CD, et al. Therapeutic vulnerability to PARP1,2 inhibition in RB1-mutant osteosarcoma. Nat Commun. (2021) 12:7064. doi: 10.1038/s41467-021-27291-8
61. Bartholf DeWitt S, Hoskinson Plumlee S, Brighton HE, Sivaraj D, Martz EJ, Zand M, et al. Loss of ATRX promotes aggressive features of osteosarcoma with increased NF-kappaB signaling and integrin binding. JCI Insight. (2022) 7:e151583. doi: 10.1172/jci.insight.151583
62. Mills LJ, Spector LG, Largaespada DA, Williams LA. Sex differences in expression of immune elements emerge in children, young adults and mice with osteosarcoma. Biol Sex Differ. (2021) 12:5. doi: 10.1186/s13293-020-00347-y
63. Aras S, Zaidi MR. TAMeless traitors: macrophages in cancer progression and metastasis. Br J Cancer. (2017) 117:1583–91. doi: 10.1038/bjc.2017.356
64. Wei J, Fang DL, Huang CK, Hua SL, Lu XS. Screening a novel signature and predicting the immune landscape of metastatic osteosarcoma in children via immune-related lncRNAs. Transl Pediatr. (2021) 10:1851–66. doi: 10.21037/tp-21-226
65. Yuan M, Zhu H, Xu J, Zheng Y, Cao X, Liu Q. Tumor-derived CXCL1 promotes lung cancer growth via recruitment of tumor-associated neutrophils. J Immunol Res. (2016) 2016:6530410. doi: 10.1155/2016/6530410
66. Hanna RN, Cekic C, Sag D, Tacke R, Thomas GD, Nowyhed H, et al. Patrolling monocytes control tumor metastasis to the lung. Science. (2015) 350:985–90. doi: 10.1126/science.aac9407
67. Kelleher FC, O’Sullivan H. Monocytes, macrophages, and osteoclasts in osteosarcoma. J Adolesc Young Adult Oncol. (2017) 6:396–405. doi: 10.1089/jayao.2016.0078
68. Wigerup C, Pahlman S, Bexell D. Therapeutic targeting of hypoxia and hypoxia-inducible factors in cancer. Pharmacol Ther. (2016) 164:152–69. doi: 10.1016/j.pharmthera.2016.04.009
69. Chung L, Maestas DR Jr., Housseau F, Elisseeff JH. Key players in the immune response to biomaterial scaffolds for regenerative medicine. Adv Drug Delivery Rev. (2017) 114:184–92. doi: 10.1016/j.addr.2017.07.006
70. Spetz J, Presser AG, Sarosiek KA. T cells and regulated cell death: kill or be killed. Int Rev Cell Mol Biol. (2019) 342:27–71. doi: 10.1016/bs.ircmb.2018.07.004
71. Jiang F, Miao XL, Zhang XT, Yan F, Mao Y, Wu CY, et al. A hypoxia gene-based signature to predict the survival and affect the tumor immune microenvironment of osteosarcoma in children. J Immunol Res. (2021) 2021:5523832. doi: 10.1155/2021/5523832
72. Argenziano M, Tortora C, Pota E, Di Paola A, Di Martino M, Di Leva C, et al. Osteosarcoma in children: not only chemotherapy. Pharm (Basel). (2021) 14:923. doi: 10.3390/ph14090923
73. Ando K, Mori K, Corradini N, Redini F, Heymann D. Mifamurtide for the treatment of nonmetastatic osteosarcoma. Expert Opin Pharmacother. (2011) 12:285–92. doi: 10.1517/14656566.2011.543129
74. Meyers PA, Chou AJ. Muramyl tripeptide-phosphatidyl ethanolamine encapsulated in liposomes (L-MTP-PE) in the treatment of osteosarcoma. Adv Exp Med Biol. (2014) 804:307–21. doi: 10.1007/978-3-319-04843-7_17
75. Georgoudaki AM, Prokopec KE, Boura VF, Hellqvist E, Sohn S, Ostling J, et al. et al: reprogramming tumor-associated macrophages by antibody targeting inhibits cancer progression and metastasis. Cell Rep. (2016) 15:2000–11. doi: 10.1016/j.celrep.2016.04.084
76. Punzo F, Bellini G, Tortora C, Pinto DD, Argenziano M, Pota E, et al. Mifamurtide and TAM-like macrophages: effect on proliferation, migration and differentiation of osteosarcoma cells. Oncotarget. (2020) 11:687–98. doi: 10.18632/oncotarget.27479
77. Bellini G, Di Pinto D, Tortora C, Manzo I, Punzo F, Casale F, et al. The role of mifamurtide in chemotherapy-induced osteoporosis of children with osteosarcoma. Curr Cancer Drug Targets. (2017) 17:650–6. doi: 10.2174/1568009616666161215163426
78. Miwa S, Yamamoto N, Hayashi K, Takeuchi A, Igarashi K, Tsuchiya H. Therapeutic targets for bone and soft-tissue sarcomas. Int J Mol Sci. (2019) 20:170. doi: 10.3390/ijms20010170
79. Mehta CR, Liu L, Theuer C. An adaptive population enrichment phase III trial of TRC105 and pazopanib versus pazopanib alone in patients with advanced angiosarcoma (TAPPAS trial). Ann Oncol. (2019) 30:103–8. doi: 10.1093/annonc/mdy464
80. Roth M, Barris DM, Piperdi S, Kuo V, Everts S, Geller D, et al. Targeting glycoprotein NMB with antibody-drug conjugate, glembatumumab vedotin, for the treatment of osteosarcoma. Pediatr Blood Cancer. (2016) 63:32–8. doi: 10.1002/pbc.25688
81. Lettieri CK, Appel N, Labban N, Lussier DM, Blattman JN, Hingorani P. Progress and opportunities for immune therapeutics in osteosarcoma. Immunotherapy. (2016) 8:1233–44. doi: 10.2217/imt-2016-0048
82. Bendell J, Saleh M, Rose AA, Siegel PM, Hart L, Sirpal S, et al. Phase I/II study of the antibody-drug conjugate glembatumumab vedotin in patients with locally advanced or metastatic breast cancer. J Clin Oncol. (2014) 32:3619–25. doi: 10.1200/JCO.2013.52.5683
83. Kolb EA, Gorlick R, Billups CA, Hawthorne T, Kurmasheva RT, Houghton PJ, et al. Initial testing (stage 1) of glembatumumab vedotin (CDX-011) by the pediatric preclinical testing program. Pediatr Blood Cancer. (2014) 61:1816–21. doi: 10.1002/pbc.25099
84. Bachelot T, Ciruelos E, Schneeweiss A, Puglisi F, Peretz-Yablonski T, Bondarenko I, et al. Preliminary safety and efficacy of first-line pertuzumab combined with trastuzumab and taxane therapy for HER2-positive locally recurrent or metastatic breast cancer (PERUSE). Ann Oncol. (2019) 30:766–73. doi: 10.1093/annonc/mdz061
85. Xiao H, Jensen PE, Chen X. Elimination of osteosarcoma by necroptosis with graphene oxide-associated anti-HER2 antibodies. Int J Mol Sci. (2019) 20:4360. doi: 10.3390/ijms20184360
86. Hingorani P, Krailo M, Buxton A, Hutson P, Sondel PM, Diccianni M, et al. Phase 2 study of anti-disialoganglioside antibody, dinutuximab, in combination with GM-CSF in patients with recurrent osteosarcoma: A report from the Children’s Oncology Group. Eur J Cancer. (2022) 172:264–75. doi: 10.1016/j.ejca.2022.05.035
87. Mody R, Yu AL, Naranjo A, Zhang FF, London WB, Shulkin BL, et al. Irinotecan, temozolomide, and dinutuximab with GM-CSF in children with refractory or relapsed neuroblastoma: A report from the children’s oncology group. J Clin Oncol. (2020) 38:2160–9. doi: 10.1200/JCO.20.00203
88. Mody R, Naranjo A, Van Ryn C, Yu AL, London WB, Shulkin BL, et al. Irinotecan-temozolomide with temsirolimus or dinutuximab in children with refractory or relapsed neuroblastoma (COG ANBL1221): an open-label, randomized, phase 2 trial. Lancet Oncol. (2017) 18:946–57. doi: 10.1016/S1470-2045(17)30355-8
89. Quinn S, Lenart N, Dronzek V, Scurti GM, Hossain NM, Nishimura MI. Genetic modification of T cells for the immunotherapy of cancer. Vaccines (Basel). (2022) 10:457. doi: 10.3390/vaccines10030457
90. Yu S, Yao X. Advances on immunotherapy for osteosarcoma. Mol Cancer. (2024) 23:192. doi: 10.1186/s12943-024-02105-9
91. Cao JW, Lake J, Impastato R, Chow L, Perez L, Chubb L, et al. Targeting osteosarcoma with canine B7-H3 CAR T cells and impact of CXCR2 Co-expression on functional activity. Cancer Immunol Immunother. (2024) 73:77. doi: 10.1007/s00262-024-03642-4
92. Li S, Zhang H, Shang G. Current status and future challenges of CAR-T cell therapy for osteosarcoma. Front Immunol. (2023) 14:1290762. doi: 10.3389/fimmu.2023.1290762
93. Meazza C, Cefalo G, Massimino M, Daolio P, Pastorino U, Scanagatta P, et al. et al: Primary metastatic osteosarcoma: results of a prospective study in children given chemotherapy and interleukin-2. Med Oncol. (2017) 34:191. doi: 10.1007/s12032-017-1052-9
94. Stern JB, Smith KA. Interleukin-2 induction of T-cell G1 progression and c-myb expression. Science. (1986) 233:203–6. doi: 10.1126/science.3523754
95. Kjaergaard J, Hokland ME, Agger R, Skovbo A, Nannmark U, Basse PH. Biodistribution and tumor localization of lymphokine-activated killer T cells following different routes of administration into tumor-bearing animals. Cancer Immunol Immunother. (2000) 48:550–60. doi: 10.1007/PL00006673
96. Errani C, Tsukamoto S, Mavrogenis AF. How safe and effective is denosumab for bone giant cell tumor? Int Orthop. (2017) 41:2397–400. doi: 10.1007/s00264-017-3536-9
97. Stadler N, Fingernagel T, Hofstaetter SG, Trieb K. A recurrent giant cell tumor of bone treated with denosumab. Clin Pract. (2015) 5:697. doi: 10.4081/cp.2015.697
98. Branstetter D, Rohrbach K, Huang LY, Soriano R, Tometsko M, Blake M, et al. RANK and RANK ligand expression in primary human osteosarcoma. J Bone Oncol. (2015) 4:59–68. doi: 10.1016/j.jbo.2015.06.002
99. Grimaud E, Soubigou L, Couillaud S, Coipeau P, Moreau A, Passuti N, et al. Receptor activator of nuclear factor kappaB ligand (RANKL)/osteoprotegerin (OPG) ratio is increased in severe osteolysis. Am J Pathol. (2003) 163:2021–31. doi: 10.1016/S0002-9440(10)63560-2
100. Gallily R, Even-Chena T, Katzavian G, Lehmann D, Dagan A, Mechoulam R. Gamma-irradiation enhances apoptosis induced by cannabidiol, a non-psychotropic cannabinoid, in cultured HL-60 myeloblastic leukemia cells. Leuk Lymphoma. (2003) 44:1767–73. doi: 10.1080/1042819031000103917
101. Gibiansky L, Sutjandra L, Doshi S, Zheng J, Sohn W, Peterson MC, et al. Population pharmacokinetic analysis of denosumab in patients with bone metastases from solid tumors. Clin Pharmacokinet. (2012) 51:247–60. doi: 10.2165/11598090-000000000-00000
102. Botter SM, Neri D, Fuchs B. Recent advances in osteosarcoma. Curr Opin Pharmacol. (2014) 16:15–23. doi: 10.1016/j.coph.2014.02.002
103. Lee JA. Osteosarcoma in Korean children and adolescents. Korean J Pediatr. (2015) 58:123–8. doi: 10.3345/kjp.2015.58.4.123
104. Punzo F, Tortora C, Argenziano M, Pinto DD, Pota E, Martino MD, et al. Can Denosumab be used in combination with Doxorubicin in Osteosarcoma? Oncotarget. (2020) 11:2763–73. doi: 10.18632/oncotarget.27669
105. Ma C, Li R, Yu R, Guo J, Xu J, Yuan X, et al. Predictive value of preoperative platelet-to-albumin ratio and apolipoprotein B-to-apolipoprotein A1 ratio for osteosarcoma in children and adolescents: a retrospective study of 118 cases. BMC Cancer. (2022) 22:113. doi: 10.1186/s12885-022-09223-x
106. Zhao F, Hong J, Zhou G, Huang T, Lin Z, Zhang Y, et al. Elucidating the role of tumor-associated ALOX5+ mast cells with transformative function in cervical cancer progression via single-cell RNA sequencing. Front Immunol. (2024) 15:1434450. doi: 10.3389/fimmu.2024.1434450
107. Li X, Lin Z, Zhao F, Huang T, Fan W, Cen L, et al. Unveiling the cellular landscape: insights from single-cell RNA sequencing in multiple myeloma. Front Immunol. (2024) 15:1458638. doi: 10.3389/fimmu.2024.1458638
108. Zhu C, Sun Z, Wang J, Meng X, Ma Z, Guo R, et al. et al: Exploring oncogenes for renal clear cell carcinoma based on G protein-coupled receptor-associated genes. Discovery Oncol. (2023) 14:182. doi: 10.1007/s12672-023-00795-z
109. Zuo D, Zhang J, Liu T, Li C, Ning G. Claudin-1 is a valuable prognostic biomarker in colorectal cancer: A meta-analysis. Gastroenterol Res Pract. (2020) 2020:4258035. doi: 10.1155/2020/4258035
110. Wang Y, Wang J, Liu Y, Wang X, Ren M. Multidimensional pan-cancer analysis of HSPA5 and its validation in the prognostic value of bladder cancer. Heliyon. (2024) 10:e27184. doi: 10.1016/j.heliyon.2024.e27184
111. Yoshida S, Celaire J, Pace C, Taylor C, Kaneuchi Y, Evans S, et al. Delay in diagnosis of primary osteosarcoma of bone in children: Have we improved in the last 15 years and what is the impact of delay on diagnosis? J Bone Oncol. (2021) 28:100359. doi: 10.1016/j.jbo.2021.100359
112. Pang ZQ, Wang JS, Wang JF, Wang YX, Ji B, Xu YD, et al. JAM3: A prognostic biomarker for bladder cancer via epithelial-mesenchymal transition regulation. Biomol BioMed. (2024) 24:897–911. doi: 10.17305/bb.2024.9979
113. Zuo D, Li C, Liu T, Yue M, Zhang J, Ning G. Construction and validation of a metabolic risk model predicting prognosis of colon cancer. Sci Rep. (2021) 11:6837. doi: 10.1038/s41598-021-86286-z
114. Wang J, Zuo Z, Yu Z, Chen Z, Tran LJ, Zhang J, et al. Collaborating single-cell and bulk RNA sequencing for comprehensive characterization of the intratumor heterogeneity and prognostic model development for bladder cancer. Aging (Albany NY). (2023) 15:12104–19. doi: 10.18632/aging.205166
115. Xiao J, Lin H, Liu B, Xia Z, Zhang J, Jin J. Decreased S1P and SPHK2 are involved in pancreatic acinar cell injury. biomark Med. (2019) 13:627–37. doi: 10.2217/bmm-2018-0404
116. Jiang S, Yang X, Lin Y, Liu Y, Tran LJ, Zhang J, et al. Unveiling Anoikis-related genes: A breakthrough in the prognosis of bladder cancer. J Gene Med. (2024) 26:e3651. doi: 10.1002/jgm.v26.1
117. Wang Y, He J, Zhao Q, Bo J, Zhou Y, Sun H, et al. Evaluating the predictive value of angiogenesis-related genes for prognosis and immunotherapy response in prostate adenocarcinoma using machine learning and experimental approaches. Front Immunol. (2024) 15:1416914. doi: 10.3389/fimmu.2024.1416914
118. Vasquez L, Silva J, Chavez S, Zapata A, Diaz R, Tarrillo F, et al. Prognostic impact of diagnostic and treatment delays in children with osteosarcoma. Pediatr Blood Cancer. (2020) 67:e28180. doi: 10.1002/pbc.28180
119. Yang B, Su Z, Chen G, Zeng Z, Tan J, Wu G, et al. Identification of prognostic biomarkers associated with metastasis and immune infiltration in osteosarcoma. Oncol Lett. (2021) 21:180. doi: 10.3892/ol.2021.12441
120. Diessner BJ, Pankratz N, Hooten AJ, Mirabello L, Sarver AL, Mills LJ, et al. Nearly half of TP53 germline variants predicted to be pathogenic in patients with osteosarcoma are de novo: A report from the children’s oncology group. JCO Precis Oncol. (2020) 4:PO.20.00087. doi: 10.1200/PO.20.00087
121. Li L, Xu C, Liu P, Huang J. Correlation study of DNA methylation of WNT6 gene with osteosarcoma in children. Oncol Lett. (2017) 14:271–5. doi: 10.3892/ol.2017.6135
122. Shulman DS, Klega K, Imamovic-Tuco A, Clapp A, Nag A, Thorner AR, et al. Detection of circulating tumor DNA is associated with inferior outcomes in Ewing sarcoma and osteosarcoma: a report from the Children’s Oncology Group. Br J Cancer. (2018) 119:615–21. doi: 10.1038/s41416-018-0212-9
123. Bahador R, Taheriazam A, Mirghasemi A, Torkaman A, Shakeri M, Yahaghi E, et al. Tissue expression levels of miR-29b and miR-422a in children, adolescents, and young adults’ age groups and their association with prediction of poor prognosis in human osteosarcoma. Tumor Biol. (2016) 37:3091–5. doi: 10.1007/s13277-015-4140-5
124. Kaplan RN, Riba RD, Zacharoulis S, Bramley AH, Vincent L, Costa C, et al. VEGFR1-positive hematopoietic bone marrow progenitors initiate the pre-metastatic niche. Nature. (2005) 438:820–7. doi: 10.1038/nature04186
Keywords: pediatric osteosarcoma, immunosuppressive environment, immunotherapy, biomarkers, ICB, PD-1
Citation: Zhang L, Jiang H and Ma H (2025) Progress in immune microenvironment, immunotherapy and prognostic biomarkers in pediatric osteosarcoma. Front. Immunol. 16:1548527. doi: 10.3389/fimmu.2025.1548527
Received: 19 December 2024; Accepted: 06 January 2025;
Published: 22 January 2025.
Edited by:
Minghua Ren, First Affiliated Hospital of Harbin Medical University, ChinaReviewed by:
Zhiheng Lin, Shanghai University of Traditional Chinese Medicine, ChinaCopyright © 2025 Zhang, Jiang and Ma. This is an open-access article distributed under the terms of the Creative Commons Attribution License (CC BY). The use, distribution or reproduction in other forums is permitted, provided the original author(s) and the copyright owner(s) are credited and that the original publication in this journal is cited, in accordance with accepted academic practice. No use, distribution or reproduction is permitted which does not comply with these terms.
*Correspondence: Haichao Ma, bWFoYWljaGFvOTNAMTYzLmNvbQ==
†These authors have contributed equally to this work and share first authorship
Disclaimer: All claims expressed in this article are solely those of the authors and do not necessarily represent those of their affiliated organizations, or those of the publisher, the editors and the reviewers. Any product that may be evaluated in this article or claim that may be made by its manufacturer is not guaranteed or endorsed by the publisher.
Research integrity at Frontiers
Learn more about the work of our research integrity team to safeguard the quality of each article we publish.