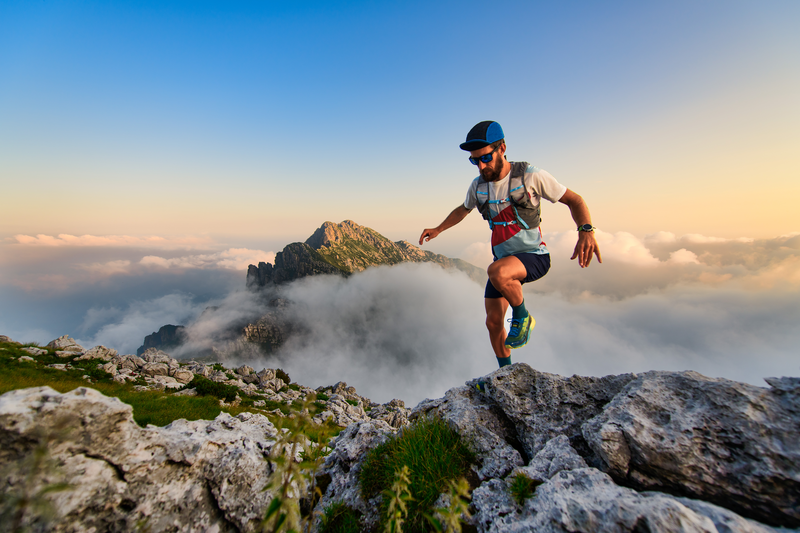
95% of researchers rate our articles as excellent or good
Learn more about the work of our research integrity team to safeguard the quality of each article we publish.
Find out more
MINI REVIEW article
Front. Immunol. , 24 February 2025
Sec. Cancer Immunity and Immunotherapy
Volume 16 - 2025 | https://doi.org/10.3389/fimmu.2025.1535930
This article is part of the Research Topic Mechanistic exploration of personalized immunotherapy for urological cancers and potential strategies for clinical translation View all 3 articles
Tissue-resident memory T (TRM) cells are a specialized subset of memory T cells that permanently reside in non-lymphoid tissues, providing localized and long-lasting immune protection. In the urinary tract, TRM cells play critical roles in defending against infections, mediating tumor immunity, and influencing the pathogenesis of chronic inflammatory diseases. Their therapeutic potential is immense, with promising avenues for vaccine development, enhanced cancer immunotherapy, and targeted treatments for chronic inflammation. However, challenges remain in harnessing their protective roles while minimizing their pathological effects, particularly in immunosuppressive or inflammatory microenvironments. This review explores the diverse roles of TRM cells in urinary tract diseases, including infections, cancer, and chronic inflammation, and discusses therapeutic strategies and future directions for leveraging TRM cells to improve clinical outcomes. By advancing our understanding of TRM cell biology, we can develop innovative interventions that balance their immune-protective and regulatory functions.
Tissue-resident memory T (TRM) cells represent a unique subset of memory T cells that reside permanently within non-lymphoid tissues without recirculating through the blood or lymphatic systems (1). Unlike central or effector memory T cells, TRM cells are characterized by the expression of markers such as CD69, which prevents egress from tissues, and CD103, which promotes adhesion to epithelial cells (2–4). These cells serve as sentinels of localized immunity, poised to rapidly respond to reinfection by previously encountered pathogens (5, 6). This unique population comprises two main subsets: CD4+ and CD8+ TRM cells, each with distinct characteristics, developmental pathways, and functional roles (7). CD8+ TRM cells act as the first line of defense against viral re-infections, rapidly eliminating infected cells upon pathogen re-encounter (8). In contrast, CD4+ TRM cells play a critical role in coordinating broader immune responses, including the support of B cell activity and the maintenance of local immunity at various tissue sites, such as skin and mucosal surfaces (9). Recent studies have shown that TRM cells comprise approximately 10-30% of the total T cell population in non-lymphoid tissues, with variations depending on tissue type and disease state (10). For example, in inflamed tissues, TRM cells can account for up to 60% of the T cell population, while in cancer tissues, their proportion typically ranges from 5% to 20%, depending on the tumor microenvironment (11–13). This variability in TRM cells frequency underscores their dynamic roles in immune responses across different conditions. Furthermore, TRM cells have been shown to persist in non-lymphoid tissues for extended periods, with some studies reporting survival times ranging from several months to years. This long-term presence highlights their critical role in maintaining tissue immunity (14, 15). These distinct functions underscore the importance of understanding the heterogeneity of TRM cells in the context of urinary tract diseases, setting the stage for a more detailed exploration of their roles in infections, tumors, and chronic inflammation that follows.
The urinary tract is a critical site for immune responses, balancing pathogen defense with tolerance of non-harmful antigens. It comprises the urinary and reproductive systems, including the kidneys, bladder, and genital organs. TRM cells, identified in bladder, ovarian, vaginal, kidney, uterine, prostate, and penile tissues, are vital for local immunity against infections and tumors and may modulate autoimmune responses (16–21). Beyond the urinary tract, TRM cells have also been implicated in other cancer types, such as melanoma, lung cancer, and breast cancer, where they contribute to tumor surveillance and immune control (22–24). For instance, in melanoma, high densities of CD8+ TRM cells are associated with improved patient survival and response to immunotherapy (25). Similarly, in lung cancer, TRM cells have been shown to enhance anti-tumor immunity and predict favorable clinical outcomes (26). These findings highlight the broader relevance of TRM cells in cancer immunity and underscore the need for further research into their roles across different tissue types and disease contexts. Their strategic localization within the epithelial barriers and ability to interact with other immune and stromal cells underline their importance as both defenders and regulators of tissue health. Understanding the unique biology of TRM cells in this context is vital for developing targeted therapeutic strategies.
TRM cells in the urinary tract share features with those in other parts of the body, such as the expression of CD69 and CD103, as well as the characteristic of tissue residency. They also display unique adaptations to their local microenvironments, including their positioning in specific tissues-like the lamina propria and basal epithelial lining in vaginal and penile tissues-and the expression of molecular markers such as CXCR3, P2RX7, CXCR6, and CD49a (27–31). Immediately upon entry, these adaptations indicate their strategic positioning in barrier tissues to intercept pathogens, including bacteria, viruses, and fungi.
Urinary tract infections (UTIs) are among the most common infections globally, characterized by frequent recurrence despite antibiotic treatment (32, 33). TRM cells play a pivotal role in the immune defense against these infections by establishing localized, long-term immunity in the urinary tract. In response to primary infection, pathogen-specific TRM cells are generated and retained in the bladder mucosa, where they can rapidly detect and respond to reinfection. However, TRM cells, including CD4+ and CD8+ subsets, do not directly recognize pathogens but instead rely on interactions with antigen-presenting cells (APCs) to initiate their immune responses. Upon reinfection, dendritic cells (DCs) and macrophages in the urinary tract rapidly process and present pathogen-derived antigens via MHC class II and class I molecules, respectively. CD4+ TRM cells recognize antigens presented on MHC class II by APCs, leading to their activation and subsequent secretion of cytokines, such as interferon-gamma (IFN-γ) and tumor necrosis factor-alpha (TNF-α), which recruit and activate additional immune cells like neutrophils and macrophages (34). Similarly, CD8+ TRM cells are activated through the recognition of antigens presented on MHC class I by APCs, enabling them to exert cytotoxic effects via the release of granzymes and perforin (35). This interaction between TRM cells and APCs ensures a rapid and localized immune response that is essential for the effective clearance of pathogens during secondary infections. These cells express markers such as CD69, which anchors them within tissues, and secrete cytokines like IFN-γ upon activation, initiating a cascade of immune responses that recruit neutrophils and other effector cells to the site of infection (18). TRM cells are particularly effective in combating uropathogenic Escherichia coli (UPEC), the most common causative agent of UTIs. CD4+ and CD8+ TRM cells play complementary roles in the immune defense against UTIs. CD4+ TRM cells primarily facilitate immune responses by secreting cytokines that help activate other immune cells, such as macrophages and B cells, promoting antibody production and maintaining immune homeostasis (36). These cells also contribute to tissue repair and inflammation regulation in the urinary tract. In contrast, CD8+ TRM cells exert direct cytotoxic effects by killing infected epithelial cells through the release of granzymes and perforin, limiting pathogen replication (37). Both CD4+ and CD8+ TRM cells provide long-term immunity by persisting in the bladder mucosa, enabling rapid and effective responses to reinfection. However, the activation and persistence of TRM cells, especially during recurrent UTIs, can also lead to chronic inflammation and tissue damage, emphasizing the need for therapeutic strategies that balance immune protection with the prevention of excessive tissue injury.
In the context of secondary infections, TRM cells are poised to rapidly exert their effector functions. They produce granzyme B and pro-inflammatory cytokines that serve to recruit and activate additional immune cells. This swift response is essential for controlling viral reactivation and combating bacterial infections. Studies have shown that UPEC-specific TRM cells remain in the bladder mucosa long after the initial infection has resolved, providing a robust immunological memory that reduces the severity of subsequent infections (18). However, the persistence and activation of TRM cells can also contribute to tissue damage and inflammation in the bladder, especially in recurrent infections, potentially exacerbating symptoms. Moreover, their interactions with other immune cells, including macrophages and DCs, further shape the local immune microenvironment (38, 39).
When TRM cells function is impaired-due to factors such as immunosuppressive environments, chronic inflammation, or aging-the immune response becomes less effective (40–42). In such scenarios, pathogen clearance is delayed, leading to prolonged infection, increased pathogen load, and heightened risk of tissue damage. The absence of immediate effector responses also results in a heavier reliance on systemic adaptive immunity, which takes more time to activate and may not effectively localize to the infection site.
TRM cells are emerging as critical players in the immune response to urinary tract tumors, including bladder, prostate, and kidney cancers (Figure 1). These cells reside within tumor tissues, where they exert localized anti-tumor effects by recognizing and responding to tumor-specific antigens. TRM cells contribute to immune surveillance, influence the tumor microenvironment (TME), and enhance responses to immune checkpoint inhibitors (ICIs). In urinary tract tumors, T cell exhaustion is a well-known feature of the tumor immune microenvironment, characterized by the upregulation of inhibitory receptors such as PD-1, TIM-3, and LAG-3 (43). Interestingly, while TRM cells are generally associated with robust immune responses, they are not immune to the effects of exhaustion in tumor settings. TRM cells within the TME can become exhausted over time due to chronic antigen stimulation and the immunosuppressive signals present in the tumor. This exhaustion is associated with a reduced capacity for cytokine production, cytotoxic activity, and overall immune surveillance (44). However, unlike recirculating T cells, TRM cells may maintain some degree of function due to their unique tissue-residency markers, such as CD69 and CD103. These markers help anchor TRM cells in the tissue, potentially allowing them to persist in the TME even when other T cells become exhausted. Recent studies suggest that enhancing the functionality of exhausted TRM cells in tumors could improve responses to immunotherapies, such as ICIs, by restoring their effector functions (45).
Figure 1. Phenotypic and functional variations of TRM cells in urinary tract tumors. (A) Bladder Cancer: TRM cells exhibit high expression of CD103 and CD69, alongside PD-1, indicating a tumor-resident yet partially exhausted state. These cells produce cytokines such as IFN-γ and TNF-α, which enhance anti-tumor immunity by recruiting CD8+ T cells and NK cells. Higher densities of CD103+CD8+ TRM cells correlate with improved prognosis and better responses to immune checkpoint inhibitors targeting PD-1/PD-L1. However, persistent antigen exposure may lead to progressive exhaustion, reducing their cytotoxic potential. (B) Prostate Cancer: TRM cells show reduced CD103 and limited effector functions due to an immunosuppressive tumor microenvironment. Elevated levels of TGF-β and myeloid-derived suppressor cells contribute to TRM cells dysfunction, reducing their ability to mount effective anti-tumor responses. As a result, TRM cells in prostate cancer are often associated with poor immune control and tumor progression. Moreover, their diminished cytotoxic potential limits the effectiveness of PD-1 blockade therapy in prostate cancer compared to other tumors. (C) Kidney Cancer: TRM cells in renal cell carcinoma express high levels of CD103 and CD69, often accompanied by PD-1, suggesting a dual role in tumor surveillance and immune suppression. Despite retaining the capacity to produce IFN-γ, TNF-α, and Granzyme B, TRM cells frequently become functionally exhausted in the TME, reducing their ability to control tumor growth. However, high TRM cells infiltration is associated with better prognosis and enhanced responses to ICIs, particularly PD-1 inhibitors. Depletion studies in murine models suggest that TRM cells play a crucial role in mediating the efficacy of immunotherapies in RCC.
In urinary cancers, tumor-infiltrating T cells display a heterogeneous phenotype. The majority of these cells express CD69 in prostate and kidney cancers, while in bladder cancers, they also commonly express CD103. Understanding the role of TRM cells in these cancers offers valuable insights into novel immunotherapeutic strategies and their potential to improve clinical outcomes.
In bladder cancer, TRM cells play a pivotal role in shaping anti-tumor immunity within the TME (46). TRM cells are localized in the bladder epithelium and tumor tissues, where they exhibit hallmark features such as CD69 and CD103 expression, enabling them to remain anchored in the tissue and maintain prolonged immune surveillance (47). These cells respond to tumor-specific antigens by producing effector cytokines like IFN-γ and tumor necrosis factor-alpha (TNF-α), which stimulate cytotoxic activity and enhance the recruitment of other immune cells, such as CD8+ T cells and natural killer cells, to the tumor site.
Recently, an in-depth analysis of CD103+CD8+ TRM cells within muscle-invasive bladder cancer (MIBC) tissues has unveiled a significant correlation with improved overall survival outcomes. The study demonstrated that patients with a high infiltration of CD103+CD8+ TRM cells, rather than CD8+ T cells alone, are more likely to benefit from both immunotherapy and adjuvant chemotherapy (ACT). These TRM cells are associated with an enhanced IFNγ-enriched and T cell-inflamed antitumor microenvironment (47). The findings underscore the pivotal role of CD103+CD8+ TRM cells in antitumor immunity and their potential as an optimal prognostic biomarker, serving as a superior companion predictor for treatment responses to PD-L1 inhibitors and ACT in MIBC patients. TRM cells may also act as a biomarker for predicting immunotherapy efficacy (48). CD103+CD8+ TRM cells despite frequently exhibiting elevated levels of immune checkpoint molecules such as PD-1, TIM-3, and LAG-3, retain their capacity to produce cytotoxic molecules and effector cytokines, which is notably different from their CD103-negative counterparts (49, 50). This characteristic is particularly relevant in the context of renal cell carcinoma, bladder, and ovarian cancer, where the high expression of PD-1 on CD103+ TRM cells is thought to be a key factor in the efficacy of anti-PD-1 therapies (51–53). Evidence from murine tumor models indicates that the depletion of CD103+ cells results in reduced effectiveness of checkpoint inhibitor treatments, highlighting the importance of these TRM cells in the response to cancer immunotherapy (54).
In prostate cancer, the role of TRM cells is less well-characterized compared to other urinary tract tumors, but emerging evidence suggests they contribute to both tumor suppression and immune modulation within the prostate microenvironment (20). However, prostate cancer is often associated with a highly immunosuppressive microenvironment that limits TRM cells functionality (55). Elevated levels of regulatory T cells, myeloid-derived suppressor cells, and inhibitory cytokines, such as transforming growth factor-beta (TGF-β), suppress TRM cells activation and reduce their anti-tumor efficacy (56). Clinical studies have confirmed that autologous active cellular immunotherapy resulted in a modest improvement in survival in prostate cancer patients, extending it by approximately 4 months (57). Additionally, androgen deprivation therapy (ADT), a common treatment for prostate cancer, has been shown to alter the immune landscape of the prostate, potentially affecting the generation and activity of TRM cells (58).
In kidney cancers, particularly renal cell carcinoma (RCC), TRM cells play a critical role in tumor immune surveillance. RCC is recognized as an immunogenic tumor, marked by significant immune cell infiltration into tumor tissues compared to adjacent normal tissues (59). Among these immune cells, CD8+ TRM cells, characterized by the expression of CD69 and CD103, are commonly found and are essential for maintaining long-term immune surveillance within the TME have shown that a high density of CD103+ TRM cells is associated with better prognostic outcomes in RCC (60). These cells retain their cytotoxic potential and can quickly respond to tumor antigens by producing effector cytokines such as IFN-γ and granzyme B. Depletion of CD103+ TRM cells in murine RCC models leads to accelerated tumor growth, highlighting their vital role in controlling tumor progression.
However, the functionality of TRM cells in RCC is often compromised by the expression of immune checkpoint molecules such as PD-1 (61). These inhibitory signals can suppress the effector functions of TRM cells. ICIs, such as anti-PD-1 therapies, can reverse this suppression, rejuvenating TRM cells activity and enhancing anti-tumor immunity. Murine studies have shown that depletion of CD103+ cells abrogates the effectiveness of ICIs, emphasizing that TRM cells are crucial mediators of therapeutic responses to checkpoint blockade in RCC.
Emerging therapies, including cancer vaccines, aim to boost the presence and activity of TRM cells within kidney tumors. By inducing tumor-specific TRM cells, these vaccines could enhance immune responses and work synergistically with ICIs to improve treatment efficacy (62). Ongoing clinical trials investigating the combination of ICIs and TRM-enhancing strategies offer promising avenues for improving RCC patient outcomes.
The roles of TRM cells in various urinary tract diseases differ significantly. As summarized in Table 1, their functions range from immune surveillance in bladder cancer to fibrosis promotion in chronic pyelonephritis. The heterogeneity of TRM cells populations, evidenced by their varied phenotypic and functional profiles within the urinary tract, offers a range of benefits. This diversity is crucial for the immune response to infections and cancers, as well as for the complex dynamics of autoimmune and inflammatory diseases affecting the urinary system. TRM cells infiltration in the kidney positively correlates with disease activity, as indicated by increased serum creatinine, proteinuria, hematuria, and histological scores in patients with lupus nephritis (LN) and antineutrophil cytoplasmic antibody (ANCA) associated glomerulonephritis (GN), as well as in murine systemic lupus erythematosus (SLE) models (61, 63–65). In murine GN models, the number of CD4+ T cells predominates over CD8+ T cells, whereas in humans, nearly equal numbers of CD4+ and CD8+ T cells are reported in many studies (66–68). Similarly, in the kidneys of patients and mice with LN, the abundance of CD8+ TRM cells was significantly increased (69). Under inflammatory conditions, renal T cells exhibit a tissue-resident phenotype, with CD69 widely expressed on both CD4+ and CD8+ T cells, indicating their significant role in local immune responses. Additionally, CD103 expression is observed on renal T cells, particularly on CD8+ T cells in patients with SLE and in SLE-prone mice (63–65). Mice studies show that both commensal and pathogenic bacteria can trigger kidney inflammation. Infection with S. aureus, and C. albicans leads to kidney CD4+ TRM cells adopting an inflammatory TH17 phenotype, exacerbating disease (64). Activation of kidney TRM cells by cytokines like IL-1β, IL-6, and IL-23 through the JAK-STAT pathway amplifies the inflammatory response. Microbiota may activate T cells with kidney-homing potential in the intestine, and fate-mapping suggests T cells migrate from the intestine to the kidney post-nephritis induction. This migration is driven by S1PR1-dependent egress from the intestine and CCL20-dependent entry into the kidney. The role of these newly infiltrated T cells in disease progression is unclear, but their presence suggests a role for microbiota-driven inflammation in promoting autoimmunity in the kidney.
In cases of chronic infections, such as chronic pyelonephritis, the formation and role of TRM cells may differ from acute infections. While TRM cells are typically associated with rapid immune responses to reinfection, their role in chronic infections is more complex. In chronic pyelonephritis, the persistent presence of pathogens and ongoing inflammation may affect the dynamics of TRM cells formation. It is possible that TRM cells are generated and persist in the kidneys during chronic infection, but their function may be impaired or dysregulated due to the prolonged inflammatory environment (70). In such conditions, TRM cells may contribute to the chronic inflammation by promoting tissue damage and fibrosis, potentially leading to a maladaptive immune response. Further research is needed to fully understand the mechanisms underlying TRM cells persistence in chronic infections and their dual role in both protective immunity and tissue pathology.
The therapeutic potential of TRM cells in urinary tract diseases, including infections, cancers, and chronic inflammatory conditions, is an area of growing interest (Table 2). Harnessing the localized immune response of TRM cells offers several promising strategies for improving treatment outcomes. In the context of infections like UTIs, vaccines designed to generate pathogen-specific TRM cells in mucosal tissues could provide long-lasting protection and reduce recurrence (71, 82). Enhancing TRM cells responses through adjuvants or immune modulators could also improve the efficacy of current vaccines and reduce the burden of recurrent infections (83). By deepening our understanding of TRM cells biology in the context of UTIs, novel interventions can be designed to improve long-term immune protection while minimizing immune-mediated pathology.
In urinary tract cancers, TRM cells have demonstrated a significant role in immune surveillance and anti-tumor immunity. Strategies that aim to boost the function of TRM cells, such as ICIs targeting PD-1/PD-L1 or enhancing their local activation, hold promise for improving response rates to immunotherapy (84, 85). In addition to PD-1/PD-L1 inhibitors, recent studies have also highlighted the importance of other immune checkpoints, such as CXCR3 and IL-15, in regulating the immune response to urinary tract tumors (86). CXCR3, a chemokine receptor, plays a crucial role in the trafficking and retention of effector T cells, including TRM cells, to tumor sites. Its expression on tumor-infiltrating lymphocytes is associated with improved immune surveillance and better responses to immunotherapy (87). Targeting CXCR3 may enhance T cell infiltration into tumors, especially in cancers such as bladder and prostate cancer, where TRM cells are critical for anti-tumor immunity. IL-15 is another key molecule involved in the activation and maintenance of TRM cells. It promotes the survival and function of memory T cells, including both CD4+ and CD8+ subsets. IL-15-based therapies are being explored as a way to boost TRM cells responses in tumors by enhancing their persistence and effector function. Recent preclinical and clinical studies suggest that IL-15 can be used to improve the efficacy of immunotherapies, particularly in cancers where TRM cells play a central role in local immunity (88). Together with PD-1 blockade, targeting these additional immune checkpoints could lead to more effective immune responses by not only reinvigorating exhausted T cells but also enhancing the recruitment and function of TRM cells within tumors. Additionally, combining these approaches with conventional therapies like chemotherapy or radiation could optimize anti-tumor responses (89).
For chronic inflammatory diseases, targeting TRM cells presents a unique challenge, as their persistent activation can contribute to tissue damage and pain. Therapies that modulate TRM cell activity, such as cytokine blockers or interventions that restore immune homeostasis (e.g. Sparsentan, the dual angiotensin II receptor and endothelin type A receptor antagonist), could reduce TRM cell responses, alleviate symptoms and reduce inflammation without compromising immune protection (69).
Looking forward, research into the specific signaling pathways that regulate TRM cell differentiation, retention, and activation will be crucial for developing targeted therapeutic approaches. While TRM cells are well recognized for their role in defending against acute reinfections, their role in chronic infections, such as tuberculosis, HIV, and chronic pyelonephritis, remains less explored. Future studies should focus on understanding how TRM cells are generated and maintained in chronic infection settings, and how their function may be altered over time in the face of persistent antigen exposure and inflammation. Targeting the modulation of TRM cells in chronic infections could lead to novel therapeutic strategies to enhance long-term immunity without causing excessive tissue damage. Immunotherapies targeting TRM cells in tumors: TRM cells have emerged as critical players in anti-tumor immunity, yet their full potential in cancer immunotherapy is still not fully realized. Future research should explore how to enhance the recruitment, persistence, and functionality of TRM cells in tumors, particularly through combination therapies that target immune checkpoints like PD-1, CXCR3, and IL-15. Investigating how TRM cells interact with the TME and their potential exhaustion during long-term antigen exposure could provide valuable insights into how to prevent or reverse TRM cells exhaustion in cancers. TRM in vaccine development: There is growing interest in developing vaccines that aim to generate long-lasting TRM cells populations at mucosal surfaces, such as the urinary tracts. These vaccines could be crucial for preventing infections like UTIs. Future research should focus on identifying the best strategies for inducing robust TRM cells responses through mucosal vaccination, as well as understanding how TRM cells contribute to vaccine-mediated immunity in both infectious and cancer settings.
In conclusion, TRM cells play a crucial role in the immune defense of the urinary tract, offering protection against infections, modulating tumor immunity, and influencing chronic inflammatory diseases. While their ability to provide long-lasting, localized immunity is promising, their dysregulation can contribute to chronic inflammation and tissue damage, especially in conditions like interstitial cystitis or chronic prostatitis. The therapeutic potential of TRM cells is immense, particularly in developing vaccines, enhancing cancer immunotherapy, and targeting chronic inflammation. Beyond urinary tract cancers, TRM cells have also been implicated in other malignancies, including lung cancer, breast cancer, and colorectal cancer. In non-small cell lung cancer, TRM cells enriched in tumor tissues have been associated with improved responses to ICIs, particularly PD-1/PD-L1 blockade. Similarly, in breast cancer, TRM cells contribute to local immune surveillance, though their exact role varies across molecular subtypes. In colorectal cancer, TRM cells have been linked to enhanced tumor control and better prognosis, especially in microsatellite instability-high tumors. Future research should explore how TRM cells contribute to tumor immunity across different cancer types and how their functional states may vary depending on the tumor microenvironment. Understanding the mechanisms regulating TRM cells exhaustion and activation in diverse cancers could provide insights into optimizing TRM-targeted immunotherapies. Expanding research beyond urinary tract cancers will be crucial for fully harnessing the therapeutic potential of TRM cells in oncology. Future research should focus on understanding the complex interactions of TRM cells in different disease contexts and exploring strategies to harness their protective functions while mitigating their pathological effects. By doing so, we can develop more effective and personalized treatments for urinary tract diseases.
GX: Writing – original draft. YL: Conceptualization, Validation, Writing – review & editing. GL: Writing – review & editing. DX: Writing – original draft, Writing – review & editing.
The author(s) declare financial support was received for the research, authorship, and/or publication of this article. This work was funded by Sichuan Science and Technology Program (2024ZYD0149), Scientific Research Startup Fund of the Affiliated Hospital of Southwest Medical University (24080), Key Research and Development Project of Deyang Science and Technology Bureau (2024SZY004, 2024SZY016), Special Research Foundation for the Postdoctoral Program of Sichuan Province (TB2024017), Natural Science Foundation of Sichuan (2023NSFSC0601), Special Fund for Incubation Projects of Deyang People’s Hospital (FHG202301, FHR202501, FHR202502) and Hospital Special Project for “Xinglin Scholar” of Chengdu University of Traditional Chinese Medicine (YYZX202258).
We would like to thank Professor Li Yang (State Key Laboratory of Biotherapy and Cancer Center, West China Hospital, Sichuan University) for technical assistance. We would like to acknowledge BioRender (www.biorender.com) for providing the platform and tools for creating the illustrations.
The authors declare that the research was conducted in the absence of any commercial or financial relationships that could be construed as a potential conflict of interest.
The author(s) declare that no Generative AI was used in the creation of this manuscript.
All claims expressed in this article are solely those of the authors and do not necessarily represent those of their affiliated organizations, or those of the publisher, the editors and the reviewers. Any product that may be evaluated in this article, or claim that may be made by its manufacturer, is not guaranteed or endorsed by the publisher.
CD4, Cluster of differentiation 4; CD8, Cluster of differentiation 8; CD69, Cluster of differentiation 69; CD103, Cluster of differentiation 103; CXCR3, C-X-C chemokine receptor 3; P2RX7, Purinergic receptor P2X7; CXCR6, C-X-C chemokine receptor 6; CD49a, Cluster of differentiation 49a; MHC, Major histocompatibility complex; IL-15, Interleukin 15; PD-1, Programmed cell death protein 1; TIM-3, T-cell immunoglobulin and mucin-domain containing-3; LAG-3, Lymphocyte-activation gene 3; IL-1β, Interleukin 1beta; IL-6, Interleukin 6; IL-23, Interleukin 23; JAK-STAT, Janus kinase-signal transducer and activator of transcription; S1PR1, Sphingosine-1-phosphate receptor 1; CCL20, C-C motif chemokine ligand 20; PD-L1, Programmed cell death ligand 1.
1. Masopust D, Soerens AG. Tissue-resident T cells and other resident leukocytes. Annu Rev Immunol. (2019) 37:521–46. doi: 10.1146/annurev-immunol-042617-053214
2. Mackay LK, Minnich M, Kragten NA, Liao Y, Nota B, Seillet C, et al. Hobit and blimp1 instruct a universal transcriptional program of tissue residency in lymphocytes. Science. (2016) 352:459–63. doi: 10.1126/science.aad2035
3. Shiow LR, Rosen DB, Brdickova N, Xu Y, An J, Lanier LL, et al. Cd69 acts downstream of interferon-alpha/beta to inhibit S1p1 and lymphocyte egress from lymphoid organs. Nature. (2006) 440:540–4. doi: 10.1038/nature04606
4. Duhen T, Duhen R, Montler R, Moses J, Moudgil T, de Miranda NF, et al. Co-expression of cd39 and cd103 identifies tumor-reactive cd8 T cells in human solid tumors. Nat Commun. (2018) 9:2724. doi: 10.1038/s41467-018-05072-0
5. Takamura S. Niches for the long-term maintenance of tissue-resident memory T cells. Front Immunol. (2018) 9:1214. doi: 10.3389/fimmu.2018.01214
6. Sun H, Sun C, Xiao W, Sun R. Tissue-resident lymphocytes: from adaptive to innate immunity. Cell Mol Immunol. (2019) 16:205–15. doi: 10.1038/s41423-018-0192-y
7. Martin MD, Badovinac VP. Defining memory cd8 T cell. Front Immunol. (2018) 9:2692. doi: 10.3389/fimmu.2018.02692
8. Wu X, Wu P, Shen Y, Jiang X, Xu F. Cd8(+) resident memory T cells and viral infection. Front Immunol. (2018) 9:2093. doi: 10.3389/fimmu.2018.02093
9. Hirahara K, Kokubo K, Aoki A, Kiuchi M, Nakayama T. The role of cd4(+) resident memory T cells in local immunity in the mucosal tissue - protection versus pathology. Front Immunol. (2021) 12:616309. doi: 10.3389/fimmu.2021.616309
10. Szabo PA. Axes of heterogeneity in human tissue-resident memory T cells. Immunol Rev. (2023) 316:23–37. doi: 10.1111/imr.13210
11. Riding RL, Harris JE. The role of memory cd8(+) T cells in vitiligo. J Immunol. (2019) 203:11–9. doi: 10.4049/jimmunol.1900027
12. Kok L, Masopust D, Schumacher TN. The precursors of cd8(+) tissue resident memory T cells: from lymphoid organs to infected tissues. Nat Rev Immunol. (2022) 22:283–93. doi: 10.1038/s41577-021-00590-3
13. Amsen D, van Gisbergen K, Hombrink P, van Lier RAW. Tissue-resident memory T cells at the center of immunity to solid tumors. Nat Immunol. (2018) 19:538–46. doi: 10.1038/s41590-018-0114-2
14. Cheon IS, Son YM, Sun J. Tissue-resident memory T cells and lung immunopathology. Immunol Rev. (2023) 316:63–83. doi: 10.1111/imr.13201
15. Yuan R, Yu J, Jiao Z, Li J, Wu F, Yan R, et al. The roles of tissue-resident memory T cells in lung diseases. Front Immunol. (2021) 12:710375. doi: 10.3389/fimmu.2021.710375
16. Kang S, Jin S, Mao X, He B, Wu C. Cd4+T and cd8+T cells in uterus exhibit both selective dysfunction and residency signatures. J Immunol Res. (2024) 2024:5582151. doi: 10.1155/2024/5582151
17. van der Putten C, Remmerswaal EBM, Terpstra ML, van der Bom ND, Kers J, Ten Berge IJM, et al. Cd8 and cd4 T cell populations in human kidneys. Cells. (2021) 10:288. doi: 10.3390/cells10020288
18. Rousseau M, Lacerda Mariano L, Canton T, Ingersoll MA. Tissue-resident memory T cells mediate mucosal immunity to recurrent urinary tract infection. Sci Immunol. (2023) 8:eabn4332. doi: 10.1126/sciimmunol.abn4332
19. Gehad A, Teague JE, Matos TR, Huang V, Yang C, Watanabe R, et al. A primary role for human central memory cells in tissue immunosurveillance. Blood Adv. (2018) 2:292–8. doi: 10.1182/bloodadvances.2017011346
20. Burbach BJ, O’Flanagan SD, Shao Q, Young KM, Slaughter JR, Rollins MR, et al. Irreversible electroporation augments checkpoint immunotherapy in prostate cancer and promotes tumor antigen-specific tissue-resident memory cd8+ T cells. Nat Commun. (2021) 12:3862. doi: 10.1038/s41467-021-24132-6
21. Oh JE, Iijima N, Song E, Lu P, Klein J, Jiang R, et al. Migrant memory B cells secrete luminal antibody in the vagina. Nature. (2019) 571:122–6. doi: 10.1038/s41586-019-1285-1
22. Byrne A, Savas P, Sant S, Li R, Virassamy B, Luen SJ, et al. Tissue-resident memory T cells in breast cancer control and immunotherapy responses. Nat Rev Clin Oncol. (2020) 17:341–8. doi: 10.1038/s41571-020-0333-y
23. Gavil NV, Cheng K, Masopust D. Resident memory T cells and cancer. Immunity. (2024) 57:1734–51. doi: 10.1016/j.immuni.2024.06.017
24. Park SL, Buzzai A, Rautela J, Hor JL, Hochheiser K, Effern M, et al. Tissue-resident memory cd8(+) T cells promote melanoma-immune equilibrium in skin. Nature. (2019) 565:366–71. doi: 10.1038/s41586-018-0812-9
25. Pizzolla A, Keam SP, Vergara IA, Caramia F, Thio N, Wang M, et al. Tissue-resident memory T cells from a metastatic vaginal melanoma patient are tumor-responsive T cells and increase after anti-pd-1 treatment. J Immunother Cancer. (2022) 10:e004574. doi: 10.1136/jitc-2022-004574
26. Corgnac S, Malenica I, Mezquita L, Auclin E, Voilin E, Kacher J, et al. Cd103(+)Cd8(+) T(Rm) cells accumulate in tumors of anti-pd-1-responder lung cancer patients and are tumor-reactive lymphocytes enriched with tc17. Cell Rep Med. (2020) 1:100127. doi: 10.1016/j.xcrm.2020.100127
27. Mackay LK, Rahimpour A, Ma JZ, Collins N, Stock AT, Hafon ML, et al. The developmental pathway for cd103(+)Cd8+ Tissue-resident memory T cells of skin. Nat Immunol. (2013) 14:1294–301. doi: 10.1038/ni.2744
28. Hombrink P, Helbig C, Backer RA, Piet B, Oja AE, Stark R, et al. Programs for the persistence, vigilance and control of human cd8+ Lung-resident memory T cells. Nat Immunol. (2016) 17:1467–78. doi: 10.1038/ni.3589
29. Stark R, Wesselink TH, Behr FM, Kragten NAM, Arens R, Koch-Nolte F, et al. Trm maintenance is regulated by tissue damage via P2rx7. Sci Immunol. (2018) 3:eaau1022. doi: 10.1126/sciimmunol.aau1022
30. Parga-Vidal L, van Aalderen MC, Stark R, van Gisbergen K. Tissue-resident memory T cells in the urogenital tract. Nat Rev Nephrol. (2022) 18:209–23. doi: 10.1038/s41581-021-00525-0
31. Sennepin A, Real F, Duvivier M, Ganor Y, Henry S, Damotte D, et al. The human penis is a genuine immunological effector site. Front Immunol. (2017) 8:1732. doi: 10.3389/fimmu.2017.01732
32. Mlugu EM, Mohamedi JA, Sangeda RZ, Mwambete KD. Prevalence of urinary tract infection and antimicrobial resistance patterns of uropathogens with biofilm forming capacity among outpatients in morogoro, Tanzania: A cross-sectional study. BMC Infect Dis. (2023) 23:660. doi: 10.1186/s12879-023-08641-x
33. Von Vietinghoff S, Shevchuk O, Dobrindt U, Engel DR, Jorch SK, Kurts C, et al. The global burden of antimicrobial resistance - urinary tract infections. Nephrol Dial Transplant. (2024) 39:581–8. doi: 10.1093/ndt/gfad233
34. Mettelman RC, Allen EK, Thomas PG. Mucosal immune responses to infection and vaccination in the respiratory tract. Immunity. (2022) 55:749–80. doi: 10.1016/j.immuni.2022.04.013
35. Iijima N. The emerging role of effector functions exerted by tissue-resident memory T cells. Oxf Open Immunol. (2024) 5:iqae006. doi: 10.1093/oxfimm/iqae006
36. Lund FE, Randall TD. Effector and regulatory B cells: modulators of cd4+ T cell immunity. Nat Rev Immunol. (2010) 10:236–47. doi: 10.1038/nri2729
37. Al Moussawy M, Abdelsamed HA. Non-cytotoxic functions of cd8 T cells: “Repentance of a serial killer. Front Immunol. (2022) 13:1001129. doi: 10.3389/fimmu.2022.1001129
38. Mora-Bau G, Platt AM, van Rooijen N, Randolph GJ, Albert ML, Ingersoll MA. Macrophages subvert adaptive immunity to urinary tract infection. PloS Pathog. (2015) 11:e1005044. doi: 10.1371/journal.ppat.1005044
39. Lacerda Mariano L, Rousseau M, Varet H, Legendre R, Gentek R, Saenz Coronilla J, et al. Functionally distinct resident macrophage subsets differentially shape responses to infection in the bladder. Sci Adv. (2020) 6:eabc5739. doi: 10.1126/sciadv.abc5739
40. Heintzman DR, Fisher EL, Rathmell JC. Microenvironmental influences on T cell immunity in cancer and inflammation. Cell Mol Immunol. (2022) 19:316–26. doi: 10.1038/s41423-021-00833-2
41. Reading JL, Galvez-Cancino F, Swanton C, Lladser A, Peggs KS, Quezada SA. The function and dysfunction of memory cd8(+) T cells in tumor immunity. Immunol Rev. (2018) 283:194–212. doi: 10.1111/imr.12657
42. Pei S, Deng X, Yang R, Wang H, Shi JH, Wang X, et al. Age-related decline in cd8(+) tissue resident memory T cells compromises antitumor immunity. Nat Aging. (2024) 4:1828-44. doi: 10.1038/s43587-024-00746-5
43. Jenkins E, Whitehead T, Fellermeyer M, Davis SJ, Sharma S. The current state and future of T-cell exhaustion research. Oxf Open Immunol. (2023) 4:iqad006. doi: 10.1093/oxfimm/iqad006
44. Jiang W, He Y, He W, Wu G, Zhou X, Sheng Q, et al. Exhausted cd8+T cells in the tumor immune microenvironment: new pathways to therapy. Front Immunol. (2020) 11:622509. doi: 10.3389/fimmu.2020.622509
45. Chow A, Perica K, Klebanoff CA, Wolchok JD. Clinical implications of T cell exhaustion for cancer immunotherapy. Nat Rev Clin Oncol. (2022) 19:775–90. doi: 10.1038/s41571-022-00689-z
46. Zheng Y, Wang X, Yang X, Xing N. Single-cell rna sequencing reveals the cellular and molecular characteristics of high-grade and metastatic bladder cancer. Cell Oncol (Dordr). (2023) 46:1415–27. doi: 10.1007/s13402-023-00820-x
47. Jin K, Yu Y, Zeng H, Liu Z, You R, Zhang H, et al. Cd103(+)Cd8(+) tissue-resident memory T cell infiltration predicts clinical outcome and adjuvant therapeutic benefit in muscle-invasive bladder cancer. Br J Cancer. (2022) 126:1581–8. doi: 10.1038/s41416-022-01725-6
48. Hartana CA, Ahlen Bergman E, Broome A, Berglund S, Johansson M, Alamdari F, et al. Tissue-resident memory T cells are epigenetically cytotoxic with signs of exhaustion in human urinary bladder cancer. Clin Exp Immunol. (2018) 194:39–53. doi: 10.1111/cei.13183
49. Komdeur FL, Prins TM, van de Wall S, Plat A, Wisman GBA, Hollema H, et al. Cd103+ Tumor-infiltrating lymphocytes are tumor-reactive intraepithelial cd8+ T cells associated with prognostic benefit and therapy response in cervical cancer. Oncoimmunology. (2017) 6:e1338230. doi: 10.1080/2162402X.2017.1338230
50. Sasson SC, Gordon CL, Christo SN, Klenerman P, Mackay LK. Local heroes or villains: tissue-resident memory T cells in human health and disease. Cell Mol Immunol. (2020) 17:113–22. doi: 10.1038/s41423-019-0359-1
51. Han L, Gao QL, Zhou XM, Shi C, Chen GY, Song YP, et al. Characterization of cd103+ Cd8+ Tissue-resident T cells in esophageal squamous cell carcinoma: may be tumor reactive and resurrected by anti-pd-1 blockade. Cancer Immunol Immunother. (2020) 69:1493–504. doi: 10.1007/s00262-020-02562-3
52. Banchereau R, Chitre AS, Scherl A, Wu TD, Patil NS, de Almeida P, et al. Intratumoral cd103+ Cd8+ T cells predict response to pd-L1 blockade. J Immunother Cancer. (2021) 9:e002231. doi: 10.1136/jitc-2020-002231
53. Laumont CM, Wouters MCA, Smazynski J, Gierc NS, Chavez EA, Chong LC, et al. Single-cell profiles and prognostic impact of tumor-infiltrating lymphocytes coexpressing cd39, cd103, and pd-1 in ovarian cancer. Clin Cancer Res. (2021) 27:4089–100. doi: 10.1158/1078-0432.CCR-20-4394
54. Stolley JM, Scott MC, Joag V, Dale AJ, Johnston TS, Saavedra F, et al. Depleting cd103+ Resident memory T cells in vivo reveals immunostimulatory functions in oral mucosa. J Exp Med. (2023) 220:e20221853. doi: 10.1084/jem.20221853
55. Stultz J, Fong L. How to turn up the heat on the cold immune microenvironment of metastatic prostate cancer. Prostate Cancer Prostatic Dis. (2021) 24:697–717. doi: 10.1038/s41391-021-00340-5
56. Garcia AJ, Ruscetti M, Arenzana TL, Tran LM, Bianci-Frias D, Sybert E, et al. Pten null prostate epithelium promotes localized myeloid-derived suppressor cell expansion and immune suppression during tumor initiation and progression. Mol Cell Biol. (2014) 34:2017–28. doi: 10.1128/MCB.00090-14
57. Kantoff PW, Higano CS, Shore ND, Berger ER, Small EJ, Penson DF, et al. Sipuleucel-T immunotherapy for castration-resistant prostate cancer. N Engl J Med. (2010) 363:411–22. doi: 10.1056/NEJMoa1001294
58. Germanos AA, Arora S, Zheng Y, Goddard ET, Coleman IM, Ku AT, et al. Defining cellular population dynamics at single-cell resolution during prostate cancer progression. Elife. (2022) 11:e79076. doi: 10.7554/eLife.79076
59. Lee MH, Laajala E, Kreutzman A, Jarvinen P, Nisen H, Mirtti T, et al. The tumor and plasma cytokine profiles of renal cell carcinoma patients. Sci Rep. (2022) 12:13416. doi: 10.1038/s41598-022-17592-3
60. Zhou J, Liu L, Yang T, Lu B. Prognostic and therapeutic value of cd103(+) cells in renal cell carcinoma. Exp Ther Med. (2018) 15:4979–86. doi: 10.3892/etm.2018.6025
61. Asada N, Ginsberg P, Gagliani N, Mittrucker HW, Panzer U. Tissue-resident memory T cells in the kidney. Semin Immunopathol. (2022) 44:801–11. doi: 10.1007/s00281-022-00927-7
62. Kim CG, Sang YB, Lee JH, Chon HJ. Combining cancer vaccines with immunotherapy: establishing a new immunological approach. Int J Mol Sci. (2021) 22:8035. doi: 10.3390/ijms22158035
63. Zhou M, Guo C, Li X, Huang Y, Li M, Zhang T, et al. Jak/stat signaling controls the fate of cd8+Cd103+ Tissue-resident memory T cell in lupus nephritis. J Autoimmun. (2020) 109:102424. doi: 10.1016/j.jaut.2020.102424
64. Krebs CF, Reimers D, Zhao Y, Paust HJ, Bartsch P, Nunez S, et al. Pathogen-induced tissue-resident memory th17 (Trm17) cells amplify autoimmune kidney disease. Sci Immunol. (2020) 5:eaba4163. doi: 10.1126/sciimmunol.aba4163
65. Tilstra JS, Avery L, Menk AV, Gordon RA, Smita S, Kane LP, et al. Kidney-infiltrating T cells in murine lupus nephritis are metabolically and functionally exhausted. J Clin Invest. (2018) 128:4884–97. doi: 10.1172/JCI120859
66. Couzi L, Merville P, Deminiere C, Moreau JF, Combe C, Pellegrin JL, et al. Predominance of cd8+ T lymphocytes among periglomerular infiltrating cells and link to the prognosis of class iii and class iv lupus nephritis. Arthritis Rheum. (2007) 56:2362–70. doi: 10.1002/art.22654
67. O’Sullivan KM, Lo CY, Summers SA, Elgass KD, McMillan PJ, Longano A, et al. Renal participation of myeloperoxidase in antineutrophil cytoplasmic antibody (Anca)-associated glomerulonephritis. Kidney Int. (2015) 88:1030–46. doi: 10.1038/ki.2015.202
68. Krebs CF, Paust HJ, Krohn S, Koyro T, Brix SR, Riedel JH, et al. Autoimmune renal disease is exacerbated by S1p-receptor-1-dependent intestinal th17 cell migration to the kidney. Immunity. (2016) 45:1078–92. doi: 10.1016/j.immuni.2016.10.020
69. Li L, Tang W, Zhang Y, Jia M, Wang L, Li Q, et al. Targeting tissue-resident memory cd8+ T cells in the kidney is a potential therapeutic strategy to ameliorate podocyte injury and glomerulosclerosis. Mol Ther. (2022) 30:2746–59. doi: 10.1016/j.ymthe.2022.04.024
70. Pauken KE, Wherry EJ. Overcoming T cell exhaustion in infection and cancer. Trends Immunol. (2015) 36:265–76. doi: 10.1016/j.it.2015.02.008
71. Muruganandah V, Sathkumara HD, Navarro S, Kupz A. A systematic review: the role of resident memory T cells in infectious diseases and their relevance for vaccine development. Front Immunol. (2018) 9:1574. doi: 10.3389/fimmu.2018.01574
72. Lacerda Mariano L, Ingersoll MA. The immune response to infection in the bladder. Nat Rev Urol. (2020) 17:439–58. doi: 10.1038/s41585-020-0350-8
73. Gitto S, Natalini A, Antonangeli F, Di Rosa F. The emerging interplay between recirculating and tissue-resident memory T cells in cancer immunity: lessons learned from pd-1/pd-L1 blockade therapy and remaining gaps. Front Immunol. (2021) 12:755304. doi: 10.3389/fimmu.2021.755304
74. Su F, Zhang W, Meng L, Zhang W, Liu X, Liu X, et al. Multimodal single-cell analyses outline the immune microenvironment and therapeutic effectors of interstitial cystitis/bladder pain syndrome. Adv Sci (Weinh). (2022) 9:e2106063. doi: 10.1002/advs.202106063
75. Fu J, Sykes M. Emerging concepts of tissue-resident memory T cells in transplantation. Transplantation. (2022) 106:1132–42. doi: 10.1097/TP.0000000000004000
76. de Leur K, Dieterich M, Hesselink DA, Corneth OBJ, Dor F, de Graav GN, et al. Characterization of donor and recipient cd8+ Tissue-resident memory T cells in transplant nephrectomies. Sci Rep. (2019) 9:5984. doi: 10.1038/s41598-019-42401-9
77. Xu X, Chen Y, Kong L, Li X, Chen D, Yang Z, et al. Potential biomarkers for immune monitoring after renal transplantation. Transpl Immunol. (2024) 84:102046. doi: 10.1016/j.trim.2024.102046
78. Dornieden T, Sattler A, Pascual-Reguant A, Ruhm AH, Thiel LG, Bergmann YS, et al. Signatures and specificity of tissue-resident lymphocytes identified in human renal peritumor and tumor tissue. J Am Soc Nephrol. (2021) 32:2223–41. doi: 10.1681/ASN.2020101528
79. Ginsberg P, Panzer U, Asada N. Tissue-resident memory T cells in renal autoimmune diseases. Front Immunol. (2023) 14:1111521. doi: 10.3389/fimmu.2023.1111521
80. Salazar FC, Martinez MS, Paira DA, Chocobar YA, Olivera C, Godoy GJ, et al. Cd8 T cells are dispensable for experimental autoimmune prostatitis induction and chronic pelvic pain development. Front Immunol. (2024) 15:1387142. doi: 10.3389/fimmu.2024.1387142
81. Lund JM, Hladik F, Prlic M. Advances and challenges in studying the tissue-resident T cell compartment in the human female reproductive tract. Immunol Rev. (2023) 316:52–62. doi: 10.1111/imr.13212
82. Saz-Leal P, Ligon MM, Diez-Rivero CM, Garcia-Ayuso D, Mohanty S, Vinuela M, et al. Mv140 mucosal vaccine induces targeted immune response for enhanced clearance of uropathogenic E. Coli in experimental urinary tract infection. Vaccines (Basel). (2024) 12:535. doi: 10.3390/vaccines12050535
83. Knight FC, Wilson JT. Engineering vaccines for tissue-resident memory T cells. Adv Ther (Weinh). (2021) 4:2000230. doi: 10.1002/adtp.202000230
84. Liu Q, Guan Y, Li S. Programmed death receptor (Pd-)1/pd-ligand (L)1 in urological cancers: the “All-around warrior” in immunotherapy. Mol Cancer. (2024) 23:183. doi: 10.1186/s12943-024-02095-8
85. Tsuji T, Matsuzaki J, Odunsi K. Tissue residency of memory cd8(+) T cells matters in shaping immunogenicity of ovarian cancer. Cancer Cell. (2022) 40:452–4. doi: 10.1016/j.ccell.2022.04.008
86. Sharma P, Goswami S, Raychaudhuri D, Siddiqui BA, Singh P, Nagarajan A, et al. Immune checkpoint therapy-current perspectives and future directions. Cell. (2023) 186:1652–69. doi: 10.1016/j.cell.2023.03.006
87. Wang X, Zhang Y, Wang S, Ni H, Zhao P, Chen G, et al. The role of cxcr3 and its ligands in cancer. Front Oncol. (2022) 12:1022688. doi: 10.3389/fonc.2022.1022688
88. Cai M, Huang X, Huang X, Ju D, Zhu YZ, Ye L. Research progress of interleukin-15 in cancer immunotherapy. Front Pharmacol. (2023) 14:1184703. doi: 10.3389/fphar.2023.1184703
Keywords: tissue-resident memory T cells, urinary tract, infection, tumor, immunotherapy
Citation: Xu G, Li Y, Lu G and Xie D (2025) Tissue-resident memory T cells in urinary tract diseases. Front. Immunol. 16:1535930. doi: 10.3389/fimmu.2025.1535930
Received: 28 November 2024; Accepted: 11 February 2025;
Published: 24 February 2025.
Edited by:
Jinze Li, Sichuan University, ChinaReviewed by:
Jin Wu, Roswell Park Comprehensive Cancer Center, United StatesCopyright © 2025 Xu, Li, Lu and Xie. This is an open-access article distributed under the terms of the Creative Commons Attribution License (CC BY). The use, distribution or reproduction in other forums is permitted, provided the original author(s) and the copyright owner(s) are credited and that the original publication in this journal is cited, in accordance with accepted academic practice. No use, distribution or reproduction is permitted which does not comply with these terms.
*Correspondence: Daoyuan Xie, eGllZGFveXVhbkBhbHUuc2N1LmVkdS5jbg==; Guanting Lu, Z3VhbnRsdkAxMjYuY29t
†These authors have contributed equally to this work
Disclaimer: All claims expressed in this article are solely those of the authors and do not necessarily represent those of their affiliated organizations, or those of the publisher, the editors and the reviewers. Any product that may be evaluated in this article or claim that may be made by its manufacturer is not guaranteed or endorsed by the publisher.
Research integrity at Frontiers
Learn more about the work of our research integrity team to safeguard the quality of each article we publish.