- 1Guangdong Provincial People’s Hospital, Zhuhai Hospital (Jinwan Central Hospital of Zhuhai), Zhuhai, Guangdong, China
- 2Sleep Medicine Center, Huai’an No.3 People’s Hospital, Huaian Second Clinical College of Xuzhou Medical University, Huaian, China
- 3Department of Pharmacy, The Fifth Affiliated Hospital, Sun Yat-sen University, Zhuhai, Guangdong, China
- 4The Affiliated Hospital of Qingdao University, Qingdao University, Qingdao Cancer Institute, Qingdao, Shandong, China
- 5School of Life Sciences, Tsinghua University, Beijing, China
CD73, an important metabolic and immune escape-promoting gene, catalyzes the hydrolysis of adenosine monophosphate (AMP) to adenosine (ADO). AMP has anti-inflammatory and vascular relaxant properties, while ADO has a strong immunosuppressive effect, suggesting that CD73 has pro-inflammatory and immune escape effects. However, CD73 also decreased proinflammatory reaction, suggesting that CD73 has a positive side to the body. Indeed, CD73 plays a protective role in diabetes, while with age, CD73 changes from anti-atherosclerosis to pro-atherosclerosis. The upregulation of CD73 with agents, including AGT-5, Aire-overexpressing DCs, Aspirin, BAFFR-Fc, CD4+ peptide, ICAs, IL-2 therapies, SAgAs, sCD73, stem cells, RAD51 inhibitor, TLR9 inhibitor, and VD, decreased diabetes and atherosclerosis development. However, the downregulation of CD73 with agents, including benzothiadiazine derivatives and CD73 siRNA, reduced atherosclerosis. Notably, many CD73 agents were investigated in clinical trials. However, no agents were used to treat diabetes and atherosclerosis. Most agents were CD73 inhibitors. Only FP-1201, a CD73 agonist, was investigated in clinical trials but its further development was discontinued. In addition, many lncRNAs, circRNAs, and genes are located at the same chromosomal location as CD73. In particular, circNT5E promoted CD73 expression. circNT5E may be a promising target for agent development. This mini-review focuses on the current state of knowledge of CD73 in diabetes, atherosclerosis, and its potential role in agent development.
1 Introduction
CD73 [also named ecto-5’-nucleotidase (5NTE), a cell surface-bound nucleotidase, is an important metabolic and immune escape-promoting gene. CD73 catalyzes the hydrolysis of adenosine monophosphate (AMP) to adenosine (ADO). AMP has anti-inflammatory and vascular relaxant properties, while ADO has a strong immunosuppressive effect by adenosine A2A receptor (A2AR) and A2BR, suggesting that CD73 promotes tumor cells to achieve immune escape (1–3). However, CD73 also has a positive side to the body. CD73 decreased proinflammatory reaction by promoting M2 macrophage phenotype (anti-inflammatory), enhancing endothelial barrier function, and inhibiting leukocyte trafficking (4). CD73 is a mesenchymal stem cell (MSC) and Breg-specific marker (5–8). CD73 is associated with a variety of diseases, including atherosclerosis, cancer, cirrhotic cardiomyopathy, diabetes, graft-versus-host disease (GVHD), periodontitis, rheumatoid arthritis, and systemic lupus erythematosus (SLE) (1–3, 9–12). Especially in cancer, the role and mechanism of CD73 have been reviewed and studied by multiple laboratories (1–3). However, few reviews exist on the agent development of CD73 and its role in diabetes and atherosclerosis. The main aims of this mini-review are to describe the current state of knowledge of CD73 in diabetes, atherosclerosis, and its potential role in agent development.
2 The role and mechanism of CD73 in diabetes and atherosclerosis
2.1 Diabetes
CD73 was increased in the kidneys of diabetic mice. The absence of CD73 was positively associated with the severity of diabetic nephropathy, suggesting that CD73 is a potential biomarker of diabetic nephropathy (13). B lymphocytes promote the development of type 1 diabetes mellitus (T1DM) by promoting the expansion of pathogenic T cells. Anti-CD20, a B lymphocyte-targeted therapy, promoted B lymphocyte depletion. However, they failed to halt β cell demise. Suppressing RAD51 with CRISPR/cas9 and inhibitors (such as 4,4’-diisothiocyanatostilbene-2, 2’-disulfonic acid) decreases the diabetes process by reducing diabetogenic T cell responses via expanding CD73+ B lymphocytes that exert regulatory activity in T1DM-susceptible nonobese diabetic (NOD) mice (14). Soluble BAFF receptor (BAFFR)-Fc (BAFFR-Fc), a fusion protein that fuses with the extracellular part of BAFFR to the Fc domain of mouse IgG1, was developed by MedImmune. BAFFR-Fc decreases T1DM procession by expanding CD73+ B lymphocytes and reduces side effects of anti-CD20 (15), suggesting that CD73 plays a key role in reducing side effects of B lymphocyte-targeted therapies. Indeed, many studies have shown that CD73 plays a protective role in diabetes. For example, AGT-5 (oral compound), a new class of fluorescent aryl hydrocarbon receptor (AHR) ligands (FluoAHRL), suppressed the severity of streptozotocin (STZ)-induced T1DM by enhancing AHR and CD73 expression in mice (16). Vitamin D3 (VD, 25-(OH)D3) reduced diabetes mellitus (DM)-related cognitive dysfunction by enhancing CD39 and CD73 expression in streptozotocin-induced T1DM rats (17). Autoimmune regulator (Aire)-overexpressing dendritic cells (DCs) delayed T1DM processing by reducing CD4+ IFN-γ+ T cells level and enhancing CD73, lymphocyte activation Gene-3 (LAG-3), and folic acid receptor 4 (FR4) expression and CD4+ T cells apoptosis in splenocytes in STZ-T1DM mouse (18). The islet-like cell aggregates (ICAs) decreased diabetes procession by expressing CD73 in STZ-induced diabetic mice (19). Interleukin-2 (IL-2) therapies with anti-IL-2 antibodies decrease the T1DM process by enhancing CD25, CD39, and CD73 expression in regulatory T cells (Treg cells) in NOD mice (20). Liposomes encapsulating the CD4+ peptide [BDC2.5mim, has a high affinity for islet autoantigen chromogranin a (ChgA)] and 1α,25-dihydroxy vitamin D3 (calcitriol) suppressed diabetes progression by activating ChgA-specific forkhead box P3 (Foxp3)+ and Foxp3- programmed cell death 1 (PD1)+ CD73+ inducible T cell costimulator (ICOS, also named CD278)+ IL-10+ peripheral regulatory T cells (21), suggesting that CD4+ peptide combination with calcitriol decreased diabetes progression by enhancing CD73+ expression. Soluble CD73 (sCD73) decreased diabetic nephropathy (13). Soluble antigen arrays (SAgAs) were able to bind more effectively to antigen-specific T cells, such as CD73, IL-10, PD-1, and killer cell lectin-like receptor G1 (KLRG1), alleviating disease progression in non-obese diabetic mouse models of T1DM (22). Toll-like receptor 9 (TLR9) deficiency and inhibitor decreases diabetes development by reducing proinflammatory cytokines and promoting anti-inflammatory cytokine release via enhancing CD73 expression in T cells in NOD mice. The increase in CD73-expressing immune cells is specific for TLR9 deficiency (23), suggesting that CD73 plays a key role in TLR9 inhibitors in reducing diabetes development. In summary, CD73 may be a promising target for treating diabetes. Overexpressing CD73 with agents, such as AGT-5, Aire-overexpressing DCs, BAFFR-Fc, CD4+ peptide, ICAs, IL-2 therapies, SAgAs, sCD73, RAD51 inhibitor, TLR9 inhibitor, and VD decreases diabetes development (Figure 1A). However, the role of CD73 knockdown (such as siRNA) and knockout in diabetes is unclear.
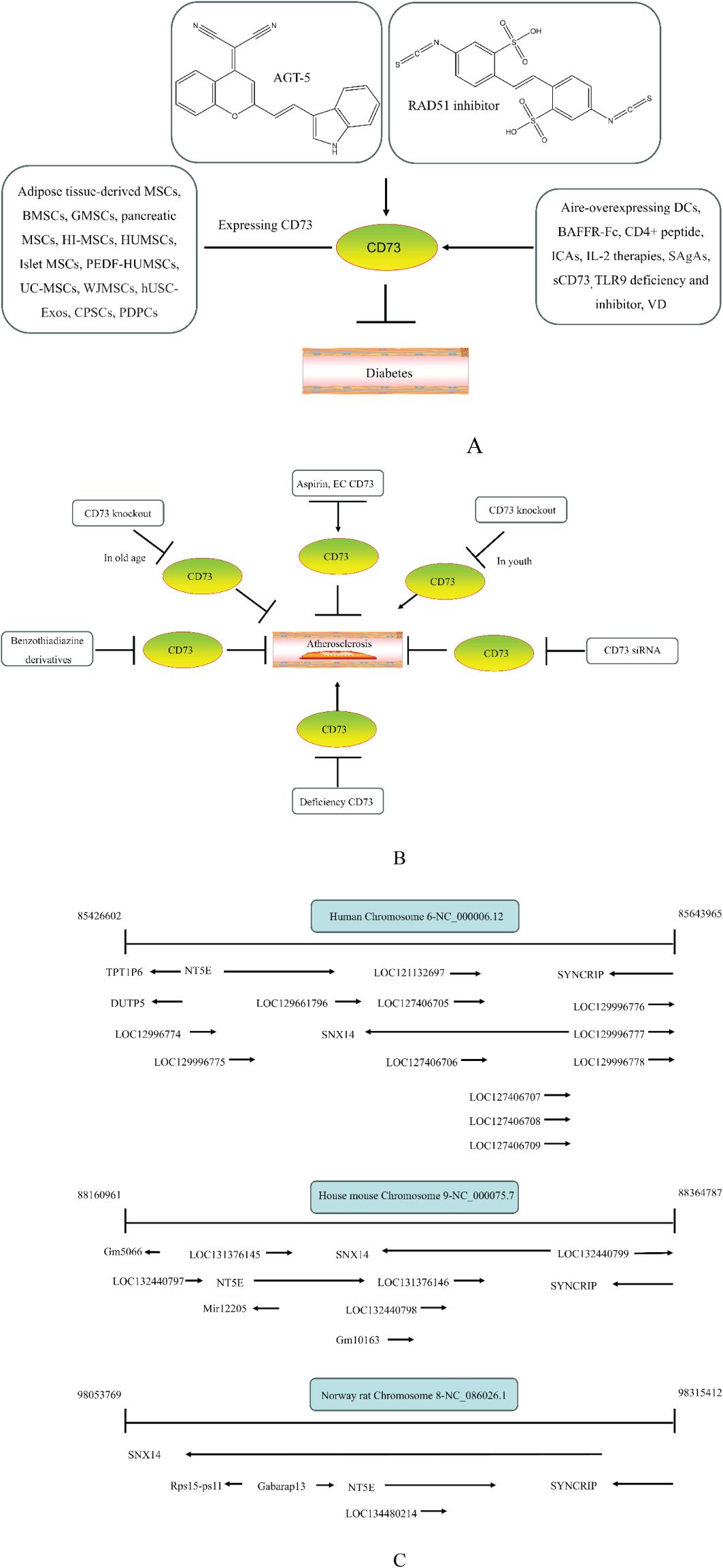
Figure 1. The role of CD73 agents in diabetes and atherosclerosis and the lncRNAs and genes at the same chromosomal location as CD73. (A) Diabetes, (B) atherosclerosis, (C) This information was modified from gene (NIH).
Many studies have shown that MSC transplantation, such as adipose tissue-derived MSCs, bone MSCs (BMSCs), human gingiva MSCs (GMSCs), pancreatic MSCs, human islet-MSCs (HI-MSCs), human umbilical cord MSCs (HUMSCs), Islet MSCs, pigment epithelial-derived factor (PEDF) gene-modified HUMSCs (PEDF-HUMSCs), umbilical cord (UC) MSCs (UC-MSCs), Wharton’s jelly-derived MSCs (WJMSCs) suppressed diabetes development (13, 16, 17, 22, 24–36). Human urine-derived stem cells (hUSCs) and their exosomes (hUSC-Exos) also suppressed diabetes development (37–39). Cow-derived placental stem cells (CPSCs) and periosteum-derived progenitor cells (PDPCs) promoted insulin secretion by differentiating into islet-producing cells (IPCs) (40, 41), suggesting that CPSCs and PDPCs have the potential to treat diabetes by differentiating IPCs. CD73 is expressed in hUSCs, hUSC-Exos, CPSCs, and PDPCs. As mentioned earlier, CD73 is a marker of MSCs. However, the role and mechanism of CD73 in MSCs, hUSCs, and USC-Exos in suppressing diabetes is unclear. Notably, MSCs, hUSCs, and USC-Exos can hydrolyze inflammatory extracellular ATP to anti-inflammatory adenosine via expressing CD73 and CD39 (42). In addition, GMSCs require CD39/CD73 signals to inhibit T1DM (43). GMSCs delayed the onset of diabetes by downregulating IL-17 and IFN-γ levels in CD4+ and CD8+ T cells in spleens, pancreatic lymph nodes (pLN), and other lymph nodes via expressing CD39 and CD73 (43). Thus, CD73 plays an anti-inflammatory role and immunoregulatory function in MSCs for treating diseases.
2.2 Atherosclerosis
Serum CD73 activity is upregulated in patients with atherosclerotic burden (44). Deficiency CD73 in patients exhibited extensive medial arterial calcification (MAC) which is an atherosclerosis risk, suggesting that CD73 may be a biomarker of MAC and atherosclerosis (45–47). Many studies have shown that CD37 is an enemy of atherosclerosis. For example, deficiency CD73 caused arterial calcification in patients, suggesting that overexpression CD73 suppressed arterial calcification (45–47). In ECs, CD73 suppressed inflammation and thrombosis and enhanced endothelial permeability by activating the adenosine/P1 receptor signaling pathway, (48). ECs CD73 can reduce leukocyte adhesion to the endothelium. Deletion of ECs connexin40 (Cx40) increases atherosclerosis by increasing CD73-dependent leukocyte adhesion via reducing CD73 expression (49), suggesting that ECs CD73 is an antiatherosclerotic factor. Aspirin reduces atherosclerotic plaque and immuno-inflammation by rebalancing Treg/Th17 cells via enhancing CD73 expression in ApoE-/- mice (50). However, CD37 is a friend of atherosclerosis. Suppressing CD73 with siRNA decreased atherosclerosis and plaque formation by reducing migration, proliferation, and foam cell transformation of vascular smooth muscle cells (VSMCs) via reducing CyclinD1 expression and serum lipid levels in ApoE-/- mice (51). However, the mechanism of CD73 on serum lipid levels is unclear. Inhibition of CD73 can reduce the increase of heart rate caused by hypoxia (52). Benzothiadiazine derivatives, the CD73 inhibitors, were investigated for treating atherosclerosis and ischemia-reperfusion injury (53). These results suggest that CD73 is double-sided in atherosclerosis. ECs CD73 is an antiatherosclerotic factor, while VSMCs CD73 is a proatherosclerotic factor. In fact, whether CD73 is a friend or foe in atherosclerosis may be age-related (Figure 1B). In early atherosclerosis, CD73 knockout promoted plaque area in apoE-/- mice at 12 weeks of age. However, the pattern shifts with age. CD73 knockout did not plaque area in apoE-/- mice at 20 weeks of age. In apoE-/- mice at 32 weeks and 52 weeks of age, CD73 knockout decreased plaque area by reducing lipolysis (54). ADO suppresses lipolysis, suggesting that CD73 promoted plaque accumulation by suppressing lipid catabolism via catalyzing the conversion of AMP to ADO with aging (54). Thus, with the increase of age, CD73 gradually changed from inhibiting atherosclerosis to promoting atherosclerosis.
3 The agent development in preclinical and clinical trials by targeting CD73
3.1 Targeting CD73
Given the important role of CD73 in diabetes mellitus and atherosclerosis, we searched for agents that target CD73 with AdisInsight, Bing, Chinadrugtrials, ClinicalTrials, Glgoo, ICTRP, Pharmacodia, Pharnexcloud, Pubmed, Yaozh, and Zhihuiya. Indeed, many agnets were developed in preclinical and clinical trials by targeting CD73 (Table 1), including A000830 (also named A-001190, A-001202, A-001421, AB-421) (55, 56), Adeno-associated viral type 5 (AAV5)-CD39/CD73 (57), ABSK051 (58–60), AG-2170 (61), AK131 (also named AK123) (62), ATN-037 (also named ATG-037, CB-708) (63–65), AP401 (67–69), APB-A2 (also named Anti-CD73 IgG4) (66), APCP (70), BB-1709 (71), BC010 (72), BP-1200 (73, 74), BPI-472372 (75, 76), BR101 (also named Ansipastobart) (77), BsAb CD73xEGFR (78), BMS-986179 (79), CBO421 (also named CBO-212, CD-421) (80–83), CC-5 (84), CD39/CD73 bifunctional fusion protein (85), CD39/CD73 transgenic exosomes and recombinant fusion protein (86, 87), CD73/PD-1 targeting DFC (88, 89), CD73 inhibitor (BioArdis) (90), CD73 inhibitor (Arcus Bios) (91), CD73 ASO (92), CHS-7304 (93), Compound 12f (94), Dalutrafusp alfa (also named AEGN-1423, GS-1423) (95), DN-018 (also named DN-019, DN-020, DN-052, DN-A1) (96), Dresbuxelimab (also named ak-119) (97), FP-1201 (also named ATC code L03AB07, Avonex, BG9418, Rebif, FP-1201-lyo, MR11A8, Traumakine) (98–101), 68GA-DOTA-dPNE, GB-7002 (also named GB-7002-01, GB-7002-04) (102), GI-108 (103, 104), HB-0039 (105), HB0045 (106), HB0046 (107), HB-0052 (106), HBM1007 (108), HLX23 (109), IBI325 (110), INCA-00186 (also named INCA-0186) (111), IOA-237 (112), IPH5301 (113), JAB-X1800 (also named CD73-STING iADC) (114, 115), JAB-BX102 (116), LY-3475070 (84), mAb19 (117), Mupadolimab (also named CPI-006, CPX-006) (118–121), Oleclumab (also named MEDI9447) (122–124), OP-5558 (125), OP-5244 (126, 152), OPN-CD73 (also named OPN-9627) (127), ORIC-533 (also named OP-5244, OR-558) (128–130), PBF2828 (131), PM-1015 (132), PSB-12379 (133), PSB-18332 (134, 135), PSB-19416 (134, 135), PT199 (136), Quemliclustat (also named AB680, A-0002396) (137–139, 152–155), S095024 (also named Sym024) (140), SHR170008 (141), siRNA-CD73 (70), SRF-373 (also named NZV930) (142), TRB-010 (143), Uliledlimab (also named TJD5, TJ004309, I-Mab Biopharma) (144–146), VE-3771 (147), VE-5953 (147), X-6350 (148), ZM514 (149), ZM552 (150), ZM553 (150), ZM557 (150), and ZS-1001 (151). However, no agents have been approved for sale. Notably, most of these agents are used to treat cancer. Whether CD73 as a target for treating atherosclerosis and diabetes is worth developing remains to be investigated. Notably, CD73 may be a delivery target for atherosclerotic plaques. As mentioned earlier, CD73 is a specific marker of MSC. Many studies have shown that umbilical cord (UC) MSC transplantation suppressed atherosclerosis development. However, MSCs have limited homing ability to atherosclerotic plaque sites. Integrin beta 3 (ITGB3)-modified MSCs successfully retained CD73 expression and enhanced the plaque-homing ability of MSCs, suggesting that ITGB3 is a good material for MSCs to deliver to plaques (7). CD73 combination with ITGB3 may be worth developing as the delivery target for atherosclerotic plaques.
3.2 Targeting CD73 antisense RNA
According to the gene (NIH), CD73 was encoded by NT5E. There are multiple long noncoding antisense RNAs (lncRNAs) and genes in the same region of NT5E. The same position of NT5E in human includes lncRNAs and genes in the same direction (inlcuding LOC121132697, LOC127406705, LOC127406706, LOC127406707, LOC127406708, LOC127406709, LOC129661796, LOC129996774, LOC129996775, LOC129996776, LOC129996777, and LOC129996778) and those in the opposite direction (including DUTP5, SNX14, SYNCRIP, and TPT1P6) (Figure 1C). The NT5E in house mouse in the same direction includes LOC131376145, LOC131376146, LOC132440797, LOC132440798, LOC132440799, and Gm10163, while the opposite direction includes miR12205, SNX14, SYNCRIP, and Gm5066. The norway rat in the same direction includes Gabarapl3 and LOC134480214, while the opposite direction includes Rps15-ps11, SNX14, and SYNCRIP. Notably, the DNA region of NT5E in humans contains both LOC129996774 and LOC129996775. The same position of NT5E in human, house mouse, and norway rat includes SNX14 and SYNCRIP. Targeting LOC129996774, LOC129996775 SNX14, and SYNCRIP may be a novel agent development strategy by regulating CD73 expression and immune homeostasis. However, the role of LOC129996774, LOC129996775, SNX14, and SYNCRIP on CD73 is unclear. In addition, the DNA region of NT5E in house mouse and norway rat did not contain LOC129996774 and LOC129996775. Notably, lncRNA NT5E (lncNT5E) was located on human chromosome 6q14.3. LncNT5E promotes pancreatic cancer (PC) development and may be a poor prognosis biomarker of PC (156). CircNT5E (also named hsa_circ_0077232), a novel circRNA derived from NT5E, promoted the development of multiple tumors, including bladder cancer (157), glioblastoma (GBM) (158), and non-small cell lung cancer (NSCLC) (159). CircNT5E promoted NT5E expression in U87 and U251 cells (158). Targeting CD73 with lncNT5E and circNT5E, specifically, circNT5E, may be a novel strategy for agent development. However, the role of lncRNA NT5E on NT5E is unclear. CircNT5E did not change NT5E expression in A549 cells (159). Research on lncNT5E and circNT5E is also scarce, with only four references in PubMed. More research is needed to confirm the feasibility of lncNT5E and circNT5E development.
4 Summary
Serum CD73 is a potential biomarker of diabetes and atherosclerosis. However, the selection of biomarkers should consider disease status, predisease status, or prognosis and should be more sensitive, specific, and easier to detect than existing markers. The upregulation of CD73 with agents, including AGT-5, Aire-overexpressing DCs, Aspirin, BAFFR-Fc, CD4+ peptide, ICAs, IL-2 therapies, SAgAs, sCD73, stem cells, RAD51 inhibitor, TLR9 inhibitor, and VD, decreased the development of diabetes and atherosclerosis in preclinical trials. However, the downregulation of CD73 with agents, including benzothiadiazine derivatives and CD73 siRNA, decreased atherosclerosis. ECs CD73 is an antiatherosclerotic factor, while VSMCs CD73 is a proatherosclerotic factor, suggesting that the role of CD73 in atherosclerosis may depend on its localization. However, CD73 may change from anti-atherosclerosis to pro-atherosclerosis with age. More studies were needed to confirm the role of CD73 in atherosclerosis. In addition, CD73 has a cardioprotective function in heart failure (HF) and myocardial infarction (MI). CD73 has a protective effect on the liver and kidney during ischemia-reperfusion injury (IR/I). The function of CD73 in multiple organ systems and cell types is reviewed by Minor et al. (160). Notably, many agents, including ABSK051, AK131, ATN-037, AP401, APB-A2, BB-1709, BPI-472372, BR101, BMS-986179, CBO421, Dalutrafusp alfa, Dresbuxelimab, FP-1201, 68GA-DOTA-dPNE, GI-108, HB0045, HB0046, HB-0052, HBM1007, HLX23, IBI325, INCA-00186, IPH5301, JAB-BX100, JAB-BX102, LY-3475070, Mupadolimab, Oleclumab, ORIC-533, PM-1015, PT199, Quemliclustat, S095024, SRF-373, and Uliledlimab, was investigated in clinical trials. However, most of these agents were CD73 inhibitors and were used to treat acute lung injury (ALI), acute respiratory distress syndrome (ARDS), cancer, and COVID-19. Only FP-1201 was a CD73 agonist and was investigated in phase 1/2/3 clinical trials. However, the further development of FP-201 was discontinued. CD39/CD73 BFP and recombinant fusion protein CD39/CD73 transgenic exosomes, the CD73 agonist were investigated for the treatment of inflammatory disease. However, more studies are needed to confirm whether clinical trials are warranted. Whether CD73 agonists are worth developing remains to be seen. In addition, many lncRNAs, circRNAs, and genes are located at the same chromosomal location as CD73. In particular, circNT5E promoted CD73 expression. circNT5E may be a promising target for agent development. However, circRNAs usually have many target genes, so how to reduce off-target effects also needs more research.
In summary, CD73 is a potential biomarker of diabetes and atherosclerosis. Targeting CD73 could improve the success rate of drug development. As research continues and technology advances, we believe that new agents will be developed to combat diseases.
Author contributions
DL: Conceptualization, Data curation, Formal analysis, Writing – original draft. JZ: Data curation, Formal analysis, Investigation, Writing – original draft, Funding acquisition. LL: Conceptualization, Investigation, Project administration, Writing – original draft. JW: Conceptualization, Formal analysis, Writing – original draft. CW: Conceptualization, Data curation, Formal analysis, Writing – original draft. YW: Conceptualization, Data curation, Formal analysis, Validation, Writing – original draft. YH: Data curation, Formal analysis, Project administration, Writing – review & editing. DX: Conceptualization, Data curation, Project administration, Writing – review & editing. WC: Funding acquisition, Project administration, Writing – review & editing.
Funding
The author(s) declare financial support was received for the research, authorship, and/or publication of this article. The authors are grateful for the financial support from the Qingdao Major Scientific and Technological Project for Distinguished Scholars (20170103), the Laoshan Major Scientific and Technological Project for Distinguished Scholars (20181030), and Huai’an natural science research program (HAB202216).
Acknowledgments
We thank the laboratory of Professor Dongming Xing for generously supplying reagents, providing technical assistance, stimulating discussions. We thank Mantao Xu (College of Information Technology, Shanghai Jian Qiao University Co Ltd, No.1111 Hucheng Ring Rd, China (Shanghai) Pilot Free Trade Zone Lin-Gang Special Area, 201315 Shanghai, China) for contributing to grammar editing.
Conflict of interest
The authors declare that the research was conducted in the absence of any commercial or financial relationships that could be construed as a potential conflict of interest.
Generative AI statement
The author(s) declare that no Generative AI was used in the creation of this manuscript.
Publisher’s note
All claims expressed in this article are solely those of the authors and do not necessarily represent those of their affiliated organizations, or those of the publisher, the editors and the reviewers. Any product that may be evaluated in this article, or claim that may be made by its manufacturer, is not guaranteed or endorsed by the publisher.
Abbreviations
A2AR, Adenosine A2A receptor; AAV5, Adeno-associated viral type 5; ADO, Adenosine; AHR, Aryl hydrocarbon receptor; Aire, Autoimmune regulator; ALI, Acute lung injury; AMP, Adenosine monophosphate; ATP, Adenosine triphosphate; ARDS, Acute respiratory distress syndrome; BAFFR, BAFF receptor; calcitriol, 1α,25-dihydroxy vitamin D3; ChgA, Chromogranin a; CPSCs, Cow-derived placental stem cells; Cx40, Connexin40; DCs, Dendritic cells; DM, Diabetes mellitus; FluoAHRL, Fluorescent AHR ligands; Foxp3, Forkhead box P3; FR4, Folic acid receptor 4; GBM, Glioblastoma; GVHD, Graft-versus-host disease; HF, Heart failure; hUSCs, Human urine-derived stem cells; ICAs, Islet-like cell aggregates; IL-2, Interleukin-2; IPCs, Islet-producing cells; IRI, Ischemia-reperfusion injury; KLRG1, Killer cell lectin-like receptor G1; LAG-3, Lymphocyte activation Gene-3; MAC, Medial arterial calcification; MI, Myocardial infarction; MSC, Mesenchymal stem cell; 5NTE, Ecto-5’-nucleotidase; NOD, Nonobese diabetic; NSCLC, Non-small cell lung cancer; PDPCs, Periosteum-derived progenitor cells; PEDF, Pigment epithelial-derived factor; SAgAs, Soluble antigen arrays; sCD73, Soluble CD73; SLE, Systemic lupus erythematosus; STZ, Streptozotocin; T1DM, Type 1 diabetes mellitus; TLR9, Toll-like receptor 9; VD, Vitamin D3; VSMCs, Vascular smooth muscle cells.
References
1. Kowash RR, Akbay EA. Tumor intrinsic and extrinsic functions of CD73 and the adenosine pathway in lung cancer. Front Immunol. (2023) 14:1130358. doi: 10.3389/fimmu.2023.1130358
2. Han K, Wang F, Ma X, Wu Y, Zhang H, Zhao Y, et al. Human placental mesenchymal stromal cells alleviate intestinal oxidative damage in mice with graft-versus-host disease via CD73/adenosine/pi3k/akt/gsk-3beta axis. Cell Signal. (2024) 123:111372. doi: 10.1016/j.cellsig.2024.111372
3. Dwyer KM, Kishore BK, Robson SC. Conversion of extracellular atp into adenosine: a master switch in renal health and disease. Nat Rev Nephrol. (2020) 16:509–24. doi: 10.1038/s41581-020-0304-7
4. Yang J, Liao X, Yu J, Zhou P. Role of CD73 in disease: promising prognostic indicator and therapeutic target. Curr Med Chem. (2018) 25:2260–71. doi: 10.2174/0929867325666180117101114
5. Nosrati S, Gheisari M, Zare S, Dara M, Zolghadri S, Razeghian-Jahromi I. The impact of diabetic glucose concentration on viability and cardiac differentiation of mesenchymal stem cells. Tissue Cell. (2024) 88:102361. doi: 10.1016/j.tice.2024.102361
6. Michelis KC, Nomura-Kitabayashi A, Lecce L, Franzen O, Koplev S, Xu Y, et al. Cd90 identifies adventitial mesenchymal progenitor cells in adult human medium- and large-sized arteries. Stem Cell Rep. (2018) 11:242–57. doi: 10.1016/j.stemcr.2018.06.001
7. Hu HJ, Xiao XR, Li T, Liu DM, Geng X, Han M, et al. Integrin beta 3-overexpressing mesenchymal stromal cells display enhanced homing and can reduce atherosclerotic plaque. World J Stem Cells. (2023) 15:931–46. doi: 10.4252/wjsc.v15.i9.931
8. Aranguren M, Doyon-Laliberte K, El-Far M, Chartrand-Lefebvre C, Routy JP, Barril JG, et al. Subclinical atherosclerosis is associated with discrepancies in BAFF and April levels and altered breg potential of precursor-like marginal zone B-cells in long-term HIV treated individuals. Vaccines (Basel). (2022) 11:81. doi: 10.3390/vaccines11010081
9. Zhao N, Shao Z, Xia G, Liu H, Zhang L, Zhao X, et al. Protective role of the CD73-A2ar axis in cirrhotic cardiomyopathy through negative feedback regulation of the nf-kappab pathway. Front Immunol. (2024) 15:1428551. doi: 10.3389/fimmu.2024.1428551
10. Ramos-Junior ES, Dawson S, Ryan W, Clinebell B, Serrano-Lopez R, Russell M, et al. The protective role of CD73 in periodontitis: preventing hyper-inflammatory fibroblasts and driving osteoclast energy metabolism. Front Oral Health. (2023) 4:1308657. doi: 10.3389/froh.2023.1308657
11. Ortiz MA, Diaz-Torne C, De Agustin JJ, Estrada P, Reina D, Hernandez MV, et al. Altered cd39 and CD73 expression in rheumatoid arthritis: implications for disease activity and treatment response. Biomolecules. (2023) 14:1. doi: 10.3390/biom14010001
12. Dong K, Wang Y, Yao Y, Yu W, Xu Z, Chen Y, et al. The reduced frequency of cd39(+)Cd73(+) B cell subsets in sle patients is correlated with disease activity. Int Immunopharmacol. (2024) 140:112743. doi: 10.1016/j.intimp.2024.112743
13. Tak E, Ridyard D, Kim JH, Zimmerman M, Werner T, Wang XX, et al. CD73-dependent generation of adenosine and endothelial adora2b signaling attenuate diabetic nephropathy. J Am Soc Nephrol. (2014) 25:547–63. doi: 10.1681/ASN.2012101014
14. Ratiu JJ, Racine JJ, Hasham MG, Wang Q, Branca JA, Chapman HD, et al. Genetic and small molecule disruption of the aid/rad51 axis similarly protects nonobese diabetic mice from type 1 diabetes through expansion of regulatory B lymphocytes. J Immunol. (2017) 198:4255–67. doi: 10.4049/jimmunol.1700024
15. Wang Q, Racine JJ, Ratiu JJ, Wang S, Ettinger R, Wasserfall C, et al. Transient BAFF blockade inhibits type 1 diabetes development in nonobese diabetic mice by enriching immunoregulatory B lymphocytes sensitive to deletion by anti-cd20 cotherapy. J Immunol. (2017) 199:3757–70. doi: 10.4049/jimmunol.1700822
16. Jonic N, Koprivica I, Kyrkou SG, Bistas VP, Chatzigiannis C, Radulovic N, et al. Novel ahr ligand agt-5 ameliorates type 1 diabetes in mice through regulatory cell activation in the early phase of the disease. Front Immunol. (2024) 15:1454156. doi: 10.3389/fimmu.2024.1454156
17. Pereira ADS, Miron VV, Castro MFV, Bottari NB, Assmann CE, Nauderer JN, et al. Neuromodulatory effect of the combination of metformin and vitamin D(3) triggered by purinergic signaling in type 1 diabetes induced-rats. Mol Cell Endocrinol. (2023) 563:111852. doi: 10.1016/j.mce.2023.111852
18. Li D, Li H, Fu H, Niu K, Guo Y, Guo C, et al. Aire-overexpressing dendritic cells induce peripheral CD4(+) T cell tolerance. Int J Mol Sci. (2015) 17:38. doi: 10.3390/ijms17010038
19. Chandravanshi B, Bhonde R. Reprogramming mouse embryo fibroblasts to functional islets without genetic manipulation. J Cell Physiol. (2018) 233:1627–37. doi: 10.1002/jcp.v233.2
20. James CR, Buckle I, Muscate F, Otsuka M, Nakao M, Oon J, et al. Reduced interleukin-2 responsiveness impairs the ability of treg cells to compete for il-2 in nonobese diabetic mice. Immunol Cell Biol. (2016) 94:509–19. doi: 10.1038/icb.2016.7
21. Bergot AS, Buckle I, Cikaluru S, Naranjo JL, Wright CM, Zheng G, et al. Regulatory T cells induced by single-peptide liposome immunotherapy suppress islet-specific T cell responses to multiple antigens and protect from autoimmune diabetes. J Immunol. (2020) 204:1787–97. doi: 10.4049/jimmunol.1901128
22. Firdessa-Fite R, Johnson SN, Leon MA, Khosravi-Maharlooei M, Baker RL, Sestak JO, et al. Soluble antigen arrays efficiently deliver peptides and arrest spontaneous autoimmune diabetes. Diabetes. (2021) 70:1334–46. doi: 10.2337/db20-0845
23. Tai N, Wong FS, Wen L. TLR9 deficiency promotes CD73 expression in T cells and diabetes protection in nonobese diabetic mice. J Immunol. (2013) 191:2926–37. doi: 10.4049/jimmunol.1300547
24. Zhang W, Duan HT, Chen S, Wang YX, Kong JH, Dong M, et al. The protective effect of pigment epithelial-derived factor modified human umbilical cord mesenchymal stem cells on rats with diabetic retinopathy. Zhonghua Yan Ke Za Zhi. (2017) 53:540–7. doi: 10.3760/cma.j.issn.0412-4081.2017.07.013
25. Zanini C, Bruno S, Mandili G, Baci D, Cerutti F, Cenacchi G, et al. Differentiation of mesenchymal stem cells derived from pancreatic islets and bone marrow into islet-like cell phenotype. PloS One. (2011) 6:e28175. doi: 10.1371/journal.pone.0028175
26. Wu D, Zou S, Chen H, Li X, Xu Y, Zuo Q, et al. Transplantation routes affect the efficacy of human umbilical cord mesenchymal stem cells in a rat gdm model. Clin Chim Acta. (2017) 475:137–46. doi: 10.1016/j.cca.2017.10.013
27. Williams MD, Joglekar MV, Satoor SN, Wong W, Keramidaris E, Rixon A, et al. Epigenetic and transcriptome profiling identifies a population of visceral adipose-derived progenitor cells with the potential to differentiate into an endocrine pancreatic lineage. Cell Transplant. (2019) 28:89–104. doi: 10.1177/0963689718808472
28. Van Pham P, Truong NC, Le PT, Tran TD, Vu NB, Bui KH, et al. Isolation and proliferation of umbilical cord tissue derived mesenchymal stem cells for clinical applications. Cell Tissue Bank. (2016) 17:289–302. doi: 10.1007/s10561-015-9541-6
29. Lin HT, Chiou SH, Kao CL, Shyr YM, Hsu CJ, Tarng YW, et al. Characterization of pancreatic stem cells derived from adult human pancreas ducts by fluorescence activated cell sorting. World J Gastroenterol. (2006) 12:4529–35. doi: 10.3748/wjg.v12.i28.4529
30. Kadam SS, Bhonde RR. Islet neogenesis from the constitutively nestin expressing human umbilical cord matrix derived mesenchymal stem cells. Islets. (2010) 2:112–20. doi: 10.4161/isl.2.2.11280
31. Gopurappilly R, Bhat V, Bhonde R. Pancreatic tissue resident mesenchymal stromal cell (Msc)-like cells as a source of in vitro islet neogenesis. J Cell Biochem. (2013) 114:2240–7. doi: 10.1002/jcb.24572
32. Gao F, Wu Y, Wen H, Zhu W, Ren H, Guan W, et al. Multilineage potential research on pancreatic mesenchymal stem cells of bovine. Tissue Cell. (2019) 56:60–70. doi: 10.1016/j.tice.2018.12.001
33. Cooper TT, Sherman SE, Bell GI, Ma J, Kuljanin M, Jose SE, et al. Characterization of a vimentin(High)/nestin(High) proteome and tissue regenerative secretome generated by human pancreas-derived mesenchymal stromal cells. Stem Cells. (2020) 38:666–82. doi: 10.1002/stem.3143
34. Chen J, Chen J, Cheng Y, Fu Y, Zhao H, Tang M, et al. Mesenchymal stem cell-derived exosomes protect beta cells against hypoxia-induced apoptosis via mir-21 by alleviating er stress and inhibiting P38 mapk phosphorylation. Stem Cell Res Ther. (2020) 11:97. doi: 10.1186/s13287-020-01610-0
35. Carlotti F, Zaldumbide A, Loomans CJ, Van Rossenberg E, Engelse M, De Koning EJ, et al. Isolated human islets contain a distinct population of mesenchymal stem cells. Islets. (2010) 2:164–73. doi: 10.4161/isl.2.3.11449
36. Ali F, Aziz F, Wajid N. Effect of type 2 diabetic serum on the behavior of Wharton’s jelly-derived mesenchymal stem cells in vitro. Chronic Dis Transl Med. (2017) 3:105–11. doi: 10.1016/j.cdtm.2017.02.006
37. Zhao T, Luo D, Sun Y, Niu X, Wang Y, Wang C, et al. Human urine-derived stem cells play a novel role in the treatment of STZ-induced diabetic mice. J Mol Histol. (2018) 49:419–28. doi: 10.1007/s10735-018-9772-5
38. Jiang ZZ, Liu YM, Niu X, Yin JY, Hu B, Guo SC, et al. Exosomes secreted by human urine-derived stem cells could prevent kidney complications from type I diabetes in rats. Stem Cell Res Ther. (2016) 7:24. doi: 10.1186/s13287-016-0287-2
39. Chen CY, Rao SS, Ren L, Hu XK, Tan YJ, Hu Y, et al. Exosomal dmbt1 from human urine-derived stem cells facilitates diabetic wound repair by promoting angiogenesis. Theranostics. (2018) 8:1607–23. doi: 10.7150/thno.22958
40. Peng SY, Chou CW, Kuo YH, Shen PC, Shaw SWS. Potential differentiation of islet-like cells from pregnant cow-derived placental stem cells. Taiwan J Obstet Gynecol. (2017) 56:306–11. doi: 10.1016/j.tjog.2017.04.007
41. Dao LT, Park EY, Lim SM, Choi YS, Jung HS, Jun HS. Transplantation of insulin-producing cells differentiated from human periosteum-derived progenitor cells ameliorate hyperglycemia in diabetic mice. Transplantation. (2014) 98:1040–7. doi: 10.1097/TP.0000000000000388
42. Burr A, Parekkadan B. Kinetics of msc-based enzyme therapy for immunoregulation. J Transl Med. (2019) 17:263. doi: 10.1186/s12967-019-2000-6
43. Zhang W, Zhou L, Dang J, Zhang X, Wang J, Chen Y, et al. Human gingiva-derived mesenchymal stem cells ameliorate streptozoticin-induced T1dm in mice via suppression of T effector cells and up-regulating treg subsets. Sci Rep. (2017) 7:15249. doi: 10.1038/s41598-017-14979-5
44. Jalkanen J, Hollmen M, Jalkanen S, Hakovirta H. Regulation of CD73 in the development of lower limb atherosclerosis. Purinergic Signal. (2017) 13:127–34. doi: 10.1007/s11302-016-9545-0
45. Ferrante EA, Cudrici CD, Rashidi M, Fu YP, Huffstutler R, Carney K, et al. Pilot study to evaluate the safety and effectiveness of etidronate treatment for arterial calcification due to deficiency of CD73 (Acdc). Vasc Med. (2024) 29:245–55. doi: 10.1177/1358863X241235669
46. Behzadi P, Cuevas RA, Crane A, Wendling AA, Chu CC, Moorhead WJ 3rd, et al. Rapamycin increases murine lifespan but does not reduce mineral volume in the matrix GLA protein (MGP) knockout mouse model of medial arterial calcification. Biorxiv. (2024). doi: 10.1101/2024.08.01.606196
47. Markello TC, Pak LK, St Hilaire C, Dorward H, Ziegler SG, Chen MY, et al. Vascular pathology of medial arterial calcifications in nt5e deficiency: implications for the role of adenosine in pseudoxanthoma elasticum. Mol Genet Metab. (2011) 103:44–50. doi: 10.1016/j.ymgme.2011.01.018
48. Zukowska P, Kutryb-Zajac B, Toczek M, Smolenski RT, Slominska EM. The role of ecto-5’-nucleotidase in endothelial dysfunction and vascular pathologies. Pharmacol Rep. (2015) 67:675–81. doi: 10.1016/j.pharep.2015.05.002
49. Chadjichristos CE, Scheckenbach KE, Van Veen TA, Richani Sarieddine MZ, De Wit C, Yang Z, et al. Endothelial-specific deletion of connexin40 promotes atherosclerosis by increasing CD73-dependent leukocyte adhesion. Circulation. (2010) 121:123–31. doi: 10.1161/CIRCULATIONAHA.109.867176
50. Bai Z, Liu Y, Zhao Y, Yan R, Yang L, Ma H, et al. Aspirin ameliorates atherosclerotic immuno-inflammation through regulating the treg/th17 axis and cd39-CD73 adenosine signaling via remodeling the gut microbiota in apoe(-/-) mice. Int Immunopharmacol. (2023) 120:110296. doi: 10.1016/j.intimp.2023.110296
51. Yang J, Jian R, Yu J, Zhi X, Liao X, Yu J, et al. CD73 regulates vascular smooth muscle cell functions and facilitates atherosclerotic plaque formation. IUBMB Life. (2015) 67:853–60. doi: 10.1002/iub.v67.11
52. Holmes AP, Ray CJ, Pearson SA, Coney AM, Kumar P. Ecto-5’-nucleotidase (CD73) regulates peripheral chemoreceptor activity and cardiorespiratory responses to hypoxia. J Physiol. (2018) 596:3137–48. doi: 10.1113/tjp.2018.596.issue-15
53. Gong YP, Wan RZ, Liu ZP. Evaluation of wo2017098421: gsk’s benzothiazine compounds as CD73 inhibitor filings. Expert Opin Ther Pat. (2018) 28:167–71. doi: 10.1080/13543776.2018.1407756
54. Sutton NR, Bouis D, Mann KM, Rashid IM, Mccubbrey AL, Hyman MC, et al. Cd73 promotes age-dependent accretion of atherosclerosis. Arterioscler Thromb Vasc Biol. (2020) 40:61–71. doi: 10.1161/ATVBAHA.119.313002
55. Jaen JC, Powers JP, Chen A, Leleti MR, Kalisiak J, Schindler U, et al. Abstract pr10: small-molecule inhibitors of CD73 promote activation of human cd8+ T cells and have profound effects on tumor growth and immune parameters in experimental tumors. Cancer Immunol Res. (2016) 4:Pr10–0. doi: 10.1158/2326-6066.IMM2016-PR10
56. Tan JB, Chen A, Leleti M, Becker A, Lindsey E, Kalisiak J, et al. Abstract B46: small-molecule inhibitors of ecto-nucleotidase CD73 promote activation of human cd8+ T cells and have profound effects on tumor growth and immune parameters in experimental tumor models. Cancer Immunol Res. (2017) 5:B46–6. doi: 10.1158/2326-6074.TUMIMM16-B46
57. Finn J, Snoek S, Van Ittersum J, Broekstra N, Braam C, Kramer E, et al. A8. 12 aav mediated expression of cd39 and CD73 is effective in reducing inflammation in the air pouch synovial inflammation model. Ann Rheum Dis. (2015) 74:A86. doi: 10.1136/annrheumdis-2015-207259.197
58. ABBISKO. Abbisko therapeutic completed the first patient dose in phase I clinical trial for novel small molecule CD73 inhibitor absk051 (2024). Available online at: https://www.abbisko.com/newsdetail/166.html (accessed January 4, 2024).
59. Deng H, Xin W, Pan W, Zhu Z, Ning M, Yu H, et al. 1441 a potent and selective small molecule antagonist of CD73 absk051 reverses immunosuppression through reduction of adenosine production. J Immunother. (2022) 10:A1–A1595. doi: 10.1136/jitc-2022-SITC2022.1441
60. Ying H, Guo F, Peng J, Wang J, Zhang J, Liu M, et al. Abstract LB316: A next-generation krasg12c inhibitor absk071 demonstrated broad synergy with other therapeutic agents in krasg12c mutated cancer models. Cancer Res. (2023) 83:Lb316–6. doi: 10.1158/1538-7445.AM2023-LB316
61. Angarus Therapeutics I. Science-pipeline (2024). Available online at: https://www.angarustx.com/science.
62. Huang Z, Pang X, Zhong T, Jin C, Chen N, Xia D, et al. AK131, an anti-pd1/CD73 bispecific antibody for cancer immune therapy. Cancer Res. (2022) 82:5526–6. doi: 10.1158/1538-7445.AM2022-5526
63. Biospace. Antennova to present latest data of atn-037 in a mini oral presentation at esmo congress 2024 (2024). Available online at: https://www.biospace.com/press-releases/antennova-to-present-latest-data-of-atn-037-in-a-mini-oral-presentation-at-esmo-congress-2024 (accessed September 9, 2024).
64. Bian G, Hua J, Tian L, Lynch K, Mei J, Shan B, et al. Atg-037, a highly potent small molecule CD73 inhibitor has superior activity of reversing immunosuppression in higher-amp environments compared with anti-CD73 antibodies. Cancer Res. (2022) 82:2576–6. doi: 10.1158/1538-7445.AM2022-2576
65. Bian G, Hua J, Lin G, Bai Y, Zhu T, Jiang B, et al. Targeting CD73-adenosine axis for the treatment of multiple myeloma. Cancer Res. (2023) 83:496–6. doi: 10.1158/1538-7445.AM2023-496
66. AdisInsight. APB A2 (2023). Available online at: https://adisinsight.springer.com/drugs/800065978 (accessed April 03, 2023).
67. Inc, A. P. Ap-401™ Ipsc-derived bi-specific car nk cells (2024). Available online at: https://alphageneron.com/ap-401-ipsc-derived-bi-specific-car-nk-cells/.
68. Inc, A. P. Pipeline (2024). Available online at: https://alphageneron.com/pipeline/.
69. Fares J, Davis ZB, Rechberger JS, Toll SA, Schwartz JD, Daniels DJ, et al. Advances in nk cell therapy for brain tumors. NPJ Precis Oncol. (2023) 7:17. doi: 10.1038/s41698-023-00356-1
70. Azambuja JH, Gelsleichter NE, Beckenkamp LR, Iser IC, Fernandes MC, Figueiro F, et al. Cd73 downregulation decreases in vitro and in vivo glioblastoma growth. Mol Neurobiol. (2019) 56:3260–79. doi: 10.1007/s12035-018-1240-4
71. AdisInsight. BB 1709 (2024). Available online at: https://adisinsight.springer.com/drugs/800077339 (accessed March 11, 2024).
72. Biopharmaceutical, D. Pipeline (2024). Available online at: http://www.dragonboatbio.com/en/innovation/pipeline.
73. Biotherapeutics, B. Pipeline-bp1200 (2024). Available online at: https://www.brightpathbio.com/english/pipeline/bp1200.html.
74. Matsumoto N, Mishima Y, Obonai T, Ban H, Mie M, Nakamura N. A novel therapeutic antibody against CD73 that ameliorates the tumor microenvironment and improves the efficacy of cancer immunotherapy. Cancer Res. (2022) 82:2905–5. doi: 10.1158/1538-7445.AM2022-2905
75. Pharmaceuticals, B. Product pipeline diagram (2024). Available online at: http://www.bettapharma.com/en.php/newdrug/projects.
76. Han H, Yang X, Zhao Y, Wang J, Yang X, Ju X, et al. Bpi-472372: a potent and orally bioavailable small molecule inhibitor of CD73 for cancer immunotherapy. Cancer Res. (2023) 83:504–4. doi: 10.1158/1538-7445.AM2023-504
77. Zhejiang BioRay Pharmaceutical Co., Ltd. BioRay's new drug br101 injection was approved in clinical trials. (2021). Available online at: https://www.bioraypharm.com/index.php?m=content&a=index&classid=5&id=13 (accessed June 21, 2021).
78. Ploeg EM, Samplonius DF, Xiong X, Ke X, Hendriks MAJM, Britsch I, et al. Bispecific antibody CD73xegfr more selectively inhibits the CD73/adenosine immune checkpoint on cancer cells and concurrently counteracts pro-oncogenic activities of CD73 and egfr. J ImmunoTher Cancer. (2023) 11:e00683. doi: 10.1136/jitc-2023-006837
79. Siu LL, Burris H, Le DT, Hollebecque A, Steeghs N, Delord J-P, et al. Abstract CT180: preliminary phase 1 profile of bms-986179, an anti-CD73 antibody, in combination with nivolumab in patients with advanced solid tumors. Cancer Res. (2018) 78:CT180–0. doi: 10.1158/1538-7445.AM2018-CT180
80. Almaguer A, Zuill D, Döhrmann S, Levin J, Dedeic N, Abelovski E, et al. Cbo421: a novel drug fc-conjugate to prevent tumor immune evasion via the CD73/adenosine pathway. Cancer Res. (2024) 84:2668–8. doi: 10.1158/1538-7445.AM2024-2668
81. Dohrmann S, Levin J, Dedeic N, Almaguer A, Zuill D, Abelovski E, et al. 1195 discovery of cbo421, a first-in-class drug fc-conjugate (DFC), targeting CD73 in cancer. BMJ Specialist Journals. (2023) 84:2668. doi: 10.1136/jitc-2023-SITC2023.1195
82. Abelovski E, Dedeic N, Döhrmann S, Levin J, Almaguer A, Zuill D, et al. Abstract lb131: cbo421, a novel drug fc-conjugate, inhibits the enzymatic activity of CD73 and triggers CD73 internalization. Cancer Res. (2024) 84:Lb131–1. doi: 10.1158/1538-7445.AM2024-LB131
83. AdisInsight. CBO 421 (2024). Available online at: https://adisinsight.springer.com/drugs/800071938 (accessed August 20, 2024).
84. Wang S, Kong Z, Shi Y, Shao C, Wang W, Su Z, et al. Discovery of small and bifunctional molecules targeting pd-L1/CD73 for cancer dual immunotherapy. J Medicinal Chem. (2024) 67(11):9447–64. doi: 10.1021/acs.jmedchem.4c00553
85. Zhong EH, Ledderose C, De Andrade Mello P, Enjyoji K, Lunderberg JM, Junger W, et al. Structural and functional characterization of engineered bifunctional fusion proteins of cd39 and CD73 ectonucleotidases. Am J Physiol Cell Physiol. (2021) 320:C15–29. doi: 10.1152/ajpcell.00430.2020
86. Finn J, Van Ittersum J, Broekstra N, Tak P, Vervoordeldonk M. A8. 14 cd39 and CD73 transgenic exosomes and recombinant fusion protein as novel therapeutics for the treatment of inflammatory disease. Ann Rheum Dis. (2015) 74:A86–7. doi: 10.1136/annrheumdis-2015-207259.199
87. Finn JD, Snoek S, Van Ittersum J, Broekstra N, Feijt C, Tak PP, et al. A1. 39 modulation of atp: adenosine balance by exosome and rain (Recombinant anti-inflammatory fusion protein) delivery of cd39 and CD73 is effective in reducing pro-inflammatory cytokine and chemokine production. Ann Rheumatic Dis. (2014) 73:A16–7. doi: 10.1136/annrheumdis-2013-205124.38
88. Inc, C. T. Pipeline (2024). Available online at: https://www.cidara.com/pipeline/.
89. Levin J, Döhrmann S, Dedeic N, Almaguer A, Zuill D, Abelovski E, et al. 153p discovery of a novel, dual CD73 and pd-1 targeting multispecific drug fc-conjugate (DFC) for the treatment of cancer. J Immuno-Oncol Technol. (2023) 20:17. doi: 10.1016/j.iotech.2023.100664
90. Bioardis. Our portfolio-small molecule pipeline (2024). Available online at: https://bioardis.com/our-portfolio/.
91. Beatty JW, Lindsey EA, Thomas-Tran R, Debien L, Mandal D, Jeffrey JL, et al. Discovery of potent and selective non-nucleotide small molecule inhibitors of CD73. J Med Chem. (2020) 63:3935–55. doi: 10.1021/acs.jmedchem.9b01713
92. Festag J, Thelemann T, Schell M, Raith S, Michel S, Jaschinski F, et al. Preventing atp degradation by aso-mediated knockdown of cd39 and CD73 results in A2ar-independent rescue of T cell proliferation. Mol Ther Nucleic Acids. (2020) 21:656–69. doi: 10.1016/j.omtn.2020.06.020
93. AdisInsight. CHS 7304 (2022). Available online at: https://adisinsight.springer.com/drugs/800068713 (accessed June 08, 2022).
94. Shi C, Chang L, Wang J, Dai J, Xu W, Tang J, et al. Discovery of novel non-nucleoside inhibitors interacting with dizinc ions of CD73. J Medicinal Chem. (2024) 67:9686—708. doi: 10.1021/acs.jmedchem.4c00825
95. Tolcher AW, Gordon M, Mahoney KM, Seto A, Zavodovskaya M, Hsueh CH, et al. Phase 1 first-in-human study of dalutrafusp alfa, an anti-CD73-TGF-beta-trap bifunctional antibody, in patients with advanced solid tumors. J Immunother Cancer. (2023) 11:e005267. doi: 10.1136/jitc-2022-005267
96. AdisInsight. Research programme: immune-mediated cancer therapeutics - shanghai de novo pharmatech (2022). Available online at: https://adisinsight.springer.com/drugs/800051798 (accessed May 28, 2022).
97. Kreuzberger N, Hirsch C, Chai KL, Tomlinson E, Khosravi Z, Popp M, et al. Sars-cov-2-neutralising monoclonal antibodies for treatment of covid-19. Cochrane Database Syst Rev. (2021) 9:Cd013825. doi: 10.1002/14651858.CD013825.pub2
98. Hakovirta H, Jalkanen J, Saimanen E, Kukkonen T, Romsi P, Suominen V, et al. Induction of CD73 prevents death after emergency open aortic surgery for a ruptured abdominal aortic aneurysm: a randomized, double-blind, placebo-controlled study. Sci Rep. (2022) 12:1839. doi: 10.1038/s41598-022-05771-1
99. Kiss J, Yegutkin GG, Koskinen K, Savunen T, Jalkanen S, Salmi M. Ifn-beta protects from vascular leakage via up-regulation of CD73. Eur J Immunol. (2007) 37:3334–8. doi: 10.1002/eji.200737793
100. Bellingan G, Maksimow M, Howell DC, Stotz M, Beale R, Beatty M, et al. The effect of intravenous interferon-beta-1a (FP-1201) on lung CD73 expression and on acute respiratory distress syndrome mortality: an open-label study. Lancet Respir Med. (2014) 2:98–107. doi: 10.1016/S2213-2600(13)70259-5
101. Ranieri VM, Pettila V, Karvonen MK, Jalkanen J, Nightingale P, Brealey D, et al. Effect of intravenous interferon beta-1a on death and days free from mechanical ventilation among patients with moderate to severe acute respiratory distress syndrome: A randomized clinical trial. Jama. (2020) 323:725–33. doi: 10.1001/jama.2019.22525
102. AdisInsight. GB 7002 (2024). Available online at: https://adisinsight.springer.com/drugs/800059638 (accessed July 28, 2024).
103. Shin K, Park M, Kim S, Lee H, Lee Y, Kim J, et al. 1072 GI-108, a novel bispecific fusion protein augments anti-tumor immunity by alleviating immunosuppressive adenosine pathway in CD8+ T cells. BMJ Specialist Journals. (2023) 11:A1181. doi: 10.1136/jitc-2023-SITC2023.1072
104. AdisInsight. GI 108 (2023). Available online at: https://adisinsight.springer.com/drugs/800075764 (accessed November 28, 2023).
105. AdisInsight. HB 0039 (2023). Available online at: https://adisinsight.springer.com/drugs/800066330 (accessed November 07, 2023).
106. Huabo Biopharm (Shanghai) Co., L. Product pipeline (2024). Available online at: https://www.huabobio.com/en/product.html.
107. Shanghai Huaota Biopharmaceutical Co., Ltd. Good news! Huaota independently developed the world's first targeted CD39/CD73 bispecific antibody was entered in clinical trails by NMPA approvation. (2024). Available online at: https://www.huaota.com/newsshow/167.html (accessed July 10, 2024).
108. Biomed, H. Harbour biomed announces ind clearance for hbm1007 targeting CD73 in the U.S (2023). Available online at: https://www.harbourbiomed.com/news/203.html.
109. Henlius. Henlius received IND approval for its novel anti-CD73 mAb HLX23 from US FDA (2021). Available online at: https://henlius.com/en/newsdetails-3094-26.html (accessed June 01, 2021).
110. Zhou Y, Shen H, Wu M, Wang J, Wu Z, Fu F, et al. Pharmacology, pharmacokinetics, and toxicity characterization of a novel anti-CD73 therapeutic antibody ibi325 for cancer immunotherapy. Int J Biol Macromol. (2023) 229:158–67. doi: 10.1016/j.ijbiomac.2022.12.258
111. AdisInsight. INCA 00186 (2023). Available online at: https://adis.springer.com/drugs/800062835 (accessed August 09, 2023).
112. Deken MA, Carruthers AM, Niewola-Staszkowska K, Gernon G, Sandig H, Shah P, et al. IOA-237-A potent anti-CD73 mAb demonstrates monotherapeutic and combination efficacy in solid tumor models. Cancer Res. (2021) 81:1877–7. doi: 10.1158/1538-7445.AM2021-1877
113. Perrot I, Michaud HA, Giraudon-Paoli M, Augier S, Docquier A, Gros L, et al. Blocking antibodies targeting the cd39/CD73 immunosuppressive pathway unleash immune responses in combination cancer therapies. Cell Rep. (2019) 27:2411–2425 E9. doi: 10.1016/j.celrep.2019.04.091
114. Kutryb-Zając B, Kawecka A, Nasadiuk K, Braczko A, Stawarska K, Caiazzo E, et al. Drugs targeting adenosine signaling pathways: a current view. BioMed Pharmacother. (2023) 165:115184. doi: 10.1016/j.biopha.2023.115184
115. Ye Y, Yang G, Wang H, Sun X, Wang Y, Yan F, et al. JAB-X1800: A potent immunostimulatory antibody-drug conjugate (IADC) targeting CD73. Cancer Res. (2023) 83:2923–3. doi: 10.1158/1538-7445.AM2023-2923
116. Wang L, Zhang J, Zhang W, Zheng M, Guo H, Pan X, et al. The inhibitory effect of adenosine on tumor adaptive immunity and intervention strategies. Acta Pharm Sin B. (2024) 14:1951–64. doi: 10.1016/j.apsb.2023.12.004
117. Wurm M, Schaaf O, Reutner K, Ganesan R, Mostbock S, Pelster C, et al. A novel antagonistic CD73 antibody for inhibition of the immunosuppressive adenosine pathway. Mol Cancer Ther. (2021) 20:2250–61. doi: 10.1158/1535-7163.MCT-21-0107
118. Mobasher M, Miller RA, Kwei L, Strahs D, Das V, Luciano G, et al. A phase I/ib multicenter study to evaluate the humanized anti-CD73 antibody, CPI-006, as A single agent, in combination with CPI-444, and in combination with pembrolizumab in adult patients with advanced cancers. Am Soc Of Clin Oncol. (2019) 37:TPS2646. doi: 10.1200/JCO.2019.37.15_suppl.TPS2646
119. Piccione E, Hotston A, Mikesell G, Daine-Matsuoka B, Gu C, Dao-Pick T, et al. Preclinical and initial phase I clinical characterization of CPI-006: an anti-CD73 monoclonal antibody with unique immunostimulatory activity. Prostate. (2018) 58:2.937.
120. Luke JJ, Powderly JD, Merchan JR, Barve MA, Hotson AN, Mobasher M, et al. Immunobiology, preliminary safety, and efficacy of CPI-006, an anti-CD73 antibody with immune modulating activity, in a phase 1 trial in advanced cancers. J Clin Oncol. (2019) 37:10.1200. doi: 10.1200/JCO.2019.37.15_suppl.2505
121. Willingham SB, Criner G, Hill C, Hu S, Rudnick JA, Daine-Matsuoka B, et al. Characterization and phase 1 trial of A B cell activating anti-CD73 antibody for the immunotherapy of covid-19. medRxiv. (2020). doi: 10.1101/2020.09.10.20191486
122. Bendell J, Lorusso P, Overman M, Noonan AM, Kim DW, Strickler JH, et al. First-in-human study of oleclumab, a potent, selective anti-CD73 monoclonal antibody, alone or in combination with durvalumab in patients with advanced solid tumors. Cancer Immunol Immunother. (2023) 72:2443–58. doi: 10.1007/s00262-023-03430-6
123. Hay CM, Sult E, Huang Q, Mulgrew K, Fuhrmann SR, Mcglinchey KA, et al. Targeting CD73 in the tumor microenvironment with medi9447. Oncoimmunology. (2016) 5:e1208875. doi: 10.1080/2162402X.2016.1208875
124. Herbst RS, Majem M, Barlesi F, Carcereny E, Chu Q, Monnet I, et al. Coast: an open-label, phase II, multidrug platform study of durvalumab alone or in combination with oleclumab or monalizumab in patients with unresectable, stage III non-small-cell lung cancer. J Clin Oncol. (2022) 40:3383–93. doi: 10.1200/JCO.22.00227
125. Ray A, Junttila MR, Ting D, Sutimantanapi D, Chen X, Warne B, et al. Cd73 inhibition overcomes immunosuppression and triggers autologous T-cell mediated multiple myeloma cell lysis in the bone marrow milieu. J Blood. (2021) 138:2675. doi: 10.1182/blood-2021-148174
126. Du X, Moore J, Blank BR, Eksterowicz J, Sutimantanapi D, Yuen N, et al. Orally bioavailable small-molecule CD73 inhibitor (Op-5244) reverses immunosuppression through blockade of adenosine production. J Med Chem. (2020) 63:10433–59. doi: 10.1021/acs.jmedchem.0c01086
127. Bio, O. Pipeline (2024). Available online at: https://www.opnabio.com/pipeline/.
128. Oric Pharmaceuticals, I. Advancing candidates designed to address resistance mechanisms in multiple cancers (2024). Available online at: https://oricpharma.com/pipeline/oric533.
129. Ray A, Du T, Wan X, Song Y, Pillai SC, Musa MA, et al. A novel small molecule inhibitor of CD73 triggers immune-mediated multiple myeloma cell death. Blood Cancer J. (2024) 14:58. doi: 10.1038/s41408-024-01019-5
130. AdisInsight. ORIC 533 (2024). Available online at: https://adisinsight.springer.com/drugs/800057354 (accessed March 14, 2024).
131. AdisInsight. PBF 2828 (2019). Available online at: https://adisinsight.springer.com/drugs/800050501 (accessed May 14, 2019).
132. Jiang K, Wu J, Wang Q, Chen X, Zhang Y, Gu X, et al. Nanoparticles targeting the adenosine pathway for cancer immunotherapy. J Mater Chem B. (2024) 12:5787–811. doi: 10.1039/D4TB00292J
133. Bhattarai S, Freundlieb M, Pippel J, Meyer A, Abdelrahman A, Fiene A, et al. Alpha,Beta-methylene-adp (Aopcp) derivatives and analogues: development of potent and selective ecto-5’-nucleotidase (Cd73) inhibitors. J Med Chem. (2015) 58:6248–63. doi: 10.1021/acs.jmedchem.5b00802
134. Schmies CC, Rolshoven G, Idris RM, Losenkova K, Renn C, Schakel L, et al. Fluorescent probes for ecto-5’-nucleotidase (CD73). ACS Med Chem Lett. (2020) 11:2253–60. doi: 10.1021/acsmedchemlett.0c00391
135. Yegutkin GG, Boison D. ATP and adenosine metabolism in cancer: exploitation for therapeutic gain. Pharmacol Rev. (2022) 74:797–822. doi: 10.1124/pharmrev.121.000528
136. Jia H, Li J, Pei F, Greenwood L, Pejza L, Long Y, et al. Pt199, a next generation anti-CD73 mAb that inhibits both membrane-bound and soluble CD73 activity to completion without “Hook effect. Cancer Res. (2022) 82:4259–9. doi: 10.1158/1538-7445.AM2022-4259
137. Bowman CE, Da Silva RG, Pham A, Young SW. An exceptionally potent inhibitor of human CD73. Biochemistry. (2019) 58:3331–4. doi: 10.1021/acs.biochem.9b00448
138. Arcus Biosciences I. Taiho pharmaceutical exercises option for an exclusive license to quemliclustat in Japan and certain territories in Asia (2024). Available online at: https://investors.arcusbio.com/investors-and-media/press-releases/press-release-details/2024/taiho-pharmaceutical-exercises-option-for-an-exclusive-license-to-quemliclustat-in-japan-and-certain-territories-in-asia/default.aspx (accessed July 08, 2024).
139. AdisInsight. Quemliclustat - arcus biosciences (2024). Available online at: https://adis.springer.com/drugs/800053357 (accessed November 08, 2024).
140. Spreafico A, Ahnert JR, Sommerhalder D, Almena-Carrasco M, Crochemore NH, Ianopulos X, et al. Molecular and early clinical characterization of the anti-CD73 antibody S095024 (Sym024). Cancer Res. (2024) 84:3737–7. doi: 10.1158/1538-7445.AM2024-3737
141. Liu S, Li D, Liu J, Wang H, Horecny I, Shen R, et al. A novel CD73 inhibitor shr170008 suppresses adenosine in tumor and enhances anti-tumor activity with pd-1 blockade in a mouse model of breast cancer. Onco Targets Ther. (2021) 14:4561–74. doi: 10.2147/OTT.S326178
142. Rizzo A, Massafra R, Fanizzi A, Rinaldi L, Cusmai A, Latorre A, et al. Adenosine pathway inhibitors: novel investigational agents for the treatment of metastatic breast cancer. Expert Opin Investig Drugs. (2022) 31:707–13. doi: 10.1080/13543784.2022.2078191
143. Biotechnology, T. Pipeline (2024). Available online at: https://www.tricanbiotech.com/.
144. Robert F, Dumbrava EE, Xing Y, Mills E, Freddo JL, Theuer CP, et al. Preliminary safety, pharmacokinetics (PK), pharmacodynamics (PD) and clinical efficacy of uliledlimab (TJ004309), a differentiated CD73 antibody, in combination with atezolizumab in patients with advanced cancer. J Clin Oncol. (2021) 39:2511. doi: 10.1200/JCO.2021.39.15_suppl.2511
145. Zhou Q, Wu L, Cui J, Jiang B, Yao Y, Zhang J, et al. Safety, efficacy, pharmacokinetics of uliledlimab alone or combined with toripalimab in advanced solid tumor: initial results of a phase I/II study. Am Soc Of Clin Oncol. (2022) 14:4561–74. doi: 10.1200/JCO.2022.40.16_suppl.e21123
146. Zhou Q, Wu L, Jiang B, Du Y, Wang B, Hu X, et al. Uliledlimab and toripalimab combination therapy in treatment naive advanced NSCLC: phase 1b/2 clinical trial results using CD73 as a potential predictive biomarker. Am Soc Of Clin Oncol. (2023) 31(7):707–13. doi: 10.1200/JCO.2023.41.16_suppl.2570
147. Verseon. Pipeline (2024). Available online at: https://www.verseon.com/pipeline-5-oncology-II.html.
148. Hupp CD, Keefe AD, Liu J, Liu Y, Monteiro M, Olszewski A, et al. Abstract 4453: novel, potent, and selective small-molecule inhibitors modulating immuno-oncology targets CD73, A2a/A2b adenosine receptors and csf1r discovered via dna-encoded library screening. Cancer Res. (2019) 79:4453. doi: 10.1158/1538-7445.AM2019-4453
149. Zhang Y, Ye S, Wang Y, Wang C, Zhu Y, Wu Y, et al. Discovery and optimization of betulinic acid derivatives as novel potent CD73 inhibitors. Bioorg Med Chem. (2022) 59:116672. doi: 10.1016/j.bmc.2022.116672
150. Zhang Y, Yang K, Ye S, Tang W, Chang X, Wang Y, et al. Application of A fluorine strategy in the lead optimization of betulinic acid to the discovery of potent CD73 inhibitors. J Steroids. (2022) 188:109112. doi: 10.1016/j.steroids.2022.109112
151. Limited GB. Product pipeline (2024). Available online at: https://en.genuine-bio.com/research.htmlproc.
152. Ballew L, Singh K, Chandra V, Mills T, Faraoni EY, Mota V, et al. Abstract ia013: preclinical testing of CD73 inhibitors for pancreatic cancer immunoprevention. J Cancer Prev Res. (2022) 15:Ia013–3. doi: 10.1158/1940-6215.TACPAD22-IA013
153. Piovesan D, Tan JBL, Becker A, Banuelos J, Narasappa N, Direnzo D, et al. Targeting CD73 with ab680 (Quemliclustat), A novel and potent small-molecule CD73 inhibitor, restores immune functionality and facilitates antitumor immunity. Mol Cancer Ther. (2022) 21:948–59. doi: 10.1158/1535-7163.MCT-21-0802
154. Jacoberger-Foissac C, Cousineau I, Bareche Y, Allard D, Chrobak P, Allard B, et al. Cd73 inhibits cgas-sting and cooperates with cd39 to promote pancreatic cancer. Cancer Immunol Res. (2023) 11:56–71. doi: 10.1158/2326-6066.CIR-22-0260
155. Chen Q, Yin H, He J, Xie Y, Wang W, Xu H, et al. Tumor microenvironment responsive cd8(+) T cells and myeloid-derived suppressor cells to trigger CD73 inhibitor ab680-based synergistic therapy for pancreatic cancer. Adv Sci (Weinh). (2023) 10:E2302498. doi: 10.1002/advs.202302498
156. Zhang P, Cao M, Zhang Y, Xu L, Meng F, Wu X, et al. A novel antisense lncRNA NT5E promotes progression by modulating the expression of syncrip and predicts a poor prognosis in pancreatic cancer. J Cell Mol Med. (2020) 24:10898–912. doi: 10.1111/jcmm.v24.18
157. Yang J, Liu X, Dai G, Qu L, Tan B, Zhu B, et al. CircNT5E promotes the proliferation and migration of bladder cancer via sponging mir-502-5p. J Cancer. (2021) 12:2430–9. doi: 10.7150/jca.53385
158. Wang R, Zhang S, Chen X, Li N, Li J, Jia R, et al. Circnt5e acts as a sponge of mir-422a to promote glioblastoma tumorigenesis. Cancer Res. (2018) 78:4812–25. doi: 10.1158/0008-5472.CAN-18-0532
159. Dong L, Zheng J, Gao Y, Zhou X, Song W, Huang J. The circular rna nt5e promotes non-small cell lung cancer cell growth via sponging microrna-134. Aging (Albany Ny). (2020) 12:3936–49. doi: 10.18632/aging.102861
Keywords: CD73, circNT5E, diabetes, atherosclerosis, agent development
Citation: Liu D, Zhao J, Li L, Wang J, Wang C, Wu Y, Huang Y, Xing D and Chen W (2024) CD73: agent development potential and its application in diabetes and atherosclerosis. Front. Immunol. 15:1515875. doi: 10.3389/fimmu.2024.1515875
Received: 23 October 2024; Accepted: 25 November 2024;
Published: 12 December 2024.
Edited by:
Vinay Kumar, The Pennsylvania State University, United StatesReviewed by:
Hina Sultana, University of North Carolina System, United StatesShwetank, University of Pennsylvania, United States
Anuradha Tyagi, Institute of Nuclear Medicine & Allied Sciences (DRDO), India
Copyright © 2024 Liu, Zhao, Li, Wang, Wang, Wu, Huang, Xing and Chen. This is an open-access article distributed under the terms of the Creative Commons Attribution License (CC BY). The use, distribution or reproduction in other forums is permitted, provided the original author(s) and the copyright owner(s) are credited and that the original publication in this journal is cited, in accordance with accepted academic practice. No use, distribution or reproduction is permitted which does not comply with these terms.
*Correspondence: Dongming Xing, eGRtX3RzaW5naHVhQDE2My5jb20=; Yucun Huang, aHljdW5AMTI2LmNvbQ==; Wujun Chen, Y3dqd3h0NTk1MUAxNjMuY29t
†These authors have contributed equally to this work