- 1Department of Pharmacy, Banasthali Vidyapith, Banasthali, India
- 2School of Health and Biomedical Sciences, RMIT University, Melbourne, VIC, Australia
- 3Discipline of Medical Biochemistry, School of Laboratory Medicine and Medical Sciences, University of KwaZulu-Natal, Durban, South Africa
Rheumatoid arthritis (RA), a condition characterized by joint deterioration through the action of matrix metalloproteinases (MMPs), is prevalent worldwide. Bee venom (BV) has traditionally been used in Chinese medicine for pain, arthritis, rheumatism, skin diseases, etc. BV is enriched with active substances, notably melittin and phospholipase A2 (PLA2), offering significant therapeutic potential. Hence, the review summarizes current insights into BV’s composition, antiarthritic mechanism and pharmacological benefits, focusing on melittin. Constituting 50-60% of BV, melittin notably downregulates nuclear factor Kappa B (NF-κB) activity, inhibits MMP-1 and MMP-8, and diminishes tumor necrosis factor (TNF-α), all of which contribute to the mitigation of type 2 collagen degradation. Despite its potential, melittin exhibits hemolytic activity and can significantly affect cell membranes, limiting its application, which poses a challenge to its therapeutic use. To overcome these challenges, delivery techniques utilizing nanocarriers and modifications in amino acid sequencing have been developed. Recent advancements in delivery systems, including nanocarriers, transdermal patches, and nanoemulsions, aim to minimize toxicity, expanding its therapeutic utility for RA. This article explores these novel strategies, underlining the evolving role of melittin in RA management.
1 Introduction
Rheumatoid arthritis (RA) is a chronic autoimmune inflammatory disease affecting up to 1% of the population in developed countries. Environmental factors, genetic predisposition, and smoking are significant contributors to the development of RA (1). RA prevalence is higher in industrialized regions, likely due to demographic factors. According to the WHO, in 2019, RA impacted around 18 million people globally, with 70% of cases occurring in women and 55% in individuals over the age of 55 (2). Of those affected, 13 million experienced moderate to severe symptoms and benefited from rehabilitation efforts (3).
In recent decades, the incidence of RA has increased significantly, and this trend is expected to continue (4). Research highlights a considerably higher prevalence of RA in women compared to men (5). The etiology of RA is complex, involving interactions between environmental factors, the microbiome, mucosal health, and host immune function (6). Typically, the disease initiates in the mucosal tissues, where various inflammatory cytokines, immune cells, and signaling pathways become involved. This process eventually leads to interactions between the mucosal immune system and dysregulated microbiota, which then migrate to the synovium and joints (1, 7).
This chronic condition is characterized by inflammation of the synovial membrane, leading to joint damage, swelling, stiffness, pain, tissue degradation, deformity, and instability. The disease usually progresses symmetrically (8–10). Key factors driving inflammation in RA include tumor necrosis factor (TNF-α), interleukin-6 (IL-6), and other cytokines (10, 11). The immune response in RA is triggered by cytokines and chemokines, which further activate B and T-cells, macrophages, and monocytes, leading to excessive swelling (12, 13). Currently, nonsteroidal anti-inflammatory drugs (NSAIDs) (13), glucocorticoids (14), and disease-modifying anti-rheumatic drug therapy (DMARDs) (8, 10, 15) are the first line of treatment for RA therapeutics (Figure 1). However, NSAIDs may lead to gastrointestinal bleeding and other adverse effects, while DMARDs can cause immune suppression, increasing the risk of infections (12, 16, 17). Recent advancements in RA therapy have introduced biologic agents targeting TNF-α and interleukins (ILs) (18–21). These biologics inhibit specific immune components, such as CXC chemokine ligand inhibitors (22, 23), anti-B-cell agents (24–26), and T-cell co-stimulation blockers (27). Combination therapies that include biologics and methotrexate are also commonly used (28–30). Additionally, synthetic agents targeting Janus-activated kinase (JAK) inhibitors and cell therapies utilizing mesenchymal stem cells (MSCs) have been incorporated into RA treatment protocols (29–31). Biologics, although targeted and effective, are often expensive, making them inaccessible for many patients (17, 32).
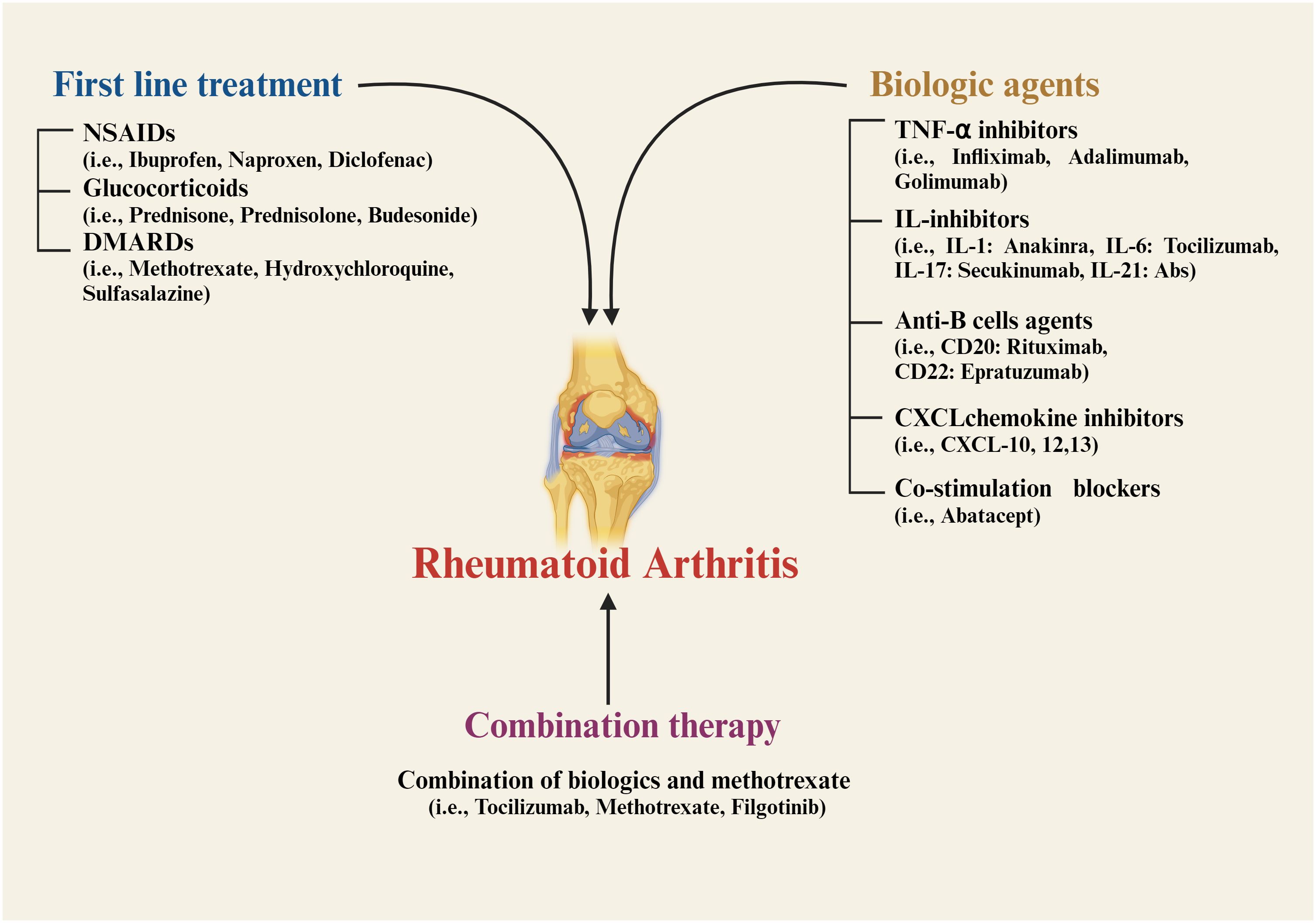
Figure 1. Current strategies for treatment of Rheumatoid arthritis. NSAIDs, nonsteroidal anti-inflammatory drugs; DMARDs, disease-modifying anti-rheumatic drug therapy; TNF, tumor necrosis factor; IL, interleukin; CXCL, CXC chemokine ligand; CD, cluster of differentiation.
Despite these treatment advancements, many RA patients face significant side effects and limited efficacy from existing therapies, leading to suboptimal treatment outcomes and continued discomfort (32, 33). In response to these challenges, researchers are exploring alternative therapies, including medicinal plants and animal-derived drugs, to provide more effective relief from this autoimmune disorder (17, 34–39). One of the traditionally used animal-derived therapies is bee venom (BV), which has been used in China for centuries to treat inflammation and pain, a practice known as ‘Apitherapy’ (40, 41). Apitherapy involves the use of bee products for the treatment or prevention of disease. BV is particularly noted for its anti-inflammatory properties, primarily due to its main active component, melittin (42). The therapeutic efficacy of BV in managing rheumatism and arthritis is thought to begin with the activation of adrenal glands that produce cortisol. Recent studies have documented various pathways through which BV and its components exert anti-inflammatory or antiarthritic effects (43, 44). Melittin, a small protein consisting of 26 amino acid residues, appears to downregulate phospholipase A2 (PLA2), cyclooxygenase (COX-2), and TNF-α expression while reducing levels of IL-1β, IL-6, nitric oxide (NO), and reactive oxygen species (ROS) (45).
2 Pathophysiology of rheumatoid arthritis
RA is primarily characterized by hyperplasia and inflammation of the synovium, leading to progressive destruction of cartilage and bone. This condition is associated with a range of systemic complications, including cardiovascular, pulmonary, and psychological disorders (46). RA typically stems from a breakdown in immune tolerance, producing a symmetric pattern of synovial inflammation. This breakdown often results from complex interactions between environmental factors, smoking, and genetic predispositions, triggering the production of autoantibodies against citrullinated antigens—a hallmark of RA (Figure 2) (47, 48).
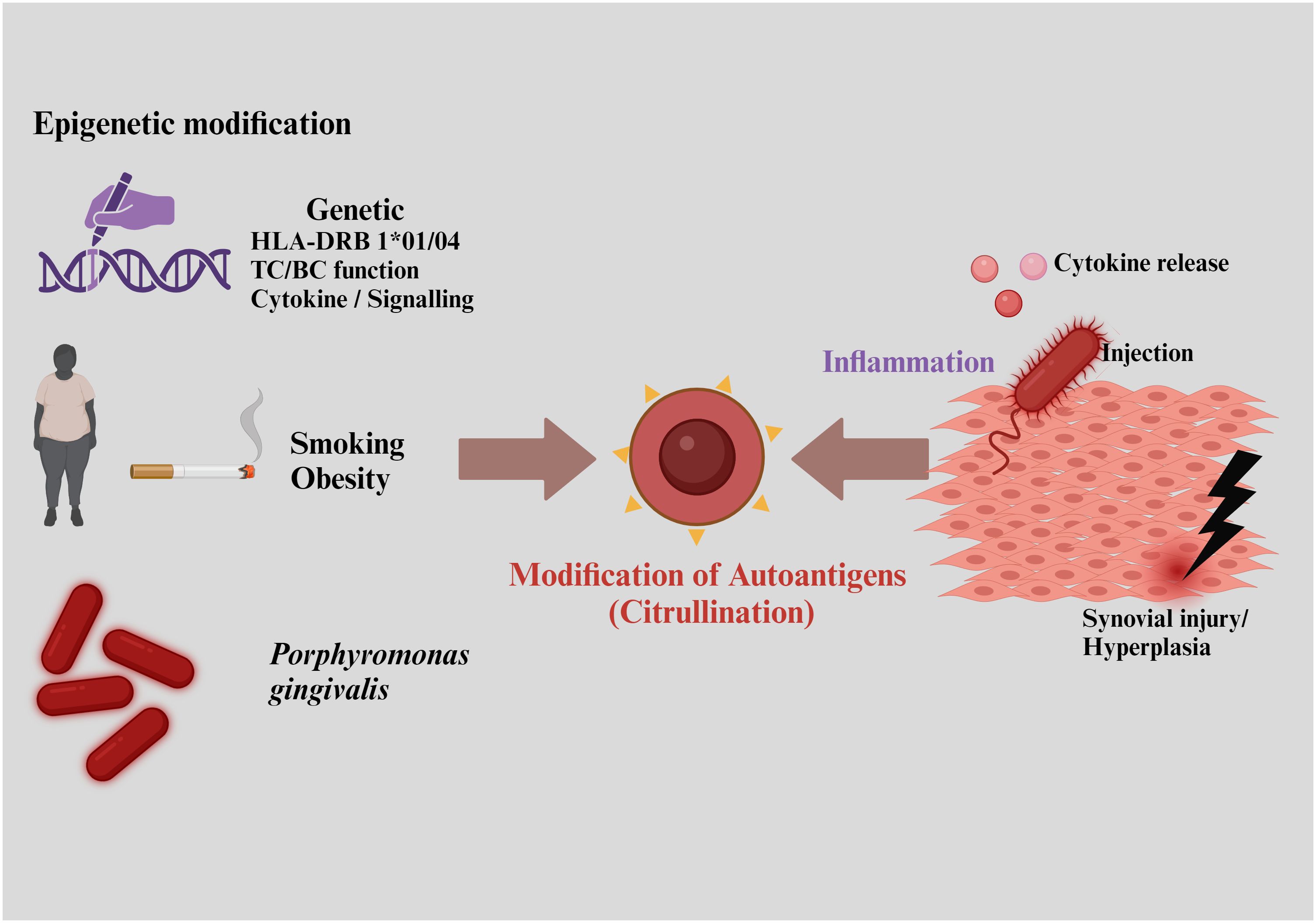
Figure 2. Risk factors responsible for rheumatoid arthritis include interactions with environmental factors, smoking, and genetic predispositions, leading to the production of autoantibodies against citrullinated antigens.
RA is classified into two major subtypes based on the presence or absence of anti-citrullinated protein antibodies (ACPAs), detectable in around 67% of patients and serving as a key diagnostic marker (49). ACPAs production is typically triggered by environmental factors and epigenetic changes, which often involve a combination of genetic and environmental influences (47). Likely trigger sites for RA include the lungs and gut, where interactions initiate autoantibody production against citrullinated peptides, marking the onset of self-protein citrullination. Lung exposure to infectious agents like Porphyromonas, Epstein-Barr virus, and gingivitis, along with noxious agents, dietary factors, and the gut microbiome, also contribute to ACPA generation (50).
Citrullination is catalyzed by the calcium-dependent enzyme protein arginine deaminases (PAD), converting neutral arginine into a polar citrulline residues in granulocytes and macrophages in RA patients (50). An abnormal antibody response produces ACPAs targeting various citrullinated proteins, including histones, type 2 collagen, Epstein-Barr nuclear antigen 1, α-enolase, vimentin, and fibronectin, contributing to the systemic nature of RA (50).
The activation of major histocompatibility complex (MHC) Class II-dependent T-cells by citrullinated neoantigens promotes B-cells production of more ACPAs and the activation of inflammatory mediators like TNF-α, IL-1β, IL-6, and NF-κB, leading to pannus formation—a thickened synovial layer that invades and destroys cartilage and bone. This stage is typically characterized by a loss of immune tolerance (Figure 3) (50, 51).
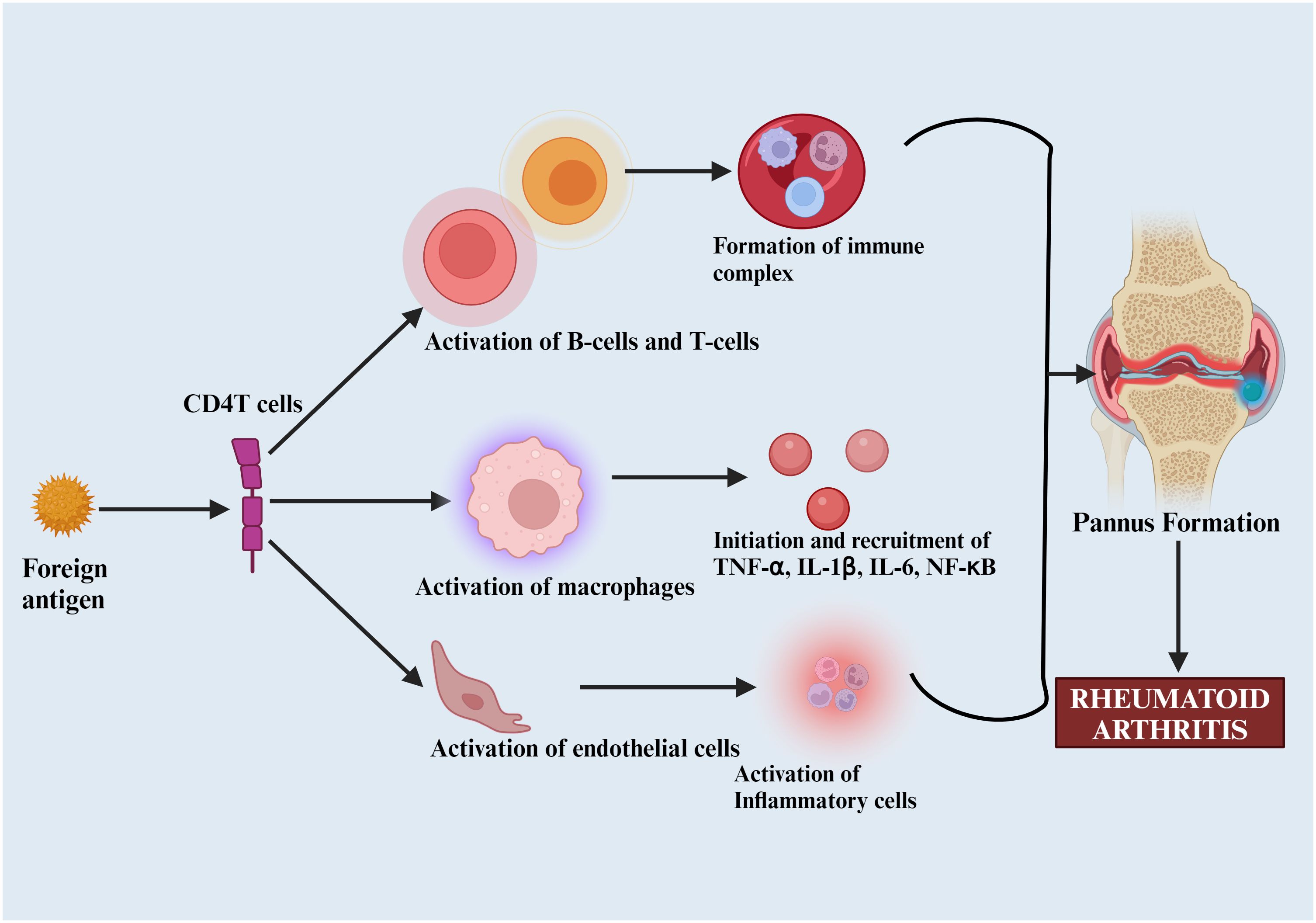
Figure 3. Pathophysiology of rheumatoid arthritis. Foreign antigens that activate B and T-cells, macrophages, and other inflammatory mediators (TNF-α, IL-1β, IL-6), leading to pannus formation and subsequent joint destruction.
Understanding the detailed pathophysiology of RA is critical for developing targeted therapies to manage and potentially alter disease progression. By elucidating these mechanisms, researchers can identify novel therapeutic targets and improve treatment strategies for RA.
3 Bee venom
BV is synthesized within the venom gland located in the bee’s abdominal cavity (52, 53). This complex mixture contains at least 18 active components, including active peptides, enzymes, amino acids, proteins, phospholipids, sugars, and other non-peptide elements (Table 1) (54, 55). Studies demonstrated the therapeutic potential of BV, highlighting its anti-atherosclerotic, antiarthritic, antimicrobial, and immunosuppressive activities, as well as its cytotoxic effects against cancer cells (56).
BV has been used as a therapeutic agent in Korea and Eastern Asia since ancient times, even before the common era, where it was administered through injections or live bee stings to treat various conditions such as back pain, tumors, arthritis, and multiple sclerosis (44).
3.1 Basic components of bee venom
BV is primarily composed of melittin, a protein that constitutes approximately 55% to 60% of its dry weight. Another significant component is the mast cell degranulation peptide (MCD), also known as peptide 401, which accounts for about 2% to 3% of BV’s weight (56).
3.2 Physical properties of bee venom
BV is a translucent liquid with a pH range of 4.5 to 5.5, characterized by an unpleasant taste and odor. It is insoluble in ammonium sulfate and ethanol but is soluble in water (57).
4 Melittin
Melittin is the key component of BV, comprising up to 52% of its dry mass. It is a basic peptide with a molecular weight of 2846.5 Da and the chemical formula C131 H229 N39 O31, consisting of 26 amino acids (58, 59) (Figure 4).
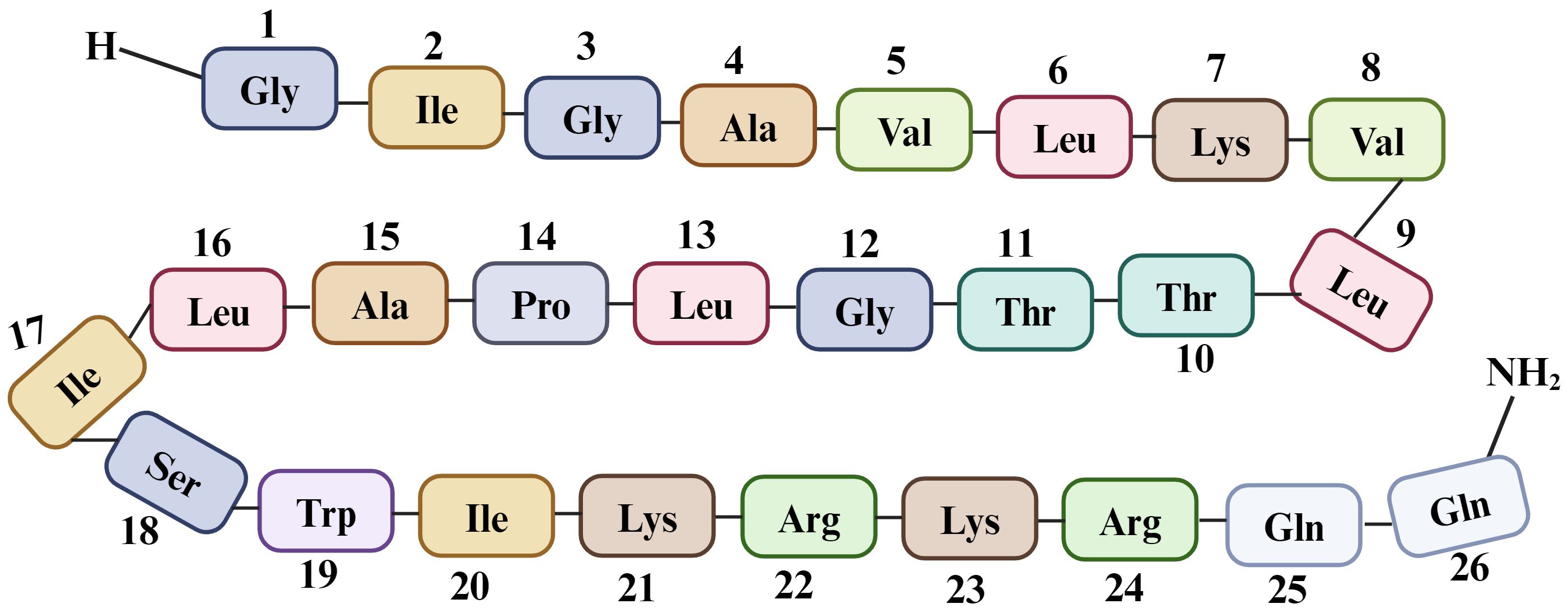
Figure 4. Sequence of 26 amino acid residues in melittin, a component of Bee venom. Melittin is synthesized as an inactive precursor within a bee’s venom gland known as “pre-pro melittin”, which consists of 70 amino acids. The active form of melittin exhibits polar characteristics, with the last six amino acids being hydrophilic and the first 20 amino acids (starting from the N-terminus) being hydrophobic (60). This arrangement of amino acids contributes to its unique three-dimensional structure and amphoteric nature, with a +6 charge at physiological pH (including a +2 charge at the C-terminal and a +4 charge at the N-terminal region). Due to this specific arrangement, melittin exhibits hemolytic activity (61).
Melittin is responsible for multiple effects, including anti-inflammatory, antibacterial, and antiviral effects, in various cell types. As a basic peptide, melittin acts as a natural detergent with high membrane surface tension, disrupting the structure of the phospholipid bilayer by forming pores and aggregates in both synthetic and natural membranes (62). In addition to causing morphological changes in membranes, melittin stimulates various enzymes such as adenylate cyclase, protein kinase, G-protein, and phospholipase C and D (63). Structurally, melittin forms a bent rod shape with two α-helical segments connected by a coiled segment containing a proline residue, enabling it to penetrate cell membranes (64).
At low concentrations, melittin exists as a monomer that can lyse cells, but it forms tetramers at concentrations typically found in BV. The pain associated with melittin is due to the depolarization of nerve endings by these tetramers. When administered intravenously, the cytolytic peptides in melittin target all lipid membranes, leading to systemic toxicity (65).
4.1 Different sources of melittin
While BV from honeybees is the primary source of melittin, it is also found in various other species, including certain insects, bacteria, amphibians, and reptiles (Figure 5) (66).
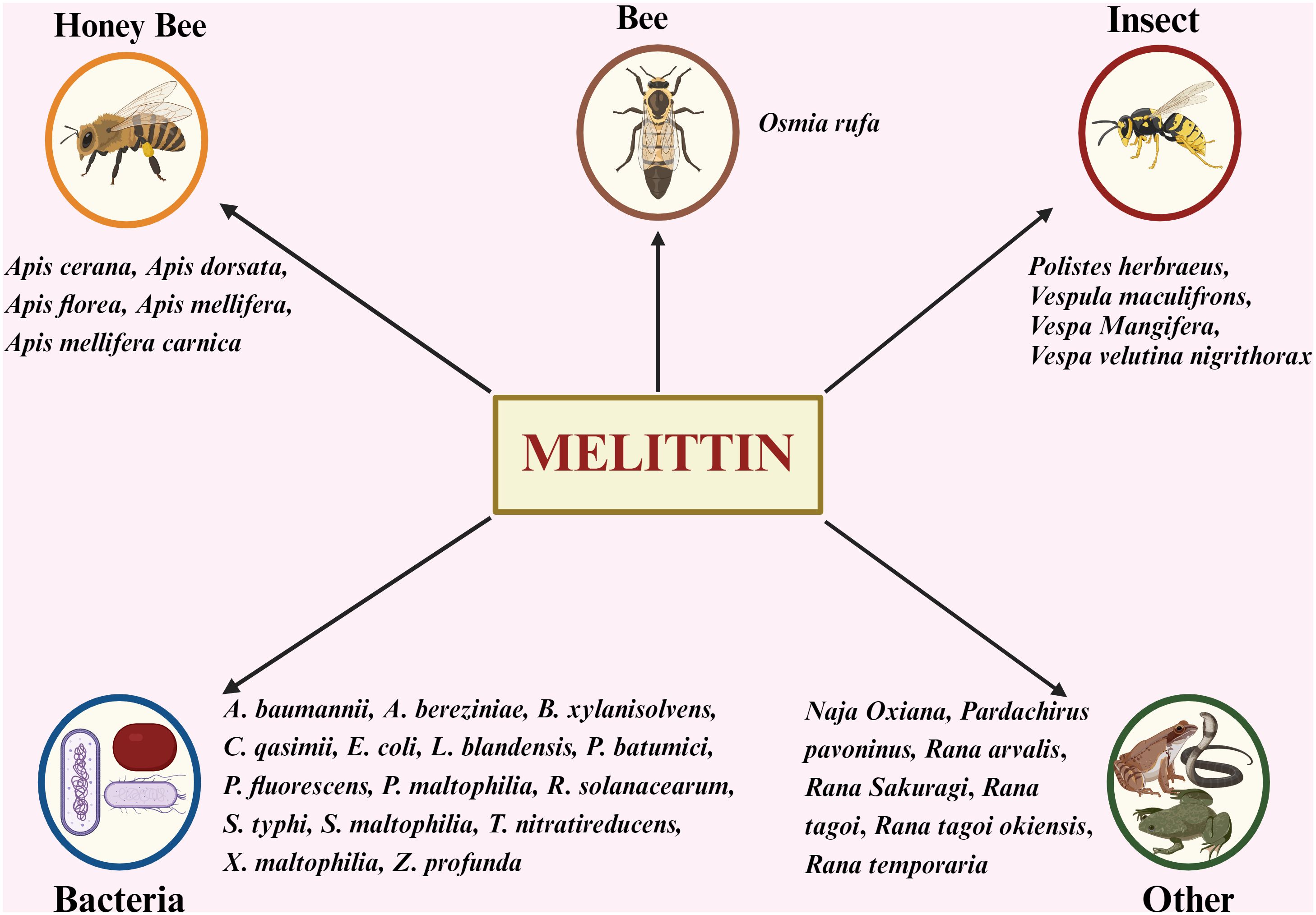
Figure 5. Exploring Diverse Origins: Sources of Melittin Beyond Honey Bees. A. baumannii, Acinetobacter baumanni; A. bereziniae, Acinetobacter bereziniae; B. xylanisolvens, Bacteroides xylanisolvens; C. qasimii, Cyclobacterium qasimii; E coli, Escherichia coli; L. blandensis, Leeuwenhoekiella blandensis; P. batumici,Pseudomonas batumici; P. fluorescens, Pseudomonas fluorescens; P. maltophilia Pseudomonas maltophilia; R. solanacearum, Ralstonia solanacearum; S. typhi, Salmonella typhi; S. maltophilia, Stenotrophomonas maltophilia; T. nitratireducens, Thioalkalivibrio nitratireducens; X. maltophilia, Xanthomonas maltophilia; Z. profunda, Zunongwangia profunda.
4.2 Various pharmacological properties of melittin
Melittin exhibits various pharmacological properties, including anti-cancer, antimicrobial (anti-fungal, anti-protozoal, antiviral, antibacterial), antiarthritic, anti-diabetic, and anti-inflammatory activities (67–70). It disrupts cancer cell membranes through endocytosis, increasing membrane permeability and intracellular Ca²+, leading to apoptosis (67, 68).
Melittin has demonstrated antimicrobial activity against various pathogens (69, 71). For instance, melittin has shown efficacy in inhibiting Mycoplasma gallisepticum infection, particularly in plasmid isolates carrying the melittin gene (72). Additionally, the BV peptide lasioglossins, known for their DNA-binding capabilities and membrane interactions, exhibit even stronger antimicrobial effects (73). In vivo studies have shown that Melittin administration reduces TNF-α and IL-1β levels, as well as the infiltration of inflammatory cells in mouse skin following a Dermatobacillus acne injection (74, 75).
Melittin-loaded nanoparticles have demonstrated the ability to destroy the human immunodeficiency virus (HIV) without harming nearby healthy cells (59, 76). Melittin also interacts with the herpes simplex virus-1 (HSV-1), blocking its replication and reducing HIV-1 expression. Moreover, PLA2, another component of BV, may serve as an adjunctive antiviral agent in HIV treatment (77, 78).
Melittin has been demonstrated to enhance insulin synthesis by reducing the inflammatory response of the pancreatic islets (79). By depolarizing the membrane of pancreatic islets, melittin facilitates the opening of Ca²+ channels, allowing calcium ions to enter more easily and triggering B-cells to produce insulin (55, 80–82). The antiarthritic effect of BV was associated with a decrease in COX-2 and PLA2 expression, as well as a lower level of IL-1, IL-6, TNF-α, ROS, and NO. This occurs through a strong protein-protein interaction (PPI) that alters the actions of IKKβ and IKKα, restricting the release of IκBβ and IκBα, which are essential for the translocation of the p50 subunit of NF-κB. As a result, the binding ability of NF-κB to DNA is reduced, leading to decreased expression of proinflammatory genes (83, 84). The anti-inflammatory effects of melittin across various disease models at different therapeutic doses are summarized in Table 2.
4.3 mechanism of the antiarthritic effect of melittin
The therapeutic potential of BV, particularly its key component melittin, has been extensively studied in both in vitro and in vivo models, revealing multiple anti-inflammatory and immunomodulatory mechanisms.
Nam et al. (100) demonstrated that the aqueous phase of BV (molecular weight <20 kDa) contains components with significant anti-inflammatory properties. In vitro studies identified that various BV extracts, including n-hexane, ethyl acetate, and the aqueous phase derived from Apis mellifera, exhibit a potent inhibitory effect on COX-2 activity while sparing COX-1. The aqueous partition was further subdivided based on molecular weight into three fractions: BV1 (>20 kDa), BV2 (10–20 kDa), and BV3 (<10 kDa). Of these, BV2 and BV3 fractions showed the most pronounced inhibition of COX-2 activity while avoiding cytotoxicity, indicating the presence of specific moieties responsible for suppressing proinflammatory cytokine production (TNF-α and IL-1β).
Further assessments in RAW 264.7 murine macrophage cell lines and synoviocytes from RA patients revealed similar anti-inflammatory effects of BV and melittin. Dose-dependent studies in macrophage models showed significant reductions in tissue inflammation, edema, and osteophyte formation with BV and melittin therapy at concentrations as low as 0.1–5 µg/kg, highlighting its therapeutic efficacy (90).
BV and melittin also effectively inhibit the production of nitric oxide (NO) and prostaglandin E2 (PGE2) in LPS-stimulated RAW 264.7 cells without cytotoxic effects. This inhibition parallels the effects observed with indomethacin, a conventional COX-2 inhibitor, and underscores BV’s capability to attenuate inflammatory mediators within synoviocytes of RA patients (84, 90).
Melittin’s mechanism of action extends to the suppression of NF-κβ activity, achieved by inhibiting IκB kinases (IKKα and IKKβ), reducing Iκβ phosphorylation, and preventing p50 translocation into the nucleus (84). These molecular actions culminate in decreased expression of proinflammatory genes, such as those encoding COX-2 and inducible nitric oxide synthase (iNOS) (Figure 6). Surface plasmon resonance analyses provide direct evidence of melittin’s interaction with these critical upstream signaling molecules, with dissociation constants of 4.6 × 10-6 M (IKKα), 1.34 × 10-9 M (IKKβ), and 1.01 × 10-9 M (p50), highlighting its specificity and potency (90, 91). This strong PPI alters the actions of IKKβ and IKKα, restricting the release of IκBβ and IκBα, which are essential for p50 translocation, thereby reducing NF-κB’s ability to bind DNA.
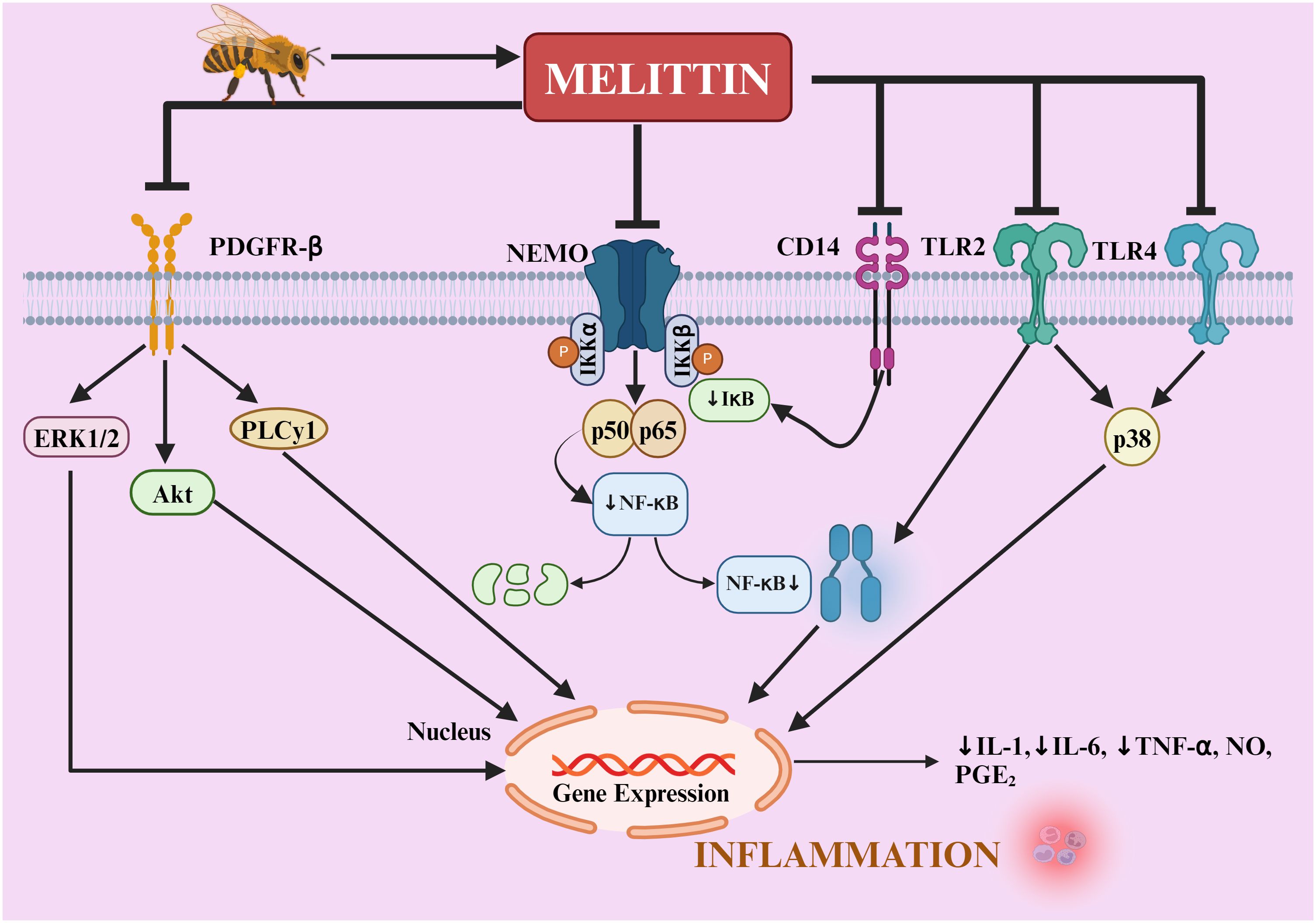
Figure 6. Mechanisms of melittin’s anti-inflammatory action in rheumatoid arthritis. melittin regulates TLR2, TLR4, CD14, NEMO, and PDGFRβ signaling pathways. Melittin blocks IKKs to prevent the release of IκB, thereby inducing NF-κB inactivation. It also decreases the activation of p38, ERK1/2, PLCγ1, and AKT, as well as the translocation of NF-κB into the nucleus, thereby reducing the inflammatory mediators (IL-1β, IL-6, TNF-α, NO, PGE2, ROS) in the liver, aorta, joints, skin, and neural tissue. NF-κB, nuclear factor-κB; IκB, inhibitor of NF-κB; IKK, IκB kinase; NEMO, NF-κB essential modulator; TNF-α, tumor necrosis factor; IL, interleukin; NO, nitric oxide; PGE2, prostaglandin E2; ERK1/2, extracellular signal-regulated protein kinases 1 and 2; CD, cluster of differentiation; TLR, toll-like receptor; PDGFR-β, platelet-derived growth factor receptor-β; PLCγ1, phospholipase Cγ1; Akt, protein kinase B.
The therapeutic effect of melittin has been further corroborated by studies showing its ability to reduce TNF-α, NO, and ROS levels—key mediators in inflammatory arthritis. These effects collectively contribute to its capacity to alleviate joint destruction and systemic inflammation (92–94, 101–103).
The potential protective mechanism of melittin against the inflammatory response is illustrated in Figure 6.
The inhibitory effects of melittin and BV on c-Jun N-terminal kinases (JNK) signaling were observed at concentrations of 5 µg/mL and within a range of 0.1–5 µg/mL, respectively. These effects were further validated by the use of SP600215, a specific JNK signal inhibitor, which suppressed the anti-inflammatory actions of melittin and BV. While other signaling pathways, such as p38 MAP kinase and ERK, may also be modulated depending on the cell type and stimulus, the specific inhibition of JNK signals appears to be a pivotal mechanism in reducing NF-κB activity and inflammatory mediator production. These findings demonstrate that melittin and BV exert their anti-inflammatory effects through a dual mechanism, targeting both NF-κB and JNK pathways. This dual inhibition contributes to the suppression of pro-inflammatory mediators, including NO and PGE2, underscoring the therapeutic potential of melittin and BV in managing RA and other inflammatory conditions (90, 91).
Synthetic melittin has been shown to bind to PLA2 and inhibit the enzymatic activity of secretory PLA2 (sPLA2) in synovial fluid taken from RA patients. This suggests that BV or its components may have a therapeutic role in disrupting key inflammatory enzymes, making the downregulation of genes that prevent inflammation crucial for its therapeutic efficacy (103, 104).
Yin et al. (105) conducted microarray analyses to investigate the global gene expression patterns in human chondrocyte-like cells exposed to BV. Their study revealed significant downregulation of key genes associated with inflammation, including the IL-6, matrix metalloproteinase-15 (MMP-15), caspase-6 and TNF-α ligand. These findings highlight BV’s ability to modulate inflammatory pathways at the transcriptional level, providing molecular evidence for its anti-inflammatory properties. The inhibition of these genes reflects BV’s potential to regulate critical processes involved in joint inflammation and destruction, further supporting its therapeutic utility in rheumatoid arthritis.
This transcriptional regulation complements the previously described suppression of pro-inflammatory mediators, such as NO, PGE2, and ROS, and the inhibition of NF-κB and JNK signaling pathways. Together, these molecular mechanisms underline melittin’s role in mitigating the systemic and localized inflammatory responses characteristic of RA. Moreover, the modulation of IL-6, MMP-15, and TNF-α ligands by BV adds another dimension to its therapeutic promise, targeting not only downstream inflammatory mediators but also upstream regulators of inflammation.
Additionally, BV-induced apoptosis in rheumatoid synovial fibroblasts has been shown to play a critical role in mitigating synovial hyperplasia, a hallmark of RA. By activating caspase-3 and modulating the balance between BAX and BCL-2 expression, BV promotes apoptosis and inhibits the proliferation of synovial cells, thereby alleviating the pathological effects of RA on joint tissues. This apoptotic mechanism not only reduces synovial cell density but also addresses one of the core drivers of joint destruction and chronic inflammation in RA (106). The dual action of melittin in targeting both inflammatory mediators and hyperplastic synovial cells highlights its potential as a multifaceted therapeutic agent for managing RA.
The collective evidence from these studies establishes melittin and BV as promising candidates for the development of novel RA treatments, particularly in cases where conventional therapies fail or cause significant side effects.
5 Melittin Formulations
5.1 Melittin transdermal delivery via polymeric microneedle for treatment of RA
Transdermal drug delivery systems offer a promising alternative for the treatment of RA, aiming to bypass the limitations associated with oral and injectable administration. Among these, melittin-loaded hyaluronic acid (HA) polymeric microneedles (Mel-HA-MN) represent a novel approach to enhance the localized and efficient delivery of melittin, a bioactive peptide known for its anti-inflammatory and immunomodulatory properties (107). Importantly, the microneedle delivery system significantly modulated the immune response in rodent models of adjuvant-induced arthritis (AIA). These Mel-HA-MN have shown potential in elevating regulatory CD4+ T cells and reducing levels of proinflammatory cytokines (TNF-α and IL-17), which may be linked to the modulation of T cells and cytokine activity (107, 108).
Mechanical characterization of the microneedles revealed a slight reduction in stress capacity upon melittin loading, with HA microneedles decreasing from 58 MPa to 38 MPa and Mel-HA-MN from 30 MPa to 27 MPa. Despite this reduction, the microneedles retained sufficient strength for effective skin penetration. The therapeutic efficacy of Mel-HA-MN was evaluated using the rat adjuvant-induced arthritis (AIA) model, where the microneedles’ penetration ability was tested by applying the patch on the rat’s abdominal skin (107).
Results demonstrated that Mel-HA-MN effectively penetrated the skin to a depth of approximately 200 µm. This depth ensures adequate delivery of melittin into the dermal layer, where it exerts its therapeutic effects. The therapeutic potential of Mel-HA-MN was further validated in AIA rodent models, where repeated applications of the microneedle patch successfully reduced paw swelling, maintained body weight and preserved cartilage integrity. By the end of the treatment, the paw thickness of treated animals decreased to less than half of that observed in the untreated control group, and clinical scores were significantly improved, matching the efficacy of subcutaneous melittin administration (107).
A key innovation in this approach is the incorporation of methacrylate-modified HA (MeHA), enabling sustained release of melittin. This modification prolonged therapeutic action and reduced administration frequency, highlighting its potential for long-term management of RA. The ability to tune drug release profiles adds an element of flexibility to this delivery method, paving the way for personalized treatment regimens.
The results of these studies emphasize the clinical relevance of Mel-HA-MN as a targeted, non-invasive treatment strategy for RA (107). By overcoming the challenges of direct administration, such as systemic toxicity and patient compliance issues (109), this technology holds significant promise for improving RA management.
5.2 Nanoemulsions of bee venom
Nanoemulsions containing BV have been developed and studied for their potential to reduce inflammation in animal models of RA. Research suggests that melittin’s antiarthritic effects can be enhanced through water-in-oil (W/O) nanoemulsion formulations containing BV (BV-NEs), which incorporate a surfactant mixture and an oil phase. These formulations have been tested on collagen-induced arthritis (CIA) in male Wistar rats (110).
A skin permeation test was conducted by applying BV-NE for up to 12 hours, revealing increased permeability with higher BV content in the formulations. Studies showed a significant decrease in serum levels of TNF-α and IL-17 from day 14 of the treatment, with further reductions observed on day 21. This demonstrates that nanoemulsions loaded with BV effectively reduce serum levels of IL-17 and TNF-α, indicating a modulation of both adaptive and innate immune responses following two weeks of topical treatment (110).
By encapsulating melittin in a nanoemulsion, BV-NEs improve the bioavailability and stability of the peptide while allowing for a controlled and sustained release. This not only enhances therapeutic efficacy but also reduces the risk of systemic side effects, making BV-NEs a safer alternative to traditional systemic therapies. In conclusion, nanoemulsions of bee venom represent an innovative and effective delivery system for the treatment of RA.
5.3 Incorporation of bee venom gel via micro-needling
In this study, BV gel was combined with a transdermal drug delivery system utilizing micro-needling to provide a precise and effective treatment option for RA. This approach leverages the ability of microneedles to create microchannels in the skin, facilitating the penetration and localized action of BV’s active components. The experiment involved inducing acute gouty inflammation in rats using monosodium urate crystal (MUC) and acute inflammation in mice using LPS. The anti-inflammatory effects were assessed by evaluating the permeability of the prepared microneedle gel.
A direct correlation was observed between the percutaneous absorption of the prepared microneedle gel and the reduction in NO levels in both the MUC and LPS-induced inflammation models.
The BV gel was prepared using carboxymethylcellulose sodium, a colorless, odorless, translucent material, as the gel matrix, along with antioxidants such as thiourea, sodium thiosulfate, glucose, and stabilizer. In patch skin tests, it was observed that melittin successfully penetrates the stratum corneum with the assistance of microneedles. Notably, the stability of melittin within the gel preparation remained intact after the addition of 0.1% stabilizer, maintaining its stability for up to 6 months. The study found that applying a force of 10 N with a 750 µm microneedle for 3 minutes produced the greatest anti-inflammatory effect (111).
The approach provides a localized and minimally invasive alternative to conventional treatments, ensuring targeted delivery while minimizing systemic exposure and associated risks.
6 Challenges with melittin as a therapeutic agent
Safety concerns have been one of the primary challenges associated with the therapeutic use of BV, particularly melittin. Despite numerous reports of adverse reactions, no comprehensive systematic analyses on safety in clinical practice have been conducted to date. The key issue with melittin is its potential to provoke the breakdown of intracellular and plasma membranes, as well as its ability to act as an allergen, inducing IgE-mediated responses that can lead to allergic reactions in several patients (112).
Melittin exhibits hemolytic activity and possesses cytotoxic and genotoxic effects (113, 114). It can disrupt lipid bilayers by acting as a natural surfactant, interacting with phospholipids, and integrating into red blood cell (RBC) membranes. This interaction with plasma membranes results in various effects, including the disruption of phospholipid packaging in the lipid bilayer, the formation of channels and pores, the aggregation of membrane proteins, and the induction of spontaneous cell lysis. When administered in high doses, melittin can cause itching, local reactions, and pain. However, at lower concentrations, it may exhibit beneficial anti-inflammatory effects, primarily due to the inhibition of PLA2 (115). Nonetheless, melittin’s administration appears to have cytotoxic effects on normal human cells, potentially raising mRNA levels of oxidative stress and apoptosis-related genes (58).
To address these challenges, several strategies have been explored to minimize the adverse effects of melittin while retaining its therapeutic potential. Asthana et al. (116) demonstrated that melittin’s hemolytic activity can be significantly reduced by substituting alanine in the leucine zipper motif. Additionally, Rayalin et al. (117) found that a melittin fusion protein with glutathione S-transferase exhibits low toxicity and retains anti-inflammatory properties.
The development of delivery techniques utilizing nanocarriers has shown promise in safely delivering melittin to specific lesions while minimizing harm to non-targeted cells. For example, Gui and colleagues (118) designed a polyelectrolyte-based nano-complex system using flash nanocomplexation technology. This system forms strong interactions between negatively charged dextran sulfate and positively charged melittin, resulting in decreased acute toxicity and enhanced pathological indicators, thereby increasing melittin’s therapeutic potential (118).
Investigating the co-treatment of PLA2 and melittin could be worthwhile, as they may complement each other well. Additionally, combining melittin with other natural products, such as curcumin or resveratrol, may provide synergistic effects and further reduce toxicity (119–121).
7 Conclusion and future aspect
BV is a complex biological mixture containing various bioactive components, such as peptides (notably melittin and PLA2), phospholipids, proteins, amino acids, enzymes, carbohydrates, minerals, and small amounts of volatile components. Historically used in traditional Chinese medicine to manage a range of conditions, like pain, arthritis, rheumatism, and skin diseases. BV and its components continue to gain attention for their therapeutic potential. Among these components, melittin has emerged as a particularly potent agent with significant anti-inflammatory effects. Its mechanism includes the inhibition of NF-κB activity by preventing IκB phosphorylation, which ultimately suppresses the expression of proinflammatory genes central to RA pathophysiology.
While existing RA treatments, such as NSAIDs, DMARDs, and biologics, offer varying degrees of symptom control, they often have limitations, including side effects, high costs, and limited efficacy for some patients. In this context, melittin offers a novel and promising approach. However, its cytotoxic potential presents challenges requiring further research to enhance its safety profile. The review highlights recent advancements in the formulation of melittin, such as transdermal patches, microneedle-delivered gels, and nanoemulsion-based topical applications, which may enhance its therapeutic delivery and minimize adverse effects.
Future research should focus on a deeper understanding of the molecular and cellular mechanisms underlying melittin’s antiarthritic activity. Continued work is essential to refine its delivery systems and assess its efficacy and safety across broader animal models, especially primates, to approximate human clinical conditions. Sustainable and standardized methods of BV extraction will also be essential to ensure consistent and reliable production.
Future studies should prioritize to investigate the key components of BV, particularly melittin and PLA2, to gain a deeper understanding of their physicochemical properties and enhance their therapeutic potential. Additionally, exploring complementary or alternative compounds—such as apamin, mast cell degranulating peptide, curcumin, and resveratrol—either individually or in combination with melittin could mitigate cytotoxicity while enhancing therapeutic efficacy. By addressing these challenges, optimizing combination therapies, and refining delivery system, melittin and other BV components may ultimately offer safer, more effective and accessible options for RA management and other inflammatory conditions. These efforts have the potential to expand treatment strategies and improve patient outcomes, presenting BV as a versatile foundation for future therapeutic advancements.
Author contributions
AsP: Conceptualization, Resources, Software, Supervision, Validation, Writing – review & editing. KM: Conceptualization, Data curation, Writing – original draft, Writing – review & editing. KT: Resources, Software, Writing – review & editing. AaP: Conceptualization, Resources, Software, Writing – review & editing. SC: Software, Visualization, Writing – review & editing. YR: Writing – review & editing. VA: Writing – review & editing. AC: Conceptualization, Writing – review & editing.
Funding
The author(s) declare that no financial support was received for the research, authorship, and/or publication of this article.
Acknowledgments
The authors express their sincere gratitude to Banasthali Vidyapith and their respective institutions for providing the essential resources and support necessary for the completion of this study. The figures included in this manuscript were created using BioRender.com.
Conflict of interest
The authors declare that the research was conducted in the absence of any commercial or financial relationships that could be construed as a potential conflict of interest.
Generative AI statement
The author(s) declare that no Generative AI was used in the creation of this manuscript.
Publisher’s note
All claims expressed in this article are solely those of the authors and do not necessarily represent those of their affiliated organizations, or those of the publisher, the editors and the reviewers. Any product that may be evaluated in this article, or claim that may be made by its manufacturer, is not guaranteed or endorsed by the publisher.
References
1. Deane KD, Demoruelle MK, Kelmenson LB, Kuhn KA, Norris JM, Holers VM. Genetic and environmental risk factors for rheumatoid arthritis. Best Pract Res Clin Rheumatol. (2017) 31:3–18. doi: 10.1016/j.berh.2017.08.003
2. Vos T, Lim SS, Abbafati C, Abbas KM, Abbasi M, Abbasifard M, et al. Global burden of 369 diseases and injuries in 204 countries and territories, 1990–2019: a systematic analysis for the Global Burden of Disease Study 2019. Lancet. (2020) 396:1204–22. doi: 10.1016/S0140-6736(20)30925-9
3. Cieza A, Causey K, Kamenov K, Hanson SW, Chatterji S, Vos T. Global estimates of the need for rehabilitation based on the Global Burden of Disease study 2019: a systematic analysis for the Global Burden of Disease Study 2019. Lancet. (2021) 396:2006–17. doi: 10.1016/S0140-6736(20)32340-0
4. Cai Y, Zhang J, Liang J, Xiao M, Zhang G, Jing Z, et al. The burden of rheumatoid arthritis: findings from the 2019 global burden of diseases study and forecasts for 2030 by bayesian age-period-cohort analysis. J Clin Med. (2023) 12:1291. doi: 10.3390/jcm12041291
5. GBD 2021 Rheumatoid Arthritis Collaborators. Global, regional, and national burden of rheumatoid arthritis, 1990-2020, and projections to 2050: a systematic analysis of the Global Burden of Disease Study 2021. Lancet Rheumatol. (2023) 5:e594–610. doi: 10.1016/S2665-9913(23)00211-4
6. Finckh A, Gilbert B, Hodkinson B, Bae SC, Thomas R, Deane KD, et al. Global epidemiology of rheumatoid arthritis. Nat Rev Rheumatol. (2022) 18:591–602. doi: 10.1038/s41584-022-00827-y
7. Zaiss MM, Joyce Wu HJ, Mauro D, Schett G, Ciccia F. The gut-joint axis in rheumatoid arthritis. Nat Rev Rheumatol. (2021) 17:224–37. doi: 10.1038/s41584-021-00585-3
8. Bullock J, Rizvi SAA, Saleh AM, Ahmed SS, Do DP, Ansari RA, et al. Rheumatoid arthritis: A brief overview of the treatment. Med principles practice. (2018) 27:501–7. doi: 10.1159/000493390
9. Sparks JA. Rheumatoid arthritis. Ann Internal Med. (2019) 170:ITC1–ITC16. doi: 10.7326/AITC201901010
10. Di Matteo A, Bathon JM, Emery P. Rheumatoid arthritis. Lancet. (2023) 402:2019–33. doi: 10.1016/S0140-6736(23)01525-8
11. Srirangan S, Choy EH. The role of interleukin 6 in the pathophysiology of rheumatoid arthritis. Ther Adv musculoskeletal disease. (2010) 2:247–56. doi: 10.1177/1759720X10378372
12. Brennan FM, McInnes IB. Evidence that cytokines play a role in rheumatoid arthritis. J Clin Invest. (2008) 118:3537–45. doi: 10.1172/JCI36389
13. Paglia MDG, Silva MT, Lopes LC, Barberato-Filho S, Mazzei LG, Abe FC, et al. CUse of corticoids and non-steroidal anti-inflammatories in the treatment of rheumatoid arthritis: Systematic review and network meta-analysis. PloS One. (2021) 16:e0248866. doi: 10.1371/journal.pone.0248866
14. Rhen T, Cidlowski JA. Anti-inflammatory action of glucocorticoids–new mechanisms for old drugs. New Engl J Med. (2005) 353:1711–23. doi: 10.1056/NEJMra050541
15. Villa-Hermosilla MC, Fernández-Carballido A, Hurtado C, Barcia E, Montejo C, Alonso M, et al. Sulfasalazine microparticles targeting macrophages for the treatment of inflammatory diseases affecting the synovial cavity. Pharmaceutics. (2021) 13:951. doi: 10.3390/pharmaceutics13070951
16. Bindu S, Mazumder S, Bandyopadhyay U. Non-steroidal anti-inflammatory drugs (NSAIDs) and organ damage: A current perspective. Biochem Pharmacol. (2020) 180:114147. doi: 10.1016/j.bcp.2020.114147
17. Nazir S, Ahmad I, Mobashar A, Sharif A, Shabbir A, Chaudhary WA. Mechanistic evaluation of antiarthritic and anti-inflammatory effect of campesterol ester derivatives in complete Freund’s adjuvant-induced arthritic rats. Front Pharmacol. (2024) 14:1346054. doi: 10.3389/fphar.2023.1346054
18. Miyazaki Y, Nakano K, Nakayamada S, Kubo S, Inoue Y, Fujino Y, et al. Efficacy and safety of tofacitinib versus baricitinib in patients with rheumatoid arthritis in real clinical practice: analyses with propensity score-based inverse probability of treatment weighting. Ann rheum diseases. (2021) 80:1130–6. doi: 10.1136/annrheumdis-2020-219699
19. Ngoufack C, Semerano L, Podglajen I, Bruneval P, Meune C, Valeyre, et al. Mitral valve granulomatosis: A paradoxical reaction complicating etanercept treatment in rheumatoid arthritis. A case report. Jt Bone Spine. (2021) 88:105183. doi: 10.1016/j.jbspin.2021.105183
20. Fleischmann R, Genovese MC, Maslova K, Leher H, Praestgaard A, Burmester GR. Long-term safety and efficacy of sarilumab over 5 years in patients with rheumatoid arthritis refractory to TNF inhibitors. Rheumatology. (2021) 60:4991–5001. doi: 10.1093/rheumatology/keab355
21. Ren HM, Lukacher AE, Rahman ZS, Olsen NJ. New developments implicating IL-21 in autoimmune disease. J autoimm. (2021) 122:102689. doi: 10.1016/j.jaut.2021.102689
22. Elemam NM, Hannawi S, Maghazachi AA. Role of chemokines and chemokine receptors in rheumatoid arthritis. ImmunoTargets Ther. (2020) 9:43–56. doi: 10.2147/ITT.S243636
23. Szekanecz Z, Koch AE. Successes and failures of chemokine-pathway targeting in rheumatoid arthritis. Nat Rev Rheumatol. (2016) 12:5–13. doi: 10.1038/nrrheum.2015.157
24. Giltiay NV, Shu GL, Shock A, Clark EA. Targeting CD22 with the monoclonal antibody epratuzumab modulates human B-cell maturation and cytokine production in response to Toll-like receptor 7 (TLR7) and B-cell receptor (BCR) signaling. Arthritis Res Ther. (2017) 19:91. doi: 10.1186/s13075-017-1284-2
25. Blair HA, Duggan ST. Belimumab: A review in systemic lupus erythematosus. Drugs. (2018) 78:355–66. doi: 10.1007/s40265-018-0872-z
26. Xu S, Lam KP. Transmembrane activator and CAML interactor (TACI): another potential target for immunotherapy of multiple myeloma? Cancers. (2020) 12:1045. doi: 10.3390/cancers12041045
27. Rubbert-Roth A, Enejosa J, Pangan AL, Haraoui B, Rischmueller M, Khan N, et al. Trial of upadacitinib or abatacept in rheumatoid arthritis. New Engl J Med. (2020) 383:1511–21. doi: 10.1056/NEJMoa2008250
28. Teitsma XM, Marijnissen AK, Bijlsma JW, Lafeber FP, Jacobs JW. Tocilizumab as monotherapy or combination therapy for treating active rheumatoid arthritis: a meta-analysis of efficacy and safety reported in randomized controlled trials. Arthritis Res Ther. (2016) 18:211. doi: 10.1186/s13075-016-1108-9
29. Westhovens R, Rigby WFC, van der Heijde DS, Ching DWT, Stohl W, Kay J, et al. Filgotinib in combination with methotrexate or as monotherapy versus methotrexate monotherapy in patients with active rheumatoid arthritis and limited or no prior exposure to methotrexate: The phase 3, randomized controlled FINCH 3 trial. Ann rheum diseases. (2021) 80:727–38. doi: 10.1136/annrheumdis-2020-219213
30. Gabay C, Riek M, Scherer A, Finckh A, SCQM collaborating physicians. Effectiveness of biologic DMARDs in monotherapy versus in combination with synthetic DMARDs in rheumatoid arthritis: data from the Swiss Clinical Quality Management Registry. Rheumatology. (2015) 54:1664–72. doi: 10.1093/rheumatology/kev019
31. Su QY, Li HC, Jiang XJ, Jiang ZQ, Zhang Y, Zhang HY, et al. Exploring the therapeutic potential of regulatory T cell in rheumatoid arthritis: Insights into subsets, markers, and signaling pathways. Biomed pharmacother. (2024) 174:116440. doi: 10.1016/j.biopha.2024.116440
32. Joensuu JT, Huoponen S, Aaltonen KJ, Konttinen YT, Nordström D, Blom M. The cost-effectiveness of biologics for the treatment of rheumatoid arthritis: a systematic review. PloS One. (2015) 10:e0119683. doi: 10.1371/journal.pone.0119683
33. Xie P, Xue W, Qi W, Li Y, Yang L, Yang Z, et al. Safety, tolerability, and pharmacokinetics of ibuprofenamine hydrochloride spray (NSAIDs), a new drug for rheumatoid arthritis and osteoarthritis, in healthy chinese subjects. Drug design Dev Ther. (2021) 15:629–38. doi: 10.2147/DDDT.S294849
34. Jantan I, Ahmad W, Bukhari SN. Plant-derived immunomodulators: an insight on their preclinical evaluation and clinical trials. Front Plant science. (2015) 6:655. doi: 10.3389/fpls.2015.00655
35. Meenakshi DU, Narde GK, Siddiqui S, Ahuja A. Marine bioactive phytoconstituents in autoimmune disorders: role and mechanism - A review. Anti-inflamm anti-allergy Agents medicinal Chem. (2023) 22:10–29. doi: 10.2174/1871523022666230731104529
36. Hannan A, Akhtar B, Sharif A, Anjum F, Pasha I, Khan A, et al. Quercetin-loaded chitosan nanoparticles ameliorate adjuvant-induced arthritis in rats by regulating anti-oxidant enzymes and downregulating pro- and inflammatory cytokines. Inflammopharmacology. (2023) 31:287–300. doi: 10.1007/s10787-022-01118-4
37. Siddique R, Muhammad F, Faisal MN, Akhtar B, Saleem A, Kousar S, et al. Gingerol nanoparticles attenuate complete Freund adjuvant-induced arthritis in rats via targeting the RANKL/OPG signaling pathway. Inflammopharmacology. (2024) 32:3311–26. doi: 10.1007/s10787-024-01537-5
38. Shen B, Cao Z, Li W, Sabatier JM, Wu Y. Treating autoimmune disorders with venom-derived peptides. Expert Opin Biol Ther. (2017) 17:1065–75. doi: 10.1080/14712598.2017.1346606
39. Ra JC, Kang SK, Shin IS, Park HG, Joo SA, Kim JG, et al. Stem cell treatment for patients with autoimmune disease by systemic infusion of culture-expanded autologous adipose tissue derived mesenchymal stem cells. J Trans Med. (2011) 9:181. doi: 10.1186/1479-5876-9-181
40. Zhang S, Liu Y, Ye Y, Wang XR, Lin LT, Xiao LY, et al. Bee venom therapy: Potential mechanisms and therapeutic applications. Toxicon. (2018) 148:64–73. doi: 10.1016/j.toxicon.2018.04.012
41. Grassberger M, Sherman RA, Gileva OS, Kim CMH. Biotherapy—History, Principles and Practice. Dordrecht, The Netherlands: Springer (2013).
42. El-Wahed AA, Khalifa SA, Sheikh BY, Farag MA, Saeed A, Larik FA, et al. Bee venom composition: from chemistry to biological activity. Stud Natural Prod Chem. (2019) 60:459–84. doi: 10.1016/B978-0-444-64181-6.00013
43. Seo BK, Han K, Kwon O, Jo DJ, Lee JH. Efficacy of bee venom acupuncture for chronic low back pain: A randomized, double-blinded, sham-controlled trial. Toxins. (2017) 9:361. doi: 10.3390/toxins9110361
44. Bava R, Castagna F, Musella V, Lupia C, Palma E, Britti D. Therapeutic use of bee venom and potential applications in veterinary medicine. Vet Sci. (2023) 10:119. doi: 10.3390/vetsci10020119
45. Jafari Z, Sadeghi S, Dehaghi MM, Bigham A, Honarmand S, Tavasoli A, et al. Immunomodulatory activities and biomedical applications of melittin and its recent advances. Archiv der Pharmazie. (2024) 357:e2300569. doi: 10.1002/ardp.202300569
46. Nygaard G, Firestein GS. Restoring synovial homeostasis in rheumatoid arthritis by targeting fibroblast-like synoviocytes. Nat Rev Rheumatol. (2020) 16:316–33. doi: 10.1038/s41584-020-0413-5
47. Chauhan K, Jandu JS, Brent LH. Rheumatoid arthritis. In: StatPearls. Treasure Island (FL): StatPearls Publishing (2023).
48. de Brito Rocha S, Baldo DC, Andrade LEC. Clinical and pathophysiologic relevance of autoantibodies in rheumatoid arthritis. Adv Rheumatol. (2019) 59:2. doi: 10.1186/s42358-018-0042-8
49. Wu CY, Yang HY, Lai JH. Anti-citrullinated protein antibodies in patients with rheumatoid arthritis: biological effects and mechanisms of immunopathogenesis. Int J Mol Sci. (2020) 21:4015. doi: 10.3390/ijms21114015
50. Guo Q, Wang Y, Xu D, Nossent J, Pavlos NJ, Xu J. Rheumatoid arthritis: pathological mechanisms and modern pharmacologic therapies. Bone Res. (2018) 6:15. doi: 10.1038/s41413-018-0016-9
51. Okada Y, Eyre S, Suzuki A, Kochi Y, Yamamoto K. Genetics of rheumatoid arthritis. Ann rheum diseases. (2019) 78:446–53. doi: 10.1136/annrheumdis-2018-213678
52. Shi P, Xie S, Yang J, Zhang Y, Han S, Su S, et al. Pharmacological effects and mechanisms of bee venom and its main components: Recent progress and perspective. Front Pharmacol. (2022) 13:1001553. doi: 10.3389/fphar.2022.1001553
53. Samanchi T, Kekeçoğlu M. Comparison of commercial and anatolian bee venom in terms of chemical composition. Uludağ Arıcılık Dergisi. (2019) 19:61–8. doi: 10.31467/uluaricilik.527986
54. Pucca MB, Cerni FA, Oliveira IS, Jenkins TP, Argemí L, Sørensen CV, et al. Bee updated: current knowledge on bee venom and bee envenoming therapy. Front Immunol. (2019) 10:2090. doi: 10.3389/fimmu.2019.02090
55. Al-Shaeli SJJ, Hussen TJ, Ethaeb AM. Effect of honey bee venom on the histological changes of testes and hormonal disturbance in diabetic mice. Vet world. (2022) 15:2357–64. doi: 10.14202/vetworld.2022.2357-2364
56. Ullah A, Aldakheel FM, Anjum SI, Raza G, Khan SA, Tlak Gajger I. Pharmacological properties and therapeutic potential of honey bee venom. Saudi Pharm J. (2023) 31:96–109. doi: 10.1016/j.jsps.2022.11.008
57. Khalil A, Elesawy BH, Ali TM, Ahmed OM. Bee venom: from venom to drug. Molecules. (2021) 26:4941. doi: 10.3390/molecules26164941
58. Chen J, Guan SM, Sun W, Fu H. Melittin, the major pain-producing substance of bee venom. Neurosci bulletin. (2016) 32:265–72. doi: 10.1007/s12264-016-0024-y
59. Guha S, Ferrie RP, Ghimire J, Ventura CR, Wu E, Sun L, et al. Applications and evolution of melittin, the quintessential membrane active peptide. Biochem Pharmacol. (2021) 193:114769. doi: 10.1016/j.bcp.2021.114769
60. Ceremuga M, Stela M, Janik E, Gorniak L, Synowiec E, Sliwinski T, et al. Melittin-A natural peptide from bee venom which induces apoptosis in human leukaemia cells. Biomolecules. (2020) 10:247. doi: 10.3390/biom10020247
61. Dabbagh Moghaddam F, Akbarzadeh I, Marzbankia E, Farid M, Khaledi L, Reihani A, et al. Delivery of melittin-loaded niosomes for breast cancer treatment: an in vitro and in vivo evaluation of anti-cancer effect. Cancer Nanotechnol. (2020) 12:1–35. doi: 10.1186/s12645-021-00085-9
62. Praphawilai P, Kaewkod T, Suriyaprom S, Panya A, Disayathanoowat T, Tragoolpua Y. Anti-Herpes Simplex Virus and Anti-Inflammatory Activities of the Melittin Peptides Derived from Apis mellifera and Apis florea Venom. Insects. (2024) 15:109. doi: 10.3390/insects15020109
63. Lubawy J, Urbański A, Mrówczyńska L, Matuszewska E, Światły-Błaszkiewicz A, Matysiak J, et al. The influence of bee venom melittin on the functioning of the immune system and the contractile activity of the insect heart-A preliminary study. Toxins. (2019) 11:494. doi: 10.3390/toxins11090494
64. Avci FG, Akbulut BS, Ozkirimli E. Membrane active peptides and their biophysical characterization. Biomolecules. (2018) 8:77. doi: 10.3390/biom8030077
65. Gajski G, Garaj-Vrhovac V. Melittin: a lytic peptide with anticancer properties. Environ Toxicol Pharmacol. (2013) 36:697–705. doi: 10.1016/j.etap.2013.06.009
66. Hossen MS, Gan SH, Khalil MI. Melittin, a potential natural toxin of crude bee venom: probable future arsenal in the treatment of diabetes mellitus. J Chem. (2017), 4035626. doi: 10.1155/2017/4035626
67. Wang A, Zheng Y, Zhu W, Yang L, Yang Y, Peng J. Melittin-based nano-delivery systems for cancer therapy. Biomolecules. (2022) 12:118. doi: 10.3390/biom12010118
68. Haque S, Hussain A, Joshi H, Sharma U, Sharma B, Aggarwal D, et al. Melittin: a possible regulator of cancer proliferation in preclinical cell culture and animal models. J Cancer Res Clin Oncol. (2023) 149:17709–26. doi: 10.1007/s00432-023-05458-8
69. El-Seedi H, Abd El-Wahed A, Yosri N, Musharraf SG, Chen L, Moustafa M, et al. Antimicrobial properties of apis mellifera’s bee venom. Toxins. (2020) 12:451. doi: 10.3390/toxins12070451
70. Lee G, Bae H. Anti-inflammatory applications of melittin, a major component of bee venom: detailed mechanism of action and adverse effects. Molecules. (2016) 21:616. doi: 10.3390/molecules21050616
71. Lee G, Bae H. Bee venom phospholipase A2: Yesterday’s enemy becomes today’s friend. Toxins. (2016) 8:48. doi: 10.3390/toxins8020048
72. Lazarev VN, Shkarupeta MM, Kostryukova ES, Levitskii SA, Titova GA, Akopian TA, et al. Recombinant plasmid constructs expressing gene for antimicrobial peptide melittin for the therapy of Mycoplasma and chlamydia infections. Bull Exp Biol Med. (2007) 144:452–6. doi: 10.1007/s10517-007-0350-1
73. Bandyopadhyay S, Lee M, Sivaraman J, Chatterjee C. Model membrane interaction and DNA-binding of antimicrobial peptide Lasioglossin II derived from bee venom. Biochem Biophys Res Commun. (2013) 430:1–6. doi: 10.1016/j.bbrc.2012.11.015
74. Memariani H, Memariani M, Shahidi-Dadras M, Nasiri S, Akhavan MM, Moravvej H. Melittin: from honeybees to superbugs. Appl Microbiol Biotechnol. (2019) 103:3265–76. doi: 10.1007/s00253-019-09698-y
75. Lee WR, Kim KH, An HJ, Kim JY, Chang YC, Chung H, et al. The protective effects of melittin on Propionibacterium acnes-induced inflammatory responses in vitro and in vivo. J Invest Dermatol. (2014) 134:1922–30. doi: 10.1038/jid.2014.75
76. Sadek KM, Shib NA, Taher ES, Rashed F, Shukry M, Atia GA, et al. Harnessing the power of bee venom for therapeutic and regenerative medical applications: an updated review. Front Pharmacol. (2024) 15:1412245. doi: 10.3389/fphar.2024.1412245
77. Uddin MB, Lee BH, Nikapitiya C, Kim JH, Kim TH, Lee HC, et al. Inhibitory effects of bee venom and its components against viruses in vitro and in vivo. J Microbiol. (2016) 54:853–66. doi: 10.1007/s12275-016-6376-1
78. Wehbe R, Frangieh J, Rima M, El Obeid D, Sabatier JM, Fajloun Z. Bee venom: overview of main compounds and bioactivities for therapeutic interests. Molecules. (2019) 24:2997. doi: 10.3390/molecules24162997
79. Al-Sarray RAH, Al-Shaeli SJJ. Metformin and bee venom: a comparative detection of histological alteration of the pancreas and systemic inflammatory markers in diabetic mice. Arch Razi Instit. (2022) 77:2335–43. doi: 10.22092/ARI.2022.358619.2269
80. Zahran F, Mohamad A, Zein N. Bee venom ameliorates cardiac dysfunction in diabetic hyperlipidemic rats. Exp Biol Med. (2021) 246:2630–44. doi: 10.1177/15353702211045924
81. Kim JY, Cho SH, Kim YW, Jang EC, Park SY, Kim EJ, et al. Effects of BCG, lymphotoxin and bee venom on insulitis and development of IDDM in non-obese diabetic mice. J Korean Med science. (1999) 14:648–52. doi: 10.3346/jkms.1999.14.6.648
82. Al-Shaeli SJJ, Ethaeb AM, Al-Zaidi EAN. Serological and histological evaluation of the effect of honeybee venom on pancreas and liver in diabetic mice. Arch Razi Instit. (2022) 77:1125–31. doi: 10.22092/ARI.2022.357385.2025
83. Son DJ, Lee JW, Lee YH, Song HS, Lee CK, Hong JT. Therapeutic application of anti-arthritis, pain-releasing, and anti-cancer effects of bee venom and its constituent compounds. Pharmacol Ther. (2007) 115:246–70. doi: 10.1016/j.pharmthera.2007.04.004
84. Park HJ, Lee SH, Son DJ, Oh KW, Kim KH, Song HS, et al. Antiarthritic effect of bee venom: inhibition of inflammation mediator generation by suppression of NF-kappaB through interaction with the p50 subunit. Arthritis rheum. (2004) 50:3504–15. doi: 10.1002/art.20626
85. Cho HJ, Kang JH, Park KK, Choe JY, Park YY, Moon YS, et al. Comparative proteome analysis of Tumor necrosis factor α-stimulated human Vascular Smooth Muscle Cells in response to melittin. Proteome science. (2013) 11:20. doi: 10.1186/1477-5956-11-20
86. Kim SJ, Park JH, Kim KH, Lee WR, Kim KS, Park KK. Melittin inhibits atherosclerosis in LPS/high-fat treated mice through atheroprotective actions. J Atheroscl thromb. (2011) 18:1117–26. doi: 10.5551/jat.8474
87. Moon DO, Park SY, Lee KJ, Heo MS, Kim KC, Kim MO, et al. Bee venom and melittin reduce proinflammatory mediators in lipopolysaccharide-stimulated BV2 microglia. Int immunopharmacol. (2007) 7:1092–101. doi: 10.1016/j.intimp.2007.04.005
88. Lee WR, Kim KH, An HJ, Kim JY, Han SM, Lee KG, et al. Protective effect of melittin against inflammation and apoptosis on Propionibacterium acnes-induced human THP-1 monocytic cell. Eur J Pharmacol. (2014) 740:218–26. doi: 10.1016/j.ejphar.2014.06.058
89. Lee SH, Choi SM, Yang EJ. Melittin ameliorates the inflammation of organs in an amyotrophic lateral sclerosis animal model. Exp neurobiol. (2014) 23:86–92. doi: 10.5607/en.2014.23.1.86
90. Park HJ, Son DJ, Lee CW, Choi MS, Lee US, Song HS, et al. Melittin inhibits inflammatory target gene expression and mediator generation via interaction with IkappaB kinase. Biochem Pharmacol. (2007) 73:237–47. doi: 10.1016/j.bcp.2006.09.023
91. Park HJ, Lee HJ, Choi MS, Son DJ, Song HS, Song MJ, et al. JNK pathway is involved in the inhibition of inflammatory target gene expression and NF-kappaB activation by melittin. J inflamm. (2008) 5:7. doi: 10.1186/1476-9255-5-7
92. Tekeoğlu İ, Akdoğan M, Çelik İ. Investigation of anti-inflammatory effects of bee venom in experimentally induced adjuvant arthritis. Reumatologia. (2020) 58:265–71. doi: 10.5114/reum.2020.99764
93. Goo B, Lee J, Park C, Yune T, Park Y. Bee venom alleviated edema and pain in monosodium urate crystals-induced gouty arthritis in rat by inhibiting inflammation. Toxins. (2021) 13:661. doi: 10.3390/toxins13090661
94. Kocyigit A, Guler EM, Kaleli S. Anti-inflammatory and antioxidative properties of honey bee venom on Freund’s Complete Adjuvant-induced arthritis model in rats. Toxicon. (2019) 161:4–11. doi: 10.1016/j.toxicon.2019.02.016
95. Duc Nguyen M, Van Tran T, Vinh Nguyen Q, Doan Ha C, Vu Phuong Dang L. Effectiveness of bee venom acupuncture for patients suffering from periarthritis humeroscapularis. J tradition Chin Med. (2023) 43:795–800. doi: 10.19852/j.cnki.jtcm.2023.04.002
96. El-Tedawy DM, Abd-Alhaseeb MM, Helmy MW, Ghoneim AI. Systemic bee venom exerts antiarthritic and anti-inflammatory properties in a rat model of arthritis. Biomed Rep. (2020) 13:20. doi: 10.3892/br.2020.1327
97. Mohamed WA, Abd-Elhakim YM, Ismail SAA. Involvement of the anti-inflammatory, anti-apoptotic, and anti-secretory activity of bee venom in its therapeutic effects on acetylsalicylic acid-induced gastric ulceration in rats. Toxicology. (2019) 419:11–23. doi: 10.1016/j.tox.2019.03.003
98. Kim KH, Sung HJ, Lee WR, An HJ, Kim JY, Pak SC, et al. Effects of melittin treatment in cholangitis and biliary fibrosis in a model of xenobiotic-induced cholestasis in mice. Toxins. (2015) 7:3372–87. doi: 10.3390/toxins7093372
99. Park JH, Lee WR, Kim HS, Han SM, Chang YC, Park KK. Protective effects of melittin on tumor necrosis factor-α induced hepatic damage through suppression of apoptotic pathway and nuclear factor-kappa B activation. Exp Biol Med. (2014) 239:1705–14. doi: 10.1177/1535370214533880
100. Nam KW, Je KH, Lee JH, Han HJ, Lee HJ, Kang SK, et al. Inhibition of COX-2 activity and proinflammatory cytokines (TNF-alpha and IL-1beta) production by water-soluble sub-fractionated parts from bee (Apis mellifera) venom. Arch pharmacal Res. (2003) 26:383–8. doi: 10.1007/BF02976695
101. Murakami M, Nakatani Y, Atsumi G, Inoue K, Kudo I. Regulatory functions of phospholipase A2. Crit Rev Immunol. (1997) 17:225–83. doi: 10.1615/CritRevImmunol.v37.i2-6.20
102. Hoffmann GE, Schneider E, Brand K, Kozumplik V, Fateh-Moghadam S. Characterization of a phospholipase A2 in human serum. Eur J Clin Chem Clin Biochem. (1992) 30:111–7.
103. Korte Suo PT, Nevalainen TJ, Buchler M, Uhl W. Characterization of two phospholipase A2 in serum of patients with sepsis and acute pancreatitis. Eur J Clin Chem Clin Biochem. (1992) 30:263–9. doi: 10.1515/cclm.1992.30.5.263
104. Saini SS, Peterson JW, Chopra AK. Melittin binds to secreto phospholipase A2 and inhibits its enzymatic activity. Biochem Biophys Res Commun. (1997) 238:436–42. doi: 10.1006/bbrc.1997.7295
105. Yin CS, Lee HJ, Hong SJ, Chung JH, Koh HG. Microarray analysis of gene expression in chondrosarcoma cells treated with bee venom. Toxicon. (2005) 45:81–91. doi: 10.1016/j.toxicon.2004.09.017
106. Hong SJ, Rim GS, Yang HI, Yin CS, Koh HG, Jang MH, et al. Bee venom induces apoptosis through caspase-3 activation in synovial fibroblasts of patients with rheumatoid arthritis. Toxicon. (2005) 46:39–45. doi: 10.1016/j.toxicon.2005.03.015
107. Du G, He P, Zhao J, He C, Jiang M, Zhang Z, et al. Polymeric microneedle-mediated transdermal delivery of melittin for rheumatoid arthritis treatment. J Controlled release. (2021) 336:537–48. doi: 10.1016/j.jconrel.2021.07.005
108. Cooles FA, Isaacs JD, Anderson AE. Treg cells in rheumatoid arthritis: an update. Curr Rheumatol Rep. (2013) 15:352. doi: 10.1007/s11926-013-0352-0
109. Prausnitz MR, Langer R. Transdermal drug delivery. Nat Biotechnol. (2008) 26:1261–8. doi: 10.1038/nbt.1504
110. Yousefpoor Y, Amani A, Divsalar A, Mousavi SE, Shakeri A, Sabzevari JT. Anti-rheumatic activity of topical nanoemulsion containing bee venom in rats. Eur J pharma biopharma. (2022) 172:168–76. doi: 10.1016/j.ejpb.2022.02.005
111. Zhao M, Bai J, Lu Y, Du S, Shang K, Li P, et al. Anti-arthritic effects of microneedling with bee venom gel. J Tradition Chin Med Sci. (2016) 3:256–62. doi: 10.1016/j.jtcms.2016.09.005
112. Sobotka AK, Franklin RM, Adkinson NF, Valentine M, Baer H, Lichtenstein LM. Allergy to insect stings. II. Phospholipase A: the major allergen in honeybee venom. J Allergy Clin Immunol. (1976) 57:29–40. doi: 10.1016/0091-6749(76)90076-2
113. Tosteson MT, Holmes SJ, Razin M, Tosteson DC. Melittin lysis of red cells. J membrane Biol. (1985) 87:35–44. doi: 10.1007/BF01870697
114. Gajski G, Domijan AM, Žegura B, Štern A, Gerić M, Novak Jovanović I, et al. Melittin induced cytogenetic damage, oxidative stress and changes in gene expression in human peripheral blood lymphocytes. Toxicon. (2016) 110:56–67. doi: 10.1016/j.toxicon.2015.12.005
115. Światły-Błaszkiewicz A, Mrówczyńska L, Matuszewska E, Lubawy J, Urbański A, Kokot ZJ, et al. The effect of bee venom peptides melittin, tertiapin, and apamin on the human erythrocytes ghosts: A preliminary study. Metabolites. (2020) 10:191. doi: 10.3390/metabo10050191
116. Asthana N, Yadav SP, Ghosh JK. Dissection of antibacterial and toxic activity of melittin: a leucine zipper motif plays a crucial role in determining its hemolytic activity but not antibacterial activity. J Biol Chem. (2004) 279:55042–50. doi: 10.1074/jbc.M408881200
117. Rayahin JE, Buhrman JS, Gemeinhart RA. Melittin-glutathione S-transferase fusion protein exhibits anti-inflammatory properties and minimal toxicity. Eur J Pharm Sci. (2014) 65:112–21. doi: 10.1016/j.ejps.2014.09.012
118. Gui Z, Zhu J, Ye S, Ye J, Chen J, Ling Y, et al. Prolonged melittin release from polyelectrolyte-based nanocomplexes decreases acute toxicity and improves blood glycemic control in a mouse model of type II diabetes. Int J pharma. (2020) 577:119071. doi: 10.1016/j.ijpharm.2020.119071
119. Gupta SC, Patchva S, Aggarwal BB. Therapeutic roles of curcumin: lessons learned from clinical trials. AAPS J. (2013) 15:195–218. doi: 10.1208/s12248-012-9432-8
120. Sheng S, Wang X, Liu X, Hu X, Shao Y, Wang G, et al. The role of resveratrol on rheumatoid arthritis: From bench to bedside. Front Pharmacol. (2022) 13:829677. doi: 10.3389/fphar.2022.829677
Keywords: rheumatoid arthritis, bee venom, melittin, anti-inflammatory, novel formulations
Citation: Pareek A, Mehlawat K, Tripathi K, Pareek A, Chaudhary S, Ratan Y, Apostolopoulos V and Chuturgoon A (2024) Melittin as a therapeutic agent for rheumatoid arthritis: mechanistic insights, advanced delivery systems, and future perspectives. Front. Immunol. 15:1510693. doi: 10.3389/fimmu.2024.1510693
Received: 13 October 2024; Accepted: 06 December 2024;
Published: 20 December 2024.
Edited by:
Shuo Wang, Shanghai Jiao Tong University, ChinaReviewed by:
Ali Sharif, Lahore College for Women University, PakistanWenbin Liu, Guangdong Pharmaceutical University, China
Copyright © 2024 Pareek, Mehlawat, Tripathi, Pareek, Chaudhary, Ratan, Apostolopoulos and Chuturgoon. This is an open-access article distributed under the terms of the Creative Commons Attribution License (CC BY). The use, distribution or reproduction in other forums is permitted, provided the original author(s) and the copyright owner(s) are credited and that the original publication in this journal is cited, in accordance with accepted academic practice. No use, distribution or reproduction is permitted which does not comply with these terms.
*Correspondence: Ashutosh Pareek, cGFzaHV0b3NoQGJhbmFzdGhhbGkuaW4=; Anil Chuturgoon, Y2h1dHVyQHVrem4uYWMuemE=