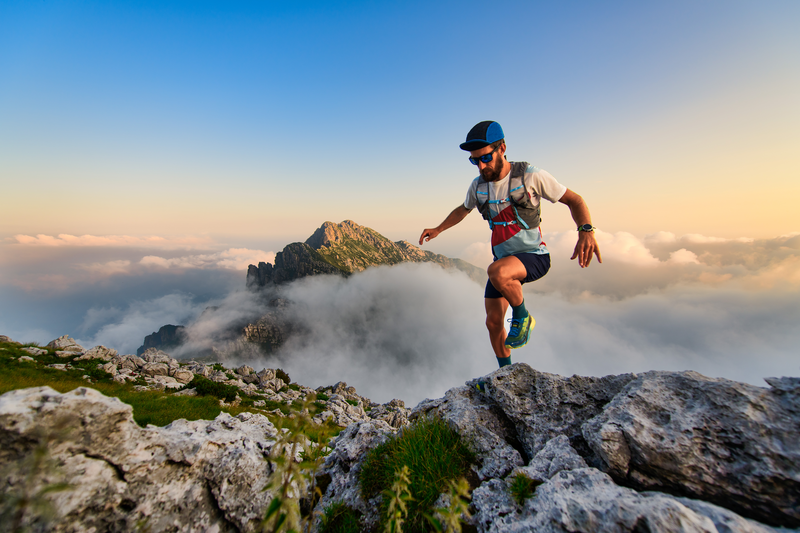
94% of researchers rate our articles as excellent or good
Learn more about the work of our research integrity team to safeguard the quality of each article we publish.
Find out more
REVIEW article
Front. Immunol. , 19 December 2024
Sec. Inflammation
Volume 15 - 2024 | https://doi.org/10.3389/fimmu.2024.1470283
This article is part of the Research Topic Immunotherapy Resistance and Chronic Inflammation View all 3 articles
Polycystic ovary syndrome (PCOS) is a prevalent endocrine and metabolic disorder affecting 6-20% of women of childbearing age worldwide. Immune cell imbalance and dysregulation of inflammatory factors can lead to systematic low-grade chronic inflammation (SLCI), which plays a pivotal role in the pathogenesis of PCOS. A significant higher infiltration of immune cells such as macrophages and lymphocytes and pro-inflammatory factors IL-6 and TNF-α has been detected in PCOS organ systems, impacting not only the female reproductive system but also other organs such as the cardiovascular, intestine, liver, thyroid, brain and other organs. Obesity, insulin resistance (IR), steroid hormones imbalance and intestinal microecological imbalance, deficiencies in vitamin D and selenium, as well as hyperhomocysteinemia (HHcy) can induce systematic imbalance between pro-inflammatory and anti-inflammatory cells and molecules. The pro-inflammatory cells and cytokines also interact with obesity, steroid hormones imbalance and IR, leading to increased metabolic imbalance and reproductive-endocrine dysfunction in PCOS patients. This review aims to summarize the dysregulation of immune response in PCOS organ system and the intrinsic mechanisms affecting SLCI in PCOS to provide new insights for the systemic inflammatory treatment of PCOS in the future.
Polycystic ovary syndrome (PCOS) is a prevalent reproductive endocrine and metabolic disorder affecting 6-20% of women of reproductive age globally (1). It is usually characterized by hyperandrogenism (HA), anovulation or oligo-ovulation, and polycystic ovary morphology (PCOM) (2). The detrimental effects of PCOS on fertility and long-term health in women have garnered considerable attention in the field of reproductive medicine. However, the etiology of PCOS remains unclear. Accumulating evidences suggested that PCOS might be a complex multigenic disorder, influenced by epigenetic and environmental factors, including lifestyle choices such as diet, exercise, rest, tobacco and alcohol consumption, psychological stress, and exposure to various pollutants like environmental endocrine disruptors (3, 4). In addition to insulin resistance(IR) and compensatory hyperinsulinemia, PCOS patients experience an elevated risk for various metabolic disorders and malignancies in the long term (5, 6). Disruptions in common regulatory mechanisms between substance-energy metabolism and reproduction may contribute to concurrent metabolic and reproductive disorders, which aligns with the evolutionary understanding of the disorder (7).
Systematic low-grade chronic inflammation (SLCI) plays a pivotal role in the pathogenesis of various chronic disorders, including PCOS. An imbalance between immune cells and inflammatory cytokines is evident in the serum, ovaries and organs of PCOS patients (8). The interplay between inflammatory state and obesity, HA and IR, leads to increased metabolic imbalance and reproductive-endocrine dysfunction in PCOS patients (9). Furthermore, SLCI contributes to PCOS-related complications of multi-organ dysfunction, including cardiovascular diseases (CVDs), non-alcoholic fatty liver disease (NAFLD), and depression (10–12). Therefore, a comprehensive understanding of SLCI in PCOS is crucial for effective prevention and management strategies.
This review elucidates the critical role of SLCI in the development of PCOS by analyzing the systemic mechanisms of chronic inflammation in PCOS from a novel perspective on the disruption of the balance between pro-inflammatory and anti-inflammatory factors. This review aims to deepen our understandings and provide new insights into the pathogenesis and treatment of PCOS (Figure 1).
Figure 1. Immune dysfunction in PCOS and the intrinsic mechanisms influencing SLCI in PCOS. Immune dysfunction in PCOS affects not only the female reproductive system but also other organ systems, including the cardiovascular, intestinal, hepatic, thyroid, pancreatic, adrenal gland,brain and other organs. Obesity, IR, hyperandrogenism, and intestinal microecological imbalance, and hyperhomocysteinemia induce the secretion of systematic pro-inflammatory cells and cytokines. Conversely, deficiencies in progesterone, vitamin D, and selenium have the exert opposite effects.
SLCI in PCOS patients plays a crucial role in disease progression (9). Compared with age-matched healthy women (controls), PCOS patients exhibited significantly increased amounts of immune cells, such as lymphocytes, neutrophils, monocytes, macrophages, and eosinophilic granulocytes in the peripheral blood (13–17). Additionally, elevated levels of inflammatory factors, such as high sensitive C-reactive protein (hs-CRP), interleukin-18 (IL-18), tumor necrosis factor α (TNF-α), interleukin-6 (IL-6), monocyte chemoattractant protein-1 (MCP-1), and macrophage inflammatory protein-1α (MIP-1α), are detected in the peripheral blood of PCOS patients (9, 18), indicating the presence of SLCI (Table 1). A significantly higher infiltration of immune cells, such as macrophages and lymphocytes, were detected in the ovaries of PCOS patients. Macrophages and immature dendritic cells (iDCs) were elevated in the endometrial tissue, and macrophages were also identified in the hearts of PCOS mice. Macrophages, neutrophils, mast cells, B cells, T cells, NKT cells infiltrate the adipose tissue. Additionally, inflammatory factors such as TNF-α, IL-6, CRP, IL-12, IFN-γ, and NLRP3 inflammasome were elevated in various tissues, including those in the ovaries and follicular fluid (FF), adipose tissue, endometrium, intestine and liver (8, 15, 17, 33). Excessive secretion of inflammatory factors TNF-α and IL-6 by immune cells activates the inflammatory signaling pathways, leading to cell damage and fibrosis within interstitial cells, ultimately causing dysfunction in these organs systems. Chronic immune response dysregulation contributes to PCOS-related immune dysregulation in the female reproductive system, cardiovascular system, cardiovascular, digestive, and endocrine systems (8). Correlation analysis indicated that increased serum inflammatory cytokine levels were strongly related to the severity of obesity, IR, ovulation disorder, and HA in PCOS patients (9, 34, 35). Taken together, the systematic imbalance between pro-inflammatory and anti-inflammatory factors in PCOS patients gives rise to the PCOS phenotypes. The pivotal role of the chronic inflammation in these organs systems and the intrinsic mechanisms of PCOS have been elaborated in the subsequent sections (Figure 2).
Figure 2. In women with PCOS, there is a discernible imbalance in the inflammatory cells and cytokines in the peripheral blood, FF, ovaries, and adipose tissue. Inflammatory mediators interact with factors such as obesity, IR, hyperandrogenism, and intestinal microecological imbalances. This interplay may exaggerate the pathophysiological features of PCOS. In adipose tissues, peripheral blood, ovary/follicle fluid of PCOS, immune cells such as T cells, B cells, and neutrophils are activated, leading to the secretion of inflammation cytokines such as TNF-α, MCP-1, IL-17, IL-23 and IL-18. Subsequently, this inflammatory milieu may induce apoptosis in the ovarian GCs and impair oocyte maturation, contributing to the reproductive dysfunctions observed in PCOS.
SLCI contributes to defects in oocyte quality, leading to ovulatory infertility, and accelerating the decline in ovarian reserve (35). Increased inflammatory response has been observed in the ovarian tissues of both PCOS patients and PCOS-like rodent models (15). Histological analysis comparing ovarian samples from of 53 PCOS patients and 48 healthy controls revealed a higher infiltration of macrophages and lymphocytes in PCOS patients. These cells secrete excessive amounts of pro-inflammatory cytokines such as TNF-α and IL-6 (9). Furthermore, inflammatory mediators such as IL-18, IL-18-binding protein (IL-18BP), pentraxin3 (PTX3), IL-1β, chemerin, hs-CRP, IFN-γ, and TNF-α were elevated in the FF from PCOS patients undergoing in vitro fertilization and embryo transfer (IVF-ET) treatment, reflecting the local function and pathological state within the PCOS ovaries (9, 36, 37). Macrophages and lymphocytes infiltration have also been detected in PCOS-like mice (38). PCOS-like rodent models exhibited increased levels of TNF-α, IFN-γ, NLRP3 and caspase-1within the ovaries (38, 39). These immune perturbations contribute to reduced fertility and metabolic comorbidities in PCOS-like models and PCOS patients. However, PCOS-like models may not fully replicate the complexity of PCOS in humans, particularly in terms of inflammatory markers and their expression levels. In PCOS patients, inflammation is influenced by factors such as obesity and IR which may not be identically represented in animal models of PCOS.
Pro-inflammatory TNF-α, primarily secreted by macrophages, was significantly increased in the ovaries and FF of PCOS patients (40, 41). TNF-α and its receptor TNFRI (TNF-a receptor 1) are expressed in mammalian oocytes and surrounding granulosa cells (GCs), indicating a direct impact of TNF-α on cells involved in reproductive function (42–44). In vitro experiments demonstrated that TNF-α can directly induce apoptosis of primary rat GCs after 24h of incubation in serum-free medium 24 (45). Furthermore, in vivo treatment with TNF‐α inhibits steroidogenesis of the thecal cells and GCs, leading to regression of the corpus luteal (21).
Another pro-inflammatory cytokine IL-6, which is produced by mononuclear (MNC) cells and adipose tissue cells. The levels of IL‐6 in the peripheral blood, ovaries, and FF in PCOS patients were higher than those in healthy control women (15, 46). IL-6 may serve as an early low-grade chronic inflammatory marker in PCOS patients with IRS-2 polymorphism (47). Higher IL-6 levels in the peripheral blood of both lean and obese women with PCOS were significantly associated with homeostasis model assessment of insulin resistance (HOMA2-IR) ratio and total testosterone ratios (48). Increased IL-6 levels in the ovary can reduce the conversion of androstenedione to estradiol by inhibiting the aromatase activity of GCs, leading to excessive androgen production (19, 49).
Inflammatory cytokines IL-1β and IL-18 present in the ovarian tissue and FF in PCOS patients alter the follicular microenvironment by binding to receptors like IL-1R and TLR4 on the GCs, activating NF-κB, which subsequently translocates into the nucleus (50). Activated NF-κB promotes gene expression of key components of the NLRP3 inflammasome, such as NLRP3, ASC and caspase-1, resulting in the death of GCs and inhibition of oocyte maturation, eventually disrupting ovarian function (50).
These results suggested that the increased pro-inflammatory profile in the ovary and FF mediates apoptosis of GCs, leading to excessive follicle atresia. This stimulates the production of excessive testosterone by thecal cells, disrupting the hypothalamic-pituitary-ovarian (HPO) axis, ultimately affecting dominant follicle generation, follicular dysfunction, and ovarian interstitial cell fibrosis (51, 52).
Successful pregnancy, encompassing embryo adhesion, implantation, growth, invasion of trophocytes, and formation and functional maintenance of the placenta, requires precise regulation of immune cells and inflammatory factors at the maternal-fetal interface to establish immune tolerance (53). This immune and inflammatory regulation in the endometrial tissue, also known as endometrial receptivity (ER), refers to the compatible state between the embryo and maternal uterus (54, 55). Reduced ER contributes significantly to early pregnancy loss, often resulting from dysregulation of immune and inflammatory responses in the endometrial tissue observed in women with autoimmune diseases (AID) or antiphospholipid syndrome (APS). Notably, the prevalence of recurrent miscarriages during early pregnancy due to an abnormal ER is higher in PCOS patients (56).
Immunohistochemical analysis of endometrial tissues from PCOS patients revealed an increase in the presence of endometrial inflammatory cells, including macrophages, iDCs, mature dendritic cells (mDCs), and CD8+ T cells (33), indicating increased chronic inflammation in the endometrial tissues. Additionally, PCOS endometrial stromal fibroblasts produce higher levels of IL-6, IL-8, MCP-1, and granulocyte-macrophage colony-stimulating factor (GM-CSF), which facilitate the maturation of endometrial DCs and macrophages, subsequently leading to progesterone resistance in the endometrium and impairing endometrial decidualization (57).
The NLRP3 inflammasome, which plays a crucial role in the processing of pro-IL-1β and pro-IL-18 into their mature forms through caspase-1, has been significantly increased in the endometrium of women with recurrent miscarriage (58), suggesting a potential dysregulation of ER mediated through inflammasome function. Therefore, NLRP3 may serve as a novel biomarker of ER dysfunction. As expected, increased expression of NLRP3 in the endometrial tissue has been implicated in the pathogenesis of higher miscarriage rates in PCOS patients (18, 59). In contrast, stanniocalcin-1 (STC-1), a glycoprotein, known for its ability to mitigate inflammatory stress is reduced in the endometrium of PCOS patients (60). This diminished expression of STC-1 may contribute to a weakened protective response to inflammatory stress (61). These findings suggested that the decrease in ER observed in PCOS is due to an imbalance between pro-inflammatory and anti-inflammatory cells and factors.
In addition to decreased ER, which contributes to adverse pregnancy outcomes, the chronic inflammation may also be associated with an increased long-term risk of endometrial carcinogenesis in PCOS patients. Simultaneously, the expression of both inflammation-related genes (CCL-2, IL-6, TNF-α, induced protein 6 [TNFAIP6] and pro-oncogenic genes (cell adhesion molecule with homology to L1CAM [CHL1]) is upregulated in the endometrium of PCOS patients than in controls (62). These findings highlight the importance of understanding and addressing the immunological and inflammatory aspects of PCOS to improve pregnancy outcomes and reduce long-term health risks for patients.
Autoimmune thyroid disease (AITD) is a common autoimmune disorder. PCOS and AITD share several clinical symptoms, including menstrual irregularities, infertility, obesity, IR, and dyslipidemia (63, 64).Therefore, screening for thyroid function and thyroid-specific autoantibodies is often recommended in the clinical diagnosis of PCOS. Furthermore, PCOS and AITD exhibit a strong clinical association. The prevalence of AITD in PCOS patients was significantly higher than that in the non-PCOS patients (65–68). Additionally, levels of thyroid-related autoantibodies, such as anti-TSH, anti-TPO, and anti-Tg, as well as T- and B-cell infiltration into the thyroid gland were higher in PCOS patients than controls (69–71). The elevated androgen levels in PCOS patients may contribute to AITD. Excessive androgens enhance the activity of T suppressor cells or promote Th1 responses, and Th1-mediated autoimmunity, resulting in thyroid cytolysis and hypothyroidism (68). Furthermore, PCOS patients exhibit compensatory increases in estrogen levels and inadequate progesterone levels. Estrogen may upregulate IL-6 expression in T cells, whereas inadequate of progesterone suppression potentially results in immune system overactivation (72). These findings indicates that the disruption of steroid hormones in PCOS patients contributes to inflammation dysregulation in the thyroid gland, leading to thyroid dysfunction. In summary, the clinical overlap between PCOS and AITD, along with the evidence of immune system dysregulation and thyroid dysfunction in PCOS patients, underscores the importance of considering thyroid health in the management of PCOS.
Pancreatic β-cell dysfunction is prevalent in PCOS patients, which significantly contributes to their abnormal glucose tolerance and the long-term risk of developing type II diabetes mellitus (T2DM) (73, 74). Malin et al. reported a direct relationship between β-cell dysfunction in PCOS patients and the MNC-derived NF-κB activation as well as an inverse correlation with IκB expression, indicating impaired inflammation regulation in the pancreas of PCOS patients (75). Conversely, nanocurcumin, a potential anti-inflammatory agent, significantly reduced oxidative markers and TNF-α levels in the pancreas, alleviated IR, restored islets integrity in PCOS models (75–77). Additionally, medications such as saxagliptin and metformin have demonstrated effectiveness in regulating β-cell function by reducing inflammation in newly diagnosed T2DM patients with PCOS (74).These findings suggest that the crucial role of pancreatic inflammation and β-cell dysfunction in PCOS-related metabolic complications and highlight the need for targeted interventions that can improve β-cell function and reduce inflammation.
Adrenal immune damage has recently been identified in patients with PCOS, coinciding with the overproduction of adrenal androgens(AA) (78, 79). Dehydroepiandrosterone (DHEA) and its sulfated form, dehydroepiandrosterone sulfate (DHEA-S), are the predominant AA in PCOS patients. DHEA synthesized in the adrenal gland is converted into DHEA-S and released into the bloodstream. Circulating DHEA-S levels has been used as a maker of adrenocortical dysfunction in PCOS (79). DHEA plays a role in maintaining ovarian immune homeostasis by modulating the balance between Th1 and Th2 immune responses within the ovary through NF-κB regulation, which decreases IL-2 and IL-10 (80). DHEA-S exerts immunomodulatory effects, reducing the T cell population with a concurrent increase in NK and T cells. These findings suggest a potential association between AA and immunological response in PCOS.
However, a retrospective cohort study identified a previously unrecognized infertile PCOS-like phenotype characterized by elevated levels of anti-Müllerian hormone (AMH) and low total testosterone, DHEA-S, and cortisol. Notably, this phenotype is also associated with increased levels of thyroid autoimmunity markers, such as thyroid autoimmunity (TPO antibodies) and the inflammatory markers CRP and IL-6. Therefore, this hypo-androgenic PCOS phenotype (HH-PCOS) may be related to the autoimmune damage in the adrenal zona reticularis (81, 82). However, further research is needed to confirm chronic inflammation in the adrenal glands and its potential effects on endocrine function in PCOS patients. These findings revealed a complex interplay between AA overproduction and immune dysfunction with adrenal gland in PCOS, Additionally, they indicate that infertile PCOS-like phenotype may be an immunoinflammatory disorder associated. This underscores the importance of reducing the AA levels and inflammation to ameliorate HH-PCOS.
Inflammatory markers, including IL-1β, IL-6, and TNF-α, were significantly upregulated within the hypothalamus of PCOS-like rats, indicating the presence of chronic low-grade neuroinflammation (83, 84). Hypothalamic inflammation contributes to the occurrence and progression of numerous metabolic disorders in PCOS, including obesity, diabetes, hypertension, and dyslipidemia, by affecting food intake homeostasis, energy balance, insulin and leptin signaling, glucose metabolism and fatty acid oxidation in the liver (85). High-fat diet (HFD)-induced overactivation and/or excessive M1-type macrophages-microglia, which is characterized by a pro-inflammatory response in the central nervous system. This M1-polarized microglia induce an inflammatory response and release a large number of inflammatory factors, such as nitric oxide, IL-6, TNF-α, and reactive oxygen species (ROS), which are key drivers of hypothalamic inflammation (86–89). Furthermore, hypothalamic inflammation alters the pulsatile secretion pattern of gonadotropin-releasing hormone (GnRH), resulting in an increase in luteinizing hormone (LH)/follicle stimulating hormone (FSH) ratios and leading to irregular menstrual cycles and ovulatory disorders (90). These findings highlight the strong association between hypothalamic inflammation and the etiology and phenotype of PCOS, suggesting that targeting hypothalamic inflammation could be a potential therapeutic strategy (91).
The risk of CVDs is higher in PCOS patients (92–94). Vascular alterations, such as endothelial dysfunction, increased arterial stiffness, enhanced intima-media thickness, and arterial wall calcification, are prevalent even in young women with PCOS (94–96). Furthermore, vascular endothelial injury and endothelial cell dysfunction in PCOS patients are independent of age, body weight, and metabolic abnormalities, suggesting that PCOS may be an independent risk factor for CVDs and could lead to an earlier onset of CVDs despite the presence of metabolic disorders (97, 98).The current consensus is that chronic inflammation of the vascular endothelium and the resulting endothelial dysfunction are fundamental to the pathogenesis of CVDs (99, 100). Additionally, pro-inflammatory factors such as CRP, IL-6 and IL-18, which are closely associated with the incidence of CVDs, are also elevated in the plasma of women with PCOS patients (18).
The critical role of macrophages in the pathogenesis of CVDs in PCOS has been recently reported, with a substantial augmentation in the number of M1-macrophages in the hearts of PCOS model mice, predominantly derived from circulating monocytes (10). Furthermore, PCOS mice with atherosclerosis and myocardial infarction exhibited a pronounced infiltration of macrophages into the myocardium (10). The adverse cardiovascular effects of PCOS could be attributed to the over-activation of the norepinephrine-NF-κB pro-inflammatory signaling pathway, contributing to increased expression of a hematopoietic progenitor retention factor, vascular cell adhesion molecule 1(Vcam1), in splenic macrophages, subsequently resulting in increased circulating total monocytes and inflammatory monocytes. These findings indicate that the accumulation of macrophages in the heart contributes to endothelial dysfunction, emphasizing the need to address chronic inflammatory state in PCOS patients for effective management of their cardiovascular health (10).
The prevalence of NAFLD is higher in PCOS patients than in healthy women (34%–70% vs. 14%–34%) (101, 102). IR, obesity, HA, chronic inflammation, genetic factors and dyslipidemia are risk factors for NAFLD development in PCOS patients (103–105). Interestingly, HA in PCOS is an independent risk factor for NAFLD, as demonstrated in a recent systematic review and meta-analysis (101, 106). Bioinformatics data identified 52 differentially expressed genes (DEGs) shared between PCOS and NAFLD. Gene ontology (GO) and Kyoto Encyclopedia of Genes and Genomes (KEGG) pathway enrichment analyses suggested that these DEGs were mostly enriched in immunity- and inflammation-related pathways (107). NAFLD-like hepatic pathological changes such as steatosis, inflammatory cell infiltration, necrotic hepatocytes, and liver fibrosis, accompanied by increased expression of inflammatory cytokines (TNF-α, IL-1β), stress-related protein urocortin-1, antioxidant gene glutathione peroxidase-1 (Gpx1), and the NLRP3 inflammasome within the hepatic tissues, have been observed in several rodent models of PCOS (108–111). Over-production of these pro-inflammatory mediators in the liver of individuals with PCOS leads to the infiltration of various immune cells, including macrophages, T lymphocytes, dendritic cells, and neutrophils (11). These cells further release additional inflammatory cytokines that interact with adipokines, such as leptin, adiponectin, vaspin, visfatin, and chimerin. This exacerbates the imbalance between the pro-inflammatory and anti-inflammatory states within the liver, ultimately contributing to the development of the NAFLD in PCOS (12).
The association between PCOS and inflammatory diseases of the lung has not been extensively investigated. Recent studies on the association between PCOS and coronavirus-induced disease 19 (COVID-19) have provided valuable insights. COVID-19 is characterized by an excess of pulmonary inflammatory cells such as macrophages, neutrophils, and dendritic cells and elevated levels of inflammatory factors, including IL-2, IL-7, IL-10, G-CSF, IP-10, MCP-1, MIP1A, and TNF-α. Overproduction of these factors can ultimately lead to damage and death of alveolar epithelial cells, potentially leading to respiratory failure and death (112). PCOS patients are more susceptible to infection by severe acute respiratory syndrome coronavirus 2(SARS-CoV-2) and exhibit more severe clinical symptoms than those of control women without PCOS (113). PCOS patients exhibit higher infection rates (28%–50%) across all age groups, leading to increased hospitalization rates and morbidity and mortality rates than those of age-matched women without PCOS. HA may be a factor in the increased susceptibility to SARS-CoV-2 in PCOS in individuals with PCOS (114). In dihydrotestosterone (DHT)-induced PCOS-like female mice, DHT up-regulates ACE2 mRNA in the lung, cecum, heart and kidney, which synergizes with host transmembrane protease serine 2 (TMPRSS2) to facilitate SARS-CoV-2 viral entry into the host cells (115). These findings suggest that PCOS patients, particularly those with HA, are more susceptible to COVID-19, which leads to increased pulmonary inflammation and damage in the lung tissues, ultimately contributing to an increased mortality rate.
Approximately 5%-10% of reproductive-age women without PCOS worldwide experience depression and anxiety, and 40% of women with PCOS suffer from depression (1, 116). Psychiatric disorders, including generalized depressive disorder, are inflammatory conditions characterized by elevated levels of inflammatory markers (117–119). Inflammatory markers such as CRP, IL-1, IL-6 and TNF-α elevated both in patients with depression and PCOS (120, 121). Inflammatory factors may penetrate the blood-brain barrier (BBB) via cytokine-specific transport mechanisms, potentially involving the active transport of saturable transporter molecules (122, 123). Subsequently, increased inflammatory factors can disrupt the metabolism of brain monoamines such as neuronal 5-hydroxytryptamine (5HT) and dopamine (DA), which are hypothesized to contribute to the pathogenesis of depressive disorders (124–126). Nevertheless, experimental animal studies to elucidate the mechanisms by which inflammatory mediators induce depressive disorders in PCOS are lacking. The complex relationship between PCOS and psychiatric disorders, particularly depression and anxiety, underscores the need for further research to better understand and address these comorbidities.
The clinical comorbidities associated with metabolic disorders in PCOS include obesity and IR. Approximately 52%-64% of women with PCOS are either overweight or obese, which is an independent risk factor for IR in PCOS. Additionally, approximately 30% of PCOS patients with a normal body mass index (BMI) also show abdominal obesity, marked by an excessive accumulation of visceral fat (127, 128). Obesity also contributes to ovarian inflammation, steroidogenesis, and ovulation (129). Visceral obesity in PCOS patients primarily contributes to systemic inflammation throughout the body. The infiltration of immune cells such as macrophages, neutrophils, mast cells, B cells, T cells, NKT cells within the adipose tissue, coupled with the overproduction of inflammatory mediators and adipokines (e.g., leptin and lipocalin), adipocyte-derived MCP-1 and hypertrophy of visceral adipocytes, leads to hypoxia, autophagy, and apoptosis (17, 130). Additionally, hypoxia adipocytes can activate the c-Jun N-terminal kinase (JNK) and NF-κB pathways, resulting in the synthesis and secretion of pro-inflammatory factors such as IL-6, TNF-α, IL-1β, IL-12, and IFN-γ, which induce a SLCI state in adipose tissue (131, 132). Specifically, adipose tissue-resident macrophages exacerbate IR by elevating the levels of TNF-α, which in turn increase the phosphorylation of insulin receptors substrate-1 (IRS-I) (phospho-IRS-I). This event, through the phosphatidylinositol 3-kinase (PI3K) pathway, which inhibits the activation of protein kinase B (PKB), a pivotal enzyme regulating the insulin-sensitive glucose transporter type 4 (GLUT-4), ultimately resulting in IR (133, 134). These additional release mediators released into the circulation can induce inflammatory responses in extra-adipose tissues, including the ovaries and peri-ovarian adipose tissues (129, 135).
IR is present in 50%-70% of PCOS patients, and high insulin levels in the FF potentially directly stimulate the LH receptors on thecal cells, increasing their sensitivity to LH or reducing the hepatic production of sex hormone binding globulin (SHBG). Elevated free testosterone levels lead to impaired follicle development in PCOS patients (136). In PCOS patients with IR, the underlying mechanisms involves a dysfunction of the PI3K pathway, whereas the MAPK pathway remains functional (137–139). Impaired mitochondrial function due to the downregulation of nuclear-encoded genes involved in oxidative phosphorylation increases ROS production, which in turn phosphorylates the serine residues of insulin receptors and IRSs, thereby resulting in SLCI and a reduction in IR. Consequently, these processes synergistically contribute to the exacerbation of SLCI in PCOS.
Obesity and IR can exacerbate the SLCI in PCOS. A causal relationship between obesity, IR, and increased pro-inflammatory activity within adipose tissue. In summary, the molecular mechanisms associated with obesity and IR play a pivotal role in the development of the SLCI in PCOS (140).
The primary etiology and symptoms of PCOS are predominantly associated with the dysregulation of steroid hormones, particularly elevated levels of androgen and decreased progesterone levels linked to luteal phase deficiency. Both androgens and progesterone play crucial roles in the inflammation response. As a pro-inflammatory steroid hormone, HA induces SLCI in the ovaries by stimulating monocyte infiltration, enhancing ROS production, and activating the NF-κB pathway, which contributes to metabolic disorders in PCOS patients (141). Additionally, HA promotes the secretion of and pro-inflammatory factors, subsequently suppressing folliculogenesis and ovulation, resulting in a cascade of events including pyroptotic death of ovarian GCs, follicular dysfunction, and ovarian interstitial cell fibrosis (15, 142). Increased endometrial cytokine synthesis and inflammation in PCOS patients induced by excess androgens through TLR4/IRF-7/NF-κB signaling contributes to inflammation in PCOS patients (143). Higher serum total testosterone and free testosterone index (FTI) were also found to be linked to an elevated risk of NAFLD in women with PCOS, independent of obesity and IR (106, 144, 145). HA induces ovarian inflammation in PCOS mice by activating the NLRP3 inflammasome, resulting in follicular dysfunction, ovarian fibrosis, and pyroptotic death (146). In vitro studies have indicated that DHEA directly inhibits the proliferation and promotes apoptosis of human ovarian granulosa tumor cell line (KGN) cells by down-regulating IFN-γ expression via the activation of the PI3K/AKT signaling pathway (147). DHEA administration directly activates the MNC cells and increases heightened sensitivity to glucose intake. In lean, healthy women, oral androgens increase mRNA expression of androgen receptors (AR) and stimulate TNF-α release from MNC in response to glucose-induced inflammation (148, 149).
Progesterone, a critical anti-inflammatory steroid hormone, has recently been studied. Although the exact anti-inflammatory mechanism of progesterone remains unclear, current evidence suggests that its effects encompass both non-specific and specific immune regulation. The non-specific regulation is hypothesized to involve the inhibition of NF-κB activation, cyclooxygenase (COX) and prostaglandin synthesis. On the other hand, specific immune regulation is thought to include the modulation of T cell activation and cytokine production by immune cells (150). Notably, a study conducted on patients with COVID-19 demonstrated that progesterone exhibited therapeutic effects that are comparable to those of glucocorticoids in preventing severe illness and mortality associated with SARS-Cov2 infection (151–153). Furthermore, progesterone decreases the production of IL-1β, IL-6, TNF-α, and IL-12, as well as MCP-1/CCL2, suggesting it as a valuable adjunct to current SARS-CoV-2 treatment regimens (154). In PCOS patients, oligo/anovulation leads to reduced progesterone levels, which may result in inadequate inhibition of inflammation at the myometrium and maternal-fetal interface (155–158). This deficiency is associated with an increased risk of adverse pregnancy outcomes (159). Moreover, progesterone enhances the release of gonadotropin-releasing hormone (GnRH) and increases the sensitivity of the pituitary gland to GnRH, thereby triggering an LH surge. This results in the normalization of hyperandrogenemia and hyperinsulinemia levels, restore the physiological balance between androgens, estradiol, and progesterone within the menstrual cycle (160). Consequently, PCOS patients with progesterone deficiency may be at an increased risk of developing multi-system diseases owing to elevated systemic inflammation.
Estrogen plays a dual role in regulating the immune system in women. During pregnancy, elevated estrogen levels inhibit the production of pro-inflammatory cytokines such as TNF-α, IL-1β, IL-6, MCP-1, iNOS, and MMPs. Concurrently, it reduces NK cell activity. Additionally, high concentrations of estradiol stimulate anti-inflammatory cytokines such as IL-4, IL-10, and TGF-β. This indicates that the sustained high levels of estrogen, in conjunction with progesterone, during gestation, play a synergistic role in suppressing the immune response and inflammation, which is crucial for the preservation of normal fetal development (161). In contrast, at the lower concentrations observed in non-pregnancy states, estrogen stimulates the production of inflammatory cytokines such as TNF-α, IFN-γ, and IL-1β, while also enhancing the activity of NK cells (161). Clinically, estrogen supplementation has shown anti-inflammatory and protective effects under certain conditions associated with chronic inflammation, such as osteoporosis, CVDs, and neurodegeneration during menopausal hormone replacement therapy (MHT) (162). Conversely, estrogen exerts pro-inflammatory effects in specific autoimmune diseases (AIDs), including rheumatoid arthritis (RA), systemic lupus erythematosus (SLE), highlighting estrogen as a risk factor for the increased incidence of various AIDs in women than in men (161, 163). PCOS patients may exhibit a lower average estrogen level owing to the absence of the fluctuations and peaks that typically occur during ovulation and the luteal phase in non-PCOS women with regular ovulation. Although estrogen levels can reach the pregnancy-like concentrations during ovulation in healthy individuals, the oligo/anovulation characteristic of PCOS leads to a deficiency in high estrogen levels, which may predispose individuals to excessive inflammation. Further investigations are necessary to clarify the potential role of estrogen in the regulation of chronic systemic inflammation associated with PCOS.
The gastrointestinal tract (GI) is inhabited by trillions of microorganisms, including bacteria, archaea, fungi, and viruses. Collectively known as the gut microbiome, these microorganisms interact with the external environment (such as nutrients), immune system of the human intestinal barrier, metabolic intermediates, and substances released from cells to establish the intestinal microecosystem (164). The gut microbiota, often referred to as the “second genome” in human beings, has significant clinical implications. Disturbances in the gut microbiota have been linked to various chronic health conditions such as metabolic syndrome, mental, and psychological diseases, and cancer (165–169). Gut microbiota disturbance is a major characteristic observed in PCOS patients and PCOS-like rodent models (170–172). Whole-genome shotgun sequencing demonstrated no significant difference in bacterial alpha diversity between PCOS patients and healthy controls; however, there was a significant increase in beta diversity in PCOS patients than that observed in healthy controls (173). DHEA-treated PCOS rats, exhibited a reduction in the relative abundances of Turicibacter, Anaerofustis and Clostridium sensustricto at the genus level. These findings underscore the potential role of gut microbiota in the pathogenesis and progression of PCOS.
The interaction between the gut immune barrier and these microbes contributes to the GI tract becoming a potential source of chronic inflammation, which is closely associated with gut microecology (174, 175). In 2012, Tremellen et al. proposed the theory of Dysbiosis of Gut Microbiota (DOGMA) in the inflammatory pathogenesis of PCOS. They suggested that diet-induced imbalances between beneficial and harmful gut bacteria lead to increased intestinal permeability involving lipopolysaccharide (LPS), LPS-binding protein (LPS-BP), and zonulin entering systemic circulation, activating the TLR-4/NF-κB-mediated inflammatory response. This interference can affect insulin receptor function, causing IR, and potentially promoting testosterone synthesis in the ovaries, contributing to PCOS (176, 177). Studies have also consistently demonstrated that androgen exposure can cause intestinal dysbiosis, forming a vicious circle in PCOS patients (9, 178–180). Furthermore, the transplantation of androgen-induced gut microbiota into pseudo germ-free recipients disrupts glucolipid metabolism, ovarian morphology, and reproductive hormone imbalance (178) (Figure 3).
Figure 3. Theory of dysbiosis of gut microbiota in PCOS. Both hyperandrogenism and high-fat diet increase the proportion of harmful gut bacteria, leading to increased intestinal permeability and the release of LPS, LPS-BP, zonulin, and activated macrophages from the colonic lumen into the circulation. SLCI disrupts glucolipid metabolism and increases insulin and testosterone secretion. Finally, SLCI induced changes in ovarian morphology, and reproductive hormone imbalances in PCOS.
The impact of intestinal microbiome dysbiosis on the inflammatory processes that affect ovarian function in PCOS has been previously demonstrated. Huang et al. observed an increased abundance of Akkermansia and desulfurization bacteria in the intestines, as well as elevated serum levels of lipopolysaccharide (LPS) and interferon IFN-γ in DHEA-induced mice. Notably, IFN-γ can trigger pyroptosis in macrophage within the ovaries, which disrupts of estrogen production and promotes apoptosis of GCs, and ultimately leading to the abnormal ovarian function in PCOS mice. However, treatment with disulfiram and metformin increased the abundance of intestinal Akkermansia bacteria, decreased serum IFN-γ levels, and inhibited the pyroptosis in ovarian macrophages, thereby improving PCOS symptoms (39). Qiao et al. found that systemic inflammation in PCOS patients may be associated with an altered abundance of intestinal Bacteroides vulgatus and changes in metabolism of bile acids glycine deoxybile acid (GDCA) and tauroursodeoxycholic acid (TUDCA). The mechanism involves the interaction of the bile acid metabolite GDCA with the intestinal inherent (innate) group 3 lymphocytes GATA binding protein 3(GATA3), resulting in reduced secretion of the anti-inflammatory regulator IL-22.The therapeutic potential of IL-22 has been demonstrated and its administration shown to ameliorates IR, ovarian dysfunction, and infertility in PCOS (173). Furthermore, in DHEA-induced female mice exhibited upregulation of the metabolite agmatine from Bacteroides vulgatus was upregulated, which activates the farnesoid X receptor (FXR) pathway to inhibit glucagon-like peptide-1 (GLP-1) secretion in intestinal epithelial L cells. Furthermore, IL-8, IL-6, IL-1β and IL-18 were upregulated, contributing to ovarian inflammation. These findings suggested that the agmatine-FXR-GLP-1 signaling axis contributes to IR and ovarian dysfunction in PCOS-like mice (181). In addition to bile acid metabolites, short-chain fatty acids (SCFAs), primarily composed of acetate (C2), propionate (C3), and butyrate (C4), are beneficial metabolites derived from the fermentation of dietary fiber and resistant starch by the gut microbiota. Furthermore, SCFAs serve as potent anti-inflammatory modulators, capable of inducing Treg differentiation and interleukin secretion in peripheral tissues (182–184). Animal experiments have demonstrated that supplementation with SCFAs, such as butyric acid, can enhance ovarian function and reduce the levels of inflammatory factors within the ovaries. Butyric acid inhibits of m6A methyltransferase METTL3 expression in KGN cells, resulting in a decrease in FOSL2m6A methylation level and mRNA expression (185). Therefore, gut microbiota dysbiosis and the alterations in its metabolites contribute to the low-grade chronic inflammation present in the peripheral blood and ovaries of PCOS patients (Figure 4).
Figure 4. Dysbiosis of the gut microbiota and bile acid metabolites in PCOS ovarian function through inflammation. (A) Bacteroides vulgatus were significantly elevated levels in the intestinal microbiota of individuals with PCOS-like mice, accompanied by reduced concentrations of bile acid metabolites GDCA and TUDCA. GDCA stimulates IL-22 secretion via GATA-binding protein 3(GATA3). The Bacteroides vulgatus metabolite agmatine activates the farnesoid X receptor (FXR) pathway, leading to the inhibition of glucagon-like peptide-1 (GLP-1) secretion in intestinal epithelial L cells. This contributes to IR and ovarian dysfunction in PCOS-like mice. (B) Elevated abundance of Akkermansia and desulfurization gut bacteria, as well as increased serum levels of LPS and interferon IFN-γ, were observed in DHEA-induced PCOS-like mice. IFN-γ has the potential to induce pyroptosis in macrophages within the ovaries, ultimately resulting in abnormal ovarian function. (C) SCFAs have the potential to modulate ovarian inflammation by reducing pro-inflammatory IL-6, TNF-α and NLRP3 inflammasome secretion in the ovaries.
Vitamin D and selenium are involved in the regulation of immunity and inflammation, and deficiencies in these essential substances are associated with an increased risk of PCOS (186). Multiple case-control studies have reported lower concentrations of 1,25-dihydroxyvitamin D, the active form of vitamin D, in women with PCOS patients (187–191). Approximately 67%-85% of PCOS patients either exhibit vitamin D deficiency or insufficiency (192), which is more prevalent in PCOS patients with HA (193). In addition to its role in regulating calcium and phosphate metabolism and maintaining skeletal structure, vitamin D also exhibits anti-inflammatory effects. Vitamin D receptors and the enzymes responsible for activating vitamin D are expressed in both innate and adaptive immune cells, including monocytes, macrophages, dendritic cells, and lymphocytes. These immune cells not only facilitate the secretion of vitamin D but also respond to activated vitamin D through the autocrine pathways (194, 195). Vitamin D deficiency has been implicated in systemic inflammation and the pathogenesis of PCOS. A meta-analysis has shown the administration of vitamin D in PCOS patients can reduce the levels of inflammatory mediators, such as serum hs-CRP, serum total testosterone (TT), and oxidative stress indices, while improving the overall antioxidant capacity. Furthermore, vitamin D replacement therapy may have a beneficial effects on IR, hormone regulation, menstruation, and ovulation disorders in PCOS patients (196–199).
A systematic review reported that plasma selenium levels were lower in PCOS patients than in healthy controls in two out of three case-control studies, with serum selenium levels were negatively correlated with androgen levels in PCOS patients. Selenium has shown therapeutic benefits owing to the its immunomodulatiory properties (200–202). Selenium, particularly the amino acid selenocysteine, exerts its biological effects primarily in the form of selenoproteins. At least 25 selenoproteins have been identified in human beings, with glutathione peroxidase (GPX) being the primary selenoprotein responsible for regulating excessive free radical production at the sites of inflammation. Apart from GPX, other selenoproteins have been recognized, including selenin-S, which modulates inflammatory cytokines, and selenin-P, which acts as an inducer of homeostasis (203–205). Selenoproteins play a crucial role in regulating inflammation and modulating clinical outcomes in various diseases including cancer, diabetes, Alzheimer’s disease, mental disorders, CVDs, fertility disorders, inflammation, and infections, including SARS-CoV-2 (206). Studies conducted on PCOS-like rat models have revealed that treatment with selenium nanoparticles, either alone or in combination with metformin, can restore the estrus cycle, reduce blood glucose, and insulin levels, improve hyperlipidemia, reduce serum testosterone levels and enhanced ovarian histopathology, accompanied by decreased levels of serum pro-inflammatory factors TNF-α and IL-6 as well as oxidative stress biomarkers MDA and GPX in the ovarian tissues (207–209). Although these animal studies have demonstrated the effectiveness of selenium in PCOS treatment. A recent systematic review concluded that selenium supplementation only positively affected total antioxidant capacity (TAC) in PCOS patients without significantly improving BMI, body weight, LDL, HDL, triglyceride, total testosterone, HOMA-IR, NO, glutathione(GSH), MDA, and FPG levels (210). Further clinical randomized controlled clinical trials are necessary to confirm the efficacy and safety of selenium supplementation or selenium-based drugs for treating of PCOS.
Preliminary investigations in observational and randomized controlled trials have indicated that women with PCOS exhibit higher serum homocysteine (Hcy) levels, along with higher levels of markers of CVDs, such as hs-CRP, soluble CD40 ligand (sCD40L), and asymmetric dimethylarginine (ADMA) than did healthy controls (211, 212). In atherosclerosis, Hcy acts as a vascular pro-inflammatory cytokine capable of activating the monocyte-macrophage system (213). Hcy stimulates the release of MCP-1 and IL-8 from peripheral blood monocytes, which are two major chemokines involved in leukocyte trafficking (214). Additionally, Hcy induces the expression and secretion of IL-8 by aortic endothelial cells and stimulates MCP-1 production by vascular smooth muscle cells (215, 216). Accumulation of MCP-1 and IL-8 has also been observed in macrophages from human atherosclerotic plaques. Furthermore, even slightly elevated levels of Hcy (10 μmol/L) can effectively stimulate the accumulation of MCP-1 and IL-8 in the injured artery wall, thereby promoting macrophage-mediated inflammation and atherosclerosis (217, 218). These studies suggested that Hcy primarily targets the monocyte-macrophage interaction.
A cross-sectional study revealed a positive correlation between HHcy and IR in PCOS patients. Compared with individuals with normal Hcy levels, individuals within the HHcy group exhibited increased numbers of CD14++CD16+ monocytes and higher levels of IL-1β, IL-6 and IL-2 in the peripheral blood in PCOS patients. These results suggested that the activation of inflammatory monocytes may be related to the Hcy-induced PCOS-IR phenotype (219). Moreover, Mondal et al. reported that female rats exposed to Hcy developed a PCOS-like reproductive-endocrinal phenotype associated with lipid metabolism disorders without involvement of other modalities used for PCOS modeling. Furthermore, this study demonstrated that HHcy and HA share a common mechanism involving the disruption of PCSK9-LDLR pathway leading to lipid homeostasis disturbances in PCOS (220). Another study showed that HHcy promotes IR and adipose tissue inflammation in PCOS mice by reducing estrogen production while inhibiting the polarization of M2 anti-inflammatory macrophages (221). This suggests that HHcy may also contribute to chronic inflammation-related metabolic disorders, potentially through its influence on monocyte-macrophage activity.
International evidence-based guidelines recommend non-pharmacological lifestyle management, including dietary modifications and regular physical activity, as the first-line treatment for infertility associated with PCOS to optimize health generally and improve fertility outcomes (222).
A healthy diet is the cornerstone of a healthy lifestyle and can significantly ameliorate the symptoms of intestinal dysbiosis, inflammatory status, and reproductive and metabolic abnormalities in PCOS. A clinical study reported that the very low-calorie ketogenic diet (VLCKD) improved ovarian function in obese women with PCOS. In this study, 25 obese patients with PCOS were enrolled to receive a VLCKD intervention for 12 weeks. Significant reductions in BMI, waist circumference (WC), and HOMA were observed. These results suggest that VLCKD may be an effective strategy for ameliorating metabolic and ovulation dysfunction in women with PCOS (223).
The Mediterranean diet (MD), known for its anti-inflammatory properties, has shown promise in managing PCOS. Barrea et al. conducted a case-controlled cross-sectional study that revealed that high adherence to the MD diet resulted in lower CRP levels, HoMA-IR, testosterone levels, and Ferriman-Gallwey scores. This preliminary evidence suggests that the MD diet reduces disease severity, IR, and hyperandrogenemia in PCOS (224).
Intermittent fasting has emerged as a viable approach for reducing weight and energy intake. The forms of intermittent fasting include three diets: alternate-day fasting (ADF), 5:2 diet, and time-restricted eating (TRE). A clinical study reported 15 anovulatory PCOS patients aged 18–31 years who completed 8-h time-restricted feeding for 6-weeks experienced substantial improvements. After TRE, body weight, BMI, HOMA-IR, and hs-CRP decreased, whereas SHBG and insulin-like growth factor 1(IGF-1) levels increased, and irregular menstrual cycles improved in 73.3% (11/15) of patients (225).
These results underscore the potential of a healthy diet in reducing weight and body fat and improving menstruation, hyperandrogenemia, IR, and chronic inflammation in PCOS patients.
Nutrients, mainly including vitamins (such as vitamin D), vitamin-like nutrients (such as α-lipoic acid), and minerals (such as magnesium), are essential components of a healthy diet. Nutrient supplementation can have a positive impact on PCOS patients (226). A randomized double-blind placebo-controlled clinical trial conducted by Bahmani et al. demonstrated that folic acid supplementation significantly reduced the plasma levels of Hcy, HOMA-B, hs-CRP, and MDA and significantly increased the plasma levels of TAC and GSH. These findings suggest that folic acid supplementation has a potential clinical role in improving metabolic conditions and reducing inflammation and oxidative stress in PCOS patients (227). In a randomized, double-blind, placebo-controlled trial involving patients with PCOS, 60 participants were randomly assigned to two groups (n = 30 in each group), one receiving a supplement of 1000 mg omega-3 and 400 IU of vitamin E, and the other receiving a placebo for a duration of 12 weeks. The study results indicated that the combined supplementation of omega-3 and vitamin E significantly reduced CIMT and serum hs-CRP levels (228). In a clinical study conducted by Stracquadanio et al., continuous administration of myo-inositol, gymnemic acid, and L-methylfolate for 6 months demonstrated substantial beneficial effects in PCOS patients, including improvement in menstrual cycle regularity and metabolic parameters, reduction in BMI and total testosterone, and increased insulin sensitivity (229). Hager et al. conducted a randomized controlled trial investigating the effects of a standardized micronutrient supplementation in PCOS patients. The supplement included omega-3 fatty acids, folic acid, selenium, vitamin E, catechin, glycyrrhizin, and coenzyme Q10, which was administered for 3 months. The study demonstrated that compared with the control group, the group receiving the micronutrient supplement experienced a significant decrease in the LH/FSH ratio, testosterone, and AMH levels (230). These findings indicated that nutrient supplementation may be beneficial for ameliorating some of the adverse health outcomes associated with PCOS. Specifically, nutritional supplements can potentially improve menstrual cycle regularity, IR, inflammation, and oxidative stress in PCOS patients.
Physical activity is recommended as a first-line approach for managing PCOS, particularly for overweight or obese women. The guidelines recommend a minimum of 150 min/week of moderate-intensity exercise, 75 min/week of vigorous-intensity exercise, or a combination of both (231). A meta-analysis by Moori et al. demonstrated that exercise training effectively lowered inflammatory markers, such as serum CRP levels (232). In a randomized clinical trial, high-intensity interval training (HIIT) elicited greater improvements in cardiometabolic and reproductive outcomes than moderate-intensity interventions in overweight women with PCOS. Patten et al. further found that HIIT considerably improved the oxygen-carrying capacity of blood than did moderate-intensity exercises. HIIT substantially increased SHBG levels and regularized menstrual cycles in PCOS patients (233). In summary, engaging in regular physical activity, particularly HIIT, is beneficial for women with PCOS, as it not only helps in managing weight but also has positive effects on inflammation, cardiometabolic health, and reproductive function. These findings emphasize the importance of incorporating exercise into the treatment plan for PCOS, highlighting its potential to improve the overall health and quality of life of affected individuals.
Metformin, a biguanide, improves insulin sensitivity, reduces androgen levels, and enhances oligo-amenorrhoea and subfertility in women with PCOS (234). Metformin increases gut Akkermansia abundance, reduces serum IFN-γ level released from T cells, and inhibits macrophage pyroptosis in ovaries in PCOS mice (234). Xue et al. revealed metformin alleviated PCOS by modulating gut microbiota, reducing plasma LPS levels, and decreasing the plasma and ovarian levels of inflammatory cytokines, including TNF-α, IL-6, and IL-17A, in PCOS patients (235).
Traditional Chinese Medicine (TCM) formulations and their active ingredients have been widely used to treat various gynecological diseases, including PCOS. Herbal medicines and active ingredients regulate gut microbiota composition and reduce systemic and ovarian inflammation in PCOS-like models (236–238). Wang et al. reported the TCM decoction Bu Shen Hua Zhuo formula (BSHZF) administration improved gut microbiota function in rats with letrozole-induced PCOS and inhibited the activation of the TLR4/NF-kB signaling pathway in PCOS-related ovarian tissue, decreasing the pro-inflammatory cytokines TNF-a, IL-6, and IL-8 (236). Zhu et al. reported Gui-zhi-Fu-ling Wan treatment reduced inflammatory markers such as hs-CRP, IL-6, and TNF-α and improved PCOS-IR by remodeling the relative abundance of multiple intestinal flora (237). Chang et al. reported that Shaoyao-Gancao Decoction (SGD), commonly used to treat multiple gynecological disorders such as dysmenorrhea, adenomyosis, and PCOS, modulated gut microbiota composition, and alleviated chronic low-grade inflammation by downregulating cytokines including IL-18, IL-1β, IL-6, and TNF-α in both serum and ovarian mRNA expression in PCOS rats (238). In summary, these studies underscore the therapeutic potential of TCM for treating PCOS by targeting gut microbiota and inflammatory pathways, which may offer a complementary approach to conventional treatments. Modulation of the gut microbiota using TCM formulations holds promise as a therapeutic strategy for PCOS.
In clinical practice, other pharmacological interventions for PCOS include combined oral contraceptives (COCs) and antiandrogens. COCs are commonly prescribed to PCOS patients with menstrual irregularities and clinical hyperandrogenism. COCs reduce free testosterone levels by increasing SHBG production in the liver, thereby alleviating hyperandrogenism (239). Anti-androgens such as finasteride, flutamide, spironolactone, or bicalutamide, along with lifestyle modifications, are more effective in improving hirsutism, SHBG, fasting insulin, and the fasting insulin:glucose ratio. Current evidence does not support the preferential use of anti-androgens over COCs for the treatment of hyperandrogenism in PCOS. However, antiandrogens are not preferred over COCs for hyperandrogenism unless COCs are contraindicated or ineffective (240). Moreover, low-dose spironolactone in PCOS rats reduces the oxidative stress markers (MDA) and inflammatory biomarkers such as NF-kB, TNF-α, and IL-6 (241).
In conclusion, SLCI plays a critical role in the pathogenesis and progression of PCOS, contributing to the manifestation of multiple symptoms and an increased risks of various long-term complications associated with PCOS. Chronic systemic inflammation observed in PCOS patients is linked to an imbalance between pro-inflammatory and anti-inflammatory intrinsic mechanisms. Targeted inflammatory regulation therapy may be an effective approach for alleviating PCOS phenotypes of PCOS and improving patient outcomes.
As for a test panel that should become standard in diagnosing PCOS patients at SLCI state, while there is no consensus on a specific “standard test panel” for diagnosing SLCI in PCOS patients, the mentioned markers could be part of a comprehensive diagnostic approach. It is important for healthcare providers to consider the individual patient’s symptoms and risk factors when determining the appropriate tests to order. Therefore, a potential test panel could include hs-CRP,IL-1 Rα,IL-6,IL-17 E/IL-25,IL-17A,IL-18,TNF-α,MIP-1α,and other markers that reflect the inflammatory state and metabolic health of the PCOS patients (242, 243).
International evidence-based guidelines recommend non-pharmacological lifestyle management, such as the ketogenic diet, the Mediterranean diet, intermittent fasting, and regular physical activity, as the first-line treatment for infertility in PCOS. These pharmacological approaches aim to reduce inflammation and optimize overall health to improve fertility (231, 244). Regarding pharmacological management of PCOS, periodic use of progesterone, including the use of COCs, can effectively regulate menstruation in PCOS patients, lower androgen levels, and protect the endometrium. Additionally, progesterone has potential to control chronic inflammation (150, 245). Metformin is an excellent regulator of inflammation and plays an important role in the treatment of PCOS by improving the metabolic disorders, reducing androgen levels and promoting follicular development and ovulation (246–248). Xue et al. found that metformin alleviates PCOS by modulating gut microbiota, resulting in reducing plasma LPS levels along with decreased plasma and ovarian inflammatory cytokines TNF-α, IL-6, and IL-17A levels in PCOS patients (235). Furthermore, statins, as antihyperlipidemic drugs, can reduce HA, improve lipid profiles, and reduce systemic inflammation in women with PCOS (249). Additionally, curcumin, inositol, CoQ10, and microelement selenium, and vitamin D is widely recommended as a fundamental intervention for PCOS owing to their ability to reduce IR and inflammation, enhance ovarian function restoration, restore hormonal balance, and regulate the menstrual cycle in PCOS. Other anti-inflammatory and antioxidant dietary supplements, such as folic acid, inositol, vitamin E, omega-3 fatty acids, alpha lipoic acid, N-acetylcysteine, have shown potential adjuvant therapeutic effects by ameliorating IR, lipid profile, reducing inflammation and oxidative stress markers of PCOS (227–229, 250–255).
Treatment options to relieve gut dysbiosis in PCOS patients include innovative approaches like fecal bacteria transplantation and “prebiotics,” which aim to improve intestinal microecology (256). Additionally, drugs targeting inflammatory cytokines have been found to ameliorates PCOS-related phenotypes. For example, Lang et al. conducted a study on the TNF-α inhibitor etanercept (ETA), which inhibited serum testosterone levels, TNF-α and MCP-1 levels, decreased excessive recruitment of lipid droplets, altered levels of pre-adipose differentiation markers, and abnormal development of follicles in letrozole-induced PCOS rat models. This suggests that anti-TNF-α therapy with ETA may have a potential ameliorative effect associated with its ability to reduce excessive androgen levels on PCOS (257). Moreover, some TCM or ingredients of Chinese herbal medicines, such as the BSHZF, Guizhi Fuling Wan, and SGD improved IR and ameliorated sex hormone disturbances in PCOS through anti-inflammatory effects activation of the PI3K/AKT pathway, and modulation of gut microbiota (236–238). In conclusion, although the etiology of PCOS remains unclear, given the important role of SLCI in PCOS, comprehensive treatment strategies involving long-term management, including non-pharmacological lifestyle interventions combined with pharmacological approaches aimed at ameliorating inflammation, should be adopted to ultimately improve the clinical phenotype of PCOS, reduce the incidence of long-term complications, and enhance overall health among individuals with PCOS.
HD: Writing – review & editing, Writing – original draft. YC: Writing – review & editing. JX: Validation, Writing – review & editing. NZ: Supervision, Validation, Writing – review & editing. LX: Writing – review & editing.
The author(s) declare financial support was received for the research, authorship, and/or publication of this article. The research was supported by the General Project of Natural science foundation project of Sichuan (2024NSFSC0599, 2023NSFSC0632), the Key Research and Development Program of Chengdu (2023-YF09-00052-SN), the Key Program of Development Fund for Clinical Disciplines of West China Second Hospital of Sichuan University (KL059), the General Project of National Natural Science Foundation of China (81971354), and the health department of Sichuan province (100377).
We would like to express our sincere thanks to all those who have lent us hands during our writing this paper.
The authors declare that the research was conducted in the absence of any commercial or financial relationships that could be construed as a potential conflict of interest.
All claims expressed in this article are solely those of the authors and do not necessarily represent those of their affiliated organizations, or those of the publisher, the editors and the reviewers. Any product that may be evaluated in this article, or claim that may be made by its manufacturer, is not guaranteed or endorsed by the publisher.
1. Azziz R, Woods KS, Reyna R, Key TJ, Knochenhauer ES, Yildiz BO. The prevalence and features of the polycystic ovary syndrome in an unselected population. J Clin Endocrinol Metab. (2004) 89:2745–9. doi: 10.1210/jc.2003-032046
2. Baracat EC, Baracat M, José M SJ Jr. Are there new insights for the definition of PCOS. Gynecol Endocrinol. (2022) 38:703–4. doi: 10.1080/09513590.2022.2121387
3. Escobar-Morreale HF. Polycystic ovary syndrome: definition, aetiology, diagnosis and treatment. Nat Rev Endocrinol. (2018) 14:270–84. doi: 10.1038/nrendo.2018.24
4. Palioura E, Diamanti-Kandarakis E. Polycystic ovary syndrome (PCOS) and endocrine disrupting chemicals (EDCs). Rev Endocr Metab Disord. (2015) 16:365–71. doi: 10.1007/s11154-016-9326-7
5. Hart R, Doherty DA. The potential implications of a PCOS diagnosis on a woman’s long-term health using data linkage. J Clin Endocrinol Metab. (2015) 100:911–9. doi: 10.1210/jc.2014-3886
6. Yin W, Falconer H, Yin L, Xu L, Ye W. Association between polycystic ovary syndrome and cancer risk. JAMA Oncol. (2019) 5:106–7. doi: 10.1001/jamaoncol.2018.5188
7. Parker J, O’Brien C, Hawrelak J, Gersh FL. Polycystic ovary syndrome: an evolutionary adaptation to lifestyle and the environment. Int J Environ Res Public Health. (2022) 19:1336. doi: 10.3390/ijerph19031336
8. Wang J, Yin T, Liu S. Dysregulation of immune response in PCOS organ system. Front Immunol. (2023) 14:1169232. doi: 10.3389/fimmu.2023.1169232
9. Zhai Y, Pang Y. Systemic and ovarian inflammation in women with polycystic ovary syndrome. J Reprod Immunol. (2022) 151:103628. doi: 10.1016/j.jri.2022.103628
10. Gao L, Zhao Y, Wu H, Lin X, Guo F, Li J, et al. Polycystic ovary syndrome fuels cardiovascular inflammation and aggravates ischemic cardiac injury. Circulation. (2023) 148:1958–73. doi: 10.1161/CIRCULATIONAHA.123.065827
11. Huby T, Gautier EL. Immune cell-mediated features of non-alcoholic steatohepatitis. Nat Rev Immunol. (2022) 22:429–43. doi: 10.1038/s41577-021-00639-3
12. Kelley CE, Brown AJ, Diehl AM, Setji TL. Review of nonalcoholic fatty liver disease in women with polycystic ovary syndrome. World J Gastroenterol. (2014) 20:14172–84. doi: 10.3748/wjg.v20.i39.14172
13. He S, Mao X, Lei H, Dong B, Guo D, Zheng B, et al. Peripheral blood inflammatory-immune cells as a predictor of infertility in women with polycystic ovary syndrome. J Inflammation Res. (2020) 13:441–50. doi: 10.2147/JIR.S260770
14. Yilmaz MA, Duran C, Basaran M. The mean platelet volume and neutrophil to lymphocyte ratio in obese and lean patients with polycystic ovary syndrome. J Endocrinol Invest. (2016) 39:45–53. doi: 10.1007/s40618-015-0335-2
15. Xiong YL, Liang XY, Yang X, Li Y, Wei LN. Low-grade chronic inflammation in the peripheral blood and ovaries of women with polycystic ovarian syndrome. Eur J Obstet Gynecol Reprod Biol. (2011) 159:148–50. doi: 10.1016/j.ejogrb.2011.07.012
16. Orio F Jr, Palomba S, Cascella T, Di Biase S, Manguso F, Tauchmanovà L, et al. The increase of leukocytes as a new putative marker of low-grade chronic inflammation and early cardiovascular risk in polycystic ovary syndrome. J Clin Endocrinol Metab. (2005) 90:2–5. doi: 10.1210/jc.2004-0628
17. Kawai T, Autieri MV, Scalia R. Adipose tissue inflammation and metabolic dysfunction in obesity. Am J Physiol Cell Physiol. (2021) 320:C375–375C391. doi: 10.1152/ajpcell.00379.2020
18. Rudnicka E, Suchta K, Grymowicz M, Calik-Ksepka A, Smolarczyk K, Duszewska AM, et al. Chronic low grade inflammation in pathogenesis of PCOS. Int J Mol Sci. (2021) 22. doi: 10.3390/ijms22073789
19. Borthakur A, Prabhu Y D, Valsala Gopalakrishnan A. Role of IL-6 signalling in Polycystic Ovarian Syndrome associated inflammation. J Reprod Immunol. (2020) 141:103155. doi: 10.1016/j.jri.2020.103155
20. Blumenfeld Z. The possible practical implication of high CRP levels in PCOS. Clin Med Insights Reprod Health. (2019) 13:1179558119861936. doi: 10.1177/1179558119861936
21. Terranova PF. Potential roles of tumor necrosis factor-alpha in follicular development, ovulation, and the life span of the corpus luteum. Domest Anim Endocrinol. (1997) 14:1–15. doi: 10.1016/s0739-7240(96)00094-x
22. Yang Y, Qiao J, Li R, Li MZ. Is interleukin-18 associated with polycystic ovary syndrome. Reprod Biol Endocrinol. (2011) 9:7. doi: 10.1186/1477-7827-9-7
23. Papalou O, Livadas S, Karachalios A, Tolia N, Kokkoris P, Tripolitakis K, et al. White blood cells levels and PCOS: direct and indirect relationship with obesity and insulin resistance, but not with hyperandogenemia. Hormones (Athens). (2015) 14:91–100. doi: 10.14310/horm.2002.1563
24. Liu Y, Li Z, Wang Y, Cai Q, Liu H, Xu C, et al. IL-15 participates in the pathogenesis of polycystic ovary syndrome by affecting the activity of granulosa cells. Front Endocrinol (Lausanne). (2022) 13:787876. doi: 10.3389/fendo.2022.787876
25. Kuang H, Duan Y, Li D, Xu Y, Ai W, Li W, et al. The role of serum inflammatory cytokines and berberine in the insulin signaling pathway among women with polycystic ovary syndrome. PloS One. (2020) 15:e0235404. doi: 10.1371/journal.pone.0235404
26. Rashid N, Nigam A, Saxena P, Jain SK, Wajid S. Association of IL-1β, IL-1Ra and FABP1 gene polymorphisms with the metabolic features of polycystic ovary syndrome. Inflammation Res. (2017) 66:621–36. doi: 10.1007/s00011-017-1045-3
27. Li L, Yu J, Zhou Z. Association between neutrophil-to-lymphocyte ratio and polycystic ovary syndrome: A PRISMA-compliant systematic review and meta-analysis. Med (Baltimore). (2022) 101:e30579. doi: 10.1097/MD.0000000000030579
28. Li L, Yu J, Zhou Z. Mean platelet volume and polycystic ovary syndrome: a systematic review and meta-analysis. J Int Med Res. (2022) 50:3000605211067316. doi: 10.1177/03000605211067316
29. Geng Y, Liu Z, Hu R, Ma W, Wu X, Dong H, et al. Opportunities and challenges: interleukin-22 comprehensively regulates polycystic ovary syndrome from metabolic and immune aspects. J Ovarian Res. (2023) 16:149. doi: 10.1186/s13048-023-01236-9
30. Diamanti-Kandarakis E, Piperi C, Kalofoutis A, Creatsas G. Increased levels of serum advanced glycation end-products in women with polycystic ovary syndrome. Clin Endocrinol (Oxf). (2005) 62:37–43. doi: 10.1111/j.1365-2265.2004.02170.x
31. Wu Z, Fang L, Li Y, Yan Y, Thakur A, Cheng JC, et al. Association of circulating monocyte chemoattractant protein-1 levels with polycystic ovary syndrome: A meta-analysis. Am J Reprod Immunol. (2021) 86:e13407. doi: 10.1111/aji.13407
32. Upadhyay N, Almeida EA, Singh A, Madhu SV, Puri D, Mehndiratta M. Evaluation of CRP/albumin ratio in polycystic ovarian syndrome. J Obstet Gynaecol India. (2024) 74:165–9. doi: 10.1007/s13224-023-01897-y
33. Liu S, Hong L, Mo M, Xiao S, Chen C, Li Y, et al. Evaluation of endometrial immune status of polycystic ovary syndrome. J Reprod Immunol. (2021) 144:103282. doi: 10.1016/j.jri.2021.103282
34. Zeng X, Xie YJ, Liu YT, Long SL, Mo ZC. Polycystic ovarian syndrome: Correlation between hyperandrogenism, insulin resistance and obesity. Clin Chim Acta. (2020) 502:214–21. doi: 10.1016/j.cca.2019.11.003
35. Boots CE, Jungheim ES. Inflammation and human ovarian follicular dynamics. Semin Reprod Med. (2015) 33:270–5. doi: 10.1055/s-0035-1554928
36. Zhang H, Wang X, Xu J, Zhu Y, Chen X, Hu Y. IL-18 and IL-18 binding protein concentration in ovarian follicular fluid of women with unexplained infertility to PCOS during in vitro fertilization. J Reprod Immunol. (2020) 138:103083. doi: 10.1016/j.jri.2020.103083
37. Kahyaoglu I, Yılmaz N, Timur H, Inal HA, Erkaya S. Granulocyte colony-stimulating factor: A relation between serum and follicular fluid levels and in-vitro fertilization outcome in patients with polycystic ovary syndrome. Cytokine. (2015) 74:113–6. doi: 10.1016/j.cyto.2014.09.002
38. Wang T, Xiong X, Xiao N, Yan Y, Liu X, Xie Q, et al. The therapeutic effect of anti-CD19 antibody on DHEA-induced PCOS mice. Int Immunopharmacol. (2024) 130:111711. doi: 10.1016/j.intimp.2024.111711
39. Huang J, Chen P, Xiang Y, Liang Q, Wu T, Liu J, et al. Gut microbiota dysbiosis-derived macrophage pyroptosis causes polycystic ovary syndrome via steroidogenesis disturbance and apoptosis of granulosa cells. Int Immunopharmacol. (2022) 107:108717. doi: 10.1016/j.intimp.2022.108717
40. Sayin NC, Gücer F, Balkanli-Kaplan P, Yüce MA, Yardim T. Elevated serum TNF-alpha levels in normal-weight women with polycystic ovaries or the polycystic ovary syndrome. J Reprod Med. (2003) 48:165–70. doi: 10.3109/01674820309042802
41. Amato G, Conte M, Mazziotti G, Lalli E, Vitolo G, Tucker AT, et al. Serum and follicular fluid cytokines in polycystic ovary syndrome during stimulated cycles. Obstet Gynecol. (2003) 101:1177–82. doi: 10.1016/s0029-7844(03)00233-3
42. Wang LJ, Brännström M, Robertson SA, Norman RJ. Tumor necrosis factor alpha in the human ovary: presence in follicular fluid and effects on cell proliferation and prostaglandin production. Fertil Steril. (1992) 58:934–40. doi: 10.1016/s0015-0282(16)55438-7
43. Roby KF, Weed J, Lyles R, Terranova PF. Immunological evidence for a human ovarian tumor necrosis factor-alpha. J Clin Endocrinol Metab. (1990) 71:1096–102. doi: 10.1210/jcem-71-5-1096
44. MadhuSudan GP, Dev R, Sharma MK, Singh D. Expression of mRNAs encoding tumor necrosis factor-alpha and its receptor-I in buffalo ovary. Indian J Exp Biol. (2007) 45:669–75.
45. Sasson R, Winder N, Kees S, Amsterdam A. Induction of apoptosis in granulosa cells by TNF alpha and its attenuation by glucocorticoids involve modulation of Bcl-2. Biochem Biophys Res Commun. (2002) 294:51–9. doi: 10.1016/S0006-291X(02)00431-X
46. Wang B, Hao M, Yang Q, Li J, Guo Y. Follicular fluid soluble receptor for advanced glycation endproducts (sRAGE): a potential protective role in polycystic ovary syndrome. J Assist Reprod Genet. (2016) 33:959–65. doi: 10.1007/s10815-016-0704-6
47. Lin YS, Tsai SJ, Lin MW, Yang CT, Huang MF, Wu MH. Interleukin-6 as an early chronic inflammatory marker in polycystic ovary syndrome with insulin receptor substrate-2 polymorphism. Am J Reprod Immunol. (2011) 66:527–33. doi: 10.1111/j.1600-0897.2011.01059.x
48. Peng Z, Sun Y, Lv X, Zhang H, Liu C, Dai S. Interleukin-6 levels in women with polycystic ovary syndrome: A systematic review and meta-analysis. PloS One. (2016) 11:e0148531. doi: 10.1371/journal.pone.0148531
49. Deura I, Harada T, Taniguchi F, Iwabe T, Izawa M, Terakawa N. Reduction of estrogen production by interleukin-6 in a human granulosa tumor cell line may have implications for endometriosis-associated infertility. Fertil Steril. (2005) 83 Suppl 1:1086–92. doi: 10.1016/j.fertnstert.2004.12.014
50. Liu Y, Liu H, Li Z, Fan H, Yan X, Liu X, et al. The release of peripheral immune inflammatory cytokines promote an inflammatory cascade in PCOS patients via altering the follicular microenvironment. Front Immunol. (2021) 12:685724. doi: 10.3389/fimmu.2021.685724
51. Lee KS, Joo BS, Na YJ, Yoon MS, Choi OH, Kim WW. Relationships between concentrations of tumor necrosis factor-alpha and nitric oxide in follicular fluid and oocyte quality. J Assist Reprod Genet. (2000) 17:222–8. doi: 10.1023/a:1009495913119
52. Tarkun I, Cetinarslan B, Türemen E, Cantürk Z, Biyikli M. Association between circulating tumor necrosis factor-alpha, interleukin-6, and insulin resistance in normal-weight women with polycystic ovary syndrome. Metab Syndr Relat Disord. (2006) 4:122–8. doi: 10.1089/met.2006.4.122
53. Deshmukh H, Way SS. Immunological basis for recurrent fetal loss and pregnancy complications. Annu Rev Pathol. (2019) 14:185–210. doi: 10.1146/annurev-pathmechdis-012418-012743
54. Ander SE, Diamond MS, Coyne CB. Immune responses at the maternal-fetal interface. Sci Immunol. (2019) 4:eaat6114. doi: 10.1126/sciimmunol.aat6114
55. Yang F, Zheng Q, Jin L. Dynamic function and composition changes of immune cells during normal and pathological pregnancy at the maternal-fetal interface. Front Immunol. (2019) 10:2317. doi: 10.3389/fimmu.2019.02317
56. Wang Q, Luo L, Lei Q, Lin MM, Huang X, Chen MH, et al. Low aneuploidy rate in early pregnancy loss abortuses from patients with polycystic ovary syndrome. Reprod BioMed Online. (2016) 33:85–92. doi: 10.1016/j.rbmo.2016.04.006
57. Piltonen TT, Chen JC, Khatun M, Kangasniemi M, Liakka A, Spitzer T, et al. Endometrial stromal fibroblasts from women with polycystic ovary syndrome have impaired progesterone-mediated decidualization, aberrant cytokine profiles and promote enhanced immune cell migration. vitro. Hum Reprod. (2015) 30:1203–15. doi: 10.1093/humrep/dev055
58. Zhou F, Li C, Zhang SY. NLRP3 inflammasome: a new therapeutic target for high-risk reproductive disorders. Chin Med J (Engl). (2020) 134:20–7. doi: 10.1097/CM9.0000000000001214
59. Tersigni C, Vatish M, D'Ippolito S, Scambia G, Di Simone N. Abnormal uterine inflammation in obstetric syndromes: molecular insights into the role of chemokine decoy receptor D6 and inflammasome NLRP3. J Int Manage. (2020) 26:111–21. doi: 10.1093/molehr/gaz067
60. Bishop A, Cartwright JE, Whitley GS. Stanniocalcin-1 in the female reproductive system and pregnancy. Hum Reprod Update. (2021) 27:1098–114. doi: 10.1093/humupd/dmab028
61. Khatun M, Arffman RK, Lavogina D, Kangasniemi M, Laru J, Ahtikoski A, et al. Women with polycystic ovary syndrome present with altered endometrial expression of stanniocalcin-1†. Biol Reprod. (2020) 102:306–15. doi: 10.1093/biolre/ioz180
62. Piltonen TT, Chen J, Erikson DW, Spitzer TL, Barragan F, Rabban JT, et al. Mesenchymal stem/progenitors and other endometrial cell types from women with polycystic ovary syndrome (PCOS) display inflammatory and oncogenic potential. J Clin Endocrinol Metab. (2013) 98:3765–75. doi: 10.1210/jc.2013-1923
63. Poppe K, Velkeniers B, Glinoer D. The role of thyroid autoimmunity in fertility and pregnancy. Nat Clin Pract Endocrinol And Metab. (2008) 4:394–405. doi: 10.1038/ncpendmet0846
64. Pergialiotis V, Konstantopoulos P, Prodromidou A, Florou V, Papantoniou N, Perrea DN. MANAGEMENT OF ENDOCRINE DISEASE: The impact of subclinical hypothyroidism on anthropometric characteristics, lipid, glucose and hormonal profile of PCOS patients: a systematic review and meta-analysis. Eur J Of Endocrinology. (2017) 176:R159. doi: 10.1530/EJE-16-0611
65. Romitti M, Fabris VC, Ziegelmann PK, Maia AL, Spritzer PM. Association between PCOS and autoimmune thyroid disease: a systematic review and meta-analysis. Endocr Connect. (2018) 7:1158–67. doi: 10.1530/EC-18-0309
66. Sen A, Kushnir VA, Barad DH, Gleicher N. Endocrine autoimmune diseases and female infertility. Nat Rev Endocrinol. (2014) 10:37–50. doi: 10.1038/nrendo.2013.212
67. Singla R, Gupta Y, Khemani M, Aggarwal S. Thyroid disorders and polycystic ovary syndrome: An emerging relationship. Indian J Endocrinol Metab. (2015) 19:25–9. doi: 10.4103/2230-8210.146860
68. Ho CW, Chen HH, Hsieh MC, Chen CC, Hsu SP, Yip HT, et al. Increased risk of polycystic ovary syndrome and it’s comorbidities in women with autoimmune thyroid disease. Int J Environ Res Public Health. (2020) 17. doi: 10.3390/ijerph17072422
69. Al-Saab R, Haddad S. Detection of thyroid autoimmunity markers in euthyroid women with polycystic ovary syndrome: a case-control study from Syria. Int J Endocrinol Metab. (2014) 12:e17954. doi: 10.5812/ijem.17954
70. Mohammed S, Awooda HA, Rayis DA, Hamdan HZ, Adam I, Lutfi MF. Thyroid function/antibodies in Sudanese women with polycystic ovarian disease. Obstet Gynecol Sci. (2017) 60:187–92. doi: 10.5468/ogs.2017.60.2.187
71. Tomer Y. Mechanisms of autoimmune thyroid diseases: from genetics to epigenetics. Annu Rev Pathol. (2014) 9:147–56. doi: 10.1146/annurev-pathol-012513-104713
72. Petríková J, Lazúrová I, Yehuda S. Polycystic ovary syndrome and autoimmunity. Eur J Intern Med. (2010) 21:369–71. doi: 10.1016/j.ejim.2010.06.008
73. Luque-Ramírez M, Alpañés M, Escobar-Morreale HF. The determinants of insulin sensitivity, β-cell function, and glucose tolerance are different in patients with polycystic ovary syndrome than in women who do not have hyperandrogenism. Fertil Steril. (2010) 94:2214–21. doi: 10.1016/j.fertnstert.2009.11.049
74. Tao T, Wu P, Wang Y, Liu W. Comparison of glycemic control and β-cell function in new onset T2DM patients with PCOS of metformin and saxagliptin monotherapy or combination treatment. BMC Endocr Disord. (2018) 18:14. doi: 10.1186/s12902-018-0243-5
75. Malin SK, Kirwan JP, Sia CL, González F. Pancreatic β-cell dysfunction in polycystic ovary syndrome: role of hyperglycemia-induced nuclear factor-κB activation and systemic inflammation. Am J Physiol Endocrinol Metab. (2015) 308:E770–7. doi: 10.1152/ajpendo.00510.2014
76. Abuelezz NZ, Shabana ME, Abdel-Mageed HM, Rashed L, Morcos G. Nanocurcumin alleviates insulin resistance and pancreatic deficits in polycystic ovary syndrome rats: Insights on PI3K/AkT/mTOR and TNF-α modulations. Life Sci. (2020) 256:118003. doi: 10.1016/j.lfs.2020.118003
77. Abuelezz NZ, Shabana M E, Rashed L, Nb Morcos G. Nanocurcumin Modulates miR-223-3p and NF-κB Levels in the Pancreas of Rat Model of Polycystic Ovary Syndrome to Attenuate Autophagy Flare, Insulin Resistance and Improve ß Cell Mass. J Exp Pharmacol. (2021) 13:873–88. doi: 10.2147/JEP.S323962
78. Yesiladali M, Yazici M, Attar E, Kelestimur F. Differentiating polycystic ovary syndrome from adrenal disorders. Diagnostics (Basel). (2022) 12:2045. doi: 10.3390/diagnostics12092045
79. Yildiz BO, Azziz R. The adrenal and polycystic ovary syndrome. Rev Endocr Metab Disord. (2007) 8:331–42. doi: 10.1007/s11154-007-9054-0
80. Zhang J, Qiu X, Gui Y, Xu Y, Li D, Wang L. Dehydroepiandrosterone improves the ovarian reserve of women with diminished ovarian reserve and is a potential regulator of the immune response in the ovaries. Biosci Trends. (2015) 9:350–9. doi: 10.5582/bst.2015.01154
81. Gleicher N, Kushnir VA, Darmon SK, Wang Q, Zhang L, Albertini DF, et al. New PCOS-like phenotype in older infertile women of likely autoimmune adrenal etiology with high AMH but low androgens. J Steroid Biochem Mol Biol. (2017) 167:144–52. doi: 10.1016/j.jsbmb.2016.12.004
82. Gleicher N, Kushnir VA, Darmon SK, Wang Q, Zhang L, Albertini DF, et al. Suspected ontogeny of a recently described hypo-androgenic PCOS-like phenotype with advancing age. Endocrine. (2018) 59:661–76. doi: 10.1007/s12020-017-1498-8
83. Gao Z, Tan H, Song X, Zhuang T, Kong R, Wang Y, et al. Troxerutin dampened hypothalamic neuroinflammation via microglial IL-22/IL-22R1/IRF3 activation in dihydrotestosterone-induced polycystic ovary syndrome rats. Phytomedicine. (2024) 124:155280. doi: 10.1016/j.phymed.2023.155280
84. Lian Y, Zhao F, Wang W. Central leptin resistance and hypothalamic inflammation are involved in letrozole-induced polycystic ovary syndrome rats. Biochem Biophys Res Commun. (2016) 476:306–12. doi: 10.1016/j.bbrc.2016.05.117
85. Jais A, Brüning JC. Hypothalamic inflammation in obesity and metabolic disease. J Clin Invest. (2017) 127:24–32. doi: 10.1172/JCI88878
86. De Souza CT, Araujo EP, Bordin S, Ashimine R, Zollner RL, Boschero AC, et al. Consumption of a fat-rich diet activates a proinflammatory response and induces insulin resistance in the hypothalamus. Endocrinology. (2005) 146:4192–9. doi: 10.1210/en.2004-1520
87. Thaler JP, Yi CX, Schur EA, Guyenet SJ, Hwang BH, Dietrich MO, et al. Obesity is associated with hypothalamic injury in rodents and humans. J Clin Invest. (2012) 122:153–62. doi: 10.1172/JCI59660
88. Mraz M, Haluzik M. The role of adipose tissue immune cells in obesity and low-grade inflammation. J Endocrinol. (2014) 222:R113–27. doi: 10.1530/JOE-14-0283
89. Lively S, Schlichter LC. Microglia responses to pro-inflammatory stimuli (LPS, IFNγ+TNFα) and reprogramming by resolving cytokines (IL-4, IL-10). Front Cell Neurosci. (2018) 12:215. doi: 10.3389/fncel.2018.00215
90. Chen X, Huang L, Cui L, Xiao Z, Xiong X, Chen C. Sodium-glucose cotransporter 2 inhibitor ameliorates high fat diet-induced hypothalamic-pituitary-ovarian axis disorders. J Physiol. (2022) 600:4549–68. doi: 10.1113/JP283259
91. Barlampa D, Bompoula MS, Bargiota A, Kalantaridou S, Mastorakos G, Valsamakis G. Hypothalamic inflammation as a potential pathophysiologic basis for the heterogeneity of clinical, hormonal, and metabolic presentation in PCOS. Nutrients. (2021) 13. doi: 10.3390/nu13020520
92. Guan C, Zahid S, Minhas AS, Ouyang P, Vaught A, Baker VL, et al. Polycystic ovary syndrome: a “risk-enhancing” factor for cardiovascular disease. Fertil Steril. (2022) 117:924–35. doi: 10.1016/j.fertnstert.2022.03.009
93. Wekker V, van Dammen L, Koning A, Heida KY, Painter RC, Limpens J, et al. Long-term cardiometabolic disease risk in women with PCOS: a systematic review and meta-analysis. Hum Reprod Update. (2020) 26:942–60. doi: 10.1093/humupd/dmaa029
94. Gomez J, VanHise K, Stachenfeld N, Chan JL, Merz NB, Shufelt C. Subclinical cardiovascular disease and polycystic ovary syndrome. Fertil Steril. (2022) 117:912–23. doi: 10.1016/j.fertnstert.2022.02.028
95. Mani H, Levy MJ, Davies MJ, Morris DH, Gray LJ, Bankart J, et al. Diabetes and cardiovascular events in women with polycystic ovary syndrome: a 20-year retrospective cohort study. Clin Endocrinol (Oxf). (2013) 78:926–34. doi: 10.1111/cen.12068
96. Caglar GS, Oztas E, Karadag D, Pabuccu R, Demirtas S. Ischemia-modified albumin and cardiovascular risk markers in polycystic ovary syndrome with or without insulin resistance. Fertil Steril. (2011) 95:310–3. doi: 10.1016/j.fertnstert.2010.06.092
97. Joham AE, Boyle JA, Zoungas S, Teede HJ. Hypertension in reproductive-aged women with polycystic ovary syndrome and association with obesity. Am J Hypertens. (2015) 28:847–51. doi: 10.1093/ajh/hpu251
98. de Groot PC, Dekkers OM, Romijn JA, Dieben SW, Helmerhorst FM. PCOS, coronary heart disease, stroke and the influence of obesity: a systematic review and meta-analysis. Hum Reprod Update. (2011) 17:495–500. doi: 10.1093/humupd/dmr001
99. Steyers CM 3rd, Miller FJ Jr. Endothelial dysfunction in chronic inflammatory diseases. Int J Mol Sci. (2014) 15:11324–49. doi: 10.3390/ijms150711324
100. Medina-Leyte DJ, Zepeda-García O, Domínguez-Pérez M, González-Garrido A, Villarreal-Molina T, Jacobo-Albavera L. Endothelial dysfunction, inflammation and coronary artery disease: potential biomarkers and promising therapeutical approaches. Int J Mol Sci. (2021) 22:3850. doi: 10.3390/ijms22083850
101. Wu J, Yao XY, Shi RX, Liu SF, Wang XY. A potential link between polycystic ovary syndrome and non-alcoholic fatty liver disease: an update meta-analysis. Reprod Health. (2018) 15:77. doi: 10.1186/s12978-018-0519-2
102. Vassilatou E, Lafoyianni S, Vryonidou A, Ioannidis D, Kosma L, Katsoulis K, et al. Increased androgen bioavailability is associated with non-alcoholic fatty liver disease in women with polycystic ovary syndrome. Hum Reprod. (2010) 25:212–20. doi: 10.1093/humrep/dep380
103. Carreau AM, Pyle L, Garcia-Reyes Y, Rahat H, Vigers T, Jensen T, et al. Clinical prediction score of nonalcoholic fatty liver disease in adolescent girls with polycystic ovary syndrome (PCOS-HS index). Clin Endocrinol (Oxf). (2019) 91:544–52. doi: 10.1111/cen.14062
104. de Zegher F, Diaz M, Ibañez L. From adolescent PCOS to adult MAFLD: opposing effects of randomised interventions. BMJ Open Gastroenterol. (2021) 8(1). doi: 10.1136/bmjgast-2020-000574
105. Brzozowska MM, Ostapowicz G, Weltman MD. An association between non-alcoholic fatty liver disease and polycystic ovarian syndrome. J Gastroenterol Hepatol. (2009) 24:243–7. doi: 10.1111/j.1440-1746.2008.05740.x
106. Rocha A, Faria LC, Guimarães T, Moreira GV, Cândido AL, Couto CA, et al. Non-alcoholic fatty liver disease in women with polycystic ovary syndrome: systematic review and meta-analysis. J Endocrinol Invest. (2017) 40:1279–88. doi: 10.1007/s40618-017-0708-9
107. Chen Y, Ma L, Ge Z, Pan Y, Xie L. Key genes associated with non-alcoholic fatty liver disease and polycystic ovary syndrome. Front Mol Biosci. (2022) 9:888194. doi: 10.3389/fmolb.2022.888194
108. Marino JS, Iler J, Dowling AR, Chua S, Bruning JC, Coppari R, et al. Adipocyte dysfunction in a mouse model of polycystic ovary syndrome (PCOS): evidence of adipocyte hypertrophy and tissue-specific inflammation. PloS One. (2012) 7:e48643. doi: 10.1371/journal.pone.0048643
109. Zhang Y, Meng F, Sun X, Sun X, Hu M, Cui P, et al. Hyperandrogenism and insulin resistance contribute to hepatic steatosis and inflammation in female rat liver. Oncotarget. (2018) 9:18180–97. doi: 10.18632/oncotarget.24477
110. Olaniyi KS, Areloegbe SE, Areola ED, Sabinari IW, Fafure AA, Agbana RD, et al. Low-dose spironolactone combats dyslipidemia and hepatic inflammation by modulating PCSK9 in rat model of polycystic ovarian syndrome. Toxicol Appl Pharmacol. (2023) 473:116604. doi: 10.1016/j.taap.2023.116604
111. Krishnan A, Muthusami S, Periyasamy L, Stanley JA, Gopalakrishnan V, Ramachandran I. Effect of DHT-induced hyperandrogenism on the pro-inflammatory cytokines in a rat model of polycystic ovary morphology. Medicina (Kaunas). (2020) 56:100. doi: 10.3390/medicina56030100
112. Yuan Y, Jiao B, Qu L, Yang D, Liu R. The development of COVID-19 treatment. Front Immunol. (2023) 14:1125246. doi: 10.3389/fimmu.2023.1125246
113. Morgante G, Troìa L, De Leo V. Coronavirus Disease 2019 (SARS-CoV-2) and polycystic ovarian disease: Is there a higher risk for these women. J Steroid Biochem Mol Biol. (2021) 205:105770. doi: 10.1016/j.jsbmb.2020.105770
114. de Medeiros SF, Yamamoto M, de Medeiros M, Yamamoto A, Barbosa BB. Polycystic ovary syndrome and risks for COVID-19 infection: A comprehensive review: PCOS and COVID-19 relationship. Rev Endocr Metab Disord. (2022) 23:251–64. doi: 10.1007/s11154-022-09715-y
115. Huffman AM, Rezq S, Basnet J, Yanes Cardozo LL, Romero DG. SARS-coV-2 viral entry proteins in hyperandrogenemic female mice: implications for women with PCOS and COVID-19. Int J Mol Sci. (2021) 22:4472. doi: 10.3390/ijms22094472
116. Kerchner A, Lester W, Stuart SP, Dokras A. Risk of depression and other mental health disorders in women with polycystic ovary syndrome: a longitudinal study. Fertil Steril. (2009) 91:207–12. doi: 10.1016/j.fertnstert.2007.11.022
117. Serafini G, Costanza A, Aguglia A, Amerio A, Trabucco A, Escelsior A, et al. The role of inflammation in the pathophysiology of depression and suicidal behavior: implications for treatment. Med Clin North Am. (2023) 107:1–29. doi: 10.1016/j.mcna.2022.09.001
118. Miller AH, Haroon E, Felger JC. Therapeutic implications of brain-immune interactions: treatment in translation. Neuropsychopharmacology. (2017) 42:334–59. doi: 10.1038/npp.2016.167
119. Felger JC. Increased inflammation and treatment of depression: from resistance to reuse, repurposing, and redesign. Adv Neurobiol. (2023) 30:387–416. doi: 10.1007/978-3-031-21054-9_16
120. Howren MB, Lamkin DM, Suls J. Associations of depression with C-reactive protein, IL-1, and IL-6: a meta-analysis. Psychosom Med. (2009) 71:171–86. doi: 10.1097/PSY.0b013e3181907c1b
121. Shelton RC, Miller AH. Inflammation in depression: is adiposity a cause. Dialogues Clin Neurosci. (2011) 13:41–53. doi: 10.31887/DCNS.2011.13.1/rshelton
122. D’Mello C, Swain MG. Immune-to-brain communication pathways in inflammation-associated sickness and depression. Curr Top Behav Neurosci. (2017) 31:73–94. doi: 10.1007/7854_2016_37
123. Quan N, Banks WA. Brain-immune communication pathways. Brain Behav Immun. (2007) 21:727–35. doi: 10.1016/j.bbi.2007.05.005
124. Haapakoski R, Ebmeier KP, Alenius H, Kivimäki M. Innate and adaptive immunity in the development of depression: An update on current knowledge and technological advances. Prog Neuropsychopharmacol Biol Psychiatry. (2016) 66:63–72. doi: 10.1016/j.pnpbp.2015.11.012
125. Zhu CB, Carneiro AM, Dostmann WR, Hewlett WA, Blakely RD. p38 MAPK activation elevates serotonin transport activity via a trafficking-independent, protein phosphatase 2A-dependent process. J Biol Chem. (2005) 280:15649–58. doi: 10.1074/jbc.M410858200
126. Zhu CB, Lindler KM, Owens AW, Daws LC, Blakely RD, Hewlett WA. Interleukin-1 receptor activation by systemic lipopolysaccharide induces behavioral despair linked to MAPK regulation of CNS serotonin transporters. Neuropsychopharmacology. (2010) 35:2510–20. doi: 10.1038/npp.2010.116
127. Goodarzi MO, Korenman SG. The importance of insulin resistance in polycystic ovary syndrome. Fertil Steril. (2003) 80:255–8. doi: 10.1016/s0015-0282(03)00734-9
128. Carmina E, Bucchieri S, Esposito A, Del Puente A, Mansueto P, Orio F, et al. Abdominal fat quantity and distribution in women with polycystic ovary syndrome and extent of its relation to insulin resistance. J Clin Endocrinol Metab. (2007) 92:2500–5. doi: 10.1210/jc.2006-2725
129. Snider AP, Wood JR. Obesity induces ovarian inflammation and reduces oocyte quality. Reproduction. (2019) 158:R79–79R90. doi: 10.1530/REP-18-0583
130. Shoelson SE, Lee J, Goldfine AB. Inflammation and insulin resistance. J Clin Invest. (2006) 116:1793–801. doi: 10.1172/JCI29069
131. Deligeoroglou E, Vrachnis N, Athanasopoulos N, Iliodromiti Z, Sifakis S, Iliodromiti S, et al. Mediators of chronic inflammation in polycystic ovarian syndrome. Gynecol Endocrinol. (2012) 28:974–8. doi: 10.3109/09513590.2012.683082
132. Spritzer PM, Lecke SB, Satler F, Morsch DM. Adipose tissue dysfunction, adipokines, and low-grade chronic inflammation in polycystic ovary syndrome. Reproduction. (2015) 149:R219–27. doi: 10.1530/REP-14-0435
133. Rui L, Aguirre V, Kim JK, Shulman GI, Lee A, Corbould A, et al. Insulin/IGF-1 and TNF-alpha stimulate phosphorylation of IRS-1 at inhibitory Ser307 via distinct pathways. J Clin Invest. (2001) 107:181–9. doi: 10.1172/JCI10934
134. Stephens JM, Pekala PH. Transcriptional repression of the C/EBP-alpha and GLUT4 genes in 3T3-L1 adipocytes by tumor necrosis factor-alpha. Regulations is coordinate and independent of protein synthesis. J Biol Chem. (1992) 267:13580–4. doi: 10.1016/S0021-9258(18)42251-X
135. Nteeba J, Ortinau LC, Perfield JW 2nd, Keating AF. Diet-induced obesity alters immune cell infiltration and expression of inflammatory cytokine genes in mouse ovarian and peri-ovarian adipose depot tissues. Mol Reprod Dev. (2013) 80:948–58. doi: 10.1002/mrd.22231
136. Chang J, Azziz R, Legro R, Dewailly D, Franks S, Fauser B, et al. Revised 2003 consensus on diagnostic criteria and long-term health risks related to polycystic ovary syndrome. Fertil Steril. (2004) 81:19–25. doi: 10.1016/j.fertnstert.2003.10.004
137. Corbould A, Zhao H, Mirzoeva S, Aird F, Dunaif A. Enhanced mitogenic signaling in skeletal muscle of women with polycystic ovary syndrome. Diabetes. (2006) 55:751–9. doi: 10.2337/diabetes.55.03.06.db05-0453
138. Rajkhowa M, Brett S, Cuthbertson DJ, Lipina C, Ruiz-Alcaraz AJ, Thomas GE, et al. Insulin resistance in polycystic ovary syndrome is associated with defective regulation of ERK1/2 by insulin in skeletal muscle in vivo. Biochem J. (2009) 418:665–71. doi: 10.1042/BJ20082176
139. Herman R, Sikonja J, Jensterle M, Janez A, Dolzan V. Insulin metabolism in polycystic ovary syndrome: secretion, signaling, and clearance. Int J Mol Sci. (2023) 24:3140. doi: 10.3390/ijms24043140
140. Stocco C. Tissue physiology and pathology of aromatase. Steroids. (2012) 77:27–35. doi: 10.1016/j.steroids.2011.10.013
141. González F, Rote NS, Minium J, Kirwan JP. Reactive oxygen species-induced oxidative stress in the development of insulin resistance and hyperandrogenism in polycystic ovary syndrome. J Clin Endocrinol Metab. (2006) 91:336–40. doi: 10.1210/jc.2005-1696
142. Luan YY, Zhang L, Peng YQ, Li YY, Liu RX, Yin CH. Immune regulation in polycystic ovary syndrome. Clin Chim Acta. (2022) 531:265–72. doi: 10.1016/j.cca.2022.04.234
143. Hu M, Zhang Y, Li X, Cui P, Sferruzzi-Perri AN, Brännström M, et al. TLR4-associated IRF-7 and NFκB signaling act as a molecular link between androgen and metformin activities and cytokine synthesis in the PCOS endometrium. J Clin Endocrinol Metab. (2021) 106:1022–40. doi: 10.1210/clinem/dgaa951
144. Cai J, Wu CH, Zhang Y, Wang YY, Xu WD, Lin TC, et al. High-free androgen index is associated with increased risk of non-alcoholic fatty liver disease in women with polycystic ovary syndrome, independent of obesity and insulin resistance. Int J Obes (Lond). (2017) 41:1341–7. doi: 10.1038/ijo.2017.116
145. Condorelli RA, Calogero AE, Di Mauro M, Mongioi’ LM, Cannarella R, Rosta G, et al. Androgen excess and metabolic disorders in women with PCOS: beyond the body mass index. J Endocrinol Invest. (2018) 41:383–8. doi: 10.1007/s40618-017-0762-3
146. Wang D, Weng Y, Zhang Y, Wang R, Wang T, Zhou J, et al. Exposure to hyperandrogen drives ovarian dysfunction and fibrosis by activating the NLRP3 inflammasome in mice. Sci Total Environ. (2020) 745:141049. doi: 10.1016/j.scitotenv.2020.141049
147. Li Y, Zheng Q, Sun D, Cui X, Chen S, Bulbul A, et al. Dehydroepiandrosterone stimulates inflammation and impairs ovarian functions of polycystic ovary syndrome. J Cell Physiol. (2019) 234:7435–47. doi: 10.1002/jcp.27501
148. González F, Sia CL, Bearson DM, Blair HE. Hyperandrogenism induces a proinflammatory TNFα response to glucose ingestion in a receptor-dependent fashion. J Clin Endocrinol Metab. (2014) 99:E848–54. doi: 10.1210/jc.2013-4109
149. González F, Nair KS, Daniels JK, Basal E, Schimke JM. Hyperandrogenism sensitizes mononuclear cells to promote glucose-induced inflammation in lean reproductive-age women. Am J Physiol Endocrinol Metab. (2012) 302:E297–306. doi: 10.1152/ajpendo.00416.2011
150. Fedotcheva TA, Fedotcheva NI, Shimanovsky NL. Progesterone as an anti-inflammatory drug and immunomodulator: new aspects in hormonal regulation of the inflammation. Biomolecules. (2022) 12. doi: 10.3390/biom12091299
151. Shah SB. COVID-19 and Progesterone: Part 2. Unraveling High Severity, Immunity Patterns, Immunity grading, Progesterone and its potential clinical use. Endocr Metab Sci. (2021) 5:100110. doi: 10.1016/j.endmts.2021.100110
152. Shah SB. COVID-19 and Progesterone: Part 1. SARS-CoV-2, Progesterone and its potential clinical use. Endocr Metab Sci. (2021) 5:100109. doi: 10.1016/j.endmts.2021.100109
153. Cai Z, Zhong J, Jiang Y, Zhang J. Associations between COVID-19 infection and sex steroid hormones. Front Endocrinol (Lausanne). (2022) 13:940675. doi: 10.3389/fendo.2022.940675
154. Mauvais-Jarvis F, Klein SL, Levin ER. Estradiol, progesterone, immunomodulation, and COVID-19 outcomes. Endocrinology. (2020) 161:bqaa127. doi: 10.1210/endocr/bqaa127
155. Lei K, Georgiou EX, Chen L, Yulia A, Sooranna SR, Brosens JJ, et al. Progesterone and the repression of myometrial inflammation: the roles of MKP-1 and the AP-1 system. Mol Endocrinol. (2015) 29:1454–67. doi: 10.1210/me.2015-1122
156. Cottrell JN, Witcher AC, Comley K, Cunningham MW Jr, Ibrahim T, Cornelius DC, et al. Progesterone-induced blocking factor improves blood pressure, inflammation, and pup weight in response to reduced uterine perfusion pressure (RUPP). Am J Physiol Regul Integr Comp Physiol. (2021) 320:R719–719R727. doi: 10.1152/ajpregu.00152.2020
157. Teraoka Y, Sugimoto J, Konishi H, Miyoshi H, Furusho H, Miyauchi M, et al. Progesterone suppresses uterine contraction by reducing odontogenic porphyromonas gingivalis induced chronic inflammation in mice. Biomolecules. (2022) 12. doi: 10.3390/biom12081029
158. Pei J, Liu Z, Wang C, Chu N, Liu L, Tang Y, et al. Progesterone attenuates SIRT1-deficiency-mediated pre-eclampsia. Biomolecules. (2022) 12. doi: 10.3390/biom12030422
159. Pařízek A, Koucký M, Dušková M. Progesterone, inflammation and preterm labor. J Steroid Biochem Mol Biol. (2014) 139:159–65. doi: 10.1016/j.jsbmb.2013.02.008
160. Nagy B, Szekeres-Barthó J, Kovács GL, Sulyok E, Farkas B, Várnagy Á, et al. Key to life: physiological role and clinical implications of progesterone. Int J Mol Sci. (2021) 22. doi: 10.3390/ijms222011039
161. Straub RH. The complex role of estrogens in inflammation. Endocr Rev. (2007) 28:521–74. doi: 10.1210/er.2007-0001
162. Trenti A, Tedesco S, Boscaro C, Trevisi L, Bolego C, Cignarella A. Estrogen, angiogenesis, immunity and cell metabolism: solving the puzzle. Int J Mol Sci. (2018) 19. doi: 10.3390/ijms19030859
163. Singh J, Wong H, Ahluwalia N, Go RM, Guerrero-Go MA. Metabolic, hormonal, immunologic, and genetic factors associated with the incidence of thyroid disorders in polycystic ovarian syndrome patients. Cureus. (2020) 12:e11681. doi: 10.7759/cureus.11681
164. Fernández L, Pannaraj PS, Rautava S, Rodríguez JM. The microbiota of the human mammary ecosystem. Front Cell Infect Microbiol. (2020) 10:586667. doi: 10.3389/fcimb.2020.586667
165. Liu R, Hong J, Xu X, Feng Q, Zhang D, Gu Y, et al. Gut microbiome and serum metabolome alterations in obesity and after weight-loss intervention. Nat Med. (2017) 23:859–68. doi: 10.1038/nm.4358
166. Qin J, Li Y, Cai Z, Li S, Zhu J, Zhang F, et al. A metagenome-wide association study of gut microbiota in type 2 diabetes. Nature. (2012) 490:55–60. doi: 10.1038/nature11450
167. Jie Z, Xia H, Zhong SL, Feng Q, Li S, Liang S, et al. The gut microbiome in atherosclerotic cardiovascular disease. Nat Commun. (2017) 8:845. doi: 10.1038/s41467-017-00900-1
168. Socała K, Doboszewska U, Szopa A, Serefko A, Włodarczyk M, Zielińska A, et al. The role of microbiota-gut-brain axis in neuropsychiatric and neurological disorders. Pharmacol Res. (2021) 172:105840. doi: 10.1016/j.phrs.2021.105840
169. Park EM, Chelvanambi M, Bhutiani N, Kroemer G, Zitvogel L, Wargo JA. Targeting the gut and tumor microbiota in cancer. Nat Med. (2022) 28:690–703. doi: 10.1038/s41591-022-01779-2
170. Lindheim L, Bashir M, Münzker J, Trummer C, Zachhuber V, Leber B, et al. Alterations in gut microbiome composition and barrier function are associated with reproductive and metabolic defects in women with polycystic ovary syndrome (PCOS): A pilot study. PloS One. (2017) 12:e0168390. doi: 10.1371/journal.pone.0168390
171. Liu R, Zhang C, Shi Y, Zhang F, Li L, Wang X, et al. Dysbiosis of gut microbiota associated with clinical parameters in polycystic ovary syndrome. Front Microbiol. (2017) 8:324. doi: 10.3389/fmicb.2017.00324
172. Torres PJ, Siakowska M, Banaszewska B, Pawelczyk L, Duleba AJ, Kelley ST, et al. Gut microbial diversity in women with polycystic ovary syndrome correlates with hyperandrogenism. J Clin Endocrinol Metab. (2018) 103:1502–11. doi: 10.1210/jc.2017-02153
173. Qi X, Yun C, Sun L, Xia J, Wu Q, Wang Y, et al. Gut microbiota-bile acid-interleukin-22 axis orchestrates polycystic ovary syndrome. Nat Med. (2019) 25:1225–33. doi: 10.1038/s41591-019-0509-0
174. Kim S, Jazwinski SM. The gut microbiota and healthy aging: A mini-review. Gerontology. (2018) 64:513–20. doi: 10.1159/000490615
175. Rizzetto L, Fava F, Tuohy KM, Selmi C. Connecting the immune system, systemic chronic inflammation and the gut microbiome: The role of sex. J Autoimmun. (2018) 92:12–34. doi: 10.1016/j.jaut.2018.05.008
176. Tremellen K, Pearce K. Dysbiosis of Gut Microbiota (DOGMA)–a novel theory for the development of Polycystic Ovarian Syndrome. Med Hypotheses. (2012) 79:104–12. doi: 10.1016/j.mehy.2012.04.016
177. Parker J, O’Brien C, Hawrelak J. A narrative review of the role of gastrointestinal dysbiosis in the pathogenesis of polycystic ovary syndrome. Obstet Gynecol Sci. (2022) 65:14–28. doi: 10.5468/ogs.21185
178. Han Q, Wang J, Li W, Chen ZJ, Du Y. Androgen-induced gut dysbiosis disrupts glucolipid metabolism and endocrinal functions in polycystic ovary syndrome. Microbiome. (2021) 9:101. doi: 10.1186/s40168-021-01046-5
179. Sherman SB, Sarsour N, Salehi M, Schroering A, Mell B, Joe B, et al. Prenatal androgen exposure causes hypertension and gut microbiota dysbiosis. Gut Microbes. (2018) 9:400–21. doi: 10.1080/19490976.2018.1441664
180. Moreno-Indias I, Sánchez-Alcoholado L, Sánchez-Garrido MÁ, Martín-Núñez GM, Pérez-Jiménez F, Tena-Sempere M, et al. Neonatal androgen exposure causes persistent gut microbiota dysbiosis related to metabolic disease in adult female rats. Endocrinology. (2016) 157:4888–98. doi: 10.1210/en.2016-1317
181. Yun C, Yan S, Liao B, Ding Y, Qi X, Zhao M, et al. The microbial metabolite agmatine acts as an FXR agonist to promote polycystic ovary syndrome in female mice. Nat Metab. (2024) 6:947–62. doi: 10.1038/s42255-024-01041-8
182. Arpaia N, Campbell C, Fan X, Dikiy S, van der Veeken J, deRoos P, et al. Metabolites produced by commensal bacteria promote peripheral regulatory T-cell generation. Nature. (2013) 504:451–5. doi: 10.1038/nature12726
183. Smith PM, Howitt MR, Panikov N, Michaud M, Gallini CA, Bohlooly-Y M, et al. The microbial metabolites, short-chain fatty acids, regulate colonic Treg cell homeostasis. Science. (2013) 341:569–73. doi: 10.1126/science.1241165
184. Haghikia A, Jörg S, Duscha A, Berg J, Manzel A, Waschbisch A, et al. Dietary fatty acids directly impact central nervous system autoimmunity via the small intestine. Immunity. (2015) 43:817–29. doi: 10.1016/j.immuni.2015.09.007
185. Liu K, He X, Huang J, Yu S, Cui M, Gao M, et al. Short-chain fatty acid-butyric acid ameliorates granulosa cells inflammation through regulating METTL3-mediated N6-methyladenosine modification of FOSL2 in polycystic ovarian syndrome. Clin Epigenetics. (2023) 15:86. doi: 10.1186/s13148-023-01487-9
186. Zhang FF, Barr SI, McNulty H, Li D, Blumberg JB. Health effects of vitamin and mineral supplements. BMJ. (2020) 369:m2511. doi: 10.1136/bmj.m2511
187. Irani M, Merhi Z. Role of vitamin D in ovarian physiology and its implication in reproduction: a systematic review. Fertil Steril. (2014) 102:460–8.e3. doi: 10.1016/j.fertnstert.2014.04.046
188. Bindayel IA. Low vitamin D level in saudi women with polycystic ovary syndrome. Front Nutr. (2021) 8:611351. doi: 10.3389/fnut.2021.611351
189. Ott J, Wattar L, Kurz C, Seemann R, Huber JC, Mayerhofer K, et al. Parameters for calcium metabolism in women with polycystic ovary syndrome who undergo clomiphene citrate stimulation: a prospective cohort study. Eur J Endocrinol. (2012) 166:897–902. doi: 10.1530/EJE-11-1070
190. Yildizhan R, Kurdoglu M, Adali E, Kolusari A, Yildizhan B, Sahin HG, et al. Serum 25-hydroxyvitamin D concentrations in obese and non-obese women with polycystic ovary syndrome. Arch Gynecol Obstet. (2009) 280:559–63. doi: 10.1007/s00404-009-0958-7
191. Hahn S, Haselhorst U, Tan S, Quadbeck B, Schmidt M, Roesler S, et al. Low serum 25-hydroxyvitamin D concentrations are associated with insulin resistance and obesity in women with polycystic ovary syndrome. Exp Clin Endocrinol Diabetes. (2006) 114:577–83. doi: 10.1055/s-2006-948308
192. He C, Lin Z, Robb SW, Ezeamama AE. Serum vitamin D levels and polycystic ovary syndrome: A systematic review and meta-analysis. Nutrients. (2015) 7:4555–77. doi: 10.3390/nu7064555
193. Davis EM, Peck JD, Hansen KR, Neas BR, Craig LB. Associations between vitamin D levels and polycystic ovary syndrome phenotypes. Minerva Endocrinol. (2019) 44:176–84. doi: 10.23736/S0391-1977.18.02824-9
194. Charoenngam N, Holick MF. Immunologic effects of vitamin D on human health and disease. Nutrients. (2020) 12:2097. doi: 10.3390/nu12072097
195. Ao T, Kikuta J, Ishii M. The effects of vitamin D on immune system and inflammatory diseases. Biomolecules. (2021) 11:1624. doi: 10.3390/biom11111624
196. Zhao JF, Li BX, Zhang Q. Vitamin D improves levels of hormonal, oxidative stress and inflammatory parameters in polycystic ovary syndrome: a meta-analysis study. Ann Palliat Med. (2021) 10:169–83. doi: 10.21037/apm-20-2201
197. Fang F, Ni K, Cai Y, Shang J, Zhang X, Xiong C. Effect of vitamin D supplementation on polycystic ovary syndrome: A systematic review and meta-analysis of randomized controlled trials. Complement Ther Clin Pract. (2017) 26:53–60. doi: 10.1016/j.ctcp.2016.11.008
198. Akbari M, Ostadmohammadi V, Lankarani KB, Tabrizi R, Kolahdooz F, Heydari ST, et al. The effects of vitamin D supplementation on biomarkers of inflammation and oxidative stress among women with polycystic ovary syndrome: A systematic review and meta-analysis of randomized controlled trials. Horm Metab Res. (2018) 50:271–9. doi: 10.1055/s-0044-101355
199. Selimoglu H, Duran C, Kiyici S, Ersoy C, Guclu M, Ozkaya G, et al. The effect of vitamin D replacement therapy on insulin resistance and androgen levels in women with polycystic ovary syndrome. J Endocrinol Invest. (2010) 33:234–8. doi: 10.1007/BF03345785
200. Huang Z, Rose AH, Hoffmann PR. The role of selenium in inflammation and immunity: from molecular mechanisms to therapeutic opportunities. Antioxid Redox Signal. (2012) 16:705–43. doi: 10.1089/ars.2011.4145
201. Hariharan S, Dharmaraj S. Selenium and selenoproteins: it’s role in regulation of inflammation. Inflammopharmacology. (2020) 28:667–95. doi: 10.1007/s10787-020-00690-x
202. Hajizadeh-Sharafabad F, Moludi J, Tutunchi H, Taheri E, Izadi A, Maleki V. Selenium and polycystic ovary syndrome; current knowledge and future directions: A systematic review. Horm Metab Res. (2019) 51:279–87. doi: 10.1055/a-0890-6823
203. Lei C, Niu X, Wei J, Zhu J, Zhu Y. Interaction of glutathione peroxidase-1 and selenium in endemic dilated cardiomyopathy. Clin Chim Acta. (2009) 399:102–8. doi: 10.1016/j.cca.2008.09.025
204. Cox AJ, Lehtinen AB, Xu J, Langefeld CD, Freedman BI, Carr JJ, et al. Polymorphisms in the Selenoprotein S gene and subclinical cardiovascular disease in the Diabetes Heart Study. Acta Diabetol. (2013) 50:391–9. doi: 10.1007/s00592-012-0440-z
205. Talbi W, Ghazouani T, Braconi D, Ben Abdallah R, Raboudi F, Santucci A, et al. Effects of selenium on oxidative damage and antioxidant enzymes of eukaryotic cells: wine Saccharomyces cerevisiae. J Appl Microbiol. (2019) 126:555–66. doi: 10.1111/jam.14150
206. Barchielli G, Capperucci A, Tanini D. The role of selenium in pathologies: an updated review. Antioxidants (Basel). (2022) 11:251. doi: 10.3390/antiox11020251
207. Butt MA, Shafique HM, Mustafa M, Moghul NB, Munir A, Shamas U, et al. Therapeutic potential of selenium nanoparticles on letrozole-induced polycystic ovarian syndrome in female wistar rats. Biol Trace Elem Res. (2023) 201:5213–29. doi: 10.1007/s12011-023-03579-2
208. Rabah HM, Mohamed DA, Mariah RA, Abd El-Khalik SR, Khattab HA, AbuoHashish NA, et al. Novel insights into the synergistic effects of selenium nanoparticles and metformin treatment of letrozole - induced polycystic ovarian syndrome: targeting PI3K/Akt signalling pathway, redox status and mitochondrial dysfunction in ovarian tissue. Redox Rep. (2023) 28:2160569. doi: 10.1080/13510002.2022.2160569
209. Abdallah A, El-Ghannam MA, Hasan AA, Mohammad LG, Mesalam NM, Alsayed RM. Selenium nanoparticles modulate steroidogenesis-related genes and improve ovarian functions via regulating androgen receptors expression in polycystic ovary syndrome rat model. Biol Trace Elem Res. (2023) 201:5721–33. doi: 10.1007/s12011-023-03616-0
210. Zhao J, Dong L, Lin Z, Sui X, Wang Y, Li L, et al. Effects of selenium supplementation on Polycystic Ovarian Syndrome: a systematic review and meta-analysis on randomized clinical trials. BMC Endocr Disord. (2023) 23:33. doi: 10.1186/s12902-023-01286-6
211. Elci E, Kaya C, Cim N, Yildizhan R, Elci GG. Evaluation of cardiac risk marker levels in obese and non-obese patients with polycystic ovaries. Gynecol Endocrinol. (2017) 33:43–7. doi: 10.1080/09513590.2016.1203893
212. Oktem M, Ozcimen EE, Uckuyu A, Esinler I, Pamuk B, Bayraktar N, et al. Polycystic ovary syndrome is associated with elevated plasma soluble CD40 ligand, a marker of coronary artery disease. Fertil Steril. (2009) 91:2545–50. doi: 10.1016/j.fertnstert.2008.03.039
213. Schini-Kerth VB. Homocysteine, a proinflammatory and proatherosclerotic factor: role of intracellular reactive oxygen species. Circ Res. (2003) 93:271–3. doi: 10.1161/01.RES.0000089561.87997.CF
214. Zeng X, Dai J, Remick DG, Wang X. Homocysteine mediated expression and secretion of monocyte chemoattractant protein-1 and interleukin-8 in human monocytes. Circ Res. (2003) 93:311–20. doi: 10.1161/01.RES.0000087642.01082.E4
215. Poddar R, Sivasubramanian N, DiBello PM, Robinson K, Jacobsen DW. Homocysteine induces expression and secretion of monocyte chemoattractant protein-1 and interleukin-8 in human aortic endothelial cells: implications for vascular disease. Circulation. (2001) 103:2717–23. doi: 10.1161/01.cir.103.22.2717
216. Zhang HS, Cao EH, Qin JF. Intracellular redox status modulates monocyte chemoattractant protein-1 expression stimulated by homocysteine in endothelial cells. J Cardiovasc Pharmacol. (2003) 42:258–65. doi: 10.1097/00005344-200308000-00016
217. Georgakis MK, van der Laan SW, Asare Y, Mekke JM, Haitjema S, Schoneveld AH, et al. Monocyte-chemoattractant protein-1 levels in human atherosclerotic lesions associate with plaque vulnerability. Arterioscler Thromb Vasc Biol. (2021) 41:2038–48. doi: 10.1161/ATVBAHA.121.316091
218. Liu Y, Hultén LM, Wiklund O. Macrophages isolated from human atherosclerotic plaques produce IL-8, and oxysterols may have a regulatory function for IL-8 production. Arterioscler Thromb Vasc Biol. (1997) 17:317–23. doi: 10.1161/01.atv.17.2.317
219. Zhang B, Qi X, Zhao Y, Li R, Zhang C, Chang HM, et al. Elevated CD14(++)CD16(+) monocytes in hyperhomocysteinemia-associated insulin resistance in polycystic ovary syndrome. Reprod Sci. (2018) 25:1629–36. doi: 10.1177/1933719118756772
220. Mondal K, Chakraborty P, Kabir SN. Hyperhomocysteinemia and hyperandrogenemia share PCSK9-LDLR pathway to disrupt lipid homeostasis in PCOS. Biochem Biophys Res Commun. (2018) 503:8–13. doi: 10.1016/j.bbrc.2018.04.078
221. Qi X, Zhang B, Zhao Y, Li R, Chang HM, Pang Y, et al. Hyperhomocysteinemia promotes insulin resistance and adipose tissue inflammation in PCOS mice through modulating M2 macrophage polarization via estrogen suppression. Endocrinology. (2017) 158:1181–93. doi: 10.1210/en.2017-00039
222. Teede HJ, Misso ML, Deeks AA, Moran LJ, Stuckey BG, Wong JL, et al. Assessment and management of polycystic ovary syndrome: summary of an evidence-based guideline. Med J Aust. (2011) 195:S65–112. doi: 10.5694/mja11.10915
223. Magagnini MC, Condorelli RA, Cimino L, Cannarella R, Aversa A, Calogero AE, et al. Does the ketogenic diet improve the quality of ovarian function in obese women. Nutrients. (2022) 14:4147. doi: 10.3390/nu14194147
224. Barrea L, Arnone A, Annunziata G, Muscogiuri G, Laudisio D, Salzano C, et al. Adherence to the mediterranean diet, dietary patterns and body composition in women with polycystic ovary syndrome (PCOS). Nutrients. (2019) 11:2278. doi: 10.3390/nu11102278
225. Li C, Xing C, Zhang J, Zhao H, Shi W, He B. Eight-hour time-restricted feeding improves endocrine and metabolic profiles in women with anovulatory polycystic ovary syndrome. J Transl Med. (2021) 19:148. doi: 10.1186/s12967-021-02817-2
226. Alesi S, Ee C, Moran LJ, Rao V, Mousa A. Nutritional supplements and complementary therapies in polycystic ovary syndrome. Adv Nutr. (2022) 13:1243–66. doi: 10.1093/advances/nmab141
227. Bahmani F, Karamali M, Shakeri H, Asemi Z. The effects of folate supplementation on inflammatory factors and biomarkers of oxidative stress in overweight and obese women with polycystic ovary syndrome: a randomized, double-blind, placebo-controlled clinical trial. Clin Endocrinol (Oxf). (2014) 81:582–7. doi: 10.1111/cen.12451
228. Talari HR, Poladchang S, Hamidian Y, Samimi M, Gilasi HR, Ebrahimi FA, et al. The effects of omega-3 and vitamin E co-supplementation on carotid intima-media thickness and inflammatory factors in patients with polycystic ovary syndrome. Oman Med J. (2018) 33:473–9. doi: 10.5001/omj.2018.88
229. Stracquadanio M, Ciotta L, Palumbo MA. Effects of myo-inositol, gymnemic acid, and L-methylfolate in polycystic ovary syndrome patients. Gynecol Endocrinol. (2018) 34:495–501. doi: 10.1080/09513590.2017.1418852
230. Hager M, Nouri K, Imhof M, Egarter C, Ott J. The impact of a standardized micronutrient supplementation on PCOS-typical parameters: a randomized controlled trial. Arch Gynecol Obstet. (2019) 300:455–60. doi: 10.1007/s00404-019-05194-w
231. Teede HJ, Misso ML, Costello MF, Dokras A, Laven J, Moran L, et al. Recommendations from the international evidence-based guideline for the assessment and management of polycystic ovary syndrome. Fertil Steril. (2018) 110:364–79. doi: 10.1016/j.fertnstert.2018.05.004
232. Hafizi Moori M, Nosratabadi S, Yazdi N, Kasraei R, Abbasi Senjedary Z, Hatami R. The effect of exercise on inflammatory markers in PCOS women: A systematic review and meta-analysis of randomized trials. Int J Clin Pract. (2023) 2023:3924018. doi: 10.1155/2023/3924018
233. Patten RK, McIlvenna LC, Levinger I, Garnham AP, Shorakae S, Parker AG, et al. High-intensity training elicits greater improvements in cardio-metabolic and reproductive outcomes than moderate-intensity training in women with polycystic ovary syndrome: a randomized clinical trial. Hum Reprod. (2022) 37:1018–29. doi: 10.1093/humrep/deac047
234. Tang T, Lord JM, Norman RJ, Yasmin E, Balen AH. Insulin-sensitising drugs (metformin, rosiglitazone, pioglitazone, D-chiro-inositol) for women with polycystic ovary syndrome, oligo amenorrhoea and subfertility. Cochrane Database Syst Rev. (2012), CD003053. doi: 10.1002/14651858.CD003053.pub5
235. Xue J, Li X, Liu P, Li K, Sha L, Yang X, et al. Inulin and metformin ameliorate polycystic ovary syndrome via anti-inflammation and modulating gut microbiota in mice. Endocr J. (2019) 66:859–70. doi: 10.1507/endocrj.EJ18-0567
236. Wang Y, Xiao H, Liu Y, Tong Q, Yu Y, Qi B, et al. Effects of Bu Shen Hua Zhuo formula on the LPS/TLR4 pathway and gut microbiota in rats with letrozole-induced polycystic ovary syndrome. Front Endocrinol (Lausanne). (2022) 13:891297. doi: 10.3389/fendo.2022.891297
237. Zhu Y, Li Y, Liu M, Hu X, Zhu H. Guizhi fuling wan, chinese herbal medicine, ameliorates insulin sensitivity in PCOS model rats with insulin resistance via remodeling intestinal homeostasis. Front Endocrinol (Lausanne). (2020) 11:575. doi: 10.3389/fendo.2020.00575
238. Chang ZP, Deng GF, Shao YY, Xu D, Zhao YN, Sun YF, et al. Shaoyao-gancao decoction ameliorates the inflammation state in polycystic ovary syndrome rats via remodeling gut microbiota and suppressing the TLR4/NF-κB pathway. Front Pharmacol. (2021) 12:670054. doi: 10.3389/fphar.2021.670054
239. Teede HJ, Tay CT, Laven J, Dokras A, Moran LJ, Piltonen TT, et al. Recommendations from the 2023 international evidence-based guideline for the assessment and management of polycystic ovary syndrome. J Clin Endocrinol Metab. (2023) 108:2447–69. doi: 10.1210/clinem/dgad463
240. Alesi S, Forslund M, Melin J, Romualdi D, Peña A, Tay CT, et al. Efficacy and safety of anti-androgens in the management of polycystic ovary syndrome: a systematic review and meta-analysis of randomised controlled trials. EClinicalMedicine. (2023) 63:102162. doi: 10.1016/j.eclinm.2023.102162
241. Areloegbe SE, Peter MU, Oyeleke MB, Olaniyi KS. Low-dose spironolactone ameliorates adipose tissue inflammation and apoptosis in letrozole-induced PCOS rat model. BMC Endocr Disord. (2022) 22:224. doi: 10.1186/s12902-022-01143-y
242. Liu W, Li S, Lou X, Li D, Wang F, Zhang Z. Assessment of neutrophil to lymphocyte ratio, C-reactive protein, mean platelet volume in obese, and nonobese patients with polycystic ovary syndrome. Med (Baltimore). (2022) 101:e29678. doi: 10.1097/MD.0000000000029678
243. Vasyukova E, Zaikova E, Kalinina O, Gorelova I, Pyanova I, Bogatyreva E, et al. Inflammatory and anti-inflammatory parameters in PCOS patients depending on body mass index: A case-control study. Biomedicines. (2023) 11:2791. doi: 10.3390/biomedicines11102791
244. Elbandrawy AM, Yousef AM, Morgan EN, Ewais NF, Eid MM, Elkholi SM, et al. Effect of aerobic exercise on inflammatory markers in polycystic ovary syndrome: a randomized controlled trial. Eur Rev Med Pharmacol Sci. (2022) 26:3506–13. doi: 10.26355/eurrev_202205_28845
245. Makedos A, Goulis DG, Papanikolaou A, Panidis D. Serum high-sensitivity C-reactive protein and homocysteine changes during hormonal therapy in women with polycystic ovary syndrome: a prospective, matched study. Angiology. (2010) 61:595–601. doi: 10.1177/0003319709361198
246. Xiao N, Wang J, Wang T, Xiong X, Zhou J, Su X, et al. Metformin abrogates pathological TNF-α-producing B cells through mTOR-dependent metabolic reprogramming in polycystic ovary syndrome. Elife. (2022) 11:e74713. doi: 10.7554/eLife.74713
247. Ala M, Ala M. Metformin for cardiovascular protection, inflammatory bowel disease, osteoporosis, periodontitis, polycystic ovarian syndrome, neurodegeneration, cancer, inflammation and senescence: what is next. ACS Pharmacol Transl Sci. (2021) 4:1747–70. doi: 10.1021/acsptsci.1c00167
248. Froment P, Plotton I, Giulivi C, Khoueiry R, Mourad NI, Horman S, et al. At the crossroads of fertility and metabolism: the importance of AMPK-dependent signaling in female infertility associated with hyperandrogenism. Hum Reprod. (2022) 37:1207–28. doi: 10.1093/humrep/deac067
249. Duleba AJ. Medical management of metabolic dysfunction in PCOS. Steroids. (2012) 77:306–11. doi: 10.1016/j.steroids.2011.11.014
250. Heidari H, Hajhashemy Z, Saneei P. A meta-analysis of effects of vitamin E supplementation alone and in combination with omega-3 or magnesium on polycystic ovary syndrome. Sci Rep. (2022) 12:19927. doi: 10.1038/s41598-022-24467-0
251. Fulghesu AM, Ciampelli M, Muzj G, Belosi C, Selvaggi L, Ayala GF, et al. N-acetyl-cysteine treatment improves insulin sensitivity in women with polycystic ovary syndrome. Fertil Steril. (2002) 77:1128–35. doi: 10.1016/s0015-0282(02)03133-3
252. Advani K, Batra M, Tajpuriya S, Gupta R, Saraswat A, Nagar HD, et al. Efficacy of combination therapy of inositols, antioxidants and vitamins in obese and non-obese women with polycystic ovary syndrome: an observational study. J Obstet Gynaecol. (2020) 40:96–101. doi: 10.1080/01443615.2019.1604644
253. Thakker D, Raval A, Patel I, Walia R. N-acetylcysteine for polycystic ovary syndrome: a systematic review and meta-analysis of randomized controlled clinical trials. Obstet Gynecol Int. (2015) 2015:817849. doi: 10.1155/2015/817849
254. Greff D, Juhász AE, Váncsa S, Váradi A, Sipos Z, Szinte J, et al. Inositol is an effective and safe treatment in polycystic ovary syndrome: a systematic review and meta-analysis of randomized controlled trials. Reprod Biol Endocrinol. (2023) 21:10. doi: 10.1186/s12958-023-01055-z
255. Di Tucci C, Di Feliciantonio M, Vena F, Capone C, Schiavi MC, Pietrangeli D, et al. Alpha lipoic acid in obstetrics and gynecology. Gynecol Endocrinol. (2018) 34:729–33. doi: 10.1080/09513590.2018.1462320
256. Giampaolino P, Foreste V, Di Filippo C, Gallo A, Mercorio A, Serafino P, et al. Microbiome and PCOS: state-of-art and future aspects. Int J Mol Sci. (2021) 22:2048. doi: 10.3390/ijms22042048
Keywords: chronic inflammation, intrinsic mechanisms, intestinal microecological, steroid hormones, polycystic ovary syndrome
Citation: Deng H, Chen Y, Xing J, Zhang N and Xu L (2024) Systematic low-grade chronic inflammation and intrinsic mechanisms in polycystic ovary syndrome. Front. Immunol. 15:1470283. doi: 10.3389/fimmu.2024.1470283
Received: 25 July 2024; Accepted: 03 December 2024;
Published: 19 December 2024.
Edited by:
Vijay Kumar, Morehouse School of Medicine, United StatesReviewed by:
Venkateswarlu Kanamarlapudi, Swansea University Medical School, United KingdomCopyright © 2024 Deng, Chen, Xing, Zhang and Xu. This is an open-access article distributed under the terms of the Creative Commons Attribution License (CC BY). The use, distribution or reproduction in other forums is permitted, provided the original author(s) and the copyright owner(s) are credited and that the original publication in this journal is cited, in accordance with accepted academic practice. No use, distribution or reproduction is permitted which does not comply with these terms.
*Correspondence: Liangzhi Xu, eHVsaWFuZ3poaUBzY3UuZWR1LmNu; Nannan Zhang, bmFubmFuNzY4N0BzY3UuZWR1LmNu
†These authors have contributed equally to this work
Disclaimer: All claims expressed in this article are solely those of the authors and do not necessarily represent those of their affiliated organizations, or those of the publisher, the editors and the reviewers. Any product that may be evaluated in this article or claim that may be made by its manufacturer is not guaranteed or endorsed by the publisher.
Research integrity at Frontiers
Learn more about the work of our research integrity team to safeguard the quality of each article we publish.