- 1Unit of Geriatric Medicine, Italian National Research Center on Aging (IRCCS INRCA), Cosenza, Italy
- 2Centre for Biostatistics and Applied Geriatric Clinical Epidemiology, Italian National Research Center on Aging (IRCCS INRCA), Ancona, Italy
- 3Independent Researcher, Messina, Italy
- 4Independent Researcher, Cosenza, Italy
- 5Territorial Office of Messina, Ministry of Health, Messina, Italy
- 6Department of Clinical and Experimental Medicine, Unit of Neurology and Neuromuscular Diseases, University of Messina, Messina, Italy
- 7Department of Pharmacy, Health and Nutritional Sciences, School of Medicine and Digital Technologies, University of Calabria, Arcavacata di Rende, Italy
- 8Dipartimento di Prevenzione, ASP di Cosenza, Cosenza, Italy
- 9Centre for Biostatistics and Applied Geriatric Clinical Epidemiology, Italian National Research Center on Aging (IRCCS INRCA), Cosenza, Italy
- 10Section of Neurology, Italian National Research Center on Aging (IRCCS INRCA), Ancona, Italy
Immunosenescence refers to the age-related progressive decline of immune function contributing to the increased susceptibility to infectious diseases in older people. Neurocryptococcosis, an infectious disease of central nervous system (CNS) caused by Cryptococcus neoformans (C. Neoformans) and C. gattii, has been observed with increased frequency in aged people, as result of the reactivation of a latent infection or community acquisition. These opportunistic microorganisms belonging to kingdom of fungi are capable of surviving and replicating within macrophages. Typically, cryptococcus is expelled by vomocytosis, a non-lytic expulsive mechanism also promoted by interferon (IFN)-I, or by cell lysis. However, whereas in a first phase cryptococcal vomocytosis leads to a latent asymptomatic infection confined to the lung, an enhancement in vomocytosis, promoted by IFN-I overproduction, can be deleterious, leading the fungus to reach the blood stream and invade the CNS. Cryptococcus may not be easy to diagnose in older individuals and, if not timely treated, could be potentially lethal. Therefore, this review aims to elucidate the putative causes of the increased incidence of cryptococcal CNS infection in older people discussing in depth the mechanisms of immunosenscence potentially able to predispose to neurocryptococcosis, laying the foundations for future research. A deepest understanding of this relationship could provide new ways to improve the prevention and recognition of neurocryptococcosis in aged frail people, in order to quickly manage pharmacological interventions and to adopt further preventive measures able to reduce the main risk factors.
1 Introduction
Aging is a gradual and irreversible physiological process characterized by declines in tissue and cell functions, thus significantly increasing the risks for chronic diseases and concomitant disabilities and comorbidities (1). Immune function also decreases with age, due to a condition known as immunosenescence, which affects both natural and acquired immunity and puts older adults at increased risk of developing infections with a more severe and protracted course (2, 3). With advancing age and especially beyond the sixth decade of life, the human immune system undergoes aging-related dysregulated processes, associated with persistent low-grade inflammation, termed “inflammaging”, that involves multiple immune and non-immune cell types (3–5).
This aging-associated basal inflammation is thought to be induced by several factors, including the reactivation of latent infections and the engagement of pathogen-associated molecular patterns (PAMPs) and/or endogenous damage-associated molecular patterns (DAMPs), together with a dysregulation of specific host pattern recognition receptors (PRRs), among which the Toll-like receptors (TLRs) play a major role (3).
The dysfunctional immune response associated with both immunosenescence and inflammaging, responsible for a dysregulation of cytokine production and a persistent low-grade immune activation, may worsen the tissue damage caused by recurrent infections often affecting older subjects (6). Moreover, immunosenescence, frailty and comorbidities are conditions frequently associated with increased susceptibility to opportunistic infections, including fungal microorganisms, which usually become virulent with immunocompromised and unhealthy individuals (7–14).
Among the opportunistic infections affecting the central nervous system (CNS), meningitis and meningoencephalitis caused by Cryptococcus neoformans and C. gattii, also indicated as neurocryptococcosis, have been observed with increased frequency in HIV-seronegative individuals > 65 years, as result of a reactivation of latent infection, or community acquisition (15).
As documented by serological and epidemiological studies, the natural exposure to Cryptococcus sp. is very common in humans, despite overt clinical manifestations of disease are rare (16).
Protective immunity against cryptococcal yeasts is dependent on recognition, control, and proper interaction by and with cells of the innate and acquired immune response. In immunocompetent humans, the yeast, entered by inhalation and reached the lung alveoli, may be either completely cleared from the respiratory tract or establish a latent asymptomatic infection in pulmonary granuloma or thoracic lymph nodes. Depending on the host immune status and fungal virulence, cryptococcal organisms may cause progressive granulomatous inflammation, or form parenchyma granulomatous masses, known as cryptococcomas, very common in the lungs and brain of immunocompetent individuals (17, 18).
As with other organ systems, the CNS vulnerability to infectious agents increases with aging, and circulating microorganisms can invade the CNS, both by crossing the blood-brain barrier (BBB), or through transneuronal routes, causing infections in the meningeal or parenchymal compartments (15).
From a general point of view, the cryptococcal disease is favored by the presence of qualitative and/or quantitative alterations of immune system (e.g. HIV disease-related or drug-induced immune dysfunctions), even if CNS infection by cryptococcus may sometimes occur also in immunocompetent individuals (19). Although often overlooked, neurocryptococcosis is extremely risky in older people, due to a hardly diagnosis and its rapidly fatal disease course without prompt treatment; moreover, the incidence of neurocryptococcosis is expected to increase with the growing trend of aged people. While progresses have been made in understanding this disease in people living with HIV, data on older populations are scarce. To the best of our knowledge, this is the first review to examine the relationship between the senescence of innate immune responses and the development of cryptococcal meningitis in the elderly.
The connections among aging, immunosenescence, and neurocryptococcosis may indeed contribute to explain the increased risk of neurocryptococcosis in aged people (15, 20–22). In light of this, the purpose of this review is to identify mechanisms of susceptibility to cryptococcal meningitis and meningoencephalitis, with emphasis on the potential role of immunosenescence in innate immune cells. A deepest understanding of this relationship could lay the foundation for future research and provide new ways to improve the prevention and recognition of neurocryptococcosis in aged frail people, in order to quickly manage pharmacological interventions and to adopt further preventive measures able to reduce the main risk factors.
2 Neurocryptococcosis and aging: epidemiological evidence
Cryptococcal organisms are facultative intracellular pathogens which may commonly cause an invasive mycosis in immunocompromised individuals. Among the more of 30 Cryptococcus species, C. neoformans and C. gatti are closely related strains that cause respiratory and neurological diseases in humans and animals (23, 24). C. neoformans, which has a ubiquitous worldwide distribution, represents a common cause of meningitis in immunocompromised hosts (23). In contrast, C. gattii, which is geographically restricted to tropical and subtropical regions and is found less frequently in temperate regions, causes disease in both immunocompetent and immunocompromised hosts (25).
The infection, initially occurring in the lungs, upon inhalation of infective particles from the environmental source, is characterized by a wide array of clinical presentations. The fungal organisms can avoid the mucociliary clearance, and directly reach the alveolar spaces, where they are phagocyted by the lung tissue-resident immune cells. In healthy immunocompetent hosts, these fungi generally can either be successfully cleared or establish long-term, latent infections (26). In most cases, despite the exposure to cryptococcal cells is common, the development of symptomatic disease is rare and usually requires immunosuppression (24, 27), since the innate immune system is generally sufficient to limit the cryptococcal infection. However, in some subjects and often months or years after the exposure, the inhaled fungus can escape the host’s defense mechanisms and disseminate from the lungs to the blood and invade the CNS, inducing likely fatal meningoencephalitis (28).
While there are several studies on cryptococcosis in immunocompromised hosts as people living with HIV, there are little data available to understand the presentation and the management of the cryptococcal infection in HIV-negative people (29–31). Generally, among patients with cryptococcosis, HIV-negative people are older than people living with HIV (32, 33). For instance, in a recent study, the median age of cryptococcal disease is 40 years in HIV people, 53 in non HIV transplanted people, and 61 in non-HIV people without history of transplantation (32). Different studies reported an higher mortality rate on HIV negative cohort compared to HIV-infected group (32, 34–37). Specifically, in a study by George et al. focused on the different epidemiology and outcomes between HIV and non-HIV patients with cryptococcosis, the authors found a lower median age (43.8 vs 58 years, p<0.001) and a lower overall mortality rate (25 vs 33.2%, p <0.001) in HIV compared to non- HIV subjects. These data support the importance of improving both diagnosis of cryptococcosis and therapeutic approach in HIV-negative patients.
This is a very relevant issue, also considering that incidence of neurocryptococcosis is increasing in HIV-negative people and in particular in the older adults (38). A recent population-based retrospective study comparing people living with HIV and HIV-negative people affected by meningoencephalitis have shown that up to 66.7% of HIV-negative patients were older than 50 years old. Furthermore, age >50 years and multiple chronic conditions were associated with higher mortality (30, 39). Another population-based study reported an increased rate of cryptococcal meningitis in the age group of 60-69 years, with a peak between 70 and 79 years (40).
Epidemiological evidence suggests that older patients (≥ 65 years), and especially those with underlying medical conditions, are more vulnerable to neurocryptococcosis than adults aged < 65 years (15, 22, 34, 36) and statistical analyses highlighted that age>60 years is a predictor of mortality (34, 41–43). In addition to older age, also impaired consciousness, hemodialysis, and previous corticosteroid usage have been associated with poor prognosis in HIV-negative patients with cryptococcal meningitis and could predict the higher mortality observed in this population compared with HIV patients (44). Furthermore, the not always overt presentation of CNS infection commonly displayed by elderly individuals makes it difficult to obtain an early diagnosis and to allow a timely therapy, thus potentially affecting the prognosis in a negative way (8, 45, 46).
Recent systematic studies focused on the identification of the risk factors mainly related to C. neoformans and/or C. gattii infections (47). According to these investigations, in addition to HIV-infected patients, the main groups of HIV-negative subjects at risk of a cryptococcal colonization and/or infection were aged people affected by immune dysregulation, lung dysfunction, kidney disease, cirrhosis, arthritis, diabetes, tuberculosis, connective tissue disorders, and patients receiving immunosuppressive therapies (47, 48). Other less commonly reported comorbidities associated to cryptococcosis are autoimmune disorders and sarcoidosis (47, 49–51).
Therefore, older age and the presence of chronic diseases are consistently associated with an increased risk of neurocryptococcosis. Nevertheless, a comparative analysis of healthy older people versus frail older people is lacking and should be further investigated. Moreover, the condition of frailty is never taken into account in any study.
3 Neurocryptococcosis and mechanisms of immune escape: the role of innate immunity
It is hypothesized that humans encounter the organism early in life, as shown by the gradual increase of cryptococcal-specific antibody response detected in humans with age (52). As most immunocompetent humans are asymptomatic and resolve the infection, there are limited observations concerning the mechanisms leading to cryptococcal clearance. However, the asymptomatic cryptococcal antigenemia, detected in the serum of HIV-infected people without signs or symptoms of meningitis or sepsis, indicates a relevant incidence of disseminated cryptococcal infection, at least in these subjects (53–55).
The natural history of human infection follows three steps: primary infection, followed by a silent phase of latency, that can last for years, and a last step consisting in the reactivation of dormant/latent fungal infection, associated with development of symptomatic disease, usually occurring in immune dysregulation conditions. Primary cryptococcosis, that initiates with lung involvement, can occur both in immunocompetent and immunocompromised hosts, as shown in Figure 1, and is triggered by inhalation of dehydrated spores, with subsequent phagocytosis of Cryptococcus by alveolar macrophages (56). The silent phase of latency can be characterized by the complete clearance of the fungus, or a latency of the disease, with the presence of the fungus in subpleural nodules and draining lymph nodes (57, 58). In any case, the survival inside macrophages and granuloma formation appears to be the predominant in latency stages (59). The reactivation, that may occur after the latency stage, is mainly dependent from two conditions: dormancy/survival of the fungi and immune dysregulation of the host, with subsequent development of secondary cryptococcosis.
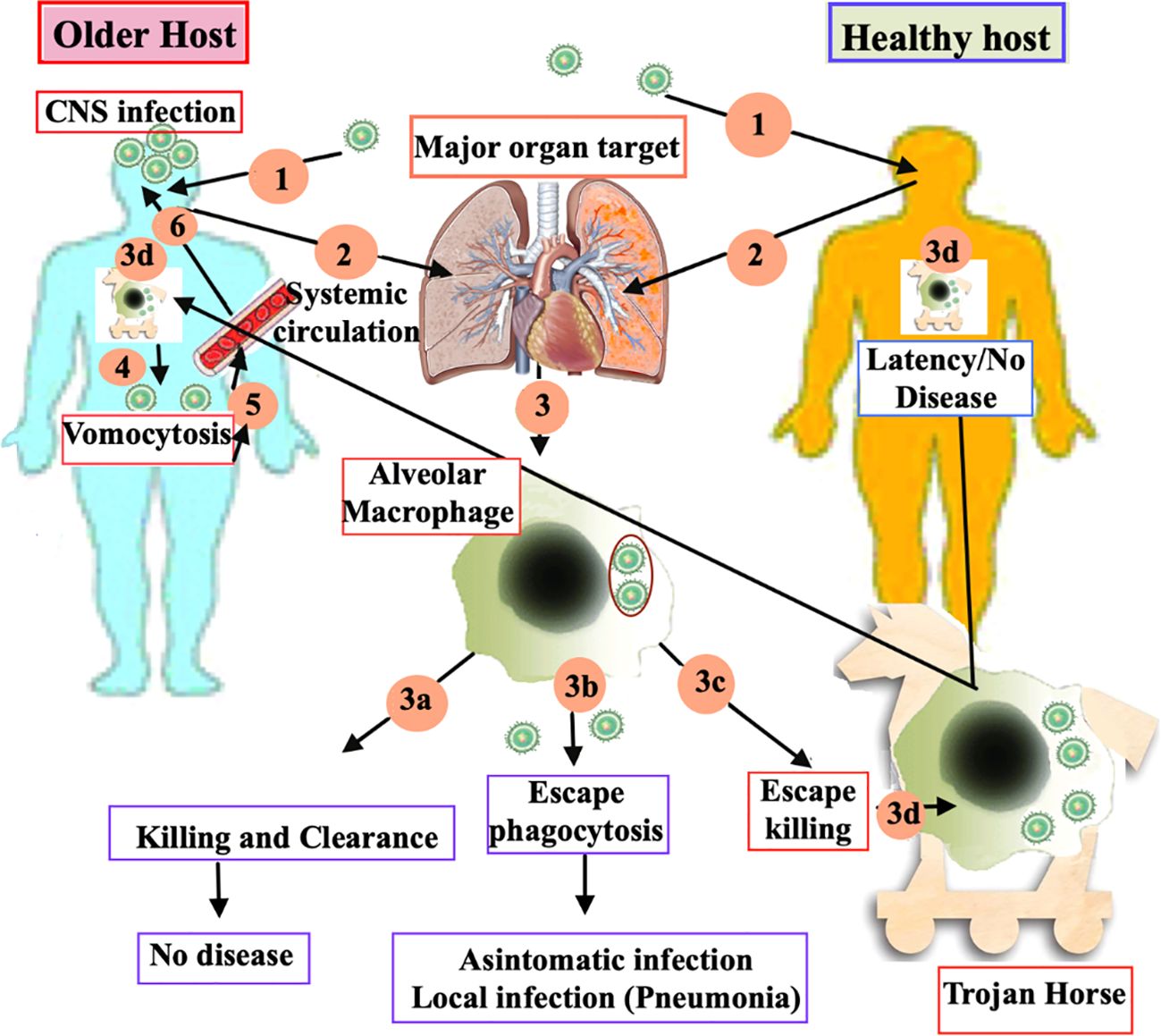
Figure 1. Current model of the immune response to Cryptococcus in older and young health host. (1) Primary cryptococcosis stars when dehydrated spores are inhaled into the lungs of both immunocompetent and immunocompromised older hosts. This is followed by the phagocytosis of the spores by the alveolar macrophages. (2)This activation triggers three different immune-responses of macrophage: (3a) successful killing and clearance, resulting in no disease; (3b) evasion of phagocytosis, leading to either an asymptomatic state or localized infection, such as pneumonia; and (3c) survival within the macrophages through evasion mechanisms, often likened to “Trojan horses” (3d). During the latency stages, survival within macrophages and granuloma formation tends to be the predominant response. (4) In older hosts, reactivation occurs more frequently due to immune dysregulation. The spread of the pathogen beyond the respiratory system via vomocytosis leads to secondary cryptococcosis. (5) Cryptococcal cells, ejected through vomocytosis, enter the bloodstream and disseminate, resulting in a blood infection called cryptococcaemia. (6) After crossing the blood brain barrier, Cryptococcus migrates to the brain parenchyma and starts to proliferate, leading to fatal meningoencephalitis.
Cryptococci can escape immune system mechanisms at several stages. One of the most important innate immune cells involved in fungal clearance are represented by dendritic cells and neutrophils; the binding of cryptococcal antigens to innate immune receptors expressed by lung dendritic cells induces the synthesis of specific cytokines able to stimulate macrophage polarization into M1 or M2 phenotypes, which guide the clinical outcome of lung infection (60); indeed, M1 cells are able to produce proinflammatory cytokines in large amount and are responsible of fungal clearance, in contrast to anti-inflammatory M2 cells which allow intracellular fungal replication; activated eosinophils contribute to macrophage polarization into M1 and cryptococcal clearance. However, multiple virulence factors, including polysaccharide capsule, melanin, and fungal proteins, allow the fungus to evade M1-mediated phagocytosis and further replicate within M2 cells (61).
Neutrophils are also important in the immune host response against the pathogen; Rocha et al. showed that neutrophils can form the neutrophil extracellular trap (NET) consisting of chromatin, cytosolic and granular proteins, in order to retain and kill the fungus (62); however, the glucuronoxylomannan capsule of C. neoformans may help the pathogen block the NET production and neutrophil migration. Indeed, in people with immune system dysfunctions, Cryptococcus may use escape mechanisms that favor its latent persistence in the lungs thanks to cell masking, N-glucan structures, and production of several enzymes and transcription factors (56).
In any case, prolonged persistence and replication of Cryptococcus within immune cells may further promote its dissemination outside of the respiratory system; indeed, the fungus uses phagocytes as reservoirs to replicate within and then be transported in the bloodstream, through a process of dissemination known as the “Trojan horse” (63). The phagocyted microorganisms, following intracellular replication, can be expelled by immune cells by both vomocytosis, that represents a mechanism of non-lytic exocytosis, and cell lysis (or rupture), due to excessive intracellular proliferation (27, 64). Vomocytosis can be also followed by phagocytosis by nearby immune cells, through a process considered a new escape mechanism and called dragotcytosis, characterized by the interaction between the donor and acceptor macrophages prior to and shortly after the pathogen transfer event (65).
Cryptococcal cells can also leave the lungs as free fungi via a number of extracellular routes, mainly including vesicular-mediated transcellular crossing, also known as transcytosis, and paracellular crossing, or paracytosis, which involves the mechanical or biochemical disruption of the tight junctions (63). Once outside of the lungs, cryptococcal cells enter the bloodstream and spread, leading to a blood infection known as cryptococcaemia, whose magnitude shows a direct correlation with severity of the infection (63). In any case, the fungi, following migration across the BBB via “Trojan horse” mechanism, transcytosis, paracytosis, and/or free entry through damaged endothelial barriers, migrate to the brain parenchyma and begin to proliferate, frequently causing fatal meningoencephalitis (66, 67).
Although C. neoformans and C. gattii enter the body through the lungs, both pathogens have indeed a strong affinity for the CNS (68). Since C. neoformans is able to survive within phagosome after being phagocytized by innate immune cells, the phagocytic cells play an essential role in the diffusion of the pathogen in the brain. Following CNS invasion, the fungal pathogens activate microglia, the brain-resident macrophages, which represent the largest population of myeloid cells in the CNS. Microglial activation induces the production of proinflammatory cytokines and chemokines, which in turn lead to neuroinflammation and promote the recruitment and accumulation of both innate and adaptive immune cells. The immune cells, along with the cytokines secreted by them, are critically involved in fighting the fungal cells.
A recent preclinical study highlighted the presence of monocytes, neutrophils, and proinflammatory cytokines in brain perivascular spaces (PVS), thus supporting the hypothesis that circulating monocytes and neutrophils can strongly contribute to the widespread dissemination of yeast cells into the CNS via a phagocyte-dependent mechanism (69). Therefore, the perivascular spaces may represent another possible entry way of C. neoformans; in particular, mice infected with this pathogen showed an increase in number of monocytes and neutrophils as well as in the number and size of cryptococcomas, with subsequent enhanced destruction of brain tissues; the proteolytic enzymes released by activated neutrophils contribute to damage the BBB, and this further facilitates neutrophil entry into PVS through a process of non-lytic exocytosis, allowing the yeast cells to disseminate within the CNS (56). In a subsequent stage of the infection, cerebral oedema and the enlargement of the PVS causes florid meningitis and cryptococcosis in the subarachnoid space (69); in contrast, the mice infected with C. gattii showed a different immunological host response with mild changes. This supports the vision according to which C. gattii has less marked neurotropism compared to C. neoformans.
Natural killer (NK) lymphocytes play also an important role in the innate immune defenses, as perforin secretion by these cells has demonstrated to enhance antifungal activity against Cryptococcus. Furthermore, the cytotoxicity against C. neoformans is increased by antibodies binding to the NK cell activating receptor CD16 (70).
Altogether, cerebral cryptococcal infection causes little or no necrosis or brain damage until later disease and, consequently, neurocryptococcosis usually presents as a subacute meningoencephalitis (71). The adult patients with neurocryptococcosis typically present neurological symptoms, including headache, altered mental status, lethargy along with fever, nausea and vomiting (71). However, in older people, these symptoms can be considered unspecific or could not to be accurately reported by patients and therefore they can be easily misdiagnosed.
4 Immunosenescence in the innate immune cells
The effectiveness of immune system against infections is challenging in the elderly. Aging induces significant changes in both innate and adaptive immune defense mechanisms, a phenomenon that has been defined as immunosenescence.
One of the first defense elements of the innate immune system is the lung surface barrier, which protects against inhaled bacteria, viruses, and fungi. The cilia motility decreases with aging leading to impaired mucociliary transit that may increase the likelihood of infection in the elderly (72, 73). Aging processes may also impair several functions of the innate immune cells, including neutrophils, macrophages, dendritic cells, and NK cells (74–76) (Figure 2).
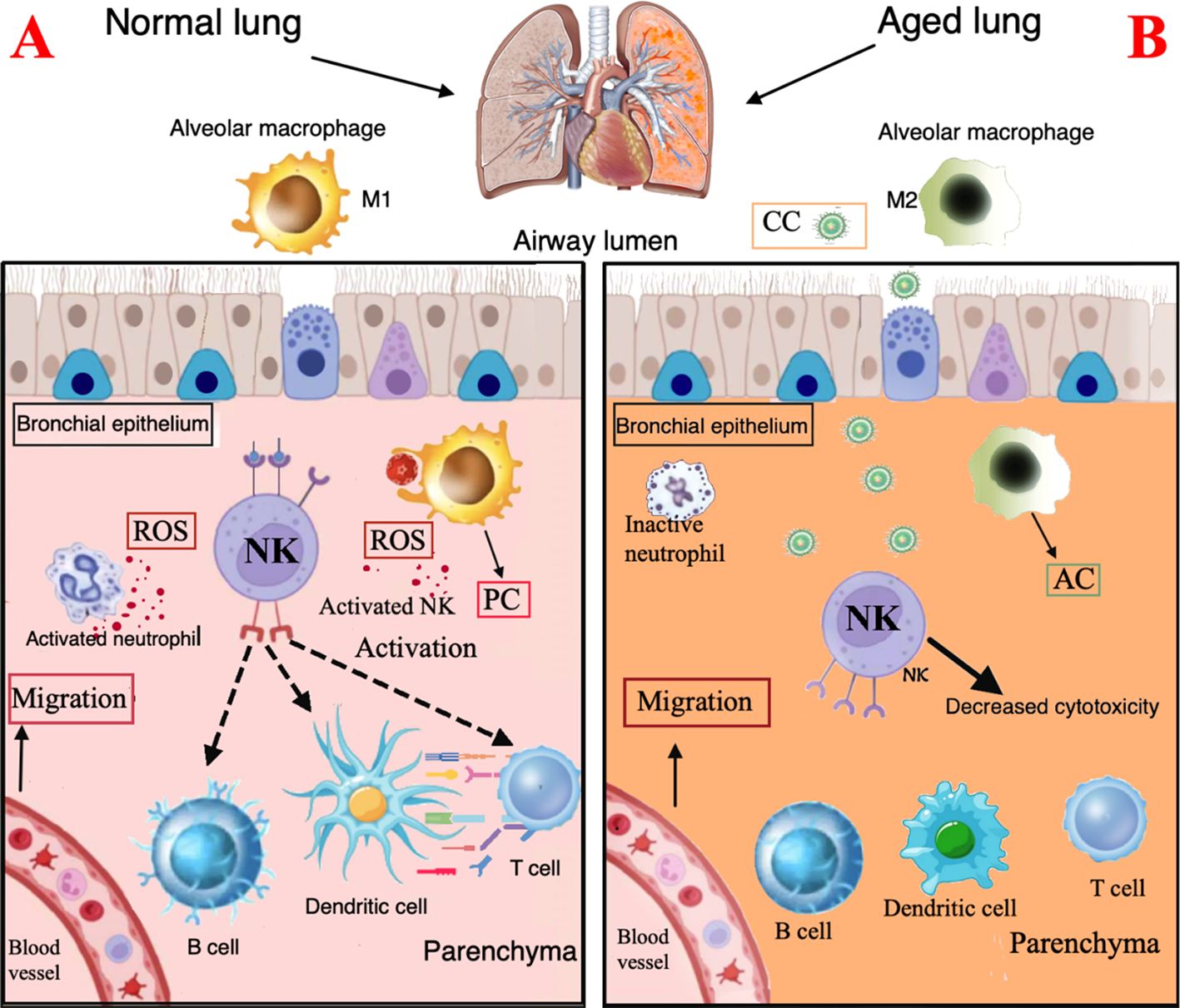
Figure 2. Immunological characteristics of the normal and the aging lung. (A). Normal lung. Surface barriers: their integrity contributes to protect against inhaled CC. Neutrophils: activated neutrophils produce ROS and contribute to recruitment and activation of macrophages and dendritic cells. Macrophages: M1 macrophages show a proinflammatory profile and express chemotactic activity and phagocytosis ability with free radical production. (B). Aging lung. Surface barriers: the decreased cilia motility is associated to higher risk of CC infection. Neutrophils, DC, and NK cells: reduced chemotactic activity, phagocytosis and ROS production. Macrophages: accumulation of M2 macrophages with anti-inflammatory profile. PC, proinflammatory cytokines; AC, anti-inflammatory cytokines; CC, Cryptococcal cells.
Neutrophils are the first responders when an infection occurs. Several studies suggest that aging reduces their chemotactic activity, phagocytosis and ROS production. Moreover, TLR signal, that activates macroautophagy, appears altered; aging itself also affects the neutrophil-mediated recruitment and activation of macrophages and dendritic cells (77).
Macrophages orchestrate the innate and adaptive responses, thanks to their role in antigen presentation. Macrophages may have two different polarization statuses: M1 phenotype, induced by lipopolysaccharide (LPS) or interferon (IFN)-γ stimulation, shows a proinflammatory profile; the M2 phenotype, induced by IL-4, has an anti-inflammatory profile. Typically aging impairs the M1/M2 balance (78, 79); in older mice, indeed, macrophages present in hepatic and adipose tissues, have shown predominantly polarization to M1 phenotype, while accumulation of M2 macrophages occurs in lung, spleen, muscle, bone marrow, and lymphoid tissues (79–83). Age-related changes in macrophages included decreased phagocytosis, autophagy, free radical production and impaired clearance of apoptotic cells. All these modifications cause a decreased ability of macrophages to fight pathogens in older people. The reduced antigen presentation cell activity is probably due to a decreased expression of major histocompatibility complex (MHC) class II molecules, which play a key role in the initiation of the adaptive immune defense of CD4 T cells; furthermore, MHC class II expression driven by IFN-γ is impaired in macrophages of aged mice (84).
Dendritic cells, another type of antigen presenting cells, are responsible for the activation of NK cells and proliferation of CD4 and CD8 T cells and produce type I and III interferon (IFN-I/III). Dendritic cell functions are reduced in older adults with decreased migration capacity and impaired ability to activate the adaptive response (85). Dendritic cells and macrophages show a low TLR expression, essential for the initiation of the inflammatory signaling cascade.
Finally, NK cell are innate immune effectors that mediate a rapid cytotoxicity through perforin exocytosis and the production of chemokines; perforin disrupts fungal membrane integrity, aiding entry of granzymes into the fungal cell, leading to apoptosis. NK cells are key regulators of other immune cells due to their presenting antigen function, which may trigger cascade of immune responses, involving T-cells, B-cells, and dendritic cells (86). Furthermore, NK cells play a role in the recognition and elimination of senescent cells. NK cells are frequently decreased in older individuals, thus contributing to impaired microbial defense and increased risk of infection (87).
In this context, the degree of immunosencesce is also influenced by the total previous immunological changes that occurred during the lifespan and involving both innate and adaptive immunity. Indeed, the concept that memory is not an exclusive attribute of adaptive immunity is now gaining ground, as it has been observed that innate immune cells also have a sort of memory, which has been termed ‘trained immunity’. The innovative concept of immunobiography proposed by Franceschi et al. suggests that the immune system, due to its memory and plasticity, is able to register all the immunological experiences and stimuli to which the organism is exposed, which induces a continuous immune adaptation (88). In summary, all the changes in the innate immune responses of older individuals, combined with senescence of lymphocytes, lead to a decrease in resilience to address infections; moreover, impaired immune cells at BBB level combined with an age-related subtle alteration of BBB place the older patient at an increased risk of infection in the CNS. Therefore, in the next section, we will also discuss BBB changes due to aging. The alteration of BBB integrity, together with senescence of the immune system, is indeed recognized as one of the most relevant mechanisms involved in the susceptibility to neurocryptococcosis in older people.
5 Aging and the blood brain barrier
The BBB is a highly selective interface that regulates communication between circulation and the brain. The BBB aims to maintain CNS homeostasis, provides nourishment, and protects from unregulated exposure to blood and its contents. The BBB acts as a selective barrier that restricts the flow of many substances into and out of the CNS, also preventing unwanted toxins and pathogens from invading the brain. Proper permeability of the BBB contributes to the maintenance of functions and brain health. The BBB is subject to changes due to natural aging processes, that affect the integrity of the barrier even in the absence of underlying pathological conditions. In fact, the vulnerability of the CNS to infectious agents increases with aging, as the stability of the BBB declines and the permeability increases, thus enhancing to an increased flow of solutes, lymphocytes, and innate immune cells (89).
Aging involves adaptive mechanisms in the BBB with alterations to various components of the BBB structure as tight junctions, transporters, microglia, astrocytes and pericytes (Figure 3).
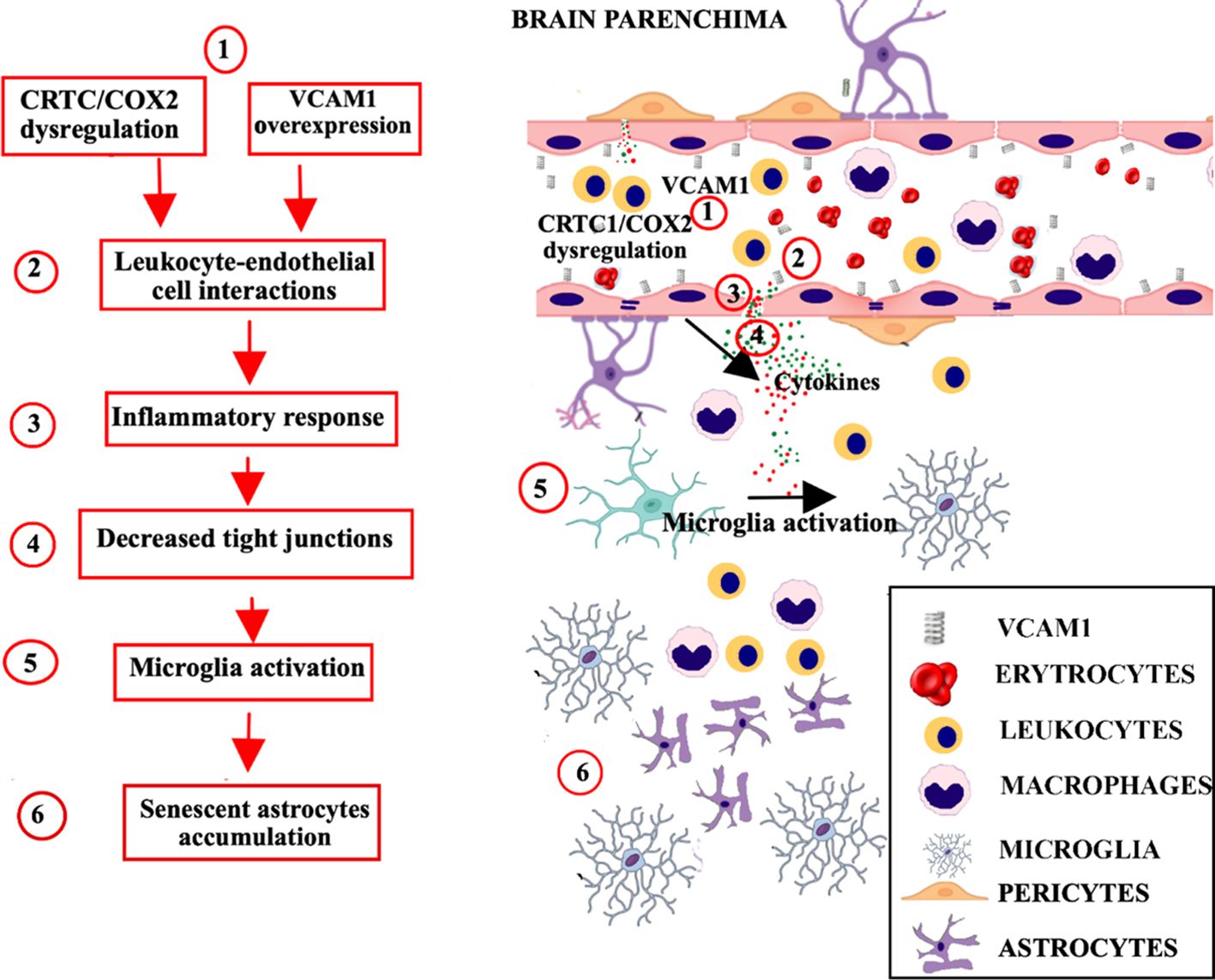
Figure 3. Age-related neurovascular changes in the brain parenchima and BBB. Progressive aging-related neurovascular changes in the BBB include mechanisms of inflammation mediated by the complement pathway (C3a/C3aR), with alterations to various components of the BBB structure as tight junctions, transporters, microglia, astrocytes and pericytes. (1). An increase in C3a/C3aR signaling promotes the over expression of VCAM1, and a dysregulation of CRTC1/cyclooxygenase-2. (2). The resulting inflammation promotes the recruitment of leukocytes across the BBB, and their interaction with endothelial cells. (3). The leukocyte-endothelial cell interaction increases the BBB permeability and induces the production of proinflammatory mediators, such as reactive oxygen species and cytokines. (4). Proinflammatory response leads to decreased tight junction proteins expression and conducts to peripheral immune cell infiltration. (5). Proinflammatory cytokines spread outside the vessels, induce the recruitment and activation of glial cells. (6). The accumulation of senescent astrocytes contributes to microglia activation, neuroinflammation and secretion of SASP factors, which communicate cellular damage to neighboring cells via autocrine/paracrine pathway. BBB, blood brain barrier; VCAM1, vascular cell adhesion molecule-1; CRTC1, CREB-regulated transcription coactivator 1.
Aging-related neurovascular changes in the BBB include mechanisms of inflammation mediated by the complement pathway. An increase in C3a/C3aR signaling promotes the expression of vascular cell adhesion molecule-1 (VCAM1), which regulates the immune cell adhesion process, and conducts to peripheral immune cell infiltration and vascular inflammation (90). Age-related inflammation and vascular cells senescence involve also CRTC1/cyclooxygenase-2 dysregulation (91). The inflammatory processes promote activation of glia cells and recruitment of leukocytes across the BBB. The leukocyte-endothelial cell interactions increase BBB permeability inducing the production of pro-inflammatory mediators such as reactive oxygen species and cytokines, which determine the alterations in paracellular and transcellular transport and decreased tight junction proteins expression (92). Also senescent erythrocytes show alterations in morphology and tightness, that consequently affects transportation and exchange of gases within the BBB and the damage mediated by reactive oxygen species (93).
Overall, several BBB modifications are favored by senescence (94). Aged pericytes show biomarkers of cellular senescence, including increased senescence-associated secretory phenotype (SASP) factors and cell cycle block (95). Accumulation of senescent astrocytes contribute to dysfunction, neuroinflammation and secretion of SASP factors, which communicate cellular damage to neighboring cells via autocrine/paracrine pathway (96).
Moreover, during aging the number of astrocytes expressing neuroinflammatory genes and the number of brain endothelial cells with high levels of senescence-related gene expression increase. Among genetic factors, the impact of ϵ4 isoform of apolipoprotein E has been associated with changes in tight-junction regulation and altered transport systems. Some BBB transporters including large neutral amino acid transporter (LAT-1), p-glycoprotein and transporters for IL-1, choline, triiodothyronine, tumor necrosis factor-alpha, glucose, Tyr-MIF-1 and enkephalins, decrease with healthy aging (97).
Furthermore, as age advances, gut microbiota dysbiosis may promote neuroinflammation causing the alteration of the brain physiopathology and BBB permeability (98). All these mechanisms may lead to BBB dysfunction and make older patients more vulnerable to neurocryptococcosis, facilitating the pathogen multiple mechanisms of barrier interaction, disruption, and CNS invasion. In this context, Cryptococcus itself is able to amplify BBB damage. Cryptococcal-mediated mechanisms of BBB permeability alteration that facilitate infection may involve the production by the fungus of urease (99), serine protease (100), metalloprotease (101), microvesicles (102), and induction of changes in the cytoskeleton of microvascular endothelial cells (103).
6 The putative link between immunosenescence and neurocryptococcosis: a comprehensive overview of current evidence
Although clinical evidence has pointed out the higher prevalence of neurocryptococcosis in older compared to younger adults, the exact mechanisms underlying this epidemiological evidence still need to be elucidated in depth.
However, it is very plausible that age-related disruption of innate and acquired immune response may increase the risk of both cryptococcaemia and neurocryptoccocis and may enhance the direct deleterious effects of cryptococcal infection. More in detail, aging-induced defective clearing activity of alveolar macrophages, along with increased polarization into pro-inflammatory M1 cells and increased activation of Th2 responses, are thought to contribute to increased cryptococcal damage (104, 105). Indeed, the high virulence of cryptococci is related to their capacity of escaping phagosome internalization or alternatively surviving within vesicles and strongly reactivating when immune defenses decrease (106, 107).
Overall, age-associated alterations in innate immune responses may increase the risk of ineffective cryptococcal clearance, severe pulmonary disease and dissemination to the CNS. Furthermore, aging is associated with increased expression of macrophage receptors with collagenous structure (MARCO), which are essentials in pathogen uptake by mononuclear phagocytes during early infection (108) but may be further exploited by the pathogen and polarize towards non-effective immune responses (109).
Aging also increases production of Th2 cells (110) thus creating a favorable environment for the survival of criptococci, mediated by the production of IL-4, IL-13, and IL-5 (105).
Furthermore, also age-related alteration of neutrophils could impact on the cryptococcal virulence. Lung neutrophils are indeed involved in degradation of pathogens as well as in the initiation of inflammation and granuloma formation against them (111), and along with dendritic cells, neutrophils also display age-driven impairment, which is more qualitative than quantitative. In fact, their number may be substantially maintained in healthy older individuals and their immune recruitment into the lungs is elevated, probably because of inflammaging (112); in contrast, the accuracy of neutrophil response towards pathogens is impaired (113–115), because of decreased specificity against the infectious stimuli, which may contribute to increased morbidity and mortality of cryptococcal infections in older hosts. Additionally, as individuals age, neutrophils produce more elastase enzymes (115), that can damage lung cells and vessels, impair macrophage immune responses (116), and potentially favoring the deleterious effects of lung infections from opportunistic microorganisms. Furthermore, decreased expression of TLR1 in aged neutrophils decrease production of IL-8 and recovery from apoptosis (117).
Overall, multiple mechanisms are involved in age-related derangement of innate immunity. In this context, several findings support the link between the higher susceptibility to infections and the increased mortality of elderly people to the emerging concept of trained innate immunity.
This theory suggests that innate immune cells possess non-specific immunological memory, whose main function is to resolve aggressions rapidly and stop the inflammatory process (118). However, after each activation, the innate immune system remains at a higher activation/readiness state (119). In older people this process is more evident, as shown by the chronic activated state of many tissue-resident and blood-born innate immune cells, including neutrophils and microglia, which display hyperactivation phenotypes in response to inflammatory stimulation, with an aberrant proinflammatory status (4, 119–121). Therefore, trained innate immunity may become hyperactivated with aging, contributing to a pro-inflammatory cytokine releasing and inflammaging. However, innate immune cells such as macrophages may also experience a decreased reactivity to different stimuli; thus, trained immunity may undergo a decline, contributing to immunosenescence. Factors that lead trained innate immunity to a weakened or hyperactivated state have not been clearly identified. However, the concept of immunobiography, which is based on the development of an immunological history, could explain the heterogeneity of responses in the elderly toward one form rather than the other (88).
The hyperactivation of inflammatory signaling pathways, associated to a dysregulation of proinflammatory mediators and defined as inflammaging, might lead to a relative immune paralysis state, characterized by markedly impaired innate immune function against pathogens (120, 122, 123).
In this framework, dysregulation of IFN-I response may represent a phenotype of late senescence and appears to contribute to the maintenance of the age-associated inflammation (124). Indeed, on one hand, aging and age-related comorbidities may impair IFN-I production thus leading to ineffective viral clearance and increased susceptibility to infections during the first contacts with the pathogens (5); on the other hand, paradoxically, various aging tissues and organs from mammalian hosts perpetually accumulate changes brought by IFN-I pathway activation (20). Therefore, IFN-I may contribute to the increased neuroinflammatory status characterizing aging of the brain (125–127), which is frequently enhanced by the presence of comorbidities (128, 129). Accordingly, IFN-I signaling has been shown to be up-activated in the choroid plexus in the aged CNS of humans and mice (130, 131).
In summary, during aging we may recognize a progressive establishment of an “interferonopathic disorder”, that is an IFN-mediated inflammatory status mainly characterized by a severe age-related neuroinflammation and IFN-I up-regulation, a phenomenon also known as IFN-aging (20, 132). In addition to aging-related impairment in IFN-I responses, many comorbidities associated with aging are known to contribute to elevated IFN-I levels. However, so far studies concerning the role of IFN-Is during fungal infections have generated conflicting results. A host protective contribution of IFN-Is to immunity against C. neoformans was highlighted by some studies showing that IFNα/β deleted mice were more susceptible to infection than wild-type mice (133–135). On the other hand, some investigations reported that the clearance of C. neoformans in the mouse lungs was accelerated at the early phase of infection, under a condition lacking IFN-I-mediated signaling, suggesting that IFN-Is may be involved in negative regulation of the early host defense (136). Probably, the role of IFN changes in the context of cryptococcal infection from a protective to a deleterious role depending on the phase of the infection, the virulence of the pathogen and the general performance status of immune system.
To this regard, it is important to take in mind that key features of cryptococcal pathogenesis are the ability of the fungus to survive and replicate within macrophages; then they are typically expelled by a mechanism of non-lytic exocytosis called vomocytosis and/or by cell lysis (64). Vomocytosis has been shown to be enhanced by IFNα and/or IFNβ, and abrogated when IFN-I signaling is blocked (137). Essential features of IFN-I signaling are shown in Figure 4.
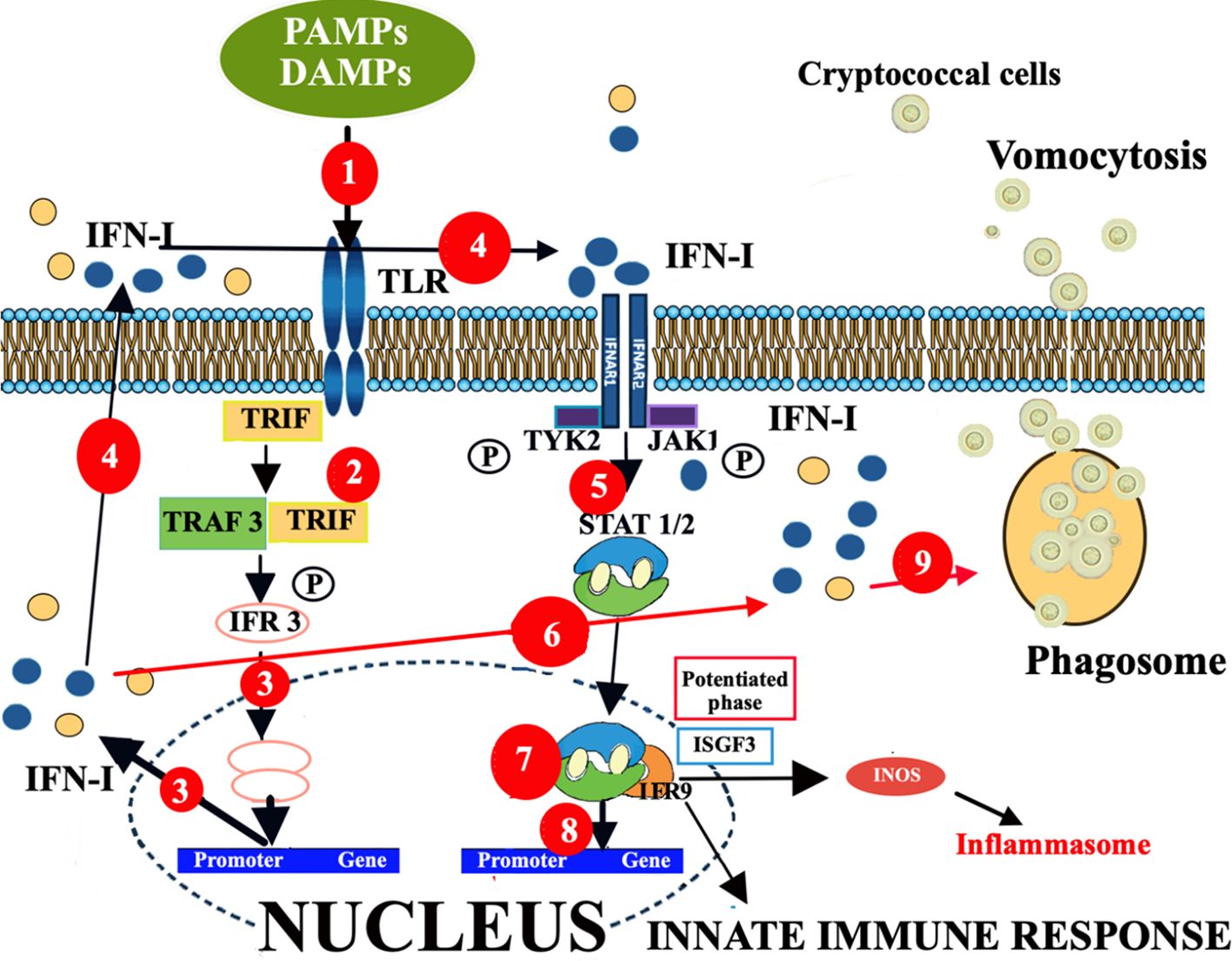
Figure 4. Enhancement of vomocytosis via IFN-I mediated pathway. The different effects of IFN-I signaling, depending on ligand, dose, and duration of exposure. (1). The TLR-PAMP/DAMP interactions induce the selective recruitment of TRIF, which binds to the TLRs and then recruits a series of downstream signaling molecules, leading to IFN-I production. (2). TLR-PAMP/DAMP interactions induce TRIF to form a signaling complex with TRAF and this leads to phosphorylation of IRF3. (3). This phosphorylation event causes IRF3 to dimerize, translocating into the nucleus, and inducing the expression of IFNα and IFNβ. (4). Both secreted IFN-Is can bind IFNAR1/2 in an autocrine or paracrine manner and activate a signaling cascade leading to expression of ISGs. (5). In particular, the c-termini of IFNAR1 and IFNAR2 are associated with TYK2 and JAK1, respectively, and activation of the receptor transduces the phosphorylation of JAK1 and TYK2 by tyrosine phosphorylation. (6). This initiates a signaling cascade composed of proteins of the STAT family. (7). STAT1 and STAT2 proteins, activated upon JAK1 phosphorylation, dimerize and rapidly translocate to the nucleus, where they, together with IRF9, form a trimolecular complex called IFN-stimulated gene factor 3 (ISGF3). ISGF3 is a critical transcription factor complex involved in the cellular response to IFN-Is, particularly IFN-α and IFN-β. (8). This complex activates the transcription of ISGs. (9). However, IFN-I responses may also induce innate immune cells to act as “vomocytes”, giving rise to an ineffective fungal clearance which leads to a latent asymptomatic lung infection. In any case, this ISGF3 engagement by IFN-I production leads to activation of the inflammatory gene expression program, responsible for activation and/or exacerbation of inflammatory responses. Altogether, ISGs may produce, via JAK-STAT/IRF signaling network, distinct biological responses and sustained IFN-I signaling leads to chronic immune activation, inflammation and, consequently, immune exhaustion and dysfunction. Consequently, during infections, the effects of IFN-I signaling can be protective or detrimental, depending on the context, including pathogen species, infection route, and tissue specific features. DAMP, damage-associated molecular patterns; IFNAR1/2, IFN-α/β receptor; IFN-I, interferon; IRF, interferon regulatory factor; ISGs, IFN-stimulated genes; ISGF3, interferon stimulated gene factor 3; ISGF9, interferon stimulated gene factor 9; JAK1, Janus kinase; PAMP, pathogen-associated molecular patterns; STAT, signal transducer and activator of transcription; TRAF, TNF receptor-associated factor; TRIF, Toll/IL-1R domain-containing adaptor-inducing IFN-β; TYK2, tyrosine kinase 2.
In brief, the PRR-PAMP/DAMP interactions induce the selective recruitment of the Toll/IL-1R domain-containing adaptor-inducing IFN-β (TRIF), which binds to the TLRs and then recruits downstream signaling molecules that ultimately induce the production of IFN-I (138). In particular, TRIF forms a signaling complex with TNF receptor-associated factor (TRAF) and this leads to phosphorylation of the transcription factor interferon regulatory factor (IRF)3 (139). This phosphorylation event causes IRF3 to dimerize, translocate into the nucleus, and induce the expression of IFNα and IFNβ. Both secreted IFN-Is can bind the IFN-α/β receptor (IFNAR1/2) in an autocrine or paracrine manner and activate a signaling cascade leading to expression of IFN-stimulated genes (ISGs), via JAK-STAT1/2 signaling (Figure 1).
It is hypothesized that in a first phase of cryptococcal infection, IFN-I responses may induce innate immune cells to act as “vomocytes”, giving rise to an ineffective fungal clearance which leads to a latent asymptomatic lung infection that may be considered as a host tolerance mechanism able to prevent or limit the tissue damage and the systemic dissemination (135). Consequently, whereas cryptococcal vomocytosis at an early stage of infection might be beneficial, since it would limit the risk of systemic fungal shedding, once the fungus has reached the blood stream or CNS, enhanced vomocytosis is likely to be deleterious, since it would allow the pathogen to escape the constraints of a phagocyte and grow rapidly in the extracellular environment.
In addition to the putative dysregulation of IFN-dependent molecular pathway, other molecular mechanisms are probably involved in the increased susceptibility to neurocrytococcosis in the older people compared to young individuals. For instance, an invertebrate model of C. neoformans and C. gattii infection suggested that defects in both longevity and innate immunity, affecting the insulin/IGF-1 signaling/DAF-16 pathway, has been linked to a raised susceptibility to Cryptococcus (140). Furthermore, the authors reported that C. neoformans or C. gattii infections reduced the host lifespan. Another study in rats showed that lung macrophages, after C. neoformans phagocytosing, induce the synthesis of monocyte chemotactic protein 1 (MCP-1), depending on the interaction of C. neoformans with CD11b/c and CD18 and, interestingly, aging appears to be related to a reduced production of MCP-1 by lung macrophages in response to cryptococcal infection (141). Another evidence suggesting this link between immunosenescence and cryptococcal infection is the increased risk for cryptococcosis associated with long-term use of fingolimod (142). Indeed, this drug induces phenotypic changes on many cells of the immune system similar to the immunosenescence observed in the older people.
In addition to systemic immunological alterations, also at CNS level, in the context of cryptococcal infection, an aberrant immune response probably contributes to the damage. To this regard, a small cohort of 17 middle-age HIV-negative adults with severe CNS cryptococcosis have shown an intrathecal expansion and activation of dendritic cells and lymphocytes (CD4+, CD8+ and NK cells) and high levels of inflammatory cytokines including IFN-γ and IL-6, leading to the idea that the increased inflammatory response in neurocryptoccocosis doesn’t represent a protective defense, but enhances markers of neuronal injury (143). These results appear to be similar to the increased immune reconstitution inflammatory syndrome (IRIS) in HIV-infected people or post-infectious inflammatory response syndrome (PIIRS) in HIV-negative people occurring occasionally with cryptococcal meningitis. A case of PIIRS was also recently described in an elderly patient, who showed increased IL-6 levels in the serial cerebrospinal fluid (CSF) (144).
Finally, immunosenescence has shown to affect also the adaptive immune response to fungal infections. In this regard, age-related T-cell dysfunctions in elderly mice have been reported to be related to an increased susceptibility to systemic cryptococcal infection compared with young adult mice (145).
Altogether, these findings underscore the importance of studying human immune responses at different stages of infection and in different immunological conditions.
7 Future directions in clinical research: diagnosis and therapy
The significant association between aging and neurocryptococcosis implies a need to adopt appropriate prevention measures.
Older populations may be more vulnerable to diagnostic error for several reasons, including an incorrect attribution of symptoms to normal aging, or to unrelated neurological findings.
Moreover, accurate diagnosis is also critical in older people, who, in addition to concomitant neurological disorders, have a slower onset of symptoms, evolving over days to a few weeks, condition that could make it difficult an early diagnosis and lead to a delay in treatment (15). Furthermore, the clinicians sometimes could focus unduly on clinical clues suggesting specific diseases, while discounting opposing clues, and sometimes they could confound meningitis with other disorders sharing similar symptoms, including achy stiffness, headache, and high fever. Misdiagnosis not only endangers the safety and health of older patients, but also incurs additional costs for health care systems due to misused clinical interventions and care of iatrogenic illness.
The clinical suspicion of neurocryptococcosis should be followed by targeted diagnostic investigations, including first-line computed tomography and magnetic resonance imaging; subsequently, lumbar puncture for CSF analysis should be performed to confirm the diagnosis; a positive CSF culture indicates active cryptococcal disease and represents the gold standard method to detect live pathogens; additional CSF analyses, including India ink preparation and Gram staining, can help quantify the degree of capsule thickness and melanization of the yeast cells cultures, which are related to fungal virulence and immunodepression of the host (146). In contexts where lumbar puncture cannot be rapidly performed, clinicians can rely on the positivity of the cryptococcal antigen in the serum, which can strongly suggest the infection, even if the diagnostic gold standard is the CSF culture. However, it is important to take in mind that, when the fungal burden is low, CSF fungal culture can produce false negative results, as well as India ink microscopy. Furthermore, the slow-growing nature of Cryptococcus as well its possible physiological viable-but-nonculturable (VBNC) status (147) may contribute to false negative CSF cultures. In any case, despite obtaining a reliable result may require several weeks, CSF fungal culture remains paramount for the definitive diagnosis of neurocryptococcosis.
The therapeutic approach also requires careful evaluation, since antifungal drugs are particularly toxic and could aggravate the basic medical conditions of frail older subjects suffering from multiple comorbidities. The principal antifungal agents classically used for the treatment of neurocryptococcosis consist of intravenous amphotericin B deoxycholate and its lipid formulations, oral flucytosine, and oral fluconazole (28). While amphotericin B and flucytosine are fungicidal, fluconazole is only fungistatic. Lipid formulations of amphotericin B are preferred on older patients often affected by renal dysfunction or at risk for renal failure. The high prevalence of multimorbidity and polypharmacy among older adults, that contributes to the relatively increased risk, associated to both the polypharmacy and the frail conditions, implies a need for a very careful evaluation also concerning the risk/benefit ratio in the different frail conditions of affected patients.
In particular, it should be taken in consideration that, older patients using antimicrobials have a higher risk of adverse side effects due to age-related changes in pharmacokinetic and pharmacodynamic, multimorbidity, and polypharmacy (148). More specifically, the use of amphotericin B is typically associated with a higher risk of nephrotoxicity, hepatotoxicity, hematological effects, and heart failure. Nephrotoxicity is the most common potential adverse effect in older patients, presenting with an increased creatinine level, hypo/hyperkalemia, and/or hypomagnesemia (148). In addition, although the lipid formulations have been shown to be substantially less toxic than conventional amphotericin B, particularly with respect to nephrotoxicity, concomitant or recent use of other nephrotoxic drugs could have an additive effect (148).
With respect to flucytosine, despite leukopenia and myelosuppression represent side effects frequently induced by high plasma levels of this drug, maintained for a long time, the current use of flucytosine is allowed for the treatment of cryptococcal meningitis, in combination with amphotericin. However, the concurrent use of flucytosine and nephrotoxic drugs should be avoided due to the risk of accumulation of flucytosine (149). Furthermore, clinicians should be aware that fluconazole, even at low doses, may cause cardiotoxicity for prolongation of the QT interval (148).
In addition to currently available anti-fungal drugs, it would be desirable for new therapeutic strategies to be available in the next future for neurocryptocococosis. To this regard, some investigations, based on pre-clinical findings, have tested commercially available drugs used for other indications, including tamoxifen and sertraline as potential treatment for cryptococcal infection, according to the so-called repurposing strategy (150, 151). However, until now these studies did not show any efficacy of these treatments against cryptococcal infection.
In our opinion, considering the putative role of immunosenesce in the increased susceptibility of older people for neurocryptococcosis, potential therapeutic approach could be based on the regulation of immune response. For instance, some strategies aimed to modulate CD22 receptor on microglia seem to be able to restore homeostatic microglial phagocytosis in ageing brain (152).
Another putative target to contrast immunosenescence is inhibition of p-38, that is a molecule strongly implicated in the aberrant inflammation due to age-related macrophagic dysfunction (153, 154).
However, all these approaches need to be tested in the context of cryptococcal infection.
8 Conclusions
Given the high complexity of neurocryptococcosis in older individuals, we suggest that a multidisciplinary and comprehensive approach to the disease to be followed; cryptococcosis presents unique challenges in older individuals due to age-related changes in immune function and increased susceptibility to infections. As our population continues to age, it is imperative that healthcare professionals remain vigilant in recognizing and managing cryptococcal infections in this vulnerable population. Early diagnosis, prompt treatment, and close monitoring are crucial for improving outcomes and reducing morbidity and mortality associated with cryptococcosis in older adults. Additionally, ongoing research is needed to better understand the epidemiology, pathogenesis, and optimal management strategies tailored specifically for older individuals.
Author contributions
LS: Conceptualization, Supervision, Writing – original draft, Writing – review & editing. AB: Writing – review & editing. MP: Writing – review & editing. EV: Visualization, Writing – review & editing. MG: Conceptualization, Supervision, Writing – original draft, Writing – review & editing. M’hA: Writing – review & editing. AC: Conceptualization, Writing – review & editing. FL: Conceptualization, Writing – review & editing. LM: Writing – review & editing. EF: Writing – review & editing. GG: Writing – review & editing. MV: Writing – review & editing. LB: Supervision, Writing – original draft, Writing – review & editing.
Funding
The author(s) declare financial support was received for the research, authorship, and/or publication of this article. This study was supported by Ricerca Corrente funding from the Italian Ministry of Health to IRCCS INRCA.
Conflict of interest
The authors declare that the research was conducted in the absence of any commercial or financial relationships that could be construed as a potential conflict of interest.
The author(s) declared that they were an editorial board member of Frontiers, at the time of submission. This had no impact on the peer review process and the final decision.
Publisher’s note
All claims expressed in this article are solely those of the authors and do not necessarily represent those of their affiliated organizations, or those of the publisher, the editors and the reviewers. Any product that may be evaluated in this article, or claim that may be made by its manufacturer, is not guaranteed or endorsed by the publisher.
References
1. Panda A, Arjona A, Sapey E, Bai F, Fikrig E, Montgomery RR, et al. Human innate immunosenescence: causes and consequences for immunity in old age. Trends Immunol. (2009) 30(7):325–33. doi: 10.1016/j.it.2009.05.004
2. Aiello A, Farzaneh F, Candore G, Caruso C, Davinelli S, Gambino CM, et al. Immunosenescence and its hallmarks: How to oppose aging strategically? A review of potential options for therapeutic intervention. Front Immunol. (2019) 10:3389/fimmu.2019.02247. doi: 10.3389/fimmu.2019.02247
3. Shaw AC, Goldstein DR, Montgomery RR. Age-dependent dysregulation of innate immunity. Nat Rev Immunol. (2013) 13:875–87. doi: 10.1038/nri3547
4. Franceschi C, Campisi J. Chronic inflammation (Inflammaging) and its potential contribution to age-associated diseases. Journals Gerontology: Ser A. (2014) 69:S4–9. doi: 10.1093/gerona/glu057
5. Feng E, Balint E, Poznanski SM, Ashkar AA, Loeb M. Aging and interferons: Impacts on inflammation and viral disease outcomes. Cells. (2021) 10(3):708. doi: 10.3390/cells10030708
6. Lu RJ, Wang EK, Benayoun BA. Functional genomics of inflamm-aging and immunosenescence. Brief Funct Genomics. (2022) 21:43–55. doi: 10.1093/bfgp/elab009
7. Esme M, Topeli A, Yavuz BB, Akova M. Infections in the elderly critically-ill patients. Front Med (Lausanne). (2019) 6:3389/fmed.2019.00118. doi: 10.3389/fmed.2019.00118
8. Lai WA, Chen SF, Tsai NW, Chang CC, Chang W, Lu CH, et al. Clinical characteristics and prognosis of acute bacterial meningitis in elderly patients over 65: a hospital-based study. BMC Geriatr. (2011) 11:91. doi: 10.1186/1471-2318-11-91
9. Liang SY, Mackowiak PA. Infections in the elderly. Clin Geriatr Med. (2007) 23:441–56. doi: 10.1016/j.cger.2007.01.010
10. Mehta JB, Dutt AK. Epidemiology and host factors. Microbiol Spectr. (2016) 4(6). doi: 10.1128/microbiolspec.TNMI7-0018-2016
11. Safdieh JE, Mead PA, Sepkowitz KA, Kiehn TE, Abrey LE. Bacterial and fungal meningitis in patients with cancer. Neurology. (2008) 70:943–7. doi: 10.1212/01.wnl.0000305960.85546.5f
12. Tannou T, Koeberle S, Manckoundia P, Aubry R. Multifactorial immunodeficiency in frail elderly patients: Contributing factors and management. Med Mal Infect. (2019) 49:167–72. doi: 10.1016/j.medmal.2019.01.012
13. Werner H, Kuntsche J. Infection in the elderly–what is different. Z Gerontol Geriatr. (2000) 33:350–6. doi: 10.1007/s003910070031
14. Vetrano DL, Triolo F, Maggi S, Malley R, Jackson TA, Poscia A, et al. Fostering healthy aging: The interdependency of infections, immunity and frailty. Ageing Res Rev. (2021) 69:101351. doi: 10.1016/j.arr.2021.101351
15. Tsai W-C, Lien CY, Lee JJ, Hsiao WC, Huang CR, Tsai NW, et al. The clinical characteristics and therapeutic outcomes of cryptococcal meningitis in elderly patients: a hospital-based study. BMC Geriatrics. (2019) 19:91. doi: 10.1186/s12877-019-1108-0
16. Shourian M, Qureshi ST. Resistance and tolerance to cryptococcal infection: an intricate balance that controls the development of disease. Front Immunol. (2019) 10:3389/fimmu.2019.00066. doi: 10.3389/fimmu.2019.00066
17. Wei J, Li X-Y, Zhang Y. Central nervous system Cryptococcoma mimicking demyelinating disease: a case report. BMC Neurol. (2020) 20:297. doi: 10.1186/s12883-020-01880-4
18. Chastain DB, Rao A, Yaseyyedi A, Henao-Martínez AF, Borges T, Franco-Paredes C, et al. Cerebral cryptococcomas: A systematic scoping review of available evidence to facilitate diagnosis and treatment. Pathogens. (2022) 11(2):205. doi: 10.3390/pathogens11020205
19. Garelnabi M, May RC. Variability in innate host immune responses to cryptococcosis. Mem Inst Oswaldo Cruz. (2018) 113:e180060. doi: 10.1590/0074-02760180060
20. Cao W. IFN-aging: Coupling aging with interferon response. Front Aging. (2022) 3:3389/fragi.2022.870489. doi: 10.3389/fragi.2022.870489
21. Frisch SM, MacFawn IP. Type I interferons and related pathways in cell senescence. Aging Cell. (2020) 19:e13234. doi: 10.1111/acel.13234
22. Zhu LP, Wu JQ, Xu B, Ou XT, Zhang QQ, Weng XH, et al. Cryptococcal meningitis in non-HIV-infected patients in a Chinese tertiary care hospital, 1997-2007. Med Mycol. (2010) 48:570–9. doi: 10.3109/13693780903437876
23. Alvarez M, Casadevall A. Phagosome extrusion and host-cell survival after Cryptococcus neoformans phagocytosis by macrophages. Curr Biol. (2006) 16:2161–5. doi: 10.1016/j.cub.2006.09.061
24. Reimão JQ, Drummond ED, Terceti MdeS, Lyon JP, Franco MC, de Siqueira AM, et al. Isolation of Cryptococcus neoformans from hollows of living trees in the city of Alfenas, MG, Brazil. Mycoses. (2007) 50:261–4. doi: 10.1111/j.1439-0507.2007.01374.x
25. Yang DH, England MR, Salvator H, Anjum S, Park YD, Marr KA, et al. Cryptococcus gattii species complex as an opportunistic pathogen: underlying medical conditions associated with the infection. mBio. (2021) 12:e0270821. doi: 10.1128/mBio.02708-21
26. Strickland AB, Shi M. Mechanisms of fungal dissemination. Cell Mol Life Sci. (2021) 78:3219–38. doi: 10.1007/s00018-020-03736-z
27. Elsegeiny W, Marr KA, Williamson PR. Immunology of cryptococcal infections: developing a rational approach to patient therapy. Front Immunol. (2018) 9:3389/fimmu.2018.00651. doi: 10.3389/fimmu.2018.00651
28. Ngan NTT, Flower B, Day JN. Treatment of cryptococcal meningitis: how have we got here and where are we going? Drugs. (2022) 82:1237–49. doi: 10.1007/s40265-022-01757-5
29. O’Halloran JA, Powderly WG, Spec A. Cryptococcosis today: It is not all about HIV infection. Curr Clin Microbiol Rep. (2017) 4:88–95. doi: 10.1007/s40588-017-0064-8
30. Guan ST, Huang YS, Huang ST, Hsiao FY, Chen YC. The incidences and clinical outcomes of cryptococcosis in Taiwan: A nationwide, population-based study, 2002-2015. Med Mycol. (2024) 62(1). doi: 10.1093/mmy/myad125
31. Toivonen A, Eriksson M, Friberg N, Hautala T, Kääriäinen S, Leppäaho-Lakka J, et al. Clinical characteristics and evaluation of the incidence of cryptococcosis in Finland 2004-2018. Infect Dis (Lond). (2021) 53:684–90. doi: 10.1080/23744235.2021.1922753
32. Hevey MA, George IA, Raval K, Powderly WG, Spec A. Presentation and mortality of cryptococcal infection varies by predisposing illness: A retrospective cohort study. Am J Med. (2019) 132:977–983.e1. doi: 10.1016/j.amjmed.2019.04.026
33. Huang SH, Chuang YC, Lee YC, Hung CC, Sheng WH, Su JJ, et al. Lumbar puncture for non-HIV-infected non-transplant patients with cryptococcosis: Should it be mandatory for all? PLoS One. (2019) 14:e0221657. doi: 10.1371/journal.pone.0221657
34. Pappas PG, Perfect JR, Cloud GA, Larsen RA, Pankey GA, Lancaster DJ, et al. Cryptococcosis in human immunodeficiency virus-negative patients in the era of effective azole therapy. Clin Infect Dis. (2001) 33:690–9. doi: 10.1086/322597
35. Shih CC, Chen YC, Chang SC, Luh KT, Hsieh WC. Cryptococcal meningitis in non-HIV-infected patients. QJM: Int J Med. (2000) 93:245–51. doi: 10.1093/qjmed/93.4.245
36. Bitar D, Lortholary O, Le Strat Y, Nicolau J, Coignard B, Tattevin P. Population-based analysis of invasive fungal infections, France, 2001-2010. Emerg Infect Dis. (2014) 20:1149–55. doi: 10.3201/eid2007.140087
37. George IA, Spec A, Powderly WG, Santos CAQ. Comparative epidemiology and outcomes of human immunodeficiency virus (HIV), non-HIV non-transplant, and solid organ transplant associated cryptococcosis: A population-based study. Clin Infect Dis. (2018) 66:608–11. doi: 10.1093/cid/cix867
38. Pyrgos V, Seitz AE, Steiner CA, Prevots DR, Williamson PR. Epidemiology of cryptococcal meningitis in the US: 1997-2009. PLoS One. (2013) 8:e56269. doi: 10.1371/journal.pone.0056269
39. Kajeekul R, Mekawichai P, Chayakulkeeree M. Clinical features of cryptococcal meningoencephalitis in HIV-positive and -negative patients in a resource-limited setting. J Fungi (Basel). (2023) 9(9):869. doi: 10.3390/jof9090869
40. Chen YY, Lai CH. Nationwide population-based epidemiologic study of cryptococcal meningitis in Taiwan. Neuroepidemiology. (2011) 36:79–84. doi: 10.1159/000323390
41. Marr KA, Sun Y, Spec A, Lu N, Panackal A, Bennett J, et al. A multicenter, longitudinal cohort study of cryptococcosis in human immunodeficiency virus-negative people in the United States. Clin Infect Dis. (2020) 70:252–61. doi: 10.1093/cid/ciz193
42. Dromer F, French Cryptococcosis Study Group. Comparison of the efficacy of amphotericin B and fluconazole in the treatment of cryptococcosis in human immunodeficiency virus-negative patients: retrospective analysis of 83 cases. Clin Infect Dis. (1996) 22 Suppl 2:S154–60. doi: 10.1093/clinids/22.Supplement_2.S154
43. Jean SS, Fang CT, Shau WY, Chen YC, Chang SC, Hsueh PR, et al. Cryptococcaemia: clinical features and prognostic factors. Qjm. (2002) 95:511–8. doi: 10.1093/qjmed/95.8.511
44. Namie H, Takazono T, Hidaka Y, Morimoto S, Ito Y, Nakada N, et al. The prognostic factors for cryptococcal meningitis in non-human immunodeficiency virus patients: An observational study using nationwide database. Mycoses. (2024) 67:e13658. doi: 10.1111/myc.13658
45. Fitch MT, van de Beek D. Emergency diagnosis and treatment of adult meningitis. Lancet Infect Dis. (2007) 7:191–200. doi: 10.1016/S1473-3099(07)70050-6
46. Nau R, Djukic M, Spreer A, Eiffert H. Bacterial meningitis: an update of new treatment options. Expert Rev Anti Infect Ther. (2015) 13:1401–23. doi: 10.1586/14787210.2015.1077700
47. van der Torre MH, Andrews RAJ, Hooker EL, Rankin A, Dodd S. Systematic review on Cryptococcus neoformans/Cryptococcus gattii species complex infections with recommendations for practice in health and care settings. Clin Infection Pract. (2022) 15:100154. doi: 10.1016/j.clinpr.2022.100154
48. Spagnolo P, Cordier JF, Cottin V. Connective tissue diseases, multimorbidity and the ageing lung. Eur Respir J. (2016) 47:1535–58. doi: 10.1183/13993003.00829-2015
49. Brennan M, Breen D. Sarcoidosis in the older person: diagnostic challenges and treatment consideration. Age Ageing. (2022) 51(9):afac203. doi: 10.1093/ageing/afac203
50. Leonhard SE, Fritz D, van de Beek D, Brouwer MC. Cryptococcal meningitis complicating sarcoidosis. Med (Baltimore). (2016) 95:e4587. doi: 10.1097/MD.0000000000004587
51. Prevel R, Guillotin V, Imbert S, Blanco P, Delhaes L, Duffau P. Central nervous system cryptococcosis in patients with sarcoidosis: comparison with non-sarcoidosis patients and review of potential pathophysiological mechanisms. (2022) 9:836886. doi: 10.3389/fmed.2022.836886
52. Wang Y, Liu Y, Jiang S, Zhao Y, Cao J, Hao W, et al. Antifungal antibodies present in intravenous immunoglobulin derived from China. Braz J Microbiol. (2023) 54:81–92. doi: 10.1007/s42770-022-00894-z
53. Oladele RO, Jordan AM, Okaa JU, Osaigbovo II, Shettima SA, Shehu NY, et al. A multicenter survey of asymptomatic cryptococcal antigenemia among patients with advanced HIV disease in Nigeria. PLoS Glob Public Health. (2023) 3:e0001313. doi: 10.1371/journal.pgph.0001313
54. Wake RM, Molloy SF, Jarvis JN, Harrison TS, Govender NP. Cryptococcal antigenemia in advanced human immunodeficiency virus disease: Pathophysiology, epidemiology, and clinical implications. Clin Infect Dis. (2023) 76:764–70. doi: 10.1093/cid/ciac675
55. Xu M, Peng Z, Xu C, Chen Y, Cheng J, Chi Y, et al. Underlying cryptococcal diseases and the correlation with serum cryptococcal antigen titers in hospitalized HIV-infected patients screened positive for cryptococcal antigenemia. Front Cell Infect Microbiol. (2020) 10:3389/fcimb.2020.00170. doi: 10.3389/fcimb.2020.00170
56. Julian J, Robiatul A, Sri W. Biomolecular activity of cryptococcus during cryptococcosis: a review of molecular interactions of cryptococcus with human immune system and blood-brain-barrier. Afr J Infect Dis. (2024) 18:11–22. doi: 10.21010/Ajidv18i1.3
57. Baker RD. The primary pulmonary lymph node complex of crytptococcosis. Am J Clin Pathol. (1976) 65:83–92. doi: 10.1093/ajcp/65.1.83
58. Bao F, Tan H, Liu W, Li Y, Li H. Pediatric cryptococcal lymphadenitis in the absence of AIDS: Case report and literature review. Case Rep Pediatr. (2013) 2013:563081. doi: 10.1155/2013/563081
59. Brunet K, Alanio A, Lortholary O, Rammaert B. Reactivation of dormant/latent fungal infection. J Infect. (2018) 77:463–8. doi: 10.1016/j.jinf.2018.06.016
60. Davis MJ, Tsang TM, Qiu Y, Dayrit JK, Freij JB, Huffnagle GB, et al. Macrophage M1/M2 polarization dynamically adapts to changes in cytokine microenvironments in Cryptococcus neoformans infection. mBio. (2013) 4:e00264–13. doi: 10.1128/mBio.00264-13
61. Wang Y, Pawar S, Dutta O, Wang K, Rivera A, Xue C, et al. Macrophage mediated immunomodulation during cryptococcus pulmonary infection. Front Cell Infect Microbiol. (2022) 12:859049. doi: 10.3389/fcimb.2022.859049
62. Rocha JD, Nascimento MTC, Decote-Ricardo D, Côrte-Real S, Morrot A, Heise N, et al. Capsular polysaccharides from Cryptococcus neoformans modulate production of neutrophil extracellular traps (NETs) by human neutrophils. Sci Rep. (2015) 5:8008. doi: 10.1038/srep08008
63. Denham ST, Brown JCS. Mechanisms of pulmonary escape and dissemination by cryptococcus neoformans. J Fungi (Basel). (2018) 4(1):25. doi: 10.3390/jof4010025
64. Pacifici N, Cruz-Acuña M, Diener A, Tu A, Senthil N, Han H, et al. Vomocytosis of Cryptococcus neoformans cells from murine, bone marrow-derived dendritic cells. PLoS One. (2023) 18:e0280692. doi: 10.1371/journal.pone.0280692
65. Dragotakes Q, Fu MS, Casadevall A. Dragotcytosis: elucidation of the mechanism for cryptococcus neoformans macrophage-to-macrophage transfer. J Immunol. (2019) 202:2661–70. doi: 10.4049/jimmunol.1801118
66. Casadevall A. Cryptococci at the brain gate: break and enter or use a Trojan horse? J Clin Invest. (2010) 120:1389–92. doi: 10.1172/JCI42949
67. May RC, Stone NR, Wiesner DL, Bicanic T, Nielsen K. Cryptococcus: from environmental saprophyte to global pathogen. Nat Rev Microbiol. (2016) 14:106–17. doi: 10.1038/nrmicro.2015.6
68. Maziarz EK, Perfect JR. Cryptococcosis. Infect Dis Clin North Am. (2016) 30:179–206. doi: 10.1016/j.idc.2015.10.006
69. Kaufman-Francis K, Djordjevic JT, Juillard PG, Lev S, Desmarini D, Grau GER, et al. The Early Innate Immune Response to, and Phagocyte-Dependent Entry of, Cryptococcus neoformans Map to the Perivascular Space of Cortical Post-Capillary Venules in Neurocryptococcosis. Am J Pathol. (2018) 188:1653–65. doi: 10.1016/j.ajpath.2018.03.015
70. Schmidt S, Zimmermann SY, Tramsen L, Koehl U, Lehrnbecher T. Natural killer cells and antifungal host response. Clin Vaccine Immunol. (2013) 20:452–8. doi: 10.1128/CVI.00606-12
71. Pescador Ruschel MA, Thapa B. Cryptococcal meningitis. Treasure Island (FL: StatPearls Publishing (2023). Available at: https://www.ncbi.nlm.nih.gov/books/NBK525986/. StatPearls.
72. Bailey KL. Aging diminishes mucociliary clearance of the lung. Adv Geriatr Med Res. (2022) 4(2):e220005. doi: 10.20900/agmr20220005
73. Torrance BL, Haynes L. Cellular senescence is a key mediator of lung aging and susceptibility to infection. Front Immunol. (2022) 13:3389/fimmu.2022.1006710. doi: 10.3389/fimmu.2022.1006710
74. Solana R, Tarazona R, Gayoso I, Lesur O, Dupuis G, Fulop T, et al. Innate immunosenescence: effect of aging on cells and receptors of the innate immune system in humans. Semin Immunol. (2012) 24:331–41. doi: 10.1016/j.smim.2012.04.008
75. Liu Z, Liang Q, Ren Y, Guo C, Ge X, Wang L, et al. Immunosenescence: molecular mechanisms and diseases. Signal Transduct Target Ther. (2023) 8:200. doi: 10.1038/s41392-023-01451-2
76. Lee KA, Flores RR, Jang IH, Saathoff A, Robbins PD. Immune senescence, immunosenescence and aging. Front Aging. (2022) 3:3389/fragi.2022.900028. doi: 10.3389/fragi.2022.900028
77. Van Avondt K, Hartl D. Mechanisms and disease relevance of neutrophil extracellular trap formation. European journal of clinical investigation. (2018) 48(2):e12919. doi: 10.1111/eci.12919
78. Mahbub S, Deburghgraeve CR, Kovacs EJ. Advanced age impairs macrophage polarization. Journal of interferon & cytokine research : the official journal of the International Society for Interferon and Cytokine Research (2012) 32(1):18–26. doi: 10.1089/jir.2011.0058
79. Liu R, Cui J, Sun Y, Xu W, Wang Z, Wu M, et al. Autophagy deficiency promotes M1 macrophage polarization to exacerbate acute liver injury via ATG5 repression during aging. Cell Death Discovery. (2021) 7:397. doi: 10.1038/s41420-021-00797-2
80. Fontana L, Zhao E, Amir M, Dong H, Tanaka K, Czaja MJ. Aging promotes the development of diet-induced murine steatohepatitis but not steatosis. Hepatology. (2013) 57(3):995–1004. doi: 10.1002/hep.26099
81. Chung EJ, Kwon S, Shankavaram U, White AO, Das S, Citrin DE, et al. Natural variation in macrophage polarization and function impact pneumocyte senescence and susceptibility to fibrosis. Aging (Albany NY). (2022) 14:7692–717. doi: 10.18632/aging.v14i19
82. Wang Y, Wehling-Henricks M, Samengo G, Tidball JG. Increases of M2a macrophages and fibrosis in aging muscle are influenced by bone marrow aging and negatively regulated by muscle-derived nitric oxide. Aging Cell. (2015) 14:678–88. doi: 10.1111/acel.12350
83. Lumeng CN, Liu J, Geletka L, Delaney C, Delproposto J, Desai A, et al. Aging is associated with an increase in T cells and inflammatory macrophages in visceral adipose tissue. J Immunol. (2011) 187:6208–16. doi: 10.4049/jimmunol.1102188
84. Herrero C, Marqués L, Lloberas J, Celada A. IFN-gamma-dependent transcription of MHC class II IA is impaired in macrophages from aged mice. J Clin Invest. (2001) 107:485–93. doi: 10.1172/JCI11696
85. Zacca ER, Crespo MI, Acland RP, Roselli E, Núñez NG, Maccioni M, et al. Aging impairs the ability of conventional dendritic cells to cross-prime CD8+ T cells upon stimulation with a TLR7 ligand. PLoS One. (2015) 10:e0140672. doi: 10.1371/journal.pone.0140672
86. Oh BLZ, Chan LWY, Chai LYA. Manipulating NK cellular therapy from cancer to invasive fungal infection: promises and challenges. Front Immunol. (2022) 13:3389/fimmu.2022.1044946. doi: 10.3389/fimmu.2022.1044946
87. Judge SJ, Murphy WJ, Canter RJ. Characterizing the dysfunctional NK cell: assessing the clinical relevance of exhaustion, anergy, and senescence. Front Cell Infect Microbiol. (2020) 10:3389/fcimb.2020.00049. doi: 10.3389/fcimb.2020.00049
88. Franceschi C, Salvioli S, Garagnani P, de Eguilor M, Monti D, Capri M, et al. Immunobiography and the heterogeneity of immune responses in the elderly: A focus on inflammaging and trained immunity. Front Immunol. (2017) 8:3389/fimmu.2017.00982. doi: 10.3389/fimmu.2017.00982
89. Galea I. The blood-brain barrier in systemic infection and inflammation. Cell Mol Immunol. (2021) 18:2489–501. doi: 10.1038/s41423-021-00757-x
90. Propson NE, Roy ER, Litvinchuk A, Köhl J, Zheng H. Endothelial C3a receptor mediates vascular inflammation and blood-brain barrier permeability during aging. J Clin Invest. (2021) 131(1):e140966. doi: 10.1172/JCI140966
91. Wang Y, Du W, Sun Y, Zhang J, Ma C, Jin X, et al. CRTC1 is a potential target to delay aging-induced cognitive deficit by protecting the integrity of the blood-brain barrier via inhibiting inflammation. J Cereb Blood Flow Metab. (2023) 43:1042–59. doi: 10.1177/0271678X231169133
92. Knox EG, Aburto MR, Clarke G, Cryan JF, O'Driscoll CM. The blood-brain barrier in aging and neurodegeneration. Mol Psychiatry. (2022) 27:2659–73. doi: 10.1038/s41380-022-01511-z
93. Amiri P, DeCastro J, Littig J, Lu HW, Liu C, Conboy I, et al. Erythrocytes, a new contributor to age-associated loss of blood–brain barrier integrity. Adv Sci (Weinh) (2021) 8(20):2101912. doi: 10.1002/advs.202101912
94. Knopp RC, Erickson MA, Rhea EM, Reed MJ, Banks WA. Cellular senescence and the blood-brain barrier: Implications for aging and age-related diseases. Exp Biol Med (Maywood). (2023) 248:399–411. doi: 10.1177/15353702231157917
95. Iwao T, Takata F, Matsumoto J, Goto Y, Aridome H, Yasunaga M, et al. Senescence in brain pericytes attenuates blood-brain barrier function in vitro: A comparison of serially passaged and isolated pericytes from aged rat brains. Biochem Biophys Res Commun. (2023) 645:154–63. doi: 10.1016/j.bbrc.2023.01.037
96. Preininger MK, Kaufer D. Blood-brain barrier dysfunction and astrocyte senescence as reciprocal drivers of neuropathology in aging. Int J Mol Sci. (2022) 23(2):e13747. doi: 10.3390/ijms23116217
97. Banks WA, Reed MJ, Logsdon AF, Rhea EM, Erickson MA. Healthy aging and the blood-brain barrier. Nat Aging. (2021) 1:243–54. doi: 10.1038/s43587-021-00043-5
98. Mou Y, Du Y, Zhou L, Yue J, Hu X, Liu Y, et al. Gut microbiota interact with the brain through systemic chronic inflammation: Implications on neuroinflammation, neurodegeneration, and aging. Front Immunol. (2022) 13:3389/fimmu.2022.796288. doi: 10.3389/fimmu.2022.796288
99. Olszewski MA, Noverr MC, Chen GH, Toews GB, Cox GM, Perfect JR, et al. Urease expression by Cryptococcus neoformans promotes microvascular sequestration, thereby enhancing central nervous system invasion. Am J Pathol. (2004) 164:1761–71. doi: 10.1016/S0002-9440(10)63734-0
100. Xu CY, Zhu HM, Wu JH, Wen H, Liu CJ, et al. Increased permeability of blood-brain barrier is mediated by serine protease during Cryptococcus meningitis. J Int Med Res. (2014) 42:85–92. doi: 10.1177/0300060513504365
101. Vu K, Tham R, Uhrig JP, Thompson GR 3rd, Na Pombejra S, Jamklang M, et al. Invasion of the central nervous system by Cryptococcus neoformans requires a secreted fungal metalloprotease. mBio. (2014) 5:e01101–14. doi: 10.1128/mBio.01101-14
102. Huang S-H, Wu CH, Chang YC, Kwon-Chung KJ, Brown RJ, Jong A, et al. Cryptococcus neoformans-derived microvesicles enhance the pathogenesis of fungal brain infection. PLoS One. (2012) 7:e48570. doi: 10.1371/journal.pone.0048570
103. Chen SHM, Stins MF, Huang SH, Chen YH, Kwon-Chung KJ, Chang Y, et al. Cryptococcus neoformans induces alterations in the cytoskeleton of human brain microvascular endothelial cells. J Med Microbiol. (2003) 52:961–70. doi: 10.1099/jmm.0.05230-0
104. Boe DM, Hulsebus HJ, Najarro KM, Mullen JE, Kim H, Tan AC, et al. Advanced age is associated with changes in alveolar macrophages and their responses to the stress of traumatic injury. J Leukoc Biol (2022) 112(6):1371–86. doi: 10.1002/JLB.3HI0620-399RR
105. Firacative C, Gressler AE, Schubert K, Schulze B, Müller U, Brombacher F, et al. Identification of T helper (Th)1- and Th2-associated antigens of Cryptococcus neoformans in a murine model of pulmonary infection. Sci Rep. (2018) 8:2681. doi: 10.1038/s41598-018-21039-z
106. Johnston SA, May RC. The human fungal pathogen Cryptococcus neoformans escapes macrophages by a phagosome emptying mechanism that is inhibited by Arp2/3 complex-mediated actin polymerisation. PLoS Pathog. (2010) 6:e1001041. doi: 10.1371/journal.ppat.1001041
107. Levitz SM, Nong SH, Seetoo KF, Harrison TS, Speizer RA, Simons ER, et al. Cryptococcus neoformans resides in an acidic phagolysosome of human macrophages. Infect Immun. (1999) 67:885–90. doi: 10.1128/IAI.67.2.885-890.1999
108. Wang L, Hong W, Zhu H, He Q, Yang B, Wang J, et al. Macrophage senescence in health and diseases. Acta Pharm Sin B. (2024) 14:1508–24. doi: 10.1016/j.apsb.2024.01.008
109. Xu J, Flaczyk A, Neal LM, Fa Z, Cheng D, Ivey M, et al. Exploitation of scavenger receptor, macrophage receptor with collagenous structure, by cryptococcus neoformans promotes alternative activation of pulmonary lymph node CD11b(+) conventional dendritic cells and non-protective th2 bias. Front Immunol. (2017) 8:3389/fimmu.2017.01231. doi: 10.3389/fimmu.2017.01231
110. Mansfield AS, Nevala WK, Dronca RS, Leontovich AA, Shuster L, Markovic SN, et al. Normal ageing is associated with an increase in Th2 cells, MCP-1 (CCL1) and RANTES (CCL5), with differences in sCD40L and PDGF-AA between sexes. Clin Exp Immunol. (2012) 170:186–93. doi: 10.1111/j.1365-2249.2012.04644.x
111. Mambula SS, Simons ER, Hastey R, Selsted ME, Levitz SM, et al. Human neutrophil-mediated nonoxidative antifungal activity against Cryptococcus neoformans. Infect Immun. (2000) 68:6257–64. doi: 10.1128/IAI.68.11.6257-6264.2000
112. Kulkarni U, Zemans RL, Smith CA, Wood SC, Deng JC, Goldstein DR, et al. Excessive neutrophil levels in the lung underlie the age-associated increase in influenza mortality. Mucosal Immunol. (2019) 12:545–54. doi: 10.1038/s41385-018-0115-3
113. Chen MM, Palmer JL, Plackett TP, Deburghgraeve CR, Kovacs EJ, et al. Age-related differences in the neutrophil response to pulmonary pseudomonas infection. Exp Gerontol. (2014) 54:42–6. doi: 10.1016/j.exger.2013.12.010
114. Sapey E, Greenwood H, Walton G, Mann E, Love A, Aaronson N, et al. Phosphoinositide 3-kinase inhibition restores neutrophil accuracy in the elderly: toward targeted treatments for immunosenescence. Blood. (2014) 123:239–48. doi: 10.1182/blood-2013-08-519520
115. Sapey E, Patel JM, Greenwood HL, Walton GM, Hazeldine J, Sadhra C, et al. Pulmonary infections in the elderly lead to impaired neutrophil targeting, which is improved by simvastatin. Am J Respir Crit Care Med. (2017) 196:1325–36. doi: 10.1164/rccm.201704-0814OC
116. Domon H, Nagai K, Maekawa T, Oda M, Yonezawa D, Takeda W, et al. Neutrophil elastase subverts the immune response by cleaving toll-like receptors and cytokines in pneumococcal pneumonia. Front Immunol. (2018) 9:3389/fimmu.2018.00732. doi: 10.3389/fimmu.2018.00732
117. Qian F, Guo X, Wang X, Yuan X, Chen S, Malawista SE, et al. Reduced bioenergetics and toll-like receptor 1 function in human polymorphonuclear leukocytes in aging. Aging (Albany NY). (2014) 6:131–9. doi: 10.18632/aging.v6i2
118. Bulut O, Kilic G, Domínguez-Andrés J, Netea MG. Overcoming immune dysfunction in the elderly: trained immunity as a novel approach. Int Immunol. (2020) 32:741–53. doi: 10.1093/intimm/dxaa052
119. Fulop T, Larbi A, Hirokawa K, Cohen AA, Witkowski JM. Immunosenescence is both functional/adaptive and dysfunctional/maladaptive. Semin Immunopathol. (2020) 42:521–36. doi: 10.1007/s00281-020-00818-9
120. Fulop T, Larbi A, Pawelec G, Khalil A, Cohen AA, Hirokawa K, et al. Immunology of aging: the birth of inflammaging. Clin Rev Allergy Immunol. (2023) 64:109–22. doi: 10.1007/s12016-021-08899-6
121. Huijser E, van Helden-Meeuwsen CG, Grashof DGB, Tarn JR, Brkic Z, Huisman JMA, et al. Trained immunity in primary sjögren's syndrome: linking type I interferons to a pro-atherogenic phenotype. Front Immunol. (2022) 13:3389/fimmu.2022.840751. doi: 10.3389/fimmu.2022.840751
122. Fulop T, Dupuis G, Baehl S, Le Page A, Bourgade K, Frost E, et al. From inflamm-aging to immune-paralysis: a slippery slope during aging for immune-adaptation. Biogerontology. (2016) 17:147–57. doi: 10.1007/s10522-015-9615-7
123. Ochando J, Mulder WJM, Madsen JC, Netea MG, Duivenvoorden R. Trained immunity - basic concepts and contributions to immunopathology. Nat Rev Nephrol. (2023) 19:23–37. doi: 10.1038/s41581-022-00633-5
124. De Cecco M, Ito T, Petrashen AP, Elias AE, Skvir NJ, Criscione SW, et al. L1 drives IFN in senescent cells and promotes age-associated inflammation. Nature. (2019) 566:73–8. doi: 10.1038/s41586-018-0784-9
125. Soraci L, Gambuzza ME, Biscetti L, Laganà P, Lo Russo C, Buda A, et al. Toll-like receptors and NLRP3 inflammasome-dependent pathways in Parkinson's disease: mechanisms and therapeutic implications. J Neurol. (2023) 270:1346–60. doi: 10.1007/s00415-022-11491-3
126. Lee J, Kim HJ. Normal aging induces changes in the brain and neurodegeneration progress: review of the structural, biochemical, metabolic, cellular, and molecular changes. Front Aging Neurosci. (2022) 14:3389/fnagi.2022.931536. doi: 10.3389/fnagi.2022.931536
127. Wyss-Coray T. Ageing, neurodegeneration and brain rejuvenation. Nature. (2016) 539:180–6. doi: 10.1038/nature20411
128. Bowman GL, Dayon L, Kirkland R, Wojcik J, Peyratout G, Severin IC, et al. Blood-brain barrier breakdown, neuroinflammation, and cognitive decline in older adults. Alzheimers Dement. (2018) 14:1640–50. doi: 10.1016/j.jalz.2018.06.2857
129. Senatorov VV Jr., Friedman AR, Milikovsky DZ, Ofer J, Saar-Ashkenazy R, Charbash A, et al. Blood-brain barrier dysfunction in aging induces hyperactivation of TGFβ signaling and chronic yet reversible neural dysfunction. Sci Transl Med. (2019) 11(521):eaaw8283. doi: 10.1126/scitranslmed.aaw8283
130. Baruch K, Deczkowska A, David E, Castellano JM, Miller O, Kertser A, et al. Aging. Aging-induced type I interferon response at the choroid plexus negatively affects brain function. Science. (2014) 346:89–93. doi: 10.1126/science.1252945
131. Roy ER, Chiu G, Li S, Propson NE, Kanchi R, Wang B, et al. Concerted type I interferon signaling in microglia and neural cells promotes memory impairment associated with amyloid β plaques. Immunity. (2022) 55:879–894.e6. doi: 10.1016/j.immuni.2022.03.018
132. McGlasson S, Jury A, Jackson A, Hunt D. Type I interferon dysregulation and neurological disease. Nat Rev Neurol. (2015) 11:515–23. doi: 10.1038/nrneurol.2015.143
133. Biondo C, Midiri A, Gambuzza M, Gerace E, Falduto M, Galbo R, et al. IFN-alpha/beta signaling is required for polarization of cytokine responses toward a protective type 1 pattern during experimental cryptococcosis. J Immunol. (2008) 181:566–73. doi: 10.4049/jimmunol.181.1.566
134. Biondo C, Yamamoto H, Nomura T, Matsumoto I, Miyasaka T, Zong T, et al. Recognition of yeast nucleic acids triggers a host-protective type I interferon response. (2011) 41(7):1969–79. doi: 10.1002/eji.201141490
135. Coelho C, Bocca AL, Casadevall A. The intracellular life of Cryptococcus neoformans. Annu Rev Pathol. (2014) 9:219–38. doi: 10.1146/annurev-pathol-012513-104653
136. Sato K, Yamamoto H, Nomura T, Matsumoto I, Miyasaka T, Zong T, et al. Cryptococcus neoformans infection in mice lacking type I interferon signaling leads to increased fungal clearance and IL-4-dependent mucin production in the lungs. PLoS One. (2015) 10:e0138291. doi: 10.1371/journal.pone.0138291
137. Cruz-Acuña M, Pacifici N, Lewis JS. Vomocytosis: too much booze, base, or calcium? mBio. (2019) 10(6):e02526-19. doi: 10.1128/mBio.02526-19
138. Duan T, Du Y, Xing C, Wang HY, Wang RF. Toll-like receptor signaling and its role in cell-mediated immunity. (2022) 13:812774. doi: 10.3389/fimmu.2022.812774
139. Yanai H, Chiba S, Hangai S, Kometani K, Inoue A, Kimura Y, et al. Revisiting the role of IRF3 in inflammation and immunity by conditional and specifically targeted gene ablation in mice. Proc Natl Acad Sci U S A. (2018) 115(20):5253–8. doi: 10.1073/pnas.1803936115
140. Brown SM, Campbell LT, Lodge JK. Cryptococcus neoformans, a fungus under stress. Curr Opin Microbiol. (2007) 10:320–5. doi: 10.1016/j.mib.2007.05.014
141. He W, Casadevall A, Lee SC, Goldman DL. Phagocytic activity and monocyte chemotactic protein expression by pulmonary macrophages in persistent pulmonary cryptococcosis. Infect Immun. (2003) 71:930–6. doi: 10.1128/IAI.71.2.930-936.2003
142. Grebenciucova E, Reder AT, Bernard JT. Immunologic mechanisms of fingolimod and the role of immunosenescence in the risk of cryptococcal infection: A case report and review of literature. Mult Scler Relat Disord. (2016) 9:158–62. doi: 10.1016/j.msard.2016.07.015
143. Panackal AA, Wuest SC, Lin YC, Wu T, Zhang N, Kosa P, et al. Paradoxical immune responses in non-HIV cryptococcal meningitis. PLoS Pathog. (2015) 11:e1004884. doi: 10.1371/journal.ppat.1004884
144. Liu J, Liu J, Qin BE, Yao S, Wang A, Yang L, et al. Post-infectious inflammatory response syndrome in an HIV-negative immunocompetent elderly patient with cryptococcal meningitis: A case report and literature review. Front Immunol. (2022) 13:3389/fimmu.2022.823021. doi: 10.3389/fimmu.2022.823021
145. Aguirre KM, Gibson GW, Johnson LL. Decreased resistance to primary intravenous Cryptococcus neoformans infection in aged mice despite adequate resistance to intravenous rechallenge. Infect Immun. (1998) 66:4018–24. doi: 10.1128/IAI.66.9.4018-4024.1998
146. de Sousa HR, de Oliveira GP Jr, Frazão SO, Gorgonha KCM, Rosa CP, Garcez EM, et al. Faster cryptococcus melanization increases virulence in experimental and human cryptococcosis. J Fungi (Basel). (2022) 8(4):393. doi: 10.3390/jof8040393
147. Hommel B, Sturny-Leclère A, Volant S, Veluppillai N, Duchateau M, Yu CH, et al. Cryptococcus neoformans resists to drastic conditions by switching to viable but non-culturable cell phenotype. PLoS Pathog. (2019) 15:e1007945. doi: 10.1371/journal.ppat.1007945
148. Soraci L, Cherubini A, Paoletti L, Filippelli G, Luciani F, Laganà P, et al. Safety and tolerability of antimicrobial agents in the older patient. Drugs Aging. (2023) 40:499–526. doi: 10.1007/s40266-023-01019-3
149. Dekkers BGJ, Veringa A, Marriott DJE, Boonstra JM, van der Elst KCM, Doukas FF, et al. Invasive candidiasis in the elderly: Considerations for drug therapy. Drugs Aging. (2018) 35:781–9. doi: 10.1007/s40266-018-0576-9
150. Rhein J, Hullsiek KH, Tugume L, Nuwagira E, Mpoza E, Evans EE, et al. “Adjunctive sertraline for HIV-associated cryptococcal meningitis: a randomised, placebo-controlled, double-blind phase 3 trial.” The Lancet. Infectious diseases. (2019) vol. 19,8:843–51. doi: 10.1016/S1473-3099(19)30127-6
151. Ngan NTT, Thanh Hoang Le N, Vi Vi NN, Van NTT, Mai NTH, Van Anh D, et al. An open label randomized controlled trial of tamoxifen combined with amphotericin B and fluconazole for cryptococcal meningitis. eLife. (2021) 10:e68929. doi: 10.7554/eLife.68929
152. Pluvinage JV, Haney MS, Smith BAH, Sun J, Iram T, Bonanno L, et al. CD22 blockade restores homeostatic microglial phagocytosis in ageing brains. Nature. (2019) 568(7751):187–92. doi: 10.1038/s41586-019-1088-4
153. Borgoni S, Kudryashova KS, Burka K, de Magalhães JP. Targeting immune dysfunction in aging. Ageing Research Reviews. (2021) 70:101410. doi: 10.1016/j.arr.2021.101410
Keywords: aging, IFN-I dysregulation, cryptococcal meningitis, vomocytosis, inflammaging
Citation: Soraci L, Beccacece A, Princiotto M, Villalta Savedra E, Gambuzza ME, Aguennouz M’H, Corsonello A, Luciani F, Muglia L, Filicetti E, Greco GI, Volpentesta M and Biscetti L (2024) The emerging links between immunosenescence in innate immune system and neurocryptococcosis. Front. Immunol. 15:1410090. doi: 10.3389/fimmu.2024.1410090
Received: 31 March 2024; Accepted: 29 July 2024;
Published: 20 August 2024.
Edited by:
Ahmed Abdellatif, American University in Cairo, EgyptReviewed by:
Maura Manion, National Institute of Allergy and Infectious Diseases (NIH), United StatesPaola Italiani, National Research Council (CNR), Italy
Copyright © 2024 Soraci, Beccacece, Princiotto, Villalta Savedra, Gambuzza, Aguennouz, Corsonello, Luciani, Muglia, Filicetti, Greco, Volpentesta and Biscetti. This is an open-access article distributed under the terms of the Creative Commons Attribution License (CC BY). The use, distribution or reproduction in other forums is permitted, provided the original author(s) and the copyright owner(s) are credited and that the original publication in this journal is cited, in accordance with accepted academic practice. No use, distribution or reproduction is permitted which does not comply with these terms.
*Correspondence: Maria Elsa Gambuzza, me.gambuzza@sanita.it