- 1Division of General Medicine, Taipei Medical University Shuang Ho Hospital, New Taipei, Taiwan
- 2Graduate Institute of Medical Sciences, College of Medicine, Taipei Medical University, Taipei, Taiwan
- 3Department of Microbiology and Immunology, School of Medicine, College of Medicine, Taipei Medical University, Taipei, Taiwan
- 4Department of Dermatology, School of Medicine, College of Medicine, Taipei Medical University, Taipei, Taiwan
- 5Department of Dermatology, Taipei Medical University Shuang Ho Hospital, New Taipei, Taiwan
- 6Department of Dermatology, Stanford University School of Medicine, Redwood City, CA, United States
Rosacea is a complex inflammatory condition characterized by papulopustular lesions and erythema on the central face for which there is no cure. The development of rosacea is influenced by both external triggers and genetics, but the common pathophysiology is overactivation of the immune system. Here, we review the current data on proinflammatory cytokines and dysregulation of the neurovascular system as targetable components of rosacea. Amelioration of cutaneous and gastrointestinal dysbiosis and other external factors impacts the immune state and has been observed to improve rosacea. While multiple treatments exist, many patients do not achieve their goals for rosacea control and highlights an unmet need for dermatologic care. Current interventions encompass topical/oral drugs, light devices, and avoidance of triggers management. Additional understanding of the underlying pathogenesis may help us develop novel targeted therapeutic strategies to improve rosacea.
1 Introduction
Rosacea is a chronic, relapsing, inflammatory skin disease characterized by symptoms such as burning, stinging, erythema, and papulopustular lesions on the central face (1). To date, rosacea is considered a multifactorial disease, but our current understand has not led to a cure, nor sufficient control of the disease in many cases. Both immune dysregulation and neuroinflammation have been implicated in the development of rosacea. Here, we review the current literature on these components (2–4) which may lead to novel targets and pathways for future clinical trials.
For instance, rosacea can worsen due to various external trigger factors of the immune system, such as ultraviolet (UV) radiation and Demodex mites (5, 6). Neuroinflammation is also significant in rosacea, triggered by factors like alcohol, spicy foods, and temperature changes, with activation of calcium channels and vasodilation manifest as skin redness, a common symptom of the condition (3).
2 Pathophysiology
The precise pathophysiology of rosacea continues to be investigated. So far, rosacea is known to be a multifactorial, chronic, inflammatory skin disease (7, 8). The contributing pathogenic mechanisms of rosacea can be categorized into four major categories which can interact with each other: external triggers (e.g., exposure to UV radiation and Demodex mites), genetic predisposition, immune dysregulation, and neurovascular dysregulation (2) (Figure 1).
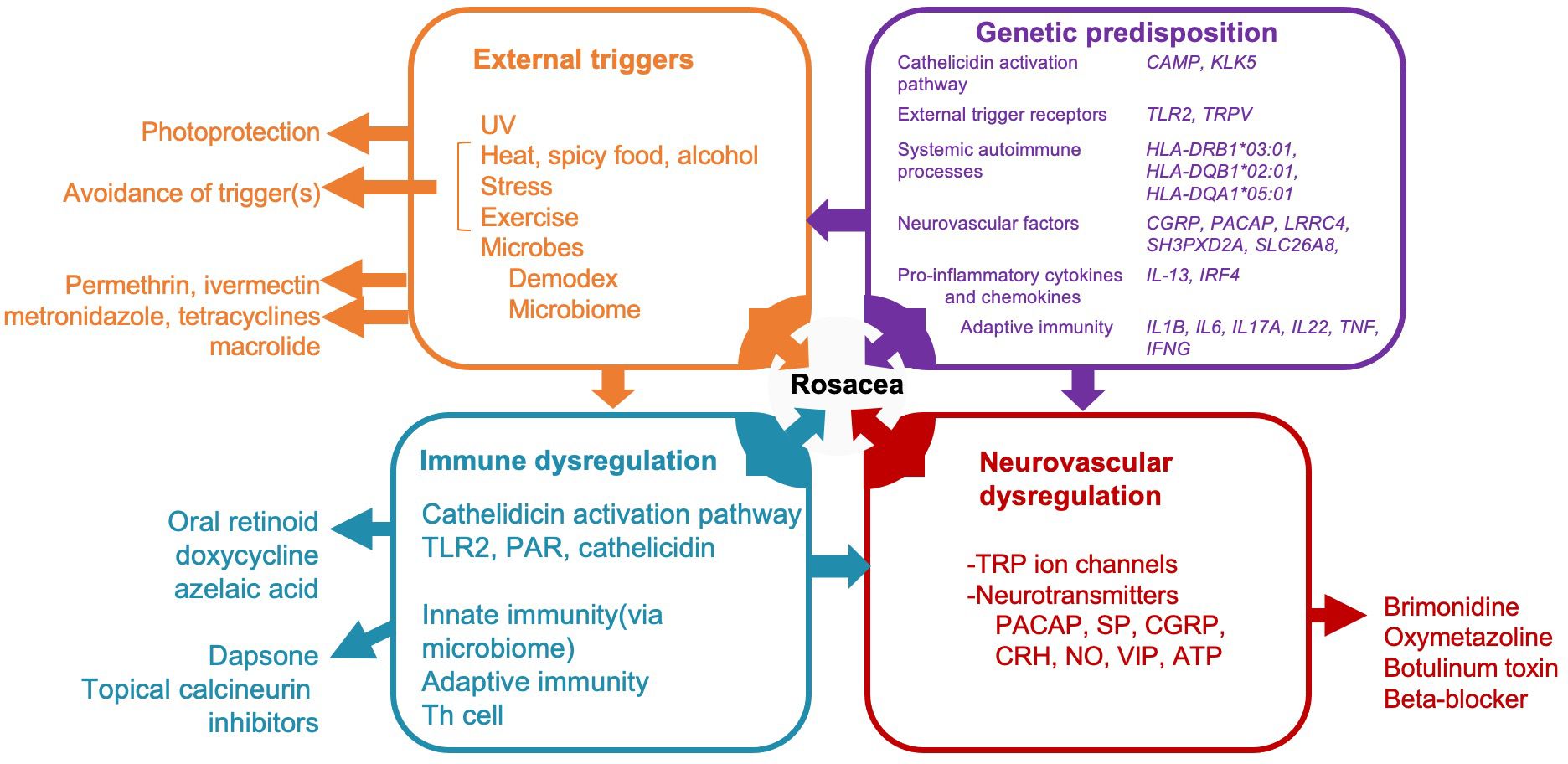
Figure 1 A diagram illustrating potential pathogenic mechanisms of rosacea categorized into four major categories and interaction with immune system. Rosacea, a multifactorial condition, involves external triggers (orange box), genetic predisposition (purple box), immune dysregulation (blue box), and neurovascular dysregulation (red box). Treatment modalities correspond to these mechanisms, as shown outside of the boxes in the diagram. Only drugs aligned with Swiss S1 and National Rosacea Society guidelines are indicated. UV, ultraviolet; CAMP, cathelicidin antimicrobial peptide; KLK-5, Kallikrein 5; TLR2, toll-like receptor 2; TRP-, transient receptor potential channel; CGRP, calcitonin gene related peptide; PACAP, pituitary adenylate cyclase-activating peptide; IL-, interleukin; IRF4, interferon regulatory factor-4; TNF, tumor necrosis factor; IFNG, Interferon gamma; PAR, protease-activated receptor; SP, substance P; CRH, corticotropin-releasing hormone; NO, Nitric oxide; VIP, vasoactive intestinal polypeptide; ATP, adenosine triphosphate.
Rosacea may be exacerbated by external factors such as ultraviolet (UV) radiation, alcohol, spicy food, extreme temperatures, and Demodex mites and other microbes (1). Exposure to UV radiation and Demodex mites activates Toll-like receptor 2 (TLR2), leading to the activation and subsequent release of cathelicidin—an antimicrobial peptide integral to the pathogenesis of rosacea (5, 6). Kallikrein 5 (KLK5) converts cathelicidin into its active form, LL-37, which has been implicated in inflammation, angiogenesis, and telangiectasis in rosacea (9).
Neuroinflammation is a major component of rosacea (4), though it is unclear if the initial trigger is immunologic, leading to stimulation of nerves that mediate itch and burning or vice versa. Some data exists that the nerves can be activated first by common triggers leading to blood vessel dilation. For instance, triggers such as alcohol and spicy food consumption and temperature changes can activate calcium channels, specifically transient receptor potential vanilloid (TRPV)1/transient receptor potential ankyrin (TRPA)1, thereby inducing the release of neurotransmitters and the dilatation of microvessels (3). These changes manifest as skin erythema, a characteristic clinical sign of rosacea.
The following sections shed more light on these mechanisms of inflammation and the components of the immune system involved in rosacea (Figure 1).
2.1 External triggers of inflammation and the immune system
Exposure to UV radiation and external pathogens activates TLR2 (5, 10), leading to the subsequent activation and release of KLK5 (11). In addition, inflammatory cells such as neutrophils and macrophages release matrix metalloproteinases (MMPs), which convert pro-KLK5 into KLK5 (12). KLK5 is a serine protease that cleaves cathelicidin, an antimicrobial peptide secreted by epidermal keratinocytes, into the human antimicrobial peptide LL-37, an immune-stimulating factor (9). This process, referred to as the cathelicidin activation pathway, plays a crucial role in the inflammatory response in rosacea (9). Given that LL-37 plays a pivotal role in rosacea-related inflammation, this pathway is frequently referenced in the subsequent discussion on immune dysregulation.
Regarding the triggers of rosacea, researchers have extensively focused on the cutaneous microbiome (13). Rosacea may be associated with certain skin microorganisms such as Demodex folliculorum, Staphylococcus epidermidis, Bacillus oleronius (a bacterium carried by Demodex mites), Bartonella quintana, and Chlamydia pneumoniae (8). However, studies examining the relationship between these microorganisms and rosacea have reported inconsistent results. Among these microorganisms, Demodex has garnered the most attention and has the largest evidence for its role in rosacea. Demodex, with its chitin exoskeleton, can increase TLR2 levels and induce a proinflammatory response (5). An in vitro study revealed that Demodex antigens can activate the human inflammasome (5). Furthermore, Demodex-associated microorganisms, such as B. oleronius, may induce the differentiation of immune cells and promote the secretion of various substances, including cathelicidin, MMP-9, tumor necrosis factor (TNF), and interleukin (IL)-8 (14). The current literature strongly supports the role of Demodex as a trigger of rosacea.
2.2 Genetic predisposition including immune associated genes
Recent epidemiological studies, family and twin studies, genetic association studies, and in vitro analyses have increasingly suggested the involvement of a genetic component in the development of rosacea (6, 15–20). By including both identical and fraternal twins, a twin study differentiated genetic susceptibility from the role of environmental factors in rosacea development; the results suggested that genetics contribute to 46% of individuals’ predisposition to rosacea (19).
The genes mentioned in the literature can be broadly classified into the following major categories:
1. Cathelicidin activation pathway: Overexpression of genes such as cathelicidin antimicrobial peptides (6), KLK5, and LL-37 (21) has been validated in molecular and functional studies using human keratinocytes and skin explants of rosacea compared to healthy skin controls.
2. External triggers: RNA sequencing revealed alteration of gene expression levels such as TLR2 and TRPV in samples from rosacea patients compared to non-lesional skins or healthy skin controls (22–25).
3. Systemic autoimmune processes: A genome wide association study (GWAS) of Caucasian rosacea patients without any autoimmune disease found single nucleotide polymorphism (SNP) in genes such as HLA-DRB1*03:01, HLA-DQB1*02:01, and HLA-DQA1*05:01, which are associated with MHC class II and antigen presentation (26).
4. Neurovascular factors: The roles of neurotransmitters such as calcitonin gene-related peptide (CGRP) and pituitary adenylate cyclase-activating peptide (PACAP) in rosacea were supported by a case-control observational study and a retrospective study (27–29). Moreover, a whole-genome sequencing in 3 large rosacea families and whole exome sequencing in 49 additional validation families from Han population which revealed rare, single deleterious variants of LRRC4, SH3PXD2A, and SLC26A8, which are genes for neural synaptic processes and cell adhesion, in large families with rosacea. Subsequent in vitro and mouse studies have revealed that these genes induced the production of vasoactive neuropeptides, thereby leading to rosacea-like skin inflammation (4).
5. Proinflammatory cytokines and chemokines: Another GWAS study of Caucasian rosacea patients found SNP in genes such as interleukin-13 (IL-13) and interferon regulatory factor-4 (IRF4) (30).
6. Altered adaptive immunity: Genes related to the Th1/Th17 pathway, specifically IL1B, IL6, IL17A, and IL22 are upregulated in rosacea patients compared to healthy skin controls (23, 24, 31).
2.3 Immune dysregulation
Data on immune dysregulation in rosacea primarily involves disturbances in the innate immune system (Figure 2). Rosacea-affected skin tissues exhibit substantially higher concentrations of antimicrobial peptides, particularly cathelicidin and LL-37, than do normal skin tissues (2, 9). In addition, rosacea upregulates the expression level of TLR2, thereby increasing the secretion of KLK5 from keratinocytes. This promotes the cathelicidin activation pathway, resulting in increased LL-37 production (2, 5). These molecules play crucial roles in regulating the innate immune system in patients with rosacea. Innate immunity–related inflammatory cells such as mast cells play important roles in rosacea. While there is limited research specifically on antihistamines or mast cell stabilizers in rosacea, some studies suggest they may have a beneficial effect in reducing inflammation and symptoms (6). The proportion of mast cells is markedly increased in affected tissues. LL-37 can induce mast cells to secrete cathelicidin, which, in turn, increases the level of LL-37 (32). LL-37 can also activate neutrophils and macrophages, leading to the release of various cytokines and MMPs (33). MMPs can convert pro-KLK5 into KLK5, further increasing the level of LL-37 in affected tissues (12). These findings indicate that the innate immune system of patients with rosacea is trapped in a cycle of activation and inflammation under the effects of various triggers.
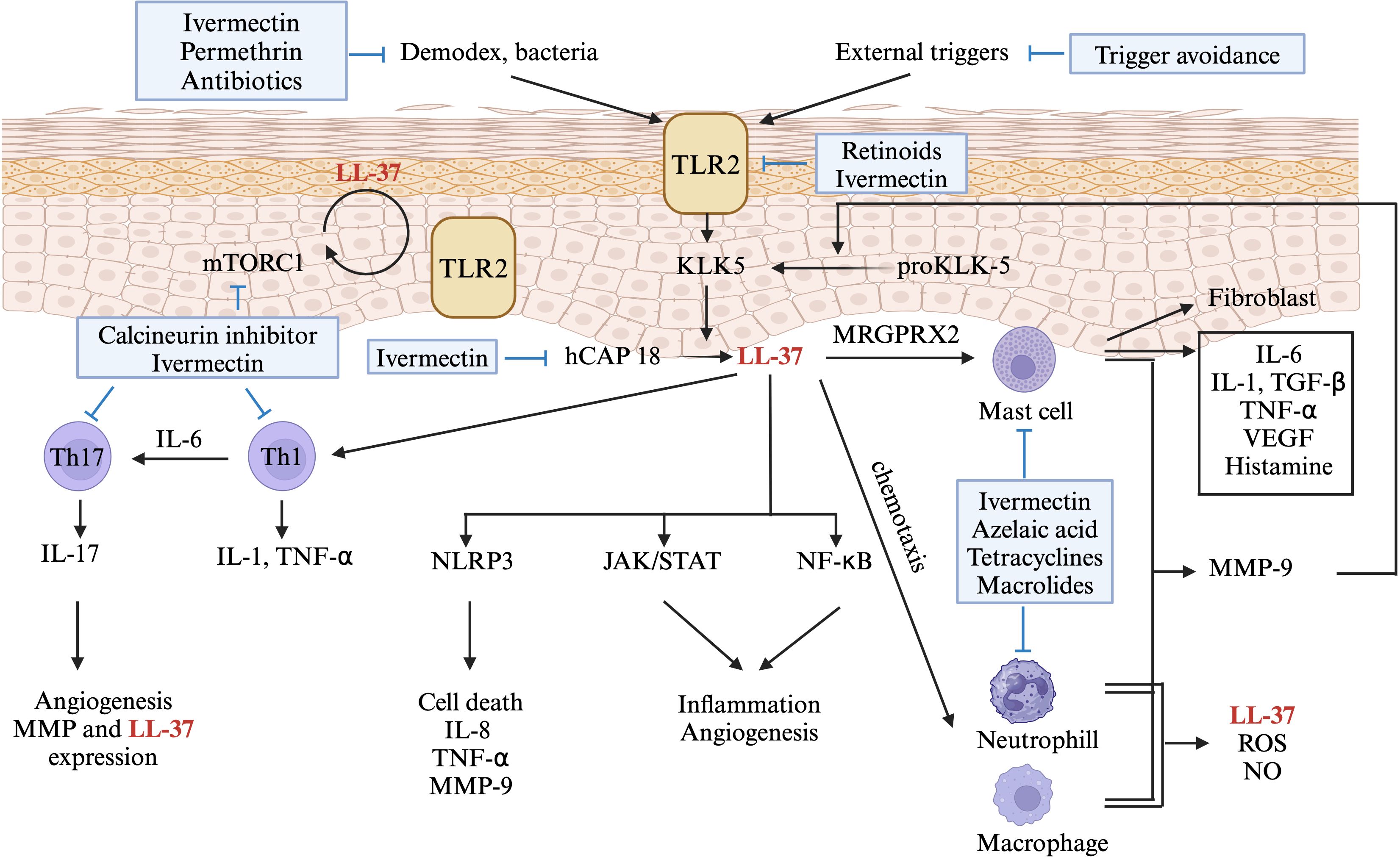
Figure 2 A diagram showing the role of immune dysregulation in the pathogenesis of rosacea. Environmental factors activate toll-like receptor (TLR)2 on keratinocytes, leading to kallikrein (KLK) 5 expression in rosacea. KLK5 cleaves human cathelicidin (hCAP), producing LL-37 (also known as hCAP18), triggering pathways like NLR family pyrin domain containing (NLRP)3 inflammasome, Janus protein tyrosine kinase/Signal Transducers and Activators of Transcription (JAK/STAT), and nuclear factor kappa-light-chain-enhancer of activated B cells (NF-kB). LL-37 stimulates mast cells via Mas-related G-protein coupled receptor member (MRGPR) X2, inducing the production of inflammatory cytokines and matrix metalloproteinase (MMP)9. MMP-9 further enhances KLK5 production or releases LL-37, creating a positive feedback loop. LL-37 also stimulates Th-1 and Th-17 cells, with IL-17 contributing to angiogenesis. Production of hCAP is regulated by LL-37-induced mammalian target of rapamycin (mTORC) 1 signaling, again creating a positive feedback loop. Immune-targeted therapies for rosacea are shown in blue boxes. Only drugs aligned with Swiss S1 and National Rosacea Society guidelines are indicated. This figure was created with BioRender.com and obtained authorization on February 11, 2024. TLR2, toll-like receptor 2; KLK-5, Kallikrein 5; hCAP, human cathelicidin; mTOR, mammalian target of rapamycin; IL-, interleukin; TGF-β, Transforming growth factor beta; TNF-α, tumor necrosis factor; VEGF, vascular endothelial growth factor; MRGPRX2, Mas-related G-protein coupled receptor member X2; Th-, T helper cell; NLRP3, NLR family pyrin domain containing 3; JAK/STAT, Janus protein tyrosine kinase/Signal Transducers and Activators of Transcription; NF-κB, nuclear factor kappa-light-chain-enhancer of activated B cells; MMP, matrix metalloproteinase; ROS, reactive oxygen species; NO, Nitric oxide.
Vitamin D plays a major role in both the innate and adaptive immune systems. In particular, this vitamin regulates the expression of cathelicidin in keratinocytes (34). Increased vitamin D levels and altered VDR single-nucleotide polymorphisms are strongly associated with rosacea (35). It is not known whether vitamin D supplementation or toxicity leads to facial redness.
Rosacea is a chronic inflammatory skin condition. The role of the antioxidative system in the regulation of oxidative stress (e.g., during inflammation) has been implicated in the pathogenesis of rosacea (36). A study revealed marked differences between patients with rosacea and individuals without this condition in the expression levels of glutathione S-transferase polymorphisms, indicating that these polymorphisms are associated with an increased risk of rosacea (37).
In addition to the innate immune system, the adaptive immune system is affected by rosacea. Abundant Th1/Th17 cells have been observed in affected tissues (31). Th17 cells secrete interleukin (IL)-17, which induces angiogenesis through vascular endothelial growth factor and affects the expression of LL-37 in keratinocytes (38).
2.4 Neurovascular dysregulation and inflammation
Transient receptor potential (TRP) channels, including TRPV1, TRPV4, and TRPA1, play key roles in neurovascular dysregulation (Figure 3). These TRPs are expressed not only in nerve endings but also in the endothelium and keratinocytes (27). External factors such as cold, heat, alcohol, spicy foods, and chemicals can theoretically activate these TRPs, leading to the release of vasoactive neurotransmitters, such as PACAP, CGRP, vasoactive intestinal peptide, and substance P (39). These neurotransmitters result in vasodilation and stimulate downstream inflammatory cells such as mast cells, macrophages, and neutrophils, thereby inducing inflammatory response (3, 27). Neuropeptides released from sensory neurons affect vascular endothelial cells, causing vasodilation and increasing capillary permeability (27, 40). In addition, these neuropeptides activate cutaneous mast cells through MRGPRX2, leading to the degranulation of these cells and the production of chemokines and cytokines (27, 40), thereby exacerbating rosacea.
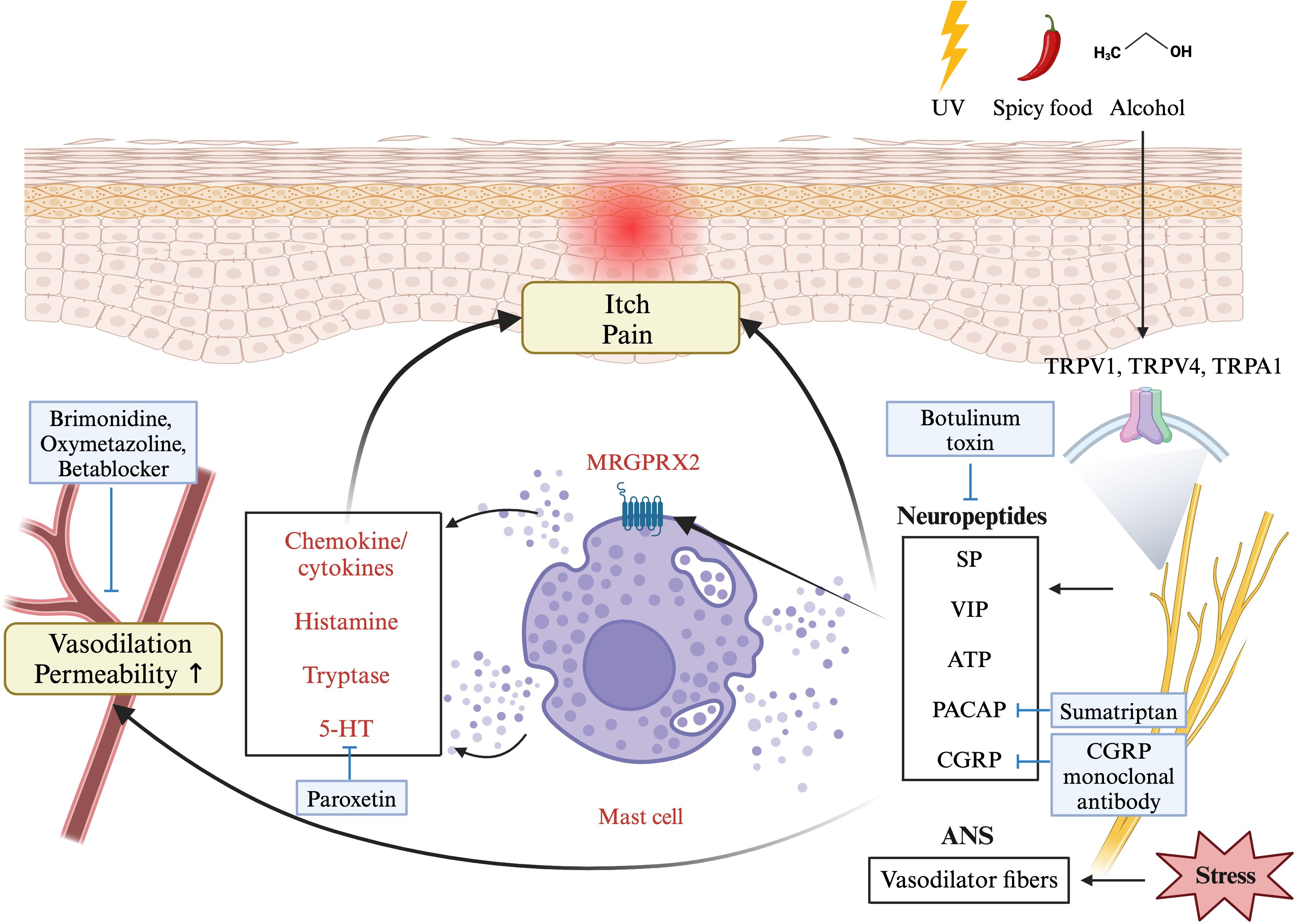
Figure 3 A diagram showing the pathophysiology of rosacea involving neurovascular dysregulation. External factors can activate sensory neurons via transient receptor potential cation channel (TRPV) receptors, leading to neuropeptide release. This activates mast cells through Mas-related G-protein coupled receptor member (MRGPR) X2, causing degranulation of histamine, serotonin, and chemokines, resulting in vasodilation, pain, and itch. Stress causes the autonomic nervous system to overstimulate vasodilator fibers, affecting the layer of smooth muscle enveloping the blood vessels, ultimately resulting in vasodilation. The figure includes medications recommended by Swiss S1 and National Rosacea Society guidelines (blue boxes) associated with this mechanism. This figure was created with BioRender.com and obtained authorization on July 03, 2024. UV, ultraviolet; TRP-, transient receptor potential channel; SP, substance P; VIP, vasoactive intestinal polypeptide; ATP, adenosine triphosphate; PACAP, pituitary adenylate cyclase-activating peptide; CGRP, calcitonin gene related peptide; ANS, autonomic nervous system.
Several studies suggest that adenosine 5’-triphosphate (ATP), a purinergic nucleotide and neurotransmitter, also participates in rosacea pathogenesis by regulating vascular tone and immune responses (41). In human microvascular endothelial cells (HMEC-1), an ATP analogue enhances the secretion of pro-inflammatory cytokines such as IL-6 and IL-8, promotes the release of chemokines like CCL-2, CCL-5, CCL-21, and CXCL1, and increases the expression of adhesion molecules (41). These actions indicate that ATP may exacerbate inflammation and vasodilation, which are key components in rosacea.
Rosacea patients exhibit heightened responses to heat or stress, leading to increased sweating and cutaneous vasodilation (42). This involves sympathetic nerve excitation, affecting vascular smooth muscle contraction in superficial peripheral vessels through multiple alpha-receptors (43). Additionally, rosacea may feature dilated blood and lymphatic vessels, contributing to erythema and edema (2). Patients with neurogenic rosacea, which resembles small fiber neuropathy, often present with gastrointestinal symptoms and dysautonomia (44). Both conditions share similar manifestations, complications, and treatments, indicating common autonomic nervous system dysregulation (44).
The aforementioned hypothesis remains to be confirmed through studies on the associations of these vasoactive neurotransmitters with rosacea. An in vitro study revealed that mast cells require prior upregulation of TRPV4 for effective LL-37-mediated stimulation and subsequent complete degranulation (45). This indicates a possible crosstalk between neurovascular dysregulation and immune dysregulation—two major pathogenic mechanisms underlying rosacea (32).
3 Current therapeutic strategies and clinical trials
Currently, treatment options for rosacea include topical and oral drugs, light devices, and appropriate skincare and lifestyle management (46).
This section provides an overview of various treatment strategies for rosacea particularly focused on modulating the immune system either directly or indirectly. To highlight key therapeutic targets, the strategies were categorized on the basis of their mechanisms of action. Although a single medication may affect multiple mechanisms, we focused on the primary target of each strategy. The following sections present commonly recommended treatment options with an overview of emerging treatment approaches based on The Oxford 2011 Levels of Evidence (47) (Supplementary Tables 1-4). Medications that have been approved by the US Food and Drug Administration (FDA) are marked with an asterisk (*).
Laser, light therapies, and surgery are essential for treating rosacea-associated telangiectasia resistant to standard therapies. Treatments such as potassium titanyl phosphate (KTP) laser, pulsed dye laser (PDL), neodymium-doped yttrium aluminum garnet (Nd : YAG) laser, and intense pulsed light (IPL) therapies address telangiectasis, erythema, and flushing (48). However, their impact on immune dysregulation and neuroinflammation lacks evidence and is beyond this article’s scope.
3.1 Immune-targeted therapy
Immune dysregulation in rosacea involves innate immune disturbances, including TLR2 activation, KLK5 release, and cathelicidin cleavage into LL-37 (9). These processes, triggered by UV radiation and pathogens, are crucial in rosacea inflammation (5, 6). Adaptive immunity, especially Th1/Th17 cells, is also affected in rosacea (31), indicating a complex immune response. Supplementary Table 2 lists selected therapeutics targeting immune dysregulation for rosacea. FDA-approved anti-inflammatory agents for rosacea include oral tetracyclines (49), topical minocycline (50), and azelaic acid (51, 52). Off-label, oral isotretinoin (53, 54) and topical calcineurin inhibitors (55, 56) are widely used. Studies have explored other potential anti-inflammatory agents, such as secukinumab, targeting IL-17, due to data indicating Th17 cells’ role in rosacea.
3.1.1 Oral tetracyclines as anti-inflammatory agents
Oral doxycycline (40 mg/day; 30 mg immediate release and 10 mg delayed release) is a well-established, FDA-approved treatment option for rosacea (57). Its benefits have been validated by a phase III randomized controlled trial (49). Submicrobial doses of doxycycline can effectively alleviate inflammation by suppressing the production of proinflammatory cytokines, reactive oxygen species, nitric oxide synthetase, and MMPs, while also avoiding antibiotic resistance or dysbiosis (58, 59). Furthermore, doxycycline can inhibit the cathelicidin activation pathway and immune cell recruitment (59). These anti-inflammatory effects of doxycycline have led to its recommendation by treatment guidelines (46, 60). While tetracyclines are commonly used in rosacea and acne for their anti-inflammatory effects, their antimicrobial properties likely alter the composition of the skin microbiome. A systematic review found that these treatments mostly decrease Cutibacterium acnes and increase the alpha diversity of the cutaneous microbiota (61).
Other tetracyclines also demonstrate significant anti-inflammatory effects, including the inhibition of chemotaxis, granuloma formation, and proteases (62). Therefore, various tetracycline drugs, formulations, and dosages are commonly used off-label in rosacea treatment (62). These alternatives include tetracycline (250–1000 mg/day), doxycycline (100–200 mg/day), and minocycline (100–200 mg/day). These agents are effective against PPR (59, 63, 64).
Other forms of oral tetracyclines have recently shown efficacy in treating rosacea. DFD-29, a minocycline extended-release oral capsule (40 mg), exhibited a significantly higher level of efficacy than did placebo and doxycycline (40 mg) in the treatment of PPR with the plasma level of drug was maintained below its antimicrobial threshold (65).
A new generation tetracycline, sarecycline, has proven effective and safe in the treatment of PPR. Compared with control, sarecycline significantly improved patients’ IGA scores, reduced inflammatory lesion counts, and alleviated rosacea’s secondary symptoms such as burning sensation, without causing any adverse effects (66). While not extensively examined in humans, sarecycline has demonstrated anti-inflammatory activity comparable to doxycycline and minocycline in a rat paw edema model (67).
Notably, the mechanism of action of tetracyclines in rosacea primarily involves modulating immune dysregulation, not neurovascular dysregulation. Therefore, when using tetracyclines in patients with ETR, significant improvement is generally not expected (46). Tetracycline may inhibit the proinflammatory process induced by the ATP analogue in vascular endothelial cells in vitro (41, 68). While there may be a slight effect on persistent erythema, the level of evidence supporting this is not strong (46).
3.1.2 Topical azelaic acid
Azelaic acid gel (15%) is a well-established, FDA-approved topical agent. According to standard guidelines and multiple randomized controlled, double-blinded multicenter trials, azelaic acid is beneficial against mild to moderate inflammatory papules or pustules (46, 57, 60, 69, 70). However, it exhibits a low level of efficacy against erythema, primarily in patients with the papulopustular phenotype (51, 52). Azelaic acid works by suppressing KLK5 and cathelicidin expression and activating peroxisome proliferator-activated receptor γ, thus reducing the degrees of inflammatory responses and the levels of proinflammatory factors such as IL-1, IL-6, and TNF-α (71, 72). Additionally, this medication can suppress the UV-induced activation of nuclear factor (NF)-κB p65 subunit (73).
3.1.3 Topical tetracyclines
Minocycline foam 1.5% (FMX103), which was approved by the US FDA in 2020, was found to be effective and safe against moderate to severe papulopustular rosacea (PPR) in two 12-week, randomized, double-blinded, vehicle-control phase III trials (50). Another topical formulation is minocycline gel, whose 1% and 3% concentrations were tested in a 12-week, randomized, double‐blinded, vehicle‐controlled phase IIb trial; the results revealed that both concentrations, particularly the 3% concentration, significantly reduced inflammatory lesion counts and improved the Investigator’s Global Assessment (IGA) scores (74). As mentioned above, oral minocycline act as anti-inflammatory agents. While topical minocycline shows promise in delivering anti-inflammatory effects with reduced risk of systemic adverse effects, further evaluation is needed.
3.1.4 Oral isotretinoin
Isotretinoin is an off-label treatment option for rosacea. It is indicated for moderate to severe PPR (46, 57, 60). Isotretinoin is typically administered at a low daily dose (0.25–0.3 mg/kg) over a 4-month period; this is followed by a gradual dose reduction (54). Mechanistically, isotretinoin works by downregulating TLR2 expression in keratinocytes (53, 54). By inhibiting the activity of the sebaceous gland and reducing the production of sebum, isotretinoin can delay the progression of inflamed phyma when used during the prefibrotic phase of PPR (54, 75). Although the anti-inflammatory effect of isotretinoin might theoretically benefit erythema by inhibiting neuro- inflammation, its efficacy in treating erythema in rosacea patients shows inconsistent results (76). Some studies indicate that low-dose (0.25 mg/kg/day) isotretinoin is not effective in treating erythema and telangiectasia (77), while others suggest that an intermediate dose (20 mg/day) effectively improves erythema within four weeks (78).
3.1.5 Oral macrolides
Macrolide drugs, such as erythromycin, clarithromycin, and azithromycin, are secondary agents used in the systemic treatment of rosacea. For azithromycin, the dosage is 500 mg three times weekly for 4 weeks, followed by 250 mg three times weekly for additional weeks. For clarithromycin, the dosage is 250 mg twice daily for 4 weeks, followed by 250 mg once daily for the next 4 weeks. They are particularly useful for patients with contraindications to tetracycline, such as those who are refractory or pregnant (57, 79). The therapeutic effects of macrolides extend beyond their antimicrobial properties to include immunomodulatory effects. For example, azithromycin can modulate transcription factors such as NF-κB, reduce the release of inflammatory cytokines, impede the migration of neutrophils, inhibit the activation of neutrophils and eosinophils, and suppress the release of reactive oxygen species (57, 80). However, despite the low level of evidence for the efficacy of azithromycin in treating rosacea, its gastrointestinal adverse effects are increasingly becoming a concern (81, 82).
3.1.6 Hydroxychloroquine
Hydroxychloroquine effectively suppresses the activation of mast cells by inhibiting Ca2+-activated K+ channels, leading to a reduction in local Ca2+ influx (83). This suppression results in the inhibition of inflammatory factor release, chemotaxis, and degranulation, ultimately reducing chemokine production and neutrophil and monocyte recruitment (83).
Patients who received hydroxychloroquine for 8 weeks exhibited improvements in their rosacea phenotypes along with improved IGA and Clinician Erythema Assessment (CEA) scores, indicating a tendency toward symptomatic relief (83). In a separate multicenter, randomized, double-blind, double-placebo pilot study, participants received either oral hydroxychloroquine (200 mg twice daily) or doxycycline (100 mg once daily) along with their respective placebos for 8 weeks; in this study, hydroxychloroquine was found to be noninferior to doxycycline in terms of rosacea-specific quality-of-life scores (84).
3.1.7 Secukinumab
IL-17, a proinflammatory cytokine produced by Th17 cells, has been implicated in rosacea-related inflammation (31, 85). Th17 activation contributes to the upregulation of LL-37 expression, which, in turn, promotes the production of cathelicidin (85). The synergistic actions of LL-37 and IL-17 result in the release of C-X-C motif chemokine ligand 8 and IL-6, facilitating neutrophil chemotaxis and Th17 differentiation, respectively (85). This Th1/Th17 polarized inflammation, often underrecognized, is common across all subtypes of rosacea (31). In an exploratory study having an open-label, rater-blinded design, secukinumab, an IL-17A inhibitor, significantly reduced papule counts and improved global severity and median RosaQOL scores after 16 weeks of systemic therapy (86). However, more comprehensive and definitive evidence from high-quality randomized controlled trials is needed to fully understand the efficacy of secukinumab in the management of rosacea.
3.1.8 Janus kinase inhibitors
The JAK-STAT signaling pathway plays a pivotal role in the proinflammatory processes within immune cells. In the pursuit of effective rosacea treatments, oral JAK inhibitors like tofacitinib and abrocitinib have been explored (87, 88). A compelling case series involving 21 patients with erythematotelangiectatic and papulopustular rosacea demonstrated promising results, with 71.4% experiencing significant regression of facial erythema (IGA ≤ 1) and a mean change of -2.24 in the IGA score (87). Additionally, a separate case series highlighted the efficacy of abrocitinib in improving steroid-induced rosacea (88). These findings underscore the substantial potential of JAK inhibitors for broader utilization in the management of rosacea.
3.1.9 Topical calcineurin inhibitors
Pimecrolimus cream proves to be an effective treatment option for those with mild to moderate inflammatory rosacea (55). Pimecrolimus exhibits anti-inflammatory activity against PPR by inhibiting the activation of T cells, suppressing the production of inflammatory cytokines, and preventing the release of cytokines from T and mast cells through the inhibition of calcineurin phosphatase (89). The calcineurin inhibitor tacrolimus can treat erythema on rosacea patients (90). However, a higher level of evidence is available for 1% pimecrolimus than for tacrolimus (55, 56, 60). Prescribing these drugs should be done cautiously due to the potential risk of causing rosacea-like eruptions (91). The potential adverse events may be attributable to their immunosuppressive effects, which results in the overgrowth of microorganisms such as D. folliculorum (91). Therefore, pimecrolimus and tacrolimus may be regarded as dual-edged swords in the treatment of rosacea (91).
3.1.10 Mammalian target of rapamycin inhibitor
Mammalian target of rapamycin complex 1 (mTORC1) can regulate cathelicidin through a positive feedback loop (92). In this loop, LL-37 activates mTORC1 signaling by binding to TLR2, thereby upregulating the expression of cathelicidin in keratinocytes (92). Excess LL-37 may induce NF-κB activation through mTORC1 signaling, leading to the production of disease-specific cytokines and chemokines (92). Overexpression of TLR7 in keratinocytes stimulates the mTORC1 pathway through NF-κB signaling (93). In a pilot study involving 18 women with rosacea, the patients were randomized to receive either a placebo (n = 8) or 0.4% topical rapamycin (sirolimus) ointment (n = 10) for 4 weeks (92). After the intervention, the level of clinical improvement was significantly higher in the rapamycin group than in the placebo group. Topical treatment with rapamycin significantly reduced the patients’ CEA and IGA scores (92).
3.1.11 Artemisinin
Artemisinin, a widely recognized antimalarial drug, effectively suppressed the infiltration of CD4+ T cells in a mouse model of LL-37-induced rosacea (94, 95). This drug also suppressed the LL-37-induced invasion of neutrophils and macrophages, thereby mitigating innate immune responses against rosacea (95). In HaCaT cells, artemisinin suppressed the release of proinflammatory factors, potentially through the NF-κB pathway (95). An open-label trial involving 130 patients with rosacea who received artemether emulsion, one of the most extensively studied lipid-based derivatives of artemisinin, revealed markedly reduced papule and pustule scores as early as 4 weeks into treatment; notably, the erythema scores of patients treated with artemether emulsion were similar to those of patients treated with standard metronidazole for 4 weeks (96).
3.1.12 ACU-D1
The 26S proteasome, a protein complex responsible for NF-κB degradation, regulates the activation of NF-κB. Therefore, inhibiting the 26S proteasome can result in the inhibition of NF-κB; this offers a novel target for rosacea treatment. A two-arm, vehicle-controlled trial evaluated the efficacy of a novel proteasome inhibitor, topical ACU-D1—pentaerythritol tetrakis (3-(3, 5-di-tert-butyl-4-hydroxyphenyl) propionate). The trial included 27 patients in the active arm and 12 patients in the vehicle arm, all presenting with moderate to severe PPR. ACU-D1 was found to be safe and well-tolerated by the patients; it effectively reduced inflammatory lesions and erythema in the patients. Although this trial lacked the statistical power to determine between-arm significance, the evaluation of both efficacy and safety yielded favorable results (97).
3.1.13 Dapsone
Dapsone exerts its anti-inflammatory effects by inhibiting the production of ROS, mitigating the effects of eosinophil peroxidase on mast cells, and suppressing inflammatory responses mediated by neutrophils (98). A case report indicated sustained remission of granulomatous rosacea after the systemic administration of dapsone (dose: 100 mg/day) (99). A double-blind, randomized clinical trial involving 56 adults with PPR demonstrated that 5% dapsone gel exhibited efficacy similar to that of 0.75% metronidazole gel in improving IGA scores and reducing the lesion counts (100). The first to study dapsone use on ETR, reported that 5% dapsone gel used alone for 12 weeks showed significant improvement in the Investigators Global Assessment score, burning sensation, pruritus, edema, and erythema (101).
3.1.14 ϵ-Aminocaproic acid
ϵ-Aminocaproic acid can effectively inhibit trypsin-like proteases, including KLK5, which regulates the cleavage of the cathelicidin precursor protein into LL-37 (102). In a randomized, double-blind, placebo-controlled study involving 11 patients with PPR, no significant differences in the IGA or CEA scores were noted between the treatment and placebo groups at any time point. Notably, in the treatment group, these scores were significantly improved in week 12 compared with baseline (102).
3.1.15 Tranexamic acid
Tranexamic acid (TXA), an antifibrinolytic drug used to treat bleeding conditions, functions by inhibiting the conversion of plasminogen to plasmin, thus suppressing plasmin-induced angiogenesis (103). This suppression is achieved through the reduction of CD31+ microvessel counts and the downregulation of vascular endothelial growth factor expression (104). In the context of skin inflammation, TXA inhibits the production of TLR2, proinflammatory cytokines (IL-6 and TNF-α), and chemokines in keratinocytes primed by LL-37 (104). In an unblinded study, TXA solution–infused wet dressing and microneedling with TXA solution ameliorated the symptoms of erythematotelangiectatic rosacea (103). In a retrospective study investigating the effects of intradermal microinjections of TXA on erythematotelangiectatic rosacea, the observed improvements in symptoms persisted for 3 months (105). In a randomized, vehicle-controlled, split-face study, topical TXA enhanced the barrier function of the epidermal layer and alleviated the clinical signs of rosacea (106). These effects are attributed to the inhibition of protease activated receptor 2 activation, which reduces the calcium influx (106). For oral TXA, one study divided patients into two groups. Seventy patients were treated with doxycycline therapy plus oral TXA or doxycycline therapy alone for 8 weeks. The results showed that the TXA group had better outcomes compared to doxycycline (107).
3.2 Targeted neurovascular therapy for inflammation
Neurovascular dysregulation in rosacea may be linked to immune dysregulation (3, 27). Activation of TRP channels and release of vasoactive neurotransmitters trigger vasodilation, inflammation, and mast cell degranulation, contributing to the symptoms (40). Supplementary Table 3 lists selected therapeutics targeting neurovascular dysregulation for rosacea. FDA-approved treatments for inflammation include topical brimonidine and oxymetazoline (46), acting as vasoconstrictors. Off-label, oral beta blockers are widely used (108). Pilot studies on agents like paroxetine (109), sumatriptan (110), and monoclonal antibodies against CGRP (28) show promise, suggesting a novel treatment approach targeting vasoactive neurotransmitters in rosacea.
3.2.1 Brimonidine
Among drugs targeting neurovascular dysfunction in rosacea, two have received FDA approval (46). The first one is brimonidine topical gel (0.33%), which is a vasoconstrictive alpha-2 adrenergic receptor agonist acting on the microvascular smooth muscles of the facial skin (46). Brimonidine specifically addresses facial erythema instead of papules and pustules (60). Clinical trials ranging in duration from 1 month to 12 months have consistently demonstrated that topical brimonidine is both safe and effective as a maintenance therapy for rosacea (111, 112). A short-term multicenter, randomized study demonstrated its efficacy in improving moderate rosacea (at least a 1-grade improvement) (113). However, brimonidine is not effective in managing telangiectasia (57, 60). Brimonidine had significant risk of developing adverse effects include local erythema, pruritus, burning sensation, and exacerbated flushing symptoms (57, 60, 114).
3.2.2 Oxymetazoline
Oxymetazoline is the second FDA-approved vasoconstrictor for the topical management of persistent erythema in patients with rosacea (46). As a sympathomimetic agent, oxymetazoline acts as a selective alpha1A adrenoreceptor agonist, exhibiting vasoconstrictive and anti-inflammatory properties (57). Multiple phase III clinical trials have reported that 1% oxymetazoline hydrochloride cream, administered for either 29 days or 52 weeks, was well-tolerated, safe, and effective in treating persistent facial erythema in patients with rosacea (115–117). Only a small proportion of patients experienced adverse effects such as apparent worsening of facial erythema, rebound effects, and occasional paradoxical erythema (115–117). A meta-analysis of adverse effects found that using oxymetazoline, compared to the vehicle, had a significantly higher risk of application-site dermatitis (RR = 8.91, 95% CI: 1.76–45.23), while other adverse effects did not show significant statistical differences (118). These findings highlight the differences between oxymetazoline and topical brimonidine in terms of safety and efficacy (114).
3.2.3 β-blocker
The potential mechanism of action of non-selective beta-blockers lies in the inhibition of beta-2 receptors on cutaneous blood vessels, reducing vasodilation (108). Additionally, systemic administration exhibits a reduction in sympathetic tone, thereby alleviating patient anxiety (119). A systematic review indicates that carvedilol and propranolol are effective for rosacea patients with persistent facial erythema and flushing (108). For carvedilol, the dosage ranges from 6.25 to 37.5 mg daily, which can be divided into doses such as 6.25 mg twice daily. For propranolol, the dosage is typically 30-40 mg daily. In a randomized controlled trial, carvedilol demonstrated superior efficacy compared to brimonidine in addressing telangiectasia and facial erythema (120). Moreover, carvedilol significantly improved patients’ depression or anxiety status (120). While beta-blockers offer alternative therapeutic options for rosacea, it is essential to be cautious about common side effects such as hypotension, bradycardia, bronchospasm, and dizziness (121).
3.2.4 Paroxetine
Treatment with paroxetine, a potent selective serotonin (5-HT) reuptake inhibitor, led to improvements in CEA scores, flushing, and burning sensation in a multicentered, randomized, double-blinded, and placebo-controlled trial involving patients with rosacea (109). Paroxetine modulates 5-HT uptake, and dysfunctional 5-HT regulation may result in abnormal blood vessel dilation and constriction (2, 122, 123). Mast cells and platelets were also proved to release 5-HT in several inflammatory skin diseases (124, 125). An association has been reported between rosacea and a single-nucleotide polymorphism of the 5-HT2A receptor (126).
3.2.5 Sumatriptan
PACAP is a potent vasodilator that directly affects smooth muscle cells in arterioles (127). A study reported elevated levels of PACAP expression in rosacea-affected tissues, suggesting its potential relevance in TRPV-mediated edema (29). Another study utilizing both clinical and experimental models confirmed that PACAP38 induces sustained flushing and facial edema (110). In a double-blind, randomized, placebo-controlled, crossover trial, sumatriptan alleviated these symptoms (110). Sumatriptan acts as a 5-HT1B/1D receptor agonist, inhibiting mast cell degranulation and reducing PACAP levels (128, 129). A case report suggested that oral sumatriptan (50 mg) successfully treated a patient with severe and painful rosacea, markedly reducing burning sensation, swelling, redness, and pain (130).
3.2.6 Monoclonal antibodies against CGRP
Erenumab, galcanezumab, fremanezumab, and eptinezumab are four FDA-approved monoclonal antibodies that target CGRP. These antibodies are primarily used for preventing migraine (131, 132). Another novel oral CGRP inhibitor in the pipeline for chronic migraine is atogepant (133). CGRP is involved in the pathophysiology of migraine through nociceptive processes in the trigeminovascular system (134). Notably, CGRP serves as a vasoactive neurotransmitter in rosacea, causing vasodilation and inducing inflammatory response (3, 135). In 2020, an exploratory trial was initiated to investigate the efficacy and tolerability of erenumab in the preventive treatment of persistent redness and flushing associated with rosacea; this trial was anticipated to conclude in 2021 (136). Furthermore, an exploratory comparative case series involving 13 patients explored the benefits of CGRP monoclonal antibodies for patients with both rosacea and migraine (28). The results revealed marked posttreatment improvements in the patients’ severity scores (28). However, two patients experienced dermatitis at the injection site (28). Although most patients in this study experienced improvements in rosacea, large-scale randomized clinical trials are still needed to confirm the efficacy and safety of CGRP monoclonal antibodies.
3.2.7 Botulinum toxin
Botulinum toxin (BTX) improves neurovascular dysfunction in rosacea by blocking the release of neurotransmitters, such as acetylcholine, calcitonin gene-related peptide, vasoactive intestinal peptide, substance P, and glutamate, or by reducing non-noxious stimulation (135, 137, 138). This action inhibits the skin’s vasodilator system (stimulated by peripheral autonomic nerves), thereby suppressing rosacea-associated neuroinflammation and vasodilation (4, 139). BTX inhibits the activation of TRPV1 by disrupting the lipid raft activity associated with TRPV1 through structural and functional interactions with it (140). Moreover, BTX directly blocks the degranulation of mast cells by cleaving SNARE protein in these cells (141). In a randomized, controlled, split-face study involving 22 patients, one side of the cheek was treated with a combination of BTX and broadband light. Compared with the pretreatment condition and control groups, the experimental group exhibited reduced global flushing symptom scores, erythema index scores, transepidermal water loss, and sebum secretion (142). The effects of using BTX typically appear within 1-2 weeks and can last for 3-6 months. The most common side effect was localized pain, while the rarer and more concerning side effect was paralysis of motor muscles, with a pooled incidence of 4.3% (95% CI: 1.8 - 7.8%). Most cases of paralysis resolved on their own (143).
3.3 Targeted antimicrobial therapy
Research on the cutaneous microbiome in rosacea focuses on Demodex folliculorum, Staphylococcus epidermidis, Bacillus oleronius, Bartonella quintana, and Chlamydia pneumoniae (5, 8, 13, 14). Demodex, with its chitin exoskeleton, triggers inflammation by increasing TLR2 levels (5, 8), and therefore becomes the major target of antimicrobial therapy for rosacea. Supplementary Table 4 lists therapeutics targeting microbiota for rosacea. FDA-approved antimicrobials include topical metronidazole (82, 144), ivermectin (145), and microencapsulated benzoyl peroxide (146). Off-label, oral metronidazole (147), oral ivermectin (148), topical sulfur preparation and topical permethrin (148) target Demodex as well. Ongoing studies investigate topical omiganan (149) and oral rifaximin (150). Despite optimism for treatments like bacterial transfer, probiotics, or prebiotics to balance the microbiome, the lack of large-scale trials hampers confirming their efficacy (151).
3.3.1 Metronidazole: oral and topical uses
Because of its dual efficacy as an antibiotic and antiparasitic agent, FDA-approved topical metronidazole is widely used for the treatment of rosacea across the globe and indirectly reduces inflammation by putative reduction of Demodex colonization. Demodex mites are implicated in the pathogenesis of rosacea, and the antiparasitic effect of metronidazole plays a key role in this context (57). Metronidazole’s efficacy in rosacea treatment is also attributed to its anti-inflammatory, immunosuppressive, and antioxidative characteristics, involving the reduction of reactive oxygen species production from neutrophils and inhibition of IL-17 (152–154). The efficacy of topical metronidazole is supported by clinical trials, including randomized, placebo-controlled double-blind studies, as highlighted in a Cochrane review (82, 144). Clinical guidelines recommend topical metronidazole for the treatment of PPR (46, 57, 60). The efficacy of oral metronidazole was evaluated in a double-blind trial, where a combination therapy comprising metronidazole (200 mg twice a day) and 1% hydrocortisone cream was administered for 6 weeks; this therapy reduced the severity of rosacea in 10 out of 14 patients (147).
3.3.2 Ivermectin: oral and topical uses
The US FDA have approved 1% ivermectin cream for the treatment of PPR (155). Clinical guidelines recommend topical ivermectin as a first-line treatment for rosacea, citing high-level evidence for its efficacy in addressing papules and pustules—the key features of rosacea (46, 60). A 2016 network meta-analysis identified ivermectin 1% cream to be the most effective treatment option for inflammatory papules and pustules (145). Regarding the Demodex decrease rate, both topical and systemic ivermectin are superior to other anti-Demodex therapies, achieving nearly a 100% decrease rate (156). Its therapeutic mechanism involves effectively reducing Demodex infestation through neurotoxic activity (157). Owing to its anti-inflammatory properties, ivermectin reduces the levels of IL-1b and TNF-α while increasing the level of IL-10. Additionally, ivermectin reduces the expression of proinflammatory genes such as IL-8, LL-37, human β-defensin 3, and TNF-α (158). At the protein level, significant reductions were observed in the levels of LL-37 and IL-8 (158).
3.3.3 Sulfur preparation
The US FDA-approved topical treatment also included sulfur preparations in various formulation such as creams, gels and cleansers. The most common formulation is sodium sulfacetamide, 10% (46). Sulfur preparations have shown positive effects on both erythematotelangiectatic rosacea (ETR) and papulopustular rosacea (PPR), similar to metronidazole (159). This effect may be due to the significant reduction in Demodex count with the sulfur-sodium combination (160). However, sulfur has a more noticeable irritating effect compared to metronidazole, permethrin, lindane, and crotamiton (161).
3.3.4 Microencapsulated benzoyl peroxide cream
Benzoyl peroxide, a potent oxidizing agent, is commonly used in acne treatment (155) and indirectly reduces inflammation through putative antibacterial effects. In 2022, the US FDA approved encapsulated cream benzoyl peroxide (E-BPO) 5% for patients with rosacea (155). The advantage of this silica-based encapsulated formulation is that it facilitates a gradual release of benzoyl peroxide into the skin. This approach enhances efficacy and prevents skin irritation associated with traditional bolus formulations of benzoyl peroxide, thereby improving tolerability (155, 162). Although E-BPO’s mechanism of action in the treatment of rosacea remains hypothetical, it primarily centers around the antibacterial effect of this drug (136, 163). In two 12-week, identical, parallel, randomized, double-blind phase III trials, a high proportion of E-BPO-treated patients achieved a clear/almost clear status in the IGA and a reduced lesion count (146). However, a few adverse events were noted; these included pain, erythema, pruritus, and edema (146). Furthermore, a 52-week, single-arm phase III trial investigating the long-term use of E-BPO indicated that prolonged E-BPO use is a safe and well-tolerated therapeutic approach (164).
3.3.5 Topical permethrin
The role of Demodex mites in the development of rosacea is well-established and its anti-inflammatory effect is indirect though putative reduction of mites that may stimulate the immune system. Researchers have explored permethrin, an antiparasitic belonging to the pyrethrin group, as a treatment choice for rosacea (154). The results indicated a notable decrease in the density of Demodex mites, indicating permethrin as a primary treatment choice for Demodex infestation (165). In a 12-week, double-blind study involving 20 patients with PPR, the application of 5% permethrin cream resulted in a marked reduction in the density of Demodex mites and an improvement in the symptoms of rosacea compared with the effects of a cream base (140). However, the use of permethrin for the treatment of rosacea is currently considered off-label.
3.3.6 Omiganan topical gel
Omiganan is an anti-microbial peptide tested against rosacea. In randomized, double-blind, vehicle-controlled, parallel-group, multicenter phase III trial involving patients with severe PPR, topical application of 1.6% omiganan gel significantly outperformed the vehicle in reducing mean inflammatory lesion counts and improving IGA scores in week 12 compared with baseline (149). The underlying mechanism of action involves the rapid bactericidal and fungicidal effects of omiganan against a wide range of pathogens (166).
3.3.7 Rifaximin
Rifaximin, a non-absorbed, gut-active antibiotic with broad-spectrum efficacy, is used to treat small intestinal bacterial overgrowth (SIBO) (155). Studies have indicated a higher prevalence of SIBO among patients with rosacea than in the normal population (167, 168). Administering rifaximin to these patients not only mitigate SIBO but also ameliorate rosacea symptoms. The underlying mechanism suggests that SIBO disrupts immunity, triggering rosacea by increasing the levels of TNF-α or other cytokines, reducing the level of IL-17, and stimulating Th1-mediated immune response (150). In a prospective trial involving 113 patients, a 10-day treatment rifaximin (1200 mg/day) significantly reduced the number of cutaneous lesions compared with the findings in the control group (150). Nonetheless, further research is needed to confirm the efficacy of rifaximin. In 2022, a phase IIa, multicenter, double-blind, placebo-controlled, randomized clinical trial (NCT 05150587) investigated the efficacy and safety of extended-release rifaximin against moderate to severe rosacea (169). The research results are yet to be published.
4 Promising therapeutic strategies in preclinical study with anti-inflammatory activity
Several therapeutic strategies for rosacea show promise in pre-clinical studies (Supplementary Table 5). Recent years have seen a growing interest in the potential of herbal medicines against rosacea. Compounds such as osthole, celastrol, Coptis chinensis Franch, and paeoniflorin have demonstrated efficacy in animal or cellular models by inhibiting inflammatory pathways, thereby alleviating rosacea symptoms (170–172). In an animal model of LL-37-induced rosacea-like lesions, the use of erythroid differentiation regulator 1 mitigated erythema, inflammatory cell infiltration, and microvessel density (173). The same model was used for benvitimod—an aryl hydrocarbon receptor agonist; upon stimulation, benvitimod inhibited TLR2-induced inflammatory responses (174). Furthermore, this agonist significantly ameliorated the rosacea-like symptoms (174).
Among antidiabetic medications, both metformin and pioglitazone have gained prominence. Pioglitazone, which is delivered using a new nan-oemulsion formulation, enhances the drug–skin contact and dermal retention while exhibiting anti-inflammatory activity (175). Metformin is effective in improving rosacea-like lesions, reducing LL-37- and TNF-α-induced reactive oxygen production, and mitigating mitogen-activated protein kinase/NF-κB signal activation in keratinocytes (176).
Melatonin, whose therapeutic potential was demonstrated through network pharmacology analysis, reduces the levels of inflammatory cytokines (177). Other compounds such as thalidomide and aspirin, known for their roles in slowing down innate immunity, reducing inflammatory cytokines, and suppressing NF-κB activation, may be effective in treating rosacea (178, 179).
5 Conclusion/discussion
The diagnosis of rosacea primarily relies on patients’ symptoms and phenotypes, as outlined by the 2018 National Rosacea Society Expert Committee guidelines (46). Given the multifactorial nature of rosacea pathophysiology and the diverse triggers and mechanisms involved, treatment approaches must be personalized, taking into account predominant symptoms, phenotypes, pathogenic mechanisms, and trigger factors. Factors such as drug efficacy, tolerability, and previous treatment history should also be considered.
In addressing inflammatory lesions such as papules and pustules, treatment selection should align with underlying pathogenic mechanisms such as immune dysregulation or the presence of Demodex mites (46, 60, 180). Recommended topical treatments include ivermectin, azelaic acid, metronidazole, or microencapsulated benzoyl peroxide cream (69, 146, 157, 181). For severe cases, FDA-approved minocycline foam 1.5% or systemic doxycycline may be appropriate (46, 50). A network meta-analysis showed that, compared to low-dose doxycycline 40 mg, topical ivermectin, and metronidazole 0.75%, oral minocycline 100 mg had the best effect on papulopustules with a low incidence of side effects (81). There are also many off-label treatment options; for refractory papulopustules, oral isotretinoin is an option (60, 182). Oral macrolides can serve as secondary agents, particularly for patients with contraindications to tetracyclines, such as those who are refractory or pregnant (79). However, azithromycin has a high risk of adverse effects (RR, 8.9, 95% CI: 1.3-83), such as gastrointestinal issues (81). Topical pimecrolimus is also mentioned in the Swiss guidelines as an anti-inflammatory agent, but care must be taken due to its immunosuppressive effect, which may cause Demodex overgrowth and pose a potential risk of rosacea-like eruptions (91). Several treatments are still under investigation. As we gain a better understanding of rosacea’s pathophysiological pathways and develop new precision medicines, targets like the pro-inflammatory cytokine IL-17, activation of NF-κB, and the JAK-STAT signaling pathway are becoming therapeutic goals. Drugs such as secukinumab, novel proteasome inhibitors, and Janus kinase inhibitors are aimed at these targets and have shown promising results in novel research (86, 87, 97).
For persistent erythema or flushing, these symptoms are highly associated with neurovascular dysregulation (3). Therefore, drug mechanisms focus on promoting vasoconstriction and inhibiting various vasoactive neuropeptides. FDA-approved medications include brimonidine and oxymetazoline (46). Off-label use of propranolol and carvedilol has also been shown to help control persistent erythema (120). Additionally, many new drugs targeting neurogenic inflammation are under investigation, such as paroxetine, sumatriptan, monoclonal antibodies against CGRP, and botulinum toxin (28, 109, 110, 135). These medications aim to reduce neuropeptide-induced vascular dysfunction. However, the side effects and cost-effectiveness of these new drugs need to be considered, and their efficacy still requires validation through more extensive clinical trials.
Many therapeutic approaches coming down the pipeline address the central role of the immune and neurovascular systems in rosacea. Controlling the immune and neurovascular dysregulations will likely lead to increased improvement in rosacea signs and symptoms, and hopefully, with less side effects. Although some of the cited studies were conducted using animal models or at the cellular level, these references were included to illustrate the breadth of ongoing research and the potential for promising new treatment options for rosacea. However, these findings need validation and rigorous evidence to support their efficacy in humans. Future large-scale clinical trials will be essential to provide such evidence and confirm the effectiveness of these treatments in clinical practice. Understanding the triggers and concomitant medications may assist the clinician in selecting the appropriate treatment regimen for particular patients to maximize chances or rosacea symptom control. Research into correlation between genotypes and phenotypes in rosacea patients may also one day lead to a personalized approach to manage rosacea.
Author contributions
K-YT: Visualization, Writing – original draft, Writing – review & editing. C-JJ: Writing – review & editing. Y-HS: Conceptualization, Writing – review & editing. AC: Conceptualization, Writing – review & editing.
Funding
The author(s) declare that no financial support was received for the research, authorship, and/or publication of this article.
Conflict of interest
The authors declare that the research was conducted in the absence of any commercial or financial relationships that could be construed as a potential conflict of interest.
AC has been an investigator for an investigator initiated rosacea study funded by Novartis.
Publisher’s note
All claims expressed in this article are solely those of the authors and do not necessarily represent those of their affiliated organizations, or those of the publisher, the editors and the reviewers. Any product that may be evaluated in this article, or claim that may be made by its manufacturer, is not guaranteed or endorsed by the publisher.
Supplementary material
The Supplementary Material for this article can be found online at: https://www.frontiersin.org/articles/10.3389/fimmu.2024.1403798/full#supplementary-material
References
2. Steinhoff M, Schauber J, Leyden JJ. New insights into rosacea pathophysiology: A review of recent findings. J Am Acad Dermatol. (2013) 69:S15–26. doi: 10.1016/j.jaad.2013.04.045
3. Marek-Jozefowicz L, Nedoszytko B, Grochocka M, Żmijewski MA, Czajkowski R, Cubała WJ, et al. Molecular mechanisms of neurogenic inflammation of the skin. Int J Mol Sci. (2023) 24(5):5001. doi: 10.3390/ijms24055001
4. Deng Z, Chen M, Zhao Z, Xiao W, Liu T, Peng Q, et al. Whole genome sequencing identifies genetic variants associated with neurogenic inflammation in rosacea. Nat Commun. (2023) 14:3958. doi: 10.1038/s41467-023-39761-2
5. Lacey N, Russell-Hallinan A, Zouboulis CC, Powell FC. Demodex mites modulate sebocyte immune reaction: possible role in the pathogenesis of rosacea. Br J Dermatol. (2018) 179:420–30. doi: 10.1111/bjd.16540
6. Melnik BC. Rosacea: the blessing of the celts - an approach to pathogenesis through translational research. Acta Derm Venereol. (2016) 96:147–56. doi: 10.2340/00015555-2220
7. Awosika O, Oussedik E. Genetic predisposition to rosacea. Dermatol Clin. (2018) 36:87–92. doi: 10.1016/j.det.2017.11.002
8. Zhu W, Hamblin MR, Wen X. Role of the skin microbiota and intestinal microbiome in rosacea. Front Microbiol. (2023) 14:1108661. doi: 10.3389/fmicb.2023.1108661
9. Yamasaki K, Gallo RL. Rosacea as a disease of cathelicidins and skin innate immunity. J Investig Dermatol Symp Proc. (2011) 15:12–5. doi: 10.1038/jidsymp.2011.4
10. Ahn CS, Huang WW. Rosacea pathogenesis. Dermatol Clin. (2018) 36:81–6. doi: 10.1016/j.det.2017.11.001
11. Yamasaki K, Kanada K, Macleod DT, Borkowski AW, Morizane S, Nakatsuji T, et al. Tlr2 expression is increased in rosacea and stimulates enhanced serine protease production by keratinocytes. J Invest Dermatol. (2011) 131:688–97. doi: 10.1038/jid.2010.351
12. Two AM, Del Rosso JQ. Kallikrein 5-mediated inflammation in rosacea: clinically relevant correlations with acute and chronic manifestations in rosacea and how individual treatments may provide therapeutic benefit. J Clin Aesthet Dermatol. (2014) 7:20–5.
13. Zaidi AK, Spaunhurst K, Sprockett D, Thomason Y, Mann MW, Fu P, et al. Characterization of the facial microbiome in twins discordant for rosacea. Exp Dermatol. (2018) 27:295–8. doi: 10.1111/exd.13491
14. Mylonas A, Hawerkamp HC, Wang Y, Chen J, Messina F, Demaria O, et al. Type I ifns link skin-associated dysbiotic commensal bacteria to pathogenic inflammation and angiogenesis in rosacea. JCI Insight. (2023) 8(4):e151846. doi: 10.1172/jci.insight.151846
15. Abram K, Silm H, Maaroos HI, Oona M. Risk factors associated with rosacea. J Eur Acad Dermatol Venereol. (2010) 24:565–71. doi: 10.1111/j.1468-3083.2009.03472.x
16. Sobolewska B, Angermair E, Deuter C, Doycheva D, Kuemmerle-Deschner J, Zierhut M. Nlrp3 A439v mutation in a large family with cryopyrin-associated periodic syndrome: description of ophthalmologic symptoms in correlation with other organ symptoms. J Rheumatol. (2016) 43:1101–6. doi: 10.3899/jrheum.150681
17. Ee HL, Tan HH, Ng SK. Autosomal dominant familial chronic mucocutaneous candidiasis associated with acne rosacea. Ann Acad Med Singap. (2005) 34:571–4.
18. Second J, Korganow AS, Jannier S, Puel A, Lipsker D. Rosacea and demodicidosis associated with gain-of-function mutation in stat1. J Eur Acad Dermatol Venereol. (2017) 31:e542–e4. doi: 10.1111/jdv.14413
19. Aldrich N, Gerstenblith M, Fu P, Tuttle MS, Varma P, Gotow E, et al. Genetic vs environmental factors that correlate with rosacea: A cohort-based survey of twins. JAMA Dermatol. (2015) 151:1213–9. doi: 10.1001/jamadermatol.2015.2230
20. Palleschi GM, Torchia D. Rosacea in a monozygotic twin. Australas J Dermatol. (2007) 48:132–3. doi: 10.1111/j.1440-0960.2007.00353.x
21. Thibaut de Ménonville S, Rosignoli C, Soares E, Roquet M, Bertino B, Chappuis JP, et al. Topical treatment of rosacea with ivermectin inhibits gene expression of cathelicidin innate immune mediators, ll-37 and klk5, in reconstructed and ex vivo skin models. Dermatol Ther (Heidelb). (2017) 7:213–25. doi: 10.1007/s13555-017-0176-3
22. Sun Y, Chen LH, Lu YS, Chu HT, Wu Y, Gao XH, et al. Identification of novel candidate genes in rosacea by bioinformatic methods. Cytokine. (2021) 141:155444. doi: 10.1016/j.cyto.2021.155444
23. Harden JL, Shih YH, Xu J, Li R, Rajendran D, Hofland H, et al. Paired transcriptomic and proteomic analysis implicates il-1beta in the pathogenesis of papulopustular rosacea explants. J Invest Dermatol. (2021) 141:800–9. doi: 10.1016/j.jid.2020.08.013
24. Shih YH, Xu J, Kumar A, Li R, Chang ALS. Alterations of immune and keratinization gene expression in papulopustular rosacea by whole transcriptome analysis. J Invest Dermatol. (2020) 140:1100–3 e4. doi: 10.1016/j.jid.2019.09.021
25. Sulk M, Seeliger S, Aubert J, Schwab VD, Cevikbas F, Rivier M, et al. Distribution and expression of non-neuronal transient receptor potential (Trpv) ion channels in rosacea. J Invest Dermatol. (2012) 132:1253–62. doi: 10.1038/jid.2011.424
26. Chang ALS, Raber I, Xu J, Li R, Spitale R, Chen J, et al. Assessment of the genetic basis of rosacea by genome-wide association study. J Invest Dermatol. (2015) 135:1548–55. doi: 10.1038/jid.2015.53
27. Helfrich YR, Maier LE, Cui Y, Fisher GJ, Chubb H, Fligiel S, et al. Clinical, histologic, and molecular analysis of differences between erythematotelangiectatic rosacea and telangiectatic photoaging. JAMA Dermatol. (2015) 151:825–36. doi: 10.1001/jamadermatol.2014.4728
28. Sia T, Webb T, Li S, Moskatel LS, Chang ALS. An exploratory comparative case series of calcitonin gene-related peptide monoclonal antibodies in patients with migraine with rosacea. Br J Dermatol. (2023) 189:776–8. doi: 10.1093/bjd/ljad277
29. Seeliger S, Buddenkotte J, Schmidt-Choudhury A, Rosignoli C, Shpacovitch V, von Arnim U, et al. Pituitary adenylate cyclase activating polypeptide: an important vascular regulator in human skin in vivo. Am J Pathol. (2010) 177:2563–75. doi: 10.2353/ajpath.2010.090941
30. Aponte JL, Chiano MN, Yerges-Armstrong LM, Hinds DA, Tian C, Gupta A, et al. Assessment of rosacea symptom severity by genome-wide association study and expression analysis highlights immuno-inflammatory and skin pigmentation genes. Hum Mol Genet. (2018) 27:2762–72. doi: 10.1093/hmg/ddy184
31. Buhl T, Sulk M, Nowak P, Buddenkotte J, McDonald I, Aubert J, et al. Molecular and morphological characterization of inflammatory infiltrate in rosacea reveals activation of Th1/Th17 pathways. J Invest Dermatol. (2015) 135:2198–208. doi: 10.1038/jid.2015.141
32. Wang L, Wang YJ, Hao D, Wen X, Du D, He G, et al. The theranostics role of mast cells in the pathophysiology of rosacea. Front Med (Lausanne). (2019) 6:324. doi: 10.3389/fmed.2019.00324
33. Margalit A, Kowalczyk MJ, Żaba R, Kavanagh K. The role of altered cutaneous immune responses in the induction and persistence of rosacea. J Dermatol Sci. (2016) 82:3–8. doi: 10.1016/j.jdermsci.2015.12.006
34. DeLuca HF. verview of general physiologic features and functions of vitamin D. Am J Clin Nutr. (2004) 80:1689s–96s. doi: 10.1093/ajcn/80.6.1689S
35. Akdogan N, Alli N, Incel Uysal P, Candar T. Role of serum 25-hydroxyvitamin D levels and vitamin D receptor gene polymorphisms in patients with rosacea: A case-control study. Clin Exp Dermatol. (2019) 44:397–403. doi: 10.1111/ced.13769
36. Baz K, Cimen MY, Kokturk A, Aslan G, Ikizoglu G, Demirseren DD, et al. Plasma reactive oxygen species activity and antioxidant potential levels in rosacea patients: correlation with seropositivity to helicobacter pylori. Int J Dermatol. (2004) 43:494–7. doi: 10.1111/j.1365-4632.2004.02137.x
37. Yazici AC, Tamer L, Ikizoglu G, Kaya TI, Api H, Yildirim H, et al. Gstm1 and gstt1 null genotypes as possible heritable factors of rosacea. Photodermatol Photoimmunol Photomed. (2006) 22:208–10. doi: 10.1111/j.1600-0781.2006.00220.x
38. Hayran Y, Şen O, Fırat Oğuz E, Yücel Ç, Eren F, Külcü Çakmak S, et al. Serum il-17 levels in patients with rosacea. J Cosmet Dermatol. (2022) 21:1147–53. doi: 10.1111/jocd.14169
39. Choi JE, Di Nardo A. Skin neurogenic inflammation. Semin Immunopathol. (2018) 40:249–59. doi: 10.1007/s00281-018-0675-z
40. Roy S, Chompunud Na Ayudhya C, Thapaliya M, Deepak V, Ali H. Multifaceted mrgprx2: new insight into the role of mast cells in health and disease. J Allergy Clin Immunol. (2021) 148:293–308. doi: 10.1016/j.jaci.2021.03.049
41. Seiffert K, Ding W, Wagner JA, Granstein RD. Atpgammas enhances the production of inflammatory mediators by a human dermal endothelial cell line via purinergic receptor signaling. J Invest Dermatol. (2006) 126:1017–27. doi: 10.1038/sj.jid.5700135
42. Metzler-Wilson K, Toma K, Sammons DL, Mann S, Jurovcik AJ, Demidova O, et al. Augmented supraorbital skin sympathetic nerve activity responses to symptom trigger events in rosacea patients. J Neurophysiol. (2015) 114:1530–7. doi: 10.1152/jn.00458.2015
43. Del Rosso JQ. Management of facial erythema of rosacea: what is the role of topical α-adrenergic receptor agonist therapy? J Am Acad Dermatol. (2013) 69:S44–56. doi: 10.1016/j.jaad.2013.06.009
44. Li M, Tao M, Zhang Y, Pan R, Gu D, Xu Y. Neurogenic rosacea could be a small fiber neuropathy. Front Pain Res (Lausanne). (2023) 4:1122134. doi: 10.3389/fpain.2023.1122134
45. Mascarenhas NL, Wang Z, Chang YL, Di Nardo A. Trpv4 mediates mast cell activation in cathelicidin-induced rosacea inflammation. J Invest Dermatol. (2017) 137:972–5. doi: 10.1016/j.jid.2016.10.046
46. Thiboutot D, Anderson R, Cook-Bolden F, Draelos Z, Gallo RL, Granstein RD, et al. Standard management options for rosacea: the 2019 update by the national rosacea society expert committee. J Am Acad Dermatol. (2020) 82:1501–10. doi: 10.1016/j.jaad.2020.01.077
47. Group OLoEW. The Oxford 2011 Levels of Evidence. In: Oxford Centre for Evidence-Based Medicine. Oxford, United Kingdom: University of Oxford (2011). Available at: http://www.cebm.net/indexaspx?o=5653.
48. Hofmann MA, Lehmann P. Physical modalities for the treatment of rosacea. J Dtsch Dermatol Ges. (2016) 14:38–43. doi: 10.1111/ddg.13144
49. Del Rosso JQ, Webster GF, Jackson M, Rendon M, Rich P, Torok H, et al. Two randomized phase iii clinical trials evaluating anti-inflammatory dose doxycycline (40-mg doxycycline, usp capsules) administered once daily for treatment of rosacea. J Am Acad Dermatol. (2007) 56:791–802. doi: 10.1016/j.jaad.2006.11.021
50. Gold LS, Del Rosso JQ, Kircik L, Bhatia ND, Hooper D, Nahm WK, et al. Minocycline 1.5% Foam for the topical treatment of moderate to severe papulopustular rosacea: results of 2 phase 3, randomized, clinical trials. J Am Acad Dermatol. (2020) 82:1166–73. doi: 10.1016/j.jaad.2020.01.043
51. Bjerke R, Fyrand O, Graupe K. Double-blind comparison of azelaic acid 20% Cream and its vehicle in treatment of papulo-pustular rosacea. Acta Derm Venereol. (1999) 79:456–9. doi: 10.1080/000155599750009906
52. Thiboutot D, Thieroff-Ekerdt R, Graupe K. Efficacy and safety of azelaic acid (15%) gel as a new treatment for papulopustular rosacea: results from two vehicle-controlled, randomized phase iii studies. J Am Acad Dermatol. (2003) 48:836–45. doi: 10.1067/mjd.2003.308
53. Dispenza MC, Wolpert EB, Gilliland KL, Dai JP, Cong Z, Nelson AM, et al. Systemic isotretinoin therapy normalizes exaggerated Tlr-2-mediated innate immune responses in acne patients. J Invest Dermatol. (2012) 132:2198–205. doi: 10.1038/jid.2012.111
54. Bagatin E, Costa CS, Rocha M, Picosse FR, Kamamoto CSL, Pirmez R, et al. Consensus on the use of oral isotretinoin in dermatology - Brazilian society of dermatology. Bras Dermatol. (2020) 95 Suppl 1:19–38. doi: 10.1016/j.abd.2020.09.001
55. Kim MB, Kim GW, Park HJ, Kim HS, Chin HW, Kim SH, et al. Pimecrolimus 1% Cream for the treatment of rosacea. J Dermatol. (2011) 38:1135–9. doi: 10.1111/j.1346-8138.2011.01223.x
56. Karabulut AA, Izol Serel B, Eksioglu HM. A randomized, single-blind, placebo-controlled, split-face study with pimecrolimus cream 1% for papulopustular rosacea. J Eur Acad Dermatol Venereol. (2008) 22:729–34. doi: 10.1111/j.1468-3083.2008.02589.x
57. Clanner-Engelshofen BM, Bernhard D, Dargatz S, Flaig MJ, Gieler U, Kinberger M, et al. S2k guideline: rosacea. J Dtsch Dermatol Ges. (2022) 20:1147–65. doi: 10.1111/ddg.14849
58. Kanada KN, Nakatsuji T, Gallo RL. Doxycycline indirectly inhibits proteolytic activation of tryptic kallikrein-related peptidases and activation of cathelicidin. J Invest Dermatol. (2012) 132:1435–42. doi: 10.1038/jid.2012.14
59. Del Rosso JQ, Webster G, Weiss JS, Bhatia ND, Gold LS, Kircik L. Nonantibiotic properties of tetracyclines in rosacea and their clinical implications. J Clin Aesthet Dermatol. (2021) 14:14–21.
60. Anzengruber F, Czernielewski J, Conrad C, Feldmeyer L, Yawalkar N, Häusermann P, et al. Swiss S1 guideline for the treatment of rosacea. J Eur Acad Dermatol Venereol. (2017) 31:1775–91. doi: 10.1111/jdv.14349
61. Lam M, Hu A, Fleming P, Lynde CW. The impact of acne treatment on skin bacterial microbiota: A systematic review. J Cutan Med Surg. (2022) 26:93–7. doi: 10.1177/12034754211037994
62. Sapadin AN, Fleischmajer R. Tetracyclines: nonantibiotic properties and their clinical implications. J Am Acad Dermatol. (2006) 54:258–65. doi: 10.1016/j.jaad.2005.10.004
63. van der Linden MMD, van Ratingen AR, van Rappard DC, Nieuwenburg SA, Spuls PI. Domino, Doxycycline 40 mg Vs. Minocycline 100 mg in the Treatment of Rosacea: A Randomized, Single-Blinded, Noninferiority Trial, Comparing Efficacy and Safety. Br J Dermatol. (2017) 176:1465–74. doi: 10.1111/bjd.15155
65. Tsianakas A, Pieber T, Baldwin H, Feichtner F, Alikunju S, Gautam A, et al. Minocycline extended-release comparison with doxycycline for the treatment of rosacea: A randomized, head-to-head, clinical trial. J Clin Aesthet Dermatol. (2021) 14:16–23.
66. Rosso JQ, Draelos ZD, Effron C, Kircik LH. Oral sarecycline for treatment of papulopustular rosacea: results of a pilot study of effectiveness and safety. J Drugs Dermatol. (2021) 20:426–31. doi: 10.36849/JDD.2021.5923
67. Bunick CG, Keri J, Tanaka SK, Furey N, Damiani G, Johnson JL, et al. Antibacterial mechanisms and efficacy of sarecycline in animal models of infection and inflammation. Antibiotics (Basel). (2021) 10(4):439. doi: 10.3390/antibiotics10040439
68. Bender A, Zapolanski T, Watkins S, Khosraviani A, Seiffert K, Ding W, et al. Tetracycline suppresses atp gamma S-induced Cxcl8 and Cxcl1 production by the human dermal microvascular endothelial cell-1 (Hmec-1) cell line and primary human dermal microvascular endothelial cells. Exp Dermatol. (2008) 17:752–60. doi: 10.1111/j.1600-0625.2008.00716.x
69. Draelos ZD, Elewski BE, Harper JC, Sand M, Staedtler G, Nkulikiyinka R, et al. A phase 3 randomized, double-blind, vehicle-controlled trial of azelaic acid foam 15% in the treatment of papulopustular rosacea. Cutis. (2015) 96:54–61.
70. Draelos ZD, Elewski B, Staedtler G, Havlickova B. Azelaic acid foam 15% in the treatment of papulopustular rosacea: A randomized, double-blind, vehicle-controlled study. Cutis. (2013) 92:306–17.
71. Coda AB, Hata T, Miller J, Audish D, Kotol P, Two A, et al. Cathelicidin, kallikrein 5, and serine protease activity is inhibited during treatment of rosacea with azelaic acid 15% Gel. J Am Acad Dermatol. (2013) 69:570–7. doi: 10.1016/j.jaad.2013.05.019
72. Searle T, Ali FR, Al-Niaimi F. The versatility of azelaic acid in dermatology. J Dermatolog Treat. (2022) 33:722–32. doi: 10.1080/09546634.2020.1800579
73. MastroFrancesco A, Ottaviani M, Aspite N, Cardinali G, Izzo E, Graupe K, et al. Azelaic acid modulates the inflammatory response in normal human keratinocytes through ppargamma activation. Exp Dermatol. (2010) 19:813–20. doi: 10.1111/j.1600-0625.2010.01107.x
74. Webster G, Draelos ZD, Graber E, Lee MS, Dhawan S, Salman M, et al. A multicentre, randomized, double-masked, parallel group, vehicle-controlled phase iib study to evaluate the safety and efficacy of 1% and 3% Topical minocycline gel in patients with papulopustular rosacea. Br J Dermatol. (2020) 183:471–9. doi: 10.1111/bjd.18857
75. Schaller M, Almeida LM, Bewley A, Cribier B, Dlova NC, Kautz G, et al. Rosacea treatment update: recommendations from the global rosacea consensus (Rosco) panel. Br J Dermatol. (2017) 176:465–71. doi: 10.1111/bjd.15173
76. Gollnick H, Blume-Peytavi U, Szabó EL, Meyer KG, Hauptmann P, Popp G, et al. Systemic isotretinoin in the treatment of rosacea - doxycycline- and placebo-controlled, randomized clinical study. J Dtsch Dermatol Ges. (2010) 8:505–15. doi: 10.1111/j.1610-0387.2010.07345.x
77. Sbidian E, Vicaut É, Chidiack H, Anselin E, Cribier B, Dréno B, et al. A randomized-controlled trial of oral low-dose isotretinoin for difficult-to-treat papulopustular rosacea. J Invest Dermatol. (2016) 136:1124–9. doi: 10.1016/j.jid.2016.01.025
78. Uslu M, Şavk E, Karaman G, Şendur N. Rosacea treatment with intermediate-dose isotretinoin: follow-up with erythema and sebum measurements. Acta Derm Venereol. (2012) 92:73–7. doi: 10.2340/00015555-1204
79. Bakar O, Demirçay Z, Gürbüz O. Therapeutic potential of azithromycin in rosacea. Int J Dermatol. (2004) 43:151–4. doi: 10.1111/j.1365-4632.2004.01958.x
80. Parnham MJ, Erakovic Haber V, Giamarellos-Bourboulis EJ, Perletti G, Verleden GM, Vos R. Azithromycin: mechanisms of action and their relevance for clinical applications. Pharmacol Ther. (2014) 143:225–45. doi: 10.1016/j.pharmthera.2014.03.003
81. Xiao W, Chen M, Wang B, Huang Y, Zhao Z, Deng Z, et al. Efficacy and safety of antibiotic agents in the treatment of rosacea: A systemic network meta-analysis. Front Pharmacol. (2023) 14:1169916. doi: 10.3389/fphar.2023.1169916
82. van Zuuren EJ, Fedorowicz Z, Carter B, van der Linden MM, Charland L. Interventions for rosacea. Cochrane Database Syst Rev. (2015) 2015:Cd003262. doi: 10.1002/14651858.CD003262.pub5
83. Li J, Yuan X, Tang Y, Wang B, Deng Z, Huang Y, et al. Hydroxychloroquine is a novel therapeutic approach for rosacea. Int Immunopharmacol. (2020) 79:106178. doi: 10.1016/j.intimp.2019.106178
84. Wang B, Yuan X, Huang X, Tang Y, Zhao Z, Yang B, et al. Efficacy and safety of hydroxychloroquine for treatment of patients with rosacea: A multicenter, randomized, double-blind, double-dummy, pilot study. J Am Acad Dermatol. (2021) 84:543–5. doi: 10.1016/j.jaad.2020.05.050
85. Amir Ali A, Vender R, Vender R. The role of Il-17 in papulopustular rosacea and future directions. J Cutan Med Surg. (2019) 23:635–41. doi: 10.1177/1203475419867611
86. Kumar AM, Chiou AS, Shih YH, Li S, Chang ALS. An exploratory, open-label, investigator-initiated study of interleukin-17 blockade in patients with moderate-to-severe papulopustular rosacea. Br J Dermatol. (2020) 183:942–3. doi: 10.1111/bjd.19172
87. Sun YH, Man XY, Xuan XY, Huang CZ, Shen Y, Lao LM. Tofacitinib for the treatment of erythematotelangiectatic and papulopustular rosacea: A retrospective case series. Dermatol Ther. (2022) 35:e15848. doi: 10.1111/dth.15848
88. Xu B, Xu Z, Ye S, Sun H, Zhao B, Wu N, et al. Jak1 inhibitor abrocitinib for the treatment of steroid-induced rosacea: case series. Front Med (Lausanne). (2023) 10:1239869. doi: 10.3389/fmed.2023.1239869
89. Weissenbacher S, Merkl J, Hildebrandt B, Wollenberg A, Braeutigam M, Ring J, et al. Pimecrolimus cream 1% for papulopustular rosacea: A randomized vehicle-controlled double-blind trial. Br J Dermatol. (2007) 156:728–32. doi: 10.1111/j.1365-2133.2006.07669.x
90. Bamford JT, Elliott BA, Haller IV. Tacrolimus effect on rosacea. J Am Acad Dermatol. (2004) 50:107–8. doi: 10.1016/s0190-9622(03)02157-1
91. Zhang H, Yang L, Wang Y, Zhang D, Tang K, Fang R, et al. Topical calcineurin inhibitors as a double-edged sword in rosacea: A systematic review. J Cosmet Dermatol. (2022) 21:1695–704. doi: 10.1111/jocd.14315
92. Deng Z, Chen M, Liu Y, Xu S, Ouyang Y, Shi W, et al. A positive feedback loop between mtorc1 and cathelicidin promotes skin inflammation in rosacea. EMBO Mol Med. (2021) 13:e13560. doi: 10.15252/emmm.202013560
93. Huang Y, Liu D, Chen M, Xu S, Peng Q, Zhu Y, et al. Tlr7 promotes skin inflammation via activating Nfκb-mtorc1 axis in rosacea. PeerJ. (2023) 11:e15976. doi: 10.7717/peerj.15976
94. Efferth T, Oesch F. The immunosuppressive activity of artemisinin-type drugs towards inflammatory and autoimmune diseases. Med Res Rev. (2021) 41:3023–61. doi: 10.1002/med.21842
95. Yuan X, Li J, Li Y, Deng Z, Zhou L, Long J, et al. Artemisinin, a potential option to inhibit inflammation and angiogenesis in rosacea. BioMed Pharmacother. (2019) 117:109181. doi: 10.1016/j.biopha.2019.109181
96. Wang GJ, Gao XY, Wu Y, He HQ, Yu Y, Qin HH, et al. Evaluation of the efficacy and tolerance of artemether emulsion for the treatment of papulopustular rosacea: A randomized pilot study. J Dermatolog Treat. (2019) 30:809–12. doi: 10.1080/09546634.2019.1610549
97. Jackson JM, Coulon R, Arbiser JL. Evaluation of a first-in-class proteasome inhibitor in patients with moderate to severe rosacea. J Drugs Dermatol. (2021) 20:660–4. doi: 10.36849/jdd.2021.5925
98. Khalilzadeh M, Shayan M, Jourian S, Rahimi M, Sheibani M, Dehpour AR. A comprehensive insight into the anti-inflammatory properties of dapsone. Naunyn Schmiedebergs Arch Pharmacol. (2022) 395:1509–23. doi: 10.1007/s00210-022-02297-1
99. Merlo G, Cozzani E, Russo R, Parodi A. Dapsone for unresponsive granulomatous rosacea. Am J Ther. (2020) 27:e304–e6. doi: 10.1097/MJT.0000000000000903
100. Faghihi G, Khosravani P, Nilforoushzadeh MA, Hosseini SM, Assaf F, Zeinali N, et al. Dapsone gel in the treatment of papulopustular rosacea: A double-blind randomized clinical trial. J Drugs Dermatol. (2015) 14:602–6.
101. Gökşin Ş, İmren IG, Kaçar N. Efficacy of topical dapsone 5% Gel for the treatment of erythematotelangiectatic rosacea: new treatment option with old drug. Dermatol Pract Concept. (2024) 14(1):e2024034. doi: 10.5826/dpc.1401a34
102. Two AM, Hata TR, Nakatsuji T, Coda AB, Kotol PF, Wu W, et al. Reduction in serine protease activity correlates with improved rosacea severity in a small, randomized pilot study of a topical serine protease inhibitor. J Invest Dermatol. (2014) 134:1143–5. doi: 10.1038/jid.2013.472
103. Bageorgou F, Vasalou V, Tzanetakou V, Kontochristopoulos G. The new therapeutic choice of tranexamic acid solution in treatment of erythematotelangiectatic rosacea. J Cosmet Dermatol. (2019) 18:563–7. doi: 10.1111/jocd.12724
104. Li Y, Xie H, Deng Z, Wang B, Tang Y, Zhao Z, et al. Tranexamic acid ameliorates rosacea symptoms through regulating immune response and angiogenesis. Int Immunopharmacol. (2019) 67:326–34. doi: 10.1016/j.intimp.2018.12.031
105. Daadaa N, Litaiem N, Karray M, Bacha T, Jones M, Belajouza Noueiri C, et al. Intradermal tranexamic acid microinjections: A novel treatment option for erythematotelangiectatic rosacea. J Cosmet Dermatol. (2021) 20:3324–9. doi: 10.1111/jocd.14209
106. Zhong S, Sun N, Liu H, Niu Y, Chen C, Wu Y. Topical tranexamic acid improves the permeability barrier in rosacea. Dermatologica Sin. (2015) 33:112–7. doi: 10.1016/j.dsi.2015.04.012
107. Xu Z, Yu B, Xu B, Ye S, Qing Y, Zhao B, et al. Oral tranexamic acid treats papulopustular rosacea by improving the skin barrier. J Cosmet Dermatol. (2024). doi: 10.1111/jocd.16339
108. Logger JGM, Olydam JI, Driessen RJB. Use of beta-blockers for rosacea-associated facial erythema and flushing: A systematic review and update on proposed mode of action. J Am Acad Dermatol. (2020) 83:1088–97. doi: 10.1016/j.jaad.2020.04.129
109. Wang B, Huang Y, Tang Y, Zhao Z, Shi W, Jian D, et al. Paroxetine is an effective treatment for refractory erythema of rosacea: primary results from the prospective rosacea refractory erythema randomized clinical trial. J Am Acad Dermatol. (2023) 88:1300–7. doi: 10.1016/j.jaad.2023.01.044
110. Wienholtz NKF, Christensen CE, Coskun H, Zhang DG, Ghanizada H, Egeberg A, et al. Infusion of pituitary adenylate cyclase-activating polypeptide-38 in patients with rosacea induces flushing and facial edema that can be attenuated by sumatriptan. J Invest Dermatol. (2021) 141:1687–98. doi: 10.1016/j.jid.2021.02.002
111. Moore A, Kempers S, Murakawa G, Weiss J, Tauscher A, Swinyer L, et al. Long-term safety and efficacy of once-daily topical brimonidine tartrate gel 0.5% for the treatment of moderate to severe facial erythema of rosacea: results of a 1-year open-label study. J Drugs Dermatol. (2014) 13:56–61.
112. Fowler J Jr., Jackson M, Moore A, Jarratt M, Jones T, Meadows K, et al. Efficacy and safety of once-daily topical brimonidine tartrate gel 0.5% for the treatment of moderate to severe facial erythema of rosacea: results of two randomized, double-blind, and vehicle-controlled pivotal studies. J Drugs Dermatol. (2013) 12:650–6.
113. Layton AM, Schaller M, Homey B, Hofmann MA, Bewley AP, Lehmann P, et al. Brimonidine gel 0.33% Rapidly improves patient-reported outcomes by controlling facial erythema of rosacea: A randomized, double-blind, vehicle-controlled study. J Eur Acad Dermatol Venereol. (2015) 29:2405–10. doi: 10.1111/jdv.13305
114. Del Rosso JQ, Tanghetti E. Topical oxymetazoline hydrochloride cream 1% for the treatment of persistent facial erythema of rosacea in adults: A comprehensive review of current evidence. J Clin Aesthet Dermatol. (2021) 14:32–7.
115. Draelos ZD, Gold MH, Weiss RA, Baumann L, Grekin SK, Robinson DM, et al. Efficacy and safety of oxymetazoline cream 1.0% for treatment of persistent facial erythema associated with rosacea: findings from the 52-week open label reveal trial. J Am Acad Dermatol. (2018) 78:1156–63. doi: 10.1016/j.jaad.2018.01.027
116. Baumann L, Goldberg DJ, Stein Gold L, Tanghetti EA, Lain E, Kaufman J, et al. Pivotal trial of the efficacy and safety of oxymetazoline cream 1.0% for the treatment of persistent facial erythema associated with rosacea: findings from the second reveal trial. J Drugs Dermatol. (2018) 17:290–8.
117. Kircik LH, DuBois J, Draelos ZD, Werschler P, Grande K, Cook-Bolden FE, et al. Pivotal trial of the efficacy and safety of oxymetazoline cream 1.0% for the treatment of persistent facial erythema associated with rosacea: findings from the first reveal trial. J Drugs Dermatol. (2018) 17:97–105.
118. Liu F, Zhou Q, Wang H, Fu H, Li Y, Tao M, et al. Efficacy and safety of oxymetazoline for the treatment of rosacea: A meta-analysis. J Cosmet Dermatol. (2023) 22:2408–19. doi: 10.1111/jocd.15747
119. Craige H, Cohen JB. Symptomatic treatment of idiopathic and rosacea-associated cutaneous flushing with propranolol. J Am Acad Dermatol. (2005) 53:881–4. doi: 10.1016/j.jaad.2005.07.021
120. Li J, Tang JY, Fu J, Zhang MW, Wan M, Chen DW, et al. Carvedilol ameliorates persistent erythema of erythematotelangiectatic rosacea by regulating the status of anxiety/depression. J Dermatol. (2022) 49:1139–47. doi: 10.1111/1346-8138.16525
121. Chen L, Tsai TF. The role of β-blockers in dermatological treatment: A review. J Eur Acad Dermatol Venereol. (2018) 32:363–71. doi: 10.1111/jdv.14566
122. Stearns V, Beebe KL, Iyengar M, Dube E. Paroxetine controlled release in the treatment of menopausal hot flashes: A randomized controlled trial. Jama. (2003) 289:2827–34. doi: 10.1001/jama.289.21.2827
123. MacLean MR. Pulmonary hypertension, anorexigens and 5-ht: pharmacological synergism in action? Trends Pharmacol Sci. (1999) 20:490–5. doi: 10.1016/S0165-6147(99)01389-9
124. Oliveira MC, Pelegrini-da-Silva A, Parada CA, Tambeli CH. 5-ht acts on nociceptive primary afferents through an indirect mechanism to induce hyperalgesia in the subcutaneous tissue. Neuroscience. (2007) 145:708–14. doi: 10.1016/j.neuroscience.2006.12.021
125. Nordlind K, Thorslund K, Lonne-Rahm S, Mohabbati S, Berki T, Morales M, et al. Expression of serotonergic receptors in psoriatic skin. Arch Dermatol Res. (2006) 298:99–106. doi: 10.1007/s00403-006-0652-6
126. Wang Z, Xie H, Gong Y, Ouyang Y, Deng F, Tang Y, et al. Relationship between rosacea and sleep. J Dermatol. (2020) 47:592–600. doi: 10.1111/1346-8138.15339
127. Dalsgaard T, Hannibal J, Fahrenkrug J, Larsen CR, Ottesen B. Vip and pacap display different vasodilatory effects in rabbit coronary and cerebral arteries. Regul Pept. (2003) 110:179–88. doi: 10.1016/S0167-0115(02)00205-7
128. Zagami AS, Edvinsson L, Goadsby PJ. Pituitary adenylate cyclase activating polypeptide and migraine. Ann Clin Transl Neurol. (2014) 1:1036–40. doi: 10.1002/acn3.113
129. Levy D, Jakubowski M, Burstein R. Disruption of communication between peripheral and central trigeminovascular neurons mediates the antimigraine action of 5ht 1b/1d receptor agonists. Proc Natl Acad Sci U.S.A. (2004) 101:4274–9. doi: 10.1073/pnas.0306147101
130. Wienholtz N, Ashina M, Thyssen JP, Egeberg A. Subtype-specific off-label treatment of rosacea. Case Rep Dermatol. (2021) 13:121–8. doi: 10.1159/000511984
131. Bagherzadeh-Fard M, Amin Yazdanifar M, Sadeghalvad M, Rezaei N. Erenumab efficacy in migraine headache prophylaxis: A systematic review. Int Immunopharmacol. (2023) 117:109366. doi: 10.1016/j.intimp.2022.109366
132. Shah T, Bedrin K, Tinsley A. Calcitonin gene relating peptide inhibitors in combination for migraine treatment: A mini-review. Front Pain Res (Lausanne). (2023) 4:1130239. doi: 10.3389/fpain.2023.1130239
133. Pozo-Rosich P, Ailani J, Ashina M, Goadsby PJ, Lipton RB, Reuter U, et al. Atogepant for the preventive treatment of chronic migraine (Progress): A randomised, double-blind, placebo-controlled, phase 3 trial. Lancet. (2023) 402:775–85. doi: 10.1016/S0140-6736(23)01049-8
134. Ho TW, Edvinsson L, Goadsby PJ. Cgrp and its receptors provide new insights into migraine pathophysiology. Nat Rev Neurol. (2010) 6:573–82. doi: 10.1038/nrneurol.2010.127
135. Scala J, Vojvodic A, Vojvodic P, Vlaskovic-Jovicevic T, Peric-Hajzler Z, Matovic D, et al. Botulin toxin use in rosacea and facial flushing treatment. Open Access Maced J Med Sci. (2019) 7:2985–7. doi: 10.3889/oamjms.2019.784
136. Okamoto K, Kanayama S, Ikeda F, Fujikawa K, Fujiwara S, Nozawa N, et al. Broad spectrum in vitro microbicidal activity of benzoyl peroxide against microorganisms related to cutaneous diseases. J Dermatol. (2021) 48:551–5. doi: 10.1111/1346-8138.15739
137. Patil S, Willett O, Thompkins T, Hermann R, Ramanathan S, Cornett EM, et al. Botulinum toxin: pharmacology and therapeutic roles in pain states. Curr Pain Headache Rep. (2016) 20:15. doi: 10.1007/s11916-016-0545-0
138. Meng J, Wang J, Lawrence G, Dolly JO. Synaptobrevin I mediates exocytosis of cgrp from sensory neurons and inhibition by botulinum toxins reflects their anti-nociceptive potential. J Cell Sci. (2007) 120:2864–74. doi: 10.1242/jcs.012211
139. Bloom BS, Payongayong L, Mourin A, Goldberg DJ. Impact of intradermal abobotulinumtoxina on facial erythema of rosacea. Dermatol Surg. (2015) 41:S9–16. doi: 10.1097/dss.0000000000000277
140. Raoufinejad K, Mansouri P, Rajabi M, Naraghi Z, Jebraeili R. Efficacy and safety of permethrin 5% Topical gel vs. Placebo for rosacea: A double-blind randomized controlled clinical trial. J Eur Acad Dermatol Venereol. (2016) 30:2105–17. doi: 10.1111/jdv.13801
141. Choi JE, Werbel T, Wang Z, Wu CC, Yaksh TL, Di Nardo A. Botulinum toxin blocks mast cells and prevents rosacea like inflammation. J Dermatol Sci. (2019) 93:58–64. doi: 10.1016/j.jdermsci.2018.12.004
142. Tong Y, Luo W, Gao Y, Liu L, Tang Q, Wa Q. A randomized, controlled, split-face study of botulinum toxin and broadband light for the treatment of erythematotelangiectatic rosacea. Dermatol Ther. (2022) 35:e15395. doi: 10.1111/dth.15395
143. Alsaati AA, Alsaadoun D, Kinkar LI, Alkhamis RS, Ahmed WA, Almathami AH. The efficacy and safety of botulinum toxin a for the treatment of rosacea: A systematic review. Cureus. (2023) 15:e51304. doi: 10.7759/cureus.51304
144. Breneman DL, Stewart D, Hevia O, Hino PD, Drake LA. A double-blind, multicenter clinical trial comparing efficacy of once-daily metronidazole 1 percent cream to vehicle in patients with rosacea. Cutis. (1998) 61:44–7.
145. Siddiqui K, Stein Gold L, Gill J. The efficacy, safety, and tolerability of ivermectin compared with current topical treatments for the inflammatory lesions of rosacea: A network meta-analysis. Springerplus. (2016) 5:1151. doi: 10.1186/s40064-016-2819-8
146. Bhatia ND, Werschler WP, Baldwin H, Sugarman J, Green LJ, Levy-Hacham O, et al. Efficacy and safety of microencapsulated benzoyl peroxide cream, 5%, in rosacea: results from two phase iii, randomized, vehicle-controlled trials. J Clin Aesthet Dermatol. (2023) 16:34–40.
147. Pye RJ, Burton JL. Treatment of rosacea by metronidazole. Lancet. (1976) 1:1211–2. doi: 10.1016/s0140-6736(76)92160-7
148. Forstinger C, Kittler H, Binder M. Treatment of rosacea-like demodicidosis with oral ivermectin and topical permethrin cream. J Am Acad Dermatol. (1999) 41:775–7. doi: 10.1016/s0190-9622(99)70022-8
149. Grada A, Van Doorn M, Lain E, Furst K, Feiss G. Lb1092 topical omiganan for severe papulopustular rosacea: A randomized, vehicle-controlled, double-blind, multicenter study. J Invest Dermatol. (2019) 139:B13. doi: 10.1016/j.jid.2019.06.057
150. Parodi A, Paolino S, Greco A, Drago F, Mansi C, Rebora A, et al. Small intestinal bacterial overgrowth in rosacea: clinical effectiveness of its eradication. Clin Gastroenterol Hepatol. (2008) 6:759–64. doi: 10.1016/j.cgh.2008.02.054
151. Knackstedt R, Knackstedt T, Gatherwright J. The role of topical probiotics in skin conditions: A systematic review of animal and human studies and implications for future therapies. Exp Dermatol. (2020) 29:15–21. doi: 10.1111/exd.14032
152. Sharma A, Kroumpouzos G, Kassir M, Galadari H, Goren A, Grabbe S, et al. Rosacea management: A comprehensive review. J Cosmet Dermatol. (2022) 21:1895–904. doi: 10.1111/jocd.14816
153. Akamatsu H, Oguchi M, Nishijima S, Asada Y, Takahashi M, Ushijima T, et al. The inhibition of free radical generation by human neutrophils through the synergistic effects of metronidazole with palmitoleic acid: A possible mechanism of action of metronidazole in rosacea and acne. Arch Dermatol Res. (1990) 282:449–54. doi: 10.1007/bf00402621
154. Koçak M, Yağli S, Vahapoğlu G, Ekşioğlu M. Permethrin 5% Cream versus metronidazole 0.75% Gel for the treatment of papulopustular rosacea. A randomized double-blind placebo-controlled study. Dermatology. (2002) 205:265–70. doi: 10.1159/000065849
155. Choe J, Barbieri JS. Emerging medical therapies in rosacea: A narrative review. Dermatol Ther (Heidelb). (2023) 13:2933–49. doi: 10.1007/s13555-023-01048-1
156. Li J, Wei E, Reisinger A, French LE, Clanner-Engelshofen BM, Reinholz M. Comparison of different anti-demodex strategies: A systematic review and meta-analysis. Dermatology. (2023) 239:12–31. doi: 10.1159/000526296
157. Trave I, Micalizzi C, Cozzani E, Gasparini G, Parodi A. Papulopustular rosacea treated with ivermectin 1% Cream: remission of the demodex mite infestation over time and evaluation of clinical relapses. Dermatol Pract Concept. (2022) 12:e2022201. doi: 10.5826/dpc.1204a201
158. Schaller M, Gonser L, Belge K, Braunsdorf C, Nordin R, Scheu A, et al. Dual anti-inflammatory and anti-parasitic action of topical ivermectin 1% in papulopustular rosacea. J Eur Acad Dermatol Venereol. (2017) 31:1907–11. doi: 10.1111/jdv.14437
159. Nobeyama Y, Aihara Y, Asahina A. Real-world evidence for the treatment of rosacea with sulfur or metronidazole preparation in Japanese patients. JMA J. (2023) 6:448–54. doi: 10.31662/jmaj.2023-0100
160. Sarac G. A comparison of the efficacy and tolerability of topical agents used in facial demodex treatment. J Cosmet Dermatol. (2019) 18:1784–7. doi: 10.1111/jocd.12986
161. Forton F, Seys B, Marchal JL, Song AM. Demodex folliculorum and topical treatment: acaricidal action evaluated by standardized skin surface biopsy. Br J Dermatol. (1998) 138:461–6. doi: 10.1046/j.1365-2133.1998.02125.x
162. Leyden JJ. Randomized, phase 2, dose-ranging study in the treatment of rosacea with encapsulated benzoyl peroxide gel. J Drugs Dermatol. (2014) 13:685–8.
163. van Zuuren EJ, Arents BWM, van der Linden MMD, Vermeulen S, Fedorowicz Z, Tan J. Rosacea: new concepts in classification and treatment. Am J Clin Dermatol. (2021) 22:457–65. doi: 10.1007/s40257-021-00595-7
164. Werschler WP, Sugarman J, Bhatia N, Baldwin H, Green LJ, Nov O, et al. Long-term efficacy and safety of microencapsulated benzoyl peroxide cream, 5%, in rosacea: results from an extension of two phase iii, vehicle-controlled trials. J Clin Aesthet Dermatol. (2023) 16:27–33.
165. Jacob S, VanDaele MA, Brown JN. Treatment of demodex-associated inflammatory skin conditions: A systematic review. Dermatol Ther. (2019) 32:e13103. doi: 10.1111/dth.13103
166. Rubinchik E, Dugourd D, Algara T, Pasetka C, Friedland HD. Antimicrobial and antifungal activities of a novel cationic antimicrobial peptide, omiganan, in experimental skin colonisation models. Int J Antimicrob Agents. (2009) 34:457–61. doi: 10.1016/j.ijantimicag.2009.05.003
167. Drago F, De Col E, Agnoletti AF, Schiavetti I, Savarino V, Rebora A, et al. The role of small intestinal bacterial overgrowth in rosacea: A 3-year follow-up. J Am Acad Dermatol. (2016) 75:e113–e5. doi: 10.1016/j.jaad.2016.01.059
168. Weinstock LB, Steinhoff M. Rosacea and small intestinal bacterial overgrowth: prevalence and response to rifaximin. J Am Acad Dermatol. (2013) 68:875–6. doi: 10.1016/j.jaad.2012.11.038
169. Alfasigma SpA, bioRasi LLC. Safety, Efficacy and Pharmacokinetics of Rifaximin in Patients with Moderate-to-Severe Papulopustular Rosacea. ClinicalTrials.gov identifier: NCT05150587. (2022). Accessed July 16, 2024. https://clinicaltrials.gov/study/NCT05150587.
170. Callahan BN, Kammala AK, Syed M, Yang C, Occhiuto CJ, Nellutla R, et al. Osthole, a natural plant derivative inhibits mrgprx2 induced mast cell responses. Front Immunol. (2020) 11:703. doi: 10.3389/fimmu.2020.00703
171. Zeng Q, Yang J, Yan G, Zhang L, Wang P, Zhang H, et al. Celastrol inhibits ll37-induced rosacea by inhibiting Ca(2+)/camkii-mtor-nf-κb activation. BioMed Pharmacother. (2022) 153:113292. doi: 10.1016/j.biopha.2022.113292
172. Roh KB, Ryu DH, Cho E, Weon JB, Park D, Kweon DH, et al. Coptis chinensis franch directly inhibits proteolytic activation of kallikrein 5 and cathelicidin associated with rosacea in epidermal keratinocytes. Molecules. (2020) 25(23):5556. doi: 10.3390/molecules25235556
173. Kim M, Kim KE, Jung HY, Jo H, Jeong SW, Lee J, et al. Recombinant erythroid differentiation regulator 1 inhibits both inflammation and angiogenesis in a mouse model of rosacea. Exp Dermatol. (2015) 24:680–5. doi: 10.1111/exd.12745
174. Sun Y, Chen L, Wang H, Zhu P, Jiang S, Qi R, et al. Activation of aryl hydrocarbon receptor ameliorates rosacea-like eruptions in mice and suppresses the tlr signaling pathway in ll-37-induced hacat cells. Toxicol Appl Pharmacol. (2022) 451:116189. doi: 10.1016/j.taap.2022.116189
175. Espinoza LC, Silva-Abreu M, Calpena AC, Rodríguez-Lagunas MJ, Fábrega MJ, Garduño-Ramírez ML, et al. Nanoemulsion strategy of pioglitazone for the treatment of skin inflammatory diseases. Nanomedicine. (2019) 19:115–25. doi: 10.1016/j.nano.2019.03.017
176. Li Y, Yang L, Wang Y, Deng Z, Xu S, Xie H, et al. Exploring metformin as a candidate drug for rosacea through network pharmacology and experimental validation. Pharmacol Res. (2021) 174:105971. doi: 10.1016/j.phrs.2021.105971
177. Zhang H, Zhang Y, Li Y, Wang Y, Yan S, Xu S, et al. Bioinformatics and network pharmacology identify the therapeutic role and potential mechanism of melatonin in ad and rosacea. Front Immunol. (2021) 12:756550. doi: 10.3389/fimmu.2021.756550
178. Deng Z, Xu S, Peng Q, Sha K, Xiao W, Liu T, et al. Aspirin alleviates skin inflammation and angiogenesis in rosacea. Int Immunopharmacol. (2021) 95:107558. doi: 10.1016/j.intimp.2021.107558
179. Chen M, Xie H, Chen Z, Xu S, Wang B, Peng Q, et al. Thalidomide ameliorates rosacea-like skin inflammation and suppresses Nf-κb activation in keratinocytes. BioMed Pharmacother. (2019) 116:109011. doi: 10.1016/j.biopha.2019.109011
180. Lazaridou E, Giannopoulou C, Fotiadou C, Vakirlis E, Trigoni A, Ioannides D. The potential role of microorganisms in the development of rosacea. J Dtsch Dermatol Ges. (2011) 9:21–5. doi: 10.1111/j.1610-0387.2010.07513.x
181. Taieb A, Ortonne JP, Ruzicka T, Roszkiewicz J, Berth-Jones J, Peirone MH, et al. Superiority of ivermectin 1% Cream over metronidazole 0·75% Cream in treating inflammatory lesions of rosacea: A randomized, investigator-blinded trial. Br J Dermatol. (2015) 172:1103–10. doi: 10.1111/bjd.13408
Keywords: rosacea, immune dysregulation, neuroinflammation, microbiota, therapeutics
Citation: Tu K-Y, Jung C-J, Shih Y-H and Chang ALS (2024) Therapeutic strategies focusing on immune dysregulation and neuroinflammation in rosacea. Front. Immunol. 15:1403798. doi: 10.3389/fimmu.2024.1403798
Received: 19 March 2024; Accepted: 09 July 2024;
Published: 29 July 2024.
Edited by:
Chun-Bing Chen, Linkou Chang Gung Memorial Hospital, TaiwanReviewed by:
Fang-Ying Wang, Chang Gung Memorial Hospital, TaiwanYi-Hua Liao, National Taiwan University, Taiwan
Copyright © 2024 Tu, Jung, Shih and Chang. This is an open-access article distributed under the terms of the Creative Commons Attribution License (CC BY). The use, distribution or reproduction in other forums is permitted, provided the original author(s) and the copyright owner(s) are credited and that the original publication in this journal is cited, in accordance with accepted academic practice. No use, distribution or reproduction is permitted which does not comply with these terms.
*Correspondence: Yi-Hsien Shih, yh.shih@tmu.edu.tw