- 1Department of Dermatology, West China Hospital, Sichuan University, Chengdu, China
- 2Laboratory of Dermatology, Clinical Institute of Inflammation and Immunology, Frontiers Science Center for Disease-Related Molecular Network, West China Hospital, Sichuan University, Chengdu, China
Rosacea is a chronic skin inflammatory disease with a global prevalence ranging from 1% to 20%. It is characterized by facial erythema, telangiectasia, papules, pustules, and ocular manifestations. Its pathogenesis involves a complex interplay of genetic, environmental, immune, microbial, and neurovascular factors. Recent studies have advanced our understanding of its molecular basis, focusing on toll-like receptor (TLR) 2 pathways, LL37 expression, mammalian target of rapamycin (mTOR) activation, interleukin (IL)-17 signaling, transient receptor potential vanilloid (TRPV) functions, and the Janus kinase-signal transducer and activator of transcription (JAK-STAT) pathways. LL37-associated signaling pathways, particularly involving TLR2 and mTORC1, are critical in the pathogenesis of rosacea. LL37 interacts with signaling molecules such as extracellular signal-regulated kinases 1 and 2 (ERK1/2), nuclear factor kappa B (NF-κB), inflammasomes, C-X-C motif chemokine ligand 8 (CXCL8), mas-related G-protein-coupled receptor X2 (MRGPRX2)-TRPV4, and vascular endothelial growth factor (VEGF). This interaction activates macrophages, neutrophils, mast cells, and vascular endothelial cells, leading to cytokine release including tumor necrosis factor-alpha (TNF-α), IL-6, IL-1β, C motif chemokine ligand (CCL) 5, CXCL9, and CXCL10. These processes contribute to immune response modulation, inflammation, and angiogenesis in rosacea pathophysiology. The IL-17 signaling pathway also plays a crucial role in rosacea, affecting angiogenesis and the production of inflammatory cytokines. In addition, recent insights into the JAK/STAT pathways have revealed their integral role in inflammatory and angiogenic mechanisms associated with rosacea. Rosacea treatment currently focuses on symptom management, with emerging insights into these molecular pathways providing more targeted and effective therapies. Biological agents targeting specific cytokines, IL-17 inhibitors, JAK inhibitors, and VEGF antagonists are promising for future rosacea therapy, aiming for enhanced efficacy and fewer side effects. This review provides a comprehensive overview of the current knowledge regarding signaling pathways in rosacea and potential targeted therapeutic strategies.
1 Introduction
Rosacea is a common chronic skin inflammatory disease affecting 1% to 20% of the global population (1). It is characterized by various signs and symptoms, including erythema, telangiectasia, papules, pustules, and flushing with burning and stinging sensations on the central face (2). Rosacea is categorized into four subtypes: erythematotelangiectatic rosacea (ETR), characterized by persistent erythema and telangiectasia on the central face; papulopustular rosacea (PPR), presenting with persistent facial erythema, papules, and pustules; phymatids rosacea (PhR), marked by thickened skin and an irregular surface texture; and ocular rosacea (3). A key hallmark of rosacea is its hypersensitivity to various stimuli like temperature changes, ultraviolet light (UV), emotional changes, and certain foods such as spicy food (4). Rosacea often impacts the facial area, significantly affecting patients’ self-esteem and mental health, and is associated with systemic diseases like hypertension, inflammatory bowel disease, autoimmune disorders, and migraines (5).
Current research indicates that the pathogenesis of rosacea is mainly due to the cross-talk of genetic and environmental factors (4, 6). This includes immune dysfunction, chronic inflammation, microbial imbalances, and vascular neurologic dysfunction (7). Recent molecular studies have identified critical signaling pathways in rosacea, highlighting the roles of toll-like receptor (TLR)2, LL37 production (8), the interleukin (IL)-17 signaling pathway (9), and the LL37- mammalian target of rapamycin (mTOR) and Janus kinase-signal transducer and activator of transcription (JAK-STAT) pathways (10, 11). These discoveries are crucial for developing targeted treatments. Currently, the treatments of rosacea are primarily symptombased, with effective solutions still under research (12). This review provides a detailed understanding of the signaling pathways involved in rosacea, as well as the emerging targeted therapeutic strategies.
2 LL37-related signaling pathways
TLRs play a crucial role in recognizing pathogen-associated molecular patterns (PAMPs) and damage-associated molecular patterns (DAMPs) (13), triggering anti-pathogen responses, including antimicrobial peptide secretion and proinflammatory cytokine and chemokine production (14). TLR2, a primary pattern recognition receptor, is significantly overexpressed in rosacea patients’ keratinocytes, contributing to heightened skin sensitivity to various stimuli (15). TLR2 is also expressed in sensory neurons, and the TLR2 signaling pathway contributes to the mechanism of neurological dysfunction in rosacea (16). Numerous studies have confirmed that TLR2 responds to environmental stimuli such as reactive oxygen species (ROS), microbial imbalance, Demodex mites, UVB radiation, and temperature changes (17–19). Glucocorticoids can increase TLR2 expression in epidermal keratinocytes, potentially leading to glucocorticoid-induced rosacea-like dermatitis (20). And these trigger factors can amplify TLR2 expression through enhanced endoplasmic reticulum (ER) stress and activating transcription factor 4 (ATF4) upregulation (16). Upon TLR2 activation, Kallikrein 5 (KLK5) and total serine protease activity are released from keratinocytes, a process reduced by TLR2-deficient mice. TLR2’s ability to release KLK5 is calcium-dependent, with TLR2 ligands triggering a calcium influx that increases KLK5 release (15, 21). KLK5 is also mediated by Metalloproteinases (MMPs), which decompose the extracellular matrix (22). MMP2 and MMP9 are associated with the pathogenesis of rosacea, with elevated MMP-9 mRNA levels in rosacea patients’ facial skin (17, 23, 24).
Cathelicidin, an antimicrobial peptide (AMP), acts as an endogenous antibiotic (25). It is initially inactive and activated by serine proteases into multiple active peptides. Specifically, KLK5, a trypsin-like serine protease, is key in converting cathelicidin into LL37 by processing its precursor, hCAP18 (human cationic antimicrobial protein of 18 kDa) (26). Research by Mylonas A. et al. have revealed that KLK5 cleaves cathelicidin, producing peptides with increased DNA binding and enhanced induction of type I interferons (IFNs) in plasmacytoid dendritic cells (pDCs) (27). Cathelicidin expression is regulated by vitamin D–dependent mechanisms involving the vitamin D receptor, controlling human cathelicidin in various cell types, as well as vitamin D-independent mechanisms that increase cathelicidin expression in response to external stressors like infections, injuries, or barrier disruption, often coinciding with ER stress (28–30). LL37 is produced via the TLR2-KLK5 pathway in response to stimuli such as temperature increase. Moreover, mTORC1, a serine/threonine protein kinase, regulates cathelicidin expression in keratinocytes through a positive feedback mechanism. LL37 binds to TLR2, activating mTORC1 signaling and increasing LL37 expression in keratinocytes, highlighting mTORC1’s vital role in LL37 amplification (10, 31, 32). LL37 is central to rosacea pathogenesis, being overexpressed in rosacea patients’ lesional skin (33–35). Intradermal injection of human LL37 in mice models induces inflammatory responses similar to rosacea, making it a key model in rosacea research (36, 37). LL37 has multiple functions, including immune response modulation, inflammation, and angiogenesis (33, 38). It activates mast cells (MCs), keratinocytes, neutrophils, and macrophages, leading to pro-inflammatory cytokine production, leukocyte chemotaxis, MMP expression, and angiogenesis (36, 39–42). LL37-associated signaling pathways are shown in Figure 1.
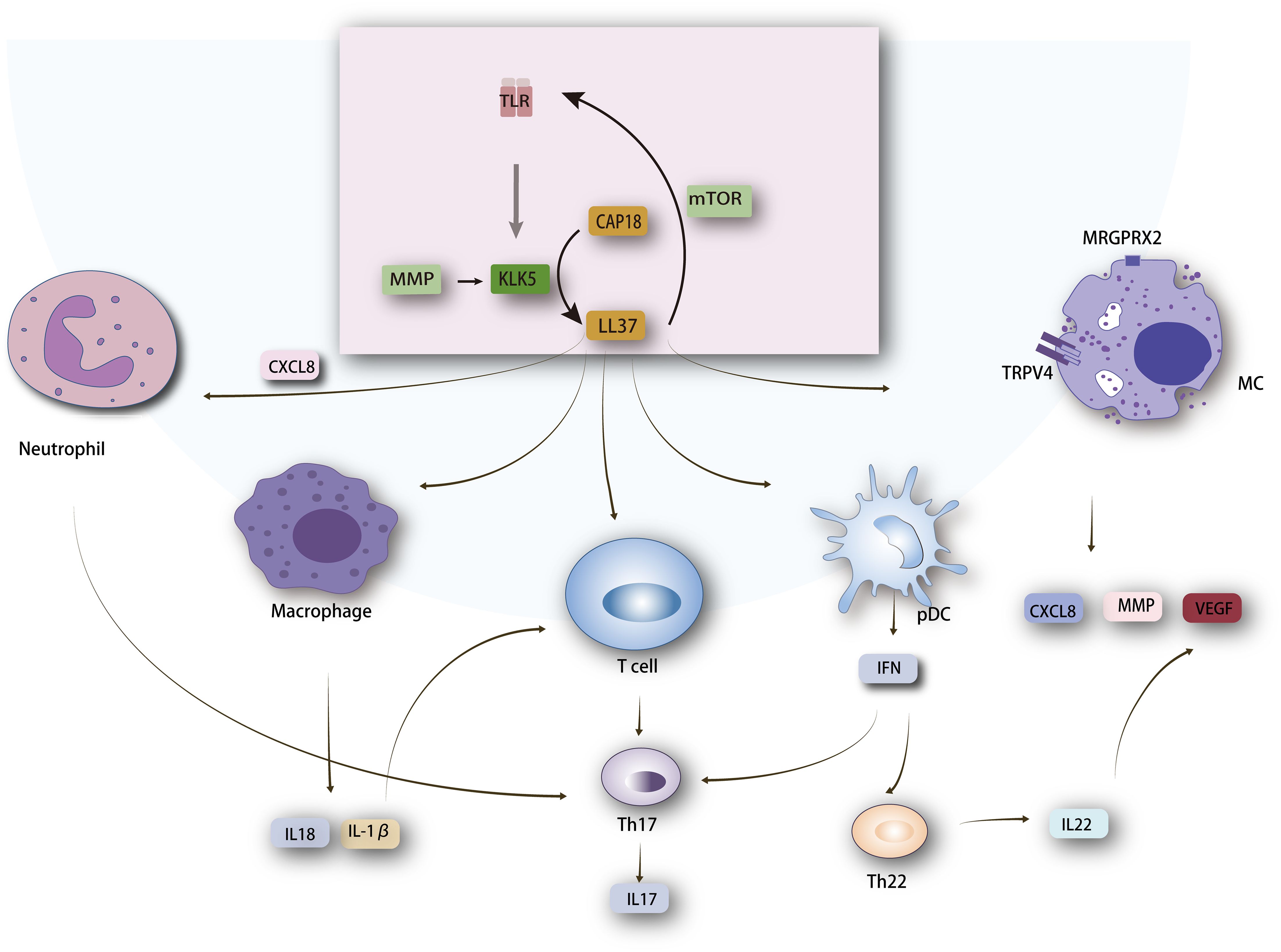
Figure 1. Mechanism of LL37 in Rosacea Pathogenesis. LL37 interacts with several key molecules, including Toll-like receptor 2 (TLR2), mechanistic target of rapamycin complex 1 (mTORC1), chemokine (C-X-C motif) ligand 8 (CXCL8), and Mas-related G-protein coupled receptor member X2 (MRGPRX2) linked to transient receptor potential vanilloid 4 (TRPV4). These interactions lead to the activation of various cell types such as macrophages, neutrophils, T cells, mast cells, and plasmacytoid dendritic cells (pDCs). Activation of these cells results in the production of cytokines, playing a critical role in inflammation, immune modulation, and angiogenesis in rosacea. The cytokines produced, such as IL-1β and TNF-α, contribute to the inflammatory responses characteristic of rosacea. The figure showcases the crucial LL37-mediated pathways and their roles in the pathogenesis of rosacea, emphasizing the complex interplay between different cell types and signaling molecules.
2.1 LL37- MRGPRX2-TRPV4 pathway in rosacea
LL-37, a potent chemoattractant, activates MCs in the inflammatory cascades. Increased MC concentration and degranulation, with a positive correlation between MC density and rosacea duration (43). In MC-deficient mice, rosacea-like symptoms are absent following LL37 dermal injection (44, 45). Subramanian H. et al. identified LL37’s induction of MCs through the Mas-related G-protein-coupled receptor-X2 (MRGPRX2) (46). β-arrestin 2 (βarr2) regulates this via extracellular Signal-Regulated Kinase 1 and 2 (ERK1/2) phosphorylation and nuclear factor kappa B (NF-κB) activation in mice, suggesting potential therapeutic targets in rosacea (47). Sulk M. et al. observed an upregulation of the transient receptor potential vanilloid (TRPV) 4 channel, co-localized with MCs in rosacea patients (48). LL37 directly increases TRPV4 expression in human MCs via MRGPRX2. This elevation in TRPV4 likely facilitates greater cation influx, raising intracellular Ca2+ levels and priming MCs for continuous degranulation or transgranulation (49, 50). Activated MCs release various cytokines, including IL-1, transforming growth factor (TGF-β), tumor Necrosis Factor-alpha (TNF-α), and vascular endothelial growth factor (VEGF) (51). Additionally, MMP9 mRNA, a key MC marker, is upregulated in rosacea-affected skin, primarily near blood vessels (45). Neutrophils, which are recruited following mast cell (MC) activation, are a significant source of LL-37. This creates a feedback loop that perpetuates MC activation and chronic cutaneous inflammation in rosacea (52). Therefore, MCs are crucial in cathelicidin-induced skin inflammation through their role in cytokine and bioactive mediator secretion upon stimulation (53).
2.2 LL37-IL1β/IL17 pathway in rosacea
The NF-κB and the mitogen-associated protein kinase (MAPK) signaling pathway are crucial in LL37mediated inflammation (54, 55). LL-37 activates MAPK, leading to phosphorylation of ERK1/2 and p38 kinases (56), and induces NF-κB-mediated gene expression (57, 58). These pathways play a central role in the pathogenesis of rosacea, as evidenced by increased p38 and ERK levels in ocular rosacea tissue (59), upregulated MAPK pathways in PPR lesional tissue (60), and elevated NF-κB activity in rosacea patients’ eyelid samples (61). Furthermore, TLR signaling pathways also converge on MAPK and NFκB-dependent gene expression (62). Importantly, the TLR2/Myeloid differentiation factor-88 adaptor protein (MyD88)/NF-κB is implicated in rosacea pathogenesis, as suggested by elevated MyD88 levels in rosacea skin biopsies (63). Moreover, dietary supplementation with n-3 PUFAs has been shown to ameliorate skin inflammation in an experimental rosacea model by inhibiting this pathway (64). Deng Z. et al. noted that LL37 initiates NF-κB activation, possibly through mTORC1 signaling (10). Additionally, UV radiation-induced ROS in keratinocytes activates MAPK and NF-κB pathways, influencing inflammatory signaling (65, 66). These pathways control inflammatory cytokine gene expression in immune cells (67, 68). Specifically, the expression of two NF-κB target genes, namely IL-1α and IL-1β, was elevated in rosacea (60, 69).
LL-37 also enhances the ability to release IL-1β by activating the inflammasome (70). NLRP3 (NOD-, LRR- and pyrin domain-containing protein 3) deficiency reduces LL37-induced rosacea-like inflammation (39). NLRP3, an intracellular sensor, is overexpressed in PPR subjects (71). The formation of the NLRP3 inflammasome subsequently leads to the caspase 1-dependent release of the pro-inflammatory cytokines IL-1β and IL-18 (72). IL-1β emerges as a critical mediator in the inflammation development in PPR (60). IL-18, an integral constituent of the IL-1 cytokine family, is heightened in rosacea patients (73). TNF-α signaling also upregulates IL-1β expression (60).
IL-1β serves as a co-stimulator of the proliferation of T-cells and is linked to Th17 lymphocyte differentiation (74). Th17 cells, active in rosacea, release proinflammatory cytokines, prominently IL-17. In rosacea, T-cell-dominated lymphocytes infiltrate affected skin (75), with consistently elevated IL-17 serum levels (76). Thus, IL-17 plays a crucial role in rosacea pathogenesis, particularly in PPR (77, 78). IL-17 has diverse functions. It activates VEGF-induced angiogenesis and expansion, as shown in both in vitro and in vivo studies (79). Obradovic´ H. et al. found that recombinant mouse IL-17 induces MMP9 expression in mouse myoblast C2C12 cells after IL-17 treatment (80).Furthermore, IL-17 stimulates vitamin-D3-induced LL37 production in keratinocytes (81, 82). Remarkably, LL37 induces genes related to Th1/Th17 polarization (83). IL-17 also prompts the production of pro-inflammatory cytokines, including TNF-α, IL-1β, IL-8, and IL-6 (84). Rosacea skin samples show increased expression of these cytokines (85). Apart from Th17 cells, Th1 cells are also involved in the pathogenesis of rosacea. Th1 cells secrete IFN-γ, a potent macrophage activator that classically activates human macrophages into a pro-inflammatory (M1) phenotype in vitro (86). This enhances the interaction between CD4+ T cells and the innate immune system in the disease.
2.3 LL37-CXCL8 interaction in rosacea
LL37 induces the release of C-X-C motif Chemokine ligand (CXCL) 8 (formerly known as IL-8) from keratinocytes, a crucial chemotactic factor for neutrophils in rosacea (57, 87). Transcriptome analyses showed increased CXCL8 expression in rosacea (88, 89). Neutrophil migration is prompted by Demodex folliculorum and its associated bacillus oleronius in rosacea (90). These neutrophil pathways and proteins are central to rosacea’s inflammation, with pustule development indicative of neutrophil infiltration (88). Neutrophils play a vital role in microbial defense, neutralizing threats through enzyme release, ROS synthesis, and inflammatory mediator production (91). This influx of neutrophils, in turn, precipitates the secretion of IL-17, thereby establishing a chronic inflammation cycle in rosacea.
2.4 LL37-VEGF axis in rosacea pathogenesis
Angiogenesis, facilitated by VEGF, is central to rosacea’s hallmark symptoms of flushing and erythema (92). VEGF serves dual roles in angiogenesis and inflammation (93). In facial redness, VEGF, VEGF-R1, and VEGF-R2 are upregulated in the granular layer and stratum corneum of keratinocytes, as well as in dermal leukocytes including lymphocytes, macrophages, and plasma cells (94, 95). The VEGF polymorphism (+405C/G) is linked to rosacea severity (96). CD31+ cells infiltrates are primary sources of VEGF, driving angiogenesis (97). VEGF production by activated T cells stimulates angiogenesis and promotes Th1 cell differentiation, creating a feedback loop (98, 99). Additionally, UVB exposure activates VEGF signaling, with VEGF-A intensifying vascular sensitivity to UVB (100).
LL37 contributes to angiogenesis in rosacea. It activates endothelial cells (ECs) and VEGF via FPRL1, promoting angiogenesis (101). mTORC1 signaling mediates LL37-induced angiogenesis, with activation noted in ECs of rosacea lesions and LL37-induced rosacea-like mouse models (102). Furthermore, LL37-induced type I IFNs from pDCs, overexpressed during rosacea flare-ups, lead to an increased Th22/Th17 cytokine response (27). Enhanced IL-22 expression and EC sensitization to IL-22 facilitate aberrant angiogenesis (27). Moreover, TLR2 pathway overexpression in keratinocytes augments proinflammatory cytokine and chemokine expression, including IL-8, IL-1β, TNF-α, and C motif chemokine ligand (CCL) 5, CXCL9, CXCL10, and CXCL11 (8). These elevated levels of cytokines and chemokines result in the induction of vascular hyper-reactivity (103).
A recent study investigated the role of Hippo signaling pathway, specifically yes-associated protein (YAP) and transcriptional coactivator with PDZ-binding motif (TAZ), in rosacea. The study found alterations in these signaling molecules in rosacea patients, suggesting their involvement in the development of new angiogenesis within the skin. Furthermore, the study showed that inhibiting YAP/TAZ reduced VEGF immunoreactivity, a marker of blood vessel formation. These findings suggest that YAP/TAZ may play a role in the mechanisms by which rosacea causes abnormal blood vessel growth (104).
3 JAK/STAT signaling pathway
The JAK/STAT pathways have a wide range of functions on immune responses, cellular proliferation, differentiation, apoptosis, and immunoregulation (105). JAK inhibitors are increasingly used in treating inflammatory skin disorders (106). In LL37-treated HaCaT cells, elevated JAK2 and STAT3 levels suggest a strong connection between JAK/STAT signaling and rosacea’s inflammatory response. JAK2/STAT3 activation interacts with TLR2 signaling (107), leading to increased production of pro-inflammatory cytokines like TNF-α, IL-6, and IL-8 (108, 109).
Rosacea’s inflammation and immune infiltration are exacerbated by skin barrier disruption, partly due to STAT3-mediated cytokine signaling in keratinocytes (110). STAT3 also regulates degranulation in human and mouse MCs (111, 112). ERK1/2-mediated mitochondrial STAT3 phosphorylation contributes to MC degranulation (113). Blazanin N.et al. observed that acute solar UV exposure activates pSTAT1-related signaling in keratinocytes (114), indicating epidermal-derived STAT1’s role in epithelial-immune communication in rosacea (115). The role of IL-17 in increasing VEGF expression via JAK/STAT signaling has been demonstrated in various contexts. IL-17 has been shown to induce reactive astrocytes and upregulate VEGF through JAK/STAT signaling, as well as up-regulate VEGF in nucleus pulposus cells via the same pathway. These findings suggest that similar mechanisms might be relevant to the inflammatory response in rosacea (116, 117).
4 Cutaneous neuroinflammation and downstream signal pathways in rosacea
Cutaneous neurogenic inflammation (CNI) is widely recognized in rosacea, involving a series of signaling cascades. Ion channels, particularly transient receptor potential (TRP) channels in skin nerve fibers, activate upon stimuli, releasing vasoactive neuropeptides that interact with keratinocytes, immune cells, and blood vessels (118, 119). These neuropeptides exacerbate inflammation and vascular dilation, translating nerve impulses into signals for immune cells. Rosacea is a classic example of CNI, which can be explained by the neurologic hypersensitivity in patients with rosacea (120).
TRPV1, a critical cation channel primarily for Ca2+, is involved in cutaneous neurogenic inflammation and pain (121). TRPV1 expression increases in rosacea, especially in keratinocytes (122), upon stimulation by factors like pH changes, high temperatures, and UVB exposure (123, 124). Activated TRPV1 stimulates sensory neuron C fibers, releasing mediators that contribute to neurogenic inflammation and pain through elevated cytosolic Ca2+ levels. This leads to increased release of neuropeptides such as pituitary adenylate cyclase-activating polypeptide (PACAP), vasoactive intestinal peptide (VIP), VEGF, adrenomedullin, calcitonin gene-related peptide (CGRP), and substance P (SP), all implicated in rosacea pathogenesis (125–127). These neuropeptides collaborate in processes like inflammation, tissue damage, vasomotor disturbances, and increased neurovascular reactivity (128, 129). Abnormal amino acid metabolism, specifically glutamic and aspartic acids, can enhance the formation of erythema and telangiectasia in rosacea-like mouse skin through vasodilatory neuropeptides in peripheral neurons and keratinocytes (130).
CGRP, a potent microvascular dilator, contributes to extensive neurogenic vasodilation and mobilizes inflammatory cells (127). It also modulates cutaneous immunity by affecting NF-κB expression in immune cells (131). SP influences the emergence of edema in rosacea through its interaction with neurokinin 1 receptors and contributes to MCs degranulation, EC proliferation, and localized vasodilation (118, 132). Intradermal PACAP38 administration increases pain perception and skin blood flow, exacerbating rosacea features like facial flushing and edema (125). Mechanistically, PACAP acts as a potent vasodilator and influences vascular responses in human skin (133). It upregulates MC proteases (MMP-1 and MMP-9) and proinflammatory cytokines, including TNF and CXCL2, and may affect the pathway converting hCAP18 into LL37 (134). VIP enhances Th17 cell differentiation, shifting the T-helper cell response towards Th17 (135). In brief, VIP, PACAP, and CGRP act as vasodilators and mediate the production of inflammatory factors through interaction with skin immune cells.
Furthermore, there is notable upregulation of TRPV expression in rosacea, affecting not only neuronal but also non-neuronal cells. Sulk M.et al. observed increased dermal immunolabeling of TRPV2 and TRPV3 and gene expression of TRPV1 in ETR. PPR shows enhanced immunoreactivity for TRPV2 and TRPV4 and increased TRPV2 gene expression (48). Zhou X. et al. identified that TPRV4 also interacts with transient receptor potential melastatin 8 (TRPM8) channels on immune cells or keratinocytes, which is strongly associated with itching in rosacea both in experimental and clinical settings (136).
5 Molecular targeted therapy in rosacea
Rosacea treatment primarily focuses on symptom management, including anti-inflammatory, immunomodulatory, microflora-regulating, and capillary dilation strategies. Common treatments include topical agents (azelaic acid, metronidazole, brimonidine, ivermectin, tacrolimus, pimecrolimus) and oral antibiotics (tetracycline, retinoids) (12, 137). However, increasing concerns over antibiotic resistance and impacts on skin flora indicate a pressing need for more effective and safer therapeutic alternatives (138). Emerging insights into the signaling pathways involved in rosacea mentioned above have led to the exploration of targeted therapies, aiming for improved efficacy and fewer side effects. Table 1 presents current therapeutic targets and corresponding treatments for rosacea. However, the efficacy of these treatments remains challenging to assess and compare due to insufficient clinical studies.
5.1 Targeting TLR2-KLK5- LL37 and mTOR-related pathways
Targeting the TLR2-KLK5-LL37 pathway is currently a key strategy for the clinical treatment of rosacea. Retinoids, azelaic acid, and doxycycline modulate this pathway, reducing KLK5 and cathelicidin expression (139–141). Azelaic acid inhibits serine protease activity, and doxycycline limits KLK5 activity by inhibiting MMP9 (139, 142). Recent studies indicate that Carvedilol and Ivermectin modulate this pathway, contributing to their efficacy in rosacea treatment (143). A vitro study demonstrated that ϵ-Aminocaproic Acid (ACA) and Superoxide Dismutase 3 (SOD3) are effective in modulating the TLR2-related pathway (65, 144). Topical Rapamycin, an inhibitor of mTOR, has shown clinical effectiveness in treating rosacea. In a controlled study, 18 female rosacea patients were randomized to receive either a placebo or 0.4% FDA-approved rapamycin ointment. The results demonstrated that the group treated with rapamycin experienced significant clinical improvement compared to the placebo group, indicating the potential of mTORC1 inhibition as a therapeutic strategy in rosacea (10). Furthermore, Celastrol and Epigallocatechin-3-gallate (EGCG) also target mTOR-related pathways, exhibiting anti-inflammatory effects (31, 32).
5.2 Targeting Th1/Th17-IL17 in rosacea
The development of biological agents targeting specific cytokines offers a promising approach to treating rosacea. Approved antibodies, including those against IL-1β and IL-17, show potential as novel treatments. Specifically, secukinumab targeting IL-17, a monoclonal antibody primarily used in psoriasis, is under investigation for its effectiveness in treating rosacea. A trial involving 24 patients with papulopustular rosacea assessed the efficacy of secukinumab. The patients received 300 mg of secukinumab weekly for 5 weeks, then monthly for 2 months, the treatment led to significant improvement in papules and overall severity in 17 of the participants, along with enhanced quality of life (145). In addition, Aspirin and Thalidomide have shown potential in moderating Th1/Th17 immune responses, further supporting the strategy of targeting specific cytokine pathways in rosacea (69, 146).
5.3 Targeting VEGF in rosacea
VEGF inhibition has emerged as an effective strategy in rosacea treatment. Topical dobesilate, known for inhibiting angiogenic factors, has been shown effective in treating erythematotelangiectatic rosacea (147). Tranexamic acid, too, has shown efficacy in reducing microvessel density, VEGF expression, and associated inflammatory markers in rosacea patients (97). Additionally, the role of erythroid differentiation regulator 1 (Erdr1) in significantly inhibiting VEGF-mediated angiogenesis has been documented (73).
5.4 JAK/STAT pathway in rosacea
The JAK/STAT pathway plays a crucial role in the pathogenesis of rosacea. Oral tofacitinib, a JAK inhibitor, has demonstrated efficacy in mitigating facial erythema in rosacea. A clinical study with 21 rosacea patients revealed that 71.4% experienced a significant reduction in facial erythema following oral tofacitinib treatment (11). Furthermore, tofacitinib’s effectiveness in a case of steroid-induced rosacea underscores its potential, particularly in cases resistant to conventional therapies (148). Additionally, Artesunate has been identified as a promising agent in reducing inflammation through its action on the JAK2/STAT3 pathway (108).
6 Conclusion
This review highlights the complex signaling pathways involved in rosacea and the advancement of targeted therapies. The targeted modulation of the TLR2-KLK5-LL37 and mTOR pathways has shown significant efficacy in clinical settings. VEGF inhibitors have proven beneficial in treating erythematotelangiectatic rosacea. Biological agents, specifically monoclonal antibodies like secukinumab targeting IL-17, have been effective in treatment. The role of the JAK/STAT pathway in rosacea’s pathology is significant, with tofacitinib notably successful in reducing facial erythema. Despite these developments, research in targeted therapies for rosacea remains incomplete. Recognizing the complexity of rosacea, which involves multiple signaling pathways, is crucial for future advancements in treatment.
Author contributions
FY: Conceptualization, Data curation, Investigation, Visualization, Writing – original draft, Writing – review & editing. LW: Funding acquisition, Investigation, Project administration, Supervision, Writing – review & editing. DS: Visualization, Writing – review & editing. LZ: Supervision, Writing – review & editing. XW: Data curation, Writing – review & editing. DD: Supervision, Writing – review & editing. XJ: Conceptualization, Funding acquisition, Supervision, Writing – review & editing.
Funding
The author(s) declare financial support was received for the author research, authorship, and/or publication of this article. This research was supported by the National Natural Science Foundation of China (82273559, 82103757), Clinical Research Innovation Project, West China Hospital Sichuan University (19HXCX010), PostDoctor Research Project, West China Hospital, Sichuan University (2020HXBH151).
Conflict of interest
The authors declare that the research was conducted in the absence of any commercial or financial relationships that could be construed as a potential conflict of interest.
Publisher’s note
All claims expressed in this article are solely those of the authors and do not necessarily represent those of their affiliated organizations, or those of the publisher, the editors and the reviewers. Any product that may be evaluated in this article, or claim that may be made by its manufacturer, is not guaranteed or endorsed by the publisher.
References
1. Gether L, Overgaard L, Egeberg A, Thyssen J. Incidence and prevalence of rosacea: a systematic review and meta-analysis. Br J Dermatol. (2018) 179:282–9. doi: 10.1111/bjd.16481
2. Schaller M, Almeida L, Bewley A, Cribier B, Del Rosso J, Dlova N, et al. Recommendations for rosacea diagnosis, classification and management: update from the global rosacea consensus 2019 panel. Br J Dermatol. (2020) 182:1269–76. doi: 10.1111/bjd.18420
3. Two AM, Wu W, Gallo RL, Hata TR. Rosacea: part i. introduction, categorization, histology, pathogenesis, and risk factors. J Am Acad Dermatol. (2015) 72:749–58. doi: 10.1016/j.jaad.2014.08.028
4. Alia E, Feng H. Rosacea pathogenesis, common triggers, and dietary role: the cause, the trigger, and the positive effects of different foods. Clinics Dermatol. (2022) 40:122–7. doi: 10.1016/j.clindermatol.2021.10.004
5. Holmes AD, Spoendlin J, Chien AL, Baldwin H, Chang ALS. Evidence-based update on rosacea comorbidities and their common physiologic pathways. J Am Acad Dermatol. (2018) 78:156–66. doi: 10.1016/j.jaad.2017.07.055
6. Deng Z, Chen M, Zhao Z, Xiao W, Liu T, Peng Q, et al. Whole genome sequencing identifies genetic variants associated with neurogenic inflammation in rosacea. Nat Commun. (2023) 14:3958. doi: 10.1038/s41467-023-39761-2
7. Hu XM, Li ZX, Zhang DY, Yang YC, Zheng SY, Zhang Q, et al. Current research and clinical trends in rosacea pathogenesis. Heliyon. (2022) 8:e10874. doi: 10.1016/j.heliyon.2022.e10874
8. Sun Y, Chen L, Wang H, Zhu P, Jiang S, Qi R, et al. Activation of aryl hydrocarbon receptor ameliorates rosacea-like eruptions in mice and suppresses the TLR signaling pathway in LL-37induced HaCaT cells. Toxicol Appl Pharmacol. (2022) 451:116189. doi: 10.1016/j.taap
9. Buhl T, Sulk M, Nowak P, Buddenkotte J, McDonald I, Aubert J, et al. Molecular and morphological characterization of inflammatory infiltrate in rosacea reveals activation of th1/th17 pathways. J Invest Dermatol. (2015) 135:2198–208. doi: 10.1038/jid.2015.141
10. Deng Z, Chen M, Liu Y, Xu S, Ouyang Y, Shi W, et al. A positive feedback loop between mTORC1 and cathelicidin promotes skin inflammation in rosacea. EMBO Mol Med. (2021) 13:e13560. doi: 10.15252/emmm.202013560
11. Sun Y, Man X, Xuan X, Huang C, Shen Y, Lao L. Tofacitinib for the treatment of erythematotelangiectatic and papulopustular rosacea: A retrospective case series. Dermatologic Ther. (2022) 35. doi: 10.1111/dth.15848
12. Delans K, Kelly K, Feldman SR. Treatment strategies, including antibiotics, to target the immune component of rosacea. Expert Rev Clin Immunol. (2022) 18:1239–51. doi: 10.1080/1744666X.2022.2128334
13. Terhorst D, Kalali BN, Ollert M, Ring J, Mempel M. The role of toll-like receptors in host defenses and their relevance to dermatologic diseases. Am J Clin Dermatol. (2010) 11:1–10. doi: 10.2165/11311110-000000000-00000
14. Fitzgerald KA, Kagan JC. Toll-like receptors and the control of immunity. Cell. (2020) 180:1044–66. doi: 10.1016/j.cell.2020.02.041
15. Yamasaki K, Kanada K, Macleod DT, Borkowski AW, Morizane S, Nakatsuji T, et al. TLR2 expression is increased in rosacea and stimulates enhanced serine protease production by keratinocytes. J Invest Dermatol. (2011) 131:688–97. doi: 10.1038/jid.2010.351
16. Melnik BC. Endoplasmic reticulum stress: key promoter of rosacea pathogenesis. Exp Dermatol. (2014) 23:868–73. doi: 10.1111/exd.12517
17. Falay Gur T, Erdemir AV, Gurel MS, Kocyigit A, Guler EM, Erdil D. The investigation of the relationships of demodex density with inflammatory response and oxidative stress in rosacea. Arch Dermatol Res. (2018) 310:759–67. doi: 10.1007/s00403-018-1857-1
18. Forton FMN. The pathogenic role of demodex mites in rosacea: A potential therapeutic target already in erythematotelangiectatic rosacea? Dermatol Ther. (2020) 10:1229–53. doi: 10.1007/s13555-020-00458-9
19. Morgado-Carrasco D, Granger C, Trullas C, Piquero-Casals J. Impact of ultraviolet radiation and exposome on rosacea: Key role of photoprotection in optimizing treatment. J Cosmetic Dermatol. (2021) 20:3415–21. doi: 10.1111/jocd.14020
20. Shibata M, Katsuyama M, Onodera T, Ehama R, Hosoi J, Tagami H. Glucocorticoids enhance toll-like receptor 2 expression in human keratinocytes stimulated with propionibacterium acnes or proinflammatory cytokines. J Invest Dermatol. (2009) 129:375–82. doi: 10.1038/jid.2008.237
21. Jang Y, Hong E, Park E, Kim K, Kim K. Immunohistochemical analysis of differences of toll-like receptor 2, mast cells, and neurofilaments between granulomatous rosacea and non-granulomatous rosacea. Indian J Dermatol. (2021) 66:343. doi: 10.4103/ijd.IJD_18_20
22. Nagase H, Visse R, Murphy G. Structure and function of matrix metalloproteinases and TIMPs. Cardiovasc Res. (2006) 69:562–73. doi: 10.1016/j.cardiores.2005.12.002
23. Jang Y, Sim J, Kang H, Kim Y, Lee E. Immunohistochemical expression of matrix metalloproteinases in the granulomatous rosacea compared with the non-granulomatous rosacea. J Eur Acad Dermatol Venereology. (2011) 25:544–8. doi: 10.1111/j.1468-3083.2010.03825
24. Fernández J, Jiménez C, Benadof D, Morales P, Astorga J, Cáceres F, et al. MMP-9 levels in the gingival crevicular fluid of Chilean rosacea patients. Int J Mol Sci. (2022) 23:9858. doi: 10.3390/ijms23179858
25. Takahashi T, Gallo RL. The critical and multifunctional roles of antimicrobial peptides in dermatology. Dermatologic Clinics. (2017) 35:39–50. doi: 10.1016/j.det.2016.07.006
26. Amagai R, Takahashi T, Terui H, Fujimura T, Yamasaki K, Aiba S, et al. The antimicrobial peptide cathelicidin exerts immunomodulatory effects via scavenger receptors. Int J Mol Sci. (2023) 24:875. doi: 10.3390/ijms24010875
27. Mylonas A, Hawerkamp HC, Wang Y, Chen J, Messina F, Demaria O, et al. Type I IFNs link skinassociated dysbiotic commensal bacteria to pathogenic inflammation and angiogenesis in rosacea. JCI Insight. (2023) 8:e151846. doi: 10.1172/jci.insight.151846
28. Park K, Elias PM, Oda Y, Mackenzie D, Mauro T, Holleran WM, et al. Regulation of cathelicidin antimicrobial peptide expression by an endoplasmic reticulum (ER) stress signaling, vitamin D receptor-independent pathway. J Biol Chem. (2011) 286:34121–30. doi: 10.1074/jbc.M111.250431
29. Schauber J, Gallo RL. The vitamin D pathway: a new target for control of the skin’s immune response? Exp Dermatol. (2008) 17:633–9. doi: 10.1111/j.1600-0625.2008.00768.x
30. Wang TT, Nestel FP, Bourdeau V, Nagai Y, Wang Q, Liao J, et al. Cutting edge: 1,25Dihydroxyvitamin D3 is a direct inducer of antimicrobial peptide gene expression. J Immunol. (2004) 173:2909–12. doi: 10.4049/jimmunol.173.5.2909
31. Zhou L, Zhong Y, Wang Y, Deng Z, Huang Y, Wang Q, et al. EGCG identified as an autophagy inducer for rosacea therapy. Front Pharmacol. (2023) 14:1092473. doi: 10.3389/fphar.2023.1092473
32. Zeng Q, Yang J, Yan G, Zhang L, Wang P, Zhang H, et al. Celastrol inhibits ll37-induced rosacea by inhibiting ca2+/camkii-mtor-nf-κb activation. Biomedicine Pharmacotherapy. (2022) 153:113292. doi: 10.1016/j.biopha.2022.113292
33. Yamasaki K, Di Nardo A, Bardan A, Murakami M, Ohtake T, Coda A, et al. Increased serine protease activity and cathelicidin promotes skin inflammation in rosacea. Nat Med. (2007) 13:975–80. doi: 10.1038/nm1616
34. Yamasaki K, Schauber J, Coda A, Lin H, Dorschner RA, Schechter NM, et al. Kallikrein-mediated proteolysis regulates the antimicrobial effects of cathelicidins in skin. FASEB J. (2006) 20:2068–80. doi: 10.1096/fj.06-6075com
35. Kim JY, Kim YJ, Lim BJ, Sohn HJ, Shin D, Oh SH. Increased expression of cathelicidin by direct activation of protease-activated receptor 2: possible implications on the pathogenesis of rosacea. Yonsei Med J. (2014) 55:1648. doi: 10.3349/ymj.2014.55.6.1648
36. Zhang C, Kang Y, Zhang Z, Liu H, Xu H, Cai W, et al. Long-term administration of LL-37 can induce irreversible rosacea-like lesion. Curr Issues Mol Biol. (2023) 45:2703–16. doi: 10.3390/cimb45040177
37. Zhang H, Zhang M, Wang Y, Zheng Q, Tang K, Liu R, et al. Murine models of rosacea: a review. J Cosmetic Dermatol. (2022) 21:905–9. doi: 10.1111/jocd.14164
38. Bals R, Wilson JM. Cathelicidins - a family of multifunctional antimicrobial peptides. Cell Mol Life Sci (CMLS). (2003) 60:711–20. doi: 10.1007/s00018-003-2186-9
39. Yoon SH, Hwang I, Lee E, Cho HJ, Ryu JH, Kim TG, et al. Antimicrobial peptide LL-37 drives rosacea-like skin inflammation in an NLRP3-dependent manner. J Invest Dermatol. (2021) 141:2885–2894.e5. doi: 10.1016/j.jid.2021.02.745
40. Kang Y, Zhang C, He Y, Zhang Z, Liu H, Wei Z, et al. Thalidomide attenuates skin lesions and inflammation in rosacea-like mice induced by long-term exposure of LL-37. Drug Design Dev Ther Volume. (2022) 16:4127–38. doi: 10.2147/DDDT.S393122
41. Kulkarni NN, Takahashi T, Sanford JA, Tong Y, Gombart AF, Hinds B, et al. Innate immune dysfunction in rosacea promotes photosensitivity and vascular adhesion molecule expression. J Invest Dermatol. (2020) 140:645–655.e6. doi: 10.1016/j.jid.2019.08.436
42. Suhng E, Kim BH, Choi YW, Choi HY, Cho H, Byun JY. Increased expression of IL-33 in rosacea skin and UVB-irradiated and LL-37-treated HaCaT cells. Exp Dermatol. (2018) 27:1023–9. doi: 10.1111/exd.13702
43. Jiang P, Liu Y, Zhang J, Liu Y, Li M, Tao M, et al. Mast cell stabilization: new mechanism underlying the therapeutic effect of intense pulsed light on rosacea. Inflammation Res. (2023) 72:75–88. doi: 10.1007/s00011-022-01635-6
44. Di Nardo A, Vitiello A, Gallo RL. Cutting edge: mast cell antimicrobial activity is mediated by expression of cathelicidin antimicrobial peptide. J Immunol. (2003) 170:2274–8. doi: 10.4049/jimmunol.170.5.2274
45. Muto Y, Wang Z, Vanderberghe M, Two A, Gallo RL, Di Nardo A. Mast cells are key mediators of cathelicidin-initiated skin inflammation in rosacea. J Invest Dermatol. (2014) 134:2728–36. doi: 10.1038/jid.2014.222
46. Subramanian H, Gupta K, Guo Q, Price R, Ali H. Mas-related gene X2 (MrgX2) is a novel G protein-coupled receptor for the antimicrobial peptide LL-37 in human mast cells. J Biol Chem. (2011) 286:44739–49. doi: 10.1074/jbc.M111.277152
47. Roy S, Alkanfari I, Chaki S, Ali H. Role of mrgprb2 in rosacea-like inflammation in mice: Modulation by β-arrestin 2. J Invest Dermatol. (2022) 142:2988–97. doi: 10.1016/j.jid.2022.05.005
48. Sulk M, Seeliger S, Aubert J, Schwab VD, Cevikbas F, Rivier M, et al. Distribution and expression of non-neuronal transient receptor potential (TRPV) ion channels in rosacea. J Invest Dermatol. (2012) 132:1253–62. doi: 10.1038/jid.2011.424
49. Mascarenhas NL, Wang Z, Chang YL, Di Nardo A. TRPV4 mediates mast cell activation in cathelicidin-induced rosacea inflammation. J Invest Dermatol. (2017) 137:972–5. doi: 10.1016/j.jid.2016.10.046
50. Chen Y, Moore CD, Zhang JY, Hall RP, MacLeod AS, Liedtke W. TRPV4 moves toward center-fold in rosacea pathogenesis. J Invest Dermatol. (2017) 137:801–4. doi: 10.1016/j.jid.2016.12.013
51. Wang L, Wang YJ, Hao D, Wen X, Du D, He G, et al. The theranostics role of mast cells in the pathophysiology of rosacea. Front Med. (2020) 6:324. doi: 10.3389/fmed.2019.00324
52. Roy S, Chompunud Na Ayudhya C, Thapaliya M, Deepak V, Ali H. Multifaceted MRGPRX2: New insight into the role of mast cells in health and disease. J Allergy Clin Immunol. (2021) 148:293–308. doi: 10.1016/j.jaci.2021.03.049
53. Woźniak E, Owczarczyk-Saczonek A, Lange M, Czarny J, Wygonowska E, Placek W, et al. The role of mast cells in the induction and maintenance of inflammation in selected skin diseases. Int J Mol Sci. (2023) 24:7021. doi: 10.3390/ijms24087021
54. Li Y, Shan Z, Yang B, Yang D, Men C, Cui Y, et al. Cathelicidin ll37 promotes epithelial and smooth-muscle-like differentiation of adipose-derived stem cells through the wnt/β-catenin and nf-κb pathways. Biochem (Moscow). (2017) 82:1336–45. doi: 10.1134/S0006297917110116
55. Kittaka M, Shiba H, Kajiya M, Ouhara K, Takeda K, Kanbara K, et al. Antimicrobial peptide ll 37 promotes vascular endothelial growth factor-a expression in human periodontal ligament cells. J periodontal Res. (2013) 48:228–34. doi: 10.1111/j.1600-0765.2012.01524.x
56. Niyonsaba F, Ushio H, Nagaoka I, Okumura K, Ogawa H. The human β-defensins (-1,-2,-3,-4) and cathelicidin ll-37 induce il-18 secretion through p38 and erk mapk activation in primary human keratinocytes. J Immunol. (2005) 175:1776–84. doi: 10.4049/jimmunol.175.3.1776
57. Kan HL, Wang CC, Cheng YH, Yang CL, Chang HS, Chen IS, et al. Cinnamtannin B1 attenuates rosacea-like signs via inhibition of pro-inflammatory cytokine production and down-regulation of the MAPK pathway. PeerJ. (2020) 8:e10548. doi: 10.7717/peerj.10548
58. Kim MS, Lim WK, Park RK, Shin T, Yoo YH, Hong SH, et al. Involvement of mitogen-activated protein kinase and NF-κB activation in Ca2+-induced IL-8 production in human mast cells. Cytokine. (2005) 32:226–33. doi: 10.1016/j.cyto.2005.10.001
59. Wladis EJ, Swamy S, Herrmann A, Yang J, Carlson JA, Adam AP. Activation of p38 and erk mitogen-activated protein kinases signaling in ocular rosacea. Invest Opthalmology Visual Sci. (2017) 58:843. doi: 10.1167/iovs.16-20275
60. Harden JL, Shih YH, Xu J, Li R, Rajendran D, Hofland H, et al. Paired transcriptomic and proteomic analysis implicates IL-1β in the pathogenesis of papulopustular rosacea explants. J Invest Dermatol. (2021) 141:800–9. doi: 10.1016/j.jid.2020.08.013
61. Wladis EJ, Lau KW, Adam AP. Nuclear factor kappa-B is enriched in eyelid specimens of rosacea: implications for pathogenesis and therapy. Am J Ophthalmol. (2019) 201:72–81. doi: 10.1016/j.ajo.2019.01.018
62. Wladis EJ, Adam AP. Immune signaling in rosacea. Ocular Surface. (2021) 22:224–9. doi: 10.1016/j.jtos.2021.08.017
63. Wladis EJ, Arunachalam T, LaJoie JE, Lau KW, Adam AP. Myeloid differentiation factor 88 expression in eyelid specimens of rosacea. Orbit. (2022) 41:329–34. doi: 10.1080/01676830.2021.1905668
64. Shen S, Yan G, Cao Y, Zeng Q, Zhao J, Wang X, et al. Dietary supplementation of n-3 PUFAs ameliorates LL37-induced rosacea-like skin inflammation via inhibition of TLR2/MyD88/NF-κB pathway. Biomedicine Pharmacotherapy. (2023) 157:114091. doi: 10.1016/j.biopha.2022.114091
65. Agrahari G, Sah SK, Nguyen CT, Choi SS, Kim HY, Kim TY. Superoxide dismutase 3 inhibits LL-37/KLK-5–mediated skin inflammation through modulation of EGFR and associated inflammatory cascades. J Invest Dermatol. (2020) 140:656–665.e8. doi: 10.1016/j.jid.2019.08.434
66. Tisma VS, Basta-Juzbasic A, Jaganjac M, Brcic L, Dobric I, Lipozencic J, et al. Oxidative stress and ferritin expression in the skin of patients with rosacea. J Am Acad Dermatol. (2009) 60:270–6. doi: 10.1016/j.jaad.2008.10.014
67. Kawai T, Akira S. Signaling to NF-κB by toll-like receptors. Trends Mol Med. (2007) 13:460–9. doi: 10.1016/j.molmed.2007.09.002
68. Liu Z, Zhang J, Jiang P, Yin Z, Liu Y, Liu Y, et al. Paeoniflorin inhibits the macrophagerelated rosacea-like inflammatory reaction through the suppressor of cytokine signaling 3-apoptosis signal-regulating kinase 1-p38 pathway. Medicine. (2021) 100:e23986. doi: 10.1097/MD.0000000000023986
69. Chen M, Xie H, Chen Z, Xu S, Wang B, Peng Q, et al. Thalidomide ameliorates rosacea-like skin inflammation and suppresses NF-κB activation in keratinocytes. Biomedicine Pharmacotherapy. (2019) 116:109011. doi: 10.1016/j.biopha.2019.109011
70. Salzer S, Kresse S, Hirai Y, Koglin S, Reinholz M, Ruzicka T, et al. Cathelicidin peptide LL-37 increases UVB-triggered inflammasome activation: Possible implications for rosacea. J Dermatol Sci. (2014) 76:173–9. doi: 10.1016/j.jdermsci.2014.09.002
71. Wang J, Sun Y, Chen L, Wang Y, Shi D, Wu Y, et al. Supramolecular salicylic acid ameliorates rosacealike eruptions by suppressing NLRP3-mediated inflammasome activation in mice. Int Immunopharmacol. (2023) 118:110057. doi: 10.1016/j.intimp.2023.110057
72. Swanson KV, Deng M, Ting JPY. The NLRP3 inflammasome: molecular activation and regulation to therapeutics. Nat Rev Immunol. (2019) 19:477–89. doi: 10.1038/s41577-019-0165-0
73. Kim M, Kim K, Jung HY, Jo H, Jeong S, Lee J, et al. Recombinant erythroid differentiation regulator 1 inhibits both inflammation and angiogenesis in a mouse model of rosacea. Exp Dermatol. (2015) 24:680–5. doi: 10.1111/exd.12745
74. Campbell L, Raheem I, Malemud C, Askari A. The relationship between NALP3 and autoinflammatory syndromes. Int J Mol Sci. (2016) 17:725. doi: 10.3390/ijms17050725
75. Cribier B. Rosacea under the microscope: characteristic histological findings. J Eur Acad Dermatol Venereology. (2013) 27:1336–43. doi: 10.1111/jdv.12121
76. Hayran Y, S¸en O, Fırat Oguz˘ E, Yucel¨ C¸, Eren F, Külcü Çakmak S, et al. Serum il-17 levels in patients with rosacea. J Cosmetic Dermatol. (2022) 21:1147–53. doi: 10.1111/jocd.14169
77. Amir Ali A, Vender R, Vender R. The role of IL-17 in papulopustular rosacea and future directions. J Cutaneous Med Surg. (2019) 23:635–41. doi: 10.1177/1203475419867611
78. Kim J, Kim K. Elucidating the potential pharmaceutical mechanism of Gyejibokryeong-hwan on rosacea using network analysis. Medicine. (2023) 102:e33023. doi: 10.1097/MD.0000000000033023
79. Numasaki M. Interleukin-17 promotes angiogenesis and tumor growth. Blood. (2003) 101:2620–7. doi: 10.1182/blood-2002-05-1461
80. Hristina O, Jelena K, Tamara K, Drenka T, Oki OI, Slavko M, et al. Doxycycline inhibits il17-stimulated mmp-9 expression by downregulating erk1/2 activation: Implications in myogenic differentiation. Mediators Inflammation. (2016) 2016:1–11. doi: 10.1155/2016/2939658
81. Sakabe J, Umayahara T, Hiroike M, Shimauchi T, Ito T, Tokura Y. Calcipotriol Increases hCAP18 mRNA Expression but Inhibits Extracellular LL37 Peptide Production in IL-17/IL-22-stimulated Normal Human Epidermal Keratinocytes. Acta Dermato Venereologica. (2014) 94:512–6. doi: 10.2340/00015555-1775
82. Peric M, Koglin S, Kim SM, Morizane S, Besch R, Prinz JC, et al. IL-17A enhances vitamin D3-induced expression of cathelicidin antimicrobial peptide in human keratinocytes. J Immunol. (2008) 181:8504–12. doi: 10.4049/jimmunol.181.12.8504
83. Minns D, Smith KJ, Alessandrini V, Hardisty G, Melrose L, Jackson-Jones L, et al. The neutrophil antimicrobial peptide cathelicidin promotes Th17 differentiation. Nat Commun. (2021) 12:1285. doi: 10.1038/s41467-021-21533-5
84. Sugaya M. The role of th17-related cytokines in atopic dermatitis. Int J Mol Sci. (2020) 21:1314. doi: 10.3390/ijms21041314
85. Casas C, Paul C, Lahfa M, Livideanu B, Lejeune O, Alvarez-Georges S, et al. Quantification of demodex folliculorum by pcr in rosacea and its relationship to skin innate immune activation. Exp Dermatol. (2012) 21:906–10. doi: 10.1111/exd.12030
86. Vogel DY, Glim JE, Stavenuiter AW, Breur M, Heijnen P, Amor S, et al. Human macrophage polarization in vitro: Maturation and activation methods compared. Immunobiology. (2014) 219:695–703. doi: 10.1016/j.imbio.2014.05.002
87. Boink MA, Roffel S, Nazmi K, Bolscher JG, Veerman EC, Gibbs S. Saliva-derived host defense peptides histatin1 and ll-37 increase secretion of antimicrobial skin and oral mucosa chemokine ccl20 in an il-1α-independent manner. J Immunol Res. (2017) 2017:3078194. doi: 10.1155/2017/3078194
88. Zhao Z, Liu T, Liang Y, Cui W, Li D, Zhang G, et al. N2-polarized neutrophils reduce inflammation in rosacea by regulating vascular factors and proliferation of CD4+ T cells. J Invest Dermatol. (2022) 142:1835–1844.e2. doi: 10.1016/j.jid.2021.12.009
89. Jiang Y, Huang Y, Ma G, Liu T, Li Q, Wu H, et al. Granulomatous rosacea in Chinese patients: Clinical-histopathological analysis and pathogenesis exploration. J Dermatol. (2023) 50:856–68. doi: 10.1111/1346-8138.16767
90. O’Reilly N, Bergin D, Reeves E, McElvaney N, Kavanagh K. Demodex-associated bacterial proteins induce neutrophil activation: Demodex-associated bacterial proteins induce neutrophil activation. Br J Dermatol. (2012) 166:753–60. doi: 10.1111/j.1365-2133.2011.10746.x
91. Liew PX, Kubes P. The neutrophil’s role during health and disease. Physiol Rev. (2019) 99:1223–48. doi: 10.1152/physrev.00012.2018
92. Lee HJ, Hong YJ, Kim M. Angiogenesis in chronic inflammatory skin disorders. Int J Mol Sci. (2021) 22:12035. doi: 10.3390/ijms222112035
93. Gomaa AHA, Yaar M, Eyada MMK, Bhawan J. Lymphangiogenesis and angiogenesis in nonphymatous rosacea. J Cutaneous Pathol. (2007) 34:748–53. doi: 10.1111/j.1600-0560.2006.00695.x
94. Smith JR, Lanier VB, Braziel RM, Falkenhagen KM, White C, Rosenbaum JT. Expression of vascular endothelial growth factor and its receptors in rosacea. Br J Ophthalmol. (2007) 91:226–9. doi: 10.1136/bjo.2006.101121
95. Kajiya K, Kajiya-Sawane M, Ono T, Sato K. Identification of an epidermal marker for reddened skin: Vascular endothelial growth factor A. J Dermatol. (2017) 44:836–7. doi: 10.1111/1346-8138.13665
96. Hayran Y, Lay I, Mocan MC, Bozduman T, Ersoy-Evans S. Vascular endothelial growth factor gene polymorphisms in patients with rosacea: A case-control study. J Am Acad Dermatol. (2019) 81:348–54. doi: 10.1016/j.jaad.2019.03.055
97. Li Y, Xie H, Deng Z, Wang B, Tang Y, Zhao Z, et al. Tranexamic acid ameliorates rosacea symptoms through regulating immune response and angiogenesis. Int Immunopharmacol. (2019) 67:326–34. doi: 10.1016/j.intimp.2018.12.031
98. Mor F, Quintana FJ, Cohen IR. Angiogenesis-inflammation cross-talk: vascular endothelial growth factor is secreted by activated T cells and induces th1 polarization. J Immunol. (2004) 172:4618–23. doi: 10.4049/jimmunol.172.7.4618
99. Chen Z, Zhang M, Liu Y, Chen Z, Wang L, Wang W, et al. VEGF-A enhances the cytotoxic function of CD4+ cytotoxic T cells via the VEGF-receptor 1/VEGF-receptor 2/AKT/mTOR pathway. J Trans Med. (2023) 21:74. doi: 10.1186/s12967-023-03926-w
100. Hirakawa S, Fujii S, Kajiya K, Yano K, Detmar M. Vascular endothelial growth factor promotes sensitivity to ultraviolet B–induced cutaneous photodamage. Blood. (2005) 105:2392–9. doi: 10.1182/blood-2004-06-2435
101. Koczulla R, Von Degenfeld G, Kupatt C, Krötz F, Zahler S, Gloe T, et al. An angiogenic role for the human peptide antibiotic LL-37/hCAP-18. J Clin Invest. (2003) 111:1665–72. doi: 10.1172/JCI17545
102. Peng Q, Sha K, Liu Y, Chen M, Xu S, Xie H, et al. mTORC1-mediated angiogenesis is required for the development of rosacea. Front Cell Dev Biol. (2021) 9:751785. doi: 10.3389/fcell.2021.751785
103. Gerber PA, Buhren BA, Steinhoff M, Homey B. Rosacea: the cytokine and chemokine network. J Invest Dermatol Symposium Proc. (2011) 15:40–7. doi: 10.1038/jidsymp.2011.9
104. Lee J, Jung Y, Jeong SW, Jeong GH, Moon GT, Kim M. Inhibition of hippo signaling improves skin lesions in a rosacea-like mouse model. Int J Mol Sci. (2021) 22:931. doi: 10.3390/ijms22020931
105. Kiu H, Nicholson SE. Biology and significance of the JAK/STAT signalling pathways. Growth Factors. (2012) 30:88–106. doi: 10.3109/08977194.2012.660936
106. Hu Q, Bian Q, Rong D, Wang L, Song J, Huang HS, et al. JAK/STAT pathway: Extracellular signals, diseases, immunity, and therapeutic regimens. Front Bioengineering Biotechnol. (2023) 11:1110765. doi: 10.3389/fbioe.2023.1110765
107. Liu YD, Yu L, Ying L, Balic J, Gao H, Deng NT, et al. Toll-like receptor 2 regulates metabolic reprogramming in gastric cancer via superoxide dismutase 2. Int J Cancer. (2019) 144:3056–69. doi: 10.1002/ijc.32060
108. Lv Y, Qi J, Babon JJ, Cao L, Fan G, Lang J, et al. The JAK-STAT pathway: from structural biology to cytokine engineering. Signal Transduct Target Ther. (2024) 9:221. doi: 10.1038/s41392-024-01934-w
109. Luu K, Greenhill CJ, Majoros A, Decker T, Jenkins BJ, Mansell A. STAT1 plays a role in TLR signal transduction and inflammatory responses. Immunol Cell Biol. (2014) 92:761–9. doi: 10.1038/icb.2014.51
110. Wang Y, Wang B, Huang Y, Li Y, Yan S, Xie H, et al. Multi-transcriptomic analysis and experimental validation implicate a central role of STAT3 in skin barrier dysfunction induced aggravation of rosacea. J Inflammation Res Volume. (2022) 15:2141–56. doi: 10.2147/JIR.S356551
111. Erlich TH, Yagil Z, Kay G, Peretz A, Migalovich-Sheikhet H, Tshori S, et al. Mitochondrial STAT3 plays a major role in IgE-antigen–mediated mast cell exocytosis. J Allergy Clin Immunol. (2014) 134:460–469.e10. doi: 10.1016/j.jaci.2013.12.1075
112. Bilotta S, Paruchuru LB, Feilhauer K, Köninger J, Lorentz A. Resveratrol is a natural inhibitor of human intestinal mast cell activation and phosphorylation of mitochondrial ERK1/2 and STAT3. Int J Mol Sci. (2021) 22:7640. doi: 10.3390/ijms22147640
113. Morales JK, Falanga YT, Depcrynski A, Fernando J, Ryan JJ. Mast cell homeostasis and the JAK–STAT pathway. Genes Immun. (2010) 11:599–608. doi: 10.1038/gene.2010.35
114. Blazanin N, Cheng T, Carbajal S, DiGiovanni J. Activation of a protumorigenic ifnγ/stat1/irf1 signaling pathway in keratinocytes following exposure to solar ultraviolet light. Mol Carcinogenesis. (2019) 58:1656–69. doi: 10.1002/mc.23073
115. Deng Z, Liu F, Chen M, Huang C, Xiao W, Gao S, et al. Keratinocyte-immune cell crosstalk in a STAT1-mediated pathway: novel insights into rosacea pathogenesis. Front Immunol. (2021) 12:674871. doi: 10.3389/fimmu.2021.674871
116. You T, Bi Y, Li J, Zhang M, Chen X, Zhang K, et al. IL-17 induces reactive astrocytes and upregulation of vascular endothelial growth factor (VEGF) through JAK/STAT signaling. Sci Rep. (2017) 7:41779. doi: 10.1038/srep41779
117. Hu B, Wang J, Wu X, Chen Y, Yuan W, Chen H. Interleukin-17 upregulates vascular endothelial growth factor by activating the JAK/STAT pathway in nucleus pulposus cells. Joint Bone Spine. (2017) 84:327–34. doi: 10.1016/j.jbspin.2016.05.014
118. Marek-Jozefowicz L, Nedoszytko B, Grochocka M, Żmijewski MA, Czajkowski R, Cubała WJ, et al. Molecular mechanisms of neurogenic inflammation of the skin. Int J Mol Sci. (2023) 24:5001. doi: 10.3390/ijms24055001
119. Choi JE, Di Nardo A. Skin neurogenic inflammation. Semin Immunopathology. (2018) 40:249–59. doi: 10.1007/s00281-018-0675-z
120. Zhang Y, Huang Y, Wang B, Shi W, Hu X, Wang Y, et al. Integrated omics reveal the molecular characterization and pathogenic mechanism of rosacea. J Invest Dermatol. (2024) 144:33–42.e2. doi: 10.1016/j.jid.2023.05.028
121. Xiao T, Sun M, Zhao C, Kang J. TRPV1: A promising therapeutic target for skin aging and inflammatory skin diseases. Front Pharmacol. (2023) 14:1037925. doi: 10.3389/fphar.2023.1037925
122. Kim HB, Na EY, Yun SJ, Lee JB. The effect of capsaicin on neuroinflammatory mediators of rosacea. Ann Dermatol. (2022) 34:261. doi: 10.5021/ad.21.223
123. Caterina MJ, Leffler A, Malmberg AB, Martin WJ, Trafton J, Petersen-Zeitz KR, et al. Impaired nociception and pain sensation in mice lacking the capsaicin receptor. Science. (2000) 288:306–13. doi: 10.1126/science.288.5464.306
124. Oh S, Son M, Park J, Kang D, Byun K. Radiofrequency irradiation modulates TRPV1-related burning sensation in rosacea. Molecules. (2021) 26:1424. doi: 10.3390/molecules26051424
125. Wienholtz NKF, Christensen CE, Coskun H, Zhang DG, Ghanizada H, Egeberg A, et al. Infusion of pituitary adenylate cyclase–activating polypeptide-38 in patients with rosacea induces flushing and facial edema that can be attenuated by sumatriptan. J Invest Dermatol. (2021) 141:1687–98. doi: 10.1016/j.jid.2021.02.002
126. Silence C, Kourosh A, Gilbert E. Placement of high dose neurotoxins for treatment-resistant rosacea. J Drugs Dermatol. (2023) 22:605–7. doi: 10.36849/JDD.7237
127. Dias M, Newton D, McLeod G, Belch J, Khan F. Vasoactive properties of calcitonin gene-related peptide in human skin. Int Angiology: J Int Union Angiology. (2011) 30:424–8.
128. Slominski AT, Slominski RM, Raman C, Chen JY, Athar M, Elmets C. Neuroendocrine signaling in the skin with a special focus on the epidermal neuropeptides. Am J Physiology-Cell Physiol. (2022) 323:C1757–76. doi: 10.1152/ajpcell.00147.2022
129. Schwab VD, Sulk M, Seeliger S, Nowak P, Aubert J, Mess C, et al. Neurovascular and neuroimmune aspects in the pathophysiology of rosacea. J Invest Dermatol Symposium Proc. (2011) 15:53–62. doi: 10.1038/jidsymp.2011.6
130. Liu T, Xiao W, Chen M, Mao R, Xu S, Peng Q, et al. Aberrant amino acid metabolism promotes neurovascular reactivity in rosacea. JCI Insight. (2022) 7:e161870. doi: 10.1172/jci.insight.161870
131. Assas BM, Pennock JI, Miyan JA. Calcitonin gene-related peptide is a key neurotransmitter in the neuro-immune axis. Front Neurosci. (2014) 8:23. doi: 10.3389/fnins.2014.00023
132. Powell FC, Corbally N, Powell D. Substance P and rosacea. J Am Acad Dermatol. (1993) 28:132–3. doi: 10.1016/S0190-9622(08)80863-8
133. Seeliger S, Buddenkotte J, Schmidt-Choudhury A, Rosignoli C, Shpacovitch V, Von Arnim U, et al. Pituitary adenylate cyclase activating polypeptide. Am J Pathol. (2010) 177:2563–75. doi: 10.2353/ajpath.2010.090941
134. Woo Y, Lim J, Cho D, Park H. Rosacea: molecular mechanisms and management of a chronic cutaneous inflammatory condition. Int J Mol Sci. (2016) 17:1562. doi: 10.3390/ijms17091562
135. Yadav M, Goetzl EJ. Vasoactive intestinal peptide–mediated th17 differentiation: an expanding spectrum of vasoactive intestinal peptide effects in immunity and autoimmunity. Ann New York Acad Sci. (2008) 1144:83–9. doi: 10.1196/annals.1418.020
136. Zhou X, Su Y, Wu S, Wang H, Jiang R, Jiang X. The temperature-sensitive receptors TRPV4 and TRPM8 have important roles in the pruritus of rosacea. J Dermatol Sci. (2022) 108:68–76. doi: 10.1016/j.jdermsci.2022.11.004
137. Sharma A, Kroumpouzos G, Kassir M, Galadari H, Goren A, Grabbe S, et al. Rosacea management: A comprehensive review. J Cosmetic Dermatol. (2022) 21:1895–904. doi: 10.1111/jocd.14816
138. Zhang Y, Zhou Y, Humbert P, Yuan D, Yuan C. Effect on the skin microbiota of oral minocycline for rosacea. Acta Dermato-Venereologica. (2023) 103:adv10331. doi: 10.2340/actadv.v103.10331
139. Coda AB, Hata T, Miller J, Audish D, Kotol P, Two A, et al. Cathelicidin, kallikrein 5, and serine protease activity is inhibited during treatment of rosacea with azelaic acid 15% gel. J Am Acad Dermatol. (2013) 69:570–7. doi: 10.1016/j.jaad.2013.05.019
140. Kanada KN, Nakatsuji T, Gallo RL. Doxycycline indirectly inhibits proteolytic activation of tryptic kallikrein-related peptidases and activation of cathelicidin. J Invest Dermatol. (2012) 132:1435–42. doi: 10.1038/jid.2012.14
141. Van Zuuren EJ, Fedorowicz Z. Low-dose isotretinoin: an option for difficult-to-treat papulopustular rosacea. J Invest Dermatol. (2016) 136:1081–3. doi: 10.1016/j.jid.2016.03.003
142. Lam-Franco L, Perfecto-Avalos Y, Patiño-Ramírez BE, Rodríguez García A. Il-1α and mmp-9 tear levels of patients with active ocular rosacea before and after treatment with systemic azithromycin or doxycycline. Ophthalmic Res. (2018) 60:109–14. doi: 10.1159/000489092
143. Zhang J, Jiang P, Sheng L, Liu Y, Liu Y, Li M, et al. A novel mechanism of carvedilol efficacy for rosacea treatment: toll-like receptor 2 inhibition in macrophages. Front Immunol. (2021) 12:609615. doi: 10.3389/fimmu.2021.609615
144. Two AM, Hata TR, Nakatsuji T, Coda AB, Kotol PF, Wu W, et al. Reduction in serine protease activity correlates with improved rosacea severity in a small, randomized pilot study of a topical serine protease inhibitor. J Invest Dermatol. (2014) 134:1143–5. doi: 10.1038/jid.2013.472
145. Kumar A, Chiou A, Shih Y, Li S, Chang A. An exploratory, open-label, investigator-initiated study of interleukin-17 blockade in patients with moderate-to-severe papulopustular rosacea. Br J Dermatol. (2020) 183:942–3. doi: 10.1111/bjd.19172
146. Deng Z, Xu S, Peng Q, Sha K, Xiao W, Liu T, et al. Aspirin alleviates skin inflammation and angiogenesis in rosacea. Int Immunopharmacol. (2021) 95:107558. doi: 10.1016/j.intimp.2021.107558
147. Cuevas P, Arrazola JM. Therapeutic response of rosacea to dobesilate. Eur J Med Res. (2005) 10:454–6.
Keywords: rosacea, pathogenesis, signaling pathways, targeted therapy, review
Citation: Yang F, Wang L, Song D, Zhang L, Wang X, Du D and Jiang X (2024) Signaling pathways and targeted therapy for rosacea. Front. Immunol. 15:1367994. doi: 10.3389/fimmu.2024.1367994
Received: 09 January 2024; Accepted: 22 August 2024;
Published: 16 September 2024.
Edited by:
Lesley Ann Bergmeier, Queen Mary University of London, United KingdomReviewed by:
Luana De Mendonça Oliveira, University of São Paulo, BrazilCraig Rohan, Wright State University, United States
Copyright © 2024 Yang, Wang, Song, Zhang, Wang, Du and Jiang. This is an open-access article distributed under the terms of the Creative Commons Attribution License (CC BY). The use, distribution or reproduction in other forums is permitted, provided the original author(s) and the copyright owner(s) are credited and that the original publication in this journal is cited, in accordance with accepted academic practice. No use, distribution or reproduction is permitted which does not comply with these terms.
*Correspondence: Xian Jiang, amlhbmd4aWFuQHNjdS5lZHUuY24=
†These authors have contributed equally to this work and share first authorship