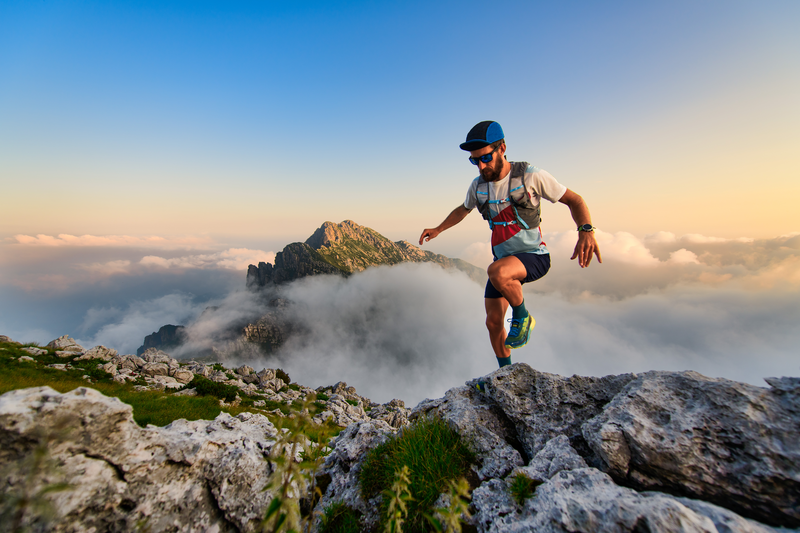
95% of researchers rate our articles as excellent or good
Learn more about the work of our research integrity team to safeguard the quality of each article we publish.
Find out more
REVIEW article
Front. Immunol. , 25 April 2024
Sec. Cancer Immunity and Immunotherapy
Volume 15 - 2024 | https://doi.org/10.3389/fimmu.2024.1384039
Chimeric antigen receptor-natural killer (CAR-NK) cell therapy is a novel immunotherapy targeting cancer cells via the generation of chimeric antigen receptors on NK cells which recognize specific cancer antigens. CAR-NK cell therapy is gaining attention nowadays owing to the ability of CAR-NK cells to release potent cytotoxicity against cancer cells without side effects such as cytokine release syndrome (CRS), neurotoxicity and graft-versus-host disease (GvHD). CAR-NK cells do not require antigen priming, thus enabling them to be used as “off-the-shelf” therapy. Nonetheless, CAR-NK cell therapy still possesses several challenges in eliminating cancer cells which reside in hypoxic and immunosuppressive tumor microenvironment. Therefore, this review is envisioned to explore the current advancements and limitations of CAR-NK cell therapy as well as discuss strategies to overcome the challenges faced by CAR-NK cell therapy. This review also aims to dissect the current status of clinical trials on CAR-NK cells and future recommendations for improving the effectiveness and safety of CAR-NK cell therapy.
Adoptive cell therapy (ACT) is a promising immunotherapy targeting cancer cells by transferring specialized or engineered immune cells which are endowed with tumor-killing ability into a cancer patient (1). Among current ACTs implemented, chimeric antigen receptor (CAR) therapy becomes increasingly significant as T cells engineered with CAR (CAR-T) have shown clinical success in hematological malignancies in clinical settings owing to their remarkable anticancer abilities. Nevertheless, CAR-T therapy possesses several limitations such as tumor antigen loss, on target off tumor side effects, cytokine release syndrome (CRS) due to overwhelmed cytokines released during CAR-T cell activation, immune effector cell-associated neurotoxicity syndrome (ICANS), difficulty in trafficking and infiltrating into solid tumor cells, and immunosuppressive tumor microenvironment (2, 3). During CAR-T cell manufacture, large T cell populations are heavily required, thus surging the cost, and lengthening the manufacturing process which further burdens cancer patients, leading to rapid late-stage cancer progression (3, 4). Moreover, graft-versus-host disease (GvHD) is a major concern when using allogenic CAR-T cell therapy due to the presence of foreign donor antigens on T cells will stimulate the patient’s immune system (5).
To resolve these obstacles, current research is moving towards the production of CAR-NK cells on account of NK cells are innate immune cells which are human leukocyte antigen (HLA)-unrestricted and able to kill infected or cancerous cells without the need of antigen priming (6). Therefore, they are safe to be used as “off-the-shelf” therapy as no GvHD cases are reported so far (7).[NO_PRINTED_FORM]. Moreover, CAR-NK cells do not cause cytokine release syndrome (CRS) and neurotoxicity when compared to CAR-T cells. Different cytokines are released by CAR-NK cells to prevent CRS from happening (8). Furthermore, the specificity of antibody-based CAR ectodomain targeting specific tumor antigen and shorter lifespan of CAR-NK cells, limit the effect of on target off tumor toxicity (9).
NK cells in the blood can be categorized into two main populations based on the expression of cell surface receptors: CD56bright CD16dim NK cells and CD56dim CD16bright NK cells (10). Theoretically, CD56bright CD16dim NK cells (5% of total NK cells) are cytokine-producing cells residing in secondary lymphoid organs while CD56dim CD16bright NK cells (95% of total NK cells) are cytotoxic cells circulating in peripheral blood (11). The activation of NK cells is regulated based on the equilibrium of ligand stimulations on both activating and inhibitory receptors of NK cells (12). The activating and inhibitory receptors of NK cells play a pivotal role in signaling and eliminating infected or cancer cells.
Naturally, NK cells eliminate cancer cells via various molecular mechanisms. The downregulation of major histocompatibility complex (MHC) in cancer cells often leads to the failure of CAR-T cell therapy. However, the loss of MHC in cancer cells reduces the inhibitory signal on NK cells. Therefore, NK cells can still be activated and able to target cancer cells via the missing-self mechanism despite MHC being shed from cancer cells (13). Moreover, the aberrant overexpression of ligands on cancer cells can activate NK cells through an induced-self mechanism when the overexpressed ligands are bound to the activating receptors of NK cells, causing the induction of cytolytic activity in cancer cells (13). Furthermore, NK cells can eradicate cancer cells by recognizing non-self-antigens presented by the MHC of cancer cells (14). In addition, NK cells can bind their CD16 (FcγRIIIA) to target cancer cells which opsonized with IgG through antibody-dependent cell-mediated cytotoxicity (ADCC) (15). NK cells can trigger apoptosis of cancer cells via the Fas/FasL signaling pathway or TNF-related apoptosis-inducing ligand (TRAIL) pathway (16). Overall, CAR-NK cells employ these NK cell killing mechanisms, thus making them more robust and superior compared to CAR-T cells.
Nonetheless, CAR-NK cell therapy faces several challenges such as lack of persistence in CAR-NK cells, inhibition of CAR-NK cells due to inhibitory receptors on NK cells, immunosuppressive tumor microenvironment, ineffective CAR-NK cell trafficking and infiltration, and immune escape. In this review, current advancements and limitations of CAR-NK cell therapy are explored to improve and leverage their ability to eradicate and kill cancer cells. Lastly, we conclude this review by discussing strategies to overcome challenges and future recommendations on CAR-NK cell therapy.
CAR-NK cells have been revolutionized over the years to explore the optimal CAR structure with enhanced cytotoxicity and better clinical responses for cancer patients. CAR-NK cells implement the concept of CAR-T cells, and thus develop into several generations. The first generation consists of an ectodomain, single chain fragment variable (scFv) linked with a transmembrane and CD3 endodomain. Nonetheless, the lack of a co-stimulating domain in the CAR structure leads to low proliferation of CAR-NK cells (12). Therefore, the second CAR generation tackles this problem by putting one co-stimulating domain such as DNAX activation protein (DAP) 10, DAP12, 2B4, and 4-1BB (CD137) into CAR structure (17). Future improvement has been done to enhance CAR structure through the addition of one more co-stimulating domain, thus generating the third CAR generation (17). To further improve the persistence and functionality of CAR-NK cells, the fourth generation of CAR is armored with cytokines such as IL-15 (18).
Optimizing CAR structure requires more novel strategies for CAR structural modification. Next generation of CAR-NK cells is the current trend to produce more effective and specific CAR, hoping to strengthen the tumor recognition ability of CAR-NK cells. Dual CAR NK cell which generates two independent receptors provides greater specificity in recognizing cancer cells. Cichocki et al. generated two independent anti-CD19 CAR and non-cleavable CD16 receptors on NK cells termed as iDuo NK cells offered higher specificity against CD19 cancer cells and prevented immune escape by targeting CD19-negative cancer cells (19). Cichocki et al. also showed that CD38 knockout CAR-NK cells which were transduced with dual targeting anti-BCMA CAR and CD16 possessed enhanced cytotoxicity and prolonged persistence against multiple myeloma cells (20). Recently, dual CAR-NK cells comprising anti-BCMA CAR and anti-GPRC5D demonstrated remarkable results in killing multiple myeloma cancer cells (21). To date, FT596, a muti-targeting CAR-NK cell which possesses anti-CD19 CAR, non-cleavable CD16 receptor, and IL15/IL-15α was developed to enhance cancer-killing ability and improve the persistence of CAR-NK cells (22). However, there are still few studies on synthesizing dual or multi-targeting CAR-NK cells to target cancer cells currently. Therefore, more preclinical studies should be done to explore dual or multi-targeting CAR-NK cells in eradicating solid tumors.
Bispecific CAR-NK cell is another advancement made for creating better CAR-NK cell immunotherapy. For instance, Kim et al. showed that bispecific anti-CD19-CD22 CAR-NK cells possessed anticancer ability to lyse B cell lymphoma effectively (23). Moreover, the synthetic Notch (synNotch) receptor which induces transcription factor upon antigen recognition is a promising approach to fine-tune the specificity of CAR-NK cells. NK cells transduced with synNotch receptor targeting GPC3 antigen of cancer cells were able to secrete IL-12 into the tumor microenvironment directly, thus aiding CAR-T cells to infiltrate into cancer cells (24). Furthermore, another approach is using inhibitory CAR to enhance CAR-NK cells in recognizing cancer cells without targeting healthy cells. For instance, a study of implementing inhibitory CAR on NK cells had successfully inhibited healthy NK cells which possessed trogocytic antigen from being killed by CAR-NK cells (25).
In addition, universal CAR shows a promising strategy to improve CAR-NK cell therapy. Mitwasi et al. developed universal CAR which could only be activated upon coupling with a specific target molecule, α-GD2 IgG4 to target GD2-positive cancer cells (26). Moreover, Kang et al. designed a universal modifiable cotinine-specific CAR which is bound to a cotinine conjugator to target multiple cancer antigens (27). Furthermore, the inducible caspase9 (iCasp9) suicide gene is used in on/off logic-gated CAR to switch off the killing mechanism of CAR-NK cells when needed (28).
There are various sources to collect NK cells for CAR-NK cell production. One of the methods is from peripheral blood. Specifically, NK cells can be obtained either from autologous or allogenic peripheral blood. Nevertheless, the limited amount of autologous or allogenic NK cells obtained from peripheral blood not only impedes NK cell expansion, but also possesses heterogenous issues and is sensitive to freeze-thaw cycles which eventually reduces the efficiency of transduction (29). Therefore, another approach such as using NK cell lines is preferred to overcome the disadvantages of primary NK cells from peripheral blood.
CAR-NK cells can be engineered from cell line such as NK-92 cells due to this cell line possesses high cytotoxicity, short cell doubling time, lack of inhibitory receptors, and is easier to be genetically modified compared to other cell lines (30, 31). However, NK-92 cells possess several disadvantages such as a lack of CD16 receptors to mediate ADCC, the requirement for IL-2 supply to proliferate, and the need for irradiation to overcome the risk of immune rejection (30). Therefore, modifying NK-92 cells is highly demanded to resolve the limitations.
Up to date, there are a lot of advancements made for NK-92 cells. For instance, NK-92 cells are modified to produce IL-2-expressing NK cell lines such as NK-92ci and NK-92mi cells (31, 32). To solve the issue of lacking CD16 receptor, high-affinity Fc receptor expressing NK-92 cells (haNK) are produced by transducing NK cells with a plasmid carrying high-affinity CD16 and IL-2 (31, 32). Furthermore, haNK cells can also be used to express CAR, thus generating other new cell lines such as targeted high-affinity NK-92 cells (t-haNK) and quadrocistronic targeted high-affinity NK-92 cells (qt-haNK) to broaden the use of NK cell lines in cancer immunotherapy (31).
Apart from NK cell lines, stem cells are used to derive CAR-NK cells due to their ability to be used as “off-the-shelf” therapy. NK cells derived from umbilical cord blood possess higher proliferation capacity and can be easily obtained or frozen compared to peripheral blood NK cells (33). Most importantly, cord blood NK cells have better homing ability and are less immunogenic due to the higher chemokine receptor expression on cord blood-derived NK cells and lesser T cells isolated from cord blood (33). Nevertheless, NK cells from cord blood are immature and express lower expression of granzyme B and activating receptors such as CD16 and DNAM-1 (34). Furthermore, the higher expression of inhibitory receptors on cord blood NK cells hinders their function to kill cancer cells effectively (34).
In another perspective, NK cells derived from induced pluripotent stem cells (iPSCs) are the current advancement made for optimizing CAR-NK cell production. Although iPSCs-derived NK cells possess tumorigenic properties and laborious transdifferentiating procedures, NK cells from iPSCs are still a promising source to produce CAR-NK cells as they are consistently homogenous, highly proliferative, and can be scaled up to produce an unlimited supply of NK cells (35). Furthermore, the risk of GvHD is low in iPSCs-derived NK cells (36). Therefore, NK cells derived from iPSCs can be used as “off-the-shelf” therapy.
Overall, CAR-NK cells can be produced from various sources, thus highlighting the importance of exploring more strategies to synthesize even better “off-the-shelf” CAR-NK cell therapy for cancer patients. Moreover, more studies on exploring other NK cell sources should be investigated for optimizing and standardizing CAR-NK cell production.
To deliver the CAR gene effectively into NK cells, various viral and non-viral methods have been implemented to produce CAR-NK cells. In fact, all methods possess pros and cons in delivering transgene. Therefore, it is hard to standardize the CAR gene delivery method and manufacture CAR-NK cells based on universal protocol. The pros and cons of respective gene delivery methods are tabulated in Table 1.
NK cells can be transduced by various viral and non-viral methods. One of the viral methods is using retrovirus. Although retrovirus is useful by integrating transgene into the host genome permanently, the risk of eliciting mutagenesis and only being able to transduce dividing NK cells limits the use of retrovirus in producing CAR-NK cells (37, 38). Therefore, other viral vectors such as lentiviral vectors are used to overcome the limitations of retroviral vectors. Lentivirus is commonly favored in delivering the CAR gene due to its ability to transduce dividing and non-dividing cells, permanently integrate transgene into the host genome, and low immunogenicity compared to other viral delivery methods (39). However, lentivirus may possess a risk of insertional mutation (39). Therefore, to ensure the safety of lentivirus in transgene delivery, second and third-generation lentiviral vectors are generated and widely used to produce CAR-NK cells. To date, one study on producing CAR-NK cells using second and third generations of lentiviral vectors showed no differences in transduction efficiency for both of them, thus highlighting both lentiviral vector generations were able to deliver CAR gene efficiency (42).
Apart from the viral method, recent studies on delivering the CAR gene via piggyBac transposons have been reported. Wang et al. used piggyBac transposons encoding NKG2D-DAP10 CAR to transfect NK cells against CD73-positive lung cancer cells (43). Wang et al. successfully demonstrated stable and sustained NKG2D expression in NK cells over the time of the study (43). Similarly, piggyBac transposon which co-expressed with IL-15 was used to generate CAR-NK cells targeting the NKG2D ligand of cancer cells (44). Therefore, these studies provide great insight into using non-viral piggyBac transposons to deliver the CAR gene which might offer safer clinical benefits for patients. Furthermore, non-viral vectors such as sleeping beauty (SB) can be used to transfect NK cells. Bexte et al. demonstrated that SB able to induce prolonged durability for CAR expression and robust cytotoxicity against acute lymphoblastic leukaemia (45). In fact, the use of piggyBac and SB transposons possess advantages such as large cargo size for transgene, low immunogenicity, and cheap manufacturing cost (40). Nonetheless, random transgene integration of piggyBac and SB transposons can lead to mutation or damage to the structure of the gene (41).
A new advancing method to deliver the CAR gene into NK cells is via clustered regularly interspaced short palindromic repeats and CRISPR-associated protein 9 (CRISPR-Cas9) technology. Using this technology, the CAR gene was carried by adeno-associated virus (AAV) and transfected into primary NK cells. The results showed that 68% of NK cells were successfully transfected to become CAR-NK cells (46, 47). CRISPR-Cas9 provides precise gene editing, but gene delivery efficiency still needs to be investigated (39).
NK cells are resistant to being transduced or transfected (37, 38). Therefore, studies have been performed to increase transduction or transfection efficiency for NK cells. Enhancing viral transduction can be achieved by alternating electrical changes on the NK cell membrane. The use of a polycationic agent, polybrene showed enhanced NK cell transduction (89.2%) when compared to other enhancers for transduction such as vectofusin 1 (70.9%) and retronectin (30.4%) (48). Similarly, Kim et al. proved polybrene improved NK cell transduction, however, they also demonstrated that high polybrene concentration and prolonged duration of polybrene exposed to NK cells could decrease NK cell viability (49).
Moreover, lentiviral transduction can be improved by upregulating low-density lipoprotein receptor (LDLR) of NK cells to bind with the vesicular stomatitis virus (VSV) of lentiviral particles carrying the CAR gene. Gong et al. demonstrated a statin known as rosuvastatin was able to upregulate LDLR of NK cells, thus promoting better CAR transduction via the increase of NK cell binding with VSV of lentiviral particles (50). Furthermore, Baboon envelope pseudotyped lentiviral vectors (BaEV-LVs) expressed higher CAR expression on NK cells compared to other pseudotyped lentiviral vectors, thus enhancing the killing effects of CAR-NK cells towards NK-resistant blood cancer cells (51). A similar result was also observed in the study done by Soldierer et al. They also explored different types of promoters in regulating CAR gene expression, suggesting that the myeloproliferative sarcoma virus (MPSV) promoter was better than other promoters used (52).
In addition, NK cell transduction can be enhanced by incorporating TBK1/IKKϵ inhibitor with VSV-pseudotyped lentiviral vector. TBK1/IKKϵ inhibitor was used to inhibit toll-like receptor (TLR) 4 antiviral signaling pathway, thus enhancing the binding of VSV receptor with NK cells and eventually enhancing viral transduction (53).
From another perspective, advancement to improve transfection efficiency for non-viral CAR delivery method is through synthesizing a novel polymer to deliver the CAR gene. For instance, oligomer such as cationic charge-altering releasable transporters (CARTs) was synthesized by Wilk et al. to bind with anti-CD19 mRNA of CAR before encapsulating this CART-anti-CD19 mRNA in a polyplex to transfect NK cells (54). The transfection efficiency shown by this CART-anti-CD19 mRNA was higher than commercial transfection agents such as lipofectamine, thus providing a new and novel strategy to increase the transfection efficiency of non-viral methods into NK cells.
To facilitate CAR gene transfection via non-viral methods, other strategy such as nanoparticles is used to enhance the transfection efficiency. Douka et al. showed that lipid nanoparticles were superior compared to polymeric nanoparticles and electroporation in delivering messenger RNA (mRNA) carrying a green fluorescent transgene and ultimately promoting higher transfection efficiency for NK cells and T cells (55). Recently, Golubovskaya et al. proved lipid nanoparticles harboring mRNA of the CAR gene were successfully transfected into NK cells and killed cancer cells in vitro and in vivo (56). Therefore, lipid nanoparticles can be used to improve the transfection efficiency of CAR-NK cells. Furthermore, magnetic nanoparticles coated with polymer also showed improved transfection efficiency towards NK cells (57). However, more studies on lipid or magnetic nanoparticles in enhancing the transfection efficiency of NK cells should be performed to produce effective CAR-NK cells from non-viral methods. The advancements of CAR-NK cell therapy and production are illustrated in Figure 1.
Figure 1 Advancements in CAR-NK cell therapy and production. CAR structure has been optimized to generate different generations of CAR as well as produce dual CAR-NK cells, bispecific CAR-NK cells, universal CAR-NK cells, and inhibitory CAR-NK cells. Moreover, exploring various new sources for NK cells such as umbilical cord blood and induced pluripotent stem cells (iPSCs) is also a new advancement made for CAR-NK cell therapy and production. Other approaches such as enhancing CAR gene delivery methods and improving CAR transduction or transfection efficiency are performed to improve CAR-NK cell production. Created with BioRender.com.
Although CAR-NK cell therapy shows promising potent anticancer results, it also possesses challenges which impair the efficacy of CAR-NK cells. One of the challenges is the lack of persistence in CAR-NK cells due to NK cells having shorter lifespans (3). Therefore, multiple rounds of CAR-NK cell administration may be required to maintain sufficient CAR-NK cells which have the potency to kill cancer cells in a patient’s body.
Moreover, NK cells express various inhibitory receptors such as killer cell immunoglobulin-like receptors (KIRs), NKG2A, PD-1, TIM-3, and TIGIT (58). Therefore, NK cells are easily inhibited and deactivated once their inhibitory receptors are bound with inhibitory ligands that are present in the tumor microenvironment.
Furthermore, CAR-NK cells are suppressed by hypoxic, nutrient-deficient, and immunosuppressive tumor microenvironment. The presence of myeloid-derived suppressor cells (MDSCs), cancer-associated fibroblasts (CAFs), regulatory T cells (Tregs), and tumor-associated macrophages (TAMs) suppress NK cell function, thus leading to inactivation of NK cells (59). NK cells are also suppressed by a nutrient-deficient environment due to a lack of glucose and amino acid supplies for them to survive (60). In addition, NK cells are hard to infiltrate into complex tumor microenvironment, thus leading to failure of CAR-NK cell therapy.
In another perspective, the loss of cancer antigens often leads to immune escape. One of the immune escape mechanisms is trogocytosis. Trogocytosis is an event involving the transfer of cancer antigens from cancer cells to NK cells, thus leading to antigen loss in cancer cells. NK cells which receive cancer antigens are targeted by CAR-NK cells (61). As a result, fratricide of NK cells occurs due to the NK cells present trogocytic antigen mediated by trogocytosis (61).
Additionally, NK cell exhaustion is also a main hurdle in CAR-NK cell therapy. The exhaustion of NK cells is mainly presented by several exhaustion phenotypes such as reduction in NK cell proliferation, activation, and cytotoxicity, downregulation of cytokines and activating receptors of NK cells, upregulation of immune checkpoints and inhibitory signals on NK cells, and dysregulation in NK cell metabolism (62). For instance, the upregulation of inhibitory receptors such as PD-1, TIGIT, TIM-3 on NK cells and increase in tumor-derived factors including prostaglandin E2 (PGE2), TGF-β, adenosine, and exosomes can eventually cause NK cell inhibition and exhaustion (62). Moreover, nutrient deprivation due to reduction in oxidative phosphorylation (OXPHOS) and glycolysis on NK cells can lead to NK cell exhaustion and cytotoxicity inhibition (63, 64). Furthermore, NK cells are exhausted by the hypoxic tumor microenvironment. Hypoxia will induce hypoxia-inducible factor 1-alpha (HIF-α) which can downregulate NK cell activating receptors and upregulate PD-L1 expression on immunosuppressive MDSCs, thus leading to NK cell inhibition (65, 66). In addition, lactate and lipid accumulation in tumor microenvironment as well as the presence of indoleamine 2,3-dioxygenase (IDO) show inhibition on NK cell proliferation, cytotoxicity release, and downregulation on NK cell activating receptors (67–69).
The activation, proliferation, and persistence of CAR-NK cells are the major barriers to sustaining and stimulating sufficient CAR-NK cells to target cancer cells in a patient’s body. One of the strategies is supplying cytokines to stimulate NK cells. Cytokine such as IL-2 provides robust stimulation on NK cells, but IL-2 also activates suppressive T regulatory cells (Treg) which in turn impede NK cells to eliminate cancer cells (70). Therefore, Bentebibel et al. tackled this problem by using a promising human recombinant IL-2, namely bempegaldesleukin (NKTR-214) to boost the activation and expansion of immune cells such as NK cells and CD8+ T cells with minimal stimulation on Treg cells during phase I human clinical trial on advanced or metastatic solid malignancies (71). To enhance anticancer effects, NKTR-214 was synergized with checkpoint inhibitors such as anti-programmed death protein-1 (PD-1) and anti-cytotoxic T-lymphocyte associated protein 4 (CTLA-4), resulting in a drastic reduction of intratumorally Treg cells and an increase in CD8+ T cell response without affecting Treg cells in peripheral blood (72). However, the synergic effects of NKTR-2214 with CAR-NK cell therapy still require further investigation.
Apart from using IL-2 to boost NK cells, IL15 is another cytokine that can improve NK cell activation and persistence. Christodoulou et al. demonstrated that anti-CD123-2B4-CD3 CAR-NK was able to increase NK cell persistence by revealing high proliferative and activated transcriptomic NK cell signatures and enhance anti-tumor ability against acute myeloid leukaemia (AML) in vitro and in vivo when co-expressed with secretory IL-15 (sIL-15) (73). However, this CAR-NK/sIL-15 should be continuously monitored as it possesses cytokine-related systemic toxicity (73). Moreover, NK-92 cell lines transduced with anti-CD19-IL15/IL-15Rα showed robust cytotoxicity against B cell malignancies in both vitro and vivo and continuously expanded without IL-2 for 21 days (74). Currently, only five clinical trials regarding CAR-NK immunotherapy with IL-15 are listed. However, three clinical trials focusing on cord blood NK cells (NCT06066424, NCT05922930, NCT05703854) and one clinical trial aiming to produce CAR-NK-T cell (NCT05487651) are still in recruiting phase. Nevertheless, one among five clinical trials has been withdrawn due to unknown reasons (NCT03579927).
In addition, IL-21 also shows promising NK cell activation, proliferation, and expansion. Initially, the K562-mIL21 feeder layer is implemented to culture NK cells. However, recent research shows other feeder layers can be used as they promote better expansion for NK cells. Preclinical data from Ojo et al. proved that NK cells cocultured with overexpressing membrane-bound IL-21 feeder layer established from OCI-AML3 cell lines were able to proliferate and expand with robust cytotoxicity against sarcoma and leukaemia in vitro and in vivo (75). IL-21 activates the STAT3/c-myc pathway in NK cells, thus increasing NK cell proliferation and metabolism (75). An alternative approach such as using an irradiated 221-mIL-21 feeder layer also showed superior advantages over to K562-mIL21 feeder layer owing to their ability to expand memory-like NK cells with less differentiated phenotypes (76). Moreover, human peripheral NK cells can be transduced with CAR-NK-IL21 to promote higher NK cell expansion. He et al. demonstrated that CAR-NK cells co-expressing IL-21 possessed higher expansion fold and produced greater cytotoxic IFN-γ and TNF-α release compared to CAR-NK cells with IL-15 co-expression against CD-19 lymphoma (77). Furthermore, the effort to improve the K562 feeder layer also provides great insight into enhancing NK cell expansion. Zhang et al. found that membrane-bound IL-21 transgene expression followed by co-expressing membrane-bound IL15/IL-15Rα on K562 feeder cells would enhance NK cell yield and anticancer properties (78). At present, there are no clinical trials on employing IL-21 in CAR-NK cell therapy.
Adopting IL-18 to stimulate NK cells is another strategy to increase NK cell persistence. By supplying IL-18 to NK cells, NK cells increased their proliferation and altered into antigen-presenting phenotype, thus mediating T cell responses to target lung cancer cells as shown by Senju et al. (79). Moreover, Gang et al. recently stimulated NK cells with IL-12, IL-15, and IL-18 to induce memory-like NK cells before transducing them into CAR-NK cells (80). Their work showed enhanced persistence and anticancer effect against AML which resists NK cell-based therapy (80). Similar results were also obtained when NK cells treated with the combination of IL-12, IL-15, and IL-18 were then used for manufacturing CAR-NK cells against CD19 of B cell cancers (81). As a result, cancer progression was suppressed along with prolonged survival of CD19 positive tumor-bearing mouse model (81). Nevertheless, none of the clinical trials show combinational therapy of various cytokines to enhance NK cell persistence for now.
Activating and inhibitory receptors of NK cells play an important role in augmenting the role of NK cells in cancer elimination. Li et al. showed that incorporation of activating receptors such as natural killer group 2 member D (NKG2D) in the transmembrane region of CAR structure and 2B4 as a co-stimulating domain was able to enhance the cytotoxicity of CAR-NK cells, thus demonstrating the highest efficacy to eradicate cancer cells compared to other CAR structures which did not use NKG2D (82). However, 2B4 cannot be used alone and should bind with CD3 for full CAR-NK cell activation (29). Parihar et al. also demonstrated that CAR-NK cells which incorporated NKG2D in their CD3 able to target NKG2D ligands on MDSCs without interfering NKG2D ligands on normal cells (83). Similarly, enhanced cytotoxicity and reduced cancer cell numbers were attained by fusing the activating receptor, NKG2D with DAP12 in CAR structure (84).
Furthermore, the CD16 activating receptor is another choice of interest to boost CAR-NK cell efficacy. Modification on activating receptors such as CD16 is needed to avoid CD16 from being cleaved by metalloproteinase, ADAM17 which is released by cancer cells (29). By implementing CRISPR technology, the ADAM17 gene was knocked out from CAR-NK cells and the results showed enhanced cytotoxicity in vitro and in vivo cancer models (85). Similarly, non-cleavable CD16a-hiPSC-NK cells created by Zhu et al. via mutation demonstrated improved survival for mouse-bearing lymphoma (86).
Reducing inhibitory signals on NK cells is another approach to increase the efficiency of CAR-NK cell therapy. A human immunoglobulin (Ig) G4 monoclonal antibody known as IPH2101 was implemented clinically to inhibit inhibitory signals on NK cells by targeting killer cell immunoglobulin-like receptors (KIRs) such as KIR2DL-1, KIR2DL-2, and KIR2DL-3 on NK cells in multiple myeloma patients (87). Conversely, IPH2101 shows unresponsive to NK cell activation due to the loss of KIR2D on NK cells from the trogocytosis event, thus leading to the termination of phase II clinical trial (88). Owing to this problem, a phase I clinical trial using another anti-KIR antibody, so-called IPH2102 (lirilumab) which is derived from IPH2101 was implemented to unlock NK cell inhibition without having dose-limiting toxicity (DLT) (89, 90). In addition, combinational therapy of IPH2102 with other therapeutic agents has been performed recently. For instance, IPH2102 was used to inhibit inhibitory signaling of NK cells by preventing inhibitory KIRs from binding to HLA-C of cancer cells, thus activating ADCC mechanisms to kill the cancer cells that had been marked with cetuximab during head and neck immunotherapy (91). IPH2102 was also coupled with other immune checkpoint inhibitors such as nivolumab and ipilimumab to synergize stronger anticancer effect towards advanced solid malignancies in a clinical trial (NCT01714739). Therefore, more studies regarding anti-KIR antibodies coupled with other therapies such as CAR-NK cells should be performed.
In addition, CD94 or NK group 2 member A receptor (NKG2A) is important in inhibiting NK cells by binding to its ligand, HLA-E on cancer cells. Monalizumab is used to target NKG2A, thus activating NK cells to exhibit cancer-killing ability (92). Moreover, monalizumab synergized with cetuximab to target head and neck squamous cell carcinoma, resulting in remarkable antic-cancer effects with a 31% response rate among patients in phase II clinical trials (93). Recently, monalizumab coupled with MEDI5752 which is a bispecific monoclonal antibody targeting checkpoint inhibitors such as PD-1 and CTLA-4 is a promising strategy to target highly immunogenic cancers in MONAMI clinical trial (NCT06152523). Nonetheless, the combination of monalizumab with trastuzumab did not achieve targeted clinical responses although the breast cancer respondents were free from DLT in the MIMOSA trial (NCT04307329), indicating that the low respondent number and scarcity of tumor infiltrating lymphocytes may inhibit anti-NKG2A therapy (94). Therefore, CAR-NK cell therapy coupled with anti-NKG2A antibodies is an urgent topic that requires further investigation to improve the overall survival of cancer patients.
NK cell engagers are another promising strategy to improve the efficacy of CAR-NK cell therapy. There are two types of NK cell engagers: BiKE (bispecific killer cell engager) and TriKE (trispecific killer cell engager) which bridge NK cells with cancer cells more effectively, thus enhancing the efficacy of NK cells to eliminate cancer cells. Various activating and inhibitory receptors on NK cells have been targeted by NK cell engagers to improve NK cells in cancer eradication, including CD16a, CD160, CD96, NKG2A, NKG2D, NKG2C, Nkp30, Nkp46, Nkp80, KIR2DS/KIR3DS, KIR2DL/KIR2DS, DNAM-1, 2B4, PD-1, IL-2/IL-15, TIGIT, and TIM-3 (95). Nonetheless, each receptor possesses pros and cons when targeted with NK cell engagers. Nikkhoi et al. showed BiKE targeting CD16 of NK cells and HER2 antigen on cancer cells was able to generate a high level of ADCC compared to conventional antibody, trastuzumab (96). Remarkable results of using BiKE are also shown in hematological malignancies (97). Moreover, TriKE armoring anti-CD16, anti-CD19, and IL-15 enhanced cancer cell lysis and cytotoxicity against CD19-positive tumors (98). In addition, anti-B7H3 CAR T cells synergized anticancer effects with BiKE which target CD16 of NK cells, thus providing attractive insight into the use of NK cell engagers with CAR-NK cell therapy (99). However, there is still lacking preclinical and clinical data on using NK cell engagers with CAR-NK cells. The idea of using NK cell engagers to bridge CAR-NK cells with other therapeutic agents such as antibodies may provide better cytotoxicity than BiKE or TriKE monotherapy, but this concept yet to be investigated.
Cancer, especially solid tumors, consists of heterogenous cell populations including immune cells which are suppressed and anergic in complex tumor microenvironments. This immunosuppressive tumor microenvironment is not only hypoxic, but it also possesses altered metabolism which supports the rapid proliferation of cancer cells.
To revitalize NK cells from the suppressive tumor microenvironment, SX-682 which is an inhibitor targeting chemokine receptor 1/2 (CXCR1/2) showed promising inhibition towards suppressive myeloid-derived suppressor cells (MDSCs), thus preventing them from infiltrating into tumor microenvironment of head and neck cancer and deactivating NK cells (100). However, the synergic effects of SX-682 with CAR-NK cell therapy should be further investigated as none of this study has been reported so far. Furthermore, cancer cells accumulate high levels of adenosine triphosphate (ATP) which in turn dephosphorylated into adenosine by CD73 of MDSCs can eventually inhibit NK cells (101). Chambers et al. transduced NK cells with anti-CD73 single chain fragment variable (scFv) CAR and successfully eliminated overexpressed CD73 lung cancer cells under hypoxic condition (101). Moreover, a drug such as nintedanib reduced IL-6 produced by cancer-associated fibroblasts (CAFs), thus enhancing the cytotoxicity of CAR-NK cells against mesothelin-positive cancer by increasing NK cell activating receptors (102).
In addition, inhibition of monocarboxylate transporter 4 (MCT4) which regulates lactate secretion and exchange led to the activation of cytotoxic NK cells to kill immunosuppressive breast cancer cells (103). In addition, high proliferative cancer cells use up the nutrients in the tumor microenvironment, leaving a nutrient-deficient hostile environment for immune cells, thus suppressing immune reactions. Therefore, Nachef et al. suggested engineering CAR-NK cells with amino acid transporters such as SLC1A5, SLC3A2, and SLC7A5 to improve amino acid uptake for CAR-NK cells and prevent them from being suppressed by nutrient-deficient tumor microenvironment (104). Nonetheless, there are no studies focusing on using CAR-NK cells directly to target MCT4 of cancer cells or enhancing CAR-NK cells with amino acids transporters currently. Furthermore, suppressive immune cells such as Tregs, tumor-associated macrophages (TAMs) and MDSCs secrete TGF-β to impede NK cell function. To tackle immunosuppressive TGF-β in the cancer microenvironment, Chaudhry et al. demonstrated that CAR-NK targeting B7H3 of glioblastoma enhanced tumor lysis even with the presence of immunosuppressive TGF-β when co-expressing this CAR-NK with TGF-β dominant negative receptor (DNR) (105). However, the in vivo model was not investigated during this study, thus more preclinical data are required to support the efficacy of CAR-NK/TGF-β DNR in targeting immunosuppressive cancers.
In addition, cancers which overexpress immune checkpoints such as PD-L1 possess poor prognosis and suppressive behavior towards NK cells in the tumor microenvironment. This could be targeted by incorporating anti-PD-L1 scFv directly into NK cells. Liu et al. proved that tumor regression in the humanized nasopharyngeal cancer (NPC) mouse model was achieved by designing CAR NK cells harboring anti-PD-L1 properties and synergized anticancer effects with a checkpoint inhibitor, nivolumab (106). Anti-PD-L1 CAR-NK was combined with anti-PD-1 and IL-15 superagonist, N-803 to boost NK cell ability in killing carcinoma in vivo while reducing T cell exhaustion and mediating T cell responses via IFN-γ release which is induced by IL-15 (107). Moreover, the combinatorial therapy of implementing anti-PD-L1 CAR-NK with anti-PD-1, pembrolizumab and N-803 is currently recruiting in the clinical trial (NCT04847466) to target refractory gastric and head and neck malignancies. From another perspectives, Lu et al. designed a chimeric costimulatory converting receptor (CCCR) in the structure of CAR to alter negative signals of PD-1 to activating signals, thus modulating, and hindering immunosuppressive tumor microenvironment via this novel CAR-NK cells (108). Furthermore, another immune checkpoint, B7H3 is a promising candidate to be targeted. Yang et al. demonstrated that anti-B7H3 CAR-NK cells increased granzyme and perforin secretion to lyse non-small lung cancer cells (109). In addition, immune checkpoint, HLA-G of cancer cells can be targeted by CAR-NK to inhibit immunosuppression. Jan et al. proved that CAR-NK cells targeting HLA-G were able to induce cytotoxicity against solid malignancies after pre-treating with chemotherapy (110). Cancer cells sensitized by chemotherapy highly expressed their HLA-G, thus promoting better activation and cancer antigen recognition for CAR-NK cells (110).
Proinflammatory cytokine such as IL-8 is produced by cancer cells during tumor development. Therefore, IL-8 becomes an attractive candidate to mediate the infiltration of CAR-NK cells into complex and immunosuppressive tumor microenvironment. By designing CXC motif chemokine receptor (CXCR) 1 with CAR construct in NK cells, these CXCR1/CAR-NK cells promoted effective trafficking and peritoneal infiltration into the ovarian cancer microenvironment (111). In hematological malignance, mRNA of CXCR4 and anti-B cell maturation antigen (BCMA) CAR were electroporated in NK cells to increase homing ability to bone marrow of multiple myeloma xenograft, thus enhancing cytotoxicity of CAR-NK cells and remarkably reducing tumor burden (112).
Chemokine receptor (CCR) is significant recruiting NK cells into the tumor microenvironment. Schomer et al. proved that CCR7-modified anti-CD19 CAR-NK cells effectively controlled the growth of CD19-positive lymphoma in mouse models by enhancing the infiltration of CAR-NK cells towards chemokine ligands, CCL9 which produced by lymphoma cells (113). Furthermore, chemokines such as CXCR2 also improved the trafficking and infiltration of NK cells into the tumor microenvironment of renal cancer, thus providing great insight into genetically engineering CXCR2 on CAR-NK cells (114). Nonetheless, more studies should be conducted to ensure the induced chemokine will not cause negative effects on cancer patients or promote tumorigenesis.
Cancer cells which shed their MHC class I chain-related protein A (MICA) and protein B (MICB) can exhibit immune escape properties and ultimately promote rapid cancer progression. Therefore, an antibody targeting MICA α3 was synthesized to prevent the proteolysis of MICA and MICB from cancer cells, thus allowing the binding of NK cell activating receptor, NKG2D with MICA/B to activate cytotoxic NK cells (115). There are also various approaches to prevent immune escape by improving antigen recognition by CAR-NK cells. As mentioned, iDuo NK cells targeting anti-CD19 and CD20 improved NK cell recognition, thus preventing immune escape (19). Similarly, dual CAR-NK cells targeting BCMA and GPRC5D also prevented immune escape for multiple myeloma cells which downregulates BCMA antigen (21).
Moreover, fratricide of NK cells which is induced by trogocytosis event becomes a major challenge for CAR-NK cell therapy as it will promote immune escape and eventually lead to cancer relapse. To resolve this issue, Li et al. developed a dual CARs system which comprised of a CAR to target cancer antigen and an inhibitory CAR to send inhibitory signals to inhibit CAR-NK cells from targeting NK cells which present trogocytic antigen (TROG) due to trogocytosis event (25). Therefore, fratricide of NK cells can be prevented by using dual CAR-NK cells and eventually prevent cancer cells from immune escape. Apart from developing dual CAR-NK cells, the use of latrunculin A (an actin inhibitor) can prevent trogocytosis since actin is important for the exchange of cancer antigens from the cancer cell surface onto NK cells (116). Moreover, the upregulation of cholesterol 25-hydroxylase (CH25H) inhibits lipid membranes from fusing. Therefore, it can be targeted by SUMOylation inhibitors such as TAK981 to prevent trogocytosis (116). Furthermore, Lu et al. proved that co-expressing CH25H in CAR structure successfully inhibited trogocytosis and improved the tumoricidal ability of CAR-T cells (117). Therefore, it will be very attracting to leverage the combinational effect of armoring CH25H in CAR-NK cells to prevent trogocytosis while exploring their synergic role in cancer elimination. In addition, immune escape could be reduced when the CD38 gene from NK cells was knocked out to reduce fratricide before transducing the CD38 knockout NK cells with anti-CD38 CAR to target CD38 of AML (118). Similarly, CD38 knockout dual CAR-NK cells targeting BCMA antigen were able to prevent trogocytosis and maintained high cytotoxicity against multiple myeloma cells (20). The strategies to overcome challenges in CAR-NK cell therapy are illustrated in Figure 2.
Figure 2 Strategies to overcome challenges in CAR-NK cell therapy. In summary, there are five major strategies which can be implemented to enhance CAR-NK cell efficacy in recognizing and killing cancer cells precisely. The five strategies include cytokine armoring, NK cell receptor modification, inhibition of immunosuppressive tumor microenvironment, improvement in CAR NK infiltration and trafficking, and immune escape prevention. Created with BioRender.com.
NK cell exhaustion can be reduced by targeting inhibitory signals induced by KIRs, PD-1, TIGIT, NKG2A, and TIM-3 of NK cells (62, 119). Moreover, various alkylating agents, proteasome inhibitors, histone deacetylation inhibitors, hyperploidy-inducing agents, and glycogen synthetase kinase-3 can be implemented to upregulate the activating receptor, NKG2D on NK cells (120). Furthermore, the activation, proliferation, and persistence of NK cells or CAR-NK cells can be boosted by supplementing various cytokines such as IL-2, IL-12 and IL-18 as mentioned previously in the section 5.1 to decrease NK cell exhaustion. However, there are still lack of studies on NK cell exhaustion on CAR-NK cell therapy. This may be due to CAR-NK cell therapy is still considered as a new promising therapy which requires more on-going preclinical and clinical data to evaluate the effectiveness and persistence of CAR-NK cells in long term studies.
To date, there are studies on the potential causes and strategies to overcome CAR-T exhaustion which can serve as an insight for CAR-NK cell therapy. CAR-T cells experience exhaustion probably due to the induction tonic signaling which continues stimulating T cells to respond and activate even without any antigen stimulation (121). Moreover, the structure of CAR-T influences T cell exhaustion. As shown by Saren et al, the different amino acid sequences in complementary determining region (CDR) could result the clustering of CAR structure, thus leading to antigen-independent tonic signaling in CAR-T cells (122). Conversely, Long et al. proved that the cause of T cell exhaustion in self-activating GD2.28z CAR-T cells was due to the clustering of framework region (FR) instead of CDR regions (123). Therefore, it is still unclear to conclude the main reason of CAR-T cell exhaustion from its structure.
CAR-T cell exhaustion is associated with upregulation of inhibitory signals. Therefore, various strategies targeting immune checkpoints or inhibitory receptors such as PD-1, CTLA-4, TIM-3, TIGIT, and LAG-3 of T cells have been extensively studied to remove T cell exhaustion (124, 125). Moreover, epigenetic activation of c-Jun axis, knockdown of transcription factors such as T-bet and Eomes, and downregulation of TOX/NR4A expression can be used to reduce T cell exhaustion (124). Similarly, cytokines can be supplied to activate and maintain T cell persistence (121). Nevertheless, there are less studies on the phenotypes and exhaustion gene profiles of CAR-NK cells, thus this part remains unknown and yet to discover.
To date, there are 49 clinical trials on CAR-NK cell therapy listed in ClinicalTrials.gov. The details for each clinical trial are tabulated in Supplementary Table 1. Among the clinical trials listed, 36 clinical trials focus on CAR-NK cells in treating hematological malignancies while only 13 CAR-NK clinical trials target solid malignancies. Therefore, more clinical studies should be conducted on solid tumors to evaluate the efficacy of CA-NK cells on different solid malignancies. Moreover, the safety of cancer patients after treating with CAR-NK cell therapy is the utmost important clinical assessment which requires prolonged monitoring.
Moreover, most NK cell sources are from allogenic NK cells although not mentioned in clinical trials. There are other sources of NK cells used such as NK-92 cell line and its derivatives, umbilical cord blood, patient peripheral blood, and induced pluripotent stem cells (iPSCs). Currently, most of the CAR-NK clinical trials are still recruiting, thus highlighting this CAR-NK cell therapy is a new promising immunotherapy to target various cancers.
In future, radiotherapy can combine with CAR-NK cell therapy to provide effective treatment for cancer patients. He et al. proposed that using radiotherapy before CAR-NK cell therapy could be a promising strategy to increase the trafficking, infiltration, and recognition of NK cells in tumors (126). However, more studies are required to seek out the optimal dose, duration, and sequence of combinational therapy, as well as to overcome immunosuppression mediated by radiotherapy (126). Moreover, chemotherapy can be used prior to CAR-NK cell therapy to increase cancer antigens released from tumor lysis, thus improving antigen recognition and activation of CAR-NK cells in tumor (127). Chemotherapy can also enhance CAR-NK cell therapy by killing suppressive immune cells such as MDSCs and Tregs in the tumor microenvironment (127). Furthermore, the combination of CAR-NK cell therapy with oncolytic virus also offers better anticancer results. For instance, anti-EFGR CAR-NK cells synergized with oncolytic virus expressing IL-15/IL15Rα sushi domain fusion protein enhanced the persistence and infiltration of CAR-NK cells and suppressed the growth of glioblastoma in vitro and in vivo (128).
In another perspective, drug-conjugated nanoparticle shows remarkable tumoricidal effects towards cancer cells. To explore the possible anticancer effects of combining drug-conjugated nanoparticles with cell-based therapy, CAR-NK cells were conjugated with cross-linked multilamellar liposomal vesicles (cMLVs) carrying chemotherapy agent, paclitaxel (PTX) to target cancer cells (129). This combinational therapy showed enhanced PTX drug delivery to cancer cells via CAR-NK cells as drug carriers and successfully eradicated cancer cells, thus highlighting the synergistic effects of nanoparticles with CAR-NK cell therapy (129).
There are also more efforts combine CAR-NK cells with monoclonal antibodies, especially immune checkpoint inhibitors. One of the examples is using PD-L1 monoclonal antibody to treat prostate cancer together with CAR-NK cells targeting prostate-specific membrane antigen (PSMA) (130). Searching for other possible combinational therapies is important to strengthen the efficacy of CAR-NK therapy against cancer cells. An approach of combining CAR-NK cells targeting epithelial cell adhesion molecule (EpCAM) with tyrosine inhibitor, regorafenib was able to boost the efficacy of CAR-NK cells against colorectal cancer (131). Moreover, romidepsin which inhibits histone deacetylase was synergized with anti-CD20 CAR-NK cells to inhibit rituximab-resistant Burkitt lymphoma with enhanced cytotoxicity induced (132). Interestingly, romidepsin increased the expression of MICA/B on lymphoma cells to activate CAR-NK cells via NKG2D activating receptor (132).
To improve the efficacy of CAR-NK cell therapy, the idea of combining CAR-NK cells with CAR-T cells has been proposed. This new concept was demonstrated by Li et al. in which CAR-T cells improved the proliferation of CAR-NK cells while CAR-NK cells reduced the risk of CRS (133). In combination, both CAR-T and CAR-NK cells showed inhibition towards cancer progression and relapse (133). Nonetheless, the side effects of implementing both CAR-T and CAR-NK therapy are still unknown for clinical study, thus this combinational therapy is still yet to be explored more.
CAR-NK cell therapy is considered a new paradigm shift for cancer immunotherapy. Future efforts on optimizing robust and tumor-specific CAR structure should be conducted actively to overcome the challenges of CAR-NK cell therapy. Moreover, combining CAR-NK cells with other therapeutic agents should be continuously explored. Currently, studies on vaccines with CAR-T cells have boosted the efficacy of CAR-T cells in eradicating cancer cells (134). Therefore, this combination provides a promising insight to implement this strategy on CAR-NK cells. Moreover, more clinical data are required to ensure the safety profile of CAR-NK cells in cancer patients.
The safety and quality of CAR-NK cell products require further monitoring and assessments. Therefore, the FDA has released guidelines to facilitate the production of CAR products so that the products will adhere to Good Manufacturing Practices (GMP). To summarize, the FDA has recommended several guidelines regarding general CAR design and development, chemistry, manufacturing, and control (CMC) of CAR products, as well as clinical and non-clinical recommendations. All the guidelines are tabulated in Table 2.
In conclusion, CAR-NK cell therapy is a new promising immunotherapy to target cancer cells due to it possesses robust cytotoxicity against cancer cells without eliciting side effects such as GvHD, CRS and neurotoxicity which are present in CAR-T cell therapy. Advancements have been made to create better CAR-NK therapy by either enhancing CAR structure or improving CAR-NK production. Enhancing CAR structure can be performed by revolutionizing the generation of CAR and increasing antigen recognition of CAR structure. While improvements on CAR-NK production can be done by exploring various NK cell sources, improving CAR gene delivery system, and enhancing CAR transduction or transfection into NK cells.
However, CAR-NK cells therapy still possesses some obstacles such as lack of persistence in NK cells, ineffective CAR-NK cell therapy, immunosuppressive tumor microenvironment, ineffective CAR-NK cell trafficking and infiltration, and immune escape. To resolve these limitations, various strategies are implemented to target the challenges respectively. Armoring cytokines to boost NK cell persistence, targeting NK cell receptors to improve efficacy of CAR-NK cells, engaging CAR-NK cell with NK cell engagers, targeting immunosuppressive tumor microenvironment to revitalize CAR-NK cells, supplying chemokine to improve CAR-NK cell infiltration, and reducing trogocytosis to prevent immune escape are the strategies used to overcome challenges of CAR-NK cell therapy. Furthermore, combinational therapy for CAR-NK cells with other therapeutic agents is the future direction of adoptive cell-based immunotherapy. Overall, clinical trials of CAR-NK therapy are moving towards solid tumor targeting. Thus, more studies should be performed to generate better and safer CAR-NK cell therapy. CAR-NK cell therapy can be tailored to become “off-the-shelf” personalized medicine which can accommodate to treat different cancers in future. In addition, FDA guidelines on CAR-NK cells should be implemented to ensure the safety and quality of CAR-based therapy.
JK: Conceptualization, Writing – original draft, Writing – review & editing. MS: Writing – review & editing. HV: Writing – review & editing. MR: Writing – review & editing. VB: Writing – review & editing. ST: Funding acquisition, Supervision, Writing – review & editing. GT: Conceptualization, Funding acquisition, Supervision, Writing – review & editing.
The author(s) declare financial support was received for the research, authorship, and/or publication of this article. The review was funded by the Ministry of Higher Education Malaysia for via the Higher Education Center of Excellence (HICoE) grant 311/CIPPM/4401005 and ALPS Global Holding, Kuala Lumpur.
MS and HV were employed by Celestialab Sdn Bhd, MR was employed by MyGenome, ALPS Global Holding, and ST was employed by ALPS Medical Centre, ALPS Global Holding.
The remaining authors declare that the research was conducted in the absence of any commercial or financial relationships that could be construed as a potential conflict of interest.
The authors declare that this study received funding from ALPS Global Holding. The authors associated to this entity where responsible for the decision to publish and preparation of the manuscript.
All claims expressed in this article are solely those of the authors and do not necessarily represent those of their affiliated organizations, or those of the publisher, the editors and the reviewers. Any product that may be evaluated in this article, or claim that may be made by its manufacturer, is not guaranteed or endorsed by the publisher.
The Supplementary Material for this article can be found online at: https://www.frontiersin.org/articles/10.3389/fimmu.2024.1384039/full#supplementary-material
1. Zhang P, Zhang G, Wan X. Challenges and new technologies in adoptive cell therapy. J Hematol Oncol. (2023) 16:1–55. doi: 10.1186/s13045-023-01492-8
2. Sterner RC, Sterner RM. CAR-T cell therapy: current limitations and potential strategies. Blood Cancer J. (2021) 11:1–11. doi: 10.1038/s41408-021-00459-7
3. Pan K, Farrukh H, Chittepu VCSR, Xu H, Pan CX, Zhu Z. CAR race to cancer immunotherapy: from CAR T, CAR NK to CAR macrophage therapy. J Exp Clin Cancer Res. (2022) 41:1–21. doi: 10.1186/s13046-022-02327-z
4. Laskowski TJ, Biederstädt A, Rezvani K. Natural killer cells in antitumour adoptive cell immunotherapy. Nat Rev Cancer. (2022) 22:557–75. doi: 10.1038/s41568-022-00491-0
5. Sanber K, Savani B, Jain T. Graft-versus-host disease risk after chimeric antigen receptor T-cell therapy: the diametric opposition of T cells. Br J Haematol. (2021) 195:660–8. doi: 10.1111/bjh.17544
6. Chan LY, Dass SA, Tye GJ, Imran SAM, Wan Kamarul Zaman WS, Nordin F. CAR-T cells/-NK cells in cancer immunotherapy and the potential of MSC to enhance its efficacy: A review. Biomedicines. (2022) 10:804. doi: 10.3390/biomedicines10040804
7. Lu H, Zhao X, Li Z, Hu Y, Wang H. From CAR-T cells to CAR-NK cells: A developing immunotherapy method for hematological Malignancies. Front Oncol. (2021) 11:720501. doi: 10.3389/fonc.2021.720501
8. Zhang X, Guo Y, Ji Y, Gao Y, Zhang M, Liu Y, et al. Cytokine release syndrome after modified CAR-NK therapy in an advanced non-small cell lung cancer patient: A case report. Cell Transplant. (2022) 31. doi: 10.1177/09636897221094244
9. Valeri A, García-Ortiz A, Castellano E, Córdoba L, Maroto-Martín E, Encinas J, et al. Overcoming tumor resistance mechanisms in CAR-NK cell therapy. Front Immunol. (2022) 13:953849. doi: 10.3389/fimmu.2022.953849
10. Poli A, Michel T, Thérésine M, Andrès E, Hentges F, Zimmer J. CD56bright natural killer (NK) cells: an important NK cell subset. Immunology. (2009) 126:458. doi: 10.1111/j.1365-2567.2008.03027.x
11. Ran GH, Lin YQ, Tian L, Zhang T, Yan DM, Yu JH, et al. Natural killer cell homing and trafficking in tissues and tumors: from biology to application. Signal Transduct Target Ther. (2022) 7:1–21. doi: 10.1038/s41392-022-01058-z
12. Khawar MB, Sun H. CAR-NK cells: from natural basis to design for kill. Front Immunol. (2021) 12:707542. doi: 10.3389/fimmu.2021.707542
13. Cruz-Muñoz ME, Valenzuela-Vázquez L, Sánchez-Herrera J, Santa-Olalla Tapia J. From the “missing self” hypothesis to adaptive NK cells: Insights of NK cell-mediated effector functions in immune surveillance. J Leukoc Biol. (2019) 105:955–71. doi: 10.1002/JLB.MR0618-224RR
14. Chu J, Gao F, Yan M, Zhao S, Yan Z, Shi B, et al. Natural killer cells: a promising immunotherapy for cancer. J Trans Med. (2022) 20:1–19. doi: 10.1186/s12967-022-03437-0
15. Nigro C, Macagno M, Sangiolo D, Bertolaccini L, Aglietta M, Merlano MC. NK-mediated antibody-dependent cell-mediated cytotoxicity in solid tumors: biological evidence and clinical perspectives. Ann Transl Med. (2019) 7:105–5. doi: 10.21037/atm.2019.01.42
16. Ramírez-Labrada A, Pesini C, Santiago L, Hidalgo S, Calvo-Pérez A, Oñate C, et al. All about (NK cell-mediated) death in two acts and an unexpected encore: initiation, execution and activation of adaptive immunity. Front Immunol. (2022) 13:896228. doi: 10.3389/fimmu.2022.896228
17. Elahi R, Heidary AH, Hadiloo K, Esmaeilzadeh A. Chimeric antigen receptor-engineered natural killer (CAR NK) cells in cancer treatment; recent advances and future prospects. Stem Cell Rev Rep. (2021) 17:2081–106. doi: 10.1007/s12015-021-10246-3
18. Biederstädt A, Rezvani K. Engineering the next generation of CAR-NK immunotherapies. Int J Hematol. (2021) 114:554–71. doi: 10.1007/s12185-021-03209-4
19. Cichocki F, Goodridge JP, Bjordahl R, Mahmood S, Davis ZB, Gaidarova S, et al. Dual antigen–targeted off-the-shelf NK cells show durable response and prevent antigen escape in lymphoma and leukemia. Blood. (2022) 140:2451–62. doi: 10.1182/blood.2021015184
20. Cichocki F, Bjordahl R, Goodridge JP, Mahmood S, Gaidarova S, Abujarour R, et al. Quadruple gene-engineered natural killer cells enable multi-antigen targeting for durable antitumor activity against multiple myeloma. Nat Commun. (2022) 13:1–15. doi: 10.1038/s41467-022-35127-2
21. Yang C, Wang Y, Liu T, Wang C, Wang H, Wang Q, et al. (2023). Dual-targeted CAR-NK cell therapy: optimized CAR design to prevent antigen escape and elicit a deep and durable response in multiple myeloma, in: Proceedings of the American Association for Cancer Research Annual Meeting 2023; Part 1 (Regular and Invited Abstracts), Orlando, FL. Philadelphia (PA, 2023 Apr 14-19, Vol. 83. AACR; Cancer Res, Abstract nr 4077.
22. Goodridge JP, Mahmood S, Zhu H, Gaidarova S, Blum R, Bjordahl R, et al. FT596: translation of first-of-kind multi-antigen targeted off-the-shelf CAR-NK cell with engineered persistence for the treatment of B cell Malignancies. Blood. (2019) 134:301. doi: 10.1182/blood-2019-129319
23. Hyori K, Mina H, Minsong K, Hyeri K, Ho JI, Nayoung K, et al. CD19/CD22 bispecific chimeric antigen receptor-NK-92 cells are developed and evaluated. Oncol lett. (2023) 25:236. doi: 10.3892/ol.2023.13822
24. Luo H, Wu X, Sun R, Su J, Wang Y, Dong Y, et al. Target-dependent expression of IL12 by synNotch receptor-engineered NK92 cells increases the antitumor activities of CAR-T cells. Front Oncol. (2019) 9:1448. doi: 10.3389/fonc.2019.01448
25. Li Y, Basar R, Wang G, Liu E, Moyes JS, Li L, et al. KIR-based inhibitory CARs overcome CAR-NK cell trogocytosis-mediated fratricide and tumor escape. Nat Med. (2022) 28:2133. doi: 10.1038/s41591-022-02003-x
26. Mitwasi N, Feldmann A, Arndt C, Koristka S, Berndt N, Jureczek J, et al. “UniCAR”-modified off-the-shelf NK-92 cells for targeting of GD2-expressing tumour cells. Sci Rep. (2020) 10:1–16. doi: 10.1038/s41598-020-59082-4
27. Kang HY, Lee SY, Kim HM, Lee SU, Lee H, Cho MY, et al. A modifiable universal cotinine-chimeric antigen system of NK cells with multiple targets. Front Immunol. (2023) 13:1089369. doi: 10.3389/fimmu.2022.1089369
28. Martín EM, Encinas J, García-Ortiz A, Castellano E, Ugalde L, Alonso R, et al. Exploring a safety switch in NKG2D and BCMA CAR NK-92MI immunotherapy. Clin Lymph Myeloma Leuk. (2021) 21:S42–3. doi: 10.1016/S2152-2650(21)02141-8
29. Schmidt D, Ebrahimabadi S, Gomes KRDS, De Moura Aguiar G, Cariati Tirapelle M, Nacasaki Silvestre R, et al. Engineering CAR-NK cells: how to tune innate killer cells for cancer immunotherapy. Immunother Adv. (2022) 2. doi: 10.1093/immadv/ltac003
30. Zhang J, Zheng H, Diao Y. Natural killer cells and current applications of chimeric antigen receptor-modified NK-92 cells in tumor immunotherapy. Int J Mol Sci. (2019) 20. doi: 10.3390/ijms20020317
31. Klingemann H. The NK-92 cell line—30 years later: its impact on natural killer cell research and treatment of cancer. Cytotherapy. (2023) 25:451–7. doi: 10.1016/j.jcyt.2022.12.003
32. Hu Z. Tissue factor as a new target for CAR-NK cell immunotherapy of triple-negative breast cancer. Sci Rep. (2020) 10:1–13. doi: 10.1038/s41598-020-59736-3
33. Zhao X, Cai L, Hu Y, Wang H. Cord-blood natural killer cell-based immunotherapy for cancer. Front Immunol. (2020) 11:584099. doi: 10.3389/fimmu.2020.584099
34. Damele L, Spaggiari GM, Parodi M, Mingari MC, Vitale M, Vitale C. Cord blood-derived natural killer cell exploitation in immunotherapy protocols: more than a promise? Cancers. (2022) 14:4439. doi: 10.3390/cancers14184439
35. Cichocki F, van der Stegen SJC, Miller JS. Engineered and banked iPSCs for advanced NK- and T-cell immunotherapies. Blood. (2023) 141:846. doi: 10.1182/blood.2022016205
36. Lu SJ, Feng Q. CAR-NK cells from engineered pluripotent stem cells: Off-the-shelf therapeutics for all patients. Stem Cells Transl Med. (2021) 10:S10. doi: 10.1002/sctm.21-0135
37. Schmidt P, Raftery MJ, Pecher G. Engineering NK cells for CAR therapy—Recent advances in gene transfer methodology. Front Immunol. (2020) 11:611163. doi: 10.3389/fimmu.2020.611163
38. Anson DS. The use of retroviral vectors for gene therapy-what are the risks? A review of retroviral pathogenesis and its relevance to retroviral vector-mediated gene delivery. Genet Vaccines Ther. (2004) 2:9. doi: 10.1186/1479-0556-2-9
39. Gong Y, Klein Wolterink RGJ, Wang J, Bos GMJ, Germeraad WTV. Chimeric antigen receptor natural killer (CAR-NK) cell design and engineering for cancer therapy. J Hematol Oncol. (2021) 14:1–35. doi: 10.1186/s13045-021-01083-5
40. Saha S, Woodard LE, Charron EM, Welch RC, Rooney CM, Wilson MH. Evaluating the potential for undesired genomic effects of the piggyBac transposon system in human cells. Nucleic Acids Res. (2015) 43:1770. doi: 10.1093/nar/gkv017
41. Wei M, Mi CL, Jing CQ, Wang TY. Progress of transposon vector system for production of recombinant therapeutic proteins in mammalian cells. Front Bioeng Biotechnol. (2022) 10:879222. doi: 10.3389/fbioe.2022.879222
42. Allan DSJ, Chakraborty M, Waller GC, Hochman MJ, Poolcharoen A, Reger RN, et al. Systematic improvements in lentiviral transduction of primary human natural killer cells undergoing ex vivo expansion. Mol Ther Methods Clin Dev. (2021) 20:559–71. doi: 10.1016/j.omtm.2021.01.008
43. Wang J, Lupo KB, Chambers AM, Matosevic S. Purinergic targeting enhances immunotherapy of CD73+ solid tumors with piggyBac-engineered chimeric antigen receptor natural killer cells. J Immunother Cancer. (2018) 6. doi: 10.1186/s40425-018-0441-8
44. Du Z, Ng YY, Zha S, Wang S. PiggyBac system to co-express NKG2D CAR and IL-15 to augment the in vivo persistence and anti-AML activity of human peripheral blood NK cells. Mol Ther Methods Clin Dev. (2021) 23:582–96. doi: 10.1016/j.omtm.2021.10.014
45. Bexte T, Botezatu L, Miskey C, Campe J, Reindl LM, Gebel V, et al. Non-viral sleeping beauty transposon engineered CD19-CAR-NK cells show a safe genomic integration profile and high antileukemic efficiency. Blood. (2021) 138:2797–7. doi: 10.1182/blood-2021-153999
46. Sabbah M, Jondreville L, Lacan C, Norol F, Vieillard V, Roos-Weil D, et al. CAR-NK cells: A chimeric hope or a promising therapy? Cancers (Basel). (2022) 14:3839. doi: 10.3390/cancers14153839
47. Naeimi Kararoudi M, Likhite S, Elmas E, Schwartz M, Sorathia K, Yamamoto K, et al. CD33 targeting primary CAR-NK cells generated by CRISPR mediated gene insertion show enhanced anti-AML activity. Blood. (2020) 136:3. doi: 10.1182/blood-2020-142494
48. Jamali A, Hadjati J, Madjd Z, Mirzaei HR, Thalheimer FB, Agarwal S, et al. Highly efficient generation of transgenically augmented CAR NK cells overexpressing CXCR4. Front Immunol. (2020) 11:2028. doi: 10.3389/fimmu.2020.02028
49. Kim Y, Lee DY, Choi JU, Park JS, Lee SM, Kang CH, et al. Optimized conditions for gene transduction into primary immune cells using viral vectors. Sci Rep. (2023) 13:12365. doi: 10.1038/s41598-023-39597-2
50. Gong Y, Klein Wolterink RGJ, Janssen I, Groot AJ, Bos GMJ, Germeraad WTV. Rosuvastatin enhances VSV-G lentiviral transduction of NK cells via upregulation of the low-density lipoprotein receptor. Mol Ther Methods Clin Dev. (2020) 17:634. doi: 10.1016/j.omtm.2020.03.017
51. Colamartino ABL, Lemieux W, Bifsha P, Nicoletti S, Chakravarti N, Sanz J, et al. Efficient and robust NK-cell transduction with baboon envelope pseudotyped lentivector. Front Immunol. (2019) 10:2873. doi: 10.3389/fimmu.2019.02873
52. Soldierer M, Bister A, Haist C, Thivakaran A, Cengiz SC, Sendker S, et al. Genetic engineering and enrichment of human NK cells for CAR-enhanced immunotherapy of hematological Malignancies. Front Immunol. (2022) 13:847008. doi: 10.3389/fimmu.2022.847008
53. Chockley P, Patil SL, Gottschalk S. Transient blockade of TBK1/IKKϵ allows efficient transduction of primary human natural killer cells with vesicular stomatitis virus G-pseudotyped lentiviral vectors. Cytotherapy. (2021) 23:787–92. doi: 10.1016/j.jcyt.2021.04.010
54. Wilk AJ, Weidenbacher NLB, Vergara R, Haabeth OAW, Levy R, Waymouth RM, et al. Charge-altering releasable transporters enable phenotypic manipulation of natural killer cells for cancer immunotherapy. Blood Adv. (2020) 4:4244. doi: 10.1182/bloodadvances.2020002355
55. Douka S, Brandenburg LE, Casadidio C, Walther J, Garcia BBM, Spanholtz J, et al. Lipid nanoparticle-mediated messenger RNA delivery for ex vivo engineering of natural killer cells. J Controlled Release. (2023) 361:455–69. doi: 10.1016/j.jconrel.2023.08.014
56. Golubovskaya V, Sienkiewicz J, Sun J, Zhang S, Huang Y, Zhou H, et al. CAR-NK cells generated with mRNA-LNPs kill tumor target cells in vitro and in vivo. Int J Mol Sci. (2023) 24. doi: 10.3390/ijms241713364
57. Sanz-Ortega L, Rojas JM, Portilla Y, Pérez-Yagüe S, Barber DF. Magnetic nanoparticles attached to the NK cell surface for tumor targeting in adoptive transfer therapies does not affect cellular effector functions. Front Immunol. (2019) 10:2073. doi: 10.3389/fimmu.2019.02073
58. Sivori S, Della Chiesa M, Carlomagno S, Quatrini L, Munari E, Vacca P, et al. Inhibitory receptors and checkpoints in human NK cells, implications for the immunotherapy of cancer. Front Immunol. (2020) 11:2156. doi: 10.3389/fimmu.2020.02156
59. Zalfa C, Paust S. Natural killer cell interactions with myeloid derived suppressor cells in the tumor microenvironment and implications for cancer immunotherapy. Front Immunol. (2021) 12:633205. doi: 10.3389/fimmu.2021.633205
60. Terrén I, Orrantia A, Vitallé J, Zenarruzabeitia O, Borrego F. NK cell metabolism and tumor microenvironment. Front Immunol. (2019) 10:2278. doi: 10.3389/fimmu.2019.02278
61. Kilgour MK, Bastin DJ, Lee SH, Ardolino M, McComb S, Visram A. Advancements in CAR-NK therapy: lessons to be learned from CAR-T therapy. Front Immunol. (2023) 14:1166038. doi: 10.3389/fimmu.2023.1166038
62. Jia H, Yang H, Xiong H, Luo KQ. NK cell exhaustion in the tumor microenvironment. Front Immunol. (2023) 14:1303605. doi: 10.3389/fimmu.2023.1303605
63. Wang Z, Guan D, Wang S, Chai LYA, Xu S, Lam KP. Glycolysis and oxidative phosphorylation play critical roles in natural killer cell receptor-mediated natural killer cell functions. Front Immunol. (2020) 11:202. doi: 10.3389/fimmu.2020.00202
64. Cong J, Wang X, Zheng X, Wang D, Fu B, Sun R, et al. Dysfunction of natural killer cells by FBP1-induced inhibition of glycolysis during lung cancer progression. Cell Metab. (2018) 28:243–255.e5. doi: 10.1016/j.cmet.2018.06.021
65. Noman MZ, Desantis G, Janji B, Hasmim M, Karray S, Dessen P, et al. PD-L1 is a novel direct target of HIF-1α, and its blockade under hypoxia enhanced MDSC-mediated T cell activation. J Exp Med. (2014) 211:781–90. doi: 10.1084/jem.20131916
66. Balsamo M, Manzini C, Pietra G, Raggi F, Blengio F, Mingari MC, et al. Hypoxia downregulates the expression of activating receptors involved in NK-cell-mediated target cell killing without affecting ADCC. Eur J Immunol. (2013) 43:2756–64. doi: 10.1002/eji.201343448
67. Della Chiesa M, Carlomagno S, Frumento G, Balsamo M, Cantoni C, Conte R, et al. The tryptophan catabolite L-kynurenine inhibits the surface expression of NKp46- and NKG2D-activating receptors and regulates NK-cell function. Blood. (2006) 108:4118–25. doi: 10.1182/blood-2006-03-006700
68. Michelet X, Dyck L, Hogan A, Loftus RM, Duquette D, Wei K, et al. Metabolic reprogramming of natural killer cells in obesity limits antitumor responses. Nat Immunol. (2018) 19:1330–40. doi: 10.1038/s41590-018-0251-7
69. Brand A, Singer K, Koehl GE, Kolitzus M, Schoenhammer G, Thiel A, et al. LDHA-associated lactic acid production blunts tumor immunosurveillance by T and NK cells. Cell Metab. (2016) 24:657–71. doi: 10.1016/j.cmet.2016.08.011
70. Ye C, Brand D, Zheng SG. Targeting IL-2: an unexpected effect in treating immunological diseases. Signal Transduct Target Ther. (2018) 3:1–10. doi: 10.1038/s41392-017-0002-5
71. Bentebibel SE, Hurwitz ME, Bernatchez C, Haymaker C, Hudgens CW, Kluger HM, et al. A first-in-human study and biomarker analysis of NKTR-214, a novel IL2Rβγ-biased cytokine, in patients with advanced or metastatic solid tumors. Cancer Discovery. (2019) 9:711–21. doi: 10.1158/2159-8290.CD-18-1495
72. Sharma M, Khong H, Fa’ak F, Bentebibel SE, Janssen LME, Chesson BC, et al. Bempegaldesleukin selectively depletes intratumoral Tregs and potentiates T cell-mediated cancer therapy. Nat Commun. (2020) 11:1–11. doi: 10.1038/s41467-020-14471-1
73. Christodoulou I, Ho WJ, Marple A, Ravich JW, Tam A, Rahnama R, et al. Engineering CAR-NK cells to secrete IL-15 sustains their anti-AML functionality but is associated with systemic toxicities. J Immunother Cancer. (2021) 9:e003894. doi: 10.1136/jitc-2021-003894
74. Silvestre RN, Eitler J, de Azevedo JTC, Tirapelle MC, Fantacini DMC, de Souza LEB, et al. Engineering NK-CAR.19 cells with the IL-15/IL-15Rα complex improved proliferation and anti-tumor effect in vivo. Front Immunol. (2023) 14:1226518. doi: 10.3389/fimmu.2023.1226518
75. Ojo EO, Sharma AA, Liu R, Moreton S, Checkley-Luttge MA, Gupta K, et al. Membrane bound IL-21 based NK cell feeder cells drive robust expansion and metabolic activation of NK cells. Sci Rep. (2019) 9:1–12. doi: 10.1038/s41598-019-51287-6
76. Yang Y, Badeti S, Tseng Hc, Ma MT, Liu T, Jiang JG, et al. Superior expansion and cytotoxicity of human primary NK and CAR-NK cells from various sources via enriched metabolic pathways. Mol Ther Methods Clin Dev. (2020) 18:428–45. doi: 10.1016/j.omtm.2020.06.014
77. He B, Chen H, Deng S, Li C, Xu N, Liu X, et al. CD19-specific CAR NK cells coexpressing IL-21 exhibit superior expansion and antitumor activity against CD19-postive lymphoma. Blood. (2023) 142:6824–4. doi: 10.1182/blood-2023-178289
78. Zhang C, Kadu S, Xiao Y, Johnson O, Kelly A, O’Connor RS, et al. Sequential exposure to IL21 and IL15 during human natural killer cell expansion optimizes yield and function. Cancer Immunol Res. (2023) 11:1524–37. doi: 10.1158/2326-6066.CIR-23-0151
79. Senju H, Kumagai A, Nakamura Y, Yamaguchi H, Nakatomi K, Fukami S, et al. Effect of IL-18 on the expansion and phenotype of human natural killer cells: application to cancer immunotherapy. Int J Biol Sci. (2018) 14:331. doi: 10.7150/ijbs.22809
80. Gang M, Marin ND, Wong P, Neal CC, Marsala L, Foster M, et al. CAR-modified memory-like NK cells exhibit potent responses to NK-resistant lymphomas. Blood. (2020) 136:2308–18. doi: 10.1182/blood.2020006619
81. He B, Mai Q, Pang Y, Deng S, He Y, Xue R, et al. Cytokines induced memory-like NK cells engineered to express CD19 CAR exhibit enhanced responses against B cell Malignancies. Front Immunol. (2023) 14:1130442. doi: 10.3389/fimmu.2023.1130442
82. Li Y, Hermanson DL, Moriarity BS, Kaufman DS. Human iPSC-derived natural killer cells engineered with chimeric antigen receptors enhance anti-tumor activity. Cell Stem Cell. (2018) 23:181–192.e5. doi: 10.1016/j.stem.2018.06.002
83. Parihar R, Rivas C, Huynh M, Omer B, Lapteva N, Metelitsa LS, et al. NK cells expressing a chimeric activating receptor eliminate MDSCs and rescue impaired CAR-T cell activity against solid tumors. Cancer Immunol Res. (2019) 7:363–75. doi: 10.1158/2326-6066.CIR-18-0572
84. Xiao L, Cen D, Gan H, Sun Y, Huang N, Xiong H, et al. Adoptive transfer of NKG2D CAR mRNA-engineered natural killer cells in colorectal cancer patients. Mol Ther. (2019) 27:1114. doi: 10.1016/j.ymthe.2019.03.011
85. Guo C, Fan Y, Aronov A, Kimura N, Juat D, Sood J, et al. (2023). Abstract 890: ADAM17 knockout NK or CAR NK cells augment antibody dependent cellular cytotoxicity (ADCC) and anti-tumor activity, in: Proceedings of the American Association for Cancer Research Annual Meeting 2023; Part 1 (Regular and Invited Abstracts), Orlando, FL. Philadelphia (PA, 2023 Apr 14-19, Vol. 83. AACR; Cancer Res, Abstract nr 890.
86. Zhu H, Blum RH, Bjordahl R, Gaidarova S, Rogers P, Lee TT, et al. Pluripotent stem cell–derived NK cells with high-affinity noncleavable CD16a mediate improved antitumor activity. Blood. (2020) 135:399. doi: 10.1182/blood.2019000621
87. Benson DM, Bakan CE, Zhang S, Collins SM, Liang J, Srivastava S, et al. IPH2101, a novel anti-inhibitory KIR antibody, and lenalidomide combine to enhance the natural killer cell versus multiple myeloma effect. Blood. (2011) 118:6387–91. doi: 10.1182/blood-2011-06-360255
88. Carlsten M, Korde N, Kotecha R, Reger R, Bor S, Kazandjian D, et al. Checkpoint inhibition of KIR2D with the monoclonal antibody IPH2101 induces contraction and hyporesponsiveness of NK cells in patients with myeloma. Clin Cancer Res. (2016) 22:5211–22. doi: 10.1158/1078-0432.CCR-16-1108
89. Vey N, Karlin L, Sadot-Lebouvier S, Broussais F, Berton-Rigaud D, Rey J, et al. A phase 1 study of lirilumab (antibody against killer immunoglobulin-like receptor antibody KIR2D; IPH2102) in patients with solid tumors and hematologic Malignancies. Oncotarget. (2018) 9:17675. doi: 10.18632/oncotarget.24832
90. Hu W, Wang G, Huang D, Sui M, Xu Y. Cancer immunotherapy based on natural killer cells: current progress and new opportunities. Front Immunol. (2019) 10:1205. doi: 10.3389/fimmu.2019.01205
91. Faden DL, Concha-Benavente F, Chakka AB, McMichael EL, Chandran U, Ferris RL. Immunogenomic correlates of response to cetuximab monotherapy in head and neck squamous cell carcinoma. Head Neck. (2019) 41:2591–601. doi: 10.1002/hed.25726
92. Van Hall T, André P, Horowitz A, Ruan DF, Borst L, Zerbib R, et al. Monalizumab: inhibiting the novel immune checkpoint NKG2A. J Immunother Cancer. (2019) 7. doi: 10.1186/s40425-019-0761-3
93. André P, Denis C, Soulas C, Bourbon-Caillet C, Lopez J, Arnoux T, et al. Anti-NKG2A mAb is a checkpoint inhibitor that promotes anti-tumor immunity by unleashing both T and NK cells. Cell. (2018) 175:1731. doi: 10.1016/j.cell.2018.10.014
94. Geurts VCM, Voorwerk L, Balduzzi S, Salgado R, Van de Vijver K, van Dongen MGJ, et al. Unleashing NK- and CD8 T cells by combining monalizumab and trastuzumab for metastatic HER2-positive breast cancer: Results of the MIMOSA trial. Breast: Off J Eur Soc Mastol. (2023) 70:76. doi: 10.1016/j.breast.2023.06.007
95. Zhang M, Lam KP, Xu S. Natural Killer Cell Engagers (NKCEs): a new frontier in cancer immunotherapy. Front Immunol. (2023) 14:1207276. doi: 10.3389/fimmu.2023.1207276
96. Nikkhoi SK, Li G, Eleya S, Yang G, Vandavasi VG, Hatefi A. Bispecific killer cell engager with high affinity and specificity toward CD16a on NK cells for cancer immunotherapy. Front Immunol. (2023) 13:1039969. doi: 10.3389/fimmu.2022.1039969
97. Reusing SB, Vallera DA, Manser AR, Vatrin T, Bhatia S, Felices M, et al. CD16xCD33 Bispecific Killer Cell Engager (BiKE) as potential immunotherapeutic in pediatric patients with AML and biphenotypic ALL. Cancer Immunol Immunother. (2021) 70:3701–8. doi: 10.1007/s00262-021-03008-0
98. Cheng Y, Zheng X, Wang X, Chen Y, Wei H, Sun R, et al. Trispecific killer engager 161519 enhances natural killer cell function and provides anti-tumor activity against CD19-positive cancers. Cancer Biol Med. (2020) 17:1026. doi: 10.20892/j.issn.2095-3941.2020.0399
99. Liu J, Yang S, Cao B, Zhou G, Zhang F, Wang Y, et al. Targeting B7-H3 via chimeric antigen receptor T cells and bispecific killer cell engagers augments antitumor response of cytotoxic lymphocytes. J Hematol Oncol. (2021) 14:1–18. doi: 10.1186/s13045-020-01024-8
100. Greene S, Robbins Y, Mydlarz WK, Huynh AP, Schmitt NC, Friedman J, et al. Inhibition of MDSC trafficking with SX-682, a CXCR1/2 inhibitor, enhances NK-cell immunotherapy in head and neck cancer models. Clin Cancer Res. (2020) 26:1420–31. doi: 10.1158/1078-0432.CCR-19-2625
101. Chambers AM, Lupo KB, Wang J, Cao J, Utturkar S, Lanman NA, et al. Engineered natural killer cells impede the immunometabolic CD73-adenosine axis in solid tumors. Elife. (2022) 11:73699. doi: 10.7554/eLife.73699
102. Lee YE, Go GY, Koh EY, Yoon HN, Seo M, Hong SM, et al. Synergistic therapeutic combination with a CAF inhibitor enhances CAR-NK-mediated cytotoxicity via reduction of CAF-released IL-6. J Immunother Cancer. (2023) 11:e006130. doi: 10.1136/jitc-2022-006130
103. Long Y, Gao Z, Hu X, Xiang F, Wu Z, Zhang J, et al. Downregulation of MCT4 for lactate exchange promotes the cytotoxicity of NK cells in breast carcinoma. Cancer Med. (2018) 7:4690–700. doi: 10.1002/cam4.1713
104. Nachef M, Ali AK, Almutairi SM, Lee SH. Targeting SLC1A5 and SLC3A2/SLC7A5 as a potential strategy to strengthen anti-tumor immunity in the tumor microenvironment. Front Immunol. (2021) 12:624324. doi: 10.3389/fimmu.2021.624324
105. Chaudhry K, Geiger A, Dowlati E, Lang H, Sohai DK, Hwang EI, et al. Co-transducing B7H3 CAR-NK cells with the DNR preserves their cytolytic function against GBM in the presence of exogenous TGF-β. Mol Ther Methods Clin Dev. (2022) 27:415–30. doi: 10.1016/j.omtm.2022.10.010
106. Liu WN, So WY, Harden SL, Fong SY, Wong MXY, Tan WWS, et al. Successful targeting of PD-1/PD-L1 with chimeric antigen receptor-natural killer cells and nivolumab in a humanized mouse cancer model. Sci Adv. (2022) 8:1187. doi: 10.1126/sciadv.add1187
107. Fabian KP, Padget MR, Donahue RN, Solocinski K, Robbins Y, Allen CT, et al. PD-L1 targeting high-affinity NK (t-haNK) cells induce direct antitumor effects and target suppressive MDSC populations. J Immunother Cancer. (2020) 8:e000450. doi: 10.1136/jitc-2019-000450
108. Lu C, Guo C, Chen H, Zhang H, Zhi L, Lv T, et al. A novel chimeric PD1-NKG2D-41BB receptor enhances antitumor activity of NK92 cells against human lung cancer H1299 cells by triggering pyroptosis. Mol Immunol. (2020) 122:200–6. doi: 10.1016/j.molimm.2020.04.016
109. Yang S, Cao B, Zhou G, Zhu L, Wang L, Zhang L, et al. Targeting B7-H3 immune checkpoint with chimeric antigen receptor-engineered natural killer cells exhibits potent cytotoxicity against non-small cell lung cancer. Front Pharmacol. (2020) 11:1089. doi: 10.3389/fphar.2020.01089
110. Jan CI, Huang SW, Canoll P, Bruce JN, Lin YC, Pan CM, et al. Targeting human leukocyte antigen G with chimeric antigen receptors of natural killer cells convert immunosuppression to ablate solid tumors. J Immunother Cancer. (2021) 9:e003050. doi: 10.1136/jitc-2021-003050
111. Ng YY, Tay JCK, Wang S. CXCR1 expression to improve anti-cancer efficacy of intravenously injected CAR-NK cells in mice with peritoneal xenografts. Mol Ther Oncol. (2020) 16:75–85. doi: 10.1016/j.omto.2019.12.006
112. Ng YY, Du Z, Zhang X, Chng WJ, Wang S. CXCR4 and anti-BCMA CAR co-modified natural killer cells suppress multiple myeloma progression in a xenograft mouse model. Cancer Gene Ther. (2021) 29:475–83. doi: 10.1038/s41417-021-00365-x
113. Schomer NT, Jiang ZK, Lloyd MI, Klingemann H, Boissel L. CCR7 expression in CD19 chimeric antigen receptor-engineered natural killer cells improves migration toward CCL19-expressing lymphoma cells and increases tumor control in mice with human lymphoma. Cytotherapy. (2022) 24:827–34. doi: 10.1016/j.jcyt.2022.02.006
114. Kremer V, Ligtenberg M, Zendehdel R, Seitz C, Duivenvoorden A, Wennerberg E, et al. Genetic engineering of human NK cells to express CXCR2 improves migration to renal cell carcinoma. J Immunother Cancer. (2017) 5:73. doi: 10.1186/s40425-017-0275-9
115. De Andrade LF, En Tay R, Pan D, Luoma AM, Ito Y, Badrinath S, et al. Antibody-mediated inhibition of MICA and MICB shedding promotes NK cell-driven tumor immunity. Sci (1979). (2018) 359:1537–42. doi: 10.1126/science.aao0505
116. Ramezani F, Panahi Meymandi AR, Akbari B, Tamtaji OR, Mirzaei H, Brown CE, et al. Outsmarting trogocytosis to boost CAR NK/T cell therapy. Mol Cancer. (2023) 22:1–14. doi: 10.1186/s12943-023-01894-9
117. Lu Z, McBrearty N, Chen J, Tomar VS, Zhang H, De Rosa G, et al. ATF3 and CH25H regulate effector trogocytosis and anti-tumor activities of endogenous and immunotherapeutic cytotoxic T lymphocytes. Cell Metab. (2022) 34:1342–1358.e7. doi: 10.1016/j.cmet.2022.08.007
118. Gurney M, Stikvoort A, Nolan E, Kirkham-McCarthy L, Khoruzhenko S, Shivakumar R, et al. CD38 knockout natural killer cells expressing an affinity optimized CD38 chimeric antigen receptor successfully target acute myeloid leukemia with reduced effector cell fratricide. Haematologica. (2022) 107:437–45. doi: 10.3324/haematol.2020.271908
119. Zhang W, Zhao Z, Li F. Natural killer cell dysfunction in cancer and new strategies to utilize NK cell potential for cancer immunotherapy. Mol Immunol. (2022) 144:58–70. doi: 10.1016/j.molimm.2022.02.015
121. Kouro T, Himuro H, Sasada T. Exhaustion of CAR T cells: potential causes and solutions. J Transl Med. (2022) 20:1–10. doi: 10.1186/s12967-022-03442-3
122. Sarén T, Saronio G, Marti Torrell P, Zhu X, Thelander J, Andersson Y, et al. Complementarity-determining region clustering may cause CAR-T cell dysfunction. Nat Commun. (2023) 14:1–11. doi: 10.1038/s41467-023-40303-z
123. Long AH, Haso WM, Shern JF, Wanhainen KM, Murgai M, Ingaramo M, et al. 4-1BB costimulation ameliorates T cell exhaustion induced by tonic signaling of chimeric antigen receptors. Nat Med. (2015) 21:581. doi: 10.1038/nm.3838
124. Poorebrahim M, Melief J, Pico de Coaña Y, L. Wickström S, Cid-Arregui A, Kiessling R. Counteracting CAR T cell dysfunction. Oncogene. (2020) 40:421–35. doi: 10.1038/s41388-020-01501-x
125. Gumber D, Wang LD. Improving CAR-T immunotherapy: Overcoming the challenges of T cell exhaustion. EBioMedicine. (2022) 77:103941. doi: 10.1016/j.ebiom.2022.103941
126. He J, Yan Y, Zhang J, Wei Z, Li H, Xing L. Synergistic treatment strategy: combining CAR-NK cell therapy and radiotherapy to combat solid tumors. Front Immunol. (2023) 14:1298683. doi: 10.3389/fimmu.2023.1298683
127. Maalej KM, Merhi M, Inchakalody VP, Mestiri S, Alam M, Maccalli C, et al. CAR-cell therapy in the era of solid tumor treatment: current challenges and emerging therapeutic advances. Mol Cancer. (2023) 22:1–54. doi: 10.1186/s12943-023-01723-z
128. Ma R, Lu T, Li Z, Teng KY, Mansour AG, Yu M, et al. An oncolytic virus expressing il15/il15ra combined with off-the-shelf egfr-car nk cells targets glioblastoma. Cancer Res. (2021) 81:3635–48. doi: 10.1158/0008-5472.CAN-21-0035
129. Siegler EL, Kim YJ, Chen X, Siriwon N, Mac J, Rohrs JA, et al. Combination cancer therapy using chimeric antigen receptor-engineered natural killer cells as drug carriers. Mol Ther. (2017) 25:2607–19. doi: 10.1016/j.ymthe.2017.08.010
130. Wang F, Wu L, Yin L, Shi H, Gu Y, Xing N. Combined treatment with anti-PSMA CAR NK-92 cell and anti-PD-L1 monoclonal antibody enhances the antitumour efficacy against castration-resistant prostate cancer. Clin Transl Med. (2022) 12:e901. doi: 10.1002/ctm2.901
131. Zhang Q, Zhang H, Ding J, Liu H, Li H, Li H, et al. Combination therapy with EpCAM-CAR-NK-92 cells and regorafenib against human colorectal cancer models. J Immunol Res. (2018) 2018:4263520. doi: 10.1155/2018/4263520
132. Chu Y, Yahr A, Huang B, Ayello J, Barth M, S. Cairo M. Romidepsin alone or in combination with anti-CD20 chimeric antigen receptor expanded natural killer cells targeting Burkitt lymphoma in vitro and in immunodeficient mice. Oncoimmunology. (2017) 6. doi: 10.1080/2162402X.2017.1341031
133. Li G, Wu X, Chan IH, Trager JB. (2020). Abstract 4235: A combination of CAR-NK and CAR-T cells results in rapid and persistent anti-tumor efficacy while reducing CAR-T cell mediated cytokine release and T-cell proliferation, in: Proceedings of the Annual Meeting of the American Association for Cancer Research 2020, Philadelphia (PA, 2020 Apr 27-28 and Jun 22-24, Vol. 80. AACR; Cancer Res, Abstract nr 4235.
134. Ma L, Hostetler A, Morgan DM, Maiorino L, Sulkaj I, Whittaker CA, et al. Vaccine-boosted CAR T crosstalk with host immunity to reject tumors with antigen heterogeneity. Cell. (2023) 186:3148–3165.e20. doi: 10.1016/j.cell.2023.06.002
135. Food and Drug Administration (FDA). Considerations for the Development of Chimeric Antigen Receptor (CAR) T Cell Products (2024). Available online at: https://www.fda.gov/regulatory-information/search-fda-guidance-documents/considerations-development-chimeric-antigen-receptor-car-t-cell-products (Accessed January 31, 2024).
Keywords: chimeric antigen receptor (CAR), natural killer (NK) cell, advancements, strategies, challenges, cancer, immunotherapy
Citation: Kong JC, Sa’ad MA, Vijayan HM, Ravichandran M, Balakrishnan V, Tham SK and Tye GJ (2024) Chimeric antigen receptor-natural killer cell therapy: current advancements and strategies to overcome challenges. Front. Immunol. 15:1384039. doi: 10.3389/fimmu.2024.1384039
Received: 08 February 2024; Accepted: 15 April 2024;
Published: 25 April 2024.
Edited by:
Zhiwei Hu, The Ohio State University, United StatesReviewed by:
Srinivas S. Somanchi, Independent Researcher, San Diego, United StatesCopyright © 2024 Kong, Sa’ad, Vijayan, Ravichandran, Balakrishnan, Tham and Tye. This is an open-access article distributed under the terms of the Creative Commons Attribution License (CC BY). The use, distribution or reproduction in other forums is permitted, provided the original author(s) and the copyright owner(s) are credited and that the original publication in this journal is cited, in accordance with accepted academic practice. No use, distribution or reproduction is permitted which does not comply with these terms.
*Correspondence: Seng Kong Tham, ZHJ0aGFtQGFscHNtZWRpY2FsLmNvbQ==; Gee Jun Tye, Z2VlanVuQHVzbS5teQ==
Disclaimer: All claims expressed in this article are solely those of the authors and do not necessarily represent those of their affiliated organizations, or those of the publisher, the editors and the reviewers. Any product that may be evaluated in this article or claim that may be made by its manufacturer is not guaranteed or endorsed by the publisher.
Research integrity at Frontiers
Learn more about the work of our research integrity team to safeguard the quality of each article we publish.