- 1Department of Proteomics and Metabolomics, MEDFUTURE Research Center for Advanced Medicine, “Iuliu Hatieganu” University of Medicine and Pharmacy, Cluj-Napoca, Romania
- 2Department of Pharmacology, Physiology and Pathophysiology, Faculty of Pharmacy, “Iuliu Hatieganu” University of Medicine and Pharmacy, Cluj-Napoca, Romania
- 3Department of Pharmaceutical Analysis, Faculty of Pharmacy, “Iuliu Hatieganu” University of Medicine and Pharmacy, Cluj-Napoca, Romania
- 4Department of Translational Medicine, MEDFUTURE Research Center for Advanced Medicine, “Iuliu Hatieganu” University of Medicine and Pharmacy, Cluj-Napoca, Romania
- 5Department of Hematology, Faculty of Medicine, “Iuliu Hatieganu” University of Medicine and Pharmacy, Cluj-Napoca, Romania
- 6Department of Stem Cell Transplantation, Fundeni Clinical Institute, Bucharest, Romania
- 7Department of Laboratory Hematology, Stem Cell Research Unit, Biomedical Center, School of Health Sciences, University Iceland, Reykjavik, Iceland
- 8Department of Immunology, University of Oslo, Oslo, Norway
- 9Department of Animal Reproduction, University of Agricultural Sciences and Veterinary Medicine, Cluj-Napoca, Romania
- 10Department of Internal Medicine, Faculty of Medicine, “Iuliu Hatieganu” University of Medicine and Pharmacy, Cluj-Napoca, Romania
Despite the numerous advantages of allogeneic hematopoietic stem cell transplants (allo-HSCT), there exists a notable association with risks, particularly during the preconditioning period and predominantly post-intervention, exemplified by the occurrence of graft-versus-host disease (GVHD). Risk stratification prior to symptom manifestation, along with precise diagnosis and prognosis, relies heavily on clinical features. A critical imperative is the development of tools capable of early identification and effective management of patients undergoing allo-HSCT. A promising avenue in this pursuit is the utilization of proteomics-based biomarkers obtained from non-invasive biospecimens. This review comprehensively outlines the application of proteomics and proteomics-based biomarkers in GVHD patients. It delves into both single protein markers and protein panels, offering insights into their relevance in acute and chronic GVHD. Furthermore, the review provides a detailed examination of the site-specific involvement of GVHD. In summary, this article explores the potential of proteomics as a tool for timely and accurate intervention in the context of GVHD following allo-HSCT.
1 Background on graft-versus-host-disease
In hematology, precision medicine has made strides, particularly in bone marrow transplants (BMT) and targeted therapies transforming blood disorder management. BMTs recent safety profile, with transplant-related mortality below 30% and a 30 to 70% risk of graft-versus-host disease (GVHD), positions it as a universal option. However, advances in genetics, molecular biology, and immunology have led to targeted therapeutics, reducing BMT necessity in some cases.
Currently, allogenic hematopoietic stem cell transplants (allo-HSCT), using bone marrow, peripheral blood stem cells (PBSCs), or cord blood, are increasingly performed globally. Allo-HSCT holds a great potential of curing many malignant and non-malignant hematologic disorders, subjected to specific conditions (1). Risk factors include human leukocyte antigen (HLA) mismatch, female donors for male recipients, and total body irradiation. Complications post-allo-HSCT involve infections, GVHD, and relapse, with risk assessment relying on clinical characteristics (2, 3).
GVHD is a life-threatening complication that can occur after the allo-HSCT. About 30 to 80% of allo-HSCT recipients develop acute GVHD (aGVHD) and around 50% chronic GVHD (cGVHD) (4). In this context, GVHD arises when immunocompetent T cells from the donated tissue (the graft) identify the recipient (the host) as foreign. This recognition triggers an immune response that activates donor T cells, enabling them to develop cytolytic capacity and attack the recipients cells carrying foreign antigens (5). In hematological malignancies, there is a fine balance between the harmful consequences of GVHD and the therapeutic effects produced when donor lymphocytes attack recipient malignant cells, in graft versus leukemia/tumor (GVL) effect.
In aGVHD, the skin, liver or gastrointestinal tract can be affected in about 2 to 12 weeks after allo-HSCT, but there are situations when aGVHD appears before or after this interval. A cascade of events initiated by the activation of innate immune cells and subsequent tissue injury induces aGVHD, which is additionally exacerbated by the engagement of adaptive immune responses. The hallmark of aGVHD is the infiltration of activated donor T cells, predominantly CD4+ cells, into host tissues, driven by their recognition of the recipients tissues as allo-antigenic. This process results in severe inflammation that further amplifies the immune response, leading to a cytokine storm. In cytokine storm, elevated levels of circulating cytokines and immune cell hyperactivation occurs. The intricate interplay between innate and adaptive immunity during aGVHD underscores the multifaceted nature of this condition, necessitating a comprehensive understanding of the underlying immunological mechanisms for the development of effective therapeutic strategies. The dynamics of aGVHD pathogenesis, particularly the contributions of specific immune cell subsets and the cytokine storm, is crucial for both understanding the disease and for identifying new biomarkers to help early diagnosis, prognosis and targeted interventions to improve clinical outcomes.
Additionally, cGVHD can develop within 4 to 6 months post-allo-HSCT, following aGVHD or in patients without any prior complications. This condition is similar to an autoimmune disease, involving, besides donor T cells, extensive B cell activation, the release of pro-inflammatory cytokines that can affect the skin, mouth, eyes, gastrointestinal tract and the liver. The pathophysiology of cGVHD involved 3 stages (1): high production of inflammatory cytokines (interleukin-1 (IL-1), IL-6, TNF-α) and expression of antigens of the major histocompatibility complex (MHC) that stimulate antigen-presenting cells to interact with the donor T cells (2), this interaction leads to the activation of T cells which then release additional inflammatory cytokines (IL-2, Interferon- γ (INF-γ)) and (3) the migration of cytotoxic T lymphocytes and natural killer cells to specific organs, producing tissue damage (6).
GVHD is an important cause of non-relapse mortality (NRM), accounting for about 11% deaths in patients receiving allo-HSCT (7). The need for risk stratification for GVHD is high, due to problems associated with immunosuppression. It is either not aggressive enough leaving patients with a high GVHD risk vulnerable, or it is too aggressive leading to infections and other complications (3, 8).
A major concern consists in the development of tools capable of (i) timely identification of patients at risk for GVHD, (ii) invasive procedures avoidance and (iii) timely intervention, one such option could be a biomarker. Currently, there is no validated specific biomarker for GVHD. In the clinical scenario the diagnosis is confirmed through analysis of the biospecimen of the involved site.
As such, fast and organ-specific diagnosis of GVHD based on biomarkers can lead to targeted interventions, and prognosis biomarkers can assist in the process of decision making for treatment. Until recently, biomarkers were identified by hypothesis-driven approaches, considering the pathophysiologic role of specific molecules in GVHD, such as miRNAs and cellular biomarkers for GVHD (2, 9).
The aim of this paper is to review the potential candidate biomarkers derived from unbiased approaches, facilitated by the recent advancements in proteomics a robust high-throughput technique. These biomarkers may or may not have a direct pathophysiologic link to GVHD; instead, they might emerge due to the condition itself or other unexplored interactions.
2 Proteomics as the key strategy for maximizing minimally invasive biopsies towards biomarker discovery
A biomarker is defined by the Food and Drug Administration - National Institute of Health (FDA-NIH) Biomarker Working Group as “a characteristic that is measured as an indicator of normal biological processes, pathogenic ones, or a response to an exposure or intervention” (10). Biomarkers have diverse applications, guiding healthcare interventions for maximum patient advantage. They are commonly utilized for disease diagnosis, risk-based population screening, evaluating disease complications and therapy effectiveness. Furthermore, they facilitate disease trajectory prediction, aid therapy selection, and assess novel drug effectiveness and safety. Thus, biomarkers can be diagnostic biomarkers, prognostic biomarkers and theranostic biomarkers (11).
An ideal biomarker should be easily quantifiable, allowing for simple procurement of biological specimens and employing a financially viable quantification method. It is crucial for a biomarker assay to demonstrate reproducibility, sensitivity (SEN), and specificity (SPE), providing accurate results for clinicians to make informed therapeutic decisions. The efficacy of a biomarker depends on its strong correlation with treatment responses. To establish clinical applicability, a comprehensive series of assays in both preclinical and clinical settings is necessary. Following the identification of a prospective biomarker candidate, validation processes, rigorous evaluation, and refinement through clinical investigations are conducted before approval and commercialization (11).
In the development of GVHD biomarkers, optimizing statistical test performance and determining cut points are critical. Bidgoli et al. (12) emphasized the importance of Receiver Operating Characteristic (ROC) curves and Area Under the Curve (AUC) in assessing assay performance. SEN and SPE, represented by ROC curves, reflect true positive and true negative rates, respectively. Positive and negative predictive values are calculated considering both cut points and GVHD incidence, with the latter being particularly relevant in HCT. Tailoring biomarker cut points for clinical use involves testing multiple values to balance intervention efficacy and toxicity. Hazard ratio (HR) is another key parameter used to gauge the impact of an intervention on a specific outcome over time. In biomarker discovery, HR helps evaluate the link between a biomarkers expression and the timing of an event, suggesting its potential as a prognostic indicator (13). Clinical trials using biomarkers for patient selection have a higher likelihood of success compared to those lacking biomarkers (14).
2.1 Proteins as a valuable biomarker source
Despite advances in genomics and technology, clinical biomarker discovery faces persistent challenges. Solely focusing on genetic abnormalities does not fully capture the complexity of disease biology, involving interactions between genetic anomalies, epigenetics, post-translational modifications, and immune responses (15). Proteins, as structural and signaling entities, are pivotal in understanding disease characteristics and serve as key drug intervention targets. Exploring the proteome, representing the entire set of proteins expressed under specific conditions, provides valuable insights into their functions, interactions, and roles in biological systems. Notably, identifying proteins and monitoring their levels in biological samples dynamically reflects the cumulative impact of genetic and epigenetic changes, crucial for understanding functional disease biology and guiding therapeutic strategies (16, 17).
Exploring biomarkers from both membrane-bound and soluble protein forms is intriguing. Membrane-bound proteins are crucial for signaling, transport, and adhesion, with changes indicating disease signatures (18). Simultaneously, measuring soluble proteins in bodily fluids offers a non-invasive and dynamic approach for biomarker discovery, providing real-time insights into physiological states. This versatile method applies across diseases, identifying diagnostic, prognostic, and therapeutic indicators. Soluble biomarkers, exemplified by soluble immune checkpoints, have emerged as dynamic molecules with significant physiological roles. The intricate network of immune checkpoints, encompassing diverse stimulatory and inhibitory pathways, plays a pivotal role in fine-tuning immune responses. Notably, molecules like Programmed Cell Death Protein 1 (PD-1), Cytotoxic T-lymphocyte associated protein 4 (CTLA4), and their ligands have emerged as focal points for anti-cancer immunotherapy, while other checkpoint pathways contribute to the immunopathogenesis of human diseases as critically analyzed by Riva A (19). Beyond the traditional view of membrane-bound systems, immune checkpoints also manifest as soluble forms (sCRs), actively regulating immune responses both locally and systemically. In a comprehensive review by Niu et al. (20), the roles of sPD-1 and sPD-L1 in cancer are explored, shedding light on their synthesis, release, and alternative mRNA splicing mechanisms. The study suggests that PD-1 may function as an immune stimulator, in contrast to its membrane-bound counterpart, while sPD-L1 retains suppressive activity. The review also proposes the intriguing idea that sCRs could act as decoy sponges for therapeutic blocking antibodies, impacting resistance to immunotherapy and disease progression. The complexity of sCR networks, disease-specific signatures, and the persistence of sCR “imprinting” post-treatment pose challenges (21–24). The articles collectively support the hypothesis that soluble isoforms of immune checkpoints may exhibit distinct functions compared to their membrane-bound counterparts. Furthermore, the existence of various isoforms for each sCR and soluble receptor-ligand complexes underscores the need for standardized measurement criteria and open comparisons of antibody clones. In conclusion, unraveling the intricacies of the sCR system holds promise for advancing both basic and translational research, providing valuable insights into their roles as disease biomarkers and potential targets for pharmacological interventions and immunotherapy. Nevertheless, analyzing both forms enhances our understanding of disease biology, supporting precision medicine. This dual approach boosts the potential for discovering robust biomarkers, influencing disease diagnosis, prognosis, and treatment (12).
2.2 Proteomics as a discovery instrument
Proteomics, an ‘omics strategy, is crucial for profiling and quantifying proteins in a biospecimen under specific conditions. This involves investigating protein abundances, protein-protein interactions, and the functional roles of proteins in diverse conditions. Proteomics methodologies are classified as low-throughput and high-throughput. Low-throughput approaches include antibody-based techniques like Enzyme-Linked Immunosorbent Assay (ELISA) and Western blotting (WB), relying on antigen-antibody interactions for protein detection. Electrophoresis methods, such as two-dimensional gel electrophoresis and sodium dodecyl-sulfate polyacrylamide gel electrophoresis (SDS-PAGE), separate proteins based on characteristics like mass, charge, or isoelectric point, followed by identification using molecular weight standards or mass spectrometry (MS). Chromatography, another separation technique, aids in isolating and purifying proteins through ion-exchange, size exclusion, and affinity chromatography. Downstream analyses, including MS, contribute to protein identification and quantification post-separation (25).
High-throughput methodologies in proteomics involve affinity binder techniques and MS. Affinity binder techniques, such as microarrays and multiplex arrays, use specific antibodies and aptamers to capture target proteins (26). Microarray research, particularly in the context of cancer and hematologic disorders, has identified candidate diagnostic biomarkers for GVHD. MS, employed in both top-down (intact protein analysis) and bottom-up (protein digestion to peptides before analysis) settings, involves ionization, separation, and detection of proteins or peptides. Ionization modes like electrospray ionization and matrix assisted laser desorption/ionization (MALDI), along with mass analyzers like time-of-flight and quadrupole, contribute to accurate protein identification, including isoforms and posttranslational modifications. MS-based protein quantification, especially with tandem MS (MS/MS), is highly accurate and relies on mass spectra matched to sequence databases (27). Best practices for protein-based biomarker discovery and validation are discussed elsewhere (28). The preferred high-throughput approaches for biomarker discovery, including those for GVHD, involve bottom-up proteomics and affinity-based methods (29). Figure 1 illustrates the advantages and disadvantages of these proteomics methods in the context of GVHD biomarker discovery. Proteomics, as a prominent ‘omics technology, has witnessed significant progress, overcoming challenges and contributing to the pursuit of protein-based biomarkers for precision medicine in recent years (30).
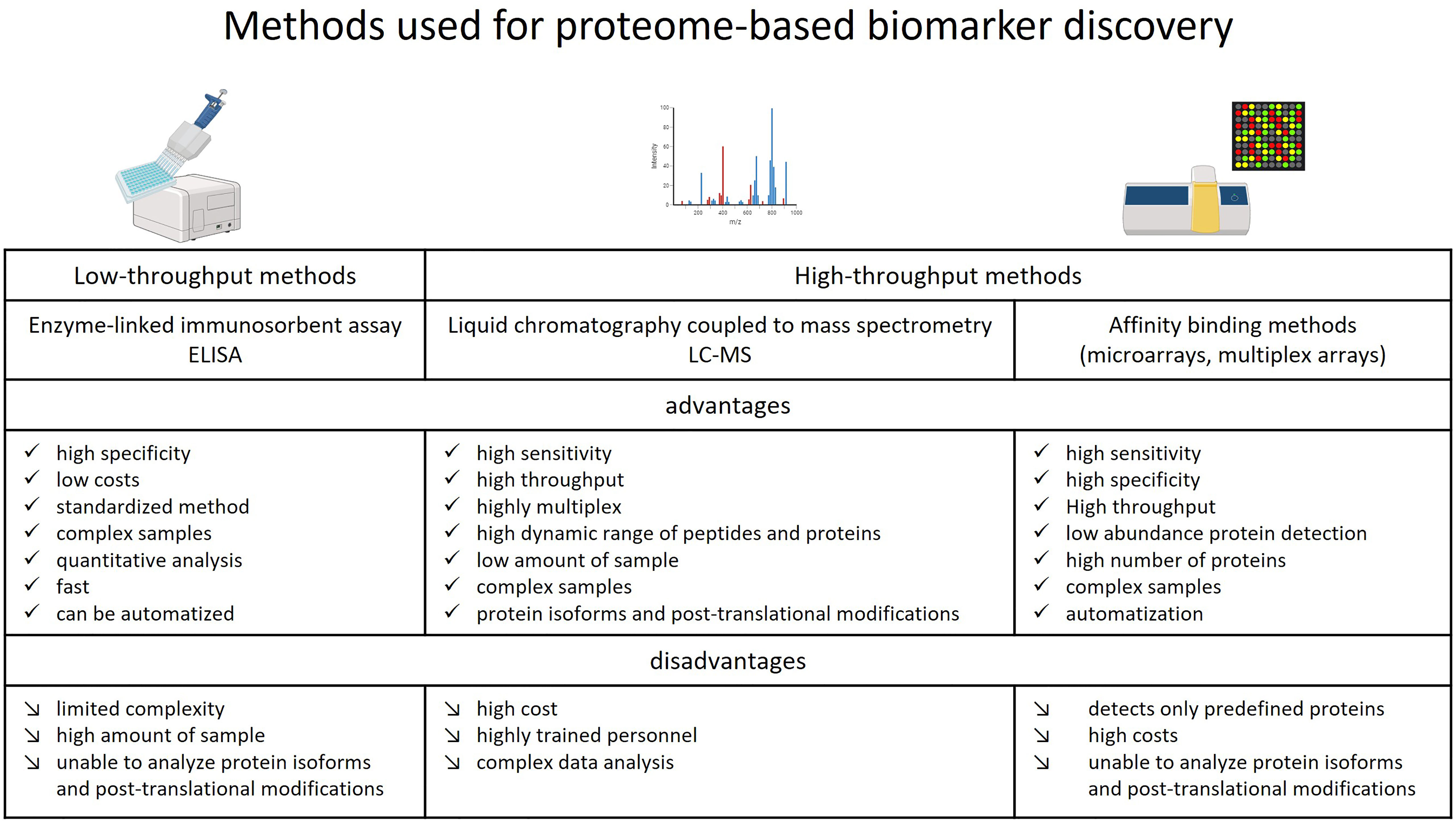
Figure 1 Advantages and disadvantages of methods used for proteome-based biomarker discovery studies for GVHD.
2.3 Biomarker panels
Efficiently measuring multiple markers can be achieved through multiplexing techniques, which allow simultaneous detection of various biomolecules in a single assay. Multiplexing enhances throughput, conserves sample volume, and reduces costs compared to running individual assays for each marker. Technologies such as multiplex immunoassays, microarrays, and MS enable the detection of multiple markers within a single experimental run. Additionally, advances in high-throughput screening methods and automation contribute to efficiency by streamlining the workflow. Choosing an appropriate multiplexing platform based on the characteristics of the markers and the research objectives ensures a comprehensive and resource-effective approach to measuring multiple biomarkers simultaneously (31).
Measurements of individual candidate levels are not always relevant or specific. That is why their use in combination of multi-marker panels can bring an increase in SEN and SPE. MS based proteome studies towards biomarker discovery for GVHD accurately distinguished GVHD samples from both posttransplant non-GVHD samples and pretransplant samples. Furthermore, distinct serum proteomic signatures were identified to distinguish pretransplant from posttransplant non-GVHD samples, opening up new insights towards biomarker panel discoveries (32). For aGVHD protein-based biomarker panel discovery, saliva and plasma were the non-invasive biofluids investigated and the identified panels are further presented.
2.4 Valuable biomarker sources
Proteome analysis of biospecimens offers insights into physiological processes, aiding the identification of actionable approaches, including biomarkers applicable at the bedside. Primary sources of protein markers include tissues and body fluids such as serum, plasma, saliva, urine, and bone marrow. While bone marrow provides heightened diagnostic precision, non-invasive biospecimens, like plasma and serum, present advantages in terms of patient compliance and reduced procedural risks.
Plasma and serum, being popular biofluids for biomarker discovery, offer easy acquisition and broad molecular representation due to systemic circulation. Challenges like sample standardization and accessing low-abundance proteins can impact proteomics studies, but the Human Proteome Organization (HUPO) provides Biomarker Discovery Protocols for addressing these issues (33). Tears, a non-invasive biospecimen, are gaining attention, particularly for ocular involvement in GVHD. In gastrointestinal GVHD, feces can serve as a useful biospecimen, providing insights into intestinal inflammation. Skin biopsies, obtained minimally invasively, are valuable for identifying specific biomarkers related to skin manifestations of GVHD. Urine, an alternative to blood samples, offers advantages such as ease of collection, larger quantities, and a less complex protein mixture with low abundance variation. However, its limitation lies in providing information primarily about diseases in the organs directly involved in its production and excretion, like the kidneys, rather than systemic diseases.
Proteomics, aiming to translate discoveries into clinical practice, focuses on uncovering minimally invasive biofluid-based biomarkers (34, 35). Examples of biospecimens for GVHD biomarker studies are detailed in the following sections.
3 Protein-based biomarkers for GVHD following allo-HSCT
Technological advancements have facilitated the comprehensive profiling of proteins within a given biological system. Unbiased investigations have yielded intriguing findings that might have been overlooked using narrower analytical approaches. Diverse biomarkers, when evaluated prior to BMT, play a pivotal role in the selection of suitable candidates for intervention, particularly in cases of unfavorable prognostics, resistance to chemotherapy, or heightened susceptibility to drug-induced toxicities. Furthermore, post- HSCT biomarkers offer valuable information regarding prognosis and the likelihood of complications. The diagnosis of GVHD is still dependent on clinical criteria in most of the part and requires the realization of skin biopsy, colonoscopy, bronchoscopy and bronchoalveolar lavage. aGVHD is the main cause of morbidity after transplant, being responsible for 15 to 40% of the post-transplant deaths. Mortality in GVHD is caused by the difficulty in giving a diagnosis and planning the optimal moment for therapy start (1, 36). As previously mentioned, GVHD markers can facilitate quick diagnosis of this complication and quick start of the therapy, which would increase the survival rate. Also, based on stratification biomarkers, the patients can get a more or less aggressive immunosuppressive regime reducing the costs and the long-term toxicity (34). Different strategies have been approached towards GVHD biomarker discovery targeting proteins. Strategies include single biomarker as well as multi-marker panel scouting and using different biospecimen. A comprehensive overview is further presented.
3.1 Protein based biomarkers for aGVHD
3.1.1 Cytokine-based biomarkers for aGVHD
Cytokines are important in both protection after HSCT, notably in graft-versus-leukemia and in disease, notably in GVHD. Acute GVHD is a complex pathologic process that involves many types of T-cells that secrete cytokines: Th1, Th2, TH17 and CD8+ T cells. Most cytokines associated with aGVHD include ILs, TNF-α and IFN-γ. Many cytokines are also important in the inflammatory cytokine storm that is specific to aGVHD, including the proinflammatory cytokines IL-12, IL-17, IL-21, IL-33, and the protective cytokines IL-10, IL-22, and TGF-β (37). Even more, the involvement of soluble interleukin-2 receptor (sIL-2R) in the physiopathology of GVHD has led to the development of targeted therapies with monoclonal antibodies, like daclizumab and conjugates like denileukin diftitox (38). The administration of anti-IL-6 receptor antibodies was associated to a drop in GVHD mortality (39). Thus, because of the role of cytokines in systemic inflammation during aGVHD, they were studied as potential biomarkers with diagnostic or predictive value. Mostly, they were studied from serum or plasma by employing ELISA methods. The cytokine-based biomarkers for aGVHD are presented in Table 1.
3.1.2 Other emerging protein-based biomarkers for aGVHD
In addition to cytokines, extensive research has been conducted on various protein-based biomarkers, and their promising utility as potential indicators of aGVHD is currently on the rise. The suppression of tumorigenicity 2 (ST2) is a receptor included in the IL-1 family, which binds specifically to IL-33. ST2 has two isoforms: a transmembrane (mST2) and a soluble form (sST2). During aGVHD, an increase in IL-33 has been observed, leading to specific inflammation and tissue damage (45). The sST2 receptors are expressed in different cells and act as decoy receptors, sequestering free IL-33, thereby preventing IL-33-mediated proinflammatory actions (46). Studies have found an increase of sST2 which could be correlated with GVHD severity in patients. A possible explanation given by earlier studies was that the release of sST2 in the serum occurs very late in the inflammatory response resulting in the inability of sST2 to sequester circulating IL-33. This could make sST2 an interesting biomarker for aGVHD and NRM (47). Also, Vander Lugt et al. found by quantitative liquid chromatography coupled with MS/MS and further ELISA validation that ST2 could be a prognostic marker for GVHD with endpoints such as treatment resistance or death 6 months after therapy, with SEN and SPE for six-month post-transplant NRM in an independent set of 70 and 64 respectively (48).
Another marker is vascular endothelial growth factor (VEGF). Studies that have evaluated the prognostic value of VEGF levels in GVHD presented with controversial results (49). Some studies have reported that high VEGF levels were associated with the development or severity of GVHD in a mouse model study (50), whereas others have found that VEGF either has a protective effect against severe aGVHD (AUC 0.69) (51) or no correlation with GVHD (52). Low VEGF levels after allo-HSCT were found to be associated with NRM due to severe exacerbation of aGVHD, implying the biomarker utility of VEGF. VEGF monitoring after allo-HSCT might identify those patients at risk of severe transplant-related mortality (53).
Another interesting molecule is T cell immunoglobulin and mucin domain 3 (TIM3). TIM3 is a transmembrane receptor protein expressed on T cells that produce IFN-gamma, Tregs, myeloid cells, natural killer cells, and mast cells, where it inhibits cytokine expression (54). Although the physiopathology concerning the involvement of TIM3 in aGVHD is not fully understood, and only preclinical studies have given hints regarding the regulation of hepatic CD8+ T cells by TIM3 (55), clinical proteomics studies have shown that the levels of the soluble extracellular domain of Tim-3 exhibited an elevation in the bloodstream of individuals preceding the clinical manifestation of aGVHD. Furthermore, these levels demonstrated a correlation with the severity of gut GVHD (AUC of 0.79) (56).
Finally, macrophage migration inhibitory factor (MIF), a pleiotropic protein, is known to participate in inflammatory and immune responses by regulating TNF-α production. Elevated peak serum MIF at acute GVHD onset and increased mean serum MIF in patients developing extensive cGVHD within 6 months suggest heightened MIF levels during active phases of both GVHD types (57).
3.1.3 Salivary protein panels for aGVHD
Towards salivary protein panel discovery, the salivary proteome was analyzed by gel electrophoresis with subsequent MS/MS analysis and ELISA. MS identified significant salivary protein fluctuations lasting at least 2 months posttransplant. High salivary levels of lactoferrin, cystatin SN, albumin and salivary amylase have been identified in patients with GVHD with oral cavity involvement. High levels of these proteins indicate pathophysiological processes that take place in GVHD with oral cavity involvement, like salivary glands infiltration, activation of immunomodulatory processes and increasing the permeability of the oral cavity mucosa and plasma extravasation (58). Also by MS, proteins from the S100 protein family was found to play a role in aGVHD diagnosis: S100A8, S100A7 and S100A9 showing SEN of 60% and SPE of 88% (59).
3.1.4 Plasma protein panels for aGVHD
Another strategy to identify biomarker panels was to screen a large set of proteins by antibody array and to validate the results by ELISA. This was done for four plasmatic diagnostic markers: IL-2-receptor-alpha (IL-2Rα), tumor-necrosis-factor-receptor-1 (TNFR1), IL-8 (IL-8), and hepatocyte growth factor (HGF). The 4 biomarkers panel confirmed their potential for diagnosis of GVHD in patients at onset of clinical symptoms and provided prognostic information independent of GVHD severity with AUC of 0.86 (60). Elafin, a skin-specific marker and regenerating islet-derived 3-α (Reg3α), a gastrointestinal tract-specific marker was added later to the 4-parameter panel. The panel was studied for its potential to discriminate between therapy responsive and nonresponsive patients and predict survival in patients receiving GVHD therapy. Even more, the 6 biomarker panel demonstrated potential to be used for early identification of patients at high or low risk for treatment non responsiveness or death, and provided opportunities for early intervention and improved survival after HSCT (61). Also in pediatric patients with aGVHD a biomarker panel of TNFR1, IL-2Rα, HGF, CCL8, IL-8 and IL-12p70 demonstrated its utility following HSCT (62). Another combination of proteins, namely a panel composed of ST2 and REG3α was found to be able to identify patients at high risk for lethal aGVHD and NRM with an AUC of 0.68, SEN of 40% and SPE of 83% (63). Including also cells to sIL-2R, a 5-parameter biomarker score based on CD4+ T cells, CD8+ T cells, CD19− CD21+ precursor B cells, CD4/CD8 T cell ratio, and sIL-2R was used to predict GVHD onset with AUC of 0.90, SEN of 88.2% and SPE of 66.7% (64). Aiming to identify and evaluate candidate biomarkers potentially predictive of response to treatment with itacitinib plus corticosteroid in aGVHD, plasma monocyte-chemotactic protein (MCP)3, pro-calcitonin/calcitonin (ProCALCA/CALCA), REG3α, ST2, and TNFR1 were found (65).
aGVHD treatment relies on corticosteroid immunosuppression, with initial response guiding further decisions. Analysis of 507 patients from 17 Mount Sinai Acute GVHD International Consortium (MAGIC) centers revealed a validated biomarker algorithm, consisting of ST2 and REG3α measured by ELISA, predicting outcomes in steroid resistant GVHD. MAGIC biomarker probabilities after 1 week of systemic GVHD treatment outperform clinical criteria (AUC 0.82), aiding in the development of improved treatment strategies (66).
3.2 Protein based biomarkers for cGVHD
cGVHD usually starts more than 3 months after a transplant and can last for as long as a lifetime (67). Preclinical studies and translational research on human biospecimen have revealed some possible biological pathways in cGvHD. This has opened the way for the exploration of diagnostic, prognostic and predictive biomarkers in both hypothesis-driven and discovery-based testing (68). Biomarkers for cGVHD are presented in Table 2.
3.2.1 Biomarker panels for cGVHD
Besides the identification of individual biomarkers for cGVHD, efforts are being made to validate panels of biomarkers (Table 3), with a much higher accuracy of diagnosis or prognosis.
3.3 Protein based biomarkers for site-specific involvement in GVHD
Site-specific involvement represents a critical facet of GVHD and manifests in distinct anatomical regions, such as the skin, gastrointestinal tract, the eyes, and the lungs, with varying degrees of severity. The skin, often the initial site of presentation, can exhibit rashes, blistering, and itching (79). Clinical aspects of skin lesions in GVHD are illustrated in Figure 2.
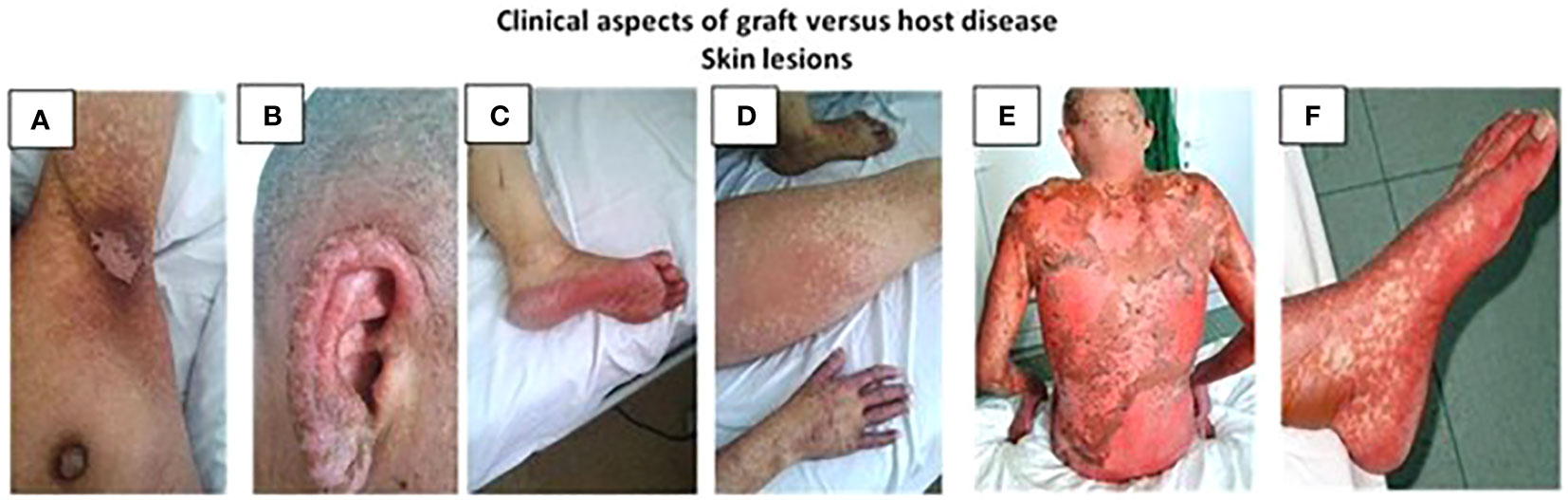
Figure 2 Clinical aspects of GVHD skin lesions (A) axillar region; (B) ear; (C) foot; (D) calfs; (E) thorax; (F) feet.
Ocular symptoms of GVHD can include dry eyes, redness, and sensitivity to light. Oral symptoms of GVHD can manifest as painful mouth sores and difficulty in swallowing (80). Gastrointestinal GVHD may lead to diarrhea, abdominal pain, and even malabsorption. Liver involvement may result in elevated liver enzymes and jaundice. Cough, shortness of breath, and lung inflammation are frequent manifestations of pulmonary site involvement of GVHD (36).
Understanding the site-specific manifestations of GVHD is essential for early diagnosis and tailored treatment strategies, as the severity and response to therapy can differ significantly depending on the affected organ. In this context, site-specific involvement represents a critical aspect of managing GVHD to optimize patient outcomes, one potential option being the protein-based biomarker deciphered below.
3.3.1 Protein biomarkers of GVHD with skin involvement
Tissue biopsies can help identify useful GVHD biomarkers, that can ultimately be sought in biofluids that can be sampled in a less invasive way. For example, skin biopsies can be used for skin GHVD biomarker identification.
Elafin, also known as skin-derived antileukoprotease (SKLP), is an inhibitor of epidermic protease induced by TNF-α and identified at the level of inflamed epidermis in autoimmune diseases such as psoriasis (1, 81). Mahabal et al. used skin biopsies and revealed that tissue elafin could be a useful biomarker of aGVHD with skin manifestations (SEN and SPE of 100% and 75%, respectively) (82). Additionally, proteomics studies have highlighted the potential of elafin as a plasmatic biomarker for skin GVHD (83), although the results may be controversial and need further evaluation in larger patient groups (84). Paczesny et al. found by quantitative liquid chromatography coupled with MS and further ELISA validation that elafin exhibited increased expression in GVHD skin biopsies. Elevated plasma elafin levels at skin GVHD onset, correlating with the maximum GVHD grade, were linked to a higher mortality risk (HR 1.78). This underscores the diagnostic and prognostic significance of elafin as a biomarker for skin GVHD (85).
3.3.2 Protein biomarkers of GVHD with ocular involvement
Another non-invasive biospecimen, the tears, is in the spotlight of researchers (86, 87). In a recent study focused on cGVHD with ocular symptoms, a team of researchers identified a tear cytokine panel comprising IL-8, IL-10, IFN-γ, CXCL9, CCL17, and CCL19 as promising biomarkers for early diagnosis of ocular GVHD. Moreover, the levels of IL-10, IFN-γ, and CXCL9 were found to be indicative of the severity of ocular GVHD following allo-HSCT (AUCs > 0.6) (88). Furthermore, in a separate investigation, the prognostic role of cytokine levels in tears prior to HSCT was examined in the context of ocular cGVHD. This pilot study included 25 patients who were prospectively monitored, with 19 cytokines measured using a multiplex bead assay. A multistate model, considering four states (HSCT, systemic cGVHD, ocular cGVHD, and death), was constructed to pinpoint cytokines linked to each transition probability. Fractalkine, IL-1Rα, and IL-6 emerged as key contributors with optimal prognostic value (AUC 67% to 80%) for predicting the development of ocular cGVHD after HSCT (89). In advancing understanding of ocular GVHD, a predictive model was developed by multiplex-bead assay at the tear molecule level. Analyzing a cytokine panels correlation with clinical features, the best model, incorporating IL-8/CXCL8, IP-10/CXCL10 tear levels, age, and sex, achieved an AUC of 0.9004, with 86.36% SEN and 95.24% SPE (87). Presence and role of autoantibodies after stem-cell transplantation and their association with cGVHD was also addressed (90) and anticardiolipin antibodies high plasmatic levels have been found to correlate with cGVHD with ocular involvement (91).
3.3.3 Protein biomarkers of GVHD with oral involvement
Because both aGVHD and cGVHD may present with oral alterations such as gingivitis, mucositis, xerostomia, mucosal atrophy, and ulcers, saliva is a target biofluid used in precision medicine approaches. Modification in salivary function is irreversible and reflects systemic GVHD, making saliva sampling and analysis a useful source of diagnostic biomarkers. Salivary proteins with potential biomarker role for oral cGVHD have been studied by MS and confirmed by ELISA or targeted label-free quantification. IL-1Rα and cystatin B distinguished oral cGVHD with a SEN of 85% and SPE of 60%. Particularly, in newly diagnosed patients assessed within 12 months of allo-HSCT, the markers exhibited enhanced discrimination (SEN 92% and SPE 73%) (92). A decline in salivary lactoperoxidase, lactotransferrin, and various proteins within the cysteine proteinase inhibitor family indicated compromised oral antimicrobial host immunity in patients with cGVHD (93). Also, high levels of lactoferrin and lactoperoxidase have been identified in the saliva of patients with GVHD with oral cavity involvement (92).
3.3.4 Protein biomarkers of GVHD with gastrointestinal involvement
GVHD with gastrointestinal involvement has been considered the major cause of morbidity, compared to all the other GVHD manifestations. Several biomarkers have been evaluated for their utility in gastrointestinal GVHD. Recently, the Reg3α has been identified as a potential biomarker for GVHD with intestinal involvement. Reg3α is a protein involved in cellular differentiation and proliferation and in defense against Gram-positive infections of the intestinal tract (1). Quantitative LC-MS analysis identified plasma Reg3α as a valuable predictor for diagnosing gastrointestinal aGVHD. Produced by Paneth cells in the gastrointestinal tract, Reg3α serves as an antimicrobial peptide with bactericidal properties against Gram-positive bacteria and antiapoptotic effects for Paneth cells (94). IL-22, secreted by lymphoid cells in the crypts of the gastrointestinal tract, stimulates Reg3α secretion. In the context of gastrointestinal GVHD, damage to the intestinal mucosa releases Reg3α into the bloodstream, making it a biomarker for gastrointestinal GVHD. Utilizing quantitative LC-MS, the predictive capability of plasma Reg3α for diagnosing gastrointestinal aGVHD was confirmed (AUC 0.80). Furthermore, combining Reg3α with clinical stage and histologic grade enhances risk stratification for patients (95).
Additionally, elevated plasma levels of TIM3, IL-6, and sTNFR1 demonstrated predictive value for the development of peak grade 3-4 GVHD (AUC 0.88). Plasma ST2 and sTNFR1 served as predictors for NRM within 1-year post-transplantation (AUC 0.90). In a landmark analysis, plasma TIM3 predicts subsequent grade 3-4 GVHD (AUC 0.76). Thus plasma levels of TIM3, sTNFR1, ST2, and IL-6 proved to be valuable in predicting more severe GVHD and NRM (96). In liver GVHD without gastrointestinal involvement, a rare occurrence (3% of GVHD patients), REG3α and HGF concentrations were elevated compared to asymptomatic patients but were like liver gastrointestinal GVHD, non-GVHD hyperbilirubinemia, and isolated skin GVHD. KRT18 concentrations were significantly higher in liver GVHD patients than in others, except those with non-GVHD liver complications. However, none of the three biomarkers effectively distinguished liver GVHD from non-GVHD liver complications. Including patients with concomitant gastrointestinal and liver involvement at GVHD onset, REG3α emerged as a stronger diagnostic biomarker for liver gastrointestinal GVHD compared to HGF and Keratin 18 (KRT18). REG3α, HGF, and KRT18 predicted day 28 nonresponse to therapy, while REG3α and HGF are good prognostic markers for 1-year NRM in liver-gastrointestinal GVHD patients (97).
The predictive value of two biomarkers, ST2 and Reg3α, in the case of NRM and GVHD, in allotransplant patients was highlighted. High concentrations of the two biomarkers tested in day 7 after transplant were associated with high GVHD related mortality and more frequent intestinal GVHD, demonstrating their usefulness in the prediction of GVHD before the clinical signs appear (63). Also, another study showed that higher levels of plasma Reg3α were found in patients with gastrointestinal-cGVHD, suggesting the utility of Reg3a as a prognostic biomarker of gastrointestinal-cGVHD (98).
Besides plasma, one more potentially useful source of biomarkers in the context of gastrointestinal GVHD is feces. Intestinal inflammation can be observed by accessing markers of leukocyte activation into the mucosa. A trial aimed to assess the dynamics of fecal biomarkers calprotectin and α1-antitrypsin (α1-AT) in GVHD. Steroid-refractory patients initially exhibited higher biomarker levels, which consistently increased throughout GVHD progression. In cortico-sensitive GvHD, calprotectin and α1-AT showed low and decreasing levels. Second-line treatment in refractory patients did not have predictive biomarker levels, but subsequent responders showed a progressive decrease in calprotectin, while non-responders maintained high levels. α1-AT values had a weaker correlation with treatment response, remaining elevated in both non-responders and responders. Monitoring calprotectin levels proved to be beneficial in managing immunosuppressive treatment for gastrointestinal GVHD (99). While there was a noticeable trend towards elevated serum calprotectin levels in GVHD development and gut involvement, statistical significance was not achieved, unlike fecal calprotectin. Therefore, fecal calprotectin, rather than serum calprotectin, may be considered a potential biomarker for gut GVHD as shown by Metafuni et al. (100).
3.3.5 Protein biomarkers of GVHD with pulmonary involvement
Pulmonary cGVHD can present with obstructive and/or restrictive disease. Severity ranges from subclinical pulmonary impairment to respiratory insufficiency with bronchiolitis obliterans, a feature of pulmonary cGVHD. Early diagnosis may improve clinical outcome, and regular post-transplant follow-ups are recommended (101).
In recent proteomics studies, regulatory proteins of the extracellular matrix have been identified as candidate biomarkers for the diagnostic and prognosis of bronchiolitis obliterans syndrome (BOS) and GVHD with pulmonary involvement. Plasmatic levels of matrix metalloproteinase 3 (MMP3) have been associated with the presence of BOS and could be correlated with disease severity (102). In another study, the diagnostic value of the lead candidate, MMP3, was evaluated by ELISA in plasma and was found to differ significantly between patients with and without BOS (AUC 0.77) (102). Moreover, in another study, the specific BOS biomarker potential of osteopontin (OPN) was explored in patients with cGVHD. Using immunohistochemistry, Williams et al. showed that elevated OPN plasma levels observed in patients with BOS, potentially originating from alveolar macrophages, correlated with disease severity. These findings, supported by lung immunohistochemistry, could be valuable for diagnosing and prognosing BOS after HSCT (103).
4 Conclusions
In summary, the exploration of protein-based biomarkers for GVHD post-allo-HSCT is crucial for improving diagnostic accuracy and treatment outcomes. Proteomics, with its ability to increase the potential of minimally invasive biopsies, serves as a powerful strategy for biomarker discovery by analyzing intricate protein profiles. Proteins, ranging from cytokines to emerging candidates, play a pivotal role as valuable biomarker sources. The strategies outlined, such as focusing on cytokine-based biomarkers, emerging protein-based markers, and biomarker panels for acute and chronic GVHD, as well as for site specific involvement of GVHD, offer a roadmap for future research, promising improved diagnostic precision and personalized treatment approaches. Nevertheless, the existing literature on GVHD protein-based biomarkers is characterized by substantial heterogeneity in reports, a lack of standardized study protocols, and inconsistent inclusion of patients. This heterogeneity and variability in methodology represent significant challenges that contribute to the observed disparity between the multitude of promising biomarkers identified in individual studies and the limited number of biomarkers poised for clinical translation. Consequently, the immediate focus may not solely be on the discovery of novel molecules and pathways, but rather on the critical task of validating or exposing the efficacy of existing methods. Addressing these challenges is imperative for bridging the gap between bench side research and clinical application in the near term.
However, the integration of proteomics into GVHD research not only enhances our understanding of the disease but also positions us at the forefront of transformative advancements in clinical practice. Collaborative efforts and technological advancements in proteomic analysis are essential for realizing the full potential of protein-based biomarkers, paving the way for a future where GVHD diagnosis and treatment are increasingly personalized and effective.
Author contributions
MI: Writing – original draft, Writing – review & editing. CP: Writing – original draft, Writing – review & editing. AU: Writing – original draft, Writing – review & editing. CM: Writing – original draft, Writing – review & editing. DC: Writing – original draft, Writing – review & editing. MZ: Writing – original draft, Writing – review & editing. AT: Writing – original draft, Writing – review & editing. JB: Writing – original draft, Writing – review & editing. VG: Writing – original draft, Writing – review & editing. MC: Writing – original draft, Writing – review & editing. CI: Writing – original draft, Writing – review & editing, Conceptualization, Formal analysis, Funding acquisition, Resources, Supervision. CT: Writing – original draft, Writing – review & editing, Conceptualization, Formal analysis, Resources, Supervision. DT: Writing – original draft, Writing – review & editing, Conceptualization, Formal analysis, Supervision.
Funding
The author(s) declare that no financial support was received for the research, authorship, and/or publication of this article.
Acknowledgments
Continuous Flow Interchange of Communication and Knowledge in Biomedical 533 University Research—FLOW”, No. 21-COP-0034.
Conflict of interest
The authors declare that the research was conducted in the absence of any commercial or financial relationships that could be construed as a potential conflict of interest.
Publisher’s note
All claims expressed in this article are solely those of the authors and do not necessarily represent those of their affiliated organizations, or those of the publisher, the editors and the reviewers. Any product that may be evaluated in this article, or claim that may be made by its manufacturer, is not guaranteed or endorsed by the publisher.
References
1. Ahmed SS, Wang XN, Norden J, Pearce K, El-Gezawy E, Atarod S, et al. Identification and validation of biomarkers associated with acute and chronic graft versus host disease. Bone Marrow Transplant. (2015) 50:1563–71. doi: 10.1038/bmt.2015.191
2. He FC, Holtan SG. Biomarkers in graft-versus-host disease: from prediction and diagnosis to insights into complex graft/host interactions. Curr Hematol Malig Rep. (2018) 13:44–52. doi: 10.1007/s11899-018-0433-2
3. Lee CJ, Wang T, Chen K, Arora M, Brazauskas R, Spellman SR, et al. Severity of chronic graft-versus-host disease and late effects following allogeneic hematopoietic cell transplantation for adults with hematologic Malignancy. Transplant Cell Ther (2023) 30(1):97.E1–97.E14. doi: 10.1016/j.jtct.2023.10.010
4. Jacobsohn DA, Vogelsang GB. Acute graft versus host disease. Orphanet J Rare Dis. (2007) 2:35. doi: 10.1186/1750-1172-2-35
5. Zeiser R, Blazar BR. Acute graft-versus-host disease — Biologic process, prevention, and therapy. N Engl J Med. (2017) 377:2167–79. doi: 10.1056/NEJMra1609337
6. Zeiser R, Blazar BR. Pathophysiology of chronic graft-versus-host disease and therapeutic targets. N Engl J Med. (2017) 377:2565–79. doi: 10.1056/NEJMra1703472
7. Presland RB. Application of proteomics to graft-versus-host disease: from biomarker discovery to potential clinical applications. Expert Rev Proteomics. (2017) 14:997–1006. doi: 10.1080/14789450.2017.1388166
8. Konuma T, Matsuda K, Shimomura Y, Tanoue S, Sugita J, Inamoto Y, et al. Effect of graft-versus-host disease on post-transplantation outcomes following single cord blood transplantation compared with haploidentical transplantation with post-transplantation cyclophosphamide for adult acute myeloid leukemia. Transplant Cell Ther. (2023) 29:365. doi: 10.1016/j.jtct.2023.03.001.
9. Tomuleasa C, Fuji S, Cucuianu A, Kapp M, Pileczki V, Petrushev B, et al. MicroRNAs as biomarkers for graft-versus-host disease following allogeneic stem cell transplantation. Ann Hematol. (2015) 94:1081–92. doi: 10.1007/s00277-015-2369-0
10. FDA-NIH Biomarker Working Group. BEST (Biomarkers, EndpointS, and other Tools) Resource [Internet]. Silver Spring (MD): Food and Drug Administration (US) (2016). Available from: https://www.ncbi.nlm.nih.gov/books/NBK326791/ Co-published by National Institutes of Health (US), Bethesda (MD).
11. Bravo-Merodio L, Acharjee A, Russ D, Bisht V, Williams JA, Tsaprouni LG, et al. Chapter Four - Translational biomarkers in the era of precision medicine. In: Makowski GSBT-A in CC. Amsterdam, The Netherlands: Elsevier (2021). p. 191–232. Available at: https://www.sciencedirect.com/science/article/pii/S0065242320300913.
12. Bidgoli A, DePriest BP, Saatloo MV, Jiang H, Fu D, Paczesny S. Current definitions and clinical implications of biomarkers in graft-versus-host disease. Transplant Cell Ther. (2022) 28:657–66. doi: 10.1016/j.jtct.2022.07.008
13. Spruance SL, Reid JE, Grace M, Samore M. Hazard ratio in clinical trials. Antimicrob Agents Chemother. (2004) 48:2787–92. doi: 10.1128/AAC.48.8.2787-2792.2004.
14. Wong CH, Siah KW, Lo AW. Estimation of clinical trial success rates and related parameters. Biostatistics [Internet]. (2019) 20:273–86. doi: 10.1093/biostatistics/kxx069
15. Wang J, Ma Z, Carr SA, Mertins P, Zhang H, Zhang Z, et al. Proteome profiling outperforms transcriptome profiling for coexpression based gene function prediction. Mol Cell Proteomics. (2017) 16:121–34. doi: 10.1074/mcp.M116.060301.
16. DAdamo GL, Widdop JT, Giles EM. The future is now? Clinical and translational aspects of “Omics” technologies. Immunol Cell Biol. (2021) 99:168–76. doi: 10.1111/imcb.12404
17. Qian L, Dima D, Berce C, Liu Y, Rus I, Raduly L-Z, et al. Protein dysregulation in graft versus host disease. Oncotarget. (2018) 9:1483–91. doi: 10.18632/oncotarget.v9i1.
18. Varady G, Cserepes J, Nemeth A, Szabo E, Sarkadi B. Cell surface membrane proteins as personalized biomarkers: where we stand and where we are headed. biomark Med. (2013) 7:803–19. doi: 10.2217/bmm.13.90.
19. Riva A. Editorial: Soluble immune checkpoints: Novel physiological immunomodulators. Front Immunol. (2023) 14:1178541. doi: 10.3389/fimmu.2023.1178541
20. Khan M, Zhao Z, Arooj S, Fu Y, Liao G. Soluble PD-1: predictive, prognostic, and therapeutic value for cancer immunotherapy. Front Immunol. (2020) 11:587460. doi: 10.3389/fimmu.2020.587460
21. Landeira-Vinuela A, Arias-Hidalgo C, Juanes-Velasco P, Alcoceba M, Navarro-Bailon A, Pedreira CE, et al. Unravelling soluble immune checkpoints in chronic lymphocytic leukemia: Physiological immunomodulators or immune dysfunction. Front Immunol. (2022) 13:965905. doi: 10.3389/fimmu.2022.965905
22. Wang Q, He Y, Li W, Xu X, Hu Q, Bian Z, et al. Soluble immune checkpoint-related proteins in blood are associated with invasion and progression in non-small cell lung cancer. Front Immunol. (2022) 13:887916. doi: 10.3389/fimmu.2022.887916
23. Noubissi Nzeteu GA, Schlichtner S, David S, Ruppenstein A, Fasler-Kan E, Raap U, et al. Macrophage differentiation and polarization regulate the release of the immune checkpoint protein V-domain ig suppressor of T cell activation. Front Immunol. (2022) 13:837097. doi: 10.3389/fimmu.2022.837097
24. Li W, Syed F, Yu R, Yang J, Xia Y, Relich RF, et al. Soluble immune checkpoints are dysregulated in COVID-19 and heavy alcohol users with HIV infection. Front Immunol. (2022) 13:833310. doi: 10.3389/fimmu.2022.833310
25. Cui M, Cheng C, Zhang L. High-throughput proteomics: a methodological mini-review. Lab Investig [Internet]. (2022) 102:1170–81. doi: 10.1038/s41374-022-00830-7
26. Chen G, Yang L, Liu G, Zhu Y, Yang F, Dong X, et al. Research progress in protein microarrays: Focussing on cancer research. Proteomics Clin Appl. (2023) 17:2200036. doi: 10.1002/prca.202200036
27. Marcus K, Eisenacher M, Sitek B. Quantitative methods in proteomics. Humana New York, NY: Springer Nature (2021). p. 2228. doi: 10.1007/978-1-0716-1024-4.
28. Nakayasu ES, Gritsenko M, Piehowski PD, Gao Y, Orton DJ, Schepmoes AA, et al. Tutorial: best practices and considerations for mass-spectrometry-based protein biomarker discovery and validation. Nat Protoc [Internet]. (2021) 16:3737–60. doi: 10.1038/s41596-021-00566-6
29. Bader JM, Albrecht V, Mann M. MS-based proteomics of body fluids: the end of the beginning. Mol Cell Proteomics. (2023) 22:100577. doi: 10.1016/j.mcpro.2023.100577
30. Mundt F, Albrechtsen NJW, Mann SP, Treit P, Ghodgaonkar-Steger M, OFlaherty M, et al. Foresight in clinical proteomics: current status, ethical considerations, and future perspectives. Open Res Eur. (2023) 3:59. doi: 10.12688/openreseurope.
31. Van Gool A, Corrales F, Colović M, Krstić D, Oliver-Martos B, Martinez-Caceres E, et al. Analytical techniques for multiplex analysis of protein biomarkers. Expert Rev Proteomics. (2020) 17:257–73. doi: 10.1080/14789450.2020.1763174
32. Srinivasan R, Daniels J, Fusaro V, Lundqvist A, Killian JK, Geho D, et al. Accurate diagnosis of acute graft-versus-host disease using serum proteomic pattern analysis. Exp Hematol. (2006) 34:796–801. doi: 10.1016/j.exphem.2006.02.013
33. Deutsch EW, Vizcaino JA, Jones AR, Binz P-A, Lam H, Klein J, et al. Proteomics standards initiative at twenty years: current activities and future work. J Proteome Res. (2023) 22:287–301. doi: 10.1021/acs.jproteome.2c00637
34. Paczesny S. Discovery and validation of graft-versus-host disease biomarkers. Blood. (2013) 121:585–94. doi: 10.1182/blood-2012-08-355990
35. Dayon L, Cominetti O, Affolter M. Proteomics of human biological fluids for biomarker discoveries: technical advances and recent applications. Expert Rev Proteomics. (2022) 19:131–51. doi: 10.1080/14789450.2022.2070477
36. Logan BR, Fu D, Howard A, Fei M, Kou J, Little MR, et al. Validated graft-specific biomarkers identify patients at risk for chronic graft-versus-host disease and death. J Clin Invest. (2023) 133:e168575. doi: 10.1172/JCI168575.
37. Kumar S, Mohammadpour H, Cao X. Targeting cytokines in GVHD therapy. J Immunol Res Ther. (2017) 2:90–9.
38. Shen M-Z, Li J-X, Zhang X-H, Xu L-P, Wang Y, Liu K-Y, et al. Meta-analysis of interleukin-2 receptor antagonists as the treatment for steroid-refractory acute graft-versus-host disease. Front Immunol. (2021) 12:749266. doi: 10.3389/fimmu.2021.749266
39. Tvedt THA, Ersvaer E, Tveita AA, Bruserud Ø. Interleukin-6 in allogeneic stem cell transplantation: its possible importance for immunoregulation and as a therapeutic target Front Immunol. (2017) 8:667. doi: 10.3389/fimmu.2017.00667
40. Dean RM, Fry T, Mackall C, Steinberg SM, Hakim F, Fowler D, et al. Association of serum interleukin-7 levels with the development of acute graft-versus-host disease. J Clin Oncol Off J Am Soc Clin Oncol. (2008) 26:5735–41. doi: 10.1200/JCO.2008.17.1314.
41. Poiret T, Rane L, Remberger M, Omazic B, Gustafsson-Jernberg A, Vudattu NK, et al. Reduced plasma levels of soluble interleukin-7 receptor during graft-versus-host disease (GVHD) in children and adults. BMC Immunol. (2014) 15:25. doi: 10.1186/1471-2172-15-25
42. Pirogova OV, Moiseev IS, Surkova EA, Lapin SV, Bondarenko SN, Kulagin AD, et al. Profiles of pro-inflammatory cytokines in allogenic stem cell transplantation with post-transplant cyclophosphamide. Cytokine. (2017) 99:148–53. doi: 10.1016/j.cyto.2017.08.016.
43. Kamel AM, Elsharkawy NM, Abdelfattah EK, Abdelfattah R, Samra MA, Wallace P, et al. IL12 and IFNγ secretion by donor mononuclear cells in response to host antigens may predict acute GVHD after HSCT. Immunobiology. (2019) 224:659–65. doi: 10.1016/j.imbio.2019.07.001.
44. Radujkovic A, Kordelas L, Dai H, Schult D, Majer-Lauterbach J, Beelen D, et al. Interleukin-18 and outcome after allogeneic stem cell transplantation: A retrospective cohort study. eBioMedicine. (2019) 49:202–12. doi: 10.1016/j.ebiom.2019.10.024
45. Liew FY, Pitman NI, McInnes IB. Disease-associated functions of IL-33: the new kid in the IL-1 family. Nat Rev Immunol. (2010) 10:103–10. doi: 10.1038/nri2692.
46. Ito S, Barrett AJ. ST2: the biomarker at the heart of GVHD severity. Blood. (2015) 125:10–1. doi: 10.1182/blood-2014-11-611780.
47. Miyazaki T, Matsumura A, Tachibana T, Ando T, Koyama M, Koyama S, et al. Predictive values of early ST2 for acute graft-versus-host disease and transplant-related complications after allogeneic stem cell transplantation. Blood. (2019) 134:1976. doi: 10.1182/blood-2019-123262
48. Vander Lugt MT, Braun TM, Hanash S, Ritz J, Ho VT, Antin JH, et al. ST2 as a marker for risk of therapy-resistant graft-versus-host disease and death. N Engl J Med. (2013) 369:529–39. doi: 10.1056/NEJMoa1213299
49. Azarpira N, Dehghani M, Darai M. The interleukin-6 and vascular endothelial growth factor in hematopoietic stem cell transplantation. Saudi J Kidney Dis Transplant an Off Publ Saudi Cent Organ Transplantation Saudi Arab. (2012) 23:521–5.
50. Riesner K, Shi Y, Jacobi A, Krater M, Kalupa M, McGearey A, et al. Initiation of acute graft-versus-host disease by angiogenesis. Blood. (2017) 129:2021–32. doi: 10.1182/blood-2016-08-736314
51. Holtan SG, Verneris MR, Schultz KR, Newell LF, Meyers G, He F, et al. Circulating angiogenic factors associated with response and survival in patients with acute graft-versus-host disease: results from blood and marrow transplant clinical trials network 0302 and 0802. Biol Blood Marrow Transplant. (2015) 21:1029–36. doi: 10.1016/j.bbmt.2015.02.018
52. Souza LN, Carneiro MA, De Azevedo WM, Gomez RS. Vascular endothelial growth factor (VEGF) and chronic graft-versus-host disease (cGVHD) in salivary glands of bone marrow transplant (BMT) recipients. J Oral Pathol Med. (2004) 33:13–6. doi: 10.1111/j.1600-0714.2004.00035.x
53. Min C-K, Kim SY, Lee MJ, Eom KS, Kim YJ, Kim HJ, et al. Vascular endothelial growth factor (VEGF) is associated with reduced severity of acute graft-versus-host disease and nonrelapse mortality after allogeneic stem cell transplantation. Bone Marrow Transplant. (2006) 38:149–56. doi: 10.1038/sj.bmt.1705410
54. Banerjee H, Kane LP. Immune regulation by tim-3. F1000Research. (2018) 7:316. doi: 10.12688/f1000research.
55. Oikawa T, Kamimura Y, Akiba H, Yagita H, Okumura K, Takahashi H, et al. Preferential involvement of Tim-3 in the regulation of hepatic CD8+ T cells in murine acute graft-versus-host disease. J Immunol. (2006) 177:4281–7. doi: 10.4049/jimmunol.177.7.4281.
56. Hansen JA, Hanash SM, Tabellini L, Baik C, Lawler RL, Grogan BM, et al. A novel soluble form of Tim-3 associated with severe graft-versus-host disease. Biol Blood marrow Transplant J Am Soc Blood Marrow Transplant. (2013) 19:1323–30. doi: 10.1016/j.bbmt.2013.06.011.
57. Toubai T, Shono Y, Nishihira J, Ibata M, Suigita J, Kato N, et al. Serum macrophage migration inhibitory factor (MIF) levels after allogeneic hematopoietic stem cell transplantation. Int J Lab Hematol. (2009) 31:161–8. doi: 10.1111/j.1751-553X.2007.01016.x.
58. Imanguli MM, Atkinson JC, Harvey KE, Hoehn GT, Ryu OH, Wu T, et al. Changes in salivary proteome following allogeneic hematopoietic stem cell transplantation. Exp. (2007) 35:184–92. doi: 10.1016/j.exphem.2006.10.009
59. Chiusolo P, Giammarco S, Fanali C, Bellesi S, Metafuni E, Sica S, et al. Salivary Proteomic Analysis and Acute Graft-versus-Host Disease after Allogeneic Hematopoietic Stem Cell Transplantation. Biol Blood Marrow Transplant. (2013) 19:888–92. doi: 10.1016/j.bbmt.2013.03.011
60. Paczesny S, Krijanovski OI, Braun TM, Choi SW, Clouthier SG, Kuick R, et al. A biomarker panel for acute graft-versus-host disease. Blood. (2009) 113:273–8. doi: 10.1182/blood-2008-07-167098
61. Levine JE, Logan BR, Wu J, Alousi AM, Bolanos-Meade J, Ferrara JLM, et al. Acute graft-versus-host disease biomarkers measured during therapy can predict treatment outcomes: a Blood and Marrow Transplant Clinical Trials Network study. Blood. (2012) 119:3854–60. doi: 10.1182/blood-2012-01-403063
62. Berger M, Signorino E, Muraro M, Quarello P, Biasin E, Nesi F, et al. Monitoring of TNFR1, IL-2Rα, HGF, CCL8, IL-8 and IL-12p70 following HSCT and their role as GVHD biomarkers in paediatric patients. Bone Marrow Transplant. (2013) 48:1230–6. doi: 10.1038/bmt.2013.41.
63. Hartwell MJ, Ozbek U, Holler E, Renteria AS, Major-Monfried H, Reddy P, et al. An early-biomarker algorithm predicts lethal graft-versus-host disease and survival. JCI Insight. (2017) 2:e89798. doi: 10.1172/jci.insight.89798.
64. Budde H, Papert S, Maas J-H, Reichardt HM, Wulf G, Hasenkamp J, et al. Prediction of graft-versus-host disease: a biomarker panel based on lymphocytes and cytokines. Ann Hematol. (2017) 96:1127–33. doi: 10.1007/s00277-017-2999-5
65. Pratta M, Paczesny S, Socie G, Barkey N, Liu H, Owens S, et al. A biomarker signature to predict complete response to itacitinib and corticosteroids in acute graft-versus-host disease. Br J Haematol. (2022) 198:729–39. doi: 10.1111/bjh.18300.
66. Major-Monfried H, Renteria AS, Pawarode A, Reddy P, Ayuk F, Holler E, et al. MAGIC biomarkers predict long-term outcomes for steroid-resistant acute GVHD. Blood. (2018) 131:2846–55. doi: 10.1182/blood-2018-01-822957.
67. Presland RB.Biology of chronic graft-vs-host disease: Immune mechanisms and progress in biomarker discovery. World J Transplant. (2016) 6:608–19. doi: 10.5500/wjt.v6.i4.608.
68. Wolff D, Greinix H, Lee SJ, Gooley T, Paczesny S, Pavletic S, et al. Biomarkers in chronic graft-versus-host disease: quo vadis? Bone Marrow Transplant. (2018) 53:832–7. doi: 10.1038/s41409-018-0092-x
69. Kitko CL, Levine JE, Storer BE, Chai X, Fox DA, Braun TM, et al. Plasma CXCL9 elevations correlate with chronic GVHD diagnosis. Blood [Internet]. (2014) 123:786–93. doi: 10.1182/blood-2013-08-520072
70. Hirayama M, Azuma E, Nakagawa-Nakazawa A, Kumamoto T, Iwamoto S, Amano K, et al. Interleukin-10 spot-forming cells as a novel biomarker of chronic graft-versus-host disease. Haematologica. (2013) 98:41–9. doi: 10.3324/haematol.2012.069815.
71. Giesen N, Schwarzbich M-A, Dischinger K, Becker N, Hummel M, Benner A, et al. CXCL9 predicts severity at the onset of chronic graft-versus-host disease. Transplantation. (2020) 104:2354–9. doi: 10.1097/TP.0000000000003108.
72. Sarantopoulos S, Stevenson KE, Kim HT, Bhuiya NS, Cutler CS, Soiffer RJ, et al. High levels of B-cell activating factor in patients with active chronic graft-versus-host disease. Clin Cancer Res an Off J Am Assoc Cancer Res. (2007) 13:6107–14. doi: 10.1158/1078-0432.CCR-07-1290.
73. Saliba RM, Sarantopoulos S, Kitko CL, Pawarode A, Goldstein SC, Magenau J, et al. B-cell activating factor (BAFF) plasma level at the time of chronic GvHD diagnosis is a potential predictor of non-relapse mortality. Bone Marrow Transplant. (2017) 52:1010–5. doi: 10.1038/bmt.2017.73
74. Cuvelier GDE, Kariminia A, Fujii H, Aslanian S, Wall D, Goldman F, et al. Anti-CD13 Abs in children with extensive chronic GVHD and their relation to soluble CD13 after allogeneic blood and marrow transplantation from a Childrens Oncology Groups Study, ASCT0031. Bone Marrow Transplant. (2010) 45:1653–7. doi: 10.1038/bmt.2010.15.
75. Inamoto Y, Martin PJ, Paczesny S, Tabellini L, Momin AA, Mumaw CL, et al. Association of plasma CD163 concentration with de novo-onset chronic graft-versus-host disease. Biol Blood marrow Transplant J Am Soc Blood Marrow Transplant. (2017) 23:1250–6. doi: 10.1016/j.bbmt.2017.04.019.
76. Fujii H, Cuvelier G, She K, Aslanian S, Shimizu H, Kariminia A, et al. Biomarkers in newly diagnosed pediatric-extensive chronic graft-versus-host disease: a report from the Childrens Oncology Group. Blood. (2008) 111:3276–85. doi: 10.1182/blood-2007-08-106286
77. Yu J, Storer BE, Kushekhar K, Abu Zaid M, Zhang Q, Gafken PR, et al. Biomarker panel for chronic graft-versus-host disease. J Clin Oncol. (2016) 34:2583–90. doi: 10.1200/JCO.2015.65.9615
78. Weissinger EM, Human C, Metzger J, Hambach L, Wolf D, Greinix HT, et al. The proteome pattern cGvHD_MS14 allows early and accurate prediction of chronic GvHD after allogeneic stem cell transplantation. Leukemia [Internet]. (2017) 31:654–62. doi: 10.1038/leu.2016.259
79. Hong J, Fraebel J, Yang Y, Tkacyk E, Kitko C, Kim TK. Understanding and treatment of cutaneous graft-versus-host-disease. Bone Marrow Transplant. (2023) 58, 1298–313. doi: 10.1038/s41409-023-02109-x.
80. Cheng X, Huang R, Huang S, Fan W, Yuan R, Wang X, et al. Recent advances in ocular graft-versus-host disease. Front Immunol. (2023) 14:1092108. doi: 10.3389/fimmu.2023.1092108.
81. Qian L, Wu Z, Shen J. Advances in the treatment of acute graft-versus-host disease. J Cell Mol Med. (2013) 17:966–75. doi: 10.1111/jcmm.12093
82. Mahabal GD, George L, Peter D, Bindra M, Thomas M, Srivastava A, et al. Utility of tissue elafin as an immunohistochemical marker for diagnosis of acute skin graft-versus-host disease: a pilot study. Clin Exp Dermatol. (2019) 44:161–8. doi: 10.1111/ced.13678
83. Paczesny S, Levine JE, Hogan J, Crawford J, Braun T, Wang H, et al. Elafin is a biomarker of graft versus host disease of the skin. Blood. (2008) 112:716. doi: 10.1182/blood.V112.11.716.716
84. George L, Mahabal G, Mohanan E, Balasubramanian P, Peter D, Pulimood S, et al. Limited utility of plasma elafin as a biomarker for skin graft-versus-host disease following allogeneic stem cell transplantation. Clin Exp Dermatol. (2021) 46:1482–7. doi: 10.1111/ced.14785
85. Paczesny S, Braun TM, Levine JE, Hogan J, Crawford J, Coffing B, et al. Elafin is a biomarker of graft-versus-host disease of the skin. Sci Transl Med. (2010) 2:13ra2–2. doi: 10.1126/scitranslmed.3000406
86. Jung JW, Han SJ, Song MK, Kim T-I, Kim EK, Min YH, et al. Tear cytokines as biomarkers for chronic graft-versus-host disease. Biol Blood marrow Transplant J Am Soc Blood Marrow Transplant. (2015) 21:2079–85. doi: 10.1016/j.bbmt.2015.08.020.
87. Cocho L, Fernandez I, Calonge M, Martinez V, Gonzalez-Garcia MJ, Caballero D, et al. Biomarkers in ocular chronic graft versus host disease: tear cytokine- and chemokine-based predictive model. Invest Ophthalmol Vis Sci. (2016) 57:746–58. doi: 10.1167/iovs.15-18615
88. Cheng X, Huang R, Fan W, Huang S, Zeng L, Wu T, et al. The tear cytokine panel is a useful biomarker for early diagnosis and severity-evaluating of ocular chronic graft-versus-host disease. Bone Marrow Transplant [Internet]. (2023) 58:732–4. doi: 10.1038/s41409-023-01952-2
89. Cocho L, Fernandez I, Calonge M, Sainz de la Maza M, Rovira M, Stern ME, et al. Prehematopoietic stem cell transplantation tear cytokines as potential susceptibility biomarkers for ocular chronic graft-versus-host disease. Invest Ophthalmol Vis Sci. (2017) 58:4836–46. doi: 10.1167/iovs.17-21670.
90. Wechalekar A, Cranfield T, Sinclair D, Ganzckowski M. Occurrence of autoantibodies in chronic graft vs. host disease after allogeneic stem cell transplantation. Clin Lab Haematol. (2005) 27:247–9. doi: 10.1111/j.1365-2257.2005.00699.x
91. Quaranta S, Shulman H, Ahmed A, Shoenfeld Y, Peter J, McDonald GB, et al. Autoantibodies in human chronic graft-versus-host disease after hematopoietic cell transplantation. Clin Immunol. (1999) 91:106–16. doi: 10.1006/clim.1998.4666.
92. Devic I, Shi M, Schubert MM, Lloid M, Izutsu KT, Pan C, et al. Proteomic analysis of saliva from patients with oral chronic graft-versus-host disease. Biol Blood Marrow Transplant. (2014) 20:1048–55. doi: 10.1016/j.bbmt.2014.03.031
93. Bassim CW, Ambatipudi KS, Mays JW, Edwards DA, Swatkoski S, Fassil H, et al. Quantitative salivary proteomic differences in oral chronic graft-versus-host disease. J Clin Immunol. (2012) 32:1390–9. doi: 10.1007/s10875-012-9738-4
94. Shin JH, Seeley RJ. Reg3 proteins as gut hormones? Endocrinology. (2019) 160:1506–14. doi: 10.1210/en.2019-00073
95. Ferrara JLM, Harris AC, Greenson JK, Braun TM, Holler E, Teshima T, et al. Regenerating islet-derived 3-alpha is a biomarker of gastrointestinal graft-versus-host disease. Blood. (2011) 118:6702–8. doi: 10.1182/blood-2011-08-375006
96. McDonald GB, Tabellini L, Storer BE, Lawler RL, Martin PJ, Hansen JA. Plasma biomarkers of acute GVHD and nonrelapse mortality: predictive value of measurements before GVHD onset and treatment. Blood. (2015) 126:113–20. doi: 10.1182/blood-2015-03-636753
97. Harris AC, Ferrara JLM, Braun TM, Holler E, Teshima T, Levine JE, et al. Plasma biomarkers of lower gastrointestinal and liver acute GVHD. Blood. (2012) 119:2960–3. doi: 10.1182/blood-2011-10-387357.
98. DePriest BP, Li H, Bidgoli A, Onstad L, Couriel D, Lee SJ, et al. Regenerating islet-derived protein 3-α is a prognostic biomarker for gastrointestinal chronic graft-versus-host disease. Blood Adv. (2022) 6:2981–6. doi: 10.1182/bloodadvances.2021005420
99. OMeara A, Kapel N, Xhaard A, Sicre de Fontbrune F, Manene D, Dhedin N, et al. Fecal calprotectin and α1-antitrypsin dynamics in gastrointestinal GvHD. Bone Marrow Transplant. (2015) 50:1105–9. doi: 10.1038/bmt.2015.109
100. Metafuni E, Giammarco S, De Ritis DG, Rossi M, De Michele T, Zuppi C, et al. Fecal but not serum calprotectin is a potential marker of GVHD after stem cell transplantation. Ann Hematol. (2017) 96:929–33. doi: 10.1007/s00277-017-2974-1
101. Hildebrandt GC, Fazekas T, Lawitschka A, Bertz H, Greinix H, Halter J, et al. Diagnosis and treatment of pulmonary chronic GVHD: report from the consensus conference on clinical practice in chronic GVHD. Bone Marrow Transplant. (2011) 46:1283–95. doi: 10.1038/bmt.2011.35
102. Liu X, Yue Z, Yu J, Daguindau E, Kushekhar K, Zhang Q, et al. Proteomic characterization reveals that MMP-3 correlates with bronchiolitis obliterans syndrome following allogeneic hematopoietic cell and lung transplantation. Am J Transplant. (2016) 16:2342–51. doi: 10.1111/ajt.13750
Keywords: allogenic stem cell transplantation, graft-versus-host-disease, biomarker, proteomics, biofluids
Citation: Iacobescu M, Pop C, Uifălean A, Mogoşan C, Cenariu D, Zdrenghea M, Tănase A, Bergthorsson JT, Greiff V, Cenariu M, Iuga CA, Tomuleasa C and Tătaru D (2024) Unlocking protein-based biomarker potential for graft-versus-host disease following allogenic hematopoietic stem cell transplants. Front. Immunol. 15:1327035. doi: 10.3389/fimmu.2024.1327035
Received: 25 October 2023; Accepted: 01 February 2024;
Published: 16 February 2024.
Edited by:
Stefan Eugen Szedlacsek, Institute of Biochemistry of the Romanian Academy, RomaniaReviewed by:
Shigeo Fuji, Osaka International Cancer Institute, JapanPranali Shah, Brigham and Womens Hospital and Harvard Medical School, United States
Copyright © 2024 Iacobescu, Pop, Uifălean, Mogoşan, Cenariu, Zdrenghea, Tănase, Bergthorsson, Greiff, Cenariu, Iuga, Tomuleasa and Tătaru. This is an open-access article distributed under the terms of the Creative Commons Attribution License (CC BY). The use, distribution or reproduction in other forums is permitted, provided the original author(s) and the copyright owner(s) are credited and that the original publication in this journal is cited, in accordance with accepted academic practice. No use, distribution or reproduction is permitted which does not comply with these terms.
*Correspondence: Cristina Adela Iuga, aXVnYWNAdW1mY2x1ai5ybw==
†These authors have contributed equally to this work and share first authorship
‡These authors have contributed equally to this work and share last authorship