- 1Students Scientific Research Center, Tehran University of Medical Sciences, Tehran, Iran
- 2Nanobiology and Nanomedicine Research Center, Shiraz University of Medical Sciences, Shiraz, Iran
- 3Laboratory of Experimental Pharmacology, IRCCS Istituto Tumori Giovanni Paolo II, Bari, Italy
- 4Cellular and Molecular Research Committee, Birjand University of Medical Sciences, Birjand, Iran
For many years, vitamin D has been acknowledged for its role in maintaining calcium and phosphate balance. However, in recent years, research has assessed its immunomodulatory role and come up with conflicting conclusions. Because the vitamin D receptor is expressed in a variety of immune cell types, study into the precise role of this molecule in diseases, notably autoimmune disorders, has been made possible. The physiologically activated version of vitamin D also promotes a tolerogenic immunological condition in addition to modulating innate and acquired immune cell responses. According to a number of recent studies, this important micronutrient plays a complex role in numerous biochemical pathways in the immune system and disorders that are associated with them. Research in this field is still relatively new, and some studies claim that patients with severe autoimmune illnesses frequently have vitamin D deficiencies or insufficiencies. This review seeks to clarify the most recent research on vitamin D’s immune system-related roles, including the pathophysiology of major disorders.
Introduction
Vitamin D is an attractive molecule that has received particular attention recently. It is a major calcium homeostasis and bone metabolism modulator, increasing phosphorus and calcium absorption from the intestine, decreasing their excretion from the kidney, and promoting osteogenesis (1). It is also one of the crucial immune system regulator hormones that affect immune responses. Vitamin D acts as a pro-survival molecule. This vitamin defends cells against damaging signals by inhibiting inflammatory responses, such as changing the pathways through which T-helper-2 (Th2), M2 macrophages, and regulatory T cells (Treg) differentiate in order to maintain energy and redox homeodynamics by supplying a tolerogenic state (2). Vitamin D insufficiency continues to be one of the most common causes of osteoporosis, muscle weakness, falling fractures (particularly in the elderly), numerous malignancies, metabolic syndrome, cardiovascular illnesses, immune-mediated diseases (including autoimmune diseases), and infections (3–8). Ultraviolet (UV) rays that reach the epidermal layer of the skin and regular diet are the two main sources of vitamin D. That suggests that it should not be viewed solely as a vitamin: pro-hormone is the proper category (9). The normal range for vitamin D is still disputed among different nations. However, it is generally acknowledged that an adult can deal with a lower limit of 50–75 nmol/L. Numerous problems, including impaired bone metabolism, falling risk, various myopathies, and immune system dysregulation, are linked to low vitamin D levels (9–12).
The amount of vitamin D the body receives from food sources is typically insufficient, and these abundant sources are also scarce. These are the main factors that contribute to vitamin D deficiency (13). Vitamin D is present in small amounts in butter, peanuts, and eggs, but is present in large quantities in some foods like fish liver oil. Additionally, both cow milk and breast milk are deficient in vitamin D (1, 14). Despite the fact that vitamin D can be gained through diet, the skin is still the primary source of this pro-hormone. Seven-dehydrogenated cholesterol undergoes spontaneous transformation into vitamin D3 under the influence of UV radiation (13). The primary molecule that carries vitamin D and its subsequent metabolites in the blood is vitamin D binding protein (DBP). This vitamin needs to be hydroxylated twice in order to be physiologically active (14). The expression of the enzyme 1-hydroxylase, which converts 25-hydroxyvitamin D3 (25-OH D3) (precursor) to 1,25-(OH)2 D3 enabling immune microenvironments to respond to this substance in antigen-presenting cells, is one of the factors contributing to the recent interest in the immune-related function of vitamin D (13). It is initially hydroxylated by hydroxylase and several cytochrome P450 isoforms on the 25th carbon. Vitamin D status is mostly tracked by measuring 25-OH D3, which has a 2-week half-life and is the primary form of the vitamin in circulation.
As a result of the CYP27B1 gene expression, 1-alpha-hydroxylase carries out the second stage of hydroxylation in the kidney, skin, and immune cells, resulting in the production of 1,25-(OH)2 D3 or calcitriol (15, 16). The binding of vitamin D to its nuclear receptors, known as vitamin D receptors or VDRs, inside target cells controls gene expression. VDR is also found in non-classical tissues like the brain, eye, heart, pancreatic islet beta cells, and immune cells. The principal mechanism of action of vitamin D in those tissues is metabolism (17). The identification of VDR in immune cells is related to the putative function of vitamin D in controlling immunological responses, cell proliferation, differentiation, and apoptosis induction. B cells, TCD4, TCD8-activated cells, neutrophils, monocytes, macrophages, NK cells, and dendritic cells all contain this receptor (18, 19). It modifies the genes of chromatin-modifying enzymes through direct and indirect interactions (20).
The VDR gene, which has 10 introns and 11 exons, is located on chromosome 12. It contains more than 900 SNPs, according to the literature. The DNA binding domain is encoded by exons 2 and 3, where the majority of these polymorphisms accumulate. Exon changes result in alterations to this domain and impact the structure of the receptor, which precludes vitamin D binding (21). Vitamin D receptors molecular signaling is somehow complex. The VDR binds to retinoid X receptors to form a complex, which is what makes up the traditional route. By attaching to the VDR-RXR, vitamin D controls the expression of many genes. VDR could, however, manifest as an intra-membranous receptor. These are the main substances that initiate the alternate routes that activate the cytochromes. Secosteroids are among the chemicals that are produced by the downstream enzymes. The metabolites modulate the function of some transcription factors and alter gene expression (22, 23).
Vitamin D and the innate immune system
The innate immune system, which is the body’s first line of defense against pathogens, is in charge of quick reactions, pathogen detection, and elimination to stop an illness from getting worse. Vitamin D has a crucial role in innate immunity by stimulating the production of pattern recognition receptors (PRRs), antimicrobial peptides, and cytokines in the cells. Additionally, it can prevent the maturation and activation of dendritic cells as well as the differentiation of monocytes into macrophages. Cathelicidins, alpha- and beta-defensins, and other cationic antimicrobial peptides make up the majority of the immune system. The primary function of vitamin D signaling is to control innate immunological responses, according to numerous studies, especially those conducted in recent decades (24). The production of antimicrobial peptides by intestinal epithelial cells, Paneth cells, monocyte/macrophages, and neutrophils is one of the key factors in this control (24). Since the middle of the nineteenth century, vitamin D has been known to induce the antibacterial activity of human monocytes and macrophages (25). The phagocytic and chemo-like activity of macrophages is enhanced by 1,25-(OH)2 D3 (26). The generation of antimicrobial cathelicidin peptides is stimulated by the activation of the toll-like receptor in monocytes and macrophages, which also results in the positive expression of the VDR and alpha 1-hydroxylase genes, killing intrathecal Mycobacterium tuberculosis (27). However, 1,25-(OH)2 D3 suppresses the expression of TLR2 and TLR4 genes in macrophages. After 72 hours, this situation frequently takes control and negatively affects TLR activation and inflammation during the late infection stage. The conversion of 25-OH D3 into 1,25-(OH)2 D3 occurs during infection as a result of increased CYP27B1 expression in activated macrophages and monocytes brought on by cytokines like interferon- γ (IFN- γ) along with toll-like receptor signaling. The 1,25-(OH)2 D3 is then used to enhance the antibacterial activity of macrophages and monocytes via the VDR-RXR pathway. The outcome is an increase in cathelicidin production. The microbial membranes of invasive bacteria and fungus are destabilized by this peptide (28). As a result, 1,25-(OH)2 D3 is immunomodulatory in Mycobacterium TB infection (29). Antimicrobial peptides including 2-defensin and cathelicidin are activated by the calcitriol complex, retinoid X receptor, and VDR (30). Human cathelicidins, such as hCAP18 (either its 17-kDa (140 amino acids) or 5-kDa (37 amino acids) forms) and Leucine-leucine-37 (LL-37), isolated from a large prepropeptide in immune cells (neutrophils) or non-immune tissues (like testis), act against bacteria, fungi, and viruses as a response to infections and destroy microbial membranes (31). Vitamin D signaling has been shown to influence the physiological intestinal tract, aid intestinal hemostasis, and regulate microbiota in healthy individuals (24). Innate lymphoid cells (ILCs) and NK cells are also affected by 1,25-(OH)2 D3. T cell and dendritic cell (DC) responses are modulated by NK cells, which are crucial components of the innate immune system (32). ILCs are a crucial component of immunity. All mucosal tissues, particularly the colon, contain these cells. They are also among the first immune cells to promote cell growth, the healing of wounds, the release of anti-inflammatory mediators in response to infections, and the secretion of antimicrobial peptides (33–35). Through their VDR receptors, 1,25-(OH)2 D3 enhances the cytotoxic activity of NK cells and ILCs. The expression of their inflammatory cytokines is similarly decreased (Figure 1) (24, 36–40) (Table 1).
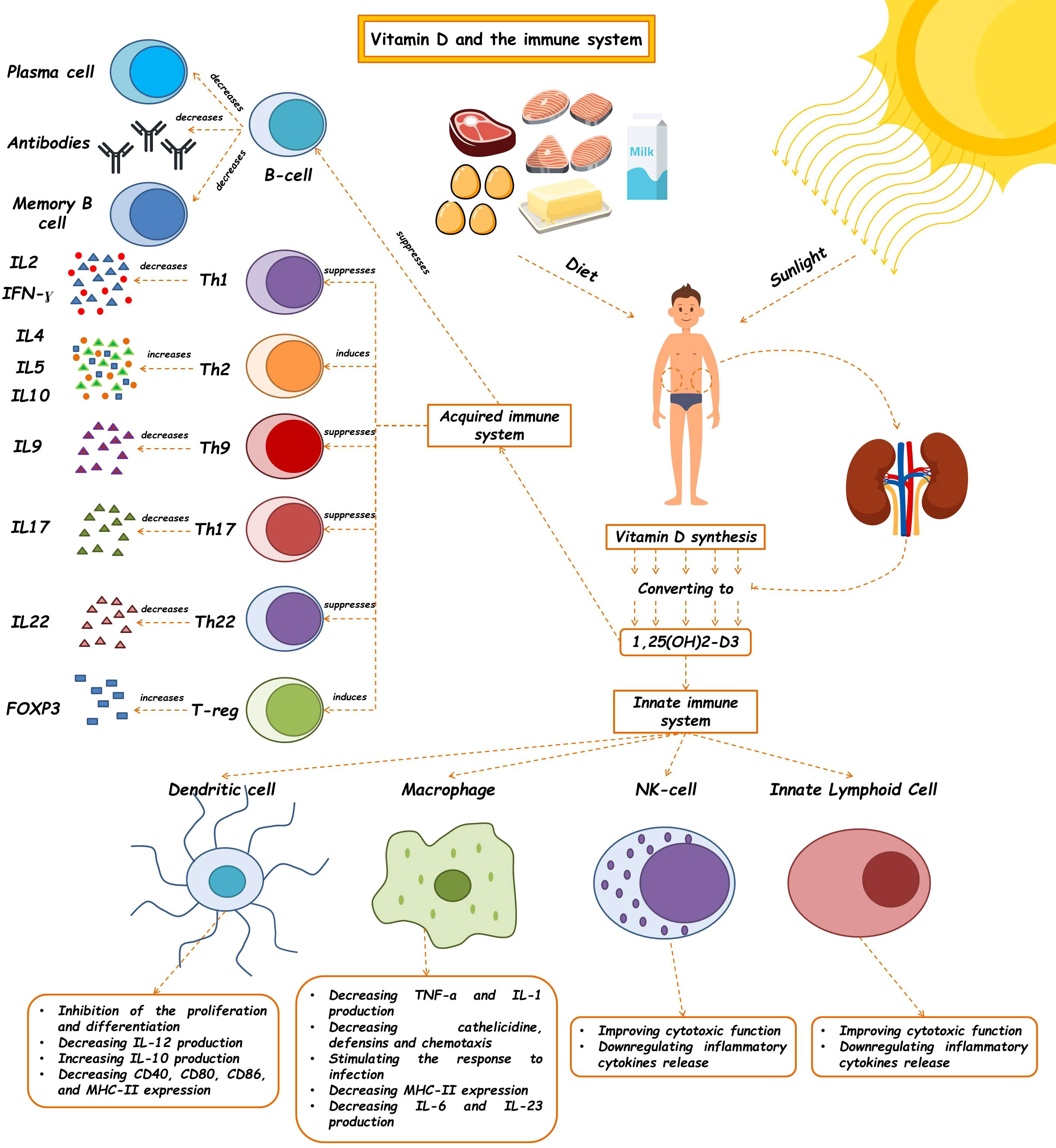
Figure 1 The effects of vitamin D on the immune system. Vitamin D and 1,25(OH)2D3 modulate the innate immune response. The regulatory role of this molecule has been shown to affect the innate immune system, such as macrophages, dendritic cells, Nk cells, and ILCs, so, as a critical molecule plays a role in many diseases' pathophysiology. This vitamin also contributes to making an acquired immune response. (See text for additional details). Th, T helper cell; IL, Interleukin; IFN-γ, Interferon-γ.
Vitamin D and the acquired immune system
Although the active form of vitamin D leads to the stimulation of innate immune responses, this vitamin causes the suppression of acquired immune responses (Table 2). The most significant secretors of IL-2 and interferon-γ (IFN-γ) are T-helper 1 (Th1) cells, induced by the activation of the T cell receptor (TCR) and CD4+ membranous protein. In fact, Th1 is a key player in stimulating other inflammatory leukocytes and serves as a marker of cellular immunity activation. 1,25-(OH)2 D3 inhibits the synthesis of the pro-inflammatory cytokines IL-2 and IFN γ as well as Th1-mediated responses. By inhibiting the nuclear factor of activated T cells (NFAT) and activator protein-1 (AP-1) factors, 1,25-(OH)2 D3 encounters this inhibitory action (49). Additionally, 1,25-(OH)2 D3 increases the production of Th2 anti-inflammatory cytokines (including IL-3, -4, -5, and -10), while decreasing the production of Th9 (IL-9) and Th22 (IL-22) pro-inflammatory cytokines (48, 50). IL-9 is essential for attracting immune cells to the sites of inflammation, particularly mast cells. It is mostly produced by Treg cells. Th9 cell release of IL-9 may be the cause of allergic reactions and inflammatory reactions. The majority of IL-22-producing body cells are still Th22 cells. This crucial interleukin promotes the proliferation and development of keratinocytes. IL-22 enhances the secretion of anti-microbial peptides in the gut epithelium, which may result in a more effective defense against encroaching microorganisms. The anti-inflammatory function of T-regulatory cells, which is crucial for autoimmune control and homeostasis, is induced by 1,25-(OH)2 D3. The primary transcription factor in this class is Forkhead Box P3 (Foxp3). It is essential for 1,25-(OH)2 D3’s transcriptional upregulation as well as for the maturation, maintenance, and function of Tregs (51). It has been suggested that 1,25-(OH)2 D3 in both in vivo and in vitro conditions increases the amount of Tregs to reduce autoimmunity (52). In general, 1,25-(OH)2 D3 suppresses the induction of T-helper 1 (Th1)-cell cytokines, particularly IFN-γ; however, it also enhances Th2-cell immunological responses, which are mediated both directly by raising IL-4 production and indirectly by suppressing IFN-γ production (53). Many autoimmune and inflammatory disorders, including systemic lupus erythematosus, multiple sclerosis, and rheumatoid arthritis, are caused by Th17 cells, a type of inflammatory lymphocytes that promote inflammation and phagocytosis, notably neutrophils. Through mechanisms such as inhibition of the NFAT and RORt transcription factors, recruitment of histone deacetylase (HDAC), Runt-related transcription factor 1 (Runx1), and a direct impact on induction of Foxp3, the complex of vitamin D/VDR would result in the suppression of IL-17 production (54). Additionally, it may result in T cell homing at inflammatory areas and in the skin via CCR5 and CCR10, respectively. The homeostasis of B cells is directly impacted by VDR expression in their membrane. The active form of vitamin D causes apoptosis in antibody-producing cells, neutralizing the production and differentiation of B cells into plasma cells and memory cells, reducing the production of antibodies, and increasing the homing of these cells through CCR10 to the skin (53). The clinical significance of this influence on B cells can be shown in autoimmune illnesses including those linked to autoreactive antibodies. B cells produced a variety of autoantibodies. These have the power to harm the organs and start an inflammatory reaction that leads to autoimmune diseases. Additionally, a large number of regulatory B cells have been found to suppress the production of autoantibodies (55). In reality, the biologically active form of vitamin D suppresses B cell proliferation and controls their responses. Through lowering stimulant molecules like CD40, CD80, CD86, and MHC class-II in cells presenting antigens like dendritic cells, this vitamin also leads to T cell suppression and immune response modification (Figure 1) (41). Let us explore the specific roles that vitamin D plays in some key immune-related disorders in the present review (Table 3).
Allergic rhinitis
Allergic rhinitis (AR) is a nasal mucosal disease characterized by an allergen reaction due to IgE production (72). The role of vitamin D is explained in multiple investigations related to this disorder. Examining the relationship between vitamin D serum levels and allergic diseases in adults using the third National Health and Nutrition Survey (NHANES III), Wjst et al. noted that AR worsened in vitamin D-deficient patients (73). Another Finnish study tried to investigate maternal intake of vitamin D in the course of pregnancy and its association with asthma and allergic rhinitis incidence in newborns. They recruited 1,669 children affected by allergic rhinitis, atopic eczema, and asthma. It was discovered that high levels of vitamin D intake in pregnant women were related to an allergic risk reduction in 5-year-old children (74). Additionally, Chen et al. suggested that prenatal vitamin D sufficiency has a protective effect on developing allergic rhinitis and aeroallergen sensitization at childhood (75).
Furthermore, pieces of evidence from clinical investigations demonstrated that AR risk can be inversely related to the serum vitamin D level (76, 77). On the contrary, Feng et al. indicated no causal association between them. This study goes against the previous claims of vitamin D supplementation’s protective effects on allergic rhinitis (78). In addition, Searing et al., in an in vitro study, explained that this important vitamin may affect dexamethasone’s stimulation on circulatory mononuclear cells’ IL-10 and mitogen-activated protein kinase phosphatase-1 (MAPKP-1). Vitamin D levels reflect a negative correlation with the dose of the inhaler, oral of steroids. The induced mRNA amount of mitogen-activated protein kinase phosphatase-1 (MKP-1) and IL-10-induced vitamin D with dexamethasone was significantly higher than those induced by dexamethasone by itself. MKP-1 and IL-10 are critical for glucocorticoid-dependent anti-inflammatory and repressive effects (79). Also, according to other in vitro studies, there is growing evidence that serum vitamin D level is correlated with inflammatory factors. Eosinophil infiltration, allergic symptom scores, and mRNA levels of IL-4 and IL-13 were all lower in the mice group under vitamin D supplementation. Moreover, CD86 expression in the cervical lymph nodes was decreased among T-helper type 2-mediated inflammation and CD11c+major histocompatibility complex II-high (MHCII high) cells. IL-4 was also conversely correlated with vitamin D levels (56, 80).
The MiR-17-92 cluster is a human miRNA located on chromosome 13. Increasing the expression of this miRNA has been reported in many immune disorders such as cancers and some allergic diseases, but the regulatory mechanism is not well defined. One way to treat allergic diseases is specific immunotherapy. It has been noted that vitamin D raises specific immunotherapy effects on MiR-17-92 cluster suppression in peripheral B cells in allergic rhinitis patients (81). This study confirmed that vitamin D improves the anti-inflammatory function of corticosteroids in patients with allergic rhinitis. Regarding the effects of vitamin D supplementation in the treatment regimen of allergic rhinitis, Bakhshaee et al. concluded that it could relatively improve symptoms of clinical AR, further emphasizing vitamin D supplementation as a therapeutic agent (82).
Asthma
The role of vitamin D in asthma is still uncertain. The CDX2 polymorphism is in the 9,913th position in the promoter region of the VDR gene emerging from the substitution of A with G. It has been mentioned that this polymorphism changes the levels of VDR transcription and its overall activity. It also subsequently modulates the genes effective in inflammation, regulation of immunity, and airways remodeling. This polymorphism in its homozygous form has been associated with the diagnosis of asthma and decreased FEV1 (21).
Some cross-sectional studies have indicated a possible relationship between asthma and vitamin D clinically (83). Studies have concluded that a drop in serum level of 1,25-(OH)2 D3 was associated with an advance in prevalence, hospitalization, the number of emergency visits, respiratory rate, and a reduction in lung function in children with asthma (84). Recent clinical trials have proved the protective effect of vitamin D supplements in asthmatic patients (85–87). One study demonstrated that weekly oral calcifediol supplementation in vitamin D-deficient adults could help control the disease course and improve patients’ quality of life (88). One study by Gapta et al. reported that the low serum levels of vitamin D in children with steroid-resistant asthma (STRAs) are characterized by decreased lung function, increased corticosteroid use, and asthma exacerbation. The possible reason was that low vitamin D levels help smooth muscles of the respiratory tract to grow and diminish lung function in severe asthma (89). Examples of vitamin D activity in asthma include improving the immune function of the lung tissue and preventing the development of respiratory infections (85, 90, 91) or overcoming resistance to steroids by increasing the production of IL -10 via CD4 + T cells (92). In contrast with the asthma prevention duty of vitamin D, it can cause the exacerbation of it. Low levels of vitamin D (<30 ng/mL) increase the risk of asthma (83, 93).
Furthermore, several studies could not show a significant correlation between vitamin D levels and the incidence of asthma in children and adults (94–96). In addition, several clinical trials failed to find a significant association between vitamin D supplementation in deficient individuals and improvement in clinical outcomes such as controlling the disease course, lessening symptoms and side effects, improving respiratory volumes and ratios, reducing exacerbation episodes, and decreasing required medications (97–99). In addition, vitamin D intake during pregnancy enhances the risk of asthma in children and adults (100, 101).
Atopic dermatitis
Among the factors involved in atopic dermatitis (AD), the growing importance of vitamin D deficiency was noted in atopic patients. Furthermore, vitamin D is correlated with the antimicrobial peptide (AMP) production by keratinocytes. Vitamin D and its analog play a vital role in treating AD, psoriasis, vitiligo, and acne (17, 102–104). Few investigations have surveyed the prevalence and severity of AD in people with vitamin D deficiency. Atopic dermatitis is associated with vitamin D levels, and vitamin D deficiency increases the risk of atopic dermatitis (105).
On the other hand, it has been noticed that vitamin D levels were higher in mild AD patients than in those with moderate to severe dermatitis (95). It seems that children born to mothers who have a low intake of fish or vitamin D during pregnancy have a higher risk of developing atopic dermatitis symptoms (106, 107). Also, as Wang et al. suggested, low breast milk vitamin D levels may influence the infant’s immune system, inversely correlated with persistent AD symptoms (108).
However, despite the positive relationship between hypovitaminosis D in the above studies and the prevalence or severity of AD, several texts have shown the reverse association (76, 109). Another investigation discovered that children of mothers with elevated serum 25-OH D3 levels had a higher risk of emerging advanced eczema at 9 months and 9 years old (100). Moreover, research carried out by Tian et al. claimed that the risk of atopic dermatitis (AD) in children at the first year of age is generally increased with higher maternal serum vitamin D levels throughout pregnancy (110). From the above considerations, it can be implied that this is a very controversial topic. Vitamin D is sometimes a protective agent and sometimes a risk factor for AD. However, it seems that at least in the majority of studies, it has been suggested that there is a reverse relationship between vitamin D serum levels (food intake or sunlight) and AD prevalence and severity. Further fueling the conflicting evidence in this matter, Lucas et al. believed that 1-hydroxylase CYP27B1 (responsible for 1,25-Vitamin D3 synthesis), the vitamin D receptor, and the vitamin D-mediated signaling target gene CYP24A1 are all upregulated as a result of allergic sensitization, which subsequently auto-downregulates vitamin D receptor-mediated signaling through reducing available ligand accumulations. This study suggested that an increase in local vitamin D-mediated signaling is a pro-allergic condition at the inflammation site, which results in low serum levels of vitamin D (111).
Vitamin D plays an essential role in regulating innate and acquired immune mechanisms. Vitamin D receptors (VDRs) have been found in various cells, including keratinocytes and multiple immune cells (112). 1,25-(OH)2 D3 prevents T cell proliferation, especially Th1s producing IL-2 and interferon-γ leading to macrophages and Th17s activation. It induces production of IL-17 and IL-22 (113–115). Moreover, 1,25-(OH)2 D3 reproduce CD4 +/CD25 + cells through stimulating IL-10 production, which ultimately impairs the Th1s and Th17s development (116, 117).
The polymorphisms of the VDR gene may cause differences in response to vitamin D in inflammatory conditions. Some of them might be discovered in severe AD patients, such as rs2228570, rs1544410, rs7975232, and rs731236. This indicates that the VDR controls AD by regulating epidermal function or affecting the local immune response (60). Langerhans and inflammatory dendritic epidermal cells (IDEC), both located in the epidermis of patients suffering from AD, expressing FcϵRI (high-affinity IgE receptor) and sensing allergens. Herrmann et al. explained that active vitamin D3 could downregulate the receptor at the protein and mRNA levels of its α-chain, impairing IgE-mediated inflammatory processes afterward (118). Cristi et al. also concluded that vitamin D sufficiency lowers the allergic phenotype of circulating DCs in AD children (119). As Mansour et al. suggested, vitamin D supplementation can be regarded as an effective treatment, decreasing the risk of severe atopic dermatitis symptoms, adding it to the existing studies already confirming vitamin D adjuvant therapy to be beneficial in mild to moderate cases (120).
Rheumatoid arthritis
Rheumatoid arthritis (RA) is an autoimmune disease of connective tissue that mainly affects synovial joints, causing grueling pain and reducing life expectancy (121). Although the exact cause is not entirely understood, researchers have suggested that genetic and environmental factors contribute to the RA pathogenesis (122). Considering the suppressant effects of vitamin D and the possible association between vitamin D deficiency and autoimmune disorders (123), it has been studied as a potential aid to the pathogenesis of autoimmune diseases, including RA (124). Studies have shown that many autoimmune disorders are correlated with lacking vitamin D (125, 126). This is confirmed that 1,25-(OH)2 D3 reduces the production of IL-12 production through NF-kB downregulation, inhibits the secretion of IFN-γ, and limits the expression of IL-6 receptor; in vivo administration of 1,25-(OH)2 D3 appears to have preventive effects on autoimmune diseases (77, 127, 128). Also, by inhibiting the expression of macrophage aromatases, vitamin D can reduce the conversion of androgens to estrogens which play a significant part in the activation of B lymphocytes and consequently the autoimmune response in RA (62).
The activity of vitamin D depends on VDR, and the activation of VDR can inhibit pro-inflammatory T cells and DC differentiation. In addition, VDR agonists induce T-regulator and NK cells and thus suppress self-immunity (63). It has been proved that VDR polymorphism accelerates RA sensitivity (129). One of these polymorphisms is TaqI or rs731236, located within Exon 9 at the 3′ end of the VDR gene. Probably the TT genotype of this polymorphism is a risk factor for RA (130). Studies have indicated that high levels of vitamin D absorption can reduce the risk of rheumatoid arthritis by 24% (131). In addition, vitamin D deficiency in these patients can lead to a more severe and active course of the disease; thus, vitamin D serum level may be a predictive factor for one-year disability and disease progression (132, 133). But not all evidence is in favor of the preventative effects of vitamin D. A recent study on RA patients concluded no significant differences between vitamin D-deficient and -non-deficient patients considering criteria such as swollen joints count (SJC), VAS-pain, tender joints count (TJC), and DAS28 scores (134). Further investigations can shed light on various features of the relationship between RA and vitamin D.
Multiple sclerosis
The pattern of MS occurrence varies throughout the world: its prevalence shows a steep slope over the equator, and the disease is more prevalent in areas near the poles (135). This can be associated with the level of UVB exposure (136) and can support the theory that environmental factors frequently influence the MS risk in early life. Studies have determined a relationship between the serum level of 25-OH D3 and MS risk development, namely, and there is a negative association between vitamin D levels and MS (137, 138). Obesity and smoking remain the two other MS risk factors correlated with vitamin D deficiency (139, 140). Although smoking and obesity may affect the MS risk by changing the vitamin D level, they may also affect the risk of MS in a vitamin D-independent way (141, 142). The association between MS and five loss-of-function mutations on the CYP27B1 gene encoding the 25-OH vit-D-1a-hydroxylase enzyme strongly indicates vitamin D’s critical role in MS etiology has been confirmed that these mutations decrease the level of vitamin D (143). However, three subsequent attempts to repeat this finding were unsuccessful. Now it seems that although these variants are associated with the risk of developing MS, their contribution to MS inheritance is small (144–146). Furthermore, the expression of HLA-DRB1*1501, the most potent genetic marker for MS, is regulated through the VDRE (vitamin D response element) in the promoter region (147, 148). Further investigations have revealed that vitamin D receptor gene polymorphisms could affect vitamin D absorption and increase MS susceptibility in various populations (148, 149). According to the meta-analysis conducted by Imani et al., Taql and Bsml polymorphisms, despite Apal polymorphism, lead to MS risk enhancement (150). Another risk reduction theory was explained in the study by Spanier et al. It stated that CTLA-4 functions as a mediator for MS prevention and, in the presence of vitamin D, its expression is significantly increased inside intracerebral myeloid cells (151).
More than 200 SNPs were detected out of the HLA region during the GWAS studies which are significantly related to MS disease. Among them, some SNPs are associated with genes responsible for vitamin D metabolisms such as rs2248359, CYP24A1, rs2248137, rs12368653, rs703842, rs10876994, CYP27B1, rs201202118, rs703842, and rs701006 (152, 153). These observations indicate that vitamin D relates to the transcription of the genes associated with MS and alters their mechanism of action (154). This important nutrient is assumed to have a key role in repairing genes expression, especially MYH, OGG1, MTH1, and NRF2 in MS patients (155).
Parkinson disease
Parkinson’s disease (PD) is a motor disorder characterized by tremor, stiffness, acne, and loss of local reflexes, leading to immobility and frequent corrosion. There were pieces of evidence that manifested vitamin D deficiency can increase the prevalence of PD (156–158). Interestingly, people with high concentrations of vitamin D in their serum show a reduction in Parkinson’s risk (159). This is also related to exposure to ultraviolet radiation (160). Many studies have proved that vitamin D is critical for the growth and function of the brain. VDRs and 1-alpha-hydroxylase, the enzyme responsible for vitamin D activation, are observed in the substantia nigra and the hypothalamus’s principal neurons and glial cells (161). Mice whose VDRs have been knocked out have developed muscular and motorized dysfunctions (162). In addition, higher levels of 1,25-(OH)2 D3 and VDR FokI CC genotypes are associated with mild forms of Parkinson’s disease (163). Different VDR polymorphisms such as the DHCR7/NADSYN1 locus and the CYP2R1 gene can affect clinical symptoms of PD through an impaired mechanism of turning vitamin D into valuable chemicals (164).
Recent studies have demonstrated the association between BsmI and FokI polymorphisms in VDR and PD sensitivity (165, 166). The BsmI bb genotype is more common in PD and causes the alteration in the VDR expression levels of mRNA. In patients with PD, FokI CC and TC genotypes are more prevalent than TT. In those regions with higher UV radiation, an association between UV and TaqI and ApaI has been found. The PD risk is reduced in the homozygous form of the TT TaqI genotype and the homozygous form of the GG ApaI genotype (167).
Infection-related disorders
Vitamin D plays an important role in regulatory actions of the immune system. This is essential for controlling infection-related disorders. Prior investigations indicated that respiratory infections, human immunodeficiency virus (HIV), and Mycobacterium tuberculosis (TB) were strongly associated with low levels of vitamin D in the circulation (168). The major antimicrobial protein, cathelicidin, is known to be necessary in the defensive actions of monocytes against the invading pathogens (66). Vitamin D receptor signaling crosstalks with the production of cathelicidin in these cells (169). Also, vitamin D-cathelicidin axis is vital in intestinal inflammation preventing gastrointestinal infections (170). The enhancement of the Paneth cells in response to the vitamin D receptor signaling was also studied very much (171). Toll-like receptors 1/2 (TLR 1/2) have been identified to be activated by 1,25-dihydroxy-vitamin D (vitamin D3) inducing the antimicrobial effects of tissue macrophages (27). By means of mammalian target of rapamycin (mToR) signaling, this micronutrient is also capable of inflammation attenuation in airway systems through enhancing cell autophagy (67).
Vitamin D supplementation and prevention of the infections such as coronavirus disease (COVID-19) has recently been investigated by many scientists (172–174). The results are still conflicting. Regarding TB infection, a large randomized clinical trial was conducted with a sample size of 8,851 children. The conclusion stated that vitamin D had no superior effect on TB infection risk than placebo (173). On the other hand, some studies demonstrated that vitamin D supplementation had a promising lowering impact on the risk of upper respiratory infections (172, 174). There remains a huge demand for larger and more accurate studies in order to assess the net effect of vitamin D on the incidence of various infection types.
Cancer
Cancers are among the most common and important immune system-related disorders in the world. They emerge from the dysfunction occurring in cell proliferation, differentiation, and apoptosis (9, 175). The association between the serum level of vitamin D and the risk of various cancers has been investigated several times in the past. Colorectal cancer (176), breast cancer (177), skin cancer (178), prostate cancer (179), liver cancer (180), and head and neck cancers (181) are some of the examples. According to Ma et al.’s study, high blood levels of vitamin D was correlated with a 33% reduction in the risk of colorectal cancer (182). Regarding head and neck neoplasms, high vitamin D serum levels decreased the incidence by 32% and also lowered the mortality risk in these patients (183).
VDR is upregulated in many tumor cells of different types of cancers. For instance, epigenetic studies indicated that in colorectal cancers, the adipose tissue overexpressed VDR. As we probably know, adipose tissue dysfunction is a vital mechanism underlying the emergence of colorectal cancer (184). This overexpression has been observed in prostate and ovarian cancers, too (179, 185). However, prior investigations have suggested that in some cases more VDR expression in tumor cells might lead to a better prognosis and treatment response (186, 187). Inflammation and immune response remained a matter of discussion in cancer progression and spreading (188). As mentioned, vitamin D has been identified to have a major impact on the regulation of inflammation, especially in microenvironments. The signaling might be activated or inhibited through two main pathways: MAP Kinase Phosphatase 5 (MKP5) and Nf-kB (9). The existing evidence revealed that vitamin D might upregulate the MKP5 in tumor cells causing MAPK inhibition and, following that, decreased production of IL-6 (189, 190). This resulted in the regulation of inflammatory response of the immune cells. Additionally, previous articles have stated that this important micronutrient could inhibit the activity of Nf-kB as the upstream compounds of another pro-inflammatory cytokine, IL-8, production (191). Also, the binding of Nf-kB to the DNA might be disturbed in the presence of vitamin D (68).
Concerning the use of vitamin D supplementation in preventing or treating distinct types of cancers, many randomized or non-randomized clinical trials have been conducted (9). A large randomized clinical trial with 25,871 patients revealed that vitamin D supplementation did not influence the incidence of invasive cancers (192). Also, another study on healthy postmenopausal women confirmed the inability of vitamin D in cancer prevention (193). On the other hand, some studies have suggested that vitamin D might improve the survival rate in patients suffering from cancer. Akiba et al. achieved favorable results regarding non-small cell lung cancers (194). Further large multicenter trials are absolutely required in order to finally build a consensus about this major issue.
Diabetes mellitus
Type 1 diabetes (T1D) is a prevalent disease across the globe with a combination of factors (genetic and environmental) as its causes. It is considered an autoimmune disease induced by a T cell-mediated mechanism in which dendritic cells (DC) and different pro-inflammatory cytokines play vital roles (195–197). On the other hand, studies are getting increasingly confident in the role of vitamin D deficiency in different autoimmune diseases (198–200). Therefore, vitamin D is discussed in many studies as a protective factor in developing T1D (201, 202).
The seasonal pattern of T1D and its various prevalence depending on latitude degree and ultraviolet B (UVB) irradiance support this association theory (203–209). Epidemiological studies show a trend of higher T1D incidents in adults and children suffering from vitamin D deficiency (196, 210–212). In addition, it is stated that there is an association between several polymorphisms of VD-related genes and T1D (196). Polymorphic expression of genes encoding vitamin D metabolizing enzymes, CYP27B1 as well as CYP2R1 and 7-dehydrocholesterol reductase (DHCR7), unlike CYP24A1 gene expression, has been suggested to be associated with T1D susceptibility (213–215). Although there is conflicting evidence regarding the association of VDR polymorphism with T1D (216, 217), Zhang et al. indicated that at least one of four VDR gene polymorphisms is linked with a higher possibility for T1D (218).
Multiple factors are involved in the vitamin D level impacting the immunopathogenesis of T1D and affecting both innate and acquired immunity (17, 195). During T1D development, islet autoantigens appear at the antigen-presenting cells (APCs) surface, including DCs and macrophages, which induce a cytotoxic T cell response (196). Vitamin D is produced by dendritic cells (DC) and also applies immunomodulatory effects toward self-tolerance in these cells (69, 219). As a matter of fact, almost all of the immune system cells express the VDR (220). The active form of vitamin D prevents the maturation of DCs. As a result, surface expression of major histocompatibility complex (MHC)-II and co-stimulatory molecules is hampered, and antigen presentation and T cell activation are stopped (70). Furthermore, this active form induces mature DCs apoptosis. Also, it differentiates them into a tolerogenic state. Therefore, regulatory T cells (Treg) are induced to a greater extent (221–223). Moreover, Mauf et al. suggested that 25-OH D3 shows its immunomodulatory effects by differentiating monocytes less into DCs and more into intermediate cells with similar phenotypes to those of tolerogenic DCs (69). Besides this indirect effect of vitamin D on T cells, they can be targeted directly, too (196). It is worth mentioning that vitamin D can also directly affect pancreatic beta cells and their insulin secretion, therefore making them more resistant (196).
As mentioned, vitamin D deficiency is considered an environmental risk factor for developing autoimmune diseases like T1D (212, 224). Therefore, many studies have evaluated its potential preventive or therapeutic effect (199, 210). It is demonstrated in animal models that the active form of vitamin D can perform immune modulation in diabetes-prone mice in several ways. Increasing Tregs, triggering a Th1/Th2 shift in the islets and the pancreatic draining lymph nodes, preserving defective T cell selection in the thymus, and eliminating apoptosis-resistant T cells are all parts of that immunomodulatory action (225–229). Apart from this non-antigen-specific immunomodulation, antigen-based immunotherapies for T1D are also reported (224). Several studies have discussed the protective immunological effect of vitamin D3 in T1D patients, especially T cell modulation (212, 230). Gabbay et al. reported a significant increase in Tregs upon 12 months of vitamin D3 supplementation in patients with recent-onset T1D (231). Also, Treiber et al. observed a significant increase in the suppressive function of Tregs after vitamin D3 supplementation for 12 months (232). Contradictory to these results, some studies show no meaningful protective effect of vitamin D (202, 233–236). That makes it crucial for future studies to better evaluate the disease intervention by assessing the administering of vitamin D, its exact compound choice, the dosage needed, and the ideal treatment regimen (224).
Inflammatory bowel disease
Inflammatory bowel diseases (IBD) are a group of chronic immune-mediated diseases, with ulcerative colitis and Crohn’s disease being the most renowned ones. Several studies have shown that the prevalence of vitamin D deficiency in patients with IBD is higher than in a healthy population (237–242). Although it has not been precisely determined that this difference is a cause or consequence of the disease, it has drawn attention to the possible impacts of vitamin D on the etiology and course of the disease. In addition to human studies, in vitro and in vivo model analysis put forward three possible mechanisms that vitamin D may affect the onset and progression of IBD.
Gut microbiota may be another target for vitamin D to ameliorate the IBD course or even prevent its onset (243, 244). These bacteria exert their effects through different metabolites. Butyrate is one of these metabolites, which, in addition to being an energy substrate for mucosal cells, accelerates epithelium healing (245, 246). Lithocholic acid, another bacterial metabolite, suppresses IL-2 production and subsequently decreases inflammation (247). It is worth noting that vitamin D and microbiota have a bidirectional interaction which means microbiota can also utilize vitamin D downstream pathways (248–250).
Vitamin D can target B cells, T cells, dendritic cells, and macrophages throughout innate and acquired immune systems, considering they all express VDR (251). In vivo and in vitro studies suggest that vitamin D is able to enhance the expression of IL-10 in dendritic cells and the production of cathelicidin by macrophages (71, 252). In contrast, toll-like receptors and IL-12 expression in macrophages and dendritic cells are inhibited by the activated form of vitamin D (29, 253). This inhibition leads to less dendritic cell-induced activation of T cells. Besides, vitamin D can directly suppress B and T cell proliferation (50). Vitamin D was able to interfere in inflammatory/anti-inflammatory production balance, resulting in reduced production of T cell-inflammatory cytokines, including IL-2, interferon (IFN)-γ, IL-17, and TNF-α, and enhanced anti-inflammatory cytokines production such as IL-10 by regulatory T cells and IL-4 by Th2 cells (50, 254–256). Overall, the induction of regulatory cells like Tregs and CD8αα was increased (255, 257).
Various human studies have suggested a positive effect on different outcomes from vitamin D supplementation in IBD patients. Reduced disease activity, decreased risk of postoperative endoscopic recurrence, lower clinical activity score, lower relapse rate, and improved odds of remission were reported by different studies (258–263). One study even reported vitamin D effects on all-cause mortality (264). However, not all evidence is in favor of the preventative effects of vitamin D (265). One RCT concluded no difference in CRP, fecal calprotectin, Crohn’s disease activity index (CDAI), and quality of life between supplemented and control groups (266). At the same time, another RCT showed only a non-significant lower relapse rate in the experimental group (267). Three meta-analyses of different RCTs were conducted. Two established that vitamin D supplementation improves clinical and biochemical disease activity scores, while the third did not report such improvements (261, 268, 269). Further investigations can reveal more aspects of the complex relationship between IBD and vitamin D.
Conclusion
Studies have shown a strong correlation between vitamin D and the innate and adaptive immune systems, suggesting that low vitamin D levels may contribute to immune response dysregulation. However, in recent years, the precise function of the alternate pathways of vitamin D and its receptor has not yet been sufficiently elucidated. We all need to be aware of what these signaling pathways do in various immune system components. Reviews of the literature revealed that, during the past 40 years, a number of studies have been conducted to suggest the protective effect of vitamin D in autoimmune illnesses. Randomized controlled clinical trials are still lacking, though, in this area. Larger clinical investigations will now be necessary to determine the precise impact of vitamin D supplementation on the pathophysiology of different diseases. Of the immunomodulatory treatments now on the market, they might be as effective as the others. Additionally, the right supplement dosage and manner of administration must be determined. But for present approaches to preventing diseases brought on by compromised immune-homeostasis, vitamin D has emerged as a potential and relatively safe supplement.
Author contributions
AR: Study design, searching databases, writing manuscript, final revise. AG: searching databases, writing manuscript, final revise. AS: writing manuscript, final revise. BS: writing manuscript, final revise. MM: writing manuscript, final revise. AD: writing manuscript, final revise. GS: study design, supervising all steps, resolving conflicts, final revise. All authors contributed to the article and approved the submitted version.
Conflict of interest
The authors declare that the research was conducted in the absence of any commercial or financial relationships that could be construed as a potential conflict of interest.
Publisher’s note
All claims expressed in this article are solely those of the authors and do not necessarily represent those of their affiliated organizations, or those of the publisher, the editors and the reviewers. Any product that may be evaluated in this article, or claim that may be made by its manufacturer, is not guaranteed or endorsed by the publisher.
References
1. Taene A, Niazi S, Bijari B, Esmaeili S, Anani sarab G. Prevalence of vitamin d deficiency and its related factors in AqQala city in 2016. J Birjand Univ Med Sci (2017) 24(2):108–16.
2. Chirumbolo S, Bjørklund G, Sboarina A, Vella A. The role of vitamin d in the immune system as a pro-survival molecule. Clin Ther (2017). doi: 10.1016/j.clinthera.2017.03.021
3. Janssen HC, Samson MM, Verhaar HJ. Vitamin d deficiency, muscle function, and falls in elderly people. Am J Clin Nutr (2002) 75(4):611–5. doi: 10.1093/ajcn/75.4.611
4. Pilz S, Tomaschitz A, Obermayer-Pietsch B, Dobnig H, Pieber TR. Epidemiology of vitamin d insufficiency and cancer mortality. Anticancer Res (2009) 29(9):3699–704.
5. Judd SE, Tangpricha V. Vitamin d deficiency and risk for cardiovascular disease. Am J Med Sci (2009) 338(1):40–4. doi: 10.1097/MAJ.0b013e3181aaee91
6. Shoenfeld N, Amital H, Shoenfeld Y. The effect of melanism and vitamin d synthesis on the incidence of autoimmune disease. Nat Clin Pract Rheumatol (2009) 5(2):99–105. doi: 10.1038/ncprheum0989
7. Adams JS, Liu PT, Chun R, Modlin RL, Hewison M. Vitamin d in defense of the human immune response. Ann N Y Acad Sci (2007) 1117:94–105. doi: 10.1196/annals.1402.036
8. Nnoaham KE, Clarke A. Low serum vitamin d levels and tuberculosis: a systematic review and meta-analysis. Int J Epidemiol (2008) 37(1):113–9. doi: 10.1093/ije/dym247
9. El-Sharkawy A, Malki A. Vitamin d signaling in inflammation and cancer: molecular mechanisms and therapeutic implications. Molecules (Basel Switzerland) (2020) 25(14). doi: 10.3390/molecules25143219
10. Valcour A, Blocki F, Hawkins DM, Rao SD. Effects of age and serum 25-OH-vitamin d on serum parathyroid hormone levels. J Clin Endocrinol Metab (2012) 97(11):3989–95. doi: 10.1210/jc.2012-2276
11. LeBlanc ES, Zakher B, Daeges M, Pappas M, Chou R. Screening for vitamin d deficiency: a systematic review for the U. S. Prev Serv Task Force. Ann Internal Med (2015) 162(2):109–22. doi: 10.7326/M14-1659
12. Gillespie LD, Robertson MC, Gillespie WJ, Sherrington C, Gates S, Clemson LM, et al. Interventions for preventing falls in older people living in the community. Cochrane Database systematic Rev (2012) 2012(9):Cd007146.
13. Harrison SR, Li D, Jeffery LE, Raza K, Hewison M. Vitamin d, autoimmune disease and rheumatoid arthritis. Calcified Tissue Int (2020) 106(1):58–75. doi: 10.1007/s00223-019-00577-2
14. Lamberg-Allardt C. Vitamin d in foods and as supplements. Prog biophysics Mol Biol (2006) 92(1):33–8. doi: 10.1016/j.pbiomolbio.2006.02.017
15. Dusso AS, Brown AJ, Slatopolsky E. Vitamin d. Am J Physiol Renal Physiol (2005) 289(1):F8–28. doi: 10.1152/ajprenal.00336.2004
16. Cheng JB, Levine MA, Bell NH, Mangelsdorf DJ, Russell DW. Genetic evidence that the human CYP2R1 enzyme is a key vitamin d 25-hydroxylase. Proc Natl Acad Sci USA (2004) 101(20):7711–5. doi: 10.1073/pnas.0402490101
17. Baeke F, Takiishi T, Korf H, Gysemans C, Mathieu C. Vitamin d: modulator of the immune system. Curr Opin Pharmacol (2010) 10(4):482–96. doi: 10.1016/j.coph.2010.04.001
18. Provvedini DM, Tsoukas CD, Deftos LJ, Manolagas SC. 1,25-dihydroxyvitamin D3 receptors in human leukocytes. Science (1983) 221(4616):1181–3. doi: 10.1126/science.6310748
19. Moossavi M, Parsamanesh N, Mohammadoo-Khorasani M, Moosavi M, Tavakkoli T, Fakharian T, et al. Positive correlation between vitamin d receptor gene FokI polymorphism and colorectal cancer susceptibility in south-khorasan of Iran. J Cell Biochem (2018) 119(10):8190–4. doi: 10.1002/jcb.26826
20. Tamari M, Tanaka S, Hirota T. Genome-wide association studies of allergic diseases. Allergology Int (2013) 62(1):21–8. doi: 10.2332/allergolint.13-RAI-0539
21. Santos HLBS, SdS S, Ed P, Pereira-Ferrari L, Mikami L, Riedi CA, et al. Vitamin d receptor gene mutations anD vitamin d serum leVels in asthmatic chilDren. Rev Paulista Pediatria (2018) 36(3):269–74. doi: 10.1590/1984-0462/;2018;36;3;00016
22. Wang Y, Zhu J, DeLuca HF. Where is the vitamin d receptor? Arch Biochem Biophysics (2012) 523(1):123–33. doi: 10.1016/j.abb.2012.04.001
23. Zmijewski MA, Carlberg C. Vitamin d receptor(s): in the nucleus but also at membranes? Exp Dermatol (2020) 29(9):876–84. doi: 10.1111/exd.14147
24. Dimitrov V, White JH. Vitamin d signaling in intestinal innate immunity and homeostasis. Mol Cell Endocrinol (2017) 453:68–78. doi: 10.1016/j.mce.2017.04.010
25. Gombart AF. The vitamin d-antimicrobial peptide pathway and its role in protection against infection. Future Microbiol (2009) 4(9):1151–65. doi: 10.2217/fmb.09.87
26. Xu H, Soruri A, Gieseler RK, Peters JH. 1,25-dihydroxyvitamin D3 exerts opposing effects to IL-4 on MHC class-II antigen expression, accessory activity, and phagocytosis of human monocytes. Scand J Immunol (1993) 38(6):535–40. doi: 10.1111/j.1365-3083.1993.tb03237.x
27. Liu PT, Stenger S, Li H, Wenzel L, Tan BH, Krutzik SR, et al. Toll-like receptor triggering of a vitamin d-mediated human antimicrobial response. Sci (New York NY) (2006) 311(5768):1770–3. doi: 10.1126/science.1123933
28. Charoenngam N, Holick MF. Immunologic effects of vitamin d on human health and disease. Nutrients (2020) 12(7):2097. doi: 10.3390/nu12072097
29. Sadeghi K, Wessner B, Laggner U, Ploder M, Tamandl D, Friedl J, et al. Vitamin D3 down-regulates monocyte TLR expression and triggers hyporesponsiveness to pathogen-associated molecular patterns. Eur J Immunol (2006) 36(2):361–70. doi: 10.1002/eji.200425995
30. Gombart AF, Borregaard N, Koeffler HP. Human cathelicidin antimicrobial peptide (CAMP) gene is a direct target of the vitamin d receptor and is strongly up-regulated in myeloid cells by 1,25-dihydroxyvitamin D3. FASEB J (2005) 19(9):1067–77. doi: 10.1096/fj.04-3284com
31. Ramanathan B, Davis EG, Ross CR, Blecha F. Cathelicidins: microbicidal activity, mechanisms of action, and roles in innate immunity. Microbes Infect (2002) 4(3):361–72. doi: 10.1016/S1286-4579(02)01549-6
32. Flodstrom-Tullberg M, Bryceson YT, Shi FD, Hoglund P, Ljunggren HG. Natural killer cells in human autoimmunity. Curr Opin Immunol (2009) 21(6):634–40. doi: 10.1016/j.coi.2009.09.012
33. Klose CS, Artis D. Innate lymphoid cells as regulators of immunity, inflammation and tissue homeostasis. Nat Immunol (2016) 17(7):765–74. doi: 10.1038/ni.3489
34. Marashian SM, Mortaz E, Jamaati HR, Alavi-Moghaddam M, Kiani A, Abedini A, et al. Role of innate lymphoid cells in lung disease. Iranian J Allergy Asthma Immunol (2015) 14(4):346.
35. Ignacio A, Breda CNS, Camara NOS. Innate lymphoid cells in tissue homeostasis and diseases. World J Hepatology (2017) 9(23):979. doi: 10.4254/wjh.v9.i23.979
36. Al-Jaderi Z, Maghazachi AA. Effects of vitamin D3, calcipotriol and FTY720 on the expression of surface molecules and cytolytic activities of human natural killer cells and dendritic cells. Toxins (2013) 5(11):1932–47. doi: 10.3390/toxins5111932
37. Mortara L, Gariboldi MB, Bosi A, Bregni M, Pinotti G, Guasti L, et al. Vitamin d deficiency has a negative impact on cetuximab-mediated cellular cytotoxicity against human colon carcinoma cells. Targeted Oncol (2018) 13(5):657–65. doi: 10.1007/s11523-018-0586-x
38. Ercolano G, Moretti A, Falquet M, Wyss T, Tran NL, Senoner I, et al. Gliadin-reactive vitamin d-sensitive proinflammatory ILCPs are enriched in celiac patients. Cell Rep (2022) 39(11):110956. doi: 10.1016/j.celrep.2022.110956
39. Zhou L, Lin Q, Sonnenberg GF. Metabolic control of innate lymphoid cells in health and disease. Nat Metab (2022), 1–10. doi: 10.1038/s42255-022-00685-8
40. Kim H, Kim J, Sa JK, Ryu B-K, Park K-J, Kim J, et al. Calcipotriol, a synthetic vitamin d analog, promotes antitumor immunity via CD4+T-dependent CTL/NK cell activation. Biomedicine Pharmacotherapy (2022) 154:113553. doi: 10.1016/j.biopha.2022.113553
41. Guillot X, Semerano L, Saidenberg-Kermanac’h N, Falgarone G, Boissier M-C. Vitamin d and inflammation. Joint Bone Spine (2010) 77(6):552–7. doi: 10.1016/j.jbspin.2010.09.018
42. Di Rosa M, Malaguarnera M, Nicoletti F, Malaguarnera L. Vitamin D3: a helpful immuno-modulator. Immunology (2011) 134(2):123–39. doi: 10.1111/j.1365-2567.2011.03482.x
43. Oh S, Chun S, Hwang S, Kim J, Cho Y, Lee J, et al. Vitamin d and exercise are major determinants of natural killer cell activity, which is age- and gender-specific. Front Immunol (2021) 12. doi: 10.3389/fimmu.2021.594356
44. Grund JC, Krammer S, Yang Z, Mitländer H, Rauh M, Zirlik S, et al. Vitamin D3 resolved human and experimental asthma via b lymphocyte–induced maturation protein 1 in T cells and innate lymphoid cells. J Allergy Clin Immunology: Global (2023) 2(3):100099. doi: 10.1016/j.jacig.2023.100099
45. Hart PH, Gorman S, Finlay-Jones JJ. Modulation of the immune system by UV radiation: more than just the effects of vitamin d? Nat Rev Immunol (2011) 11(9):584–96. doi: 10.1038/nri3045
46. Wei R, Christakos S. Mechanisms underlying the regulation of innate and adaptive immunity by vitamin d. Nutrients (2015) 7(10):8251–60. doi: 10.3390/nu7105392
47. Maruotti N, Cantatore FP. Vitamin d and the immune system. J Rheumatol (2010) 37(3):491–5. doi: 10.3899/jrheum.090797
48. Szymczak I, Pawliczak R. The active metabolite of vitamin D3 as a potential immunomodulator. Scandinavian J Immunol (2016) 83(2):83–91. doi: 10.1111/sji.12403
49. Mrad MF, El Ayoubi NK, Esmerian MO, Kazan JM, Khoury SJ. Effect of vitamin d replacement on immunological biomarkers in patients with multiple sclerosis. Clin Immunol (2017). doi: 10.1016/j.clim.2017.05.017
50. Cantorna MT, Snyder L, Lin YD, Yang L. Vitamin d and 1,25(OH)2D regulation of T cells. Nutrients (2015) 7(4):3011–21. doi: 10.3390/nu7043011
51. Kitz A, de Marcken M, Gautron AS, Mitrovic M, Hafler DA, Dominguez-Villar M. AKT isoforms modulate Th1-like treg generation and function in human autoimmune disease. EMBO Rep (2016):e201541905. doi: 10.15252/embr.201541905
52. Cantorna MT. Mechanisms underlying the effect of vitamin d on the immune system. Proc Nutr Society (2010) 69(3):286–9. doi: 10.1017/S0029665110001722
53. Mora JR, Iwata M, von Andrian UH. Vitamin effects on the immune system: vitamins a and d take centre stage. Nat Rev Immunol (2008) 8(9):685–98. doi: 10.1038/nri2378
54. Joshi S, Pantalena L-C, Liu XK, Gaffen SL, Liu H, Rohowsky-Kochan C, et al. 1, 25-dihydroxyvitamin D3 ameliorates Th17 autoimmunity via transcriptional modulation of interleukin-17A. Mol Cell Biol (2011) 31(17):3653–69. doi: 10.1128/MCB.05020-11
55. Ding T, Su R, Wu R, Xue H, Wang Y, Su R, et al. Frontiers of autoantibodies in autoimmune disorders: crosstalk between Tfh/Tfr and regulatory b cells. Front Immunol (2021) 12:641013. doi: 10.3389/fimmu.2021.641013
56. Cho SW, Zhang YL, Ko YK, Shin JM, Lee JH, Rhee CS, et al. Intranasal treatment with 1, 25-dihydroxyvitamin D3 alleviates allergic rhinitis symptoms in a mouse model. Allergy Asthma Immunol Res (2019) 11(2):267–79. doi: 10.4168/aair.2019.11.2.267
57. Zhu DC, Feng Y, Wang BQ. [Research progress on the relevance between serum vitamin d and IL-33/ST2 levels and allergic rhinitis]. Lin chuang er bi yan hou tou jing wai ke za zhi = J Clin otorhinolaryngology Head Neck Surgery (2019) 33(9):898–900.
58. Chen YH, Cheadle CE, Rice LV, Pfeffer PE, Dimeloe S, Gupta A, et al. The induction of alpha-1 antitrypsin by vitamin d in human T cells is TGF-β dependent: a proposed anti-inflammatory role in airway disease. Front Nutr (2021) 8:667203. doi: 10.3389/fnut.2021.667203
59. Kalicki B, Wawrzyniak A, Lipińska-Opałka A, Lewicki S, Zdanowski R. Influence of vitamin d and cotinine on T-regulatory cells and asthma severity in children. Adv Exp Med Biol (2017) 1021:27–36. doi: 10.1007/5584_2017_27
60. Heine G, Hoefer N, Franke A, Nöthling U, Schumann RR, Hamann L, et al. Association of vitamin d receptor gene polymorphisms with severe atopic dermatitis in adults. Br J Dermatol (2013) 168(4):855–8. doi: 10.1111/bjd.12077
61. Hallau J, Hamann L, Schumann RR, Worm M, Heine G. A promoter polymorphism of the vitamin d metabolism gene Cyp24a1 is associated with severe atopic dermatitis in adults. Acta dermato-venereologica (2016) 96(2):169–72. doi: 10.2340/00015555-2226
62. Cutolo M, Straub RH. Sex steroids and autoimmune rheumatic diseases: state of the art. Nat Rev Rheumatol (2020) 16(11):628–44. doi: 10.1038/s41584-020-0503-4
63. Wen H, Baker JF. Vitamin d, immunoregulation, and rheumatoid arthritis. J Clin Rheumatol Pract Rep Rheumatic Musculoskeletal Dis (2011) 17(2):102–7. doi: 10.1097/RHU.0b013e31820edd18
64. Agliardi C, Guerini FR, Saresella M, Caputo D, Leone MA, Zanzottera M, et al. Vitamin d receptor (VDR) gene SNPs influence VDR expression and modulate protection from multiple sclerosis in HLA-DRB1*15-positive individuals. Brain Behav Immun (2011) 25(7):1460–7. doi: 10.1016/j.bbi.2011.05.015
65. Niu MY, Wang L, Xie AM. ApaI, BsmI, FokI, and TaqI polymorphisms in the vitamin d receptor gene and parkinson's disease. Chin Med J (2015) 128(13):1809–14. doi: 10.4103/0366-6999.159358
66. Chung C, Silwal P, Kim I, Modlin RL, Jo EK. Vitamin d-cathelicidin axis: at the crossroads between protective immunity and pathological inflammation during infection. Immune Network (2020) 20(2):e12. doi: 10.4110/in.2020.20.e12
67. Zha L, Wu G, Xiao H, Xiao Y. Vitamin d attenuates airway inflammation in asthmatic Guinea pigs using mammalian target of rapamycin-mediated autophagy. J Interferon Cytokine Res Off J Int Soc Interferon Cytokine Res (2022) 42(4):170–9. doi: 10.1089/jir.2021.0189
68. Tse AK, Zhu GY, Wan CK, Shen XL, Yu ZL, Fong WF. 1alpha,25-dihydroxyvitamin D3 inhibits transcriptional potential of nuclear factor kappa b in breast cancer cells. Mol Immunol (2010) 47(9):1728–38. doi: 10.1016/j.molimm.2010.03.004
69. Mauf S, Penna-Martinez M, Jentzsch T, Ackermann H, Henrich D, Radeke HH, et al. Immunomodulatory effects of 25-hydroxyvitamin D3 on monocytic cell differentiation and influence of vitamin D3 polymorphisms in type 1 diabetes. J Steroid Biochem Mol Biol (2015) 147:17–23. doi: 10.1016/j.jsbmb.2014.11.001
70. van Etten E, Dardenne O, Gysemans C, Overbergh L, Mathieu C. 1,25-dihydroxyvitamin D3 alters the profile of bone marrow-derived dendritic cells of NOD mice. Ann New York Acad Sci (2004) 1037:186–92. doi: 10.1196/annals.1337.030
71. Verma R, Kim JY. 1,25-dihydroxyvitamin D3 facilitates M2 polarization and upregulates TLR10 expression on human microglial cells. Neuroimmunomodulation (2016) 23(2):75–80. doi: 10.1159/000444300
73. Wjst M, Hyppönen E. Vitamin d serum levels and allergic rhinitis. Allergy (2007) 62(9):1085–6. doi: 10.1111/j.1398-9995.2007.01437.x
74. Erkkola M, Kaila M, Nwaru B, Kronberg-Kippilä C, Ahonen S, Nevalainen J, et al. Maternal vitamin d intake during pregnancy is inversely associated with asthma and allergic rhinitis in 5-year-old children. Clin Exp Allergy (2009) 39(6):875–82. doi: 10.1111/j.1365-2222.2009.03234.x
75. Chen Y-CS, Mirzakhani H, Lu M, Zeiger RS, O'Connor GT, Sandel MT, et al. The association of prenatal vitamin d sufficiency with aeroallergen sensitization and allergic rhinitis in early childhood. J Allergy Clin Immunology: In Practice (2021) 9(10):3788–96.e3. doi: 10.1016/j.jaip.2021.06.009
76. Hyppönen E, Sovio U, Wjst M, Patel S, Pekkanen J, Hartikainen AL, et al. Infant vitamin d supplementation and allergic conditions in adulthood: northern Finland birth cohort 1966. Ann New York Acad Sci (2004) 1037(1):84–95. doi: 10.1196/annals.1337.013
77. Bener A, Ehlayel MS, Bener HZ, Hamid Q. The impact of vitamin d deficiency on asthma, allergic rhinitis and wheezing in children: an emerging public health problem. J Family Community Med (2014) 21(3):154. doi: 10.4103/2230-8229.142967
78. Feng Q, Bønnelykke K, Ek W, Chawes B, Yuan S, Cheung C-L, et al. Null association between serum 25-hydroxyvitamin d levels with allergic rhinitis, allergic sensitization and non-allergic rhinitis: a mendelian randomization study. Clin Exp Allergy J Br Soc Allergy Clin Immunol (2020) 51.
79. Searing DA, Zhang Y, Murphy JR, Hauk PJ, Goleva E, Leung DY. Decreased serum vitamin d levels in children with asthma are associated with increased corticosteroid use. J Allergy Clin Immunol (2010) 125(5):995–1000. doi: 10.1016/j.jaci.2010.03.008
80. Fang X, Xie Q, Zhang X. Serum vitamin d level in mice with allergic rhinitis is correlated with inflammatory factors. Am J Trans Res (2021) 13(4):3351–6.
81. Yu Z-J, Zeng L, Luo X-Q, Geng X-R, Xu R, Chen K, et al. Vitamin D3 inhibits micro RNA-17-92 to promote specific immunotherapy in allergic rhinitis. Sci Rep (2017) 7(1):546. doi: 10.1038/s41598-017-00431-1
82. Bakhshaee M, Sharifian M, Esmatinia F, Rasoulian B, Mohebbi M. Therapeutic effect of vitamin d supplementation on allergic rhinitis. Eur Arch Oto-Rhino-Laryngology (2019) 276(10):2797–801. doi: 10.1007/s00405-019-05546-x
83. Brehm JM, Celedón JC, Soto-Quiros ME, Avila L, Hunninghake GM, Forno E, et al. Serum vitamin d levels and markers of severity of childhood asthma in Costa Rica. Am J Respir Crit Care Med (2009) 179(9):765–71. doi: 10.1164/rccm.200808-1361OC
84. Devereux G, Wilson A, Avenell A, McNeill G, Fraser W. A case–control study of vitamin d status and asthma in adults. Allergy (2010) 65(5):666–7. doi: 10.1111/j.1398-9995.2009.02220.x
85. Urashima M, Segawa T, Okazaki M, Kurihara M, Wada Y, Ida H. Randomized trial of vitamin d supplementation to prevent seasonal influenza a in schoolchildren. Am J Clin Nutr (2010) 91(5):1255–60. doi: 10.3945/ajcn.2009.29094
86. Majak P, Olszowiec-Chlebna M, Smejda K, Stelmach I. Vitamin d supplementation in children may prevent asthma exacerbation triggered by acute respiratory infection. J Allergy Clin Immunol (2011) 127(5):1294–6. doi: 10.1016/j.jaci.2010.12.016
87. Yadav M, Mittal K. Effect of vitamin d supplementation on moderate to severe bronchial asthma. Indian J Pediatrics (2014) 81(7):650–4. doi: 10.1007/s12098-013-1268-4
88. Andújar-Espinosa R, Salinero-González L, Illán-Gómez F, Castilla-Martínez M, Hu-Yang C, Ruiz-López FJ. Effect of vitamin d supplementation on asthma control in patients with vitamin d deficiency: the ACVID randomised clinical trial. Thorax (2021) 76(2):126. doi: 10.1136/thoraxjnl-2019-213936
89. Gupta A, Dimeloe S, Richards DF, Chambers ES, Black C, Urry Z, et al. Defective IL-10 expression and in vitro steroid-induced IL-17A in paediatric severe therapy-resistant asthma. Thorax (2014) 69(6):508–15. doi: 10.1136/thoraxjnl-2013-203421
90. Brunetti L, Colazzo D, Francavilla R, Tesse R, De Sario V, Lore M, et al. The role of pulmonary infection in pediatric asthma. In: Allergy and asthma proceedings. OceanSide Publications, Inc (2007).
91. Ginde AA, Mansbach JM, Camargo CA. Vitamin d, respiratory infections, and asthma. Curr Allergy Asthma Rep (2009) 9(1):81–7. doi: 10.1007/s11882-009-0012-7
92. Xystrakis E, Kusumakar S, Boswell S, Peek E, Urry Z, Richards DF, et al. Reversing the defective induction of IL-10–secreting regulatory T cells in glucocorticoid-resistant asthma patients. J Clin Invest (2006) 116(1):146.
93. Brehm JM, Schuemann B, Fuhlbrigge AL, Hollis BW, Strunk RC, Zeiger RS, et al. Serum vitamin d levels and severe asthma exacerbations in the childhood asthma management program study. J Allergy Clin Immunol (2010) 126(1):52–8. e5. doi: 10.1016/j.jaci.2010.03.043
94. Cheng HM, Kim S, Park G-H, Chang SE, Bang S, Won CH, et al. Low vitamin d levels are associated with atopic dermatitis, but not allergic rhinitis, asthma, or IgE sensitization, in the adult Korean population. J Allergy Clin Immunol (2014) 133(4):1048–55. doi: 10.1016/j.jaci.2013.10.055
95. Gergen PJ, Teach SJ, Mitchell HE, Freishtat RF, Calatroni A, Matsui E, et al. Lack of a relation between serum 25-hydroxyvitamin d concentrations and asthma in adolescents. Am J Clin Nutr (2013) 97(6):1228–34. doi: 10.3945/ajcn.112.046961
96. Wawro N, Heinrich J, Thiering E, Kratzsch J, Schaaf B, Hoffmann B, et al. Serum 25 (OH) d concentrations and atopic diseases at age 10: results from the GINIplus and LISAplus birth cohort studies. BMC Pediatrics (2014) 14(1):286. doi: 10.1186/s12887-014-0286-3
97. Forno E, Bacharier LB, Phipatanakul W, Guilbert TW, Cabana MD, Ross K, et al. Effect of vitamin D3 supplementation on severe asthma exacerbations in children with asthma and low vitamin d levels: the VDKA randomized clinical trial. JAMA (2020) 324(8):752–60. doi: 10.1001/jama.2020.12384
98. Jat K, Goel N, Gupta N, Gupta C, Datta S, Lodha R, et al. Efficacy of vitamin d supplementation in asthmatic children with vitamin d deficiency: a randomized controlled trial (ESDAC trial). Pediatr Allergy Immunol (2020) 32.
99. Dodamani M, Muthu V, Thakur R, Pal A, Sehgal I, Dhooria S, et al. A randomized trial of vitamin d in acute-stage allergic bronchopulmonary aspergillosis complicating asthma. Mycoses (2018) 62.
100. Gale CR, Robinson SM, Harvey NC, Javaid MK, Jiang B, Martyn CN, et al. Maternal vitamin d status during pregnancy and child outcomes. Eur J Clin Nutr (2008) 62(1):68–77. doi: 10.1038/sj.ejcn.1602680
101. Hollams E, Hart P, Holt B, Serralha M, Parsons F, De Klerk N, et al. Vitamin d and atopy and asthma phenotypes in children: a longitudinal cohort study. Eur Respir J (2011) 38(6):1320–7. doi: 10.1183/09031936.00029011
102. Peroni DG, Piacentini GL, Cametti E, Chinellato I, Boner AL. Correlation between serum 25-hydroxyvitamin d levels and severity of atopic dermatitis in children. Br J Dermatol (2011) 164(5):1078–82. doi: 10.1111/j.1365-2133.2010.10147.x
103. van Etten E, Mathieu C. Immunoregulation by 1,25-dihydroxyvitamin D3: basic concepts. J Steroid Biochem Mol Biol (2005) 97(1-2):93–101. doi: 10.1016/j.jsbmb.2005.06.002
104. Mutgi K, Koo J. Update on the role of systemic vitamin d in atopic dermatitis. Pediatr Dermatol (2013) 30(3):303–7. doi: 10.1111/j.1525-1470.2012.01850.x
105. Oren E, Banerji A, Camargo CA Jr. Vitamin d and atopic disorders in an obese population screened for vitamin d deficiency. J Allergy Clin Immunol (2008) 121(2):533–4. doi: 10.1016/j.jaci.2007.11.005
106. Miyake Y, Sasaki S, Tanaka K, Hirota Y. Dairy food, calcium and vitamin d intake in pregnancy, and wheeze and eczema in infants. Eur Respir J (2010) 35(6):1228–34. doi: 10.1183/09031936.00100609
107. Willers S, Devereux G, Craig L, McNeill G, Wijga A, El-Magd WA, et al. Maternal food consumption during pregnancy and asthma, respiratory and atopic symptoms in 5-year-old children. Thorax (2007) 62(9):773–9. doi: 10.1136/thx.2006.074187
108. Wang L-C, Chiang B-L, Huang Y-M, Shen P-T, Huang H-Y, Lin B-F. Lower vitamin d levels in the breast milk is associated with atopic dermatitis in early infancy. Pediatr Allergy Immunol (2020) 31(3):258–64. doi: 10.1111/pai.13179
109. Bäck O, Blomquist HK, Hernell O, Stenberg B. Does vitamin d intake during infancy promote the development of atopic allergy? Acta dermato-venereologica (2009) 89(1):28–32. doi: 10.2340/00015555-0541
110. Tian Y, Ye Y, Zhang Y, Dou L, Dou Y, Zhao P, et al. Maternal serum 25-hydroxyvitamin d levels and infant atopic dermatitis: a prospective cohort study. Pediatr Allergy Immunol (2021). n/a(n/a). doi: 10.1111/pai.13582
111. Lucas R, Mihály J, Gericke J, Törőcsik D, Rühl R. Vitamin d signaling in a mouse allergic sensitization model. Int J Vitamin Nutr Res (2020) 90(5-6):385–8. doi: 10.1024/0300-9831/a000633
112. Amestejani M, Salehi BS, Vasigh M, Sobhkhiz A, Karami M, Alinia H, et al. Vitamin d supplementation in the treatment of atopic dermatitis: a clinical trial study. J Drugs dermatology: JDD (2012) 11(3):327–30.
113. Rigby W, Stacy T, Fanger MW. Inhibition of T lymphocyte mitogenesis by 1, 25-dihydroxyvitamin D3 (calcitriol). J Clin Invest (1984) 74(4):1451.
114. Lemire JM, Archer DC, Beck L, Spiegelberg HL. Immunosuppressive actions of 1, 25-dihydroxyvitamin D3: preferential inhibition of Th1 functions. J Nutr (1995) 125(6 Suppl):1704S–8S. doi: 10.1016/0960-0760(95)00106-A
115. Daniel C, Sartory NA, Zahn N, Radeke HH, Stein JM. Immune modulatory treatment of trinitrobenzene sulfonic acid colitis with calcitriol is associated with a change of a T helper (Th) 1/Th17 to a Th2 and regulatory T cell profile. J Pharmacol Exp Ther (2008) 324(1):23–33. doi: 10.1124/jpet.107.127209
116. Penna G, Adorini L. 1α, 25-dihydroxyvitamin D3 inhibits differentiation, maturation, activation, and survival of dendritic cells leading to impaired alloreactive T cell activation. J Immunol (2000) 164(5):2405–11. doi: 10.4049/jimmunol.164.5.2405
117. Griffin MD, Xing N, Kumar R. Vitamin d and its analogs as regulators of immune activation and antigen presentation. Annu Rev Nutr (2003) 23(1):117–45. doi: 10.1146/annurev.nutr.23.011702.073114
118. Herrmann N, Nümm T, Iwamoto K, Leib N, Koch S, Majlesain Y, et al. Vitamin d 3 –induced promotor dissociation of PU.1 and YY1 results in FcϵRI reduction on dendritic cells in atopic dermatitis. J Immunol (2020) 206:ji2000667.
119. Cristi F, Perez-Mateluna G, Vera-Kellet C, Silva-Valenzuela S, Iturriaga C, Hoyos-Bachiloglu R, et al. Vitamin d modulates the allergic phenotype of dendritic cells in children with atopic dermatitis. Exp Dermatol (2019) 28(3):308–11. doi: 10.1111/exd.13873
120. Mansour NO, Mohamed AA, Hussein M, Eldemiry E, Daifalla A, Hassanin S, et al. The impact of vitamin d supplementation as an adjuvant therapy on clinical outcomes in patients with severe atopic dermatitis: a randomized controlled trial. Pharmacol Res Perspectives (2020) 8(6):e00679. doi: 10.1002/prp2.679
121. Harris ED Jr. Rheumatoid arthritis: pathophysiology and implications for therapy. New Engl J Med (1990) 322(18):1277–89.
122. Choi S, Rho Y, Ji J, Song G, Lee Y. Genome scan meta-analysis of rheumatoid arthritis. Rheumatology (2005) 45(2):166–70.
123. Szodoray P, Nakken B, Gaal J, Jonsson R, Szegedi A, Zold E, et al. The complex role of vitamin d in autoimmune diseases. Scandinavian J Immunol (2008) 68(3):261–9. doi: 10.1111/j.1365-3083.2008.02127.x
124. Gatenby P, Lucas R, Swaminathan A. Vitamin d deficiency and risk for rheumatic diseases: an update. Curr Opin Rheumatol (2013) 25(2):184–91. doi: 10.1097/BOR.0b013e32835cfc16
125. Kamen D, Aranow C. Vitamin d in systemic lupus erythematosus. Curr Opin Rheumatol (2008) 20(5):532–7. doi: 10.1097/BOR.0b013e32830a991b
126. Cutolo M, Otsa K, Paolino S, Yprus M, Veldi T, Seriolo B. Vitamin d involvement in rheumatoid arthritis and systemic lupus erythaematosus. Ann Rheumatic Diseases (2009) 68(3):446–7. doi: 10.1136/ard.2008.093476
127. Koizumi T, Nakao Y, Matsui T, Nakagawa T, Matsuda S, Komoriya K, et al. Effects of corticosteroid and 1, 24R-dihydroxy-vitamin D3 administration on lymphoproliferation and autoimmune disease in MRL/MP-lpr/lpr mice. Int Arch Allergy Immunol (1985) 77(4):396–404. doi: 10.1159/000233815
128. Zhou L, Wang J, Li J, Li T, Chen Y, June RR, et al. 1,25-dihydroxyvitamin D3 ameliorates collagen-induced arthritis via suppression of Th17 cells through miR-124 mediated inhibition of IL-6 signaling. Front Immunol (2019) 10(178). doi: 10.3389/fimmu.2019.00178
129. Lee YH, Bae S-C, Choi SJ, Ji JD, Song GG. Associations between vitamin d receptor polymorphisms and susceptibility to rheumatoid arthritis and systemic lupus erythematosus: a meta-analysis. Mol Biol Rep (2011) 38(6):3643–51. doi: 10.1007/s11033-010-0477-4
130. Khoja SO, Al-Jehani RF, Bahlas SM, Algamdi SA, Attar S. EVALUATION OF RELATIONSHIP BETWEEN VITAMIN d RECEPTOR GENE POLYMORPHISMS AND RHEUMATOID ARTHRITIS IN SAUDI POPULATION. PHARMACOPHORE (2018) 9(1):85–94.
131. Song GG, Bae S-C, Lee YH. Association between vitamin d intake and the risk of rheumatoid arthritis: a meta-analysis. Clin Rheumatol (2012) 31(12):1733–9. doi: 10.1007/s10067-012-2080-7
132. Mouterde G, Gamon E, Rincheval N, Lukas C, Seror R, Berenbaum F, et al. Association between vitamin d deficiency and disease activity, disability and radiographic progression in early rheumatoid arthritis. the ESPOIR cohort. J Rheumatol (2019). jrheum.190795.
133. Nakayama M, Furuya T, Inoue E, Tanaka E, Ikari K, Yamanaka H, et al. Vitamin d deficiency is a risk factor for new fractures in Japanese postmenopausal women with rheumatoid arthritis: results from the IORRA cohort study. Arch Osteoporosis (2021) 16:119. doi: 10.1007/s11657-021-00982-x
134. Miler M, Nikolac Gabaj N, Grazio S, Vahtarić A, Vrtaric A, Grubišić F, et al. Lower concentration of vitamin d is associated with lower DAS28 and VAS-pain scores in patients with inflammatory rheumatic diseases treated with infliximab: a pilot study. Rheumatol Int (2020) 40. doi: 10.1007/s00296-020-04607-3
135. Koch-Henriksen N, Sørensen PS. The changing demographic pattern of multiple sclerosis epidemiology. Lancet Neurology (2010) 9(5):520–32. doi: 10.1016/S1474-4422(10)70064-8
136. Sloka S, Silva C, Pryse-Phillips W, Patten S, Metz L, Yong VW. A quantitative analysis of suspected environmental causes of MS. Can J Neurological Sci (2011) 38(1):98–105. doi: 10.1017/S0317167100011124
137. Salzer J, Hallmans G, Nyström M, Stenlund H, Wadell G, Sundström P. Vitamin d as a protective factor in multiple sclerosis. Neurology (2012) 79(21):2140–5. doi: 10.1212/WNL.0b013e3182752ea8
138. Munger KL, Levin LI, Hollis BW, Howard NS, Ascherio A. Serum 25-hydroxyvitamin d levels and risk of multiple sclerosis. Jama (2006) 296(23):2832–8. doi: 10.1001/jama.296.23.2832
139. Vimaleswaran KS, Berry DJ, Lu C, Tikkanen E, Pilz S, Hiraki LT, et al. Causal relationship between obesity and vitamin d status: bi-directional mendelian randomization analysis of multiple cohorts. PloS Med (2013) 10(2):e1001383. doi: 10.1371/journal.pmed.1001383
140. Brot C, Rye Jørgensen N, Helmer Sørensen O. The influence of smoking on vitamin d status and calcium metabolism. Eur J Clin Nutr (1999) 53(12):920–6. doi: 10.1038/sj.ejcn.1600870
141. Hedström AK, Olsson T, Alfredsson L. High body mass index before age 20 is associated with increased risk for multiple sclerosis in both men and women. Multiple Sclerosis J (2012) 18(9):1334–6. doi: 10.1177/1352458512436596
142. Ascherio A, Munger KL. Environmental risk factors for multiple sclerosis. Part II: Noninfectious factors. Ann Neurol (2007) 61(6):504–13.
143. Ramagopalan SV, Dyment DA, Cader MZ, Morrison KM, Disanto G, Morahan JM, et al. Rare variants in the CYP27B1 gene are associated with multiple sclerosis. Ann Neurology (2011) 70(6):881–6. doi: 10.1002/ana.22678
144. Ban M, Caillier S, Mero IL, Myhr KM, Celius EG, Aarseth J, et al. No evidence of association between mutant alleles of the CYP27B1 gene and multiple sclerosis. Ann Neurology (2013) 73(3):430–2. doi: 10.1002/ana.23833
145. Barizzone N, Pauwels I, Luciano B, Franckaert D, Guerini FR, Cosemans L, et al. No evidence for a role of rare CYP27B1 functional variations in multiple sclerosis. Ann Neurology (2013) 73(3):433–7. doi: 10.1002/ana.23834
146. Bufalino C, Hepgul N, Aguglia E, Pariante CM. The role of immune genes in the association between depression and inflammation: a review of recent clinical studies. Brain Behav Immun (2013) 31:31–47. doi: 10.1016/j.bbi.2012.04.009
147. Ramagopalan SV, Maugeri NJ, Handunnetthi L, Lincoln MR, Orton S-M, Dyment DA, et al. Expression of the multiple sclerosis-associated MHC class II allele HLA-DRB1* 1501 is regulated by vitamin d. PloS Genet (2009) 5(2):e1000369. doi: 10.1371/journal.pgen.1000369
148. Narooie-Nejad M, Moossavi M, Torkamanzehi A, Moghtaderi A. Positive association of vitamin d receptor gene variations with multiple sclerosis in south East Iranian population. BioMed Res Int (2015) 2015. doi: 10.1155/2015/427519
149. Narooie-Nejad M, Moossavi M, Torkamanzehi A, Moghtaderi A, Salimi S. Vitamin d receptor gene polymorphism and the risk of multiple sclerosis in south Eastern of Iran. J Mol Neurosci (2015) 56(3):572–6. doi: 10.1007/s12031-015-0513-x
150. Imani D, Razi B, Motallebnezhad M, Rezaei R. Association between vitamin d receptor (VDR) polymorphisms and the risk of multiple sclerosis (MS): an updated meta-analysis. BMC Neurology (2019) 19(1):339. doi: 10.1186/s12883-019-1577-y
151. Spanier JA, Nashold FE, Nelson CD, Praska CE, Hayes CE. Vitamin D3-mediated resistance to a multiple sclerosis model disease depends on myeloid cell 1,25-dihydroxyvitamin D3 synthesis and correlates with increased CD4+ T cell CTLA-4 expression. J Neuroimmunology (2020) 338. doi: 10.1016/j.jneuroim.2019.577105
152. Carlberg C. Vitamin d genomics: from in vitro to in vivo. Front Endocrinol (2018) 9. doi: 10.3389/fendo.2018.00250
153. Sezer E, Can Demirdöğen B, Demirkaya Ş, Bulut G, Akkulak M, Evin E, et al. Association of cholesterol 7α-hydroxylase (CYP7A1) promoter polymorphism (rs3808607) and cholesterol 24S-hydroxylase (CYP46A1) intron 2 polymorphism (rs754203) with serum lipids, vitamin d levels, and multiple sclerosis risk in the Turkish population. Neurological Sci Off J Ital Neurological Soc Ital Soc Clin Neurophysiol (2021). doi: 10.1007/s10072-021-05597-1
154. Davey Smith G, Ebrahim S. ‘Mendelian randomization’: can genetic epidemiology contribute to understanding environmental determinants of disease? Int J Epidemiol (2003) 32(1):1–22. doi: 10.1093/ije/dyg070
155. Amirinejad R, Shirvani-Farsani Z, Naghavi Gargari B, Sahraian MA, Mohammad Soltani B, Behmanesh M. Vitamin d changes expression of DNA repair genes in the patients with multiple sclerosis. Gene (2021) 781:145488. doi: 10.1016/j.gene.2021.145488
156. Sato Y, Kikuyama M, Oizumi K. High prevalence of vitamin d deficiency and reduced bone mass in parkinson's disease. Neurology (1997) 49(5):1273–8. doi: 10.1212/WNL.49.5.1273
157. Evatt ML, DeLong MR, Khazai N, Rosen A, Triche S, Tangpricha V. Prevalence of vitamin d insufficiency in patients with Parkinson disease and Alzheimer disease. Arch Neurology (2008) 65(10):1348–52. doi: 10.1001/archneur.65.10.1348
158. Evatt ML, DeLong MR, Kumari M, Auinger P, McDermott MP, Tangpricha V. High prevalence of hypovitaminosis d status in patients with early Parkinson disease. Arch Neurology (2011) 68(3):314–9. doi: 10.1001/archneurol.2011.30
159. Knekt P, Kilkkinen A, Rissanen H, Marniemi J, Sääksjärvi K, Heliövaara M. Serum vitamin d and the risk of Parkinson disease. Arch Neurology (2010) 67(7):808–11. doi: 10.1001/archneurol.2010.120
160. Kenborg L, Lassen CF, Ritz B, Schernhammer ES, Hansen J, Gatto NM, et al. Outdoor work and risk for Parkinson9s disease: a population-based case–control study. Occup Environ Med (2010) oem:2010.057448.
161. Eyles DW, Smith S, Kinobe R, Hewison M, McGrath JJ. Distribution of the vitamin d receptor and 1α-hydroxylase in human brain. J Chem Neuroanatomy (2005) 29(1):21–30. doi: 10.1016/j.jchemneu.2004.08.006
162. Burne TH, McGrath JJ, Eyles DW, Mackay-Sim A. Behavioural characterization of vitamin d receptor knockout mice. Behav Brain Res (2005) 157(2):299–308. doi: 10.1016/j.bbr.2004.07.008
163. Liu Y, Zhang B-S. Serum 25-hydroxyvitamin d predicts severity in parkinson’s disease patients. Neurological Sci (2014) 35(1):67–71. doi: 10.1007/s10072-013-1539-x
164. Alaylıoğlu M, Dursun E, Genç G, Şengül B, Bilgiç B, Gündüz A, et al. Genetic variants of vitamin d metabolism-related DHCR7/NADSYN1 locus and CYP2R1 gene are associated with clinical features of parkinson’s disease. Int J Neurosci (2020), 1–11.
165. Gao J, Teng J, Liu Z, Cai M, Xie A. Association between vitamin d receptor polymorphisms and susceptibility to parkinson's disease: an updated meta-analysis. Neurosci Letters (2020) 720:134778. doi: 10.1016/j.neulet.2020.134778
166. Lv L, Tan X, Peng X, Bai R, Xiao Q, Zou T, et al. Vitamin d receptor gene polymorphisms, and vitamin d supplementation with parkinson's disease. Trans Neurodegeneration (2020) 9(1):34.
167. Gatto NM, Sinsheimer JS, Cockburn M, Escobedo LA, Bordelon Y, Ritz B. Vitamin d receptor gene polymorphisms and parkinson's disease in a population with high ultraviolet radiation exposure. J Neurological Sci (2015) 352(1-2):88–93. doi: 10.1016/j.jns.2015.03.043
168. Dini C, Bianchi A. The potential role of vitamin d for prevention and treatment of tuberculosis and infectious diseases. Annali dell'Istituto superiore di sanita (2012) 48(3):319–27. doi: 10.4415/ANN_12_03_13
169. Xu G, Wang J, Gao GF, Liu CH. Insights into battles between mycobacterium tuberculosis and macrophages. Protein Cell (2014) 5(10):728–36. doi: 10.1007/s13238-014-0077-5
170. Clark A, Mach N. Role of vitamin d in the hygiene hypothesis: the interplay between vitamin d, vitamin d receptors, gut microbiota, and immune response. Front Immunol (2016) 7:627. doi: 10.3389/fimmu.2016.00627
171. Wu S, Yoon S, Zhang YG, Lu R, Xia Y, Wan J, et al. Vitamin d receptor pathway is required for probiotic protection in colitis. Am J Physiol Gastrointestinal liver Physiol (2015) 309(5):G341–9. doi: 10.1152/ajpgi.00105.2015
172. Annweiler C, Beaudenon M, Simon R, Guenet M, Otekpo M, Célarier T, et al. Vitamin d supplementation prior to or during COVID-19 associated with better 3-month survival in geriatric patients: extension phase of the GERIA-COVID study. J Steroid Biochem Mol Biol (2021) 213:105958. doi: 10.1016/j.jsbmb.2021.105958
173. Ganmaa D, Uyanga B, Zhou X, Gantsetseg G, Delgerekh B, Enkhmaa D, et al. Vitamin d supplements for prevention of tuberculosis infection and disease. New Engl J Med (2020) 383(4):359–68. doi: 10.1056/NEJMoa1915176
174. Arihiro S, Nakashima A, Matsuoka M, Suto S, Uchiyama K, Kato T, et al. Randomized trial of vitamin d supplementation to prevent seasonal influenza and upper respiratory infection in patients with inflammatory bowel disease. Inflamm Bowel Dis (2019) 25(6):1088–95. doi: 10.1093/ibd/izy346
175. Dolgin E. Cancer's new normal. Nat cancer (2021) 2(12):1248–50. doi: 10.1038/s43018-021-00304-7
176. Kim H, Lipsyc-Sharf M, Zong X, Wang X, Hur J, Song M, et al. Total vitamin d intake and risks of early-onset colorectal cancer and precursors. Gastroenterology (2021) 161(4):1208–17.e9. doi: 10.1053/j.gastro.2021.07.002
177. Estébanez N, Gómez-Acebo I, Palazuelos C, Llorca J, Dierssen-Sotos T. Vitamin d exposure and risk of breast cancer: a meta-analysis. Sci Rep (2018) 8(1):9039.
178. Martin-Gorgojo A, Gilaberte Y, Nagore E. Vitamin d and skin cancer: an epidemiological, patient-centered update and review. Nutrients (2021) 13(12). doi: 10.3390/nu13124292
179. Kim JS, Roberts JM, Bingman WE 3rd, Shao L, Wang J, Ittmann MM, et al. The prostate cancer TMPRSS2:ERG fusion synergizes with the vitamin d receptor (VDR) to induce CYP24A1 expression-limiting VDR signaling. Endocrinology (2014) 155(9):3262–73. doi: 10.1210/en.2013-2019
180. Markotić A, Kelava T, Markotić H, Silovski H, Mrzljak A. Vitamin d in liver cancer: novel insights and future perspectives. Croatian Med J (2022) 63(2):187–96. doi: 10.3325/cmj.2022.63.187
181. Mäkitie A, Tuokkola I, Laurell G, Mäkitie O, Olsen K, Takes RP, et al. Vitamin d in head and neck cancer: a systematic review. Curr Oncol Rep (2020) 23(1):5. doi: 10.1007/s11912-020-00996-7
182. Ma Y, Zhang P, Wang F, Yang J, Liu Z, Qin H. Association between vitamin d and risk of colorectal cancer: a systematic review of prospective studies. J Clin Oncol Off J Am Soc Clin Oncol (2011) 29(28):3775–82. doi: 10.1200/JCO.2011.35.7566
183. Pu Y, Zhu G, Xu Y, Zheng S, Tang B, Huang H, et al. Association between vitamin d exposure and head and neck cancer: a systematic review with meta-analysis. Front Immunol (2021) 12:627226. doi: 10.3389/fimmu.2021.627226
184. Castellano-Castillo D, Morcillo S, Clemente-Postigo M, Crujeiras AB, Fernandez-García JC, Torres E, et al. Adipose tissue inflammation and VDR expression and methylation in colorectal cancer. Clin Epigenetics (2018) 10:60. doi: 10.1186/s13148-018-0493-0
185. Czogalla B, Deuster E, Liao Y, Mayr D, Schmoeckel E, Sattler C, et al. Cytoplasmic VDR expression as an independent risk factor for ovarian cancer. Histochem Cell Biol (2020) 154(4):421–9. doi: 10.1007/s00418-020-01894-6
186. Kim SH, Chen G, King AN, Jeon CK, Christensen PJ, Zhao L, et al. Characterization of vitamin d receptor (VDR) in lung adenocarcinoma. Lung Cancer (Amsterdam Netherlands) (2012) 77(2):265–71. doi: 10.1016/j.lungcan.2012.04.010
187. Hendrickson WK, Flavin R, Kasperzyk JL, Fiorentino M, Fang F, Lis R, et al. Vitamin d receptor protein expression in tumor tissue and prostate cancer progression. J Clin Oncol Off J Am Soc Clin Oncol (2011) 29(17):2378–85. doi: 10.1200/JCO.2010.30.9880
189. Nonn L, Peng L, Feldman D, Peehl DM. Inhibition of p38 by vitamin d reduces interleukin-6 production in normal prostate cells via mitogen-activated protein kinase phosphatase 5: implications for prostate cancer prevention by vitamin d. Cancer Res (2006) 66(8):4516–24. doi: 10.1158/0008-5472.CAN-05-3796
190. Kim EK, Choi EJ. Pathological roles of MAPK signaling pathways in human diseases. Biochim Biophys Acta (2010) 1802(4):396–405. doi: 10.1016/j.bbadis.2009.12.009
191. Bao BY, Yao J, Lee YF. 1alpha, 25-dihydroxyvitamin D3 suppresses interleukin-8-mediated prostate cancer cell angiogenesis. Carcinogenesis (2006) 27(9):1883–93. doi: 10.1093/carcin/bgl041
192. Manson JE, Cook NR, Lee IM, Christen W, Bassuk SS, Mora S, et al. Vitamin d supplements and prevention of cancer and cardiovascular disease. New Engl J Med (2019) 380(1):33–44. doi: 10.1056/NEJMoa1809944
193. Lappe J, Watson P, Travers-Gustafson D, Recker R, Garland C, Gorham E, et al. Effect of vitamin d and calcium supplementation on cancer incidence in older women: a randomized clinical trial. Jama (2017) 317(12):1234–43. doi: 10.1001/jama.2017.2115
194. Akiba T, Morikawa T, Odaka M, Nakada T, Kamiya N, Yamashita M, et al. Vitamin d supplementation and survival of patients with non-small cell lung cancer: a randomized, double-blind, placebo-controlled trial. Clin Cancer Res an Off J Am Assoc Cancer Res (2018) 24(17):4089–97. doi: 10.1158/1078-0432.CCR-18-0483
195. Rose K, Penna-Martinez M, Klahold E, Kärger D, Shoghi F, Kahles H, et al. Influence of the vitamin d plasma level and vitamin d-related genetic polymorphisms on the immune status of patients with type 1 diabetes: a pilot study. Clin Exp Immunol (2013) 171(2):171–85. doi: 10.1111/cei.12013
196. Wolden-Kirk H, Overbergh L, Christesen HT, Brusgaard K, Mathieu C. Vitamin d and diabetes: its importance for beta cell and immune function. Mol Cell endocrinology (2011) 347(1):106–20. doi: 10.1016/j.mce.2011.08.016
197. Zhou LJ, Tedder TF. Human blood dendritic cells selectively express CD83, a member of the immunoglobulin superfamily. J Immunol (Baltimore Md 1950) (1995) 154(8):3821–35. doi: 10.4049/jimmunol.154.8.3821
198. Bivona G, Agnello L, Pivetti A, Milano S, Scazzone C, Sasso BL, et al. Association between hypovitaminosis d and systemic sclerosis: true or fake? Clinica Chimica Acta (2016) 458:115–9. doi: 10.1016/j.cca.2016.04.026
199. Dankers W, Colin EM, Van Hamburg JP, Lubberts E. Vitamin d in autoimmunity: molecular mechanisms and therapeutic potential. Front Immunol (2017) 697. doi: 10.3389/fimmu.2016.00697
200. Scazzone C, Agnello L, Bivona G, Lo Sasso B, Ciaccio M. Vitamin d and genetic susceptibility to multiple sclerosis. Biochem Genet (2021) 59(1):1–30. doi: 10.1007/s10528-020-10010-1
201. Hou Y, Song A, Jin Y, Xia Q, Song G, Xing X. A dose–response meta-analysis between serum concentration of 25-hydroxy vitamin d and risk of type 1 diabetes mellitus. Eur J Clin Nutr (2021) 75(7):1010–23. doi: 10.1038/s41430-020-00813-1
202. Infante M, Ricordi C, Sanchez J, Clare-Salzler MJ, Padilla N, Fuenmayor V, et al. Influence of vitamin d on islet autoimmunity and beta-cell function in type 1 diabetes. Nutrients (2019) 11(9):2185. doi: 10.3390/nu11092185
203. Chen Y-L, Huang Y-C, Qiao Y-C, Ling W, Pan Y-H, Geng L-J, et al. Climates on incidence of childhood type 1 diabetes mellitus in 72 countries. Sci Rep (2017) 7(1):1–17. doi: 10.1038/s41598-017-12954-8
204. Gerasimidi Vazeou A, Kordonouri O, Witsch M, Hermann JM, Forsander G, de Beaufort C, et al. Seasonality at the clinical onset of type 1 diabetes–lessons from the SWEET database. Pediatr Diabetes (2016) 17:32–7. doi: 10.1111/pedi.12433
205. Mohr S, Garland C, Gorham E, Garland F. The association between ultraviolet b irradiance, vitamin d status and incidence rates of type 1 diabetes in 51 regions worldwide. Diabetologia (2008) 51(8):1391–8. doi: 10.1007/s00125-008-1061-5
206. Moltchanova E, Schreier N, Lammi N, Karvonen M. Seasonal variation of diagnosis of type 1 diabetes mellitus in children worldwide. Diabetic Med (2009) 26(7):673–8. doi: 10.1111/j.1464-5491.2009.02743.x
207. Sloka S, Grant M, Newhook LA. The geospatial relation between UV solar radiation and type 1 diabetes in Newfoundland. Acta Diabetologica (2010) 47(1):73–8. doi: 10.1007/s00592-009-0100-0
208. Staples JA, Ponsonby A-L, Lim LL, McMichael AJ. Ecologic analysis of some immune-related disorders, including type 1 diabetes, in Australia: latitude, regional ultraviolet radiation, and disease prevalence. Environ Health Perspectives (2003) 111(4):518–23. doi: 10.1289/ehp.5941
209. Kalliora MI, Vazeou A, Delis D, Bozas E, Thymelli I, Bartsocas CS. Seasonal variation of type 1 diabetes mellitus diagnosis in Greek children. Hormones (2011) 10(1):67–71. doi: 10.14310/horm.2002.1294
210. Hyppönen E, Läärä E, Reunanen A, Järvelin M-R, Virtanen SM. Intake of vitamin d and risk of type 1 diabetes: a birth-cohort study. Lancet (2001) 358(9292):1500–3. doi: 10.1016/S0140-6736(01)06580-1
211. Janner M, Ballinari P, Mullis P-E, Flück C. High prevalence of vitamin d deficiency in children and adolescents with type 1 diabetes. Swiss Med Weekly (2010) 140:w13091. doi: 10.4414/smw.2010.13091
212. Pinheiro MM, Pinheiro FMM, Diniz SN, Fabbri A, Infante M. Combination of vitamin d and dipeptidyl peptidase-4 inhibitors (VIDPP-4i) as an immunomodulation therapy for autoimmune diabetes. Int Immunopharmacology (2021) 95:107518. doi: 10.1016/j.intimp.2021.107518
213. Bailey R, Cooper JD, Zeitels L, Smyth DJ, Yang JH, Walker NM, et al. Association of the vitamin d metabolism gene CYP27B1 with type 1 diabetes. Diabetes (2007) 56(10):2616–21. doi: 10.2337/db07-0652
214. Cooper JD, Smyth DJ, Walker NM, Stevens H, Burren OS, Wallace C, et al. Inherited variation in vitamin d genes is associated with predisposition to autoimmune disease type 1 diabetes. Diabetes (2011) 60(5):1624–31. doi: 10.2337/db10-1656
215. Ramos-Lopez E, Brück P, Jansen T, Herwig J, Badenhoop K. CYP2R1 (vitamin d 25-hydroxylase) gene is associated with susceptibility to type 1 diabetes and vitamin d levels in germans. Diabetes/metabolism Res Rev (2007) 23(8):631–6. doi: 10.1002/dmrr.719
216. Kahles H, Morahan G, Todd J, Badenhoop K. Association analyses of the vitamin d receptor gene in 1654 families with type I diabetes. Genes Immunity (2009) 10(1):S60–S3. doi: 10.1038/gene.2009.93
217. Nejentsev S, Cooper JD, Godfrey L, Howson JM, Rance H, Nutland S, et al. Analysis of the vitamin d receptor gene sequence variants in type 1 diabetes. Diabetes (2004) 53(10):2709–12. doi: 10.2337/diabetes.53.10.2709
218. Zhang J, Li W, Liu J, Wu W, Ouyang H, Zhang Q, et al. Polymorphisms in the vitamin d receptor gene and type 1 diabetes mellitus risk: an update by meta-analysis. Mol Cell endocrinology (2012) 355(1):135–42. doi: 10.1016/j.mce.2012.02.003
219. Sigmundsdottir H, Pan J, Debes GF, Alt C, Habtezion A, Soler D, et al. DCs metabolize sunlight-induced vitamin D3 to 'program' T cell attraction to the epidermal chemokine CCL27. Nat Immunol (2007) 8(3):285–93. doi: 10.1038/ni1433
220. Veldman CM, Cantorna MT, DeLuca HF. Expression of 1, 25-dihydroxyvitamin D3 receptor in the immune system. Arch Biochem Biophysics (2000) 374(2):334–8. doi: 10.1006/abbi.1999.1605
221. Adorini L, Penna G, Giarratana N, Uskokovic M. Tolerogenic dendritic cells induced by vitamin d receptor ligands enhance regulatory T cells inhibiting allograft rejection and autoimmune diseases. J Cell Biochem (2003) 88(2):227–33. doi: 10.1002/jcb.10340
222. Penna G, Amuchastegui S, Giarratana N, Daniel KC, Vulcano M, Sozzani S, et al. 1, 25-dihydroxyvitamin D3 selectively modulates tolerogenic properties in myeloid but not plasmacytoid dendritic cells. J Immunol (2007) 178(1):145–53. doi: 10.4049/jimmunol.178.1.145
223. van Halteren AG, Tysma OM, van Etten E, Mathieu C, Roep BO. 1α, 25-dihydroxyvitamin D3 or analogue treated dendritic cells modulate human autoreactive T cells via the selective induction of apoptosis. J Autoimmunity (2004) 23(3):233–9. doi: 10.1016/j.jaut.2004.06.004
224. Takiishi T, Van Belle T, Gysemans C, Mathieu C. Effects of vitamin d on antigen-specific and non-antigen-specific immune modulation: relevance for type 1 diabetes. Pediatr Diabetes (2012) 14(2):81–9.
225. Decallonne B, van Etten E, Overbergh L, Valckx D, Bouillon R, Mathieu C. 1α, 25-dihydroxyvitamin D3 restores thymocyte apoptosis sensitivity in non-obese diabetic (NOD) mice through dendritic cells. J autoimmunity (2005) 24(4):281–9. doi: 10.1016/j.jaut.2005.03.007
226. Driver J, Foreman O, Mathieu C, Van Etten E, Serreze D. Comparative therapeutic effects of orally administered 1, 25-dihydroxyvitamin D3 and 1alpha-hydroxyvitamin D3 on type-1 diabetes in non-obese diabetic mice fed a normal-calcaemic diet. Clin Exp Immunol (2008) 151(1):76–85.
227. Gregori S, Giarratana N, Smiroldo S, Uskokovic M, Adorini L. A 1α, 25-dihydroxyvitamin D3 analog enhances regulatory T-cells and arrests autoimmune diabetes in NOD mice. Diabetes (2002) 51(5):1367–74. doi: 10.2337/diabetes.51.5.1367
228. Mathieu C, Waer M, Laureys J, Rutgeerts O, Bouillon R. Prevention of autoimmune diabetes in NOD mice by 1, 25 dihydroxyvitamin D3. Diabetologia (1994) 37(6):552–8. doi: 10.1007/BF00403372
229. Zella JB, McCary LC, DeLuca HF. Oral administration of 1, 25-dihydroxyvitamin D3 completely protects NOD mice from insulin-dependent diabetes mellitus. Arch Biochem Biophysics (2003) 417(1):77–80. doi: 10.1016/S0003-9861(03)00338-2
230. Rak K, Bronkowska M. Immunomodulatory effect of vitamin d and its potential role in the prevention and treatment of type 1 diabetes mellitus–a narrative review. Molecules (2018) 24(1):53. doi: 10.3390/molecules24010053
231. Gabbay MA, Sato MN, Finazzo C, Duarte AJ, Dib SA. Effect of cholecalciferol as adjunctive therapy with insulin on protective immunologic profile and decline of residual β-cell function in new-onset type 1 diabetes mellitus. Arch Pediatr Adolesc Med (2012) 166(7):601–7. doi: 10.1001/archpediatrics.2012.164
232. Treiber G, Prietl B, Fröhlich-Reiterer E, Lechner E, Ribitsch A, Fritsch M, et al. Cholecalciferol supplementation improves suppressive capacity of regulatory T-cells in young patients with new-onset type 1 diabetes mellitus–a randomized clinical trial. Clin Immunol (2015) 161(2):217–24. doi: 10.1016/j.clim.2015.08.002
233. Bizzarri C, Pitocco D, Napoli N, Di Stasio E, Maggi D, Manfrini S, et al. No protective effect of calcitriol on β-cell function in recent-onset type 1 diabetes: the IMDIAB XIII trial. Diabetes Care (2010) 33(9):1962–3. doi: 10.2337/dc10-0814
234. Brekke HK, Ludvigsson J. Vitamin d supplementation and diabetes-related autoimmunity in the ABIS study. Pediatr Diabetes (2007) 8(1):11–4. doi: 10.1111/j.1399-5448.2006.00223.x
235. Perchard R, Magee L, Whatmore A, Ivison F, Murray P, Stevens A, et al. A pilot interventional study to evaluate the impact of cholecalciferol treatment on HbA1c in type 1 diabetes (T1D). Endocrine Connections (2017) 6(4):225–31. doi: 10.1530/EC-17-0045
236. Walter M, Kaupper T, Adler K, Foersch J, Bonifacio E, Ziegler A-G. No effect of the 1α, 25-dihydroxyvitamin D3 on β-cell residual function and insulin requirement in adults with new-onset type 1 diabetes. Diabetes Care (2010) 33(7):1443–8. doi: 10.2337/dc09-2297
237. Fletcher J. Vitamin d deficiency in patients with inflammatory bowel disease. Br J Nurs (Mark Allen Publishing) (2016) 25(15):846–51. doi: 10.12968/bjon.2016.25.15.846
238. Fletcher J, Swift A. Vitamin d screening in patients with inflammatory bowel disease. Gastrointestinal Nursing (2017) 15(9):16–23. doi: 10.12968/gasn.2017.15.9.16
239. Vernia P, Burrelli Scotti G, Dei Giudici A, Chiappini A, Cannizzaro S, Afferri MT, et al. Inadequate sunlight exposure in patients with inflammatory bowel disease. J Dig Dis (2018) 19(1):8–14. doi: 10.1111/1751-2980.12567
240. Burrelli Scotti G, Afferri MT, De Carolis A, Vaiarello V, Fassino V, Ferrone F, et al. Factors affecting vitamin d deficiency in active inflamm Bowel Dis. Digestive liver Dis Off J Ital Soc Gastroenterol Ital Assoc Study Liver (2019) 51(5):657–62.
241. Del Pinto R, Pietropaoli D, Chandar AK, Ferri C, Cominelli F. Association between inflammatory bowel disease and vitamin d deficiency: a systematic review and meta-analysis. Inflamm Bowel Dis (2015) 21(11):2708–17. doi: 10.1097/MIB.0000000000000546
242. Fletcher J, Brown M, Hewison M, Swift A, Cooper SC. Prevalence of vitamin d deficiency and modifiable risk factors in patients with crohn's disease: a prospective observational study. J Advanced Nurs (2022). doi: 10.1111/jan.15476
243. Lagishetty V, Misharin AV, Liu NQ, Lisse TS, Chun RF, Ouyang Y, et al. Vitamin d deficiency in mice impairs colonic antibacterial activity and predisposes to colitis. Endocrinology (2010) 151(6):2423–32. doi: 10.1210/en.2010-0089
244. Ooi JH, Li Y, Rogers CJ, Cantorna MT. Vitamin d regulates the gut microbiome and protects mice from dextran sodium sulfate-induced colitis. J Nutr (2013) 143(10):1679–86. doi: 10.3945/jn.113.180794
245. D'Argenio G, Cosenza V, Sorrentini I, De Ritis F, Gatto A, Delle Cave M, et al. Butyrate, mesalamine, and factor XIII in experimental colitis in the rat: effects on transglutaminase activity. Gastroenterology (1994) 106(2):399–404. doi: 10.1016/0016-5085(94)90598-3
246. Wu S, Zhang YG, Lu R, Xia Y, Zhou D, Petrof EO, et al. Intestinal epithelial vitamin d receptor deletion leads to defective autophagy in colitis. Gut (2015) 64(7):1082–94. doi: 10.1136/gutjnl-2014-307436
247. Pols TWH, Puchner T, Korkmaz HI, Vos M, Soeters MR, de Vries CJM. Lithocholic acid controls adaptive immune responses by inhibition of Th1 activation through the vitamin d receptor. PloS One (2017) 12(5):e0176715. doi: 10.1371/journal.pone.0176715
248. Vernia F, Valvano M, Longo S, Cesaro N, Viscido A, Latella G. Vitamin d in inflamm Bowel Dis. mechanisms of action and therapeutic implications. Nutrients (2022) 14(2). doi: 10.3390/nu14020269
249. Szaleniec M, Wojtkiewicz AM, Bernhardt R, Borowski T, Donova M. Bacterial steroid hydroxylases: enzyme classes, their functions and comparison of their catalytic mechanisms. Appl Microbiol Biotechnol (2018) 102(19):8153–71. doi: 10.1007/s00253-018-9239-3
250. Bora SA, Kennett MJ, Smith PB, Patterson AD, Cantorna MT. The gut microbiota regulates endocrine vitamin d metabolism through fibroblast growth factor 23. Front Immunol (2018) 9:408. doi: 10.3389/fimmu.2018.00408
251. Mahon BD, Wittke A, Weaver V, Cantorna MT. The targets of vitamin d depend on the differentiation and activation status of CD4 positive T cells. J Cell Biochem (2003) 89(5):922–32. doi: 10.1002/jcb.10580
252. Korf H, Wenes M, Stijlemans B, Takiishi T, Robert S, Miani M, et al. 1,25-dihydroxyvitamin D3 curtails the inflammatory and T cell stimulatory capacity of macrophages through an IL-10-dependent mechanism. Immunobiology (2012) 217(12):1292–300. doi: 10.1016/j.imbio.2012.07.018
253. Griffin MD, Lutz W, Phan VA, Bachman LA, McKean DJ, Kumar R. Dendritic cell modulation by 1alpha,25 dihydroxyvitamin D3 and its analogs: a vitamin d receptor-dependent pathway that promotes a persistent state of immaturity in vitro and in vivo. Proc Natl Acad Sci USA (2001) 98(12):6800–5. doi: 10.1073/pnas.121172198
254. Mathieu C, Adorini L. The coming of age of 1,25-dihydroxyvitamin D(3) analogs as immunomodulatory agents. Trends Mol Med (2002) 8(4):174–9. doi: 10.1016/S1471-4914(02)02294-3
255. Cantorna MT, McDaniel K, Bora S, Chen J, James J. Vitamin d, immune regulation, the microbiota, and inflammatory bowel disease. Exp Biol Med (Maywood NJ) (2014) 239(11):1524–30. doi: 10.1177/1535370214523890
256. Ochs HD, Gambineri E, Torgerson TR. IPEX, FOXP3 and regulatory T-cells: a model for autoimmunity. Immunologic Res (2007) 38(1-3):112–21. doi: 10.1007/s12026-007-0022-2
257. Bruce D, Cantorna MT. Intrinsic requirement for the vitamin d receptor in the development of CD8αα-expressing T cells. J Immunol (Baltimore Md 1950) (2011) 186(5):2819–25. doi: 10.4049/jimmunol.1003444
258. Gubatan J, Chou ND, Nielsen OH, Moss AC. Systematic review with meta-analysis: association of vitamin d status with clinical outcomes in adult patients with inflammatory bowel disease. Alimentary Pharmacol Ther (2019) 50(11-12):1146–58. doi: 10.1111/apt.15506
259. Yamada A, Komaki Y, Komaki F, Haider H, Micic D, Pekow J, et al. The correlation between vitamin d levels and the risk of postoperative recurrence in crohn's disease. Digestion (2021) 102(5):767–75. doi: 10.1159/000513589
260. Garg M, Rosella O, Rosella G, Wu Y, Lubel JS, Gibson PR. Evaluation of a 12-week targeted vitamin d supplementation regimen in patients with active inflammatory bowel disease. Clin Nutr (Edinburgh Scotland) (2018) 37(4):1375–82. doi: 10.1016/j.clnu.2017.06.011
261. Li J, Chen N, Wang D, Zhang J, Gong X. Efficacy of vitamin d in treatment of inflammatory bowel disease: a meta-analysis. Medicine (2018) 97(46):e12662. doi: 10.1097/MD.0000000000012662
262. Winter RW, Collins E, Cao B, Carrellas M, Crowell AM, Korzenik JR. Higher 25-hydroxyvitamin d levels are associated with greater odds of remission with anti-tumour necrosis factor-α medications among patients with inflamm Bowel Dis. Alimentary Pharmacol Ther (2017) 45(5):653–9. doi: 10.1111/apt.13936
263. Sohouli MH, Farahmand F, Alimadadi H, Rahmani P, Motamed F, da Silva Magalhães EI, et al. Vitamin d therapy in pediatric patients with inflammatory bowel disease: a systematic review and meta-analysis. World J Pediatr WJP (2022). doi: 10.1007/s12519-022-00605-6
264. Dan L, Chen X, Xie Y, Sun Y, Hesketh T, Wang X, et al. Nonlinear association between serum 25-hydroxyvitamin d and all-cause mortality in adults with inflammatory bowel disease in a prospective cohort study. J Nutr (2022) 152(9):2125–34. doi: 10.1093/jn/nxac148
265. Ratajczak AE, Szymczak-Tomczak A, Michalak M, Rychter AM, Zawada A, Dobrowolska A, et al. The associations between vitamin d, bone mineral density and the course of inflammatory bowel disease in polish patients. Polish Arch Internal Med (2022). doi: 10.20452/pamw.16329
266. Raftery T, Martineau AR, Greiller CL, Ghosh S, McNamara D, Bennett K, et al. Effects of vitamin d supplementation on intestinal permeability, cathelicidin and disease markers in crohn's disease: results from a randomised double-blind placebo-controlled study. United Eur Gastroenterol J (2015) 3(3):294–302. doi: 10.1177/2050640615572176
267. Jørgensen SP, Agnholt J, Glerup H, Lyhne S, Villadsen GE, Hvas CL, et al. Clinical trial: vitamin D3 treatment in crohn's disease - a randomized double-blind placebo-controlled study. Alimentary Pharmacol Ther (2010) 32(3):377–83. doi: 10.1111/j.1365-2036.2010.04355.x
268. Guzman-Prado Y, Samson O, Segal JP, Limdi JK, Hayee B. Vitamin d therapy in adults with inflammatory bowel disease: a systematic review and meta-analysis. Inflamm Bowel Dis (2020) 26(12):1819–30. doi: 10.1093/ibd/izaa087
Keywords: Vitamin D, autoimmunity, innate immunity, acquired immunity, autoimmune disorders
Citation: Ghaseminejad-Raeini A, Ghaderi A, Sharafi A, Nematollahi-Sani B, Moossavi M, Derakhshani A and Sarab GA (2023) Immunomodulatory actions of vitamin D in various immune-related disorders: a comprehensive review. Front. Immunol. 14:950465. doi: 10.3389/fimmu.2023.950465
Received: 22 May 2022; Accepted: 27 June 2023;
Published: 14 July 2023.
Edited by:
Chafia Touil-Boukoffa, University of Science and Technology Houari Boumediene, AlgeriaReviewed by:
Rachida Raache, University of Science and Technology Houari Boumediene, AlgeriaLorenzo Mortara, University of Insubria, Italy
Houda Belguendouz, University of Science and Technology Houari Boumediene, Algeria
Copyright © 2023 Ghaseminejad-Raeini, Ghaderi, Sharafi, Nematollahi-Sani, Moossavi, Derakhshani and Sarab. This is an open-access article distributed under the terms of the Creative Commons Attribution License (CC BY). The use, distribution or reproduction in other forums is permitted, provided the original author(s) and the copyright owner(s) are credited and that the original publication in this journal is cited, in accordance with accepted academic practice. No use, distribution or reproduction is permitted which does not comply with these terms.
*Correspondence: Gholamreza Anani Sarab, ghansa@yahoo.com