- 1First College of Clinical Medicine, Shandong University of Traditional Chinese Medicine, Jinan, China
- 2Institute of Chinese Orthopedics and Traumatology, Shandong Wendeng Osteopathic Hospital, Weihai, China
- 3Experimental Center, Shandong University of Traditional Chinese Medicine, Jinan, China
- 4Department of Rheumatology, Affiliated Hospital of Shandong University of Traditional Chinese Medicine, Jinan, China
- 5Science and Technology Department, Affiliated Hospital of Shandong University of Traditional Chinese Medicine, Jinan, China
Transient receptor potential cation channel subfamily V member 1 (TRPV1) is a Ca2+permeable, non-selective cation channel that is found primarily in sensory nerve fibres. Previous studies focused on pain transmission. However, recent studies have found that the TRPV1 channel, in addition to being associated with pain, also plays a role in immune regulation and their dysregulation frequently affects the development of rheumatoid arthritis (RA). A thorough understanding of the mechanism will facilitate the design of new TRPV1-targeted drugs and improve the clinical efficacy of RA. Here, we provide an updated and comprehensive overview of how the TRPV1 channel intrinsically regulates neuronal and immune cells, and how alterations in the TRPV1 channel in synoviocytes or chondrocytes extrinsically affect angiogenesis and bone destruction. Rapid progress has been made in research targeting TRPV1 for the treatment of inflammatory arthritis, but there is still much-uncharted territory regarding the therapeutic role of RA. We present a strategy for targeting the TRPV1 channel in RA therapy, summarising the difficulties and promising advances in current research, with the aim of better understanding the role of the TRPV1 channel in RA pathology, which could accelerate the development of TRPV1-targeted modulators for the design and development of more effective RA therapies.
1 Introduction
Rheumatoid arthritis (RA) is a chronic autoimmune disease with morning stiffness, swelling, pain, and functional impairment of the joints as the main clinical manifestations (1). The pathological process of RA involves immune cell infiltration, excessive cytokine production, angiogenesis, and cartilage destruction, which can lead to joint ankylosis, destruction, and deformity, resulting in disability and affecting the patient’s quality of life (2).
Inflammation and pain are prominent problems in the treatment of RA. Glucocorticoids, non-steroidal anti-inflammatory drugs (NSAIDs), and disease-modifying anti-rheumatic drugs (DMARDs) are currently available to improve the patient’s condition, and bio DMARDs or targeted synthetic DMARDs can be used when improvement is not evident (1, 3, 4). Despite the many drugs currently available, the response to treatment remains unsatisfactory, usually between 50% and 70%, and a significant number of patients have poor treatment outcomes (5).In clinical practice, if arthritis does not respond to initial treatment, we should change the treatment strategy as soon as possible. Some DMARDs suppress the immune system, resulting in an added risk of infection in RA patients and further aggravating the disease condition (6–8). Therefore, there is a real requirement to develop therapeutic agents for new targets to improve the outcome of RA treatment and prognosis.
TRPV1 is the first identified member of the vanilloid receptor subfamily of the TRP ion channel.TRPV1 is not only involved in the sensation of heat and pain but is also associated with abnormal immune cell function and the production of inflammation in the body, among other mechanisms (9). It was revealed that synovial fibroblasts from RA patients express TRPV1 (10). Compared to TRPV1+/+ animals, TRPV1-/- animals exhibited reduced pain and reduced joint inflammation following complete Freund’s adjuvant (CFA)-mediated induction of arthritis (11). Injection of TRPV1 antagonists A-889425 and JNJ-17203212 systemically reduces pain behaviour and decreases peripheral A and C fibre joint afferent nerve and injury receptor firing in a model of arthritis (12, 13).In this review, we summarise the TRPV1 channel expression associated with RA reported to date, and the impact of channel alterations on the pathogenesis of RA inflammation, pain, angiogenesis, and cartilage destruction. The impact of interfering with the TRPV1 channel on the above mechanisms is also listed in detail to further assess the therapeutic potential of targeting TRPV1 in RA to guide future research efforts.
2 Structure and function of TRPV1
TRPV1 is a non-selective cation channel, which was characterized as a receptor for capsaicin (CAP) by the Julius laboratory in 1997 (14). TRPV1 has a tetrameric structure, similar in structure to most TRP channels, and consists of three parts: the N- and C-termini located in the cell, and six transmembrane regions (S1-S6), with the pore loop region located between S5 and S6 (15). TRPV1 has a long N-terminal containing an anchor protein repeat domain and a C-terminal containing a TRP-box close to the sixth transmembrane structural domain (16), the N-terminal plays a role in the sensitivity of the channel to activators, and the C-terminal TRP-box mainly affects channel stability and function. Binding sites on the repeat structural domain of TRPV1-anchored proteins can bind to nucleotide triphosphates like ATP and calmodulin at the identical site (17, 18), upon binding, these molecules modify the sensitization of TRPV1 and modulate its function (19). The structure of the TRPV1 channel is summarized in Figure 1.
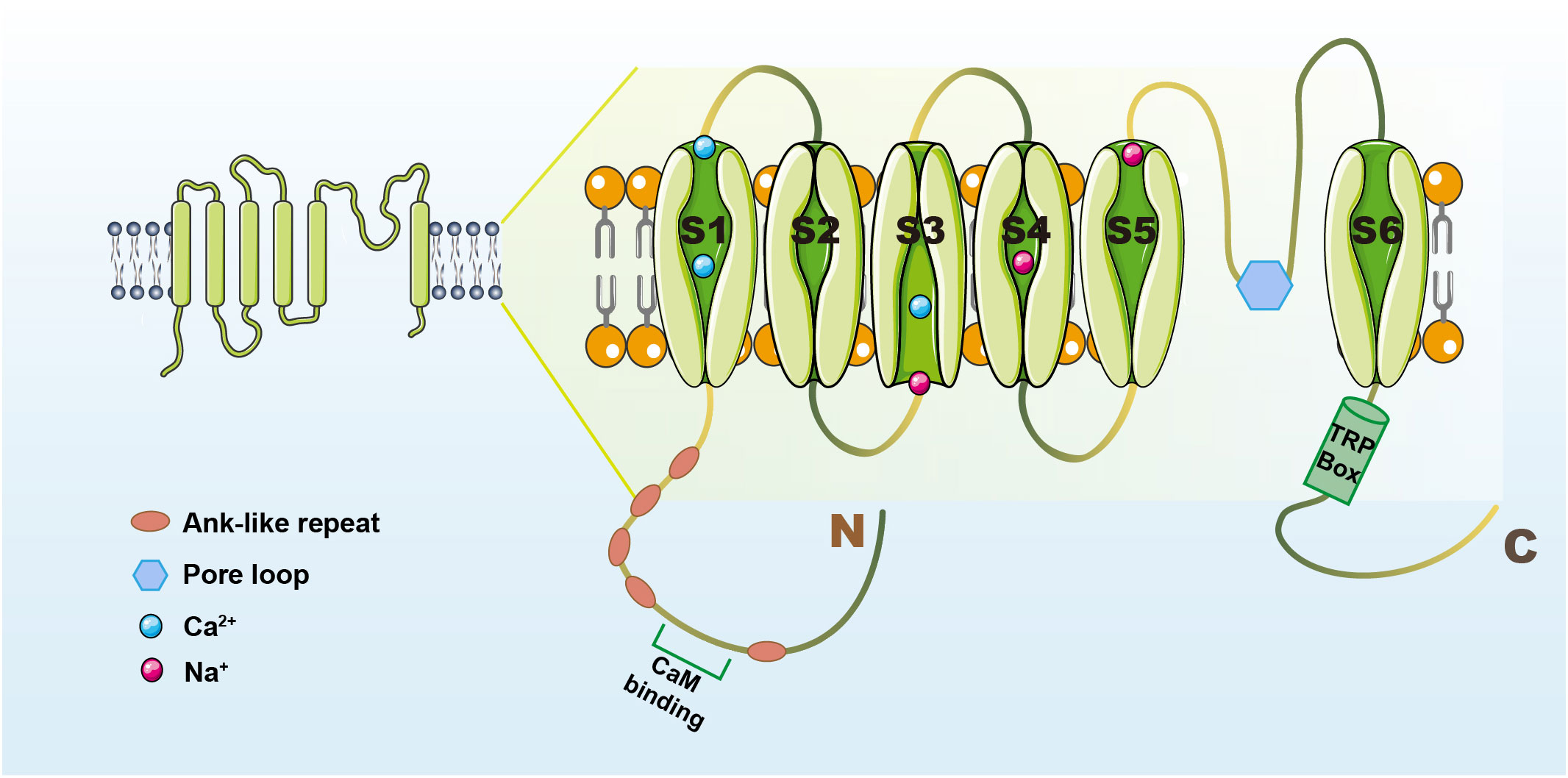
Figure 1 The structure of the TRPV1 channel. TRPV1 contains N- and C-termini and intermediate transmembrane structural domains (S1-S6). The N-terminus has a cam-binding site and an anchor protein repeat structural domain (16). The C-terminus has a TRP-box near the sixth transmembrane structural domain that can influence channel function (16). The pore loop region is located between S5 and S6, and when activators act on TRPV1, the structure of S5-6 is altered and contributes to Ca2+-based cation inward flow (15).
TRPV1 is mainly expressed in sensory nerve fibres, including unmyelinated C nerve fibres and small-diameter myelinated A nerve fibres. As a result, early studies focused on pain transmission, including thermal and inflammatory pain. However, as research progressed, it was discovered that TRPV1 was not only found in neuronal cells but also in other cell types, including RA synovial fibroblasts and human immune cells such as dendritic cells(DCs), macrophages or T lymphocytes (20). TRPV1 is tightly correlated with autoimmune diseases. TRPV1 knockout (TRPV1 KO) mice confirm a key role for TRPV1 in pain and inflammation (21). Therefore, understanding its anti-inflammatory and analgesic mechanisms and how TRPV1-targeted therapies work in joints and other tissues may provide new options for the treatment of inflammation and pain in RA patients.
3 The potential role of TRPV1 in RA
3.1 TRPV1 is involved in organismal inflammation
Inflammation is a key pathological manifestation in the pathogenesis of RA, and there is increasing interest in the mechanisms involved, mainly related to the over-activation of immune cells and dysregulation of inflammatory cell secretion (22–25). TRPV1 has been reported to show increased expression at the mRNA and protein levels in synovial fibroblasts from RA patients, correlating with RA inflammation and pain (10). The use of TRPV1 antagonists or knockouts relieves joint inflammation (26), again confirming its key role. Further studies should focus on the cell types expressing TRPV1 and the regulatory role of TRPV1 on cells, in relation to cytokine release, to elucidate the true function of TRPV1 in the pro-inflammatory process.
3.1.1 Immune cell infiltration
The site of RA is mainly confined to synovial joints and is closely associated with immune cell infiltration (27). TRPV1 was identified as present in cells of either the innate or adaptive immune system, regulating immune cell activation and influencing their function (28–32). TRPV1 is involved in Ca2+ signalling and the transduction of external stimuli (e.g. temperature or pH). Ca2+ is a well-known second messenger and plays a pivotal role in the activation of immune cells, proliferation, cytokine secretion and other functions (33, 34). Therefore, TRPV1 appears to be an essential participant in the regulation of immune cells.
3.1.1.1 T lymphocytes
The immunopathogenesis of RA spans decades, with T-cell dysregulation found in the asymptomatic autoimmune abnormal phase of RA (a period of autoantibody production to post-translational modified proteins), the acute synovitis phase, and the chronic destructive synovitis phase (35). Aberrant T cell differentiation is fundamental in promoting the remodelling of the immune system prior to the disease (36). Another way in which pathogenic T cells drive chronic inflammation is associated with the formation of organised lymphoid structures (37), which provide strength and persistence to inflammatory immunity. Moreover, synovial T cells are an important source of inflammatory factors such as IFN-γ (38), IL-17 (39), and TNF-α (40), and are a key bridge between cytokines and adaptive immune abnormalities and tissue remodelling.
Expression of TRPV1 was detected in human primary T cells, Jurkat T cell line, and mouse spleen T cells (29, 41–43). TRPV1 is positioned on the T cell plasma membrane and is one of the pivotal components of the T cell receptor (TCR) signalling cascade. TRPV1 activation and function can be regulated through TCR-induced signalling pathways (44). TRPV1 is rapidly recruited to the TCR cluster in an Src-dependent manner after TCR stimulation (42), which is an important way to increase Ca2+ concentration in CD4+ T cells. Resiniferatoxin, a specific agonist of TRPV1, leads to increased Ca2+ influx in T cells (29). Elevated intracellular Ca2+ is imperative for promoting T cell functions, such as activation, proliferation, differentiation and exerting effector functions (45). Genetic deletion or pharmacological inhibition of TRPV1 can attenuate the pro-inflammatory phenotype of CD4+ T cells (42, 46). Knockdown of TRPV1 in human primary CD4+ T cells reduces the expression of CD25 and shared epitope-positive HLA-DR alleles and diminishes the generation of anti-citrullinated protein antibodies (ACPA) in RA patients (42).In addition, TRPV1 antagonists can reduce the production of inflammatory factors. Compared to wild-type(WT), TRPV1-/-CD4+ T cells secreted fewer cytokines (IFN-γ, IL-17A, IL-2, IL-10, IL-4 and TNF) following anti-CD3 + 28 stimulation, and their reduced cytokine production may be due to a decrease in TCR-induced the TRPV1 channel-mediated Ca2+ influx (42). Although the role of TRPV1 and T cell activation in the pathogenesis of RA remains to be clarified, the role of TRPV1-mediated Ca2+ influx in T cell proliferation and activation is undisputed. Considering the important pathological role of T-cell activation in RA, it can be inferred that targeting and regulating TRPV1 to restore normal physiological functions of T-cells holds great promise in the treatment of RA.
3.1.1.2 Macrophages
TRPV1 has been reported to be involved in macrophage-related immune mechanisms (47). Abnormal activation of pro-inflammatory macrophages in synovial tissue is detected in early RA (48, 49). When pro-inflammatory macrophage M1 are over-polarized, the secretion of cytokines such as IL-1β, IL-6 and TNF-α increases in vivo (48, 50), inducing inflammation production and leading to high disease activity in RA. TRPV1 hyperactivation promotes M1-type pro-inflammatory macrophage activation by altering macrophage status (51). An imbalance in the M1/M2 ratio of articular synovial macrophages is a distinctive feature of the acute inflammatory phase of RA (52, 53). Not only are macrophages themselves a source of inflammation, but they are also associated with the activation of T cells. Macrophages can present non-self antigens to nascent T cells, by releasing cytokines and growth factors that elicit Th1 or Th2-mediated immune responses and modulate inflammation in vivo (54). Pretreatment of mouse macrophages with TRPV1 inhibitor AMG9810 or CPZ significantly inhibited the expression of pro-inflammatory cytokines IL-6, IL-1β and IL-18, as well as cyclooxygenase 2 (COX-2) (55). An opposite result has been shown, with TRPV1 agonist treatment significantly reducing macrophage polarisation, attenuating synovial inflammation and minimising cartilage destruction and bone formation (31).
Macrophage polarization derangement is a key mechanism of bone destruction in RA. The degree of synovial macrophage infiltration is closely related to the degree of joint erosion (49). An interesting subpopulation of macrophages called arthritis-associated osteoclast macrophages, has been discovered in the synovial fluid and tissues of RA patients (56). These macrophages are characterised by a high osteoclastogenic potential (56). TRPV1 activation facilitates the mobilization of M1-type pro-inflammatory macrophages. Inflammatory macrophages are involved in joint surface erosion through the production and release of matrix metalloproteinases (57). M1-type macrophages are intimately associated with osteoblasts. During the erosive phase of the disease, chemokine CCL21 enhances RA osteoclastogenesis by driving Th17 polarization through M1-type macrophages. Thus, CCL21-mediated differentiation of M1-type macrophages linked to Th17 cells extends joint inflammation into bone erosion (57). The macrophage-osteoclast axis may be an important mechanism of bone destruction in RA (58). TRPV1 can reduce inflammation and osteoclast production by regulating the M1/M2 macrophage imbalance and is expected to be a new therapeutic target for RA.
TRPV1 and macrophages are not limited to their involvement in inflammation and bone destruction but are also associated with mechanical pain and burning sensations. IL-23/IL-17A/TRPV1 axis activation contributes to macrophage-sensory neuron crosstalk, creating mechanical pain (59). Radiofrequency irradiation lowers TRPV1 activation, diminishes neuropeptide expression, attenuates neuropeptide-induced macrophage activation, and ultimately reduces inflammatory factor expression and burning pain in vitro models (60). The mechanism by which TRPV1 is involved in macrophages to regulate inflammation and bone destruction in the body has been well clarified. Yet, the mechanism of TRPV1-induced neuropeptide expression and macrophage activation in RA patients with burning joint pain remains to be further elucidated.
3.1.1.3 Dendritic cells
TRPV1 has been proven to be expressed in mouse and human DCs at the protein and gene levels (28, 61, 62). The TRPV1 agonist CAP exhibits a dose-dependent effect of inducing DCs differentiation and prompting DCs activation. TRPV1 binds to immature DCs and promotes DCs maturation through antigen presentation and upregulation of co-stimulatory molecules (61). Substantial amounts of DCs are recruited in the synovial fluid and tissues of RA patients and are integral in the pathogenesis of RA (63, 64). DCs specialized antigen-presenting cells bridge innate and adaptive immunity (65), drive Th1/Th2 imbalance, activate B cells and follicular helper T cells (Tfh) (66, 67), and stimulate the body to generate high levels of autoantibodies, resulting in an inflammatory response.
TRPV1 regulates DCs function, and sustained opening of the TRPV1 channel promotes activation of calcium-regulated neuro phosphatase/NFATc2 signalling in DCs, impairing Ca2+ homeostasis in DCs, enhancing Th17 cell differentiation, inducing cytokine secretion and strengthening susceptibility to inflammatory factors (68). Excessive secretion of chemokines by synovial DCs in RA patients and recruitment of immune cells with pro-inflammatory functions, such as macrophages and neutrophils (69, 70). Overexpression of NF-κB was found in RA synovial DCs, causing upregulation of nuclear RelB, a binding protein for NF-κB, and promoting inflammation. Similar manifestations were found in inflammatory bowel disease (IBD) mice, where activation of TRPV1 promoted DCs recruitment and activation compared to TRPV1-/-, exacerbating the colitis manifestations of the model (68).
However, studies have suggested the opposite, that the failure of CAP to induce changes in intracellular Ca2+ or membrane currents in DCs does not support the expression of the TRPV1 channel in DCs (71). The reason for this may be that although CAP can agonize the TRPV1 channel, numerous studies have shown that TRPV1 receptors may not be the only target of CAP. The specific role of TRPV1 in the regulation of DCs by CAP remains unclear. Although several studies have supported the idea that TRPV1 regulates DCs and influences the inflammatory response of the body. Further validation at the cellular level using TRPV1-/- or agonists and inhibitors to elucidate the mechanisms of TRPV1 regulation of inflammation in DCs and RA patients is an important next step in the research.
3.1.2 Cytokine production
3.1.2.1 IL-1
Overproduction of IL-1β causes vasodilation, promotes granulocyte recruitment to inflamed tissues and induces prostaglandin (PG) expression, contributing to acute joint inflammation and pain (72, 73). IL-1β also stimulates osteoclast formation through the induction of osteoclast genesis in regulatory T-cells (Tregs), contributing to bone erosion and joint function reduction (74, 75). TRPV1 is an integral pathway for IL-1β release. Studies have shown that injurious TRPV1+ axons co-express IL-1R1 and are tightly correlated with infiltrating IL-1β+ cells. In both mouse and human models, IL-1R1 was found to be highly expressed through a subpopulation of TRPV1+ dorsal root ganglion neurons (76). TRPV1 channel induces Ca2+ influx in the extracellular medium and increases cytosolic Ca2+ concentration, possibly through increased nuclear NF-κB phosphorylation leading to increased IL-1β release (77). Pretreatment with TRPV1 antagonist significantly eliminates IL-1β-induced pain (78). No elevation of IL-1β was seen in the TRPV1 KO mouse model compared to WT mice (79, 80). Inhibition of IL-1 secretion through modulation of the TRPV1 channel to attenuate joint inflammation and pain merits further exploration.
3.1.2.2 IL-6
Elevated levels of synovial TRPV1 in RA patients are accompanied by abnormal secretion of IL-6 (81). TRPV1-induced IL-6 secretion plays an instrumental role in patients’ pain, inflammation, and joint destruction. Activation of TRPV1 operates as an inducer of inflammatory signalling and collaborates with neuropeptides to augment the production of the cytokine IL-6. However, this synergistic mechanism does not affect healthy synovial cells, and only inflammation-initiated cells can generate IL-6 through the activation of the TRPV1 channel (81). IL-6 plays a checkpoint role in the differentiation pathway of naive T cells to pro-inflammatory Th17 cells or Tregs (82). IL-6 acts as a chemoreceptor for monocytes at the site of inflammation, affecting signalling molecules such as Toll-like receptors (TLR) and promoting angiogenesis (83). IL-6 mediates the induction of bone resorption by TNF-α and IL-1. IL-6 stimulates the release of osteoblast nuclear factor-κB receptor activator (RANK) ligand (RANKL) and destroys bone via the RANK/RANKL/osteoprotegerin (OPG) pathway (84). The simultaneous destruction of the vascular opacification leads to irreversible damage to the joint (85). The use of TRPV1 inhibitors reduces the body’s IL-6 levels (86). TRPV1 KO mice secreted less IL-6 compared to WT mice (87). Thus, TRPV1-induced IL-6 secretion has an essential role in inflammatory, neurogenic and pressure pain in the body.
3.1.2.3 IL-8
TRPV1 has a regulatory effect on IL-8. It was revealed that activation of TRPV1 often induces IL-8 formation. Elevated levels of TRPV1 in the hyperosmotic state and response to dramatic temperature changes stimulate MAPK and NF-κB activation and mediate an increase in the chemokine IL-8 (88). IL-8 is a key chemokine that promotes neutrophil migration (89). IL-8 activates neutrophils, stimulates neutrophil extracellular trap formation, promotes their degranulation, produces respiratory bursts, releases superoxide and lysosomal enzymes, and facilitates the activation and recruitment of neutrophils at sites of inflammation (90). IL-8 also has the capability to appeal to and activate monocytes, and large quantities of inflammatory cells infiltrate the interstitial matrix of articular cartilage and bone (91, 92). IL-8 can also stimulate angiogenesis, resulting in the formation of RA vascular opacities (91). The use of the TRPV1 agonist CAP confirms the regulatory function of TRPV1 on IL-8 (93). Reduced local pH is an essential pathological feature of RA. Acidic conditions can contribute to elevated IL-8 mRNA expression (94). Intriguingly, the selective TRPV1 antagonist 5’-iodoresiniferatoxin reduced the production of IL-8 under acidic conditions and attenuated the inflammatory state of the organism (95).
IL-8 also has osteoclastogenic activity. IL-8 promotes the formation of pro-osteoblast-like cells (OCL) in an environment with anti-RANKL antibodies, demonstrating that IL-8 may compensate for RANKL function during the induction of OCL in a low-RANKL environment and accelerate the process of RA bone destruction (96). TRPV1 may have an impact on bone destruction in RA patients by mediating IL-8 secretion.
3.1.2.4 IL-17
It was revealed that TRPV1+ injury receptors are closely related to the production of IL-17. IL-17 can induce aggregation of neutrophils and monocytes in the circulatory system, activates a series of inflammatory cascades and plays a fundamental role in RA (97). TRPV1 activates the IL-23/IL-17 axis, which mediates inflammation through macrophage-neuron crosstalk drive (98). TRPV1 KO abrogates IL-23/IL-17 axis-induced inflammation. At the same time, IL-17, in turn, acts on TRPV1, the two interact through neural-immune interactions, contributing to the body’s mechanical pain (59). The use of TRPV1 agonists CAP, SA13353 [1-[2-(1-adamantyl)ethyl]-1-pentyl-3-[3-(4-pyridyl)propyl]urea] also demonstrated anti-inflammatory effects. CAP administration inhibited the expression of the IL-23/IL-17 pathway in psoriasis models, alleviated the microscopic appearance of lesions and reduced the secretion of various cytokines (99). SA13353 suppresses the recruitment and production of IL-17-producing cells and decreases inflammation in the body (100). TRPV1 KO confirmed its induction of IL-17 production causing inflammation in the body, but its agonist use also reduced inflammation in the body, suggesting that both agonists and inhibitors of TRPV1 can exert anti-inflammatory effects via IL-17 secretion, and the mechanism of action requires further exploration.
3.1.2.5 TNF-α
TNF-α induces inflammation and promotes osteoclastogenesis, which is critical in the pathogenesis of RA. TNF-α inhibitors are widely used to treat RA and can mitigate RA symptoms by preventing the pro-inflammatory signalling pathway mediated by TNF receptor 1 (TNFR1) (101). TRPV1 promotes TNF-α production and is also induced by TNF-α, both of which exert synergistic inflammatory effects. Pretreatment of isolated rat vagus nerves with TNF-α enhances the response of sensory neurons to TRPV1 agonists and mediates increased TRPV1 expression and Ca2+ influx (102). In TNF-α overexpressing mice, elevated TRPV1 levels and elevated Ca2+ influx levels were demonstrated, indicating that TNF-α overexpression induced TRPV1 sensitization (103). TNF-α-triggered thermal and mechanical hypersensitivity in the body is mediated by TRPV1 signalling downstream of TNFR1 receptor activation, respectively, and sensitization of injury receptors is dependent on TNFR1 expression (104).
Also, TRPV1 mediates the production of TNF-α. Paeoniflorin inhibits TRPV1 expression in foot pad tissue samples, suppresses inflammatory cytokine TNF-α production and ameliorates inflammation and pain in mice in an LPS-induced acute pain model (105). However, the analgesic effect of paeoniflorin can be significantly reversed by CAP, suggesting that TRPV1 channel activation mediates the release of inflammatory factors such as TNF-α, leading to inflammatory pain.
TRPV1 and TNF-α exhibit a close reciprocal relationship and their mutual regulatory role cannot be ignored. Of interest is that in two studies, TRPV1 agonists showed a dual anti-inflammatory and pro-inflammatory effect and inflammatory cytokines such as TNF-α also showed a paradoxical elevation and inhibition (106, 107). The mechanism of TRPV1’s role in this requires further clarification. It is undeniable that TRPV1 is tightly associated with TNF-α, and blocking the reciprocal relationship may reduce the inflammatory response in RA patients, and may also delay bone destruction, diminish disability and improve the quality of patient survival.
3.1.2.6 IFN-γ
Overproduction of IFN-γ in RA patients drives the recruitment of synovial neutrophils, which is accompanied by high disease activity and leads to persistent inflammation (108, 109). TRPV1 mediates IFN-γ production. In a model of inflammation induction, both inflammatory genes IFN-γ and TRPV1 channel were upregulated, and TRPV1 agonists systematically increased T cell counts, enhanced CD8+ T cell recruitment and induced overproduction of IFN-γ in healthy mice (110, 111). Nevertheless, a novel TRPV1 agonist, SA13353, attenuated IFN-γ cytokine levels, possibly associated with the desensitization of TRPV1 (100). Although TRPV1 channel opening correlates with IFN-γ secretion, there is still a lack of strong evidence to support this. Whether TRPV1 KO affects the secretion of IFN-γ and thus the development of RA still needs to be further explored.
Activation of the TRPV1 channel is known to cause inflammation in vivo, but there is growing evidence that it has anti-inflammatory effects. TRPV1 is expressed in a variety of immune cell subpopulations, and activation of the channel is closely synergistic with the release of regulatory proteins, and inflammatory factors. These synergistic relationships may influence the final outcome of TRPV1 activation.
3.2 TRPV1 regulates nociplastic pain
Pain is a distinctive feature of RA and a major source of poor prognosis and low quality of life for patients. As pain caused by RA is traditionally considered to be a direct result of peripheral inflammation, doctors have traditionally considered pain to be a marker of inflammation. However, despite the success of DMARDs in suppressing inflammation, many people with RA still have pain. Pain in inflammatory arthritis has a variety of causes; peripheral inflammatory triggers, structural damage, psychosocial factors, etc. These factors are entwined with the central mechanisms of pain (112). Pain management in RA is an increasing challenge for rheumatologists. Comprehending the underlying biological mechanisms of pain is essential to improving treatment, disease management and patient health. The pathogenesis of pain can be divided into 3 categories (113): 1) Nociceptive pain, which is the response of the somatosensory system to an injurious stimulus; 2) Neuropathic pain, defined as a consequence of direct neurological damage; 3) Nociplastic pain, defined as a dysfunctional neurological response to pain management in the absence of peripheral tissue injury, somatosensory system damage or injury receptor engagement, is manifested as neuro sensitisation. Cutting-edge research confirms nociplastic pain as an essential cause of non-inflammatory pain in RA (113).
Excessive release of neuropeptides (including substance P (SP) and calcitonin gene-related peptide (CGRP) and microglia dysfunction were revealed to be crucial mechanisms in the collagen-induced arthritis (CIA) model (114). There is substantial evidence that TRPV1 is widely expressed in the CNS and that TRPV1 agonist-endocannabinoids can be used to treat pain caused by nerve sensitization. TRPV1 affects neuropeptide secretion, contributes to microglia activation and is closely associated with neuron sensitization, which may play an essential role in non-inflammatory pain in RA (115). Therefore, starting with TRPV1 and inhibiting abnormal activation of neuropeptides and microglia overproliferation may be a potential mechanism to inhibit central and peripheral sensitization and treat non-inflammatory pain in RA.
3.2.1 TRPV1 affects neuropeptide secretion
TRPV1 mediates CGRP release. Immunohistochemistry showed that TRPV1 receptors and CGRP co-localized in a considerable proportion of neurons (116). Decreased calcium-regulated neuro phosphatase activity in sensory neurons leads to activation of the TRPV1 channel and increased intracellular Ca2+ concentration, which can cause increased CGRP secretion. Drugs reduce the pain-induced phosphorylation state of the TRPV1 channel by enhancing calcium-regulated neuro phosphatase activity, diminishing Ca2+ inward flow, and mediating neuro calmodulin-dependent desensitization of TRPV1 in sensory neurons, reducing subsequent CGRP neuropeptide transmitter release (117). TRPV1 KO or pretreatment with GCRP receptor antagonists significantly reduced the mechanosensitization induced by C5a, a component of the complement system, confirming that TRPV1 and CGRP receptors are key steps in the mechanosensitization process (118). Overexpression of αCGRP found in RA patients (119). Elevated TRPV1 mRNA and increased Ca2+ influx with the subsequent increased neuronal release of CGRP were detected in the CFA-induced pain model compared to the control group (120). As mechanical hypersensitivity develops in the disease, innervated neurons exhibit enhanced CGRP expression, as well as stronger pain manifestations (121).
TRPV1 is not just essential for promoting the synthesis and release of CGRP (122), but SP is also regulated by TRPV1. SP activation was observed in cultured RA synovial cells to stimulate the release of PGE2 and collagenase from synovial cells and promote synovial cell proliferation (123). Sensory neurons of TRPV1+ release SP upon stimulation in an allergic mouse model (124). In a chronic compression injury model, SP rises with TRPV1 levels. Treatment with ferulic acid reduces TRPV1 levels and subsequently reduces serum SP levels, inhibits peripheral sensitization and alleviates sciatica (125). The TRPV1 antagonist capsazepine significantly attenuates TRPV1 expression and SP release (126). Consistent with this, CAP treatment activates the TRPV1 channel and dose-dependently promotes the release of neuropeptides SP and CGRP (127). Interestingly, neuropeptides interact with TRPV1, with neuropeptides, in turn, activating TRPV1 in RA synovial fibroblasts and promoting IL-6 and IL-8 production, promoting synovial peripheral inflammation (81).
TRPV1 and the neuropeptides SP and CGRP have been shown to be abnormally elevated in RA patients. Multiple pain models demonstrate that TRPV1 mediates SP and CGRP secretion. However, the effect of TRPV1 KO or antagonists on downstream SP and GCRP neuropeptide secretion, on central and peripheral sensitization in RA models remains to be further investigated. While most anti-inflammatory drugs can target the immune cellular component of neuroinflammation, they usually fail to interfere with the neuronal component (117). Interfering neuropeptides have great potential value for the treatment of non-inflammatory pain in RA.
3.2.2 TRPV1 and microglia activation
Nociplastic pain is associated with microglia overactivation (128). Various humoral factors released by microglia (such as IL-10, IL-1β), contribute to synapse formation and mediate pain caused by nerve sensitization, which may be a key mechanism connecting synaptic plasticity and pain (129). Pharmacological inhibition of microglia reverses region-specific synaptic plasticity in a pain model, reaffirming the key role of microglia in nociplastic pain (130).
Notably, TRPV1 is highly expressed in microglia. Activation of the TRPV1 channel regulates microglia function and microglia influence synaptic transmission and plasticity in neurons (131). Chronic pain model studies provide ample evidence (131): (a) Pain model cortical microglia exhibit high expression of TRPV1 mRNA and protein compared to negative controls; (b) The TRPV1 agonist CAP induces the shedding of microvesicles from the surface of microglia, increases glutamatergic synaptic activity and regulates synaptic transmission within the central nervous system; (c) Microglia change both morphologically and phenotypically upon TRPV1 activation, demonstrating an activation phenotype; (d) the TRPV1 channel is highly permeable to Ca2+ primarily in microglia. activation of microglial TRPV1 by CAP drives up intracellular Ca2+ and promotes the release of mitochondrial cytochrome c, leading to increased microglial apoptosis and autophagy (132); (e) Application of TRPV1 agonist to elicit concentration-dependent migration and chemotaxis of microglia (133). Several studies have shown that microglia exhibit hyperproliferation and increased reactivity in the CIA model. Progressive increase in microglia with escalating activation and sensitization to injurious neurons in CIA joints, closely associated with pain (121, 134). Peripheral inflammatory signals can stimulate CNS-resident microglia, prompting rapid conversion to an activated phenotype that perpetuates neuroinflammation (135).
This differential TRPV1 expression pattern in chronic pain conditions places microglia TRPV1 at the centre of a new and important mechanism, providing a link between physiological and pathological states. Demonstrating a key role for TRPV1 regulating microglia in nociplastic pain. AMG9810, a TRPV1-specific inhibitor, attenuates microglia activation, effectively attenuates mechanical hypersensitivity and reduces pain (136). The use of the TRPV1 blocker SB366791 significantly inhibited microglia migration and attenuated the development of mechanically abnormal pain and nociceptive hyperalgesia, with the same results observed in TRPV1 KO mice (133).
The above studies support the vital role of abnormal microglia activation in RA nociplastic pain. Focusing on sensitization and pain caused by abnormal microglia activation, modulating the TRPV1 channel, inhibiting microglia overactivation, and repairing neural sensitization may be a promising strategy for treating nociplastic pain in RA.
3.3 TRPV1 mediates angiogenesis
Angiogenesis in synovial tissue is a key pathological event in the progression of RA (137). Angiogenesis recruits inflammatory cells from the circulatory system, leading to persistent synovitis and the formation of invasive vascular opacities that further lead to cartilage destruction and exacerbate the progression of RA (137). Endothelial Ca2+ signalling plays a crucial role in angiogenesis. Various pro-angiogenic factors, such as Vascular endothelial growth factor (VEGF) and transforming growth factor β1 (TGF-β1), are involved in regulating endothelial cell proliferation and angiogenesis by increasing intracellular Ca2+ concentration (138, 139). TRPV1, a multimodal cation channel that mediates Ca2+ influx, is an important player in vascular endothelial cell migration, proliferation and angiogenesis (140). It was found that intraperitoneal injection of the TRPV1 ligand wogonin (a TRPV1 agonist) promoted angiogenesis in WT mice (141). The same results were observed in in vitro experiments (142). In contrast, TRPV1 antagonists eliminated drug-induced angiogenesis (143). TRPV1 KO and siRNA-interfered animals show a significant reduction in induced angiogenesis, and VEGF and TGF-β1 expression is inhibited in TRPV1 KO mice (144). Not only that but interestingly, recent findings suggest that TRPV1 triggers angiogenic activity independently of VFGF and that blocking the TRPV1 channel has no effect on VEGF-stimulated angiogenesis or Ca2+ signalling in vitro (142). The TRPV1 agonist CAP exhibits an inhibitory effect on angiogenesis. Retinal microvasculature in diabetic rats exhibits increased retinal neovascularization and CAP ameliorates diabetic retinopathy by activating TRPV1 (145). Another study also demonstrated that CAP inhibits VEGF-induced endothelial cell proliferation, migration and angiogenesis (146). It is worth noting that TRPV1 is not the only channel activated by CAP, and the above studies did not explore the role of the TRPV1 channel in CAP anti-angiogenesis, which may be related to other mechanisms of CAP action in vivo. The role of TRPV1 in angiogenesis has been repeatedly demonstrated through technical means such as gene knockout, interference and inhibitors. Thus, modulation of the TRPV1 channel may be a possible means of inhibiting RA angiogenesis.
3.4 Potential role of TRPV1 in joint destruction
Cartilage destruction in the affected joints is the main pathological feature of RA that causes disability. Cartilage destruction occurs rapidly from the onset of RA and can lead to joint deformation and functional deterioration. Therefore, controlling cartilage destruction is an important part of treatment to reduce the disability rate of RA (147). Abnormal activation of osteoclasts leads to increased bone resorption and insufficient production of osteoblasts leads to impaired bone formation. Osteoclast/osteoblast imbalance underlies bone loss in RA, including bone erosion, periarticular bone loss and systemic osteoporosis. RANKL is an important mediator of osteoclast production and the key role of osteoclasts in bone erosion has been demonstrated in basic research and the clinical efficacy of antibodies targeting RANKL (148).
It was found that TRPV1 was expressed in chondrocytes (149). TRPV1 channel activation enhances RANKL-mediated differentiation of bone marrow-derived macrophages (BMM) to osteoblasts. TRPV1 channel inhibition reduces RANKL-mediated osteoclast formation. This suggests that although TRPV1 activation by itself does not induce osteoclastogenesis, it has a critical synergistic effect on RANKL-mediated signalling events. In vivo, experiments showed the same results, with mice in the TRPV1 agonist (curcumin or CAP) group having a higher degree of trabecular osteoclast formation, trabecular microstructure erosion, bone loss and reduced vertebral bone density, comparable to the ovariectomized group (150). Capsazepine, a TRPV1 ion channel antagonist, inhibits osteoclast bone resorption and prevents ovariectomy-induced bone loss in mice (151). The reduced osteoclast formation in TRPV1 KO mice is a strong indication of the important role of TRPV1 in bone destruction. In vitro, experiments have further explored the mechanism of action of the TRPV1 channel in regulating bone destruction. In bone marrow-osteoblast co-cultures and RANKL-generated osteoblast cultures, capsazepine inhibited osteoclast formation and bone resorption in a dose-dependent manner. The TRPV1 agonist CAP enhances RANKL-stimulated osteoclast formation. CAP also inhibits RANKL-induced phosphorylation of IkappaB and ERK1/2 and causes apoptosis in mature osteoblasts, and inhibits alkaline phosphatase activity and bone nodule formation in calcified osteoblast cultures (151). The use of glucocorticoids is also known to be an important cause of joint destruction and bone loss in RA. TRPV1 can be induced to dysregulate by glucocorticoids and promote osteoclastogenesis. The pharmacology of TRPV1 significantly inhibited the over-activation of osteoclasts, suggesting a therapeutic use of this channel in protecting against glucocorticoid-induced bone loss (152).
In summary, the TRPV1 channel regulates osteoclast activation and apoptosis and also affects osteoblasts, playing an important role in RA articular bone/chondral destruction. Modulation of the TRPV1 channel may be a promising therapeutic idea to mitigate bone destruction in RA.
4 TRPV1-targeted therapy
The above studies shed light on the key role of TRPV1 in the mechanisms of inflammation and pain in RA(Figure 2). In recent years, TRPV1-targeted drugs have received much attention in inflammatory arthritis, e.g. RA, OA, gouty arthritis, etc. Data on the modulation of the TRPV1 channel for arthritis are summarised in Table 1. Many clinical trials are also being conducted to treat arthritis and pain by targeting TRPV1(Table 2).
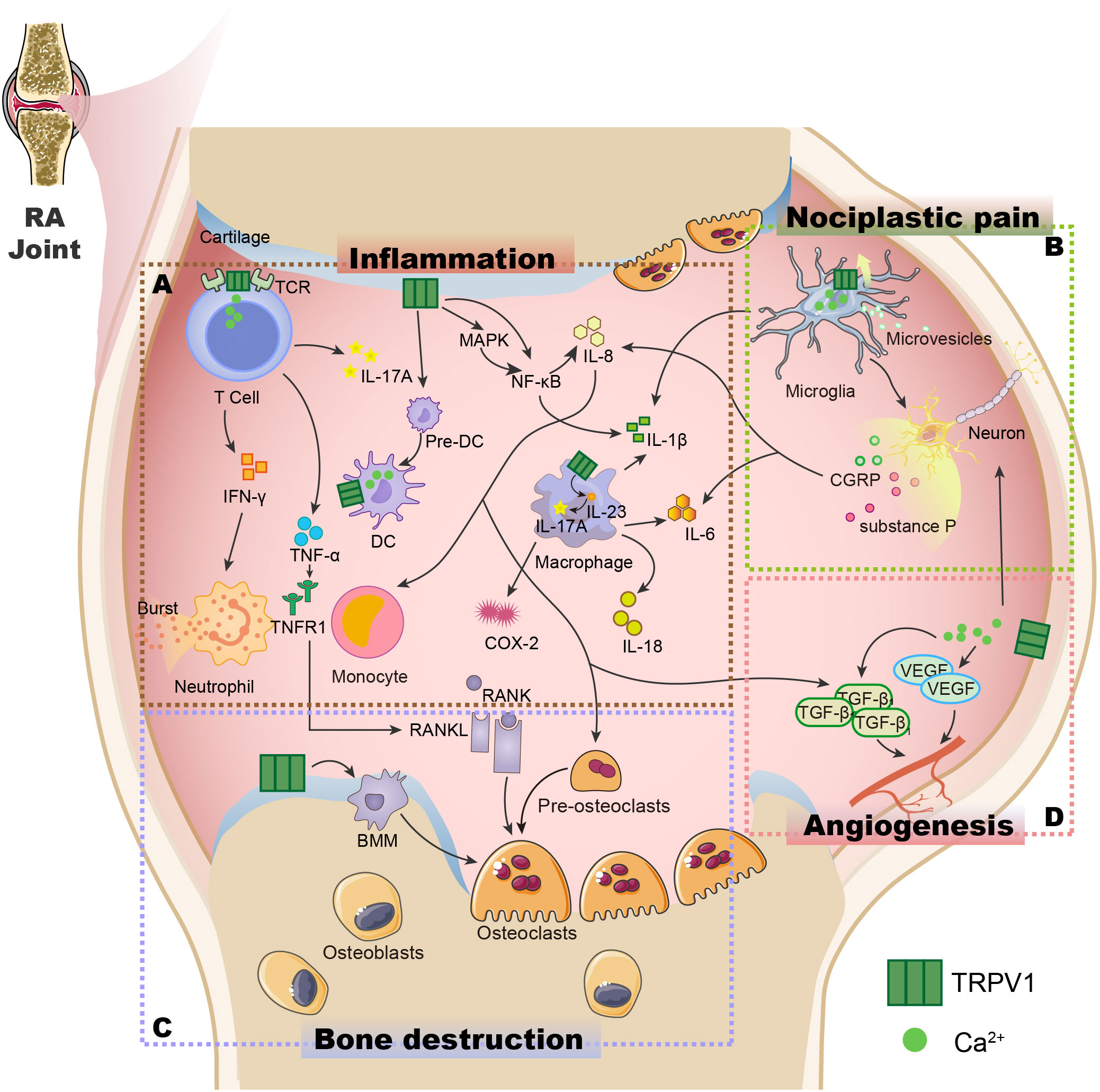
Figure 2 The potential role of the TRPV1 channel in the pathogenesis of RA. (A) TRPV1 is expressed not just in synovial cells but also in immune cells such as T cells (44), macrophages (47), and dendritic cells (68), controlling immune cell activity, influencing cytokine release, and causing inflammation in the body. (B) The opening of the TRPV1 channel induces increased Ca2+ influx, activates microglia (131), promotes the release of the neuropeptide substance P and CGRP from sensory nerve fibres (116, 126), causes neuron sensitization and the release of cytokines, and results in nociplastic pain. (C) TRPV1 is expressed in chondrocytes, induces RANK/RANKL production, promotes the maturation of pre-osteoclasts, and mediates the formation of osteoclasts from BMM, leading to bone destruction (150, 151). (D) The opening of the TRPV1 channel can cause an increase in Ca2+ influx, promoting VEGF and TGF-β1 generation (144), and inducing angiogenesis. TCR, T-cell receptors; IFN-γ, Interferon-γ; IL-17A, Interleukin-17A; Pre-DC, Immature dendritic cells; DC, Dendritic cells; TNF-α, Tumor Necrosis Factor-α; TNFR1, Tumor necrosis factor receptor 1; MAPK, Mitogen-activated protein kinase; NF-κB, Nuclear factor kappa-B; IL-8, Interleukin-8; IL-1β, Interleukin-1β; IL-23, Interleukin-23; COX-2, Cyclooxygenase-2; RANK, receptor activator of NF-κB; RANKL, Receptor activator of NF-κB ligand; CGRP: Calcitonin gene-related peptide; VEGF, Vascular endothelial growth factor; TGF-β1,Transforming growth factor-β1.
It is clear from the above that TRPV1 is a highly druggable target. The development of TRPV1-targeted drugs for arthritis is actively pursued worldwide. There is considerable evidence in the table that TRPV1 channel inhibition plays an important role in reducing joint oedema and destruction, as well as relieving inflammation and pain. TRPV1 channel agonists can also target intractable pain and chronic pain after inflammatory remission through desensitization, suggesting that targeting TRPV1 has great potential for the treatment of RA. However, the development of TRPV1-targeted drugs has not always been smooth. TRPV1 has complex regulatory functions and is essential for the maintenance of normal body temperature (117). Systemic antagonism of TRPV1 can damage harmful heat sensations in human skin, increasing the harmful heat threshold and leading to accidents such as burns and scalds (177). Antagonizing TRPV1 also interferes with body thermoregulation, leading to excessive body temperature rise (172). The use of TRPV1 agonists such as CAP causes a strong initial pain response and induces vasodilation and the desensitisation and toxicity doses are relatively close to each other, making adverse effects difficult to control (178).
Although these issues have lowered expectations, there has been some promising progress. Region-specific antagonism of TRPV1 can exert analgesic effects without causing hyperthermia (179). “Non-stimulatory” TRPV1 agonists have been developed, such as Olvanil (NE19550) and MRD-652, both of which have shown promise in inflammatory pain models (178, 180), its clinical value, as yet, remains to be proven. In addition, we can also look for natural products that have a regulatory effect on the TRPV1 channel. Given the complexity of TRPV1 function, keeping TRPV1 activity within the physiological range and reducing its sensitizing effects that occur in pathophysiological pain states may be a more promising research and development Strategy.
5 Conclusion
The therapeutic role of TRPV1 is a topic that cannot be ignored in the field of inflammation and pain, with some scholars even calling it the “holy grail of pain management” (181). Based on the evidence reviewed, it is clear that TRPV1 plays a central role in the pathology of RA. Excessive activation of TRPV1 leads to immune cell dysfunction and excessive release of inflammatory factors that mediate inflammation in the body. Activation of the TRPV1 channel mediated by neuropeptide release and microglia activation induces nociplastic pain after inflammation control. TRPV1 also plays an important role in angiogenesis and cartilage destruction. Pain and inflammation, angiogenesis, and cartilage destruction are all important parts of the treatment of RA. Thus, TRPV1 is a remarkably promising target for RA therapy, especially in pain management. This is because interfering with its activity alters the function of multiple signalling pathways in the pathogenesis of RA, thereby slowing its progression. Research on TRPV1 channel-targeting drugs is complex and demanding, and a better understanding of TRPV1 function and post-antagonism in the RA paradigm should accelerate the development of TRPV1-targeted modulators.
Author contributions
This article is mainly written by YQ. YF and YL wrote part of the manuscript and proofread the manuscript. CL, BX, and QZ helped us collect literature information and draw pictures. PJ reviewed the manuscript and proposed final revisions. All authors contributed to the article and approved the submitted version.
Funding
This work was supported by the National Natural Science Foundation of China [grant number 82274481] and by the Natural Science Foundation of Shandong Province [grant number ZR2022LZY004].
Acknowledgments
We acknowledge the support of the National Natural Science Foundation of China and the Natural Science Foundation of Shandong Province for this project.
Conflict of interest
The authors declare that the research was conducted in the absence of any commercial or financial relationships that could be construed as a potential conflict of interest.
Publisher’s note
All claims expressed in this article are solely those of the authors and do not necessarily represent those of their affiliated organizations, or those of the publisher, the editors and the reviewers. Any product that may be evaluated in this article, or claim that may be made by its manufacturer, is not guaranteed or endorsed by the publisher.
References
1. Huang J, Fu X, Chen X, Li Z, Huang Y, Liang C. Promising therapeutic targets for treatment of rheumatoid arthritis. Front Immunol (2021) 12:686155. doi: 10.3389/fimmu.2021.686155
2. Klareskog L, Catrina AI, Paget S. Rheumatoid arthritis. Lancet (London England) (2009) 373(9664):659–72. doi: 10.1016/s0140-6736(09)60008-8
3. Rubbert-Roth A, Szabó MZ, Kedves M, Nagy G, Atzeni F, Sarzi-Puttini P. Failure of anti-tnf treatment in patients with rheumatoid arthritis: the pros and cons of the early use of alternative biological agents. Autoimmun Rev (2019) 18(12):102398. doi: 10.1016/j.autrev.2019.102398
4. Liu S, Ma H, Zhang H, Deng C, Xin P. Recent advances on signaling pathways and their inhibitors in rheumatoid arthritis. Clin Immunol (Orlando Fla) (2021) 230:108793. doi: 10.1016/j.clim.2021.108793
5. Chandrupatla D, Molthoff CFM, Lammertsma AA, van der Laken CJ, Jansen G. The folate receptor B as a macrophage-mediated imaging and therapeutic target in rheumatoid arthritis. Drug Deliv Trans Res (2019) 9(1):366–78. doi: 10.1007/s13346-018-0589-2
6. Sotoudehmanesh R, Anvari B, Akhlaghi M, Shahraeeni S, Kolahdoozan S. Methotrexate hepatotoxicity in patients with rheumatoid arthritis. Middle East J digestive Dis (2010) 2(2):104–9.
7. Bombardier C, Laine L, Reicin A, Shapiro D, Burgos-Vargas R, Davis B, et al. Comparison of upper gastrointestinal toxicity of rofecoxib and naproxen in patients with rheumatoid arthritis. Vigor Study Group N Engl J Med (2000) 343(21):1520–8. doi: 10.1056/nejm200011233432103
8. Gautam R, Singh M, Gautam S, Rawat JK, Saraf SA, Kaithwas G. Rutin attenuates intestinal toxicity induced by methotrexate linked with anti-oxidative and anti-inflammatory effects. BMC Complement Altern Med (2016) 16:99. doi: 10.1186/s12906-016-1069-1
9. Abbas MA. Modulation of trpv1 channel function by natural products in the treatment of pain. Chem-Biol Interact (2020) 330:109178. doi: 10.1016/j.cbi.2020.109178
10. Engler A, Aeschlimann A, Simmen BR, Michel BA, Gay RE, Gay S, et al. Expression of transient receptor potential vanilloid 1 (Trpv1) in synovial fibroblasts from patients with osteoarthritis and rheumatoid arthritis. Biochem Biophys Res Commun (2007) 359(4):884–8. doi: 10.1016/j.bbrc.2007.05.178
11. Hsieh WS, Kung CC, Huang SL, Lin SC, Sun WH. Tdag8, trpv1, and asic3 involved in establishing hyperalgesic priming in experimental rheumatoid arthritis. Sci Rep (2017) 7(1):8870. doi: 10.1038/s41598-017-09200-6
12. Chu KL, Chandran P, Joshi SK, Jarvis MF, Kym PR, McGaraughty S. Trpv1-related modulation of spinal neuronal activity and behavior in a rat model of osteoarthritic pain. Brain Res (2011) 1369:158–66. doi: 10.1016/j.brainres.2010.10.101
13. Kelly S, Chapman RJ, Woodhams S, Sagar DR, Turner J, Burston JJ, et al. Increased function of pronociceptive trpv1 at the level of the joint in a rat model of osteoarthritis pain. Ann Rheum Dis (2015) 74(1):252–9. doi: 10.1136/annrheumdis-2013-203413
14. Caterina MJ, Schumacher MA, Tominaga M, Rosen TA, Levine JD, Julius D. The capsaicin receptor: A heat-activated ion channel in the pain pathway. Nature (1997) 389(6653):816–24. doi: 10.1038/39807
15. Zhao R, Tsang SY. Versatile roles of intracellularly located trpv1 channel. J Cell Physiol (2017) 232(8):1957–65. doi: 10.1002/jcp.25704
16. Benítez-Angeles M, Morales-Lázaro SL, Juárez-González E, Rosenbaum T. Trpv1: structure, endogenous agonists, and mechanisms. Int J Mol Sci (2020) 21(10):3421. doi: 10.3390/ijms21103421
17. Zheng J. Molecular mechanism of trp channels. Compr Physiol (2013) 3(1):221–42. doi: 10.1002/cphy.c120001
18. Szolcsányi J, Sándor Z. Multisteric trpv1 nocisensor: A target for analgesics. Trends Pharmacol Sci (2012) 33(12):646–55. doi: 10.1016/j.tips.2012.09.002
19. Phelps CB, Wang RR, Choo SS, Gaudet R. Differential regulation of trpv1, trpv3, and trpv4 sensitivity through a conserved binding site on the ankyrin repeat domain. J Biol Chem (2010) 285(1):731–40. doi: 10.1074/jbc.M109.052548
20. Omari SA, Adams MJ, Geraghty DP. Trpv1 channels in immune cells and hematological Malignancies. Adv Pharmacol (San Diego Calif) (2017) 79:173–98. doi: 10.1016/bs.apha.2017.01.002
21. Caterina MJ, Leffler A, Malmberg AB, Martin WJ, Trafton J, Petersen-Zeitz KR, et al. Impaired nociception and pain sensation in mice lacking the capsaicin receptor. Sci (New York NY) (2000) 288(5464):306–13. doi: 10.1126/science.288.5464.306
22. Sharma P. Inflammation and the metabolic syndrome. Indian J Clin biochemistry: IJCB (2011) 26(4):317–8. doi: 10.1007/s12291-011-0175-6
23. Murata M. Inflammation and cancer. Environ Health Prev Med (2018) 23(1):50. doi: 10.1186/s12199-018-0740-1
24. Pohl D, Benseler S. Systemic inflammatory and autoimmune disorders. Handb Clin Neurol (2013) 112:1243–52. doi: 10.1016/b978-0-444-52910-7.00047-7
25. Chen WW, Zhang X, Huang WJ. Role of neuroinflammation in neurodegenerative diseases (Review). Mol Med Rep (2016) 13(4):3391–6. doi: 10.3892/mmr.2016.4948
26. Barton NJ, McQueen DS, Thomson D, Gauldie SD, Wilson AW, Salter DM, et al. Attenuation of experimental arthritis in trpv1r knockout mice. Exp Mol Pathol (2006) 81(2):166–70. doi: 10.1016/j.yexmp.2006.04.007
27. Chemin K, Gerstner C, Malmström V. Effector functions of cd4+ T cells at the site of local autoimmune inflammation-lessons from rheumatoid arthritis. Front Immunol (2019) 10:353. doi: 10.3389/fimmu.2019.00353
28. Assas MB, Wakid MH, Zakai HA, Miyan JA, Pennock JL. Transient receptor potential vanilloid 1 expression and function in splenic dendritic cells: A potential role in immune homeostasis. Immunology (2016) 147(3):292–304. doi: 10.1111/imm.12562
29. Majhi RK, Sahoo SS, Yadav M, Pratheek BM, Chattopadhyay S, Goswami C. Functional expression of trpv channels in T cells and their implications in immune regulation. FEBS J (2015) 282(14):2661–81. doi: 10.1111/febs.13306
30. Kim HS, Kwon HJ, Kim GE, Cho MH, Yoon SY, Davies AJ, et al. Attenuation of natural killer cell functions by capsaicin through a direct and trpv1-independent mechanism. Carcinogenesis (2014) 35(7):1652–60. doi: 10.1093/carcin/bgu091
31. Lv Z, Xu X, Sun Z, Yang YX, Guo H, Li J, et al. Trpv1 alleviates osteoarthritis by inhibiting M1 macrophage polarization via ca(2+)/camkii/nrf2 signaling pathway. Cell Death Dis (2021) 12(6):504. doi: 10.1038/s41419-021-03792-8
32. Güzel M, Akpınar O. Hydroxychloroquine attenuates acute inflammation (Lps)-induced apoptosis via inhibiting trpv1 channel/ros signaling pathways in human monocytes. Biology (2021) 10(10):967. doi: 10.3390/biology10100967
33. Feske S, Wulff H, Skolnik EY. Ion channels in innate and adaptive immunity. Annu Rev Immunol (2015) 33:291–353. doi: 10.1146/annurev-immunol-032414-112212
34. Toldi G. The regulation of calcium homeostasis in T lymphocytes. Front Immunol (2013) 4:432. doi: 10.3389/fimmu.2013.00432
35. Weyand CM, Goronzy JJ. The immunology of rheumatoid arthritis. Nat Immunol (2021) 22(1):10–8. doi: 10.1038/s41590-020-00816-x
36. Waase I, Kayser C, Carlson PJ, Goronzy JJ, Weyand CM. Oligoclonal T cell proliferation in patients with rheumatoid arthritis and their unaffected siblings. Arthritis Rheum (1996) 39(6):904–13. doi: 10.1002/art.1780390606
37. Takemura S, Braun A, Crowson C, Kurtin PJ, Cofield RH, O’Fallon WM, et al. Lymphoid neogenesis in rheumatoid synovitis. J Immunol (Baltimore Md: 1950) (2001) 167(2):1072–80. doi: 10.4049/jimmunol.167.2.1072
38. Dolhain RJ, van der Heiden AN, ter Haar NT, Breedveld FC, Miltenburg AM. Shift toward T lymphocytes with a T helper 1 cytokine-secretion profile in the joints of patients with rheumatoid arthritis. Arthritis Rheum (1996) 39(12):1961–9. doi: 10.1002/art.1780391204
39. Pène J, Chevalier S, Preisser L, Vénéreau E, Guilleux MH, Ghannam S, et al. Chronically inflamed human tissues are infiltrated by highly differentiated th17 lymphocytes. J Immunol (Baltimore Md: 1950) (2008) 180(11):7423–30. doi: 10.4049/jimmunol.180.11.7423
40. Zhang F, Wei K, Slowikowski K, Fonseka CY, Rao DA, Kelly S, et al. Defining inflammatory cell states in rheumatoid arthritis joint synovial tissues by integrating single-cell transcriptomics and mass cytometry. Nat Immunol (2019) 20(7):928–42. doi: 10.1038/s41590-019-0378-1
41. Wenning AS, Neblung K, Strauss B, Wolfs MJ, Sappok A, Hoth M, et al. Trp expression pattern and the functional importance of trpc3 in primary human T-cells. Biochim Biophys Acta (2011) 1813(3):412–23. doi: 10.1016/j.bbamcr.2010.12.022
42. Bertin S, Aoki-Nonaka Y, de Jong PR, Nohara LL, Xu H, Stanwood SR, et al. The ion channel trpv1 regulates the activation and proinflammatory properties of Cd4+ T cells. Nat Immunol (2014) 15(11):1055–63. doi: 10.1038/ni.3009
43. Samivel R, Kim DW, Son HR, Rhee YH, Kim EH, Kim JH, et al. The role of trpv1 in the cd4+ T cell-mediated inflammatory response of allergic rhinitis. Oncotarget (2016) 7(1):148–60. doi: 10.18632/oncotarget.6653
44. Bertin S, de Jong PR, Jefferies WA, Raz E. Novel immune function for the trpv1 channel in T lymphocytes. Channels (Austin Tex) (2014) 8(6):479–80. doi: 10.4161/19336950.2014.991640
45. Hogan PG, Lewis RS, Rao A. Molecular basis of calcium signaling in lymphocytes: stim and orai. Annu Rev Immunol (2010) 28:491–533. doi: 10.1146/annurev.immunol.021908.132550
46. Bertin S, Aoki-Nonaka Y, Lee J, de Jong PR, Kim P, Han T, et al. The trpa1 ion channel is expressed in Cd4+ T cells and restrains T-cell-mediated colitis through inhibition of trpv1. Gut (2017) 66(9):1584–96. doi: 10.1136/gutjnl-2015-310710
47. Bujak JK, Kosmala D, Szopa IM, Majchrzak K, Bednarczyk P. Inflammation, cancer and immunity-implication of trpv1 channel. Front Oncol (2019) 9:1087. doi: 10.3389/fonc.2019.01087
48. Ross EA, Devitt A, Johnson JR. Macrophages: the good, the bad, and the gluttony. Front Immunol (2021) 12:708186. doi: 10.3389/fimmu.2021.708186
49. Udalova IA, Mantovani A, Feldmann M. Macrophage heterogeneity in the context of rheumatoid arthritis. Nat Rev Rheumatol (2016) 12(8):472–85. doi: 10.1038/nrrheum.2016.91
50. Arango Duque G, Descoteaux A. Macrophage cytokines: involvement in immunity and infectious diseases. Front Immunol (2014) 5:491. doi: 10.3389/fimmu.2014.00491
51. Jiang X, Wang C, Ke Z, Duo L, Wu T, Wang W, et al. The ion channel trpv1 gain-of-function reprograms the immune microenvironment to facilitate colorectal tumorigenesis. Cancer Lett (2022) 527:95–106. doi: 10.1016/j.canlet.2021.12.012
52. Yang X, Chang Y, Wei W. Emerging role of targeting macrophages in rheumatoid arthritis: focus on polarization, metabolism and apoptosis. Cell Prolif (2020) 53(7):e12854. doi: 10.1111/cpr.12854
53. Ambarus CA, Noordenbos T, de Hair MJ, Tak PP, Baeten DL. Intimal lining layer macrophages but not synovial sublining macrophages display an il-10 polarized-like phenotype in chronic synovitis. Arthritis Res Ther (2012) 14(2):R74. doi: 10.1186/ar3796
54. Wynn TA, Chawla A, Pollard JW. Macrophage biology in development, homeostasis and disease. Nature (2013) 496(7446):445–55. doi: 10.1038/nature12034
55. Ninomiya Y, Tanuma SI, Tsukimoto M. Differences in the effects of four trpv1 channel antagonists on lipopolysaccharide-induced cytokine production and cox-2 expression in murine macrophages. Biochem Biophys Res Commun (2017) 484(3):668–74. doi: 10.1016/j.bbrc.2017.01.173
56. Hasegawa T, Kikuta J, Sudo T, Matsuura Y, Matsui T, Simmons S, et al. Identification of a novel arthritis-associated osteoclast precursor macrophage regulated by foxm1. Nat Immunol (2019) 20(12):1631–43. doi: 10.1038/s41590-019-0526-7
57. Van Raemdonck K, Umar S, Palasiewicz K, Volkov S, Volin MV, Arami S, et al. Ccl21/ccr7 signaling in macrophages promotes joint inflammation and th17-mediated osteoclast formation in rheumatoid arthritis. Cell Mol Life sciences: CMLS (2020) 77(7):1387–99. doi: 10.1007/s00018-019-03235-w
58. Yao Y, Cai X, Ren F, Ye Y, Wang F, Zheng C, et al. The macrophage-osteoclast axis in osteoimmunity and osteo-related diseases. Front Immunol (2021) 12:664871. doi: 10.3389/fimmu.2021.664871
59. Luo X, Chen O, Wang Z, Bang S, Ji J, Lee SH, et al. Il-23/il-17a/trpv1 axis produces mechanical pain via macrophage-sensory neuron crosstalk in female mice. Neuron (2021) 109(17):2691–706.e5. doi: 10.1016/j.neuron.2021.06.015
60. Oh S, Son M, Park J, Kang D, Byun K. Radiofrequency irradiation modulates trpv1-related burning sensation in rosacea. Molecules (Basel Switzerland) (2021) 26(5):1424. doi: 10.3390/molecules26051424
61. Basu S, Srivastava P. Immunological role of neuronal receptor vanilloid receptor 1 expressed on dendritic cells. Proc Natl Acad Sci USA (2005) 102(14):5120–5. doi: 10.1073/pnas.0407780102
62. Tóth BI, Benko S, Szöllosi AG, Kovács L, Rajnavölgyi E, Bíró T. Transient receptor potential vanilloid-1 signaling inhibits differentiation and activation of human dendritic cells. FEBS Lett (2009) 583(10):1619–24. doi: 10.1016/j.febslet.2009.04.031
63. Lebre MC, Jongbloed SL, Tas SW, Smeets TJ, McInnes IB, Tak PP. Rheumatoid arthritis synovium contains two subsets of cd83-dc-lamp- dendritic cells with distinct cytokine profiles. Am J Pathol (2008) 172(4):940–50. doi: 10.2353/ajpath.2008.070703
64. Thomas R, Davis LS, Lipsky PE. Rheumatoid synovium is enriched in mature antigen-presenting dendritic cells. J Immunol (Baltimore Md: 1950) (1994) 152(5):2613–23. doi: 10.4049/jimmunol.152.5.2613
65. Wehr P, Purvis H, Law SC, Thomas R. Dendritic cells, T cells and their interaction in rheumatoid arthritis. Clin Exp Immunol (2019) 196(1):12–27. doi: 10.1111/cei.13256
66. Pan F, Xiang H, Yan J, Hong L, Zhang L, Liu Y, et al. Dendritic cells from rheumatoid arthritis patient peripheral blood induce th17 cell differentiation via mir-363/integrin Av/tgf-B; Axis. Scandinavian J Immunol (2017) 85(6):441–9. doi: 10.1111/sji.12550
67. Lebre MC, Tak PP. Dendritic cell subsets: their roles in rheumatoid arthritis. Acta reumatologica portuguesa (2008) 33(1):35–45.
68. Duo L, Wu T, Ke Z, Hu L, Wang C, Teng G, et al. Gain of function of ion channel trpv1 exacerbates experimental colitis by promoting dendritic cell activation. Mol Ther Nucleic Acids (2020) 22:924–36. doi: 10.1016/j.omtn.2020.10.006
69. Prevosto C, Goodall JC, Hill Gaston JS. Cytokine secretion by pathogen recognition receptor-stimulated dendritic cells in rheumatoid arthritis and ankylosing spondylitis. J Rheumatol (2012) 39(10):1918–28. doi: 10.3899/jrheum.120208
70. Yu MB, Langridge WHR. The function of myeloid dendritic cells in rheumatoid arthritis. Rheumatol Int (2017) 37(7):1043–51. doi: 10.1007/s00296-017-3671-z
71. O’Connell PJ, Pingle SC, Ahern GP. Dendritic cells do not transduce inflammatory stimuli via the capsaicin receptor trpv1. FEBS Lett (2005) 579(23):5135–9. doi: 10.1016/j.febslet.2005.08.023
72. Firestein GS, Berger AE, Tracey DE, Chosay JG, Chapman DL, Paine MM, et al. Il-1 receptor antagonist protein production and gene expression in rheumatoid arthritis and osteoarthritis synovium. J Immunol (Baltimore Md: 1950) (1992) 149(3):1054–62. doi: 10.4049/jimmunol.149.3.1054
73. Schett G, Dayer JM, Manger B. Interleukin-1 function and role in rheumatic disease. Nat Rev Rheumatol (2016) 12(1):14–24. doi: 10.1038/nrrheum.2016.166
74. Levescot A, Chang MH, Schnell J, Nelson-Maney N, Yan J, Martínez-Bonet M, et al. Il-1β-driven osteoclastogenic tregs accelerate bone erosion in arthritis. J Clin Invest (2021) 131(18):e141008. doi: 10.1172/jci141008
75. Nakamura I, Jimi E. Regulation of osteoclast differentiation and function by interleukin-1. Vitamins hormones (2006) 74:357–70. doi: 10.1016/s0083-6729(06)74015-8
76. Mailhot B, Christin M, Tessandier N, Sotoudeh C, Bretheau F, Turmel R, et al. Neuronal interleukin-1 receptors mediate pain in chronic inflammatory diseases. J Exp Med (2020) 217(9):e20191430. doi: 10.1084/jem.20191430
77. Li C, Bo L, Liu Q, Liu W, Chen X, Xu D, et al. Activation of trpv1-dependent calcium oscillation exacerbates seawater inhalation-induced acute lung injury. Mol Med Rep (2016) 13(3):1989–98. doi: 10.3892/mmr.2016.4804
78. Gao Y, Liu Y, Zhu K, Zhang Z, Qiao H, Lu Z, et al. Blocking of trpv-1 in the parodontium relieves orthodontic pain by inhibiting the expression of trpv-1 in the trigeminal ganglion during experimental tooth movement in rats. Neurosci Lett (2016) 628:67–72. doi: 10.1016/j.neulet.2016.06.007
79. Marshall NJ, Liang L, Bodkin J, Dessapt-Baradez C, Nandi M, Collot-Teixeira S, et al. A role for trpv1 in influencing the onset of cardiovascular disease in obesity. Hypertension (Dallas Tex: 1979) (2013) 61(1):246–52. doi: 10.1161/hypertensionaha.112.201434
80. Yang XL, Wang X, Shao L, Jiang GT, Min JW, Mei XY, et al. Trpv1 mediates astrocyte activation and interleukin-1β Release induced by hypoxic ischemia (Hi). J Neuroinflamm (2019) 16(1):114. doi: 10.1186/s12974-019-1487-3
81. Terenzi R, ROmano E, Manetti M, Peruzzi F, Nacci F, Matucci-Cerinic M, et al. Neuropeptides activate trpv1 in rheumatoid arthritis fibroblast-like synoviocytes and foster il-6 and il-8 production. Ann Rheumatic Dis (2013) 72(6):1107–9. doi: 10.1136/annrheumdis-2012-202846
82. Tanaka T, Narazaki M, Kishimoto T. Il-6 in inflammation, immunity, and disease. Cold Spring Harbor Perspect Biol (2014) 6(10):a016295. doi: 10.1101/cshperspect.a016295
83. Favalli EG. Understanding the role of interleukin-6 (Il-6) in the joint and beyond: A comprehensive review of Il-6 inhibition for the management of rheumatoid arthritis. Rheumatol Ther (2020) 7(3):473–516. doi: 10.1007/s40744-020-00219-2
84. De Benedetti F, Rucci N, Del Fattore A, Peruzzi B, Paro R, Longo M, et al. Impaired skeletal development in interleukin-6-transgenic mice: A model for the impact of chronic inflammation on the growing skeletal system. Arthritis Rheum (2006) 54(11):3551–63. doi: 10.1002/art.22175
85. Devlin RD, Reddy SV, Savino R, Ciliberto G, Roodman GD. Il-6 mediates the effects of Il-1 or tnf, but not pthrp or 1,25(Oh)2d3, on osteoclast-like cell formation in normal human bone marrow cultures. J Bone mineral Res (1998) 13(3):393–9. doi: 10.1359/jbmr.1998.13.3.393
86. Sappington RM, Calkins DJ. Contribution of trpv1 to microglia-derived Il-6 and Nfkappab translocation with elevated hydrostatic pressure. Invest Ophthalmol Visual Sci (2008) 49(7):3004–17. doi: 10.1167/iovs.07-1355
87. Zhou Y, Follansbee T, Wu X, Han D, Yu S, Domocos DT, et al. Trpv1 mediates inflammation and hyperplasia in imiquimod (Imq)-induced psoriasiform dermatitis (Psd) in mice. J Dermatol Sci (2018) 92(3):264–71. doi: 10.1016/j.jdermsci.2018.11.009
88. Son GY, Hong JH, Chang I, Shin DM. Induction of Il-6 and Il-8 by activation of thermosensitive trp channels in human pdl cells. Arch Oral Biol (2015) 60(4):526–32. doi: 10.1016/j.archoralbio.2014.12.014
89. Zhu X, Xiao L, Huo R, Zhang J, Lin J, Xie J, et al. Cyr61 is involved in neutrophil infiltration in joints by inducing Il-8 production by fibroblast-like synoviocytes in rheumatoid arthritis. Arthritis Res Ther (2013) 15(6):R187. doi: 10.1186/ar4377
90. Sung HJ, Choi S, Lee JW, Ok CY, Bae YS, Kim YH, et al. Inhibition of human neutrophil activity by an rna aptamer bound to interleukin-8. Biomaterials (2014) 35(1):578–89. doi: 10.1016/j.biomaterials.2013.09.107
91. Koch AE, Polverini PJ, Kunkel SL, Harlow LA, DiPietro LA, Elner VM, et al. Interleukin-8 as a macrophage-derived mediator of angiogenesis. Sci (New York NY) (1992) 258(5089):1798–801. doi: 10.1126/science.1281554
92. An Q, Yan W, Zhao Y, Yu K. Enhanced neutrophil autophagy and increased concentrations of Il-6, Il-8, Il-10 and mcp-1 in rheumatoid arthritis. Int Immunopharmacol (2018) 65:119–28. doi: 10.1016/j.intimp.2018.09.011
93. Kim HB, Na EY, Yun SJ, Lee JB. The effect of capsaicin on neuroinflammatory mediators of rosacea. Ann Dermatol (2022) 34(4):261–9. doi: 10.5021/ad.21.223
94. Sušjan P, Benčina M, Hafner-Bratkovič I. Differential effect of extracellular acidic environment on Il-1β Released from human and mouse phagocytes. Int J Mol Sci (2020) 21(19):7229. doi: 10.3390/ijms21197229
95. Nakanishi M, Morita Y, Hata K, Muragaki Y. Acidic microenvironments induce lymphangiogenesis and Il-8 production via trpv1 activation in human lymphatic endothelial cells. Exp Cell Res (2016) 345(2):180–9. doi: 10.1016/j.yexcr.2016.06.006
96. Morita T, Shima Y, Fujimoto K, Tsuboi H, Saeki Y, Narazaki M, et al. Anti-receptor activator of nuclear factor Kb ligand antibody treatment increases osteoclastogenesis-promoting Il-8 in patients with rheumatoid arthritis. Int Immunol (2019) 31(5):277–85. doi: 10.1093/intimm/dxz009
97. Peng H, Xing J, Wang X, Ding X, Tang X, Zou J, et al. Circular rna circnup214 modulates the T helper 17 cell response in patients with rheumatoid arthritis. Front Immunol (2022) 13:885896. doi: 10.3389/fimmu.2022.885896
98. Riol-Blanco L, Ordovas-Montanes J, Perro M, Naval E, Thiriot A, Alvarez D, et al. Nociceptive sensory neurons drive interleukin-23-mediated psoriasiform skin inflammation. Nature (2014) 510(7503):157–61. doi: 10.1038/nature13199
99. Chan TC, Lee MS, Huang WC, Chang WY, Krueger JG, Tsai TF. Capsaicin attenuates imiquimod-induced epidermal hyperplasia and cutaneous inflammation in a murine model of psoriasis. Biomed pharmacother = Biomedecine pharmacotherapie (2021) 141:111950. doi: 10.1016/j.biopha.2021.111950
100. Tsuji F, Murai M, Oki K, Seki I, Ueda K, Inoue H, et al. Transient receptor potential vanilloid 1 agonists as candidates for anti-inflammatory and immunomodulatory agents. Eur J Pharmacol (2010) 627(1-3):332–9. doi: 10.1016/j.ejphar.2009.10.044
101. Chu X, Du X, Yang L, Wang Z, Zhang Y, Wang X, et al. Targeting tumor necrosis factor receptor 1 with selected aptamers for anti-inflammatory activity. ACS Appl Mater interfaces (2023) 15(9):11599–608. doi: 10.1021/acsami.3c00131
102. Hsu CC, Lin YS, Lin RL, Lee LY. Immediate and delayed potentiating effects of tumor necrosis factor-A on trpv1 sensitivity of rat vagal pulmonary sensory neurons. Am J Physiol Lung Cell Mol Physiol (2017) 313(2):L293–l304. doi: 10.1152/ajplung.00235.2016
103. Rozas P, Lazcano P, Piña R, Cho A, Terse A, Pertusa M, et al. Targeted overexpression of tumor necrosis factor-A Increases cyclin-dependent kinase 5 activity and trpv1-dependent ca2+ Influx in trigeminal neurons. Pain (2016) 157(6):1346–62. doi: 10.1097/j.pain.0000000000000527
104. Barker KH, Higham JP, Pattison LA, Taylor TS, Chessell IP, Welsh F, et al. Sensitization of colonic nociceptors by tnfα Is dependent on tnfr1 expression and P38 mapk activity. J Physiol (2022) 600(16):3819–36. doi: 10.1113/jp283170
105. Yin N, Gao Q, Tao W, Chen J, Bi J, Ding F, et al. Paeoniflorin relieves lps-induced inflammatory pain in mice by inhibiting nlrp3 inflammasome activation via transient receptor potential vanilloid 1. J leukocyte Biol (2020) 108(1):229–41. doi: 10.1002/jlb.3ma0220-355r
106. Chung YC, Baek JY, Kim SR, Ko HW, Bok E, Shin WH, et al. Capsaicin prevents degeneration of dopamine neurons by inhibiting glial activation and oxidative stress in the mptp model of parkinson’s disease. Exp Mol Med (2017) 49(3):e298. doi: 10.1038/emm.2016.159
107. Huang WX, Yu F, Sanchez RM, Liu YQ, Min JW, Hu JJ, et al. Trpv1 promotes repetitive febrile seizures by pro-inflammatory cytokines in immature brain. Brain Behav Immun (2015) 48:68–77. doi: 10.1016/j.bbi.2015.01.017
108. Grieshaber-Bouyer R, Exner T, Hackert NS, Radtke FA, Jelinsky SA, Halyabar O, et al. Ageing and interferon gamma response drive the phenotype of neutrophils in the inflamed joint. Ann Rheum Dis (2022) 81(6):805–14. doi: 10.1136/annrheumdis-2021-221866
109. Maggi J, Carrascal M, Soto L, Neira O, Cuéllar MC, Aravena O, et al. Isolation of hla-dr-naturally presented peptides identifies T-cell epitopes for rheumatoid arthritis. Ann Rheum Dis (2022) 81(8):1096–105. doi: 10.1136/annrheumdis-2021-220371
110. Erin N, Akman M, Aliyev E, Tanrıöver G, Korcum AF. Olvanil activates sensory nerve fibers, increases T cell response and decreases metastasis of breast carcinoma. Life Sci (2022) 291:120305. doi: 10.1016/j.lfs.2022.120305
111. Erin N, Akman M. Effects of in-vitro modulation of trpv1 activity on immune response of mice bearing metastatic breast carcinoma: enhanced inflammatory response may hinder therapeutic potentials of trpv1 agonists. Life Sci (2021) 287:120115. doi: 10.1016/j.lfs.2021.120115
112. Lee YC. Effect and treatment of chronic pain in inflammatory arthritis. Curr Rheumatol Rep (2013) 15(1):300. doi: 10.1007/s11926-012-0300-4
113. Cohen SP, Vase L, Hooten WM. Chronic pain: an update on burden, best practices, and new advances. Lancet (London England) (2021) 397(10289):2082–97. doi: 10.1016/s0140-6736(21)00393-7
114. Nieto FR, Clark AK, Grist J, Hathway GJ, Chapman V, Malcangio M. Neuron-immune mechanisms contribute to pain in early stages of arthritis. J Neuroinflamm (2016) 13(1):96. doi: 10.1186/s12974-016-0556-0
115. Wang Y. The functional regulation of trpv1 and its role in pain sensitization. Neurochem Res (2008) 33(10):2008–12. doi: 10.1007/s11064-008-9750-5
116. Rosta J, Tóth M, Friedrich N, Sántha P, Jancsó G, Dux M. Insulin sensitizes neural and vascular trpv1 receptors in the trigeminovascular system. J headache Pain (2022) 23(1):7. doi: 10.1186/s10194-021-01380-x
117. Wedel S, Mathoor P, Rauh O, Heymann T, Ciotu CI, Fuhrmann DC, et al. Safit2 reduces neuroinflammation and ameliorates nerve injury-induced neuropathic pain. J Neuroinflamm (2022) 19(1):254. doi: 10.1186/s12974-022-02615-7
118. Warwick CA, Shutov LP, Shepherd AJ, Mohapatra DP, Usachev YM. Mechanisms underlying mechanical sensitization induced by complement C5a: the roles of macrophages, trpv1, and calcitonin gene-related peptide receptors. Pain (2019) 160(3):702–11. doi: 10.1097/j.pain.0000000000001449
119. Maleitzke T, Hildebrandt A, Weber J, Dietrich T, Appelt J, Jahn D, et al. Proinflammatory and bone protective role of calcitonin gene-related peptide alpha in collagen antibody-induced arthritis. Rheumatol (Oxford England) (2021) 60(4):1996–2009. doi: 10.1093/rheumatology/keaa711
120. Fattori V, Pinho-Ribeiro FA, Staurengo-Ferrari L, Borghi SM, Rossaneis AC, Casagrande R, et al. The specialised pro-resolving lipid mediator maresin 1 reduces inflammatory pain with a long-lasting analgesic effect. Br J Pharmacol (2019) 176(11):1728–44. doi: 10.1111/bph.14647
121. Nieto FR, Clark AK, Grist J, Chapman V, Malcangio M. Calcitonin gene-related peptide-expressing sensory neurons and spinal microglial reactivity contribute to pain states in collagen-induced arthritis. Arthritis Rheumatol (Hoboken NJ) (2015) 67(6):1668–77. doi: 10.1002/art.39082
122. Ma J, Chen L, Fan J, Cao W, Zeng G, Wang Y, et al. Dual-targeting rutaecarpine-no donor hybrids as novel anti-hypertensive agents by promoting release of cgrp. Eur J Med Chem (2019) 168:146–53. doi: 10.1016/j.ejmech.2019.02.037
123. Lotz M, Carson DA, Vaughan JH. Substance P activation of rheumatoid synoviocytes: neural pathway in pathogenesis of arthritis. Sci (New York NY) (1987) 235(4791):893–5. doi: 10.1126/science.2433770
124. Cheadle CE, Glencross DA, Hawrylowicz CM. Allergens trp a switch to initiate type 2 immunity. Immunity (2020) 53(5):900–2. doi: 10.1016/j.immuni.2020.10.019
125. Zhang D, Jing B, Chen Z, Li X, Shi H, Zheng Y, et al. Ferulic acid alleviates sciatica by inhibiting peripheral sensitization through the rhoa/P38mapk signalling pathway. Phytomed: Int J phytother phytopharmacol (2022) 106:154420. doi: 10.1016/j.phymed.2022.154420
126. Lian Z, Qi H, Liu X, Zhang Y, Xu R, Yang X, et al. Ambient ozone, and urban pm(2.5) co-exposure, aggravate allergic asthma via transient receptor potential vanilloid 1-mediated neurogenic inflammation. Ecotoxicol Environ Saf (2022) 243:114000. doi: 10.1016/j.ecoenv.2022.114000
127. Xiang Q, Tang X, Cui S, Zhang Q, Liu X, Zhao J, et al. Capsaicin, the spicy ingredient of chili peppers: effects on gastrointestinal tract and composition of gut microbiota at various dosages. Foods (Basel Switzerland) (2022) 11(5):686. doi: 10.3390/foods11050686
128. Hiraga SI, Itokazu T, Nishibe M, Yamashita T. Neuroplasticity related to chronic pain and its modulation by microglia. Inflamm Regen (2022) 42(1):15. doi: 10.1186/s41232-022-00199-6
129. Huang L, Jin J, Chen K, You S, Zhang H, Sideris A, et al. Bdnf produced by cerebral microglia promotes cortical plasticity and pain hypersensitivity after peripheral nerve injury. PloS Biol (2021) 19(7):e3001337. doi: 10.1371/journal.pbio.3001337
130. Liu Y, Zhou LJ, Wang J, Li D, Ren WJ, Peng J, et al. Tnf-A Differentially regulates synaptic plasticity in the hippocampus and spinal cord by microglia-dependent mechanisms after peripheral nerve injury. J neuroscience: Off J Soc Neurosci (2017) 37(4):871–81. doi: 10.1523/jneurosci.2235-16.2016
131. Marrone MC, Morabito A, Giustizieri M, Chiurchiù V, Leuti A, Mattioli M, et al. Trpv1 channels are critical brain inflammation detectors and neuropathic pain biomarkers in mice. Nat Commun (2017) 8:15292. doi: 10.1038/ncomms15292
132. Kim SR, Kim SU, Oh U, Jin BK. Transient receptor potential vanilloid subtype 1 mediates microglial cell death in vivo and in vitro via ca2+-mediated mitochondrial damage and cytochrome C release. J Immunol (Baltimore Md: 1950) (2006) 177(7):4322–9. doi: 10.4049/jimmunol.177.7.4322
133. Miyake T, Shirakawa H, Nakagawa T, Kaneko S. Activation of mitochondrial transient receptor potential vanilloid 1 channel contributes to microglial migration. Glia (2015) 63(10):1870–82. doi: 10.1002/glia.22854
134. Matsushita T, Otani K, Oto Y, Takahashi Y, Kurosaka D, Kato F. Sustained microglial activation in the area postrema of collagen-induced arthritis mice. Arthritis Res Ther (2021) 23(1):273. doi: 10.1186/s13075-021-02657-x
135. Süß P, Hoffmann A, Rothe T, Ouyang Z, Baum W, Staszewski O, et al. Chronic peripheral inflammation causes a region-specific myeloid response in the central nervous system. Cell Rep (2020) 30(12):4082–95.e6. doi: 10.1016/j.celrep.2020.02.109
136. Hu Q, Wang Q, Wang C, Tai Y, Liu B, Shao X, et al. Trpv1 channel contributes to the behavioral hypersensitivity in a rat model of complex regional pain syndrome type 1. Front Pharmacol (2019) 10:453. doi: 10.3389/fphar.2019.00453
137. Guo D, Lin C, Lu Y, Guan H, Qi W, Zhang H, et al. Fabp4 secreted by M1-polarized macrophages promotes synovitis and angiogenesis to exacerbate rheumatoid arthritis. Bone Res (2022) 10(1):45. doi: 10.1038/s41413-022-00211-2
138. Savage AM, Kurusamy S, Chen Y, Jiang Z, Chhabria K, MacDonald RB, et al. Tmem33 is essential for vegf-mediated endothelial calcium oscillations and angiogenesis. Nat Commun (2019) 10(1):732. doi: 10.1038/s41467-019-08590-7
139. Simons M, Gordon E, Claesson-Welsh L. Mechanisms and regulation of endothelial vegf receptor signalling. Nat Rev Mol Cell Biol (2016) 17(10):611–25. doi: 10.1038/nrm.2016.87
140. Negri S, Faris P, Rosti V, Antognazza MR, Lodola F, Moccia F. Endothelial trpv1 as an emerging molecular target to promote therapeutic angiogenesis. Cells (2020) 9(6):1341. doi: 10.3390/cells9061341
141. Ching LC, Kou YR, Shyue SK, Su KH, Wei J, Cheng LC, et al. Molecular mechanisms of activation of endothelial nitric oxide synthase mediated by transient receptor potential vanilloid type 1. Cardiovasc Res (2011) 91(3):492–501. doi: 10.1093/cvr/cvr104
142. O’Leary C, McGahon MK, Ashraf S, McNaughten J, Friedel T, Cincolà P, et al. Involvement of trpv1 and trpv4 channels in retinal angiogenesis. Invest Ophthalmol Visual Sci (2019) 60(10):3297–309. doi: 10.1167/iovs.18-26344
143. Su KH, Lin SJ, Wei J, Lee KI, Zhao JF, Shyue SK, et al. The essential role of transient receptor potential vanilloid 1 in simvastatin-induced activation of endothelial nitric oxide synthase and angiogenesis. Acta physiologica (Oxford England) (2014) 212(3):191–204. doi: 10.1111/apha.12378
144. Perna A, Sellitto C, Komici K, Hay E, Rocca A, De Blasiis P, et al. Transient receptor potential (Trp) channels in tumor vascularization. Int J Mol Sci (2022) 23(22):14253. doi: 10.3390/ijms232214253
145. Liu K, Gao X, Hu C, Gui Y, Gui S, Ni Q, et al. Capsaicin ameliorates diabetic retinopathy by inhibiting poldip2-induced oxidative stress. Redox Biol (2022) 56:102460. doi: 10.1016/j.redox.2022.102460
146. Min JK, Han KY, Kim EC, Kim YM, Lee SW, Kim OH, et al. Capsaicin inhibits in vitro and in vivo angiogenesis. Cancer Res (2004) 64(2):644–51. doi: 10.1158/0008-5472.can-03-3250
147. Yari S, Kikuta J, Shigyo H, Miyamoto Y, Okuzaki D, Furusawa Y, et al. Jak inhibition ameliorates bone destruction by simultaneously targeting mature osteoclasts and their precursors. Inflamm Regen (2023) 43(1):18. doi: 10.1186/s41232-023-00268-4
148. Komatsu N, Takayanagi H. Mechanisms of joint destruction in rheumatoid arthritis - immune cell-fibroblast-bone interactions. Nat Rev Rheumatol (2022) 18(7):415–29. doi: 10.1038/s41584-022-00793-5
149. Gavenis K, Schumacher C, Schneider U, Eisfeld J, Mollenhauer J, Schmidt-Rohlfing B. Expression of ion channels of the trp family in articular chondrocytes from osteoarthritic patients: changes between native and in vitro propagated chondrocytes. Mol Cell Biochem (2009) 321(1-2):135–43. doi: 10.1007/s11010-008-9927-x
150. Khan K, Singh A, Mittal M, Sharan K, Singh N, Dixit P, et al. [6]-gingerol induces bone loss in ovary intact adult mice and augments osteoclast function via the transient receptor potential vanilloid 1 channel. Mol Nutr Food Res (2012) 56(12):1860–73. doi: 10.1002/mnfr.201200200
151. Idris AI, Landao-Bassonga E, Ralston SH. The trpv1 ion channel antagonist capsazepine inhibits osteoclast and osteoblast differentiation in vitro and ovariectomy induced bone loss in vivo. Bone (2010) 46(4):1089–99. doi: 10.1016/j.bone.2010.01.368
152. Bellini G, Torella M, Manzo I, Tortora C, Luongo L, Punzo F, et al. Pkcβii-mediated cross-talk of trpv1/cb2 modulates the glucocorticoid-induced osteoclast overactivity. Pharmacol Res (2017) 115:267–74. doi: 10.1016/j.phrs.2016.11.039
153. Jolliffe VA, Anand P, Kidd BL. Assessment of cutaneous sensory and autonomic axon reflexes in rheumatoid arthritis. Ann Rheum Dis (1995) 54(4):251–5. doi: 10.1136/ard.54.4.251
154. Borbély É, Botz B, Bölcskei K, Kenyér T, Kereskai L, Kiss T, et al. Capsaicin-sensitive sensory nerves exert complex regulatory functions in the serum-transfer mouse model of autoimmune arthritis. Brain Behav Immun (2015) 45:50–9. doi: 10.1016/j.bbi.2014.12.012
155. Iadarola MJ, Sapio MR, Raithel SJ, Mannes AJ, Brown DC. Long-term pain relief in canine osteoarthritis by a single intra-articular injection of resiniferatoxin, a potent trpv1 agonist. Pain (2018) 159(10):2105–14. doi: 10.1097/j.pain.0000000000001314
156. Lowin T, Apitz M, Anders S, Straub RH. Anti-inflammatory effects of N-acylethanolamines in rheumatoid arthritis synovial cells are mediated by trpv1 and trpa1 in a cox-2 dependent manner. Arthritis Res Ther (2015) 17:321. doi: 10.1186/s13075-015-0845-5
157. Gauldie SD, McQueen DS, Pertwee R, Chessell IP. Anandamide activates peripheral nociceptors in normal and arthritic rat knee joints. Br J Pharmacol (2001) 132(3):617–21. doi: 10.1038/sj.bjp.0703890
158. Murai M, Tsuji F, Nose M, Seki I, Oki K, Setoguchi C, et al. Sa13353 (1- 2-(1-adamantyl)Ethyl -1-pentyl-3- 3-(4-pyridyl)Propyl urea) inhibits tnf-alpha production through the activation of capsaicin-sensitive afferent neurons mediated via transient receptor potential vanilloid 1 in vivo. Eur J Pharmacol (2008) 588(2-3):309–15. doi: 10.1016/j.ejphar.2008.04.037
159. Trevisan G, Hoffmeister C, Rossato MF, Oliveira SM, Silva MA, Ineu RP, et al. Transient receptor potential ankyrin 1 receptor stimulation by hydrogen peroxide is critical to trigger pain during monosodium urate-induced inflammation in rodents. Arthritis Rheum (2013) 65(11):2984–95. doi: 10.1002/art.38112
160. Wu YW, Hao T, Kou XX, Gan YH, Ma XC. Synovial trpv1 is upregulated by 17-beta-estradiol and involved in allodynia of inflamed temporOmandibular joints in female rats. Arch Oral Biol (2015) 60(9):1310–8. doi: 10.1016/j.archoralbio.2015.05.011
161. Hoffmeister C, Trevisan G, Rossato MF, de Oliveira SM, Gomez MV, Ferreira J. Role of trpv1 in nociception and edema induced by monosodium urate crystals in rats. Pain (2011) 152(8):1777–88. doi: 10.1016/j.pain.2011.03.025
162. Cathcart CJ, Johnston SA, Reynolds LR, Al-Nadaf S, Budsberg SC. Efficacy of abt-116, an antagonist of transient receptor potential vanilloid type 1, in providing analgesia for dogs with chemically induced synovitis. Am J Vet Res (2012) 73(1):19–26. doi: 10.2460/ajvr.73.1.19
163. Yin C, Liu B, Wang P, Li X, Li Y, Zheng X, et al. Eucalyptol alleviates inflammation and pain responses in a mouse model of gout arthritis. Br J Pharmacol (2020) 177(9):2042–57. doi: 10.1111/bph.14967
164. Malek N, Mrugala M, Makuch W, Kolosowska N, Przewlocka B, Binkowski M, et al. A multi-target approach for pain treatment: dual inhibition of fatty acid amide hydrolase and trpv1 in a rat model of osteoarthritis. Pain (2015) 156(5):890–903. doi: 10.1097/j.pain.0000000000000132
165. Okun A, Liu P, Davis P, Ren J, Remeniuk B, Brion T, et al. Afferent drive elicits ongoing pain in a model of advanced osteoarthritis. Pain (2012) 153(4):924–33. doi: 10.1016/j.pain.2012.01.022
166. Logashina YA, Palikova YA, Palikov VA, Kazakov VA, Smolskaya SV, Dyachenko IA, et al. Anti-inflammatory and analgesic effects of trpv1 polypeptide modulator aphc3 in models of osteo- and rheumatoid arthritis. Mar Drugs (2021) 19(1):39. doi: 10.3390/md19010039
167. Puttfarcken PS, Han P, Joshi SK, Neelands TR, Gauvin DM, Baker SJ, et al. A-995662 [(R)-8-(4-methyl-5-(4-(Trifluoromethyl)Phenyl)Oxazol-2-ylamino)-1,2,3,4-tetrahydronaphthalen-2-ol], a novel, selective trpv1 receptor antagonist, reduces spinal release of glutamate and cgrp in a rat knee joint pain model. Pain (2010) 150(2):319–26. doi: 10.1016/j.pain.2010.05.015
168. Lowin T, Ren TT, Zurmahr J, Classen T, Schneider M, Pongratz G. Cannabidiol (Cbd): A killer for inflammatory rheumatoid arthritis synovial fibroblasts. Cell Death Dis (2020) 11(8):714. doi: 10.1038/s41419-020-02892-1
169. Horváth Á, Tékus V, Bencze N, Szentes N, Scheich B, Bölcskei K, et al. Analgesic effects of the novel semicarbazide-sensitive amine oxidase inhibitor szv 1287 in mouse pain models with neuropathic mechanisms: involvement of transient receptor potential vanilloid 1 and ankyrin 1 receptors. Pharmacol Res (2018) 131:231–43. doi: 10.1016/j.phrs.2018.02.006
170. Veigas JM, Williams P, Halade G, Rahman MM, Yoneda T, Fernandes G. Fish oil concentrate delays sensitivity to thermal nociception in mice. Pharmacol Res (2011) 63(5):377–82. doi: 10.1016/j.phrs.2011.02.004
171. Wang J, Wen W, Gong DY, Chen Q, Li P, Liu PW, et al. Szap exerts analgesic effects on rheumatalgia in cia rats by suppressing pain hyperalgesia and inhibiting trpv1 and P2x3. J Ethnopharmacol (2022) 284:114780. doi: 10.1016/j.jep.2021.114780
172. Stevens RM, Ervin J, Nezzer J, Nieves Y, Guedes K, Burges R, et al. Randomized, double-blind, placebo-controlled trial of intraarticular trans-capsaicin for pain associated with osteoarthritis of the knee. Arthritis Rheumatol (Hoboken NJ) (2019) 71(9):1524–33. doi: 10.1002/art.40894
173. Arendt-Nielsen L, Harris S, Whiteside GT, Hummel M, Knappenberger T, O’Keefe S, et al. A randomized, double-blind, positive-controlled, 3-way cross-over human experimental pain study of a trpv1 antagonist (V116517) in healthy volunteers and comparison with preclinical profile. Pain (2016) 157(9):2057–67. doi: 10.1097/j.pain.0000000000000610
174. Sjögren E, Ståhle L, Quiding H, Jonzon B, Halldin MM, Sundgren AK. The effect of intradermal microdosing of a transient receptor potential cation channel subfamily V member 1 antagonist on heat evoked pain and thermal thresholds in normal and ultraviolet-C exposed skin in healthy volunteers. Eur J Pain (London England) (2019) 23(10):1767–79. doi: 10.1002/ejp.1451
175. Chizh BA, O’Donnell MB, Napolitano A, Wang J, Brooke AC, Aylott MC, et al. The effects of the trpv1 antagonist sb-705498 on trpv1 receptor-mediated activity and inflammatory hyperalgesia in humans. Pain (2007) 132(1-2):132–41. doi: 10.1016/j.pain.2007.06.006
176. Gavva NR, Treanor JJ, Garami A, Fang L, Surapaneni S, Akrami A, et al. Pharmacological blockade of the vanilloid receptor trpv1 elicits marked hyperthermia in humans. Pain (2008) 136(1-2):202–10. doi: 10.1016/j.pain.2008.01.024
177. Li T, Chung MK. Striving toward hyperthermia-free analgesia: lessons from loss-of-function mutations of human trpv1. J Clin Invest (2023) 133(3):e167338. doi: 10.1172/jci167338
178. Koivisto AP, Belvisi MG, Gaudet R, Szallasi A. Advances in trp channel drug discovery: from target validation to clinical studies. Nat Rev Drug Discov (2022) 21(1):41–59. doi: 10.1038/s41573-021-00268-4
179. He S, Zambelli VO, Sinharoy P, Brabenec L, Bian Y, Rwere F, et al. A human trpv1 genetic variant within the channel gating domain regulates pain sensitivity in rodents. J Clin Invest (2023) 133(3):e163735. doi: 10.1172/jci163735
180. Ann J, Kim HS, Thorat SA, Kim H, Ha HJ, Choi K, et al. Discovery of nonpungent transient receptor potential vanilloid 1 (Trpv1) agonist as strong topical analgesic. J Med Chem (2020) 63(1):418–24. doi: 10.1021/acs.jmedchem.9b01046
Keywords: TRPV1, rheumatoid arthritis, pathogenesis, pain, inflammation, treatment, ion channels
Citation: Qu Y, Fu Y, Liu Y, Liu C, Xu B, Zhang Q and Jiang P (2023) The role of TRPV1 in RA pathogenesis: worthy of attention. Front. Immunol. 14:1232013. doi: 10.3389/fimmu.2023.1232013
Received: 31 May 2023; Accepted: 28 August 2023;
Published: 08 September 2023.
Edited by:
Vaibhavkumar S. Gawali, Charles River Laboratories, United StatesReviewed by:
Camila Rodrigues Ferraz, University of Maryland, United StatesEva Reali, University of Ferrara, Italy
Copyright © 2023 Qu, Fu, Liu, Liu, Xu, Zhang and Jiang. This is an open-access article distributed under the terms of the Creative Commons Attribution License (CC BY). The use, distribution or reproduction in other forums is permitted, provided the original author(s) and the copyright owner(s) are credited and that the original publication in this journal is cited, in accordance with accepted academic practice. No use, distribution or reproduction is permitted which does not comply with these terms.
*Correspondence: Ping Jiang, bG1kbG1kNjYxN0AxNjMuY29t