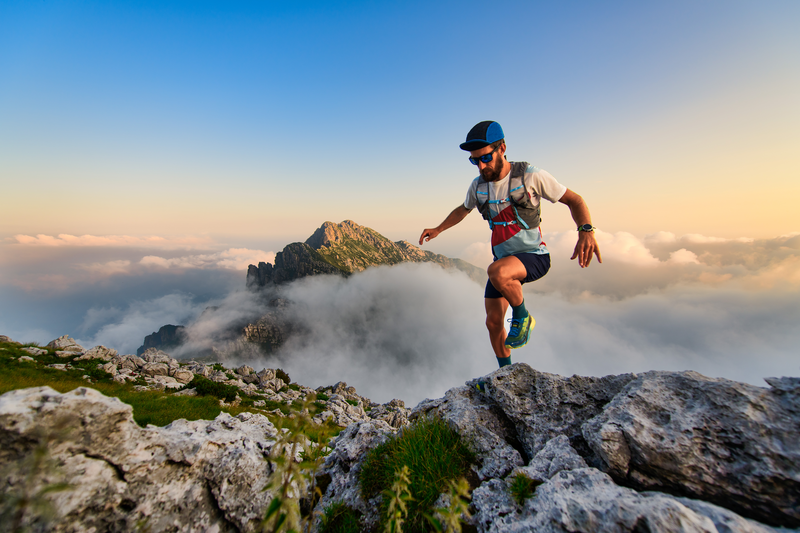
94% of researchers rate our articles as excellent or good
Learn more about the work of our research integrity team to safeguard the quality of each article we publish.
Find out more
REVIEW article
Front. Immunol. , 12 September 2023
Sec. Autoimmune and Autoinflammatory Disorders : Autoimmune Disorders
Volume 14 - 2023 | https://doi.org/10.3389/fimmu.2023.1230264
This article is part of the Research Topic Treat-to-target in systemic lupus erythematosus: Cytokine Transduction Pathways in SLE View all 7 articles
Systemic lupus erythematosus (SLE) is a heterogeneous multisystem inflammatory disease with wide variability in clinical manifestations. Natural arising CD4+ regulatory T cells (Tregs) play a critical role in maintaining peripheral tolerance by suppressing inflammation and preventing autoimmune responses in SLE. Additionally, CD8+ regulatory T cells, type 1 regulatory T cells (Tr1), and B regulatory cells also have a less well-defined role in the pathogenesis of SLE. Elucidation of the roles of various Treg subsets dedicated to immune homeostasis will provide a novel therapeutic approach that governs immune tolerance for the remission of active lupus. Diminished interleukin (IL)-2 production is associated with a depleted Treg cell population, and its reversibility by IL-2 therapy provides important reasons for the treatment of lupus. This review focuses on the pathogenesis and new therapeutics of human Treg subsets and low-dose IL-2 therapy in clinical benefits with SLE.
Systemic lupus erythematosus (SLE) is a chronic multisystem systemic inflammatory disorder characterized by an overactivated innate immune response, uncontrolled autoantibody production, and the presence of self-antigens that induce the deposition of immune complexes (1, 2). Endogenous self-antigen and nucleosomes from apoptotic cells in SLE will activate plasmacytoid dendritic cells (DCs) through the Toll-like receptor (TLR) 7 and TLR 9 to interact with pathogenic self-reactive T cells for type 1 interferon production, and with B cells for the manufacture of autoantibodies (2–5). The inappropriate stimulation of Th1 and Th17 inflammatory cytokines and the loss of self-tolerance will contribute to immune dysfunction and tissue inflammation in SLE (1, 6, 7). As shown in Figure 1, we summarize the pathogenesis of SLE in the overactivation of Th1 and Th17 responses with lower regulatory T cells (Tregs) resulting in a high expression of type 1 interferon cytokines and adhesion molecules that increase neutrophil netosis and tissue damage and apoptosis with nuclear DNA exposure for the induction of anti-DNA autoantibodies. Skewed lower Treg cells attributed to inappropriate immune homeostasis and proper targeting of imbalanced Treg function and/or low-dose IL-2 the administration could rescue the progress of SLE.
Figure 1 Schematic diagram showing the pathogenic mechanism of overactivated T cell responses and the therapeutic potential of regulatory cells with low-dose IL-2 therapy in systemic lupus erythematosus. (A) SLE is characterized by increased apoptosis and defective clearance mechanisms leading to the formation of immune complexes against the self-antigen. These immune complexes cause neutrophil netosis and activate plasmacytoid dendritic cells to interact with self-reactive pathogenic T cells. (B) Overactivation of Th1 and Th17 responses results in high expression of type 1 interferon, cytokines, and adhesion molecules that increase tissue damage and induction of anti-DNA autoantibodies. (C) Subsets of skewed lower Treg cells with inappropriate immune homeostasis will contribute to the worsening of lupus. (D) IL-2 induces Tregs differentiation in association with STAT5 phosphorylation with sustained expression of FoxP3. IL-2 directly inhibits expression of RORγt and blocks Th17 development. IL-2 also induces Blimp-1 to decrease Bcl-6 expression in T follicular helper cells. Low dose IL-2 therapy restores Treg suppressive function mainly by secreting inhibitory cytokines with IL-10, TGF-β, IL-35, and cytolysis with granzyme B and perforin, and deprivation of effector T cell growth with IL-2 and suppression of dendritic cell function. (created with BioRender.com). Agreement number: QI25RDVKPZ.
Tregs are vital for maintaining immune self-tolerance and regulation of immune tolerance by inhibiting autoimmune and inflammatory responses (8–11). Natural CD4+ Tregs (nTregs) are derived from thymic precursors, consisting of approximately 5% to 10% of the circulating population of CD4+ T cells (12, 13). The nTregs had high expression of CD25 and low expression of CD127 in CD4+ T cells, and the transcription factor forkhead box P3 (FoxP3) served as the best known Treg hallmarks (12, 14, 15). It is important to note that genetic factors contribute to the risk of developing SLE and immune dysregulation (16). Certain genes involved in T-cell activation, regulation, and type I interferons have been identified as risk factors for SLE susceptibility (17). For example, variations in the FoxP3 gene, which encodes the critical transcription factor for Treg development and function, have been linked to impaired Treg function in SLE patients. FoxP3 induces the expression of IL-2, CD25, CTLA-4, and miR-155 in Tregs (18). GDF7, a secreted ligand of the TGF-β superfamily of proteins, exhibited positive regulatory effects on Tregs via increasing the expression of FoxP3 and CTLA4. Recent research showed that the downregulation of GDF7 in CD4+ T cells could lead to impaired suppressive functions of lupus Tregs (17, 19). Natural CD4+ Tregs are rendered unstable by loss of FoxP3 expression, which can ultimately lead to autoimmunity. FoxP3 mutation has been reported as a rare X-linked recessive (IPEX) syndrome with aggressive autoimmunity with the clinical feature of immune dysregulation, polyendocrinopathy, and enteropathy in humans (9, 20). Epigenetic modifications, which can be influenced by both genetic and environmental factors, also impact T-cell function in SLE. The interaction between genetics, T cell abnormalities, and environmental factors also plays a critical role in in the onset and exacerbation of the disease (16).
Natural Tregs-mediated immune regulation is mediated primarily by cell contact and suppressive cytokines (Table 1A). The immunoregulatory mechanism of nTregs includes the secretion of inhibitory cytokines with IL-10, IL35, TGF-β, and cell-contact inhibition with perforin and granzyme B, and the competition of IL-2 with effector T cell growth, and suppression of DC function (8). The tolerance of DCs can be induced by Tregs through positive feedback loops to reinforce the suppressive environment, and CTLA-4 expressed by Tregs can inhibit the expression of CD80 and CD86 on DCs to down-regulate effector T cells via the costimulatory cell surface protein CD28. Tolerogenic DCs can express inhibitory molecules such as indoleamine 2,3-dioxygenase (IDO) and retinoic acid, to improve Treg survival and proliferation to suppress autoimmunity (21).
Numerical and functional deficits of abnormalities in Treg subtypes have been reported to have a significant impact on the pathogenesis and outcome from patients with autoimmune disorders (22–26). Elucidation of the roles of various subsets of Treg (Table 1) dedicated to immune balance will provide a novel therapeutic approach that governs immune tolerance for SLE remission (27, 28). However, no definitive phenotypic markers or functions have been recognized to distinguish between these Treg subtype populations in SLE. In this mini-review, we summarize the current literature on the function and diversity of Treg subsets, focusing mainly on CD4+ Tregs, CD8+ Tregs, B regulatory cells, and type 1 regulatory T cells (Tr1), which provide immunotherapeutic modulation of SLE (Table 2). Furthermore, we discuss the clinical trials and therapeutic potential of regulatory cell-based therapies and lower-dose IL-2 therapy to restore immune tolerance by Tregs manipulation in patients with SLE.
Table 2 Characteristics and main findings of regulatory T cell subsets and regulatory B cells in systemic lupus erythematosus.
Controversial results were reported whether decreased and increased Tregs cells frequencies were reported in SLE. This discrepancy may arise from the difference in phenotypic analyses and the composition of study cohorts. Control groups in clinical trials are made up of patients who receive a standardized treatment or placebo. The baseline characteristics and ongoing treatments of these patients can affect Treg populations. For example, high-dose glucocorticoid therapy has been found to increase the percentage of circulating Tregs in active lupus patients (61). Although circulating CD4+Treg levels have concluded inconsistent results, there is increasing evidence to specify that the numbers of CD4+Tregs were substantially reduced in patients with lupus (Table 2A). A meta-analysis showed that a lower percentage of Tregs/CD4+T cells was observed in patients with active lupus compared to those with inactive SLE (31). A recent meta-analysis showed that SLE patients had a decreased Treg/PBMC ratio, which Treg is defined by CD4+CD25+, CD4+FoxP3+ and CD4+CD25+FoxP3+ compared to healthy subjects. However, no significant differences were found between the percentage of CD4+Tregs and the activity of SLE disease due to the sample size or inclusion criteria (29). Another meta-analysis showed that patients with active SLE have a decreased absolute number of CD4+Tregs and the results were not affected by the gating strategies (30). However, considering that most patients with lupus received glucocorticoid, CD4+Treg has been reported to be unaffected by drug therapy (62). Furthermore, lupus patients have an increased rate of CD4+ Tregs apoptosis and a decrease in total Tregs count, and CD4+Tregs apoptosis which was positively associated with lupus disease activity (63).
Th17 cells play a critical role in the pathogenesis of SLE and several evidences have confirmed that refining the balance of CD4+Treg/Th17 cells could benefit from reducing disease activity (32, 64). The disturbance between Th17 and CD4+Treg proliferation was highlighted by an imbalance in FoxP3 and RORγt gene expression in the development of SLE (6). Dysregulated Th17/CD4+ Treg cell balance was associated with miR-19b gene expression. Exosomes from human umbilical cord blood-derived mesenchymal stem cells could regulate the expression of inflammatory factors through in vitro miR-19b/KLF13 experiments in patients with lupus (32) (Table 2A). In lupus murine models, CD4+Foxp3+ Treg cells reduced the suppressive capacity against T cell proliferation, and adoptive transfer of Treg cells leads to lupus disease suppression and remission (33). Administration of recombinant IL-2 could restore the imbalance of effector T cells and proliferation of Treg cells (34, 35) (Table 2A).
The T follicular helper cells (TFh), which are defined by a phenotype of Bcl6+CXCR5highPD-1high, in the germinal center had the ability to stimulate the follicular B cells for the production of high-affinity autoantibodies. Several studies discovered an increase in the number of peripheral TFh cells in lupus patients, which is positively correlated with disease activity and autoantibody titers (65). Circulating lymphoid tissue follicular Treg cells (TFr), which expressed PD-1, ICOS, CD25 and CXCR5, can suppress TFh cell activity by inhibiting IL-10, IL-35, and TGF-β cytokine production (66). An increase in circulating TFr cell numbers was observed with successful treatments in patients with SLE, suggesting that restoring TFr cells may provide new therapeutic methods in SLE (38) (Table 2B). In lupus murine models, the percentage of Tfh cells increased and the Tfr (CD4+CXCR5+FoxP3+) cells decreased in 16-week-old MRL/lpr mice (39) (Table 2B).
The disruption of CD4+Treg cell homeostasis triggered by the lack of IL-2 is a critical event in the pathogenesis of SLE (67). The number and function of CD4+Treg can be controlled by the IL-2/STAT5 signaling pathway with sustained expression of FoxP3. IL-2 has also been reported to increase TFr proliferation which is dependent on the STAT5/Blimp-1 dependent mechanism (68). Impaired CD4+CXCR5+CD45RA-FoxP3high TFr cells with defective IL-2 production could be found in patients with SLE (69). Furthermore, T cells from SLE patients exhibit abnormal microRNAs (for example, miR-200a-3p) and enzymatic activity of protein phosphatase 2A (PP2A), resulting in downregulation of IL-2 by inactivation of the phosphorylated cAMP response element-binding protein (pCREB) (16, 70).
Cytotoxic Tregs are heterogeneous in periphery circulation and retain the ability to destroy T cell activation and proliferation to ensure immune homeostasis (71). CD8+ FoxP3+ Treg cells were first recognized in human tonsils, and CD8+ Treg cell subsets based on CD25+ expression may share similar suppressive capacity typically associated with CD4+ Treg (28, 71, 72). Isolated human CD8+ Tregs frequently express several cell surface molecules that include CD8, CD103, CD25, CD39, CD28, ICOS, CD122, CD39, CTLA-4, CXCR3, 4-1BB and lymphocyte activation gene 3 (LAG-3) (73). Suppressive CD8+FoxP3+ Treg cells could decrease the proliferation of CD4+ effector T cells through the above mechanism, including cell-cell contact lysis through the granzyme/perforin, Fas/FasL signaling pathways and secreting inhibitory cytokines, and deprivation effector T cell growth by metabolic competition of IL-2 (28, 71, 74–76) (Table 1B).
Loss of homeostatic balance is observed in lupus patients and restored human CD8+ Treg cells can be vital for the development of therapies in SLE (77). A decrease in circulating CD8+ FoxP3+ Treg cells has been observed in SLE patients, and autologous hemopoietic stem cell transplantation could increase CD8+Treg cells, which are related to better control (42). High-dose methylprednisolone therapy could increase circulating CD8+CD25+FoxP3+Tregs and decrease disease activity in patients with lupus nephritis (40). All-trans retinoic acid has been shown to increase the CD4+ and CD8+ regulatory T Cells, which have therapeutic potential in the treatment of SLE and other autoimmune diseases (78) Furthermore, a nucleosomal histone peptide could improve human CD8+CD25+FoxP3+ T cells to decrease the production of pathogenic autoantibody via TGF-β/ALK-5/pSmad 2/3 signaling pathway (41) (Table 2C).
Defective CD8+ Treg cells and function were found in murine lupus nephritis models (28), and adaptive transfer of suppressive CD8+CD103+CD39+ Tregs could inhibit chronic graft versus host disease in murine lupus nephritis model (43). Transfection plasmid DNA vectors encoding epitopes activated CD8+ cytotoxic T lymphocytes (CTL) to kill autoantibody-producing B cells and ameliorated the severity of lupus (44). Immune tolerance induced by anti-DNA-based peptides (pConsensus, pCons peptide) could improve human CD8+ Tregs to decrease proliferation of naïve CD4+ T cells and B cells (73). The suppressive properties of CD8+ Tregs could be manipulated by administering pCons peptides to suppress lupus nephritis with anti-dsDNA autoantibodies in the lupus murine model (NZB x NZW F1) (45, 46) (Table 2C).
SLE is associated with abnormal activation of B lymphocytes and excessive autoantibodies production. Regulatory B (Breg) cells have an important role in the maintenance of immune tolerance and attenuating systemic inflammation (47). Human Bregs are generally recognized to have a phenotype of CD24highCD27+ or CD19+CD24highCD38high (52, 53). Human B10 cells, which constitute approximately 25% of B cells in the blood, are activated by the B-cell receptor (BCR) of progenitor B10 cells (79, 80). B10 cells as a functional Bregs subtype have a negative immunoregulatory function, and CD40 and CD40L signaling, apoptotic cells, and TLR signaling were able to increase the number of B10 cells (53, 80, 81). pDCs can induce Breg differentiation via an IFN-r-dependent pattern by secreting IL-10 and IL-35 (49, 51) (Table 1C).
Emerging evidence has revealed that Breg numerical deficiency and/or dysfunction play a critical role in the pathogenesis of lupus and other autoimmune diseases (47, 82, 83). Identification and characterization of Bregs facilitate new approaches to targeting pathogenic B cells in patients with SLE (84) (Table 2D). Impaired regulatory B cells producing granzyme B were found in patients with SLE (82). Patients with lupus nephritis had a significantly decreased in circulating IL-10+ Bregs levels compared to health controls (51). Patients with new-onset lupus nephritis had a decrease in CD19+CD5+CD1dhighIL-10+ Bregs and restored Breg deficiency in patients who respond to immunosuppressive medications (48). The percentage of peripheral CD19+CD24highCD27+ B cells with IL-10 and IL-35 production was low in patients with new-onset SLE (47, 52). Granzyme B-producing Bregs, which play a negative regulatory role in immunity, and Granzyme B-producing B cell frequencies decreased in patients with SLE (82). During CD40 stimulation, decreased CD19+CD24highCD38high B cells with IL-10 production and inhibitory capacity was observed in SLE patients (85). pDCs initiative the differentiation of CD19+CD24high CD38high (immature) B cells into IL-10-producing CD24+CD38high Breg cells. Defective pDC-mediated expansion of CD24+CD38high Breg cells was associated with decreased activation of the STAT1 and STAT3 signaling pathway in patients with SLE. STAT1-STAT3 activation between pDC and CD24+CD38high Breg cell interaction was restored in SLE patients responding to rituximab, a chimeric monoclonal antibody targeted against the CD20 marker (50).
Bregs and T cells can influence each other on the functions through direct cell-cell interactions and the production of cytokines. Dysfunctional Bregs may provide inadequate regulatory signals to T cells, leading to unchecked pro-inflammatory responses. Bregs exert profound impacts on the differentiation, function, and distribution of Tfh cells in the immune microenvironment. Human Breg cells control IL-21 receptor expressions in Tfh cell maturation, and inhibit Tfh cell–mediated antibody secretion through IL-10 and TGF-β (86). Human CD19+CD25high Bregs can inhibit the expansion and function of autologous CD4+ T cells, and promote Treg differentiation and activity by secreting IL-10 (87). The IL-10-independent regulatory mechanisms of Bregs to inhibit effector T cell differentiation are primarily mediated by IL-35, TGF-β, and granzyme B (88). Programmed death-ligand 1 high (PD-L1hi) Bregs inhibit Tfh cell expansion through elevated expression of PD-L1 (89). Furthermore, specific IgG4 antibodies produced by human CD73−CD25+CD71+IL-10-producing regulatory B cells could suppress antigen-specific CD4+ T cell proliferation (90). Enhancing Breg function or adoptive transfer of Bregs in animal models of SLE has shown promising results in reducing disease severity and ameliorating T-cell abnormalities. The development and function of Bregs are likely to be regulated by Tfh cells, and the percentage of B10 cells in the spleens had a positive correlation with Tfh cell-derived IL-21 from a MRL/lpr mice model (54). IL-17 deficiency led to an increased number of CD19+IL-10+Breg in the spleen of a murine model of lupus (55). The gut microbiota DNA has been shown to expand the Bregs in a murine lupus model (56) (Table 2D). Collectively, all these data support the fact that Breg cells are critical in T cells homoeostasis to ensure central tolerance and prevent autoantibodies production, and may have implications for the regulation of autoimmune diseases.
Periphery Tregs developed in conventional CD4+ T cells after stimulation with tolerogenic antigens are termed iTregs (58, 60, 91, 92). IL-10 producing T regulatory type 1 (Tr1) cells are induced in peripheral CD4+ T cells after antigen through the secretion of IL-10 and TGF-β (91, 92) (Table 1D). Tr1 cells secreting IL-10 are important in the regulation of immune tolerance to reduce tissue damage in SLE (58, 60). The aryl hydrocarbon receptor (AHR) and Blimp-1 are mandatory for Tr1 differentiation with IL-10 production (91, 93). IL-10R signals through the STAT3 and p38/MAPK signaling pathway will result in expansion of peripheral blood Tr1 cells (94). Furthermore, tolerogenic DCs producing IL-10 (DC-10) isolated from human peripheral blood are potent inducers of adaptive Tr1 cells through the IL-10–dependent ILT4/HLA-G signaling pathway (91, 95).
Tr1-like cells play a crucial role in suppressing antibody-driven immune responses. The function with inhibition of IgG production from Tr1-like cells was deficiency in SLE patients (57) (Table 2E). CD46, the complement regulatory protein, will be expressed in activated immune cells to prevent autologous complement-mediated lysis of cells by binding to complement components C3b and C4b in an inflammatory situation (59, 96). The uncontrolled immune response of Th1 contributes to a wide range of lupus, and CD46 activation has emerged as a powerful controller of T cell-mediated immunity to contraction of Th1 immunity (59). Activation of CD46 proteins with IL-2 in activated CD4+ T cells will enhance the secretion of IL-10 and granzyme B, a phenotype shared with Tr1 cells (60, 96, 97) (Table 1E). Costimulation by CD3/CD46 proteins leads to IL-2 dependent Tr1-like cells with high IL-10 and low IFN-γ level production, which is associated with disease activity in lupus patients (59, 98). Furthermore, we observed that the functional defect of IL-10+ Tr1-like cells in pediatric patients with lupus nephritis can be restored by corticosteroid treatment (58) (Table 2F).
Regulatory cell-based therapy can restore the homeostatic balance in the immune system (3, 99, 100). A variety of target regulatory cell-based therapies are currently being explored for SLE (Table 3). Conventional immunotherapy for SLE is mainly based on corticosteroids or/and immunosuppressive therapy (66, 77, 108). Restoration and maintenance of the Treg cell population have the therapeutic potential to improve immune tolerance by manipulating suppressive Tregs in patients with SLE (109–111). High-dose corticosteroid pulse therapy has been shown to induce monocytes to produce TGF-β and improve Treg differentiation. Furthermore, steroids play a role in activating Tregs through the miR-342-3p-mTOR complex 2 axis (22, 40, 101, 112) (Table 3A). Furthermore, antigen-specific Tregs can be triggered and expanded by administering autologous tolerogenic DCs with autoantigenic peptides and can be considered as a type of Treg therapies targeted against autoimmunity (22, 27) (Table 3B).
Several studies have also investigated the mammalian target-of-rapamycin (mTOR) signaling pathway in Tregs and its impact on SLE pathogenesis (102). Rapamycin alone or in combination with all-trans retinoic acid reduced disease activity and the glucocorticoid requirement, which can regulate the balance of Th17/Treg cells (102, 103) (Table 3C). The tolerance of histone peptide was shown to be a novel therapy suitable for the treatment of lupus nephritis and restored Th17/Treg cell balance (64). Potent Treg cells are activated by histone peptide epitopes in vitro from patients with lupus, which can inhibit interferon and anti-dsDNA autoantibody production (41, 73, 104) (Table 3D). Treg therapy can be further enhanced by administering antigen-specific nanoparticles, or engineering Treg cells to be autoantigen-specific T cell receptor (TCR) or chimeric antigen receptor (CAR)-specific Tregs (22, 105) (Table 3E).
CD25 is persistently expressed in CD4+ FoxP3+ Tregs, when IL-2 activated CD25 forming high affinity IL-2R to compete for available IL-2 in the circulation (113). The decrease in IL-2 level was found to be associated with a depleted Treg cell population, and its reversibility by IL-2 therapy provides important reasons for the treatment of lupus (114, 115). High doses administration of recombinant human IL-2 (rIL-2) can aid conventional T cells and naïve CD8+ T cells that produce perforin, granzymes, IL-5, IL-13, and IFN-γ (116). However, low-dose rIL-2 management preferentially promoted Treg cell-mediated beneficial activities in disease manifestations (105, 106).
The benefit of low-dose IL-2 therapy was associated with restored Tfr/Tfh cell balance. In CD4+ T cells, IL-2 can directly activate Blimp-1 and suppress Bcl-6 to antagonize Tfh cell differentiation (106). Furthermore, IL-2 therapy also directly prevents the expression of RORγt, thereby repressing Th17 development, which plays a vital role in the development of autoimmune disorders (107) (Table 3F). Tfr cells from SLE patients had reduced suppressive function. PD-1+ Tfr cells in SLE were positively correlated with disease activity and anti-DNA antibody levels. With in vitro low-dose IL-2 stimulation, the expression of PD-1 in Tfr cells decreased significantly, together with the increased expression of CTLA-4 and FoxP3 (36). Low-dose IL-2 therapy increases the circulating Tfr/Tfh ratio, which was accompanied by reduced anti-dsDNA titers and improved kidney damage in mice and lupus patients (117). A randomized cohort study showed that patients with lupus who received prolonged low-dose IL-2 therapy recoveed the immune balance Tfr/Tfh in lupus (65, 118).
Humrich et al. (119) first reported that a 36-year-old patient with SLE who received human IL-2 recombinant (aldesleukin) 1.5 or 3.0 million IU subcutaneous injections daily for five days can improve arthritis and the increase of CD25+FoxP3+CD127lo Treg cells. Clinical and preclinical studies demonstrate that low-dose rIL-2 can be administered safely to humans and the potential benefits in the induction of remission in active lupus patients as shown in Table 4. The low-dose recombinant human rIL-2 regimens contain 3-4 cycles of 7-10 million IU and are separated by rest periods of 9-16 days (37, 118, 120–124). Recent two randomized trials have shown that low-dose IL-2 therapy can restore the Treg population in active lupus patients. A randomized, double-blind, placebo-controlled clinical trial was designed to treat active lupus patients who received IL-2 at a dose of 1 million IU or placebo with standard treatment for 3 months and were followed for another 3 months. In the IL-2 group, the SRI-4 response rates were 55.17% compared to 30.00% in the control group. Although the SRI-4 response did not achieve a significant difference at 3 months, the IL-2 group had significantly high SRI-4 response rate after 6 months follow-up. In the IL-2 group, patients with lupus nephritis had a complete remission rate of 53.85% compared to 16.67% in the placebo group. No obvious systemic side effect was observed in the IL-2 group. Low-dose IL-2 therapy can expand regulatory T cells and also maintain cellular immunity with improved natural killer cells (118). An international, multicenter, double-blind, randomized, placebo-controlled phase II clinical trial (LUPIL-2) to enroll hundred lupus patients with moderate to severe activity. The patients were randomly assigned 1:1 to receive 1.5 million IU/day of subcutaneous IL-2 (ILT-101) or placebo for five days and followed by weekly injections of 3 months. In the intention-to-treat population, the SRI-4 response was not met (ILT-101: 68% vs. placebo: 58%; P =0.34), due to a 100% SRI-4 response rate at two sites in the control group. After excluded patients from these two sites, the SRI-4 response rate showed a statistically significant difference (ILT-101: 83.3% vs placebo: 51.7%; P =0.02). ILT-101 was tolerated without systemic side effects and there was no detection of antidrug antibodies. Robust and sustained increases in the numbers and frequencies of the highly suppressive CD4+FoxP3+CD127loCD25hi Treg subset were evident in the IL-2 group. Assessing the characteristics of control groups and the changes of Treg cells could provide a more comprehensive understanding of the observed therapeutic effects. To compare the Treg responses between clinical responders and non-responders of the ILT-101 group, a significant increase in Treg numbers was detectable in the responding patients, suggesting that the clinical outcome is associated with the increased Treg response (120). These results provide strong support for further testing of lower-dose IL-2 therapy in patients with SLE.
There are new modified forms of IL-2 which exhibit an extended half-life or a higher affinity for trimeric IL-2R in clinical trials (125). IL-2 mutein NKTR-358 with an extended half-life between 7 and 13 days had clinical effects on skin lupus and increased the number and percentage of CD25hi Tregs in a randomized, placebo-controlled phase 1 trial (121). Low-dose IL-2 therapy has been associated with improved lupus disease activity, reduced autoantibody production, and the steroid-sparing effect. The ongoing research is focuses on further understanding the mechanism of low-dose IL-2 therapy, optimizing dosing protocols, and identifying specific patient subgroups that may benefit the most from this treatment approach. Furthermore, long-term safety and efficacy data from large-scale clinical trials are needed to establish the role of low-dose IL-2 therapy as an alternative regimen for SLE (118). In summary, clinical studies have consistently demonstrated that low-dose IL-2 therapy in SLE is well tolerated, and capable of increasing suppressive Treg population that appears to provide complementary immunomodulatory effects on the treatment of SLE.
Elucidation of the roles of various subsets of Treg dedicated to immune homeostasis and the restoration of the balance between Tregs and autoreactive T cells will provide a novel therapeutic potential that governs immune tolerance for the remission of SLE. Although more data on the manipulation and propagation of Tregs subsets and their therapeutic application are still needed, promising clinical responses were observed in patients with SLE who received low-dose IL-2 therapy to balancing Th17 versus Tregs. Future research is warranted to decipher new immunotherapeutics of the balance between Th1/Th17 overactivation and the development of nTregs and peripheral Tregs in SLE.
Y-GT: Conceptualization, literature search, original draft preparation, visualization, editing. P-FL and H-MW: critical review, editing and commentary. K-HH: critical review, editing, commentary and funding acquisition. C-YL: supervision, critical review, editing commentary and funding acquisition. KY: supervision, conceptualization, critical review, editing, commentary, and funding acquisition. All authors contributed to the article and approved the submitted version.
This study was supported by the Ministry of Science and Technology (grant number MOST 111-2314-B-371-006, NSTC 112-2314-B-371-006) in Taiwan, Changhua Christian Hospital (112-CCH-IRP-022; 112-CCH-ICO-152; 111-CCH-IRP-018; 110-CCH-IRP-030, 110-CCH-ICO-152), and Academia Sinica, Taiwan (AS-SS-111-02-1).
The authors declare that the research was conducted in the absence of any commercial or financial relationships that could be construed as a potential conflict of interest.
All claims expressed in this article are solely those of the authors and do not necessarily represent those of their affiliated organizations, or those of the publisher, the editors and the reviewers. Any product that may be evaluated in this article, or claim that may be made by its manufacturer, is not guaranteed or endorsed by the publisher.
1. Alforaih N, Whittall-Garcia L, Touma Z. A review of lupus nephritis. J Appl Lab Med (2022) 7(6):1450–67. doi: 10.1093/jalm/jfac036
2. Cui M, Wang C, Shen Q, Ren H, Li L, Li S, et al. Integrative analysis of omics summary data reveals putative mechanisms linked to different cell populations in systemic lupus erythematosus. Genomics (2022) 114(4):110435. doi: 10.1016/j.ygeno.2022.110435
3. Bradford HF, Haljasmagi L, Menon M, McDonnell TCR, Sarekannu K, Vanker M, et al. Inactive disease in patients with lupus is linked to autoantibodies to type I interferons that norMalize blood IFNalpha and B cell subsets. Cell Rep Med (2023) 4(1):100894. doi: 10.1016/j.xcrm.2022.100894
4. Infante B, Mercuri S, Dello Strologo A, Franzin R, Catalano V, Troise D, et al. Unraveling the link between interferon-alpha and systemic lupus erythematosus: From the molecular mechanisms to target therapies. Int J Mol Sci (2022) 23(24). doi: 10.3390/ijms232415998
5. Sieber J, Daridon C, Fleischer SJ, Fleischer V, Hiepe F, Alexander T, et al. Active systemic lupus erythematosus is associated with a reduced cytokine production by B cells in response to TLR9 stimulation. Arthritis Res Ther (2014) 16(6):477. doi: 10.1186/s13075-014-0477-1
6. Ali HN, Alubaidi GT, Gorial FI, Jasim IA. Disturbance in serum levels of IL-17 and TGF-beta1 and in gene expression of ROR-gammat and FOX-P3 is associated with pathogenicity of systematic lupus erythematosus. Prague Med Rep (2022) 123(3):166–80. doi: 10.14712/23362936.2022.15
7. Tenbrock K, Rauen T. T cell dysregulation in SLE. Clin Immunol (2022) 239:109031. doi: 10.1016/j.clim.2022.109031
8. Skartsis N, Muller YD, Ferreira LMR. Regulatory T cell homeostasis: Requisite signals and implications for clinical development of biologics. Clin Immunol (2023) 246:109201. doi: 10.1016/j.clim.2022.109201
9. Li HT, Zhang SX, Zhang JQ, Cheng T, Liu Y, Liu HQ, et al. A decreased number of circulating regulatory T cells is associated with adverse pregnancy outcomes in patients with systemic lupus erythematosus. Immun Inflammation Dis (2022) 10(12):e731. doi: 10.1002/iid3.731
10. Giang S, La Cava A. Regulatory T cells in SLE: Biology and use in treatment. Curr Rheumatol Rep (2016) 18(11):67. doi: 10.1007/s11926-016-0616-6
11. Jeffery HC, Braitch MK, Brown S, Oo YH. Clinical potential of regulatory T cell therapy in liver diseases: An overview and current perspectives. Front Immunol (2016) 7:334. doi: 10.3389/fimmu.2016.00334
12. Tao JH, Cheng M, Tang JP, Liu Q, Pan F, Li XP. Foxp3, regulatory T cell, and autoimmune diseases. Inflammation (2017) 40(1):328–39. doi: 10.1007/s10753-016-0470-8
13. Hatzioannou A, Boumpas A, Papadopoulou M, Papafragkos I, Varveri A, Alissafi T, et al. Regulatory T cells in autoimmunity and cancer: A duplicitous lifestyle. Front Immunol (2021) 12:731947. doi: 10.3389/fimmu.2021.731947
14. La Cava A. Tregs in SLE: an update. Curr Rheumatol Rep (2018) 20(2):6. doi: 10.1007/s11926-018-0714-8
15. Zhao Z, Zhang X, Su L, Xu L, Zheng Y, Sun J. Fine tuning subsets of CD4(+) T cells by low-dosage of IL-2 and a new therapeutic strategy for autoimmune diseases. Int Immunopharmacol (2018) 56:269–76. doi: 10.1016/j.intimp.2018.01.042
16. Yin X, Kim K, Suetsugu H, Bang SY, Wen L, Koido M, et al. Biological insights into systemic lupus erythematosus through an immune cell-specific transcriptome-wide association study. Ann Rheum Dis (2022) 81(9):1273–80. doi: 10.1136/annrheumdis-2022-222345
17. Li Z, Wang Z, Sun T, Liu S, Ding S, Sun L. Identifying key genes in CD4(+) T cells of systemic lupus erythematosus by integrated bioinformatics analysis. Front Genet (2022) 13:941221. doi: 10.3389/fgene.2022.941221
18. Corte-Real BF, Arroyo Hornero R, Dyczko A, Hamad I, Kleinewietfeld M. Dissecting the role of CSF2RB expression in human regulatory T cells. Front Immunol (2022) 13:1005965. doi: 10.3389/fimmu.2022.1005965
19. Ding M, Malhotra R, Ottosson T, Lundqvist M, Mebrahtu A, Brengdahl J, et al. Secretome screening reveals immunomodulating functions of IFNalpha-7, PAP and GDF-7 on regulatory T-cells. Sci Rep (2021) 11(1):16767.
20. Roach T, Morel L. Genetic variations controlling regulatory T cell development and activity in mouse models of lupus-like autoimmunity. Front Immunol (2022) 13:887489. doi: 10.3389/fimmu.2022.887489
21. Scheinecker C, Goschl L, Bonelli M. Treg cells in health and autoimmune diseases: New insights from single cell analysis. J Autoimmun (2020) 110:102376. doi: 10.1016/j.jaut.2019.102376
22. Doglio M, Alexander T, Del Papa N, Snowden JA, Greco R, Autoimmune Diseases Working Party of the European Society for B, et al. New insights in systemic lupus erythematosus: From regulatory T cells to CAR-T-cell strategies. J Allergy Clin Immunol (2022) 150(6):1289–301. doi: 10.1016/j.jaci.2022.08.003
23. Wang X, Zhang Q, Luo S, Zhang H, Lu Q, Long H. Advances in therapeutic targets-related study on systemic lupus erythematosus. Zhong Nan Da Xue Xue Bao Yi Xue Ban (2021) 46(11):1267–75. doi: 10.11817/j.issn.1672-7347.2021.200056
24. Mizui M, Tsokos GC. Targeting regulatory T cells to treat patients with systemic lupus erythematosus. Front Immunol (2018) 9:786. doi: 10.3389/fimmu.2018.00786
25. Ghali JR, Wang YM, Holdsworth SR, Kitching AR. Regulatory T cells in immune-mediated renal disease. Nephrol (Carlton) (2016) 21(2):86–96. doi: 10.1111/nep.12574
26. Kaneko M, Jackson SW. Recent advances in immunotherapies for lupus nephritis. Pediatr Nephrol (2023) 38(4):1001–12. doi: 10.1007/s00467-022-05670-7
27. Ghobadinezhad F, Ebrahimi N, Mozaffari F, Moradi N, Beiranvand S, Pournazari M, et al. The emerging role of regulatory cell-based therapy in autoimmune disease. Front Immunol (2022) 13:1075813. doi: 10.3389/fimmu.2022.1075813
28. Singh RP, Bischoff DS, Hahn BH. CD8(+) T regulatory cells in lupus. Rheumatol Immunol Res (2021) 2(3):147–56. doi: 10.2478/rir-2021-0021
29. Zhu Y, Huang Y, Ming B, Wu X, Chen Y, Dong L. Regulatory T-cell levels in systemic lupus erythematosus patients: a meta-analysis. Lupus (2019) 28(4):445–54. doi: 10.1177/0961203319828530
30. Li W, Deng C, Yang H, Wang G. The regulatory T cell in active systemic lupus erythematosus patients: A systemic review and meta-analysis. Front Immunol (2019) 10:159. doi: 10.3389/fimmu.2019.00159
31. Zhang SX, Ma XW, Li YF, Lai NL, Huang ZH, Fan K, et al. The proportion of regulatory T cells in patients with systemic lupus erythematosus: A meta-analysis. J Immunol Res 2018 (2018) p:7103219. doi: 10.1155/2018/7103219
32. Tu J, Zheng N, Mao C, Liu S, Zhang H, Sun L. UC-BSCs Exosomes Regulate Th17/Treg Balance in Patients with Systemic Lupus Erythematosus via miR-19b/KLF13. Cells (2022) 11(24). doi: 10.3390/cells11244123
33. Divekar AA, Dubey S, Gangalum PR, Singh RR. Dicer insufficiency and microRNA-155 overexpression in lupus regulatory T cells: an apparent paradox in the setting of an inflammatory milieu. J Immunol (2011) 186(2):924–30. doi: 10.4049/jimmunol.1002218
34. Scalapino KJ, Tang Q, Bluestone JA, Bonyhadi ML, Daikh DI. Suppression of disease in New Zealand Black/New Zealand White lupus-prone mice by adoptive transfer of ex vivo expanded regulatory T cells. J Immunol (2006) 177(3):1451–9. doi: 10.4049/jimmunol.177.3.1451
35. Humrich JY, Morbach H, Undeutsch R, Enghard P, Rosenberger S, Weigert O, et al. Homeostatic imbalance of regulatory and effector T cells due to IL-2 deprivation amplifies murine lupus. Proc Natl Acad Sci U.S.A. (2010) 107(1):204–9. doi: 10.1073/pnas.0903158107
36. Kurata I, Mikami N, Ohyama A, Osada A, Kondo Y, Tsuboi H, et al. Impaired function of PD-1(+) follicular regulatory T cells in systemic lupus erythematosus. Clin Exp Immunol (2021) 206(1):28–35. doi: 10.1111/cei.13643
37. Hao H, Nakayamada S, Yamagata K, Ohkubo N, Iwata S, Inoue Y, et al. Conversion of T follicular helper cells to T follicular regulatory cells by interleukin-2 through transcriptional regulation in systemic lupus erythematosus. Arthritis Rheumatol (2021) 73(1):132–42. doi: 10.1002/art.41457
38. Xu B, Wang S, Zhou M, Huang Y, Fu R, Guo C, et al. The ratio of circulating follicular T helper cell to follicular T regulatory cell is correlated with disease activity in systemic lupus erythematosus. Clin Immunol (2017) 183:46–53. doi: 10.1016/j.clim.2017.07.004
39. Yang J, Yang X, Yang J, Li M. Baicalin ameliorates lupus autoimmunity by inhibiting differentiation of Tfh cells and inducing expansion of Tfr cells. Cell Death Dis (2019) 10(2):140. doi: 10.1038/s41419-019-1315-9
40. Tsai YG, Lee CY, Lin TY, Lin CY. CD8(+) Treg cells associated with decreasing disease activity after intravenous methylprednisolone pulse therapy in lupus nephritis with heavy proteinuria. PloS One (2014) 9(1):e81344. doi: 10.1371/journal.pone.0081344
41. Zhang L, Bertucci AM, Ramsey-Goldman R, Harsha-Strong ER, Burt RK, Datta SK. Major pathogenic steps in human lupus can be effectively suppressed by nucleosomal histone peptide epitope-induced regulatory immunity. Clin Immunol (2013) 149(3):365–78. doi: 10.1016/j.clim.2013.08.008
42. Zhang L, Bertucci AM, Ramsey-Goldman R, Burt RK, Datta SK. Regulatory T cell (Treg) subsets return in patients with refractory lupus following stem cell transplantation, and TGF-beta-producing CD8+ Treg cells are associated with immunological remission of lupus. J Immunol (2009) 183(10):6346–58. doi: 10.4049/jimmunol.0901773
43. Zhang X, Ouyang X, Xu Z, Chen J, Huang Q, Liu Y, et al. CD8+CD103+ iTregs inhibit chronic graft-versus-host disease with lupus nephritis by the increased expression of CD39. Mol Ther (2019) 27(11):1963–73. doi: 10.1016/j.ymthe.2019.07.014
44. Fan GC, Singh RR. Vaccination with minigenes encoding V(H)-derived major histocompatibility complex class I-binding epitopes activates cytotoxic T cells that ablate autoantibody-producing B cells and inhibit lupus. J Exp Med (2002) 196(6):731–41. doi: 10.1084/jem.20020223
45. Singh RP, La Cava A, Hahn BH. pConsensus peptide induces tolerogenic CD8+ T cells in lupus-prone (NZB x NZW)F1 mice by differentially regulating Foxp3 and PD1 molecules. J Immunol (2008) 180(4):2069–80. doi: 10.4049/jimmunol.180.4.2069
46. Singh RP, La Cava A, Wong M, Ebling F, Hahn BH. CD8+ T cell-mediated suppression of autoimmunity in a murine lupus model of peptide-induced immune tolerance depends on Foxp3 expression. J Immunol (2007) 178(12):7649–57. doi: 10.4049/jimmunol.178.12.7649
47. Xiong H, Tang Z, Xu Y, Shi Z, Guo Z, Liu X, et al. CD19(+)CD24(high)CD27(+) B cell and interleukin 35 as potential biomarkers of disease activity in systemic lupus erythematosus patients. Adv Rheumatol (2022) 62(1):48.
48. Girimaji N, Kaundal U, Rathi M, Sharma A, Nada R, Ramachandran R, et al. Regulatory B and T cells and their association with clinical response in new-onset lupus nephritis patients. Kidney Int Rep (2020) 5(7):1081–6. doi: 10.1016/j.ekir.2020.04.019
49. Ye Z, Jiang Y, Sun D, Zhong W, Zhao L, Jiang Z. The plasma interleukin (IL)-35 level and frequency of circulating IL-35(+) regulatory B cells are decreased in a cohort of Chinese patients with new-onset systemic lupus erythematosus. Sci Rep (2019) 9(1):13210. doi: 10.1038/s41598-019-49748-z
50. Menon M, Blair PA, Isenberg DA, Mauri C. A regulatory feedback between plasmacytoid dendritic cells and regulatory B cells is aberrant in systemic lupus erythematosus. Immunity (2016) 44(3):683–97. doi: 10.1016/j.immuni.2016.02.012
51. Heinemann K, Wilde B, Hoerning A, Tebbe B, Kribben A, Witzke O, et al. Decreased IL-10(+) regulatory B cells (Bregs) in lupus nephritis patients. Scand J Rheumatol (2016) 45(4):312–6. doi: 10.3109/03009742.2015.1126346
52. Jin L, Weiqian C, Lihuan Y. Peripheral CD24hi CD27+ CD19+ B cells subset as a potential biomarker in naive systemic lupus erythematosus. Int J Rheum Dis (2013) 16(6):698–708. doi: 10.1111/1756-185X.12229
53. Blair PA, Norena LY, Flores-Borja F, Rawlings DJ, Isenberg DA, Ehrenstein MR, et al. CD19(+)CD24(hi)CD38(hi) B cells exhibit regulatory capacity in healthy individuals but are functionally impaired in systemic Lupus Erythematosus patients. Immunity (2010) 32(1):129–40. doi: 10.1016/j.immuni.2009.11.009
54. Yang X, Yang J, Chu Y, Wang J, Guan M, Zhu X, et al. T follicular helper cells mediate expansion of regulatory B cells via IL-21 in Lupus-prone MRL/lpr mice. PloS One (2013) 8(4):e62855. doi: 10.1371/journal.pone.0062855
55. Lee SY, Lee SH, Seo HB, Ryu JG, Jung K, Choi JW, et al. Inhibition of IL-17 ameliorates systemic lupus erythematosus in Roquin(san/san) mice through regulating the balance of TFH cells, GC B cells, Treg and Breg. Sci Rep (2019) 9(1):5227. doi: 10.1038/s41598-019-41534-1
56. Mu Q, Edwards MR, Swartwout BK, Cabana Puig X, Mao J, Zhu J, et al. Gut microbiota and bacterial DNA suppress autoimmunity by stimulating regulatory B cells in a murine model of lupus. Front Immunol (2020) 11:593353. doi: 10.3389/fimmu.2020.593353
57. Facciotti F, Gagliani N, Haringer B, Alfen JS, Penatti A, Maglie S, et al. IL-10-producing forkhead box protein 3-negative regulatory T cells inhibit B-cell responses and are involved in systemic lupus erythematosus. J Allergy Clin Immunol (2016) 137(1):318–321 e5. doi: 10.1016/j.jaci.2015.06.044
58. Tsai YG, Chien JW, Chiu YM, Su TC, Chiu PF, Hsiao KH, et al. Lupus nephritis with corticosteroid responsiveness: molecular changes of CD46-mediated type 1 regulatory T cells. Pediatr Res (2022) 92(4):1099–107. doi: 10.1038/s41390-021-01882-z
59. Ellinghaus U, Cortini A, Pinder CL, Le Friec G, Kemper C, Vyse TJ. Dysregulated CD46 shedding interferes with Th1-contraction in systemic lupus erythematosus. Eur J Immunol (2017) 47(7):1200–10. doi: 10.1002/eji.201646822
60. Le Buanec H, Gougeon ML, Mathian A, Lebon P, Dupont JM, Peltre G, et al. IFN-alpha and CD46 stimulation are associated with active lupus and skew natural T regulatory cell differentiation to type 1 regulatory T (Tr1) cells. Proc Natl Acad Sci U.S.A. (2011) 108(47):18995–9000. doi: 10.1073/pnas.1113301108
61. Lee HY, Hong YK, Yun HJ, Kim YM, Kim JR, Yoo WH. Altered frequency and migration capacity of CD4+CD25+ regulatory T cells in systemic lupus erythematosus. Rheumatol (Oxford) (2008) 47(6):789–94. doi: 10.1093/rheumatology/ken108
62. Mellor-Pita S, Citores MJ, Castejon R, Tutor-Ureta P, Yebra-Bango M, Andreu JL, et al. Decrease of regulatory T cells in patients with systemic lupus erythematosus. Ann Rheum Dis (2006) 65(4):553–4. doi: 10.1136/ard.2005.044974
63. Li M, Luo L, Wu Y, Song Z, Ni B, Hao F, et al. Elevated apoptosis and abnormal apoptosis signaling of regulatory T cells in patients with systemic lupus erythematosus. Lupus (2022) 31(12):1441–55. doi: 10.1177/09612033221119455
64. Fu D, Senouthai S, Wang J, You Y. Vasoactive intestinal peptide ameliorates renal injury in a pristane-induced lupus mouse model by modulating Th17/Treg balance. BMC Nephrol (2019) 20(1):350. doi: 10.1186/s12882-019-1548-y
65. Miao M, Xiao X, Tian J, Zhufeng Y, Feng R, Zhang R, et al. Therapeutic potential of targeting Tfr/Tfh cell balance by low-dose-IL-2 in active SLE: a post hoc analysis from a double-blind RCT study. Arthritis Res Ther (2021) 23(1):167. doi: 10.1186/s13075-021-02535-6
66. Wei X, Zhang J, Zhou X. Ex-TFRs: A missing piece of the SLE puzzle? Front Immunol (2021) 12:662305. doi: 10.3389/fimmu.2021.662305
67. Ballesteros-Tato A, Papillion A. Mechanisms of action of low-dose IL-2 restoration therapies in SLE. Curr Opin Immunol (2019) 61:39–45. doi: 10.1016/j.coi.2019.07.003
68. Burchill MA, Yang J, Vogtenhuber C, Blazar BR, Farrar MA. IL-2 receptor beta-dependent STAT5 activation is required for the development of Foxp3+ regulatory T cells. J Immunol (2007) 178(1):280–90. doi: 10.4049/jimmunol.178.1.280
69. Hao H, Nakayamada S, Ohkubo N, Yamagata K, Zhang M, Shan Y, et al. Involvement of lncRNA IL21-AS1 in interleukin-2 and T follicular regulatory cell activation in systemic lupus erythematosus. Arthritis Res Ther (2021) 23(1):302. doi: 10.1186/s13075-021-02682-w
70. Tan W, Sunahori K, Zhao J, Deng Y, Kaufman KM, Kelly JA, et al. Association of PPP2CA polymorphisms with systemic lupus erythematosus susceptibility in multiple ethnic groups. Arthritis Rheum (2011) 63(9):2755–63. doi: 10.1002/art.30452
71. Dempsey LA. Human regulatory CD8(+) T cells. Nat Immunol (2022) 23(5):645. doi: 10.1038/s41590-022-01206-1
72. Tsai YG, Yang KD, Wen YS, Hung CH, Chien JW, Lin CY. Allergen-specific immunotherapy enhances CD8(+) CD25(+) CD137(+) regulatory T cells and decreases nasal nitric oxide. Pediatr Allergy Immunol (2019) 30(5):531–9. doi: 10.1111/pai.13061
73. Singh RP, Hahn BH, Bischoff DS. Cellular and molecular phenotypes of pConsensus peptide (pCons) induced CD8(+) and CD4(+) regulatory T cells in lupus. Front Immunol (2021) 12:718359. doi: 10.3389/fimmu.2021.718359
74. Liston A, Aloulou M. A fresh look at a neglected regulatory lineage: CD8(+)Foxp3(+) Regulatory T cells. Immunol Lett (2022) 247:22–6. doi: 10.1016/j.imlet.2022.05.004
75. Levescot A, Cerf-Bensussan N. Regulatory CD8(+) T cells suppress disease. Science (2022) 376(6590):243–4. doi: 10.1126/science.abp8243
76. Olson BM, Jankowska-Gan E, Becker JT, Vignali DA, Burlingham WJ, McNeel DG. Human prostate tumor antigen-specific CD8+ regulatory T cells are inhibited by CTLA-4 or IL-35 blockade. J Immunol (2012) 189(12):5590–601. doi: 10.4049/jimmunol.1201744
77. Horwitz DA, Bickerton S, La Cava A. Strategies to use nanoparticles to generate CD4 and CD8 regulatory T cells for the treatment of SLE and other autoimmune diseases. Front Immunol (2021) 12:681062. doi: 10.3389/fimmu.2021.681062
78. Ma J, Liu Y, Li Y, Gu J, Liu J, Tang J, et al. Differential role of all-trans retinoic acid in promoting the development of CD4+ and CD8+ regulatory T cells. J Leukoc Biol (2014) 95(2):275–83.
79. Chun W, Tian J, Zhang Y. Transplantation of mesenchymal stem cells ameliorates systemic lupus erythematosus and upregulates B10 cells through TGF-beta1. Stem Cell Res Ther (2021) 12(1):512. doi: 10.1186/s13287-021-02586-1
80. Wu H, Su Z, Barnie PA. The role of B regulatory (B10) cells in inflammatory disorders and their potential as therapeutic targets. Int Immunopharmacol (2020) 78:106111. doi: 10.1016/j.intimp.2019.106111
81. Mauri C. Novel frontiers in regulatory B cells. Immunol Rev (2021) 299(1):5–9. doi: 10.1111/imr.12964
82. Bai M, Xu L, Zhu H, Xue J, Liu T, Sun F, et al. Impaired granzyme B-producing regulatory B cells in systemic lupus erythematosus. Mol Immunol (2021) 140:217–24. doi: 10.1016/j.molimm.2021.09.012
83. Cencioni MT, Ali R, Nicholas R, Muraro PA. Defective CD19+CD24(hi)CD38(hi) transitional B-cell function in patients with relapsing-remitting MS. Mult Scler (2021) 27(8):1187–97. doi: 10.1177/1352458520951536
84. Szelinski F, Lino AC, Dorner T. B cells in systemic lupus erythematosus. Curr Opin Rheumatol (2022) 34(2):125–32. doi: 10.1097/BOR.0000000000000865
85. Oleinika K, Mauri C, Salama AD. Effector and regulatory B cells in immune-mediated kidney disease. Nat Rev Nephrol (2019) 15(1):11–26. doi: 10.1038/s41581-018-0074-7
86. Achour A, Simon Q, Mohr A, Seite JF, Youinou P, Bendaoud B, et al. Human regulatory B cells control the T(FH) cell response. J Allergy Clin Immunol (2017) 140(1):215–22. doi: 10.1016/j.jaci.2016.09.042
87. Hong M, Liao Y, Liang J, Chen X, Li S, Liu W, et al. Immunomodulation of human CD19(+)CD25(high) regulatory B cells via Th17/Foxp3 regulatory T cells and Th1/Th2 cytokines. Hum Immunol (2019) 80(10):863–70. doi: 10.1016/j.humimm.2019.05.011
88. Ding T, Su R, Wu R, Xue H, Wang Y, Su R, et al. Frontiers of autoantibodies in autoimmune disorders: Crosstalk between tfh/tfr and regulatory B cells. Front Immunol (2021) 12:641013. doi: 10.3389/fimmu.2021.641013
89. Khan AR, Hams E, Floudas A, Sparwasser T, Weaver CT, Fallon PG. PD-L1hi B cells are critical regulators of humoral immunity. Nat Commun (2015) 6:5997. doi: 10.1038/ncomms6997
90. van de Veen W, Stanic B, Yaman G, Wawrzyniak M, Sollner S, Akdis DG, et al. IgG4 production is confined to human IL-10-producing regulatory B cells that suppress antigen-specific immune responses. J Allergy Clin Immunol (2013) 131(4):1204–12. doi: 10.1016/j.jaci.2013.01.014
91. Sayitoglu EC, Freeborn RA, Roncarolo MG. The yin and yang of type 1 regulatory T cells: From discovery to clinical application. Front Immunol (2021) 12:693105. doi: 10.3389/fimmu.2021.693105
92. Freeborn RA, Strubbe S, Roncarolo MG. Type 1 regulatory T cell-mediated tolerance in health and disease. Front Immunol (2022) 13:1032575. doi: 10.3389/fimmu.2022.1032575
93. Gandhi R, Kumar D, Burns EJ, Nadeau M, Dake B, Laroni A, et al. Activation of the aryl hydrocarbon receptor induces human type 1 regulatory T cell-like and Foxp3(+) regulatory T cells. Nat Immunol (2010) 11(9):846–53. doi: 10.1038/ni.1915
94. Schmetterer KG, Neunkirchner A, Wojta-Stremayr D, Leitner J, Steinberger P, Pickl WF. STAT3 governs hyporesponsiveness and granzyme B-dependent suppressive capacity in human CD4+ T cells. FASEB J (2015) 29(3):759–71. doi: 10.1096/fj.14-257584
95. Gregori S, Tomasoni D, Pacciani V, Scirpoli M, Battaglia M, Magnani CF, et al. Differentiation of type 1 T regulatory cells (Tr1) by tolerogenic DC-10 requires the IL-10-dependent ILT4/HLA-G pathway. Blood (2010) 116(6):935–44. doi: 10.1182/blood-2009-07-234872
96. Cardone J, Le Friec G, Vantourout P, Roberts A, Fuchs A, Jackson I, et al. Complement regulator CD46 temporally regulates cytokine production by conventional and unconventional T cells. Nat Immunol (2010) 11(9):862–71. doi: 10.1038/ni.1917
97. Kemper C, Chan AC, Green JM, Brett KA, Murphy KM, Atkinson JP. Activation of human CD4+ cells with CD3 and CD46 induces a T-regulatory cell 1 phenotype. Nature (2003) 421(6921):388–92. doi: 10.1038/nature01315
98. Alegretti AP, Schneider L, Piccoli AK, Xavier RM. The role of complement regulatory proteins in peripheral blood cells of patients with systemic lupus erythematosus: review. Cell Immunol (2012) 277(1-2):1–7. doi: 10.1016/j.cellimm.2012.06.008
99. Zhang B, Sun J, Yuan Y, Ji D, Sun Y, Liu Y, et al. Proximity-enabled covalent binding of IL-2 to IL-2Ralpha selectively activates regulatory T cells and suppresses autoimmunity. Signal Transduct Target Ther (2023) 8(1):28. doi: 10.1038/s41392-022-01208-3
100. Li Y, Liu H, Yan H, Xiong J. Research advances on targeted-Treg therapies on immune-mediated kidney diseases. Autoimmun Rev (2023) 22(2):103257. doi: 10.1016/j.autrev.2022.103257
101. Sun JL, Lyu TB, Chen ZL, Lian CF, Liu SY, Shao TH, et al. Methylprednisolone pulse therapy promotes the differentiation of regulatory T cells by inducing the apoptosis of CD4(+) T cells in patients with systemic lupus erythematosus. Clin Immunol (2022) 241:109079. doi: 10.1016/j.clim.2022.109079
102. Zhao X, Wang S, Wang S, Xie J, Cui D. mTOR signaling: A pivotal player in Treg cell dysfunction in systemic lupus erythematosus. Clin Immunol (2022) 245:109153. doi: 10.1016/j.clim.2022.109153
103. Chu Y, Zhao C, Zhang B, Wang X, Wang Y, An J, et al. Restoring T-helper 17 cell/regulatory T-cell balance and decreasing disease activity by rapamycin and all-trans retinoic acid in patients with systemic lupus erythematosus. Lupus (2019) 28(12):1397–406. doi: 10.1177/0961203319877239
104. Datta SK. Harnessing tolerogenic histone peptide epitopes from nucleosomes for selective down-regulation of pathogenic autoimmune response in lupus (Past, present, and future). Front Immunol (2021) 12:629807. doi: 10.3389/fimmu.2021.629807
105. Giang S, Horwitz DA, Bickerton S, La Cava A. Nanoparticles engineered as artificial antigen-presenting cells induce human CD4(+) and CD8(+) tregs that are functional in humanized mice. Front Immunol (2021) 12:628059. doi: 10.3389/fimmu.2021.628059
106. Papillion A, Ballesteros-Tato A. The potential of harnessing IL-2-mediated immunosuppression to prevent pathogenic B cell responses. Front Immunol (2021) 12:667342. doi: 10.3389/fimmu.2021.667342
107. Laurence A, Tato CM, Davidson TS, Kanno Y, Chen Z, Yao Z, et al. Interleukin-2 signaling via STAT5 constrains T helper 17 cell generation. Immunity (2007) 26(3):371–81. doi: 10.1016/j.immuni.2007.02.009
108. Robinson S, Thomas R. Potential for antigen-specific tolerizing immunotherapy in systematic lupus erythematosus. Front Immunol (2021) 12:654701. doi: 10.3389/fimmu.2021.654701
109. Scherlinger M, Pan W, Hisada R, Boulougoura A, Yoshida N, Vukelic M, et al. Phosphofructokinase P fine-tunes T regulatory cell metabolism, function, and stability in systemic autoimmunity. Sci Adv (2022) 8(48):eadc9657. doi: 10.1126/sciadv.adc9657
110. Li H, Boulougoura A, Endo Y, Tsokos GC. AbnorMalities of T cells in systemic lupus erythematosus: new insights in pathogenesis and therapeutic strategies. J Autoimmun (2022) 132:102870. doi: 10.1016/j.jaut.2022.102870
111. Venkatadri R, Sabapathy V, Dogan M, Sharma R. Targeting regulatory T cells for therapy of lupus nephritis. Front Pharmacol (2021) 12:806612. doi: 10.3389/fphar.2021.806612
112. Schloder J, Shahneh F, Schneider FJ, Wieschendorf B. Boosting regulatory T cell function for the treatment of autoimmune diseases - That's only half the battle! Front Immunol (2022) 13:973813. doi: 10.3389/fimmu.2022.973813
113. Rajendeeran A, Tenbrock K. Regulatory T cell function in autoimmune disease. J Transl Autoimmun (2021) 4:100130. doi: 10.1016/j.jtauto.2021.100130
114. Ohl K, Tenbrock K. [Regulatory T-cells in systemic lupus erythematosus. IL-2 is decisive for loss of tolerance]. Z Rheumatol (2016) 75(3):253–64. doi: 10.1007/s00393-016-0060-z
115. Fathman CG, Yip L, Gomez-Martin D, Yu M, Seroogy CM, Hurt CR, et al. How GRAIL controls Treg function to maintain self-tolerance. Front Immunol (2022) 13:1046631. doi: 10.3389/fimmu.2022.1046631
116. Kato H, Perl A. Double-Edged Sword: Interleukin-2 Promotes T Regulatory Cell Differentiation but Also Expands Interleukin-13- and Interferon-gamma-Producing CD8(+) T Cells via STAT6-GATA-3 Axis in Systemic Lupus Erythematosus. Front Immunol (2021) 12:635531. doi: 10.3389/fimmu.2021.635531
117. Liang K, He J, Wei Y, Zeng Q, Gong D, Qin J, et al. Sustained low-dose interleukin-2 therapy alleviates pathogenic humoral immunity via elevating the Tfr/Tfh ratio in lupus. Clin Transl Immunol (2021) 10(6):e1293. doi: 10.1002/cti2.1293
118. He J, Zhang R, Shao M, Zhao X, Miao M, Chen J, et al. Efficacy and safety of low-dose IL-2 in the treatment of systemic lupus erythematosus: a randomised, double-blind, placebo-controlled trial. Ann Rheum Dis (2020) 79(1):141–9. doi: 10.1136/annrheumdis-2019-215396
119. Humrich JY, von Spee-Mayer C, Siegert E, Alexander T, Hiepe F, Radbruch A, et al. Rapid induction of clinical remission by low-dose interleukin-2 in a patient with refractory SLE. Ann Rheum Dis (2015) 74(4):791–2. doi: 10.1136/annrheumdis-2014-206506
120. Humrich JY, Cacoub P, Rosenzwajg M, Pitoiset F, Pham HP, Guidoux J, et al. Low-dose interleukin-2 therapy in active systemic lupus erythematosus (LUPIL-2): a multicentre, double-blind, randomised and placebo-controlled phase II trial. Ann Rheum Dis (2022) 81(12):1685–94. doi: 10.1136/ard-2022-222501
121. Fanton C, Furie R, Chindalore V, Levin R, Diab I, Dixit N, et al. Selective expansion of regulatory T cells by NKTR-358 in healthy volunteers and patients with systemic lupus erythematosus. J Transl Autoimmun (2022) 5:100152. doi: 10.1016/j.jtauto.2022.100152
122. Shao M, He J, Zhang R, Zhang X, Yang Y, Li C, et al. Interleukin-2 deficiency associated with renal impairment in systemic lupus erythematosus. J Interferon Cytokine Res (2019) 39(2):117–24. doi: 10.1089/jir.2018.0016
123. Zhao C, Chu Y, Liang Z, Zhang B, Wang X, Jing X, et al. Low dose of IL-2 combined with rapamycin restores and maintains the long-term balance of Th17/Treg cells in refractory SLE patients. BMC Immunol (2019) 20(1):32. doi: 10.1186/s12865-019-0305-0
124. Humrich JY, Riemekasten G. Low-dose interleukin-2 therapy for the treatment of systemic lupus erythematosus. Curr Opin Rheumatol (2019) 31(2):208–12. doi: 10.1097/BOR.0000000000000575
Keywords: systemic lupus erythematosus, lupus nephritis, regulatory T cells, interleukin-2, B regulatory cells
Citation: Tsai Y-G, Liao P-F, Hsiao K-H, Wu H-M, Lin C-Y and Yang KD (2023) Pathogenesis and novel therapeutics of regulatory T cell subsets and interleukin-2 therapy in systemic lupus erythematosus. Front. Immunol. 14:1230264. doi: 10.3389/fimmu.2023.1230264
Received: 28 May 2023; Accepted: 15 August 2023;
Published: 12 September 2023.
Edited by:
Yuji Nozaki, Kindai University Hospital, JapanReviewed by:
Reza Akbarzadeh, University of Lübeck, GermanyCopyright © 2023 Tsai, Liao, Hsiao, Wu, Lin and Yang. This is an open-access article distributed under the terms of the Creative Commons Attribution License (CC BY). The use, distribution or reproduction in other forums is permitted, provided the original author(s) and the copyright owner(s) are credited and that the original publication in this journal is cited, in accordance with accepted academic practice. No use, distribution or reproduction is permitted which does not comply with these terms.
*Correspondence: Ching-Yuang Lin, Y3lsaW5AbWFpbC5jbXVoLm9yZy50dw==; Kuender D. Yang, eWFuZ2tkLnllaEBnbWFpbC5jb20=
†These authors have contributed equally to this work
Disclaimer: All claims expressed in this article are solely those of the authors and do not necessarily represent those of their affiliated organizations, or those of the publisher, the editors and the reviewers. Any product that may be evaluated in this article or claim that may be made by its manufacturer is not guaranteed or endorsed by the publisher.
Research integrity at Frontiers
Learn more about the work of our research integrity team to safeguard the quality of each article we publish.