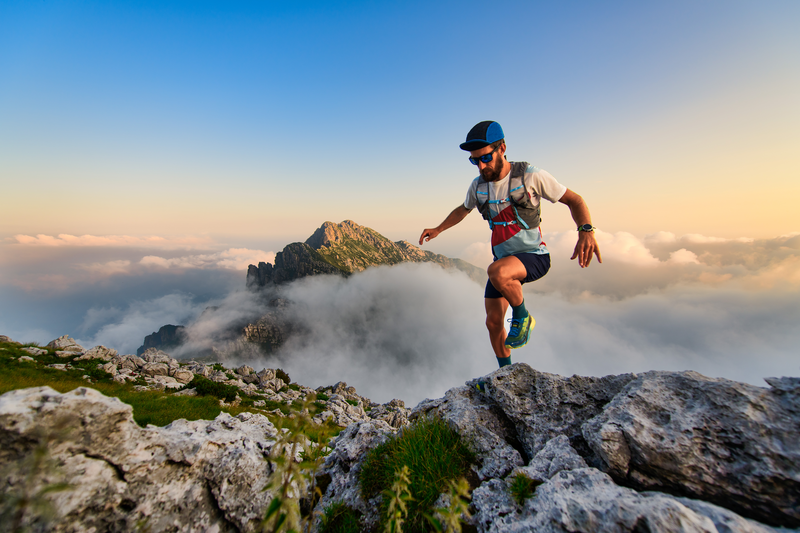
94% of researchers rate our articles as excellent or good
Learn more about the work of our research integrity team to safeguard the quality of each article we publish.
Find out more
REVIEW article
Front. Immunol. , 28 July 2023
Sec. Autoimmune and Autoinflammatory Disorders : Autoimmune Disorders
Volume 14 - 2023 | https://doi.org/10.3389/fimmu.2023.1215216
This article is part of the Research Topic Practical recommendations and consensus for the management of immune mediated hematologic diseases View all 6 articles
The pathophysiology of immune thrombocytopenia (ITP) is complex and encompasses innate and adaptive immune responses, as well as megakaryocyte dysfunction. Rituximab is administered in relapsed cases and has the added benefit of inducing treatment-free remission in over 50% of patients. Nevertheless, the responses to this therapy are not long-lasting, and resistance development is frequent. B cells, T cells, and plasma cells play a role in developing resistance. To overcome this resistance, targeting these pathways through splenectomy and novel therapies that target FcγR pathway, FcRn, complement, B cells, plasma cells, and T cells can be useful. This review will summarize the pathogenetic mechanisms implicated in rituximab resistance and examine the potential therapeutic interventions to overcome it. This review will explore the efficacy of established therapies, as well as novel therapeutic approaches and agents currently in development.
Immune thrombocytopenia (ITP) is a rare hematologic disorder with an incidence rate of 2.25 per 100,000 and slight female predomince (1). Diagnosis of ITP is based on isolated thrombocytopenia lower than 100×109/L with the exclusion of other causes (2). Manifestations of ITP range from asymptomatic thrombocytopenia to severe life-threatening bleeding depending on the platelet level. In the recent update of the international consensus on the investigation and management of ITP, the management of ITP should commence when the platelet count drops below 20-30×109/L to minimize the likelihood of bleeding. Other factors such as quality of life, existence of other hemostatic defects, impending surgeries, and susceptibility to trauma from occupation or lifestyle should also be considered to individualize therapy (2). Corticosteroids are the first-line therapy with an initial response rate of 75%, however 80% of patients eventually relapse or become corticosteroid-dependent (3). The second-line treatments are thrombopoietin receptor agonist (TPO-RA), splenectomy, rituximab and fostamatinib (3). Choosing between medications and agents should employ shared decision-making with the patient among the second-line treatment options. If the patient values treatment-free remission and avoidance of surgery, rituximab is the preferred treatment (4). However, the long-term response of rituximab is not sustained (5). In this review, we will discuss potential mechanisms of rituximab resistance in ITP, treatment strategies with available agents and new therapies in development which can potentially overcome the resistance.
While the cause of ITP was initially identified as a humoral factor by observing that the infusion of citrated whole blood or plasma from ITP patients into healthy recipients would result in a decrease in platelet count (6), more recently, the mechanism of ITP has been better understood. Circulating B cells and autoreactive plasma cells are responsible for the overproduction of autoantibodies (7, 8). Autoantibody binding with glycoproteins (GPs) IIb/IIIa, Ib, IV and Ia/IIa (9, 10) on the platelet surface is the crucial element in the pathogenesis of ITP. Autoantibody (singular) coated platelets are destroyed by a number of mechanisms. In the spleen, anti-GP IIb/IIIa autoantibody interacts with Fcγ receptors I and III on the macrophages to trigger phagocytosis (11) Platelet autoantibodies, particularly those targeting GP IIb/IIIa and Ib/IX (12), trigger the activation of the classical complement pathway, leading to the deposition and fixation of C1q and C4d on the platelet surface. This process facilitates the generation of the membrane attack complex (MAC), which leads to increased clearance of platelets (13, 14). Additionally, phagocytosis is also mediated by C3b interaction with macrophage complement receptor 1 (CR1) (15). Moreover, autoantibody is also observed to inhibit megakaryocyte maturation and production (16).
Desialylation of platelets is a physiologic mechanism responsible for removal of senescent platelets via hepatic Ashwell–Morell receptor (AMR), also stimulating TPO production and generation of new platelets (17). Murine models demonstrate that anti-GPIb/IX antibodies induce Fc-independent platelet activation and desialylation of platelets, which leads to platelet clearance via AMR (18). Patients with GpIb antibodies have been reported to have inferior response to Fc-dependent therapies such as corticosteroids and IVIG (19, 20), and multi-refractory patients with ITP have higher incidence of GPIbα antibodies and increased platelet activation and desialylation (21).
Apart from the humoral response, T cell dysregulation also plays a role in ITP pathogenesis. In ITP patients, it has been demonstrated that clones of CD4 positive T cells that can recognize GPIIb/IIIa are present in both the blood and spleen (22). Increase in CD4+ T helper 1 (Th1) cell activation (23, 24) and upregulation of Th17 cells (25) is associated with deficiency of CD4+CD25+ regulatory T cells (Treg), which are responsible for maintaining peripheral immune tolerance (26). CD3+CD8+ T cells are also increased in ITP patients and mediate cell-mediated cytotoxicity and cell-mediated platelet lysis (27). In addition, CD8+ T cells induce platelet desialylation leading to increase the clearance in the liver (28).
Megakaryocyte dysfunction also contributes to thrombocytopenia in ITP. Autoantibody IgG binding to the surface of megakaryocyte has been reported (29), associated with suppressed megakaryocyte production and impaired maturation (16) and decreased release of platelet from megakaryocyte (30). Autophagy is the process of eliminating old, damaged, or abnormal cytoplasmic proteins constituents and believed to be impaired in various of autoimmune disorders including ITP. The abnormal of autophagy in ITP can affect the differentiation of megakaryocytes into platelets (31). Disruption of megakaryocyte apoptosis by cytotoxic lymphocyte cytotoxicity also plays a role in megakaryocyte dysfunction leading to impaired platelet production (32, 33).
Rituximab, the first monoclonal antibody to be approved for therapeutic use, is a chimeric monoclonal antibody that targets CD20 (34). Rituximab’s Fab region has a specific binding affinity for the CD20+ target antigen. On the other hand, its Fc region can bind to various receptors, including the Fcγ receptors on immune effector cells, the neonatal Fc receptor, and the head of C1q (35). The binding and interaction leads to antigen-dependent cell-mediated cytotoxicity (ADCC), phagocytosis and complement-induced cell lysis (36). Due to the depletion of B cells, rituximab was used in various autoimmune disorders (37). Rituximab was first implemented in treatment of ITP around 2000 with responses, including complete, seen in patients refractory to steroids, splenectomy and other immune suppressants (38–40). Currently rituximab one of the recommended second-line therapies after steroid failure (2). Response to therapy is seen as soon as in the first week with median time to achieve response around 1 month (38, 41). Rituximab 375mg/m2 weekly for four doses is considered the standard dose based on the original clinical trial adopted from lymphoma therapy (38). In contrast, a low dose of 100mg/m2 weekly for four doses showed similar efficacy (42). Despite an initial response rate of nearly 60%, with half of the responses being complete, the long-term effectiveness of rituximab is not maintained. In fact, only 21% of adults and 26% of children were able to remain treatment-free based on a five-year outcome analysis (5). A number of predictive factors of response to rituximab has been evaluated. Several studies reported higher responses associated with female gender, younger age (40, 43), although this was not confirmed by other studies (5, 38, 44). Another study demonstrated that adolescent females with an ITP duration of less than 12 months had the longest response duration (45). In both pediatric and adult patients with ITP, the response to rituximab was not predicted by immunologic markers, such as antinuclear antibody, direct antiglobulin testing, immunoglobulin levels, and lymphocyte subsets, or primary versus secondary ITP (46). Although patients with anti-GPIIb/IIIa antibodies appear to have a higher response rate of 75% compared to those without at 46% (47), this finding is not sufficient to base treatment decisions regarding the clinical use of rituximab.
Resistance to rituximab in ITP patients can be mediated by a number of mechanisms.
As rituximab directly targets CD20+ B cells, the incomplete depletion of B cells has been linked to nonresponse to rituximab, and B cell reconstitution can predict relapse (48). During a 5-year follow-up study, patients who experienced a relapse had a faster reconstitution of B cells compared to those who maintained a response lasting over 2.5 years (5).
In a study evaluating B cell populations in splenectomy specimens of patients who did not respond to rituximab, two distinct groups of B cells were responsible for rituximab relapse. The germinal center B cell population was significantly expanded compared to the healthy donors along with increase in CD19+ naïve B cells, suggesting B cell reconstitution in the spleen after the clearance of rituximab. In addition, a population of preexisting mutated memory B cells characterized by up-regulation of several prosurvival genes and down-regulation of B-cell receptor (BCR) complex surface expression was identified, accounting for the escape of rituximab depletion (49). These groups of B cells can reactivate and differentiate into plasma cells once rituximab is cleared.
Autoreactive anti-GpIIbIIIa antibody-secreting plasma cells have been observed in the patient’s plasma and bone marrow following the development of resistance to rituximab, suggesting their involvement in rituximab refractoriness (22). In a study analyzing the spleens of ITP patients, it was demonstrated that those who experienced rituximab failure had a greater number of autoreactive anti-GpIIbIIIa antibody-secreting long-lived plasma cells (LLPCs), despite near-complete B cell depletion in peripheral blood. Patients who responded to rituximab were also found to have lower levels of antibody-secreting plasma cells. Gene expression profiling of these plasma cells revealed a long-lived pattern characterized by overexpression of antiapoptotic genes while responders displayed a short-lived program (50). The results suggest that the long-lived plasma cells in the spleen could explain rituximab resistance. These pathogenic plasma cells have been demonstrated throughout the spleen, peripheral blood, and bone marrow, potentially leading to treatment failure (8). Higher concentration of B-cell-activating factor (BAFF), a member of the tumor necrosis factor family which promotes B-cell survival was detected in the supernatant of spleen cell cultures of rituximab refractory patients (50). In a subsequent study by the same group, using mouse model depletion of B cells led to elevated levels of BAFF and the appearance of splenic LLPCs. A combination of rituximab and BAFF-neutralizing antibody reduced the amount of LLPCs (51), suggesting that BAFF targeted therapy can potentially overcome the rituximab resistance by eliminating long-lived plasma cells.
In addition to its direct effect on B cells, rituximab also has an effect on the T cell population. As Treg cells are generally suppressed in ITP patients, infusion of rituximab has been shown to increase number and percentage of polyclonal Treg cells, thereby aiding in the suppression of Th1 cell function and decreasing inflammation (52). In rituximab non-responders, there was oligoclonal expansion of T cells with increased Th1/Th2 and Tc1 (IFN-γ single-positive CD8 cells)/Tc2 (IL-4 single-positive CD8 cells) ratio as well as the expression of Fas ligand on Th1 and Th2 cells (53). One potential reason for the lack of response to rituximab may be related to the specific role of CTLs in targeting and damaging platelets or megakaryocytes. This is supported by evidence indicating that rituximab non-responsive ITP patients exhibit an increase in splenic effector memory CTLs that produce large quantities of interferon-γ and display clonal restriction (54).
In a mouse model, CD4 positive T cells were also shown to play a role in extending plasma cell survival after rituximab treatment. Combination of CD4+ depleting therapy and anti-CD20 antibody led to a significant reduction in splenic plasma cells (51). Additionally, a separate study demonstrated that rituximab-resistant patients had significantly higher levels of CD4+CD45RO+ memory T lymphocytes in their peripheral blood compared to rituximab-responsive patients (55). T follicular helper cells (TFH) is a major T cell involving in B cell differentiation and proliferation in lymphoid organ. In chronic ITP patients who responded to treatment, the percentage of TFH is decreased significantly (56). Another study suggested that the follicular helper T cells can increase the level of BAFF in germinal center (57, 58).
Historically, splenectomy as well as rituximab were utilized in second line therapy for ITP (2). There is no head to head comparison of the efficacy studies. The results from retrospective studies vary from similar efficacy (59) to longer response duration with splenectomy (60, 61) and a higher response rate with splenectomy (62). A review of 16 clinical studies suggested that rituximab carries a lower response rate and response duration than splenectomy (63) whereas meta-analysis in pediatric population suggested higher CR rates of 52% with 43% of responses persisting (64).
As discussed previously, pathogenic lymphocytes and plasma cells primarily residing in the spleen play a role in rituximab resistance. In that case, splenectomy could theoretically be the potential treatment for ITP beyond rituximab by eliminating the immune effector cells in the spleen. One retrospective study suggested that in rituximab non-responders who were treated with splenectomy the response rate was 100%. A retrospective study from Mayo Clinic found that patients receiving sequential splenectomy-rituximab or rituximab-splenectomy had similar 2-year freedom from relapse and were superior to those who received rituximab treatment alone (61).
The main mechanism of platelet destruction in ITP is phagocytosis of antibody coated platelets. FcγR signaling inhibition interferes with phagocytosis preventing platelet destruction. Syk and BTK are key tyrosine kinases that play a role in both B cell development and function as well as FcγR-mediated phagocytosis in macrophages (65–67). The neonatal crystallizable fragment receptor (FcRn) is involved in recycling of endogenous IgG including pathogenic autoantibodies (68). Agents targeting FcγR function present an alternative therapeutic approach in refractory ITP patients.
Fostamatinib, an oral potent and selective small molecule inhibitor of spleen tyrosine kinase (SyK), inhibits signal transduction of B-cell receptors and FcR-triggered Syk-dependent cytoskeletal rearrangement during phagocytosis, degranulation, and cytokine production. In the ITP model it been shown to decrease antibody-mediated platelet destruction (67). In two multicenter, double-blind, placebo-controlled, phase 3 clinical trials in patients with persistent/chronic ITP treated with fostamatinib 100mg twice a day with dose escalation to 150mg twice daily after 4 weeks in non-responders demonstrated overall response rate (ORR) of 43% compared to 14% on placebo (69), which was maintained in the long term follow up (70). Based on that, fostamatinib was approved by Food and Drug Administration (FDA) on April 2018 to treat ITP.
Bruton’s tyrosine kinase (BTK) is crucial for B cell development as well as cytokine and antibody production. Increased production of autoantibody associated with BTK expression is thought to be one of the mechanisms involved in the pathogenesis of systemic autoimmune diseases (65). In addition, BTK is also involved in FcγR-mediated phagocytosis in macrophages (71). The inhibition of BTK provides a potential target for autoimmune diseases including ITP. Rilzabrutinib, a covalent reversible BTK inhibitor, has demonstrated potent and durable inhibition of BTK. The reversible covalent inhibitors can maintain inhibition of a target proteins even after washout, potentially reducing off-target effects by minimizing drug exposure (72). BTK is homologous to Tec (tyrosine kinase expressed in hepatocellular carcinoma), which is expressed in platelets and plays a role in platelet aggregation through GPVI activation upon collagen stimulation. Rilzabrutinib is more selective for BTK than Tec compared to irreversible covalent BTK inhibitors, which is thought to result in fewer bleeding-related adverse effects (73). In a preclinical study, rilzabrutinib inhibited the activation and inflammatory activities of B cells and innate cells, reducing autoantibody mediated FcγR signaling (74). In a phase 1b/2 study of 60 heavily pre-treated including rituximab refractory ITP patients, rilzabrutinib demonstrated dose dependent improvement in platelet count with 40% of patients achieving the primary endpoint of platelet response (two consecutive platelet counts separated by ≥5 days of at least 50×103/mm3 and an increase from baseline of at least 20×103/mm3 without the use of rescue medication) at the highest dose of 400mg twice a day with median time to response of 12.5 days. Toxicity was low and no treatment-related adverse effect above grade 3 was observed (75). Currently rilzabrutinib 400mg twice daily is being evaluated in a phase 3 multicenter, randomized, double-blind clinical trial (NCT04562766).
The neonatal crystallizable fragment receptor (FcRn) functions as intracellular shield from catabolism for immunoglobulin G (IgG), binding IgG and albumin in lysosomes under acidic conditions, protecting them from degradation, and recycling them to the cell surface (68). Blocking FcRn-IgG binding could facilitate lysosomal degradation of endogenous IgG, reducing the half-life of pathogenic IgG as the therapeutic target in antibody-mediated autoimmune diseases (76).
In a phase 2 clinical trial involving 66 patients with relapsed persistent or chronic ITP, the monoclonal anti-FcRn antibody Rozamolixizumab produced rapid and significant increases in platelet counts, along with substantial reductions in IgG levels, especially with single higher dose subcutaneous infusion (77). A phase 3 open-label extension study to investigate the long-term safety, efficacy and tolerability was recently completed (NCT04596995).
Efgartigimoid is a a human IgG1 antibody Fc-fragment with a high affinity for FcRn. In a randomized double blinded placebo controlled phase 2b trial of ITP patients refractory to previous lines of therapy four weekly IV infusions of efgartigimod induced a dose dependent rapid reduction of total IgG levels, which was associated with clinically relevant increases in platelet counts with almost half the patients achieving platelet count of ≥50 × 109/L on at least two occasions, and a reduced proportion of patients with bleeding (78). In a multicenter, randomized, double-blinded, placebo-controlled trial in adults with persistent or chronic ITP recently reported (79), 51.2% of participants on efgartigimoid 10 mg IV weekly for 4 weeks then every 2 weeks achieved IWG response criteria versus 20% on placebo.
Sirolimus is macrocyclic lactone with antifungal, antitumor and immunosuppressive activity and is a potent inhibitor of antigen-induced proliferation of T cells, B cells, and antibody production. Sirolimus complexes with family of intracellular binding proteins termed FKBPs (FK binding proteins) targeting mTOR and inhibiting the mTOR-mediated signal-transduction pathways, which results in cell cycle arrest in G1 phase (80). Activation of the mTOR protein is thought to play a significant role in the disruption of hematopoiesis in individuals with autoimmune disease (81). Sirolimus has been used successfully in children with autoimmune lymphoproliferative syndrome (ALPS) (82), as well as other primary or secondary autoimmune cytopenias (83), and has been particularly effective in managing autoimmune cytopenias in the setting of primary immunodeficiency and immune dysregulation. In a multicenter prospective study involving 30 children and young adults with relapsed/refractory autoimmune cytopenias, including 16 patients previously treated with rituximab, all 12 children with ALPS achieved a lasting complete response (CR). Additionally, CRs were observed in 8 out of 12 patients with multilineage cytopenias associated with common variable immunodeficiency, Evans syndrome, or systemic lupus erythematosus (84). In a preliminary report of a prospective multi-center clinical trial in patients with ITP, 66 patients who failed the second-line therapy, including 30% who received rituximab, 46/66 (70%) responded to sirolimus 2mg orally every day at 3 months with 45% responses being complete (85). In a longer follow-up, the overall response rate (ORR) was 70% and 65% at 6 months and 12 months, respectively. Responders demonstrated a decrease in the proportion of Th2 and Th17 cells, along with an increase in the percentage of M-MDSCs and Tregs, suggesting that sirolimus could potentially restore peripheral tolerance (86). In a prospective randomized observation trial of 43 patients with chronic ITP who relapsed after multiple prior therapies including rituximab, combination of low dose prednisone with sirolimus was compared to cyclosporine and sirolimus. Although ORR was similar (58% versus 62%), treatment with sirolimus and prednisone was associated with a higher rate of sustained response (68% versus 39%, P < 0.05) and a increase in Treg cell levesl (87).
Given role of plasma cells in ITP relapse, plasma cell inhibition is a therapeutic target, particularly in rituximab relapsed patients.
Bortezomib, first proteasome inhibitor approved for treating multiple myeloma, inhibits the ubiquitin-proteasome proteolytic pathway responsible for intracellular protein turnover, disrupting the cell cycle, inducing apoptosis, altering the bone marrow microenvironment and inhibiting nuclear factor kappa B to cause plasma cell depletion (88). By inducing apoptosis in antibody-secreting cells such as plasma cells and memory B cells, bortezomib leads to a reduction in antibody secretion (89, 90). Bortezomib also has been shown to have both immunosuppressive and immunostimulatory effects which is widely used in immune mediated disorders. It reduces the number of CD4 T cells and decreases their production of Th1 cytokines while also increasing the population of regulatory T cells (Tregs) (88). Bortezomib has been successfully used in several antibody mediated disorders, including thrombotic cytopenic purpura, warm autoimmune hemolytic anemia, and cold agglutinin disease (91–94). Bortezomib use in ITP treatment is limited in case reports. Therapy was successful in three out of four cases reported in the literature (95–98). However, use of additional immunosuppressive therapies could have a confounding effect on response to bortezomib. Bortezomib is currently being evaluated in refractory ITP, alone and in combination with rituximab in two clinical trials (NCT05599880, NCT03443570).
CD38 is a membrane glycoprotein which is present in hematopoietic cells, including plasma cells which is involved in cell adhesion, migration, and signal transduction. Daratumumab is a high-affinity IgG1 monoclonal antibody against a unique CD38 epitope, it clears CD38-positive plasma cells via antibody-dependent cellular cytotoxicity and complement-dependent cytotoxicity (99). Due to the plasma depletion effect, daratumumab has been used in autoimmune hemolytic anemia and lupus (100–103). In case reports and case series, daratumumab has shown efficacy in the context of post-allogeneic bone marrow transplant associated autoimmune thrombocytopenia (104–106) as well as other causes of secondary ITP (107, 108) and most recently in a relapsed multi refractory primary ITP (109, 110). A phase 2 clinical trial is currently evaluating the efficacy and safety of daratumumab in patients with ITP who did not respond to at least two prior therapies (NCT04703621). During the safety run-in, two out of three enrolled patients responded to the treatment at week 12, with one patient experiencing a relapse by week 24 (111). Another anti-CD38 monoclonal antibody, mezagitamab (TAK-079), a fully human IgG1 is currently being studied in chronic and persistent ITP (NCT04278924).
Given the role of BAFF in failure of B cell depleting therapy with rituximab in ITP, BAFF inhibition is emerging as an important therapeutic target to mitigate rituximab resistance. Belimumab is a human IgG1λ monoclonal antibody directed against BAFF which causes reversibly decrease of B lymphocyte production (112). Belimumab already shows its efficacy in autoimmune disorders including lupus (113). Based on the assumption of the synergistic effect of anti-CD20 and anti-BAFF, belimumab was administered in conjunction with rituximab in a phase 2b trial in 15 non-splenectomized patients with chronic and persistent ITP who failed at least one prior line of therapy. Patients received two doses of rituximab 1000mg 2 weeks apart combined with 5 infusions of belimumab 10mg/kg at weeks 0, 2, 4, 8 and 12. Overall response was 80% at 1 year follow up, including 66.7% complete responses. The combination arm resulted in similar B cell repopulation compared to patients who received rituximab alone, but there was a significant decrease in T-follicular helper cells (114). Addition of subcutaneous belimumab to rituximab is currently investigated in a randomized placebo-controlled phase 3 trial (NCT05338190).
Ianalumab is an afucosylated humanized IgG1 monoclonal antibody directed against the BAFF receptor. In addition to BAFF receptor blockade that interrupts BAFF-mediated important signaling for B-cell maturation, proliferation, and survival, ianalumab induces direct lysis of B cells by antibody-dependent cellular cytotoxicity, resulting in a rapid and profound B cell depletion of long-lasting duration (115–117). The efficacy and safety of lanalumab in addition to standard of care for first line and second line treatment of ITP are currently evaluated by phase 3 trials (NCT05653349, NCT05653219).
CD19 is expressed on B cells as well as plasma cells. Inebilizumab is a humanized anti-CD19 monoclonal antibody that targets and depletes CD19-expressing cells via antibody-dependent cell-mediated cytotoxicity. It is currently approved for treatment of neuromyelitis optica spectrum disorder (118) and is being evaluated in other antibody mediated diseases including IgG4-related Disease (NCT04540497), myasthenia gravis (NCT04524273), systemic sclerosis (NCT05198557) and autoimmune encephalitis (NCT04372615). Hypogammaglobinemia with associated infectious complications have been reported in 20% of patients along with 12% risk of infusion reactions (118).
Obexelimab (XmAb5871) is an anti-CD19 non-depleting B cell monoclonal antibody that co-engages B cell antigen receptor complex and Fcγ receptor IIb inhibitory receptor resulting in inhibition of B cell proliferation, antibody secretion and plasma cell differentiation (119, 120) and is currently being investigated in SLE (NCT02725515) and IgG4-related disease (NCT05662241).
CD19 inhibition can be a potential therapeutic targets in patients who fail to respond to rituximab due to reconstitution of CD19+ B cells (49).
Decitabine, a DNA methylation inhibitor with antimetabolite effect approved in myeloid hematologic disorders which induces hypomethylation when incorporated into DNA (121). In a preclinical study, low-dose decitabine increased the Treg cells and enhanced their immunosuppressive function, decreased the Th1 and Th17 cells and proinflammatory cytokines and inhibited STAT3 activation (122). Low dose decitabine has also demonstrated to restore the methylation level and expression of the programmed cell death protein 1 (PD-1) promoter, activating PD-1 signaling pathway and resulting in decreased number and cytotoxicity of CD8+ T cells in ITP patients (123). A multi-center prospective study evaluated low dose of decitabine 3.5mg/m2 for 3 consecutive days every 4 weeks for 3 cycles in 45 refractory ITP patients, 23 were previously treated with rituximab. The ORR was 51% (17.8% CR) at the end of therapy and 31% at 12 months with responses seen on retreatment (124).
Mycophenolate mofetil (MMF) is a prodrug of mycophenolic acid which is an inhibitor of inosine-5-monophosphate dehydrogenase, which leads to l depletion of guanosine nucleotides in T and B lymphocytes, inhibition of proliferation, suppression of antibody production and cell-mediated immune response (125). In patients with ITP treatment with MMF was associated with changed in T cell lymphocytes, decreased inflammatory markers (126), decreased cytotoxic CD8+ T cells (127), suggesting that MMF works via T cell regulation. Among the case reports and case series, MMF is effective either as a single agent (128–130) or combined with other agents (131) in relapsed/refractory ITP. MMF was previously widely used as the second-line therapy in pre-rituximab era in Europe and Asia. Several clinical studies suggest the efficacy and effectiveness of MMF after steroids and splenectomy (127, 129, 132–136), with ORR ranging 38.9%-80%, although platelet response can take 4 to 6 weeks. MMF also demonstrated efficacy after rituximab therapy. In a retrospective study of 46 patients with severe ITP, all requiring second-line therapy, approximately one-third of them having received rituximab, the ORR was 52% with 33% of patients achieving complete response. There was no difference in response rates between patients who had previously received rituximab and those who had not (135). MMF has been evaluated in the first-line setting in a multi-center, open-label, randomized trial in United Kingdom as addition to corticosteroids in the first-line. Compared to steroids alone, patients who received steroids plus MMF achieved superior treatment response and experienced less treatment failure, albeit at the cost of reporting lower quality-of-life outcomes (136).
Cyclosporin is an immunosuppressant that inhibits the phosphatase activity of calcineurin regulating gene expression in activated T cells and blocking signaling pathways triggered by antigen recognition (137). Cyclosporin was used sporadically pre-rituximab era as second-line therapy after failure of steroids and splenectomy, with evidence level limited to case reports, retrospective case series and one small prospective study (138–143). The ORR ranged from 55% to 100% with majority of responses sustained. A four week course of high-dose dexamethasone, low-dose rituximab 100mg on days 7, 14, 21 and 28 plus oral cyclosporin 2.5 to 3mg/kg daily was prospectively evaluated in a single-arm phase 2b study of 20 patients most of whom received at least three lines of therapy. Although overall response rate was 60%, relapse-free survival at 12 and 24 months was 92% and 76% respectively among the responders, suggesting that dual B and T cell inhibition can provide durable remissions with a short course of therapy (144). Successful use of cyclosporin post rituximab has been described in case report and case series (141, 142). In a recent meta-analysis cyclosporine-based combinations improved ORR and CR rates while reducing the rate of relapse (145). Importantly, this improvement was achieved without increasing the rate of adverse events. These findings suggest that cyclosporine alone or in combination with other agents can provide deeper and more durable responses in patients with refractory ITP.
Multi-refractory ITP patients have a higher proportion of anti-GPIb/IX antibodies, which can cause platelet desialylation, leading to accelerated clearance of platelets via the hepatic AMR (18) and an elevated number of desialylated platelets (21).
Oseltamivir, is an inhibitor of neuraminidase, the enzyme involved in the desialylation of platelets. In a prospective case series of seven patients with persistent, chronic, or refractory ITP treated with oral oseltamivir 75 mg twice daily for 5 days initial responses were seen in all patients but were not sustained (146). In an open-label randomized phase 2 trial in newly diagnosed ITP addition of oseltamivir (75 mg twice a day for 10 days) to dexamethasone (40 mg/day for 4 days) produced significantly higher 6-month sustained response rate (53 vs. 30%; OR 2.17; P = 0.032). Interestingly, patients with anti-GPIb/IX did not achieve better responses with oseltamivir (147).
Thrombopoietin receptor agonists (TPO-RA) interact with the thrombopoietin (TPO) receptor, induce a conformational change, triggering activation of the JAK2/STAT5 pathway, enhancing proliferation of megakaryocyte progenitors which leads to increased platelet production (148). TPO-RAs have been successfully used in ITP since 2006 (149).
Currently there are 3 TPO-RAs including romiplostim, eltrombopag, avatrombopag approved by FDA based on several prospective randomized phase 3 clinical trials with response seen in 60–90% of the cases, including rituximab refractory patients.
Due to their favorable safety profile TPO-RAs have increasingly been used in second line setting. Thrombosis is a complication seen in 6% cases and while previously a concern, bone marrow fibrosis reported in 1.4 to 6% of cases, is reversible on discontinuation of the agent. Eltrombopag has dietary restrictions and risk of hepatotoxicity, while most recently approved avatrombopag can be administered in patients with hepatic dysfunction (150–154). Romiplostim is subcutaneously administered peptibody that directly binds to the TPO binding site in a competitive manner, whereas eltrombopag and avatrombopag are oral small molecules that binds to a trans-membrane site. Eltrombopag also exhibits off-target effects, acting as a chelator of both extra- and intra-cellular calcium and iron, and facilitating the transport of iron out of cells. This iron-chelating property of eltrombopag results in a TPO-independent stimulation of stem cells and megakaryocyte precursors (155). Lusutrombopag is currently approved for the treatment of thrombocytopenia in patients with chronic liver disease (156). However, a study evaluating its effectiveness in treating ITP was terminated prematurely due to the inability to achieve the study objectives (NCT01054443). Hetrombopag is a TPO-RA approved in China with similar response rate in patients with relapsed or refractory ITP (157).
Head-to-head comparison between TPO-Ras are lacking. Options of the agent should take into consideration the way of administration (158). Switching between TPO-Ras can be considered based on lack of efficacy, platelet fluctuations, safety, and tolerability. If switching was due to lack of efficacy, the response rate to the second TPO-RA could be still as high as 65%. Almost every patient switched TPO-RA due to reasons other than lack of efficacy continued to respond after the switch (93%) (159). In a recent multicenter observational study of 44 patients with chronic ITP who had not responded or were intolerant to either romiplostim or eltrombopag, 41 patients (93%) achieved a platelet response (platelet count of ≥ 50 × 109/L) after switching to avatrombopag (160).
Due to their role as growth factors and the observation that most patients experienced relapse soon after discontinuing TPO-RA treatment (148, 153). TPO-RAs were traditionally viewed as chronic supportive therapy with no effect on the immune system. However, retrospective studies have indicated that 15-20% of patients may achieve long term remissions off therapy, suggesting that these agents may also have an immunomodulatory effect in ITP (161). Restoration of Tregs levels and function in peripheral blood and spleen has been proposed as a potential mechanism (162). In a prospective multicenter study of 48 patients with persistent or chronic ITP and complete response to TPO-RA therapy, sustained response off-treatment and sustained complete response off-treatment were achieved in 56% and 31% of patients at week 24 and 52% and 29% at week 52, respectively, suggesting that TPO-RA discontinuation can be considered in ITP patients in long-term CR (163).
Complement plays an important role in ITP pathogenesis, with classical complement pathway activated by antibodies binding to platelets leading to complement-dependent cytotoxicity (CDC) as well as phagocytosis by macrophages which recognize C3 via complement receptor 1 (CR1) (13–15).
In an vitro study increased complement activation was demonstrated in 47% patients with ITP with increased C1q deposition in 42% of patients and was inhibited by TNT003, a murine monoclonal antibody that targets C1s and inhibits classical complement pathway (164).
Sutimlimab, a humanized C1s IgG4 monoclonal antibody, was evaluated in a Phase I trial in 12 patients with chronic severe ITP who failed at least two prior therapies, including 8 patients with insufficient response to rituximab and administered as an infusion Days 0 and 7, then every 2 weeks for up to 21 weeks with an option to enter a long term extension after 9 week washout (165). Durable overall response (platelet ≥50 G/L in ≥50% of follow-up visits) was seen in 42% of patients, with 33% of patients achieving CR (platelet count ≥100 G/L) with the median time to response of 2 days. The median platelet count returned to baseline during the washout, suggesting need for ongoing therapy to maintain response. Complement inhibition is a promising therapeutic target in a subset of patients with complement activation which underscores the importance of identifying biomarkers that can help determine which patients will respond to treatment. A phase 2 study evaluating BIVV020, a C1s inhibitor which can be self-administered subcutaneously, has been recently completed but results are not yet available (NCT04669600). Inhibition of the alternative complement pathway with iptacopan, a Factor B inhibitor is currently being evaluated in a phase 2 basket study in ITP and cold agglutinin disease (NCT05086744).
Most adult patients with ITP relapse after initial therapy and require subsequent treatment. Rituximab therapy has been utilized in relapsed and refractory ITP with high response rates with short treatment duration. However, remission duration is limited, and many patients become refractory to therapy. In addition, immunosuppressive effect and impairment of response to vaccination has become a concern, particularly in the era of COVID-19 pandemic. There is an unmet need for novel therapeutic approaches that are safe, tolerable and can overcome rituximab resistance. Potential escape mechanisms include expansion of CD19+ B cells, T cells, plasma cells and complement. Based on the mechanism, several available approaches are proposed to overcome rituximab resistance. Surgical intervention splenectomy after rituximab is more effective than rituximab alone. Novel agents that interfere with FcγR dependent phagocytosis including fostamatinib, rilzabrutinib and inhibition of FcRn mediated pathogenic antibody recycling, and complement inhibitors are demonstrating their efficacy when patients relapse after rituximab. Plasma cell directed therapies and BAFF inhibitors could be used either simultaneously in addition to rituximab to provide synergy or solely after rituximab. Last but not least, conventional immune-suppressant agents which target T cells should also be considered given the potential synergistic effect of B and T cell depletion. TPO-Ra’s remain an attractive therapy with potential of treatment free remission after a stable response.
All authors listed have made a substantial, direct and intellectual contribution to the work, and approved it for publication.
IM has served as a consultant for Novartis, Janssen, Sanofi, Alexion, Apellis, Rigel.
The remaining author declares that the research was conducted in the absence of any commercial or financial relationships that could be construed as a potential conflict of interest.
All claims expressed in this article are solely those of the authors and do not necessarily represent those of their affiliated organizations, or those of the publisher, the editors and the reviewers. Any product that may be evaluated in this article, or claim that may be made by its manufacturer, is not guaranteed or endorsed by the publisher.
1. Frederiksen H, Schmidt K. The incidence of idiopathic thrombocytopenic purpura in adults increases with age. Blood (1999) 94:909–13. doi: 10.1182/blood.V94.3.909.415k02_909_913
2. Provan D, Arnold DM, Bussel JB, Chong BH, Cooper N, Gernsheimer T, et al. Updated international consensus report on the investigation and management of primary immune thrombocytopenia. Blood Adv (2019) 3:3780–817. doi: 10.1182/bloodadvances.2019000812
3. Ghanima W, Gernsheimer T, Kuter DJ. How I treat primary ITP in adult patients who are unresponsive to or dependent on corticosteroid treatment. Blood (2021) 137:2736–44. doi: 10.1182/blood.2021010968
4. DeSouza S, Angelini D. Updated guidelines for immune thrombocytopenic purpura: Expanded management options. Cleve Clin J Med (2021) 88:664–8. doi: 10.3949/ccjm.88a.20201
5. Patel VL, Mahevas M, Lee SY, Stasi R, Cunningham-Rundles S, Godeau B, et al. Outcomes 5 years after response to rituximab therapy in children and adults with immune thrombocytopenia. Blood (2012) 119:5989–95. doi: 10.1182/blood-2011-11-393975
6. Harrington WJ, Minnich V, Hollingsworth JW, Moore CV. Demonstration of a thrombocytopenic factor in the blood of patients with thrombocytopenic purpura. J Lab Clin Med (1951) 38:1–10.
7. Chen JF, Yang LH, Chang LX, Feng JJ, Liu JQ. The clinical significance of circulating B cells secreting anti-glycoprotein IIb/IIIa antibody and platelet glycoprotein IIb/IIIa in patients with primary immune thrombocytopenia. Hematology (2012) 17:283–90. doi: 10.1179/1607845412Y.0000000014
8. Canales-Herrerias P, Crickx E, Broketa M, Sokal A, Chenon G, Azzaoui I, et al. High-affinity autoreactive plasma cells disseminate through multiple organs in patients with immune thrombocytopenic purpura. J Clin Invest (2022) 132. doi: 10.1172/JCI153580
9. McMillan R, Tani P, Millard F, Berchtold P, Renshaw L, Woods VL Jr. Platelet-associated and plasma anti-glycoprotein autoantibodies in chronic ITP. Blood (1987) 70:1040–5. doi: 10.1182/blood.V70.4.1040.1040
10. He R, Reid DM, Jones CE, Shulman NR. Spectrum of Ig classes, specificities, and titers of serum antiglycoproteins in chronic idiopathic thrombocytopenic purpura. Blood (1994) 83:1024–32. doi: 10.1182/blood.V83.4.1024.1024
11. Norris PAA, Segel GB, Burack WR, Sachs UJ, Lissenberg-Thunnissen SN, Vidarsson G, et al. FcgammaRI and FcgammaRIII on splenic macrophages mediate phagocytosis of anti-glycoprotein IIb/IIIa autoantibody-opsonized platelets in immune thrombocytopenia. Haematologica (2021) 106:250–4. doi: 10.3324/haematol.2020.248385
12. Najaoui A, Bakchoul T, Stoy J, Bein G, Rummel MJ, Santoso S, et al. Autoantibody-mediated complement activation on platelets is a common finding in patients with immune thrombocytopenic purpura (ITP). Eur J Haematol (2012) 88:167–74. doi: 10.1111/j.1600-0609.2011.01718.x
13. Castelli R, Lambertenghi Delilliers G, Gidaro A, Cicardi M, Bergamaschini L. Complement activation in patients with immune thrombocytopenic purpura according to phases of disease course. Clin Exp Immunol (2020) 201:258–65. doi: 10.1111/cei.13475
14. Peerschke EI, Andemariam B, Yin W, Bussel JB. Complement activation on platelets correlates with a decrease in circulating immature platelets in patients with immune thrombocytopenic purpura. Br J Haematol (2010) 148:638–45. doi: 10.1111/j.1365-2141.2009.07995.x
15. Kurata Y, Curd JG, Tamerius JD, McMillan R. Platelet-associated complement in chronic ITP. Br J Haematol (1985) 60:723–33. doi: 10.1111/j.1365-2141.1985.tb07477.x
16. McMillan R, Wang L, Tomer A, Nichol J, Pistillo J. Suppression of in vitro megakaryocyte production by antiplatelet autoantibodies from adult patients with chronic ITP. Blood (2004) 103:1364–9. doi: 10.1182/blood-2003-08-2672
17. Grozovsky R, Begonja AJ, Liu K, Visner G, Hartwig JH, Falet H, et al. The Ashwell-Morell receptor regulates hepatic thrombopoietin production via JAK2-STAT3 signaling. Nat Med (2015) 21:47–54. doi: 10.1038/nm.3770
18. Li J, van der Wal DE, Zhu G, Xu M, Yougbare I, Ma L, et al. Desialylation is a mechanism of Fc-independent platelet clearance and a therapeutic target in immune thrombocytopenia. Nat Commun (2015) 6:7737. doi: 10.1038/ncomms8737
19. Zeng Q, Zhu L, Tao L, Bao J, Yang M, Simpson EK, et al. Relative efficacy of steroid therapy in immune thrombocytopenia mediated by anti-platelet GPIIbIIIa versus GPIbalpha antibodies. Am J Hematol (2012) 87:206–8. doi: 10.1002/ajh.22211
20. Webster ML, Sayeh E, Crow M, Chen P, Nieswandt B, Freedman J, et al. Relative efficacy of intravenous immunoglobulin G in ameliorating thrombocytopenia induced by antiplatelet GPIIbIIIa versus GPIbalpha antibodies. Blood (2006) 108:943–6. doi: 10.1182/blood-2005-06-009761
21. Revilla N, Corral J, Minano A, Mingot-Castellano ME, Campos RM, Velasco F, et al. Multirefractory primary immune thrombocytopenia; targeting the decreased sialic acid content. Platelets (2019) 30:743–51. doi: 10.1080/09537104.2018.1513476
22. Kuwana M, Iki S, Urabe A. The role of autoantibody-producing plasma cells in immune thrombocytopenic purpura refractory to rituximab. Am J Hematol (2007) 82:846–8. doi: 10.1002/ajh.20951
23. Semple JW, Milev Y, Cosgrave D, Mody M, Hornstein A, Blanchette V, et al. Differences in serum cytokine levels in acute and chronic autoimmune thrombocytopenic purpura: relationship to platelet phenotype and antiplatelet T-cell reactivity. Blood (1996) 87:4245–54. doi: 10.1182/blood.V87.10.4245.bloodjournal87104245
24. Semple JW, Freedman J. Increased antiplatelet T helper lymphocyte reactivity in patients with autoimmune thrombocytopenia. Blood (1991) 78:2619–25. doi: 10.1182/blood.V78.10.2619.2619
25. Zhang J, Ma D, Zhu X, Qu X, Ji C, Hou M. Elevated profile of Th17, Th1 and Tc1 cells in patients with immune thrombocytopenic purpura. Haematologica (2009) 94:1326–9. doi: 10.3324/haematol.2009.007823
26. Sakakura M, Wada H, Tawara I, Nobori T, Sugiyama T, Sagawa N, et al. Reduced Cd4+Cd25+ T cells in patients with idiopathic thrombocytopenic purpura. Thromb Res (2007) 120:187–93. doi: 10.1016/j.thromres.2006.09.008
27. Olsson B, Andersson PO, Jernas M, Jacobsson S, Carlsson B, Carlsson LM, et al. T-cell-mediated cytotoxicity toward platelets in chronic idiopathic thrombocytopenic purpura. Nat Med (2003) 9:1123–4. doi: 10.1038/nm921
28. Qiu J, Liu X, Li X, Zhang X, Han P, Zhou H, et al. CD8(+) T cells induce platelet clearance in the liver via platelet desialylation in immune thrombocytopenia. Sci Rep (2016) 6:27445. doi: 10.1038/srep27445
29. Arnold DM, Nazi I, Toltl LJ, Ross C, Ivetic N, Smith JW, et al. Antibody binding to megakaryocytes in vivo in patients with immune thrombocytopenia. Eur J Haematol (2015) 95:532–7. doi: 10.1111/ejh.12528
30. Iraqi M, Perdomo J, Yan F, Choi PY, Chong BH. Immune thrombocytopenia: antiplatelet autoantibodies inhibit proplatelet formation by megakaryocytes and impair platelet production in vitro. Haematologica (2015) 100:623–32. doi: 10.3324/haematol.2014.115634
31. Sun RJ, Shan NN. Megakaryocytic dysfunction in immune thrombocytopenia is linked to autophagy. Cancer Cell Int (2019) 19:59. doi: 10.1186/s12935-019-0779-0
32. Olsson B, Ridell B, Carlsson L, Jacobsson S, Wadenvik H. Recruitment of T cells into bone marrow of ITP patients possibly due to elevated expression of VLA-4 and CX3CR1. Blood (2008) 112:1078–84. doi: 10.1182/blood-2008-02-139402
33. Li S, Wang L, Zhao C, Li L, Peng J, Hou M. CD8+ T cells suppress autologous megakaryocyte apoptosis in idiopathic thrombocytopenic purpura. Br J Haematol (2007) 139:605–11. doi: 10.1111/j.1365-2141.2007.06737.x
34. Smith MR. Rituximab (monoclonal anti-CD20 antibody): mechanisms of action and resistance. Oncogene (2003) 22:7359–68. doi: 10.1038/sj.onc.1206939
35. Golay J, Semenzato G, Rambaldi A, Foa R, Gaidano G, Gamba E, et al. Lessons for the clinic from rituximab pharmacokinetics and pharmacodynamics. MAbs (2013) 5:826–37. doi: 10.4161/mabs.26008
36. Cooper N, Arnold DM. The effect of rituximab on humoral and cell mediated immunity and infection in the treatment of autoimmune diseases. Br J Haematol (2010) 149:3–13. doi: 10.1111/j.1365-2141.2010.08076.x
37. Randall KL. Rituximab in autoimmune diseases. Aust Prescr (2016) 39:131–4. doi: 10.18773/austprescr.2016.053
38. Cooper N, Stasi R, Cunningham-Rundles S, Feuerstein MA, Leonard JP, Amadori S, et al. The efficacy and safety of B-cell depletion with anti-CD20 monoclonal antibody in adults with chronic immune thrombocytopenic purpura. Br J Haematol (2004) 125:232–9. doi: 10.1111/j.1365-2141.2004.04889.x
39. Braendstrup P, Bjerrum OW, Nielsen OJ, Jensen BA, Clausen NT, Hansen PB, et al. Rituximab chimeric anti-CD20 monoclonal antibody treatment for adult refractory idiopathic thrombocytopenic purpura. Am J Hematol (2005) 78:275–80. doi: 10.1002/ajh.20276
40. Stasi R, Pagano A, Stipa E, Amadori S. Rituximab chimeric anti-CD20 monoclonal antibody treatment for adults with chronic idiopathic thrombocytopenic purpura. Blood (2001) 98:952–7. doi: 10.1182/blood.v98.4.952
41. Penalver FJ, Jimenez-Yuste V, Almagro M, Alvarez-Larran A, Rodriguez L, Casado M, et al. Rituximab in the management of chronic immune thrombocytopenic purpura: an effective and safe therapeutic alternative in refractory patients. Ann Hematol (2006) 85:400–6. doi: 10.1007/s00277-005-0073-1
42. Zaja F, Battista ML, Pirrotta MT, Palmieri S, Montagna M, Vianelli N, et al. Lower dose rituximab is active in adults patients with idiopathic thrombocytopenic purpura. Haematologica (2008) 93:930–3. doi: 10.3324/haematol.12206
43. Marangon M, Vianelli N, Palandri F, Mazzucconi MG, Santoro C, Barcellini W, et al. Rituximab in immune thrombocytopenia: gender, age, and response as predictors of long-term response. Eur J Haematol (2017) 98:371–7. doi: 10.1111/ejh.12839
44. Khellaf M, Charles-Nelson A, Fain O, Terriou L, Viallard JF, Cheze S, et al. Safety and efficacy of rituximab in adult immune thrombocytopenia: results from a prospective registry including 248 patients. Blood (2014) 124:3228–36. doi: 10.1182/blood-2014-06-582346
45. Chapin J, Lee CS, Zhang H, Zehnder JL, Bussel JB. Gender and duration of disease differentiate responses to rituximab-dexamethasone therapy in adults with immune thrombocytopenia. Am J Hematol (2016) 91:907–11. doi: 10.1002/ajh.24434
46. Harris EM, Hillier K, Al-Samkari H, Berbert L, Grace RF. Response to rituximab in children and adults with immune thrombocytopenia (ITP). Res Pract Thromb Haemost (2021) 5:e12587. doi: 10.1002/rth2.12587
47. Feng Y, Xiao Y, Yan H, Wang P, Zhu W, Cassady K, et al. Sirolimus as rescue therapy for refractory/relapsed immune thrombocytopenia: results of a single-center, prospective, single-arm study. Front Med (Lausanne) (2020) 7:110. doi: 10.3389/fmed.2020.00110
48. Kado R, Sanders G, McCune WJ. Suppression of normal immune responses after treatment with rituximab. Curr Opin Rheumatol (2016) 28:251–8. doi: 10.1097/BOR.0000000000000272
49. Crickx E, Chappert P, Sokal A, Weller S, Azzaoui I, Vandenberghe A, et al. Rituximab-resistant splenic memory B cells and newly engaged naive B cells fuel relapses in patients with immune thrombocytopenia. Sci Transl Med (2021) 13. doi: 10.1126/scitranslmed.abc3961
50. Mahevas M, Patin P, Huetz F, Descatoire M, Cagnard N, Bole-Feysot C, et al. B cell depletion in immune thrombocytopenia reveals splenic long-lived plasma cells. J Clin Invest (2013) 123:432–42. doi: 10.1172/JCI65689
51. Thai LH, Le Gallou S, Robbins A, Crickx E, Fadeev T, Zhou Z, et al. BAFF and CD4(+) T cells are major survival factors for long-lived splenic plasma cells in a B-cell-depletion context. Blood (2018) 131:1545–55. doi: 10.1182/blood-2017-06-789578
52. Stasi R, Cooper N, Del Poeta G, Stipa E, Laura Evangelista M, Abruzzese E, et al. Analysis of regulatory T-cell changes in patients with idiopathic thrombocytopenic purpura receiving B cell-depleting therapy with rituximab. Blood (2008) 112:1147–50. doi: 10.1182/blood-2007-12-129262
53. Stasi R, Del Poeta G, Stipa E, Evangelista ML, Trawinska MM, Cooper N, et al. Response to B-cell depleting therapy with rituximab reverts the abnormalities of T-cell subsets in patients with idiopathic thrombocytopenic purpura. Blood (2007) 110:2924–30. doi: 10.1182/blood-2007-02-068999
54. Audia S, Samson M, Mahevas M, Ferrand C, Trad M, Ciudad M, et al. Preferential splenic CD8(+) T-cell activation in rituximab-nonresponder patients with immune thrombocytopenia. Blood (2013) 122:2477–86. doi: 10.1182/blood-2013-03-491415
55. Ma H, Wang Y, Sun L, Gong H. [Absence of response to rituximab in patients with refractory primary immune thrombocytopenia]. Zhonghua Yi Xue Za Zhi (2014) 94:3011–3. doi: 10.3760/cma.j.issn.0376-2491.2014.38.014
56. Dai L, He L, Wang Z, Bai X, He Y, Cao L, et al. Altered circulating T follicular helper cells in patients with chronic immune thrombocytopenia. Exp Ther Med (2018) 16:2471–7. doi: 10.3892/etm.2018.6508
57. Goenka R, Matthews AH, Zhang B, O'Neill PJ, Scholz JL, Migone TS, et al. Local BLyS production by T follicular cells mediates retention of high affinity B cells during affinity maturation. J Exp Med (2014) 211:45–56. doi: 10.1084/jem.20130505
58. Audia S, Rossato M, Santegoets K, Spijkers S, Wichers C, Bekker C, et al. Splenic TFH expansion participates in B-cell differentiation and antiplatelet-antibody production during immune thrombocytopenia. Blood (2014) 124:2858–66. doi: 10.1182/blood-2014-03-563445
59. Al Askar AS, Shaheen NA, Al Zahrani M, Al Otaibi MG, Al Qahtani BS, Ahmed F, et al. Splenectomy vs. rituximab as a second-line therapy in immune thrombocytopenic purpura: a single center experience. Int J Hematol (2018) 107:69–74. doi: 10.1007/s12185-017-2325-y
60. Moulis G, Sailler L, Sommet A, Lapeyre-Mestre M, Derumeaux H, Adoue D. Rituximab versus splenectomy in persistent or chronic adult primary immune thrombocytopenia: an adjusted comparison of mortality and morbidity. Am J Hematol (2014) 89:41–6. doi: 10.1002/ajh.23580
61. Hammond WA, Vishnu P, Rodriguez EM, Li Z, Dholaria B, Shreders AJ, et al. Sequence of splenectomy and rituximab for the treatment of steroid-refractory immune thrombocytopenia: does it matter? Mayo Clin Proc (2019) 94:2199–208. doi: 10.1016/j.mayocp.2019.05.024
62. Ay Y, Karapinar TH, Oymak Y, Toret E, Demirag B, Ince D, et al. Retrospective analysis of rituximab therapy and splenectomy in childhood chronic and refractory immune thrombocytopenic purpura. Blood Coagul Fibrinolysis (2016) 27:431–5. doi: 10.1097/MBC.0000000000000488
63. Cooper N, Evangelista ML, Amadori S, Stasi R. Should rituximab be used before or after splenectomy in patients with immune thrombocytopenic purpura? Curr Opin Hematol (2007) 14:642–6. doi: 10.1097/MOH.0b013e3282c8ca50
64. Qu M, Zhou J, Yang SJ, Zhou ZP. Efficacy and safety of rituximab for minors with immune thrombocytopenia: a systematic review and meta-analysis. J Int Med Res (2020) 48:300060520962348. doi: 10.1177/0300060520962348
65. Rip J, van der Ploeg EK, Hendriks RW, Corneth OBJ. The role of bruton's tyrosine kinase in immune cell signaling and systemic autoimmunity. Crit Rev Immunol (2018) 38:17–62. doi: 10.1615/CritRevImmunol.2018025184
66. Crofford LJ, Nyhoff LE, Sheehan JH, Kendall PL. The role of Bruton's tyrosine kinase in autoimmunity and implications for therapy. Expert Rev Clin Immunol (2016) 12:763–73. doi: 10.1586/1744666X.2016.1152888
67. Newland A, McDonald V. Fostamatinib: a review of its clinical efficacy and safety in the management of chronic adult immune thrombocytopenia. Immunotherapy (2020) 12:1325–40. doi: 10.2217/imt-2020-0215
68. Blumberg LJ, Humphries JE, Jones SD, Pearce LB, Holgate R, Hearn A, et al. Blocking FcRn in humans reduces circulating IgG levels and inhibits IgG immune complex-mediated immune responses. Sci Adv (2019) 5:eaax9586. doi: 10.1126/sciadv.aax9586
69. Bussel J, Arnold DM, Grossbard E, Mayer J, Trelinski J, Homenda W, et al. Fostamatinib for the treatment of adult persistent and chronic immune thrombocytopenia: Results of two phase 3, randomized, placebo-controlled trials. Am J Hematol (2018) 93:921–30. doi: 10.1002/ajh.25125
70. Bussel JB, Arnold DM, Boxer MA, Cooper N, Mayer J, Zayed H, et al. Long-term fostamatinib treatment of adults with immune thrombocytopenia during the phase 3 clinical trial program. Am J Hematol (2019) 94:546–53. doi: 10.1002/ajh.25444
71. Nasillo V, Lagreca I, Vallerini D, Barozzi P, Riva G, Maccaferri M, et al. BTK inhibitors impair platelet-mediated antifungal activity. Cells (2022) 11. doi: 10.3390/cells11061003
72. Owens TD, Brameld KA, Verner EJ, Ton T, Li X, Zhu J, et al. Discovery of reversible covalent bruton's tyrosine kinase inhibitors PRN473 and PRN1008 (Rilzabrutinib). J Med Chem (2022) 65:5300–16. doi: 10.1021/acs.jmedchem.1c01170
73. Duan R, Goldmann L, Brandl R, Spannagl M, Weber C, Siess W, et al. Effects of the btk-inhibitors remibrutinib (LOU064) and rilzabrutinib (PRN1008) with varying btk selectivity over tec on platelet aggregation and in vitro bleeding time. Front Cardiovasc Med (2021) 8:749022. doi: 10.3389/fcvm.2021.749022
74. Langrish CL, Bradshaw JM, Francesco MR, Owens TD, Xing Y, Shu J, et al. Preclinical efficacy and anti-inflammatory mechanisms of action of the bruton tyrosine kinase inhibitor rilzabrutinib for immune-mediated disease. J Immunol (2021) 206:1454–68. doi: 10.4049/jimmunol.2001130
75. Kuter DJ, Efraim M, Mayer J, Trneny M, McDonald V, Bird R, et al. Rilzabrutinib, an oral BTK inhibitor, in immune thrombocytopenia. N Engl J Med (2022) 386:1421–31. doi: 10.1056/NEJMoa2110297
76. Kuo TT, Aveson VG. Neonatal Fc receptor and IgG-based therapeutics. MAbs (2011) 3:422–30. doi: 10.4161/mabs.3.5.16983
77. Robak T, Kazmierczak M, Jarque I, Musteata V, Trelinski J, Cooper N, et al. Phase 2 multiple-dose study of an FcRn inhibitor, rozanolixizumab, in patients with primary immune thrombocytopenia. Blood Adv (2020) 4:4136–46. doi: 10.1182/bloodadvances.2020002003
78. Newland AC, Sanchez-Gonzalez B, Rejto L, Egyed M, Romanyuk N, Godar M, et al. Phase 2 study of efgartigimod, a novel FcRn antagonist, in adult patients with primary immune thrombocytopenia. Am J Hematol (2020) 95:178–87. doi: 10.1002/ajh.25680
79. Broome CM, McDonald V, Miyakawa Y, Carpenedo M, Kuter DJ, Al-Samkari H, et al. Efficacy and safety of intravenous efgartigimod in adults with primary immune thrombocytopenia: results of a phase 3, multicenter, double-blinded, placebo-controlled, randomized clinical trial (ADVANCE IV). Blood (2022) 140:6–8. doi: 10.1182/blood-2022-167838
80. Sehgal SN. Sirolimus: its discovery, biological properties, and mechanism of action. Transplant Proc (2003) 35:7S–14S. doi: 10.1016/s0041-1345(03)00211-2
81. Zheng P, Chang X, Lu Q, Liu Y. Cytopenia and autoimmune diseases: a vicious cycle fueled by mTOR dysregulation in hematopoietic stem cells. J Autoimmun (2013) 41:182–7. doi: 10.1016/j.jaut.2012.12.011
82. Teachey DT, Greiner R, Seif A, Attiyeh E, Bleesing J, Choi J, et al. Treatment with sirolimus results in complete responses in patients with autoimmune lymphoproliferative syndrome. Br J Haematol (2009) 145(1):101–6. doi: 10.1111/j.1365-2141.2009.07595.x
83. Miano M, Scalzone M, Perri K, Palmisani E, Caviglia I, Micalizzi C, et al. Mycofenolate mofetil and sirolimus as second or further line treatment in children with chronic refractory primary or secondary autoimmune cytopenias: a single center experience. Br J Haematol (2015) 171(2):247–253. doi: 10.1111/bjh.13533
84. Bride KL, Vincent T, Smith-Whitley K, Lambert MP, Bleesing JJ, Seif AE, et al. Sirolimus is effective in relapsed/refractory autoimmune cytopenias: results of a prospective multi-institutional trial. Blood (2016) 127(1):17–28. doi: 10.1182/blood-2015-07-657981
85. Gao L, Yan H, Wang S, Liu F, Wang J, Huang X, et al. Sirolimus is effective in refractory immune thrombocytopenia: results of an open-label prospective multicenter trial in china. Blood (2017) 130:4728–8. doi: 10.1182/blood.V130.Suppl_1.4728.4728
86. Miano M, Rotulo GA, Palmisani E, Giaimo M, Fioredda F, Pierri F, et al. Sirolimus as a rescue therapy in children with immune thrombocytopenia refractory to mycophenolate mofetil. Am J Hematol (2018) 93:E175–7. doi: 10.1002/ajh.25119
87. Li J, Wang Z, Dai L, Cao L, Su J, Zhu M, et al. Effects of rapamycin combined with low dose prednisone in patients with chronic immune thrombocytopenia. Clin Dev Immunol (2013) 2013:548085. doi: 10.1155/2013/548085
88. Field-Smith A, Morgan GJ, Davies FE. Bortezomib (Velcadetrade mark) in the treatment of multiple myeloma. Ther Clin Risk Manag (2006) 2:271–9. doi: 10.2147/tcrm.2006.2.3.271
89. Pellom ST Jr., Dudimah DF, Thounaojam MC, Sayers TJ, Shanker A. Modulatory effects of bortezomib on host immune cell functions. Immunotherapy (2015) 7:1011–22. doi: 10.2217/imt.15.66
90. Li G, Wang S, Li N, Liu Y, Feng Q, Zuo X, et al. Proteasome inhibition with bortezomib induces apoptosis of long-lived plasma cells in steroid-resistant or relapsed immune thrombocytopaenia. Thromb Haemost (2018) 118:1752–64. doi: 10.1055/s-0038-1669921
91. Mazepa MA, Raval JS, Moll S, Ma A, Park YA. Bortezomib induces clinical remission and reduction of ADAMTS13 inhibitory antibodies in relapsed refractory idiopathic thrombotic thrombocytopenic purpura. Br J Haematol (2014) 164:900–2. doi: 10.1111/bjh.12696
92. Shortt J, Oh DH, Opat SS. ADAMTS13 antibody depletion by bortezomib in thrombotic thrombocytopenic purpura. N Engl J Med (2013) 368:90–2. doi: 10.1056/NEJMc1213206
93. Pasquale R, Giannotta JA, Barcellini W, Fattizzo B. Bortezomib in autoimmune hemolytic anemia and beyond. Ther Adv Hematol (2021) 12:20406207211046428. doi: 10.1177/20406207211046428
94. Xiao Z, Murakhovskaya I. Development of new drugs for autoimmune hemolytic anemia. Pharmaceutics (2022) 14. doi: 10.3390/pharmaceutics14051035
95. Beckman JD, Rollins-Raval MA, Raval JS, Park YA, Mazepa M, Ma A. Bortezomib for refractory immune-mediated thrombocytopenia purpura. Am J Ther (2018) 25:e270–2. doi: 10.1097/MJT.0000000000000517
96. Itoh T. Multiple myeloma developing in a patient with immune thrombocytopenia. Rinsho Ketsueki (2016) 57:630–3. doi: 10.11406/rinketsu.57.630
97. Yao H, Zhang X, Liu J, Zhu L, Chen G, Wu S, et al. Multiple myeloma developing during long-term clinical course of refractory immune thrombocytopenic purpura: a case report and review of literature. Int J Clin Exp Pathol (2015) 8:15429–32.
98. Conti F, Gottardi F, Moratti M, Belotti T, Ferrari S, Selva P, et al. Refractory immune thrombocytopenia successfully treated with bortezomib in a child with 22q11.2 deletion syndrome, complicated by Evans syndrome and hypogammaglobulinemia. Platelets (2022) 33:801–6. doi: 10.1080/09537104.2021.2002835
99. de Weers M, Tai YT, van der Veer MS, Bakker JM, Vink T, Jacobs DC, et al. Daratumumab, a novel therapeutic human CD38 monoclonal antibody, induces killing of multiple myeloma and other hematological tumors. J Immunol (2011) 186:1840–8. doi: 10.4049/jimmunol.1003032
100. Barcellini W, Fattizzo B. The changing landscape of autoimmune hemolytic anemia. Front Immunol (2020) 11:946. doi: 10.3389/fimmu.2020.00946
101. Jain A, Gupta DK. Daratumumab for refractory warm autoimmune hemolytic anemia. Ann Hematol (2021) 100:1351–3. doi: 10.1007/s00277-020-04063-w
102. Zaninoni A, Giannotta JA, Galli A, Artuso R, Bianchi P, Malcovati L, et al. The immunomodulatory effect and clinical efficacy of daratumumab in a patient with cold agglutinin disease. Front Immunol (2021) 12:649441. doi: 10.3389/fimmu.2021.649441
103. Ostendorf L, Burns M, Durek P, Heinz GA, Heinrich F, Garantziotis P, et al. Targeting CD38 with daratumumab in refractory systemic lupus erythematosus. N Engl J Med (2020) 383:1149–55. doi: 10.1056/NEJMoa2023325
104. Migdady Y, Ediriwickrema A, Jackson RP, Kadi W, Gupta R, Socola F, et al. Successful treatment of thrombocytopenia with daratumumab after allogeneic transplant: a case report and literature review. Blood Adv (2020) 4:815–8. doi: 10.1182/bloodadvances.2019001215
105. Driouk L, Schmitt R, Peters A, Heine S, Girschick HJ, Strahm B, et al. Daratumumab therapy for post-HSCT immune-mediated cytopenia: experiences from two pediatric cases and review of literature. Mol Cell Pediatr (2021) 8:5. doi: 10.1186/s40348-021-00114-y
106. Blennerhassett R, Sudini L, Gottlieb D, Bhattacharyya A. Post-allogeneic transplant Evans syndrome successfully treated with daratumumab. Br J Haematol (2019) 187:e48–51. doi: 10.1111/bjh.16171
107. Khandelwal P, Teusink-Cross A, Kumar AR, Bleesing JJ, Mehta PA, Jordan MB, et al. Daratumumab for the management of autoimmune cytopenias in children and young adults: a case series. Br J Haematol (2021) 194:e84–9. doi: 10.1111/bjh.17565
108. Crickx E, Audia S, Robbins A, Boutboul D, Comont T, Cheminant M, et al. Daratumumab, an original approach for treating multi-refractory autoimmune cytopenia. Haematologica (2021) 106:3198–201. doi: 10.3324/haematol.2021.279232
109. Vernava I, Schmitt CA. Daratumumab as a novel treatment option in refractory ITP. Blood Cells Mol Dis (2023) 99:102724. doi: 10.1016/j.bcmd.2023.102724
110. Strussmann T, Jung J, Heinz J, Duque Afonso J, Wasch R, Engelhardt M, et al. Long-term complete remission of refractory severe idiopathic immune thrombocytopenia (ITP) treated with daratumumab. Ann Hematol (2023) 102:245–7. doi: 10.1007/s00277-022-05035-y
111. Tsykunova G, Holme PA, Thi Thyuet Tran H, Tvedt THA, Munthe L, Michel M, et al. PB2313: DARATUMUMAB AS A TREATMENT FOR ADULT IMMUNE THROMBOCYTOPENIA: A PHASE II STUDY WITH SAFETY RUN-IN (THE DART STUDY). HemaSphere (2022) 6:2182–3. doi: 10.1097/01.Hs9.0000852076.33574.89
112. Halpern WG, Lappin P, Zanardi T, Cai W, Corcoran M, Zhong J, et al. Chronic administration of belimumab, a BLyS antagonist, decreases tissue and peripheral blood B-lymphocyte populations in cynomolgus monkeys: pharmacokinetic, pharmacodynamic, and toxicologic effects. Toxicol Sci (2006) 91:586–99. doi: 10.1093/toxsci/kfj148
113. Dubey AK, Handu SS, Dubey S, Sharma P, Sharma KK, Ahmed QM. Belimumab: First targeted biological treatment for systemic lupus erythematosus. J Pharmacol Pharmacother (2011) 2:317–9. doi: 10.4103/0976-500X.85930
114. Mahevas M, Azzaoui I, Crickx E, Canoui-Poitrine F, Gobert D, Languille L, et al. Efficacy, safety and immunological profile of combining rituximab with belimumab for adults with persistent or chronic immune thrombocytopenia: results from a prospective phase 2b trial. Haematologica (2021) 106:2449–57. doi: 10.3324/haematol.2020.259481
115. Dorner T, Posch MG, Li Y, Petricoul O, Cabanski M, Milojevic JM, et al. Treatment of primary Sjogren's syndrome with ianalumab (VAY736) targeting B cells by BAFF receptor blockade coupled with enhanced, antibody-dependent cellular cytotoxicity. Ann Rheum Dis (2019) 78:641–7. doi: 10.1136/annrheumdis-2018-214720
116. McWilliams EM, Lucas CR, Chen T, Harrington BK, Wasmuth R, Campbell A, et al. Anti-BAFF-R antibody VAY-736 demonstrates promising preclinical activity in CLL and enhances effectiveness of ibrutinib. Blood Adv (2019) 3:447–60. doi: 10.1182/bloodadvances.2018025684
117. Bowman SJ, Fox R, Dorner T, Mariette X, Papas A, Grader-Beck T, et al. Safety and efficacy of subcutaneous ianalumab (VAY736) in patients with primary Sjogren's syndrome: a randomised, double-blind, placebo-controlled, phase 2b dose-finding trial. Lancet (2022) 399:161–71. doi: 10.1016/S0140-6736(21)02251-0
118. Frampton JE. Inebilizumab: first approval. Drugs (2020) 80:1259–64. doi: 10.1007/s40265-020-01370-4
119. Szili D, Cserhalmi M, Banko Z, Nagy G, Szymkowski DE, Sarmay G. Suppression of innate and adaptive B cell activation pathways by antibody coengagement of FcgammaRIIb and CD19. MAbs (2014) 6:991–9. doi: 10.4161/mabs.28841
120. Chu SY, Yeter K, Kotha R, Pong E, Miranda Y, Phung S, et al. Suppression of rheumatoid arthritis B cells by XmAb5871, an anti-CD19 antibody that coengages B cell antigen receptor complex and Fcgamma receptor IIb inhibitory receptor. Arthritis Rheumatol (2014) 66:1153–64. doi: 10.1002/art.38334
121. Bates SE. Epigenetic therapies for cancer. N Engl J Med (2020) 383:650–63. doi: 10.1056/NEJMra1805035
122. Han P, Hou Y, Zhao Y, Liu Y, Yu T, Sun Y, et al. Low-dose decitabine modulates T-cell homeostasis and restores immune tolerance in immune thrombocytopenia. Blood (2021) 138:674–88. doi: 10.1182/blood.2020008477
123. Han P, Yu T, Hou Y, Zhao Y, Liu Y, Sun Y, et al. Low-dose decitabine inhibits cytotoxic T lymphocytes-mediated platelet destruction via modulating PD-1 methylation in immune thrombocytopenia. Front Immunol (2021) 12:630693. doi: 10.3389/fimmu.2021.630693
124. Zhou H, Qin P, Liu Q, Yuan C, Hao Y, Zhang H, et al. A prospective, multicenter study of low dose decitabine in adult patients with refractory immune thrombocytopenia. Am J Hematol (2019) 94:1374–81. doi: 10.1002/ajh.25646
125. Allison AC. Mechanisms of action of mycophenolate mofetil. Lupus (2005) 14 Suppl 1:s2–8. doi: 10.1191/0961203305lu2109oa
126. Xu J, Wang G, Tan S, Ge Y, Liu J, Rao M, et al. The clinical effect of Prednisone in combination with Mycophenolate mofetil on idiopathic thrombocytopenic purpura (ITP) and its influence on the level of peripheral blood T lymphocytes and NK lymphocytes. Saudi J Biol Sci (2019) 26:2108–12. doi: 10.1016/j.sjbs.2019.09.013
127. Zhang WG, Ji L, Cao XM, Chen YX, He AL, Liu J, et al. Mycophenolate mofetil as a treatment for refractory idiopathic thrombocytopenic purpura. Acta Pharmacol Sin (2005) 26:598–602. doi: 10.1111/j.1745-7254.2005.00088.x
128. Bruserud O, Havardstein K. Should low-dose mycophenolate mofetil be used to prolong the response after rituximab therapy in patients with immune thrombocytopenic purpura? A case report. Hematology (2009) 14:224–6. doi: 10.1179/102453309X439782
129. Provan D, Moss AJ, Newland AC, Bussel JB. Efficacy of mycophenolate mofetil as single-agent therapy for refractory immune thrombocytopenic purpura. Am J Hematol (2006) 81:19–25. doi: 10.1002/ajh.20515
130. Howard J, Hoffbrand AV, Prentice HG, Mehta A. Mycophenolate mofetil for the treatment of refractory auto-immune haemolytic anaemia and auto-immune thrombocytopenia purpura. Br J Haematol (2002) 117:712–5. doi: 10.1046/j.1365-2141.2002.03430.x
131. Arnold DM, Nazi I, Santos A, Chan H, Heddle NM, Warkentin TE, et al. Combination immunosuppressant therapy for patients with chronic refractory immune thrombocytopenic purpura. Blood (2010) 115:29–31. doi: 10.1182/blood-2009-06-222448
132. Hou M, Peng J, Shi Y, Zhang C, Qin P, Zhao C, et al. Mycophenolate mofetil (MMF) for the treatment of steroid-resistant idiopathic thrombocytopenic purpura. Eur J Haematol (2003) 70:353–7. doi: 10.1034/j.1600-0609.2003.00076.x
133. Colovic M, Suvajdzic N, Colovic N, Tomin D, Vidovic A, Palibrk V. Mycophenolate mophetil therapy for chronic immune thrombocytopenic purpura resistant to steroids, immunosuppressants, and/or splenectomy in adults. Platelets (2011) 22:153–6. doi: 10.3109/09537104.2010.520372
134. Kotb R, Pinganaud C, Trichet C, Lambotte O, Dreyfus M, Delfraissy JF, et al. Efficacy of mycophenolate mofetil in adult refractory auto-immune cytopenias: a single center preliminary study. Eur J Haematol (2005) 75:60–4. doi: 10.1111/j.1600-0609.2005.00437.x
135. Taylor A, Neave L, Solanki S, Westwood JP, Terrinonive I, McGuckin S, et al. Mycophenolate mofetil therapy for severe immune thrombocytopenia. Br J Haematol (2015) 171:625–30. doi: 10.1111/bjh.13622
136. Bradbury CA, Pell J, Hill Q, Bagot C, Cooper N, Ingram J, et al. Mycophenolate mofetil for first-line treatment of immune thrombocytopenia. N Engl J Med (2021) 385:885–95. doi: 10.1056/NEJMoa2100596
137. Matsuda S, Koyasu S. Mechanisms of action of cyclosporine. Immunopharmacology (2000) 47:119–25. doi: 10.1016/s0162-3109(00)00192-2
138. Kappers-Klunne MC, van't Veer MB. Cyclosporin A for the treatment of patients with chronic idiopathic thrombocytopenic purpura refractory to corticosteroids or splenectomy. Br J Haematol (2001) 114:121–5. doi: 10.1046/j.1365-2141.2001.02893.x
139. Emilia G, Luppi M, Morselli M, Forghieri F, Potenza L, Torelli G. A possible role for low-dose cyclosporine in refractory immune thrombocytopenic purpura. Haematologica (2008) 93:1113–5. doi: 10.3324/haematol.12741
140. Zver S, Zupan IP, Cernelc P. Cyclosporin A as an immunosuppressive treatment modality for patients with refractory autoimmune thrombocytopenic purpura after splenectomy failure. Int J Hematol (2006) 83:238–42. doi: 10.1532/IJH97.05149
141. Hlusi A, Szotkowski T, Indrak K. Refractory immune thrombocytopenia. Successful treatment with repeated cyclosporine A: two case reports. Clin Case Rep (2015) 3:337–41. doi: 10.1002/ccr3.182
142. Liu AP, Cheuk DK, Lee AH, Lee PP, Chiang AK, Ha SY, et al. Cyclosporin A for persistent or chronic immune thrombocytopenia in children. Ann Hematol (2016) 95:1881–6. doi: 10.1007/s00277-016-2791-y
143. Fattizzo B, Cantoni S, Giannotta JA, Bandiera L, Zavaglia R, Bortolotti M, et al. Efficacy and safety of cyclosporine A treatment in autoimmune cytopenias: the experience of two Italian reference centers. Ther Adv Hematol (2022) 13:20406207221097780. doi: 10.1177/20406207221097780
144. Choi PY-I, Roncolato F, Badoux X, Ramanathan S, Ho S-J, Chong BH. A novel triple therapy for ITP using high-dose dexamethasone, low-dose rituximab, and cyclosporine (TT4). Blood (2015) 126:500–3. doi: 10.1182/blood-2015-03-631937
145. Li X, Zhu W, Bao J, Li J, Zhou Y. Efficacy and safety of cyclosporine-based regimens for primary immune thrombocytopenia: a systematic review and meta-analysis. J Int Med Res (2023) 51:3000605221149870. doi: 10.1177/03000605221149870
146. Colunga-Pedraza PR, Pena-Lozano SP, Sanchez-Rendon E, de la Garza-Salazar F, Colunga-Pedraza JE, Gomez-De Leon A, et al. Oseltamivir as rescue therapy for persistent, chronic, or refractory immune thrombocytopenia: a case series and review of the literature. J Thromb Thrombolysis (2022) 54:360–6. doi: 10.1007/s11239-022-02651-3
147. Sun L, Wang J, Shao L, Yuan C, Zhao H, Li D, et al. Dexamethasone plus oseltamivir versus dexamethasone in treatment-naive primary immune thrombocytopenia: a multicentre, randomised, open-label, phase 2 trial. Lancet Haematol (2021) 8:e289–98. doi: 10.1016/S2352-3026(21)00030-2
148. Ghanima W, Cooper N, Rodeghiero F, Godeau B, Bussel JB. Thrombopoietin receptor agonists: ten years later. Haematologica (2019) 104:1112–23. doi: 10.3324/haematol.2018.212845
149. Bussel JB, Kuter DJ, George JN, McMillan R, Aledort LM, Conklin GT, et al. AMG 531, a thrombopoiesis-stimulating protein, for chronic ITP. N Engl J Med (2006) 355:1672–81. doi: 10.1056/NEJMoa054626
150. Kuter DJ, Bussel JB, Lyons RM, Pullarkat V, Gernsheimer TB, Senecal FM, et al. Efficacy of romiplostim in patients with chronic immune thrombocytopenic purpura: a double-blind randomised controlled trial. Lancet (2008) 371:395–403. doi: 10.1016/S0140-6736(08)60203-2
151. Cheng G, Saleh MN, Marcher C, Vasey S, Mayer B, Aivado M, et al. Eltrombopag for management of chronic immune thrombocytopenia (RAISE): a 6-month, randomised, phase 3 study. Lancet (2011) 377:393–402. doi: 10.1016/S0140-6736(10)60959-2
152. Jurczak W, Chojnowski K, Mayer J, Krawczyk K, Jamieson BD, Tian W, et al. Phase 3 randomised study of avatrombopag, a novel thrombopoietin receptor agonist for the treatment of chronic immune thrombocytopenia. Br J Haematol (2018) 183:479–90. doi: 10.1111/bjh.15573
153. Bussel JB, Provan D, Shamsi T, Cheng G, Psaila B, Kovaleva L, et al. Effect of eltrombopag on platelet counts and bleeding during treatment of chronic idiopathic thrombocytopenic purpura: a randomised, double-blind, placebo-controlled trial. Lancet (2009) 373:641–8. doi: 10.1016/S0140-6736(09)60402-5
154. Kuter DJ, Rummel M, Boccia R, Macik BG, Pabinger I, Selleslag D, et al. Romiplostim or standard of care in patients with immune thrombocytopenia. N Engl J Med (2010) 363:1889–99. doi: 10.1056/NEJMoa1002625
155. Olnes MJ, Scheinberg P, Calvo KR, Desmond R, Tang Y, Dumitriu B, et al. Eltrombopag and improved hematopoiesis in refractory aplastic anemia. N Engl J Med (2012) 367:11–9. doi: 10.1056/NEJMoa1200931
156. Kim ES. Lusutrombopag: first global approval. Drugs (2016) 76:155–8. doi: 10.1007/s40265-015-0525-4
157. Mei H, Liu X, Li Y, Zhou H, Feng Y, Gao G, et al. A multicenter, randomized phase III trial of hetrombopag: a novel thrombopoietin receptor agonist for the treatment of immune thrombocytopenia. J Hematol Oncol (2021) 14:37. doi: 10.1186/s13045-021-01047-9
158. Gilreath J, Lo M, Bubalo J. Thrombopoietin receptor agonists (TPO-RAs): drug class considerations for pharmacists. Drugs (2021) 81:1285–305. doi: 10.1007/s40265-021-01553-7
159. Gonzalez-Porras JR, Godeau B, Carpenedo M. Switching thrombopoietin receptor agonist treatments in patients with primary immune thrombocytopenia. Ther Adv Hematol (2019) 10:2040620719837906. doi: 10.1177/2040620719837906
160. Al-Samkari H, Jiang D, Gernsheimer T, Liebman H, Lee S, Wojdyla M, et al. Adults with immune thrombocytopenia who switched to avatrombopag following prior treatment with eltrombopag or romiplostim: A multicentre US study. Br J Haematol (2022) 197:359–66. doi: 10.1111/bjh.18081
161. Mahevas M, Fain O, Ebbo M, Roudot-Thoraval F, Limal N, Khellaf M, et al. The temporary use of thrombopoietin-receptor agonists may induce a prolonged remission in adult chronic immune thrombocytopenia. Results of a French observational study. Br J Haematol (2014) 165:865–9. doi: 10.1111/bjh.12888
162. Bao W, Bussel JB, Heck S, He W, Karpoff M, Boulad N, et al. Improved regulatory T-cell activity in patients with chronic immune thrombocytopenia treated with thrombopoietic agents. Blood (2010) 116:4639–45. doi: 10.1182/blood-2010-04-281717
163. Guillet S, Crickx E, Azzaoui I, Chappert P, Boutin E, Viallard JF, et al. Prolonged response after TPO-RA discontinuation in primary ITP: results of a prospective multicenter study. Blood (2023) 171(23):2867–77. doi: 10.1182/blood.2022018665
164. Peerschke EI, Panicker S, Bussel J. Classical complement pathway activation in immune thrombocytopenia purpura: inhibition by a novel C1s inhibitor. Br J Haematol (2016) 173:942–5. doi: 10.1111/bjh.13648
Keywords: ITP, immune thrombocytopenia, rituximab, rituximab refractory, rituximab resistance, novel therapies
Citation: Xiao Z and Murakhovskaya I (2023) Rituximab resistance in ITP and beyond. Front. Immunol. 14:1215216. doi: 10.3389/fimmu.2023.1215216
Received: 01 May 2023; Accepted: 10 July 2023;
Published: 28 July 2023.
Edited by:
David Gomez-almaguer, Autonomous University of Nuevo León, MexicoReviewed by:
Natasa Stanisavljevic, University Hospital Medical Center Bezanijska kosa, SerbiaCopyright © 2023 Xiao and Murakhovskaya. This is an open-access article distributed under the terms of the Creative Commons Attribution License (CC BY). The use, distribution or reproduction in other forums is permitted, provided the original author(s) and the copyright owner(s) are credited and that the original publication in this journal is cited, in accordance with accepted academic practice. No use, distribution or reproduction is permitted which does not comply with these terms.
*Correspondence: Irina Murakhovskaya, aW11cmFraG9AbW9udGVmaW9yZS5vcmc=
Disclaimer: All claims expressed in this article are solely those of the authors and do not necessarily represent those of their affiliated organizations, or those of the publisher, the editors and the reviewers. Any product that may be evaluated in this article or claim that may be made by its manufacturer is not guaranteed or endorsed by the publisher.
Research integrity at Frontiers
Learn more about the work of our research integrity team to safeguard the quality of each article we publish.