- Department of Internal Medicine, Section of Immunomodulation and Regenerative Cell Therapy, Leiden University Medical Center, Leiden, Netherlands
The current standard of care for type 1 diabetes patients is limited to treatment of the symptoms of the disease, insulin insufficiency and its complications, not its cause. Given the autoimmune nature of type 1 diabetes, immunology is critical to understand the mechanism of disease progression, patient and disease heterogeneity and therapeutic action. Immune monitoring offers the key to all this essential knowledge and is therefore indispensable, despite the challenges and costs associated. In this perspective, I attempt to make this case by providing evidence from the past to create a perspective for future trials and patient selection.
Introduction
The type 1 diabetes (T1D) community has been blessed with an impressive gain in insight into the immunopathogenesis of T1D in recent years. Detection of islet autoantibodies has been standard of practice to confirm a diagnosis of T1D or to predict future development of this disease (1). Islet autoantibodies have proven unambiguous, robust and specific immune correlates of risk and development of T1D (2). With the autoantibody targets (pro)insulin, zinc-transporter 8 (ZnT8), glutamic acid decarboxylase 65kDa (GAD65) and insulinoma antigen-2 (IA-2) being intracellular and thus being inaccessible, the conundrum of autoantibodies in relation with T1D has not yet been resolved and a pathogenic role remains elusive. Accordingly, their role as correlates of successful immune intervention has been disappointing: preserved beta-cell function so far does not associate with changes in islet autoantibody appearance in immune intervention trials of T1D, despite many efforts (3). This notion sets the bar high to define immune correlates of immune intervention therapy: we need to define suitable leukocyte profiles or markers of islet-specific T-cells that could help to assess mechanism of therapeutic action and/or beta-cell preservation.
Immunopathogenesis of type 1 diabetes
Islet autoreactive CD4 and CD8 T-cells can be detected in blood of T1D patients (4–12). Since their presence is not limited to T1D patients (6, 13, 14), islet autoreactive T-cells cannot be used diagnostically on the individual basis, but changes in their profiles, reactivity and frequency have been shown to correlate with disease and recurrence of T1D after beta-cell replacement therapy. The targets of islet autoreactive T-cells are numerous and include insulin, proinsulin, preproinsulin, GAD65, 38kDa insulin granule protein, ZnT8 and IA-2 (15). Recently, a new universe of modified beta-cell proteins targets was identified as neoantigens in T1D that include post-translational (chemical and enzymatic changes) (16–18) and post-transcriptional modifications (alternative splicing of mRNA and misreads resulting from ribosomal infidelity) (19, 20). Given that these neoantigens are not expected to contribute to thymic education and central immune tolerance, their immunogenicity is high, and their terms of engagement differ from T-cell responses against native self-proteins: T-cells against native autoantigens tend to have lower TCR avidity and docking abnormalities, while their epitope binding affinity to HLA often is low (11, 12, 18, 21–25); T-cells reactive with neoantigens resemble those against viruses, bacteria and alloantigens (17–19, 26, 27). This renders neoantigens strong candidates provoking the immune system and contributing to loss of immune tolerance and epitope spreading that both precede and follow diagnosis of T1D. Neoantigens also point to a role of the target tissue in its own demise, since these proteins changes tend to follow metabolic, inflammatory or infectious stress of pancreatic islets (15).
Efforts to standardize robust immune assays to detect islet-specific autoreactive T-cells have been challenging (28–39). Decades of attempts to standardize T-cells assays by the Immunology of Diabetes Society have led us to appreciate that T-cell autoreactivity does not equal serology in terms of opportunities and expectations to run these assays routinely to robustly measure islet autoimmunity as diagnostics. The frequencies of islet autoreactive CD8 and in particular CD4 T-cells in circulation is very low. Together with the wide range of candidate islet proteins, epitopes and HLA restriction elements, this affects the feasibility to detects islet autoreactive T-cells comprehensively. In case of CD8 T-cell autoreactivity, combinatorial assays were developed to reduce blood volume needs and allow simultaneous detection of different T-cell specificities (7, 28). HLA class II tetramers have rarely been used, and with few HLA-DR polymorphisms and epitopes only (40). No consensus has yet been reached on cryopreservation, ELISPOT and proliferation protocols, but cryopreservation does affect islet autoreactive T-cell responses, notably in case of IL-10 production (41–44). Functional T-cell have been regarded as ‘boutique’ assays that require particular skills and expertise to be executed, thus limiting their use in multicenter clinical trials (3). Detection of islet autoantigen-specific regulatory T-cells (Treg) in clinical blood samples is particularly challenging (45, 46). There are specific Treg phenotypes. Indirectly, the presence of immune regulation of islet autoimmunity can be detected either by IL-10 production by PBMC in response to stimulation with islet autoantigens identified by ELISA (47, 48) or cytokine capture by FACS (10), by cell sorting (10, 49) or limiting dilution analyses (50) showing both effector and regulatory T-cell subpopulations responding to insulin, and by blocking T-cell responses to insulin or GAD65 with anti-HLA-DQ antibodies (51).
This being stated, it does not exclude benefit of T-cell studies to understand disease heterogeneity and progression, and therapeutic or clinical efficacy of immune intervention strategies (8). Given the disappointing outcomes of clinical trials in T1D and the growing awareness of patient and disease heterogeneity, there is an unmet need to define this heterogeneity, mechanism of therapeutic action, responsiveness and clinical efficacy of such trials (52–54). I propose that immune monitoring may offer measures to stratify patients to participate in trials based on immune signatures and genetic barcodes.
Disease endotypes?
Genetic diversity between T1D patients is one of the signs that the disease may differ between patients (Figure 1) (9, 52, 55). HLA polymorphisms correlate with islet serology and T-cell responses, which is related to the role of HLA in thymic education and antigen presentation. In addition, genetic polymorphisms in for instance IL-2 signaling or vitamin D3 metabolism have implications for efficacy of related immune intervention strategies (56–60). So-called genetic risk scores differ between ancestries and ethnicities, which may reflect different corresponding disease endotypes, as suggested by disease acceleration by abatacept in patients ‘of color’ (ethnicity was not further specified by the authors) versus preservation of beta-cell function in patients of European descent (61–64). Perhaps to most visible correlate of disease variation is age: T1D often presents more aggressively and acutely in infants, whereas disease progression and loss of beta-cell function is more moderate in T1D diagnosed in adults. Insulin autoantibodies are most frequent to first appear in infants below the age of 2, and if they carry HLA-DR4, whereas older children show GAD65 antibodies first before converting to T1D, with HLA-DR3 as genetic correlate (65). The lesion also shows differences with age, with insulitis being moderate in older T1D patients (66). Even in children, two patterns were identified, with children diagnosed before 7 years of age often showed a high rate of inflammation with leukocytes that even included some B cells, whereas children diagnosed beyond 12 years of age show less inflammation and rarely any B-cells (9, 66). This difference in insulitis was mirrored by abnormalities in the remaining beta-cells, with younger cases showing co-localization of proinsulin with insulin, possibly pointing to impaired beta-cell biosynthesis, function and stress. Importantly, T-cell autoreactivity in the pancreatic lesion appears to reflect what is seen in circulation but the reverse is not always true (6, 14, 26, 67–70). Islet autoreactive T-cells isolated from circulation can lyse beta-cells (i.e., diabetogenic) (19, 71), home to pancreatic tissue (72) and cause T1D upon adoptive transfer into humanized mice (73). In terms of T-cell reactivity, distinct profiles of CD4 T-cell autoimmunity could be identified in children with T1D, where some showed immune reactivities to all four tested islet autoantigens (PPI, IA-2, DRiP and GAD65), whereas others responded to none, and half of the children reacted with 2-3 islet autoantigens. Curiously, epitope spreading was most pronounced in children with the longest disease duration, implying activation of new T-cells to new islet autoantigens even after diagnosis. Comparison of T-cell autoreactivity to insulin before and after diagnosis and initiation of insulin replacement therapy revealed a loss of autoreactivity to insulin rather than exacerbation of this response (74). Yet, no autoimmune T-cell correlates could be identified that associated with a temporary clinical remission and reduced insulin need (‘honeymoon’) often seen in the first year after diagnosis of T1D, even though serum cytokine profiles relating to remission were reported (75–77). Autoimmune phenotypes also differed between children and adults, while half of the T1D patients showed signs of IL-17 in response to islet autoantigens (78), underscoring the use of T-cell assays to determine disease heterogeneity and possibly point to endotypes more or less likely to respond to particular intervention therapies. While frequencies of islet autoreactive T-cells often overlap between T1D cases and non-diabetic control subjects, their functionality may differ (48). Intriguingly, T-cell reactivity to islet epitopes was characterized by proinflammatory responses (i.e., IFNγ) in diabetic cases versus anti-inflammatory or regulatory responses (IL-10) in age- and HLA-matched controls. Curiously, patients showing both IFNg and IL-10 in response to islet epitopes manifested disease significantly later than those only producing IFNγ. These observations illustrate the presence of favorable and unfavorable immune signatures that may help identify disease endotypes with faster or slower progression and guide patient selection for distinct therapeutics.
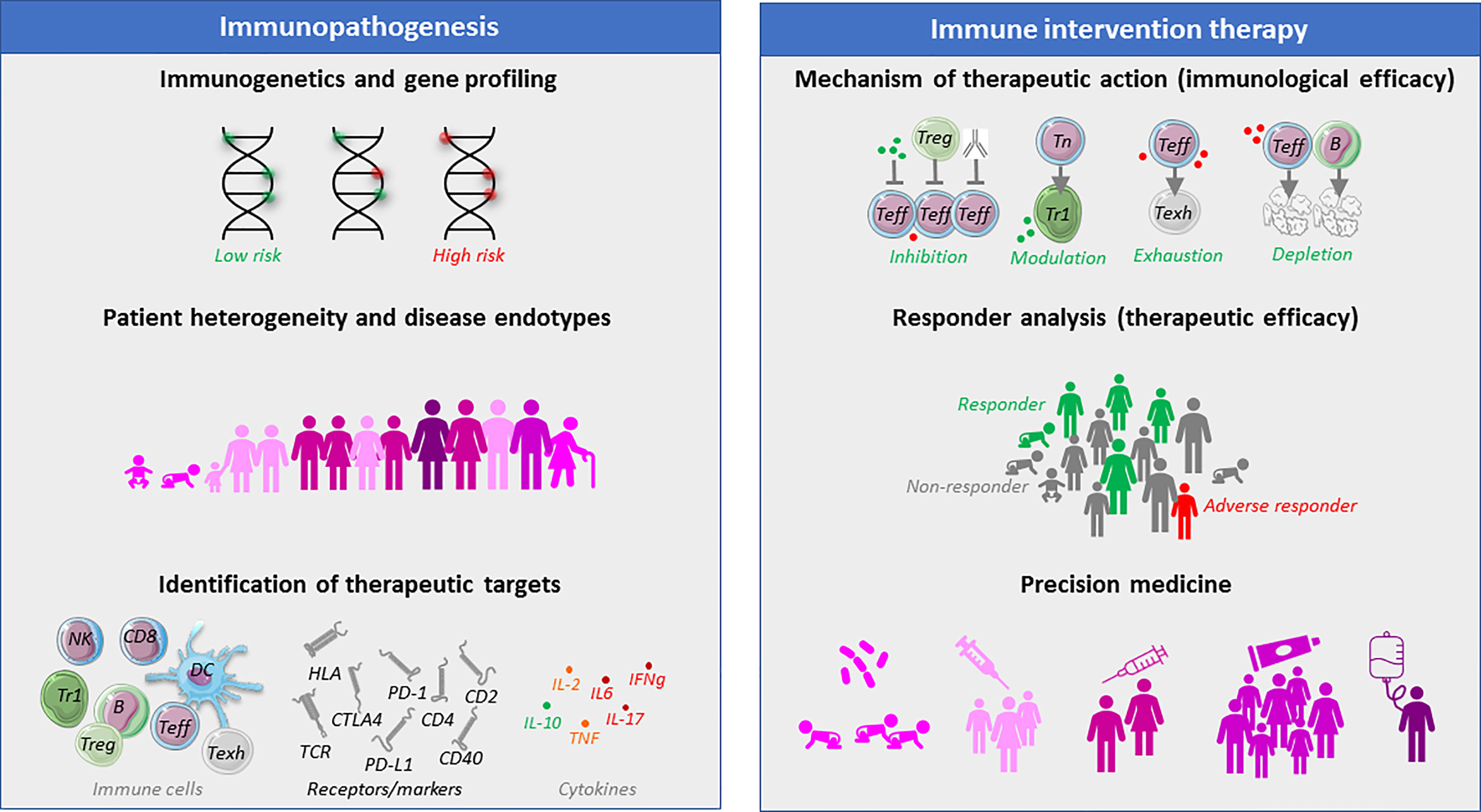
Figure 1 The need and benefit of immune monitoring of T1D patients before and during therapeutic immune intervention. Different aspects of immune monitoring with regard to defining disease heterogeneity, that may lead to the identification of targets of therapeutic intervention. Once such immune intervention is explored in clinical trials, immune monitoring can help define the mechanism of immunological action of an intervention strategy, as well as define baseline characteristics or immunological correlates that may act as endpoints of clinical therapeutic efficacy. It is believed that immune assays may help determine patient subgroups with particular disease entities (endotypes) with particularly favorable chances of clinical benefit (precision medicine), which will avoid subjecting other patients with unlikely clinical benefit to a particular therapeutic intervention strategy.
Identification of therapeutic targets
Immunological monitoring studies have also pointed to potential targets of therapy. For instance, the cytokine production patterns in response to stimulation with islet epitopes has led to the development of several therapeutic strategies aiming to convert a proinflammatory response into an anti-inflammatory one, either with peptides injection in solution, or loaded into tolerogenic dendritic cells or nanoparticles (8, 22, 47, 79–90). The discovery of impaired IL-2 signaling has led to assessment of ultra-low dose IL-2 to selectively stimulate regulatory T-cells (60, 91), while IL-17 production by some patients in response to islet epitopes has made pharma consider to test blockade of this cytokine as intervention strategy (78). The expression of the chemokine receptor CXCR3 by islet infiltrating autoreactive T-cells and the production of the corresponding ligand, chemokine CXCL10 (IP-10), by distressed beta-cells pointed to the opportunity to interfere in leukocyte migration to pancreatic islets (92, 93). A therapeutic monoclonal antibody against B-cells (rituximab) has been tested to preserve beta-cell function shortly after diagnosis (94). Not surprisingly, given my exposé above, this drug only had some effect in the youngest patients. Most importantly, the undisputed role of T-cells in the pathogenesis of T1D has led to the development of several anti-T-cell therapeutic strategies that showed benefit in subsets of patients to either preserve beta-cell function or delay clinical manifestation of the disease (95–97). Additional candidates for targeting by immunotherapy are shown in Figure 1.
Mechanism of therapeutic action (immunological efficacy)
Immunological assays are ideally suited to determine the mechanism of action and potential identify subsets of patients better suited for certain strategies. For instance, in-vitro studies of inhibitors of the co-stimulatory checkpoint CTLA4 that is important in activation and regulation of T-cells responses by antigen-presenting cells proved to have differential effects on naïve T-cells versus autoreactive memory T-cells, impairing activation of the first but sparing reactivation of the latter and implying that this strategy is best suited to prevent priming of naïve T-cells and epitope spreading (63, 98, 99). The effects of anti-thymocyte globulin (ATG) and humanized monoclonal antibodies against CD3 (ChAglyCD3) or CD25 (daclizumab) were tested on prediabetic islet antigen-specific autoreactive T-cells with regard to downmodulation of the target protein, proliferation, cytokine production, complement-dependent cytotoxicity (CDC), antibody-dependent cell cytotoxicity (ADCC), and survival (100). ATG leads to depletion of autoreactive CD4+ T-cells by ADCC, CDC, and apoptosis, whereas anti-CD3 and anti-CD25 inhibited T-cell autoreactivity in a nondepleting fashion. ATG treatment led to a cytokine burst of Th1- and Th2-associated cytokines. Modulation of cytokine release through humanized monoclonal antibodies was moderate and selective: anti-CD25 led to increased release of IL-2 and reduced production of TNF, whereas anti-CD3 decreased release of IFNγ and IL-5 and increased secretion of IL-10. Thus, ATG and the humanized monoclonal antibodies displayed contrasting mechanisms of action.
Ex-vivo analyses of blood samples from participants of immune intervention trials also helped to define the therapeutic mechanism of action. CD19+ B lymphocytes were depleted in patients in receiving rituximab (94). In a beautiful side experiment, the authors demonstrated that this B-cell depletion impaired the response to a neo-vaccine, but a subsequent vaccination with the same neoantigen led to immune responses that were indistinguishable from untreated patients, demonstrating that rituximab is a temporary immune suppressant but does not induce immune tolerance (94). Early B cell reconstitution in multiple sclerosis patients treated with rituximab was not associated with a risk of relapse or progression, but instead could reflect regulatory immunological phenomena in subgroup of patients (101). Similarly, T-cell levels temporarily dropped in T1D patients receiving anti-CD3 monoclonal antibodies, abatacept or anti-thymocyte globulin (96, 97, 102, 103). In case of the latter, effector T-cells were hit harder than Tregs, favoring immune regulation. Immunological monitoring of autoimmune and anti-vaccine responses in blood of T1D patients treated with Otelixizumab demonstrated that recall immunity is preserved despite high-dose anti-CD3 treatment, adding to the safety of anti-CD3 treatment as an immune-modulatory agent in the treatment of T1D (104). Reactivation of Epstein-Barr virus and self-limiting mononucleosis-like symptoms were regarded as adverse side-effects but could be seen as evidence of mechanistic efficacy, as this points to the inhibition of active effector T-cells (97). Indeed, CD8 T-cell responses against EBV quickly increased after treatment blunting the clinical symptoms of viral reactivation (102). Blood samples from participants of various islet-antigen specific immune intervention studies showed that injection of islet autoantigen does not exacerbate disease progression but instead induced antigen-specific regulatory T-cells that in some cases corresponded with improved metabolic outcome (8, 79, 81, 83, 105–107). Most mentioned immune modifying intervention therapies caused delays in the fall in C-peptide in subsets of patients but they did not appear to fundamentally alter the immune phenotypes and reactivities durably or change underlying pathophysiology of the disease. This is in line with results from immune monitoring that only showed temporal changes.
Responder analysis (therapeutic efficacy)
Most value from immunological monitoring came from studies comparing patients clinically responding to certain immune intervention strategies versus those that did not (Figure 1). This so-called responder analysis in the rituximab trial revealed that an increased T-cell proliferative responses to islet antigens identified clinical responders to rituximab therapy. In the Teplizumab trial in new-onset T1D a partially exhausted T-cell type (KLRG1+TIGIT+) was identify, functionally characterize and associated with response to teplizumab therapy (108). Changes in this CD8+ T-cell subset of partially exhausted effector cells were associated with clinical response in a trial attempting to delay clinical onset of T1D; these cells showed reduced secretion of IFNγ and TNF (95). While the authors propose that pathways regulating T-cell exhaustion may play a role in successful immune interventions for T1D, biomarkers do not necessarily equal mechanism: the increases in lymphocyte subsets may be a consequence rather than a cause of immunotherapy and reflect relative reductions in other subsets that led to this increase. The teplizumab trial also revealed baseline characteristics that, next to metabolic features, involved a lower frequency of CD4+CCR4+ memory and naïve T-cells, CD4+CCR6+ naïve CD4+ T-cells, naïve CCR4+ CD8+ T-cells, and IFN-γ-producing CD8+ T-cells, versus higher numbers of activated CD8+ terminally differentiated effector and CD8+ effector memory T-cells at baseline in responders versus non-responders. These immune correlates warrant exploring these as predictors of clinical efficacy. Similarly, exhausted-like CD8+ T-cell phenotypes were linked to C-peptide preservation in alefacept-treated (LFA3-Ig) T1D subjects (109), with CD4+CD25+CD127hi T-cell frequencies at baseline as potential predictor of disease progression and therapeutic efficacy in T1D (110). Autologous hematopoietic stem cell transplantation (aHSCT) is the only therapeutic intervention thus far resulting in complete and sometimes durable remission (insulin independence) in new-onset T1D patients (111, 112). Patients with lower frequencies of autoreactive islet-specific T-cells remained insulin-free longer and presented greater C-peptide levels than those with higher frequencies of these cells (111). In the prolonged-remission-group, baseline islet-specific T-cell autoreactivity persisted after transplantation, but regulatory T-cell counts increased. For the entire follow-up, CD3+CD8+ T-cell levels did not change, whereas CD3+CD4+ T-cell numbers remained lower than baseline in both groups, resulting in a CD4/CD8 ratio inversion. Thus, immune monitoring identified a subgroup of patients with superior clinical outcome of aHSCT.
In the context of beta-cell therapies (pancreas, isolated islets or stem cell derived beta-cells), immune monitoring provided seminal proof to determine the in-vivo fate of islet allografts implanted into type 1 diabetic recipients (4, 7, 113–130). In this case, two distinct immune reactions should be monitored: recurrent islet autoimmunity and allograft rejection. Again, baseline immune profiles were highly predictive of clinical outcome: lack of CD4 T-cell autoreactivity to islets resulted in insulin-independence in 86% of the cases, whereas pre-existent T-cell responses to both GAD65 and IA-2 never led to this favored outcome. General T- and B-cell counts also predicted outcome (123, 127). Induction immunotherapy (thymoglobulin, basiliximab or alemtuzumab) led to temporal reductions in T-cell counts and islet autoimmunity that recured in relapsing patients either as isolated phenomenon or followed by allograft rejection, pointing to the pre-existent islet autoimmunity being the principal hurdle in beta-cell replacement therapy in T1D, in spite of continuous immune suppression (tacrolimus, mycomofetyl phenolate and/or sirolimus) that was dictated to prevent induction of alloimmunity to the islet graft. Persistence of recall T-cell immunity against vaccine antigens (e.g., tetanus toxoid) was indicative of sub-maximal or insufficient immune suppression regimes. Immune monitoring also identified recurrent islet autoimmunity as cause of rare and late loss pancreas allograft function in the case of whole pancreas transplantation, underscoring the value of immunological studies (120, 131).
Future perspectives
The complexity and diversity of the immune system and the range and diversity of immunological features and mechanisms that are believed to be involved in the selective beta-cell destruction leading to T1D put a daunting but indispensable task on the shoulders of immunologists. Despite the many challenges involved, including the shortage of robust and reproducible immune assays and limited access to both blood samples and the lesion, it has proven excessively rewarding to define and apply immune correlates to further define patient and disease heterogeneity, to identify, monitor and validate candidate immune intervention strategies and to define T1D subgroups or endotypes that may most favorable outcome on the one side (precision medicine), and to avoid offering immunotherapies to those unlikely to benefit (‘imprecision medicine’). Immunomonitoring of T1D patients receiving islet allografts defined the immunological fate of the islet grafts in vivo, and guided choice of transplant immune suppression, islet encapsulation and gene-editing protocols stem cells and beta-cell progenitors aiming to reduce islet immunogenicity. Given the remaining knowledge gaps in immunopathogenesis and heterogeneity of T1D, it should not be a matter whether, but rather how, to monitor the immune system of T1D patients and at-risk individuals. Bio sampling and storage is critical to determine immunological and possibly even therapeutic efficacy of immune intervention trials. New robust, high through-put and reproducible technologies including multiplex phenotyping and single cell transcriptomics are welcome for the definition of high-resolution immune correlates but given that non-adaptive immune parameters rarely delivered as informative in terms of disease progression, heterogeneity and therapeutic response, focus on the lymphocyte compartment is justifiable. Given that the single endpoint accepted by FDA or EMA as primary outcome remains beta-cell function, whereas the currently explored therapeutic interventions target the immune system rather than beta-cells, there is a growing but largely unmet need to define immune correlates of both mechanistic and clinical efficacy (132). The first steps have been made in this direction and the future looks promising.
Data availability statement
The original contributions presented in the study are included in the article/Supplementary Material. Further inquiries can be directed to the corresponding author.
Ethics statement
This report is based on published articles that are referenced. Written informed consent to participate in this study was provided by the participants’ legal guardian/next of kin.
Author contributions
BR conceived and wrote this perspective. The author confirms being the sole contributor of this work and has approved it for publication.
Funding
BR was supported by the Dutch Diabetes Research Foundation, The European Commission, the International Juvenile Diabetes Research Foundation, INNODIA and Stichting DON (Diabetes Research Netherlands). The author also declares that this study received funding from the Wanek family. The funder was not involved in the study design, collection, analysis, interpretation of data, the writing of this article, or the decision to submit it for publication.
Conflict of interest
The author declares that the research was conducted in the absence of any commercial or financial relationships that could be construed as a potential conflict of interest.
Publisher’s note
All claims expressed in this article are solely those of the authors and do not necessarily represent those of their affiliated organizations, or those of the publisher, the editors and the reviewers. Any product that may be evaluated in this article, or claim that may be made by its manufacturer, is not guaranteed or endorsed by the publisher.
References
1. Insel RA, Dunne JL, Atkinson MA, Chiang JL, Dabelea D, Gottlieb PA, et al. Staging presymptomatic type 1 diabetes: a scientific statement of JDRF, the endocrine society, and the American diabetes association. Diabetes Care (2015) 38(10):1964–74. doi: 10.2337/dc15-1419
2. Bloem SJ, Roep BO. The elusive role of b lymphocytes and islet autoantibodies in (human) type 1 diabetes. Diabetologia (2017) 60(7):1185–9. doi: 10.1007/s00125-017-4284-5
3. Roep BO, Peakman M. Surrogate end points in the design of immunotherapy trials: emerging lessons from type 1 diabetes. Nat Rev Immunol (2010) 10(2):145–52. doi: 10.1038/nri2705
4. Pinkse GG, Tysma OH, Bergen CA, Kester MG, Ossendorp F, van Veelen PA, et al. Autoreactive CD8 T cells associated with beta cell destruction in type 1 diabetes. Proc Natl Acad Sci U S A (2005) 102(51):18425–30. doi: 10.1073/pnas.0508621102
5. Roep BO. The role of T-cells in the pathogenesis of type 1 diabetes: from cause to cure. Diabetologia (2003) 46(3):305–21. doi: 10.1007/s00125-003-1089-5
6. Roep BO, Kallan AA, Duinkerken G, Arden SD, Hutton JC, Bruining GJ, et al. T-Cell reactivity to beta-cell membrane antigens associated with beta-cell destruction in IDDM. Diabetes (1995) 44(3):278–83. doi: 10.2337/diab.44.3.278
7. Velthuis JH, Unger WW, Abreu JR, Duinkerken G, Franken K, Peakman M, et al. Simultaneous detection of circulating autoreactive CD8+ T-cells specific for different islet cell-associated epitopes using combinatorial MHC multimers. Diabetes (2010) 59(7):1721–30. doi: 10.2337/db09-1486
8. Arif S, Gomez-Tourino I, Kamra Y, Pujol-Autonell I, Hanton E, Tree T, et al. GAD-alum immunotherapy in type 1 diabetes expands bifunctional Th1/Th2 autoreactive CD4 T cells. Diabetologia (2020) 63(6):1186–98. doi: 10.1007/s00125-020-05130-7
9. Arif S, Leete P, Nguyen V, Marks K, Nor NM, Estorninho M, et al. Blood and islet phenotypes indicate immunological heterogeneity in type 1 diabetes. Diabetes (2014) 63(11):3835–45. doi: 10.2337/db14-0365
10. Tree TI, Lawson J, Edwards H, Skowera A, Arif S, Roep BO, et al. Naturally arising human CD4 T-cells that recognize islet autoantigens and secrete interleukin-10 regulate proinflammatory T-cell responses via linked suppression. Diabetes (2010) 59(6):1451–60. doi: 10.2337/db09-0503
11. Abreu JR, Martina S, Verrijn Stuart AA, Fillie YE, Franken KL, Drijfhout JW, et al. CD8 T cell autoreactivity to preproinsulin epitopes with very low human leucocyte antigen class I binding affinity. Clin Exp Immunol (2012) 170(1):57–65. doi: 10.1111/j.1365-2249.2012.04635.x
12. Unger WW, Velthuis J, Abreu JR, Laban S, Quinten E, Kester MG, et al. Discovery of low-affinity preproinsulin epitopes and detection of autoreactive CD8 T-cells using combinatorial MHC multimers. J Autoimmun (2011) 37(3):151–9. doi: 10.1016/j.jaut.2011.05.012
13. Gonzalez-Duque S, Azoury ME, Colli ML, Afonso G, Turatsinze JV, Nigi L, et al. Conventional and neo-antigenic peptides presented by beta cells are targeted by circulating naive CD8+ T cells in type 1 diabetic and healthy donors. Cell Metab (2018) 28(6):946–60 e6. doi: 10.1016/j.cmet.2018.07.007
14. Culina S, Lalanne AI, Afonso G, Cerosaletti K, Pinto S, Sebastiani G, et al. Islet-reactive CD8(+) T cell frequencies in the pancreas, but not in blood, distinguish type 1 diabetic patients from healthy donors. Sci Immunol (2018) 3(20):eaao4013. doi: 10.1126/sciimmunol.aao4013
15. Roep BO, Thomaidou S, van Tienhoven R, Zaldumbide A. Type 1 diabetes mellitus as a disease of the beta-cell (do not blame the immune system)? Nat Rev Endocrinol (2021) 17(3):150–61. doi: 10.1038/s41574-020-00443-4
16. Mannering SI, Harrison LC, Williamson NA, Morris JS, Thearle DJ, Jensen KP, et al. The insulin a-chain epitope recognized by human T cells is posttranslationally modified. J Exp Med (2005) 202(9):1191–7. doi: 10.1084/jem.20051251
17. van Lummel M, Duinkerken G, van Veelen PA, de Ru A, Cordfunke R, Zaldumbide A, et al. Posttranslational modification of HLA-DQ binding islet autoantigens in type 1 diabetes. Diabetes (2014) 63(1):237–47. doi: 10.2337/db12-1214
18. Delong T, Wiles TA, Baker RL, Bradley B, Barbour G, Reisdorph R, et al. Pathogenic CD4 T cells in type 1 diabetes recognize epitopes formed by peptide fusion. Science (2016) 351(6274):711–4. doi: 10.1126/science.aad2791
19. Kracht MJ, van Lummel M, Nikolic T, Joosten AM, Laban S, van der Slik AR, et al. Autoimmunity against a defective ribosomal insulin gene product in type 1 diabetes. Nat Med (2017) 23(4):501–7. doi: 10.1038/nm.4289
20. de Jong VM, Abreu JR, Verrijn Stuart AA, van der Slik AR, Verhaeghen K, Engelse MA, et al. Alternative splicing and differential expression of the islet autoantigen IGRP between pancreas and thymus contributes to immunogenicity of pancreatic islets but not diabetogenicity in humans. Diabetologia (2013) 56(12):2651–8. doi: 10.1007/s00125-013-3034-6
21. Ouyang Q, Standifer NE, Qin H, Gottlieb P, Verchere CB, Nepom GT, et al. Recognition of HLA class I-restricted beta-cell epitopes in type 1 diabetes. Diabetes (2006) 55(11):3068–74. doi: 10.2337/db06-0065
22. Beringer DX, Kleijwegt FS, Wiede F, van der Slik AR, Loh KL, Petersen J, et al. T Cell receptor reversed polarity recognition of a self-antigen major histocompatibility complex. Nat Immunol (2015) 16(11):1153–61. doi: 10.1038/ni.3271
23. Hahn M, Nicholson MJ, Pyrdol J, Wucherpfennig KW. Unconventional topology of self peptide-major histocompatibility complex binding by a human autoimmune T cell receptor. Nat Immunol (2005) 6(5):490–6. doi: 10.1038/ni1187
24. Bulek AM, Cole DK, Skowera A, Dolton G, Gras S, Madura F, et al. Structural basis for the killing of human beta cells by CD8(+) T cells in type 1 diabetes. Nat Immunol (2012) 13(3):283–9. doi: 10.1038/ni.2206
25. Gomez-Tourino I, Kamra Y, Baptista R, Lorenc A, Peakman M. T Cell receptor beta-chains display abnormal shortening and repertoire sharing in type 1 diabetes. Nat Commun (2017) 8(1):1792. doi: 10.1038/s41467-017-01925-2
26. Anderson AM, Landry LG, Alkanani AA, Pyle L, Powers AC, Atkinson MA, et al. Human islet T cells are highly reactive to preproinsulin in type 1 diabetes. Proc Natl Acad Sci U.S.A. (2021) 118(41):e2107208118. doi: 10.1073/pnas.2107208118
27. Wen X, Yang J, James E, Chow IT, Reijonen H, Kwok WW. Increased islet antigen-specific regulatory and effector CD4(+) T cells in healthy individuals with the type 1 diabetes-protective haplotype. Sci Immunol (2020) 5(44):eaax8767. doi: 10.1126/sciimmunol.aax8767
28. James EA, Abreu JRF, McGinty JW, Odegard JM, Fillie YE, Hocter CN, et al. Combinatorial detection of autoreactive CD8(+) T cells with HLA-A2 multimers: a multi-centre study by the immunology of diabetes society T cell workshop. Diabetologia (2018) 61(3):658–70. doi: 10.1007/s00125-017-4508-8
29. Tree TI, Roep BO, Peakman M. A mini meta-analysis of studies on CD4+CD25+ T cells in human type 1 diabetes: report of the immunology of diabetes society T cell workshop. Ann N Y Acad Sci (2006) 1079:9–18. doi: 10.1196/annals.1375.002
30. Nagata M, Kotani R, Moriyama H, Yokono K, Roep BO, Peakman M. Detection of autoreactive T cells in type 1 diabetes using coded autoantigens and an immunoglobulin-free cytokine ELISPOT assay: report from the fourth immunology of diabetes society T cell workshop. Ann N Y Acad Sci (2004) 1037:10–5. doi: 10.1196/annals.1337.002
31. Schloot NC, Meierhoff G, Karlsson Faresjo M, Ott P, Putnam A, Lehmann P, et al. Comparison of cytokine ELISpot assay formats for the detection of islet antigen autoreactive T cells. report of the third immunology of diabetes society T-cell workshop. J Autoimmun (2003) 21(4):365–76. doi: 10.1016/S0896-8411(03)00111-2
32. Peakman M, Tree TI, Endl J, van Endert P, Atkinson MA, Roep BO, et al. Characterization of preparations of GAD65, proinsulin, and the islet tyrosine phosphatase IA-2 for use in detection of autoreactive T-cells in type 1 diabetes: report of phase II of the second international immunology of diabetes society workshop for standardization of T-cell assays in type 1 diabetes. Diabetes (2001) 50(8):1749–54. doi: 10.2337/diabetes.50.8.1749
33. Roep BO, Atkinson MA, van Endert PM, Gottlieb PA, Wilson SB, Sachs JA. Autoreactive T cell responses in insulin-dependent (Type 1) diabetes mellitus. report of the first international workshop for standardization of T cell assays. J Autoimmun (1999) 13(2):267–82. doi: 10.1006/jaut.1999.0312
34. James EA, Mallone R, Schloot NC, Gagnerault MC, Thorpe J, Fitzgerald-Miller L, et al. Immunology of diabetes society T-cell workshop: HLA class II tetramer-directed epitope validation initiative. Diabetes Metab Res Rev (2011) 27(8):727–36. doi: 10.1002/dmrr.1244
35. Mallone R, Scotto M, Janicki CN, James EA, Fitzgerald-Miller L, Wagner R, et al. Immunology of diabetes society T-cell workshop: HLA class I tetramer-directed epitope validation initiative T-cell workshop report-HLA class I tetramer validation initiative. Diabetes Metab Res Rev (2011) 27(8):720–6. doi: 10.1002/dmrr.1243
36. Mallone R, Mannering SI, Brooks-Worrell BM, Durinovic-Bello I, Cilio CM, Wong FS, et al. Isolation and preservation of peripheral blood mononuclear cells for analysis of islet antigen-reactive T cell responses: position statement of the T-cell workshop committee of the immunology of diabetes society. Clin Exp Immunol (2011) 163(1):33–49. doi: 10.1111/j.1365-2249.2010.04272.x
37. Britten CM, Janetzki S, Butterfield LH, Ferrari G, Gouttefangeas C, Huber C, et al. T Cell assays and MIATA: the essential minimum for maximum impact. Immunity (2012) 37(1):1–2. doi: 10.1016/j.immuni.2012.07.010
38. Britten CM, Janetzki S, van der Burg SH, Huber C, Kalos M, Levitsky HI, et al. Minimal information about T cell assays: the process of reaching the community of T cell immunologists in cancer and beyond. Cancer Immunol Immunother (2011) 60(1):15–22. doi: 10.1007/s00262-010-0940-z
39. Ahmed S, Cerosaletti K, James E, Long SA, Mannering S, Speake C, et al. Standardizing T-cell biomarkers in type 1 diabetes: Challenges and recent advances. Diabetes (2019) 68(7):1366–79. doi: 10.2337/db19-0119
40. Reijonen H, Kwok WW, Nepom GT. Detection of CD4+ autoreactive T cells in T1D using HLA class II tetramers. Ann N Y Acad Sci (2003) 1005:82–7. doi: 10.1196/annals.1288.009
41. Huurman VA, Decochez K, Mathieu C, Cohen IR, Roep BO. Therapy with the hsp60 peptide DiaPep277 in c-peptide positive type 1 diabetes patients. Diabetes Metab Res Rev (2007) 23(4):269–75. doi: 10.1002/dmrr.691
42. Roep BO. Standardization of T-cell assays in type I diabetes. immunology of diabetes society T-cell committee. Diabetologia (1999) 42(5):636–7. doi: 10.1007/s001250051209
43. Musthaffa Y, Hamilton-Williams EE, Nel HJ, Bergot AS, Mehdi AM, Harris M, et al. Proinsulin-specific T-cell responses correlate with estimated c-peptide and predict partial remission duration in type 1 diabetes. Clin Transl Immunol (2021) 10(7):e1315. doi: 10.1002/cti2.1315
44. Musthaffa Y, Nel HJ, Ramnoruth N, Patel S, Hamilton-Williams EE, Harris M, et al. Optimization of a method to detect autoantigen-specific T-cell responses in type 1 diabetes. Front Immunol (2020) 11:587469. doi: 10.3389/fimmu.2020.587469
45. Roep BO, Tree TI. Immune modulation in humans: implications for type 1 diabetes mellitus. Nat Rev Endocrinol (2014) 10(4):229–42. doi: 10.1038/nrendo.2014.2
46. Roep BO, Buckner J, Sawcer S, Toes R, Zipp F. The problems and promises of research into human immunology and autoimmune disease. Nat Med (2012) 18(1):48–53. doi: 10.1038/nm.2626
47. Huurman VA, van der Meide PE, Duinkerken G, Willemen S, Cohen IR, Elias D, et al. Immunological efficacy of heat shock protein 60 peptide DiaPep277 therapy in clinical type I diabetes. Clin Exp Immunol (2008) 152(3):488–97. doi: 10.1111/j.1365-2249.2008.03656.x
48. Arif S, Tree TI, Astill TP, Tremble JM, Bishop AJ, Dayan CM, et al. Autoreactive T cell responses show proinflammatory polarization in diabetes but a regulatory phenotype in health. J Clin Invest (2004) 113(3):451–63. doi: 10.1172/JCI19585
49. Peterson LD, van der Keur M, de Vries RR, Roep BO. Autoreactive and immunoregulatory T-cell subsets in insulin-dependent diabetes mellitus. Diabetologia (1999) 42(4):443–9. doi: 10.1007/s001250051177
50. Naik RG, Beckers C, Wentwoord R, Frenken A, Duinkerken G, Brooks-Worrell B, et al. Precursor frequencies of T-cells reactive to insulin in recent onset type 1 diabetes mellitus. J Autoimmun (2004) 23(1):55–61. doi: 10.1016/j.jaut.2004.04.002
51. Tree TI, Duinkerken G, Willemen S, de Vries RR, Roep BO. HLA-DQ-regulated T-cell responses to islet cell autoantigens insulin and GAD65. Diabetes (2004) 53(7):1692–9. doi: 10.2337/diabetes.53.7.1692
52. Battaglia M, Ahmed S, Anderson MS, Atkinson MA, Becker D, Bingley PJ, et al. Introducing the endotype concept to address the challenge of disease heterogeneity in type 1 diabetes. Diabetes Care (2020) 43(1):5–12. doi: 10.2337/dc19-0880
53. den Hollander NHM, Roep BO. From disease and patient heterogeneity to precision medicine in type 1 diabetes. Front Med (Lausanne) (2022) 9:932086. doi: 10.3389/fmed.2022.932086
54. Woittiez NJ, Roep BO. Impact of disease heterogeneity on treatment efficacy of immunotherapy in type 1 diabetes: different shades of gray. Immunotherapy (2015) 7(2):163–74. doi: 10.2217/imt.14.104
55. Claessens LA, Wesselius J, van Lummel M, Laban S, Mulder F, Mul D, et al. Clinical and genetic correlates of islet-autoimmune signatures in juvenile-onset type 1 diabetes. Diabetologia (2020) 63(2):351–61. doi: 10.1007/s00125-019-05032-3
56. Waldron-Lynch F, Kareclas P, Irons K, Walker NM, Mander A, Wicker LS, et al. Rationale and study design of the adaptive study of IL-2 dose on regulatory T cells in type 1 diabetes (DILT1D): a non-randomised, open label, adaptive dose finding trial. BMJ Open (2014) 4(6):e005559. doi: 10.1136/bmjopen-2014-005559
57. Vanherwegen AS, Eelen G, Ferreira GB, Ghesquiere B, Cook DP, Nikolic T, et al. Vitamin d controls the capacity of human dendritic cells to induce functional regulatory T cells by regulation of glucose metabolism. J Steroid Biochem Mol Biol (2019) 187:134–45. doi: 10.1016/j.jsbmb.2018.11.011
58. Eerligh P, Koeleman BP, Dudbridge F, Jan Bruining G, Roep BO, Giphart MJ. Functional genetic polymorphisms in cytokines and metabolic genes as additional genetic markers for susceptibility to develop type 1 diabetes. Genes Immun (2004) 5(1):36–40. doi: 10.1038/sj.gene.6364036
59. Koeleman BP, Valdigem G, Eerligh P, Giphart MJ, Roep BO. Seasonality of birth in patients with type 1 diabetes. Lancet (2002) 359(9313):1246–7; author reply 7-8. doi: 10.1016/S0140-6736(02)08228-4
60. Rosenzwajg M, Salet R, Lorenzon R, Tchitchek N, Roux A, Bernard C, et al. Low-dose IL-2 in children with recently diagnosed type 1 diabetes: a phase I/II randomised, double-blind, placebo-controlled, dose-finding study. Diabetologia (2020) 63(9):1808–21. doi: 10.1007/s00125-020-05200-w
61. Redondo MJ, Geyer S, Steck AK, Sharp S, Wentworth JM, Weedon MN, et al. A type 1 diabetes genetic risk score predicts progression of islet autoimmunity and development of type 1 diabetes in individuals at risk. Diabetes Care (2018) 41(9):1887–94. doi: 10.2337/dc18-0087
62. Kaddis JS, Perry DJ, Vu AN, Rich SS, Atkinson MA, Schatz DA, et al. Improving the prediction of type 1 diabetes across ancestries. Diabetes Care (2022) 45(3):e48–50. doi: 10.2337/dc21-1254
63. Orban T, Bundy B, Becker DJ, DiMeglio LA, Gitelman SE, Goland R, et al. Co-Stimulation modulation with abatacept in patients with recent-onset type 1 diabetes: a randomised, double-blind, placebo-controlled trial. Lancet (2011) 378(9789):412–9. doi: 10.1016/S0140-6736(11)60886-6
64. Roep BO. Immune intervention therapy in type 1 diabetes: safety first. Lancet Diabetes Endocrinol (2013) 1(4):263–5. doi: 10.1016/S2213-8587(13)70124-4
65. Sims EK, Besser REJ, Dayan C, Geno Rasmussen C, Greenbaum C, Griffin KJ, et al. Screening for type 1 diabetes in the general population: A status report and perspective. Diabetes (2022) 71(4):610–23. doi: 10.2337/dbi20-0054
66. Leete P, Oram RA, McDonald TJ, Shields BM, Ziller C, Team TS, et al. Studies of insulin and proinsulin in pancreas and serum support the existence of aetiopathological endotypes of type 1 diabetes associated with age at diagnosis. Diabetologia (2020) 63(6):1258–67. doi: 10.1007/s00125-020-05115-6
67. Velthuis JH, Unger WW, van der Slik AR, Duinkerken G, Engelse M, Schaapherder AF, et al. Accumulation of autoreactive effector T cells and allo-specific regulatory T cells in the pancreas allograft of a type 1 diabetic recipient. Diabetologia (2009) 52(3):494–503. doi: 10.1007/s00125-008-1237-z
68. Coppieters KT, Dotta F, Amirian N, Campbell PD, Kay TW, Atkinson MA, et al. Demonstration of islet-autoreactive CD8 T cells in insulitic lesions from recent onset and long-term type 1 diabetes patients. J Exp Med (2012) 209(1):51–60. doi: 10.1084/jem.20111187
69. Babon JA, DeNicola ME, Blodgett DM, Crevecoeur I, Buttrick TS, Maehr R, et al. Analysis of self-antigen specificity of islet-infiltrating T cells from human donors with type 1 diabetes. Nat Med (2016) 22(12):1482–7. doi: 10.1038/nm.4203
70. Bender C, Rodriguez-Calvo T, Amirian N, Coppieters KT, von Herrath MG. The healthy exocrine pancreas contains preproinsulin-specific CD8 T cells that attack islets in type 1 diabetes. Sci Adv (2020) 6(42):eabc5586. doi: 10.1126/sciadv.abc5586
71. Skowera A, Ellis RJ, Varela-Calvino R, Arif S, Huang GC, Van-Krinks C, et al. CTLs are targeted to kill beta cells in patients with type 1 diabetes through recognition of a glucose-regulated preproinsulin epitope. J Clin Invest (2008) 118(10):3390–402. doi: 10.1172/JCI35449
72. Unger WW, Pearson T, Abreu JR, Laban S, van der Slik AR, der Kracht SM, et al. Islet-specific CTL cloned from a type 1 diabetes patient cause beta-cell destruction after engraftment into HLA-A2 transgenic NOD/scid/IL2RG null mice. PloS One (2012) 7(11):e49213. doi: 10.1371/journal.pone.0049213
73. Tan S, Li Y, Xia J, Jin CH, Hu Z, Duinkerken G, et al. Type 1 diabetes induction in humanized mice. Proc Natl Acad Sci U S A (2017) 114(41):10954–9. doi: 10.1073/pnas.1710415114
74. Schloot NC, Roep BO, Wegmann D, Yu L, Chase HP, Wang T, et al. Altered immune response to insulin in newly diagnosed compared to insulin-treated diabetic patients and healthy control subjects. Diabetologia (1997) 40(5):564–72. doi: 10.1007/s001250050716
75. Kaas A, Pfleger C, Kharagjitsingh AV, Schloot NC, Hansen L, Buschard K, et al. Association between age, IL-10, IFNgamma, stimulated c-peptide and disease progression in children with newly diagnosed type 1 diabetes. Diabetes Med (2012) 29(6):734–41. doi: 10.1111/j.1464-5491.2011.03544.x
76. Pfleger C, Mortensen HB, Hansen L, Herder C, Roep BO, Hoey H, et al. Association of IL-1ra and adiponectin with c-peptide and remission in patients with type 1 diabetes. Diabetes (2008) 57(4):929–37. doi: 10.2337/db07-1697
77. Schloot NC, Hanifi-Moghaddam P, Aabenhus-Andersen N, Alizadeh BZ, Saha MT, Knip M, et al. Association of immune mediators at diagnosis of type 1 diabetes with later clinical remission. Diabetes Med (2007) 24(5):512–20. doi: 10.1111/j.1464-5491.2007.02096.x
78. Arif S, Moore F, Marks K, Bouckenooghe T, Dayan CM, Planas R, et al. Peripheral and islet interleukin-17 pathway activation characterizes human autoimmune diabetes and promotes cytokine-mediated beta-cell death. Diabetes (2011) 60(8):2112–9. doi: 10.2337/db10-1643
79. Alhadj Ali M, Liu YF, Arif S, Tatovic D, Shariff H, Gibson VB, et al. Metabolic and immune effects of immunotherapy with proinsulin peptide in human new-onset type 1 diabetes. Sci Transl Med (2017) 9(402):eaaf7779. doi: 10.1126/scitranslmed.aaf7779
80. Gibson VB, Nikolic T, Pearce VQ, Demengeot J, Roep BO, Peakman M. Proinsulin multi-peptide immunotherapy induces antigen-specific regulatory T cells and limits autoimmunity in a humanized model. Clin Exp Immunol (2015) 182(3):251–60. doi: 10.1111/cei.12687
81. Nikolic T, Zwaginga JJ, Uitbeijerse BS, Woittiez NJ, de Koning EJ, Aanstoot HJ, et al. Safety and feasibility of intradermal injection with tolerogenic dendritic cells pulsed with proinsulin peptide-for type 1 diabetes. Lancet Diabetes Endocrinol (2020) 8(6):470–2. doi: 10.1016/S2213-8587(20)30104-2
82. Dul M, Nikolic T, Stefanidou M, McAteer MA, Williams P, Mous J, et al. Conjugation of a peptide autoantigen to gold nanoparticles for intradermally administered antigen specific immunotherapy. Int J Pharm (2019) 562:303–12. doi: 10.1016/j.ijpharm.2019.03.041
83. Bonifacio E, Ziegler AG, Klingensmith G, Schober E, Bingley PJ, Rottenkolber M, et al. Effects of high-dose oral insulin on immune responses in children at high risk for type 1 diabetes: the pre-POINT randomized clinical trial. JAMA (2015) 313(15):1541–9. doi: 10.1001/jama.2015.2928
84. Hannelius U, Beam CA, Ludvigsson J. Efficacy of GAD-alum immunotherapy associated with HLA-DR3-DQ2 in recently diagnosed type 1 diabetes. Diabetologia (2020) 63(10):2177–81. doi: 10.1007/s00125-020-05227-z
85. Kleijwegt FS, Roep BO. Infectious tolerance as candidate therapy for type 1 diabetes: transfer of immunoregulatory properties from human regulatory T cells to other T cells and proinflammatory dendritic cells. Crit Rev Immunol (2013) 33(5):415–34. doi: 10.1615/CritRevImmunol.2013006782
86. Kleijwegt FS, Jansen DT, Teeler J, Joosten AM, Laban S, Nikolic T, et al. Tolerogenic dendritic cells impede priming of naive CD8(+) T cells and deplete memory CD8(+) T cells. Eur J Immunol (2013) 43(1):85–92. doi: 10.1002/eji.201242879
87. Ferreira GB, Kleijwegt FS, Waelkens E, Lage K, Nikolic T, Hansen DA, et al. Differential protein pathways in 1,25-dihydroxyvitamin d(3) and dexamethasone modulated tolerogenic human dendritic cells. J Proteome Res (2012) 11(2):941–71. doi: 10.1021/pr200724e
88. Kleijwegt FS, Laban S, Duinkerken G, Joosten AM, Koeleman BP, Nikolic T, et al. Transfer of regulatory properties from tolerogenic to proinflammatory dendritic cells via induced autoreactive regulatory T cells. J Immunol (2011) 187(12):6357–64. doi: 10.4049/jimmunol.1101638
89. Kleijwegt FS, Laban S, Duinkerken G, Joosten AM, Zaldumbide A, Nikolic T, et al. Critical role for TNF in the induction of human antigen-specific regulatory T cells by tolerogenic dendritic cells. J Immunol (2010) 185(3):1412–8. doi: 10.4049/jimmunol.1000560
90. Suwandi JS, Nikolic T, Roep BO. Translating mechanism of regulatory action of tolerogenic dendritic cells to monitoring endpoints in clinical trials. Front Immunol (2017) 8:1598. doi: 10.3389/fimmu.2017.01598
91. Hartemann A, Bensimon G, Payan CA, Jacqueminet S, Bourron O, Nicolas N, et al. Low-dose interleukin 2 in patients with type 1 diabetes: a phase 1/2 randomised, double-blind, placebo-controlled trial. Lancet Diabetes Endocrinol (2013) 1(4):295–305. doi: 10.1016/S2213-8587(13)70113-X
92. Roep BO, Kleijwegt FS, van Halteren AG, Bonato V, Boggi U, Vendrame F, et al. Islet inflammation and CXCL10 in recent-onset type 1 diabetes. Clin Exp Immunol (2010) 159(3):338–43. doi: 10.1111/j.1365-2249.2009.04087.x
93. Rigby MR, Harris KM, Pinckney A, DiMeglio LA, Rendell MS, Felner EI, et al. Alefacept provides sustained clinical and immunological effects in new-onset type 1 diabetes patients. J Clin Invest (2015) 125(8):3285–96. doi: 10.1172/JCI81722
94. Pescovitz MD, Greenbaum CJ, Krause-Steinrauf H, Becker DJ, Gitelman SE, Goland R, et al. Rituximab, b-lymphocyte depletion, and preservation of beta-cell function. N Engl J Med (2009) 361(22):2143–52. doi: 10.1056/NEJMoa0904452
95. Sims EK, Bundy BN, Stier K, Serti E, Lim N, Long SA, et al. Teplizumab improves and stabilizes beta cell function in antibody-positive high-risk individuals. Sci Transl Med (2021) 13(583):eabc8980. doi: 10.1126/scitranslmed.abc8980
96. Haller MJ, Schatz DA, Skyler JS, Krischer JP, Bundy BN, Miller JL, et al. Low-dose anti-thymocyte globulin (ATG) preserves beta-cell function and improves HbA1c in new-onset type 1 diabetes. Diabetes Care (2018) 41(9):1917–25. doi: 10.2337/dc18-0494
97. Keymeulen B, Vandemeulebroucke E, Ziegler AG, Mathieu C, Kaufman L, Hale G, et al. Insulin needs after CD3-antibody therapy in new-onset type 1 diabetes. N Engl J Med (2005) 352(25):2598–608. doi: 10.1056/NEJMoa043980
98. de Jong VM, Zaldumbide A, van der Slik AR, Laban S, Koeleman BP, Roep BO. Variation in the CTLA4 3’UTR has phenotypic consequences for autoreactive T cells and associates with genetic risk for type 1 diabetes. Genes Immun (2016) 17(1):75–8. doi: 10.1038/gene.2015.51
99. de Jong VM, Zaldumbide A, van der Slik AR, Persengiev SP, Roep BO, Koeleman BP. Post-transcriptional control of candidate risk genes for type 1 diabetes by rare genetic variants. Genes Immun (2013) 14(1):58–61. doi: 10.1038/gene.2012.38
100. van de Linde P, Tysma OM, Medema JP, Hale G, Waldmann H, Roelen DL, et al. Mechanisms of antibody immunotherapy on clonal islet reactive T cells. Hum Immunol (2006) 67(4-5):264–73. doi: 10.1016/j.humimm.2006.02.027
101. Dorcet G, Migne H, Biotti D, Bost C, Lerebours F, Ciron J, et al. Early b cells repopulation in multiple sclerosis patients treated with rituximab is not predictive of a risk of relapse or clinical progression. J Neurol (2022) 269(10):5443–53. doi: 10.1007/s00415-022-11197-6
102. Keymeulen B, van Maurik A, Inman D, Oliveira J, McLaughlin R, Gittelman RM, et al. A randomised, single-blind, placebo-controlled, dose-finding safety and tolerability study of the anti-CD3 monoclonal antibody otelixizumab in new-onset type 1 diabetes. Diabetologia (2021) 64(2):313–24. doi: 10.1007/s00125-020-05317-y
103. Herold KC, Hagopian W, Auger JA, Poumian-Ruiz E, Taylor L, Donaldson D, et al. Anti-CD3 monoclonal antibody in new-onset type 1 diabetes mellitus. N Engl J Med (2002) 346(22):1692–8. doi: 10.1056/NEJMoa012864
104. Alkemade GM, Hilbrands R, Vandemeulebroucke E, Pipeleers D, Waldmann H, Mathieu C, et al. Preservation of recall immunity in anti-CD3-treated recent onset type 1 diabetes patients. Diabetes Metab Res Rev (2011) 27(8):925–7. doi: 10.1002/dmrr.1273
105. Ludvigsson J, Faresjo M, Hjorth M, Axelsson S, Cheramy M, Pihl M, et al. GAD treatment and insulin secretion in recent-onset type 1 diabetes. N Engl J Med (2008) 359(18):1909–20. doi: 10.1056/NEJMoa0804328
106. Thrower SL, James L, Hall W, Green KM, Arif S, Allen JS, et al. Proinsulin peptide immunotherapy in type 1 diabetes: report of a first-in-man phase I safety study. Clin Exp Immunol (2009) 155(2):156–65. doi: 10.1111/j.1365-2249.2008.03814.x
107. Roep BO, Wheeler DCS, Peakman M. Antigen-based immune modulation therapy for type 1 diabetes: the era of precision medicine. Lancet Diabetes Endocrinol (2019) 7(1):65–74. doi: 10.1016/S2213-8587(18)30109-8
108. Long SA, Thorpe J, DeBerg HA, Gersuk V, Eddy J, Harris KM, et al. Partial exhaustion of CD8 T cells and clinical response to teplizumab in new-onset type 1 diabetes. Sci Immunol (2016) 1(5):eaai7793. doi: 10.1126/sciimmunol.aai7793
109. Diggins KE, Serti E, Muir V, Rosasco M, Lu T, Balmas E, et al. Exhausted-like CD8+ T cell phenotypes linked to c-peptide preservation in alefacept-treated T1D subjects. JCI Insight (2021) 6(3):e142680. doi: 10.1172/jci.insight.142680
110. Narsale A, Lam B, Moya R, Lu T, Mandelli A, Gotuzzo I, et al. CD4+CD25+CD127hi cell frequency predicts disease progression in type 1 diabetes. JCI Insight (2021) 6(2):e136114. doi: 10.1172/jci.insight.136114
111. Malmegrim KC, de Azevedo JT, Arruda LC, Abreu JR, Couri CE, de Oliveira GL, et al. Immunological balance is associated with clinical outcome after autologous hematopoietic stem cell transplantation in type 1 diabetes. Front Immunol (2017) 8:167. doi: 10.3389/fimmu.2017.00167
112. Voltarelli JC, Couri CE, Stracieri AB, Oliveira MC, Moraes DA, Pieroni F, et al. Autologous nonmyeloablative hematopoietic stem cell transplantation in newly diagnosed type 1 diabetes mellitus. JAMA (2007) 297(14):1568–76. doi: 10.1001/jama.297.14.1568
113. Van Hulle F, De Groot K, Hilbrands R, Van de Velde U, Suenens K, Stange G, et al. Function and composition of pancreatic islet cell implants in omentum of type 1 diabetes patients. Am J Transpl (2022) 22(3):927–36. doi: 10.1111/ajt.16884
114. Roep BO. Improving clinical islet transplantation outcomes. Diabetes Care (2020) 43(4):698–700. doi: 10.2337/dci19-0080
115. Maffi P, Nano R, Monti P, Melzi R, Sordi V, Mercalli A, et al. Islet allotransplantation in the bone marrow of patients with type 1 diabetes: A pilot randomized trial. Transplantation (2019) 103(4):839–51. doi: 10.1097/TP.0000000000002416
116. Odorico J, Markmann J, Melton D, Greenstein J, Hwa A, Nostro C, et al. Report of the key opinion leaders meeting on stem cell-derived beta cells. Transplantation (2018) 102(8):1223–9. doi: 10.1097/TP.0000000000002217
117. van der Torren CR, Suwandi JS, Lee D, Van’t Wout ET, Duinkerken G, Swings G, et al. Identification of donor origin and condition of transplanted islets In situ in the liver of a type 1 diabetic recipient. Cell Transpl (2017) 26(1):1–9. doi: 10.3727/096368916X693437
118. Demeester S, Balke EM, van der Auwera BJ, Gillard P, Hilbrands R, Lee D, et al. HLA-A*24 carrier status and autoantibody surges posttransplantation associate with poor functional outcome in recipients of an islet allograft. Diabetes Care (2016) 39(6):1060–4. doi: 10.2337/dc15-2768
119. van der Torren CR, Verrijn Stuart AA, Lee D, Meerding J, van de Velde U, Pipeleers D, et al. Serum cytokines as biomarkers in islet cell transplantation for type 1 diabetes. PloS One (2016) 11(1):e0146649. doi: 10.1371/journal.pone.0146649
120. Ringers J, van der Torren CR, van de Linde P, van der Boog PJ, Mallat MJ, Bonifacio E, et al. Pretransplantation GAD-autoantibody status to guide prophylactic antibody induction therapy in simultaneous pancreas and kidney transplantation. Transplantation (2013) 96(8):745–52. doi: 10.1097/TP.0b013e3182a012cc
121. Huurman VA, van der Torren CR, Gillard P, Hilbrands R, van der Meer-Prins EP, Duinkerken G, et al. Immune responses against islet allografts during tapering of immunosuppression–a pilot study in 5 subjects. Clin Exp Immunol (2012) 169(2):190–8. doi: 10.1111/j.1365-2249.2012.04605.x
122. Harlan DM, Kenyon NS, Korsgren O, Roep BO. Immunology of diabetes s. current advances and travails in islet transplantation. Diabetes (2009) 58(10):2175–84. doi: 10.2337/db09-0476
123. Hilbrands R, Huurman VA, Gillard P, Velthuis JH, De Waele M, Mathieu C, et al. Differences in baseline lymphocyte counts and autoreactivity are associated with differences in outcome of islet cell transplantation in type 1 diabetic patients. Diabetes (2009) 58(10):2267–76. doi: 10.2337/db09-0160
124. Gillard P, Huurman V, van der Auwera B, Decallonne B, Poppe K, Roep BO, et al. Graves hyperthyroidism after stopping immunosuppressive therapy in type 1 diabetic islet cell recipients with pretransplant TPO autoantibodies. Diabetes Care (2009) 32(10):1817–9. doi: 10.2337/dc08-2339
125. Roelen DL, Huurman VA, Hilbrands R, Gillard P, Duinkerken G, van der Meer-Prins PW, et al. Relevance of cytotoxic alloreactivity under different immunosuppressive regimens in clinical islet cell transplantation. Clin Exp Immunol (2009) 156(1):141–8. doi: 10.1111/j.1365-2249.2008.03812.x
126. Huurman VA, Velthuis JH, Hilbrands R, Tree TI, Gillard P, van der Meer-Prins PM, et al. Allograft-specific cytokine profiles associate with clinical outcome after islet cell transplantation. Am J Transpl (2009) 9(2):382–8. doi: 10.1111/j.1600-6143.2008.02479.x
127. Huurman VA, Hilbrands R, Pinkse GG, Gillard P, Duinkerken G, van de Linde P, et al. Cellular islet autoimmunity associates with clinical outcome of islet cell transplantation. PloS One (2008) 3(6):e2435. doi: 10.1371/journal.pone.0002435
128. Bouwman LH, Ling Z, Duinkerken G, Pipeleers DG, Roep BO. HLA incompatibility and immunogenicity of human pancreatic islet preparations cocultured with blood cells of healthy donors. Hum Immunol (2005) 66(5):494–500. doi: 10.1016/j.humimm.2005.01.018
129. Stobbe I, Duinkerken G, van Rood JJ, Lernmark A, Keymeulen B, Pipeleers D, et al. Tolerance to kidney allograft transplanted into type I diabetic patients persists after in vivo challenge with pancreatic islet allografts that express repeated mismatches. Diabetologia (1999) 42(11):1379–80. doi: 10.1007/s001250051456
130. Roep BO, Stobbe I, Duinkerken G, van Rood JJ, Lernmark A, Keymeulen B, et al. Auto- and alloimmune reactivity to human islet allografts transplanted into type 1 diabetic patients. Diabetes (1999) 48(3):484–90. doi: 10.2337/diabetes.48.3.484
131. Vendrame F, Pileggi A, Laughlin E, Allende G, Martin-Pagola A, Molano RD, et al. Recurrence of type 1 diabetes after simultaneous pancreas-kidney transplantation, despite immunosuppression, is associated with autoantibodies and pathogenic autoreactive CD4 T-cells. Diabetes (2010) 59(4):947–57. doi: 10.2337/db09-0498
Keywords: immunotherapy, type 1 diabetes, immune intervention, precision medicine, immune monitoring, autoimmune disease, disease endotypes, mechanism of action
Citation: Roep BO (2023) The need and benefit of immune monitoring to define patient and disease heterogeneity, mechanisms of therapeutic action and efficacy of intervention therapy for precision medicine in type 1 diabetes. Front. Immunol. 14:1112858. doi: 10.3389/fimmu.2023.1112858
Received: 30 November 2022; Accepted: 04 January 2023;
Published: 17 January 2023.
Edited by:
Sefina Arif, King’s College London, United KingdomReviewed by:
Hugo Barcenilla, Karolinska Institutet (KI), SwedenCopyright © 2023 Roep. This is an open-access article distributed under the terms of the Creative Commons Attribution License (CC BY). The use, distribution or reproduction in other forums is permitted, provided the original author(s) and the copyright owner(s) are credited and that the original publication in this journal is cited, in accordance with accepted academic practice. No use, distribution or reproduction is permitted which does not comply with these terms.
*Correspondence: Bart O. Roep, boroep@lumc.nl